- State Key Laboratory of Estuarine and Coastal Research, East China Normal University, Shanghai, China
Phytoplankton blooms, including the diatom-to-dinoflagellate succession in coastal regions, are frequently observed by researchers through incubation experiments, albeit mainly in nutrient-enrichment conditions. This study, complementary to such previous experiments, aims at the dynamics of nutrients and organic matter during the natural community shift in a nutrient-constraint condition. A nutrient-constraint incubation experiment is conducted herein to investigate nutrient dynamics during a diatom-to-dinoflagellate succession and evaluate its effects on carbon cycling. The incubation successfully induced a transition from a diatom bloom dominated by Skeletonema costatum to a dinoflagellate bloom dominated by Prorocentrum donghaiense. Results showed that the DIP limitation triggers the succession. This experiment provides an excellent opportunity to examine how senescent bloom under nutrient stress affects the amount and bioavailability of dissolved organic matter (DOM) that is produced. Under the nutrient limitation, organic carbon production was significantly influenced by nutrient availability. Particulate organic carbon (POC) production is closely related to phytoplankton growth phases, while dissolved organic carbon (DOC) is related to nutrient structure. The relative partitioning of net production to POC is higher in the dinoflagellate-dominant stage than that in the diatom-dominant stage, which is driven by quick turnover of DOM related to nutrient structure in different stages. In terms of C:Chl a ratio, it was strongly dependent on growth phases and nutrient conditions. From the signatures of chromophoric dissolved organic matter (CDOM), the bioavailability of DOM decreased during the succession. In different phases, DOM composition varied, containing more protein-like components in the degradation phase than in the growth phases. Differences of DOM composition among varying community dominance were far smaller than different growth phases during this succession. Such an observation of a diatom-to-dinoflagellate succession and the related dynamics of nutrients and organic matter benefits the prediction of organic carbon export during community shifts in ecological models.
Introduction
A seasonal succession from diatoms to dinoflagellates has been widely observed in mid- to high-latitude marginal seas (Spilling et al., 2018; Zhang et al., 2019). This transition significantly influences the sustainability of ecosystems and biogeochemical processes, as well as carbon cycling in oceans (Irwin et al., 2015; Basu and Mackey, 2018). Response of the phytoplankton community to variable nutrient supplies (Behrenfeld et al., 2021) or organic carbon dynamics in different communities (Richardson and Jackson, 2007; Leblanc et al., 2018) have been investigated. However, nutrients and organic carbon dynamics during the phytoplankton community succession process remain unclear due to difficulties involved in field observations.
Phytoplankton communities are influenced by the interplay of constituent species traits and the environment conditions (Lima-Mendez et al., 2015; Lin et al., 2021). The nutrient inputs have a significant impact on the composition of phytoplankton communities and influence the subsequent nutrient dynamic (Altman and Paerl, 2012). Both field investigations and incubation experiments suggest that phytoplankton abundance and community structure can be modulated by nutrient inputs (Domingues et al., 2005; Furnas et al., 2005; Paerl, 2006). The competition of phytoplankton for nutrients not only drives the shift of community structure, but also generates corresponding changes in phytoplankton ecological stoichiometry (Tréguer et al., 2018; Zhou et al., 2021). As mentioned above, the shift of community composition is hardly observed in field campaigns. Incubation experiments, on the other hand, provide alternative opportunities to examine the details of such a succession. To study phytoplankton community structure and biodiversity responses to increasing nutrient disturbances, a series of incubation experiments with nutrient amendments have been designed and copious amounts of data were collected. For example, dissolved inorganic nitrogen (DIN) supply can alter the phytoplankton community structure and stimulate a regime shift from cyanobacteria to diatoms (Zhou et al., 2018). The results of the Prorocentrum donghaiense and Skeletonema costatum bi-algal culture experiments indicate that S. costatum dominates over P. donghaiense under high-nutrient conditions (Wang et al., 2013). Up to now, the majority of incubation experiments assume a high-nutrient input condition despite the relatively oligotrophic character of sea water. Such an idealistic approach is not conducive to numerical simulations due to the overestimated reaction rates from those high-nutrient incubation systems.
The community composition and nutrient availability are known as the main factors that influence the organic carbon production (Casareto et al., 2012). Production and partitioning of phytoplankton-derived organic matter are varied among species and growing phases (Kinsey et al., 2018). Previous studies in field and laboratory experiments show that a large fraction of organic carbon produced during phytoplankton blooms is partitioned into POC when diatoms dominate the community (Wetz and Wheeler, 2003; Hasegawa et al., 2010), and is partitioned into DOC when pico-plankton dominates (Teira et al., 2001). However, there are also studies indicate that the relative partitioning of net production to POC and DOC is not related to phytoplankton communities but related to nutrients available (Conan et al., 2007). Investigations into the coupling between nutrients and organic carbon during these community shifts remain limited.
Dissolved organic matter (DOM) in the marine environment is a key component in the global carbon cycle (Carlson and Hansell, 2014). The optical properties of DOM (absorbance and fluorescence) have been widely used to determine the origin and fate of DOM, as they are linked to the inherent chemical characteristics of the DOM pool (Reader et al., 2015). One of the major sources of DOM is the autochthonous production by phytoplankton (Stedmon and Markager, 2005). Previous incubation experiments have relied on high nutrients to observe DOM formation by phytoplankton (Romera-Castillo et al., 2010), which demonstrate different components exudation by different phytoplankton species. Organic matter produced and released by phytoplankton is processed by heterotrophic bacterial communities that transform DOM into biomass and recycle inorganic nutrients (Kinsey et al., 2018S). The DOM characteristics in different phytoplankton growth phases are quite different due to the microbial reprocessing (Shields et al., 2019). Asmala et al. (2018) suggests that nutrient status is the major driver for DOM dynamic, whereas the characteristics of phytoplankton community seemed to have a minor role. Our understanding of DOM characteristics and transformation during the natural continuous process of diatom-to-dinoflagellate succession is still limited.
A seasonal shift of phytoplankton composition from a diatom- to a dinoflagellate-dominant community is observed in coastal regions influenced by the Changjiang Diluted Water (CDW) (Zhang et al., 2015). Many studies have discussed the influence of nutrient structure on the diatom-to-dinoflagellate succession. Dissolved inorganic phosphorus (DIP) limitation is regarded as the main limiting factor (Wang et al., 2003; Huang et al., 2012; Liu et al., 2016). However, the characteristics of organic matter dynamics in natural community succession influenced by nutrient structure is less studied in this area. A clear understanding of nutrient and organic carbon dynamics and their relationship in a diatom-to-dinoflagellate succession is still missing.
We present a hypothesis stating that depleted DIP triggers the diatom-to-dinoflagellate succession, and thereby significantly influences the organic carbon partitioning and composition. A nutrient-constraint incubation experiment (low DIP concentration) was conducted in this study, which successfully reproduced the transition from a diatom bloom dominated by S. costatum to a dinoflagellate bloom dominated by P. donghaiense in the observation field. The nutrient and organic carbon dynamics during this succession are determined and discussed in detail, and their biogeochemical implications are addressed.
Materials and Methods
Blooms were often observed around the Changjiang Estuary from April to July, with community succession from diatoms to dinoflagellates especially during the summer period. Upon sampling on 8 July during the cruise of R/V Zheyuke in 2017, the dominant phytoplankton was S. costatum at the blooming station (122.67°E, 31.37°N, Chang et al., 2021). Approximately 120 L of surface water was collected with a pump and filtered with a 200 μm mesh to remove the meso-zooplankton. The filtered water was then shipped to the laboratory immediately for the incubation experiments. The initial water chemical parameters are shown in Table S1 (day 0), characterized as brackish water (salinity 14) with depleted DIP.
Experiment Design
The experimental design is shown in Figure S1. The filtered water is mixed and transferred into twelve 10 L acid-washed transparent polycarbonate bottles (Nalgene, USA). This experiment was aimed to adjust nutrient concentrations by adding sodium silicate as the DSi source, and potassium dihydrogen phosphate as the DIP source. These 12 incubation bottles were then divided into four groups for testing the impact of increased nutrients in four different parametric cases, each with three bottles to be treated identically: (1) group A (control), the control group in which the water remains unaltered; (2) group B (+DSi), with an increase in DSi concentration by 16 μM; (3) group C (+DIP), with an increase in DIP concentration by 1 μM; (4) group D (+DSi, +DIP), with increases in DSi and DIP concentrations by 16 μM and 1 μM, respectively. More detailed nutrient structure information of the four groups is described in Table S2. The incubation bottles are exposed to natural light irradiation in an outdoor tank filled with tap water. Circulating water is used to keep the temperature at about 27~29°C consistent with offshore conditions (Jiang et al., 2021). The incubation experiments are simplified cultured systems without gas exchange most of the time, but are opened daily and gently shaken twice a day. The duration of the incubation experiments is 13 days. Aside from the initial gathering, samples are collected on days 1, 3, 6, 9, and 13.
Samples Collection
Prior to sampling, the incubation bottles were stirred to ensure an even distribution of phytoplankton. The dissolved oxygen (DO) was directly measured in situ with a multiparameter probe (Multi 340i/SET, WTW, GmbH, Germany).
In the laboratory, the samples were filtered within several hours of collection. The samples for DOC, nutrients (inorganic nutrients, , DSi; organic nutrients, dissolved organic nitrogen (DON) and phosphorous (DOP)) were filtered through 0.45 μm nylon filters (Rephile, Shanghai, China). And then, saturated HgCl2 solution was added in each inorganic nutrient sample for further analysis. The samples for chromophoric dissolved organic matter (CDOM) were filtered through 0.22 μm polyethersulfone filters (Millipore, Darmstadt, Germany). The samples for POC, total suspended particles (TSM) and chlorophyll a (Chl a) were filtered through pre-combusted (at 500°C for 5h) GF/F filters (pore size: 0.7 μM, Whatman). The phytoplankton samples were preserved with formaldehyde solution. Nutrient samples and phytoplankton samples were room temperature preservation, while the remaining samples were stored in a -20°C freezer prior to analyses.
Chemical Analyses
Chlorophyll a (Chl a) concentration was measured using a Cary 100 Ultrviolet-visible (UV-Vis) spectrophotometer (Varian, CA, USA) after extraction with 90% acetone at 4°C for 24h in the dark with a precision of ± 0.1 μg/L (Wang et al., 2017).
Dissolved oxygen (DO) was directly measured in situ with a multiparameter probe (WTW Multi 350i, Munich, Germany).
The phytoplankton species and abundance were identified using an imaging flow cytometer (FlowCAM 8400, Fluid Imaging Technologies, USA).
The concentrations of nutrients were determined by performing a Continuous Flow Analysis (CFA) using a Skalar Sanplus system in the laboratory with colorimetric methods described by (Grasshoff et al., 1999). The analytical precision was 0.06 μM for , 0.01 μM for , 0.09 μM for , 0.03 μM for , and 0.15 μM for . Dissolved organic nitrogen (DON) and phosphorous (DOP) were quantified after alkalinous digestion using potassium persulfate (Jiang et al., 2019).
Dissolved organic carbon (DOC) samples were assessed using a total organic carbon analyzer (Shimadzu TOC L-CPH, Japan) with the high-temperature catalytic oxidation method. The concentrations of POC were determined using an elemental analyzer (Flash EA 1112, Thermo Fisher, USA) after removing the carbonate fraction by acid fumigation with concentrated HCl. Repeated analyses of the intra-laboratory standard samples revealed a variation of < 5% (Wu et al., 2003).
The absorption spectra of chromophoric dissolved organic matter (CDOM) were measured with a Cary 100 Ultraviolet-visible (UV-Vis) spectrophotometer (Varian, CA, USA) with a wavelength range from 200 to 800 nm. Samples were measured in a 1 cm quartz cuvette, and Milli-Q water was used as the blank. The absorbance at each wavelength (Aλ) was corrected for instrument drift by subtracting the mean absorbance for the 700-710 nm wavelength interval. The absorption coefficients (aλ, in Napierian form, per meter) were calculated as described by Del Vecchio and Blough (2004):
Where l is the path length in meters.
The CDOM concentration was quantified using the absorption coefficient at 350 nm (a350). The absorption spectra were characterized by fitting an exponential model with a nonlinear regression as described in Stedmon et al. (2000). A slope of these spectra, S coefficient for wavelengths 275-295 nm (S275-295) were calculated. SUVA254 is defined as the UV absorbance at 254 nm divided by the DOC concentration (Weishaar et al., 2003).
The fraction of CDOM that emits induced fluorescent light is called fluorescent dissolved organic matter (FDOM). The emission excitation matrices (EEMs) for FDOM were measured with an F-4500 fluorescence spectrophotometer (Hitachi, Tokyo, Japan). The excitation wavelength was scanned from 250 to 478 nm (at 6 nm intervals), and the emission spectra were collected every 1 nm from 300 to 550 nm. The scan speed was 1200 nm/min, and the excitation and emission slits were 5 nm. The spectra of Milli-Q water were subtracted as a blank. The fluorescence intensity was calibrated using Milli-Q water and reported in Raman units (R.U.) (Lawaetz and Stedmon, 2009). In brief, the calibration was performed by diving the fluorescence intensity at a given emission wavelength by the water Raman scatter band collected at 350 nm excitation and integrated over 380–420 nm. PARAFAC was applied to decompose the EEMs into individual fluorescent components using the DOMFluor toolbox according to the method established by Stedmon and Bro (2008). OpenFluo was applied to search for matches of the spectra modeled by PARAFAC (Murphy et al., 2014). In our experiments, four fluorescent components were identified using a PARAFAC model with a split-half validation producer: three humic-like components (C1, C2, and C4) and one protein-like components (C3). Due to the complex characteristics of dissolved organic matter (DOM), each component represented a group of fluorophores with similar fluorescence character. The details of their spectral properties are shown in Table S3.
Data Analysis
The ratio of the net consumption rates for DSi and DIN (v(DSi)/v(DIN) ratio) was calculated based on the following equation:
Here, C(DSi)t, C(DIN)t are the DSi, DIN concentrations at day t, respectively. C(DSi)t-1, C(DIN)t-1 are the DSi, DIN concentrations at day t – 1, respectively.
Results
The Initial Conditions of the Sampling Water
The initial concentrations for nutrient and organic carbon were shown in Table S1. In the initial water, a high Chl a concentration (16.31 μg L-1) revealed that the phytoplankton bloom (Chl a > 10 μg L-1, Zhu et al., 2009) occurred in the sampling site. The DIP concentration was below the detection limit, indicating that the sampling water was a typical phosphorus limitation environment. The DSi and DIN concentration were 64.53 ± 1.12 and 68.75 ± 1.71 μM, respectively, resulting in a low DSi/DIN ratio (0.94). Our nutrient treatments did not change the phosphorus limitation condition and the nutrient structure, as shown by the initial nutrient structures of the four groups. The DOC concentration was 95.70 ± 3.10 μM, the POC was 0.53 mg/L.
Biological Response During the Incubation Experiments
The dissolved oxygen (DO) variation among the four groups was similar (Figure 1A), where they were observed to increase during the first 3 days and last 4 days, while observed to decrease during the middle period, from day 3 to day 9. In terms of Chl a concentration (Figure 1B), nutrient amendments increased the Chl a concentration to its peak value at day 3 in the incubation experiments of all groups, among which the values of groups with the DIP treatment (group C and D) always had higher concentrations than another two groups. The response of Chl a concentration suggested the transition of the phytoplankton community from growth to decline, and then to regrowth. Adding DIP significantly stimulates phytoplankton growth.
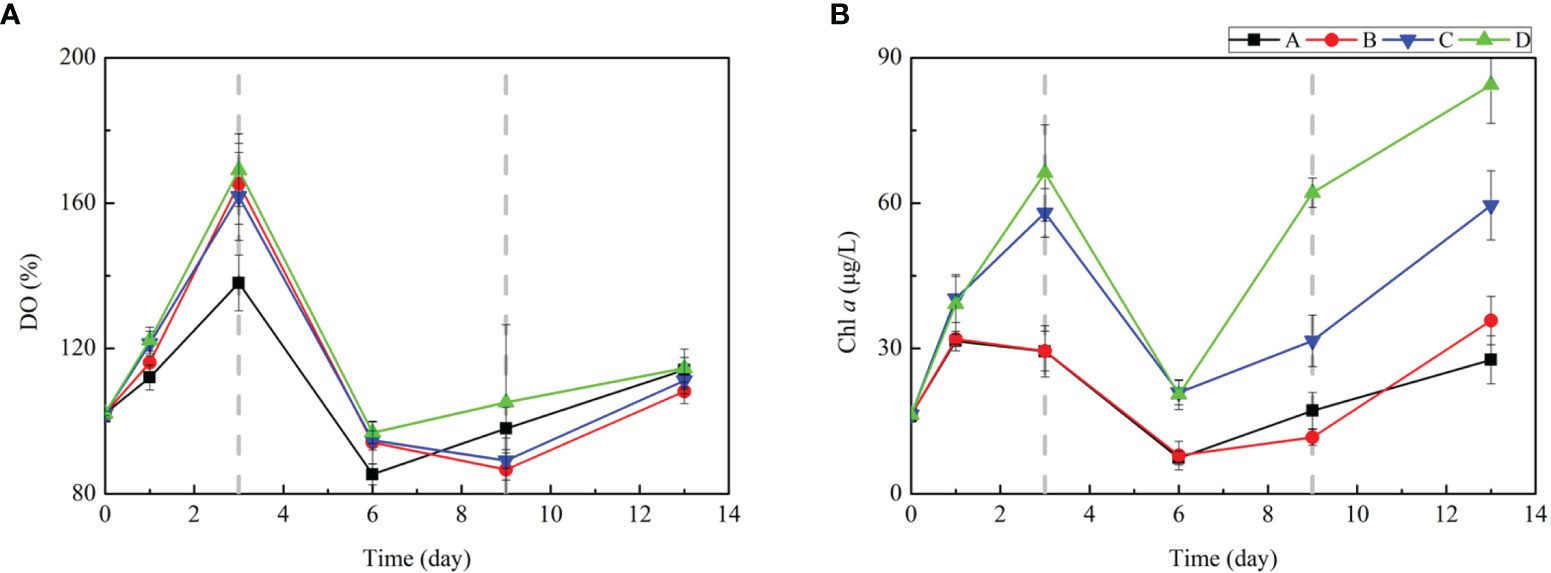
Figure 1 Changes in (A) DO, (B) Chl a during the incubation experiment. The error bars represent the standard deviation from triplicate incubations.
The phytoplankton community composition in four groups showed a similar succession (Figure S2 and Table 1), but the biomass responses are different which are characterized by Chl a concentrations. In the first 3 days, the dominant phytoplankton community was S. cotatum. In the 3–9 day interval, diatoms still dominated the community despite the decline of S. cotatum and the significant increase of Cyclotella. In the period 9–13 day interval, the dominant phytoplankton community changed to dinoflagellate, to be P. donghainese. The succession process reproduced the realistic algae succession in the Changjiang Estuary. By combining the Chl a and DO concentration variation with the transform of phytoplankton community composition, we defined three phases in our incubation experimental period: the growth phase (GP) from day 0 to day 3, the declining phase (DP) from day 3 to day 9, and the regrowth phase (RP) from day 9 to day 13, together with two stages: the diatom-dominant stage, which includes the GP and DP, and the dinoflagellate-dominant stage which refers to the RP.
Nutrient Variation During the Incubation Experiments
concentrations showed similar trends in the four groups (Table S1), which decreased in the GP, held relatively stable in the DP, and then decreased again in RP. Notably, the groups with the DIP treatment showed higher uptake rates compared to other groups. The concentration was relatively low, approaching 1.2 μM in our experiment. The concentration began to increase at incubations until the peak value at day 9, except for group D that peaked at day 6, after which they all decreased again. DON accumulated in the GP, and then decreased in the DP. In the RP, DON concentrations were relatively stable.
The DSi concentration decreased in the GP and reached its minimum value on day 3. The groups with DIP treatment showed the most consumption of all, which were ca. 27 µM in groups without DIP treatment (A and B) and 38 μM in groups with DIP treatment (C and D). Thereafter, the DSi concentration increased in the DP, and the concentration increases mainly occurred in the early stage of DP among all groups. The concentration variation of DSi indicated its reuse in the RP, and the amounts were much lower than those found in the GP.
The DIP concentrations were below the detection limit in all bottles, except the groups with the DIP treatment at day 0. The DOP showed different characteristics in four groups. For the DIP addition groups, the DOP concentrations were much higher, in which DOP increased in the GP along with the algae growth, and decreased in the DP. In the RP, DOP was relatively stable. In contrast, in the groups without the DIP addition, DOP had minimal accumulate over the study period.
Organic Carbon Variation During the Incubation Experiments
The organic carbon variation was also linked to the phases of phytoplankton. POC concentration variation showed similar trend in four groups, which increased in the GP, decreased in the DP and then increased again in the RP (Figure S3A). The groups with DIP treatment (groups C and D) showed relatively higher values than that in groups without DIP treatment (groups A and B) in the late of the experiment (Table S1). However, there was no significant difference among the different nutrient treatments in the GP (ANOVA, p > 0.05).
The DOC concentration averaged 95.70 μM at the start of the experiment. The increasing trends of DOC were observed in all four groups and showed an insignificant difference (ANOVA, p > 0.05). The accumulation rates in different stages varied (Figure S3B). In the diatom stage, there was a significantly higher accumulation rate of DOC than in the dinoflagellate stage.
The a350 value showed an increasing trend, with insignificant difference among groups. The increasing trends of humic-like components (C1, C2, and C4) were observed in the growth phase of phytoplankton (GP and RP). The fluorescence of protein-like components (C3) in all groups increased steadily until day 6. After a slight decrease in the DP, it continuously increased in the RP.
Discussion
Nutrient Dynamics in the DIP Depleted Condition During the Diatom-to-Dinoflagellate Succession
Previous studies usually consider the DIP limitation to be the major limiting factor in initiating a change in the major species from diatoms to dinoflagellates in the Changjiang Estuary (Wang et al., 2003; Ou et al., 2008; Huang et al., 2012; Wang et al., 2013; Liu et al., 2016; Mo et al., 2020). Our results also revealed the dominant role that DIP limitation played during the succession. In our DIP limitation incubation, increased and DSi net consumption in all DIP treatment groups was associated with significant increases in diatom biomass (characterized by Chl a, Figure 1B), while the Chl a concentrations showed insignificant responses to the DSi treatment by the comparison between groups A and B (Figure 1B). This result indicated that DIP deficiency was the main limiting factor during this succession. However, small amounts of DIP enrichment did not change the phytoplankton community succession or delay the diatom community decline in our incubation experiments. Meanwhile, the DIP depletion in the initial did not stop the diatom blooms. This suggested that there may be another phosphate source to support phytoplankton growth. The DOP variation in our experiment also showed quick recycling of phosphate, which attests to the DOP’s function in the incubation process as a phosphate source, especially among the groups without DIP treatment (Table S1). It has been confirmed that both S. costatum and P. donghainese can use DOP efficiently, especially P. donghainese in DIP depletion (Ou et al., 2015). Dinoflagellates are typically able to hydrolyze a variety of organic compounds and sustain their growth via the recycling of metabolic products under conditions of inorganic nutrient limitation (Glibert et al., 2012). Meanwhile, organic nutrients released into the water in the diatom phases can also stimulate the growth of dinoflagellate (Glibert et al., 2012). The advantage of P. donghainese in utilizing DOP for growth is what allowed it to become abundant or even dominant in natural communities when DIP is depleted (Ou et al., 2015). This indicated that in natural diatom-to-dinoflagellate succession under DIP limitation, the role which DOP plays was indispensable (Huang et al., 2005; Nicholson et al., 2006; Fitzsimons et al., 2020).
The nutrient consumption during the succession process may be related to the ambient nutrient structure. The nutrient consumption structure was highly related to the nutrient structure in water (Figure 2). In the GP, the ratio of the consumption rates for DSi and DIN (v(DSi)/v(DIN) ratio) are positively correlated to the DSi/DIN ratio in all groups (Figure 2).The relative higher demands for DSi than DIN is owing to physiological change in diatoms under nutrient stress during photosynthesis (Sarmiento, 2006). Diatom cell growth under nutrient limitation have DSi/DIN ratios that can be many times higher than those from adequate nutrients (Claquin et al., 2002; Leynaert et al., 2004). This may cause a high v(DSi)/v(DIN) ratio which is higher than the Redfield ratio. On day 3 (the peak day of Chl a in the GP), the S. costatum biomass began to decline and gradually became an opportunistic species. Meanwhile, the v(DSi)/v(DIN) ratio was less than 1 as the DSi/DIN ratio declined. In the DP, extensive DSi regenerated from diatom detritus owing to the rapid and intense colonization of diatom detritus by bacteria (Amin et al., 2012). Large amounts of DSi were released into the water and the DSi/DIN ratio increased. However, due to the greater adaptability of dinoflagellates compared to diatoms under DIP limitation and low DIN concentrations (Wang et al., 2013; Olofsson et al., 2019), dinoflagellates can easily become the dominant species when diatoms decay. Therefore, the P. donghainese began to increase and started to dominate the phytoplankton community. It can be seen from the trend of DSi concentration that the DSi was re-used by residual diatoms in the RP. However, there was no significant relationship between the v(DSi)/v(DIN) ratio and DSi/DIN ratio, while the v(DSi)/v(DIN) ratio was always below 1 (Figure 2). Our results indicate that the v(DSi)/v(DIN) ratio tends to decrease during the succession. The significant variation of v(DSi)/v(DIN) ratio can be used to distinguish phytoplankton community dominated by diatoms from those dominated by non-siliceous species, with decreased ratios indicative of a succession from diatoms to non-siliceous species.
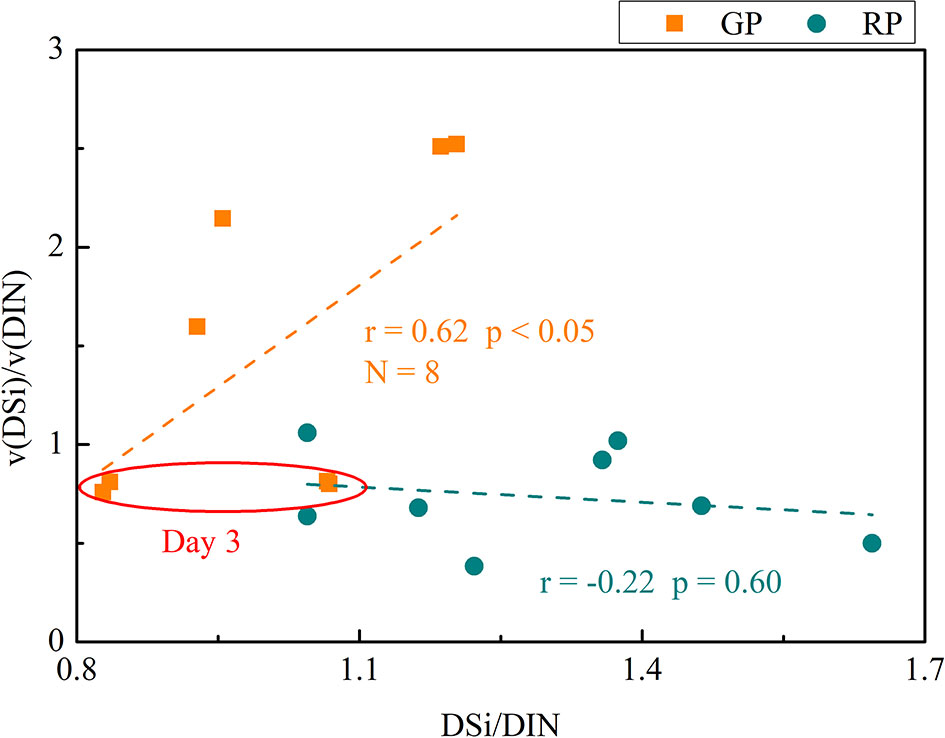
Figure 2 The relationship between the ratio of DSi and DIN consumption rate and DSi/DIN ratio. The points in red circle were the ratios of four group at the day 3.
Organic Carbon Dynamics During the Diatom-to-Dinoflagellate Succession on Bulk Level
Unlike wide studies on organic carbon dynamics which observe the phytoplankton community during the shift from a nutrient-replete to a nutrient-constrained condition, our experiments are designed under a nutrient-constrained condition throughout the community succession, which may obtain the new knowledge. Differences in predominant phytoplankton community members may lead to differences in organic carbon production (Conan et al., 2007). Our results also showed the different production of POC and DOC in varied communities (Figure S3). The accumulation rate of POC and DOC in the diatom-dominant stage was significantly higher than that in the dinoflagellate-dominant stage except group D during the succession process (Figures S3A, B). Meanwhile, the POC/DOC ratio (indicated by the slopes in Figure 3) indicated a significantly higher partition of net production into POC in the dinoflagellate-dominant stage than that in the diatom-dominant stage. These results were quite different from other results suggesting that DOC accounts for major fraction of organic carbon under nutrient depletion (Casareto et al., 2012). Many studies have suggested that the relative partitioning of net production to POC and DOC is not related to phytoplankton communities but related to nutrients available (Conan et al., 2007). The higher partitioning to DOC occurs in nutrient-limited condition (Conan et al., 2007; Casareto et al., 2012; Wyatt et al., 2014). These findings indicate that organic carbon dynamics is significantly different under different nutrient conditions. With the knowledge of either or both phytoplankton community composition and signatures of nutrient limitation, we can determine the environmental conditions that control the partitioning of organic carbon production. Although we cannot exclude the influence of phytoplankton communities, the inconsistency with previous studies may be related to nutrient-constraint conditions.
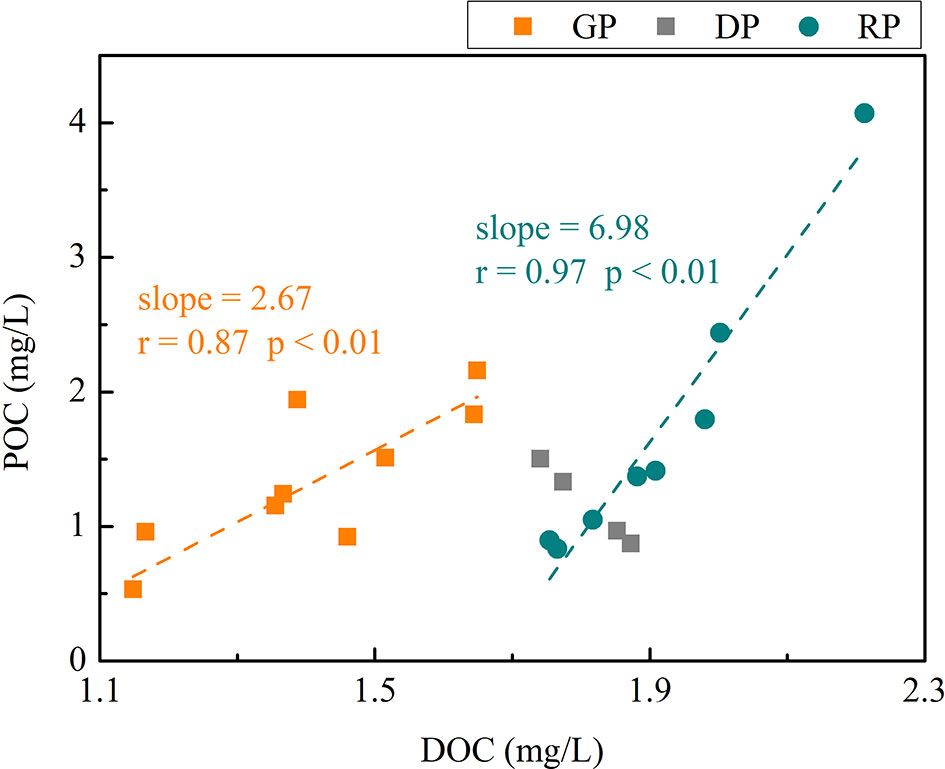
Figure 3 The relationship between DOC concentration and POC concentration in different algae phases of the four groups.
DOC production was related to nutrient conditions (Figure 4). Whereas our results indicated an association between DIP enrichment to the significant increase of phytoplankton biomass in groups B and D, the resulting DOC production associated therewith is insignificant (Figure 1 and Table S1). This result indicated that the increased biomass did not stimulate the DOC production. Enhanced production was likely closely coupled to the removal of organic carbon. The positive correlation between POC concentration and Chl a (r = 0.78, p < 0.01) indicates the POC production was related to phytoplankton growth. Combined with the weak relationship between DOC and Chl a (r = 0.33, p > 0.05), we suggested that the different partition of organic carbon production may be caused by the DOC recycling. Previous study has suggested that DOM plays an important role in nutrient regeneration (Zheng et al., 2021). Hence, the quick turnover of DOM may be the reason for the less pronounced organic carbon accumulation and the lower DOC portioning of organic carbon in dinoflagellate-dominant stage.
To evaluate the DOC production in different dominant algae species stages, the values of DOC production in different stages of diatoms and dinoflagellates were normalized by the initial day of each state (day 0 and day 6 for the diatom and dinoflagellate states, respectively). We marked this value as ΔDOC. The ΔDOCs were strongly related to nitrogen structure, although they had significantly different behaviors in different stages (Figure 4). In the diatom-dominant stage (GP and DP), the ΔDOC showed a positive correlation with the /DIN (Figure 4). The increase in /DIN indicated a rapid turnover of nitrogen compounds and the recycling of nitrogen, especially in the DP. In this stage, relatively abundant nutrients supported large amounts of regenerated from bacterial remineralization of DOM in waters (Killops and Killops, 2005). Along with the development of the algae succession, the DIN concentration gradually decreased and became an important factor modulating the growth of dinoflagellates. In the dinoflagellate-dominant stage (RP), although ΔDOC showed a similar positive correlation with /DIN, the utilization of nitrogen was quite different (Figure 4). While DOC accumulated, /DIN decreased. Due to the low concentration and the obvious dinoflagellate preference for (Li et al., 2010; Yamamoto et al., 2017), the /DIN decreased. Low DON concentrations in the RP suggested quick DON recycling and subsequent difficulty in the accumulation due to the lack of nitrogen (Table S1). This also caused a lower accumulation rate of DOC in the RP. Our results show that the nutrient is the main driver for the organic carbon dynamics during the diatom-to-dinoflagellate succession.
The accumulation of POC in algal cells are influenced by phytoplankton growth phases and nutrient conditions, leading to the dynamics of carbon-to-chlorophyll ratios (C: Chl a) (Figure 5). C: Chl a ratios in our experiments showed that the highest ratios were presented in bloom decay phases (day 6). While C: Chl a ratios are relatively low during the phytoplankton growth phases, and the community composition had a minor effect (ANOVA, p > 0.05). This result indicates that the C: Chl a is significantly influenced by phytoplankton growth phases but not species dominant stages under the nutrient limitation (Figure 5). The C: Chl a ratios in groups without DIP treatment are significantly higher than those in groups with DIP treatment during the late of the experiments (day 3-13) (ANOVA, p < 0.05). This indicated the C: Chl a ratio increased under more restricted nutrient conditions, and the physiological base is the accumulation of excess carbon at low nutrient conditions (Jakobsen and Markager, 2016). C: Chl a ratio is governed by a combination of growth phases and nutrient availability effects. These transient, magnitude changes during the diatom-to-dinoflagellate succession within several days present so-far-overlooked challenges for models of phytoplankton acclimation and geographically extensive production estimates based on satellite remote sensing (Spilling et al., 2014).
Bioavailability of DOM During the Diatom-to-Dinoflagellate Succession
Our experiments provide an excellent opportunity to examine how senescent bloom under nutrient stress affects the amount, bioavailability and fate of DOM that is produced. DOC accumulated in all phases during the incubation period, with compositional shifts indicated by CDOM parameters. The strong increase in DOC combined with insignificant trend in DON over RP suggests that the accumulated DOM became increasing C-rich as nutrients were depleted (Table S1). As the succession occurs, an increase in a350 as well as decreases in SUVA254 and S275-295 are observed (Table S1 and Figures 6A, B). This indicates a shift in DOM composition, specifically, an input of relatively fresh, high molecular weight (HMW) DOM (Del Vecchio and Blough, 2004; Berggren et al., 2009). In the Changjiang Estuary, substantial microbes are identified as heterotrophic functions (Wei et al., 2022). Heterotrophic bacteria responded quickly to consume the DOC to replenish nutrients (Zheng et al., 2021). In the GP, the values of SUVA254 and S275-295 were higher than that in DP and RP, which suggested the less bioavailability of DOM produced. Previous studies have presented that less labile DOM largely originates during the nutrient-stressed and senescent phases of diatoms (Wear et al., 2015). In both the DP and RP, there were significant decreases of SUVA254 and S275-295, indicating the production of a large amount of less aromatic and HMW DOC. The molecular size of DOM has implications for bacterial utilization of the DOM, as generally larger molecules suggest a high bioavailability of DOM and support higher bacterial growth and DOM utilization (Amon and Benner, 1996). This observation suggested that the newly produced DOM have a high potential for bacterial utilization of fresh allochthonous material (Asmala et al., 2013). The biological availability of DOM is a combination of the functioning of the microbes and environmental conditions, such as inorganic nutrient availability (Marín-Spiotta et al., 2014). The difference in DOM bioavailability in different phases may related to the nutrient condition. The more abundant nutrient condition (in the GP) allowed microbes to utilize CDOM more effectively. In the later stages of the experiments, the limitation of nutrients affected the microbial uptake of DOC due to a reduced assimilation of dissolved organic substrates when phosphorous was not available for microbes metabolism (Thingstad et al., 2008). On the other hand, the microbes shifts during the succession may also influence the DOM availability. Heterotrophic bacteria are the main consumers of the DOM produced by phytoplankton, and both diatoms and dinoflagellates have been associated to different bacterial communities (Camarena-Gómez et al., 2018). The growth of copiotrophic bacteria with high production rates in diatom-dominant communities may favor the recycling of carbon through the microbial loop (Camarena-Gómez et al., 2021), which may cause the low DOM bioavailability. In contrast, the increasing dinoflagellates seem to shift the bacterial community towards more oligotrophic generalist and reduce the bacterial production rates (Camarena-Gómez et al., 2021). Hence, the DOM bioavailability variation is the result of the interaction of phytoplankton community, nutrient availability and bacterial community.
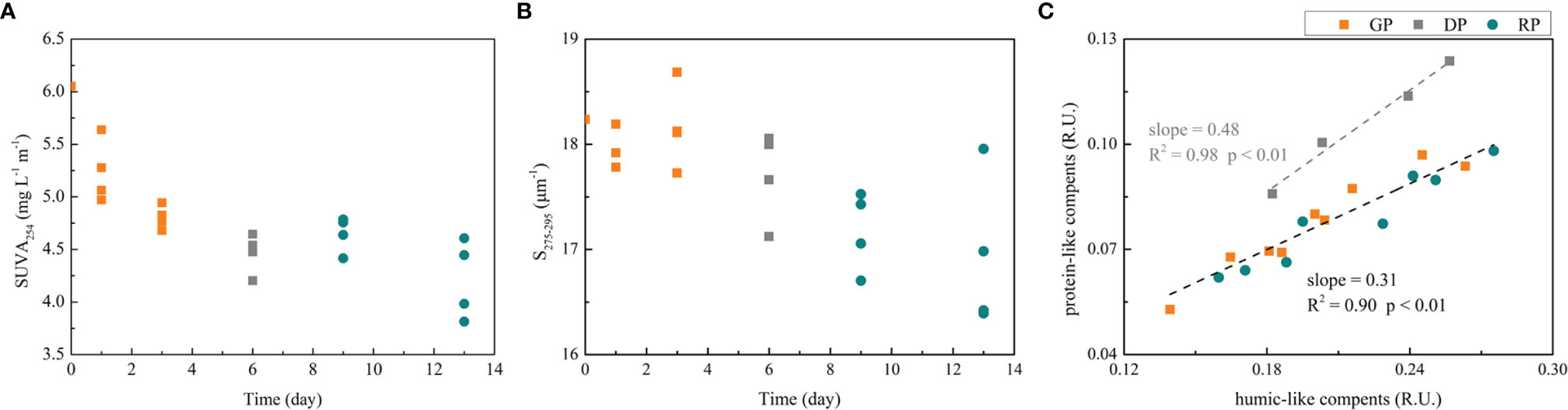
Figure 6 (A) The variation of SUVA254 in different algae phases of the four groups; (B) The variation of S275-295 in different algae phases of the four groups; (C) The relationship between protein-like components and humic-like components of the four groups.
The FDOM components showed no significant differences in the two different community growth phases (GP and RP), but contained more protein-like components in the degradation phase (DP). The higher ratio of protein-like components to humic-like components in the DP implied a higher bioavailability of DOM upon its release during diatom degradation (Figure 6C). In the DP, the DOC increase was associated with cell lysis and the subsequent release of intracellular DOC into the water. On the contrary, in the GP and RP, the DOC was mainly attributed to the extracellular release from live phytoplankton cells. Intracellular DOC is more biolabile than extracellular DOC (Bittar et al., 2015). There was no significant difference in the GP and RP, suggesting that the difference in DOM dynamics and bioavailability was not directly linked to group-specific phytoplankton extracellular release (Asmala et al., 2018). This observation was also evident in Spilling et al. (2014), where it was concluded that during the buildup of the bloom, differences between varying community dominance were far smaller than anticipated from monoculture studies. The quantity and characteristics of DOM varied differently in different phytoplankton incubation systems due to the nature of the bacterial community (Zhang et al., 2013; Kinsey et al., 2018; Shields et al., 2019). The observation of bacteria is not involved in this research; however, as it may provide more details to this phenomenon, such an observation is worthy of consideration in future research.
This study documents a novel relationship between instant nutrients and organic carbon responses derived from a natural diatom-to-dinoflagellate succession under nutrient-constrained condition in coastal regions as presented by a conceptual model in Figure 7. In coastal ecosystems, the coupling nutrients and organic carbon are important for ecosystem functioning. Changes in the phytoplankton communities may consequently lead to differences in the quantity and quality of organic matter export to the sea water, which in turn influences the biogeochemical cycling of nutrients. To have a better understanding of the seasonal phytoplankton community succession mechanisms, numerical modelling is an important approach to extract information from the complex field investigation data (Mutshinda et al., 2013). This study, which demonstrates synchronous shifts in organic carbon and nutrients responses over the diatom-to-dinoflagellate succession in detail, will provide new insight to improve the numerical modelling. For example, the significant effect of direct DOP assimilation in supporting the phytoplankton community, especially in DIP depletion condition of late bloom, is rarely considered in many ecology models. The changing bioavailability of DOM in different growth phases makes it unreasonable to use a constant rate for DOM mineralization in ecological modelling, which may cause an inaccurate estimate of organic carbon dynamics.
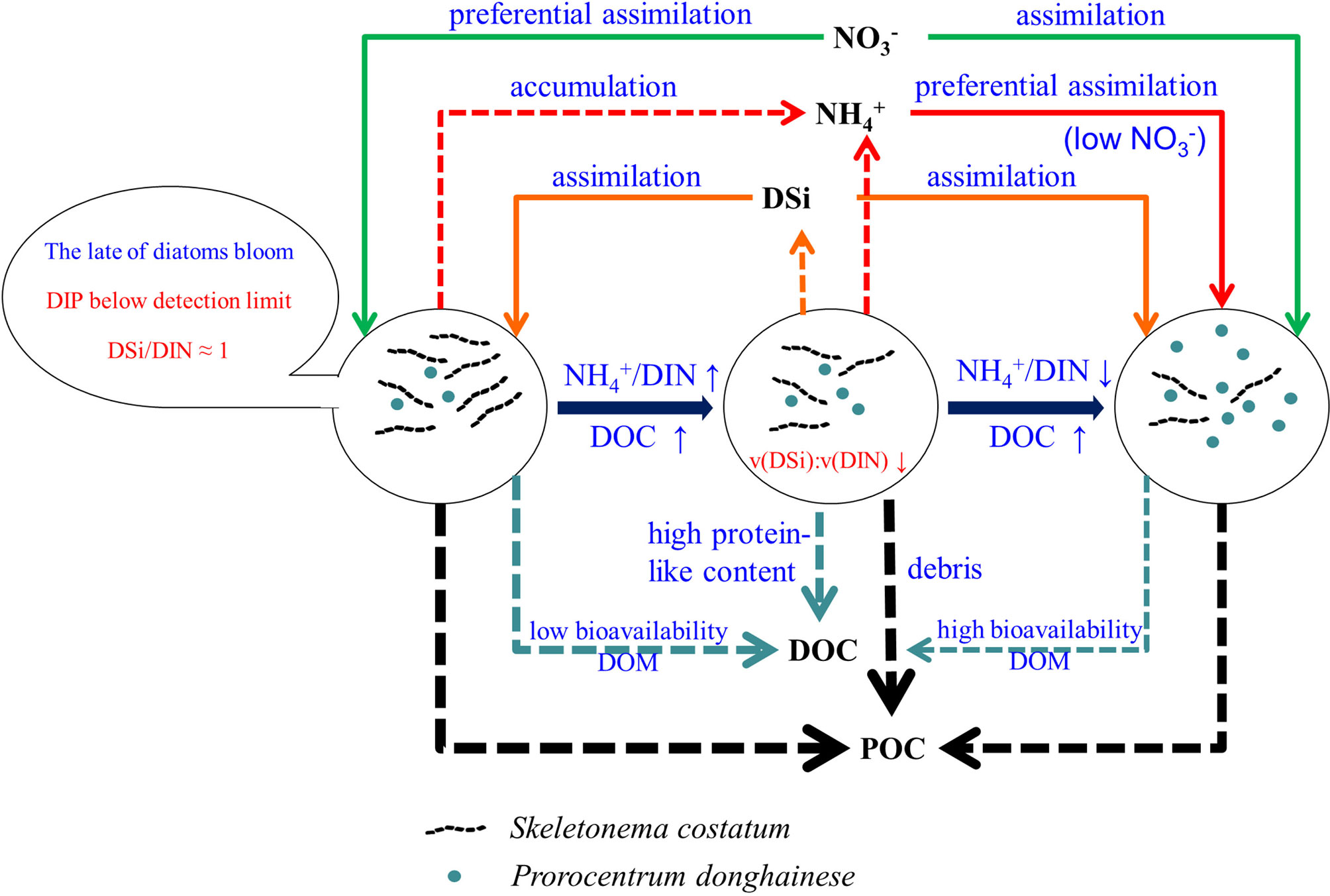
Figure 7 A conceptual model of phytoplankton community succession process in natural Changjiang estuary. The dotted line means an increase of matter and the solid line means a decrease of matter in the incubation system. Line thickness of arrows indicate relative amount of matter.
Conclusion
This incubation experiment successfully reproduced the succession of dominant phytoplankton species from diatoms to dinoflagellates in coastal regions receiving CDW. Nutrients and organic carbon, with concentrations of different chemical forms, in this process were well evaluated. The DIP limitation is the major factor of the diatom-to-dinoflagellate succession. In terms of consumption rates, the v(DSi)/v(DIN) ratio tends to decrease with the diatom decay. The organic carbon production was related to the phytoplankton community and nutrient structure. Due to the influence of nutrient availability, the relative partitioning of net production to DOC is higher in the diatom-dominant stage than that in the dinoflagellate-dominant stage. The relative partitioning of net production to POC is higher in the dinoflagellate-dominant stage than that in the diatom-dominant stage, which is driven by quick turnover of DOM related to nutrient structure in different stages. The production of DOC was influenced by nutrient structure. The accumulation of DOC was more closely related to the /DIN ratio and its fractional abundance in different phases was quite different. C: Chl a ratio is governed by a combination of growth phases and nutrient availability effects. As the succession occurs, DOM became more bioavailable as the result of the interaction of phytoplankton community, nutrient availability and bacterial community. DOM composition varied in different phases. The DOM released during diatom degradation containing more protein-like components than the growth phases. Differences of organic carbon production among varying community dominance were far smaller than different growth phases.
Data Availability Statement
The original contributions presented in the study are included in the article/Supplementary Material. Further inquiries can be directed to the corresponding author.
Author Contributions
XW: conceptualization, investigation, formal analysis, data curation, writing - original draft, and visualization. SJ: conceptualization, investigation, and writing - review & editing. YWu: conceptualization, resources, writing - review & editing, supervision, funding acquisition, and project administration. YWa: investigation. All authors contributed to the article and approved the submitted version.
Funding
This study was supported by the National Natural Science Foundation of China (grant numbers 41876074).
Conflict of Interest
The authors declare that the research was conducted in the absence of any commercial or financial relationships that could be construed as a potential conflict of interest.
Publisher’s Note
All claims expressed in this article are solely those of the authors and do not necessarily represent those of their affiliated organizations, or those of the publisher, the editors and the reviewers. Any product that may be evaluated in this article, or claim that may be made by its manufacturer, is not guaranteed or endorsed by the publisher.
Acknowledgments
Analytical measurements were carried out at the State Key Laboratory of Estuarine and Coastal Research. We acknowledge the technical support by the Zheyuke crew during the cruise. We thank Lijun Qi, Jinlong Dai and Dr. Yan Chang for helping collect the incubation water on board. We thank Kun Zhu for assisting with the sampling during the incubation experiment. We thank Anqiang Yang for helping identify the algae species. The assistance of all members of the organic geochemical group in sampling and daily experiments is appreciated.
Supplementary Material
The Supplementary Material for this article can be found online at: https://www.frontiersin.org/articles/10.3389/fmars.2022.845372/full#supplementary-material
References
Altman J. C., Paerl H. W. (2012). Composition of Inorganic and Organic Nutrient Sources Influences Phytoplankton Community Structure in the New River Estuary, North Carolina. Aquat. Ecol. 46, 269–282. doi: 10.1007/s10452-012-9398-8
Amin S. A., Parker M. S., Armbrust E. V. (2012). Interactions Between Diatoms and Bacteria. Microbiol. Mol. Biol. R 76, 667–684. doi: 10.1128/MMBR.00007-12
Amon R. M. W., Benner R. (1996). Bacterial Utilization of Different Size Classes of Dissolved Organic Matter. Limnol. Oceanogr. 41, 41–51. doi: 10.4319/lo.1996.41.1.004
Asmala E., Autio R., Kaartokallio H., Pitkänen L., Stedmon C. A., Thomas D. N. (2013). Bioavailability of Riverine Dissolved Organic Matter in Three Baltic Sea Estuaries and the Effect of Catchment Land Use. Biogeosciences 10, 6969–6986. doi: 10.5194/bg-10-6969-2013
Asmala E., Haraguchi L., Jakobsen H. H., Massicotte P., Carstensen J. (2018). Nutrient Availability as Major Driver of Phytoplankton-Derived Dissolved Organic Matter Transformation in Coastal Environment. Biogeochemistry 137, 93–104. doi: 10.1007/s10533-017-0403-0
Basu S., Mackey K. R. (2018). Phytoplankton as Key Mediators of the Biological Carbon Pump: Their Responses to a Changing Climate. Sustainability-Basel 10, 869. doi: 10.3390/su10030869
Behrenfeld M. J., Boss E. S., Halsey K. H. (2021). Phytoplankton Community Structuring and Succession in a Competition-Neutral Resource Landscape. SME Commun. 1, 12. doi: 10.1038/s43705-021-00011-5
Berggren M., Laudon H., Jansson M. (2009). Aging of Allochthonous Organic Carbon Regulates Bacterial Production in Unproductive Boreal Lakes. Limnol. Oceanogr. 54 (4), 1333–1342. doi: 10.4319/lo.2009.54.4.1333
Bittar T. B., Vieira A. A. H., Stubbins A., Mopper K. (2015). Competition Between Photochemical and Biological Degradation of Dissolved Organic Matter From the Cyanobacteria Microcystis Aeruginosa. Limnol. Oceanogr. 60, 1172–1194. doi: 10.1002/lno.10090
Camarena-Gómez M. T., Lipsewers T., Piiparinen J., Eronen-Rasimus E., Perez-Quemaliños D., Hoikkala L. (2018). Shifts in Phytoplankton Community Structure Modify Bacterial Production, Abundance and Community Composition. Aquat. Microb. Ecol. 81 (2), 149–170. doi: 10.3354/ame01868
Camarena-Gómez M. T., Ruiz-González C., Piiparinen J., Lipsewers T., Sobrino C., Logares R. (2021). Bacterioplankton Dynamics Driven by Interannual and Spatial Variation in Diatom and Dinoflagellate Spring Bloom Communities in the Baltic Sea. Limnol. Oceanogr. 66 (1), 255–271. doi: 10.1002/lno.11601
Carlson C. A., Hansell D. A. (2014). “DOM Sources, Sinks, Reactivity, and Budgets,” in Biogeochemistry of Marine Dissolved Organic Matter, 2nd ed. (Oxford: Elsevier Inc).
Casareto B. E., Niraula M. P., Suzuki Y. (2012). Dynamics of Organic Carbon Under Different Inorganic Nitrogen Levels and Phytoplankton Composition. Estuar. Coast. Shelf. S 102, 84–94. doi: 10.1016/j.ecss.2012.03.019
Chang Y., Wu Y., Zhang J., Wang X., Jiang S., Cao W., et al. (2021). Effects of Algal Blooms on Selenium Species Dynamics: A Case Study in the Changjiang Estuary, China. Sci. Total Environ. 768, 144235. doi: 10.1016/j.scitotenv.2020.144235
Claquin P., Martin Jézéquel V., Kromkamp J. C., Veldhuis M. J., Kraay G. W. (2002). Uncoupling of Silicon Compared With Carbon and Nitrogen Metabolisms and the Role of the Cell Cycle in Continuous Cultures of Thalassiosira Pseudonana (Bacillariophyceae) Under Light, Nitrogen, and Phosphorus Control1. J. Phycol. 38, 922–930. doi: 10.1046/j.1529-8817.2002.t01-1-01220.x
Conan P., Søndergaard M., Kragh T., Thingstad F., Pujo-Pay M., Williams P. J. L. B., et al. (2007). Partitioning of Organic Production in Marine Plankton Communities: The Effects of Inorganic Nutrient Ratios and Community Composition on New Dissolved Organic Matter. Limnol. Oceanogr. 52, 753–765. doi: 10.4319/lo.2007.52.2.0753
Del Vecchio R., Blough N. V. (2004). Spatial and Seasonal Distribution of Chromophoric Dissolved Organic Matter and Dissolved Organic Carbon in the Middle Atlantic Bight. Mar. Chem. 89, 169–187. doi: 10.1016/j.marchem.2004.02.027
Domingues R. B., Barbosa A., Galvao H. (2005). Nutrients, Light and Phytoplankton Succession in a Temperate Estuary (the Guadiana, South-Western Iberia). Estuar. Coast. Shelf. S 64, 249–260. doi: 10.1016/j.ecss.2005.02.017
Fitzsimons M. F., Probert I., Gaillard F., Rees A. P. (2020). Dissolved Organic Phosphorus Uptake by Marine Phytoplankton is Enhanced by the Presence of Dissolved Organic Nitrogen. J. Exp. Mar. Biol. Ecol. 530-531, 151434. doi: 10.1016/j.jembe.2020.151434
Furnas M., Mitchell A., Skuza M., Brodie J. (2005). In the Other 90%: Phytoplankton Responses to Enhanced Nutrient Availability in the Great Barrier Reef Lagoon. Mar. Pollut. Bull. 51, 253–265. doi: 10.1016/j.marpolbul.2004.11.010
Glibert P. M., Burkholder J. M., Kana T. M. (2012). Recent Insights About Relationships Between Nutrient Availability, Forms, and Stoichiometry, and the Distribution, Ecophysiology, and Food Web Effects of Pelagic and Benthic Prorocentrum Species. Hamful ALGAE 14, 231–259. doi: 10.1002/lno.10203
Grasshoff K., Kremling K., Ehrhardt M. (1999). Methods of Seawater Analysis, 3rd Edition. Weinheim, New York, Chichester, Brisbane, Singapore, Toronto: Wiley-VCH Verlag GmbH.
Hasegawa T., Kasai H., Ono T., Tsuda A., Ogawa H. (2010). Dynamics of Dissolved and Particulate Organic Matter During the Spring Bloom in the Oyashio Region of the Western Subarctic Pacific Ocean. Aquat. Microb. Ecol. 60, 127–138. doi: 10.3354/ame01418
Huang B., Ou L., Hong H., Luo H., Wang D. (2005). Bioavailability of Dissolved Organic Phosphorus Compounds to Typical Harmful Dinoflagellate Prorocentrum Donghaiense Lu. Mar. Pollut. Bull. 51, 838–844. doi: 10.1016/j.marpolbul.2005.02.035
Huang W., Zhu X., Zeng J., He Q., Xu X., Liu J., et al. (2012). Responses in Growth and Succession of the Phytoplankton Community to Different N/P Ratios Near Dongtou Island in the East China Sea. J. Exp. Mar. Biol. Ecol. 434, 102–109. doi: 10.1016/j.jembe.2012.08.008
Irwin A. J., Finkel Z. V., Müller-Karger F. E., Troccoli Ghinaglia L. (2015). Phytoplankton Adapt to Changing Ocean Environments. P Natl. Acad. Sci. U. S. A. 112, 5762. doi: 10.1073/pnas.1414752112
Jakobsen H. H., Markager S. (2016). Carbon-To-Chlorophyll Ratio for Phytoplankton in Temperate Coastal Waters: Seasonal Patterns and Relationship to Nutrients. Limnol. Oceanogr. 61 (5), 1853–1868. doi: 10.1002/lno.10338
Jiang S., Jin J., Zhang G., Chang Y., Zhang Z., Zhou M., et al. (2021). Nitrate in the Changjiang Diluted Water: An Isotopic Evaluation on Sources and Reaction Pathways. Chin. J. Oceanol. LIMN 39 (3), 830–845. doi: 10.1007/s00343-020-0149-8
Jiang S., Müller M., Jin J., Wu Y., Zhu K., Zhang G., et al. (2019). Dissolved Inorganic Nitrogen in a Tropical Estuary in Malaysia: Transport and Transformation. Biogeosciences 16, 2821–2836. doi: 10.5194/bg-16-2821-2019
Killops S., Killops V. (2005). Introduction to Organic Geochemistry, 2nd Edn (Paperback) (Oxford, UK: Blackwell Science Ltd).
Kinsey J. D., Corradino G., Ziervogel K., Schnetzer A., Osburn C. L. (2018). Formation of Chromophoric Dissolved Organic Matter by Bacterial Degradation of Phytoplankton-Derived Aggregates. Front. Mar. Sci. 4. doi: 10.3389/fmars.2017.00430
Lawaetz A. J., Stedmon C. A. (2009). Fluorescence Intensity Calibration Using the Raman Scatter Peak of Water. Appl. Spectrosc. 63, 936–940. doi: 10.1366/000370209788964548
Leblanc K., Quéguiner B., Diaz F., Cornet V., Michel-Rodriguez M., Durrieu De Madron X., et al. (2018). Nanoplanktonic Diatoms are Globally Overlooked But Play a Role in Spring Blooms and Carbon Export. Nat. Commun. 9, 953. doi: 10.1038/s41467-018-03376-9
Leynaert A., Bucciarelli E., Claquin P., Dugdale R. C., Martin Jézéquel V., Pondaven P., et al. (2004). Effect of Iron Deficiency on Diatom Cell Size and Silicic Acid Uptake Kinetics. Limnol. Oceanogr. 49, 1134–1143. doi: 10.4319/lo.2004.49.4.1134
Li J., Glibert P. M., Zhou M. (2010). Temporal and Spatial Variability in Nitrogen Uptake Kinetics During Harmful Dinoflagellate Blooms in the East China Sea. Harmful ALGAE 9, 531–539. doi: 10.1016/j.hal.2010.03.007
Lima-Mendez G., Faust K., Henry N., Decelle J., Colin S., Carcillo F., et al. (2015). Determinants of Community Structure in the Global Plankton Interactome. Science 348, 1262073. doi: 10.1126/science.1262073
Lin Y., Moreno C., Marchetti A., Ducklow H., Schofield O., Delage E., et al. (2021). Decline in Plankton Diversity and Carbon Flux With Reduced Sea Ice Extent Along the Western Antarctic Peninsula. Nat. Commun. 12, 1–9. doi: 10.1038/s41467-021-25235-w
Liu S., Qi X., Li X., Ye H., Wu Y., Ren J., et al. (2016). Nutrient Dynamics From the Changjiang (Yangtze River) Estuary to the East China Sea. J. Marine Syst. 154, 15–27. doi: 10.1016/j.jmarsys.2015.05.010
Marín-Spiotta E., Gruley K. E., Crawford J., Atkinson E. E., Miesel J. R., Greene S., et al. (2014). Paradigm Shifts in Soil Organic Matter Research Affect Interpretations of Aquatic Carbon Cycling: Transcending Disciplinary and Ecosystem Boundaries. Biogeochemistry 117, 279–297. doi: 10.1007/s10533-013-9949-7
Mo Y., Ou L., Lin L., Huang B. (2020). Temporal and Spatial Variations of Alkaline Phosphatase Activity Related to Phosphorus Status of Phytoplankton in the East China Sea. Sci. Total Environ. 731, 139192. doi: 10.1016/j.scitotenv.2020.139192
Murphy K. R., Stedmon C. A., Wenig P., Bro R. (2014). OpenFluor–an Online Spectral Library of Auto-Fluorescence by Organic Compounds in the Environment. Anal. Methods-UK 6, 658–661. doi: 10.1039/C3AY41935E
Mutshinda C. M., Finkel Z. V., Irwin A. J. (2013). Which Environmental Factors Control Phytoplankton Populations? A Bayesian Variable Selection Approach. Ecol. Model 269, 1–8. doi: 10.1016/j.ecolmodel.2013.07.025
Nicholson D., Dyhrman S., Chavez F., Paytan A. (2006). Alkaline Phosphatase Activity in the Phytoplankton Communities of Monterey Bay and San Francisco Bay. Limnol. Oceanogr. 51, 874–883. doi: 10.4319/lo.2006.51.2.0874
Olofsson M., Robertson E. K., Edler L., Arneborg L., Whitehouse M. J., Ploug H. (2019). Nitrate and Ammonium Fluxes to Diatoms and Dinoflagellates at a Single Cell Level in Mixed Field Communities in the Sea. Sci. Rep-UK 9, 1–12. doi: 10.1038/s41598-018-38059-4
Ou L., Huang X., Huang B., Qi Y., Lu S. (2015). Growth and Competition for Different Forms of Organic Phosphorus by the Dinoflagellate Prorocentrum Donghaiense With the Dinoflagellate Alexandrium Catenella and the Diatom Skeletonema Costatum Sl. Hydrobiologia 754 (1), 29–41. doi: 10.1007/s10750-014-1994-2
Ou L., Wang D., Huang B., Hong H., Qi Y., Lu S. (2008). Comparative Study of Phosphorus Strategies of Three Typical Harmful Algae in Chinese Coastal Waters. J. Plankton Res. 30, 1007–1017. doi: 10.1093/plankt/fbn058
Paerl H. W. (2006). Assessing and Managing Nutrient-Enhanced Eutrophication in Estuarine and Coastal Waters: Interactive Effects of Human and Climatic Perturbations. Ecol. Eng. 26, 40–54. doi: 10.1016/j.ecoleng.2005.09.006
Reader H. E., Stedmon C. A., Nielsen N. J., Kritzberg E. S. (2015). Mass and UV-Visible Spectral Fingerprints of Dissolved Organic Matter: Sources and Reactivity. Front. Mar. Sci. 2. doi: 10.3389/fmars.2015.00088
Richardson T. L., Jackson G. A. (2007). Small Phytoplankton and Carbon Export From the Surface Ocean. Science 315, 838–840. doi: 10.1126/science.1133471
Romera-Castillo C., Sarmento H., Alvarez-Salgado X. A., Gasol J. M., Marraséa C. (2010). Production of Chromophoric Dissolved Organic Matter by Marine Phytoplankton. Limnol. Oceanogr. 55, 446–454. doi: 10.4319/lo.2010.55.1.0446
Shields M. R., Bianchi T. S., Osburn C. L., Kinsey J. D., Ziervogel K., Schnetzer A., et al. (2019). Linking Chromophoric Organic Matter Transformation With Biomarker Indices in a Marine Phytoplankton Growth and Degradation Experiment. Mar. Chem. 214, 103665. doi: 10.1016/j.marchem.2019.103665
Spilling K., Kremp A., Klais R., Olli K., Tamminen T. (2014). Spring Bloom Community Change Modifies Carbon Pathways and C: N: P: Chl a Stoichiometry of Coastal Material Fluxes. Biogeosciences 11, 7275–7289. doi: 10.5194/bg-11-7275-2014
Spilling K., Olli K., Lehtoranta J., Kremp A., Tedesco L., Tamelander T., et al. (2018). Shifting Diatom—Dinoflagellate Dominance During Spring Bloom in the Baltic Sea and its Potential Effects on Biogeochemical Cycling. Front. Mar. Sci. 5. doi: 10.3389/fmars.2018.00327
Stedmon C. A., Bro R. (2008). Characterizing Dissolved Organic Matter Fluorescence With Parallel Factor Analysis: A Tutorial. Limnol. Oceanogr-Meth 6, 572–579. doi: 10.4319/lom.2008.6.572
Stedmon C. A., Markager S. (2005). Resolving the Variability in Dissolved Organic Matter Fluorescence in a Temperate Estuary and its Catchment Using PARAFAC Analysis. Limnol. Oceanogr. 50 (2), 686–697. doi: 10.4319/lo.2005.50.2.0686
Stedmon C. A., Markager S., Kaas H. (2000). Optical Properties and Signatures of Chromophoric Dissolved Organic Matter (CDOM) in Danish Coastal Waters. Estuar. Coast. Shelf. S 51, 267–278. doi: 10.1006/ecss.2000.0645
Teira E., Serret P., Fernández E. (2001). Phytoplankton Size-Structure, Particulate and Dissolved Organic Carbon Production and Oxygen Fluxes Through Microbial Communities in the NW Iberian Coastal Transition Zone. Mar. Ecol. Prog. Ser. 219, 65–83. doi: 10.3354/meps219065
Thingstad T. F., Bellerby R., Bratbak G., Børsheim K. Y., Egge J. K., Heldal M., et al. (2008). Counterintuitive Carbon-to-Nutrient Coupling in an Arctic Pelagic Ecosystem. Nature 455, 387–390. doi: 10.1038/nature07235
Tréguer P., Bowler C., Moriceau B., Dutkiewicz S., Gehlen M., Aumont O., et al. (2018). Influence of Diatom Diversity on the Ocean Biological Carbon Pump. Nat. Geosci. 11, 27–37. doi: 10.1038/s41561-017-0028-x
Wang B., Wang X., Zhan R. (2003). Nutrient Conditions in the Yellow Sea and the East China Sea. Estuar. Coast. Shelf. S 58, 127–136. doi: 10.1016/S0272-7714(03)00067-2
Wang X., Wu Y., Jiang Z., Ma Q., Zhang J., Liu S. (2017). Quantifying Aquaculture-Derived Dissolved Organic Matter in the Mesocosms of Sanggou Bay Using Excitation-Emission Matrix Spectra and Parallel Factor Analysis. J. World Aquacult. Soc. 48, 909–926. doi: 10.1111/jwas.12409
Wang J., Zhang Y., Li H., Cao J. (2013). Competitive Interaction Between Diatom Skeletonema Costatum and Dinoflagellate Prorocentrum Donghaiense in Laboratory Culture. J. Plankton Res. 35, 367–378. doi: 10.1093/plankt/fbs098
Wear E. K., Carlson C. A., James A. K., Brzezinski M. A., Windecker L. A., Nelson C. E. (2015). Synchronous Shifts in Dissolved Organic Carbon Bioavailability and Bacterial Community Responses Over the Course of an Upwelling-Driven 10.1111/Jwas.12409phytoplankton Bloom. Limnol. Oceanogr. 60 (2), 657–677. doi: 10.1002/lno.10042
Wei Y., Jiang S., Tian L., Wei L., Jin J., Ibánhez J. S. P., et al. (2022). Benthic Microbial Biogeography Along the Continental Shelf Shaped by Substrates From the Changjiang River Plume. Acta Oceanol. Sin. 41, 1–13. doi: 10.1007/s13131-021-1861-8
Weishaar J. L., Aiken G. R., Bergamaschi B. A., Fram M. S., Fujii R., Mopper K. (2003). Evaluation of Specific Ultraviolet Absorbance as an Indicator of the Chemical Composition and Reactivity of Dissolved Organic Carbon. Environ. Sci. Technol. 37 (20), 4702–4708. doi: 10.1021/es030360x
Wetz M. S., Wheeler P. A. (2003). Production and Partitioning of Organic Matter During Simulated Phytoplankton Blooms. Limnol. Oceanogr. 48, 1808–1817. doi: 10.4319/lo.2003.48.5.1808
Wu Y., Zhang J., Li D. J., Wei H., Lu R. X. (2003). Isotope Variability of Particulate Organic Matter at the PN Section in the East China Sea. Biogeochemistry 65, 31–49. doi: 10.1023/A:1026044324643
Wyatt K. H., Tellez E., Woodke R. L., Bidner R. J., Davison I. R. (2014). Effects of Nutrient Limitation on the Release and Use of Dissolved Organic Carbon From Benthic Algae in Lake Michigan. Freshw. Sci. 33, 557–567. doi: 10.1086/675453
Yamamoto T., Kishigami T., Nakagawa H. (2017). Uptake Kinetics of Nitrate, Ammonia and Phosphate by the Dinoflagellate Heterocapsa Circularisquama Isolated From Hiroshima Bay, J Apan. Phycol. Res. 65, 333–339. doi: 10.1111/pre.12193
Zhang G., Liang S., Shi X., Han X. (2015). Dissolved Organic Nitrogen Bioavailability Indicated by Amino Acids During a Diatom to Dinoflagellate Bloom Succession in the Changjiang River Estuary and its Adjacent Shelf. Mar. Chem. 176, 83–95. doi: 10.1016/j.marchem.2015.08.001
Zhang Y., Lin X., Shi X., Lin L., Luo H., Li L., et al. (2019). Metatranscriptomic Signatures Associated With Phytoplankton Regime Shift From Diatom Dominance to a Dinoflagellate Bloom. Front. Microbiol. 10. doi: 10.3389/fmicb.2019.00590
Zhang Y., Liu X., Wang M., Qin B. (2013). Compositional Differences of Chromophoric Dissolved Organic Matter Derived From Phytoplankton and Macrophytes. Org Geochem. 55, 26–37. doi: 10.1016/j.orggeochem.2012.11.007
Zheng Q., Lin W., Wang Y., Li Y., He C., Shen Y., et al. (2021). Highly Enriched N-Containing Organic Molecules of Synechococcus Lysates and Their Rapid Transformation by Heterotrophic Bacteria. Limnol. Oceanogr. 66, 335–348. doi: 10.1002/lno.11608
Zhou Y., Hu B., Zhao W., Cui D., Tan L., Wang J. (2018). Effects of Increasing Nutrient Disturbances on Phytoplankton Community Structure and Biodiversity in Two Tropical Seas. Mar. Pollut. Bull. 135, 239–248. doi: 10.1016/j.marpolbul.2018.07.033
Zhou Y., Yang X., Wang Y., Li F., Wang J., Tan L. (2021). Exogenous Nutrient Inputs Restructure Phytoplankton Community and Ecological Stoichiometry of Eastern Indian Ocean. Ecol. Indic. 127, 107801. doi: 10.1016/j.ecolind.2021.107801
Keywords: diatom-to-dinoflagellate succession, nutrient structure, organic carbon dynamic, dissolved organic matter characteristics, Changjiang Estuary
Citation: Wang X, Jiang S, Wu Y and Wang Y (2022) Synchronous Shifts in Nutrients and Organic Carbon Responses Over the Diatom-to-Dinoflagellate Succession. Front. Mar. Sci. 9:845372. doi: 10.3389/fmars.2022.845372
Received: 29 December 2021; Accepted: 07 March 2022;
Published: 24 March 2022.
Edited by:
Jie Xu, University of Macau, ChinaReviewed by:
Yuyuan Xie, University of South Florida, United StatesSarat Chandra Tripathy, National Centre for Polar and Ocean Research (NCPOR), India
Copyright © 2022 Wang, Jiang, Wu and Wang. This is an open-access article distributed under the terms of the Creative Commons Attribution License (CC BY). The use, distribution or reproduction in other forums is permitted, provided the original author(s) and the copyright owner(s) are credited and that the original publication in this journal is cited, in accordance with accepted academic practice. No use, distribution or reproduction is permitted which does not comply with these terms.
*Correspondence: Ying Wu, wuying@sklec.ecnu.edu.cn