- 1Department of Marine Science, Incheon National University, Incheon, South Korea
- 2Research Institute of Basic Sciences, Incheon National University, Incheon, South Korea
- 3College of Marine Ecology and Environment, Shanghai Ocean University, Shanghai, China
- 4Department of Ecology and Evolutionary Biology, University of Connecticut, Stamford, CT, United States
- 5Fisheries Seed and Breeding Research Institute, National Institute of Fisheries Science, Jeonnam, South Korea
Climate change directly influences species composition and distribution of macroalgae on a global scale. Meanwhile, eutrophication and heavy metal pollution continue to be major concerns in coastal areas. The objective of this study was to explore interactive effects of these complex environmental conditions (high temperature, eutrophication and heavy metal pollution) in the bloom forming green alga, Ulva prolifera. This study evaluated the physiological characteristics of two strains of U. prolifera (Korean strain and Chinese strain) at two temperature levels (20 and 25°C), two nutrient concentrations (low nutrient: 50 μM of N and 5 μM of P; high nutrient: 250 μM of N and 25 μM of P) and three copper concentrations [Control (0.03 μM); 0.1 μM; 1 μM]. Under the 20°C and low nutrient condition, the Chinese strain grew significantly slower at 1μM copper group compared to copper control group. Whereas there was no significant change in the growth rate of the Korean strain. High nutrient significantly increased the growth rate, photosynthetic and respiration rates, soluble protein and tissue N in both strains at all copper groups under 20°C compared to copper groups under 20°C and low nutrient condition. Both strains grew significantly slower at 1 μM copper group compare to copper control group under high nutrient and 20°C condition. 25°C significantly reduced the growth rate of both strains at each nutrient condition. Under 25°C and low nutrient condition, 1 μM copper had no effects on growth rate and all photosynthetic parameters of both strains. Meanwhile, under 25°C and high nutrient conditions, 1 μM copper significantly inhibited growth and photosynthetic rates and increased soluble protein content in the Chinese strain compared to copper control treatment. These results suggest that the Korean strain had higher tolerance to the copper stress than the Chinese strain. Higher nutrient levels enhanced the temperature tolerance and the copper stress in U. prolifera. Increased temperature reduced the negative effects caused by copper stress in U. prolifera.
Introduction
Recent years, increased anthropogenic activities and climate change have resulted in the formation of massive blooms of macroalgae, which have caused huge environmental problems in coastal areas (Smetacek and Zingone, 2013; Wei et al., 2018; Wu H. L. et al., 2018). Green tides have received attention since 1970s when green algae grew excessively and became a disaster along coastal areas worldwide (Briand, 1987; Sfriso et al., 1987; Fletcher, 1990; Song et al., 2019; Sun et al., 2021). Since 2007, the world’s largest green tides (Cui et al., 2018; Zhang J. H. et al., 2019), have been observed every summer in the Yellow Sea with distribution areas ranging from 13,000 to 30,000 km2 (Liu et al., 2009; Huo et al., 2016, 2021; Zhang J. H. et al., 2019). Green tides have also occurred in the southwest sea of Korea during the same period (Kang et al., 2014). The amount of annual biomass produced from green tides in Jeju Island, Korea was about 10,000 tons (Cho et al., 2019). This is much smaller than those in the Yellow Sea, China, where the biomass was as high as about 3.5 × 106 tons (Zhang J. H. et al., 2019). The successive occurrence of green tides in these regions have posed significant threats to the health of marine ecosystems and lead to serious economic losses in aquaculture and coastal managements (Zhao et al., 2018, 2021). For example, in 2008, massive U. prolifera floated onto the coasts of Qingdao right before the Olympic games. The estimated cost of removing the green tides was more than US$100 million (Wang et al., 2009; Ye et al., 2011; Zhang et al., 2014). The massive outbreak of Ulva spp. has also changed marine community structures and functions due to shading, biomass decomposition and anoxia (Nelson et al., 2008).
The growth of macroalgae is strongly controlled by temperature (Breeman, 1990; Lüning, 1991; Sunday et al., 2012; Wu H. L. et al., 2018; Zhang Y. Y. et al., 2019). Seawater temperatures are expected to rise 3–7°C by the end of century (Stocker et al., 2014). Generally, the increase in temperature is expected to enhance growth and photosynthetic activities of macroalgae within a certain range below thermal tolerance limits (Pereira et al., 2006; Gao et al., 2016; Wu H. L. et al., 2018). However, macroalgae from different locations may be adapted to different temperature ranges (Lüning, 1991). Therefore, the responses to global warming may be species specific and even population specific (Poloczanska et al., 2013; Ji et al., 2016; Wiens, 2016).
The estuarine and coastal areas are the regions with active land-ocean interactions. In recent decades, with the development of the coastal economies and the increase in populations, marine pollutants, such as inorganic nutrients have flowed into these areas causing coastal eutrophication and even harmful algal blooms (Smith et al., 1999; Li et al., 2013; Wang et al., 2018). Heavy metals and copper (Cu) in particular enter marine ecosystems by air, domestic and industrial wastewater and natural run-off (Sharp et al., 1988; Moenne et al., 2016). Copper is a required trace element for marine macroalgae but can become toxic if it occurs at a high concentration (Gao et al., 2017; Xu et al., 2021). The photosynthetic apparatus is the primary target of copper, resulting in reduced algal growth (Rocchetta and Küpper, 2009; Kumar et al., 2014; Moenne et al., 2016).
With the increase of marine pollution, high concentrations of various pollutants, such as nutrients and heavy metals may appear simultaneously in certain coastal areas. While global warming is a constant environmental stress. Thus to better manage and control the green tides in the Yellow Sea, it is critical to understand interactive effects of the environmental stressors (c.f. nutrients and heavy metals) and the global warming on macroalgae, especially the green tide forming Ulva prolifera.
Materials and Methods
Sample Collection and Acclimation
The Korean strain of Ulva prolifera was collected from Anmogseom, Jawoldo, Korea in June, 2018. The Chinese strain of U. prolifera was originally collected from a Neopyropia yezoensis farm at Rudong, Jiangsu Province, China in May, 2011. This site is known to be a source of the green tides bloom in the Yellow Sea (Zhang et al., 2013). Both strains were propagated at Marine Ecology and Green Aquaculture Laboratory, Incheon National University, Korea. To induce spore release, both strains were pre-cultivated in artificial seawater with von Stosch enriched (VSE) medium (Ott, 1965) at 30 psu of salinity, 150 ± 10 μmol photons m–2 s–1 of photosynthetically active radiation (PAR) provided by light-emitting diode (LED) lamps, and the photoperiod of 12:12 h light: dark. The temperature was maintained at 20°C. The medium was renewed every 5 days during the period of pre-cultivation. After spores were released and seedlings grew approximately 2–3 cm long, the Ulva samples were placed in the experimental conditions.
Experimental Setup
Approximately 0.2 g fresh weight of Ulva prolifera were cultivated in a 250 mL conical flask containing 200 mL of artificial seawater enriched with VSE. This stocking density is known to be optimal for the growth of this species (Sun et al., 2021). Ulva prolifera was exposed to two levels of temperature (20°C; 25°C), two levels of nutrient (low nutrient: 50 μM nitrate and 5 μM phosphate; high nutrient: 250 μM nitrate and 25 μM phosphate) and three levels of copper (Control; 0.1 μM; 1 μM). The temperature, nutrients and copper levels were determined according to the environmental data in different regions and seasons that U. prolifera blooms occurred (Wu et al., 2015; Gao et al., 2017). Ulva prolifera were cultivated in von Stosch-enriched (VSE) medium (Ott, 1965), with adjustment of nitrogen and phosphorus to the experimental levels. The seawater without the addition of CuSO4 (Sigma) was considered as the control. The background concentration of copper was 0.03 μM in the artificial seawater, which was measured by Inductively Coupled Plasma Mass Spectrometer (Thermo Scientific iCAP Q ICP-MS; US). All other conditions were the same as mentioned above. Each treatment had three replicates, and the culture medium was replaced every 2 days during the cultivation period of 2 weeks.
Growth Rate
The relative growth rate of Ulva prolifera was determined by measuring thallus weight. Fresh weight (FW) was measured every 2 days after removing surface water from thalli with paper towels when the medium was changed. Relative growth rate (RGR) was calculated according to the formula as follows:
RGR(%day–1) = (lnWt2–lnWt1)/t × 100,
Where Wt2 and Wt1 are the fresh weight at day t2 and t1, respectively. t represents the cultivation period.
Photosynthesis and Respiration
Net photosynthetic and dark respiration rates of Ulva prolifera were measured using optical DO sensors at the end of the experiment (ProODO-BOD, YSI, United States). Approximately 0.2 g FW of U. prolifera from each treatment were placed in a 100 mL BOD bottle containing cultivation medium. The temperature was maintained at the cultivation temperature, 20 or 25°C, respectively. The dissolved oxygen (DO) sensors were inserted into the bottles, and no bubbles were trapped. Water in the bottle was stirred continuously during the period of measurement. DO contents (mg L–1) were recorded every minute during dark and light (150 μmol photons m–2 s–1) conditions. The net photosynthetic and dark respiration rates were determined as mg O2 L–1 FW–1 h–1.
Pigment Contents
Chlorophyll a (chl a), b (chl b) and carotenoids from Ulva prolifera were extracted and estimated based on the method reported by Wellburn (1994). Briefly, approximately 0.02 g FW of U. prolifera thalli was placed in 5 mL of methanol (100%) solution and kept at 4°C for 24 h at dark (Gao and Xu, 2008). The absorbance was measured using a spectrophotometer (Orion AquaMate 8000, Thermo fisher Scientific Solutions LLC, Korea) at 470, 653 and 666 nm, respectively. Pigment contents were expressed as mg g–1 FW.
Soluble Protein
The soluble protein contents of the Ulva prolifera thalli were measured following Bradford protein assay (Bradford, 1976). Briefly, approximately 0.05 g of FW algal thalli from each treatment was ground on ice with 5 mL extraction buffer (0.1 mol L–1, potassium phosphate buffer, pH 7.0) containing 0.25% Triton X-100 and 1% polyvinylpyrrolidone, and then centrifuged for 20 min at 12,000 g. The supernatant was mixed with Bradford’s reagent and allowed for 5 min prior to measuring absorbance using a spectrophotometer (Orion AquaMate 8000, Thermo Fisher Scientific Solutions LLC, Korea) at 595 nm. Soluble protein content was calculated with bovine serum albumin as a standard and expressed as mg g–1FW.
Tissue Carbon and Nitrogen Contents
Approximately 0.05 g of FW algal samples were collected from each treatment at the end of the experiment to measure tissue carbon and nitrogen contents. Ulva prolifera samples were oven-dried at 60°C until constant weight, and then ground to powder by MM400 Ball Mill (Retsch, Germany). About 2–3 mg dry weight (DW) sample was made into capsule, and tissue carbon and nitrogen contents were analyzed using a CHN analyzer (Thermo Scientific™ Flash 2000 CHNS/O Analyzers, United States).
Data Analysis
The results were expressed as the mean of triplicate ± standard deviation. Data were processed using Origin 9.0 and SPSS 25.0 software. The data conformed to a normal distribution from each treatment (Shapiro–Wilk, P > 0.05) and the variances could be considered equal (Levene’s test, P > 0.05). Three-way multivariate ANOVAs (M-ANOVAs) were conducted to assess the effects of temperature, nutrient and copper levels on relative growth rate, net photosynthetic and dark respiration rates, pigment contents, soluble protein contents, tissue carbon and nitrogen, and C:N. While three-way analysis of variance (Three-way ANOVA) was used to analyze the interactive effects of temperature, nutrient and copper levels, Tukey’s honest significant difference (Tukey test) was used for post hoc investigation. In addition, the Pearson correction coefficients were conducted between all variables to determine statistical relationship between physiological and biochemical parameters. A confidence interval of 95% was set for all analyses.
Results
Growth Rates
Relative growth rates (RGR) of both strains of Ulva prolifera were significantly affected by temperature (P < 0.001), nutrients (P < 0.001), copper (P < 0.001), and the interactions between temperature and nutrients (P < 0.001), nutrients and copper (P < 0.05), and temperature and copper (P < 0.05). RGR of the Chinese strain was also significantly affected by the interactive effects of temperature, nutrients and copper (P < 0.001) (Supplementary Table 1). In terms of copper, 0.1 μM copper has no significant effect on RGR in both strains at each nutrient and temperature conditions (P > 0.05) (Figures 1A,B). However, the effects varied at the 1 μM copper condition. Under 20°C and low nutrients condition, 1 μM copper only significantly decreased RGR in the Chinese strain (P < 0.05) (Figure 1B). High nutrients significantly enhanced RGR in both strains among all copper conditions under 20°C (P < 0.001). Under 20°C and high nutrient condition, 1 μM copper significantly decreased RGR of both strains compared to copper control treatment (P < 0.05) (Figures 1A,B). RGR of both strains was decreased in the 25°C treatment at each nutrient condition (P < 0.05), and no differences among these three copper treatments was observed under 25°C and low nutrient condition (P > 0.05) (Figures 1A,B). RGR f both strains were significantly higher at 25°C and high nutrient condition than that under 20°C and low nutrient condition (P < 0.05) (Figures 1A,B). Meanwhile, 1 μM copper significantly inhibited RGR of Chinese strain compared to control under 25°C and high nutrient condition (P < 0.05) (Figure 1B).
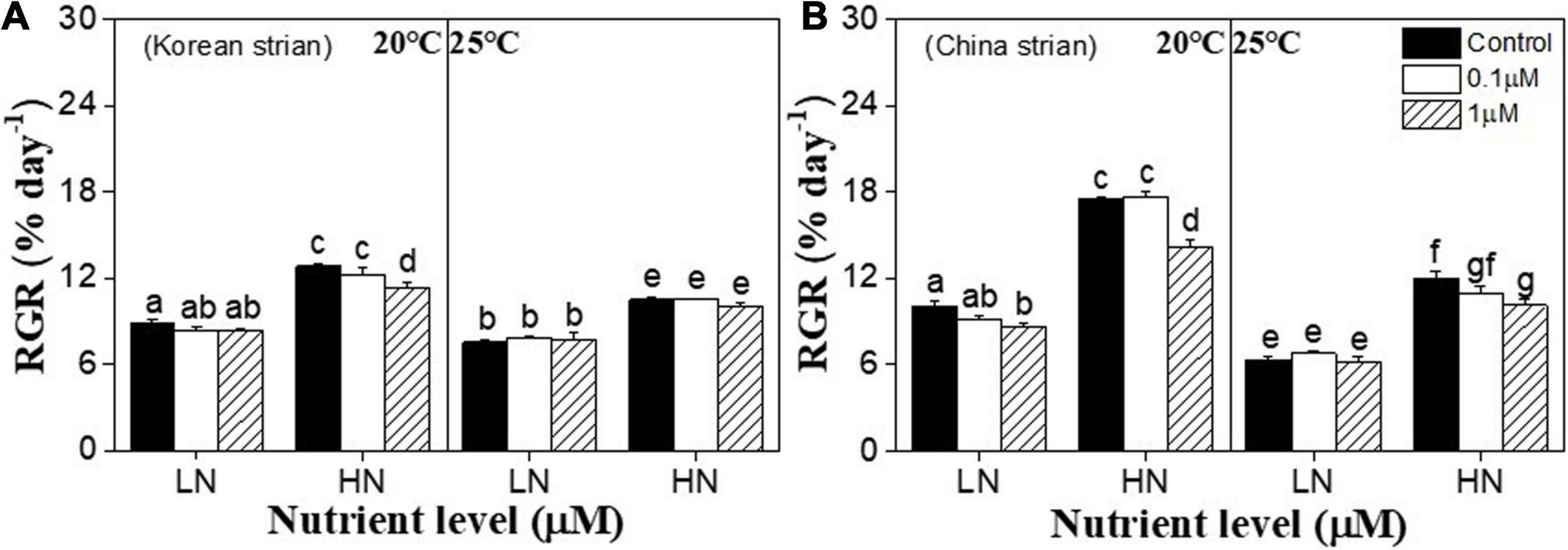
Figure 1. Relative growth rate (RGR) in a Korean strain (A) and Chinese strain (B) of Ulva prolifera cultivated under various experimental conditions. The error bars represent the standard deviation (n = 3). Low nutrient = 50 μM nitrate and 5 μM phosphate (LN); high nutrient = 250 μM nitrate and 25 μM phosphate (HN). Different letters above the error bars represent statistical significance (P < 0.05) among different treatments.
Photosynthesis and Respiration
Net photosynthetic rates (NPR) of both strains of Ulva prolifera were significantly affected by temperature (P < 0.001), nutrients (P < 0.001). NPR of Chinese strain was also significantly affected by copper (P = 0.001), the interactions between temperature and nutrient (P < 0.001), and the interactions of temperature, nutrients and copper (P = 0.004) (Supplementary Table 2). Under 20°C, 1 μM copper had no effect on NPR in both strains under each nutrient condition (P > 0.05) and high nutrients significantly increased NPR in both strains (P < 0.05) (Figures 2A,C). Meanwhile, compared to 20°C and low nutrients, NPR of both strains were not affected by high temperature (25°C) and copper stresses under low nutrient (P > 0.05) (Figures 2A,C). No extra enhancing effect was observed in the interactions between 25°C and high nutrient in the Korean strain (P > 0.05) (Figure 3A). The highest NPR in the Chinese strain was observed at 25°C and high nutrient groups (P < 0.05) (Figure 2C). Meanwhile, under 25°C and high nutrient condition, net photosynthetic rate decreased by 0.1 and 1 μM copper in Chinese strain (P < 0.05; Figure 2C).
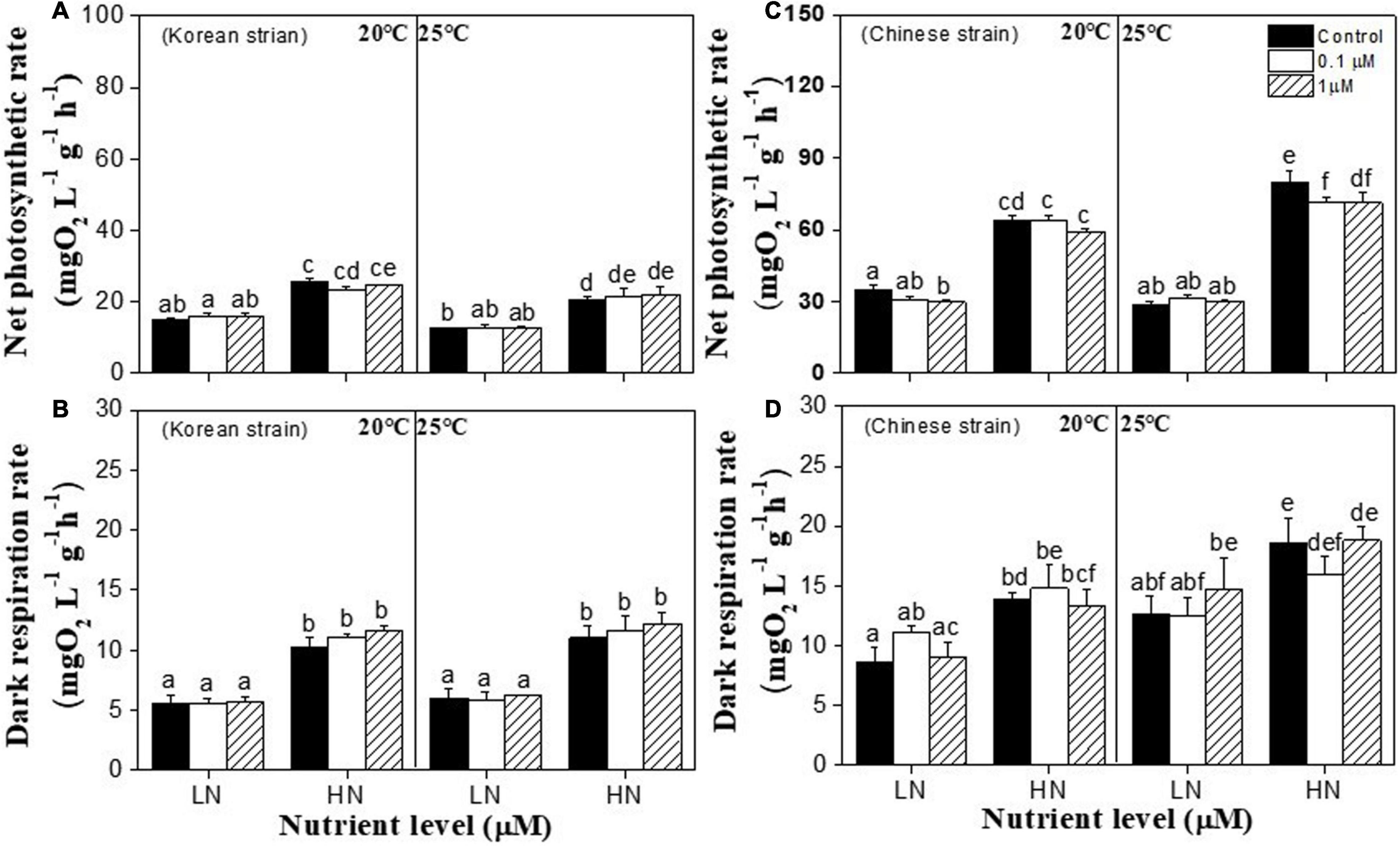
Figure 2. Net photosynthetic rate (NPR) in a Korea strain (A) and Chinese strain (C), and dark respiration rate (Rd) in a Korea strain (B) and Chinese strain (D) of Ulva prolifera cultivated under various experimental conditions. The error bars represent the standard deviation (n = 3). Low nutrient = 50 μM nitrate and 5 μM phosphate (LN); high nutrient = 250 μM nitrate and 25 μM phosphate (HN). Different letters above the error bars represent statistical significance (P < 0.05) among different treatments.
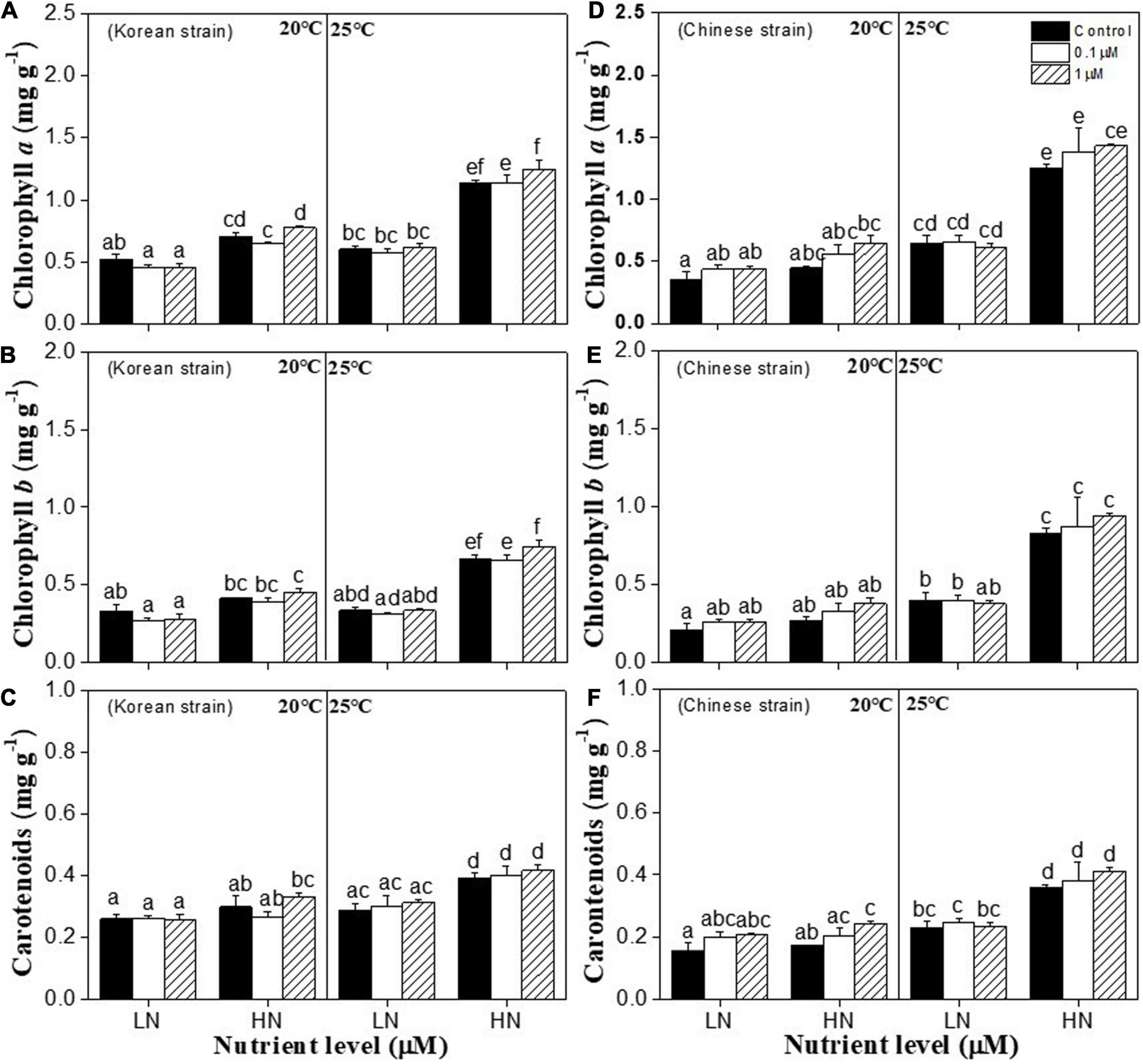
Figure 3. Pigments contents in a Korean strain [Chlorophyll a (A); Chlorophyll b (B); Carotenoids (C)] and a Chinese strain [Chlorophyll a (D); Chlorophyll b (E); Carotenoids (F)] and of Ulva prolifera cultivated under various experimental conditions. The error bars represent the standard deviation (n = 3). Low nutrient = 50 μM nitrate and 5 μM phosphate (LN); high nutrient = 250 μM nitrate and 25 μM phosphate (HN). Different letters above the error bars represent statistical significance (P < 0.05) among different treatments.
Dark respiration rates (Rd) of both strains of Ulva prolifera were significantly affected by (P < 0.001), Rd of the Chinese strain was also significantly affected by temperature (P < 0.05) and the interactions between temperature and copper (P < 0.05) (Supplementary Table 2). In the Korean strain, high nutrients increased Rd at each temperature (P < 0.05) while temperature had no effect on Rd (P > 0.05) (Figure 2B). However, compared to low nutrient and 20°C, Rd of the Chinese strain was increased by high nutrient or 25°C conditions alone. But no extra enhancing effect was observed in the interactions between 25°C and high nutrients (P < 0.05) (Figure 2D).
Pigment Contents
Chlorophyll a (chl a) content was significantly affected by temperature (P < 0.001), nutrients (P < 0.001), copper (P < 0.05), the interactions between nutrients and temperature (P < 0.001), and interactions between nutrients and copper (P < 0.05) in both strains (Supplementary Table 3). Copper treatments had no significant effect on chl a contents of both strains compared to the copper control at each nutrient and temperature conditions (P > 0.05) (Figures 3A,D). High nutrients and 25°C alone induced higher chl a contents in both strains (Figures 3A,D). The highest chl a content in both strains were found at 25°C and high nutrient conditions, which were significantly higher than those at other three nutrient and temperature conditions (P < 0.05) (Figures 3A,D). Chlorophyll b (chl b) and carotenoids contents were significantly affected by temperature (P < 0.001), nutrients (P < 0.001), and the interactions between temperature and nutrient (P < 0.001) in both strains, and carotenoids were affected by copper (P < 0.05) (Supplementary Table 3). Same to chl a, the highest chl b and carotenoids contents in both strains were found at 25°C and high nutrient conditions (Figures 3B,C,E). Besides, copper only increased carotenoids content of Chinese strains at the copper concentration of 1 μM under 20°C and high nutrient conditions (P < 0.01) (Figure 3F).
Soluble Protein
Soluble protein contents were significantly affected by nutrients (P < 0.001) in both strains (Supplementary Table 4). Soluble protein content of Chinese strain was also significantly affected by temperature (P < 0.001), the interactions between nutrients and copper (P = 0.039), temperature and copper (P = 0.028), and the interactions of temperature, nutrients and copper (P = 0.014) (Supplementary Table 4). In the Korean strain, high nutrient increased soluble protein content at each temperature (P < 0.05), while temperature and copper had no effect on it (P > 0.05) (Figure 4A). Similar to the Korean strain, under 20°C condition, high nutrient increased soluble protein content of the Chinese strain (P < 0.05), and copper had no effects on soluble protein content under each nutrient conditions (P > 0.05) (Figure 4B). High temperature increased soluble protein content at all copper conditions under low nutrient (P < 0.05), but no difference was found among these three copper treatments (P > 0.05) (Figure 4B). Soluble protein content of the Chinese strain was enhanced by 25°C and high nutrient condition under both 0.1 and 1 μM copper conditions (P < 0.05). Meanwhile, soluble protein content was significantly higher at 1 μM copper group compare to copper control group under 25°C and high nutrient (P < 0.05) (Figure 4B).
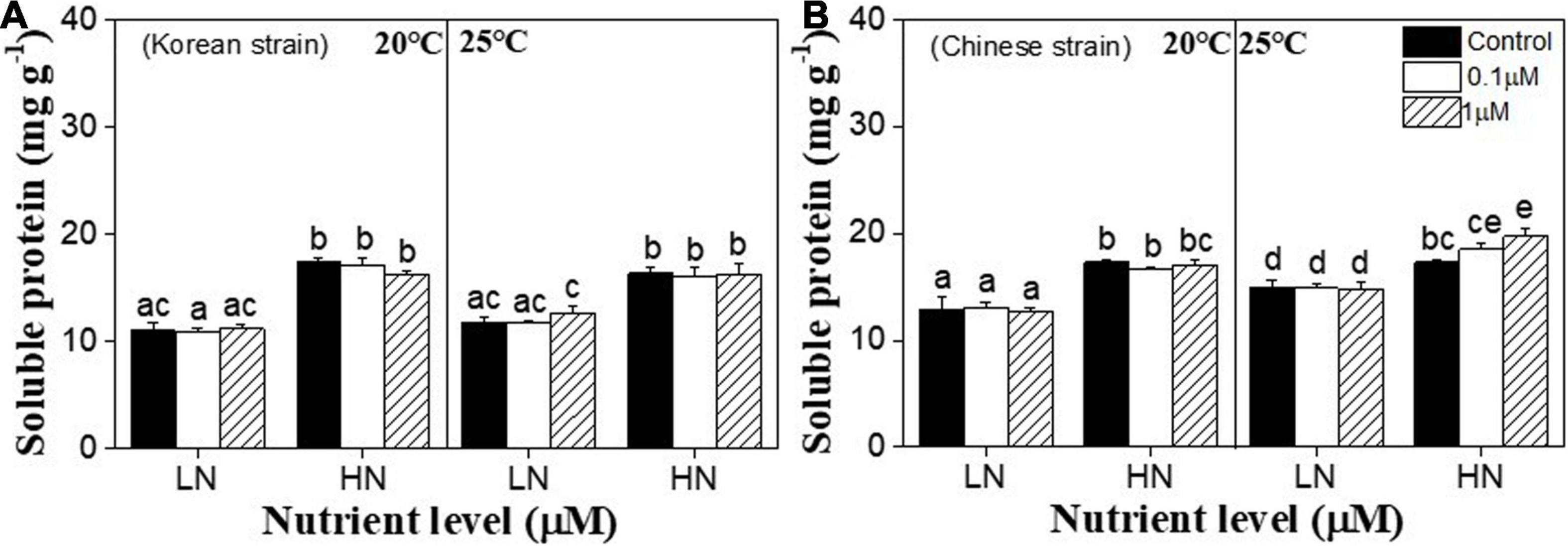
Figure 4. Soluble protein contents in a Korean strain (A) and a Chinese strain (B) of Ulva prolifera cultivated under various experimental conditions. The error bars represent the standard deviation (n = 3). Low nutrient = 50 μM nitrate and 5 μM phosphate (LN); high nutrient = 250 μM nitrate and 25 μM phosphate (HN). Different letters above the error bars represent statistical significance (P < 0.05) among different treatments.
Tissue Carbon and Nitrogen Contents
Tissue C content of both strains showed no difference at any one of temperature, nutrients and copper treatments compared to 20°C, low nutrient and copper control group (P > 0.05) (Figures 5A,D). Tissue nitrogen (N) content was significantly affected by temperature (P < 0.001), nutrients (P < 0.001), and the interactions between temperature and nutrients (P < 0.05), and nutrients and copper (P < 0.05) in both strains (Supplementary Table 5). High nutrients or 25°C alone significantly increased tissue N content in both strains at all copper conditions compared to 20°C and low nutrient condition (P < 0.05) (Figures 5B,E). The highest tissue N content in both strains was observed at 25°C and high nutrient condition (P < 0.05) (Figures 5B,E). 1 μM copper only significantly increased tissue N in the Chinese strain compared to control under high nutrient and 25°C conditions (P < 0.05) (Figure 5E).
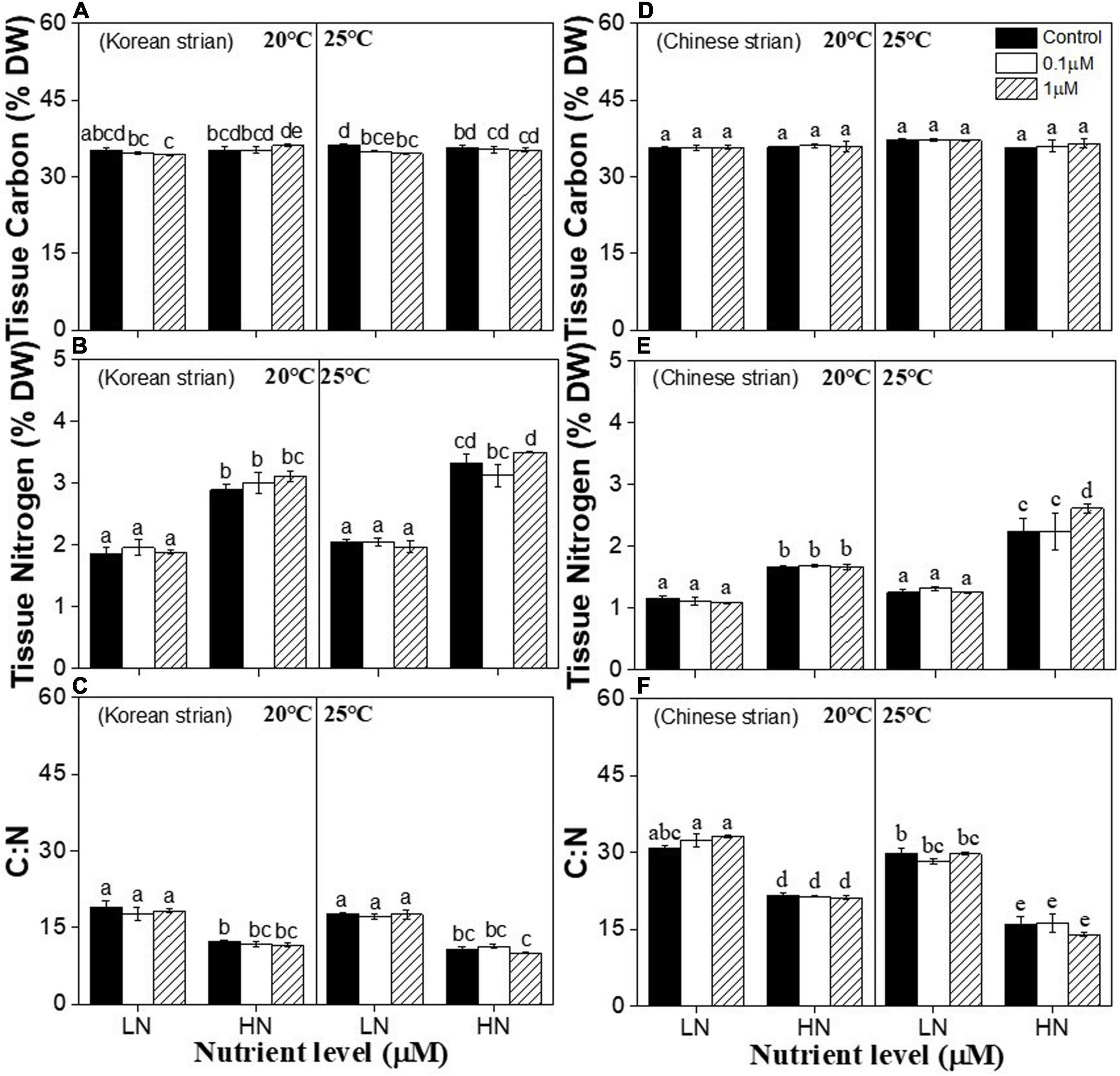
Figure 5. Tissue contents in a Korean strain [Tissue Carbon (A); Tissue Nitrogen (B); C:N (C)] Chinese strain [Tissue Carbon (D); Tissue Nitrogen (E); C:N (F)] and of Ulva prolifera cultivated under various experimental conditions. The error bars represent the standard deviation (n = 3). Low nutrient = 50 μM nitrate and 5 μM phosphate (LN); high nutrient = 250 μM nitrate and 25 μM phosphate (HN). Different letters above the error bars represent statistical significance (P < 0.05) among different treatments.
C:N ratio was significantly affected by temperature (P < 0.001) and nutrients in both strains (P < 0.001). C:N ratio of the Chinese strain was also significantly affected by the interactions between temperature and nutrients (P < 0.001), nutrients and copper (P < 0.05), and temperature and copper (P < 0.05) (Supplementary Table 5). Copper had no significant effects on C:N in both strains at each temperature and nutrient conditions (P > 0.05) (Figures 5C,F). High nutrient significantly decreased C:N ratio in both strains at all copper conditions under 20°C condition (P < 0.05) (Figures 5C,F). High temperature had no effect on C:N ratio in the Korean strain (P > 0.05) (Figure 5C). While, high temperature significantly decreased C:N ratio of Chinese strain at high nutrient condition (P < 0.05) (Figure 5F).
Pearson Correlation Coefficients
Pearson correlation coefficients between physiological and biochemical parameters were varied in both strains of Ulva prolifera (Table 1). Net photosynthetic rates, soluble protein and tissue N contents were positively correlated with growth (Table 1). Additionally, pigment contents (chlorophyll a and chlorophyll b) were positively related with growth only in the Korean strain, not in the Chinese strain. Correlation analysis demonstrated that photosynthetic activities were positively related with pigment levels (chlorophyll a, chlorophyll b, and carotenoids), soluble protein, and tissue N, but negatively related with C:N ratio in both strains (P < 0.01) (Table 1). Pigment levels were positively related with soluble protein and tissue N, but negatively related with C:N ratio. Soluble protein contents were positively related with tissue N (P < 0.01), and negatively related with C:N ratio (P < 0.01) in both strains (Table 1).
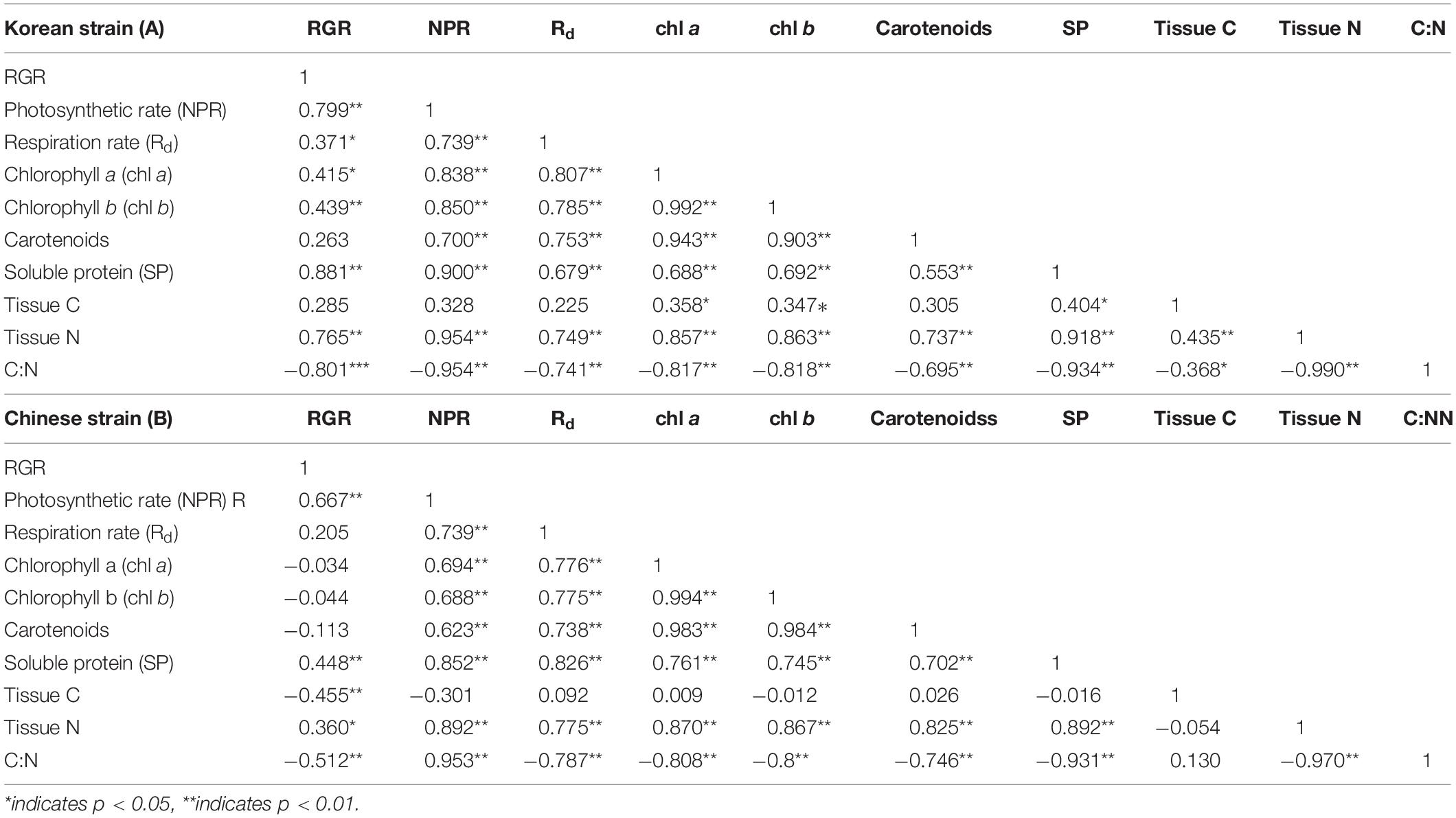
Table 1. Pearson correction coefficients (n = 36) between physiological and biochemical parameters between a Korean strain (A) and Chinese strain (B) of Ulva prolifera.
Discussion
The biological role of copper is concentration dependent. Elevated copper (Cu2+) concentrations are toxic to algal cells and inhibit their growth (Han et al., 2008; Gao et al., 2017). In this study, two Ulva prolifera strains had different physiological responses to the high concentration copper stress. Under the 20°C and low nutrient group, the growth rate of the Chinese strain was significantly reduced by 1 μM copper treatment compared to copper control treatment. While there was no significant change in the growth rate of the Korean strain, suggesting that the Korean strain had higher tolerance to the copper stress than the Chinese strain. The previous study reported that the adaptive characteristics are genetically determined and have not been obliterated by acclimatization to seawater (Russell and Bolton, 1975). Thus, the different tolerance of the Chinese and Korean strains should be linked with the conditions in the original habitats. Additionally, the result of net photosynthetic rate measurement also showed the similar trend with growth rate, indicating that copper stress affected the growth of U. prolifera by inhibiting photosynthesis. This result is consistent with the observation in a previous study, in which copper stress significantly reduced the photosynthesis rate in other two Ulva species: U. pertusa and U. armoricana (Han et al., 2008).
Nevertheless, high nutrients significantly increased the photosynthetic rates in all copper treatments of two strains compared to copper treatments under 20°C and low nutrient condition, as well as respiration rates, soluble protein, growth rates and tissue N content. This result suggests that high nutrient enhanced the tolerance to copper stress in both strains. The enhancement of growth by high nutrients has also been previously observed in different algal species (Luo et al., 2012; Li et al., 2016; Wu H. et al., 2018; Lee and Kang, 2020). For instance, high nitrogen and phosphorus concentration increased the nutrient uptake and growth in two Ulva species: U. prolifera and U. linza (Luo et al., 2012). However, among all groups under 20°C and high nutrient condition, the enhancement of growth by 1 μM copper was the lowest in both strains. These results suggest that 1 μM copper negatively affected growth of U. prolifera under high nutrients. High nutrient conditions enhance the assimilation and accumulation of heavy metal ions (Wang and Dei, 2001). For example, high concentrations of nitrate, ammonium, and phosphate have been shown to increase the accumulation of trace metals (Cd, Cr, Zn and Se) in Ulva fasciata (Lee and Wang, 2001). Furthermore, the growth rate of the Chinese strain increased 75.7% under a high nutrient condition compared with a low nutrient condition, while the Korean strain increased only 44.2%, suggesting a high capacity of nutrient assimilation in the Chinese strain. A previous study also reported that the rapid growth ability of U. prolfiera was due to the high nutrient uptake efficiency (Luo et al., 2012). The high capacity of the nutrient assimilation is associated to the efficient nutrient transport through membranes, which also causes the influx of non-essential heavy metal ions due to the non-selective ion transport (Clarkson and Lüttge, 1989; Lee and Wang, 2001). These results suggest that the low tolerance to copper stress in Chinese strain might be due to its high nutrient assimilation capacity.
In this study, high temperature significantly reduced the growth rate of two Ulva prolifera strains. It has been reported in several studies that a temperature higher than 20°C inhibits the growth of U. prolifera (Cui et al., 2015; Wu H. L. et al., 2018). These results suggest that the increasing temperature in marine environment caused by global warming may reduce the U. prolifera blooms. Meanwhile, a series of studies reported a higher temperature enhanced the sensitivity of algae to metals and promoted the inhibition effects of metal ions (Lewis and Horning, 1991; Wang and Wang, 2008; Zhong et al., 2021). The increased metabolic rate caused by higher temperature may induce more metal ions transfer across membranes, causing more accumulation of metal ions inside of cells (Miao and Wang, 2004; Sarı and Tuzen, 2008; Zhong et al., 2021). For example, the growth of Fucus serratus was reduced by 50% under a high temperature (22°C) and high copper (1 μM) conditions (Nielsen et al., 2014), and the growth of Gelidium floridanum was reduced by 85% with high temperature (30°C) and high copper concentration (3 μM) (Kreusch et al., 2018). Interestingly, in this study, no negative effect on growth, photosynthetic rate and other metabolic activities caused by copper stress was observed in groups exposed to 25°C and low nutrient condition. In U. prolifera, high temperature stress increases the gene expression and enzyme activities related to antioxidative responses (Yang et al., 2019). High temperature can also increase the rate of metabolism and regeneration of photosynthetic system (Huner et al., 1996; Zeng and Wang, 2011; Nielsen et al., 2014). Therefore, the possible explanation to this result is that high temperature activated some responses of stress resistance, which reduced the negative effects caused by copper stress.
When the nutrient concentration was elevated, the enhancement of growth rate was also observed even under the high temperature conditions. Among all treatment groups, the pigment contents and photosynthetic rates were the highest in groups under 25°C and high nutrient conditions, suggesting that high nutrient condition might enhance the high temperature tolerance in U. prolifera by increasing pigments and photosynthesis. Similar results have been observed for U. conglobata in which the pigments and proteins synthesis were enhanced by higher temperature (25°C) and high nutrient (200 μM NO3–) (Zou and Gao, 2014). Among the three groups under 25 °C and high nutrient conditions, the growth rate of the Chinese strain at 1 μM copper condition was the lowest, because high nutrient enhanced the negative effect of copper stress on growth, but, photosynthetic rate was significantly decreased in 0.1 and 1 μM copper treatment groups. The results indicate that the interaction of high temperature and copper stress inhibited photosynthesis of the Chinese strain under a high nutrient treatment. On the other hand, the content of soluble protein was significantly increased by 1 μM copper under 25°C and high nutrient condition. Similarly, increased soluble proteins due to heavy metal treatment has been reported in other green alga, Chlorella vulgaris (Afkar et al., 2010). Soluble proteins are related to various metabolic processes and the previous study reported that the accumulation of protein may be one of the ways to abolish the metal toxic effects on algae (Osman et al., 2004; Afkar et al., 2010). In this study, the soluble protein content had a positive correlation with copper conditions under the 25°C and high nutrient condition, suggesting that the combination of a higher temperature and a high nutrient level might maximize the toxicity of copper in U. prolifera. However, under 25°C and high nutrient condition, growth and photosynthetic rates in the Korean strain were not affected by 1 μM copper compared to control copper treatment. The result suggests that the Korean strain has high tolerance to copper stress under 25°C and high nutrient condition.
In summary, the results of this study indicate that as global warming continues to increase and approach the upper thermal limits, Ulva prolifera blooms will decline. However, this decline may be moderated by higher levels of eutrophication. Under laboratory conditions, high nutrient concentration enhanced the thermal tolerance and copper stress in U. prolifera. Furthermore, two U. prolifera strains had different nutrient assimilation capacities, suggesting that the different response of geographically separated populations to environmental factors might be due to genetic differentiation, which still needs further exploration in the future.
Data Availability Statement
The original contributions presented in the study are included in the article/Supplementary Material, further inquiries can be directed to the corresponding author/s.
Author Contributions
MB: conceptualization, data curation, formal analysis, methodology, and writing—original draft. J-SP: conceptualization, data curation, and methodology. QX, PH, JZ, and CY: conceptualization, writing—review, and editing. HY: conceptualization, writing—review, and editing, funding acquisition. JK: conceptualization, methodology, writing—review and editing, supervision, project administration, funding acquisition. All authors contributed to the article and approved the submitted version.
Funding
This study was supported by the Basic Science Research Program through the National Research Foundation of Korea (NRF) funded by the Ministry of Education (NRF- 2017R1A6A1A06015181) and a grant from the National Institute of Fisheries of Science (R2022013).
Conflict of Interest
The authors declare that the research was conducted in the absence of any commercial or financial relationships that could be construed as a potential conflict of interest.
Publisher’s Note
All claims expressed in this article are solely those of the authors and do not necessarily represent those of their affiliated organizations, or those of the publisher, the editors and the reviewers. Any product that may be evaluated in this article, or claim that may be made by its manufacturer, is not guaranteed or endorsed by the publisher.
Supplementary Material
The Supplementary Material for this article can be found online at: https://www.frontiersin.org/articles/10.3389/fmars.2022.863918/full#supplementary-material
References
Afkar, E., Ababna, H., and Fathi, A. A. (2010). Toxicological response of the green alga Chlorella vulgaris, to some heavy metals. Am. J. Environ. Sci. 6, 230–237.
Bradford, M. M. (1976). A rapid and sensitive method for the quantitation of microgram quantities of protein utilizing the principle of protein-dye binding. Anal. Biochem. 72, 248–254. doi: 10.1016/0003-2697(76)90527-3
Breeman, A. M. (1990). “Expected effects of changing seawater temperatures on the geographic distribution of seaweed species,” in Expected Effects of Climatic Change on Marine Coastal Ecosystems, eds J. J. Beukema, W. J. Wolff, and J. J. W. M. Brouns (Dordrecht: Springer), 69–76. doi: 10.1016/j.marpolbul.2014.04.032
Briand, X. (1987). “Stranded algae and disposable biomass. Aquatic primary biomass (marine macroalgae): Biomass conversion, removal and use of nutrients,” in Proc. 1st Workshop COST 48 subgroup 3, (Brussels: Commission of the European Communities), 13–16.
Cho, H. M., Kim, G., and Shin, K. H. (2019). Tracing nitrogen sources fueling coastal green tides off a volcanic island using radon and nitrogen isotopic tracers. Sci. Total Environ. 665, 913–919. doi: 10.1016/j.scitotenv.2019.02.212
Clarkson, D. T., and Lüttge, U. (1989). “Mineral nutrition: divalent cations, transport and compartmentation,” in Progress in Botany, eds H. D. Behnke, K. Esser, K. Kubitzki, M. Runge, and H. Ziegler (Berlin: Springer), 93–112.
Cui, J. J., Monotilla, A. P., Zhu, W. R., Takano, Y., Shimada, S., Ichihara, K., et al. (2018). Taxonomic reassessment of Ulva prolifera (Ulvophyceae, Chlorophyta) based on specimens from the type locality and Yellow Sea green tides. Phycologia 57, 692–704. doi: 10.2216/17-139.1
Cui, J. J., Zhang, J. H., Huo, Y. Z., Zhou, L. J., Wu, Q., Chen, L. P., et al. (2015). Adaptability of free-floating green tide algae in the Yellow Sea to variable temperature and light intensity. Mar. Pollut. Bull. 101, 660–666. doi: 10.1016/j.marpolbul.2015.10.033
Fletcher, R. (1990). “The “green tide”problem: a review,” in Proceedings from a Second Workshop: Marine Biotechnology on Eutrophication and Biotransformation in Coastal Waters, (Sorrento: Ecolmare), 18–24.
Gao, G., Liu, Y. M., Li, X. S., Feng, Z. H., Xu, Z. G., Wu, H. L., et al. (2017). Expected CO2-induced ocean acidification modulates copper toxicity in the green tide alga Ulva prolifera. Environ. Exp. Bot. 135, 63–72. doi: 10.1016/j.envexpbot.2016.12.007
Gao, G., Zhong, Z. H., Zhou, X. H., and Xu, J. T. (2016). Changes in morphological plasticity of Ulva prolifera under different environmental conditions: a laboratory experiment. Harmful Algae 59, 51–58. doi: 10.1016/j.hal.2016.09.004
Gao, K. S., and Xu, J. T. (2008). Effects of solar UV radiation on diurnal photosynthetic performance and growth of Gracilaria lemaneiformis (Rhodophyta). Eur. J. Phycol. 43, 297–307. doi: 10.1080/09670260801986837
Han, T. J., Kang, S. H., Park, J. S., Lee, H. K., and Brown, M. T. (2008). Physiological responses of Ulva pertusa and U. armoricana to copper exposure. Aquat. Toxicol. 86, 176–184. doi: 10.1016/j.aquatox.2007.10.016
Huner, N. P. A., Maxwell, D. P., Gray, G. R., Savitch, L. V., Krol, M., Ivanov, A. G., et al. (1996). Sensing environmental temperature change through imbalances between energy supply and energy consumption: redox state of photosystem II. Physiol. Plant. 98, 358–364. doi: 10.1034/j.1399-3054.1996.980218.x
Huo, Y. Z., Han, H. B., Hua, L., Wei, Z. L., Yu, K. F., Shi, H. H., et al. (2016). Tracing the origin of green macroalgal blooms based on the large scale spatio-temporal distribution of Ulva microscopic propagules and settled mature Ulva vegetative thalli in coastal regions of the Yellow Sea, China. Harmful Algae 59, 91–99. doi: 10.1016/j.hal.2016.09.005
Huo, Y. Z., Kim, J. K., Yarish, C., Augyte, S., and He, P. M. (2021). Responses of the germination and growth of Ulva prolifera parthenogametes, the causative species of green tides, to gradients of temperature and light. Aquat. Bot. 170:103343. doi: 10.1016/j.aquabot.2020.103343
Ji, Y., Xu, Z. G., Zou, D. H., and Gao, K. S. (2016). Ecophysiological responses of marine macroalgae to climate change factors. J. Appl. Phycol. 28, 2953–2967. doi: 10.1007/s10811-016-0840-5
Kang, E. J., Kim, J. H., Kim, K. Y., Choi, H. G., and Kim, K. Y. (2014). Re-evaluation of green tide-forming species in the Yellow Sea. Algae 29, 267–277. doi: 10.4490/algae.2014.29.4.267
Kreusch, M., Poltronieri, E., Bouvie, F., Batista, D., Pereira, D. T., Ramlov, F., et al. (2018). Ocean warming and copper pollution: implications for metabolic compounds of the agarophyte Gelidium floridanum (Gelidiales, Rhodophyta). J. Phycol. 54, 870–878. doi: 10.1111/jpy.12793
Kumar, K. S., Dahms, H. U., Lee, J. S., Kim, H. C., Lee, W. C., and Shin, K. H. (2014). Algal photosynthetic responses to toxic metals and herbicides assessed by chlorophyll a fluorescence. Ecotoxicol. Environ. Saf. 104, 51–71. doi: 10.1016/j.ecoenv.2014.01.042
Lee, J. E., and Kang, J. W. (2020). The interactive effects of elevated temperature and nutrient concentrations on the physiological responses of Ulva linza Linnaeus (Ulvales, Chlorophyta). J. Appl. Phycol. 32, 2459–2467. doi: 10.1007/s10811-019-02031-0
Lee, W. Y., and Wang, W. X. (2001). Metal accumulation in the green macroalga Ulva fasciata: effects of nitrate, ammonium and phosphate. Sci. Total Environ. 278, 11–22. doi: 10.1016/S0048-9697(00)00884-6
Lewis, P. A., and Horning, W. B. (1991). Differences in acute toxicity test results of three reference toxicants on Daphnia at two temperatures. Environ. Toxicol. Chem. 10, 1351–1357. doi: 10.1002/etc.5620101014
Li, R. H., Liu, S. M., Zhang, G. L., Ren, J. L., and Zhang, J. (2013). Biogeochemistry of nutrients in an estuary affected by human activities: the Wanquan River estuary, eastern Hainan Island, China. Cont. Shelf Res. 57, 18–31. doi: 10.1016/j.csr.2012.02.013
Li, S. X., Yu, K. F., Huo, Y. Z., Zhang, J. H., Wu, H. L., Cai, C., et al. (2016). Effects of nitrogen and phosphorus enrichment on growth and photosynthetic assimilation of carbon in a green tide-forming species (Ulva prolifera) in the Yellow Sea. Hydrobiologia 776, 161–171. doi: 10.1007/s10750-016-2749-z
Liu, D. Y., Keesing, J. K., Xing, Q. G., and Shi, P. (2009). World’s largest macroalgal bloom caused by expansion of seaweed aquaculture in China. Mar. Pollut. Bull. 58, 888–895. doi: 10.1016/j.marpolbul.2009.01.013
Lüning, K. (1991). Seaweeds: Their Environment, Biogeography, and Ecophysiology. Hoboken: John Wiley & Sons.
Luo, M. B., Liu, F., and Xu, Z. L. (2012). Growth and nutrient uptake capacity of two co-occurring species, Ulva prolifera and Ulva linza. Aquat. Bot. 100, 18–24. doi: 10.1016/j.aquabot.2012.03.006
Miao, A. J., and Wang, W. X. (2004). Relationships between cell-specific growth rate, and uptake rate of cadmium and zinc by a coastal diatom. Mar. Ecol. Prog. Ser. 275, 103–113. doi: 10.3354/meps275103
Moenne, A., Gonzalez, A., and Saez, C. A. (2016). Mechanisms of metal tolerance in marine macroalgae, with emphasis on copper tolerance in Chlorophyta and Rhodophyta. Aquat. Toxicol. 176, 30–37. doi: 10.1016/j.aquatox.2016.04.015
Nelson, T. A., Haberlin, K., Nelson, A. V., Ribarich, H., Hotchkiss, R., Van Alstyne, K. L., et al. (2008). Ecological and physiological controls of species composition in green macroalgal blooms. Ecology 89, 1287–1298. doi: 10.1890/07-0494.1
Nielsen, S. L., Nielsen, H. D., and Pedersen, M. F. (2014). Juvenile life stages of the brown alga Fucus serratus L. are more sensitive to combined stress from high copper concentration and temperature than adults. Mar. Biol. 161, 1895–1904. doi: 10.1007/s00227-014-2471-1
Osman, M. E., El-Naggar, A. H., El-Sheekh, M. M., and El-Mazally, E. E. (2004). Differential effects of Co2+ and Ni2+ on protein metabolism in Scenedesmus obliquus and Nitzschia perminuta. Environ. Toxicol. Pharmacol. 16, 169–178. doi: 10.1016/j.etap.2003.12.004
Ott, F. D. (1965). Synthetic media and techniques for the xenic cultivation of marine algae and flagellate. Va. J. Sci. 16, 205–218.
Pereira, R., Yarish, C., and Sousa-Pinto, I. (2006). The influence of stocking density, light and temperature on the growth, production and nutrient removal capacity of Porphyra dioica (Bangiales, Rhodophyta). Aquaculture 252, 66–78. doi: 10.1016/j.aquaculture.2005.11.050
Poloczanska, E. S., Brown, C. J., Sydeman, W. J., Kiessling, W., Schoeman, D. S., Moore, P. J., et al. (2013). Global imprint of climate change on marine life. Nat. Clim. Change 3, 919–925. doi: 10.1038/nclimate1958
Rocchetta, I., and Küpper, H. (2009). Chromium-and copper-induced inhibition of photosynthesis in Euglena gracilis analysed on the single-cell level by fluorescence kinetic microscopy. New Phytol. 182, 405–420. doi: 10.1111/j.1469-8137.2009.02768.x
Russell, G., and Bolton, J. J. (1975). Euryhaline ecotypes of Ectocarpus siliculosus (Dillw.) Lyngb. Estuar. Coast. Mar. Sci. 3, 91–94. doi: 10.1016/0302-3524(75)90008-0
Sarı, A., and Tuzen, M. (2008). Biosorption of Pb (II) and Cd (II) from aqueous solution using green alga (Ulva lactuca) biomass. J. Hazard. Mater. 152, 302–308. doi: 10.1016/j.jhazmat.2007.06.097
Sfriso, A., Marcomini, A., and Pavoni, B. (1987). Relationships between macroalgal biomass and nutrient concentrations in a hypertrophic area of the Venice Lagoon. Mar. Environ. Res. 22, 297–312. doi: 10.1016/0141-1136(87)90005-5
Sharp, G. J., Samant, H. S., and Vaidya, O. C. (1988). Selected metal levels of commercially valuable seaweeds adjacent to and distant from point sources of contamination in Nova Scotia and New Brunswick. Bull. Environ. Contam. Toxicol. 40, 724–730. doi: 10.1007/BF01697522
Smetacek, V., and Zingone, A. (2013). Green and golden seaweed tides on the rise. Nature 504, 84–88. doi: 10.1038/nature12860
Smith, V. H., Tilman, G. D., and Nekola, J. C. (1999). Eutrophication: impacts of excess nutrient inputs on freshwater, marine, and terrestrial ecosystems. Environ. Pollut. 100, 179–196. doi: 10.1016/S0269-7491(99)00091-3
Song, W., Wang, Z. L., Li, Y., Han, H. B., and Zhang, X. L. (2019). Tracking the original source of the green tides in the Bohai Sea, China. Estuar. Coast. Shelf Sci. 219, 354–362. doi: 10.1016/j.ecss.2019.02.036
Stocker, T. F., Qin, D., Plattner, G. K., Tignor, M. M., Allen, S. K., Boschung, J., et al. (2014). “Climate Change 2013: The physical science basis,” in Contribution of Working Group I to the Fifth Assessment Report of IPCC the Intergovernmental Panel on Climate Change, eds T. F. Stocker, D. Qin, G.-K. Plattner, M. Tignor, S. K. Allen, J. Boschung, et al. (New York: Cambridge university press).
Sun, J. Y., Bao, M. L., Xu, T. P., Li, F. T., Wu, H. L., Li, X. S., et al. (2021). Elevated CO2 influences competition for growth, photosynthetic performance and biochemical composition in Neopyropia yezoensis and Ulva prolifera. Algal Res. 56:102313. doi: 10.1016/j.algal.2021.102313
Sunday, J. M., Bates, A. E., and Dulvy, N. K. (2012). Thermal tolerance and the global redistribution of animals. Nat. Clim. Change 2, 686–690. doi: 10.1038/NCLIMATE1539
Wang, B. D., Xin, M., Wei, Q. S., and Xie, L. P. (2018). A historical overview of coastal eutrophication in the China Seas. Mar. Pollut. Bull. 136, 394–400. doi: 10.1016/j.marpolbul.2018.09.044
Wang, M. J., and Wang, W. X. (2008). Temperature-dependent sensitivity of a marine diatom to cadmium stress explained by subcelluar distribution and thiol synthesis. Environ. Sci. Technol. 42, 8603–8608. doi: 10.1021/es801470w
Wang, W. X., and Dei, R. C. H. (2001). Effects of major nutrient additions on metal uptake in phytoplankton. Environ. Pollut. 111, 233–240. doi: 10.1016/S0269-7491(00)00071-3
Wang, X. H., Li, L., Bao, X., and Zhao, L. D. (2009). Economic cost of an algae bloom cleanup in China’s 2008 Olympic sailing venue. Eos 90, 238–239. doi: 10.1029/2009EO280002
Wei, Q. S., Wang, B. D., Yao, Q. Z., Fu, M. Z., Sun, J. C., Xu, B. C., et al. (2018). Hydro-biogeochemical processes and their implications for Ulva prolifera blooms and expansion in the world’s largest green tide occurrence region (Yellow Sea, China). Sci. Total Environ. 645, 257–266. doi: 10.1016/j.scitotenv.2018.07.067
Wellburn, A. R. (1994). The spectral determination of chlorophylls a and b, as well as total carotenoids, using various solvents with spectrophotometers of different resolution. J. Plant Physiol. 144, 307–313. doi: 10.1016/S0176-1617(11)81192-2
Wiens, J. J. (2016). Climate-Related Local Extinctions Are Already Widespread among Plant and Animal Species. PLoS Biol. 14:e2001104. doi: 10.1371/journal.pbio.2001104
Wu, H. L., Gao, G., Zhong, Z. H., Li, X. S., and Xu, J. T. (2018). Physiological acclimation of the green tidal alga Ulva prolifera to a fast-changing environment. Mar. Environ. Res. 137, 1–7. doi: 10.1016/j.marenvres.2018.02.018
Wu, H., Shin, S. K., Jang, S., Yarish, C., and Kim, J. (2018). Growth and nutrient bioextraction of Gracilaria chorda, G. vermiculophylla, Ulva prolifera, and U. compressa under hypo-and hyper-osmotic conditions. Algae 33, 329–340. doi: 10.4490/algae.2018.33.11.13
Wu, H. L., Huo, Y. Z., Zhang, J. H., Liu, Y. Y., Zhang, Y. T., and He, P. M. (2015). Bioremediation efficiency of the largest scale artificial Porphyra yezoensis cultivation in the open sea in China. Mar. Pollut. Bull. 95, 289–296. doi: 10.1016/j.marpolbul.2015.03.028
Xu, T. P., Cao, J. Y., Qian, R., Song, Y. J., Wang, W., Ma, J., et al. (2021). Ocean acidification exacerbates copper toxicity in both juvenile and adult stages of the green tide alga Ulva linza. Mar. Environ. Res. 170:105447. doi: 10.1016/j.marenvres.2021.105447
Yang, J. J., Yu, D. C., Ma, Y. F., Yin, Y., and Shen, S. D. (2019). Antioxidative defense response of Ulva prolifera under high or low-temperature stimulus. Algal Res. 44:101703. doi: 10.1016/j.algal.2019.101703
Ye, N. H., Zhang, X. W., Mao, Y. Z., Liang, C. W., Xu, D., Zou, J., et al. (2011). ‘Green tides’ are overwhelming the coastline of our blue planet: taking the world’s largest example. Ecol. Res. 26, 477–485. doi: 10.1007/s11284-011-0821-8
Zeng, J., and Wang, W. X. (2011). Temperature and irradiance influences on cadmium and zinc uptake and toxicity in a freshwater cyanobacterium, Microcystis aeruginosa. J. Hazard. Mater. 190, 922–929. doi: 10.1016/j.jhazmat.2011.04.009
Zhang, J. H., Huo, Y. Z., Wu, H. L., Yu, K. F., Kim, J. K., Yarish, C., et al. (2014). The origin of the Ulva macroalgal blooms in the Yellow Sea in 2013. Mar. Pollut. Bull. 89, 276–283. doi: 10.1016/j.marpolbul.2014.09.049
Zhang, J. H., Huo, Y. Z., Zhang, Z. L., Yu, K. F., He, Q., Zhang, L. H., et al. (2013). Variations of morphology and photosynthetic performances of Ulva prolifera during the whole green tide blooming process in the Yellow Sea. Mar. Environ. Res. 92, 35–42. doi: 10.1016/j.marenvres.2013.08.009
Zhang, J. H., Shi, J. T., Gao, S., Huo, Y. Z., Cui, J. Y., Shen, H., et al. (2019). Annual patterns of macroalgal blooms in the Yellow Sea during 2007–2017. PLoS One 14:e0210460. doi: 10.1371/journal.pone.0210460
Zhang, Y. Y., He, P. M., Li, H. M., Li, G., Liu, J. H., Jiao, F. L., et al. (2019). Ulva prolifera green-tide outbreaks and their environmental impact in the Yellow Sea, China. Natl. Sci. Rev. 6, 825–838. doi: 10.1093/nsr/nwz026
Zhao, J., Jiang, P., Qiu, R., Ma, Y. Y., Wu, C. H., Fu, H. H., et al. (2018). The Yellow Sea green tide: a risk of macroalgae invasion. Harmful Algae 77, 11–17. doi: 10.1016/j.hal.2018.05.007
Zhao, S. B., Xu, B. C., Yao, Q. Z., Burnett, W. C., Charette, M. A., Su, R. G., et al. (2021). Nutrient-rich submarine groundwater discharge fuels the largest green tide in the world. Sci. Total Environ. 770:144845. doi: 10.1016/j.scitotenv.2020.144845
Zhong, Z. H., Wang, Y., Qin, S., Song, W. L., Zhuang, L. C., Zhao, Z. F., et al. (2021). High temperature promotes the inhibition of Zn2+ to physiological performance of green tide-forming seaweed Ulva prolifera. Acta Ecol. Sin. 41, 424–431. doi: 10.1016/j.chnaes.2020.10.009
Keywords: copper, eutrophication, global warming, green tides, Ulva prolifera
Citation: Bao M, Park J-S, Xing Q, He P, Zhang J, Yarish C, Yoo HI and Kim JK (2022) Comparative Analysis of Physiological Responses in Two Ulva prolifera Strains Revealed the Effect of Eutrophication on High Temperature and Copper Stress Tolerance. Front. Mar. Sci. 9:863918. doi: 10.3389/fmars.2022.863918
Received: 27 January 2022; Accepted: 25 February 2022;
Published: 18 March 2022.
Edited by:
Guang Gao, Xiamen University, ChinaReviewed by:
Craig Steven Young, Stony Brook University, United StatesDi Zhang, Yantai University, China
Copyright © 2022 Bao, Park, Xing, He, Zhang, Yarish, Yoo and Kim. This is an open-access article distributed under the terms of the Creative Commons Attribution License (CC BY). The use, distribution or reproduction in other forums is permitted, provided the original author(s) and the copyright owner(s) are credited and that the original publication in this journal is cited, in accordance with accepted academic practice. No use, distribution or reproduction is permitted which does not comply with these terms.
*Correspondence: Jang K. Kim, amFuZy5raW1AaW51LmFjLmty