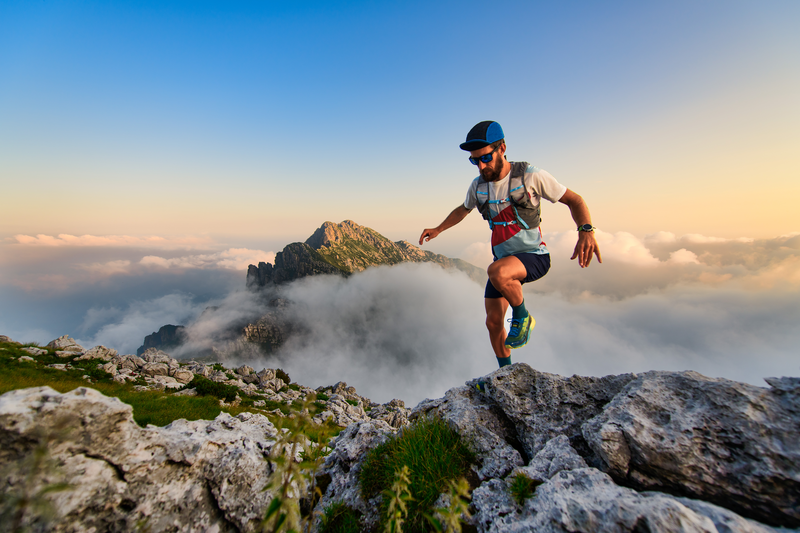
94% of researchers rate our articles as excellent or good
Learn more about the work of our research integrity team to safeguard the quality of each article we publish.
Find out more
ORIGINAL RESEARCH article
Front. Mar. Sci. , 18 May 2022
Sec. Marine Fisheries, Aquaculture and Living Resources
Volume 9 - 2022 | https://doi.org/10.3389/fmars.2022.872577
This article is part of the Research Topic New Progresses and Effects of Functional Feed Additives on Marine Aquatic Animals View all 13 articles
This study aimed to evaluate the effects of soluble and insoluble non-starch polysaccharides (NSPs) on growth performance, digestive enzyme activity, antioxidant capacity, and intestinal flora of juvenile GIFT tilapia (Oreochromis niloticus). Four isonitrogenous and isolipidic experimental diets were formulated, including the FM diet (0% NSPs, FM), INSP diet (5.8% insoluble NSPs, INSP), SNSP diet (12.2% soluble NSPs, SNSP), and NSP diet (5.8% INSP and 12.2% SNSP, NSPs). Each diet was fed to triplicate groups of 40 fish (2.14 ± 0.04 g) per repetition for 10 weeks. Results showed that dietary supplementation with different types of NSPs significantly affected the digestive enzyme activity, antioxidant capacity, and intestinal flora of juvenile GIFT tilapia. Specifically, dietary supplementation with INSP significantly increased pepsin and intestinal lipase activity, while dietary supplementation with SNSP significantly decreased intestinal lipase activity and resulted in a significant decrease in plasma catalase, glutathione peroxidase, and total antioxidant capacity activity. Meanwhile, dietary supplementation with SNSP significantly increased the concentration of urea nitrogen and high-density lipoprotein cholesterol in plasma and alanine aminotransferase activity in plasma and hepatic. Moreover, LEfSe analysis showed that dietary supplementation with SNSP significantly increased the abundance of Bacteroidales, Bacteroidia, and Cellulosilyticum, while dietary supplementation with NSPs significantly decreased the abundance of Mycobacteriaceae, Mycobacterium_neoaurum, Corynebacteriales, and Mycobacterium. These results suggest that dietary INSP is an inert ingredient with limited effect, while dietary SNSP inhabited antioxidant capacity, induced liver damage, and altered the abundance of anaerobic/aerobic bacteria in the intestine of GIFT tilapia.
Fish meal (FM) is widely used in aquafeed production as an ideal protein source due to its good palatability and high nutrition value (FAO, 2014; Deng et al., 2017). In recent years, the limited supply of FM has made it increasingly expensive (Zhang et al., 2019), leading to a rapid increase in aquafeed production costs. Feed inputs have been reported to account for more than 50% of production costs in intensive aquaculture, which severely limits aquaculture development (Medard et al., 2018; Yannis et al., 2018). Hence, it is essential to find suitable protein sources to replace FM for the sustainable development of aquaculture (Li et al., 2006; Tacon et al., 2010; Altan and Korkut, 2011). Currently, conventional plant protein sources (PPS) have become the main alternative sources of FM with the advantages of relatively abundant supplementation, lower price, and stable quality (Deng et al., 2015; Zhang et al., 2018). However, PPS has an unbalanced amino acid profile, lacks essential nutrients, and carries anti-nutritional factors (ANFs) (Lee et al., 2010; Gao et al., 2018), making it extremely difficult to guarantee the normal growth and health of fish when FM is largely or completely replaced by PPS (Sales, 2009; Collins et al., 2013). Several studies have confirmed that ANFs disrupt intestine and liver morphology and function, upregulate inflammation-related gene expression, alter intestinal flora structure, and ultimately reduce fish feeding rate, nutrient digestibility, feed conversion rate, and growth performance (Merrifield et al., 2009; Mansfield et al., 2010; Gao et al., 2011; Król et al., 2016) Also, an excessive addition level of PPS even induced enteritis in fish (Refstie et al., 2000; He et al., 2020). Hence, ANFs are considered as the main factors restricting the use of PPS in aquafeed (Collins et al., 2013; Azeredo et al., 2017).
As the main components of plant cell walls, non-starch polysaccharides (NSPs) are highly abundant in PPS (Ma et al., 2017), which reach 10-70% of plant ingredients (varies with plant species) (Choct, 2015). Consequently, increasing the use of PPS ultimately significantly increases the content of NSPs in aquafeed (Deng et al., 2021). NSPs are indigestible carbohydrates for fish due to the scarcity or absence of endogenous NSPs-degrading enzymes in monogastric animals (including fish) (Kuz Mina, 1996), and thus NSPs are generally considered as a low- or non-nutritional feed ingredient for fish (Sinha et al., 2011). Indeed, although some components of NSPs have been observed to have beneficial effects on fish [e.g., β-glucan enhancing non-specific cellular defense mechanisms and reducing inflammation on fish (Kumar et al., 2005; Suchecka et al., 2015)], dietary NSPs are still generally considered to be one of the major anti-nutritional components of the fish diets. As previous studies reported, dietary NSPs tend to reduce the absorption of feed nutrients (Amirkolaie et al., 2005; Kraugerud et al., 2007; Glencross, 2009; Lekva et al., 2010; Glencross et al., 2012), and dietary inclusion of a high level of NSPs even causes liver lesions in fish (Ren et al., 2020). Specifically, NSPs can be divided into insoluble NSPs (INSP) and soluble NSPs (SNSP) according to solubility in neutral buffer. INSP is mainly cellulose, while SNSP consists of a range of hemicelluloses including arabinoxylan, β-glucan, mannans, and pectin, which have different physicochemical properties and thus produce different effects on fish (Dalsgaard et al., 2016; Deng et al., 2021). At present, limited studies have been conducted to evaluate the differential effects of dietary INSP and SNSP on fish (Glencross et al., 2012).
GIFT tilapia (Genetic improvement of farmed tilapia, Oreochromis niloticus), which is considered one of the most important aquaculture species in China, is widely cultivated due to its enormous advantages, such as fast growth, strong disease resistance and delicious meat quality (Jiang et al., 2014; Deng et al., 2020). As a typical omnivorous fish, tilapia has a high capacity to utilize dietary carbohydrates (Wilson, 1994). The digestibility of dietary NSPs ranging from 2.8% to 73% in tilapia, with an average of 24.3% (Amirkolaie et al., 2005; Haidar et al., 2016; Maas et al., 2020). Previous studies have focused on the effects of different NSP types on nutrient digestibility (Amirkolaie et al., 2005; Leenhouwers et al., 2007), while limited studies have been conducted to evaluate the effects of different NSP types on the physiology and intestinal flora of tilapia. Intestinal flora is an integral part of the organism (Bi et al., 2015), which extensively participates in the physiological processes of fish, including food digestion and absorption, nutrient metabolism, and immunity response (Dan et al., 2021). The structure and function of intestinal flora are influenced by dietary components (Du et al., 2018). NSPs are quantitatively the most important substrates for intestinal flora (Macfarlane and Macfarlane, 2002), which alters the structure and function of intestinal flora (Sinha et al., 2011). Several studies have confirmed that different dietary NSP sources have different effects on the intestinal flora of pigs, due to the different INSP/SNSP ratio of different dietary NSPs sources (Chen et al., 2013; Chen et al., 2014). This phenomenon may be associated with the inconsistent effects of dietary INSP and SNSP on the intestinal flora (Yang et al., 2020). However, studies on the effects of different types of NSPs on intestinal flora in fish are still lacking. Thus, this study evaluated the effects of different NSP types on growth performance, digestive enzyme activity, antioxidant capacity, and intestinal flora of juvenile GIFT tilapia.
Fish meal was used as the primary protein source and soybean oil and soybean lecithin were the primary lipid sources used to prepare the basic feed (control diet, FM). By adding different types of NSPs to basic feed, four experimental diets were formulated to contain NSP levels of 0% NSPs, 5.8% INSP (cellulose), 12.2% SNSP (including 3.48% arabinoxylan, 0.82% β-glucan, 0.94% mannan and 6.96% pectin), and 18.0% NSPs (5.8% INSP and 12.2% SNSP), respectively. The inclusion levels of different types of NSPs were referred to in previous reports (Deng et al., 2021), based on their contents present in wheat bran and soybean meal as the sole protein and carbohydrate source for GIFT tilapia.
Each diet ingredient was ground into powder and passed through a 300-μm mesh (SFSP series, produced by Kunming Huaming grain, oil and feed equipment factory, Yunnan, China). Soybean lecithin was first dissolved in soybean oil (mixed oil), then mixed with each ingredient evenly, and then 30% pure water was added to produce a stiff dough. The dough was pelleted using an extruder (KS-18, Produced by Jiangsu Jinggu rice machine Co. Ltd, Yunnan, China) through a 1.0-mm die. The moist pellets were dried at 40°C for 12 h, and then stored at -20°C until used. The formulation and proximate composition of experimental diets are shown in Table 1 and the amino acid profiles of experimental diets are shown in Supplementary Table 1.
Juvenile GIFT tilapias were obtained from the Yunnan Xishuangbanna Aquatic Technology Promotion Station (Yunnan, China), and the experiment was completed in the aquaculture laboratory of Yunnan Agriculture University. Juvenile GIFT tilapia were placed in a net cage (0.9 m * 0.9 m * 1.0 m) and fed twice daily (08:00 and 17:00) with a commercial feed (provided by Tongwei Co. Ltd, China) for 2 weeks to acclimatize to the new cultural environment. Tilapias were fasted 24 h after 2 weeks of acclimatization, then weighed and grouped. A total of 480 healthy juvenile tilapia with uniform size (2.14 ± 0.04 g) were selected and randomly assigned to 12 net cages with 40 fish per cage (all net cages are placed in the same cement pond with a circulating water system and the culture water used was tap water with a flow rate of 100 L/min after aeration and dichlorination).The culture experiments lasted 10 weeks and were conducted under natural photoperiod (14 h light/10 h dark at the end of July and 12 h light/12 h dark at the beginning of October). The water temperature was maintained at 28 ± 1°C with NH4+-N < 0.02 mg/L and dissolved oxygen >5 mg/L. The test fish were fed twice daily (08:00 and 17:00) to apparent satiation and the feed consumption of each net cage was recorded during the experiment period.
After the breeding experiment, fish were fasted for 24 h before collecting samples. The total number and weight of tilapia in each cage were checked and recorded to calculate weight gain rate (WGR), daily growth coefficient (DGC), metabolic body weight (MBW), feed intake (FI), feed conversion rate (FCR), and protein efficiency ratio (PER).
After counting and weighing, fish were anesthetized with eugenol (1: 12000, Macklin, Shanghai, China) and then randomly selected for sampling. Five fish were taken from each cage, stored at -20°C for measure the proximate composition of the whole-body. The dorsal muscles of another three fish were taken from each cage and placed in sealed bags, stored at -20°C immediately for amino acid profiles analysis. Blood from the tail vein of ten fish per cage was half drawn with a 1-ml pretreated syringe (moistened by heparin sodium) and the rest drawn using a normal 1-ml syringe. Blood drawn by pretreated syringe were collected in heparin sodium anti-coagulation centrifuge tubes and stored at 4°C for 3 h and centrifuged at 4000 rpm/min for 10 min to obtain plasma samples, while blood drawn by normal syringe was transferred to 1.5 mL Eppendorf tubes and stored at 4°C for 12 h, and then centrifuged at 3000 rpm/min for 10 min at 4°C to prepare serum samples. Both plasma and serum samples were stored at -80°C immediately for subsequent analysis. Another four fish per cage were dissected, and the liver, stomach, and intestine were harvested and stored at -80°C until used. Four fish were randomly selected after drawing blood and sterile dissected and the hindgut was quickly gathered to avoid pollution. Four mixed samples were prepared in each group, with each mixed sample consisting of three separate hindguts (one fish per tank in the same group was provided). The mixed samples were quickly frozen in the liquid nitrogen tank and transferred to an ultra-low-temperature refrigerator immediately, stored at -80°C for subsequent analysis.
The proximate composition of experimental diets and whole-body were analyzed by using the standard of AOAC (AOAC, 2005). The sample was dried to a constant weight at 105°C to determine moisture content and combusted at 550°C for 6 h to determine the ash content. The crude protein content was determined using the Kjeldahl method (N*6.25), the crude lipid content was estimated using the chloroform-methanol method. Starch + sugar in each experimental diets was determined by an enzymatic digestion method according to a previous study (Staessen et al., 2020).
The amino acid profiles of diets and muscles were measured by an automatic amino acid analyzer (Hitachi L-8800, Japan). All samples were freeze-dried at low temperature and the weight of each tested sample was in the range of 50 to 200 mg (accuracy is 0.01mg). The contents of amino acids were analyzed after the sample being hydrolyzed with HCl (6 mol/L) at 110°C for 24 h.
The content of total amino acids (TAA), total protein (TP), blood urea nitrogen (BUN), ammonia nitrogen (AN) and malondialdehyde (MDA), and the activity of γ-glutamyl transferase (GGT), alanine aminotransferase (ALT), aspartate aminotransferase (AST), superoxide dismutase (SOD), catalase (CAT), peroxidase (POD), glutathione peroxidase (GPx), and total antioxidant capacity (TAC) in plasma were measured by commercially available kits (Nanjing Jiancheng Bioengineering Institute Co. Ltd, Nanjing, China). Hepatic GGT, ALT, AST, SOD, CAT, POD, GPx activity, and MDA content were measured following the method used in plasma. The contents of total cholesterol (TC), free cholesterol (FC), cholesterol ester (CE), low-density lipoprotein cholesterol (LDL-C), high-density lipoprotein cholesterol (HDL-C) and triglyceride (TG) were also measured using commercially available kits (Nanjing Jiancheng Bioengineering Institute Co. Ltd, Nanjing, China). The protein concentration of tissue samples (stomach, intestine, liver) was also determined using commercially available kits (Nanjing Jiancheng Bioengineering Institute Co. Ltd, Nanjing, China). Sample processing, reagent preparation, and determination processes were performed in strict accordance with the methods provided in the kit instructions.
Intestinal flora DNA was extracted using the SDS method. The DNA samples that passed the quality test were used for further amplification and sequencing. The V3-V4 region of the 16S rRNA gene was amplified using universal primers 27F (5’-AGAGTTTGATCCTGGCTCAG-3’) and 1492R (5’-GGTTACCTTGTTACGACTT-3’). The detection and recovery of PCR products were performed by 0.2% agarose gel electrophoresis and MinElute Gel Extraction Kit (Qiagen, Germany), respectively. The prepared library was sequenced on the Hiseq2500 PE250 pla-tform (Illumina, USA). After removing barcodes and primers, FLASH was used to splice the reads of each sample to obtain the original data tags (raw Tags). The quality control of raw tags was carried out with reference to the QIIME tags quality control standard. Raw Tags were truncated at the first low mass base site where the base number of continuous low-quality values (default quality threshold ≤ 19) reached the set length (the default length value 3). In addition, tags with consecutive high-quality bases less than 75% of the tag length were excluded from further analysis. USEARCH 7.0 software was used to remove the chimeric sequence and the final effective tags were obtained for further analysis.
All effective tags of all samples were clustered and the sequences were clustered into Operational Taxonomic Units (OTUs) with 97% consistency by default using Uparse software. Taxonomic annotation analysis was obtained and the community composition of each sample was counted at each classification level using the Mothur method and the SSUrRNA database of Silva. QIIME was used to calculate α-diversity and R software was used to perform PCA analysis, species accumulation boxplot, rarefaction curve, and difference significance analysis of inter-group community structure. In addition, the Venn diagram was drawn based on the data processed by homogenization and LEfSe analysis was used to identify species with significant differences between the groups.
Growth performance and feed utilization were calculated by the following formulas:
Where Wi and Wt (g) are mean initial and final body weight of the experimental fish, respectively; Wf and Wp (Wf * crude protein content of the diets, g) are mean dry feed and protein intake of the experimental fish, respectively; t represents the duration of the feeding experiment (days). All data are shown as means ± standard error of measurement (Means ± SEM) and analyzed by SPSS (v20.0) software (Chicago, IL, USA) for one-way variance (ANOVA). P < 0.05 indicates a significant difference between the groups, and in this case, Tukey’s HSD test was used for multiple comparison analysis.
Dietary supplementation with different types of NSPs had no significant effect on the WGR, DGC, FI, FCR, and PER of GIFT tilapia (P > 0.05; Table 2).
Table 2 Growth performance and feed utilization of GIFT tilapia fed diets with different types of non-starch polysaccharides.
Dietary supplementation with different types of NSPs significantly increased the pepsin activity of tilapias (P < 0.05). In addition, dietary supplementation with INSP significantly increased intestinal lipase activity, while dietary supplementation with SNSP significantly decreased intestinal lipase activity (P < 0.05; Table 3).
Table 3 Digestive enzyme activities in GIFT tilapias fed diets with different types of non-starch polysaccharides.
Dietary supplementation with different types of NSPs had no significant effect on serum TC, TAG, LDL-C, FC, CE contents, and LDL-C/HDL-C, FC/TC ratio (P > 0.05) and dietary supplementation with SNSP significantly increased serum HDL-C content (P < 0.05; Table 4).
Table 4 Serum lipoprotein profile of GIFT tilapia fed diets with different types of non-starch polysaccharides.
Dietary supplementation with different types of NSPs had no significant effect on plasma TP, TAA, AN contents, hepatic AST, and GGT activity (P > 0.05). Dietary supplementation with SNSP or 18.0% NSPs significantly increased plasma BUN content, plasma, and hepatic ALT activity (P < 0.05). Conversely, dietary supplementation with INSP significantly decreased hepatic ALT activity (P < 0.05; Table 5).
Table 5 Protein metabolism-related parameters in GIFT tilapia fed diets with different types of non-starch polysaccharides.
Dietary supplementation with different types of NSPs had no significant effect on plasma SOD, POD activity, and MDA content (P > 0.05) but significantly decreased plasma CAT activity (P < 0.05). Dietary supplementation with SNSP significantly decreased the activity of plasma GPx, TAC and hepatic SOD, CAT, POD, GPx and TAC, but dietary supplementation with INSP significantly increased hepatic CAT activity (P < 0.05). Moreover, dietary supplementation with 18.0% NSPs significantly decreased hepatic SOD and GPx activity (P < 0.05; Table 6).
Table 6 Antioxidant capacity of GIFT tilapia fed diets with different types of non-starch polysaccharides.
Dietary supplementation with SNSP or 18.0% NSPs significantly decreased the crude protein and lipid content of the whole-body (P < 0.05). Moreover, dietary supplementation with 18.0% NSPs significantly increased the ash content of the whole-body (P < 0.05; Table 7).
Table 7 The whole-body composition of GIFT tilapia fed diets with different types of non-starch polysaccharides.
The contents of muscular histidine, methionine, phenylalanine, threonine, valine, glycine, tyrosine, proline, cysteine, and EAA/NEAA ratio were not significantly affected by the experimental diets (P > 0.05). Dietary supplementation with 18.0% NSPs significantly decreased the contents of muscular arginine (Arg), essential amino acids (EAA), glutamate (Glu), aspartate (Asp), serine (Ser), non-essential amino acids (NEAA), and total amino acids (TAA) (P < 0.05; Table 8).
Table 8 Amino acids profiles (% dry matter) in the whole-body of GIFT tilapia fed diets with different types of non-starch polysaccharides.
A total of 978,800 effective tags were obtained from 16 samples, with an average of 61,175 per sample. The species accumulation boxplot and rarefaction curve indicate that the number of samples and tags were sufficient to identify almost all microbial species (Figure 1). Meanwhile, the Good’s coverage indices of each sample was as high as 99%, which also indicated that the depth of sequencing was sufficient (Table 9). The Alpha diversity of intestinal flora was calculated by the Sobs, Shannon, Simpson, Chao1, ACE, and PD-whole-tree diversity indices and these results indicated that there was no significant difference in Alpha diversity of intestinal flora between groups (P > 0.05, Table 9). A total of 3,474 OTUs were obtained in all samples, of which 1,064 OTUs were shared with all four groups. The number of unique OTUs in the FM group, INSP group, SNSP group, and NSP group were 239, 262, 314, and 159, respectively (Figure 2).
Figure 1 Determine whether the number of tags and samples in this study were sufficient for subsequent data analysis. (A) Rarefaction curves were constructed based on the amount of sequencing data extracted in this study and the corresponding number of species. (B) Species accumulation boxplot was used to describe the increasing trend of species diversity as the number of samples increased.
Figure 2 Venn diagram of OTUs in each group. Venn graph was drawn by analyzing the common and unique OTUs among different groups according to the results of OTU clustered analysis with 97% identification based on 16S rRNA sequencing data.
Intestinal flora composition analysis showed that Actinobacteria, Firmicutes, Planctomycetes, Proteobacteria, Fusobacteria, Chlamydiae, TM6, and Bacteroidetes were the dominant phyla in all groups (Figure 3A). In the FM group, the four dominant phyla were Firmicutes (16.83%), Proteobacteria (15.11%), Fusobacteria (12.99%), and TM6 (12.42%). In the SNSP group, the four dominant phyla were Proteobacteria (23.53%), Fusobacteria (19.96%), Actinobacteria (19.34%), and Firmicutes (16.27%). In the INSP group, Proteobacteria (22.58%) and Planctomycetes (15.87%) were the most abundant phyla, followed by Fusobacteria (14.94%) and Firmicutes (14.64%). In the NSP group, Firmicutes (39.56%), Proteobacteria (17.21%), Fusobacterium (9.78%), and Planctomycetes (7.96%) were the four dominant phyla.
Figure 3 Microbial community structure of different groups. (A) Microbial community structure at the phylum level in different groups of FM, SNSP, INSP and NSP. (B) Microbial community structure at the genera level in different groups of FM, SNSP, INSP and NSP.
Intestinal flora community structure at the genus level in different groups is shown in Figure 3B. In the FM group, the four dominant genera were Cetobacterium (12.84%), Mycobacterium (6.78%), Pirellula (6.16%), and Alpinimonas (2.98%). While in the SNSP group, Cetobacterium (18.99%), Alpinimonas (12.49%), Bacteroides (5.18%), and Cellulosilytium (2.27%) were the most prevalent genera. In the INSP group, the four dominant genera were successively Cetobacterium (15.66%), Alpinimonas (8.14%), Bacteroides (6.84%), and Pirellula (5.54%). Turicibacter (15.62%), Cetobacterium (9.71%), Alpinimonas (6.00%), and Pirellula (4.92%) were the most abundant genera in the NSP group. Moreover, the heatmap presented the flora distribution at the genus level in different groups more intuitively (Supplementary Figure 1).
Anosim inter-group difference analysis showed that there were significant differences in the community structure between the FM group and the SNSP group (P < 0.05, Table 10). LEfSe analysis results showed that the abundance of Bacteroidales, Bacteroidia, and Cellulosilyticum in the SNSP group were significantly higher than that in the FM group (P < 0.05, Figure 4A), and the abundance of Mycobacteriaceae, Mycobacterium_neoaurum, Corynebacteriales, and Mycobacterium in the FM group were significantly higher than that in the NSP groups (P < 0.05, Figure 4B).
Figure 4 LEfSe analysis of intestine microbial compositions among different groups. (FM, SNSP, INSP and NSP). Significant differences (LDA score ≥ 2, P < 0.05) between FM and SNSP (A), FM and NSP, (B). There was no significant difference between the other groups (P > 0.05).
Fish have limited tolerance to dietary fiber, which at a low level (30-50 g/kg), improves fish growth (Zhou et al., 2005; Altan and Korkut, 2011) but excessive dietary fiber content inhibits fish digestion and absorption of feed nutrients (Watanabe et al., 1994). In addition, the effect of dietary fiber on fish is related to the fiber type (Adorian et al., 2016; Goulart et al., 2017). (Amirkolaie et al., 2005) reported that dietary supplementation with 8% INSP (cellulose) had non negative effects on Nile tilapia (Oreochromis niloticus L), while dietary supplementation with 8% SNSP (guar gum) significantly reduced the growth and feed conversion rate on Nile tilapia. However, dietary supplementation with different types of NSPs (5.8% INSP, 12.2% SNSP, and 18.0% NSPs) had no significant effects on the growth and FCR of GIFT tilapia in this study. These results indicate that the antinutritional effect of dietary SNSP is closely related to its composition.
Digestive enzymes could effectively metabolize proteins, lipids, and carbohydrates (Zokaeifar et al., 2012) and their activities are closely related to the characteristic and quantity of feed nutrients (Zhang et al., 2021). The significant increase of pepsin and intestinal lipase activity in the INSP group may be related to the physicochemical properties (e.g., insolubility, water absorption) of INSP and dietary INSP expanded the digesta, thereby increasing the contact area between digestive enzymes and substrates, leading to an increase in digestive activity (Li et al., 2017). While dietary SNSP form a high-viscosity environment in the digestive tract and provide a high-quality reproduction condition for microorganisms, these microorganisms produce a large amount of short-chain fatty acid through a fermentation process that lowers the pH of the digestive tract (Engberg et al., 2009; Niba et al., 2009), and thus participated in the regulation of digestive enzyme activity, which may explain the pepsin activity significant increase in SNSP and NSP groups. However, digestive enzymes activity may also be decreased by complexation with NSPs or by the encapsulation of substrates by NSPs (Sinha et al., 2011), which may explain the significant decrease in intestinal lipase activity in the SNSP group. Similarly, dietary supplementation with 16.8% SNSP significantly decreased the intestinal trypsin activity of rainbow trout (Deng et al., 2021). Moreover, considering that dietary supplementation with 18.0% NSPs (5.8% INSP + 12.2% SNSP) had no significant effect on intestinal lipase activity of GIFT tilapia in this study, this phenomenon also indicated that dietary INSP was able to mitigate the adverse effects on digestive enzymes activities of dietary SNSP.
ALT, AST, and GGT are enzymes involved in amino acid metabolism which are mainly distributed in hepatocytes and released into the blood when hepatocytes are damaged. Therefore, the activity of ALT, AST, and GGT in plasma can effectively reflect the status of liver function (Habte-Tsion et al., 2015; Hanim et al., 2015; Talaveron et al., 2019). In this study, dietary supplementation with SNSP significantly increased plasma ALT activity, indicating that dietary SNSP induced liver function impairment in GIFT tilapia. Similarly, largemouth bass (Micropterus salmoides) suffered severe liver damage with dietary supplementation of 6% SNSP (carboxymethyl cellulose) (Shi et al., 2019), and dietary supplementation of 15% SNSP (pectin) induced liver damage in yellow catfish (Pelteobagrus fulvidraco) (Cai et al., 2019).
The significant increase of plasma BUN level and hepatic ALT activity of GIFT tilapia in the SNSP group suggests that dietary SNSP may enhance protein catabolism, thus causing a decrease in the whole-body crude protein and amino acid content in both the SNSP and NSP groups. The activation of protein catabolism may be associated with the reduced intake of lipids and carbohydrates, with protein being used more for catabolism to supply energy as dietary SNSP interferes with the absorption of carbohydrates and lipids (Choct et al., 1996), which may also explain the significant decrease in the content of some amino acids in muscle. Moreover, dietary fiber has been reported to stimulate mucus secretion from the digestive tract and accelerate the loss of endogenous nitrogen (Larsen et al., 1993; Sales, 2009),while Ser, Thr, Gly, Glu, and Asp account for more than 50% of the total amino acid content of mucin (Sales, 2009), which means that these amino acids are easily lost with the excretion of mucin. Additionally, Ala, Arg, Ile, Lys, Ser, Glu, and Asp are all important glucogenic amino acids that can be converted into glucose by the gluconeogenic pathway when animals are deficient in carbohydrates. This may explain the significant decrease in Ala, Arg, Ile, Lys, Ser, Glu, and Asp of the muscles in GIFT tilapia with the dietary supplementation of 18.0% NSP in this study.
Previous studies have shown that dietary SNSP can further influence lipid metabolism, as Hossain et al. (Hossain et al., 2001; Hossain et al., 2003) reported that dietary supplementation with SNSP (sesbania endosperm, Sesbania aculeate) significantly reduced serum TC concentrations in carp and tilapia; (Liu et al., 2021) also reported that soluble fiber had the ability to reduce serum TC concentrations. However, in contrast to these above studies, dietary supplementation with SNSP had no significant effect on serum TC concentrations but significantly increased serum HDL concentrations in this study. The difference in these results suggest that the impact of dietary SNSP on serum TC concentrations may vary with the test species and the composition of dietary SNSP. The hypolipidemic effect of dietary SNSP may be related to the viscosity change of chyme, and (Pasquier et al., 1996) suggested that high viscosity conditions would inhibit the fat emulsification process and lipolysis. This may explain the significant decrease in whole-body crude lipid content in the SNSP and NSP groups in this study. Similarly, dietary supplementation with SNSP (endosperm, separated from Sesbania seeds) significantly decreased the whole-body crude protein and lipid contents in common carp (Hossain et al., 2001), and the whole-body lipid content of largemouth bass also significantly decreased with dietary supplementation of 6% SNSP (carboxymethyl cellulose) or 6% INSP (microcrystalline cellulose) (Shi et al., 2019). However, dietary supplementation with either INSP or SNSP had no significant effect on the body composition of Chinese mitten crab (Eriocheir sinensis) (Wu et al., 2015). The differences in these results may be related to the differences in digestive physiology between fish and crustaceans, which requires further study.
The antioxidant capacity of plasma and the liver significantly decreased in the SNSP group, indicating dietary SNSP induced oxidative stress in GIFT tilapia. Similarly, dietary supplementation with SNSP also induced oxidative stress in largemouth bass and rainbow trout (Shi et al., 2019; Deng et al., 2021). Conversely, (Enes et al., 2012) suggested that dietary supplementation with low doses of SNSP (4% guar gum) may have a preventive effect on intestinal oxidative stress in white seabream (Diplodus sargus). Moreover, several recent studies have shown that dietary SNSP from natural resources possess strong reducing power and antioxidant properties (Amamou et al., 2020), which may promote antioxidant enzyme activity or modulate antioxidant signaling pathways by scavenging free radicals (Chen et al., 2021). These results suggest that the effect of dietary SNSP on the antioxidant capacity of fish was type- and dose-dependent.
A recent study reported that dietary SNSP (xylan and pectin) had no significant effects on the Alpha diversity of intestinal flora in yellow catfish (Pelteobagrus fulvidraco) (Zhang, 2014). In this study, diets containing different types of NSPs also had no significant effect on the Alpha diversity of intestinal flora in GIFT tilapia. Contrary to our results, dietary soluble xylan significantly increased the Alpha diversity and richness of intestinal flora in Chinese mitten crab (Eriocheir sinensis) (Wu, 2015). These different results may be caused by the types of dietary NSPs and the differences of digestive physiology between fish and crustaceans, which needs to be further studied. Noteworthy, different diets significantly changed the composition of dominant bacteria in GIFT tilapia.
At the phylum level, although the abundance of dominant phylum in each group was different, the species of dominant phylum were relatively consistent and all groups have Firmicutes, Proteobacteria, Fusobacteria, Actinobacteria, Planctomycetes, and Bacteroidetes as the core flora. This result was consistent with the intestinal flora of the cichlid (Baldo et al., 2015) and partially similar with the wild and cultured Nile tilapia (Zheng et al., 2018; Beneberu, 2020), which proved that our results were reliable.
At the genus level, Cetobacterium was the dominant genus in the intestine of GIFT tilapia in all groups in this study. Cetobacterium is the core flora in the intestinal tract of fish (Ni et al., 2012), and it has been identified in carp (Cyprinus carpio) (Kessel et al., 2011), Nile tilapia (Yun et al., 2018), giant amazonian fish (Arapaima gigas) (Ramírez et al., 2018) and some other freshwater fish. The metabolism of Cetobacterium in the intestine of freshwater fish can produce vitamin B12 (VB12) and acetic acid (Sugita et al., 1991; Lin et al., 2019) and these metabolites have important functions. The VB12 participates in the regulation of intestinal ecology and promotes intestinal health (Degnan et al., 2014), while acetic acid can promote sugar and fat metabolism or protein synthesis and can synthesize proteins, carbohydrates, and fats for the host’s use (Lin et al., 2019). Host species are a key factor affecting the composition of the intestinal flora (Egerton et al., 2018), Cetobacterium can be the dominant intestinal microbiota of many freshwater fish, which may be due to its important physiological functions. Mycobacterium has been proven to be associated with pathogenic bacteria (Novotny et al., 2004; Shanmugham and Pan, 2013) and can even cause the infection of fish (Hashish et al., 2018). Mycobacterium is a kind of aerobic bacteria, while the dietary SNSP decreases the oxygen tension of the intestine (Sinha et al., 2011), and this may explain why the abundance of Mycobacterium was significantly decreased in the SNSP and NSP groups. This phenomenon suggests that dietary SNSP possesses a potential mechanisms to reduce the aboudance of aerobic bacteria in the intestine of fish, which needs to be further studied.
Early studies have shown that the abundance of Bacteroides increases when inflammatory bowel disease occurs (Prindiville et al., 2000; Bloom et al., 2011) and so Bacteroides may serve as a marker bacteria for the development of intestinal diseases. Moreover, Bacteroides is one of the main bacteria involved in the fermentation process of intestinal contents and its metabolic production of short-chain fatty acids plays an important role in resisting host enteritis (Besten et al., 2013). Increasing the abundance of Bacteroides may be one of the strategies for host resistance to enteritis but this hypothesis needs to be further explored. Bacteroides is a kind of anaerobic bacteria and its relative abundance increased significantly in the SNSP group, which may be associated with the reduction of oxygen tension in the intestine via the SNSP fermentation process.
In summary, this study showed that dietary INSP had limited effects on the growth and intestinal flora of GIFT tilapia, whereas dietary SNSP inhibited intestinal lipase activity, reduced antioxidant capacity, and induced liver damage of GIFT tilapia. Additionally, dietary SNSP reduced intestinal aerobic microbial abundance but elevated anaerobic microbial abundance.
The datasets presented in this study can be found in online repositories. The names of the repository/repositories and accession number(s) can be found below: NCBI [accession: PRJNA806406].
The animal study was reviewed and approved by Animal Ethics Committee of Guangdong Ocean University.
JD and BT directed the completion of the experimental design, and YL completed the breeding of test fish, sample collection and measurement, data analysis and manuscript writing. SX and WZ directed the manuscript revision. All authors contributed to the article and approved the submitted version.
This study was financially supported by the National Natural Science Foundation of China (31760761); National Key R&D Program of China (2019YFD0900200); Foundation of Tongwei Co., Ltd. (TA2019A003); Program for Scientific Research Startup Funds of Guangdong Ocean University (060302022007).
The authors declare that the research was conducted in the absence of any commercial or financial relationships that could be construed as a potential conflict of interest.
This study received funding from Foundation of Tongwei Co., Ltd. The funder was not involved in the study design, collection, analysis, interpretation of data, the writing of this article or the decision to submit it for publication.
All claims expressed in this article are solely those of the authors and do not necessarily represent those of their affiliated organizations, or those of the publisher, the editors and the reviewers. Any product that may be evaluated in this article, or claim that may be made by its manufacturer, is not guaranteed or endorsed by the publisher.
The authors thank the Department of Aquaculture, College of Animal Science and Technology, Yunnan Agricultural University for providing the experimental sites and breeding tools.
The Supplementary Material for this article can be found online at: https://www.frontiersin.org/articles/10.3389/fmars.2022.872577/full#supplementary-material
Adorian T. J., Goulart F. R., Mombach P. I., Naglezi D. M. L., Dalcin M., Mabel M, et al. (2016). Effect of Different Dietary Fiber Concentrates on the Metabolism and Indirect Immune Response in Silver Catfish. Anim. Feed. Sci. Technol. 215, 124–132. doi: 10.1016/j.anifeedsci.2016.03.001
Altan O., Korkut A. (2011). Appearent Digestibility of Plant Protein-Based Diets by European Sea Bass Dicentrarchus Labrax L. 1758. Turkish. J. Fish. Aquat. Sci. 11, 87–92. doi: 10.4194/trjfas.2011.0112
Amamou S., Lazreg H., Hafsa J., Majdoub H., Achour L. (2020). Effect of Extraction Condition on the Antioxidant, Antiglycation and α-Amylase Inhibitory Activities of Opuntia Macrorhiza Fruit Peels Polysaccharides. Lwt-. Food Sci. Technol. 127, 109411. doi: 10.1016/j.lwt.2020.109411
Amirkolaie A. K., Leenhouwers J. I., Verreth J. A. J., Schrama J. W. (2005). Type of Dietary Fibre (Soluble Versus Insoluble) Influences Digestion, Faeces Characteristics and Faecal Waste Production in Nile Tilapia (Oreochromis Niloticus L.). Aquacul. Res. 36, 1157–1166. doi: 10.1111/j.1365-2109.2005.01330.x
AOAC (2005). Official Methods of Analysis of Aoac International, 18th Ed (Gaithersburg, Md, USA: Association of Official Analytical Chemists).
Azeredo R., Machado M., Guardiola F. A., Cerezuela R., Afonso A., Peres H., et al. (2017). Local Immune Response of Two Mucosal Surfaces of the European Seabass, Dicentrarchus Labrax, Fed Tryptophan- or Methionine-Supplemented Diets. Fish. Shellf. Immunol. 70, 76–86. doi: 10.1016/j.fsi.2017.09.016
Baldo L., Riera J., Tooming-Klunderud A., Albà M., Salzburger W. (2015). Gut Microbiota Dynamics During Dietary Shift in Eastern African Cichlid Fishes. PLoS One 10, e0127462. doi: 10.1371/journal.pone.0127462
Beneberu G. (2020). Metabarcoding Analyses of Gut Microbiota of Nile Tilapia (Oreochromis Niloticus) From Lake Awassa and Lake Chamo, Ethiopia. Microorganisms 8, 1040. doi: 10.3390/microorganisms8071040
Besten G., van Eunen K., Groen A., Venema K., Reijngoud D., Bakker B. (2013). The Role of Short-Chain Fatty Acids in the Interplay Between Diet, Gut Microbiota, and Host Energy Metabolism. J. Lipid Res. 54, 2325–2340. doi: 10.1194/jlr.R036012
Bi Y., Qin N., Yang R. (2015). Human Microbiota: A Neglected " Organ " in Precision Medicine. Infect. Dis. Trans. Med. 1, 1–10. doi: 10.11979/idtm.2015
Bloom S., Bijanki V., Nava G., Sun L., Malvin N., Donermeyer D., et al. (2011). Commensal Bacteroides Species Induce Colitis in Host-Genotype-Specific Fashion in a Mouse Model of Inflammatory Bowel Disease. Cell Host Microbe 9, 390–403. doi: 10.1016/j.chom.2011.04.009
Cai C., Ren S., Cui G., Ni Q., Cao X. (2019). Short-Term Stress Due to Dietary Pectin Induces Cholestasis, and Chronic Stress Induces Hepatic Steatosis and Fibrosis in Yellow Catfish, Pelteobagrus Fulvidraco. Aquaculture 516, 734607. doi: 10.1016/j.aquaculture.2019.734607
Chen H., Mao X. B., Che L., Yu B., He J., Yu J., et al. (2014). Impact of Fibre Types on Gut Microbiota, Gut Environment and Gut Function in Fattening Pigs. Anim. Feed. Sci. Technol. 195, 101–111. doi: 10.1016/j.anifeedsci.2014.06.002
Chen H., Mao X., He J., Yu B., Huang Z.. (2013). Dietary Fibre Affects Intestinal Mucosal Barrier Function and Regulates Intestinal Bacteria in Weaning Piglets. Br. J. Nutr. 110, 1–12. doi: 10.1017/S0007114513001293
Chen X., Wang Y., Shen M., Yu Q., Xie J. (2021). The Water-Soluble Non-Starch Polysaccharides From Natural Resources Against Excessive Oxidative Stress: A Potential Health-Promoting Effect and Its Mechanisms. Int. J. Biol. Macromol. 171, 320–330. doi: 10.1016/j.ijbiomac.2021.01.022
Choct M. (2015). Feed Non-Starch Polysaccharides for Monogastric Animals: Classification and Function. Anim. Product. Sci. 55, 1360–1366. doi: 10.1071/AN15276
Choct M., Hughes R. J., Wang J., Bedford M. R., Morgan A. J., Annison G. (1996). Increased Small Intestinal Fermentation Is Partly Responsible for the Anti-Nutritive Activity of Non-Starch Polysaccharides in Chickens. Br. Poult. Sci. 37, 609–621. doi: 10.1080/00071669608417891
Collins S. A., Mansfield G. S., Desai A. R., Van Kessel A. G., Hill J. E., Drew M.D. (2013). Structural Equation Modeling of Antinutrients in Rainbow Trout Diets and Their Impact on Feed Intake and Growth. Aquaculture 416–417, 219–227. doi: 10.1016/j.aquaculture.2013.09.020
Dalsgaard J., Bach Knudsen K. E., Verlhac V., Ekmann K. S., Pedersen P. B. (2016). Supplementing Enzymes to Extruded, Soybean-Based Diet Improves Breakdown of Non-Starch Polysaccharides in Rainbow Trout (Oncorhynchus Mykiss). Aquacul. Nutr. 22, 419–426. doi: 10.1111/anu.12258
Dan F., Xiao-di G., Yun-kai L. (2021). Research Progress and Application Prospect of Gut Microbiota of Marine Fish. Chin. J. Ecol. 40, 255–265. doi: 10.13292/j.1000-4890.202101.026
Degnan P. H., Taga M. E., Goodman A. L. (2014). Vitamin B 12 as a Modulator of Gut Microbial Ecology. Cell Metab. 20, 769–778. doi: 10.1016/j.cmet.2014.10.002
Deng J. M., Lin B. B., Zhang X. D., Guo L., Chen L. S., Li G.B., et al. (2020). Effects of Dietary Sodium Humate on Growth, Antioxidant Capacity, Non-Specific Immune Response, and Resistance to Aeromonas Hydrophila in Genetic Improvement of Farmed Tilapia (Gift, Oreochromis Niloticus). Aquaculture 520, 734788. doi: 10.1016/j.aquaculture.2019.734788
Deng J. M., Wang Y., Chen L. Q., Mai K. S., Wang Z., Zhang X. (2015). Effects of Replacing Plant Proteins With Rubber Seed Meal on Growth, Nutrient Utilization and Blood Biochemical Parameters of Tilapia (Oreochromis Niloticus × O.Aureus). Aquacul. Nutr. 23, 30–39. doi: 10.1111/anu.12355
Deng J., Wang K., Mai K., Chen L., Zhang L., Mi H., et al. (2017). Effects of Replacing Fish Meal With Rubber Seed Meal on Growth, Nutrient Utilization, and Cholesterol Metabolism of Tilapia (Oreochromis Niloticus × O. Aureus). Fish. Physiol. Biochem. 43, 941–954. doi: 10.1007/s10695-016-0313-4
Deng J., Zhang X., Sun Y., Mi H., Zhang L. (2021). Effects of Different Types of Non-Starch Polysaccharides on Growth, Digestive Enzyme Activity, Intestinal Barrier Function and Antioxidant Activity of Rainbow Trout (Oncorhynchus Mykiss). Aquacul. Rep. 21, 100864. doi: 10.1016/j.aqrep.2021.100864
Du P., Jun-cai H., Chang-bao S., Zhi-jing L. (2018). Impact of Dietary Components on Gut Microbiota Structure. Food Res. Dev. 39, 178–182. doi: 10.3969/j.issn.1005-6521.2018.09.034
Egerton S., Culloty S., Whooley J., Stanton C., Ross R. (2018). The Gut Microbiota of Marine Fish. Front. Microbiol. 9, 873. doi: 10.3389/fmicb.2018.00873
Enes P., Pérez-Jiménez A., Peres H., Couto A., Pous O-Ferreira P., Oliva-Teles A. (2012). Oxidative Status and Gut Morphology of White Sea Bream, Diplodus Sargus Fed Soluble Non-Starch Polysaccharide Supplemented Diets. Aquaculture 358–359, 79–84. doi: 10.1016/j.aquaculture.2012.06.020
Engberg R. M., Hammershøj M., Johansen N. F., Abousekken M. S., Steenfeldt S., Jensen B. B. (2009). Fermented Feed for Laying Hens: Effects on Egg Production, Egg Quality, Plumage Condition and Composition and Activity of the Intestinal Microflora. Br. Poult. Sci. 50, 228–239. doi: 10.1080/00071660902736722
FAO. (2014). The State of World Fisheries and Aquaculture: Opportunities and Challenges. State. World Fish. Aquacul. 78, 40–41. doi: 10.1016/0302-184X(78)90006-9
Gao Y., Li X., Dong Y., Zhu X., Li W., Wu X. (2018). Replacement of Fishmeal by Mixed Animal and Plant Protein Sources in Diets for Juvenile Mangrove Red Snapper (Lutjanus Argentimaculatus) (Forskal 1775). The Israeli. J. Aquaculture-. Bamidgeh. 1462, 8. doi: 10.46989/001c.20950
Gao Y., Storebakken T., Shearer K. D., Penn M., Øverland M. (2011). Supplementation of Fishmeal and Plant Protein-Based Diets for Rainbow Trout With a Mixture of Sodium Formate and Butyrate. Aquaculture 311, 233–240. doi: 10.1016/j.aquaculture.2010.11.048
Glencross B. (2009). The Influence of Soluble and Insoluble Lupin Non-Starch Polysaccharides on the Digestibility of Diets Fed to Rainbow Trout (Oncorhynchus Mykiss). Aquaculture 294, 256–261. doi: 10.1016/j.aquaculture.2009.06.010
Glencross B., Rutherford N., Bourne N. (2012). The Influence of Various Starch and Non-Starch Polysaccharides on the Digestibility of Diets Fed to Rainbow Trout (Oncorhynchus Mykiss). Aquaculture 356–357, 141–146. doi: 10.1016/j.aquaculture.2012.05.023
Goulart F. R., Silva L. P., Loureiro B. B., Adorian T. J., Mombach P. I., Petkowicz C. L. O. (2017). Effects of Dietary Fibre Concentrates on Growth Performance and Digestive Enzyme Activities of Jundiá (Rhamdia Quelen). Aquacul. Nutr. 23, 1–9. doi: 10.1111/anu.12400
Habte-Tsion H. M., Ge X., Liu B., Xie J., Ren M., Zhou Q., et al. (2015). A Deficiency or an Excess of Dietary Threonine Level Affects Weight Gain, Enzyme Activity, Immune Response and Immune-Related Gene Expression in Juvenile Blunt Snout Bream (Megalobrama Amblycephala). Fish. Shellf. Immunol. 42, 439–446. doi: 10.1016/j.fsi.2014.11.021
Haidar M. N., Petie M., Heinsbroek L. T. N., Verreth J. A. J., Schrama J. W. (2016). The Effect of Type of Carbohydrate (Starch vs. Nonstarch Polysaccharides) on Nutrients Digestibility, Energy Retention and Maintenance Requirements in Nile Tilapia. Aquaculture 463, 241–247. doi: 10.1016/j.aquaculture.2016.05.036
Hanim S. F., Azrina A., Khoo H. E., Amin I. (2015). Protective Effects of Pulp and Kernel Oils From Canarium Odontophyllum Fruit in Normal and Hypercholesterolemic Rabbits. Int. Food Res. J. 22, 1318–1326.
Hashish E., Merwad A., Elgaml S., Amer A., Kamal H., Fakhr A., et al. (2018). Mycobacterium Marinum Infection in Fish and Man: Epidemiology, Pathophysiology and Management; A Review. Veterinary. Q. 38, 1–34. doi: 10.1080/01652176.2018.1447171
He Y., Ye G., Chi S., Tan B., Dong X., Yang Q., et al. (20201502). Integrative Transcriptomic and Small Rna Sequencing Reveals Immune-Related Mirna–Mrna Regulation Network for Soybean Meal-Induced Enteritis in Hybrid Grouper, Epinephelus Fuscoguttatus♀ × Epinephelus Lanceolatus♂. Front. Immunol. 11, 1501. doi: 10.3389/fimmu.2020.01502
Hossain M. A., Focken U., Becker K. (2001). Galactomannan-Rich Endosperm of Sesbania (Sesbania Aculeata) Seeds Responsible for Retardation of Growth and Feed Utilisation in Common Carp, Cyprinus Carpio L. Aquaculture 203, 121–132. doi: 10.1016/S0044-8486(01)00617-2
Hossain M. A., Focken U., Becker K. (2003). Antinutritive Effects of Galactomannan-Rich Endosperm of Sesbania (Sesbania Aculeata) Seeds on Growth and Feed Utilization in Tilapia, Oreochromis Niloticus. Aquacul. Res. 34, 1171–1179. doi: 10.1046/j.1365-2109.2003.00924.x
Jiang M., Huang F., Wen H., Yang C., Wu F., Liu W., et al. (2014). Dietary Niacin Requirement of Gift Tilapia, Oreochromis Niloticus, Reared in Freshwater. J. World Aquacul. Soc. 45, 333–341. doi: 10.1111/jwas.12119
Kessel M., Dutilh B., Neveling K., Kwint M., Veltman J., Flik G., et al. (2011). Pyrosequencing of 16s Rrna Gene Amplicons to Study the Microbiota in the Gastrointestinal Tract of Carp (Cyprinus Carpio L.). Amb. Express. 1, 41. doi: 10.1186/2191-0855-1-41
Kraugerud O. F., Penn M., Storebakken T., Refstie S. L., Krogdahl S., Svihus B. (2007). Nutrient Digestibilities and Gut Function in Atlantic Salmon (Salmo Salar) Fed Diets With Cellulose or Non-Starch Polysaccharides From Soy. Aquaculture 273, 96–107. doi: 10.1016/j.aquaculture.2007.09.013
Król E., Douglas A., Tocher D. R., Crampton V. O., Speakman J. R., Secombes C.J., et al. (2016). Differential Responses of the Gut Transcriptome to Plant Protein Diets in Farmed Atlantic Salmon. BMC Genomics 17, 56–72. doi: 10.1186/s12864-016-2473-0
Kumar V., Saurabh, Sahu, Pal A. K. (2005). β-Glucan, a Feed Additive to Manage Aquatic Animal Health. Aqua. Feed.: Formulat. Beyond. 3, 9–11.
Kuz Mina V. V. (1996). Influence of Age on Digestive Enzyme Activity in Some Freshwater Teleosts. Aquaculture 148, 25–37. doi: 10.1016/S0044-8486(96)01370-1
Larsen F. M., Moughan P. J., Wilson M. N. (1993). Dietary Fiber Viscosity and Endogenous Protein Excretion at the Terminal Ileum of Growing Rats. J. Nutr. 123, 1898–1904. doi: 10.1093/jn/123.11.1898
Leenhouwers J. I., Ortega R. C., Verreth J. A. J., Schrama J. W. (2007). Digesta Characteristics in Relation to Nutrient Digestibility and Mineral Absorption in Nile Tilapia (Oreochromis Niloticus L.) Fed Cereal Grains of Increasing Viscosity. Aquaculture 273, 556–565. doi: 10.1016/j.aquaculture.2007.10.044
Lee K. J., Powell M. S., Barrows F. T., Smiley S., Bechtel P., Hardy R. W. (2010). Evaluation of Supplemental Fish Bone Meal Made From Alaska Seafood Processing Byproducts and Dicalcium Phosphate in Plant Protein-Based Diets for Rainbow Trout (Oncorhynchus Mykiss). Aquaculture 302, 248–255. doi: 10.1016/j.aquaculture.2010.02.034
Lekva A., Hansen A., Rosenlund G., Karlsen Ø., Hemre G. (2010). Energy Dilution With α-Cellulose in Diets for Atlantic Cod (Gadus Morhua L.) Juveniles — Effects on Growth, Feed Intake, Liver Size and Digestibility of Nutrients. Aquaculture 300, 169–175. doi: 10.1016/j.aquaculture.2010.01.001
Li E., Chen L., Cai Y., Gu S., Hong M., Zhang L. (2006). Effects of Soy Protein Concentrate as a Dietary Protein Source on Digestive Enzyme Activities of Eriocheir Sinensis. J. Guangdong. Ocean. Univ. 26, 14–21. doi: 10.3969/j.issn.1673-9159.2006.04.004
Lin M., Zeng C. X., Jia X. Q., Zhai S. W., Li Z. Q., et al. (2019). The Composition and Structure of the Intestinal Microflora of Anguilla Marmorata at Different Growth Rates: A Deep Sequencing Study. J. Appl. Microbiol. 126,14174–14210. doi: 10.1111/jam.14174
Liu H., Zeng X., Huang J., Yuan X., Wang Q., Ma L. (2021). Dietary Fiber Extracted From Pomelo Fruitlets Promotes Intestinal Functions, Both In Vitro and In Vivo. Carbohydr. Polymer. 252, 117186. doi: 10.1016/j.carbpol.2020.117186
Li E., Zhao J., Liu L., Zhongchao L., Shuai Z. (2017). Effects of Insoluble Raw Fibre Concentrate Addition on Weanling Piglets and Growing Pigs. Chin. J. Anim. Sci. 53, 80–85. doi: 10.19556/j.0258-7033.2017-09-080
Ma J., Adler L., Srzednicki G., Arcot J. (2017). Quantitative Determination of Non-Starch Polysaccharides in Foods Using Gas Chromatography With Flame Ionization Detection. Food Chem. 220, 100–107. doi: 10.1016/j.foodchem.2016.09.206
Maas R. M., Verdegem M., Wiegertjes G. F., Schrama J. W. (2020). Carbohydrate Utilisation by Tilapia: A Meta-Analytical Approach. Rev. Aquacul., 12413, 1–16. doi: 10.1111/raq.12413
Macfarlane G. T., Macfarlane S. (2002). Diet and Metabolism of the Intestinal Flora. Biosci. Microflora. 21, 199–208. doi: 10.12938/bifidus1996.21.199
Mansfield G. S., Desai A. R., Nilson S. A., Van Kessel A. G., Drew M. D., Hill J. E. (2010). Characterization of Rainbow Trout (Oncorhynchus Mykiss) Intestinal Microbiota and Inflammatory Marker Gene Expression in a Recirculating Aquaculture System. Aquaculture 307, 95–104. doi: 10.1016/j.aquaculture.2010.07.014
Medard G., Ouattara N’Golo, Yacouba B., Mamadou O., Allassane O., Kouakou Y. (2018). Substitution of the Fish Meal by the Earthworm and Maggot Meal in the Feed of Nile Tilapia Oreochromis Niloticus Reared in Freshwater. Int. J. Fish. Aquacul. 10, 77–85. doi: 10.5897/IJFA2018.0682
Merrifield D. L., Dimitroglou A., Bradley G., Baker R. T. M., Davies S. J. (2009). Soybean Meal Alters Autochthonous Microbial Populations, Microvilli Morphology and Compromises Intestinal Enterocyte Integrity of Rainbow Trout, Oncorhynchus Mykiss (Walbaum). J. Fish. Dis. 32, 755–766. doi: 10.1111/j.1365-2761.2009
Niba A. T., Beal J. D., Kudi A. C., Brooks P. H. (2009). Potential of Bacterial Fermentation as a Biosafe Method of Improving Feeds for Pigs and Poultry. Afr. J. Biotechnol. 8, 1758–1767. doi: 10.1186/1471-2105-10-130
Ni J., Yu Y., Zhang T., Gao L. (2012). Comparison of Intestinal Bacterial Communities in Grass Carp, Ctenopharyngodon Idellus, From Two Different Habitats. Chin. J. Oceanol. Limnol. 30,757–765. doi: 10.1007/s00343-012-1287-4
Novotny L., Dvorska L., Lorencova A., Beran V., Pavlik I. (2004). Fish: A Potential Source of Bacterial Pathogens for Human Beings. Veterinarni. Med. 49, 343–358. doi: 10.17221/5715-VETMED
Pasquier B., Armand M., Castelain C., Guillon F., Borel P., Lafont H., et al. (1996). Emulsification and Lipolysis of Triacylglycerols Are Altered by Viscous Soluble Dietary Fibres in Acidic Gastric Medium In Vitro. J. Nutr. Biochem. 7, 293–302. doi: 10.1042/bj3140269
Prindiville T. P., Sheikh R. A., Cohen S. H., Tang Y. J., Cantrell M. C., Silva J. J. (2000). Bacteroides Fragilis Enterotoxin Gene Sequences in Patients With Inflammatory Bowel Disease. Emerg. Infect. Dis. 6, 171–174. doi: 10.3201/eid0602.000210
Ramírez C., Coronado J., Silva A., Romero J. (2018). Cetobacterium Is a Major Component of the Microbiome of Giant Amazonian Fish (Arapaima Gigas) in Ecuador. Animals 8, 189–195. doi: 10.3390/ani8110189
Refstie S., Korsøen Ø.J., Storebakken T., Baeverfjord G., Lein I., Roem A. J. (2000). Differing Nutritional Responses to Dietary Soybean Meal in Rainbow Trout (Oncorhynchus Mykiss) and Atlantic Salmon (Salmo Salar). Aquaculture 190, 49–63. doi: 10.1016/S0044-8486(00)00382-3
Ren S., Cai C., Cui G., Ni Q., Jiang R., Su X., et al. (2020). High Dosages of Pectin and Cellulose Cause Different Degrees of Damage to the Livers and Intestines of Pelteobagrus Fulvidraco. Aquaculture 514, 734445. doi: 10.1016/j.aquaculture.2019.734445
Sales J. (2009). The Effect of Fish Meal Replacement by Soyabean Products on Fish Growth: A Meta-Analysis. Br. J. Nutr. 102, 1709–1722. doi: 10.1017/S0007114509991279
Shanmugham B., Pan A. (2013). Identification and Characterization of Potential Therapeutic Candidates in Emerging Human Pathogen Mycobacterium Abscessus: A Novel Hierarchical in Silico Approach. PLoS One 8, e59126. doi: 10.1371/journal.pone.0059126
Shi C., Jiang Y., Zhong Y., Chen Y., Luo L., Lin S. (2019). Effects of Dietary Fiber Sources on Growth, Plasma Biochemical Indexes, Intestinal Antioxidant Capacity and Histology of Largemouth Bass (Micropterus Salmoides). J. Fish. China 43, 2485–2493. doi: 10.11964/jfc.20190511814
Sinha A. K., Kumar V., Makkar H. P. S., De Boeck G., Becker K. (2011). Non-Starch Polysaccharides and Their Role in Fish Nutrition – A Review. Food Chem. 127, 1409–1426. doi: 10.1016/j.foodchem.2011.02.042
Staessen T. W. O., Verdegem M. C. J., Weththasinghe P., Schrama J. W. (2020). The Effect of Dietary Non-Starch Polysaccharide Level and Bile Acid Supplementation on Fat Digestibility and the Bile Acid Balance in Rainbow Trout (Oncorhynchus Mykiss). Aquaculture 523, 735174. doi: 10.1016/j.aquaculture.2020.735174
Suchecka D., Harasym J. P., Wilczak J., Gajewska M., Oczkowski M., Gudej S., et al. (2015). Antioxidative and Anti-Inflammatory Effects of High Beta-Glucan Concentration Purified Aqueous Extract From Oat in Experimental Model of Lps-Induced Chronic Enteritis. J. Funct. Food. 14, 244–254. doi: 10.1016/j.jff.2014.12.019
Sugita H., Miyajima C., Deguchi Y. (1991). The Vitamin B12-Producing Ability of the Intestinal Microflora of Freshwater Fish. Aquaculture 92, 267–276. doi: 10.1016/0044-8486(91)90028-6
Tacon A. G. J., Hasan M. R., Allan G., El-Sayed A. F. M., Jackson A., Kaushik S.J., et al. (2010). Aquaculture Feeds: Addressing the Long-Term Sustainability of the Sector. Farming Waters People Food. 193–231.
Talaveron J. L., Badía-Tahull M. B., Lozano-Andreu T., Rigo-Bonin R., Leiva-Badosa E. (2019). Phytosterolemia and Gamma Glutamyltransferase in Adults With Parenteral Nutrition: Fish Versus Vegetal Lipids, Randomized Clinical Trial. Nutrition 70, 1–19. doi: 10.1016/j.nut.2019.110587
Watanabe T., Pongmaneerat J., Sato S., Takeuchi T. (1994). Replacement of Fish Meal by Alternative Protein Sources in Rainbow Trout Diets. Nipp. Suisan. Gakkaishi. 59, 1573–1579. doi: 10.2331/suisan.59.1573
Wilson R. P. (1994). Utilization of Dietary Carbohydrate by Fish. Aquaculture 124, 67–80. doi: 10.1016/0044-8486(94)90363-8
Wu T. (2015). Effect of Different Soluble Pectin and Xylan on Growth, Digestive Physiology and Intestinal Flora of Chinese Mitten Crab, Eriocheir Sinensis (Suzhou, China: Soochow University).
Wu T., Zhu J., Li T., Cai C., Ye Y., Wu P., et al. (2015). Effect of Pectin and Xylan With Different Solubility on Growth and Feed Utilization of Chinese Mitten Crab, Eriocheir Sinensis. J. Shanghai. Ocean. Univ. 24, 862–868.
Yang L., Zeng X., Qiao S. (2020). Research Advances on Non-Starch Polysaccharide in the Regulation of Intestinal Microflora in Pigs. Biotechnol. Bull. 36, 9–16. doi: 10.13560/j.cnki.biotech.bull.1985.2019-1224
Yannis K., Emmanouil K., Vassiliki I., John H., Nikos P., Kantham P., et al. (2018). Effects of High-Level Fishmeal Replacement by Plant Proteins Supplemented With Different Levels of Lysine on Growth Performance and Incidence of Systemic Noninfectious Granulomatosis in Meagre (Argyrosomus Regius). Aquacul. Nutr. 24, 1738–1751. doi: 10.1111/anu.12814
Yun X., Maixin L., Gang C., Jianmeng C., Fengying G., Miao W., et al. (2018). Effects of Dietary Lactobacillus Rhamnosus Jcm1136 and Lactococcus Lactis Subsp. Lactis Jcm5805 on the Growth, Intestinal Microbiota, Morphology, Immune Response and Disease Resistance of Juvenile Nile Tilapia, Oreochromis Niloticus. Fish. Shellf. Immunol. 76, 368–379. doi: 10.1016/j.fsi.2018.03.020
Zhang Z. (2014). The Effects of Pectin and Xylan on Growth, Digestion and Intestinal Flora in Chinese Mitten Crab (Eriocheir Sinensis) and Yellow Catfish (Pelteobagrus Fulvidraco) (Soochow University), Suzhou, China.
Zhang Y., Chen P., Liang X. F., Han J., Wu X. F., Yang Y.H., et al. (2019). Metabolic Disorder Induces Fatty Liver in Japanese Seabass, Lateolabrax Japonicas Fed a Full Plant Protein Diet and Regulated by Camp-Jnk/Nf-Kb-Caspase Signal Pathway. Fish. Shellf. Immunol. 90, 223–234. doi: 10.1016/j.fsi.2019.04.060
Zhang M., Pan L., Fan D., He J., Su C., Gao S., et al. (2021). Study of Fermented Feed by Mixed Strains and Their Effects on the Survival, Growth, Digestive Enzyme Activity and Intestinal Flora of Penaeus Vannamei. Aquaculture 530, 735703. doi: 10.1016/j.aquaculture.2020.735703
Zhang H., Pu X., Yang Q., Tan B., Dong X. H., Chi S.Y., et al. (2018). Effects of Replacing Fish Meal With High-Protein Cottonseed Meal on Growth Performance, Non-Specific Immune Index and Disease Resistance for Litopenaeus Vannamei. J. Guangdong. Ocean. Univ. 038, 20–26. doi: 10.3969/j.issn.1673-9159.2018.04.003
Zheng Y., Wu W., Hu G., Qiu L., Shunlong M., Chao S., et al. (2018). Gut Microbiota Analysis of Juvenile Genetically Improved Farmed Tilapia (Oreochromis Niloticus) by Dietary Supplementation of Different Resveratrol Concentrations. Fish. Shellf. Immunol. 77, 200–207. doi: 10.1016/j.fsi.2018.03.040
Zhou Q., Mai K., Liu Y., Tan B. (2005). Advances in Animal and Plant Protein Sources in Place of Fish Meal. J. Fish. China 29, 404–410. doi: 10.3321/j.issn:1000-0615.2005.03.021
Zokaeifar H., Balcázar J. L., Saad C. R., Kamarudin M. S., Sijam K., Arshad A., et al. (2012). Effects of Bacillus Subtilis on the Growth Performance, Digestive Enzymes, Immune Gene Expression and Disease Resistance of White Shrimp, Litopenaeus Vannamei. Fish. Shellf. Immunol. 33, 683–689. doi: 10.1016/j.fsi.2012.05.027
Keywords: non-starch polysaccharides, GIFT tilapia, antioxidant capacity, growth performance, intestinal flora
Citation: Liu Y, Deng J, Tan B, Xie S and Zhang W (2022) Effects of Soluble and Insoluble Non-Starch Polysaccharides on Growth Performance, Digestive Enzyme Activity, Antioxidant Capacity, and Intestinal Flora of Juvenile Genetic of Improvement of Farmed Tilapia (Oreochromis niloticus). Front. Mar. Sci. 9:872577. doi: 10.3389/fmars.2022.872577
Received: 09 February 2022; Accepted: 19 April 2022;
Published: 18 May 2022.
Edited by:
Johan Verreth, Wageningen University and Research, NetherlandsReviewed by:
Chunxiao Zhang, Jimei University, ChinaCopyright © 2022 Liu, Deng, Tan, Xie and Zhang. This is an open-access article distributed under the terms of the Creative Commons Attribution License (CC BY). The use, distribution or reproduction in other forums is permitted, provided the original author(s) and the copyright owner(s) are credited and that the original publication in this journal is cited, in accordance with accepted academic practice. No use, distribution or reproduction is permitted which does not comply with these terms.
*Correspondence: Junming Deng, ZGp1bm1pbmdAMTYzLmNvbQ==; Beiping Tan, YnB0YW5AMTI2LmNvbQ==
Disclaimer: All claims expressed in this article are solely those of the authors and do not necessarily represent those of their affiliated organizations, or those of the publisher, the editors and the reviewers. Any product that may be evaluated in this article or claim that may be made by its manufacturer is not guaranteed or endorsed by the publisher.
Research integrity at Frontiers
Learn more about the work of our research integrity team to safeguard the quality of each article we publish.