- 1Key Laboratory of Marine Ecology and Environmental Science, Institute of Oceanology, Chinese Academy of Sciences, Qingdao, China
- 2Key Laboratory of Sustainable Development of Marine Fisheries, Ministry of Agriculture, Yellow Sea Fisheries Research Institute, Chinese Academy of Fishery Sciences, Qingdao, China
- 3University of Chinese Academy of Sciences, Beijing, China
- 4College of Fisheries and Life Science, Dalian Ocean University, Dalian, China
Marine Heatwaves (MHWs) events have been increasing, causing severe impacts on marine ecosystems and aquaculture. In this study, the effects of temperature on changes in sediment dissolved oxygen (DO) and hydrogen sulfide (H2S) concentrations and their effects on the behavioral and physiological responses of a bivalve were investigated by simulating different sudden summer temperature change conditions. The results showed that temperature was an important factor affecting DO consumption and H2S release in sediments, and sediment type also played a key role. At higher temperatures, DO was consumed more rapidly and H2S release increased in sediments, and the DO was consumed and H2S released in sandy sediments was less than in silty sand sediments. The response of Manila clam’s exercise behavior under environmental pressure was also rapid. The excavation index of the Manila clam decreased with the DO consumption, indicating that the Manila clam could alter its burial depth and move toward the sediment-water interface when stressed. Compared with phenol oxidases (PO) activity being activated under experimental conditions, succinate concentrations only increased slightly at 32°C, and did not surpass the threshold indicative of anaerobic metabolism. However, when toxic substances such as H2S start to accumulate, they may damage the immune system and tissues of the Manila clam, thereby affecting its future survival. Therefore, when temperatures are high for long periods in summer, it is necessary to take timely action to prevent and guard against harm caused by DO and H2S to aquaculture organisms.
Introduction
Global climate change is responsible for increased frequency, intensity, and duration of extreme events, such as marine heatwaves (MHWs) are having far-reaching impacts on marine ecosystems (Gall et al., 2021; Mcpherson et al., 2021). MHWs are driving temperature increases at rates and levels well above projected warming scenarios (Babcock et al., 2019; Holbrook et al., 2019). The coupled impacts of acute temperature change and species thermal tolerance are causing havoc across the aquaculture industry as seen in the mass mortality of bivalves and seaweeds (Smale et al., 2019; He et al., 2021; Plecha et al., 2021; Xu et al., 2022). Increasing temperatures appear to be the more important contemporary stressor for many marine organisms (Hughes et al., 2017; Smale et al., 2019; Scanes et al., 2020a,b).
Increasing temperatures are not the only environmental source of stress to marine organisms. Increases in temperature are naturally accompanied by decreases in dissolved oxygen (DO) concentration. Globally, the prevalence of hypoxic areas caused by high temperatures in summer continues to increase, especially in offshore eutrophic areas (Diaz and Rosenberg, 2008; Suikkanen et al., 2013; Smith et al., 2020). Following the excessive reproduction of phytoplankton, massive amounts of organic matter sink to the seafloor where they are degraded via processes that consume oxygen and produce hypoxia or anoxia regions (Li et al., 2018). Especially in summer, haloclines and thermoclines weaken the vertical exchange of water, hindering the vertical mixing of DO, and aggravating the hypoxic or anoxic conditions of bottom waters (Wei et al., 2007). Additionally, biological sedimentation resulting from benthic shellfish culture has also led to increased sediment oxygen consumption, which exacerbated the risk of hypoxia or anoxia in the sediment environment of culture areas (Gilbert et al., 1997; Yang et al., 2007). In hypoxic or anoxic environments, the sedimentary environment shifts from an oxidative state to a reductive state (Nagasoe et al., 2011), which accelerates the process of acid volatile sulfur (AVS) reduction to produce hydrogen sulfide (H2S) (Kodama et al., 2018). H2S can be harmful, and even lethal, to benthic organisms (Smith et al., 1977; Joyner-Matos et al., 2010; Soldatov et al., 2018). To date, many studies regarding the environmental impact on shellfish have focused on the variation of DO concentration and the content of AVS in the water column, little attention has been paid to sedimental DO concentration and the H2S content which is confirmed to be the only sulfide that causes harm to aquatic animals (Kodama et al., 2018; Liang, 2019). Therefore, the variation of DO and H2S concentrations in the sedimental environment under high summertime water temperature are currently unknown.
Changes in environmental factors significantly affect the behavioral and physiological responses of marine organisms (Long et al., 2008; Li et al., 2019). When benthic shellfish experience hypoxia, they often seek access to additional oxygen by altering their digging behavior to reduce their burial depth (Lee et al., 2011). Bivalves mainly rely on glycolysis to meet their energy requirements when exposed to hypoxic conditions, and when the DO concentration continues to decrease, the dissimilation of glycolysis and the accumulation of succinate or propionate will be observed (Lee et al., 2011). Therefore, succinate concentrations can be used as an indicator of hypoxia stress in bivalve molluscs (Lee et al., 2011). Additionally, phenol oxidases (PO) and hydrolases, which are usually involved in detoxification, inflammation, and digestion, are considered to be the immune functions of bivalve molluscs that are most susceptible to environmental stressors (Hellio et al., 2006). Meanwhile, PO also plays an important role in the melanization of soft tissues and shells of bivalves (Cerenius et al., 2008). Even though the multiple environmental pressures often overlap in practical cases, most studies focus on individual pressures and few have considered the relationships between multiple pressure sources and how they affect marine organisms (Parisi et al., 2017).
The Manila clam Ruditapes philippinarum aquacultured in America and Europe was originated from Asia, and is now one of the most popular clams worldwide. However, Manila clam is threatened with mass death every summer (Jonsson and Andé, 1992; Wootton et al., 2003). Although there is evidence that environmental factors play a decisive role in the survival of Manila clam (Gestal et al., 2008; Matozzo and Marin, 2011), it does not seem possible to pin the cause of a large-scale Manila clam die-offs in summer on a single environmental pressure (Gajbhiye and Khandeparker, 2019). This is because any change in an environmental factor will trigger a series of environmental chain reactions. Therefore, a single change can drive changes in other environmental factors that may jointly affect the survival of marine organisms in an environment, resulting in large-scale mortality in conditions that would normally be considered tolerable (Parisi et al., 2017; Kim et al., 2018). In this study, temperature-controlled experiments were conducted to simulate sudden temperature changes in summer, to identify the characteristics of variation of DO and H2S in different substrate types of sediments under different temperature conditions, and to clarify the environmental chain reactions brought about by temperature, as well as the effects on the behavioral and physiological responses of Manila clam. This study will serve as a reference for future research on artificial breeding, resource conservation, behavior, and early warning mechanisms of the Manila clam and related molluscs.
Materials and Methods
Method
In June 2020, following previous studies, box-corer and water sampler were used to collect sediments and bottom seawater from high-density and low-density breeding areas in the Weifang Longwei group breeding area in the Xiaoqing River Estuary (37°16.290′N, 119°04.248′E) in Shandong Province, China. Sediment samples were firstly filtered to remove macrobenthic organisms. After the samples were collected, kept separate according to different sites and put into a refrigerator, and the experiment was initiated immediately after returning to the laboratory. The environmental characteristics of the sampling sites are shown in Table 1.
Manila clams were provided by the Shandong Longwei Group. To avoid any transportation/transition effect on the shellfish in this experiment, the clams were acclimatized to the laboratory for 1 week before the experiment. During acclimation, the water temperature was 24°C, salinity was 30, the clams were fed chlorella every day, and oxygen was maintained and water changed daily.
The sediments of different types were spread evenly in experimental tanks [20 cm (L) × 15 cm (W) × 25 cm (H)] to a depth of approximately 10 cm. The bottom seawater collected in situ was added (water depth of about 10 cm), and the experimental tanks were placed in the thermostatic water baths at 24 28, and 32°C. The water level in the water bath exceeded the height of the overlying water in the experiment tanks. There were two different sediment types in each temperature water bath, making 6 experimental groups, and each experimental group had three replicate tanks. The experiment lasted for 12 h (one tidal cycle).
A particularly designed cylindrical collector (5 cm in diameter and 20 cm in depth) was inserted in each experimental tank to avoid the interference of experimental organisms. The sedimental DO and H2S content at different depths were measured in the collector using a microelectrode system (Unisense, Aarhus, Denmark). The experimental monitoring parameters were measured every 3 h. The measurements were conducted to 10 cm below the surface sediment with microelectrode steps of 5 mm.
At the beginning of the experiment, the Manila clams in the holding tank were directly placed into the experimental tanks to simulate sudden temperature change in natural conditions. 10 clams (5.3 ± 0.27 g, 30.38 ± 1.23 mm shell length) were placed in each experimental tank and 30 Manila clams with the same specifications as the experimental clams were selected from the temporary culture flume to serve as the control (3 groups). The experiment lasted for 12 h. The succinate concentration in the muscle and PO activity in the hepatopancreas in the Manila clams were measured before and after the experiment, and the excavation index of Manila clam was measured after the experiment.
Sample Measurement
H2S at different depths were measured using the manual propeller paired with the microelectrode system. The overlying water-sediment interface was recorded as the depth of 0 cm. The change of DO was quantified based on the overlying water DO concentration, penetration depth, and average DO concentration of the penetration layer. Specific operational parameters: the DO penetration depth referred to the depth from the sediment water interface to the depth where the DO concentration was lower than 0.1 mg/L, and the average concentration of DO in the penetration layer was recorded (Liang, 2019).
After the experiment, the digging scores of all Manila clams in each experimental group were recorded. The specific scores were graded as follows: 1, shells were completely buried in the sediment; 0.5, shells were partially exposed; 0, shells were entirely exposed on the sediment surface (Lee et al., 2007, 2011). The digging scores of all shellfish were added together and divided by the number of shellfish to obtain the digging index for the Manila clam. The higher the index was, the deeper the Manila clam was. After that, the Manila clams from each tank were collected and the hepatopancreas and soft tissues were dissected, sampled, and stored in liquid nitrogen for later use.
Succinate was measured in clams exposed to different temperatures and compared to the control clams measured before exposure. After freeze-drying the muscle tissue, the samples were ground into a powder with a freeze grinder. 100 mg dried sample powder was weighed and 1 mL of 50% methanol/water solution was added, this solution was vortexed for 30 min, centrifugated at 12000 rpm for 5 min, and 50 μL of supernatant was collected. 50 μL of propionic acid isotope standard (5 μg/ml), 50 μL of 3-nitrophenylhydrazine (3-NPH) (250 mM, prepared with 50% methanol/water solution), and 50 μL of EDC [150 mM, prepared with 75% methanol/water solution (containing 7.5% pyridine), i.e., methanol: water: pyridine = 69.375: 23.125: 7.5] were added to the supernatant and it was placed in a shaking mixer for derivatization at 30°C for 30 min. After that, 50 μL of 2,6-di-tert-butyl-p-cresol (BHT) methanol solution (2 mg/mL) and 250 μL of 75% methanol aqueous solution were added. The sample was centrifuged at 12000 rpm for 5 min at 4°C. 200 μl of the supernatant was extracted and analyzed by mass spectrometry.
The separation was performed using a Waters UPLC BEH C8 column (2.1 × 100 mm, 1.7 μm) with the mobile phases of A-phase (water, 0.01% formic acid) and B-phase (methanol: isopropanol = 8: 2) at a flow rate of 0.3 ml/min. The column temperature was 45°C. Mass spectrometry was performed using a Waters XEVO TQ-S Micro tandem quadrupole mass spectrometer system. The ion source voltage was 3.0 kV, the temperature was 150°C, the desolvation temperature was 350°C, the desolvation gas flow rate was 1000 L/h, and the cone well gas flow rate was 10 L/h.
The PO activity was measured in the hepatopancreas of each clam. The frozen samples were ground into powder using a cold grinder (SPEX, United States) then 500 μL of lysate was added and the solution was homogenized before centrifugation at 1000 r/min at 4°C for 45 min. The supernatant was collected and the PO activity was determined by a previously reported method. Specifically, 50 μL of supernatant and 50 μL of Tris-HCl buffer were incubated at 25°C for 10 min, then 100 μL of 0.04 M L-DOPA was added and the absorbance value at 492 nm was read 30 min later with a microplate reader at 25°C. Meanwhile, the spontaneous oxidation of L-dopa was measured using the same method, but pure water was used as the reactant instead of supernatant and any spontaneous oxidation results were removed from the measured values during calculation.
The PO specific activities were calculated as:
PO specific activities (U/mg prot) = (ΔA/min × dilution factor)/total protein concentration
where, ΔA/min is the value of the increase in absorbance per minute; dilution factor is the sample’s dilution factor; and total protein concentration is the sample’s protein concentration assayed with Bradford’s method.
Data Analysis
Three-factor analysis of variance was used to explore the influences of temperature, time, and geological type on the variables. One-way analysis of variance was used to explore the influences of temperature, DO, and H2S on behavior and physiological indicators. The above analysis is completed in the R language program using the “vegan” package.
Results
Characteristics of Environmental Factor Changes in Sediments
Dissolved Oxygen Content in Sediment
At 24°C, the average DO content of the penetration layer, penetration depth of the penetration layer, and DO content of overlying water in silty sand sediments and sandy sediments difference was not significant (p > 0.05, Figure 1). At 28°C, DO-related parameters began to decline as the experiment progressed. The average DO content of the penetration layer, penetration depth of the penetration layer, and DO content of overlying water in silty sand and sandy sediments decreased from 3.58 mg/L, 1.50 cm, and 6.51 mg/L and 3.91 mg/L, 1.75 cm, and 6.75 mg/L at the beginning of the experiment to 0.79 mg/L, 0.75 cm, and 2.88 mg/L and 1.75 mg/L, 1.14 cm, and 4.78 mg/L at the end of the experiment, respectively. Likewise, at 32°C, all parameters of both sediment substrate types showed significantly decreases (p < 0.05) after 3 h. The average DO content of the penetration layer, penetration depth of the penetration layer, and DO content of the overlying water in the silty sand and sandy sediments at 32°C decreased from 3.37 mg/L, 1.65 cm, and 6.71 mg/L and 3.92 mg/L, 1.59 cm, and 6.71 mg/L at the beginning of the experiment to 0.32 mg/L, 0.25 cm, and 2.62 mg/L and 0.63 mg/L, 0.75 cm, and 3.82 mg/L at the end, respectively. In comparison, the decrease in DO concentration of the penetration layer (55–84%) was much higher than that of the overlying water (29–43%).
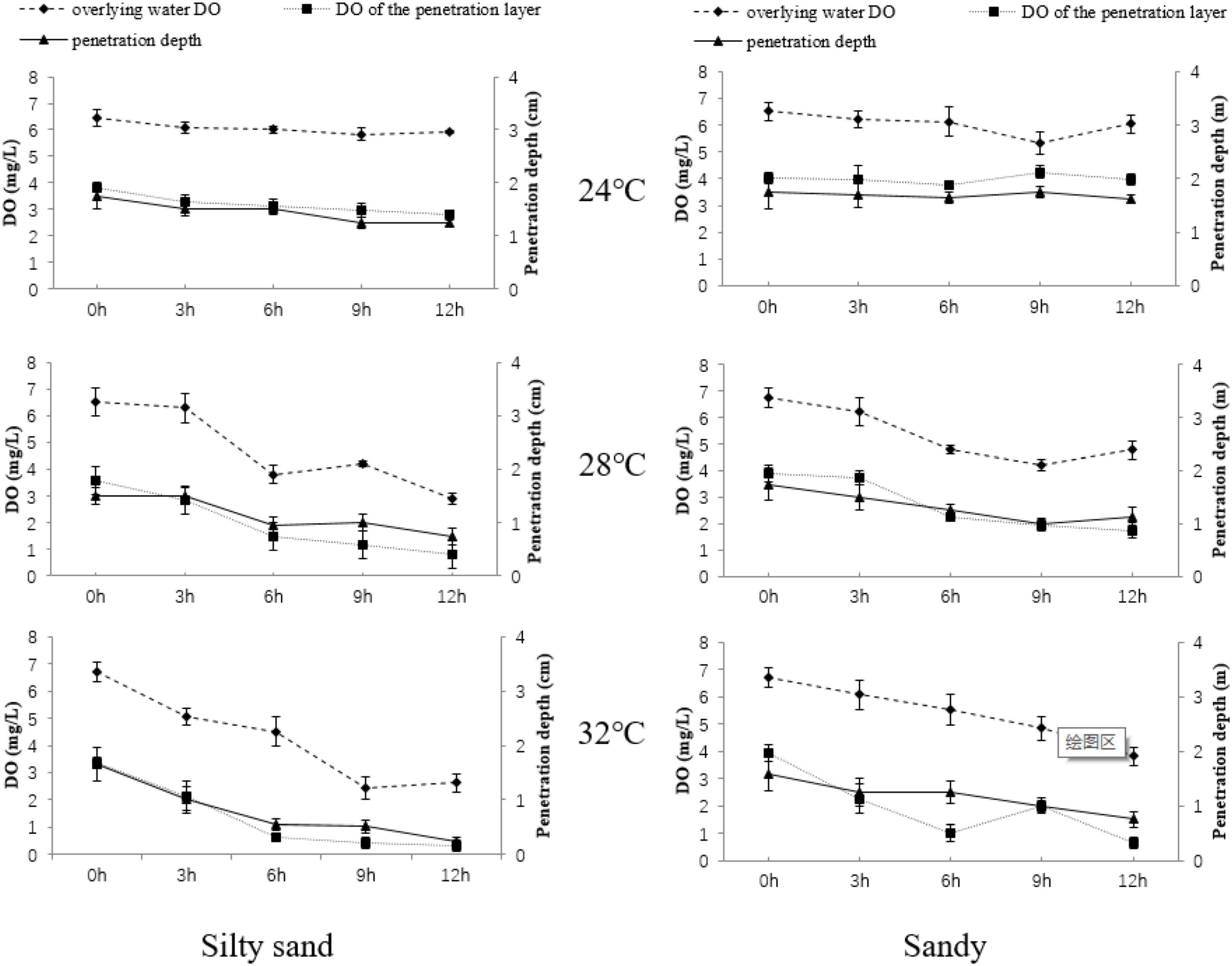
Figure 1. Changes in the overlying water DO concentration, penetration depth of the penetration layer, and average DO concentration of the penetration layer in different sediment substrate types over time.
The multivariate analysis of variance showed that the DO-related parameters were significantly affected by temperature, substrate, and time. The DO content of the overlying water was significantly affected by the interaction between temperature and substrate, as well as temperature and time individually. The average DO content of the penetration layer was significantly affected by the interaction between temperature and time, and the penetration depth was significantly affected by the interaction between temperature and time as well as substrate and time individually (Table 2).
H2S Content in Sediment
As shown in Figure 2, at the beginning of the experiment, there were no significant differences in H2S concentrations among the experimental groups (p > 0.05). After the experiment started, there were still no significant differences in H2S concentration at different measurement time points in silty sand or sandy sediment substrates at 24°C (p > 0.05), but there was a significant change with depth (p < 0.05). The change characteristics were small in the middle and high in the surface and bottom layers. In the other temperature groups, the H2S concentrations gradually increased as time progressed, and the H2S concentration changed significantly with depth (p < 0.05), but there was no obvious change law. At 28°C, the H2S concentrations in both sediment types increased drastically after 6 h (p < 0.05), and the maximum H2S concentrations in silty sand and sandy sediments were 16.90 and 10.41 μmol/L, respectively. At 32 °C, H2S concentrations in both sediments showed a significant increase (p < 0.05) after 3 h and the maximum H2S concentrations in silty sand and sandy sediments were 18.10 μmol/L and 12.32 μmol/L, at the end of the experiment, respectively.
The multivariate analysis of variance showed that the H2S concentrations in sediments were significantly affected by temperature, substrate, and time (temperature: F = 358.458, p < 0.01; substrate: F = 86.124, p < 0.01; time: F = 352.653, p < 0.01). There were significant interactions between temperature, time, and substrate (temperature × time: F = 89.948, p < 0.01; temperature × substrate: F = 44.648, p < 0.01; time × substrate: F = 13.553, p < 0.01). At high temperatures, the H2S concentrations in silty sand sediments were significantly higher than those in sandy sediments (p < 0.05).
Behavioral and Physiological Responses of the Manila Clam
While none of the Manila clams died during the experiment, their digging behavior was affected. With increased temperature, the digging index of Manila clam decreased, and the number of Manila clam that appeared on the sediment surface began to increase. Temperature and the concentrations of DO and H2S at the sediment-water interface at the end of the experiment all exhibited significant effects on the digging index of Manila clams (temperature: F = 6.104, p < 0.05; DO: F = 5.54, p < 0.05; H2S: F = 6.275, p < 0.05) (Figure 3).
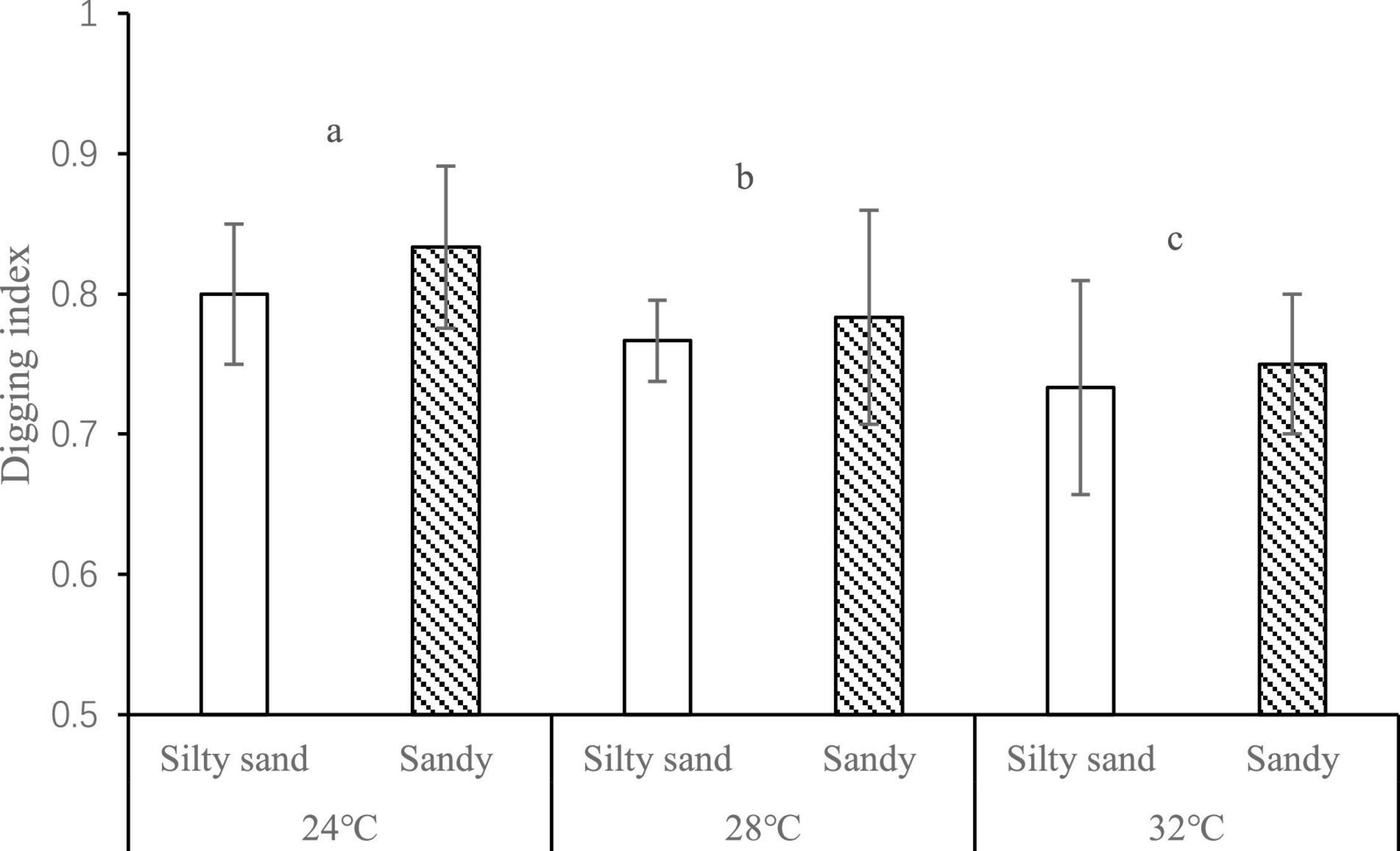
Figure 3. Digging index of Manila clam at different temperatures and in different sediment types. The different letters indicate significant difference of digging index at different temperatures.
After the experiment, the concentrations of succinate in the soft tissues of the Manila clams exhibited significantly accumulation, but only at 32°C (p < 0.01), with a concentration of about 2 μmol/g, which was 60% higher than that at the beginning of the experiment (Figure 4). However, the concentration was far lower than the anoxic stress index of bivalves reported in the literature (4.4 μmol/g; Lee et al., 2011), therefore, the Manila clams were not utilizing anaerobic metabolism under the experimental conditions of this study.
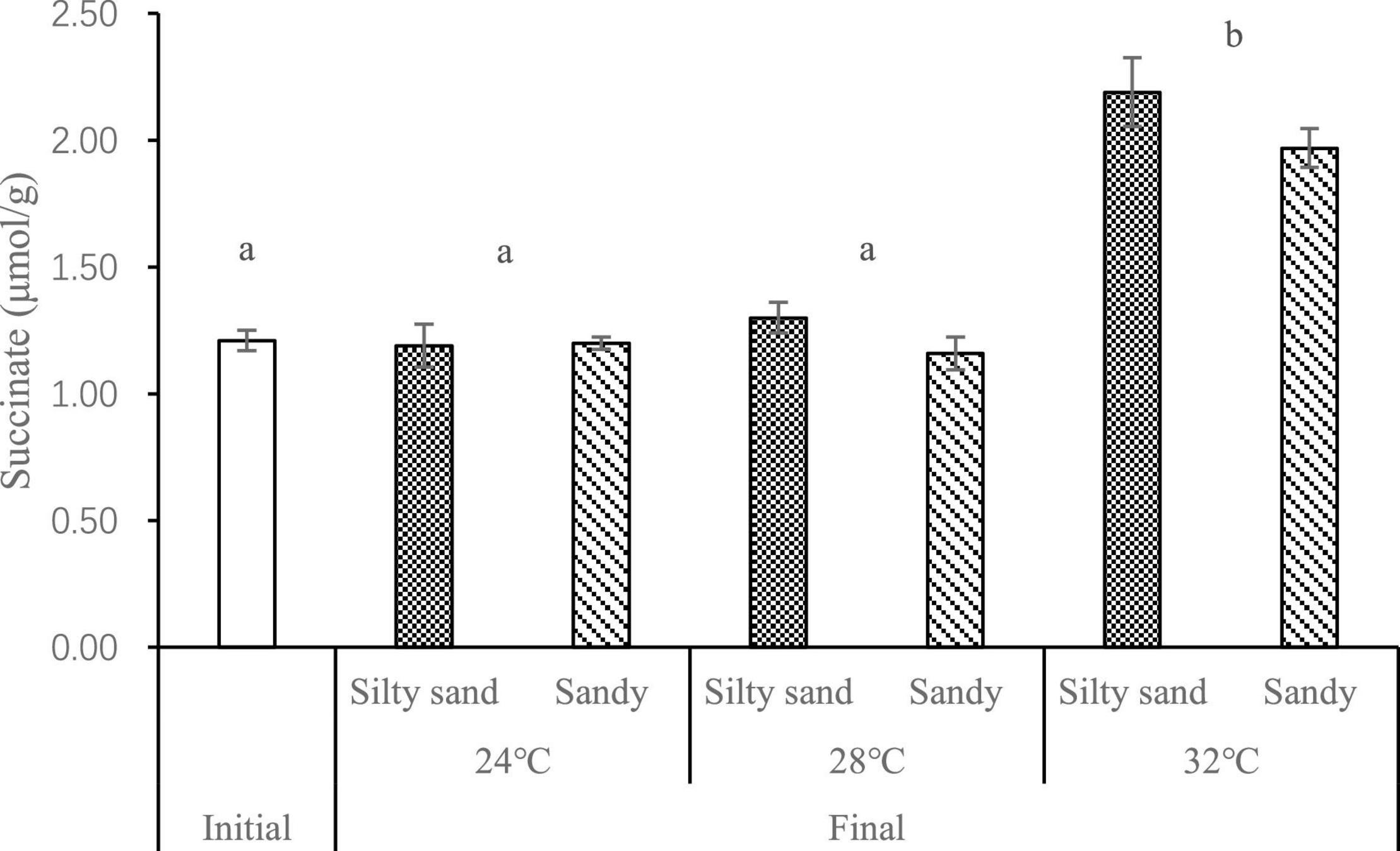
Figure 4. Concentrations of succinate in Manila clam soft tissue at different temperatures and in different sediment types. The different letters indicate significant difference of succinate at different temperatures.
The largest difference was seen in the activity of PO in Manila clam hepatopancreas, which was activated as the temperature rose (Figure 5). The temperature and concentrations of DO and H2S at the sediment-water interface at the end of the experiment had a significant impact on the PO activity of the Manila clam (temperature: F = 145.9, p < 0.001; DO: F = 54.23, p < 0.001; H2S: F = 123.6, p < 0.001), and the interaction between H2S and DO also significantly affected PO activity (DO × H2S: F = 6.381, p < 0.05).
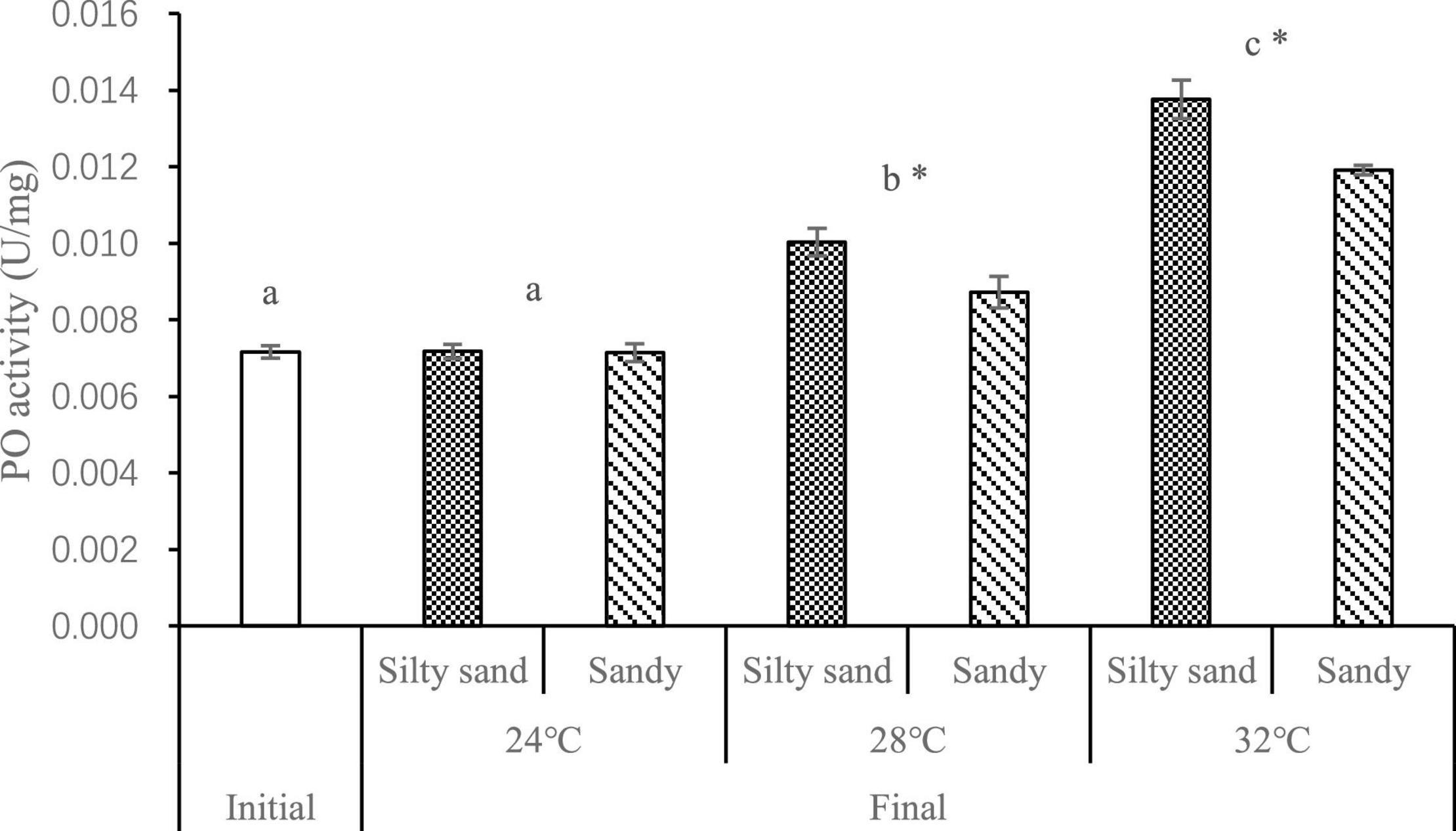
Figure 5. The activity of PO in Manila clam hepatopancreas at different temperatures and in different sediment types. The different letters indicate significant difference of PO at different temperatures. * indicates significant difference of the activity of PO in manila clam in different sediment types under the same temperature conditions.
Discussion
Typically, global pressure sources (e.g., global warming and ocean acidification) tend to vary at large time scales. Indeed, while climatic events are playing an important in marine organisms and the ecosystems they support, extreme environmental changes (e.g., MHWs) on a short time scale, in some cases, may generate even more profound impacts. This impact is more apparent for mudflat bivalves living in estuarine which are more likely to be exposed to drastic temperature change. These rapid temperature change (e.g., within a single tidal cycle) over small spatial scales may trigger unexpected environmental chain reactions (Helmuth et al., 2014). In this study, increased temperature and more prolonged duration resulted in a significant decrease in the DO of the sediment. The higher the temperature, the more dramatic the effect. Therefore, hypoxia or anoxic conditions are more likely to occur in summer with higher temperatures (Diaz, 2001). Furthermore, the effect of temperature on sedimental DO is much higher than that on the overlying water. Likely, the DO concentration of overlying water has not been reduced to hypoxic conditions (DO < 4 mg/L) when the sediment is already in anoxic condition (DO < 2 mg/L). So it may not be accurate to focus only on the DO concentration in the bottom water in benthic shellfish culture area.
When high temperatures and low DO conditions co-occur, the increase in H2S release also becomes obvious. At the end of this study, the H2S concentrations in the sediments under normal conditions (24°C) were about 3∼5 μmol/L, while at higher temperatures, the maximum concentration of H2S in sediment samples was 18.10 μmol/L. However, even this high concentration was still far lower than the concentration of AVS in sampling area sediments (45∼180 mg/kg) (Liu et al., 2009; Yang et al., 2018), but it still approached or exceeded the half lethal concentration for many polychaetes with strong tolerances to H2S (Wang and Chapman, 1999). So, it is important to accurately measure H2S concentrations (Liang, 2019). In addition, H2S is very easy to oxidize and hardly exists in oxygen enriched water, so increases of H2S concentration have usually been associated with hypoxic or anoxic conditions (Nagasoe et al., 2011). H2S concentration would increase under the condition of decreasing DO concentration in the sediment. The increased H2S will also be oxidized by DO, which will accelerate the consumption of the remaining DO and lead to more serious hypoxia phenomenon. In summary, temperature changes may bring about a correlated environmental change of DO and H2S anomalies in the sediment, resulting in benthic organisms that can be exposed to three stresses simultaneously.
In addition, we note that the effects of DO depletion and H2S release vary significantly among substrate types in this study, and the environmental indicators of sediments with larger grain diameters are relatively better. Because the water is more easily exchanged, which prevents the accumulation of pollutants (Ichimi et al., 2019) and can effectively increase the oxidation intensity in sandy sediments (Yang et al., 1999). The finer the sediment particles are, the more quickly they accumulate organic matter (Hatcher et al., 1994; Mayer, 1994), which drives sediments to shift to a reductive state. Indeed, the phenomenon where DO concentrations decrease and H2S concentrations increase does not occur every summer (Kodama et al., 2018). Since the increase of H2S was also related to the consumption of DO in the bottom waters. Therefore, in a natural environment, under the influence of tidal and other hydrodynamic effects, the exchange of water might disassociate the effect of temperature from the increase in H2S concentration. Because of this, it’s likely that sediment DO and H2S concentrations in summer were related substrate in addition to magnitude and duration of elevated temperatures. It also reminds us that in summer, when there is a continuous high temperature, it is necessary to monitor H2S concentrations in addition to changes in the sediment DO.
In this study, increasing temperature and decreasing DO levels did not affect the survival on Manila clams. While there is evidence that increasing temperature and decreasing DO did appear to influence Manila clam mortality additively because temperature increases narrow the tolerable DO range (Kim et al., 2018). However, this conclusion was based on prolonged stress conditions in the laboratory. In a natural environment, under the influence of tides and other hydrodynamic effects, benthic organisms rarely have the opportunity to be exposed to prolonged environmental stress. Manila clam is highly tolerant of temperature or DO variations. The results of succinate proved that Manila clam did not utilize anaerobic metabolism during the experiment. Therefore, the increasing temperature and decreasing DO within a specific range is not the direct cause of the massive mortality of Manila clam. Nevertheless, even increasing temperature and decreasing DO level within know tolerable ranges affected on the behavior of Manila clam. When faced with environmental pressure, marine organisms have various coping strategies, including behavioral and physiological responses (Wu, 2002). This study found that when the sedimental DO decreased, Manila clam will reduce their burial depth to be closer to the sediment-water interface. It also seemed that the Manila clam’s locomotory response to environmental pressure was relatively rapid. Firstly, Manila clam may emerge from sediment to breathe more efficiently to cope with stress and then through regulating physiological metabolism, energy supply strategy, and the other physiological processes (Kang et al., 2016). However, the shallower burial depth may increase the risk of predation, indirectly increasing the likelihood of high mortality (Lee et al., 2011; Howard et al., 2017).
Dissolved oxygen, rather than other environmental factors, is a significant stressor in the digging behavior of Manila clam (Kim et al., 2018). Due to the release of H2S along with increased temperature and decreased DO, Manila clam may be harmed by higher H2S concentrations in the sediment surface while seeking more oxygen. Indeed, changes in behavioral characteristics of Manila clam also increase the concentration of H2S. Because the lack of movement (excavation) decreased the irrigation and penetration of DO into the sediments and, therefore, increased H2S production. In this study, PO activity did change significantly. Our results showed that the release of H2S might activate PO activity and damage the immune system of the Manila clam. In mollusks, PO is an important substance involved in immune defense that can be activated by external factors and is a key enzyme for melanin formation in the soft tissues and shells of bivalves (Asokan et al., 1997). Changes in the activity of this key component of the immune system may directly affect the survival of mollusks under environmental stress (Cajaraville et al., 1996; Xing et al., 2008). Nagasoe et al. (2011) reported that that exposure to high-H2S concentrations can cause tissue melanization and damage of the Manila clam, including damage to the siphon, gills, adductor muscle, etc., and further affect physiological processes involved in breathing and feeding. Therefore, even though several environmental factors had no significant effect on the survival of the Manila clam during the initial exposure, as the environmental stress frequency or duration increased, damage to immune system could occur, potentially leading to significantly reduced performance in the future (Kozuki et al., 2013). MHWs induced elevated water temperatures are not the unique source of stress for marine organisms, and the environmental effects on the behavior and physiology of organisms should be considered in an integrated manner.
Conclusion
At higher temperatures, DO was consumed more rapidly and H2S release increased in sediments. Sediment type also affected oxygen consumption and H2S release rates. When Manila clams face environmental pressure, they respond quickly with locomotory behaviors (e.g., digging). Manila clams shows a strong tolerance to both high temperature and low DO and their physiological metabolism may not be affected by temperature increases or DO reduction within a certain range. However, when toxic substances such as H2S start to accumulate, they may damage the immune system and tissues of the Manila clam, thereby affecting its future survival. Therefore, when temperatures are high for long periods in summer, and especially when the bottom water DO begins to decrease, it is necessary to take timely action to prevent and guard against harm caused by H2S to aquaculture organisms. Therefore, areas that experience weak hydrodynamic exchange or that have small sediment particle sizes should be avoided in when selecting benthic bivalves culture locations.
Data Availability Statement
The original contributions presented in the study are included in the article/supplementary material, further inquiries can be directed to the corresponding author.
Author Contributions
YL and JZ conceptualized the study. WW and QK were responsible for the experimental operation. XW was in charge of the data curation. YL prepared and wrote the original draft. CL reviewed and edited the manuscript. All authors read and approved the final manuscript.
Funding
This study was funded by the National Natural Science Foundation of China (41776155), Strategic Priority Research Program of the Chinese Academy of Science (XDA23050402), and Ministry of agriculture national outstanding agricultural talents and innovative team “shallow aquaculture capacity and healthy aquaculture.”
Conflict of Interest
The authors declare that the research was conducted in the absence of any commercial or financial relationships that could be construed as a potential conflict of interest.
Publisher’s Note
All claims expressed in this article are solely those of the authors and do not necessarily represent those of their affiliated organizations, or those of the publisher, the editors and the reviewers. Any product that may be evaluated in this article, or claim that may be made by its manufacturer, is not guaranteed or endorsed by the publisher.
References
Asokan, R., Arumugam, M., and Mullainadhan, P. (1997). Activation of prophenoloxidase in the plasma and haemocytes of the marine mussel Perna viridis Linnaeus. Dev. Comp. Immunol. 21, 1–12. doi: 10.1016/S0145-305X(97)00004-9
Babcock, R. C., Bustamante, R. H., Fulton, E. A., Fulton, D. J., Haywood, M. D. E., Hobday, A. J., et al. (2019). Severe continental-scale impacts of climate change are happening now: extreme climate events impact marine habitat forming communities along 45% of Australia’s coast. Front. Mar. Sci. 6:411. doi: 10.3389/fmars.2019.00411
Cajaraville, M. P., Olabarrieta, I., and Marigomez, I. (1996). In vitro activities in mussel haemocytes as biomarkers of environmental quality: a case of study in the Abra estuary (Biscay Bay). Ecotoxicol. Environ. Saf. 35, 253–260. doi: 10.1006/eesa.1996.0108
Cerenius, L., Lee, B. L., and Soderhall, K. (2008). The proPO-system: pros and cons for its role in invertebrate immunity. Trends. Immunol. 29, 263–271. doi: 10.1016/j.it.2008.02.009
Diaz, R. J. (2001). Overview of hypoxia around the world. J. Environ. Qual. 30, 275–281. doi: 10.2134/jeq2001.302275x
Diaz, R. J., and Rosenberg, R. (2008). Spreading dead zones and consequences for marine ecosystems. Science 321, 926–929. doi: 10.1126/science.1156401
Gajbhiye, D. S., and Khandeparker, L. (2019). Immunoecology of the short neck clam Paphia malabarica (Chemnitz, 1782) in a tropical monsoon-influenced estuary. Mar. Environ. Res. 143, 60–70. doi: 10.1016/j.marenvres.2018.11.004
Gall, M. L., Holmes, S. P., Campbell, H., and Byrne, M. (2021). Effects of marine heatwave conditions across the metamorphic transition to the juvenile sea urchin (Heliocidaris erythrogramma). Mar. Pollut. Bull. 163:111914. doi: 10.1016/j.marpolbul.2020.111914
Gestal, C., Roch, P., Renault, T., Pallavivini, A., Paillard, C., and Novoa, B. (2008). Study of diseases and the immune system of bivalves using molecular biology and genomics. Rev. Fish. Sci. Aquac. 16, 133–156. doi: 10.1080/10641260802325518
Gilbert, F., Souchu, P., Bianchi, M., and Bonin, P. (1997). Influence of shellfish farming activities on nitrification, nitrate reduction to ammonium and denitrification at the water-sediment interface of the Thau lagoon, France. Mar. Ecol. Prog. Ser. 151, 143–153. doi: 10.3354/meps151143
Hatcher, A., Grant, J., and Schofield, B. (1994). Effects of suspended mussel culture (Mytilus spp.) on sedimentation, benthic respiration and sediment nutrient dynamics in a coastal bay. Mar. Ecol. Prog. Ser. 115, 219–235. doi: 10.3354/meps115219
He, G., Liu, X., Xu, Y., Liang, J., Deng, Y., Zhang, Y., et al. (2021). Repeated exposure to simulated marine heatwaves enhances the thermal tolerance in pearl oysters. Aquat. Toxicol. 239:105959. doi: 10.1016/j.aquatox.2021.105959
Hellio, C., Bado-Nilles, A., Gagnaire, B., Renault, T., and Thomas-Guyon, H. (2006). Demonstration of a true phenoloxidase activity and activation of a ProPO cascade in Pacific oyster, Crassostrea gigas (Thunberg) in vitro. Fish. Shellfish. Immun. 22, 433–440. doi: 10.1016/j.fsi.2006.06.014
Helmuth, B., Russell, B. D., Connell, S. D., Dong, Y., Harley, C., Lima, F. P., et al. (2014). Beyond long-term averages: making biological sense of a rapidly changing world. Clim. Chang. Responses 1:6. doi: 10.1186/s40665-014-0006-0
Holbrook, N. J., Scannell, H. A., Gupta, A. S., Benthuysen, J. A., Feng, M., Oliver, E. C. J., et al. (2019). A global assessment of marine heatwaves and their drivers. Nat. Commun. 10:2624. doi: 10.1038/s41467-019-10206-z
Howard, A. C., Poirrier, M. A., and Caputo, C. E. (2017). Exposure of rangia clams to hypoxia enhances blue crab predation. J. Exp. Mar. Biol. Ecol. 489, 32–35. doi: 10.1016/j.jembe.2017.01.012
Hughes, T. P., Kerry, J. T., Alvarez-Noriega, M., Alvarez-Romero, J. G., Anderson, K. D., Baird, A. H., et al. (2017). Global warming and recurrent mass bleaching of corals. Nature 543, 373–377. doi: 10.1038/nature21707
Ichimi, K., Honda, M., Okada, Y., Tsuzuki, K., and Yamaguchi, H. (2019). Importance of shingle beaches as habitat for Manila clam Ruditapes philippinarum. Fish. Sci. 85, 417–427. doi: 10.1007/s12562-019-01300-8
Jonsson, P. R., and Andé, C. (1992). Mass mortality of the bivalve Cerastoderma edule on the Swedish west coast caused by infestation with the digenean trematode Cercaria cerastodermae I. Ophelia 36, 151–157. doi: 10.1080/00785326.1992.10430365
Joyner-Matos, J., Predmore, B. L., Stein, J. R., Leeuwenburgh, C., and Julian, D. (2010). Hydrogen sulfide induces oxidative damage to RNA and DNA in a sulfide-tolerant marine invertebrate. Physiol. Biochem. Zool. 83, 356–365. doi: 10.1086/597529
Kang, H. Y., Lee, Y., Choi, K., Park, H. J., Yun, S., and Kang, C. (2016). Combined effects of temperature and Seston concentration on the physiological energetics of the Manila clam Ruditapes philippinarum. PLoS One 11:3. doi: 10.1371/journal.pone.0152427
Kim, T. W., Park, S., and Sin, E. (2018). At the tipping point: differential influences of warming and deoxygenation on the survival, emergence, and respiration of cosmopolitan clams. Ecol. Evol. 8, 4860–4866. doi: 10.1002/ece3.4041
Kodama, K., Waku, M., Sone, R., Miyawaki, D., Ishida, T., Akatsuka, T., et al. (2018). Ontogenetic and temperature-dependent changes in tolerance to hypoxia and hydrogen sulfide during the early life stages of the Manila clam Ruditapes philippinarum. Mar. Environ. Res. 137, 177–187. doi: 10.1016/j.marenvres.2017.12.019
Kozuki, Y., Yamanaka, R., Matsushige, M., Saiton, A., Otani, S., and Ishida, T. (2013). The after-effects of hypoxia exposure on the clam Ruditapes philippinarum in Omaehama beach, Japan. Estuar. Coast. Shelf Sci. 116, 50–56. doi: 10.1016/j.ecss.2012.08.026
Lee, A. C., Lee, Y. C., and Chin, T. S. (2011). Effects of low dissolved oxygen on the digging behavior and metabolism of the hard clam (Meretrix lusoria). Aquac. Res. 43, 1–13. doi: 10.1111/j.1365-2109.2010.02785.x
Lee, A. C., Lin, Y. H., Lin, C. R., Lee, M. C., and Chen, Y. P. (2007). Effects of components in sea water on the digging behavior of the hard clam (Meretrix lusoria). Aquaculture 272, 636–643. doi: 10.1016/j.aquaculture.2007.06.013
Li, Q., Sun, S., Zhang, F., Wang, M., and Li, M. (2019). Effects of hypoxia on survival, behavior, metabolism and cellular damage of Manila clam (Ruditapes philippinarum). PLoS One 14:4. doi: 10.1371/journal.pone.0215158
Li, Z., Song, S., Li, C., and Yu, Z. (2018). The sinking of the phytoplankton community and its contribution to seasonal hypoxia in the Changjiang (Yangtze River) estuary and its adjacent waters. Estuar. Coast. Shelf Sci. 208, 170–179. doi: 10.1016/j.ecss.2018.05.007
Liang, Y. (2019). Impacts of Scallop Farming on Nutrient Limitation in the Zhangzi Island Area. Ph.D. thesis. Huairou District: University of Chinese academy of sciences.
Liu, Y. F., Wu, S., Sun, S., and Sun, Y. (2009). Comparison between sedimental contamination in intertidal zone of Jiaozhou Bay and the counterpart of Laizhou Bay. Coast. Eng. 8, 61–68. doi: 10.3969/j.issn.1002-3682.2009.02.008
Long, W. C., Brylawski, B. J., and Seitz, R. D. (2008). Behavioral effects of low dissolved oxygen on the bivalve Macoma balthica. J. Exp. Mar. Biol. Ecol. 359, 34–39. doi: 10.1016/j.jembe.2008.02.013
Matozzo, V., and Marin, M. G. (2011). Bivalve immune responses and climate changes: is there a relationship? Invertebrate Surviv. J. 2011, 70–77.
Mayer, L. M. (1994). Surface area control of organic carbon accumulation in continental shelf sediments. Geochim. Cosmochim. Acta 58, 1271–1284. doi: 10.1016/0016-7037(94)90381-6
Mcpherson, M. L., Finger, D. J. I., Houskeeper, H. F., Bell, T. W., Carr, M. H., Rogers-Bennett, L., et al. (2021). Large-scale shift in the structure of a kelp forest ecosystem co-occurs with an epizootic and marine heatwave. Commun. Biol. 4:298. doi: 10.1038/s42003-021-01827-6
Nagasoe, S., Yurimoto, T., Suzuki, K., Maeno, Y., and Kimoto, K. (2011). Effects of hydrogen sulfide on the feeding activity of Manila clam Ruditapes philippinarum. Aquat. Biol. 13, 293–302. doi: 10.3354/ab00374
Parisi, M. G., Mauro, M., Sara, G., and Cammarata, M. (2017). Temperature increases, hypoxia, and changes in food availability affect immunological biomarkers in the marine mussel Mytilus galloprovincialis. J. Comp. Physiol. B. 187, 1117–1126. doi: 10.1007/s00360-017-1089-2
Plecha, S. M., Soares, P. M. M., Silva-Fernandes, S. M., and Cabos, W. (2021). On the uncertainty of future projections of Marine Heatwave events in the North Atlantic Ocean. Clim. Dynam. 56, 2027–2056. doi: 10.1007/s00382-020-05529-3
Scanes, E., Scanes, R. P., and Ross, P. M. (2020a). Climate change rapidly warms and acidifies Australian estuaries. Nat. Commun. 11:1803. doi: 10.1038/s41467-020-15550-z
Scanes, E., Parker, L. M., O’Connor, W. A., Dove, M. C., and Ross, P. M. (2020b). Heatwaves alter survival of the Sydney rock oyster, Saccostrea glomerata. Mar. Poll. Bull. 158:11138. doi: 10.1016/j.marpolbul.2020.111389
Smale, D. A., Wernberg, T., Oliver, E. C. J., Thomsen, M. S., Harvey, B. P., Straub, S. C., et al. (2019). Marine heatwaves threaten global biodiversity and the provision of ecosystem services. Nat. Clim. Chang. 9, 306–312. doi: 10.1038/s41558-019-0412-1
Smith, J. S., Winston, R. J., Tirpak, R. A., Wituszynski, D. M., Boening, K. M., and Martin, J. F. (2020). The seasonality of nutrients and sediment in residential stormwater runoff: implications for nutrient-sensitive waters. J. Environ. Manage. 276:111248. doi: 10.1016/j.jenvman.2020.111248
Smith, L., Kruszyna, H., and Smith, R. P. (1977). The effect of methemoglobin on the inhibition of cytochrome c oxidase by cyanide, sulfide or azide. Biochem. Pharmacol. 26, 2247–2250. doi: 10.1016/0006-2952(77)90287-8
Soldatov, A. A., Kukhareva, T. A., Andreeva, A. Y., and Efremova, E. S. (2018). Erythroid Elements of Hemolymph in Anadara kagoshimensis (Tokunaga, 1906) under conditions of the combined action of hypoxia and hydrogen sulfide contamination. Russ. J. Mar. Biol. 44, 452–457. doi: 10.1134/S1063074018060111
Suikkanen, S., Pulina, S., Engstrom-Ost, J., Lehtiniemi, M., Lehtinen, S., and Brutemark, A. (2013). Climate change and eutrophication induced shifts in northern summer plankton communities. PLoS One 8:6. doi: 10.1371/journal.pone.0066475
Wang, F., and Chapman, P. M. (1999). Biological implications of sulfide in sediment: a review focusing on sediment toxicity. Environ. Toxicol. Chem. 18, 2526–2532. doi: 10.1897/1551-50281999018<2526:BIOSIS<2.3.CO;2
Wei, H., He, Y., Li, Q., Liu, Z., and Wang, H. (2007). Summer hypoxia adjacent to the Changjiang Estuary. J. Marine. Syst. 67, 292–303. doi: 10.1016/j.jmarsys.2006.04.014
Wootton, E. C., Dyrynda, E. A., and Ratcliffe, N. A. (2003). Bivalve immunity: comparisons between the marine mussel (Mytilus edulis), the edible cockle (Cerastoderma edule) and the razor-shell (Ensis siliqua). Fish Shellfish Immunol. 15, 195–210. doi: 10.1016/S1050-4648(02)00161-4
Wu, R. S. (2002). Hypoxia: from molecular responses to ecosystem responses. Mar. Pollut. Bull. 45, 35–45. doi: 10.1016/S0025-326X(02)00061-9
Xing, J., Lin, T., and Zhan, W. (2008). Variations of enzyme activities in the haemocytes of scallop Chlamys farreri after infection with the acute virus necrobiotic virus (AVNV). Fish Shellfish Immunol. 25, 847–852. doi: 10.1016/j.fsi.2008.09.008
Xu, Y., Wang, Z., Zhang, Y., Liang, J., He, G., Liu, X., et al. (2022). Transcriptome analysis reveals acclimation responses of pearl oysters to marine heatwaves. Sci. Total. Environ. 810:151189. doi: 10.1016/j.scitotenv.2021.151189
Yang, J., Wu, X., Li, X., and Cui, L. (2018). Quality evaluation of surface sediments in scallop culture area in Laizhou Bay. Fish. Sci. 37, 361–367. doi: 10.16378/j.cnki.1003-1111.2018.03.012
Yang, Q., Jiang, Y., Zhang, X., and Yang, Y. (1999). Study on the effects of decomposition of the bait in a shrimp-pond on the maricultural environment II. Effects of decomposition of the subbottom bair in a shrimp-pond on the sediment environment. Mar. Environ. Sci. 18, 11–15. doi: 10.3969/j.issn.1007-6336.1999.03.003
Keywords: Ruditapes philippinarum, temperature, dissolved oxygen, hydrogen sulfide, behavioral characteristic, physiological response
Citation: Liu Y, Zhang J, Wang X, Wu W, Kang Q and Li C (2022) Temperature-Induced Environmental Chain Reaction in Marine Sedimentation and Its Impact on Manila Clam Ruditapes philippinarum. Front. Mar. Sci. 9:845768. doi: 10.3389/fmars.2022.845768
Received: 30 December 2021; Accepted: 25 February 2022;
Published: 01 April 2022.
Edited by:
Dapeng Liu, Georgia Institute of Technology, United StatesReviewed by:
Fang Wang, Ocean University of China, ChinaMartin F. Soto-Jimenez, National Autonomous University of Mexico, Mexico
Xin Shen, Jiangsu Ocean University, China
Liqiang Zhao, Guangdong Ocean University, China
Copyright © 2022 Liu, Zhang, Wang, Wu, Kang and Li. This is an open-access article distributed under the terms of the Creative Commons Attribution License (CC BY). The use, distribution or reproduction in other forums is permitted, provided the original author(s) and the copyright owner(s) are credited and that the original publication in this journal is cited, in accordance with accepted academic practice. No use, distribution or reproduction is permitted which does not comply with these terms.
*Correspondence: Chaolun Li, bGNsQHFkaW8uYWMuY24=