- 1Simon F.S. Li Marine Science Laboratory, School of Life Sciences, The Chinese University of Hong Kong, Hong Kong, Hong Kong SAR, China
- 2Key Laboratory of the Ministry of Education for Coastal and Wetland Ecosystems, College of the Environment and Ecology, Xiamen University, Xiamen, China
Sesarmid crabs play an important role in mangrove biogeochemical processes due to their leaf-eating and burrowing activities. How leaf-eating mangrove crabs meet their nitrogen (N) needs remains a puzzle, as N-poor leaf litter (<1% dry weight) cannot support the growth of most macrofauna. Several strategies for overcoming this challenge have been proposed, but the actual mechanisms remain unknown. We identified two categories of leaf-eating crabs according to their feeding habits (1): species active outside their burrows during low tide - we hypothesize that they meet their N needs through consuming the microphytobenthos (MPB) (hypothesis H1); and (2) species spending most of their time inside burrows - we hypothesize that they meet their N needs through N-fixation by associated microbes (H2). To test H1, we conducted a series of feeding experiments on the sesarmid crab Parasesarma affine (model species for category 1) with 13C- and 15N-enriched MPB and mangrove leaves. P. affine relied mainly on mangrove leaves as their C source and MPB as their N source, supporting H1. Two feeding experiments on Neosarmatium smithi (model species for category 2) showed that N limitation could be ameliorated by selecting seasonally available higher-quality food items such as floral parts, or by supplements from the associated nitrogen-fixing bacteria, as identified by genomic analysis. The strategy by which leaf-eating crabs meet their N needs may make significant contribution both to the growth of crabs and to their ecosystem functions of regulating the cycling of significant nutrient elements. The stoichiometric regulations by leaf-eating crabs to meet their N needs, such as food selection, can enhance trophic efficiency and nutrient transfer rate at the community level and ultimately increase nutrient turnover rate at the ecosystem level.
Introduction
Mangrove forests are amongst the most productive ecosystems on Earth (Bouillon et al., 2008), and they support diverse associated faunas (Nagelkerken et al., 2008; Lee et al., 2017). Sesarmid crabs are some of the most diverse and abundant macrofauna in mangroves throughout the Indo-west-Pacific, and their importance to mangrove trophic ecology is unequivocal [see Lee (2008) for a review]. They are regarded as keystone species or ecological engineers in tropical mangroves because their feeding and burrowing activities regulate ecosystem functioning, such as modifying biogeochemical processes of sediment, altering plant zonation, and enhancing primary productivity (Kristensen, 2008). Being one of the initial processors of mangrove leaf litter, the sesarmid crabs play an important role in nutrient cycling in mangroves.
Mangrove leaves, low in N (<1% N by dry weight) and high in carbon (C) with C/N ratios at 46~92 (Micheli, 1993), are also difficult to digest since they contain structural polymers such as cellulose and hemicellulose (Giddens et al., 1986). Secondary metabolites such as tannins may further disrupt digestion (Linton and Greenaway, 2007). Recent studies have shed light on how leaf-eating crabs may assimilate structural C (Bui and Lee, 2015a; Bui and Lee, 2015b) but how they meet their nitrogen (N) needs remains a puzzle. This is because nutrient-poor leaf litter cannot support the growth of most macrofauna, as the C/N ratio of mangrove leaf litter is far higher than the general maximum C/N value of 17 for sustainable animal nutrition (Russell-Hunter, 1970). The need of leaf-eating crabs to maintain body homeostasis while on a low-nutrient diet can affect trophic interactions and trophic efficiency they mediate (Welti et al., 2017), which will determine the rates of nutrient turnover and thus affect ecosystem functional processes such as primary production. Therefore, clarifying how leaf-eating crabs meet their N needs is important for assessing their role in mediating biogeochemical processes of essential nutrients (e.g., C and N), and how they help sustain the function and services of mangrove ecosystems.
(Linton and Greenaway 2007) described several strategies how semi-terrestrial herbivorous crabs may meet their N requirement on a N-poor diet, but some strategies (e.g., leaf-aging) are not supported by experimental studies and field observations (Skov and Hartnoll, 2002; Harada and Lee, 2016), while others lack direct evidence (e.g., selective consumption of higher quality food items). By selected consumption of higher quality foods such as floral parts the crabs might partly meet their nutrient requirement on a N-poor diet. However, this opportunity is limited due to their small foraging ranges (< 1 m), seasonal availability of food items, and intraspecific competition (Wilde et al., 2004; Linton and Greenaway, 2007). Digestive strategies and other adaptations for a low-nutrient diet may be present in leaf-eating crabs, such as increased food consumption, slow growth and extended longevity, larger body size and low frequency of reproduction (Linton and Greenaway, 2000; Nordhaus, 2004; Green, 2004a; Green, 2004b; Pinheiro et al., 2005). Parasesarma messa, a primary consumer of mangrove litter, exhibited slow growth and individuals took more than four years to reach maximum size (Robertson, 2021). A N mass balance analysis showed that the population of P. messa ingested twice the amount of N that could be supplied by leaf litter, suggesting that mangrove leaves are unlikely their only N source (Robertson, 2021).
Until recently, the prevailing hypothesis is that herbivorous crabs can partly meet their N requirement by occasional consumption of animal tissue, through predation or cannibalism (Thongtham et al., 2008; Nordhaus et al., 2011; Pereira et al., 2019). Additional N may be supplied by consuming the microphytobenthos (MPB), fungi, meiofauna and particular organic matter in surface sediment (Bouillon et al., 2002; Oakes et al., 2010; De Lima-Gomes et al., 2011; Tue et al., 2012; MacKenzie et al., 2020) or macroalgae on mangrove roots (Gao et al., 2018). (Kristensen et al. 2017) investigated the food partitioning (mangrove leaves, animal tissue, and MPB) of sesarmid and ucidid mangrove leaf-eating crabs and reassessed the role of MPB as a N source in their diet. As MPB has a much lower C/N ratio (15~25) than mangrove leaf litter, they are more easily assimilated by animals compared to mangrove leaf litter, which has a low N content of <1% but high concentration of secondary metabolites (Lee, 1993). MPB could be one of the most important nitrogen sources for mangrove crabs; however, direct evidence is still lacking to date. Some observations on chelal morphology also questions the trophic significance of MPB in the diet of sesarmid crabs (Lee, 2008). For example, the absence of spoon-tipped ‘fingers’ similar to those of typical deposit-feeding species (e.g. Uca) may reduce foraging efficiency on MPB from mangrove sediments.
Nitrogen fixation by symbionts as a supplement to host nutrition has long been hypothesized, but this contribution has not yet been demonstrated in leaf-eating crabs. Waterbury et al. (1983) isolated a cellulolytic N-fixing bacteria from the gland of Deshayes in six species of shipworms. Its presence in large numbers may account for the ability of shipworms to digest cellulose and meet their N needs. Active N fixation by the symbionts of the lucinid clam Loripes lucinalis were verified using both molecular and stable isotope techniques (Petersen et al., 2016). The N-fixation rates of symbiotic microbes in the hindguts of arthropods could reach 10-40 kg ha-1 y-1, which may contribute significantly both to growth of the hosts and ecosystem function (Nardi et al., 2002). A diverse microbial community was found on the carapace or in the gut of deposit-feeding ocypodid crabs (Cuellar-Gempeler and Leibold, 2018). Zilius et al. (2020) investigated the associated microbial biofilm on the carapace of fiddler crabs and suggested that active microbial N fixation on their carapace played an important role in N cycling.
A number of studies have examined the food composition of leaf-eating crabs using the natural abundance stable isotope analysis approach (Nordhaus et al., 2011; Kristensen et al., 2017; MacKenzie et al., 2020). This approach is strongly dependent on preliminary identification of the potential food choices, and the application of suitable trophic discrimination factors in mixing models, which are difficult to verify unless they are specifically tested by laboratory experiments. Apart from the trophic discrimination factor, a precondition for using the mixing model is that there are significant differences in stable isotope values (e.g., δ13C and δ15N) among the different food sources. Species-specific isotope fractionation values for accurately identification of important food resources may help (MacKenzie et al., 2020) but this approach effectively defeats the purpose of using the isotope tracers. Isotope labeling is a useful tool to complement natural abundance stable isotope analysis in food web studies (Michener and Kaufman, 2007).
This study aimed to evaluate the significance of different pathways through which the N requirement of leaf eating crabs may be met. Mangrove leaf-eating sesarmid crabs may be classified into two main categories according to their feeding habits: (1) species spending most of their time outside burrows during low tide - we hypothesize that these crabs meet their N needs through consuming the microphytobenthos (MPB) in surface sediment (H1); (2) species spending most of their time inside burrows - we hypothesize that they meet their N need through N-fixation by the associated microbes in their gut or habitat, such as the sediment (H2). These hypotheses were tested by a series of feeding experiments utilizing both natural-abundance and enriched stable isotope analyses.
Materials and Methods
Selection of Animal Models
We identified two categories of leaf-eating crabs according to their feeding habits: (1) species active outside their burrows during low tide; and (2) species spending most of their time inside burrows. For the first category, we used Parasesarma affine, a common sesarmid associated with mangrove forests in Hong Kong, as the animal model. This crab is distributed in the mid intertidal zone, spending most of their foraging time (45~83% of observation time) consuming mangrove leaves or scraping the surface sediment during the low tide (Kwok, 1999). For the second category, we used Neosarmatium smithi as the model species, which is a large species (maximum carapace width about 5 cm) specifically inhabiting the high-intertidal Bruguiera sexangula mangrove forests on tropical Hainan Island, China. Feeding activities of N. smithi in the field monitored using an infrared camera for 24 hours during the active season of the crabs (June 2016) within the site where the crabs were sampled in this study. It showed that they spent most of their time in the burrows (75~85% of observation time) even without any disturbance and only 15 to 25% of observation time searching and walking. Neither of the two species showed readiness to consume animal material when provided under captive conditions in this study.
Sample Collection
Individuals of P. affine of carapace width (CW) ranging from 11 to 20 mm were collected from the mangrove forest (Kandelia obovata) at Mai Po (22°29’38”N,114°01’56”E), Hong Kong. Individuals of N. smithi of CW ranging from 20 to 25 mm were collected from the Bruguiera sexangula forest at Dongzhai Bay (19°57’01”N,110°34’45”E), Hainan Island. Only male crabs were collected for experiment to reduce the effect on the crab populations as female crabs were often pregnant, and the female crabs may also have different physiology during egg production. All the crabs, fresh litter (yellow leaves), fallen calyx, and MPB (surface sediment) were collected from the same sites in the mangrove forests. Crabs for the feeding experiment were allocated to individual containers and starved for two days and “field” samples were rinsed with MilliQ water and sacrificed by freezing. Sediments were collected by scraping the top 1 cm surface layer, homogenized with a hand trowel before incubation with isotopically enriched media or used for MBP extraction.
MPB Extraction
MPB was extracted from sediment by density gradient centrifugation in colloidal silica (Bui and Lee, 2014). The sediment sample was washed through a series of decreasing mesh sizes: 2-mm, 500μm, 250μm, 45μm and 5μm. The material retained on the 5μm sieve was then transferred to 50ml falcon tubes. After settling at 4°C overnight, the clear supernatant was removed carefully to avoid disturbing the sediment at the bottom. 23ml of colloidal silica (LUDOX™) solution (1.340g ml-1 density) was then added to each tube containing the sample, mixed and centrifuged (4000 rpm at 4°C) for 10 mins. The entire top layer (MPB was in this layer) was isolated and washed with MilliQ water and then transferred into a tin capsule and dried for stable isotope analysis.
Preparation of 13C- and 15N-Enriched Leaves
Two enrichment solutions were prepared following Putz et al. (2011) with some modification: 13C-urea solution and 15N-urea solution by dissolving 100 mg 99-atom% 13C urea and 2 mg 98-atom% 15N urea in 50 mL MilliQ water, respectively. 12.5µl of wetting agent was added to each solution for good contact of the labeling solution with leaf surface. The two urea solutions were applied with a small paint brush on the upper and lower surfaces of the mangrove leaves on two Kandelia obovata trees in the field. Two trees separated by >100 m were selected for the two respective enrichments to avoid cross-contamination. Ten branches (each with 10 to 15 leaves) on each tree were selected for enrichment. Labelling was applied once a day over five consecutive days for 15N enrichment and 15 days for 13C enrichment.
Preparation of 15N-Enriched and 13C-Enriched MPB
Surface sediment from the study sites was incubated with F/2 medium (Guillard and Ryther, 1962; Guillard, 1975). The N source (NaNO3) in F/2 medium was replaced by 15NH4Cl (99-atom% 15N), incubated for 10 days with an irradiance at 150 to 200 μmol m-2 s-1 (same level as in the field), and ambient outdoor temperature at 25°C to 35°C. 13C-enriched MPB preparation followed the same method for 15N-enriched MPB, with the N source being NaNO3 and NaH13CO3 (99-atom% 13C, 2.5mM) was added to F/2 medium. MPB incubated with non labelled F/2 medium was used as a control (IMPB).
Stable Isotope Analysis
C and N content as well as stable isotope values (δ13C, δ15N and δ34S) of leaf, crab, and MPB samples were measured with a Thermo Analytical elemental analyzer, Flash EA 1112 Series coupled via a ConFlo IV interface to a Thermo Delta V Plus isotope ratio mass spectrometer. Stable isotope ratios are expressed as δ values (in per mil notation, ‰) relative to conventional standards (Vienna Pee Dee Belemnite for C and atmospheric N2 for N), according to:
where X = 13C, 15N or 34S, and R = 13C/12C, 15N/14N or 34S/32S. Measurement precision was better than 0.3‰ for δ13C, δ15N and δ34S.
Abundance of N-Fixing Bacteria
Sediment inside crab burrows in the field and faeces of crabs fed yellow leaves (for 30 days, 60 days and 210 days) were sampled for functional bacteria analysis. Using qPCR and amplicon sequencing technology, the special functional genes could be identified directly, and the universality and diversity of information for key functional genes was also provided. Here our target functional gene was the N-fixing gene nif H. According to the trait of the amplification area, based on the IonS5TMXL sequencing platform, a small fragment library was constructed for single-end sequencing. By cutting and filtering reads, clustering operational taxonomic units (OTUs), and annotating species and abundance analysis, the microbial community structure of the samples was assessed, and the difference among samples was further compared by alpha and beta diversity analyses.
N Mass Balance
N mass balance was assessed for the field population of P. affine to address the question of whether sole dependence on MPB N could sustain the crab’s need. The sesarmid crabs usually collect MPB at the surface sediment using their chelae, with a length of the dactyl and propodus at about 4 mm. However, they usually do not insert the whole dactyl and propodus into the sediment, so we assumed the depth of the sediment they could have access to MPB was 2 mm. MPB biomass (g m-2) in the top 2 mm surface sediment was estimated by the concentration of chlorophyll-a (by aqueous acetone extraction method, Johan et al., 2014), assuming a carbon to chlorophyll-a ratio (C: Chl-a) of 40 (De Jonge, 1980). The amount of MPB N available (g m-2) was determined according to their biomass and N content (%) obtained from elemental analysis.
The amount of N needed (g m-2 day-1) for P. affine growth was estimated according to their tissue N turnover rate (NTR):
where DW = total dry weight of crabs m-2 (g DW m-2); N% = N content of crab tissues; and D = turnover time (days) for tissue N, estimated to be 70 days for sesarmid crabs (Bui and Lee, 2014).
In the K. obovata mangrove forest at Mai Po, the mean population density of P. affine was 1.39 individuals m-2, with the mean carapace width (CW) and the mean dry weight at 18.3 mm and 1.5 g m-2, respectively (Lee and Kwok, 2002). The N content of the crab tissues was about 6.5% of dry mass estimated according to the equation %N = 7.536 – 0.059 CW established for the congener P. messa (Robertson, 2021). Therefore, assuming that no other significant N loss pathways exists, the amount of N needed for sustaining the biomass of P. affine at field density was taken to be their tissue N turnover rate (NTR). This demand was then compared with the amount of N available from MPB in the top 2 mm of the sediment.
Feeding Experiments
Five feeding experiments (E1 to E5) were designed to test the hypotheses H1 and H2. Experiments E1~E3 were aimed to test H1, experiments E4~E5 were to test H2, with details of each experiment summarized in Table 1. For E1~E4 and treatments T2 and T3 in E5, the crabs were kept in natural atmosphere. For the treatment T1 in E5 crabs were fed in an air-tight container (size: 70.5cm × 48 cm ×38.5 cm) with an atmosphere enriched with 15N2, and 1L 15N2 was added to the container at the beginning of experiment (δ15N of the atmosphere in the experimental container was about 3100‰) and was replenished with the same volume after each weekly sampling. All the crabs were sacrificed by freezing and the muscle tissue was taken from their claw for stable isotope analysis.
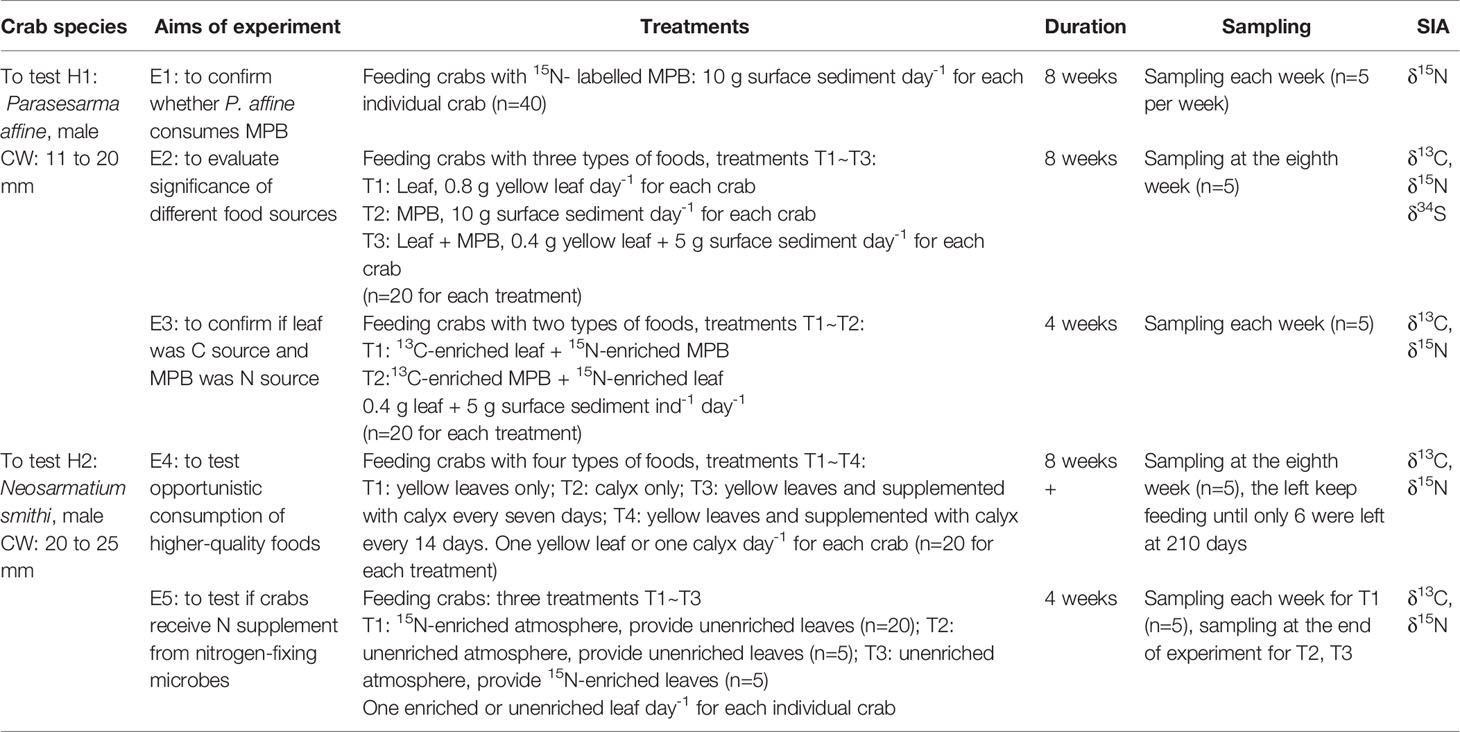
Table 1 Aims and details of the experiments to test hypotheses H1 and H2. CW, carapace width; SIA, stable isotope analysis.
Statistical Analyses
One-way ANOVA followed by post hoc Tukey’s test were used to test differences (α=0.05) in stable isotopic values among different groups or treatments. Nonparametric Kruskal-Wallis test followed by all pairwise multiple comparisons was conducted if the assumption of homogeneity of variance was violated. All data analyses were performed using SPSS 28.0.
Results
Experiment 1 (E1)
After incubation for ten days with F/2 medium (N: 15NH4Cl), the δ15N level of MPB reached 262.2 ± 51.3 ‰ (mean ± SD). Tissue δ15N value of crabs fed 15N-enriched MPB increased significantly with experiment time, from 12.6 ± 0.1‰ (mean ± SD) at the beginning to 183.1 ± 89.2‰ (mean ± SD) at the end of experiment, which was almost approaching the enrichment level of the MPB (Figure 1).
Experiment 2 (E2)
Crabs on a sole diet (leaf or MPB) showed a higher mortality than crabs fed a mixture of MPB and leaves (Figure 2). δ15N values separated crabs from food sources, while δ34S values were able to separate crabs on different food types. The δ34S value of the crabs fed both MPB and yellow leaves (M+YL) was intermediate between those fed MPB (M) and yellow leaves (YL) (Figure 3A), so the contribution of each of the two foods was approximately 50%. For δ13C there was significant difference between crabs and foods, but no significant difference among crabs fed different foods (Figure 3B).
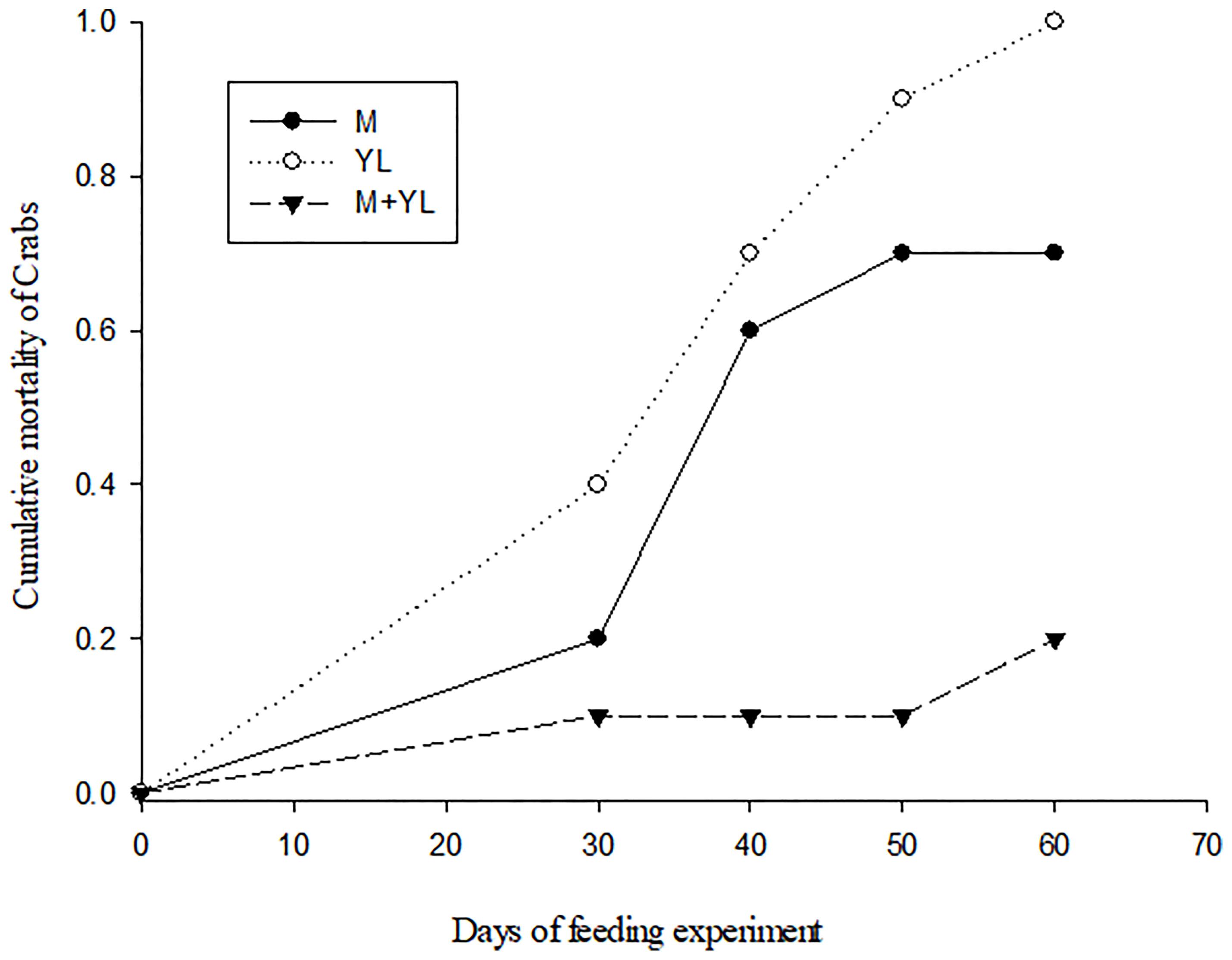
Figure 2 Cumulative mortality (proportion of individuals dead) of P. affine plotted against days of feeding experiment on different food sources. M, Microphytobenthos (MPB) only; L, Yellow leaf of Kandelia obovata only; M+L, MPB and yellow leaf of K. obovata.
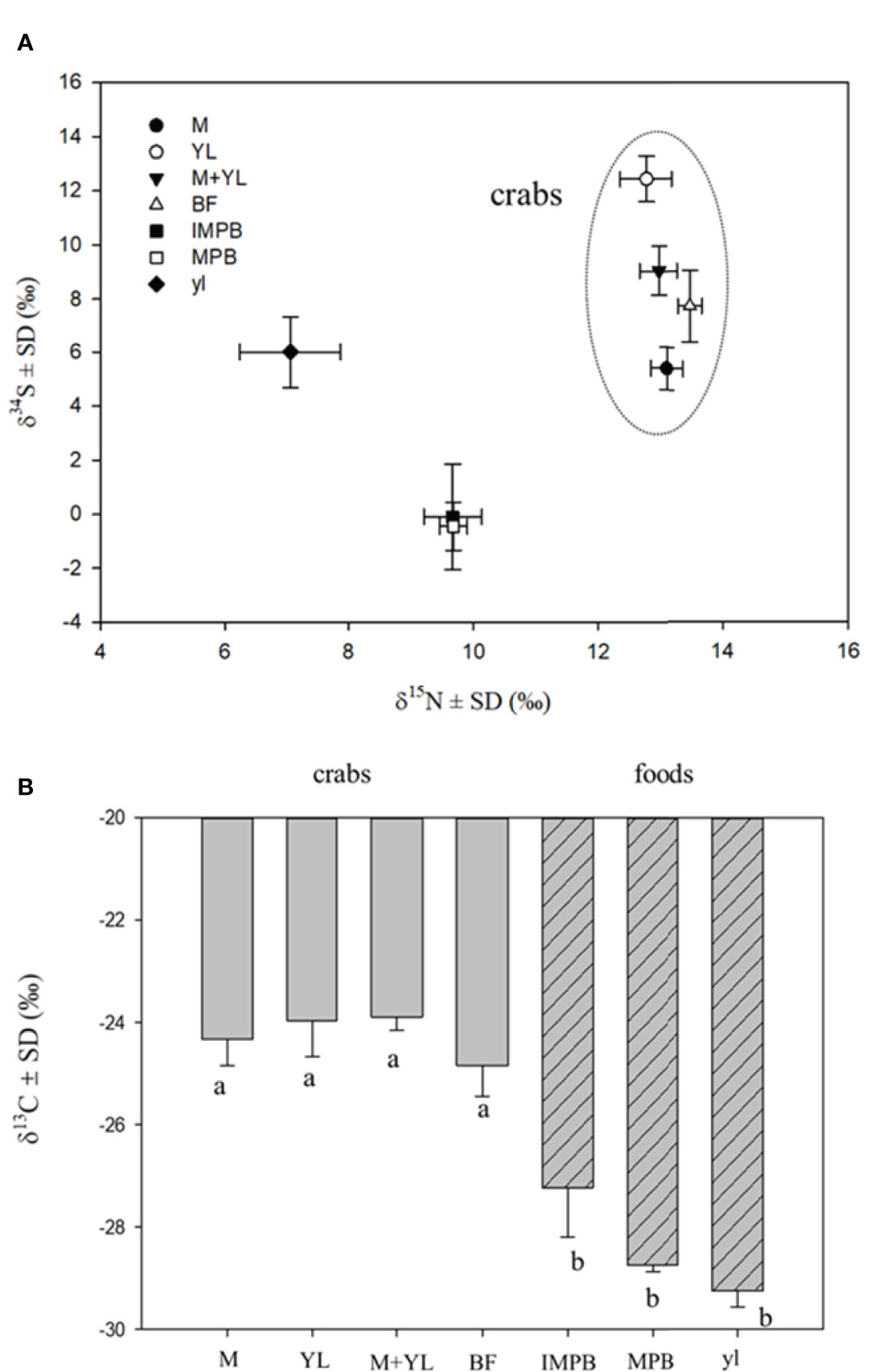
Figure 3 Dual stable isotope plots (A) of mean δ15N and δ34S values and mean δ13C (B) of crabs fed different food types and their food sources, different lowercase letters denote significant differences (p<0.05). M, crabs fed MPB; YL, crabs fed yellow leaves; M+YL, crabs fed MPB and yellow leaves; BF, before feeding; MPB, microphytobenthos; IMPB, incubated MPB with non labelled F/2 medium; yl, yellow leaves.
Experiment 3 (E3)
For crabs fed 13C-enriched leaf + 15N-enriched MPB (treatment 1), the tissue δ13C values increased with leaf δ13C, whereas the δ15N values increased with MPB δ15N from week 1 to week 4 (W1 to W4, respectively). There were significant differences between the start date (δ13C= -24.9 ± 06 ‰, δ15N = 13.5 ± 0.2 ‰), and end date values (δ13C = -17.2 ± 2.7 ‰, δ15N = 174 ± 77.1 ‰) for both δ13C and δ15N (p<0.05). For crabs fed 13C-enriched MPB + 15N-enriched leaf (treatment 2), the δ13C and δ15N of crab tissue did not show any significant change (Figure 4).
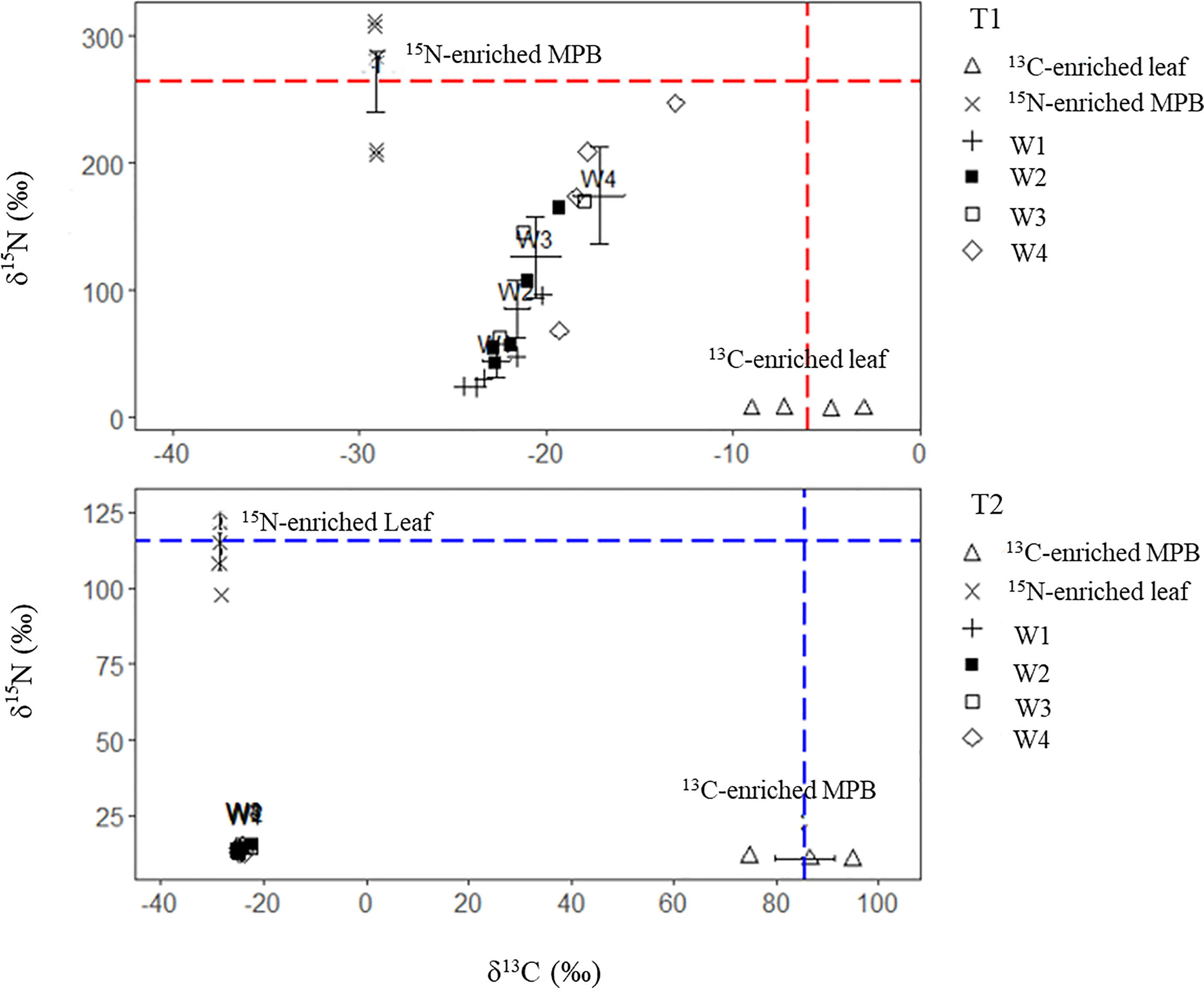
Figure 4 Variations in δ13C and δ15N values (both individual and mean ± SD values) of muscle tissues of crabs fed 13C- and 15N-enriched foods in two different treatments (T1, 13C-enriched leaf + 15N-enriched MPB; T2, 13C-enriched MPB + 15N-enriched leaf) over the four-week experiment (W1-W4). The dash lines indicate the enrichment level of the respective food sources.
N Mass Balance
Conversion from the chlorophyll a content in the top 2 mm of surface sediment (9.42 mg m-2) using a 40x conversion factor (De Jonge, 1980) suggests that the C biomass of MPB was 377 mg C m-2. As the C/N of MPB is ~15, the amount of MPB N in the top 2 mm of the surface sediment was 25.1 mg N m-2. Based on the NTR formula, the amount of N needed for sustaining the biomass of P. affine at field density was 1.5 g m-2 × 6.5%/70 days = 1.4 mg N m-2 day-1. The N requirement for crab sustenance is therefore 5.58% of the total MPB N standing crop in the surface sediment.
Experiment 4 (E4)
There is a significant difference in δ13C and δ15N values as well as their C and N contents (p<0.05) between the calyx and yellow leaves of B. sexangula. The calyx was more depleted in 15N than the yellow leaf, but less depleted in 13C (Figure 5). The calyx has a higher N content (0.83 ± 0.13%) and lower C content (C/N = 48.3) than the yellow leaf (0.44 ± 0.08%) (C/N= 99.5). After feeding for 60 days, the group that was supplied with only yellow leaves showed a significant increase in δ15N compared with those before feeding (p<0.05), but no change in δ13C value. The group supplied with only calyx showed no significant change in both their δ13C and δ15N values. The group fed with yellow leaves and supplemented with calyx (YL+C7) showed a significant change in both δ13C and δ15N values (p<0.05): lower δ15N but higher δ13C values, with the change in the YL+C7 group being much larger than that of the YL+C14 group (Figure 5).
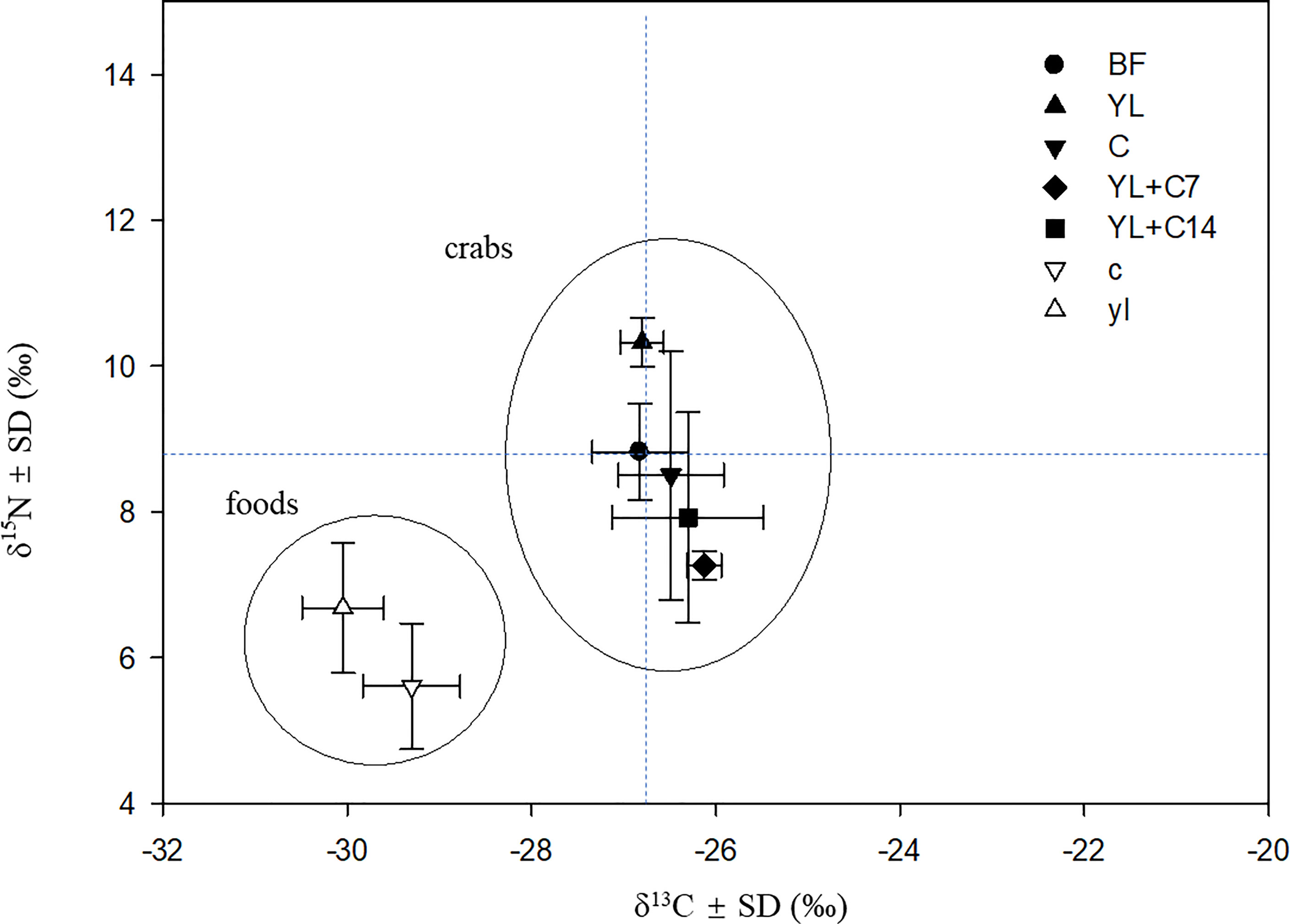
Figure 5 δ13C and δ15N plot of food sources and N. smithi before and after feeding for 60 days on different food items. BF, before feeding experiment (just after collection from the field); YL, fed yellow leaves; C, fed calyx; YL+C7, fed yellow leaves and supplemented with calyx every 7 days; YL+C14, fed yellow leaves and supplemented with calyx every 14 days; yl, yellow leaves; c, calyx.
After feeding on yellow leaves only for 60 days, the δ15N value of N. smithi showed a significant increase of 1.51‰ compared with that before feeding (p<0.001). However, 210 days later, the δ15N of N. smithi decreased by approximately 2‰. The δ13C of N. smithi showed no significant change with feeding time (Figure 6).
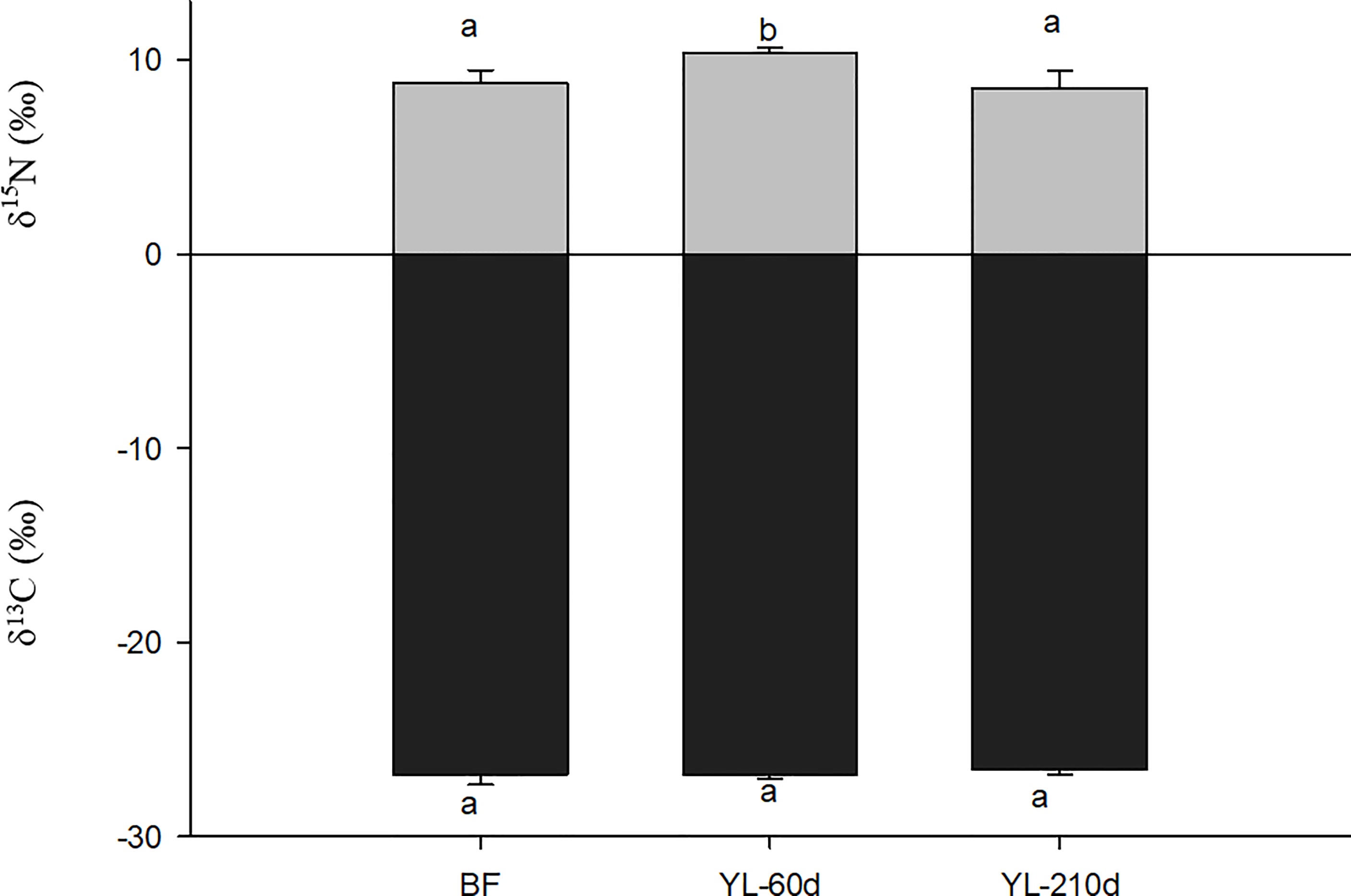
Figure 6 Variation of δ13C and δ15N (mean ± SD) of N. smithi after 60 days and 210 days in experiment 4. BF, before feeding; YL-60D (210D), fed yellow leaves for 60 (210) days. Different letters denote significant differences (p<0.001).
Experiment 5 (E5)
The crabs started to become enriched from 6.3 ± 1.4 ‰ (mean ± SD) in the field to 25.6 ± 16.1‰ (mean ± SD) after three weeks in a 15N-enriched atmosphere. At the fourth week, their tissue δ15N had enriched significantly (p<0.05) to 67.0 ± 33.4‰ (mean ± SD) compared with the start date values (Figure 7A). The crabs fed normal mangroves in an unenriched atmosphere showed no significant change in their δ15N after four weeks (Figure 7B). The crabs fed enriched mangrove leaves (δ15N (mean ± SD) = 3395 ± 2464 ‰) in an unenriched atmosphere also got enriched significantly (p<0.05) compared with the start date values after feeding for four weeks, and their δ15N enriched from 6.3 ± 1.4 ‰ (mean ± SD) before feeding to 166.7 ± 71.4 ‰ (mean ± SD) at the end of the experiment (Figure 7C), δ15N of crabs fed only unenriched yellow leaves changed to 7.4 ± 1.1 ‰ (mean ± SD) after four weeks.
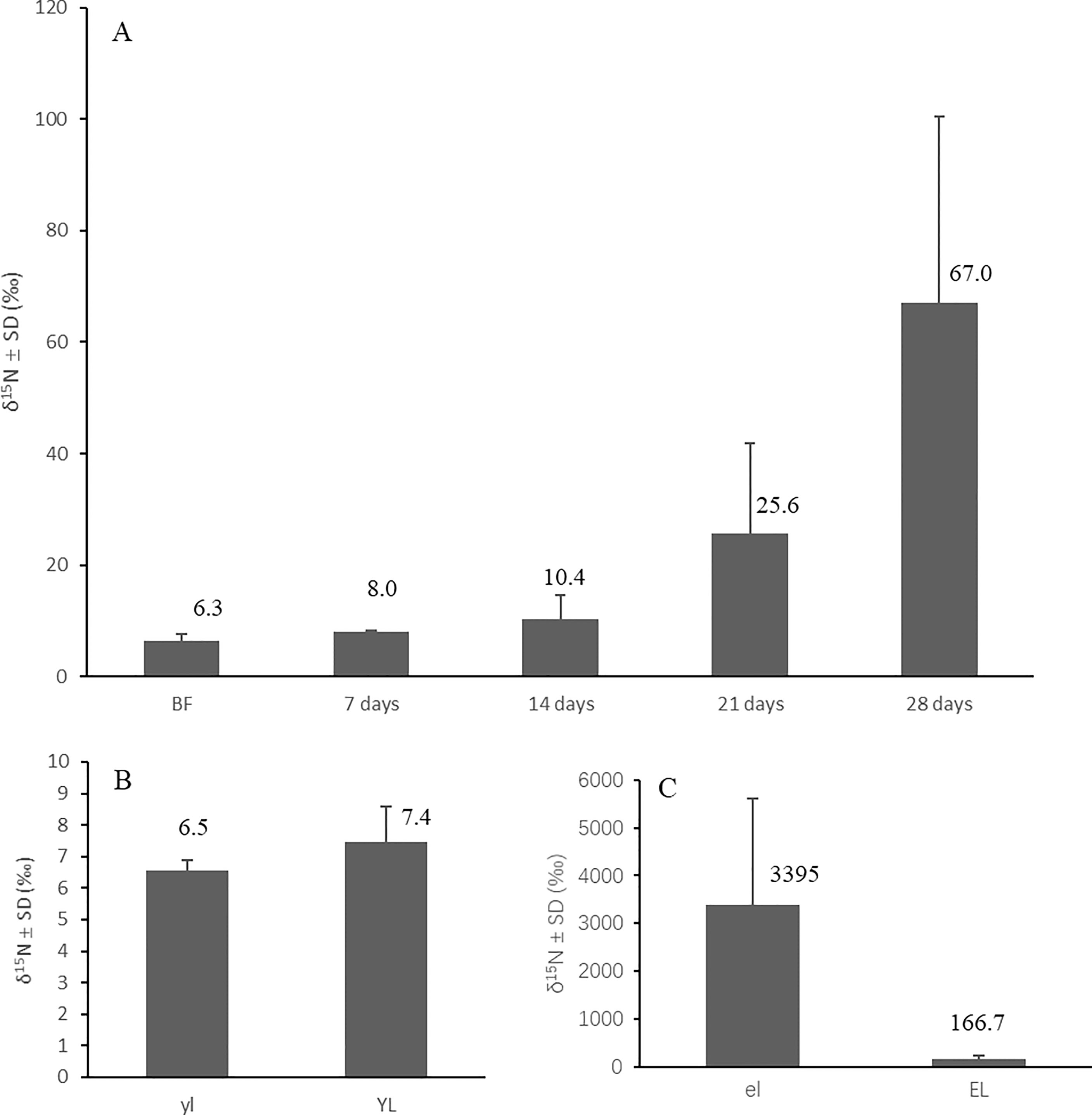
Figure 7 Variation of tissue δ15N of N. smithi with different treatments and experiment days, feeding on unenriched leaves in a 15N-enriched atmosphere (A), feeding in an unenriched atmosphere on unenriched leaves for 28 days (B) and 15N-enriched leaves for 28 days (C), respectively. BF, before feeding; el, 15N-enriched leaves; EL, crabs feeding on 15N-enriched leaves; yl, unenriched yellow leaves; YL, crabs feeding on unenriched yellow leaves.
Abundance of N-Fixing Bacteria in Burrow Sediment
Genomic analysis of functional bacteria groups showed a high relative abundance of nitrogen-fixing bacteria in the living environment (sediment from crab burrows) (~20%) of N. smithi, also their fecal material (~10 to 15%) (Figure 8). And also a high relative abundance in the fecal material of the crabs fed only mangrove leaves in experiment 4.
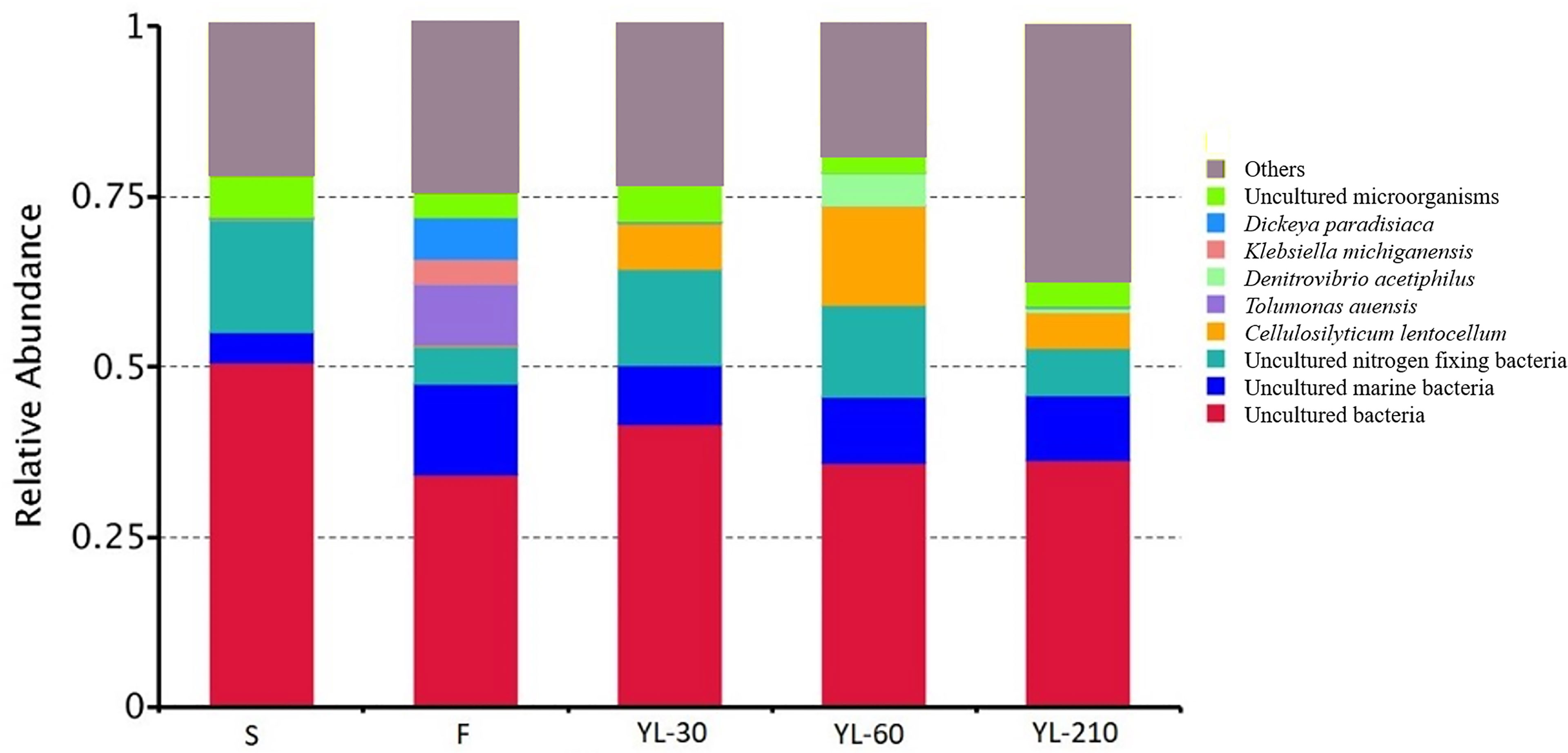
Figure 8 Relative abundance of different bacterial assemblages as indicated by gene sequencing. S, sediment from burrows; F, faeces of crab before feeding with specific diet; YL-30, YL-60, YL-210, feces of crab after feeding on yellow leaves for 30, 60, and 210 days, respectively.
Discussion
N Source of Parasesarma affine
In our study, the higher mortality of crabs on a sole diet (MPB or mangrove leaves) than on a mixed diet (both MPB and mangrove leaves, Experiment 2) suggests that P. affine achieved better physiological performance on a mixture of available food items. The δ34S value of the crab tissues suggested that the contributions of the two food sources were each ~50%. Results of our enrichment experiment (Experiment 1) indicate that P. affine consumed MPB as a N source, which is the first study to provide direct evidence of MPB dependence using 15N-tracer. The results of experiment 3 in a differential labelling design using dual isotope tracers (13C-MPB + 15N-leaf and 13C-leaf + 15N-MPB) also suggest that P. affine relied on mangrove leaves as their C source and MPB as their N source. The N mass balance calculations indicate that sole dependence on MPB N is possible to sustain the crab’s need. As the N requirement for crab sustenance at field density and biomass is only 5.58% of the total MPB standing crop, it is possible for MPB to recover to original concentration quickly enough to sustain future extraction. In the field, P. affine spends 45%~ 83% of their time scraping material from the surface sediment (Kwok, 1999). All these findings suggest that consumption of MPB could be one way P. affine meets its N needs. However, there are still questions concerning the efficacy of this approach. In order to obtain this amount of N from MPB consumption, each crab needs to consume or process ~110 ml day-1 of surface sediment, which is quite impossible to ingest as it is >100 times the stomach volume, unless they can separate the MPB from sediment effectively before ingestion. The mechanism by which the crab may extract MPB effectively from the sediment to make MPB a feasible food source is worth further investigation.
Several studies have highlighted the prominent trophic roles of MPB in coastal ecosystems (Miller et al., 1996; O'Meara et al., 2017; Pinckney, 2018; Hope et al., 2020). Even in the high Antarctic ice sheets with little light availability (<0.1%), MPB account for a significant proportion of the marine primary production (Dayton et al., 1986; Lohrer et al., 2013). Earlier data from stable isotope and other tracers suggest an important contribution of MPB to mangrove macrobenthos as a carbon source (Alongi, 2009; Oakes et al., 2010). However, only recently did Kristensen et al. (2017) first suggest that MPB was an important N source using the IsoConc mixing model on isotopic data to assess the food partitioning of mangrove leaf-eating crabs. These authors also suggested that mangrove litter was the dominant carbon source (contribution ratio >50%) for all the leaf-eating crabs.
Previous studies, however, have noted that several mangrove crab species spent most of their feeding time scraping material from the mud surface, e.g. Parasesarma messa (Robertson, 1986), P. erythodactyla (Camilleri, 1992), P. bidens and P. affine (Kwok and Lee, 1995), P. guttatum (Skov and Hartnoll, 2002), and Neosarmatium meinerti (Dahdouh-Guebas et al., 1997). These crabs occur in different intertidal zones and are associated with different mangrove communities. These crabs may rely on MPB as their N source to different extents, responding to local conditions such as forest light penetration and sediment nutrient levels that influence MPB abundance. The trophic role of MPB as the principal N source for leaf-eating sesarmid crabs in general needs to be further assessed in the future using a wider range of species covering different geographic and biogeographic locations.
Nitrogen Source of Neosarmatium smithi
Study of foraging behavior using remotely operated infrared cameras showed that this species spent the majority of time inside their burrow. In our observation, N. smithi did not feed on surface sediment. Our data agree with previous studies that the congener N. trispinosum actively collects and promptly feeds on mangrove leaf litter freshly available on the forest floor, and spends 97.5% of time remaining inside its burrow (Harada and Lee, 2016). This behaviour also excluded the possibility that they meet their N needs through consuming MPB. The stable isotope values also showed that the crabs assimilated N from the calyx food. Therefore, problems associated with low-nutrient vascular plant diets may be ameliorated by selecting higher-quality food items such as calyx, when they become available, which may be only for restricted periods. For example, calyx of B. sexangula are only available during the blossom season, which only lasts for less than 1 month (personal observation).
The δ15N value of the muscle tissue of N. smithi increased significantly after feeding on yellow leaves for 60 days. Previous studies showed that animals feeding on a diet with a low nitrogen content might recycle their internal N to sustain their metabolism and excrete the isotopically lighter 14N, which leads to an increase in 15N in their tissues (McCutchan et al., 2003; Herbon and Nordhaus, 2013). 15N enrichment in Daphnia magna also occurs when individuals suffered from starvation (Adams and Sterner, 2000).
Our results indicate that N deficiency occurred on N. smithi after feeding only on leaves after 60 days. After continued feeding on leaves only for 210 days, the δ15N value decreased significantly, suggesting that the N supply may be sourced from a lighter N source, which could be atmospheric N2 (δ15N =0). Genomic analysis of functional bacteria groups showed a high relative abundance of nitrogen-fixing bacteria in their living environment (sediment from crab burrows), also the crab faecal material. The results of the crab feeding experiment on a sole diet of mangrove leaves in 15N-enriched air (experiment 5) showed that crab tissue became enriched from the third week of experiment, suggesting that N fixed from the atmosphere may be assimilated by the crabs through ingesting the bacteria directly or the organic matter they produced. However, the actual mechanism and processes need to be clarified in the future. Recently, nitrogen fixation was also found in the intestine of two sesarmid crabs (N. smithi and Episesarma versicolor) and their habitat sediments (by determining the nitrogenase activity using acetylene reduction activity) but no evidence for the contribution of the fixed N to the crabs’ tissues was provided (Tongununui et al., 2021). Crabs feeding on 15N-enriched mangroves leaves also became enriched in 15N after feeding for four weeks. All these findings suggest that N. smithi can get their N from both mangrove leaves and N-fixation by the associated microbes, and they can also meet their N need by selecting high-quality food sources when available.
Ecological Role of Sesarmid Crabs in Mangrove N Dynamics
The ecological role of crabs in mangrove ecosystems has been addressed in many studies (Lee, 1998; Welsh, 2003; Werry and Lee, 2005; Kristensen, 2008; Laverock et al., 2011; Gilbertson et al., 2012). The trophic significance of leaf-eating crabs is two-fold: (1) removal of leaves from the forest floor and therefore reduction in tidal export to the estuary, leading to N retention; and (2) processing of leaf litter to fine sized particles, which can more readily be mineralized by microbes and utilized by detritivores (Werry and Lee, 2005). Leaf-eating crabs digesting/assimilating mangrove detritus also play an important role in acting as a trophic intermediate between hard-to-digest detritus and higher-level consumers in mangrove ecosystems, as they are preyed upon by higher consumers such as fish (Sheaves and Molony, 2000; Lee, 2008; Kawaida et al., 2021; Robertson, 2021).
Previous studies have demonstrated that animals can play integral roles in the storage and remineralization of elements, thus they also play an important role in the biogeochemical cycles of both terrestrial and aquatic ecosystems (Augustine and McNaughton, 2006; Vanni et al., 2006; Vaughn, 2010; Coetsee et al., 2011). Strategies by which some species meet their nutrient requirement on low-nutrient diets may mediate a keystone role in nutrient cycling and overall ecosystem stoichiometry (Small et al., 2011). Like other organisms, leaf-eating crabs often face stoichiometrically imbalanced diets as mangrove leaves are high in C and low in N. They can meet their nutrient demands by both pre-ingestion (e.g. food selection) and post-ingestion regulation (e.g. egestion or excretion). These stoichiometric regulations influence the amount of nutrients retained or released by the individual organismal-level processes, such as food choice or the selective release of nutrients, ultimately driving higher ecosystem-level dynamics, such as ecosystem elemental cycling (Schade et al., 2011; Sperfeld et al., 2016). The strategy by which sesarmid crabs meet their N needs is important, as it can affect the growth rate and population size of these primary nutrient processors at community levels, ultimately affect trophic efficiency and nutrient fluxes in ecosystem levels.
The strategy by which leaf-eating crabs meet their N needs represents a significant contribution both to the growth of crabs and to their ecosystem functions of processing biologically significant elements such as carbon and nitrogen. We hypothesize that the consequence of this consumer-driven nutrient dynamic (CND) is more rapid nutrient turnover and higher primary productivity in ecosystems with large populations of leaf-eating crabs. CND also has been identified as an important function contributing to ecosystem services (Cardinale et al., 2012; Doughty et al., 2016; Atkinson et al., 2017). This role of mangrove leaf-eating crabs in regulating essential nutrients cycling has important implications for community structure and ecosystem functioning (Allgeier et al., 2013). Further work on the effects of leaf-eating crabs on ecosystem-level nutrient dynamics will help develop a comprehensive and mechanistic understanding of the functional role of sesarmid crabs, and how their diversity and abundance may influence mangrove ecosystem characteristics such as stability and resilience.
Conclusion
Different leaf-eating crab species may adopt different strategies to meet their N needs depending on their feeding habit and also food availability. For species spending most of their time outside burrows during the low tide, consuming the N-rich microphytobenthos (MPB) in surface sediment may be an important way to balance their nitrogen budget. For species spending most of their time inside burrows, N-fixation by the associated microbes or consumption of N-fixing cyanobacteria may help to meet their N requirement. Problems associated with a regular diet of low-grade vascular plant diets may also be ameliorated by selecting seasonally available higher-quality food items such as floral parts. The strategy by which leaf-eating mangrove crabs meet their N needs represent a significant contribution both to the growth of crabs and to their ecosystem functions of processing carbon and nitrogen in this dynamic habitat.
Data Availability Statement
The raw data supporting the conclusions of this article will be made available by the authors, without undue reservation.
Author Contributions
Both authors conceived the ideas, designed the experiments, wrote the manuscript and gave final approval for publication.
Funding
Part of this work was funded by a grant from the National Natural Science Foundation of China (No. 31600436) and a General Research Funds grant from the Research Grants Council Hong Kong (14302420). XG is supported by a PhD scholarship from The Chinese University of Hong Kong.
Conflict of Interest
The authors declare that the research was conducted in the absence of any commercial or financial relationships that could be construed as a potential conflict of interest.
Publisher’s Note
All claims expressed in this article are solely those of the authors and do not necessarily represent those of their affiliated organizations, or those of the publisher, the editors and the reviewers. Any product that may be evaluated in this article, or claim that may be made by its manufacturer, is not guaranteed or endorsed by the publisher.
Acknowledgments
We would like to thank Leo Chiu-Leung and Yan Ping Loo for help with field sampling, and Dr Fen Guo for helping with data analysis.
References
Adams T. S., Sterner R. W. (2000). The Effect of Dietary Nitrogen Content on Trophic Level 15n Enrichment. Limnol. Oceanogr. 45, 601–607. doi: 10.4319/lo.2000.45.3.0601
Allgeier J. E., Yeager L. A., Layman C. A. (2013). Consumers Regulate Nutrient Limitation Regimes and Primary Production in Seagrass Ecosystems. Ecology 94, 521–529. doi: 10.1890/12-1122.1
Atkinson C. L., Capps K. A., Rugenski A. T., Vanni M. J. (2017). Consumer-Driven Nutrient Dynamics in Freshwater Ecosystems: From Individuals to Ecosystems. Biol. Rev. 92, 2003–2023. doi: 10.1111/brv.12318
Augustine D. J., McNaughton S. J. (2006). Interactive Effects of Ungulate Herbivores, Soil Fertility, and Variable Rainfall on Ecosystem Processes in a Semi-Arid Savanna. Ecosystems 9, 1242–1256. doi: 10.1007/s10021-005-0020-y
Bouillon S., Borges A. V., Castañeda-Moya E., Diele K., Dittmar T., Duke N. C., et al. (2008). Mangrove Production and Carbon Sinks: A Revision of Global Budget Estimates. Global Biogeochem. Cycle 22. doi: 10.1029/2007GB003052
Bouillon S., Koedam N., Raman A., Dehairs F. (2002). Primary Producers Sustaining Macro-Invertebrate Communities in Intertidal Mangrove Forests. Oecologia 130, 441–448. doi: 10.1007/s004420100814
Bui T. H. H., Lee S. Y. (2014). Does ‘You Are What You Eat’Apply to Mangrove Grapsid Crabs? PloS One 9 (2), e89074. doi: 10.1371/journal.pone.0089074
Bui H. T. H., Lee S. Y. (2015a). Endogenous Cellulase Production in the Leaf Litter Foraging Mangrove Crab, Parasesarma Erythodactyla. Comp. Biochem. Physiol. B. 179, 27–36. doi: 10.1016/j.cbpb.2014.09.004
Bui H. T. H., Lee S. Y. (2015b). Potential Contributions of Gut Microbiota to the Nutrition of the Detritivorous Sesarmid Crab Parasesarma Erythodactyla. Mar. Biol. 162, 1969–1981. doi: 10.1007/s00227-015-2723-8
Camilleri J. C. (1992). Leaf-Litter Processing by Invertebrates in a Mangrove Forest in Queensland. Mar. Biol. 114, 139–145. doi: 10.1007/BF00350863
Cardinale B. J., Duffy J. E., Gonzalez A., Hooper D. U., Perrings C., Venail P., et al. (2012). Biodiversity Loss and Its Impact on Humanity. Nature 486, 59–67. doi: 10.1038/nature11148
Coetsee C., Stock W. D., Craine J. M. (2011). Do Grazers Alter Nitrogen Dynamics on Grazing Lawns in a South African Savannah? Afr. J. Ecol. 49, 62–69. doi: 10.1111/j.1365-2028.2010.01236.x
Cuellar-Gempeler C., Leibold M. A. (2018). Multiple Colonist Pools Shape Fiddler Crab-Associated Bacterial Communities. ISME. J. 12, 825–837. doi: 10.1038/s41396-017-0014-8
Dahdouh-Guebas F., Verneirt M., Tack J. F., Koedam N. (1997). Food Preferences of Neosarmatium Meinerti De Man (Decapoda: Sesarminae) and Its Possible Effect on the Regeneration of Mangroves. Hydrobiologia 347, 83–89. doi: 10.1023/A:1003015201186
Dayton P. K., Watson D., Palmisano A., Barry J. P., Oliver J. S., Rivera D. (1986). Distribution Patterns of Benthic Microalgal Standing Stock at McMurdo Sound, Antarctica. Polar. Biol. 6, 207–213. doi: 10.1007/BF00443397
De Jonge V. N. (1980). Fluctuations in the Organic Carbon to Chlorophyll a Ratios for Estuarine Benthic Diatom Populations. Mar. Ecol. Prog. Ser. 2, 345–353. doi: 10.3354/meps002345
De Lima-Gomes R. C., Cobo V. J., Fransozo A. (2011). Feeding Behaviour and Ecosystem Role of the Red Mangrove Crab Goniopsis Cruentata (Latreille 1803) (Decapoda, Grapsoidea) in a Subtropical Estuary on the Brazilian Coast. Crustaceana. 84, 735–747. doi: 10.1163/001121611X579141
Doughty C. E., Roman J., Faurby S., Wolf A., Haque A., Bakker E. S., et al. (2016). Global Nutrient Transport in a World of Giants. Proc. Natl. Acad. Sci. 113, 868–873. doi: 10.1073/pnas.1502549112
Gao X., Wang M., Wu H., Wang W., Tu Z. (2018). Effects of Spartina Alterniflora Invasion on the Diet of Mangrove Crabs (Parasesarma Plicata) in the Zhangjiang Estuary, China. J. Coast. Res. 34, 106–113. doi: 10.2112/JCOASTRES-D-17-00002.1
Giddens R. L., Lucas J. S., Neilson M. J., Richards G. N. (1986). Feeding Ecology of the Mangrove Crab Neosarmatium Smithi (Crustacea: Decapoda: Sesarmidae). Mar. Ecol. Prog. Ser. 33, 147–155. doi: 10.3354/meps033147
Gilbertson W. W., Solan M., Prosser J. I. (2012). Differential Effects of Microorganism-Invertebrate Interactions on Benthic Nitrogen Cycling. FEMS Microbiol. Ecol. 82, 11–22. doi: 10.1111/j.1574-6941.2012.01400.x
Green P. T. (2004a). Burrow Dynamics of the Red Land Crab Gecarcoidea Natalis (Brachyura, Gecarcinidae) in Rain Forest on Christmas Island (Indian Ocean). J. Crust. Biol. 24, 340–349. doi: 10.1651/C-2447
Green P. T. (2004b). Field Observations of Moulting and Moult Increment in the Red Land Crab, Gecarcoidea Natalis (Brachyura, Gecarcinidae), on Christmas Island (Indian Ocean). Crustaceana 77, 125–128. doi: 10.1163/156854004323037937
Guillard R. R. (1975). “Culture of Phytoplankton for Feeding Marine Invertebrates,” in Culture of Marine Invertebrate Animals. Eds. Smith W. L., Chanley M. H. (New York, USA: Plenum Press), pp 26–pp 60.
Guillard R. R., Ryther J. H. (1962). Studies of Marine Planktonic Diatoms: I. Cyclotella Nana Hustedt, and Detonula Confervacea (Cleve). Gran. Can. Microbiol. 8, 229–239. doi: 10.1139/m62-029
Harada Y., Lee S. Y. (2016). Foraging Behavior of the Mangrove Sesarmid Crab Neosarmatium Trispinosum Enhances Food Intake and Nutrient Retention in a Low-Quality Food Environment. Estuar. Coast. Shelf. Sci. 174, 41–48. doi: 10.1016/j.ecss.2016.03.017
Herbon C. M., Nordhaus I. (2013). Experimental Determination of Stable Carbon and Nitrogen Isotope Fractionation Between Mangrove Leaves and Crabs. Mar. Ecol. Prog. Ser. 490, 91–105. doi: 10.3354/meps10421
Hope J. A., Paterson D. M., Thrush S. F. (2020). The Role of Microphytobenthos in Soft-Sediment Ecological Networks and Their Contribution to the Delivery of Multiple Ecosystem Services. J. Ecol. 108, 815–830. doi: 10.1111/1365-2745.13322
Johan F., Jafri M. Z., Lim H. S., Maznah W. W. (2014). “Laboratory Measurement: Chlorophyll-A Concentration Measurement With Acetone Method Using Spectrophotometer,” in In 2014 IEEE International Conference on Industrial Engineering and Engineering Management.(Selangor: IEEE) 744–748. doi: 10.1109/IEEM.2014.7058737
Kawaida S., Nanjo K., Ohtsuchi N., Kohno H., Sano M. (2021). Crabs Assimilating Cellulose Materials Drive the Detritus Food Chain in a Mangrove Estuary. Food Web. 26, e00180. doi: 10.1016/j.fooweb.2020.e00180
Kristensen E. (2008). Mangrove Crabs as Ecosystem Engineers: With Emphasis on Sediment Processes. J. Sea. Res. 59, 30–43. doi: 10.1016/j.seares.2007.05.004
Kristensen E., Lee S. Y., Mangion P., Quintana C. O., Valdemarsen T. (2017). Trophic Discrimination of Stable Isotopes and Potential Food Source Partitioning by Leaf-Eating Crabs in Mangrove Environments. Limnol. Oceanogr. 62, 2097–2112. doi: 10.1002/lno.10553
Kwok P. W. (1999). “Time Activity Budget of Perisesarma Bidens and Parasesarma Affinis (Brachyura: Sesarminae) at the Mai Po Marshes Mangrove, Hong Kong,” in The Mangrove Ecosystem of Deep Bay and the Mai Po Marshes, Hong Kong. Ed. Lee S. Y. (Hong Kong: Hong Kong University Press), 137–151.
Kwok P. W., Lee S. Y. (1995). The Growth Performances of Two Mangrove Crabs, Chiromanthes Bidens and Parasesarma Plicata Under Different Leaf Litter Diets. Hydrobiologia 295, 141–148. doi: 10.1007/BF00029121
Laverock B., Gilbert J. A., Tait K., Osborn A. M., Widdicombe S. (2011). Bioturbation: Impact on the Marine Nitrogen Cycle. Biochem. Soc Trans. 39, 315–320. doi: 10.1042/BST0390315
Lee S. Y. (1993). “Leaf Choice of the Sesarmid Crabs Chiromanthes Bidens and C. Plicata in a Hong Kong Mangal,” in Proceedings of the International Conference on Marine Biology of Hong Kong and the South China Sea, University of Hong Kong, October 1990, 597– 604 (Hong Kong: Hong Kong University Press).
Lee S. Y. (1998). Ecological Role of Grapsid Crabs in Mangrove Ecosystems: A Review. Mar. Freshwater. Res. 49, 335–343. doi: 10.1071/MF97179
Lee S. Y. (2008). Mangrove Macrobenthos: Assemblages, Services, and Linkages. J. Sea. Res. 59, 16–29. doi: 10.1016/j.seares.2007.05.002
Lee S. Y., Jones G., Diele K., Castellanos-Galindo G. A., Nordhaus I., Lee S. Y., et al. (2017). “Chapter 3 Biodiversity,” in Mangrove Ecosystem Structure and Function: A Biogeographic Perspective. Ed. Rivera-Monroy V. (New York: Springer), Pp. 55–Pp. 86.
Lee S. Y., Kwok P. W. (2002). The Importance of Mangrove Species Association to the Population Biology of the Sesarmine Crabs Parasesarma Affinis and Perisesarma Bidens. Wetl. Ecol. Manage. 10, 215–226. doi: 10.1023/A:1020175729972
Linton S. M., Greenaway P. (2000). The Nitrogen Requirements and Dietary Nitrogen Utilization for the Gecarcinid Land Crab Gecarcoidea Natalis. Physiol. Biochem. Zool. 73, 209–218. doi: 10.1086/316735
Linton S. M., Greenaway P. (2007). A Review of Feeding and Nutrition of Herbivorous Land Crabs: Adaptations to Low Quality Plant Diets. J. Comp. Physiol. B. 177, 269–286. doi: 10.1007/s00360-006-0138-z
Lohrer A. M., Cummings V. J., Thrush S. F. (2013). Altered Sea Ice Thickness and Permanence Affects Benthic Ecosystem Functioning in Coastal Antarctica. Ecosystems 16, 224–236. doi: 10.1007/s10021-012-9610-7
MacKenzie R. A., Cormier N., Demopoulos A. W. (2020). Estimating the Value of Mangrove Leaf Litter in Sesarmid Crab Diets: The Importance of Fractionation Factors. Bull. Mar. Sci. 96, 501–520. doi: 10.5343/bms.2019.0026
McCutchan J. H. Jr., Lewis W. M. Jr., Kendall C., McGrath C. C. (2003). Variation in Trophic Shift for Stable Isotope Ratios of Carbon, Nitrogen, and Sulfur. Oikos 102, 378–390. doi: 10.1034/j.1600-0706.2003.12098.x
Micheli F. (1993). Feeding Ecology of Mangrove Crabs in North Eastern Australia: Mangrove Litter Consumption by Sesarma Messa and Sesarma Smithii. J. Exp. Mar. Biol. Ecol. 171, 165–186. doi: 10.1016/0022-0981(93)90002-6
Michener R. H., Kaufman L. (2007). “Stable Isotope Ratios as Tracers in Marine Food Webs: An Update,” in Stable Isotopes in Ecology and Environmental Science. Eds. Michener R., Lajtha K. (UK: Blackwell, Oxford), pp.238–pp.282.
Miller D. C., Geider R. J., MacIntyre H. L. (1996). Microphytobenthos: The Ecological Role of the “Secret Garden” of Unvegetated, Shallow-Water Marine Habitats. II. Role in Sediment Stability and Shallow-Water Food Webs. Estuar. Coast. 19, 202–212. doi: 10.2307/1352225
Nagelkerken I., Blaber S. J. M., Bouillon S., Green P., Haywood M., Kirton L. G., et al. (2008). The Habitat Function of Mangroves for Terrestrial and Marine Fauna: A Review. Aquat. Bot. 89, 155–185. doi: 10.1016/j.aquabot.2007.12.007
Nardi J. B., Mackie R. I., Dawson J. O. (2002). Could Microbial Symbionts of Arthropod Guts Contribute Significantly to Nitrogen Fixation in Terrestrial Ecosystems? J. Insect Physiol. 48, 751–763. doi: 10.1016/S0022-1910(02)00105-1
Nordhaus I. (2004). Feeding Ecology of the Semi-Terrestrial Crab Ucides Cordatus (Decapoda: Brachyura) in a Mangrove Forest in Northern Brazil (Bremen: University of Bremen). PhD Thesis.
Nordhaus I., Salewski T., Jennerjahn T. C. (2011). Food Preferences of Mangrove Crabs Related to Leaf Nitrogen Compounds in the Segara Anakan Lagoon, Java, Indonesia. J. Sea. Res. 65, 414–426. doi: 10.1016/j.seares.2011.03.006
O'Meara T. A., Hillman J. R., Thrush S. F. (2017). Rising Tides, Cumulative Impacts and Cascading Changes to Estuarine Ecosystem Functions. Sci. Rep. 7, 1–7. doi: 10.1038/s41598-017-11058-7
Oakes J. M., Connolly R. M., Revill A. T. (2010). Isotope Enrichment in Mangrove Forests Separates Microphytobenthos and Detritus as Carbon Sources for Animals. Limnol. Oceanogr. 55, 393–402. doi: 10.4319/lo.2010.55.1.0393
Pereira T. M., Nóbrega G. N., Ferreira T. O., Ogawa C. Y., de Camargo P. B., Silva J. R. F., et al. (2019). Does Food Partitioning Vary in Leaf-Eating Crabs in Response to Source Quality? Mar. Environ. Res. 144, 72–83. doi: 10.1016/j.marenvres.2018.12.005
Petersen J. M., Kemper A., Gruber-Vodicka H., Cardini U., van der Geest M., Kleiner M., et al. (2016). Chemosynthetic Symbionts of Marine Invertebrate Animals are Capable of Nitrogen Fixation. Nat. Microbiol. 2, 1–11. doi: 10.1038/nmicrobiol.2016.195
Pinckney J. L. (2018). A Mini-Review of the Contribution of Benthic Microalgae to the Ecology of the Continental Shelf in the South Atlantic Bight. Estuar. Coast. 41, 2070–2078. doi: 10.1007/s12237-018-0401-z
Pinheiro M. A. A., Fiscarelli A. G., Hattori G. Y. (2005). Growth of the Mangrove Crab Ucides Cordatus (Brachyura, Ocypodidae). J. Crust. Biol. 25, 293–301. doi: 10.1651/C-2438
Putz B., Drapela T., Wanek W., Schmidt O., Frank T., Zaller J. G. (2011). A Simple Method for in Situ-Labelling With 15N and 13C of Grassland Plant Species by Foliar Brushing. Methods Ecol. Evol. 2, 326–332. doi: 10.1111/j.2041-210X.2010.00072.x
Robertson A. I. (1986). Leaf-Burying Crabs: Their Influence on Energy Flow and Export From Mixed Mangrove Forests (Rhizophora Spp.) in Northeastern Australia. J. Exp. Mar. Biol. Ecol. 102, 237–248. doi: 10.1016/0022-0981(86)90179-6
Robertson A. I. (2021). Leaf-Litter Consumption Slows Crab Growth But Transforms Mangrove Food Chains. Mar. Ecol. Prog. Ser. 667, 83–98. doi: 10.3354/meps13709
Russell-Hunter W. D. (1970). Aquatic Productivity: An Introduction to Some Basic Aspects of Biological Oceanography and Limnology (New York: Macmillan).
Schade J. D., MacNEILL K., Thomas S. A., Camille McNeely F., Welter J. R., Hood J., et al. (2011). The Stoichiometry of Nitrogen and Phosphorus Spiraling in Heterotrophic and Autotrophic Streams. Freshwater. Biol. 56, 424–436. doi: 10.1111/j.1365-2427.2010.02509.x
Sheaves M., Molony B. (2000). Short-Circuit in the Mangrove Food Chain. Mar. Ecol. Prog. Ser. 199, 97–109. doi: 10.3354/meps199097
Skov M. W., Hartnoll R. G. (2002). Paradoxical Selective Feeding on a Low-Nutrient Diet: Why Do Mangrove Crabs Eat Leaves? Oecologia 131, 1–7. doi: 10.1007/s00442-001-0847-7
Small G. E., Pringle C. M., Pyron M., Duff J. H. (2011). Role of the Fish Astyanax Aeneus (Characidae) as a Keystone Nutrient Recycler in Low-Nutrient Neotropical Streams. Ecology 92, 386–397. doi: 10.1890/10-0081.1
Sperfeld E., Halvorson H. M., Malishev M., Clissold F. J., Wagner N. D. (2016). Woodstoich III: Integrating Tools of Nutritional Geometry and Ecological Stoichiometry to Advance Nutrient Budgeting and the Prediction of Consumer-Driven Nutrient Recycling. Oikos 125, 1539–1553. doi: 10.1111/oik.03529
Thongtham N., Kristensen E., Puangprasan S. Y. (2008). Leaf Removal by Sesarmid Crabs in Bangrong Mangrove Forest, Phuket, Thailand; With Emphasis on the Feeding Ecology of Neoepisesarma Versicolor. Estuar. Coast. Shelf. Sci. 80, 573–580. doi: 10.1016/j.ecss.2008.09.017
Tongununui P., Kuriya Y., Murata M., Sawada H., Araki M., Nomura M., et al. (2021). Mangrove Crab Intestine and Habitat Sediment Microbiomes Cooperatively Work on Carbon and Nitrogen Cycling. PloS One 16, e0261654. doi: 10.1371/journal.pone.0261654
Tue N. T., Hamaoka H., Sogabe A., Quy T. D., Nhuan M. T., Omori K. (2012). Food Sources of Macro-Invertebrates in an Important Mangrove Ecosystem of Vietnam Determined by Dual Stable Isotope Signatures. J. Sea. Res. 72, 14–21. doi: 10.1016/j.seares.2012.05.006
Underwood G. J. C., Kromkamp J. (1999). Primary Production by Phytoplankton and Microphytobenthos in Estuaries. Adv. Ecol. Res. 29, 93–153. doi: 10.1016/S0065-2504(08)60192-0
Vanni M. J., Bowling A. M., Dickman E. M., Hale R. S., Higgins K. A., Horgan M. J., et al. (2006). Nutrient Cycling by Fish Supports Relatively More Primary Production as Lake Productivity Increases. Ecology 87, 1696–1709. doi: 10.1890/0012-9658(2006)87[1696:NCBFSR]2.0.CO;2
Vaughn C. C. (2010). Biodiversity Losses and Ecosystem Function in Freshwaters: Emerging Conclusions and Research Directions. BioScience 60, 25–35. doi: 10.1525/bio.2010.60.1.7
Waterbury J. B., Calloway C. B., Turner R. D. (1983). A Cellulolytic Nitrogen-Fixing Bacterium Cultured From the Gland of Deshayes in Shipworms (Bivalvia: Teredinidae). Science 221, 1401–1403. doi: 10.1126/science.221.4618.1401
Welsh D. T. (2003). It's a Dirty Job But Someone has to do it: The Role of Marine Benthic Macrofauna in Organic Matter Turnover and Nutrient Recycling to the Water Column. Chem. Ecol. 19, 321–342. doi: 10.1080/0275754031000155474
Welti N., Striebel M., Ulseth A. J., Cross W. F., DeVilbiss S., Glibert P. M., et al. (2017). Bridging Food Webs, Ecosystem Metabolism, and Biogeochemistry Using Ecological Stoichiometry Theory. Front. Microbiol. 8. doi: 10.3389/fmicb.2017.01298
Werry J., Lee S. Y. (2005). Grapsid Crabs Mediate Link Between Mangrove Litter Production and Estuarine Planktonic Food Chains. Mar. Ecol. Prog. Ser. 293, 165–176. doi: 10.3354/meps293165
Wilde J. E., Linton S. M., Greenaway P. T. (2004). Dietary Assimilation and the Digestive Strategy of the Omnivorous Anomuran Land Crab Birgus Latro (Coenobitidae). J. Comp. Physiol. B. 174, 299–308. doi: 10.1007/s00360-004-0415-7
Keywords: mangrove trophodynamics, sesarmid crabs, nitrogen requirement, isotope tracer, nutrient cycling, enriched isotope experiments
Citation: Gao X and Lee SY (2022) Feeding Strategies of Mangrove Leaf-Eating Crabs for Meeting Their Nitrogen Needs on a Low-Nutrient Diet. Front. Mar. Sci. 9:872272. doi: 10.3389/fmars.2022.872272
Received: 09 February 2022; Accepted: 30 March 2022;
Published: 27 April 2022.
Edited by:
Alex S. J. Wyatt, Hong Kong University of Science and Technology, Hong Kong SAR, ChinaReviewed by:
Anirban Akhand, Port and Airport Research Institute (PARI), JapanRichard MacKenzie, USDA Forest Service, United States
Tiago Osório Ferreira, University of São Paulo, Brazil
Copyright © 2022 Gao and Lee. This is an open-access article distributed under the terms of the Creative Commons Attribution License (CC BY). The use, distribution or reproduction in other forums is permitted, provided the original author(s) and the copyright owner(s) are credited and that the original publication in this journal is cited, in accordance with accepted academic practice. No use, distribution or reproduction is permitted which does not comply with these terms.
*Correspondence: Shing Yip Lee, joesylee@cuhk.edu.hk