- 1National Reference Laboratory for the Monitoring of Marine Biotoxins, Unit Contaminants, Department of Safety in the Food Chain, German Federal Institute for Risk Assessment, Berlin, Germany
- 2Department of Pharmacy, School of Medicine and Surgery, University of Naples Federico II, Naples, Italy
- 3Department of Exposure, German Federal Institute for Risk Assessment, Berlin, Germany
- 4Bavarian State Office for Health and Food Safety (LGL), Erlangen, Germany
Ciguatera poisoning (CP) is a prevalent food related health risk, caused by the consumption of seafood contaminated with ciguatoxins (CTXs). Seafood is the most traded food commodity worldwide, and since 2012, imported snapper fish (Lutjanidae) were the leading cause of CP in Germany. Following a Germany wide CP outbreak in 2017, a product trace-back investigation was conducted for imported fish labeled as “Red Snapper” (Lutjanus malabaricus). Forty-five fish muscle-tissue portions from the implicated batch and two meal remnants were analyzed for CTXs. All samples were positive for “CTX-like toxicity” containing a range of 0.23–11.4 ng CTX3C equivalents per gram of wet tissue, determined by an in vitro cell assay [N2a-3-(4,5-dimethylthiazol-2-yl)-2,5-diphenyltetrazolium bromide (MTT)]. Liquid chromatography with tandem mass spectrometry (LC-MS/MS) chromatograms revealed the (potential) presence of several marine biotoxins of the class CTX in all batch samples. All samples exceeded current multi-national product legal requirements and recommended guidelines for CTXs. DNA barcoding confirmed the fish sold was mislabeled and was identified as L. bohar, a species frequently involved in CP. Consequently, the mislabeled food and contaminants risk focuses attention on the importance of correct food labeling. Processes for food authentication and CTX contaminant analysis exist and can be used to potentially prevent, stop, and remove foods from commerce for further evaluation to ensure consumer safety. This study further demonstrates their necessity.
Introduction
Ciguatoxin (CTX)-group compounds, when present in seafood, can cause a mild to severe intoxication in humans, described as ciguatera poisoning (CP). Marine biotoxins in the class CTX (comprising > 30 congeners) are classified as a human health hazard and therefore, within the European Union’s (EU) commercial jurisdiction, products containing CTXs must not be placed on the market (European Commission [EC], 2004a,2019; EFSA, 2010b). CTXs are produced by cosmopolitan tropical and sub-tropical species of microalgae, dinoflagellates in the genus Gambierdiscus and Fukuyoa. When the habitat and conditions are conducive (generally, shallow < 200 m, warm > 16°C, marine waters (Kibler et al., 2012, 2017; Xu et al., 2016) these microalgae can occur in the benthic and epi-benthic dinoflagellate community.
After CTXs are produced by microalgae, the process of an animal ingesting and acquiring CTXs at concentrations capable of causing CP is dependent upon a multitude of complex species and region-specific, biotic, abiotic, temporal, and geographic factors. Currently, the human health relevant information for a consumer regarding CP is knowing whether the species and region the seafood product was harvested from has a historic association with CP. Together the species-region information is intended to provide a measure of consumer protection for CP (Olsen et al., 1984; Chinain et al., 2010, 2020a; Dickey and Plakas, 2010; Sydney Fish Market, 2015; Loeffler et al., 2018, 2019, 2022; U.S. Food Drug Administration, 2020; André et al., 2021; Holmes et al., 2021). No consumer oriented pre-market commercially available test for CTXs yet exists and the species-region consumer guidance information that does exist is knowledge-limited by data gaps regarding the predictive accuracy for CTXs and CP. Individual fish can feed or behave differently which can change their CTX accumulation. Consequently, not all members of a species contain CTXs and the free movement of fish between areas of high and low CP associations complicates regional CP correlations. Informed risk evaluation efforts with regard to CP can be further complicated by broad seafood marketing descriptions of products that can include large classes of fish (e.g., snapper) or large oceanic catch regions. For instance, snapper is a commonly marketed product description for a commercially recognized family of fish (Lutjanidae order Perciformes). There are about 105 species that fit this snapper description (Britannica, 2015) and there is a wide range of variability among these species for their recognized CP risk. Customers relying on accurate product labeling for a species description must also face the reality that high levels of non-compliance in product labeling for snappers have been reported (Di Muri et al., 2018; Kappel and Schröder, 2020; Kusche and Hanel, 2021). Regardless of whether a product was unintentionally or illicitly mislabeled, the act of species substitution can result in misbranding violations or economic fraud, depending on the jurisdiction (Calosso et al., 2020). But because CP can be species-associated, mislabeling a species can represent a potential CP food safety risk. Consequently, species substitution can bypasses the recognition process relied upon by food processors, consumers, or regulators for identifying a CP hazard by species, making this a particular concern for CP (Kappel and Schröder, 2020; Kusche and Hanel, 2021). In these situations, DNA-testing can be a useful tool for authenticating a species and it can serve as a precautionary product verification method that can discourage operators from falsely labeling catches (and bypassing CP species-association scrutiny). Furthermore, species authentication for products involved in CP outbreaks can provide additional consumer or producer awareness as well as inform future CP-species risk assessments (European Commission [EC], 2002; European Union [EU], 2011, 2013a,b; Handy et al., 2011; Deeds et al., 2014; Friedman et al., 2017; Friedemann, 2019; U.S. Food Drug Administration, 2020).
For newly emerging endemic CP regions occurring in the EU the causative benthic microalgae, related toxins, and CP intoxications have been identified, particularly in the Canary Islands and Madeira (Aligizaki and Nikolaidis, 2008; Otero et al., 2010; Fraga et al., 2011; Costa et al., 2018, 2021; Reverté et al., 2018; Estevez et al., 2019a,b, 2020; Rossignoli et al., 2020; Sanchez-Henao et al., 2020; Diogène et al., 2021; Gago-Martinez et al., 2021). With the intention of precautionary consumer protection, these areas now contain CP-induced fishing restrictions and have monitoring protocols in place (Sanchez-Henao et al., 2019; Canals et al., 2021). These measures are not only relevant for the endemic regions, but also for other EU member states involved in the unrestricted trade of seafood, as the risk to a non-CP endemic territory (e.g., Germany) is either travel-related, import, or trade induced. The EU regulations regarding health standards for fisher products (EC No. 853/2004) states that food business operators must ensure that fishery products placed on the market for human consumption do not contain CTXs. Furthermore EC No. 2019/627 Ch. I.G.3 states that controls are to take place to ensure fishery products containing ‘ciguatera’ are not placed on the market (European Commission [EC], 2004a,b, 2019; EFSA, 2010b). However, beginning in 2012, a series of six outbreaks of CP were documented in Germany whose exclusive economic zone (EEZ) for fishing does not border a CP endemic oceanic area (Strehlow et al., 2012). Consequently, the source was identified as imported snappers (Lutjanidae) in all cases (Friedemann, 2019) and this represents the primary uncontrolled pathway of CP risk to consumers. Herein, we present comprehensive epidemiological details on an outbreak of CP that occurred in Germany in 2017 regarding the cases (clinical aspects), clusters (epidemic aspects), causes (CTX analysis), food authentication (DNA-barcoding), and the entire trace-back-process including a discussion concerning the environmental origin of the fish (endemic aspects), all based on portions of the available commercial product and CP related meal remnants.
The current census on Gambierdiscus spp. and Fukuyoa spp. indicates that many species are geographically isolated (Litaker et al., 2010; Tester et al., 2018; FAO and WHO, 2020). This regional presence and absence extends to the respective toxin profiles and biomarkers found in the species that feed on them (FAO and WHO, 2020). These region-specific toxin profiles can be useful for trace-back efforts when attempting to identify a source region or when investigating a species from a region with a known toxin profile for a targeted toxin analysis. However, a meal remnant from a CP outbreak without identifiable characteristics could theoretically be from any CP endemic region, complicating a targeted technique for CTX profile analysis. Currently, among all regions there are over 30 CTX congeners identified which are categorized into four groups [CTX4A and CTX3C for the Pacific region, C-CTX, and I-CTX for the Caribbean and Indian Ocean region, respectively (FAO and WHO, 2020)]. Of these, only two CTX standards are commercially available, CTX1B and CTX3C (both are from the group associated with the Pacific region), which further complicates CTX analysis methods targeting the toxin profile of a specific region.
The methods available to investigate CTXs from algal source (toxin generation) to products targeted for human consumption (toxin ingestion), are tailored according to the user’s intents, purposes, and infrastructure. The simplest efforts are those of the lowest cost and highest accessibility, e.g., crude bioassays using domesticated or semi-wild animals. The mouse bioassay is a more controlled approach than a crude bioassay (Banner et al., 1960; Hoffman et al., 1983), however, directive 2010/63/EU of the European Parliaments legislation ‘on the protection of animals used for scientific purposes’ directs the replacement of the mouse bioassay by using a scientifically satisfactory method or testing strategy in routine analyses (European Parliament [EU], 2010). In vitro cell-based assays as well as other biological or biochemical based methods (e.g., enzyme-linked immunosorbent assay, receptor binding assay) are therefore used as a “screening-type” method. They provide semi-quantitative capabilities where a positive “CTX-like toxicity” result is based on the CTX molecular mechanism of action (activating effect on sodium channels). Following the screening method, a confirmatory method is used for identifying the presence of a CTX-group compound. Identification is based on mass and retention time equivalency to a standard or reference material. The European Food Safety Authority (EFSA) recommended that methods should be developed to test for CTXs in products. However, current legislation does not contain official methods for CTX contaminant analysis (EFSA, 2010a). Therefore, a two-tiered analysis method was adapted for this investigation, incorporating both a screening-type method and a targeted method. The adaptation was based on the CTX analysis methodology applied by the US Food and Drug Administration (FDA) for official CP case sample analysis (in use since 1999) (Dickey, 2008), and is applicable to cooked or raw product (Abraham et al., 2012). Within tier two of CTX identification, no a priori assumption concerning the region of sample origin (for targeting a specific CTX class) was used due to the recognized potential for investigating a mislabeled fish product. Therefore, an unbiased liquid chromatography with tandem mass spectrometry (LC-MS/MS) analysis approach which included all currently described CTXs was used in the investigation by the National Reference Laboratory for the Monitoring of Marine Biotoxins at the German Federal Institute for Risk Assessment (BfR) in Berlin.
Ciguatera poisoning outbreaks often seem sporadic because many factors are involved in their events, from CTX production, fish to fork, followed by diagnosis and medical advice, trace-back, and reporting (also confounded by un-reported illnesses). The global seafood trade, responsible for many non-endemic CP events, provides nearly 20% of the animal protein intake for more than 3.3 billion people, and nearly 40% of total fisheries and aquaculture production are traded internationally every year (FAO, 2020). The outbreak reported herein is a culmination of this CP reality involving a globalized market, novel clinical features (in non-endemic regions), and required coordinated responses coupled with new analytical approaches. Concurrently, this is the first demonstration in Germany of an established method for toxicity evaluation of CTXs in suspected and outbreak-related fish.
Materials and Methods
Trace-Back and Sample Collection
The first CP case(s) reported in this outbreak were from the south of Germany and occurred in March 2017. The event became generally known when the reports from the regional food safety authorities were consolidated as an alert notification in the European Rapid Alert System for Food and Feed (RASFF) by the German Federal Office of Consumer Protection (BVL). This RASFF alert notification was made concerning the serious risk on human health due to the potential presence of the food contaminant “ciguatoxin” in a seafood product described as “Red Snapper” (RASFF alert notification number 2017.0345) (European Commission [EC], 2017). Through the progression of this RASFF process, other Member States of the EU identified related products from the two implicated lots, indicating a wider distribution of the product beyond the informing country where the CP cases were initially reported. The lot numbers were VN/385/III/122 and VN/385/III/124. Lot VN/385/III/122 was exported from Vietnam to Hamburg, Germany and landed in September 2016. Lot number VN/385/III/124 was exported in August 2016 from the Cam Lam district of the Khanh Hoa province, Vietnam, and imported into Hamburg, Germany in September 2016. The product distributor informed customers of the issuance of a recall on the two seafood lots and provided investigators with a list of customers. In response to the alert from the EU, the Vietnamese Agro-Forestry-Fisheries Quality Assurance Department issued an official letter (Ref. # 451/QLCL-CL1, 28th March 2017) to the seafood processing plant. This letter required the processing plant to investigate the cause of the CTX contamination and to apply corrective actions if possible, however no follow-up to this letter regarding actions taken by the processing plant were provided. Consequently, the product remaining in commerce was recalled from the market by the wholesaler, voluntarily, due to the concern that it could also contain CTXs. The Bavarian State Office for Health and Food Safety (LGL) sent 10 kg (total gross weight) of the recalled product (European Commission [EC], 2017) for CTX analysis by the BfR. A subset of samples from both implicated lots were sent for DNA-barcoding for product authenticity testing and species attribution. At the request of the BfR, the FDA Gulf Coast Seafood Laboratory (Dauphin Island, AL, United States) was provided two meal remnants, pieces of cooked fish with the skin on, for bio-toxicity assessments and LC-MS/MS analysis. The two samples were shipped on dry ice on the 18th of July 2017 and were received July 20th 2017 frozen on dry ice and in good condition at the FDA. The sample weights were 22.6 g (FDA 1) and 28 g (FDA 2).
Species Authentication and Confirmation
Species identification by DNA barcoding was performed using cytochrome oxidase c subunit 1 gene specific fish cocktail primers VF2_t1, FishF2_t1, FishR2_t1 and FR1d_t1 according to Ivanova et al. (2007) with modifications as described by Spielmann et al. (2018). Amplification by polymerase chain reaction (PCR) and sequencing were performed in 50 μL reactions in a Mastercycler gradient cycler (Eppendorf, Hamburg, Germany). Amplicons were sequenced using the ABI Prism 3130 Genetic Analyzer (Applied Biosystems, Foster City, CA, United States). Sequence chromatograms and alignments were checked visually using the Integrated Database Network System (IDNS) Software (SmartGene Inc., Lausanne, Switzerland) and queried against the publicly available nucleotide database GenBank of the National Center for Biotechnology Information (NCBI1) using Basic Local Alignment Search Tool (BLAST).
Sample Extraction and Purification
Forty-five fish muscle-tissue portions were received at the BfR for analysis, frozen and in good condition. Muscle tissue (5 g) was excised from each sample, without bones or skin, to facilitate the extraction. The tissue samples were processed for toxin extraction using previously published methods (Dickey, 2008). The muscle tissue was homogenized by ultra turrax and extracted twice with 15 and 10 mL acetone, respectively. The extract was dried under a stream of nitrogen at 40°C. The residue was reconstituted in 5 mL of 80 vol% methanol in water and defatted twice with 5 mL n-hexane. The aqueous methanol phase was reduced to dryness under a stream of nitrogen at 40°C. The dry residue was reconstituted in 5 mL HPLC-grade water, and CTXs were extracted twice with chloroform at 5 mL for each extraction. The extracts were combined and dried. The dry residue was reconstituted in 50 μL chloroform and applied to a pre-conditioned (5 vol% water in methanol, 100% methanol, and chloroform) Bond Elute SI cartridge (Bond Elut SI, 500 mg 3 mL, Agilent, Waldbronn, Germany). The glass vessel was rinsed three times with 200 μL chloroform and each solvent rinse step was applied to the column. The cartridge was washed with one column volume of chloroform and the wash was discarded. Elution was carried out with two column volumes of 10 vol% methanol in chloroform. The eluate was dried and reconstituted in 1 mL methanol and stored in a glass vial at –20°C until usage.
For the two meal remnants (FDA 1 and 2), the skin was removed, and the tissue of each piece was homogenized separately. For each sample, homogenates were extracted with acetone, and the acetone extracts were subjected to solvent partitioning and cleaned up by solid-phase extraction, using the same procedure as described above, based on Dickey (2008).
Toxicity Evaluation by in vitro Neuro-2a Cytotoxicity 3-(4,5-Dimethylthiazol-2-yl)-2,5-Diphenyltetrazolium Bromide-Assay
The sample extracts from the 45 processed fish were analyzed for CTXs by a semi-quantitative in vitro neuro-2a cytotoxicity assay as described in Manger et al. (1995) and Dickey (2008), and with modifications, as described in Loeffler et al. (2021a). In short, mouse (Mus musculus) neuroblastoma cells (N2a CCL-131, American Type Culture Collection, Rockville, MD, United States, passage number 185) were propagated and subjected to the ouabain and veratridine lower sensitivity (OV-LS) method as described in Loeffler et al. (2021a). Cells were harvested for assay, when cultures were approximately 80–90% confluent, and seeded at 4 × 104 cells/well (200 μl volume) into sterile 96-well polystyrene plates (Corning™ 3596). Sodium channel activity in N2a cells is dependent on ouabain and veratridine, and these compounds are used to sensitize cells for the detection of sodium channel-specific effects. Conversely, N2a cytotoxicity caused by any other sample constituent can be evaluated using non-sensitized cells. Full dose-response curves (8-dilutions) of sample extracts were prepared with sensitized and non-sensitized cells to determine the concentration at which cell viability was reduced by 50% (EC50) compared with a CTX3C standard (Lot #TWJ6482, Wako chemicals GmbH, Neuss, Germany) (Dickey, 2008). Samples, standards, and relevant controls were assayed in triplicate. Results were expressed as ng CTX3C per gram tissue equivalent (TE), a wet-weight measurement.
Sample analysis on the two meal remnants was performed by the N2a-MTT assay at the FDA (Dickey, 2008). Caribbean and Pacific Ciguatoxin-1 [C-CTX-1 and P-CTX-1 (CTX1B)] reference standards were used for CTX-like activity comparison. C-CTX-1 standards used in the cytotoxicity assay were prepared at the FDA and purity was verified by LC-MS/MS before use. CTX1B standard was provided to the FDA by Dr. Richard J. Lewis, The Queensland University, Australia.
Fractionation Method
Bioassay-guided fractionation was performed for one sample showing CTX-like activity in the N2a assay. The tissue extract (section “Sample extraction and purification”) was directly utilized for HPLC fractionation, using the same setup as for low-resolution LC-MS/MS analysis (section “LC-MS/MS”). A total volume of 20 μL was applied, split into three consecutive runs consisting of two times 7 μL and one time 6 μL per injection, respectively. Higher injection volumes were avoided due to increasing peak broadening. Fractions were collected in glass vials in intervals of 30 seconds over a run time of 11 min (0 to 11 min), resulting in 22 fractions. Collected fractions were reduced to dryness in a stream of nitrogen and reconstituted in 50 μL 5% FBS RPMI and utilized for the N2a-assay (20 mg wet TE applied per well). Reconstituted samples were utilized undiluted and after 1:2 dilution with 5% FBS RPMI.
Liquid Chromatography With Tandem Mass Spectrometry
Analyses were conducted using a UHPLC-MS/MS system consisting of an Agilent 1290 Infinity II UHPLC (Agilent, Waldbronn, Germany) and a Sciex QTrap 6500 + (Sciex, Darmstadt, Germany). High-resolution measurements were performed with an Agilent 1260 Infinity II LC connected to a Sciex TripleTOF 6600+. Details regarding chromatographic setups are provided in Spielmeyer et al. (2021). The LC-MS/MS method for analysis of the sodium adducts covered over 30 congeners by applying twenty different ion transitions (multiple reaction monitoring, MRM). Both in Q1 and Q3, the m/z of the respective sodium adduct was selected. Product ions of the ammonium adducts were analyzed using two different methods. For low-resolution analysis (UHPLC-QTrap system), four MRM transitions were monitored for each congener, with fragments corresponding to the [M + H]+, [M + H-H2O]+, [M + H-2H2O]+, and [M + H-3H2O]+ of the respective congener. For the high-resolution analysis (UHPLC-TripleTOF system), product ion scans were performed and the aforementioned fragment ions were extracted from the chromatograms with an extraction window of ±25 mDa. All details about the m/z and MS-parameters are provided in Spielmeyer et al. (2021).
LC-MS/MS analysis was performed on the meal remnants by the FDA. The analysis was conducted using an Agilent 1260 LC and ABSciex 4000 QTrap mass spectrometer (Abraham et al., 2012). Extracts were examined for the presence of C-CTX-1 and CTX1B using C-CTX-1 and CTX1B reference materials.
Results and Discussion
Ciguatera Poisoning Outbreak in Germany, 2017
According to the RASFF-notification 2017.0345, the index case occurred on the 3rd of March 2017 in Garmisch-Partenkirchen, part of South Germany, the patient was admitted to the local hospital (Supplementary Table 1). According to the supplier distribution list, 20 kg was delivered to this town (Table 1). Ten additional cases were also reported in the same district in Bavaria (South Germany). Consequently, the CP outbreak was initially presumed to be restricted to this South German region. Due to the magnitude of the outbreak a public recall campaign was conducted by the wholesaler, and because of this effort other affected people self-reported symptoms to German Public Health Authorities. Furthermore, patients of three different regions (later defined as clusters) self-reported to a specifically established hotline at the German Federal Office of Consumer Protection and Food Safety (BVL). The clusters were named according to the geographic places where the fish were purchased by the victims, following Friedemann (2019). Clusters linked by a common batch of imported fish define the geographical dimension of a CP outbreak. In total, 16 CP patients were known to have suffered symptoms consistent with CP after the consumption of fish labeled as “Red Snapper” in March 2017 (here the name Red Snapper within quotation marks ‘ ‘ is to indicate a false or mislabeled name). Patients were from four districts in Germany which fall within four German Federal States, namely Bavaria, Baden-Württemberg, North Rhine-Westphalia, and Mecklenburg-Western Pomeranian. The places of CP intoxication were four restaurants and two households, forming seven distinct outbreak clusters (the fish for postal code cluster 82275 was purchased at a restaurant, but consumed at home) (Table 1). Additionally, an unknown number of people were similarly affected in at least two hotels (personal communication), therefore, this outbreak could be considered as another example of the underreporting problem facing the accurate accounting of CP patients, clusters, and outbreaks.
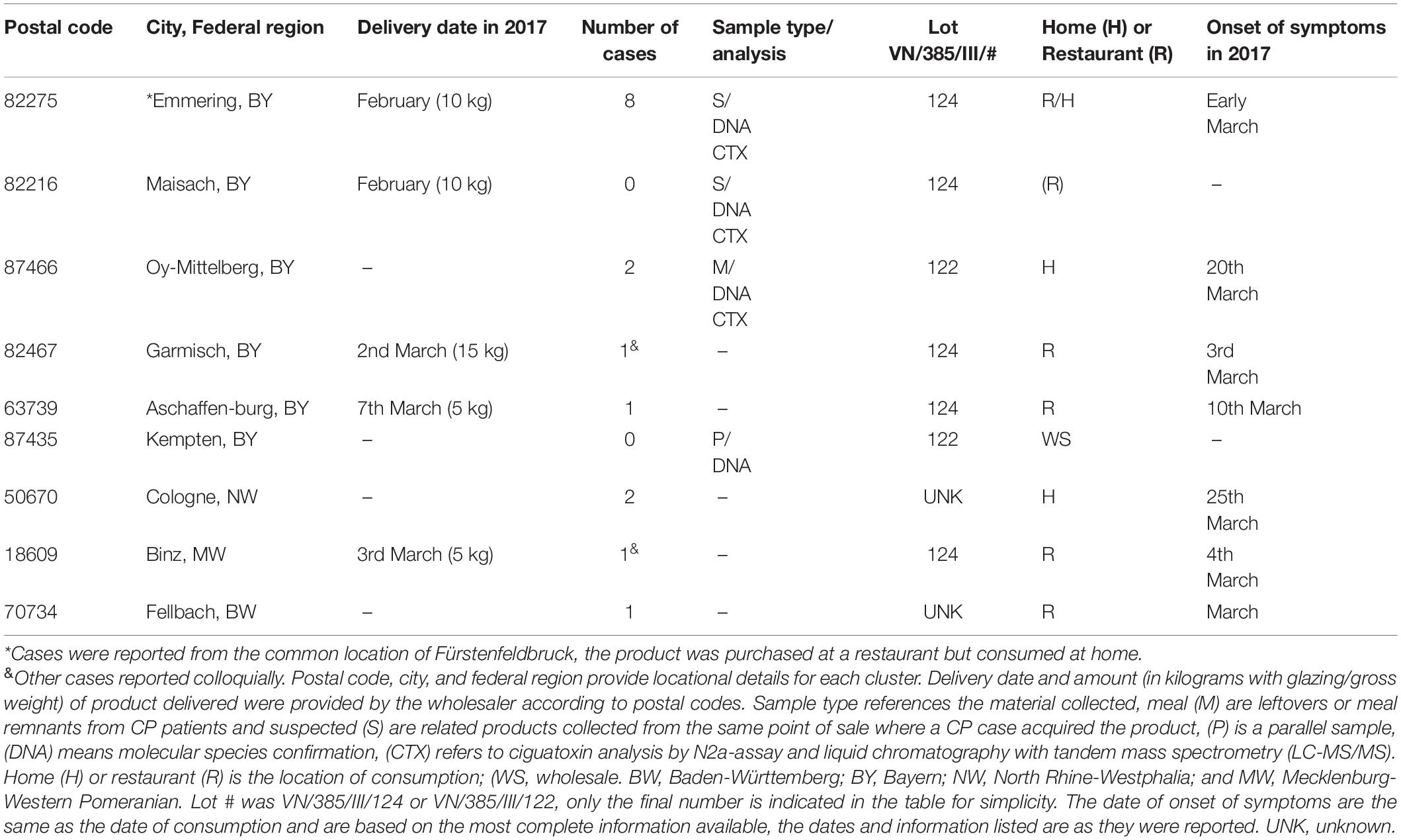
Table 1. Ciguatera poisoning outbreak clusters that occurred in Germany in 2017 from imported fish labeled as “Red Snapper”.
Data collected during the investigation at that time included two medical reports provided by the German Poisoning Information Centers to the BfR. These reports were provided following section §16e of the German Chemicals Act (ChemG), directing physicians to report poisonings to the BfR. Three other patients returned questionnaires directly to the BfR, Department Exposure. In total, detailed information is available for nine patients of the outbreak, regarding their sex (5 female/4 male), age (median 49 years, minimum 26 years, maximum 60 years), and interval time after ingestion of the fish before the onset of symptoms became evident (median 2 h, minimum 1 h, maximum 4 h). Five patients (4 female/1 male) with a mean age of 42.5 years showed a medium degree of severity, three of whom were hospitalized, and one woman (37 years old) was treated by an emergency medicine physician.
Symptoms in the acute phase started with gastrointestinal (GI) disorders (nausea, abdominal pain, vomiting, and diarrhea). The strong vomiting in some cases was a noticeable deviation from the previous CP outbreaks that occurred in Germany which did not include strong vomiting (fish origin from the previous cases was the Indian Ocean and Pacific Ocean region). All patients reported cold allodynia, i.e., an increased sensitivity to normally non-painful cool temperatures. Other neurological symptoms were pruritus mainly of the extremities, numbness of oral skin and mucosa, muscle pain, and headache. Three patients reported enduring burning pain of genital mucosa. A 48-year-old woman showed respiratory and cardiovascular disorders, high sensitivity to pain, and a burning sensation of the oral mucosa lasting for several weeks. One patient reported taste disorders and the aggravation of symptoms during stress.
From one of the meal remnants a laboratory staff individual (age: 60 years, sex: male) conducted (out of his own volition) a self-administered dab sensory test with a 90 mg piece of the sample (personal communication). The test person dabbed the portion on the left side of the lip, tongue, and incisors and held it in place for 2 min. During the placement, the subject felt a sensation described as a “mild sunburn” which within one hour spread to the skin around the mouth, cheeks, and nose. Immediately following this short perioral dermal and mucosal contact, the individual developed tingling and numbness in the mouth. After one hour a sensation of “coolness” was perceptible in the mucous membranes of both nasal passages, described as a sensation similar to inhalation after peppermint oil. These sensations were perceptible daily for several months. This “coolness” sensation was also reported from a patient involved in a CP outbreak from the area of export, also involving L. bohar, that occurred in the same month as the packaging date for the lot that was associated with the meal remnant used in the dab sensory test (Lot #122) (Ha et al., 2018). This method of “contact reaction,” where a small piece of suspected fish is rubbed on the mouth or skin, has been reportedly used in folk tests for CP in endemic regions (Darius et al., 2013).
Source-Food Trace-Back Sample Collection and Product Description
The ciguatera outbreak that occurred in Germany in 2017, as indicated in the RASFF report notification reference 2017.0345, was associated with the consumption of imported tropical fish or ‘Snapper product’ from lot numbers VN/385/III/122 and VN/385/III/124. Lot VN/385/III/122 was exported from Vietnam to Hamburg, Germany and landed in September 2016. According to the commercial invoice, 250 boxes were delivered. Each box contained ten package bags, each bag had a net weight of 800 g fish (listed at 20% glazing), and the total lot weight was reported at 2,500 kg. The focus of this study was on the lot VN/385/III/124 comprising 5,400 kg (net weight without glazing) (Figure 1), as this was the main set of products available for the CTX laboratory analysis described herein. Lot number VN/385/III/124 was exported in August 2016 from the Cam Lam district of the Khanh Hoa province, Vietnam, and imported into Hamburg, Germany in September 2016 with a content listed at 6,000 kg split into 1,200 boxes (5 kg per box). Without glazing (listed at 10%), the product was described as weighing 5,400 kg. Shipments were made to regional wholesalers throughout Germany between the 9th of November 2016 and the 13th of March 2017. The wholesaler distributed 4,260 kg (net after recalled product) of the frozen fish product throughout Germany, among 191 unique postal codes and 138 population centers. The consignment was also distributed to Austria (220 kg – product returned or destroyed), Czech Republic (35 kg – all returned), Netherlands (380 kg – none was distributed), and Poland (70 kg – some consumed, the remaining product was withdrawn, and a statement was provided that read “any complaints were reported”). The best before/expiration dates for lot #122 and #124 were the 31st of December 2018 and the 16th of July 2018, respectively.
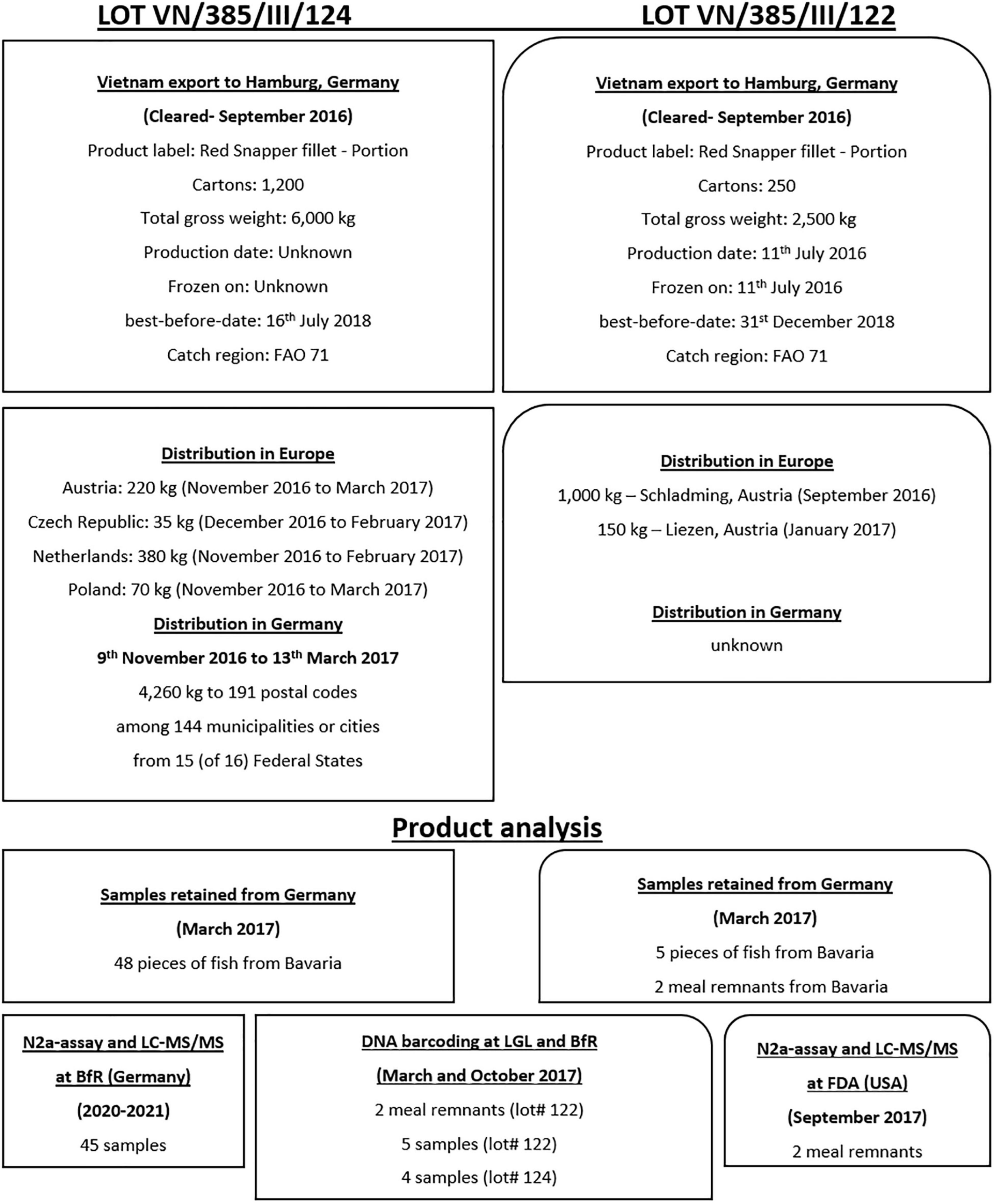
Figure 1. Description of both lots involved in the CP outbreaks in Germany. Import descriptions, distribution, and product analysis information for both lots are shown.
In March 2017, following the report of the first CP cases, the LGL collected two meal remnants (22.6 and 28.9 g) along with one bag from the implicated lot (#122) consisting of 5 fish pieces (800 g net weight) for DNA-barcoding (Figures 1, 2). Additionally, two original boxes of frozen product from the other implicated lot number (#124, 5 kg each) (Figure 2) were collected for DNA-barcoding and toxin analysis. The two boxes each contained twenty-four individual pieces of snapper filets of varying sizes and were described on the product label as weighing 170–230 g (Figure 2). The individual pieces were in the shape of 3- to 4-cornered filet cut, and the skin color was described as partly orange, partly carmine to blood-colored skin, which ran light to dark brown toward the back (Figure 2). According to the characteristics of the cuts, it is assumed that during handling, processing, and packaging at least six filet pieces were obtained per fish (Figure 2), which were probably distributed unequally among the individual boxes of 5 kg each during product packaging, as was observed from the collected material (e.g., 16 pieces cut from the tail section, 13 from the middle, 15 from the forward end of the fish and 4 pieces were from either the middle or forward sections, see Figure 2 for an example t sections). The original catch weight of each fish, with an assumed fileting yield of 35%, was estimated to be approximately 3.5 kg. Therefore, lot number VN/385/III/124 with a processed net weight of 5,400 kg may have required an original (whole fish) catch landing size of approximately 15,500 kg (i.e., 4,428 fish). In total, the two boxes contained 48 filets weighing (frozen and glazed in packaging) an estimated 10 kg, this represented approximately 0.2% of the net 4,260 kg (minus returned) of product distributed by the wholesaler throughout Germany.
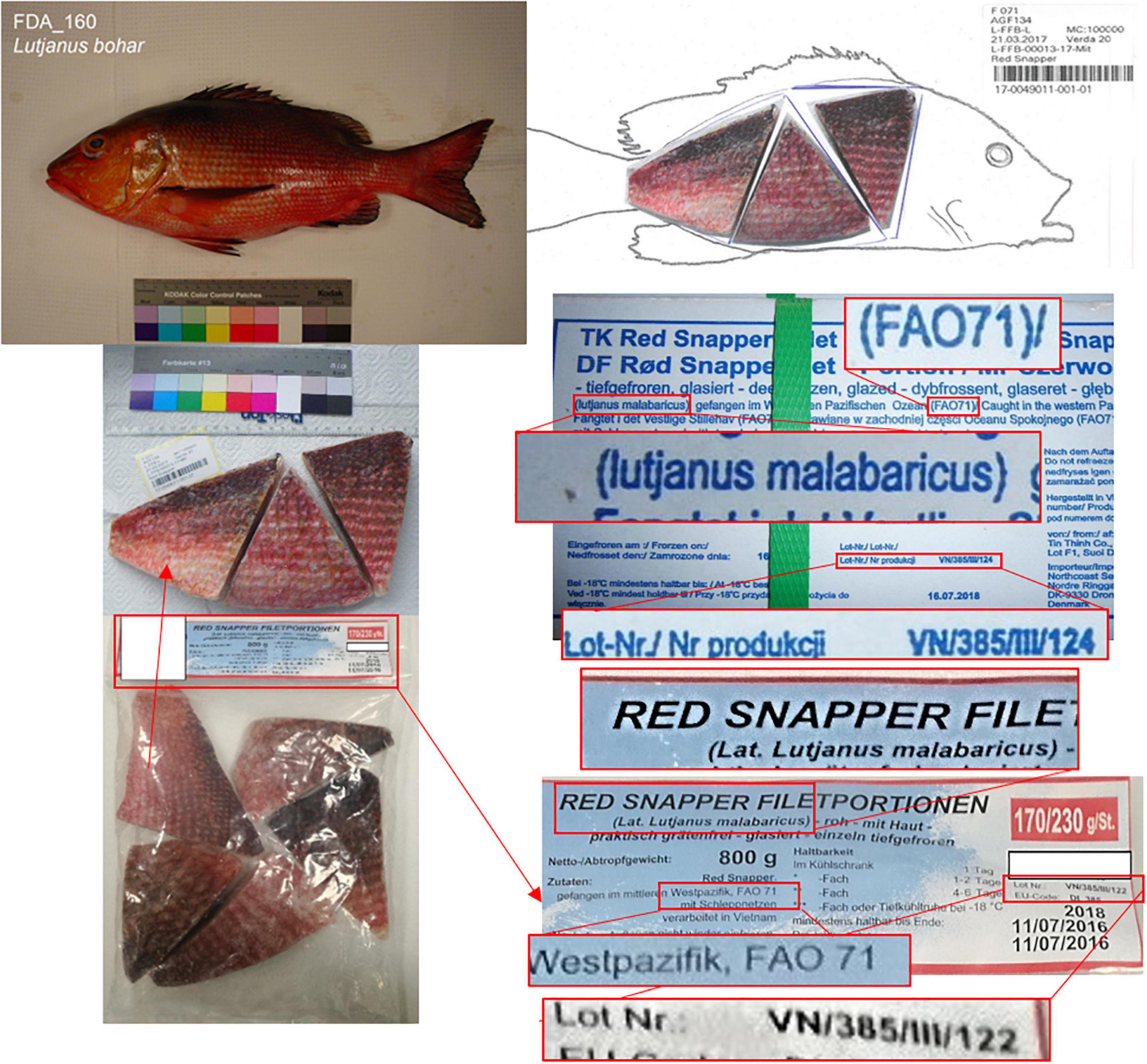
Figure 2. Reference fish photo for L. bohar from the US Smithsonian National Fish Collection (USNMNH 395518) and US Food and Drug Administration reference standard sequence library for seafood identification, specimen ID (FDA 160) (Smithsonian National Fish Collection, 2021) (top left). Processed fish filets of the confiscated product (middle and bottom left)and the purported filet source from the whole fish (top right), relevant trace-back information were obtained from the boxes and packaging of the product (bottom right). Highlighted information includes catch region [Food and Agricultural Organization of the United Nations (FAO) zone 71], lot numbers VN/385/III/122 and 124, and marketed species name on the packaging. Samples from both lots were investigated by DNA barcoding for species authentication and CTX analysis (see sections “DNA-barcoding and labeling of the imported fishes,” “Evaluation of samples by in vitro cytotoxicity assay,” and “Evaluation of samples for ciguatoxins by liquid chromatography with tandem mass spectrometry (LC-MS/MS)”).
From the original 48 filets of lot #124, three were retained for records (DNA-barcoding), and 45 were transferred (frozen) to the BfR for contaminant analysis. Filets weighed between 141 and 209 g (wet weight) with a median of 175 ± 16.1 g. The difference between sample analysis weight and the weight description on the box (170–230 g) may have been due to water loss. At the BfR, frozen water i.e., “glazing” was removed from the surface of the samples before weighing.
DNA-Barcoding and Labeling of the Imported Fishes
Seafood consumption habits in Germany indicate that 61 and 15% of respondents eat fishery and aquaculture products ≥ 1x per month at home and outside the home, respectively, and 64% of respondents thought the common name and species should be included on the label to authenticate the product (European Commission [EC], 2021). Identifying and authenticating hundreds of fish species circulating in the international fish trade is a major challenge. It is estimated that 25–41% of seafood in commerce may be mislabeled by species (Miller et al., 2012; Aung and Chang, 2014; Khaksar et al., 2015; Di Muri et al., 2018), this inaccuracy and non-compliance in labeling negatively impacts global trade. Accurately labeling products is necessary to ensure compliance with regulations for protecting fish stocks (i.e., sustainability) (Calosso et al., 2020), product quality, and enabling the accurate valuation of fish products (Ardura et al., 2010; Di Pinto et al., 2015; Di Muri et al., 2018).
Species substitution can also present a direct risk to consumers via foodborne illnesses (Kusche and Hanel, 2021). The documents for the description of the imported fishes indicated the product was ‘Red Snapper filet’ (Lutjanus malabaricus) (Figure 2). Within the German seafood market, the trade name Red Snapper may only be applied to L. malabaricus, specifically originating from the Indo-Pacific region (Federal Agency for Agriculture and Food, 2021; Kusche and Hanel, 2021). The market name Red Snapper is an inordinately broad category encompassing many cosmopolitan species with a high commercial value that have a conserved morphology and coloration (O’Neill et al., 2011; Gold et al., 2015), but possess various inherent CP risks (Kusche and Hanel, 2021). Globally, the relationship of CP to products sold as Red Snapper is as broad as the potential species the name encompasses, from “high-risk” species banned in certain markets (e.g., L. bohar, Darius et al., 2007; Mak et al., 2013; Sydney Fish Market, 2015) to species with “no known risk” (e.g., L. malabaricus), and many have overlapping habitats. All outbreaks of CP in Germany from 2012 to 2017 were caused by imported snappers (Lutjanidae) (Friedemann, 2019).
To confirm the species attributable to the 2017 CP outbreaks, DNA-barcoding was performed by LGL Oberschleißheim, Germany. The Unit of Effect-based Analytics and Toxicogenomics, Department Food Safety at the BfR also performed an additional authentication test via DNA-barcoding on a filet from the samples received for toxin investigation (lot #124). The RASFF notification reports the DNA-barcoding analyses of ten samples from three outbreak-involved cluster regions in Germany, performed by the LGL. All independent molecular biological examinations of individual fish filets revealed the species to be L. bohar (base pair agreement of 100% in nine samples and 99% in two samples), four were from lot number #124 (including one analysis at the BfR), seven from lot #122 (including both meal remnants). The raw fish filets were labeled and placed onto the market under the agreement of Annex 1 (a) Regulation (EU) 1379/2013 (CN code 0304) (European Union [EU], 2013a) which allows the sale to final consumers or caterers if the commercial designation of the species and its scientific name is indicated and in conjunction with national law (FischEtikettG, 2015; FischEtikettV, 2015). Conferring with the list of trade names for products sold in Germany, the name Red Snapper may only be used for L. malabaricus, therefore this product was inaccurately labeled (Bundesanstalt für Landwirtschaft und Ernährung, 2021). Permissible names for L. bohar for sale in Germany are “Snapper,” “Lutjanus spp.,” or “Doppelfleckschnapper” (Bundesanstalt für Landwirtschaft und Ernährung, 2021). The designation Red Snapper for sale in the German market, therefore, is not applicable for the examined fish filets and consequently judged as misleading according to European legislation [Regulation (EU) No 1169/2011; Article 7, 1(a); Regulation (EU) No 178/2002; Article 16] (European Union [EU], 2011). These regulations are not without warrant, as L. bohar is recognized by the FDA (FDA fish/fisheries handbook) as a potential species-related risk for natural toxins related to CP and is also recognized as a “high-risk” species for CP throughout its native range (Food and Agricultural Organization of the United Nations fishing zones 51, 57, 61, 71, 77, 81). L. bohar has also been confirmed as responsible for CP outbreaks in Vietnam (i.e., the country of export) (Ha et al., 2018) and in the wider region of South East Asia (Ha et al., 2018; Dao et al., 2021). In contrast to that, L. malabaricus is not considered as a potential for species-related CP hazard even though they co-inhabit the same Food and Agricultural Organization of the United Nations fishing (FAO) zones.
Different CP risk associations for species occupying the same habitats are understood to be due to the feeding behavior or food web association of the individual species, because CTXs are accumulated through diet. L. bohar subsists mostly on fish (70–80% of diet) (Randall, 1995; Ali et al., 2016) feeding predominantly on coral reef-associated species in shallow water (4–180 m depth) (Froese and Pauly, 2020), environments that coincide with Gambierdiscus and Fukuyoa spp. In the North West of Australia, Takahashi et al. (2020) conducted a study to identify and distinguish cryptic Lutjanus species based on their diet. Adult L. malabaricus was found to be unique in comparison to three other studied groups having the highest prey richness (19 prey taxa) with 68% of the prey taxa being malacostracans (crustaceans) (Takahashi et al., 2020). Diet composition is strongly linked to habitat utilization and diet patterns can be a reflection of what habitats are being utilized, as well as fine-scale partitioning of habitats between similar species hunting different prey in the same space (Takahashi et al., 2020). Therefore, even fish that share the same habitat can have divergent CP risks due to their niche prey selection (i.e., diet). The life history of L. bohar feeding at a high trophic level in an endemic region for CTXs has consistently led to tissue concentrations of CTXs in the fish that have resulted in this species being implicated in cases of CP illnesses. Over time this reliable CP association has resulted in L. bohar being widely regarded as a high-risk species for CP (Banner et al., 1966; Oshiro et al., 2010; Gaboriau et al., 2014; Yogi et al., 2014; Rajisha et al., 2017; Ha et al., 2018).
In general, food must be labeled in such a way that an informed consumer can make an appropriate purchase decision based on the information provided. Substitution with an inferior product (e.g., price or quality) or one with an unequal risk constitutes inaccurate labeling. The latter was the case here, where a product with an inherent or known CP risk (L. bohar) was labeled and placed onto the market as a product that does not carry a known risk for CP (L. malabaricus) (Kusche and Hanel, 2021). Therefore, this CP event demonstrates that regular surveillance regarding the labeled species of Lutjanidae could reduce the risk of mislabeled products entering the market. Reinforcing the recognition that CP prevention can be strengthened through multi-disciplinary and inter-agency recognition and coordination (FAO and WHO, 2020; Canals et al., 2021; Loeffler et al., 2021b).
Source Product Location of Capture
The exclusive fishing area, or catch region, indicated on the product packaging was the FAO region 71 (Figure 2). This region is described as Pacific, Western Central, which comprises the Western Pacific Ocean and includes Oceania and Australia. Small-scale fisheries are prominent in FAO 71, indicating nearshore and shallow water catch efforts (Pomeroy et al., 2009; Zeller et al., 2015; Teh and Pauly, 2018). In this catch region, fish that are discarded at sea due to potentially being a risk for “ciguatera” (among other non-desirable species) is considered “insignificant” (0.5%) (Kelleher, 2005). The FAO reports that this area practices CP self-governance, where “fishing in areas known for ‘ciguatera’ is prohibited or avoided” (Kelleher, 2005). Vietnam is not currently listed as a high-risk area for CP.
The location of export, identified in the RASFF report, was Khanh Hoa Cam Lam, Vietnam (Black square, Figure 3). A precedent for CP in this region, specifically from L. bohar, was described in 2014 Phan Thiet City (left gray square, Figure 3) and on the 22nd of June, 2016 in Nha Trang City (right gray square, Figure 3; Ha et al., 2018). Both areas in Vietnam were implicated as the point of origin for CP outbreaks involving 10 cases of CP from L. bohar fish weighing 2–5 kg (a similar size range estimated for the product investigated here, see section “Source-food trace-back sample collection and product description 31”). Meal remnants were recovered and CTX1B, 54-deoxyCTX1B, and 52-epi-54-deoxyCTX1B were identified as the CTXs ascribed to the outbreaks (Ha et al., 2018). While a connection cannot be confirmed, there were similarities noted between lot #122 and one of the CP outbreaks in Vietnam (Ha et al., 2018), similarities such as the general region of processing, the same species, and that both the CP outbreak and the processing and packaging for lot #122 occurred in June 2016. CP information in Vietnam is extremely limited, however, Ha et al. (2018) indicated that since 2007 outbreaks related to Red Snappers have been increasingly reported. Therefore, if CP events are becoming more frequent it may indicate a shift in the local environment toward a more CTX prone state where snappers might be accumulating higher levels of CTXs. In the wider region of Southeast Asia CP has been reported. In neighboring Thailand, un-authenticated fish described as Red Snapper was implicated in the poisoning of four individuals who shared a meal of fish curry (Saraya et al., 2014) and L. bohar was confirmed in a CP outbreak involving a family of four that occurred in Malaysia in 2017 (Dao et al., 2021).
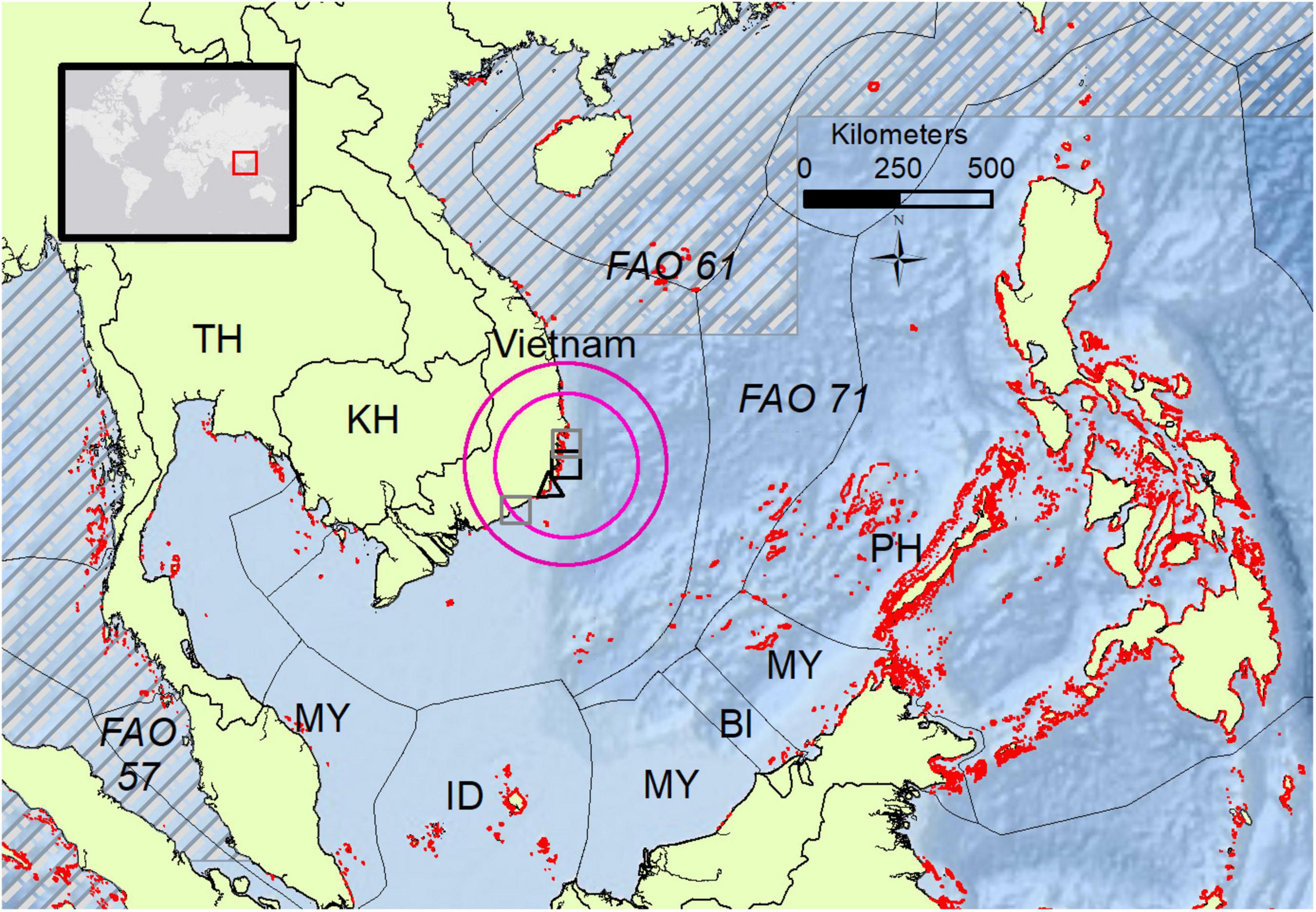
Figure 3. Map displaying the country of export (Vietnam) and surrounding waters (Flanders Marine Institute [VLIZ], 2021), exclusive economic zones (EEZ) of neighboring countries (Flanders Marine Institute [VLIZ], 2014), FAO recognized fishing zones (FAO, 2014), known coral reefs (Institute for Marine Remote Sensing et al., 2011), and symbols representing locations of interest. The World map in the upper left corner contains a red square that indicates the regional area depicted in the main map figure. FAO major fishing zones are indicated with italicized font and dashed horizontal gray bars for zone 57 and 61, whereas FAO fishing zone 71 (catch region for the implicated product) is without horizontal hashing. The estimated catch area (based on the factors discussed in Section “Source product location of capture”) for the product implicated in CP is within the two pink rings, inner and outer pink rings represent approximately 220 and 330 km distance from the coastal area of the processing plant for the exported product (black square). Pink circles represent a “best guess” area for where the implicated fish were likely harvested from based on the EEZ (black dotted line) surrounding the exporting country, the ecology of L. bohar, and distance restrictions of the modern-large class local fishing vessels (Tun et al., 2005). Gray squares are locations of CP outbreaks attributed to L. bohar in 2014 and 2016, black triangle is the region of Cau Island Binh Thuan, Vietnam, where Gambierdiscus toxicus was collected (Roeder et al., 2010). Country codes (BI, Burundi; ID, Indonesia; KH, Cambodia; MY, Malaysia; PH, Philippines; TH, Thailand) were based on the International Organization for Standardization (Patrias and Wendling, 2007).
Vietnam has 3,260 km of coastline, 3,000 islands and islets, and 1,270 km2 of coral reef area (red areas on Figure 3; Tun et al., 2005). To date, three species of Gambierdiscus have been identified around Vietnam (G. toxicus, G. pacificus, and G. polynesiensis) (Rhodes et al., 2017). G. toxicus was collected from Cau Island, Binh Thuan, Vietnam (Figure 3, black triangle), and in culture, it was described as producing CTXs (Roeder et al., 2010). Biologically and ecologically, the habitat around the processing plant is suitable for a CTX producing source algae and the implicated vector (L. bohar). Therefore, the encircled area in Figure 3 encompasses a location where CTX-producing dinoflagellates were collected (black triangle) and includes a preferred habitat of the captured implicated species (coral reefs, red areas Figure 3).
In Vietnam (product origin), the fishery consists of medium-sized modern-style boats with a 5–7 person crew that conduct day fishing 9–13 km offshore, while larger boats can reach fishing grounds 110–240 km away (Pham, 2015). The descriptions of the fishing industry and the vessel distance to shore capabilities imply a higher likelihood that the product catch region was located within the western portion of FAO 71, within the exclusive economic zone of the exporting country (Figure 3), rather than being from the distant north-eastern portion of FAO 71. The description on the export item list indicated that the fish were caught in the Western Central Pacific and caught by a trawl fishing method. Based on this assumption and guided by the convergence of CP records, biological and ecological information a specific location as a “best guess” for the collection source of the implicated fish was encircled (Figure 3). The smaller pink and larger pink circles in Figure 3 represent a distance of 220 and 330 km from the coastal region of the CP implicated processing plant (black square, Figure 3), respectively. The circles were generated to represent a distance to shore that was restricted by several factors including, the fishing vessel distance limit (e.g., larger local fishing vessels travel distance plus a conservative 90 km additional distance buffer), the FAO 71 fishing zone (described on the packaging), and the exclusive economic zone of Vietnam (adhering to international fishing rights) (Pho Hoang Han, 2007).
Trace-back information provided by the packaging and information obtained from the literature enabled the narrowing down of the potential region where capture occurred and drawing conclusions regarding CTXs in the region and the species history of CP association. However, additional uncertainty for CP prediction and trace-back can arise through the method of fish processing and packaging employed, specifically, a box of fish for sale that consists of mixed-fish and/or fish from mixed-locations. Because of the variability in size and shape of the pieces of fish in the packages described here (Figure 2), a mixed type processing (either, mixed-fish, mixed-location, or both) was likely used (Dickey and Plakas, 2010). Lot #124 was estimated at 15,500 kg total original fish weight (see Section “Source-food trace-back sample collection and product description”) and based on an average 3.5 kg fish size, this would require approximately 4,400 fish. Because many red snapper-type resources in the region have been effectively exploited (Badrudin and Aisyah, 2017), this lot was likely sourced from multiple catch locations. Alternatively, all fish could have been captured at a spawning aggregation site, as fish that form spawning aggregations (as reported for L. bohar, De Mitcheson et al., 2008) can concentrate individual populations into a large mass. However, precautionary management measures are often recommended to be put in place to restrict harvesting fish at these events, as this method of harvesting fish has historically resulted in overfishing and the collapse of various fisheries (De Mitcheson et al., 2008; Chollett et al., 2020). Mixed-batch and/or mixed-source processing reduces the confidence in a CP trace-back regarding the origin of the product, whether it is made up of region-wide or representative of a specific reef. Fish sourced from a single location or multiple locations are distinct in their food web association, and because CTX producing algae are site attached, and CP risk can vary among locations, even around a single island (Loeffler et al., 2018, 2022; Chinain et al., 2020a,b; Oshiro et al., 2021a). Therefore, when drawing conclusions from an individual fish implicated in an outbreak to make an assumption regarding the larger lot (Dickey and Plakas, 2010), these two harvest practices should be considered (i.e., is the fish indicative of one reef, or is it a random selection from a region). The trace-back method, while useful and informative (when accurate) regarding the species and location, still falls short of providing information about the wider lot. Only the implicated fish may be contaminated (of which there may be no remaining meal remnant to verify CTXs) or all fish in the lot may have some level of contamination (a potential “hot-spot” harvest). While the latter scenario is rarer (Dickey, 2008; Dickey and Plakas, 2010), both extreme scenarios are theoretically plausible and therefore should be considered (European Commission [EC], 2002).
Evaluation of Samples by in vitro Cytotoxicity Assay
N2a cells used in this study were OV-LS modified and had a survival of 60–80% when exposed to 0.22/0.022 mM O/V. Cell line passage numbers for utilization were between 190 and 245 (original passage number 185 from manufacturer). The range of raw absorbance values recorded after the MTT-colorimetric assay evaluation was between 0.08 and 1.43 nm (minimum to maximal survival). The effective concentration causing a reduction in cell viability at 50% (EC50) for CTX3C standard, calculated from the dose-response curve with a regression factor (R2 = 0.99), was 1.35 ± 0.28 pg mL–1 (n = 25), as described in Loeffler et al. (2021a). The fish TE mL–1 range used for analysis among all samples was 0.0085–130.0 mg TE mL–1, determined by the individual samples’ assay response. All samples tested exceeded our determined limit of detection (>0.001 ppb CTX3C) and therefore required less tissue than the 150 mg (wet TE) mL–1 applicability previously demonstrated (Loeffler et al., 2021a).
All 45 fish samples tested were deemed ‘positive’ by the N2a-MTT assay for the presence of CTX-like activity. Toxin estimates per gram of fish wet tissue equivalents (eq.) ranged from 0.23 to 11.4 ng CTX3C eq. g–1 (n = 45) (Table 2, Figure 4, and Figure 5A). Thus, all samples exceeded the official controls of fishery products in the EU. The total amount of toxin per fish portion (toxin concentration × portion weight) ranged from 39 to 2148 ng CTX3C eq. (n = 45) (Table 2). Regarding a total CTX intake, 70 ng has been proposed as a recommended limit for human health seafood-consumption safety (Yasumoto, 2005). With two exceptions, this 70 ng level was exceeded by all samples (Figure 5B). CTXs can bio-accumulate and the human health implications for low-dose long-term exposure are not yet understood. Therefore, samples containing less than 70 ng would still constitute an exposure to CTXs with unknown health implications. Two meal remnants were analyzed at the US FDA Gulf Coast Seafood Lab and both tested positive for CTX-like activity by the N2a-MTT assay (samples #46 and 47 in Figure 4B). Composite cytotoxicity for the two meal remnants was 3.67 and 2.88 ng CTX3C eq. per gram wet tissue equivalent. When compared with the C-CTX-1 and CTX1B standards these two meal remnants contained 4.29 and 3.23 ng C-CTX-1 eq. and 2.71 and 2.03 ng CTX1B eq. per gram wet tissue equivalent. Therefore, both meal remnants exceeded the FDA guidance levels of 0.1 ng C-CTX-1 eq. per g and 0.01 ng CTX1B eq. per g (U.S. Food Drug Administration, 2020).

Table 2. Toxin content description for recalled fish filet samples (n = 45) in CTX3C equivalent (eq.).
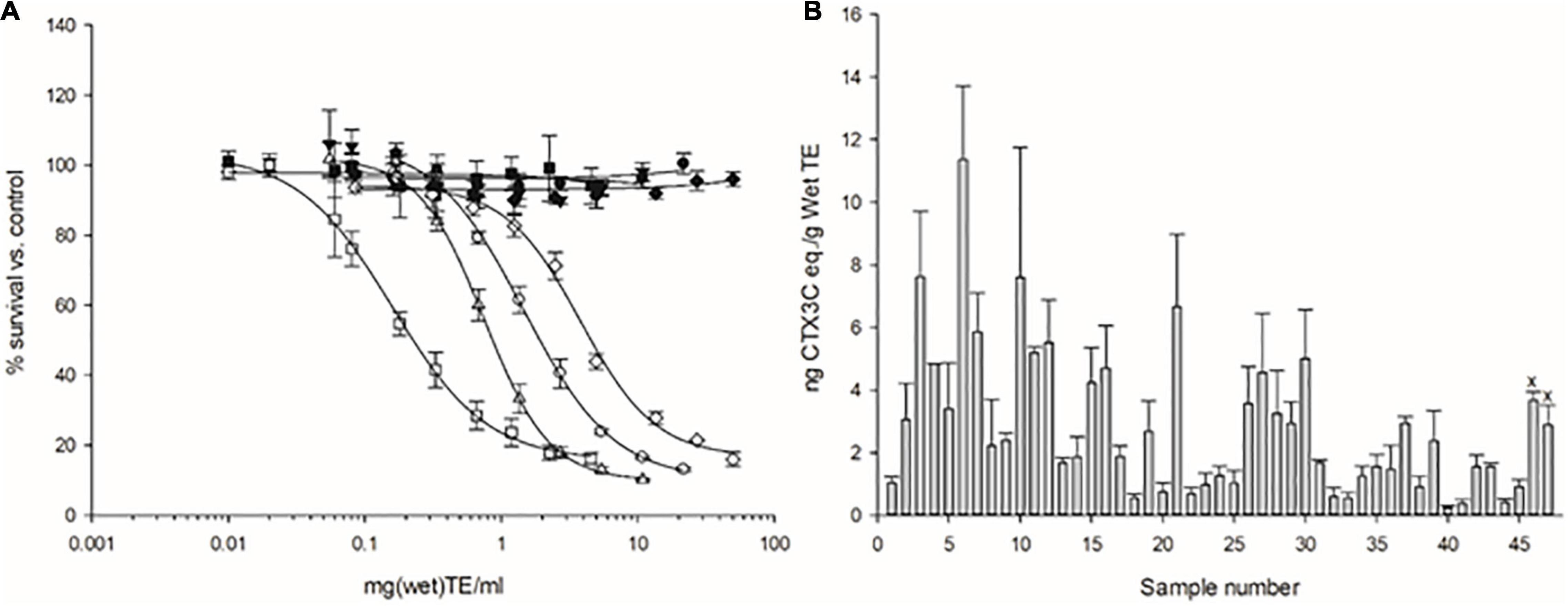
Figure 4. (A) Combined concentration-response curves of N2a cells without (–) the addition of ouabain (O) and veratridine (V) (– OV, solid symbols) and with the addition of OV (+OV, open symbols), when exposed to various concentrations of semi-purified extracts of fish. Lines are high (square, sample #21), medium (triangle, sample #35), medium-low (circle, sample #1), and lowest (diamond, sample #40) toxicity based on the dose-response curves representing high to low toxin concentration estimates among the sampled lot. OV-LS N2a cells were exposed to 0.22/0.022 mM O/V. Dose-response curves were used to measure the effective concentration (EC) for 50% reduction in cell survival. (B) Bar graphs with error bars representing the toxin concentration (based on the mean EC50’s measured) expressed as ng CTX3C eq. per gram wet tissue eq., generated from the dose-response curves: data display all samples tested and all replicates. Symbol ‘x’ above the error bar indicates samples from meal remnants from lot # 122 (samples # 46 and 47). Error bars represent the standard deviation from all independent 96-well plate analyses performed for each sample (minimum three independent assays, each assay includes three replicate points).
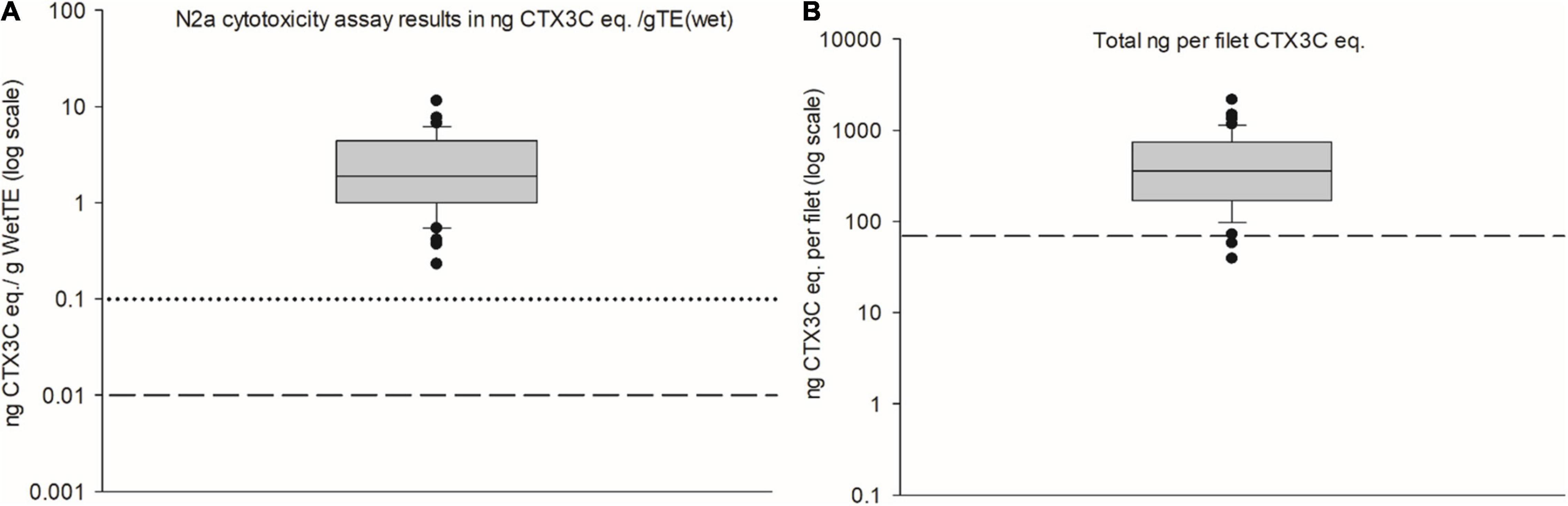
Figure 5. (A) Box-and-whisker plot of the toxin concentration of CTX3C eq. per gram of fish tissue eq. for the 45 fish samples, the box represents the upper to lower quartile range, the median is marked by the horizontal line in the box, and whiskers indicate variability outside the upper and lower quartiles, outside points are outliers. Health guidance levels for CTX1B eq. (dashed line at 0.01 ppb) and C-CTX-1 eq. (dotted line at 0.1 ppb) are indicated for the context of human health seafood-consumption safety levels used by the US Food and Drug Administration. (B) Box-and-whisker plot of the total toxin content per filet (ng CTX3C eq.), with a 70 ng health guidance level (dashed horizontal line), based on the recommendation by Yasumoto regarding a total ng CTX intake for human health seafood-consumption safety (Yasumoto, 2005).
Results of toxin quantitation presented in this study refer to wet tissue equivalents. Frozen fish filets can contain additional water due to glazing (i.e., net weight versus gross weight) and freeze-thaw cycles can result in water loss (e.g., due to freeze-drying during prolonged storage). Both factors can alter the weight of the fish (see also Section “Source-food trace-back sample collection and product description”). As toxin eq. is in reference to the weight initially used for extraction, subsequently these weight changes can alter the estimated toxin content. This is particularly problematic when attempting to establish a guidance level or when comparing a current CTX result with a legal limit or guideline.
Therefore, when reporting toxin equivalents of a sample, a consensus on the sample weight description would help normalize methods, reports, and comparisons among studies. Specifically, whether to report either wet or dry tissue equivalents. Ultimately, we chose to report our results in wet tissue equivalents, because this is the most commonly reported sample description and value. The condition of a product will influence the water content (by up to 80%, raw filet versus freeze-dried material), therefore if a product is cooked, raw, frozen, or dry the condition (i.e., water content) should be interpreted accordingly when reporting a final toxin per tissue eq. value. Removing the confounding factor of water weight and starting with a dry product (or simply reporting the wet versus the dry weight of the sample) could simplify inter-laboratory toxin equivalency comparisons. The EU policy for CTXs makes this a semi-moot point because a sample that tests positive for CTXs (irrespective of toxin concentration or CTX-group) is not permitted for sale. However, in regions where guidance levels represent actionable values (which can indicate the suitability of a seafood product’s wholesomeness for sale or consumption), this issue of reporting values based on an original tissue weight could be a confounding factor worthy of further discussion.
Evaluation of Samples for Ciguatoxins by Liquid Chromatography With Tandem Mass Spectrometry
Forty-five samples removed from commerce, in addition to the two meal remnants, were deemed positive in the N2a-MTT assay and investigated further by LC-MS/MS. The two meal-remnants were investigated for the presence of the confirmatory compounds for Caribbean and Pacific ciguatoxins (C-CTX-1 and CTX1B, respectively) by LC-MS/MS at the US FDA Gulf Coast Seafood Laboratory, however, both samples were deemed negative for these compounds. The FDA provided the following summary statement in an analysis report to the BfR: “…Based on the species of the fish samples reported by the German Federal Institute for Risk Assessment (L. bohar) and the presence of sodium channel-specific cytotoxicity, the samples analyzed may contain uncharacterized ciguatera-like toxins.”
To investigate the possible presence of other CTXs, an HPLC fractionation of a sample extract followed by the N2a-MTT analysis was conducted (i.e., bio-assay guided fractionation). For this, sample #21 was chosen due to its high degree of measured toxicity (Figure 4B). Multiple fractions (5–7, 11–13, 19 for undiluted samples) of the extract showed a high degree of sodium channel (Nav) specific activity indicating the presence of several distinct Nav activating compounds (CTX-like toxicity) (Figure 6A). Thus, the dose-response curves observed in the N2a-MTT assay (Figure 4A) were likely the result of the composite toxicity effect due to a mixture of CTX-like congeners.
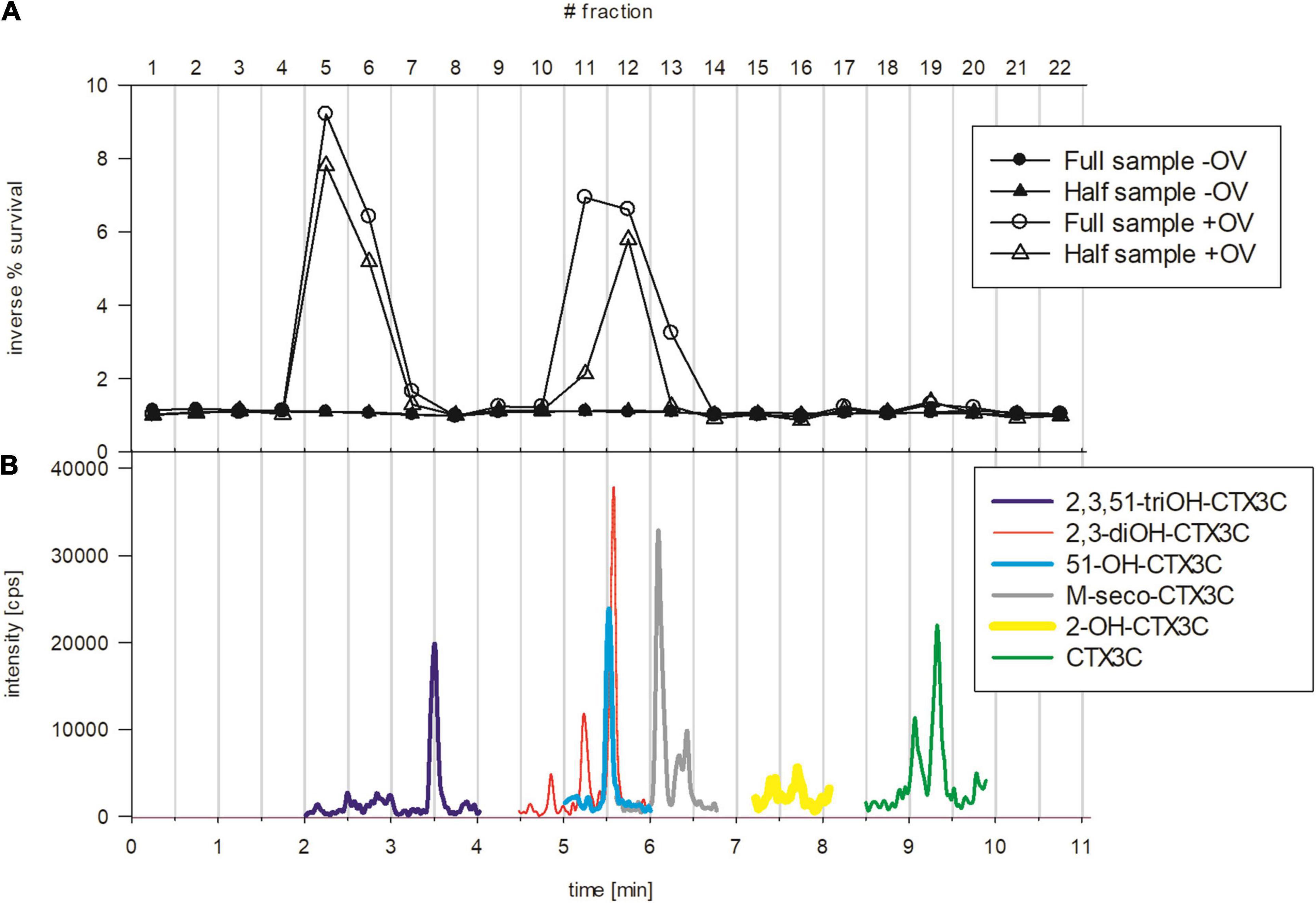
Figure 6. (A) Bioassay-guided fractionation using the extract of sample #21 with fractions showing CTX-like toxicity in the N2a-3-(4,5-dimethylthiazol-2-yl)-2,5-diphenyltetrazolium bromide (MTT) assay. The assay was performed with fractions collected according to Section “Fractionation method,” the circle and triangle represent undiluted and 1:2 diluted samples, respectively. The black symbols correspond to control wells (–OV) and open symbols correspond to +OV conditions (0.22/0.022 mM O/V) (see legend of Figure 4A for details). (B) LC-MS/MS analysis of sample extract #21 (non-fractionated) with Extracted Ion Chromatograms of potential CTX congeners detected in the sample. The colored lines represent the m/z of the sodium adducts of 2,3,51-trihydroxyCTX3C (dark blue, min 2-4), 2,3-dihydroxyCTX3C (red, min 4.5-6), 51-hydroxyCTX3C (light blue, min 5-6), M-seco-CTX3C (gray, min 6–6.45), 2-hydroxyCTX3C (bold yellow min 7.2–8.1), and CTX3C (green, min 8.5–9.8); OH, hydroxy. Vertical lines indicate the collection period of the fractions during bioassay-guided fractionation.
The same sample extract was utilized for LC-MS/MS analysis, the analytical method included the >30 CTX congeners reported in the literature (FAO and WHO, 2020; Spielmeyer et al., 2021). Using this broad compound approach, several potential CTX congeners of the CTX3C group were identified based on their sodium adducts ([M + Na]+, left column in Figure 7). Compound identification was further supported by low- and high-resolution analyses of ammonium adducts’ fragmentation (middle and right column in Figure 7). At least six potential congeners plus their putative epimeric forms were detected, including 2,3,51-trihydroxyCTX3C (3.50 min), 2,3-dihydroxyCTX3C (5.23 and 5.57 min), 51-hydroxyCTX3C (5.54 min), M-seco-CTX3C (6.13 and 6.46 min), 2-hydroxyCTX3C (7.47 and 7.74 min), and CTX3C (9.05 and 9.33 min) (additional details regarding compound assignment are provided in Spielmeyer et al., 2021). Congeners of the CTX3C group (2,3,51-trihydroxyCTX3C, 2,3-dihydroxyCTX3C, 51-hydroxyCTX3C, 2-hydroxyCTX3C) were previously reported by other groups for L. bohar and other fish species caught in the Pacific (Yogi et al., 2011, 2014; Oshiro et al., 2021b), supporting the peak annotation. The identification of multiple CTX candidates by MS further supports the conclusion that composite cytotoxicity was observed in the N2a-MTT assay, likely influenced by the presence of a mixture of congeners. However, of all the congeners labeled within the samples, none have an attributed specific guidance value, and there are no regulatory limits for CTX3C-group toxins in the EU. The official controls of fishery products in the EU states that “fishery products containing biotoxins such as ciguatera [sic] or other toxins dangerous to human health are not placed on the market.”
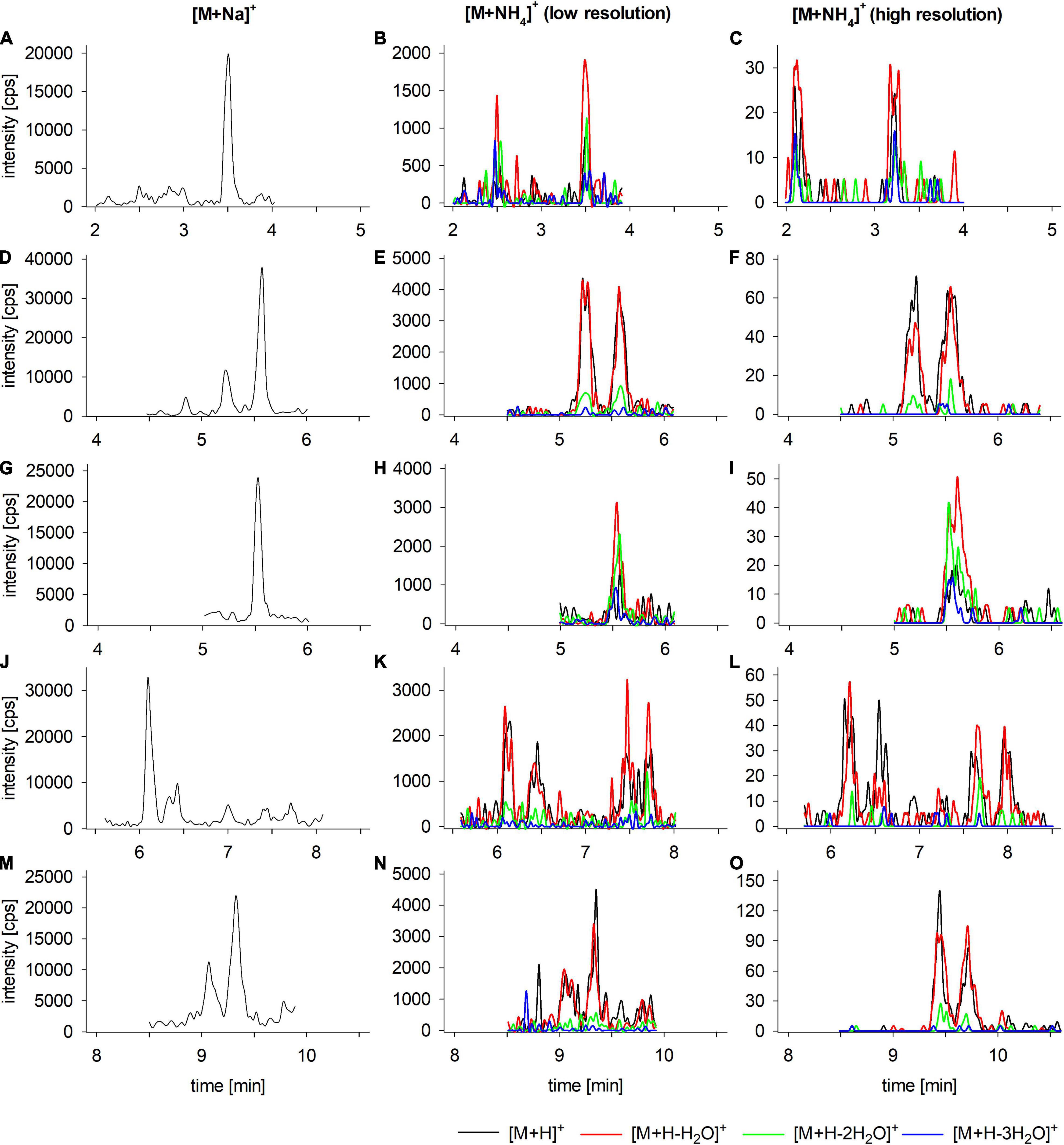
Figure 7. Extracted Ion Chromatograms (EIC) of the LC-MS/MS analyses of the CTX-like positive sample #21 (toxicity determined by the N2a-MTT-assay, Section “Toxicity evaluation by in vitro Neuro-2a cytotoxicity MTT-assay”), the panels show the EIC of the respective m/z of (A–C) 2,3,51-trihydroxyCTX3C, (D–F) 2,3,-dihydroxyCTX3C, (G–I) 51-hydroxyCTX3C, (J–L) M-seco-CTX3C and 2-hydroxyCTX3C, and (M–O) CTX3C. Analyses were performed for the sodium adducts (left column) as well as the low-resolution (middle column) and high-resolution analyses (right column) of the fragments of the respective ammonium adducts. For ammonium adducts, fragments correspond to [M + H]+ (black), [M + H-H2O]+ (red), [M + H-2H2O]+ (green), and [M + H-3H2O]+ (blue) (for color the reader is referred to the online version of the manuscript), low and high-resolution analysis was performed with two different setups, leading to slightly different retention times, setup details are provided in Spielmeyer et al. (2021).
To further aid the identification of compounds, results of the bioassay-guided fractionation and LC-MS/MS analyses were compared. The toxicity in fractions 11–13 may be ascribed to 51-hydroxyCTX3C, 2,3,-dihydroxyCTX3C and/or M-seco-CTX3C and in fraction 19 ascribed to CTX3C. Although CTX3C and its potential epimer, 49-epi-CTX3C (CTX3B), showed an intense signal in the LC-MS/MS chromatogram, only slight toxicity was observed in fraction 19 (Figure 6). Matrix effects on the cell assay, such as enhanced cell growth that can obscure results have previously been described (Caillaud et al., 2012; Viallon et al., 2020). However, no growth was observed in the control (–OV) portion of the assay (Figure 6A), therefore a matrix effect is considered less likely in this case. The peak intensity of CTX3C/B was comparable to other congeners present in the sample (Figure 6B). Varying ionization efficiencies can result in remarkable differences concerning peak intensities of equimolar compounds. Although showing a distinct signal in the LC-MS/MS analysis, the corresponding CTX3C/B concentration might have been too low to deliver a pronounced response in the isolated fraction within the N2a-MTT assay. Alternatively, similarly structured ladder-shaped cyclic polyether marine toxins (e.g., brevetoxin) have shown varying affinities on the N2a-MTT assay. Therefore, CTX3C/B and the other congeners may possess similar concentrations, but differ in their affinity to the Nav and resulting in a lower effect on the cells. Unfortunately, due to the lack of analytical standards, single compound toxicity has not been systematically evaluated so far. Toxicity equivalent factors (TEFs) are available, however, these were determined by the mouse bioassay. Based on those data, the TEF of CTX1B was set to 1.0, and the potency of other congeners was related to this compound and value. TEFs of other CTXs described therein are as follows: CTX3C 0.2, 51-hydroxyCTX3C 1.3, 2,3-dihydroxyCTX3C 0.1 (FAO and WHO, 2020). According to the TEFs, 51-hydroxyCTX3C might be considered up to six times more toxic than CTX3C and over ten times more toxic than 2,3-dihydroxyCTX3C. This could explain the higher toxicity observed in fractions 11/12 compared to fraction 19 (Figure 6A), although CTX3C/B showed peak intensities comparable to the other congeners (Figures 6B, 7). A better understanding of the function of these toxins by their form (i.e., Nav affinity) will enhance the interpretation of bioassay-guided fractionation results as well as present an opportunity for pharmaceutical investigations.
No toxicity was observed in fractions 15 and 16 in the N2a-MTT assay, although peaks of potential 2-hydroxyCTX3C were detected in that time window (Figures 7J–L). This congener was not included as being evaluated concerning its TEF, thus no statement was provided about the toxicity of this specific congener compared to the others (EFSA, 2010b). However, it is expected that 2-hydroxyCTX3C possesses Nav activity as well. As discussed for CTX3C/B the concentration in the fractions may have been too low for detection in the N2a-MTT assay. The assumption of “generally low concentrations” in the collected fractions is supported by results for fractions 11 and 13 which showed a remarkably reduced or no toxicity after diluting samples by factor 2 (Figure 6A). Concentrations in the fractions might be close to the limit of detection within the N2a-MTT assay, therefore in future studies additional collections can be made to increase the compounds availability, or the number of fractions can be reduced to ensure an accurate collection window (e.g., collection of one congener per fraction versus one congener spread/diluted over two fractions).
Toxicity in fraction 7 might be caused by 2,3,51-trihydroxyCTX3C. The high toxicity in fractions 5 and 6 cannot be explained so far. Unidentified matrix compounds, eluting at the beginning of the chromatographic run could contribute to cell toxicity. However, no adverse impact on cell survival was observed in the control portion of the plate (–OV) in the presence of fractions 5–7. An adverse effect on cell survival was only observed when in the presence of +O/V, indicating the fractions contained a compound with a specific interaction on the Nav, similar to CTXs. At 2.51 min, a putative CTX congener was observed showing the same m/z like 2,3,51-trihydroxyCTX3C (Figures 7B,C). It might be an epimeric form, however, epimers of the other congeners were found to elute close to the parent compound (time window < 0.5 min, Figure 7). In the case of 2,3,51-trihydroxyCTX3C, the retention times of the two peaks differed by 1 min. Due to the observed fragmentation (similar to 2,3,51-trihydroxyCTX3C, Figures 7B,C) and the toxicity of the respective fractions, the peak is considered as a potential CTX congener. Based on the MS data it might be an epimer or isomer of 2,3,51-trihydroxyCTX3C which is currently un-described. Besides this compound, the observed toxicity in fractions 5 and 6 (both undiluted and diluted) could derive from other unknown CTX congeners.
Public Health Implications
The incidence rates of CP in the EU/European Economic Area countries are extremely low (0.0054 per 100,000 inhabitants per year, excluding travel-related cases) (Canals et al., 2021). It is believed that only 10–20% of CP cases are reported worldwide (Canals et al., 2021). However, this percentage has never been estimated for non-endemic countries. It is evident that additional people were involved in this outbreak who suffered adverse effects, but were not counted if they did not seek medical advice, medical care, or self-report to a Public Health institution (see Section “CP outbreak in Germany, 2017”). In non-endemic countries (e.g., Germany), people and even health care providers are generally not aware of CP. However, efforts are underway to raise awareness of CP. In 2017, CP was part of a pilot monitoring program bringing together institutions responsible in the field of poisonings (e.g., German Association of Clinical Toxicologists, the German Poisoning Information Centers, and the German Federal Institute for Risk Assessment). Hopefully, with the methods for CTX analysis now established at the BfR, awareness of the diagnosis of CP will increase in Germany.
All samples tested here were positive for CTX-like activity by the N2a-MTT assay and several (potential) congeners from the CTX3C-group were identified by LC-MS/MS, providing additional evidence for the risk L. bohar can pose for CP. There are no specific guidance levels for congeners of the CTX3C-group, but the demonstration here of this potential CTX hazard in seafood products provides further evidence that this toxin group should be included as part of future assessment efforts for CTXs. Given the congeners of CTXs identified, our findings agree with the literature regarding CTXs found in the tropical western Pacific catch region (Oshiro et al., 2021a) and within L. bohar (Yogi et al., 2011).
Based on the package description of 170 g minimum weight per filet, the total weight of the sixteen portions (representing the known sixteen cases of CP reported) was assumed to be 2.7 kg. This 2.7 kg represents 0.032% of the total weight of the two original lots (2,500 kg lot #122 and 6,000 kg lot # 124) in Germany (irrespective of glazing weight or recalled product, i.e., as originally distributed). The sampling size of this study was 0.14% (7.5 kg net weight measured from lot # 124 with a net weight of 5,400 kg), and 100% of the samples were deemed CTX-positive. All samples contained CTX toxin equivalents that exceeded EU product legal requirements and recommended guidelines for CTXs. Therefore, the 45 samples deemed positive for CTX-like toxicity, examined in this recall effort, represent potential CP cases that were demonstrably avoided.
Not all fish of the same species (in a mixed batch) or from a given area are toxic. Consequently, negative results for CTX detection in replicate samples collected from the same lot that intoxicated patients have been documented (Klekamp et al., 2015), and a negative result can be consistent with the sporadic distribution of CTX among individual fish. Because of these reasons, food recalls were considered inefficient public health tools in response to CP outbreaks, and that the testing of a commercial lot may not help identify the source of toxic fish (Dickey and Plakas, 2010; Klekamp et al., 2015). Therefore, conclusions based on the CTX content of the meal remnant or extrapolated from tests conducted on the related product can be inconclusive. However, testing the wider lot or related product can still provide coverage information about the implicated species from the processor or region of capture. Currently, basic information for most species and their habitats regarding CTXs (qualitatively and quantitatively), spatially and temporally is lacking and in need of further investigation. Therefore, in the least, investigating associated lots can fill research data gaps but if more fish in the lot are toxic, then a meal remnant can also serve as a warning for hazards contained within the larger lot. Varriale (2021) described an outbreak that occurred in 2017 in the United Kingdom involving a family (n = 3) where one uncooked frozen Red Snapper filet was recovered (species data not available). A preliminary positive toxin analysis by LC-MS/MS and the N2a-MTT assay for CTXs on this sample led to the consignment of the whole production batch of the product (total weight of entire withdrawn production batch 1230 kg). Subsequent testing by LC-MS/MS and N2a-MTT assay on 24% (467 packets) of the entire lot (99 boxes that contained 1973 packets and each packet contained 550 g of “Red Snapper”) revealed that all samples tested by LC-MS/MS contained CTXs with chromatographic peaks attributed to potential C/I- CTX-1 and -2 (Varriale, 2021). The fish samples were from India and reportedly caught in the FAO major fishing area 51 (Indian Ocean). Because CP is chronically underreported, when a victim or patient comes forward with a legitimate complaint the event should be taken seriously. A fast recall effort can remove the additional product from the market before the remaining product is sold, potentially removing any remaining product which could pose a serious and significant health threat to the fish consuming public, as was demonstrated by Varriale (2021).
In the present case, Table 1 illustrates that in the context of CTX outbreaks it may be necessary, in terms of precautionary consumer protection, to apply the so-called “batch suspicion” according to Regulation (EC) No 178/2002; Art. 14 (6) (20) “where any food which is unsafe is part of a batch, lot or consignment of food of the same class or description, it shall be presumed that all the food in that batch, lot or consignment is also unsafe, unless following a detailed assessment there is no evidence that the rest of the batch, lot or consignment is unsafe.” The example of the CP outbreak involving a family (n = 3) in the United Kingdom in 2017 mentioned above, provides strong additional evidence for the incentive to investigate the wider commercial lot when implicated in a CP outbreak. The two boxes of the product tested in this investigation, which were demonstrated to contain CTXs, are another example of a successful and clear protectionary recall measure.
A positive rate of 100% for both detection methods for all 45 filet samples is remarkable for CP lot investigations, however, it remains unknown whether the samples investigated in this study are anomalous to the larger lot, the CTX levels in the other portions were below an adverse effect level, or more cases went unreported. The analyzed quantity is most likely not representative for both causative lots of fish, which could be comprised of fish from different locations with different concentrations of various CTX congeners. The risk by mixed lots with different fish species from different locations remains incalculable in large volume fish imports.
The case- and cluster-related approach for trace-back examinations including the combination of CTX and fish-DNA analysis was demonstrated here as an important workflow for identifying the key aspects of a CP outbreak. In addition to information about patients and their symptoms, the fish species and the CTX content should be determined in meal remnants in addition to the analysis of additional lot-related products, which was achieved for the first time here in Germany.
Conclusion
In the complex CP outbreak documented here (see also Supplementary Table 1), consumers faced many uncertainties beginning with an internationally traded, mislabeled species with a history of carrying a health risk that is unfamiliar to non-endemic consumers. The fish was widely distributed with a large geographic impact, and because CTXs are stable the frozen products’ risk for CP was long-term as well. All filet portions measured from these lots had CTX contamination that exceeded the EU recommendations and the two meal remnants exceeded the FDA guidance for CTXs in seafood products. This ostensibly high fail rate for samples from a large lot is concerning. Taken together, the consumer lacked adequate information to apply the known self-protection measures against CP (e.g., CP is a known hazard for L. bohar, and guidance on this species is available, U.S. Food Drug Administration, 2020). From the supply side of preventing CTX contaminated products from reaching the market, studies are needed to provide additional information about CTXs in the harvest region and CP outbreaks. There is a paucity of data regarding the identification of CTX producing algal species, the environmental factors driving their growth/distribution, frequency of CTXs in commercial species, and regions of concern (i.e., hot-spots). Furthermore, the context of expected changes and adaptations to climate changes (Hung et al., 2017; Dung, 2020; Tester et al., 2020) should be accounted for in any assessment to understand the current and future risk of CP to local consumers and those vulnerable to CP via the international seafood trade. For the response for human health, the exported lots of fish were large (combined ∼8,500 kg) and because CP events are classically underreported, the results obtained for our comparatively small sampling size suggest a potential underestimation of the health impact of these lots in Germany. Public outreach and education, in particular, are needed for consumers, restaurants, and physicians to help to identify patients and to provide samples for analyses in case of a potential CP event. If not for the public outreach efforts following the recall, several of the cases would have been missed, emphasizing the importance of awareness campaigns. The various competent authorities involved in a CP event could benefit from a coordinated procedure, given the response complexity and rarity of these events in non-endemic regions. Particularly, where to send samples for toxin analysis, that fish species should be determined, or that physicians and patients have an available resource to contact if CP is suspected (i.e., the regional Poisoning Information Centers and the regional Public Health authorities, or directly to the BfR). Taken together, this study adds evidence to the various important response efforts to a CP outbreak: (i) immediate action for consumer health protection and legal aspects (recognition of the symptoms of CP, reporting an outbreak to the responsible authorities, initializing a trace-back effort, removing the potentially hazardous product from the market) and (ii) follow up action (authenticating the responsible product, testing for CTXs, and identifying the toxin source). The awareness of this outbreak was dependent upon the recognition of CP symptoms especially by the physicians of the German Poisoning Centers, and by the competent Food Safety Authorities to create the RASFF reporting process. Otherwise, the responsible lot of fish (if not reclaimed) demonstrably could have created more harm. The two-tiered semi-targeted CTX analysis approach utilized herein was important for the successful identification of CTXs. Standards covering the range of CTXs are not yet available, therefore this tandem approach can prevent a false negative sample analysis conclusion. This study unveiled a high level of mislabeling in these lots and this non-compliance indicates that the seafood global market (e.g., source, exporters, fish-importers, wholesalers, as well as inspectors and governments) may not be fully aware of CP as a risk potential in mislabeled products. Therefore, the seafood supply chain should follow Reg. (EC) No 178/2002; art. 14 (6) that states “where any part of a batch, lot, or consignment of food is found unsafe then it shall be presumed that all the food in that batch, lot, or consignment is also unsafe unless following a detailed assessment there is no evidence that the rest of the batch, lot or consignment is unsafe” (European Commission [EC], 2002). Compliance with the existing regulatory framework for labeling legislation can protect consumers, and therefore, indicates a need for stronger enforcement of the EU labeling legislations. This study showed a direct connection between CP, mislabeled fish, and the detection of the causative substance therein, which was demonstrated for the first time in Germany.
Data Availability Statement
The original contributions presented in the study are included in the article/Supplementary Material, further inquiries can be directed to the corresponding author/s.
Author Contributions
CL: conceptualization, methodology, formal analysis, investigation, writing-original draft, writing-review and editing, and visualization. AS: methodology, formal analysis, investigation, writing-review and editing, and visualization. MF: methodology, formal analysis, data curation, and writing-review and editing. KK: investigation, data curation, and visualization. US: methodology, investigation, and data curation. OK: resources, writing-review and editing. DB: resources, writing-review and editing, and supervision. All authors contributed to the article and approved the submitted version.
Conflict of Interest
The authors declare that the research was conducted in the absence of any commercial or financial relationships that could be construed as a potential conflict of interest.
Publisher’s Note
All claims expressed in this article are solely those of the authors and do not necessarily represent those of their affiliated organizations, or those of the publisher, the editors and the reviewers. Any product that may be evaluated in this article, or claim that may be made by its manufacturer, is not guaranteed or endorsed by the publisher.
Acknowledgments
The late Ann Abraham for her contribution of LC-MS/MS analysis at the FDA GCSL. LGL staff Ingrid Huber for DNA-barcoding analysis and sample collection. BfR staff V. Blaschke for DNA barcoding. L. Stegmaier and S. Zinke for laboratory technical assistance.
Supplementary Material
The Supplementary Material for this article can be found online at: https://www.frontiersin.org/articles/10.3389/fmars.2022.849857/full#supplementary-material
Footnotes
References
Abraham, A., Jester, E. L. E., Granade, H. R., Plakas, S. M., and Dickey, R. W. (2012). Caribbean ciguatoxin profile in raw and cooked fish implicated in ciguatera. Food Chem. 131, 192–198. doi: 10.1016/j.foodchem.2011.08.059
Ali, M. K. H., Belluscio, A., Ventura, D., and Ardizzone, G. (2016). Feeding ecology of some fish species occurring in artisanal fishery of Socotra Island (Yemen). Mar. Pollut. Bull. 105, 613–628. doi: 10.1016/j.marpolbul.2016.01.051
Aligizaki, K., and Nikolaidis, G. (2008). Morphological identification of two tropical dinoflagellates of the genera Gambierdiscus and Sinophysis in the Mediterranean Sea. J. Biol. Res. 9, 75–82.
André, L. V., Van Wynsberge, S., Chinain, M., Gatti, C. M. I., Dempsey, A., and Andréfouët, S. (2021). A framework for mapping local knowledge on ciguatera and artisanal fisheries to inform systematic conservation planning. ICES J. Mar. Sci. 78, 1357–1371.
Ardura, A., Linde, A. R., Moreira, J. C., and Garcia-Vazquez, E. (2010). DNA barcoding for conservation and management of Amazonian commercial fish. Biol. Conserv. 143, 1438–1443.
Aung, M. M., and Chang, Y. S. (2014). Traceability in a food supply chain: safety and quality perspectives. Food Control 39, 172–184.
Badrudin, B., and Aisyah, A. (2017). Separate stocks of red snapper exploitation and management in the Indonesian sector of the Arafura Sea. Indones. Fish. Res. J. 15, 81–88.
Banner, A. H., Helfrich, P., and Piyakarnchana, T. (1966). Retention of Ciguatera Toxin by the Red Snapper, Lutjanus bohar. Copeia 1966, 297–301. doi: 10.2307/1441137
Banner, A. H., Scheuer, P. J., Sasaki, S., Helfrich, P., and Alender, C. B. (1960). Observations on Ciguatera-Type Toxin in Fish. Ann. N. Y. Acad. Sci. 90, 770–787. doi: 10.1111/j.1749-6632.1960.tb26421.x
Britannica (2015). ‘snapper’ [Online]. Available Online at: https://www.britannica.com/animal/snapper (accessed 26 January 2022)
Bundesanstalt für Landwirtschaft und Ernährung (2021). Verzeichnis der Handelsbezeichnungen für Erzeugnisse der Fischerei und Aquakultur (List of commercial designations for fishery and aquaculture products. Germany: Bundesanstalt für Landwirtschaft und Ernährung
Caillaud, A., Eixarch, H., de La Iglesia, P., Rodriguez, M., Dominguez, L., Andree, K., et al. (2012). Towards the standardisation of the neuroblastoma (neuro-2a) cell-based assay for ciguatoxin-like toxicity detection in fish: application to fish caught in the Canary Islands. Food Addit. Contam. A 29, 1000–1010. doi: 10.1080/19440049.2012.660707
Calosso, M. C., Claydon, J. A. B., Mariani, S., and Cawthorn, D.-M. (2020). Global footprint of mislabelled seafood on a small island nation. Biol. Conserv. 245:108557. doi: 10.1016/j.biocon.2020.108557
Canals, A., Martínez, C. V., Diogène, J., Gago-Martínez, A., Cebadera-Miranda, L., de Vasconcelos, F. M., et al. (2021). Risk characterisation of ciguatera poisoning in Europe. EFSA Support. Publ. 18:6647E. doi: 10.2903/sp.efsa.2021.EN-6647
Chinain, M., Darius, H. T., Ung, A., Fouc, M. T., Revel, T., Cruchet, P., et al. (2010). Ciguatera risk management in French Polynesia: the case study of Raivavae Island (Australes Archipelago). Toxicon 56, 674–690. doi: 10.1016/j.toxicon.2009.05.032
Chinain, M., Gatti, C. M. I., Darius, H. T., Quod, J. P., and Tester, P. A. (2020a). Ciguatera poisonings: a global review of occurrences and trends. Harmful Algae 102:101873. doi: 10.1016/j.hal.2020.101873
Chinain, M., Gatti, C. M. I., Ung, A., Cruchet, P., Revel, T., Viallon, J., et al. (2020b). Evidence for the Range Expansion of Ciguatera in French Polynesia: a Revisit of the 2009 Mass-Poisoning Outbreak in Rapa Island (Australes Archipelago). Toxins 12:759. doi: 10.3390/toxins12120759
Chollett, I., Priest, M., Fulton, S., and Heyman, W. D. (2020). Should we protect extirpated fish spawning aggregation sites? Biol. Conserv. 241:108395. doi: 10.1016/j.biocon.2019.108395
Costa, P. R., Estevez, P., Castro, D., Soliño, L., Gouveia, N., Santos, C., et al. (2018). New Insights into the Occurrence and Toxin Profile of Ciguatoxins in Selvagens Islands (Madeira, Portugal). Toxins 10:524. doi: 10.3390/toxins10120524
Costa, P. R., Estévez, P., Soliño, L., Castro, D., Rodrigues, S. M., Timoteo, V., et al. (2021). An update on ciguatoxins and CTX-like toxicity in fish from different trophic levels of the Selvagens Islands (NE Atlantic, Madeira, Portugal). Toxins 13:580. doi: 10.3390/toxins13080580
Dao, H. V., Uesugi, A., Uchida, H., Watanabe, R., Matsushima, R., Lim, Z. F., et al. (2021). Identification of Fish Species and Toxins Implicated in a Snapper Food Poisoning Event in Sabah, Malaysia, 2017. Toxins 13:657. doi: 10.3390/toxins13090657
Darius, H. T., Drescher, O., Ponton, D., Pawlowiez, R., Laurent, D., Dewailly, E., et al. (2013). Use of folk tests to detect ciguateric fish: a scientific evaluation of their effectiveness in Raivavae Island (Australes, French Polynesia). Food Addit. Contam. A 30, 550–566. doi: 10.1080/19440049.2012.752581
Darius, H. T., Ponton, D., Revel, T., Cruchet, P., Ung, A., Tchou Fouc, M., et al. (2007). Ciguatera risk assessment in two toxic sites of French Polynesia using the receptor-binding assay. Toxicon 50, 612–626. doi: 10.1016/j.toxicon.2007.05.007
De Mitcheson, Y. S., Cornish, A., Domeier, M., Colin, P. L., Russell, M., et al. (2008). A Global Baseline for Spawning Aggregations of Reef Fishes. Conserv. Biol. 22, 1233–1244. doi: 10.1111/j.1523-1739.2008.01020.x
Deeds, J. R., Handy, S. M., Fry, F. Jr., Granade, H., Williams, J. T., Powers, M., et al. (2014). Protocol for building a reference standard sequence library for DNA-based seafood identification. J. AOAC Int. 97, 1626–1633. doi: 10.5740/jaoacint.14-111
Di Muri, C., Vandamme, S. G., Peace, C., Barnes, W., and Mariani, S. (2018). Biodiversity defrosted: unveiling non-compliant fish trade in ethnic food stores. Biol. Conserv. 217, 419–427. doi: 10.1016/j.biocon.2017.11.028
Di Pinto, A., Marchetti, P., Mottola, A., Bozzo, G., Bonerba, E., Ceci, E., et al. (2015). Species identification in fish fillet products using DNA barcoding. Fish. Res. 170, 9–13. doi: 10.1016/j.fishres.2015.05.006
Dickey, R. W., and Plakas, S. M. (2010). Ciguatera: a public health perspective. Toxicon 56, 123–136. doi: 10.1016/j.toxicon.2009.09.008
Diogène, J., Rambla, M., Campàs, M., Fernández, M., Andree, K., Tudó, A., et al. (2021). Evaluation of ciguatoxins in seafood and the environment in Europe. EFSA Support. Publ. 18:6648E. doi: 10.2903/sp.efsa.2021.EN-6648
Dung, L. D. (2020). The status of coral reefs in central Vietnam’s coastal water under climate change. Aquat. Ecosyst. Health Manag. 23, 323–331. doi: 10.1080/14634988.2020.1819715
EFSA (2010a). Panel on Contaminants in the Food Chain; Scientific Opinion on marine biotoxins in shellfish–Emerging toxins: ciguatoxin group. EFSA J. 8:1627.
EFSA (2010b). Scientific Opinion on marine biotoxins in shellfish – Emerging toxins: ciguatoxin group. EFSA J. 8:1627. doi: 10.2903/j.efsa.2010.1627
Estevez, P., Castro, D., Manuel Leao, J., Yasumoto, T., Dickey, R., and Gago-Martinez, A. (2019a). Implementation of liquid chromatography tandem mass spectrometry for the analysis of ciguatera fish poisoning in contaminated fish samples from Atlantic coasts. Food Chem. 280, 8–14. doi: 10.1016/j.foodchem.2018.12.038
Estevez, P., Castro, D., Pequeño-Valtierra, A., Leao, J. M., Vilariño, O., Diogène, J., et al. (2019b). An Attempt to Characterize the Ciguatoxin Profile in Seriola fasciata Causing Ciguatera Fish Poisoning in Macaronesia. Toxins 11:221. doi: 10.3390/toxins11040221
Estevez, P., Sibat, M., Leão-Martins, J. M., Reis Costa, P., Gago-Martínez, A., and Hess, P. (2020). Liquid chromatography coupled to high-resolution mass spectrometry for the confirmation of Caribbean ciguatoxin-1 as the main toxin responsible for ciguatera poisoning caused by fish from European Atlantic coasts. Toxins 12:267. doi: 10.3390/toxins12040267
European Commission [EC] (2002). Regulation (EC) No 178/2002 of the European Parliament and of the Council of 28 January 2002 laying down the general principles and requirements of food law, establishing the European Food Safety Authority and laying down procedures in matters of food safety. OJ L 031 1.2.2002. Brussels: European Commission, 1.
European Commission [EC] (2004a). Regulation (EC) No 853/2004 of the European Parliament and of the Council of 29 April 2004 laying down specific hygiene rules for food of animal origin”, in: official Journal of the European Union. L 139/55. Brussels: European Commission.
European Commission [EC] (2004b). Regulation (EC) No 854/2004 of the European Parliament and of the Council of 29 April 2004 laying down specific rules for the organisation of official controls on products of animal origin intended for human consumption. Brussels: European Commission.
European Commission [EC] (2017). RASFF Window Version 2.0.5 No. 2017.0345 [Online]. © European Union, 1995-2021. Available Online at: https://webgate.ec.europa.eu/rasff-window/screen/search (accessed August 2021 2021).
European Commission [EC] (2019). Commission Implementing Regulation (EU) 2019/627 of 15 March 2019”, in: 02019R0627 — EN — 01.01.2021 — 001.001 — 1. Brussels: European Commission.
European Commission [EC] (2021). EU consumer habits regarding fishery and aquaculture products, Fishery and Aquaculture Products. Maritime Affairs and Fisheries. Brussels: European Commission
European Parliament [EU] (2010). Directive 2010/63/EU of the European Parliament and of the Council of 22 September 2010 on the protection of animals used for scientific purposes Text with EEA relevance. Strasbourg: European Parliament.
European Union [EU] (2011). Regulation (EU) No 1169/2011 of the European Parliament and of the Council of 25 October 2011 on the provision of food information to consumers, amending Regulations (EC) No 1924/2006 and (EC) No 1925/2006 of the European Parliament and of the Council, and repealing Commission Directive 87/250/EEC, Council Directive 90/496/EEC, Commission Directive 1999/10/EC, Directive 2000/13/EC of the European Parliament and of the Council, Commission Directives 2002/67/EC and 2008/5/EC and Commission Regulation (EC) No 608/2004 Text with EEA relevance”. Brussels: European Union, 18–63.
European Union [EU] (2013a). Regulation (EU) No 1379/2013 of the European Parliament and of the Council of 11 December 2013 on the Common Fisheries Policy, amending Council Regulations (EC) No 1954/2003 and (EC) No 1224/2009 and repealing Council Regulations (EC) No 2371/2002 and (EC) No 639/2004 and Council Decision 2004/585/EC. Brussels: European Union.
European Union [EU] (2013b). Regulation (EU) No 1380/2013 of the European Parliament and of the Council of 11 December 2013 on the Common Fisheries Policy, amending Council Regulations (EC) No 1954/2003 and (EC) No 1224/2009 and repealing Council Regulations (EC) No 2371/2002 and (EC) No 639/2004 and Council Decision 2004/585/EC. Brussels: European Union.
FAO (2014). FAO Statistical Areas for Fishery Purposes. In: fAO Fisheries and Aquaculture Department [online]. Rome: FAO.
FAO, and WHO (2020). Report of the expert meeting on ciguatera poisoning. Rome, 19–23 November 2018 Food Safety and Quality No. 9. Rome: World Health Organization, doi: 10.4060/ca8817en
Federal Agency for Agriculture and Food (2021). Verzeichnis der Handelsbezeichnungen für Erzeugnisse der Fischerei und Aquakultur. Bonn: Bundesanstalt für Landwirtschaft und Ernährung.
FischEtikettG (2015). Gesetz zur Durchführung, der Rechtsakte der Europäischen Gemeinschaft über die Etikettierung von Fischen und Fischereierzeugnissen, (Fischetikettierungsgesetz - FischEtikettG) FischEtikettG Ausfertigungsdatum: 01.08.2002 Vollzitat:”Fischetikettierungsgesetz vom 1. August 2002 (BGBl. I S. 2980), das zuletzt durch Artikel 1 des Gesetzes vom 20. Oktober 2015 (BGBl. I S. 1736) geändert worden ist. Germany: Bundesgesetzblatt.
FischEtikettV (2015). Verordnung zur Durchführung des Fischetikettierungsgesetzes,(Fischetikettierungsverordnung-FischEtikettV) Fisch Etikett V Ausfertigungsdatum: 15.08.2002Vollzitat:”Fischetikettierungsverordnung vom 15. August 2002 (BGBl. I S. 3363), die zuletzt durch Artikel 1 derVerordnung vom 5. November 2015 (BGBl. I S. 1926) geändert worden ist. Germany: Bundesgesetzblatt.
Flanders Marine Institute [VLIZ] (2014). Maritime Boundaries Geodatabase, version 8 in conjunction with NOAA. Ostend: Flanders Marine Institute.
Flanders Marine Institute [VLIZ] (2021). MarineRegions.org. Available online at: www.marineregions.org (accessed January 1, 2022).
Fraga, S., Rodríguez, F., Caillaud, A., Diogène, J., Raho, N., and Zapata, M. (2011). Gambierdiscus excentricus sp. nov. (Dinophyceae), a benthic toxic dinoflagellate from the Canary Islands (NE Atlantic Ocean). Harmful Algae 11, 10–22. doi: 10.1016/j.hal.2011.06.013
Friedemann, M. (2019). Ciguatera fish poisoning outbreaks from 2012 to 2017 in Germany caused by snappers from India, Indonesia, and Vietnam. J. Consum. Prot. Food Saf. 14, 71–80. doi: 10.1007/s00003-018-1191-8
Friedman, M. A., Fernandez, M., Backer, L. C., Dickey, R. W., Bernstein, J., Schrank, K., et al. (2017). An Updated Review of Ciguatera Fish Poisoning: clinical, Epidemiological, Environmental, and Public Health Management. Mar. Drugs 15:72. doi: 10.3390/md15030072
Froese, R., and Pauly, D. (2020). FishBase [Online]. Available Online at: www.fishbase.org (accessed 11.20.2020 2016)
Gaboriau, M., Ponton, D., Darius, H. T., and Chinain, M. (2014). Ciguatera fish toxicity in French Polynesia: size does not always matter. Toxicon 84, 41–50. doi: 10.1016/j.toxicon.2014.03.006
Gago-Martinez, A., Leão, J. M., Estevez, P., Castro, D., Barrios, C., Hess, P., et al. (2021). Characterisation of ciguatoxins. EFSA Support. Publ. 18:6649E. doi: 10.2903/sp.efsa.2021.EN-6649
Gold, J. R., Willis, S. C., Renshaw, M. A., Buentello, A., Walker, H. J., Puritz, J. B., et al. (2015). Phylogenetic relationships of tropical eastern Pacific snappers (Lutjanidae) inferred from mitochondrial DNA sequences. Syst. Biodivers. 13, 596–607. doi: 10.1080/14772000.2015.1078857
Ha, D. V., Uesugi, A., Uchida, H., Ky, P. X., Minh, D. Q., Watanabe, R., et al. (2018). Identification of Causative Ciguatoxins in Red Snappers Lutjanus bohar Implicated in Ciguatera Fish Poisonings in Vietnam. Toxins 10:420. doi: 10.3390/toxins10100420
Handy, S. M., Deeds, J. R., Ivanova, N. V., Hebert, P. D., Hanner, R. H., Ormos, A., et al. (2011). A single-laboratory validated method for the generation of DNA barcodes for the identification of fish for regulatory compliance. J. AOAC Int. 94, 201–210.
Hoffman, P. A., Granade, H. R., and McMillan, J. P. (1983). The mouse ciguatoxin bioassay: a dose - response curve and symptomatology analysis. Toxicon 21, 363–369. doi: 10.1016/0041-0101(83)90092-2
Holmes, M. J., Venables, B., and Lewis, R. J. (2021). Critical Review and Conceptual and Quantitative Models for the Transfer and Depuration of Ciguatoxins in Fishes. Toxins 13:515. doi: 10.3390/toxins13080515
Hung, N. Q., Chieu, H. D., Dung, D. T., and Duc, V. T. (2017). “Climate Change Impacts on Marine Ecosystems in Vietnam,” in Environmental Management of Marine Ecosystems, eds M. D. N. Islam and S. E. Jorgensen (Boca Raton: CRC Press), 209–235.
Institute for Marine Remote Sensing, University of South Florida [IMaRS/USF], Institut de Recherche pour le Développement [IRD], UNEP-WCMC, The WorldFish Center, and WRI (2011). Global Coral Reefs Composite Dataset Compiled from Multiple Sources for Use in the Reefs at Risk Revisited Project Incorporating Products from the Millennium Coral Reef Mapping Project prepared by IMaRS/USF and IRD. St. Petersburg, FL: IMaRS/USF.
Ivanova, N. V., Zemlak, T. S., Hanner, R. H., and Hebert, P. D. N. (2007). Universal primer cocktails for fish DNA barcoding. Mol. Ecol. Notes 7, 544–548. doi: 10.1111/j.1471-8286.2007.01748.x
Kappel, K., and Schröder, U. (2020). Difficulties in DNA barcoding-based authentication of snapper products due to ambiguous nucleotide sequences in public databases. Food Control 118:107348. doi: 10.1016/j.foodcont.2020.107348
Kelleher, K. (ed.) (2005). Discards in the world’s marine fisheries. An update. Rome, Italy: Food and agriculture organization of the united nations.
Khaksar, R., Carlson, T., Schaffner, D. W., Ghorashi, M., Best, D., Jandhyala, S., et al. (2015). Unmasking seafood mislabeling in US markets: DNA barcoding as a unique technology for food authentication and quality control. Food Control 56, 71–76.
Kibler, S. R., Davenport, E. D., Tester, P. A., Hardison, D. R., Holland, W. C., and Litaker, R. W. (2017). Gambierdiscus and Fukuyoa species in the greater Caribbean: regional growth projections for ciguatera-associated dinoflagellates. Ecol. Model. 360, 204–218. doi: 10.1016/j.ecolmodel.2017.07.007
Kibler, S. R., Litaker, R. W., Holland, W. C., Vandersea, M. W., and Tester, P. A. (2012). Growth of eight Gambierdiscus (Dinophyceae) species: effects of temperature, salinity and irradiance. Harmful Algae 19, 1–14. doi: 10.1016/j.hal.2012.04.007
Klekamp, B. G., Bodager, D., and Matthews, S. D. (2015). Use of Surveillance Systems in Detection of a Ciguatera Fish Poisoning Outbreak - Orange County, Florida, 2014. MMWR Morb. Mortal. Wkly. Rep. 64, 1142–1144. doi: 10.15585/mmwr.mm6440a3
Kusche, H., and Hanel, R. (2021). Consumers of mislabeled tropical fish exhibit increased risks of ciguatera intoxication: a report on substitution patterns in fish imported at Frankfurt Airport, Germany. Food Control 121:107647. doi: 10.1016/j.foodcont.2020.107647
Litaker, R. W., Vandersea, M. W., Faust, M. A., Kibler, S. R., Nau, A. W., Holland, W. C., et al. (2010). Global distribution of ciguatera causing dinoflagellates in the genus Gambierdiscus. Toxicon 56, 711–730. doi: 10.1016/j.toxicon.2010.05.017
Loeffler, C. R., Abraham, A., Stopa, J. E., Flores Quintana, H. A., Jester, E. L. E., La Pinta, J., et al. (2022). Ciguatoxin in Hawai’i: fisheries forecasting using geospatial and environmental analyses for the invasive Cephalopholis argus (Epinephelidae). Environ. Res. 207:112164. doi: 10.1016/j.envres.2021.112164
Loeffler, C. R., Bodi, D., Tartaglione, L., Dell’Aversano, C., and Preiss-Weigert, A. (2021a). Improving in vitro ciguatoxin and brevetoxin detection: selecting neuroblastoma (Neuro-2a) cells with lower sensitivity to ouabain and veratridine (OV-LS). Harmful Algae 103:101994. doi: 10.1016/j.hal.2021.101994
Loeffler, C. R., Tartaglione, L., Friedemann, M., Spielmeyer, A., Kappenstein, O., and Bodi, D. (2021b). Ciguatera Mini Review: 21st Century Environmental Challenges and the Interdisciplinary Research Efforts Rising to Meet Them. Int. J. Environ. Res. Public Health 18:3027. doi: 10.3390/ijerph18063027
Loeffler, C. R., Handy, S. M., Flores Quintana, H. A., and Deeds, J. R. (2019). Fish hybridization leads to uncertainty regarding ciguatera fish poisoning risk: confirmation of hybridization and ciguatoxin accumulation with implications for stakeholders. J. Mar. Sci. Eng. 7:105.
Loeffler, C. R., Robertson, A., Flores Quintana, H. A., Silander, M. C., Smith, T. B., and Olsen, D. (2018). Ciguatoxin prevalence in 4 commercial fish species along an oceanic exposure gradient in the US Virgin Islands. Environ. Toxicol. Chem. 37, 1852–1863. doi: 10.1002/etc.4137
Mak, Y. L., Wai, T.-C., Murphy, M. B., Chan, W. H., Wu, J. J., Lam, J. C., et al. (2013). Pacific Ciguatoxins in Food Web Components of Coral Reef Systems in the Republic of Kiribati. Environ. Sci. Technol. 47, 14070–14079. doi: 10.1021/es403175d
Manger, R. L., Leja, L. S., Lee, S. Y., Hungerford, J. M., Hokama, Y., Dickey, R. W., et al. (1995). Detection of sodium channel toxins: directed cytotoxicity assays of purified ciguatoxins, brevetoxins, saxitoxins, and seafood extracts. J. AOAC Int. 78, 521–527.
Miller, D., Jessel, A., and Mariani, S. (2012). Seafood mislabelling: comparisons of two western European case studies assist in defining influencing factors, mechanisms and motives. Fish Fish. 13, 345–358. doi: 10.1111/j.1467-2979.2011.00426.x
Olsen, D. A., Nellis, D. W., and Wood, R. S. (1984). Ciguatera in the Eastern Caribbean. Mar. Fish. Rev. 46, 13–18.
O’Neill, M. F., Leigh, G. M., Martin, J. M., Newman, S. J., Chambers, M., Dichmont, C. M., et al. (2011). Sustaining productivity of tropical red snappers using new monitoring and reference points. Queensland: Department of Employment, Economic Development and Innovation.
Oshiro, N., Tomikawa, T., Kuniyoshi, K., Ishikawa, A., Toyofuku, H., Kojima, T., et al. (2021a). LC–MS/MS Analysis of Ciguatoxins Revealing the Regional and Species Distinction of Fish in the Tropical Western Pacific. J. Mar. Sci. Eng. 9:299. doi: 10.3390/jmse9030299
Oshiro, N., Tomikawa, T., Kuniyoshi, K., Kimura, K., Kojima, T., Yasumoto, T., et al. (2021b). [Detection of Ciguatoxins from Fish Introduced into a Wholesale Market in Japan]. Shokuhin Eiseigaku Zasshi 62, 8–13. doi: 10.3358/shokueishi.62.8
Oshiro, N., Yogi, K., Asato, S., Sasaki, T., Tamanaha, K., Hirama, M., et al. (2010). Ciguatera incidence and fish toxicity in Okinawa, Japan. Toxicon 56, 656–661. doi: 10.1016/j.toxicon.2009.05.036
Otero, P., Peìrez, S., Alfonso, A., Vale, C., Rodriìguez, P., Gouveia, N. N., et al. (2010). First Toxin Profile of Ciguateric Fish in Madeira Arquipelago (Europe). Anal. Chem. 82, 6032–6039. doi: 10.1021/ac100516q
Patrias, K., and Wendling, D. (2007). Citing medicine: the NLM style guide for authors, editors, and publishers. Bethesda, Maryland: National Library of Medicine (US).
Pham, C. M. H. (2015). “Boats of Vietnam,” in Encyclopaedia of the History of Science, Technology, and Medicine in Non-Western Cultures, ed. H. Selin (Dordrecht: Springer).
Pho Hoang Han, M. M. M. (2007). Fisheries development in Vietnam: a case study in the exclusive economic zone. Ocean Coast. Manag. 50, 699–712. doi: 10.1016/j.ocecoaman.2002.06.001
Pomeroy, R., Thi Nguyen, K. A., and Thong, H. X. (2009). Small-scale marine fisheries policy in Vietnam. Mar. Policy 33, 419–428. doi: 10.1016/j.marpol.2008.10.001
Rajisha, K., Kishore, P., Panda, S. K., Harikrishnan, G., Ajitha, K., Suresh, M., et al. (2017). Incidence of ciguatoxin fish poisoning in Trivandrum, India. Indian J. Fish. 64, 129–133.
Reverté, L., Toldrà, A., Andree, K. B., Fraga, S., de Falco, G., Campàs, M., et al. (2018). Assessment of cytotoxicity in ten strains of Gambierdiscus australes from Macaronesian Islands by neuro-2a cell-based assays. J. Appl. Phycol. 30, 2447–2461. doi: 10.1007/s10811-018-1456-8
Rhodes, L. L., Smith, K. F., Murray, S., Harwood, D. T., Trnski, T., and Munday, R. (2017). The epiphytic genus Gambierdiscus (Dinophyceae) in the Kermadec Islands and Zealandia regions of the southwestern Pacific and the associated risk of ciguatera fish poisoning. Mar. Drugs 15:219. doi: 10.3390/md15070219
Roeder, K., Erler, K., Kibler, S., Tester, P., Van The, H., Nguyen-Ngoc, L., et al. (2010). Characteristic profiles of Ciguatera toxins in different strains of Gambierdiscus spp. Toxicon 56, 731–738. doi: 10.1016/j.toxicon.2009.07.039
Rossignoli, A. E., Tudó, A., Bravo, I., Díaz, P. A., Diogène, J., and Riobó, P. (2020). Toxicity Characterisation of Gambierdiscus Species from the Canary Islands. Toxins 12:134. doi: 10.3390/toxins12020134
Sanchez-Henao, A., García-Álvarez, N., Silva Sergent, F., Estévez, P., Gago-Martínez, A., Martín, F., et al. (2020). Presence of CTXs in moray eels and dusky groupers in the marine environment of the Canary Islands. Aquat. Toxicol. 221:105427. doi: 10.1016/j.aquatox.2020.105427
Sanchez-Henao, J. A., García-Álvarez, N., Fernández, A., Saavedra, P., Silva Sergent, F., Padilla, D., et al. (2019). Predictive score and probability of CTX-like toxicity in fish samples from the official control of ciguatera in the Canary Islands. Sci. Total Environ. 673, 576–584. doi: 10.1016/j.scitotenv.2019.03.445
Saraya, A., Sintunawa, C., Wacharapluesadee, S., Swangpun, K., Dumrongchua, S., Wilde, H., et al. (2014). Marine Fish Toxins in Thailand: report of 6 Suspected Ciguatera Cases. Case Rep. Clin. Med. 3, 286–292. doi: 10.4236/crcm.2014.35065
Smithsonian National Fish Collection (2021). Fish Collections Data at National Museum of Natural History [Online]. Available: Online at: https://collections.nmnh.si.edu/search/fishes/ (accessed April 11th 2021).
Spielmann, G., Gerdes, L., Miller, A., Verhaelen, K., Schlicht, C., Schalch, B., et al. (2018). Molecular biological species identification of animal samples from Asian buffets. J. Consum. Prot. Food Saf. 13, 271–278. doi: 10.1007/s00003-018-1168-7
Spielmeyer, A., Loeffler, C. R., and Bodi, D. (2021). Extraction and LC-MS/MS Analysis of Ciguatoxins: a Semi-Targeted Approach Designed for Fish of Unknown Origin. Toxins 13:630. doi: 10.3390/toxins13090630
Strehlow, H. V., Schultz, N., Zimmermann, C., and Hammer, C. (2012). Cod catches taken by the German recreational fishery in the western Baltic Sea, 2005–2010: implications for stock assessment and management. ICES J. Mar. Sci. 69, 1769–1780. doi: 10.1093/icesjms/fss152
Sydney Fish Market (2015). Sydney fish market seafood handing guidelines [Online]. Locked Bag 247, PYRMONT NSW 2009. Australia: Sydney Fish Market Pty Ltd.
Takahashi, M., DiBattista, J. D., Jarman, S., Newman, S. J., Wakefield, C. B., Harvey, E. S., et al. (2020). Partitioning of diet between species and life history stages of sympatric and cryptic snappers (Lutjanidae) based on DNA metabarcoding. Sci. Rep. 10, 1–13. doi: 10.1038/s41598-020-60779-9
Teh, L. C. L., and Pauly, D. (2018). Who Brings in the Fish? The Relative Contribution of Small-Scale and Industrial Fisheries to Food Security in Southeast Asia. Front. Mar. Sci. 5:44. doi: 10.3389/fmars.2018.00044
Tester, P., Wickliffe, L., Jossart, J., Rhodes, L., Enevoldsen, H., Adachi, M., et al. (2018). Global distribution of the genera Gambierdiscus and Fukuyoa. HARMFUL ALGAE 2018–FROM ECOSYSTEMS TO SOCIO-ECOSYSTEMS, 138. New York: OBIS.
Tester, P. A., Litaker, R. W., and Berdalet, E. (2020). Climate change and harmful benthic microalgae. Harmful Algae 91:101655. doi: 10.1016/j.hal.2019.101655
Tun, K., Chou, L. M., Cabanban, A., Tuan, V., Suharsono, T. Y., Sour, K., et al. (2005). “Status of coral reefs, coral reef monitoring and management in Southeast Asia 2004,” in Status of Coral Reefs of the World, 2004 (Vol 1), ed. C. Wilkinson (Townsville, Australia: Australian Institute of Marine Science), 235–275.
U.S. Food Drug Administration (2020). Fish and Fishery Products Hazards and Controls Guidance Fourth Edition - March 2020. Available Online at: https://www.fda.gov/media/80637/download (accessed 15 October 2020)
Varriale, F. (2021). Emerging toxins of European concern: identification, development of reference material and methods for their detection. Italy: University of Naples Federico II
Viallon, J., Chinain, M., and Darius, H. T. (2020). Revisiting the Neuroblastoma Cell-Based Assay (CBA-N2a) for the Improved Detection of Marine Toxins Active on Voltage Gated Sodium Channels (VGSCs). Toxins 12:281. doi: 10.3390/toxins12050281
Xu, Y., Richlen, M. L., Liefer, J. D., Robertson, A., Kulis, D., Smith, T. B., et al. (2016). Influence of Environmental Variables on Gambierdiscus spp.(Dinophyceae) Growth and Distribution. PLoS One 11:e0153197. doi: 10.1371/journal.pone.0153197
Yasumoto, T. (2005). Chemistry, etiology, and food chain dynamics of marine toxins. Proc. Jpn. Acad. Ser. B 81, 43–51. doi: 10.2183/pjab.81.43
Yogi, K., Oshiro, N., Inafuku, Y., Hirama, M., and Yasumoto, T. (2011). Detailed LC-MS/MS Analysis of Ciguatoxins Revealing Distinct Regional and Species Characteristics in Fish and Causative Alga from the Pacific. Anal. Chem. 83, 8886–8891. doi: 10.1021/ac200799j
Yogi, K., Sakugawa, S., Oshiro, N., Ikehara, T., Sugiyama, K., and Yasumoto, T. (2014). Determination of Toxins Involved in Ciguatera Fish Poisoning in the Pacific by LC/MS. J. AOAC Int. 97, 398–402. doi: 10.5740/jaoacint.sgeyogi
Keywords: ciguatera poisoning, outbreak, mass spectrometry analysis, cell assay, food labeling, public health action
Citation: Loeffler CR, Spielmeyer A, Friedemann M, Kapp K, Schwank U, Kappenstein O and Bodi D (2022) Food Safety Risk in Germany From Mislabeled Imported Fish: Ciguatera Outbreak Trace-Back, Toxin Elucidation, and Public Health Implications. Front. Mar. Sci. 9:849857. doi: 10.3389/fmars.2022.849857
Received: 06 January 2022; Accepted: 07 February 2022;
Published: 17 March 2022.
Edited by:
Panagiota Katikou, Greek Ministry of Rural Development and Food, GreeceReviewed by:
Pedro R. Costa, Portuguese Institute for Sea and Atmosphere (IPMA), PortugalMònica Campàs, IRTA Sant Carles de la Ràpita, Spain
Wayne Litaker, CSS Inc., for NOAA National Centers for Coastal Ocean Science, United States
Carmen Vale, University of Santiago de Compostela, Spain
Copyright © 2022 Loeffler, Spielmeyer, Friedemann, Kapp, Schwank, Kappenstein and Bodi. This is an open-access article distributed under the terms of the Creative Commons Attribution License (CC BY). The use, distribution or reproduction in other forums is permitted, provided the original author(s) and the copyright owner(s) are credited and that the original publication in this journal is cited, in accordance with accepted academic practice. No use, distribution or reproduction is permitted which does not comply with these terms.
*Correspondence: Christopher R. Loeffler, Q2hyaXN0b3BoZXIubG9lZmZsZXJAYmZyLmJ1bmQuZGU=