- 1Laboratory of Aquatic Nutrition and Feed, College of Fisheries, Guangdong Ocean University, Zhanjiang, China
- 2Key Laboratory of Aquatic, Livestock and Poultry Feed Science and Technology in South China, Ministry of Agriculture, Zhanjiang, China
- 3Aquatic Animals Precision Nutrition and High Efficiency Feed Engineering Research Center of Guangdong Province, Zhanjiang, China
- 4Institute of Animal Science, Guangdong Academy of Agricultural Sciences, Guangzhou, China
This study evaluated the effects of lipid sources on the replacement of fishmeal with Clostridium autoethanogenum protein (CAP) in the diet of Pacific white shrimp (Litopenaeus vannamei). By using CAP to replace 40% fishmeal in the basal diet (contains 25% fishmeal), four diets (PC as the control diet, LSO as the low soybean oil level, MSO as the middle soybean oil level, and HSO as the high soybean oil level) were formulated by adding different proportions of fish oil and soybean oil. Each diet was assigned to four replicates (40 shrimps per replicate, initial weight = 1.79 ± 0.02 g). A 7-day Vibrio parahaemolyticus challenge test was conducted at the end of the 50-day feeding experiment. Weight gain was significantly higher and the cumulative mortality after challenge was lower in HSO treatment. The total antioxidant capacity (T-AOC) in hepatopancreas was significantly raised with increasing dietary soybean oil based on CAP substitution. After challenge, Pen3 mRNA expression was significantly higher in hepatopancreas and NF-κB pathway-related mRNA (dorsal, relish, and IKKβ) expression was higher in the intestine in HSO treatment. The height of mucosal folds and the thickness of the muscle layer were positively correlated with the level of dietary soybean oil. Transmission electron microscopy (TEM) analysis showed that the microvilli were damaged and the endoplasmic reticulum was swollen in shrimp fed the LSO diet. Diversity of intestinal microbiota was increased in shrimp fed the LSO diet, with a significant increase in beneficial bacteria such as Halocynthiibacter and Ruegeria as well as less harmful bacteria such as Vibrio. These results suggested that the replacement of fishmeal with CAP and the high proportion of soybean oil in the diet could improve the growth performance, disease resistance, and intestinal structure of Pacific white shrimp, and high fish oil in a low-FM diet raised the percentage of beneficial bacteria in the intestine of shrimp.
Introduction
Pacific white shrimp (Litopenaeus vannamei) is widely farmed in the world due to its rapid growth rate and great economic value (National Research Council, 2011). The global production of Pacific white shrimp is 496 million tons, accounting for 52.9% of the culture production of crustaceans (FAO, 2018). Fishmeal (FM) is an important source of protein in aquafeed especially in shrimp feed owing to the abundant essential amino acids, minerals, and nucleotides (Oliva-Teles et al., 2015; Froehlich et al., 2018). However, with the increasing demand, it is urgent to find alternatives to FM in feed (Henry et al., 2015).
In recent years, there have been many studies about replacing FM with different protein ingredients, including animal-derived protein sources, plant-derived protein sources, and single-cell proteins (SCPs). SCP generally includes bacterial protein, microalgae, and yeast. Some industrial Methanotroph (Methylococcus capsulatus, Bath) bacteria and Clostridium autoethanogenum could use methane, carbon monoxide, and carbon dioxide to produce proteins and active substances (Berge et al., 2005; Aas et al., 2006). Bacterial protein contains less anti-nutritional factors compared with plant-derived proteins, and has less biogenic amine content and lower infection by Salmonella compared with animal-derived proteins (Yao et al., 2022). Nowadays, more and more studies have evaluated the effects of replacing FM with bacterial protein. It is reported that adding 180 g/kg of bacterial protein powder (Methylococcus capsulatus, Alcaligenes acidovorans, Bacillus brevis, and B. firmus) in the diet increased the weight gain of Atlantic salmon by 34% (Aas et al., 2006). After using Methanotroph bacteria meal to replace 45% FM, no significant difference was found in the growth performance of Pacific white shrimp; moreover, the mucosal fold height and disease resistance were increased (Chen et al., 2021b).
C. autoethanogenum was first isolated from rabbit feces under a carbon dioxide, carbon monoxide, and nitrogen atmosphere in 1994, and was identified as a facultative chemotactic bacteria whose only energy source is carbon monoxide (Abrini et al., 1994). C. autoethanogenum protein (CAP) is a by-product of C. autoethanogenum. With high production efficiency and nutrition value, CAP was considered to be a renewable energy and environmentally friendly ingredient. The previous FM replacement studies in black bream (Acanthopagrus schlegelii) (Chen et al., 2020b), Jian carp (Cyprinus carpio var. Jian) (Li et al., 2021), grass carp (Ctenopharyngodon idellus) (Wei et al., 2018), and GIFT have shown the safety and high efficacy of CAP. Replacement of 20% dietary soybean meal with CAP had a positive effect on growth performance and antioxidant capacity of Jian carp and had no significant effects on morphology of liver and midgut (Li et al., 2021). CAP (150–200 g/kg) replacing FM significantly increased weight gain rate and lipid synthesis response in GIFT (Maulu et al., 2021). Replacement of 58.20% dietary FM with CAP did not affect the growth performance of black seabream and phosphorus retention efficiency significantly increased with increasing CAP substitution levels (Chen et al., 2020b). CAP substitution of 75% FM improved the antioxidant capacity of largemouth bass (Zhu et al., 2022). Low level of CAP substitution (< 30%) did not adversely affect growth, gut morphology, and immunity of Pacific white shrimp, but substitution level >50% caused adverse results (Jiang et al., 2021). Overall, the application of CAP as a protein ingredient in aquatic feeds is still at an early stage, with reports on Pacific white shrimp only hovering at gradient replacement of FM.
Lipids constitute the cellular component, provide energy, are involved in the absorption and transport of lipid-soluble vitamins, and also have a protein-sparing effect and improve the utilization of feed protein. Lipids are involved in many physiological mechanisms in the metabolism of aquatic animals and are essential nutrients (Turchini et al., 2009). In addition to being a source of energy for crustaceans, lipids also provide essential fatty acids, sterols, phospholipids, and fat-soluble vitamins for crustaceans to grow (Lim et al., 1997). One of the features of CAP is the extremely low lipid content (approximately one-fiftieth of FM), which is the reason why extra dietary lipid needs to be supplemented based on FM replacement with CAP. In general, fish oil is the most common source of lipids used in aquafeeds. However, even the most optimistic estimates suggest that global fish oil production may not be sufficient to meet the growing demand of the aquaculture industry in the coming years (Bimbo, 1990). Soybean oil is cheaper than fish oil in these years owing to the developing industry. So, the appropriate ratio of fish oil and soybean oil with FM replacement by CAP in the diet of Pacific white shrimp needs to be evaluated. So far, Hu’s et al. (2011) and Kumar’s et al. (2018) studies have shown the feasibility on soybean oil substitution of dietary fish oil in L. vannamei in terms of biochemistry parameters or body composition.
In this study, we examined the effects of dietary lipid sources on the utilization of CAP by Pacific white shrimp in terms of growth performance, disease resistance, antioxidant capacity, intestinal morphology, and intestinal microbial community.
Materials and Methods
Diet Preparation
Four isonitrogenous and isolipidic diets (PC, LSO, MSO, and HSO) were prepared to feed the shrimp. The basal diet was formulated to contain 25% FM as the positive control diet (PC) in this study. Based on a previous study (Jiang et al., 2021), 40% of the FM protein was replaced with CAP under uniform conditions for the trial diets. With the replacement of FM by CAP, MSO diet contains 25 g/kg fish oil (FO) and 25 g/kg soybean oil (SO). LSO diet contains 40 g/kg FO and 10 g/kg SO and HSO diet contains 10 g/kg FO and 40 g/kg SO. The ingredients and approximate nutrient compositions of the diets in this study are shown in Table 1. The essential amino acid and fatty acid profiles of the diets are shown in Tables 2, 3.
CAP was provided by Hebei Shoulang New Energy Technology Co., Ltd. (Tangshan, China). FM, soybean meal, CAP, peanut meal, shrimp shell meal, beer yeast, and wheat flour were used as protein sources. Lipids were provided in the form of fish oil, soybean oil, and soybean lecithin. All ingredients were crushed using a hammer mill (SF-320, Suzhong Pharmaceutical Machinery Co., Ltd., Jiangsu, China), passed through a 250-μm sieve, and mixed thoroughly in a Hobart-type mixer (M-256, South China University of Technology, Guangzhou, China) before adding oil and water. After mixing the lipid and water evenly into the feed for each group, the 1-mm- and 1.5-mm-diameter pellets were prepared by a twin-screw extruder (F-26, South China University of Technology, Guangzhou, China). Then, the diets were heated in an oven at 60°C for 30 min, dried at room temperature to approximately 10% moisture, sealed in ziplock bags, and stored at −20°C until used.
Trial Shrimp and Experimental Conditions
Juvenile L. vannamei were obtained from the breeding base of Guangdong HAID Group Co., Ltd. (Zhanjiang, China). Commercial feed (Guangdong HAID Group, 48.0% crude protein, 8.0% crude fat) was fed to the shrimp for 1 week to domesticate them. After 24 h of starvation treatment, a total of 640 shrimp (initial body weight = 1.79 ± 0.02 g) were randomly divided into four trial groups (four replicates in each group), feeding in 300-L glass fiber tanks (40 shrimp in each tank). Shrimp were fed four times daily at 07:00, 12:00, 17:00, and 22:00 for 50 days. In the first 25 days, shrimp were fed the 1.0-mm-diameter diet, and in the last 25 days, they were fed the 1.5-mm-diameter diet. At the first day of the experiment, shrimp would be fed 8% of initial body weight. When shrimp finished eating within 30 min, 0.2 g of feed was added to the amount of feed per tank per day. During the experiment, approximately 60% of the water disinfected with chlorine dioxide was exchanged daily to maintain water quality. The temperature and salinity of water measured daily during the experiment were 25–28°C and 28–30‰ respectively.
Sample Collection
Sampling was carried out after 50 days of feeding. After fasting for 24 h, shrimp were weighed and the survival rates were calculated. A random sample of 20 shrimp was taken from each tank for sampling. Samples were placed in liquid nitrogen for rapid freezing and then transferred to −80°C storage for subsequent analysis. The trial material was collected from each group and crushed and determined for moisture, crude protein, crude fat, crude ash, and amino acid composition. Moisture content was determined by drying at 105°C for 24 h; crude protein content was determined by Dumas nitrogen determination using a Primacs100 analyzer (Skalar, Netherlands); crude fat was determined by ether extraction using an XT15 extractor (Ankom, USA); crude ash was determined by burning at 550°C for 4 h in a muffle furnace. Amino acid profiles were determined according to the national standard of P.R. China (GB/T 18246-2019): samples were hydrolyzed in HCl at 110°C for 22 h, then separated by ion exchange columns and reacted with ninhydrin solution to obtain amino acid concentrations by spectrophotometry. Fatty acids profiles were also determined by the national standard of the P.R. China (GB/T 5009.168-2016). The amino acid and fatty acid profiles of FM and CAP as well as the trial diet are listed in Table 2. In each tank, 6 shrimp were taken and hemolymph was extracted from the pericardial cavity using a 1-ml syringe and left at 4°C for 10 h. After centrifuging at 4°C and 3,500 rpm for 10 min, the hemolymph supernatant was collected and stored in a −80°C refrigerator for subsequent biochemical analysis. The hepatopancreas of 4 shrimp were collected, washed with saline (0.9% NaCl solution), and then rapidly submerged in liquid nitrogen before being transferred and stored in a −80°C refrigerator for subsequent biochemical analysis. Hepatopancreas and intestine of 4 shrimp were collected, submerged in RNAlater (Ambion®, ThermoFisher), and stored at −80°C in the refrigerator for subsequent mRNA expression analysis. The mid-intestine of 2 shrimp were collected and submerged in fixative solution (4% formaldehyde solution and 2.5% glutaraldehyde solution) for intestinal morphology analysis. The intestine of 3 shrimp were collected, immersed in liquid nitrogen, and transferred to the −80°C refrigerator for subsequent microbiota analysis.
Challenge Test
Before the challenge test, shrimp reared by commercial feed for 50 days were divided into 3 groups (10 shrimp per group) and injected with 50 μl of different concentrations of Vibrio parahaemolyticus: 107, 108, and 109 cfu ml−1 and monitored for 3 days to determine the median lethal concentration (LC50).
At the end of the 50-day feeding trial, 33 shrimp per experimental group were taken and divided equally into 3 transparent containers for the challenge test. Each shrimp was injected with 50 μl of the bacterial solution (concentration = 8.90×108 cfu ml−1) and closely monitored. Survival rates was recorded every 8 h from the injection until the time of half lethality. Meanwhile, the cumulative mortality was counted. Hepatopancreas and intestine were collected after the injection and immediately placed in RNAlater and stored at −80°C for subsequent mRNA expression analysis.
Biochemical Parameters
In this study, immune-related and antioxidant parameters were determined in hemolymph supernatant and hepatopancreas. Before determining, the hepatopancreas was homogenized 1:9 with physiological saline (concentration = 0.9%), centrifuged at 4°C and 3,500 rpm for 10 min, and the supernatant was taken for the determination of biochemical parameters. The total protein content of the hepatopancreas homogenate supernatant was determined by the BCA method using a total protein quantitative assay kit, as a prerequisite for the calculation of biochemical parameters of the hepatopancreas.
All the parameters except phenol oxidase (PO) were determined using kits (Nanjing Jiancheng Bioengineering Institute, China) and detected using a microplate photometer (MultiskanTMGo, Thermo Fisher Scientific, Waltham, MA, United States) or UV spectrophotometer. Alanine transaminase (ALT) and aspartate transaminase (AST) were determined by Lai’s method; malondialdehyde (MDA) was determined by the thiobarbituric acid method; alkaline phosphatase (AKP) was determined according to the kit instructions; catalase (CAT) was determined by the ammonium molybdate method; total antioxidant capacity (T-AOC) was determined by the ABTS method; glutathione peroxidase (GSH-Px) was determined by the colorimetric method.
Phenoloxidase (PO) activity in hemolymph supernatant was determined by spectrophotometer based on the formation of dopachrome produced from L-dihydroxyphenylalanine (L-DOPA). Hemolymph supernatant (10 μl) was mixed with 200 μl of phosphate buffer (0.1 mol L−1, pH 6.0) and 10 μl of L-DOPA (0.01 mol L−1) in a 96-pore ELISA plate. Absorption was recorded immediately every 2 min at 490 nm for 20 min. One unit of PO enzyme activity was defined as a linear increase in absorbance of 0.001 per minute per ml hemolymph supernatant.
mRNA Relative Expression
In this study, the relative expression of mRNA in the hepatopancreas and intestine was determined. Total RNA was extracted from the hepatopancreas and intestine using the TriZol Up Plus RNA kit (TransGen Biotech Co., Ltd, China). Agarose gel electrophoresis and Nanodrop 2000 were used to check the purity and concentration of RNA (A260:A280). The cDNA was synthesized using the Evo M-MLV RT Kit. The steps included gDNA removal and reverse transcription reactions. Briefly, gDNA was eliminated using gDNA Clean Reagent, 5×gDNA Clean Buffer and RNase free water at 42°C. RNA (1,000 ng) was reversely transcribed using RT Primer Mix, Evo M-MLV RTase Enzyme Mix, 5×RTase Reaction Buffer, and RNase-free water at 37°C, followed by inactivation at 85°C for 5 s. The reverse transcription reaction was carried out in a 20-μl volume.
Real-time PCR reactions were performed on a LightCycler 480 (Roche Applied Science, Switzerland) using the SYBR®Green Premix Pro Taq HS qPCR Kit II (Accurate Biotechnology Hunan Co., Ltd, China) by the following procedure: 0.4 μl of forward primer, 0.4 μl of reverse primers, 1 μl of cDNA template (<50 ng), and 5 μl of 2× SYBR®Green Premix Pro Taq HS Premix II and RNase-free water to a final volume of 10 μl. Denaturation was carried out at 95°C for 30 s, followed by 40 amplification cycles, denaturation at 95°C for 5 s, annealing at 60°C for 30 s, followed by melting curve analysis to verify whether a single PCR product was produced, and finally cooled to 4°C. The expressions of the arget gene were normalized to β-actin in this study. Real-time PCR primers in this study are listed in Table 4. The 2−ΔΔCT method was used to calculate mRNA expression according to the method of Dvinge and Bertone (2009).
Intestinal Morphology
Intestinal samples that temporarily present in 4% formaldehyde solution were transferred to Bouin’s solution for 24 h to fix and then transferred to 70% ethanol. The samples were dehydrated in a series of graded ethanol and then embedded in paraffin. Sections were stained with hematoxylin-eosin stain and viewed under a microscope (Nikon Ni-U, Japan). Four additional midguts were collected for transmission electron microscopy (TEM) analysis. According to the method of Xie et al. (2020b), intestinal samples were fixed at 4°C, in a 2.5% glutaraldehyde solution for 24 h, post-fixed in 1% osmium tetroxide (OsO4) for 1 h, dehydrated in a series of graded ethanol, and finally embedded in resin. Ultrathin sections (90 nm) were placed on copper grids, stained with saturated uranyl acetate solution for 30 min, rinsed with distilled water, and then stained with lead citrate for 30 min. The ultrathin sections made were observed with a TEM (Hitachi HT7700, Japan). Subsequently, three parameters of intestinal morphology were measured by ImageJ software, including the height of the mucosal folds as well as the muscle layer thickness. Six parallel data per parameter in each section were measured randomly using one-way ANOVA followed by a Tukey’s multiple-range test to determine significant differences among trial groups. The probability value of p < 0.05 indicates statistical significance.
Intestinal Microbiota
Intestinal microbial DNA from 3 shrimp per tank was extracted using the E.Z.N.A. Fecal DNA Kit (Omega Bio-tek, Inc., United States). To analyze microbial populations, through using primers 341F: CCTACGGGNGGCWGCAG and 806R: GGACTACHVGGGTATCTAAT, the V3–V4 variable region of the 16S ribosomal RNA gene was amplified by PCR, which was run as follows: initial denaturation at 94°C for 2 min, denaturation at 98°C for 10 s, denaturation at 62°C for 30 s, denaturation at 68°C for 30 s, and finally extension at 68°C for 5 min. Amplicons were separated on a 2% agarose gel and then purified using the AxyPrep DNA Gel Extraction Kit (Axygen Biosciences, Union City, CA, USA), and quantified using an ABI StepOnePlus real-time PCR instrument (Life Technologies, Carlsbad). Subsequently, purified amplicons were mixed in equimolar amounts and paired-end sequencing (2×250) was performed on an Illumina platform (HiSeq 2,500, Illumina, San Diego, CA, United States) according to standard protocols. After filtering for noisy sequences and checking for the presence of chimeras, data were clustered at a 97% homology level threshold (VSEARCH, version 10.0) (Edgar, 2013). Data were assigned to the same operational taxonomic units (OTUs) (Bokulich et al., 2013). To assess sequencing depth, sparsity curves were plotted by plotting the number of OTUs versus the total number of sequences. Intergroup Venn analysis was performed to identify endemic and shared OTUs using the microbial indicative analysis function of the Omicshare platform (https://www.omicshare.com/). The alpha diversity index (e.g., coverage, ACE, Chao1, Shannon and Simpson index) was calculated using Mothur software (version v.1.30, http://www.mothur.org/) (Schloss et al., 2009; Aβhauer et al., 2015). In addition, the overall microbial composition was conducted with beta analysis through partial least squares discriminant analysis in the Omicshare platform to model the relationships between sample categories and reduce the dimensionality of the data. Microbial species annotation (version 2.2, http://sourceforge.net/projects/rdpclassifier/) was performed using the RDP classifier software based on the SILVA database (https://www.arb-silva.de/) (Wang et al., 2007).
Calculation and Statistical Methods
The growth performance was calculated as follows: weight gain (WG, %) = (final body weight − initial body weight)/initial body weight×100; specific growth rate (SGR, %day-1) = [ln(final body weight) − ln(initial body weight)]/days of feeding trial×100; survival rate (SR, %) = final number of shrimp/initial number of shrimp×100; feed conversion ratio (FCR) = feed consumed/(final body weight − initial body weight); feed intake (FI) = feed consumed/final number of shrimp; protein efficiency rate (PER) = (final body weight − initial body weight)/feed consumed×crude protein of feed.
All raw data were counted and validated using one-way ANOVA followed by Tukey’s multiple test to compare means among treatments. All statistical analyses were performed using SPSS 24.0 software. The data are presented as means ± standard deviations (SD) and differences were considered significant at p < 0.05.
Results
Growth Performance and Cumulative Mortality After Challenge
As shown in Table 5, the shrimp fed the HSO diet had significantly higher FBW, WG, and SGR than those fed the LSO and MSO diets (p < 0.05), which was not significantly different from the shrimp fed PC (p > 0.05). SR among four groups was higher than 92% in this trial. However, there were no significant differences in SR, FCR, and PER among four groups (p > 0.05). The FI of shrimp fed the PC diet was significantly higher than those fed CAP-containing diets (p < 0.05). Moreover, the shrimp fed the HSO diet had the significantly lowest FCR and highest PER among four groups (p < 0.05).
The result of the challenge test injected by V. parahaemolyticus is shown in Figure 1. At the 96th hour after injection, the cumulative mortality in shrimp fed HSO were significantly lower than that those fed the LSO diet (p < 0.05).
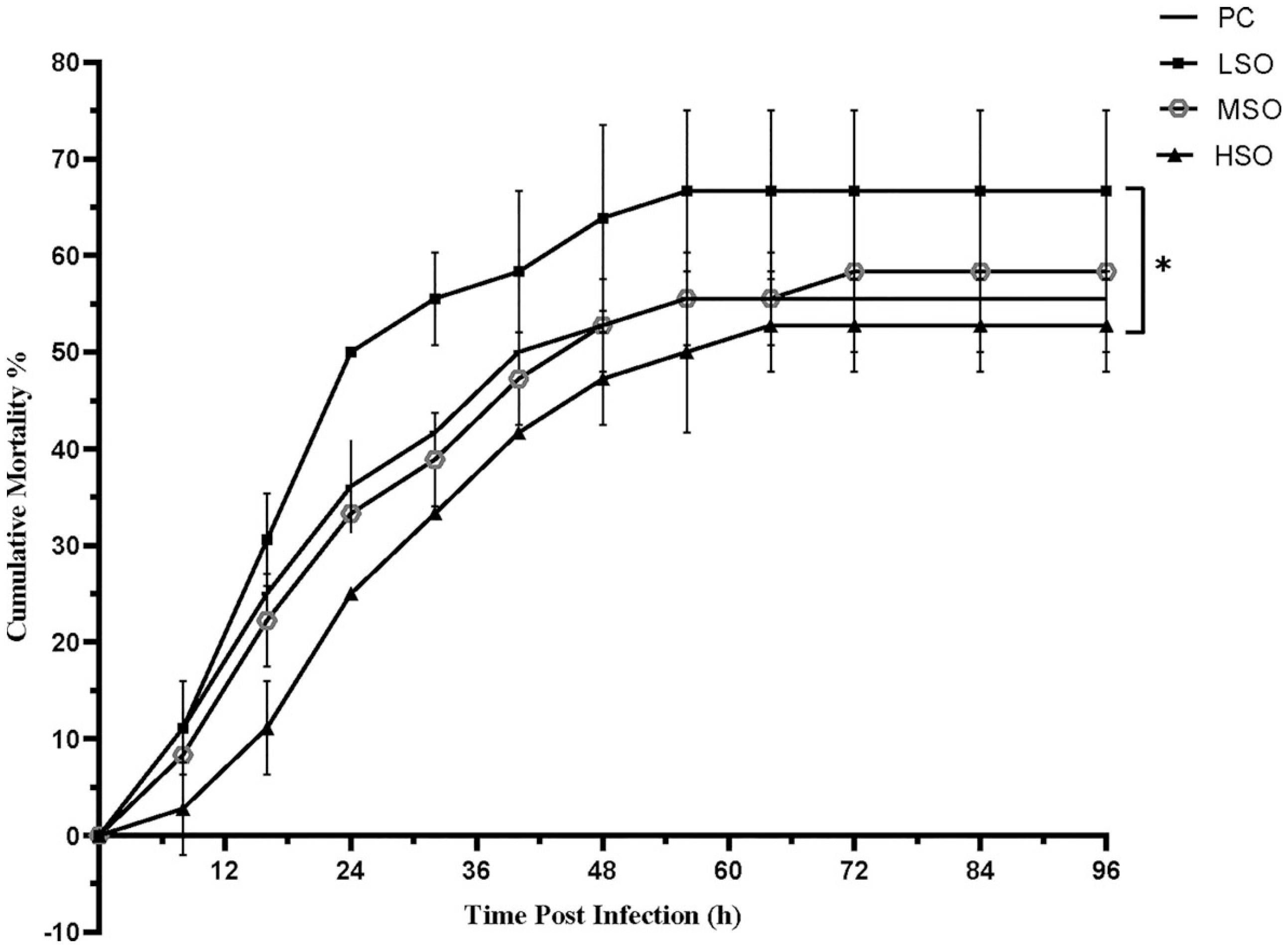
Figure 1 Cumulative mortality of shrimp after infection of V. parahaemolyticus in this study. Asterisk (*) indicates significantly different (p < 0.05) among groups based on Tukey’s multiple-test.
Antioxidant and Non-Specific Immunity Parameters in Hemolymph and Hepatopancreas
The results are shown in Table 6. Among the biochemical parameters of the hemolymph, the level of ALT and AST of shrimp fed HSO were both significantly higher than other groups (p < 0.05), while the AST of shrimp fed the LSO diet was significantly lower than other groups (p < 0.05). The MDA content was significantly higher in shrimp fed the LSO and MSO diets than those fed the PC diet. However, the PO activity in shrimp fed the MSO diet was significantly higher than other groups (p < 0.05). AKP activity was significantly higher in shrimp fed the LSO diet than other groups (p < 0.05), and there was no significant difference between the shrimp fed PC and HSO diets (p > 0.05). Shrimp fed the LSO diet showed higher CAT activities in hepatopancreas than those fed the PC diet (p < 0.05). However, T-AOC in shrimp fed the PC diet was significantly higher than the other three groups, and shrimp fed the HSO diet was significantly lower than those fed the LSO and MSO diets (p < 0.05). GSH-Px was not significantly different in any of the four groups (p > 0.05).
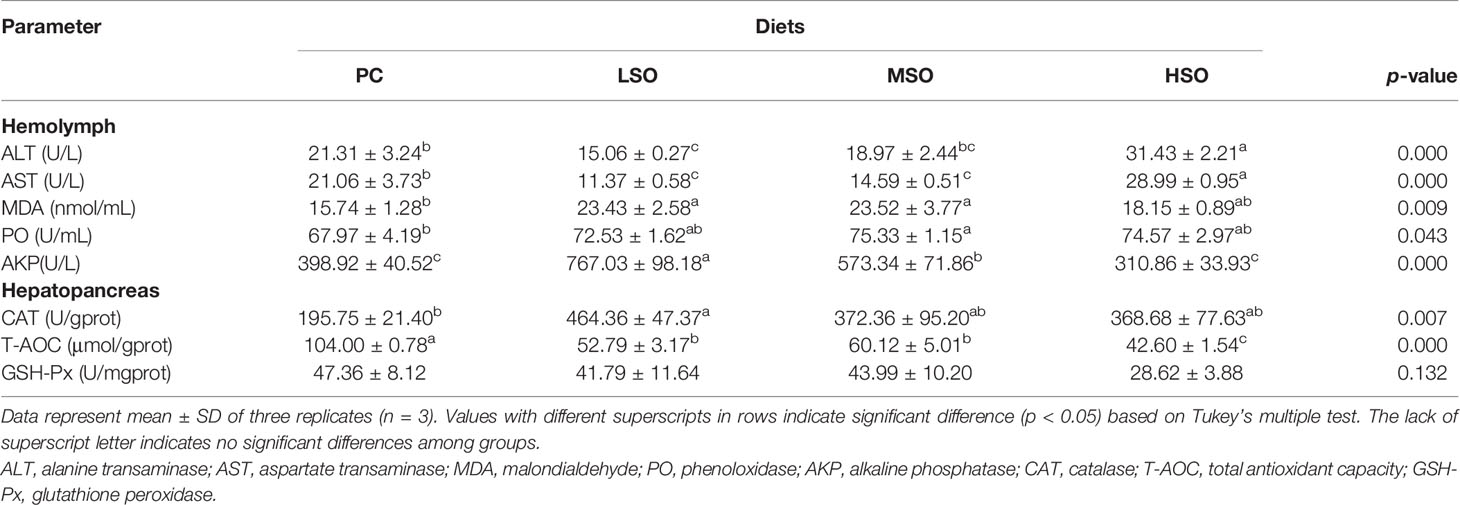
Table 6 Antioxidant and non-specific immunity parameters on hemolymph and hepatopancreas of shrimp in this study.
Expression of Immune-Related Genes Before and After the Challenge
The mRNA expression of immune-related genes in the hepatopancreas of shrimp is shown in Figure 2A. Before the bacteria injection, the mRNA expressions of PPAF and lysozyme were significantly higher in shrimp fed the MSO diet than other groups, and the mRNA expressions of proPO and LGBP in shrimp fed the MSO diet were significantly higher than those fed the PC diet (p < 0.05). The mRNA expression of pen3 was significantly lower in shrimp fed the HSO diet than other groups, and the lysozyme was significantly lower than those fed the PC diet (p < 0.05). After injection, the mRNA expression of proPO and lysozyme decreased significantly in shrimp fed MSO and HSO diets compared to the shrimp fed the PC diet (p < 0.05), while the trend of LGBP was the opposite. In contrast to the results before injection, the mRNA expression of PPAF was significantly lower in shrimp fed the MSO diet than those fed the PC diet, while the pen3 was significantly higher in shrimp fed the HSO diet than other groups (p < 0.05).
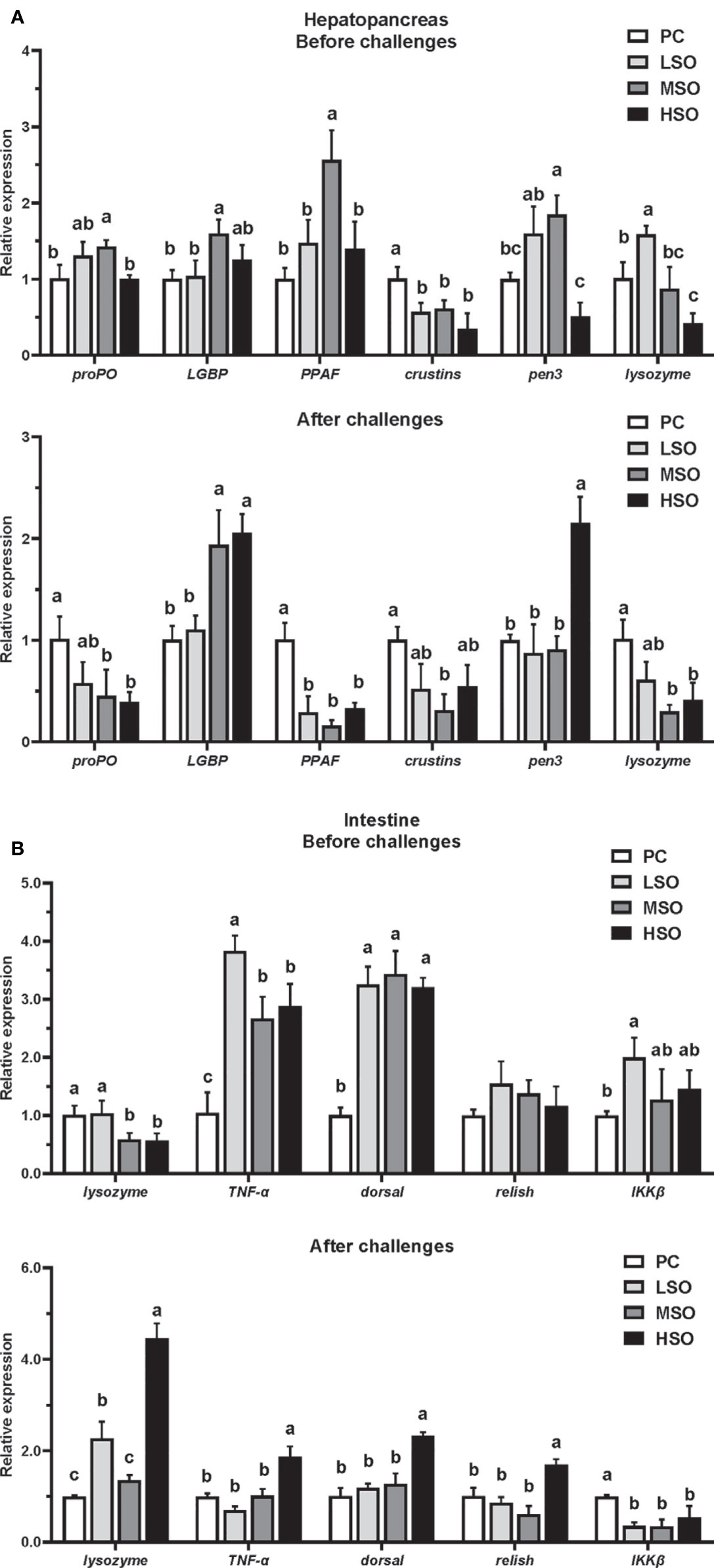
Figure 2 Immune-related gene mRNA relative expression (A) in hepatopancreas of shrimp in this study; (B) in intestine of shrimp in this study. Note: Vertical bars represent the mean ± SD (n = 3). Data marked with different letters differ significantly (p < 0.05) among groups based on Tukey’s multiple test. proPO, prophenoloxidase; LGBP, Beta-1,3-glucan-binding Protein; PPAF, prophenoloxidase activating factor; Pen3, penaeidins3. TNF-α, tumor necrosis factor-α; IKKβ, inhibitor of nuclear factor kappa-B kinase β.
The mRNA expression of immune-related genes in the intestine of shrimp is shown in Figure 2B. Before the injection, the mRNA expression of TNF-α was significantly higher in shrimp fed the LSO diet than other groups and the IKKβ was significantly higher than those fed the PC diet (p < 0.05). The mRNA expression of dorsal in three low-FM treatments was significantly higher than the PC group (p < 0.05). After injection, the mRNA expression of lysozyme, TNF-α, dorsal, and relish were all significantly higher in shrimp fed the HSO diet than other treatments (p < 0.05). The mRNA expression of IKKβ was significantly lower in three low-FM treatments than PC treatment (p < 0.05).
Intestinal Morphology
The intestinal morphological parameters of the four groups are shown in Table 7. The results showed that the height of the mucosal folds in shrimp fed the LSO diet was significantly lower than those fed the PC diet (p < 0.05). The thickness of the muscle layer was increased with the increasing dietary soybean oil level, and it was significantly higher in shrimp fed the HSO diet than those fed PC and LSO diets (p < 0.05). Figure 3 has shown that the intestinal epithelial cells in shrimp fed the MSO diet were slightly separated from the basement membrane (pointed by arrows). The results of TEM revealed that, as the dietary soybean oil level increased in the low FM treatments, the mitochondria of shrimp fed LSO, MSO, and HSO diets showed varying degrees of swelling and irregular arrangement of mitochondrial cristae. In particular, there was a separation between the outside and inside nuclear membrane and a slight degree of swelling in the endoplasmic reticulum in shrimp fed the LSO diet. Furthermore, the microvilli in shrimp fed LSO and HSO diets were apparently shorter than those fed PC and MSO diets.
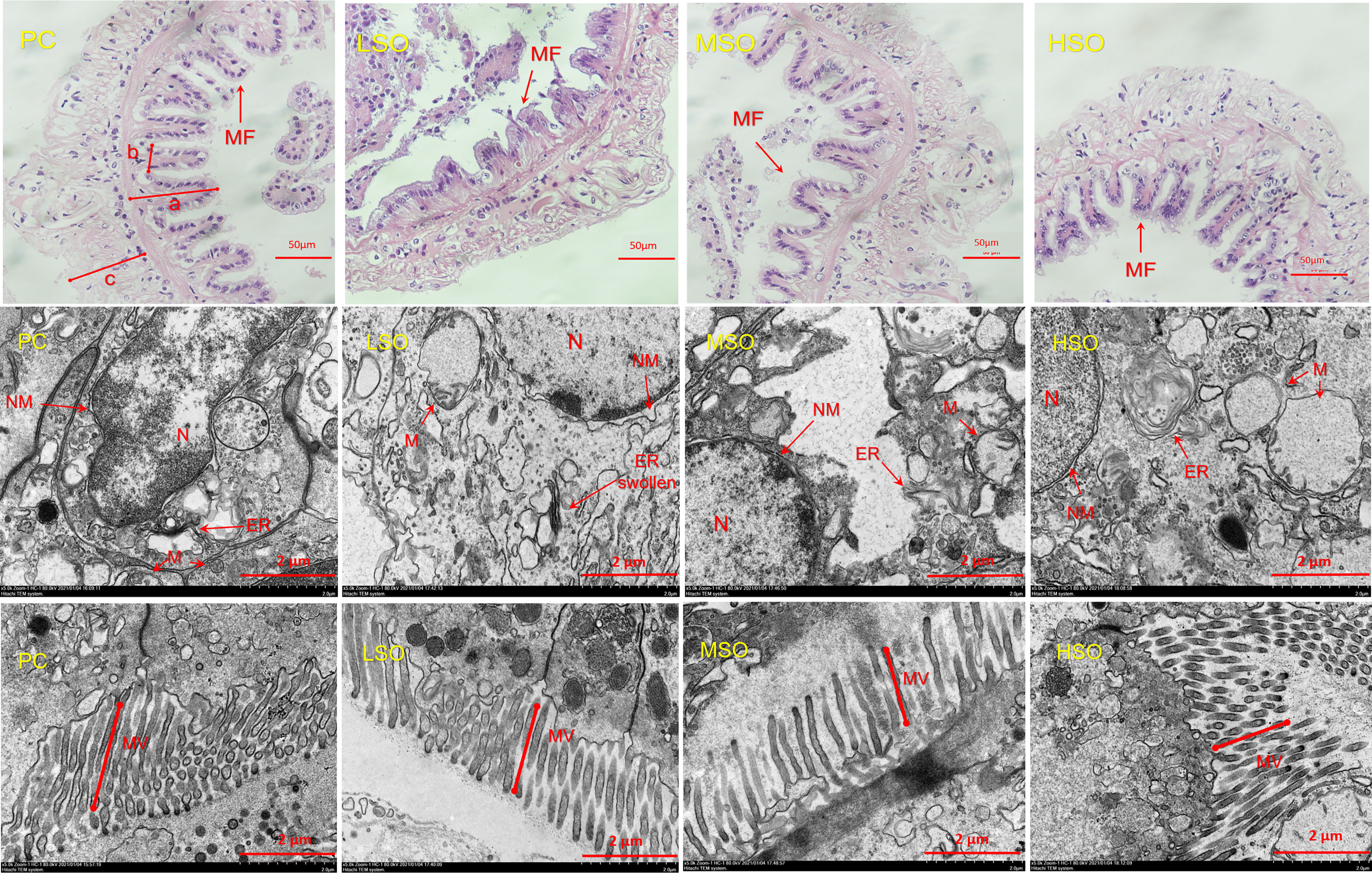
Figure 3 Mid-intestinal morphology (H&E staining and TEM) of shrimp in this study. Note: MF, mucosal folds; a shows height of mucosal folds; b shows width of mucosal folds; c shows thickness of muscle layer; N, cell nucleus; NM, nucleus membrane; M, mitochondria; ER, endoplasmic reticulum; MV, microvilli. Pictures in the same row are considered as the same group.
Intestinal Microbiota
The 16s gene rRNA sequence analysis indicated that a sum of 1,384,444 raw tags (115,370 raw tags per sample) were obtained from the intestinal samples of shrimp in the study. After filtering, a sum of 1,295,308 effective tags (107,942 effective tags per sample).
Sequences with more than 97% similarity were clustered into OTUs. Figure 4A shows that the highest number (196) of unique OTUs was obtained in shrimp fed the PC diet, and the lowest number (26) of unique OTUs was observed in shrimp fed the MSO diet. Alpha diversity analysis is shown in Table 8; the Simpson index was significantly higher in shrimp fed the LSO diet than those fed PC and MSO diets, and the Shannon index showed a similar trend, but no significant differences among the four groups. The community richness (Ace and Chao1 index) was not significantly different among the four groups. This indicated that the low dietary soybean oil after replacement could increase the microbial diversity in the intestine of shrimp but did not affect the richness of the microbiota. Beta diversity analysis is shown in Figure 4B, the analysis of PCoA showed that the first two principal components explained 86.50% of the variations, and there was a clear separation among the four groups. As shown in Figure 4B, the intestinal microbiota of shrimp fed the PC diet was clustered closer to shrimp fed MSO and HSO diets rather than shrimp fed the LSO diet. The results of R > 0 and p < 0.05 in Anosim test indicated that the microbial composition of shrimp fed the LSO diet was significantly different from those fed PC, MSO, and HSO diets (p < 0.05) (Supplementary Figure). Figure 5 suggested the relative abundance of intestinal microbiota in each group at the phylum level. Proteobacteria, Bacteroidetes, Firmicutes, Verrucomicrobia, Actinobacteria, Planctomycetes, and Cyanobacteria were the dominant phyla in four groups. The relative abundance of Proteobacteria exceeded 75% in each of the groups. The relative abundance of Verrucomicrobia and Actinobacteria in shrimp fed the LSO diet was significantly higher than those in other groups (p < 0.05). In the phyla Planctomycetes, the relative abundance in shrimp fed the HSO diet was higher significantly than other groups (p < 0.05). The relative abundance of intestinal microbiota at the genus level is shown in Figure 6. The dominant genera in four groups were Vibrio (phylum of Proteobacteria), Halocynthiibacter (phylum of Proteobacteria), Motilimonas (phylum of Proteobacteria), Pseudoalteromonas (phylum of Proteo-bacteria), Haloferula (phylum of Verrucomicrobia), Ruegeria (phylum of Proteobacte-ria), and Demequina (phylum of Actinobacteria). The relative abundance of Vibrio and Pseudoalteromonas was lower in shrimp fed the LSO diet than other groups (p < 0.05), while the abundance of Halocynthiibacter and Ruegeria was higher in shrimp fed the LSO diet than other treatments (p < 0.05). Meanwhile, there were no significant differences in the abundance of Motilimonas and Demequina in all groups (p > 0.05). The abundance of Pseudoalteromonas and Haloferula showed a relatively increasing trend with increasing dietary soybean oil (p < 0.05).
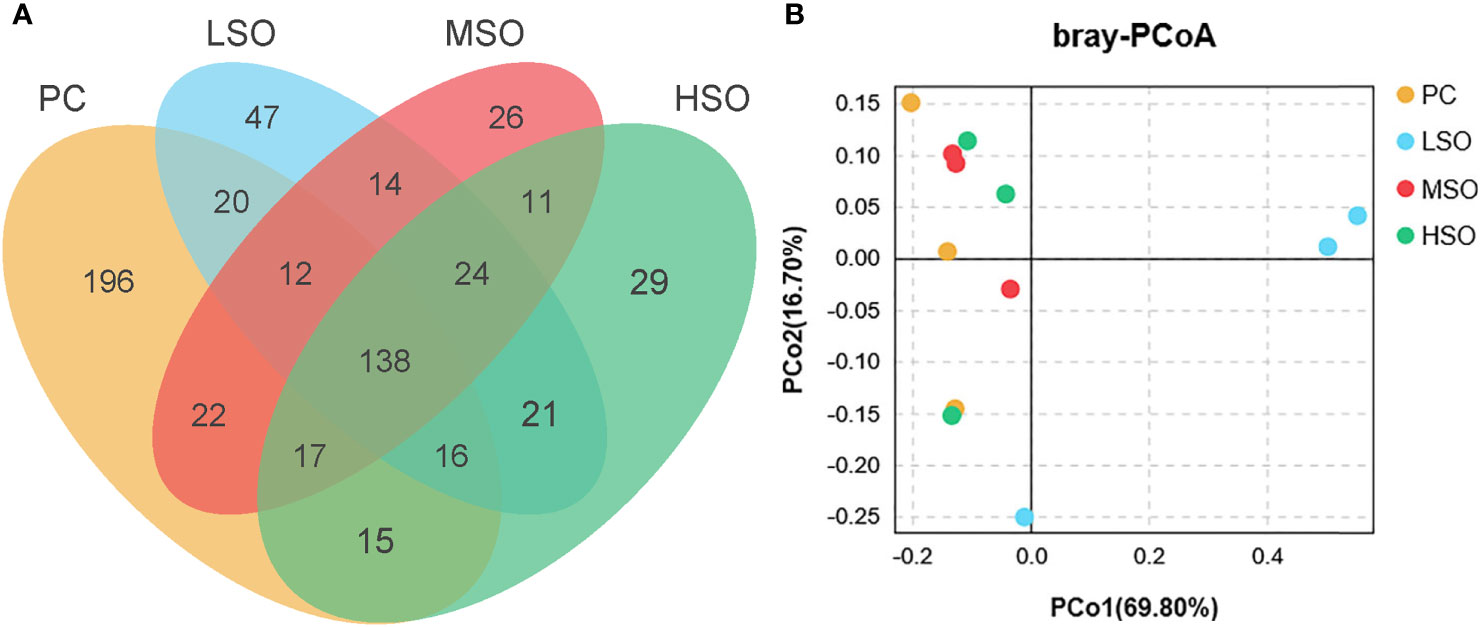
Figure 4 Mid-intestinal microbiota responses of L. vannamei fed with experimental diets. (A) Venn diagram of shared and unique OTUs of shrimp in this study. (B) Principal coordinates analysis (PCoA) based on Bray analysis of shrimp in this study. Note: Vertical bars represent the mean ± SD (n = 3). Data marked with different letters differ significantly (p < 0.05) among groups based on Tukey’s multiple test.
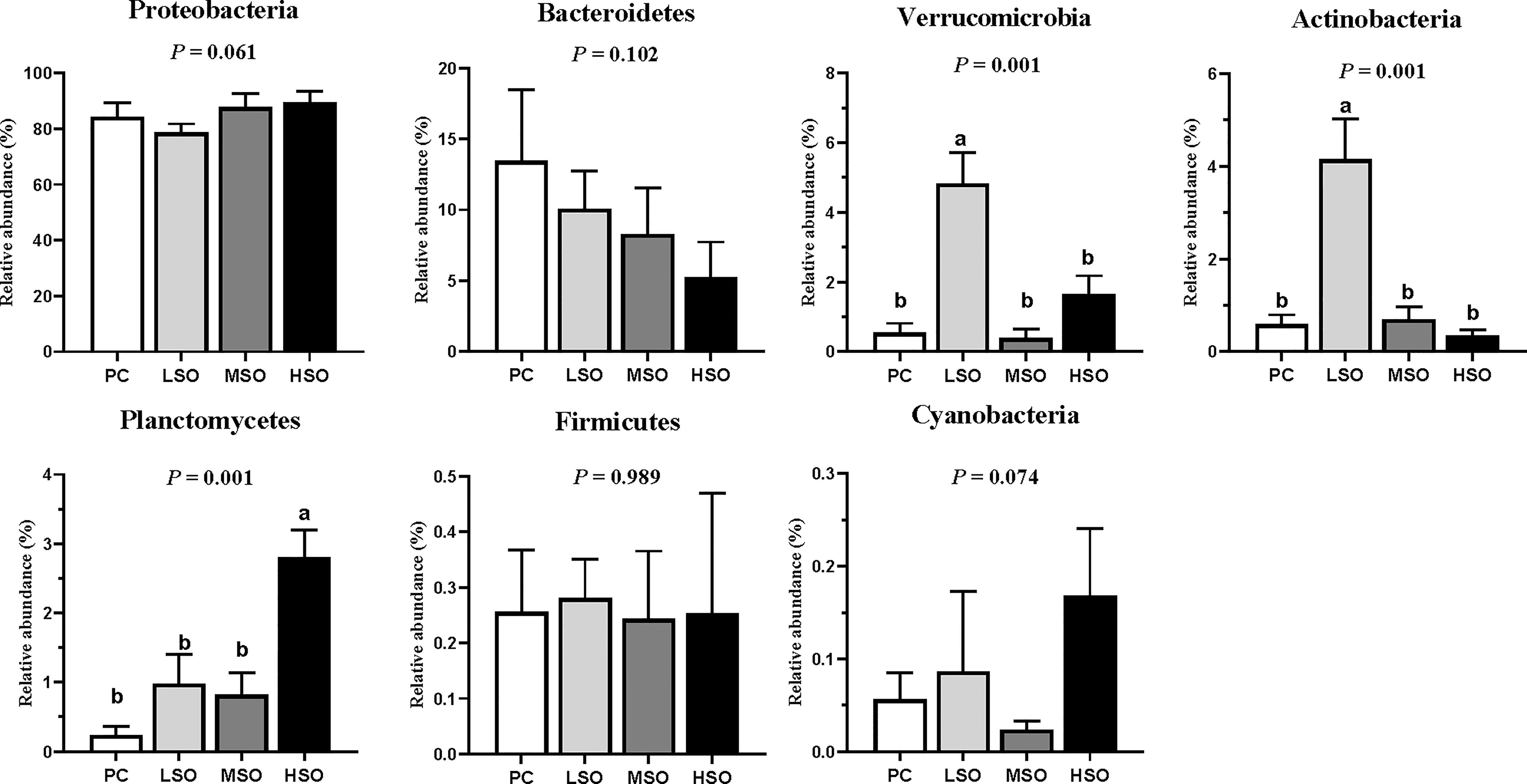
Figure 5 Microbial relative abundance on the top 7 phylum level of shrimp in this study. Note: Vertical bars represent the mean ± SD (n = 3). Data marked with different letters differ significantly (p < 0.05) among groups based on Tukey’s multiple test.
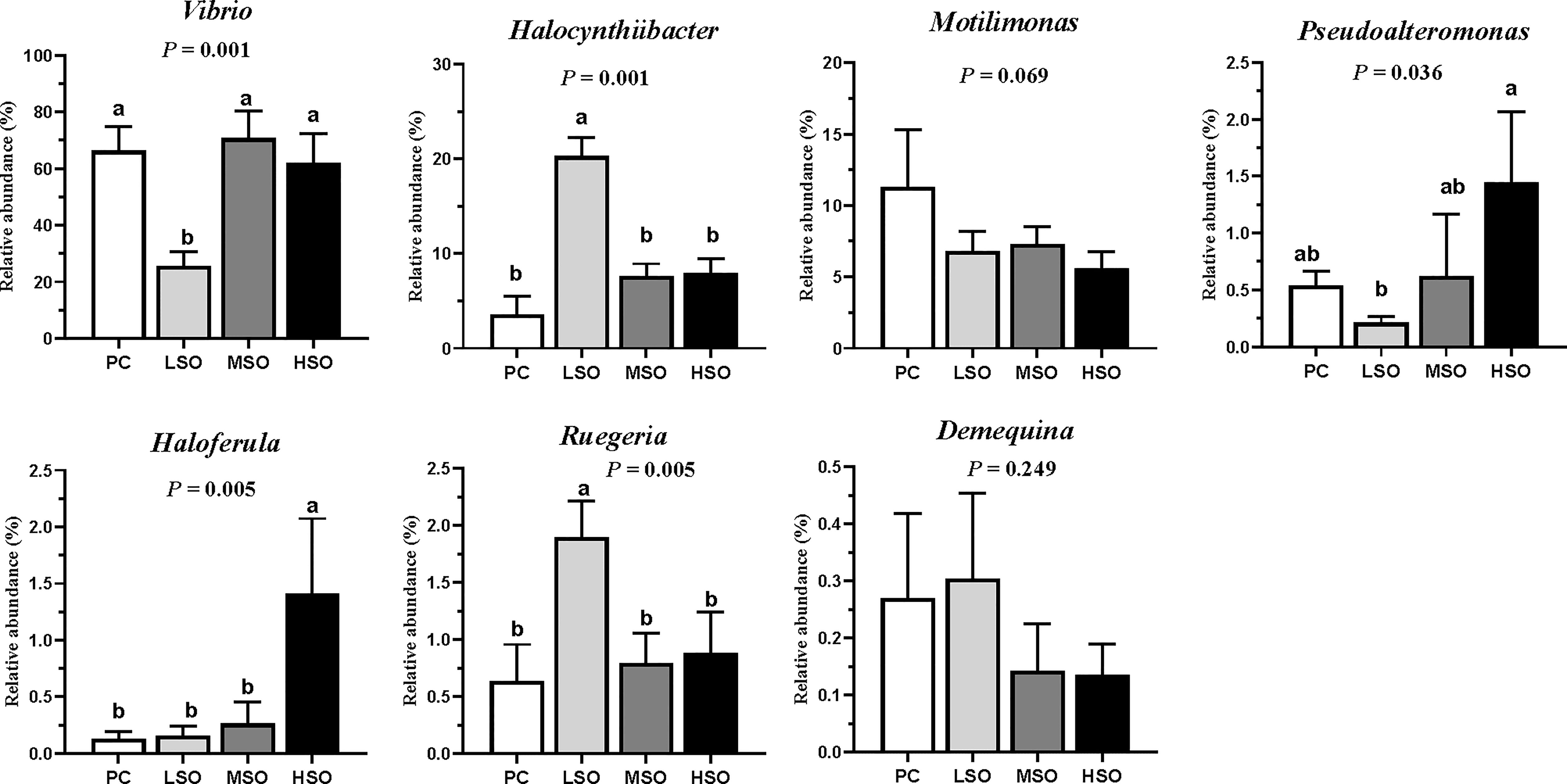
Figure 6 Microbial relative abundance on the top 7 genus level of shrimp in this study. Vertical bars represent the mean ± SD (n = 3). Data marked with different letters differ significantly (p < 0.05) among groups based on Tukey’s multiple test.
Discussion
Growth Performance
Many studies about CAP in recent years reflected the availability of bacterial protein in aquafeeds. In this study, 40% FM was replaced with CAP in the low-FM diets, and total fish oil level and fatty acid profiles in the MSO diet was similar to the PC diet. The results showed that replacing 40% of feed FM with CAP would significantly reduce the WG and FI of Pacific white shrimp, as seen in shrimp fed PC and MSO diets. This is consistent with the results of Jiang et al. (2021), which reported that feeding a diet with substitution of above 45% CAP had a significant negative effect on WG, SGR, and FI of L. vannamei. Similar results were found in black bream fed 58.2% CAP (Chen et al., 2020b). Since CAP might contain flavor compounds that affect the palatability of the feed compared to FM, the reduced growth performance might be due to the reduced feed intake (Hauptman et al., 2014). In addition, results from GIFT fed 20% CAP (Maulu et al., 2021) and Jian carp fed 20% CAP (Li et al., 2021) show that CAP substitution for soybean meal resulted in lower FCR and higher PER in aquatic animals. This indicates that low substitution level of CAP has a positive effect on the nutrient utilization of aquatic animals, while a high level can have a negative effect on growth performance.
On the other hand, high level of soybean oil in a low-FM diet significantly increased the weight gain of Pacific white shrimp. Suitable ratio of fish oil and soybean oil in the diet usually leads to better growth performance of aquatic animals. Improved growth performance and feed utilization were shown on marine herbivorous teleost (Siganus canaliculatus) for 67% fish oil replacement by soybean oil (Xu et al., 2012). Replacement for half of fish oil content with soybean oil could still enhance the weight gain rate of juvenile largemouth bass (Micropterus salmoides) compared with the control treatment (Chen et al., 2020c). Besides, some studies have shown that partial replacement of fish oil with soybean oil did not exhibit negative effects of growth performance in juvenile white grouper (Argyrosomus regius) (Emre et al., 2016) and grouper (Epinephelus coioides) (He et al., 2021). In addition to growth performance, the effects of replacement fish oil with soybean oil on the immunity of Pacific white shrimp have been still rarely reported.
Hemolymph and Hepatopancreas Biochemical Parameters
There is a close relationship among metabolism, nutritional status disease, and hemolymph. When shrimp are affected by external factors, pathological or physiological changes are reflected in hemolymph indices. Therefore, hemolymph biochemical indices are widely used to evaluate the health, nutrition, and environmental adaptation of shrimp (Brum et al., 2018). Alanine aminotransferase (AST) and aspartate aminotransferase (ALT) usually exist in hepatocytes; they would be released into the hemolymph when the hepatopancreas was damaged and the cell membrane permeability was increased. Therefore, serum AST and ALT activities are commonly used as indicators of liver function (Boone et al., 2005). In previous research, excessive CAP replacement of dietary FM impaired the liver health of shrimp (Jiang et al. 2021). In this study, ALT and AST showed a significant increase with the raise of soybean oil ratio in low FM groups. These suggested that a high proportion of soybean oil in the diet might lead to hepatopancreatic damage in Pacific white shrimp. Similar results were also found in large yellow croaker (Larimichthys crocea) fed a high soybean oil diet (Mu et al., 2018).
Studies have shown that MDA, a product of lipid peroxidation reactions, can be used as an indicator of the extent of oxygen radical damage in body cells (Koruk et al., 2004) and is widely used to evaluate the health status of animals (Xie et al., 2016). In the present study, the addition of CAP resulted in an increase in MDA content. It was consistent with the results of Pacific white shrimp fed Methanotroph bacteria meal (Chen et al., 2021b) and largemouth black bass fed CAP (Yang et al., 2021). Results from a study of replacing fish oil with dietary soybean oil in large yellow croaker have shown that MDA decreased as the replacement level increased (Mu et al., 2018), but similar results were not found in our study. These studies seemed to suggest that CAP increases the chances of oxidative damage in Pacific white shrimp, whereas dietary lipid sources had no effects on the oxidative damage of shrimp. GSH-Px and CAT are crucial antioxidant enzymes responsible for scavenging oxygen free radicals and hydrogen peroxide. In this study, there was no significant difference in CAT activity among the PC and low-FM treatments (MSO and HSO). In addition, with increasing soybean oil content, CAT activity tended to decrease but was not statistically significant (p > 0.05). These results indicated that partial replacement with CAP did not affect CAT activity in shrimp. Similarly, CAT activity would not be influenced by replacing 38.2% dietary FM with CAP in black seabream (Chen et al., 2020b). T-AOC is an important indicator to evaluate the overall antioxidant status of animals (Xie et al., 2016). Results of this study have shown that both CAP and dietary soybean oil could decrease T-AOC. It was demonstrated that tilapia (Oreochromis niloticus) fed a diet with fish oil replaced by soybean oil could harm the capacity of T-AOC and SOD (Peng et al., 2016). In conclusion, FM replacement by CAP increased oxidative damage and was harmful to the antioxidant capacity of shrimp.
Phenoloxidase (PO) is an important immune factor in crustaceans and is usually present in the hemolymph in the form of a zymogen. When it is stimulated by a foreign body, it undergoes a cascade reaction, which then induces a variety of factors that mediate agglutination and coagulation and produce bactericidal substances (Shen et al., 2007; Wu et al., 2021). In this study, the replacement of FM by CAP in the diet caused an increase in PO activity in Pacific white shrimp, which is consistent with the findings of Jiang et al. (2021). This suggested that the phenoloxidase immune system of shrimp was activated when fed a low-FM diet. AKP is a phosphomonoesterase that could help to detoxify crustacean lysosomal enzymes (Lee et al., 2018). In this study, the activity of the AKP decreased as the proportion of soybean oil increased, suggesting that the fish oil replaced by soybean oil might reduce the immune ability of the phosphatase.
Challenge Test
Survival after a challenge by certain pathogens is commonly used as a measure of disease resistance (Deng et al., 2013). Most pathogenic bacteria of the genus Vibrio have been reported to be major causative agents of aquatic animals, particularly V. parahaemolyticus (Zhu et al., 2006; Duan et al., 2016). Early mortality syndrome (EMS) or acute hepatopancreatic necrosis syndrome (AHPNS) is a serious disease caused by V. parahaemolyticus that often occurs in L. vannamei and Penaeus monodon, causing huge economic losses to the aquaculture industry (Dhar et al., 2019). In this study, the V. parahaemolyticus injection test was used to assess the resistance of shrimp to the disease. At the 96-h post-injection, the shrimp fed the LSO diet showed the highest cumulative mortality, which was significantly higher than shrimp fed the HSO diet. There was no significant difference between the mortality of shrimp fed PC and MSO diets, indicating that 40% FM replacement with CAP substitution has not caused a significant effect on the disease resistance of shrimp. In contrast to the present study, 15% FM replacement with Methanotroph bacteria meal significantly improved the resistance of L. vannamei to V. parahaemolyticus (Chen et al., 2021b). The resistance to a natural infestation of parasites of large yellow croaker decreased significantly with increasing n-3HUFA in the diet (Zuo et al., 2012), similar to the results of this study. It has been suggested that the moderate substitution of CAP would not weaken the resistance of L. vannamei and increasing the proportion of soybean oil in the diet could help to improve disease resistance.
Immune-Related mRNA Expression in the Hepatopancreas and Intestine Before and After Challenge
Crustaceans lack acquired specific immunity, but they have a relatively well-developed non-specific immune system that can rapidly recognize and clear invading microorganisms (Jin et al., 2018). The prophenoloxidase (proPO) activating system is one of the major innate immune systems of crustaceans (Söderhäll and Cerenius, 1998). The proPO system can be activated by harmful substances such as bacterial lipopolysaccharides and then converts into active phenoloxidase (PO). Those harmful substances are activated by serine proteases to activate the proPO system and react to produce large amounts of melanin and quinone peroxides to kill bacteria (Vargas-Albores and Yepiz-Plascencia, 2000). In this study, high level of proPO expression was activated in shrimp fed the MSO diet. Similarly, yeast hydrolysate, a type of unicellular protein, was added to the feed to upregulate proPO expression in L. vannamei (Jin et al., 2018). LGBP is an important pattern recognition protein in the proPO system that binds to lipopolysaccharide and β-1.3-glucan in the fungal cell wall (Zokaeifar et al., 2012). It was shown that the MSO and HSO diets activated the expression of LGBP in hepatopancreas of shrimp. The activation has become more significant after disease challenge (p < 0.05), which might cause the lower cumulative mortality in shrimp fed MSO and HSO diets. The PPAF is another important factor in the proPO system (Lai et al., 2005). It was seen that the gene expressions of proPO, LGBP, and PPAF in shrimp fed the MSO diet were significantly higher than those in shrimp fed the PC diet, suggesting that CAP substitution of FM appears to have potential to activate proPO system in Pacific white shrimp. Crustins and Penaeidin3 (Pen3) are two major antimicrobial peptides in shrimp with antimicrobial and chitin-binding properties that coordinate immune function with exoskeletal synthesis (Destoumieux et al., 2000). Antimicrobial peptide is a category of active substances produced by animals under induced conditions to combat the pathogenic effects of exogenous pathogens (Song et al., 2010). The upregulation of Pen3 expression suggested that the substitution of CAP might be involved in the antimicrobial response of L. vannamei. This is also consistent with Jin’s et al. (2018) study of Pacific white shrimp fed yeast hydrolysate. Furthermore, black soldier fly larvae meal replacing FM has been reported to decrease n-6 PUFA content of feed and downgrade the pen3 expression of L. vannamei (Chen et al., 2021a). It was consistent with our study that pen3 expression was largely activated in shrimp fed by high soybean oil treatment after the V. parahaemolyticus challenge. Consequently, these results have implied that the n-6 PUFA rich in soybean oil might be a factor in activating the pen3 antimicrobial response.
The immune function of the hepatopancreas is an important barrier against invading harmful substances in Pacific white shrimp, but the immune function of the intestine cannot be ignored, as pathogens can pass through the intestine (Pilotto et al., 2020). As a member of the tumor necrosis factor superfamily, TNF-α is a key gene on the immune response that regulates inflammatory and apoptotic cascades in aquatic animals (Aggarwal, 2003; Akira, 2009). In this work, dietary CAP greatly activated this inflammatory factor before the challenge. NF-κB is a key regulator of the immune and inflammatory response. The dorsal and relish genes are transcription factors of the NF-κB pathway, which could regulate the expressions of antimicrobial peptides (AMPs) to protect the organism from diseases (Tassanakajon et al., 2013). NF-κB is usually inhibited in its activity by its inhibitor IκBα, whereas IκBα kinase β (IKKβ) can phosphorylate IκBα and thus activate the NF-κB pathway (Ko et al., 2017). Furthermore, IKKβ is also a prerequisite for the endoplasmic reticulum stress response (Tam et al., 2012), and the mRNA expression of IKKβ in the four groups was compatible with the intestinal TEM analysis. In the present study, CAP substitution of FM resulted in upregulation of intestinal dorsal, relish, and IKKβ, indicating CAP possibly activates the intestinal NF-κB pathway of Pacific white shrimp. Previous studies have also shown that feeding Pacific white shrimp with yeast hydrolysate significantly elevated mRNA expression of dorsal and relish (Jin et al., 2018), and low-FM diets activated transcript level of IKKβ in shrimp intestine (Xie et al., 2020a). After the challenge, the expressions of dorsal and relish were significantly stimulated in shrimp fed the HSO diet, while the expression was suppressed to control level in shrimp fed LSO and MSO diets. These have suggested that soybean oil could improve the immune response of shrimp by activating the NF-κB pathway. A study has supported this notion that dietary bile acid addition stimulated the expressions of AMPs through the NF-κB-mediated signaling pathway intestine of L. vannamei (Su et al., 2021). In addition, the lysozyme in shrimp fed the HSO diet was significantly upregulated after the challenge, which might explain the lower cumulative mortality in shrimp fed the HSO diet.
Mid-Intestinal Morphology (H&E Staining and TEM Analysis)
The intestinal barrier in animals has an immune function and a physiological function. If the structural integrity of the intestine is compromised, pathogens, and toxins can penetrate the intestinal epithelium and disrupt host immunity (Rungrassamee et al., 2016). Also, the shrimp intestine is an important organ for nutrient absorption, so the morphology and structure of the intestine are critical to the growth and immunity of the animal. Generally, the height of mucosal folds and the muscle layer thickness are two basic indicators (Manzanilla et al., 2004). In H&E-staining sections, the height of mucosal folds was significantly lower in shrimp fed the LSO diet compared to shrimp fed the PC diet, and the intestinal epithelial cells were not tightly connected to the basement membrane in shrimp fed the MSO diet. Damage to the mid-intestine affects the digestion and absorption of nutrients, which might be one of the reasons for poor growth in shrimp fed LSO and MSO diets. This result was similar to previous studies (Bansemer et al., 2015; Xie et al., 2021). Also, 45% FM replaced by CAP also caused adverse impacts on intestine morphology of shrimp (Jiang et al. 2021). Adjusting the proportion of fish oil to soybean oil in diet did not affect the height of mucosal folds. This contrasted with the findings of Zhu et al. (2020), in which dietary soybean oil substitution for fish oil resulted in a reduction in height of mucosal folds in juvenile yellow drum (Nibea albiflora), with no effect on muscle layer thickness. However, in this study, the muscle layer thickness increased significantly with increasing dietary soybean oil level, likely to elevate better digestion and absorption of feed. It was demonstrated that thicker muscle layer thickness is beneficial to strengthen intestinal contraction and facilitates food mixing and digestion (Huang et al., 2018). TEM analysis showed that the length of microvilli in shrimp fed the PC diet was significantly higher than that in shrimp fed LSO and HSO diets, and their quantity was more than those fed the MSO diet. Similarly, 1,200 mg kg−1 of dietary nucleotides significantly reduced the height of microvilli (Guo et al., 2016), while replacing fish oil with dietary soybean oil reduced the length and density of microvilli (Zhu et al., 2020). Shrimp fed the LSO diet showed swelling of the nuclear membrane and endoplasmic reticulum, and mitochondria showed an abnormal state. It could be seen that the shrimp fed the LSO diet (dietary n-3 HUFA level of 1.80%) suffered from intestinal cell damage in this study. These might be related to the inflammatory response caused by the LSO diet containing excessive n-3 HUFA content. An’s et al. (2020) study has shown that dietary n-3 HUFA level above 1.58% would cause blurred edges and structural damage to the hepatopancreas cells of L. vannamei. Thus, it was indicated that high fish oil level in a low-FM diet might cause stress damage to the intestine of Pacific white shrimp. However, the effects of n-3HUFA on the aquatic intestine in TEM analyses have been rarely reported yet and further studies are needed.
Intestinal Microbiota
The microbiota that hosts in the intestine is the biological barrier of the intestine and is a key factor in intestinal health. Intestinal microbiota is known to benefit the host by improving their immune response, nutrient absorption, and homeostatic maintenance (Li et al., 2018). It is important to maintain the dynamic balance of intestinal microbiota for controlling shrimp disease (He et al., 2017). Numerous studies have been conducted to assess the effects of different protein sources on the composition of the intestinal microbiota of aquatic animals (Zhou et al., 2018; Niu et al., 2019). In Pacific white shrimp, only a few characteristic genera can be used to evaluate the health status of the shrimp’s internal environment. For example, Vibrio, Tenacibaculum, Photobacterium, Kangiella, and Spongiimonas are known as opportunistic pathogens, and Acetobacter, Bacillus, Bacteroides, Bdellovibrio, Lactococcus, Rhodopseudomonas, and Streptococcus are reported as the beneficial bacteria of L. vannamei (Sun et al., 2016). In our study, the alpha diversity analysis revealed that the Simpson and Shannon index showed that the diversity of the intestinal microbiota in the shrimp fed the LSO diet was improved by dietary CAP and high fish oil content, while the beta diversity analysis also indicated that the microbiota composition of the LSO treatment was significantly far from the other three groups. No matter how diet changes, the phylum Proteobacteria and Bacteroidetes were the most stable bacteria located at the shrimp intestine, which was consistent with the findings of Amoh et al. (2019) and Chen et al. (2021a). The shrimp fed the LSO diet was detected with a high level of the phylum Actinobacteria. Usually, Actinobacteria are mainly composed of saprophytic bacteria and generally distributed in the soil. There are some parasitic bacteria from Actinobacteria that can possibly cause disease. It was reported that Actinobacteria and Proteobacteria were conditionally pathogenic (Chen et al., 2020a). Vibrio is a typical harmful genus (Xie et al., 2020b). In the present study, high fish oil level in diet has increased the diversity of shrimp by decreasing the microbial relative abundance of the genus Vibrio and increasing the microbial relative abundance of Halocynthiibacter and Ruegeria. The other three groups of shrimp had significantly higher levels of Vibrio than the shrimp fed the LSO diet. This might be a result of the high fish oil content, which is usually rich in n-3 LC-PUFA, and some active substances that play a positive role in the physiological function of the animal. Ruegeria is involved in protein utilization (Duan et al., 2019) and may be responsible for mitigating the effects of low weight gain rates associated with CAP replacement of FM. Pseudoalteromonas is regarded as a kind of probiotics in shrimp, to produce extracellular antibacterial compounds, which could enhance the resistance of shrimp to V. parahaemolyticus (Sorieul et al., 2018; Wang et al., 2018). This is consistent with the results of this study’s challenge test, where the cumulative mortality rate was lower in shrimp fed the HSO diet that is rich in Pseudoalteromonas. It has been reported that Haloferula in sea cucumbers (Apostichopus japonicus) was decreased by the high content of selenium and vitamin C supplementation, indicating that Haloferula is related to the antioxidant capacity of the intestine (Zeng et al., 2021). The increase of genus Pseudoalteromonas and Haloferula in shrimp fed the HSO diet and genus Ruegeria in shrimp fed the LSO diet might enhance intestinal health. Although the level of Vibrio in the intestinal environment was not low, they did not cause adverse effects such as reduced disease resistance in shrimp fed the HSO diet, suggesting that increasing the dietary soybean oil level with CAP replacement of dietary FM enhanced intestinal stability and did not cause disorders even with higher pathogen abundance.
Conclusions
This study evaluated the effects of lipid sources on FM replacement with CAP in the diet of Pacific white shrimp (L. vannamei). Results have shown that high soybean oil in the diet could relieve the negative effects caused by CAP substitution on growth performance, immune response, bacterial resistance, and intestinal damage of the shrimp, while high fish oil in a low-FM diet improved the intestinal microbiota of shrimp. Therefore, a diet with partial CAP substitution and high soybean oil level could be considered for the FM replacement of Pacific white shrimp.
Data Availability Statement
The datasets presented in this study can be found in online repositories. The names of the repository/repositories and accession number(s) can be found at: https://www.ncbi.nlm.nih.gov/ PRJNA809478.
Ethics Statement
This study was carried out in accordance with the requirements of Care and Use of Laboratory Animals in China, Animal Ethical and Welfare Committee of China Experimental Animal Society. The protocol was approved by the Animal Ethical and Welfare Committee of Guangdong Ocean University, China.
Author Contributions
CZ: Conceptualization, Investigation, Formal analysis, and Writing—Original Draft. SX: Conceptualization, Writing—Review and Editing, Supervision, and Project administration. BT: Methodology, Resources, Supervision, and Funding acquisition. SG: Experiment Assistance. JC: Methodology, Resources, and Funding acquisition. XD: Methodology and Resources. SC: Methodology and Resources. QY: Methodology and Resources. HL: Methodology and Resources. SZ: Methodology and Resources. All authors contributed to the article and approved the submitted version.
Funding
This work was supported by the National Key R&D Program of China (2019YFD0900200), the National Natural Science Foundation of China (32002402), and the Guangdong Basic and Applied Basic Research Foundation (2019A1515011970, 2021A1515010428).
Conflict of Interest
The authors declare that the research was conducted in the absence of any commercial or financial relationships that could be construed as a potential conflict of interest.
Publisher’s Note
All claims expressed in this article are solely those of the authors and do not necessarily represent those of their affiliated organizations, or those of the publisher, the editors and the reviewers. Any product that may be evaluated in this article, or claim that may be made by its manufacturer, is not guaranteed or endorsed by the publisher.
Acknowledgments
We thank Hebei Shoulang New Energy Technology Co., Ltd. for providing Clostridium autoethanogenum protein and Guangzhou Genedenovo Biotechnology Co., Ltd (Guangdong, China) for the services of sequencing and bioinformatics analysis (http://www.omicsmart.com).
Supplementary Material
The Supplementary Material for this article can be found online at: https://www.frontiersin.org/articles/10.3389/fmars.2022.879364/full#supplementary-material
References
Aβhauer K. P., Wemheuer B., Daniel R., Meinicke P. (2015). Tax4Fun: Predicting Functional Profiles From Metagenomic 16S rRNA Data. Bioinformatics 31 (17), 2882–2884. doi: 10.1093/bioinformatics/btv287
Aas T. S., Grisdale-Helland B., Terjesen B. F., Helland S. J. (2006). Improved Growth and Nutrient Utilisation in Atlantic Salmon (Salmo Salar) Fed Diets Containing a Bacterial Protein Meal. Aquaculture 259 (1-4), 365–376. doi: 10.1016/j.aquaculture.2006.05.032
Abrini J., Naveau H., Nyns E.-J. (1994). Clostridium Autoethanogenum, Sp. Nov., an Anaerobic Bacterium That Produces Ethanol From Carbon Monoxide. Arch. Microbiol. 161 (4), 345–351. doi: 10.1007/bf00303591
Aggarwal B. B. (2003). Signalling Pathways of the TNF Superfamily: A Double-Edged Sword. Nat. Rev. Immunol. 3 (9), 745–756. doi: 10.1038/nri1184
Akira S. (2009). Pathogen Recognition by Innate Immunity and its Signaling. Proc. Japan Academy Ser. B 85 (4), 143–156. doi: 10.2183/pjab.85.143
Amoah K., Huang Q.-C., Tan B.-P., Zhang S., Chi S.-Y., Yang Q.-H., et al. (2019). Dietary Supplementation of Probiotic Bacillus Coagulans ATCC 7050, Improves the Growth Performance, Intestinal Morphology, Microflora, Immune Response, and Disease Confrontation of Pacific White Shrimp, Litopenaeus Vannamei. Fish Shellfish Immunol. 87, 796–808. doi: 10.1016/j.fsi.2019.02.029
An W., He H., Dong X., Tan B., Yang Q., Chi S., et al. (2020). Regulation of Growth, Fatty Acid Profiles, Hematological Characteristics and Hepatopancreatic Histology by Different Dietary N-3 Highly Unsaturated Fatty Acids Levels in the First Stages of Juvenile Pacific White Shrimp (Litopenaeus Vannamei). Aquaculture Rep. 17, 100321. doi: 10.1016/j.aqrep.2020.100321
Bansemer M., Forder R., Howarth G., Suitor G., Bowyer J., Stone D. (2015). The Effect of Dietary Soybean Meal and Soy Protein Concentrate on the Intestinal Mucus Layer and Development of Subacute Enteritis in Y Ellowtail K Ingfish (S Eriola Lalandi) at Suboptimal Water Temperature. Aquaculture Nutr. 21 (3), 300–310. doi: 10.1111/anu.12160
Berge G. M., Baeverfjord G., Skrede A., Storebakken T. (2005). Bacterial Protein Grown on Natural Gas as Protein Source in Diets for Atlantic Salmon, Salmo Salar, in Saltwater. Aquaculture 244 (1-4), 233–240. doi: 10.1016/j.aquaculture.2004.11.01
Bimbo A. P. (1990). “"Fish Meal and Oil,",” in The Seafood Industry (Washington, DC: Springer), 325–350. doi: 10.1007/978-1-4615-2041-2_20
Bokulich N. A., Subramanian S., Faith J. J., Gevers D., Gordon J. I., Knight R., et al. (2013). Quality-Filtering Vastly Improves Diversity Estimates From Illumina Amplicon Sequencing. Nat. Methods 10 (1), 57–59. doi: 10.1038/nmeth.2276
Boone L., Meyer D., Cusick P., Ennulat D., Bolliger A. P., Everds N., et al. (2005). Selection and Interpretation of Clinical Pathology Indicators of Hepatic Injury in Preclinical Studies. Vet. Clin. Pathol. 34 (3), 182–188. doi: 10.1111/j.1939-165x.2005.tb00041
Brum A., Pereira S. A., Cardoso L., Chagas E. C., Chaves F. C. M., Mouriño J. L. P., et al. (2018). Blood Biochemical Parameters and Melanomacrophage Centers in Nile Tilapia Fed Essential Oils of Clove Basil and Ginger. Fish Shellfish Immunol. 74, 444–449. doi: 10.1016/j.fsi.2018.01.021
Chen Y., Chi S., Zhang S., Dong X., Yang Q., Liu H., et al. (2021a). Evaluation of the Dietary Black Soldier Fly Larvae Meal (Hermetia Illucens) on Growth Performance, Intestinal Health, and Disease Resistance to Vibrio Parahaemolyticus of the Pacific White Shrimp (Litopenaeus Vannamei). Front. Marine Sci. 8, 1072. doi: 10.3389/fmars.2021.706463
Chen Y., Chi S., Zhang S., Dong X., Yang Q., Liu H., et al. (2021b). Replacement of Fish Meal With Methanotroph (Methylococcus Capsulatus, Bath) Bacteria Meal in the Diets of Pacific White Shrimp (Litopenaeus Vannamei). Aquaculture 541, 736801. doi: 10.1016/j.aquaculture.2021.736801
Chen Y., Sagada G., Xu B., Chao W., Zou F., Ng W. K., et al. (2020b). Partial Replacement of Fishmeal With Clostridium Autoethanogenum Single-Cell Protein in the Diet for Juvenile Black Sea Bream (Acanthopagrus Schlegelii). Aquaculture Res. 51 (3), 1000–1011. doi: 10.1111/are.14446
Chen Y., Sun Z., Liang Z., Xie Y., Su J., Luo Q., et al. (2020c). Effects of Dietary Fish Oil Replacement by Soybean Oil and L-Carnitine Supplementation on Growth Performance, Fatty Acid Composition, Lipid Metabolism and Liver Health of Juvenile Largemouth Bass, Micropterus Salmoides. Aquaculture 516, 734596. doi: 10.1016/j.aquaculture.2019.734596
Chen G., Yin B., Liu H., Tan B., Dong X., Yang Q., et al. (2020a). Effects of Fishmeal Replacement With Cottonseed Protein Concentrate on Growth, Digestive Proteinase, Intestinal Morphology and Microflora in Pearl Gentian Grouper (♀ Epinephelus Fuscoguttatus×♂ Epinephelus Lanceolatu). Aquaculture Res. 51 (7), 2870–2884. doi: 10.1111/are.14626
Deng J., Kang B., Tao L., Rong H., Zhang X. (2013). Effects of Dietary Cholesterol on Antioxidant Capacity, non-Specific Immune Response, and Resistance to Aeromonas Hydrophila in Rainbow Trout (Oncorhynchus Mykiss) Fed Soybean Meal-Based Diets. Fish Shellfish Immunol. 34 (1), 324–331. doi: 10.1016/j.fsi.2012.11.008
Destoumieux D., Muñoz M., Cosseau C., Rodriguez J., Bulet P., Comps M., et al. (2000). Penaeidins, Antimicrobial Peptides With Chitin-Binding Activity, are Produced and Stored in Shrimp Granulocytes and Released After Microbial Challenge. J. Cell Sci. 113 (3), 461–469. doi: 10.1242/jcs.113.3.461
Dhar A. K., Piamsomboon P., Caro L. F. A., Kanrar S., Adami R. Jr., Juan Y.-S. (2019). First Report of Acute Hepatopancreatic Necrosis Disease (AHPND) Occurring in the USA. Dis. Aquat. Organisms 132 (3), 241–247. doi: 10.3354/dao03330
Duan Y., Wang Y., Liu Q., Dong H., Li H., Xiong D., et al. (2019). Changes in the Intestine Microbial, Digestion and Immunity of Litopenaeus Vannamei in Response to Dietary Resistant Starch. Sci. Rep. 9 (1), 1–10. doi: 10.1038/s41598-019-42939-8
Duan Y., Zhang J., Dong H., Wang Y., Liu Q., Li H. (2016). Effect of Desiccation and Resubmersion on the Oxidative Stress Response of the Kuruma Shrimp Marsupenaeus Japonicus. Fish Shellfish Immunol. 49, 91–99. doi: 10.1016/j.fsi.2015.12.018
Dvinge H., Bertone P. (2009). HTqPCR: High-Throughput Analysis and Visualization of Quantitative Real-Time PCR Data in R. Bioinformatics 25 (24), 3325–3326. doi: 10.1093/bioinformatics/btp578
Edgar R. C. (2013). UPARSE: Highly Accurate OTU Sequences From Microbial Amplicon Reads. Nat. Methods 10 (10), 996–998. doi: 10.1038/nmeth.2604
Emre Y., Kurtoğlu A., Emre N., Güroy B., Güroy D. (2016). Effect of Replacing Dietary Fish Oil With Soybean Oil on Growth Performance, Fatty Acid Composition and Haematological Parameters of Juvenile Meagre, A Rgyrosomus Regius. Aquaculture Res. 47 (7), 2256–2265. doi: 10.1111/are.12677
FAO. (2018). Food and Agriculture Organization of the United Nations. The State of World Fisheries and Aquaculture 2018 - Meeting the sustainable development goals. Rome. Licence: CC BY-NC-SA 3.0 IGO
Froehlich H. E., Jacobsen N. S., Essington T. E., Clavelle T., Halpern B. S. (2018). Avoiding the Ecological Limits of Forage Fish for Fed Aquaculture. Nat. Sustainability 1 (6), 298–303. doi: 10.1038/s41893-018-0077-1
Guo J., Guo B., Zhang H., Xu W., Zhang W., Mai K. (2016). Effects of Nucleotides on Growth Performance, Immune Response, Disease Resistance and Intestinal Morphology in Shrimp Litopenaeus Vannamei Fed With a Low Fish Meal Diet. Aquaculture Int. 24 (4), 1007–1023. doi: 10.1007/s10499-015-9967-7
Hauptman B. S., Barrows F. T., Block S. S., Gaylord T. G., Paterson J. A., Rawles S. D., et al. (2014). Evaluation of Grain Distillers Dried Yeast as a Fish Meal Substitute in Practical-Type Diets of Juvenile Rainbow Trout, Oncorhynchus Mykiss. Aquaculture 432, 7–14. doi: 10.1016/j.aquaculture.2014.03.026
He Y., Chi S., Tan B., Zhang H., Dong X., Yang Q., et al. (2017). Effect of Yeast Culture on Intestinal Microbiota of Litopenaeus Vannamei. J. Guangdong Ocean Univ. 37 (4), 21–27. doi: 10.3969/j.issn.1673-9159.2017.04.004
Henry M., Gasco L., Piccolo G., Fountoulaki E. (2015). Review on the Use of Insects in the Diet of Farmed Fish: Past and Future. Anim. Feed Sci. Technol. 203, 1–22. doi: 10.1016/j.anifeedsci.2015.03.001
He L., Qin Y., Wang Y., Li D., Chen W., Ye J. (2021). Effects of Dietary Replacement of Fish Oil With Soybean Oil on the Growth Performance, Plasma Components, Fatty Acid Composition and Lipid Metabolism of Groupers Epinephelus Coioides. Aquaculture Nutr. doi: 10.1111/anu.13292
Huang Q., Tan B., Dong X., Zhang S., Yang Q., Chi S. (2018). Effect of Chitosan Oligosaccharide and Mycotoxin Adsorbents on Intestinal Mucosa Structure and Gut Flora of Litopenaeus Vannamei. J. Fishery Sci. China 25 (2), 373–383. doi: 10.3724/SP.J.1118.2018.17236
Hu Y., Tan B., Mai K., Ai Q., Zhang L., Zheng S. (2011). Effects of Dietary Menhaden Oil, Soybean Oil and Soybean Lecithin Oil at Different Ratios on Growth, Body Composition and Blood Chemistry of Juvenile Litopenaeus Vannamei. Aquaculture Int. 19 (3), 459–473. doi: 10.1007/s10499-010-9361-4
Jiang X., Yao W., Yang H., Tan S., Leng X., Li X. (2021). Dietary Effects of Clostridium Autoethanogenum Protein Substituting Fish Meal on Growth, Intestinal Histology and Immunity of Pacific White Shrimp (Litopenaeus Vannamei) Based on Transcriptome Analysis. Fish Shellfish Immunol. 119, 635–644. doi: 10.1016/j.fsi.2021.10.005
Jin M., Xiong J., Zhou Q.-C., Yuan Y., Wang X.-X., Sun P. (2018). Dietary Yeast Hydrolysate and Brewer's Yeast Supplementation Could Enhance Growth Performance, Innate Immunity Capacity and Ammonia Nitrogen Stress Resistance Ability of Pacific White Shrimp (Litopenaeus Vannamei). Fish Shellfish Immunol. 82, 121–129. doi: 10.1016/j.fsi.2018.08.020
Ko E.-Y., Cho S.-H., Kwon S.-H., Eom C.-Y., Jeong M. S., Lee W., et al. (2017). The Roles of NF-κb and ROS in Regulation of Pro-Inflammatory Mediators of Inflammation Induction in LPS-Stimulated Zebrafish Embryos. Fish Shellfish Immunol. 68, 525–529. doi: 10.1016/j.fsi.2017.07.041
Koruk M., Taysi S., Savas M. C., Yilmaz O., Akcay F., Karakok M. (2004). Oxidative Stress and Enzymatic Antioxidant Status in Patients With Nonalcoholic Steatohepatitis. Ann. Clin. Lab. Sci. 34 (1), 57–62.
Kumar V., Habte-Tsion H. M., Allen K. M., Bowman B. A., Thompson K. R., El-Haroun E., et al. (2018). Replacement of Fish Oil With Schizochytrium Meal and its Impacts on the Growth and Lipid Metabolism of Pacific White Shrimp (Litopenaeus Vannamei). Aquaculture Nutr. 24 (6), 1769–1781. doi: 10.1111/anu.12816
Lai C.-Y., Cheng W., Kuo C.-M. (2005). Molecular Cloning and Characterisation of Prophenoloxidase From Haemocytes of the White Shrimp, Litopenaeus Vannamei. Fish Shellfish Immunol. 18 (5), 417–430. doi: 10.1016/j.fsi.2004.10.004
Lee S., Katya K., Hamidoghli A., Hong J., Kim D.-J., Bai S. C. (2018). Synergistic Effects of Dietary Supplementation of Bacillus Subtilis WB60 and Mannanoligosaccharide (MOS) on Growth Performance, Immunity and Disease Resistance in Japanese Eel, Anguilla Japonica. Fish Shellfish Immunol. 83, 283–291. doi: 10.1016/j.fsi.2018.09.031
Li M., Liang H., Xie J., Chao W., Zou F., Ge X., et al. (2021). Diet Supplemented With a Novel Clostridium Autoethanogenum Protein Have a Positive Effect on the Growth Performance, Antioxidant Status and Immunity in Juvenile Jian Carp (Cyprinus Carpio Var. Jian). Aquaculture Rep. 19, 100572. doi: 10.1016/j.aqrep.2020.100572
Lim C., Ako H., Brown C. L., Hahn K. (1997). Growth Response and Fatty Acid Composition of Juvenile Penaeus Vannamei Fed Different Sources of Dietary Lipid. Aquaculture 151 (1-4), 143–153. doi: 10.1016/S0044-8486(96)01500-1
Li E., Xu C., Wang X., Wang S., Zhao Q., Zhang M., et al. (2018). Gut Microbiota and its Modulation for Healthy Farming of Pacific White Shrimp Litopenaeus Vannamei. Rev. Fisheries Sci. Aquaculture 26 (3), 381–399. doi: 10.1080/23308249.2018.1440530
Manzanilla E., Perez J., Martin M., Kamel C., Baucells F., Gasa J. (2004). Effect of Plant Extracts and Formic Acid on the Intestinal Equilibrium of Early-Weaned Pigs. J. Anim. Sci. 82 (11), 3210–3218. doi: 10.2527/2004.82113210
Maulu S., Liang H., Ge X., Yu H., Huang D., Ke J., et al. (2021). Effect of Dietary Clostridium Autoethanogenum Protein on Growth, Body Composition, Plasma Parameters and Hepatic Genes Expression Related to Growth and AMPK/TOR/PI3K Signaling Pathway of the Genetically Improved Farmed Tilapia (GIFT: Oreochromis Niloticus) Juveniles. Anim. Feed Sci. Technol. 276, 114914. doi: 10.1016/j.anifeedsci.2021.114914
Mu H., Shen H., Liu J., Xie F., Zhang W., Mai K. (2018). High Level of Dietary Soybean Oil Depresses the Growth and Anti-Oxidative Capacity and Induces Inflammatory Response in Large Yellow Croaker Larimichthys Crocea. Fish Shellfish Immunol. 77, 465–473. doi: 10.1016/j.fsi.2018.04.017
National Research Council (2011). Nutrient Requirements of Fish and Shrimp (Washington, DC: National Academies Press 2011).
Niu J., Xie J.-J., Guo T.-Y., Fang H.-H., Zhang Y.-M., Liao S.-Y., et al. (2019). Comparison and Evaluation of Four Species of Macro-Algaes as Dietary Ingredients in Litopenaeus Vannamei Under Normal Rearing and WSSV Challenge Conditions: Effect on Growth, Immune Response, and Intestinal Microbiota. Front. Physiol. 9. doi: 10.3389/fphys.2018.01880
Oliva-Teles A., Enes P., Peres H. (2015). Replacing Fishmeal and Fish Oil in Industrial Aquafeeds for Carnivorous Fish. Feed Feeding Practices Aquaculture, 203–233. doi: 10.1016/b978-0-08-100506-4.00008-8
Peng X., Li F., Lin S., Chen Y. (2016). Effects of Total Replacement of Fish Oil on Growth Performance, Lipid Metabolism and Antioxidant Capacity in Tilapia (Oreochromis Niloticus). Aquaculture Int. 24 (1), 145–156. doi: 10.1007/s10499-015-9914-7
Pilotto M. R., Argenta N., Forte J. M., Hostins B., Menezes F. G. R., Maggioni R., et al. (2020). Environmental Rearing Conditions are Key Determinants of Changes in Immune Gene Expression Patterns in Shrimp Midgut. Dev. Comp. Immunol. 106, 103618. doi: 10.1016/j.dci.2020.103618
Rungrassamee W., Klanchui A., Maibunkaew S., Karoonuthaisiri N. (2016). Bacterial Dynamics in Intestines of the Black Tiger Shrimp and the Pacific White Shrimp During Vibrio Harveyi Exposure. J. Invertebrate Pathol. 133, 12–19. doi: 10.1016/j.jip.2015.11.004
Schloss P. D., Westcott S. L., Ryabin T., Hall J. R., Hartmann M., Hollister E. B., et al. (2009). Introducing Mothur: Open-Source, Platform-Independent, Community-Supported Software for Describing and Comparing Microbial Communities. Appl. Environ. Microbiol. 75 (23), 7537–7541. doi: 10.1128/AEM.01541-09
Shen L., Chen Z., Chen C., He X. (2007). Growth and Immunities of Shrimp, Litopenaeus Vannamei (Boone) Exposed to Different Salinity Levels. J. Jimei Univ. (Natural Science) 12 (2), 108–113. doi: 10.19715/j.jmuzr.2007.02.003
Söderhäll K., Cerenius L. (1998). Role of the Prophenoloxidase-Activating System in Invertebrate Immunity. Curr. Opin. Immunol. 10 (1), 23–28. doi: 10.1016/s0952-7915(98)80026-5
Song L., Hu B., Wang A., Mao S., Zhang Y., Pan X. (2010). Effects of Antibacterial Peptide on Growth and Immunity of Penaeus Vannamei. J. Guangdong Ocean Univ. 30 (03), 28–32.
Sorieul L., Wabete N., Ansquer D., Mailliez J.-R., Pallud M., Zhang C., et al. (2018). Survival Improvement Conferred by the Pseudoalteromonas Sp. NC201 Probiotic in Litopenaeus Stylirostris Exposed to Vibrio Nigripulchritudo Infection and Salinity Stress. Aquaculture 495, 888–898. doi: 10.1016/j.aquaculture.2018.06.058
Su C., Liu X., Li J., Zhang M., Pan L., Lu Y., et al. (2021). Effects of Bile Acids on the Growth Performance, Lipid Metabolism, non-Specific Immunity and Intestinal Microbiota of Pacific White Shrimp (Litopenaeus Vannamei). Aquaculture Nutr. 27 (6), 2029–2041. doi: 10.1111/anu.13338
Sun Z., Xuan Y., Zhang H., Jiang M., Pan Y., Zhang Y., et al. (2016). Bacterial Diversity in the Penaeus Vannamei Boone Intestine and Aquaculture Environment. J. Fish Sci. China 32, 594–605. doi: 10.3724/SP.J.1118.2016.15255
Tam A. B., Mercado E. L., Hoffmann A., Niwa M. (2012). ER Stress Activates NF-κb by Integrating Functions of Basal IKK Activity, IRE1 and PERK. PloS One 7 (10), e45078. doi: 10.1371/journal.pone.0045078
Tassanakajon A., Somboonwiwat K., Supungul P., Tang S. (2013). Discovery of Immune Molecules and Their Crucial Functions in Shrimp Immunity. Fish Shellfish Immunol. 34 (4), 954–967. doi: 10.1016/j.fsi.2012.09.021
Turchini G. M., Torstensen B. E., Ng W. K. (2009). Fish Oil Replacement in Finfish Nutrition. Rev. Aquaculture 1 (1), 10–57. doi: 10.1111/j.1753-5131.2008.01001.x
Vargas-Albores F., Yepiz-Plascencia G. (2000). Beta Glucan Binding Protein and its Role in Shrimp Immune Response. Aquaculture 191 (1-3), 13–21. doi: 10.1016/s0044-8486(00)00416-6
Wang Q., Garrity G. M., Tiedje J. M., Cole J. R. (2007). Naive Bayesian Classifier for Rapid Assignment of rRNA Sequences Into the New Bacterial Taxonomy. Appl. Environ. Microbiol. 73 (16), 5261–5267. doi: 10.1128/AEM.00062-07
Wang H., Wang C., Tang Y., Sun B., Huang J., Song X. (2018). Pseudoalteromonas Probiotics as Potential Biocontrol Agents Improve the Survival of Penaeus Vannamei Challenged With Acute Hepatopancreatic Necrosis Disease (AHPND)-Causing Vibrio Parahaemolyticus. Aquaculture 494, 30–36. doi: 10.1016/j.aquaculture.2018.05.020
Wei H., Huanhuan Y. U., Chen X., Chao W., Zou F., Chen P., et al. (2018). Effects of Soybean Meal Replaced by Clostridium Autoethanogenum Protein on Growth Performance,Plasma Biochemical Indexes and Hepatopancreas and Intestinal Histopathology of Grass Carp(Ctenopharyngodon Idllus). Chin. J. Anim. Nutr. 30, 4190–4201 doi: 10.3969/j.issn.1006-267x.2018.10.045
Wu Y., Li R., Shen G., Huang F., Yang Q., Tan B., et al. (2021). Effects of Dietary Small Peptides on Growth, Antioxidant Capacity, Nonspecific Immunity and Ingut Microflora Structure of Litopenaeus Vannamei. J. Guangdong Ocean Univ. 41 (5), 1–9. doi: 10.3969/j.issn.1673-9159.2021.05.001
Xie S., Liu Y., Tian L., Niu J., Tan B. (2020a). Low Dietary Fish Meal Induced Endoplasmic Reticulum Stress and Impaired Phospholipids Metabolism in Juvenile Pacific White Shrimp, Litopenaeus Vannamei. Front. Physiol. 11. doi: 10.3389/fphys.2020.01024
Xie S., Wei D., Tan B., Liu Y., Tian L., Niu J. (2020b). Schizochytrium Limacinum Supplementation in a Low Fish-Meal Diet Improved Immune Response and Intestinal Health of Juvenile Penaeus Monodon. Front. Physiol. 11. doi: 10.3389/fphys.2020.00613
Xie S., Wei D., Tian L., Liu Y. (2021). Dietary Supplementation of Chenodeoxycholic Acid Improved the Growth Performance, Immune Response and Intestinal Health of Juvenile Penaeus Monodon Fed a Low Fish-Meal Diet. Aquaculture Rep. 20, 100773. doi: 10.1016/j.aqrep.2021.100773
Xie S., Zhou W., Tian L., Niu J., Liu Y. (2016). Effect of N-Acetyl Cysteine and Glycine Supplementation on Growth Performance, Glutathione Synthesis, Anti-Oxidative and Immune Ability of Nile Tilapia, Oreochromis Niloticus. Fish Shellfish Immunol. 55, 233–241. doi: 10.1016/j.fsi.2016.05.033
Xu S., Wang S., Zhang L., You C., Li Y. (2012). Effects of Replacement of Dietary Fish Oil With Soybean Oil on Growth Performance and Tissue Fatty Acid Composition in Marine Herbivorous Teleost S Iganus Canaliculatus. Aquaculture Res. 43 (9), 1276–1286. doi: 10.1111/j.1365-2109.2011.02931.x
Yang P., Li X., Song B., He M., Wu C., Leng X. (2021). The Potential of Clostridium Autoethanogenum, a New Single Cell Protein, in Substituting Fish Meal in the Diet of Largemouth Bass (Micropterus Salmoides): Growth, Feed Utilization and Intestinal Histology. Aquaculture Fisheries. doi: 10.1016/j.aaf.2021.03.003
Yao W., Yang P., Zhang X., Xu X., Zhang C., Li X., et al. (2022). Effects of Replacing Dietary Fish Meal With Clostridium Autoethanogenum Protein on Growth and Flesh Quality of Pacific White Shrimp (Litopenaeus Vannamei). Aquaculture 549, 737770. doi: 10.1016/j.aaf.2021.03.003
Zeng F., Rabbi M. H., Hu Y., Li Z., Ren X., Han Y., et al. (2021). Synergistic Effects of Dietary Selenomethionine and Vitamin C on the Immunity, Antioxidant Status, and Intestinal Microbiota in Sea Cucumber (Apostichopus Japonicus). Biol. Trace Element Res. 199 (10), 3905–3917. doi: 10.1007/s12011-020-02483-3
Zhou Z., Ringø E., Olsen R., Song S. (2018). Dietary Effects of Soybean Products on Gut Microbiota and Immunity of Aquatic Animals: A Review. Aquaculture Nutr. 24 (1), 644–665. doi: 10.1111/anu.12532
Zhu S., Gao W., Wen Z., Chi S., Shi Y., Hu W., et al. (2022). Partial Substitution of Fish Meal by Clostridium Autoethanogenum Protein in the Diets of Juvenile Largemouth Bass (Micropterus Salmoides). Aquaculture Rep. 22, 100938. doi: 10.1016/j.aqrep.2021.100938
Zhu W., Tan P., Lou B., Chen R., Wang L., Xu D. (2020). Supplementation of a Soybean Oil-Based Diet With Tributyrin Influences Growth, Muscle Composition, Intestinal Morphology, and Expression of Immune-Related Genes of Juvenile Yellow Drum (Nibea Albiflora Richardson 1846). Aquaculture Int. 28 (5), 2027–2043. doi: 10.1007/s10499-020-00572-7
Zhu H., Yu D., Wang G., Xie J. (2006). Effects of Vibrio Parahaemolyticus, LPS and Pediococcus Acidilactici on Nitric Oxide Synthase Activity of Litopenaeus Vannamei. J. Trop. Oceanography 25, 27–32.
Zokaeifar H., Balcázar J. L., Saad C. R., Kamarudin M. S., Sijam K., Arshad A., et al. (2012). Effects of Bacillus Subtilis on the Growth Performance, Digestive Enzymes, Immune Gene Expression and Disease Resistance of White Shrimp, Litopenaeus Vannamei. Fish Shellfish Immunol. 33 (4), 683–689. doi: 10.1016/j.fsi.2012.05.027
Zuo R., Ai Q., Mai K., Xu W., Wang J., Xu H., et al. (2012). Effects of Dietary N-3 Highly Unsaturated Fatty Acids on Growth, Nonspecific Immunity, Expression of Some Immune Related Genes and Disease Resistance of Large Yellow Croaker (Larmichthys Crocea) Following Natural Infestation of Parasites (Cryptocaryon Irritans). Fish Shellfish Immunol. 32 (2), 249–258. doi: 10.1016/j.fsi.2011.11.005
Keywords: Clostridium autoethanogenum protein, lipid sources, Pacific white shrimp (Litopenaeus vannamei), growth, immunity, intestinal morphology, intestinal microbiota
Citation: Zheng C, Gong S, Cao J, Dong X, Chi S, Yang Q, Liu H, Zhang S, Xie S and Tan B (2022) Effects of Dietary Lipid Sources on Alleviating the Negative Impacts Induced by the Fishmeal Replacement With Clostridium autoethanogenum Protein in the Diet of Pacific White Shrimp (Litopenaeus vannamei). Front. Mar. Sci. 9:879364. doi: 10.3389/fmars.2022.879364
Received: 19 February 2022; Accepted: 11 March 2022;
Published: 31 March 2022.
Edited by:
Seyed Hossein Hoseinifar, Gorgan University of Agricultural Sciences and Natural Resources, IranReviewed by:
Hongyan Tian, Yancheng Institute of Technology, ChinaXiaodan Wang, East China Normal University, China
Copyright © 2022 Zheng, Gong, Cao, Dong, Chi, Yang, Liu, Zhang, Xie and Tan. This is an open-access article distributed under the terms of the Creative Commons Attribution License (CC BY). The use, distribution or reproduction in other forums is permitted, provided the original author(s) and the copyright owner(s) are credited and that the original publication in this journal is cited, in accordance with accepted academic practice. No use, distribution or reproduction is permitted which does not comply with these terms.
*Correspondence: Shiwei Xie, eHN3enNkeEAxNjMuY29t; Beiping Tan, YnB0YW5AMTI2LmNvbQ==