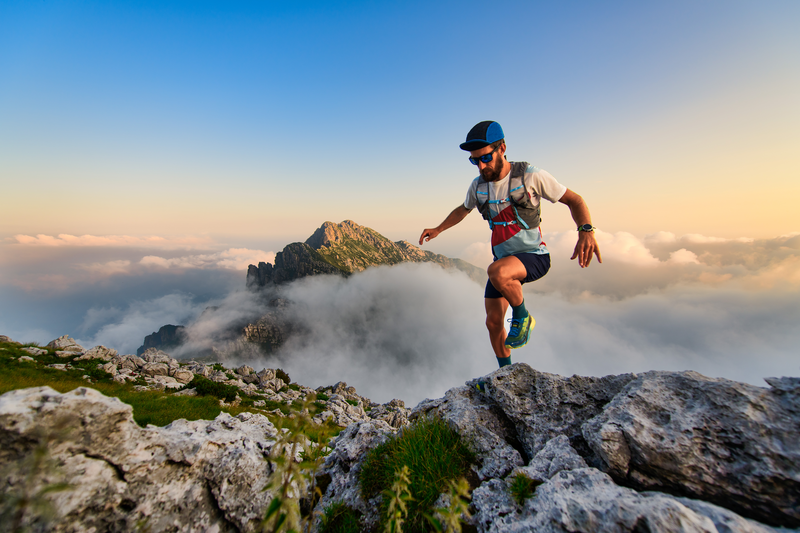
95% of researchers rate our articles as excellent or good
Learn more about the work of our research integrity team to safeguard the quality of each article we publish.
Find out more
ORIGINAL RESEARCH article
Front. Mar. Sci. , 10 May 2022
Sec. Marine Conservation and Sustainability
Volume 9 - 2022 | https://doi.org/10.3389/fmars.2022.877325
The need for habitat recovery in coastal areas, especially those subjected to intensive exploitation, has increased because of significant historical loss and alteration of habitats and, therefore, adverse ecological impacts. The present study defines a stepwise approach to mitigate habitat loss in deep coastal environments, describing the planning, intervention, and monitoring phases following the Trans Adriatic Pipeline (TAP) laying along the Apulian coast (Adriatic Sea, Italy). Preliminary field activities encompassed morpho-bathymetric data (MBES and SSS), SCUBA and ROV observations to accurately map and characterize the mesophotic reefs built by invertebrates found in the area. The pipeline route interfered with 30 outcrops between 50 and 80 m depth, mostly colonized by 15 taxa/morphological groups. A functional/conservative approach was adopted to recognize the taxa/morphological groups on which to focus the removal and following relocation activities based on their abundance, conservation status, and functional traits. Saturation divers teams, ROV pilot technicians, and researchers collaborated to minimize the physical impact and the loss of organisms due to the pipeline installation. They relocated a total of 899 living portions (nuclei) from the 30 interfered reefs on the top of the pipeline. The following monitoring activities, carried out after fourteen months since the intervention, revealed a high mean survival rate (88.1%) and slight variations in the structure of the nuclei assemblages. This study represents a paradigmatic case of involvement and support of the private oil and gas sector to mitigate habitat loss in the Mediterranean Sea, and stresses the need for integrated management involving different stakeholders to mitigate the effects of the exploitation of marine resources through ante operam assessment and active restoration actions.
The synergic pressures of human activities and climate change threaten the structure and functioning of marine ecosystems, leading to habitats depletion and endangering all life in the worldwide seas. Cumulative impact assessment studies on key taxa or ecosystems have dramatically updated information for the Mediterranean Sea, where up to the 99% of the territorial waters of EU member states are subjected to high impact, and less than 1% of the surface is relatively unaffected by human activities (Micheli et al., 2013; Gerovasileiou et al., 2019). The effects of the multiple drivers that lead to the degradation of habitats within the European seas will increase in the next few decades without a sustainable approach to using natural resources, large-scale actions to mitigate human impacts through ecosystem-based management, and effective restoration programs (Danovaro et al., 2021).
The restoration ecology encompasses a wide range of concepts driving different actions to improve the recovery of habitats and ecosystems after natural or anthropogenic changes (Elliott et al., 2007). Within the restoration ecology, the mitigation aims to reduce and offset the environmental impacts deriving from development projects through conservation or active measures. It refers to in situ actions to make an impact less severe and represents a way of reconciling economic development and biodiversity conservation (Jacob et al., 2018). On the other hand, when the rehabilitation occurs in another system rather than the impacted one (i.e., ex situ creation of habitats) or after the habitat or ecosystem loss due to disturbances we should refer to compensation (Elliott et al., 2007). Following the Impact Assessment Directive (2014/52/EU) and national laws of EU members, marine development projects are subject to Environmental Impact Assessment (EIA) or Strategic Environmental Assessment (SEA). When the works are expected to cause environmental impacts on priority habitats, EIA often requests mitigation measures that permit or consent for any projects carried out at sea (Elliott and Cutts, 2004; Wood, 2014). The current Italian legislative framework imposes mitigation and compensation measures within certain limits of authorized impact, following the cost-benefit analysis of a given project. Under this scenario, transplantations of Posidonia oceanica (L.) Delile, 1813 shoots have been carried out in Italian waters as a suitable measure to mitigate or compensate for the impacts caused by coastal maritime works (Mancini et al., 2021; Zenone et al., 2021).
The Mediterranean Sea is relatively poorly affected by the oil and gas industry’s impacts if compared to other basins (Micheli et al., 2013; Fraschetti et al., 2016), although the explorations and exploitations of the deep sea are increasing concerns worldwide (Cordes et al., 2016). If the major threats are related to episodic and unpredictable oil spills, the installation of structures on the seafloor (i.e., oil rigs, pipelines, and anchors) is harmful to marine benthic communities due to physical disturbance and sediment resuspension. Along the Italian coasts, the installation of methane pipelines was reported as impacting the shallow water habitats by removing seagrass meadows and modifying the substrate features and sedimentary processes (Di Carlo et al., 2005; Badalamenti et al., 2006). Consequently, several interventions to recover the meadows impacted by these coastal infrastructures have been carried out (Alagna et al., 2019; Alagna et al., 2020; Calvo et al., 2020). Subsea pipelines are a crucial component of oil and gas installations, being the pathways used for natural gas and liquid petroleum transportation. Pipelines are made of steel tube sections - generally 12 m long - with concrete coatings joined together to cover long distances; they can be installed directly on the seafloor or within trenches and tunnels. Several studies highlighted how submerged oil and gas installations act as artificial reefs, providing hard substrates for benthic organisms colonization and attracting fish fauna (Jensen, 2002; Consoli et al., 2013; Rouse et al., 2018; Rouse et al., 2019; Redford et al., 2021). Such evidence offers alternatives for the decommissioning process of subsea platforms and pipelines that should be carefully evaluated, considering opportunities given by in situ decommissioning and the potential impacts of removal activities (Macreadie et al., 2011; Burdon et al., 2018).
The Trans Adriatic Pipeline (TAP), the Trans-Anatolian Natural Gas Pipeline (TANAP), and SCP (South Caucasus Pipeline) are the three segments of the Southern Gas Corridor, a 3,500‐kilometer-long gas value chain to transport natural gas from the Caspian basin to Europe (Figure 1; https://www.tap-ag.com/). It has been named a Project of Common Interest for Europe’s energy future, and it has several implications in the geopolitical scenario involving the EU, USA, and Russia. TAP crosses Greece, Albania, and the Adriatic Sea: natural hazards (Marinos et al., 2019; Slejko et al., 2021), local conflicts (Mocavini, 2019), archaeological findings (Bejko, 2020), and environmental matters arose during planning (2008-2016) and construction phase (2016-2020). As for the latter issue, the presence of protected and vulnerable marine ecosystems along the Apulian coasts, where the final section of the pipeline was located, imposed the development of biodiversity conservation measures to avoid or mitigate impacts on natural habitats. In particular, mesophotic reefs structured by hexacorals, bivalves, serpulids, and bryozoans create a mosaic of bioconstructions in the area at depths between 30 and 70 m (Bracchi et al., 2017; Corriero et al., 2019; Piazzi et al., 2019; Cardone et al., 2020; Giampaoletti et al., 2020). These invertebrates can build structurally complex and taxonomically rich bioconstructions by playing the role of primary builders, such as calcareous red algae belonging to the Corallinales and Peyssonneliales in the coralligenous reefs. The heterotrophic dominance shown by these reefs contributes to diversifying the panorama of coastal Mediterranean bioconstructions (Ingrosso et al., 2018), attracting increasing interest from scientists, and stressing the need for their protection and conservation. Following the EIA emitted for TAP, the Italian Ministry of the Environment imposed ad hoc mitigation measures, also based on improvement proposals directly made by TAP, encompassing both monitoring and active actions to reduce the impact on mesophotic reefs,.
Figure 1 The upper figure shows the whole route of the Southern Gas Corridor with the TAP, TANAP and SCP segments highlighted in yellow-blue, violet, and green respectively (modified from https://www.tap-ag.com/), and the location of the intervention site. The lower map shows the shallower TAP section along the Italian coast: the pipeline entry point in the microtunnel is indicated by a blue asterisk, the pipeline’s footprint is highlighted in red. The seabed morphology up to 80 m depth has been obtained through MBES data.
The present work examines the approach, decision-making, tools, and technologies applied to mitigate the interference between TAP pipeline and mesophotic reefs built by invertebrates, stressing the policy relevance of mitigation interventions following human underwater constructions/installations. Through a detailed description, this unique case study outlines the planning, intervention, and monitoring phases that constitute a paradigmatic example of oil and gas industry involvement, together with scientific researchers, in mitigating the loss of Mediterranean benthic habitats. The scope of the intervention was to avoid the loss of organisms relocating living portions of the bioconstructions, certainly affected during the installation works, on the concrete coating of the pipeline. We expected that the proposed approach will reduce the impact on the benthic habitats (mitigating the impact of man-made structure without considering a donor site except for those affected by the pipeline installation), reflect the ecological complexity of the mesophotic reefs, and facilitate the presence of slow-growing species of high ecological/conservation concern as fouling on the pipeline.
The study area was located a few kilometers north of the little harbor of San Foca, along the southern Adriatic coast of Italy (Figure 1; 18°24’29.726”E 40°19’10.829”N): the site represents the pipeline’s landfall on the Italian coast. The intervention site was chosen in 2012 from among twelve design alternatives, also due to the narrow distance among Italian and Albanian shores and seafloor morphological features. Furthermore, the absence of continuous P. oceanica meadows and rocky reefs, and therefore the absence of Sites of Community Interest (SCIs), contributed to the choice of the site (Regione Puglia, 2006; Costantino et al., 2010). Preliminary field activities for the construction phase were carried out between winter and spring of 2017 along the Apulian coasts to comply with the prescription of the Italian Ministry of the Environment that imposed the mapping of benthic habitats (biogenic reefs in particular) in the proximity of the pipeline laying area. High-resolution (0.2 m) multibeam sonar imagery and side-scan sonar data (Kongsberg EM 2040 MBES and Edgetech DSS2000 SSS, respectively) were collected over a corridor of 800 m along the whole pipeline route, 400 m on both north and south sides, to gain the morpho-bathymetric map of the area covering 2.65 km2. The present work considers this shallowest TAP section along the Italian coast up to 80 m depth. The digital terrain model (DTM, 0.5 × 0.5 m grid size, projection WGS84, UTM Zone 34N), obtained from the bathymetric profiles, and the sonar mosaics were then imported into the geographic information system (GIS ESRI, ArcMap 10.4). The geomorphological map was validated through both scuba-diving (performed up to 40 m depth by scientific divers equipped with HD cameras) and Remotely Operated Vehicle (ROV, Panther Seaeye equipped with High-Definition camera with resolution of 1920×1080 pixels) surveys, to draw a bionomic map of the area of interest between 5 and 80 m depth and detect the habitats and the species of conservation concern colonizing the seafloor.
Several biogenic outcrops were found within the investigated area deeper than 30 m. The characterization of the assemblages was carried out by dividing the outcrops into three depth layers identified according to the distribution (visually assessed from DTM), number, area, perimeter, and elevation of the outcrops (data and methods are provided as Supplementary materials, Table S1): 30-49 m (hereafter called A), 50-69 m (B), and 70-80 m (C). Within each depth layer, 25 outcrops were selected as representative of the whole bathymetric range to be photographed during ROV surveys, for a total of 75 outcrops. A photographic sample of approximately 1 m2 of surface was acquired for each of the 75 outcrops; the samples were selected and analyzed to assess the percentage cover of the main taxonomical units. Photographic samples were analyzed by freehand region drawing technique through ImageJ software (Piazzi et al., 2019).
The map of the benthic habitats of the area was adopted to identify the portion of the seabed where the TAP pipeline route interfered with the outcrops: we considered as interfered those outcrops located within the pipeline designed route, therefore, that would have been physically impacted by the TAP laying operations. It should be noted that the restrictions given by the pipeline design implied the impossibility of working around each biogenic outcrop. The modern pipeline laying technologies allowed for a laying error extremely low. Furthermore, the TAP pipeline was laid slower than the average laying speed, and guided by ROV, to fit as much as possible within the designed footprint. This produced a maximum deviance of 0.8 m measured at the end of the installation operations compared to the project route.
For a fine-scale analysis of the assemblages colonizing the biogenic reefs, ROV video footage was carried out on all the sides of the outcrops interfered by the pipeline installation. ROV surveys were carried out in the spring of 2018 through a Work Class ROV installed onboard the IEVOLI COBALT (Offshore Tug/Supply vessel). The Work Class ROV was equipped with HD cameras and laser lines spaced 1 m. Videos were analyzed through a visual assessment (VA) approach to characterize the outcrop assemblages (Casoli et al., 2017), measuring the percentage cover of the main conspicuous taxa/morphological groups. The organisms easily recognizable by VA were reported at the species level, whereas the others were categorized into taxonomical or morphological groups following Piazzi et al. (2017, 2019). The percentage cover of the taxa/morphological groups was visually estimated through the semi-quantitative Braun–Blanquet categories (Westhoff and van der Maarel, 1978): 0 = absent; 1 = occasionally present, <5%; 2 = 5–25% of coverage; 3 = 26–50%; 4 = 51–75%; 5 = >75%.
We collected and assigned information on the conservation status of each of the identified taxa/morphological groups, comparing those reported in the Habitat Directive (HD), the IUCN Red Lists of Threatened Species, and additional agreements (Berne and Barcelona Conventions, SPA/BD Protocol, CITES) to consider the most significant number of taxa, and assess the relevance of the intervention focusing on the taxa of conservation concern.
Furthermore, we estimate the functional traits of each taxon found on the interfered outcrops to assess their importance in the growth and three-dimensionality of the bioconstruction. Although no a priori information exists on the functional role of the taxa/morphological groups within the reefs, as well as on the traits that are related to these functions, we followed the approach reported by Doxa et al. (2016) when considering a set of traits of the coralligenous species accounting for morphology, size, role within the reefs (Fagerstrom, 1991), aggregation, and distribution. The list of traits taken into consideration is reported in the Supplementary material Table S2. The information used to fill the functional database were obtained from the existing bibliography (Garrabou, 1999; Ballesteros, 2006; Coppari et al., 2014; Casoli et al., 2016; Gerovasileiou et al., 2018; Ingrosso et al., 2018; Longo et al., 2018; Corriero et al., 2019; Cardone et al., 2020; Cardone et al., 2020; Giampaoletti et al., 2020; Azzola et al., 2021; Canessa et al., 2021; Gravina et al., 2021), the expertise of the team members, and by consulting other experts. Finally, a relocation score was assigned to each taxon/morphological group summing the most frequent abundance category obtained through VA, its inclusion in directives, agreements, or protocols to enhance its conservation status, and the values calculated through functional trait analysis. The relocation score allowed the identification of the taxa for which the relocation activities were of primary concern, according to their frequency of occurrence, conservation status, and role in the growth and functioning of the reef.
All the technologies usually employed in the oil and gas industry were applied for the removal and relocation activities. The targets of the interventions were bioconstruction portions (hereafter referred to as nuclei) mostly colonized by taxa/morphological groups of primary ecological/conservation concern defined through the application of the relocation score. Saturation divers teams, ROV pilots and technicians, and marine ecologists were boarded on EDT PROTEA research vessel during the following operational phases:
i) December 2019 – February 2020. The nuclei were manually removed from the 30 interfered outcrops by using hammer and chisel to achieve a high degree of accuracy during the removal and reduce as much as possible damages to the benthic organisms. All the activities were followed remotely by onboard researchers that chose the nuclei to be removed by watching real-time diver and ROV cameras, leading saturation divers activities.
ii) February 2020 – March 2020. The nuclei were temporarily placed on underwater iron grid tables to allow water flows to ensure filter-feeding, waiting for pipeline laying and the following relocation. To avoid interferences during the installation, the iron tables were positioned at the same depths as the outcrops from which the nuclei were removed, a few tens of meters away from the pipeline’s route.
iii) March 2020 – April 2020. After the pipeline laying occurred between February 2020 and March 2020, the nuclei were relocated on the top of the pipeline using epoxy resin Milliput Standard Yellow-Grey type, which has been recently reported as effective and biocompatible epoxy putty to attach benthic colonial organisms (Casoli et al., 2022). The nuclei were relocated on 17 pipeline segments measuring 10 m in length close to the interfered bioconstruction from which they were removed.
A non-destructive sampling method was used to monitor the relocated nuclei assessing i) survival rate and health status and ii) diversity and structure of the nuclei assemblages. Survival rate was defined as the ratio, calculated as a percentage, between the number of living (i.e., colonized by living organisms) and attached nuclei and the total number of nuclei relocated in April 2020. Health status was assessed by calculating the number of colonies affected by necrosis phenomena and the maximum extent of injury reported, following the procedures defined in monitoring protocols for the coralligenous community (Garrabou et al., 2014). Finally, in order to characterize the diversity and the structure of the assemblages over time, we considered taxa/morphological groups richness and abundance: the former was defined as the number of taxa/morphological groups found on the nuclei during each monitoring event, and the latter was expressed as the frequency of occurrence of each taxon/morphological group on the relocated nuclei.
Monitoring activities were carried out in three separate events (times): at the end (April 2020), one month after (May 2020), and fourteen months after (July 2021) the relocation activities. The monitoring covered 170 m over the 760 m of pipeline length between 50 and 80 m depth (within B and C depth layers), corresponding to the 17 pipeline segments where the nuclei were relocated.
Monitoring activities were carried out using the ROV Falcon (SAAB - Seaeye) equipped with the underwater positioning system Ultra Short Base Line System (USBL), depth sensor, compass, and High-Definition (HD) camera. The ROV’s equipment allowed us to obtain accurate georeferenced positions every second during the surveys. As for video acquisition, ROV moved at a constant speed of less than 0.3 m sec-1 and a distance from the pipeline lower than 1.0 m, as recommended for the ROV exploration and health status assessment of megabenthic communities up to 200 m depth (Enrichetti et al., 2019a; Enrichetti et al., 2019b). The monitoring descriptors explained above were calculated for the 10 m sampling areas representing the pipeline sections where nuclei were relocated. No monitoring was performed in the A depth layer due to the absence of interfered outcrops.
The differences in the biogenic reefs assemblages according to depth layers were visualized through a Principal Component Analysis (PCA) that was carried out on taxonomical groups percentage coverage estimated during ROV preliminary survey. An Analysis of Similarities (ANOSIM) was carried out on a Bray-Curtis similarity matrix calculated on the above-mentioned data to test differences among the depth layers.
As for the monitoring of relocation activities, the variation in the structure of the nuclei assemblages among time (sampling events, as fixed factor: April 2020, May 2020, and July 2021), depth layers (B and C, as fixed factor), and the interaction of these two factors was tested using Multivariate permutational analyses of variance (PERMANOVA) on the frequency of occurrence data of each taxon/morphological group on the relocated nuclei. Furthermore, pairwise tests were used to discriminate differences among time (the three monitoring events: April 2020, May 2020, and July 2021). Finally, a similarity percentage—species contribution analysis (SIMPER) was carried out to identify the taxa/morphological groups that mainly contributed to the dissimilarity among nuclei assemblages relocated in the two depth layers.
In both ANOSIM and PERMANOVA, a level of significance of 0.05 (p-value < 0.05) was chosen. All statistical analyses were performed in the R platform (version 4.1.0).
The 2.65 km2 of seabed surface mapped during the preliminary surveys (Figure 2) was characterized by P. oceanica meadows colonizing a small area up to 15 m depth, in proximity to the two separated rocky ridges developing perpendicularly to the coastline. Few isolated patches of P. oceanica on dead matte were also localized close to the lower limit of the Cymodocea nodosa meadow which formed a continuous belt parallel to the coast on soft sandy bottoms from 5 up to 25 m depth. Deeper than 25 m depth, coarse detritic sediments cover the seabed with sparse outcrops between 30 to 80 m depth. A total of 4,507 outcrops with variable sizes (mean size ± SD of 6.41 ± 10.80 m2) were spread over the coarse detritic sediment bottoms and covered approximately 0.78 km2. These outcrops reflected the mosaic of coralligenous habitats on coarse detritic bottom as described by Bracchi et al. (2017). From 30 to 49 m depth (A depth layer), the assemblages found on the outcrops were mainly composed of encrusting red algae (belonging to the genera Lithophyllum, Mesophyllum, and Peyssonnelia) together with benthic invertebrates acting as secondary builders, such as serpulids, solitary and colonial scleractinians, and encrusting bryozoans.
Figure 2 Map of the benthic habitats identified along the TAP shallower section in front of the Italian coast, from 5 up to 80 m depth. The pipeline entry point in the microtunnel is indicated by a blue asterisk, the pipeline’s footprint is highlighted in red.
In this bathymetric layer, the erect bryozoan Schizoretepora serratimago, the aggregations of the serpulids Salmacina/Filograna complex, and the erect sponges of the genus Axinella structured the upper layer of the bioconstruction. Between 50 and 69 m depth (B depth layer), the assemblages were characterized by the decrease or disappearance of encrusting red algae and erect bryozoans, whereas, Axinella verrucosa, Parazohantus axinellae, scleractinians, hydrozoans, and the encrusting ascidian Diplosoma spongiforme dominated the assemblages. Sedimentation increased according to depth; the reduction of the surface covered by organisms was higher on the deep outcrops if compared to those developing in A depth layer. Fine sediment affected outcrops deeper than 70 m depth (C depth layer), reducing the richness of the assemblage and the portion of the seabed covered: this was favored by the relatively small size and height (only a few centimeters or tens of centimeters) of the outcrops. In this bathymetric layer, the higher coverage values were reported for erect sessile invertebrates, such as the serpulids belonging to the Salmacina/Filograna complex, hydrozoans, and erect sponges of the genus Axinella. The described differences were evident on the factorial plane of the PCA (39.69% of the total variance explained) analysis, where samples were clearly separated according to the three depth layers (Figure 3). The ANOSIM revealed significant differences in the composition of the outcrops assemblages among the three depth layers (R = 0.700; p < 0.01).
Figure 3 Results of the Principal Component Analysis (PCA) carried out on the structure of the assemblages colonizing outcrops over the study area: (A) Biplot showing sampling points and taxonomical units (solid lines with arrows); (B) sampling points grouped according to depth layers; (C) Pictures acquired during ROV preliminary surveys showing the outcrop assemblages in each of the three depth layers (scale bars refer to 10 cm).
The pipeline laying interfered with 30 outcrops, 21 located in the B depth layer and 9 in the C depth layer, respectively (Figure 4). No segments of the pipeline route interfered with the outcrops in the A depth layer. A total of 15 taxa/morphological groups were recorded through HD video surveys on the 30 interfered outcrops. Hydrozoans, Diplosoma spongiforme, scleractinians, Axinella verrucosa, and P. axinellae were the most abundant taxa/morphological groups, showing cover percentages ranging from 5 to 50% (Supplementary Figure S1). The ascidians D. spongiforme was reported as the only taxon exceeding the percentage cover of 50% in two outcrops located in the B depth layer. From an operational point of view, not all the taxa found on the 30 outcrops interfered by the pipeline were suitable for relocation activities due to the size, consistency of their structures, and abundance. A functional/conservative approach was adopted to recognize the taxa/morphological groups on which to focus the interventions based on their abundance, conservation status, and functional traits. Furthermore, the approach had to reflect the diversity and composition of the outcrop assemblages. The information gathered was summarized in Table 1. A total of 7 taxa/morphological groups showed a relocation score equal to or greater than 10 (Figure 5). This value has been conventionally designated as a threshold to distinguish taxa of high ecological/conservation concern on which focusing efforts. This means that each portion of the interfered outcrops colonized by A. verrucosa, A. polypoides, A. cannabina, P. axinellae, scleractinians, and S. serratimago had to be removed and subsequently relocated on the pipeline, to reduce as much as possible the losses of these taxa due to the laying of the TAP pipeline. All the other taxa were only secondarily included in the relocation operations, as they colonized concretion portions close to the taxa of primary ecological/conservation concern.
Figure 4 Maps of the 30 interfered outcrops (highlighted in yellow) distributed along the TAP pipeline route, from shallower to deeper parts.
Table 1 List of the values assigned to each of the taxa/morphological groups found on the 30 interfered outcrops and summed to calculate the relocation score used to identify the primary ecological/conservation concern organisms.
Figure 5 Relocation score assigned to the taxa/morphological groups composing the interfered outcrop assemblages. The dashed line marks the threshold value of 10; the taxa of high ecological/conservation concern on which focusing efforts have been highlighted in green.
During March and April 2020, a total of 899 nuclei with variable dimensions, ranging from 100 cm2 up to 1600 cm2, were removed and then relocated on the top of the pipeline during the operational phases (showed in Figure 6). The 10 m pipeline segments, where the nuclei were relocated, were distributed as follows: 11 in the B depth layer and 6 in the C depth layer reflecting the position of the interfered outcrops.
Figure 6 Photo-table showing the three different phases of the removal and relocation intervention, and the following monitoring: (A, B) saturation divers removed nuclei from the interfered outcrops; (C, D) the nuclei were temporarily placed on the iron grid tables; (E, F) saturation divers relocated the nuclei on the top of the pipeline by using epoxy resin on concrete coatings; (G, H) images acquired during monitoring activities.
Monitoring activities immediately followed the relocation phases to assess the effectiveness of the intervention over a short-time scale.
After fourteen months since the relocation interventions (July 2021), 816 of the 899 transplanted nuclei were found. The losses were due to the detachment of the nuclei from the resin. The mean survival rate was 88.1%: the losses were 9.4% and 17.4% for the B and C depth layers, respectively (Figure 7). No partial or total necrosis events were reported for the relocated organisms that remained attached to the pipeline.
Figure 7 Survival rate (expressed as a percentage of the total number of nuclei relocated) of the transplanted nuclei measured at the end (April 2020), one month after (May 2020), and fourteen months after (July 2021) the relocation activities. Bars color refers to the depth layers: orange for B (50-69 m), and blue for C (70-80) (same tones applied in Figure 3).
After fourteen months since the nuclei relocation, the number of taxa increased from 13 to 15 due to the colonization of two new species: the gregarious polychaetes of the Salmacina/Filograna complex and the erect bryozoan Reteporella sp. The former created dense and thick aggregations on the pipeline connecting several nuclei. Salmacina/Filograna complex and Reteporella sp. settled both on the pipeline concrete coatings and on the relocated nuclei. As for the analysis of the structure of the assemblages, the Salmacina/Filograna complex was included in the polychaetes group. Assemblages significantly differed according to monitoring time and depth layer (Table 2): such differences were highlighted after fourteen months since the relocation intervention exclusively (pairwise test, Table 2). However, the interaction among monitoring time and depth layer did not show significant differences.
Table 2 The output of PERMANOVA (number of permutations = 999) and pairwise tests on relocated nuclei assemblages according to monitoring time, depth layer, and their interaction.
Parazoanthus axinellae, serpulids, A. polypoides, hydrozoans, A. verrucosa, and scleractinians were the taxa/morphological groups mostly responsible (76.1%) for the dissimilarities between the two depth layers (Supplementary material Table S3). The nuclei maintained the structure of the assemblages unchanged over time (Figure 8). Decrease in mean frequency of occurrence was reported for P. axinellae, A. verrucosa, A. polypoides, H. papillosa, and scleractinians in both the depth layers; on the contrary, the number of nuclei colonized by serpulids, Reteporella sp., and encrusting sponges increased after fourteen months. The lost nuclei were mostly colonized by P. axinellae, A. verrucosa, H. papillosa, and scleractinians; whereas, serpulids (mainly Salmacina/Filograna complex), the erect bryozoan Reteporella sp., and encrusting sponges showed settlement on the pipeline and the relocated nuclei, as specified above.
Figure 8 Structure of the assemblage at the end (April 2020), one month after (May 2020), and fourteen months after (July 2021) the relocation activities. The nuclei assemblages have been separated to visualize differences among depth layers better. Furthermore, to improve the comprehension of the temporal trends, the lost nuclei have been excluded from the analysis.
The present study sets up a stepwise approach for the mitigation of the impacts on mesophotic reefs deriving from the laying of the TAP pipeline, based on the accurate investigation of the area, the choice of the taxa on which to focus the interventions, and the following monitoring activities, by means of the employment of technologies and tools of the oil and gas industry. Each phase is propaedeutic for the next one and outlines the workflow of decision-making and operational phases that represent the first intervention of relocation of portions from the mesophotic reefs. Such workflow is summarized in Figure 9.
Figure 9 The framework synthesis the intervention’s workflow, with assessment and planning, intervention, and monitoring representing the main steps to mitigate the interference between TAP and mesophotic reefs built by invertebrates.
As highlighted by Fraschetti et al. (2021) in their recent analysis, the knowledge of the structure and state of ecosystems affected by anthropogenic disturbances, and the understanding of the disturbances, play a key role in the development (and effectiveness) of actions aiming at the recovery of biological communities. We accurately mapped and characterized the benthic assemblages within the area affected by the pipeline installation through the preliminary surveys carried out in 2017. The assemblages showed different structures and compositions according to depth. The reefs dominated by encrusting red algae belonging to the coralligenous reefs were located between 30 - 50 m depth. The assemblages of the biogenic outcrops between 50 and 80 m depth share the features of the mesophotic reefs described in studies carried out along the Apulian coasts at similar bathymetric ranges (Longo et al., 2018; Corriero et al., 2019; Piazzi et al., 2019; Cardone et al., 2020; Giampaoletti et al., 2020), forming a unique environment in the Mediterranean basin. In these temperate reefs, the dominance of encrusting red algae typical of the coralligenous is shifted in favor of invertebrate taxa (mainly scleractinians), showing similar growth and functioning patterns if compared to the deep cold-water coral habitats (Roberts et al., 2009). Despite the importance of the development project due to the geopolitical and energy context, and the small number of interfered outcrops, these findings highlighted the need to plan an intervention to mitigate the impacts related to the laying of the pipeline. TAP pipeline represented a physical disturbance for the outcrops located within its footprint (i.e., the area with direct impact on the seafloor), as well as the placement of these infrastructures on the seabed may cause a transient increase in local sedimentation (Cordes et al., 2016). Mitigation measures have been implemented in situ to avoid the loss of organisms and facilitate the presence of species of high ecological/conservation concern as fouling on the pipeline. We adopted a hybrid method that reflects two different approaches:
- The use of artificial structures (namely the cause of the disturbance, TAP pipeline), as in cold-water corals or deep-sea restoration interventions due to operational issues (O’Connor et al., 2020; Montseny et al., 2021);
- The relocation of the portions of the reefs that would have been lost during the laying of the TAP pipeline, following the transplanting fragmented dislodged colonies widespread in coral reef environments (Henry and Hart, 2005; Forrester et al., 2011).
The experience and methods developed in shallow coral active restoration represent a great opportunity to successfully address the challenges of restoring deeper similar ecosystems, such as cold-water coral habitats (Montseny et al., 2021). The advent of mitigation interventions on deeper ecosystems, broadly known as deep-sea restoration, poses new challenges related to habitat features, operational difficulties, and high costs (Van Dover et al., 2014). Albeit the TAP mitigation activities were carried out in deep coastal habitats, and not properly in the deep-sea, we managed operational issues and efforts on those habitats with low restoration potential (Bekkby et al., 2020).The relocated nuclei have the function of recreating the morphology and complexity of the biogenic outcrops which, together with the functional traits of the dominant species and the duration of the immersion of the substrate, have been recognized as the main factors accounting for the similarities (or dissimilarities) between artificial and natural reefs (Perkol-Finkel et al., 2005; Perkol-Finkel et al., 2006). The nuclei were designed as expansion patches that will colonize the pipeline themselves (Rouse et al., 2019). Furthermore, the presence of several taxa on the nuclei reflected the complexity of the ecological interaction within the mesophotic reefs, overcoming the limitations of an intervention conducted on a single species (Fraschetti et al., 2021) and transferring to the pipeline, as less altered as possible, the assemblages found on the interfered outcrops.
To preserve the richness and complexity of the assemblages found on the outcrops, we focused the efforts on those taxa of most significant ecological (or functional) and conservation interest. On the one hand, this reflects the general features of the restoration actions, but on the other, it constitutes one of the novelties of the study. The application of species functional trait-based approaches is receiving growing attention in the restoration ecology and can represent significant progress for maintaining or recovering ecosystem services (Laughlin, 2014; Laughlin et al., 2018; Carlucci et al., 2020). Using these approaches, some species are selected instead of others based on their functional traits and the expected outcomes. While this is widely recognized for terrestrial habitats, one of the main issues in applying these metrics in the marine environment is the lack of taxonomic and functional information. The lack of knowledge is exacerbated in poorly explored and recently defined habitats, such as mesophotic reefs, in which it is urgent to define or update the information associated with the taxa structuring the assemblages. In this context, the relocation score was conceived as a synthetic tool, simple but effective in selecting the key species whose loss had to be minimized without altering the original assemblages. Therefore, we selected the set of the most abundant taxa responsible for the growth and the three-dimensional structure of the reef. Due to the impossibility of entirely relocating or moving the outcrops, this approach encompassed the need to mitigate the loss of species and habitats. Furthermore, the relocation score contributed to selecting the taxa whose size and consistency took into account operational limitations and the possibility of handling the organisms for relocation purposes.
The relocation of the nuclei allowed the presence of taxa on the pipeline that would otherwise have taken decades to colonize it (i.e., A. cannabina, A. polypoides, A. verrucosa, and scleractinians) due to their low dynamics and slow growth features (Teixidó et al., 2011; Montero-Serra et al., 2018). On the other hand, the monitoring of the nuclei provided information on fast-growing species that rapidly colonized both the nuclei and the pipeline concrete coating after one year of immersion (i.e., encrusting corallinales, encrusting sponges, Reteporella sp., and serpulids). These taxa have been reported as opportunistic or characteristic of the first stage of community development on coralligenous reefs (Fava et al., 2016; Sartoretto et al., 2017; Casoli et al., 2020b; Turicchia et al., 2021). Albeit not analyzed in the present work, fast-growing species settled on the pipeline sections not interested by nuclei relocation, so we can argue that their settlement was not influenced by the relocation activities. Ad-hoc sampling methods will be conceived in the future to identify and quantify the occurrence of competition phenomena among fast and slow-growing taxa and assess the influence of the relocated nuclei on the colonization of the TAP pipeline.
Effectiveness and success are key concepts in restoration ecology, but difficult to quantify because they are strictly related to the period of monitoring, which often has a limited duration, and due to the absence of univocal indicators to assess what was expected (Bayraktarov et al., 2016; Abelson et al., 2020; Basconi et al., 2020). The results gained through the monitoring activities are extremely limited in time; they must be evaluated with caution, assessing the survival rate of the nuclei on the pipeline and the structure of the assemblages after one year from the intervention exclusively. The high survival rate of the nuclei, as well as the settlement of new taxa, are encouraging data for future measures. However, these results represent the first efforts of a long-term and annual monitoring that will last 10 years. At the end of this period, the same measurement will be acquired, with particular attention to assessing if the nuclei will increase in size and will influence the biological colonization along the traits of the pipeline interested by the relocation activities.
One of the main novelties of this study is the involvement and support (economic, technological, and operational) of the private oil and gas sector, which made the intervention possible. This study represents a paradigmatic case of involvement of the oil and gas industry in the Mediterranean to mitigate habitat loss utilizing vessels, ROV’s, and saturation commercial diving technologies. The activities followed the Ministry for the Environment requirements, and were continuously monitored and shared with the Italian Institute for Environmental Protection and Research (ISPRA) and the Regional Environmental Protection Agency of the Apulia Region (ARPA Puglia). After the accurate preliminary surveys along the pipeline route, the activities required extensive seafloor monitoring and mitigation measures for the negative impacts that could not be avoided. The recovery of degraded or damaged habitats represents a crucial aid for management strategies to provide biodiversity conservation and improving services for human health and well-being (Possingham et al., 2015). However, active marine habitat restoration has extremely high costs (Bayraktarov et al., 2016) that in deep habitats are estimated to rise by two or three orders of magnitude per hectare if compared to the costs of activities carried out in coastal marine ecosystems (Van Dover et al., 2014; Danovaro et al., 2021; Montseny et al., 2021). The potential of recovery actions, proven by positive outcomes of worldwide efforts carried out both on land and underwater, together with the need to reverse the trend of ecosystems degradation, are the pillars of the UN Decade on Ecosystem Restoration 2021–2030: a global rallying cry to heal our planet (https://www.decadeonrestoration.org/). Both costs and operational issues are not fully manageable by the sole scientific community, especially when dealing with deep-sea environments. The sole restoration actions, albeit carried out over large spatial scales, have a low chance of success without the support of different spheres of our society (Waltham et al., 2020): a further step should be adopted to achieve the goals we hope, involving governments, industries, and finances.
Despite the scope of the study, we stress the importance that the mitigation actions, such as those described in this study, should not be used to tolerate impacts created by development projects and not be taken as a “license to trash “. Furthermore, among the criteria that guide the choice of the route of a pipeline in the projecting phase, environmental issues must have the same weight as economic (including the length of the pipeline) and operational factors (Hamid-Mosaku et al., 2020). In the specific case of TAP, the number of affected outcrops was relatively low to allow the development of actions to mitigate the impacts; such an approach should be carefully evaluated case by case. In parallel, it is necessary and imperative to create a policy framework that drives decisions on the exploitation of marine resources and take into consideration i) to avoid irreversible impacts, ii) impose mitigation interventions, iii) assess costs and iv) identifies who takes responsibility for financing (Da Ros et al., 2019).
According to FAO’s guidelines, mesophotic reefs represent vulnerable marine ecosystems: their knowledge and distribution are far from being wholly achieved. However, they are receiving growing attention worldwide as they may offer refuge to shallow species exposed to unstable environmental conditions and climate change effects (Cerrano et al., 2019). In the light of their role in the functioning of the marine ecosystems, as well as the provision of essential goods and services for human populations, conservation and restoration measures should be developed in the future to decrease human impacts and guarantee the sustainable use of natural resources (Chimienti et al., 2019; Bevilacqua et al., 2021).
This study represents the first intervention in deep Mediterranean coastal habitats to actively mitigate impacts linked to human economic activities, such as pipeline laying, using oil and gas industry technologies and tools. The support of the private sector can be fundamental for recovery actions to operate in environments that would otherwise be difficult (due to depth or spatial constraints) and cover expensive costs. However, a correct evaluation during the project phase (i.e., ante operam) should drive the choice of the site in light of its environmental features, economic and political reasons.
Policymakers, industry, and researchers should work side by side in the next future, developing practical projects and interventions to mitigate the effects of the exploitation of marine resources. This is particularly relevant with the upcoming development of offshore wind farms in the Mediterranean Sea. Integrated management involving all the stakeholders is urgently needed to identify feasible solutions or avoid irreversible degradation.
The raw data supporting the conclusions of this article will be made available by the authors, without undue reservation.
EC: conceptualization, data curation, investigation, methodology, formal analysis, writing—original draft, writing—review and editing; DV: data curation, investigation, formal analysis, writing—review and editing; GM: data curation, formal analysis, writing—review and editing; AB: investigation, methodology, writing—review and editing; GA: supervision, conceptualization, writing—review and editing. All authors contributed to the article and approved the submitted version.
All the authors, as scientists of the Sapienza University of Rome and CIBM, were commissioned by TAP to assess the environmental risks of the pipeline laying operations, to monitor the environmental conditions during the works, and to follow the relocation activities to mitigate the impacts. As mentioned in the text, the authors’ work was controlled and shared with the Italian Institute for Environmental Protection and Research (ISPRA) and the Regional Environmental Protection Agency of the Apulia Region (ARPA Puglia).
All claims expressed in this article are solely those of the authors and do not necessarily represent those of their affiliated organizations, or those of the publisher, the editors and the reviewers. Any product that may be evaluated in this article, or claim that may be made by its manufacturer, is not guaranteed or endorsed by the publisher.
The Supplementary Material for this article can be found online at: https://www.frontiersin.org/articles/10.3389/fmars.2022.877325/full#supplementary-material
Abelson A., Reed D. C., Edgar G. J., Smith C. S., Kendrick G. A., Orth R. J., et al (2020). Challenges for Restoration of Coastal Marine Ecosystems in the Anthropocene. Front. Mar. Sci. 7. doi: 10.3389/fmars.2020.544105
Alagna A., D'Anna G., Musco L., Fernández T. V., Gresta M., Pierozzi N., et al (2019). Taking Advantage of Seagrass Recovery Potential to Develop Novel and Effective Meadow Rehabilitation Methods. Mar. Pollut. Bull. 149, 110578. doi: 10.1016/j.marpolbul.2019.110578
Alagna A., D'Anna G., Musco L., Fernández T. V., Gresta M., Pierozzi N., et al (2020). Reply to “Letter to the Editor Regarding the Article ‘Taking Advantage of Seagrass Recovery Potential to Develop Novel and Effective Meadow Rehabilitation Methods’ by Alagna Et Al., Published in Marine Pollution Bulletin, 149: 2019 (110578)” by Calvo Et Al. Marine Pollution Bulletin, 158: 2020 (111395). Mar. Pollut. Bull. 161, 111754. doi: 10.1016/j.marpolbul.2020.111754
Azzola A., Bavestrello G., Bertolino M., Bianchi C. N., Bo M., Enrichetti F., et al (2021). You Cannot Conserve a Species That has Not Been Found: The Case of the Marine Sponge Axinella Polypoides in Liguria, Italy. Aquat. Conserv. Mar. Freshw. Ecosyst. 31, 737–747. doi: 10.1002/aqc.3492
Badalamenti F., Carlo G. D., D’Anna G., Gristina M., Toccaceli M. (2006). Effects of Dredging Activities on Population Dynamics of Posidonia Oceanica (L.) Delile in the Mediterranean Sea: The Case Study of Capo Feto (SW Sicily, Italy). Hydrobiologia 555, 253–261. doi: 10.1007/s10750-005-1121-5
Ballesteros E. (2006). Mediterranean Coralligenous Assemblages: A Synthesis of Present Knowledge. Oceanogr. Mar. Biol. Annu. Rev. 44, 123–195. doi: 10.1201/9781420006391.ch4
Basconi L., Cadier C., Guerrero-Limón G. (2020). “Challenges in Marine Restoration Ecology: How Techniques, Assessment Metrics, and Ecosystem Valuation Can Lead to Improved Restoration Success,” in YOUMARES 9 - The Oceans: Our Research, Our Future (Cham: Springer), 83–99. doi: 10.1007/978-3-030-20389-4_5
Bayraktarov E., Saunders M. I., Abdullah S., Mills M., Beher J., Possingham H. P., et al (2016). The Cost and Feasibility of Marine Coastal Restoration. Ecol. Appl. 26, 1055–1074. doi: 10.1890/15-1077.1
Bejko L. (2020). Emerging Needs for Creative Mitigation: Recent Trends in Archaeology and Historic Preservation in Albania. Adv. Archaeol. Pract. 8, 288–298. doi: 10.1017/aap.2020.19
Bekkby T., Papadopoulou N., Fiorentino D., McOwen C. J., Rinde E., Boström C., et al (2020). Habitat Features and Their Influence on the Restoration Potential of Marine Habitats in Europe. Front. Mar. Sci. 7. doi: 10.3389/fmars.2020.00184
Bevilacqua S., Airoldi L., Ballesteros E., Benedetti-Cecchi L., Boero F., Bulleri F., et al (2021). Mediterranean Rocky Reefs in the Anthropocene: Present Status and Future Concerns. Adv. Mar. Biol. 89, 1–51. doi: 10.1016/bs.amb.2021.08.001
Bracchi V. A., Basso D., Marchese F., Corselli C., Savini A. (2017). Coralligenous Morphotypes on Subhorizontal Substrate: A New Categorization. Cont. Shelf Res. 144, 10–20. doi: 10.1016/j.csr.2017.06.005
Burdon D., Barnard S., Boyes S. J., Elliott M. (2018). Oil and Gas Infrastructure Decommissioning in Marine Protected Areas: System Complexity, Analysis and Challenges. Mar. Pollut. Bull. 135, 739–758. doi: 10.1016/j.marpolbul.2018.07.077
Calvo S., Pirrotta M., Tomasello A. (2020). Letter to the Editor Regarding the Article “Taking Advantage of Seagrass Recovery Potential to Develop Novel and Effective Meadow Rehabilitation Methods” by Alagna Et Al., Published in Marine Pollution Bulletin, 149: 2019 (110578). Mar. Pollut. Bull. 158, 111395. doi: 10.1016/j.marpolbul.2020.111395
Canessa M., Bavestrello G., Trainito E., Bianchi C. N., Morri C., Navone A., et al (2021). A Large and Erected Sponge Assemblage on Granite Outcrops in a Mediterranean Marine Protected Area (NE Sardinia). Reg. Stud. Mar. Sci. 44, 101734. doi: 10.1016/j.rsma.2021.101734
Cardone F., Corriero G., Longo C., Mercurio M., Onen Tarantini S., Gravina M. F., et al (2020). Massive Bioconstructions Built by Neopycnodonte Cochlear (Mollusca, Bivalvia) in a Mesophotic Environment in the Central Mediterranean Sea. Sci. Rep. 10, 6337. doi: 10.1038/s41598-020-63241-y
Carlucci M. B., Brancalion P. H. S., Rodrigues R. R., Loyola R., Cianciaruso M. V. (2020). Functional Traits and Ecosystem Services in Ecological Restoration. Restor. Ecol. 28, 1372–1383. doi: 10.1111/rec.13279
Casoli E., Bonifazi A., Ardizzone G., Gravina M. F. (2016). How Algae Influence Sessile Marine Organisms: The Tube Worms Case of Study. Estuar. Coast. Shelf Sci. 178, 12–20. doi: 10.1016/j.ecss.2016.05.017
Casoli E., Mancini G., Ventura D., Pace D. S., Belluscio A., Ardizzone G. D. (2020b). Reteporella Spp. Success in the Re-Colonization of Bare Coralligenous Reefs Impacted by Costa Concordia Shipwreck: The Pioneer Species You did Not Expect. Mar. Pollut. Bull. 161, 111808. doi: 10.1016/j.marpolbul.2020.111808
Casoli E., Piazzi L., Nicoletti L., Jona-Lasinio G., Cecchi E., Mancini G., et al (2020a). Ecology, Distribution and Demography of Erect Bryozoans in Mediterranean Coralligenous Reefs. Estuar. Coast. Shelf Sci. 235, 106573. doi: 10.1016/j.ecss.2019.106573
Casoli E., Ventura D., Cutroneo L., Capello M., Jona-Lasinio G., Rinaldi R., et al (2017). Assessment of the Impact of Salvaging the Costa Concordia Wreck on the Deep Coralligenous Habitats. Ecol. Indic. 80, 124–134. doi: 10.1016/j.ecolind.2017.04.058
Casoli E., Ventura D., Mancini G., Cardone S., Farina F., Donnini L., et al (2022). Rehabilitation of Mediterranean Animal Forests Using Gorgonians From Fisheries by-Catch. Restor. Ecol. 30 (1), e13465. doi: 10.1111/rec.13465
Cerrano C., Bastari A., Calcinai B., Di Camillo C., Pica D., Puce S., et al (2019). Temperate Mesophotic Ecosystems: Gaps and Perspectives of an Emerging Conservation Challenge for the Mediterranean Sea. Eur. Zool. J. 86, 370–388. doi: 10.1080/24750263.2019.1677790
Chimienti G., Mastrototaro F., D’Onghia G. (2019). “Mesophotic and Deep-Sea Vulnerable Coral Habitats of the Mediterranean Sea: Overview and Conservation Perspectives,” in Advances in the Studies of the Benthic Zone, ed. Soto L. A. (London, UK: IntechOpen) p. 20. doi: 10.5772/intechopen.90024
Consoli P., Romeo T., Ferraro M., Sarà G., Andaloro F. (2013). Factors Affecting Fish Assemblages Associated With Gas Platforms in the Mediterranean Sea. J. Sea Res. 77, 45–52. doi: 10.1016/j.seares.2012.10.001
Coppari M., Gori A., Rossi S. (2014). Size, Spatial, and Bathymetrical Distribution of the Ascidian Halocynthia Papillosa in Mediterranean Coastal Bottoms: Benthic–Pelagic Coupling Implications. Mar. Biol. 161, 2079–2095. doi: 10.1007/s00227-014-2488-5
Cordes E. E., Jones D. O. B., Schlacher T. A., Amon D. J., Bernardino A. F., Brooke S., et al (2016). Environmental Impacts of the Deep-Water Oil and Gas Industry: A Review to Guide Management Strategies. Front. Environ. Sci. 4. doi: 10.3389/fenvs.2016.00058
Corriero G., Pierri C., Mercurio M., Nonnis Marzano C., Onen Tarantini S., Gravina M. F., et al (2019). A Mediterranean Mesophotic Coral Reef Built by non-Symbiotic Scleractinians. Sci. Rep. 9, 3601. doi: 10.1038/s41598-019-40284-4
Costantino G., Mastrototaro F., Tursi A., Torchia G., Pititto F., Salerno G., et al (2010). Distribution and Bio-Ecological Features of Posidonia Oceanica Meadows Along the Coasts of the Southern Adriatic and Northern Ionian Seas. Chem. Ecol. 26, 91–104. doi: 10.1080/02757541003705484
Danovaro R., Aronson J., Cimino R., Gambi C., Snelgrove P. V. R., Van Dover C. (2021). Marine Ecosystem Restoration in a Changing Ocean. Restor. Ecol. 29, 1–8. doi: 10.1111/rec.13432
Da Ros Z., Dell’Anno A., Morato T., Sweetman A. K., Carreiro-Silva M., Smith C. J., et al (2019). The Deep Sea: The New Frontier for Ecological Restoration. Mar. Policy 108, 103642. doi: 10.1016/j.marpol.2019.103642
Di Carlo G., Badalamenti F., Jensen A. C., Koch E. W., Riggio S. (2005). Colonisation Process of Vegetative Fragments of Posidonia Oceanica (L.) Delile on Rubble Mounds. Mar. Biol. 147 (6), 1261–1270. doi: 10.1007/s00227-005-0035-0
Doxa A., Holon F., Deter J., Villéger S., Boissery P., Mouquet N. (2016). Mapping Biodiversity in Three-Dimensions Challenges Marine Conservation Strategies: The Example of Coralligenous Assemblages in North-Western Mediterranean Sea. Ecol. Indic. 61, 1042–1054. doi: 10.1016/j.ecolind.2015.10.062
Elliott M., Burdon D., Hemingway K. L., Apitz S. E. (2007). Estuarine, Coastal and Marine Ecosystem Restoration: Confusing Management and Science - A Revision of Concepts. Estuar. Coast. Shelf Sci. 74, 349–366. doi: 10.1016/j.ecss.2007.05.034
Elliott M., Cutts N. D. (2004). Marine Habitats: Loss and Gain, Mitigation and Compensation. Mar. Pollut. Bull. 9, 671–674. doi: 10.1016/j.marpolbul.2004.08.018
Enrichetti F., Bo M., Morri C., Montefalcone M., Toma M., Bavestrello G., et al (2019a). Assessing the Environmental Status of Temperate Mesophotic Reefs: A New, Integrated Methodological Approach. Ecol. Indic. 102, 218–229. doi: 10.1016/j.ecolind.2019.02.028
Enrichetti F., Dominguez-Carrió C., Toma M., Bavestrello G., Betti F., Canese S., et al (2019b). Megabenthic Communities of the Ligurian Deep Continental Shelf and Shelf Break (NW Mediterranean Sea). PloS One 14, e0223949. doi: 10.1371/journal.pone.0223949
Fagerstrom J. A. (1991). Reef-Building Guilds and a Checklist for Determining Guild Membership - A New Approach for Study of Communities. Coral Reefs 10, 47–52. doi: 10.1007/BF00301908
Fava F., Ponti M., Abbiati M. (2016). Role of Recruitment Processes in Structuring Coralligenous Benthic Assemblages in the Northern Adriatic Continental Shelf. PloS One 11 (10), e0163494. doi: 10.1371/journal.pone.0163494
Forrester G. E., O’Connell-Rodwell C., Baily P., Forrester L. M., Giovannini S., Harmon L., et al (2011). Evaluating Methods for Transplanting Endangered Elkhorn Corals in the Virgin Islands. Restor. Ecol. 19, 299–306. doi: 10.1111/j.1526-100X.2010.00664.x
Fraschetti S., Guarnieri G., Gambi C., Bevilacqua S., Terlizzi A., Danovaro R. (2016). Impact of Offshore Gas Platforms on the Structural and Functional Biodiversity of Nematodes. Mar. Environ. Res. 115, 56–64. doi: 10.1016/j.marenvres.2016.02.001
Fraschetti S., McOwen C., Papa L., Papadopoulou N., Bilan M., Boström C., et al (2021). Where Is More Important Than How in Coastal and Marine Ecosystems Restoration. Front. Mar. Sci. 8. doi: 10.3389/fmars.2021.626843
Garrabou J. (1999). Life-History Traits of Alcyonium Acaule and Parazoanthus Axinellae (Cnidaria, Anthozoa), With Emphasis on Growth. Mar. Ecol. Prog. Ser. 178, 193–204. doi: 10.3354/meps178193
Garrabou J., Kipson S., Kaleb S., Kruzic P., Jaklin A., Zuljevic A., et al (2014). Monitoring Protocol for Reefs-Coralligenous Community. MedMPAnet Project, pp. 1–42. doi: 10.13140/2.1.1266.8482
Gerovasileiou V., Dailianis T., Sini M., Del Mar Otero M., Numa C., Katsanevakis S., et al (2018). Assessing the Regional Conservation Status of Sponges (Porifera): The Case of the Aegean Ecoregion. Mediterr. Mar. Sci. 19 (3), 526–537. doi: 10.12681/mms.14461
Gerovasileiou V., Smith C. J., Sevastou K., Papadopoulou N., Dailianis T., Bekkby T., et al (2019). Habitat Mapping in the European Seas - is it Fit for Purpose in the Marine Restoration Agenda? Mar. Policy 106, 103521. doi: 10.1016/j.marpol.2019.103521
Giampaoletti J., Cardone F., Corriero G., Gravina M. F., Nicoletti L. (2020). Sharing and Distinction in Biodiversity and Ecological Role of Bryozoans in Mediterranean Mesophotic Bioconstructions. Front. Mar. Sci. 7. doi: 10.3389/fmars.2020.581292
Gravina M. F., Pierri C., Mercurio M., Nonnis Marzano C., Giangrande A. (2021). Polychaete Diversity Related to Different Mesophotic Bioconstructions Along the Southeastern Italian Coast. Diversity 13 (6), 239. doi: 10.3390/d13060239
Hamid-Mosaku I. A., Oguntade O. F., Ifeanyi V. I., Balogun A. L., Jimoh O. A. (2020). Evolving a Comprehensive Geomatics Multi-Criteria Evaluation Index Model for Optimal Pipeline Route Selection. Struct. Infrastruct. Eng. 16, 1382–1396. doi: 10.1080/15732479.2020.1712435
Henry L. A., Hart M. (2005). Regeneration From Injury and Resource Allocation in Sponges and Corals - A Review. Int. Rev. Hydrobiol. 90, 125–158. doi: 10.1002/iroh.200410759
Ingrosso G., Abbiati M., Badalamenti F., Bavestrello G., Belmonte G., Cannas R., et al (2018). Mediterranean Bioconstructions Along the Italian Coast. Adv. Mar. Biol. 79, 61–136. doi: 10.1016/bs.amb.2018.05.001
Jacob C., Buffard A., Pioch S., Thorin S. (2018). Marine Ecosystem Restoration and Biodiversity Offset. Ecol. Eng. 120, 585–594. doi: 10.1016/j.ecoleng.2017.09.007
Jensen A. (2002). Artificial Reefs of Europe: Perspective and Future. ICES J. Mar. Sci. 59, S3–S13. doi: 10.1006/jmsc.2002.1298
Laughlin D. C. (2014). Applying Trait-Based Models to Achieve Functional Targets for Theory-Driven Ecological Restoration. Ecol. Lett. 17, 771–784. doi: 10.1111/ele.12288
Laughlin D. C., Chalmandrier L., Joshi C., Renton M., Dwyer J. M., Funk J. L. (2018). Generating Species Assemblages for Restoration and Experimentation: A New Method That Can Simultaneously Converge on Average Trait Values and Maximize Functional Diversity. Methods Ecol. Evol. 9, 1764–1771. doi: 10.1111/2041-210X.13023
Longo C., Cardone F., Pierri C., Mercurio M., Mucciolo S., Marzano C. N., et al (2018). Sponges Associated With Coralligenous Formations Along the Apulian Coasts. Mar. Biodivers. 48, 2151–2163. doi: 10.1007/s12526-017-0744-x
Macreadie P. I., Fowler A. M., Booth D. J. (2011). Rigs-to-Reefs: Will the Deep Sea Benefit From Artificial Habitat? Front. Ecol. Environ. 9, 455–461. doi: 10.1890/100112
Mancini G., Casoli E., Ventura D., Jona Lasinio G., Belluscio A., Ardizzone G. D. (2021). An Experimental Investigation Aimed at Validating a Seagrass Restoration Protocol Based on Transplantation. Biol. Conserv. 264, 109397. doi: 10.1016/j.biocon.2021.109397
Marinos V., Stoumpos G., Papazachos C. (2019). Landslide Hazard and Risk Assessment for a Natural Gas Pipeline Project: The Case of the Trans Adriatic Pipeline, Albania Section. Geosciences 9, 61. doi: 10.3390/geosciences9020061
Micheli F., Halpern B. S., Walbridge S., Ciriaco S., Ferretti F., Fraschetti S., et al (2013). Cumulative Human Impacts on Mediterranean and Black Sea Marine Ecosystems: Assessing Current Pressures and Opportunities. PloS One 8, e79889. doi: 10.1371/journal.pone.0079889
Mocavini G. (2019). “The Trans-Adriatic Pipeline and the Nimby Syndrome,” (Roma Tre: University of Rome).
Montero-Serra I., Garrabou J., Doak D. F., Figuerola L., Hereu B., Ledoux J. B., et al (2018). Accounting for Life-History Strategies and Timescales in Marine Restoration. Conserv. Lett. 11, 1–9. doi: 10.1111/conl.12341
Montseny M., Linares C., Carreiro-Silva M., Henry L. A., Billett D., Cordes E. E., et al (2021). Active Ecological Restoration of Cold-Water Corals: Techniques, Challenges, Costs and Future Directions. Front. Mar. Sci. 8. doi: 10.3389/fmars.2021.621151
O’Connor E., Hynes S., Chen W. (2020). Estimating the non-Market Benefit Value of Deep-Sea Ecosystem Restoration: Evidence From a Contingent Valuation Study of the Dohrn Canyon in the Bay of Naples. J. Environ. Manage. 275, 111180. doi: 10.1016/j.jenvman.2020.111180
Perkol-Finkel S., Shashar N., Barneah O., Ben-David-Zaslow R., Oren U., Reichart T., et al (2005). Fouling Reefal Communities on Artificial Reefs: Does Age Matter? Biofouling 21, 127–140. doi: 10.1080/08927010500133451
Perkol-Finkel S., Shashar N., Benayahu Y. (2006). Can Artificial Reefs Mimic Natural Reef Communities? The Roles of Structural Features and Age. Mar. Environ. Res. 61, 121–135. doi: 10.1016/j.marenvres.2005.08.001
Piazzi L., Gennaro P., Cecchi E., Serena F., Bianchi C. N., Morri C., et al (2017). Integration of ESCA Index Through the Use of Sessile Invertebrates. Sci. Mar 81 (2), 283–290. doi: 10.3989/scimar.04565.01B
Piazzi L., Kaleb S., Ceccherelli G., Montefalcone M., Falace A. (2019). Deep Coralligenous Outcrops of the Apulian Continental Shelf: Biodiversity and Spatial Variability of Sediment-Regulated Assemblages. Cont. Shelf Res. 172, 50–56. doi: 10.1016/j.csr.2018.11.008
Possingham H. P., Bode M., Klein C. J. (2015). Optimal Conservation Outcomes Require Both Restoration and Protection. PloS Biol. 13, 1–16. doi: 10.1371/journal.pbio.1002052
Redford M., Rouse S., Hayes P., Wilding T. A. (2021). Benthic and Fish Interactions With Pipeline Protective Structures in the North Sea. Front. Mar. Sci. 8. doi: 10.3389/fmars.2021.652630
REGIONE PUGLIA (2006). Inventario e Cartografia Delle Praterie di Posidonia nei Compartimenti Marittimi di Manfredonia, Molfetta, Bari, Brindisi, Gallipoli e Taranto. POR Puglia 2000–2006, 105. Available online: https://www.yumpu.com/it/document/view/15363912/relazione-generale-file-pdf-28-mb-pugliamareit (Accessed on 5 January 2022).
Roberts J. M., Wheeler A., Freiwald A., Cairns S. (2009). Cold-Water Corals: The Biology and Geology of Deep-Sea Coral Habitats (Cambridge, UK: Cambridge University Press).
Rouse S., Kafas A., Catarino R., Peter H. (2018). Commercial Fisheries Interactions With Oil and Gas Pipelines in the North Sea: Considerations for Decommissioning. ICES J. Mar. Sci. 75, 279–286. doi: 10.1093/icesjms/fsx121
Rouse S., Lacey N. C., Hayes P., Wilding T. A. (2019). Benthic Conservation Features and Species Associated With Subsea Pipelines: Considerations for Decommissioning. Front. Mar. Sci. 6. doi: 10.3389/fmars.2019.00200
Sartoretto S., Schohn T., Bianchi C. N., Morri C., Garrabou J., Ballesteros E., et al (2017). An Integrated Method to Evaluate and Monitor the Conservation State of Coralligenous Habitats: The INDEX-COR Approach. Mar. Pollut. Bull. 120 (1-2), 222–231. doi: 10.1016/j.marpolbul.2017.05.020
Slejko D., Rebez A., Santulin M., Garcia-Pelaez J., Sandron D., Tamaro A., et al (2021). Seismic Hazard for the Trans Adriatic Pipeline (TAP). Part 1: Probabilistic Seismic Hazard Analysis Along the Pipeline. Bull. Earthq. Eng. 19 (9), 3349–3388. doi: 10.1007/s10518-021-01111-2
Teixidó N., Garrabou J., Harmelin J. G. (2011). Low Dynamics, High Longevity and Persistence of Sessile Structural Species Dwelling on Mediterranean Coralligenous Outcrops. PloS One 6, e23744. doi: 10.1371/journal.pone.0023744
Turicchia E., Abbiati M., Bettuzzi M., Calcinai B., Morigi M. P., Summers A. P., et al (2021). Bioconstruction and Bioerosion in the Northern Adriatic Coralligenous Reefs Quantified by X-Ray Computed Tomography. Front. Mar. Sci. 8. doi: 10.3389/fmars.2021.790869
Van Dover C. L., Aronson J., Pendleton L., Smith S., Arnaud-Haond S., Moreno-Mateos D., et al (2014). Ecological Restoration in the Deep Sea: Desiderata. Mar. Policy 44, 98–106. doi: 10.1016/j.marpol.2013.07.006
Waltham N. J., Elliott M., Lee S. Y., Lovelock C., Duarte C. M., Buelow C., et al (2020). UN Decade on Ecosystem Restoration 2021–2030—What Chance for Success in Restoring Coastal Ecosystems? Front. Mar. Sci. 7. doi: 10.3389/fmars.2020.00071
Westhoff V., van der Maarel E. (1978). “The Braun-Blanquet Approach,” in Classification of Plant Communities (Dordrecht: Springer), 287–399.
Wood C. (2014). Environmental Impact Assessment: A Comparative Review (London, UK: Routledge). doi: 10.4324/9781315838953
Zenone A., Pipitone C., D’anna G., La Porta B., Bacci T., Bertasi F., et al (2021). Stakeholders’ Attitudes About the Transplantations of the Mediterranean Seagrass Posidonia Oceanica as a Habitat Restoration Measure After Anthropogenic Impacts: A Q Methodology Approach. Sustain 13 (21), 12216. doi: 10.3390/su132112216
Keywords: human impact mitigation, restoration ecology, mesophotic reefs, reef relocation, oil and gas industry, Mediterranean Sea
Citation: Casoli E, Ventura D, Mancini G, Belluscio A and Ardizzone G (2022) When Scientists and Industry Technologies Mitigate Habitat Loss: The First Bioconstruction Relocation in the Mediterranean Sea. Front. Mar. Sci. 9:877325. doi: 10.3389/fmars.2022.877325
Received: 16 February 2022; Accepted: 11 April 2022;
Published: 10 May 2022.
Edited by:
Romuald Lipcius, College of William & Mary, United StatesReviewed by:
Luigi Musco, University of Salento, ItalyCopyright © 2022 Casoli, Ventura, Mancini, Belluscio and Ardizzone. This is an open-access article distributed under the terms of the Creative Commons Attribution License (CC BY). The use, distribution or reproduction in other forums is permitted, provided the original author(s) and the copyright owner(s) are credited and that the original publication in this journal is cited, in accordance with accepted academic practice. No use, distribution or reproduction is permitted which does not comply with these terms.
*Correspondence: Edoardo Casoli, ZWRvYXJkby5jYXNvbGlAdW5pcm9tYTEuaXQ=
Disclaimer: All claims expressed in this article are solely those of the authors and do not necessarily represent those of their affiliated organizations, or those of the publisher, the editors and the reviewers. Any product that may be evaluated in this article or claim that may be made by its manufacturer is not guaranteed or endorsed by the publisher.
Research integrity at Frontiers
Learn more about the work of our research integrity team to safeguard the quality of each article we publish.