- 1Key Laboratory of Exploration and Utilization of Aquatic Genetic Resources, Ministry of Education, Shanghai Ocean University, Shanghai, China
- 2Shanghai Engineering Research Center of Aquaculture, Shanghai Ocean University, Shanghai, China
- 3National Demonstration Center for Experimental Fisheries Science Education, Shanghai Ocean University, Shanghai, China
Vitellogenin (VTG) plays a very important role in the development of oocytes. This study aims to explore the effect of swimming on the VTG synthesis of conger eel (Conger myriaster). Circulating water flow and hormone injection regimen were two factors set in the following trials: A1 (no injection, no water flow), A2 (no injection, water flow), B1 (injection, no water flow), and B2 (injection, water flow). The flow velocity of the flowing water was 0.4 m/s (12 h, 60 days). We examined VTG, estrogen receptor (Er) gene expression, and VTG content in the liver and serum on the 30th and 60th days. VTG gene expression in A1 and A2 gradually decreased and was not significantly different between the two groups. The expression of Er gene in A1 was significantly higher than that in A2. The expression of VTG gene in group B1 was significantly higher than that in group B2, whereas the expression level of Er gene in group B1 was slightly higher than that in group B1. The VTG content in the liver in group B1 reached 1,396.93 μg/L, which was significantly higher than that in group B2 (1,302.06 μg/L). Results showed that the flowing water factor can inhibit the expression of VTG and Er genes in the liver and reduce the synthesis of VTG in the liver. Stimulation of flowing water can inhibit the yolk accumulation during the ovarian development of conger eel.
Introduction
Vitellogenin (VTG), a large-molecular-weight glycolipoprotein (Xu and Quan, 2016), is synthesized in the liver and transported to egg cells during the artificial reproduction of the Japanese eel (Anguilla japonica) (Wang and Lou, 2006). The gene expression and VTG synthesis are promoted by estrogen from the estrogen receptor in the liver (Williams et al., 2013; Hara et al., 2016). After being absorbed by the egg cell, VTG is converted into yolk protein (Yp), which is an important nutrient for the development of seedling embryos (Reading et al., 2017). In striped bass (Morone saxatilis), VTG gene is weakly expressed in organs, such as the heart and intestine, but is considerably expressed in the liver; different types of VTG are deposited in egg cells with different proportions (Williams et al., 2014). So far, two VTG genes were cloned from the European eel (Anguilla anguilla), three from the Japanese eel, and only one VTG gene from the conger eel (Conger myriaster); conger eel VTG is the most similar to Japanese eel VTG-1 (80% identity at the amino acid level) (GenBank accession no. AY423445) (Mikawa et al., 2006). The VTG receptor in the ovary of the European eel is expressed in juvenile eels, so the VTG receptor is not a limiting factor for the uptake of VTG by the egg (Palstra et al., 2010c; Morini et al., 2020b). The synthesis of VTG has a very important effect on ovarian development.
The conger eel is a deep-sea migratory fish (Hao et al., 2020). The ovaries of the conger eel gradually mature during migration and lay eggs near the spawning grounds (Mingkun et al., 2018; Xiaolong et al., 2021). In 2008, the spawning sites of the conger eel were found in the Kyushu–Palau ridge region, 380 km west of Okinotori Island (Miller et al., 2011). The conger eel has experienced a long migration time during its gonad development, but the current artificial reproduction process is in still water and is not influenced by the external environment during migration. In 2003, female eels were first induced to mature and lay eggs by adjusting the water temperature; this study confirmed that the external environment is important for the development of ovaries (Utoh et al., 2013). A long-distance swim could promote the oocyte development of the European eel (Ginneken et al., 2007). Male European eels can mature their gonads by swimming, and female eels can promote lipid accumulation in oocytes by swimming (Palstra et al., 2007; Palstra et al., 2008). Therefore, swimming has a very important effect on the development of the eels’ gonads.
During migration, the lipids and VTG in the ovaries of the conger eel gradually accumulate until maturity, but they could not spontaneously mature under cultured conditions. Based on previous experiments, the conger eel can mature under the conditions of artificial injection of hormones (Meng et al., 2021). However, the artificial breeding of the conger eel has problems and difficulties, such as poor egg quality, low fertilization rate, and high seedling mortality (Horie et al., 2003; Horie et al., 2010). Eels cannot naturally develop to sexual maturity under artificial culture due to the insufficient secretion of gonadotropin-releasing hormone and dopamine inhibition (Burgerhout et al., 2013). In contrast to the European eel, the conger eel does not undergo the transition from freshwater to saltwater (Shimizu, 2001). We hypothesize that swimming is an important condition for the development of the eel ovary. In this study, the effects of swimming stimulation on the development of the ovary and the expression of VTG and Er genes were investigated with or without hormone injection to understand the regulatory role of swimming in artificial reproduction.
Materials and Methods
Experimental Eels
The conger eel (n = 86, 303.64 ± 10.02 g) samples, whose oocytes represent the oil droplet stage and primary yolk globule stage, were obtained from Shenghang Aquatic Technology Co., Ltd. (Weihai, China). The experiment was begun in May 2021 at the company’s fish farm. The eels were acclimated in a cement tank (30.0 ± 0.5 psu, 18°C ± 0.5°C) for 2 weeks before the experiment.
Swimming Tank System and Conditions
A cylindrical glass fiber tank (diameter = 0.75 m, height = 1.5 m, 1,000 L) with a built-in pump was designed to provide circulating water flow for fish swimming (Figure 1). According to a previous study on flow velocity (0.4 ± 0.05 m/s) and the findings of the eel migration route, the swimming duration was set up at 12 h per day (Hammer, 1995; Miller et al., 2011; Meng, 2020).
Experiment Design
Healthy eels were selected and randomly distributed into four cylindrical glass fiber tanks (n = 20, 30.0 ± 0.5 psu, 18°C ± 0.5°C). Six acclimated eels were sampled and measured as a control group on the first day. Circulating water flow and hormone injection regimen were two factors set in the following trials: A1 (no injection, no water flow), A2 (no injection, water flow), B1 (injection, no water flow), and B2 (injection, water flow). All experimental groups were placed in a dark environment.
The mixture of carp pituitary extract (CPE; 20 mg/kg) and human chorionic gonadotropin (HCG; 100 IU/kg) was intraperitoneally injected weekly to induce eel development until the end of the experiment.
Measurement and Sampling
On the 30th and 60th days of the trial, six eels in each group were anesthetized using MS-222 (0.05 g/L). The weight and body morphological parameters of each eel were measured. Blood samples were collected from the bulbus arteriosus using a syringe and centrifuged at 3,000 rpm for 15 min at 4°C. The serum was frozen at −80°C. The liver, digestive tract, and ovarian were fixed in Bouin’s solution or stored at −80°C after weighing until analysis.
Histology
The ovarian tissue fixed in Bouin’s solution was dehydrated gradient with 30%–100% ethanol, xylene transparent, paraffin-embedded, cut into 6–8-μm-thick paraffin sections (Kodi 1508A, China), and stained with H&E. The oocytes were studied visually under the microscope (Nikon Eclipse 80i, Nikon, Tokyo, Japan), and overview pictures were taken (Nikon Coolpix 4500). Per section, oocytes that were cut through the nucleus were randomly selected. For each oocyte, the developmental stage was determined.
Quantitative Real-Time PCR
After the gene sequences of VTG (GenBank accession no. AB185334.1) and Er (GenBank accession no. AB117930.1) of the conger eel were obtained at the National Center for Biotechnology Information (NCBI), specific primers were designed by primer software and used for quantitative real-time PCR to analyze gene expression. The primer for β-actin was designed from the sequences of A. japonica (GenBank accession no. GU001950.1). Before the experiment, the specificity and amplification efficiency of β-actin primers were verified.
Total RNA was obtained from the liver by using a kit (Tiangen Biotech Co., Ltd., Beijing, China) according to the manufacturer’s instructions. About 1 μg of total RNA was reverse transcribed with Hifair® II 1st Strand cDNA Synthesis SuperMix for qPCR (gDNA digester plus) (CAT: 11123ES). Diluted cDNA (1:10) was used in all qPCR experiments. The qRT-PCR experiments were carried out in triplicate on CFX96 Touch Real-time PCR Detection System (Applied Biosystems®, Bio-Rad, Hercules, CA, USA) using 1 μl of diluted cDNA as a template for each reaction with SYBR Green PCR Master Mix (Bio-Rad). Thermal cycling conditions included an initial heat denaturation step at 95°C for 30 s, 40 cycles at 95°C for 5 s, 60°C for 30 s, and 95°C for 15 s. Melting curves of the PCR products were determined from 60°C to 95°C to ascertain the specificity of the amplification. Relative gene expression was calculated through the 2−ΔΔCt method (Livak and Schmittgen, 2002).
Vitellogenin Content
VTG content in the liver and serum was determined using an ELISA kit (Shanghai Enzyme-linked Biotechnology Co., Ltd., Shanghai, China) in accordance with the instructions of the kit.
Statistical Analysis
Gonadosomatic index (GSI), digestive tract somatic index (DTSI), eye index (EI), and hepatosomatic index (HIS) were determined according to the following formulas:
1) DTSI = (DTW/BW) × 100% ; DTW indicates digestive tract weight (g).
2) GSI = (GW/BW) × 100%; GW indicates gonad weight (g), and BW body weight (g).
3) EI = [((EDh + EDv)/4)2 × л/TL] × 100; TL indicates total length (cm), EDv eye diameter vertical (cm), and Edh eye diameter horizontal (cm).
4) HIS = (LW/BW) × 100%; LW indicates liver weight (g).
All statistical analyses were performed using SPSS Statistics 12.0 (SPSS Inc., Chicago, IL, USA). Data were expressed as average ± SE (av ± se). Differences at p ≤ 0.05 were considered statistically significant.
Results
Swimming Behavior and Distance
The eels from groups A2 and B2 swam around 518.4 and 1,036.8 km over 30 and 60 days, respectively. Few eels were observed resting at the bottom of the tanks during water flow.
Oocyte Developmental Status
The ovaries of the control group, group A1, and group A2 did not develop. However, the ovaries of groups B1 and B2 were further developed. Eels of the control group (Figure 2A), group A1 (Figures 2B, C), and group A2 (Figures 2D, E) contained oocytes representing the oil droplet stage and primary yolk globule stage according to Tomoko et al. (Tomoko et al., 2003). The ovaries of eels in groups B1 (Figure 2F) and B2 (Figure 2H) were at the secondary pharmaceutical globule stage and tertiary pharmaceutical globule stage on the 30th day. The ovaries of eels in groups B1 (Figure 2G) and B2 (Figure 2I) are in the tertiary globule stage and migratory nucleus stage on the 60th day.
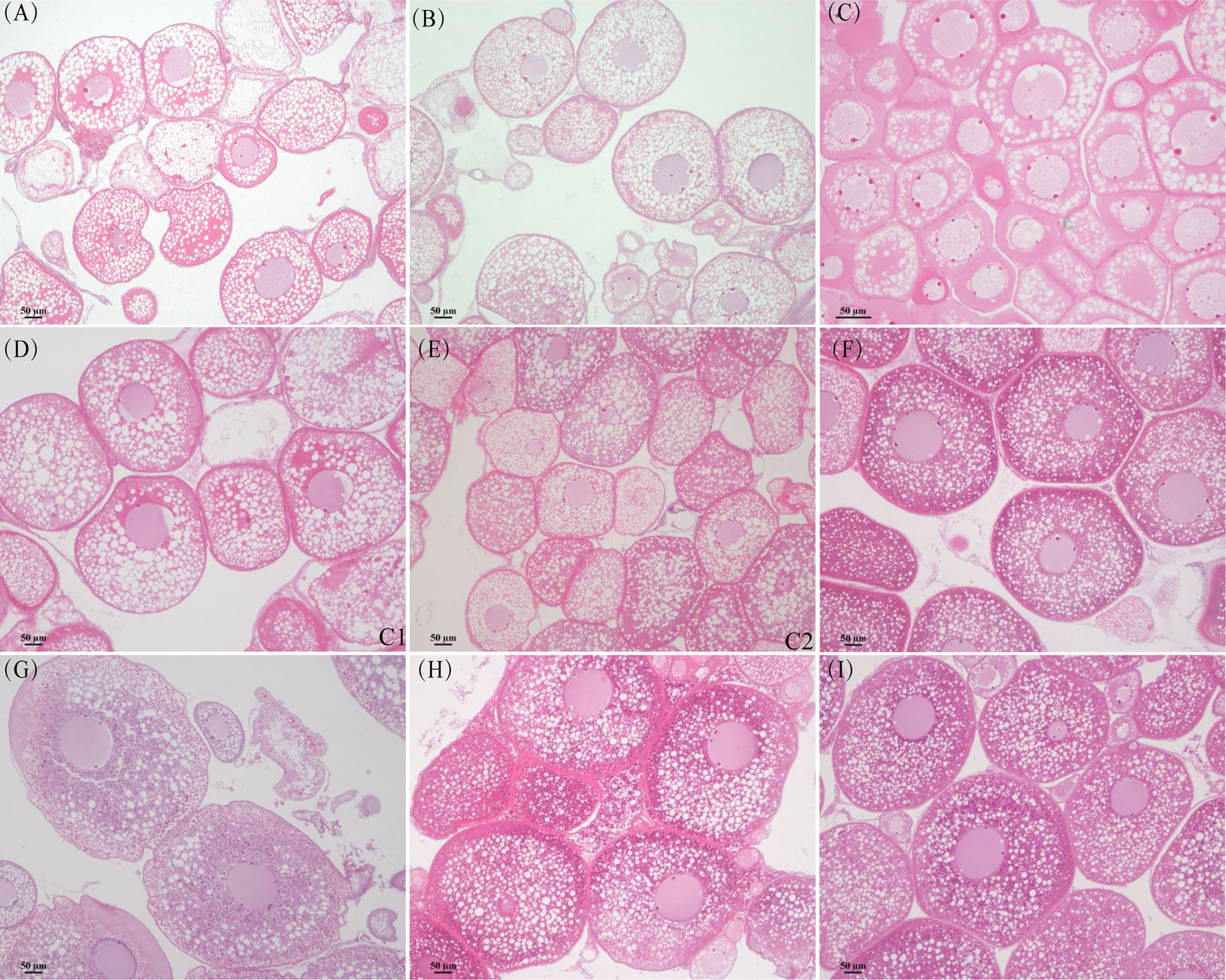
Figure 2 Histology section of ovary in different groups. (A) Control group. (B) Group A1 (30 days). (C) Group A1 (60 days). (D) Group A2 (30 days). (E) Group A2 (30 days). (F) Group B1 (30 days). (G) Group B1 (60 days). (H) Group B2 (30 days). (I) Group B2 (60 days).
Effect of Swimming on Ovarian Development
Two groups (A1 and A2) without injection presented no significant difference in HIS and GSI and had no ovaries grown during the experiment (Table 1). The BW of eels in A2 declined fast (BW, −21.78%) and was significantly lower than that in A1 when sampled on the 30th day (250.67 ± 23.05 vs. 284 ± 34.98; Table 1). The average value of TL in A2 was significantly lower than that in A1 on the 60th day (54.67 ± 2.4 vs. 59.02 ± 2.9; Table 1). The DTSI in group A2 showed a downward trend (DTSI, −48.94%) and was significantly lower than that in A1 on the 30th day (0.0021 ± 0.0003 vs. 0.0028 ± 0.0006; Table 1).
The expression of VTG in A1 and A2 was gradually reduced compared with that in the control group (Figure 3A). Although the expression in A1 was slightly higher than that in A2 on the 30th day, the difference between the two groups was not significant. The expression of Er gene in both groups did not change significantly compared with that in the control group (Figure 3B), but the expression in group A1 was significantly higher than that in A2 through the 60-day treatment (p < 0.05).
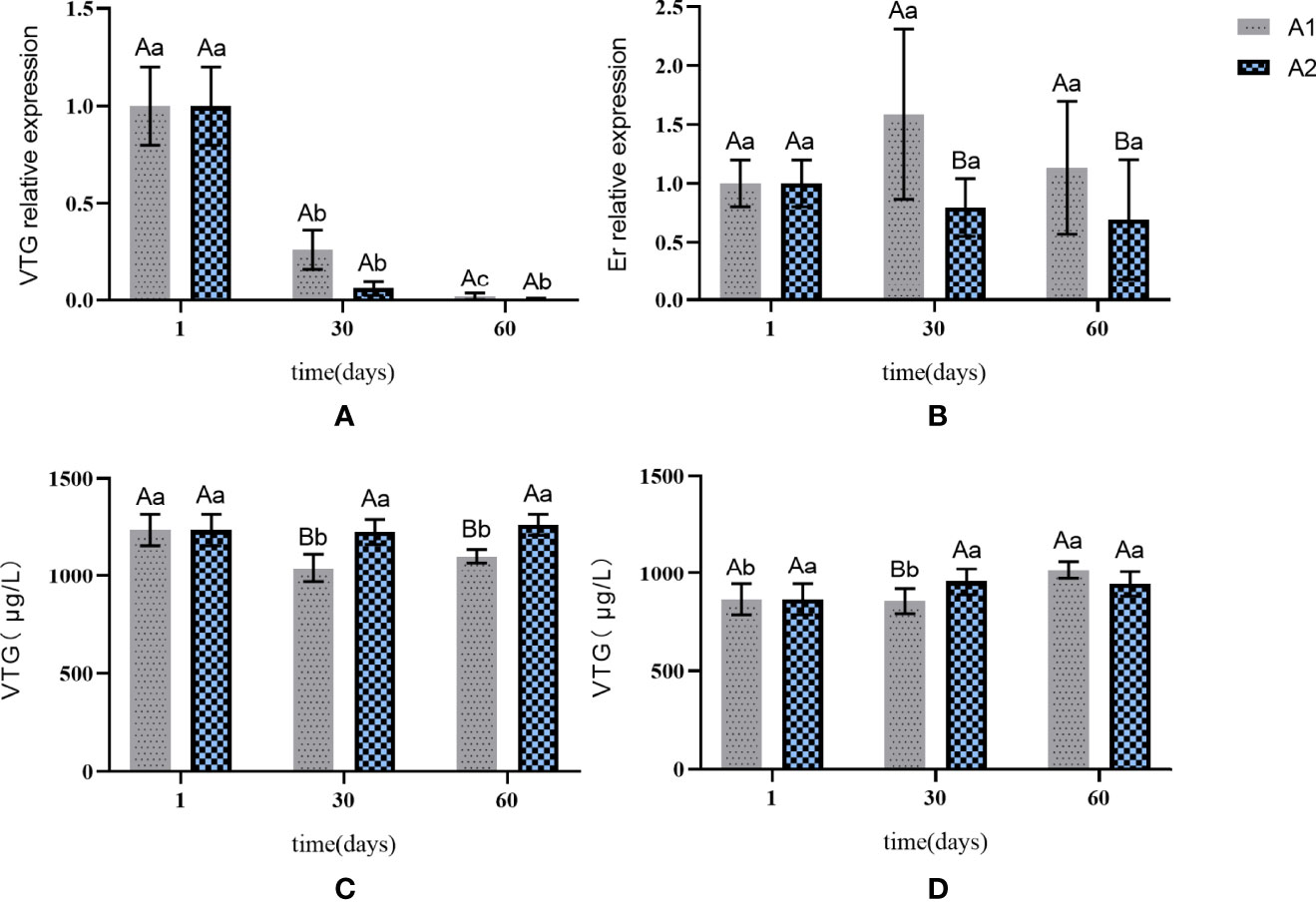
Figure 3 The expression of VTG and Er genes in liver and the content of VTG in liver and serum in A1 and A2. (A) VTG gene expression in the liver. (B) Er gene expression in the liver. (C) changes in the content of VTG in the liver. (D) changes in the content of VTG in the serum. The capital letters marked in the chart indicate significant differences between the swimming group and the resting group (p < 0.05). Lowercase letters indicate differences within the same group (p < 0.05). VTG, vitellogenin; Er, estrogen receptor.
The VTG concentration in serum was not significantly different between A1 and A2 (Figure 3D). The VTG content in the liver in group A1 showed a downward trend, while that in A2 remained stable (Figure 3C). No obvious change in the VTG content was observed in the liver and serum in the two groups.
Effect of Swimming Under Hormone Injection on Ovarian Development
The GSI of eels in groups B1 and B2 increased gradually during the experiment (Table 1). The GSI in B1 was significantly higher than that in B2 on the 30th day (0.1762 ± 0.042 vs. 0.1141 ± 0.0437), but no significant difference was observed on the 60th day. The HIS in groups B1 and B2 increased on the 30th day (HIS, +24.64% and +21.74%, respectively). On the 60th day, the HIS in B1 decreased and that in B2 remained unchanged, but the difference between the two groups was not significant (Table 1). The body weight in groups B1 and B2 increased gradually (weight, +21.30% and +18.07%, respectively) and had no significant difference between the two groups (Table 1). The DTSI of eels in B1 was significantly lower than that in B2 on the 30th day (p < 0.05, Table 1).
The expression levels of VTG and Er increased gradually in B1 and B2 compared with those in the control group (Figures 4A, B). The VTG expression was significantly higher in B1 than in B2 on the 30th day but was not significantly different on the 60th day. The Er gene expression showed no significant difference between B1 and B2 during the entire experiment (Figure 4B).
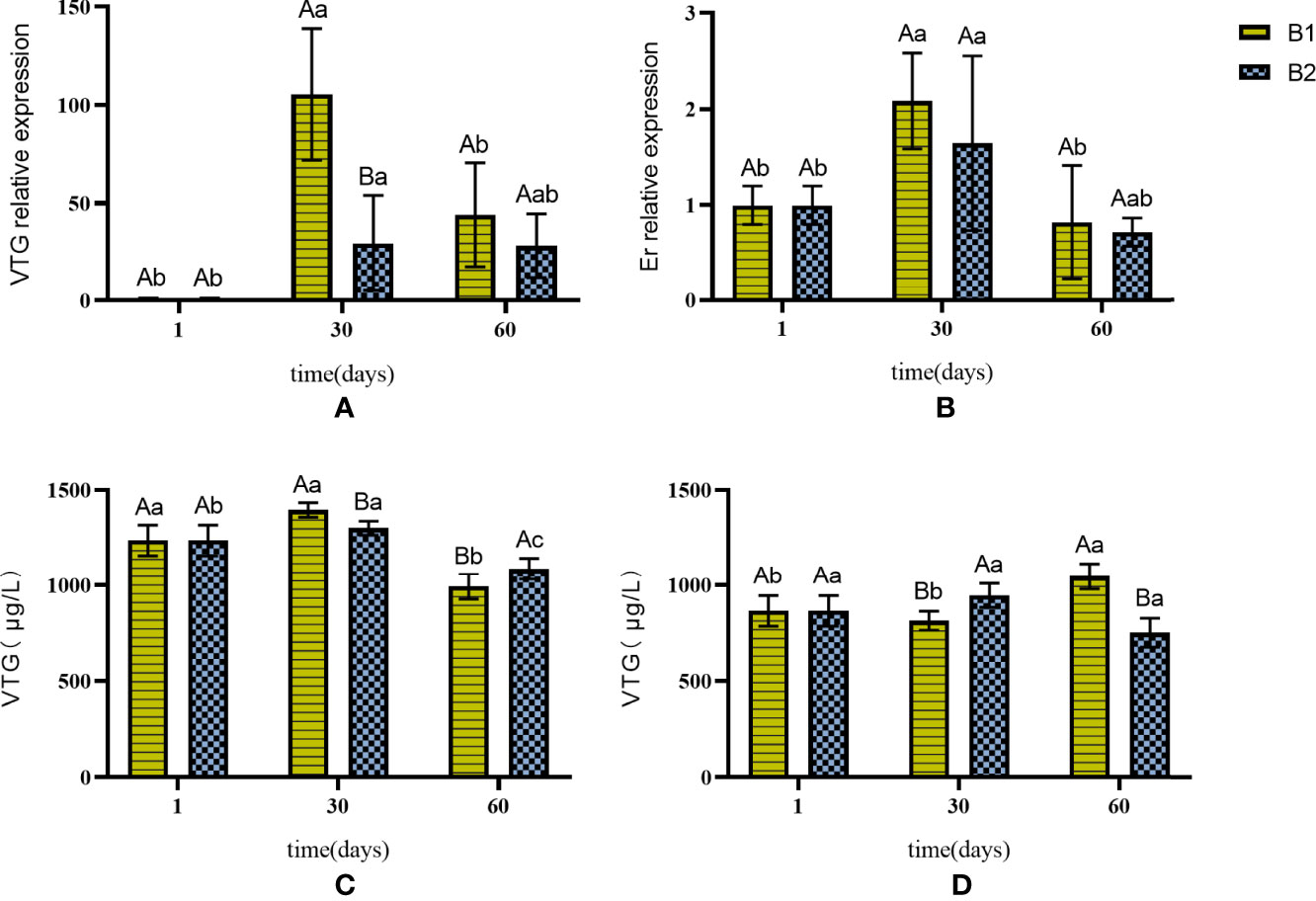
Figure 4 The expression of VTG and Er genes in liver and the content of VTG in liver and serum in B1 and B2. (A) VTG gene expression in the liver. (B) Er gene expression in the liver. (C) changes in the content of VTG in the liver. (D) changes in the content of VTG in the serum. The capital letters marked in the chart indicate significant differences between the swimming group and the resting group (p < 0.05). Lowercase letters indicate differences within the same group (p < 0.05). VTG, vitellogenin; Er, estrogen receptor.
The VTG content in the liver increased first and then decreased in B1 and B2 (Figure 4C). The VTG content in B1 was significantly higher than that in B2 on the 30th day (Figure 4C). However, changes in the VTG content in the serum of the two groups had no obvious regularity (Figure 4D).
Discussion
In this experiment, the conger eel presented a very high swimming efficiency, similar to the European eel, which swam a maximum of 1,000 km without eating and adverse reactions, such as illness or death (Burgerhout et al., 2013). Swimming consumed much energy mainly from fat and protein, leading to a more significant reduction in the BW of the conger eel in A2 than in A1 (Nowosad et al., 2014). The European eel consumes 32% fat for its migration to spawning grounds (Clevestam et al., 2011). The body weight and GSI increased gradually in B1 and B2. The abdomen expanded during ovarian development, and no weight loss was found in eels in group B2. Hydration may be the main reason for counteracting the depleting effects of swimming (Qi, 2010). During gonadal development, various nutrients, such as fatty acids and proteins, are transferred from the muscle to the gonad (Nowosad et al., 2014). In the European eel, swimming is believed to be key to the activation of lipid metabolism (Palstra and van den Thillart, 2010). Therefore, sufficient nutrient reserve is one of the necessary conditions for eel migration.
Swimming reduced the DTSI in the conger eel but had no significant effect on EI. Scholars believe that EI and other external morphological changes are important markers of ovarian development in fish. Changes in DTSI and EI were found during the ovarian development of the Japanese eel and the European eel. In particular, in the Japanese eel, the EI increased and the DTSI decreased with the gradual development of the ovary (Fontaine et al., 1995; Meng et al., 2021). In the present study, swimming reduced the DTSI in eels without hormone injection; however, no significant difference in DTSI was found between swimming and resting eels with injection. Exogenous hormones play a key role in reducing variations in DTSI caused by swimming. As another important marker, the EI in the European eel would increase through silver plating, which can be promoted by swimming until it reaches the silver eel stage (Ginneken et al., 2007; Palstra et al., 2007). In the present experiment, swimming did not accelerate the growth of EI in the conger eel, but long-term swimming decreased the DTSI. Fish coping with environmental pressure during long-term swimming among different species is a complicated system (Rousseau K et al., 2020).
Swimming inhibits the gene expression of VTG and Er in the liver. The ovary of eels in B1 and B2 developed gradually compared with that in the control group; meanwhile, swimming inhibited the VTG expression in B2 compared with that in B1. Similar to the present experiment, previous work reported that swimming inhibits the expression of two VTGs in the European eel (Palstra et al., 2010b). However, the VTG expression was not inhibited by swimming in A1 and A2 when the ovary did not develop. During the ovarian development of eels, the Er in the liver combines with estradiol and promotes the synthesis of VTG (Morini et al., 2020a). Swimming inhibited Er expression in group A without hormone injection. The expression was slightly higher in B1 than in B2, but the difference was not significant. The inhibition of VTG expression could be due to exogenous hormones and the suppression of Er expression. In our previous experiment, swimming increased the serum cortisol level (unpublished results). In the European eel, swimming and salinity changes can increase the cortisol content (Cutler et al., 2007; Palstra et al., 2009). In response to environmental stress, the ACTH axis is activated and regulated by secreting corresponding hormones, such as cortisol (Ganesh, 2020). Cortisol inhibited hepatic Er expression in rainbow trout (Lethimonier, 2000). The inhibitory effect of cortisol could be related to the inhibition of VTG and Er genes. Changes in salinity during swimming are similar to migration conditions for eels, so the migration process may be accompanied by increased cortisol levels. The inhibition of VTG expression by swimming delayed the accumulation of yolk. The GSI was higher in B1 than in B2, suggesting that fluid stimulation slowed down the rate of pre-ovarian development. Prolonged swimming can delay ovulation in rainbow trout (Oncorhynchus mykiss) (Contreras, 1998). Meanwhile, in the European eel, swimming promoted lipid deposition in egg cells (Palstra et al., 2008). In the conger eel, the inhibition of VTG expression by swimming may promote lipid accumulation.
The VTG content in the liver and serum was also detected. The change in the serum VTG content is similar to that in the European eel under the two conditions with and without hormone injection, and no obvious change rule existed. The same phenomenon was observed in swimming experiments in rainbow trout (Palstra et al., 2007; Palstra et al., 2010a). The inhibition of VTG by swimming may be responsible for the difference in the serum VTG content (Palstra et al., 2010a). Although the amount of VTG in the liver in group B1 was significantly higher than that in group B2 for the first 30 days, the exogenous hormone plays an important factor than swimming through the continuous treatment. A higher VTG content was observed in the liver of group B2 than B1 on the 60th day. The impact of both swimming and exogenous hormone in conger eel liver remains to be further studied.
The results showed that swimming could significantly reduce DTSI in the conger eel without affecting EI. During artificial reproduction, swimming inhibited the expression of VTG gene and slowed down the early development of the conger eel.
Data Availability Statement
The original contributions presented in the study are included in the article/supplementary material. Further inquiries can be directed to the corresponding authors.
Ethics Statement
All fish samples were handled in accordance with the Animal Ethics Committee of Shanghai Ocean University (2016NO.4) and the Regulations for the Administration of Affairs Concerning Experimental Animals approved and authorized by the State Council of the People’s Republic of China.
Author Contributions
RL carried out the experiment, analyzed the data, and wrote the manuscript. KL and LL were responsible for guiding the experiment and modifying the article. GW, XB, and ZJ helped RL to collect and analyze the experimental samples. All authors contributed to the article and approved the submitted version.
Funding
This study was funded by the Shanghai Agriculture Applied Technology Development Program, China (2020-02-08-00-10-F01471), National Natural Science Foundation of China (32072994), Special Fund for Science and Technology Development of Shanghai Ocean University, and Startup Foundation for Young Teachers of Shanghai Ocean University.
Conflict of Interest
The authors declare that the research was conducted in the absence of any commercial or financial relationships that could be construed as a potential conflict of interest.
Publisher’s Note
All claims expressed in this article are solely those of the authors and do not necessarily represent those of their affiliated organizations, or those of the publisher, the editors and the reviewers. Any product that may be evaluated in this article, or claim that may be made by its manufacturer, is not guaranteed or endorsed by the publisher.
Acknowledgments
Thanks to X. L. Yue from Shandong Weihai Shenghang Aquatic Science and Technology Co., Ltd., for supporting the fish culturing during the study.
References
Burgerhout E., Brittijn S. A., Tudorache C., Wijze D. D., Dirks R. P., van den Thillart G.E.E.J.M. (2013). Male European Eels are Highly Efficient Long Distance Swimmers: Effects of Endurance Swimming on Maturation. Comp. Biochem. Physiol. A Mol. Integr. Physiol. 166 (3), 522–527. doi: 10.1016/j.cbpa.2013.08.002
Clevestam P. D., Ogonowski M., SjoBerg N. B., WickstroM H. (2011). Too Short to Spawn? Implications of Small Body Size and Swimming Distance on Successful Migration and Maturation of the European Eel Anguilla Anguilla. J. Fish. Biol. 78 (4), 1073–1089. doi: 10.1111/j.1095-8649.2011.02920.x
Contreras W. M. (1998). Effects of Stress on the Reproductive Performance of Rainbow Trout (Oncorhynchus Mykiss). Biol. Reprod. 58 (2), 439. doi: 10.1095/biolreprod58.2.439
Cutler C. P., Phillips C., Hazon N., Cramb G. (2007). Cortisol Regulates Eel (Anguilla Anguilla) Aquaporin 3 (AQP3) mRNA Expression Levels in Gill. Gen. Comp. Endocrinol. 152 (2-3), 310–313. doi: 10.1016/j.ygcen.2007.01.031
Fontaine Y. A., Pisam M., Moal C., Rambourg A. (1995). Silvering and Gill "Mitochondria-Rich" Cells in the Eel, Anguilla Anguilla. Cell Tissue Res. 281 (3), 465–471. doi: 10.1007/BF00417863
Ganesh C. B. (2020). The Stress – Reproductive Axis in Fish: The Involvement of Functional Neuroanatomical Systems in the Brain. J. Chem. Neuroanat. 112 (101904), 1–13. doi: 10.1016/j.jchemneu.2020.101904
Ginneken V., Dufour S., Sbaihi M., Balm P., Noorlander K., de Bakker M., et al. (2007). Does a 5500-Km Swim Trial Stimulate Early Sexual Maturation in the European Eel (Anguilla Anguilla L.)? Comp. Biochem. Physiol. A Mol. Integr. Physiol. 147 (4), 1095–1103. doi: 10.1016/j.cbpa.2007.03.021
Hammer C. (1995). Fatigue and Exercise Tests With Fish. Comp.e Biochem.Physiol. Part A Physiol. 112, 1, 1–20. doi: 10.1016/0300-9629(95)00060-K
Hao Y., Bao S., Hua xin N., Dai qiang Z., Jing L. (2020). Advances and Future Prospects in Conger Myriaster Research. Mar. Sci. No.372 (06), 154–160.
Hara A., Hiramatsu N., Fujita T. (2016). Vitellogenesis and Choriogenesis in Fishes. Fish. Sci. 82 (2), 187–202. doi: 10.1007/s12562-015-0957-5
Horie N., Utoh T., Yama Da Y., Okamura A., Zhang H., Mikawa N., et al. (2010). Development of Embryos and Larvae in the Common Japanese Conger Conger Myriaster. Fish. Ence. 68 (5), 972–983. doi: 10.1046/j.1444-2906.2002.00521.x
Horie N., Yamada Y., Okamura A., Tanaka S., Utoh T., Mikawa N., et al. (2003). Effective Hormonal Treatments for Induction of Ovarian Maturation and Ovulation in the Common Japanese Conger Conger Myriaster. NIPPON. SUISAN. GAKKAISHI. 69 (3), 337–346. doi: 10.2331/suisan.69.337
Lethimonier C. (2000). Transcriptional Interference Between Glucocorticoid Receptor and Estradiol Receptor Mediates the Inhibitory Effect of Cortisol on Fish Vitellogenesis. Biol. Reprod. 62 (6), 1763–1771. doi: 10.1095/biolreprod62.6.1763
Livak K. J., Schmittgen T. D. (2002). Analysis of Relative Gene Expression Data Using Real-Time Quantitative PCR. Methods 25 (4), 402–408. doi: 10.1006/meth.2001.1262
Meng W. (2020). The Impact of Flowing Water and Starvation on Gonad Development During Artificial Ripening of the Conger Eel (Conger Myriaster) (Shanghai: Shanghai Ocean University).
Meng W., Qiang L., Rucong L., Xinglong J., Zongcheng S., Kang L., et al. (2021). Changes of Individual Morphology and Oocyte Characteristics and Analysis of Tissue Nutrient Content During the Ovary Development of Conger Eel (Conger Myriaster). J. Shanghai. Ocean. Univ. 30 (03), 442–453. doi: 10.12024/jsou.20200503026
Mikawa N., Utoh T., Horie N., Okamura A., Aoki T. (2006). Cloning and Characterization of Vitellogenin cDNA From the Common Japanese Conger (Conger Myriaster) and Vitellogenin Gene Expression During Ovarian Development. Comp. Biochem. Physiol. Part B. Biochem. Mol. Biol. 143 (4), 404–414. doi: 10.1016/j.cbpb.2005.12.013
Miller M. J., Yoshinaga T., Aoyama J., Otake T., Mochioka N., Kurogi H., et al. (2011). Offshore Spawning of Conger Myriaster in the Western North Pacific: Evidence for Convergent Migration Strategies of Anguilliform Eels in the Atlantic and Pacific. Die. Naturwissenschaften. 98 (6), 537–543. doi: 10.1007/s00114-011-0787-y
Mingkun L., Chongliang Z., Min L., Xiuxia M., Yiping R. (2018). Relationship Between the Spatiotemporal Distribution of Conger Myriaster and Environmental Factors in the Southern Waters Off the Shandong Peninsula During Autumn and Winter. J. Fish. Sci. China 25 (05), 1115–1122. doi: 10.11964/jfc.20181011514
Morini M., Lafont A. G., Maugars G., Baloche S., Dufour S., Asturiano J. F., et al. (2020a). Identification and Stable Expression of Vitellogenin Receptor Through Vitellogenesis in the European Eel. Animal 14 (6), 1213–1222. doi: 10.1017/S1751731119003355
Morini M., Lafont A. G., Maugars G., Baloche S., Pérez L. (2020b). Identification and Stable Expression of Vitellogenin Receptor Through Vitellogenesis in the European Eel. Animal 1-10. doi: 10.1017/S1751731119003355
Nowosad J., Kucharczyk D., Luczyńska J., Targońska K., Czarkowski T. K., Bilas ,. M., et al. (2014). Changes in European Eel Ovary Development and Body and Ovary Chemistry During Stimulated Maturation Under Controlled Conditions: Preliminary Data. Aquacult. Int. 23 (1), 13–27. doi: 10.1007/s10499-014-9794-2
Palstra A. P., Crespo D., van den Thillart G.E.E.J.M., Planas J. V. (2010a). Saving Energy to Fuel Exercise: Swimming Suppresses Oocyte Development and Downregulates Ovarian Transcriptomic Response of Rainbow Trout Oncorhynchus Mykiss. Am. J. Physiol. Regul. Integr. Comp. Physiol. 299 (2), R486. doi: 10.1152/ajpregu.00109.2010
Palstra A., Curiel D., Fekkes M., Bakker M., Székely C., Ginneken V. V., et al. (2007). Swimming Stimulates Oocyte Development in European Eel(Anguilla Anguilla). Aquaculture 270 (1-4), 321–332. doi: 10.1016/j.aquaculture.2007.04.015
Palstra A., Ginneken V. V., Thillart G. (2009). “Effects of Swimming on Silvering and Maturation of the European Eel, Anguilla Anguilla L,” in Spawning Migration of the European Eel. 10, 229–251. doi: 10.1007/978-1-4020-9095-0_10
Palstra A. P., Schnabel D., Nieveen M. C., Spaink H. P., Thillart G. (2010b). Swimming Suppresses Hepatic Vitellogenesis in European Female Silver Eels as Shown by Expression of the Estrogen Receptor 1, Vitellogenin1 and Vitellogenin2 in the Liver. Reprod. Biol. Endocrinol. 8 (1), 27. doi: 10.1186/1477-7827-8-27
Palstra A. P., Schnabel D., Nieveen M. C., Spaink H. P., Thillart G. (2010c). Temporal Expression of Hepatic Estrogen Receptor 1, Vitellogenin1 and Vitellogenin2 in European Silver Eels. Gen. Comp. Endocrinol. 166 (1), 1–11. doi: 10.1016/j.ygcen.2009.09.006
Palstra A. P., Schnabel D., Nieveen M. C., Spaink H. P., van den Thillart G. E. (2008). Male Silver Eels Mature by Swimming. BMC Physiol. 8, 14. doi: 10.1186/1472-6793-8-14
Palstra A. P., van den Thillart G. (2010). Swimming Physiology of European Silver Eels (Anguilla Anguilla L.): Energetic Costs and Effects on Sexual Maturation and Reproduction. Fish. Physiol. Biochem. 36 (3), 297–322. doi: 10.1007/s10695-010-9397-4
Qi X. (2010). Researches on the Artificial Induced Maturation of Female Giant Mottled Eel (Anguilla Marmorata) (Hainan: College of Maritime Hainan University).
Reading B. J., Sullivan C. V., Schilling J. (2017). Vitellogenesis in Fishes. Ref. Mod. Life Sci. 635–646. doi: 10.1016/B978-0-12-809633-8.03076-4
Rousseau K A., Prunet P B., Sd A. (2020). Special Features of Neuroendocrine Interactions Between Stress and Reproduction in Teleosts. Gen. Comp. Endocrinol. 300:1–23. doi: 10.1016/j.ygcen.2020.113634
Shimizu T. (2001). Conger-Eel Fisheries and Fisheries Management in Tokyo Bay (II. Conger-Eel Fisheries and Fisheries Management)(Fisheries Biology and Fisheries of White-Spotted Conger-Eel Conger Myriaster). Nip. Suisan. Gakkaishi. 67 (1), 121–122. doi: 10.2331/suisan.67.121
Tomoko U., Noriyuki H., Akihiro O., Yoshiaki Y., Satoru T., Naomi M., et al. (2003). Oogenesis in the Common Japanese Conger Conger Myriaster. Fish. Sci. 69 (1), 181–188. doi: 10.1046/j.1444-2906.2003.00604.x
Utoh T., Horie N., Okamura A., Mikawa N., Yamada Y., Tanaka S., et al. (2013). Water Temperature Manipulation can Induce Oocyte Maturation and Ovulation in the Common Japanese Conger, Conger Myriaster. Aquaculture 392, 120–127. doi: 10.1016/j.aquaculture.2013.02.007
Wang Y. S., Lou S. W. (2006). Structural and Expression Analysis of Hepatic Vitellogenin Gene During Ovarian Maturation in Anguilla Japonica. J. Steroid Biochem. Mol. Biol. 100 (4-5), 193–201. doi: 10.1016/j.jsbmb.2006.04.011
Williams V. N., Reading B. J., Amano H., Hiramatsu N., Schilling J., Salger S. A., et al. (2014). Proportional Accumulation of Yolk Proteins Derived From Multiple Vitellogenins is Precisely Regulated During Vitellogenesis in Striped Bass (Morone Saxatilis). J. Exp. Zool. Part A Ecol. Genet. Physiol. 321 (6), 301–315. doi: 10.1002/jez.1859
Williams V. N., Reading B. J., Hiramatsu N., Amano H., Sullivan C. V. (2013). Multiple Vitellogenins and Product Yolk Proteins in Striped Bass, Morone Saxatilis: Molecular Characterization and Processing During Oocyte Growth and Maturation. Fish. Physiol. Biochem. 40 (2), 395–415. doi: 10.1007/s10695-013-9852-0
Xiaolong L., Kang L., Gaomeng R. U., Xinglong J., Liping L. (2021). Comparative Analysis of the Effects of HCG and CPE Combinations on the Sexual Maturation of Common Japanese Conger Conger Myriaster. J. Shanghai. Ocean. Univ. 30 (01), 29–38. doi: 10.12024/jsou.20190502657
Keywords: conger eel, swimming, ovarian development, vitellogenin, estrogen receptor
Citation: Liu R, Li K, Wang G, Jiang Z, Ba X and Liu L (2022) Effect of Swimming on the Induction of Vitellogenin in Conger Eel (Conger myriaster). Front. Mar. Sci. 9:887074. doi: 10.3389/fmars.2022.887074
Received: 01 March 2022; Accepted: 26 April 2022;
Published: 30 May 2022.
Edited by:
Wei Huang, Ministry of Natural Resources, ChinaCopyright © 2022 Liu, Li, Wang, Jiang, Ba and Liu. This is an open-access article distributed under the terms of the Creative Commons Attribution License (CC BY). The use, distribution or reproduction in other forums is permitted, provided the original author(s) and the copyright owner(s) are credited and that the original publication in this journal is cited, in accordance with accepted academic practice. No use, distribution or reproduction is permitted which does not comply with these terms.
*Correspondence: Kang Li, bGszMTI3QGhvdG1haWwuY29t; Liping Liu, bHAtbGl1QHNob3UuZWR1LmNu
†These authors have contributed equally to this work