- 1Centro Oceanográfico de Málaga, Instituto Español de Oceanografía (IEO), Consejo Superior de Investigaciones Científicas (CSIC), Puerto Pesquero s/n Fuengirola, Malaga, Spain
- 2Instituto Mediterráneo de Estudios Avanzados, IMEDEA Consejo Superior de Investigaciones Científicas-Universidad de las Islas Baleares (CSIC-UIB), Esporles, Spain
- 3Grupo en Biodiversidad y Conservación, Instituto Universitario de Investigación en Acuicultura Sostenible y Ecosistemas Marinos (IU-ECOAQUA), Universidad de Las Palmas de Gran Canaria, Las Palmas, Spain
The introduction and successful expansion of tropical species into temperate systems is being exacerbated by climate change, and it is particularly important to identify the impacts that those species may have, especially when habitat-forming species are involved. Seagrass meadows are key shallow coastal habitats that provide critical ecosystem services worldwide, and they are threatened by the arrival of non-native macroalgae. Here, we examined the effects of Halimeda incrassata, a tropical alga that has recently colonized the Mediterranean Sea, on epifaunal assemblages associated with Cymodocea nodosa seagrass meadows of Mallorca Island (Western Mediterranean Sea). This invasive macroalga is an ecological engineer and thus has a high potential of modifying native habitats. A seagrass meadow colonized by H. incrassata exhibited important changes on associated epifaunal assemblages, with an increase in abundance and diversity, particularly driven by higher abundances of Gammaridae, Polychaeta, Copepoda and Caprellidae. Given the key ecological contribution of epifauna to food webs, these alterations will likely have important implications for overall food web structure and ecosystem functioning of native ecosystems.
Introduction
Climate change and globalization are exacerbating the introduction and spread of tropical species into temperate systems (Bianchi and Morri, 2003; Raitsos et al., 2010; Essla et al., 2011; Vergés et al., 2014; Canning-Clode and Carlton, 2017). Many of the species that can become invasive are macroalgae, which are especially relevant because they exhibit fast spread and a high capacity to establish large populations (Anton et al., 2019 and references therein). The presence of these species in native macrophyte-dominated habitats usually has negative effects on the existing primary producers (macroalgae and seagrasses) affecting the survivorship, cover, biomass, size, and species richness, often leading to an homogenization of assemblages (Anton et al., 2019; Ballesteros et al., 2007; Thomsen et al., 2012; Thomsen et al., 2014Streftaris and Zenetos, 2006; Thomsen et al., 2009; Williams and Smith, 2007, but see García-Gómez et al., 2020; Thomsen, 2020). In contrast, the effects of invasive macroalgae on an invaded habitat may be negative or even positive on the abundance and diversity of associated faunal assemblages (e.g., fish and invertebrates) because of the additional habitat provided by these new structuring species (Thomsen, 2010; Willette and Ambrose, 2012; Thomsen et al., 2014; Navarro-Barranco et al., 2018; ; Anton et al., 2019; Navarro-Barranco et al., 2019; Vivó-Pons et al., 2020). For example, while the presence of Gracilaria vermiculophylla reduces the aboveground biomass of eelgrass (Z. marina), it favors epifaunal assemblages associated with this seagrass (Thomsen et al., 2013). Similarly, the invasive seagrass Halophila stipulacea was able to displace the native seagrass Syringodium filiforme in 10-12 weeks, but conversely it supported significantly more epibiotic invertebrates (Willette and Ambrose, 2012). Invasions by habitat-forming macrophytes could thus lead to changes in the composition, species interactions and trophic structure of the associated faunal assemblages (Vázquez-Luis et al., 2008; Alós et al., 2018; Anton et al., 2019). Indeed, the effects of invasive macroalgae on native ecosystems are multiple, complex and not easy to predict (e.g. Vivó-Pons et al., 2020), so it is necessary to further study the interactions with native habitats in order to properly manage them, as pursued by different legislations, such as the European Marine Strategy Framework Directive.
The Mediterranean Sea is suffering a change in seagrass extension, while some seagrass species (e.g. Zostera marina and Posidonia oceanica) have declined, at least locally, other species are progressing with the help of seawater warming (e.g. Cymodocea nodosa and the non-native H. stipulacea) (Boudouresque et al., 2021). This seawater warming is also accelerating the tropicalization of the Mediterranean Sea (Vergés et al., 2014; Hoffman, 2014), and several non-native macroalgae from tropical and subtropical areas have become invasive in the Mediterranean, including Caulerpa cylindracea, Lophocladia lallemandii, Womersleyella setacea or Acrothamnion preissii, among others (Hoffman, 2014; Zenetos and Galandi, 2020). These non-native macroalgae can generate important negative effects on native macrophytes. For example, L. lallemandii produces a significant reduction in Posidonia oceanica shoot size, leaf biomass, and percentage of living shoots (Ballesteros et al., 2007). Other species such as Asparagopsis armata, or Codium fragile subsp. fragile form monospecific coverages dominating many algal assemblages (Streftaris and Zenetos, 2006; Mancuso et al., 2022). Moreover, the recent case of Rugulopteryx okamurae has marked an unprecedented milestone on the coastal ecosystems of north Africa and south Spain. This species is transforming native habitats of macroalgae beds and coralligenous habitats, producing a homogenization and inflicting heavy losses on ecosystem services in a record time (García-Gómez et al., 2018; García-Gómez et al., 2020; Navarro-Barranco et al., 2021). The biotic impoverishment on coralligenous epifaunal produced by the spreading of R. okamurae is especially notable, whith a reduction in number of species and a taxonomical and functional homogenization (Navarro-Barranco et al., 2021).
The effects on epifaunal assemblages are usually modulated by the structural complexity and chemical traits of novel macrophytes (Schmidt and Scheibling, 2006; Thomsen et al., 2013; Navarro-Barranco et al., 2019). When the novel species have a higher complexity compared to the native macrophytes, there is frequently an increase in epifaunal species richness (Schmidt and Scheibling, 2006; Belattmania et al., 2018) and/or in their abundances (Willette and Ambrose, 2012; Thomsen et al., 2013). Conversely, if the new macrophyte is less structurally complex than the native species, it can lead to a decrease in the abundance and/or richness of epifaunal assemblages (Guerra-García et al., 2012; Navarro-Barranco et al., 2018; Mancuso et al., 2022). However, novel macrophytes may still enhance epifaunal assemblages even when co-inhabiting or replacing a native species of equal structural complexity (Navarro-Barranco et al., 2019). Thus, the arrival of non-native macrophyte species to the Mediterranean Sea will likely have a negative impact on the native macrophytes, but the consequences for epifaunal assemblage remain inconclusive.
Recently, a new siphonous macroalga, Halimeda incrassata (J. Ellis) J. V. Lamouroux, has appeared in the Mediterranean Sea (Alós et al., 2016). This is the first recorded invasion of a species of the genus Halimeda for the Mediterranean Sea. The native distribution of this tropical seaweed encompasses the Western Atlantic and Indo-Pacific Oceans (Guiry and Guiry, 2016). In 2005, its presence was reported on the island of Madeira (NE Atlantic) for the first time (Wirtz and Kaufmann, 2005). At the end of 2008, H. incrassata was detected in La Palma (Canary Islands) (Sangil et al., 2018) and four years later, in 2011, H. incrassata was found colonizing sandy bottoms in the south-western coast of Mallorca Island (NW Mediterranean) (Alós et al., 2016), rapidly spreading into different areas of Mallorca since then (Tomas et al., unpublished).
Halimeda incrassata has a high invasion potential, since it can colonize soft bottoms (e.g., sandy habitats and seagrass beds) by attaching to the substrate through its bulbous rhizoidal holdfasts (van Tussenbroek and Barba Santos, 2011). In addition, not only does H. incrassata reproduce sexually, but it also exhibits asexual reproduction by rhizoidal extension and fragmentation, thereby displaying a high expansion potential (van Tussenbroek and Barba Santos, 2011). Furthermore, its calcified thallus and the presence of chemical defenses provide Halimeda species a considerable resistance to herbivory pressure (Hay et al., 1987; Paul and Van Alstyne, 1988), suggesting that native herbivores in the Mediterranean may be unable to feed on it. However, some native herbivores (e.g. the sea urchin Paracentrotus lividus and the fish Sarpa salpa) are able to consume invasive macroalgae (mainly C. racemosa), but their capacity to control the invasion is somehow limited (Cebrian et al., 2011; Tomas et al., 2011; Santamaría et al., 2021). Thus, the combination of defense and reproductive traits provide H. incrassata with a strong potential of invasion and ecosystem modification (Alós et al., 2016; Sangil et al., 2018). Importantly, because H. incrassata is a habitat-forming species with an important ecological role on tropical sandy-bottom habitats (e.g. it provides microhabitat for numerous species of epifaunal invertebrates; Verbruggen et al., 2006), it may have major ecological effects on native ecosystems.
Currently, information about the effects of H. incrassata on invaded habitats is scant. The few existing studies have mainly focused on impacts on fish assemblages on invaded sandy habitats, and have led to ambiguous results. While some fish species are not affected by the presence of H. incrassata (e.g., the Starry weever Trachinus radiatus and the Atlantic lizardfish Synodus saurus), other fish are deterred (e.g., the flatfish Bothus podas), while other species seem to be attracted (i.e. the Razorfish Xyrichtys novacula) (Alós et al., 2018; Vivó-Pons et al., 2020). Significant effects on fish have been hypothesized to be related to a direct loss of suitable habitat (e.g., burrowing space for flatfish), but also to indirect positive effects (e.g., potential increase of invertebrate prey) resulting from the presence of the new three-dimensional structure provided by this habitat-forming alga (Alós et al., 2018; Vivó-Pons et al., 2020). In addition to invading sandy bottoms, H. incrassata has recently been detected on meadows of the seagrass C. nodosa (Tomas et al., 2020), without impacts on this native seagrass having thus far been observed. Since seagrasses already provide habitat for numerous associated invertebrates and fish (Hemminga and Duarte, 2000; Boström et al., 2006; Thomsen, 2010; Nordlund et al., 2016; Orth et al., 2020), including C. nodosa (Espino et al., 2011; Espino et al., 2015), it is unclear how an additional macrophyte may alter such habitat-provisioning function.
Given the current global scenario of expansion of tropical macrophyte species (Anton et al., 2019; Beca-Carretero et al., 2020; Winter et al., 2020), the global and local regression of seagrass meadows in the Mediterranean Sea (Waycott et al., 2009; Boudouresque et al., 2021) and the accelerated tropicalisation of the Mediterranean Sea (Bianchi and Morri, 2003; Vergés et al., 2014), understanding the community effects that tropical habitat-forming species have on native seagrass habitats is key for adopting meaningful conservation and management practices. Studying associated epifaunal assemblages is particularly relevant because many of these organisms play a critical role in food webs dynamics and structure and can also be key grazers determining competitive outcomes amongst primary producers in seagrass beds (e.g., Taylor, 1998; Heck et al., 2000; Heck and Valentine, 2006; Duffy et al., 2015).
The aim of the present work was to study the effects that the invasive tropical alga H. incrassata may have on epifaunal assemblages (composition, diversity and abundances) associated with the seagrass C. nodosa. By using an ‘ACI’ (After-Control/Impact) design, we tested the hypothesis that H. incrassata has a positive effect on epifaunal assemblages (in terms of diversity and abundances).
Material and Methods
Study Area and Sampling Design
To compare epifaunal assemblages on Cymodocea nodosa meadows invaded and not invaded by H. incrassata, we selected five (one invaded, four uninvaded) shallow-water C. nodosa meadows (sites) along Mallorca (Balearic Islands; Western Mediterranean). Three of the uninvaded meadows (Formentor, Es Barcares and Aucanada) were located on the Northeast coast of Mallorca, between ca. 1.5 to 3 m depth. The two other meadows (uninvaded Santa Maria “henceforth, St Maria”, at ca. 8 m depth, and the invaded Sa Platgeta, at ca. 2 m deep) were located at the National Park of Cabrera (a small island south of Mallorca; Figure 1). Mallorca and Cabrera share the same island shelf and geo-morphological context (Acosta et al., 2003). Halimeda incrassata has recently (September of 2016) colonized the latter meadow (i.e. Sa Platgeta), and it has been expanding since then (Tomas et al., 2020), whereas St. Maria remains uninvaded. All meadows are located in small beaches in protected bays, undergoing similar environmental conditions but with temporal variability (Supplementary Figure S1).
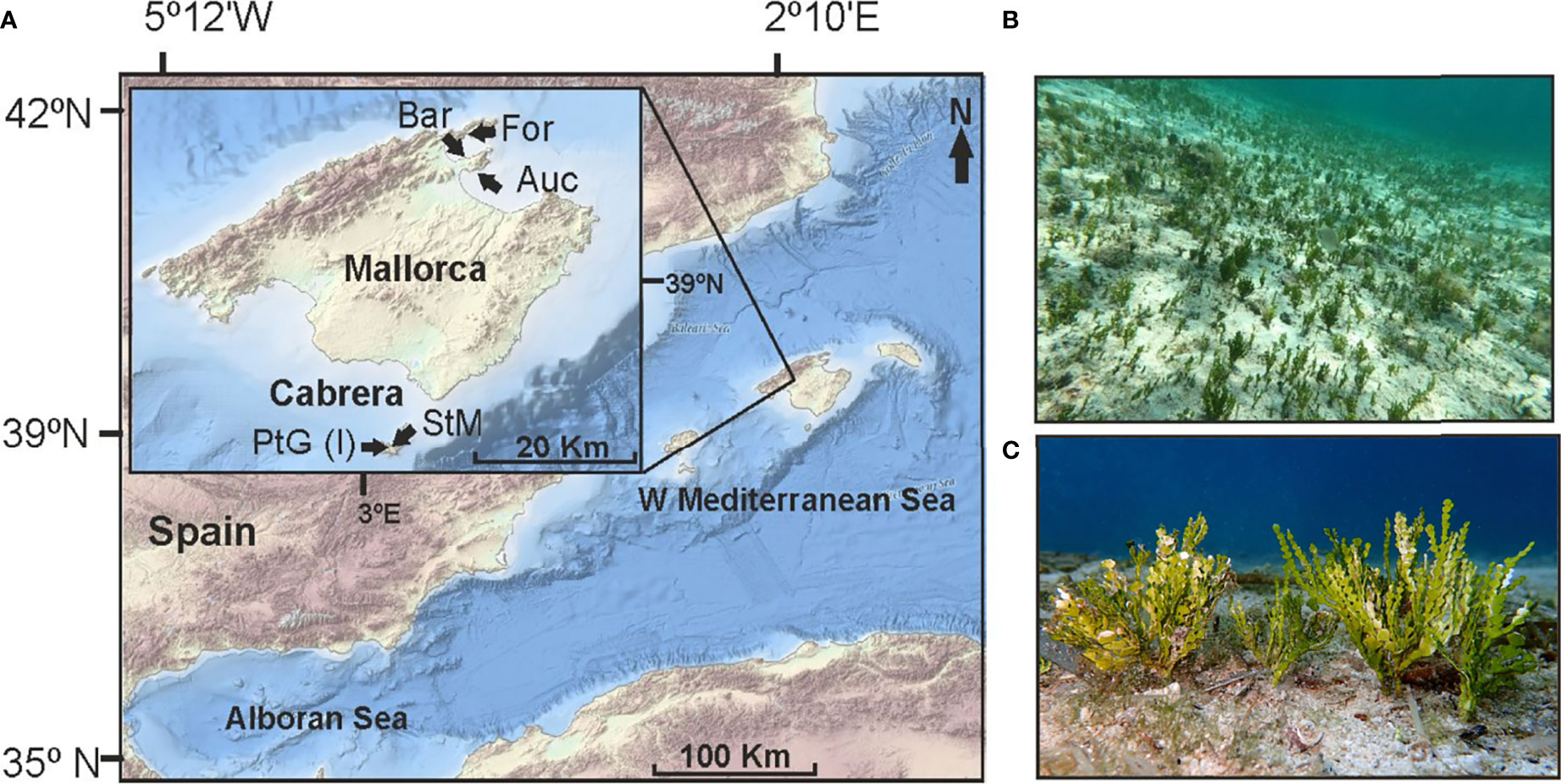
Figure 1 (A) Location of the Cymodocea nodosa meadows: (For) Formentor; (Bar) Es Barcares; (Auc) Aucanada; (StM) Santa María; (PtG) Sa Platgeta. (B) Aspect of the invasion in summer (picture by F. Tomas). (C) Detail of the structural complexity of Halimeda incrassata thalli (picture by Enric Ballesteros).
Sample Collection and Processing
Temperature was monitored in situ at 4 sites (Es Barcares, Aucanada, St Maria and Sa Platgeta) using HOBO loggers that saved measurements every 15 minutes, in order to assess for potential differences in environmental conditions between meadows.
Epifaunal samples of invaded and uninvaded C. nodosa meadows were collected three times; in April 2017, June 2017 and February 2018 by SCUBA diving. At each site and time, five replicates were taken by haphazardly placing a 0.0314 m-2 cylinder attached to a fine-meshed (125 µm) bag onto the substrate. All aboveground material and associated epifauna were covered and C. nodosa shoots and H. incrassata thalli were cut using scissors and harvested within the bag (as in Tuya et al., 2019). In addition, densities of C. nodosa shoots and cover of H. incrassata thalli were recorded using a 20 x 20 cm quadrat subdivided into 25 sections (n = 10 replicates for density and n= 20 replicates for cover; cover of H. incrassata was only taken in Sa Platgeta). The number of C. nodosa shoots in each quadrant was counted to estimate density. To estimate the cover of H. incrassata, we recorded the number of sections occupied by H. incrassata thalli inside each quadrant (Boudouresque, 1971). In the laboratory, shoots and thalli were carefully washed in freshwater to remove epifauna, which was sieved through a 500 µm mesh size and conserved in 70° ethanol. Afterwards, epiphytes were carefully scraped off the seagrass leaves using a microscope slide and placed in a previously weighed filter. Epiphytes, seagrass leaves and H. incrassata thalli were dried for ca. 48 hours (until constant weight) at 60°C, and subsequently weighed and standardized to g DW 0.0314 m-2. The epiphytes of H. incrassata were weighed together with their thalli. Additionally, subsequent sampling using 0.0314 m-2 cylinder (5 replicates per time) was carried out (October 2017, April 2018 and July 2018) to assess epiphyte and H. incrassata biomasses separately. Small mobile invertebrates (epifauna) were separated, counted and identified at coarse levels of taxonomic resolution under a stereo microscope. Epifaunal individuals included a total of 22 different taxa: Acari, Anomura, Bivalvia, Brachyura, Caprellidae, Caridea, Copepoda, Cumacea, Echinoidea, Gammaridae, Gastropoda, Isopoda, Mysidacea, Nematoda, Ophiuroidea, Heterobranchia, Ostracoda, Turbellaria, Polychaeta, Pycnogonidae, Sipunculidae and Tanaidacea.
Data and Statistical Analyses
Site and time differences in seawater temperature were analyzed using ANOVA and Tukey’s Post Hoc comparisons. Prior to performing ANOVAs and PERMANOVAs (see below), Levene’s tests were used to test for heterogeneity of variances. When homogeneity of the variance was not achieved, square root transformed data was analyzed, to reach linearity assumptions.
Differences in C. nodosa shoot density and H. incrassata cover between meadows and times were analyzed based on Euclidean distances and following a two-way PERMANOVA (2 factors, Meadows: 5 levels, fixed and Time: 3 levels, random), and one-way PERMANOVA (Time: 3 levels, random), respectively (Table 1, Figure 2). One-way PERMANOVA (Time: 3 levels, random) was also used to analyze the biomass evolution of C. nodosa, H. incrassata and their epiphytes in the invaded meadow of Sa Platgeta (Table 1, Figure 3).
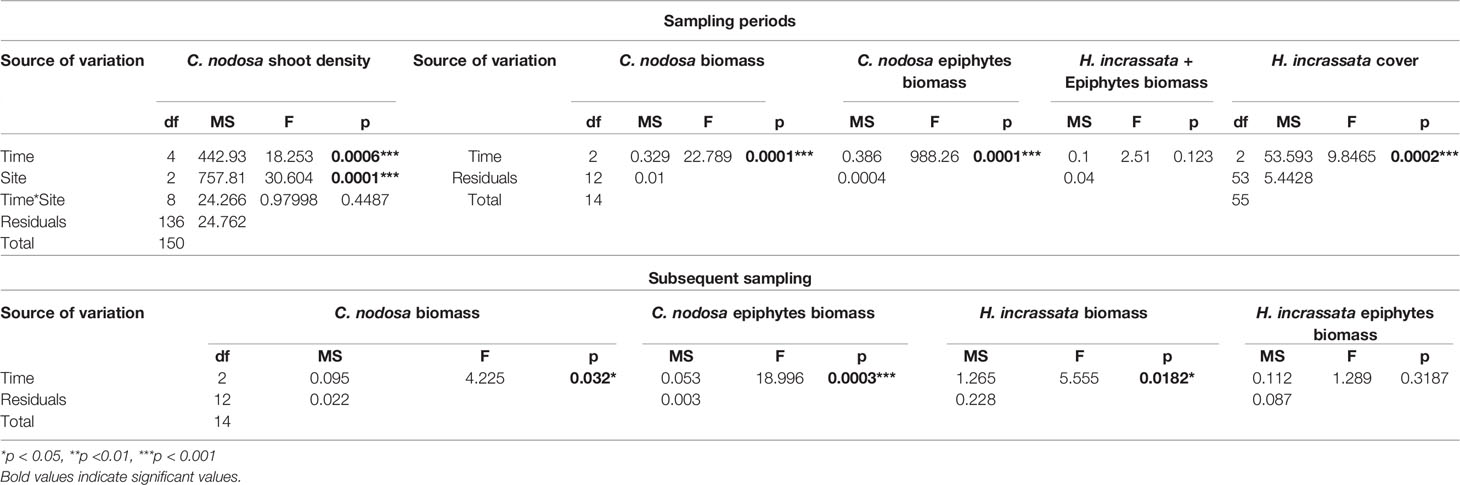
Table 1 Results of the one and two-way PERMANOVAs examining spatial and temporal patterns of macrophyte (Cymodocea nodosa and Halimeda incrassata) and their epiphyte abundances.
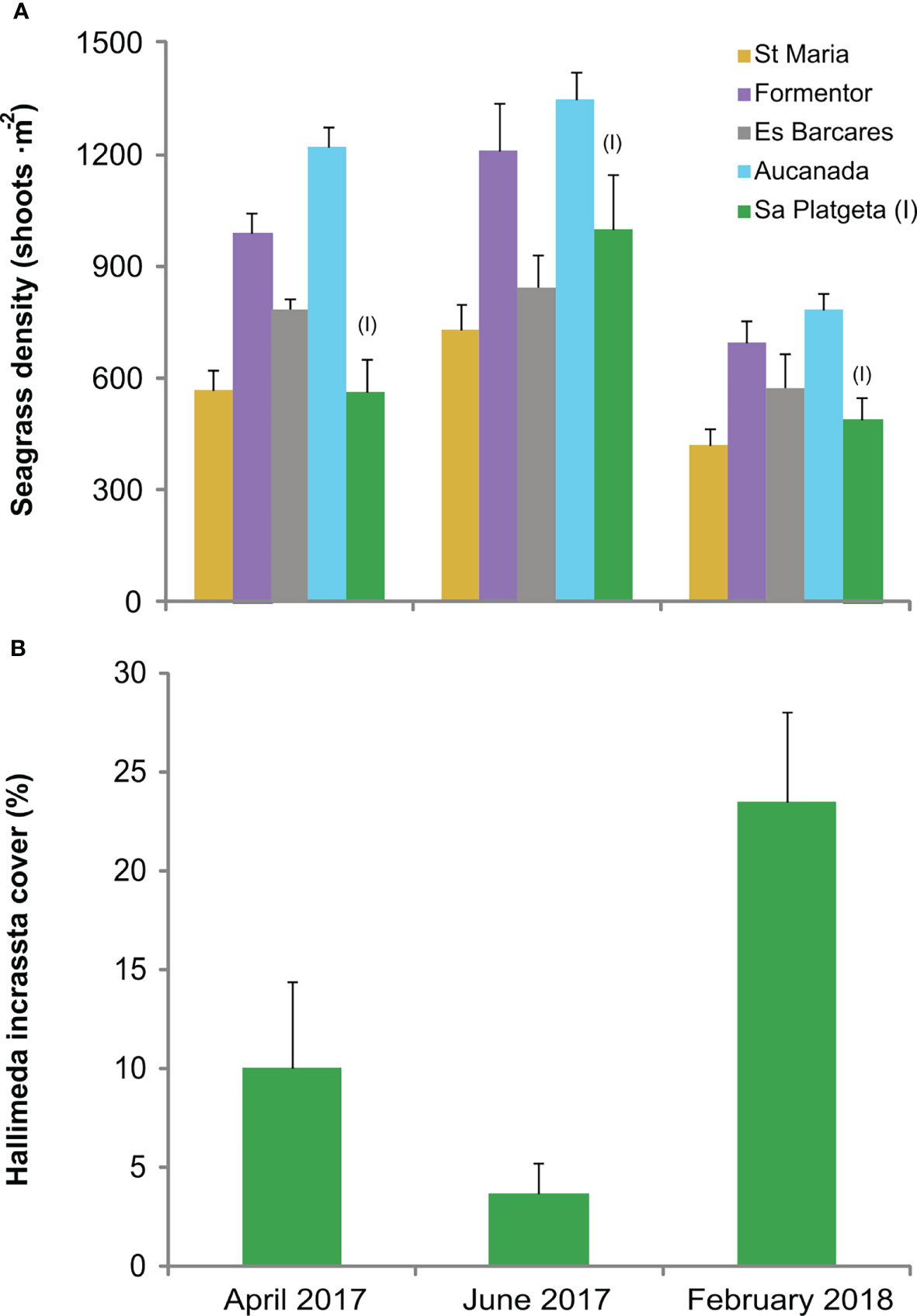
Figure 2 (A) Temporal variation in seagrass shoot density of the five Cymodocea nodosa meadows. (B) Temporal variation in Halimeda incrassata cover. Mean ± SE. I = Invaded.
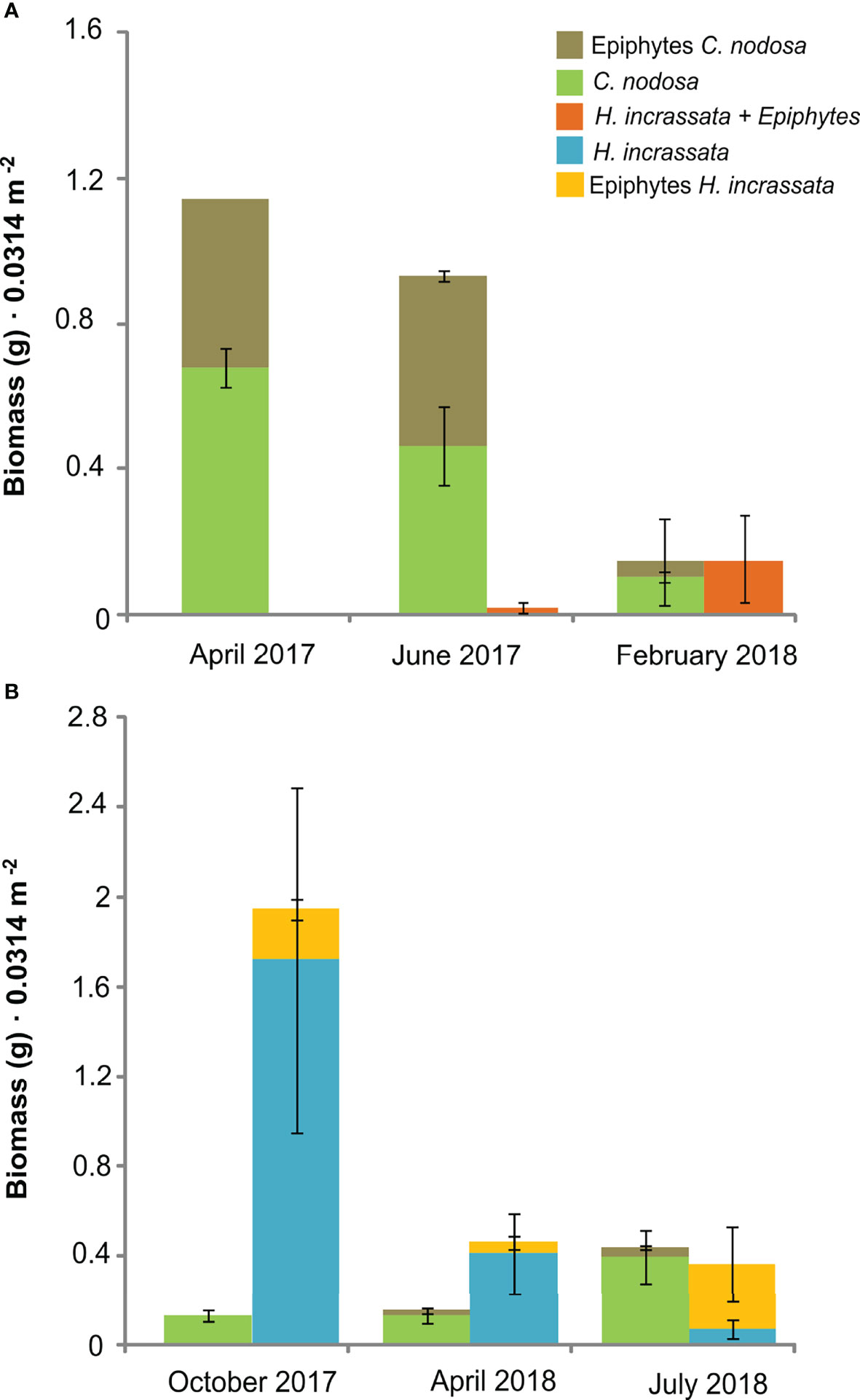
Figure 3 Temporal changes in biomass of Cymodocea nodosa, Halimeda incrassata and their epiphytes (mean ± SE) in Sa Platgeta. (A) Data considering H. incrassata and its epiphytes together (from epifauna samples). (B) Data with values of H. incrassata and its epiphytes (additional sampling).
Epifaunal assemblages were examined by considering several ecological variables: abundance of individuals (ind.·0.0314 m-2), taxon richness (number of taxonomic groups; taxa·0.0314 m-2), the Shannon-Wiener diversity index (H´log2), the Pielou evenness index, and assemblage structure (composition and abundances of all taxa).
Data was analyzed according to an “After-Control-Impact” (ACI) design (Glasby, 1997; Terlizzi et al., 2005), using two-way asymmetrical uni- (for total abundance, taxa richness, Shannon-Wiener and Pielou indexes) and multivariate (for assemblage structure) PERMANOVAs (Anderson, 2001), which tested for differences in the ecological variables between the invaded meadow (Impact, hereafter indicated as I) and uninvaded meadows (Controls, hereafter indicated as Cs) through the three sampling times, with seagrass and epiphyte biomass used as covariables (Table 2). The model consisted of 2 factors: meadows (with 1 impacted and 4 control meadows; I vs. Cs term was considered fixed, while the term between Cs was random) and time (3 levels, random). Subsequently, the data were re-analysed while omitting the I location, i.e. only Cs. The asymmetrical components were then calculated by subtractions and additions of components (Underwood, 1993; Terlizzi et al., 2005; Tuya et al., 2006). Differences between taxon richness and abundance between I and Cs meadows (mean values of the 4 meadows) at each sampling time were tested using Euclidean distances and following a one-way PERMANOVA (Factor I vs Cs).
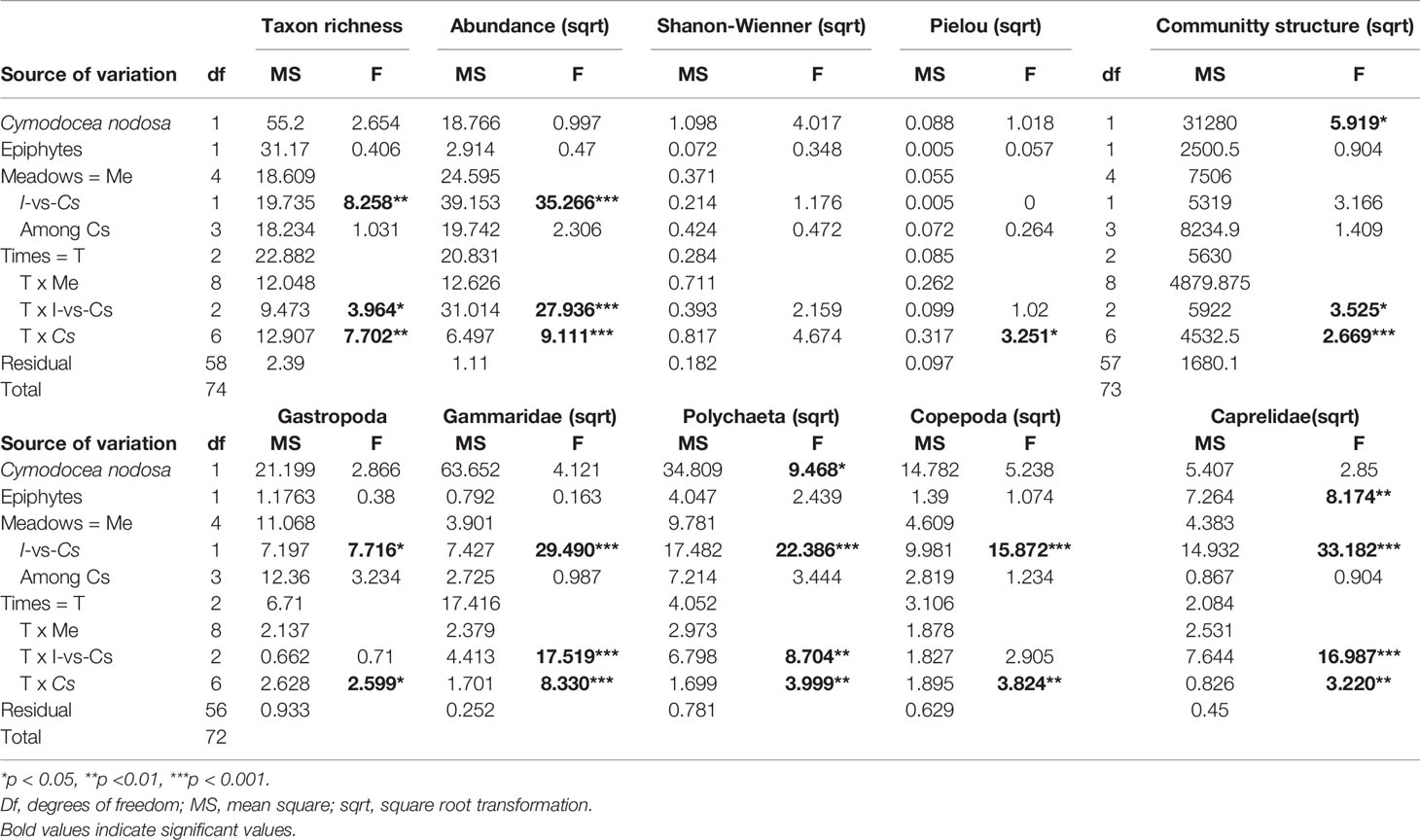
Table 2 Results of two-way PERMANOVAs examining spatial and temporal patterns of epifaunal abundance, diversity and assemblage structure.
Pairwise tests based on Euclidean distances were carried out on the ecological variables with significant differences between times and I vs Cs term. Visualization of differences in the epifaunal multivariate structure between I and Cs meadows (mean values of the 5 samples for each time and meadow) was performed through a non-metric multidimensional scaling (nMDS) ordination, from square root transformed abundance data and Bray-Curtis similarities. The SIMPER procedure was used to identify, for each time, those taxa that most contributed to differences between I and Cs meadows, through measures of the Bray-Curtis dissimilarity between the average of the Cs versus I. Taxa were selected as “important”, if they exceeded a threshold value of their percent dissimilarity of ≥3%. From these taxa identified as important, we only selected as significant those with a ratio dissimilarity/standard deviation (Diss/SD) > 1 (Terlizzi et al., 2005). Higher values of ratio Diss/SD than 1 indicate that the contribution of this taxon to the average dissimilarity is relatively consistent across the majority of the samples in the comparison between I and Cs (Clarke, 1993). Using the same ACI design previously explained, we tested the changes in abundance of the five most important taxa between I and Cs meadows, as well as between sampled times. We implemented univariate and multivariate routines in PRIMER 6.0 + PERMANOVA (Clarke and Warwick, 2001).
Results
Habitat Variables
Seagrass shoot density exhibited significant spatial and temporal differences (but no interaction between time and meadow; Table 1, Figure 2A), with maximum densities in Aucanada (mean ± SE, 1117.5 ± 77.2 shoot·m-2) and Formentor (965 ± 62.3 shoot·m-2), and minimum densities in St Maria (573.3 ± 39.4 shoot·m-2). Seagrass shoot density exhibited the highest values in April 2017 and the lowest values in February 2018 (Table 1, Figure 2A). Halimeda incrassata cover in Sa Platgeta also exhibited temporal differences, with an increase from April 2017 (10%) to February 2018 (23%) (Table 1, Figure 2B). The biomass of C. nodosa and its epiphytes showed a decrease from April 2017 (0.68 ± 0.05 g and 0.46 ± 0 g, respectively), to February 2018 (0.1 ± 0.01 g and 0.04 ± 0.01 g), whereas H. incrassata biomass (including its epiphytes) presented an increase (from 0 g to 0.15 ± 0.12 g) although this was not significant (Table 1, Figure 3A). Regarding the additional subsequent sampling done to assess epiphyte and H. incrassata biomass separately, a decrease on H. incrassata biomass was detected from October 2017 (1.74 ± 0.78 g) to July 2018 (0.07 ± 0.04 g), which coincides with its dynamics in this zone (Tomas et al., 2020). The epiphytes of H. incrassata did not present significant differences during this period (Table 1, Figure 3B), with values ranging between 0.05 ± 0.03 g and 0.29 ± 0.17 g. Conversely, the biomass of C. nodosa (from 0.13 ± 0.02 g to 0.39 ± 012 g) and its epiphytes increased between October 2017 and July 2018 (from 0 to 0.04 ± 0.01 g) (Table 1, Figure 3B).
Epifaunal Assemblages
We identified 22 taxonomic groups from a total of 1,326 epifaunal organisms. The most abundant taxa were Gastropoda (354 ind.), Gammaridae (243 ind.), Polychaeta (234 ind.), Copepoda (132 ind.) and Caprellidae (117 ind.).
Taxon richness and epifaunal abundance exhibited significant differences between I and Cs meadows, reaching the highest values at I with 5.4 ± 0.75 taxa·0.0314 m-2 and 35.9 ± 7.9 ind.·0.0314 m-2, respectively (Table 2, Figure 4). The absence of H. incrassata biomass in April 2017 and its presence in the subsequent months appear to be driving the T x I-vs-Cs interactions. Indeed, the pair-wise comparisons between I and Cs meadows were higher during the months when H. incrassata biomass was present (June 2017 and February 2018). The I meadow was the one that presented the highest abundance (66 ± 8.9 ind.·0.0314 m-2) and richness (7.8 ± 0.5 taxa·0.0314 m-2) values during February 2018 (Table 2, Figure 4). Shannon-Wiener diversity and evenness indexes did not exhibit significant differences, either for the factor I vs Cs or T x I-vs-Cs interactions (Table 2).
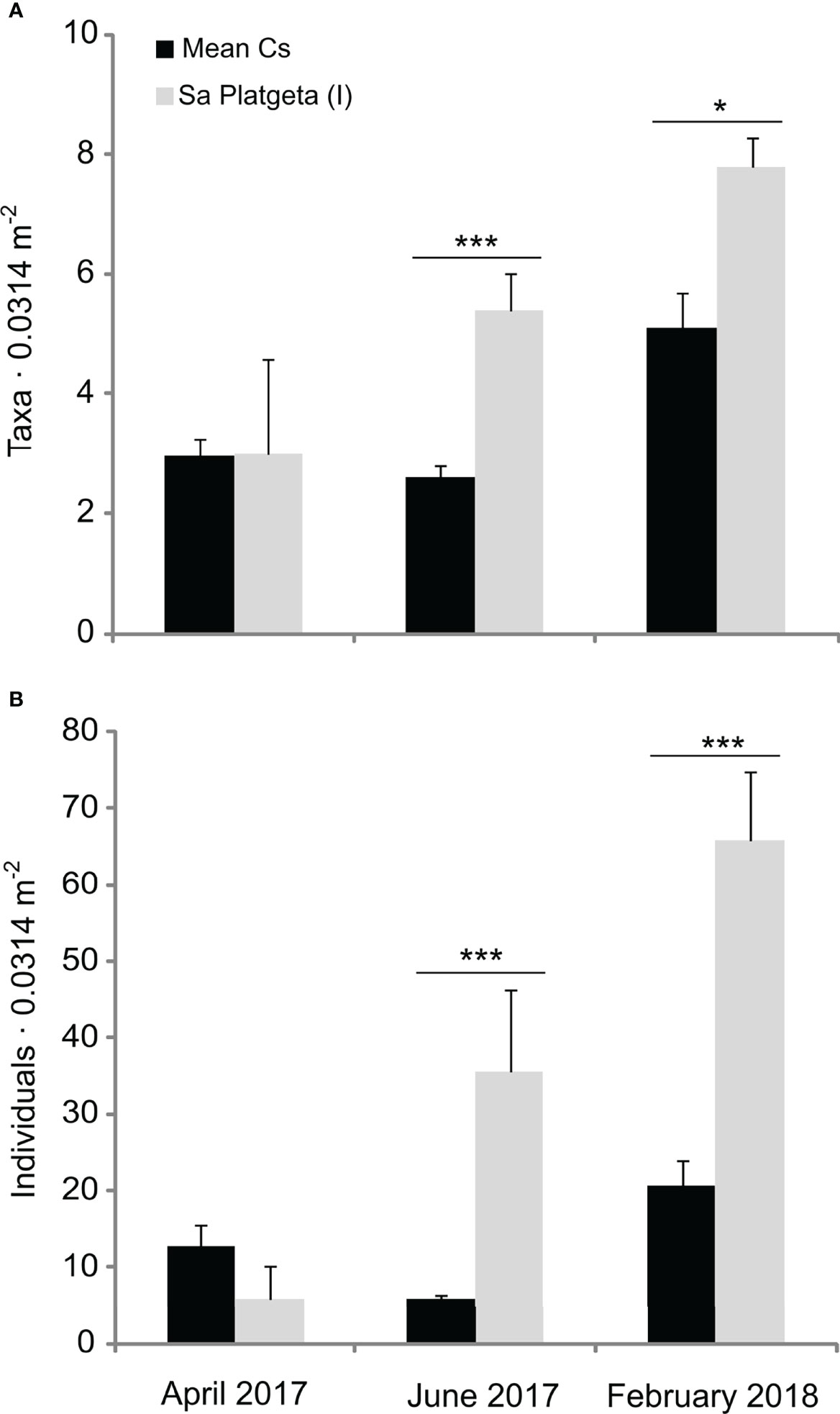
Figure 4 (A) Taxon richness and (B) epifaunal abundances (mean ± SE) through the three sampled times for control meadows (Cs) and the invaded meadow (I). *p < 0.05, ***p < 0.001.
Multivariate analysis provided evidence for statistically significant differences in the epifaunal assemblage structures associated with I meadow compared with the Cs meadows. However, these changes in epifaunal structure had a temporal dependence (T x I-vs-Cs) (Table 2, Figure 5). Similarly to taxon richness and epifaunal abundance, these differences were higher when H. incrassata biomass was present, although those were only statistically significant in June 2017 (F1,19 = 4.45; p < 0.05) (Table 2, Figure 5).The SIMPER routine identified 10 taxa as responsible for the differences between assemblages associated with I and Cs meadows during June 2017 (Table 3). Of these taxa, Caprellidae, Polychaeta, Gammaridae, Gastropoda and Copepoda were the most important contributors to discriminate between I and Cs, and they exhibited higher abundances at the I meadow than at Cs (Table 3, Figure 6). Other contributing groups were Ostracoda and Mysidacea, which were present almost exclusively at the Cs (Table 3). Regarding temporal evolution of abundance, Gammaridae, Polychaeta, and Caprellidae were the ones among the most abundant taxa that exhibited T x I-vs-Cs interactions. These taxa presented their highest abundances in June 2017 and February 2018 (again in relation to the presence of H. incrassata biomass) at I meadow, with abundances of 21.2 ± 3.2, 16.8 ± 2.2 and 12.4 ± 5.4 ind.·0.0314 m-2, respectively (Table 2, Figure 6).
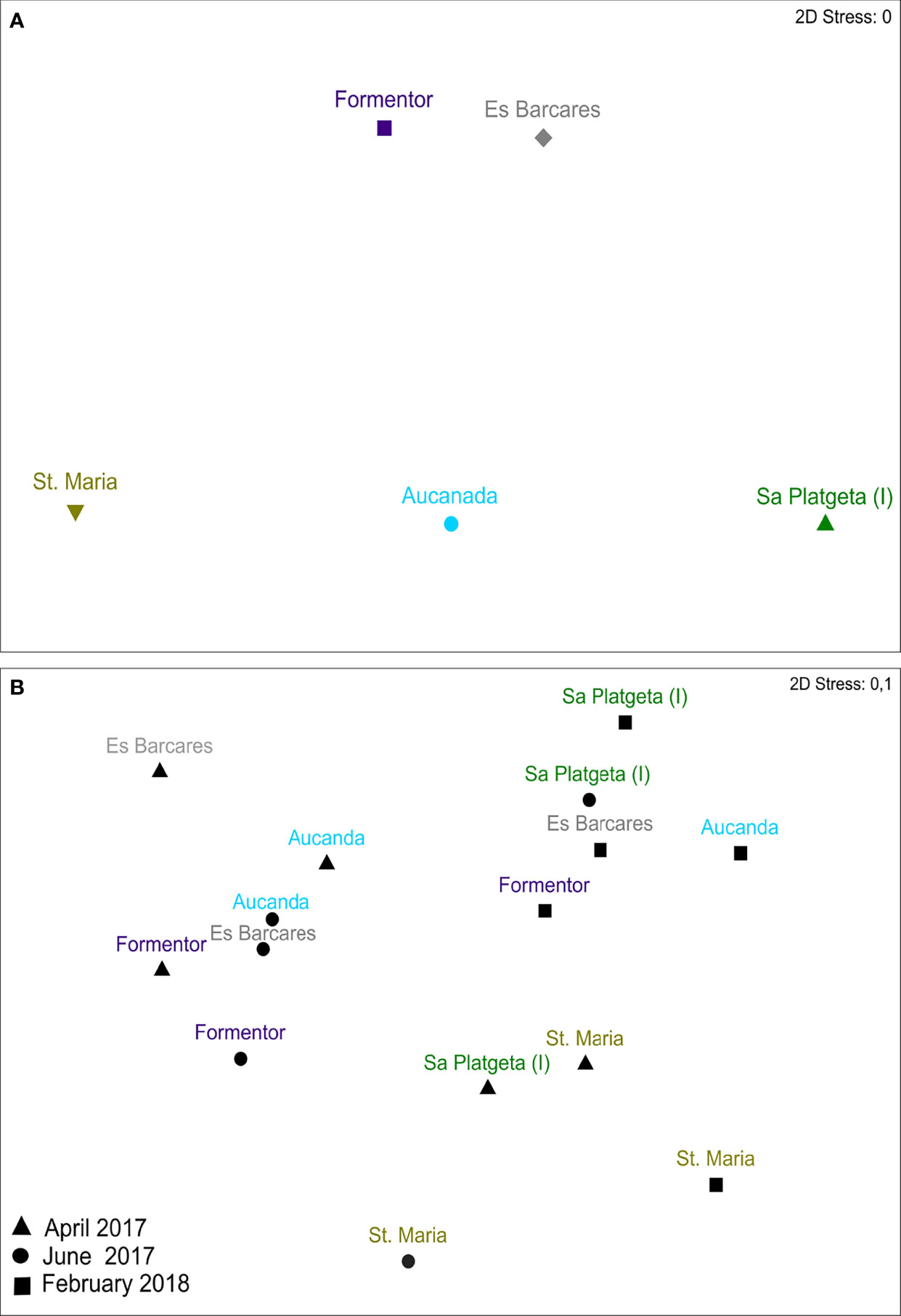
Figure 5 nm-MDS based on quantitative (square root transformed abundance data) similarities (Bray Curtis index) showing similarities in epifaunal communities from the studied Cymodocea nodosa meadows, controls and invaded. (A) Samples of each location (n = 15, mean values of the three times of sampling). (B) Samples of each location at each time of sampling (n = 5). I = Invaded.
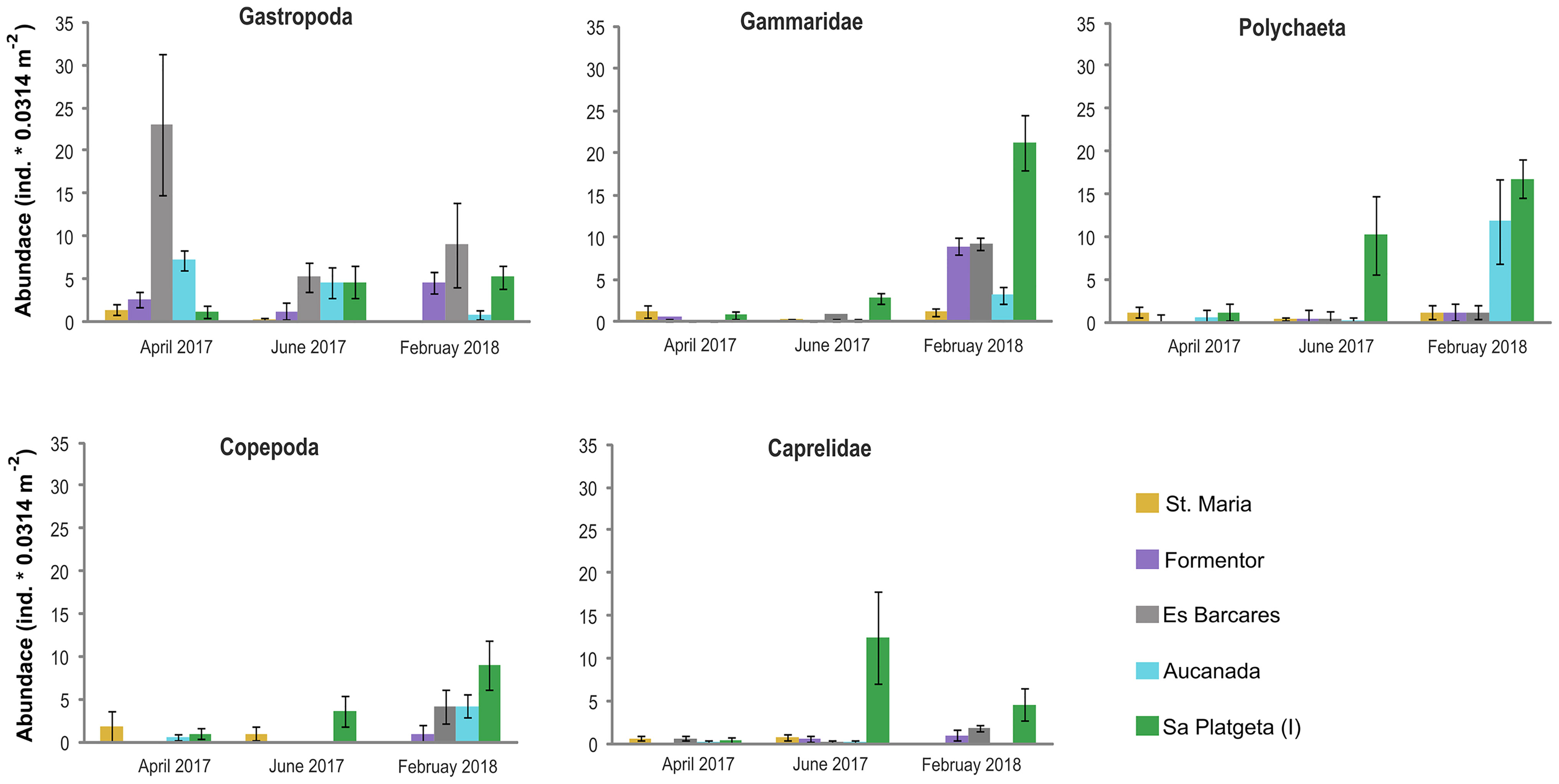
Figure 6 Abundance (mean ± SE) of the main epifaunal taxa through time that presented an increase in the invaded meadow of Sa Platgeta (I) compared to uninvaded Cymodocea nodosa meadows.
Discussion
Our study provides further support of a novel macrophyte enhancing the abundance and diversity of epifaunal assemblages. Indeed, most taxa increased their abundances across sampling times in the seagrass bed colonized with H. incrassata, with certain groups, such as Gammaridae, Polychaeta, Copepoda and Caprellidae, undergoing particularly relevant changes as the invasion progressed. This trend matches the composition of the epifauna associated with H. incrassata beds in its native distribution area. Naim (1988) found that the mobile fauna inhabiting the beds of H. incrassata from Tiathura (French Polynesia) was mainly composed by a highly diverse assemblage of microcrustaceans (mainly isopods, tanaids and amphipods) and a diversified assemblage of Syllidae species (Polychaeta). We also found that Syllidae was the most abundant family of polychaetes in the invaded C. nodosa meadow. The response of Gastropoda to the presence of H. incrassata is also interesting, given that their abundance trajectories were opposite in the invaded meadow (where they increased), vs. the monospecific (Cs) seagrass meadows, where they decreased, especially in February 2018, when C. nodosa presented its lowest shoot density.
The increase in epifaunal abundances observed in the I seagrass meadow seems to be related with a higher availability of additional habitat and microhabitat provided by this novel species and its epiphytes. Higher availability of habitat, not only implies more inhabitable space, but also likely a decrease in predation pressure and enhanced food availability (Orth et al., 1984; Heck and Crowder, 1991; Moore and Hovel 2010; Reynolds et al., 2018; Jiménez-Ramos et al., 2019). Indeed, some epifaunal groups (e.g. amphipods), which can be highly susceptible to predation (Reynolds et al., 2018), exhibited higher abundances in the seagrass meadow that harbored H. incrassata. Furthermore, the positive effects of the presence of this tropical alga may be especially relevant in winter, when C. nodosa exhibit its lowest shoot density (this work, Máñez-Crespo et al., 2020), as well as shoot size (Máñez-Crespo et al., 2020), and thus when habitat availability provided by the seagrass is reduced.
Most of the taxa that increased their abundance in the invaded C. nodosa meadow are species within detritivorous or omnivorous trophic guilds and, in addition, many species of some of these groups (Polychaeta, Gammaridae and Caprellidae) are often opportunistic, with a high adaptive potential and reproductive capacity (López and Viéitez, 1999; Vázquez-Luis et al., 2009; Woods, 2009; Vázquez-Luis et al., 2008; Casu et al., 2009; Brun et al., 2017; Ribeiro et al., 2018). For example, Casu et al. (2009) showed that in an assemblage of photophilic algae invaded by the macroalga C. cylindracea, the polychaeta Silly prolifera and the gammarid Corophium sextonae can amplify their food sources and incorporate detritus provided by this novel species. This flexibility could benefit the aforementioned taxa over more specialized taxa in this new environment by being able to take advantage of the extra resources provided by H. incrassata, the associated epiphytes, and their detritus. We also found a high abundance of herbivores, such as snails, isopods, and opisthobranchs, suggesting that these taxa may feed on H. incrassata or its epiphytes, as it has been suggested for other invasive algae (e.g. G. vermiculophylla; Thomsen et al., 2013). Therefore, in our study area, it seems that the presence of the tropical alga H. incrassata favors both opportunistic taxa with high capacity of adaptation and with detritivorous or omnivorous trophic guilds as well as herbivorous species.
Crustaceans and polychaetes are important food sources for higher trophic groups, such as fish (Castriota et al., 2005; Valls, 2017), and these groups act as key trophic links contributing to the transfer of energy to higher trophic levels (Taylor, 1998). Consequently, changes on epifaunal assemblage structure would likely affect the abundance and distribution of higher trophic levels, thus ultimately altering food webs (e.g. Lazzari and Stone, 2006; Dijkstra et al., 2017). In fact, in the Marine Protected Area (MPA) of Palma Bay (South Mallorca Island), the occurrence and fast spread of H. incrassata on shallow sandy bottoms has produced a redistribution of the populations of the Razorfish Xyrichtys novacula (Alós et al., 2018; Vivó-Pons et al., 2020). This species, which is very important in local fisheries (Morales-Nin et al., 2005), is shifting its populations towards the newly created H. incrassata beds present in this MPA (Alós et al., 2018; Vivó-Pons et al., 2020), which could be explained by a higher amount of food available, since the diet of this fish mainly consists of small invertebrates, such as polychaetes, amphipods, copepods and molluscs (Castriota et al., 2005).
Conclusions
Our study reinforces the notion that the arrival of novel species does not always cause net negative species losses on native ecosystems. Indeed, the colonization of the tropical alga H. incrassata has produced positive effects, in terms of increases in the abundance and diversity of epifaunal assemblages. The most abundant taxa were those with opportunistic species and with trophic guilds able to exploit the new resources offered by the new habitat. It could be expected that, as the habitat stabilizes (the invasion is still very recent), the epifaunal associations will tend to stabilize as well, and create a new assemblage with a more stable food web, in the same way as observed in other invertebrates assemblages (e.g. polychaetes and amphipods) altered by an “impact” (Del-Pilar-Ruso et al., 2015; de-la-Ossa-Carretero et al., 2016). However, our study was limited to one invaded meadow, so more meadows should be studied in the future for a longer time to unambiguously infer causality resulting from the invasion. So far, we have not observed apparent negative effects on C. nodosa density, but perhaps a longer temporal data series may be necessary. Indeed, H. incrassata beds are important producers of organic carbon and calcium carbonate (CaCO3) in tropical areas (Hillis-Colinvaux, 1980), and thus, their capacity to modify sediments could compromise the development of C. nodosa meadows in the future.
Data Availability Statement
The raw data supporting the conclusions of this article will be made available by the authors, without undue reservation.
Author Contributions
ÁM-R and FiT contributed to conceptualization and design of the article. ÁM-R, FiT, JM-C, LR, FeT, IC-S, GH, LP-B and JT contributed to acquisition of the data. AM-R, FiT, JM-C and FeT contributed to analysis and interpretation of the data. All authors contributed to the writing of the article and approved the final version.
Funding
This work was supported by a Margalida Comas post-doctoral fellowship (2017) to AMR funded by the Balearic Island Govern, Department of Innovation, Research and Tourism. Funding was provided by RESIGRASS (CGL2014-58829-C2-1-R) and DIVCYMOGEN (OAPN1623/2015) to FiT.
Conflict of Interest
The authors declare that the research was conducted in the absence of any commercial or financial relationships that could be construed as a potential conflict of interest.
Publisher’s Note
All claims expressed in this article are solely those of the authors and do not necessarily represent those of their affiliated organizations, or those of the publisher, the editors and the reviewers. Any product that may be evaluated in this article, or claim that may be made by its manufacturer, is not guaranteed or endorsed by the publisher.
Acknowledgments
We would like to thank Albert Sasplugas, Paula Bonet and Cayetana Casas for their help in the field and in sample processing and the Cabrera National Park staff for logistical support.
Supplementary Material
The Supplementary Material for this article can be found online at: https://www.frontiersin.org/articles/10.3389/fmars.2022.886009/full#supplementary-material
Supplementary Figure 1 | Temperature (mean ± SE) through times in the invaded meadow of Sa Platgeta compared to uninvaded Cymodocea nodosa meadows. The meadow of Formentor was not included due to problems in data collection. The data were obtained using Hobo data loggers taking data every 15 minutes (n=2304). Different letters indicate differences between meadows inside each time (Post Hoc comparison, Tukey).
References
Acosta J. D., Canals M., López-Martínez J., Muñoz A., Herranz P., Urgeles R., et al. (2003). The Balearic Promontory Geomorphology (Western Mediterranean): Morphostructure and Active Processes. Geomorphology 49, 177–204. doi: 10.1016/S0169-555X(02)00168-X
Alós J., Bujosa-Homar E., Terrados J., Tomas F. (2018). Spatial Distribution Shifts in Two Temperate Fish Species Associated to a Newly-Introduced Tropical Seaweed Invasion. Biol. Invasions 20, 3193. doi: 10.1007/s10530-018-1768-2
Alós J., Tomas F., Terrados J., Verbruggen H., Ballesteros E. (2016). Fast-Spreading Green Beds of Recently Introduced Halimeda Incrassata Invade Mallorca Island (NW Mediterranean Sea). Mar. Ecol. Prog. Ser. 558, 153–158. doi: 10.3354/meps11869
Anderson M.J.. (2001). A New Method for Non-Parametric Multivariate Analysis of Variance. Austral. Ecol. 26, 32–46. doi: 10.1111/j.1442-9993.2001.01070.pp.x
Anton A., Geraldi N., Lovelock C., Apostolaki E. T., Bennett S., Cebrian J., et al. (2019). Global Ecological Impacts of Marine Exotic Species. Nat. Ecol. Evol. 3, 787–800. doi: 10.1038/s41559-019-0851-0
Ballesteros E., Cebrian E., Alcoverro T. (2007). Mortality of Shoots of Posidonia Oceanica Following Meadow Invasion by the Red Alga Lophocladia Lallemandii. Bot. Mar. 50 (1), 8–13. doi: 10.1515/BOT.2007.002
Beca-Carretero P., Teichberg M., Winters G., Procaccini G., Reuter H. (2020). Projected Rapid Habitat Expansion of Tropical Seagrass Species in the Mediterranean Sea as Climate Change Progresses. Front. Plant Sci. 11. doi: 10.3389/fpls.2020.555376
Belattmania Z., Chaouti A., Reani A., Engelen A. H., Machado M., Serrão E. A., et al. (2018). The Introduction of Sargassum Muticum Modifies Epifaunal Patterns in a Moroccan Seagrass Meadow. Mar. Ecol. 39 (3), e12507. doi: 10.1111/maec.12507
Bianchi C. N., Morri C. (2003). Global Sea Warming and ‘Tropicalization’ of the Mediterranean Sea: Biogeographic and Ecological Aspects. Biogeographia 24, 319–327. doi: 10.21426/B6110129
Boström C., Jackson E. L., Simenstand C. A. (2006). Seagrass Landscapes and Their Effects on Associated Fauna: A Review. Estuar. Coast. Shelf. Sci. 68 (3-4), 383–403. doi: 10.1016/j.ecss.2006.01.026
Boudouresque C. F. (1971). Méthodes D ‘ Étude Qualitative Et Quantitative Du Benthos (En Particulier Du Phytobenthos). Téthys 3, 79–104.
Boudouresque C. F., Blanfuné A., Pergent G., Thibaut T. (2021). Restoration of Seagrass Meadows in the Mediterranean Sea: A Critical Review of Effectiveness and Ethical Issues. Water. 13, 1034. doi: 10.3390/w13081034Boström
Brun P., Payne M. R., Kiørboe T. (2017). A Trait Database for Marine Copepods. Earth Syst. Sci. Data 9, 99–113. doi: 10.5194/essd-9-99-2017
Canning-Clode J., Carlton J. T. (2017). Refining and Expanding Global Climate Change Scenarios in the Sea: Poleward Creep Complexities, Range Termini, and Setbacks and Surges. Diver. Distrb. 23, 463–473. doi: 10.1111/ddi.12551
Castriota L., Scarabello M. P., Finoia M. G., Sinopoli M., Andaloro F. (2005). Food and Feeding Habits of Pearly Razorfish, Xyrichtys Novacula (Linnaeus 1758), in the Southern Tyrrhenian Sea: Variation by Sex and Size. Environ. Biol. Fishes 72, 123–133. doi: 10.1007/s10641-004-6576-0
Casu D., Ceccherelli G., Sechi N., Rumolo P., Sarà G. (2009). Caulerpa Racemosa Var. Cylindracea as a Potential Source of Organic Matter for Benthic Consumers: Evidences From a Stable Isotope Analysis. Aquat. Ecol. 43 (4), 1032–1029. doi: 10.1007/s10452-008-9223-6
Cebrian E., Ballesteros E., Linares C., Tomas F. (2011). Do Native Herbivores Provide Resistance to Mediterranean Marine Bioinvasions? A Seaweed Example. Biol. Invasions 13, 1397–1408. doi: 10.1007/s10530-010-9898-1
Clarke K. R. (1993). Non-Parametric Multivariate Analyses of Changes in Community Structure. Aust. J. Ecol. 18, 117–143. doi: 10.1111/j.1442-9993.1993.tb00438.x
Clarke K. R., Warwick R. M. (2001). Change in Marine Communities: An Approach to Statistical Analysis and Interpretation. 2nd ed. (Plymouth: PRIMER-E).
de-la-Ossa-Carretero J. A., Del-Pilar-Ruso Y., Loya-Fernández A., Ferrero-Vicente L. M., Marco-Menéndez C., Martínez-García E., et al. (2016). Response of Amphipod Assemblages to Desalination Brine Discharge: Impact and Recovery. Estuar. Coast. Shelf Sci. 172, 13–23. doi: 10.1016/j.ecss.2016.01.035
Del-Pilar-Ruso Y., Martínez-García E., Giménez-Casalduero F., Loya-Fernández A., Ferrero-Vicente L. M., Marco-Menéndez C., et al. (2015). Benthic Community Recovery From Brine Impact After the Implementation of Mitigation Measures. Water Res. 70, 325–336. doi: 10.1016/j.watres.2014.11.036
Dijkstra J. A., Harris L. G., Mello K., Littere A., Wells C., Ware C. (2017). Invasive Seaweeds Transform Habitat Structure and Increase Biodiversity of Associated Species. J. Ecol. 105, 1668–1678. doi: 10.1111/1365-2745.12775
Duffy J. E., Reynolds P. L., Boström C., Coyer J. A., Cusson M., Donadi S., et al (2015). Biodiversity Mediates Top-Down Control in Eelgrass Ecosystems: A Global Comparative-Experimental Approach. Ecol. Lett. 18, 696–705. doi: 10.1111/ele.12448
Espino F., González J. A., Haroun R., Tuya F. (2015). Abundance and Biomass of the Parrotfish Sparisoma Cretense in Seagrass Meadows: Temporal and Spatial Differences Between Seagrass Interiors and Seagrass Adjacent to Reefs. Environ. Biol. Fishes 98 (1), 121–133. doi: 10.1007/s10641-014-0241-z
Espino F., Tuya F., Brito A., Haroun R. (2011). Ichthyofauna Associated With Cymodocea Nodosa Meadows in the Canarian Archipelago (Central Eastern Atlantic): Community Structure and Nursery Role. Cienc. Mar. 37 (2), 157–174. doi: 10.7773/cm.v37i2.1720
Essla F., Dullingerc S., Rabitscha W. (2011). Socioeconomic Legacy Yields an Invasion Debt. PNAS 108 (1), 203–207. doi: 10.1073/pnas.1011728108
García-Gómez J. C., Sempere-Valverde J., Ostalé-Valriberas E., Martínez M., Olaya-Ponzone L., Roi A., et al. (2018). Rugulopteryx Okamurae (E.Y. Dawson) I.K. Hwang, W. J. Lee & H.S. Kim (Dictyotales, Ochrophyta), Alga Exótica “Explosiva” En El Estrecho De Gibraltar. Observaciones Preliminares De Su Distribución E Impacto. Almoraima 49, 97–113.
García-Gómez J. C., Sempere-Valverde J., Roi A., Martínez-Chacón M., Oloya-Ponzone L., Sánchez-Moyano E., et al. (2020). From Exotic to Invasive in Record Time: The Extreme Impact of Rugulopteryx Okamurae (Dictyotales, Ochrophyta) in the Strait of Gibraltar. Sci. Total Environ. 704, 135408. doi: 10.1016/j.scitotenv.2019.135408
Glasby T. M. (1997). Analysing Data From Post-Impact Studies Using Asymmetrical Analyses of Variance: A Case Study of Epibiota on Marinas. Aust. J. Ecol. 22, 448–459. doi: 10.1111/j.1442-9993.1997.tb00696.x
Guerra-García J. M., Ros M., Izquierdo D., Soler M. (2012). The Invasive Asparagopsis Armata Versus the Native Corallina Elongata: Differences in Associated Peracarid Assemblages. J. Exp. Mar. Biol. Eco.l 416–417, 121–128. doi: 10.1016/j.jembe.2012.02.018
Guiry M. D., Guiry G. M. (2016) AlgaeBase (Galway: World-Wide Electronic Publication, National University of Ireland) (Accessed 19 March 2019).
Hay M. E., Duffy J. E., Pfister C. A., Fenical W. (1987). Chemical Defense Against Different Marine Herbivores: Are Amphipods Insect Equivalents? Ecology 68, 1567–1580. doi: 10.2307/1939849
Heck K., Crowder L. B. (1991). Habitat Structure and Predator—Prey Interactions in Vegetated Aquatic Systems. In: Bell S. S., McCoy E. D., Mushinsky H. R. (eds) Habitat Structure. Population and Community Biology Series, vol 8. Dordrecht, Springer. https://doi.org/10.1007/978-94-011-3076-9_14
Heck K., Pennock J. R., Valentine J., Coen L. D., Sklenar S. A. (2000). Effects of Nutrient Enrichment and Small Predator Density on Seagrass Ecosystems: An Experimental Assessment. Limnol. Oceanogr. 45 (5), 1041–1057. doi: 10.4319/lo.2000.45.5.1041
Heck K., Valentine J. F. (2006). Plant–herbivore Interactions in Seagrass Meadows. J. Exp. Mar. Biol. Ecol. 330 (1), 420–436. doi: 10.1016/j.jembe.2005.12.044
Hemminga M., Duarte C. (2000). Seagrass Ecology. (Cambridge: Cambridge University Press). doi: 10.1017/CBO9780511525551
Hillis-Colinvaux L. (1980). Ecology and Taxonomy of Halimeda: Primary Producer of Coral Reefs. Adv. Mar. Biol. 17, 1–327. doi: 10.1016/S0065-2881(08)60303-X
Hoffman R. (2014). “Alien Benthic Algae and Seagrasses in the Mediterranean Sea and Their Connection to Global Warming,” in The Mediterranean Sea: Its History and Present Challenges. Eds. Goffredo S., Dubinsky Z. (Dordrecht: Springer).
Jiménez-Ramos R., Egea L. G., Vergara J. J., Brun G. (2019). The Role of Flow Velocity Combined With Habitat Complexity as a Top–Down Regulator in Seagrass Meadows. Oikos 128, 64–76. doi: 10.1111/oik.05452
Lazzari M. A., Stone B. Z. (2006). Use of Submerged Aquatic Vegetation as Habitat by Young-of-the-Year Epibenthic Fishes in Shallow Maine Nearshore Waters. Estuar. Coast. Shelf. Sci. 69, 591–606. doi: 10.1016/j.ecss.2006.04.025
López E., Viéitez J. (1999). Polychaete Assemblages on non-Encrusting Infralittoral Algae From the Chafarinas Islands (SW Mediterranean). Cah. Biol. Mar. 10, 375–384.
Mancuso F. P., D’Agostaro R., Milazzo M., Badalamenti F., Musco L., Mikac B., et al. (2022). The Invasive Seaweed Asparagopsis Taxiformis Erodes the Habitat Structure and Biodiversity of Native Algal Forests in the Mediterranean Sea. Mar. Environ. Res. 173, 105515. doi: 10.1016/j.marenvres.2021.105515
Máñez-Crespo J., Tuya F., Fernández-Torquemada Y., Royo L., del Pilar-Ruso Y., Espino F., et al. (2020). Seagrass Cymodocea Nodosa Across Biogeographical Regions and Times: Differences in Abundance, Meadow Structure and Sexual Reproduction. Mar. Environ. Res. 162, 105159. doi: 10.1016/j.marenvres.2020.105159
Moore E. C., Hovel K. A. (2010). Relative Influence of Habitat Complexity and Proximity to Patch Edges on Seagrass Epifaunal Communities. Oikos 119, 1299–1311. doi: 10.1111/j.1600-0706.2009.17909.x
Morales-Nin B., Moranta J., Garcia C., Tugores M. P., Grau A. M., Riera F., et al. (2005). The Recreational fishery Off Majorca Island (Western Mediterranean): Some Implications for Coastal Resource Management. ICES J. Mar. Sci. 62, 727–739. doi: 10.1016/j.icesjms.2005.01.022
Naim O. (1988). Distributional Patterns of Mobile Fauna Associated With Halimeda on the Tiahura Coral-Reef Complex. Coral Reefs 6 (3), 237–250. doi: 10.1007/BF00302020
Navarro-Barranco C., Florido M., Ros M., González-Romero P., Guerra-García J. M. (2018). Impoverished Mobile Epifaunal Assemblages Associated With the Invasive Macroalga Asparagopsis Taxiformis in the Mediterranean Sea. Mar. Environ. Res. 1, 1. doi: 10.1016/j.marenvres.2018.07.016
Navarro-Barranco C., Moreira J., Espinosa F., Ros M., Rallis I., Semper-Valverde J., et al. (2021). Evaluating the Vulnerability of Coralligenous Epifauna to Macroalgal Invasions. Aquat. Conserv. 31 (9), 2305–2319. doi: 10.1002/aqc.3633
Navarro-Barranco C., Muñoz-Gómez B., Saiz D., Ros M., Guerra-García J. M., Altamirano M., et al. (2019). Can Invasive Habitat-Forming Species Play the Same Role as Native Ones? The Case of the Exotic Marine Macroalga Rugulopteryx Okamurae in the Strait of Gibraltar. Biol. Invasions 21, 3319–3334. doi: 10.1007/s10530-019-02049-y
Nordlund L. M., Koch E. W., Barbier E. B., Creed J. C. (2016). Seagrass Ecosystem Services and Their Variability Across Genera and Geographical Regions. PloS One 12 (1), e0169942. doi: 10.1371/journal.pone.0169942
Orth R. J., Heck K. L., van Montfrans J. (1984). Faunal Communities in Seagrass Beds: A Review of the Influence of Plant Structure and Prey Characteristics on Predator: Prey Relationships. Estuaries 7 (4), 339–350. doi: 10.2307/1351618
Orth R. J., Lefcheck J. S., McGlathery K. S., Aoki L., Luckenbach M. W., Moore K. A., et al. (2020). Restoration of Seagrass Habitat Leads to Rapid Recovery of Coastal Ecosystem Services. Sci. Adv. 6 (41), eabc6434. doi: 10.1126/sciadv.abc6434
Paul V. J., Van Alstyne K. L.. (1988). Chemical defense and chemical variation in some tropical Pacific species of Halimeda (Halimedaceae; Chlorophyta). Coral Reefs 6, 263–269. doi: 10.1007/BF00302022
Raitsos D. E., Beaugrand G., Georgopoulos D., Zenetos A., Pancucci-Papadopoulou A. M., Theocharis A., et al. (2010). Global Climate Change Amplifies the Entry of Tropical Species Into the Eastern Mediterranean Sea. Limnol. Oceanogr. 55 (4), 1478–1484. doi: 10.4319/lo.2010.55.4.1478
Reynolds P. I., Stachowicz J. J., Hovel K., Boström C., Boyer K., Cusson M., et al. (2018). Latitude, Temperature, and Habitat Complexity Predict Predation Pressure in Eelgrass Beds Across the Northern Hemisphere. Ecology 99 (1), 29–35. doi: 10.1002/ecy.2064
Ribeiro R. P., Bleidorn C., Aguado M. T. (2018). Regeneration Mechanisms in Syllidae (Annelida). Regeneration 5, 26–42. doi: 10.1002/reg2.98
Sangil C., Martín-García L., Afonso-Carrillo J., Barquín J., Sansón M. (2018). Halimeda Incrassata (Bryopsidales, Chlorophyta) Reaches the Canary Islands: Mid- and Deep-Water Meadows in the Eastern Subtropical Atlantic Ocean. Bot. Mar. 61 (2), 103–110. doi: 10.1515/bot-2017-0104
Santamaría J., Tomas F., Ballesteros E., Ruiz J. M., Bernardeau-Esteller J., Terrados J., et al. (2021). The Role of Competition and Herbivory in Biotic Resistance Against Invaders: A Synergisticeffect. Ecology 102 (9), e03440. doi: 10.1002/ecy.3440
Schmidt A. L., Scheibling R. E. (2006). A Comparison of Epifauna and Epiphytes on Native Kelps (Laminaria Species) and an Invasive Alga (Codium Fragile Ssp. Tomentosoides) in Nova Scotia, Canada. Bot. Mar. 49 (4), 315 330. doi: 10.1515/BOT.2006.039
Streftaris N., Zenetos A. (2006). Alien Marine Species in the Mediterranean the 100 ‘Worst Invasives’ and Their Impact. Mediterr. Mar. Sci. 7 (1), 87–118. doi: 10.12681/mms.180
Taylor R. B (1998). Density, Biomass and Productivity of Animals in Four Subtidal Rocky Reef Habitats: The Importance of Small Mobile Invertebrates. Mar. Ecol. Prog. Ser. 172, 37–51. doi: 10.3354/meps172037
Terlizzi A., Benedetti-Cecchi L., Bevilacqua S., Fraschetti S., Guidetti P., Anderson M.J. (2005). Multivariate and Univariate Asymmetrical Analyses in Environmental Impact Assessment: A Case Study of Mediterranean Subtidal Sessile Assemblages. Mar. Ecol. Prog. Ser 289, 27–42. doi: 10.3354/meps289027
Thomsen M. S. (2010). Experimental Evidence for Positive Effects of Invasive Seaweed on Native Invertebrates via Habitat-Formation in a Seagrass Bed. Aquat. Invasions 5 (4), 341–346. doi: 10.3391/ai.2010.5.4.02
Thomsen M. S. (2020). Indiscriminate Data Aggregation in Ecological Meta-Analysis Underestimates Impacts of Invasive Species. Nat. Ecol. Evol. 4, 312–314. doi: 10.1038/s41559-020-1117-6
Thomsen M. S., Byers J. E., Schiel1 R., Bruno J. F., Olden J. D., Werberg T., et al. (2014). Impacts of Marine Invaders on Biodiversity Depend on Trophic Position and Functional Similarity. Mar. Ecol. Prog. Ser. 495, 39–47. doi: 10.3354/meps10566
Thomsen M. S., Stæhr P. A., Nejruo L., Schiel D. (2013). Effects of the Invasive Macroalgae Gracilaria Vermiculophylla on Two Co-Occurring Foundation Species and Associated Invertebrates. Aquat. Invasions 8 (2), 133–145. doi: 10.3391/ai.2013.8.2.02
Thomsen M. S., Wernberg T., Engelen A. H., Tuya F., Vanderklift M. A., Holmer M., et al. (2012). A Meta-Analysis of Seaweed Impacts on Seagrasses: Generalities and Knowledge Gaps. PloS One 7 (1), e28595. doi: 10.1371/journal.pone.0028595
Thomsen M. S., Wernberg T., Tuya F., Silliam B. R. (2009). Evidence for Impacts of non Indigenous Macroalgae: A Meta-Analysis of Experimental Field Studies. J. Phycol. 45, 812–819. doi: 10.1111/j.1529-8817.2009.00709.x
Tomas F., Box A., Terrados J. (2011). Effects of Invasive Seaweeds on Feeding Preference and Performance of a Keystone Mediterranean Herbivore. Biol. Invasions 13, 1559–1570. doi: 10.1007/s10530-010-9913-6
Tomas F., Royo L., Mateo-Ramírez A., Máñez-Crespo J., Curbelo L., Amigo M., et al. (2020). “Cymodocea Nodosa Meadows of Cabrera National Park: The Role of Plant Genetic Diversity in Determining Ecosystem Functioning and Implications for Conservation,” in Monografía Del Programa De Investigación Del Organismo Autónomo De Parques Nacionales 2015-2020 (Spain: Ministerio para la Transición Ecológica y el Reto Demográfico), pp 1–pp56.
Tuya F., Fernández-Torquemada Y., Zarcero J., del Pilar R.Y., Csenteri I., Espino F., et al. (2019). Biogeographical Scenarios Modulate Seagrass Resistance to Small-Scale Perturbations. J. Ecol. 107(12), 1263–75. doi: 10.1111/1365-2745.13114
Tuya F., Sanchez-Jerez P., Dempster T., Boyra A., Haroun R. J. (2006). Changes in Demersal Wild Fish Aggregations Beneath a Sea-Cage Fish Farm After the Cessation of Farming. J. Fish Biol. 69, 682–697. doi: 10.1111/j.1095-8649.2006.01139.x
Underwood A. J. (1993). The Mechanisms of Spatially Replicated Sampling Programmes to Detect Environmental Impact in Avariable World. Aust. J. Ecol. 18, 99–116. doi: 10.1111/j.1442-9993.1993.tb00437.x
Valls M. M. (2017). Trophic Ecology in Marine Ecosystems From the Balearic Sea (Western Mediterranean) (PhD Thesis). Spain: Universitat de les Illes Balears. http://hdl.handle.net/10803/461496
van Tussenbroek B. I., Barba Santos M. G. (2011). Demography of Halimeda Incrassata (Bryopsidales, Chlorophyta) in a Caribbean Reef Lagoon. Mar. Biol. 158, 1461–1471. doi: 10.1007/s00227-011-1662-2
Vázquez-Luis M., Guerra-García J. M., Sanchez-Jerez P., Bayle-Sempere J. T. (2009). Caprellid Assemblages (Crustacea: Amphipoda) in Shallow Waters Invaded by Caulerpa Racemosa Var. Cylindracea From Southeastern Spain. Helgol. Mar. Res. 63, 107. doi: 10.1007/s10152-008-0129-4
Vázquez-Luis M., Sanchez-Jerez P., Bayle-Sempere J. T. (2008). Changes in Amphipod (Crustacea) Assemblages Associated With Shallow-Water Algal Habitats Invaded by Caulerpa Racemosa Var. Cylindracea in the Western Mediterranean Sea. Mar. Environ. Res. 65, 16–426. doi: 10.1016/j.marenvres.2008.01.006
Verbruggen H., De Clerck O., N’Yeurt A. D. R., Splading H., Vroom P. S. (2006). Phylogeny and Taxonomy of Halimeda Incrassata, Including Descriptions of H. Kanaloana and H. Heteromorpha Spp. Nov. (Bryopsidales, Chlorophyta). Eur. J. Phycol. 41, 337–362. doi: 10.1080/09670260600709315
Vergés A., Steinberg P. D., Hay M. E., Poore A. G. B., Campbell A. H., Ballesteros E., et al. (2014). The Tropicalization of Temperate Marine Ecosystems: Climate-Mediated Changes in Herbivory and Community Phase Shifts. Proc. R. Soc B. 281, 20140846. doi: 10.1098/rspb.2014.0846
Vivó-Pons A., Alós J., Tomas F. (2020). Invasion by an Ecosystem Engineer Shifts the Abundance and Distribution of Fish But Does Not Decrease Diversity. Mar. pollut. Bull. 160, 11586. doi: 10.1016/j.marpolbul.2020.111586
Willette D. A., Ambrose R. F. (2012). Effects of the Invasive Seagrass Halophila Stipulacea on the Native Seagrass, Syringodium Filiforme, and Associated Fish and Epibiota Communities in the Eastern Caribbean. Aquat. Bot. 103, 74–82. doi: 10.1016/j.aquabot.2012.06.007
Waycott M., Duarte C. M., Carruthers T. J.B., Orth R. J., Dennison W. C., Olyarnik S., et al (2009). Accelerating Loss of Seagrasses Across the Globe Threatens Coastal Ecosystems. Proc. Natl. Acad. Sci. 106, 12377–81. doi: 10.1073/pnas.0905620106
Williams S. L., Smith J. E. (2007). A Global Review of the Distribution, Taxonomy, and Impacts of Introduced Seaweeds. Annu. Rev. Ecol. Evol. Syst. 38, 327–359. doi: 10.1146/annurev.ecolsys.38.091206.095543
Winters G., Beer S., Willette D. A., Viana I. G., Chiquillo K. L., Beca-Carretero P., et al (2020). The Tropical Seagrass Halophila Stipulacea: Reviewing What We know From Its Native and Invasive Habitats, Alongside Identifying Knowledge Gaps. Front. Mar. Sci. 7, 300. doi: 10.3389/fmars.2020.00300
Wirtz P., Kaufmann M. (2005). Pfennigalgen: Neu Für Madeira Und Den Ostatlantik: Halimeda Incrassata. Das Aquarium 431, 48–50.
Woods C. M. C. (2009). Caprellid Amphipods: An Overlooked Marine Finfish Aquaculture Resource? Aquaculture 289, 199–211. doi: 10.1016/j.aquaculture.2009.01.018
Keywords: tropicalization, habitat-forming species, food web, invertebrate assemblages, Cymodocea nodosa
Citation: Mateo-Ramírez Á, Máñez-Crespo J, Royo L, Tuya F, Castejón-Silvo I, Hernan G, Pereda-Briones L, Terrados J and Tomas F (2022) A Tropical Macroalga (Halimeda incrassata) Enhances Diversity and Abundance of Epifaunal Assemblages in Mediterranean Seagrass Meadows. Front. Mar. Sci. 9:886009. doi: 10.3389/fmars.2022.886009
Received: 28 February 2022; Accepted: 24 May 2022;
Published: 23 June 2022.
Edited by:
Glenn Hyndes, Edith Cowan University, AustraliaReviewed by:
Candela Marco-Mendez, Center for Advanced Studies of Blanes, Spanish National Research Council (CSIC), SpainJohn F. Valentine, Dauphin Island Sea Lab, United States
Copyright © 2022 Mateo-Ramírez, Máñez-Crespo, Royo, Tuya, Castejón-Silvo, Hernan, Pereda-Briones, Terrados and Tomas. This is an open-access article distributed under the terms of the Creative Commons Attribution License (CC BY). The use, distribution or reproduction in other forums is permitted, provided the original author(s) and the copyright owner(s) are credited and that the original publication in this journal is cited, in accordance with accepted academic practice. No use, distribution or reproduction is permitted which does not comply with these terms.
*Correspondence: Ángel Mateo-Ramírez, angel.mateo@ieo.csic.es; angel.mateo@ieo.es; a.mateoramirez@gmail.com; Fiona Tomas, fiona@imedea.uib-csic.es
†ORCID: Ángel Mateo-Ramírez, orcid.org/0000-0002-3825-3279
Julia Máñez-Crespo, orcid.org/0000-0001-5998-1366
Fernando Tuya, orcid.org/0000-0001-8316-5887
Inés Castejón-Silvo, orcid.org/0000-0003-1247-787X
Gema Hernan, orcid.org/0000-0002-0806-9729
Laura Pereda-Briones, orcid.org/0000-0002-4028-0188
Jorge Terrados, orcid.org/0000-0002-0921-721X
Fiona Tomas, orcid.org/0000-0001-8682-2504