- 1Nature Coast Biological Station, University of Florida Institute of Food and Agricultural Sciences, Cedar Key, FL, United States
- 2Dauphin Island Sea Lab, Dauphin Island, AL, United States
A warming climate is driving the poleward expansion of tropical, subtropical, and temperate plant and animal distributions. These changes have and continue to lead to the colonization of novel organisms into areas beyond their historical ranges. While the full scope of ecological impacts remains unclear, these expansions could alter density-dependent interactions, habitat occupancy patterns, and food web dynamics– similar to exotic species impacts in invaded ecosystems. Seagrasses are habitats of particular interest, given their widespread distribution and ecosystem services. While multiple recent studies report on the effects of the return of larger tropical herbivores in seagrass beds in warming subtropical waters, less is known about the addition of mid-trophic level consumers. These consumers are often key determinants of energy and nutrient transfers from basal resources to higher order predators. Here, we discuss the potential impacts of these distribution changes on temperate and subtropical seagrass communities using information derived from invasive species studies. Notably, we outline several scenarios and generate predictions about how their establishment might occur and speculate on impacts of warmer water consumers as they move poleward. We also discuss potential confounding factors of detecting changes in these consumer distributions. Following the invasive species literature, we offer a framework for generating hypotheses and predicting effects from these range-expanding organisms. Given that climates are predicted to continue to warm into the future, thus facilitating additional species expansions, our goal is to guide future research efforts and provide information for rapid dissemination and utility for this growing subdiscipline of marine ecology.
Introduction
Globally, temperatures are projected to increase by as much as 4.5°C this century, with the “overwhelming majority of regional climate effects on terrestrial species revealing consistent responses to warming trends, including poleward and elevational range shifts of flora and fauna’’ (Bernstein et al., 2008; Sorte et al., 2010). Ocean ecologists have similarly documented the poleward expansion of species (often referred to as “tropicalization,” although expansion across other climate zones occur). With the elimination of allopatric thermal dispersal barriers, new records of non-endemic occurrences in subtropical, temperate, and polar waters are now common (Figure 1). An extensive survey of marine range extensions along the Pacific coast of the United States, for example, documented the poleward movement of some 128 (primarily invertebrate) species (Sorte et al., 2010), with 11 negatively impacting newly colonized ecosystems. Since then, additional studies have highlighted examples of expanding distributions poleward (Fodrie et al., 2010, e Costa et al., 2014; Vergés et al., 2016). We, and others (Dunstan and Bax, 2007; Sorte et al., 2010), find movement of warmer water species into new areas analogous to introductions of non-native species.
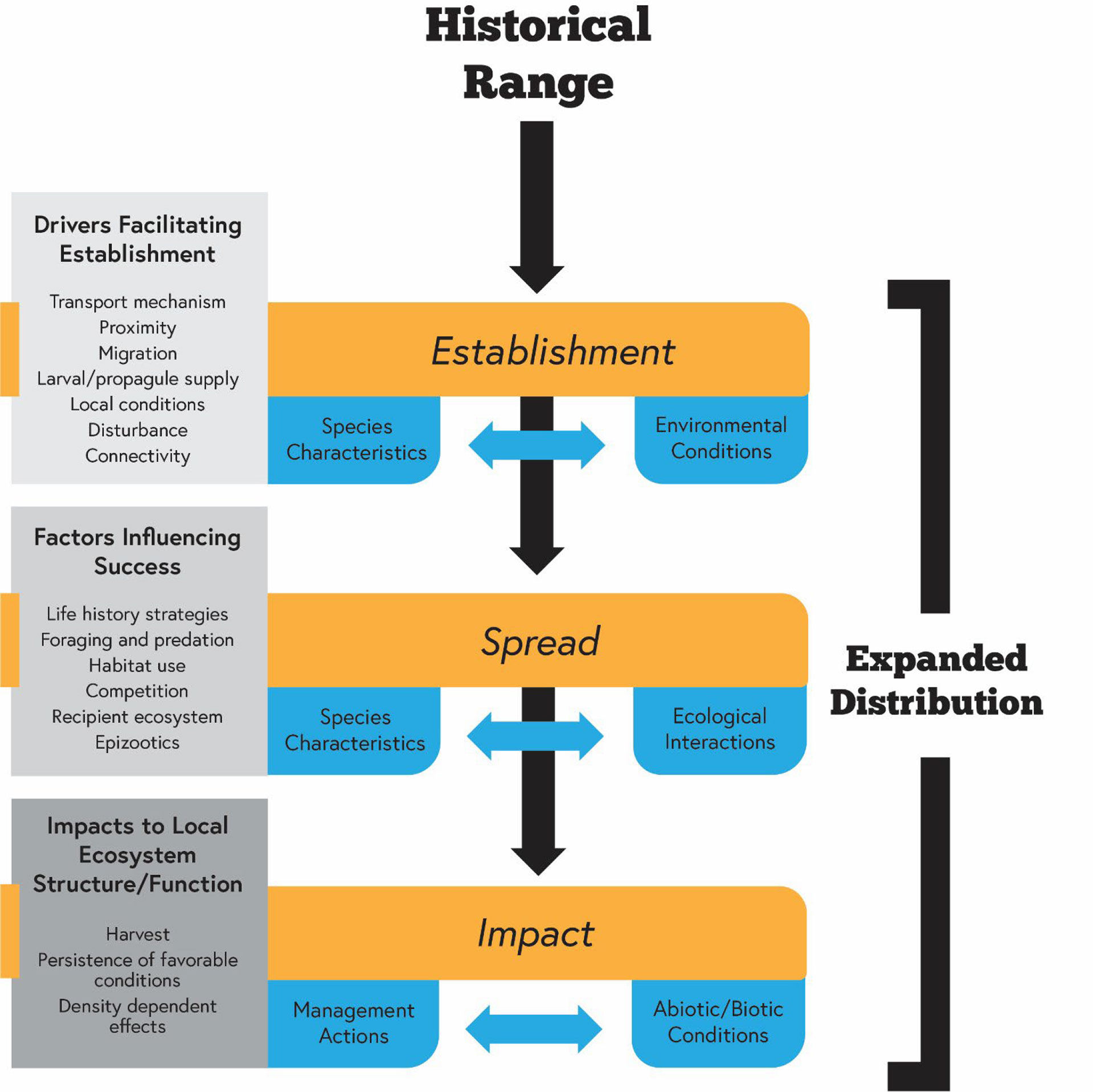
Figure 1 Conceptual diagram highlighting a subset of the important factors influencing the poleward geographic expansion and effects of mesopredators in seagrass ecosystems. Specific attention is given to drivers facilitating establishment, factors influencing success once established, and impacts within the expanded geographic range.
Losses of biological diversity continue to be driven by the effects of multiple historical stressors, chief among these is the introduction (intentional or accidental) of invasive species into areas outside their historical geographic ranges (also known as exotic species and/or nonnative species). As with invasive species, historical reductions of endemic biodiversity may facilitate the poleward expansion of warm-water organisms no longer limited by thermal barriers. In each case, this should lead to the mixing of the endemic species assemblages (“Wallace’s Realm’s,” Elton, 1958) with new species for which no evolutionary history exists. This lack of a shared history between invasive and endemic species is hypothesized to negatively impact native species.
The potential impacts of novel species in critical nursery areas are of special concern for many habitats. Seagrasses, for example, are a widespread foundation species that provide ecosystem services ranging from nutrient and carbon dioxide sequestration and erosion protection to predation refuge and sustenance (Duarte, 2000; Heck et al., 2003; Orth et al., 2020). These plants are not immune to anthropogenic disturbances, with decreasing areal coverage globally (Orth et al., 2006; Waycott et al., 2009). While mechanisms of decline vary, chronic disturbances make these habitats, and their faunal constituents, prone to establishment by invasive and range-expanding species. Indeed, seagrasses now support a number of novel, warm-water consumers: Sea Bream Archosargus rhomboidalis (Seyoum et al., 2020), blue crab Callinectes sapidus (Johnson, 2015), Common Snook Centropomus undecimalus (Purtlebaugh et al., 2020), Grey Snapper Lutjanus griseus (Hare et al., 2012), Tarpon Megalops atlanticus ((Mace et al., 2020), Ornate Wrasse Thallasoma pavo (Encarnação et al., 2019), among others.
Here, we use concepts developed in the invasion literature to predict how mid-level consumers (hereafter referred to as mesopredators) might become established and impact subtropical and temperate seagrass ecosystems. We focus on mesopredators, primarily fishes and macroinvertebrates, as the return of tropical herbivores in seagrasses are now well recognized (Heck et al., 2015; Hyndes et al., 2017; Zarco-Perello et al., 2019). Importantly, mesopredators can be strong interactors (i.e., having disproportionate impacts on food web structure) and we predict that over time their poleward expansion will alter density-dependent interactions, probably in unexpected ways. These consumers are key trophic intermediates and may utilize unrecognized foraging behaviors to capture endemic, naive prey who do not perceive these new consumers to be threats (Martin, 2014; Anton et al., 2016). Moreover, we concentrate on mesopredators as higher-order, larger predators already have extensive home ranges that integrate multiple habitats and energy sources (Keppeler et al., 2021).
Predictions
Based on invasive species literature, we propose a framework for predicting favorable environmental requirements and natural history characteristics that facilitates establishment and subsequent impacts of mesopredators on subtropical and temperate seagrass communities (Figure 1). Specifically, we predict that:
1. Movement of warmer water species into subtropical and temperate seagrasses will depend on reproductive and recruitment strategies that provide persistent supplies of propagules/recruits (Leung and Mandrak, 2007),
2. Subsequent recruit survival will be contingent on matching habitats of origin with newly colonized habitats with similar abiotic conditions (sensu Stohlgren et al., 2005),
3. Initially, warm water mesopredators may rely on winter temperature refugia, including groundwater discharge or offshore habitats for overwintering (sensu Peterson et al., 2005),
4. Marine heatwaves are increasing in frequency and intensity (Oliver et al., 2021), thus rapidly making conditions tolerable to warmer water species,
5. Colonization will be more successful in seagrass ecosystems characterized by frequent disturbances that decrease endemic biodiversity, opening vacant niches for exploitation (sensu With, 2004),
6. Mesopredators must avoid detection by larger, endemic predators, at least until they can adapt to a new suite of predators,
7. If colonizers are strong interactors, they will dramatically restructure food web interactions (due to prey naivete, trait/behaviorally mediated indirect interactions, etc.),
8. Density dependence, in the form of intraguild predation, cannibalism, disease/epizootics, or parasites, may limit naïve/undefended mesopredator establishment in invaded regions, and
9. Additional export of warm-water species into historically cooler areas will progress as temperatures warm and expansions will continue in a stepwise fashion.
Consumer Establishment in Novel Ecosystems
The high biological diversity of successionally-mature communities exists at, or near, a saturation point (Sax and Gaines, 2008). This saturation point is hypothesized to limit the successful colonization of new species (“invasion resistance,” Stachowicz et al., 1999). As such, undisturbed subtropical and temperate seagrass communities may resist the poleward movement of warm water mesopredators. Interactions between resident organisms and warm-water colonizers, with no evolutionary history, may further limit their persistence and facilitate endemic species adapted to the frequency and magnitude of local disturbance regimes. Given the intensification of anthropogenic impacts in marine ecosystems, however, resistance to invasions/expansions will likely wane over time. Reductions in the incidences of extreme winter temperatures that once limited the distribution of these mesopredators may make colonization sustainable (Fodrie et al., 2010; Purtlebaugh et al., 2020) and ultimately lead to the competitive exclusion and/or predation of endemic species (sensu Martin et al., 2010). When added to other historical stressors (e.g., overharvesting, introduced species, habitat loss, pollution, etc.), temperature increases may represent a catastrophic threat to resident stenothermic fauna and seagrass resilience, allowing warmer water species to expand poleward.
Guidance on how the initial establishment of mesopredators will occur can be found in the invasive species and metacommunity literature. As thermal barriers degrade, years of propagule settlement from established source populations should lead to subsequent survival in newly colonized areas (albeit sinks, initially), as documented for invasions (Bhaud et al., 1995; Lockwood et al., 2005). Establishment of “pseudo-populations” will initially occur, especially during favorable climate conditions, but may not be sustainable if elevated mortality occurs during future episodic cold events (Sanford et al., 2006). Invasive species models assume the distance that invasive species propagules spread (e.g., seed shadows, plant dispersal, and mark-recapture) and dispersal distances are typically leptokurtic (i.e., dispersal occurs slowly and decreases exponentially with increasing distance from a source population) (Marchetti et al., 2004; Leung and Mandrak, 2007). Survival will be low initially thus rendering newly-colonized seagrass sinks (Figures 1, 2), and this early phase of colonization may go undetected/unnoticed as few nations conduct sustained or extensive seagrass or faunal monitoring programs (Figure 2). This implies that mesopredator populations will establish gradually, perhaps initially restricted to deeper, temperature-stable locations (where little/no monitoring, for the most part, occurs). As mesopredator biomass increases, recruitment should intensify, and warming will lead to successful establishment.
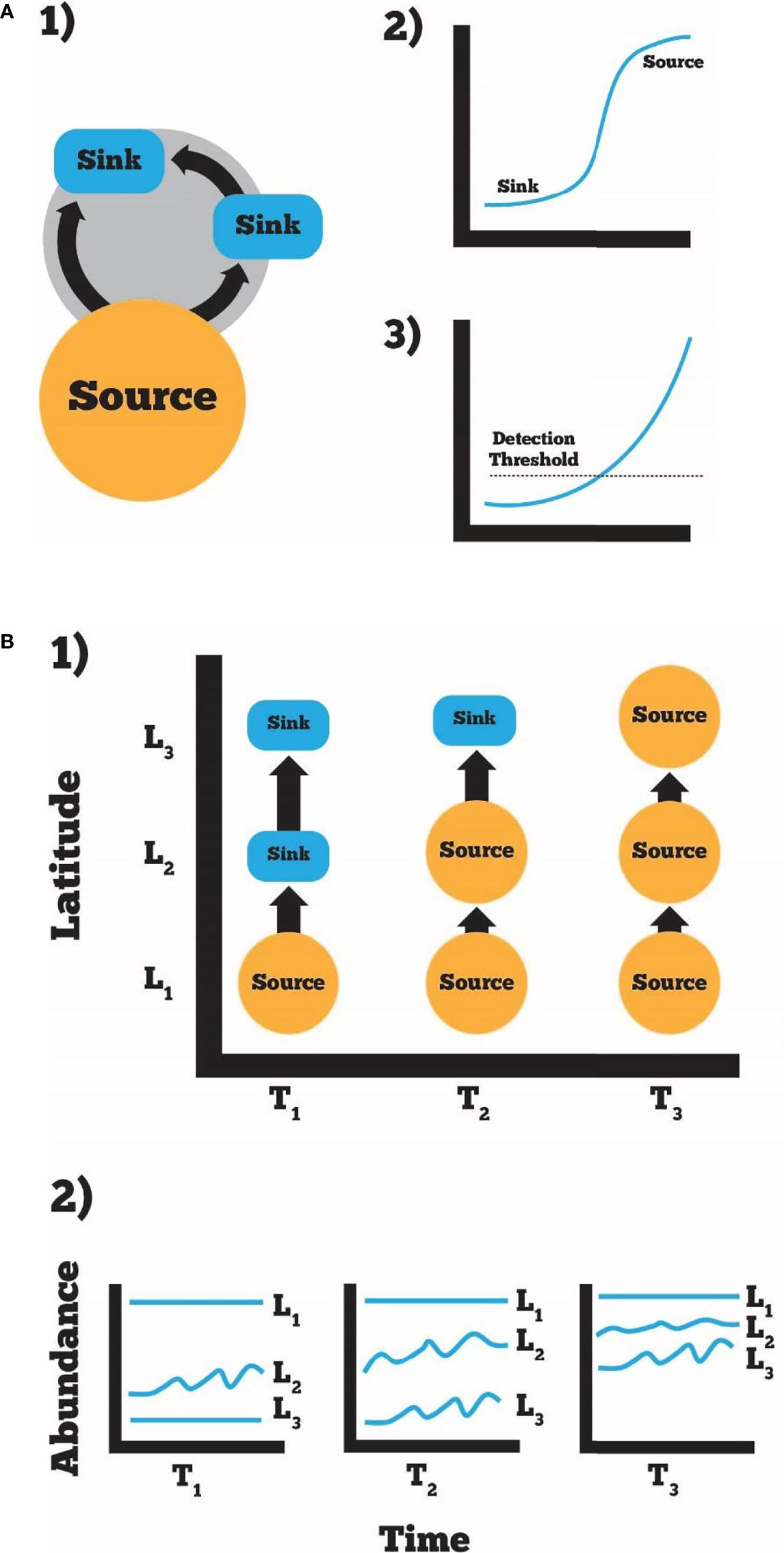
Figure 2 Source-sink metacommunity ecology can inform the spread of warmer water species into areas not previously tolerable (Lockwood et al., 2013). We predict that early in the process, source populations may exist that fuel sinks during short-term, favorable conditions (A, 1) and as temperatures continue to warm over time these sinks become sources (A, 2). Novel organisms will be below a detectable threshold for many gear types and monitoring strategies early and as abundance increases become more detectable (A, 3). Latitudinal abundance (L) will also change over time (T), as conditions change there will be less occurrences below a species thermal tolerance and sinks become source populations (B, 1). In this way, we predict abundance will increase over time and species can move poleward in a stepwise fashion (B, 2).
Numerous other factors may also influence mesopredator establishment in seagrasses. The three-dimensional structure provided by seagrass may prevent the extirpation of new recruits via reduced foraging success and encounter rates, enhancing establishment of expanding species (Heck et al., 2003). Warm-water species may require unique, trait-mediated defenses to remain undetected by endemic predators. Thus, predictions about the successful invasion of future climate vagrants should include detailed assessments of natural history, including species-specific life history, reproductive, and behavioral information. Assuming that populations can survive repeated episodic bouts of cold/mild weather in new habitats and reproduce locally, populations should eventually increase exponentially, similar to invasive species such as lionfish (Scyphers et al., 2015), and poleward expansion will continue to progress (Figure 2).
Geographic considerations are an influential consideration for successful expansion, including proximity to thermal refuges and optimal habitats. To better understand how mesopredators establish initially, the identification and distance to source populations and the proximity to various sources of thermal refugia are critical. These include anthropogenic sources (such as power plants, Carr and Milstein, 2018), offshore/deep waters (Lamont et al., 2018), or groundwater vents/seeps (Laist et al., 2013), all of which may allow initial winter survival. Unlike shallow coastal waters that experience periodic winter storms, climate-expanding mesopredators may need thermally-stable, deeper water seagrasses initially, such as those found along western Australia, the Mediterranean Sea, and the Big Bend area of Florida (USA). Again, however, little/no monitoring occurs in such deeper waters, and early stages of establishment may be missed. Similar geographic concerns including connectivity within the seascape may also limit the successful poleward movement of mesopredators if their life history requires multiple habitats across life stages (i.e, ontogenetic niche requirements). As such, seagrasses occurring near hardened substrates that mimic the live bottoms and coral reefs, assuming these alternative habitats are buffered from low temperatures, could importantly facilitate poleward expansion.
Finally, mesopredators will not be the only trophic group affected by temperature changes in a future ocean. Seagrasses may also experience climate effects, and previous estimates of herbivore increases and impacts (Heck et al., 2015), combined with conservation successes of historically depleted turtles and manatees (Hays, 2004; Runge et al., 2017), mean that the lush, three-dimensional structural refuge that seagrasses currently provide may be diminished and both native and expanding species will be exposed to higher levels of predation in the future. Warming will further alter the seasonality and timing of endemic organism phenologies (Poloczanska et al., 2013) with ensuing effects on resident organisms. Thus, there is a need for animals to adapt to future environmental conditions and colonization success will be determined in many cases by plasticity in species traits (Sih et al., 2011). These critical traits will influence individual fitness and species success, and if expanding species can respond rapidly to changing environmental conditions, and changes in the endemic ecosystem (including local community, diseases/epizootics, etc.), they may persist. If evolutionary history has also produced traits that facilitate a rapid response to such challenges (short generation times, high reproduction, suitable genetic heterozygosity in key traits, etc.; see Hendry et al., 2011), then expanding mesopredators should respond favorably to future stochastic environments. In general, these hypotheses align with traditional disturbance theory, with one exception: responses to disturbance need to closely parallel the frequency of environmental changes and concurrent impacts on the endemic community.
Impacts of Mesopredators Post-Establishment
Concerns about potential impacts to resident fauna and flora by expanding mesopredators distributions are, in many cases, driven by documented negative impacts from invasive species. While invasive species are known to impact food webs (Deudero et al., 2011; David et al., 2017), others highlight the idiosyncratic impacts of these species on recipient ecosystems (Davis, 2003). Specifically, the trophic level of introduction often determines the severity of ecosystem impacts, with higher order consumers negatively impacting endemic biodiversity more than primary producers (primarily via predation; Sax and Gaines, 2008; David et al., 2017). Invasive basal resources have unexpectedly led to local increases in species richness (Cleland et al., 2004; Smith et al., 2004; Capers et al., 2007; Martin and Valentine, 2012). However, top-down consumption of lower order organisms can exert strong controlling influences over food web structure (Heck and Valentine, 2007). The indirect effects (trait and behaviorally mediated indirect interactions) of higher trophic levels can also exacerbate mesopredator’s negative impacts (“the ecology of fear,” Brown and Kotler, 2007). Even when comprehensive monitoring is in place (which often lacks invertebrates, larval stages, or epizootics), seldom are these processes or impacts documented.
It is widely recognized in the biodiversity literature that consumer identity drives the intensity of many ecological processes (Bruno et al., 2005; Duffy, 2006). As such, life history and morphological species traits will be an important determinant of species success in novel environments (Tabak et al., 2018). Of these traits, behavioral plasticity is critically important (Sih et al., 2004, (Hendry et al., 2011). Assuming novel predators establish themselves in seagrass habitats with a rich prey abundance, and that they recognize resident species as prey, the response of endemic fauna may represent a novel aspect of this interaction (Sih et al., 2010) and it seems probable that revisiting historical predator-prey theory could provide productive insights into future investigations. Not all colonizing mesopredators will be strong interactors, but even the addition of weakly-interacting species could increase biodiversity, amplifying density-dependent interactions and stimulating more complete use of basal resources. It is also possible that weak interactors can become strong interactors in their new range.
Antipredator strategies are a fundamental function of perceptual and cognitive capabilities of prey (Sih et al., 2011; Carthey and Banks, 2014; Greggor et al., 2015). In general, most prey possess behavioral strategies that allow them to avoid detection and/or capture by predators. In most marine ecosystems, prey risk attack from multiple consumers whose interactions are not always additive, and novel mesopredators could render historically effective antipredator behaviors useless (Sih et al., 2011). We predict that one of two scenarios could occur: 1) a “general predator response” in which many endemic fauna may recognize these new mesopredators as being similar to endemic predators for which they have a long evolutionary histories (thus, effects will be minimal), or 2) a “specific predator response” in which prey recognize species-specific traits for use as antipredator cues (thus effects will be large). This, however, may be an oversimplification, as Carthey and Blumstein (2018) outlined 11 different responses from complete naivete to various levels of recognition and reactions when describing novel predator-prey co-occurrences (Banks and Dickman, 2007). Should the top-down effects of introductions become additive, prey would be forced to survive in a food web with unprecedented consumer richness, unless intraguild predation or cannibalism among these consumer’s self-limit.
Finally, it is important to note that contemporary organisms descended from ancestors that weathered similar environmental and evolutionary changes during the Mesozoic Revolution and other warmer periods. As a result, predators and prey have evolved a diverse array of attack and defense strategies that have altered the intensity of predator-prey and life history strategies. This suggests it is possible this could happen again in a warming ocean.
Discussion
Climate-induced environmental changes will continue to drive the poleward expansions of consumers into subtropical and temperate seagrasses. Recent examples highlight the impacts of the rapid expansion of vertebrate herbivores (Heck et al., 2015; Hyndes et al., 2017; Zarco-Perello et al., 2019). Prior to targeted overfishing, top predators have always had large home ranges with effects dispersed over time and space, although there is some evidence these patterns are changing (Hammerschlag et al., 2022). In contrast, mesopredators have strong, local effects that can determine ecological patterns via direct and indirect top-down processes (Heck et al., 2000). In this perspective, we discussed the possibilities for future colonization of poleward seagrass beds by warmer water mesopredators and the potential and mechanisms for trophic alterations based on invasive literature.
We offer several suggestions for future conservation, research, and management priorities. Future efforts will be well-served to establish comprehensive monitoring plans that consider both natural history and ecosystem properties and compile all available information to document historical assemblages and forecast future seagrass communities. It is likely that mesopredator expansions have been historically underestimated (Sorte et al., 2010) or relegated to obscure gray literature or natural history reports. An absence of published baseline studies on various aspects of seagrass communities (e.g., environmental variables, seagrass metrics, seasonal dynamics, etc.) hamper our ability to predict the effects of future disturbances. This was clearly illustrated after the Deepwater Horizon oil spill in the Gulf of Mexico (Martin et al., 2020), when an absence of historical data precluded traditional before-after-control-impact designs from impact assessments. We suggest that agencies consider additional funding to: 1) scour publicly available databases and obscure literature to generate georeferenced species inventories, 2) enhance monitoring efforts to supplement limited historical information for comparisons with current information on species distributions, 3) develop additional predictions for seagrass based on other, nearby (and perhaps better studied) ecosystems such as reefs or kelp beds, 4) maintain strong conservation efforts to minimize confounding effects from unrelated disturbances (described below), and 5) carefully consider future management and regulatory actions for novel species. Regarding the last point, many agencies are reluctant to suggest management changes or control efforts for expanding warmer water species; however, we posit that a priori guidelines are needed to properly control populations using best available information if populations pose threats to resident ecosystems. Without changes to ambient temperatures driving expansion, any control efforts may be futile. Preparation for future short-duration, intense colder periods may be fruitful; although long-term trends in rising temperatures (and specifically minimum temperatures) are apparent, evidence exists that jet streams driven by the anomaly between polar and temperate regions are becoming less stable resulting in greater oscillation. This weakening has produced strong cold fronts into lower latitudes (“polar vortexes,” Francis et al., 2017). With more warmer water species expanding into historically cooler regions, these fronts have catastrophic potential for expanding species (Foley et al., 2007).
Finally, we caution attributing changes in species composition to climate change without applying appropriate scientific verification. Ecosystem structure and function can change for a variety of reasons, including, but not limited to, changes in recruitment/year class strength of resident organisms, the frequency and intensity local disturbances, larger scale patterns and stressors unrelated to climate change, and successful conservation efforts for species such as turtles. Most notably, confounding factors (e.g., concurrent hydrological changes, overharvesting, etc.) should be considered to the extent possible. The successful conservation of seagrass ecosystems and the ecosystem services they provide depends on rigorous and replicable scientific approaches, and we postulate that mesopredators will play a large role in future climate-driven changes in seagrass beds.
Data Availability Statement
The original contributions presented in the study are included in the article/supplementary material. Further inquiries can be directed to the corresponding author.
Author Contributions
CM and JV conceived, designed, wrote, and critically revised text for this perspective. All authors contributed to the article and approved the submitted version.
Funding
This project was paid for with federal funding from the Department of the Treasury under the Resources and Ecosystems Sustainability, Tourist Opportunities, and Revived Economies of the Gulf Coast States Act of 2012 (RESTORE Act). The statements, findings, conclusions, and recommendations are those of the author(s) and do not necessarily reflect the views of the Department of the Treasury, ADCNR. or MESC/Dauphin Island Sea Lab.
Conflict of Interest
The authors declare that the research was conducted in the absence of any commercial or financial relationships that could be construed as a potential conflict of interest.
Publisher’s Note
All claims expressed in this article are solely those of the authors and do not necessarily represent those of their affiliated organizations, or those of the publisher, the editors and the reviewers. Any product that may be evaluated in this article, or claim that may be made by its manufacturer, is not guaranteed or endorsed by the publisher.
Acknowledgments
We acknowledge Emily Colson for assistance with figures and Ken Heck, Scott Alford, and Ashley McDonald for discussions that assisted in the construction of this final document.
References
Anton A., Cure K., Layman C. A., Puntila R., Simpson M. S., Bruno J. F. (2016). Prey Naiveté to Invasive Lionfish Pterois Volitans on Caribbean Coral Reefs. Marine Ecol. Prog. Ser. 544, 257–269. doi: 10.3354/meps11553
Banks P. B., Dickman C. R. (2007). Alien Predation and the Effects of Multiple Levels of Prey Naiveté. Trends Ecol. Evol. 22 (5), 229–230. doi: 10.1016/j.tree.2007.02.006
Bernstein L., Bosch P., Canziani O., Chen Z., Christ R., Riahi K. (2008). IPCC 2007: Climate Change 2007: Synthesis Report. Geneva: IPCC.
Bhaud M., Cha J. H., Duchene J. C., Nozais C. (1995). Influence of Temperature on the Marine Fauna: What can be Expected From a Climatic Change. J. Thermal Biol. 20 (1-2), 91–104. doi: 10.1016/0306-4565(94)00031-D
Brown J. S., Kotler B. P. (2007). Foraging and the Ecology of Fear. Foraging: Behaviour and Ecology. Chicago, Illinois, USA: Universtiy the Chicago Press 437–480.
Bruno J. F., Boyer K. E., Duffy J. E., Lee S. C., Kertesz J. S. (2005). Effects of Macroalgal Species Identity and Richness on Primary Production in Benthic Marine Communities. Ecol. Lett. 8 (11), 1165–1174. doi: 10.1111/j.1461-0248.2005.00823.x
Capers R. S., Selsky R., Bugbee G. J., White J. C. (2007). Aquatic Plant Community Invasibility and Scale-Dependent Patterns in Native and Invasive Species Richness. Ecology 88 (12), 3135–3143. doi: 10.1890/06-1911.1
Carr J., Milstein T. (2018). Keep Burning Coal or the Manatee Gets it: Rendering the Carbon Economy Invisible Through Endangered Species Protection. Antipode 50 (1), 82–100. doi: 10.1111/anti.12355
Carthey A. J., Banks P. B. (2014). Naïveté in Novel Ecological Interactions: Lessons From Theory and Experimental Evidence. Biol. Rev. 89 (4), 932–949. doi: 10.1111/brv.12087
Carthey A. J., Blumstein D. T. (2018). Predicting Predator Recognition in a Changing World. Trends Ecol. Evol. 33 (2), 106–115. doi: 10.1016/j.tree.2017.10.009
Cleland E. E., Smith M. D., Andelman S. J., Bowles C., Carney K. M., Claire Horner-Devine M., et al. (2004). Invasion in Space and Time: non-Native Species Richness and Relative Abundance Respond to Interannual Variation in Productivity and Diversity. Ecol. Lett. 7 (10), 947–957. doi: 10.1111/j.1461-0248.2004.00655.x
David P., Thebault E., Anneville O., Duyck P. F., Chapuis E., Loeuille N. (2017). Impacts of Invasive Species on Food Webs: A Review of Empirical Data. Adv. Ecol. Res. 56, 1–60. doi: 10.1016/bs.aecr.2016.10.001
Davis M. A. (2003). Biotic Globalization: Does Competition From Introduced Species Threaten Biodiversity? Bioscience 53 (5), 481–489. doi: 10.1641/0006-3568(2003)053[0481:BGDCFI]2.0.CO;2
Deudero S., Box A., Alós J., Arroyo N. L., Marbà N. (2011). Functional Changes Due to Invasive Species: Food Web Shifts at Shallow Posidonia Oceanica Seagrass Beds Colonized by the Alien Macroalga Caulerpa Racemosa. Estuarine Coastal Shelf Sci. 93 (2), 106–116. doi: 10.1016/j.ecss.2011.03.017
Duarte C. M. (2000). Marine Biodiversity and Ecosystem Services: An Elusive Link. J. Exp. Marine Biol. Ecol. 250 (1-2), 117–131. doi: 10.1016/S0022-0981(00)00194-5
Duffy J. E. (2006). Biodiversity and the Functioning of Seagrass Ecosystems. Marine Ecol. Prog. Ser. 311, 233–250. doi: 10.3354/meps311233
Dunstan P. K., Bax N. J. (2007). How Far can Marine Species Go? Influence of Population Biology and Larval Movement on Future Range Limits. Marine Ecol. Prog. Ser. 344, 15–28. doi: 10.3354/meps06940
e Costa B. H., Assis J., Franco G., Erzini K., Henriques M., Gonçalves E. J., et al. (2014). Tropicalization of Fish Assemblages in Temperate Biogeographic Transition Zones. Marine Ecology Progress Series 504, 241–252. doi: 10.3354/meps10749
Elton C. S. (2020). The Ecology of Invasions by Animals and Plants (Methuen, London: Springer Nature).
Encarnação J., Morais P., Baptista V., Cruz J., Teodósio M. A. (2019). New Evidence of Marine Fauna Tropicalization Off the Southwestern Iberian Peninsula (Southwest Europe). Diversity 11 (4), 48. doi: 10.3390/d11040048
Fodrie F. J., Heck K. L. Jr., Powers S. P., Graham W. M., Robinson K. L. (2010). Climate-Related, Decadal-Scale Assemblage Changes of Seagrass-Associated Fishes in the Northern Gulf of Mexico. Global Change Biol. 16 (1), 48–59. doi: 10.1111/j.1365-2486.2009.01889.x
Foley A. M., Singel K. E., Dutton P. H., Summers T. M., Redlow A. E., Lessman J. (2007). Characteristics of a Green Turtle (Chelonia Mydas) Assemblage in Northwestern Florida Determined During a Hypothermic Stunning Event. Gulf Mexico Sci. 25 (2), 4. doi: 10.18785/goms.2502.04
Francis J. A., Vavrus S. J., Cohen J. (2017). Amplified Arctic Warming and Mid-Latitude Weather: New Perspectives on Emerging Connections. Wiley Interdiscip. Rev.: Climate Change 8 (5), e474. doi: 10.1038/s41558-019-0662-y
Greggor A. L., Thornton A., Clayton N. S. (2015). Neophobia is Not Only Avoidance: Improving Neophobia Tests by Combining Cognition and Ecology. Curr. Opin. Behav. Sci. 6, 82–89. doi: 10.1016/j.cobeha.2015.10.007
Hammerschlag N., McDonnell L. H., Rider M. J., Street G. M., Hazen E. L., Natanson L. J., et al. (2022). Ocean Warming Alters the Distributional Range, Migratory Timing, and Spatial Protections of an Apex Predator, the Tiger Shark (Galeocerdo Cuvier). Global Change Biol. doi: 10.1111/gcb.16045
Hare J. A., Wuenschel M. J., Kimball M. E. (2012). Projecting Range Limits With Coupled Thermal Tolerance-Climate Change Models: An Example Based on Gray Snapper (Lutjanus Griseus) Along the US East Coast. PloS One 7 (12), e52294. doi: 10.1371/journal.pone.0052294
Hays G. C. (2004). Good News for Sea Turtles. Trends Ecol. Evol. 19 (7), 349–351. doi: 10.1016/j.tree.2004.05.009
Heck J. K.L., Fodrie F. J., Madsen S., Baillie C. J., Byron D. A. (2015). Seagrass Consumption by Native and a Tropically Associated Fish Species: Potential Impacts of the Tropicalization of the Northern Gulf of Mexico. Marine Ecol. Prog. Ser. 520, 165–173. doi: 10.3354/meps11104
Heck J. K.L., Hays G., Orth R. J. (2003). Critical Evaluation of the Nursery Role Hypothesis for Seagrass Meadows. Marine Ecol. Prog. Ser. 253, 123–136. doi: 10.3354/meps253123
Heck J. K.L., Pennock J. R., Valentine J. F., Coen L. D., Sklenar S. A. (2000). Effects of Nutrient Enrichment and Small Predator Density on Seagrass Ecosystems: An Experimental Assessment. Limnol. Oceanography 45 (5), 1041–1057. doi: 10.4319/lo.2000.45.5.1041
Heck K. L., Valentine J. F. (2007). The Primacy of Top-Down Effects in Shallow Benthic Ecosystems. Estuaries Coasts 30 (3), 371–381. doi: 10.1007/BF02819384
Hendry A. P., Kinnison M. T., Heino M., Day T., Smith T. B., Fitt G., et al. (2011). Evolutionary Principles and Their Practical Application. Evol. Appl. 4 (2), 159–183. doi: 10.1111/j.1752-4571.2010.00165.x
Hyndes G. A., Heck K. L. Jr., Vergés A., Harvey E. S., Kendrick G. A., Lavery P. S., et al. (2017). Accelerating Tropicalization and the Transformation of Temperate Seagrass Meadows. Bioscience 66 (11), 938–948. doi: 10.1093/biosci/biw111
Johnson D. S. (2015). The Savory Swimmer Swims North: A Northern Range Extension of the Blue Crab Callinectes Sapidus? J. Crustacean Biol. 35 (1), 105–110. doi: 10.1163/1937240X-00002293
Keppeler F. W., Olin J. A., López-Duarte P. C., Polito M. J., Hooper-Bùi L. M., Taylor S. S., et al. (2021). Body Size, Trophic Position, and the Coupling of Different Energy Pathways Across a Saltmarsh Landscape. Limnol. Oceanography Lett. 6 (6), 360–368. doi: 10.1002/lol2.10212
Laist D. W., Taylor C., Reynolds J. E. III (2013). Winter Habitat Preferences for Florida Manatees and Vulnerability to Cold. PloS One 8 (3), e58978. doi: 10.1371/journal.pone.0058978
Lamont M. M., Seay D. R., Gault K. (2018). Overwintering Behavior of Juvenile Sea Turtles at a Temperate Foraging Ground. Ecology 99 (11), 2621–2624. doi: 10.1002/ecy.2439
Leung B., Mandrak N. E. (2007). The Risk of Establishment of Aquatic Invasive Species: Joining Invasibility and Propagule Pressure. Proc. R. Soc. B.: Biol. Sci. 274 (1625), 2603–2609. doi: 10.1098/rspb.2007.0841
Lockwood J. L., Cassey P., Blackburn T. (2005). The Role of Propagule Pressure in Explaining Species Invasions. Trends Ecol. Evol. 20 (5), 223–228. doi: 10.1016/j.tree.2005.02.004
Lockwood J. L., Hoopes M. F., Marchetti M. P. (2013). Invasion Ecology (Malden, Massachusetts, USA: John Wiley & Sons).
Mace M. M., Kimball M. E., Elmo G. M., Crane D. P. (2020). Overwinter Survival, Age, and Growth of Juvenile Tarpon (Megalops Atlanticus) in a Shallow, Tidally-Restricted Habitat in South Carolina. Environ. Biol. Fishes 103 (8), 965–972. doi: 10.1007/s10641-020-00998-1
Marchetti M. P., Moyle P. B., Levine R. (2004). Invasive Species Profiling? Exploring the Characteristics of non-Native Fishes Across Invasion Stages in California. Freshwater Biol. 49 (5), 646–661. doi: 10.1111/j.1365-2427.2004.01202.x
Martin C. W. (2014). Naïve Prey Exhibit Reduced Antipredator Behavior and Survivorship. PeerJ 2, e665. doi: 10.7717/peerj.665
Martin C. W., Lewis K. A., McDonald A. M., Spearman T. P., Alford S. B., Christian R. C., et al. (2020). Disturbance-Driven Changes to Northern Gulf of Mexico Nekton Communities Following the Deepwater Horizon Oil Spill. Marine Poll. Bull. 155, 111098. doi: 10.1016/j.marpolbul.2020.111098
Martin C. W., Valentine J. F. (2012). Eurasian Milfoil Invasion in Estuaries: Physical Disturbance can Reduce the Proliferation of an Aquatic Nuisance Species. Marine Ecol. Prog. Ser. 449, 109–119. doi: 10.3354/meps09515
Martin C. W., Valentine M. M., Valentine J. F. (2010). Competitive Interactions Between Invasive Nile Tilapia and Native Fish: The Potential for Altered Trophic Exchange and Modification of Food Webs. PloS One 5 (12), e14395. doi: 10.1371/journal.pone.0014395
Oliver E. C., Benthuysen J. A., Darmaraki S., Donat M. G., Hobday A. J., Holbrook N. J., et al (2021). Marine heatwaves. Annual Rev Marine Sci. 13, 313–342. doi: 10.1146/annurev-marine-032720-095144
Orth R. J., Carruthers T. J., Dennison W. C., Duarte C. M., Fourqurean J. W., Heck K. L., et al. (2006). A Global Crisis for Seagrass Ecosystems. Bioscience 56 (12), 987–996. doi: 10.1641/0006-3568(2006)56[987:AGCFSE]2.0.CO;2
Orth R. J., Lefcheck J. S., McGlathery K. S., Aoki L., Luckenbach M. W., Moore K. A., et al. (2020). Restoration of Seagrass Habitat Leads to Rapid Recovery of Coastal Ecosystem Services. Sci. Adv. 6 (41), eabc6434. doi: 10.1126/sciadv.abc6434
Peterson M. S., Slack W. T., Woodley C. M. (2005). The Occurrence of non-Indigenous Nile Tilapia, Oreochromis Niloticus (Linnaeus) in Coastal Mississippi, USA: Ties to Aquaculture and Thermal Effluent. Wetlands 25 (1), 112–121. doi: 10.1672/0277-5212(2005)025[0112:TOONNT]2.0.CO;2
Poloczanska E. S., Brown C. J., Sydeman W. J., Kiessling W., Schoeman D. S., Moore P. J., et al. (2013). Global Imprint of Climate Change on Marine Life. Nat. Climate Change 3 (10), 919–925. doi: 10.1038/nclimate1958
Purtlebaugh C. H., Martin C. W., Allen M. S. (2020). Poleward Expansion of Common Snook Centropomus Undecimalis in the Northeastern Gulf of Mexico and Future Research Needs. PloS One 15 (6), e0234083. doi: 10.1371/journal.pone.0234083
Runge M. C., Sanders-Reed C. A., Langtimm C. A., Hostetler J. A., Martin J., Deutsch C. J., et al. (2017). Status and Threats Analysis for the Florida Manatee (Trichechus Manatus Latirostris), 2016 (US Geological Survey), 40.
Sanford E., Holzman S. B., Haney R. A., Rand D. M., Bertness M. D. (2006). Larval Tolerance, Gene Flow, and the Northern Geographic Range Limit of Fiddler Crabs. Ecology 87 (11), 2882–2894. doi: 10.1890/0012-9658(2006)87[2882:LTGFAT]2.0.CO;2
Sax D. F., Gaines S. D. (2008). Species Invasions and Extinction: The Future of Native Biodiversity on Islands. Proc. Natl. Acad. Sci. 105 (Supplement 1), 11490–11497. doi: 10.1073/pnas.0802290105
Scyphers S. B., Powers S. P., Akins J. L., Drymon J. M., Martin C. W., Schobernd Z. H., et al. (2015). The Role of Citizens in Detecting and Responding to a Rapid Marine Invasion. Conserv. Lett. 8 (4), 242–250. doi: 10.1111/conl.12127
Seyoum S., Adams D. H., Matheson R. E., Whittington J. A., Alvarez A. C., Sheridan N. E., et al. (2020). Genetic Relationships and Hybridization Among Three Western Atlantic Sparid Species: Sheepshead (Archosargus Probatocephalus), Sea Bream (A. Rhomboidalis) and Pinfish (Lagodon Rhomboides). Conserv. Genet. 21 (1), 161–173. doi: 10.1007/s10592-019-01244-7
Sih A., Bell A., Johnson J. C. (2004). Behavioral Syndromes: An Ecological and Evolutionary Overview. Trends Ecol. Evol. 19 (7), 372–378. doi: 10.1016/j.tree.2004.04.009
Sih A., Bolnick D. I., Luttbeg B., Orrock J. L., Peacor S. D., Pintor L. M., et al. (2010). Predator–prey Naïveté, Antipredator Behavior, and the Ecology of Predator Invasions. Oikos 119 (4), 610–621. doi: 10.1111/j.1600-0706.2009.18039.x
Sih A., Ferrari M. C., Harris D. J. (2011). Evolution and Behavioural Responses to Human-Induced Rapid Environmental Change. Evol. Appl. 4 (2), 367–387. doi: 10.1111/j.1752-4571.2010.00166.x
Smith M. D., Wilcox J. C., Kelly T., Knapp A. K. (2004). Dominance Not Richness Determines Invasibility of Tallgrass Prairie. Oikos 106 (2), 253–262. doi: 10.1111/j.0030-1299.2004.13057.x
Sorte C. J., Williams S. L., Carlton J. T. (2010). Marine Range Shifts and Species Introductions: Comparative Spread Rates and Community Impacts. Global Ecol. Biogeography 19 (3), 303–316. doi: 10.1111/j.1466-8238.2009.00519.x
Stachowicz J. J., Whitlatch R. B., Osman R. W. (1999). Species Diversity and Invasion Resistance in a Marine Ecosystem. Science 286 (5444), 1577–1579. doi: 10.1126/science.286.5444.1577
Stohlgren T. J., Crosier C., Chong G. W., Guenther D., Evangelista P. (2005). Life-History Habitat Matching in Invading non-Native Plant Species. Plant Soil 277 (1), 7–18. doi: 10.1007/s11104-005-4893-5
Tabak M. A., Webb C. T., Miller R. S. (2018). Propagule Size and Structure, Life History, and Environmental Conditions Affect Establishment Success of an Invasive Species. Sci. Rep. 8 (1), 1–9. doi: 10.1038/s41598-018-28654-w
Vergés A., Doropoulos C., Malcolm H. A., Skye M., Garcia-Pizá M., Marzinelli E. M., et al. (2016). Long-Term Empirical Evidence of Ocean Warming Leading to Tropicalization of Fish Communities, Increased Herbivory, and Loss of Kelp. Proc. Natl. Acad. Sci. 113 (48), 13791–13796. doi: 10.1073/pnas.1610725113
Waycott M., Duarte C. M., Carruthers T. J., Orth R. J., Dennison W. C., Olyarnik S., et al. (2009). Accelerating Loss of Seagrasses Across the Globe Threatens Coastal Ecosystems. Proc. Natl. Acad. Sci. 106 (30), 12377–12381. doi: 10.1073/pnas.0905620106
With K. A. (2004). Assessing the Risk of Invasive Spread in Fragmented Landscapes. Risk Analysis: Int. J. 24 (4), 803–815. doi: 10.1111/j.0272-4332.2004.00480.x
Keywords: climate change, exotic species, range expansions, tropical, plant-animal interactions, submerged aquatic vegetation
Citation: Martin CW and Valentine JF (2022) Climate-Induced Expansion of Consumers in Seagrass Ecosystems: Lessons From Invasion Ecology. Front. Mar. Sci. 9:867173. doi: 10.3389/fmars.2022.867173
Received: 31 January 2022; Accepted: 07 April 2022;
Published: 02 May 2022.
Edited by:
Jessie Campbell Jarvis, University of North Carolina Wilmington, United StatesReviewed by:
Mat Vanderklift, Commonwealth Scientific and Industrial Research Organisation (CSIRO), AustraliaCopyright © 2022 Martin and Valentine. This is an open-access article distributed under the terms of the Creative Commons Attribution License (CC BY). The use, distribution or reproduction in other forums is permitted, provided the original author(s) and the copyright owner(s) are credited and that the original publication in this journal is cited, in accordance with accepted academic practice. No use, distribution or reproduction is permitted which does not comply with these terms.
*Correspondence: Charles W. Martin, martin.charles.w@gmail.com