- 1Departamento de Oceanografía Biológica, Centro de Investigación Científica y de Educación Superior de Ensenada (CICESE), Ensenada, Mexico
- 2Laboratorio de Mamíferos Marinos (LabMMar, IIB-ICIMAP), Universidad Veracruzana, Xalapa, Mexico
- 3Instituto de Investigaciones Biológicas, Universidad Veracruzana, Xalapa, Mexico
Competition between fisheries and bottlenose dolphins is a globally relevant conflict given its socioeconomic and ecological implications. Understanding the factors driving the interactions between dolphins and fishery activities is key to the development of appropriate mitigation strategies. Our study aimed to assess whether these interactions are related to the ecological, trophic, and nutritional characteristics of the catch. We used 117 gillnet sets from 48 fishing trips during 2009 – 2010 and 2015 – 2019, which were classified based on the presence or absence of dolphin interactions. These interactions occurred year-round and were documented in 46.1% of the sets, with 14.5% of those showing signs of depredation. The passive acoustic predatory hypothesis, which states that fish species that generate sound are subject to a higher predation intensity by dolphins, was not supported by our data. Also, with the exception of species diversity, ecological parameters such as richness, biomass and CPUE were slightly higher, although not significant in sets with dolphin interaction. Furthermore, during 2015 – 2016, we sampled 123 organisms of 25 representative fish species in the catches and determined the whole fish isotopic composition (δ13C and δ15N), and estimated the nutritional value (i.e., lipid, protein, and energy content) of each species. Isotopic values showed no differences between net settings (with and without interaction), fish habitat, or prey type (potential prey, n= 11 species, vs. non-potential prey, n= 14). However, a preference towards fish from a certain range of thropic levels was evident. All the fish (N= 123) showed significantly higher protein values during the Rainy period, which may be attributed to their reproductive cycles and higher primary productivity. Interestingly, energy contents of the dolphins’ potential prey were also significantly higher during this period. Unexpectedly, protein and energy contents were significantly higher in the fishes caught in the sets without dolphin interaction, but only during the Dry and Windy periods, respectively. Opportunistic feeding habits are well known for bottlenose dolphins, and our results showed that “easy access” to prey will likely prompt interaction with gillnets, regardless of the species composition, biomass, seasonality, preferred habitat, sound production capacity, or nutritional value of the captured fish.
1 Introduction
Expansion of human populations and local fisheries have led to increasing opportunities for competition with coastal marine predators. A recen review by Jog et al. (2022) shows that the number of published studies of marine mammal interactions with fisheries is rising and is largely dominated by cetaceans in both commercial and small-scale fisheries. Depredation of catches in at least 214 fisheries covering almost all of FAO’s (Food and Agriculture Administration) fishing areas has been reported since 1979 (Northridge, 1991; Tixier et al., 2020). Marine mammals such as cetaceans and pinnipeds are the most frequently reported taxonomic groups that exhibit this behavior (Tixier et al., 2020; Jog et al., 2022). Depredation of small-scale fisheries is less frequently reported for pinnipeds due to the distributions of this type of fisheries; within the odontocetes, killer whales (Orcinus orca) and common bottlenose dolphins (Tursiops truncatus, hereafter referred to as “bottlenose dolphin”), possess the highest number of depredation reports, but only bottlenose dolphin has been documented to interact with different types of fishing gear used by the recreational, commercial, and artisanal sectors (Northridge, 1984; Tixier et al., 2020).
Competition has been recognized by FAO as one of the most relevant conflicts faced by coastal bottlenose dolphins at a global level, given the socioeconomic and ecological importance for man and marine predators, respectively (Northridge, 1984; Northridge, 1991; Bearzi, 2002; Read, 2008; Tixier et al., 2020; Jog et al., 2022). Socioeconomic impacts include damage to fishing equipment and reduction of the catch, threatening the economic security and food acquisition for fishers, especially those that operate at subsistence level (Bearzi, 2002; Bearzi et al., 2019; Guerra, 2019). Depredation on fishery catches may have positive and negative effects on dolphins, on the one hand, it reduces foraging effort and/or allows access to additional food resources, and on the other hand it increases the risk of bycatch or intentional death due to violent retaliation by fishers and may also result in more rapid depletion of fish stocks (Beddington et al., 1985; Fertl and Leatherwood, 1997; Bearzi, 2002; Bearzi et al., 2006; Bearzi et al., 2019; Tixier et al., 2020). Additionally, dolphin populations may change their feeding behavior and/or distribution, when interacting with different types of fisheries (Blasi et al., 2015), either to avoid them (Morteo et al., 2012) or to spend more time feeding in areas with gillnets (Díaz-López, 2006; Morales-Rincon et al., 2019).
Coastal bottlenose dolphins are generally considered opportunistic predators (Northridge, 1991; Fertl and Leatherwood, 1997; Bearzi, 2002; Lauriano et al., 2004), and have adapted to prey upon accessible resources within their habitats (Lauriano et al., 2004; Tixier et al., 2020; Pardalou and Tsikliras, 2020). However, research on their feeding ecology (Rossman et al., 2015a; Rossman et al., 2015b), shows that some populations and different demographic groups (i.e., males, females, and juveniles) may develop foraging specializations in particular habitats (e.g., seagrass) and prey upon certain trophic levels. Also, bottlenose dolphins may show preference towards prey that produce sounds (McCabe et al., 2010; Dunshea et al., 2013), as this may cue their predatory behavior when fish are captured in the gear, thus facilitating information on the type, number and/or location of the species involved (Rocklin et al., 2009; Rechimont et al., 2018). Additionally, a significant relationship between the prey quality of some cetacean species, including the bottlenose dolphin, and their species-especific energetic requirements has been proposed (Spitz et al., 2010a; Spitz et al., 2012). In particular, bottlenose dolphins consume medium quality prey with an energy content from 4 to 5.5 kJ g-1 (Spitz et al., 2012). However, the underlying factors driving the interactions between this species and the fisheries are still largely unknown.
It is widely accepted that wherever the natural distribution of bottlenose dolphin’s prey overlaps with that of artisanal fisheries, there is potential for competition (Lauriano et al., 2004). Numerous studies have been developed on the interactions between marine mammals and fisheries (e.g., Lauriano et al., 2004; Rocklin et al., 2009; Rechimont et al., 2018; Pardalou and Tsikliras, 2020), with the aim of improving the understanding of such events by identifying the potential factors related to the causes and assessing the impact on the economic yield and perception of fishers (Arias-Zapata, 2019).
Bottlenose dolphins in Mexican waters, are widely distributed, but studies on their interaction with fisheries remain scarce (Vidal et al., 1994; Zavala-González et al., 1994) and are mostly limited to one population (Morteo et al., 2012; Rechimont et al., 2018; Morales-Rincon et al., 2019), despite the socioeconomic importance that fishing represents in this region, and the chronic and acute conflict reported in some areas, such as the coastal waters of Alvarado, Veracruz (Morteo et al., 2012; Morteo et al., 2017; Rechimont et al., 2018; Morales-Rincon et al., 2019).
Alvarado constitutes an important feeding area for bottlenose dolphins within the Gulf of Mexico (Morteo et al., 2012; Morteo et al., 2017; Rechimont et al., 2018; Morales-Rincon et al., 2019). A high rate of interactions has been documented with local artisanal gillnet fisheries (in up to 80% of net settings; Rechimont et al., 2018), and during which the dolphins capture prey that are part of their diet (Chávez-Martínez, 2017; Rechimont et al., 2018). Previous research by Rechimont et al. (2018), suggests that the probability of depredation is related to fishing techniques (i.e., set gillnet), catch per unit effort (CPUE) and the presence of species that are common prey items (i.e., Scomberomorus maculatus). Recently, Morales-Rincon et al. (2019) documented individual behavioral traits that showed reductions in the frequency of surface behaviors and smaller group sizes for bottlenose dolphins that interact with artisanal gillnets (i.e.,<5 individuals), possibly as a strategy to reduce the risk of injuries while feeding on the nets, suggesting an apparent habituation to fishing activities.
This study aims to assess whether these interactions are related to the ecological, trophic, and nutritional characteristics of the prey caught by the artisanal gillnet fishery of Alvarado, Veracruz. Specifically, our goals were (1) to test for differences in biomass, richness, diversity and CPUE between net settings with and without interactions with bottlenose dolphins, (2) to determine whether depredation was related to fish trophic level based on the nitrogen isotopic composition (δ15N values) of the catch, (3) their nutritional value estimated from proximate analyses of lipids and proteins and estimates of energy content, and (4) ecological characteristics, including feeding regions inferred from fish δ13C values and whether the sound production capacity of fish species is related to the depredation.
2 Materials and methods
2.1 Study area
The coastal waters off Alvarado are a highly productive area located along the central state of Veracruz, in the southwestern Gulf of Mexico (Figure 1). They are influenced by the Alvarado estuarine-lagoon system, the third-largest in Mexico, which receives continental water input from different rivers (~40 million m3 d-1) (Contreras, 1985; Morán-Silva et al., 2005), thus the high amount of suspended particulate matter causes low visibility underwater (Morteo, 2011). There are three well-defined climatic conditions (Dry= March to May, Rainy= June to October, and Windy= November to February; the latter period corresponds to winter in other geographic regions), and there is a broad variation in freshwater inflow, sea surface temperature, salinity, and nutrient concentrations in the estuarine-lagoon system and adjacent coastal waters, leading to ecological changes influencing the abundance and reproduction of fish species (Morán-Silva et al., 2005).
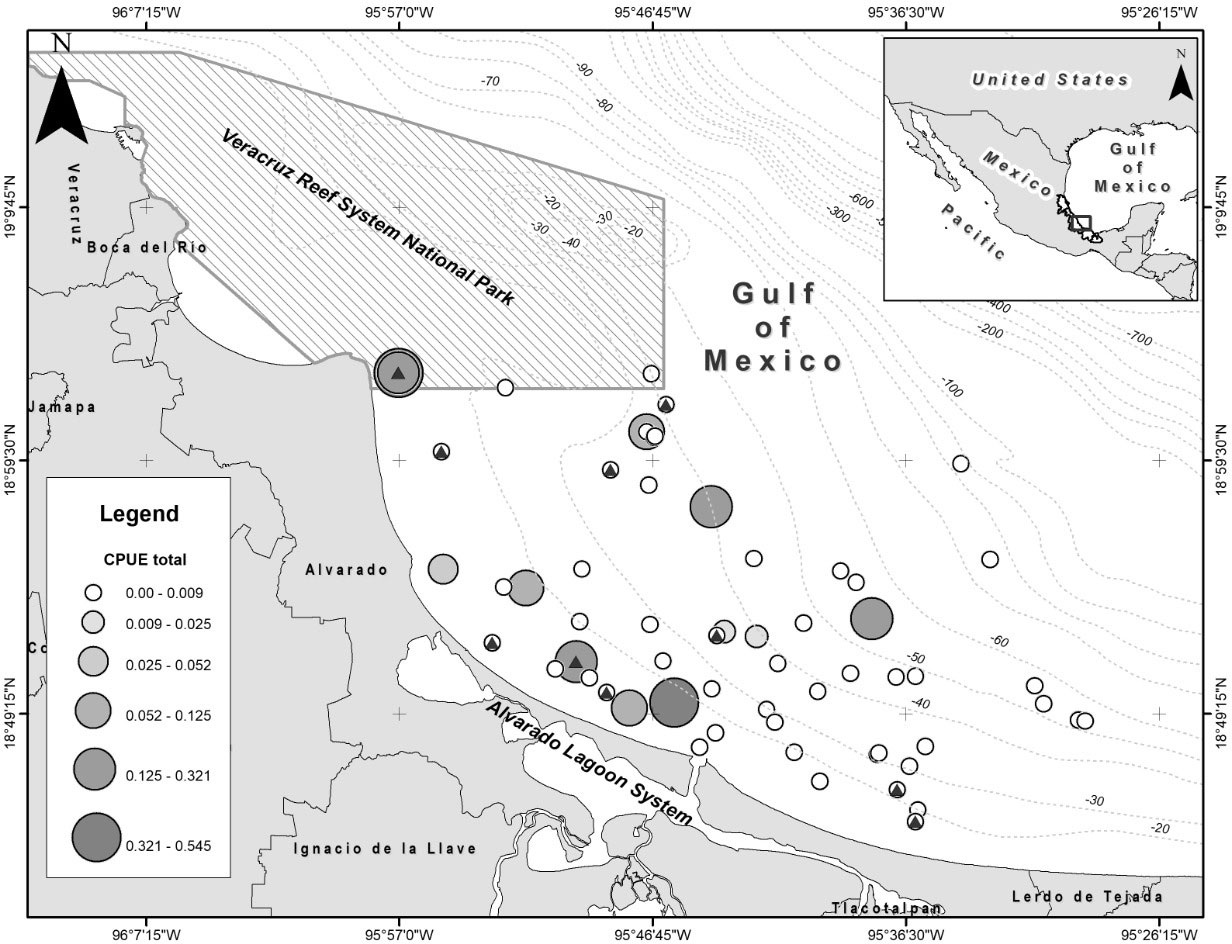
Figure 1 Geographic location of the study area: coastal waters off Alvarado, Veracruz in the southwestern Gulf of Mexico. Circles show the position of sets and the size/shade the correspondent CPUE value. Triangles show sets with interaction/depredation. Dashed lines show depth contours (m).
The main economic activity in Alvarado is artisanal fishing, which takes place throughout the year and sustains the economy of around 2000 fishers (Morteo et al., 2012; Carrillo-Alejandro et al., 2014). The taxonomic composition of the catches based on their commercial importance includes crabs, shrimp, prawns, clams, and several demersal fishes (Carrillo-Alejandro et al., 2014; Villanueva-Fortanelli, 2015), of which at least 17 species have been documented as part of the diet of T. truncatus (Chávez-Martínez, 2017; Rechimont et al., 2018). Artisanal fishing activities are carried out on board small vessels known as “pangas” (2.9 – 8.2 m in length with one or two 40 – 75 hp outboard motors), and with different types of fishing gear depending on the type, abundance, and seasonality of the resources and target species (Morteo et al., 2012; Carrillo-Alejandro et al., 2014; Rechimont et al., 2018). However, gillnets are the most frequently used gear, and the main instance for dolphin-fisheries interactions (Morteo et al., 2012; Rechimont et al., 2018; Morales-Rincon et al., 2019).
2.2 Data collection
Data were collected following Rechimont et al. (2018) and Morales-Rincon et al. (2019) during normal operations of the artisanal fleet using their fishing boats (≈7 m length) that are typically equipped with one outboard motor of 40 or 60 hp. Observations and sampling were performed during the three climatic conditions and under sea conditions lower than Beaufort 3 (wind speed between 12 to 19 km h-1). A total of 48 surveys were carried out periodically over 7 years, from August 2009 to May 2010, November 2015 to September 2016, January 2017 to July 2018, and March to August 2019 (Table 1). The route, distance from shore, and type of fishing gear used during the operations were chosen by the fishers, thus the research crew acted only as observers, where each “set” was considered as an independent experiment (Lauriano et al., 2004; Rocklin et al., 2009; Rechimont et al., 2018; Morales-Rincon et al., 2019). During each net set, operational and environmental variables were recorded, and whenever interactions with bottlenose dolphins were observed, their group size, presence or absence of depredation, damage to fishing gear, and the duration of the events were also documented (Table 2). Only gillnet sets were depredated and thus considered in the following analyses.
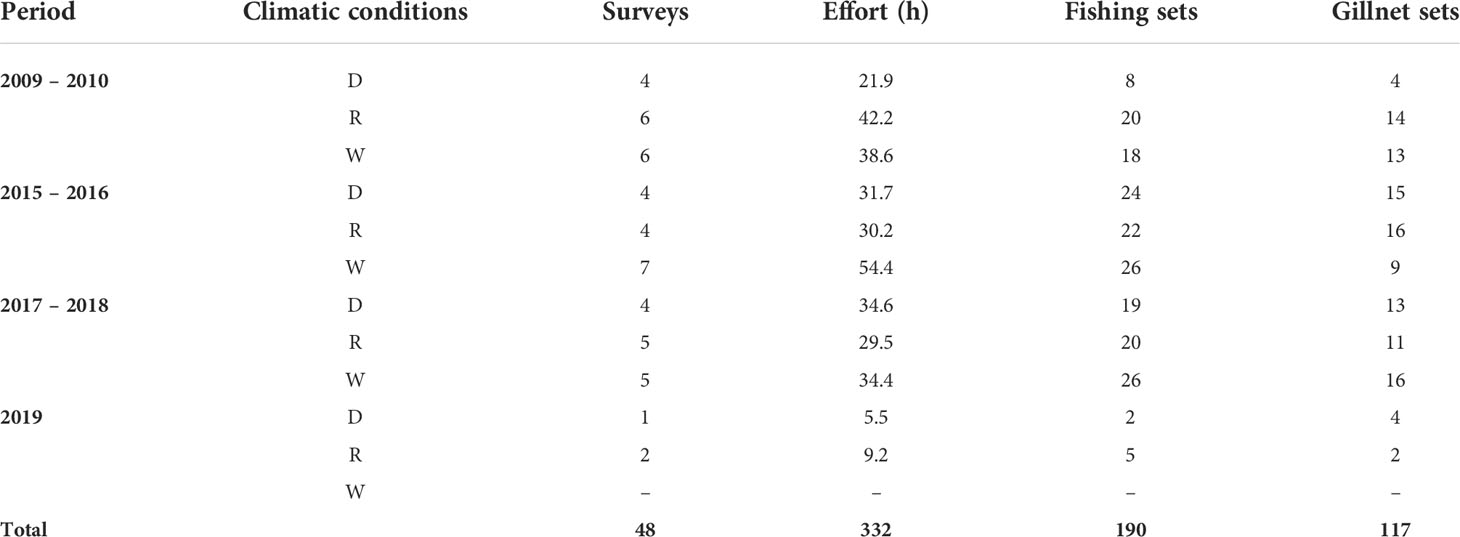
Table 1 Survey effort carried out during the Dry (D), Rainy (R) and Windy (W) climatic conditions on the coast of Alvarado, Veracruz.
Interactions were defined as the simultaneous observation of bottlenose dolphins and gillnets within a 200 m radius (Morteo et al., 2012; Rechimont et al., 2018; Morales-Rincon et al., 2019). Also, depredation was recorded as bottlenose dolphins feeding directly on the catch, and/or by the presence of at least one fish with evidence of physical damage (e.g., one or more parts removed) (Rocklin et al., 2009). We recorded sudden and frequent movements of the gear as it was being pulled from below, and these were deemed indicative of dolphin interacting directly with the net. Furthermore, hydrophones were used in the surveys for a separate study, where screeching and crunching noises were always consistent with the recorded movements of the gear. Whenever possible, five fish of each species were selected (with or without evidence of physical damage) when their sizes fell within the range reported in the stomach contents of bottlenose dolphins (total length=100 – 1027 mm; Barros and Odell, 1990; Barros and Wells, 1998; McCabe et al., 2010; Hernandez-Milian et al., 2015; Milmann et al., 2016). Subsequently, each specimen was individually labeled and refrigerated (≈4° C) until arrival to the laboratory, where it was frozen (-20° C) pending further analysis.
2.3 Taxonomy and identification of potential prey
In the laboratory, all fishes were identified to species using taxonomic keys and open access databases (Jiménez-Badillo et al., 2006; Froese and Pauly, 2021). Based on the literature and the evidence of physical damage, species were grouped into potential or non-potential prey of bottlenose dolphins. Due to the low underwater visibility, we tested the “passive acoustic hypothesis” (Gannon et al., 2005), thus the species were subsequently classified based on their ability to produce sound (Jiménez-Badillo et al., 2006; McCabe et al., 2010; Froese and Pauly, 2021; IUCN (International Union for Conservation of Nature), 2021) as follows: produces sound, produces no sound, and not determined. This was also based on the possibile distress noises by sonorous prey while being caught in gear (Rocklin et al., 2009; Knight and Ladich, 2014; Rechimont et al., 2018).
2.4 Stable isotopic analyses
Samples of fish were obtained during 2015 – 2019; however, budget restrictions only allowed for stable isotope and proximate analyses for samples collected in the Dry, Rainy and Windy periods from 2015 – 2016, which are considered representative of the artisanal fisheries in Alvarado. Fishes were processed whole, since they reflect the food ingested and assimilated by the dolphins (i.e., feeding on complete prey); however, the stomachs were removed to avoid bias due to the fishes’ prey (process described in detail in Chávez-Martínez, 2017). Samples were processed in the Fisheries Ecology Laboratory of the Department of Biological Oceanography at the Center for Scientific Research and Higher Education of Ensenada (CICESE), as well as in the Food Quality Laboratory of the Faculty of Nutrition at Universidad Veracruzana (UV) and in the Microscopy Laboratory at the Faculty of Biology (UV). All fish were blended wet, dried, powdered with a stainless-steel blender and homogenized thoroughly (see Chávez-Martínez, 2017). Aproximately ~1 mg samples were weighed into tin capsules and sent for analysis to the Stable Isotope Facility of the University of California Davis (SIF UC Davis), USA, using a Carlo-Erba NC 2500 elemental analyzer coupled to Finnigan Delta Plus XL mass spectrometer. Carbon and nitrogen isotope composition are expressed in delta (δ) notation in parts per thousand (‰) relative to PeeDee Belemnite limestone (PDB) and atmospheric N2, respectively:
Where: Rsample is the ratio of the heavy to the light isotope of the sample, and Rstandard is the ratio of the heavy to the light isotope of the standard. According to SIF UC Davis, the secondary standards employed during the analysis of the samples where bovine liver, nylon 5, peach leaves and glutamic acid, which had a SD of 0.03, 0.04, 0.05 and 0.09‰ for δ13C values, respectively, whereas for δ15N values the SD was 0.1, 0.1, 0.7 and 0.7‰, respectively. Therefore, our isotopic analyses had a precision of at least 0.1‰ for carbon and nitrogen.
2.5 Proximate analyses
These were carried out in the Food Quality Laboratory of the Faculty of Nutrition at UV and in the Food and Nutrition Laboratory of CICESE’s Aquaculture Department. Duplicates of the processed and homogenized samples that were used for the stable isotope analysis were used to measure water, lipid and protein content of the samples following the methods of the “Manual of laboratory techniques for fish and crustacean nutrition” (FAO (Food and Agriculture Organization), 1993), as described by Chávez-Martínez (2017). All analyses were performed in triplicate whenever the number of fish caught allowed for it. Since the lipid and protein content was initially determined on a dry weight basis, a correction was applied to obtain a wet weight with which subsequently the energy content of each fish was estimated. This correction was:
Where: A= proportion of nutrient in dry weight, and B= proportion of water in the sample, which was estimated emperically. Finally, the proportion of protein and lipids were obtained by gram of wet weight for each fish, and then multiplied by their respective energy conversion factors (5.6 and 9.4 kcal g-1, respectively), and both values were then added and converted to kJ g-1 (1 kcal= 4.184 kJ) to obtain the energetic content in 1 g of the whole wet fish.
2.6 Data analyses
2.6.1 Characterization of the gillnet fisheries
First, we quantified the number of gillnet sets with and without depredation, the number and species of the fishes caught in each set, and the species with physical evidence of feeding (i.e., bite marks) and the type of damage (body parts removed; Table 2).
Set data were then grouped based on the presence or absence of interactions with bottlenose dolphins, and total biomass and capture per unit effort (CPUE) for each type of setting were computed. Due to variations in gillnet lengths for each study period, CPUE was defined as the total catch weight (kg) divided by the length of the net (m) and soak time (h) and standardized to 1000 m of net (kg m-1 h-1). Species richness and diversity were determined for sets with and without dolphin interactions using the Shannon-Weiner diversity index (H’). Differences in biomass, CPUE, richness and diversity of species were assessed between both types of sets using Mann-Whitney (M-W) and Kolmogorov-Smirnov (K-S) tests.
The classification of the species caught based on their capacity to produce sound (Fish and Mowbray, 1970; Jiménez-Badillo et al., 2006; Froese and Pauly, 2021; IUCN (International Union for Conservation of Nature), 2021), was used to test their degree of association as a potential prey (Mora-Manzano, 2018) of bottlenose dolphins through a non-metric correspondence analysis. We also used this approach to search for correspondence between the sound producing capacity and the occurrence of interaction between dolphins and gillnets.
2.6.2 Feeding areas and estimates of trophic level
Fish species were grouped according to their predominant habitat type in: estuarine, demersal, pelagic and reef associated (Jiménez-Badillo et al., 2006; Froese and Pauly, 2021; IUCN (International Union for Conservation of Nature), 2021). The latter would aid in determining whether dolphin interactions with gillnets was related to the presence of prey from a particular habitat (i.e., preferred feeding area), which should be reflected in carbon isotope ratios. Differences in δ13C values of the species by type of habitat were examined with a Kruskal-Wallis test (K-W). Also, differences were sought in the habitat type of potential and non-potential prey.
Pearson correlations were used to examine variation in δ15N values as a function of fish standard length SL; n= 123). Isotope compositions were averaged (± SD in ‰) by species, and differences in δ15N and δ13C values (± SD in ‰) were assessed between potential and non-potential prey, and between sets with and without dolphin interactions using M-W and K-S tests.
The trophic position (TP) of the species fished in gillnets were calculated to search for relationships with the occurrence of dolphin interactions. We used the δ15N values of primary consumers as indicators of the base of the food web using the formula proposed by Post (2002):
Where, λ = average of the trophic level of Cetengraulis edentulus and Opisthonema oglinum (2.3), δ15Nbase is the average δ15N value of these two species, and Δδ15N is the isotopic discrimination factor for nitrogen in fish (2.3‰; McCutchan et al., 2003). Weighted averages of the trophic level were estimated for each set based on the number of individuals of each species caught and their estimates of trophic position. The averages of trophic levels per species were then grouped by type of prey (potential and non-potential) and type of sets (with and without interaction). Differences in the weighted average for the trophic level between set types were assessed using M-W and/or t-Student tests. Finally, we searched for differences in the mean trophic level between types of prey through M-W tests.
2.6.3 Proximal composition and energy content
Average lipids, proteins (± SD in %), and energy content estimates (± SD in kJ g-1) were determined for each fish species and compared using K-W and M-W or t-Student tests when they were caught in different climatic periods (i.e., Scomberomorus maculatus, S. cavalla and Conodon nobilis). Also, seasonal differences in these nutritional values were calculated for all the fish data for each climatic period and examined using ANOVA or K-W tests. The same process was applied to search for seasonal differences in these variables between gillnet sets with and without dolphin interaction (M-W and/or t-Student tests). Since not all captured fish species were available for analysis due to limited catches or because fishers sold all the fish, missing data were recorded based on averaged values (only in 31 sets) for the missing species (only 11 species), and according to the climatic period.
3 Results
Sampling effort totaled 48 surveys in 332 h, carried out during 2009 – 2010 and 2015 – 2019. We recorded 190 fishing operations (46 in 2009 – 2010, 72 in 2015 – 2016, 65 in 2017 – 2018, and 7 in 2019), from which only 117 involved gillnets, thus only these were considered for the study. Gillnet fishing operations captured 56 different species of 30 families, including bony and cartilaginous fish, as well as crustaceans (Suplementary material). At least 41.7% of these species have commercial value in the region.
3.1 Interactions with bottlenose dolphins and depredation on gillnets
Dolphin-fisheries interactions were recorded in 54 net sets (46.1% of total, n= 117), which cummulatively were characterized by a higher species richness (43) than that sets with no interaction (40). However, both types of sets presented a similar per set average richness (mean ± SD) (interaction 2.6 ± 2.0 vs. no interaction 3.0 ± 2.3), with no significant differences (M-W: U(54,63) = 1540.5, P ≥ 0.05). Both types of sets presented medium diversity values (H’ with interaction= 2.1, H’ without interaction= 2.3) and there were no significant differences (M-W: U(43,40)= 681, P ≥ 0.05). Therefore, the composition and abundance of captured species were similar (Figure 2).
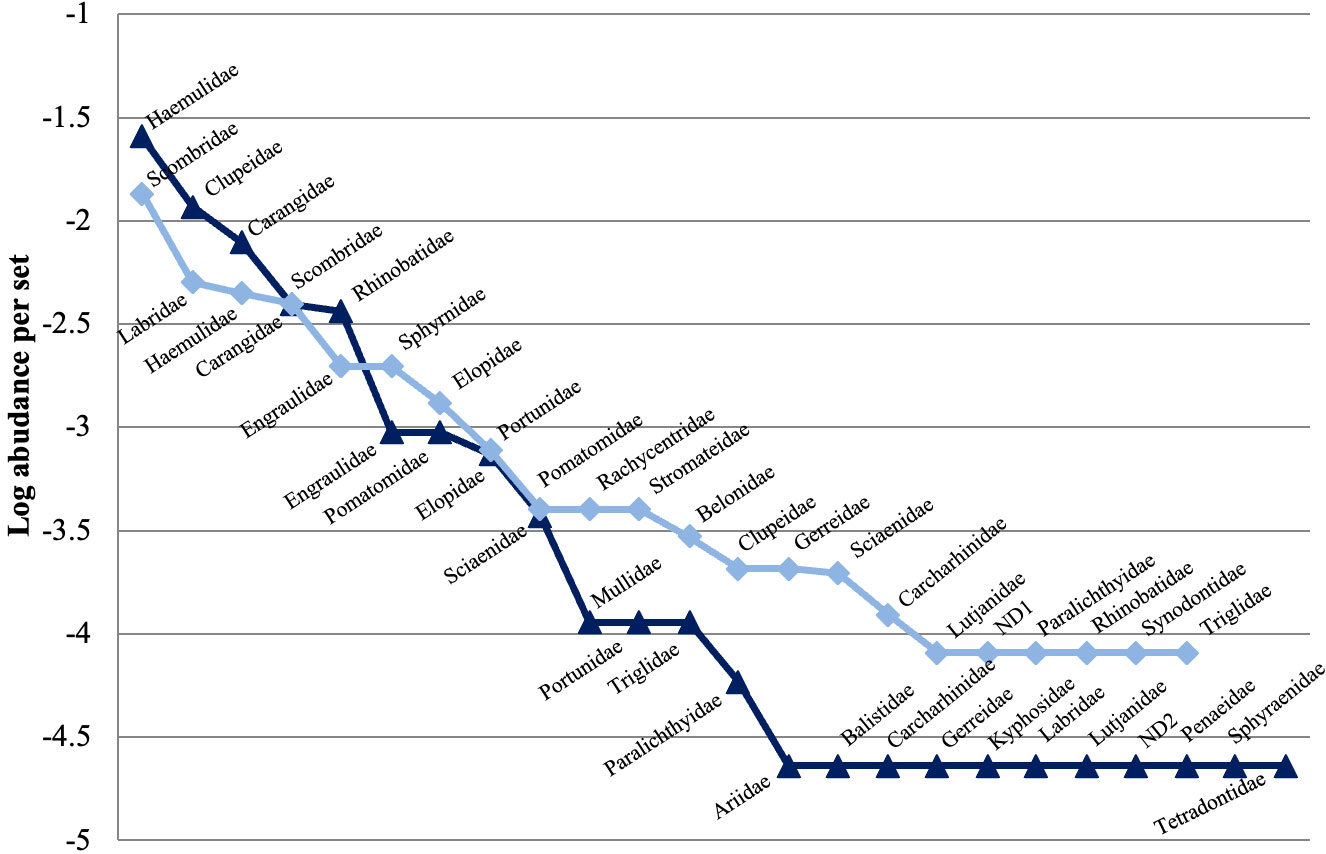
Figure 2 Abundance per set of families captured by the artisanal fishery from Alvarado, Veracruz during fishing operations with (triangles) and without interaction (rhombus) with bottlenose dolpins. ND1: Undetermined family number 1 (registered in the data base as “banderita”); ND2: Undetermined family number 2 (registered in the data base as “jurelito”).
Average biomass for sets with interaction (22.0 ± 79.7; range 0 – 551 kg set-1) was 3.3 times higher than sets without interaction (6.6 ± 10.2; 0 – 42.7 kg set-1); however, differences were not significant (M-W: U(54,63)= 1564.5, P ≥ 0.05) (Figure 3A). This was also true for CPUE values between sets with interaction (77.4 ± 205.5; Min= 0, Max= 1302.4 kg m-1 h-1), and without interaction (48.5 ± 156.6; Min= 0, Max= 1150.1 kg m-1 h-1) (M-W: U(54,63)= 1532.0, P ≥ 0.05) (Figure 3B). On the other hand, evidence of depredation was observed in 17 sets, resulting in a 14.5% depredation rate. In total, 25 fish of 12 species showed evidence of damage: Cetengraulis edentulus was the most frequent (n=5), followed by S. cavalla (n=4), S. maculatus (n=4), Chloroscombrus chrysurus (n=3) and C. nobilis (n=2). Of the remaining species (Ariopsis felis, Caranx crysos, Caranx spp., Hemicaranx amblyrhynchus, Eucinostomus argenteus, Portunidae spp. And Euthynnus alletteratus) only one individual was caught that showed evidence of depredation. Only half of these species (i.e., A. felis, C. crysos, Caranx spp., C. nobilis, S. cavalla and S. maculatus) are considered potential prey of the bottlenose dolphins. Records of physical damage to the fish included types a, c, d, and e, where type c (one or more parts removed) was the most frequent (i.e., 66.6% of damaged species).
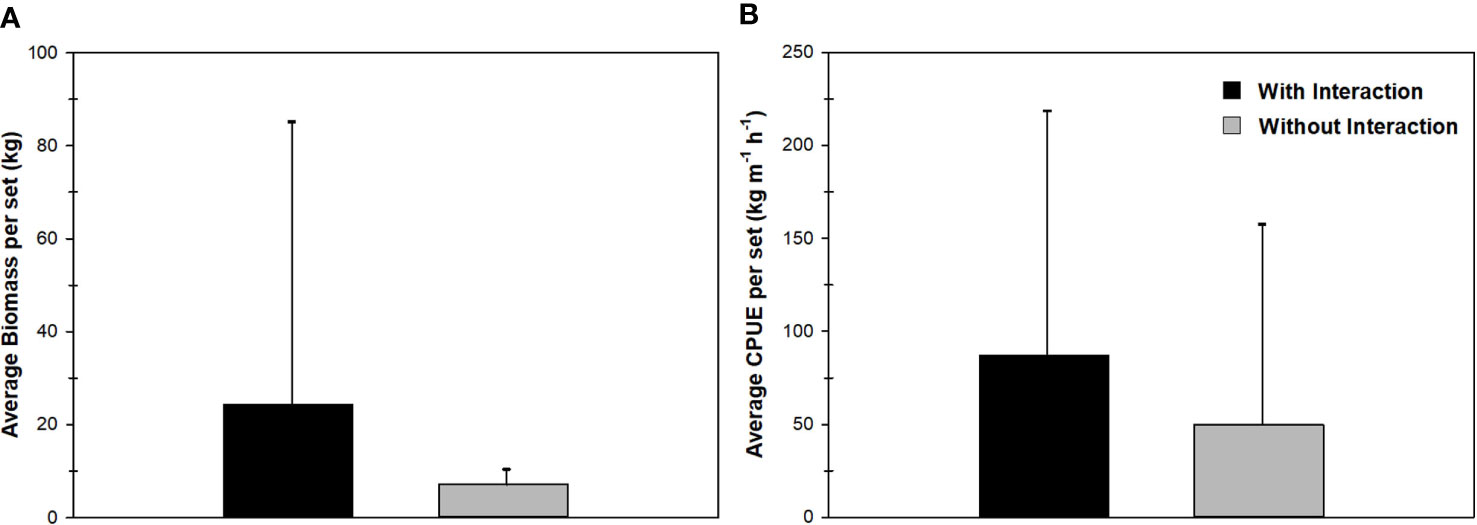
Figure 3 (A) Median values of Biomass (kg) and (B) CPUE (kg m-1 h-1) captured in sets with (black) and without (gray) interactions by bottlenose dolphins (T. truncatus) in gillnets from the artisanal fishery of Alvarado, Veracruz. Boxes show the interquartile ranges, bars represent the minimum and maximum values, and the blank points are outliers.
3.2 Fish characteristics
A total of 160 fish were collected during 2015 – 2016, of which 123 were processed and correspond to 25 different species, 12 are of economic importance, 11 are considered potential prey for the bottlenose dolphin, including a non-commercial species (Cetengraulis edentulus) (Table 4). Fishes analyzed for stable isotope and proximate analyses (n= 123) covered a size range of 10.5 to 47.5 cm SL (22.7 ± 7.7); individual species-specific weights within the catches ranged between 0.03 and 1.1 kg (0.3 ± 0.1) (Table 3).
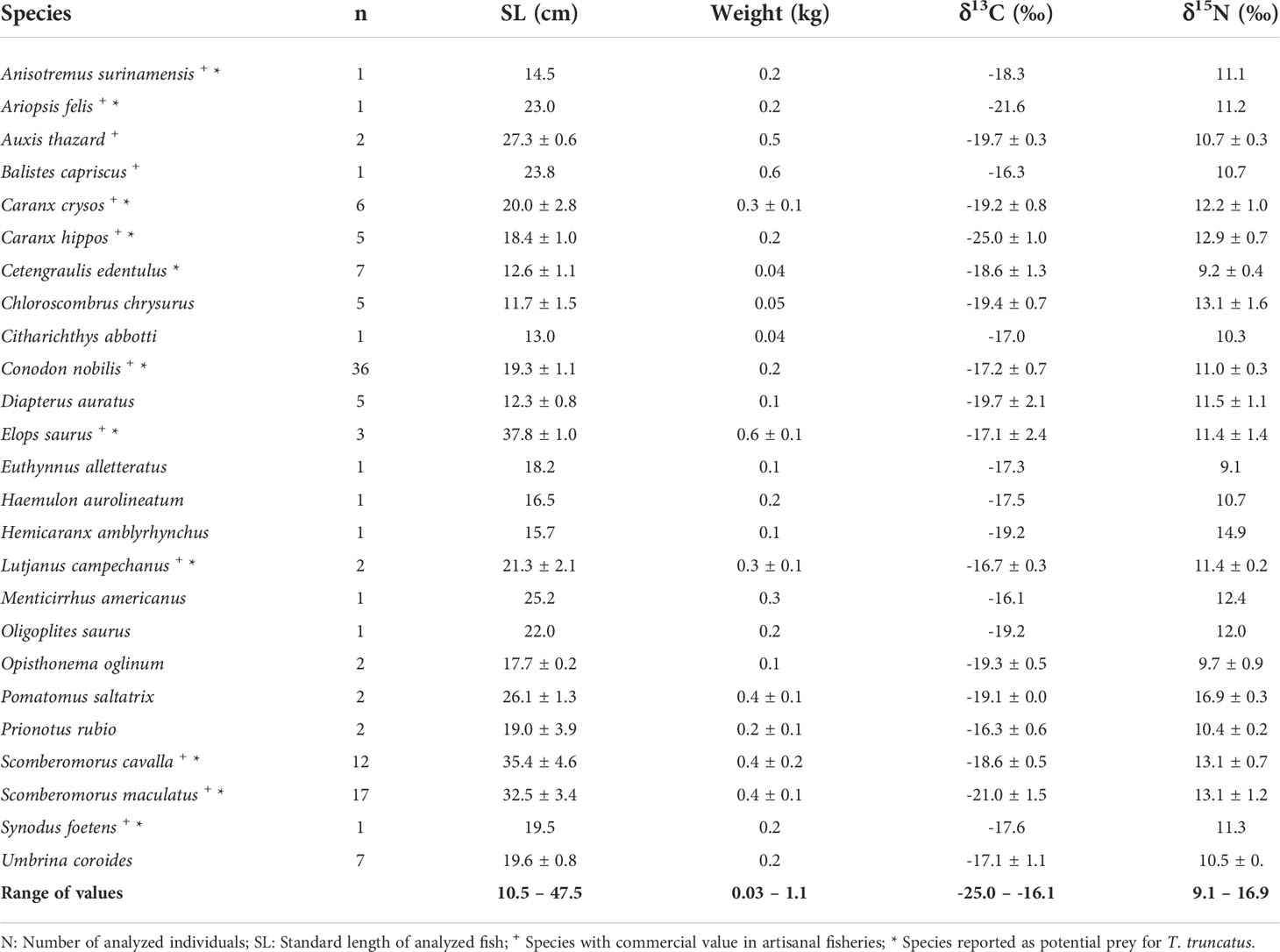
Table 3 δ13C and δ15N values (average ± SD) for the captured species in gillnets from the artisanal fishery of Alvarado, Veracruz for the period 2015 – 2016.
3.2.1 Passive acoustic hypothesis
Of the 25 species caught by gillnets during 2015 – 2019, six (24%) were sonorous, eight were not (32%), and information for the remaining (44%) was unavailable. There was no significant relationship between the type of set (with and without interaction; χ2 = 1.6, P ≥ 0.05), nor the type of prey (potential and non-potential; χ2 = 1.2, P ≥ 0.05) and the sound production of the species captured in gillnets.
3.2.2. Feeding areas and trophic level
Species-specific average δ13C values ranged between -25.0 and -16.1‰ (for C. hippos and M. americanus, respectively), and 9.1 and 16.9‰ for δ15N values (for E. alletteratus and P. saltatrix, respectively) (Table 3). As expected, a low but highly significant correlation was found for δ15N values and fish length (SL) (r2 = 0.21, F1, 121 = 31.74, P< 0.001). Only three species (i.e., C. nobilis, S. maculatus and S. cavalla) were captured in all climatic conditions allowing for the assessment of seasonal variations in isotopic composition, and no significant differences were observed for either carbon (K-W, P ≥ 0.05; t= 0.47, P ≥ 0.05, respectively) nor nitrogen isotope ratios (K-W, P ≥ 0.05; t= 0.98, P ≥ 0.05, respectively). Thus, all isotopic data was grouped by species.
Regarding habitat type (i.e., fish feeding areas), seven species were classified as potentially estuarine, seven demersal, six were reef-associated and five were pelagic. This was reflected in significant differences in average δ13C values (K-W, P= 0.002). However, it did not affect dolphin preference, since both potential and non-potential prey are typical of all types of habitats.
Comparisons of the average δ13C and δ15N values showed no significant differences between potential and non-potential prey (M-W: U(11,14)= 64, P > 0.05; U(11,14)= 63, P > 0.05, respectively). Also, no significance difference was found in the frequency distribution of mean δ15N values between both prey types (K-S, P > 0.05)). As shown in Figure 4, the isotopic biplot of all prey indicates that the range of δ13C values was broader for potential prey (mostly influenced by one species, C. hippos), compared with those that have not been described as potential prey. On the other hand, the average δ15N values of potential prey (n= 11) were significantly lower than for non-potential prey (n= 14) species (i.e., 1.8‰ vs. 7.8‰, respectively), the latter were also influenced by one species (P. saltatrix); however, this indicates preference toward fish from a certain range of trophic levels (i.e., 3 to 4, see below). Additionally, no significant differences were found in the frequency distribution of δ13C and δ15N values for the fish caught in sets with and without dolphin interaction (K-S, P ≥ 0.05).
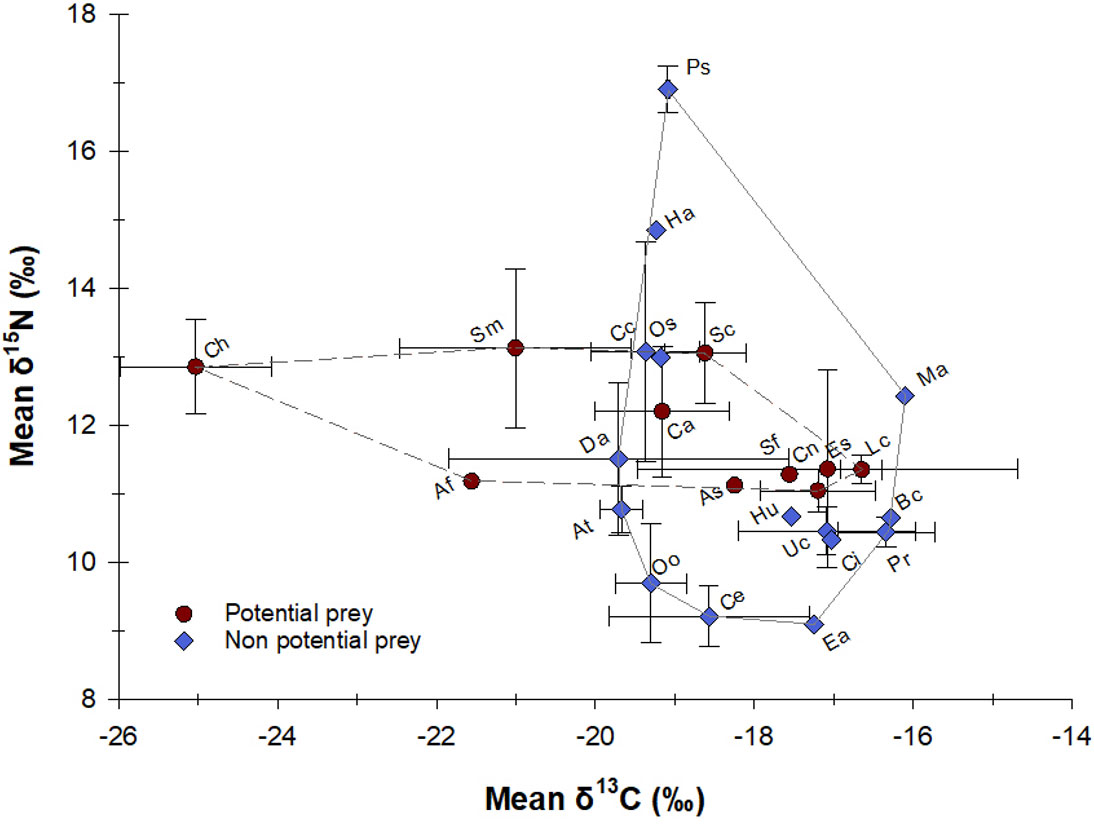
Figure 4 Mean δ13C and δ15N values (± SD, in ‰) of potential and non potential prey captured in gillnets from the artisanal fishery of Alvarado, Veracruz for the period 2015 – 2016. Ch: C. hippos; Af: A. felis; Sm: S. maculatus; Da: D. auratus; At: A. thazard; Cc: C. chrysurus; Oo: O. oglinum; Ha: H. amblyrhynchus; Ps: P. saltatrix; Os: O. saurus; Ca: C. crysos; Sc: S. cavalla; Ce: C. edentulus; As: A. surinamensis; Sf: S. foetens; Hu: H. aurolineatum; Ea: E. alletteratus; Cn: C. nobilis; Uc: U. coroides; Es: E. saurus; Ci: C. abbotti; Lc: L. campechanus; Pr: P. rubio; Bc: B. capriscus; Ma: M. americanus.
As for the trophic position, nine of the analyzed species were classified as primary consumers (TP= 2 ≤ 3), 14 were secondary consumers (TP= 3 ≤ 4), only one was a tertiary consumer (TP= 4 ≤ 5), and we had a single top predator (TP > 5). Comparisons of the weighted trophic level for all gillnets yielded no significant differences between set types (with interaction: 3.2 ± 0.3 vs. without interaction: 3.3 ± 0.5; t= -0.48, P ≥ 0.05); the same was true for prey types (potential: 3.3 ± 0.5 vs. non-potential: 3.3 ± 0.9; t=-0.11, P > 0.05) M-W: U(11,15)= 49, P ≥ 0.05).
3.2.3. Proximal composition and energy content
Average content of lipids, proteins, and energy by prey species and climatic period is shown in Table 4. In general, lipids had the greatest variation (0.7 to 32.8%), followed by proteins (55.2 to 81.2%) and energy (4.1 to 8.5 kJ g-1) considering the three climatic conditions.
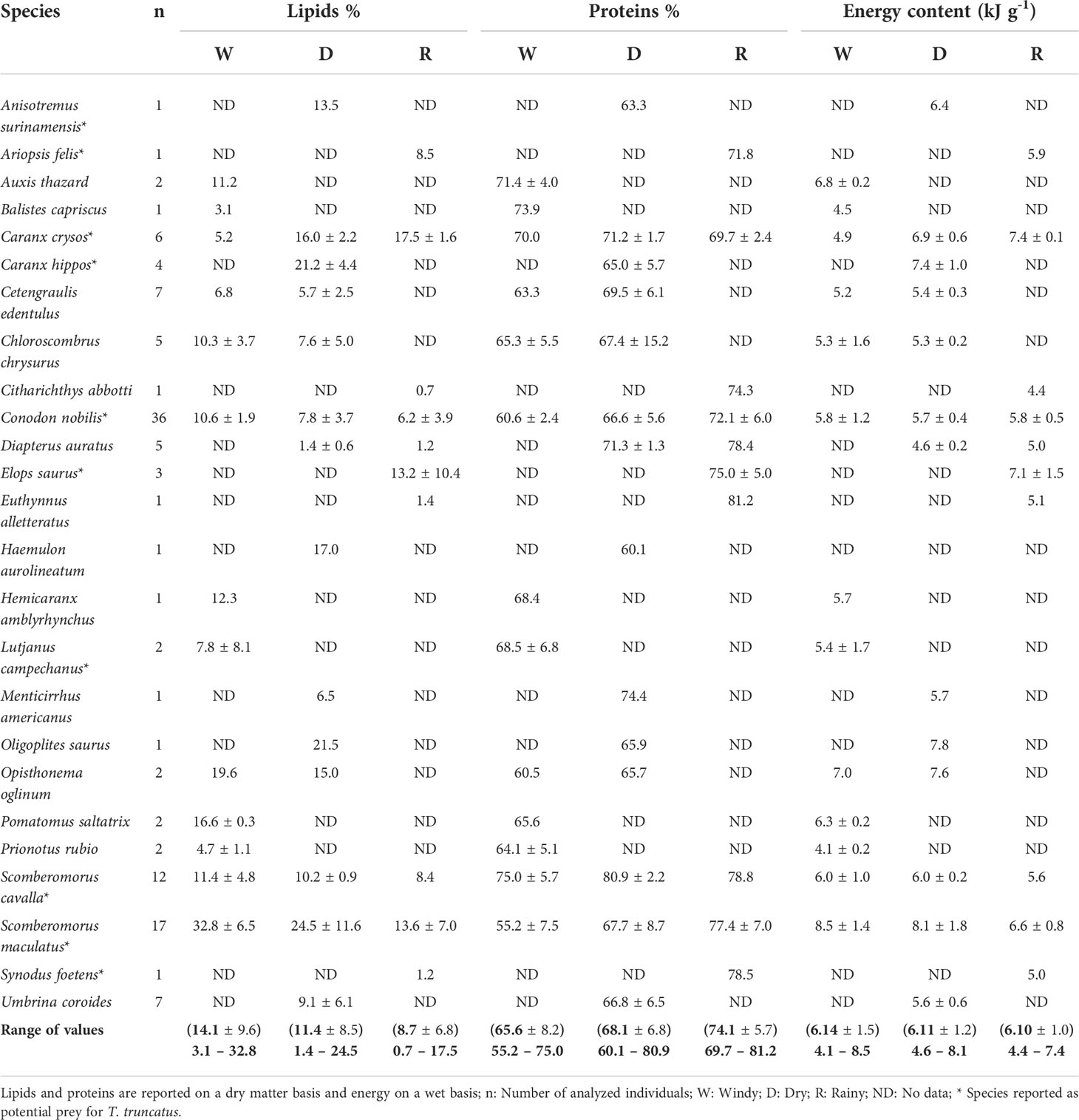
Table 4 Lipids, proteins, and energy content (average ± SD) for the captured species in gillnets from the artisanal fishery of Alvarado, Veracruz for the period 2015 – 2016.
3.2.3.1. Lipids
The species with the lowest lipid content (i.e., 0.7%) was C. abbotti, whereas S. maculatus a potential prey for the bottlenose dolphin had the highest content (i.e., 32.8%). Although this component tended to decrease from the Windy to the Rainy period, more than half of the analyzed species (68.0%, n= 25) maintained a lipid content with values higher than 6.0% throughout the three climatic conditions (Table 4). Only S. cavalla, S. maculatus and C. nobilis had sufficient data to assess seasonal differences in lipids, but significant differences were detected only for the latter (K-W, P = 0.008).
3.2.3.2. Proteins
Contrary to observations for lipid content, this proximate component increased as the Rainy period approached; however, all species exhibited proteic values over 55.0% during the three climatic conditions (Table 4). Surprisingly, S. maculatus had the lowest protein content (i.e., 55.25%), whereas the highest (i.e., 81.2%) was documented for E. alleterattus. Of the three species with sufficient temporal data, significant differences were only detected for S. maculatus (K-W, P= 0.016) and C. nobilis (K-W, P= 0.0007).
3.2.3.3. Energy content
The average energy content of the analyzed species fluctuated from 4.1 kJ g-1 for P. rubio to 8.5 kJ g-1 for S. maculatus. In some cases, (i.e., C. nobilis, S. maculatus and S. cavalla) the energy content of the species fluctuated throughout the year (Table 4), but no significant differences were detected (K-W, P ≥ 0.05; M-W: U(3,8)= 6, P ≥ 0.05).
3.2.3.4. Seasonal variation in fish proximate composition and energy
When data was polled by each climatic condition, proximal composition values for all fish (n= 123) showed a decreasing trend towards the Rainy period for lipids, and energy, whereas proteins showed the opposite pattern (Table 4). Thus, highly significant seasonal differences were only detected for protein content (F= 11.78, P= 0.00002).
3.2.3.5. Proximate composition and energy content by prey and set types
Comparisons of mean contents of lipids, proteins and energy showed that in general, potential prey (n= 11) possessed higher values than non-potential prey (n= 14), during all three climatic conditions, except for the Windy and Rainy periods when protein content was higher in non-potential prey. We found significant differences in the energy content of the potential prey among climatic conditions, specifically during the Rainy period when the averge values were higher (t= 2.61, P= 0.03). Conversely, mean lipid and protein content were higher for sets without dolphin interactions, for the Windy and Dry periods, respectively, but significant differences were only detected in the Dry period (t= -2.33, P= 0.024). Also, these sets showed a higher energy content during the Windy and Dry periods, but significant differences were found only in the Windy period (M-W: U(3,27)= 7, P= 0.02) (Table 5).

Table 5 Lipids, proteins, and energy contents (average ± SD) for the captured species in gillnets with and without dolphin interactions with the artisanal fishery of Alvarado, Veracruz for the period 2015 – 2016.
4 Discussion
Understanding of the factors (e.g., presence of target species, CPUE, gear type, etc.) related to the occurrence of depredation on fishing gear is difficult, despite of being key elements in the development of mitigation strategies (Tixier et al., 2020). To the best of our knowledge, our work is the first to assess the interaction of bottlenose dolphins with gillnets, using the ecological, trophic, and nutritional characteristics of the catch. Although our results support that interaction with gillnets is opportunistic, we found interesting evidence suggesting consistency in the trophic level of the preferred prey, as well as potential seasonal variations related to their nutritional value (i.e., protein content and energy), and also a potential selection of preferred or avoided items, as seen in other populations of T. truncatus (Hanson and Defran, 1993).
4.1 Interactions between bottlenose dolphins and depredation in gillnets
Dolphin-fisheries interactions were common (46.1%, n= 117), but less frequent than previous estimates by Rechimont et al. (2018) (i.e., 80%) for the region; such differences may be attributed to spatial and temporal variations in fishing effort (Morteo et al., 2012). Also, the depredation rate in this study (14.5%) was 5.5 times lower than that reported by Rechimont et al. (2018), but consistent with other fishing areas in the Mediterranean (i.e., 12.4%: Lauriano et al., 2004; 10%: Rocklin et al., 2009). Such striking difference may be attributed to the effect of sample size (24 vs. 117 sets in this study) and fishing techniques (i.e., gillnet traversed vs. purse seine, respectively).
The number of species (12) with evidence of depredation was lower than those reported in other areas (e.g., Lauriano et al., 2004: 14; Rocklin et al., 2009: 23); however, direct comparisons are difficult not only because of the differences in habitat biodiversity among study areas, but also since these values must always be considered minimum estimates due to survivor bias, since counts may only reflect the inability of the predators to successfully remove the whole fish from the gear. For instance, only 50% of the fish species showing evidence of depredation in our study have been reported as part of the diet of the bottlenose dolphin (Gunter, 1942; Barros and Wells, 1998; Zollett and Read, 2006; Pansard et al., 2011; Chávez-Martínez, 2017; Rechimont et al., 2018). Since the dolphins were targeting those components of the catch, these species were also considered potential prey. It is noteworthy that, despite of being one of the species with the highest signs of depredation, C. edentulus is considered an occasional prey (Naranjo-Ruiz et al., 2019)
Species richness in catches was slightly lower than previously reported, but diversity values were not different between sets with and without dolphin interaction, as was also described by Rechimont et al. (2018). The number of captured fish species is likely the result of the differences between fishing areas, since mesh sizes were the same (22.5 – 30 mm). Also, bottlenose dolphins predate on fish caught on gear that may or may not be targeting species that are part of their diet (Mitra et al., 2001; Lauriano et al., 2004; Pennino et al., 2015), but whose mesh size is small enough (i.e., 27 mm) to capture a wide variety of species including those that these dolphins may also prey upon opportunistically (Pardalou and Tsikliras, 2020).
Moreover, previous studies have exhibited varying results regarding the utility of richness and diversity, as well as biomass and CPUE, to predict depredation on gear. For instance, lower richness and diversity reportedly increased the probability of dolphin interactions with gillnets in our study area (Rechimont et al., 2018), contrasting with studies in the Mediterranean Sea, in which there was no relationship (Rocklin et al., 2009). Also, Rocklin et al. (2009) found significantly higher CPUE values in sets that showed evidence of depredation and observed that for a particular prey species (i.e., Pagellus erythrinus) the catch biomass was significantly and positively correlated with observed depredated biomass (r= 0.34, P= 0.02). Conversely, Lauriano et al. (2004) and Pennino et al. (2015) observed that CPUE values and biomass of the catch were significantly lower in fishing gear with which bottlenose dolphins interacted. It is noteworthy that we did detect higher values of biomass and CPUE in the presence of bottlenose dolphin interactions (Figures 3A, B), but there was a lack of significant differences between set types that may be due to the high variability that is inherent to fishing operations. Thus, CPUE values can vary for different reasons (e.g., availability and catchability of fishing resources, changes in fishing areas, techniques, experience and hability of the crew) (Cubillos et al., 1998; Gassman and López-Rojas, 2015), including temporal changes (e.g., climatic, and oceanographic conditions, reproductive cycles, fishing moratoriums) as occurs in our study area (Chávez-Martínez, 2017). Thus, standardized fishery data using independent experiments are warranted.
4.2 Fish characteristics
4.2.1 Passive acoustic hypothesis, feeding areas and thropic level
The passive acoustic hypothesis was not supported by our data. Hurtado-Mejorada (2021) already found that bottlenose dolphin echolocation trains were significantly more frequent during fishing manouvers in the Alvarado area. Also, García-Vital et al. (2015) found lower group sizes as a possible adaptative response to lower the risk of human retaliation during interactions with fisheris (Morales-Rincon et al., 2019). Therefore, hearing interference due to high marine traffic (e.g. Morteo et al., 2012), and/or feeding specializations should not be ruled out yet.
Bottlenose dolphins are known to feed in a great variety of habitats (Reeves et al., 2002; Niño-Torres et al., 2011; Hernandez-Milian et al., 2015). Here, both potential and non-potential fish prey seem to occupy all habitat types within the study area, supporting the opportunistic feeding nature of the dolphins. The isotopic composition of coastal fish communities is usually variable due to the different potential sources of carbon and nitrogen (Nyunja et al., 2009; Cherel et al., 2011; Rossman et al., 2015a). The fish analyzed here showed 8.9 and 7.8‰ differences between the lightest and heaviest values of δ13C and δ15N, respectively (Table 4). The broad range of isotopic compositions likely reflects different food preferences, trophic levels, and carbon and nitrogen sources (Post, 2002; Nyunja et al., 2009; Cherel et al., 2011). δ13C values were consistent with feeding in coastal and marine areas; pelagic and reef-associated species averaged -19.7 and -17.4‰, respectively, which is within the range of coastal marine phytoplankton (-22‰; France, 1995). Estuarine species with lighter isotopic composition (ca. -20‰), possibly reflect freshwater inputs that usually transport organic matter of terrestrial origin (C3) that has lower isotopic composition (ca. -27‰). Also, demersal species such as the ones found in our study have a higher isotopic composition (-17.3‰) and are more enriched in δ13C than pelagic fish (France, 1995; Cherel et al., 2011).
On the other hand, δ15N values had a positive correlation with fish SL, showing the expected increase in trophic level with age/size (Beaudoin et al., 1999; Overman and Parrish, 2001; Melville and Connolly, 2003; Fernández et al., 2011). However, due to the high variability in nitrogen isotope ratios, no significant differences in trophic level were found between prey types, nor between set types, which supports the interpretation of an opportunistic feeding behavior of bottlenose dolphins. However, potential prey species showed lower variation in δ15N (i.e., an absolute range of 2.1‰ vs. 7.8‰ for non-potential prey); the latter suggests a certain consistency in the range of trophic levels on which they feed (i.e., 3 to 4), as found in other studies where feeding specialization has been observed (Rossman et al., 2015a; Rossman et al., 2015b; Hernandez-Milian et al., 2015). This could be at least partially related to the size of prey if dolphin tend to select fish of a certain size interval, but addressing this possibility requires more detailed research on the size distribution of ingested prey. Our TL estimates are deemed reasonably reliable because the isotopic composition of the primary consumers was used as indicator of the base of the food chain, which are concordant with their diet (i.e., filter species with low trophic level). Also, the isotopic discrimination factor for nitrogen used here (Δδ15N) is appropriate for fish (McCutchan et al., 2003).
The lack of seasonal differences in isotopic composition for both elements in the few species with sufficient data (i.e., S. maculatus, S. cavalla y C. nobilis), may be attributed to small sample sizes, but also to the ability of these species to use different habitat types, or differences on the feeding habits of the fish (Simenstad and Wissmar, 1985; Vizzini and Mazzola, 2002; Vizzini and Mazzola, 2003).
4.2.2 Proximal composition and energy content
Lipid contents of fishes were highly variable as reported elsewhere (Bernard and Ullrey, 1989; Corse et al., 1999; Vollenweider et al., 2011). Nevertheless, the average content of lipids and proteins were within the ranges of known food resources for marine predators such as the bottlenose dolphin (Bernard and Allen, 1997; Spitz et al., 2010b; Vollenweider et al., 2011; Slifka et al., 2013), as well as their energy values (Spitz et al., 2012).
4.2.2.1 Lipids and proteins
Lipid and protein content, which reflects the nutritional value of a prey for consumers, typically shows strong seasonal variations in fishes (Krzynowek and Murphy, 1987; Hislop et al., 1991; Huss et al., 1998; Vollenweider et al., 2011), and are attributed to differences in size, their reproductive cycle, changes in food availability, body condition, and migratory behavior (Huss et al., 1998; Kitts et al., 2004; González et al., 2009; Vollenweider et al., 2011). Fishes can often experience periods of starvation (Huss et al., 1998), and thus rely on lipid and protein reserves for survival (Huss et al., 1998; González et al., 2009; Vollenweider et al., 2011; Martin et al., 2017).
Significant seasonal differences were detected in two species (i.e., lipids in C. nobilis, and proteins in C. nobilis and S. maculatus); both showed a decrease in lipid contents between the Windy and Rainy periods, whereas the opposite pattern was observed for proteins. For adult fish of C. nobilis (>246 mm TL: AUNAP (Autoridad Nacional de Acuicultura y Pesca), 2013) (41%, n= 36), these differences are mainly attributed to their reproductive cycle (Hislop et al., 1991; Pangle and Sutton, 2005; Pombo et al., 2014). In contrast, for young individuals (59%, n= 36) of this species, these changes seem to be related to differences in the abundance and availability of food (Huss et al., 1998; Vollenweider et al., 2011; Martin et al., 2017).
As for S. maculatus, 10 of the 17 analyzed fish (58.8%) were juveniles (<330 mm FL; Mendoza, 1968; Jiménez-Badillo et al., 2006) and the seasonal variation in their protein content may be attributed to differences in their feeding habits during their annual migration in spring and early summer (Sutherland and Fable, 1980; Colette and Nauen, 1983; Valeiras and Abad, 2006). Also, seasonal variations in seven adult fish from the 17 analyzed (41.2%) were probably related to their reproductive cycle (Vasconcelos Perez, 1976; Valeiras and Abad, 2006), as after migration or spawning, fish usually regain their protein and lipid content through feeding (Huss et al., 1998; González et al., 2009). However, these results should be treated with caution, due to the low sample size in our study.
4.2.2.2 Energy content as an indicator of gear interaction and depredation by bottlenose dolphins
Potential prey for bottlenose dolphins had a higher content of lipids, proteins and energy compared to the non-potential ones. Specifically, seasonal differences were detected for the potential prey during the Rainy period, altough with low sample sizes. Higher primary productivity caused by the runoff of continental water during this period and hence food availability within the study area (Morán-Silva et al., 2005), may produce such differences in fish species (Párraga et al., 2010). Also, higher average CPUE values (Pilling et al., 2007; Gassman and López-Rojas, 2015) are common in the area during this period (Chávez-Martínez, 2017), which suggests an increase in fish abundance.
Higher proximal composition (i.e., proteins) and energy content in prey captured in sets without dolphin interaction during some periods may be also supported by seasonal upwellings close to the coast during the dry months (Zavala-Hidalgo et al., 2003); this should produce a higher abundance of prey (Chávez-Martínez, 2017; Rechimont et al., 2018; Tixier et al., 2020). However, energy requirements for bottlenose dolphins are moderate, and they mostly target medium quality prey (4 – 5.5 kJ g-1) (Spitz et al., 2012). All the species captured by the gillnets were either within this range or higher, which would therefore meet their energy requirements. Thus, in terms of energy supply, the opportunity cost of feeding on prey captured in the gillnet is very low for this marine predator, and these will be the preferred choice regardless the energetic and nutritional value of the captured prey (Shane et al., 1986; Spitz et al., 2010a; Spitz et al., 2012; Rossman et al., 2015a).
The high proportion of interactions with gillnet sets (46.1%), and the high depredation rate (14.5%) found in this study suggest that this marine predator will likely interact with local fisheries, despite the challenges and/or hazards described for these encounters between bottlenose dolphins and artisanal fisheries (Chilvers and Corkeron, 2001; Spitz et al., 2010a; Spitz et al., 2012; Laporta et al., 2016), specifically in this area (Morteo et al., 2012; Morteo et al., 2017; Rechimont et al., 2018; Morales-Rincon et al., 2019). However, this may not be the case for the entire dolphin population inhabiting the Alvarado, Veracruz, region. For instance, Morteo et al. (2014) found that this population is sexually segregated; also, Morteo et al. (2012) and La Fauci (2017) showed reciprocal evasion between dolphins and fisheries, and this was especially true within dolphin nursing groups. In addition, as noted by Morteo et al. (2017) and Bolaños-Jiménez et al. (2021), individual dolphins that are “new” to the area (the majority of which were presumed to be male; Morales-Rincon et al., 2019), have been found to have a higher tendency to interact with local artisanal fisheries than local residents. Furthermore, dolphins in the region reduce their group sizes and reduce their surface behaviors when interacting with gillnet fisheries (García-Vital et al., 2015; Morales-Rincon et al., 2019), presumably to lower the risk of being spotted, since they often face retaliation while interacting with local artisanal fisheries (Morteo et al., 2017; Rechimont et al., 2018). In addition, dolphin’s groups may reduce their size possibly to lower intraspecific competition, and also because obtaining the resource in fishing nets is easier, so they do not need to employ cooperative hunting strategies, which also reduces interindividual competition (Methion and Díaz López, 2020). Eitherway, the latter points to feeding specializations and possibly towards a social strategy or culturally transmitted behavior (Chilvers and Corkeron, 2001; McCabe et al., 2010; Hernandez-Milian et al., 2015; Rossman et al., 2015a; Rossman et al., 2015b; Milmann et al., 2016). It is also important to notice that individual variability in highly adaptable species such as dolphins plays a key role in the evolution of foraging strategies, as these can be taught to other individuals (e.g. sponging, conching, lateral stranding, mud rings, cooperative fishing with humans, etc.) (Pryor and Lindbergh, 1990; Kopps et al., 2014). However, here we were interested in the drivers of depredation events at a population level.
Finally, we acknowledge that our sample has limited geographic and temporal coverage; however, our work enphazizes the use of ecological, trophic and nutritional data to aid in the identification of the mechanisms that prompt dolphin-fisheries interactions, which may be applied and studied elsewhere. The variables analized here are far from covering entire scope of possibilities (which is unlikely due to logistic and financial restrictions), but we selected those that have been deemed accurate predictors of these interactions (i.e., biomass, CPUE, species composition), and added a few more (i.e., δ13C, δ15N, lipid, protein, prey sound capacity, and energy content), to have a better understanding on the factors involved in depredation events.
Data availability statement
The raw data supporting the conclusions of this article will be made available by the authors, without undue reservation.
Ethics statement
Ethical review and approval was not required for the animal study because Samples involved collection of dead fish from artisanal fishers. Also, information on bottlenose dolphins was collected on board of legal fishers’ boats, thus no direct interaction with live organisms was involved in our research, which could require ethic reviews or permissions from any authority.
Author contributions
KC-M - Data collection, Sample processing, Data analysis, Manuscript Writing. EM - Conceptualization, Funds acquisition, Project manager, Methodology, Data collection, Manuscript writing and reviewing. SH - Conceptualization, Methodology, Sample processing, Manuscript reviewing. CD-A - Methodology, Data collection, Manuscript reviewing. IH-C - Methodology, Manuscript reviewing. All authors contributed to the article and approved the submitted version.
Funding
This study was part of the Master’s thesis of the first author at CICESE (Centro de Investigación Cientifica y de Educacion Superior de Ensenada), which was supported by a CONACYT scholarship (No. 339291). Field work carried out through a CONACYT grant (No. 221750) for the project “Ecología trófica de las interacciones entre delfines costeros (Tursiops truncatus) y la pesca artesanal en aguas veracruzanas”.
Acknowledgments
This study was part of the Master’s thesis of the first author at CICESE (Centro de Investigación Cientifica y de Educacion Superior de Ensenada), wich was supported by a CONACYT scholarship (No. 339291). Field work carried out through a CONACYT grant (No. 221750) for the project “Ecología trófica de las interacciones entre delfines costeros (Tursiops truncatus) y la pesca artesanal en aguas veracruzanas”. We are grateful to the three anonymous reviewers that provided detailed observations that greatly enhanced our manuscript. We also thank fisher Roberto Tiburcio† and his family, which allowed us to tag along in their fishing activities. We also want to thank Mariely Daniel-Presa, Pilar Gonzalez-Barroso, and Nataly Morales-Rincón for their help in data collection, as well as Erick Bolaños and Pamela García for processing fish samples. We are particurlary greatful to Marcela Rosas-Nexticapa, and Lol Ki I. López-Galindo at Facultad de Nutrición Xalapa (UV), and J. Gaudencio Barreda-Herrera at Facultad de Biología Xalapa (UV), also, to Juan Pablo Lazo of the Department of Aquaculture (CICESE) and Luis Eduardo Calderon at Deparment of Biological Oceanograpy (CICESE) for granting access to their laboratories and equipment for the proximate analyses. Many thanks also to Maria Teresa Nuche for her help with the lipid extraction equipment, and in the determination of the proximate components.
Conflict of interest
The authors declare that the research was conducted in the absence of any commercial or financial relationships that could be construed as a potential conflict of interest.
Publisher’s note
All claims expressed in this article are solely those of the authors and do not necessarily represent those of their affiliated organizations, or those of the publisher, the editors and the reviewers. Any product that may be evaluated in this article, or claim that may be made by its manufacturer, is not guaranteed or endorsed by the publisher.
Supplementary material
The Supplementary Material for this article can be found online at: https://www.frontiersin.org/articles/10.3389/fmars.2022.870012/full#supplementary-material
References
Arias-Zapata A. (2019). Percepción de pescadores artesanales ante la interacción con tursiones costeros (Tursiops truncatus). ([Veracruz, México]: Universidad Veracruzana).
AUNAP (Autoridad Nacional de Acuicultura y Pesca). (2013)Evaluación de la información biológico pesquera y estado de aprovechamiento de las especies avaluadas. In: Convenio 0005 AUNAP-UNIMAGDALENA (Universidad del Magdalena). Available at: https://1library.co/document/yjj8196y-evaluacion-informacion-biologico-pesquera-aprovechamiento-especies-evaluadas-unimagdalena.html (Accessed November 17, 2021).
Barros N. B., Odell D. K. (1990). “"Food habits of bottlenose dolphins in the southeastern united states,",” in The bottlenose dolphin. Eds. Leatherwood S., Reeves R. R. (San Diego, CA: Academic Press, Inc), 309–328.
Barros N. B., Wells R. S. (1998). Prey and feeding patterns of resident bottlenose dolphins (Tursiops truncatus) in Sarasota bay, Florida. J. Mammal. 79, 1045–1059. doi: 10.2307/1383114
Bearzi G. (2002). “"Interactions between cetaceans and fisheries in the Mediterranean sea," in cetaceans of the Mediterranean and black seas,” in State of knowledge and conservation strategies. Ed. Notarbartolo di Sciara G. (Rome, Italy: A report to the ACCOBAMS Secretariat, Monaco), 78–97.
Bearzi G., Piwetz S., Reeves R. R. (2019). “"Odontocete adaptations to human impact, and vice-versa,",” in Ethology and behavioral ecology of odontocetes. Ed. Würsig B. (Cham: Springer, Heidelberg), 211–235.
Bearzi G., Politi E., Agazzi S., Azzellino A. (2006). Prey depletion caused by overfishing and the decline of marine megafauna in the eastern Ionian Sea coastal waters (central Mediterranean). Biol. Conserv. 127, 373–382. doi: 10.1016/j.biocon.2005.08.017
Beaudoin C. P., Tonn W. M., Prepas E. E., Wassenaar L. I. (1999). Individual specialisation and trophic adaptability of northern pike (Exos lucius): an isotope and dietary analysis. Oecologia 120, 386–396. doi: 10.1007/s004420050871
Beddington J. R., Beverton R. J. H., Lavigne D. M. (1985). Marine mammals and fisheries (London: George Allen and Unwin).
Bernard J. B., Allen M. A. (1997). “"Feeding captive piscivorous animals: nutritional aspects of fish as food,",” in Nutrition advisory group handbook. Eds. Baer D., Crissey S., Ullrey D. (American Zoo and Aquarium Association, Brookfield, IL), 1–12. Fact Sheet, 5.
Bernard J. B., Ullrey D. E. (1989). Evaluation of dietary husbandry of marine mammals at two major zoological parks. J. Zoo Wildl. Med. 20, 45–52. https://www.jstor.org/stable/20094916
Blasi M. F., Giuliani A., Boitani L. (2015). Influence of trammelnets on the behaviour and spatial distribution of bottlenose dolphins (Tursiops truncatus) in the aeolian archipelago, southern Italy. Aquat. Mamm. 41, 295–310. doi: 10.1578/AM.41.3.2015.295
Bolaños-Jiménez J., Morteo E., Delfín-Alfonso C., Fruet P. F., Secchi E. R., Bello-Pineda J. (2021). Population dynamics reveal a core community of the common bottlenose dolphin (Tursiops truncatus) in open waters of the south-Western gulf of Mexico. Front. Mar. Sci. 8. doi: 10.3389/fmars.2021.753484
Carrillo-Alejandro P., Beléndez-Moreno L. F. J., Quiroga-Brahms C., Lorán-Núñez R.M., Martínez Isunza F.R., Pech-Paat J.A., et al. (2014). “"Plan de manejo pesquero del sistema lagunar de alvarado, veracruz",” in Sustentabilidad y pesca responsable en méxico (CDMX, México: Instituto Nacional de pesca), 359–463. Evaluación y manejo, ed. Instituto Nacional de Pesca.
Chávez-Martínez K. (2017). Interacción entre el tursión (Tursiops truncatus) y la pesca artisanal de alvarado, veracruz, en función de la captura, calidad nutricional y composición isotópica de la comunidad de peces (Baja California, México: Centro de Investigación Científica y de Educación Superior de Ensenada, Baja California).
Cherel Y., Koubbi P., Giraldo C., Penot F., Tavernier E., Moteki M., et al. (2011). Isotopic niches of fishes in coastal, neritic and oceanic waters off adélie land, Antarctica. Polar Sci. 5, 286–297. doi: 10.1016/j.polar.2010.12.004
Chilvers B. L., Corkeron P. J. (2001). Trawling and bottlenose dolphins' social structure. Proc. R. Soc London Ser. B: Biol. Sc. 268, 1901–1905. doi: 10.1098/rspb.2001.1732
Colette B. B., Nauen C. E. (1983). “FAO (Food and agriculture organization) species catalogue. vol. 2. scombrids of the world,” in An annotated and illustrated catalogue of tunas, mackerels, bonitos and related species known to date (Rome, Italy: FAO).
Contreras E. F. (1985). Lagunas costeras mexicanas (México: Centro de Ecodesarrollo, Secretaria de Pesca).
Corse M., Glick-Bauer M., Saul B., Dierenfeld E. S. (1999). “Nutrient composition of locallyobtained native fishes (St. catherine’s island wildlife survival center, GA, USA) compared with fish commonly purchased for north American zoo feeding programs,” in Proceedings of the 3rd nutrition advisory group conference (Columbus, Ohio, AZA Nutrition Advisory Group).
Cubillos L., Canales M., Hernández A., Bucarey D., Vilugrón L., Miranda L. (1998). Poder de pesca, esfuerzo de pesca y cambios estacionales e interanuales en la abundancia relativa de strangomera bentincki y Engraulis ringens en el área frente a talcahuano, Chile (1990-1997). Invest. Mar. Valparaiso. 26, 3–14.
Díaz-López B. (2006). Interactions between Mediterranean bottlenose dolphins (Tursiops truncatus) and gillnets off Sardinia, Italy. ICES J. Mar. Sci. 63 (5), 946–951. doi: 10.1016/j.icesjms.2005.06.012
Dunshea G., Barros N. B., McCabe E. J. B., Gales N. J., Hindell N. A., Jarman S. N., et al. (2013). Stranded dolphin stomach contents represent the free-ranging population´s diet. Biol. Lett. 9, 20121036. doi: 10.1098/rsbl.2012.1036
FAO (Food and Agriculture Organization). (1993). Manual de técnicas para laboratorio de nutrición de peces y crustáceos (México: Programa cooperativo gubernamental FAO–Italia).
Fernández R., García-Tiscar S., Santos M. B., López A., Martínez-Cedeira J. A., Newton J., et al. (2011). Stable isotope analysis in two sympatric populations of bottlenose dolphins Tursiops truncatus: Evidence of resource partitioning? Mar. Biol. 158, 1043–1055.
Fertl D., Leatherwood S. (1997). Cetacean interactions with trawls: A preliminary review. J. Northwest Atl. Fish. Sci. 22, 219–248. doi: 10.2960/J.v22.a17
Fish M. P., Mowbray W. H. (1970). “Sounds of Western North Atlantic fishes: a reference file of biological underwater sounds,” (Baltimore: Johns Hopkins Press).
France R. (1995). Stable nitrogen isotopes in fish: literature synthesis on the influence of ecotonal coupling. Estuar. Coast. Shelf Sci. 41, 737–742. doi: 10.1006/ecss.1995.0087
Froese R., Pauly D. (2021) FishBase (World Wide Web electronic publication). Available at: www.fishbase.org (Accessed September 12, 2021).
Gannon D. P., Barros N. B., Nowacek D. P., Read A. J., Waples D. M., Wells R. S. (2005). Prey detection by bottlenose dolphins, Tursiops truncatus: An experimental test of the passive listening hypothesis. Anim. Behav. 69, 709–720. doi: 10.1016/j.anbehav.2004.06.020
García-Vital M., Morteo E., Martínez-Serrano I., Delgado-Estrella A., Bazúa-Durán C. (2015). Inter-individual association levels correlate to behavioral diversity in coastal bottlenose dolphins (Tursiops truncatus) from the southwestern gulf of Mexico. Therya 6, 337–350. doi: 10.12933/therya-15-270
Gassman J., López-Rojas H. (2015). Variación de la abundancia de los recursos pesqueros en el parque nacional Laguna de tacarigua, Venezuela. Acta Biol. Venez. 35, 11–25 http://saber.ucv.ve/ojs/index.php/revista_abv/article/view/10307.
González Á., Márquez A., Senior W., Martínez G. (2009). Contenido de grasa y proteina en pygocentrus cariba, prochilodus mariae, Plagioscion squamosissimus, piaractus brachypomus e Hypostomus plecostomus en una laguna de inundacion del orinoco medio. Rev. Cient. 19, 15–21 http://www.redalyc.org/articulo.oa?id=95911638003.
Guerra A. S. (2019). Wolves of the Sea: Managing human-wildlife conflicto in an increasingly tense ocean. Mar. Policy. 99, 369–373. doi: 10.1016/j.marpol.2018.11.002
Gunter G. (1942). Contributions to the natural history of the bottlenose dolphin, Tursiops truncatus (Montague), on the Texas coast, with particular reference to food habits. J. Mammal. 23, 267–276. doi: 10.2307/1374993
Hanson M. T., Defran R. H. (1993). The behaviour and feeding ecology of the pacific coast bottlenose dolphin, tursiops truncatus. Aquat. Mamm. 19, 127–142.
Hernandez-Milian G., Berrow S., Santos M. B., Reid D., Rogan E. (2015). Insights into the trophic ecology of bottlenose dolphins (Tursiops truncatus) in Irish waters. Aquat. Mamm. 41, 226–239. doi: 10.1578/AM.41.2.2015.226
Hislop J. R. G., Harris M. P., Smith J. G. M. (1991). Variation in the calorific value and total energy content of the lesser sandeel (Ammodytes marinus) and other fish preyed on by seabirds. J. Zool. 224, 501–517. doi: 10.1111/j.1469-7998.1991.tb06039.x
Hurtado-Mejorada O. (2021). “Actividad vocal y contexto conductual de tursiones que interactúan con la pesca artesanal en Alvarado, Veracruz, México,” (Veracruz, México: Universidad Veracruzana).
Huss H. H., Boerresen T., Dalgaard P., Gram L., Jensen B. (1998). “El Pescado fresco: su calidad y cambios de su calidad,” in FAO (Food and agriculture organization) documento técnico de pesca no. 348 (Rome, Italy: Programa cooperativo gubernamental FAO–Italia).
IUCN (International Union for Conservation of Nature). (2021) The IUCN red list of threatened species. version 2021-3. Available at: https://www.iucnredlist.org (Accessed September 12, 2021).
Jiménez-Badillo M. D. L., Pérez-España H., Vargas-Hernández J. M., Cortéz-Salinas J. C., Flores-Pineda P. A. (2006). Catálogo de especies y artes de pesca del parque nacional sistema arrecifal veracruzano (México: Comisión Nacional para el Conocimiento y Uso de la Biodiversidad/Universidad Veracruzana).
Jog K., Sutaria D., Diedrich A., Grech A., Marsh H. (2022). Marine mammal interactions with fisheries: Review of research and management trends across commercial and small-scale fisheries. Front. Mar. Sci. 9, 758013. doi: 10.3389/fmars.2022.758013
Kitts D. D., Huynh M. D., Hu C., Trites A. W. (2004). Season variation in nutrient composition of alaskan walleye pollock. Can. J. Zool. 82, 1408–1415. doi: 10.1139/z04-116
Knight L., Ladich F. (2014). Distress sound of thorny catfishes emitted underwater and in air: characteristics and potential significance. J. Exp. Biol. 217, 4068–4078. doi: 10.1242/jeb.110957
Kopps A. M., Krützen M., Allen S. J., Bacher K., Sherwin W. B. (2014). Characterizing the socially transmitted foraging tactic “sponging” by bottlenose dolphins (Tursiops sp.) in the western gulf of shark bay, Western Australia. Mar. Mam Sci. 30, 847–863. doi: 10.1111/mms.12089
Krzynowek J., Murphy J. (1987). Proximate composition, energy, fatty acid, sodium, and cholesterol content of finfish, shellfish, and their products (Maryland: US Department of Commerce, National Oceanic and Atmospheric Administration, National Marine Fisheries Service).
La Fauci D. (2017). Spatial segregation by age class in the bottlenose dolphin tursiops truncatus (Montagü, 1821) of the central coast of veracruz ([Veracruz, México]: Universidad Veracruzana).
Laporta P., Fruet P. F., Siciliano S., Flores P. A., Loureiro J. D. (2016). Report of the working group on the biology and ecology of tursiops truncatus in the southwest Atlantic ocean. Lat. Am. J. Aquat. Mamm. 11, 62–70. doi: 10.5597/00216
Lauriano G., Fortuna C. M., Moltedo G., Notarbartolo Di Sciara G. (2004). Interactions between bottlenose dolphins (Tursiops truncatus) and the artisanal fishery in asinara island national park (Sardinia): Assessment of catch damage and economic loss. J. Cetacean Res. Manage. 6, 165–173. https://www.researchgate.net/publication/229180774_Interactions_between_bottlenose_dolphins_and_the_artisanal_fishery_in_Asinara_Island_National_Park_Sardinia_assessment_of_catch_damage_and_economic_loss
Martin B. T., Heintz R., Danner E. M., Nisbet R. M. (2017). Integrating lipid storage into general representations of fish energetics. J. Anim. Ecol. 86, 812–825. doi: 10.1111/1365-2656.12667
McCabe E. J. B., Gannon D. P., Barros N. B., Wells R. S. (2010). Prey selection by resident common bottlenose dolphins (Tursiops truncatus) in Sarasota bay, Florida. Mar. Biol. 157, 931–942. doi: 10.1007/s00227-009-1371-2
McCutchan J. H., Lewis W. M., Kendall C., Mcgrath C. C. (2003). Variation in trophic shift for stable isotope ratios of carbon, nitrogen, and sulfur. Oikos 102, 378–390. doi: 10.1034/j.1600-0706.2003.12098.x
Melville A. J., Connolly R. M. (2003). Spatial analysis of stable isotope data to determine primary sources of nutrition for fish. Oecologia 136, 499–507. doi: 10.1007/s00442-003-1302-8
Mendoza N. A. (1968). Consideraciones sobre la biología pesquera de la sierra, scomberomorus maculatus (Mitchill), en el estado de veracruz. Bios 1, 11–22.
Methion S., Díaz López B. (2020). Individual foraging variation drives social organization in bottlenose dolphins. Behav. Ecol. 31 (1), 97–106. doi: 10.1093/beheco/arz160
Milmann L., Danilewicz D., Machado R., Santos R. A. D., Ott P. H. (2016). Feeding ecology of the common bottlenose dolphin, Tursiops truncatus, in southern Brazil: analyzing its prey and the potential overlap with fisheries. Braz. J. Oceanogr. 64, 415–422. doi: 10.1590/s1679-87592016116406404
Mitra S., Koutrakis E., Clark T., Milani C. (2001). “Cetacean interaction with small scale coastal fisheries: Implications for conservation and damage limitation in the northern Aegean, Greece,” in Abstract book of the 15th annual conference of the European cetacean society, (6–10).
Morales-Rincon N., Morteo E., Delfín-Alfonso C. A. (2019). Influence of artisanal fisheries on the behaviour and social structure of tursiops truncatus in the south-western gulf of Mexico. J. Mar. Biolog. Assoc. 99, 1–9. doi: 10.1017/S002531541900078X
Mora-Manzano M. (2018). “Análisis de la riqueza y diversidad taxonómica de la dieta del tursión, tursiops truncatus (Montagu 1821), con base en literatura publicada,” in Centro universitario de ciencias biológicas y agropecuarias (Guadalajara, Jalisco, México: Universidad de Guadalajara), 55 pp.
Morán-Silva Á., Franco L. A. M., Chávez-López R., Franco-López J., Bedia-Sanchez C., Espinosa C., et al. (2005). Seasonal and spatial patterns in salinity, nutrients, and chlorophyll a in the alvarado lagoonal system, veracruz, Mexico. Gulf Caribb. Res. 17, 133–143. doi: 10.18785/gcr.1701.14
Morteo E. (2011). Ecología social de delfines tursiops truncatus en aguas costeras de alvarado, veracruz, méxico (Boca del Río, Veracruz, México: Instituto de Ciencias Marinas y Pesquerías, Universidad Veracruzana), 129 pp. doi: 10.13140/2.1.1030.048
Morteo E., Rocha-Olivares A., Abarca-Arenas L. G. (2014). Sexual segregation in coastal bottlenose dolphins (Tursiops truncatus) in the south-western gulf of Mexico. Aquat. Mamm. 40, 375–385. doi: 10.1578/AM.40.4.2014.375
Morteo E., Rocha-Olivares A., Abarca-Arenas L. G. (2017). Abundance, residency and potential hazards for coastal bottlenose dolphins (Tursiops truncatus) off a productive lagoon in the gulf of Mexico. Aquat. Mamm. 43, 308–319. doi: 10.1578/AM.43.3.2017.308
Morteo E., Rocha-Olivares A., Arceo-Briseño P., Abarca-Arenas L. G. (2012). Spatial analyses of bottlenose dolphin-fisheries interactions reveal human avoidance off a productive lagoon in the western gulf of Mexico. J. Mar. Biol. Assoc. U. K. 92, 1893–1900. doi: 10.1017/S0025315411000488
Naranjo-Ruiz K. L., Delgado-Estrella A., Morquecho-León M. R. K., Torres-Rojas Y. E. (2019). Determinación de peces presas consumidos por toninas (Tursiops truncatus) que vararon en la isla del Carmen, campeche. Rev. Mex. Biodivers. 90, e902513.
Niño-Torres C. A., Urbán Ramírez J., Vidal O. (2011). “Mamíferos marinos del golfo de California,” in Guía ilustrada (México: Alianza WWF México-Telcel).
Northridge S. P. (1984). “FAO (Food and agriculture organization) fisheries technical paper. no. 251,” in World review of interactions between marine mammals and fisheries (Rome, Italy: FAO).
Northridge S. P. (1991). “FAO (Food and agriculture organization) fisheries technical paper. no. 251, suppl. 1,” in An updated world review of interactions between marine mammals and fisheries (Rome, Italy: FAO).
Nyunja J., Ntiba M., Onyari J., Mavuti K., Soetaert K., Bouillon S. (2009). Carbon sources supporting a diverse fish community in a tropical coastal ecosystem (Gazi bay, Kenya). Estuar. Coast. Shelf Sci. 83, 333–341. doi: 10.1016/j.ecss.2009.01.009
Overman N. C., Parrish D. L. (2001). Stable isotope composition of walleye: 15N accumulation with age and area-specific differences in δ13C. Can. J. Fish. Aquat. Sci. 58, 1253–1260. doi: 10.1139/f01-072
Pangle K. L., Sutton T. M. (2005). Temporal changes in the relationship between condition indices and proximate composition of juvenile coregonus artedi. J. Fish Biol. 66, 1060–1072. doi: 10.1111/j.0022-1112.2005.00660.x
Pansard K. C. A., Gurgel H. d. C.B., Andrade L.C. d. A., Yamamoto M. E. (2011). Feeding ecology of the estuarine dolphin (Sotalia guianensis) on the coast of Rio grande do norte, Brazil. Mar. Mamm. Sci. 27, 673–687. doi: 10.1111/j.1748-7692.2010.00436.x
Pardalou A., Tsikliras A. C. (2020). Factors influencing dolphin depredation in coastal fisheries of the northern Aegean Sea: Implications on defining mitigation measures. Mar. Mamm. Sci. 36, 1126–1149. doi: 10.1111/mms.12702
Párraga D. P., Cubillos L. A., Correa-Ramirez M. A. (2010). Variaciones espacio-temporales de la captura por unidad de esfuerzo en la pesquería artesanal costera del pargo rayado lutjanus synagris, en el caribe colombiano y su relación con variables ambientales. Rev. Biol. Mar. Oceanogr. 45, 77–88. doi: 10.4067/S0718-19572010000100007
Pennino M. G., Rotta A., Pierce G. J., Bellido J. M. (2015). Interaction between bottlenose dolphin (Tursiops truncatus) and trammel nets in the archipelago de la maddalena, Italy. Hydrobiologia 747, 69–82. doi: 10.1007/s10750-014-2127-7
Pilling G. M., Cotter A. J. R., Metcalfe J. D. (2007). “ICCAT field manual: Chapter 4,” in Data for assessment and research (Lowestoft, UK: ICCAT Publications (on-line).
Pombo M., Denadai M. R., Bessa E., Santos F. B., Faria V. H., Turra A. (2014). The barred grunt Conodon nobilis (Perciformes: Haemulidae) in shallow areas of a tropical bight: spatial and temporal distribution, body growth and diet. Helgol. Mar. Res. 68, 271–279. doi: 10.1007/s10152-014-0387-2
Post D. M. (2002). Using stable isotopes to estimate trophic position: models, methos, and assumptions. Ecology 83, 703–718. doi: 10.1890/0012-9658(2002)083[0703:USITET]2.0.CO;2
Pryor K., Lindbergh J. (1990). A dolphin-human fishing cooperative in Brazil. Mar. Mamm. Sci. 6, 77–82. doi: 10.1111/j.1748-7692.1990.tb00228.x
Read A. J. (2008). The looming crisis: Interactions between marine mammals and fisheries. J. Mammal. 89, 541–548. doi: 10.1644/07-MAMM-S-315R1.1
Rechimont M. E., Lara-Domínguez A. L., Morteo E., Martínez-Serrano I., Equihua M. (2018). Depredation by coastal bottlenose dolphins (Tursiops truncatus) in the southwestern gulf of Mexico in relation to fishing techniques. Aquat. Mamm. 44, 469–481. doi: 10.1578/AM.44.5.2018.469
Reeves R. R., Stewartt B. S., Clapham P. J., Powell J. A., Folkens P. A. (2002). Guide to marine mammals of the world (New York: National Audubon Society).
Rocklin D., Santoni M.-C., Culioli J.-M., Tomasini J.-A., Pelletier D., Mouillot D. (2009). Changes in the catch composition of artisanal fisheries attributable to dolphin depredation in a Mediterranean marine reserve. ICES J. Mar. Sci. 66, 699–707. doi: 10.1093/icesjms/fsp036
Rossman S., Berens-Mccabe E., Barros N. B., Gandhi H., Ostrom P. H., Stricker C. A., et al. (2015a). Foraging habits in a generalist predator: Sex and age influence habitat selection and resource use among bottlenose dolphins (Tursiops truncatus). Mar. Mamm. Sci. 31, 155–168. doi: 10.1111/mms.12143
Rossman S., Ostrom P. H., Stolen M., Barros N. B., Gandhi H., Stricker C. A., et al. (2015b). Individual specialization in the foraging habits of female bottlenose dolphins living in a trophically diverse and habitat rich estuary. Oecologia 178, 415–425. doi: 10.1007/s00442-015-3241-6
Shane S. H., Wells R. S., Würsig B. (1986). Ecology, behavior and social organization of the bottlenose dolphin: A review. Mar. Mamm. Sci. 2, 34–63. doi: 10.1111/j.1748-7692.1986.tb00026.x
Simenstad C. A., Wissmar R. C. (1985). δ¹³C evidence of the origins and fates of organic carbon in estuarine and nearshore food webs. Mar. Ecol. Prog. Ser. 22, 141–152. doi: 10.3354/meps022141
Slifka K. A., Wells R. S., Ardente A. J., Crissey S. (2013). Comparative diet analysis of fish species commonly consumed by managed and free-ranging bottlenose dolphins (Tursiops truncatus). J. Veterin. Med. 10, 1–6.
Spitz J., Mourocq E., Leauté J.-P., Quéro J.-C., Ridoux V. (2010a). Prey selection by the common dolphin: Fulfilling high energy requirements with high quality food. J. Exp. Mar. Biol. Ecol. 390, 73–77. doi: 10.1016/j.jembe.2010.05.010
Spitz J., Mourocq E., Schoen V., Ridoux V. (2010b). Proximate composition and energy content of forage species from the bay of Biscay: high-or low-quality food? ICES J. Mar. Sci. 67, 909–915. doi: 10.1093/icesjms/fsq008
Spitz J., Trites A. W., Becquet V., Brind’Amour A. (2012). Cost of living dictates what whales, dolphins and porpoises eat: the importance of prey quality on predator foraging strategies. PloS One 7, 1–7. doi: 10.1371/journal.pone.0050096
Sutherland D. F., Fable W. A. (1980). Results of a king mackerel (Scomberomorus cavalla) and Atlantic Spanish mackerel (Scomberomorus maculatus) migration study 1975-79. Technical Memorandum. National Oceanic and Atmospheric Administration/National Marine Fisheries ServiceSEFC 1–24.
Tixier P., Lea M. A., Hindell M. A., Welsford D., Mazé C., Gourguet S., et al. (2020). When large marine predators feed on fisheries catches: Global patterns of the depredation conflict and directions for coexistence. Fish Fish. 22, 31–53. doi: 10.1111/faf.12504
Valeiras J., Abad E. (2006). “Manual de ICCAT: Capítulo 2.1.10.6,” in Carita atlántico (Lowestoft, UK: ICCAT Publications).
Vasconcelos Perez M. J. (1976). “Observations on reproduction, fecundity and condition factor of the Spanish mackerel scomberomorus maculatus off the coast of the veracruz state,” in Memoirs (Veracruz, Mexico: Meeting on the Coastal Fishery Resources of Mexico).
Vidal O., Van Waerebeek K., Findley L. T. (1994). “Cetaceans and gillnet fisheries in Mexico, central America and the wider Caribbean: a preliminary review,” in Gillnets and Cetaceans Perrin W. F., Donovan G. P., Barlow J. (Cambridge: Report of the international whaling commission), 221–233. doi: 10.13140/RG.2.2.31459.76326
Villanueva-Fortanelli J. J. (2015). Estudio socieconómico de los pescadores de jaiba en la Laguna de alvarado, veracruz, méxico, para contribuir al manejo integral de la pesquería. Cienc. Pesq. 23, 101–113 https://www.gob.mx/cms/uploads/attachment/file/194914/11_Villanueva_2015__23_especial_.pdf.
Vizzini S., Mazzola A. (2002). Stable carbon and nitrogen ratios in the sand smelt from a Mediterranean coastal area: feeding habits and effect of season and size. J. Fish Biol. 60, 1498–1510. doi: 10.1111/j.1095-8649.2002.tb02443.x
Vizzini S., Mazzola A. (2003). Seasonal variations in the stable carbon and nitrogen isotope ratios (13C/12C and 15N/14N) of primary producers and consumers in a western Mediterranean coastal lagoon. Mar. Biol. 142, 1009–1018. doi: 10.1007/s00227-003-1027-6
Vollenweider J. J., Heintz R. A., Schaufler L., Bradshaw R. (2011). Seasonal cycles in whole-body proximate composition and energy content of forage fish vary with water depth. Mar. Biol. 158, 413–427. doi: 10.1007/s00227-010-1569-3
Zavala-González A., Urbán-Ramírez J., Esquivel-Macías C. (1994). “A note on artisanal fisheries interactions with small cetaceans in Mexico,” in Gillnets and Cetaceans Perrin W. F., Donovan G. P., Barlow J. (Cambridge: Report of the international whaling commission), 235–237.
Zavala-Hidalgo J., Morey S. L., O’Brien J. J. (2003). Seasonal circulation on the western shelf of the gulf of Mexico using a high-resolution numerical model. J. Geophys. Res. 108, 3389. doi: 10.1029/2003JC001879
Zollett E. A., Read A. J. (2006). Depredation of catch by bottlenose dolphins (Tursiops truncatus) in the Florida king mackerel (Scomberomorus cavalid) troll fishery. Fish Bull. 104, 343–349 https://spo.nmfs.noaa.gov/sites/default/files/pdf-content/2006/1043/zollett.pdf.
Keywords: artisanal fishery, energy content, biomass, CPUE, protein, lipid, isitopic carbon and nitrogen
Citation: Chávez-Martínez K, Morteo E, Hernández-Candelario I, Herzka SZ and Delfín-Alfonso CA (2022) Opportunistic gillnet depredation by common bottlenose dolphins in the southwestern Gulf of Mexico: Testing the relationship with ecological, trophic, and nutritional characteristics of their prey. Front. Mar. Sci. 9:870012. doi: 10.3389/fmars.2022.870012
Received: 05 February 2022; Accepted: 05 July 2022;
Published: 28 July 2022.
Edited by:
Jeremy Kiszka, Florida International University, United StatesReviewed by:
Severine Methion, Bottlenose Dolphin Research Institute (BDRI), SpainVictor Hugo Valiati, University of the Rio dos Sinos Valley, Brazil
Copyright © 2022 Chávez-Martínez, Morteo, Hernández-Candelario, Herzka and Delfín-Alfonso. This is an open-access article distributed under the terms of the Creative Commons Attribution License (CC BY). The use, distribution or reproduction in other forums is permitted, provided the original author(s) and the copyright owner(s) are credited and that the original publication in this journal is cited, in accordance with accepted academic practice. No use, distribution or reproduction is permitted which does not comply with these terms.
*Correspondence: Eduardo Morteo, ZWR1YXJkby5tb3J0ZW9AZ21haWwuY29t