- 1Frontiers Science Center for Deep Ocean Multispheres and Earth System, and Key Laboratory of Marine Chemistry Theory and Technology, Ministry of Education, Ocean University of China, Qingdao, China
- 2Laboratory of Marine Ecology and Environmental Science, Qingdao National Laboratory for Marine Science and Technology, Qingdao, China
- 3College of Environmental Science and Engineering, Ocean University of China, Qingdao, China
- 4First Institute of Oceanography, Ministry of Natural Resources, Qingdao, China
The concentration and structure of nutrients play a key role in sustaining marine ecosystems. In recent decades, the change in nutrient input from land has led to significant changes in nutrient concentration and structure in the marginal sea and has had an important impact on the coastal ecosystem. Based on historical data from 1984 to 2016, the long-term variations in nutrients and their potential influencing factors, were analyzed in the Changjiang River Estuary. The concentration of dissolved inorganic nitrogen (DIN) increased gradually from 1984 to 2003, but after 2003 the concentration began to decrease. Compared with DIN, the change in dissolved inorganic phosphate (DIP) had a delayed effect and has exhibited a slow downward trend since 2005. The decreases in DIN and DIP are mainly due to the improvement of wastewater treatment efforts and chemical fertilizer use efficiency in recent years. The retention of nutrients by the Three Gorges Reservoir (TGR) has been another important factor since its completion in 2003, especially because the large amount of particulate phosphorus retained by the TGR has led to a significant decrease in the total phosphorus flux into the sea, which is the main reason for the decrease in DIP. The vatiations of DIP and DIN have been instrumental in causing the observed changes of phytoplankton community in the Changjiang River Estuary.
Introduction
Estuaries are key systems where nutrient fluxes from land and sea are modified by biogeochemical processes. Both dissolved and particulate matter experience transformations within the freshwater-estuarine continuum. Rivers transport suspended and dissolved material from the land to the coastal ocean (Skoulikidis and Amaxidis, 2009) and strongly influence aquatic ecology, especially in estuaries (Statham, 2012). Globally, anthropogenic activities have caused large increases in fluvial nutrient fluxes (e.g., from wastewater and fertilizer inputs) and have caused a worldwide increase in the river transport of nitrogen (N) and phosphorus (P) to coastal seas (Anderson et al., 2008), leading to eutrophication; this eutrophication causes an increase in the frequency and extent of unusual and/or noxious algal blooms and hypoxia (Anderson et al., 2008; Conley et al., 2009; Rabalais et al., 2009).
The construction of riverine impoundments such as dams and reservoirs modified the hydrology and, as a consequence (Anderson et al., 2008; Conley et al., 2009; Rabalais et al., 2009), the fluvial transport and retention of nutrients and sediments (Humborg and Ittekkot, 1997). The changes in riverine material loads and their associated impacts on the functioning of large estuaries and coastal seas are a cause of environmental concern. Nutrient loading is not the only concern: the ratios between nutrients are also changing, for example, in many European rivers (Romero et al., 2013) and in Changjiang (Liu et al., 2018). High N/P ratios have important impacts on the functioning of coastal marine ecosystems and may increase the frequency, extent and toxicity of algal blooms (Romero et al., 2013). At the same time, dissolved silica (DSi) concentrations declined after the construction of dams, for example, in the Nile (Wahby and Bishara, 1980) and lower Danube Rivers (Humborg and Ittekkot, 1997).
The Changjiang River basin is the third largest river basin in the world, with a drainage area of 1.8 Mkm2, a length of 6300 km, a water discharge level of 900 km3 and a sediment discharge level of 470 Mton (Liu et al., 2007; Fan and Song, 2014). In Changjiang River, there has been a recent dramatic increase in the number of water conservation projects and reservoir volume (Li et al., 2007), population growth, development of important economic zones such as Chongqing, Wuhan, Nanjing and Shanghai, and agricultural production (producing 40% of China’s food) (Li et al., 2007). These changes have had a major impact on water quality (Shen and Liu, 2009), thus affecting the aquatic ecosystems of the Changjiang River Estuary and adjacent coastal waters (Li et al., 2007). Dissolved inorganic nitrogen and phosphorus (DIN and DIP) concentrations increased by a factor of five from the 1960s to the end of the 1990s in Changjiang (Li et al., 2007). These changes have caused a dramatic increase in microalgal biomass (cell density and chlorophyll-a) along with a reduced proportion of siliceous diatoms to the proliferation of red tides, especially after the 1990s (Wang, 2006). There have been many surveys of nutrient changes in the Changjiang River Estuary (Shen and Liu, 2009; Liu et al., 2013; Fan and Song, 2014; Liu et al., 2018), but these surveys covered different time periods, areas and station locations. In this paper, we analyze the long-term trends of the DIN and DIP concentrations and ratios in the Changjiang River at Datong station (~600 km upstream) and in the Changjiang River Estuary using a 32-year (1984 to 2016) dataset to analyze the factors causing the changing DIN and DIP concentrations. This paper constitutes a considerable expansion of previous work, and we also explored potential influencing factors to reveal the typical regional response of the Changjiang River Estuary and its relationship to changes occurring in the ecosystem during this period.
Study Area And Methods
The Changjiang River Estuary is the pathway through which the Changjiang River discharges into the East China Sea. In addition, the Changjiang River Estuary is a mesotidal estuary with regular semidiurnal tides outside the mouth and nonregular semidiurnal tides inside the mouth. The average tidal range is 2.7 m with a maximum of 4.7 m (Tian et al., 1993). To analyze a long time series of DIN and DIP, a limited area within the mixing zone of the Changjiang River Estuary with 12 fixed stations with long-term data were chosen for this study (Figure 1).
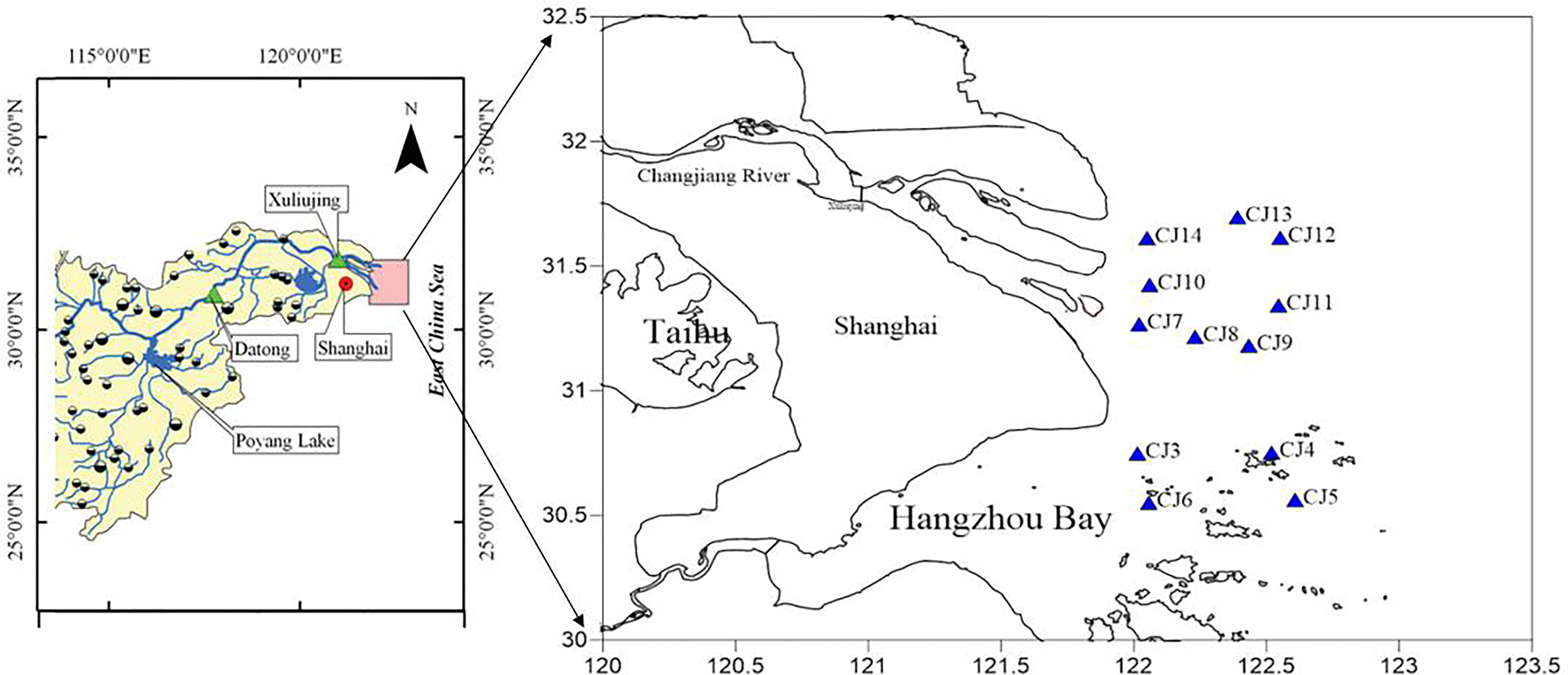
Figure 1 Location of the study area (121.5°-122.8°E, 30.5°-31.8°N) in the Changjiang River Estuary and adjacent coastal waters with monitoring stations CJ3-CJ14 the mixing zone. representative sampling point; representative survey region.
This dataset includes seasonally monitored surface DIN (DIN is the sum of ammonium (NH4+), nitrite (NO2-) and nitrate (NO3-)) and DIP (PO43-) concentration data (Table 1), which are maintained by the State Oceanic Administration of China (1984-2006) (The State Oceanic Administration of China). The observations were carried out in April, July and November during 1984-1999 and in February, May, August, and November during 2000-2006. Further DIN and DIP data from various sources for the period 2007-2016 are listed in Table 1.
For all stations of the mixing zone (CJ3-CJ14), we calculated the surface mean values of the nutrient concentrations for each sampling cruise, and the mean values of the all stations for each sampling cruise were calculated as the annual means. The sampling frequency ranged from two to four times (different seasons) a year, and the temporal series of nutrient data spanned from 1984 to 2016. Thus, the interannual variations in DIN and DIP were obtained, and generalized additive models and change-point analysis were used to evaluate the variation trends. However, during the period from 2008 to 2010, no relevant survey data was available.
Results
DIN and DIP Concentrations in the Mixing Zone of the Changjiang River Estuary
The DIN concentrations varied within a wide range, from 0.70 to 84.2 μmol/L, during the period of 1984 to 2016 (Figure 2A), and the average value for all years was 43.7 μmol/L. The DIP concentrations varied between 0.01 and 1.60 μmol/L (Figure 2B), and the average for all years was 0.70 μmol/L. From 1984 to 2016, the DIN concentrations increased sharply from the lowest concentration of 0.70 μmol/L in November 1984 to the peak concentration of 94.6 μmol/L in May 2003, whereas or even declined after 2003. Similar to DIN, between 1984 and 2016, the DIP concentrations increased sharply from the lowest value of 0.01 μmol/L in November 1984 to the highest value of 1.56 μmol/L in November 2004; however, the growth rate of the DIP concentration slowed or even declined after 2005. Both nutrients tended to increase in the initial period until 2005, and then the growth rate slowed and tends to be stable, or even declined, but the observed change for DIP slightly lagged behind that of DIN.
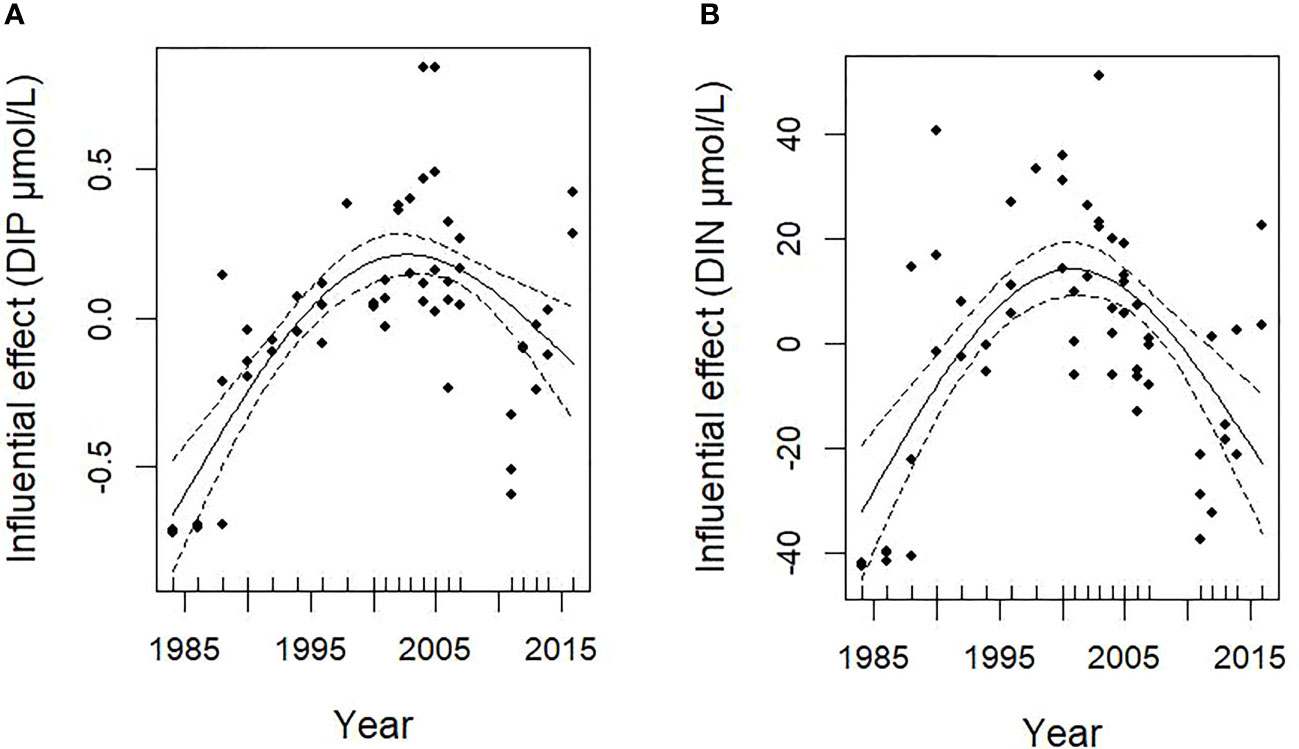
Figure 2 Analysis of nutrients concentrations for (A) DIN and (B) DIP in the mixing zone of the Changjiang River Estuary during 1984 to 2016 based on generalized additive model. For the location of the mixing zone see Figure 1.
N/P Ratios in the Mixing Zone of the Changjiang River Estuary
The N/P ratios fluctuated significantly, from 17.1 to 114.1, during the period of 1984 to 2016 (Figure 3A), and the average value for all years was 61.9. During the period from 1984 to 2016, the N/P ratio in the mixing zone of the Changjiang River Estuary presented an obvious trend of first increasing and then decreasing. The change-point analysis method is used to analyze the location of N/P shifts in the time series of the Changjiang River Estuary, and the results show a breakpoint occurred in approximately 2002 (Figure 3B).
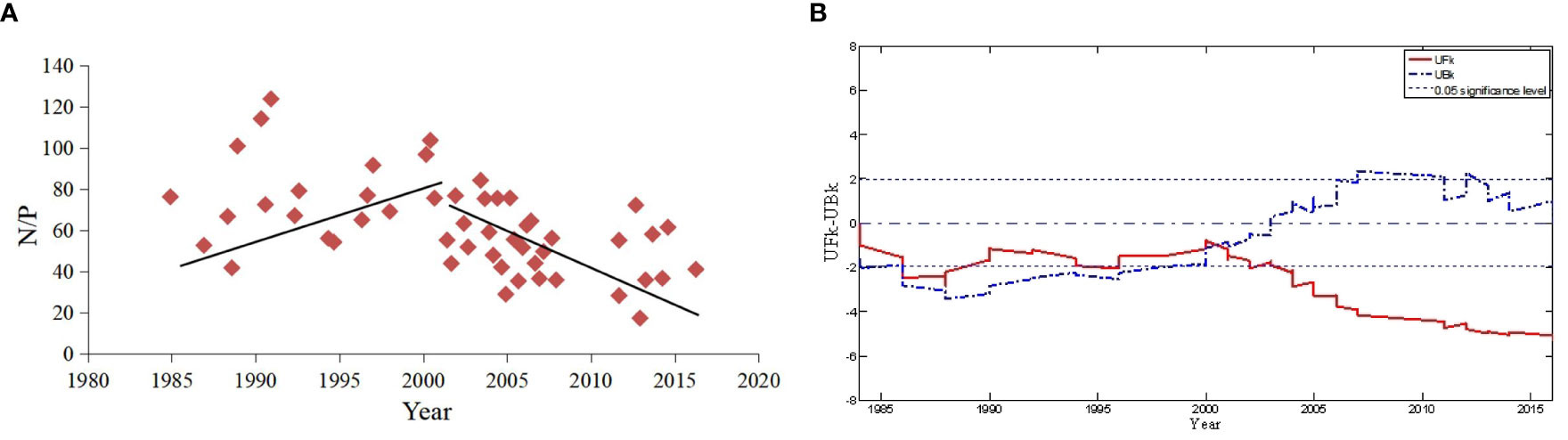
Figure 3 (A) N/P in the mixing zone of the Changjiang River Estuary during 1984 to 2016. (B) N/P change-point analysis of the Changjiang River Estuary during 1984 to 2016.
Input From the Changjiang River
The Datong Station is located in the main stream of the lower reaches of the Changjiang River. It is the upper boundary of the river that can be reached by the ocean tides of the East China Sea. The long-term trends of the DIN and DIP concentrations and fluxes were analyzed at Datong Station using 46-year (1970-2016) data. The DIN concentration and flux at Datong Station increased from 1970 to 2003 (Figures 4A, B), but the more recent data showed the growth rate slowed and tends to be stabilized, or even declined in the DIN concentrations and flux at Datong Station after 2003, with a particularly notable changes in the flux; in contrast, the DIP concentration and flux gradually increased, there was no obvious downward trend, and the concentration stabilized after 2003 (Figures 4C, D).
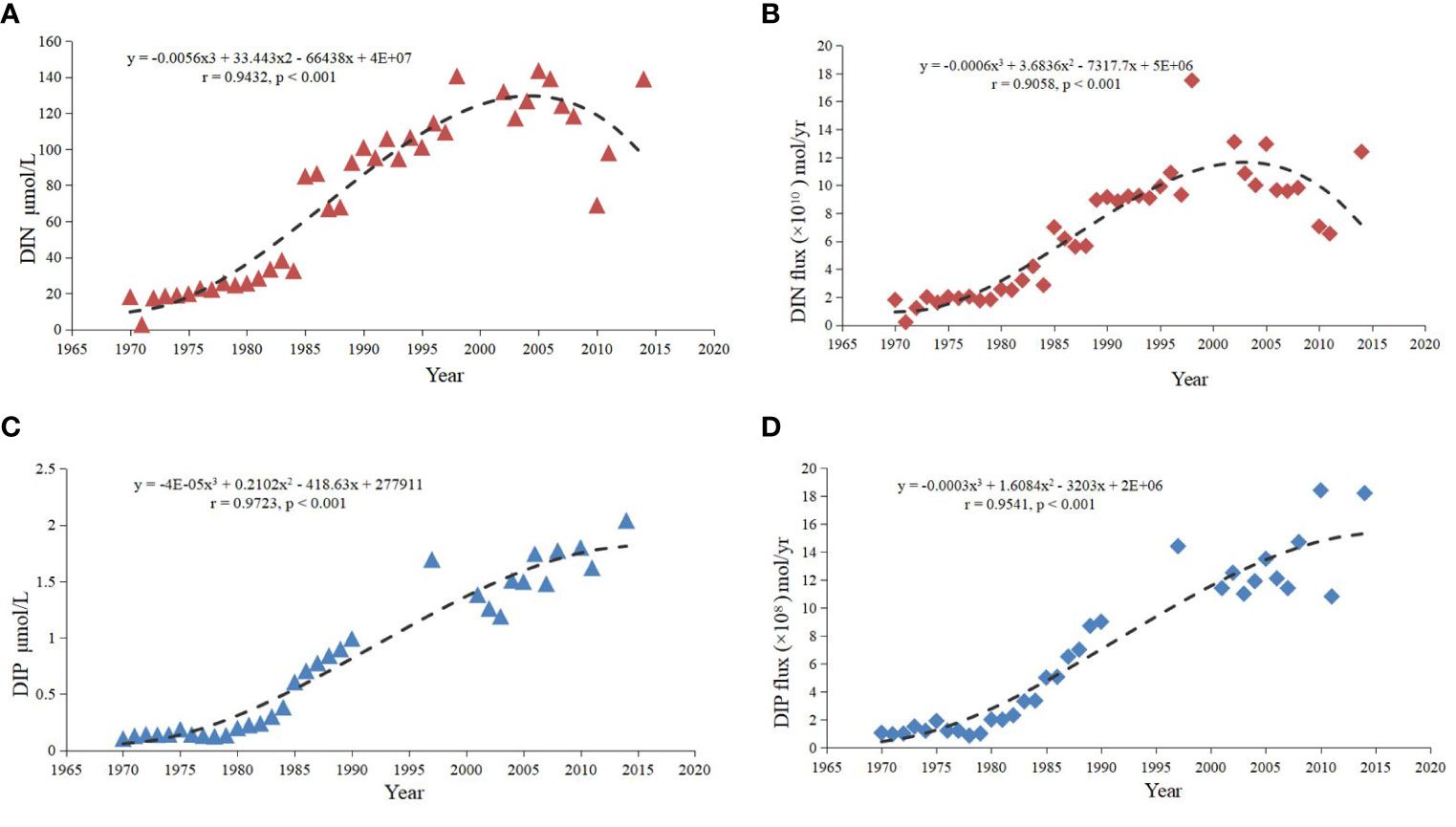
Figure 4 Long-term changes in nutrient concentration (A): DIN; (C): DIP and flux (B): DIN; (D) DIP) at Datong Station in 1970-2014.
Discussion
Effect of Anthropogenic Input
Rivers play an important role in nutrient transport from land to ocean. The Changjiang River is the third largest river in the world and the largest river in China, and it has a drainage area of 1.8×106 square kilometers. It flows 6,300 kilometers eastward and finally injects into the East China Sea. Currently, humans account for more than one-third of the production in terrestrial ecosystems and use approximately half of the available fresh water. As a result of population growth and increased demands for food, large quantities of fertilizer have been produced and released globally, doubling the supply of terrestrial N and P (Tilman et al., 2001). Human disturbances lead to a significant worldwide increase in river nutrient flux (Wang, 2006; Conley et al., 2009), and the amounts of N and P input by rivers into the ocean have tripled between the 1970s and the 1990s (Wang, 2006). All kinds of human activities (e.g., crop cultivation, animal husbandry, sewage, industrial drainage) produce N and P (Anderson et al., 2008; Conley et al., 2009; Rabalais et al., 2009; Statham, 2012). The supply of chemical fertilizers from domestic sources has increased by four percent annually since 1980, and the average national per hectare application of chemical fertilizers in China reached 280 kilograms in 2000, which is three times the world average (Williams, 2005). From 1980 to 2015, the use of N and P fertilizers in China increased steadily and showed a steady trend after 2011 (Figure 5B). More than 90% of the N discharged into the Changjiang River originates from agriculture (Müller et al., 2012). In recent years, the utilization efficiency of chemical fertilizer in China has been greatly improved (He et al., 2019). According to statistics, in rice, wheat and corn production, the efficiency of N fertilizer in China (kg yield/kg N) decreased from 164 to 10, from 44 to 6, and from 93 to 9 from 1961 to 1998, respectively (Tong et al., 2003). It is expected that fertilizer application could be reduced by 30-60% without any loss of crop yields (Ju et al., 2009). The increase in fertilizer utilization efficiency leading to a decrease in the use of fertilizers in agricultural activities may be one of the reasons for the stable or declining concentration and flux of DIN in the Changjiang River (Figures 2A, 4A, B).
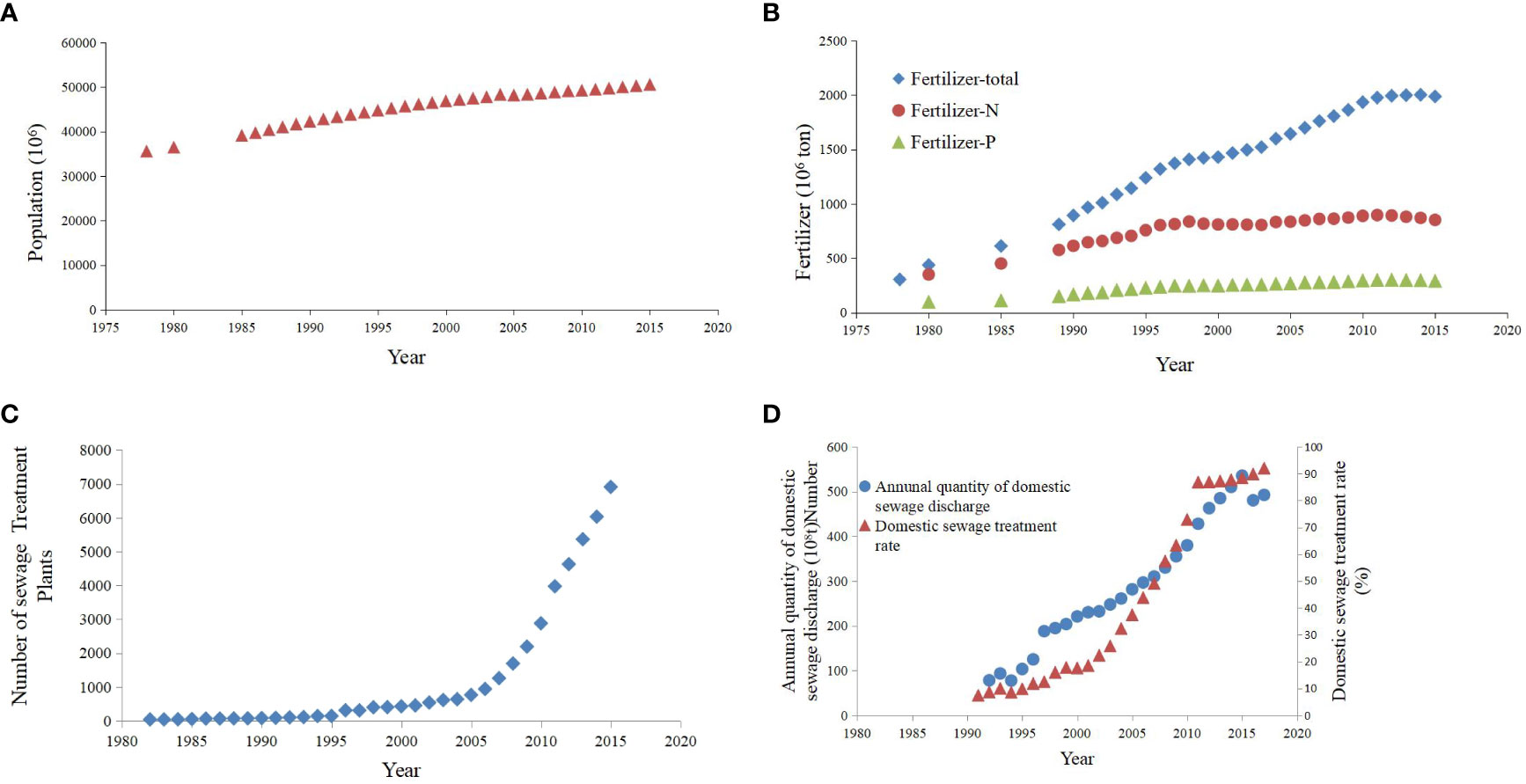
Figure 5 Long-term changes in population (A) and fertilizer use (B) in the Changjiang River Basin; Long term variation of number of sewage treatment plants (C), annual quantity of domestic sewage discharge (D), domestic sewage treatment rate (D) in China.
Although chemical P fertilizer use in the Changjiang River basin also increased substantially in the study period (Figure 5B), P fertilizer was more likely to be absorbed onto soil particles, without much leaching from croplands (Duan et al., 2007). In fact, riverine DIP was more likely to be derived from industrial wastewater and urban effluent from urban areas along rivers (Duan et al., 2007). The continuous increases in DIN and DIP in the Changjiang River correspond with economic development in China (Shen and Liu, 2009) and the discharge of wastewater has also increased. Over 10 million tons of industrial wastewater and domestic sewage were discharged into the Changjiang River and its tributaries each day (Heming and Rees, 2000). The long-term variations in P concentration and flux in the lower reaches of the Changjiang River are closely related to the discharge of wastewater, which indicates that the flux of DIP in the estuary is mainly affected by wastewater emissions.
From 1980 to the present, the population of the Changjiang River basin has been increasing (Figure 5A). Due to the increase in population, the amount of domestic sewage produced has also increased significantly. The discharge of domestic sewage in China has been increasing rapidly since 1992, although the value decreased slightly after 2015 (Figure 5D). However, China has a long history of sewage treatment, and the first large sewage treatment plant dates back to the 1980s. Since the implementation of corresponding sewage treatment in China in 1999, sewage treatment plants have increasingly been used to control nutrient inputs into China’s water, and the number of sewage treatment plants increased nearly 80 times from 1982 to 2017, increasing from 87 to 6910 (Figure 5C). More importantly, in recent years, China’s sewage treatment technology has developed rapidly, the domestic sewage treatment rate increased from 8% in 1991 to 92% in 2017 (Figure 5D), and the amounts of TN and TP discharged in China by urban and industrial sewage have shown downward trends, with decreases of nearly 50% and 75%, respectively (National Bureau of Statistics of People’s Republic of China). Sewage treatment has negatively affected the increases in TP and TN inputs in the Changjiang River basin to a certain extent. In addition, the sewage treatment capacity of China is concentrated on the coastal areas and middle reaches of the Changjiang River, which are economically developed zones (Jin et al., 2014). Although sewage discharge has continued to increase in recent years, due to the large-scale shift in sewage treatment in approximately 2005, the discharge of N and P in sewage of China has decreased. The annual discharge of total N and total P in sewage of China decreased sharply from 461.3 and 54.68 tons in 2015 to 212.1 and 13.49 tons in 2016, respectively (National Bureau of Statistics of People’s Republic of China). The decrease in total N and P in sewage would thus lead to a decrease in the amount of nutrients transported to the estuary.
Retention of the TGR
The retention effect of dams on N and P can also be reflected in other dams in the world. The Iron Gates Reservoir, the largest reservoir in the lower Danube, traps a large amount of silicate and a small amount of N and P in the form of deposited diatoms (Humborg and Ittekkot, 1997). The Lake Diefenbaker Reservoir in Canada intercepted 70% of the TN and 90% of the TP in the waters (Morales-Marín et al., 2017). The well-known “Three Gorges Project” (TGP) of the Changjiang River started in 1994, and two cofferdams of the TGD were closed on November 8, 1997, with the first filling stage of the reservoir completed in June 2003, and the water level reached 135 m. The second impoundment of the TGP was carried out, and the water level reached 156 m in September 2006. The construction of the dam was completed in 2008, at which point all of the generation units were fully operational. Furthermore, the dam achieved the goal of raising the water level in the reservoir to 175 m. The TGP began full-scale operations in 2009. The construction of the TGR in the Changjiang River has played a positive role in reducing the N and P loads to the East China Sea (Chai et.al., 2009). The TN retention rate in the TGR increased from 1 Gg N yr-1 in 2003 to 90 Gg N yr-1 in 2004, and the TP retention rate increased from 0.2 Gg P yr-1 in 2003 to 20 Gg P yr-1 in 2004 (Liu et al., 2018). After the impoundment of the TGR reached 135 m in 2003, approximately 18% of TN, 15% of TP and 20% of DSi were trapped in the reservoir (Ran, 2009). When the TGR launched its 175 m experimental water storage in 2009, approximately 21% of TDN, 24% of TP and 3% of DSi were retained in the TGR, and the amount of retained nutrients accounted for 15%, 12% and 1% of the TDN, TP and DSi fluxes in the estuary, respectively (Ding et al., 2019).
DIN was the most abundant N form in the Changjiang River (Liu et al., 2003). After dam completion, the decreased flow velocity and the increased residence time may have stimulated the growth of phytoplankton; thus, the growth of phytoplankton and the removal of particulate matter have played an important role in the reduction of the N flux (Ran et al., 2017). The construction of the TGR has slowed the water flow, leading to regional eutrophication, which is responsible for the spring proliferation of phytoplankton in Xiangxi Bay of the TGR, as reported by Zhou et al. (2011). There is significant DIN consumption and weak P consumption during the algal blooms in the TGR (Ye et al., 2007). Actually, phytoplankton mass production consumes 6.84×104 t/a of DIN in the TGR (Ding et al., 2019). Thus, the consumption of DIN by the algal blooms of the TGR has caused some of the DIN to be trapped in the reservoir, which may also be an important cause of the decrease in DIN concentration in the mixing zone of the Changjiang River Estuary (Figure 2A).
Furthermore, the retention of particulate phosphorus (PP) and particulate nitrogen (PN) caused by the TGR may be significant. Unlike N, P exists mainly in the particulate form. PP (>80% of total phosphorus (TP)) is a dominant component of TP in the Changjiang River (Zhou et al., 2013), while PN accounts for approximately 10% of total nitrogen (TN) (Liu et al., 2003). In general, the concentration of TP in the Changjiang River has increased mainly in the upper reaches and decreased in the middle and lower reaches (Shen and Liu, 2009). On a global scale, fluvial particulates could transport 1.4 to 14×1010 mol yr-1 of reactive phosphate to the sea, which is approximately 2-5 times more than that in the dissolved load alone (Froelich, 1988). The loads of P downstream reach of the Changjiang River are predominantly from the upstream reaches in the form of PP, and a majority of the P loads are deposited in the TGR and other reservoirs (Zhou et al., 2013). After the completion of the TGR, due to the large interception effect of the TGD on the sediment, the sediment load greatly decreased, resulting in a reduction in the amounts of PP and PN imported into the river. As a result, the PP supplies, which are either from upstream or from riverbed resuspension, to the downstream reaches will further decline (Chai et al., 2009; Zhou et al., 2013). Additionally, the PP in the downstream declined by 86% after the construction of the TGR in 2003 (Gong et al., 2006), which resulted in a reduced TP load to the estuary.
P transport in the Changjiang River mainly depends on suspended matter, and the TP and PP concentrations in the lower reaches are closely related to sediment discharges (Shen and Liu, 2009). Although the annual water discharge at Datong Station has changed little in the past 50 years, the sediment discharge has decreased significantly, especially after 2003 (Figure 6). After the construction of the TGR in 2003, the amount of sediment entering the sea has decreased steadily; for example, from 2002 to 2011, the amount of sediment entering the sea decreased by 203 million tons, a 74% reduction compared to the value in 2002. According to statistics, the sedimentation of the TGR was 124 million tons in 2003 (Changjiang Water Resources Commission), and the value in the post-TGR period (2003-2012) is equivalent to a reduction of 56% compared with that just before construction of the TGR (Wang et al., 2016). In fact, the retention effect of dams on sediment has been confirmed in some other rivers. After the completion of the dam, the sediment fluxes in the Sebou and Molouyuya Rivers were reduced by nearly 95% and 93%, respectively (Snoussi et al., 2002), and the amount of P trapped in sediments in the semi-closed bay of the Seto inland sea in Japan was 1.7 times higher than the previous value (Jin et al., 2013). More importantly, dams can even impact marine ecosystems by changing the nutrient concentrations and ratios in the coastal zone (Gong et al., 2006).
Biogeochemical Process in the Estuary Mixing Zone
Estuaries are key sites where nutrient fluxes from land are modified by biogeochemical processes. Both dissolved and particulate matter experience changes within the freshwater-estuarine continuum. The estuarine processes of nutrients are generally expressed by the salinity-nutrient relation during the mixing of nutrient-rich freshwater and nutrient-poor seawater (Yao et al., 2014). Important estuarine processes for PO43- include adsorption on or desorption from Fe oxy-hydroxide-bearing suspended matter or underlying sediments (Froelich, 1988). This kind of behavior is well known for dissolved P and is explained by the adsorption on and desorption from Fe (oxy) hydroxides (Froelich, 1988). Thus, when heavily PO43–loaded Fe particles reach seawater with a PO43- concentration below the equilibrium concentration, PO43- will be desorbed and released to the surrounding water. This process represents the estuarine buffering of phosphate (Froelich, 1988). The internal estuarine fluxes associated with the abovementioned buffering effect play an important role in the nutrient estuarine fluxes, which can account for 45% of the P flux (Yao et al., 2014).
Particulate organic N and particulate inorganic P were the major forms of particulate N and P, respectively. Particulate inorganic P mainly exists in the mineral phase and as adsorbed to particles, whereas particulate organic N primarily comprises living and detrital organic compounds, which can be returned to seawater through the remineralization process (Zuo et al., 2016). Particulate N and P account for a large proportion of the N and P pools and are potential sources of dissolved nutrients (Lipizer et al., 2012). It is assumed that the retained mineralized nitrogen is totally released from the sediments into the water column, contributing 12-15% of the total DIN sources (Lin et al., 2016). The drastic decline in the sediment load from the Changjiang River reduced turbidity in the Changjiang River Estuary (Wang et al., 2016) An overall declining trend in surface sediment concentrations has been identified in the Changjiang River Estuary. Comparing the surface sediment concentration just before the impoundment of the TGD (62 mg/L in 1997-2002) with the current concentration (35 mg/L in 2006-2011), a sharper decrease in surface sediment concentrations was observed, reflecting the impact of the TGD (Wang et al., 2016). Due to the retention of sediment by the TGR, the PP and PN inputs to the estuary have been reduced, correspondingly resulting in a reduction in the amounts of DIP and DIN released during the estuary process. However, because the proportion of PN in TN is not as important as that of PP in TP, the retention effect of TGR on PN is not as obvious as that in PP in the mixing zone. This result is because of the estuarine processes in the mixing zone of the Changjiang River Estuary, in which the trend of the DIP concentration decreased slightly later than that of the DIN (Figure 2).
Response of Phytoplankton Community
Eutrophication in coastal waters during the 20th and 21st centuries is associated with excessive nutrient loading, which can stimulate the growth of phytoplankton (Rabalais et al., 2009), leading to red tides or hypoxia. Seawater eutrophication is the main cause of red tides (Anderson et al., 2008), and increases in nutrient concentrations, especially increases in DIN and DIP concentrations, will lead to eutrophication and have serious impacts on coastal ecosystems (Conley et al., 2009). In the second half of the 20th century, due to the increases in population size and fertilizer use, many estuaries and coastal ecosystems have experienced intensified eutrophication, which has led to the development of hypoxia in the ecosystem (Wang, 2006; Li et al., 2007; Rabalais et al., 2009). Eutrophication may be intensified in the context of increased river discharge and fertilizer use (Rabalais et al., 2009), and this eutrophication has a significant impact on ecosystems.
The increase in nutrient concentrations in the Changjiang River has led to severe nutrient loading in the Changjiang River Estuary, which has led to the proliferation of red tide algae. The Changjiang River Estuary and its adjacent waters are red tide-prone areas; in the nearly 30 years since the 1990s, large-scale red tides and extensive hypoxic areas have been reported in coastal waters near the Changjiang River Estuary (Liu et al., 2013). Red tides in the Changjiang River Estuary and adjacent coastal areas were rare before 1985 but have increased rapidly thereafter; for example, 58 red tide events were found in the Changjiang River Estuary and adjacent coastal areas in 2003, and this value was 10 times higher than the average number in the 1990s (Wang, 2006). The frequency of red tides increased sharply from the 1980s to 2000 with the rapid growth of China’s economy (Li et al., 2007). The outbreak frequency of red tides increased rapidly from the 1990s to 2003 but then decreased significantly since 2004; there appeared to be a lower number in 2015, which was less than the average frequency from 2006 to 2014 (Figure 7). Similar to the changes in DIN and DIP concentrations (Figure 2), a stable trend in the frequency of red tides appeared in approximately 2003. The occurrence frequency of red tides began to stabilize after 2000. Moreover, the HAB species changed, in particular, P. donghaiense bloomed most frequently since 2000 (>50% of the total frequency), although the HABs caused by Skeletonema spp. also increased dramatically (Jiang et al., 2014). This finding is consistent with the variation in the concentrations of nutrients in the Changjiang River Estuary, which slowed or even declined after the completion of the TGR. It has also been proven that the bloom of red tide algae depends on nutrients.
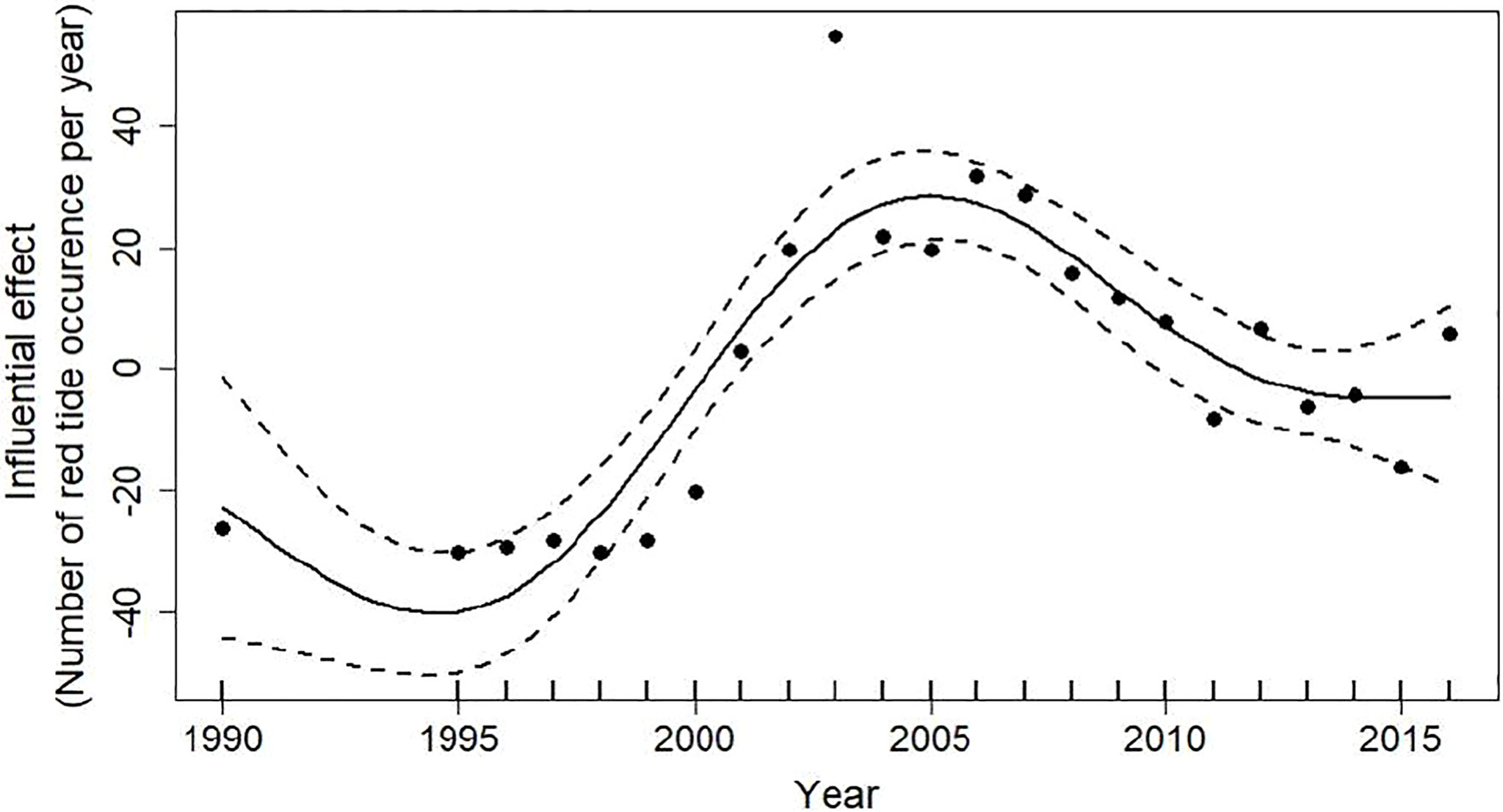
Figure 7 Analysis of number of occurrences of red tides in the East China Sea in 1990-2017 based on generalized additive model.
Changes in nutrient structure affect phytoplankton growth and community structure (Romero et al., 2013). In recent years, the decline of the N/P ratio in the mixed zone of the Changjiang River Estuary (Figure 3) indicates that the area of potential P limitation of phytoplankton growth weakened after 2003 and potential N limitation appeared. The abundance and production of phytoplankton in the Changjiang River Estuary are closely related to nitrogen concentration (Jiang et al., 2010). Research has shown that diatom species in the Changjiang River Estuary has decreased from 84.6% during 1980s to 69.8% during 2005, while the abundance of dinoflagellates has increased dramatically, from 0.7% to 25.4% (Jiang et al., 2010). Before 2000, red tide outbreaks were largely caused by Skeletonema costatum and Nactiluca scientillans, with some contribution from Prorocentrum micans Ehrenberg and Oscillatoria; however, after 2003, the most significant causative species was Prorocentrum dong-haiense, which causes the red tides that now occur every year in the Changjiang River Estuary (Liu et al., 2013; Jiang et al., 2014). Second, in addition to nutrient limitations, the reproduction of algae is affected by light and hydrology. Due to the construction of the TGR, the sediment flux and its natural seasonal variation in the waters along the Changjiang River have been greatly reduced (Xu and Milliman, 2009). The TGR retention of sediment has led to the decrease in turbidity in the estuary (Wang et al., 2016) and a decrease the abundance of nutrients required for phytoplankton reproduction; therefore, nutrients may no longer be a limiting factor for phytoplankton growth, especially in spring, where improved light conditions may lead to more frequent and intense algal reproduction in the freshwater-estuary continuum (Jiang et al., 2014).
The Changjiang River Estuary and its adjacent waters, one of the largest and most complex environments among Chinese estuaries, have been seriously polluted due to the heavy input of waste loads from watershed and human activities (Liu et al., 2013). In 2016, the National Environmental Protection Supervisor work was fully started, several small livestock and poultry farms were closed, and all large-scale livestock and poultry farms were required to be equipped with sewage treatment facilities. These measures significantly reduced the amounts of TN and TP discharged in sewage. In 2016, the amount of TN was reduced by half compared with that in 2015, while the amount of TP was reduced by 4/5. In addition, the amount of chemical fertilizer was reduced and fertilizer use efficiency increased, and the amounts of TN and TP discharged into the environment have been reduced accordingly. Therefore, the concentrations of DIN and DIP in the Changjiang River Estuary may decrease significantly in the future, and their impacts on the estuarine ecosystem require further study.
Conclusions
From the 1980s to the beginning of the 21st century, the concentrations of DIN and DIP in the Changjiang River Estuary increased significantly. However, after 2003, the concentration of DIN shifted, and the concentration tends to be stable, or even declined. The turning point of DIP concentration obviously lagged behind that of DIN. The changes in the DIN concentration can be attributed to large-scale wastewater treatment, increased chemical fertilizer efficiency, consumption of DIN by phytoplankton in the TGR, and weak retention of PN by the TGR. The increase in sewage treatment and chemical fertilizer efficiency also contributes to the stability of the estuary’s DIP concentration. Moreover, the high retention of PP in the TGR has made an important contribution to the change in DIP concentration in the mixing zone of the Changjiang River Estuary. In addition, the estuary P process may be the main reason for the change in DIP being later than that of DIN. The decrease in the outbreak frequency of red tides after 2003 may be related to the variations in the concentrations of DIN and DIP, which have slowed or even declined. The long-term nutrient variations in the Changjiang River Estuary have potential ecological impacts on the frequencies and diversity of the dominant species in the local red tide blooms, and their impacts on the estuarine ecosystem require further study.
Data Availability Statement
The raw data supporting the conclusions of this article will be made available by the authors, without undue reservation.
Author Contributions
All authors contributed to the study conception and design. JL: Methodology, Writing-original draft. QZY: Investigation, Review and editing, Visualization, Supervision, Funding acquisition. TZM: Investigation, Review. QW: Review. HTC: Investigation, Review, Funding acquisition. ZGY: Review, Funding acquisition. All authors contributed to the article and approved the submitted version.
Conflict of Interest
The authors declare that the research was conducted in the absence of any commercial or financial relationships that could be construed as a potential conflict of interest.
Publisher’s Note
All claims expressed in this article are solely those of the authors and do not necessarily represent those of their affiliated organizations, or those of the publisher, the editors and the reviewers. Any product that may be evaluated in this article, or claim that may be made by its manufacturer, is not guaranteed or endorsed by the publisher.
Acknowledgments
This study was funded by The Strategic Priority Research Program of the Chinese Academy of Sciences (XDA19060201), The Joint Fund between NSFC and Shandong Province (U1906210), The National Natural Science Foundation of China (42130410, 41876116), The Natural Science Foundation of Shandong Province (ZR2019MD035). We thank our laboratory colleagues for their collaboration in sampling and data acquisition.
References
Anderson D. M., Burkholder J. M., Cochlan W. P., Glibert P. M., Gobler C. J., Heil C. A., et al. (2008). Harmful Algal Blooms and Eutrophication: Examining Linkages From Selected Coastal Regions of the United States. Harmful Algae 8, 39–53. doi: 10.1016/j.hal.2008.08.017
Chai C., Yu Z. M., Shen Z. L., Song X. X., Cao X. H., Yao Y. (2009). Nutrient Characteristics in the Yangtze River Estuary and the Adjacent East China Sea Before and After Impoundment of the Three Gorges Dam. Sci. Total Environ. 407, 4687–4695. doi: 10.1016/j.scitotenv.2009.05.011
Changjiang Water Resources Commission (CWRC) (1970-2016; 1962-1999). (1962). Changjiang Sediment Bulletin. 1970-2016 (Wuhan: Changjiang Press).
Conley D. J., Paerl H. W., Howarth R. W., Boesch D. F., Seitzinger S. P., Havens K. E., et al. (2009). Controlling Eutrophication: Nitrogen and Phosphorus. Science 323, 1014–1015. doi: 10.1126/science.1167755
Dai Z. J., Du J. Z., Zhang X. L., Su N., Li J. F. (2011). Variation of Riverine Material Loads and Environmental Consequence on the Changjiang (Yangtze) Estuary in Recent Decades-2008. Environ. Sci. Technol. 45, 223–227. doi: 10.1021/es103026a
Ding X., Chen P. P., Liu S. M., Zhang G. L., Zhang J., Dan S. F. (2019). Nutrient Dynamics in the Changjiang and Retention Effect in the Three Gorges Reservoir. J. Hydrol 574, 96–109. doi: 10.1016/j.jhydrol.2019.04.034
Duan S. W., Xu F., Wang L. J. (2007). Long-Term Changes in Nutrient Concentrations of the Changjiang River and Principal Tributaries. Biogeochemistry 85, 215–234. doi: 10.1007/s10533-007-9130-2
Fan W., Song J. (2014). A Numerical Study of the Seasonal Variations of Nutrients in the Changjiang River Estuary and its Adjacent Sea Area. Ecol. model 291, 69–81. doi: 10.1016/j.ecolmodel.2014.07.026
Froelich P. N. (1988). Kinetic Control of Dissolved Phosphate in Natural Rivers and Estuaries: A Primer on the Phosphate Buffer Mechanism. Limnol Oceanogr 33, 649–68. doi: 10.4319/lo.1988.33.4part2.0649
Gong G. C., Chang J., Chiang K. P., Hsiung T. M., Hung C. C., Duan S. W., et al. (2006). Reduction of Primary Production and Changing of Nutrient Ratio in the East China Sea: Effect of the Three Gorges Dam? Geophys Res. Lett. 33, L07610. doi: 10.1029/2006GL025800
Heming L., Rees P. (2000). Population Displacement in the Three Gorges Reservoir Area of the Yangtze River, Central China: Relocation Policies and Migrant Views. Int. J. Popul Geogr 6, 439–462. doi: 10.1002/1099-1220(200011/12)6:6<439::AID-IJPG198>3.0.CO;2-L
He Y., Qi Y. B., Tang J. Q. (2019). Regional Difference and Convergence Analysis on Fertilizer Application Efficiency of Grain Production in China (in Chinese). Jiangsu J. Agric. Sci. 35, 729–735. doi: is10.3969/j.issn.1000-4440.2019.03.032
Humborg C., Ittekkot V. (1997). Effect of Danube River Dam on Black Sea Biogeochemistry and Ecosystem Structure. Nature 386, 385–388. doi: 10.1038/386385a0
Jiang Z. B., Liu J. J., Chen J. ,. F., Chen Q. Z., Yan X. J., Xuan J. L., et al. (2014). Responses of Summer Phytoplankton Community to Drastic Environmental Changes in the Changjiang (Yangtze River) Estuary During the Past 50 Years. Water Res. 54, 1–11. doi: 10.1016/j.watres.2014.01.032
Jiang Z., Liu J., Chen J., Chen Q., Yan X., Xuan J., et al. (2014). Response of Summer Phytoplankton Community to Drastic Environmental Changes in the Changjiang (Yangtze River) Estuary During the Past 50 Years. Water Res. 54, 1–11. doi: 10.1016/j.watres.2014.01.032
Jiang T., Yu Z. M., Song X. X., Cao X. H., Yuan Y. Q. (2010). Long-Term Ecological Interactions Between Nutrient and Phytoplankton Community in the Changjiang Estuary. Chin. J. Oceanol Limnol 28, 887–894. doi: 10.1007/s00343-010-9059-5
Jin G. Z., Onodera S., Amano A., Saito M., Shimizu Y., Satou T. (2013). Effects of Dam Construction on Sediment Phosphorus Variation in a Semi-Enclosed Bay of the Seto Inland Sea, Japan. Estuar Coastal Shelf Sci. 135, 191–220. doi: 10.1016/j.ecss.2013.10.009
Jin L. Y., Zhang G. M., Tian H. F. (2014). Current State of Sewage Treatment in China. Water Res. 66, 85–98. doi: 10.1016/j.watres.2014.08.014
Ju X. T., Xing G. X., Chen X. P. (2009). Reducing Environmental Risk by Improving N Management in Intensive Chinese Agricultural Systems. Proc. Natl. Acad. Sci. U States America 106, 3041–3046. doi: 10.1073/pnas.0813417106
Lin X. B., Hou L. J., Liu M., Li X. F., Yin G. Y. (2016). Gross Nitrogen Mineralization in Surface Sediments of the Yangtze Estuary. Public Library Sci. 11, 1–16. doi: 10.1371/journal.pone.0151930
Lipizer M., Vittor C. D., Comici C., Tamberlich F., Giani M. (2012). Effects of Intense Physical and Biological Forcing Factors on CNP Pools in Coastal Waters (Gulf of Trieste, Northern Adriatic Sea). Estuar coastal shelf Sci. 115, 40–50. doi: 10.1016/j.ecss.2012.03.024
Liu X. C., Beusen A. H. W., Van Beek L. P. H., Mogollon J. M., Ran X. B., Bouwman A. F. (2018). Exploring Spatiotemporal Changes of the Yangtze River (Changjiang) Nitrogen and Phosphorus Sources, Retention and Export to the East China Sea and Yellow Sea. Water Res. 142, 246–255. doi: 10.1016/j.watres.2018.06.006
Liu J. P., Xu K. H., Li A. E., Milliman J. D., Velozzi D. M., Xiao S. B., et al. (2007). Flux and Fate of Yangtze River Sediment Delivered to the East China Sea. Geomorphology 85, 208–224. doi: 10.1016/j.geomorph.2006.03.023
Liu S. M., Zhang J., Chen H. T., Wu Y., Xiong H., Zhang Z. F. (2003). Nutrients in the Changjiang and its Tributaries. Biogeochemistry 62, 1–18. doi: 10.1023/A:1021162214304
Liu L. S., Zhou J., Zheng B. H., Cai W. Q., Lin K. X., Tang J. L. (2013). Temporal and Spatial Distribution of Red Tide Outbreaks in the Yangtze River Estuary and Adjacent Waters, China. Mar Pollut Bull. 73, 213–221. doi: 10.1016/j.marpolbul.2013.04.002
Li M. T., Xu K. Q., Watanabe M., Chen Z. Y. (2007). Long-Term Variations in Dissolved Silicate, Nitrogen, and Phosphorus Flux From the Yangtze River Into the East China Sea and Impacts on Estuarine Ecosystem. Estuar Coastal Shelf Sci 71, 3–12. doi: 10.1016/j.ecss.2006.08.013
Morales-Marín L. A., Wheater H. S., Lindenschmidt K. E. (2017). Assessment of Nutrient Loadings of a Large Multipurpose Prairie Reservoir. J. Hydrol 550, 166–185. doi: 10.1016/j.jhydrol.2017.04.043
Müller B., Berg M., Pernet-Coudrier B., Qi W. X., Liu H. J. (2012). The Geochemistry of the Yangtze River: Seasonality of Concentrations and Temporal Trends of Chemical Loads. Global Biogeochem Cycles 26, 2. doi: 10.1029/2011GB004273
National Bureau of Statistics of People's Republic of China (2019). “China Statistical Yearbook,” in China Statistical Yearbook on Environmen -2018) (Beijing: China Statistical Yearbook).
Rabalais N. N., Turner R. E., Diaz R. J., Justic D. (2009). Global Change and Eutrophication of Coastal Waters. ICES J. Mar Sci. 66, 1528–1537. doi: 10.1093/icesjms/fsp047
Ran X. B. (2009). Nutrient Distributions and Retention of Three Gorges Reservoir (Qingdao: Ocean University of China).
Ran X. B., Bouwman L., Yu Z. G., Beusen A., Chen H. T., Yao Q. Z. (2017). Nitrogen Transport, Transformation, and Retention in the Three Gorges Reservoir: A Mass Balance Approach. Limnol Oceanogr 62, 2323–2337. doi: 10.1002/lno.10568
Romero E., Garnier J., Lassaletta L., Garnier J., Lassaletta L., Billen G., et al. (2013). Large-Scale Patterns of River Inputs in Southwestern Europe: Seasonal and Interannual Variations and Potential Eutrophication Effects at the Coastal Zone. Biogeochemistry 113, 481–505. doi: 10.1007/s10533-012-9778-0
Shen Z. L., Liu Q. (2009). Nutrients in the Changjiang River. Environ. Monit Assess. 153, 27–44. doi: 10.1007/s10661-008-0334-2
Skoulikidis N., Amaxidis Y. (2009). Origin and Dynamics of Dissolved and Particulate Nutrients in a Minimally Disturbed Mediterranean River With Intermittent Flow. J. hydrol (Amsterdam) 373, 218–229. doi: 10.1016/j.jhydrol.2009.04.032
Snoussi M., Haïda S., Imassi S. (2002). Effects of the Construction of Dams on the Water and Sediment Fluxes of the Moulouya and the Sebou Rivers, Morocco. Reg Environ. Change 3, 5–12. doi: 10.1007/s10113-001-0035-7
State Oceanic Administration People’s Republic of China (2009-2014) Marine Disasters Bulletin (in Chinese). Available at: http://www.soa.gov.cn/zwgk/hygb/zghyzhgbChina (Accessed 30 July 2020).
Statham P. J. (2012). Nutrients in Estuaries an Overview and the Potential Impacts of Climate Change. Sci. Total Environ. 434, 213–227. doi: 10.1016/j.scitotenv.2011.09.088
The State Oceanic Administration of China (1984-1999; 2000-2006). (1964). China Marine Statistical Yearbook (Beijing: Ministry of Natural Resources,People’s Republic of China).
Tian R. C., Hu F. C., Martin J. M. (1993). Summer Nutrient Fronts in the Changjiang (Changjiang River) Estuary. Estuar Coastal Shelf Sci. 37, 27–41. doi: 10.1006/ecss.1993.1039
Tilman D., Fargione J., Wolff B., D'Antonio C., Dobson A., Howarth R., et al. (2001). Forecasting Agriculturally Driven Global Environmental Change. Science 292, 281–284. doi: 10.1126/science.1057544
Tong C. L., Hall C. A. S., Wang H. Q. (2003). Land Use Change in Rice, Wheat and Maize Production in China, (1961-1998). Agricult Ecosyst Environ. 95, 523–536. doi: 10.1016/S0167-8809(02)00182-2
Wahby S. D., Bishara N. F. (1980). “The Effect of the River Nile on Mediterranean Water, Before and After the Construction of the High Dam at Aswan,” in River Inputs to Ocean Systems. Eds. Martin J. M., Burton J. D., Eisma D. (New York: UNEP, IOC, SCOR, United Nations), 311–318.
Wang B. D. (2006). Cultural Eutrophication in the Changjiang (Yangtze River) Plume: History and Perspective. Estuar Coastal Shelf Sci. 69, 471–477. doi: 10.1016/j.ecss.2006.05.010
Wang B. D., Xin M., Sun X., Wei Q. S., Zhang X. L. (2016). Does Reduced Sediment Load Contribute to Increased Outbreaks of Harmful Algal Blooms Off the Changjiang Estuary? Acta Oceanol Sin. 35, 16–21. doi: 10.1007/s13131-016-0846-5
Wang J. N., Yan W. J., Chen N. W., Li X. Y., Liu L. S. (2015). Modeled Long-Term Changes of DIN:DIP Ratio in the Changjiang River in Relation to Chl-A and DO Concentrations in Adjacent Estuary. Estuar Coastal Shelf Sci. 166, 153–160. doi: 10.1016/j.ecss.2014.11.028
Williams J. (2005)Understanding the Overuse of Chemical Fertilizer in China. In: A Synthesis of Historic Trends, Recent Studies, and Field Experiences (Accessed 5 June 2019).
Xu K. H., Milliman J. D. (2009). Seasonal Variations of Sediment Discharge From the Yangtze River Before and After Impoundment of the Three Gorges Dam. Geomorphology 104, 276–283. doi: 10.1016/j.geomorph.2008.09.004
Yao Q. Z., Yu Z. G., Li L. L., Chen H. T., Mi T. Z. (2014). Transformation and Source of Nutrients in the Changjiang River Estuary. Sci. China Chem. 57, 779–790. doi: 10.1007/s11426-013-5040-4
Ye L., Han X. Q., Xu Y. Y., Cai Q. H. (2007). Spatial Analysis for Spring Bloom and Nutrient Limitation in Xiangxi Bay of Three Gorges Reservoir. Environ. Monit Assess. 127, 135–145. doi: 10.1007/s10661-006-9267-9
Zhou G. J., Zhao X. M., Bi Y. H., Liang Y. B., Hu J. L., Yang M., et al. (2011). Phytoplankton Variation and its Relationship With the Environment in Xiangxi Bay in Spring After Damming of the Three-Gorges, China. Environ. Monit Assess. 176, 125–141. doi: 10.1007/s10661-010-1571-8
Zhou G. J., Zhao X. M., Bi Y. H., Liang Y. B., Hu J. L., Yang M., et al. (2011). The Effect of Dams on Phosphorus in the Middle and Lower Yangtze River. Water Resour. Res. 49, 3659–3669. doi: 10.1002/wrcr.20283
Keywords: Changjiang River Estuary, DIP, DIN, long-term variation, Three Gorges Reservoir
Citation: Liu J, Yao Q, Mi T, Wei Q, Chen H and Yu Z (2022) Change of the Long-Term Nitrogen and Phosphorus in the Changjiang (Yangtze) River Estuary. Front. Mar. Sci. 9:885311. doi: 10.3389/fmars.2022.885311
Received: 27 February 2022; Accepted: 03 May 2022;
Published: 02 June 2022.
Edited by:
Jun Sun, China University of Geosciences Wuhan, ChinaReviewed by:
Jianfeng Feng, Nankai University, ChinaYongquan Yuan, Institute of Oceanology (CAS), China
Copyright © 2022 Liu, Yao, Mi, Wei, Chen and Yu. This is an open-access article distributed under the terms of the Creative Commons Attribution License (CC BY). The use, distribution or reproduction in other forums is permitted, provided the original author(s) and the copyright owner(s) are credited and that the original publication in this journal is cited, in accordance with accepted academic practice. No use, distribution or reproduction is permitted which does not comply with these terms.
*Correspondence: Qingzhen Yao, cXpoeWFvQG91Yy5lZHUuY24=; Zhigang Yu, WmhpZ2FuZ1l1QG91Yy5lZHUuY24=
†Present Address: Qingzhen Yao, Key Laboratory of Marine Chemistry Theory and Technology, Ministry of Education, Ocean University of China, Qingdao, China
Zhigang Yu, Key Laboratory of Marine Chemistry Theory and Technology, Ministry of Education, Ocean University of China, Qingdao, China