- 1Engineering Research Center of Mindong Aquatic Product Deep-Processing, Fujian Province University; School of Life Sciences, Ningde Normal University, Ningde, China
- 2Key Laboratory of Healthy Mariculture for the East China Sea, Ministry of Agriculture, Fisheries College, Jimei University, Xiamen, China
- 3Fisheries Technical Extension Station of Zhangzhou, Zhangzhou, China
- 4Fujian Agriculture and Forestry University, Fuzhou, China
Ubiquitination is an essential post-translational modification of proteins and involved in many physiological processes, including sperm differentiation, oocyte maturation, and embryonic development. Among them, the ubiquitin-conjugating enzyme E2 plays a crucial role in gametogenesis. However, the participation of E2 in the regulation of the gonad development of crustaceans is still very limited, especially for oogenesis. In this research, we isolated and identified full-length complementary DNA of ubiquitin-conjugating enzyme E2 from mud crab (Scylla paramamosain) (Sp-Ubc2), detected distribution in different tissues by real-time quantitative PCR, and described expression patterns during oogenesis by in situ hybridization. The results demonstrated that the deduced proteins with a ubiquitin-conjugating (UBC) catalytic domain was clustered with other arthropods. In addition, the Sp-Ubc2 was widely distributed in different tissues of female individuals and kept the highest expression level in the ovary. Moreover, the expression level of Sp-Ubc2 changed significantly during oogenesis and reached peak in secondary and tertiary vitellogenesis stages. At the same time, the Sp-Ubc2 signal in the ovary gradually concentrated in nuclei. These results indicated that Sp-Ubc2 might play important roles in the cell cycle of oogenesis. This study would provide insights into the regulatory mechanism of gonad development in S. paramamosain, and it also enriched the theoretical basis of ubiquitin family genes involved in crustacean gonad development.
Introduction
Ubiquitin is a highly conserved protein composed of 76 amino acid residues, which is widely present in eukaryotes (Swatek and Komander, 2016). The ubiquitin can be covalently attached to a target protein to influence protein stability and biological function, which is called ubiquitination (Choo and Zhang, 2009). As an important type of post-translational modification of proteins, ubiquitination is mainly involved in the intracellular translocation of proteins, chromosomal organization, DNA repair, cell cycle control, and apoptosis (Dye and Schulman, 2007; Gao et al., 2017). At present, there are at least three enzymes of ubiquitin-activating enzyme E1, ubiquitin-conjugating enzyme E2, and ubiquitin ligase E3 that are necessary for ubiquitination (Neutzner and Neutzner, 2012). In the process of ubiquitination, E1, E2, and E3 cooperate to transfer ubiquitin molecules to target proteins, in which E1 activates the ubiquitin in an ATP-dependent manner and delivers to E2. Then, E3 transfers the E2-bound activated ubiquitin to the target protein (Hershko et al., 1983).
For ubiquitin enzymes, E2 contains a ubiquitin-conjugating (UBC) domain, spanning 140–200 amino acids in length, which mediates the interaction between E2 and E3 (Gao et al., 2017). In ubiquitination, E2 plays a key role in regulating the fate and function of target proteins (Li et al., 2020). E2 genes exist as a multigene family that has been identified in model organisms, including Saccharomyces cerevisiae (Seufert et al., 1990), Caenorhabditis elegans (Jones et al., 2002), Homo sapiens (Jiang and Beaudet, 2004), Arabidopsis thaliana (Kraft et al., 2005), and Drosophila melanogaster (Michelle et al., 2009; Nagy et al., 2012). Currently, E2 genes in more and more economic species are cloned and researched. In plants, E2 responds to environmental stress and influence development. For example, E2 is involved in abiotic stresses and hormone treatments in rice (Zhiguo et al., 2015) and maize (Jue et al., 2015), while E2 changes the fruit-ripening process and response to cold and heat stress in tomato (Wang et al., 2014) and grape (Gao et al., 2017). In animals, the levels of E2 are increased when the fish or crustaceans are exposed to pesticide and an oxidative state (Horst et al., 2007; Sanz et al., 2012). However, limited information can describe the specific function and mechanism of E2 in these species.
Additionally, the ubiquitin enzymes, especially for E2, were found to possibly regulate the development process in Drosophila (Ohlmeyer and Schüpbach, 2003; Chen et al., 2009; Lu et al., 2013). The ubiquitination, a highly selective proteolysis pathway, is also the basis of cell cycle regulation. In addition, the eukaryotic cell cycle depends on precise and rigorous regulation of various factors, the core of which is the periodic synthesis and destruction of cyclins (Johnson and Walker, 1999). Cyclin plays a crucial role in mitosis and meiosis and is an important regulator of egg or sperm development (Jeong et al., 2011). Therefore, cyclin ubiquitination can mediate germ cell proliferation, differentiation, and apoptosis. In a previous study, the Drosophila E2-encoding gene–mediated degradation of Cyclin A was essential for the maintenance of germline stem cells (Chen et al., 2009). Subsequently, the conserved E2 polyubiquitin gene was proven that was essential for male meiotic cell cycle progression and germ cell differentiation in Drosophila (Lu et al., 2013). Like Drosophila, E2 was identified and characterized in the testes of the giant tiger shrimp (Penaeus monodon) as other sex-related genes and suggested that this gene involved gonadal development (Leelatanawit et al., 2008). Subsequently, the UBE2r showed significantly different expression levels in developing the testis and ovary of penaeid shrimp (Marsupenaeus japonicus), suggesting that UBE2r had an important role in oogenesis and spermatogenesis (Shen et al., 2009). Therefore, E2 is also related to the development and regulation of aquatic organisms.
The mud crab (Scylla paramamosain) is one of economically important marine crabs with commercial and nutritional values (Wan et al., 2019). S. paramamosain has the advantages of a large body size and rapid growth rate, which has been one of artificial cultivation crabs in China and South Asia (Chen and Wang, 2019; Wan et al., 2021). In recent years, the demand for S. paramamosain has been increasing, while the amount of wild-caught resources has been decreasing, which significantly restricts the healthy and sustainable development of S. paramamosain aquaculture. Therefore, the breeding techniques related to improving spawning and the breeding survival rate and decreasing the cost have become the current main tasks in the aquaculture production of the mud crab. Additionally, a better understanding of the molecular regulatory mechanisms involved in gonad development, sex determination, and gametogenesis would be helpful for crab aquaculture. In a previous study, we identified and described the expression profiles of ubiquitin C-terminal hydrolases Sp-uchl3 and Sp-uchl5 during the gonad of S. paramamosain; it indicated that these genes participated in the development process (Han et al., 2018). In this study, we aimed to identify the E2 of S. paramamosain (Sp-Ubc2). Then, we detected the distribution of Sp-Ubc2 in different tissues of female individuals and the expression level of Sp-Ubc2 at different development stages of ovary by real-time quantitative PCR (RT-qPCR). At the same time, we described the specific expression patterns of Sp-Ubc2 during oogenesis by in situ hybridization (ISH). This results of this project would lay a foundation for further research about the molecular mechanism of the gonad development of crab, especially for oogenesis.
Materials and Methods
Animals and Tissue Collection
S. paramamosain was purchased from a local jimei market (Xiamen, China), and a total of 25 were used for this study. According to the external morphology, color, gonadosomatic index (GSI), and histological feature of previous studies, the ovary at different development stages was classified into five periods: O1 (proliferation, GSI=0.57 ± 0.47), O2 (pre-vitellogenesis, GSI=2.19 ± 0.21), O3 (primary vitellogenesis, GSI=3.68 ± 0.20), O4 (secondary vitellogenesis, GSI=7.81 ± 0.94), and O5 (tertiary vitellogenesis, GSI=10.49 ± 0.49) (Jia et al., 2013; Wang et al., 2021). At least three crabs were used for the experiments at each developmental stage. The various tissues including muscle, testis, ovary, brain, eyestalk, gill, heart, hepatopancreas, intestines, and stomach were dissected and stored in liquid nitrogen for future use. In addition, some ovaries at different developmental stages were preserved in liquid nitrogen for RNA extraction, and the others were fixed in 4% paraformaldehyde solution for ISH.
RNA Isolation and cDNA Synthesis
The total RNA of different tissues was isolated as described in the previous study (Han et al., 2018). The residual DNA contamination was eliminated using RNase-free DNase I at 37°C for 30 min. Additionally, the first-strand cDNA was reverse-transcribed using the oligo-dT-adaptor primer and Moloney Murine Leukemia Virus (M-MLV) reverse transcriptase (Promega, Madison, WI, USA), following the instruction.
Cloning of Sp-Ubc2
Based on our Expressed Sequence Tags (EST) database from our laboratory, the partial fragment of Sp-Ubc2 cDNA sequences was obtained. According to the above fragment, the rapid-amplification of cDNA ends (RACE) specific primers were designed. Following the manual, 5’- and 3’-RACE were used to isolate the full-length cDNA sequence using the SMARTer RACE cDNA Amplification Kit (BD Biosciences Clontech, Palo Alto, CA, USA). Then, the open reading frame (ORF) was validated by head-to-toe PCR. The PCR products were separated by electrophoresis on 1% agarose gel, then purified and subcloned into pMD™19-T Vector (Takara, Beijing, China). Finally, the bacteria solution was sent for sequencing at Sangon (Shanghai, China). All primers used in this study are listed in Table 1.
Bioinformatics Analysis
The bioinformatics of sequence was done as described in the previous study (Han et al., 2018), including the analysis of signal peptide, isoelectric point, molecular weight, cellular localization, phosphorylation sites, multiple alignments, three-dimensional structure, and phylogenic tree.
Real-Time Quantitative PCR and In Situ Hybridization
The expression of Sp-Ubc2 in different tissues of female individuals and different ovary development stages was quantified by RT-qPCR as described in the previous study (Han et al., 2018). The results were analyzed by the comparative Ct method. In this method, the expression of Sp-Ubc2 was normalized against 18S expression, generating a ΔCt. Relative expression was calculated according to the equation, 2-ΔΔCt.
The Sp-Ubc2 mRNA distribution was detected in different ovary development stages by ISH. The ovary samples were dehydrated through a graded series of ethanol, embedded in paraffin wax, and cut into 7 μm for H&E staining or in situ hybridization. In addition, the protocols of ISH followed the methods described previously (Zhang et al., 2003), with a few modifications as detailed below. Briefly, approximately 600-bp Sp-Ubc2 fragment was inserted into the pGEM-T Easy vector (Promega, Madison, WI, USA). Sense and antisense RNA probes were synthesized by in vitro transcription from a vector under the drive of the T7 or SP6 promoter with the Digoxigenin (DIG) RNA Labeling Kit (Roche, Mannheim, Germany). The sections were dewaxed, rehydrated, and digested, then performed with the probes at 65°C for 14 h. Stained samples were observed and photographed through a Nikon Eclipse E600 microscope, using a Nikon DC50NN imaging system.
Statistical Analysis
The software SPSS 26 was used for statistical analyses. The data of RT-qPCR expression were subjected to one-way ANOVA, followed by Tukey’s test. All data are presented as mean ± standard error (SE) of the different tissues or development stages. Differences were considered significant at P < 0.05.
Results
Molecular Characterization of Sp-Ubc2cDNA
A part fragment of Sp-Ubc2 was obtained from the EST library constructed by our laboratory, and the remaining unknown regions were isolated through 3’- and 5’-RACE. The full-length 971-bp Sp-Ubc2 cDNA (Genbank : FJ265877) was produced by overlapping the sequences of all fragments, which consisted of a 5’UTR of 69 bp, an ORF of 483 bp, and a 3’UTR of 419-bp (Figure 1). Then, the ORF was validated by head-to-toe PCR, which encodes 160 amino acids. Additionally, the molecular mass and theoretical isoelectric point (pI) of deduced protein sequence were 18.3kDa and 8.64, respectively. Sequence analysis by ProScan revealed that Sp-Ubc2 protein has 8 motifs, including 1 cAMP- and cGMP-dependent protein kinase phosphorylation site (K48-T51), 1 protein kinase C site (T108-K110), 2 casein kinase II phosphorylation sites(T51-E54, S95-D98), 1 N-myristoylation phosphorylation site(G3-R8), 1 N-myristoylation sites (P46-K49), and 2 ubiquitin conjugation enzyme active sites (F82-L96, F82-L97) (Figure 1).
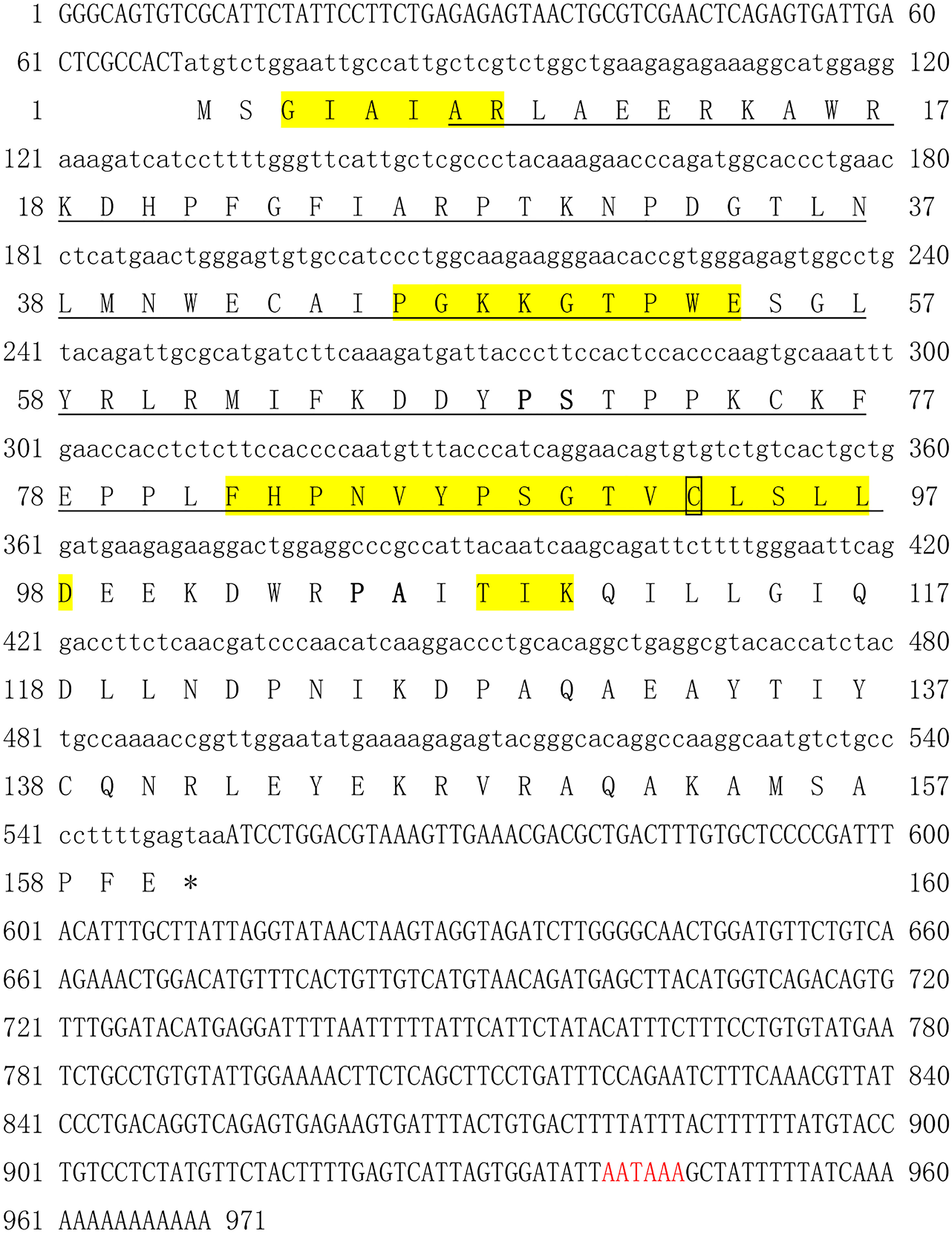
Figure 1 The full length of Sp-Ubc2 cDNA and deduced amino acid sequence. The underlined sequences showed the E2 catalytic domain; 8 motifs in the domain were labeled with yellow shading; the polyadenylation signal (AATAA), active cysteine, and amino acids interacted with E3 were marked red, box, and bold, respectively.
Structure and Phylogenetic Analysis of Sp-UBC 2 Protein
The result predicted by the software of PSORT II indicated that Sp-UBC 2 protein may be mainly located in the nucleus (60.9%). The two-dimensional (2D) structure of Sp-UBC 2 showed 4-α helix, 5 extended strands, and several random coils (Figure 2A). In addition, the three-dimensional (3D) structure of Sp-UBC 2 was at the SWISS-MODEL server using an automatically matched template (protein data bank code : 2pe6A); the structure of α-helix and β-sheet was clearly observed in this picture (Figure 2B). Moreover, a Cys-site was also found in the domain (Figure 2B).
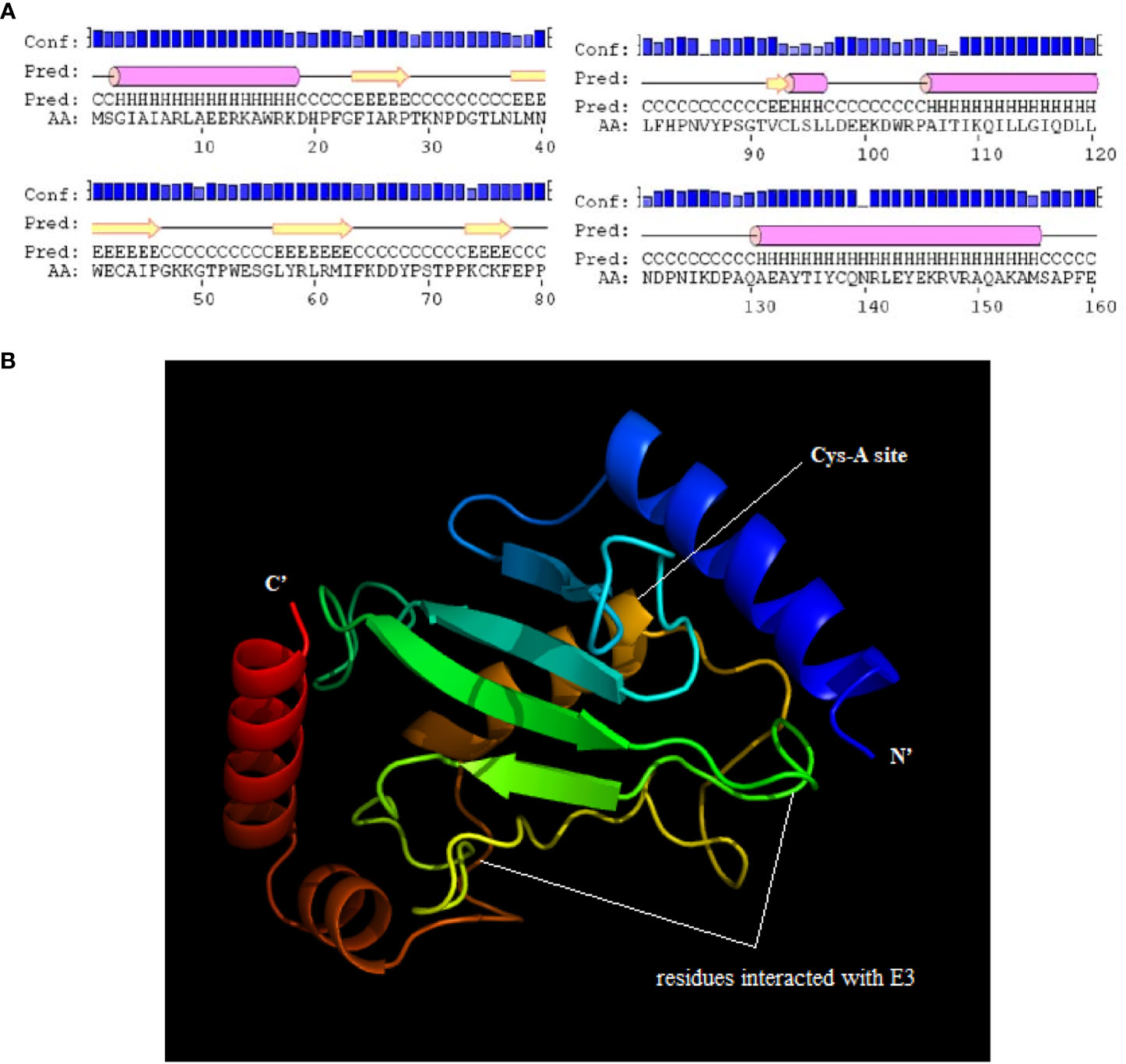
Figure 2 The predicted two-dimensional (2D) and three-dimensional (3D) structure of Sp-UBC 2. (A) the Pred showed the predicted 2D structure. (B) The predicted 3D structure of Sp-UBC 2. Conf: credibility; AA: amino acid sequence; cylinder:α helix; arrow: extended strand; line: random coil.
The homology analysis was conducted using Sp-UBC 2 with UBC 2 from other organisms, which indicated that the catalytic domain and active Cys-A site were evolutionarily conserved across mammals, arthropods, fishes, birds, echinozoa, and fungi (Figure 3). The phylogenetic analysis of UBC 2 protein demonstrated that the above species were clustered into three clusters: arthropod, cnidarian, and vertebrate. The Sp-UBC 2 was clustered in the first cluster with other arthropods, such as Procambarus clarkii, Hyalella Azteca, and Penaeus vannamei (Figure 4).
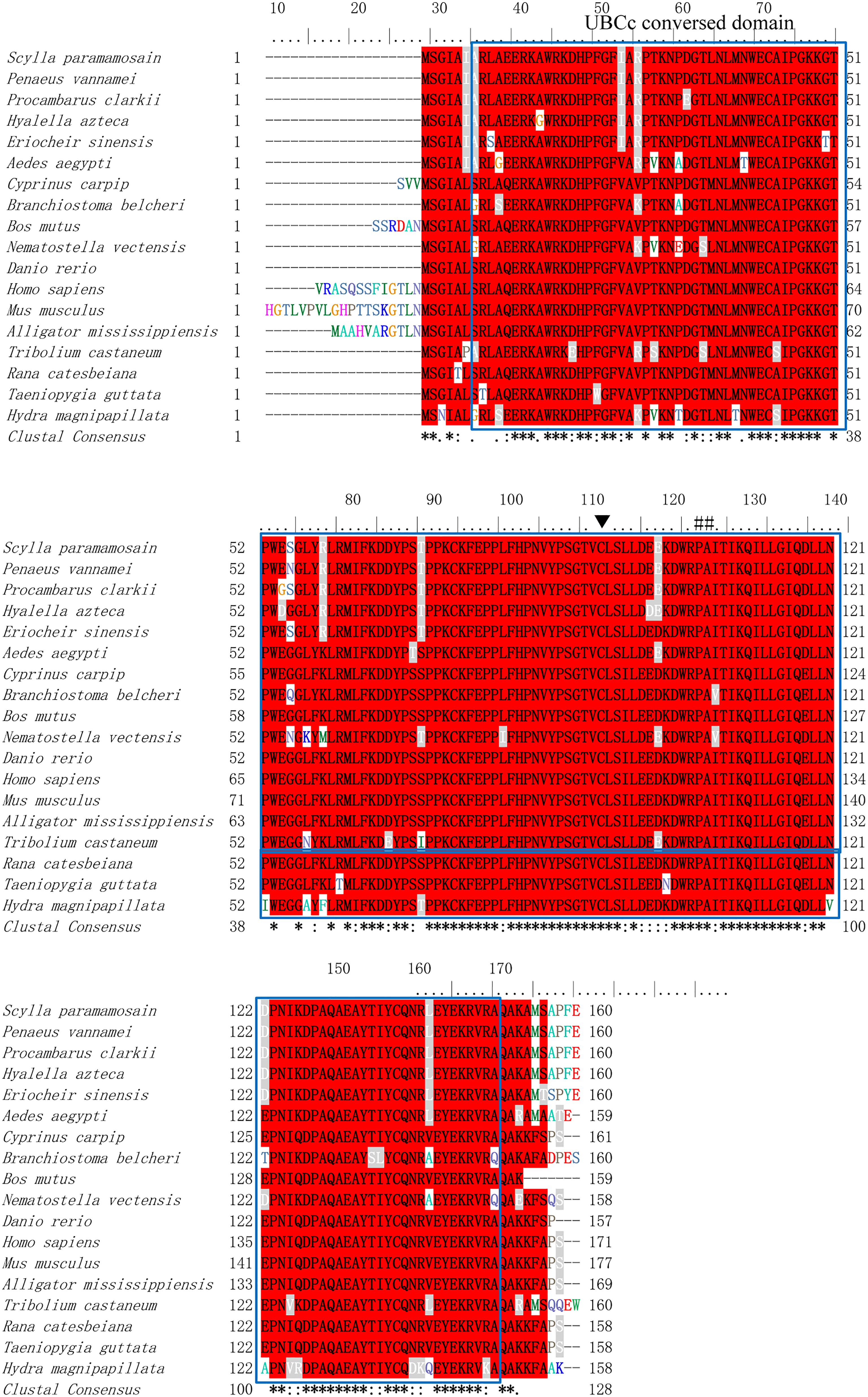
Figure 3 The homology analysis of Sp-UBC 2 in S. paramamosain and other organisms. The sequence used in homology analysis was as follows: Scylla paramamosain: ACJ03792; Penaeus vannamei: AFU93453; Procambarus clarkii: AFV69238; Hyalella azteca: XP_018014422; Eriocheir sinensis: ADW82097; Ceratosolen solmsi marchali: XP_011500210; Aedes aegypti: XP_001654033; Cyprinuscarpip: KTG33102; Cyprinuscarpip: KTG33102; Bos mutus: ELR62878; Nematostella vectensis: XP_001640489; Danio rerio: NP_571908; Homo sapiens: BAD92225; Mus musculus: EDL22414; Alligator mississippiensis: XP_019355948. blue box: the conversed UBCc domain; ▼: the conversed Cys site; ##: the residue of E2 interaction with E3.
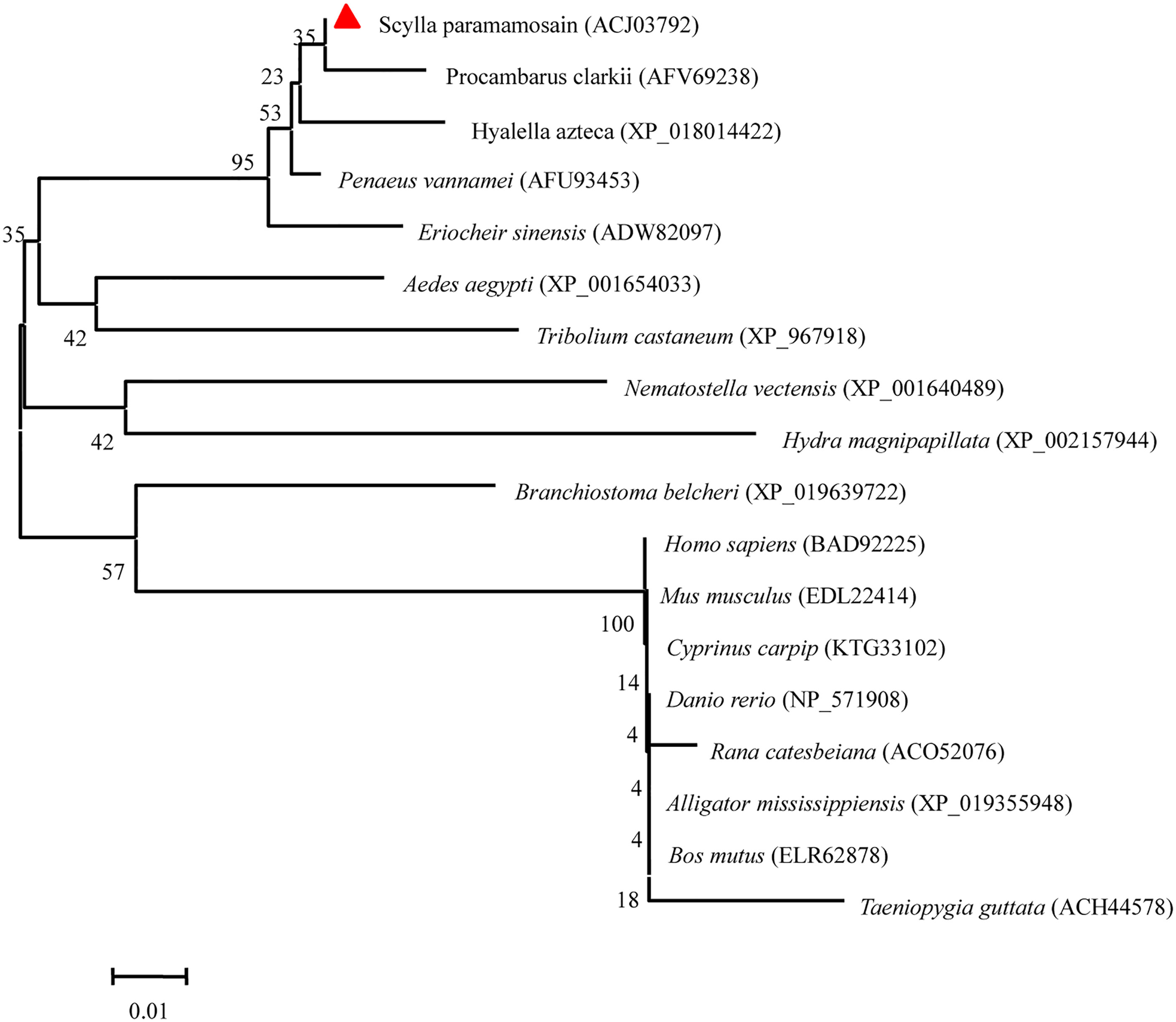
Figure 4 The phylogenetic analysis of Sp-UBC 2 in S. paramamosain and other organisms. The GenBank codes of species were described in brackets. The Sp-UBC 2 was marked by a red triangle. The numbers adjacent to nodes indicated the bootstrap percentage value for 1,000 replicates.
The Distribution of Sp-Ubc2 in Female Different Tissues
RT-qPCR analysis showed that the Sp-Ubc2 distributed various tissues of female individuals, including the heart, brain, muscle, and ovary (Figure 5A). However, the expression level of Sp-Ubc2 in the ovary was the highest, compared with other tissues (Figure 5A). There was a significant difference between the ovary and other tissues, which revealed that the high expression of Sp-Ubc2 might play crucial roles in ovary development in S. paramamosain.
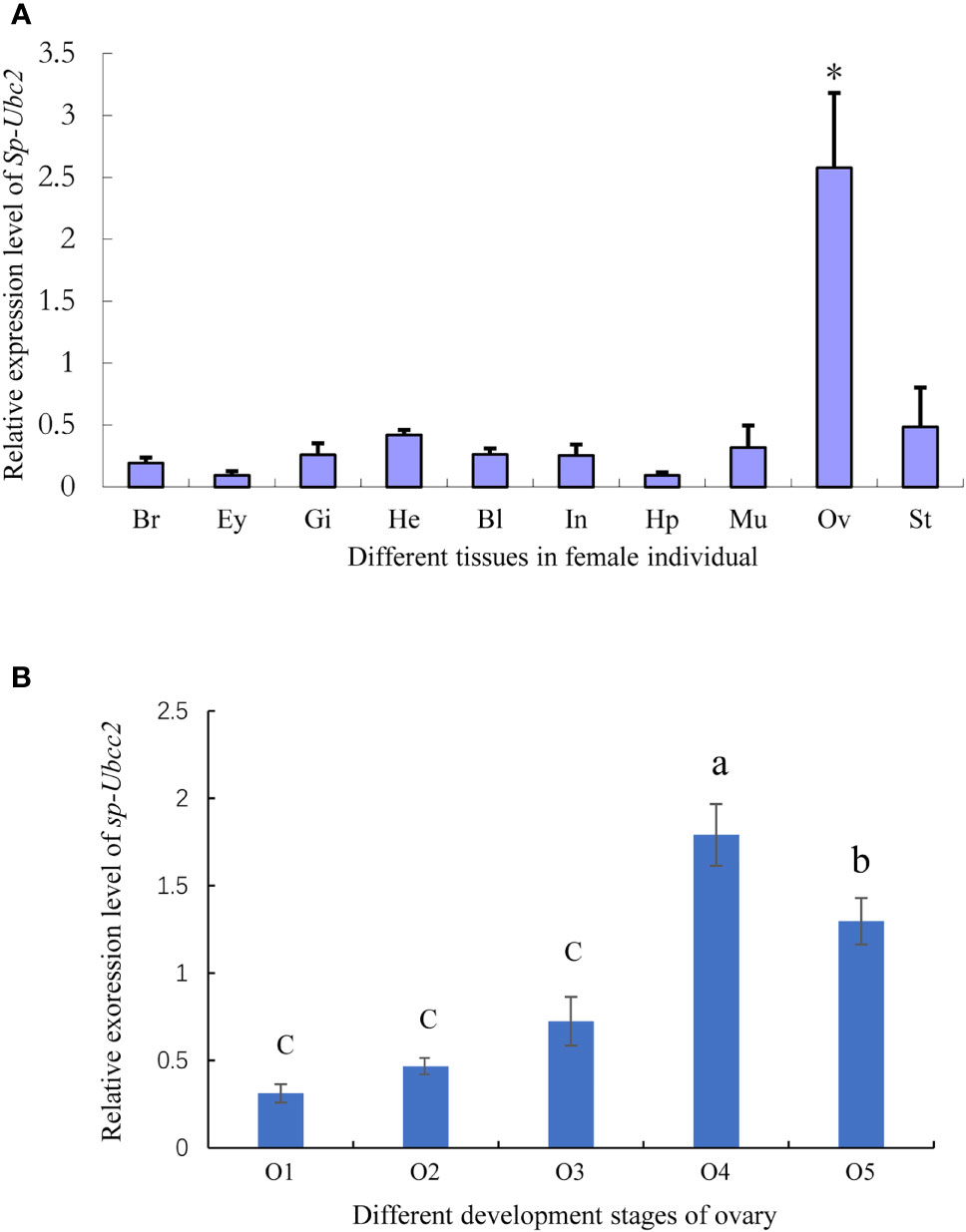
Figure 5 The expression level of Sp-Ubc2 mRNA in female tissues. (A) The distribution of Sp-Ubc2 in different female tissues. (B) The expression pattern of Sp-Ubc2 during oogenesis. Br, brain; Ey, eye; Gi, gill; He, heart; Ha, hemolymph; Bl, blood; In: intestine; Hp, hepatopancreas; Mu, muscle; Ov, ovary; St, stomach; O1, O2, O3, O4, and O5 represented different developmental ovaries; * in (A) indicated the significant difference (p < 0.05); different letters in (B) indicated statistically significant differences (P < 0.05).
The Expression Pattern of Sp-Ubc2 During Oogenesis
According to the RT-qPCR, the ovary was found to have the highest level of Sp-Ubc2. For the specific expression level of Sp-Ubc2 at various development stages, further RT-qPCR detection demonstrated that during the ovary oogenesis, the expression level was highest in the O4 stage, followed by the O5 stage and lower and stable between O1 and O3 (Figure 5B).
Moreover, the localization of Sp-Ubc2 was investigated in the ovary by ISH during oogenesis. In accordance with histology, the stages of stained cells were identified (Figures 6K–O). As control, the tissue section hybridized with the sense probe was not stained (Figures 6F–J). From proliferation to pre-vitellogenesis, a positive signal could be observed in the nuclei and cytoplasm of oogonia, so was the result in oocytes (Figures 6A, B). In primary vitellogenesis, Sp-Ubc2 mRNA was almost distributed evenly in whole oocytes (Figure 6C). Meanwhile, from secondary vitellogenesis to tertiary vitellogenesis, a positive signal was mainly observed in the nuclei (Figures 6D, E).
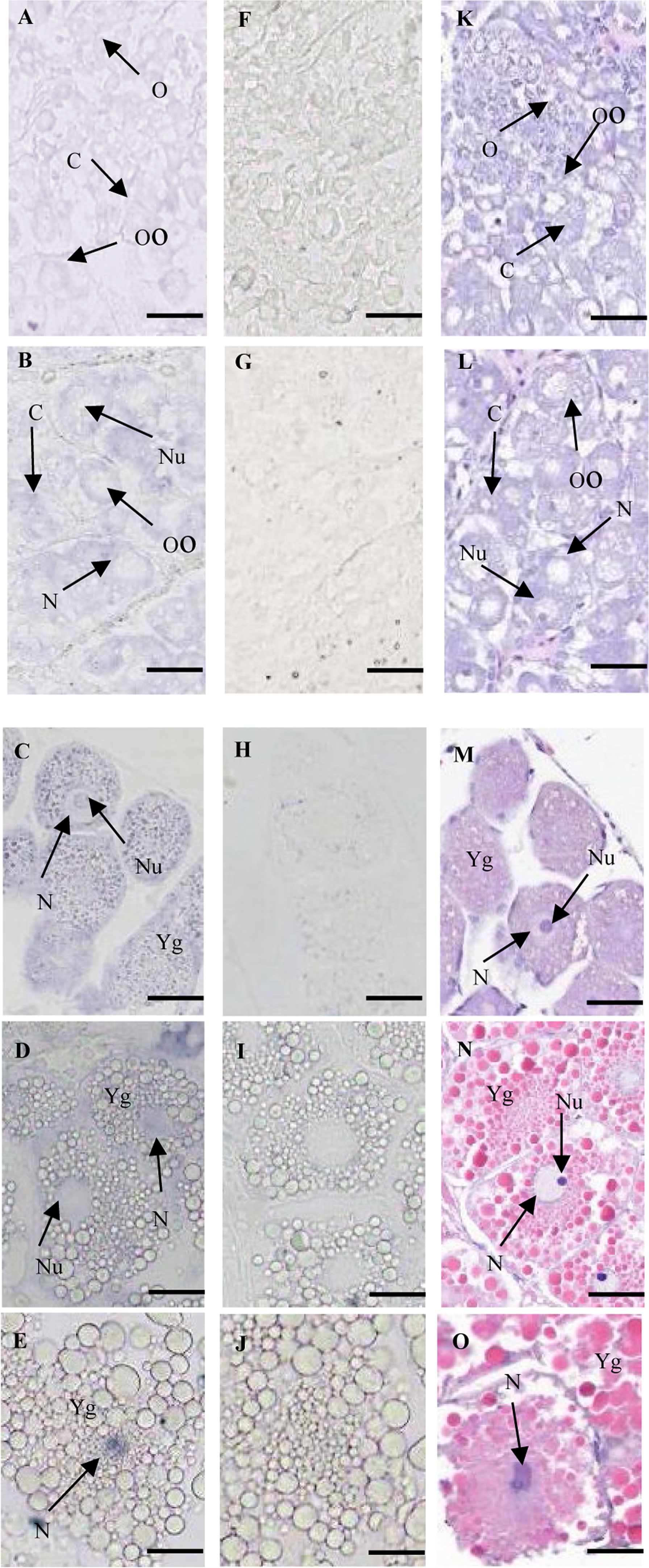
Figure 6 The expression pattern of Sp-Ubc2 mRNA during oogenesis. (A–E) The paraffin section of ovary was hybridized with the Sp-Ubc2 antisense probe. (F–J) The paraffin section of ovary was hybridized with the Sp-Ubc2 sense probe. (F–J) The paraffin section and (H, E) of ovary. (A, F, and K): proliferation stage; (B, G, and L): pre-vitellogenesis; (C, H,M), primary vitellogenesis, (D, I,N), secondary vitellogenesis, (E, J,O), tertiary vitellogenesis; O, oogonia, Oo, oocytes, Cy, cytoplasm, N, nuclei, Nu, nucleolus, Yg, vitellogenin. scale bar, 100 μm.
Discussion
The present study successfully isolated and identified the Sp-Ubc2 homologue of S. paramamosain. The deduced Sp-UBC 2 proteins had the UBC catalytic domain containing 8 motifs, as observed in penaeid shrimp (Shen et al., 2009) and grape (Gao et al., 2017). In addition, phylogenetic analysis revealed that the Sp-UBC 2 was clustered with other arthropods. The 2D and 3D structure of Sp-UBC 2 further predicted that the 4-α helix, β-sheet, and Cys-site presented in the UBC catalytic domain, which might be involved in various processes such as cell cycle progression, organelle biogenesis, and transcriptional regulation (DiAntonio et al., 2001).
Regarding the tissue distribution of Sp-Ubc2, RT-qPCR analysis showed that the Sp-Ubc2 mRNA is widely distributed in various tissues of female individuals. This suggested that Sp-Ubc2 participated in many physiological processes such as chromosomal organization, DNA repair, and cell cycle control, similar to Homo sapiens (Jiang and Beaudet, 2004) and D. melanogaster (Michelle et al., 2009; Nagy et al., 2012). Surprisingly, the Sp-Ubc2 demonstrated the highest expression level in the ovary, which was significantly different from other tissues. Moreover, the expression level of Sp-Ubc2 changed prominently as the developing ovary. In secondary and tertiary vitellogenesis stages, the expression level of Sp-Ubc2 reached its peak. The results were consistent with the previous research in penaeid shrimp, which all indicated that the expression of Sp-Ubc2 plays a more important role in the development process of the ovary (Shen et al., 2009). For the specific expression pattern of Sp-Ubc2 during oogenesis, the Sp-Ubc2 mRNA was observed in the nuclei and cytoplasm of oogonia and oocytes at proliferation, pre-vitellogenesis, and primary vitellogenesis stages, while the positive signal was gradually concentrated in nuclei from secondary vitellogenesis to tertiary vitellogenesis. According to some reports, ubiquitination contributed to several regulatory mechanisms of gametogenesis. In ascidians, the ubiquitin system participated in the elimination of defective sperm in epididymis and paternal mitochondria in fertilized eggs (Sakai et al., 2004). During early Drosophila oogenesis, the ubiquitin ligase complex was essential for cell cycle control that ensured proper transport of centrosomes into the oocyte for maintaining the development fate (Braun et al., 2021). Therefore, this change of Sp-Ubc2 in the distribution position of ovary further suggested that the crucial function of Sp-Ubc2 was related to the cell cycle.
In addition, a number of other small proteins can be covalently attached to target proteins in a manner similar to ubiquitination, such as SUMO (small ubiquitin-like modifier) (Dohmen, 2004). In recent years, SUMOylation has emerged in almost all aspects of cellular physiology (Liu et al., 2017). Like ubiquitination, there are three corresponding enzymes (E1, E2, and E3) involved in SUMOylation. Among them, the Ubc9 is only an E2-conjugating enzyme currently found, which could mediate SUMOylation through directly binding SUMO to target proteins (Sakaguchi et al., 2007). It has been proven that the Ubc9 is associated with various processes during spermatogenesis and oogenesis. In Chinese mitten crab (Eriocheir sinensis), the EsUbc9 expression in the ovary were low in the early stage, reached the highest level at stage III, and then gradually decreased in stage IV, which suggested that SUMOylation might play an important role during oogenesis (Wang et al., 2012). Ubiquitination and SUMOylation are all important ways of protein post-translational modification, which are widely involved in regulating protein function and various aspects of cell life activities. Polyubiquitination degrades proteins, while SUMOylation mainly regulates protein interaction and localization (Bossis and Melchior, 2006). Under certain circumstances, SUMOylation and ubiquitination can both synergistically regulate protein function and antagonize each other (Choi et al., 2008; Kang et al., 2008). Therefore, more work may be required in the future to research the regulation of ubiquitination and SUMOylation during the oogenesis of S. paramamosain.
In conclusion, we firstly reported of Sp-Ubc2 in S. paramamosain. We obtained the full-length cDNA of Sp-Ubc2 with UBC domain. Then, we detected that the Sp-Ubc2 was widely distributed in different tissues of female individuals and kept the highest expression level in the ovary. Furthermore, we found that during oogenesis, the expression level of Sp-Ubc2 was higher in O4 and O5 stages than other stages, and the Sp-Ubc2 signal was gradually concentrated in nuclei, which indicated that Sp-Ubc2 might play important roles in the cell cycle. This study would provide insights into the regulatory mechanism of gonad development in S. paramamosain, and it also enriched the theoretical basis of ubiquitin family genes involved in crustacean gonad development.
Data Availability Statement
The datasets presented in this study can be found in online repositories. The names of the repository/repositories and accession number(s) can be found in the article/supplementary material.
Ethics Statement
All of the study design and animal experiments were conducted in accordance with guidelines of Jimei University’s Animal Care and Use Committee (2011-59).
Author Contributions
YW conceived and designed the study. KH and YD contributed to the experimental work and original data preparation. LZ contributed to the manuscript writing and revision. ZZ and CR contributed with the experimental materials. All authors read and approved the final manuscript.
Funding
The work was supported by the Natural Science Foundation of Fujian (2020J02011), the United Front Special project of Good Strategies for the Construction of New Fujian (No.JAT21028), the National Natural Science Foundation of China, China (41676161, 31672681), and special funds provided by the Ministry of Science and Technology of the People’s Republic of China to Guide the Development of Science and Technology in Fujian Province, China (2020L3011).
Conflict of Interest
The authors declare that the research was conducted in the absence of any commercial or financial relationships that could be construed as a potential conflict of interest.
Publisher’s Note
All claims expressed in this article are solely those of the authors and do not necessarily represent those of their affiliated organizations, or those of the publisher, the editors and the reviewers. Any product that may be evaluated in this article, or claim that may be made by its manufacturer, is not guaranteed or endorsed by the publisher.
References
Bossis G., Melchior F. (2006). Regulation of SUMOylation by Reversible Oxidation of SUMO Conjugating Enzymes. Mol. Cell. 21, 349–357. doi: 10.1016/j.molcel.2005.12.019
Braun A. L., Meghini F., Villa-Fombuena G., Guermont M., Fernandez-Martinez E., Qian Z., et al. (2021). The Careful Control of Polo Kinase by APC/C-Ube2C Ensures the Intercellular Transport of Germline Centrosomes During Drosophila Oogenesis. Open Biol. 11, 200371. doi: 10.1098/rsob.200371
Chen F., Wang K. (2019). Characterization of the Innate Immunity in the Mud Crab Scylla Paramamosain. Fish. Shellfish. Immunol. 93, 436–448. doi: 10.1016/j.fsi.2019.07.076
Chen D., Wang Q., Huang H., Xia L., Jiang X., Kan L. (2009). Effete-Mediated Degradation of Cyclin A is Essential for the Maintenance of Germline Stem Cells in Drosophila. Development. 136, 4133–4142. doi: 10.1242/dev.039032
Choo Y. S., Zhang Z. (2009). Detection of Protein Ubiquitination. J. Vis. Exp. 19, 1293. doi: 10.3791/1293
DiAntonio A., Haghighi A. P., Portman S. L., Lee J. D., Amaranto A. M., Goodman C. S. (2001). Ubiquitination-Dependent Mechanisms Regulate Synaptic Growth and Function. Nature. 412, 449–452. doi: 10.1038/35086595
Dohmen R. J. (2004). SUMO Protein Modification. Biochim. Biophys. Acta 1695, 113–131. doi: 10.1016/j.bbamcr.2004.09.021
Dye B. T., Schulman B. A. (2007). Structural Mechanisms Underlying Posttranslational Modification by Ubiquitin-Like Proteins. Annu. RevBiophys. Biomol. Struct. 36, 131–150. doi: 10.1146/annurev.biophys.36.040306.132820
Gao Y., Wang Y., Xin H., Li S., Liang Z. (2017). Involvement of Ubiquitin-Conjugating Enzyme (E2 Gene Family) in Ripening Process and Response to Cold and Heat Stress of Vitis Vinifera. Sci. Rep. 27, 13290. doi: 10.1038/s41598-017-13513-x
Han K., Dai Y., Zhang Z., Zou Z., Wang Y. (2018). Molecular Characterization and Expression Profiles of Sp-Uchl3 and Sp-Uchl5 During Gonad Development of Scylla Paramamosain. Molecules. 23, 213. doi: 10.3390/molecules23010213
Hershko A., Heller H., Elias S., Ciechanover A. (1983). Components of Ubiquitin-Protein Ligase System. Resolution, Affinity Purification, and Role in Protein Breakdown. J. Biol. Chem. 258, 8206–8214. doi: 10.1016/S0021-9258(20)82050-X
Horst M. N., Walker A. N., Bush P., Wilson T., Chang E. S., Miller T., et al. (2007). Pesticide Induced Alterations in Gene Expression in the Lobster, Homarus Americanus. Comp. Biochem. Physiol. Part D Genomics Proteomics. 2, 44–52. doi: 10.1016/j.cbd.2006.11.004
Jeong J., Verheyden J. M., Kimble J. (2011). Cyclin E and Cdk2 Control GLD-1, the Mitosis/Meiosis Decision, and Germline Stem Cells in Caenorhabditis Elegans. PloS Genet. 7, e1001348. doi: 10.1371/journal.pgen.1001348
Jia X., Chen Y., Zou Z., Lin P., Wang Y., Zhang Z. (2013). Characterization and Expression Profile of Vitellogenin Gene From Scylla Paramamosain. Gene. 15, 119–130. doi: 10.1016/j.gene.2013.02.035
Jiang Y. H., Beaudet A. L. (2004). Human Disorders of Ubiquitination and Proteasomal Degradation. Curr. Opin. Pediatr. 16, 419–426. doi: 10.1097/01.mop.0000133634.79661.cd
Johnson D. G., Walker C. L. (1999). Cyclins and Cell Cycle Checkpoints. Annu. Rev. Pharmacol. Toxicol. 39, 295–312. doi: 10.1146/annurev.pharmtox.39.1.295
Jones D., Crowe E., Stevens T. A., Candido E. P. (2002). Functional and Phylogenetic Analysis of the Ubiquitylation System in Caenorhabditis Elegans: Ubiquitin-Conjugating Enzymes, Ubiquitin-Activating Enzymes, and Ubiquitin-Like Proteins. Genome. Biol. 3, RESEARCH0002. doi: 10.1186/gb-2001-3-1-research0002
Jue D., Sang X., Lu S., Dong C., Zhao Q., Chen H., et al. (2015). Genome-Wide Identification, Phylogenetic and Expression Analyses of the Ubiquitin-Conjugating Enzyme Gene Family in Maize. PLoS. One 10, e0143488. doi: 10.1371/journal.pone.0143488
Kang S. I., Choi H. W., Kim I. Y. (2008). Redox-Mediated Modification of PLZF by SUMO-1 and Ubiquitin. Biochem. Biophys. Res. Commun. 369, 1209–1214. doi: 10.1016/j.bbrc.2008.03.037
Kraft E., Stone S. L., Ma L., Su N., Gao Y., Lau O. S., et al. (2005). Genome Analysis and Functional Characterization of the E2 and RING-Type E3 Ligase Ubiquitination Enzymes of Arabidopsis. Plant Physiol. 139, 1597–1611. doi: 10.1104/pp.105.067983
Leelatanawit R., Sittikankeaw K., Yocawibun P., Klinbunga S., Roytrakul S., Aoki T., et al. (2008). Identification, Characterization and Expression of Sex-Related Genes in Testes of the Giant Tiger Shrimp Penaeus Monodon. Comp. Biochem. Physiol. A Mol. Integ.r Physiol. 152, 66–76. doi: 10.1016/j.cbpa.2008.09.004
Liu F. Y., Liu Y. F., Yang Y., Luo Z. W., Xiang J. W., Chen Z. G., et al. (2017). SUMOylation in Neurological Diseases. Curr. Mol. Med. 16, 893–899. doi: 10.2174/1566524017666170109125256
Li Y., Zhou Z., She M., Ge L., Liu F. (2020). Ubiquitin-Conjugating Enzyme E2 E Inhibits the Accumulation of Rice Stripe Virus in Laodelphax Striatellus (Fallén). Viruses. 12, 908. doi: 10.3390/v12090908
Lu C., Kim J., Fuller M. T. (2013). The Polyubiquitin Gene Ubi-P63e is Essential for Male Meiotic Cell Cycle Progression and Germ Cell Differentiation in Drosophila. Development. 140, 3522–3531. doi: 10.1242/dev.098947
Michelle C., Vourc’h P., Mignon L., Andres C. R. (2009). What was the Set of Ubiquitin and Ubiquitin-Like Conjugating Enzymes in the Eukaryote Common Ancestor? J. Mol. Evol. 68, 616–628. doi: 10.1007/s00239-009-9225-6
Nagy O., Pál M., Udvardy A., Shirras C. A., Boros I., Shirras A. D., et al. (2012). lemmingA Encodes the Apc11 Subunit of the APC/C in Drosophila Melanogaster That Forms a Ternary Complex With the E2-C Type Ubiquitin Conjugating Enzyme, Vihar and Morula/Apc2. Cell. Div. 7, 9. doi: 10.1186/1747-1028-7-9
Neutzner M., Neutzner A. (2012). Enzymes of Ubiquitination and Deubiquitination. Essays. Biochem. 52, 37–50. doi: 10.1042/bse0520037
Ohlmeyer J. T., Schüpbach T. (2003). Encore Facilitates SCF-Ubiquitin-Proteasome-Dependent Proteolysis During Drosophila Oogenesis. Development. 130, 6339–6349. doi: 10.1242/dev.00855
Sakaguchi K., Koshiyama A., Iwabata K. (2007). Meiosis and Small Ubiquitin-Related Modifier (SUMO)-Conjugating Enzyme, Ubc9. FEBS. J. 274, 3519–3531. doi: 10.1111/j.1742-4658.2007.05905.x
Sakai N., Sawada M. T., Sawada H. (2004). Non-Traditional Roles of Ubiquitin-Proteasome System in Fertilization and Gametogenesis. Int. J. Biochem. Cell Biol. 36, 776–784. doi: 10.1016/S1357-2725(03)00263-2
Sanz A., Furné M., Trenzado C. E., Haro C. D., Sánchez-Muros M. J. (2012). Study of the Oxidative State, as a Marker of Welfare, on Gilthead Sea Bream, Sparus Aurata, Subjected to Handling Stress. J. Word Aquacult. Soc 43, 707–715. doi: 10.1111/j.1749-7345.2012.00602.x
Seufert W., McGrath J. P., Jentsch S. (1990). UBC1 Encodes A Novel Member of an Essential Subfamily of Yeast Ubiquitin-Conjugating Enzymes Involved in Protein Degradation. EMBO J. 9, 4535–4541. doi: 10.1002/j.1460-2075.1990.tb07905.x
Shen B., Zhang Z., Wang Y., Wang G., Chen Y., Lin P. (2009). Differential Expression of Ubiquitin-Conjugating Enzyme E2r in the Developing Ovary and Testis of Penaeid Shrimp Marsupenaeus Japonicus. Mol. Biol. Rep. 36, 1149–1157. doi: 10.1007/s11033-008-9291-7
Swatek K. N., Komander D. (2016). Ubiquitin Modifications. Cell. Res. 26, 399–422. doi: 10.1038/cr.2016.39
Wang Y., Wang W., Cai J., Zhang Y., Qin G., Tian S. (2014). Tomato Nuclear Proteome Reveals the Involvement of Specific E2 Ubiquitin-Conjugating Enzymes in Fruit Ripening. Genome. Biol. 15, 548. doi: 10.1186/s13059-014-0548-2
Wang Q., Wang Y., Chen L., He L., Li W., Jiang H. (2012). Expression Characteristics of the SUMOylation Genes SUMO-1 and Ubc9 in the Developing Testis and Ovary of Chinese Mitten Crab, Eriocheir Sinensis. Gene. 501, 135–143. doi: 10.1016/j.gene.2012.04.016
Wan H., Jia X., Zou P., Zhang Z., Wang Y. (2019). The Single-Molecule Long-Read Sequencing of Scylla Paramamosain. Sci. Rep. 9, 12401. doi: 10.1038/s41598-019-48824-8
Wan H., Liao J., Zhang Z., Zeng X., Liang K., Wang Y. (2021). Molecular Cloning, Characterization, and Expression Analysis of a Sex-Biased Transcriptional Factor Sox9 Gene of Mud Crab Scylla Paramamosain. Gene. 774, 145423. doi: 10.1016/j.gene.2021.145423
Zhang Z., Wu R. S., Mok H. O., Wang Y., Poon W. W., Cheng S. H., et al. (2003). Isolation, Characterization and Expression Analysis of a Hypoxia-Responsive Glucose Transporter Gene From the Grass Carp, Ctenopharyngodon Idellus. Eur. J. Biochem. 270, 3010–3017. doi: 10.1046/j.1432-1033.2003.03678.x
Keywords: Scylla paramamosain, Sp-Ubc2, oogenesis, tissue expression, ubiquitination
Citation: Han K, Dai Y, Zhang Z, Rao C, Zhou L and Wang Y (2022) Molecular Characteristics of Scylla paramamosain Ubiquitin-Conjugating Enzyme E2 and Expression Analysis During Oogenesis. Front. Mar. Sci. 9:855523. doi: 10.3389/fmars.2022.855523
Received: 15 January 2022; Accepted: 22 June 2022;
Published: 22 July 2022.
Edited by:
Yangfang Ye, Ningbo University, ChinaReviewed by:
Xianliang Meng, Chinese Academy of Fishery Sciences (CAFS), ChinaHanafiah Fazhan, Shantou University, China
Copyright © 2022 Han, Dai, Zhang, Rao, Zhou and Wang. This is an open-access article distributed under the terms of the Creative Commons Attribution License (CC BY). The use, distribution or reproduction in other forums is permitted, provided the original author(s) and the copyright owner(s) are credited and that the original publication in this journal is cited, in accordance with accepted academic practice. No use, distribution or reproduction is permitted which does not comply with these terms.
*Correspondence: Li Zhou, zhoulisdta@163.com; Yilei Wang, 1428763881@qq.com