- 1Biology Laboratory, Istituto Centrale per il Restauro (ICR), Ministry of Culture (MIC), external collaborator, Rome, Italy
- 2Bio.Co.Ré. Laboratory, Scurcola Marsicana, Italy
- 3Department of Life and Environmental Sciences - DiSVA, Università Politecnica delle Marche, Ancona, Italy
- 4Department of Environmental Biology, Sapienza University of Rome, Rome, Italy
- 5Department of Biology, “Tor Vergata” University of Rome, Rome, Italy
- 6National Inter-University Consortium for Marine Sciences (CoNISMa), Rome, Italy
- 7Biology Laboratory, Istituto Centrale per il Restauro (ICR), Ministry of Culture (MIC), Rome, Italy
Bioerosion is the destruction of hard substrates resulting from biological activity, and plays a relevant role in the ecological interactions and coastal dynamics processes. Several organisms have evolved structures and behaviors allowing them to perforate biotic and abiotic surfaces, transforming hard surfaces into particles, and contributing significantly to sediment production in the coastal and marine environment. Due to the large geographical diffusion of marine borers, bioerosion is relevant in many scientific and applied fields of interest. Most bioerosion studies have hitherto been conducted in tropical areas, where borers are a critical component of coral reef destruction. Comparatively, little information is available for the bioerosion of submerged archeological heritage. This review focuses on the bioerosion of archeological calcareous artifacts in the Mediterranean Sea, summarizing studies concerning the colonization of statues, shipwrecks, cargo, and the remains of submerged cities. The paper includes the first comprehensive listing of the archeological sites in the Mediterranean Sea where bioerosion has been assessed. The diversity of boring organisms affecting marine archeological remains and their boring patterns, the various types of bored materials, and the severity of the damage caused to heritage artifacts are also included. Both microborers (algae, fungi, and cyanobacteria) and macroborers (sponges, bivalves, polychaetes, sipunculids, and echinoids) are considered, and their roles in the structuring of endolithic assemblages are also covered. The experimental techniques currently employed to analyze bioerosion traces, helping to identify particular species and ichnospecies and their ecological dynamics, are also considered. Finally, a discussion of the current strategies proposed for the in situ protection and conservation of Underwater Cultural Heritage is provided.
Introduction
The Mediterranean Sea represents one of the most peculiar basins of the world, due to its geographical location, its geomorphological features, and its shape. It is the largest and deepest semi-closed sea on Earth, and thus historically identified as “the Sea between the lands”. Due to morphology, historical patterns, as well as favorable climatic and hydrodynamic conditions, the Mediterranean basin has been colonized by humans since ancient times. The foundation of Jericho dates back to almost 9,000 years BC, and it is considered one of the oldest cities in the world. However, other ancient settlements have been found all along the Mediterranean coasts giving rise to magnificent civilizations. The shape and the hydrodynamic regime of the Mediterranean Sea facilitated trades and commercial routes, which allowed for easier connection and communication among the different populations around its basins. Given the long history of such marine traffic in the Mediterranean, several shipwrecks have occurred in the past. Parker (1992) reported 1,189 shipwrecks and abandoned hulls dating back to before AD 1500, discovered all over the Mediterranean Sea. In the past twenty years, however, the number of discovered shipwrecks has increased by the hundreds, due to technological progress helping underwater archeology and marine exploration (Ballard et al., 2000; Bingham et al., 2010; Williams et al., 2016). Therefore, an accurate quantification of ancient artifacts and materials lying on the Mediterranean Sea bottoms is not easily achievable. These underwater archeological remains are a significant portion of the worldwide cultural heritage, as well as high-value historical finds. They provide information on ancient technologies, exchanges of material and resources, contributing to the reconstruction of the origins of important historical events among Mediterranean civilizations (Davidde Petriaggi and Petriaggi, 2015; Ricca and La Russa, 2020). Therefore, their conservation has been promoted since 2001 through the UNESCO Convention on the Protection of the Underwater Cultural Heritage (UCH), which defends such remains from destruction and looting, encourages in situ conservation, prohibits commercial exploitation, and promotes cooperation and information exchange among States Parties (UNESCO, 2001).
All submerged solid surfaces are subjected to seawater physicochemical aggression and to colonization by marine organisms, which transform the aesthetic and functional features of their material, altering its composition and structure, and causing its decay (Crisci et al., 2010). The action of hydrological (i.e., salinity, temperature, oxygen content, and ionic concentration) and hydrodynamic (i.e., waves, currents, and sedimentation rate) processes physico-chemically modifies materials through oxidation, corrosion, and physical damages. The erosion of the material can also be biologically mediated, through the activity of marine organisms. They exhibit two main alteration patterns as they interact with underwater substrates: epilithics settle on the material’s surface by fixing their calcareous structures (i.e., shells, skeletons), thus encrusting solid substrates with carbonate deposits; endolithics are able to settle in or actively perforate cavities, and burrows in both natural and artificial substrates.
Bioerosion: Essential Bibliographic Review
The word “bioerosion” was first used by Neumann (1966) to describe the complex erosive processes resulting from the activity of various organisms and causing the destruction of hard substrates. Since the degradation of rocks is caused by organisms which produce permanent traces, bioerosion represents an across-the-board topic, embracing several different fields of science: biology, geology, ecology, paleontology, sedimentology, as well as archeology. The study of bioerosion phenomena has also involved various aspects of scientific and historical knowledge (Ekdale et al., 1984) by using different approaches.
In the marine environment bioerosion acts on different materials. Rocks containing soluble minerals such as carbonates, phosphates, and sulfates are affected by: a) chemical processes promoting the dissolution of substrates and b) mechanical processes, such as abrasion, caused by boring and rasping organisms (Golubic and Schneider, 1979; Tribollet et al., 2011; Schönberg et al., 2017a). As for the carbonates, marine organisms cause both precipitation and erosion of calcium carbonate. Bioerosion is the antagonist reaction of the bioconstruction process. Epilithic bioconstructors and borers play opposing roles: the former create new encrusting calcareous layers through their skeletal elements (Ingrosso et al., 2018), the latter dissolve rocks and produce new fine sediments. This new carbonate deposit represents a suitable material used as growth substrate for bioeroders (Spencer and Viles, 2002). Carbonate accretion and bioerosion rate affect the habitat structure of both tropical coral reefs (Hutchings, 1986) and coralligenous reefs in the Mediterranean Sea (Turicchia et al., 2022), with remarkable effects on biodiversity and biogeochemical cycling. Bioerosion acts over a wide range of natural substrates, such as encrusting coralline algal thalli and calcareous skeletons of living and dead organisms (Le Campion-Alsumard et al., 1995; Tribollet and Payri, 2001; Hutchings and Peyrot-Clausade, 2002; Bick, 2006; Smith et al., 2015; Meyer et al., 2021; Ramírez-Viaña et al., 2021). Bioerosion processes occur at different depths, from coastal to bathyal rocks in tropical, temperate (Coombes et al., 2011; Wisshak et al., 2011; Weinstein et al., 2019), and polar ecosystems (Meyer et al., 2021). The removal of calcium carbonate carried out by bioeroders has a pivotal role in the carbonate cycle, and therefore has far-reaching ecological implications, especially in coral reef ecosystems. The recycle of materials, accretion and formation of different habitats create suitable environments for the settlement of other taxa, facilitating larval recruitment, and producing sediment (Hutchings, 2011; Tribollet and Golubic, 2011; Perry and Harborne, 2016; Weinstein et al., 2019).
Golubic et al. (1981) proposed a revision of the terminology previously used by Friedmann et al. (1967) to describe the lithobiontic ecological niches of endolithic organisms defining: i) euendoliths the organisms that penetrate actively into the interior of rocks, forming tunnels modeled on the shapes of their bodies; ii) cryptoendoliths, which colonize the structural cavities of porous rocks, including the spaces produced and vacated by euendoliths; iii) chasmoendoliths, those organisms colonizing fissures and cracks in the rock. There have been also several other categorization proposals for euendoliths based on the scope of their boring activity (e.g., food or shelter) or on which part of the substrate is attacked (e.g., internal or external bioeroders). Commonly, borers are divided into three main functional groups, according to the size and the way they bore hard substrates: 1) microborers (< 100 μm diameter traces); 2) grazers (or external bioeroders, as defined by Schönberg et al., 2017a); and 3) macroborers (≥ 100 μm diameter traces). Although they have been divided into three separate guilds, they all interact with each other and with the environment (Schönberg et al., 2017a). In fact, endolithic communities are controlled by abiotic (temperature, depth, light, nutrients, water chemistry, sedimentation, and substrate features) and biotic factors (competition, predation, and succession). Several articles offer in-depth analyses of how these factors shape endolithic communities in both tropical and temperate environments (Hutchings, 2011; Tribollet et al., 2011; Wisshak et al., 2011; Schönberg et al., 2017a; Schönberg et al., 2017b; Weinstein et al., 2019).
This review aims to provide the current state of the art on the bioerosion of submerged archeological lapideous artifacts in the Mediterranean Sea, specifically focusing on bioerosion of calcareous materials. In particular, are reported: a) a list of the archeological sites with bioerosion evidences in the Mediterranean Sea; b) the different activities of micro and macroborers, including the methods employed; c) the impact of bioerosion on Underwater Cultural Heritage; d) the appropriate procedures and strategies for the conservation of submerged archeological artifacts.
Archeological Study Areas in the Mediterranean Sea
Underwater archeological sites are classified in relation to the heritage they hold, such as architectural structures (e.g., submerged cities, villas, nymphaea, harbors), ancient and modern shipwrecks (cargoes), and natural caves. The Mediterranean Sea guards hundreds of different sites. Table 1 and Figure 1 resume and illustrate environmental and geographical features of the sites, the historical and archeological information, and biological studies performed.
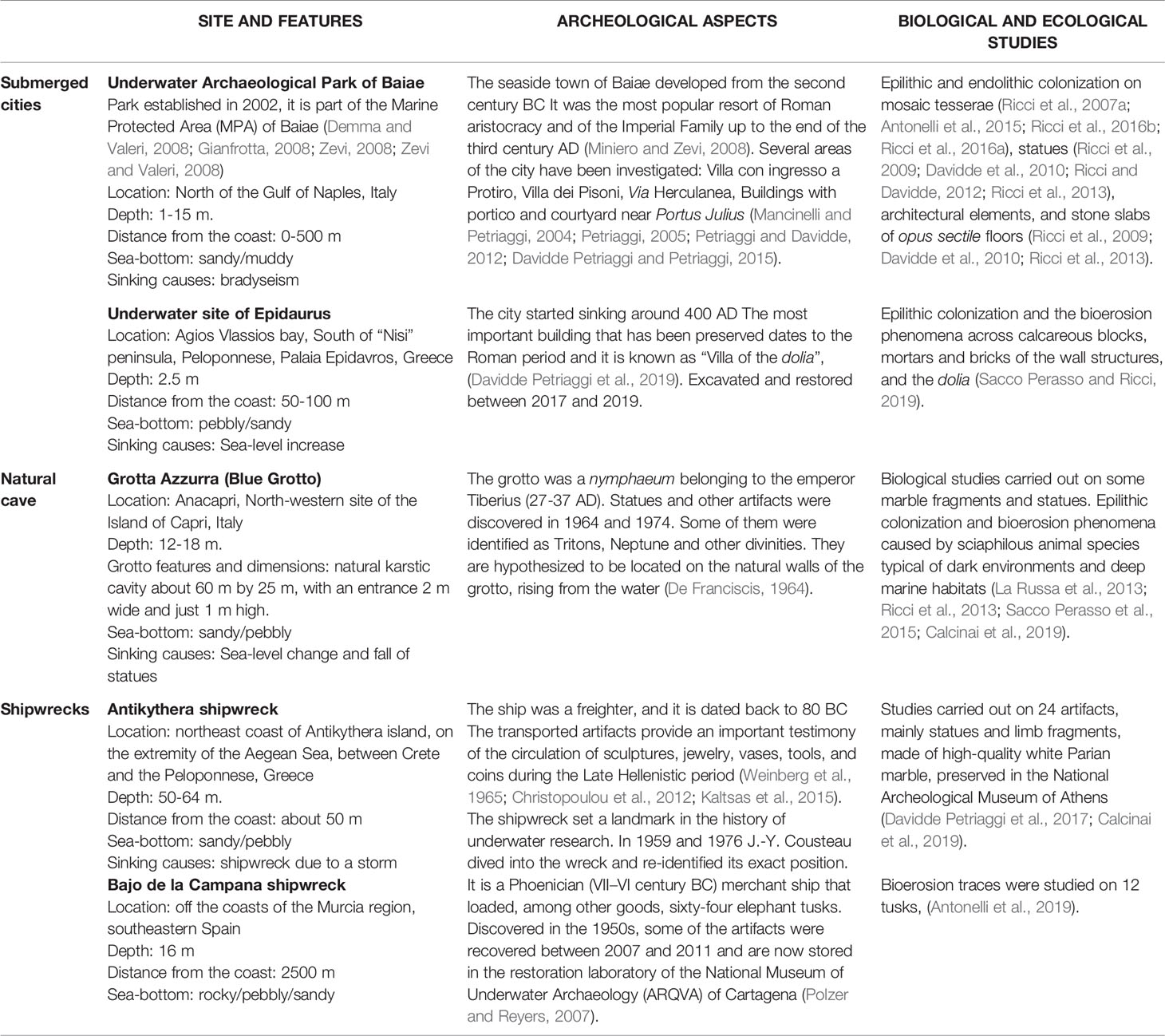
Table 1 General, archeological, biological and ecological features of the sites described in the review.
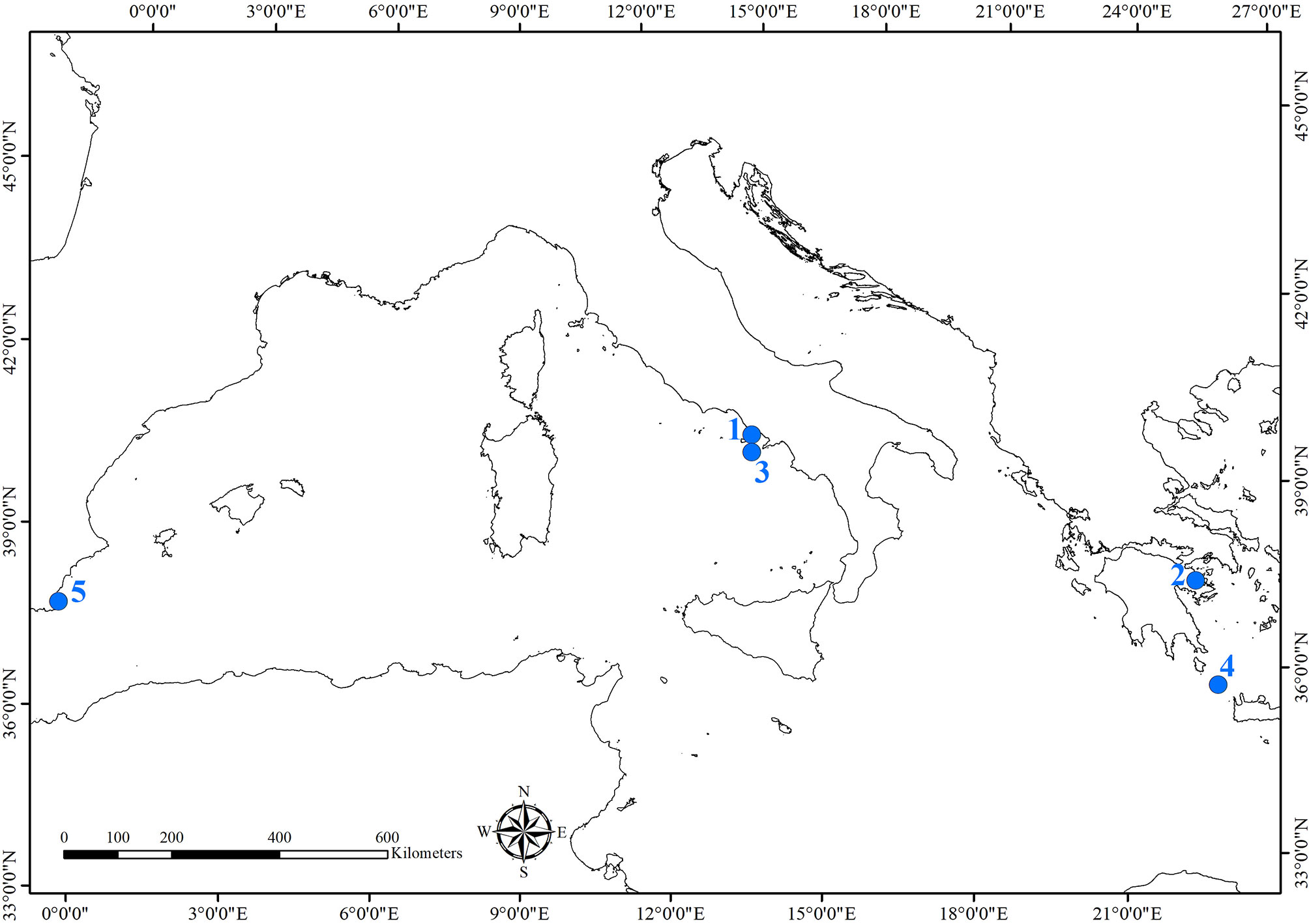
Figure 1 Map of the archeological sites where studies of the bioerosion on cultural heritage were carried out. Sites were numbered as they are cited in the text: 1) Underwater Archaeological Park of Baiae; 2) Underwater archeological site of Epidaurus; 3) Grotta Azzurra, Capri; 4) Antikythera shipwreck; 5) Bajo de la Campana shipwreck.
Bioerosion Impact on Underwater Cultural Heritage
The words “archeological remains’’ encompass all the man-made objects, structures or burials, animal or vegetal parts that have been preserved through time, independently from their origin or nature (organic or inorganic). In submerged sites, the most frequently found remains are architectural structures (i.e., walls, mosaic or opus sectile floors, decorative elements), ships parts, and their loads (i.e., wooden hulls, rams, ceramics, statues, pieces of stones to be used for architectural or decorative purposes, glass, ivory, metals). Once submerged, all these materials are subjected to biological colonization by micro and macroorganisms, which compromises their state of preservation and can generically be defined as biodeterioration. While organic materials like wood and textiles are a food source for several organisms, inorganics represent growth substrates used to anchor or take shelter. Obviously, the composition of communities colonizing the artifacts is highly influenced by the environmental features of the site (e.g., depth, light penetration, pH, salinity, hydrodynamism); anyway, a key role in the development of biodeteriogens is represented by the nature of the material. Ceramics, glass, and metals are not susceptible to boring organisms, and thus they are only subjected to superficial colonization (Ricci and Bartolini, 2005; Gravina et al., 2021a). On the other hand, ivory and stone materials (in particular those with a high carbonate concentration like calcitic marbles) can be easily perforated by chemical and mechanical action.
Boring organisms produce holes and galleries, whose shape reflects the morphology of the bioeroder. This feature is helpful in studies characterizing the bioerosion of archeological artifacts, since sometimes inside the bores there are no biological remains useful for species identification left. This can happen when the colonization had occurred in past times and for some reason the bioeroder died (for example as the artifact moved on the seabed or was covered by sediment), or when the studies have been carried out on artifacts recovered from the sea in the past and now exposed in museums. In these cases, the study of trace morphology can be carried out through optical microscope and SEM observations, or resin and silicone casting techniques (Golubic et al., 1970; Ricci et al., 2013; Antonelli et al., 2015). Several works concerning the bioerosion of UCH have turned to the paleontological approach based on the identification of ichnotaxa. Even if ichnotaxa are defined as taxa “based on the fossilized work of an organism, including fossilized trails, traces, or burrows (trace fossils) made by an animal” (ICZN, Ride et al., 1999), in the last years the use of this classification was also widely applied to modern traces, allowing to define the associations ichnospecies/biospecies. For this reason, the identification of ichnospecies found on archeological artifacts helps in the identification of bioeroders, even if no biological remains are present.
It is worth mentioning that in most cases the study of bioerosion traces implies sampling pieces of the material necessary for microscope observations and casting techniques. In the case of UCH, only a small amount of stone can be sampled, because the sampling operations cannot compromise artifact integrity.
The study of the biological colonization of archeological artifacts not only allows to define the state of preservation of the material, but can also provide important information on colonization and degradation dynamics, on the succession of time phases, and help identify the pioneer species and those that have settled later. Such information is of great importance for the knowledge of the submerged life of the artifacts and for reconstructing the features of the environment in which the objects have laid.
Bioeroders and Bioerosion Patterns
Microborers
Marine microborers are conditioned in their endolithic growth by microenvironmental, environmental, and edaphic/trophic factors (e.g., depth, light, lithotype, dimensions of the artifact, position on the seabed). Phototrophic microorganisms mainly colonize the outer part of the substrate, from the surface down to the depth of light penetration. Furthermore, sciaphilous species can grow on artifacts lying at great depths or even in shallow and shaded environments, such as caves. Chemotrophics, such as fungi, are light-independent but need organic sources such as other micro- and macro-organisms remains (Golubic et al., 2005; Tribollet et al., 2011). They are able to bore into the substrate up to several millimeters, also colonizing tunnels previously dug by macroborers like bivalves or sponges.
Cyanobacteria, microalgae, and microfungi are the main groups of microborers. They mainly bore calcareous stones and organogenic structures such as corals, shells, tests, algal thalli (Nielsen, 1987; Raghukumar and Lande, 1988; Radtke and Golubic, 2005), from the supratidal coastal spray zone to the abyssal depths (Le Campion-Alsumard, 1979; Golubic et al., 1984; Tribollet and Payri, 2001; Ghirardelli, 2002; Tribollet and Golubic, 2005). Microborers live inside the bored cavities and tunnels whose morphology varies in relation to the morphological features of the species. The bored cavities range from 1 to 200-300 µm and the boring process is carried out by acid metabolic substances (Golubic et al., 1981).
Cyanobacteria
These prokaryotic microorganisms are largely present within the endolithic assemblages responsible for the bioerosion of submerged stone artifacts. Many species of marine cyanobacteria have epilithic, endolithic or strictly euendolithic behavior. The species can grow inside the stone showing different patterns and levels of penetration, in relation to the microenvironmental features and their peculiar ecological needs. Although small in size (only a few micrometers), they form dense layers or large colonies (Figure 2A), with a considerable destructive action (Radtke and Golubic, 2005).
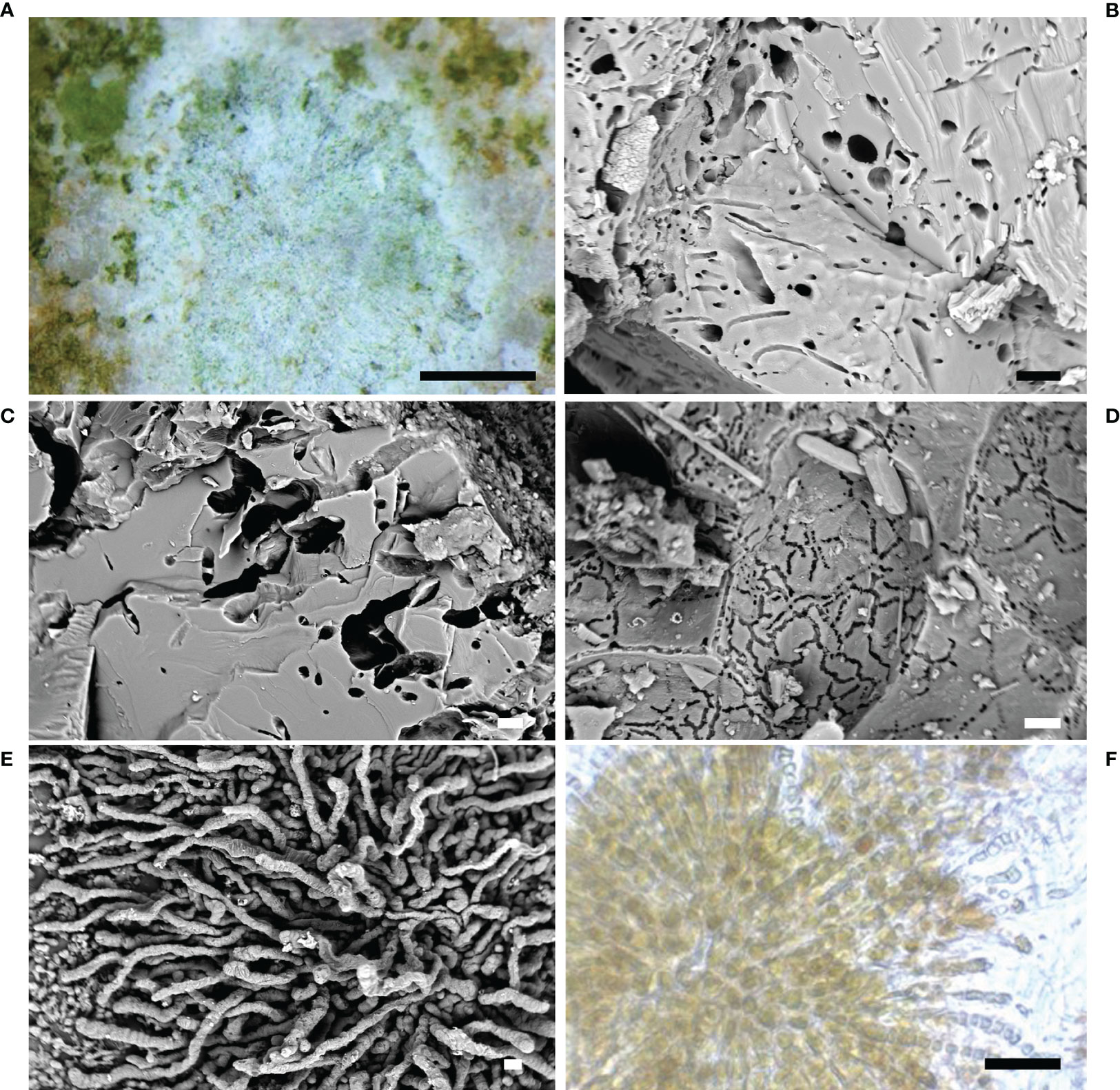
Figure 2 Cyanobacteria. (A) Cyanobacteria colonization on calcareous substrate (stereomicroscope image); (B–D) microboring caused by endolithic cyanobacteria in the stone (SEM image); (E) the ichnospecies Fascichnus dactylus (SEM image); (F) the radiated bundles of the species Hyella caespitosa (optical microscope image). Scale bars: (A) = 0.5 mm; (B–D) = 10 µm; (E, F) = 20 µm.
Endolithic cyanobacteria and their traces (i.e., holes, cavities and tunnels) (Figures 2B–D) were largely found in the submerged Archaeological Park of Baiae on statues, architectural and sculptural elements, mosaics and opus sectile pavements (Ricci, 2004; Ricci et al., 2009; Davidde et al., 2010; Ricci et al., 2013; Ricci et al., 2016a). This group was also observed on the marble statues of the decorative apparatus of the Grotta Azzurra of Capri (Sacco Perasso et al., 2015) and on several statues and stone artifacts recovered from the wreck of Antikythera (Davidde Petriaggi et al., 2017).
The ichnospecies and related biospecies found in the studied sites are listed below.
Scolecia filosa Radtke, 1991 is the trace of Plectonema terebrans Bornet and Flahault, 1889 (Ord. Oscillatoriales).
Scolecia filosa was observed on statues and architectural structures collected from the Underwater Park of Baiae (Ricci et al., 2013; Ricci et al., 2016a). This ichnospecies was mainly constituted by unbranched galleries penetrated into the stone up to one millimeter. The filaments, 1-1.5 µm in diameter, were long, flexuous, forming dense interwoven networks.
Eurygonum nodosum Schmidt, 1992 is the trace produced by the cyanobacterium Mastigocoleus testarum Lagerheim, 1886 (Ord. Stigonematales).
This ichnospecies showed curved or straight tunnels, 5-10 µm in diameter, with lateral branches and nodular swellings, 4-5 µm thick. It formed dense carpets with orthogonal branching, and lateral/terminal swellings, 6–10 µm in diameter, as traces of heterocysts. The network of tunnels was located orthogonally to the surface, up to 200- 300 µm in depth, decreasing in density in the inner parts of the substratum. Eurygonum nodosum was observed on statues and architectural structures from the underwater park of Baiae (Ricci et al., 2013; Ricci et al., 2016a).
Fascichnus dactylus Radtke, 1991 is the trace of Hyella caespitosa Bornet & Flahault, 1888 (Ord. Pleurocapsales).
The ichnospecies showed thick and straight branched tunnels, 5-8 µm in diameter, forming radiating bundles or expanded carpets (Figures 2E, F). The tunnels were slightly undulated with rounded ends. Fascichnus dactylus was observed on limestone tesserae collected from mosaic floors of the Villa dei Pisoni in Baiae (Ricci et al., 2016a).
Green Algae
This group of photosynthetic microorganisms played an important role in the bioerosion of stone artifacts. Several species dug tunnels and cavities up to the depth that allowed them to carry out photosynthesis. In many cases green algae bored deeper in the stone using the walls of cavities eroded by macroorganisms, such as sponges and bivalves.
The ichnospecies and related biospecies identified in the studied sites are listed below.
Ichnoreticulina elegans Radtke 1991 is produced by the siphonal green alga Ostreobium quekettii Bornet and Flahault, 1889 (Ord. Bryopsidales).
This ichnospecies showed several morphologies according to the different life stages of the biospecies O. quekettii. The first one was characterized by straight or winding filaments, 4-5 µm in diameter, narrowing gradually in distal direction. In the second stage, the filaments developed branches, 2-5 µm in diameter, forming zig-zag shaped networks (Figures 3A, B). The presence of numerous branched filaments produced dense horizontal networks of dorso-ventrally flattened borings (typical zig-zag pattern). Arch-like branches connected with the substrate surface were also observed. Several widenings and swellings with different shape and size, typical of the mature Ichnoreticulina, were frequently observed.
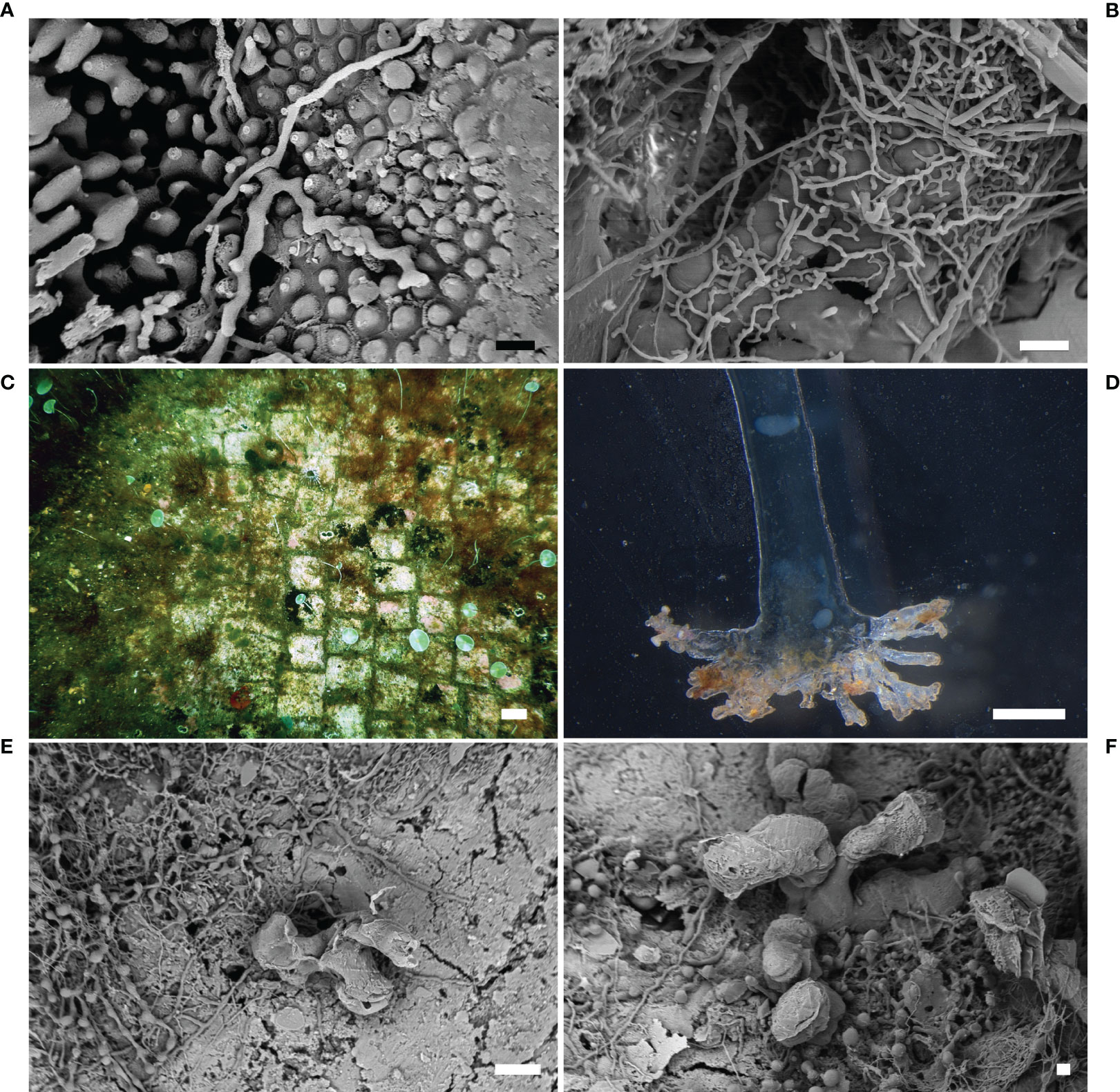
Figure 3 Green algae. (A) The ichnospecies Ichnoreticulina elegans (SEM image); (B) the typical zig-zag shaped networks of I. elegans (SEM image); (C) specimens of Acetabularia acetabulum on the calcareous marble tesserae of the Villa dei Pisoni Mosaic floor in Baiae, Naples (underwater photo); (D) endolithic rhizoid of the green alga A. acetabulum (stereomicroscope image); (E) the branched rhizoidal apparatus of the green alga A. acetabulum (SEM image); (F) detail of the branches of the endolithic rhizoid of A. acetabulum (SEM image) Scale bars: (A) = 10 µm; (B) = 20 µm; (C) = 1 cm; (D) = 0.5 mm; (E) = 100 µm; (F) = 20 µm.
The ichnospecies was found in Lunense marble statues, in slabs of opus sectile floors (Cipollino, Penthelic, Paros, Docimio, Lunense, and Ancient Red Tenario marbles) collected from the site of Baiae (Ricci et al., 2009; Davidde et al., 2010; Ricci et al., 2013), and in limestone tesserae from Baiae (Ricci et al., 2016b). Its traces were also found in marble statues of the Grotta Azzurra, Capri (Sacco Perasso et al., 2015). Since Ichnoreticulina elegans is a key-ichnospecies for deep and dark habitats, its presence on the statues of the Grotta Azzura confirms the scarce lighting of the bottom of the cave in which artifacts were exposed
Fascichnus grandis Radtke, 1991 is the ichnospecies of Acetabularia acetabulum (Linnaeus) P.C. Silva, 1952 (Ord. Dasycladales).
Acetabularia acetabulum is a unicellular eukaryotic green alga which grows in sheltered environments on hard substrates. It lives in shallow waters in the Mediterranean Sea, Red Sea, eastern Atlantic, and the Indian Ocean (Guiry and Guiry, 2013). The life cycle has an adult stadium consisting of a rhizoidal part, with the nucleus in one of its branches, a thin stalk, and a reproductive cap, shaped like an umbrella, containing gametangial cysts (Van den Hoek et al., 1995).
The species has a semi-endolithic habitus and settles by means of branched rhizoids which can penetrate carbonate substrates probably due to chemical actions, as for cyanobacteria (Garcia-Pichel, 2006; Garcia-Pichel et al., 2010). The endolithic penetration of rhizoids was well documented in works that described the bioerosive activity through the resin embedding-casting technique (Radtke et al., 1997; Perry and Macdonald, 2002; Radtke and Golubic, 2005; Radtke and Golubic, 2011; Casoli et al., 2015).
The boring role of A. acetabulum was observed on numerous artifacts in Baiae (Figure 3C) (Ricci et al., 2016a; Ricci et al., 2016b), underlining its ability to bore stones with different chemical composition. In particular, it was observed a semi-endolithic habitus of the rhizoidal apparatus (Figures 3D–F), in marble samples with a lower depth of penetration and a smaller number of branches than other calcareous stones, less hard like limestone. These results confirmed that the boring pattern is strongly influenced by the characteristics of the substrate, as already highlighted by Golubic et al. (1975).
Fungi
Marine fungi are chemotrophic microorganisms that live independently of light. Their growth in the submerged lapideous artifacts is linked to the presence of organic matter inside the stone, as food source. For this reason, fungi are an euendolithic group able to penetrate mineral substrates (Golubic et al., 2005). Gleason et al. (2017) reported an exhaustive review on the role of endolithic fungi in bioerosion and disease in marine ecosystems. They described the importance of fungi in natural substrates as components of the marine calcium carbonate cycle and bioerosion phenomena, because of their contribution to the biodegradation of calcium carbonate shells/skeletons and calcareous geological substrates.
Fungi traces are produced by the thin exploratory hyphae, more or less uniform in diameter, quite similar to of the filaments of cyanobacteria, and bag-shaped swellings with spores dispersion function (Figure 4A), whose shape and dimension are useful for the identification (Wisshak et al., 2019).
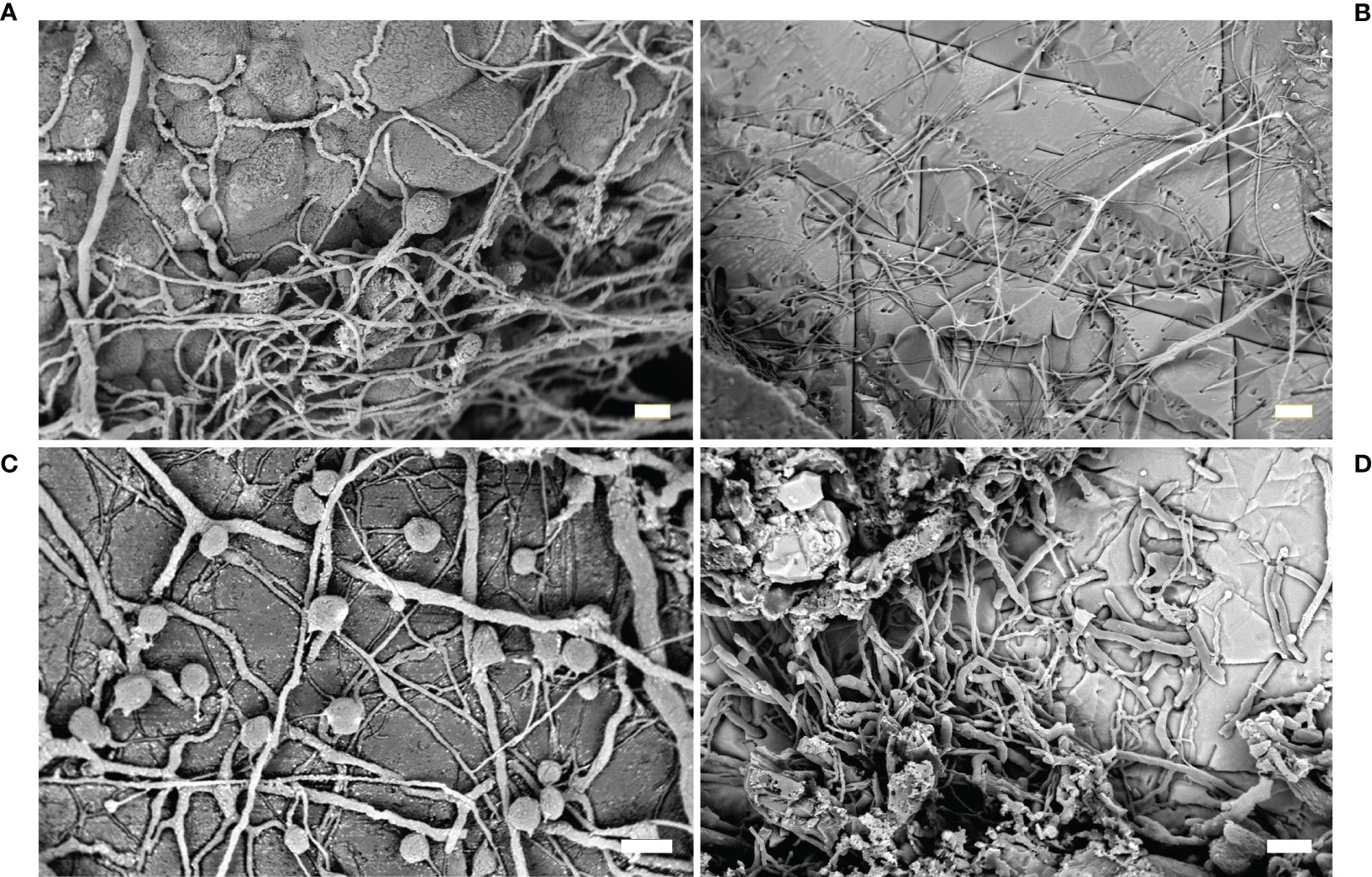
Figure 4 Fungi. (A) Fungi traces with typical thin exploratory hyphae and bag-shaped swellings (SEM image); (B) traces of the ichnospecies Orthogonum fusiferum (SEM image); (C) resin casts of the ichnospecies Saccomorpha sphaerula showing typical globular swellings and filaments (SEM image); (D) O. fusiferum mixed with other cyanobacterial traces spreading parallel to the substrate surface (SEM image). Scale bars: (A, C) = 10 µm; (B) = 30 µm; (D) = 20 µm.
Traces of euendolithic fungi were often found in stone artifacts in association with cavities produced by other microorganisms and boring sponges, which had provided a food source and had modified the petrographic features of the stone, favoring their settlement (Ricci et al., 2013; Sacco Perasso et al., 2015; Ricci et al., 2016a). Studies on microboring fungi showed that these microorganisms are generally slow in colonizing newly exposed substrata, becoming more abundant only after one year of exposure (Kiene et al., 1995; Gektidis, 1999; Casoli et al., 2015).
The ichnospecies and related biospecies identified in the studied sites are listed and described below.
Orthogonum fusiferum Radtke, 1991, is the ichnospecies produced by the fungus Ostracoblabe implexa Bornet and Flahault, 1891.
This ichnospecies is characterized by straight and branched filaments, more than 100 µm long, with diameter ranging from 1 to 4 µm, spreading parallel to the substrate surface, with bean-shaped swellings (Figures 4B, D). The ichnospecies was very frequently found in marble artifacts in Baiae and Capri (Ricci et al., 2013; Sacco Perasso et al., 2015; Ricci et al., 2016a). In particular, the study carried out on the Grotta Azzurra statues showed the presence of O. fusiferum in association with boring phototrophics (i.e., I. elegans) and sponges (Entobia isp.) (Sacco Perasso et al., 2015). The relevant presence of fungal traces was related to the micro environmental conditions present on the sea bottom of the grotto. The fungal colonization can be considered a phase successive to the colonization by other micro- and macro-organisms.
Saccomorpha clava Radtke, 1991, ichnospecies produced by the fungus Dodgella priscus Zebrowski, 1936.
This ichnospecies was observed in the ichnocoenosis observed on some marble statues of the Grotta Azzurra (Sacco Perasso et al., 2015). The traces were single club-shaped sacs, with diameter ranging from 25 to 40 µm, penetrated vertically into the material (Figure 4C). Globular swellings were connected to the surface by a narrow neck, isolated or together in small groups, referable to the fungal reproductive structures.
Saccomorpha sphaerula Radtke, 1991, ichnospecies of the biospecies Lithopythium gangliforme Bornet and Flahault, 1889.
This ichnospecies was frequently observed in association with Ichnoreticulina elegans in some marble statues of the Grotta Azzurra (Sacco Perasso et al., 2015). The traces were sphere/pear-shaped swellings, 7-9 µm wide, placed perpendicularly to the substrate surface. Several thin tubes, originating at the base, spread radially to interlink the sacs.
Macroborers
Many boring organisms responsible for biodegradation of carbonate are macroborers, characterized by diameter equal to or greater than 0.1 mm. They include sponges, worms mostly polychaetes and a few sipunculids, mollusc bivalves, and echinoderms that affect calcareous natural and man-made substrates by means of chemical and physical aggression. As a result, macroborers have a significant influence on the structure and dynamics of the communities colonizing calcareous surfaces that lie on the seabed: indeed, macroborers play a relevant role in the destruction or creation of secondary porosity in the substrate, and in the creation of fine sediments resulting from their boring activity (Warme, 1975; Tapanila, 2008; Flügel, 2010).
Macroborers are not to be confused with other groups of organisms capable of eroding the calcareous substrates, such as grazers and scrapers. These latter (e.g., chitons, gastropods, echinoids, and fishes) remove carbonate while feeding on microbial cover or epilithic algae found on limestone surfaces and thus they leave marks of teeth, jaws and beaks on the surfaces. Notwithstanding, they are unable to penetrate deep into the carbonate. On the contrary, the true macroborers excavate from simple pits or holes to complex tunnel systems branching into the substrate. Size and morphology of the burrows are typical of individual groups of organisms or can be ascribed to different groups. The morphology and formal nomenclature of borings were extensively described by paleontologists for their interest as traces of the activity of fossil organisms (Bromley and D’Alessandro, 1983; Bromley and D’Alessandro, 1987; Bromley, 2004; Costa de Almeida, 2007), but their typology is also well suited to the borings produced by the current species. Thus, the distinctive borings of sponges exhibit a series of external, rounded holes connected deeply into the substratum with largely cavities and tunnels. Such typical borings are attributable to the ichnogenus Entobia (Bromley and D’Alessandro, 1984). Some polychaetes and the sipunculans produce single-entrance cylindrical excavations in some cases distally enlarged to protrude outward with their anterior end; other polychaetes form double-entrance U-shaped excavations and large meanders through the substrate with parallel or irregular loops. The burrows of worms mostly belong to different ichnospecies of the ichnogenera Maeandropolydora and Caulostrepsis. Bivalve borers produce typical flask- or pouch-shaped excavations with a single circular or 8-shaped opening, Gastrochaenolites (Kelly and Bromley, 1984), which are characteristic of individual species.
Sponges
Sponges are among the most common and destructive boring organisms affecting archeological artifacts (Figures 5A–E). Any type of calcareous substrates immersed for long periods (i.e., archeological structures) can be colonized by sponges which typically characterize the late successional phase of borer communities (Chazottes et al., 1995). The damage caused by sponges is superficially extended but often produces deep cavities in the substratum thus affecting a large part of the archeological remains (Figures 5A–C) (e.g., La Russa et al., 2013; Davidde Petriaggi et al., 2017).
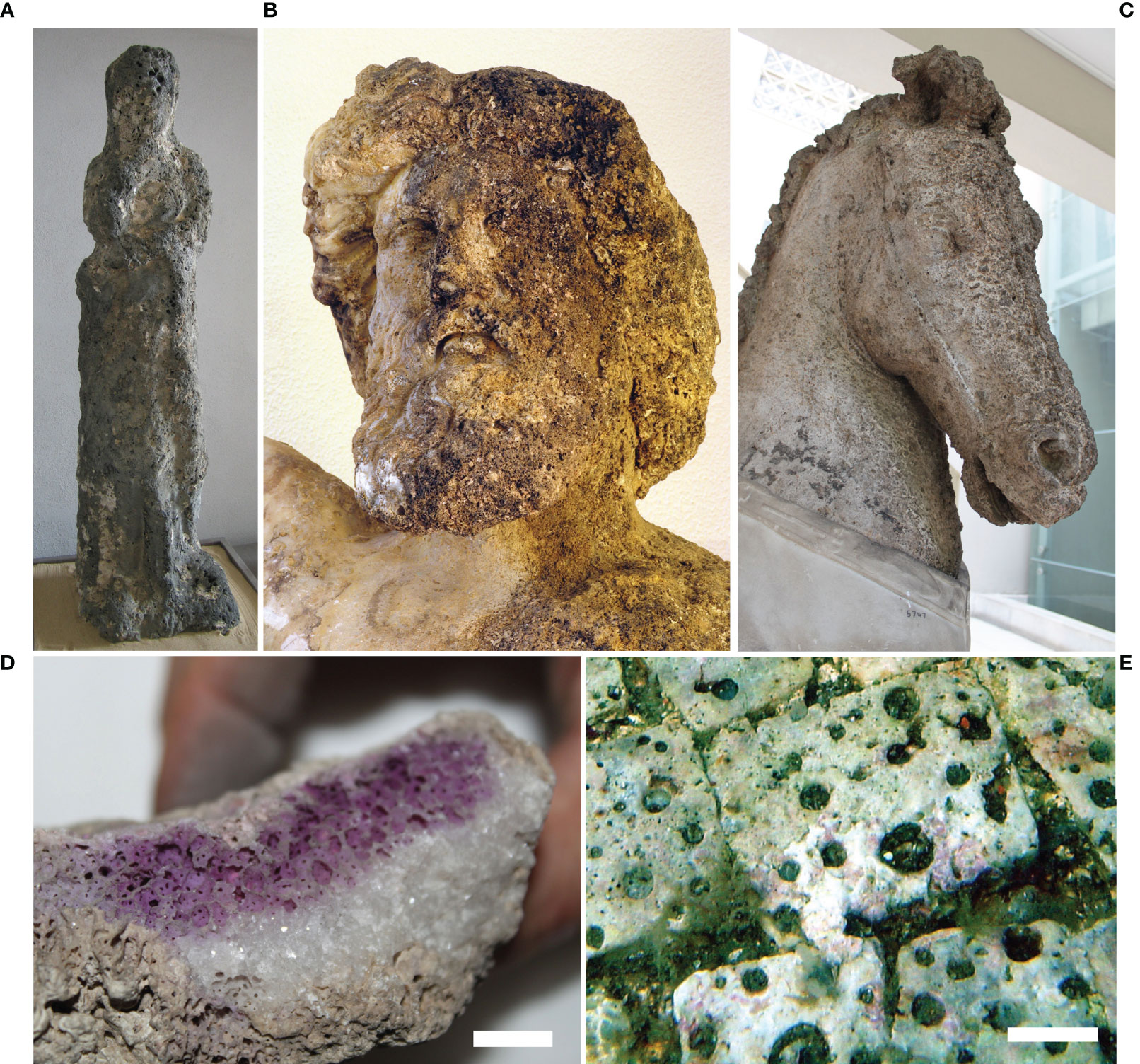
Figure 5 Sponges. (A) Statue of the Pudicitia type from submerged Baiae (Archaeological Museum of Campi Flegrei); (B) head of the statue of Poseidon from the Grotta Azzurra (Casa Rossa, Anacapri); (C) horse statue from the Antikythera shipwreck (National Archaeological Museum of Athens); (D) boring chambers produced by the species Cliona schmidtii in marble statue fragment; this species is characterized by a purple color which persisted in the samples (macro photo, scale bar: 1 cm); (E) mosaic tesserae of the submerged floor of the Villa dei Pisoni in the Underwater Archaeological Park of Baiae excavated by C. celata (underwater photo). Scale bars: (D) = 1 cm; (E) = 0.5 cm.
Endolithic sponges are often difficult to be detected in situ due to their cryptic habit (Marlow et al., 2019). On the surface, they produce papillae which represent the openings of their aquiferous system; through these circular holes the water enters carrying oxygen and food inside the sponge, and it exits transporting waste products outside. These papillae appear as small dots (often only a few mm in diameter), thus difficult to be detected, even though sometimes they can be vividly colored (yellow, red, purple or green), and more easily visible. This type of organization is generally called “alpha stage”, a typical growth organization of most of the Mediterranean boring species. Conversely, some species may also grow in beta and/or gamma and delta stages. In the beta stage, the sponge completely covers the substrate developing a thin ectosomal layer connecting papillae, while in the following gamma stage, the sponge completely erodes the substrate and grows freely like a non-endolithic sponge, with a massive shape; sponges in delta stage live partially buried in sediments and incorporate particles. Although the last kinds of organizations are mainly typical of tropical species, in the Mediterranean beta and gamma stages are characteristic of the sponges Cliona viridis (Schmidt, 1862) and C. celata Grant, 1826 (Cerrano et al., 2007; Evcen and Çinar, 2015).
In the Mediterranean Sea, the identification of boring sponges at the genus level can be relatively easy, just basing on their macroscleres (i.e. larger skeletal elements) (Figure 6A–D); for example, Siphonodictyon spp. present only oxeas, Cliona spp. only tylostyles, Pione spp. tylostyles and microxeas, etc. However, the identification at the species level is more problematic and additional features, such as microscleres (i.e., smaller skeletal elements), are mandatory for their recognition. Microscleres, in some cases, may be very rare and even if microscleres and macroscleres of boring species can be found in the etched chambers (Figures 6C, D), other not-boring species can exploit existing holes: in this case, the observed spicule set may actually belong to several different sponge species.
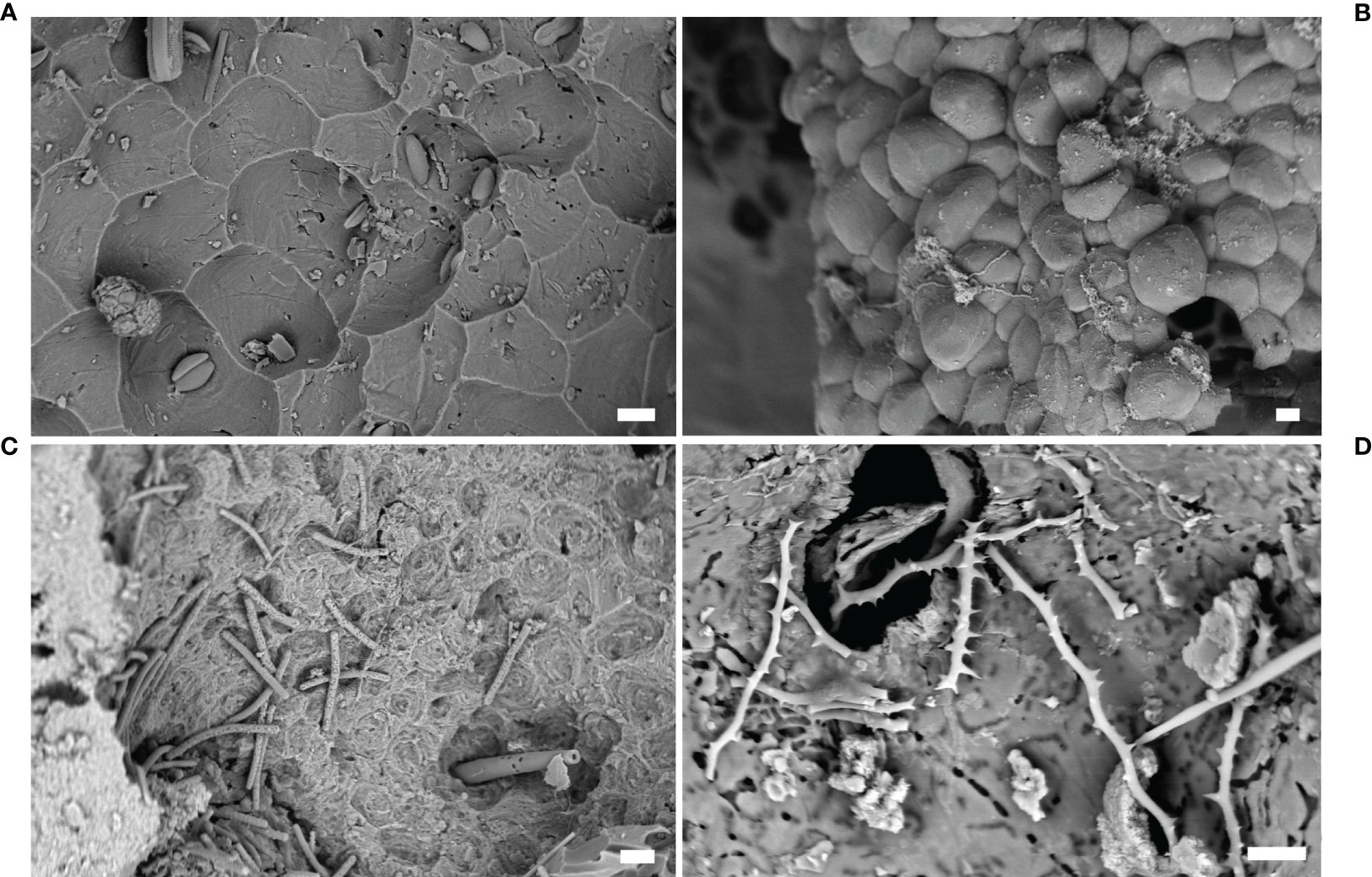
Figure 6 Sponges. (A) Microbioerosion pattern produced by Cliona celata, marble statue from Baiae (SEM image); (B) resin cast (Entobia isp.) of erosion chambers produced by Cliona sp. (SEM image); (C) spicules and pits in an erosion chamber produced by Dotona pulchella mediterranea (SEM image); (D) spicules of C. schmidtii inside a eroded chamber (SEM image). Scale bars: (A–C) = 20 µm; (D) =10 µm.
Bioerosion traces can be attributed to different perforating sponge taxa, based on their morphology and organization. Most of Porifera, being modular organisms, typically produce numerous serial erosion chambers (Figure 5D) connected to each other, creating a macro pattern of bioerosion, and to the surface of the substrate through the papillae. These features can be useful diagnostic elements since many species are characterized by cavities with a peculiar shape and/or size (Bromley, 1978; Calcinai et al., 2007). In fact, many erosion traces are attributed to different ichnospecies belonging to the ichnogenus Entobia (biogenus Cliona) (Figure 6B) considering the morphological characteristics of the cavity networks (Bromley and D’Alessandro, 1984).
Nevertheless, it is important to keep in mind that sponge erosion patterns may vary with the type of substrate (Bromley and D’Alessandro, 1984; Schönberg, 2000), the proximity of other boring organisms, or when the available substrate for the boring agent is spatially limited (Bromley and D’Alessandro, 1984). Morevover, the same erosion pattern may be produced by different species (Färber et al., 2016). For these reasons, the taxonomic identification of boring sponges should be conducted considering not only the erosion pattern but also the skeleton characteristics. On the contrary, the attribution of a cavity to the taxon Porifera is possible in light of the presence of the typical microbioerosion signs (pits) on the chamber walls (Figures 6A–D). These scars are exclusively produced by sponges and, in some cases, can be attributed to specific genera according to the different pattern (Calcinai et al., 2003; Calcinai et al., 2004). In this way, an erosion cavity can be traced back to the sponge action even if no sponge (and so no diagnostic spicules) are longer present inside the cavity, as happens in fossil structures.
The family Clionaidae is the taxon including most of the excavating sponges. Cliona celata and other species belonging to this genus are the most frequently reported sponges into archeological artifacts (Ricci et al., 2007a; Ricci et al., 2007b; Ricci et al., 2009; Ricci and Davidde, 2012; Ricci et al., 2016a). In Baiae, this species was responsible for intense and extensive damages on statues (Figure 5A) and other artifacts, destroying in many cases the original sculptural model (Ricci et al., 2009; Davidde et al., 2010; Aloise et al., 2014). Its growth was also observed on mosaic floors of the same site (Figure 5E). In addition, some studies carried out on tesserae showed that C. celata eroded the limestone, causing a loss up to 80% of the stone material (Ricci et al., 2007b).
Among the excavating taxon of Clionaidae, Dotona pulchella mediterranea Rosell and Uriz, 2002 (Figure 6C), Cliona janitrix Topsent. 1932, Cliona schmidtii (Ridley, 1881) (Figures 5D, 6D), Cliona vermifera Hancock, 1867, Pione vastifica (Hancock, 1848), and Spiroxya levispira (Topsent, 1898) were identified into archeological artifacts recovered from Grotta Azzurra and Antikythera shipwreck (Sacco Perasso et al., 2015; Davidde Petriaggi et al., 2017; Calcinai et al., 2019). These endolithic species were often found in association with other unidentified species belonging to the genera Alectona and Siphonodictyon in marble statues from Antikythera (Calcinai et al., 2019).
Sacco Perasso et al. (2015) and Calcinai et al. (2019) documented how erosion chambers in archeological material from Antikythera and the Grotta Azzurra have been secondarily occupied by non-boring sponges and the assignment of the agents responsible for the erosion of the artifact may be uncertain. When the artifact crosses several phases of cover-up, often the chambers and cavities may be empty, devoid of sponge tissue and so devoid of diagnostic characters; it happens when the original sponge died and the tissue with the spicules has been washed away, depriving us of important taxonomic characters. Another problem compromising species identification is related to the cultural value of the material which prevents us from sampling large quantities. In these cases, the species identification can be impossible and only the observations of the micro-erosion pattern (type of pits) and of the spicule set directly under the SEM (bypassing the traditional method for spicule preparation) may support the identification, but often only at the genus level (Calcinai et al., 2019). For perforating sponges, in fact not only the microscopic pattern, but also the macroscopic boring pattern (i.e., shape and organization of the erosion chambers) represent additional characters useful for taxonomy (Figure 5D, Figure 6B).
For this reason, the embedding casting procedure is useful in sponge erosion studies, allowing for the accurately reproducing of the erosion traces (Figure 6B), also because they can be observed using SEM, highlighting the superficial ornamentation produced by the sponges themelves.
Bivalves
Mollusca is one of the most species-rich and widely distributed phylum in the Mediterranean Sea, encompassing around 2000 species (Coll et al., 2010): such biodiversity reflects several adaptations to different environmental features and food sources. Bivalves, which among Mollusca are represented mainly by sessile or sedentary benthic organisms, show a variety of relationships with the hard substrates colonized. For example, they adhere to the rock as epilithics, actively perforate the substrate as euendolithics, creep into pre-existing cavities produced by other organisms or vacated by boring bivalves as nestlers (such as Coralliophaga lithophagella, Lamarck 1819, Barbatia barbata, Linnaeus 1758) not acting as borers (Morton, 1980; Morton, 1982; Morton, 2014).
Endolithic bivalves have adapted to boring into hard substrata such as consolidated clay, calcareous rocks, and wood (Clapp and Kenk, 1963; Turner, 1971; Perry, 1998; Devescovi, 2009; Bagur et al., 2013) and play a significant role in the degradation of the submerged archeological artifacts (Ricci et al., 2015). Calcareous rocks are highly susceptible to boring bivalves’ colonization, although lithotype (limestone, calcitic or dolomitic marble, travertine), surface inclination, morphology of the rocky bottom, and depth reflect the different degree of substrate aggression (Kleemann, 1973a; Kleemann, 1974a; Fanelli et al., 1994). Furthermore, both abiotic and biotic factors affect colonization patterns and growth of boring bivalves, such as substrate composition, hydrodynamic conditions, physical features of the habitat, nutrient concentration, and intraspecific competition for food and space (Kleemann, 1973a; Kleemann, 1973b; Kleemann, 1974a; Kleemann, 1974b; Devescovi and Ivesa, 2008; Devescovi, 2009).
Bivalves are characterized by slow growth rates and long lifespan (Montero-Serra et al., 2018) and dominate the final stages of the ecological succession of endolithic communities (Hutchings, 1986; Chazottes et al., 1995; Casoli et al., 2016).
The highest number of boring bivalves has been reported in tropical waters, where the bioerosion processes and borers’ biodiversity greatly influence the morphology and functioning of carbonate coral reefs; on the contrary, few species occur in temperate regions (Valentich-Scott and Dinesen, 2004; Valentich-Scott and Tongkerd, 2008). Boring bivalves mostly belong to Mytilidae, Pholadidae, Gastrochaenidae, and Petricolidae. In the Mediterranean Sea, the most widespread species of boring bivalves are Lithophaga lithophaga Linnaeus, 1758 (Colletti et al., 2020), Petricola lithophaga Retzius, 1888 (Donnarumma et al., 2018; Casoli et al., 2019a), Pholas dactylus Linnaeus, 1758 (Voultsiadou et al., 2010; Danovaro et al., 2018), and Rocellaria dubia Pennant, 1777 (Schiaparelli et al., 2005; Fava et al., 2016; Casoli et al., 2019a). These species share chemical and mechanical erosion processes through which they penetrate the rock by secreting acids and rotating the shells (Kühnelt, 1933; Kleemann, 1990; Kleemann, 1996).
Some of the species mentioned above colonized underwater archeological calcareous artifacts (mosaic floors, statues, and architectural elements) and were defined as responsible for the biological degradation (Ricci et al., 2015; Ricci et al., 2016a; Casoli et al., 2016). In addition, boring bivalves are important biological sea level indicators. The boreholes of boring bivalves in Puteoli, Naples (e.g., Serapis temple - Macellum) indicate the ancient sea levels and give information on the bradyseism phenomena and related movements that affected the area (Morhange et al., 2001; Morhange et al., 2006).
Lithophaga lithophaga is protected by the EU Habitats Directive and several international directives and conventions in all Mediterranean countries. It is distributed along the whole Mediterranean coastline (Fisher et al., 1987), throughout the Atlantic Ocean from Portugal to the northern coast of Angola (Gonzalez et al., 2000), and it is also reported on the coast of Mozambique (Macnae and Kalk, 1958), albeit Indo-Pacific records of the species should be possibly carefully revised (Huber, 2010). It is an edible endolithic bivalve illegally harvested by breaking the rocks that it colonizes: because of such exploitation, the rocky reefs turn into a biological desert, with dramatic biodiversity and habitat loss (Colletti et al., 2020 and references therein; Bevilacqua et al., 2021).
This species is commonly called Mediterranean date mussel because of its shell morphology and color. The shell is elongate-elliptical, and it can exceed 90 mm in length (Kefi et al., 2014; Peharda et al., 2015). Lithophaga lithophaga generally inhabits galleries bored in calcareous rocks by attaching its antero-ventral shell margin to the inner wall of the holes with byssus (Owada, 2007). Mechanical boring mechanisms are excluded due to the absence of erosion marks on the shell; neutral mucoproteins with calcium-binding ability, secreted by pallial glands, chemically mediate the calcareous excavation (Jaccarini et al., 1968). Date mussels start boring rocks after about 5-10 years from the larval settlement (Pierotti et al., 1966; sub Lithodomus lithophagus), producing deep club-shaped cavities that accommodate the shell, showing dumbbell-shaped openings at the rock surface corresponding to inhalant and exhalant siphons of the organism (Kázmér and Taborosi, 2012).
This species exhibits a slow growth rate and long lifespan: the application of von Bertalanffy growth function shows one of the lowest growth rates among bivalves (k = 0.03 year -1, Peharda et al., 2015). Shell length of 10 mm is reached at three years of age (Kleemann, 1973b; Galinou-Mitsoudi and Sinis, 1995; Kleemann, 1996), while a specimen measuring 30-50 mm has about 21-35 years, and regarded 80 mm are related to the age of 54-80 years (Kleemann, 1973b; Galinou-Mitsoudi and Sinis, 1995; Devescovi and Ivesa, 2008).
Lithophaga lithophaga was reported in most of the archeological artifacts (marble statues and architectural elements) submerged in Baiae, Capri, Antikythera, and Epidaurus (Figure 7A) and described by Ricci et al. (2015) and Davidde Petriaggi et al. (2019). Holes were round and oval, ranging from 0.5 to 3 cm in diameter. In many cases, two confluent holes forming the dumbbell-shaped elongate aperture were observed, as well as three or more holes confluent were also reported (Ricci et al., 2015; Ricci et al., 2017). The cavity orientation, indicating the shell position, can help the hypothetical reconstruction of the artifact lying conditions on the sea bottom (Figures 7B, C).
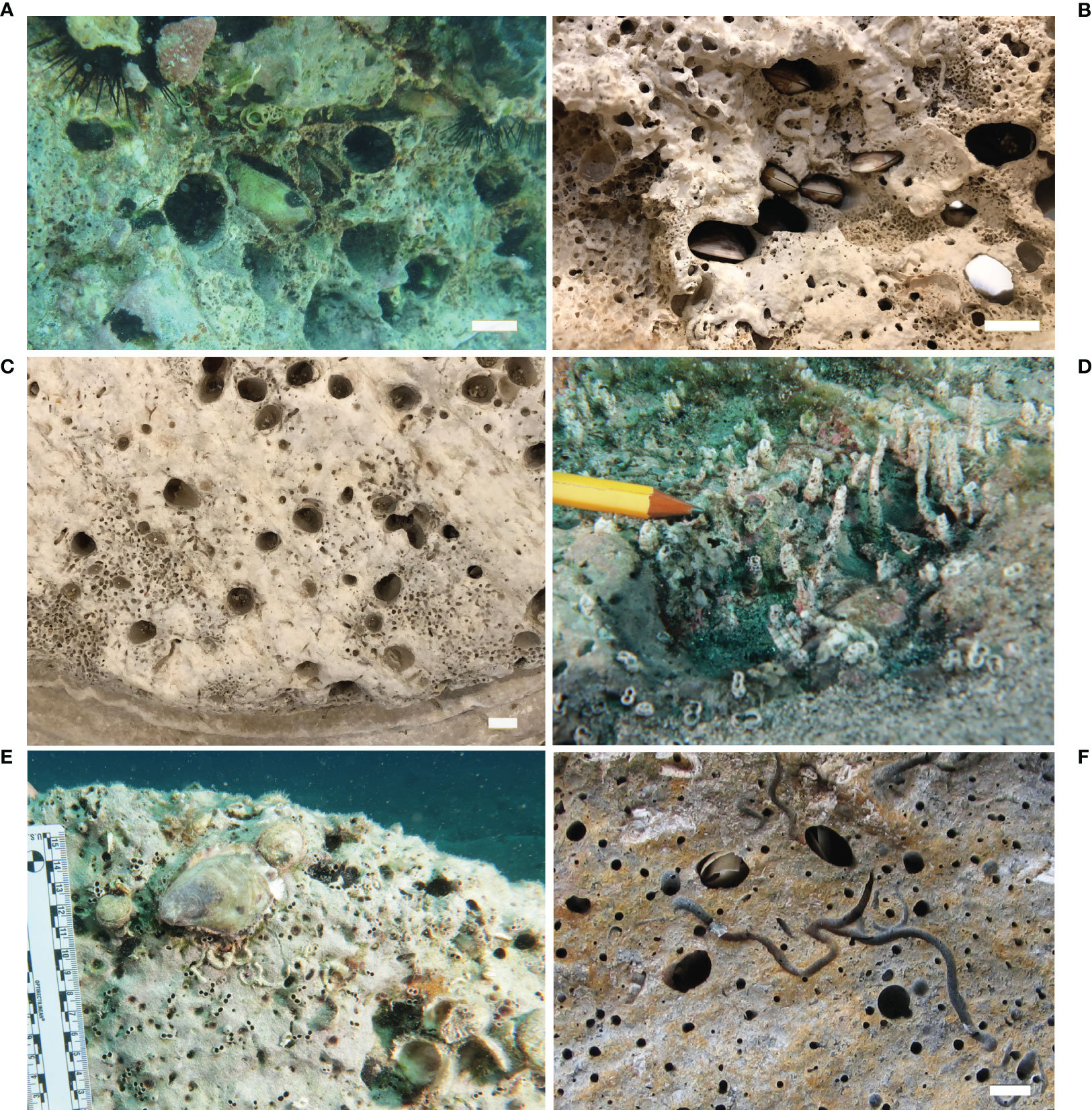
Figure 7 Bivalves. (A) Specimen of Lithophaga lithophaga inside a calcarenite block in the underwater site of Epidaurus (underwater photo); (B) holes produced by L. lithophaga specimens in a marble sarcophagus in the National Archaeological Museum of Capo Colonna, Crotone*; (C) holes produced by L. lithophaga in a marble column base in the National Archaeological Museum of Capo Colonna, Crotone*; (D) aragonite tubes secrete by the bivalve Rocellaria dubia on a marble block in the ancient Kaulon submerged site, Monasterace (underwater photo)*; (E) 8-shape holes of R. dubia on a column drum in the ancient Kaulon submerged site, Monasterace, (underwater photo)*; (F) Petricola lithophaga: specimens and holes in a marble statue, Archaeological Museum of Campi Flegrei, Baiae. Scale bars: (A–C) = 2 cm; (F) = 1 cm. * = Photos produced in the frame of MUSAS Project - Museums of Underwater Archaeology - protection, enhancement and networking of the underwater archaeological heritage, Calabria-Campania-Puglia, of the Central Institute for Restoration, Italian Ministry of Culture (www.progettomusas.eu).
Rocellaria dubia colonizes both infralittoral and circalittoral hard bottoms and has been reported as a borer of the skeleton of living bivalves and echinoderm lying on soft sediments (El-Menif et al., 2005; La Perna, 2005; Morton et al., 2011; Belaustegui et al., 2013; Donnarumma et al., 2018; Casoli et al., 2019a; Mikac et al., 2021).
Rocellaria dubia is distributed in the Mediterranean Sea, Black Sea, British islands, and the eastern Atlantic Ocean (Tebble, 1966), preferentially boring horizontal or slightly inclined substrates and being able to cope with high sedimentation rates thanks to its long siphons that rise from the bottom, allowing feeding and avoiding suffocation (Kleemann, 1974b; Schiaparelli et al., 2003). Larval settlement occurs in holes and crevices in the substrate where they metamorphose into a juvenile and start to penetrate the substrate. The boring activity was described as a combination of chemical and mechanical processes due to the acid secretion produced by pallial glands (Morton and Tseng, 1982) and the contraction of pedal retraction muscles (Carter, 1978; Morton et al., 2011). It shows a variable boring pattern, changing the perforation direction to avoid competition or direct contact with other endoliths, or selecting the most suitable parts of the rock (Schiaparelli et al., 2003; Morton et al., 2011). This bivalve secretes an aragonitic chimney encircling siphonal tube that appears as an external “8” shaped borehole (Figures 7D, E), well distinguishable through the observation of substrates (Morton et al., 2011; Casoli et al., 2016; Mikac et al., 2021). Schiaparelli et al. (2005) defined the correlation between the size of the “8” shaped siphonal tube and the shell dimensions: the equation they provided represents an effective method to quantify the bioerosive activity of this species by measuring the volume of the chamber.
Although R. dubia was found in different artificial substrates (concrete, travertine tiles, and limestone) in the Mediterranean Sea (Nicoletti et al., 2007; Spagnolo et al., 2014; Fava et al., 2016), it was reported for the first time by Davidde et al. (2010) and later by Ricci et al. (2009 sub Gastrochaena dubia; Ricci et al., 2015; Ricci et al., 2016a) as a component of the endolithic assemblages on archeological artifacts recovered in Baiae and the Gulf of Puteoli. Dense colonization of living specimens was observed in situ on mosaic floors (Ricci et al., 2016a) in the Underwater Archaeological Park of Baiae. The shells were found inside the limestone, developing vertical and sub-horizontal cavities, and affected adjacent tiles: the specimens ranged from 11 to 13 mm in length. Casoli et al. (2016) described the colonization dynamic highlighting the role of the bivalve in the bioerosion process of artificial limestone panels submerged in the Underwater Archaeological Park of Baiae. The results showed that the colonization was fast, and the bioerosion rate depended on the interactions with epibenthic assemblage. The presence of elliptical/spherical-shaped holes indicated that the specimens have settled on the encrusting layer formed by epilithics (i.e., barnacles and bryozoans), partially reducing the amount of eroded calcareous material.
Petricola lithophaga is a small bivalve living in shallow holes in calcareous rocks, likely excavated by acid secretion (Morton and Scott, 1988). This rock-borer bivalve is distributed in the Boreo-Atlantic littoral zone: the Atlantic coasts of Europe and North Africa, from Great Britain in the north to Morocco in the south, as well as in the Mediterranean, Aegean, Marmara, and Black seas (Kovalyova, 2017). Despite the cryptic mode of life, studies carried out in the Black Sea showed that P. lithophaga is widely distributed in limestone rocks, where it is present as dominant species showing a high abundance and biomas (Kovalyova, 2012; Kovalyova, 2015). Nevertheless, it is the lesser-known and studied among the boring bivalves found in the Mediterranean Sea. The shell is rounded or elongated-oval; shell length ranged from 4 mm to 24 mm. The valves are gray to white in color, thin, with c. 60 radial ridges on the surface (Kovalyova, 2015).
Petricola lithophaga was rarely reported as a bioeroder of Cultural Heritage. Davidde et al. (2010) and Ricci et al. (2015) found several specimens inside marble statues and architectural elements recovered from Baiae and the Port of Puteoli (Figure 7F). The dimension of the valves ranged from 11 to 19 mm, and the external holes had a diameter varying from 4 to 13 mm. As for the conservation of submerged archeological remains, P. lithophaga seems to play a minor role as abioerosion agent due to the smaller shell size and less frequency of occurrence on the artifacts if compared to L. lithophaga and R. dubia.
Polychaetes
Polychaetes contribute greatly to internal bioerosion through their ability to bore calcareous substrates by digging cavities, chambers, and tunnels, via either through secretion of acid compounds, mechanical actions or combination of both. Chemical dissolution of carbonate is due to secretion of acid mucopolysaccharides that are produced by the ventral epithelium and segmental mucus glands; meanwhile the substratum is physically eroded using hard structures, such as jaw teeth or specialized chaetae (Blake, 1969; Hutchings, 1986; Hutchings, 2008).
Boring polychaetes belong to the families Spionidae, Cirratulidae, Eunicidae, Dorvilleidae, and Sabellidae and their bioerosive activity is known in different types of calcareous substrates, such as rocks, corals reefs, calcareous algae, shells, and experimental substrates (Hutchings et al., 1992; Martin and Britayev, 1998; Pari et al., 1998; Pari et al., 2002; Hutchings, 2008; Gibson, 2017; Çinar and Dagli, 2021). Boring polychaetes has been studied mainly in tropical coral reefs (Hutchings and Murray, 1982; Hutchings and Peyrot-Clausade, 2002; Osorno et al., 2005; Hutchings, 2008), where eunicids, spionids, cirratulids, and sabellids actively perforate corals (Bromley, 1978; Hutchings, 1986; Le Grand and Fabricius, 2011). Boring polychaetes belonging to spionids (Polydora and related species) are particularly investigated in commercially valued shellfish species because of the consequence of such infestation on the economy (Blake and Evans, 1973; Diez et al., 2011; Diez et al., 2016; Waser et al., 2020). In contrast, compared to tropical areas, little data is available from the Mediterranean Sea particularly with respect to archeological artifacts. Despite the limitations imposed by the sampling of archeological remains, the micritic limestone tesserae collected from the submerged Roman mosaic floor of Portus Julius represent a unique substrate exposed for centuries to marine organism colonization and bioerosion processes (Antonelli et al., 2015). Here the cirratulid Dodecaceria concharum Örsted, 1843 (Figure 8A) dominates the endobenthic polychaete assemblage having a substantial role in bioerosion, while the spionids Polydora ciliata (Johnston, 1838) (Figure 8B) and Pseudopolydora antennata (Claparède, 1869) have a secondary role; in addition, the eunicid Lysidice unicornis (Grube, 1840) is found as nestler and possible borer (Gravina et al., 2019). The mechanisms of perforating and type of borings reflect the morphology and ecology of the various species. Spionids use their modified chaetae of the fifth chaetiger, which are assisted by the acid mucus produced by ventral glands (Dorsett, 1961), then enter a variety of substrates, including limestone and bivalve shells, as well as tropical coral skeletons. The burrows of the Polydora species are V- or U-shaped tunnels with small diameter entrances; here, the worms are folded in half and emerge from the openings with the head and the pygidium. By protruding the pair of long tentacles through the openings, Polydora individuals collect food particles from both the substrate and water column. As the individuals grow by elongating, the burrows are branched and result in a complex boring system of well-developed sinuous, cylindrical tunnels. In cultured bivalve species, such as oysters, scallops, mussels (Blake and Evans, 1973; Diez et al., 2011) and gastropods (Guţu and Marinescu, 1976), Polydora worms settle between the mantle and shell and induce the oyster to produce a mud blister around the worm’s body, as a mechanism to isolate the worm (Zottoli and Carriker, 1974; Lauckner, 1983). Consequently, depending on the intensity of infestation, the commercial value of the damaged oysters is reduced. The cirratulid D. concharum, in the Mediterranean Sea, together with D. fimbriata (Verrill, 1879) in northern Europe coast, burrows both in natural calcareous substrates, mollusc shells, corals, algae (Lithothamnion, Lithophyllum), and in man-made artifacts (Hartman, 1951; Gibson, 2008; Gravina et al., 2019). Dodecaceria species use their spoon-shaped chaetae present along the body to perforate the carbonate (Gibson, 2017), but chemical dissolution is an additional method to enter limestone substrates (Hutchings, 2008). Such mechanism is confirmed by the characteristic behavior of the worms, which exhibit close contact with the substrate during forward and backward movements and stop for periods of rest preceding the boring activity. The burrows of Dodecaceria are pouch-like, flattened in cross-section, distally enlarged and characterized by an 8-shaped single entrance, where the individuals are folded on themselves with the head and anus facing the opening. As deposit-feeders, these worms protrude their long palps through the opening for collecting sediment particles. Sabellids have no hard structures, thus their eroding ability is attributable to acid content of the mucus, which is produced by the glands that open at the base of the branchial crown (Chughtau and Wyn Knight-Jones, 1988). Unfortunately, this mechanism has not been demonstrated, although sabellids are well known to produce mucous material (Giangrande et al., 2014). Sabellids produce narrow, unbranched tunnels of circular section; here the worms remain with the pygidium at the blind end and keep their branchial crown expanded through the opening, thus ensuring respiration and filter-feeding. The tunnels are considerably longer than the animals and are lined with a chitinous coating that isolates the worms from the cavity wall. Potamilla sp. is the only sabellid that is known to bore in the Mediterranean Sea (Çinar and Dagli, 2021), but no sabellids have so far been found responsible for boring archeological artifacts (Gravina et al., 2019). Eunicids produce intricate branching tunnels of circular and oval sections of large diameter, where the animals remain folded in on themselves. The burrows differentiate from those of sabellids because they have unlined walls (Hutchings, 2008). Moreover, the tunnel of eunicids show evident teeth marks as signs of the bioerosive activity especially on corals, due to developed jaws equipped with mandible and maxillary toothed plates, as an efficient mechanical erosive system. Acid secretions are also hypothesized to occur. Some large eunicids, Eunice dubitata Fauchald, 1974, E. floridana (Pourtalès, 1867), and Leodice torquata (Quatrefages, 1866) live associated among the scleractinian corallites and the valve of the deep-oyster Neopycnodonte cochlear in the mesophotic zone of the Mediterranean Sea; although they are not clearly identified as opportunistic nestlers or true bioeroders (Gravina et al., 2021b). The same bioerosion mechanism is reported for the boring species of the related family Dorvillaeidae (Hutchings, 2008).
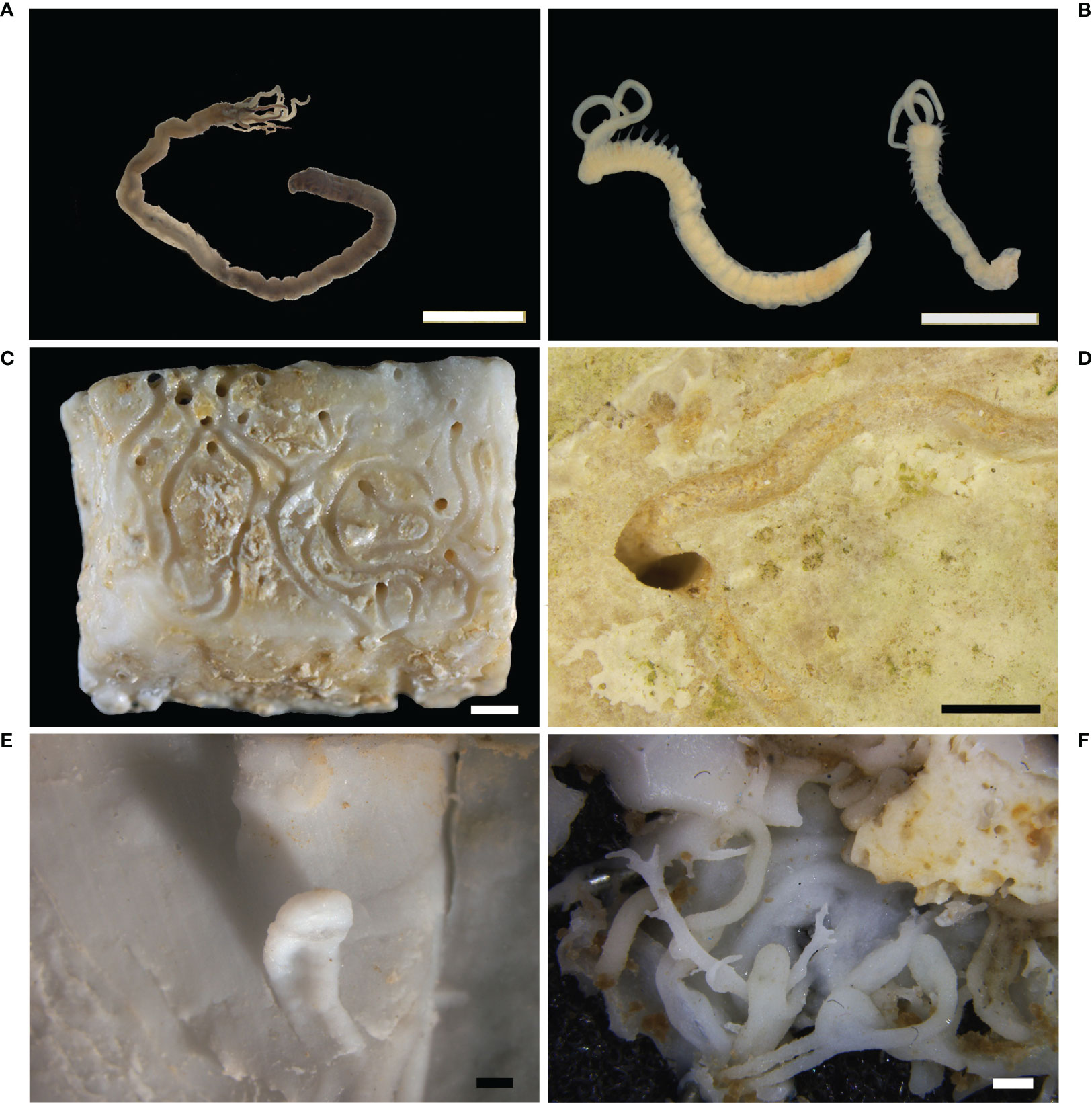
Figure 8 Polychaetes. (A) The cirratulid Dodecaceria concharum from Portus Julius, Baiae; (B) the spionids Polydora ciliata: specimens from the tesserae of the Roman mosaic floor of Baiae; (C) circular holes and a 8-shaped hole associated to sinuous and semi-spiraled furrows on a limestone mosaic tessera, Baiae; (D) elliptic hole associated to sinuous furrows on the surface of a limestone tessera, Baiae; (E) silicone cast of Caulostrepsis taeniola showing the limbs interconnected by a distinct vane; (F) silicone cast of Meandropolydora decipiens showing irregularly disposed cylindrical galleries, also bent into lobes enlarged as pouches. Scale bars: 1 mm.
Concerning the archeological artifacts, the tesserae of the Roman mosaic floor are largely bored on their surfaces, showing holes and furrows different in arrangement, shape and extension (Gravina et al., 2019). Single or paired furrows with U-shaped and sinuous arrangement highlight that polychaete galleries develop both in the tesserae and in the space between the tesserae and the epilithic layer encrusting them (Figures 8C, D). At the same time, circular and elliptical holes, isolated or paired in an 8 shape, represent the entry and exit points of the galleries (Gravina et al., 2019). Similar morphologies of traces are noticeable on the experimental panels submerged in the Archaeological Marine Park of Baiae and confirmed that the previous cover by encrusting organisms favors the settlement and the subsequent bioerosive action of polychaetes (Casoli et al., 2019b). Due to its dominance, D. concharum appears to be a secondary borer, together with L. unicornis. Meanwhile, P. ciliata and P. antennata, present in low abundance, are consistent with the hypothesis of early colonization of these species. Such spionids continue to settle as long as new substratum remains available, but with reduced density. Experimental studies have been based on artificial calcareous panels placed in the same archeological site of the Roman mosaic floor of Portus Julius and allowed repeated observations on different time scales (Casoli et al., 2015; Casoli et al., 2019b). The endolithic polychaete assemblage that occurred in the panels confirmed the findings reported for the tesserae of the Roman mosaic floor (Gravina et al., 2019), where P. ciliata and P. antennata are the first colonizers and are dominant species after 1 year of underwater exposure. Then, their density declines in the following 2-3 years, in correspondence with the arrival of D. concharum, which thrives in the mature community; also, the eunicid L. unicornis is a component of this phase. Interactions between the encrusting and boring organisms are other relevant information supported by these studies. They highlight that encrusting epibenthos provides a new substratum available for the boring polychaetes, where the worms can complete their life cycles and find their feeding resources (Casoli et al., 2019b). Consequently, the worms bore the encrusting stratum and dig different holes and furrows in the surface of the panels, which remain as a trace of the interaction between encrusting and boring organisms (Casoli et al., 2019b). The same sequence of settlement has been highlighted on the valves of sea scallops, where spionids are the main colonizers in the early stage, while D. concharum is the secondary borer which settles in previously formed holes (Evans, 1969). Other data from tropical coral reefs show Polydora spp., dorvilleids (Schistomeringos) and sabellids (Notaulux) to be the early colonizers, while Dodecaceria spp. and some eunicids the component of the mature boring community (Hutchings, 2008).
Internal bioerosion has been studied with various techniques used to detect the type and distribution of burrows, recognize their burrowers, and reconstruct the bioerosion pattern. In natural substrates, as bivalve shells, the X-ray radiography has been used (Evans, 1969); while the micro-computed tomography has been employed for both the investigation on fossil bioerosion traces in Cretaceous belemnite (Wisshak et al., 2017) and traces present in experimental blocks (Färber et al., 2016). The internal bioerosion of archeological artifacts, as the Roman mosaic floor of the Underwater Archaeological Park of Baiae, has been studied with the technique of silicone casts, which highlighted six different ichnospecies (Gravina et al., 2019). The present borings clearly match to the fossil traces belonging to both ichnogenera Caulostrepsis and Meandropolydora, described by Bromley and D’Alessandro (1983; 1987), Costa de Almeida (2007), Voigt (1965; 1971; 1975), and Wisshak (2006). The traces associated to the ichnospecies Caulostrepsis taeniola and C. cretacea are identified by the tongue- and ribbon-shape of borings and differ in the presence of a vane interconnecting the limbs (Figure 8E). The traces ascribed to the ichnospecies Maeandropolydora barocca, M. decipiens, M. elegans, M. sulcans are characterized by a more complex system of tunnels, which differ in the arrangement of single, parallel U-shaped or spiral furrows (Figure 8F), as well as in the shape of the external holes. The complex arrangement of polychaete boring traces occurring in the mosaic tesserae results in the high ichnospecies’ diversity. In accordance with the dominance of their inhabitants in the artifacts and the succession pattern on experimental substrates (Casoli et al., 2019b), these variations show that the traces correspond to various bioerosive stages. They all correspond to the action of D. concharum: Caulostrepsis spp. corresponds to its primary bioerosion activity, while Maeandropolydora spp. corresponds to its secondary boring activity, when D. concharum uses and modifies primary borings made by spionids (Gravina et al., 2019).
Sipunculids
The phylum Sipuncula comprises species adapted to live in a wide range of temperatures and depths, often nestling inside vacant shells or polychaetes tubes or burrowing sandy bottoms (Pancucci-Papadopoulou et al., 2014). Many literature works report about the ability of some Sipuncula species (belonging to the genera Phascolosoma and Aspidosiphon) of actively boring hard carbonatic substrates (e.g., corals, shells, rocks, and bones) mainly by mechanical action of the caudal shield (Rice, 1969; Rice and Macintyre, 1972; Williams and Margolis, 1974). However, evidences proved that the epidermal glands distributed on the worm’s body produce substances, like chelating agents or acids, able to chemically modify calcareous substrata (Williams and Margolis, 1974). Once burrowed a flask shaped tunnel, the organism settles inside it, with the anterior end directed toward the opening, and periodically extends the introvert outside the burrow scraping the surface of the substrate to collect food. The presence of hooks on the surface of the introvert and its movement along the surface can also contribute to the superficial erosion of the material (Rice, 1969).
The bioerosive role of sipunculids on archeological artifacts has been reported for the first time by Antonelli et al. (2015). Two specimens belonging to the species Aspidosiphon muelleri Diesing, 1851 were observed in erratic mosaic fragments recovered from the Underwater Archaeological Park of Baiae. This is the most widespread species of the genus Aspidosiphon, distributed between 5 and 2900 m in depth, from the north-eastern Atlantic to Chile, from Japan to the Mediterranean Sea (Cutler, 1994; Hong and Fenglu, 1998; Dean, 2001; Ferrero-Vicente et al., 2011; Pancucci-Papadopoulou et al., 2014). Sipunculids belonging to this species are characterized by an elliptical dark brown anal shield, a caudal shield with papillae separated by tangential furrows, and an introvert with compressed uni- or bi-dentate hooks arranged in rings in the distal part and pyramidal, uni-dentate hooks randomly arranged in the proximal part (Stephen and Edmonds, 1972; Cutler and Cutler, 1989; Pancucci-Papadopoulou et al., 2014). The two reported specimens had penetrated the mosaic boring both limestone tesserae and mortar, producing tunnels up to 5 mm in diameter (Figure 9A). After this report several A. muelleri specimens were observed in the mosaics of Portus Julius (Underwater Archaeological Park of Baiae; Figure 9B). As for the previous report, the organisms were observed both inside the tesserae and mortar and had produced tunnels up to 4 mm in diameter.
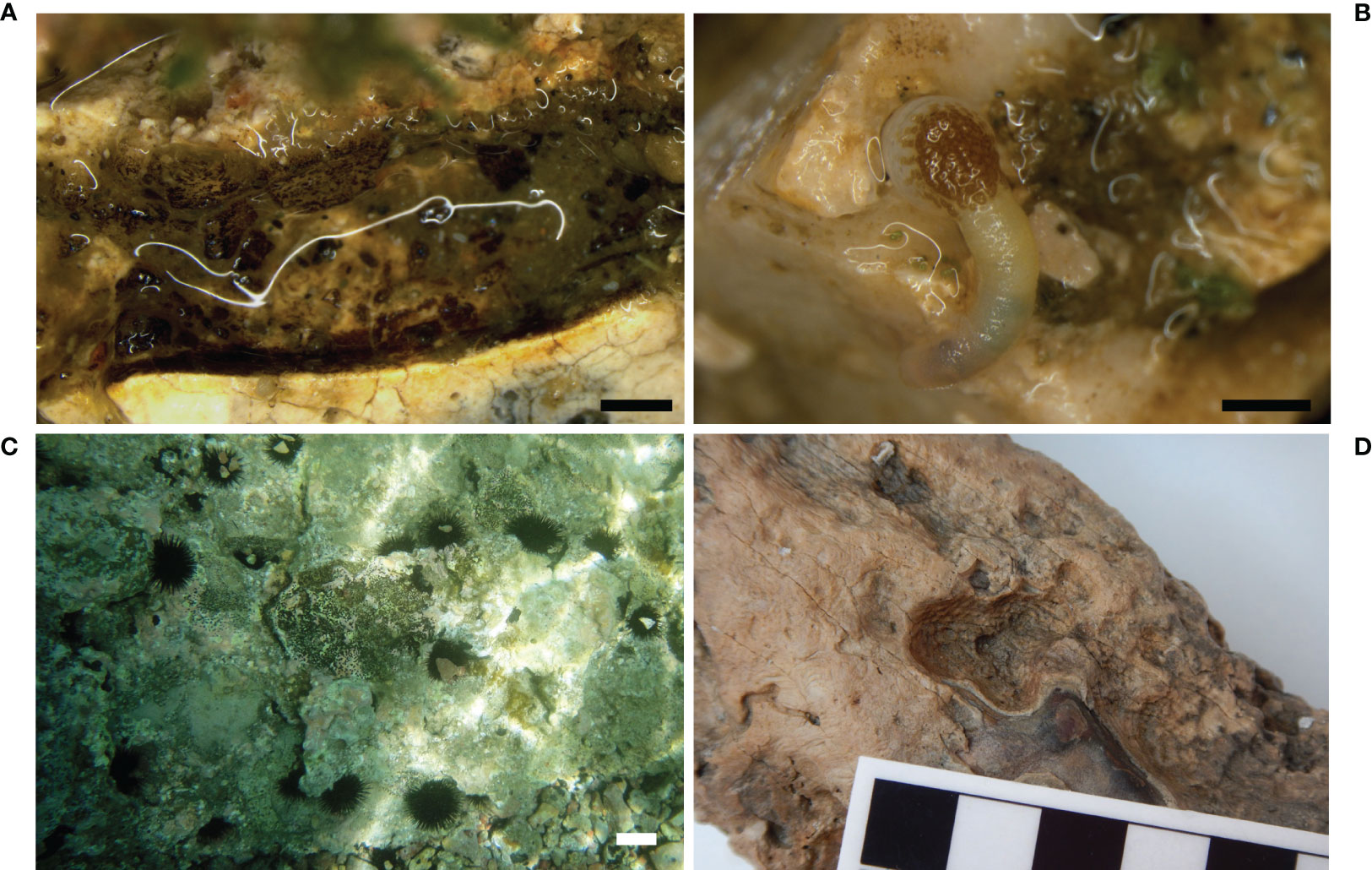
Figure 9 Sipunculids and echinoids. (A) Tunnel produced by the sipunculid Aspidosiphon muelleri in the bedding mortar of a mosaic floor in the Underwater Archaeological Park of Baiae (stereomicroscope photo); (B) specimen of A. muelleri inside an erratic tessera of a mosaic floor in the area of Portus Julius, Baiae (stereomicroscope photo); (C) sea urchins on architectural elements of the submerged Villa of the dolia, Epidaurus (underwater photo); (D) bioerosion traces attributed to sea urchins on an ivory tusk recovered from the Bajo de la Campana shipwreck, Cartagena. Scale bars: (A, B) = 1 mm; (C) = 5 cm.
Echinoids
Differently from all the other bioeroder groups discussed in the present paper, echinoids belong to the vagile epifauna and their boring action is usually associated with their grazing activity, leading to the formation of superficial scars on rocks and other hard substrates. However, some species developed a rock burrowing behavior to overcome environmental stresses (e.g., waves and tidal action) that can cause severe damages to hard substrates. For example, species of the family Echinometridae are considered the most destructive borers of tropical coasts (Asgaard and Bromley, 2008; Glynn and Manzello, 2015). Through the action of the teeth of Aristotle’s lantern, echinoids produce cup-shaped pits or, more frequently, elongated grooves in which they settle more or less permanently. As said, echinoids are vagile animals, so they are not imprisoned in their burrows but are free to leave them, if necessary, by lowering their spines to pass the narrow entrances (Asgaard and Bromley, 2008).
Until now, in the field of UCH, the boring action of echinoids has been observed only on the architectural structures of the roman “Villa of the dolia’’ in Palaia Epidaurus as superficial sub-circular traces produced by the species Paracentrotus lividus Lamarck, 1816 (Figure 9C). In this site, echinoids reduced the presence of the algal turf, common on horizontal surfaces in shallow waters, due to their grazing activity. On the contrary, echinoids were often observed in association with encrusting red algae (Corallinales), because they are not able to feed on them, due to the calcified thallus.
Furthermore, it has been hypothesized that the sub-circular or ovoid furrows (0.5- 3 cm in diameter, up to 1 cm in depth) observed on some of the tusks recovered from the shipwreck of Bajo de la Campana (Figure 9D) could be attributed to echinoid action (Antonelli et al., 2019). Unfortunately, in this case no body’s elements (e.g., teeth, spines) were present inside the furrows, so the attribution of these bioerosion traces to their activity can be only hypothesized.
Discussion
The present overview collects studies concerning the bioerosion of underwater archeological calcareous artifacts, carried out in the last two decades. They are the result of a pioneering field of research developed by the Istituto Centrale per il Restauro, Rome (Italian Ministry of Culture), involving marine biology applied to the conservation of UCH.
The case studies reported here are particularly relevant due to their still unique character. Beside the two important shipwrecks of Antikythera and Bajo de la Campana, the site of Baiae is the result of the distinctive patterns of Phlegraean bradyseism, which brought the entire city underwater, while the submersion of the Greek sites of Epidaurus and Agios Vlassios in the Peloponnese is the result of a local rise of the sea level. The case of the Roman Nymphaeum of the Grotta Azzurra in Capri, in which the cave habitat produced a peculiar lying site for its artifacts, is also unique.
The studies carried out on the archeological materials submerged in these sites have highlighted the high degree of complexity and diversity within bioerosion phenomena, focusing on the main bioeroders, from micro to macroborers, on the results of their boring activity in different environmental contexts, and on the ecological successions occurring on stone substrates. This complexity is the result of the constantly evolving character of bioerosion, a process unfolding along mutable environmental conditions and composition of boring communities. Observing the bioerosion of submerged archeological artifacts and lithoid formations worldwide has shown how microorganisms and marine organisms can produce significant destructive effects on stone calcareous materials. The susceptibility to degradation is influenced by many factors, but it is evident that how stone is more subject to degradation when it lies underwater, without being protected by the seabed sediment.
The chief hindrance in the study of bioerosion of archeological artifacts is the progressive quality of erosive action, which makes difficult to clearly identify individual phases of its process. In the case of precious artifacts such as statues, mosaics, and other decorative elements, the unavailability of a representative number and size of samples prevents an overall knowledge of the complex conditions of the bioerosive phenomena at the time of the recovery or during the in situ study. The successive colonizations of the artifacts in different times and lying conditions, e.g. covered/uncovered by sands, sea bottom movements, position changes, can be inferred only by observing the traces remained in the material, or through the use of experimental substrates miming the UCH. Moreover, early restoration procedures remove the biotic concretions settled on the artifacts, and so hinder the study of epibionts as well as of the underlying boring traces. Another limitation in the study of bioerosion is the impossibility of knowing the state of the archeological artifacts at the time of their immersion: the evolution of conservation cannot therefore be described in detail from the early colonization by bioeroders.
Fortunately, considerable information on the time-scale bioerosion activity can be gathered from the studies carried out on experimental substrates and shell samples (among others Wisshak et al., 2011; Casoli et al., 2015), which highlight how interspecies relationships play a relevant role in substrate modifications. Indeed, the stone material initially dug by the pioneer boring species is subsequently used by other species characterizing the mature stages of community development. In addition, some epilithic organisms, as corallinales algae, barnacles and polychaete tube worms, create protective calcareous layers on the substrate, capable of inhibiting or influencing the settlement of endolithic organisms, hampering bioerosion and abrasion (Casoli et al., 2019b; Ricci et al., 2019).
In short, the extent of degradation and the diversity of colonizers increase with the exposure time available for the settlement and growth of the organisms. At the time of their recovery, artifacts exhibit a level of bioerosion, that is the result of a long colonization process, characterized by different phases. Within this process, bioeroders played a specific role in degradation of both the substrate and superimposed biogenic layers (Ricci et al., 2019).
Both epilithic and endolithic communities associated with UCH can be informative of environmental features, especially when organisms commonly used as bioindicators are studied (e.g., polychaetes; Gravina et al., 2019). The study of the community structure and composition can provide valuable information regarding the sedimentary dynamics affecting the UCH that have pivotal implications for conservation actions.
Bioerosion-related problems are well known, while the strategies for addressing them represent an open challenge. Especially in the case of archeological artifacts, the choice of most suitable strategies for in situ conservation is difficult. While the UNESCO Convention recommends in situ protection of the artifacts in their original submerged sites, the evidence of irreversible damage can require the recovery of fragile and particularly at-risk objects for their preservation. Indeed, the exposure to marine agents and boring micro and macroorganisms causes irreversible damage, as biologists, restorers, conservators, and archeologists widely agree. Underwater permanence in conditions favoring bioerosion phenomena can lead to the complete loss of artifacts or to their partial destruction. For this reason, the necessity of promptly intervening in the case of in situ preservation of the artifacts is a priority. The protective methods employed included covering the artifacts with sediments and other materials, such as geotextiles, and using artificial seagrasses, mainly acting on the current flow and favoring the sediment deposition on the archeological structures (Petriaggi, 2005; Petriaggi and Davidde, 2012; Davidde Petriaggi and Petriaggi, 2015; Davidde Petriaggi et al., 2016; Ricci et al., 2017; Davidde Petriaggi et al., 2018).
On the other hand, the necessity of protecting both the archeological heritage and the surrounding ecosystems presents new challenges. From an ecological point of view, a submerged stone artifact provides a new substrate suitable for benthic species colonization and increases the biodiversity of the area when lying on soft bottoms. At the same time, operations meant to protect the artifacts, even when limited and carefully carried out, could alter the features of the associated community. Every choice must therefore be performed by assessing case by case the appropriate conservation strategies, through the cooperation of different professionals, i.e. archeologists, biologists, conservators, and restorers, in order to address such multifaceted issues. A successful example of this strategy can be found in the comparative study of bioerosion of the Roman mosaic floor and the benthic community in the Archaeological Park of Baiae (Naples, Italy). Here the polychaete assemblage proved to be a good indicator of the ecological conditions of the mosaic, highlighting the low hydrodynamics as the main driving factor of the habitat. In this condition, the best practices for the in situ preservation of the artifacts resulted in the covering of the mosaics with sand or geotextiles (Gravina et al., 2019).
The studies of biological colonization are not yet routinely performed on underwater artifacts, and they are still limited to few archeological sites in the Mediterranean basin, as highlighted in this review and other works (Cámara et al., 2017). This is partly due to the difficulty of operating on archeological artifacts bound and protected by law. A wider involvement of biologists and conservation scientists in the activities concerning underwater archeological sites would greatly increase the records on the biological colonization of UCH, allowing to evaluate the effects of biodegradation on different materials and in different contexts.
Far from claiming to be exhaustive, this overview presents the first state of the art concerning the main organisms responsible for bioerosion and the results of their activity on submerged archeological artifacts hitherto studied in the Mediterranean Sea. In this context, our review constitutes a valuable baseline for future investigations on most of the still submerged artifacts. Further efforts should be made to consider more archeological remains lying on the Mediterranean Sea bottom: these would likely produce a more complete list of the boring species. Lastly, discussing of the issues and problems that emerged through these studies contributes to the design and implementation of appropriate conservation strategies concerning the environmental and cultural heritage, as the latter deserves to be preserved for its intrinsic values and as a resource for the human identity and future generations.
Author Contributions
All the authors conceived the paper, extensively contributing to the conceptualization, collection and analysis of data, writing-original draft, writing-review and editing. In detail, FA sipunculids and echinoids; BC sponges; EC and CSP bivalves; MFG polychaetes, supervision; SR microborers, archeological aspects, supervision. All the authors read and approved the final manuscript.
Conflict of Interest
The authors declare that the research was conducted in the absence of any commercial or financial relationships that could be construed as a potential conflict of interest.
Publisher’s Note
All claims expressed in this article are solely those of the authors and do not necessarily represent those of their affiliated organizations, or those of the publisher, the editors and the reviewers. Any product that may be evaluated in this article, or claim that may be made by its manufacturer, is not guaranteed or endorsed by the publisher.
Acknowledgments
This review is based on published studies carried out in the framework of several national and international projects conducted by the Istituto Centrale per il Restauro (Italian Ministry of Culture). The authors express their gratitude to all the colleagues with whom they worked together in the Restoring Underwater Project, BlueMed Project (Plan/test/coordinate Underwater Museums, Diving Parks and Knowledge Awareness Centres in order to support sustainable and responsible tourism development and promote Blue growth in coastal areas and islands of the Mediterranean), MUSAS Project - Museums of Underwater Archaeology (protection, enhancement and networking of the underwater archaeological heritage, Calabria-Campania-Puglia).
The authors would also like to thank Lorenzo Marinucci, Ph.D for his support in the editing of English form.
References
Aloise P., Ricca M., La Russa M. F., Ruffolo S. A., Belfiore C. M., Padeletti G., et al. (2014). Diagnostic Analysis of Stone Materials From Underwater Excavations: The Case Study of the Roman Archaeological Site of Baia (Naples, Italy). Appl. Phys. A 114, 655–662. doi: 10.1007/s00339-013-7890-1
Antonelli F., Sacco Perasso C., Ricci S., Davidde Petriaggi B. (2015). Impact of the Sipunculan Aspidosiphon muelleri Diesing 1851 on Calcareous Underwater Cultural Heritage. Int. Biodeterior. Biodegradation 100, 133–139. doi: 10.1016/j.ibiod.2015.02.025
Antonelli F., Ricci S., Davidde Petriaggi B., Ortuño M. B. (2019). Study of the Bioerosion of Phoenician Elephant Tusks From the Shipwreck of Bajo De La Campana: Lots of Hypotheses, Few Certainties. Facies 65, 10. doi: 10.1007/s10347-019-0553-8
Asgaard U., Bromley R. G. (2008). “Echinometrid Sea Urchins, Their Trophic Styles and Corresponding Bioerosion,” in Current Developments in Bioerosion. Eds. Wisshak M., Tapanila L. (Berlin Heidelberg: Springer), 279–303.
Bagur M., Richardson C. A., Gutiérrez J. L., Arribas L. P., Doldan M. S., Palomo M. G. (2013). Age, Growth and Mortality in Four Populations of the Boring Bivalve Lithophaga patagonica From Argentina. J. Sea Res. 81, 49–56. doi: 10.1016/j.seares.2013.04.003
Ballard R. D., McCann A. M., Yoerger D., Whitcomb L., Mindell D., Oleson J., et al. (2000). The Discovery of Ancient History in the Deep Sea Using Advanced Deep Submergence Technology. Deep Sea Res. Part I Oceanogr. Res. Pap. 47, 1591–1620. doi: 10.1016/S0967-0637(99)00117-X
Belaustegui Z., De Gibert J. M., Nebelsick J. H., Domenech R., Martinell J. (2013). Clypeasteroid Echinoid Tests as Benthic Islands for Gastrochaenid Bivalve Colonization: Evidence From the Middle Miocene of Tarragona, North-East Spain. Palaeontology 56, 783–796. doi: 10.1111/pala.12015
Bevilacqua S., Airoldi L., Ballesteros E., Benedetti-Cecchi L., Boero F., Bulleri F., et al. (2021). Mediterranean Rocky Reefs in the Anthropocene: Present Status and Future Concerns. Adv. Mar. Biol. 89, 1–51. doi: 10.1016/bs.amb.2021.08.001
Bick A. (2006). Polychaete Communities Associated With Gastropod Shells Inhabited by the Hermit Crabs Clibanarius erythropus and Calcinus tubularis From Ibiza, Mediterranean Sea. J. Mar. Biol. Assoc. UK 86, 83–92. doi: 10.1017/S0025315406012884
Bingham B., Foley B., Singh H., Camilli R., Delaporta K., Eustice R., et al. (2010). Robotic Tools for Deep Water Archaeology: Surveying an Ancient Shipwreck With an Autonomous Underwater Vehicle. J. F. Robot. 27, 702–717. doi: 10.1002/rob.20350
Blake J. A. (1969). Systematics and Ecology of Shell-Boring Polychaetes From New England. Am. Zool. 9, 813–820. doi: 10.1093/icb/9.3.813
Blake J. A., Evans J. D. (1973). Polydora and Related Genera (Polychaeta: Spionidae) as Borers in Mollusk Shells and Other Calcareous Substrata. Veliger 15, 235–249.
Bromley R. G. (1978). Bioerosion of Bermuda Reefs. Palaeogeogr. Palaeoclimatol. Palaeoecol. 23, 169–197. doi: 10.1016/0031-0182(78)90093-7
Bromley R. G. (2004). “A Stratigraphy of Marine Bioerosion,” in The Application of Ichnology to Paleoenvironmental and Stratigraphic Analysis. Ed. McIlroy D. (London: Geological Society of London), 455–479.
Bromley R. G., D’Alessandro A. (1983). Bioerosion in the Pleistocene of Southern Italy: Ichnogenera Caulostrepsis and Maeandropolydora. Riv. Ital. di Paleontol. e Stratigr. 89, 283–309.
Bromley R. G., D’Alessandro A. (1984). The Ichnogenus Entobia From the Miocene, Pliocene and Pleistocene of Southern Italy. Riv. Ital. di Paleontol. e Stratigr. 90(2), pp. 227–296.
Bromley R. G., D’Alessandro A. (1987). Bioerosion of the Plio-Pleistocene Transgression of Southern Italy. Riv. Ital. di Paleontol. e Stratigr. 93, 379–442.
Calcinai B., Arillo A., Cerrano C., Bavestrello G. (2003). Taxonomy-Related Differences in the Excavating Micro-Patterns of Boring Sponges. J. Mar. Biol. Assoc. UK 83, 37–39. doi: 10.1017/S0025315403006775h
Calcinai B., Bavestrello G., Cerrano C. (2004). Bioerosion micro-patterns as diagnostic characteristics in boring sponges. Bollettino dei Musei e degli Istituti Biologici dell'Università di Genova 68, 229–238.
Calcinai B., Cerrano C., Bavestrello G. (2007). Three New Species and One Re-Description of Aka. J. Mar. Biol. Assoc. UK 87, 1355–1365. doi: 10.1017/S0025315407058377
Calcinai B., Sacco Perasso C., Petriaggi Davidde B., Ricci S. (2019). Endolithic and Epilithic Sponges of Archaeological Marble Statues Recovered in the Blue Grotto, Capri (Italy) and in the Antikythera Shipwreck (Greece). Facies 65, 1–18. doi: 10.1007/s10347-019-0562-7
Cámara B., Álvarez de Buergo M., Bethencourt M., Fernández-Montblanc T., La Russa M. F., Ricca M., et al. (2017). Biodeterioration of Marble in an Underwater Environment. Sci. Total Environ. 609, 109–122. doi: 10.1016/j.scitotenv.2017.07.103
Carter J. G. (1978). Ecology and Evolution of the Gastrochaenacea (Mollusca, Bivalvia) With Notes on the Evolution of the Endolithic Habitat. Bull. Peabody Mus. Nat. Hist. Yale Univ 41, 1–92.
Casoli E., Ricci S., Belluscio A., Gravina M. F. F., Ardizzone G. D. (2015). Settlement and Colonization of Epi-Endobenthic Communities on Calcareous Substrata in an Underwater Archaeological Site. Mar. Ecol. 36, 1060–1074. doi: 10.1111/maec.12201
Casoli E., Ricci S., Antonelli F., Sacco Perasso C., Belluscio A., Ardizzone G. D. (2016). Impact and Colonization Dynamics of the Bivalve Rocellaria dubia on Limestone Experimental Panels in the Submerged Roman City of Baiae (Naples, Italy). Int. Biodeterior. Biodegrad. 108, 9–15. doi: 10.1016/j.ibiod.2015.11.026
Casoli E., Bonifazi A., Ardizzone G., Gravina M. F. M. F., Russo G. F. G. F., Sandulli R., et al. (2019a). Comparative Analysis of Mollusc Assemblages From Different Hard Bottom Habitats in the Central Tyrrhenian Sea. Diversity 11:74. doi: 10.3390/d11050074
Casoli E., Ricci S., Antonelli F., Sacco Perasso C., Ardizzone G. D., Gravina M. F. (2019b). Colonization Dynamic on Experimental Limestone Substrata: The Role of Encrusting Epilithics Favouring Boring Polychaetes. Hydrobiologia 842, 101–112. doi: 10.1007/s10750-019-04028-9
Cerrano C., Sambolino P., Azzini F., Calcinai B., Bavestrello G. (2007). Growth of the Massive Morph of Cliona nigricans (Schmidt 1862) (Porifera, Clionaidae) on Different Mineral Substrata. Ital. J. Zool. 74, 13–19. doi: 10.1080/11250000600929370
Chazottes V., Le Campion-Alsumard T., Peyrot-Clausade M. (1995). Bioerosion Rates on Coral Reefs: Interactions Between Macroborers, Microborers and Grazers (Moorea, French Polynesia). Palaeogeogr. Palaeoclimatol. Palaeoecol. 113, 189–198. doi: 10.1016/0031-0182(95)00043-L
Christopoulou A., Gaolou A., Bougia P., Fowler M. A. (2012). The Antikythera Shipwreck: The Technology of the Ship, the Cargo, the Mechanism. Natl. Archaeological Museum. 109.
Chughtau I., Wyn Knight-Jones E. (1988). Burrowing Into Limestone by Sabellid Polychaetes. Zool. Scr. 17, 231–238. doi: 10.1111/j.1463-6409.1988.tb00098.x
Çinar M. E., Dagli E. (2021). Bioeroding (Boring) Polychaete Species (Annelida: Polychaeta) From the Aegean Sea (Eastern Mediterranean). J. Mar. Biol. Assoc. UK 101, 309–318. doi: 10.1017/S002531542100031X
Clapp W. F., Kenk R. (1963). Marine Borers. An Annotated Bibliography. Office Naval Research Dept. Navy. 1136.
Colletti A., Savinelli B., Di Muzio G., Rizzo L., Tamburello L., Fraschetti S., et al. (2020). The Date Mussel Lithophaga lithophaga: Biology, Ecology and the Multiple Impacts of its Illegal Fishery. Sci. Total Environ. 140866:140866. doi: 10.1016/j.scitotenv.2020.140866
Coll M., Piroddi C., Steenbeek J., Kaschner K., Ben Rais Lasram F., Aguzzi J, et al. (2010). The Biodiversity of the Mediterranean Sea: Estimates, Patterns, and Threats. PloS One 5:e11842. doi: 10.1371/journal.pone.0011842
Coombes M. A., Naylor L. A., Thompson R. C., Roast S. D., Gómez-Pujol L., Fairhurst R. J. (2011). Colonization and Weathering of Engineering Materials by Marine Microorganisms: An SEM Study. Earth Surf. Process. Landf. 36 (5), 582–593. doi: 10.1002/esp.2076
Costa de Almeida J. A. (2007). Icnofósseis De Macrobioerosão Na Bacia Da Paraíba (Cretáceo Superior Paleógeno), Nordeste do Brasil. [Phd’s Thesis] (Recife:Universidade Federal de Pernambuco, Recife), 264.
Crisci G. M., La Russa M. F., Macchione M., Malagodi M., Palermo A. M., Ruffolo S. A. (2010). Study of Archaeological Underwater Finds: Deterioration and Conservation. Appl. Phys. A 100, 855–863. doi: 10.1007/s00339-010-5661-9
Cutler E. B. (1994). The Sipuncula: Their Systematics, Biology, and Evolution (New York: Cornell University Press).
Cutler E. B., Cutler N. J. (1989). A Revision of the Genus Aspidosiphon (Sipuncula: Aspidosiphonidae). Proc. Biol. Soc Washingt. 102, 826–865.
Danovaro R., Nepote E., Lo Martire M., Ciotti C., De Grandis G., Corinaldesi C., et al. (2018). Limited Impact of Beach Nourishment on Macrofaunal Recruitment/Settlement in a Site of Community Interest in Coastal Area of the Adriatic Sea (Mediterranean Sea). Mar. Pollut. Bull. 128, 259–266. doi: 10.1016/j.marpolbul.2018.01.033
Davidde B., Bartolini M., Poggi D., Ricci S. (2010). Marine Bioerosion of Stone Artefacts Preserved in the Museo Archeologico dei Campi Flegrei in the Castle of Baia (Naples). Archaeol. Maritima Mediterr. 7, 75–115. doi: 10.1400/143102
Davidde Petriaggi B., Petriaggi R. (2015). Archeologia Sott’acqua: Teoria e Pratica. Fabrizio Serra. 272.
Davidde Petriaggi B., Ricci S., Poggi D. (2016). “The Restoration in Situ of a Pavement in Opus Sectile in the Underwater Archaeological Park of Baiae (Naples, Italy),” in Actas del V Congreso Internacional de Arqueología Subacuática (IKUWA V) (Cartagena, Spain: Subdirección General de Documentación y Publicaciones), 293–301.
Davidde Petriaggi B., Ricci S., Sacco Perasso C., Antonelli F., Vlachogianni E. (2017). An Overview of the State of Conservation of the Marble Artefacts From the Antikythera Shipwreck. Archaeol. Maritima Mediterr. 14, 13–74. doi: 10.19272/201704501002
Davidde Petriaggi B., Ricci S., Antonelli F., Sacco Perasso C., Gregory . D. J (2018). “Protezione in Situ di Strutture Architettoniche Sommerse Con L’impiego di Fronde Artificiali: Il Caso di Studio di Baia,” in Il Patrimonio Culturale Sommerso. Ricerche e Proposte per il Futuro dell’archeologia Subacquea in Italia. Ed. Capulli M. (Udine, Italy: Forum Editore).
Davidde Petriaggi B., Galiatsatou P., Medaglia S. (2019). “The Submerged ‘Villa of the Dolia’ Near Ancient Epidaurus. The Preliminary Results of the First Excavation and Conservation Campaign,” in Annuario della Scuola Archeologica di Atene e delle Missioni Italiane in Oriente (Atene: Scuola Archeologica Italiana di Atene).
Dean H. K. (2001). Marine Biodiversity of Costa Rica: The Phyla Sipuncula and Echiura. Rev. Biol. Trop. 49(2), 85–90.
De Franciscis A. (1964). “Le statue della Grotta Azzurra nell’isola di Capri,” in Conferenza tenuta il 22 agosto 1964 presso il" Centro Caprense di vita e di studi Ignazio Cerio" (Capri: ESI).
Demma F., Valeri C. (2008). “Baia”, in Gli edifici severiani, Museo Archeologico dei Campi Flegrei, Catalogo Generale 3, Liternum, Baia, Miseno. Eds. Miniero P., Zervi F. (Naples, Italy: Electa Napoli Spa).
Devescovi M. (2009). Biometric Differences Between Date Mussels Lithophaga lithophaga Colonizing Artificial and Natural Structures. Acta Adriat. Int. J. Mar. Sci. 50, 129–138.
Devescovi M., Ivesa L. (2008). Colonization Patterns of the Date Mussel Lithophaga lithophaga (L. 1758) on Limestone Breakwater Boulders of a Marina. Period. Biol. 110, 339–345.
Diez M. E., Radashevsky V. I., Orensanz J. M., Cremonte F. (2011). Spionid Polychaetes (Annelida: Spionidae) Boring Into Shells of Molluscs of Commercial Interest in Northern Patagonia, Argentina. Ital. J. Zool. 78, 497–504. doi: 10.1080/11250003.2011.572565
Diez M. E., Vázquez N., da Cunha Lana P., Cremonte F. (2016). Biogenic Calcareous Growth on the Ribbed Mussel Aulacomya atra (Bivalvia: Mytilidae) Favours Polydorid Boring (Polychaeta: Spionidae). Hydrobiologia 766, 349–355. doi: 10.1007/s10750-015-2467-y
Donnarumma L., Sandulli R., Appolloni L., Sánchez-Lizaso J. L., Russo G. F. (2018). Assessment of Structural and Functional Diversity of Mollusc Assemblages Within Vermetid Bioconstructions. Diversity 10, 96. doi: 10.3390/d10030096
Dorsett D. A. (1961). The Behaviour of Polydora ciliata (Johnst.). Tube-Building and Burrowing. J. Mar. Biol. Assoc. UK 41, 577–590. doi: 10.1017/S0025315400016167
Ekdale A. A., Bromley R. G., Pemberton S. G. (1984). “Bioerosion,” in Ichnology: The Use of Trace Fossils in Sedimentology and Stratigraphy. Eds. Ekdale A. A., Bromley R. G., Pemberton S. G. (Tulsa: Society for Sedimentary Geology), 108–128.
El-Menif N. T., Guezzi Y., Le Pennec M., Boumaiza M., Le Pennec G. (2005). Infestation of the Clam Venus verrucosa by Sipunculoidea and the Lithophagus Bivalve, Gastrochaena dubia. Acta Adriat. Int. J. Mar. Sci. 46, 83–90.
Evans J. W. (1969). Borers in the Shell of the Sea Scallop, Placopecten magellanicus. Am. Zool. 9, 775–782. doi: 10.1093/icb/9.3.775
Evcen A., Çinar M. E. (2015). Bioeroding Sponge Species (Porifera) in the Aegean Sea (Eastern Mediterranean). J. Black Sea/Mediterranean Environ. 21 (3), 285–306.
Fanelli G., Piraino S., Belmonte G., Geraci S., Boero F. (1994). Human Predation Along Apulian Rocky Coasts (SE Italy): Desertification Caused by Lithophaga lithophaga (Mollusca) Fisheries. Mar. Ecol. Prog. Ser. 110 (1), 1–8. doi: 10.3354/meps110001
Färber C., Titschack J., Schönberg C. H. L., Ehrig K., Boos K., Baum D., et al. (2016). Long-Term Macrobioerosion in the Mediterranean Sea Assessed by Micro-Computed Tomography. Biogeosciences 13, 3461–3474. doi: 10.5194/bg-13-3461-2016
Fava F., Ponti M., Abbiati M. (2016). Role of Recruitment Processes in Structuring Coralligenous Benthic Assemblages in the Northern Adriatic Continental Shelf. PloS One 11: e0163494. doi: 10.1371/journal.pone.0163494
Ferrero-Vicente L. M., Loya-Fernández A., Marco-Méndez C., Martinez-Garcia E., Sánchez-Lizaso J. L. (2011). Soft–Bottom Sipunculans From San Pedro Del Pinatar (Western Mediterranean): Influence of Anthropogenic Impacts and Sediment Characteristics on Their Distribution. Anim. Biodivers. Conserv. 34, 101–111. doi: 10.32800/abc.2011.34.0101
Fisher W., Bauchot M. L., Schneider M. (1987). “Fiches FAO D’identification Des Espèces Pour Les Besoins De La Pêche (Révision 1),” in Méditerranée Et Mer Noire - Zone De Pêche 37 (Rome: Végétaux et invertébrés).
Flügel E. (2010). “Limestones are Biological Sediments,” in Microfacies of Carbonate Rocks (Berlin: Springer Berlin Heidelberg), 369–398. doi: 10.1007/978-3-642-03796-2_9
Friedmann I., Lipkin Y., Ocampo-Paus R. (1967). Desert Algae of the Negev (Israel). Phycologia 6, 185–200. doi: 10.2216/i0031-8884-6-4-185.1
Galinou-Mitsoudi S., Sinis A. I. (1995). Age and Growth of Lithophaga lithophaga (Linnaeus 1758) (Bivalvia: Mytilidae), Based on Annual Growth Lines in the Shell. J. Molluscan Stud. 61, 435–453. doi: 10.1093/mollus/61.4.435
Garcia-Pichel F. (2006). Plausible Mechanisms for the Boring on Carbonates by Microbial Phototrophs. Sediment. Geol. 185, 205–213. doi: 10.1016/j.sedgeo.2005.12.013
Garcia-Pichel F., Ramírez-Reinat E., Gao Q. (2010). Microbial Excavation of Solid Carbonates Powered by P-Type ATPase-Mediated Transcellular Ca2+ Transport. Proc. Natl. Acad. Sci. 107, 21749–21754. doi: 10.1073/pnas.1011884108
Gektidis M. (1999). Development of Microbial Euendolithic Communities: The Influence of Light and Time. Bull. Geol Soc. Denmark 45, 147–150. doi: 10.37570/bgsd-1998-45-18
Ghirardelli L. A. (2002). Endolithic Microorganisms in Live and Dead Thalli of Coralline Red Algae (Corallinales, Rhodophyta) in the Northern Adriatic Sea. Acta Geol. Hisp. 37, 53–60.
Gianfrotta P. A. (2008). “Lo Scavo Subacqueo Del Ninfeo,” in Museo Archeologico dei Campi Flegrei, Catalogo Generale. Eds. Miniero P., Zevi F. (Naples, Italy:Electa Napoli Spa), 145–151.
Giangrande A., Licciano M., Schirosi R., Musco L., Stabili L. (2014). Chemical and Structural Defensive External Strategies in Six Sabellid Worms (Annelida). Mar. Ecol. 35, 36–45. doi: 10.1111/maec.12053
Gibson P. H. (2008). Speciation of Dodecaceria fimbriata and Dodecaceria berkeleyi: Convergent Evolution or Continental Drift. Porcup. Mar. Nat. Hist. Soc Newsl. 24, 31–33.
Gibson P. H. (2017). A Search for Trace Fossils of the Burrowing Cirratulid Polychaetes Dodecaceria fimbriata and D. concharum. Ichnos 24, 83–90. doi: 10.1080/10420940.2015.1137215
Gleason F. H., Gadd G. M., Pitt J. I., Larkum A. W. D. (2017). The Roles of Endolithic Fungi in Bioerosion and Disease in Marine Ecosystems. I. General Concepts. Mycology 8, 205–215. doi: 10.1080/21501203.2017.1352049
Glynn P. W., Manzello D. P. (2015). “Bioerosion and Coral Reef Growth: A Dynamic Balance,” in Coral Reefs in the Anthropocene (Dordrecht: Springer), 67–97.
Golubic S., Brent G., Le Campion-Alsumard T. (1970). Scanning Electron Microscopy of Endolithic Algae and Fungi Using a Multipurpose Casting Embedding Technique. Lethaia 3, 203–209. doi: 10.1111/j.1502-3931.1970.tb01858.x
Golubic S., Perkins R. D., Lukas K. J. (1975). “Boring Microorganisms and Microborings in Carbonate Substrates,” in The Study of Trace Fossils (Berlin, Heidelberg: Springer), 229–259.
Golubic S., Schneider J. (1979). “Carbonate Dissolution,” in Studies in Environmental Science (Amsterdam-Oxford-New York: Elsevier), 107–129.
Golubic S., Friedmann E. I., Schneider J. (1981). The Lithobiontic Ecological Niche, With Special Reference to Microorganisms. J. Sediment. Res. 51, 475–478.
Golubic S., Campbell S. E., Drobne K., Cameron B., Balsam W. L., Cimerman F., et al. (1984). Microbial Endoliths: A Benthic Overprint in the Sedimentary Record, and a Paleobathymetric Cross-Reference With Foraminifera. J. Paleontol. 58 (2), 351–361.
Golubic S., Radtke G., Le Campion-Alsumard T. (2005). Endolithic Fungi in Marine Ecosystems. Trends Microbiol. 13, 229–235. doi: 10.1016/j.tim.2005.03.007
Gonzalez J. T., Halcon R. M. A., Barrajon A., Calvo M., Frias A., Morreno D., et al. (2000). “Estudio Sobre La Biologia, Conservación Y Problemática Del Dátil De Mar (Lithophaga lithophaga) En Espana,” in Madrid, Ministerio De Medio Ambiente, Dirección General De Conservación De La Naturaleza, (Madrid, Spain:Ministerio de Medio Ambiente, Dirección General de Conservación de la Naturaleza) vol. 66.
Gravina M. F., Antonelli F., Sacco Perasso C., Cesaretti A., Casoli E., Ricci S. (2019). The Role of Polychaetes in Bioerosion of Submerged Mosaic Floors in the Underwater Archaeological Park of Baiae (Naples, Italy). Facies 65:19. doi: 10.1007/s10347-019-0563-6
Gravina M. F., Casoli E., Donnarumma L., Giampaoletti J., Antonelli F., Sacco Perasso C., et al. (2021a). First Report on the Benthic Invertebrate Community Associated With a Bronze Naval Ram From the First Punic War: A Proxy of Marine Biodiversity. Front. Mar. Sci. 8. doi: 10.3389/fmars.2021.772499
Gravina M. F., Pierri C., Mercurio M., Nonnis Marzano C., Giangrande A. (2021b). Polychaete Diversity Related to Different Mesophotic Bioconstructions Along the Southeastern Italian Coast. Diversity 13, 239. doi: 10.3390/d13060239
Guiry M. D., Guiry G. M. (2013) AlgaeBase (Galway: World-wide electronic publication, National University of Ireland). Available at: http://www.algaebase.org (Accessed February 01, 2022).
Guţu M., Marinescu A. (1976). Polydora ciliata (Polychaeta) Perfore Le Gastéropode Rapana thomasiana De La Mer Noire. Trav. du Muséum d′Histoire Nat. “Grigore Antipa” 20, 35–41.
Hartman O. (1951). The Littoral Marine Annelids of the Gulf of Mexico. Publ. Inst. Geol. Sci. Technol. 2, 92–93.
Hong Z., Fenglu L. (1998). “Sipunculans From the South China Sea,” in Proceedings of the Third International Conference on the Marine Biology of the South China Sea (Hong Kong: Hong Kong University Press), 129–140.
Huber M. (2010). Compendium of Bivalves. A Full-Color Guide to 3,300 of the World’s Marine Bivalves. A Status on Bivalvia After 250 Years of Research (Hackenheim:ConchBooks).
Hutchings P. A., Murray A. (1982). Patterns of Recruitment of Polychaetes to Coral Substrates at Lizard Island, Great Barrier Reef–an Experimental Approach. Mar. Freshw. Res. 33, 1029–1037. doi: 10.1071/MF9821029
Hutchings P. A. (1986). Biological Destruction of Coral Reefs. Coral reefs 4, 239–252. doi: 10.1007/BF00298083
Hutchings P. A., Kiene W. E., Cunningham P. B., Donnelly C. (1992). Spatial and Temporal Patterns of non-Colonial Boring Organisms (Polychaetes, Sipunculans and Bivalve Molluscs) in Porites at Lizard Island, Great Barrier Reef. Coral Reefs 11, 23–31. doi: 10.1007/BF00291931
Hutchings P. A., Peyrot-Clausade M. (2002). The Distribution and Abundance of Boring Species of Polychaetes and Sipunculans in Coral Substrates in French Polynesia. J. Exp. Mar. Bio. Ecol. 269, 101–121. doi: 10.1016/S0022-0981(02)00004-7
Hutchings P. A. (2008). “Role of Polychaetes in Bioerosion of Coral Substrates,” in Current Developments in Bioerosion. Eds. Wisshak M., Tapanila L. (Berlin: Springer).
Hutchings P. A. (2011). “Bioerosion,” in Encyclopedia of Modern Coral Reefs-Structure, Form and Processes (Hiedelberg, Germany: Springer-Verlag), 139–156.
Ingrosso G., Abbiati M., Badalamenti F., Bavestrello G., Belmonte G., Cannas R., et al. (2018). Mediterranean Bioconstructions Along the Italian Coast. Adv. Mar. Biol. 79, 61–136. doi: 10.1016/bs.amb.2018.05.001
Jaccarini V., Bannister W. H., Micallef H. (1968). The Pallial Glands and Rock Boring in Lithophaga lithophaga (Lamellibranchia, Mytilidae). J. Zool. 154, 397–401. doi: 10.1111/j.1469-7998.1968.tb01672.x
Kaltsas N., Vlachogianni E., Bouyia P. (2015). “The Antikythera Shipwreck,” in The Ship, the Treasures, the Mechanism 2 (Athens: National Archaeological Museum).
Kázmér M., Taborosi D. (2012). Bioerosion on the Small Scale–Examples From the Tropical and Subtropical Littoral. Hantkeniana 7, 37–94.
Kefi F. J., Boubaker S., El Menif N. T. (2014). Relative Growth and Reproductive Cycle of the Date Mussel Lithophaga lithophaga (Linnaeus 1758) Sampled From the Bizerte Bay (Northern Tunisia). Helgol. Mar. Res. 68, 439–450. doi: 10.1007/s10152-014-0400-9
Kelly S. R. A., Bromley R. G. (1984). Ichnological Nomenclature of Clavate Borings. Palaeontology 27, 793–807.
Kiene W. E., Radtke G., Gektidis M., Golubic S., Vogel K. (1995). Factors Controlling the Distribution of Microborers in Bahamian Reef Environments. Facies 32, 176–188.
Kleemann K. H. (1973a). Der Gesteinsabbau Durch Ätzmuscheln an Kalkküsten. Oecologia 13, 377–395. doi: 10.1007/BF01825527
Kleemann K. H. (1973b). Lithophaga lithophaga (L.)(Bivalvia) in Different Limestone. Malacologia 14, 345–347.
Kleemann K. H. (1974a). Beitrag Zur Kenntnis Des Verhaltens Von Lithophaga lithophaga (L.) (Bivalvia) Im Bohrloch. Sitz Ber Österr Akad Math naturwiss 182, 201–210.
Kleemann K. H. (1974b). Raumkonkurrenz Bei Ätzmuscheln. Mar. Biol. 26, 361–364. doi: 10.1007/BF00391519
Kleemann K. H. (1990). Boring and Growth in Chemically Boring Bivalves From the Caribbean, Eastern Pacific and Australia’s Great Barrier Reef. Senckenbergiana Maritima 21, 101–154.
Kleemann K. H. (1996). Biocorrosion by Bivalves. Mar. Ecol. 17, 145–158. doi: 10.1111/j.1439-0485.1996.tb00496.x
Kovalyova M. A. (2012). Preliminary Data on the Distribution of the Rock-Boring Mollusc Petricola lithophaga (Philippson 1788) Along the Western Coast of Crimea. Proc. V. Gnatyuk Ternopol Natl. Pedagogic University. Biol. 2, 132–136.
Kovalyova M. A. (2015). Morphometric Features of the Shell and Distribution of Petricola lithophaga (Retzius 1788) (Mollusca: Veneridae) Along the Crimean Coast (Black Sea). Acta Zool. Bulg. 67, 587–590.
Kovalyova M. A. (2017). Reproductive Biology of the Rock-Borer Petricola lithophaga (Retzius 1788) (Bivalvia: Veneridae) in the Black Sea. Molluscan Res. 37, 159–166. doi: 10.1080/13235818.2017.1279475
La Perna R. (2005). Tube-Dwelling in Gastrochaena dubia (Bivalvia): Ecological Requirements, Functional Morphology and Structure of the Crypt. Boll. della Soc Paleontol. Ital. 44, 145.
La Russa M. F., Ruffolo S. A., Ricci S., Davidde B., Barca D., Ricca M., et al. (2013). A Multidisciplinary Approach for the Study of Underwater Artefacts: The Case of Tritone Barbato Marble Statue (Grotta Azzurra, Island of Capri, Naples). Period. di Mineral. 82:101–111.
Lauckner G. (1983). “Diseases of Mollusca: Bivalvia,” in Diseases of Marine Animals. Ed. Kinne O. (Hamburg:Biologische Anstalt Helgoland Hamburg), 477–961.
Le Campion-Alsumard T. (1979). Les Cyanophycées Endolithes Marines. Systématique, Ultrastructure, Ecologie Et Biodestruction. Oceanol. Acta 2, 143–156.
Le Campion-Alsumard T., Golubic S., Hutchings P. (1995). Microbial Endoliths in Skeletons of Live and Dead Corals: Porites lobata (Moorea, French Polynesia). Mar. Ecol. Prog. Ser. 117, 149–157. doi: 10.3354/meps117149
Le Grand H. M., Fabricius K. E. (2011). Relationship of Internal Macrobioeroder Densities in Living Massive Porites to Turbidity and Chlorophyll on the Australian Great Barrier Reef. Coral Reefs 30, 97–107. doi: 10.1007/s00338-010-0670-x
Macnae W., Kalk M. (1958). A Natural History of Inhaca Island, Mozambique Vol. I-IV (Johannesburg: Witwatersrand Univ. Press).
Mancinelli R., Petriaggi R. (2004). An Experimental Conservation Treatment on the Mosaic Floor and Perimeter Walls of Room N. 1 of the So-Called "Villa Con Ingresso a Protiro" in the Underwater Archaeological Park of Baia (Naples). Archaeol. Maritima Mediterr. 1, 109–126.
Marlow J., Schönberg C. H. L., Davy S. K., Haris A., Jompa J., Bell J. J. (2019). Bioeroding Sponge Assemblages: The Importance of Substrate Availability and Sediment. J. Mar. Biol. Assoc. UK 99, 343–358. doi: 10.1017/S0025315418000164
Martin D., Britayev T. A. (1998). Symbiotic Polychaetes: Review of Known Species. Ocean. Mar. Biol. Annu. Rev. 36, 217–340.
Meyer N., Wisshak M., Freiwald A. (2021). Bioerosion Ichnodiversity in Barnacles From the Ross Sea, Antarctica. Polar Biol. 44, 667–682. doi: 10.1007/s00300-021-02825-4
Mikac B., Tarullo A., Colangelo M. A., Abbiati M., Costantini F. (2021). Shell Infestation of the Farmed Pacific Oyster Magallana gigas by the Endolith Bivalve Rocellaria dubia. Diversity 13, 526. doi: 10.3390/d13110526
Miniero P., Zevi F. (2008). “Museo Archeologico Dei Campi Flegrei,” in Catalogo Generale, vol. I, II, III. Ed. Napoli E.. (Naples, Italy: Electa).
Montero-Serra I., Linares C., Doak D. F., Ledoux J. B., Garrabou J. (2018). Strong Linkages Between Depth, Longevity and Demographic Stability Across Marine Sessile Species. Proc. R. Soc B Biol. Sci. 285:20172688. doi: 10.1098/rspb.2017.2688
Morhange C., Laborel J., Hesnard A. (2001). Changes of Relative Sea Level During the Past 5000 Years in the Ancient Harbor of Marseilles, Southern France. Palaeogeogr. Palaeoclimatol. Palaeoecol. 166, 319–329. doi: 10.1016/S0031-0182(00)00215-7
Morhange C., Marriner N., Laborel J., Todesco M., Oberlin C. (2006). Rapid Sea-Level Movements and Noneruptive Crustal Deformations in the Phlegrean Fields Caldera, Italy. Geology 34, 93–96. doi: 10.1130/G21894.1
Morton B. (1980). “Some Aspects of the Biology and Functional Morphology of I Coralliophaga (Coralliophaga) coralliophaga (Gmelin 1791)(Bivalvia: Arcticacea): A Coral Associated Nestler in Hong Kong,” in Proceedings of the First International Workshop on the Malacofauna of Hong Kong and Southern China 1977 (Hong Kong: Hong Kong University Press), 311–330.
Morton B. (1982). The Mode of Life and Functional Morphology of Gregariella coralliophaga (Gmelin 1791) (Bivalvia: Mytilacea) With a Discussion on the Evolution of the Boring Lithophaginae and Adaptive Radiation in the Mytilidae. Proc. First Int. Marine Biol. Workshop: marine fauna flora Hong Kong South. China 1980, 875–895.
Morton B., Tseng C. K. (1982). “Pallial Specializations in Gastrochaena (Cucurbitula) cymbium Spengler 1783 (Bivalvia: Gastrochaenacea),” in Proceedings of the First International Marine Biological Workshop: The Marine Flora and Fauna of Hong Kong and Southern China (Hong Kong: Hong Kong University Press), 859–873.
Morton B., Scott P. J. B. (1988). Evidence for Chemical Boring in Petricola lapicida (Gmelin 1791)(Bivalvia: Petricolidae). J. Molluscan Stud. 54, 231–237. doi: 10.1093/mollus/54.2.231
Morton B., Peharda M., Petric M. (2011). Functional Morphology of Rocellaria dubia (Bivalvia: Gastrochaenidae) With New Interpretations of Crypt Formation and Adventitious Tube Construction, and a Discussion of Evolution Within the Family. Biol. J. Linn. Soc 104, 786–804. doi: 10.1111/j.1095-8312.2011.01763.x
Morton B. (2014). The Biology and Functional Morphology of Coralliophaga lithophagella (Bivalvia: Arcticoidea: Trapezidae): An Abyssate, Deep-Water, Nestler From the Açores. With Comparative Notes on the Estuarine Trapezium liratum From Hong Kong. Estuar. Coast. Shelf Sci. 142, 50–59. doi: 10.1016/j.ecss.2014.03.003
Neumann A. C. (1966). Observations on Coastal Erosion in Bermuda and Measurements of the Boring Rate of the Sponge, Cliona lampa. Limnol. Oceanogr. 11, 92–108. doi: 10.4319/lo.1966.11.1.0092
Nicoletti L., Marzialetti S., Paganelli D., Ardizzone G. D. D. (2007). Long-Term Changes in a Benthic Assemblage Associated With Artificial Reefs. Hydrobiologia 580, 233–240. doi: 10.1007/s10750-006-0450-3
Nielsen R. (1987). Marine Algae Within Calcareous Shells From New Zealand. New Zeal. J. Bot. 25, 425–438. doi: 10.1080/0028825X.1987.10413359
Osorno A., Peyrot-Clausade M., Hutchings P. A. (2005). Patterns and Rates of Erosion in Dead Porites Across the Great Barrier Reef (Australia) After 2 Years and 4 Years of Exposure. Coral Reefs 24, 292–303. doi: 10.1007/s00338-005-0478-2
Owada M. (2007). Functional Morphology and Phylogeny of the Rock-Boring Bivalves Leiosolenus and Lithophaga (Bivalvia: Mytilidae): A Third Functional Clade. Mar. Biol. 150, 853–860. doi: 10.1007/s00227-006-0409-y
Pancucci-Papadopoulou M. A., Murina G. V. V., Zenetos A. (2014). The Phylum Sipuncula in the Mediterranean Sea. Monogr. Mar. Sci. 2, 1–109.
Pari N., Peyrot-Clausade M., Le Campion-Alsumard T., Hutchings P. A., Chazottes V., Golubic S., et al. (1998). Bioerosion of Experimental Substrates on High Islands and on Atoll Lagoons (French Polynesia) After Two Years of Exposure. Mar. Ecol. Prog. Ser. 166, 119–130. doi: 10.3354/meps166119
Pari N., Peyrot-Clausade M., Hutchings P. A. (2002). Bioerosion of Experimental Substrates on High Islands and Atoll Lagoons (French Polynesia) During 5 Years of Exposure. J. Exp. Mar. Bio. Ecol. 276, 109–127. doi: 10.1016/S0022-0981(02)00243-5
Parker A. J. (1992). Ancient Shipwrecks of the Mediterranean and the Roman Provinces (Oxford: BAR Inter. Ser).
Peharda M., Puljas S., Chauvaud L., Schöne B. R., Ezgeta-Balić D., Thébault J. (2015). Growth and Longevity of Lithophaga lithophaga: What Can We Learn From Shell Structure and Stable Isotope Composition? Mar. Biol. 162, 1531–1540. doi: 10.1007/s00227-015-2690-0
Perry C. T. (1998). Grain Susceptibility to the Effects of Microboring: Implication for the Preservation of Skeletal Carbonates. Sedimentology 45, 39–51. doi: 10.1046/j.1365-3091.1998.00134.x
Perry C. T., Macdonald I. A. (2002). Impacts of Light Penetration on the Bathymetry of Reef Microboring Communities: Implications for the Development of Microendolithic Trace Assemblages. Palaeogeogr. Palaeoclimatol. Palaeoecol. 186, 101–113. doi: 10.1016/S0031-0182(02)00446-7
Perry C. T., Harborne A. R. (2016). “Bioerosion on Modern Reefs: Impacts and Responses Under Changing Ecological and Environmental Conditions,”, in Coral Reefs at the Crossroads. Eds. Hubbard D. K., Rogers C. S., Lipps J. H., Stanley G. D. J. (Dordrecht: Springer), 69–101.
Petriaggi R. (2005). Nuove Esperienze di Restauro Conservativo nel Parco Sommerso di Baia. Archaeol. Maritima Mediterr. 2, 135–147. doi: 10.1400/52978
Petriaggi R., Davidde B. (2012). The ISCR Project ‘Restoring Underwater’: An Evaluation of the Results After Ten Years. Conserv. Manage. Archaeol. sites 14, 193–200. doi: 10.1179/1350503312Z.00000000016
Pierotti P., Lo Russo R., Sivieri Buggiani S. (1966). Il Dattero di Mare, Lithodomus lithophagus, nel Golfo della Spezia. Ann. Fac. Med. Vet. Univ. di Pisa 18, 157–174.
Polzer M. E., Reyers J. P. (2007). Phoenicians in the West: The Ancient Shipwreck Site of Bajo de la Campana, Spain. The Ina Annu. Proj. and Res.. Institute of Nautical Archaeology, University of Texas, 57–61.
Radtke G., Gektidis M., Golubic S., Hofmann K., Kiene W. E., Le Campion-Alsumard T. (1997). The Identity of an Endolithic Alga: Ostreobium brabantium Weber-Van Bosse is Recognized as Carbonate-Penetrating Rhizoids of Acetabularia (Chlorophyta, Dasycladales). Cour. Forschungsinstitut Senckenb. 201, 341–347.
Radtke G., Golubic S. (2005). Microborings in Mollusk Shells, Bay of Safaga, Egypt: Morphometry and Ichnology. Facies 51, 118–134. doi: 10.1007/s10347-005-0016-02
Radtke G., Golubic S. (2011). “Microbial Euendolithic Assemblages and Microborings in Intertidal and Shallow Marine Habitats: Insight in Cyanobacterial Speciation,” in Advances in Stromatolite Geobiology (Berlin Heidelberg: Springer), 233–263.
Raghukumar C., Lande V. (1988). Shell Disease of Rock Oyster Crassostrea cucullata. Dis. Aquat. Organ. 4, 77–81. doi: 10.3354/dao004077
Ramírez-Viaña A., Diaz-Pulido G., García-Urueña R. (2021). Bioerosion of Reef-Building Crustose Coralline Algae by Endolithic Invertebrates in an Upwelling-Influenced Reef. Coral Reefs 40, 651–662. doi: 10.1007/s00338-021-02065-2
Ricca M., La Russa M. F. (2020). Challenges for the Protection of Underwater Cultural Heritage (UCH), From Waterlogged and Weathered Stone Materials to Conservation Strategies: An Overview. Heritage 3, 402–411. doi: 10.3390/heritage3020024
Ricci S. (2004). La Colonizzazione Biologica Di Strutture Archeologiche Sommerse: I Casi Di Torre Astura E Baia. Archaeol. Maritima Mediterr. 1, 127–135.
Ricci S., Bartolini M. (2005). “Il Biodeterioramento Del Satiro”, in Il Satiro Danzante Di Mazara del Vallo, Il Restauro E L’immagine (Naples, Italy: Electa Napoli Edizioni Napoli), 49–57.
Ricci S., Priori G. F., Bartolini M. (2007a). Bioerosione Di Pavimentazioni Musive Sommerse ad Opera della Spugna Endolitica Cliona celata. Boll. ICR Nuova Ser. 15, 7–18.
Ricci S., Priori G. F., Bartolini M. (2007b). Il Degrado Biologico dei Manufatti Archaeologici dell’area Marina Protetta di Baia. Boll. ICR Nuova Ser. 14, 116–126.
Ricci S., Priori G. F., Bartolini M., Davidde B. (2009). Bioerosion of Lapideous Artefacts Found in the Underwater Archaeological Site of Baia (Naples). Archaeol. Maritima Mediterr. 6, 167–188.
Ricci S., Davidde B. (2012). Some Aspects of the Bioerosion of Stone Artefact Found Underwater: Significant Case Studies. Conserv. Manage. Archaeol. Sites 14, 28–34. doi: 10.1179/1350503312Z.0000000003
Ricci S., Pietrini A. M., Bartolini M., Sacco Perasso C. (2013). Role of the Microboring Marine Organisms in the Deterioration of Archaeological Submerged Lapideous Artifacts (Baia, Naples, Italy). Int. Biodeterior. Biodegradation 82, 199–206. doi: 10.1016/j.ibiod.2013.03.016
Ricci S., Sacco Perasso C., Antonelli F., Davidde Petriaggi B. (2015). Marine Bivalves Colonizing Roman Artefacts Recovered in the Gulf of Pozzuoli and in the Blue Grotto in Capri (Naples, Italy): Boring and Nestling Species. Int. Biodeterior. Biodegradation 98, 89–100. doi: 10.1016/j.ibiod.2014.12.001
Ricci S., Antonelli F., Davidde Petriaggi B., Poggi D., Sacco Perasso C. (2016a). Observations of Two Mosaic Fragments From the Underwater City of Baiae (Naples, Italy): Archaeological, Geological and Biological Investigations. Int. J. Conserv. Sci. 7, 415–430.
Ricci S., Antonelli F., Sacco Perasso C., Poggi D., Casoli E. (2016b). Bioerosion of Submerged Lapideous Artefacts: Role of Endolithic Rhizoids of Acetabularia acetabulum (Dasycladales, Chlorophyta). Int. Biodeterior. Biodegrad. 107, 10–16. doi: 10.1016/j.ibiod.2015.10.024
Ricci S., Davidde Petriaggi B., Priori G. F., Sacco Perasso C., Lucci F., Gomez de Ayala G. (2017). “In Situ Conservation and Presentation of Submerged Mosaic Pavements Located in the Underwater Archaeological Park of Baiae (Naples),” in The Conservation and Presentation of Mosaics: At What Cost?: Proceedings of the 12th Conference of the International Committee for the Conservation of Mosaics, Sardinia (Sassari-Alghero:Getty Publications), 145.
Ricci S., Sanfilippo R., Basso D., Sacco Perasso C., Antonelli F., Rosso A. (2019). Benthic Community Formation Processes of the Antikythera Shipwreck Statues Preserved in the National Archaeological Museum of Athens (Greece). J. Marit. Archaeol. 14, 81–106. doi: 10.1007/s11457-018-9205-3
Rice M. E. (1969). Possible Boring Structures of Sipunculids. Am. Zool. 9, 803–812. doi: 10.1093/icb/9.3.803
Rice M. E., Macintyre I. G. (1972). A Preliminary Study of Sipunculan Burrows in Rock Thin Sections. Carib. J. Sci. 12, 41–44.
Ride W. D. L., Cogger H. G., Dupuis C., Kraus O., Minelli A., Thompson F. C., et al. (1999). International Code of Zoological Nomenclature (London, UK:International Trust for Zoological Nomenclature).
Sacco Perasso C., Ricci S., Davidde Petriaggi B., Calcinai B. (2015). Marine Bioerosion of Lapideous Archaeological Artifacts Found in the Grotta Azzurra (Capri, Naples, Italy): Role of Microbiota and Boring Porifera. Int. Biodeterior. Biodegradation 99, 146–156. doi: 10.1016/j.ibiod.2014.08.010
Sacco Perasso C., Ricci S (2019). “Biological Aspects,” in The Submerged “Villa of the Dolia” Near Ancient Epidaurus. The Preliminary Results of the First Excavation and Conservation Campaign. Annuario Della Scuola Di Archeologica Italiana Di Atene E Delle Missioni Italiane in Oriente, vol. 545. Eds. Davidde Petriaggi D., Galiatsatou P., Medaglia S.. (Rome-Athens:SAIA).
Schiaparelli S., Franci G., Albertelli G., Cattaneo-Vietti R. (2003). Autoecologia del Bivalve Perforatore Gastrochaena dubia Lungo la Falesia del Promontorio di Portofino. Atti Assoc. Ital. Oceanol. e Limnol. 16, 29–36.
Schiaparelli S., Franci G., Albertelli G., Cattaneo-Vietti R. (2005). A non Destructive Method to Evaluate Population Stucture and Bioerosion Activity of the Boring Bivalve Gastrochaena dubia. J. Coast. Res. 21, 383–386. doi: 10.2112/03-0054.1
Schönberg C. H. L. (2000). Bioeroding Sponges Common to the Central Australian Great Barrier Reef: Descriptions of Three New Species, Two New Records, and Additions to Two Previously Described Species. Senckenbergiana maritima 30, 161–221. doi: 10.1007/BF03042965
Schönberg C. H. L., Fang J. K. H., Carreiro-Silva M., Tribollet A., Wisshak M. (2017a). Bioerosion: The Other Ocean Acidification Problem. ICES J. Mar. Sci. 74, 895–925. doi: 10.1093/icesjms/fsw254
Schönberg C. H. L., Fang J. K. H., Carballo J. L. (2017b). “Bioeroding Sponges and the Future of Coral Reefs,”, in Climate Change, Ocean Acidification and Sponges (Cham: Springer), 179–372.
Smith C. R., Glover A. G., Treude T., Higgs N. D., Amon D. J. (2015). Whale-Fall Ecosystems: Recent Insights Into Ecology, Paleoecology, and Evolution. Ann. Rev. Mar. Sci. 7, 571–596. doi: 10.1146/annurev-marine-010213-135144
Spagnolo A., Cuicchi C., Punzo E., Santelli A., Scarcella G., Fabi G. (2014). Patterns of Colonization and Succession of Benthic Assemblages in Two Artificial Substrates. J. Sea Res. 88, 78–86. doi: 10.1016/j.seares.2014.01.007
Spencer T., Viles H. (2002). Bioconstruction, Bioerosion and Disturbance on Tropical Coasts: Coral Reefs and Rocky Limestone Shores. Geomorphology 48 (1-3), 23–50. doi: 10.1016/S0169-555X(02)00174-5
Stephen A. C., Edmonds S. J. (1972). The Phyla Sipuncula and Echiura (British Museum of Natural History,London).
Tapanila L. (2008). “The Endolithic Guild: An Ecological Framework for Residential Cavities in Hard Substrates,” in Current Developments in Bioerosion (Berlin, Heidelberg:Springer Berlin Heidelberg), 3–20.
Tebble N. (1966). “British Bivalve Seashells; a Handbook for Identification,” in British Museum (Natural History) (London: Edinburgh (UK) Her Majesty’s Stationery Office).
Tribollet A., Payri C. (2001). Bioerosion of the Coralline Alga Hydrolithon onkodes by Microborers in the Coral Reefs of Moorea, French Polynesia. Oceanol. Acta 24, 329–342. doi: 10.1016/S0399-1784(01)01150-1
Tribollet A., Golubic S. (2005). Cross-Shelf Differences in the Pattern and Pace of Bioerosion of Experimental Carbonate Substrates Exposed for 3 Years on the Northern Great Barrier Reef, Australia. Coral Reefs 24, 422–434. doi: 10.1007/s00338-005-0003-7
Tribollet A., Golubic S. (2011). “Reef Bioerosion: Agents and Processes,” in Coral Reefs: An Ecosystem in Transition (Dordrecht: Springer), 435–449.
Tribollet A., Golubic S., Radtke G. (2011). “Bioerosion,”, in Encyclopedia of Geobiology. Eds. Reitner J., Thiel J. (Netherlands: Springer), 117–134.
Turicchia E., Abbiati M., Bettuzzi M., Calcinai B., Morigi M. P., Summers A. P., et al. (2022). Bioconstruction and Bioerosion in the Northern Adriatic Coralligenous Reefs Quantified by X-Ray Computed Tomography. Front. Mar. Sci. 8. doi: 10.3389/fmars.2021.790869
Turner R. D. (1971). “Identification of Marine Wood-Boring Molluscs,” in Marine Borers, Fungi and Fouling Organisms of Wood. Eds. Jones E. B. G., Eltringham S. K. (Paris: Organisation for economic co-operation and development), 17–64.
UNESCO. (2001). Convention on the Protection of the Underwater Cultural Heritage. Available at: http://www.unesco.org/new/en/culture/%0Athemes/underwater-cultural-heritage/2001-convention/.
Valentich-Scott P., Dinesen G. E. (2004). Rock and Coral Boring Bivalvia (Mollusca) of the Middle Florida Keys, USA. Malacologia 46, 339–354.
Valentich-Scott P., Tongkerd P. (2008). Coral-Boring Bivalve Molluscs of Southeastern Thailand, With the Description of a New Species. Raffles Bull. Zool. 18, 191–216.
Van den Hoek C., Mann D. G., Jahns H. M. (1995). Algae: An Introduction to Phycology (Cambridge, UK: Cambridge University Press).
Voigt E. (1965). Über Parasitische Polychaeten in Kreide-Austern Sowie Einige Andere in Muschelschalen Bohrende Würmer. Paläontologische Z. 39, 193–211. doi: 10.1007/BF02990164
Voigt E. (1971). Fremdskulpturen an Steinkernen Von Polychaeten-Bohrgängen Aus Der Maastrichter Tuffkreide. Paläontologische Z. 45 (3), 144–153. doi: 10.1007/BF02989572
Voigt E. (1975). Tunnelbaue Rezenter Und Fossiler Phoronidea. Paläontologische Z. 49, 135–167. doi: 10.1007/BF02988072
Voultsiadou E., Koutsoubas D., Achparaki M. (2010). Bivalve Mollusc Exploitation in Mediterranean Coastal Communities: An Historical Approach. J. Biol. Res. 13, 35–45.
Warme J. E. (1975). “Borings as Trace Fossils, and the Processes of Marine Bioerosion,”, in The Study of Trace Fossils (Berlin, Heidelberg:Springer Berlin Heidelberg), 181–227.
Waser A. M., Lackschewitz D., Knol J., Reise K., Wegner K. M., Thieltges D. W. (2020). Spread of the Invasive Shell-Boring Annelid Polydora websteri (Polychaeta, Spionidae) Into Naturalised Oyster Reefs in the European Wadden Sea. Mar. Biodivers. 50:63. doi: 10.1007/s12526-020-01092-6
Weinberg G. D., Grace V. R., Edwards G. R., Robinson H. S., Throckmorton P., Ralph E. K. (1965). The Antikythera Shipwreck Reconsidered. Trans. Am. Philos. Soc 55, 3–48. doi: 10.2307/1005929
Weinstein D. K., Maher R. L., Correa A. M. (2019). “Bioerosion,” in Mesophotic Coral Ecosystems (Cham: Springer), 829–847.
Williams J. A., Margolis S. V. (1974). Sipunculid Burrows in Coral Reef: Evidence for Chemical and Mechanical Excavation. Pacific Sci. 28, 357–359.
Williams S. B., Pizarro O., Foley B. (2016). “Return to Antikythera: Multi-Session SLAM Based AUV Mapping of a First Century BC Wreck Site”, in Field and Service Robotics (Cham: Springer), 45–49.
Wisshak M. (2006). “High-Latitude Bioerosion: The Kosterfjord Experiment,” in Lecture Notes in Earth Sciences, vol. 109. (Heidelberg: Springer Berlin).
Wisshak M., Tribollet A., Golubic S., Jakobsen J., Freiwald A. (2011). Temperate Bioerosion: Ichnodiversity and Biodiversity From Intertidal to Bathyal Depths (Azores). Geobiology 9, 492–520. doi: 10.1111/j.1472-4669.2011.00299.x
Wisshak M., Titschack J., Kahl W.-A., Girod P. (2017). Classical and New Bioerosion Trace Fossils in Cretaceous Belemnite Guards Characterised via Micro-CT. Foss. Rec. 20, 173–199. doi: 10.5194/fr-20-173-2017
Wisshak M., Knaust D., Bertling M. (2019). Bioerosion Ichnotaxa: Review and Annotated List. Facies 65, 1–39. doi: 10.1007/s10347-019-0561-8
Zevi F. (2008). “Pozzuoli Come ‘Delus Minor’. La Città Cosmopolita E L’emporio,”, in Museo Archeologico Dei Campi Flegrei. Catalogo Generale. Liternum, Baia, Miseno. Eds. Zevi F., Demma F., Nuzzo E., Rescigno C., Valeri C. (Naples, Italy: Electa Napoli Spa), 52–79.
Zevi F., Valeri C. (2008). “Sculture e Arredi Marmorei Del Ninfeo,”, in Museo Archeologico dei Campi Flegrei, Catalogo Generale, Liternum, Baia, Miseno. Eds. Zevi F., Demma F., Nuzzo E., Rescigno C., Valeri C. (Napoli: Electa Napoli Spa), 152–164.
Keywords: microborers, macroborers, boring traces, calcareous substrates, Underwater Cultural Heritage, bioerosion succession, silicone casts, resin casts
Citation: Sacco Perasso C, Antonelli F, Calcinai B, Casoli E, Gravina MF and Ricci S (2022) The Bioerosion of Submerged Archeological Artifacts in the Mediterranean Sea: An Overview. Front. Mar. Sci. 9:888731. doi: 10.3389/fmars.2022.888731
Received: 03 March 2022; Accepted: 19 April 2022;
Published: 26 May 2022.
Edited by:
Ralf Schwamborn, Federal University of Pernambuco, BrazilReviewed by:
Martin Coombes, University of Oxford, United KingdomMelih Ertan ÇINAR, Ege University, Turkey
Copyright © 2022 Sacco Perasso, Antonelli, Calcinai, Casoli, Gravina and Ricci. This is an open-access article distributed under the terms of the Creative Commons Attribution License (CC BY). The use, distribution or reproduction in other forums is permitted, provided the original author(s) and the copyright owner(s) are credited and that the original publication in this journal is cited, in accordance with accepted academic practice. No use, distribution or reproduction is permitted which does not comply with these terms.
*Correspondence: Edoardo Casoli, edoardo.casoli@uniroma1.it
†These authors have contributed equally to this work and share first authorship