- 1Fisheries and Oceans Canada, Arctic and Aquatic Research Division, Winnipeg, MB, Canada
- 2Fisheries and Oceans Canada, Maurice Lamontagne Institute, Mont-Joli, QC, Canada
- 3Department of Biology, ArcticNet, Québec Océan, Takuvik, Laval University, Quebec City, QC, Canada
Arctic ecosystems are changing rapidly due to global warming, industrial development, and economic growth. However, the ecological consequences for these ecosystems are difficult to predict due to limited knowledge on species abundance, distribution, and biodiversity patterns. This study evaluated the diversity and assemblage composition of epibenthic macrofauna in shallow coastal areas from five Eastern Arctic locations with increasing economic and shipping activity. Benthic trawls (n=198) were conducted in nearshore coastal habitats of Anaktalak Bay (Labrador), Churchill (Manitoba), Deception Bay (Quebec), Iqaluit (Nunavut), and Milne Inlet (Nunavut), at depths between 3 and 30 m. Diversity and assemblage composition were compared at various taxonomic levels from phylum to species and correlations with broad oceanographic variables were investigated to identify potential drivers of biodiversity. The spatial variability of benthic assemblages was also assessed within each study location. A total of 297,417 macroinvertebrates was identified, belonging to 900 taxa. Abundance and taxonomic richness were highest in Anaktalak Bay. Shannon-Wiener diversity was higher in Anaktalak Bay, Iqaluit, and Milne Inlet than in Churchill and Deception Bay. Churchill showed the lowest diversity metrics among locations. No relationships were observed between diversity and depth, chlorophyll-a, particulate organic carbon, sea surface temperature, or sea ice duration. Assemblages differed among locations at all taxonomic levels, with the highest dissimilarities at the species level; however, dispersion of samples within-groups was significant, suggesting that factors other than locations (e.g., habitat type) influence assemblage composition. While Churchill, Deception Bay, and Iqaluit showed distinct local spatial patterns in diversity metrics and assemblage composition, no patterns were detected in Anaktalak Bay and Milne Inlet. This study represents one of the largest systematic assessments of coastal epibenthic biodiversity in the Canadian Arctic. It identifies patterns of biodiversity and assemblage composition and provides a baseline for studies of community change and the development of informed management and conservation strategies for Arctic coastal ecosystems.
1 Introduction
Arctic costal ecosystems are one of most dynamic and complex marine environments on Earth (McMeans et al., 2013). They are home to numerous species and are an important component of the structural and functional integrity of Arctic biodiversity. Coastal habitats serve as nursery areas and year-round habitats to several commercially and culturally important species of fish and invertebrates (e.g., capelin, sculpin, clams) and provide feeding grounds for migratory birds, seals, polar bears, and whales; some of which are crucial for the subsistence of many people in Arctic communities (Rapinski et al., 2018). However, the Arctic is undergoing drastic transformation due to climate change and increasing industrial development. Since the late 19th century, the Arctic has warmed by 3°C (Post et al., 2019), causing earlier snow melt (Derksen and Brown, 2012), increased precipitation and freshwater runoff (Bintanja and Andry, 2017), and a significant reduction of perennial (i.e., multi-year) sea ice cover (Stroeve et al., 2012; Overland et al., 2014). Climate simulations predict an ice-free summer in the Arctic within the next three decades as temperature continues to rise (Wang and Overland, 2012; Overland et al., 2019). The retreat of Arctic sea ice opens new opportunities for the intensification of anthropogenic pressures (e.g., shipping, tourism, fishing, mining and hydrocarbon exploration/extraction) (Lasserre and Têtu, 2015), with the potential for habitat alteration and introductions of non-indigenous species (Goldsmit et al., 2020; Saebi et al., 2020). Additionally, sea level rise, ocean acidification, pollution, costal erosion, and fishing operations have intensified throughout the Arctic in recent years (Jørgensen et al., 2019; Niemi et al., 2019; Overland et al., 2019; Terhaar et al., 2020). The rapid transformation of Arctic ecosystems can significantly alter the distribution and abundance of species, affect species interaction and food webs, and reshape community structure, thus impacting ecosystem services. Understanding the impacts of global warming and anthropogenic activities requires comprehensive information on biodiversity and assemblage composition as a first step to develop long-term monitoring programs and sustainable conservation strategies for Arctic biodiversity (Archambault et al., 2010; Piepenburg et al., 2011; CAFF, 2019).
Coastal benthic communities play a crucial role in Arctic food webs, trophic pathways, and nutrient cycling. They are also particularly vulnerable to environmental changes and can serve as indicators of immediate and long-term consequences of global warming (Kröncke et al., 2001; Kortsch et al., 2012; Al-Habahbeh et al., 2020) and other anthropogenic stressors (Kaiser et al., 2006). The impact of human-induced environmental changes on higher latitude coastal areas has been documented in only a few areas of Northern Europe, where quantitative baseline data exists and monitoring programs have been in place for decades. In the North Sea (Germany), benthic assemblages and macrofauna biomass of Arctic species increased in the late 1970s and early 1980s, likely due to unusual cold winter sea surface temperatures associated with low anomalies in the North Atlantic Oscillation Index (NAOI) (Kröncke et al., 2001). However, as water temperature increased in the late 1980s due to high NAOI, macrofaunal communities shifted towards temperate species (Kröncke et al., 2001). Gulliksen and Bahr (2001) observed increased taxonomic richness and diversity of epibenthic assemblages on pier-pilings (3-4 m deep) in Tromsø (northern Norway) during years with warmer temperatures. More recently, a reorganization of the epibenthos (<15 m) was documented in Svalbard (Norway), where an abrupt increase in macroalgal cover due to increased sea water temperatures likely altered local habitat characteristics (i.e., reduced water movement, increased sedimentation, and food availability), leading to changes in epibenthic macrofauna (Kortsch et al., 2012). The shift in assemblage composition following macroalgae takeover led to an epibenthic community dominated by small and fast growing organisms (Al-Habahbeh et al., 2020). In the context of rapidly changing environment, it is fundamental to establish baseline indices to monitor future impacts on Arctic coastal areas and meet legislative and policy strategies for conservation of coastal biodiversity.
Currently, no comprehensive large-scale baseline data on taxonomy, species distribution, and assemblage composition exist for shallow coastal (<30 m) areas of the Canadian Arctic, representing one of the largest knowledge gaps for Arctic coastal ecosystems (Archambault et al., 2010; CAFF, 2019; Wei et al., 2020). Many reasons can be attributed to the deficiency in information on Arctic coastal assemblages, including difficulty in accessibility of shallow waters by large ships equipped for benthic sampling, lack of accurate bathymetric charts, geographic remoteness, long persistence of sea ice, highly variable weather condition, and financial constraints generally related to Arctic research.
Despite the challenges, information on shallow epifauna assemblages is scattered in government reports and published literature. The identification of shallow water organisms in the Canadian Arctic started in the early 19th century during multiple exploratory expeditions; e.g., John Ross (1818), Willian Perry (1819-1820), Diana (1897), Fram (1898-1902), followed by Neptune (1903-1904), the Hudson Bay Expedition (1920), and the Calanus (1947-1952). However, benthic macrofauna were only collected on a casual basis as the primary goal was to search for the Northwest Passage, establish military bases, and characterize ocean currents, navigability, and the geomorphology of the Arctic coast (Curtis, 1975). It was not until the Canadian Arctic Expedition (1913-1918) that information on taxonomy and species distribution for several locations was made available through a series of reports. However, samples were not collected in a systematic way and were limited to presence-only data (Dall, 1919; Chamberlin, 1920; Clark, 1920). A more systematic collection was conducted later by the Fisheries Research Board of Canada in southern Beaufort Sea (Wacasey et al., 1977), around Victoria Island (Atkinson and Wacasey, 1989a), James and Hudson bays (Wacasey et al., 1976; Atkinson and Wacasey, 1989b), and Frobisher Bay (Wacasey et al., 1979; Wacasey et al., 1980), although the focus was on deep (>50 m) infaunal organisms. While a few shallow (<30 m) sites were trawled, small epibenthos were not identified and the data was either not quantified or combined with that from deeper sites (Atkinson and Wacasey, 1989a; Atkinson and Wacasey, 1989b), hindering any quantitative assessment of nearshore communities. More recently, a benthic survey identified 57 genera of macrobenthic nematodes in coastal areas of Churchill, Deception Bay, Iqaluit, and Steensby Inlet (Gianasi et al., 2022). Additionally, image-based surveys (e.g., video or photo transects) of the seafloor have been used to assess shallow macrofauna assemblages in Baffin Island (Ellis, 1960), Lancaster and Eclipse sounds (Thomson, 1982), Resolute Bay (Conlan et al., 1998; Conlan and Kvitek, 2005), and Banks and King William islands (Brown et al., 2011), although they did not fully document the extent of nearshore benthic biodiversity due to the coarse taxonomic resolution associated with these methods.
The establishment of quantitative baseline data on benthic assemblages is fundamental to identifying potential ecological shifts and predicting how Arctic ecosystems may respond to increasing anthropogenic stressors (Archambault et al., 2010; Piepenburg et al., 2011). Coastal areas provide crucial habitats for Arctic wildlife and food security for people in northern communities, thus ecological assessment of coastal ecosystems is increasingly a central topic for the management and conservation of Arctic biodiversity (CAFF, 2019). The present study compares biodiversity and assemblage composition, providing a baseline inventory of epibenthic macrofauna, in shallow (<30 m) coastal areas in five Arctic locations of increasing economic interest. More specifically, diversity and assemblage compositions were compared among locations and correlated with depth and broad oceanographic parameters in an attempt to elucidate ecological processes that govern Arctic coastal biodiversity. This study represents one of the largest systematic surveys of epibenthic macrofauna in shallow waters of the Canadian Arctic. It provides quantitative baseline data to monitor future changes in benthic assemblages and promotes the development of a framework for the management and conservation of Arctic coastal biodiversity.
2 Methods
2.1 Study locations
Benthic surveys were conducted in five Arctic locations during the ice-free season in Anaktalak Bay (Labrador, July 2018), Churchill (Manitoba, August 2015), Deception Bay (Quebec, August 2016), Iqaluit (Nunavut, July 2016), and Milne Inlet (Nunavut, August 2017; Figure 1). These locations were chosen due to the increasing industrial development and shipping activities in the area in recent years, their economic importance for the Arctic, and the general lack of information on benthic macrofauna in shallow coastal waters (<30 m). All samples from a given location were collected in the same year to avoid temporal variability within locations.
Anaktalak Bay (56.43°N 62.13°W), located in central Labrador, was the southernmost surveyed location. The port of Anaktalak Bay has supported the extraction and transport of nickel-copper-cobalt and copper concentrates from the Vale Voisey’s Bay mine since the early 2000s (Têtu et al., 2015). Concentrates are usually shipped to Long Harbour (Placentia Bay, Newfoundland) for further processing and refining (Têtu et al., 2015). Anaktalak Bay is influenced by the Atlantic Ocean, in particular the Labrador Current, although it is protected by numerous islands.
Churchill (58.78°N 94.21°W) is located on the southwest coast of Hudson Bay. The port began operating in 1930s and is connected to the Hudson Bay Railway system, which facilitates the transport of grains, minerals, forest, mining, and petroleum products from across Canada for export overseas (Pahl and Kaiser, 2018). The port briefly closed in 2016 due to management and ownership changes but recommenced international shipping and Arctic community resupply operations in 2019 (Lin et al., 2020).
Deception Bay (62.18°N 74.73°W) is situated in Hudson Strait, which connects the Labrador Sea to Hudson Bay (Figure 1). The port is an important hub for nickel concentrate exports from the Glencore Raglan Mine destined for ports along the St. Lawrence Seaway and ultimately smelters in Sudbury, Ontario (Pronovost, 1999).
Iqaluit (63.73°N 68.50°W) is located on the southeast coast of Baffin Island adjacent to the Sylvia Grinnell River. Several industrial activities have developed in Iqaluit since the 1980s, including shipping of dry products and petroleum across the Canadian Arctic, offshore fisheries (e.g., shrimp, Greenland halibut), and tourism (Johnston et al., 2019). Due to the high tidal amplitude (~10 m) and limited port facilities, ships are typically offloaded offshore and the cargo transported on barges to the beach by tugboats. A deep-water port is under construction to provide all-tide access and port facilities for Canadian and international cargo and cruise ships (Aarluk et al., 2005).
Milne Inlet (71.94°N 80.80°W), the northernmost location surveyed, is situated on the northeast coast of Baffin Island (Figure 1). This port provides dock support, stockpile, maintenance facilities, and associated infrastructure for the operation of Mary River mine (Baffinland Iron Mines Corporation), facilitating docking and loading of iron ore carriers (Baffinland, 2020). Milne Inlet is connected to Baffin Bay and Lancaster Sound via Eclipse Sound and Admiralty Inlet and it is relatively well-protected by several irregularly shaped islands off the coast of Baffin Island.
2.2 Bottom trawl sampling
Bottom trawls were done in 39 sites at Anaktalak Bay, Churchill, Deception Bay, and Iqaluit, and 42 sites at Milne Inlet, for a total of 198 trawls (Figure 1). Sites were haphazardly chosen to cover different shallow subtidal habitats (<30 m) and maximize the collection of coastal biodiversity for species inventories. Trawls were done between 3 and 30 m depth and parallel to shore. The initial goal was to conduct trawls in groups of 3 replicates in the same depth range. However, due to irregular bottom morphology combined with inaccuracies in the bathymetric charts of Arctic coastal waters, it was not to possible to maintain a constant depth during replicate trawls. Each trawl was considered an independent sample and average trawl depths were calculated as (end depth + start depth)/2. Depth values were standardized to chart datum.
The trawl consisted of a benthic sledge designed to collect macrofauna from shallow subtidal areas (McNeill and Bell, 1992). It comprised a rigid rectangular mouth (1 m × 0.5 m) and two skids of ~1.2 m long attached to the bottom of the frame with a rope bridle. The body of the net measured ~1.8 m long with a mesh size of 10 mm, while the codend measured ~1.2 m in length with a mesh size of 6 mm. A chain at the bottom of the net mouth helped maintain contact with the bottom while weights (~30 kg in total) attached to the skids helped the frame sink to and remain on the seafloor. Depth and coordinates were collected at the beginning and end of each trawl. The trawl was towed for 2 or 3 minutes (after making contact with the seafloor) at an approximately constant speed of 1-2 knots using a line (with a length 2-3 times the estimated depth) attached to the bridle, covering an area of ~270 m2.
Upon completing each trawl, the catch was placed in fish totes (81 × 48 × 30 cm; ~120 L) for sorting and documentation. A photograph was taken with a digital camera (Olympus tough TG-6) positioned ~1.5 m above the totes to help identify macroalgae species (when present). At times, the catch was split and only a fraction (1/2 to 1/4) of the total was sorted and preserved to focus on ensuring wide coverage rather than exhaustive sampling within a given site. Macroinvertebrates (> 1 mm) were then separated from the macroalgae and fish and preserved with 95% ethanol. To avoid damaging organisms attached to substrates (e.g., rocks, shells), individual specimens were often preserved with them. The ethanol was replaced in each container 24 h post sampling to ensure proper preservation of the specimens. Species identifications and counts were performed using a stereo-microscope to the lowest taxonomic level possible. All taxonomic nomenclature was verified using the World Register of Marine Species (https://www.marinespecies.org/).
2.3 Statistical analyses
Rarefaction curves were calculated to visualize species diversity among Arctic locations and provide estimates of the expected number of species based on sample size (i.e., number of trawls). Sample-size based rarefaction curves with 95% confidence intervals were quantified using bootstrap methods with 200 replications. The extrapolation of curves was limited to 80 trawls; about double the number of trawls performed in each location (see above for sample size) to avoid a sampling bias (Chao et al., 2015). Instead, the non-parametric Chao2 index was quantified to estimate the total number of taxa expected in each location (Chao, 1987). Variation in benthic biodiversity indices was assessed by comparing abundance (individuals m-2), taxonomic richness (S¸ number of taxa), Shannon-Wiener diversity (H’, loge), and Pielou’s evenness (J’: H’/logS) among locations with depth as a co-variable using negative binomial generalized linear models. Values for S, H’, and J’ were calculated per trawl. Biodiversity indices were also compared between Churchill Estuary and Hudson Bay as these locations present distinct oceanography conditions; Churchill Estuary receives freshwater inputs during the spring and summer months, while Hudson Bay is an exclusively marine environment. Within locations, Spearman’s rank correlations were used to examine the relationship between diversity and depth.
Drivers of benthic biodiversity were evaluated by constructing scatter plots of abundance, S, H’, and J’ with sea surface temperature (SST), chlorophyll-a (Chl-a), particulate organic carbon (POC), and duration of the sea-ice cover averaged over 5 years preceding sample collection in each location. Data on SST, Chl-a, and POC was obtained from remote sensing imagery (satellite MODIS-Aqua, 4 km grid resolution) available on the Giovanni platform (GES-DISC Interactive Online Visualization and Analysis Infrastructure, NASA, https://giovanni.gsfc.nasa.gov/giovanni/). Data on the duration of sea ice was obtained from the Canadian Ice Service (CIS, https://www.canada.ca/en/environment-climate-change/services/ice-forecasts-observations/latest-conditions.html) website and considered the number of days that sea ice with thickness ≥1 cm was present in each area.
Multivariate analyses of assemblage composition were performed on both abundance and presence/absence data. Data on abundance was either square-root transformed to reduce the effect of dominant taxa or transformed to presence/absence. Presence/absence analyses were incorporated in this study to determine if assemblages differ among locations with the minimum amount of taxonomic information (presence-only) as this is often the only information available on historical data for the Canadian Arctic (1880s and earlier) and on public available data repositories (e.g., Ocean Biodiversity Information System). Non-metric multidimensional scaling ordinations (nMDS) were used to visualize variation in assemblages among locations and between Churchill Estuary and Hudson Bay at the phylum, class, order, family, genus, and species levels. Resemblance matrices were calculated using zero-adjusted Bray-Curtis similarity coefficients (dummy value = 1) (Clarke et al., 2014).
Variations in assemblage composition were evaluated for all taxonomic levels (as described above) to determine the influence of taxonomic resolution (e.g., level of identification) on distinguishing coastal benthic communities. Variation among ports was evaluated using permutational multivariate analysis of variance (PERMANOVA) based on 9999 permutations. Since PERMANOVA is sensitive to data dispersion and may confuse within-group variation with among-group variation, analysis of multivariate homogeneity (PERMDISP) with 9999 permutations was used to test if average within-group dispersion differed among locations. Similarity percentage analysis (SIMPER) was used on square-root transformed abundance data at the phylum and species level to determine the overall (dis)similarity of benthic assemblages among Arctic locations at the highest and lowest taxonomic levels. SIMPER was also used to the determine which species contributed the most for the overall assemblage in each location. Circos plots (i.e., circular charts of high-dimensional data) were then created with SIMPER results to illustrate similarities in assemblage composition.
Finally, data on abundance, H’, and assemblage composition were mapped in ArcGIS Pro v2.9 to assess location-specific patterns in biodiversity and benthic assemblages at various sites within sampled bays. Assemblages were using class as the default taxonomic level to provide a representation of the different taxonomic groups in each location. Classes that best represented the contribution to the overall assemblage structure were chosen based on SIMPER analysis (70% cut off). Statistical analyses were conducted using the software R and routines within the PRIMER statistical package (Primer-e, Plymouth, UK). Data in the text are expressed as mean ± standard error (se) using critical α=0.05.
3 Results
3.1 Biodiversity patterns among Arctic locations
3.1.1 Biodiversity indices
A total of 297,417 macroinvertebrates was collected in the present study. Although the majority of individuals were identified to species levels, some were only possible to identify at higher taxonomic levels. Nevertheless, a total of 507 species was identified and the remaining individuals were classified into a further 224 genera, 117 families, and 52 orders, for a total of 900 unique taxa. In all locations, rarefaction curves did not reach an asymptote, suggesting that sampling effort was insufficient to characterise the full extent of benthic biodiversity (Figure 2A). Interestingly, the rate of species accumulation in Anaktalak Bay and Iqaluit was about twice that at Churchill, where the lowest rate was observed. Intermediate rates of taxa accumulation were observed in Deception Bay and Milne Inlet (Figure 2A). The Chao2 index exceeded the number of observed taxa for all locations and indicated that this study documented between 62% (Deception Bay) to 78% (Anaktalak Bay) of the expected taxonomic richness at a given location (Table 1).
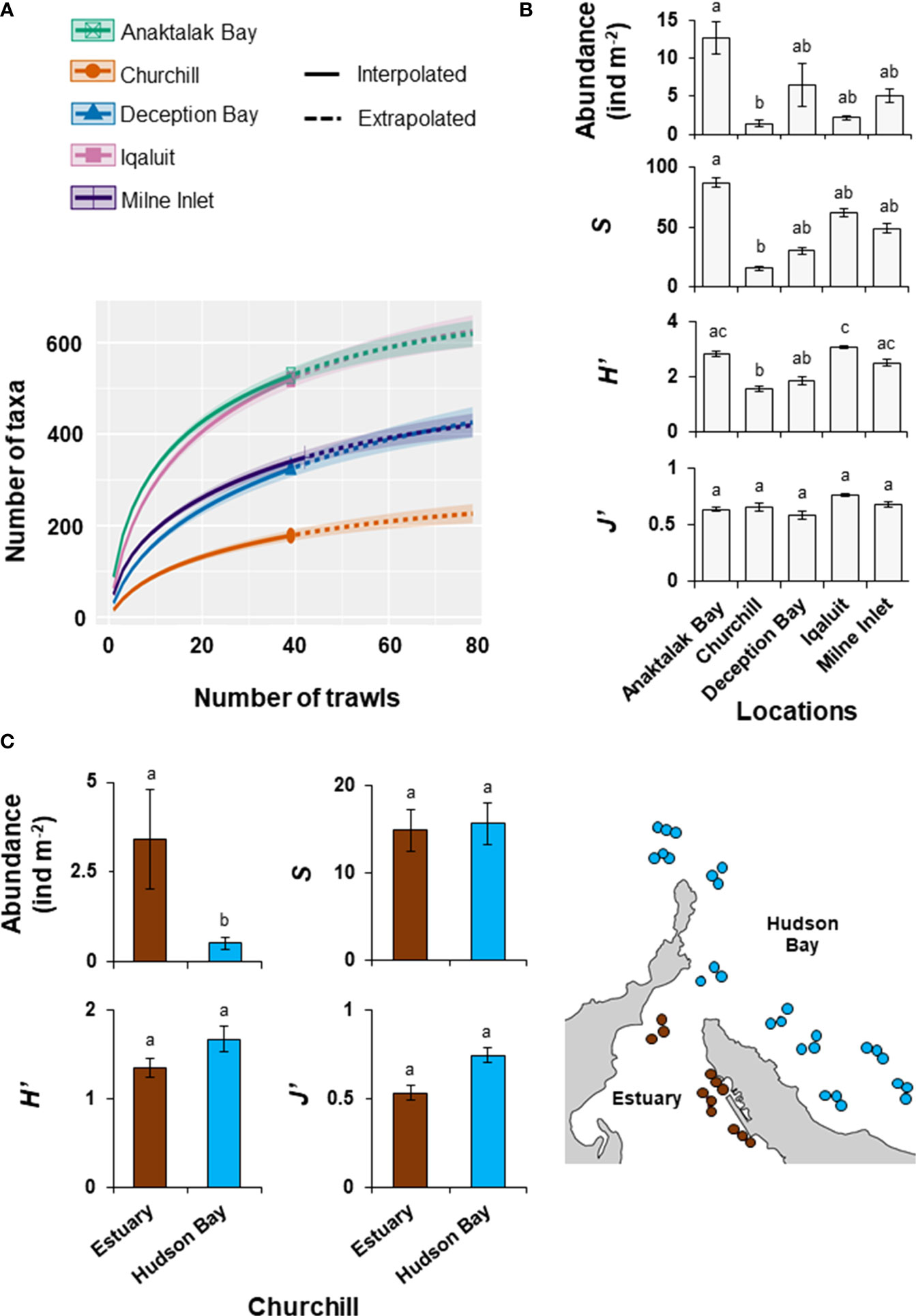
Figure 2 Diversity metrics in shallow coastal areas of the Canadian Arctic. (A) Sample-size based rarefaction curves with 95% confidence intervals for each Arctic locations. Comparison of abundance (ind m-2), taxonomic richness (S, number of taxa), Shannon-Wiener diversity (H’), and Pielou’s evenness (J’) among (B) Arctic ports and (C) between Churchill Estuary and Hudson Bay. Data is shown as mean ± se. Means with different letters are significantly different. See Table 2 for full statistics and Figure 1 for geographic locations.
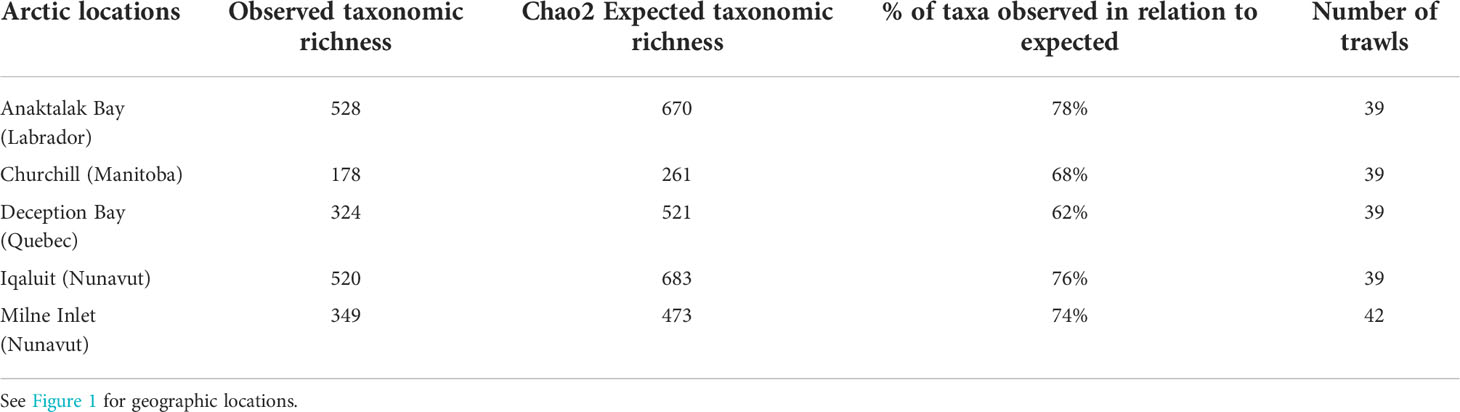
Table 1 Number of distinct taxonomic groups observed and expected in each location of the Canadian Arctic and total number of trawls.
Abundance and taxonomic richness (S) were highest in Anaktalak Bay (Figure 2B; Table 2). High Shannon-Wiener diversity (H’) was observed in Anaktalak Bay, Iqaluit, and Milne Inlet, whereas Deception Bay showed intermediate H’ values. Churchill showed the lowest abundance, S, and H’ among locations. Pielou’s evenness was slightly higher in Iqaluit and Milne Inlet than in Anaktalak Bay, Churchill, and Deception Bay (Figure 2B, Table 2). Depth showed no relationship with diversity indices across locations (Table 2) nor did it when correlated with indices within locations (Table S1, Supplementary Material). Additionally, there was no relationship between abundance, S, H’, and J’ with chlorophyll-a, particulate organic carbon, sea surface temperature and sea ice duration (Figure S1, Supplementary Material).
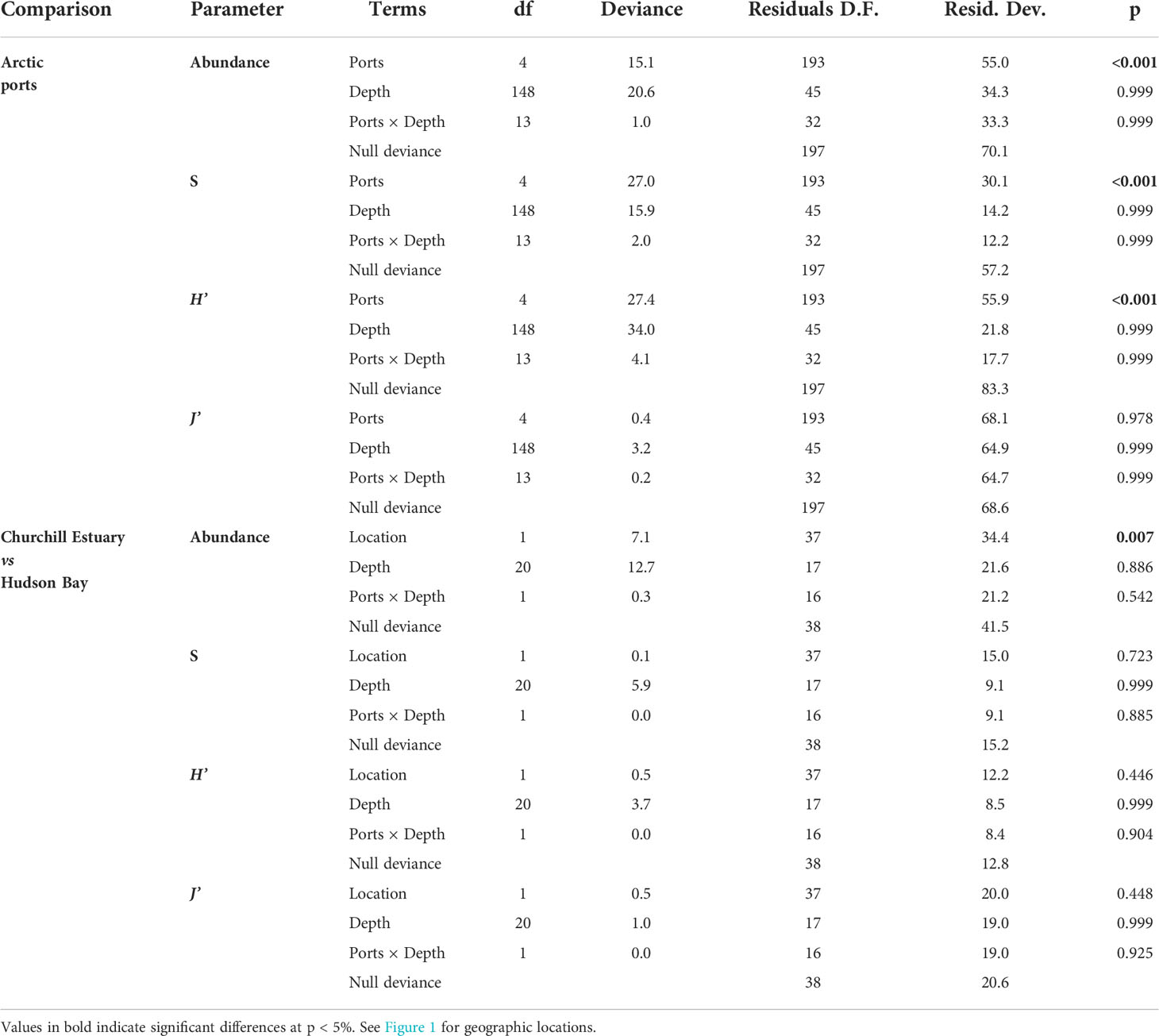
Table 2 Results of generalized linear models to examine the effect of ports and Churchill Estuary and Hudson Bay on the abundance (ind m-2), taxonomic richness (S), Shannon-Wiener diversity (H’), and Pielou’s evenness (J’).
3.1.2 Assemblage composition
PERMANOVA analyses showed that benthic assemblages differed significantly among locations at all taxonomic level and similar results were obtained for both abundance and presence/absence data (Table 3). This indicates that locations were characterized by different assemblages even with a significant reduction in taxonomic resolution from species to phylum and no effect of data transformation was observed. These results were strongly supported by ordination plots (Figure 3A) and similarity percentage analyses (Figure 4A). However, the PERMANOVA results could be affected by non-homogeneous dispersion of the data. Indeed, samples from all locations showed heterogeneous dispersion at all taxonomic levels (Table 4). Nevertheless, the ordination plots (particularly at the genus and species levels) highlight the importance of location driving assemblage composition.
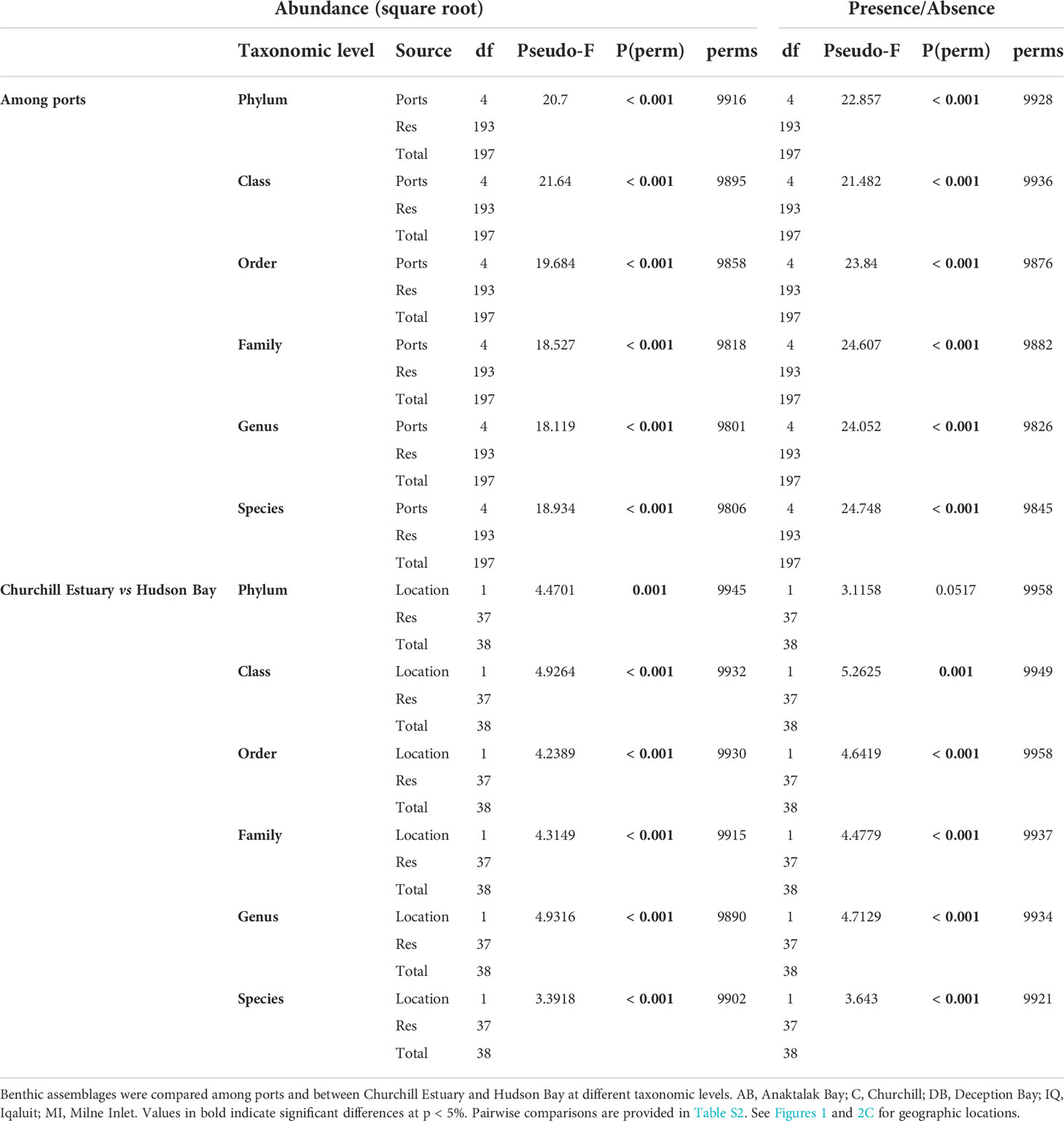
Table 3 Results of PERMANOVA analysis based on similarity matrices derived from square-root transformed abundance and presence/absence data.
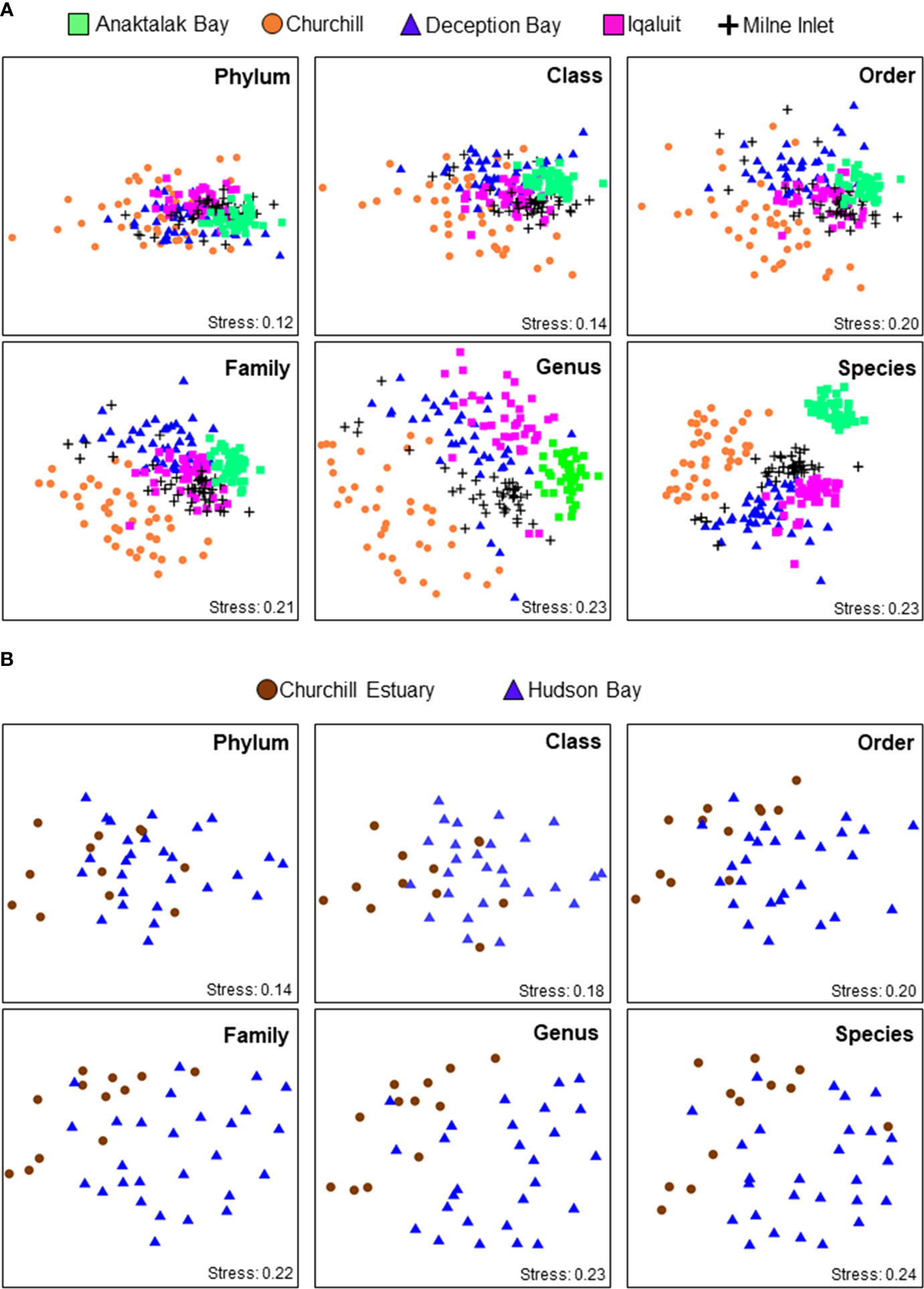
Figure 3 non-Metric multidimensional scaling (nMDS) ordinations of assemblage composition based on Bray-Curtis similarities matrix from square-root transformed abundance data among (A) Arctic ports and between (B) Churchill Estuary and Hudson Bay at various taxonomic levels. See Table 3 for full statistics and Figures 1 and 2C for geographic locations.
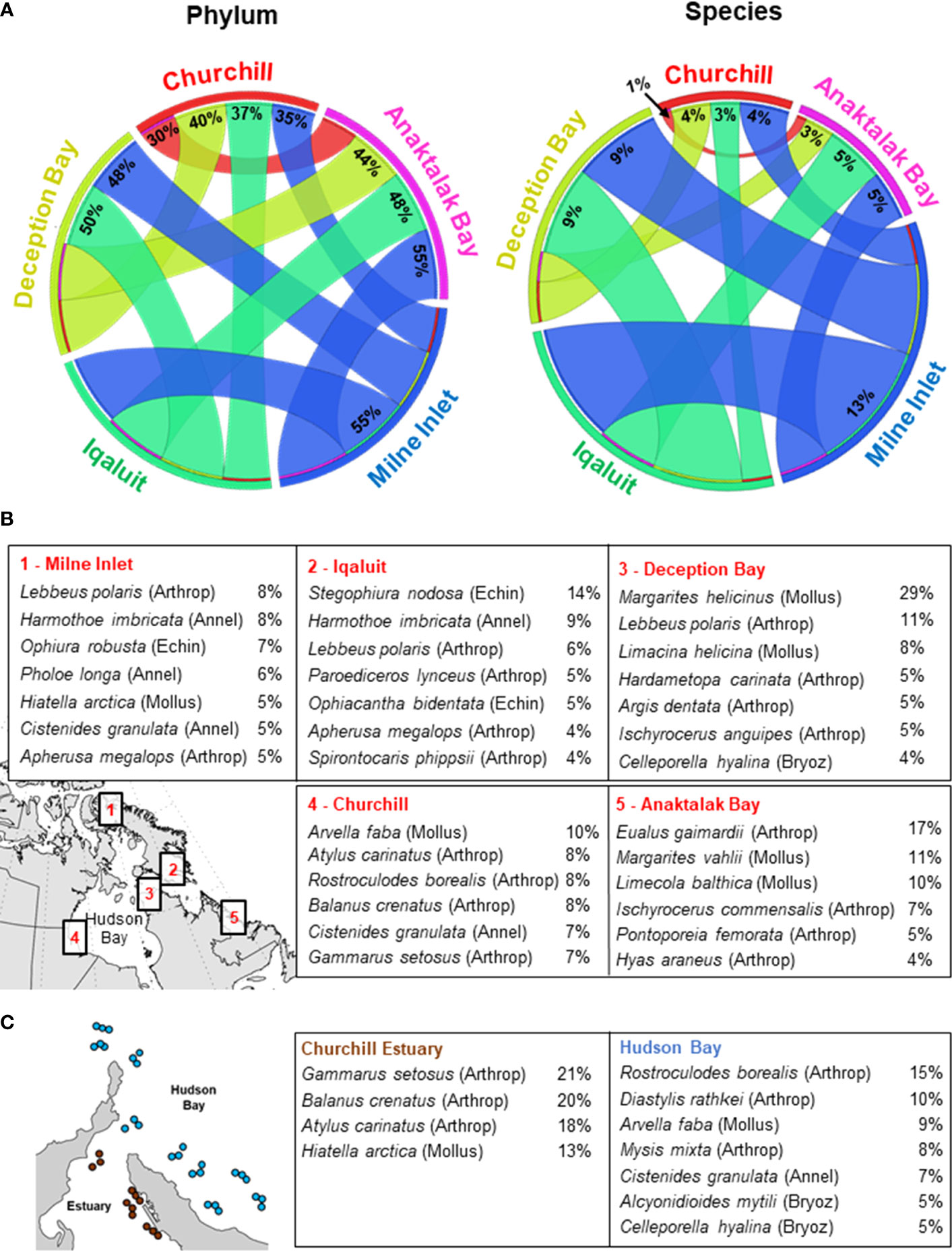
Figure 4 Similarity (%) of benthic assemblages among Arctic locations. (A) Circos plots showing similarities in assemblage composition among Arctic locations at the phylum and species levels. Values range from 0 (complete dissimilarity) to 100% (complete similarity) and are represented by the width of the lines between locations. Contribution of each species to the overall assemblage composition of (B) each Arctic location and (C) between Churchill Estuary and Hudson Bay based on SIMPER analyses. Phyla are shown in parenthesis as Arthrop, Arthropoda; Mollus, Mollusca; Annel, Annelida; Bryoz, Bryozoa; Echin, Echinodermata.
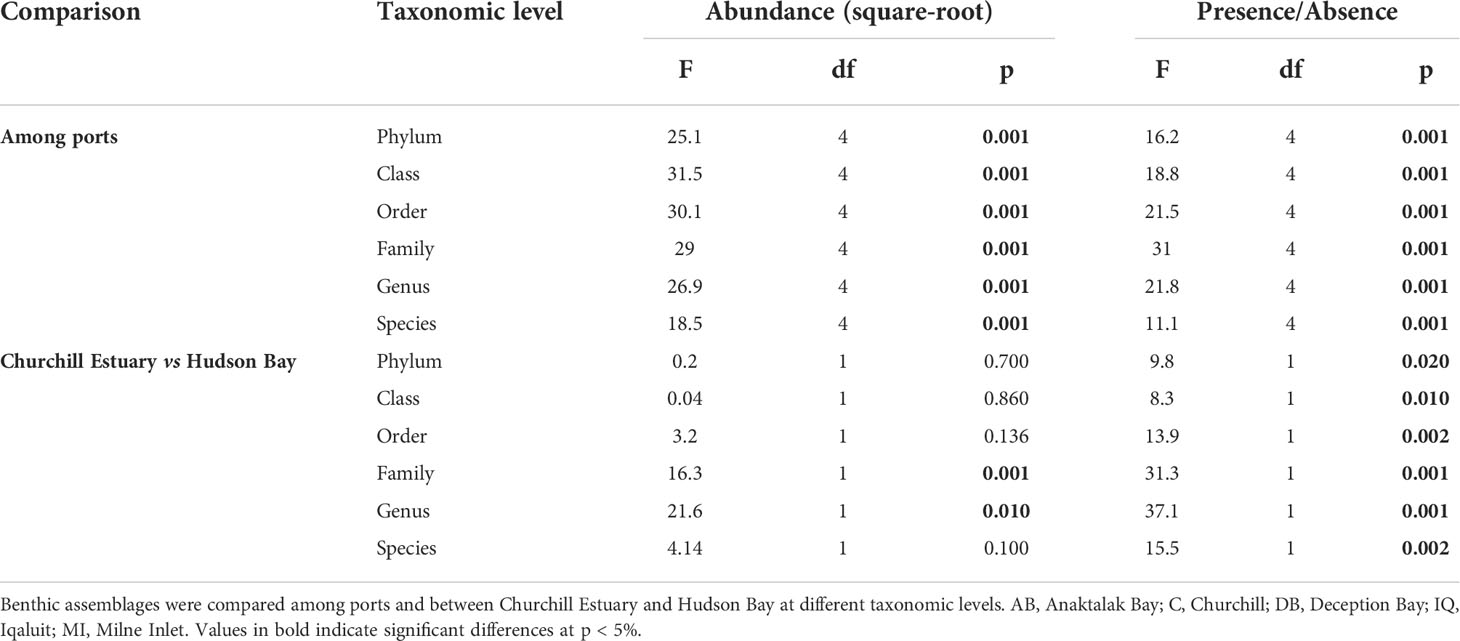
Table 4 Results of PERMISP on similarity matrices derived from square-root transformed abundance and presence/absence data.
Overall, benthic assemblages showed low similarities among locations at both the phylum and species levels (Figure 4A). For instance, Churchill exhibited the lowest similarities at both phylum and species level with other locations and had a relatively unique community dominated by Arthropoda (43%, mainly Atylus carinatus, Rostroculodes borealis, Balanus crenatus), Mollusca (23%, Arvella faba), and Annelida (13%, Cistenides granulata; Figure 4B). Assemblages in Milne Inlet, Iqaluit, and Anaktalak Bay exhibited higher similarity at the species level, ranging from 5 to 13%. The community in Milne Inlet was composed mainly of Arthropoda (38%, Lebbeus polaris), Annelida (23%, Harmothoe imbricata, Pholoe longa, Cistenides granulata), and Mollusca (21%, Hiatella arctica), whereas in Anaktalak Bay the community was dominated by Arthropoda (47%, Eualus gaimardii, Ischyrocerus commensalis, Pontoporeia femorata) followed by Mollusca (22%, Margarites vahlii, Limecola balthica). Iqaluit showed a diverse community represented by Arthropoda (28%, Lebbeus polaris, Paroediceros lynceus, Apherusa megalops), Annelida (21%, H. imbricata), and Echinodermata (18%, Stegophiura nodosa, Ophiacantha bidentata; (Figure 4B). The assemblage in Deception Bay was more similar to that of Iqaluit, which is relatively closer (Figure 4A), and was dominated by Arthropoda (36%, Lebbeus polaris, Hardametopa carinata, Argis dentata), Mollusca (33%, Margarites helicinus, Limacina helicina), and Bryozoa (11%, Celleporella hyalina; Figure 4B).
3.2 Location-specific biodiversity patterns
3.2.1 Anaktalak Bay
In Anaktalak Bay, trawls were done at an average depth between 5.5 and 22.7 m. Abundance varied from 1.8 to 83.0 ind m-2, S ranged from 4 to 137, while H’ and J’ varied from 2.1 and 0.01 to 4.0 and 0.9, respectively. When community metrics were plotted on a map, no large discrepancies were observed in abundance and H’ among sites, indicating relatively similar distribution in the abundance and diversity of organisms in the bay (Figure 5). The assemblage composition showed low variability among sites. While crustaceans were the most abundant group, polychaeta was dominant in a few locations in the northwest portion of the bay, where the trawl collected a considerable amount of soft sediment. Bivalves, gastropods, and ascidians showed relatively low contribution to the overall assemblage structure (Figure 5).
3.2.2 Churchill
In Churchill, trawls were done between 5.0 and 15.0 m deep. Abundance varied between 0.03 and 15.0 ind m-2, S between 0 and 49, and H’ and J’ from 0 to 3.2 and 1.0, respectively. An overall low abundance and H’ were observed in Churchill (Figure 6). Of 39 trawls, only four sites showed abundance >6 ind m-2, and only one site exhibited high diversity (H’ = 4; Figure 6). When sites in Churchill Estuary were compared with sites in Hudson Bay, only abundance differed significantly; S, H’, and J’ had similar values (Figure 2C, Table 2). In fact, abundance was ~7 times higher in Churchill Estuary (~3.4 ind m-2) than in Hudson Bay (0.5 ind m-2; Figure 2C). Assemblage composition differed between these locations at almost all taxonomic levels when using abundance data (Figure 3B, Table 3); however, no difference in assemblages was detected between Churchill Estuary and Hudson Bay at the phylum level when data was transformed to presence/absence, indicating that this taxonomic level is insufficient to determine differences in assemblages between these two locations (Table 3).
Assemblages in Churchill Estuary showed somewhat high abundance, low H’, and were dominated by Arthropoda (Gammarus setosus, B. crenatus, A. carinatus) and Mollusca (H. arctica) (Figures 4C and 6). Conversely, assemblages in Hudson Bay exhibited relatively low abundance, slightly higher H’, and a community composed by various taxonomic groups, including Arthropoda (R. borealis, Diastylis rathkei, Mysis mixta), Mollusca (Arvella faba), Annelida (Cistenides granula), and Bryozoa (Alcyonidioides mytili, C. hyalina; Figures 4C and 6).
3.2.3 Deception Bay
In Deception Bay, trawls were done at an average depth between 3.0 and 26.1 m. Abundance of organisms varied from 0.04 to 95.0 ind m-2, S ranged from 2.8 to 73.0, while H’ and J’ varied from 0.1 and 0.03 to 3.5 and 0.9, respectively. A clear distinction in community metrics and composition was observed between the outer and inner bay (Figure 7). The outer bay showed relatively low abundance and H’, and a community composed mainly by gastropods and crustaceans. In contrast, the inner bay exhibited relatively high abundance and H’ and a community comprised mainly by crustaceans and polychaetes, followed by a small contribution of gastropods and bivalves (Figure 7).
3.2.4 Iqaluit
In Iqaluit, trawls were done at an average depth between 5.5 and 27.3 m. Abundance of organisms was relatively low and ranged from 0.3 to 9.2 ind m-2; however, high values for S and H’ were observed throughout the bay varying from 3.4 and 2.0 to 144 and 3.9, respectively. Pielou’s evenness (J’) varied between 0.01 and 0.9. Although sites displayed relatively similar diversity metrics, assemblage composition clearly varied between inshore (i.e., sites close to the shoreline) and offshore areas (e.g., sites relatively far away from the shoreline; Figure 8). Inshore assemblages were composed mainly by crustaceans and ophiuroids, with a small contribution of polychaetes and gastropods. Conversely, offshore assemblages were dominated by polychaetes, followed by a small contribution of crustaceans, ophiuroids, gastropods, and cirripeds (Figure 8).
3.2.5 Milne Inlet
In Milne Inlet, trawls were done at depths averaging between 5.3 and 20.4 m. Overall, the abundance of organisms varied between 0.07 and 28.7 ind m-2 and S ranged from 3.0 to 105. Shannon-Wiener diversity (H’) and Pielou’s evenness (J’) varied from 0.4 and 0.02 to 3.9 and 0.9, respectively. When community metrics were mapped, no clear patterns in abundance and H’ were observed among sites, indicating an evenly distributed community within the bay (Figure 9). Assemblages were composed mainly by crustaceans, polychaetes, bivalves, and cirripeds. While no major differences in assemblage composition was detected among sites, bivalves were more dominant in the inner bay assemblages than those in the outer bay (Figure 9).
4 Discussion
Arctic coastal habitats provide essential services to the overall functioning of marine ecosystems and are particularly sensitive to changes caused by global warming and anthropogenic activities. The assessment of human-induced environmental stressors on Arctic coastal habitats requires a detailed baseline inventory of the current status of the ecosystem (Piepenburg et al., 2011; Wei et al., 2020). This study represents one of the largest systematic and quantitative trawl surveys of epibenthic assemblages in shallow coastal waters (<30 m) of the Canadian Arctic. A total of 297,417 macroinvertebrates belonging to 900 taxa were collected and identified, providing an extensive baseline on biodiversity metrics and assemblage compositions. In contrast to previous works in shallow waters (Thomson, 1982; Brown et al., 2011), this study showed that Arctic coastal areas have highly diverse epibenthic communities and a rich biodiversity comparable to that of lower latitudes. The lack of relationship between biodiversity metrics and large-scale oceanographic variables suggests that shallow epibenthic communities are likely shaped by local environmental conditions. Assemblages differed among locations at all taxonomic levels. Thematic maps displaying abundance, H’, and assemblage composition revealed distinct local spatial patterns in diversity metrics and epibenthic communities in Churchill, Deception Bay, and Iqaluit, whereas no patterns were detected in Anaktalak Bay and Milne Inlet. This study also presents the first step to characterize and assess benthic assemblages in the Canadian Arctic where industrial and economic activities are expected to development and expand (Lasserre and Têtu, 2015; Baffinland, 2020).
4.1 Arctic coastal biodiversity and sampling methods
Taxonomic richness was ~3-fold higher that reported from trawl surveys in coastal Alaska and ~10-fold higher than image-based surveys in the Canadian and European Arctic. Bluhm et al. (2009) used a modified beam trawl to quantify epibenthic biodiversity in the Chukchi Sea (western Alaska) and observed 165 taxa between 31 and 100 m deep. Ravelo et al. (2015) used two trawl types (a modified and a standard beam trawl) and identified 133 taxa at depths between 13 and 220 m in the Beaufort Shelf (eastern Alaska). Apart from a much wider depth range, a few other reasons can be attributed to the large discrepancies in observed taxonomic richness among studies. While the present study included all the specimens collected in the trawl and identified the majority of them to species/genus level in laboratory, trawl surveys in Alaska did not include all taxonomic groups; e.g., polychaetes were excluded (Ravelo et al., 2015), and many rare species that could conceivably contribute significantly to the taxonomic richness of shallow coastal ecosystems may have been missed or overlooked. Additionally, the coarse taxonomy (i.e., phylum or class) applied to Amphipoda, Bryozoa, and Hydrozoa likely resulted in lower values for richness (Bluhm et al., 2009; Ravelo et al., 2015). Although trawls had similar mesh sizes in both studies in the coastal Alaska and present studies (7-10 mm for net body and 4-6 mm for codend), gear configuration can also influence the observed taxonomic richness. The present study used a beam trawl that was specifically designed to catch macroinvertebrates in shallow coastal areas where macroalgae is generally abundant and maximize the collection of a wide range of both soft and hard bottom organisms for biodiversity inventories (McNeill and Bell, 1992). Conversely, a modified plumb trawl initially designed to sample juvenile crabs and flatfishes was used in Alaska (Gunderson and Ellis, 1986). Although it is difficult to compare the performance of sampling gear based on design and configuration, differences in catchability of different trawls, specifically for rare species, may reflect variations in taxonomic richness. This is particularly true for many studies in polar ecosystems where the focus is generally on common and widespread species, whereas rare species are often discarded, overlooked, or down-weighted for either being under-sampled or requiring high taxonomic skills to be identified correctly (Ellingsen et al., 2007).
Considering the high costs and logistical difficulties associated with trawling in remote shallow locations that are not accessible by large vessels, photographic/video sampling is commonly used to provide a rapid and easy-to-obtain estimate of megafauna biodiversity. For instance, image-based surveys identified 13 taxa in Laptev Sea (14 - 45 m), Northern Russia (Piepenburg and Schmid, 1997), 47 in Kongsfjordern (15 - 30 m), Svalbard (Sahade et al., 2004), and 52 in Vestmann Islands (10 - 15 m), southern Iceland (Witman et al., 2008). In the Canadian Arctic, Brown et al. (2011) observed 64 taxa at Banks Island and 39 at King William Island between depths of 1 and 40 m. While there are several advantages of using images (e.g., relatively low cost and environmental impact), the number of taxa obtained is comparatively low and represents approximately one tenth of the taxa observed in the present study. A quantitative comparison of taxonomic richness from a benthic trawl and a towed camera system on seamounts off southern Tasmania (Australia) revealed that the data collected by trawls yielded >3 times more taxa (190 taxa) than were identified using towed cameras (57 taxa) (Williams et al., 2015). The inability of image-based surveys to fully document benthic biodiversity is likely a function of many epibenthic groups, as those in shallow polar habitats, are of small size and exhibit cryptic behaviour by living either in rock crevices or among macroalgae foliage (Al-Habahbeh et al., 2020) and are not easily visible on images, leading to relatively low richness (Williams et al., 2015). Additionally, many benthic species require the use of a microscope for identification to low taxonomic levels (e.g., genus or species) which limits the utility of image-based methods. Thus, taxonomic richness collected by different sampling methods are often not comparable and particular attention should be paid to method-induced biases when comparing studies and locations. Since the main goal of the present study was to create a comprehensive species inventory, benthic trawls were selected to help maximize the collection of epibenthic biodiversity in shallow coastal habitats and likely explain the substantially higher taxonomic richness observed in this study.
Although this study reports one of the highest levels of taxonomic richness among shallow polar ecosystems, these values are still conservative estimates of the total richness for this part of the Canadian Arctic coast (i.e., at depths <30 m). Rarefaction curves did not approach asymptotes and the expected taxonomic richness (Chao2) exceeded the observed taxonomic richness obtained from all surveyed locations, indicating that sampling effort was insufficient to characterize the full extent of epibenthic biodiversity. This situation is not uncommon in benthic surveys and many other studies have also reported curves without reaching a plateau (Brown et al., 2011; Ravelo et al., 2015; Roy et al., 2015). A plausible explanation for the incomplete taxa inventory, despite the relatively large sample size (39 to 42 trawls in each location), is that trawls were done in different coastal habitats with varying sediment types and related assemblage compositions. High habitat heterogeneity of shallow coastal waters is believed to increase taxonomic richness through partitioned niche space, facilitate speciation, and avoid competition through spatial segregation (Hewitt et al., 2008; Rufino et al., 2017). Additionally, species rarity is often associated with habitat specificity, and thus, an increase in rare species is expected as habitats become more dissimilar (Ellingsen et al., 2007). As the present study did not consider fine-scale habitat characteristics (these were not previously known for surveyed locations), trawls were likely distributed unevenly among coastal habitats and failed to fully document epibenthic biodiversity. Future surveys to expand epibenthic taxonomic inventories in shallow coastal waters of the Canadian Arctic should consider habitat mapping and stratification of samples by habitat types to maximize the collection of abundant and rare species from different environments.
4.2 Arctic shallow epibenthic communities
4.2.1 Latitudinal gradient
The hypothesis of declining biodiversity with increasing latitude (Tittensor et al., 2010; McClain and Schlacher, 2015; Costello and Chaudhary, 2017) remains strongly debated as increasing evidence suggests that this relationship does not seem to apply to all taxonomic groups and locations (Wei et al., 2020). In the present study, similar Shannon-Wiener diversity (H’) was observed in both the southernmost and northernmost surveyed locations (Anaktalak Bay and Milne Inlet, respectively) and there was no relationship between diversity and large-scale oceanographic variables. Additionally, the observed diversity is within the range detected for shallow epibenthic assemblages of southern Portugal (Rufino et al., 2017) and South Africa (Griffiths et al., 2010). Although this study covered a wider latitudinal range (56 to 72°N) than that typically seen for benthic studies, the data is limited to provide more comprehensive analyses as only one location was surveyed at each latitude. Nevertheless, these results corroborate previous studies and provide further evidence that Arctic coastal ecosystems hold comparable benthic biodiversity to that observed at lower latitudes (Kendall and Aschan, 1993; Cusson et al., 2007; Wei et al., 2020).
4.2.2 Influence of local variables
The lack of latitudinal cline and relationship with large scale oceanographic variables begs the question of whether local environmental conditions that are specific to each Arctic location (e.g., frequency and intensity of ice scouring, variation in salinity, and habitat heterogeneity) act synergistically to govern patterns in nearshore epibenthic biodiversity and assemblage composition (Barnes, 1995). Arctic coastal waters are characterized by extreme seasonality in physical-chemical parameters that are known to impact benthic communities (Gutt, 2001; Piepenburg et al., 2011). For instance, ice scouring is a major structuring agent of Arctic coastal ecosystems that occurs when floating sea ice or icebergs touch, plough, or penetrate the seafloor, causing mortality and displacement of organisms, sediment reworking, and the creation and destruction of habitats (Conlan et al., 1998). However, the damaging effect of ice scouring depends on a number of factors, including the frequency of scouring, ice thickness, hydrological regime, and coastal geomorphology (e.g., slope) (Conlan et al., 1998; Gutt, 2001). In Iqaluit and Milne Inlet (Nunavut), for example, the sea ice persists for ~7-8 months of the year with an average thickness of ~2 m, whereas it occurs only for ~4 months in Labrador with a thickness of ~0.9 m (NSIDC, 2021). While there was no correlation between community metrics and sea ice duration in the present study, the shorter ice season combined with thinner sea ice in Anaktalak Bay (Labrador) likely reduces physical impacts and disturbance on benthic communities and may help explain the relatively high abundance of epibenthic organisms compared to more northern locations.
A gradient of ice scour with depth is usually observed on the Arctic continental shelf where shallow areas are frequently scoured by abundant drift ice, whereas deep waters are sporadically scoured only by large icebergs (Lewis and Blasco, 1990). Thus, it is expected that benthic biodiversity follows the gradient of disturbance with relatively lower abundance in shallow than deep waters (Carey, 1991; Conlan and Kvitek, 2005; Smale, 2008). However, the assumption that benthic community metrics vary across a depth gradient in coastal environments is still unclear and studies have provided mixed results. In the present study, depth showed no effect on epibenthic diversity metrics between 3 and 30 m at both large (among locations) and small (within location) scales. However, benthic biodiversity paralleled the disturbance regime of ice scour in Resolute (Canadian Archipelago) between 5 and 50 m, where shallow communities were associated with a highly disturbed assemblage compared to deeper areas (Conlan et al., 1998). In southern Beaufort Sea, mollusc biodiversity was relatively similar between 5 and 25 m (Carey et al., 1984), whereas megafauna biodiversity increased with increasing depth between 3 and 100 m (Ravelo et al., 2015). On the Laptev Sea Shelf and in Kongsfjorden (Svalbard), coastal areas showed low epibenthic diversity (Piepenburg and Schmid, 1997; Sahade et al., 2004). The lack of a consistent results may be due to the effect of depth usually being confounded with other environmental variables, such as substrate type, wave action, turbidity, and frequency of ice scouring, making it difficult to draw general conclusions on biodiversity-depth relationships (Piepenburg and Schmid, 1997). Further studies encompassing detailed correlations of these environmental variables and biodiversity would be needed to better evaluate the drivers of Arctic shallow benthic biodiversity.
While coastal epibenthic communities in Churchill also experience physical disturbance by sea ice, the low biodiversity metrics observed in this location may be the result of salinity stress imposed by freshwater discharge from the Churchill River. In contrast to other surveyed Arctic locations where river runoff is low (e.g., Iqaluit and Deception Bay) and coastal areas are characterized as marine, Churchill is located at the mouth of Churchill River and the area is characterized as estuarine. The Churchill River is a major contributor of freshwater input into western Hudson Bay with annual average discharge of ~1,200 m3 s-1, causing variable salinity and water stratification in the estuary and adjacent areas (Déry et al., 2005). During summer, the water column is highly stratified with relatively low salinity (5-10 psu), whereas in the winter, freshwater discharge is substantially reduced, creating a more saline (33-35 psu) and homogenous environment (Kuzyk et al., 2008). Additionally, the river transports high quantities of terrestrial materials (e.g., terrestrial carbon) that is incorporated into estuarine and marine food webs (Kuzyk et al., 2008). Salinity stress and the ability of organisms to utilise terrestrial material as food sources exert an additional pressure that shapes benthic communities (Van Diggelen and Montagna, 2016). Thus, these variables likely explain the low biodiversity metrics in this location. Moreover, Churchill showed the lowest similarity in assemblage composition among locations at all taxonomic levels (from phylum to species) as well as a distinct community between Churchill Estuary and Hudson Bay. Similar results were observed in a previous study where epibenthic assemblages on the continental shelf of the Canadian Arctic were most dissimilar near the Mackenzie River (Roy et al., 2014). In Hudson Bay, assemblages showed low biodiversity values and high dissimilarity near the Nelson River Estuary (Pierrejean et al., 2020). A metadata analysis by Cusson et al. (2007) also identified salinity as one of the most important variables that explained patterns in benthic biodiversity and assemblage composition in the Canadian Arctic. Additionally, previous work in the intertidal zone of Churchill observed distinct benthic assemblages between Churchill Estuary and Hudson Bay (Cypihot, 2018). The effect of salinity was not only observed on the overall assemblage, but was also reflected in the distribution of certain taxa. For instance, tunicates have low tolerance to low salinity (<10 ppt) (Rocha et al., 2017), such that only Molgula sp. was detected in Churchill Estuary, whereas Molgula sp., Molgula retortiformis, Boltenia echinata, Styela rustica and, Chelyosoma macleayanum were detected in Hudson Bay. The stress imposed by salinity variance due to river runoff on nearshore benthic communities has also been documented in many other Arctic and subarctic locations (Jørgensen et al., 1999; Denisenko et al., 2003; Witman et al., 2008) and highlights the importance of salinity variance as one of the drivers of local benthic communities in Arctic shallow ecosystems.
Coastal ecosystems support structurally complex habitats that have a critical influence on species distribution and benthic assemblages. Habitat heterogeneity is considered a major structuring factor of benthic communities and significantly contributes to nearshore biodiversity (Hewitt et al., 2008; Leps et al., 2015). At a local scale, habitat heterogeneity is predicted to support diverse biological assemblages due to spatial variation in substrate types, vegetation, food sources, and seafloor topography, providing multiple niches and contributing to the coexistence of numerous species (Leps et al., 2015). While it was not possible to characterize benthic habitats in the present study, differences in community metrics and assemblage composition within Arctic locations may reflect differences in benthic habitats, as indicated by the high level of dispersion among samples from one location. For example, habitat heterogeneity seemed to be more important in Churchill, Deception Bay, and Iqaluit, where substantial spatial differences in community metrics and assemblage composition were observed, whereas it may be less relevant in Anaktalak Bay and Milne Inlet as relatively similar metrics and assemblages were detected among sample sites. Thus, future characterization of benthic habitats in coastal environments of the Canadian Arctic could provide essential information on the spatial variability of nearshore benthic biodiversity and assemblage composition.
4.3 Implications and future directions
Arctic coastal ecosystems are facing drastic changes due to global warming and anthropogenic activities. Such modifications of the natural environment impose substantial pressure on Arctic coastal communities potentially leading to loss of biodiversity and changes in assemblage composition. However, the lack of quantitative data on Arctic coastal waters hinders assessment of the impacts of climate and human-induced changes on coastal biodiversity. The present study provides the most comprehensive baseline information on biodiversity metrics and assemblage composition on nearshore (<30 m) benthic communities in the Eastern Canadian Arctic. It represents a significant contribution to the understanding of local and regional Arctic benthic biodiversity and fills important gaps in knowledge of coastal communities in five locations with increasing industrial development. These results provide valuable baselines for environmental monitoring, detection of rare, new, and non-indigenous species, and to help establish conversation strategies for Arctic costal ecosystems.
The high taxonomic richness observed in all surveyed locations remain conservative estimates of the total number of epibenthic taxa and provides further evidence that Arctic coastal ecosystems host communities with a biodiversity comparable to that seen at temperate and tropical latitudes. As sampling effort was insufficient to characterize the full epibenthic biodiversity, future sampling is needed to describe the actual number of species. Comparison of biodiversity metrics in Arctic coastal waters must be done cautiously due to methodological differences among studies. However, recent development in molecular markers, particularly eDNA, will certainly help comparison of biodiversity metrics (e.g., presence/absence) and expand species inventories. Additionally, further investigations should incorporate information on environmental variables to help explain variation in local biodiversity metrics and assemblage composition. Arctic coastal ecosystems are characterized by a variety of physically diverse and biologically distinct habitats that collectively add to the local biodiversity. As many environmental variables do not occur in isolation, but rather interact to each other to yield intricate responses, multidisciplinary studies should be undertaken to assess the synergic effect of ice scouring, salinity variance, and habitat heterogeneity (i.e., substrate type) on Arctic coastal biodiversity. A final consideration concerns temporal variability. Although the present study provided the most comprehensive information on community metrics and assemblage composition, the maps generated here represent a snapshot in time and do not account for seasonal or inter-annual variation. As coastal ecosystems experience considerable variation in physical-chemical parameters throughout the year and between years, the potential influence of temporal dynamics in species distribution and assemblage composition need to be further investigated. The establishment of temporal baseline information and long-term time series can significantly contribute to understanding the effect of natural variability vs climate/human-induced impacts on biodiversity metrics, species distributions, and assemblage composition of Arctic coastal ecosystems.
Data availability statement
The original contributions presented in the study are included in the article/Supplementary Material. Further inquiries can be directed to the corresponding author.
Author contributions
KH and CM conceived and designed the study. KH led the field program. KH, NS, and CM contributed to field data collection. Data analysis and interpretation were performed by BG, CM, KH, PA, and NS. BG wrote the manuscript. All authors contributed to the article and approved the submitted version.
Funding
This project was funded by POLAR Knowledge, ArcticNet, Fisheries and Oceans Canada (Aquatic Invasive Species Monitoring Program, Strategic Program for Ecosystem-Based Research and Advice and Arctic Science Funds), Nunavut Wildlife Management Board (NWMB), Nunavik Marine Region Wildlife Board (NMRWB) and World Wildlife Fund (WWF). This work benefited from feedback received during project development through presentations to the International Council for Exploration of the Seas (ICES), Expert Working Groups on Ballast Water and Other Shipping Vectors, and Introductions and Transfers of Marine Organisms.
Acknowledgments
We thank taxonomists Laure De Montety and Lisa Treau De Coeli for identification of organisms. We acknowledge the Churchill Northern Studies Centre, Glencore-Raglan, Baffinland and Nunatsiavut Government, Vale Mines, Environment and Climate Change Canada and Government of Nunavut MV Nuliajuk for providing field accommodations and/or logistic support. We are especially grateful for help with field coordination and/or assistance from the following: Adamie Keatanik, Anais Lacoursiere, Andrew Arreak, Antoine Dispas, Austin MacLeod, Chris Idlaut, Colin Gallagher, Daniel Gibson, Dick Hunter, Emmanuelle Porter, Eric Solomon, Fatma Dhifallah, Frédéric Hartog, Frederic Lemire, Heather Clark, LeeAnn Fishback, Lillian Angnatok, Markusie Jaaka, Melissa Nacke, Noemie Leduc, Ken Cameron, Rachel Smale, Rory McDonald, Shelley Elverum, Sylvia Pewatoalook, Thomas Whittle, Valérie Cypihot, Willie Keatanik, Willie Alaku, and Zoya Martin. Finally, we thank two anonymous reviewers for their helpful comments on this manuscript.
Conflict of interest
The authors declare that the research was conducted in the absence of any commercial or financial relationships that could be construed as a potential conflict of interest.
Publisher’s note
All claims expressed in this article are solely those of the authors and do not necessarily represent those of their affiliated organizations, or those of the publisher, the editors and the reviewers. Any product that may be evaluated in this article, or claim that may be made by its manufacturer, is not guaranteed or endorsed by the publisher.
Supplementary material
The Supplementary Material for this article can be found online at: https://www.frontiersin.org/articles/10.3389/fmars.2022.873608/full#supplementary-material.
References
Aarluk C., Gartner L., Anderson C. (2005). Strategic plan for the Iqaluit deepwater port project (Aarluk Consulting Inc., Gartner Lee Limited and Chris Anderson), 95 p.
Al-Habahbeh A. K., Kortsch S., Bluhm B. A., Beuchel F., Gulliksen B., Ballantine C., et al. (2020). Arctic Coastal benthos long-term responses to perturbations under climate warming. Philos. Trans. R. Soc A 378, 20190355. doi: 10.1098/rsta.2019.0355
Archambault P., Snelgrove P. V. R., Fisher J. A. D., Gagnon J.-M., Garbary D. J., Harvey M., et al. (2010). From sea to sea: Canada's three oceans of biodiversity. PLoS One 5, e12182. doi: 10.1111/ddi.13013
Atkinson E. G., Wacasey J. W. (1989a). Benthic invertebrates collected from the western Canadian Arctic 1951 to 1985. Can. Data Rep. Fish. Aquat. Sci. 745, 138.
Atkinson E. G., Wacasey J. W. (1989b). Benthic invertebrates from Hudson bay, Canada 1953 to 1965. Can. Data Rep. Fish. Aquat. Sci. 745, 138.
Baffinland (2020). Community roundtable, phase 2 proposal, September 28-30, 2020. powerpoint presentation. Nunavut impact review board, public registry file: 200923-08MN053.
Barnes D. K. A. (1995). Sublittoral epifaunal communities at signy island, antarctica. II. below the ice-foot zone. Mar. Biol. 121, 565–572. doi: 10.1007/BF00349467
Bintanja R., Andry O. (2017). Towards a rain-dominated Arctic. Nat. Clim. Change 7, 263–267. doi: 10.1038/nclimate3240
Bluhm B. A., Iken K., Hardy S. M., Sirenko B.I., Holladay B.A.. (2009). Community structure of epibenthic megafauna in the chukchi Sea. Aquat. Biol. 7, 269–293. doi: 10.3354/ab00198
Brown T. M., Edinger E. N., Hooper R. G., Belliveau K. (2011). Benthic marine fauna and flora of two nearshore coastal locations in the western and central Canadian Arctic. Arctic 64, 281–301. doi: 10.14430/arctic4119
CAFF (2019). “Arctic Coastal biodiversity monitoring plan,” in Conservation of Arctic flora and fauna, vol. v29. (Akureyri, Iceland), p72.
Carey A. G. Jr (1991). Ecology of north American Arctic continental shelf benthos: a review. Cont. Shelf Res. 11, 865–883. doi: 10.1016/0278-4343(91)90083-I
Carey J., Scott P. H., Walter K. R. (1984). Distributional ecology of shallow southwestern Beaufort Sea (Arctic ocean) bivalve Mollusca. Mar. Ecol. Prog. Ser. 17, 125–134. doi: 10.3354/meps017125
Chamberlin R. V. (1920). The polychaetes collected by the Canadian Arctic expedition 1913-18. Ottawa. vol IX, annelids, parasitic worms, protozoans, etc. part b: Polychaeta. 46p. (FA Acland, Ottawa, ON)
Chao A. (1987). Estimating the population size for capture-recapture data with unequal catchability. Biometrics, 43:783–791. doi: 10.2307/2531532
Chao A., Chiu C. H., Hsieh T. C., Davis T., Nipperess D. A., Faith D. P., et al. (2015). Rarefaction and extrapolation of phylogenetic diversity. Methods Ecol. Evol. 6, 380–388. doi: 10.1111/2041-210X.12247
Clark A. H. (1920). The echinoderms of the Canadian Arctic expedition 1913-18. vol VIII, mollusks, echinoderms, coelenterates, etc. part c: Echinoderms (FA Acland, Ottawa, ON), 20p.
Clarke K. R., Gorley R. N., Somerfield P. J., Warwick R. M. (2014). Change in marine communities: an approach to statistical analysis and interpretation. 3rd edition. PRIMER-e (PRIMER-E: Plymouth, UK).
Conlan K. E., Kvitek R. G. (2005). Recolonization of soft-sediment ice scours on an exposed Arctic coast. Mar. Ecol. Prog. Ser. 286, 21–42. doi: 10.3354/meps286021
Conlan K. E., Lenihan H. S., Kvitek R. G., Oliver J. S. (1998). Ice scour disturbance to benthic communities in the Canadian high Arctic. Mar. Ecol. Prog. Ser. 166, 1–16. doi: 10.3354/meps331291
Costello M. J., Chaudhary C. (2017). Marine biodiversity, biogeography, deep-sea gradients, and conservation. Curr. Biol. 27, R511–R527. doi: 10.1016/j.cub.2017.04.060
Curtis M. A. (1975). The marine benthos of the Arctic and sub-Arctic continental shelves. Polar Rec. 17, 595–626. doi: 10.1017/S0032247400032691
Cusson M., Archambault P., Aitken A. (2007). Biodiversity of benthic assemblages on the Arctic continental shelf: historical data from Canada. Mar. Ecol. Prog. Ser. 331, 291–304. doi: 10.3354/meps331291
Cypihot V. (2018). Validation des caractéristiques biologiques et fonctionnelles des communautés benthiques associées à des habitats côtiers dans l’Arctique canadien (Rimouski: Université du Québec à Rimouski), 108.
Dall W. H. (1919). Report of the Canadian Arctic expedition 1913-18. the Mollusca of the Arctic coast of America collected by the Canadian Arctic expedition west from bathurst inlet with an appended report on a collection of pleistocene fossil mollusca. vol VIII. mollusks, echinoderms, coelenterates, etc. part a: Mollusks, recent and pleistocene (FA Acland, Ottawa, ON), 42p.
Denisenko S. G., Denisenko N. V., Lehtonen K. K., Andersin A.-B., Laine A.O.. (2003). Macrozoobenthos of the pechora Sea (SE barents sea): community structure and spatial distribution in relation to environmental conditions. Mar. Ecol. Prog. Ser. 258, 109–123. doi: 10.3354/meps258109
Derksen C., Brown R. (2012). Spring snow cover extent reductions in the 2008–2012 period exceeding climate model projections. Geophys. Res. Lett. 39, 1–6. doi: 10.1029/2012GL053387
Déry S. J., Stieglitz M., McKenna E. C., Wood E. F. (2005). Characteristics and trends of river discharge into Hudson, James, and ungava bays 1964–2000. J. Clim. 18, 2540–2557. doi: 10.1175/JCLI3440.1
Ellingsen K. E., Hewitt J. E., Thrush S. F. (2007). Rare species, habitat diversity and functional redundancy in marine benthos. J. Sea Res. 58, 291–301. doi: 10.1016/j.seares.2007.10.001
Ellis D. V. (1960). Marine infaunal benthos in arctic north America (Arctic Institute of North America), 53 pp.
Gianasi B. L., Goldsmit J., Archambault P., McKindsey C. W., Holovachov O., Howland K. L.. (2022). Biodiversity of macrobenthic nematodes in the intertidal and shallow subtidal zones in the Eastern Canadian Arctic. Polar Biol. 45:225–242. doi: 10.1007/s00300-021-02989-z. 1-.
Goldsmit J., McKindsey C. W., Schlegel R. W., Stewart D. B., Archambault P., Howland K. L.. (2020). What and where? predicting invasion hotspots in the Arctic marine realm. Global Change Biol. 26, 4752–4771. doi: 10.1111/gcb.15159
Griffiths C. L., Robinson T. B., Lange L., Mead A. (2010). Marine biodiversity in south Africa: an evaluation of current states of knowledge. PLoS One 5, e12008. doi: 10.1371/journal.pone.0012008
Gulliksen B., Bahr G. (2001). Variation of the epifauna on pier-pilings between 1980 and 1992 near the city of tromsø, northern Norway. Polar Biol. 24, 282–291. doi: 10.1007/s003000000212
Gunderson D. R., Ellis I. E. (1986). Development of a plumb staff beam trawl for sampling demersal fauna. Fish. Res. 4, 35–41. doi: 10.1016/0165-7836(86)90026-3
Gutt J. (2001). On the direct impact of ice on marine benthic communities, a review. Polar Biol. 24, 553–564. doi: 10.1007/s003000100262
Hewitt J. E., Thrush S. F., Dayton P. D. (2008). Habitat variation, species diversity and ecological functioning in a marine system. J. Exp. Mar. Biol. Ecol. 366, 116–122. doi: 10.1016/j.jembe.2008.07.016
Jørgensen L. L., Pearson T. H., Anisimova N. A., Gulliksen B., Dahle S., Denisenko S. G., et al. (1999). Environmental influences on benthic fauna associations of the kara Sea (Arctic Russia). Polar Biol. 22, 395–416. doi: 10.1007/s003000050435
Jørgensen L. L., Primicerio R., Ingvaldsen R. B., Fossheim M., Strelkova N., Thangstad T. H., et al. (2019). Impact of multiple stressors on sea bed fauna in a warming Arctic. Mar. Ecol. Prog. Ser. 608, 1–12. doi: 10.3354/meps12803
Johnston M., Dawson J., Stewart E. (2019). “Marine tourism in Nunavut: Issues and opportunities for economic development in Arctic Canada,” in Perspectives on rural tourism geographies. Eds. Koster R., Carson D. (Springer, Cham), pp 115–pp 136. doi: 10.1007/978-3-030-11950-8_7
Kaiser M. J., Clarke K. R., Hinz H., Austen M. C. V., Somerfield P. J., Karakassis I., et al. (2006). Global analysis of response and recovery of benthic biota to fishing. Mar. Ecol. Prog. Ser. 311, 1–14. doi: 10.3354/meps311001
Kendall M. A., Aschan M. (1993). Latitudinal gradients in the structure of macrobenthic communities: a comparison of Arctic, temperate and tropical sites. J. Exp. Mar. Biol. Ecol. 172, 157–169. doi: 10.14430/arctic1411
Kortsch S., Primicerio R., Beuchel F., Renaud P. E., Rodrigues J., Lønne O. J., et al. (2012). Climate-driven regime shifts in Arctic marine benthos. Proc. Natl. Acad. Sci. U.S.A. 109, 14052–14057. doi: 10.1073/pnas.1207509109
Kröncke I., Zeiss B., Rensing C. (2001). Long-term variability in macrofauna species composition off the island of norderney (East frisia, Germany) in relation to changes in climatic and environmental conditions. Senckenb Marit 31, 65–82. doi: 10.1007/BF03042837
Kuzyk Z. A., Macdonald R. W., Granskog M. A., Scharien R. K., Galley R. J., Michel C., et al. (2008). Sea Ice, hydrological, and biological processes in the Churchill river estuary region, Hudson bay. Estuar. Coast. Shelf Sci. 77, 369–384. doi: 10.1016/j.ecss.2007.09.030
Lasserre F., Têtu P.-L. (2015). The cruise tourism industry in the Canadian Arctic: analysis of activities and perceptions of cruise ship operators. Polar Rec. 51, 24–38. doi: 10.1017/S0032247413000508
Leps M., Tonkin J. D., Dahm V., Haase P., Sundermann A.. (2015). Disentangling environmental drivers of benthic invertebrate assemblages: the role of spatial scale and riverscape heterogeneity in a multiple stressor environment. Sci. Total Environ. 536, 546–556. doi: 10.1016/j.scitotenv.2015.07.083
Lewis C. F. M., Blasco S. M. (1990). “Character and distribution of sea-ice and iceberg scour,” in Proceedings of the workshop on ice scouring and the design of offshore pipelines. Ed. Clark J. I. (Canada, Calgary, Alberta: Canada Oil and Gas Lands Administration, Energy, Mines and Resources Canada and Indian and Northern Affairs), p 57–p101.
Lin Y., Ng A. K. Y., Afenyo M. (2020). “Climate change, a double-edged sword: The case of Churchill on the Northwest passage,” in Maritime transport and regional sustainability. Eds. Ng A. K. Y., Monios J., Jiang C. (Elsevier), p 223–p 235.
McClain C. R., Schlacher T. A. (2015). On some hypotheses of diversity of animal life at great depths on the sea floor. Mar. Ecol. 36, 849–872. doi: 10.1111/maec.12288
McMeans B. C., Rooney N., Arts M. T., Fisk A. T. (2013). Food web structure of a coastal Arctic marine ecosystem and implications for stability. Mar. Ecol. Prog. Ser. 482, 17–28. doi: 10.3354/meps10278
McNeill S. E., Bell J. D. (1992). Comparison of beam trawls for sampling macrofauna of posidonia seagrass. Estuaries 15, 360–367. doi: 10.2307/1352783
Niemi A., Ferguson S., Hedges K., Melling H., Michel C., Ayles B., et al. (2019). State of canada’s Arctic seas. Can. Tech. Rep. Fish. Aquat. Sci. 3344, xv + 189.
NSIDC (2021) (National Snow & Ice Data Center). Available at: https://nsidc.org/data/G02171 (Accessed July 16, 2021).
Overland J., Dunlea E., Box J. E., Corell R., Forsius M., Kattsov V., et al. (2019). The urgency of Arctic change. Polar Sci. 21, 6–13. doi: 10.1016/j.polar.2018.11.008
Overland J., Wang M., Walsh J. E., Stroeve J. C. (2014). Future Arctic climate changes: Adaptation and mitigation time scales. Earth's Future 2, 68–74. doi: 10.1002/2013EF000162
Pahl J., Kaiser B. A. (2018). “Arctic Port development,” in Arctic Marine resource governance and development. Eds. Vestergaard N., Kaiser B. A., Fernandez L., Nymand Larsen J. (Cham: Springer Polar Sciences), pp 139–pp 184. doi: 10.1007/978-3-319-67365-3_8
Piepenburg D., Archambault P., Ambrose W. G., Blanchard A. L., Bluhm B. A., Carroll M. L., et al. (2011). Towards a pan-Arctic inventory of the species diversity of the macro-and megabenthic fauna of the Arctic shelf seas. Mar. Biodiv. 41, 51–70. doi: 10.1007/s12526-010-0059-7
Piepenburg D., Schmid M. K. (1997). A photographic survey of the epibenthic megafauna of the Arctic laptev Sea shelf: distribution, abundance, and estimates of biomass and organic carbon demand. Mar. Ecol. Prog. Ser. 147, 63–75. doi: 10.3354/meps147063
Pierrejean M., Babb D. G., Maps F., Nozais C., Archambault P.. (2020). Spatial distribution of epifaunal communities in the Hudson bay system: Patterns and drivers. Elem. Sci. Anth. 8. doi: 10.1525/elementa.00044
Post E., Alley R. B., Christensen T. R., Macias-Fauria M., Forbes B. C., Gooseff M. N., et al. (2019). The polar regions in a 2°C warmer world. Sci. Adv. 5, eaaw9883. doi: 10.1126/sciadv.aaw9883
Pronovost F. (1999). “Arctic Mining in raglan,” in Mining in the Arctic. Eds. Udd J. E., Keen A. J. (London: CRC Press), pp 29–pp 42. doi: 10.1201/9781003078555
Rapinski M., Cuerrier A., Harris C., Lemire M. (2018). Inuit Perception of marine organisms: From folk classification to food harvest. J. Ethnobiol. 38, 333–355. doi: 10.2993/0278-0771-38.3.333
Ravelo A. M., Konar B., Bluhm B. A. (2015). Spatial variability of epibenthic communities on the Alaska Beaufort shelf. Polar Biol. 38, 1783–1804. doi: 10.1007/s00300-015-1741-9
Rocha R. M., Castellano G. C., Freire C. A. (2017). Physiological tolerance as a tool to support invasion risk assessment of tropical ascidians. Mar. Ecol. Prog. Ser. 577, 105–119. doi: 10.3354/meps12225
Roy V., Iken K., Archambault P. (2014). Environmental drivers of the Canadian Arctic megabenthic communities. PLoS One 9, e100900. doi: 10.1371/journal.pone.0100900
Roy V., Iken K., Archambault P. (2015). Regional variability of megabenthic community structure across the Canadian Arctic. Arctic 68, 180–192. doi: 10.14430/arctic4486
Rufino M. M., Pereira A. M., Pereira F., Moura P., Vasconcelos P., Gaspar M. B.. (2017). Habitat structure shaping megabenthic communities inhabiting subtidal soft bottoms along the Algarve coast (Portugal). Hydrobiologia 784, 249–264. doi: 10.1007/s10750-016-2879-3
Saebi M., Xu J., Curasi S. R., Grey E. K., Chawla N.V., Lodge D. M.. (2020). Network analysis of ballast-mediated species transfer reveals important introduction and dispersal patterns in the Arctic. Sci. Rep. 10, 1–15. doi: 10.1038/s41598-020-76602-4
Sahade R., Stellfeldt A., Tatián M., Laudien J. (2004). “Macro-epibenthic communities and diversity of Arctic kongsforden, Svalbard, in relation to depth and substrate,” in The coastal ecosystem of kongsfjorden, Svalbard: synopsis of biological research performed at the koldewey station in the years 1991-2003. Ed. Wiencke C. (Alfred-Wegener-Institut für Polar-und Meeresforschung) (Bremerhaven, Germany).
Smale D. A. (2008). Ecological traits of benthic assemblages in shallow Antarctic waters: does ice scour disturbance select for small, mobile, secondary consumers with high dispersal potential? Polar Biol. 31, 1225–1231. doi: 10.1007/s00300-008-0461-9
Stroeve J. C., Serreze M. C., Holland M. M., Kay J. E., Malanik J., Barrett A. P.. (2012). The arctic’s rapidly shrinking sea ice cover: a research synthesis. Clim. Change 110, 1005–1027. doi: 10.1007/s10584-011-0101-1
Terhaar J., Kwiatkowski L., Bopp L. (2020). Emergent constraint on Arctic ocean acidification in the twenty-first century. Nature 582, 379–383. doi: 10.1038/s41586-020-2360-3
Têtu P.-L., Pelletier J.-F., Lasserre F. (2015). The mining industry in Canada north of the 55th parallel: a maritime traffic generator? Polar Geogr. 38, 107–122. doi: 10.1080/1088937X.2015.1028576
Thomson D. H. (1982). Marine benthos in the eastern Canadian high arctic: multivariate analyses of standing crop and community structure. Arctic 35, 61–74. doi: 10.14430/arctic2308
Tittensor D. P., Mora C., Jetz W., Lotze H. K., Ricard D., Berghe E. V., et al. (2010). Global patterns and predictors of marine biodiversity across taxa. Nature 466, 1098–1101. doi: 10.1038/nature09329
Van Diggelen A. D., Montagna P. A. (2016). Is salinity variability a benthic disturbance in estuaries? Estuaries Coast. 39, 967–980. doi: 10.1007/s12237-015-0058-9
Wacasey J. W., Atkinson E. G., Derick L., Weinstein A. (1977). Zoobenthos data from the southern Beaufort Sea 1971- 1975. Can. Data Rep. Fish. Aquat. Sci. 41, 193.
Wacasey J. W., Atkinson E. G., Glasspoole L. (1979). Zoobenthos data from upper Frobisher bay 1967 - 1973. Can. Data Rep. Fish. Aquat. Sci. 164, 107.
Wacasey J. W., Atkinson E. G., Glasspoole L. (1980). Zoobenthos data from inshore stations of upper Frobisher bay 1969 - 1976. Can. Data Rep. Fish. Aquat. Sci. 205, 48.
Wacasey J. W., Atkinson E. G., Kinlough L. (1976). Zoobenthos data from James bay 1959, 1974. Can. Data Rep. Fish. Aquat. Sci. 661, 73.
Wang M., Overland J. E. (2012). A sea ice free summer Arctic within 30 years: An update from CMIP5 models. Geophys. Res. Lett. 39, 1–6. doi: 10.1029/2012GL052868
Wei C. L., Cusson M., Archambault P., Belley R., Brown T., Burd B. J., et al. (2020). Seafloor biodiversity of canada's three oceans: Patterns, hotspots and potential drivers. Divers. Distrib. 26, 226–241. doi: 10.1111/ddi.13013
Williams A., Althaus F., Schlacher T. A. (2015). Towed camera imagery and benthic sled catches provide different views of seamount benthic diversity. Limnol. Oceanogr. Methods 13, 62–73. doi: 10.1002/lom3.10007
Keywords: Arctic, biogeography, bottom trawling, community analyses, coastal habitat, taxonomy, species distribution, survey
Citation: Gianasi BL, McKindsey CW, Archambault P, Simard N and Howland KL (2022) Biodiversity of coastal epibenthic macrofauna in Eastern Canadian Arctic: Baseline mapping for management and conservation. Front. Mar. Sci. 9:873608. doi: 10.3389/fmars.2022.873608
Received: 11 February 2022; Accepted: 30 June 2022;
Published: 27 July 2022.
Edited by:
Susana Carvalho, King Abdullah University of Science and Technology, Saudi ArabiaReviewed by:
Henning Reiss, Nord University, NorwayAldo S. Pacheco, National University of San Marcos, Peru
Copyright © 2022 Gianasi, McKindsey, Archambault, Simard and Howland. This is an open-access article distributed under the terms of the Creative Commons Attribution License (CC BY). The use, distribution or reproduction in other forums is permitted, provided the original author(s) and the copyright owner(s) are credited and that the original publication in this journal is cited, in accordance with accepted academic practice. No use, distribution or reproduction is permitted which does not comply with these terms.
*Correspondence: Bruno L. Gianasi, bruno.gianasi@dfo-mpo.gc.ca