- 1Research Center of Harmful Algae and Marine Biology, and Key Laboratory of Eutrophication and Red Tide Prevention of Guangdong Higher Education Institutes, Jinan University, Guangzhou, China
- 2Southern Marine Science and Engineering Guangdong Laboratory, (Zhuhai), Zhuhai, China
Xincun Lagoon and Li’an Lagoon are typical tropical lagoons containing unique seagrass meadow and mangrove forest ecosystems. However, nutrient pollution from human activities threatens ecosystem health in these areas. A year-long bimonthly survey was carried out to study the bioavailability of organic nutrients through hydrolysis of the extracellular enzymes β-glucosidase (GLU), leucine aminopeptidase (LAP), and alkaline phosphatase (AP) in the two lagoons. The results showed that the nutrient statuses in Xincun Lagoon and Li’an Lagoon were highly variable and enriched with organic nutrients, especially nitrogen. Organic nitrogen and phosphorus were actively recycled in the lagoons through active LAP and AP hydrolysis. The enzyme activities were mostly produced by particulate-associated bacteria and were positively regulated by the existence of organic nutrients. The enzymatic stoichiometry results indicated the importance of dissolved proteins and aminopolysaccharides as sources of both carbon and nitrogen and a potential phosphorus limitation on microbial growth in the lagoons. Our results emphasized the importance of EEAs in the recycling of organic nutrients and the potential influence of enriched organic nutrients on both microorganisms and plants in Xincun Lagoon and Li’an Lagoon.
Introduction
Seagrass meadows, mangrove forests, and salt marshes are highly effective at sequestering and storing carbon dioxide and are thus considered coastal blue carbon ecosystems (Nellemann et al., 2009; Mcleod et al., 2011). In addition to the storage of carbon and thus the mitigation of climate change, blue carbon ecosystems also play important roles in protecting coastal communities from harmful impacts, such as rising seas, flooding and water pollution, and provide important habitats for marine organisms (Pendleton et al., 2012). Unfortunately, these blue carbon ecosystems have been degraded due to direct or indirect anthropogenic impacts, such as reclamation, deforestation, engineering and urbanization, transformation to aquaculture ponds, and climate change, at a critical rate; an estimated one-third of the global total area has been lost over the past several decades (Mcleod et al., 2011; Pendleton et al., 2012). Given the importance of carbon sequestration and storage in vegetated coastal ecosystems, many countries and areas are beginning to implement blue carbon restoration projects (Wylie et al., 2016).
Xincun Lagoon and Li’an Lagoon are located on the coast of southern Hainan Island in the South China Sea and are typical tropical lagoons. Due to the species abundance, wide distribution and optimal growth of seagrass in these lagoons, the first provincial seagrass-type specific nature reserve was established for the lagoons in 2007. In addition, large-scale mangrove forests and coral reefs were distributed in these lagoons (Fang et al., 2018). However, with the rapid development of aquaculture in recent decades, large-scale mangrove areas have been converted to shallow-water fish farms and cages (Richards and Friess, 2016; Herbeck et al., 2021). The existing mangrove forest area in the two lagoons was only approximately 20% of that in the 1980s. Large amounts of untreated aquaculture sewage are directly discharged into the sea and pollute adjacent environments (Fang et al., 2020; Herbeck et al., 2020). The disappearance of mangrove forests further weakens the self-purification ability of water bodies. Moreover, runoff from terrestrial agriculture and domestic wastewater activities has intensified the pollution problem in lagoons (Fang et al., 2020). The ecosystems in Xincun Lagoon and Li’an Lagoon suffer from continuous and serious degradation, and many habitats in the lagoons are strongly affected; seagrass meadows suffer reductions in their areas and biomass, mangrove forests are fragmented into patches, and coral reefs no longer exist (Fang et al., 2018).
Eutrophication is the most concerning ecological problem in Xincun Lagoon and Li’an Lagoon (Liu et al., 2017; Fang et al., 2019). It is clear that nutrient pollution has put great pressure on seagrass meadows and mangrove forests in the two lagoons (Liu et al., 2020; Fang et al., 2020). Nutrients from shallow-sea cage aquaculture activities, which are enriched with high amounts of NH4+ and DON, and runoff mainly from agricultural activities and untreated domestic wastewater in the basin which are enriched with DIN, especially NO3−, account for almost all of the total nutrient input to Xincun Lagoon and Li’an Lagoon (Zhang et al., 2018; Fang et al., 2020). The two lagoons are enriched with both inorganic and organic nutrients, and the amount of organic nutrients might be times that of inorganic nutrients (Liu et al., 2016; Liu et al., 2020). Organic nutrients have often been correlated with an increase in harmful algal blooms in recent years (Glibert and Burford, 2017). Xincun Lagoon was chosen as the only national red tide monitoring area on Hainan Island due to frequent pelagic blooms (Lu et al., 2016). A toxic benthic dinoflagellate bloom attached to seagrass was reported in Xincun Lagoon in 2019 (Zou et al., 2020). Liu et al. (2020) suggested that increasing organic nutrient loads promoted blooms of epiphyte, phytoplankton, and macroalgae in seagrass meadows in lagoons. The potential influence of organic nutrients on primary production in Xincun Lagoon and Li’an Lagoon is worth noting. It is well-known that organic nutrients should be hydrolyzed by extracellular enzymes into low-molecules (< 600 Da) before they can be taken up directly by microorganisms and plants (Obayashi and Suzuki, 2005). Liu et al. (2017) observed active extracellular enzyme activities (EEAs) associated with carbon cycling (polyphenol oxidase, invertase, and cellulose) in the sediment of Xincun Lagoon. However, the lability and bioavailability of organic nutrients through EEAs in water bodies of coastal lagoons are largely unknown.
In the present study, the activities of three extracellular enzymes, β-glucosidase (GLU), leucine aminopeptidase (LAP), and alkaline phosphatase (AP), which play crucial roles in the hydrolysis of dissolved organic carbon (C) (DOC), dissolved organic nitrogen (N) (DON), and dissolved organic phosphorus (P) (DOP) (Sinsabaugh and Follstad Shah, 2012; Ayo et al., 2017) were analyzed along with their contributions from different size fractions. In the present study, enzyme-hydrolysable DOP was further studied to analyze its bioavailability. The objective of this study was to provide a comprehensive view of the activities of extracellular enzyme activities (EEAs) and their potential regulating factors in lagoons. The findings of the present study aim to contribute to a better understanding of bioavailability of organic nutrients and their possible influence on both microorganisms and plants in Xincun Lagoon and Li’an Lagoon.
Materials and Methods
Study Area and Sampling
Xincun Lagoon and Li’an Lagoon are located in southeastern Lingshui County along the coast of Hainan Island, covering the area from N 18°22’–18°47’ and from E 109°45’–110°08’ (Figure 1). Xincun Lagoon and Li’an Lagoon are approximately 22.5 and 7.9 square kilometers, respectively, and are nearly enclosed and connected with the South China Sea through narrow mouths. Xincun Lagoon has an average water depth of 4.2 m with a tidal range of 0.69 m, whereas Li’an Lagoon has an average water depth of 5.5 m with a tidal range of 0.65 m (Gu et al., 2016; Huang et al., 2020). In Xincun Lagoon, mangroves are mainly distributed in the northern part, with an area of 1.48 km2, whereas seagrasses are mainly distributed in the southern part, with an area of 3.28 km2. In Li’an Lagoon, seagrasses are distributed around the lagoon with an area of 0.93 km2. There are fish cages near the mouth of Xincun Lagoon and at the eastern section of Li’an Lagoon. The aquaculture areas in Xincun Lagoon and Li’an Lagoon are more than 3.2 km2 and 2.4 km2 respectively. The sediments of the lagoons are sand-mud type (Gu et al., 2016). Located on the southern side of Xincun Lagoon, Nanwan Monkey Island is the only island nature reserve of macaque primates in the world and hosts nearly 400 tropical plant species and nearly 100 animal species.
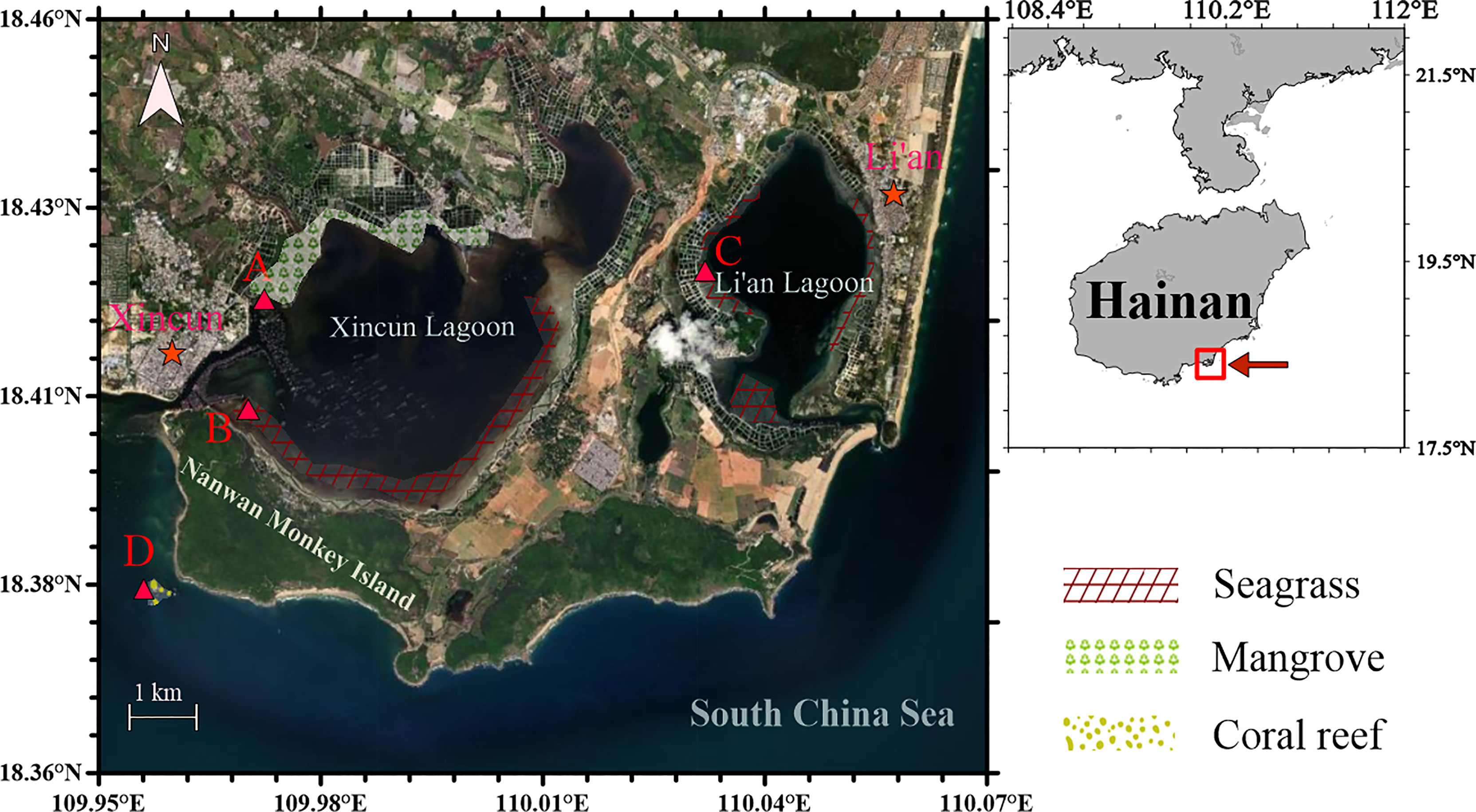
Figure 1 Sampling stations (A–D) in the coastal lagoons of southeastern Hainan Island in the South China Sea. Sampling was conducted once every two months from December 2018 to December 2019.
In the present study, station A was established in the northern mangrove forest, and station B was set at the southern seagrass meadow in Xincun Lagoon. Station C was set at the eastern seagrass meadow in Li’an Lagoon. Station D, as a control, was set outside the lagoons within a coral reef area near the southeastern coast of Nanwan Monkey Island. Sampling was carried out every two months during the neap tide period (normally during 10:00 a.m.–16:00 p.m.) of each sample month from December 2018 to December 2019. Surface water (at a depth of 0.5 m, and approximately 0.5–1 m above the sediment) was collected using 5-L Niskin samplers.
Hydrological Parameters and Nutrient Analysis
A YSI 6600 Multiparameter Water Quality Sonde (YSI Incorporated, Ohio, USA) was used to obtain the temperature, salinity, dissolved oxygen (DO), and pH at each station. The machine was effectively calibrated before each sampling.
The samples used for the nutrient analysis were filtered through Whatman GF/F filters (450°C, 2 h) and frozen at ‒20°C until they were analyzed in the laboratory in the following month. The NO3‒, NO2‒, NH4+, and dissolved inorganic silicon (DSi) concentrations were analyzed colorimetrically using an FIA automated ion analyzer (QC 8500 Series 2, Lachat, Colorado, USA; Fiz et al., 1998). The concentration of dissolved inorganic N (DIN) was calculated as the sum of NO3‒, NO2‒, and NH4+. The concentration of total dissolved N (TDN) was determined after wet oxidation with alkaline K2S2O8 (Hansen and Koroleff, 1999). The concentration of DON was calculated by subtracting DIN from TDN.
The P nutrient concentrations were measured manually in triplicate. The concentration of dissolved inorganic P (DIP) was determined using the molybdenum blue method described by Valderrama (1995). Total dissolved P (TDP) was analyzed according to Jeffries et al. (1979) by employing acid potassium persulfate (K2S2O8). The DOP concentration was calculated by subtracting DIP from TDP. Alkaline phosphatase (AP)-hydrolysable DOP (also named labile DOP, DOPL) was measured according to the method of Hashihama et al. (2013). In triplicate, 10-ml samples were incubated with 100 µl of 100 U mL−1 purified bovine intestine AP and 100 µl of 0.5 mol l−1 sodium azide in the dark for 4 h at 35°C. The DOPL concentration was calculated as the difference between the DIP concentrations released before and after the addition of purified AP. All P nutrient analyses were measured by a spectrophotometer (Hitachi U-2910, Tokyo, Japan) at 882 nm.
Extracellular Enzyme Activity Assays
The seawater was filtered through a 200-µm filter to remove large zooplankton. The GLU activity (GLUA), LAP activity (LAPA), and AP activity (APA) were measured in triplicate according to the method of Hoppe (1993), and modified as described by Chróst & Velimirov (1991). The seawater was unfiltered or filtered through 0.22-µm and 2-µm polycarbonate filters under < 100-mmHg pressure; thus, the enzymes were divided into free (< 0.22 µm), pico- (0.22–2 µm), and nano- (> 2 µm) size fractions. The seawater samples (1.8 ml) were introduced to 200 µl of 4-methylumbelliferyl β-D-glucopyranoside, L-leucine-7-amino-4-methylcoumarin hydrochloride or 4-methylumbellifery phosphate substrate at final concentrations of 100 µmol l−1, 200 µmol l−1, and 25 µmol l−1 and incubated in the dark at 30°C for 2 h. The samples were frozen at ‒20°C until the analyses of fluorescence using a HITACHI (F–4600) spectrofluorometer (Tokyo, Japan) in the laboratory. The excitation and emission wavelengths corresponding to LAPA were set at 380 nm and 440 nm, respectively. For both GLUA and APA, the excitation and emission wavelengths were set at 365 nm and 455 nm, respectively. Sterile seawater supplemented with the corresponding substrate was used as the control. Calibration was performed with 7-amino-4-methyoxyethanol ranging from 0–1000 nmol l−1 for LAPA and 4-methylumbelliferone ranging from 0–500 nmol l−1 for GLUA and APA.
Data Analysis
One-way ANOVA was performed to compare the differences among the parameter sets obtained at different stations using Tukey’s test, where a p value of 0.05 was regarded as significant. Spearman correlation and multiple stepwise linear regression tests were also applied to analyze the relationships between EEAs and environmental parameters.
Results
Hydrological Parameters
The temperature measurements were lowest in December (22.6–25.3°C), increasing gradually until April and then fluctuating near 28.0–31.3°C during April and October (Table 1 and Figure S1A).
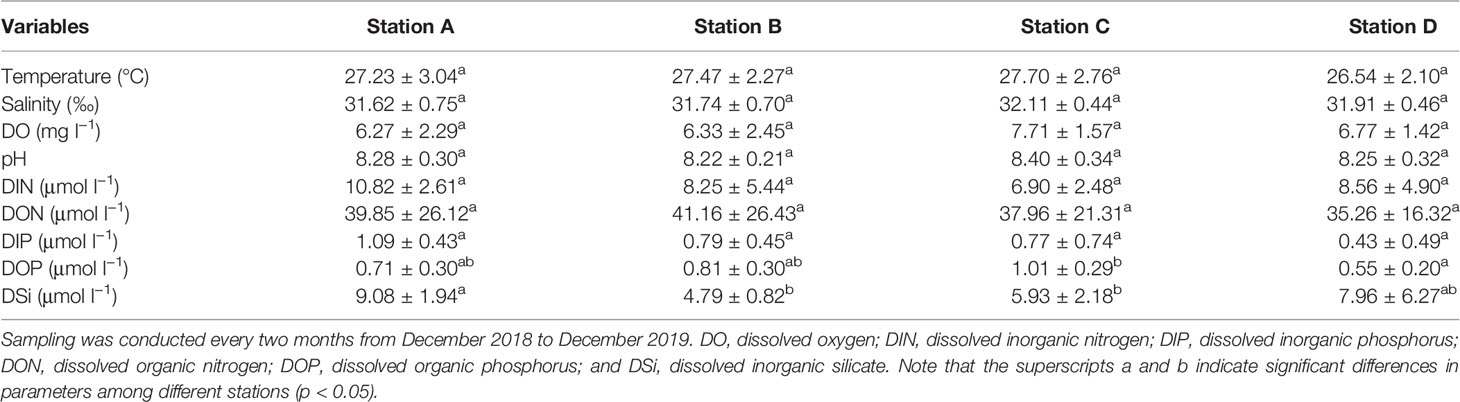
Table 1 Environmental variables measured during a survey round of one year in the coastal lagoons of southeastern Hainan Island.
The salinity measurements varied from 30.29–32.70 in the study area (Figure S1B). Relatively low salinity values (< 31) were observed at Stations A, B, and D in June and/or August.
The DO measurements at Stations A and B varied greatly among different months, being lowest in June (at 3.53 mg l−1 and 3.48 mg l−1, respectively) and highest in August (at 10.67 mg l−1 and 10.76 mg l−1, respectively) (Figure S1C). The DO measurements at Stations C and D did not change extensively, and the lowest values were also observed in June at 5.28 mg l−1 and 5.33 mg l−1, respectively.
The pH measurements varied from 7.80 to 8.88, with relatively low values observed in the last two observed months in the study area (Figure S1D).
Nutrients
The DIN varied from 2.27 µmol l−1 to 18.30 µmol l−1 in the study area (Table 1 and Figures 2A–D). The DIN was relatively high in June, August, and/or December. The DON varied from 7.03 µmol l−1 to 93.47 µmol l−1 (Figures 2E–H). At Stations A and B, the DON increased greatly in August. DIP varied between 0.08 µmol l−1 and 1.98 µmol l−1 (Figures 2I–L). The DIP values lower than 0.1 µmol l−1 were observed at Station C in August and at Station D in February and April. The DOP and DOPL varied between 0.21–1.33 µmol l−1 (Figures 2M–P) and 0.03–0.63 µmol l−1 (Figures 2Q–T), respectively. Relatively low organic phosphorus values were observed in the last two observed months. The DOP value at Station C was significantly higher than that at Station D (p < 0.05). The DSi values were in the range of 2.98–21.58 µmol l−1, and the value at Station A was significantly higher than those at Stations B and C (p < 0.05) (Figures 2U–X).
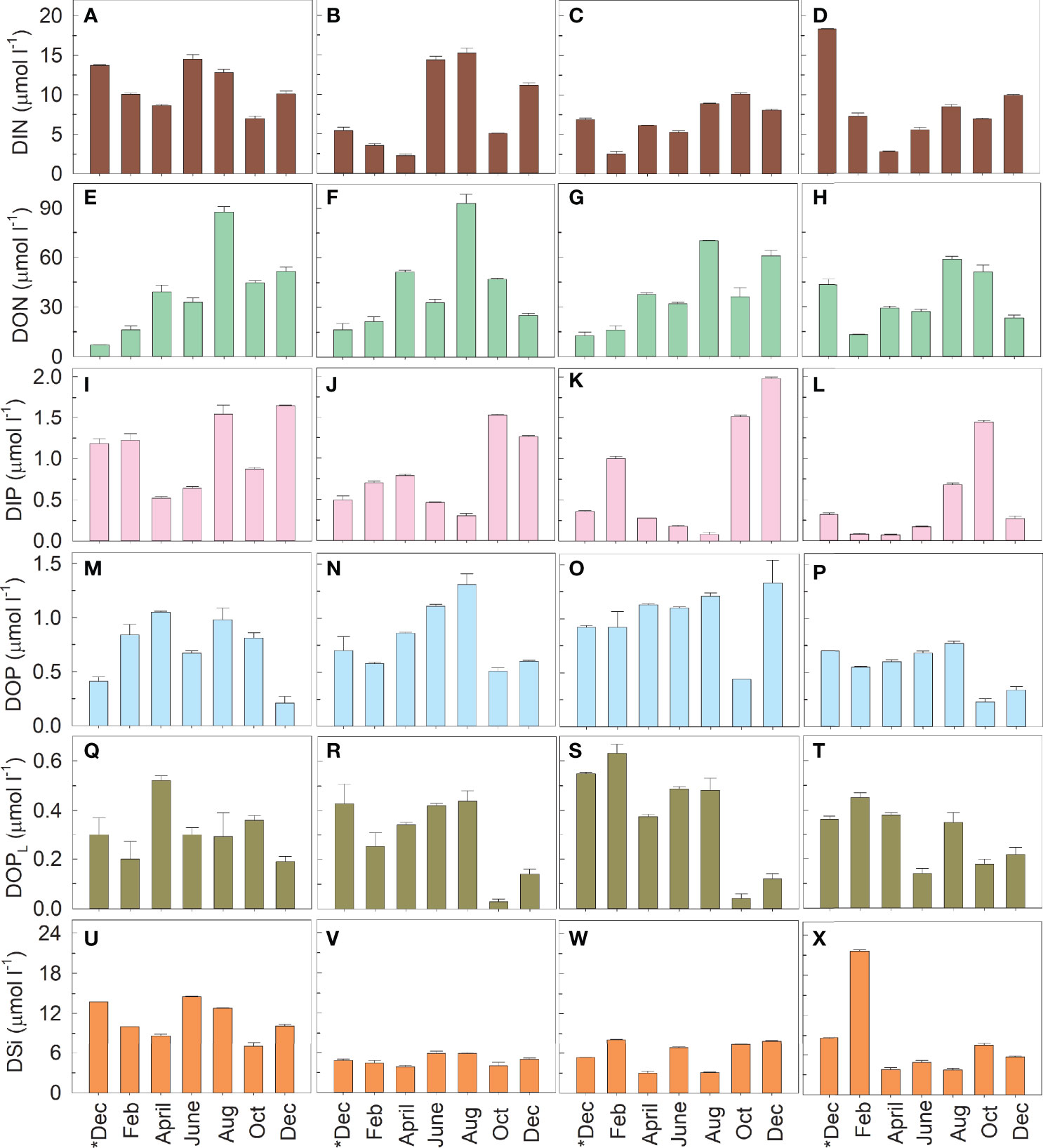
Figure 2 Variations in the concentrations of dissolved inorganic nitrogen (DIN) (A–D), dissolved organic nitrogen (DON) (E–H), dissolved inorganic phosphorus (DIP) (I–L), dissolved organic phosphorus (DOP) (M–P), dissolved labile organic phosphorus (DOPL) (Q–T), and dissolved inorganic silicon (DSi) (U–X) at Stations A–D. DOPL is the hydrolyzable form of DOP derived by commercial alkaline phosphatase. Sampling was conducted once every two months from December 2018 (*Dec) to December 2019 (Dec).
At most times, NH4+ was the main form of inorganic N (Figure 3A), but NO3− increased significantly and became the major inorganic N form in August (Figure 3B). On average, DON and DOP represented 72.3–82.3% (Figure 3C) and 40.4–63.9% (Figure 3D) of TDN and TDP, respectively, and DOPL contributed 34.9–57.9% to DOP (Figure 3E). The DIN : DSi ratios varied from 0.7 to 2.9 (Figure 3F). The DIN : DIP ratios at Stations A and B were lower than 12 at most times, whereas those at Station D were higher than 32 at most times (Figure 3G). The DIN : DIP ratio with an extremely high value of 141.1 ± 73.1 was observed at Station C in August. The TDN : TDP ratios varied from 8.2 to 55.3, with most values higher than 22, especially at Station D (Figure 3H).
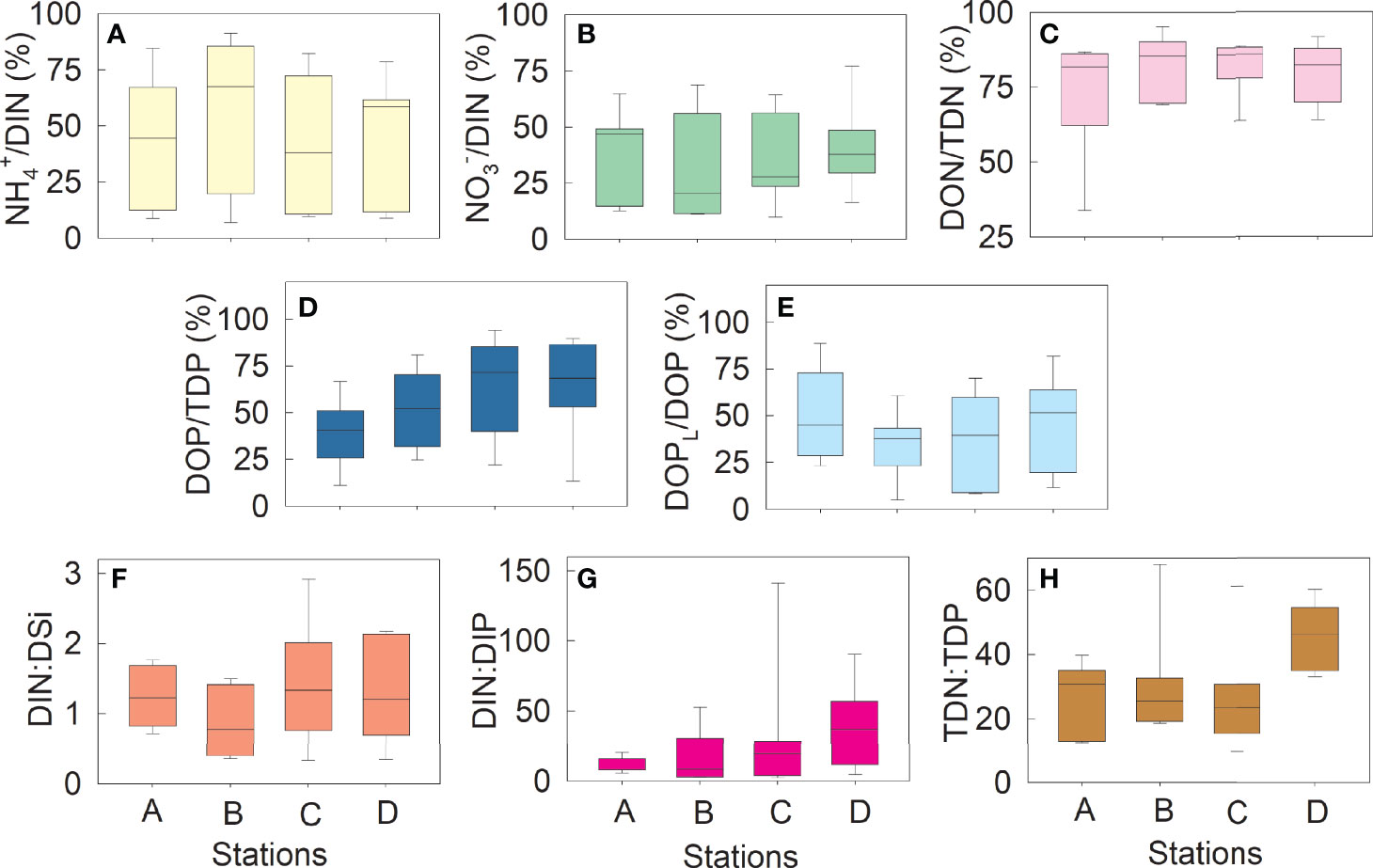
Figure 3 Variations in the nutrient ratios at Stations A–D Contribution (%) of NH4+ to dissolved inorganic nitrogen (DIN) (A); contribution (%) of NO3− to DIN (B); contribution of dissolved organic nitrogen (DON) to total dissolved nitrogen (TDN) (C); contribution (%) of dissolved organic phosphorus (DOP) to total dissolved phosphorus (TDP) (D); contribution (%) of dissolved labile organic phosphorus (DOPL) to DOP (E); DIN: dissolved inorganic silicon (DSi) ratio (F); DIN : DIP ratio (G); and TDN : TDP ratio (H). DOPL is the hydrolyzable form of DOP derived by commercial alkaline phosphatase. Sampling was conducted once every two months from December 2018 to December 2019.
Extracellular Enzyme Activities
The total GLUA varied from undetectable to 37.85 nmol l−1 h−1 in the study area, and most values at Station D were significantly lower than those at Stations A, B, and C (p < 0.01) (Figure 4A). Most high total GLUA values (> 10 nmol l−1 h−1) were observed at Stations A, B, and C in February, April, and August. GLUA in different size fractions varied with time and with stations. At Station C, the total GLUA was contributed most by the picosized fraction (72.3%) in February, but this role changed to the nanosize fraction (75.8%) in April. In most cases, the nanosized GLUA was the main contributor to the total GLUA, with average bimonthly percentages of 66.8 ± 24.0%, 66.0 ± 29.0%, 62.8 ± 25.8%, and 84.4 ± 16.4% at Stations A, B, C, and D, respectively.
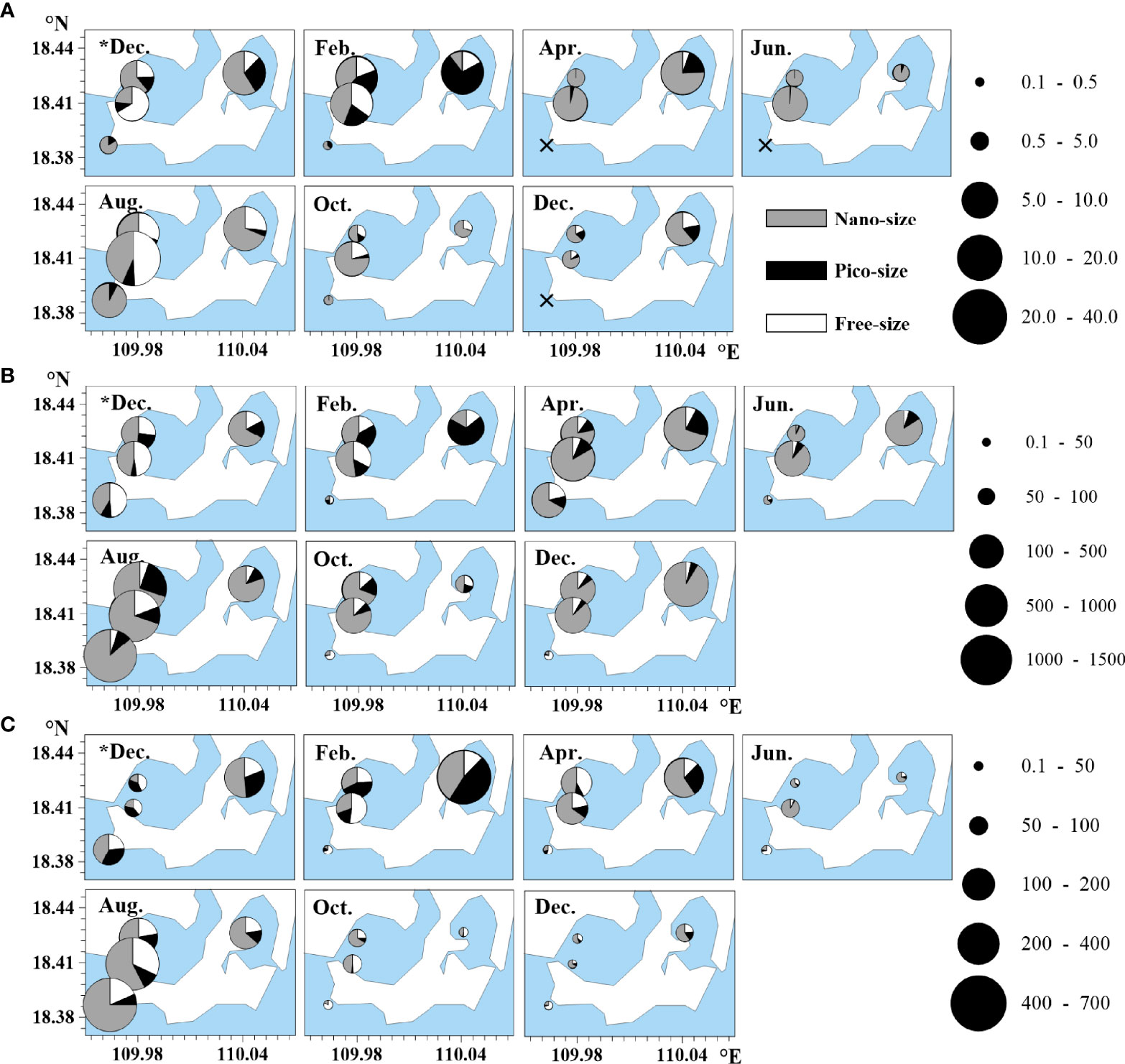
Figure 4 Temporal distributions of (A) β-glucosidase activity, (B) leucine aminopeptidase activity, and (C) alkaline phosphatase activity (unit: nmol l−1 h−1) in the coastal lagoons of southeastern Hainan Island. Nanosized, > 2 µm; picosized, 0.22–2 µm; and free-sized, < 0.22 µm. Sampling was conducted once every two months from December 2018 (*Dec.) to December 2019 (Dec.). The × symbol denotes stations with undetectable activity. Filled black circles denote values of the enzyme activities in five levels.
The total LAPA ranged from 35.84–1328.28 nmol l−1 h−1 in the study area (Figure 4B), with bimonthly averages of 346.64 ± 325.05, 470.48 ± 476.95, 384.99 ± 181.65, and 244.63 ± 480.04 nmol l−1 h−1 at Stations A, B, C, and D, respectively. The total LAPA at Station D was significantly lower than that at any other station in the same month except December of the first study year and August (p < 0.01). The total LAPA values at Stations A, B, and D were maximized in August, at 1040.59 ± 6.39, 1426.78 ± 5.87, and 1328.28 ± 2.30 nmol l−1 h−1, respectively, whereas that at Station C was maximized in December of the second study year, with a value of 596.78 ± 0.45 nmol l−1 h−1. The nanosized LAPA contributed most to the total LAPA (> 60%) most of the time at Stations A, B, and C.
The total APA varied between 3.91–632.66 nmol l−1 h−1 in the study area (Figure 4C), with bimonthly averages of 98.08 ± 74.45, 165.24 ± 187.06, 191.45 ± 214.38, and 85.19 ± 157.74 nmol l−1 h−1 at Stations A, B, C, and D, respectively. Additionally, the total APA at Station D was significantly lower than that at any other station at all times except in December of the first study year (p < 0.01). The total APA at all stations was relatively low in June, October, and December of the second study year. The highest total APA values were observed in August for Stations A, B, and D, with values of 211.09 ± 3.25, 575.59 ± 2.52, and 432.45 ± 9.68 nmol l−1 h−1, respectively; the corresponding value occurred in February for Station C, with a value of 632.66 ± 13.21 nmol l−1 h−1. The nanosized APA was the main contributor to the total APA, with bimonthly average percentages of 49.0 ± 17.2%, 56.4 ± 20.7%, 57.7 ± 10.4%, and 34.0 ± 22.6% at Stations A, B, C, and D, respectively.
All the GLUA : LAPA and GLUA : APA ratios were lower than 0.05 and 0.11, respectively (Figure S2A, B). The LAPA : APA ratios varied between 0.70 and 10.42 in the study area, with bimonthly averages of 4.36 ± 2.64, 3.17 ± 1.78, 4.30 ± 3.74, and 3.97 ± 1.37 at Stations A, B, C, and D, respectively (Figure S2C).
Relationships Between EEAs and Environmental Parameters
Contents of DO, DON, and DOP played the most important roles in regulating EEAs with different size fractions (Table S1). Total GLUA was positively correlated with DO (p < 0.05) and DOP (p < 0.01), and these two parameters explained 40.5% of the variation in total GLUA (p < 0.01) (Table 2). Total LAPA showed positive correlations with DO, NO3−, DON, and DOP (p < 0.05 or 0.01), and the nanosized LAPA showed positive correlations with DON and DOP (p < 0.01), among which DON was the most important and explained 45.5% and 50.4% of the total LAPA and the nanosized LAPA, respectively (p < 0.01). Different size fractions of APA were positively correlated with DOP and/or DOPL (p < 0.05 or 0.01). In addition, NO3− regulated 41.1% and 20.7% of the variations in free GLUA and LAPA, respectively, and pH seemed to play a role in regulating picosized GLUA and APA.
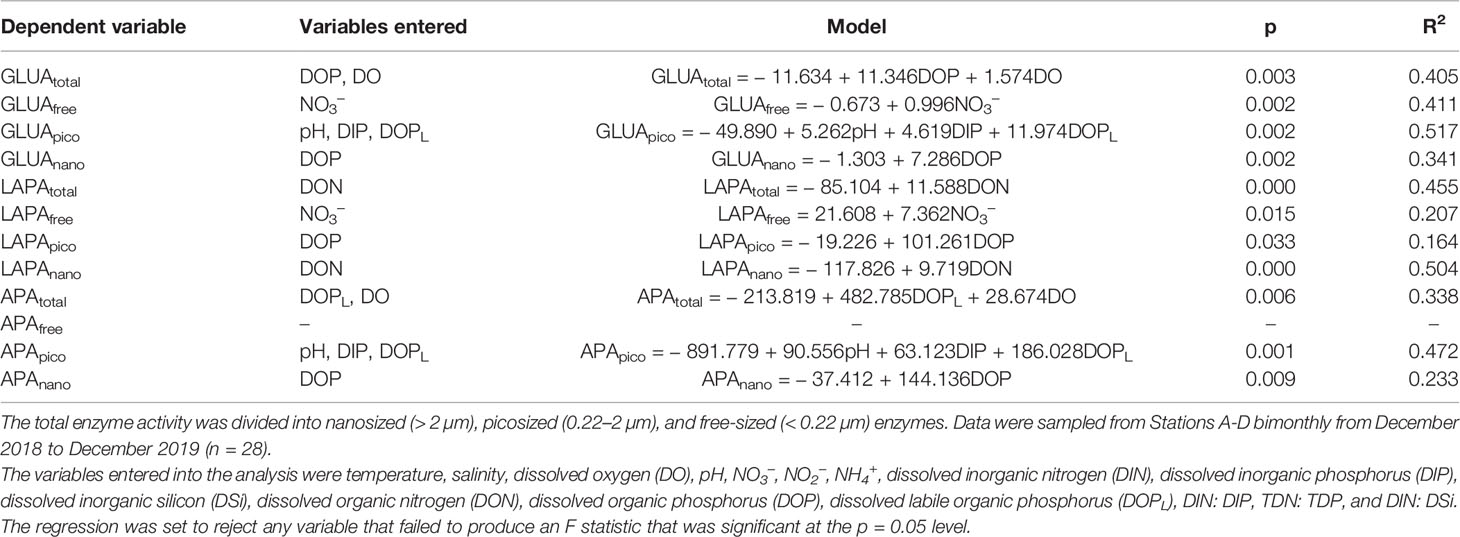
Table 2 Stepwise linear regression analysis of β-glucosidase activity (GLUA), leucine aminopeptidase activity (LAPA), and alkaline phosphatase (APA) with environmental variables in the coastal lagoons of southeastern Hainan Island.
Discussion
Nutrient Characteristics in Xincun Lagoon and Li’an Lagoon
Hainan Island is characterized by a tropical monsoon climate with a rainy season from May to October, especially from July to September (Herbeck et al., 2021). It rained on the sampling dates in June, August, and October, and especially heavy rain occurred on the sampling date of August; thus, the environmental data on these days might have been more affected by terrestrial inputs than the data gathered on other days. The nutrient data utilized herein varied greatly and irregularly among different months; even the parameters measured in the month of December changed extensively between 2018 and 2019, suggesting highly anthropogenic impacts in the two lagoons. In our experimental design, station D, which is located within the coral reef area outside Xincun Lagoon and is thus less affected by humans, was chosen as a control and was thus compared to the stations located in the lagoons (Figure 1). However, to our surprise, although they were slightly lower, the nutrient concentrations at Station D showed no significant differences from those measured at the stations within the lagoons (Figure 2, Table 1), indicating that the supposedly clean coral reef area also suffered nutrient pollution from Xincun Lagoon.
It is conceivable that high concentrations of DON exist in Xincun Lagoon and Li’an Lagoon because high amounts of organic matter are exported into the sea due to aquaculture activities (Li et al., 2014; Boudouresque et al., 2020). Meanwhile, organic nutrient contents in the water column are normally high in shallow lagoons (Sundbäck et al., 2004). The DON levels measured in the present study were comparable to or even higher than those in previous studies (Liu et al., 2020). Considering bioavailability of the high amount of DON, the nutrient conditions in the two lagoons seemed to favor the growth of dinoflagellates rather than diatoms (Glibert and Burford, 2017; Huang et al., 2020). There are reasons to believe that the risk of harmful dinoflagellate blooms is increasing in the two lagoons.
The DOPL results showed more accurate bioavailable DOP data in the lagoons. On average, 35–58% of the DOP was hydrolysable by AP in four hours (Figure 3E), and these data were comparable to or even higher than those of previous studies (Suzumura et al., 1998; Boǵe et al., 2014). In this study, the average DOPL : DIP ratios were as high as 2 at Stations C and D, indicating the importance of highly bioavailable DOP in these areas, especially when phosphate becomes limiting.
Extracellular Enzyme Activities and Their Roles in Organic Matter
β-Glucosidase, LAP and AP are the most widely studied extracellular enzymes that catalyze the degradation of the largest environmental sources of organic C, N, and P, respectively (Sinsabaugh and Follstad Shah, 2012; Ayo et al., 2017; Ou et al., 2018). The EEAs measured in the study area, especially those of LAPA and APA, were several times greater than those observed in other coastal waters of China (Ou et al., 2018; Mo et al., 2020), even during a dinoflagellate bloom period (Qin et al., 2021), indicating active organic nutrient recycling through enzyme hydrolysis in the lagoons. In contrast to the nutrient conditions at Station D, which were similar to those in the lagoons, all the EEAs at Station D were significantly lower than those in the lagoons (Figure 4). Bacteria are the main producers of EEAs (Chróst et al., 1989). Although not measured in the present study, it could be inferred that the low EEAs at Station D were due to low bacterial production and activity in the open outside waters compared to those in the lagoons. In addition, all of the EEAs were attributed mostly to the nanosized fraction (Figure 4), indicating that EEAs were contributed mainly by bacteria attached to particulates in the lagoons. These results coincided with the findings of previous studies showing that the degradation of organic matter by enzymes is likely dependent on the microbial communities attached to particles in coastal and estuarine waters (Allison et al., 2012; Labry et al., 2016).
According to economic theories of microbial metabolism, enzyme production should increase when available nutrients are scarce and complex nutrients are abundant (Koch, 1985). In the present study, although inorganic nutrients were abundant, EEAs were highly expressed (Figure 4) and showed positive correlations with organic matter (Table S1), suggesting that the existence of abundant complex organic substrates induces enzyme production. Normally, different enzymes are expressed by microorganisms to hydrolyze different types of organic matter into low-molecular-weight (< 600 Da) products that can be directly taken up (Obayashi and Suzuki, 2005). β-Glucosidase catalyzes the final step in the breakdown of cellulose, thus mediating the subsequent release of monomeric glucose. leucine aminopeptidase hydrolyzes the most abundant amino acids from the N-terminus of polypeptides, and AP hydrolyzes phosphate from phosphomonoesters and some phosphodiesters (Millar et al., 2015; Pastor et al., 2019). In the present study, DOC was not measured. All three total EEAs and the most contributing nanosized EEAs showed significant correlations with DON and/or DOP (Table 2 and Table S1), indicating more complicated and interactive hydrolysis mechanisms on organic matter by EEAs, as has been suggested by some studies (Anderson, 2018; Fitzsimons et al., 2020). DO also seemed to play a role in regulating EEAs in the lagoons. Hypoxia occurred from time to time in the lagoons due to high-density mariculture activities and enriched organic matter (Fang et al., 2019). The positive correlation between EEAs and DO indicated that DO plays an essential role in regulating the recycling of organic matter by EEAs in ecosystems.
The present results showed that EEAs in various size fractions were regulated by different environmental parameters (Table S1). In addition to organic matter and DO, NO3− and pH also played roles in regulating free and picosized EEAs, respectively. Generally, free extracellular enzymes lose control from the microorganisms that release them (Sinsabaugh and Follstad Shah, 2012). In a marine environment, the activity of free-sized extracellular enzymes is affected by changes in environmental factors, such as UV radiation, temperature, pH, heavy metals, and humic acids (Steen and Arnosti, 2011; Ayo et al., 2017). In the present study, free-sized extracellular enzymes might be affected by materials from terrestrial runoff together with NO3−. It seems that the picosized EEAs were affected by pH, which might have been due to the more distinct influence of pH on free-living bacteria rather than on particle-associated bacteria. Krause et al. (2012) suggested that moderate changes in pH might cause changes in bacterial composition rather than abundance.
Enzymatic stoichiometry can be used to indicate microbial nutrient acquisition with the relatively available C, N, and P from environmental sources (Sinsabaugh et al., 2009). The GLUA : LAPA, GLUA : APA and LAPA : APA ratios refer to microbial C:N, C:P and N:P acquisition. When GLUA : LAPA and/or GLUA : APA < 1.0, it implies microbial N and/or P limitation relative to C, and when LAPA : APA < 1.0, it suggests microbial P limitation relative to N (Sinsabaugh et al., 2009; Luo et al., 2017). Sinsabaugh and Follstad Shah (2012) summarized that in different marine systems, the GLUA: LAPA and GLUA : APA ratios varied between 0.003 and 0.792 and between 0.020 and 0.759, with averages of 0.125 and 0.327, respectively. In the present study, the GLUA : LAPA, GLUA : APA, and LAPA : APA ratios (Figure S2) indicated the importance of dissolved proteins and aminopolysaccharides as sources of both C and N and a potential P limitation on microbial growth that coincided with the elemental stoichiometry.
Conclusion
Xincun Lagoon and Li’an Lagoon are enriched with organic nutrients. EEAs, especially LAP and AP, were highly expressed to hydrolyze the organic nutrients. All three total EEAs were positively correlated with DON and/or DOP, suggesting more complicated and interactive hydrolysis mechanisms on organic matter by EEAs. Meanwhile, EEAs in various size fractions were regulated by different environmental parameters, such as NO3− and pH. Ratios between the EEAs indicated the importance of dissolved proteins and aminopolysaccharides as sources of both C and N and a potential P limitation on microbial growth. The results showed the high bioavailability of organic nutrients through active EEAs and suggested a potential influence of the enriched organic nutrients on microorganisms and plants.
Data Availability Statement
The original contributions presented in the study are included in the article/Supplementary Material. Further inquiries can be directed to the corresponding author.
Author Contributions
JL, ZW, and JZ conducted the sampling and measurements. LO, JL, and XZ analyzed the results. LO wrote the original and revised manuscripts. SL designed the research and revised the manuscript. All authors contributed to the article and approved the submitted version.
Funding
This work was supported by the National Science Foundation of China [grant numbers 42076144, 41776121, and 41576162], and the Innovation Group Project of Southern Marine Science and Engineering Guangdong Laboratory (Zhuhai) (No. 311021004).
Conflict of Interest
The authors declare that the research was conducted in the absence of any commercial or financial relationships that could be construed as a potential conflict of interest.
Publisher’s Note
All claims expressed in this article are solely those of the authors and do not necessarily represent those of their affiliated organizations, or those of the publisher, the editors and the reviewers. Any product that may be evaluated in this article, or claim that may be made by its manufacturer, is not guaranteed or endorsed by the publisher.
Supplementary Material
The Supplementary Material for this article can be found online at: https://www.frontiersin.org/articles/10.3389/fmars.2022.864579/full#supplementary-material
Abbreviations
AP, Alkaline phosphatase; APA, AP activity; GLU, β-Glucosidase; GLUA, GLU activity; DIN, dissolved inorganic nitrogen; DIP, dissolved inorganic phosphorus; DOC, dissolved organic carbon; DON, dissolved organic nitrogen; DOP, dissolved organic phosphorus; DO, dissolved oxygen; EEA, extracellular enzyme activity; DOPL, labile DOP; LAP, leucine aminopeptidase; LAPA, LAP activity; TDN, total dissolved nitrogen; TDP, total dissolved phosphorus.
References
Allison S., Chao Y., Farrara J., Hatosy S., Martiny A. (2012). Fine-Scale Temporal Variation in Marine Extracellular Enzymes of Coastal Southern California. Front. Microb. 50. doi: 10.3389/fmicb.2012.00301
Anderson O. R. (2018). Evidence for Coupling of the Carbon and Phosphorus Biogeochemical Cycles in Freshwater Microbial Communities. Front. Mar. Sci. 5. doi: 10.3389/fmars.2018.00020
Ayo B., Abad N., Artolozaga I., Azua I., Baña Z., Unanue M., et al. (2017). Imbalanced Nutrient Recycling in a Warmer Ocean Driven by Differential Response of Extracellular Enzymatic Activities. Global Change Biol. 23, 4084–4093. doi: 10.1111/gcb.13779
Bogé G., Lespilette M., Jamet D., Jamet J. L. (2014). Analysis of the Role of DOP on the Particulate Phosphatase Activity in Toulon Bay (N.W. Mediterranean Sea, France). Mar. Pollut. Bull. 86, 342–348. doi: 10.1016/j.marpolbul.2014.06.045
Boudouresque C. F., Blanfuné A., Pergent G., Pergent-Martini C., Perret-Boudouresque M., Thibaut T. (2020). Impacts of Marine and Lagoon Aquaculture on Macrophytes in Mediterranean Benthic Ecosystems. Front. Mar. Sci. 7. doi: 10.3389/fmars.2020.00218
Chróst R. J., Münster U., Rai H., Albrecht D., Witzel P. K., Overbeck J. (1989). Photosynthetic Production and Exoenzymatic Degradation of Organic Matter in the Euphotic Zone of a Eutrophic Lake. J. Plankton Res. 11, 223–242. doi: 10.1093/plankt/11.2.223
Chróst R. J., Velimirov B. (1991). Measurement of Enzyme Kinetics in Water Samples: Effect of Freezing and Soluble Stabilizer. Mar. Ecol. Prog. Ser. 70, 93–100. doi: 10.3354/meps070093
Fang X., Hao C., Cheng C., Ni W., Wang J., Zhang Y. (2018). Pressure Analysis and Spatial Quantitative Assessment of Impact of Human Activities on Lagoon Ecosystem: A Case Study in Xincun and Li’an Lagoons, Hainan, China. Appl. Ecol. Environ. Res. 16, 6253–6266. doi: 10.15666/aeer/1605_62536266
Fang X., Li X., Hao C., Zhang D., Wang J., Zhang Y. (2019). Suitability Evaluation of Shallow Net Cage Culture Based on Interaction Matrix–A Case Study of Xincun Lagoon, Hainan, China. Appl. Ecol. Environ. Res. 17, 6669–6679. doi: 10.15666/aeer/1703_66696679
Fang X., Li X., Xiang Y., Hao C., Zhao Y., Zhang Y. (2020). Cumulative Impact of Anthropogenic Nutrient Inputs on Lagoon Ecosystems–A Case Study of Xincun Lagoon, Hainan, China. Reg. Stud. Mar. Sci. 35, 101213. doi: 10.1016/j.rsma.2020.101213
Fitzsimons M. F., Probert I., Gaillard F., Rees A. P. (2020). Dissolved Organic Phosphorus Uptake by Marine Phytoplankton is Enhanced by the Presence of Dissolved Organic Nitrogen. J. Exp. Mar. Biol. Ecol. 530–531, 151434. doi: 10.1016/j.jembe.2020.151434
Fiz F. P., Aida F. R., Carmen G. C. (1998). Mixing Analysis of Nutrient, Oxygen and Dissolved Inorganic Carbon in the Upper and Middle North Atlantic Ocean East of the Azores. J. Mar. Syst. 16, 219–233. doi: 10.1016/S0924-7963(97)00108-5
Glibert P. M., Burford M. A. (2017). Globally Changing Nutrient Loads and Harmful Algal Blooms: Recent Advances, New Paradigms, and Continuing Challenges. Oceanography 30, 58–69. doi: 10.5670/oceanog.2017.110
Gu Z., Jia P., Li G., Xu W., Yang Y., Zhou L. (2016). Research on a Technique of Extracting Coastline Shoreline Information Extraction Using the Canny Edge Detection Operator for Two Coastal Lagoons in Lingshui, Hainan Province. Quat. Sci. 36, 113e120. doi: 10.11928/j.issn.1001-7410.2016.11
Hansen H. P., Koroleff F. (1999). “Determination of Nutrients,” in Methods of Seawater Analysis. Eds. Grasshoff K., Kremling K., Ehrhardt M. (Weinheim: Wiley-VCH), 159–228.
Hashihama F., Kinouchi S., Suwa S., Suzumura M., Kanda J. (2013). Sensitive Determination of Enzymatically Labile Dissolved Organic Phosphorus and its Vertical Profiles in the Oligotrophic Western North Pacific and East China Sea. J. Oceanogr. 69, 357–367. doi: 10.1007/s10872-013-0178-4
Herbeck L. S., Krumme U., Andersen T. J., Jennerjahn T. C. (2020). Decadal Trends in Mangrove and Pond Aquaculture Cover on Hainan (China) Since 1966: Mangrove Loss, Fragmentation and Associated Biogeochemical Changes. Estuar. Coast. Shelf Sci. 233, 106531. doi: 10.1016/j.ecss.2019.106531
Herbeck L. S., Krumme U., Nordhaus I., Jennerjahn T. C. (2021). Pond Aquaculture Effluents Feed an Anthropogenic Nitrogen Loop in a SE Asian Estuary. Sci. Total Environ. 756, 144083. doi: 10.1016/j.scitotenv.2020.144083
Hoppe H. G. (1993). “Use of Fluorogenic Model Substrate for Extracellular Enzyme Activity (EEA) Measurement of Bacteria,” in Handbook of Methods in Aaquatic Microbial Ecology. Eds. Kemp P. F., Sherr B. F., Sherr E. B., Cole J. J. (Boca Raton: Lewis Publishers), 423–431.
Huang K., Feng Q., Zhang Y., Ou L., Cen J., Lu S., et al. (2020). Comparative Uptake and Assimilation of Nitrate, Ammonium, and Urea by Dinoflagellate Karenia Mikimotoi and Diatom Skeletonema Costatum s.L. In the Coastal Waters of the East China Sea. Mar. Pollut. Bull. 155, 111200. doi: 10.1016/j.marpolbul.2020.111200
Jeffries D. S., Dieken F. P., Jones D. E. (1979). Performance of the Autoclave Digestion Method for Total Phosphorus Analysis. Water Res. 13, 275–279. doi: 10.1016/0043-1354(79)90206-9
Koch A. L. (1985). “The Macroeconomics of Bacterial Growth,” in Bacteria in Their Natural Environments. Eds. Fletcher M., Floodgate G. D. (London: Academic Press), 1–42.
Krause E., Wichels A., Giménez L., Lunau M., Schilhabel M. B., Gerdts G. (2012). Small Changes in pH Have Direct Effects on Marine Bacterial Community Composition: A Microcosm Approach. PloS One 7 (10), e47035. doi: 10.1371/journal.pone.0047035
Labry C., Delmas D., Youenou A., Quere J., Leynaert A., Fraisse S., et al. (2016). High Alkaline Phosphatase Activity in Phosphate Replete Waters: The Case of Two Macrotidal Estuaries. Limnol. Oceanogr. 61, 1513–1529. doi: 10.1002/lno.10315
Li R. H., Liu S. M., Li Y. W., Zhang G. L., Ren J. L., Zhang J. (2014). Nutrient Dynamics in Tropical Rivers, Lagoons, and Coastal Ecosystems of Eastern Hainan Island, South China Sea. Biogeosciences 11, 481–506. doi: 10.5194/bg-11-481-2014
Liu S., Deng Y., Jiang Z., Wu Y., Huang X., Macreadie P. I. (2020). Nutrient Loading Diminishes the Dissolved Organic Carbon Drawdown Capacity of Seagrass Ecosystems. Sci. Total Environ. 740, 140185. doi: 10.1016/j.scitotenv.2020.140185
Liu S., Jiang Z., Wu Y., Zhang J., Arbi I., Ye F., et al. (2017). Effects of Nutrient Load on Microbial Activities Within a Seagrass-Dominated Ecosystem: Implications of Changes in Seagrass Blue Carbon. Mar. Pollut. Bull. 117, 214–221. doi: 10.1016/j.marpolbul.2017.01.056
Liu S., Jiang Z., Zhou C., Wu Y., Gan M., Zhang J., et al. (2016). Temporal and Spatial Variation of Dissolved Organic Matter and Its Controlling Factors in Seagrass Bed Ecosystem of Xincun Bay, Hainan Island. Chin. J. Ecol. 35, 2144–2151. doi: 10.13292/j.1000-4890.201608.004
Luo L., Meng H., Gu J. D. (2017). Microbial Extracellular Enzymes in Biogeochemical Cycling of Ecosystems. J. Environ. Manage. 197, 539–549. doi: 10.1016/j.jenvman.2017.04.023
Lu S. G., Tang D. L., Guan X. B., Cen J. Y., Lu S. H. (2016). Identification of Susceptibility of Harmful Algal Blooms and Determining the Key Monitoring Zones in the Coastal Water of Hainan Island. Mar. Environ. Sci. 35, 580–586. doi: 10.13634/j.cnki.mes.2016.04.016
Mcleod E., Chmura G. L., Bouillon S., Salm R., Björk M., Duarte C. M., et al. (2011). A Blueprint for Blue Carbon: Toward an Improved Understanding of the Role of Vegetated Coastal Habitats in Sequestering CO2. Front. Ecol. Environ. 9, 552–560. doi: 10.1890/110004
Millar J. J., Payne J. T., Ochs C. A., Jackson C. R. (2015). Particle-Associated and Cell-Free Extracellular Enzyme Activity in Relation to Nutrient Status of Large Tributaries of the Lower Mississippi River. Biogeochemistry 124, 255–271. doi: 10.1007/s10533-015-0096-1
Mo Y., Ou L., Lin L., Huang B. (2020). Temporal and Spatial Variations of Alkaline Phosphatase Activity Related to Phosphorus Status of Phytoplankton in the East China Sea. Sci. Total Environ. 731, 139192. doi: 10.1016/j.scitotenv.2020.139192
Nellemann C., Corcoran E., Duarte C. M., Valdes L., DeYoung C., Fonseca L., et al. (2009) Blue Carbon: The Role of Healthy Oceans in Binding Carbon. A Rapid Response Assessment (United Nations Environment Programme, GRID-Arendal website). Available at: www.grida.no (Accessed 2011 Nov 11).
Obayashi Y., Suzuki S. (2005). Proteolytic Enzymes in Coastal Surface Seawater: Significant Activity of Endopeptidases and Exopeptidases. Limnol. Oceanogr. 50, 722–726. doi: 10.4319/lo.2005.50.2.0722
Ou L., Liu X., Li J., Qin X., Cui L., Lu S. (2018). Significant Activities of Extracellular Enzymes From a Brown Tide in the Coastal Waters of Qinhuangdao, China. Harmful Algae 74, 1–9. doi: 10.1016/j.hal.2018.03.005
Pastor A., Freixa A., Skovsholt L. J., Wu N., Romaní A. M., Riis T. (2019). Microbial Organic Matter Utilization in High-Arctic Streams: Key Enzymatic Controls. Microb. Ecol. 78, 539–554. doi: 10.1007/s00248-019-01330-w
Pendleton L., Donato D. C., Murray B. C., Crooks S., Jenkins W. A., Sifleet S., et al. (2012). Estimating Global ‘‘Blue Carbon’’ Emissions From Conversion and Degradation of Vegetated Coastal Ecosystems. PloS One 7, e43542. doi: 10.1371/journal.pone.0043542
Qin X., Shi X., Gao Y., Dai X., Ou L., Guan W., et al. (2021). Alkaline Phosphatase Activity During a Phosphate Replete Dinoflagellate Bloom Caused by Prorocentrum Obtusidens. Harmful Algae 103, 101979. doi: 10.1016/j.hal.2021.101979
Richards D. R., Friess D. A. (2016). Rates and Drivers of Mangrove Deforestation in Southeast Asia 2000–2012. P. Natl. Acad. Sci. U.S.A. 113, 344. doi: 10.1073/pnas.1510272113
Sinsabaugh R. L., Follstad Shah J. J. (2012). Ecoenzymatic Stoichiometry and Ecological Theory. Annu. Rev. Ecol. Evol. S. 43, 313–343. doi: 10.1146/annurev-ecolsys-071112-124414
Sinsabaugh R. L., Hill B. H., Follstad Shah J. J. (2009). Ecoenzymatic Stoichiometry of Microbial Organic Nutrient Acquisition in Soil and Sediment. Nature 462, 795–798. doi: 10.1038/nature08632
Steen A. D., Arnosti C. (2011). Long Lifetimes of β-Glucosidase, Leucine Aminopeptidase, and Phosphatase in Arctic Seawater. Mar. Chem. 123, 127–132. doi: 10.1016/j.marchem.2010.10.006
Sundbäck K., Linares F., Larson F., Wulff A. (2004). Benthic Nitrogen Fluxes Along a Depth Gradient in a Microtidal Fjord: The Role of Denitrification and Microphytobenthos. Limnol. Oceanogr. 49 (4), 1095–1107. doi: 10.4319/lo.2004.49.4.1095
Suzumura M., Ishikawa K., Ogawa H. (1998). Characterization of Dissolved Organic Phosphorus in Coastal Seawater Using Ultrafiltration and Phosphohydrolytic Enzymes. Limnol. Oceanogr. 43, 1553–1564. doi: 10.4319/lo.1998.43.7.1553
Valderrama J. C. (1995). “Methods of Nutrient Analysis,” in Manual on Harmful Marine Microalga. Eds. Hallegraeff G. M., Anderson D. M., Cembella A. D. (Paris: UNESCO Publ), 251–568.
Wylie L., Sutton-Grier A. E., Moore A. (2016). Keys to Successful Blue Carbon Projects: Lessons Learned From Global Case Studies. Mar. Policy 65, 76–84. doi: 10.1016/j.marpol.2015.12.020
Zhang J., Zhu Z., Mo W. Y., Liu S. M., Wang D. R., Zhang G. S. (2018). Hypoxia and Nutrient Dynamics Affected by Marine Aquaculture in a Monsoon-Regulated Tropical Coastal Lagoon. Environ. Monit. Assess. 190, 656. doi: 10.1007/s10661-018-7001-z
Keywords: Dissolved organic matter (DOM), coastal lagoon, aquaculture, eutrophication, extracellular enzyme activity (EEA), seagrass, Hainan Island
Citation: Ou L, Li J, Zhang X, Wang Z, Zou J and Lu S (2022) Dissolved Organic Matter and Activities of Extracellular Enzymes in Two Lagoons (South China Sea) Affected by Aquaculture. Front. Mar. Sci. 9:864579. doi: 10.3389/fmars.2022.864579
Received: 28 January 2022; Accepted: 23 March 2022;
Published: 13 April 2022.
Edited by:
Christian Joshua Sanders, Southern Cross University, AustraliaCopyright © 2022 Ou, Li, Zhang, Wang, Zou and Lu. This is an open-access article distributed under the terms of the Creative Commons Attribution License (CC BY). The use, distribution or reproduction in other forums is permitted, provided the original author(s) and the copyright owner(s) are credited and that the original publication in this journal is cited, in accordance with accepted academic practice. No use, distribution or reproduction is permitted which does not comply with these terms.
*Correspondence: Songhui Lu, lusonghui1963@163.com