- 1School of Naval Architecture and Maritime, Zhejiang Ocean University, Zhoushan, China
- 2School of Marine Engineering Equipments, Zhejiang Ocean University, Zhoushan, China
Artificial reefs are beneficial to restore fishery resources and increase fishery production. Meanwhile, they play a significant role in improving ocean ecology and accelerating the evolution of fishery industries. Since they are generally affected by currents, waves, and other hydrological factors, the flow field around artificial reefs and their stabilities have become a research hotspot in recent years. Research on artificial reefs is a systematic process consisting of four aspects: Firstly, the significance, the definition, the mechanism, and the present research progress were introduced for artificial reefs in detail. Secondly, the development trend of the sit-bottom artificial reef and that of the floating artificial reef were summarized, respectively. Thirdly, it was found that the combination of traditional artificial reefs and emerging ocean engineering has a great development potential in practical engineering. Finally, the existing problems related to the hydrodynamic characteristics of the artificial reefs in China were summarized, and the prospects of artificial reefs were proposed. The purpose of this study is to provide a scientific reference for the ecological and sustainable development of the large-scale construction of artificial reefs in the ocean.
1 Significance of Artificial Reef Construction
With the increasingly severe pollution, the ocean environment is gradually deteriorating. At the same time, modern fishing modes led to the rapid decline in fishery resources (Mansfield, 2010). According to one of the latest surveys conducted by a coupled climate simulation, the average fishery production in the Seven Oceans will decline by more than 20% by 2300. In addition, the fishery production in the North Atlantic will reduce by nearly 60% and the fishery production in the Western Pacific will decrease by more than 50% (Moore et al., 2018). In response to critical problems in the sustainable development of marine fisheries, most developed countries regard the artificial reefs construction as public welfare, and have incorporated it into their national economic plans since the 1970s. Public welfare mainly includes three parts: (1) the protection of fishery resources, (2) the promotion of marine biodiversity, and (3) the improvement of marine ecology. The current research on artificial reefs worldwide is shown in Figure 1 (Lima et al., 2019).
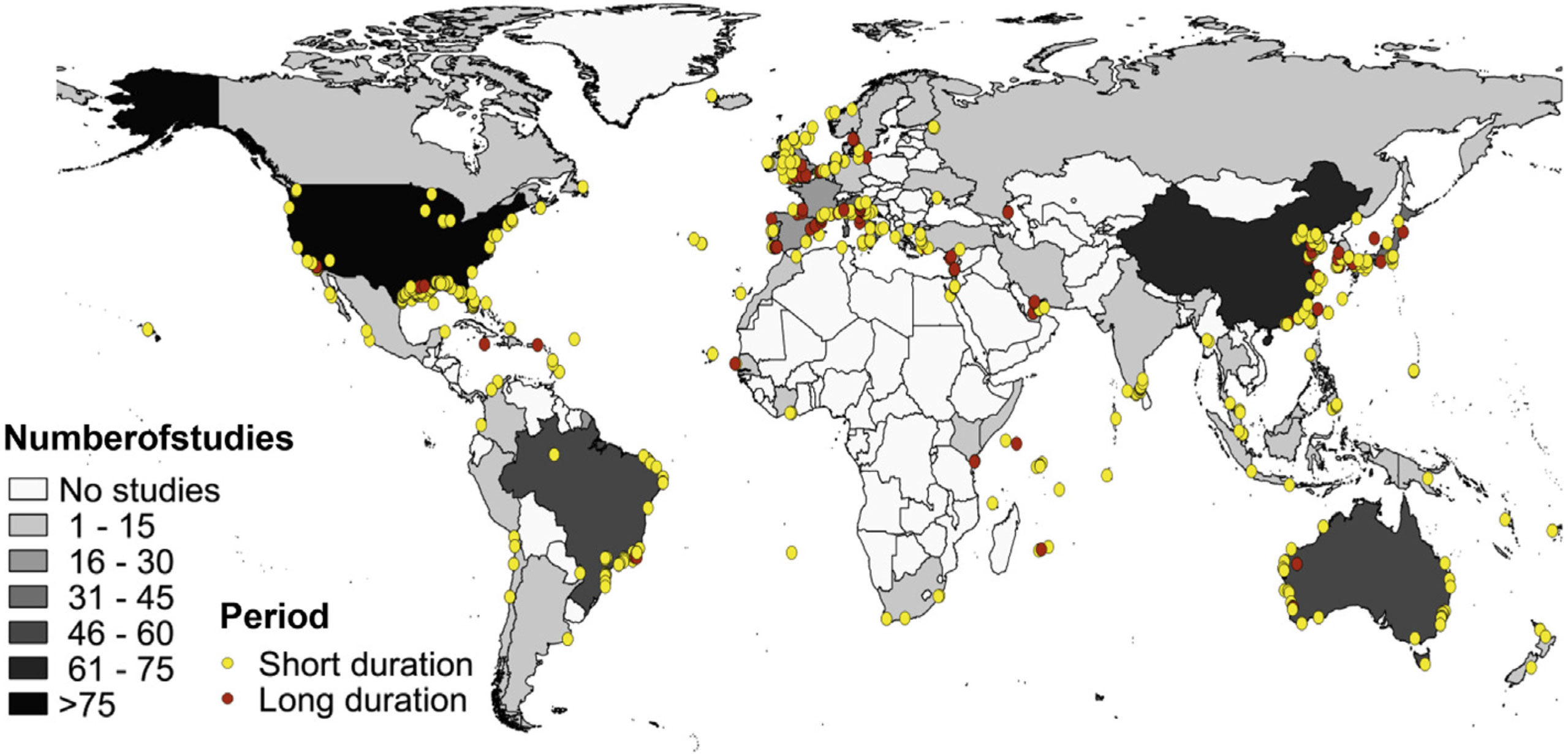
Figure 1 Distribution of artificial reef’s research worldwide and the development of study period: short duration (≤2 years) and long duration (>2 years) (Lima et al., 2019).
China is one of the earliest countries to develop artificial reefs engineering. Records of reefs appeared in the Spring and Autumn period and the Warring State period (a dynasty in Chinese history, 770–221 BC), but the construction of artificial reefs was slow. It was not until the 1980s that China began to construct artificial reefs on a large scale. With remarkable effects, coastal cities began to accelerate the construction of reefs (Li et al., 2014). By 2016, China has deployed more than 20 million cubic meters of artificial reefs, covering an area of more than 1.1×103 km2 (Zhou et al., 2019). Nowadays, developments in artificial reef engineering in China have already taken shape, whose benefits have become considerably prominent. China plans to deploy over 50 million cubic meters of artificial reefs by 2025, covering an area of more than 1200 km2. It is obvious that China attaches great importance to the progress of artificial reef engineering in coastal areas from Figure 1. However, the selection of its type, the distribution of its place, the safety of structure and materials, and the effect of fish aggregation and shelter are the essential prerequisites for fish spawning and conservation zones. Further understanding of the hydrodynamic characteristics around artificial reefs is beneficial to analyze the above effects and proliferation (Jiangtao et al, 2014; Lokesha et al., 2019). Generally, the flow field effect and the stability of artificial reefs are the representative factors reflecting their performance. The flow field effect refers to local upwelling in front of artificial reef after it is placed on the seabed. Local upwelling can bring the bottom sediments and the dissolved inorganic salts related to nutrients into the water of the upper layers. Therefore, the effectiveness for aggregating fish is achieved due to the increase in baits in the water of the upper layers. Additionally, the wake flow around the back side of the artificial reef characterized by a vortex with slower velocities provides habitat, shelter, and breeding areas for fish (Liu and Su, 2013). Furthermore, the artificial reefs on the seabed will be affected by the combination of waves and currents resulting in an unbalanced resultant force, leading to their failure (Zhao et al., 2015b). It is necessary to study the hydrodynamic characteristics of an artificial reef to ensure their long-term operational safety. After more than 30 years of exploration, the hydrodynamic characteristics of artificial reefs have been studied by numerous researchers and fruitful results have been obtained (Tamura et al., 2007; Zheng et al., 2015; Yaakob et al., 2016; Wang et al., 2018; Jiang et al., 2020). Nevertheless, most studies have focused on the single reef or artificial reef cluster, which is mainly based on numerical and experimental results. The relationship between the hydrodynamic characteristics and the ecological environment construction of artificial reefs is still unclear. With the severe erosion of the global coastline and the shortage of renewable energy, some researchers began to combine artificial reefs with the underwater breakwaters, the wave-dissipating structures, and the renewable energy devices (Srisuwan and Rattanamanee, 2015; De Almeida, 2017; Jiao et al., 2017; Kim et al., 2020; Ghiasian et al., 2021; Marin-Diaz et al., 2021). These multi-functional artificial reefs can not only fulfill the functions of ocean structures, but also enrich the diversity of marine organism. Studies have shown that the effects of protecting fishery resources can also be addressed with pile foundation of offshore wind turbine and oil platforms. It was further stated that the governments around the world should transform abandoned platforms and equipment from offshore structures into artificial reefs and put them into the ocean. The benefits lie in reducing the cost of dismantling and the impact on the ecological environment of the ocean (Gallaway et al., 2009; Krone et al., 2017; Bull and Love, 2019; van Elden et al., 2019).
Depending on the water depth of the layout location, the artificial reef can be divided into sit-bottom artificial reef and floating artificial reef. As an essential component of marine ranching, sit-bottom artificial reefs are mostly arranged in shallow waters to proliferate fisheries, repair the marine environment, and create habitats. However, the hydrodynamic characteristics of artificial reefs need to be considered comprehensively from the aspects of site selection, type, structural design, and habitat construction. The hydrodynamic properties of artificial reefs in waves and currents are of great importance as they are closely associated with the flow field effect and the physical stability of artificial reefs (Seaman, 2000; Jiang et al., 2019). The hydrodynamic characteristics of floating artificial reefs are more complicated than those of the sit-bottom reefs, because the hydrodynamic characteristics of the buoyancy system, fishing net system, mooring system, and their coupling responses need to be considered.
2 Definition and Mechanism of Artificial Reef
2.1 Definition
An artificial reef was originally defined as one or more artificial or natural reefs placed on the seabed by humans. In the past, many different objects have been used to create artificial reefs including culvert pipes, concrete pilings, cars, liberty ships, and tires. Moreover, offshore platforms, floating structures, and flotsam have been shown to attract local reef and pelagic fishes. In some cases, these fish aggregations supported recreational and commercial fisheries (Howe, 2003).
The artificial reefs in China originated in the Spring and Autumn period as well as in the Warring State period. Artificial reefs at that time were mainly made of tree branches or stones. During the Ming Dynasty, fishermen put bamboo fences filled with branches, stones, and weeds into the sea to attract fish. During the Qing Dynasty, it was reported that broken boats, stones, fences, and other obstacles were intentionally thrown into the sea, which became the prototype of artificial reefs (Lima et al, 2019).
(I)n the middle of the 20th century, sunken shipwrecks were included in the ranks of artificial reefs, and the materials of reefs became diverse. In addition, pipelines, tires, abandoned cars and ships, and defunct offshore platforms have been turned into artificial reefs. With the development of artificial reef engineering, the concept and applicability of artificial reefs are constantly enriched and expanded. By the end of the 20th century, the definition of artificial reefs was no longer limited to artificial structures and fishing facilities. Its connotation extended to the category of artificial habitats for marine organisms. After the 21st century, the principal aims of artificial reefs, as an important part of modern marine ranching, were transformed from fishing to protecting marine resources, proliferating marine resources, and improving the marine environment (Yu and Zhang, 2020).
(T)he current definition of artificial reef refers to the intentional arrangement of structures in specific waters to improve the ecological environment. It provides a suitable place for marine organisms to prey, avoid enemies, gather, reproduce, and grow, which greatly improves fishery resources and production (Seaman and Jensen, 2000). In recent years, coastal countries such as South Korea, Japan, and the United States have begun to combine artificial reefs with offshore structures for coastline protection, port stability, renewable energy utilization, and recreation. This can reduce the interference of manufactured structures in the marine environment and improve the utilization of resources. In the future, the function of artificial reefs can be more diversified. Meanwhile, the artificial reef groups with multiple functions are environment-friendly for marine ecology.
2.2 Mechanism
The basic objectives of artificial reefs include biological attraction, water quality improvement, and ecosystem restoration (Paxton et al., 2020). Firstly, biological attraction is significantly attributed to the formation of an environment that is conducive to the reproduction and survival of marine organisms through the usage of artificial reefs. It is jointly determined by the impact of reefs on the marine environment and the behavioral characteristics of fish (Mablouké et al., 2013). The effects of artificial reefs on the marine environment include two aspects: physical and biological environmental impacts. On the one hand, physical environmental impacts refer to the flow field effect, the shadow effect, and the water temperature effect around the artificial reef. On the other hand, the changes in the physical environment around the artificial reef can promote the varying progress of the biological environment. The formation of food, the shelter effect, and the reef-seeking instinct may attract marine organisms to gather in reef areas and increase fishery resources (Lima et al., 2019). Secondly, McKindsey et al. (2011) found that the surface of an artificial reef could not only attract algae and filter-feeding benthic macro invertebrate, but also rapidly absorb inorganic and dissolved nutrients in the seabed. As a result, the enrichment of organic matter was reduced to improve degraded water quality (Miller, 2002). Many scholars confirmed this argument by comparing the water quality around artificial reefs before and after its usage (Chojnacki, 2000; Marsden and Chotkowski, 2001; Tang and Yu, 2016). Thirdly, the ecological restoration mainly promotes the formation of newly distributed biological community around the reefs by reconstructing ocean seabed material, the flow field effect, the food effect, and habitat rehabilitation, so as to restore and reconstruct the marine ecosystem. Figure 2 shows the specific framework (Guo et al., 2019).
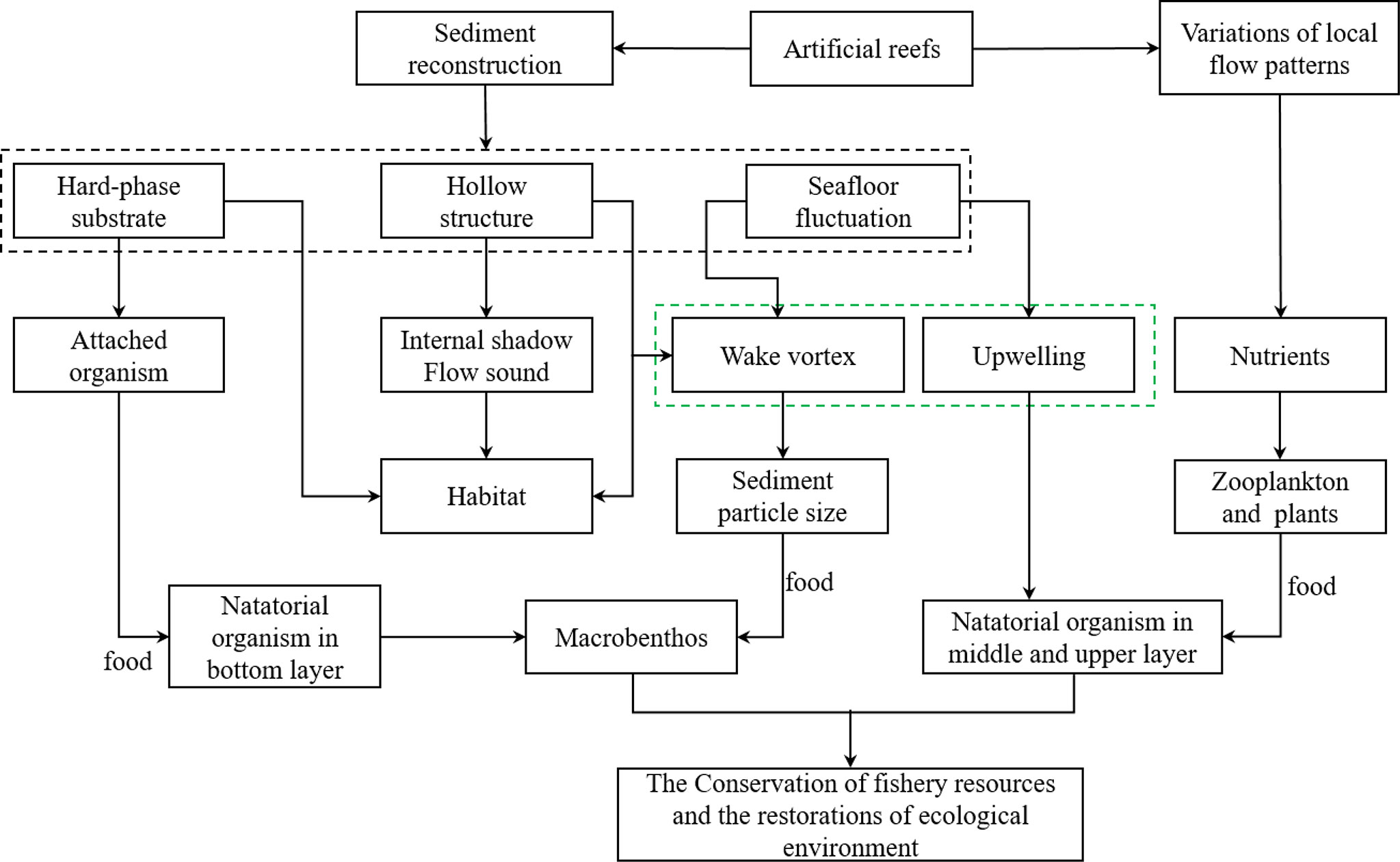
Figure 2 The framework of the ecological restoration of artificial reef (Guo et al., 2019).
3 Development Trend of Artificial Reef
The types and classification of artificial reefs vary with their functions, design methods, and structural materials. There are mainly four methods for classifying artificial reefs: the waters distribution, the purpose of construction, the production of materials, and the operating water depth. As shown in Table 1, firstly, the waters distribution can be divided into the type of ecological public welfare, definitely ecological public welfare and non-ecological public welfare. Secondly, the purpose of construction can be divided into different kinds in order to proliferate resources and improve environment and fishery harvesting. Thirdly, the production of materials can be divided into concrete, steel, and hybrid. Finally, the artificial reef can be divided into sit-bottom and floating artificial reefs regarding the water depth. In addition, the floating artificial reefs are further divided into the middle layer and surface layer (Perkol-Finkel et al., 2006).
3.1 Modern Sit-Bottom Artificial Reef
The sit-bottom artificial reefs are widely used in coastal countries to develop marine ranching projects. It generally depends on the reef weight to sink into the specified position (Lima et al., 2019). At present, the studies on sit-bottom artificial reefs in China mainly focus on the construction materials, the structural design, the distribution of biological community, and the hydrodynamic characteristics of artificial reefs (Zhang et al., 2010).
In the early days, Japanese and American governments used abandoned tires to build sit-bottom artificial reefs. However, this approach presented shortcomings such as the high cost of modification, the structural instability, and the release of toxic substances from tires (Sherman and Spieler, 2006). Due to its welding technology, the artificial reef made of metal materials can make more complex structures. Iron ions released by the structure during oxidation can be utilized by algae and provide primary productivity (Layman et al., 2016). Benefiting from the advantages of substantial biological attachment rates, stable structures, low costs, and simple moldings, the concrete material has become one of the most widely used types of artificial reefs (Dennis et al., 2018). In the 21st century, the concept of ecological concrete was proposed. Compared to traditional concrete, the ecological concrete, which has a better performance in terms of construction, viscosity, and mechanical properties, is more beneficial for the sustainable economic development and ecological environment. Many researchers have developed materials for new types of ecological concrete for artificial reefs, such as low alkalinity and high strength of concrete composed of cementitious materials and marine sand (Margheritini et al., 2021), concrete composed of polymer modified cementitious material with high porosity and permeability (Ly et al., 2021), concrete composed of steel and mineral slag (Wang et al., 2012), and concrete composed of aluminate cement, marine sand, and seawater (Xu et al., 2019). Different materials are required to achieve the best results for various target species of fish or aquatic organisms.
The structure of reefs includes the single reef structure and the laying structure. The laying structure consists of the unitary reef, the reef group, and the reef belt, as shown in Figure 3A (Yoon et al., 2016). On the one hand, the geometry, opening, internal structure and void volume (Sherman et al., 2002; Scheffers et al., 2003; Costa et al., 2016; Zheng et al., 2022) should be considered for the structure of the single reef. On the other hand, it is necessary for the reef group to consider the interval of arrangement and the accumulation height (Armono and Hall, 2003; Yoon et al., 2016; Kim et al., 2017; Callaway, 2018). Some researchers have summarized research on the structural types of reefs commonly used in different countries over the past few years, as shown in Figure 3B (Lemoine et al., 2019), Figure 3C (Tang et al., 2017), and Figure 3D (Kim et al., 2014). Through the comparison, meaningful findings emerge: (1) China pays more attention to the geometric and advanced materials of the reef structure, (2) South Korea pays more attention to the spatial complexity and heterogeneity of reefs, and (3) The United States focuses on investigating large reef structures and integrated reef materials and their impact on biological communities.
The composition of the biological community around artificial reefs plays a crucial role in the proliferation of fishery resources. The material, structure, deployment time, and ocean environment of artificial reefs closely affect the distribution of biological communities. The benthic community of artificial reefs in the Double-Islands Bay of the Yellow Sea was analyzed by Liu et al. (2017). It was found that the benthic community of artificial reefs varies with their deployment time. As the artificial reefs age, mollusks and saprophytic organisms will increase significantly. Moreover, water temperature, seasonal variation, and reef materials have significant effects on algae growth, affecting the distribution of the fish community (Burkepile et al., 2013; Noh et al., 2017). Therefore, the heterogeneity of the marine environment and the complexity of reef structure promoted biodiversity (Cardoso et al., 2020). However, it is said that artificial reefs change the composition of biological communities and maintain the balance of food chain around reef areas. Moreover, specific impacts on the natural environment and overall implications for nutrient dynamics around reef areas need to be considered when deploying artificial reefs (Einbinder et al., 2006). The influences of artificial reefs on hydrodynamic characteristics are described in the next two sections.
3.1.1 The Effect of Flow Field
As the water flow passes through the artificial reef, the local upwelling region is formed in front of the reef body, the turbulence fluctuations is formed inside the reefs, and the wake region with vortex is formed in the downstream of the artificial reef. In the sea area without artificial reefs, the vertical movement of water flow is usually negligible under normal climatic conditions. However, as the flow interacts with the artificial reefs, a distinct local upwelling can be located in front of the reef body, with an amplitude equivalent to the horizontal flow (Eidens et al., 2015). A more quantitative definition of the upwelling is 120% of the incoming flow velocity (Wang et al., 2018). This local upwelling can effectively promote the exchange of seawater at different depths. Tang et al. (2019) claimed that the flow field effect of reefs body is proportional to the range of upwelling region, i.e., the larger the range of upwelling area, the better the flow field effect, and the more obvious the fish aggregation effect. Many researchers have studied the structural factors of the artificial reef, dramatically affecting the height, length, and dimension of the upwelling. The research results are divided into two aspects. The upwelling region of a single reef is significantly affected by the flow velocity, the cut-opening properties of the reef body, the structural type, and the angle of the guide plate (Fulton and Bellwood, 2005; Preker and Lawn, 2012; Jiang et al., 2016; Wang et al., 2018; Zhao et al., 2019). On the other hand, the stacking height, the arrangement patterns, and the separation distances among multiple reef are factors affecting different heights and areas of upwelling (Jiao et al., 2017; Shu et al., 2021a; Wang et al., 2021).
The fish aggregation effects of artificial reefs are not only related to the time-averaged flow field around the reef body, but also closely related to the turbulence fields. Due to the reef edge blocking the flow, turbulence fluctuations can be seen around the inner, on both sides, and at the edge of the wake region. There is a low turbulent region inside the reef body, which is conducive to the adsorption and retention of marine organisms. The dramatic turbulence region on both sides of the reef body can also provide water space for fish to swim. Different marine organisms have different tendencies towards the strong or weak turbulence fields. Such turbulence intensities around artificial reefs can meet the needs of different biological characteristics (Kim et al., 2014b; Shu et al., 2021b). The affected regions of the turbulent area produced by different types of reefs are varied, which has been discussed by Howe (2003). The height ratio and the length ratio of the turbulent area produced by the permeable reef are less than 1 and less than 4, respectively, while the height ratio and the length ratio of the turbulent area produced by the non-permeable reef are greater than 1 and may reach 14, respectively. The combination of pyramidal artificial reefs can produce more complex turbulent areas, which has been investigated by Tamura et al. (2007). The dominant factor affecting the range of the turbulent area is the upstream regions, but not the current velocity.
The wake region with vortex that provides fish space for shelter, foraging, spawning, habitat, and temporary relaxation is often formed at the back of the reef body. In addition, vortexes and eddy currents in the wake region can also attract fish. As shown in Figure 4 (Kim et al., 2014b), the wake region is characterized by the circulating flow of fluid in the defined region. It is defined as the region occupied by the flow vector in the opposite direction to that of incoming flow. Therefore, it is quite crucial to quantify the size of the wake region for studying the implications of artificial reefs. According to the length of the wake region, many researchers have quantified the size, area, and volume of the wake region (Liu et al., 2013; Jung et al., 2016; Jiao et al., 2017; Huang et al., 2017). The three-dimensional wake volume is more representative compared to other properties of the wake region, such as areas and lengths of the wake region (Kim et al., 2014a). The artificial reefs have significant influences on the position, size, and direction of the vortex in the wake region. Following the published PIV and the numerical studies for the frame-type artificial reefs with square openings by Jiang et al. (2013), the clockwise vortex was formed at the bottom of artificial reefs and the counterclockwise vortex was formed at the top of the reef body. Based on the PIV and the numerical simulation for the semi-spherical floating artificial reefs, the vortex direction in the wake region is counterclockwise and the vortex position is related to the height of the wake region (Kim et al., 2021).
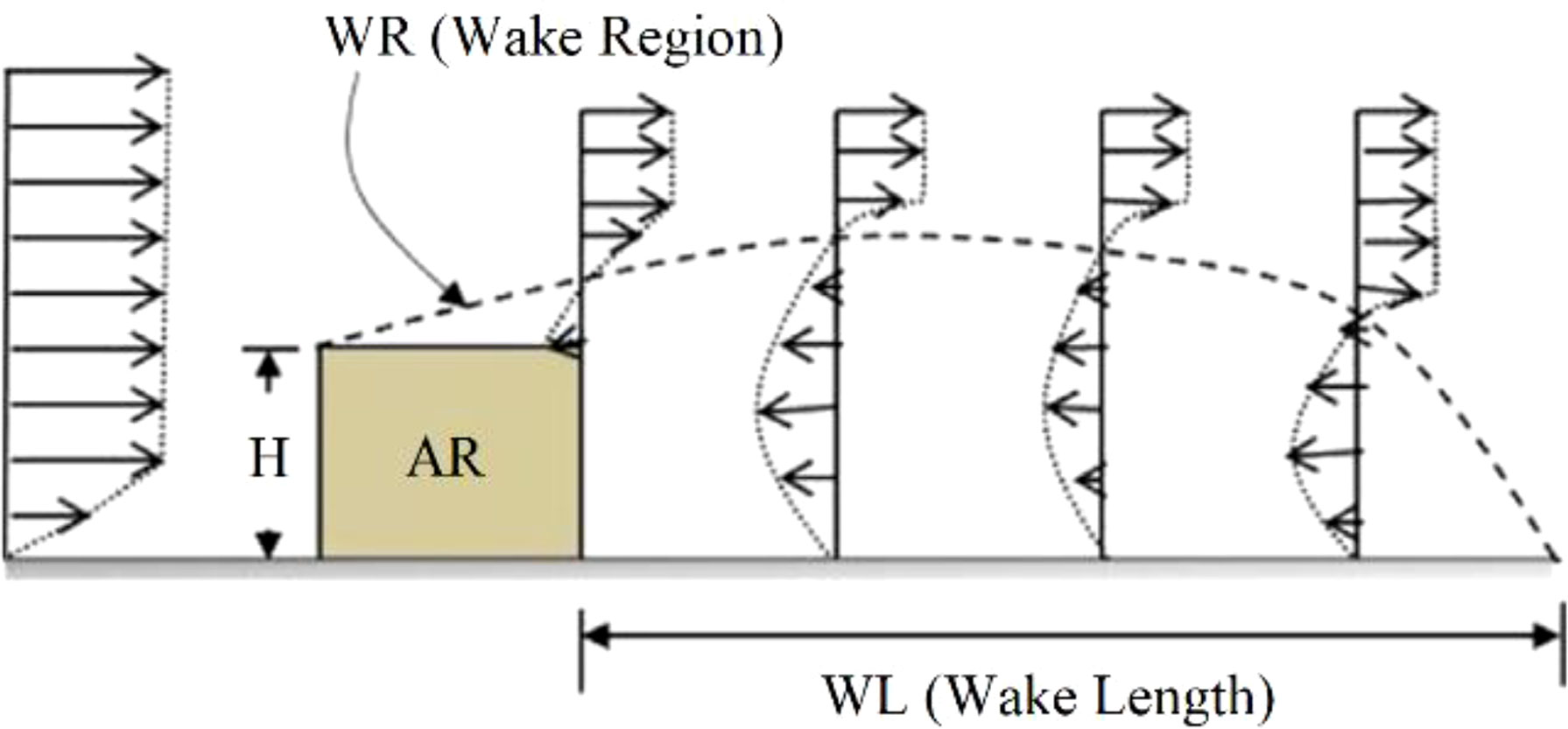
Figure 4 Definition of wake region and wake length (Kim et al., 2014).
3.1.2 The Stability
The sit-bottom artificial reefs placed on the seabed are subject to the combined currents and waves. The artificial reefs would slip if the horizontal forces (mainly hydrodynamic drags) on the reef body were greater than the friction between the reef body and the seabed. Similarly, the reef structure would be overturned when the overturn moment generated by the forces is greater than the anti-overturned moment (Zhao et al., 2015b). The stability of the sit-bottom artificial reef requires that the reef body cannot slip or overturn to ensure that the absolute sink does not occur after the reef body is put into the sea. Within the design stage, besides the hydrodynamic features in waves and currents, the loading capacity of the pile foundation, the stability of anti-slipping, the stability of anti-overturn, and the impact resistance, the situation of seabed erosion around the reef body should also be considered (Kim et al., 2016).
There are roughly two ways to deploy sit-bottom artificial reefs: one is sunk into the sea by free-falling and the other is installed on the seabed with the help of divers. When the artificial reefs were put into the sea by free-falling, the reef body experienced collisions with the seabed. Therefore, the initial subsidence happened. The characteristics of the initial subsidence of artificial reefs on seabed with different soil components were analyzed by Han et al. (2020). They suggested that researchers should avoid the seabed containing clay when installing artificial reefs through free-falling. The long axis of the reef body should remain parallel to the direction of the integrated velocity of the wave current to minimize the force of the wave current on the launched reef body, which was pointed out by Zhao et al. (2006).
Since the length scale of a single artificial reef is generally smaller than the wave length, the Morison equation can be used to calculate the wave forces. The formula is as follows (Zhao et al., 2015b):
where Cd and Cmrepresent the drag coefficient and the inertia coefficient, respectively, ρ is the seawater density, and A and V are the incoming front projected area and the volume of reef body, respectively.
It can be seen from the formula that the drag coefficient Cd and the inertia coefficient Cm are the key factors to calculate the wave–current force on the reef body. The drag coefficient of 24 artificial reefs commonly used in South Korea was investigated in Woo et al. (2014), and the linear equations were fitted to estimate the relationship between the shape of reef bodies and the drag coefficient. In addition, the relationship between flow velocity and reef body height was also studied. The artificial reef with triangular structure was included by Tamura et al. (2007), and they found that the drag coefficient of the reef body would decrease with the increase in Reynolds number and Keulegan–Carpenter number. However, the drag coefficient fluctuates with the increase of Reynolds number and Keulegan–Carpenter number. At the same time, some researchers pointed out that the flow velocity, the angle of flow, the mode of cut-opening, and the cut-ratio opening ratios of reef bodies will affect the drag coefficient (Tang et al., 2010; Zhang et al., 2016; Tang et al., 2021). However, the Morison equation is not applicable to calculate the wave force for large-scale reef bodies or artificial reef groups. Therefore, it is necessary to determine the forces on the reef body under the action of waves and currents through physical model experiments or computational fluid dynamics (CFD) methods.
The main contents of stability study of the sit-bottom artificial reefs are the anti-slip coefficient, the anti-overturn coefficient, the bearing capacity of the pile foundation, and the sluicing around the reef body. In addition, its research methods are primarily theoretical calculation, physical experiments, and CFD modelings. Based on the theory of the wave–current dynamics, the anti-slip coefficient and anti-overturn coefficient of the square steel platform reef body were calculated by Zhong et al. (2006). The average anti-slip and anti-overturn coefficients are 1.44 and 2.23, respectively. The cut-opening ratio of reef bodies also affects the safety coefficient, which was reported by Kim (2018). With the increase in the cut-opening ratio of reef bodies, the safety coefficient shows a decreasing trend.
(T)he deployment of sit-bottom artificial reefs leads to the local variations of flow fields around the reef structure. Since the fine-grained soil at the bottom of the reef body in the area with relatively fast flow velocity is easy to be carried away by the water, the seabed soil around the reef body gradually becomes thicker and the reef begins to sink slowly. In contrast, the fine-grained soil will form sedimentation in the area with the relatively slow flow velocity. As a result, the local shape of the seabed in the reef area has been changed. The artificial reefs on soft ground are more likely to fail due to seabed sedimentation and erosion. Considerable flume experiments and measurements in the field have proved that the main reasons for the seabed sedimentation and erosion around reef bodies are the change of flow field, the disturbance, and the wave forces. The physical experiments of seabed sedimentation and erosion of artificial reefs with different soil components were performed by Yun and Kim (2018). The results showed that strengthening the bottom of artificial reefs could significantly reduce seabed sedimentation and erosion. Based on the flume experiments, artificial reefs can block the flow and reduce the sediment-carrying capacity (Hou et al. (2017). As a result, it provides a favorable dynamic environment for depositing the suspended and bed load sediments behind the reefs. The sinking of artificial reefs placed on muddy and sandy coasts in the Jiangling area of eastern South Korea was measured by Kim et al. (2010). As a result, he found that the reefs installed on soft seabed had sunk more than 50%.
3.2 Modern Floating Artificial Reef
Although floating reefs appear earlier, the use of floating reefs as ecological restoration is still in its infancy. Because the floating reef is a flexible structure with a mooring system, it has good characteristics under strong wind and wave conditions, is less restricted by seabed geology, and is easy to cluster. Therefore, the combination of floating reefs and sit-bottom reefs has complemented each other and has the effect of comprehensive utilization. In addition, the floating artificial reef can become an important part of the coastal ecological corridor. The large-scale construction of floating reefs deployed by optimizing mooring methods can effectively improve the ecological effects of coastal projects. The body of the floating artificial reefs is arranged in the upper- and middle-layer waters and fixed with anchor blocks in specific sea areas to attract migratory fishes. The floating artificial reef can be divided into the floating fish trapping reef and the floating fishery reef proliferation according to their usage (Zhang et al., 2016; Palo et al., 2021). On the one hand, the floating artificial reef of fish trapping, so-called FAD (Fish Aggregating Devices), often carries the fish monitoring system, the sonar system, and the luminescence system. It can attract a large number of fish gathered near and under the reef, significantly increasing the catch. Because of the regulation of floating artificial reefs for trapping only, FAD was currently used for monitoring fishery resources and evaluating biomass (Eighani et al., 2019). On the other hand, the floating artificial reefs for fishery proliferation are shown in the structure in Figure 5A. A few of the floating artificial reefs were put into sea and evaluated with fish aggregation. However, relatively few previous studies have been carried out on marine hydrodynamic characteristics, which include the safety, stability, and deployment of floating artificial reefs, and other fundamental engineering problems or techniques. The survival performance of floating artificial reefs mainly refers to safety under ultimate loads. The operational performance refers to the effect of fish gathering and sheltering in a year, which is related to the motion state of floating reefs in the actual sea conditions (Palo et al., 2021; Pan et al., 2021). The floating artificial reefs deployed in the field were observed by Frank (2018), and he found that the fastened floating structures often attract larger fish stocks, while the dragged floating structures are not. The reason for this needs further research. In addition, the smaller the fish is, the closer it is to the structure, and the fish stock is close to the structure at night. However, if the structure oscillates with waves for a long time, the fish stocks will be dispersed. Therefore, the floating artificial reef fastened by a mooring cable has good stability to ensure the effect of fish gathering and sheltering under wave action.
In summary, the existing floating artificial reefs have not been widely used in the construction of marine ranch in China primarily due to the following: (1) Early in Japan, the United States, Britain, and other countries, the floating artificial reefs in the upper and middle layer carry meteorological monitoring and fish monitoring systems used in more than 100 m water depth. Therefore, the application in shallow water needs further research. (2) The existing design of the structure of floating artificial reefs, which cannot be used in shallow waters with 10–20 m depth, has the shortcomings of weak flexibility and strong swaying, because the aggregation effect is inversely proportional to the swaying degree of the floating artificial reef. (3) Due to the large volume of the traditional floating artificial reefs, it is only used for a single launch. (4) Because typhoons and cold waves have a significant impact on the East China Sea, especially on the coast of Zhejiang, scholars should not only study the effect of characteristic waves on the existing floating artificial reefs but also pay attention to the effect of extreme random waves.
3.2.1 Hydrodynamic Characteristics
A floating artificial reef is principally composed of the buoyancy system, the fishing net system, and the mooring system. Compared with the sit-bottom artificial reef, the hydrodynamic characteristics of the floating artificial reef are more complicated.
As shown in Figure 5A, the buoyancy system of the floating artificial reef is mainly composed of floating buoys or floating tubes. The main body of the reef is woven by mooring cables and fishing net. The structure components are very similar to traditional fish cages. The research of the structure type, the movement of floating frame, the mode of mooring, and the volume change of fish cage were studied by Zhao et al. (2015a); Moe-Føre et al. (2016); Hou et al. (2018); Liu et al. (2019); Chu et al. (2020); Guo et al. (2020) and Strand et al. (2016). They studied the volume reduction and structural safety of the flexible fish cage in waves and tidal currents, ensuring that fish cages could meet the design requirements for different environmental conditions. The floating artificial reefs also need to maintain a certain height and attitude in order to act on the fish stocks in the surrounding waters (Pan et al., 2021). With a vertical dimension 5–10 times larger than the horizontal dimension, artificial reefs can be deployed in groups and they are usually fastened by a single mooring cable. Therefore, as shown in Figure 5A, the floating artificial reef in the middle and upper layers reduces the volume easily, is greatly deformed, and stacks layers of waves (especially random waves), thus causing the reef’s failure. At present, the current research about floating artificial reefs is not correlated with the dynamic response of floating structures in random waves (Liu et al., 2021) and deformed waves (Harris et al., 2015), compared with other typical offshore platforms and wave energy devices fastened by a mooring system. However, this is the content that needs to be highlighted during the operation of floating artificial reefs.
(I)n recent years, the concept of floating fish cages was used by Kim et al. (2001) to design a flexible floating artificial reef. It can only be used offshore, and the cost is relatively high. Based on static theory and the Morison equation, the distribution of the flow forces and the wave forces on floating artificial reefs with a cylindrically rigid frame was statically discussed by Miao and Xie (2007). However, due to the use of rigid rods in floating artificial reefs, large buoyancy and high cost are needed to form a large floating artificial reef volume. By referring to the gravity fish cage, a newly floating artificial reef, which consists of 4 cylindrical frames with tied fishing nets, was proposed by Zhang et al. (2016). It integrated rigidity and flexibility and has achieved remarkable results in the marine ranching of Xiangshan Port. Nevertheless, all designs were based on static calculations, and more floating spheres are still needed at the top of floating reefs to provide buoyancy. Since high-density polyethylene (HDPE) pipes have many advantages such as large buoyancy, high corrosion resistance, strong rigidity, and no marine pollution, the hydrodynamic features of the floating artificial reefs composed of HDPE pipes in waves were studied by Pan et al. (2021), as shown in Figures 5B, C. In addition, by analyzing the trajectory of floating artificial reefs and the tension of mooring cables, it was found that the vertical height of the floating artificial reef significantly affects its safety and operation performance, and the optimal ratio of horizontal length to vertical height of floating artificial reefs and the optimal tying position were given. In the latest research, it was found that the rigidly floating frame of floating artificial reef yawing was obviously under diagonal wave action. Although the rotation group can be designed, the mooring system is also a potential safety hazard.
3.2.2 Component of the Floating Reef
In order to show the research methods of the hydrodynamic characteristics of the floating reef better, the hydrodynamic characteristics of each component of the floating reef were introduced as follows.
(1) The hydrodynamic characteristics of the buoyancy system
The buoyancy system is mainly composed of floating spheres or floating tubes. As shown in Figure 5D, an array of floating spheres fastened by a mooring cable is used in reef bodies. On the one hand, sufficient buoyancy can be provided evenly with a floating array. On the other hand, there are many other advantages of the floating array such as the small hydrodynamic drags, the changeable distances between the floating spheres, and the good permeability of the floating artificial reef. The reason why floating artificial reefs have good permeability is that algae adhere to the mooring cables in the limited space. As the reserve buoyancy, the large-diameter floating spheres can effectively regulate the buoyancy required by floating artificial reefs in different water depths and different algae and shellfish attachments. Since the ratio of the characteristic length of the structure to the wavelength of the buoyancy system (the diameter of floating sphere and floating tube) of floating artificial reefs is less than 0.2, that is, a small-scale structure, the finite element method and the lumped mass point method can be used to establish the model of floating artificial reefs. The drag and inertia forces in waves and currents can be determined following the Morison equation (Sohn et al., 2011; Zhao et al., 2015; Hou et al., 2018; Liu et al., 2019; Chu et al., 2020; Guo et al., 2020). However, the critical issue is the determination of the hydrodynamic coefficients. At present, the hydrodynamic coefficients of floating structures are analyzed by regression analysis under a submerged state, and the obtained wave forces are consistent with the experimental value. However, the above results are not fully applicable to the hydrodynamic calculation of flexible floating artificial reefs. This is because the characteristic length of the structure of the floating artificial reef as a whole is much larger than 0.2 times of the incident wave length, and the interference and shelter of the movement of water particle between the floating sphere and the floating tube are generated. Therefore, it is unreasonable to estimate the dynamic response of the floating artificial reef only by empirical formulas.
To the best of our knowledge, there are three methods to calculate the wave forces of the small-scale and non-submerged floating spheres and floating tubes: Firstly, the overall geometric configuration of the structure was drawn, then the polygonal mesh was generated and tightly adhered to the geometric configuration. However, the calculation is complex because the calculation of hydrodynamic load needs to capture the free surface in the two-phase flow domain to determine whether each grid is submerged and to determine the normal vector of the grid surface (Wang et al., 2017; Palm and Eskilsson, 2020). This method is called the volume of fluids. Secondly, based on the smoothed particle hydrodynamics (SPH) method, the structure is described as a solid particle, and then the fluid–solid coupling method is used to calculate the forces and motions (Gunn et al., 2018; Domínguez et al., 2019; Altomare et al., 2020; Ropero-Giralda et al., 2020). Thirdly, the geometric relations between non-submerged floating spheres or tubes and wave surface were solved (Zhu et al., 2016a; Zhu et al., 2020; Feng et al., 2021). The Morison equation has the advantage of high efficiency and accuracy in calculating wave force. However, it does not consider the interaction of the structure to the fluid. Thus, it is only applicable to a single or to a certain interval distance of the floating spheres or floating tubes. To overcome this, an improved Morison hydrodynamics model for knotless nets based on CFD and metamodeling methods was developed (Huang et al., 2022). The SPH method was used by Gunn et al. (2018); Altomare et al. (2020); Ropero-Giralda et al. (2020) and Domínguez et al. (2019) to investigate the flow interactions between waves and floating spheres or floating blocks of a single mooring system through numerical simulations, and validated it with the physical experimental data. This has provided a theoretical basis for studying floating spheres in the system of the flexible floating artificial reef in three-dimensional wave field. Since the fastened floating sphere or fastened floating tube moves with 6 degrees of freedom and interacts with nonlinear surface waves, special requirements are required for the modeling method: (1) the description of mechanical properties of mooring materials (rope, cable, and chain), (2) the calculation of the 6 degrees of freedom response motions of the fastened floating sphere and floating tube, and (3) the description of the interaction between the fluid and the floating structures.
(2) The hydrodynamic characteristics of the fishing net
The fishing net is woven by the twines with a diameter on the order of millimeters, and its outline length scale reaches the 10-m level. In addition, the porosity properties of the fishing net are unique compared to the typical offshore structures. The study of the hydrodynamic characteristics of the fishing net can be divided into two aspects: On the one hand, computational cost can be dramatically increased if the physical net meshes were directly modeled with the numerous small-scale rigid rods (Cornejo et al., 2014; Winthereig-Rasmussen et al., 2016; Yao et al., 2016; Touzon et al., 2021). On the other hand, the porous medium method to describe the small-scale rigid rod was adopted (Bi et al., 2014; Zhao et al., 2015); as a result, the real influences of the topology as well as the structural details of net meshes are overlooked. Currently, some researchers described the fishing net and fluid as spherical particles (Agarwal and Jain, 2003). The deformation of the fishing net was solved by the lumped mass point method and the N–S equation is solved by the SPH method. The deformation of the fishing net considering fluid–solid coupling can be simulated more precisely.
(3) The hydrodynamic characteristics of the mooring system
The floating structure fastened by mooring cables has been widely utilized in marine resource restoration and offshore engineering such as ocean meteorology, water quality and ecological monitoring, navigation system, oil and gas exploitation, and marine aquaculture facilities. To ensure the long-term stability of the mooring system, it is necessary to calculate the forces and state of the system in viscous fluids. The primary methods are the static and dynamic analysis (Tu et al., 2020). The lumped mass point model is a common method to study the dynamic response of mooring systems (Zhu et al., 2016a; Pan et al., 2021; Palo et al., 2021; Feng et al., 2021a; Zhu et al., 2020; Touzon et al., 2021). The dynamic safety performance of the floating platform with iron chain was studied by Gobat and Grosenbaugh (2001); Agarwal and Jain (2003), and Umar and Datta (2003). The hydrodynamic characteristics of the floating Spar platform fastened by single mooring or a multi-mooring system was studied by Kim et al. (2013) and Zhu et al. (2016b). They unanimously pointed out that the motions of the floating structure were related to the tension and the restoring effects of mooring lines. Therefore, the influence of the mooring system cannot be overlooked. The physical and mechanical properties of the materials of the mooring system will affect the motion of the floating structure. The diameter, density, elastic modulus, and damping coefficient of the mooring system are the most important factors. The materials of the mooring system are divided into rope, cable, and chain. Except for the obvious difference in density, the damping coefficients of the rope, the cable, and the chain are 102 N•m/s, 104 N•m/s, and 105 N•m/s, respectively. Therefore, the hydrodynamic characteristics of the above three components are also quite different. The single mooring system of floating structures, such as buoys, platforms, and ships, is a combination of the upper mooring ropes and the lower mooring chains. This is because using only tight mooring ropes will greatly reduce the stability and operation performance of the upper floating structure. The single mooring system has the advantages of having a simple structure, a good motion performance, and a low cost. However, due to the motion in all directions, single mooring cables cause the device to rotate around a single point (Ma et al., 2022). Because of its advantages (low cost, simple deployment, and the large-scale construction of reefs), single-point mooring is the preferred mooring method for the middle and upper floating artificial reefs. However, the safety and operational performance of middle and upper floating reefs need to be ensured firstly. It can be improved by reasonably optimizing the gravity and buoyancy of the single mooring system.
3.3 Ecological Artificial Reef and Multifunctional Artificial Reef
3.3.1 Artificial Reefs Combined With Offshore Oil and Gas Platforms
At the beginning of 1920, the industry infrastructures of offshore oil began to be established. After 50 years, these infrastructures have gradually lost their functions. By 1990, even relatively complex marine infrastructures cannot be reused. So far, except for a series of seabed infrastructures, pipelines, and oil wells, there are thousands of offshore oil and gas facilities and platforms worldwide. Considering the engineering and economic aspects, these decommissioned facilities are still not demolished (Hamzah, 2003). The hard substrates of the pile foundation of offshore oil and gas platforms play the role of artificial reefs to provide habitats for marine organisms such as mussels, barnacles, scallops, sponges, tunicate, corals, oysters, crabs, and shrimps. In addition, the invertebrate layers provide food and habitats for fish (Bull and Love, 2019). Therefore, researchers from many coastal countries have appealed that local governments should not abolish offshore oil and gas platforms directly, as artificial reefs have been formed around these structures. The removal may cause significant harm to local ecosystems (Love and York, 2005; Love et al., 2006).
The construction of oil and gas platforms in the Gulf of Mexico has reduced the mortality of red snapper, which was clarified in Gallaway et al. (2009). An interesting phenomenon is that younger and smaller fish occupy the deeper water of the pile foundation of oil and gas platforms, and larger and older fish occupy the lower water. The pile foundation provides shelter and feeding opportunities for red snapper in the whole water. Therefore, the removal of oil and natural gas platforms may lead to a large reduction in the number of red snappers. From the perspective of engineering, Chandler et al. (2017) believed that the consolidation and the sediment transportation caused the changes in seabed strength and water depth. Infrastructure stability for oil and gas platforms has been enhanced recently; thus, re-using them as floating artificial reefs can maximize their performance of proliferating fisheries. Three options for transforming abandoned oil and gas platforms into artificial reefs were described by Bull and Love (2019) (as shown in Figure 6): (a) the relocation by drag, (b) the overturning of a platform in place, and (3) the partial removal by non-blasting method. Compared with the other two methods, partial removal can produce higher profiles for artificial reefs and reduce damage to the existing marine organisms.
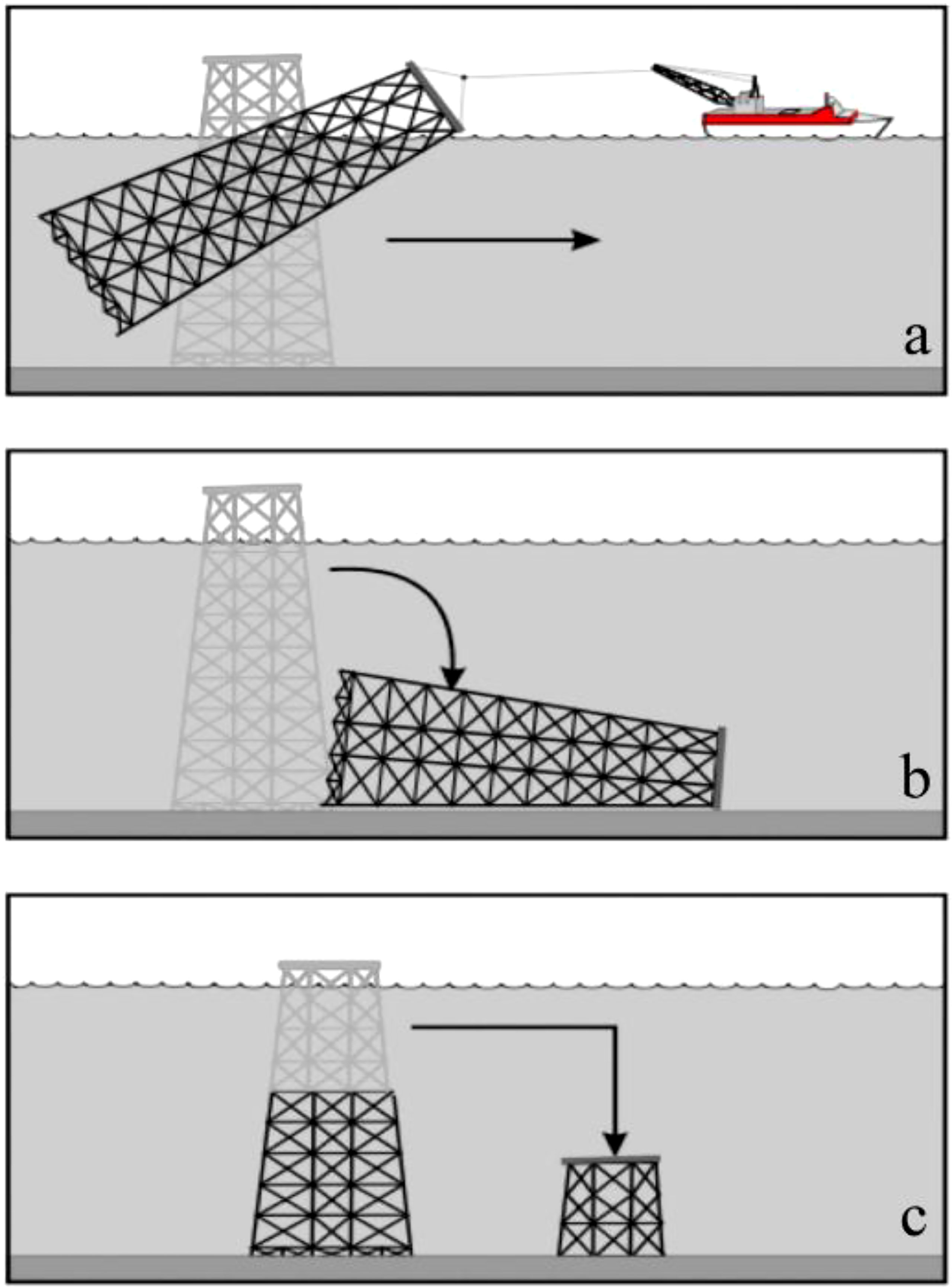
Figure 6 Transformation scheme for oil and natural gas platforms (Bull and Love, 2019).
3.3.2 Artificial Reefs and Coastal Protection Engineering
The coastlines of more than 163 coastal countries worldwide are suffering from varying degrees of erosion. In the United States, the average annual coastline erosion is 1.8 m (Lawrence, 1996). In addition, due to the threat of coastal erosion, Thailand has lost more than $156 million annually, which is considerable for a developing country (Schulze et al., 2020). Inevitably, 70% of sandy beaches and the majority of muddy tidal flats in China have also suffered from varying degrees of erosion (Hinkel et al., 2013). Researchers in various countries have tried to use different coastal protection structures to reduce coastline erosion. In terms of physical appearance and the interaction with waves or currents, the underwater breakwater is similar to the artificial reef originally used for ecological enhancement. For this reason, some artificial reefs are also designed as multi-function coastal protection structures. This enables more economical and practical materials and environmentally friendly construction (Ranasinghe and Turner, 2006).
A new multifunctional artificial reef named Seadome was designed by Srisuwan and Rattanamanee (2015), as shown in Figure 7. Based on physical experiments and field tests, the ability of Seadome array to attenuate wave height was verified. Therefore, this kind of artificial reef effectively prevents coastline erosion. A hybrid artificial reef with a trapezoidal structure was designed by Ghiasian et al. (2021). In addition, the model experiment showed that it can reduce wave height up to 35% and dissipate wave energy up to 63%. Therefore, it effectively reduces wave energy and protects the coastline from erosion. A 630-m large-scale biodegradable artificial reef was installed by Marin-Diaz et al. (2021) on the tidal flats of the Walden Sea in the Netherlands to monitor the wave, sediment dynamics, and sediment characteristics. The results showed that artificial reefs can weaken waves and capture sediments on tidal flats.
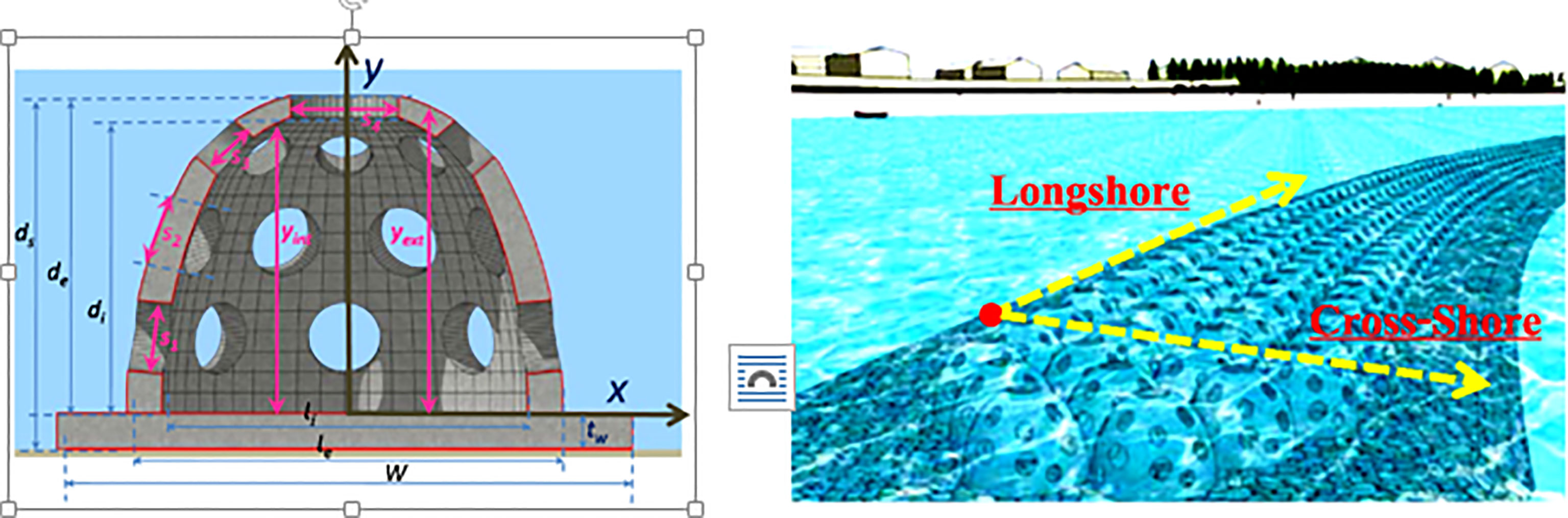
Figure 7 Single structure of Seadome and the array of Seadome (Marin-Diaz et al., 2021).
Due to the issues resulting from the large scale of the model (the high cost of the experiments and the lack of suitable sea area for field tests), little research was carried out on the multi-functional artificial reefs for coastal protections in China. In order to further promote the sustainable development of marine fishery resources and environment, the multifunctional artificial reef should be promoted to play different roles in the future. As a result, it will reduce the construction cost and improve the ecological effect.
3.3.3 Artificial Reefs and Renewable Energy Projects
With the world shifting to a low-carbon economy, renewable energy has gradually become a research hotspot in different countries. Offshore wind turbines are more sufficient than land, and they have great potential. Researchers hold different attitudes toward the installation of renewable energy equipment, which can be roughly divided into the following two categories: On the one hand, as renewable marine energy has become a rapidly growing industry, some researchers believe that over-installation of renewable energy equipment in the sea may lead to the loss or degradation of marine habitats (Inger et al., 2009). On the other hand, some researchers believe that the protection zone of erosion of the pile foundation of offshore wind turbines can act as artificial reefs to create new habitats to compensate for the habitat loss caused by the installation of offshore energy devices. At the same time, prohibiting fishery zones, which are set around renewable energy facilities, can also avoid fishing. To some extent, it reduces the pressure of over-exploitation of fishery species (Wilhelmsson and Langhamer, 2014). In the long run, offshore renewable energy devices and the protection zone of erosion have great potential to create a certain number of marine habitats. This will have a profound impact on the marine environment and local fisheries. The large wind turbine foundation with the scour protection function as artificial reef was found by Krone et al. (2017). It can significantly increase marine bioaccumulation and also enrich species populations. Langhamer (2012) noted that the construction of renewable energy installations in offshore regions could not mitigate the loss of marine habitats, but it can create new habitats to compensate for them. For example, renewable wind turbines and their associated scour protection can be seen as an artificial reef. Because their surfaces are easily settled by the typical and predictable biological communities, they would gradually become a new habitat for marine organisms. Yang et al. (2019) proposed an idea of integrating artificial reefs with offshore wind turbines to form a new industry from the intensive use of the sea. The organic integration of the base piles of wind turbines and artificial reefs can achieve the functional integration of resource conservation and environmental restoration, as shown in Figure 8.
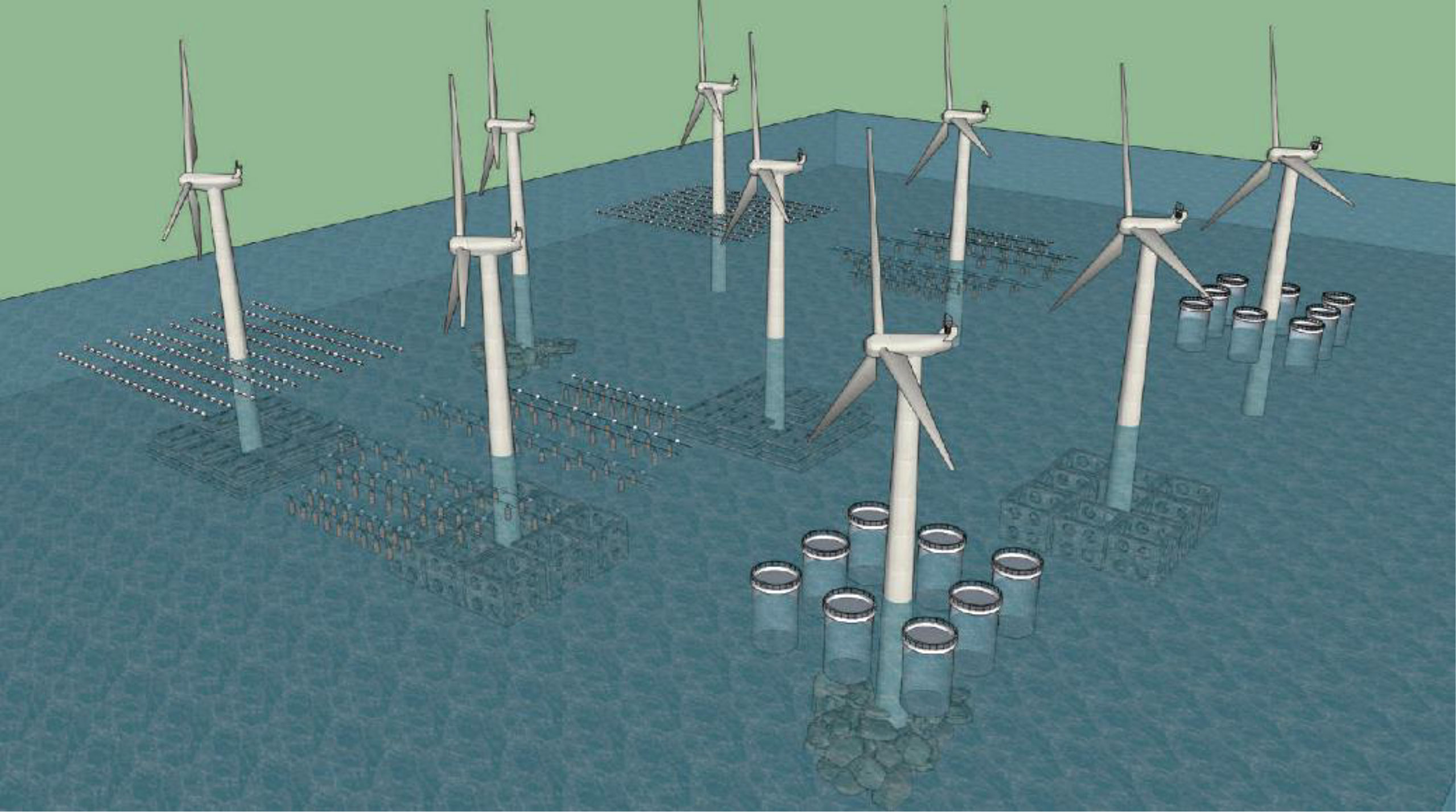
Figure 8 Integration of offshore wind turbines and artificial reefs (Yang et al., 2019).
The combination of artificial reefs and offshore wind turbines can improve the utilization efficiency of marine resources and achieve sustainable, green, and efficient development. In addition, countries such as the Netherlands, Germany, South Korea, and Norway (Buck and Langan, 2017) have integrated offshore wind turbines with artificial reefs. However, there are no examples of integrated development of artificial reefs and offshore wind turbines in China. At present, it is crucial to study the mechanism of artificial reefs and offshore wind turbines through experiments and explore the influence of offshore wind turbines on artificial reefs, so as to achieve the production of clean energy as well as the protection of marine environments.
4 Conclusions and Prospects
Artificial reefs combined with emerging floating and fixed structures in ocean engineering play an essential role in repairing and conserving fishery resources and enhancing the fish stock; thus, the purpose of both habitat construction and new functions or protection can be achieved to some extent. The hydrodynamic characteristics around artificial reefs are correlated with the selection of artificial reef sites, types, design, and the ecological effects. The variation of hydrodynamics properties has a considerable impact on flow fields and stabilities of bottom artificial reefs. The evolution of PIV technology and numerical modeling has made it possible to accurately describe the surrounding flow patterns of bottom reefs, and the relationship between the structural optimized results with upwelling regions can be established; thereby, the transportations of nutrients in oceans are expected to be adapted. Nevertheless, the existing hydrodynamics models that calculate the hydrodynamic forces on the bottom reef structures neglect the significance of wave forces, and the roughness and porosity of the bottom-layer reef surfaces are overlooked as well. The consideration of the various parameters of artificial reefs into the wave model is still a major issue that needs to be solved urgently in the field of hydrodynamics modeling of artificial reefs.
Given the development from the bottom type, floating type, to the novel ecological reefs, the concentration of research has shifted from designs, performances, applications, and managements of artificial reefs to artificial reef ecological community structures and compositions. Previous studies related to the reef structures in the bottom layer focused on the improvement of structures, the usage of materials, the surrounding flow fields, and stability. Numerous papers have clarified that the complexity and heterogeneity of the spatial structure of the bottom reef can significantly improve the biomass abundance of biological species, and there is no unified scientific quantification standard for the description of spatial complexity and heterogeneity yet. In order to improve the efficiencies of artificial reef deployments in the future, the standardized method to quantify the structural complexity and heterogeneity of different artificial reefs needs to be proposed. Artificial reef materials have varied from the original rubber, rock, to concrete and gel materials, with more and more countries actively developing new environmentally friendly synthetic bottom reef materials. In the future, the degree of bio-fouling issues of artificial reefs needs to be justified and the long-term impact on the marine environment needs to be monitored.
Even though the floating-type artificial reefs have many advantages such as the excellent anti-wave and current performances, less geological constraints on the seabed, and feasible grouping arrangement patterns of multiple reefs, the usage of floating reefs to repair the ecological environment is still in the initial stage. Thereby, the research and development of floating reefs with high safety performance and operational performance still has technical limitations in the construction of marine pelagic habitats. Additionally, the latest research about floating reefs focused on the effects of characteristic waves; however, the impact of extreme random waves such as typhoons and cold waves should be further studied.
Lastly, the development of artificial reefs combined with emerging offshore structures, the so-called the ecological artificial reef, can be improved following these novel ideas:
(1) Under the strategic objectives of carbon emission reductions, floating photovoltaic and floating wind turbines are becoming hot topics in the future. To further develop the upper and middle waters, floating artificial reefs combined with aquaculture rafts or fish cages can be built with renewable energy projects. In addition, the sit-bottom artificial reef is combined with the pile foundation of emerging structures in ocean engineering, such as wind turbines, aquaculture platforms, and landscape platforms. This way, they can prevent sediment erosion around pile foundations.
(2) At present, submarine pipelines, as the fourth essential mode of transportation in the world, can realize the collection and transportation of marine resources such as oil, gas, and water. Therefore, studies on artificial reefs with submarine pipeline protection or ecological environment construction are promising.
(3) The study on multifunctional artificial reefs mainly includes the following: firstly, the research on the structure type aims to meet the developing requirements of the flow field around traditional artificial reefs and ocean engineering protection. Secondly, the study on the accumulation mode and the scale effect of the multifunctional artificial reef on the marine environment was proposed.
Although remarkable achievements have been made in the research on artificial reefs in China, the hydrodynamic effect of artificial facilities as a key component of marine ranching remains unclear. In addition, existing studies are single studies and lack continuity and systematisms in reef bodies, layout patterns, and material selections. Until now, the studies on artificial reefs in China are mainly targeted at sit-bottom artificial reefs. This way, only the bottom marine layer is developed, and most of the upper-middle layers cannot be effectively utilized. However, floating artificial reefs can better utilize marine space, which needs further research. In the future, the organic integration of artificial reefs and emerging structures in ocean engineering can achieve the functional integration of environmental restoration and resource conservation to explore an ecological and sustainable development path for the efficient exploitation of marine space.
Author Contributions
Conceptualization, methodology, and writing—original draft preparation: YP and DX. Writing—review and check: HT and WX. Project administration and funding acquisition: DW. Visualization: DX. All authors have read and agreed to the published version of the manuscript.
Funding
This study is financially supported by the National Natural Science Foundation of China (Nos. 52101330 and 42006175) and the Zhejiang Provincial Natural Science Foundation of China (No. LQ21E090008). The authors would like to express profound thanks to them.
Conflict of Interest
The authors declare that the research was conducted in the absence of any commercial or financial relationships that could be construed as a potential conflict of interest.
Publisher’s Note
All claims expressed in this article are solely those of the authors and do not necessarily represent those of their affiliated organizations, or those of the publisher, the editors and the reviewers. Any product that may be evaluated in this article, or claim that may be made by its manufacturer, is not guaranteed or endorsed by the publisher.
Acknowledgments
Thank you very much to all the teachers and students who have revised the study and put forward their opinions. Thank you very much to Dr. Wang Gang of Ocean University of China for your valuable comments on the paper.
References
Agarwal A. K., Jain A. K. (2003). Dynamic Behavior of Offshore Spar Platforms Under Regular Sea Waves. Ocean Engineering 30 (4), 487–516. doi: 10.1016/S0029-8018(02)00034-3
Altomare C., Tafuni A., Domínguez J. M., Crespo A. J., Gironella X., Sospedra J. (2020). SPH Simulations of Real Sea Waves Impacting a Large-Scale Structure. J. Mar. Sci. Engineering 8 (10), 826. doi: 10.3390/jmse8100826
Armono H. D., Hall K. R. (2003). “Wave Transmission on Submerged Breakwaters Made of Hollow Hemispherical Shape Artificial Reefs,” in Canadian Coastal Conference (Kingston, Ontario, Canada), 313–322.
Bi C. W., Zhao Y. P., Dong G. H., Xu T. J., Gui F. K. (2014). Numerical Simulation of the Interaction Between Flow and Flexible Nets. J. Fluids Structures. 45, 180–201. doi: 10.1016/j.jfluidstructs.2013.11.015
Buck B. H., Langan R. (2017). Aquaculture Perspective of Multi-Use Sites in the Open Ocean: The Untapped Potential for Marine Resources in the Anthropocene (Cham, Switzerland: Springer Nature).
Bull A. S., Love M. S. (2019). Worldwide Oil and Gas Platform Decommissioning: A Review of Practices and Reefing Options. Ocean Coast. Manag. 168, 274–306. doi: 10.1016/j.ocecoaman.2018.10.024
Burkepile D. E., Allgeier J. E., Shantz A. A., Pritchard C. E., Lemoine N. P., Bhatti L. H., et al. (2013). Nutrient Supply From Fishes Facilitates Macroalgae and Suppresses Corals in a Caribbean Coral Reef Ecosystem. Sci. Rep. 3 (1), 1–9. doi: 10.1038/srep01493
Callaway R. (2018). Interstitial Space and Trapped Sediment Drive Benthic Communities in Artificial Shell and Rock Reefs. Front. Mar. Science 5, 288. doi: 10.3389/fmars.2018.00288
Cardoso A. P., Matos M. R., Rosa R. S., Alvarado F., Medeiros A. P., Santos B. A. (2020). Increased Fish Diversity Over Day and Night in Structurally Complex Habitats of Artificial Reefs. J. Exp. Mar. Biol. Ecol. 522, 151244. doi: 10.1016/j.jembe.2019.151244
Chandler J., White D., Techera E. J., Gourvenec S., Draper S. (2017). Engineering and Legal Considerations for Decommissioning of Offshore Oil and Gas Infrastructure in Australia. Ocean Engineering 131, 338–347. doi: 10.1016/j.oceaneng.2016.12.030
Chojnacki J. C. (2000). “Environmental Effects of Artificial Reefs in the Southern Baltic (Pomeranian Bay),” in Artificial Reefs in European Seas (Dordrecht: Springer), 307–317.
Chu Y. I., Wang C. M., Park J. C., Lader P. F. (2020). Review of Cage and Containment Tank Designs for Offshore Fish Farming. Aquaculture. 519, 734928. doi: 10.1016/j.aquaculture.2020.734928
Cornejo P., Sepúlveda H. H., Gutiérrez M. H., Olivares G. (2014). Numerical Studies on the Hydrodynamic Effects of a Salmon Farm in an Idealized Environment. Aquaculture. 430, 195–206. doi: 10.1016/j.aquaculture.2014.04.015
Costa M. B., Araújo M., Araújo T. C., Siegle E. (2016). Influence of Reef Geometry on Wave Attenuation on a Brazilian Coral Reef. Geomorphology. 253, 318–327. doi: 10.1016/j.geomorph.2015.11.001
De Almeida J. L. (2017). REEFS: An Artificial Reef for Wave Energy Harnessing and Shore Protection–A New Concept Towards Multipurpose Sustainable Solutions. Renewable energy. 114, 817–829. doi: 10.1016/j.renene.2017.07.076
Dennis H. D., Evans A. J., Banner A. J., Moore P. J. (2018). Reefcrete: Reducing the Environmental Footprint of Concretes for Eco-Engineering Marine Structures. Ecol. Engineering 120, 668–678. doi: 10.1016/j.ecoleng.2017.05.031
Domínguez J. M., Crespo A. J., Hall M., Altomare C., Wu M., Stratigaki V., et al. (2019). SPH Simulation of Floating Structures With Moorings. Coast. Engineering 153, 103560. doi: 10.1016/j.coastaleng.2019.103560
Eidens C., Hauffe T., Bayraktarov E., Wild C., Wilke T. (2015). Multi-Scale Processes Drive Benthic Community Structure in Upwelling-Affected Coral Reefs. Front. Mar. Science 2, 2. doi: 10.3389/fmars.2015.00002
Eighani M., Paighambari S. Y., Taquet M., Gaertner J. C. (2019). Introducing Nearshore Fish Aggregation Devices (FAD) to Artisanal Persian Gulf Fisheries: A Preliminary Study. Fish. Res. 212, 35–39. doi: 10.1016/j.fishres.2018.12.005
Einbinder S., Perelberg A., Ben-Shaprut O., Foucart M. H., Shashar N. (2006). Effects of Artificial Reefs on Fish Grazing in Their Vicinity: Evidence From Algae Presentation Experiments. Mar. Environ. Res. 61 (1), 110–119. doi: 10.1016/j.marenvres.2005.07.001
Feng D., Meng A., Wang P., Yao Y., Gui F. (2021). Effect of Design Configuration on Structural Response of Longline Aquaculture in Waves. Appl. Ocean Res. 107, 102489. doi: 10.1016/j.apor.2020.102489
Frank M. (2018). Artificial Reefs: Marine and Freshwater Applications (Boca Raton, FL, USA: CRC Press).
Fulton C. J., Bellwood D. R. (2005). Wave-Induced Water Motion and the Functional Implications for Coral Reef Fish Assemblages. Limnol. Oceanogr. 50 (1), 255–264. doi: 10.4319/lo.2005.50.1.0255
Gallaway B. J., Szedlmayer S. T., Gazey W. J. (2009). A Life History Review for Red Snapper in the Gulf of Mexico With an Evaluation of the Importance of Offshore Petroleum Platforms and Other Artificial Reefs. Rev. Fish. Science 17 (1), 48–67. doi: 10.1080/10641260802160717
Ghiasian M., Carrick J., Rhode-Barbarigos L., Haus B., Baker A. C., Lirman D. (2021). Dissipation of Wave Energy by a Hybrid Artificial Reef in a Wave Simulator: Implications for Coastal Resilience and Shoreline Protection. Limnol. Oceanogr.: Methods 19 (1), 1–7. doi: 10.1002/lom3.10400
Gobat J. I., Grosenbaugh M. A. (2001). A Simple Model for Heave-Induced Dynamic Tension in Catenary Moorings. Appl. Ocean Res. 23 (3), 159–174. doi: 10.1016/S0141-1187(01)00013-X
Gunn D. F., Rudman M., Cohen R. C. (2018). Wave Interaction With a Tethered Buoy: SPH Simulation and Experimental Validation. Ocean Engineering 156, 306–317. doi: 10.1016/j.oceaneng.2018.03.001
Guo Y. C., Mohapatra S. C., Soares C. G. (2020). Review of Developments in Porous Membranes and Net-Type Structures for Breakwaters and Fish Cages. Ocean Engineering 200, 107027. doi: 10.1016/j.oceaneng.2020.107027
Guo Y., Zhang S., Lin J. (2019). Flow Field Efficiency of Mi-Zi Artificial Reefs in Different Construction Modes Based on Numerical Experiments. J. Fish. China 43 (9), 2025–2038. doi: 10.11964/jfc.20190511800
Hamzah B. A. (2003). International Rules on Decommissioning of Offshore Installations: Some Observations. Mar. Policy 27 (4), 339–348. doi: 10.1016/S0308-597X(03)00040-X
Han S., Jung S., Na W. B. (2020). Estimation of Seabed Settlement During Initial Installation of a Box-Type Artificial Reef Considering Different Seabed Soil Compositions and Incident Angles. Ocean Engineering 218, 108269. doi: 10.1016/j.oceaneng.2020.108269
Harris D. L., Vila-Concejo A., Webster J. M., Power H. E. (2015). Spatial Variations in Wave Transformation and Sediment Entrainment on a Coral Reef Sand Apron. Mar. Geol. 363, 220–229. doi: 10.1016/j.margeo.2015.02.010
Hinkel J., Nicholls R. J., Tol R. S., Wang Z. B., Hamilton J. M., Boot G., et al. (2013). A Global Analysis of Erosion of Sandy Beaches and Sea-Level Rise: An Application of DIVA. Global Planet Change 111, 150–158. doi: 10.1016/j.gloplacha.2013.09.002
Hou H. M., Dong G. H., Xu T. J., Zhao Y. P., Bi C. W., Gui F. K. (2018). Fatigue Reliability Analysis of Mooring System for Fish Cage. Appl. Ocean Res. 71, 77–89. doi: 10.1016/j.apor.2017.12.008
Hou Q., Wang Z., Lu Y., Mo S. (2017). The Morphodynamic Responses to Deposition-Promoting Projects in Island and Reef Coasts of the Zhoushan Archipelago, China. Int. J. Sediment. Res. 32 (3), 351–363. doi: 10.1016/j.ijsrc.2017.07.001
Howe J. C. (2003). Artificial Reef Evaluation With Application to Natural Marine Habitats. Fish. Res. 2 (63), 297–298. doi: 10.1016/S0165-7836(03)00126-7
Huang L., Cheng H., Tang Y., Yang Q., Wang X. (2017). Comparison of Three Ways to Assess the Influence Range of Different Artificial Reefs. Int. J. Eng. Technol. 9 (2), 105. doi: 10.7763/IJET.2017.V9.953
Huang L., Li Y., Wang G., Wang Y., Wu Q., Jia M., et al. (2022). An Improved Morison Hydrodynamics Model for Knotless Nets Based on CFD and Metamodelling Methods. Aquacult. Engineering 96, 102220. doi: 10.1016/j.aquaeng.2021.102220
Inger R., Attrill M. J., Bearhop S., Broderick A. C., James Grecian W., Hodgson D. J., et al. (2009). Marine Renewable Energy: Potential Benefits to Biodiversity? An Urgent Call for Research. J. Appl. Ecol. 46 (6), 1145–1153. doi: 10.1111/j.1365-2664.2009.01697.x
Jiang Z., Liang Z., Liu Y., Tang Y., Huang L. (2013). Particle Image Velocimetry and Numerical Simulations of the Hydrodynamic Characteristics of an Artificial Reef. Chin. J. Oceanol. Limnol. 31 (5), 949–956. doi: 10.1007/s00343-013-2241-9
Jiang Z., Liang Z., Tang Y. (2019). Numerical Analysis of the Effect of an Inner Structure of a Cubic Frame Reef on Flow Field. Mar. Technol. Soc. J. 53 (2), 83–92. doi: 10.4031/MTSJ.53.2.8
Jiang Z., Liang Z., Zhu L., Guo Z., Tang Y. (2020). Effect of Hole Diameter of Rotary-Shaped Artificial Reef on Flow Field. Ocean Engineering 197, 106917. doi: 10.1016/j.oceaneng.2020.106917
Jiang Z., Liang Z., Zhu L., Liu Y. (2016). Numerical Simulation of Effect of Guide Plate on Flow Field of Artificial Reef. Ocean Engineering 116, 236–241. doi: 10.1016/j.oceaneng.2016.03.005
Jiangtao F., Pimao C., Xue F., Guobao C. (2014). Research Advances in Hydrodynamics of Artificial Reef. Anim. Husband. Feed Science 6 (3), 154. doi: 10.19578/j.cnki.ahfs.2014.03.016
Jiao L., Yan-Xuan Z., Pi-Hai G., Chang-Tao G. (2017). Numerical Simulation and PIV Experimental Study of the Effect of Flow Fields Around Tube Artificial Reefs. Ocean Engineering 134, 96–104. doi: 10.1016/j.oceaneng.2017.02.016
Jung S., Kim D., Na W. B. (2016). Wake Volume Characteristics Considering Artificial Reef Canyon Intervals Constructed by Flatly Distributed Artificial Reef Set. J. Ocean Eng. Technol. 30 (3), 169–176. doi: 10.5574/KSOE.2016.30.3.169
Kim Y. H. (Ed.). (2018). Progress in Civil, Architectural and Hydraulic Engineering IV: Proceedings of the 2015 4th International Conference on Civil, Architectural and Hydraulic Engineering (ICCAHE 2015), Guangzhou, China, June 20-21, 2015. Boca Raton, FL, USA: CRC Press
Kim T., Baek S., Kwon Y., Lee J., Cha S. M., Kwon S. (2020). Improved Coastal Erosion Prevention Using a Hybrid Method With an Artificial Coral Reef: Large-Scale 3d Hydraulic Experiment. Water 12 (10), 2801. doi: 10.3390/w12102801
Kim D., Jung S., Na W. B. (2021). Evaluation of Turbulence Models for Estimating the Wake Region of Artificial Reefs Using Particle Image Velocimetry and Computational Fluid Dynamics. Ocean Engineering 223, 108673. doi: 10.1016/j.oceaneng.2021.108673
Kim C. G., Kim H. S., Kim T. H., Baik C. I. (2001). Monitoring of Floating Fish Reef Installed in Koje Coastal Waters. Ocean Polar. Res. 23 (3), 305–310. doi: 10.1111/j.1447-0756.2010.01395.x
Kim D. K., Suh S. H., Cho J. K., Kim C. G., Choi I. H., Kim B. S. (2010). Settlement Characteristics of Square Reefs Installed on Soft Seafloor Ground. J. Korean Soc. Mar. Engineering 34 (1), 163–167. doi: 10.5916/jkosme.2010.34.1.163
Kim B. W., Sung H. G., Kim J. H., Hong S. Y. (2013). Comparison of Linear Spring and Nonlinear FEM Methods in Dynamic Coupled Analysis of Floating Structure and Mooring System. J. Fluids Structures 42, 205–227. doi: 10.1016/j.jfluidstructs.2013.07.002
Kim D., Woo J., Na W. B. (2017). Intensively Stacked Placement Models of Artificial Reef Sets Characterized by Wake and Upwelling Regions. Mar. Technol. Soc. J. 51 (3), 60–70. doi: 10.4031/MTSJ.51.3.7
Kim D., Woo J., Na W. B., Yoon H. S. (2014b). Flow and Structural Response Characteristics of a Box-Type Artificial Reef. J. Korean Soc. Coast. Ocean Engineers 26 (3), 113–119. doi: 10.9765/KSCOE.2014.26.3.113
Kim D., Woo J., Yoon H. S., Na W. B. (2014a). Wake Lengths and Structural Responses of Korean General Artificial Reefs. Ocean Engineering 92, 83–91. doi: 10.1016/j.oceaneng.2014.09.040
Kim D., Woo J., Yoon H. S., Na W. B. (2016). Efficiency, Tranquillity and Stability Indices to Evaluate Performance in the Artificial Reef Wake Region. Ocean Engineering 122, 253–261. doi: 10.1016/j.oceaneng.2016.06.030
Krone R., Dederer G., Kanstinger P., Krämer P., Schneider C., Schmalenbach I. (2017). Mobile Demersal Megafauna at Common Offshore Wind Turbine Foundations in the German Bight (North Sea) Two Years After Deployment-Increased Production Rate of Cancer Pagurus. Mar. Environ. Res. 123, 53–61. doi: 10.1016/j.marenvres.2016.11.011
Langhamer O. (2012). Artificial Reef Effect in Relation to Offshore Renewable Energy Conversion: State of the Art. Sci. World J. 2012, 386713. doi: 10.1100/2012/386713
Lawrence P. L. (1996). An Introduction to Coastal Zone Management. Ocean Coast. Manag. 1 (32), 67–68. doi: 10.1016/S0964-5691(96)00034-8
Layman C. A., Allgeier J. E., Montaña C. G. (2016). Mechanistic Evidence of Enhanced Production on Artificial Reefs: A Case Study in a Bahamian Seagrass Ecosystem. Ecol. Engineering 95, 574–579. doi: 10.1016/j.ecoleng.2016.06.109
Lemoine H. R., Paxton A. B., Anisfeld S. C., Rosemond R. C., Peterson C. H. (2019). Selecting the Optimal Artificial Reefs to Achieve Fish Habitat Enhancement Goals. Biol. Conserv. 238, 108200. doi: 10.1016/j.biocon.2019.108200
Li J., Guan C., Cui Y., Chen J., Wang J., Yuan W., et al. (2014). Progress on Research and Construction of Marine Ranching Along the Coast of Shandong Province of China. J. Shipping. Ocean Engineering 4, 338–347.
Lima J. S., Zalmon I. R., Love M. (2019). Overview and Trends of Ecological and Socioeconomic Research on Artificial Reefs. Mar. Environ. Res. 145, 81–96. doi: 10.1016/j.marenvres.2019.01.010
Liu H. Y., Huang X. H., Wang S. M., Hu Y., Yuan T. P., Guo G. X. (2019). Evaluation of the Structural Strength and Failure for Floating Collar of a Single-Point Mooring Fish Cage Based on Finite Element Method. Aquacult. Engineering 85, 32–48. doi: 10.1016/j.aquaeng.2018.12.007
Liu Y., Li S., Liao Z., Liu K. (2021). Physical and Numerical Modeling of Random Wave Transformation and Overtopping on Reef Topography. Ocean Engineering 220, 108390. doi: 10.1016/j.oceaneng.2020.108390
Liu G., Li W. T., Zhang X. (2017). Assessment of the Benthic Macrofauna in an Artificial Shell Reef Zone in Shuangdao Bay, Yellow Sea. Mar. Pollut. Bullet. 114 (2), 778–785. doi: 10.1016/j.marpolbul.2016.11.004
Liu T. L., Su D. T. (2013). Numerical Analysis of the Influence of Reef Arrangements on Artificial Reef Flow Fields. Ocean Engineering 74, 81–89. doi: 10.1016/j.oceaneng.2013.09.006
Liu Y., Zhao Y. P., Dong G. H., Guan C. T., Cui Y., Xu T. J. (2013). A Study of the Flow Field Characteristics Around Star-Shaped Artificial Reefs. J. Fluids Structures 39, 27–40. doi: 10.1016/j.jfluidstructs.2013.02.018
Lokesha, Sannasiraj S. A., Sundar V. (2019). Hydrodynamic Characteristics of a Submerged Trapezoidal Artificial Reef Unit. Proc. Institution Mechanical Engineers Part M. 233 (4), 1226–1239. doi: 10.1177/1475090218825178
Love M. S., Schroeder D. M., Lenarz W., MacCall A., Bull A. S., Thorsteinson L. (2006). Potential Use of Offshore Marine Structures in Rebuilding an Overfished Rockfish Species, Bocaccio (Sebastes Paucispinis). Fish Bulletin 104 (3), 383–390. doi: 10.1016/j.fishres.2006.03.027
Love M. S., York A. (2005). A Comparison of the Fish Assemblages Associated With an Oil/Gas Pipeline and Adjacent Seafloor in the Santa Barbara Channel, Southern California Bight. Bull. Mar. Science 77 (1), 101–118.
Ly O., Yoris-Nobile A. I., Sebaibi N., Blanco-Fernandez E., Boutouil M., Castro-Fresno D., et al. (2021). Optimisation of 3D Printed Concrete for Artificial Reefs: Biofouling and Mechanical Analysis. Construction. Building Materials 272, 121649. doi: 10.1016/j.conbuildmat.2020.121649
Mablouké C., Kolasinski J., Potier M., Cuvillier A., Potin G., Bigot L., et al. (2013). Feeding Habits and Food Partitioning Between Three Commercial Fish Associated With Artificial Reefs in a Tropical Coastal Environment. Afr. J. Mar. Science 35 (3), 323–334. doi: 10.2989/1814232X.2013.829790
Mansfield B. (2010). “Modern” Industrial Fisheries and the Crisis of Overfishing,” in Global Political Ecology (London: Routledge), 98–113.
Margheritini L., Møldrup P., Jensen R. L., Frandsen K. M., Antonov Y. I., Kawamoto K., et al. (2021). Innovative Material can Mimic Coral and Boulder Reefs Properties. Front. Mar. Science 8, 750. doi: 10.3389/fmars.2021.652986
Marin-Diaz B., Fivash G. S., Nauta J., Temmink R. J., Hijner N., Reijers V. C., et al. (2021). On the Use of Large-Scale Biodegradable Artificial Reefs for Intertidal Foreshore Stabilization. Ecol. Engineering 170, 106354. doi: 10.1016/j.ecoleng.2021.106354
Marsden J. E., Chotkowski M. A. (2001). Lake Trout Spawning on Artificial Reefs and the Effect of Zebra Mussels: Fatal Attraction? J. Great Lakes Res. 27 (1), 33–43. doi: 10.1016/S0380-1330(01)70621-1
Ma C., Zhao Y. P., Bi C. W. (2022). Numerical Study on Hydrodynamic Responses of a Single-Point Moored Vessel-Shaped Floating Aquaculture Platform in Waves. Aquacult. Engineering 96, 102216. doi: 10.1016/j.aquaeng.2021.102216
McKindsey C. W.,, Archambault P.,, Callier M. D., Olivier F. (2011). Influence of Suspended and Off-Bottom Mussel Culture on the Sea Bottom and Benthic Habitats: a review. Can. J. Zool. 89 (7), 622–646. doi: 10.1139/z11-037
Miao Z. Q., Xie Y. H. (2007). Effects of Water-Depth on Hydrodynamic Force of Artificial Reef. J. Hydrodynam Ser. B. 19 (3), 372–377. doi: 10.1016/S1001-6058(07)60072-9
Miller M. W. (2002). Using Ecological Processes to Advance Artificial Reef Goals. ICES J. Mar. Science 59 (suppl), S27–S31. doi: 10.1006/jmsc.2001.1162
Moe-Føre H., Lader P. F., Lien E., Hopperstad O. S. (2016). Structural Response of High Solidity Net Cage Models in Uniform Flow. J. Fluids Structures 65, 180–195. doi: 10.1016/j.jfluidstructs.2016.05.013
Moore J. K., Fu W., Primeau F., Britten G. L., Lindsay K., Long M., et al. (2018). Sustained Climate Warming Drives Declining Marine Biological Productivity. Science 359 (6380), 1139–1143. doi: 10.1126/Scienceaao637
Noh J., Ryu J., Lee D., Khim J. S. (2017). Distribution Characteristics of the Fish Assemblages to Varying Environmental Conditions in Artificial Reefs of the Jeju Island, Korea. Mar. Pollut. Bullet 118 (1-2), 388–396. doi: 10.1016/j.marpolbul.2017.02.066
Palm J., Eskilsson C. (2020). Mooring Systems With Submerged Buoys: Influence of Buoy Geometry and Modelling Fidelity. Appl. Ocean Res. 102, 102302. doi: 10.1016/j.apor.2020.102302
Palo M., Marimba A. A., Jurdillah N. A. (2021). “Catching Reef Fish Using Floating Traps,” in IOP Conference Series: Earth and Environmental Science, vol. Vol. 860, No. 1. (England: IOP Publishing), 012103.
Pan Y., Tong H., Zhou Y., Liu C., Xue D. (2021). Numerical Simulation Study on Environment-Friendly Floating Reef in Offshore Ecological Belt Under Wave Action. Water 13 (16), 2257. doi: 10.3390/w13162257
Paxton A. B., Shertzer K. W., Bacheler N. M., Kellison G. T., Riley K. L., Taylor J. C. (2020). Meta-Analysis Reveals Artificial Reefs can be Effective Tools for Fish Community Enhancement But are Not One-Size-Fits-All. Front. Mar. Science 7, 282. doi: 10.3389/fmars.2020.00282
Perkol-Finkel S., Zilman G., Sella I., Miloh T., Benayahu Y. (2006). Floating and Fixed Artificial Habitats: Effects of Substratum Motion on Benthic Communities in a Coral Reef Environment. Mar. Ecol. Prog. Ser. 317, 9–20. doi: 10.3354/meps317009
Preker M., Lawn I. D. (2012). Hydroids From the Heron Island Reef Flat. Queensland. Natural. 50 (4/5/6), 32–58.
Ranasinghe R., Turner I. L. (2006). Shoreline Response to Submerged Structures: A Review. Coast. Engineering 53 (1), 65–79. doi: 10.1016/j.coastaleng.2005.08.003
Ropero-Giralda P., Crespo A. J., Tagliafierro B., Altomare C., Domínguez J. M., Gómez-Gesteira M., et al. (2020). Efficiency and Survivability Analysis of a Point-Absorber Wave Energy Converter Using DualSPHysics. Renewable Energy 162, 1763–1776. doi: 10.1016/j.renene.2020.10.012
Scheffers S. R., de Goeij J., van Duyl F. C., Bak R. P. (2003). The Cave-Profiler: A Simple Tool to Describe the 3-D Structure of Inaccessible Coral Reef Cavities. Coral. Reefs. 22 (1), 49–53. doi: 10.1007/s00338-003-0285-6
Schulze A., Erdner D. L., Grimes C. J., Holstein D. M., Miglietta M. P. (2020). Artificial Reefs in the Northern Gulf of Mexico: Community Ecology Amid the “Ocean Sprawl”. Front. Mar. Science 7, 447. doi: 10.3389/fmars.2020.00447
Seaman W. (2000). Artificial Reef Evaluation: With Application to Natural Marine Habitats. Boca Raton, FL, USA: CRC Press.
Seaman W., Jensen A. C. (2000). Purposes and Practices of Artificial Reef Evaluation (Boca Raton, FL: CRC Press LLC), 2–19.
Sherman R. L., Gilliam D. S., Spieler R. E. (2002). Artificial Reef Design: Void Space, Complexity, and Attractants. ICES J. Mar. Science 59 (suppl), S196–S200. doi: 10.1006/jmsc.2001.1163
Sherman R. L., Spieler R. E. (2006). Tires: Unstable Materials for Artificial Reef Construction. Trans. Ecol. Environment 88, 215–223. doi: 10.2495/CENV060211
Shu A., Qin J., Rubinato M., Sun T., Wang M., Wang S., et al. (2021a). An Experimental Investigation of Turbulence Features Induced by Typical Artificial M-Shaped Unit Reefs. Appl. Sci. 11 (4), 1393. doi: 10.3390/app11041393
Shu A., Rubinato M., Qin J., Zhu J., Sun T., Yang W., et al. (2021b). The Hydrodynamic Characteristics Induced by Multiple Layouts of Typical Artificial M-Type Reefs With Sea Currents Typical of Liaodong Bay, Bohai Sea. J. Mar. Sci. Engineering 9 (11), 1155. doi: 10.3390/jmse9111155
Sohn B. K., Yi B. H., Yoon H. S. (2011). Hydraulic Characteristics of Train Carriage Artificial Reef in Wave and Current Field Conditions. J. Korean. Soc. Mar. Engineering 35 (1), 108–117. doi: 10.5916/jkosme.2011.35.1.108
Srisuwan C., Rattanamanee P. (2015). Modeling of Seadome as Artificial Reefs for Coastal Wave Attenuation. Ocean Engineering 103, 198–210. doi: 10.1016/j.oceaneng.2015.04.069
Strand I. M., Sørensen A. J., Volent Z., Lader P. (2016). Experimental Study of Current Forces and Deformations on a Half Ellipsoidal Closed Flexible Fish Cage. J. Fluids Structures 65, 108–120. doi: 10.1016/j.jfluidstructs.2016.05.011
Tamura H., Nadaoka K., Paringit E. C. (2007). Hydrodynamic Characteristics of a Fringing Coral Reef on the East Coast of Ishigaki Island, Southwest Japan. Coral Reefs 26 (1), 17–34. doi: 10.1007/s00338-006-0164-z
Tang Y., Huang L., Ge C., Liang Z., Sun P. (2010). A New Algorithm for Estimating Gillnet Selectivity. Chin. J. Oceanol. Limnol. 28 (2), 274–279. doi: 10.1007/s00343-010-9256-2
Tang Y., Hu Q., Wang X., Zhao F., Huang L., Xie T. (2019). “Evaluation of Flow Field in the Layouts of Cross-Shaped Artificial Reefs,” in International Conference on Offshore Mechanics and Arctic Engineering, vol. Vol. 58882. (Glasgow, Scotland, UK: American Society of Mechanical Engineers), V009T13A002.
Tang Y., Long X., Wang X., Jiang Z., Cheng H., Zhang T. (2017). Comparative Analysis on Flow Field Effect of General Artificial Reefs in China. Trans. Chin. Soc. Agric. Engineering 33 (8), 97–103. doi: 10.11975/j.issn.1002-6819.2017.08.013
Tang Y., Wei S., Yang M., Wang X., Zhao F. (2021). Experimental Investigation of Local Scour Around Artificial Reefs in Steady Currents. J. Ocean Univ. China 21 (2), 445–456. doi: 10.1002/stc.1937
Tang Y. L., Yu Q. (2016). An Integrative Evaluation of Ecological Effect of Artificial Reefs With Entropy-Weighted Fuzzy Matter-Element Method. Periodical. Ocean Univ. China 46 (1), 18–26. doi: 10.16441/j.cnki.hdxb.20150011
Touzon I., Nava V., Gao Z., Petuya V. (2021). Frequency Domain Modelling of a Coupled System of Floating Structure and Mooring Lines: An Application to a Wave Energy Converter. Ocean Engineering 220, 108498. doi: 10.1016/j.oceaneng.2020.108498
Tu G., Liu H., Ru Z., Shao D., Yang W., Sun T., et al. (2020). Numerical Analysis of the Flows Around Fishing Plane Nets Using the Lattice Boltzmann Method. Ocean Engineering 214, 107623. doi: 10.1016/j.oceaneng.2020.107623
Umar A., Datta T. K. (2003). Nonlinear Response of a Moored Buoy. Ocean Engineering 30 (13), 1625–1646. doi: 10.1016/S0029-8018(02)00144-0
van Elden S., Meeuwig J. J., Hobbs R. J., Hemmi J. M. (2019). Offshore Oil and Gas Platforms as Novel Ecosystems: A Global Perspective. Front. Mar. Science 6, 548. doi: 10.3389/fmars.2019.00548
Wang S., Hang X., Yuan G. (2017). A Pyramid Scheme for Three-Dimensional Diffusion Equations on Polyhedral Meshes. J. Comput. Physics 350, 590–606. doi: 10.1016/j.jcp.2017.08.060
Wang X., Liu X., Tang Y., Zhao F., Luo Y. (2021). Numerical Analysis of the Flow Effect of the Menger-Type Artificial Reefs With Different Void Space Complexity Indices. Symmetry 13 (6), 1040. doi: 10.3390/sym13061040
Wang Z., Ni W., Cheng-hong F., Shu-jie G., Ying L. (2012). Preparation of Green Artificial Reefs Concrete Used Steel Slag and Blast Furnace Slag. Multipurp. Util. Miner. Resour. 5, 12. doi: 10.3969/j.issn.1000-6532.2012.05.011
Wang G., Wan R., Wang X., Zhao F., Lan X., Cheng H., et al. (2018). Study on the Influence of Cut-Opening Ratio, Cut-Opening Shape, and Cut-Opening Number on the Flow Field of a Cubic Artificial Reef. Ocean Engineering 162, 341–352. doi: 10.1016/j.oceaneng.2018.05.007
Wilhelmsson D., Langhamer O. (2014). “The Influence of Fisheries Exclusion and Addition of Hard Substrata on Fish and Crustaceans,” in Marine Renewable Energy Technology and Environmental Interactions (Dordrecht: Springer), 49–60.
Winthereig-Rasmussen H., Simonsen K., Patursson Ø. (2016). Flow Through Fish Farming Sea Cages: Comparing Computational Fluid Dynamics Simulations With Scaled and Full-Scale Experimental Data. Ocean Engineering 124, 21–31. doi: 10.1016/j.oceaneng.2016.07.027
Woo J., Kim D., Yoon H. S., Na W. B. (2014). Characterizing Korean General Artificial Reefs by Drag Coefficients. Ocean Engineering 82, 105–114. doi: 10.1016/j.oceaneng.2014.02.025
Xu Q., Ji T., Yang Z., Ye Y. (2019). Steel Rebar Corrosion in Artificial Reef Concrete With Sulphoaluminate Cement, Sea Water and Marine Sand. Construction. Building. Materials. 227, 116685. doi: 10.1016/j.conbuildmat.2019.116685
Yaakob O. B., Ahmed Y. M., Jalal M. R., Faizul A. A., Koh K. K., Zaid T. J. (2016). “Hydrodynamic Design of New Type of Artificial Reefs,” in Applied Mechanics and Materials, vol. Vol. 819. (Switzerland: Trans Tech Publications Ltd), 406–419.
Yang H., Ru X., Zhang L., Lin C. (2019). Industrial Convergence of Marine Ranching and Offshore Wind Power: Concept and Prospect. China Acad. Sci. 6, 700–707. doi: 10.16418/j.issn.1000-3045.2019.06.011
Yao Y., Chen Y., Zhou H., Yang H. (2016). Numerical Modeling of Current Loads on a Net Cage Considering Fluid–Structure Interaction. J. Fluids Structures 62, 350–366. doi: 10.1016/j.jfluidstructs.2016.01.004
Yoon H. S., Kim D., Na W. B. (2016). Estimation of Effective Usable and Burial Volumes of Artificial Reefs and the Prediction of Cost-Effective Management. Ocean Coast. Manag. 120, 135–147. doi: 10.1016/j.ocecoaman.2015.12.007
Yun D. H., Kim Y. T. (2018). Experimental Study on Settlement and Scour Characteristics of Artificial Reef With Different Reinforcement Type and Soil Type. Geotextiles. Geomembranes 46 (4), 448–454. doi: 10.1016/j.geotexmem.2018.04.005
Yu J., Zhang L. (2020). Evolution of Marine Ranching Policies in China: Review, Performance and Prospects. Sci. Total Environment 737, 139782. doi: 10.1016/j.scitotenv.2020.139782
Zhang L., Wang J., Hu Q., Shen T. (2016). Design and Application of Offshore Middle-Upper-Layer Flexible Floating Reefs. J. Shanghai. Ocean University 25 (4), 613–619.
Zhang S. Y., Xu M., Wang Z. H. (2010). Review of Artificial Reef and Stock Enhancement. Fish. Modernization 37 (3), 55–58. doi: 10.27314/d.cnki.yyxdh.2010.3703
Zhao Y. P., Bai X. D., Dong G. H., Bi C. W., Gui F. K. (2015a). Numerical Analysis of the Elastic Response of a Floating Collar in Waves. Ocean Engineering 95, 175–182. doi: 10.1016/j.oceaneng.2014.12.015
Zhao F., Ong M. C., Tang Y., Wang X. (2019). “Experimental Study on the Hydrodynamic Characteristics of Artificial Reefs,” in International Conference on Offshore Mechanics and Arctic Engineering, vol. Vol. 58882. (Glasgow, Scotland, UK: American Society of Mechanical Engineers), V009T13A014.
Zhao Y. P., Wang X. P., Dong G. H. (2015b). Numerical Simulation and Experimental Validation of Hydrodynamic Characteristics of Submerged Artificial Reef in Waves. Ocean Engineering 33 (6), 52–61. doi: 10.1016/j.oceaneng.2014.12.015
Zhao H., Zhang Y., Hao C., LI Q. (2006). Sitting and Designing of Artificial Reefs. J. Mar. Sci. 24 (4), 69–76. doi: 10.3969/j.issn.1001-909X.2006.04.008
Zheng Y., Kuang C., Zhang J., Gu J., Chen K., Liu X. (2022). Current and Turbulence Characteristics of Perforated Box-Type Artificial Reefs in a Constant Water Depth. Ocean Engineering 244, 110359. doi: 10.1016/j.oceaneng.2021.110359
Zheng Y. X., Liang Z. L., Guan C. T., Song X. F., Li J., Cui Y., et al. (2015). Numerical Simulation and Experimental Study of the Effects of Disposal Space on the Flow Field Around the Combined Three-Tube Reefs. China Ocean Engineering 29 (3), 445–458. doi: 10.1007/s13344-015-0031-1
Zhong S., Sun M., Zhang S., Zhong S. (2006). Study on the Design and Stability of the Artificial Steel Prism Reef. Mar. Fish. 28 (3), 234–240.
Zhou X., Zhao X., Zhang S., Lin J. (2019). Marine Ranching Construction and Management in East China Sea: Programs for Sustainable Fishery and Aquaculture. Water 11 (6), 1237. doi: 10.3390/w11061237
Zhu X., Wang Y., Yu K., Pei Y., Wei Z., Zong L. (2020). Dynamic Analysis of Deep-Towed Seismic Array Based on Relative-Velocity-Element-Frame. Ocean Engineering 218, 108243. doi: 10.1016/j.oceaneng.2020.108243
Zhu X., Yoo W. S. (2016a). Dynamic Analysis of a Floating Spherical Buoy Fastened by Mooring Cables. Ocean Engineering 121, 462–471. doi: 10.1016/j.oceaneng.2016.06.009
Keywords: artificial reefs, floating artificial reefs, hydrodynamic characteristics, flow field effect, stability of artificial reef, offshore engineering
Citation: Pan Y, Tong H, Wei D, Xiao W and Xue D (2022) Review of Structure Types and New Development Prospects of Artificial Reefs in China. Front. Mar. Sci. 9:853452. doi: 10.3389/fmars.2022.853452
Received: 12 January 2022; Accepted: 09 June 2022;
Published: 11 July 2022.
Edited by:
Edgar Mendoza, National Autonomous University of Mexico, MexicoReviewed by:
Francisco Taveira-Pinto, University of Porto, PortugalXizeng Zhao, Zhejiang University, China
Copyright © 2022 Pan, Tong, Wei, Xiao and Xue. This is an open-access article distributed under the terms of the Creative Commons Attribution License (CC BY). The use, distribution or reproduction in other forums is permitted, provided the original author(s) and the copyright owner(s) are credited and that the original publication in this journal is cited, in accordance with accepted academic practice. No use, distribution or reproduction is permitted which does not comply with these terms.
*Correspondence: Dawen Xue, xuedw@zjou.edu.cn