- 1The Swire Institute of Marine Sciences and Area of Ecology and Biodiversity, School of Biological Sciences, The University of Hong Kong, Hong Kong, Hong Kong SAR, China
- 2Biosciences Institute, São Paulo State University (UNESP), São Vicente, Brazil
- 3Department of Biology, University of Florence, Sesto Fiorentino, Italy
Global temperature increases are predicted to have pronounced negative effects on the metabolic performance of both terrestrial and aquatic organisms. These metabolic effects may be even more pronounced in intertidal organisms that are subject to multiple, abruptly changing abiotic stressors in the land-sea transition zone. Of the available studies targeting the intertidal environment, emphasis has largely been on water-breathing model organisms and this selective focus resulted in limited reliable forecasts on the impact of global warming on primarily air-breathing intertidal species. We investigated the thermal sensitivity of six phylogenetically related fiddler crab species that occupy different microhabitats on intertidal shores from south America and east Asia to test how bimodal-breathing intertidal ectotherms cope with thermal stress. We examined the metabolic physiology and thermal limits of the crabs by measuring their cardiac function and oxygen consumption along a thermal gradient. Their specific thermal microhabitat was also appraised. We found that subtropical fiddler crab species inhabiting vegetated microhabitats have lower upper lethal temperatures and therefore greater thermal sensitivity in comparison to their tropical counterparts. Additionally, females exhibited higher oxygen consumption and lower lethal temperatures in comparison to males. Our results contradict previous predictions that species from higher latitudes that experience greater temperature variability have broader latitudinal distributions, greater phenotypic plasticity and lower thermal sensitivity. Furthermore, the higher thermal sensitivity demonstrated by female fiddler crabs with respect to males strongly suggests a role of both gametogenesis and physiological dimorphism on the thermal performance of tropical and subtropical intertidal organisms. These observations ultimately, advocates for further studies on sex-biased and development-biased thermal sensitivity before drawing any generalizations based on a single sex or life stage.
Introduction
Intertidal habitats are challenging environments as organisms that colonize them must cope with tidal cycles and high thermal variability (Denny and Paine, 1998; Raffaelli and Hawkins, 1999; Denny et al., 2011). Due to the tides, for instance, organisms occupying the mid-high intertidal zone experience longer periods of emersion and greater thermal fluctuations compared to species present at the low intertidal zone (Williams and Morritt, 1995; Finke et al., 2007). These differences in inundation time often result in spatial patterns of species distribution along a mosaic of specific microhabitats (Peterson, 1991), mainly driven by species-specific thermal tolerances linked to evolutionary plasticity (Stillman and Somero, 1996; Stillman and Somero, 2000; Somero, 2002; Stillman, 2002). In these extremely variable habitats, resident organisms have evolved diverse strategies to mitigate the stress posed by fluctuating environmental conditions over different temporal scales (Viña et al., 2018). These strategies often involve the evolution of plastic morphological (Helmuth et al., 2006b; Harley et al., 2009), physiological (Somero, 2010) and behavioral (Chou et al., 2019) modifications, but local adaptation can play a role in coping with conditions fluctuating at small spatial and temporal scales (Jahnke et al., 2022).
Intertidal shores are also spatially diverse environments that range from bare rock surfaces and mud/sand flats to more structurally complex habitats, such as mangrove forests (Beck, 1998; Vorsatz et al., 2021b). Tidal ranges and local abiotic conditions interact to form complex thermal mosaics across the intertidal zone (Helmuth and Hofmann, 2001; Helmuth, 2002; Helmuth et al., 2006a). Thus, adjacent intertidal microhabitats can have variable microclimates, with areas directly exposed to solar radiation reaching higher temperatures than shaded habitat underneath mangrove trees (Seabra et al., 2011; Chou et al., 2019). This microclimate variability has been shown to influence the thermal tolerance of organisms, with species living in warmer areas showing higher thermal limits but lower physiological plasticity to cope with further warming (Stillman and Somero, 2000).
Temperature is a major factor linked to the life traits and geographic distribution of ectotherms (Roy et al., 1998; Helmuth et al., 2002; Brayard et al., 2005; Somero, 2010). Experienced extreme temperatures, in particular, are regarded as the driving factor that influences their physiological tolerance to heat stress (Pither, 2003; Gaston et al., 2009). Phylogenetic constraints can also drive tolerance traits of species, with phylogenetically related organisms exhibiting similar tolerance to heat stress (Bennett et al., 2021). These phylogenetic signals, also termed niche conservatism (Wiens and Graham, 2005), proved to be particularly important in setting the upper thermal limits of species (Hoffmann et al., 2013), ultimately limiting their phenotypic plasticity and response to climate change (Bennett et al., 2021). Thus, phylogenetic history can play a role in the differential response of intertidal species to fluctuating conditions, but this role was never tested in detail.
Intertidal ectotherms often live close to their thermal limits making these species vulnerable to further temperature increases linked to climate change. Many intertidal crustaceans, mollusks and some fish are bimodal breathers and are able to extract oxygen from both air and water (Mcmahon, 1988; Henry, 1994; Graham, 1997). Due to their thermal physiology and unique respiratory strategies, these organisms are interesting models to investigate the vulnerability of ectotherms to temperature changes. There is, however, a dearth of information on the response of primarily air-breathing intertidal ectotherms to environmental change, with the literature predominantly focused on water-breathing organisms inhabiting rocky shores (e.g. Stillman and Somero, 1996; Stillman and Somero, 2000; Przeslawski et al., 2005; Marshall et al., 2015).
Fiddler crabs (Ocypodidae: Gelasiminae) are represented by an Atlantic-East Pacific (AEP) and an Indo-West Pacific (IWP) clade (Shih et al., 2016a). Crabs from both clades can be found in a wide range of intertidal habitats, such as mud/sand flats and mangrove forests (Crane, 1975), where they experience different thermal environments (Alongi, 1990). Fiddler crabs have functional lungs and are true air breathers (Paoli et al., 2015). They show strong morphological and behavioral differences between sexes. Males have one enlarged cheliped used to court females and fight competitors, which is also useful for thermoregulation (Windsor et al., 2005; Darnell and Munguia, 2011; Darnell et al., 2013). Females have two small chelipeds that they use for feeding (Levinton et al., 1995). This difference in cheliped characteristics cause sex-related constraints on their time-energy budget (Valiela et al., 1974; Darnell et al., 2013), which, ultimately, reflect on the individual thermal physiology (Pörtner, 2012). During the reproductive season female fiddler crabs invest more energy than males to develop their gonads (Yamaguchi, 2003; Hartnoll, 2006; Colpo and López-greco, 2018). The higher energetic investment in reproduction by females is known to constrain their energy budgets, limit growth, increase mortality rates, reduce feeding and hinder other functions (Hartnoll, 2006; Guderley and Pörtner, 2010).
Here, we tested the physiological responses to temperature of six fiddler crab species with tropical and subtropical distributions belonging to two separate phylogenetic clades and from two geographic realms, the IWP and the AEP. We investigated whether fiddler crab species that occupy intertidal habitats with contrasting environmental characteristics could display differences in their thermal limits across sexes. Our hypotheses were: (1) the habitat occupied by ectotherms in physiologically challenging intertidal zones is reflected in their thermal limits, with species living in more thermally stressful environments being better adapted to cope with heat stress; (2) during their extended reproductive season, male and female fiddler crabs have different physiological responses to increasing temperature, with females being more vulnerable due to higher reproductive investment and (3) the phylogenetic distance and evolutionary history of the selected fiddler crabs result in different adaptations in thermal tolerance. Understanding the physiological responses of fiddler crabs to thermal stress can improve our comprehension on the consequences of climate change effects on intertidal ectotherms. Furthermore, investigating the physiological responses to temperature increase of closely related species from different geographical regions can inform which species are most at risk to climate change.
Materials and Methods
Study Areas and Model Species
Two localities, Tung Chung, Hong Kong Special Administrative Region, People’s Republic of China and the Itaguaré river mouth, Bertioga, São Paulo State, Brazil, were selected for crab collection. These mangrove areas placed in the IWP and AEP regions, respectively, have very similar thermal and environmental characteristics (Table S1).
The Hong Kong fiddler crabs were Gelasimus borealis, Austruca lactea, and Paraleptuca splendida; while the species from Brazil were Leptuca thayeri, L. leptodactyla, and L. uruguayensis. The model species were selected to represent different distributions along a latitudinal and intertidal habitat gradient (Figure 1; Table 1).
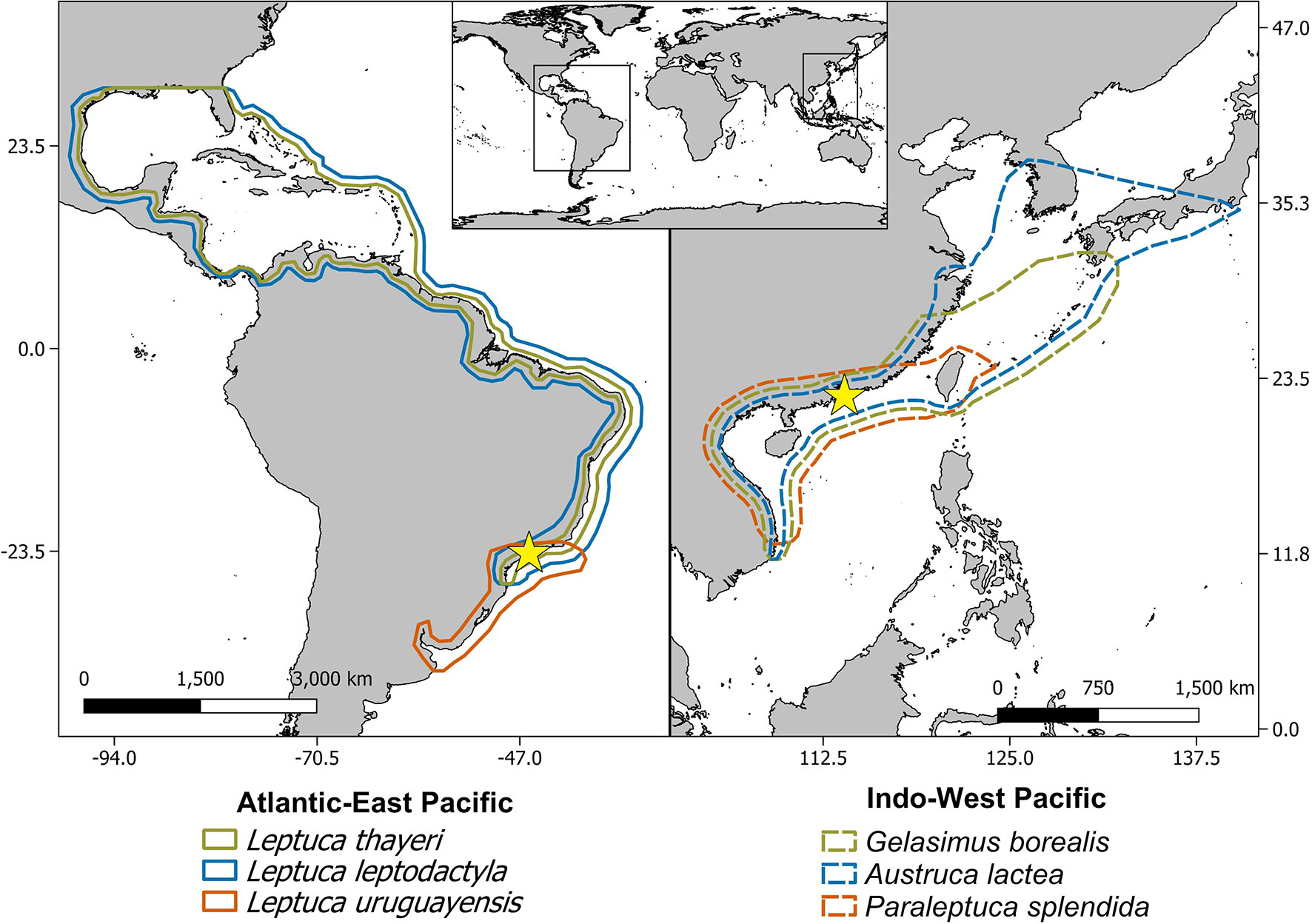
Figure 1 Map showing the latitudinal range of the model species (based on Shih, 2012; Shih et al., 2012; Thurman et al., 2013; Kostina et al., 2016; Shih et al., 2016b; Yuhara et al., 2017). Yellow stars represent the sampling areas: Itaguaré river mouth, Bertioga, São Paulo, Brazil in the Atlantic-East Pacific, and Tung Chung, Hong Kong SAR, PR China in the Indo-West Pacific.
Thirty adult males and 30 females of each species were collected by hand on the mud/sandflats and under the mangrove canopy at both regions. Sampling occurred during the reproductive season, with ovigerous females present in all the sampled fiddler crab populations. Only non-ovigerous females were used in the experiments, because brood care is an energetically costly activity that affects the crabs’ physiology (Fernández et al., 2000; Baeza and Fernández, 2002). For each species, the experimental crabs collected belonged to similar size classes, to reduce the variance in size related differences. The carapace width (CW) of the crabs ranged from 17.5 to 23.9 mm in G. borealis males and 13.6 to 18.6 mm in females; 15.0 to 18.0 mm in A. lactea males and 12.1 to 15.8 mm in females; 14.7 to 19.3 mm in P. splendida males and 12.8 to 19.5 in females; 17.7 to 22.6 in L. thayeri males and 16.3 to 23.4 in females; 10.6 to 14.4 mm in L. leptodactyla males and 8.8 to 11.4 in females; and 8.7 to 11.4 mm in L. uruguayensis male and 8.7 to 10.4 mm in females. The collected males had integral chelipeds and collected individuals of both sexes lacked no more than two walking legs, to reduce any effect of crab mutilation on the results. Crabs collected in Hong Kong and Brazil were transported in boxes and buckets with sediment to the facilities of the Swire Institute of Marine Sciences (SWIMS), the University of Hong Kong, and to the Laboratory of Ecology and Animal Behavior (LABECOM), Sao Paulo State University - Coastal Campus, respectively. At each institution, crabs were conditioned in three separated outdoor stock tanks, one for each species, measuring 41 × 56 × 78 cm (height × width × length), prepared with a layer of 10 cm of sediment from the sampling sites. In the tanks, water influx was controlled to simulate a semi diurnal tidal regime. Sea water (salinity ~ 34‰) was used for the tide simulation. Fiddler crabs are osmoregulators capable of maintaining their blood osmolarity over a large range of salinities (Lin et al., 2002). These crabs tolerate great variations in salinity across short temporal scales due to the tidal influence, with sea water being well within their tolerance range (Lin et al., 2002; Thurman et al., 2010; Faria et al., 2017). The stock tanks were kept under temperatures similar to the sampling sites, to avoid effects of acclimation in the crabs’ physiological responses. No more than 20 crabs were held in each stock tank at once. Since fiddler crabs can have fast acclimation to different thermal conditions (Darnell et al., 2015), experiments were performed within two to 14 days from the sampling date, to avoid effects of long-term captivity on their physiology.
Thermal Characterization of Microhabitats
To measure the soil surface temperature (°C) of the natural environment of the tested species, four iButton® (Maxim Integrated®) thermologgers, waterproofed in silicon glue, were deployed at the two sampling sites, in areas dominated by the different species. In Tung Chung, the thermologgers were deployed during September, at the unshaded intertidal flats, where G. borealis and A. lactea are found in mixed populations, and at the vegetated area dominated by P. splendida. At the Itaguaré river mouth, the thermologgers were deployed in January/February, at the sand flat dominated by L. leptodactyla, the vegetated area dominated by L. uruguayensis, and at the lower intertidal zone dominated by L. thayeri.
Phylogenetic Relationships and Phylogenetic Signal on Thermal Limits
A phylogenetic tree was constructed to investigate phylogenetic relationship between model fiddler crabs. Mitochondrial gene sequences for subunits 16S and 28S ribosomal and cytochrome oxidase subunit I (COI) were obtained from Genbank (Clark et al., 2016) (accession numbers are in Table S2) for each of the tested species and for Ocypode ceratophthalmus, selected as an outgroup because it belongs to the same family of the fiddler crabs, Ocypodidae, but not to the Gelasiminae subfamily.
Determination of Cardiac Function
Experiments were conducted to determine the heart rate of males and females of the model species in response to temperature (n = 10 for each experimental group, N = 120 specimens). The specimens were placed in ‘blacked-out’ containers to reduce exposure to visual stimuli. A layer of seawater (salinity 30 ‰) that extended to the height of the crab’s ventral surface was added to each holding container to avoid the potential influence of water loss on crab physiology (Burggren et al., 1990; Burggren, 1992). The heart function was measured using a method adapted from Depledge and Andersen (1990). An infrared sensor (Vishay Semiconductors®, CNY70) was attached to each crab’s carapace, above the cardiac region, using Blu Tack® adhesive together with cyanoacrylate glue. The sensor was used to record heartbeat signals, which were filtered (Burnett et al., 2013), sent to an oscilloscope (PicoScope® 2204) connected to a computer and recorded with PicoScope 7 software. Individual crabs were placed in individual holding containers positioned in a programmable water bath (Grant® Optima TXF-200 heated circulation bath, Cambridge, UK). The crabs were allowed to recover from handling stress and to acclimate to the initial experimental temperature for 30 min at 25°C. Then, the crabs were exposed to an increasing thermal ramp, starting at 25°C, with a 1°C increase every 20 min until heart function ceased. The initial temperature of 25°C was chosen because it is close to the average minimum air temperature experienced by the crabs at Tung Chung and Itaguaré river mouth during summer The temperature increase was based on the 95th percentile for the temperature variation rate in the vegetated habitats occupied by L. uruguayensis and P. splendida, 1.2 and 0.8°C each 20 min respectively, thus, mimicking actual conditions and avoiding possible effects of extreme heating rates on the crabs.
Crab body temperatures were corrected in relation to the water bath temperature by employing a method similar to (Levinton et al., 2020). We recorded the body temperature of crabs subjected to the same temperature-ramp used for the heart rate experiments by means of a temperature probe. For these tests, ten male and female individuals of each model species (N = 120), of comparable size to those in the heart function experiments, were subjected to an identical thermal ramp as previously described. A k-type thermocouple was inserted and fixed in their branchial chambers using Blu Tack® adhesive and cyanoacrylate glue. The thermocouples were connected to a thermometer (Lutron® TM-947SD) that logged the crab body temperature (± 0.1°C) every minute for the duration of the thermal ramp. The temperature inside the water bath was also recorded every minute. Body temperature was then plotted against the water temperature and sex-specific linear regressions were fitted to describe the body/water temperature relationships for each species. The linear regressions were then used to correct body temperature in relation to the temperature within the water bath in the heart function experiment.
Determination of Metabolic Rate
To investigate the basal metabolic rate of the model species in response to increasing temperature, oxygen consumption (MO2) experiments were conducted on crabs subjected to a thermal ramp. Before the experiments commenced, the carapace and appendages of ten males and females of each of the six species (N = 120) were blotted dry and cleaned with a cotton swab using distilled water and absolute ethanol. The crabs were then allocated to individual ethanol sterilized glass chambers (130 mL), fitted with an oxygen spot sensor (Loligo Systems®, Viborg, Denmark) glued to its inner wall. The respirometry chambers containing the smaller species (L. leptodactyla and L. uruguayensis) had glass marbles inserted in them, to reduce the volume and ensure an adequate drawdown of oxygen. The total volume of the glass marbles was subsequently subtracted from the chamber volume. All MO2 measurements were recorded in air, as fiddler crabs are subjected to intense heat stress during their activity periods at low tides (Powers and Cole, 1976; Allen and Levinton, 2014). The chambers were sealed and placed in a water bath (Grant® Optima TXF-200 heated circulation bath). Before the experiment started, the crabs within the chambers were left in the water bath for 30 min at 25°C, to recover from handling stress and acclimate to the initial experimental temperature. Subsequently, the crabs were exposed to a thermal ramp, starting from 25°C up to 50°C, with an increase of 1°C every 20 min. Two oxygen saturation measurements were recorded at each one-minute interval, at every 1°C stepwise increase along the thermal ramp, using a Witrox® oxygen meter (Loligo Systems®), through a fiber optic cable connected to the oxygen spots. The data were then logged on a computer with the Witrox® View software. The MO2 was then calculated using the difference in oxygen saturation of air between the two points in time. To record the temperature inside the containers, a temperature probe was inserted into an empty and sealed container subjected to the same thermal ramp in parallel with the ones containing the crabs. The recorded temperature was used in the subsequent MO2 analyses. Chambers without animals (N = 10) were used as blanks, to control for background changes in oxygen saturation in each trial. The absolute values for oxygen saturation changes obtained in the control chambers were then subtracted from the experimental values. Crab volume was also measured using water displacement in graduate cylinders and subtracted from the volume of the chamber upon completion of experiments. Oxygen measurements were taken up to the upper lethal temperatures (LT) obtained for each species from the heart rate experiments. During the experiments, the oxygen saturation inside the chambers never fell below 70%, avoiding hypoxic conditions (Burke, 1979). The crabs used in the experiment were weighted on a precision scale (± 0.001g), and the oxygen consumption was expressed as mg O2 g-1 h-1.
For the cardiac function, body temperature and metabolic rates experiments, crabs were weighed with a precision scale (± 0.001 g) and measured with a dial caliper (CW; ± 0.1 mm). The sizes of the animals used in the experiments are summarized in Table S3.
Data Analyses
For the data analyses, the model species were grouped according to their geographic region (factor cluster, IWP and AEP) and the microhabitat they occupy. Thus, G. borealis and L. thayeri were classified as lower intertidal flat (LI) species, A. lactea and L. leptodactyla as sand flat (SF) species, and P. splendida and L. uruguayensis as vegetated area (VG) species. Data analyses were performed in Primer7 and R version 4.0.3 (R core team, 2021).
The temperatures (average, average daily maxima, and minima) were compared among sites (N = 11 days in Hong Kong and N = 14 days in Itaguaré river mouth). To check for differences in temperature, models were constructed with cluster (IWP and AEP) and habitat (unshaded intertidal flat, which comprise lower intertidal and sand flat where A. lactea and G. borealis coexist, vs vegetated area for Hong Kong, and lower intertidal, sand flat and vegetated area for Brazil) as fixed factors and the temperatures as response variables. The data were not normally distributed (Shapiro-Wilk test, p < 0.05) and did not meet the assumptions of homogeneity of variances (Levene test, p < 0.05). Euclidean distance matrices were built for each of the response variables. The matrices showed homogeneity of the multivariate dispersion (Betadisper, p > 0.05) and were analyzed using a PERMANOVA routine (9999 permutations). When necessary, post-hoc pairwise PERMANOVA tests were used to check for differences among experimental groups, with Holm adjusted p values (Holm, 1979). The significance level for all statistical analysis was set as α = 0.05.
For the phylogenetic analyses, the mitochondrial gene sequences were aligned with the software mega X (Kumar et al., 2018), using the MUSCLE method (Edgar, 2004). The phylogenetic relationships were estimated using a Bayesian tree. The phylogenetic tree was obtained through conducting a Bayesian Markov Chain Monte Carlo simulation with 100,000,000 generations, sampling parameters every 1000 generations in BEAST v.1.10.4 (Drummond and Rambaut, 2007; Suchard et al., 2018), using a GTR substitution model (Tavaré, 1986), with Γ site heterogeneity model (4 Γ categories) to account for invariable sites (Yang, 1994), using a uncorrelated relaxed clock with log-normal distribution (Drummond et al., 2006) and a tree prior based on Yule Process speciation model (Yule, 1924; Gernhard, 2008). The GTR+I+ Γ model was used because it leads to accurate phylogenies and ancestral sequences (Abadi et al., 2019). The sampled parameters were examined in Tracer 1.7.1 (Rambaut et al., 2018), where effective sampling size was found to be higher than 1000 for all parameters. The first 10% of the Bayesian analysis were discarded as burn-in trees, and the remaining trees were summarized by Maximum Clade Credibility using the software TreeAnnotator 1.10.4. To account for non-independence of the data that could arise from phylogenetic relationships, a Pagel’s λ approach, which assumes a Brownian motion model of trait evolution (Pagel, 1997; Pagel, 1999), was applied to investigate phylogenetic constraints in the recorded physiological traits. Values of Pagel’s λ approaching 1 indicate a Brownian model of evolution, where the evolution of a trait is proportional to branch lengths in the phylogenetic tree (Pagel, 1999), while lower values indicate a star phylogeny model of evolution. Pagel’s λ was calculated using the package “phytools” (Revell, 2012).
The heart rate (BPM, beats min-1) was determined at one-minute intervals along a thermal ramp and plotted against the temperature. The endpoint of heart function, i.e., when the heart stops beating, was taken as a proxy for LT (Marshall et al., 2010). Then, the LT50, i.e., the median temperature at which 50% of the organisms died was obtained for each species. The Optimum Performance Temperature (OPT), i.e., the temperature where the heart rate is at its maximum, was obtained by applying Lowess smoothers to the heart rate thermal performance curves obtained (smoother span = 0.05, robustifying iterations = 3). The temperature at which the heart rates were at their maximum in the smoothed curve was taken as the OPT. The Arrhenius Breakpoint temperature (ABT), i.e., the temperature at which there is a discontinuity in the slope of the Arrhenius plot and the heart rate rapidly drops (Stillman and Somero, 1996; Marshall et al., 2010; Marshall et al., 2011) was also calculated by plotting the natural log of the heart rates BPM against the inverse Kelvin temperature in Arrhenius form. The ABT was calculated for every individual by piece-wise regression using the package ‘segmented’ (Muggeo, 2003) on R. As an estimate of vulnerability to climate warming of the studied fiddler crabs, we used the thermal safety margin (TSM) concept (Deutsch et al., 2008). The TSM’s of terrestrial and marine ectotherms have been defined and calculated in various ways in the literature and we calculated this margin by subtracting the LT of each species from their maximum experienced habitat temperature, following the definition of Sunday et al. (2014). The data describing OPT, ABT and LT were non-normally distributed (Shapiro-Wilk test, p < 0.05). To check for differences in OPT, ABT and LT among experimental groups, Euclidean distance matrices were constructed for each response variable and PERMANOVA (9999 permutations) routines were computed with cluster (IWP and AEP), habitat (LI, SF and VG) and sex (male and female) as fixed factors. The matrices for ABT and LT showed homogeneity of the multivariate dispersion (Betadisper, p > 0.05) but not the matrix for OPT (Betadisper, p < 0.05). Nonetheless, PERMANOVA was still used, as this routine is robust with non-homogeneous data in balanced designs (Anderson and Walsh, 2013).
Thermal performance curves for MO2 were fitted for each sex of each species using the R packages ‘nls.multstart’ (Padfield and Matheson, 2020) and ‘rTPC’ (Padfield and O’Sullivan, 2020; Padfield et al., 2020). Twenty-one non-linear regression models were constructed to determine the best-fitting TPC for our data (Table S4). Using measures of relative fit, Pawar’s model for fitting TPC’s (Kontopoulos et al., 2018) was selected as the best-fitting model through the Akaike information criterion (AIC) (Table S4). For further details about the selected model parameters see Equation S1. Confidence intervals were computed using bootstrapping (case resampling method, 999 iterations). Parameters obtained from the fitted TPC and LT50 are shown in Table S5.
The MO2 in response to temperature were compared among model species for each 1°C interval ranging from 26°C until the LT for each species. The differences in MO2 among species were tested according to four fixed factors: cluster (AEP and IWP), temperature, habitat (LI, SF and VG) and sex (male and female), and individual as a random factor. The data showed a non-normal distribution (Shapiro-Wilk test, p < 0.05), therefore a PERMANOVA (9999 permutations) was applied to test the design (Euclidean distances matrix with homogeneity of the multivariate dispersion, Betadisper, p > 0.05).
Results
Microhabitats Thermal Characterization
Average daily soil surface temperature variability at the Itaguaré river mouth ranged from 19.6°C to 36.8°C at the L. thayeri area, 20.1°C to 45.3°C at the L. leptodactyla area, and 20.7°C to 39.7°C at the L. uruguayensis area (Figure 2A). The temperature at Tung Chung was less variable, ranging from 25.1°C to 37.6°C at the P. splendida area and 24.4°C to 44.7°C at the G. borealis and A. lactea area (Figure 2B).
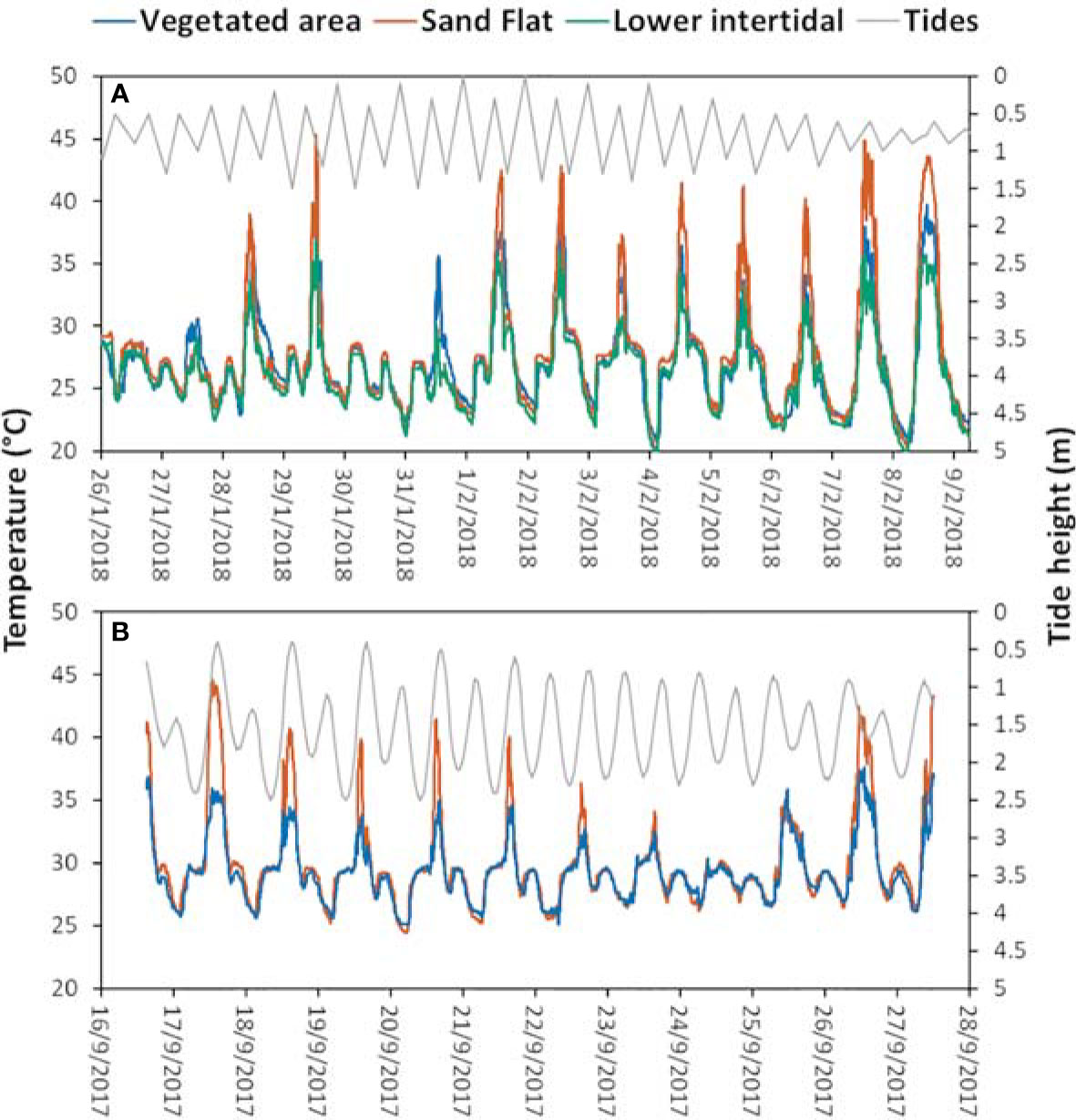
Figure 2 Temperature and tidal regimes recorded at the collection sites during the sampling period. (A) Itaguaré river mouth, (B) Tung Chung. The orange line in (B) represents the mud-sand flat in Tung Chung where both A. lactea and G. borealis are found in mixed populations.
The average soil surface temperature differed between sites and was higher at Tung Chung (PERMANOVA, pseudo-F(1,18795) = 2225.201, p < 0.005). Soil surface temperature also differed among habitats (PERMANOVA, pseudo-F(1,18795) = 187.954, p < 0.005), with the sand flat experiencing higher temperatures at both sites, followed by the vegetated areas. The lower intertidal shore at Itaguaré river mouth experienced the lowest temperatures among the three habitats (p < 0.05, PERMANOVA post-hoc test). The average daily soil surface minimum temperature was lower at the Itaguaré river mouth than at Tung Chung (PERMANOVA, pseudo-F(1,59) = 51.398, p < 0.005), but was not different among habitats. The average daily maximum soil surface temperature did not differ between the two collection sites (PERMANOVA, pseudo-F(2,59) = 1.350, p > 0.05). The maximum temperature however differed among habitats (PERMANOVA, pseudo-F(2,59) = 8.828, p < 0.005), with higher temperatures in the sand flats compared to the vegetated areas and lower shores (p < 0.05, PERMANOVA post-hoc test).
Phylogenetic Relationships and Phylogenetic Signal on Thermal Limits
As expected, the phylogenetic tree constructed to investigate phylogenetic relationship between model fiddler crabs showed that the fiddler crabs from AEP and IWP form two distinct clades (Figure 3). The results related to the possible phylogenetic signal on the physiological traits of the different species are shown in Table S6. As there was no significant effect of phylogeny on cardiac function and metabolic rate patterns, the data were treated as independent and phylogeny was not accounted for in further analyses.
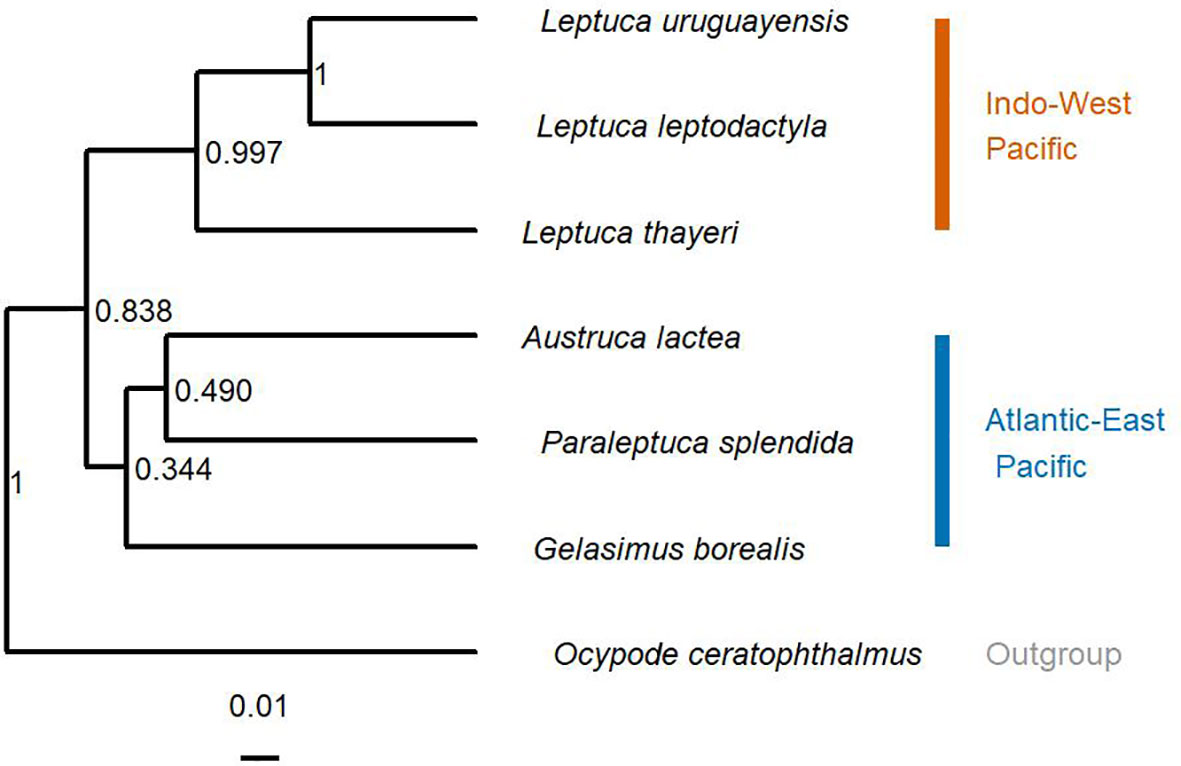
Figure 3 Phylogenetic relationships among species of fiddler crabs from the Atlantic-East Pacific and Indo-West Pacific. Ocypode ceratophthalmus is an outgroup. Node numbers are the posterior probability from the Bayesian analysis.
Cardiac Function
There was no significant difference in size when comparing animals of the same sex and species in the heart rate and body temperature experiments (data non-normally distributed, Shapiro-Wilks, p < 0.05; and non-homogeneous, Levene Test, p < 0.05; Mann-Whitney comparisons, p > 0.05).
There was a significant effect of the interaction between the factors cluster and habitat on OPT, ABT and LT among the model species (Tables S7, S8). The OPT was similar among the species within clusters and habitat types although L. uruguayensis had a lower OPT in comparison to A. lactea and G. borealis (Figure 4A). Leptuca uruguayensis had lower ABT and LT compared to the other model species (Figure 4B). Austruca lactea, had a higher LT compared to G. borealis, the lower intertidal species in Hong Kong (Figure 4C). Furthermore, there was a significant difference in ABT and LT between sexes (Table S7). The model also shows higher LT and ABT in males compared to females (Figure 5).
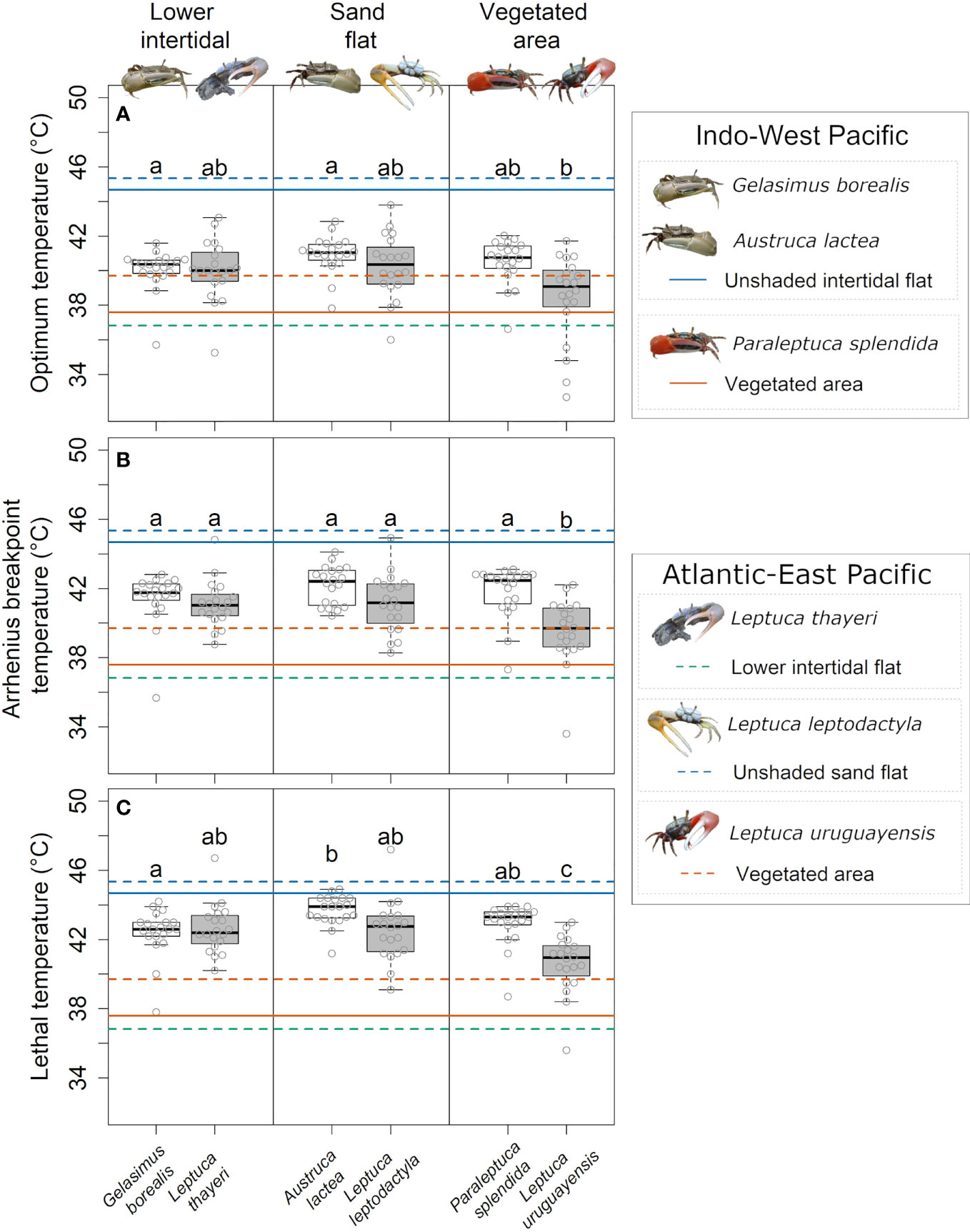
Figure 4 Comparison of: (A) Optimum temperature (OPT); (B) Arrhenius Breakpoint Temperature (ABT); and (C) Lethal temperature (LT) among the model species across habitats. Boxplots show the median, 25th and 75th percentiles, and whiskers are 1.5 times the spread beyond the hinge. White boxes represent Hong Kong species, grey boxes represent Brazilian ones. Circles are the raw data points. Dashed lines and continuous lines represent the maximum temperatures experienced by the model species at the collection sites in Brazil and Hong Kong, respectively. Different letters represent significant differences among species (PERMANOVA pairwise, p < 0.05).
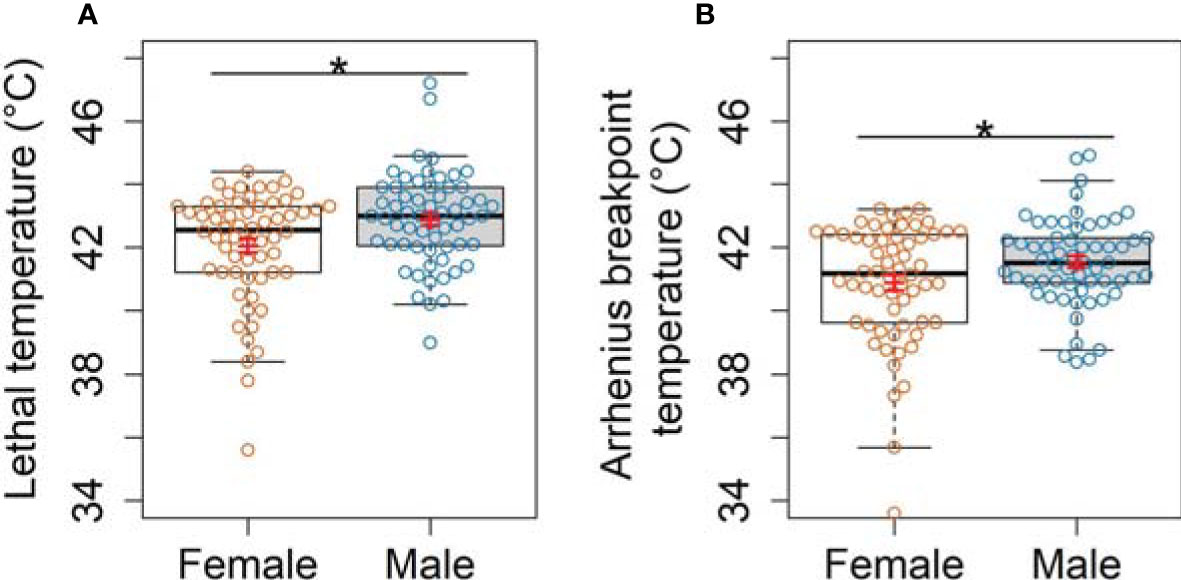
Figure 5 Comparison of: (A) Lethal temperature and (B) Arrhenius Breakpoint temperature between male and female fiddler crabs. Boxplots show the median, 25th and 75th percentiles, and whiskers are 1.5 times the spread beyond the hinge. White and grey boxes represent females and males, respectively. Orange and blue circles characterize raw data points for females and males, respectively. Red lines show mean and standard errors. Asterisks denote significant differences (PERMANOVA, p < 0.05).
Thermal safety margins were negative for crabs inhabiting exposed areas (Figure 6; Table S8). Leptuca leptodactyla, which inhabits the mid-intertidal zone in the AEP, had the lowest TSM, followed by G. borealis and A. lactea that occupy the low- and mid-intertidal zone, respectively, in the IWP (Figure 6). In the AEP, L. thayeri, which occupy the low-intertidal shores, had the highest TSM, followed by P. splendida and L. uruguayensis that both inhabit vegetated areas at the high-intertidal shore in the IWP and AEP, respectively. Furthermore, females showed consistently lower TSM’s for the majority of studied species.
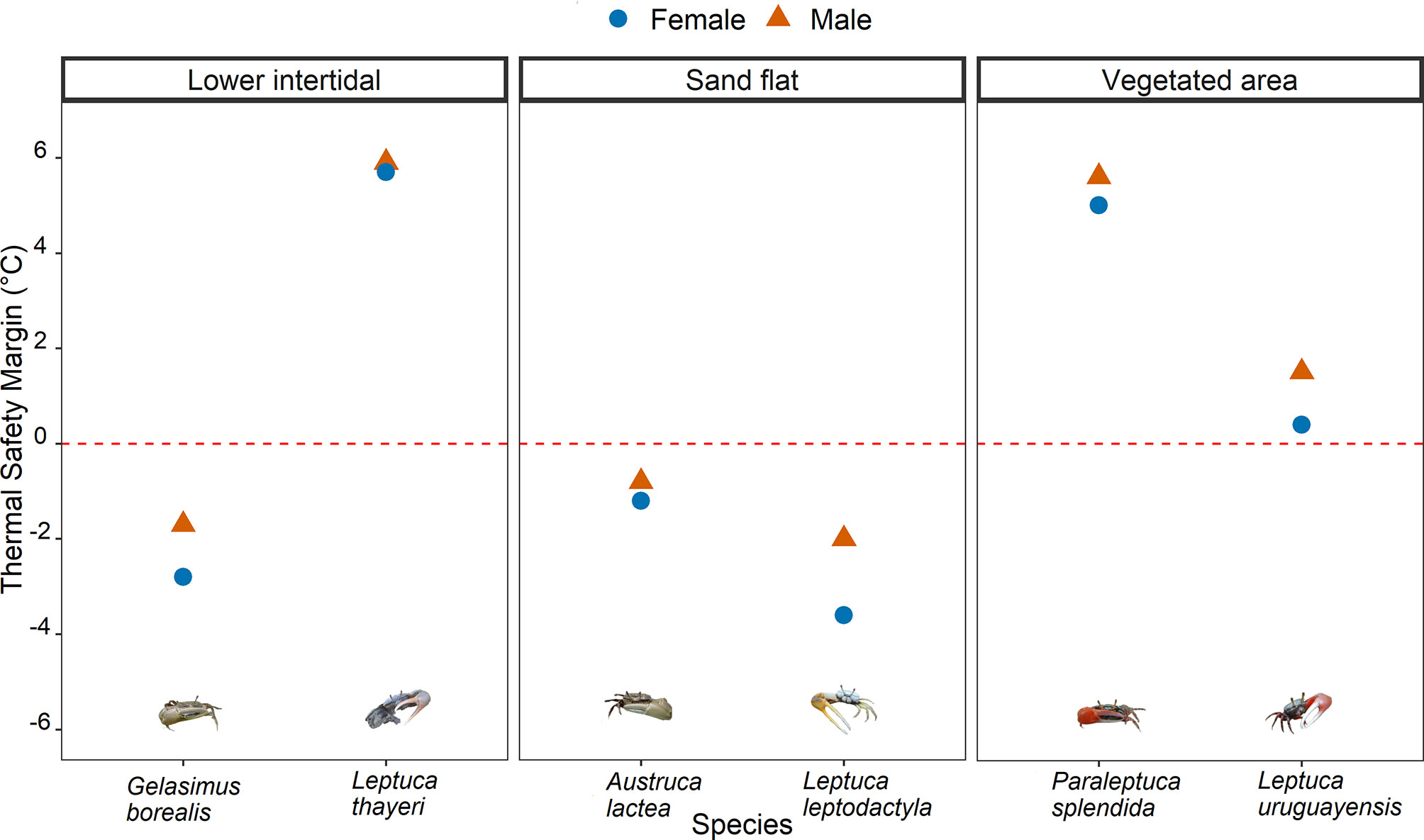
Figure 6 Thermal safety margins of each species across habitats in the present study. Females are represented by blue circles and males are represented by orange triangles. Species on the left of each facet represent the Indo-West Pacific, species on the right of each facet represent the Atlantic East Pacific.
Metabolic Rate
There was a significant effect of the interaction between temperature, cluster, and habitat on MO2 (Table S9). Leptuca uruguayensis, showed the highest MO2, followed by L. leptodactyla. These species had higher rates of MO2 compared to crabs from Hong Kong (Figure 7). There was also an effect of the interaction between cluster, habitat, and sex on MO2 (Table S9). The MO2 differed between males and females in all species (post-hoc PERMANOVA, p < 0.05). The fitted TPCs for the MO2 showed lower activation energy in both male and female L. uruguayensis indicating a higher temperature sensitivity in this species.
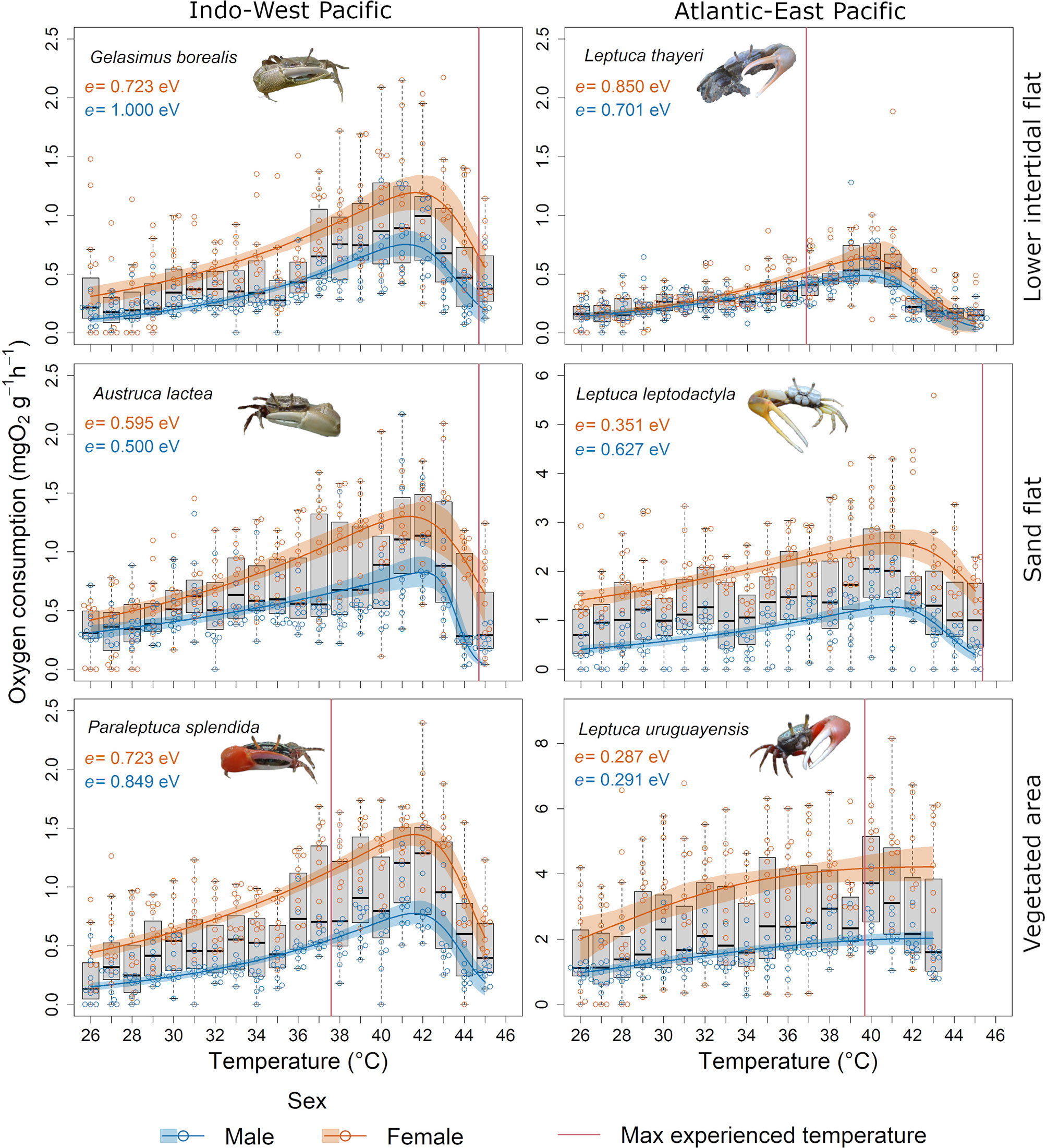
Figure 7 Thermal performance curves and activation energy (e) for the oxygen consumption of the studied fiddler crab species. Boxplots show the median, 25th and 75th percentiles, and whiskers are 1.5 times the spread beyond the hinge of MO2 for each species. Orange and blue circles characterize raw data points for females and males, respectively. Orange and blue lines characterize raw data points for females and males, respectively. The shading represents the bootstrapped 95% confidence interval around the thermal performance curves.
Discussion
As a transitional zone between the land and the sea, intertidal habitats are subjected to strong thermal variations and represent a challenging environment that requires adaptations to withstand variable milieus and high thermal stress. Here, using six species of fiddler crabs inhabiting similar thermal habitats along a very wide geographical range, we showed that primarily air-breathing intertidal organisms are indeed adapted to cope with high temperatures. Additionally, we showed that gross latitudinal temperature gradients may in part explain the thermal vulnerability of intertidal species. This macrophysiological conformity is signified by the lower thermal tolerance of species distributed in the subtropics when compared to those in the tropics, even when colonizing similar habitats. More importantly, our data demonstrate that species living in areas where the temperatures exceed their thermal limits are still thriving, casting doubt over the universal use of TSM as predictors for thermal vulnerability in ectotherms, at least for intertidal habitats. We also found differences in thermal sensitivity between sexes of fiddler crabs. This notion strongly suggests refraining from generalizing species-specific patterns of thermal sensitivity based solely on data related to one sex or a single ontogenetic stage.
The physiological performance of ectotherms is governed by temperature dependent processes that either enhance or impair metabolic functions (Brown et al., 2004). Species occupying different habitats in this study had similar thermal limits, indicating that the temperatures experienced by fiddler crabs in their habitats alone do not satisfactorily explain their thermal performance. The high thermal limits of the species inhabiting the exposed area of the shore (such as A. lactea, G. borealis and L. leptodactyla) seem congruent with the Climate Extremes Hypothesis, which postulates that thermal limits are set by the temperature extremes that organisms face in their specific habitats (Pither, 2003; Sunday et al., 2019). The conservation of thermal limits in ectotherm evolutionary history, however, could explain the high thermal tolerance showed by P. splendida and L. thayeri, despite occupying areas where the upper limit temperatures are lower (Hoffmann et al., 2013; Gutiérrez-Pesquera et al., 2016).
Intertidal crabs derived from marine ancestors maintain functional gills, which enable gaseous exchanges in water (Burggren, 1988). However, fiddler crabs have also evolved an efficient mechanism to breath in air through the development of a functional lung within the gill chambers (Bliss, 1968; Días and Rodríguez, 1977; Farrelly and Greenaway, 1993; Farrelly and Greenaway, 1994; Paoli et al., 2015). Oxygen is less limiting, and gaseous exchange less costly, in air than in water (Giomi et al., 2014), regardless of temperature. Thus, at high temperatures, air-breathing intertidal ectotherms are less constrained by oxygen limitation and show wider thermal tolerances than water breathing ectotherms (Fusi et al., 2016). The broader thermal window allows the maintenance of the animals’ aerobic scope at higher temperatures (Fusi et al., 2016) and it is probably a major factor allowing the high thermal tolerance of air-breathing intertidal ectotherms.
The acclimatization of our model crabs to their localized thermal extremes is demonstrated by the variability of their TSM, which was independent of their latitudinal ranges (see, for example, L. leptodactyla vs. L. thayeri and A. lactea vs. P. splendida). Even at negative TSM, the Brazilian species with a more tropical latitudinal range colonizing bare intertidal flats actively thrived in thermally stressful conditions, even reproducing during the warmest months of the year (Costa et al., 2006; Bezerra and Matthews-Cascon, 2007; Masunari, 2012). This indicates that TSM; usually calculated by subtracting a proxy for upper thermal limit from a constant metric of experienced temperatures (Sunday et al., 2014; Marshall et al., 2015; Rezende and Bozinovic, 2019; Vinagre et al., 2019; Dahlke et al., 2020), although useful to predict the vulnerability of species to temperature increases, may fail to account for relevant factors involved in thermal tolerance. Moreover, the methods used to estimate TSM are often inconsistent throughout the literature and in many instances ignore local thermal heterogeneity. Additionally, ectotherms often live at supra-lethal temperatures, and behavioral thermoregulation is an essential trait in these animals (Sunday et al., 2014). Fiddler crabs, for example, can thermoregulate by retreating to their burrows (Munguia et al., 2017). Our data confirm that the full understanding of the thermal vulnerability of a particular group of organisms requires a deep knowledge on their behavioral and physiological adaptations, as well as information on possible thermal refugia.
The comparison of species belonging to separate, but closely related, clades revealed similar thermal limits between the two taxa. Our observations do not support the hypothesis that the phylogenetic distance and evolutionary history of fiddler crabs led to different thermal adaptations. Our data, instead, show that the thermal vulnerability of species may not only be related to the experienced temperatures, but also to their latitudinal distribution, since the only truly subtropical species we tested, L. uruguayensis, showed significantly lower thermal limits than the other species tested. This indicates that L. uruguayensis populations inhabiting the lower latitudinal ranges of its distribution are highly vulnerable to extreme temperature events. Accordingly, this species is more prone to latitudinal shifts or local extinctions at the lower edges of its latitudinal distribution (Thomas, 2010; Hoffmann et al., 2013; Kingsolver et al., 2013). Their populations are also vulnerable to invasions by low latitude species due to temperature increases (Murphy, 2020). Poleward distribution shifts are already occurring in Gelasiminae species (e.g. Perry et al., 2005; Castiglioni et al., 2010; Thurman et al., 2013; Johnson, 2014; Arakaki et al., 2020), and the invasion of low latitude species is projected to affect native populations, due to the very similar ecological characteristics shared by fiddler crab species.
Of course, future latitudinal distribution of these fiddler crabs, and local extinction events of their populations, will not be determined by their thermal vulnerability alone. Being part of the mangrove associated fauna, their vulnerability to the ongoing climatic changes is inherently linked to the resilience of such intertidal forests to global warming and ocean acidification. Mangrove ecosystems showed to be rather resilient to the ongoing decrease in pH of coastal waters (Alongi, 2008) and their resident fauna proved to be well adapted to the periodic events of hypoxia regularly affecting this habitat, mainly though the evolution of semi-terrestrial adaptations (Fusi et al., 2015; Fusi et al., 2017). At present, the most impacting consequence of climate change for mangrove ecosystems proved to be sea level rise (Gilman et al., 2008; Ellison, 2012), which will also strongly affect the microhabitat of the fiddler crab species we studied. A more holistic approach, taking into account the influence of climate change on their micro-habitat and their food sources is thus necessary to model future distribution of these crabs.
In the present study, sex-specific responses to temperature increases were evident, with females showing greater MO2 than males. These metabolic differences can be both a consequence of the strong sexual dimorphism of fiddler crabs and of the differential sex-related energy allocation in reproduction common in most animals. During the reproductive season, female fiddler crabs produce large quantities of eggs (Yamaguchi, 2001; Koch et al., 2005; Litulo, 2005; Castiglioni et al., 2007), at energetic costs higher than the ones involved in sperm production (Hartnoll, 2006; Rennie et al., 2008). A large allocation of energy on reproduction reduces the available aerobic scope of organisms (Sokolova, 2013) and it is assumed to reduce their thermal window (Pörtner and Farrell, 2008; Vorsatz et al., 2021a). If female fiddler crabs are more thermally sensitive, they may be the first to succumb to temperature increases, thereby reducing the viability and resilience of populations. Similar results have been observed in spawning fishes (Dahlke et al., 2020) and mangrove crabs (Vorsatz et al., 2021a), suggesting that reproductively active females may constitute an ontogenetic thermal bottleneck in climate change scenarios.
Our data confirmed that bimodal-breathing ectotherms are able to cope with ambient temperatures that regularly reach levels above their thermal limits, showing the efficiency of their thermoregulatory and respiratory mechanisms. These mechanisms allow ectotherms to conform and acclimatize to the conditions they experience, thus supporting the Climate Extremes Hypothesis (Pither, 2003; Bozinovic et al., 2011; Sunday et al., 2019) whereby an ectotherms’ thermal tolerance reflects the thermal extremes they face in their habitat along their geographical distribution. Differences in the thermal vulnerability between males and female, however, might also be an important factor in predicting species distributions and the resilience of populations of ectotherms to temperature rises. Indeed, reliable models on the impact of global warming on intertidal species distribution and resilience should include data on both sexes, reproductive biology and different ontogenetic stages, as well as information on local thermal environment. Based on our data, we advocate for the inclusion of physiological traits such as breathing mode and sex-related differences in energy allocation to reproduction in general models aimed to describe and forecast the relationship between thermal sensitivity and the present and future distribution of intertidal species.
Data Availability Statement
All data needed to support the conclusions are present in the article/Supplementary Material. Further inquiries can be directed to the corresponding author.
Author Contributions
PJ and SC conceived the original idea and designed the methodology. PJ collected the data. PJ and SC analyzed the data. PJ and LV wrote the manuscript. SC and TC revised and edited the manuscript. SC and TC provided funding for the study. All authors contributed critically to the drafts and gave final approval for publication.
Funding
This study was funded by the Travel Support for Research Postgraduate Students for International Academic Training/Research Activities from The University of Hong Kong, by the Faculty of Science Seed fund #104005887.088562.26000.301.01, from the University of Hong Kong, and by the São Paulo Research Foundation (FAPESP) (Costa, TM - #2015/50300-6).
Conflict of Interest
The authors declare that the research was conducted in the absence of any commercial or financial relationships that could be construed as a potential conflict of interest.
Publisher’s Note
All claims expressed in this article are solely those of the authors and do not necessarily represent those of their affiliated organizations, or those of the publisher, the editors and the reviewers. Any product that may be evaluated in this article, or claim that may be made by its manufacturer, is not guaranteed or endorsed by the publisher.
Acknowledgments
We thank the members of the Integrated Mangrove and Ecology lab of the University of Hong Kong (IMEco Lab) of the Laboratory of Ecology and Animal Behavior of the state University of Sao Paulo (LABECOM) for the field work assistance. We also thank the two reviewers for comments that helped improve the quality of the manuscript.
Supplementary Material
The Supplementary Material for this article can be found online at: https://www.frontiersin.org/articles/10.3389/fmars.2022.858280/full#supplementary-material
References
Abadi S., Azouri D., Pupko T., Mayrose I. (2019). Model Selection may Not be a Mandatory Step for Phylogeny Reconstruction. Nat. Commun. 10, 934. doi: 10.1038/s41467-019-08822-w
Allen B. J., Levinton J. S. (2014). Sexual Selection and the Physiological Consequences of Habitat Choice by a Fiddler Crab. Oecologia 176, 25–34. doi: 10.1007/s00442-014-3002-y
Alongi D. M. (1990). Community Dynamics of Free-Living Nematodes in Some Tropical Mangrove and Sandflat Habitats. Bull. Marine Sci. 46, 358–373.
Alongi D. M. (2008). Mangrove Forests: Resilience, Protection From Tsunamis, and Responses to Global Climate Change. Estuarine Coastal Shelf Sci. 76, 1–13. doi: 10.1016/j.ecss.2007.08.024
Anderson M. J., Walsh D. C. I. (2013). PERMANOVA, ANOSIM, and the Mantel Test in the Face of Heterogeneous Dispersions: What Null Hypothesis Are You Testing? Ecol. Monogr. 83, 557–574. doi: 10.1890/12-2010.1
Arakaki J. Y., Grande F. R., Arvigo A. L., Pardo J. C. F., Fogo B. R., Sanches F. H. C., et al. (2020). Battle of the Borders: Is a Range-Extending Fiddler Crab Affecting the Spatial Niche of a Congener Species? J. Exp. Marine Biol. Ecol. 532, 151445. doi: 10.1016/j.jembe.2020.151445
Baeza J. A., Fernández M. (2002). Active Brood Care in Cancer Setosus (Crustacea: Decapoda): The Relationship Between Female Behaviour, Embryo Oxygen Consumption and the Cost of Brooding. Funct. Ecol. 16, 241–251. doi: 10.1046/j.1365-2435.2002.00616.x
Beck M. W. (1998). Comparison of the Measurement and Effects of Habitat Structure on Gastropods in Rocky Intertidal and Mangrove Habitats. Marine Ecol. Prog. Ser. 169, 165–178. doi: 10.3354/meps169165
Bennett J. M., Sunday J., Calosi P., Villalobos F., Martínez B., Molina-Venegas R., et al. (2021). The Evolution of Critical Thermal Limits of Life on Earth. Nat. Commun. 12, 1–9. doi: 10.1038/s41467-021-21263-8
Bezerra L. E. A., Matthews-Cascon H. (2007). Population and Reproductive Biology of the Fiddler Crab Uca Thayeri Rathbu(Crustacea: Ocypodidae) in a Tropical Mangrove From Northeast Brazil. Acta Oecologica 31, 251–258. doi: 10.1016/j.actao.2006.10.003
Bliss D. E. (1968). Transition From Water to Land in Decapod Crustaceans. Integr. Comp. Biol. 8, 355–392. doi: 10.1093/icb/8.3.355
Bozinovic F., Calosi P., Spicer J. I. (2011). Physiological Correlates of Geographic Range in Animals. Annu. Rev. Ecol. Evol. Systematics 42, 155–179. doi: 10.1146/annurev-ecolsys-102710-145055
Brayard A., Escarguel G., Bucher H. (2005). Latitudinal Gradient of Taxonomic Richness: Combined Outcome of Temperature and Geographic Mid-Domains Effects? J. Zoological Systematics Evol. Res. 43, 178–188. doi: 10.1111/j.1439-0469.2005.00311.178
Brown J. H., Gillooly J. F., Allen A. P., Savage V. M., West G. B. (2004). Toward a Metabolic Theory of Ecology. Ecology 85, 1771–1789. doi: 10.1890/03-9000
Burggren W., Mcmahon B. R. (1988). Biology of the Land Crabs (Cambridge: Cambridge University Press). doi: 10.1017/CBO9780511753428
Burggren W. W. (1992). Respiration and Circulation in Land Crabs: Novel Variations on the Marine Design. Am. Zoologist 32, 417–427. doi: 10.1093/icb/32.3.417
Burggren W. W., Pinder A., McMahon B., Doyle M., Wheatly M. (1990). Heart Rate and Hemolymph Pressure Responses to Hemolymph Volume Changes in the Land Crab Cardisoma Guanhumi: Evidence for “Baroreflex” Regulation. Physiol. Zool. 63, 167–181. doi: 10.1086/physzool.63.1.30158159
Burke E. M. (1979). Aerobic and Anaerobic Metabolism During Activity and Hypoxia in Two Species of Intertidal Crabs. Biol. Bull. 156, 157–168. doi: 10.2307/1541040
Burnett N. P., Seabra R., de Pirro M., Wethey D. S., Woodin S. A., Helmuth B., et al. (2013). An Improved Noninvasive Method for Measuring Heartbeat of Intertidal Animals. Limnol. Oceanography: Methods 11, 91–100. doi: 10.4319/Lom.2013.11.91
Castiglioni D., da S., Almeida A. O., Bezerra L. E. A. (2010). More Common Than Reported: Range Extension, Size–Frequency and Sex-Ratio of Uca (Minuca) Victoriana (Crustacea: Ocypodidae) in Tropical Mangroves, Brazil. Marine Biodiversity Records 3, 1–7. doi: 10.1017/S1755267210000874
Castiglioni D., da S., Negreiros-Fransozo M. L., Cardoso R. C. F. (2007). Breeding Season and Molt Cycle of the Fiddler Crab Uca Rapax (Brachyura, Ocypodidae) in a Subtropical Estuary, Brazil, South America. Gulf Caribbean Res. 19, 11–20. doi: 10.18785/gcr.1901.02
Chou C. C., Perez D. M., Johns S., Gardner R., Kerr K. A., Head M. L., et al. (2019). Staying Cool: The Importance of Shade Availability for Tropical Ectotherms. Behav. Ecol. Sociobiol. 73, 106. doi: 10.1007/s00265-019-2721-9
Clark K., Karsch-Mizrachi I., Lipman D. J., Ostell J., Sayers E. W. (2016). Genbank. Nucleic Acids Res. 44, D67–D72. doi: 10.1093/nar/gkv1276
Colpo K. D., López-greco L. S. (2018). Dynamics of Energy Reserves and the Cost of Reproduction in Female and Male Fiddler Crabs. Zoology 126, 11–19. doi: 10.1016/j.zool.2018.01.004
Costa T. M., Silva S. M. J., Negreiros-Fransozo M. L. (2006). Reproductive Pattern Comparison of Uca Thayeri Rathbuand U. Uruguayensis Nobil(Crustacea, Decapoda, Ocypodidae). Braz. Arch. Biol. Technol. 49, 117–123. doi: 10.1590/S1516-89132006000100014
Crane J. (1975). Fiddler Crabs of the World Ocypodidae: Genus (Uca. Princeton, New Jersey: Princeton University Press). doi: 10.1016/0003-3472(78)90130-6
Dahlke F. T., Wohlrab S., Butzin M., Pörtner H.-O. (2020). Thermal Bottlenecks in the Life Cycle Define Climate Vulnerability of Fish. Science 369, 65–70. doi: 10.1126/science.aaz3658
Darnell M. Z., Fowler K. K., Munguia P. (2013). Sex-Specific Thermal Constraints on Fiddler Crab Behavior. Behav. Ecol. 24, 997–1003. doi: 10.1093/beheco/art006
Darnell M. Z., Munguia P. (2011). Thermoregulation as an Alternate Function of the Sexually Dimorphic Fiddler Crab Claw. Am. Nat. 178, 419–428. doi: 10.1086/661239
Darnell M. Z., Nicholson H. S., Munguia P. (2015). Thermal Ecology of the Fiddler Crab Uca Panacea: Thermal Constraints and Organismal Responses. J. Thermal Biol. 52, 157–165. doi: 10.1016/j.jtherbio.2015.06.004
Denny M. W., Dowd W. W., Bilir L., Mach K. J. (2011). Spreading the Risk: Small-Scale Body Temperature Variation Among Intertidal Organisms and Its Implications for Species Persistence. J. Exp. Marine Biol. Ecol. 400, 175–190. doi: 10.1016/j.jembe.2011.02.006
Denny M. W., Paine R. T. (1998). Celestial Mechanics, Sea-Level Changes, and Intertidal Ecology. Biol. Bull. 194, 108–115. doi: 10.2307/1543040
Depledge M. H., Andersen B. B. (1990). A Computer-Aided Physiological Monitoring-System for Continuous, Long-Term Recording of Cardiac Activity in Selected Invertebrates. Comp. Biochem. Physiol. 96A, 473–477. doi: 10.1016/0300-9629(90)90664-E
Deutsch C. A., Tewksbury J. J., Huey R. B., Sheldon K. S., Ghalambor C. K., Haak D. C., et al. (2008). Impacts of Climate Warming on Terrestrial Ectotherms Across Latitude. Proc. Natl. Acad. Sci. U. S. A. 105, 6668–6672. doi: 10.1073/pnas.0709472105
Días H., Rodríguez G. (1977). The Branchial Chamber in Terrestrial Crabs: A Comparative Study. Biol. Bull. 153, 485–504. doi: 10.2307/1540602
Diele K., Koch V., Abrunhosa F. A., de Farias Lima J., de Jesus de Brito Simith D., Lima J. D. F., et al. (2010). “The Brachyuran Crab Community of the Caete Estuary, North Brazil: Species Richness, Zonation and Abundance,” in Mangrove Dynamics and Management in North Brazil. Eds. Saint-Paul U., Schneider H. (Berlin: Springer-Verlag Berlin Heidelberg), 251–263. doi: 10.1007/978-3-642-13457-9
Drummond A. J., Ho S. Y. W., Phillips M. J., Rambaut A. (2006). Relaxed Phylogenetics and Dating With Confidence. PloS Biol. 4, 699–710. doi: 10.1371/journal.pbio.0040088
Drummond A. J., Rambaut A. (2007). BEAST: Bayesian Evolutionary Analysis by Sampling Trees. BMC Evol. Biol. 7, 1–8. doi: 10.1186/1471-2148-7-214
Edgar R. C. (2004). MUSCLE: Multiple Sequence Alignment With High Accuracy and High Throughput. Nucleic Acids Res. 32, 1792–1797. doi: 10.1093/nar/gkh340
Ellison J. C. (2012). “Mangrove Swamps: Causes of Decline and Mortality,” in Forest Decline: Causes and Impacts. Ed. Jenkins J. A. (New York: Nova Science Publishers), 39–68.
Faria S. C., Provete D. B., Thurman C. L., Mcnamara J. C. (2017). Phylogenetic Patterns and the Adaptive Evolution of Osmoregulation in Fiddler Crabs (Brachyura, Uca). PloS One 2, 1–19. doi: 10.1371/journal.pone.0171870
Farrelly C. A., Greenaway P. (1993). Land Crabs With Smooth Lungs: Grapsidae, Gecarcinidae, and Sundathelphusidae Ultrastructure and Vasculature. J. Morphol. 215, 245–260. doi: 10.1002/jmor.1052150306
Farrelly C. A., Greenaway P. (1994). Gas Exchange Through the Lungs and Gills in Air-Breathing Crabs. J. Exp. Biol. 187, 113–130. doi: 10.1242/jeb.187.1.113
Fernández M., Bock C., Pörtner H.-O. (2000). The Cost of Being a Caring Mother: The Ignored Factor in the Reproduction of Marine Invertebrates. Ecol. Lett. 3, 487–494. doi: 10.1046/j.1461-0248.2000.00172.x
Finke G. R., Navarrete S. A., Bozinovic F. (2007). Tidal Regimes of Temperate Coasts and Their Influences on Aerial Exposure for Intertidal Organisms. Marine Ecol. Prog. Ser. 343, 57–62. doi: 10.3354/meps06918
Fusi M., Babbini S., Giomi F., Fratini S., Daniele F. D., Christopher D., et al. (2017). Thermal Sensitivity of the Crab Neosarmatium Africanum in Tropical and Temperate Mangroves on the East Coast of Africa. Hydrobiologia 803, 251–263. doi: 10.1007/s10750-017-3151-1
Fusi M., Cannicci S., Daffonchio D., Mostert B., Pörtner H.-O., Giomi F. (2016). The Trade-Off Between Heat Tolerance and Metabolic Cost Drives the Bimodal Life Strategy at the Air-Water Interface. Sci. Rep. 6, 19158. doi: 10.1038/srep19158
Fusi M., Giomi F., Babbini S., Daffonchio D., Mcquaid C. D., Porri F., et al. (2015). Thermal Specialization Across Large Geographical Scales Predicts the Resilience of Mangrove Crab Populations to Global Warming. Oikos 124, 784–795. doi: 10.1111/oik.01757
Gaston K. J., Chown S. L., Calosi P., Bernardo J., Bilton D. T., Clarke A., et al. (2009). Macrophysiology: A Conceptual Reunification. Am. Nat. 174, 595–612. doi: 10.1086/605982
Gernhard T. (2008). The Conditioned Reconstructed Process. J. Theor. Biol. 253, 769–778. doi: 10.1016/j.jtbi.2008.04.005
Gilman E. L., Ellison J., Duke N. C., Field C. (2008). Threats to Mangroves From Climate Change and Adaptation Options: A Review. Aquat. Bot. 89, 237–250. doi: 10.1016/j.aquabot.2007.12.009
Giomi F., Fusi M., Barausse A., Mostert B., Pörtner H.-O., Cannicci S. (2014). Improved Heat Tolerance in Air Drives the Recurrent Evolution of Air-Breathing. Proc. R. Soc. B 281, 20132927. doi: 10.1098/rspb.2013.2927
Graham J. B. (1997). Air-Breathing Fishes. 1st Ed (London: Academic Press). doi: 10.1016/B978-0-12-294860-2.X5000-4
Guderley H., Pörtner H. O. (2010). Metabolic Power Budgeting and Adaptive Strategies in Zoology: Examples From Scallops and Fish. Can. J. Zool. 88, 753–763. doi: 10.1139/Z10-039
Gutiérrez-Pesquera L. M., Tejedo M., Olalla-Tárraga M. A., Duarte H., Nicieza A., Solé M. (2016). Testing the Climate Variability Hypothesis in Thermal Tolerance Limits of Tropical and Temperate Tadpoles. J. Biogeography 43, 1166–1178. doi: 10.1111/jbi.12700
Harley C. D. G., Denny M. W., MacH K. J., Miller L. P. (2009). Thermal Stress and Morphological Adaptations in Limpets. Funct. Ecol. 23, 292–301. doi: 10.1111/j.1365-2435.2008.01496.x
Hartnoll R. G. (2006). Reproductive Investment in Brachyura. Hydrobiologia 557, 31–40. doi: 10.1007/s10750-005-9305-6
Helmuth B. (2002). How do We Measure the Environment? Linking Intertidal Thermal Physiology and Ecology Through Biophysics. Integr. Comp. Biol. 42, 837–845. doi: 10.1093/icb/42.4.837
Helmuth B., Broitman B. R., Blanchette C., Gilman S., Halpin P., Harley C. D., et al. (2006a). Mosaic Patterns of Thermal Stress in the Rocky Intertidal Zone: Implications for Climate Change. Ecol. Monogr. 76, 461–479. doi: 10.1890/0012-9615(2006)076[0461:MPOTSI]2.0.CO;2
Helmuth B., Harley C. D. G., Halpin P. M., O’Donnell M., Hofmann G. E., Blanchette C. a (2002). Climate Change and Latitudinal Patterns of Intertidal Thermal Stress. Science 298, 1015–1017. doi: 10.1126/science.1076814
Helmuth B. S. T. T., Hofmann G. E. (2001). Microhabitats, Thermal Heterogeneity, and Patterns of Physiological Stress in the Rocky Intertidal Zone. Biol. Bull. 201, 374–384. doi: 10.2307/1543615
Helmuth B., Mieszkowska N., Moore P., Hawkins S. J. (2006b). Living on the Edge of Two Worlds: Forecasting the Responses of Rocky Intertidal Ecosystems to Climate Change. Annu. Rev. Ecol. Systematics 37, 373–404. doi: 10.2307/annurev.ecolsys.37.091305.30000015
Henry R. P. (1994). Morphological, Behavioral, and Physiological Characterization of Bimodal Breathing Crustaceans. Integr. Comp. Biol. 34, 205–215. doi: 10.1093/icb/34.2.205
Hoffmann A. A., Chown S. L., Clusella-Trullas S. (2013). Upper Thermal Limits in Terrestrial Ectotherms: How Constrained Are They? Funct. Ecol. 27, 934–949. doi: 10.1111/j.1365-2435.2012.02036.x
Holm S. (1979). A Simple Sequentially Rejective Multiple Test Procedure. Scandinavian J. Stat 6, 65–70.
Jahnke M., Moknes P., le Moan A., Martens G. A., Jonsson P. R. (2022). Seascape Genomics Identify Adaptive Barriers Correlated to Tidal Amplitude in the Shore Crab Carcinus Maenas. Mol. Ecol. 1–15. doi: 10.1111/mec.16371
Johnson D. S. (2014). Fiddler on the Roof: A Northern Range Extension for the Marsh Fiddler Crab Uca Pugnax. J. Crustacean Biol. 34, 671–673. doi: 10.1163/1937240X-00002268
Kingsolver J. G., Diamond S. E., Buckley L. B. (2013). Heat Stress and the Fitness Consequences of Climate Change for Terrestrial Ectotherms. Funct. Ecol. 27, 1415–1423. doi: 10.1111/1365-2435.12145
Koch V., Wolff M., Diele K. (2005). Comparative Population Dynamics of Four Fiddler Crabs (Ocypodidae, Genus Uca) From a North Brazilian Mangrove Ecosystem. Marine Ecol. Prog. Ser. 291, 177–188. doi: 10.3354/meps291177
Kontopoulos D. G., García-Carreras B., Sal S., Smith T. P., Pawar S. (2018). Use and Misuse of Temperature Normalisation in Meta-Analyses of Thermal Responses of Biological Traits. PeerJ 2018, 1–18. doi: 10.7717/peerj.4363
Kostina E. E., Tsurpalo A. P., Gulbin V. v. (2016). “The Species Composition and Distribution of Macrobenthic Communities in the Intertidal Zone of Vietnam,” in Biodiversity of the Western Part of the South China Sea, vol. 502. Eds. v Adrianov A., Lutaenko K. A. (Vladivostok: Dalnauka).
Kumar S., Stecher G., Li M., Knyaz C., Tamura K. (2018). Mega X: Molecular Evolutionary Genetics Analysis Across Computing Platforms. Mol. Biol. Evol. 35, 1547–1549. doi: 10.1093/molbev/msy096
Kwok W. P. W., Tang W. (2006). Fiddler Crabs in Hong Kong – An Overview. Hong Kong Biodiversity 12, 1–7.
Levinton J. S., Judge M. L., Kurdziel J. P. (1995). Functional Differences Between the Major and Minor Claws of Fiddler Crabs (Uca, Family Ocypodidae, Order Decapoda, Subphylum Crustacea): A Result of Selection or Developmental Constraint? J. Exp. Marine Biol. Ecol. 193, 147–160. doi: 10.1016/0022-0981(95)00115-8
Levinton J. S., Volkenborn N., Gurr S., Correal K., Villacres S., Seabra R., et al. (2020). Temperature-Related Heart Rate in Water and Air and a Comparison to Other Temperature-Related Measures of Performance in the Fiddler Crab Leptuca Pugilator (Bosc 1802). J. Thermal Biol. 88, 102502. doi: 10.1016/j.jtherbio.2019.102502
Lin H.-C., Su Y., Su S.-H. (2002). A Comparative Study of Osmoregulation in Four Fiddler Crabs (Ocypodidae: Uca). Zoological Sci. 19, 643–650. doi: 10.2108/zsj.19.643
Litulo C. (2005). Population Structure and Reproductive Biology of the Fiddler Crab Uca Urvillei (Brachyura: Ocypodidae) in Maputo Bay (South Mozambique). J. Natural History 39, 2307–2318. doi: 10.1080/00222930502005688
Marshall D. J., Dong Y., Mcquaid C. D., Williams G. A. (2011). Thermal Adaptation in the Intertidal Snail Echinolittorina Malaccana Contradicts Current Theory by Revealing the Crucial Roles of Resting Metabolism. J. Exp. 214, 3649–3657. doi: 10.1242/jeb.059899
Marshall D. J., McQuaid C. D., Williams G. A. (2010). Non-Climatic Thermal Adaptation: Implications for Species’ Responses to Climate Warming. Biol. Lett. 6, 669–673. doi: 10.1098/rsbl.2010.0233
Marshall D. J., Rezende E. L., Baharuddin N., Choi F., Helmuth B. (2015). Thermal Tolerance and Climate Warming Sensitivity in Tropical Snails. Ecol. Evol. 5, 5905–5919. doi: 10.1002/ece3.1785
Masunari S. (2012). Hood Construction as an Indication of the Breeding Period of the Fiddler Crab Uca (Leptuca) Leptodactyla Rathbu(Decapoda, Ocypodidae) From Guaratuba Bay, Southern Brazil. Crustaceana 85, 1153–1169. doi: 10.1163/156854012X651277
Mcmahon R. F. (1988). Respiratory Response to Periodic Emergence in Intertidal Molluscs. Integr. Comp. Biol. 28, 97–114. doi: 10.1093/icb/28.1.97
Muggeo V. M. R. (2003). Estimating Regression Models With Unknown Break-Points. Statist. Med. 22, 3055–3071. doi: 10.1002/sim.1545
Munguia P., Backwell P. R. Y., Darnell M. Z. (2017). Thermal Constraints on Microhabitat Selection and Mating Opportunities. Anim. Behav. 123, 259–265. doi: 10.1016/j.anbehav.2016.11.004
Murphy J. T. (2020). Climate Change, Interspecific Competition, and Poleward vs. Depth Distribution Shifts: Spatial Analyses of the Eastern Bering Sea Snow and Tanner Crab (Chionoecetes Opilio and C. Bairdi). Fisheries Res. 223, 105417. doi: 10.1016/j.fishres.2019.105417
Padfield D., Matheson G. (2020) Nls.Multstart: Robust Non-Linear Regression Using AIC Scores. Available at: https://cran.r-project.org/package=nls.multstart.
Padfield D., O’Sullivan H. (2020) Rtpc: Functions for Fitting Thermal Performance Curves. Available at: http://github.com/padpadpadpad/rTPC.
Padfield D., O’Sullivan H., Pawar S. (2020). Rtpc and Nls.Multstart: A New Pipeline to Fit Thermal Performance Curves in R. bioRxiv 12, 16. doi: 10.1101/2020.12.16.423089
Pagel M. (1997). Inferring Evolutionary Processes From Phylogenies. Zool. Scripta 26, 331–348. doi: 10.1111/j.1463-6409.1997.tb00423.x
Pagel M. (1999). Inferring the Historical Patterns of Biological Evolution. Nature 401, 877–884. doi: 10.1038/44766
Paoli F., Wirkner C. S., Cannicci S. (2015). The Branchiostegal Lung of Uca Vocans (Decapoda: Ocypodidae): Unreported Complexity Revealed by Corrosion Casting and Microct Techniques. Arthropod Structure Dev. 44, 622–629. doi: 10.1016/j.asd.2015.09.006
Perry A. L., Low P. J., Ellis J. R., Reynolds J. D. (2005). Climate Change and Distribution Shifts in Marine Fishes. Science 308, 1912–1915. doi: 10.1126/science.1111322
Peterson C. H. (1991). Intertidal Invertebrates Zonation in Sand and Mud. Am. Scientist 79, 236–249.
Pither J. (2003). Climate Tolerance and Interspecific Variation in Geographic Range Size. Proc. R. Soc. B: Biol. Sci. 270, 475–481. doi: 10.1098/rspb.2002.2275
Pörtner H.-O. (2012). Integrating Climate-Related Stressor Effects on Marine Organisms: Unifying Principles Linking Molecule to Ecosystem-Level Changes. Marine Ecol. Prog. Ser. 470, 273–290. doi: 10.3354/meps10123
Pörtner H.-O., Farrell A. P. (2008). Physiology and Climate Change. Science 322, 690–692. doi: 10.1126/science.1163156
Powers L. W., Cole J. F. (1976). Temperature Variation in Fiddler Crab Microhabitats. J. Exp. Marine Biol. Ecol. 21, 141–157. doi: 10.1016/0022-0981(76)90035-6
Przeslawski R., Davis A. R., Benkendorff K. (2005). Synergistic Effects Associated With Climate Change and the Development of Rocky Shore Molluscs. Global Change Biol. 11, 515–522. doi: 10.1111/j.1365-2486.2005.00918.x
Rambaut A., Drummond A. J., Xie D., Baele G., Suchard M. A. (2018). Posterior Summarization in Bayesian Phylogenetics Using Tracer 1.7. Systematic Biol. 67, 901–904. doi: 10.1093/sysbio/syy032
R core team. (2021). R: A Language and Environment for Statistical Computing. Available at: https://www.r-project.org/.
Rennie M. D., Purchase C. F., Lester N., Collins N. C., Shuter B. J., Abrams P. A. (2008). Lazy Males? Bioenergetic Differences in Energy Acquisition and Metabolism Help to Explain Sexual Size Dimorphism in Percids. J. Anim. Ecol. 77, 916–926. doi: 10.1111/j.1365-2656.2008.01412.x
Revell L. J. (2012). Phytools: An R Package for Phylogenetic Comparative Biology (and Other Things). Methods Ecol. Evol. 3, 217–223. doi: 10.1111/j.2041-210X.2011.00169.x
Rezende E. L., Bozinovic F. (2019). Thermal Performance Across Levels of Biological Organization. Philos. Trans. R. Soc. B: Biol. Sci. 374, 20180549. doi: 10.1098/rstb.2018.0549
Ribeiro P. D., Christy J. H., Nuñez J. D., Iribarne O. O. (2016). Hood Building Dynamics and Mating Mode in the Temperate Fiddler Crab Uca Uruguayensis Nobil. J. Crustacean Biol. 36, 507–514. doi: 10.1163/1937240X-00002440
Roy K., Jablonski D., Valentine J. W., Rosenberg G. (1998). Marine Latitudinal Diversity Gradients: Tests of Causal Hypotheses. Proc. Natl. Acad. Sci. U. S. A. 95, 3699–3702. doi: 10.1073/pnas.95.7.3699
Seabra R., Wethey D. S., Santos A. M., Lima F. P. (2011). Side Matters: Microhabitat Influence on Intertidal Heat Stress Over a Large Geographical Scale. J. Exp. Marine Biol. Ecol. 400, 200–208. doi: 10.1016/j.jembe.2011.02.010
Shih H.-T. (2012). Distribution of Fiddler Crabs in East Asia, With a Note on the Effect of the Kuroshio Current. Kuroshio Sci. 6, 83–89.
Shih H.-T., Lee J. H., Ho P. H., Liu H. C., Wang C. H., Suzuki H., et al. (2016b). Species Diversity of Fiddler Crabs, Genus Uca Leach 1814 (Crustacea: Ocypodidae), From Taiwan and Adjacent Islands, With Notes on the Japanese Species. Zootaxa 4083, 57–82. doi: 10.11646/zootaxa.4083.1.3
Shih H.-T., Ng P. K. L., Davie P. J. F., Schubart C. D., Türkay M., Naderloo R., et al. (2016a). Systematics of the Family Ocypodidae Rafinesqu(Crustacea: Brachyura), Based on Phylogenetic Relationships, With a Reorganization of Subfamily Rankings and a Review of the Taxonomic Status of Uca Leach 1814, Sensu Lato and Its Subgenera. Raffles Bull. Zool. 64, 139–175.
Shih H.-T., Ng P. K. L., Wong K. J. H., Chan B. K. K. (2012). Gelasimus Splendidus Stimpson, 1858 (Crustacea: Brachyura: Ocypodidae), a Valid Species of Fiddler Crab From the Northern South China Sea and Taiwan Strait. Zootaxa 3490, 30–37. doi: 10.11646/zootaxa.3490.1.2
Sokolova I. M. (2013). Energy-Limited Tolerance to Stress as a Conceptual Framework to Integrate the Effects of Multiple Stressors. Integr. Comp. Biol. 53, 597–608. doi: 10.1093/icb/ict028
Somero G. N. (2002). Thermal Physiology and Vertical Zonation of Intertidal Animals: Optima, Limits, and Costs of Living. Integr. Comp. Biol. 42, 780–789. doi: 10.1093/Icb/42.4.780
Somero G. N. (2010). The Physiology of Climate Change: How Potentials for Acclimatization and Genetic Adaptation Will Determine “Winners” and “Losers.” J. Exp. Biol. 213, 912–920. doi: 10.1242/jeb.037473
Stillman J. H. (2002). Causes and Consequences of Thermal Tolerance Limits in Rocky Intertidal Porcelain Crabs, Genus Petrolisthes. Integr. Comp. Biol. 42, 790–796. doi: 10.1093/icb/42.4.790
Stillman J. H., Somero G. N. (1996). Adaptation to Temperature Stress and Aerial Exposure in Congeneric Species of Intertidal Porcelain Crabs (Genus Petrolisthes): Correlation of Physiology, Biochemistry and Morphology With Vertical Distribution. J. Exp. Biol. 1855, 1845–1855. doi: 10.1073/pnas.93.20.10855
Stillman J. H., Somero G. N. (2000). A Comparative Analysis of the Upper Thermal Tolerance Limits of Eastern Pacific Porcelain Crabs, Genus Petrolisthes: Influences of Latitude, Vertical Zonation, Acclimation, and Phylogeny. Physiol. Biochem. Zool. 73, 200–208. doi: 10.1086/316738
Suchard M. A., Lemey P., Baele G., Ayres D. L., Drummond A. J., Rambaut A. (2018). Bayesian Phylogenetic and Phylodynamic Data Integration Using BEAST 1.10. Virus Evol. 4, vey016. doi: 10.1093/ve/vey016
Sunday J. M., Bates A. E., Kearney M. R., Colwell R. K., Dulvy N. K., Longino J. T., et al. (2014). Thermal-Safety Margins and the Necessity of Thermoregulatory Behavior Across Latitude and Elevation. Proc. Natl. Acad. Sci. U. S. A. 111, 5610–5615. doi: 10.1073/pnas.1316145111
Sunday J. M., Bennett J. M., Calosi P., Clusella-Trullas S., Gravel S., Hargreaves A. L., et al. (2019). Thermal Tolerance Patterns Across Latitude and Elevation. Philos. Trans. R. Soc. B: Biol. Sci. 374, 20190036. doi: 10.1098/rstb.2019.0036
Tavaré S. (1986). Some Probabilistic and Statistical Problems in the Analysis of DNA Sequences. Am. Math. Society: Lectures Mathematics Life Sci. 17, 57–86.
Thomas C. D. (2010). Climate, Climate Change and Range Boundaries. Diversity Distributions 16, 488–495. doi: 10.1111/j.1472-4642.2010.00642.x
Thurman C. L., Faria S. C., McNamara J. C. (2013). The Distribution of Fiddler Crabs (Uca) Along the Coast of Brazil: Implications for Biogeography of the Western Atlantic Ocean. Marine Biodiversity Records 6, e1. doi: 10.1017/S1755267212000942
Thurman C. L., Hanna J., Bennett C. (2010). Ecophenotypic Physiology: Osmoregulation by Fiddler Crabs (Uca Spp.) From the Northern Caribbean in Relation to Ecological Distribution. Marine Freshwater Behav. Physiol. 43, 339–356. doi: 10.1080/10236244.2010.526407
Valiela I., Babiec D. F., Atherton W., Seitzinger S., Krebs C. (1974). Some Consequences of Sexual Dimorphism: Feeding in Male and Female Fiddler Crabs, Uca Pugnax (Smith). Biol. Bull. 147, 652–660. doi: 10.2307/1540748
Viña N., Bascur M., Guzmán F., Riera R., Paschke K., Urzúa Á. (2018). Interspecific Variation in the Physiological and Reproductive Parameters of Porcelain Crabs From the Southeastern Pacific Coast: Potential Adaptation in Contrasting Marine Environments. Comp. Biochem. Physiol. Part A: Mol. Integr. Physiol. 226, 22–31. doi: 10.1016/j.cbpa.2018.07.006
Vinagre C., Dias M., Cereja R., Abreu-Afonso F., Flores A. A. V., Mendonça V. (2019). Upper Thermal Limits and Warming Safety Margins of Coastal Marine Species – Indicator Baseline for Future Reference. Ecol. Indic. 102, 644–649. doi: 10.1016/j.ecolind.2019.03.030
Vorsatz L. D., Mostert B. P., McQuaid C. D., Cannicci S., Porri F. (2021a). Thermal Sensitivity in Dual-Breathing Ectotherms: Embryos and Mothers Determine Species’ Vulnerability to Climate Change. Limnol. Oceanography Lett. doi: 10.1002/lol2.10225
Vorsatz L. D., Pattrick P., Porri F. (2021b). Quantifying the in Situ 3-Dimensional Structural Complexity of Mangrove Tree Root Systems: Biotic and Abiotic Implications at the Microhabitat Scale. Ecol. Indic. 121, 107154. doi: 10.1016/j.ecolind.2020.107154
Wiens J. J., Graham C. H. (2005). Niche Conservatism: Integrating Evolution, Ecology, and Conservation Biology. Annu. Rev. Ecol. Evol. Systematics 36, 519–539. doi: 10.1146/annurev.ecolsys.36.102803.095431
Williams G. A., Morritt D. (1995). Habitat Partitioning and Thermal Tolerance in a Tropical Limpet, Cellana Grata. Marine Ecol. Prog. Ser. 124, 89–103. doi: 10.3354/meps124089
Windsor A., Crowe M., Bishop J. (2005). Determination of Temperature Preference and the Role of the Enlarged Cheliped in Thermoregulation in Male Sand Fiddler Crabs, Uca Pugilator. J. Thermal Biol. 30, 37–41. doi: 10.1016/j.jtherbio.2004.06.006
Yamaguchi T. (2001). The Breeding Period of the Fiddler Crab, Uca Lactea (Decapoda, Brachyura, Ocypodidae) in Japan. Crustaceana 74, 285–293. doi: 10.1163/156854001505523
Yamaguchi T. (2003). Seasonal Changes in the Energy Content of Females of the Fiddler Crab, Uca Lactea, Especially During the Reproductive Period. Crustaceana 76, 1371–1397. doi: 10.1163/156854003323009867
Yang Z. (1994). Maximum Likelihood Phylogenetic Estimation From Dna Sequences With Variable Rates Over Sites: Approximate Methods. J. Mol. Evol. 39, 306–314. doi: 10.1007/BF00160154
Yuhara T., Yokooka H., Taru M. (2017). Range Extension of the Sesarmid Crab Clistocoeloma Villosum Along the Eastern Pacific Coast of the Izu Peninsula, Japan. Marine Biodiversity Records 10, 1–5. doi: 10.1186/s41200-017-0122-1
Keywords: thermal adaptations, fiddler crabs, intertidal organisms, bimodal breathers, thermal physiology, latitudinal gradient, habitat temperature
Citation: Jimenez PJ, Vorsatz LD, Costa TM and Cannicci S (2022) Temperature Extremes and Sex-Related Physiology, Not Environmental Variability, Are Key in Explaining Thermal Sensitivity of Bimodal-Breathing Intertidal Crabs. Front. Mar. Sci. 9:858280. doi: 10.3389/fmars.2022.858280
Received: 19 January 2022; Accepted: 02 March 2022;
Published: 25 March 2022.
Edited by:
Christian Pansch, Åbo Akademi University, FinlandReviewed by:
Carolina Madeira, NOVA University Lisbon, PortugalLaura Ramajo, Universidad Católica del Norte, Chile
Copyright © 2022 Jimenez, Vorsatz, Costa and Cannicci. This is an open-access article distributed under the terms of the Creative Commons Attribution License (CC BY). The use, distribution or reproduction in other forums is permitted, provided the original author(s) and the copyright owner(s) are credited and that the original publication in this journal is cited, in accordance with accepted academic practice. No use, distribution or reproduction is permitted which does not comply with these terms.
*Correspondence: Stefano Cannicci, cannicci@hku.hk