- 1Institute for Terrestrial and Aquatic Wildlife Research (ITAW), University of Veterinary Medicine Hannover, Foundation, Buesum, Germany
- 2DW-ShipConsult GmbH, Schwentinental, Germany
Large rivers like the Elbe or the Weser are periodically entered by harbor porpoises of the North Sea. They may even move 97 km upstream to the port of Hamburg, where their presence is highest in spring. This migration is believed to be related to important anadromous prey species travelling upstream for spawning. An acoustic flowmeter in the port of Hamburg emits signals in the hearing range of harbor porpoises. The pulses have a duration of 0.2 ms, a peak frequency of 28 kHz, a source level of 210 dB re 1 µPa and an inter-pulse interval of 4.2 s. The signals are continuously emitted from both sides of the river at a location where the river is 400 m wide. We evaluated the potential of these signals to induce temporary threshold shift (TTS) in harbor porpoise hearing. Hearing tests with a harbor porpoise in human care were conducted to determine TTS onset. We modelled the acoustic field based on underwater noise measurements. The acoustic flowmeters emit pulses in a highly directional beam with a source level high enough for inducing TTS in harbor porpoises by a single exposure up to a distance of approximately 72 m. The received cumulative sound exposure levels for harbor porpoises travelling along the flowmeters are mainly dependent on the timing and distance to the sound source. Accordingly, a close approach to the flowmeter at the time of transmission should be prevented. This could be the case, if vessels force harbor porpoises to displace closer to the flowmeters. We therefore suggest to decrease acoustic flowmeter source levels. This case study emphasizes the need for a mandatory authorization process prior to the use of underwater sound for any purpose with potential effects on aquatic life. Such an authorization process should carefully consider potential effects for target and non-target species.
Introduction
Anthropogenic noise is omnipresent in almost all terrestrial and aquatic ecosystems and has been recognized as one of the most hazardous forms of pollution (Andrew et al., 2002; World Health Organization, 2017). It’s environmental effects are considered as a key threat to biodiversity at an unpredictable scale (Kunc et al., 2016). The heavy reliance on acoustic perception across marine mammal, bird and fish species results from adaptations to living in an environment where light propagation is poor but sound propagation is excellent. Numerous anthropogenic activities generate underwater noise, including industry, e.g. during the construction and operation of offshore wind farms (Dähne et al., 2013; Brandt et al., 2018; Graham et al., 2019), shipping (e.g. reviewed in Erbe et al., 2019) or the clearance of unexploded ordnance (von Benda-Beckmann et al., 2015; Siebert et al., 2022). Intense sound is also emitted during seismic explorations of the seafloor (e.g. Sarnocińska et al., 2020), by military sonars (Filadelfo et al., 2009; Curé et al., 2016; Isojunno et al., 2016) or for the deterrence of animals (Brandt et al., 2013a; Brandt et al., 2013b; Götz and Janik, 2013).
Biological consequences of noise exposure are reflected on multiple scales, reaching from displacement (Brandt et al., 2018), physiological stress (Rolland et al., 2012), disturbances in communication (Erbe et al., 2016; Kragh et al., 2019) or foraging (Wisniewska et al., 2018) up to hearing impairment (Reichmuth et al., 2019; Schaffeld et al., 2019). Anthropogenic noise is widely recognized as a pervasive and significant threat to wildlife (Francis and Barber, 2013), which can be particularly detrimental for vulnerable animals with high metabolic rates (Hoekendijk et al., 2018; Rojano-Doñate et al., 2018), like the harbor porpoise (Phocoena phocoena). Short disturbances that affect foraging time (Wisniewska et al., 2018) may accumulate over repeated noise events and affect the long-term fitness of harbor porpoises, which forage nearly continuously to meet energy demands (Wisniewska et al., 2016).
For harbor porpoises, the key to successful hunting is a well-functioning hearing system, since they sense their environment acoustically by echolocation. Harbor porpoises are considered to be very sensitive to effects of noise and are therefore regarded as an indicator species in noise impact evaluations (Tougaard et al., 2015; Southall et al., 2019). Being critically dependent on sound perception for orientation, communication and foraging (Villadsgaard et al., 2007; Wisniewska et al., 2016; Sørensen et al., 2018) makes harbor porpoises vulnerable to noise disturbance.
In European waters, harbor porpoises are protected amongst others within the framework of the Habitats Directive (listed in annexes II and IV European Union, 1992) and Council Regulation 812/2004 (European Union, 2004) (Council Directive 92/43/EEC). Damage to hearing is to be regarded as injury within the meaning of Section §44 (1) of the Federal Nature Conservation Act. Its prevention therefore demonstrates the most important tool for the regulation of underwater noise in German waters. While a permanent threshold shift (PTS) is generally considered as an injury, Germany has the strictest definitions and considers even temporary threshold shifts (TTS) as injury [e.g. ASCOBANS Advisory Committee (2014)]. A TTS represents the limit where noise affects hearing and can be measured without damaging the individual being tested. TTS assessments are therefore a perfect tool for estimating effects on hearing. Affected hearing can potentially affect the survival of an individual (Mann et al., 2010; Morell et al., 2017; Morell et al., 2021a; Morell et al., 2021b), decreasing fitness and possibly leading to long-term population consequences (King et al., 2015). Temporarily affected hearing thresholds have been found in harbor porpoises after the exposure to single noise exposures with high received levels (Lucke et al., 2009), but also after an exposure to multiple events with lower received levels (Kastelein et al., 2016).
Historic reports prove that harbor porpoises not only inhabited coastal waters (Gilles et al., 2016; Hammond et al., 2017), but also lower stretches of large rivers e.g. as shown by sightings or strandings in the Weser River south of Bremen (Häpke, 1880) or even up to Magdeburg, which is upstream the Elbe River at a distance of 400 km from the coast (Kölmel, 1998). The number of harbor porpoises in the southern North Sea strongly declined between 1950 and 1970 (Addink and Smeenk, 1999). Since the late 1990s harbor porpoise sightings increased again in estuaries and rivers running into the North Sea (Camphuysen, 2004; Wenger and Koschinski, 2012; Taupp, 2021). These ecosystems are highly threatened and modified by anthropogenic activities and disturbances (Kennish, 2002; Mahoney and Bishop, 2017). Moving upstream rivers despite the continuous presence of vessels and limited space to evade is believed to be prey driven (Wenger and Koschinski, 2012; Leopold, 2015). Spatial and temporal occurrence of harbor porpoises in this area correlates with the annual migration of anadromous fish species like smelt (Osmerus eperlanus) and twaite shad (Allosa fallax), which are known to spawn in this area (Wenger and Koschinski, 2012; Leopold, 2015; Thiel, 2015).
Although great effort is made in Germany to mitigate noise related to the construction of Offshore Windfarms ASCOBANS Advisory Committee (2014), some noise sources exist that have been overlooked. This study aims to estimate the potential to induce TTS by an acoustic flowmeter, which is regularly applied in rivers to measure the water flow velocity. This was achieved by making underwater noise measurements along the flowmeter and performing hearing tests with a harbor porpoise in human care, which was exposed to comparable acoustic signals. This study sheds light on gaps in noise regulation and raises attention for the naive application of acoustic devices.
Methods
Study Area and Underwater Noise Measurements
An acoustic flowmeter exists 97 kilometers upstream the Elbe (Portal Tideelbe, accessed: 2022-05-12) in an area with regular harbor porpoise sightings (Wenger et al., 2016). The flowmeter (Quantum Hydromet, Germany) emits signals at 28 kHz (Frequency tolerance ±1.4kHz) with a peak source level of 218 dB re 1 µPa and a -6 dB beamwidth of 26°, according to the spec sheet. It is located in the Elbe River close to the port of Hamburg (Figure 1) and adjacent to the Natura 2000 conservation area “Neßsand”. The water depth at this site is an almost constant 18 m with a very steep slope in the area close to the riverbanks. The ultrasonic aqua flowmeter system consists of two pairs of hydroacoustic transducers and receivers, which are located at the north and south riverbank, transmitting acoustic signals from both sides of the Elbe River to obtain the flow speed from the measured difference in travel time between upstream and downstream direction.
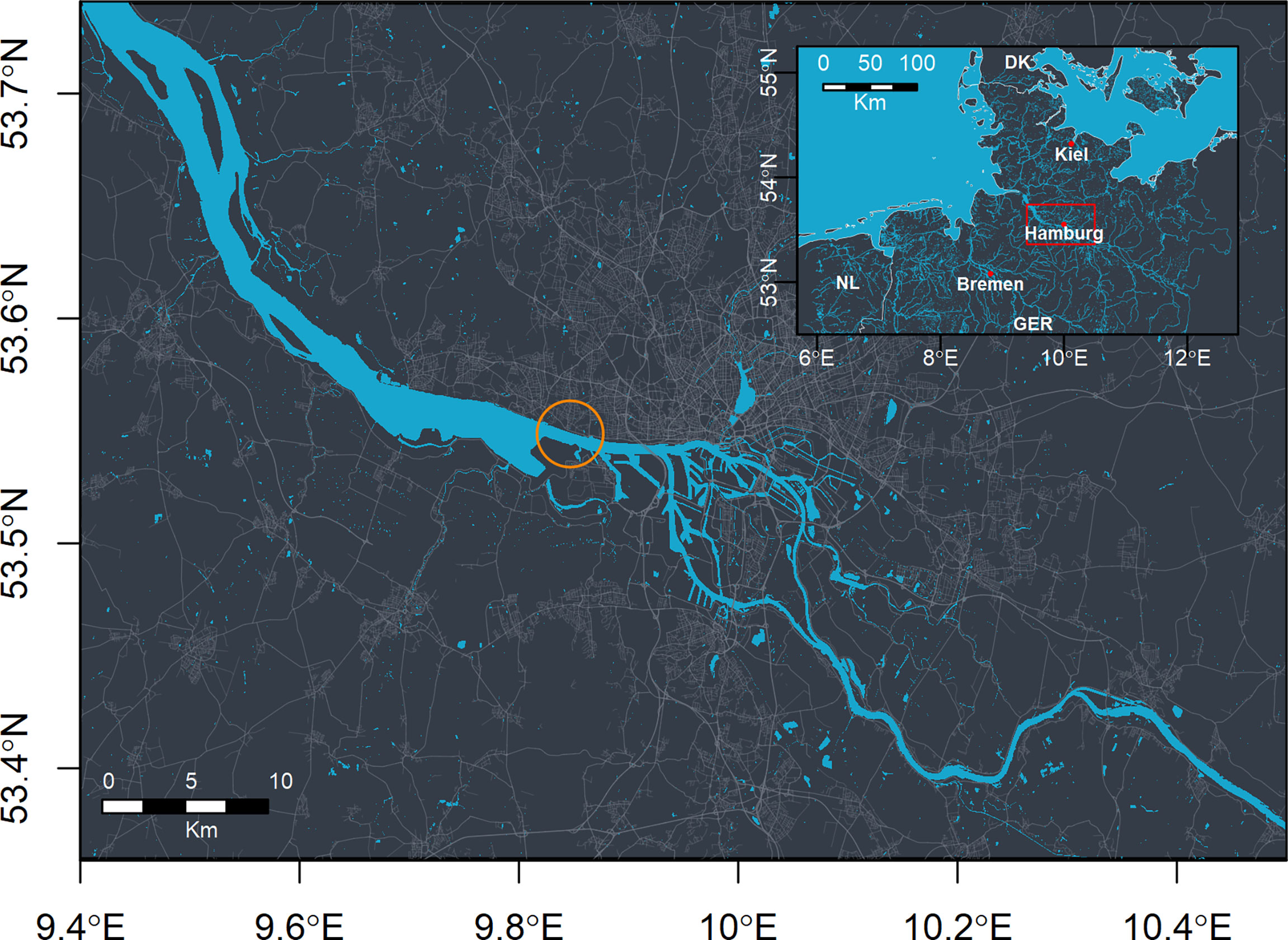
Figure 1 Overview of the study area (top-right corner). The red rectangle marks the area shown in the detailed map. The orange circle marks the position of the acoustic flowmeters.
We recorded underwater sound at three depth levels (2, 5, 8 m below water surface) by a linear vertical hydrophone array, which was fixed with snap hooks to a steel guidance cable on board of a vessel. At each water depth, underwater noise was recorded by two hydrophones with different sensitivities, to cover a wide dynamic range a) TC4014, sensitivity= -180.3 and -185.5 dB re 1V µPa-1, Reson Teledyne, DK, b) TC4013, sensitivity= -210.7 to -212.8 dB re 1V µPa-1, Reson Teledyne, DK, and c) d/140/h, sensitivity= -169.0 dB re 1V µPa-1, Neptune Sonar Ltd., UK). Underwater noise was recorded using the Avisoft Sound Recording Software (Avisoft Bioacoustics, Germany). Signals were pre-amplified by 0-20 dB with an eight-channel power amplifier (B2008APC – SN1212000, ETEC, DK) with a passband from 1 to 300 kHz. Recordings were digitized with a data acquisition card (NI USB 6366, National Instruments, USA) sampling at 750 ksamples s-1 and saved as wav files with a 16-bit resolution.
Acoustic signals from the flowmeter on both sides of the Elbe River were recorded on transects along the river. Therefore the vessel was steered upstream of the flowmeter, where the engine was stopped. The vessel drifted on transects at different distances to the flowmeters downstream, while the hydrophone array was in the water. Additionally, underwater noise was recorded during one transect from one flowmeter to the other by diagonally crossing the Elbe River. During this transect the engine was running. A mobile GPS device (Garmin 72H, CH) on top of the hydrophone array recorded simultaneously GPS positions at a sample rate of 1 Hz.
The underwater noise recordings, which were gathered on the different transects were analyzed with custom written manuscripts in MATLAB (MathWorks, USA). The sound pressure level (SPL, RMS) and sound exposure level (SEL) were calculated for each detected signal and correlated to the corresponding GPS position on the track.
Sound Propagation Model
The sound propagation was modelled based on the determined sound exposure levels for single acoustic signals and the corresponding recording locations. The aim of the sound propagation model was to generate a high-resolution spatial array with modelled SELs of single signals for the entire area of investigation. The sound transmission in the area is dependent on the propagation loss but also on the directivity of the sound source. Therefore, the propagation loss was estimated based on the recordings from the direct transect between the two flowmeters only, as this was assumed to be in the center of the beam. The source level was estimated from these results by the use of a logarithmic regression. Additional signals were used to determine the directional characteristic of the transmission beam.
Sound propagation was modelled for a three-dimensional area reaching 1000 m (x-axis) down- and upstream of the acoustic flowmeter, a width of 391 m between the two flowmeters (y-axis) and a depth of 18 m (z-axis). The received level was calculated with a resolution of 1×1×0.1 m (x×y×z). This calculation was based on a simplified ray tracing model and the passive sonar equation (Urick, 1983).The model assumes a spherical propagation loss of 20×log10(distance) and considers a three-way-propagation, taking the direct path and the first reflection from the surface and the bottom into account. The reflection coefficient is dependent on sediment type and angle of the ray. Based on a sample, the sediment was determined as muddy sand, which has a density of 1,782 kg m-3 and a sound velocity of 1,599 m s-1 (Urban, 2002). The loss by reflection was calculated for each indirect path considering the angle, the given speed of sound and density. The estimated source level, propagation loss over distance and the directivity index were taken into account. The estimated SEL for each cube within the array corresponds to the sum of the direct path and the first reflection from the surface and the bottom. Absorption was not considered, as this was assumed negligible at 28 kHz in freshwater and over short distances.
Effects on Hearing Thresholds
The effect of a comparable stimulus like the acoustic flow meter on harbor porpoise hearing was assessed by hearing tests with an animal in human care. The experiments were conducted in 2014 at the Fjord & Bælt Centre in Kerteminde (DK). The study animal was a healthy adult female harbor porpoise born in 1995. The animal and the experimental setup were the same as in Schaffeld et al. (2019). All trials were conducted adhering to the respective ethical principles as well as to the relevant international and national guidelines for animal experiments and under constant supervision of experienced biologists and animal trainers. Experienced animal trainers monitored animal condition and signs of stress of the animal throughout all experiments. In case of an observed stress response the experiments would have been stopped immediately. The harbor porpoise has been trained 2 times a day through standard operant conditioning and positive reinforcement techniques by a team of professional animal trainers.
Hearing thresholds were determined by measuring auditory evoked potentials (AEPs) of the brainstem, a commonly used non-invasive electrophysiological technique (Nachtigall et al., 2016; Ruser et al., 2016; Schaffeld et al., 2019). AEPs were recorded by silver plated electrodes, gently attached to the body surface by suction cups, while the animals hearing was stimulated. A rugged notebook (Panasonic Toughbook CF30) computer was used to digitally generate stimuli, which were converted to analogue by a USB multifunction data acquisition card (NI USB 6251, National Instruments, Austin, TX). The stimuli were updated at a 1MHz rate with a 16 bit resolution and bandpass filtered (100 Hz–250 kHz, 24 dB/octave, Krohn Hite, Brockton, MA) before emission by the transducer. The generated stimuli were emitted by a TC4033 transducer (Teledyne Reson, Denmark), placed at depth of 0.8m and a distance of 1m in front of the animal. Generated stimuli consisted of 1024 tone pips for each tested sound intensity. Each pip consisted of five cycles of the stimulus waveform with a cosine envelope, leading to a plateau of one cycle. The epoch length was 17 ms (Figure 2). Received sound pressure levels of test stimuli were calibrated by averaging 1024 stimuli prior to each trial with a TC4013 hydrophone (Teledyne Reson, DK).
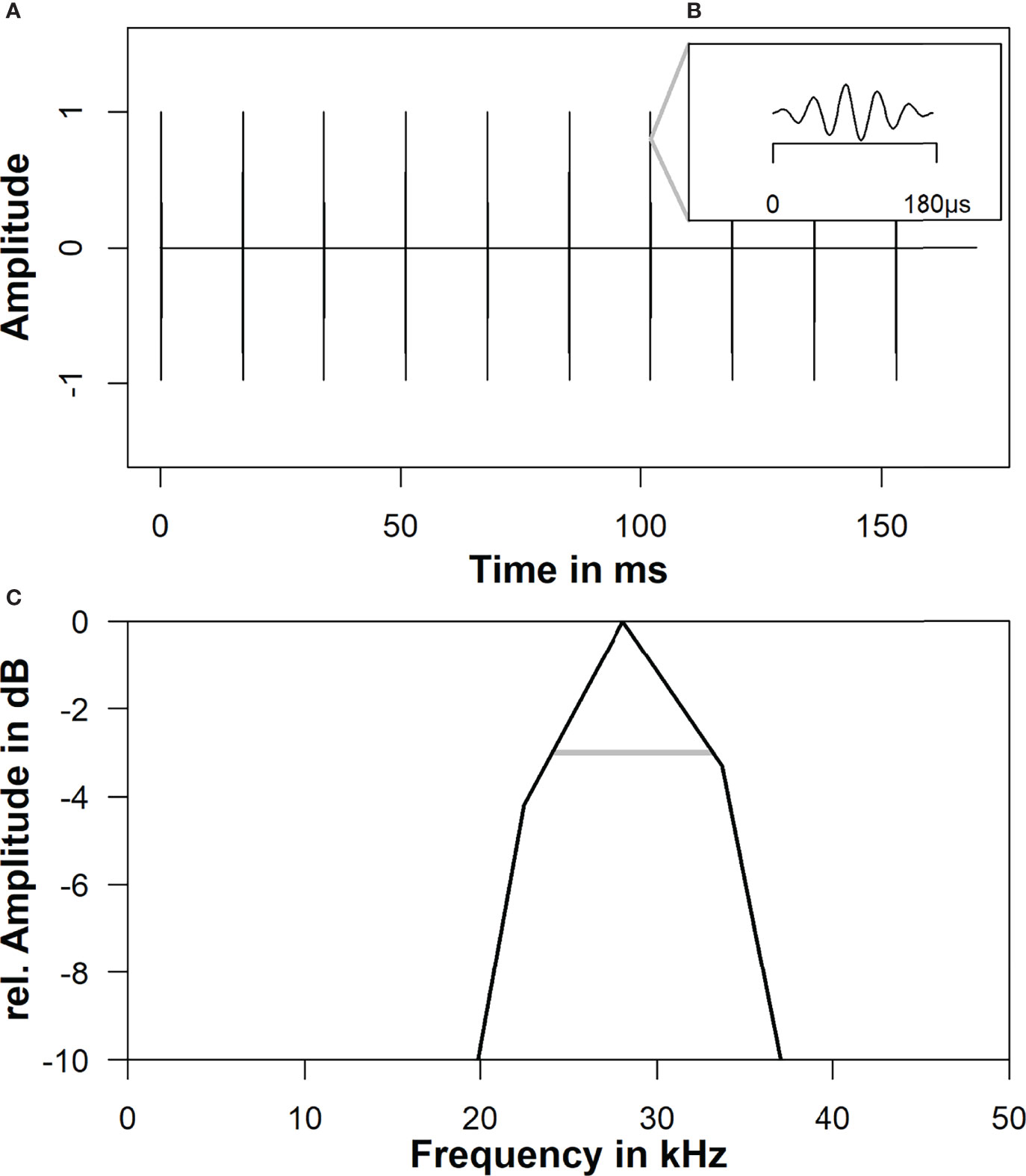
Figure 2 Tone pips, used as hearing stimuli. (A) Section with ten tone pips out of a complete train consisting of 1024 repetitions. (B) Detail view of one pip (five cycles of 28 kHz carrier) within the pip train. (C) Frequency spectra of the 28 kHz tone pips with ±3 dB bandwidth levels (gray horizontal lines).
Baseline hearing threshold at 28 kHz was determined and tested for temporary threshold shifts (TTS) after the exposure to a fatiguing stimulus. A tone at 28 kHz with a duration of 3.5 s was used for the exposure trials, which was emitted by a HS17 transducer (Neptune Sonar Limited, UK). The harbor porpoise was exposed once a day. Sound exposure levels were changed every day. All playbacks of hearing test stimuli and recordings of auditory evoked potentials were conducted with custom written software (Evoked Response Study Tool, EVREST, (Finneran et al., 2008; Finneran, 2009).
The sound exposure level (SEL; dB re 1 µPa²s) associated with the onset of TTS (TTSonset) was defined as a significant shift from baseline hearing. This TTSonset was estimated by a linear regression of sound exposure levels and the determined hearing threshold shifts (in dB) relative to the baseline.
Simulation of Received Levels of Travelling Harbor Porpoises Along Flow Meter
We simulated swimming tracks through the area to evaluate potential effects of the flow meter on the hearing. We defined transects in the direction of the river with a constant y-axis position, passing the flowmeters. We simulated such transects for each of the 391 possible y-axis positions over the complete width of the river. For each of these 391 simulated tracks along the x-axis, we calculated harbor porpoise positions at the time of the reception of the acoustic flowmeter signals. We calculated the positions of the harbor porpoise on each track from 1,000 m before up to the distance of 1,000 m behind the flowmeters. The first reception was assumed at the starting position at a distance of 1,000 m to the flowmeter. Each following reception was obtained from the inter-pulse interval×swim speed. We applied swim speeds between 0.25 and 8 m s-1. This broad range was chosen to represent harbor porpoises at all life stages and to include slowly swimming individuals, like mother and calf pairs. Data on the swim speed of harbor porpoises are rare due to their inconspicuous lifestyle and poor accessibility. Maximum swim speed for animals in human care were determined at 2 m s-1 (Kastelein et al., 2018), 4.3 m s-1 (Otani et al., 2001), and in the wild at 4.3 and 6.1 m s-1 (Gaskin et al., 1974; Otani et al., 2000). A mean swim speed of a free-ranging harbor porpoise was determined at 0.9 m s-1 (Otani et al., 2000). Since the authors are not aware of any publication of swimming speed of harbor porpoises in river systems, the effect of flow velocity on swim speed was estimated as the sum of the maximum swim speed and assumed maximum flow velocity. A maximum swim speed of 7.7 m s-1 was estimated, considering an estimated maximum flow velocity of 1.6 m s-1 (pers. comm. Nino Ohle in 2017, HPA).
Large variations in received levels occur at close distances to the flowmeters as signals are emitted in a very narrow beam. This may result in uncertainties when estimating the cumulative received levels, since these are dependent on the timing when the harbor porpoise enters the main beam with regards to the inter-pulse interval. We addressed this time-related variability by shifting the start positions along the travelled distance for a given swim speed between two pulses. Therefore, we tested multiple starting positions corresponding to the distance a harbor porpoise can travel for the tested swim speed between two consecutive pulses. The received level for each position on the track was obtained from the modelled acoustic array. Since we do not know at which water depth a harbor porpoise would most likely migrate through the area around the acoustic flowmeters, we assumed a continuous diving depth of 5 m for practical reasons. However, the sound field does not show substantial differences in received sound exposure levels over depth. The estimated received levels from both acoustic flowmeters were accumulated for each simulated track and presented as the mean for all tested starting positions. We only considered received signals with single pulse SEL exceeding an effective quiet threshold. Single events below a certain single signal sound exposure level (SELSS) have never been shown to induce a TTS or affect recovery and are therefore regarded as “effective quiet” (Ward et al., 1976; Finneran, 2015). In absence of an effective quiet threshold we regard the lowest SELSS, capable of inducing a TTS by multiple exposure. This was determined at 145 dB re 1 µPa²s for pile-driving noise (Kastelein et al., 2016). The 145 dB re 1 µPa²s derives from exposures to pile-driving noise with a peak frequency between 500 and 800 Hz. Harbor porpoise hearing at 28 kHz is better than at 500 to 800 Hz. Differences between hearing thresholds account for approximately 40 dB (Kastelein et al., 2002; Ruser et al., 2016). In the absence of an empirically derived effective quiet threshold, we defined instead a threshold which is “still higher than effective quiet threshold” (SHEQ) as a proxy. Accordingly, we adjusted the SHEQ to 105 dB re 1 µPa²s and considered received levels on the track only if this value was exceeded.
Evaluation of Potential to Induce a TTS
The potential to induce a TTS in harbor porpoises from exposure to signals of the flowmeter was evaluated by using the modelled acoustic field and simulated harbor porpoise travel. A TTS can be induced in three different scenarios. Single pulses (1) can induce a TTS. For this scenario, we used the SEL-TTSonset, which derives from the hearing tests with a harbor porpoise in human care exposed to a 28 kHz tone. A TTS can be induced also by multiple exposures (2). The single SEL-TTSonset cannot be directly transferred to a multiple exposure, as the same SEL of exposure does not automatically induce the same shift, if the SEL is received over a longer duration. The assumption that an equal cumulative SEL induces an equal hearing shift is only valid for continuous fatiguing noises or for exposures with similar duty cycles (e.g. Mooney et al., 2009; Finneran et al., 2010a; Finneran et al., 2010b; Kastelein et al., 2014b; Popov et al., 2014; Kastelein et al., 2015a). We followed instead the suggested noise exposure criteria of Southall et al. (2019, Table 6), who suggested a frequency weighted cumulative SEL-TTSonset of 153 dB re 1 µPa²s. Since this SEL-TTSonset is frequency weighted, based on the audiogram of harbor porpoises (National Oceanic and Atmospheric Administration; National Marine Fisheries Service, 2016; Southall et al., 2019), this onset cannot be transferred directly to the single pulse exposure levels of the modelled sound field. According to the proposed frequency weighting, the amplitude of a 28 kHz signal is effectively decreased by 0.3 dB. This means, that the SEL-TTSonset for multiple pulses accounts for 153.3 dB re 1 µPa²s. Flight transects were simulated in order to estimate the TTS potential for a multiple exposure. We tested, if the cumulative received SEL for the entire track along the x-axis and at a constant y-axis position exceeds the cumulative SEL-TTSonset suggested by Southall et al. (2019). This approach does not regard potential avoidance reactions of the animals, where animals identify the position of the flowmeter and stay out of the area with highest noise levels. Potential reactions of harbor porpoises are assumed to be highly variable. Therefore we chose a further approach to assess the TTS potential as a function of the location within the sound field. We evaluated the potential to induce a TTS for harbor porpoises, which do not show a flight response but rather stay in the area (3). Based on the sound field of the flowmeter and the determined inter-pulse interval, we calculated the time for each position, needed to exceed the cumulative SEL-TTSonset of 153 dB re 1 µPa²s (frequency weighted).
Results
Underwater Noise Recordings
We analyzed 839 recorded signals from the flowmeter in the North at distances between 3 and 763 m. Sound pressure levels (RMS) were determined between 98 and 164 dB re 1 µPa. Sound exposure levels ranged from 61 to 132 dB re 1 µPa²s. 750 signals were analyzed from the flowmeter in the south at distances between 19 and 663 m. Sound pressure levels ranged from 94 to 164 dB re 1 µPa. The SEL were determined between 86 and 134 dB re 1 µPa²s (Figure 3). The source levels of the flow meters were determined from the received levels in the main beam at distances up to 150 m. The best fit for the logarithmic regression was determined at 20 × log10 (distance). The source level for both flowmeters was estimated at 210 dB re 1 µPa at 1 m with a peak frequency of 28 kHz (Figure 4A). The signal duration (T90, time interval with 90 % of energy) was determined as 0.2 ms. The signal consisted of five cycles with a rise and fall in amplitude (Figure 4B). Signals from both flowmeters were recorded with an inter-pulse interval of 4.2 s (Figure 4C). The emission of signals alternated between the north and south flowmeter, leading to an effective inter-pulse interval of 2 s. Sound exposure levels ranged from 77 to 164 dB re 1 µPa²s for the flowmeter in the north and from 78 and 165 dB re 1 µPa²s for the flowmeter in the south within the modelled sound field (2000×390×18 m, Figure 5). The variability of modelled sound exposure levels over depth was low (median standard deviation <0.1 dB). The single arrays of modelled sound fields for the two flowmeters were combined in a shared array (Figure 6), which was used in all further simulations.
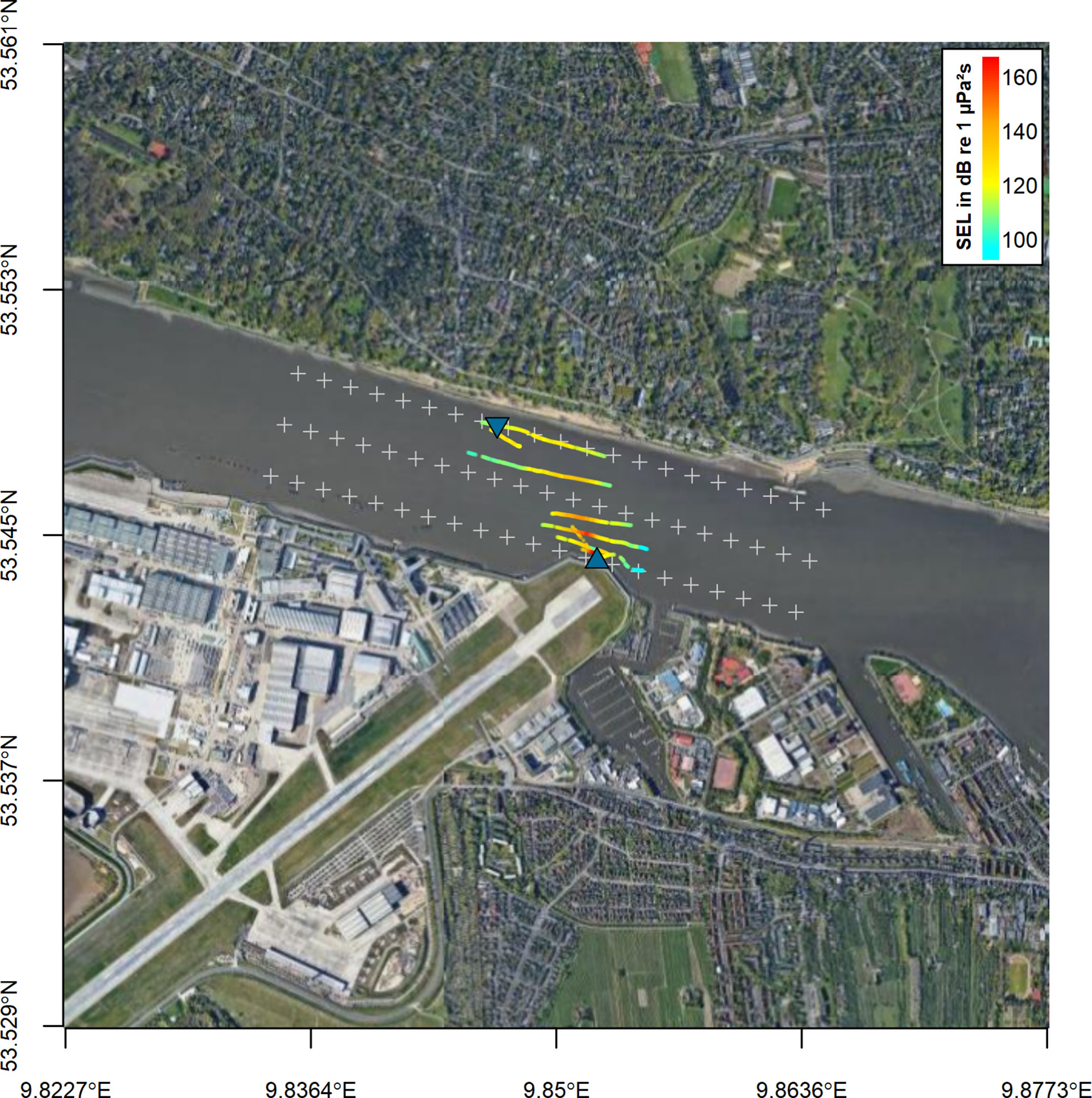
Figure 3 Study area in the Elbe River on the West side of Hamburg. The positions of the underwater flowmeters are shown by blue triangles. The positions of recorded signals from the flowmeter in the South are marked by points, color coded for the received SEL (RMS in dB re 1 µPa²s). Signals from the flowmeter in the North are not shown. Grey crosses mark the extent of the modelled sound field with a width of 391 m and a length of 2001 m. The distance between two crosses on a line is 100 m. The map was generated using the R-package “RgoogleMaps” (Loecher and Ropkins, 2015).
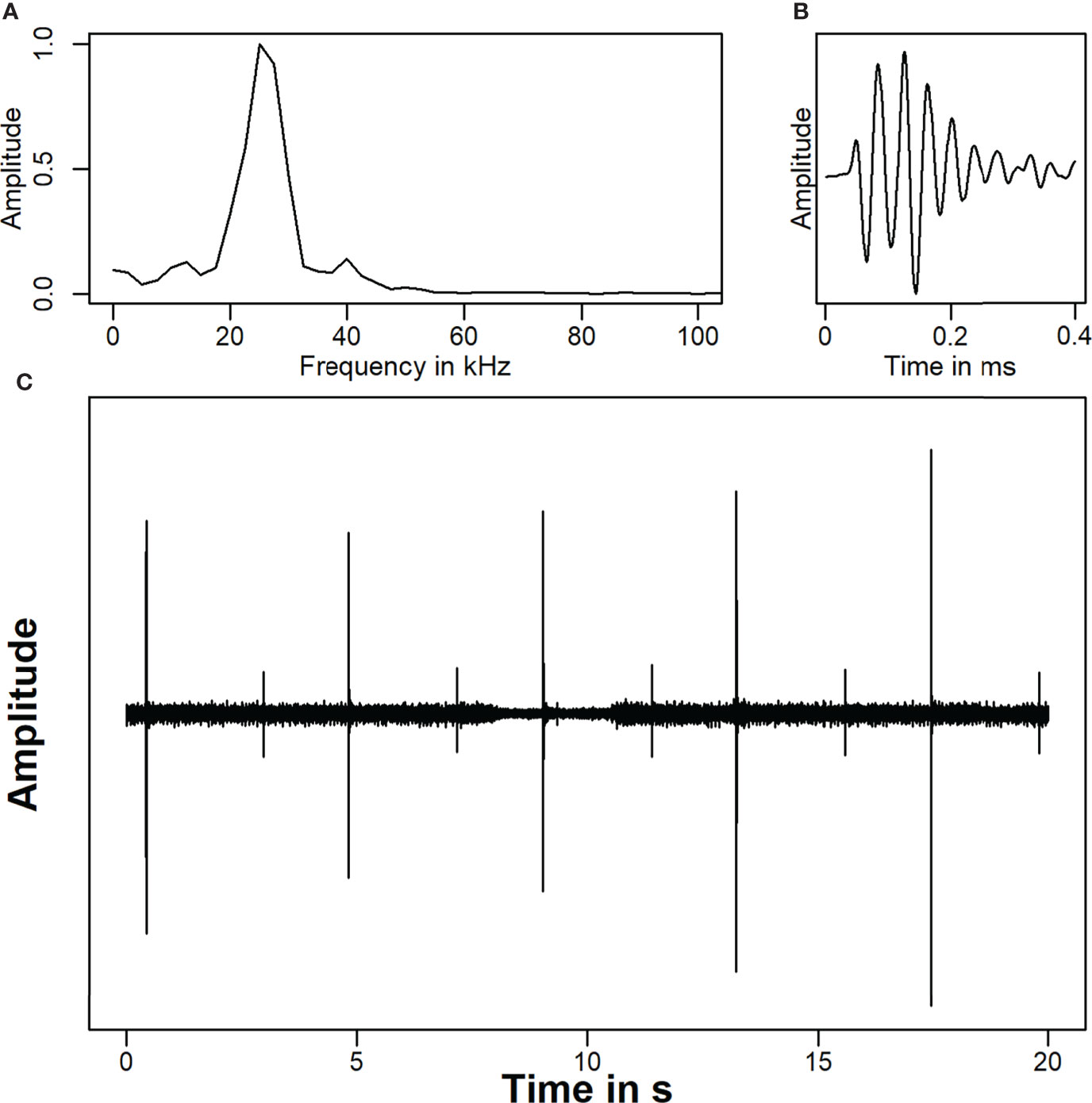
Figure 4 Underwater noise recording at a water depth of 5 m during a vessel passage close to the flow meter in the north. (A) The spectrogram of the time wave was generated for one flow meter signal in a time window of 0.4 ms by the function “spec” from the package “seewave” (Sueur et al., 2008) (B). The time representation of the signal show that the signal consists of five cycles (C). The 20 s recording shows each five signals from the flow meter in the North and in the South. The signals from the North have a higher amplitude, as recordings were made closer to this flowmeter.
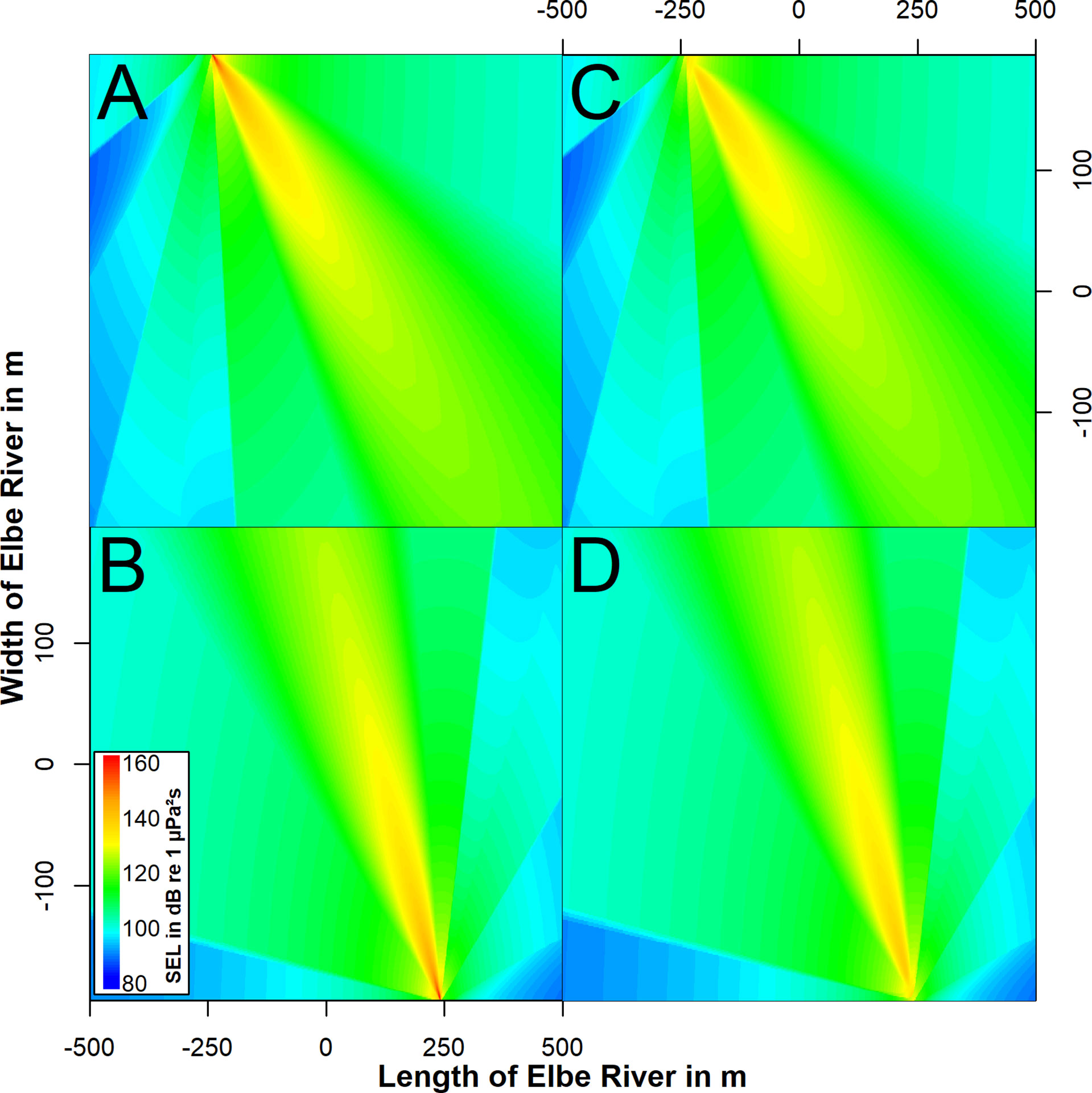
Figure 5 Top view on the modelled sound field of the acoustic flowmeter on the north (top row) and south (bottom row) riverbank. The sound field is shown for depths of 5 (A, B) and 12 m (C, D). The sound exposure level of a single pulse is shown by color-coding in dB re 1 µPa²s.
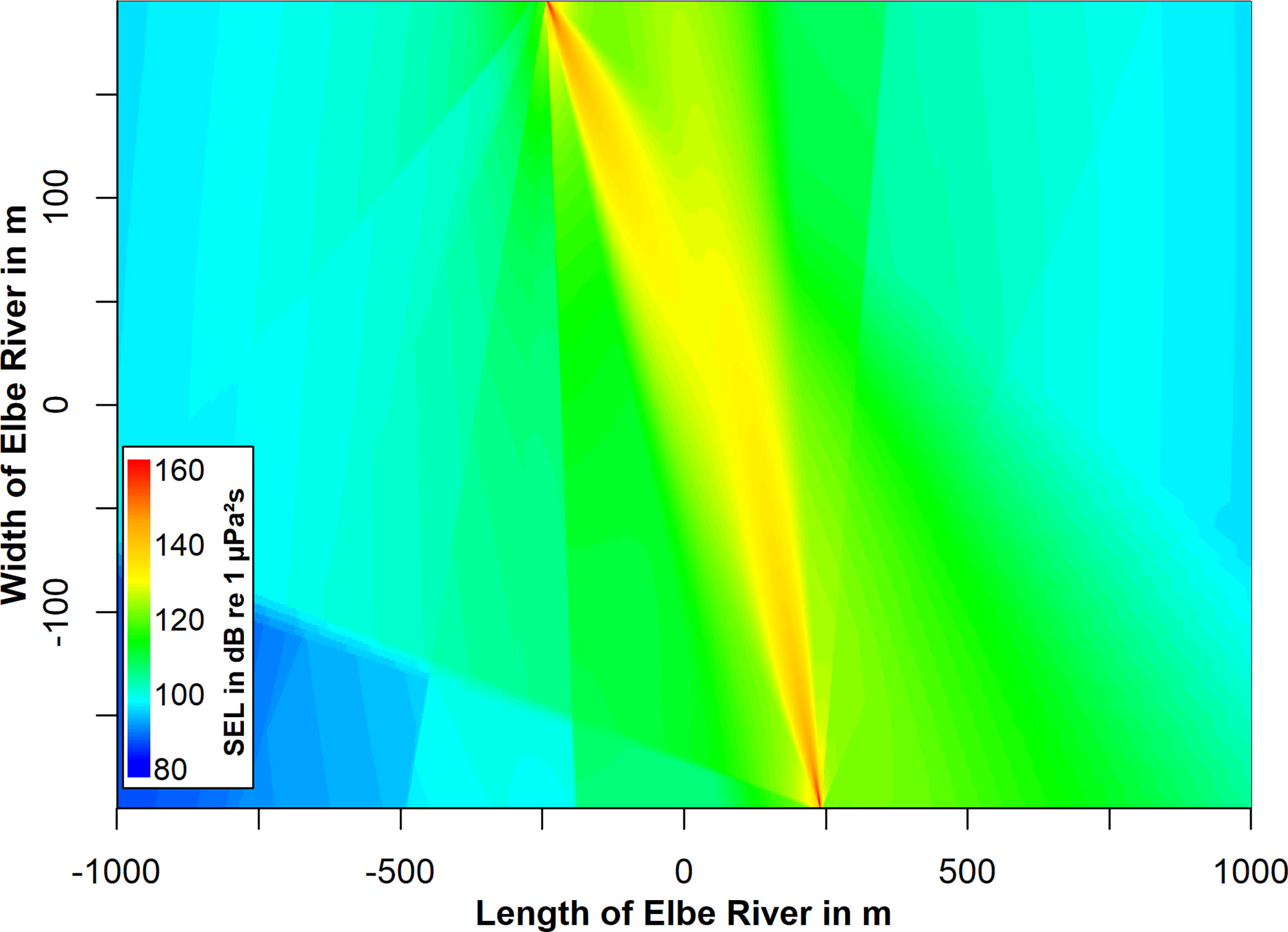
Figure 6 Top view on the combined sound field of the two flowmeters at a depth of 5 m. This combination is derived from summing up the single arrays of the two flow meters. The flowmeters alternately emit pulses with a combined inter-pulse interval of 2 s. The inter-pulse interval of each flowmeter is 4.2 s. The color coded sound exposure level represents a double pulse, meaning a pulse of each flowmeter emitted simultaneously.
Baseline Hearing Thresholds
Baseline hearing at 28 kHz was measured over 14 trials resulting in a mean hearing threshold of 82.0 dB re 1 µPa ± 2.4 dB (range 78.2-88.1 dB re 1 µPa). We obtained a critical value (p ≤ 0.05) of 85.7 dB re 1 µPa as the threshold for a significant hearing elevation. Accordingly, any hearing shift larger than 3.8 dB was regarded as a TTS.
Post-Exposure Thresholds
Hearing thresholds after an exposure to a 3.5 s fatiguing tone at 28 kHz were conducted in eleven trials. The animal was exposed to SELs between 139.7 and 156.3 dB re 1 µPa²s. Post-exposure hearing thresholds were between 86.7 and 97.6 dB re 1 µPa, accounting for elevated thresholds between 4.8 and 15.7 dB. Accordingly, all post-exposure thresholds exceeded the critical value of 3.8 dB. The TTSonset was estimated by a linear regression of threshold shifts versus sound exposure levels. According to the linear regression (intercept=-83.95, slope=0.64), the critical value of 3.8 dB was exceeded at an SEL of 137.4 dB re 1 µPa²s (Figure 7). This TTSonset was subsequently used to evaluate the TTS potential for single exposures to the flowmeter signals.
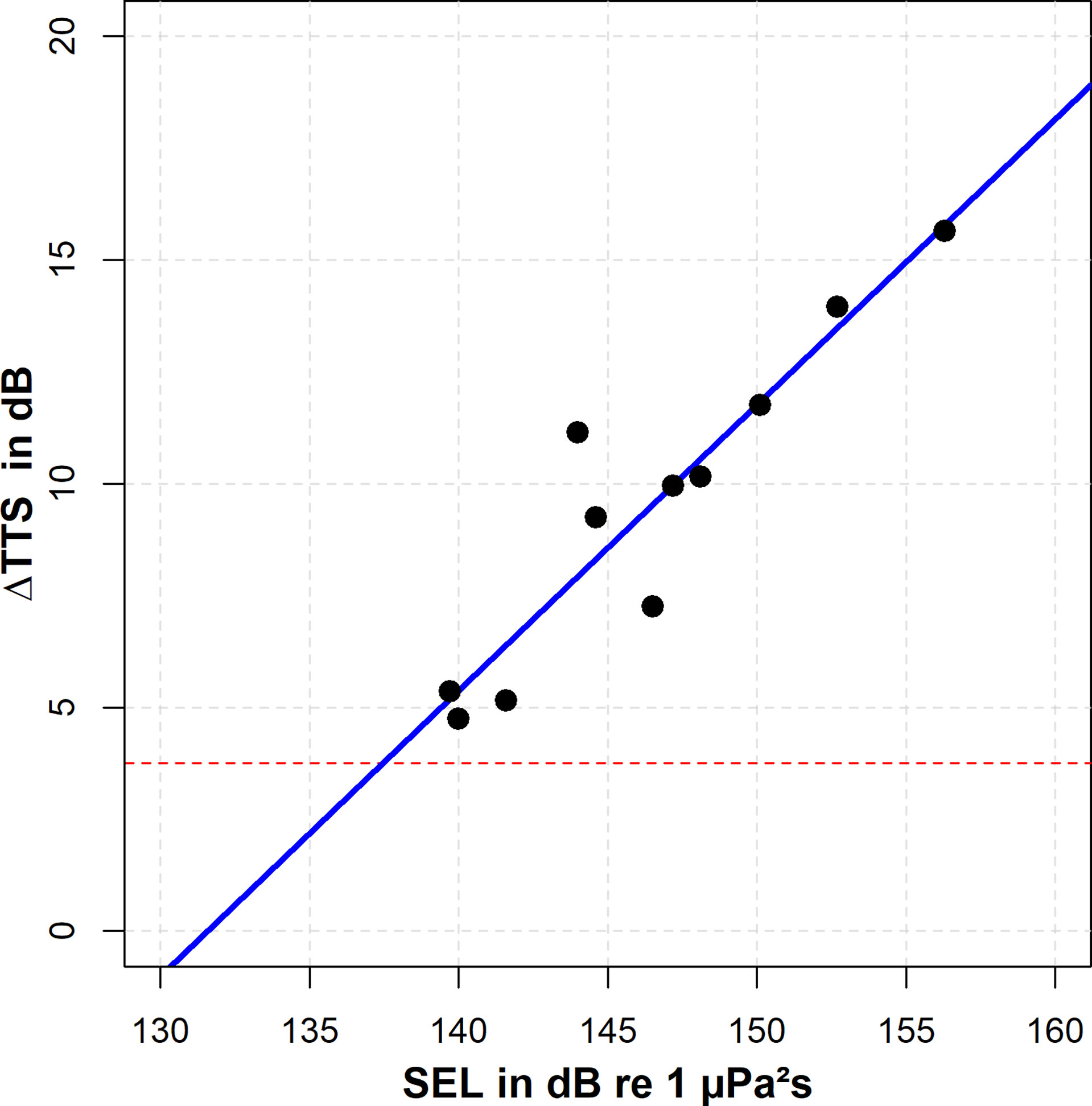
Figure 7 Effects of a 28 kHz tone exposure with a duration of 3.5 s on hearing thresholds at 28 kHz (black circles). The horizontal red dashed line represents the critical value of 3.8 dB shift, which indicates the threshold of significance. A linear regression was calculated in order to estimate the TTSonset, using only exposures which led to a TTS. The SEL-TTSonset at 28 kHz was determined as 137.4 dB re 1 µPa²s.
Evaluation of TTS Potential After Exposure to Single Flowmeter Pulses
The SEL-TTSonset for a single exposure was determined at 137.4 dB re 1 µPa²s. The underwater noise measurements and the following sound propagation model show that the source level of the underwater flowmeter exceeds this TTSonset. Single pulses therefore contain sufficient SEL to induce a TTS in a harbor porpoise. The flowmeters are highly directional leading to a narrow beam, in which amplitudes are highest. Within the beam of highest amplitude, the modelled sound fields at a depth of 5 m show single SELs above the TTSonset up to a distance of approximately 72 m from the flowmeter in the north and 74 m from the flowmeter in the south. Since the flowmeters are not aligned in a 90° angle to the x-axis these distances in the beam do not correspond to the distance in the y-axis, meaning the position in the width of the river. Harbor porpoises which travel along a transect need to keep a distance of 40 m to the flowmeter in the north and 60 m to the flowmeter in the south in order to avoid TTS from a single exposure.
Evaluation of TTS Potential for Harbor Porpoises, Which Stay Around the Flowmeters
Based on the constant inter-pulse intervals, we calculated the duration until the SEL-TTSonset was exceeded for every position in the modelled sound field. This was done to estimate the TTS potentialfor harbor porpoises, which do not show a flight response but rather stay within the area (Figure 8). The area of highest potential to induce TTS is between the two flowmeters. If a harbor porpoise stays within this area for 1 to 30 minutes, TTS can be induced. The risk for TTS strongly decreases, when leaving the narrow beam of highest amplitude.
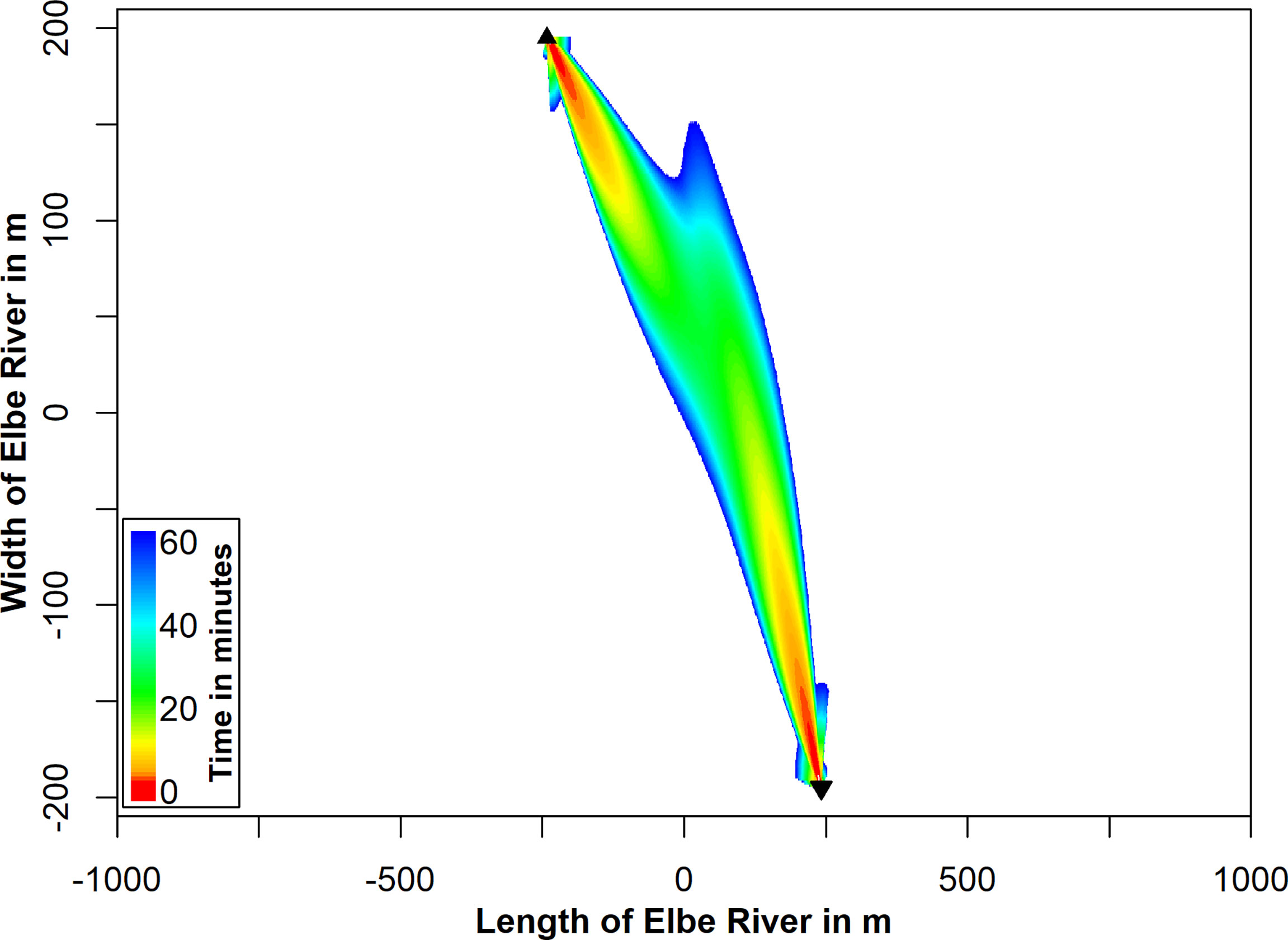
Figure 8 The time in minutes is presented (color-coded), which is needed to exceed the cumulative SEL-TTSonset of 153.3 dB re 1 µPa²s. Only the areas, where a TTS can be induced within 60 minutes for an inter-pulse interval of 4.2 s are marked. The flowmeter positions are marked by black triangles.
Received Levels on Simulated Flights
The received SEL for harbor porpoises travelling along the acoustic flow meters were simulated, assuming a swim speed between 0.25 and 8 m s-1 (Figure 9). We accumulated the received sound exposure levels for each transect line. We simulated swimming path along the x-axis without any simulated zigzag courses. Travelling harbor porpoises with a swimming speed above 2 m s-1 would not receive sufficient SEL from multiple pulses to induce TTS. The TTS-potential is comparably low. However, harbor porpoises swimming with a speed of 0.9 m s-1 could receive cumulative sound exposure levels exceeding the SEL-TTSonset up to distances of about 20 m. The received SELcum is strongly dependent on the timing of pulses. If the harbor porpoises is within the narrow beam of highest amplitude at the moment a pulse is emitted, the received cumulative SEL can be as high as 165 dB re 1 µPa²s for all swimming speeds and a TTS would be likely.
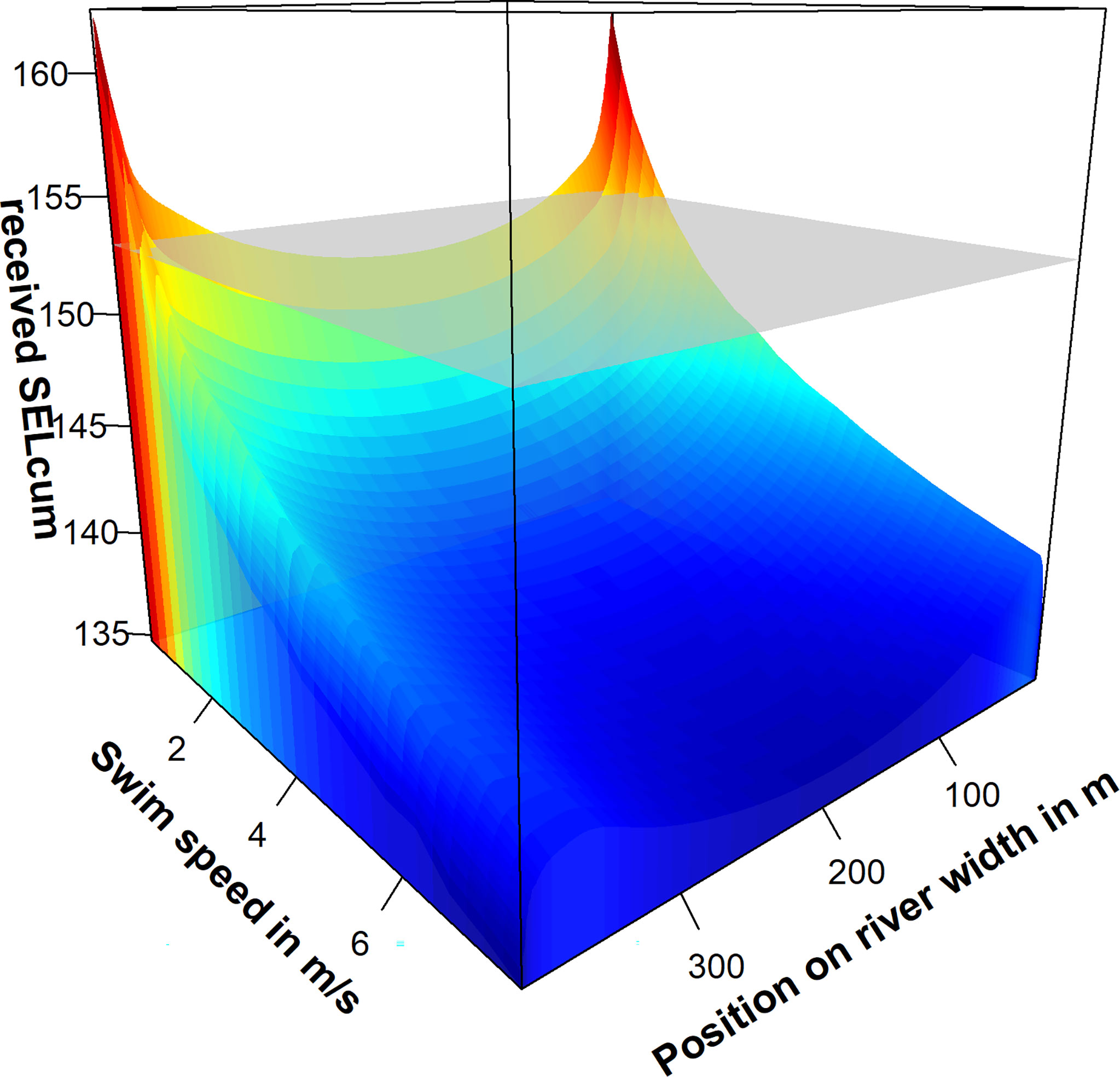
Figure 9 Received cumulative sound exposure levels (SELcum) on simulated transects passing the acoustic flowmeters. The simulation was performed for a swim speed between 0.25 and 8 m s-1) and a continuous depth of 5 m. The received SELcum (z-axis, color-coded) represents the cumulative SEL a harbor porpoise would receive, when passing the flowmeters and keeps a constant distance to the riverbanks. The grey grid represents the cumulative SEL-TTSonset suggested by Southall et al. (2019).
Discussion
Evaluation of Potential Effects of the Flowmeter on Harbor Porpoise Hearing
The acoustic flowmeter has the potential to temporarily affect harbor porpoise hearing. Sound exposure levels exceeding 137.4 dB re 1 µPa²s can induce TTS at 28 kHz, as shown by the exposure experiments with animals under human care. A much higher SEL-TTSonset of 166 dB re 1µPa²s was found for an exposure of harbor porpoises to 32 kHz band noise (Kastelein et al., 2019a). This difference of 29 dB in the TTSonset could be explained by the much higher SPL and much shorter exposure duration in this study. The flow meters in the Elbe River are believed to induce TTS at close distances up to 74 m after the reception of a single pulse. Received cumulative SELs exceed the TTS-onset for travelling transect at close distances with less than 2 m s-1 (Figure 9). Although harbor porpoises are capable of swimming faster, slower swim speed levels have been measured for mother calf pairs (Gaskin et al., 1974; Otani et al., 2000; Otani et al., 2001). It is reasonable to assume that a swim speed > 2m s-1 cannot be maintained if the health of animals is affected by diseases or animals have to swim against the current.
Following the recommend noise exposure criteria for marine mammals (Southall et al., 2019), a PTS is expected at a cumulative SEL of 173 dB re 1 µPa²s. The highest SEL within the acoustic field was estimated at a SEL of 165 dB re 1 µPa²s. To exceed the PTS-SELonset of 173 dB re 1 µPa²s, seven pulses with a SEL of 165 dB 1 µPa²s would be needed. Accordingly, a harbor porpoise would need to stay at close distance to the flowmeter for 28.7 s.
The presence of harbor porpoises in the port of Hamburg is believed to be driven by the migration of prey species (Wenger et al., 2016). Very limited fish species show good hearing abilities at frequencies as high as the 28 kHz flowmeter signals (Ladich and Fay, 2013). Effects on fish distribution are therefore believed to be negligible. If for some reason prey species aggregate close to the flowmeter, harbor porpoises could try to get access to this important food source despite high sound exposure levels. If the energetic benefit is high enough, harbor porpoises could tolerate the exposure to these signals. We found a period of less than 60 minutes being sufficient to induce a TTS along the entire width of the Elbe River as well as areas close to the flowmeter where a TTS can be induced in minutes.
Effects on Behavior
Effects of anthropogenic noise are numerous and must not be restricted to injuries and death. The introduction of underwater noise within the hearing range of harbor porpoises can also elicit behavioral changes. An increased swim speed and breathing rate but also aversive behavior with jumping out of the water was shown for harbor porpoises in human care, when exposed to comparable pinger signals (Kastelein et al., 2014a; Kastelein et al., 2015c). Accordingly, it is likely that harbor porpoises are likewise disturbed by the flowmeters in the port of Hamburg and try to avoid close approaches to the flowmeters. Such disturbance effects may even result in decreased individual fitness and could lead to long-term population consequences (King et al., 2015).
It is reasonable to assume, that entering the sound field elicits discomfort or stress in the animals. In this case, the acoustic flowmeter could create a barrier effect and could lead to habitat loss. This could slow down or prevent the return of harbor porpoises. Future research should focus on such a potential barrier effect and habitat exclusion. The Elbe River is highly exposed to ship traffic, which contributes significantly to the underwater soundscape. However, the Elbe River is most likely of high ecological importance for harbor porpoises with strong biological drivers, as harbor porpoises regularly occur in this noisy area. Future studies should investigate the habitat usage of harbor porpoises in this area to determine its ecological importance. Currently no systematic monitoring of harbor porpoises in large river systems exists, although sightings are regularly reported (Wenger and Koschinski, 2012; Wenger et al., 2016). Monitoring is needed for harbor porpoises, which are protected throughout their range, to better understand the driving factors for entering rivers. This possible barrier with a TTS potential should also be addressed for harbor seals, since these animals also occur in this area (Taupp, 2019) and are capable of hearing the acoustic flowmeter signal (Kastelein et al., 2009a; Kastelein et al., 2019b). Seals may also suffer from habitat exclusion by the acoustic signals of the flowmeter as acoustic signals with a 28 kHz component are even used for deterring seals (Kastelein et al., 2015b). Potential effects on seals should be considered in future research.
Management Approach for Noise Mitigation
The understanding of effects of noise on marine life evolved over the last decades and is still scarce, since the field of research regarding potential effects of anthropogenic underwater noise is relatively new. This could be the reason why underwater acoustic devices are still applied without considering potential biological effects. Likewise, acoustic deterrent devices are used around aquacultures in Scotland to prohibit depredation by seals causing economic loss. However, this happens unregulated to such an extent that up to 37 % of a Special Area of Conservation for harbor porpoises could exceed the threshold for TTS within a day (Findlay et al., 2021).
The simulation of harbor porpoises travelling along the acoustic flow meter could reveal a high variability in the received cumulative SEL. This large variability is due to the inter-pulse interval of 4.2 s, the narrow beam of the flowmeter and the swimming speed of harbor porpoises. Although more pulses could be received on the complete track for a slower swimming speed, the highest possible received levels do not differ significantly between different speeds. This clearly demonstrates that the timing and the distance to the sound source are the most important factors for the received cumulative SEL.
Our results demonstrate that the risk for TTS is highest in a comparably small area up to 74 m from the source. Due to the porpoises’ good hearing abilities at 28 kHz (Kastelein et al., 2002; Ruser et al., 2016), the flowmeter signals might be heard from larger distances and could keep animals at a distance from the sound source. Travelling harbor porpoises could possibly choose to travel in the middle of the Elbe River when passing the flowmeter. The high ship traffic in the Elbe might affect harbor porpoise distribution, as well. Accordingly, harbor porpoises might have to choose between high vessel and flowmeter noise exposure. We believe that following approaches for noise mitigation could help to reduce such conflicts between anthropogenic activities and wildlife.
Switching off the flowmeter in the presence of vessels could be an option to mitigate potential effects on harbor porpoise hearing. Vessel presence could be derived in real-time from AIS data or background noise levels. We assume that the presence of vessels negatively affects the flow measurement. Switching off the flowmeter in such situations would therefore have only minor effects on the recorded data. Furthermore, there is no need for such a high temporal resolution of flow measurements. A much lower temporal resolution could still be sufficient to ascertain flow speed trends. Emissions could also start with a lower source level and increase gradually so that harbor porpoises are not surprised by high level signals at close range. The advantage of the currently used high source level and short inter-pulse interval is that travelling harbor porpoises can hear the signal at sufficient distances and are aware of them in time to react. In theory, this reduces the probability that harbor porpoises enter the area of highest received levels. A possible adjustment could be measuring the flow speed on an hourly basis. A measurement could start with signals with reduced source levels, which are gradually increased in steps of 3 dB, giving the harbor porpoise time to react. For instance, the SEL could start at 102 dB re 1 µPa²s and could be increased by 3 dB each 4.2 s. After the emission of 13 signals, corresponding to 50 s, the TTSonset for a single exposure could be exceeded. This would give the harbor porpoise sufficient time to leave the area of highest intensity, until the actual flow velocity measurement starts. Such an adjustment represents an effective noise mitigation and should be considered, if technically feasible. The underwater measurements revealed that the acoustic flowmeters on the northern and southern bank of the Elbe River were misaligned. Accordingly, an adjustment of the orientation could allow for lower source levels, without any loss in quality of the results. A source level reduction of the flowmeter was already instructed by the HPA to improve the current situation.
Due to the lack of an established monitoring program of harbor porpoises in the Elbe River, we do not have any information on whether harbor porpoises regularly occur close to the acoustic flowmeters. Harbor porpoises frequently pass the sound field of the acoustic flowmeters as they are sighted up- and downstream (Wenger et al., 2016). An exposure to high received levels could be tolerated, if the expected benefit is high. We do not have detailed information on the overall application of these devices in large river systems. The HPA runs two more flowmeters upstream the Elbe River in addition to the flowmeter in the port of Hamburg (pers. comm. HPA). The presence of harbor porpoise in this area is assumed lower, but the current database is insufficient for reliable estimates. Additional flowmeters could be applied along the Elbe River or other river systems by different operators, as well. It is reasonable to assume that harbor porpoises have to pass other acoustic barriers during their annual upstream travel. A mandatory authorization process for acoustic devices should be carefully considered, if effects on the hearing of marine mammals can be expected.
Conclusion
The distribution of harbor porpoises largely overlaps with areas where anthropogenic activities take place. A perfect management of these activities would prevent any negative impact on harbor porpoise health or behavior but simultaneously maintain anthropogenic activities. This case study casts a new light on the application of acoustic devices without considering biological consequences. The acoustic flowmeter has the potential to induce TTS in harbor porpoises. Decreasing the source level and increasing the inter-pulse intervals could help to mitigate potential effects on harbor porpoise hearing. Effects on spatial distribution of present harbor porpoises and seals are likely. The occurrence of harbor porpoise in this area suggests that good reasons for a 97 km upstream travel exists. Information on harbor porpoise presence and habitat usage is missing, which underscores the need for a monitoring program along the Elbe River. Unfortunately, further examples of incautious noise emission exist (Findlay et al., 2021). This emphasizes the need for a mandatory authorization process prior to the use of underwater sound for any purpose with potential effects on aquatic life. Such an authorization process should carefully consider potential effects for target and non-target species.
Data Availability Statement
The raw data supporting the conclusions of this article will be made available by the authors, without undue reservation.
Ethics Statement
The animal study was reviewed and approved by Danish Ministry of Food, Agriculture and Fisheries.
Author Contributions
AR, MS and US were involved in designing the project and applying for the project grant. AR and TS prepared the underwater noise measurements, which were conducted by AR, TS and MS. The underwater noise recordings were analysed by MS. Hearing tests with a harbour porpoise in human care were performed by AR. All analyses to evaluate the TTS potential for a harbour porpoise in the sound field of the acoustic flowmeters were performed by TS under supervision of AR, JS and US. All authors provided critical feedback and helped to shape the research and analysis. The manuscript was written by TS with supportive contributions to the final version of all authors. All authors contributed to the article and approved the submitted version.
Funding
Underwater noise measurements and biological impact assessments within this study were funded by the Hamburg Port Authority (HPA). The investigations on harbor porpoise hearing were part of the research carried out during the project “Impacts of underwater noise on marine vertebrates—Cluster 7” (Grant No. Z 1.2–53302/2010/14), funded by the Federal Agency for Nature Conservation (BfN). This Open Access publication was funded by the Deutsche Forschungsgemeinschaft (DFG, German Research Foundation) - 491094227 "Open Access Publication Costs" and the University of Veterinary Medicine Hannover, Foundation.
Conflict of Interest
Author MS was employed by company DW-ShipConsult GmbH.
The remaining authors declare that the research was conducted in the absence of any commercial or financial relationships that could be construed as a potential conflict of interest.
Publisher’s Note
All claims expressed in this article are solely those of the authors and do not necessarily represent those of their affiliated organizations, or those of the publisher, the editors and the reviewers. Any product that may be evaluated in this article, or claim that may be made by its manufacturer, is not guaranteed or endorsed by the publisher.
Acknowledgments
We would like to thank the Hamburg Port Authority for vessel time and the crew of the vessel “Reinhard Woltman” (GER) for great support during noise recordings. We would like to express our gratitude to the team of animal trainers of the Fjord & Bælt Centre for the huge effort in training and handling the harbor porpoise prior to and during experiments. We are grateful to Magnus Wahlberg from the Marine Biological Research Center of the University of Southern Denmark for continuous support in the experiments with harbor porpoises.
References
Addink M. J., Smeenk C. (1999). The Harbour Porpoise (Phocoena Phocoena) in Dutch Coastal Waters: Analysis of Stranding Records for the Period 1920-1994. Lutra 41, 55–80.
Andrew R. K., Howe B. M., Mercer J. A., Dzieciuch M. A. (2002). Ocean Ambient Sound: Comparing the 1960s With the 1990s for a Receiver Off the California Coast. Acoust. Res. Lett. Online 3, 65–70. doi: 10.1121/1.1461915
ASCOBANS Advisory Committee (2014). Review of New Information on Threats to Small Cetaceans. in 21st ASCOBANS Advisory Committee Meeting, Gothenburg, Sweden, 29 September - 1 October 2014 AC21/Inf.3.2.2.a (P) (ASCOBANS). Available at: https://www.ascobans.org/sites/default/files/document/AC21_Inf_3.2.2.a_German_Sound_Protection_Concept.pdf
Brandt M. J., Dragon A. C., Diederichs A., Bellmann M. A., Wahl V., Piper W., et al. (2018). Disturbance of Harbour Porpoises During Construction of the First Seven Offshore Wind Farms in Germany. Mar. Ecol. Prog. Ser. 596, 213–232. doi: 10.3354/meps12560
Brandt M. J., Höschle C., Diederichs A., Betke K., Matuschek R., Nehls G. (2013a). Seal Scarers as a Tool to Deter Harbour Porpoises From Offshore Construction Sites. Mar. Ecol. Prog. Ser. 475, 291–302. doi: 10.3354/meps10100
Brandt M. J., Höschle C., Diederichs A., Betke K., Matuschek R., Witte S., et al. (2013b). Far-Reaching Effects of a Seal Scarer on Harbour Porpoises, Phocoena Phocoena. Aquat. Conserv. Mar. Freshw. Ecosyst. 23, 222–232. doi: 10.1002/aqc.2311
Camphuysen K. (2004). The Return of the Harbour Porpoise (Phocoena Phocoena) in Dutch Coastal Waters. Lutra 47, 135–144.
Curé C., Isojunno S., Visser F., Wensveen P., Sivle L., Kvadsheim P., et al. (2016). Biological Significance of Sperm Whale Responses to Sonar: Comparison With Anti-Predator Responses. Endanger. Species Res. 31, 89–102. doi: 10.3354/esr00748
Dähne M., Gilles A., Lucke K., Peschko V., Adler S., Krügel K., et al. (2013). Effects of Pile-Driving on Harbour Porpoises (Phocoena Phocoena) at the First Offshore Wind Farm in Germany. Environ. Res. Lett. 8, 025002 doi: 10.1088/1748-9326/8/2/025002. 025002.
Erbe C., Marley S. A., Schoeman R. P., Smith J. N., Trigg L. E., Embling C. B. (2019). The Effects of Ship Noise on Marine Mammals—A Review. Front. Mar. Sci. 6. doi: 10.3389/fmars.2019.00606
Erbe C., Reichmuth C., Cunningham K., Lucke K., Dooling R. (2016). Communication Masking in Marine Mammals: A Review and Research Strategy. Mar. pollut. Bull. 103, 15–38. doi: 10.1016/j.marpolbul.2015.12.007
European Union (1992). Council Directive 92/43/EEC of 21 May 1992. Off. J. Eur. Communities 35, 7–51. Available at: http://data.europa.eu/eli/dir/1992/43/oj
European Union (2004) Council Regulation (EC) No 812/2004 of 26.4.2004 Laying Down Measures Concerning Incidental Catches of Cetaceans in Fisheries and Amending Regulation (EC) No 88/89. Available at: https://eur-lex.europa.eu/legal-content/DE/TXT/?uri=CELEX:32004R0812
Filadelfo R., Mintz J., Michlovich E., D’Amico A., Tyack P. L., Ketten D. R. (2009). Correlating Military Sonar Use With Beaked Whale Mass Strandings: What Do the Historical Data Show? Aquat. Mamm. 35, 435–444. doi: 10.1578/AM.35.4.2009.435
Findlay C. R., Aleynik D., Farcas A., Merchant N. D., Risch D., Wilson B. (2021). Auditory Impairment From Acoustic Seal Deterrents Predicted for Harbour Porpoises in a Marine Protected Area. J. Appl. Ecol. 58, 1631–42. doi: 10.1111/1365-2664.13910. 1365-2664.13910.
Finneran J. J. (2009). Evoked Response Study Tool: A Portable, Rugged System for Single and Multiple Auditory Evoked Potential Measurements. J. Acoust. Soc Am. 126, 491–500. doi: 10.1121/1.3148214
Finneran J. J. (2015). Noise-Induced Hearing Loss in Marine Mammals : A Review of Temporary Threshold Shift Studies From 1996 to 2015. J. Acoust. Soc Am. 138, 1702–1726. doi: 10.1121/1.4927418
Finneran J. J., Carder D. A., Schlundt C. E., Dear R. L. (2010a). Growth and Recovery of Temporary Threshold Shift at 3 kHz in Bottlenose Dolphins: Experimental Data and Mathematical Models. J. Acoust. Soc Am. 127, 3256–3266. doi: 10.1121/1.3372710
Finneran J. J., Carder D. A., Schlundt C. E., Dear R. L. (2010b). Temporary Threshold Shift in a Bottlenose Dolphin (Tursiops Truncatus) Exposed to Intermittent Tones. J. Acoust. Soc Am. 127, 3267–3272. doi: 10.1121/1.3377052
Finneran J. J., Houser D. S., Blasko D., Hicks C., Hudson J., Osborn M. (2008). Estimating Bottlenose Dolphin (Tursiops Truncatus) Hearing Thresholds From Single and Multiple Simultaneous Auditory Evoked Potentials. J. Acoust. Soc Am. 123, 542–551. doi: 10.1121/1.2812595
Francis C. D., Barber J. R. (2013). A Framework for Understanding Noise Impacts on Wildlife: An Urgent Conservation Priority. Front. Ecol. Environ. 11, 305–313. doi: 10.1890/120183
Gaskin D. E., Arnold P. W., Blair B. A. (1974). Phocoena Phocoena. Mamm. Species, 42, 1–8. https://www.jstor.org/stable/3504041. Available at: https://www.jstor.org/stable/3504041
Gilles A., Viquerat S., Becker E. A., Forney K. A., Geelhoed S. C. V., Haelters J., et al. (2016). Seasonal Habitat-Based Density Models for a Marine Top Predator, the Harbor Porpoise, in a Dynamic Environment. Ecosphere 7, e01367. doi: 10.1002/ecs2.1367
Götz T., Janik V. M. (2013). Acoustic Deterrent Devices to Prevent Pinniped Depredation: Efficiency, Conservation Concerns and Possible Solutions. Mar. Ecol. Prog. Ser. 492, 285–302. doi: 10.3354/meps10482
Graham I. M., Merchant N. D., Farcas A., Barton T. R., Cheney B., Bono S., et al. (2019). Harbour Porpoise Responses to Pile-Driving Diminish Over Time. R. Soc Open Sci. 6, 190335. doi: 10.1098/rsos.190335
Hammond P. S., Lacey C., Gilles A., Viquerat S., Börjesson P., Herr H., et al. (2017) Estimates of Cetacean Abundance in European Atlantic Waters in Summer 2016 From the SCANS-III Aerial and Shipboard Surveys. Available at: https://synergy.st-andrews.ac.uk/scans3/files/2017/04/SCANS-III-design-based-estimates-2017-04-28-final.pdf.
Häpke L. (1880). Fische Und Fischerei Im Wesergebiete. Abhandlungen Des. Naturwissenschaftlichen Vereins zu Bremen 6, 577–616. Available at: https://www.zobodat.at/pdf/Abh-natwiss-Verein-Bremen_6_0577-0616.pdf
Hoekendijk J. P. A., Spitz J., Read A. J., Leopold M. F., Fontaine M. C. (2018). Resilience of Harbor Porpoises to Anthropogenic Disturbance: Must They Really Feed Continuously? Mar. Mammal Sci. 34, 258–264. doi: 10.1111/mms.12446
Isojunno S., Curé C., Kvadsheim P. H., Lam F. P. A., Tyack P. L., Wensveen P. J., et al. (2016). Sperm Whales Reduce Foraging Effort During Exposure to 1-2 kH Z Sonar and Killer Whale Sounds. Ecol. Appl. 26, 77–93. doi: 10.1890/15-0040.1/suppinfo
Kastelein R. A., Bunskoek P., Hagedoorn M., Au W. W. L., de Haan D. (2002). Audiogram of a Harbor Porpoise (Phocoena Phocoena) Measured With Narrow-Band Frequency-Modulated Signals. J. Acoust. Soc Am. 112, 334–344. doi: 10.1121/1.1480835
Kastelein R., Gransier R., Schop J., Hoek L. (2015a). Effects of Exposure to Intermittent and Continuous 6–7 kHz Sonar Sweeps on Harbor Porpoise (Phocoena Phocoena) Hearing. J. Acoust. Soc Am. 137, 1623–1633. doi: 10.1121/1.4916590
Kastelein R. A., Helder-Hoek L., Cornelisse S., Huijser L. A. E., Gransier R. (2019a). Temporary Hearing Threshold Shift in Harbor Porpoises (Phocoena Phocoena) Due to One-Sixth-Octave Noise Band at 32 kHz. Aquat. Mamm. 45, 549–562. doi: 10.1578/AM.45.5.2019.549
Kastelein R. A., Helder-Hoek L., Covi J., Gransier R. (2016). Pile Driving Playback Sounds and Temporary Threshold Shift in Harbor Porpoises (Phocoena Phocoena): Effect of Exposure Duration. J. Acoust. Soc Am. 139, 2842–2851. doi: 10.1121/1.4948571
Kastelein R. A., Helder-Hoek L., Gransier R. (2019b). Frequency of Greatest Temporary Hearing Threshold Shift in Harbor Seals (Phoca Vitulina) Depends on Fatiguing Sound Level. J. Acoust. Soc Am. 145, 1353–1362. doi: 10.1121/1.5092608
Kastelein R., Helder-Hoek L., Gransier R., Terhune J. M., Jennings N., Jong C. A. F. (2015b). Hearing Thresholds of Harbor Seals (Phoca Vitulina) for Playbacks of Seal Scarer Signals, and Effects of the Signals on Behavior. Hydrobiologia 756, 75–88. doi: 10.1007/s10750-014-2152-6
Kastelein R., Hoek L., Gransier R., de Jong C. A. F., Terhune J. M., Jennings N. (2014a). Hearing Thresholds of a Harbor Porpoise (Phocoena Phocoena) for Playbacks of Seal Scarer Signals, and Effects of the Signals on Behavior. Hydrobiologia 756, 89–103. doi: 10.1007/s10750-014-2035-x
Kastelein R. A., Hoek L., Gransier R., Rambags M., Claeys N. (2014b). Effect of Level, Duration, and Inter-Pulse Interval of 1-2 kHz Sonar Signal Exposures on Harbor Porpoise Hearing. J. Acoust. Soc Am. 136, 412–422. doi: 10.1121/1.4883596
Kastelein R., van den Belt I., Helder-Hoek L. (2015c). Behavioral Responses of a Harbor Porpoise (Phocoena Phocoena) to 25-kHz FM Sonar Signals. Aquat. Mamm. 41, 311–326. doi: 10.1578/AM.41.3.2015.311. 2015, U.
Kastelein R. A., Van de Voorde S., Jennings N. (2018). Swimming Speed of a Harbor Porpoise (Phocoena Phocoena) During Playbacks of Offshore Pile Driving Sounds Title. Aquat. Mamm., 44, 92–99. doi: 10.1578/AM.44.1.2018.92
Kastelein R. A., Wensveen P. J., Hoek L., Terhune J. M. (2009a). Underwater Hearing Sensitivity of Harbor Seals (Phoca Vitulina) for Narrow Noise Bands Between 0.2 and 80 kHz. J. Acoust. Soc Am. 126, 476–483. doi: 10.1121/1.3132522
Kastelein R. A., Wensveen P. J., Hoek L., Verboom W. C., Terhune J. M. (2009b). Underwater Detection of Tonal Signals Between 0 . 125 and 100 kHz by Harbor Seals (Phoca Vitulina). J. Acoust. Soc Am. 125, 1222–1229. doi: 10.1121/1.3050283
Kennish M. J. (2002). Environmental Threats and Environmental Future of Estuaries. Environ. Conserv. 29, 78–107. doi: 10.1017/S0376892902000061
King S. L., Schick R. S., Donovan C., Booth C. G., Burgman M., Thomas L., et al. (2015). An Interim Framework for Assessing the Population Consequences of Disturbance. Methods Ecol. Evol. 6, 1150–1158. doi: 10.1111/2041-210X.12411
Kölmel R. (1998). Wale Aus Der Elbe, 340 Jahre Funde Und Fang. Schriftreihen Natureum Niederelbe 2, 48–49.
Kragh I. M., McHugh K., Wells R. S., Sayigh L. S., Janik V. M., Tyack P. L., et al. (2019). Signal-Specific Amplitude Adjustment to Noise in Common Bottlenose Dolphins (Tursiops Truncatus). J. Exp. Biol. 22, jeb216606. doi: 10.1242/jeb.216606. jeb216606.
Kunc H. P., McLaughlin K. E., Schmidt R. (2016). Aquatic Noise Pollution: Implications for Individuals, Populations, and Ecosystems. Proc. R. Soc B Biol. Sci. 283, 20160839 doi: 10.1098/rspb.2016.0839. 20160839.
Ladich F., Fay R. R. (2013). Auditory Evoked Potential Audiometry in Fish. Rev. Fish Biol. Fish. 23, 317–64 doi: 10.1007/s11160-012-9297-z
Leopold M. F. (2015) Eat and be Eaten - Porpoise Diet Studies. Available at: https://www.wur.nl/upload_mm/6/a/a/8ba64cfe-3209-4f3f-a6dc-08d2647b11c4_Leopold.2015 PhD thesis Eat and be eaten-Porpoise diet studies.pdf.
Loecher M., Ropkins K. (2015)RgoogleMaps and Loa: Unleashing R Graphics Power on Map Tiles (Accessed June 10, 2021).
Lucke K., Siebert U., Lepper P. A., Blanchet M.-A. (2009). Temporary Shift in Masked Hearing Thresholds in a Harbor Porpoise (Phocoena Phocoena) After Exposure to Seismic Airgun Stimuli. J. Acoust. Soc Am. 125, 4060–4070. doi: 10.1121/1.3117443
Mahoney P. C., Bishop M. J. (2017). Assessing Risk of Estuarine Ecosystem Collapse. Ocean Coast. Manage. 140, 46–58. doi: 10.1016/J.OCECOAMAN.2017.02.021
Mann D., Hill-Cook M., Manire C., Greenhow D., Montie E., Powell J., et al. (2010). Hearing Loss in Stranded Odontocete Dolphins and Whales. PLos One 5, e13824. doi: 10.1371/journal.pone.0013824
Mooney T. A., Nachtigall P. E., Breese M., Vlachos S., Au W. W. L. (2009). Predicting Temporary Threshold Shifts in a Bottlenose Dolphin (Tursiops Truncatus): The Effects of Noise Level and Duration. J. Acoust. Soc Am. 125, 1816–1826. doi: 10.1121/1.3068456
Morell M., Brownlow A., McGovern B., Raverty S. A., Shadwick R. E., André M. (2017). Implementation of a Method to Visualize Noise-Induced Hearing Loss in Mass Stranded Cetaceans. Sci. Rep. 7, 41848. doi: 10.1038/srep41848
Morell M., Ijsseldijk L. L., Berends A. J., Gröne A., Siebert U., Raverty S. A., et al. (2021a). Evidence of Hearing Loss and Unrelated Toxoplasmosis in a Free - Ranging Harbour Porpoise (Phocoena Phocoena). Animals 11, 3085. doi: 10.3390/ani11113058
Morell M., IJsseldijk L. L., Piscitelli-Doshkov M., Ostertag S., Estrade V., Haulena M., et al. (2021b). Cochlear Apical Morphology in Toothed Whales: Using the Pairing Hair Cell-Deiters’ Cell as a Marker to Detect Lesions. Anat. Rec., 305, 1–46. doi: 10.1002/ar.24680
Nachtigall P. E., Supin A. Y., Pacini A. F., Kastelein R. A. (2016). Conditioned Hearing Sensitivity Change in the Harbor Porpoise (Phocoena Phocoena). J. Acoust. Soc Am. 140, 960–967. doi: 10.1121/1.4960783
National Oceanic and Atmospheric Administration, National Marine Fisheries Service (2016). Technical Guidance for Assessing the Effects of Anthropogenic Sound on Marine Mammal Hearing: Underwater Acoustic Thresholds for Onset of Permanent and Temporary Threshold Shifts (Silver Spring). U.S. Dept. of Commer., Available at: http://www.nmfs.noaa.gov/pr/acoustics/Acoustic Guidance Files/opr-55_acoustic_guidance_tech_memo.pdf.
Otani S., Naito Y., Kato A., Kawamura A. (2000). Diving Behavior and Swimming Speed of a Free-Ranging Harbor Porpoise, Phocoena Phocoena. Mar. Mammal Sci. 16, 811–814. doi: 10.1111/j.1748-7692.2000.tb00973.x
Otani S., Naito Y., Kato A., Kawamura A. (2001). Oxygen Consumption and Swim Speed of the Harbor Porpoise Phocoena Phocoena. Fish. Sci. 67, 894–898. doi: 10.1046/j.1444-2906.2001.00338.x
Popov V. V., Supin A. Y., Rozhnov V. V., Nechaev D. I., Sysueva E. V. (2014). The Limits of Applicability of the Sound Exposure Level (SEL) Metric to Temporal Threshold Shifts (TTS) in Beluga Whales, Delphinapterus Leucas. J. Exp. Biol. 217, 1804–1810. doi: 10.1242/jeb.098814
Reichmuth C., Sills J. M., Mulsow J., Ghoul A. (2019). Long-Term Evidence of Noise-Induced Permanent Threshold Shift in a Harbor Seal (Phoca Vitulina). J. Acoust. Soc Am. 146, 2552–2561. doi: 10.1121/1.5129379
Rojano-Doñate L., McDonald B. I., Wisniewska D. M., Johnson M., Teilmann J., Wahlberg M., et al. (2018). High Field Metabolic Rates of Wild Harbour Porpoises. J. Exp. Biol. 221, jeb185827 doi: 10.1242/jeb.185827. jeb185827.
Rolland R. M., Parks S. E., Hunt K. E., Castellote M., Corkeron P. J., Nowacek D. P., et al. (2012). Evidence That Ship Noise Increases Stress in Right Whales. Proc. R. Soc B Biol. Sci. 279, 2363–2368. doi: 10.1098/rspb.2011.2429
Ruser A., Dähne M., van Neer A., Lucke K., Sundermeyer J., Siebert U., et al. (2016). Assessing Auditory Evoked Potentials of Wild Harbor Porpoises (Phocoena Phocoena). J. Acoust. Soc Am. 140, 442–452. doi: 10.1121/1.4955306
Sørensen P. M., Wisniewska D. M., Jensen F. H., Johnson M., Teilmann J., Madsen P. T. (2018). Click Communication in Wild Harbour Porpoises (Phocoena Phocoena). Sci. Rep. 8, 9702. doi: 10.1038/s41598-018-28022-8
Sarnocińska J., Teilmann J., Balle J. D., van Beest F. M., Delefosse M., Tougaard J. (2020). Harbor Porpoise (Phocoena Phocoena) Reaction to a 3D Seismic Airgun Survey in the North Sea. Front. Mar. Sci. 6. doi: 10.3389/fmars.2019.00824
Schaffeld T., Ruser A., Woelfing B., Baltzer J., Kristensen J. H., Larsson J., et al. (2019). The Use of Seal Scarers as a Protective Mitigation Measure can Induce Hearing Impairment in Harbour Porpoises. J. Acoust. Soc Am. 146, 4288–4298. doi: 10.1121/1.5135303
Siebert U., Stürznickel J., Schaffeld T., Oheim R., Rolvien T., Prenger-Berninghoff E., et al. (2022). Blast Injury on Harbour Porpoises (Phocoena Phocoena) From the Baltic Sea After Explosions of Deposits of World War II Ammunition. Environ. Int. 159, 107014. doi: 10.1016/j.envint.2021.107014. 107014.
Southall B. L., Finneran J. J., Reichmuth C., Nachtigall P. E., Ketten D. R., Bowles A. E., et al. (2019). Marine Mammal Noise Exposure Criteria: Updated Scientific Recommendations for Residual Hearing Effects. Aquat. Mamm. 45, 125–232. doi: 10.1578/am.45.2.2019.125
Sueur J., Aubin T., Simonis C. (2008). Seewave: A Free Modular Tool for Sound Analysis and Synthesis. Bioacoustics 18, 213–226. doi: 10.1080/09524622.2008.9753600
Taupp T. (2019). Seehunde in Der Tideelbe Zwischen Hamburg Und Cuxhaven 2018/2019. Koblenz: Bundesanstalt für Gewässerkunde. 31p doi: 10.5675/BfG-1996
Taupp T. (2021). Against All Odds: Harbor Porpoises Intensively Use an Anthropogenically Modified Estuary. Mar. Mammal Sci., 1–16. doi: 10.1111/mms.12858
Thiel R. (2015). City: Hamburg, publisher: Freie und Hansestadt Hamburg, Behörde für Stadtentwicklung und Umwelt, Amt für Natur- und Ressourcenschutz, Abteilung Naturschutz. https://epub.sub.uni-hamburg.de/epub/volltexte/2015/38650/pdf/download_fischgutachten_2015.pdf
Tougaard J., Wright A. J., Madsen P. T. (2015). Cetacean Noise Criteria Revisited in the Light of Proposed Exposure Limits for Harbour Porpoises. Mar. pollut. Bull. 90, 196–208. doi: 10.1016/j.marpolbul.2014.10.051
Villadsgaard A., Wahlberg M., Tougaard J. (2007). Echolocation Signals of Wild Harbour Porpoises, Phocoena Phocoena. J. Exp. Biol. 210, 56–64. doi: 10.1242/jeb.02618
von Benda-Beckmann A. M., Aarts G., Sertlek H.Ö., Lucke K., Verboom W. C., Kastelein R. A., et al. (2015). Assessing the Impact of Underwater Clearance of Unexploded Ordnance on Harbour Porpoises (Phocoena Phocoena) in the Southern North Sea. Aquat. Mamm. 41, 503–523. doi: 10.1578/AM.41.4.2015.503
Ward W. D., Cushing E. M., Burns E. M. (1976). Effective Quiet and Moderate TTS: Implications for Noise Exposure Standards. J. Acoust. Soc Am. 59, 160–165. doi: 10.1121/1.380835
Wenger D., Koschinski S. (2012). Harbour Porpoise (Phocoena Phocoena Linnaeus 1758) Entering the Weser River After Decades of Absence. Mar. Biol. Res. 8, 737–745. doi: 10.1080/17451000.2012.676184
Wenger D., Siebert U., Hennig V. (2016). “The Return of Harbour Porpoises (Phocoena Phocoena) to the Lower Elbe and Weser Rivers , Following Anadromous Fish Shoals , Foraging in Hamburg Harbour,” in Conference of the European Cetacean Society, Poster Presentation. Available at: https://www.researchgate.net/publication/318317169_The_return_of_harbour_porpoises_Phocoena_phocoena_to_the_lower_Elbe_and_Weser_rivers_following_anadromous_fish_shoals_foraging_in_Hamburg_harbour.
Wisniewska D. M. M., Johnson M., Teilmann J., Rojano-Doñate L., Shearer J., Sveegaard S., et al. (2016). Ultra-High Foraging Rates of Harbor Porpoises Make Them Vulnerable to Anthropogenic Disturbance. Curr. Biol. 26, 1441–1446. doi: 10.1016/j.cub.2016.03.069
Wisniewska D. M., Johnson M., Teilmann J., Siebert U., Galatius A., Dietz R., et al. (2018). High Rates of Vessel Noise Disrupt Foraging in Wild Harbour Porpoises (Phocoena Phocoena). Proc. R. Soc B Biol. Sci. 285, 20172314 doi: 10.1098/rspb.2017.2314. 20172314.
Keywords: harbor porpoise Phocoena phocoena, noise pollution, temporary threshold shift (TTS), noise mitigation, hearing
Citation: Schaffeld T, Schnitzler JG, Ruser A, Baltzer J, Schuster M and Siebert U (2022) A Result of Accidental Noise Pollution: Acoustic Flowmeters Emit 28 kHz Pulses That May Affect Harbor Porpoise Hearing. Front. Mar. Sci. 9:892050. doi: 10.3389/fmars.2022.892050
Received: 08 March 2022; Accepted: 06 June 2022;
Published: 07 July 2022.
Edited by:
Dorian S. Houser, National Marine Mammal Foundation, United StatesCopyright © 2022 Schaffeld, Schnitzler, Ruser, Baltzer, Schuster and Siebert. This is an open-access article distributed under the terms of the Creative Commons Attribution License (CC BY). The use, distribution or reproduction in other forums is permitted, provided the original author(s) and the copyright owner(s) are credited and that the original publication in this journal is cited, in accordance with accepted academic practice. No use, distribution or reproduction is permitted which does not comply with these terms.
*Correspondence: Tobias Schaffeld, dG9iaWFzLnNjaGFmZmVsZEB0aWhvLWhhbm5vdmVyLmRl