- 1Instituto de Ciencias Marinas de Andalucía (ICMAN), Consejo Superior de Investigaciones Científicas (CSIC), Puerto Real, Spain
- 2Lamont-Doherty Earth Observatory, Columbia University, Palisades, NY, United States
- 3Department of Earth and Environmental Science, Columbia University, Palisades, NY, United States
- 4Institute of Carbon Cycles, Helmholtz-Zentrum Hereon, Max-Planck Straße, Geesthacht, Germany
Inputs of new nitrogen by cyanobacterial diazotrophs are critical to ocean ecosystem structure and function. Relative to other ocean regions, there is a lack of data on the distribution of these microbes in the western South Atlantic. Here, the abundance of six diazotroph phylotypes: Trichodesmium, Crocosphaera, UCYN-A, Richelia associated with Rhizosolenia (Het-1) or Hemiaulus (Het-2), and Calothrix associated with Chaetoceros (Het-3) was measured by quantitative PCR (qPCR) of the nifH gene along a transect extending from the shelf-break to the open ocean along the Vitória-Trindade seamount chain (1200 km). Using nifH gene copies as a proxy for phylotype abundance, Crocosphaera signals were the most abundant, with a broad distribution throughout the study region. Trichodesmium signals were the second most abundant, with the greatest numbers confined to the warmer waters closer to the coast, and a significant positive correlation with temperature. The average signals for the host-associated diazotrophs (UCYN-A, Het-1, and Het-2) were consistently lower than for the other phylotypes. These findings expand measurements of cyanobacterial diazotroph distribution in the western South Atlantic, and provide a new resource to enhance modeling studies focused on patterns of nitrogen fixation in the global ocean.
Introduction
Diazotrophic cyanobacteria are critical for driving inputs of new nitrogen (N) into the oligotrophic ocean and supporting carbon fixation (Gruber and Galloway, 2008; Zhang et al., 2019), particularly where marine primary production is limited by nitrogen (Moore et al., 2013). However, the composition and distribution of diazotrophs, as well as their contribution to dinitrogen (N2) fixation, in the western South Atlantic remain largely unknown, and this presents a gap in global nitrogen fixation estimates and hampers related modeling efforts (Coles and Hood, 2007; Sohm et al., 2011; Snow et al., 2015; Landolfi et al., 2018; Tang and Cassar, 2019; Wang et al., 2019).
Much of what is known about marine nitrogen fixation is based on studies from the North and South Pacific (e.g., Church et al., 2009; Benavides et al., 2016; Zhang et al., 2019; Zehr and Capone, 2020), and the tropical and subtropical regions of the North Atlantic (e.g., Orcutt et al., 2001; Capone et al., 2005; Montoya et al., 2019; Tang et al., 2019; Palter et al., 2020), with comparatively few observations in the South Atlantic (e.g., Fernández et al., 2010; Sohm et al., 2011; Snow et al., 2015; Wasmund et al., 2015). Visible surface “slicks” of Trichodesmium have been observed in the southwestern Atlantic Ocean (Carvalho et al., 2008; Detoni et al., 2016), which are usually associated with warm surface water of the poleward-flowing Brazil Current (BC), and its meanders at the shelf-break region. Nitrogen fixation is thought to be largely attributable to Trichodesmium in this region (Moore et al., 2014), yet nitrogen fixation by unicellular diazotrophic cyanobacteria Crocosphaera watsonii, host-associated groups like UCYN-A, and diatom-diazotroph associations (DDAs) in other areas of the global ocean can be equal to or greater than those of Trichodesmium (Church et al., 2005a; Foster et al., 2007; Church et al., 2009; Fernández et al., 2010; Wu et al., 2018; Zehr and Capone, 2020).
The distribution and abundance of diazotrophic cyanobacteria can be tracked with measurements of nifH gene copies for different phylotypes, among other approaches (e.g., Karlusich et al., 2021). Database compilations of diazotroph distributions (Luo et al., 2012; Tang and Cassar, 2019) provide critical resources for studying the factors which drive these organisms’ distribution and for modeling nitrogen fixation. However, these observations are regionally skewed, and Tang and Cassar (2019) noted the limitations posed by the lack of observations in the South Atlantic. In the present study, we addressed this knowledge gap by examining diazotroph community structure in the western South Atlantic.
Materials and Methods
Samples were obtained from 18 stations during a cruise aboard the R/V Alpha Crucis (February 2 – 17, 2017) along the Vitória-Trindade Ridge (21°S, 1200 km transect), extending from the continental slope to the western limb of the South Atlantic subtropical gyre (Figure 1). The first three stations were located in meridional positions, following the 1000 m isobath, and subsequent stations followed the deeper isobaths along approximately the same latitude (around 20°S).
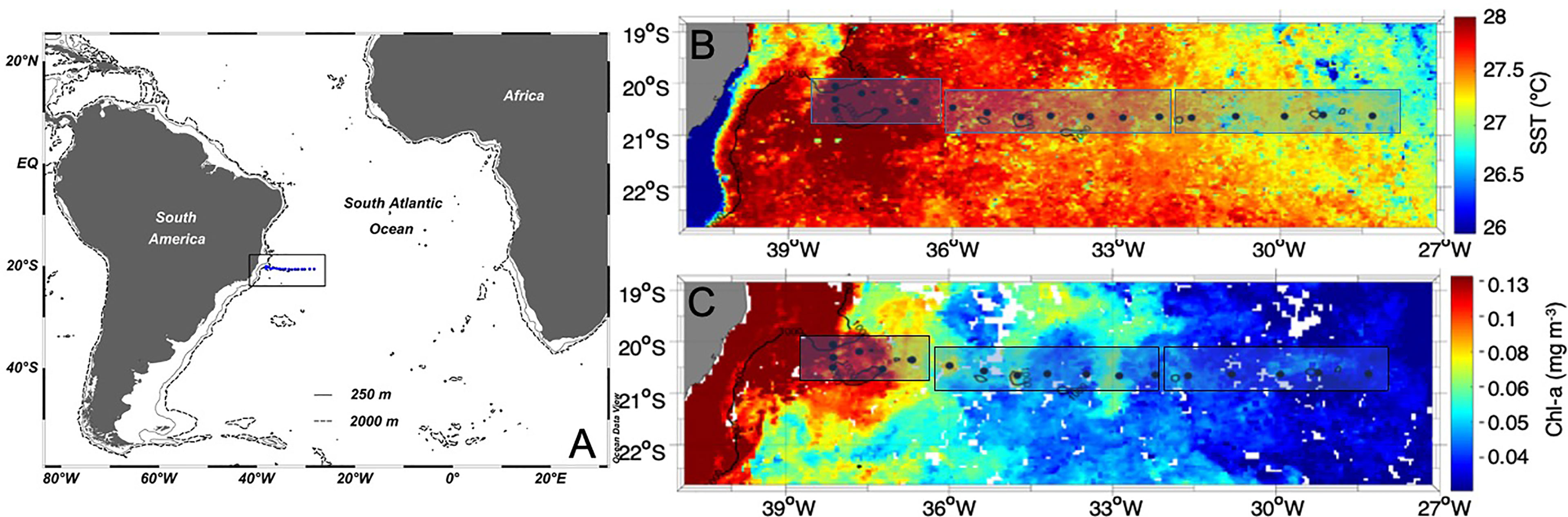
Figure 1 (A) Map of the South Atlantic Ocean and the study area (black rectangle). The blue dots indicate the locations of the sampling stations. (B) Sea surface temperature average (°C) from two 8-day composite images of MODIS-Aqua (4 km) (February 2 – 17, 2017). (C) Chlorophyll-a concentration average (mg m-3) from two 8-day composite images of MODIS-Aqua (L3, 4 km) (February 2 – 17, 2017). The black rectangles indicate the three sectors (West, Middle, and East) derived from a hierarchical cluster analysis of the physical parameters.
Physical Parameters
Temperature, salinity, and fluorescence profiles were measured from the CTD downcast profiles (SeaBird CTD/Carrousel 911+system® with fluorescence sensor – WetLabs ECO-AFL/FL®) that were then used to compute the potential density of seawater and the mixed-layer depth (MLD). The MLD was calculated using the finite difference criteria method (Glover and Brewer, 1988, and Kara et al., 2000), with a temperature difference (dT) that best fit the station profiles (dT=0.1). Water column stability was estimated by the Brunt-Väisälä frequency (BV) (× 10-5 rad2 s-2), which considers the atmospheric gravity (m s-2) and the potential density of seawater (kg m-3). Sea surface temperature (SST) and chlorophyll a (Chl-a) concentration were derived from MODIS-Aqua satellite images (http://oceancolor.gsfc.nasa.gov). Sea Level Anomaly (SLA) products were obtained from Archiving, Validation, and Interpretation of Satellite Oceanographic Data (AVISO) (spatial resolution 1/8° × 1/8°). The water masses found in the study area were Tropical Water (TW) (Silveira et al., 2000), and West South Atlantic Central Water (WSACW) (Liu and Tanhua, 2019).
DNA Sampling, Extraction and qPCR Assays
Water samples were collected from the surface using a bucket, and sub-surface (10 m), DCM, and bDCM samples were collected with niskin bottles on the CTD rosette. Seawater (between 2.5 to 6 L) was filtered through polycarbonate filters (47 mm, 0.2 μm) (Millipore Sigma) using a peristaltic pump. Volumes were variable to keep the total filtration time to less than 2 h. Filters were flash frozen in liquid nitrogen and then stored at -80° C until extraction. DNA was extracted using the DNeasy Plant Mini Kit (Qiagen), with a minor modification to the lysis procedure. Lysis was performed by adding ~ 250 µL zirconium/silica beads (0.5 mm) to each sample with the lysis reagent, Buffer AP1. Extracted DNA was quantified spectrophotometrically using a Take3 micro-volume plate in a Synergy-H1 Hybrid Reader (Biotek).
The abundance of six nitrogen-fixing target phylotypes in the western South Atlantic were assayed by qPCR using primer and probe sequences for nifH (Table S1). This study targeted the unicellular diazotroph, Crocosphaera watsonii (also known as UCYN-B; Moisander et al., 2010); filamentous, Trichodesmium sp. (Church et al., 2005a); UCYN-A (Church et al., 2005a) and three diatom-diazotroph associations (DDAs), Richelia associated with Rhizosolenia (Het-1; Church et al., 2005b), Richelia-associated with Hemiaulus (Het-2; Foster et al., 2007), and Calothrix-associated with Chaetoceros (Het-3; Foster et al., 2007) (Table S1). All analyses were performed in triplicate in a CFX96 Touch Real-Time PCR detection system (Bio-Rad) in a total volume of 20 μL. The reaction mix contained 10 μL SsoAdvanced Universal Probes Supermix (Bio-Rad), 0.6 μM (final concentration) forward and reverse primers, 0.3 μM (final concentration) fluorogenic probe, and 6 μL DNA template. No template (water only) control samples were included in quadruplicate for all qPCR assays. Inhibition controls were included on all plates, where a 105 standard was added to one well of each sample to verify the quantitation cycle (Cq) values were the same as the 105 standard. When inhibition was detected, samples were re-run at a 1:10 dilution. Thermal cycling conditions were 50°C for 2 min, 95°C for 10 min, and 45 cycles of 95°C for 15s followed by 60°C for 1 min. The nifH gene copy abundance for each phylotype was determined from the mean Cq value (n = 3), and standard curves (linear regression) generated for the appropriate primer and probe set. Each primer and probe set spanned 109 to 103 gene copies per reaction. The relationship between Cq values and gene copy number was linear from 103 to 109 target diazotrophs for all of the TaqMan primer and probe sets, and r2 values for linear regressions were > 0.98. The standard curves were prepared from synthesized gBlocks gene fragments (IDT) of the target nifH. Amplification efficiencies were > 90% for all phylotype targets. Gene copy values were normalized by the sample volume to determine nifH copies L-1.
Statistical Analyses
A hierarchical clustering analysis was applied (Euclidean distance and Ward method) (Legendre and Legendre, 1998) using surface physical variables (temperature, salinity, and SLA) to geographically sector the stations. Significant differences in nifH gene copy number were determined using the ANOVA with Tukey test (honestly significant difference – HSD) to compare all the averages across each depth. Pairwise Spearman rank correlations (Spearman’s rho) were calculated between temperature and the nifH gene abundance for each phylotype.
Results
Physical Parameters
The mixed layer depth (MLD) ranged from 20 to 66 m [average of 40 m (±10)], and the average BV above the MLD ranged from 0.1 to 2.7 × 10-5 rad2 s-2, indicating neutral stability of the water column (Pond and Pickard, 1983). Hierarchical clustering analysis divided the transect stations in this region of the western South Atlantic into three sectors, (West, Middle, and East) with temperature and salinity as follows: West [28.3°C ( ± 0.1), 37.5 (± 0.05), n = 6), Middle [27.6°C ( ± 0.2), 37.5 ( ± 0.09), n = 7], and East (27.3°C ( ± 0.2), 37.45 ( ± 0.05), n = 5]. Average temperature was significantly different between sectors (Tukey test (HSD), p-value < 0.02). Temperatures were highest in the West sector, likely associated with the influence of Brazil Current (BC) water (Silveira et al., 2000) (Figure 2 and Figure S1).
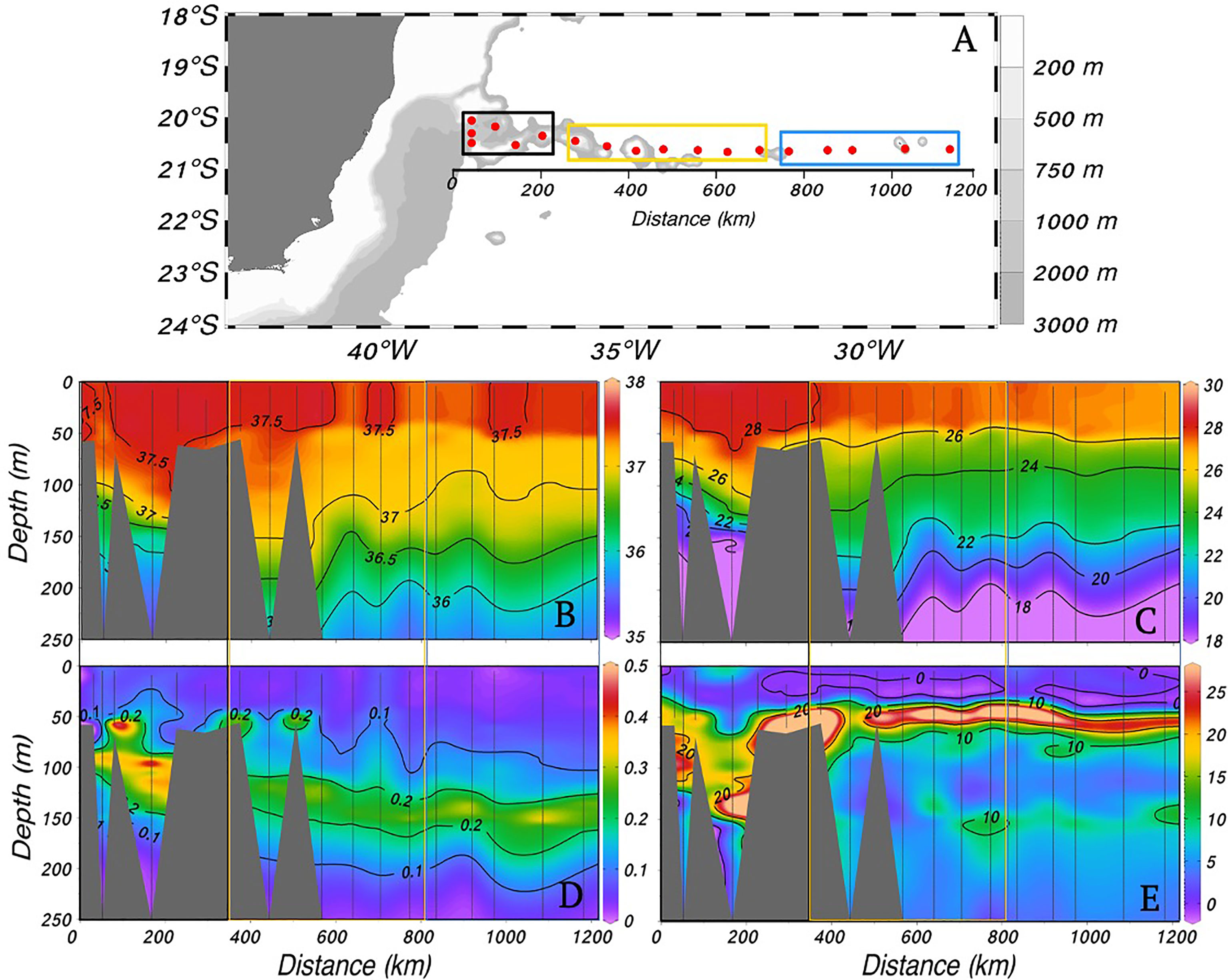
Figure 2 (A) Map of station locations in the western South Atlantic. (B-E) Depth profiles in the upper 250 m along a transect (approximately 20°S) during February 2017: (B) salinity, (C) temperature (°C), (D) chlorophyll concentration from the CTD fluorometer (mg m-3), and (E) Brunt-Vaisala frequency (× 10-5 rad2 s-2). The rectangles show the three sectors (West, Middle, and East) derived from a hierarchical cluster analysis of the physical parameters. In panels B-E, vertical black lines indicate the station locations.
Diazotroph Community Composition
The nifH gene copy number can be used as a proxy for the relative abundance of each phylotype, providing data on community composition. Recent studies have highlighted some of the potential caveats associated with this approach, including the lack of nifH genes in members of a genus previously considered to all be diazotrophic (Delmont, 2021), and the presence of “pseudo”-nifH genes in microbial genomes (primarily anaerobes) that do not carry the full complement of genes for nitrogen fixation (Mise et al., 2021). However, the approach applied here has been extensively validated (e.g. Table S1) and broadly applied for decades (Tang and Cassar, 2019; Karlusich et al., 2021), which makes its application valuable despite the potential caveats.
In the mixed layer (surface and 10 m samples), the diazotroph community in this dataset was dominated by nifH gene copies belonging to the unicellular diazotroph Crocosphaera watsonii (Table 1). In fact, comparing the entire transect average at each depth for each phylotype, the Crocosphaera nifH gene copies were significantly higher than the other phylotypes at each depth (p-value < 0.001) (Table 1). The abundance of Trichodesmium nifH gene copies varied from below the detection limit (BDL) to 209 × 103 nifH copies L-1 with the highest average abundances also observed in the mixed layer (Table S2; Figure 3). The average transect nifH gene copy number from host-associated diazotrophs (Het-1, Het-2, and UCYN-A) was consistently lower than that from the free-living diazotrophs in the mixed layer (Table 1). The UCYN-A nifH gene copies ranged from BDL to 63 × 103 nifH copies L-1 (Table S2). The Het-1 and Het-2 nifH gene copy number in the dataset (all depths and stations) ranged from BDL to 42.6 × 103 nifH copies L-1 and BDL to 8.6 × 103 nifH copies L-1, respectively (Table S2). Het-3 nifH genes were not detected in any sample. The average nifH gene copy number decreased with depth in all groups except Het-1 and Het-2 (Table 1; Figure 3). While the concentration of nifH genes for these two host-associated groups was low and variable overall, the average nifH gene copy number was highest in bDCM samples (Table 1; Figure 3). The Trichodesmium (Spearman’s rho ≥ 0.74 p > 0.001), Crocosphaera (Spearman’s rho ≥ 0.57 p > 0.001), and Het-2 (Spearman’s rho ≥ 0.43 p > 0.001) phylotype abundances significantly positively co-varied with temperature.
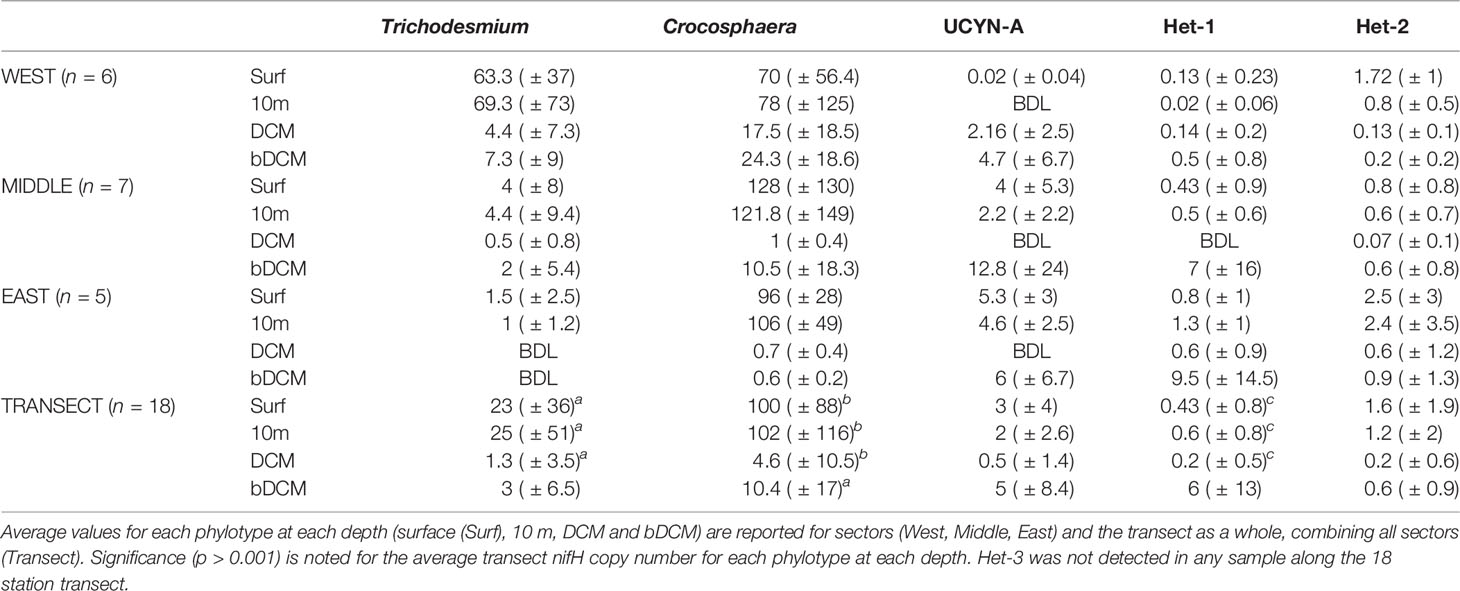
Table 1 The nifH abundances (× 103 copies L-1 ± standard deviation) for each nitrogen-fixing phylotype (Trichodesmium, Crocosphaera, UCYN-A, Het-1 and Het-2).
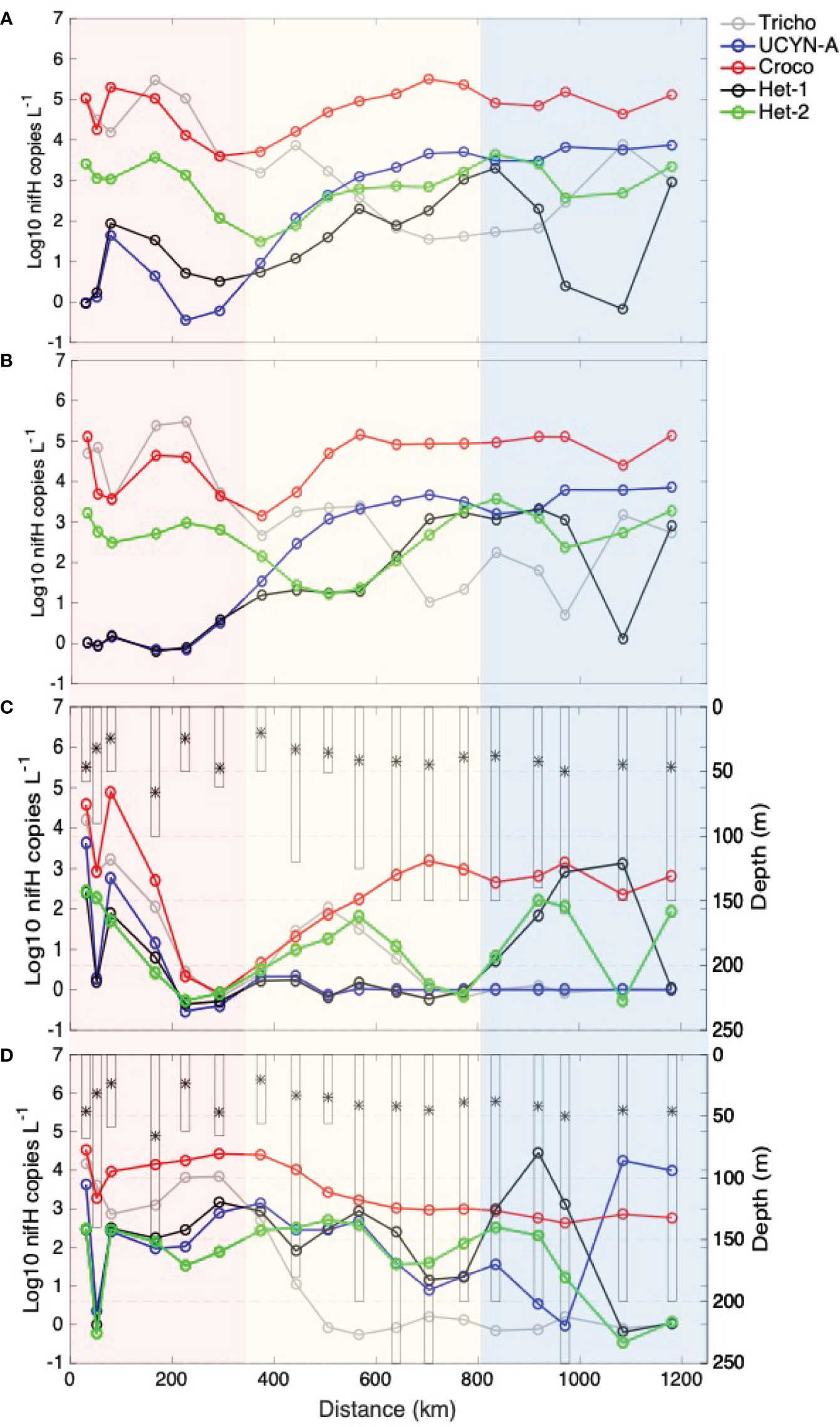
Figure 3 Spatial distribution of nifH gene copies for each nitrogen-fixing phylotype [log10 (copies L-1)]: Trichodesmium (Tricho) (grey), UCYN-A (blue), Crocosphaera (Croco) (red), Het-1 (black), and Het-2 (green), along the sampled transect through the three sectors: West (shaded in pink), Middle (shaded in yellow), and East (shaded in blue) derived from a hierarchical cluster analysis of the physical parameters. Data from each sample depth are plotted as follows: (A) surface, (B) 10 m, (C) DCM, where the DCM depths for each station are shown with bars (D) bDCM, where the bDCM depths for each station are shown with bars. The asterisk (*) denotes the mixed layer depth.
Discussion
Recent synthesis and modeling efforts (Tang and Cassar, 2019; Karlusich et al., 2021) have highlighted that diazotroph distribution and community structure are under-sampled in the South Atlantic. Herein, we addressed this knowledge gap with a western South Atlantic survey of nifH genes representing six diazotroph phylotypes. The transect extended 1200 km, roughly along 20°S, traversing three sectors (West, Middle and East). These sectors were distinguished by temperature, which was significantly higher in the West relative to the other sectors. This likely reflects the influence of tropical water associated with the BC. Temperature significantly positively co-varied with the Trichodesmium, Crocosphaera and Het-2 nifH gene copy abundances, and average Trichodesmium nifH gene copies were highest in the West sector. Water temperature is a well-known factor controlling the distribution of diazotrophs (Luo et al., 2014), and typically their distribution has been thought to be largely restricted to warm, stratified, high solar radiation regions in the tropics and subtropics.
The average nifH gene distribution along the transect was dominated by Crocosphaera signals, and the average mixed layer nifH gene copies (~100 x 103 nifH copies L-1) were in the general range of Crocosphaera nifH gene abundance found in other regions such as the North Pacific (103 to 104 nifH copies L-1; Church et al., 2005a) and the western tropical South Pacific (102 to 106 nifH copies L−1; Stenegren et al., 2018). Recent imaging work by Karlusich et al. (2021) identified Crocosphaera sp. that were clustered together, or associated with large diatoms (e.g., Climacodium), in addition to solitary cells. This highlights the potential for disparate ecologies within the phylotype which may, in part, support the broad distribution observed here. The apparent dominance of the Crocosphaera phylotype in this dataset suggests that it is an important contributor to nitrogen fixation in this region. However, the activity of the phylotypes cannot be accurately extrapolated from these results.
The average mixed layer Trichodesmium nifH gene copies were the second most abundant on the transect (~20 x 103 nifH copies L-1). This average is within the range observed in other systems such as the western tropical South Pacific (102 to 106 nifH gene copies L-1; Stenegren et al., 2018). The distribution of Trichodesmium is known to be highly variable, ranging from concentrations so high they are visible in pigmented surface slicks to non-detectable in this region (Lima et al., 2019). Here, the concentration of Trichodesmium nifH gene copies was significantly positively correlated with temperature, with a stronger correlation coefficient than that observed for Crocosphaera. Crocosphaera occupies a broader temperature range (15-30°C) than Trichodesmium (Fu et al., 2014), which might, in part, underpin the differences in the distribution of these two phylotypes in this study.
A recent study identified the widespread occurrence of a non-diazotrophic Trichodesmium species reconstructed from a global metagenomic survey (Delmont, 2021). By tracking nifH genes, this, and other studies that use this approach, may under-count Trichodesmium spp. Although the presence of the nifH gene for this phylotype assay suggests these Trichodesmium species have the ability to fix nitrogen, we can’t exclude the possibility that some portion of the Trichodesmium population lacks the full complement of genes required for nitrogen fixation. Thus, the values reported here could be an underestimate of Trichodesmium abundance.
The host-associated diazotrophs (UCYN-A, Het-1, and Het-2) had lower average abundances of nifH genes than Crocosphaera or Trichodesmium in the mixed layer. Although UCYN-A is increasingly recognized as a widely-distributed and abundant diazotroph (Martínez-Pérez et al., 2016), the UCYN-A nifH gene copy number observed here was not the dominant signal. This pattern is consistent with a study by Stenegren et al. (2018) in the western tropical South Pacific, where UCYN-A1 and A2 ecotypes were low to non-detectable and the system was dominated by Trichodesmium and Crocosphaera signals. Also, Karlusich et al. (2021) found low abundances of the UCYN-A nifH gene in the South Atlantic Ocean, making up only 3-4% of the bacterioplankton population.
The low copy number, or lack of detection, of nifH genes from the other host-associated diazotrophs Richelia (Het-1 and Het-2) and Calothrix (Het-3) may be, in part, driven by the silica requirement and physiological ecology of their diatom hosts. These DDAs are often observed in the transitional waters of river plumes (Foster et al., 2009; Montoya et al., 2019), although they can be observed in other open ocean systems (Karl et al., 2012; Stenegren et al., 2018). Overall, the low abundances of Het-1 nifH gene copies (102-103 nifH gene copies L-1) among samples over the transect were consistent with studies from the North Atlantic, which have generally found low abundances of Het-1 (~102 nifH copies L-1) (Benavides et al., 2016), and are in the range of Het-1 nifH gene copies found in the western tropical South Pacific (101-105 nifH gene copies L-1). We note that the lack of Het-3 nifH gene copies detected in this study is also consistent with the findings of previous work in the South Pacific and North Atlantic where Het-3 signals were low or non-detectable (Foster et al., 2007; Stenegren et al., 2018). In sum, the community composition of nifH phylotypes observed here is in the range of other systems, but requires more information across a broader swath of the South Atlantic to determine whether the patterns observed here are representative of this region.
A recent study by Tang and Cassar (2019) used a broad compilation of nifH phylotype data to model and then extrapolate phylotype distribution in the global ocean. They predicted that the western South Atlantic would transition from Trichodesmium dominance to UCYN-A dominance at roughly 30°S, but acknowledged the lack of data for this region (Tang and Cassar, 2019). With the dominance of the Crocosphera nifH phylotype, the observations in this study do not support this model output, but are limited in number. Expanding on the data presented here with a larger set of observations and related chemical and physical data would be valuable for future synthesis and modeling efforts.
Here we observed mixed diazotrophic assemblages with the distribution of colonial, free-living, and host-associated forms along the transect. Tracing diazotroph abundance, and the factors that drive both abundance and community structure, are critical to building robust models of marine nitrogen and carbon cycling. This is only becoming more pressing in a changing ocean. The data presented herein provides valuable information on the composition and abundance of the diazotrophic community in an under-sampled region in the western South Atlantic. Further expanding studies such as this in the South Atlantic would help to validate models of diazotroph biogeography and their effects on ecosystem processes such as primary production.
Data Availability Statement
The original contributions presented in the study are included in the article/Supplementary Materials. Further inquiries can be directed to the corresponding author.
Author Contributions
AMSD collected and processed samples. AMSD and AS analyzed the data. AMSD and PHRC contributed to the study implementation. STH and STD supported and advised the molecular methods. All authors discussed the results and contributed to the final manuscript.
Funding
We are grateful for the project support from the Conselho Nacional de Desenvolvimento Científico e Tecnológico (CNPq, Projeto ILHAS Processes: 458583/2013-8). Postdoctoral support to AD was funded by the Coordination for the Improvement of Higher Education Personnel Foundation (CAPES, Proejto REMARSUL processes: 23038.004299/2014-53) for one year at the Lamont-Doherty Earth Observatory – Columbia University. This project was partly supported by the Gordon and Betty Moore Foundation Grant #4886 and US National Science Foundation grant 1737128 to AS.
Conflict of Interest
The authors declare that the research was conducted in the absence of any commercial or financial relationships that could be construed as a potential conflict of interest.
Publisher’s Note
All claims expressed in this article are solely those of the authors and do not necessarily represent those of their affiliated organizations, or those of the publisher, the editors and the reviewers. Any product that may be evaluated in this article, or claim that may be made by its manufacturer, is not guaranteed or endorsed by the publisher.
Supplementary Material
The Supplementary Material for this article can be found online at: https://www.frontiersin.org/articles/10.3389/fmars.2022.856643/full#supplementary-material
References
Benavides M., Moisander P. H., Daley M. C., Bode A., Arístegui J. (2016). Longitudinal Variability of Diazotroph Abundances in the Subtropical North Atlantic Ocean. J. Plankton Res. 38, 662–672. doi: 10.1093/plankt/fbv121
Capone D. G., Burns J. A., Montoya J. P., Subramaniam A., Mahaffey C., Gunderson T., et al. (2005). Nitrogen Fixation by Trichodesmium Spp.: An Important Source of New Nitrogen to the Tropical and Subtropical North Atlantic Ocea. Global Biogeochem. Cycles. 19, 1–17. doi: 10.1029/2004GB002331
Carvalho M., Gianesella S. M. F., Saldanha-Corrêa F. M. P. (2008). Trichodesmium Erythraeum Bloom on the Continental Shelf Off Santos, Southeast Brazil. Braz. J. Oceanogr. 56, 307–311. doi: 10.1590/S1679-87592008000400006
Church M. J., Jenkins B. D., Karl D. M., Zehr J. P. (2005a). Vertical Distribution of Nitrogen-Fixing Phylotypes at Stn ALOHA in the Oligotrophic North Pacific Ocean. Aquat. Microb. Ecol. 38, 3–14. doi: 10.3354/ame038003
Church M. J., Mahaffey C., Letelier R. M., Lukas R., Zehr J. P., Karl D. M. (2009). Physical Forcing of Nitrogen Fixation and Diazotroph Community Stricture in the North Pacific Subtropical Gyre. Global Biogeochem. Cycles. 23, 1–19. doi: 10.1029/2008GB003418
Church M. J., Short C. M., Jenkins B. D., Karl D. M., Zehr J. P. (2005b). Temporal Patterns of Nitrogenase Gene (Nifh) Expression in the Oligotrophic North Pacific Ocean. Appl. Environ. Microbiol. 71, 5362–5370. doi: 10.1128/aem.71.9.5362-5370.2005
Coles V. J., Hood R. R. (2007). Modeling the Impact of Iron and Phosphorus Limitations on Nitrogen Fixation in the Atlantic Ocean. Biogeosciences 4, 455–479. doi: 10.5194/bg-4-455-2007
Delmont T. O. (2021). Discovery of Nondiazotrophic Trichodesmium Species Abundant and Widespread in the Open Ocean. Proc. Natl. Acad. Sci. U.S.A. 118, 1–10. doi: 10.1073/pnas.2112355118
Detoni A. M. S., Ciotti A. M., Calil P. H. R., Tavano V. M., Yunes J. S. (2016). Trichodesmium Latitudinal Distribution on the Shelf Break in the Southwestern Atlantic Ocean During Spring and Autumn. Global Biogeochem. Cycles. 30, 1738–1753. doi: 10.1002/2016GB005431
Fernández A., Mouriño-Carballido B., Bode A., Varela M., Marañón E. (2010). Latitudinal Distribution of Trichodesmium Spp. And N2 Fixation in the Atlantic Ocean. Biogeosciences 7, 3167–3176. doi: 10.5194/bg-7-3167-2010
Foster R. A., Subramaniam A., Mahaffey C., Carpenter E. J., Capone D. G., Zehr J. P. (2007). Influence of the Amazon River Plume on Distributions of Free-Living and Symbiotic Cyanobacteria in the Western Tropical North Atlantic Ocean. Limnol. Oceanogr. 52, 517–532. doi: 10.4319/lo.2007.52.2.0517
Foster R. A., Subramaniam A., Zehr J. P. (2009). Distribution and Activity of Diazotrophs in the Eastern Equatorial Atlantic. Environ. Microbiol. 11, 741–750. doi: 10.1111/j.1462-2920.2008.01796.x
Fu F.-X., Yu E., Garcia N. S., Gale J., Luo Y., Webb E. A., et al. (2014). Differing Responses of Marine N2 Fixers to Warming and Consequences for Future Diazotroph Community Structure. Aquat. Microb. Ecol. 72, 33–46. doi: 10.3354/ame01683
Glover D. M., Brewer P. G. (1988). Estimates of Wintertime Mixed Layer Nutrient Concentrations in the North Atlantic. Deep-Sea Res. 35 (9), 1525–1546. doi: 10.1016/0198-0149(88)90101-X
Gruber N., Galloway J. N. (2008). An Earth-System Perspective of the Global Nitrogen Cycle. Nature 451 (17), 293–296. doi: 10.1038/nature06592
Kara A. B., Rochford P. A., Hurlburt H. E. (2000). An Optimal Definition for Ocean Mixed Layer Depth. J. Geophys. Res. 105, 16,803–16,821. doi: 10.1029/2000JC900072
Karl D. M., Church M. J., Dore J. E., Letelier R. M., Mahaffey C. (2012). Predictable and Efficient Carbon Sequestration in the North Pacific Ocean Supported by Symbiotic Nitrogen Fixation. Proc. Natl. Acad. Sci. U.S.A. 109, 1842–1849. doi: 10.1073/pnas.1120312109
Karlusich J. J. P., Pelletier E., Lombard F., Carsique M., Dvorak E., Colin S., et al. (2021). Global Distribution Patterns of Marine Nitrogen-Fixers by Imaging and Molecular Methods. Nat. Commun. 12:4160, 1–18. doi: 10.1038/s41467-021-24299-y
Landolfi A., Kahler P., Koeve W., Oschlies A. (2018). Global Marine N2 Fixation Estimates: From Observations to Models. Front. Microbiol. 9, 2112. doi: 10.3389/fmicb.2018.02112
Legendre P., Legendre L. (1998). “Multiple Linear Regression,” in Numerical Ecology. Eds. Legendre P., Legendre L. (Amsterdam: Elsevier Science B. V), 517–525.
Lima C. R., Mendes C. R. B., Tavano V. M., Detoni A. M. S., Secchi E. R. (2019). Chemotaxonomy-Based Mapping of Phytoplankton Communities in the Subtropical Southwestern Atlantic Ocean, With Emphasis on the Marine Cyanobacterium Trichodesmium. Prog. Oceanogr. 172, 77–88. doi: 10.1016/j.pocean.2019.01.008
Liu M., Tanhua T. (2019). Characteristics of Water Masses in the Atlantic Ocean Based on GLODAPv2 Data. J. Ocean Sci. Discuss. 139, 139–181. doi: 10.5194/os-2018-139
Luo Y.-W., Doney S. C., Anderson L. A., Benavides M., Berman-Frank I., Bode A., et al. (2012). Database of Diazotrophs in Global Ocean: Abundance, Biomass and Nitrogen Fixation Rates. Earth Syst. Sci. Data 4, 47–73. doi: 10.5194/essd-4-47-2012
Luo Y.-W., Lima I. D., Karl D. M., Deutsch C. A., Doney S. C. (2014). Data-Based Assessment of Environmental Controls on Global Marine Nitrogen Fixation. Biogeosciences 11, 691–708. doi: 10.5194/bg-11-691-2014
Martínez-Pérez C., Mohr W., Loscher C. R., Dekaezemacker J., Littmann S., Yilmaz P., et al. (2016). The Small Unicellular Diazotrophic Symbiont, UCYN-A, is a Key Player in the Marine Nitrogen Cycle. Nat. Microbiol. 1, 16163. doi: 10.1038/nmicrobiol.2016.163
Mise K., Masuda Y., Senoo K., Itoh H. (2021). Undervalued pseudo-nifH Sequences in Public Databases Distort Metagenomic Insights Into Biological Nitrogen Fixers. AMS mSphere. 6 (6), e00785-21. 1-12. doi: 10.1128/msphere.00785-21
Moisander P. H., Beinart R. A., Hewson I., White A. E., Johnson K. S., Carlson C. A., et al. (2010). Unicellular Cyanobacterial Distributions Broaden the Oceanic N2 Fixation Domain. Science 327, 1512–1514. doi: 10.1126/science.1185468
Montoya J. P., Landrum J. P., Weber S. C. (2019). Amazon River Influence on Nitrogen Fixation in the Western Tropical North Atlantic. J. Mar. Res. 77, 191–213. doi: 10.1357/002224019828474278
Moore R. M., Kienast M., Fraser M., Cullen J. J., Deutsch C., Dutkiewicz S., et al. (2014). Extensive Hydrogen Supersaturations in the Western South Atlantic Ocean Suggest Substantial Underestimation of Nitrogen Fixation. J. Geophys. Res. Oceans 119, 4340–4350. doi: 10.1002/2014JC010017
Moore C. M., Mills M. M., Arrigo K. R., Berman-Frank I., Bopp L., Boyd P. W., et al. (2013). Processes and Patterns of Oceanic Nutrient Limitation. Nat. Geosci. 6, 701–710. doi: 10.1038/NGEO1765
Orcutt K. M., Lipschultz F., Gundersen K., Arimoto R., Michaels A. F., Knap A. H., et al. (2001). A Seasonal Study of the Significance of N2 Fixation by Trichodesmium Spp. At the Bermuda Atlantic Time-Series Study (BATS) Site. Deep-Sea Res. Pt II 48, 1583–1608. doi: 10.1016/S0967-0645(00)00157-0
Palter J. B., Ames E. J., Benavides M., Neto A. G., Granger J., Moisander P. H., et al. (2020). High N2 Fixation in and Near the Gulf Stream Consistent With a Circulation Control on Diazotrophy. J. Geophys. Res. Lett. 47, e2020GL089103. doi: 10.1029/2020GL089103
Silveira I. C., Schmidt A. C. K., Campos E. J. D., de Godoi S. S., Ikeda Y. (2000). A Corrente do Brasil Ao Largo Da Costa Leste Brasileira. Rev. Bras. Oceanogr. 48, 171–183. doi: 10.1590/S1413-77392000000200008
Snow J. T., Schlosser C., Woodward E. M. S., Mills M. M., Achterberg E. P., Mahaffey C., et al. (2015). Environmental Controls on the Biogeography of Diazotrophy and Trichodesmium in the Atlantic Ocean. Global Biogeochem. Cycles. 29, 865–884. doi: 10.1002/2015GB005090
Sohm J. A., Hilton J. A., Noble A. E., Zehr J. P., Saito M. A., Webb E. A. (2011). Nitrogen Fixation in the South Atlantic Gyre and the Benguela Upwelling System. J. Geophys. Res. Lett. 38, L16608. doi: 10.1029/2011GL048315
Stenegren M., Caputo A., Berg C., Bonnet S., Foster R. A. (2018). Distribution and Drives of Symbiotic and Free-Living Diazotrophic Cyanobacteria in the Western Tropical South Pacific. Biogeosciences 15, 1559–1578. doi: 10.5194/bg-15-1559-2018
Tang W., Cassar N. (2019). Data-Driven Modeling of the Distribution of Diazotrophs in the Global Ocean. J. Geophys. Res. Lett. 46, 12,258–12,269. doi: 10.1029/2019GL084376
Tang W., Wang S., Fonseca-Batista D., Dehairs F., Gifford S., Gonzalez A. G., et al. (2019). Revisiting the Distribution of Oceanic N2 Fixation and Estimating Diazotrophic Contribution to Marine Production. Nat. Commun. 10:831, 1–10. doi: 10.1038/s41467-019-08640-0
Wang W. L., Moore J. K., Martiny A. C., Primeau F. W. (2019). Convergent Estimates of Marine Nitrogen Fixation. Nature 566, 205–211. doi: 10.1038/s41586-019-0911-2
Wasmund N., Struck U., Hansen A., Flohr A., Nausch G., Gruttmuller A., et al. (2015). Missing Nitrogen Fixation in the Benguela Region. Deep Sea Res. I 106, 30–41. doi: 10.1016/j.dsr.2015.10.007
Wu C., Fu F., Sun J., Thangaraj S., Pujari L. (2018). Nitrogen Fixation by Trichodesmium and Unicellular Diazotrophs in the Northern South China Sea and the Kuroshio in Summer. Sci. Rep. 8, 2415. doi: 10.1038/s41598-018-20743-0
Zehr J. P., Capone D. G. (2020). Changing Perspectives in Marine Nitrogen Fixation. Science 368, eaay9514. doi: 10.1126/science.aay9514
Keywords: nifH gene abundance, Crocosphaera, UCYN-A, diatom diazotroph association, diazotroph, western South Atlantic, Trichodesmium
Citation: Detoni AMS, Subramaniam A, Haley ST, Dyhrman ST and Calil PHR (2022) Cyanobacterial Diazotroph Distributions in the Western South Atlantic. Front. Mar. Sci. 9:856643. doi: 10.3389/fmars.2022.856643
Received: 17 January 2022; Accepted: 04 April 2022;
Published: 03 May 2022.
Edited by:
Punyasloke Bhadury, Indian Institute of Science Education and Research Kolkata, IndiaReviewed by:
Taketoshi Kodama, Japan Fisheries Research and Education Agency (FRA), JapanSubhajit Basu, Max Planck Society, Germany
Copyright © 2022 Detoni, Subramaniam, Haley, Dyhrman and Calil. This is an open-access article distributed under the terms of the Creative Commons Attribution License (CC BY). The use, distribution or reproduction in other forums is permitted, provided the original author(s) and the copyright owner(s) are credited and that the original publication in this journal is cited, in accordance with accepted academic practice. No use, distribution or reproduction is permitted which does not comply with these terms.
*Correspondence: Amália Maria Sacilotto Detoni, YW1hbGlhLmRldG9uaUBjc2ljLmVz