- 1Research Institute for Global Change, Japan Agency for Marine-Earth Science and Technology (JAMSTEC), Yokosuka, Japan
- 2Atmosphere and Ocean Research Institute, The University of Tokyo, Kashiwa, Japan
- 3Institute for Space-Earth Environmental Research, Nagoya University, Nagoya, Japan
- 4School of Marine Science and Technology, Tokai University, Shizuoka, Japan
- 5Research Group for Environmental Sciences, Nuclear Science and Engineering Center, Japan Atomic Energy Agency, Ibaraki, Japan
We studied seasonal variations of the mesozooplankton swimmer community collected by a sediment trap moored at 873 m in the Kuroshio–Oyashio Transition region off the east coast of Japan from 5 August 2011 to 23 June 2013, with sampling bottles rotating at 26-day intervals. The total flux of mesozooplankton swimmers varied between 0 and 11.1 individuals m–2 d–1, with a mean of 3.1 individuals m–2 d–1 during the sampling period. In total, 89 taxa were found in the trap material, of which copepods comprised 87.1% of all swimmers on average. Among the Copepoda, Neocalanus cristatus was the most dominant taxon (76.2% of copepods on average during the sampling period), and all of them were stage C5 copepodite to adult. The species composition of the swimmers closely reflected the mesopelagic mesozooplankton of the Oyashio region. Because all N. cristatus observed in the trap were stage C5 to adult, its flux represents a time series of variations in life history and response to environmental changes at the depth of the sediment trap. The fluxes of Neocalanus species (N. cristatus, N. flemingeri, and N. plumchrus) reflected ontogenetic vertical migration, but may have been overestimates of active fluxes if they included dormant individuals that accidentally entered the sediment trap. The apparent active carbon flux of Neocalanus species ranged from 0 to 22.3 mg C m–2 d–1 during the sampling period, with a mean value of 4.9 mg C m–2 d–1.
Introduction
In the western North Pacific Ocean, the sea around Japan is roughly divided into north and south by the Kuroshio Front (around 35°N in the sea around Japan), which is caused by the Kuroshio Extension, a current that continues eastward from the Kuroshio Current south of the Japanese Islands (Figure 1). In addition, there is the subarctic front around 40°N at 50–200 m depth, which is caused by subarctic circulation represented by the Oyashio Current (Kawai, 1972). The subtropical region is south of the Kuroshio Front, and the subarctic region is north of the subarctic front (Kawai, 1972). The area between the Kuroshio Front and the Subarctic Front is called the Kuroshio–Oyashio Transition region, where subtropical and subarctic waters mix. The cold, dense Oyashio Current moving southward reaches the Japanese coast in spring and early summer, submerges into the transition region at 300–500 m depth, and forms North Pacific Intermediate Water (Yasuda et al., 1996; Yasuda, 1997; Yasuda, 2003). The North Pacific Intermediate Water circulates in the ocean interior along the Subtropical Gyre and extends to depths of 300–800 m in the mid-latitudes of the North Pacific (Reid, 1965; Talley, 1993).
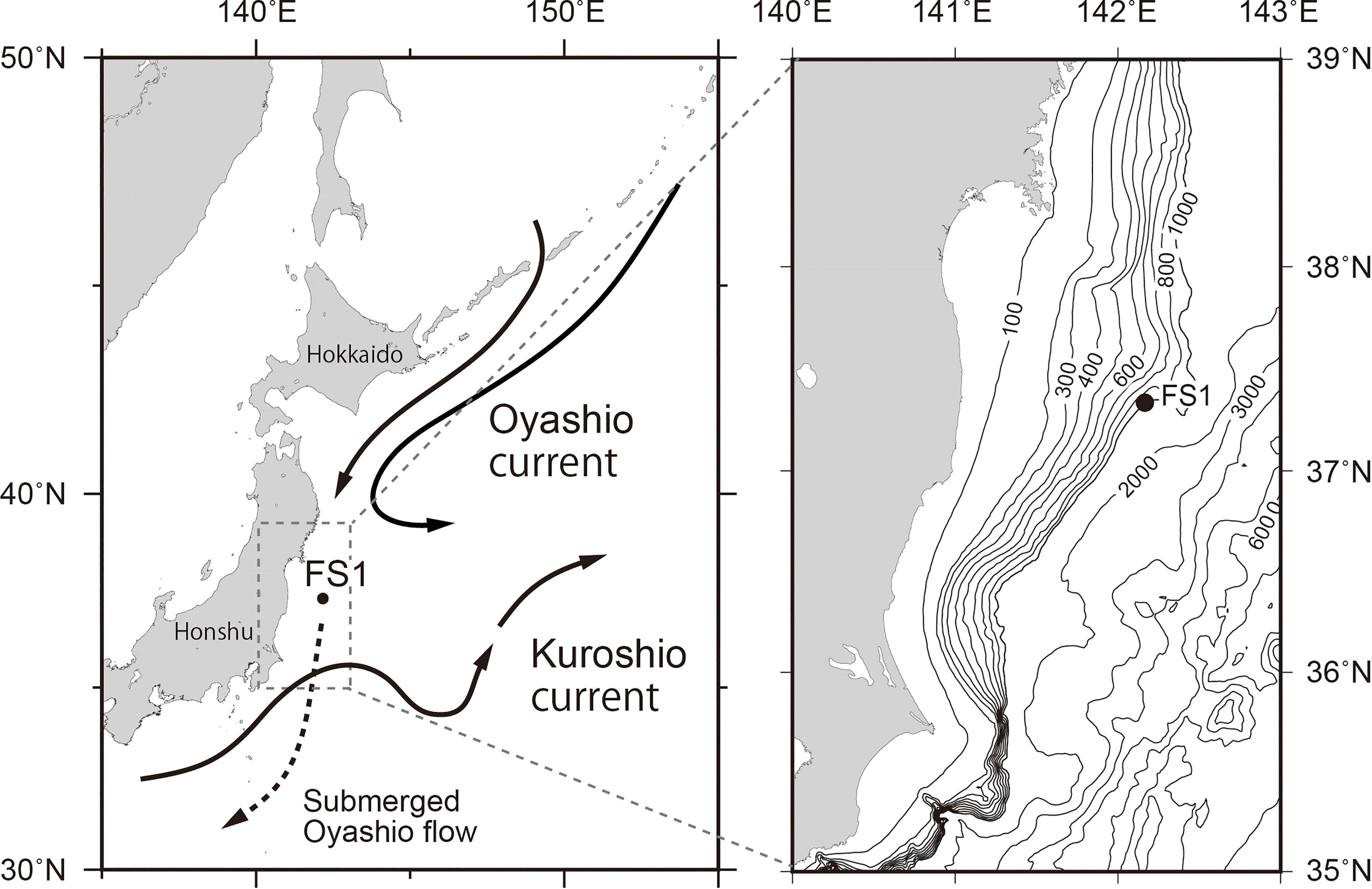
Figure 1 Map of the study area. The solid circles represent the location of the sediment-trap mooring at station FS1. The solid arrows represent surface currents. The dotted arrow represents submerged flow.
The Kuroshio–Oyashio Transition region is an important foraging area for many pelagic fishes and is important for fishery production, because many species visit the area from early summer to autumn (Noto and Yasuda, 1999; Tian et al., 2004). Fluctuations in mesozooplankton abundance and catches of Pacific saury are suggested to be synchronized in the transition region (Odate, 1994; Sugisaki and Kurita, 2004). Thus, it is important to monitor the time-series variation of mesozooplankton, the food of small pelagic fish, in the transition region. In addition, mesozooplankton can play a crucial role in vertical material export within the pelagic food web via ontogenetic vertical migration (active flux) and sinking carcasses and fecal pellets (passive flux) (Steinberg et al., 2001; Kobari et al., 2003). Among these roles, the contribution of ontogenetic vertical migration of mesozooplankton to carbon sequestration in the deep ocean is thought to be important (Boyd et al., 2019). However, it is difficult to observe vertical distributions of mesozooplankton, even at one-month intervals, by water sampling and/or net collection from ships, and studies showing time-series variations in marine ecosystem structure at a monthly scale are rare (Tsuda et al., 2004; Shoden et al., 2005).
The analysis of swimmers in samples collected by time-series sediment traps deployed in the shallow layer (<250-m depth), where mesozooplankton abundance is high and swimmers are collected in large numbers, has received much attention, even in recent years (Willis et al., 2008; Makabe et al., 2016; Tokuhiro et al., 2019). Swimmers are living mesozooplankton that are thought to actively enter sediment-trap collection bottles and augment the trap contents, and they have been traditionally separated from the sinking particles collected in most sediment-trap studies (Steinberg et al., 1998; Ikenoue et al., 2019). Swimmers in sediment-trap samples can be used to analyze seasonal variability of mesozooplankton at continuous intervals over time, albeit qualitatively, at fixed depths in inaccessible ocean (or pelagic) areas (Makabe et al., 2010; Matsuno et al., 2014). However, few studies have analyzed the time-series variability of swimmers in the open ocean of the western North Pacific (Ohashi et al., 2011; Yokoi et al., 2018; Amei et al., 2020), and this is the first to analyze those in the hemipelagic waters around Japan.
In this study, we used time-series sediment traps moored in the Kuroshio–Oyashio Transition region to reveal the temporal variation of mesozooplankton swimmers in the samples. We focused on Neocalanus cristatus, a large copepod that is outstanding in terms of both population and biomass among mesozooplankton in the subarctic region. We also examined the usefulness of swimmers collected in deep traps for ecological and biogeochemical studies and estimated the amount of carbon export by ontogenetic vertical migration of Neocalanus species (N. cristatus, N. flemingeri, and N. plumchrus).
Materials and Methods
Field Sampling
A bottom-tethered time-series sediment-trap mooring was deployed at station FS1 in the Kuroshio–Oyashio Transition region (37°20.1′N, 142°10.0′E; seafloor depth, 992 m) (Figure 1). The sediment trap (SMD6000-13W, NiGK; aperture area, 0.5 m2) was installed at 873-m depth (119 m above the bottom) and collected samples with sampling bottles that rotated at consecutive intervals of 26 d from 5 August 2011 to 23 June 2013. Because of replacement of the mooring array, sampling was interrupted from 8–20 July 2012. The sampling bottles of the sediment trap were filled with a 5% formalin neutral buffer solution with salinity controlled at 38‰ to prevent biological degradation of collected material. The sea surface temperature (SST) around station FS1 from National Oceanic and Atmospheric Administration (NOAA) local area coverage data (multi-channel sea surface temperature, MCSST) was obtained from the Agriculture, Forestry and Fisheries Research Information Center, Tsukuba, Japan (http://rms1.agsearch.agropedia.affrc.go.jp/sidab/index-ja.html. Accessed 10 April 2014). The sea surface chlorophyll-a concentration data were obtained from MODIS-Aqua from the National Aeronautics and Space Administration (NASA) Ocean Color website (https://oceancolor.gsfc.nasa.gov. Accessed 17 Feburuary 2021), and the daily averaged values of chlorophyll-a concentration for an area 27 km square centered at station FS1 were calculated. The winter monsoon index was obtained from the Japan Meteorological Agency website (https://www.data.jma.go.jp/kaiyou/data/db/obs/knowledge/stmw/moi.txt. Accessed 22 January 2022).
Analysis of Mesozooplankton Swimmers
After recovery of the sediment trap, mesozooplankton swimmers were collected with tweezers from each sediment-trap sample. Identification and enumeration of mesozooplankton swimmers were made under a dissecting microscope. In this study, swimming mesozooplankton with no physical defects due to decay or predation were defined as swimmers according to Ikenoue et al. (2019). Identification of mesozooplankton swimmers mainly followed Chihara and Murano (1997). Species identification of copepods mainly followed Brodsky (1967) and Miller (1988) for Neocalanus spp. The mesozooplankton swimmers were divided into six groups by habitat according to Chihara and Murano (1997): Oyashio species (cold water), Kuroshio–Oyashio Transition species, Kuroshio species (warm water), mesopelagic species, benthic species, and others. For species with high biomass, such as Neocalanus and Eucalanus species, we counted them by copepodite stage. For other species, such as Gaetanus species, it was difficult to identify each copepodite stage, so stages C1 to C5 were collectively categorized as C. Adult male, and female copepods were represented as M and F, respectively. Adult females of Neocalanus cristatus, the most dominant copepod species, were discriminated into two stages according to the criteria of Miller et al. (1984): Fnm (newly mature) and Fspw (spawning). The stage 5 copepodite of N. cristatus contains a large oil sac in the prosome. To evaluate the seasonal variation in lipid storage of the C5 stage, the oil sac length relative to prosome length (PL) was scored into three groups: C5a (oil sac length less than 4% of PL), C5b (4–40% of PL), and C5c (>40% of PL).
The ontogenetic vertical migration observed in copepods is expressed as active flux, whereas the total flux of swimmers is expressed as flux, since it is the total amount of mesozooplankton collected in the trap because of various mesozooplankton behaviors near the mooring depth of the sediment-trap. The flux of mesozooplankton swimmers (no. of individuals m–2 d–1) was calculated from the counted number of swimmers, the aperture area of the sediment trap (0.5 m2), and the sampling interval (days). The flux of Neocalanus species is expressed as the apparent active flux, taking into account the possible overestimation due to factors detailed in the discussion section below. The apparent active carbon flux of Neocalanus species (mg C m–2 d–1) was calculated based on their dry weight according to the growth stage (stage C5 to adult), the ratio of carbon to dry weight (45.4%) (Kobari et al., 2003), and the apparent active flux of Neocalanus species observed in this study.
Chemical Analysis of Sinking Particles
After the collection of “swimmers” described above, sediment-trap samples were collected on a pre-weighed 0.6-μm pore size membrane filter (Millipore Co.). Samples were rinsed with deionized water (>18.2 MΩ) during the filtration procedures and were dried under a vacuum at 60°C. After the dried samples were weighed to calculate the total mass flux, the sinking particles were crushed and powdered. Aliquots of powdered samples were used for chemical analysis. The powdered samples were used to analyze the bulk components (lithogenic matter, biogenic carbonate, biogenic opal, organic matter). Concentrations of lithogenic matter and biogenic carbonate were calculated from Al and Ca concentrations measured by ICP atomic emission spectrometry. Concentrations of biogenic opal were analyzed using a modified alkaline extraction method. The loss of ignition method was used to determine the organic-matter content. Details of the chemical analysis methods and bulk component data can be found in Otosaka et al. (2014). For analysis of organic carbon content, a portion of a dried sample was fumigated with concentrated hydrochloric acid for about 5 h and then placed in a vacuum desiccator for 24 h, followed by measurement of carbon content using an elemental analyzer (vario PYRO cube, Elementar, Germany).
Results
Hydrography
The SST at station FS1 exceeded 20°C from June to November in summer and autumn and decreased below 10°C in February and March in winter (Figure 2A). The sea surface chlorophyll-a concentration around station FS1 began to rise after the decrease of SST in winter, and peaks above 10 mg m–3 were observed at the end of April in 2012 and at the end of March in 2013 (Figure 2B). The winter monsoon index in 2012 (24.4) was higher than that in 2013 (21.7). The total mass flux of sinking particles was higher than 500 mg-dry m–2 d–1 during January–May 2012 and January–April 2013, and there were two maxima during each of these periods (Figure 2C). Similar seasonal changes were observed for biogenic-opal flux and organic-matter flux. Detailed results of the chemical components of sinking particles have been described by Otosaka et al. (2014).
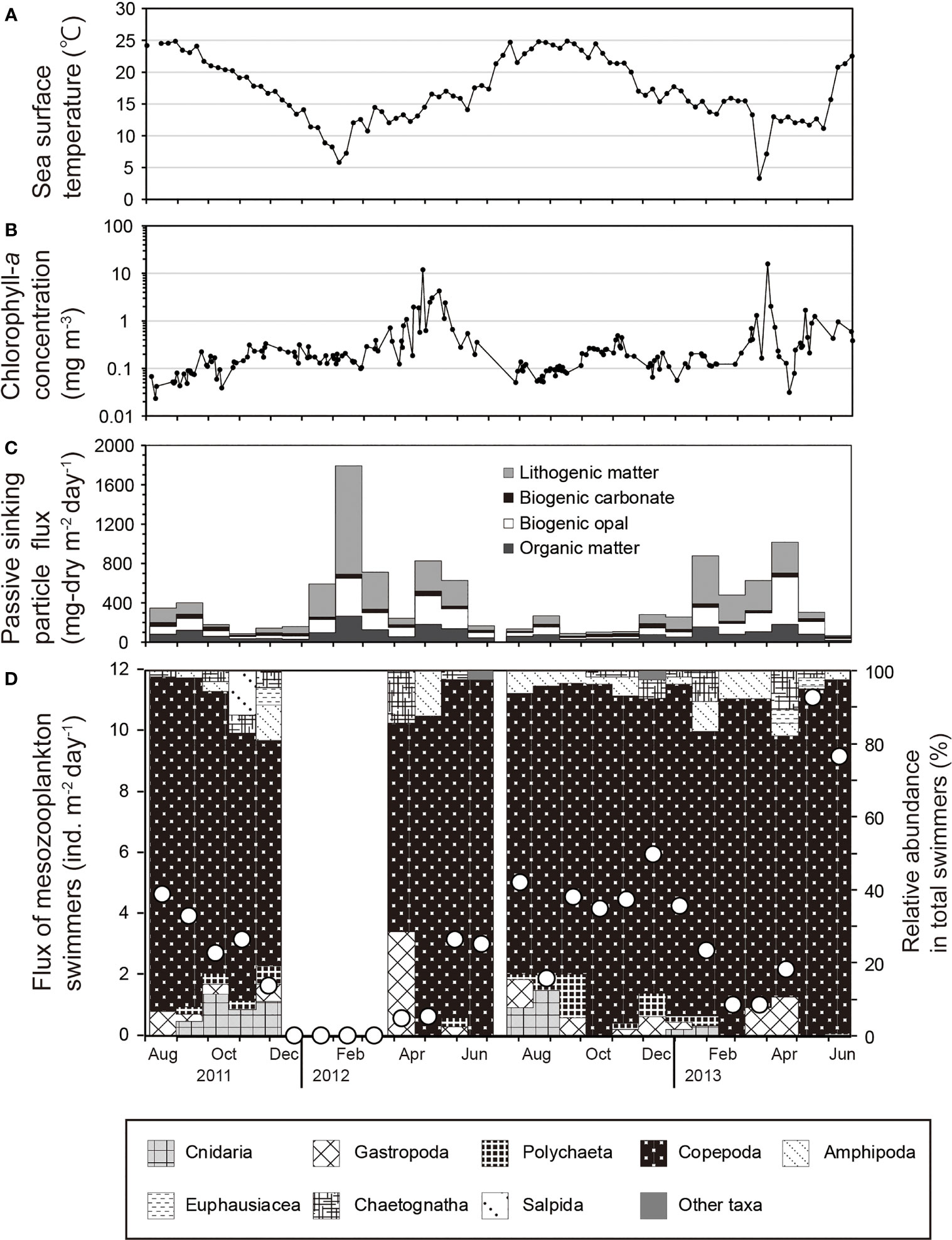
Figure 2 Seasonal variations at station FS1 of (A) sea surface temperature, (B) average surface chlorophyll-a concentration for a 27-km-square area centered at the station, (C) passive sinking particle total mass flux and flux of main components, and (D) flux of zooplankton swimmers. The line with open circles in (D) indicates the total flux of zooplankton swimmers, and the fill patterns indicate the percentages of the flux by taxon.
Mesozooplankton Swimmer Community
Eighty-nine taxa of swimmers were collected in the sediment trap during the sampling period. The total flux of mesozooplankton swimmers varied between 0 and 11.1 ind. m–2 d–1, with a mean value of 3.1 ind. m–2 d–1 during the sampling period (Figure 2D). The flux was higher than the mean value during August–November 2011, May 2012–January 2013, and May–June 2013, with minimum values observed in December 2011–March 2012 and February–March 2013 during winter. For December 2011–March 2012, there is no evidence of problems collecting sinking particles, and the fact that swimmers were not collected at all during this period is probably neither a sampling error nor an artificial error.
Among the higher order taxa, Copepoda was the most dominant taxon (87.1% of all swimmers on average during the sampling period), followed by Gastropoda (2.7%) and Euphausiacea (2.6%) (Figure 2D). In terms of zooplankton habitat, on average, cold water species belonging to the Oyashio Current accounted for 72% of total mesozooplankton swimmers during the sampling period, mesopelagic species for 9.6%, warm water species belonging to the Kuroshio Current for 6.1%, transition region species for 0.5%, benthic species for 0.4%, and others for 11.4% (Figure 3).
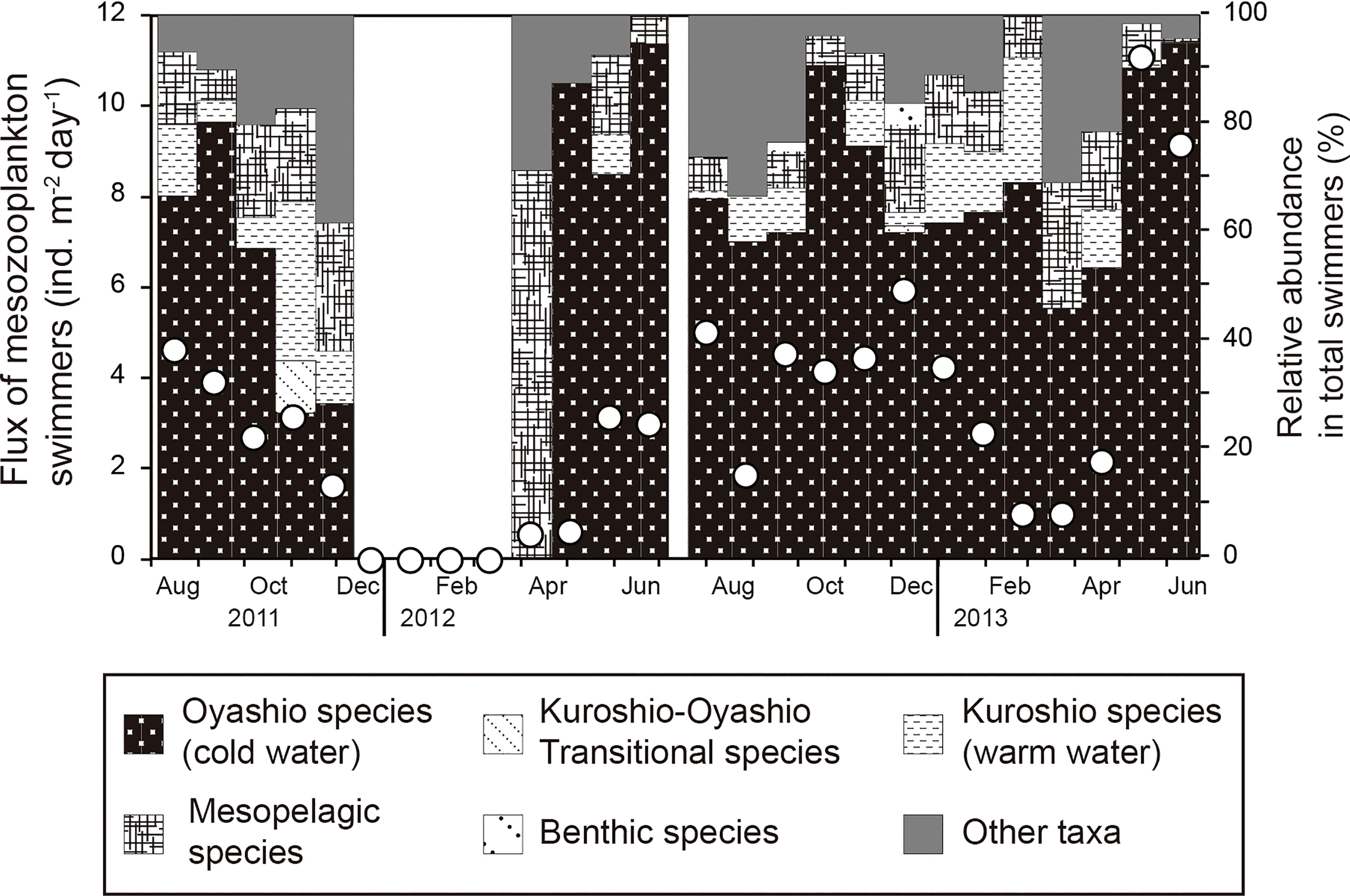
Figure 3 Seasonal variations in the percentage composition by habitat group of zooplankton swimmers collected in a moored sediment trap at station FS1. The open circles indicate the total flux of zooplankton swimmers.
Copepod Community
Among the Copepoda, Neocalanus cristatus was the most dominant taxon (76.2% in the abundance of Copepoda on average during the sampling period), followed by Rhincalanus nasutus (4.6%), Neocalanus plumchrus (2.4%), and Eucalanus bungii (2.1%) (Supplementary Table S1). Most of the Copepoda occurred as stage C5 copepodite to adult. The flux of N. cristatus varied between 0 and 9.6 ind. m–2 d–1, with a mean value of 2.1 ind. m–2 d–1 during the sampling period (Figure 4). Their flux was higher than 2.0 ind. m–2 d–1 during August–September 2011, May–January 2012, and May–June 2013, with minimum values observed in winter. Neocalanus cristatus occurred as stage C5 copepodite to adult; stage C5c appeared from April to August, C5b throughout the year, and C5a from May to February. The adult M stage appeared mainly from May to August, Fspw mainly from August to December, and Fnm from March to April. The flux of R. nasutus showed no clear seasonality; fluxes of N. plumchrus and E. bungii showed generally similar seasonal changes as N. cristatus, but fluxes of E. bungii showed little increase during May–June 2013 (Supplementary Table S1).
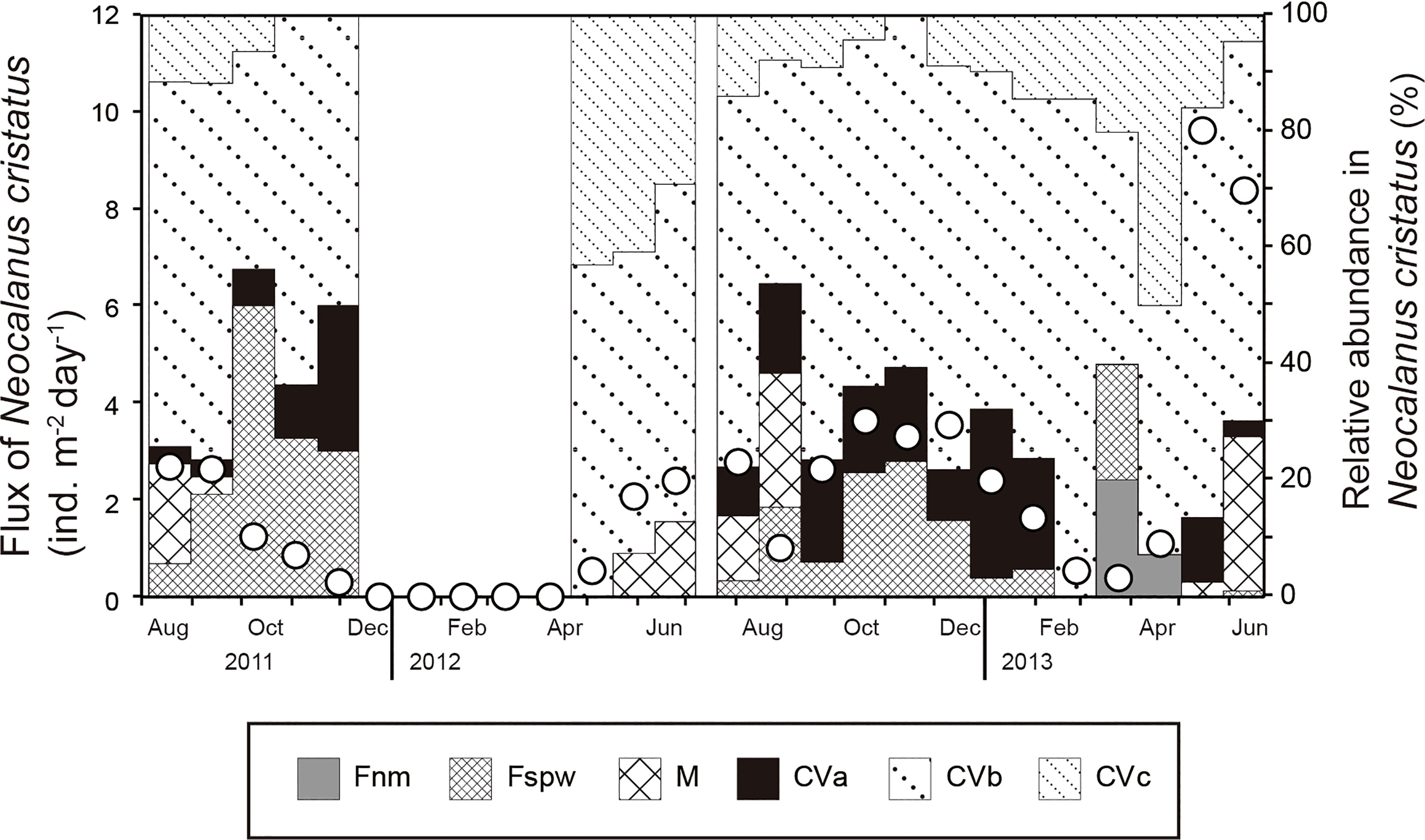
Figure 4 Seasonal variations in the flux and copepodite-stage composition of Neocalanus cristatus collected in a moored sediment trap at station FS1. The open circles indicate the total flux of N. cristatus. Fnm, female (newly mature); Fspw, female (spawning); M, male (adult); CVa, copepodite stage C5a (oil sac length less than 4% of prosome length [PL]); CVb, (4–40% of PL); CVc (>40% of PL).
Apparent Active Carbon Flux of Neocalanus Species
The apparent active carbon flux of Neocalanus species at station FS1 ranged from 0 to 22.3 mg C m–2 d–1 during the sampling period, with a mean value of 4.9 mg C m–2 d–1. Organic carbon fluxes by passive sinking particles at station FS1 varied from 4.5 to 61.1 mg C m–2 d–1, with a mean value of 20.3 mg C m–2 d–1 (Supplementary Table S2, Figure 5). The apparent active carbon flux of Neocalanus species was comparable to the passive organic carbon flux during ontogenetic vertical migration in spring and early summer, and in autumn when the proportion of adults increased, but the passive carbon flux was higher most of the time. Thus, the mean value of the apparent active carbon flux of Neocalanus species at station FS1 is 24% that of organic carbon flux by sinking particles.
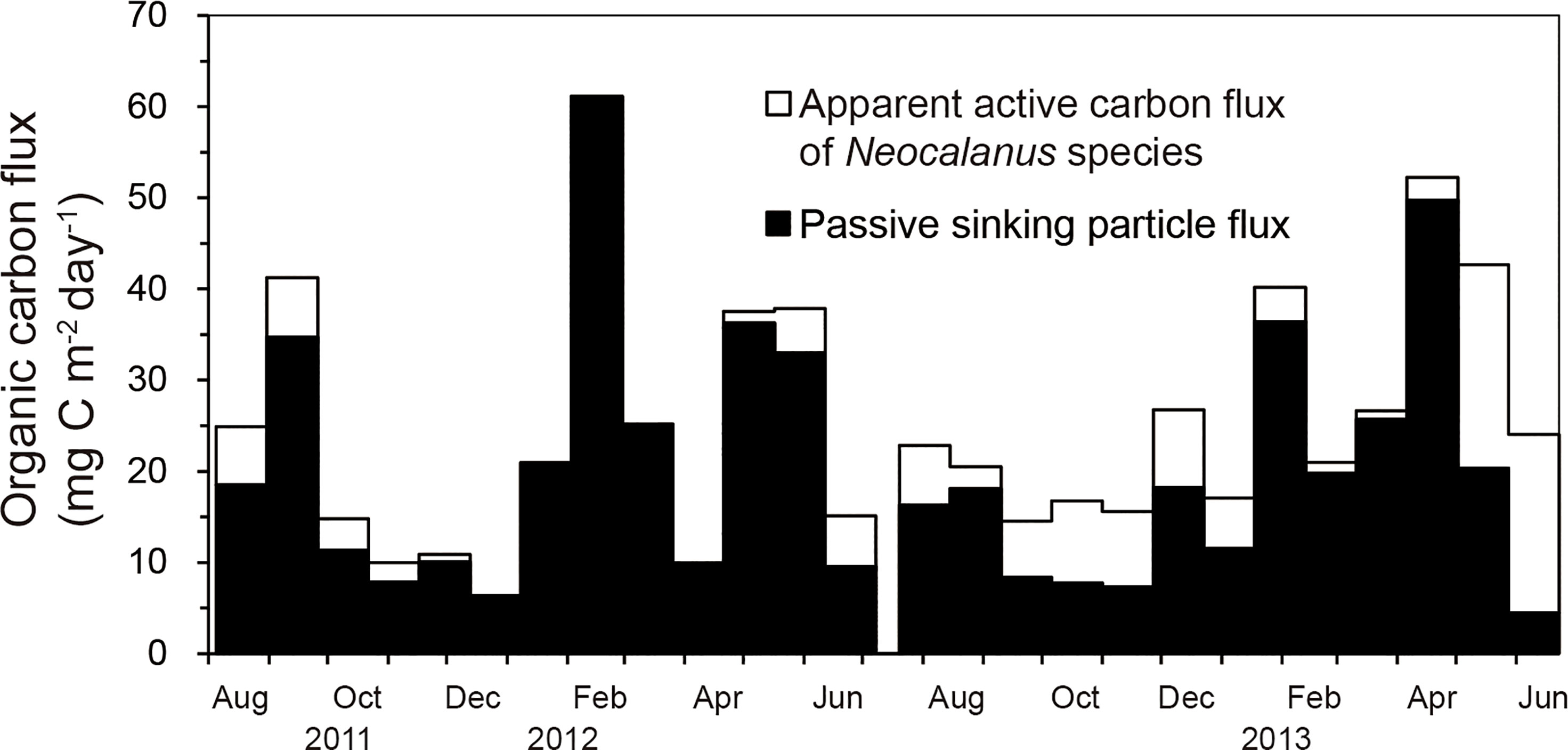
Figure 5 Seasonal variations of apparent active carbon flux of Neocalanus species and passive organic carbon flux by sinking particles at station FS1.
Discussion
Swimmers Collected in Deep Trap
On average, over 80% of the swimmers collected by the sediment trap at station FS1 were Oyashio species and mesopelagic species (Figure 3), indicating that the Oyashio water mass is more dominant than the Kuroshio at station FS1. The absence of any coastal species indicates that the zooplankton of this area are pelagic in nature (Figure 3). Although the Oyashio is a surface current, most of the Oyashio species observed in this study were Neocalanus species and Eucalanus bungii (Supplementary Table S1), which are known to be species that undergo ontogenetic vertical migration, descent from the surface to the mesopelagic layer (>200 m depth) for overwintering (Miller et al., 1984; Tsuda et al., 1999; Tsuda et al., 2001a; Tsuda et al., 2004). The Neocalanus species collected were all stage C5 to adult and are life history stages that inhabit the mesopelagic layer and never return to the surface after descent to the mesopelagic layer. Although E. bungii is also one of the major species in the Oyashio region (Tsuda et al., 2004), it was rarely collected in the sediment trap at station FS1, probably because of its life cycle, in which it returns to the surface to spawn and its preferred overwintering depth, which is shallower than the trap mooring depth. N. flemingeri and E. bungii prefer to overwinter at depths of 300–500 m due to ontogenetic vertical migration, whereas N. cristatus and N. plumchrus prefer to overwinter at depths greater than 500 m (Shimizu et al., 2009). Therefore, the smaller percentage of N. flemingeri and E. bungii in the flux in this study compared to N. cristatus and N. plumchrus is probably because their ontogenetic vertical migrations rarely reached the depth of the sediment trap. Rhincalanus nasutus, also rarely collected in the sediment trap, is a relatively common species in the Kuroshio Current and is known to be a species showing ontogenetic vertical migration, with adults inhabiting the mesopelagic depths (Shimode et al., 2012a). Other than R. nasutus, four Kuroshio species, Eucalanus californicus, Eucalanus hyalinus, Pleuromamma xiphias, and Paraeuchaeta elongate, were observed very rarely in the sediment trap (Supplementary Table S1). Of these, E. californicus and P. elongate have been reported to undergo ontogenetic vertical migration in their life cycles (Shimode et al., 2012b; Yamaguchi et al., 2019), but for E. hyalinus and P. xiphias, it is unknown so far whether they undergo ontogenetic vertical migration. Considering the mooring depth of the trap, it is possible that these four species also invaded the sediment trap by ontogenetic vertical migration.
It is noteworthy that the number of swimmers and the sinking particle flux showed opposite seasonal variation, which means that the change in the number of swimmers collected in the trap is not primarily a result of seasonal changes in shallow zooplankton abundance (which, like changes in sinking particle flux, depends in general on primary and secondary production in the surface layer), but rather a result of ontogenetic vertical migration. These facts suggest that the species composition of the mesozooplankton swimmers collected in the trap reflects variations in the species composition of mesozooplankton at the mooring depth of the trap. The fact that no swimmers were observed from December 2011 to March 2012, while fewer were observed during the same period in 2013, could be due to changes in hydrography, such as currents in the upper layer of the trap mooring depth, but the factors could not be clearly identified.
Seasonal Variation in Flux of Neocalanus cristatus
In the Oyashio (subarctic) region, water temperature, nutrients, phytoplankton density, and zooplankton density show large seasonal variations with the spring bloom (Saito et al., 2002). Increases in fluxes of organic matter and biogenic opal were observed at station FS1 from January to March and from April to June, suggesting production by siliceous-shelled plankton (e.g., diatoms, silicoflagellates, radiolaria and phaeodaria) and an associated increase in biogenic sinking particles during this period. The winter peak in biogenic sinking particles would be due to contributions from siliceous Rhizaria (radiolaria and phaeodaria) rather than silicious phytoplankton (e.g., diatoms or silicoflagellates) because it does not coincide with the increase in chlorophyll-a concentration (Figures 2B, C). On the other hand, the spring peak would be mainly derived from silicious phytoplankton because it coincides with the increase in chlorophyll-a concentration. The spring increase in chlorophyll-a concentration and sinking particles at station FS1 coincides with the general peak of chlorophyll-a concentrations in the Oyashio region (April–May; Saito et al., 2002).
The peak of the lithogenic-matter flux in February 2012 was about 2.3 times that in 2013 and is possibly weather-related. The winter monsoon index is expressed as the pressure difference between Irkutsk (east-central Russia) and Nemuro (Hokkaido, Japan); the stronger the index, the stronger the monsoon and the deeper the mixed layer (Hanawa et al., 1988). The winter monsoon index in 2012 was higher than that in 2013, so the enhanced 2012 monsoon and deeper mixed layer would have caused an increase in lithogenic-matter flux.
Neocalanus species have a lifespan of one or two years, growing in the surface layer during winter to early summer and migrating from the surface layer to the mesopelagic layer as they grow (Miller et al., 1984). Among Neocalanus species, N. cristatus ascends to the surface as stage C1 for feeding in January and migrates to the deeper layers as stage C5 in July–August in the Oyashio region (Tsuda et al., 2004). The period when the N. cristatus flux reaches a minimum coincides with the peak fluxes of organic matter and biogenic opal (Figures 2, 4). This means that N. cristatus was not collected in the deep sediment-trap at station FS1 during this period because it inhabits the surface layer as a copepodite in winter as in the Oyashio region (Tsuda et al., 2004). In addition, the following two observations point to the beginning of ontogenetic vertical migration in April–May: 1) the peak N. cristatus flux was observed in May 2013, and 2) a high percentage of this flux consisted first of stage C5c containing much oil, followed by an increase in stage C5a without oil, and spawning Fspw. It is unclear how spawning Fspws reached the sediment trap, but they might be precocious individuals. On the other hand, the appearance of stage C5c in the autumn of 2012 and the slight appearance of Fnm during the winter flux minimum may be related to the delayed ontogenetic vertical migration of individuals carried by the submerged Oyashio Current (August–December), which will be discussed in the next section.
The flux of stage C5 to adult N. cristatus began to increase in May–June, about two months earlier than its vertical migration in the Oyashio region (Figure 4). In the eastern North Pacific, N. plumchrus is known to grow faster in coastal waters where sea surface temperatures are higher than in the open ocean, and the timing of ontogenetic vertical migration near the coast is accelerated (Goldblatt et al., 1999; Mackas et al., 2007). In the Oyashio region, SST is below 5°C during winter (Saito et al., 2002), but at station FS1 in the transition region, SST rarely drops below 10°C, even in winter, and is warmer than in the Oyashio region (Figure 2A). Therefore, the earlier onset of ontogenetic vertical migration of N. cristatus in this study than in the Oyashio region may be related to the difference of SST between the two areas.
The earlier vertical migration of N. cristatus may also be related to the increase in winter food availability. Siliceous Rhizaria are reportedly a major component of the vertical flux in the eastern North Pacific along the North American coast (Gutierrez-Rodriguez et al., 2019). Although we did not perform microscopic examination of sinking particles in this study, siliceous Rhizaria may contribute to the increase in opal flux in sinking particles during winter when chlorophyll-a concentrations are low. Therefore, N. cristatus at station FS1, located in the transition region, might have fed not only on phytoplankton, which increases in spring, but also on siliceous Rhizaria and other zooplankton that increase during the same period in winter, resulting in faster growth at the surface than in Oyashio waters.
Previous studies of swimmers collected in mid-depth to deep-sea sediment traps have sometimes described new species by taking advantage of the frequency of collection at depths that are difficult to survey (Ivanenko et al., 2011). However, there are few examples of ecological studies using deep-trap swimmers (e.g., Gislason and Astthorsson, 1992; Kraft et al., 2011) because of the small number of organisms that can be collected compared to plankton net tows. In this study we used swimmer samples collected in the trap to clarify the relationship between flux variations and the life cycle of N. cristatus at the mooring depth of the trap. Therefore, the analysis of surface and mid- to deep-water swimmer samples from sediment traps at multiple depths could be used to study the ecology of species whose life cycles are still unknown.
Carbon Export Through Neocalanus Active Flux
There are several cases whereby swimmers are collected in sediment traps: passively sinking, actively moving downward, following a sinking prey, or falling in a straight line after contacting an obstacle (e.g., Alldredge, 1972; Gilmer, 1974). Therefore, depending on the behavioral pattern of a species, the flux may be underestimated or overestimated, and it is difficult to quantitatively assess the active flux by swimmers. It may however be possible to perform quantitative studies for specific species where the growth stage and life history of the specimens can be confirmed.
The Neocalanus species collected in this study were stage C5 to adult, and they were clearly collected either during the process of ontogenetic vertical migration or as dormant individuals drifting after migration. There are two sources for the Neocalanus populations appearing in the sediment-trap samples: one is the population that migrates southward with the Oyashio Current during surface development and then undergoes ontogenetic vertical migration; the other is the population undergoing ontogenetic vertical migration and dormancy that is transported to the transition region by the submerged Oyashio flow (Kobari et al., 2008). The submerged Oyashio flow in the transition region reaches 400-m depth in November–December (Kobari et al., 2008). Therefore, of these Neocalanus populations, only individuals that were collected through ontogenetic vertical migration and those that were dormant near the sediment-trap depth after migration and were collected incidentally during their residence reached the sediment trap at station FS1.
It is difficult to determine whether individuals were transported by the submerged Oyashio flow or not, but, considering the time of arrival of the flow, those transported by the flow were probably collected in the sediment trap later than those during ontogenetic vertical migration in spring. Because of the residence time of dormant individuals after migration, the flux of dormant individuals per sampling period cannot be regarded as an accurate active flux. Therefore, in this study, assuming that Neocalanus species do not avoid the sediment-trap structure during their migration, the flux of Neocalanus species is expressed as the apparent active flux, taking into account the possible overestimation due to the entry of dormant individuals into the sediment trap.
The mean value of the apparent active carbon flux of Neocalanus species at station FS1 is 42–175% of the range of 2.8–11.7 mg C m–2 d–1 for organic carbon in the sinking particle flux of the North Pacific open ocean at water depths of around 1000 m, as reported by Honda et al. (2002). Note that for convenience, many studies, including that by Honda et al. (2002), have treated the fractions of sediment-trap samples larger than 1 mm as swimmers and fractions smaller than 1 mm as passive sinking particles. Therefore, the values for passive organic carbon fluxes reported by Honda et al. (2002) may be overestimates if the <1-mm fraction included active fluxes. On the other hand, if they do not include passive sinking particles in the >1-mm fraction as reported by Ikenoue et al. (2019), the passive organic carbon fluxes would have been underestimated. The possible active flux in the <1-mm fraction is due to oncaeid copepods. They are widely distributed in the world ocean with an adult body length of 0.2–1.6 mm, and they have a tendency to attach to large sinking particles while swimming in the water column (Alldredge, 1972). At a depth of 1000 m in the western North Pacific, passive sinking particles >1 mm account for an average of 12% of the passive organic carbon fluxes and cannot be ignored (Ikenoue et al., 2019). In this study, we did not use a 1-mm sieve, but rather picked out swimmers from the bulk sediment-trap samples using tweezers, so the passive-sinking-particle fraction at station FS1 includes passive sinking particles >1 mm.
Among the Copepoda collected in this study, the three Neocalanus species, Eucalanus bungii and Rhincalanus nasutus, which undergo ontogenetic vertical migration during their life histories, are all larger than 1 mm in body size from stage C5 to adult (Bradford-Grieve,1994; Tsuda et al., 2001b; Shimode et al., 2012a). There were no copepod species smaller than 1 mm in our swimmer samples. Therefore, we assumed that the active flux in particles <1 mm was negligible at station FS1. However, the method used in this study to pick out only the swimmers with tweezers makes it difficult to divide the passive sinking particles evenly with a rotary splitter (e.g., wet sample divider WSD-10; McLane, East Falmouth, Massachusetts, USA) when the sediment sample includes many passively sinking particles >1 mm. Furthermore, if the <1-mm fraction includes a large number of swimmers, it is difficult to remove them from the bulk sediment-trap sample. Therefore, it would be more efficient to follow the conventional method using a 1-mm stainless-steel sieve together with tweezers to pick out the swimmers. In other words, after sieving, for the <1mm fraction, the residue after removing small swimmers with tweezers should be treated as <1mm passive sinking particles, and for the >1mm fraction, the residue after removing large swimmers with tweezers (e.g., large detrital particles or aggregates) should be treated as >1mm passive sinking particles. Accurate separation of swimmers and passive sinking particles is very important to correctly evaluate passive sinking particle fluxes, and separation methods should continue to be devised according to sample composition and inter-compared with previous studies (Buesseler et al., 2007).The apparent annual active carbon flux of Neocalanus species in the transition region at station FS1 was 1.8 g C m–2 yr–1, which is 36% of the value of 5.0 g C m–2 yr–1 reported by Kobari et al. (2003) for 1000-m depth in the Oyashio region. Because the abundance of Copepoda decreases with increasing distance south of the Oyashio region (Omori, 1967; Omori and Tanaka, 1967), it is reasonable that the apparent active carbon flux of Neocalanus species in this study is lower than that of Kobari et al. (2003) for the Oyashio region. On the other hand, the value of Kobari et al. (2003) may be overestimated because it was calculated assuming that the entire Neocalanus population descended from the surface to 1000-m depth. Therefore, the difference between the two areas may be smaller than that estimated in this study.
Estimating the irreversible carbon sequestration of active fluxes into the deep ocean is important for understanding the biologically mediated ocean carbon cycle (Boyd et al., 2019). In the Oyashio region, 14% of the Neocalanus active flux returns to the surface as eggs in winter and spring (Kobari et al., 2003). Thus, 86% of the Neocalanus active flux is sequestered in the deep ocean. Applying this ratio from the Oyashio region to the present study, we estimate that 1.6 g C m–2 yr–1 of organic carbon would be irreversibly sequestered by the active flux of Neocalanus species around station FS1. If Neocalanus species avoid sediment-trap structures during ontogenetic vertical migration, then this may be an underestimate of the active flux of Neocalanus. It would therefore be necessary to observe Neocalanus species’ behavior using a deep-sea camera and acoustic Doppler current profiler (ADCP) at the mooring depth of the trap to properly evaluate the active flux of Neocalanus using a deep sediment-trap (De Leo et al., 2018).
Concluding Remarks
We investigated the flux variations of N. cristatus at different copepodite stages collected in a deep sediment trap in the Kuroshio–Oyashio Transition region. The flux variations represent a time series of stages during the life history of N. cristatus at the sediment-trap depth and its response to environmental changes. The study of swimmer fluxes collected in sediment traps at multiple depths could provide a qualitative but higher-resolution view of the time series variation of zooplankton with unknown life histories. On the other hand, it is currently difficult to guarantee the accuracy of the estimated swimmer flux. Although we attempted to quantify the active flux of Neocalanus species, we could not distinguish between individuals collected in the sediment trap during ontogenetic vertical migration and those collected by chance during dormancy. Therefore, we may have overestimated the true active flux. To ensure the accurate quantification of the flux of Neocalanus species collected by sediment traps, it is necessary to conduct supplementary surveys on their vertical distribution in the study area using plankton nets, as well as develop methods to observe their behavior near sediment traps using video imagery combined with ADCP backscatter time-series data and to identify dormant individuals.
Data Availability Statement
The original contributions presented in the study are included in the article/Supplementary Material. Further inquiries can be directed to the corresponding author.
Author Contributions
TI and SO conceived the study. TI analyzed the data, wrote the manuscript, and prepared the figures. TI, SO, MH, MK, and YM participated in discussions and contributed to the manuscript. TI conducted analysis of mesozooplankton swimmers. SO conducted chemical analysis of sinking particles. TK and HN planned for data Acquisition. All authors contributed to the article and approved the submitted version.
Funding
This study was supported by a Grant-in-Aid for Scientific Research from the Japan Society for the Promotion of Science (JSPS; 20H04984) to TI.
Conflict of Interest
The authors declare that the research was conducted in the absence of any commercial or financial relationships that could be construed as a potential conflict of interest.
Publisher’s Note
All claims expressed in this article are solely those of the authors and do not necessarily represent those of their affiliated organizations, or those of the publisher, the editors and the reviewers. Any product that may be evaluated in this article, or claim that may be made by its manufacturer, is not guaranteed or endorsed by the publisher.
Acknowledgments
We are grateful to the captains, crews, and scientists aboard R/V Hakuho Maru (cruise KH-11-07), R/V Soyo Maru (SY1203), and R/V Mirai (MR-13-04). We also thank Amane Fujiwara for technical support in obtaining chlorophyll-a concentration data.
Supplementary Material
The Supplementary Material for this article can be found online at: https://www.frontiersin.org/articles/10.3389/fmars.2022.884320/full#supplementary-material
References
Alldredge A. L. (1972). Abandoned Larvacean Houses: A Unique Food Source in the Pelagic Environment. Science 177, 885–887. doi: 10.1126/science.177.4052.885
Amei K., Jimi N., Kitamura M., Yokoi N., Yamaguchi A. (2020). Community Structure and Seasonal Changes in Population Structure of Pelagic Polychaetes Collected by Sediment Traps Moored in the Subarctic and Subtropical Western North Pacific Ocean. Zoosymposia 19, 41–50. doi: 10.11646/zoosymposia.19.1.9
Boyd P. W., Claustre H., Levy M., Siegel D. A., Weber T. (2019). Multi-Faceted Particle Pumps Drive Carbon Sequestration in the Ocean. Nature 568, 327–335. doi: 10.1038/s41586-019-1098-2
Bradford-Grieve J. M. (1994). The Marine Fauna of New Zealand: Pelagic Calanoid Copepoda: Megacalanidae, Calanidae, Paracalanidae, Mecynoceridae, Eucalanidae, Spinocalanidae, Clausocalanidae. N. Z. Oceanogr. Inst. Mem. 102, 1–160.
Brodsky K. A. (1967). Calanoida of the Far Eastern Seas and Polar Basin of the USSR. (in Russian, Translated by Israel Program for Scientific Translations) (Jerusalem: Keter Press).
Buesseler K. O., Antia A. N., Chen M., Fowler S. W., Gardner W. D., Gustafsson O., et al. (2007). An Assessment of the Use of Sediment Traps for Estimating Upper Ocean Particle Fluxes. J. Mar. Res. 65, 345–416. doi: 10.1357/002224007781567621
Chihara M., Murano M. (1997). An Illustrated Guide to Marine Plankton in Japan (Tokyo: Tokai University Press).
De Leo F. C., Ogata B., Sastri A. R., Heesemann M., Mihály S., Galbraith M., et al. (2018). High-Frequency Observations From a Deep-Sea Cabled Observatory Reveal Seasonal Overwintering of Neocalanus Spp. In Barkley Canyon, NE Pacific: Insights Into Particulate Organic Carbon Flux. Prog. Oceanog. 169, 120–137. doi: 10.1016/j.pocean.2018.06.001
Gilmer R. W. (1974). Some Aspects of Feeding in Thecosomatous Pteropod Molluscs. J. Exp. Mar. Bio. Ecol. 15, 127–144. doi: 10.1016/0022-0981(74)90039-2
Gislason A., Astthorsson O. S. (1992). Zooplankton Collected by Sediment Trap Moored in Deep Water South of Iceland. Sarsia 77, 219–224. doi: 10.1080/00364827.1992.10413507
Goldblatt R. H., Mackas D. L., Lewis A. G. (1999). Mesozooplankton Community Characteristics in the NE Subarctic Pacific. Deep Sea Res. Part II Top. Stud. Oceanogr. 46, 2619–2644. doi: 10.1016/S0967-0645(99)00078-8
Gutierrez-Rodriguez A., Stukel M. R., Dos Santos A. L., Biard T., Scharek R., Vaulot D., et al. (2019). High Contribution of Rhizaria (Radiolaria) to Vertical Export in the California Current Ecosystem Revealed by DNA Metabarcoding. ISME J. 13, 964–976. doi: 10.1038/s41396-018-0322-7
Hanawa K., Watanabe T., Iwasaka N., Suga T., Toba Y. (1988). Surface Thermal Conditions in the Western North Pacific During the ENSO Events. J. Meteorol. Soc Japan Ser. II. 66, 445–456. doi: 10.2151/jmsj1965.66.3_445
Honda M. C., Imai K., Nojiri Y., Hoshi F., Sugawara T., Kusakabe M. (2002). The Biological Pump in the Northwestern North Pacific Based on Fluxes and Major Components of Particulate Matter Obtained by Sediment-Trap Experiment–2000). Deep Sea Res. Part II Top. Stud. Oceanogr. 49, 5595–5625. doi: 10.1016/S0967-0645(02)00201-1
Ikenoue T., Kimoto K., Okazaki Y., Sato M., Honda M. C., Takahashi K., et al. (2019). Phaeodaria: An Important Carrier of Particulate Organic Carbon in the Mesopelagic Twilight Zone of the North Pacific Ocean. Glob. Biogeochem. Cycles 33, 1146–1160. doi: 10.1029/2019GB006258
Ivanenko V. N., Defaye D., Segonzac M., Khripounoff A., Sarrazin J., Ferrari F. D. (2011). A New Species of Exrima, Synonymy of Four Species of Aphotopontius, Stygiopontius and Rhogobius, and Record of First Copepodid Stage of Dirivultidae (Copepoda: Siphonostomatoida) From Deep-Sea Hydrothermal Vents of the East Pacific Rise (13 N). J. Mar. Biol. Assoc. U. K. 91, 1547–1559. doi: 10.1017/S0025315411000178
Kawai H. (1972). Hydrography of the Kuroshio and the Oyashio (in Japanese). Physical Oceanography II, Fundamental Lectures of Oceanography (Tokyo: Tokai University Press), 129–320.
Kobari T., Moku M., Takahashi K. (2008). Seasonal Appearance of Expatriated Boreal Copepods in the Oyashio–Kuroshio Mixed Region. ICES J. Mar. Sci. 65, 469–476. doi: 10.1093/icesjms/fsm194
Kobari T., Shinada A., Tsuda A. (2003). Functional Roles of Interzonal Migrating Mesozooplankton in the Western Subarctic Pacific. Prog. Oceanog. 57, 279–298. doi: 10.1016/S0079-6611(03)00102-2
Kraft A., Bauerfeind E., Nöthig E. M. (2011). Amphipod Abundance in Sediment Trap Samples at the Long-Term Observatory HAUSGARTEN (Fram Strait,∼79°N/4°E). Variability in Species Community Patterns. Mar. Biodivers. 41, 353–364. doi: 10.1007/s12526-010-0052-1
Mackas D. L., Batten S., Trudel M. (2007). Effects on Zooplankton of a Warmer Ocean: Recent Evidence From the Northeast Pacific. Prog. Oceanog. 75, 223–252. doi: 10.1016/j.pocean.2007.08.010
Makabe R., Hattori H., Sampei M., Darnis G., Fortier L., Sasaki H. (2016). Can Sediment Trap-Collected Zooplankton be Used for Ecological Studies? Polar Biol. 39, 2335–2346. doi: 10.1007/s00300-016-1900-7
Makabe R., Hattori H., Sampei M., Ota Y., Fukuchi M., Fortier L., et al. (2010). Regional and Seasonal Variability of Zooplankton Collected Using Sediment Traps in the Southeastern Beaufort Sea, Canadian Arctic. Polar Biol. 33, 257–270. doi: 10.1007/s00300-009-0701-7
Matsuno K., Yamaguchi A., Fujiwara A., Onodera J., Watanabe E., Imai I., et al. (2014). Seasonal Changes in Mesozooplankton Swimmers Collected by Sediment Trap Moored at a Single Station on the Northwind Abyssal Plain in the Western Arctic Ocean. J. Plankton Res. 36, 490–502. doi: 10.1093/plankt/fbt092
Miller C. B. (1988). Neocalanus Flemingeri, a New Species of Calanidae (Copepoda: Calanoida) From the Subarctic Pacific Ocean, With a Comparative Redescription of Neocalanus Plumchrus (Marukawa) 1921. Prog. Oceanog. 20, 223–273. doi: 10.1016/0079-6611(88)90042-0
Miller C. B., Frost B. W., Batchelder H. P., Clemons M. J., Conway R. E. (1984). Life History of Large, Grazing Copepods in a Subarctic Ocean Gyre: Neocalanus Plumchrus, N. Cristatus, and Eucalanus Bungii in the Northeast Pacific. Prog. Oceanog. 13, 201–243. doi: 10.1016/0079-6611(84)90009-0
Noto M., Yasuda I. (1999). Population Decline of the Japanese Sardine, Sardinops Melanostictus, in Relation to Sea Surface Temperature in the Kuroshio Extension. Can. J. Fish. Aquat. Sci. 56, 973–983. doi: 10.1139/f99-028
Odate K. (1994). Zooplankton Biomass and its Long-Term Variation in the Western North Pacific Ocean, Tohoku Sea Area, Japan. Bull. Tohoku. Natl. Fish. Res. Inst. 56, 115–173.
Ohashi R., Ishii K., Fujiki T., Kitamura M., Matsumoto K., Honda M. C., et al. (2011). Short-Term Changes in the Planktonic Community of the Western Subarctic Pacific During Early Summer: Analysis of Sediment Trap Samples. Bull. Plankton Soc Jpn. 58, 123–135.
Omori M. (1967). Calanus Cristatus and Submergence of the Oyashio Water. Deep-Sea Res. 14A, 525–532. doi: 10.1016/0011-7471(67)90062-9
Omori M., Tanaka O. (1967). Distribution of Some Cold-Water Species of Copepods in the Pacific Water Off East-Central Honshu, Japan. J. Oceanogr. Soc Japan 23, 63–73. doi: 10.5928/kaiyou1942.23.63
Otosaka S., Nakanishi T., Suzuki T., Satoh Y., Narita H. (2014). Vertical and Lateral Transport of Particulate Radiocesium Off Fukushima. Environ. Sci. Technol. 48, 12595–12602. doi: 10.1021/es503736d
Reid J. L. Jr (1965). “Intermediate Waters of the Pacific Ocean,” in Johns Hopkins Oceanogr. Stud., 2, Baltimore: Johns Hopkins press. 85pp.
Saito H., Tsuda A., Kasai H. (2002). Nutrient and Plankton Dynamics in the Oyashio Region of the Western Subarctic Pacific Ocean. Deep Sea Res. Part II Top. Stud. Oceanogr. 49, 5463–5486. doi: 10.1016/S0967-0645(02)00204-7
Shimizu Y., Takahashi K., ITO S. I., Kakehi S., Tatebe H., Yasuda I., et al. (2009). Transport of Subarctic Large Copepods From the Oyashio Area to the Mixed Water Region by the Coastal Oyashio Intrusion. Fish. Oceanogr. 18, 312–327. doi: 10.1111/j.1365-2419.2009.00513.x
Shimode S., Takahashi K., Shimizu Y., Nonomura T., Tsuda A. (2012a). Distribution and Life History of Two Planktonic Copepods, Rhincalanus Nasutus and Rhincalanus Rostrifrons, in the Northwestern Pacific Ocean. Deep Sea Res. Part I Oceanogr. Res. Pap. 65, 133–145. doi: 10.1016/j.dsr.2012.03.008
Shimode S., Takahashi K., Shimizu Y., Nonomura T., Tsuda A. (2012b). Distribution and Life History of the Planktonic Copepod, Eucalanus Californicus, in the Northwestern Pacific: Mechanisms for Population Maintenance Within a High Primary Production Area. Prog. Oceanog. 96, 1–13. doi: 10.1016/j.pocean.2011.08.002
Shoden S., Ikeda T., Yamaguchi A. (2005). Vertical Distribution, Population Structure and Lifecycle of Eucalanus Bungii (Copepoda: Calanoida) in the Oyashio Region, With Notes on its Regional Variations. Mar. Biol. 146, 497–511. doi: 10.1007/s00227-004-1450-3
Steinberg D. K., Carlson C. A., Bates N. R., Johnson R. J., Michaels A. F., Knap A. H. (2001). Overview of the US JGOFS Bermuda Atlantic Time-Series Study (BATS): A Decade-Scale Look at Ocean Biology and Biogeochemistry. Deep Sea Res. Part II Top. Stud. Oceanogr. 48, 1405–1447. doi: 10.1016/S0967-0645(00)00148-X
Steinberg D. K., Pilskaln C. H., Silver M. W. (1998). Contribution of Zooplankton Associated With Detritus to Sediment Trap ‘Swimmer’ Carbon in Monterey Bay, California, USA. Mar. Ecol. Prog. Ser. 164, 157–166. doi: 10.3354/meps164157
Sugisaki H., Kurita Y. (2004). Daily Rhythm and Seasonal Variation of Feeding Habit of Pacific Saury (Cololabis Saira) in Relation to Their Migration and Oceanographic Conditions Off Japan. Fish. Oceanogr. 13, 63–73. doi: 10.1111/j.1365-2419.2004.00310.x
Talley L. D. (1993). Distribution and Formation of North Pacific Intermediate Water. J. Phys. Oceanogr. 23, 517–517. doi: 10.1175/1520-0485(1993)023<0517:DAFONP>2.0.CO;2
Tian Y., Akamine T., Suda M. (2004). Modeling the Influence of Oceanic-Climatic Changes on the Dynamics of Pacific Saury in the Northwestern Pacific Using a Life Cycle Model. Fish. Oceanogr. 13 (sup.1), 125–137. doi: 10.1111/j.1365-2419.2004.00314.x
Tokuhiro K., Abe Y., Matsuno K., Onodera J., Fujiwara A., Harad N., et al. (2019). Seasonal Phenology of Four Dominant Copepods in the Pacific Sector of the Arctic Ocean: Insights From Statistical Analyses of Sediment Trap Data. Polar Sci. 19, 94–111. doi: 10.1016/j.polar.2018.08.006
Tsuda A., Saito H., Kasai H. (1999). Life Histories of Neocalanus Flemingeri and Neocalanus Plumchrus (Calanoida: Copepoda) in the Western Subarctic Pacific. Mar. Biol. 135, 533–544. doi: 10.1007/s002270050654
Tsuda A., Saito H., Kasai H. (2001a). Life History Strategies of Subarctic Copepods Neocalanus Flemingeri and N. Plumchrus, Especially Concerning Lipid. Plankton Biol. Ecol. 48, 52–58.
Tsuda A., Saito H., Kasai H. (2001b). Geographical Variation of Body Size of Neocalanus Cristatus, N. Plumchrus and N. Flemingeri in the Subarctic Pacific and Its Marginal Seas: Implications for the Origin of Large Form of N. Flemingeri in the Oyashio Area. J. Oceanogr. 57, 341–352. doi: 10.1023/A:1012490730792
Tsuda A., Saito H., Kasai H. (2004). Life Histories of Eucalanus Bungii and Neocalanus Cristatus (Copepoda: Calanoida) in the Western Subarctic Pacific Ocean. Fish. Oceanogr. 13, 10–20. doi: 10.1111/j.1365-2419.2004.00315.x
Willis K. J., Cottier F. R., Kwaśniewski S. (2008). Impact of Warm Water Advection on the Winter Zooplankton Community in an Arctic Fjord. Polar Biol. 31, 475–481. doi: 10.1007/s00300-007-0373-0
Yamaguchi A., Ashjian C. J., Campbell R. G., Abe Y. (2019). Ontogenetic Vertical Migration of the Mesopelagic Carnivorous Copepod Paraeuchaeta Spp. Is Related to Their Increase in Body Mass. J. Plankton Res. 41, 791–797. doi: 10.1093/plankt/fbz051
Yasuda I. (1997). The Origin of the North Pacific Intermediate Water. J. Geophys. Res. 102, 893–909. doi: 10.1029/96JC02938
Yasuda I. (2003). Hydrographic Structure and Variability in the Kuroshio-Oyashio Transition Area. J. Oceanogr. 59, 389–402. doi: 10.1023/A:1025580313836
Yasuda I., Okuda K., Shimizu Y. (1996). Distribution and Modification of North Pacific Intermediate Water in the Kuroshio-Oyashio Interfrontal Zone. J. Phys. Oceanogr. 26, 448–465. doi: 10.1175/1520-0485(1996)026<0448:DAMONP>2.0.CO;2
Keywords: swimmer, Copepoda, ontogenetic vertical migration, sediment trap, Kuroshio–Oyashio transition region
Citation: Ikenoue T, Otosaka S, Honda MC, Kitamura M, Mino Y, Narita H and Kobayashi T (2022) Neocalanus cristatus (Copepoda) From a Deep Sediment-Trap: Abundance and Implications for Ecological and Biogeochemical Studies. Front. Mar. Sci. 9:884320. doi: 10.3389/fmars.2022.884320
Received: 26 February 2022; Accepted: 27 April 2022;
Published: 20 May 2022.
Edited by:
Philip Boyd, University of Tasmania, AustraliaReviewed by:
Atsushi Yamaguchi, Hokkaido University, JapanAkash R. Sastri, Institute of Ocean Sciences, Canada
Copyright © 2022 Ikenoue, Otosaka, Honda, Kitamura, Mino, Narita and Kobayashi. This is an open-access article distributed under the terms of the Creative Commons Attribution License (CC BY). The use, distribution or reproduction in other forums is permitted, provided the original author(s) and the copyright owner(s) are credited and that the original publication in this journal is cited, in accordance with accepted academic practice. No use, distribution or reproduction is permitted which does not comply with these terms.
*Correspondence: Takahito Ikenoue, ikenouet@jamstec.go.jp