- 1Centre for Marine Socioecology, Institute for Marine and Antarctic Studies, University of Tasmania, Hobart, TAS, Australia
- 2South Atlantic Environmental Research Institute, Stanley, Falkland Islands
- 3Tasmanian Museum and Art Gallery (TMAG), Hobart, TAS, Australia
- 4British Antarctic Survey (BAS), Cambridge, United Kingdom
- 5Alfred Wegener Institute Helmholtz Centre for Polar and Marine Research (AWI), Bremerhaven, Germany
- 6Geological Survey of Norway, Trondheim, Norway
- 7Australian National University, Canberra, ACT, Australia
- 8Environment Centre Wales, UK Centre for Ecology and Hydrology (UKCEH), Wallingford, United Kingdom
- 9UK Centre for Ecology and Hydrology, Bangor, United Kingdom
- 10School of Biological Sciences, University of Aberdeen, Aberdeen, United Kingdom
- 11Duke University, Nicholas School of the Environment, Durham, NC, United States
- 12Shallow Marine Surveys Group, Stanley, Falkland Islands
- 13Centre for Biodiversity & Environment Research, University College London, Bloomsbury, London, United Kingdom
Ecosystem-based conservation that includes carbon sinks, alongside a linked carbon credit system, as part of a nature-based solution to combating climate change, could help reduce greenhouse gas levels and therefore the impact of their emissions. Blue carbon habitats and pathways can also facilitate biodiversity retention, aiding sustainable fisheries and island economies. However, robust blue carbon research is often limited at the scale of regional governance and management, lacking both incentives and facilitation of policy-integration. The remote and highly biodiverse coastal ecosystems and surrounding continental shelf can be used to better inform long-term ecosystem-based management in the vast South Atlantic Ocean and sub-Antarctic, to synergistically protect both unique biodiversity and inform on the magnitude of nature-based benefits they provide. Understanding key ecosystem information such as their location, extent, and condition of habitat types, will be critical in understanding carbon pathways to sequestration, threats to this, and vulnerability. This paper considers the current status of blue carbon data and information available, and what is still required before blue carbon can be used as a conservation management tool integrated in national Marine Spatial Planning (MSP) initiatives. Our research indicates that the data and information gathered has enabled baselines for a number of different blue carbon ecosystems, and indicated potential threats and vulnerability that need to be managed. However, significant knowledge gaps remain across habitats, such as salt marsh, mudflats and the mesophotic zones, which hinders meaningful progress on the ground where it is needed most.
Introduction
To synergistically tackle nature loss and climate change, the few remaining intact carbon-rich habitats need to be prioritised in conservation efforts (Smith et al., 2022). By protecting these carbon- and species-rich habitats, there is a reduction in carbon release and subsequent contribution to the climate change crisis (Pörtner et al., 2021). The cumulative consequences of anthropogenic climate change, habitat destruction and marine resource extraction are evident globally, even in the most isolated oceans and coastlines (Halpern et al., 2019; Bergstrom et al., 2021; Ward et al., 2022). The comparative isolation and reduced exposure from human pressures on islands and offshore shelf habitats imparts some level of biodiversity protection, but even these are impacted by climate change, marine debris, and non-native species threats (e.g. Barnes et al., 2018a; Hughes et al., 2020). Isolation does not absolve the need for management, nor does it guarantee the long-term sustainability of ecosystem services such as marine carbon capture to sequestration (‘blue carbon’; Bax et al., 2021; Bayley et al., 2021). Indeed, remote islands present unique physical and economic barriers to conservation planning. These constraints are evident in a lack of governmental capacity and ecosystem-based knowledge (Queirós et al., 2021), due in part to constraints on financial resourcing for science and marine management (Bax et al., 2022). A comparatively high carbon footprint and associated ‘risk footprint’ (e.g., fuel transport, site contamination/remediation and oil spill mitigation), pose additional logistical complexities for carbon off-setting in remote environments (Brooks et al., 2018; Brooks et al., 2019). Overcoming these limitations is particularly pressing as CO2 emissions continue to rise, and biodiversity continues to decline globally (Pörtner et al., 2021). Identifying locations with multiple intact blue carbon ecosystems such as seagrass, saltmarsh, mangrove, macroalgae and deeper seabed habitats is only one part of the challenge. Once these locations are identified, the additional tasks exist of securing adequate resourcing to support their conservation and ensuring this best mitigates threats.
Incorporating blue carbon as a conservation management tool is timely, as the global United Nations Framework Convention on Climate Change (UNFCCC) recommendations for the Conference of the Parties (COP26) in November 2021 noted that ‘marine ecosystems’ are also recognised as ‘carbon sinks’ in Article 21 of the final decision. This UNFCCC recommendation emphasises the importance of the protection, conservation, and restoration of terrestrial and marine ecosystems alongside the urgent and considerable reduction of greenhouse gas (GHG) emissions (UNFCCC, 2021a). Article 6 of the Paris Agreement outlines how governments can assist in achieving their Nationally Determined Contributions (NDCs) to carbon reduction and removal targets. This legislation includes international transactions in carbon reduction credits, and could encourage the private sector to contribute to GHG emissions reductions through “non-market approaches” under the Paris Climate Agreement (UNFCCC, 2021b). If such approaches are embraced, economists predict that the revenue from carbon markets could exceed USD 1 trillion by 2050 (IETA and the University of Maryland, 2021). These economic incentives pave the way for carbon credits linked to blue carbon and marine biodiversity conservation management in the near future. Momentum is therefore growing for robust blue carbon research to facilitate policy-climate-integration and inform accurate carbon accounting across multiple habitats through for example, an understanding of the distribution of carbon sequestering habitat for Marine Spatial Planning (MSP) that addresses ocean climate-driven change (‘climate-smart MSP’) (see Queirós et al., 2021).
A Broader Blue Carbon Definition
Many marine ecosystems are officially recognised as highly efficient carbon sinks. However, when Nellemann et al. (2009) originally defined blue carbon as carbon captured by marine living organisms, they put the spotlight on shallower, relatively accessible mangroves, salt marshes, and seagrasses. Blue carbon research continues to narrowly focus on these coastal ecosystem types because they are actionable; many nearshore systems which remove significant amounts of CO2 and store fixed carbon long-term, are subjected to undesirable anthropogenic impacts, and can be managed to maintain or enhance carbon stocks. Such management aligns with climate change mitigation and adaptation policies (Lovelock and Duarte, 2019). Other potential blue carbon ecosystem types have only been considered recently, such as macroalgae (Krause-Jensen and Duarte, 2016), and seafloor blue carbon (Barnes, 2015; Bax et al., 2021). However, significant knowledge gaps currently preclude them from being considered as actionable blue carbon ecosystems. These important overlooked blue carbon ecosystems should be reassessed as actionable or non-actionable, once knowledge gaps have been filled.
Here we consider the situation in the Falkland Islands (FI), as an exemplar of global challenges faced. In order to identify and fill key knowledge gaps, this paper discusses the major ecosystems, from terrestrial (green carbon) sequestration and fluvial loss in the shallow sea across the water column, until its potential burial in seabed sediment to provide a framework across the carbon cycle (Figure 1). Proposed Marine Management Areas (MMAs) are being consulted upon in 2022 and would encompass 15% of FI marine waters if implemented. This paper provides a foundation for future work on these important ecosystems.
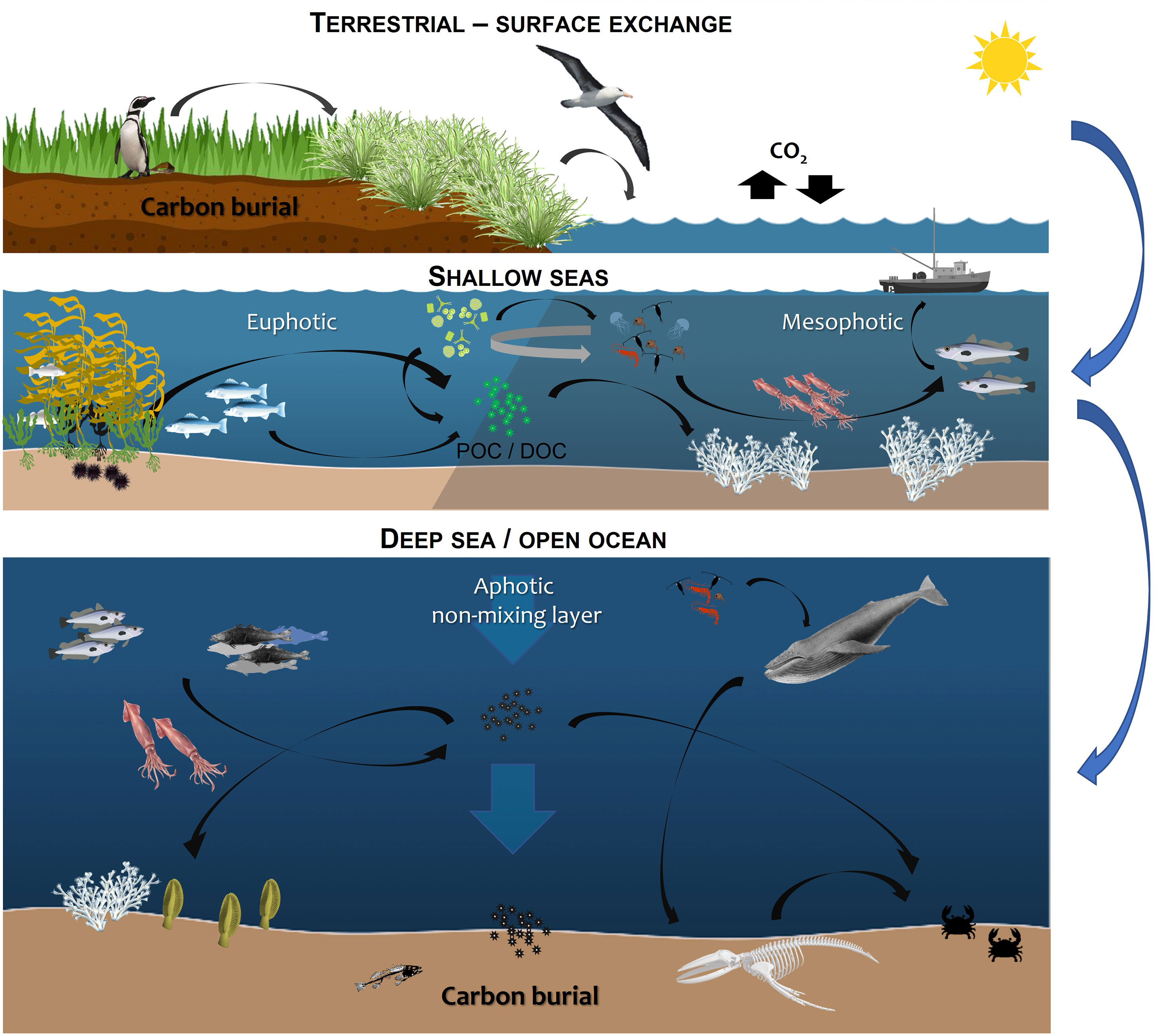
Figure 1 Overview of blue carbon habitats considered here including tidal marsh, mud flats and terrestrial input, the mesophotic zone (30 - 150 metres) and seafloor (carbon storage and sequestration potential) (see SI for an overview of the habitat data available and limitations of the methodology).
Incorporating ‘Blue Carbon’ Habitats Into Marine Spatial Planning in the Falklands Islands
The FI in the South West Atlantic, are positioned as a unique ecotone between the southern tip of South America and the Sub-Antarctic. The two main islands (East and West Falkland) are surrounded by over 700 smaller outer islands, and the FI coastline represents some of the world’s remaining pristine kelp ecosystems (van Tussenbroek, 1993; Friedlander et al., 2020; Mora-Soto et al., 2021), with higher biodiversity and species richness than neighbouring South America or the Sub-Antarctic island South Georgia (Figuerola et al., 2017; Beaton et al., 2020). The Falklands Interim Conservation and Management Zone (FICZ; established in 1986) and the Falklands Outer Conservation Zone (FOCZ; established in 1990) extending to 200 nautical miles (~370 km), gave the FI sovereignty over its fisheries and marine waters (Harte and Barton, 2007). The total resident human population is 3203 (Census preliminary results, Falkland Islands Government press statement, 2021), and the Falkland Islands Government launched an Environment Strategy 2021 – 2040, recognising ‘‘the central and universal role that the natural environment plays in the sustainable development of our health and wellbeing, our economy and our nation as a whole’’ (Falkland Islands Government, 2021).
To balance the need for regional economic security and biodiversity conservation, a holistic approach to MSP was initiated in 2014 (Brickle et al., 2019). The MSP process in the FI included an Assessment of Fishing Closure Areas as Sites for wider management (Golding et al., 2017). This process prioritised marine wilderness areas as potential MMAs (Brickle et al., 2019). The proposed MMAs represent an ecosystem-based approach to management planning objectives. The proposed MMAs, if designated, will include protection of kelp forests in nearshore waters, where they are recognised as structurally complex habitats, providing important ecosystem services (Bayley et al., 2021). In deeper waters, the proposed MMAs encompass the eastern slope of the Burdwood Bank, which is rich in unique and fragile Vulnerable Marine Ecosystems (VME) indicators (Auster et al., 2011; Brewin et al., 2020). Consequently, the MMAs could help to ensure the long-term resilience of multiple habitats and dependent species, as well as the sustainability of economically important fisheries by protecting connectivity between neighbouring biodiversity refugia (Briggs and Bowen, 2013). However, the data gaps common to highly biodiverse, but funding-limited remote island locations hinder the inclusion of management tools, such as the incorporation of blue carbon into MSP presently.
The First Annual Flux Measurements of Dissolved Organic Carbon in Falklands Rivers
The FI terrestrial ecosystems have amongst the highest proportional peat cover recorded anywhere in the world (Carter et al., 2020), making them extraordinarily carbon rich. It is estimated that its peatlands hold an organic carbon stock of 934 Mt C (Upson et al., 2016), although this is possibly a slight overestimate (SAERI unpublished data). These terrestrial carbon stocks are unlikely to be uniformly distributed, and global soil maps suggest that the South West Atlantic region has variable stocks that are up to 100% peat cover (Poggio et al., 2021 and FAO mapping respectively). Peat run-off impacts neighbouring coastal ecosystems via natural processes of organic carbon (OC), from soils to rivers, and subsequent transport into estuaries and then ocean basins (Dinsmore et al., 2010; Evans et al., 2016). These natural processes are sensitive to climate, seasonality and hydrology, and can also be altered or intensified by land-management impacts, including peatland degradation. Recent data from four East Falkland rivers show very high inputs of dissolved organic carbon (DOC) to estuaries (Evans et al., unpublished data), with DOC concentrations approaching those observed in tropical ‘blackwater’ rivers (Moore et al., 2011). Assuming that measured DOC concentrations in these rivers (which drain a substantial part of the landmass of East FI) are representative of the overall land area, we calculated that the annual flux of DOC carbon in Falkland rivers may be around 0.05 Mt C yr-1 (equivalent to 0.17 Mt CO2 yr-1 if subsequently oxidised). It appears that the majority of DOC exported from the terrestrial ecosystems is exported to the coastal ocean, but its subsequent fate and impact currently remains unclear. Visual observations have also documented the covering of seabed floors with particulate organic matter (POM) from eroded peat areas and peaty runoff discolouring the intertidal zone after rainfall events, but neither have been quantified (SMSG/SAERI unpublished data). The introduction of grazing animals following European settlement in the 1760s has changed the natural peatlands significantly, causing widespread soil erosion and drainage (Wilson et al., 1993; Otley et al., 2008), which climate change could be exacerbating (Upson et al., 2016). This can be devastating as peat fires alter carbon accumulation potential, can burn for months, producing peat-fire derived emissions (Turetsky et al., 2015). These combined impacts could increase dissolved and particulate export of terrestrial OC to the ocean, with implications such as altered biogeochemical cycles and aquatic light regimes. Research into the land-ocean carbon cycle is therefore required to understand the current impact of fluvial OC transport on the near-shore blue carbon cycle.
Undescribed Coastal Blue Carbon Habitats
Globally, salt marshes and mudflats are recognised as important blue carbon habitats (e.g. Newton et al., 2021), and conservation measures for carbon accounting and accreditation can be quantifiably applied in some locations (Burden et al., 2019). In the FI, these habitats are limited in extent for two reasons: 1) the tidal range is generally small – a maximum of 1.90 m during spring tides at Stanley, East Falkland, (National Oceanography Centre, 2022) with lower amplitudes south of East Falkland and higher ones west of West Falkland (Glorioso and Flather, 1995); and 2) coastal areas tend to be steep, so the effect of tides are therefore not visible across large areas. Consequently, salt marsh and mudflat extent is neither mapped nor quantified, limiting any inclusion in conservation management. The application of high-resolution satellite imagery is essential to map these small habitats scattered around the coastline - considering the global loss of these important ecosystems due to ongoing climate change, their disproportionate contribution to blue carbon stocks, and the potential for cross-habitat subsidies in estuaries (Bulmer et al., 2020), baseline information is urgently needed.
The First Sediment Organic Carbon Content Estimates Across the Falklands Conservation Zones
Estimates of organic carbon stocks are required to gauge the importance of a system in the marine organic carbon cycle. Additionally, organic carbon stocks are a measure of the potential vulnerability to human-induced disturbance (Jennerjahn, 2020). Existing seabed sediment maps provide hints to where sizable organic carbon stocks might be expected, as organic carbon content scales with grain size. There are no maps available for this specific geographic region. However, predictive mapping of carbonates and grain size are available (Brewin et al., 2020) and comparative datasets from neighbouring geographies for the Argentinian and FI Island continental shelves do provide insight. For example, Parker et al. (1997) describe most of the continental shelf as either sand or gravel, and open-access seafloor type datasets support this assessment (Petschick et al., 1996; Servicio de Hidrografia Naval 2022). This is clearly an area of much needed future research to inform assessments on organic carbon stocks in surface sediments (upper 10 cm of the sediment column).
A more direct way to estimate organic is to spatially predict them with geostatistical or machine learning methods. This requires observations and suitable predictor variables and has been successfully demonstrated at regional scales (e.g., Diesing et al., 2017; Wilson et al., 2018; Diesing et al., 2021; Pace et al., 2021). The amount of suitable observations within the Falklands Conservation Zones (FCZs) that could be retrieved from relevant databases (PANGAEA, https://www.pangaea.de/, MOSAIC, http://mosaic.ethz.ch/ and dbSEABED http://instaar.colorado.edu/~jenkinsc/dbseabed/) is, however, very limited. To derive a first-order approximation of the size of the organic carbon pool in FCZs surface sediments, we utilise recently published global maps of organic carbon content (Lee et al., 2019) and stock (Atwood et al., 2020).
Atwood et al. (2020) provide a global map of organic carbon stocks, measured in Mg C km-2, that can be clipped to the FCZs which covers close to half a million km2 (SI.1 Table 1). Mean values are then derived for the inner and outer zones with zonal statistics in ESRI ArcGIS, and these are multiplied by the surface area, yielding a pool size of 167 Tg and 138 Tg for the inner and outer zones, respectively. Conversely, using global predictions of organic carbon content and porosity (Lee et al., 2019) gives pool sizes of 98 Tg and 89 Tg for the inner and outer zones, respectively. These differing estimates indicate that dedicated predictive models based on newly collected data will be necessary to narrow down the uncertainty in the organic carbon pool size (see SI for limitations of this methodology).
Seafloor ecosystems store and sequester from external sources across pelagic food chains with important linkages to macroalgal beds onshore (Bayley et al., 2021). In this manner, seafloor biota do not only bury their own carbon, but trap carbon from multiple sources (Laffoley and Grimsditch, 2009). Knowledge on source-to-sink pathways, organic carbon reactivity (Smeaton and Austin, 2022) and burial rates is essential to understand long-term storage and sequestration capacity. Therefore, without the collection and analysis of new sample data and investment, it is difficult to know how vulnerable the FI carbon stores are to depletion, or where additional carbon sequestration might be possible.
Carbon Storage in Falkland Islands Kelp Ecosystems
Kelp forests dominate the FI coastal zone (Beaton et al., 2020), creating habitat for commercially important species and rapidly cycling carbon from the surrounding waters. Existing worldwide, kelps boast high growth rates and productivity (Wernberg et al., 2018). Despite disagreement in the literature on macroalgae’s status as ‘blue carbon’, there is growing evidence for their ability to sequester biomass to surrounding deeper waters by drifting to stable sediment beyond the turbulent mixing layer, and potentially having a substantial role in global carbon cycles (Krause-Jensen and Duarte, 2016; Smale et al., 2018; Froehlich et al., 2019; Filbee-Dexter and Wernberg, 2020).
As knowledge around macroalgal distribution and potential sequestration rates becomes more robust (Queirós et al., 2019; Blain et al., 2021; Pedersen et al., 2021; Smale et al., 2021), kelp’s likely role in global carbon storage becomes evident. Bayley et al. (2021), conservatively estimated that kelp in the FI may sequester in the order of 0.299 Mt of CO2 annually (equating to ~£31.07 million per year at 2020 carbon price). However, a range of assumptions are included in such estimates, particularly relating to total carbon sequestered and the appropriate valuation of carbon credits. The financial value of carbon credits are only eligible for trading if they result from a management activity that illustrates net sequestration gain. For example, through the identification of a degraded location and its restoration. Limiting a nascent area of the blue carbon market, which is not currently accredited.
Due to the large size of blades that extend to the sea surface or sub-surface, it is possible to map the extent of Macrocystis pyrifera presence with good confidence. This is a method which has been applied to FI coasts (Golding et al., 2019; Mora-Soto et al., 2020; Houskeeper et al., 2022). However, this method is still limited by factors such as water clarity, wave action, and water depth. Alongside M. pyrifera, smaller kelp species found around the archipelago include the two ‘tree kelps’, Lessonia vadosa and Lessonia flavicans, and the ‘bull kelp’ Durvillaea antarctica (Beaton et al., 2020; Mora-Soto et al., 2021). Smaller Lessonia species do not raft at the surface and often occur in deeper water or are obscured within the understory, making them difficult to map accurately (Golding et al., 2019). The larger D. antarctica can raft, however, its distribution is restricted to a thin band along exposed coasts, which can be difficult to access. Additionally, kelps undergo changes in extent and biomass according to annual/seasonal cycles and wave exposure, making survey timing important (Graham et al., 2007; Beaton et al., 2020). However, estimates of typical biomass, carbon storage per thali, and average net primary production are currently highly uncertain, and include regional taxonomic uncertainty for the Lessonia species (Bayley et al., 2021).
With these limiting factors in mind, confidence in kelp distribution may be improved through the collection of acoustic data (multibeam bathymetry/backscatter, side-scan sonar) (Golding et al., 2019). These data will help identify presence and type of vegetated benthic habitat, and the environmental factors which drive their distribution (Kenny et al., 2003; Rattray et al., 2013; Rattray et al., 2015). Combining above-water instrumentation with systematic in-water benthic surveys and use of remote-sensing tools will improve species distribution modelling estimates (Jayathilake and Costello, 2020; Mora-Soto et al., 2020). This will be particularly useful for quantifying vertical distribution of kelps, which can extend to ~60 m depth (Graham et al., 2007).
Once kelp source extent and condition are parameterised, mapping of source-to-sink pathways and quantification of carbon already sequestered within deep sea sediments will be necessary. Sediment depth, type, rate of sedimentation, bioturbation and average anthropogenic or natural disturbance will all influence the amount of carbon stored locally, now and in the future (Macreadie et al., 2017; Green et al., 2018; van de Velde et al., 2018; Atwood et al., 2020).
Blue Carbon and Planktonic and Pelagic Organisms
Pelagic ecosystem function is integral to global biogeochemical cycling and plays a major role in modulating atmospheric CO2 concentrations (Howard et al., 2017). The southern Patagonian Shelf, a component of the Patagonian Large Marine Ecosystem, is one of the most productive marine ecosystems globally (Marrari et al., 2017). However, our understanding of regional phytoplankton, zooplankton and bacteria is hampered by a lack of knowledge of the taxa dynamics across the Patagonian Shelf, and particularly within the FCZs (e.g. Sabatini et al., 2004), where there are no oceanographic circulation models available to date. Consequently taxa dynamics are only inferred by proxies such as chlorophyll, which is a measure of phytoplankton biomass in surface waters (NASA satellite data). In contrast, pelagic fish communities in this region are relatively well known (Arkhipkin et al., 2012; Arkhipkin et al., 2013a; Arkhipkin et al., 2013b), due to a long history of fishing pressure across the region. For example, the squid (Illex argentinus) represents the world’s second largest cephalopod fishery, and during peak intensity it is a visible feature from space (NASA Earth Observatory 2022). Illex argentinus is primarily harvested by squid jigging in the FCZs, the high seas of the Southwest Atlantic, and Argentine Exclusive Economic Zone (to a value of $597 million USD; range $301-2,396 million USD based on 2005-2016 data, see Harte et al., 2018). Species at mid to low trophic positions play a key role in the trophodynamics of this ecosystem (e.g. Sprattus fuegensis, Patagonotothen ramsayi, Micromesistius australis, and Munida gregaria) and are considered to be a “wasp waist” structured ecosystem (Laptikhovsky et al., 2013; Riccialdelli et al., 2020). Therefore, there is an increasing awareness of the contribution of these fisheries’ to important large-scale processes, such as carbon storage, and how ecosystem-based management can help sustain them. However, more knowledge and increased fisheries-science collaboration is required to quantify the specific trophic pathways and fate of carbon transferred through passive settlement to deep marine sediments, or transported via active migration of fish to neighbouring regions (Saba et al., 2021; Wing et al., 2021).
Carbon transported to the deep sea through these trophic pathways can remain sequestered for millennia (Howard et al., 2017). However, overfishing and the use of sediment-disturbing bottom trawl nets in particular prevent and reverse blue carbon sequestration over large time scales (van de Velde et al., 2018; Mariani et al., 2020). For example, Mariani et al. (2020) show that through historical extraction and fuel consumption, ocean fisheries have released ≥0.73 billion metric tons of CO2 (GtCO2) into the atmosphere since 1950. Globally, 43.5% of that blue carbon extracted by these fisheries in the high seas comes from areas that would be economically unprofitable without subsidies (not a component in FI Fisheries). Maintaining well managed fisheries will reduce blue carbon loss to the atmosphere by sustaining the natural carbon pump through healthy fish stocks and increase natural carcass deadfall to the deep sea (Higgs et al., 2014; Honjo et al., 2014). Roughly 60% of the FICZ has fishing restrictions in place presently (SI.1 Table 3) and future monitoring could inform on the effectiveness of these restrictions and closures for protecting carbon stores. Whilst, the proposed FI MMAs could preserve existing carbon storage and stock densities through conserving local marine ‘wilderness’ areas.
Blue Carbon Potential of Habitats Extending Into the Mesophotic Zone
Mesophotic ecosystems are largely unexplored in the FI and the lower depth limit of most light-dependent species is unknown (Goodwin et al., 2011; Goodwin et al., 2012; Brewin et al., 2020). These habitats constitute complex biodiversity at depths between 30 m to 150 m (Hinderstein et al., 2010) and we calculate that the projected areal extent of this habitat is over c. 50,000 km2 (SI.1 Table 3). However, despite this substantial area, only incidental nearshore surveys have been conducted to explore these depths to date. For example, surveys in 2020 and 2021 recorded the presence of stylasterid coral (hydrocoral) gardens, rhodolith beds (coralline algae nodules), and reef-like aggregations of parchment worms below 40 m during Remote Operated Vehicle (ROV) and drop cameras surveys (SAERI unpublished data). These observations constitute the discovery of carbon rich taxa in the mesophotic zone. Without investments in time and resources, observational data cannot be meaningfully quantified or included in distribution models and conservation planning at present. For example, a search of the Ocean Biodiversity Information System (OBIS) database for VME indicator taxa (FAO, 2008; CCAMLR, 2009) to identify potential benthic sequesters across the FCZs, showed that 26% of all the OBIS VME records are found in the mesophotic zone (Figure 2). However, the upper mesophotic zone (30 - 60 m) has only 0.5% of these, and 29% (c. 700 records) of OBIS VME records do not include depth information at all. These gaps highlight that there is much to be discovered in the FI marine environment, and much that needs to be done to progress the available information into broader application. Investments in nearshore marine science voyages and technology to survey the seafloor through multibeam echo sounder and backscatter data and sampling by means such as with Remote Operated Vehicles (ROVs) are needed to gain a baseline understanding. This will be an important first step to gain an understanding of undiscovered blue carbon habitats and biodiversity in areas where fisheries do and do not operate. Sampling in areas where fisheries operate could be undertaken in collaboration with existing ship-based data collections, and would aid in validating onshore to offshore carbon pathways and natural fluctuations through time. However, demonstrating permanence (removal of carbon from the biological to the geological carbon cycle), and identifying an intervention to increase carbon drawdown at scale is a substantial research effort, across much of the FCZs and central plateau region of the southern proposed Burdwood Bank MMA to understand mesophotic blue carbon (c. 11% SI.1. Table 3).
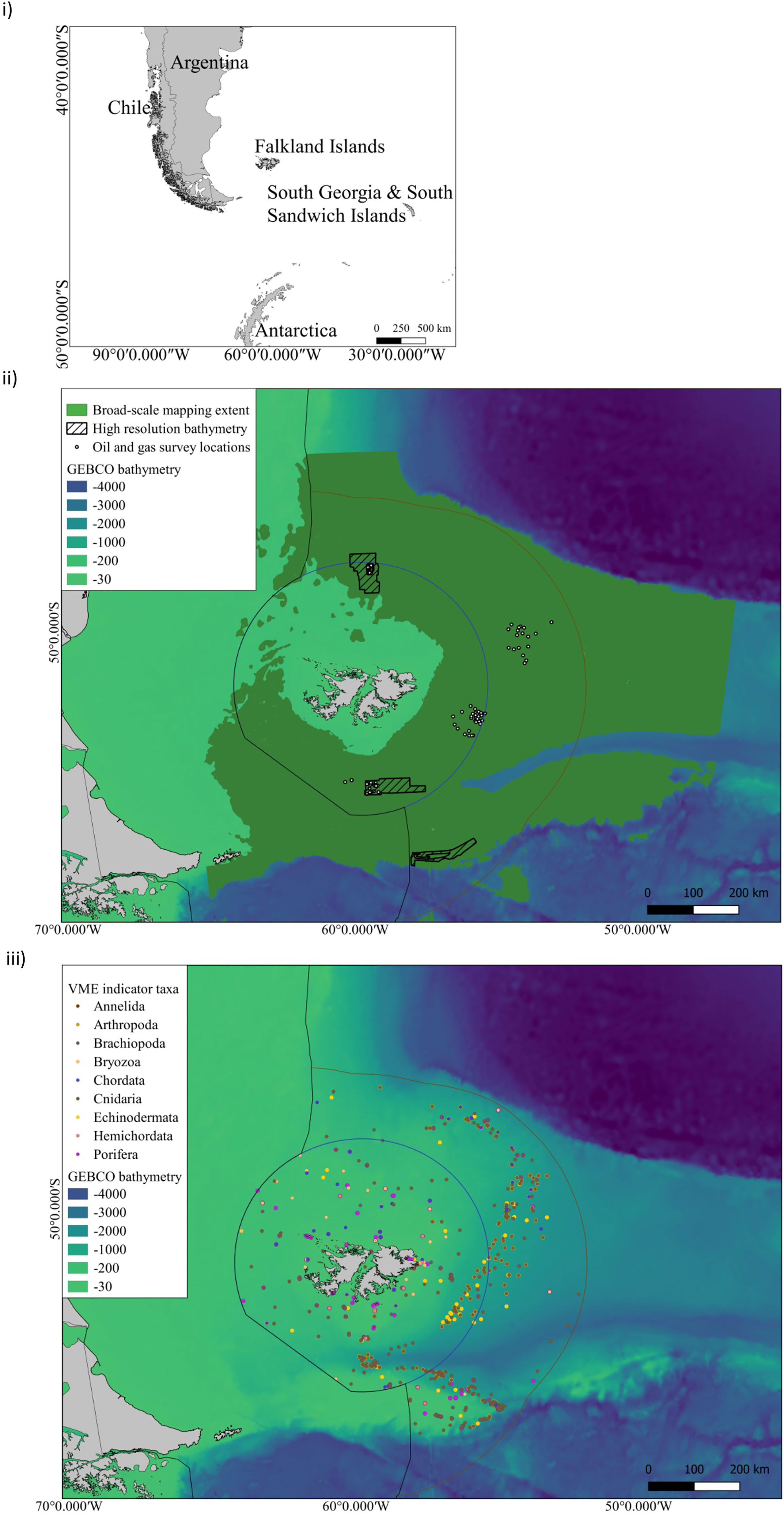
Figure 2 i) The Falkland Islands geographic location in the South West Atlantic ii) Distribution of offshore surveys in FCZs: Broad-scale classification habitat maps and predictive maps of VME taxa as proxies of VMEs have been produced over the spatial extent indicated in dark green. These broad-scale maps have been based upon GEBCO bathymetry. Higher resolution mapping has been undertaken at oil and gas survey point locations shown by white circles (2x2km2). High-resolution bathymetry has been collected at these sites and within the hashed boxes. ii) VME indicator taxa compiled from OBIS, SAERI unpublished data and Brewin et al., 2020 (SI.1 Table 2) for the following taxa grouped by Phylum - Annelida - Serpulidae; Arthropoda - Bathylasmatidae; Brachiopoda; Bryozoa; Chordata- Ascidiacea; Cnidaria- Actiniaria, Alcyonacea, Pennatulacea, Scleractinia, Antipathraia, Hydroidolina, Stylasteridae; Echinodermata- Crinoidea, Ophiurida, Cidaroida; Hemichordata; Porifera.
Benthic Habitats Offshore With Long-Term Carbon Storage and Sequestration Potential
The FI benthic slope habitats share close affinities with the Sub-Antarctic, one of the world’s most important geographic regions for the sequestration of anthropogenic CO2 by marine ecosystems (Thomalla et al., 2011; Swart et al., 2015; Pörtner et al., 2021). Marine organic carbon accumulation is predicted to be primarily on shelf and slope habitats globally (e.g., Muller-Karger et al., 2005), where biodiversity is greatest, and where it is often vulnerable to the impacts of fishing gear and biomass extraction (Mariani et al., 2020). The biota on continental shelves at high southern latitudes are effective at sequestering carbon and unlike other carbon sinks, they are increasing with climate change (for example around Antarctica: Barnes et al., 2018a; Bax et al., 2021; Cavanagh et al., 2021). A better understanding of these potentially rare and sizable (in terms of area) negative feedbacks on climate change, is valuable in social carbon costs. Seabed biomass shallower than 1000 m in some South Atlantic habitats can accumulate carbon of considerable mitigation potential (Barnes et al., 2021). The eastern Burdwood Bank (adjacent to the Namuncurá MPA), is a proposed MMA, and preliminary research suggests it hosts high carbon storage and sequestration potential (Bax and Cairns, 2014). Furthermore, carbon and biodiversity rich habitats including abundant stylasterid and scleractinian coral assemblages add to the conservation significance of this region (Bax et al., 2014; Cairns and Polonio, 2013). Cold water corals are debated as ‘blue carbon’ habitats as they re-emit some CO2 when building their CaCO3 skeletons (Laffoley and Grimsditch, 2009). Much seafloor life has importance as ecosystem engineers and sediment creators, but their vulnerability to climate change (Figuerola et al., 2021, Rogers et al., 2021) and bottom fishing mean a focus on maintaining ecosystem function at the site of sequestration - where it is most crucial to long-term climate mitigation - includes conservation of VME indicator taxa (such as corals) and the ecosystem services a biodiverse seafloor habitat can provide.
If research on ecosystem function at the site of sequestration is prioritised, a quantification and forecasting approach to blue carbon in locations like the Burdwood Bank could potentially lead to future investments in biodiversity conservation offshore, which includes economic gains by sustaining ecosystem services to inform national emission targets. However, there are a number of limitations to ground truthing long term carbon sequestration estimates on the seafloor (100s - 1000s of years), and the climate mitigation potential of benthic ecosystems in a policy-specific context (Bax et al., 2021). Primarily, this ground-truthing relies on seabed mapping technology with the capacity to discriminate between benthic habitats and model species distributions at multiple spatial scales. Investment at this scale is lacking, and large swaths of seafloor have never been sampled, or examined by multibeam echosounder, and in some cases these data are under commercial licence and not openly available for use (Figure 2). The limited spatial extent of ground-truthed data, coupled with the low resolution of environmental data used in these models, is the main limitation of habitat maps (Guisan and Thuiller, 2005; Ross et al., 2015), and we present a synthesis of high resolution bathymetry available to date (Figure 2 limitations outlined in SI).
The wealth of VME indicator taxa recorded from across the FI (Figure 2), including high sponge diversity (Goodwin et al., 2011), scleractinian coral reefs (Cairns and Polonio, 2013; Brewin et al., 2020), sea pen fields, coral gardens (Brewin et al., 2020), chemosynthetic communities (Noble, 2014 unpublished data) and field-like aggregations of deep-sea stylasterid (lace) corals across the plateau (Bax and Cairns, 2014; Bax, 2014); highlight that there is much to discover by investing in seafloor science in the FI. In an attempt to inform protection of these blue carbon habitats, spatial overlap between functional group records and VME indicator taxa were compared (SI.1 Table 2). Considering the proportion of soft sediment on the FCZs (Petschick et al., 1996; Parker et al., 1997; Servicio de Hidrografia Naval 2022; Gerdes and Montiel, 1999), and the areal extent of VME indicator taxa of the FCZs c. 175, 000 km2 (SI.1 Table 2), future work could align these groupings with geographic data sets in the Southern Ocean where core estimates have been contrasted against seabed images to clarify blue carbon gains (Zwerschke et al., 2022). However, whilst an imaging research approach is non-invasive and helps to clarify seafloor community ecology, it can fail to include infaunal species data. These data are often only available based on generalised biomass estimates (e.g., Brey and Gerdes, 1999) and species lists from adjacent regions (e.g., Gerdes and Montiel, 1999; Rios et al., 2003) limiting our capacity to understand basic seafloor biodiversity composition.
Future Seafloor Blue Carbon Priority Research Areas
Future work would be most informative if bathymetric data were ground-truthed with seabed core sampling to clarify carbon content and production estimates, combined with carbon dating technology, through time (Atwood et al., 2020). Data from the Magellan and Beagle Channel region (including shelf estimates) provide comparable carbon content and production estimates to the eastern Weddell Sea (Brey and Gerdes, 1999). Ideally this information can be overlayed with VME and infaunal assemblage mapping, biogeochemistry, and population genetics to estimate blue carbon connectivity from key model sequesters (e.g., corals, sponges and echinoderms). This type of information would validate carbon storage and sequestration estimates based on functional groups at finer temporal and spatial scales (e.g., per population, per area, per year over time) both offshore (e.g., Barnes and Sands, 2017; Barnes et al., 2021) and nearshore (e.g., Morley et al., 2022). However, such estimates also rely on knowledge of a taxon’s traits such as feeding strategy, mobility and lifestyle, which is lacking for most benthic taxa (www.SCAR-MarBIN.be). Therefore, an accurate estimation of carbon storage rates (such as annual increments) across areas for the purpose of carbon accreditation is currently difficult at the scales needed by policy (unless undertaken on a taxon by taxon basis (e.g. bryozoans, see Barnes, 2015).
Forward Look
The preservation of nature as a functional carbon sink, is based upon the long-term burial capacity of seafloor biota and the continued efficiency of external sources of carbon such as macroalgal beds and pelagic ecosystems. This recognition imparts the need for a multi-tiered approach to blue carbon research that includes fisheries management and terrestrial blue carbon pathways. This approach also focuses on ecosystem based preservation, as it is far more efficient and less costly to protect habitats while healthy than to restore once degraded, with compromised carbon storage and sequestration capabilities (Barnes et al., 2021; Bax et al., 2021; Bayley et al., 2021). In the context of blue carbon, understanding key ecosystem information like the location, extent, and condition of broad scale habitat types, will be critical in understanding sequestration pathways and capacity. In turn, blue carbon habitats and pathways can facilitate strategic and adaptive planning aimed at retaining biodiversity, maintaining sustainable fisheries, and preserving ecosystem services and economies for generations to come (Bertram et al., 2021). However, before this utility of blue carbon as a MSP tool can be realised, a substantial knowledge and understanding base is required.
MPAs and spatial management are an important part of sustainable futures, especially if MSP can keep pace with climate change and remain both locally and globally relevant (Queirós et al., 2021). As the UN Ocean Decade for Sustainable Development (2021 - 2030) progresses, there is an increasing emphasis on negotiations for a new UN treaty on Biodiversity Beyond National Jurisdictions (BBNJ), where greater than 50% of earth’s biodiversity resides (FAO, 2020). The total area of fishing footprint determined by Brewin et al. (2020) for both the FCZs and ABNJ is 36 924km2, and the total footprint of actual fished ground in the ABNJ is almost twice as large as the FICZ (23 928km2) and FOCZ (12 997 km2). Therefore, whilst this paper focuses on knowledge gaps within the FCZs, as extractive industries expand globally, the FI also provides a test-case for the necessity of protection and sustainable practice in jurisdictional waters - to mitigate the threat of unregulated fleets in Areas Beyond National Jurisdiction (ABNJ). Furthermore, by filling the common knowledge gaps outlined here, all conservation and monitoring strategies south of 40° would benefit and could potentially replicate multi-tier approaches. In this manner, the inclusion of blue carbon research into MSP must also grapple with a contiguous managed area that includes ABNJ fisheries across a vast area extending into the Scotia arc in the sub-Antarctic. Given the diversity of habitats and high endemism to consider (Morley et al., 2022), a precautionary and informed management approach to conservation will be imperative.
Data Availability Statement
The datasets presented in this study can be found in online repositories. The names of the repository/repositories and accession number(s) can be found in the article/Supplementary Material.
Author Contributions
NB, DTIB, and KL formed the original concept for a blue carbon synthesis paper. NB wrote the draft manuscript. NB, DKAB, MD, SP-M and DTIB contributed to the introduction and manuscript framing. AB and NB contributed to the main text on FI policy and MSP. SC and CE contributed text on terrestrial carbon and fluvial input. TP and RD provided maps and compiled available bathymetry, VME and OBIS data for this publication. RD calculated areal extent estimates. MD provided the first marine carbon stock estimates for the FICZs. DTIB, AG and AA contributed text on FI kelp ecosystems. NB and RD contributed text on FI mesophotic ecosystems. DTIB, PB, NB and SP-M contributed text on pelagic blue carbon and fisheries. NB, TP, RD, MD, DKAB and SP-M contributed text on seafloor blue carbon. DTIB created the visual abstract and paper figure. All co-authors were invited to review and edit content prior to manuscript submission. NB formed this multi-disciplinary collaboration as part of the Sub-Antarctic Blue Carbon and Natural Archives Network and as part of her research agenda as MCPC at SAERI to support research on the proposed MMAs in the FI. All authors contributed to the article and approved the submitted version.
Funding
We are grateful to the John Ellerman foundation for funding the Marine and Coastal Program Coordinator (NB) at the South Atlantic Environmental Research Institute (SAERI), SAERI is a registered charity in England and Wales. All authors contributed their time in kind to progress research and conservation. RD is funded by an Australian Government Research Training Program for her PhD research. DOC flux measurements reported were supported by the UK Natural Environment Research Council LOCATE project (NE/N018087/1). The author contributions (SC, CDE) on terrestrial carbon were supported by the Darwin Initiative through UK Government funding for projects DPLUS083 and DPLUS116. AG is funded by the Falkland Islands Government CDS Fund, John Cheek Trust, RBC Ltd for her PhD program. Funding for open access publication was provided by the British Antarctic Survey.
Conflict of Interest
The authors declare that the research was conducted in the absence of any commercial or financial relationships that could be construed as a potential conflict of interest.
Publisher’s Note
All claims expressed in this article are solely those of the authors and do not necessarily represent those of their affiliated organizations, or those of the publisher, the editors and the reviewers. Any product that may be evaluated in this article, or claim that may be made by its manufacturer, is not guaranteed or endorsed by the publisher.
Acknowledgments
We gratefully acknowledge our collaborators and colleagues, the organisations and individuals who contributed their time and expertise to marine spatial planning in the Falkland Islands, Alex Blake and Jorge Ramos at Falkland Island Fisheries for spatial estimates of fisheries in FCZs, Jack Ingledew for providing GIS and metadata assistance, and Chester Sands for providing input on an earlier draft of the manuscript. This paper is dedicated to the Falklands’ future generations and the important marine biodiversity they will inherit.
Supplementary Material
The Supplementary Material for this article can be found online at: https://www.frontiersin.org/articles/10.3389/fmars.2022.872727/full#supplementary-material
References
Arkhipkin A., Barton J., Wallace S., Winter A. (2013a). Close Cooperation Between Science, Management and Industry Benefits Sustainable Exploitation of the Falkland Islands Squid Fisheries. J. Fish Biol. 83 (4), 905–920. doi: 10.1111/jfb.12098
Arkhipkin A., Brickle P., Laptikhovsky V. (2013b). Links Between Marine Fauna and Oceanic Fronts on the Patagonian Shelf and Slope. Arquipelago Life Mar. Sci. 30, 19–37. doi: 10.1111/jfb.12098
Arkhipkin A., Brickle P., Laptikhovsky V., Winter A. (2012). Dining Hall at Sea: The Eastern Patagonian Shelf is a Destination for Feeding Migrations of Nektonic Predators. J. Fish Biol. 81, 882–902. doi: 10.1111/j.1095-8649.2012.03359.x
Atwood T. B., Witt A., Mayorga J., Hammill E., Sala E. (2020). Global Patterns in Marine Sediment Carbon Stocks. Front. Mar. Sci. 7. doi: 10.3389/fmars.2020.00165
Auster P. J., Gjerde K., Heupel E., Watling L., Grehan A., Rogers A. D. (2011). Definition and Detection of Vulnerable Marine Ecosystems on the High Seas: Problems With the “Move-on” Rule. ICES J. Mar. Sci. 68 (2), 254–264. doi: 10.1093/icesjms/fsq074
Barnes D. K. A. (2015). Antarctic Sea Ice Losses Drive Gains in Benthic Carbon Drawdown. Curr. Biol. 25 (18), R789–R790. doi: 10.1016/j.cub.2015.07.042
Barnes D. K. A., Bell J. B., Bridges A. E., Ireland L., Howell K. L., Martin S. M., et al. (2021). Climate Mitigation Through Biological Conservation: Extensive and Valuable Blue Carbon Natural Capital in Tristan Da Cunha’s Giant Marine Protected Zone. Biology 10, 1339. doi: 10.3390/biology10121339
Barnes D. K. A., Fleming A., Sands C. J., Quartino M. L., Deregibus D., Chester J., et al. (2018a). Icebergs, Sea Ice, Blue Carbon and Antarctic Climate Feedbacks. Philos. Trans. R. Soc A 376 (2122), 20170176. doi: 10.1098/rsta.2017.0176
Barnes D. K. A., Morlet S. A., Bell J., Brewin P., Brigden K., Collins M., et al. (2018b). Marine Plastics Threaten Giant Atlantic Marine Protected Areas. Curr. Biol. 28, R1137–R1138. doi: 10.1016/j.cub.2018.08.064
Barnes D. K. A., Sands C. J. (2017). Functional Group Diversity is Key to Southern Ocean Benthic Carbon Pathways. PloS One 12 (6), 1–14. doi: 10.1371/journal.pone.0179735
Bax N., Cairns S. D. (2014). Stylasteridae (Cnidaria; Hydrozoa). Biogeographic Atlas of the Southern Ocean.
Bax N. (2014). Deep-Sea Stylasterid Corals in the Antarctic, Sub-Antarctic and Patagonian Benthos: Biogeography, Phylogenetics, Connectivity and Conservation (Doctoral dissertation, University of Tasmania).
Bax N., Novaglio C., Maxwell K. H., Meyers K., McCann J., Jennings S., et al. (2022). Ocean Resource Use: Building the Coastal Blue Economy. Rev. Fish Biol. Fish. 32 (1), 189–207. doi: 10.1007/s11160-021-09636-0
Bax N., Sands C. J., Gogarty B., Downey R. V., Moreau C. V. E., Moreno B., et al. (2021). Perspective: Increasing Blue Carbon Around Antarctica is an Ecosystem Service of Considerable Societal and Economic Value Worth Protecting. Glob. Change Biol. 27 (1), 5–12. doi: 10.1111/gbc.15392
Bayley D. T. I., Brickle P., Brewin P. E., Golding N., Pelembe T. (2021). Valuation of Kelp Forest Ecosystem Services in the Falkland Islands: A Case Study Integrating Blue Carbon Sequestration Potential. One Ecosyst. 6, e62811. doi: 10.3897/oneeco.6.e62811
Beaton E. C., Küpper F. C., van West P., Brewin P. E., Brickle P. (2020). The Influence of Depth and Season on the Benthic Communities of a Macrocystis Pyrifera Forest in the Falkland Islands. Polar Biol. 43, 573–586. doi: 10.1007/s00300-020-02662-x
Bergstrom D. M., Wienecke B. C., van den Hoff J., Hughes L., Lindenmayer D. B., Ainsworth T. D., et al. (2021). Combating Ecosystem Collapse From the Tropics to the Antarctic. Glob. Change Biol. 27 (9), 1692–1703. doi: 10.1111/gbc.15539
Bertram C., Quaas M., Reusch T. B., Vafeidis A. T., Wolff C., Rickels W. (2021). The Blue Carbon Wealth of Nations. Nat. Climate Change 11 (8), 704–709. doi: 10.1038/s41558-021-01089-4
Blain C. O., Hansen S. C., Shears N. T. (2021). Coastal Darkening Substantially Limits the Contribution of Kelp to Coastal Carbon Cycles. Glob. Change Biol. 27, 5547–5563. doi: 10.1111/gcb.15837
Brewin P. E., Farrugia T. J., Jenkins C., Brickle P. (2020). Straddling the Line: High Potential Impact on Vulnerable Marine Ecosystems by Bottom-Set Longline Fishing in Unregulated Areas Beyond National Jurisdiction. ICES J. Mar. Sci. 78 (6), 2132–2145. doi: 10.1093/icesjms/fsaa106
Brey T., Gerdes D. (1999). Benthic Community Productivity in the Magellan Region and in the Weddell Sea. Sci. Mar. 63 (1), 145–148. doi: 10.3989/scimar.1999.63s1145
Brickle P., Barton J., Simsovic D., Golding N., de Lecea A., Brewin P. (2019). Marine Ecosystem Protection in the Falkland Islands. Mar. Biol. Mag. 13, 20–21.
Briggs J. C., Bowen B. W. (2013). Marine Shelf Habitat: Biogeography and Evolution. J. Biogeogr. 40 (6), 1023–1035. doi: 10.1111/jbi.12082
Brooks S. T., Jabour J., Bergstrom D. M. (2018). What is ‘Footprint’in Antarctica: Proposing a Set of Definitions. Antarct. Sci. 30 (4), 227–235. doi: 10.1017/S0954102018000172
Brooks S. T., Jabour J., Van Den Hoff J., Bergstrom D. M. (2019). Our Footprint on Antarctica Competes With Nature for Rare Ice-Free Land. Nat. Sustain. 2 (3), 185–190. doi: 10.1038/s41893-019-0237-y
Bulmer R. H., Stephenson F., Jones H. F., Townsend M., Hillman J. R., Schwendenmann L., et al. (2020). Blue Carbon Stocks and Cross-Habitat Subsidies. Front. Mar. Sci. 7, 380. doi: 10.3389/fmars.2020.00380
Burden A., Garbutt A., Evans C. D. (2019). Effect of Restoration on Saltmarsh Carbon Accumulation in Eastern England. Biol. Lett. 15 (1), 20180773. doi: 10.1098/rsbl.2018.0773
Cairns S. D., Polonio V. (2013). New Records of Deep-Water Scleractinia Off Argentina and the Falkland Islands. Zootaxa 3691 (1), 58–86. doi: 10.11646/zootaxa.3691.1.2
Carter S., Aitkenhead M., Evans C., Jungblut A., McAdam J., McNee M., et al. (2020). Soil Map and Online Database as Climate Change Mitigation Tools. Darwin Plus, 083.
Cavanagh R. D., Melbourne-Thomas J., Grant S. M., Barnes D. K., Hughes K. A., Halfter S., et al. (2021). Future Risk for Southern Ocean Ecosystem Services Under Climate Change. Front. Mar. Sci. 7, 1224. doi: 10.3389/fmars.2020.615214
CCAMLR (2009). VME Taxa Classification Guide (Australia: Commission for the Conservation of Antarctic Marine Living Resources).
Census preliminary results, Falkland Islands Government press statement (2021). Available at: www.falklands.gov.fk/census (Accessed February 1, 2022).
Diesing M., Kröger S., Parker R., Jenkins C., Mason C., Weston K. (2017). Predicting the Standing Stock of Organic Carbon in Surface Sediments of the North-West European Continental Shelf. Biogeochemistry 135, 183–200. doi: 10.1007/s10533-017-0310-4
Diesing M., Thorsnes T., Bjarnadóttir L. R. (2021). Organic Carbon Densities and Accumulation Rates in Surface Sediments of the North Sea and Skagerrak. Biogeosciences 18, 2139–2160. doi: 10.5194/bg-18-2139-2021
Dinsmore K. J., Billett M. F., Skiba U. M., Rees R. M., Drewer J., Helfter C. (2010). Role of the Aquatic Pathway in the Carbon and Greenhouse Gas Budgets of a Peatland Catchment. Glob. Change Biol. 16 (10), 2750–2762. doi: 10.1111/j.1365-2486.2009.02119.x
Evans C. D., Renou-Wilson F., Strack M. (2016). The Role of Waterborne Carbon in the Greenhouse Gas Balance of Drained and Re-Wetted Peatlands. Aquat. Sci. 78 (3), 573–590. doi: 10.1007/s00027-015-0447-y
Falkland Islands Government (2021) Falkland Islands Environment Strategy 2021-2040 (Stanley, Falkland Islands). Available at: https://www.fig.gov.fk/policy/environment/environment-strategy (Accessed February 10, 2022).
FAO. (2008). International Guidelines for the Management of Deep-Sea Fisheries in the High-Seas. Food and Agriculture Organization of the United Nations. Adopted in Rome, Italy on 29 August 2008.
FAO (2020) Common Oceans, A Partnership for Sustainability in the ABNJ (Food and Agriculture Organization of the United Nations). Available at: http://www.fao.org/in- action/commonoceans/en/ (Accessed April 01, 2022).
Figuerola B., Barnes D. K. A., Brickle P., Brewin P. E. (2017). Bryozoan Diversity Around the Falkland and South Georgia Islands: Overcoming Antarctic Barriers. Mar. Environ. Res. 126, 81–94. doi: 10.1016/j.marenvres.2017.02.005
Figuerola B., Hancock A. M., Bax N., Cummings V. J., Downey R., Griffiths H. J., et al. (2021). A Review and Meta-Analysis of Potential Impacts of Ocean Acidification on Marine Calcifiers From the Southern Ocean. Front. Mar. Sci. 8. doi: 10.3389/fmars.2021.584445
Filbee-Dexter K., Wernberg T. (2020). Substantial Blue Carbon in Overlooked Australian Kelp Forests. Sci. Rep. 10, 12341. doi: 10.1038/s41598-020-69258-7
Friedlander A. M., Ballesteros E., Bell T. W., Caselle J. E., Campagna C., Goodell W., et al. (2020). Kelp Forests at the End of the Earth: 45 Years Later. PloS One 15 (3), e0229259. doi: 10.1371/journal.pone.0229259
Froehlich H. E., Afflerbach J. C., Frazier M., Halpern B. S. (2019). Blue Growth Potential to Mitigate Climate Change Through Seaweed Offsetting. Curr. Biol. 29, 3087–3093.e3. doi: 10.1016/j.cub.2019.07.041
Gerdes D., Montiel A. (1999). Distribution Patterns of Macrozoobenthos: A Comparison Between the Magellan Region and the Weddell Sea (Antarctica). Sci. Mar. 63 (1), 149–153. doi: 10.3989/scimar.1999.63s1149
Glorioso P. D., Flather R. A. (1995). A Barotropic Model of the Currents Off SE South America. J. Geophys. Res. Oceans 100 (C7), 13427–13440. doi: 10.1029/95JC00942
Golding N., Black B., Blake D., Brewin P., Harte M., Havercroft H., et al. (2019). Long-Term Coastal Habitat Mapping & Monitoring Handbook. Examples Based on Work Undertaken in the Falkland Islands & South Georgia (DPLUS065 Coastal Habitat Mapping project). Available at: https://www.south-atlantic-research.org/wp-content/uploads/2020/05/DPLUS065_MonitoringHandbook_Final.pdf.
Goodwin C., Brewin P. E., Brickle P. (2012). Sponge Biodiversity of South Georgia Island With Descriptions of Fifteen New Species. Zootaxa 3542, 1–48. doi: 10.11646/zootaxa.3542.1.1
Goodwin C., Jones J., Neely K., Brickle P. (2011). Sponge Biodiversity of the Jason Islands and Stanley, Falkland Islands With Descriptions of Twelve New Species. J. Mar. Biolog. Assoc. UK 91 (2), 275–301. doi: 10.1017/S0025315410001542
Graham M. H., Vásquez J. A., Buschmann A. H. (2007). Global Ecology of the Giant Kelp Macrocystis: From Ecotypes to Ecosystems. Oceanogr. Mar. Biol. 45, 39–88. doi: 10.1201/9781420050943.ch2
Green A., Chadwick M. A., Jones P. J. S. (2018). Variability of UK Seagrass Sediment Carbon: Implications for Blue Carbon Estimates and Marine Conservation Management. PloS One 13, 1–18. doi: 10.1371/journal.pone.0204431
Guisan A., Thuiller W. (2005). Predicting Species Distribution: Offering More Than Simple Habitat Models. Ecol. Lett. 8 (9), 993–1009. doi: 10.1111/j.1461-0248.2005.00792
Halpern B. S., Frazier M., Afflerbach J., Lowndes J. S., Micheli F., O’Hara C., et al. (2019). Recent Pace of Change in Human Impact on the World’s Ocean. Sci. Rep. 9, 11609. doi: 10.1038/s41598-019-47201-9
Harte M., Barton J. (2007). Balancing Local Ownership With Foreign Investment in a Small Island Fishery. Ocean Coast Manage. 7, 523–537. doi: 10.1016/j.ocecoaman.2007.03.001
Harte M., Borberg J., Sylvia G. (2018). Argentine Shortfin Squid (Illex Argentines) Value Chain Analysis With an Emphasis on the Falkland Islands. Final Report for the South Atlantic Overseas Territories Natural Capital Assessment (Joint Nature Conservation Committee (JNCC)). Available at: https://data.jncc.gov.uk/data/3d406e7f-3bb0-4bed-9bfc-584398a2aafe/ot-nca-sup-sat-28-fi-mar2019.pdf.
Higgs N. D., Gates A. R., Jones D. O. B. (2014). Fish Food in the Deep Sea: Revisiting the Role of Large Food-Falls. PloS One 9, e96016. doi: 10.1371/journal.pone.0096016
Hinderstein L. M., Marr J. C. A., Martinez F. A., Dowgiallo M. J., Puglise K. A., Pyle R. K., et al. (2010). Theme Section on “Mesophotic Coral Ecosystems: Characterization, Ecology, and Management”. Coral Reefs 29, 247–251. doi: 10.1007/s00338-010-0614-5
Honjo S., Eglinton T., Taylor C., Ulmer K., Sievert S., Bracher A., et al. (2014). Understanding the Role of the Biological Pump in the Global Carbon Cycle: An Imperative for Ocean Science. Oceanography 27, 10–16. doi: 10.5670/oceanog.2014.78
Houskeeper H. F., Rosenthal I. S., Cavanaugh K. C., Pawlak C., Trouille L., Byrnes J. E. K., et al. (2022). Automated Satellite Remote Sensing of Giant Kelp at the Falkland Islands (Islas Malvinas). PloS One 17, e0257933. doi: 10.1371/journal.pone.0257933
Howard J., Sutton-Grier A., Herr D., Kleypas J., Landis E., Mcleod E., et al. (2017). Clarifying the Role of Coastal and Marine Systems in Climate Mitigation. Front. Ecol. Environ. 15, 42–50. doi: 10.1002/fee.1451
Hughes K. A., Pescott O. L., Peyton J., Adriaens T., Cottier-Cook E. J., Key G., et al. (2020). Invasive non-Native Species Likely to Threaten Biodiversity and Ecosystems in the Antarctic Peninsula Region. Glob. Change Biol. 26, 2702–2716. doi: 10.1111/gcb.14938
IETA and the University of Maryland (2021) The Potential Role of Article 6 Compatible Carbon Markets in Reaching Net-Zero. Available at: https://www.ieta.org/resources/Resources/Net-Zero/Final_Net-zero_A6_working_paper.pdf (Accessed February 7, 2022).
Jayathilake D. R. M., Costello M. J. (2020). A Modeled Global Distribution of the Kelp Biome. Biol. Conserv. 252, 108815. doi: 10.1016/j.biocon.2020.108815
Jennerjahn T. C. (2020). Relevance and Magnitude of “Blue Carbon” Storage in Mangrove Sediments: Carbon Accumulation Rates vs. Stocks, Sources vs. Sinks. Estuar. Coast Shelf Sci. 247, 107027. doi: 10.1016/j.ecss.2020.107027
Kenny A., Cato I., Desprez M., Fader G., Schuttenhelm R., Side J. (2003). An Overview of Seabed-Mapping Technologies in the Context of Marine Habitat Classification. ICES J. Mar. Sci. 60, 411–418. doi: 10.1016/S1054-3139(03)00006-7
Krause-Jensen D., Duarte C. M. (2016). Substantial Role of Macroalgae in Marine Carbon Sequestration. Nat. Geosci. 9, 737–742. doi: 10.1038/ngeo2790
Laffoley D., Grimsditch G. D. (2009). The Management of Natural Coastal Carbon Sinks. Gland (SwissPrinters IRL). Available at: https://books.google.com/books?hl=en&lr=&id=NZzlOHYvvO4C&oi=fnd&pg=PR5&dq=Laffoley,+D.,+and+Grimsditch,+G.+D.+ (2009).+The+Management+of+Natural+Coastal+Carbon+Sinks.+Gland+(SwissPrinters+IRL).&ots=8fBTkOtmpw&sig=VxLB3iHVFCX1ivnwNNFtwUjgBEM.
Laptikhovsky V., Arkhipkin A., Brickle P. (2013). From Small Bycatch to Main Commercial Species: Explosion of Stocks of Rock Cod Patagonotothen Ramsayi (Regan) in the Southwest Atlantic. Fish. Res. 147, 399–403. doi: 10.1016/j.fishres.2013.05.006
Lee T. R., Wood W. T., Phrampus B. J. (2019). A Machine Learning (kNN) Approach to Predicting Global Seafloor Total Organic Carbon. Glob. Biogechem. Cycles 33, 37–46. doi: 10.1029/2018GB005992
Lovelock C. E., Duarte C. M. (2019). Dimensions of Blue Carbon and Emerging Perspectives. Biol. Lett. 15, 20180781. doi: 10.1098/rsbl.2018.0781
Macreadie P. I., Nielsen D. A., Kelleway J. J., Atwood T. B., Seymour J. R., Petrou K., et al. (2017). Can We Manage Coastal Ecosystems to Sequester More Blue Carbon? Front. Ecol. Environ. 15, 206–213. doi: 10.1002/fee.1484
Mariani G., Cheung W. W. L., Lyet A., Sala E., Mayorga J., Velez L., et al. (2020). Let More Big Fish Sink: Fisheries Prevent Blue Carbon Sequestration-Half in Unprofitable Areas. Sci. Adv. 6, 1–9. doi: 10.1126/sciadv.abb4848
Marrari M., Piola A. R., Valla D. (2017). Variability and 20-Year Trends in Satellite-Derived Surface Chlorophyll Concentrations in Large Marine Ecosystems Around South and Western Central America. Front. Mar. Sci. 4, 372. doi: 10.3389/fmars.2017.00372
Moore S., Gauci V., Evans C. D., Page S. E. (2011). Fluvial Organic Carbon Losses From a Bornean Blackwater River. Biogeosciences 8, 901–909. doi: 10.5194/bg-8-901-2011
Mora-Soto A., Capsey A., Friedlander A. M., Palacios M., Brewin P. E., Golding N., et al. (2021). One of the Least Disturbed Marine Coastal Ecosystems on Earth: Spatial and Temporal Persistence of Darwin’s Sub-Antarctic Giant Kelp Forests. J. Biogeogr. 48, 2562–2577. doi: 10.1111/jbi.14221
Mora-Soto A., Palacios M., Macaya E. C., Gómez I., Huovinen P., Pérez-Matus A., et al. (2020). A High-Resolution Global Map of Giant Kelp (Macrocystis Pyrifera) Forests and Intertidal Green Algae (Ulvophyceae) With Sentinel-2 Imagery. Remote Sens. 12, 694. doi: 10.3390/rs12040694
Morley S. A., Souster T. A., Vause B. J., Gerrish L., Peck L. S., Barnes D. K. (2022). Benthic Biodiversity, Carbon Storage and the Potential for Increasing Negative Feedbacks on Climate Change in Shallow Waters of the Antarctic Peninsula. Biology 11 (2), 320. doi: 10.3390/biology11020320
Muller-Karger F. E., Varela R., Thunell R., Luerssen R., Hu C., Walsh J. J. (2005). The Importance of Continental Margins in the Global Carbon Cycle. Geophys. Res. Lett. 32, 1–4. doi: 10.1029/2004GL021346
NASA Earth Observatory (2022) Something Fishy in the Atlantic Night. Available at: https://earthobservatory.nasa.gov/features/Malvinas (Accessed February 7, 2022).
Nellemann C., Corcoran E., Duarte C. M., Valdeís L., De Young C., Fonseca L., et al. (2009). Blue Carbon: The Role of Healthy Oceans in Binding Carbon: A Rapid Response Assessment (UN Environment, GRID-Arendal). Available at: https://ccom.unh.edu/sites/default/files/publications/Nellemann_2010_BlueCarbon_book.pdf.
Newton T. L., Gehrels W. R., Fyfe R. M., Daley T. J. (2021). Reconstructing Sea-Level Change in the Falkland Islands (Islas Malvinas) Using Salt-Marsh Foraminifera, Diatoms and Testate Amoebae. Mar. Micropaleont. 162, 101923. doi: 10.1016/j.marmicro.2020.101923
Otley H., Munro H., Clausen A., Ingham B. (2008). Falkland Islands State of the Environment Report 2008 (Stanley: Falkland Islands Government and Falklands Conservation).
Pace M. C., Bailey D. M., Donnan D. W., Narayanaswamy B. E., Smith H. J., Speirs D. C., et al. (2021). Modelling Seabed Sediment Physical Properties and Organic Matter Content in the Firth of Clyde. Earth Syst. Sci. Data 13, 5847–5866. doi: 10.5194/essd-13-5847-2021
Parker G., Paterlini C. M., Violante R. A. (1997). “El Fondo Marino,” in El Mar Argentino Y Sus Recursos Marinos. Ed. Boschi E. E. (Mar del Plata: INIDEP), 65–87.
Pedersen M., Filbee-Dexter K., Frisk N., Sárossy Z., Wernberg T. (2021). Carbon Sequestration Potential Increased by Incomplete Anaerobic Decomposition of Kelp Detritus. Mar. Ecol. Prog. Ser. 660, 53–67. doi: 10.3354/meps13613
Petschick R., Kuhn G., Gingele F. (1996). Data From: Grain Size Distribution of Surface Sediments of the South Atlantic (PANGAEA). doi: 10.1594/PANGAEA.51464
Poggio L., de Sousa L. M., Batjes N. H., Heuvelink G. B. M., Kempen B., Ribeiro E., et al. (2021). SoilGrids 2.0: Producing Soil Information for the Globe With Quantified Spatial Uncertainty. SOIL 7, 217–240. doi: 10.5194/soil-7-217-2021
Pörtner H.-O., Scholes R. J., Agard J., Archer E., Bai X., Barnes D. K. A., et al. (2021). IPBES-IPCC Co-Sponsored Workshop Report on Biodiversity and Climate Change (Version 2) (IPBES-IPCC). doi: 10.5281/ZENODO.4782538
Queirós A. M., Stephens N., Widdicombe S., Tait K., McCoy S. J., Ingels J., et al. (2019). Connected Macroalgal-Sediment Systems: Blue Carbon and Food Webs in the Deep Coastal Ocean. Ecol. Monogr. 89, 1–21. doi: 10.1002/ecm.1366
Queirós A. M., Talbot E., Beaumont N. J., Somerfield P. J., Kay S., Pascoe C., et al. (2021). Bright Spots as Climate-Smart Marine Spatial Planning Tools for Conservation and Blue Growth. Global Change Biol. 27 (21), 5514–5531. doi: 10.1111/gcb.15827
Rattray A., Ierodiaconou D., Monk J., Versace V. L., Laurenson L. J. B. (2013). Detecting Patterns of Change in Benthic Habitats by Acoustic Remote Sensing. Mar. Ecol. Prog. Ser. 477, 1–13. doi: 10.3354/meps10264
Rattray A., Ierodiaconou D., Womersley T. (2015). Wave Exposure as a Predictor of Benthic Habitat Distribution on High Energy Temperate Reefs. Front. Mar. Sci. 2. doi: 10.3389/fmars.2015.00008
Riccialdelli L., Becker Y. A., Fioramonti N. E., Torres M., Bruno D. O., Rey A. R., et al. (2020). Trophic Structure of Southern Marine Ecosystems: A Comparative Isotopic Analysis From the Beagle Channel to the Oceanic Burdwood Bank Area Under a Wasp-Waist Assumption. Mar. Ecol. Prog. Ser. 655, 1–27. doi: 10.3354/meps13524
Rios C., Mutschke E., Morrison E. (2003). Benthic Sublittoral Diversity in the Strait of Magellan. Chile. Rev. Biol. Mar. Oceanogr. 38 (1), 1–12. doi: 10.4067/S0718-19572003000100001
Rogers A. D., Baco A., Escobar-Briones E., Gjerde K., Gobin J., Jaspars M., et al. (2021). Marine Genetic Resources in Areas Beyond National Jurisdiction: Promoting Marine Scientific Research and Enabling Equitable Benefit Sharing. Front. Mar. Sci. 8, 600.
Ross L. K., Ross R. E., Stewart H. A., Howell K. L. (2015). The Influence of Data Resolution on Predicted Distribution and Estimates of Extent of Current Protection of Three ‘Listed’ Deep-Sea Habitats. PloS One 10 (10), e0140061. doi: 10.1371/journal.pone.014006
Saba G. K., Burd A. B., Dunne J. P., Hernández-León S., Martin A. H., Rose K. A., et al. (2021). Toward a Better Understanding of Fish-Based Contribution to Ocean Carbon Flux. Limnol. Oceanogr. 66, 1639–1664. doi: 10.1002/lno.11709
Sabatini M., Reta R., Matano R. (2004). Circulation and Zooplankton Biomass Distribution Over the Southern Patagonian Shelf During Late Summer. Cont. Shelf Res. 24, 1359–1373. doi: 10.1016/j.csr.2004.03.014
Servicio de Hidrografía Naval (2022) Calidad De Fondo. Available at: http://www.hidro.gob.ar/DA/SHNCfondo.asp (Accessed 24.01.2022).
Smale D. A., Moore P. J., Queirós A. M., Higgs N. D., Burrows M. T. (2018). Appreciating Interconnectivity Between Habitats is Key to Blue Carbon Management. Front. Ecol. Environ. 16, 71–73. doi: 10.1002/fee.1765
Smale D. A., Pessarrodona A., King N., Moore P. J. (2021). Examining the Production, Export, and Immediate Fate of Kelp Detritus on Open-Coast Subtidal Reefs in the Northeast Atlantic. Limnol. Oceanogr., 1–14. doi: 10.1002/lno.11970
Smeaton C., Austin W. E. N. (2022). Quality Not Quantity: Prioritizing the Management of Sedimentary Organic Matter Across Continental Shelf Seas. Geophys. Res. Lett. 49, e2021GL097481. doi: 10.1029/2021GL097481
Smith P., Arneth A., Barnes D. K. A., Ichii K., Marquet P. A., Popp A., et al. (2022). How do We Best Synergize Climate Mitigation Actions to Co-Benefit Biodiversity? Glob. Change Biol. 28, 1–8. doi: 10.1111/gcb.16056
Swart S., Thomalla S. J., Monteiro P. M. S. (2015). The Seasonal Cycle of Mixed Layer Dynamics and Phytoplankton Biomass in the Sub-Antarctic Zone: A High-Resolution Glider Experiment. J. Mar. Syst. 147, 103–115. doi: 10.1016/j.jmarsys.2014.06.002
Thomalla S. J., Fauchereau N., Swart S., Monteiro P. M. S. (2011). Regional Scale Characteristics of the Seasonal Cycle of Chlorophyll in the Southern Ocean. Biogeosciences 8, 2849–2866. doi: 10.5194/bg-8-2849-2011
Turetsky M. R., Benscoter B., Page S., Rein G., van der Werf G. R., Watts A. (2015). Global Vulnerability of Peatlands to Fire and Carbon Loss. Nat. Geosci. 8 (1), 11–14. doi: 10.1038/ngeo2325
UNFCCC. (2021a). Nationally Determined Contributions Under the Paris Agreement. Synthesis Report by the Secretariat. Available at: https://unfccc.int/documents/306848 (Accessed February 7, 2022).
UNFCCC. (2021b). Negotiations on Action for Climate Empowerment. Available at: https://unfccc.int/topics/education-and-outreach/the-big-picture/education-and-outreach-in-the-negotiations (Accessed February 7, 2022).
Upson R., McAdam J., Clubbe C. (2016). Climate Change Risk Assessment for Plants and Soils of the Falkland Islands and the Services They Provide (Royal Botanic Gardens Kew and UK Falkland Islands Trust). doi: 10.13140/RG.2.2.15660.67203
van de Velde S., Van Lancker V., Hidalgo-Martinez S., Berelson W. M., Meysman F. J. R. (2018). Anthropogenic Disturbance Keeps the Coastal Seafloor Biogeochemistry in a Transient State. Sci. Rep. 8, 1–10. doi: 10.1038/s41598-018-23925-y
van Tussenbroek B. I. (1993). Plant and Frond Dynamics of the Giant Kelp, Macrocystis Pyrifera, Forming a Fringing Zone in the Falkland Islands. Eur. J. Phycol. 28 (3), 161–165. doi: 10.1080/09670269300650251
Ward D., Melbourne-Thomas J., Pecl G. T., Evans K., Green M., McCormack P. C., et al. (2022). Safeguarding Marine Life: Conservation of Biodiversity and Ecosystems (Reviews in Fish Biology and Fisheries), 1–36. Available at: https://link.springer.com/article/10.1007/s11160-022-09700-3.
Wernberg T., Krumhansl K., Filbee-Dexter K., Pedersen M. F. (2018). Status and Trends for the World’s Kelp Forests. 2nd ed. (Elsevier Ltd). doi: 10.1016/B978-0-12-805052-1.00003-6
Wilson P., Clark R., McAdam J. H., Cooper E. A. (1993). Soil Erosion in the Falkland Islands: An Assessment. Appl. Georg. 13 (4), 329–352. doi: 10.1016/0143-6228(93)90036-Z
Wilson R. J., Speirs D. C., Sabatino A., Heath M. R. (2018). A Synthetic Map of the North-West European Shelf Sedimentary Environment for Applications in Marine Science. Earth Syst. Sci. Data 10, 109–130. doi: 10.5194/essd-10-109-2018
Wing S. R., Durante L. M., Connolly A. J., Sabadel A. J., Wing L. C. (2021). Overexploitation and Decline in Kelp Forests Inflate the Bioenergetic Costs of Fisheries (Global Ecology and Biogeography). Available at: https://onlinelibrary.wiley.com/doi/abs/10.1111/geb.13448.
Keywords: Falkland Islands, kelp, land-ocean carbon, mesophotic biodiversity, Marine Managed Areas, blue carbon, marine spatial planning, sub-Antarctic
Citation: Bax N, Barnes DKA, Pineda-Metz SEA, Pearman T, Diesing M, Carter S, Downey RV, Evans CD, Brickle P, Baylis AMM, Adler A, Guest A, Layton KKS, Brewin PE and Bayley DTI (2022) Towards Incorporation of Blue Carbon in Falkland Islands Marine Spatial Planning: A Multi-Tiered Approach. Front. Mar. Sci. 9:872727. doi: 10.3389/fmars.2022.872727
Received: 09 February 2022; Accepted: 29 April 2022;
Published: 10 June 2022.
Edited by:
Joanne S Porter, Heriot-Watt University, United KingdomReviewed by:
Lida Teneva, Independent Researcher, Sacramento, CA, United StatesCraig Smeaton, University of St Andrews, United Kingdom
Copyright © 2022 Bax, Barnes, Pineda-Metz, Pearman, Diesing, Carter, Downey, Evans, Brickle, Baylis, Adler, Guest, Layton, Brewin and Bayley. This is an open-access article distributed under the terms of the Creative Commons Attribution License (CC BY). The use, distribution or reproduction in other forums is permitted, provided the original author(s) and the copyright owner(s) are credited and that the original publication in this journal is cited, in accordance with accepted academic practice. No use, distribution or reproduction is permitted which does not comply with these terms.
*Correspondence: Narissa Bax, nbax@saeri.ac.fk
†These authors share first authorship