- 1Experimental Ecology and Aquaculture Laboratory, Department of Biology, University of Rome Tor Vergata, Rome, Italy
- 2PhD Program in Evolutionary Biology and Ecology, Department of Biology, University of Rome Tor Vergata, Rome, Italy
- 3National Inter-University Consortium for Marine Sciences-CoNISMa, Rome, Italy
- 4Department of Earth and Marine Sciences, University of Palermo, Palermo, Italy
- 5Aix Marseille Univ., Université de Toulon, CNRS, IRD, MIO UM 110, Marseille, France
Investigations on trophic requirements of different life cycle stages of Paracentrotus lividus are crucial for the comprehension of species ecology and for its artificial rearing. The future success of echinoculture depends heavily on the development of suitable and cost-effective diets that are specifically designed to maximize somatic growth during the early life stages and gonadal production in the later stages. In this context, a considerable number of studies have recommended animal sources as supplements in sea urchin diets. However, with the exception of Fernandez and Boudouresque (2000), no studies have investigated the dietary requirements over the different life stages of the sea urchin. In the present study, the growth and nutrition of three life stages of P. lividus (juveniles: 15-25 mm; subadults: 25-35 mm; adults: 45-55 mm) were analyzed over a 4-month rearing experiment. Three experimental diets, with 0%, 20% and 40% of animal sourced enrichments, were tested in parallel in sea urchin three size classes. The food conversion ratio, somatic and gonadal growth were assessed in each condition in order to evaluate the optimal level of animal-sourced supplements for each life stage. A general growth model covering the full post-metamorphic P. lividus life cycle was defined for each condition. During the juvenile stage P. lividus requires higher animal supply (40%), while a feeding requirement shift takes place toward lower animal supply (20%) in sub-adult and adult stages. Our results evidenced that the progressive increase in size after the metamorphosis led to a consequent variation of trophic requirements and food energy allocation in the sea urchin P. lividus. Macronutrient requirements varied widely during the different life stages, in response to changes in the energy allocation from somatic growth to reproductive investment. This study sheds light on P. lividus trophic ecology, broadening our basic knowledge of the dietary requirements of juveniles, sub-adults and adults as a function of their behavior also in the natural environment.
Introduction
The sea urchin Paracentrotus lividus (Lamarck, 1816) is an edible echinoid, which is widespread throughout the Mediterranean coasts and in the northeastern Atlantic, from Scotland to southern Morocco (Tortonese, 1965). Since the time of ancient Greece, its gonads (sea urchin roe), which are the edible part of this organism, have been considered a seafood delicacy in the Mediterranean Sea. Indeed, in some Italian localities, the reddish-orange gonads of P. lividus are still called “red gold” to indicate a luxury sea food of an intense color and unique flavor (Sartori and Gaion, 2016). Nowadays, P. lividus is the most commercially exploited and high market value echinoid in Europe and its gonads can be sold for up to 150 € kg-1 (Carboni et al., 2012). The increasing market value of this species, however, has caused the species to be targeted in the huge spread of illegal, unreported and unregulated fishing that is leading to the fast decline or in some cases even the collapse of local stocks (Guidetti et al., 2004; Ceccherelli et al., 2009; Rakaj et al., 2021). This phenomenon is very harmful also for the biodiversity of the benthic communities where this species inhabits since this sea urchin has an important ecological role, strictly connected to its trophic strategy (Tortonese, 1965; Yeruham et al., 2015). P. lividus is, in fact, a voracious and generalist herbivore, as well as a prey for a number of fish, starfish, and mollusks, and hence it is a key species in the dynamics of sublittoral ecosystems (Boudouresque and Verlaque, 2020).
In this context, aquaculture is a promising solution, offering a sustainable alternative to wild exploitation for meeting the current market demand (McBride, 2005; Bartley and Bell, 2008). However, the economic effectiveness of this solution, which is a crucial factor for the feasibility of industrial plants, is still hindered by the lack of excellent feeds, able to guarantee sea urchin fast growth and gonadal maturation (Spirlet et al., 1998; Cook and Kelly, 2009; Shpigel et al., 2018; Zupo et al., 2019). In fact, food quality and quantity to administrate at each developmental stages are still among the main challenges in echinoculture research to overcome the bottlenecks in commercial scale productions (Kelly, 2004). The development of successful sea urchin aquaculture therefore requires a basic understanding of the dietary requirements during both planktonic and benthonic life stages. More specifically, after the metamorphosis, the progressive variation in sea urchin size leads to a consequent variation in dietary requirements and food energy allocation (McCarron et al., 2009; Boudouresque and Verlaque, 2020). Sea urchins may require different levels of dietary protein and/or carbohydrate at different life stages (Heflin et al., 2012) and the level of energy allocated for reproduction increases with the increase in size, with a concurrent decrease in the allocation to the test and lantern (Fernandez and Boudouresque, 2000). Several studies have already demonstrated that formulated feeds can better satisfy specific nutrient requirements when compared with the food sources traditionally used in echinoculture (e.g. macroalgae) (Pearce et al., 2002; Daggett et al., 2005; Schlosser et al., 2005; Sartori and Gaion, 2016; Prato et al., 2018; Ruocco et al., 2018; Santos et al., 2020a). For this reason, modern aquaculture is turning to formulated diets with more sustainable, low-cost and constantly available feedstuffs than natural sources. Vegetable and animal ingredients mixed in a formulated diet can, in fact, maximize sea urchin growth performance, reducing time for the production of high-quality marketable gonads (Vizzini et al., 2019). To date, several attempts have been made to obtain a good market product using a balanced mix of vegetable and animal sources, as alternative diets to traditional ones (Vizzini et al., 2015; Sartori and Gaion, 2016; Cirino et al., 2017; Fabbrocini et al., 2019; Raposo et al., 2019; Zupo et al., 2019; Ciriminna et al., 2020; Santos et al., 2020b; Ciriminna et al., 2021; Lourenço et al., 2021). However, as far as we know, only Fernandez and Boudouresque (2000) focused on a comparative investigation of the dietary requirements of the sea urchin during different life stages. In addition, they analyzed the effects of a wide range of fishmeal enrichment, testing vegetable, mixed and animal feed (respectively with 0.0%, 44.7% and 89.4% of fish meal). Although levels of animal-sourced supplements between 0 and 50% are recommended, no investigations have so far defined more specifically the requirements of these supplements in the different life stages of P. lividus. Hence, this issue is still crucial in order to reduce food waste in echinoculture activities, whilst maximizing growth performance and reducing the environmental footprint.
To address this gap, the present study aimed to evaluate the effects of a narrow range of animal supply (0%; 20%; 40%) in a vegetable based diet, on three size-classes of P. lividus (15-25 mm; 25-35 mm; 45-55 mm). Maize, carrots, and soy, were selected as vegetable ingredients for the diet base due to their complementary profiles in terms of macronutrients (Hammer et al., 2012; Sartori and Gaion, 2016). Fish-meal was chosen as animal supply to be tested among the different life stages because of its large literature background on P. lividus rearing (Fernandez and Boudouresque, 2000; Spirlet et al., 2001; Fabbrocini et al., 2012; Sartori and Gaion, 2016; Ruocco et al., 2018; Volpe et al., 2018; Fabbrocini et al., 2019; Zupo et al., 2019; Ciriminna et al., 2021; Lourenço et al., 2021).
The division of sea urchins into discrete life stages is a controversial topic in literature. Several studies, in fact, have adopted heterogeneous criteria such as test diameter, age, reproductive maturity or trophic behavior to classify juveniles, sub-adults and adults in P. lividus (Sala, 1997; Grosjean et al., 1998; Cook and Kelly, 2007; McCarron et al., 2010; Boudouresque and Verlaque, 2020). In this study sea urchins were allocated into three size groups (test diameter) according to Fernandez and Boudouresque (2000). Based on their growth phase and gonads maturity, small specimens with Test Diameter TD < 25 mm were termed “juveniles” as they belong to the exponential growth phase and are not reproductively active; intermediate specimens with TD between 25 and 35 mm were termed “sub-adults” since they belong to the end of the exponential growth phase, but their gonads may be able to produce small amount of gametes; large specimens with TD > 35 mm were termed “adults”, since their gonads are fully reproductively active producing large quantities of healthy gametes. On the basis of this division, the effect of each experimental diet was evaluated in terms of somatic growth for the three sea urchin size classes and in terms of gonad yield for sub-adults and adults. In addition, these investigations were associated with the study of the Feed Conversion Ratio (FCR), in order to identify the best performing animal-sourced supplement for each sea urchin size-class, able to guarantee the highest growth with lowest food input.
Materials and Methods
Specimen Collection and Maintenance
Three size classes of P. lividus: 15 – 25 mm (juveniles); 25 – 35 mm (sub-adults) and 45 – 55 mm (adults) were reared in a four-month experiment (15 May - 20 September 2020). Sea urchins of the smallest size-class (15 – 25 mm, juveniles) were obtained by artificial reproduction, carried out in 2019 at the Laboratory of Experimental Ecology and Aquaculture (LESA, University of Rome “Tor Vergata”) in the frame of an echinoderm hatchery production project (PO FEAMP 2.1 2014/2020; See Supplementary Material). The other two size classes were collected by snorkeling (1–8 m depth) at Santa Marinella in the central Tyrrhenian Sea, Italy (42°03′00″ North, 11°49′09″ East) in May 2020. Specimens were then transported to the LESA inside 30 L tanks equipped with aerators and dry ice (Rakaj et al., 2018; Rakaj et al., 2019). Once in the lab, the specimens were acclimatized in floating baskets inside 600 L indoor tanks (Morroni et al., 2020). Water temperature was kept at 20 ± 0.5°C (Spirlet et al., 1998), which was the rearing temperature also used during the experiment. Sea urchins of each size class were starved for two weeks in the maintaining system before undergoing the experimental trials (Prato et al., 2018)
Experimental Design
The experiment was run in the same recirculating aquaculture system (RAS) made up of 40 L aquaria each connected in tandem (Grosso et al., 2021). This RAS was specifically designed for feeding investigations, as it was equipped with an efficient waste removal system. The water leaving the aquaria was firstly subjected to a mechanical filtration phase in a drum filter, in order to remove waste particles greater than 20 μm. In the second step, organic compounds, such as proteins and amino acids, were removed from a strong protein skimming. In the next step, the residual particles (such as nitrogen compounds) were retained and oxidized in fine sand filters (first) and biofilters with moving bed media (after). In the final filtration phase, 5 μm cartridge filter allowed fine particle removal and a UV sterilizer eliminated bacteria load (See Supplementary material). This rearing system guaranteed a flow rate of 120 L h-1 (in each aquarium) and ensured similar physico-chemical parameters: temperature (20 ± 1°C); salinity (36 ± 1‰); pH (7.95-8.25), and natural photoperiod. Water exchange of 50% of the whole volume was undertaken at least twice a week using 5 μm filtered and UV-sterilized seawater. Ammonia, nitrate and nitrite concentration were monitored every two days by means of spectrophotometer (Hach Lange D3900), to maintain the rearing condition within a healthy range (Huguenin and Colt, 2002; Mortensen et al., 2012). Additionally, pH, temperature and salinity were monitored daily through a probe (EUTECH PCD 650).
18 sub-adults and 18 adults were sacrificed prior to the experiment in order to assess the baseline of the Gonad Index (GI %) at time T0. After starvation, 108 specimens of P. lividus were sorted into the three size classes - 36 in each size class - by measuring their test diameters with a digital calliper ( ± 0.01 mm accuracy). Then each size class was reared separately, with juveniles, sub-adults and adults allocated in different 40 L aquaria, inside perforated single floating baskets (Figure 1). The mean test diameter and wet weight of the three size classes at the beginning of the experiment are shown in Table 1.
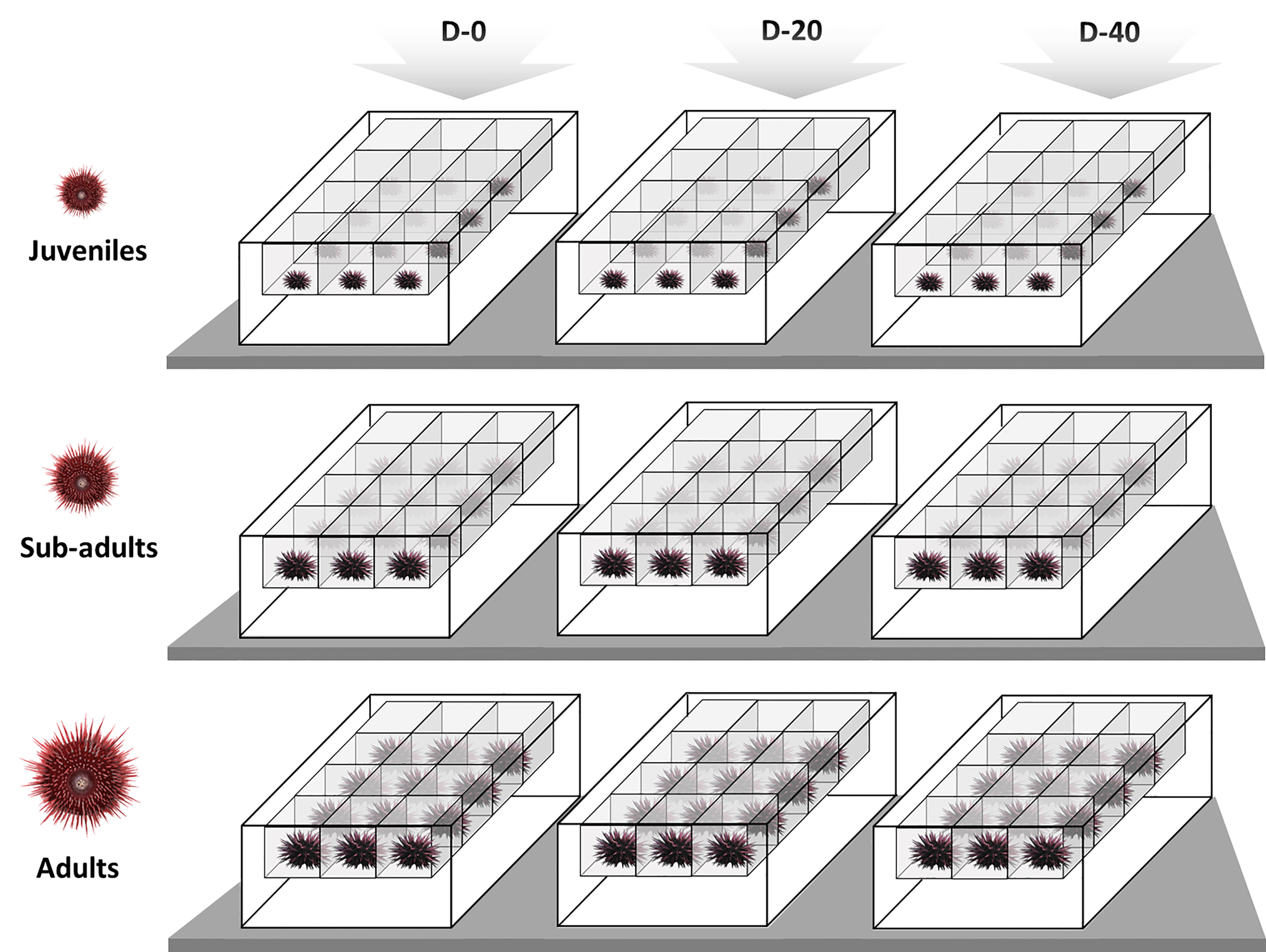
Figure 1 Representation of the experimental design: above the three experimental diets administrated, D-0 (100% vegetables, 0% fish meal); D-20 (80% vegetables, 20% fish meal); D-40 (60% vegetables, 40% fish meal), and at each level the respective P. lividus size classes, juveniles (15-25 mm); sub-adults (25-35 mm); adults (45-55 mm). Twelve sea urchins were employed in each condition, individually placed in feeding boxes within same RAS.
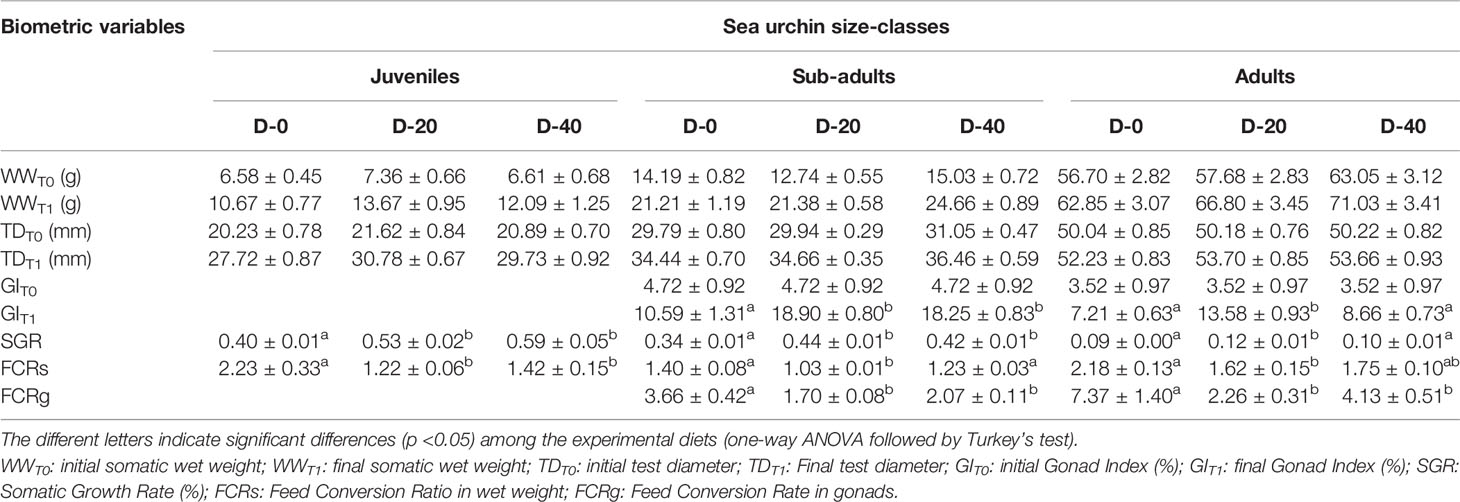
Table 1 Biometric measures (mean ± SE) and growth performances of P. lividus size classes fed with the three experimental diets (D-0, D-20 and D-40).
Diet Preparation and Feeding Parameters
Three experimental diets were tested in this study:
● D-0 (0% fish meal, 100% vegetables)
● D-20 (20% fish meal, 80% vegetables)
● D-40 (40% fish meal, 60% vegetables)
Carrots, maize and soy were employed to make up the vegetable base of all the experimental diets. In each diet, 119.80 g (carrots), 33.73 g (maize) and 46.46 g (soy) respectively, were used to obtain the same proportion (1: 1: 1) of vegetables in dry weight (Table 2) (Grosso et al., 2021). The vegetables were steamed, blended and extruded with different levels of fish-meal enrichment (0%, 20% and 40%) using agar-agar (Nature et Plantes - St Paul Les Dax, France) as a binder to minimize food dispersion (Fabbrocini et al., 2012; Volpe et al., 2018). All diet ingredients were certified for human consumption complying the HACCP principles. The mixtures were rolled out into moist blocks (1 cm x side) and administered fresh to the sea urchins. Each week, the diets were freshly prepared and stored at 4°C to preserve the nutritional profile and avoid bacteria growth. Diets were administered once a day ad libitum (around 1% of sea urchins weight in food dry weight) to sea urchins of each size-class. Over a period of four weeks (one week for each month), the ingestion was calculated for each specimen by weighing the administered food (wet weight) and leftover food produced after 24 h (in dry weight). The conversion of administrated food from wet to dry weight was obtained weekly by drying 5 replicates for each diet. Finally, the Feed Conversion Ratio (FCR) was calculated by combining sea urchin ingestion and growth data.
Growth Rates
During the experiment, diets performances were investigated through different measurements for each life stage: in juveniles, since gonads were immature, we focused on somatic growth performances (Somatic Growth Rates SGR and Feed Conversion Ration in wet weight FCRs); in sub-adults and adults, in terms of both somatic growth (SGR and FCRs) and gonad yield (Gonad Index GI and Feed Conversion Ratio in gonads FCRg). The somatic growth of juvenile, sub-adult and adult sea urchins was followed, measuring the wet weight of specimens, as described above. At the end of the experiment, the sea urchins were starved for 48 h in order to void their digestive tract before being weighed to calculate the Somatic Growth Rate SGR % (Grosso et al., 2021) as follows:
•
Where Wf and Wi are the final and initial wet weights (g) of juvenile or subadult sea urchins and t represents time in days of the experiment.
The Gonad Index GI % (Vizzini et al., 2015) was calculated for sub-adult and adult sea urchins, as follows:
•
Where Wg is the wet weight (g) of the gonad and Wt is the total wet weight (g) of the sea urchin.
Finally, combining the data collected regarding the ingestion and growth of the three sea urchin size-classes, Feed Conversion Ratio in gonads (FCRg) and Feed Conversion Ratio in somatic weight (FCRs) were calculated:
•
Where DWi is the total dry weight of feed added to the aquaria and DWf is the total dry weight of the uneaten feed; WWfg and WWig are the final and initial wet weights of sub-adult and adult P. lividus gonads.
•
Where DWi is the total dry weight of feed added to the aquaria and DWf is the total dry weight of the uneaten feed; WWfs and WWis are the final and initial wet weights of juvenile and sub-adult P. lividus.
Statistical Analysis
Prior to analysis, raw data were diagnosed for normality of distribution and homogeneity of variance by means of a Levene’s test and a Kolmogorov-Smirnov test respectively (Whitlock and Schluter, 2010). Samples were close to a normal distribution (Kolmogorov-Smirnov p > 0.05) with similar variances (Levene’s p > 0.05). One-way analysis of variance ANOVA was carried out to analyze the effects of experimental diets on somatic and gonadic growth performances and the feeding parameters of each sea urchin size-class. Where p values were generated in ANOVA, Turkey multiple comparison tests were used to evaluate differences among pair-wise means (p < 0.05). Local regression analysis (LOESS) was then performed in order to estimate the Somatic Growth Rate (%) for a continuous distribution of sea urchin test diameters in relation to each experimental diet (Figure 3). Statistical analyses were performed with PAST 3.0 (Hammer et al., 2013).
Results
All the experimental diets were found to be suitable to sustain the breeding of juvenile, sub-adult and adult P. lividus. In fact, during the experiment specimens from all the sea urchin size-classes showed a 100% survival rate and positive somatic or gonadal growth in all the feeding conditions. However, the cross-comparison analysis highlighted significant differences in growth performances among the sea urchin size classes related to the three diets administrated.
Somatic Growth
The results of this experiment showed an overall reduction in the somatic growth rate with the increase in the size class. In fact, in all feeding trials (D-0, D-20, D-40), juveniles obtained the highest values of Somatic Growth Rate (SGR%) among the sea urchin size classes, followed by sub-adults and adults. Maximal growth was observed for juveniles fed with the 40% fishmeal-enriched diet (D-40; SGR = 0.59%) and the 20% fishmeal-enriched diet (D-20, SGR = 0.53%), which did not differ each other but were higher than SGR value obtained with the entirely vegetable diet (D-0, SGR = 0.40%) (Figure 2). Similarly, in sub-adults the maximal growth was obtained for D-20 (SGR = 0.44%) and D-40 (SGR = 0.42%), while D-0 yielded a lower growth rate (SGR = 0.34%) (Figure 2). Finally, for adult sea urchins the highest SGR value was obtained for D-20 (0.12%), which was higher than that obtained for D-40 and D-0 (0.10% and 0.09% respectively) (Figure 2).
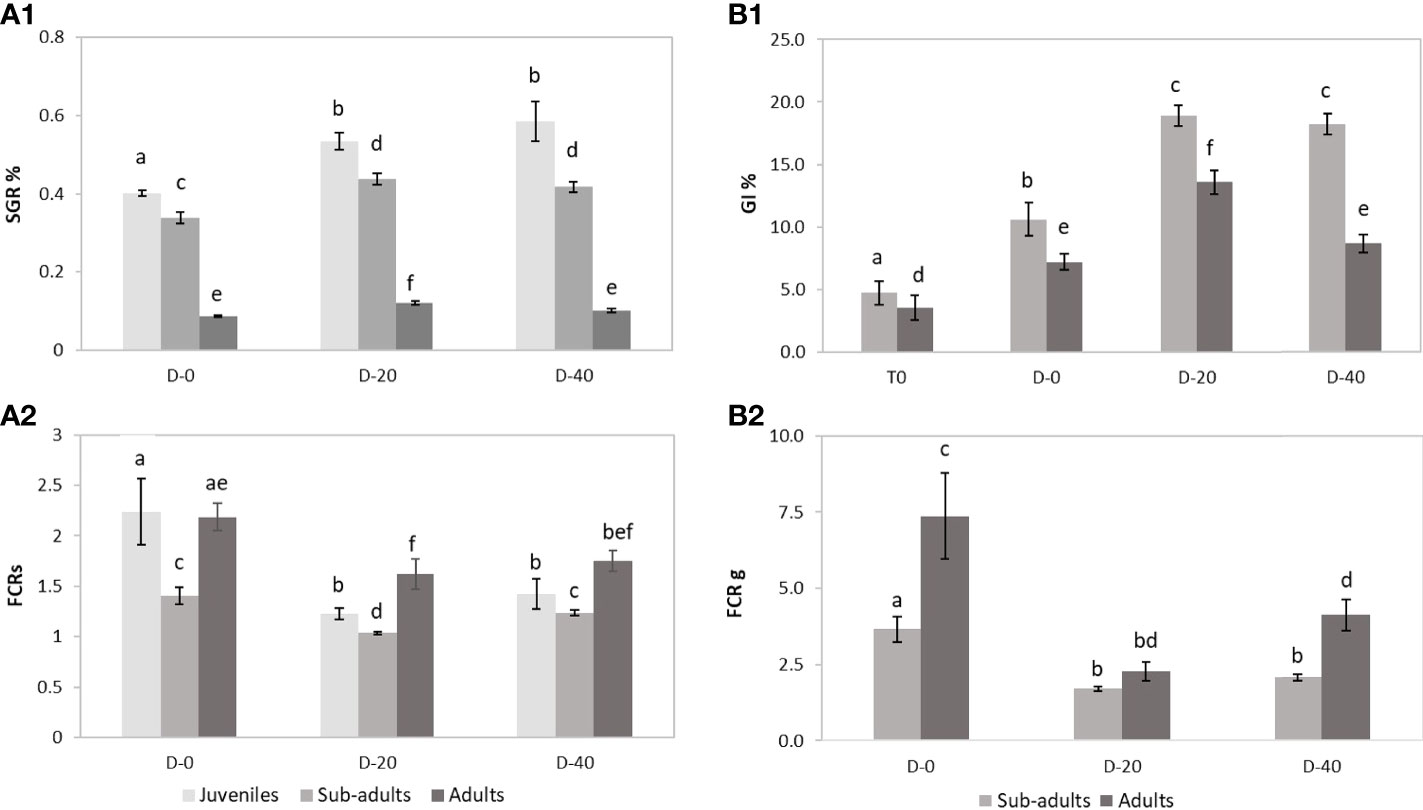
Figure 2 Somatic and gonadal growth performances in a four-month experiment across three P. lividus life stages (juveniles, sub-adults and adults) fed with three different diets (D-0, D-20 and D-40) (n=12 sea urchins for each condition). (A1) Somatic growth rate (SGR); (A2) Feed conversion ratio in somatic weight (FCRs), based in dry weight; (B1) Gonad index (GI%); (B2) Feed conversion ratio in gonads (FCRg), based in dry weight. Error bars indicate standard errors of the means. Columns with the same letter are not significantly different (p> 0.05).
In addition, the estimation of the maximum growth of P. lividus within a continuous distribution of test diameter for each of the three experimental diets was carried out through local regression analysis (LOESS) (Figure 3). The highest growth level in the sea urchins with smaller test diameters was obtained for the D-40 diet. However, the increase in test diameter was related to a decrease in the requirement of animal-source in the diet, as shown by the progressive slope inversion between the equation-lines of D-20 and D-40 (Figure 3). In fact, the sub-adult sea urchins obtained similar SGR % when fed with D-20 and D-40. Instead, the adults showed higher SGR values when fed with D-20. Finally, the sea urchin of all test diameters fed with D-0 showed the lowest growth.
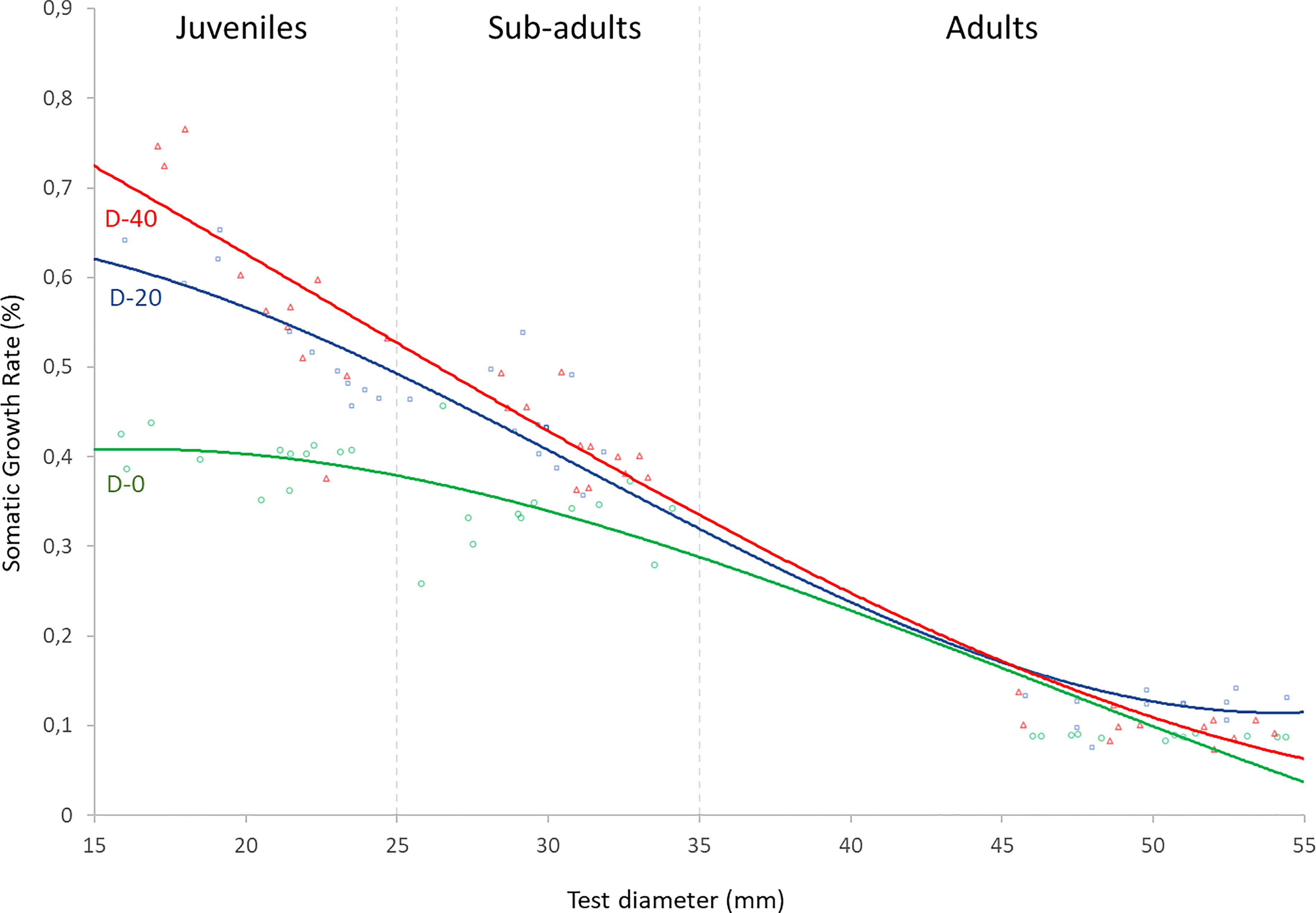
Figure 3 LOESS curves describe the relationship between weight gain and diameter of P. lividus fed with three experimental diets. Y axis: Somatic Growth Rate of sea urchins during the four months of the experiment; X axis: diameter of sea urchins at the beginning of the experiment.
These results were also confirmed by the FCRs (Figure 2). In fact, for all the three sea urchin size classes, the lowest FCRs values were obtained for D-20 (1.23, 1.04, 1.62, respectively for juveniles, sub-adults and adults) and D-40 (1.42, 1.23, 1.75 respectively), which did not differ each other, while values for D-0 were significantly higher (2.24, 1.40, 2.18 respectively).
Comparison analysis across size classes evidenced that sub-adults yielded lower FCRs in all feeding conditions (Figure 2B). Juveniles, on the other hand, obtained lower FCRs than adults in enriched animal diets (D-20 and D-40), although for the entirely vegetable diet (D-0), FCRs were similar between the two groups (Figure 2B).
Gonadal Growth
At the end of the experiment, only sub-adult and adult specimens showed gonadal production. In fact, for these two sea urchin size classes the final Gonad Index was significantly higher than the initial values (Figure 2). However, ANOVA highlighted significant differences in gonadal growth performances among the diets administrated for each size class. Sub-adults showed higher GI values when fed with D-20 (18.90) and D-40 (18.25) than with D-0 (10.60) (Figure 2). Instead, the maximal value for the adults was recorded for D-20 (13.58), which was higher than those for D-40 (8.66) and D-0 (7.21) (Figure 2).
These results were also confirmed by the FCRg (Figure 2), which was lower for sub-adults fed with D-20 (1.70) and D-40 (2.08) than with D-0 (3.66) and for adults fed with D-20 (2.26) than both those fed with D-40 (4.12) and D-0 (7.36).
Finally, cross-comparison analysis evidenced that in all feeding condition (D-0, D-20 and D-40) the GI and FCRg detected for sub-adults were respectively higher and lower than values obtained in adults (Figure 3).
Discussion
Experimental Setting
Many animal species change their feeding habits during their various life cycle stages, in relation to variations in their dietary requirements. High dietary variations may occur in the transition from the growth to the reproductive phases. In this context, animal protein sources are very important macronutrients for omnivorous species such as P. lividus since they provide essential amino acids for growth and reproduction (Scheibling and Hatcher, 2013). Several investigations have suggested the pivotal role of animal proteins, underlining how the somatic and gonadal growth of sea urchins fed with animal-source enriched diets were higher than those fed with entirely vegetable diets (Fernandez and Pergent, 1998; Fernandez and Boudouresque, 2000). However, in these studies the highest growth performances, in terms of somatic and gonadal growth, were obtained with the mix of animal and vegetal sources, whereas the sole animal food had no beneficial effects. These results, although highlighting the importance of animal supply in formulated diets, have also suggested the existence of a specific threshold which must be still investigated in P. lividus. Moreover, excessive amounts of animal protein may substantially increase the costs of diet formulation and of the treatment of waste in farming. These protein sources are, in fact, the most expensive nutrients in aquafeeds and their use produces a high level of nitrogenous pollution, which has a heavy ecological footprint and decreases the quality of the rearing seawater. For this reason, finding an optimal integration level of animal supply in formulated diets is a key issue for the future development of echinoculture.
This study evaluated the adequate provision level of animal supply in three sea urchin life stages, broadening basic knowledge on the dietary requirements of P. lividus post-metamorphic rearing. The experimental diets were made up of the same vegetable base in order to investigate exclusively the effects of animal supply variation. Vegetable ingredients employed in this study (carrots, maize and soy) were selected for their market availability, low price and their nutritional profiles which can cover a wide range of dietary requirements. Carrots were chosen for their notable β-carotene content, which plays an important role in promoting egg production and gonad development (Sartori and Gaion, 2016). Soy, instead, is one of the most valuable vegetable protein sources in animal farming (Hammer et al., 2012). Finally, maize is a rich source of carbohydrates, which are the preferred energy source for sea urchins, as it is for most herbivores and omnivores (Hammer et al., 2012).
The present study was set up within a single RAS in order to standardize the environmental conditions and allow the evaluation of the experimental hypothesis without random variations among the tested conditions. This investigation approach is largely shared in current aquaculture literature and also in echinoculture (Spirlet et al., 2001; Fabbrocini and D’Adamo, 2010; Cirino et al., 2017; Baião et al., 2019; Ciriminna et al., 2021; Grosso et al., 2021). Conversely, in separate RASs a random variation in environmental factors (such as a different microbial proliferation, anomalies in the filtering systems, different exposure, etc.) is much more likely than within the same recirculating system.
Furthermore, since the waste residual signature usually represents the main bias in this type of investigation, we adopted strict solutions to avoid their influence on experimental conditions: i) lower biomass (< 5%) than the actual carrying capacity of the RAS was employed; ii) gelatin agar-agar was employed as the binder for the experimental diets to reduce food dissolving in seawater; iii) the leftover food and feces were removed daily from the system; iiii) a water change was carried out at least twice a week, although the water parameters never exceeded the healthy range during the experimental period.
Growth Performances
During the experiment, overall somatic growth (SGR) was higher in sea urchin juveniles than in sub-adults and adults (Figure 2). These results are in agreement with the asymptotic model elaborated by Grosjean et al. (2003), which gives a good description of the growth of P. lividus. Positive results in survivorship and growth were obtained with all the experimental diets. However, statistical analysis highlighted that variations in the level of animal-sourced food in the experimental diets differentially affect sea urchin growth in the three life stages. In fact, the cross-comparison analysis showed significant differences in growth performance among juveniles, sub-adults and adults, confirming that each sea urchin size-class have specific dietary requirements.
In juveniles, animal enriched diets (D-40 and D-20) performed better than the entirely vegetal diet (D-0), as highlighted by both growth rate and FCRs values, lower and higher respectively in D-0. These results suggest that P. lividus requires a rich animal diet to promote somatic growth during the juvenile stage, suggesting that animal supply provides essential amino acids and fatty acids. Accordingly, Fernandez and Boudouresque (2000) observed the highest weight gain of the smallest size classes of P. lividus (TD: 20-25 mm) fed with an animal-diet; and Cook and Kelly (2007) observed that P. lividus in an IMTA experiment with Salmo salar may benefit from a high animal-enriched diet, up to a test diameter of 25 mm.
SGR value in D-0 resulted to be similar to that achieved by Vizzini et al. (2018), when testing L. sativa based diet. In D-20, we obtained SGR values comparable to Lourenço et al. (2021), who tested similar dietary animal supply (12,5%). Both these SGR values were, however, lower than that observed in D-40 in the present study, suggesting the better suitability of a high amount of animal supply (around 40%) to meet the dietary requirements of sea urchin juveniles. According to previous investigations on different sea urchin species, juvenile growth may be maximized with total protein levels around 20% or greater in the diets (Akiyama et al., 2001; Pearce et al., 2002; Kennedy et al., 2005; Heflin et al., 2016). This dietary requirement was highlighted in our study also for P. lividus juveniles, since the highest dietary protein levels tested (around 37% in D-40) promoted the maximum weight gain in this life stage. Furthermore, a protein/carbohydrate ratio of around 1:1 in D-40 yielded the best results, indicating that in this condition juveniles may have sufficient energy (from carbohydrates) to utilize protein mainly for growth with a consequent protein sparing.
With regards to sub-adults, SGR, although lower than in juveniles, was higher than in adults in each diet. This is in accordance with general growth theory, for which energy allocation (EA) changes with the variation in animal size. Therefore, the proportion of food energy allocated to somatic growth decreases with the increase in sea urchin size, whereas a higher allocation for reproduction is observed with size growth (Giese et al., 1966; Fernandez and Boudouresque, 2000; McCarron et al., 2009).
Our results, moreover, showed that the best somatic growth values in sub-adults were achieved in both animal enriched diets (D-20 and D-40). No difference was highlighted between the two enriched diets but both cases were significantly higher than the value obtained with the entirely vegetable diet (D-0). These results are comparable with those of Fernandez and Boudouresque (2000) which suggested that the limited amount of proteins versus the insoluble carbohydrates in vegetable meals negatively affects the nutrient uptake of P. lividus, causing growth to be lower with a purely vegetable diet. However, from our investigation, it emerged that sub-adult sea urchins require less animal supply than juveniles. This was also confirmed by the lower FCRs data for D-20, highlighting the high efficiency of sub-adult sea urchins consuming the intermediate enriched animal diet (20% of animal supply).
For this size class, the diet yields were investigated also in terms of gonadal production. Indeed, although most of the energy is allocated to somatic growth in this life stage, the sub-adult sea urchins show a trade-off in resource allocation between reproduction and growth (Grosjean et al., 1998). The GI values obtained with fishmeal enriched diets resulted higher than those observed in D-0, highlighting the benefits of animal supply on sub-adult growth. These results were also confirmed by comparison with the current literature. In fact, previous studies that administrated entirely vegetable diets to similar sea urchin size class (Vizzini et al., 2015; Santos et al., 2020b), obtained comparable GI values to those obtained in this study with D-0, but lower GI compared to D-20 and D-40. Furthermore, among the animal enriched diets tested in the present study, D-20 yielded the highest value of gonad index, as well as the lowest value of FCRg. The gonad growth in this feeding condition was also higher than the values obtained with 44.7% and 88.9% of fishmeal supply by Fernandez and Boudouresque (2000). These results, therefore, suggested the better suitability of a low amount of animal supply (around 20%) to meet the dietary requirements for somatic and gonadic growth in this sea urchin size class.
Considering the macronutrient proximate composition of the experimental diets, the best growth performances in sub-adults were achieved with dietary proteins ranging between 29.92 - 37.01%, in D-20 and D-40 respectively. This is in line with the optimal protein range found by Fernandez and Boudouresque (2000) and Fabbrocini et al. (2019) for this sea urchin size class. Finally, conversely to juveniles, sub-adults benefitted from high levels of dietary carbohydrates (42.68% in D-20). This could be related to the different food allocation in these life stages. In fact, sub-adults begin to be reproductively active, and most dietary carbohydrates are generally stored in the gonads (Marsh et al., 2013).
In adult sea urchins, the best measure of well-being is the ability to produce healthy and large reproductive organs (Vadas, 1977). Since the adult gonads are of high market value and can be traded, the most important goal for aquaculture production is to guarantee high gonad quality and yields. During this life stage, sea urchins allocate the highest level of food energy to reproduction, and only a small part to somatic growth. In fact, during our experiment, adult sea urchins showed the lowest somatic growth rates of all the three size classes, although still in line with the SGR values already found in other studies (Baião et al., 2019; Lourenço et al., 2020). Hence, the diet which promoted the highest gonadal production (in terms of GI%) in our study was assumed to be the best to satisfy the dietary requirements of adult sea urchins, providing key information on its feeding behaviour and nutritional needs. D-20 (20% of animal supply) gave the best result in terms of P. lividus GI% (13,58%) and FCRg, suggesting a better capacity of the sea urchins to transform food input into gonad production with this diet. Hence, 20% animal supply emerged as a threshold level for adult sea urchins at which gonad growth is maximized, and over which only disadvantages in terms of cost and water pollution were observed. This finding is also corroborated by the results we obtained with the entirely vegetal diet. Indeed, in adults, contrary to what is observed in sub-adults, D-0 showed similar GI values to those achieved with D-40, evidencing that adult sea urchins need a lower animal supply than in the previous life stages. Raposo et al. (2019) obtained comparable GI to ours in D-0, by feeding sea urchins with maize and spinach. However, adopting the same diet, Sartori and Gaion (2016) achieved a higher value after a four-month experiment in RAS. The different gonad growth detected among these studies may be related to specific experimental conditions, such as the rearing temperature. In our study, the GI value in D-20 resulted higher than in previous investigations with predominantly vegetable diets (Prato et al., 2018; Ruocco et al., 2018), but comparable to those achieved with similar fishmeal supply (Fernandez and Boudouresque, 2020; Ciriminna et al., 2021). Conversely, Cirino et al. (2017) evidenced higher gonad growth than we found in D-20, applying a similar animal supply (25%). This difference is probably due to the smaller size of the sea urchin specimens employed by the authors and/or to the macronutrient levels and ratios in the different experimental feeding conditions. The lipid/protein ratio in the diet tested by Cirino et al. (2017), in fact, was significantly lower than in ours and Hammer et al. (2010) have shown that high dietary levels of lipids (> 9%) may negatively influence growth. Hence, further investigations are still needed to clarify the optimal lipid/protein ratio to adopt in adult sea urchin diets.
Summarizing our results, as far as we know, Fernandez and Boudouresque (2000) were the only authors who compared in parallel the effects of formulated diets on different P. lividus life stages. Our study therefore expands on their previous investigation, identifying more specifically the possible optimal level of animal-sourced supplements for the three P. lividus life stages. The diet containing 40% animal supply showed high benefits for the juveniles, while levels around 20% performed very well for sub-adults and adults. In addition, by apply a Local regression analysis (LOESS) we were able to determine the most effective diets in terms of the highest growth performances for the full post-metamorphic P. lividus life cycle. These findings provide a practical tool for developing efficient and sustainable commercial feeds for P. lividus rearing, representing a guideline on how and when to change the level of animal source enrichment in diets during the sea urchin production phases.
General Considerations on P. lividus Ecology and Trophic Behavior
This controlled-environment experiment, evidencing the animal protein requirement of P. lividus, shed light also on its feeding ecology and local-scale migratory movements. Crook et al. (2000) suggested that daily migration between the sides, interstices, and the upper surface of rocks is size dependent. Previous investigations have claimed that sheltering from predators is the driver of the migratory behavior in the different life stages (Boudouresque and Verlaque, 2020). However, Rico (1989) showed that the percentage of time P. lividus spends in motion, depends on the type of food available, although the authors didn’t find evidence of a clear relationship with food choice. Our results corroborated this theory, revealing that sea urchin spatial behavior may also reflect the variations in the dietary requirements. Indeed, the juvenile specimens live under rocks and refuges, where they can rely on protein-rich animal food sources (i.e., sponges, bryozoans and polychaetes). The sub-adults, instead, start performing foraging excursions on the photic zone where both algae and animal food sources become available. Adult sea urchins become dominant in algal beds where they graze on predominantly vegetal sources. This micro-habitat migration in P. lividus closely matches the feeding requirement shift that emerged in our study, leading us to hypothesize that this may be the root cause or a contributing cause of the sheltering behavior. Then, stable isotope analysis may be a promising tool in order to further investigate this topic as a function of resource availability and habitat features in the natural environment (Boncagni et al., 2019; Camps-Castellà et al., 2020; Rumolo et al., 2020)
Conclusion
A balanced mix of vegetal and animal sources in the diets is highly recommended to maximize the growth performance of the sea urchin species, reducing time to market and the production of high quality gonads (Vizzini et al., 2019). However, sea urchins change their feeding habits during their life cycle in relation to variations in dietary requirement. Therefore, finding the optimum level of animal-source supplements in each stage of the sea urchin life cycle is crucial in echinoculture research. Indeed, animal proteins, despite being a very important nitrogen and amino acid source, are also one of the most expensive nutrients in aquafeeds and their metabolism as an energy source is highly inefficient, producing a huge ecological footprint as a result of nitrogenous pollution.
The results of this study provide another step towards the development of a full life-cycle aquaculture of the sea urchin P. lividus. More specifically, this 4-month rearing experiment identified the optimal level of animal-sourced supplements in the diets for three P. lividus life stages, expanding on the investigation of Fernandez and Boudouresque (2000). Moreover, a general growth model covering the full post-metamorphic P. lividus life cycle was defined for each experimental diet, providing a practical tool for the identification of a better level of animal-sourced supplement in each of the sea urchin rearing phases.
Data Availability Statement
The original contributions presented in the study are included in the article/supplementary material. Further inquiries can be directed to the corresponding author.
Author Contributions
LG and AR conceived the experimental design and carried out the investigation, performed the statistical analysis, wrote the first draft of the manuscript. AF, SV, and MS wrote sections of the manuscript. All authors contributed to manuscript revision, read, and approved the submitted version.
Funding
The research and publication materials was supported by FEAMP project: ”E84I19000640002” and PON project: “E86C18001440008”.
Conflict of Interest
The authors declare that the research was conducted in the absence of any commercial or financial relationships that could be construed as a potential conflict of interest.
The reviewer CS declared a past co-authorship with one of the authors C-FB to the handling Editor.
Publisher’s Note
All claims expressed in this article are solely those of the authors and do not necessarily represent those of their affiliated organizations, or those of the publisher, the editors and the reviewers. Any product that may be evaluated in this article, or claim that may be made by its manufacturer, is not guaranteed or endorsed by the publisher.
Supplementary Material
The Supplementary Material for this article can be found online at: https://www.frontiersin.org/articles/10.3389/fmars.2022.865450/full#supplementary-material
References
Akiyama T., Unuma T., Yamamoto T. (2001). Optimum Protein Level in a Purified Diet for Young Red Sea Urchin Pseudocentrotus Depressus. Fish. Sci. 67 (2), 361–363. doi: 10.1046/j.1444-2906.2001.00252.x
Baião L. F., Rocha F., Costa M., Sá T., Oliveira A., Maia M. R. G., et al. (2019). Effect of Protein and Lipid Levels in Diets for Adult Sea Urchin Paracentrotus Lividus (Lamarck 1816). Aquaculture 506, 127–138. doi: 10.1016/j.aquaculture.2019.03.005
Bartley D. M., Bell J. D. (2008). Restocking, Stock Enhancement, and Sea Ranching: Arenas of Progress. Rev. Fish. Sci. 16, 357–365. doi: 10.1080/10641260701678058
Boncagni P., Rakaj A., Fianchini A., Vizzini S. (2019). Preferential Assimilation of Seagrass Detritus by Two Coexisting Mediterranean Sea Cucumbers: Holothuria Polii and Holothuria Tubulosa. Estua. Coast. Shelf. Sci. 231, 106464. doi: 10.1016/j.ecss.2019.106464
Boudouresque C. F., Verlaque M. (2020). “Paracentrotus Lividus,” in Developments in Aquaculture and Fisheries Science (London, United Kingdom: Elsevier B.V), pp. 447–485. doi: 10.1016/B978-0-12-819570-3.00026-3
Camps-Castellà J., Romero J., Prado P. (2020). Trophic Plasticity in the Sea Urchin Paracentrotus Lividus, as a Function of Resource Availability and Habitat Features. Mar. Ecol. Prog. Ser. 637, 71–85. doi: 10.3354/meps13235
Carboni S., Vignier J., Chiantore M., Tocher D. R., Migaud H. (2012). Effects of Dietary Microalgae on Growth, Survival and Fatty Acid Composition of Sea Urchin Paracentrotus Lividus Throughout Larval Development. Aquaculture 324–325, 250–258. doi: 10.1016/j.aquaculture.2011.10.037
Ceccherelli G., Pinna S., Sechi N. (2009). Evaluating the Effects of Protection on Paracentrotus Lividus Distribution in Two Contrasting Habitats. Estua. Coast. Shelf. Sci. 81, 59–64. doi: 10.1016/j.ecss.2008.10.007
Ciriminna L., Signa G., Vaccaro A. M., Messina C. M., Mazzola A., Vizzini S. (2020). Formulation of a New Sustainable Feed From Food Industry Discards for Rearing the Purple Sea Urchin Paracentrotus Lividus. Aquacult. Nutr. 26, 1046–1057. doi: 10.1111/anu.13063
Ciriminna L., Signa G., Vaccaro A. M., Visconti G., Mazzola A., Vizzini S. (2021). Turning Waste Into Gold: Sustainable Feed Made of Discards From the Food Industries Promotes Gonad Development and Colouration in the Commercial Sea Urchin Paracentrotus Lividus (Lamarck 1816). Aquacult. Rep. 21, 100881. doi: 10.1016/J.AQREP.2021.100881
Cirino P., Ciaravolo M., Paglialonga A., Toscano A. (2017). Long-Term Maintenance of the Sea Urchin Paracentrotus Lividus in Culture. Aquacult. Rep. 7, 27–33. doi: 10.1016/j.aqrep.2017.04.003
Cook E. J., Kelly M. S. (2007). Effect of Variation in the Protein Value of the Red Macroalga Palmaria Palmata on the Feeding, Growth and Gonad Composition of the Sea Urchins Psammechinus Miliaris and Paracentrotus Lividus (Echinodermata). Aquaculture 270, 207–217. doi: 10.1016/j.aquaculture.2007.01.026
Cook E. J., Kelly M. S. (2009). Co-Culture of the Sea Urchin Paracentrotus Lividus and the Edible Mussel Mytilus Edulis L. On the West Coast of Scotland, United Kingdom. J. Shellf. Res. 28 (3), 553–559. doi: 10.2983/035.028.0318
Crook A. C., Long M., Barnes D. K. (2000). Quantifying Daily Migration in the Sea Urchin Paracentrotus Lividus. J. Mar. Biol. Assoc. Unit. Kingd. 80 (1), 177–178. doi: 10.1017/S0025315499001721
Daggett T. L., Pearce C. M., Tingley M., Robinson S. M. C., Chopin T. (2005). Effect of Prepared and Macroalgal Diets and Seed Stock Source on Somatic Growth of Juvenile Green Sea Urchins (Strongylocentrotus Droebachiensis). Aquaculture 244, 263–281. doi: 10.1016/j.aquaculture.2004.11.030
Fabbrocini A., D’Adamo R.. (2010). Gamete Maturation and Gonad Growth in Fed and Starved Sea Urchin Paracentrotus lividus (Lamarck, 1816). J. Shellfish Res. 29 (4), 1051–1059. doi: 10.2983/035.029.0407
Fabbrocini A., D’Adamo R.. (2012). Gamete Maturation and Gonad Growth in Fed and Starved Sea Urchin Paracentrotus Lividus (Lamarck, 1816). J. Shellfish Res. 29 (4), 1051–59. doi: 10.2983/035.029.0407
Fabbrocini A., Volpe M. G., di Stasio M., D’Adamo R., Maurizio D., Coccia E., et al. (2012). Agar-Based Pellets as Feed for Sea Urchins (Paracentrotus Lividus): Rheological Behaviour, Digestive Enzymes and Gonad Growth. Aquacult. Res. 43, 321–331. doi: 10.1111/j.1365-2109.2011.02831.x
Fabbrocini A., Volpe M. G., Hoseinifar S. H., Siano F., Coccia E., Scordella G., et al. (2019). Paracentrotus Lividus Roe Enhancement by a Short-Time Rearing in Offshore Cages Using Two Agar-Based Experimental Feed. Int. J. Aquat. Biol. 7, 155–165. doi: 10.22034/ijab.v7i3.626
Fernandez C., Boudouresque C. (2000). Nutrition P. Lividus Artificial Food. Mar. Ecol. Prog. Ser. 204, 131–141. doi: 10.3354/meps204131
Fernandez C., Pergent G. (1998). Effect of Different Formulated Diets and Rearing Conditions on Growth Parameters in the Sea Urchin Paracentrotus Lividus. J. Shellf. Res. 17, 1571–1581.
Giese A. C., Farmanfarmaian A., Hilden S., Doezema P. (1966). Respiration During the Reproductive Cycle in the Sea Urchin, Strongylocentrotus Purpuratus. Biol. Bull. 130, 192–201. doi: 10.2307/1539696
Grosjean P., Spirlet C., Gosselin P., Vaïtilingon V., Jangoux M. (1998). Land-Based, Closed-Cycle Echiniculture of Paracentrotus Lividus (Lamarck)(Echinoidea: Echinodermata): A Long-Term Experiment at a Pilot Scale. J. Shellf. Res. 17 (5), 1523–1531.
Grosjean P., Spirlet C., Jangoux M. (2003). A Functional Growth Model With Intraspecific Competition Applied to a Sea Urchin, Paracentrotus Lividus. Can. J. Fish. Aquat. Sci. 60, 237–246. doi: 10.1139/F03-017
Grosso L., Rakaj A., Fianchini A., Morroni L., Cataudella S., Scardi M. (2021). Integrated Multi-Trophic Aquaculture (IMTA) System Combining the Sea Urchin Paracentrotus Lividus, as Primary Species, and the Sea Cucumber Holothuria Tubulosa as Extractive Species. Aquaculture 534, 736268. doi: 10.1016/J.AQUACULTURE.2020.736268
Guidetti P., Terlizzi A., Boero F. (2004). Effects of the Edible Sea Urchin, Paracentrotus Lividus, Fishery Along the Apulian Rocky Coast (SE Italy, Mediterranean Sea). Fish. Res. 66, 287–297. doi: 10.1016/S0165-7836(03)00206-6
Hammer Ø., Harper D. A. T., Ryan P. D. (2013). “PAST: Paleontological Statistics,” in Version 3.0. National History Museum (Oslo, Norway: University of Oslo).
Hammer H., Powell M., Gibbs V., Jones W., Watts S., Lawrence A., et al. (2010). Effect of Dietary Menhaden Oil and Soy Oil on Consumption,Somatic Growth and Gonad Production of the Sea Urchin, Lytechinus Variegatus. Echinoderms, 377–383.
Hammer H. S., Powell M. L., Jones W. T., Gibbs V. K., Lawrence A. L., Lawrence J. M., et al. (2012). Effect of Feed Protein and Carbohydrate Levels on Feed Intake, Growth, and Gonad Production of the Sea Urchin, Lytechinus Variegatus. J. World Aquacult. Soc. 43, 145–158. doi: 10.1111/j.1749-7345.2012.00562.x
Heflin L. E., Gibbs V. K., Powell M. L., Makowsky R., Lawrence J. M., Lawrence A. L., et al. (2012). Effect of Dietary Protein and Carbohydrate Levels on Weight Gain and Gonad Production in the Sea Urchin Lytechinus Variegatus. Aquaculture 358–359, 253–261. doi: 10.1016/j.aquaculture.2012.06.009
Heflin L. E., Makowsky R., Taylor J. C., Williams M. B., Lawrence A. L., Watts S. A. (2016). Production and Economic Optimization of Dietary Protein and Carbohydrate in the Culture of Juvenile Sea Urchin Lytechinus Variegatus. Aquaculture 463, 51–60. doi: 10.1016/j.aquaculture.2016.05.023
Huguenin J. E., Colt J. (2002). Design and Operating Guide for Aquaculture Seawater Systems (Amsterdam, Netherlands: Elsevier).
Kelly M. S. (2004). Sea Urchin Aquaculture: A Review and Outlook. Echinoderms (London: Taylor & Francis Group), 283–289.
Kennedy E. J., Robinson S. M., Parsons G. J., Castell J. D. (2005). Effect of Protein Source and Concentration on Somatic Growth of Juvenile Green Sea Urchins Strongylocentrotus Droebachiensis. J. World Aquacult. Soc. 36 (3), 320–336. doi: 10.1111/j.1749-7345.2005.tb00336.x
Lourenço S., Cunha B., Raposo A., Neves M., Santos P. M., Gomes A. S., et al. (2021). Somatic Growth and Gonadal Development of Paracentrotus Lividus (Lamarck 1816) Fed With Diets of Different Ingredient Sources. Aquaculture 539, 736589. doi: 10.1016/J.AQUACULTURE.2021.736589
Lourenço S., José R., Andrade C., Valente L. M. P. (2020). Growth Performance and Gonad Yield of Sea Urchin Paracentrotus Lividus (Lamarck 1816) Fed With Diets of Increasing Protein: Energy Ratios. Anim. Feed. Sci. Technol. 270, 114690. doi: 10.1016/j.anifeedsci.2020.114690
Marsh A. G., Powell M., Watts S. (2013). “Energy Metabolism and Gonad Development,” in Sea Urchins: Biology and Ecology, Third Ed. Ed. Lawrence J. (Amsterdam, The Netherlands: Elsevier BV), 35–50.
McBride S. C. (2005). Sea Urchin Aquaculture. Am. Fish. Soc. 46, 179–208. doi: 10.1201/9780203970881.ch48
McCarron E., Burnell G., Kerry J., Mouzakitis G. (2010). An Experimental Assessment on the Effects of Photoperiod Treatments on the Somatic and Gonadal Growth of the Juvenile European Purple Sea Urchin Paracentrotus Lividus. Aquacult. Res. 41 (7), 1072–1081. doi: 10.1111/j.1365-2109.2009.02392.x
McCarron E., Burnell G., Mouzakitis G. (2009). Growth Assessment on Three Size Classes of the Purple Sea Urchin Paracentrotus Lividus Using Continuous and Intermittent Feeding Regimes. Aquaculture 288, 83–91. doi: 10.1016/j.aquaculture.2008.11.025
Morroni L., Rakaj A., Grosso L., Fianchini A., Pellegrini D., Regoli F. (2020). Sea Cucumber Holothuria Polii (Delle Chiaje 1823) as New Model for Embryo Bioassays in Ecotoxicological Studies. Chemosphere 240, 124819. doi: 10.1016/j.chemosphere.2019.124819
Mortensen A., Siikavuopio I., James P. (2012). Water Quality Requirements for Culture of the Green Sea Urchin, Strongylocentrotus Droebachiensis. World Aquacult. 46–50.
Pearce C. M., Daggett T. L., Robinson S. M. C. (2002). Effect of Protein Source Ratio and Protein Concentration in Prepared Diets on Gonad Yield and Quality of the Green Sea Urchin, Strongylocentrotus Droebachiensis. Aquaculture 214, 307–332. doi: 10.1016/S0044-8486(02)00041-8
Prato E., Fanelli G., Biandolino F., Chiantore M., Secci M., Angioni A., et al. (2018). Influence of a Prepared Diet and a Macroalga ( Ulva Sp.) on the Growth, Nutritional and Sensory Qualities of Gonads of the Sea Urchin Paracentrotus Lividus. Aquaculture 493, 240–250. doi: 10.1016/j.aquaculture.2018.05.010
Rakaj A., Fianchini A., Boncagni P., Lovatelli A., Scardi M., Cataudella S. (2018). Spawning and Rearing of Holothuria Tubulosa: A New Candidate for Aquaculture in the Mediterranean Region. Aquacult. Res. 49 (1), 557–568. doi: 10.1111/are.13487
Rakaj A., Fianchini A., Boncagni P., Scardi M., Cataudella S. (2019). Artificial Reproduction of Holothuria Polii: A New Candidate for Aquaculture. Aquaculture 498, 444–453. doi: 10.1016/j.aquaculture.2018.08.060
Rakaj A., Morroni L., Grosso L., Fianchini A., Pensa D., Pellegrini D., et al. (2021). Towards Sea Cucumbers as a New Model in Embryo-Larval Bioassays: Holothuria Tubulosa as Test Species for the Assessment of Marine Pollution. Sci. Total. Environ. 787, 147593. doi: 10.1016/j.scitotenv.2021.147593
Raposo A. I. G., Ferreira S. M. F., Ramos R., Santos P. M., Anjos C., Baptista T., et al. (2019). Effect of Three Diets on the Gametogenic Development and Fatty Acid Profile of Paracentrotus Lividus (Lamarck 1816) Gonads. Aquacult. Res. 50, 2023–2038. doi: 10.1111/are.14051
Rico V. (1989). “Contribution a`L’E ́Tude Des Preferenda Alimentaires Et Du Comportement Moteur De L’Oursin Re ́Gulierparacentrotus Lividus,” in Advenced Degree Dissertation Oceanography (Marseille: Universite ́Aix-Marseille 2).
Rumolo P., Zappes I. A., Fabiani A., Barra M., Rakaj A., Palozzi R., et al. (2020). The Diet of Weddell Seals (Leptonychotes Weddellii) in Terra Nova Bay Using Stable Isotope Analysis. Eur. Zool. J. 87 (1), 94–104. doi: 10.1080/24750263.2020.1720832
Ruocco N., Zupo V., Caramiello D., Glaviano F., Polese G., Albarano L., et al. (2018). Experimental Evaluation of the Feeding Rate, Growth and Fertility of the Sea Urchins Paracentrotus Lividus. Invertebr. Reprod. Dev. 62, 209–220. doi: 10.1080/07924259.2018.1504125
Sala E. (1997). Fish Predators and Scavengers of the Sea Urchin Paracentrotus Lividus in Protected Areas of the North-West Mediterranean Sea. Mar. Biol. 129 (3), 531–539. doi: 10.1007/s002270050194
Santos P. M., Albano P., Raposo A., Ferreira S. M. F., Costa J. L., Pombo A. (2020a). The Effect of Temperature on Somatic and Gonadal Development of the Sea Urchin Paracentrotus Lividus (Lamarck 1816). Aquaculture 528, 735487. doi: 10.1016/J.AQUACULTURE.2020.735487
Santos P. M., Ferreira S. M. F., Albano P., Raposo A., Costa J. L., Pombo A. (2020b). Can Artificial Diets be a Feasible Alternative for the Gonadal Growth and Maturation of the Sea Urchin Paracentrotus Lividus (Lamarck 1816)? J. World Aquacult. Soc. 51, 463–487. doi: 10.1111/jwas.12656
Sartori D., Gaion A. (2016). Can Sea Urchins Benefit From an Artificial Diet? Physiological and Histological Assessment for Echinoculture Feasibility Evaluation. Aquacult. Nutr. 22, 1214–1221. doi: 10.1111/anu.12326
Scheibling R. E., Hatcher B. G. (2013). “Strongylocentrotus Droebachiensis,” in Developments in Aquaculture and Fisheries Science, vol. Vol. 38. (London, United Kingdom: Elsevier), pp. 381–412.
Schlosser S. C., Lupatsch I., Lawrence J. M., Lawrence A. L., Shpigel M. (2005). Protein and Energy Digestibility and Gonad Development of the European Sea Urchin Paracentrotus Lividus (Lamarck) Fed Algal and Prepared Diets During Spring and Fall. Aquacult. Res. 36, 972–982. doi: 10.1111/j.1365-2109.2005.01306.x
Shpigel M., Shauli L., Odintsov V., Ben-Ezra D., Neori A., Guttman L. (2018). The Sea Urchin, Paracentrotus Lividus, in an Integrated Multi-Trophic Aquaculture (IMTA) System With Fish (Sparus Aurata) and Seaweed (Ulva Lactuca): Nitrogen Partitioning and Proportional Configurations. Aquaculture 490, 260–269. doi: 10.1016/j.aquaculture.2018.02.051
Spirlet C., Grosjean P., Jangoux M. (1998). Reproductive Cycle of the Echinoid Paracentrotus Lividus: Analysis by Means of the Maturity Index. Invertebr. Reprod. Dev. 34, 69–81. doi: 10.1080/07924259.1998.9652355
Spirlet C., Grosjean P., Jangoux M. (2001). Cultivation of Paracentrotus Lividus (Echinodermata: Echinoidea) on Extruded Feeds: Digestive Efficiency, Somatic and Gonadal Growth. Aquacult. Nutr. 7 (2), 91–100.
Vadas R. L. (1977). Preferential Feeding : An Optimization Strategy in Sea Urchins. Ecol. Soc. America Stable. 47, 337–371. doi: 10.2307/1942173
Vizzini S., Miccichè L., Vaccaro A., Mazzola A. (2015). Use of Fresh Vegetable Discards as Sea Urchin Diet: Effect on Gonad Index and Quality. Aquacult. Int. 23, 127–139. doi: 10.1007/s10499-014-9803-5
Vizzini S., Visconti G., Signa G., Romano S., Mazzola A. (2019). A New Sustainable Formulated Feed Based on Discards From Food Industries for Rearing the Sea Urchin Paracentrotus Lividus (Lmk). Aquacult. Nutr. 25, 691–701. doi: 10.1111/anu.12890
Vizzini S., Visconti G., Vaccaro A., Mazzola A. (2018). Experimental Rearing of the Sea Urchin Paracentrotus Lividus Fed With Discards of the Lettuce Lactuca Sativa in a Sea-Based System. Aquacult. Res. 49 (2), 631–636. doi: 10.1111/are.13492
Volpe M. G., Fabbrocini A., Siano F., Coccia E., Scordella G., Licchelli C., et al (2018). Gonad Quality of Sea Urchin Paracentrotus Lividus Cultured in an Offshore Pilot-Scale Trial on the South-East Italian Coast. Aquacult. Nutr 24(5), 1444–1455. doi: 10.1111/anu.12681
Yeruham E., Rilov G., Shpigel M., Abelson A. (2015). Collapse of the Echinoid Paracentrotus Lividus Populations in the Eastern Mediterranean - Result of Climate Change? Sci. Rep. 5 (1), 1–6. doi: 10.1038/srep13479
Keywords: Paracentrotus lividus, sea urchin, echinoculture, life stages, ecology and behavior, aquaculture, feeding requirements
Citation: Grosso L, Rakaj A, Fianchini A, Tancioni L, Vizzini S, Boudouresque C-F and Scardi M (2022) Trophic Requirements of the Sea Urchin Paracentrotus lividus Varies at Different Life Stages: Comprehension of Species Ecology and Implications for Effective Feeding Formulations. Front. Mar. Sci. 9:865450. doi: 10.3389/fmars.2022.865450
Received: 29 January 2022; Accepted: 09 May 2022;
Published: 24 June 2022.
Edited by:
Sílvia Lourenço, Instituto Politécnico de Leiria, PortugalReviewed by:
Coleen Suckling, University of Rhode Island, United StatesAdele Fabbrocini, Istituto di Scienze Marine (ISMAR) (CNR), Italy
Victoria Gibbs, Birmingham–Southern College, United States
Copyright © 2022 Grosso, Rakaj, Fianchini, Tancioni, Vizzini, Boudouresque and Scardi. This is an open-access article distributed under the terms of the Creative Commons Attribution License (CC BY). The use, distribution or reproduction in other forums is permitted, provided the original author(s) and the copyright owner(s) are credited and that the original publication in this journal is cited, in accordance with accepted academic practice. No use, distribution or reproduction is permitted which does not comply with these terms.
*Correspondence: Arnold Rakaj, arnold.rakaj@uniroma2.it
†These authors have contributed equally to this work