- 1Chaire de recherche en géosciences côtières, Université du Québec à Rimouski, Rimouski, QC, Canada
- 2Québec-Océan, Université Laval, Québec, QC, Canada
- 3Centre d’études Nordiques (CEN), Université du Québec à Rimouski, Rimouski, QC, Canada
- 4Laboratoire de la STEPPE-ETS, École de technologie supérieure, Montréal, QC, Canada
Coastal socio-ecological systems are complex adaptive systems with nonlinear changing properties and multi-scale dynamics. They are influenced by unpredictable coastal hazards accentuated by the effects of climate change, and they can quickly be altered if critical thresholds are crossed. Additional pressures come from coastal activities and development, both of which attracting stakeholders with different perspectives and interests. While coastal defence measures (CDMs) have been implemented to mitigate coastal hazards for centuries, a lack of knowledge and tools available to make informed decision has led to coastal managers favouring the choice of seawalls or rock armours with little consideration for socio-ecological systems features, and stakeholders’ priorities. Though it is not currently widely applied in coastal zone management, multicriteria decision analysis (MCDA) is a tool that can be useful to facilitate decision making. PROMETHEE, an outranking method, was chosen to support the multicriteria decision analysis for the evaluation of CDMs in the context of four study sites characterized by distinct environmental features. The aim was to determine the relevance and benefits of a MCDA by integrating coastal zone stakeholders in a participatory decision-making process in order to select CDMs that are better adapted to the whole socio-ecological system. First, in a series of five workshops, stakeholders were asked to identify and weigh criteria that were relevant to their local conditions. Second and third, CDMs were evaluated in relation to each criterion within the local context, then, hierarchized. Initial results show that vegetation came first in three of the four sites, while rock armour ranked first in the fourth site. A post-evaluation of the participatory process indicated that the weighting phase is an effective way to integrate local knowledge into the decision-making process, but the identification of criteria could be streamlined by the presentation of a predefined list from which participants could make a selection. This would ensure criteria that are standardized, and in a format that is compatible with the MCDA. Coupled with a participatory process MCDA proved to be a flexible methodology that can synthetize multiple aspects of the problem, and contribute in a meaningful way to the coastal engineering and management decision-making process.
1 Introduction
In most regions of the world, the economic and social benefits in coastal zones tend to increase when population grows together with industrial and recreational activities (Airoldi et al., 2005; Dugan et al., 2011; Gittman et al., 2015). This attracts stakeholders with different perspectives and interests. As any socio-ecological system, coastal environments are complex adaptive systems with nonlinear changing properties and multi-scale dynamics that can be quickly and even irreversibly altered if critical thresholds are crossed. They are affected by multiple drivers of change, and subject to reciprocal feedbacks between social and natural components (Holling and Gunderson, 2002; Gallopín, 2006; Folke, 2016). Coastal systems are also influenced by uncertain coastal hazards, such as erosion and flooding, which are accentuated by the effects of climate change (Nicholls and Cazenave, 2010; Church and White, 2011; Wong et al., 2014; Ranasinghe, 2016).
Coastal defence measures (CDMs) have been implemented to mitigate coastal hazards ever since the establishment of human settlements in coastal zones (Charlier et al., 2005). In the last decades, coastal zone development and the effects of climate change have led to an increase in shoreline armouring, mainly through the implementation of hard coastal defence structures (seawalls and rock armours) (Bernatchez and Fraser, 2012; Sauvé et al., 2020), which can have a significant impact on coastal socio-ecological systems (Moschella et al., 2005; Dugan et al., 2011). In the Canadian province of Quebec, on the coasts of the St. Lawrence Estuary and Gulf, coastal defence structures were implemented as emergency measures, between the 1980s and the early 2000s, and it is still the case today, though to a lesser extent (Boyer-Villemaire et al., 2015). A lack of knowledge and tools available to make informed decisions has led to coastal managers favouring the choice of seawalls or rock armours over other types of CDMs (Friesinger and Bernatchez, 2010; Drejza et al., 2011; Marie et al., 2017). In addition, incomplete scientific knowledge with regard to CDMs effects on different types of coasts, an imbalance in scientific studies worldwide (Sauvé et al., 2022), and uncertainties brought about by varying climate projections (Polasky et al., 2011), all add complexity to the decision-making process, and most certainly play a part in the reluctance by coastal managers to explore lesser known alternatives.
Knowledge and learning process are key to improving the resilience of coastal socio-ecological systems, and overcoming difficulties and uncertainties associated with the complex, and sometimes conflictual environmental management issues (Folke et al., 2005; Garmendia et al., 2010; Koontz et al., 2015). Decision-making, traditionally the sole responsibility of scientists and experts, today tends to involve different stakeholders in an attempt to improve the transparency and flexibility of the process, and to implement measures that are better adapted to the whole socio-ecological system (Reed, 2008; Garmendia et al., 2010; Jones et al., 2014; Marttunen et al., 2017). A participatory process, implemented at an early stage by combining local interests and needs with scientific knowledge, leads to interventions that are better adapted to local socio-cultural and environmental conditions, and allows easier and more accurate monitoring and managing of environmental changes by local communities (Reed, 2008; Jacob et al., 2021).
The decision-making process can also be enhanced by the use of decision support systems which are developed to improve the understanding of complex problems (Westmacott, 2001; National Research Council, 2009). Multicriteria decision analysis (MCDA) is one of the support systems used to facilitate decision making in cases where a variety of alternatives are possible, and depend on multiple and sometimes conflicting criteria. It is based on a pairwise comparison between different alternatives rated against every criterion from a set of pre-defined decision criteria (Scott et al., 2012; Marttunen et al., 2017). Criteria are parameters used to assess how each scenario would contribute to the achievement of a project objective (André et al., 2010). The criteria cover multiple aspects of the issue, while taking into consideration the needs and expectations of local stakeholders (Garmendia et al., 2010). Within the decision-making process, once the problem, the context, and the objective have been established, MCDA is applied, and generally consists of three steps: criteria identification and weighting; scenario evaluation according to each criterion; and scenarios hierarchization (Dodgson et al., 2009). The aim of criteria identification is to select a complete set of criteria that are mutually independent, without duplication, applicable to the local context, and consistent with effects occurring over time (De Bruin et al., 2009). In the scientific literature, criteria identification is typically undertaken by scientists or experts (e.g. Monterroso et al., 2011; Trutnevyte et al., 2011; Chang et al., 2012) or, in more advanced participatory processes, by stakeholders through questionnaires, workshops, etc. (e.g. Stagl, 2006; Garmendia et al., 2010; Antunes et al., 2011; Garmendia and Gamboa, 2012; Trutnevyte et al., 2012). Criteria weighting can be defined as the measure of the importance of criteria according to stakeholders, experts, and scientists (Stagl, 2006; Garmendia and Gamboa, 2012). A group of stakeholders can agree on a set of criteria without attaching the same importance to each criterion (Garmendia and Gamboa, 2012). For the evaluation of scenarios according to each criterion, and for the scenarios hierarchization, MCDA can be divided into three types of methodology: complete aggregation methods, outranking methods, and iterative, trial-error methods (Maystre et al., 1994; Gamper and Turcanu, 2007; André et al., 2010). Complete aggregation methods allow the comparison of scenarios by aggregating all criteria into a single, synthetized, and exhaustive performance vector (André et al., 2010). Outranking methods, through a preferential reference system, compare scenarios against a set of predefined criteria (Gamper and Turcanu, 2007; André et al., 2010). Iterative, trial-error methods are based on a process that explores the feasibility of scenarios as discussed in successive dialogues with decision-makers (Gamper and Turcanu, 2007). Outranking methods are best suited to holistic land management because they take into account all stakeholders concerns, and integrate them into the analysis (Garmendia and Gamboa, 2012). MCDA outranking methods, such as PROMETHEE and ELECTRE, are generally applied to solve discrete choice problems by focusing on pairwise comparisons between different options (Belton and Stewart, 2002).
While MCDA has been used in many environmental management contexts (Gamper and Turcanu, 2007; Ananda and Herath, 2009) and in engineering problem solving in a marine context (Tavra et al., 2017; Zafirakou et al., 2018; Jajac et al., 2019; Abdel-Basset et al., 2021), to the authors’ knowledge, it has not been applied to the evaluation and hierarchization of CDMs. From the review of scientific literature, it appears that cost-benefit analysis (CBA) has been the only decision support system used in such context (Polomé et al., 2005). CBA is based on the evaluation of alternatives in terms of monetary units. It is fairly intuitive and straightforward for some aspects of the coastal system, but it is not appropriate or sophisticated enough when intangible and non-monetary characteristics, like aesthetic values or ecological impacts, are criteria identified as important factors in the decision-making process. The process of monetization leads to giving a monetary value to social or environmental non-market components (McCauley, 2006; Chan et al., 2012; Bryce et al., 2016). In contrast, MCDA is based on evaluation units that are specific to each of the selected criterion, which is one of the reasons why several European Union countries and United Nations’ documents recommend the use of MCDA rather than CBA (Gamper and Turcanu, 2007).
Based on the results of an extensive participatory process held in Eastern Quebec, the aim of the present study is to determine the relevance of a multi-criteria decision analysis (MCDA) as a tool to structure and analyze a complex problem related to the selection of CDMs, while taking into account their effect on the socio-ecological system. The study also aims at assessing the benefits of a participatory decision-making process, which involves coastal zone managers and professionals in the identification and weighting of criteria used for the selection of coastal defence measures.
2 Methods
2.1 Study Sites
The studies were carried out in the Canadian province of Quebec, on the coasts of the Estuary and Gulf of St. Lawrence (EGSL). Four municipalities, characterised by distinct geomorphological, hydrodynamic, ecological or socio-economic features (Figure 1), were selected as study sites: Pessamit, Gallix (Sept-Îles), Cap-des-Rosiers (Gaspé), and Baie-des-Capucins (Cap-Chat) (Figure 2).
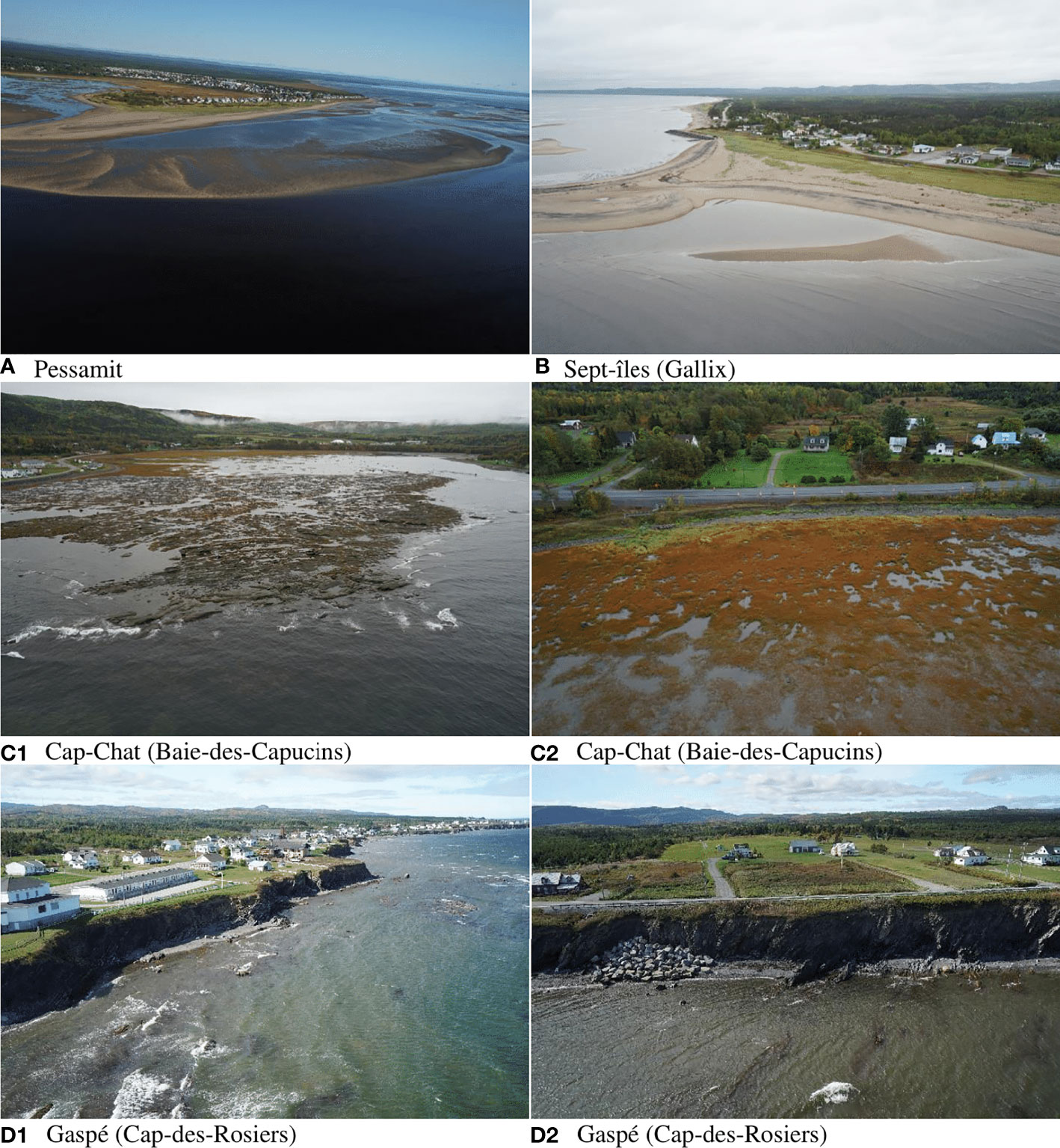
Figure 1 Study sites. (A) Pessamit: indigenous community located on a sandy littoral spit with wide unvegetated sandy foreshore. (B) Sept-Îles (Gallix): sandy beach terrace with a narrow band of sea cabbage characterised by the formation and disappearance of a sandy triangular salient. (C1, C2) Cap-Chat (Baie-des-Capucins): beach terrace of coarse sand, cobbles and pebbles, fronted by a wide vegetated salt marsh. (D1, D2) Gaspé (Cap-des-Rosiers): rocky cliff with an unconsolidated top and a lower foreshore of cobbles partially covered by rockweeds.
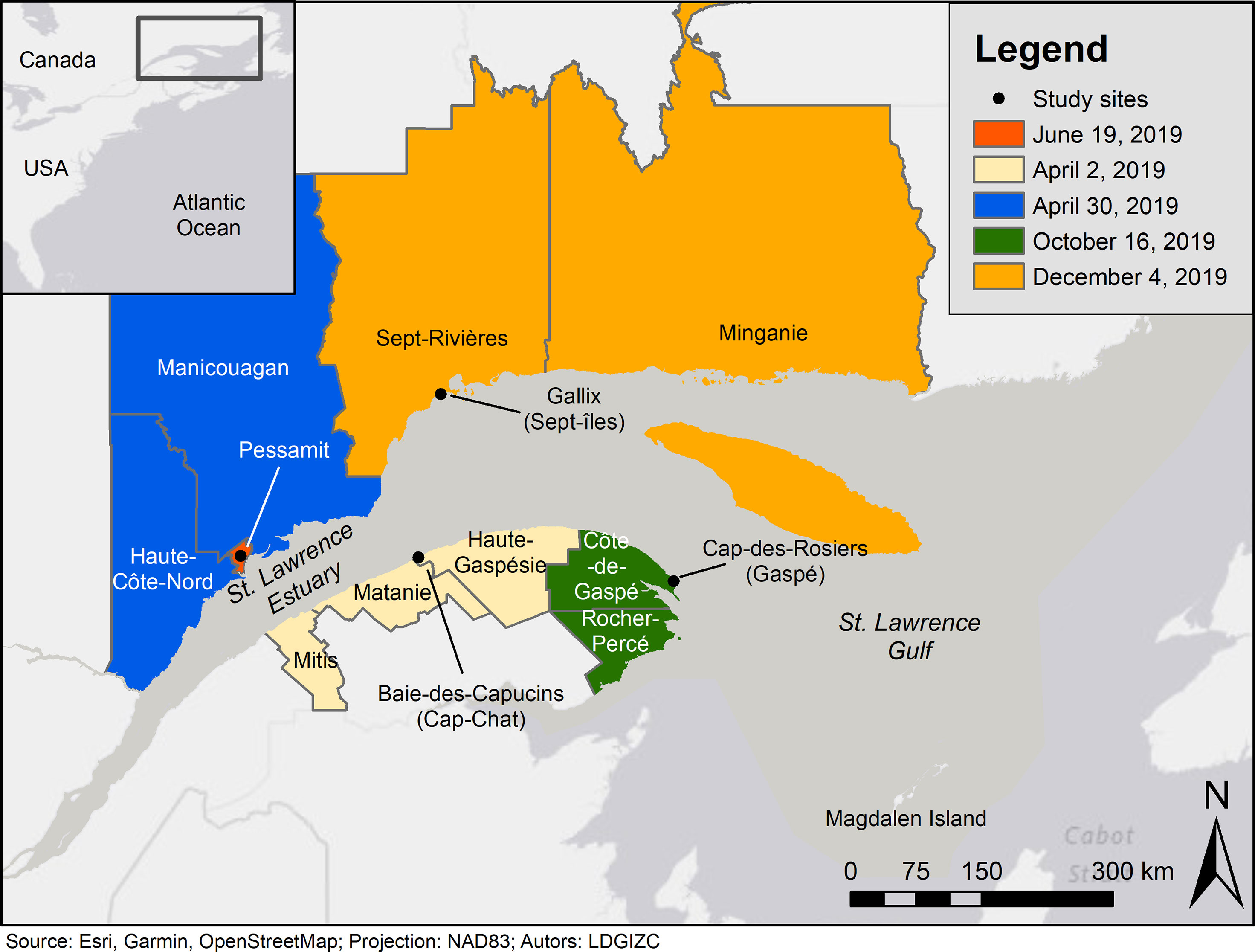
Figure 2 Sites and MRCs from Eastern Quebec included in the study, with the workshops’ dates in the legend.
2.2.1 Pessamit
Pessamit is an indigenous community located on the North Shore of the St. Lawrence maritime estuary. Pessamit’s coast, which extends over a 12 km span, is mainly composed of unconsolidated cliffs (53.7%), littoral spit (21.0%), salt marsh (20.7%), and beach terrace (4.6%). The tidal range is mesotidal and the offshore significant wave height (95th percentile) is 0.85 m (depth of 132 m). The study site is located on a sandy littoral spit. A wide unvegetated sandy foreshore is present in front of the coast. The study site is a part residential, and part public sector, highly frequented by the local community for a variety of uses and activities: off-road vehicles, boat launching, gatherings, walking, beach activities, archeological site, waterfowl concentration area, etc.
2.1.2 Gallix (Sept-Îles)
Sept-Îles is located on the North Shore of the Gulf of St. Lawrence. The study site, Gallix, is located in the Sainte-Marguerite Bay, which extends along 26.90 km, and is mainly composed of littoral spit (10.4 %), unconsolidated cliff (47.0 %), beach terrace (41.8 %), and rocky shore (0.8 %). The tidal range is mesotidal and the offshore significant wave height (95th percentile) is 0.75 m (depth of 90 m) (Corriveau et al., 2021). The study site is located on a sandy beach terrace, and is characterised by the formation and disappearance of a sandy triangular salient (Figure 3). This dynamic is generated by estuarian currents and littoral drift. Storm events, between 2009 and 2017, have caused high shoreline retreat (7.96 m/yr), leading to a shoreline enlargement on the west side of the coastal sector (Figure 3). A new salient slightly to the east was formed between 2013 and 2016, and was still present in 2017. That new salient modifies the longitudinal sediment transport processes, leading to an offshore sediment deviation. Sediments are redirected towards the coast, further to the West, resulting in a sediment progradation in the coastal sectors (Corriveau et al., 2019). A rock armour structure is present between the salient formed in 2013-2016, and the high shoreline retreat sector (Figure 3). The sandy lower foreshore is partially covered (0-25 %) by a narrow band of macroalgua (mainly laminaria longicruris). A bar system is also present in front of the study site. The study site is a residential sector, with high scenic and socio-cultural values, and is mainly used by the local community for fishing, nautical and beach activities, gatherings, walking, etc. It is also a waterfowl concentration area, and spawning ground for capelin.
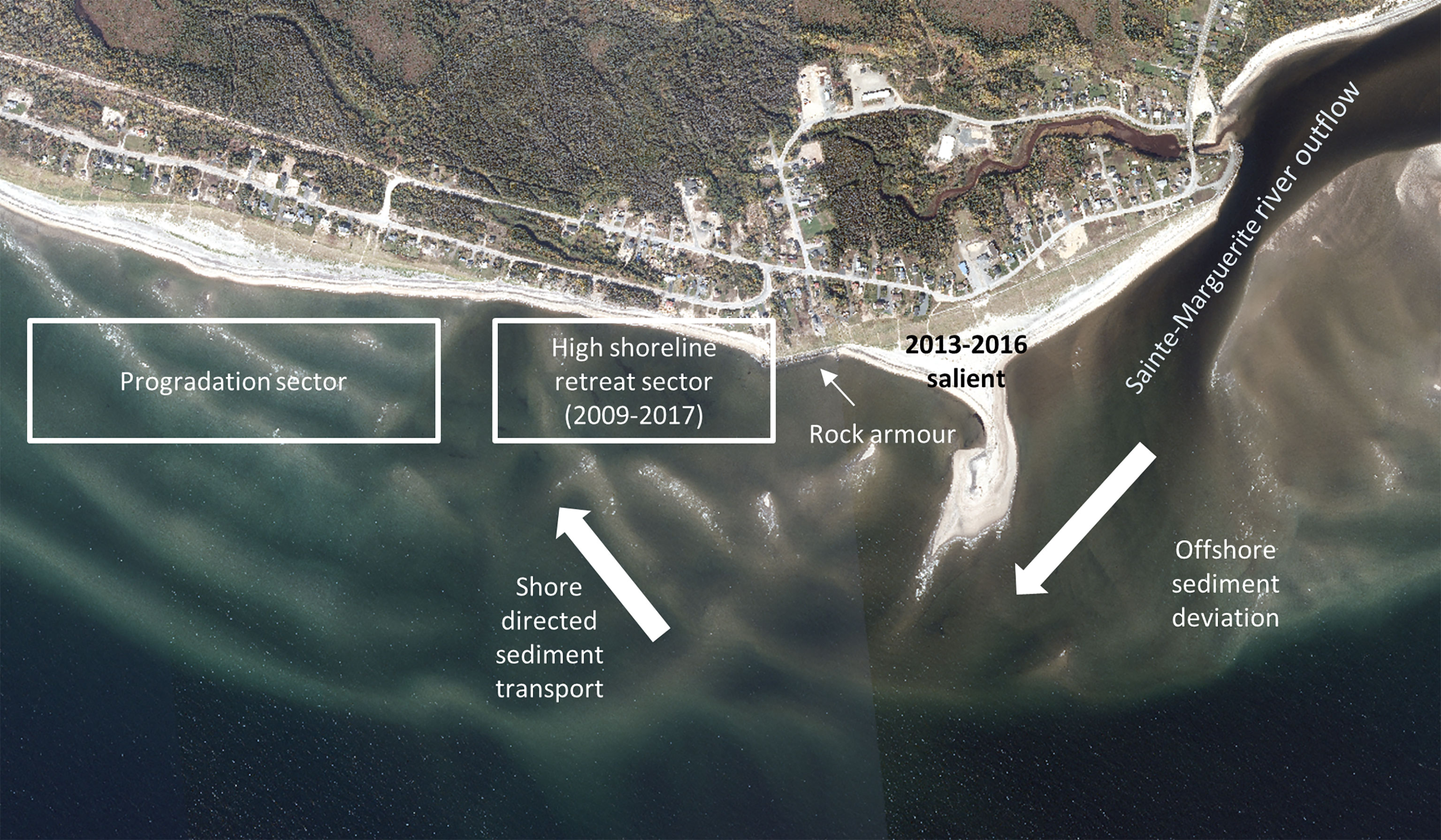
Figure 3 Coastal dynamic illustration from the Gallix study site [modified from Corriveau et al. (2019)].
2.1.3 Baie-des-Capucins (Cap-Chat)
Cap-Chat is located on the Gaspé Peninsula on the south shore of the St. Lawrence river. Baie-des-Capucins, a bay located east of Cap-Chat, extends over approximately 3.2 km, and is mainly composed of beach terrace (54.4 %), unconsolidated cliff (15.0 %), rocky cliff (10.3 %), and salt marsh (8.1 %). The study site is located on a beach terrace composed of a mixture of coarse sand, cobbles and pebbles, and by a wide salt marsh vegetated with spartina alterniflora. The bay’s entrance is partially (1-25%) vegetated with Zostera marina and Fucus sp. The tidal range is mesotidal and the wave energy is low. The littoral drift is directed towards the inside of the bay. The site is a biodiversity hotspot, and the main activities are nautical activities, walking and fishing. The national road 132 is, in some areas, less than 5 metres from the shoreline.
2.1.4 Cap-des-Rosiers (Gaspé)
Gaspé is located on the Gaspé Peninsula on the south shore of the Gulf of St. Lawrence. Cap-des-Rosiers, a former village annexed to the town of Gaspé, is located at the far north-east end of the Gaspé peninsula. The study site is located on a rocky cliff stretch of coast with an unconsolidated lower (cobbles) foreshore and a rocky infralittoral zone. The lower foreshore is partially (0-25%) covered by macroalgua (mainly fucaceae). The tidal range is mesotidal and the offshore significant wave height from ESE-SE reached more than 3 m between November 2017 and 2019 (Savoie-Ferron et al., 2020). The study site is a residential sector with low density activities. Still, tourism infrastructures, such as motels, are present in Cap-des-Rosiers due to the proximity of the Forillon National Park, which brings a high volume of tourism during the summer season. The main activities are gatherings and relaxation. Cap-des-Rosiers is a biodiversity hotspot, and has a high socio-cultural value with, among others, the Cap-des-Rosiers lighthouse, the tallest in Canada. The national road 132 is, in some areas, less than 5 metres from the cliff.
2.2 Selection of Coastal Defence Measures to be Evaluated
CDMs that were suitable for each of the four study sites were pre-selected, either by a comity of experts, or by using a coastal defence measure identification algorithm (CDMIA) developed by Sauvé et al. (2022). The number of CDMs can vary from site to site (Table 1). The CDMIA processed information that was drawn from 411 published scientific case studies, which included 1709 statements on the effects of CDMs on the environment as observed by the authors of the studies. It then established a correspondence between user-selected environmental features, and those stocked in the database, and it evaluated user-selected CDMs in relation to the specified coastal characteristics by identifying, collating, and rating their effects as observed in similar contexts. Since few CDMs studies have been conducted on rocky cliffs, the CDMs deemed suitable for the terrain at Cap-des-Rosiers, were selected by a comity of experts, instead of through the CDMIA. In the case of Baie-des-Capucins which is characterized by two types of coasts, a beach terrace and a salt marsh, the results of the CDMIA from both types of coasts were combined to select CDMs adapted to such conditions. Also, in Baie-des-Capucins, the low-crested breakwater scenario was based on a living shoreline rock sill concept (Bilkovic et al., 2017). The selected CDMs were then evaluated with the use of a MCDA methodology.
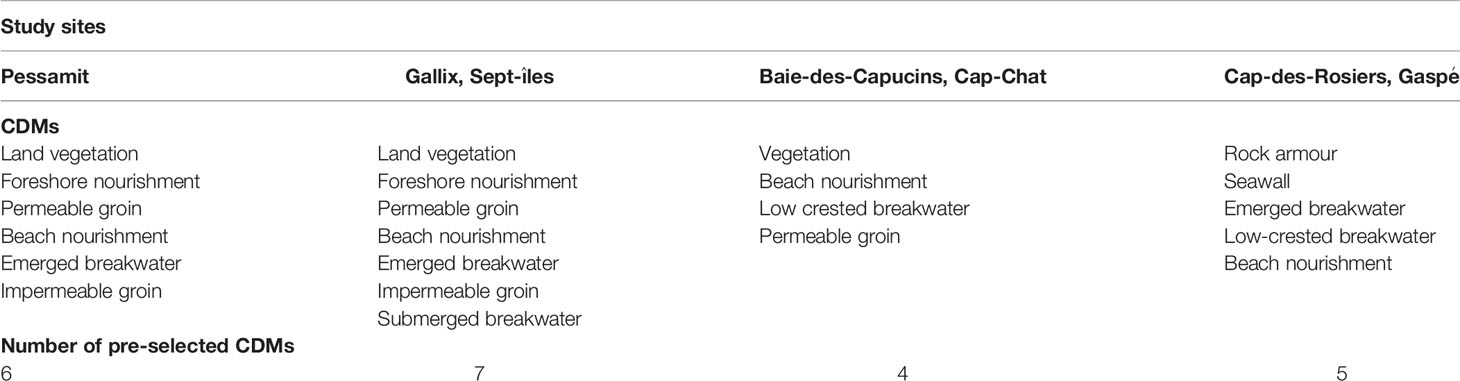
Table 1 CDMs pre-selected for 3 of the study sites using Sauvé et al., (2022) CDMIA, and in the case of Cap-des-Rosiers, selected by a comity of experts, with the number of CDMs per site.
2.3 Multi-Criteria Decision Analysis
For the multi-criteria decision analysis, an outranking method was preferred, as it allows an evaluation between scenarios that initially do not appear to be comparable with each other, and it maintains ranking units that are specific to each criterion (Gamper and Turcanu, 2007; Garmendia and Gamboa, 2012). The PROMETHEE method was chosen as multi-criteria decision-making tool, using the VISUAL PROMETHEE software (VPSolutions, 2013). It was preferred for its stability (Brans et al., 1986), and because it is widely used in environmental management contexts (Behzadian et al., 2010).
2.3.1 PROMETHEE Method
The PROMETHEE method is based on a pairwise comparison between different alternatives, following their assessment A = {a1, a2, …, an} against each criterion ck (Δk(ai, aj)) from a defined set of criteria C = {c1, c2, …, cm} (equation 1). The variations in the results of the assessments Δk(ai, aj) associated with criterion ck, are translated into a preference index P[Δk(ai, aj)] through a preference function, which lies between 0 and 1, 1 being a strong preference, and 0 meaning no preference. In this study, the usual preference function was used; it corresponds to the optimization of values without threshold, that is, larger values are better than lower ones. The multicriteria index π(ai, aj) is the weighted sum of the preference index P, and is calculated by dividing the preference index by the weight wk, which is a measure of the importance of the criterion ck determined by workshop participants (equation 2). The leaving Φ+(ai) and entering Φ-(ai) flows are then calculated in relation to the multicriteria index. The leaving flow expresses the extent to which ai outranks all other alternatives (equation 3), and the entering flow expresses how much ai is outranked by all other alternatives (equation 4). Thus, the best alternative has the highest leaving flow and the lowest entering flow. The net flow is the sum of the leaving and entering flows and represents an overall ranking. All criteria were set as maximum with the exception of the criteria whose evaluation was based on the 5 points impact qualitative scale (geomorphological effects, ecological effects and aesthetics). The specificities of the PROMETHEE method are described in the works of Brans et al. (1986) and in the Visual PROMETHEE software’s manual (VPSolutions, 2013).
2.3.2 Criteria Identification and Weighting
Coastal zone stakeholders were consulted in the course of two action research projects, with the aim of developing tools to improve coastal planning and protection, and to facilitate the choice of solutions adapted to climate change, in the short, medium and long terms. First, four workshops were organized between April and December 2019, in Eastern Quebec, in the context of the Coastal Resilience project. Stakeholders who were invited to the workshops included administrative personnel and professionals from local municipalities and coastal MRCs (regional county municipalities), relevant ministries, local and regional organizations, and members of the First Nations (Table 2). Second, in June 2019, in the context of a project entitled Identification de solutions d’adaptation aux aléas côtiers pour augmenter la résilience des communautés des Premières Nations dans un contexte de changements climatiques, a workshop was organized in Pessamit. Participants to this workshop comprised administrative personnel and professionals from the community. Through various activities, one of the aims was to integrate stakeholders into the decision-making process leading to the implementation of coastal defence measures, by asking them to identify and weigh the CDMs selection criteria (Figure 4). The locations of the four study sites and five workshops are shown in Figure 2.
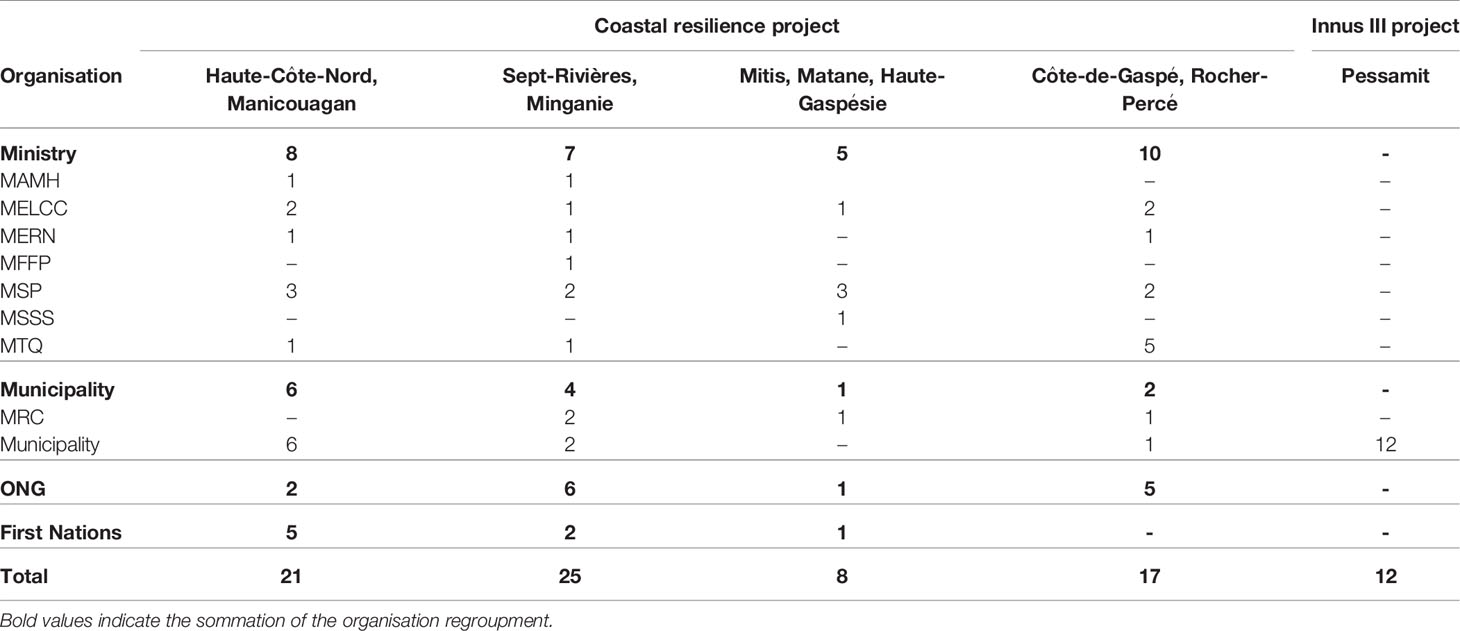
Table 2 Number of participants per organisation (MAMH, Ministry of Municipal Affairs and Housing; MELCC, Ministry of Environment and Climate Change; MERN, Ministry of Energy and Natural Resources; MFFP, Ministry of Forests, Wildlife and Parks; MSP, Ministry of Public Security; MSSS, Ministry of Health and Social Services; MTQ, Ministry of Transports; MRC, regional county municipality).
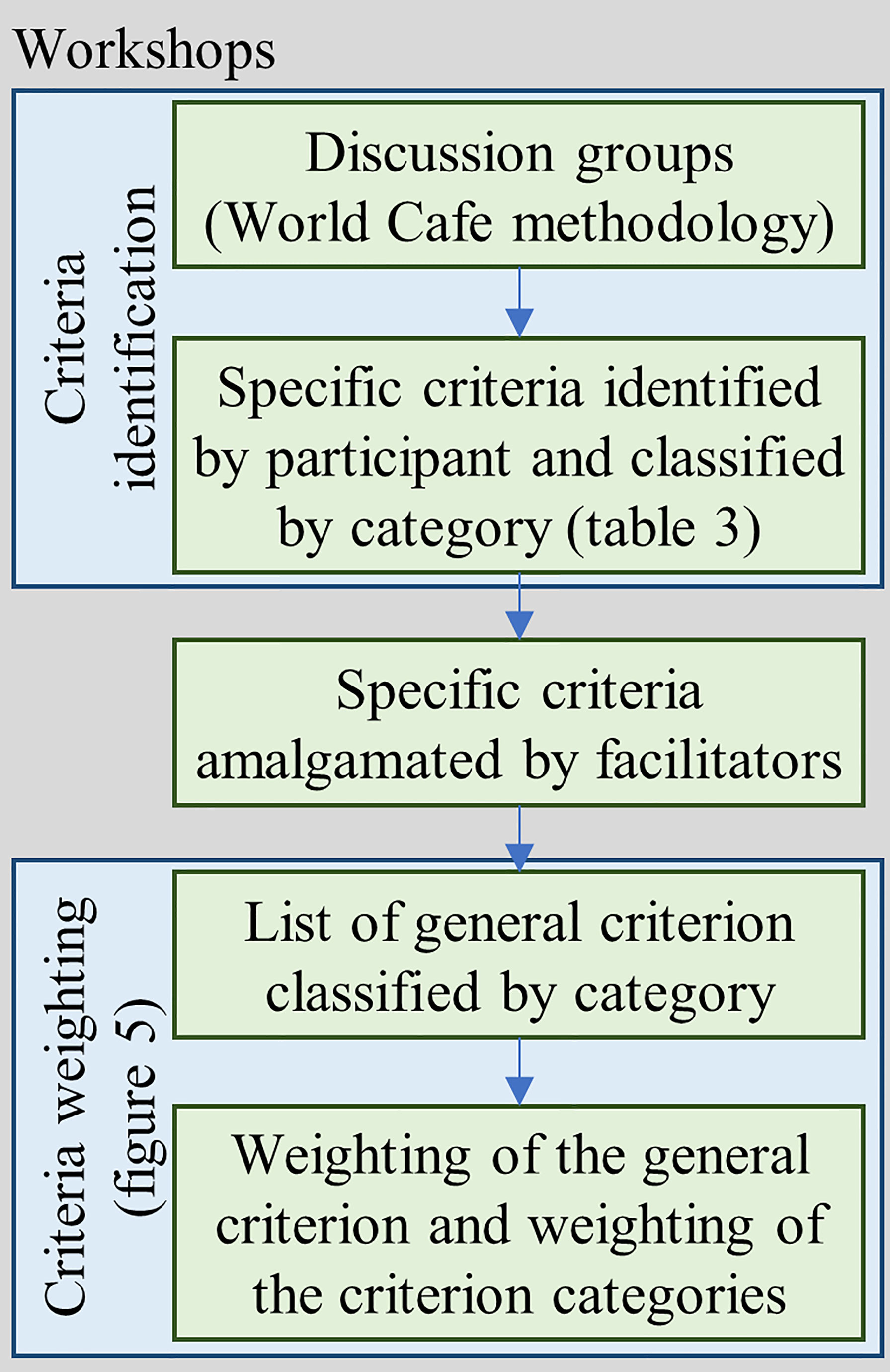
Figure 4 Graphical representation of the method used for the implication of workshop participants in the identification and weighting of the CDMs selection criteria.
For Pessamit, all participants were employees of the municipality, many of them, members of the First Nations; they were all recorded under Municipality rather than First Nations to avoid duplication.
Using an adaptation of the World Cafe methodology (Brown et al., 2005), the participants were separated into rotating discussions groups of between five and fifteen people. The aim was to allow participants to express their views on different subjects related to coastal management, each within a 25-minute time frame. With two facilitators in charge of the discussion, one table was dedicated to discussing relevant criteria to be integrated in the decision-making process for the selection of coastal defence measures.
At the beginning of the discussion, participants were presented with a brief description of factors affecting the CDMs decision-making process, in order for them to understand the context before identifying the CDMs selection criteria. The question “In your opinion, which are the criteria to be considered when a coastal defence measure must be selected?” was asked of participants to start the discussion. Five criteria categories written on cards were presented to participants as a guide (Table 3). Specific criteria identified by participants were written on post-it notes and affixed to their corresponding category. The selection criteria identified by participants of one table served as a basis for discussion in the following rotating groups.
A weighting method was established to allow participants to assess the importance of the criteria they previously identified. A three steps classification system was created: first, a list of criteria, as identified by the participants, was compiled; second, from that list, criteria that were similar in nature were amalgamated by the facilitators under a maximum of five general criteria per criteria category; third, the general criteria were grouped under five criteria categories.
Each criteria category was limited to a maximum of five general criteria to limit the total number of criteria, and because it was deemed sufficient to capture and adequately represent all of the criteria identified by the participants. Each general criterion was written on a card with the list of similar criteria originally identified by the participants. The weighting of the general criteria was carried out in two steps. The voting table was divided into six sections: one for each criteria category with its list of general criterion, and one which listed the five criteria categories (Figure 5). As a first step, each general criterion card was placed on the voting table under its respective category, with a corresponding voting box. Each participant was given ten tokens per criteria category to weight each general criterion according to the question “For each criteria category, how important is each criterion when selecting a coastal defence measure?”. As a second step, the five criteria categories’ cards were placed in the sixth section, each with a corresponding voting box. As for the previous exercise, participants were given ten tokens to weight the criteria categories according to the question “How important is each criteria category for the selection of a coastal defence measure?”.
2.3.3 CDMs Evaluation According to Each Criterion
The evaluation of CDMs in relation to each criterion was based on experts’ judgement, and on different sources of data such as literature reviews, reports on CDMs, etc. In the PROMETHEE method, different rating scales can be defined, depending on the nature of the criterion. Here, two scales were used: a 9 points qualitative scale for the criteria that lead to the comparison of CDMs’ performance relative to each other [very good (9), very good – good, good, good – average, average, average - bad, bad, bad – very bad, and very bad (1)]; and a 5 points impact qualitative scale for the criteria that lead to the evaluation of the direct effects of CDMs on the coastal system [very low (5), low, moderate, high, very high (1)]. Two qualitative scales were used for the evaluation of criteria due to a lack of quantitative local data. For example, the evaluation for the cost related criteria was based on the cost of previous projects at a national scale.
3 Results
3.1 Identified and Weighted CDM Criteria
Following the five workshops, the criteria identified by the participants were standardized with uniform wording, and classified under five categories (Table 4). A comprehensive list of sixteen criteria that were mutually independent, without duplication, relevant to the context, and consistent with effects occurring over time, was thus established (Figure 6). The list of criteria built during the five workshops was used in the analysis of the four study sites described above, even though only one of the workshops was specific to a study site (Pessamit). Of the remaining workshops, three drew participants from regions around and including a study site, and one was held in a region that did not include a study site (the MRC of Haute-Côte-Nord and Manicouagan).
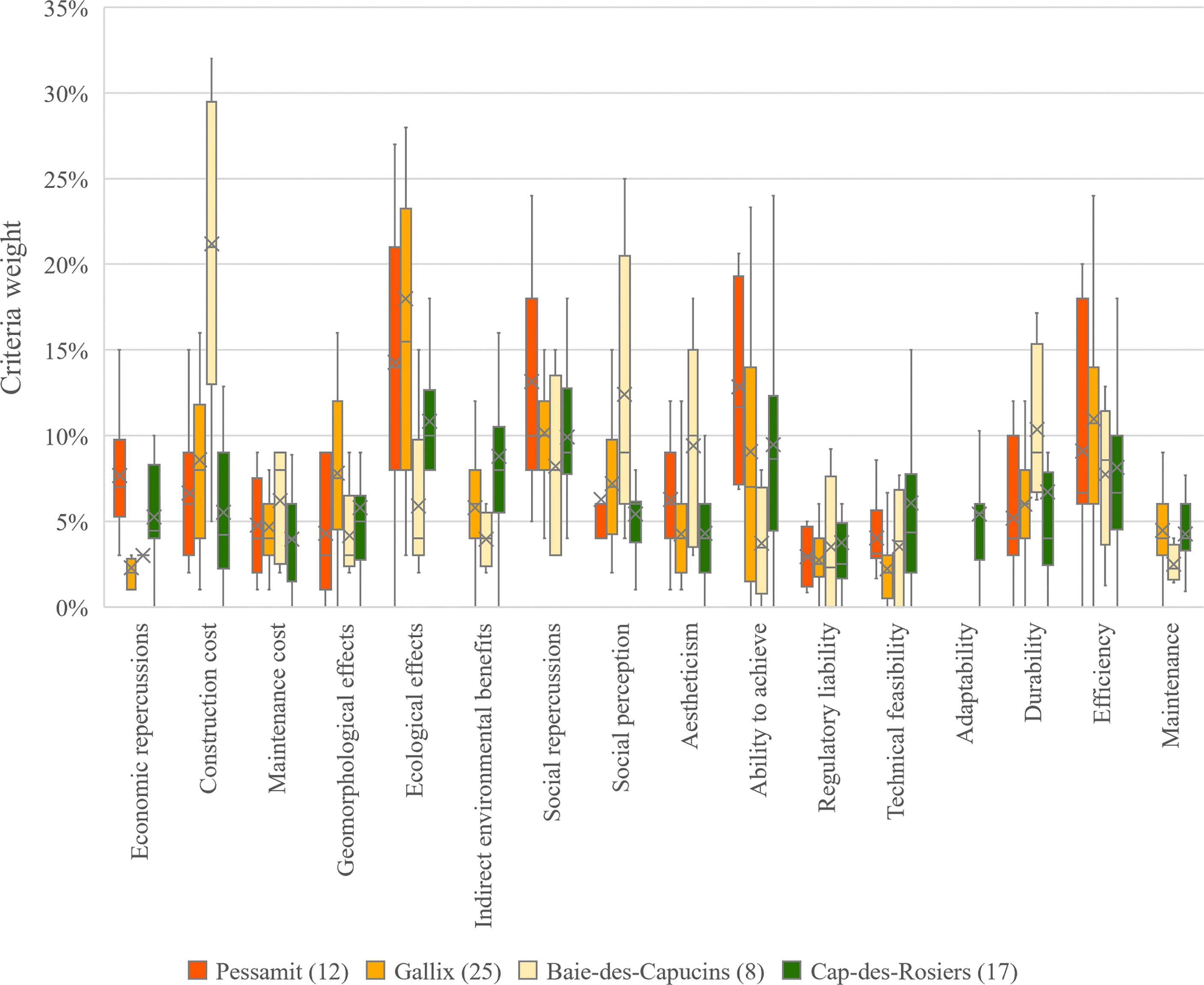
Figure 6 Standardized criteria identified by participants and their weighting range for each workshop. Number of participants per site is indicated in parenthesis.
As shown in Figure 6, the identification and weighting of criteria vary between workshops. First, not all criteria were selected in every workshop, as some may not have been relevant or important to the particular context. The adaptability criterion for instance was only identified in the case of Cap-des-Rosiers, while the maintenance and indirect environmental effects were not selected at the Pessamit workshop. Second, the weight assigned to different criteria varied between workshops, and the data range is wide. In general, the average weight is highest for ecological effects and social repercussions. In the case of Baie-des-Capucins, the weight given to the construction cost criterion is the highest among all criteria for all workshops.
For the purpose of weighting analysis, an average baseline can be calculated by dividing 100 percent by the number of identified criteria. For example, in Cap-des-Rosiers, 16 criteria were identified by workshop participants for an average baseline of 6.25%. While the majority of the criteria weight values are close to 6.25%, the ecological effects and social repercussions criteria are nearly twice the average value, which is an indication of the high importance accorded to these criteria by local stakeholders.
3.2 CDMs Evaluation
CDMs evaluation was carried out in two phases: (1) weighting of each CDM in relation to each criterion; (2) final hierarchization.
3.2.1 CDMs Evaluation in Relation to Each Criterion
The CDMs evaluation is dependent on each criterion (Table 5), and on the socio-ecological features associated with each site. The evaluation scale was adapted to the nature of each criterion.
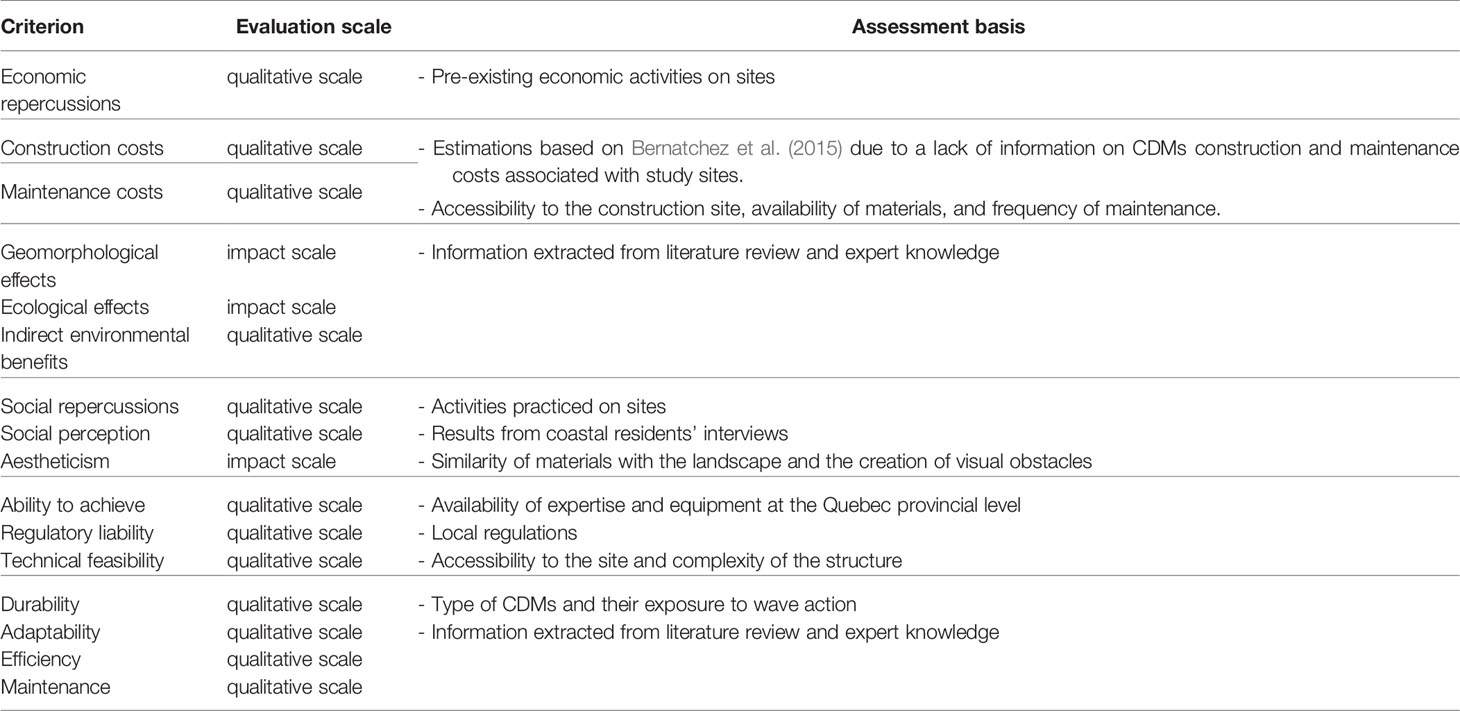
Table 5 Assessment basis and evaluation scale for the evaluation of CDMs in relation to each criterion.
The evaluation of the CDMs in relation to each criterion and to each study site’s socio-ecological features is presented in Table 6, with rationale where relevant.
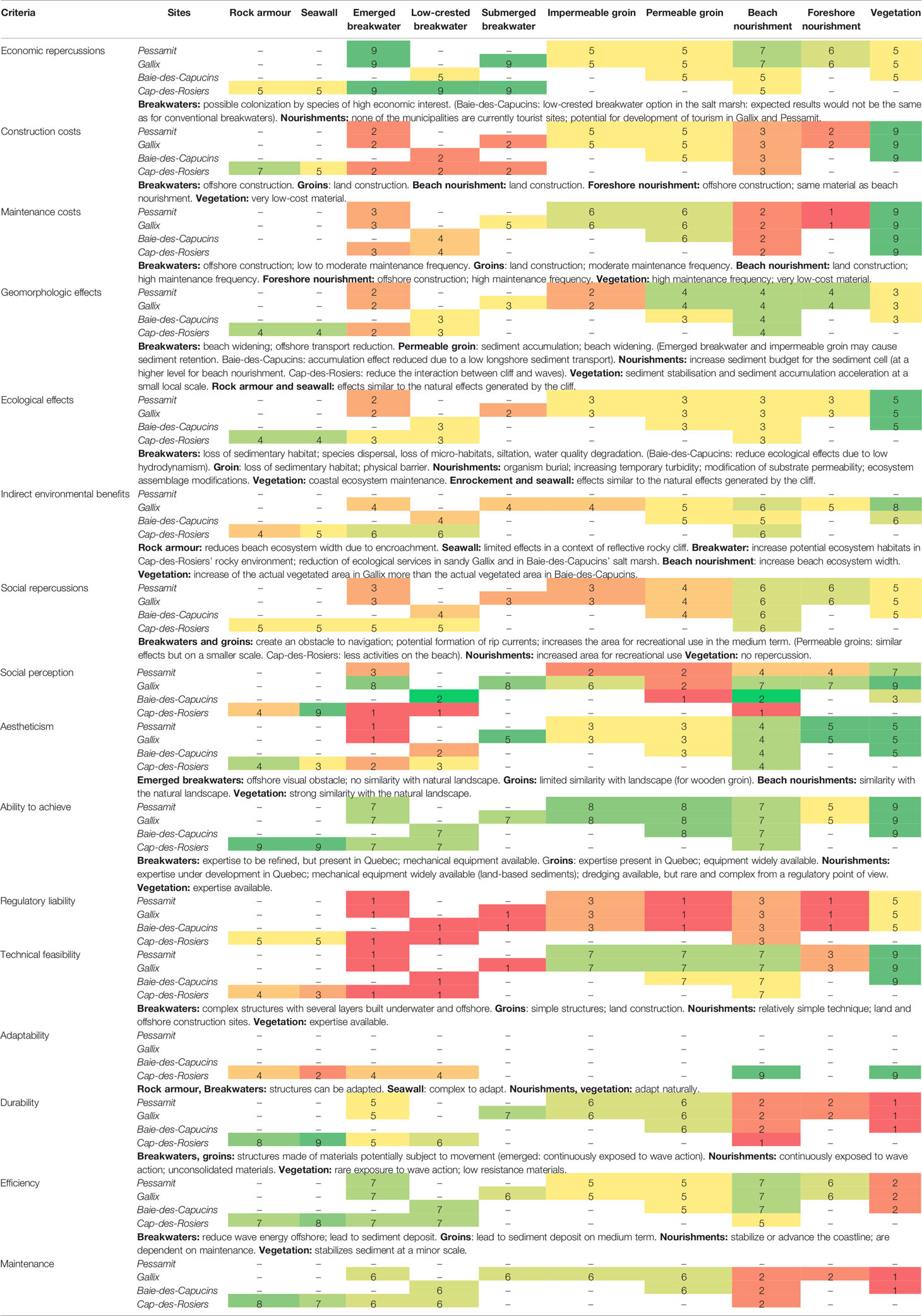
Table 6 Weighting of CDMs in relation to each criterion and sites’ characteristics, with relevant rationale.
3.2.2 CDMs Hierarchization
The CDMs hierarchization is thereafter presented in diamond shape figures for each study site (Figure 7). This shape shows the overall ranking (Φ net) along the vertical axis, as well as the leaving Φ+ and entering Φ- flows along the left edges of the diamond. Each CDM is represented by a grey dot. For one CDM to be ranked higher than another, it must outperform it on both the leaving and entering flow axes.
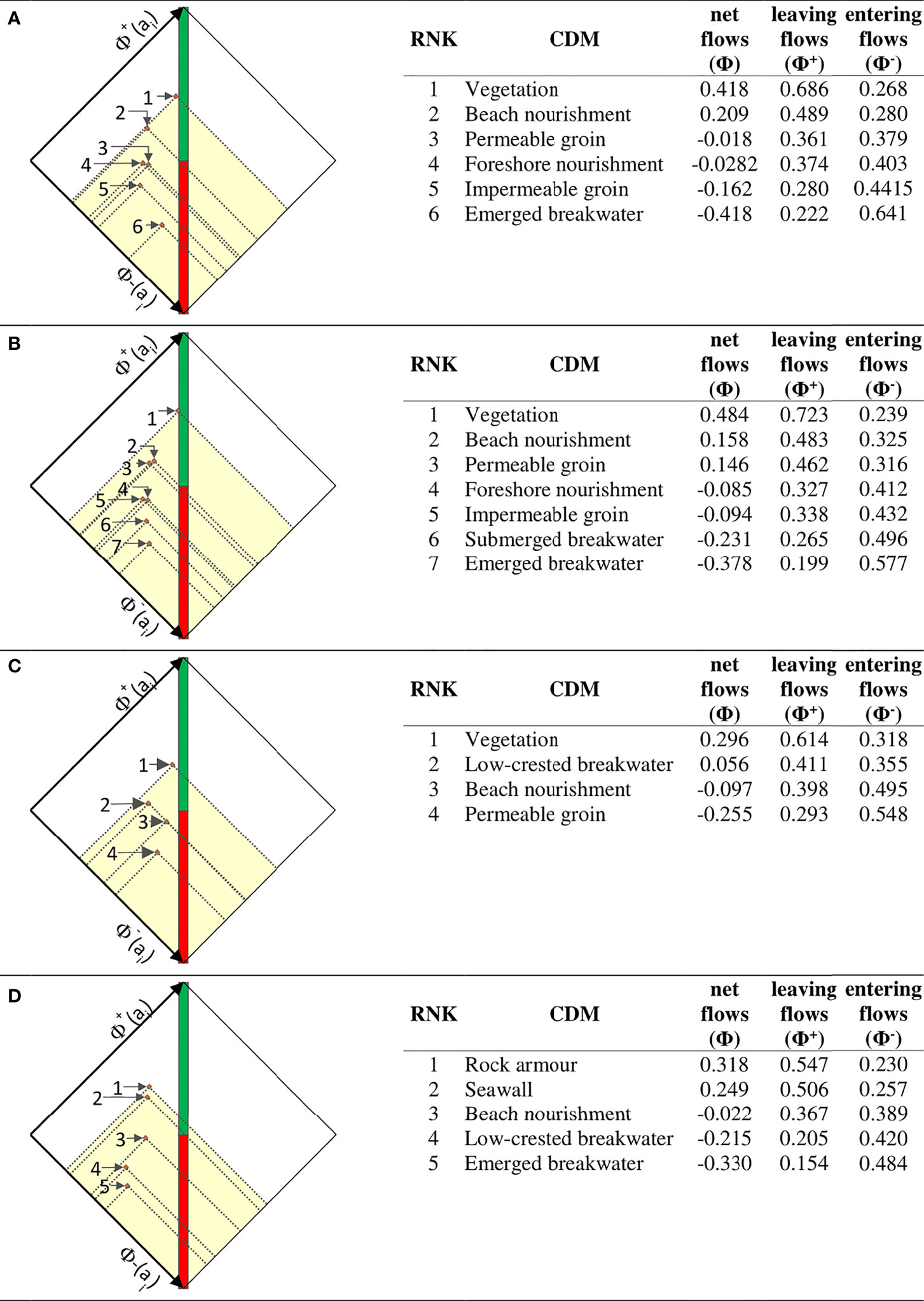
Figure 7 CDMs hierarchization presented in a diamond figure with the net, leaving and entering flows for each study site; (A) Pessamit; (B) Gallix; (C) Baie-des-Capucins; (D) Cap-des-Rosiers.
For the Pessamit site, the analysis of the results shows that, according to the workshop participants’ preferences, vegetation is ranked first followed by beach nourishment. Despite the large outperformance of vegetation over beach nourishment on the net and leaving flows, their respective entering flows are quite close together, thus weakening the general outperformance of the vegetation option. In third and fourth ranks, while permeable groin outperforms foreshore nourishment on the leaving flow axis, foreshore nourishment outperforms permeable groin on the entering flow. This results in a quasi-tie on the net flow axis. Meanwhile, impermeable groin and emerged breakwater come last, in fifth and sixth ranks, respectively.
For the Gallix site, the vegetation option is ranked first as shown by its significant outperformance over all other CDMs. In second and third ranks, a quasi-tie between beach nourishment and permeable groin on the net flow axis is explained by an outperformance of foreshore nourishment on the leaving flow, and an outperformance of permeable groin on the entering flow. Impermeable groin and foreshore nourishment are also on a quasi-tie in fourth and fifth ranks, respectively, while submerged breakwater and emerged breakwater are ranked sixth and seventh.
The top five CDMs for the Pessamit and Gallix sites are in the same order. Two reasons can explain the similarity. First, their socio-ecological systems are comparable. The environmental characteristics of both sites are low-lying sandy coast with a sandy lower foreshore, mesotidal shoreline, and a low energy environment (<1 m). Also, both sites are part residential part public localities, mostly frequented by the local community for a variety of uses and activities. Due to these similarities, the evaluation of the CDMs in relation to each criterion is equivalent for all criteria with the exception of social perception (Table 6). Second, the difference in average weight is below 3 % for seven of the 13 and 15 criteria identified, respectively, in Pessamit and Gallix (Figure 7). The local stakeholders’ preferences were quite similar in nearly half of the identified criteria, though variances explain differences in the net, leaving, and entering flows between the two sites (Figure 7). While the final ranking for the top 5 options is equivalent in both sites, inner differences shown by the above-mentioned three indicators, provide information that is relevant to the decision-makers.
For the Baie-des-Capucins site, the vegetation alternative also outperformed all other CDMs. In second and third ranks, while low-crested breakwater outperformed beach nourishment on the net, and entering flow axes, both are on a quasi-tie on the leaving flows axis. It is to be noted here that, because of the presence of salt marsh, the low-crested breakwater scenario was actually based on a living shoreline rock sill concept (Bilkovic et al., 2017). Finally, permeable groin was ranked fourth.
As for Cap-des-Rosiers, rock armour outperformed all other CDMs, but seawall is a close second. Beach nourishment, low-crested breakwater and emerged breakwater were ranked third, fourth and fifth, respectively. The evaluated CDMs and the final ranking is quite different from the other three sites, which is explained by their significantly dissimilar local conditions.
3.2.3 Robustness Analysis
An analysis was performed to evaluate the robustness of the CDMs hierarchization method in relation to each criterion (Figure 8). The circles and triangles show the highest and lowest thresholds at which there is a change in ranking of the top 3 CDMs for a given criterion. For example, in Pessamit under the criterion Economic repercussions, the CDM ranked first is outperformed by the CDM ranked second above 21.06%, but it is never outperformed by the CDM ranked third. Also, in Pessamit with regard to the three criteria Ecological effects, Regulatory liability, and Technical feasibility, the circles representing CDMs 1, 2, and 3 are all at 100%. This means that no change occurs in the top 3 CDMs’ ranking, no matter the weight attributed to those criteria. An empty criterion cell indicates that the criterion was not identified at the site’s workshop.
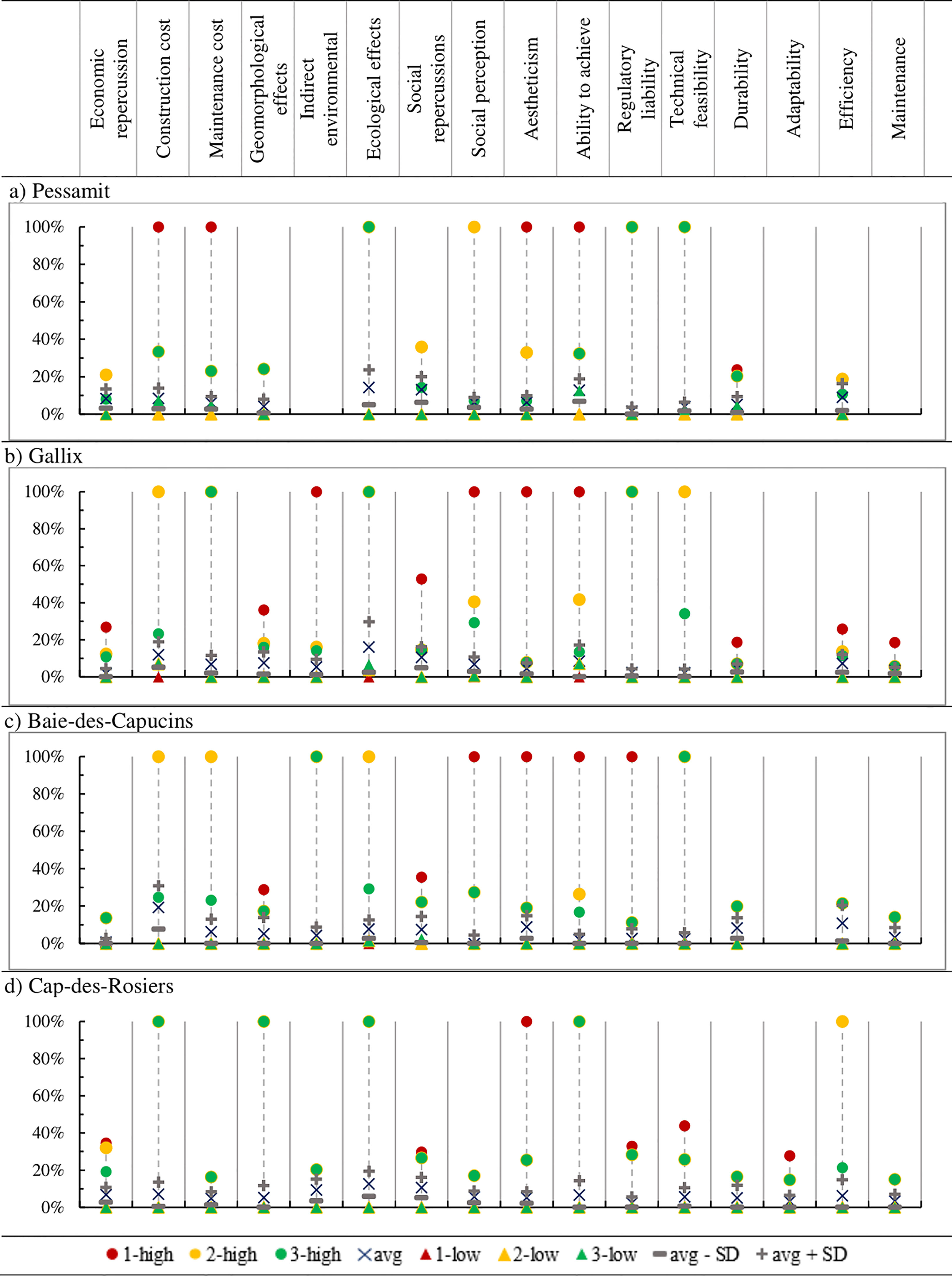
Figure 8 Robustness analysis of CDMs ranked 1 (red), 2 (yellow), and 3 (green), in relation to each criterion for each site (A) Pessamit; (B) Gallix; (C) Baie-des-Capucins; (D) Cap-des-Rosiers). The vertical axis shows the average criteria weight in percentage. The circles and triangles show the highest and lowest thresholds at which there is a change in the top 3 ranked CDMs for a given criterion. The blue X shows the criterion weighted average. The grey plus and minus signs show the weighted average plus and minus the standard deviation.
The grey plus and minus signs show the weighted average plus or minus the standard deviation. For example, if there is no circle or triangle within the range of the standard deviation (between the plus and minus signs), any change to the weight of the criteria would not affect the ranking, which is an indication that the results are robust. In Pessamit and Gallix, the CDMs ranked 1 and 2 are outside the standard deviation range for all criteria, which indicates that the results are robust for the first two CDMs. On the other hand, because CDM ranked 3 sometimes falls within the standard deviation range, it may be outperformed by the CDM ranked 4 if a change occurs in some of the criteria weight values. This shows that the results for CDM ranked 3 are not as robust as the ones for the first two CDMs. In Cap-des-Rosiers, all of the CDMs fall outside of the standard deviation range. This indicates that, in the case of the first three CDMs, the results would not be affected by any change in the criteria weights. In Baie-des-Capucins, the CDM ranked 3 falls within the standard deviation range for the criteria Construction costs, and Social repercussions. In this case, two out of sixteen criteria would not be enough to change the ranking.
Considering Figures 6 and 7, even though the results are not equally robust in all cases, vegetation, beach nourishment, and permeable groin were ranked first, second, and third, respectively, for the three study sites with a sandy low coast (Pessamit, and Gallix). As for Cap-des-Rosiers, with a cliff coast that is naturally reflective, rock armour and seawall were solidly ranked first and second. These results are in line with those obtained through the CDMIA developed by Sauvé et al., (2022), but the MCDA adds a layer of refinement to the assessment.
In Pessamit, the quasi-tie between permeable groin and foreshore nourishment in third and fourth ranks (a) is validated by the robustness analysis, which shows that the lowest and highest thresholds of the CDM ranked 3 (represented by a green triangle and a green dot, respectively), each fall within the standard deviation range in 5 of the criteria (a). In Gallix, the quasi-tie between beach nourishment and permeable groin (b, ranks 2 and 3, respectively) is confirmed by the robustness analysis, which shows the permeable groin’s lowest threshold falling within the standard deviation in 3 of the criteria, and the highest threshold, in 6 of the criteria (b). In Baie-des-Capucins, there is no quasi-tie in the CDMs hierachization (c). Indeed, the robustness analysis shows that the thresholds for CDMs ranked 1 and 2 all fall outside of the standard deviation, and even though, in one criterion, the highest threshold falls within the standard deviation for the CDMs ranked three (permeable groin) (c), any change in the criteria weighting would not be enough to affect the final hierarchization. In Cap-des-Rosiers, the CDMs hierarchization is unambiguous (d), and is validated by the robustness analysis which shows that, for all criteria, the top 3 CDMs fall outside the standard deviation (d).
4 Discussion
In order to solve a predefined coastal erosion or flooding problem with a solution that is adapted to the specific socio-ecological context, a variety of scenarios must be considered in a multiphase process before the design and construction phases are undertaken (USACE, 2006). In most cases, decision-making has traditionally been limited to engineers, experts and scientists (Garmendia et al., 2010; Sauvé et al., 2020), and have led to a high rate of shoreline artificiality worldwide, the majority consisting of hard coastal defense structures (Koike, 1996; Valloni et al., 2003; EEA, 2006; Gittman et al., 2015; Cooper et al., 2020; Sauvé et al., 2020). In the past decade, a trend reversal has been observed with the implementation of soft techniques like beach nourishment or vegetation Sauvé et al., (2022), ecological approaches (Morris et al., 2018; Morris et al., 2019) such as Engineering With Nature in the U.S.A. (Bridges et al., 2018) or Building With Nature in The Netherlands (de Vriend and Van Koningsveld, 2012; van Slobbe et al., 2013), and the use of ecological or socio-economic enhancements in the design of hard structures (Evans et al., 2017; Schoonees et al., 2019; Vuik et al., 2019). Still, the decisions regarding the selection of CDMs are not systematically being made through a participatory process (O’Riordan, 2005; Sauvé et al., 2020).
In recent years, decision support tools have been increasingly used in the field of environmental management (Walling and Vaneeckhaute, 2020; Wong-Parodi et al., 2020; Barzehkar et al., 2021). One of the main reasons being the need for a framework to support the meaningful integration of multiple stakeholders in the decision-making process (Wong-Parodi et al., 2020). Such tools help decision-makers address complex and inherently uncertain problems related to socio-ecological systems (Baquerizo and Losada, 2008; Polasky et al., 2011) by objectively structuring and analyzing the information, and by offering multiple solutions for consideration (Walling and Vaneeckhaute, 2020; Wong-Parodi et al., 2020).
4.1 Relevance of MCDA as a Tool to Evaluate CDMs
Cost benefit analysis (CBA) and multicriteria decision analysis (MCDA) are both used for different purposes in environmental and coastal zone management, and in the evaluation of ecosystem services (Horstman et al., 2009; Saarikoski et al., 2016). CBA is often used to analyze CDMs, but rarely through a process of prioritization (Polomé et al., 2005; Boyer-Villemaire et al., 2016; Chow et al., 2017; Thi Oanh et al., 2020). CBA has been used more frequently to assess the cost of CDMs’ maintenance, to compare the pros and cons, and the costs of scenarios with or without a CDM (Maia et al., 2015; Ha et al., 2021) or to evaluate a single given solution in monetary terms (Lima et al., 2020). There are two schools of thought regarding CBA and MCDA. The selection of one over the other depends on the project objectives. While CBA can be useful in some contexts (Gamper and Turcanu, 2007; Horstman et al., 2009), MCDA are better suited to the processing of tangible and intangible information obtained when, among others, multiple stakeholders are involved, when all aspects of communities’ well-being are taken into consideration, and when scientific uncertainty and spatiotemporal ecological impacts are significant factors in the decision-making process (Wegner and Pascual, 2011; Saarikoski et al., 2016; Alves et al., 2018). CDMs can have different effects on the components of the socio-ecological system, and these effects are usually measured in incommensurable scales and units (Choo et al., 1999). MCDA are best adapted to the evaluation and comparison of CDMs because they allow the simultaneous analysis of dissimilar measurement units that are specific to each criterion (Horstman et al., 2009).
The output reports from the three phases of the MCDA PROMETHEE method combined with the robustness analysis (Figure 9) provide the decision-makers with a structured, transparent and integrated analysis, and, as an end result, present alternatives that take stakeholders’ preferences into account, and are more likely to be acceptable to all parties (Saaty, 2008; McIntosh et al., 2011; Saarikoski et al., 2016). First, the criteria, identified and weighted by workshop participants, give an indication of the local stakeholders’ overall priorities and, more specifically, identifies conflicts and agreements within the consulted group, with regard to each criterion. Decision-makers can use that information, and manage conflictual issues by eliciting further discussion and exploring trade-offs in order to build a consensus around the most suitable solution. Second, the effects of each CDM are evaluated in relation to each criterion. This can be used in the design process to identify conflicts and synergies between CDMs, and to make end users (decision-makers, coastal managers or coastal engineers) aware of the effect of a CDM on individual criteria. Moreover, as mentioned in section 2.3.3, the PROMETHEE method allows the use of different rating scales depending on the nature of each criterion. Thus, when relevant data is available, the accuracy of the results could be improved by using quantitative scale for some of the criteria (e.g. cost related criteria). Third, the CDMs hierarchization, in the diamond figure, shows the interpretation of the results on three axes, and establishes equivalencies between scenarios. For example, in Pessamit and Gallix, there was a quasi-tie between two CDMs. In such cases, the decision-makers should consider both options on the same level. Finally, the robustness analysis gives an indication of how trustworthy the CDMs hierarchization results are. Reflected in the results is the objective of a MCDA, which is not necessarily to point to a unique solution. Rather, it provides a layered structure to facilitate the evaluation of different alternatives, suitable to answer a complex problem. In doing so, MCDA helps the decision-makers examine all aspects of the problem, understand the consequences surrounding the choice of one or a combination of CDMs, and choose the best alternative in accordance with their priorities and preferences.
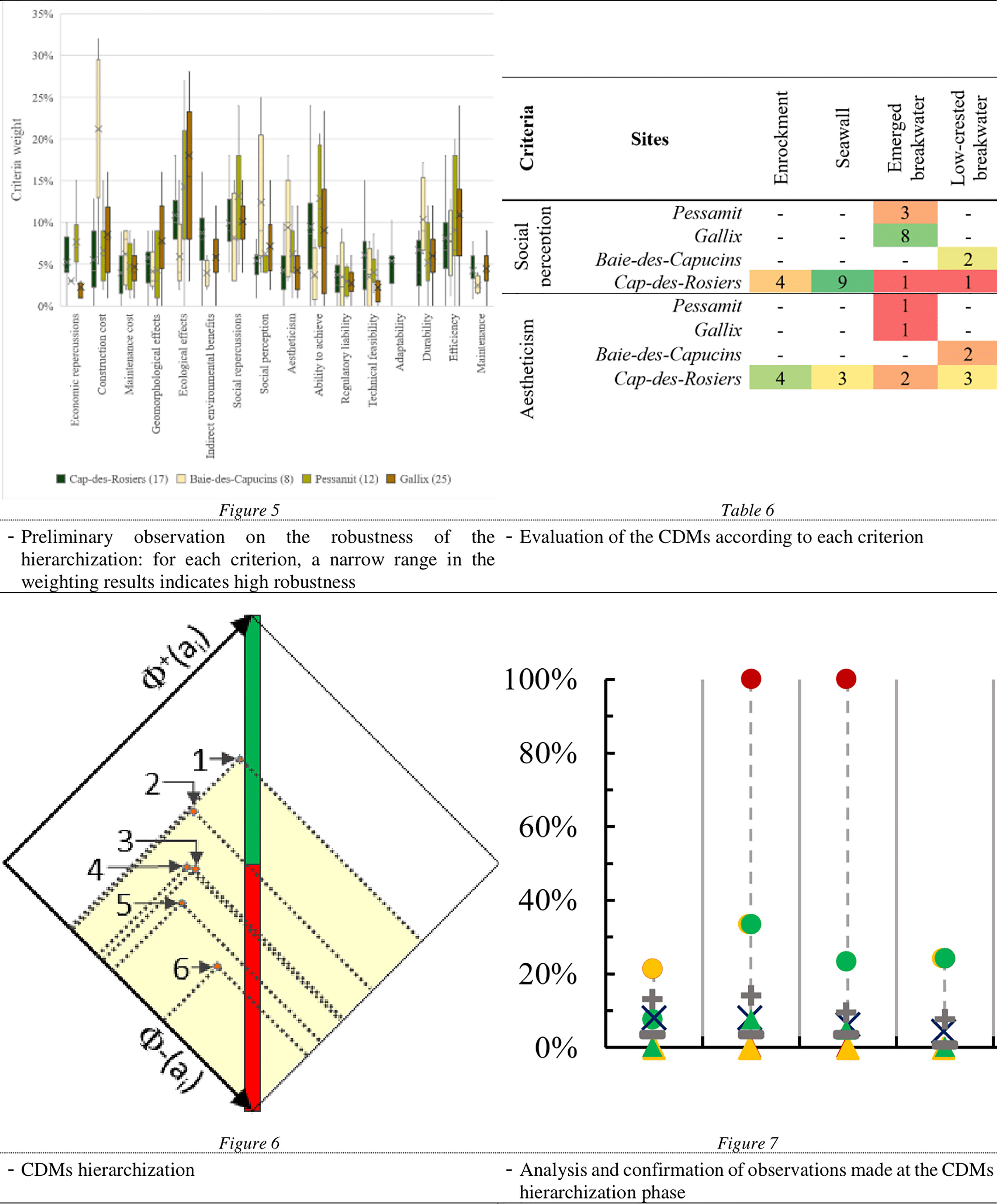
Figure 9 Illustration of the three phases of the MCDA process and robustness analysis in different report formats.
In comparison, while CBA, like MCDA, is conducted in a process involving a few sequential steps (Saarikoski et al., 2016; Boardman et al., 2017), these are only related to solving the economic efficiency of scenarios (Wegner and Pascual, 2011). The monetary units used in CBA for the valuation of environmental features can limit the stakeholders in the expression of their preferences (Wegner and Pascual, 2011; Saarikoski et al., 2016). Decision-making based on CBA is focused on an economic perspective, and the analyzed components of the socio-ecological system do not provide as much information to decision-makers, coastal managers and coastal engineers, as MCDA does.
4.2 Analysis of the Participatory Process Involving Criteria Identification and Weighting
Following the uniformization process, a standardized list of 16 criteria was established (Table 4), based on the criteria identified by participants during the course of five workshops (Figure 5). The identification exercise and subsequent discussions were useful for participants to enhance their understanding of the potential effects of CDMs on socio-ecological systems, and to learn how the relative values attached to each criterion, by different stakeholders, can influence the final decision [e.g. Garmendia and Stagl (2010); Grêt-Regamet et al,. (2017), and Reed (2008)].
However, the exercise led to an over specificity of the criteria, which happens when different criteria statements have a similar meaning, and when the theoretical basis used for the evaluation cannot reflect the accuracy of each statement. Another issue was the process of synthetizing the criteria identified by participants into a concise standardized list, which had to be achieved in a short time to avoid slowing the pace of the workshop, and to allow enough time for the participants to weigh the criteria. The lack of time may have resulted in the presence of inconsistencies in the categories and groupings created during the workshop. These observations were made during the first workshop of the series. However, the same approach was maintained for reasons of methodology and consistency between workshops. The uniformization of the criteria, identified by the participants, certainly influences the interpretation of the results. Subsequent work regarding the use of multicriteria analysis for the evaluation of CDMs should consider taking advantage of the established list of 16 criteria, in order to avoid a repetition of this phase of the process, and allow more time for the weighting phase. Prior to the workshops, the list can be modified or expanded, depending on the context and environmental conditions.
Despite these drawbacks, the use of multicriteria analysis is advantageous, especially when dealing with complex problems. Its capacity to integrate and process subjective information obtained from local stakeholders in participatory processes, is invaluable to an inclusive decision-making process. In the future, the participatory process could be improved by allowing more time for discussion on the values and weighting of the criteria. For instance, participants could be asked to select from a pre-established list of criteria, following a thorough presentation describing the meaning, the scope, and the limits of each criterion. A better understanding of each criterion by participants, would result in a set of criteria that is more accurate, and more representative of their local socio-ecological contexts. Allowing more time to the weighting phase would also possibly result in more accuracy, and narrower ranges in the weighted values.
4.3 Contribution to Coastal Engineering and Management
The proposed MCDA approach is a flexible tool that could contribute to coastal engineering and management by its capacity to structure and analyze the multiple dimensions of a complex problem, that are not necessarily easy to quantify. The method helps compare alternatives in relation to all relevant criteria (Choo et al., 1999; Horstman et al., 2009), while taking into consideration their relative importance, as rated by different local stakeholders. The end result is a better knowledge of the specific context, and is more likely to lead to a solution that is well adapted to the environment in question. This would answer a need raised, in the past, by coastal decision-makers for the necessity to make better decisions related to CDMs (Friesinger and Bernatchez, 2010; Drejza et al., 2011; Marie et al., 2017). The inclusion of coastal managers and professionals in the criteria identification and weighting phases, is in line with a trend to involve more social stakeholders in environmental management (Reed, 2008; Garmendia et al., 2010; Jones et al., 2014; Marttunen et al., 2017). It gives decision-makers a better understanding of local conditions, priorities and interests, and it enhances the stakeholders’ comprehension of all issues related to the interaction between CDMs and socio-ecological systems. The approach is conducive to supporting discussions in a group of stakeholders from different disciplines and functions. Therefore, the solutions emerge from an interdisciplinary exchange process (Gamper and Turcanu, 2007), which contributes to increasing the resilience of the socio-ecological system (Folke, 2016). In three of the four study sectors presented in this article, the MCDA approach made it possible to consider solutions other than rock armour and seawalls which have, up until now, often been implemented in Quebec, regardless of the type of coastal environment (Bernatchez and Fraser, 2012; Sauvé et al., 2020).
5 Conclusion
Coastal zone managers and professionals were involved in a participatory process, which led to the identification and weighting of criteria for the purpose of selecting CDMs that are suitable to specific conditions. A multicriteria decision analysis approach was used to evaluate and hierarchize CDMs. The methodology was applied to four study sites in the province of Quebec, Canada: Pessamit, Gallix, Baie-des-Capucins, and Cap-des-Rosiers. The study sites have distinct geomorphological, hydrodynamic, ecological or socio-economic characteristics. PROMETHEE, an outranking method, was chosen to carry out the multicriteria analysis. First, a set of 16 criteria were identified and weighted by participants of five workshops. Second, CDMs were evaluated in relation to each criterion, and according to local socio-ecological features. Third, CDMs were hierarchized using the information obtained in the first two steps. Results show that vegetation holds the first rank in the Pessamit, Gallix, and Baie-des-Capucins sites, while rock armour is first in Cap-des-Rosiers. Still, deeper analysis indicates that, because of their high ranking, beach nourishment and permeable groin are options that are worthwhile considering. Finally, the results are supported by a robustness analysis. The Pessamit, and Gallix sites have similar results, which are explained by the comparability of their environmental characteristics and coastal activities. Findings regarding criteria identification and weighting as a participatory process can be divided in two parts. First, the criteria as identified by workshop participants were too specific, making it difficult to synthetize into a concise and comprehensive list useful for the evaluation of CDMs. To alleviate this problem, this phase of the process could be substituted by a detailed presentation of a pre-established list of criteria, followed by a discussion leading to the selection and weighting, by participants, of relevant criteria from the predefined list. Second, the weighting process was found to be a highly effective way to integrate local knowledge into the decision-making process. The three stages of the multicriteria decision analysis facilitate the decision-making process by presenting the results in a structured, transparent and integrated way, while taking stakeholders’ preferences into account. Ultimately, the multicriteria decision analysis coupled with a participatory process is a flexible methodology that structures multiple aspects related to the selection of a CDM. It is a tool that can appreciably improve the coastal engineering and management decision-making process, and contribute to a better understanding of the socio-ecological systems.
Data Availability Statement
The raw data supporting the conclusions of this article will be made available by the authors, without undue reservation.
Ethics Statement
The studies involving human participants were reviewed and approved by Comité d’éthique de la recherche avec des êtres humains de l’UQAR (CÉR-101-748). The patients/participants provided their written informed consent to participate in this study
Author Contributions
PS defined the state of knowledge, developed and executed the methodology, analyzed the data, and wrote the manuscript. PB contributed to the execution of the methodology and the editing of the manuscript. MG contributed to the development and execution of the methodology, the analysis of the results, and the editing of the manuscript. All authors contributed to the article and approved the submitted version.
Funding
We are grateful to the Ministère de la Sécurité publique du Québec from his program for natural risks prevention 2013-2020 (CPS 16-17-07 project), to the Ministère de l’Environnement et de la Lutte contre les changements climatiques from the Fonds vert and the Climate Change Action Plan (PACC 2013-2020) of the Government of Québec, and to Natural Resources Canada for its programme on Climate change adaptation (AP660 V205 2017-12-28) for financing the project.
Conflict of Interest
The authors declare that the research was conducted in the absence of any commercial or financial relationships that could be construed as a potential conflict of interest.
Publisher’s Note
All claims expressed in this article are solely those of the authors and do not necessarily represent those of their affiliated organizations, or those of the publisher, the editors and the reviewers. Any product that may be evaluated in this article, or claim that may be made by its manufacturer, is not guaranteed or endorsed by the publisher.
Acknowledgments
The authors would like to thank all the members of the Research Chair in Coastal Geoscience who participated in consultations organisation and execution, particularly Maud Touchette, Catherine Paul-Hus, Stéphanie Friesinger, and Christian Fraser. We would also like to thank all the workshops participants. Finally, a special thank you to Danielle Allard for the English revision.
References
Abdel-Basset M., Gamal A., Chakrabortty R. K., Ryan M. (2021). A New Hybrid Multi-Criteria Decision-Making Approach for Location Selection of Sustainable Offshore Wind Energy Stations: A Case Study. J. Clean. Prod. 280, 124462. doi: 10.1016/j.jclepro.2020.124462
Airoldi L., Abbiati M., Beck M. W., Hawkins S. J., Jonsson P. R., Martin D., et al. (2005). An Ecological Perspective on the Deployment and Design of Low-Crested and Other Hard Coastal Defence Structures. Coast. Eng. 52, 1073–1087. doi: 10.1016/j.coastaleng.2005.09.007
Alves A., Gersonius B., Sanchez A., Vojinovic Z., Kapelan Z. (2018). Multi-Criteria Approach for Selection of Green and Grey Infrastructure to Reduce Flood Risk and Increase CO-Benefits. Water Resour. Manage. 32, 2505–2522. doi: 10.1007/s11269-018-1943-3
Ananda J., Herath G. (2009). A Critical Review of Multi-Criteria Decision Making Methods With Special Reference to Forest Management and Planning. Ecol. Econ. 68, 2535–2548. doi: 10.1016/j.ecolecon.2009.05.010
André P., Delisle C. E., Revéret J.-P. (2010). L’évaluation Des Impacts Sur L’environnement: Processus, Acteurs Et Pratique Pour Un Développement Durable. 3e ed (Montréal, QC, Canada: Presses internationales Polytechnique).
Antunes P., Karadzic V., Santos R., Beça P., Osann A. (2011). Participatory Multi-Criteria Analysis of Irrigation Management Alternatives: The Case of the Caia Irrigation District, Portugal. Int. J. Agric. Sustain. 9, 334–349. doi: 10.1080/14735903.2011.582358
Baquerizo A., Losada M. A. (2008). Human Interaction With Large Scale Coastal Morphological Evolution. An Assessment of the Uncertainty. Coast. Eng. 55, 569–580. doi: 10.1016/j.coastaleng.2007.10.004
Barzehkar M., Parnell K. E., Mobarghaee Dinan N., Brodie G. (2021). Decision Support Tools for Wind and Solar Farm Site Selection in Isfahan Province, Iran. Clean. Technol. Environ. Policy 23, 1179–1195. doi: 10.1007/s10098-020-01978-w
Behzadian M., Kazemzadeh R. B., Albadvi A., Aghdasi M. (2010). PROMETHEE: A Comprehensive Literature Review on Methodologies and Applications. Eur. J. Oper. Res. 200, 198–215. doi: 10.1016/j.ejor.2009.01.021
Belton V., Stewart T. (2002). Multiple Criteria Decision Analysis: An Integrated Approach (Boston, USA: Kluwer Academic Publishers, Springer Science & Business Media).
Bernatchez P., Dugas S., Fraser C., Da Silva L. (2015) Évaluation Economique Des Impacts Potentiels De L’érosion Des Côtes Du Québec Maritime Dans Un Contexte De Changements Climatiques. Available at: https://www.ouranos.ca/publication-scientifique/RapportBernatchez2015_FR.pdf%5Cnhttps://www.ouranos.ca/programmes/evaluation-economique/.
Bernatchez P., Fraser C. (2012). Evolution of Coastal Defence Structures and Consequences for Beach Width Trends, Québec, Canada. J. Coast. Res. 28, 1550–1566. doi: 10.2112/JCOASTRES-D-10-00189.1
Bilkovic D. M., Mitchell M. M., La Peyre M. K., Toft J. D. (2017). Living Shorelines: The Science and Management of Nature-Based Coastal Protection (Boca Raton: CRC Press).
Boardman A. E., Greenberg D. H., Vining A. R., Weimer D. L. (2017). Cost-Benefit Analysis: Concepts and Practice (Cambridge, United Kingdom: Cambridge University Press).
Boyer-Villemaire U., Circé M., Da Silva L., Desjarlais C., Morneau F. (2016). Analyses Coûts-Avantages Des Options D’adaptation En Zone Côtière Au Québec Et Dans Les Provinces Atlantiques (Montréal: Ouranos), pp. 36.
Boyer-Villemaire U., Lamari M., Bernatchez P., Jacob J. L., Nouwodjro K. (2015). ““Analyse Institutionnelle De La Trajectoire D’adaptation Aux Changements Climatiques Dans Le Québec Maritime,” in Adaptation Aux Changements Climatiques En Zones Côtières: Politiques Publiques Et Indicateurs De Suivi Des Progrès Dans Sept Pays Occidentaux. Eds. Lamari M., Jacob J. L. (Québec, Canada: Presses de l’Université du Québec), 77–126.
Brans J. P., Vincke P., Mareschal B. (1986). How to Select and How to Rank Projects: The PROMETHEE Method. Eur. J. Oper. Res. 24, 228–238. doi: 10.1016/0377-2217(86)90044-5
Bridges T. S., Bourne E. M., King J. K., Kuzmitski H. K., Moynihan E. B., Suedel B. C. (2018). Engineering With Nature: An Atlas (Vicksburg, MS: U.S. Army).
Brown J., Isaacs D., World Café community. (2005). The World Café: Shaping Our Futures Through Conversations That Matter (Oakland, CA: Berrett-Koehler Publishers, Inc).
Bryce R., Irvine K. N., Church A., Fish R., Ranger S., Kenter J. O. (2016). Subjective Well-Being Indicators for Large-Scale Assessment of Cultural Ecosystem Services. Ecosyst. Serv. 21, 258–269. doi: 10.1016/j.ecoser.2016.07.015
Chang H.-K., Liou J.-C., Chen W.-W. (2012). Protection Priority in the Coastal Environment Using a Hybrid AHP-TOPSIS Method on the Miaoli Coast, Taiwan. J. Coast. Res 28, 369–374. doi: 10.2112/JCOASTRES-D-10-00092.1
Chan K. M. A., Satterfield T., Goldstein J. (2012). Rethinking Ecosystem Services to Better Address and Navigate Cultural Values. Ecol. Econ. 74, 8–18. doi: 10.1016/j.ecolecon.2011.11.011
Charlier R. H., Chaineux M. C. P., Morcos S. (2005). Panorama of the History of Coastal Protection. J. Coast. Res. 21, 79–111. doi: 10.2112/03561.1
Choo E. U., Schoner B., Wedley W. C. (1999). Interpretation of Criteria Weights in Multicriteria Decision Making. Comput. Ind. Eng. 37, 527–541. doi: 10.1016/S0360-8352(00)00019-X
Chow A., Leung T., Lee F. (2017). Benefit-Cost Analysis on Coastal Structures Design for Climate Change Adaptation in Hong Kong. Coast. Eng. J. 59, 1–25. doi: 10.1142/S0578563417400058
Church J. A., White N. J. (2011). Sea-Level Rise From the Late 19th to the Early 21st Century. Surv. Geophys. 32, 585–602. doi: 10.1007/s10712-011-9119-1
Cooper J. A. G., O’Connor M. C., McIvor S. (2020). Coastal Defences Versus Coastal Ecosystems: A Regional Appraisal. Mar. Policy 111, 102332. doi: 10.1016/j.marpol.2016.02.021
Corriveau M., Bernatchez P., Dugas S. (2019). “Mise a Jour De La Cartographie Des Marges De Sécurité En Erosion Côtière Pour Le Secteur De Gallix, Municipalité De Sept-Îles: Analyse De L’évolution Côtière Et De La Dynamique,” in Chaire de recherche en géoscience côtière, Laboratoire de dynamique et de gestion intégrée des zones côtières (Rimouski, Qc, Canada: Université du Québec à Rimouski), pp. 108.
Corriveau M., Caulet C., Bernatchez P. (2021). “Modélisation Et Cartographie Du Risque De Submersion Côtière De La Région De Sept-Îles, Rapport Intermédiaire,” in Chaire de recherche en géoscience côtière, Laboratoire de dynamique et de gestion intégrée des zones côtières (Rimouski, Qc, Canada: Université du Québec à Rimouski), pp. 91
De Bruin K., Dellink R. B., Ruijs A., Bolwidt L., van Buuren A., Graveland J., et al. (2009). Adapting to Climate Change in the Netherlands: An Inventory of Climate Adaptation Options and Ranking of Alternatives. Clim. Change 95, 23–45. doi: 10.1007/s10584-009-9576-4
de Vriend H. J., Van Koningsveld M. (2012). Building With Nature: Thinking, Acting and Interacting Differently. (Dordrecht, the Netherlands: EcoShape, Building with Nature). doi: 10.1080/02513625.2014.925714
Dodgson J. S., Spackman M., Pearman A. D., Phillips L. D. (2009). Multi-Criteria Analysis: A Manual (London: Department for Communities and Local Government).
Drejza S., Bernatchez P., Dugas C. (2011). Effectiveness of Land Management Measures to Reduce Coastal Georisks, Eastern Québec, Canada. Ocean. Coast. Manage. 54, 290–301. doi: 10.1016/j.ocecoaman.2011.01.001
Dugan J. E., Airoldi L., Chapman M. G., Walker S. J., Schlacher T. A. (2011). ““Estuarine and Coastal Structures: Environmental Effects, A Focus on Shore and Nearshore Structures,” in Treatise on Estuarine and Coastal Science. Eds. Wolanski E., McLusky D. S. (Elsevier Inc), 17–41. doi: 10.1016/B978-0-12-374711-2.00802-0
Evans A. J., Garrod B., Firth L. B., Hawkins S. J., Morris-Webb E. S., Goudge H., et al. (2017). Stakeholder Priorities for Multi-Functional Coastal Defence Developments and Steps to Effective Implementation. Mar. Policy 75, 143–155. doi: 10.1016/j.marpol.2016.10.006
Folke C., Hahn T., Olsson P., Norberg J. (2005). Adaptive Governance of Social-Ecological Systems. Annu. Rev. Environ. Resour., 441–473. doi: 10.1146/annurev.energy.30.050504.144511
Friesinger S., Bernatchez P. (2010). Perceptions of Gulf of St. Lawrence Coastal Communities Confronting Environmental Change: Hazards and Adaptation, Québec, Canada. Ocean. Coast. Manage. 53, 669–678. doi: 10.1016/j.ocecoaman.2010.09.001
Gallopín G. C. (2006). Linkages Between Vulnerability, Resilience, and Adaptive Capacity. Glob. Environ. Change 16, 293–303. doi: 10.1016/j.gloenvcha.2006.02.004
Gamper C. D., Turcanu C. (2007). On the Governmental Use of Multi-Criteria Analysis. Ecol. Econ. 62, 298–307. doi: 10.1016/j.ecolecon.2007.01.010
Garmendia E., Gamboa G. (2012). Weighting Social Preferences in Participatory Multi-Criteria Evaluations: A Case Study on Sustainable Natural Resource Management. Ecol. Econ. 84, 110–120. doi: 10.1016/j.ecolecon.2012.09.004
Garmendia E., Gamboa G., Franco J., Garmendia J. M., Liria P., Olazabal M. (2010). Social Multi-Criteria Evaluation as a Decision Support Tool for Integrated Coastal Zone Management. Ocean. Coast. Manage. 53, 385–403. doi: 10.1016/j.ocecoaman.2010.05.001
Garmendia E., Stagl S. (2010). Public Participation for Sustainability and Social Learning: Concepts and Lessons From Three Case Studies in Europe. Ecol. Econ. 69, 1712–1722. doi: 10.1016/j.ecolecon.2010.03.027
Gittman R. K., Fodrie F. J., Popowich A. M., Keller D. A., Bruno J. F., Currin C. A., et al. (2015). Engineering Away Our Natural Defenses: An Analysis of Shoreline Hardening in the US. Front. Ecol. Environ. 13, 301–307. doi: 10.1890/150065
Grêt-Regamey A., Sirén E., Brunner S. H., Weibel B. (2017). Review of Decision Support Tools to Operationalize the Ecosystem Services Concept. Ecosyst. Serv. 26, 306–315. doi: 10.1016/j.ecoser.2016.10.012
Ha S., Tatano H., Mori N., Fujimi T., Jiang X. (2021). Cost–benefit Analysis of Adaptation to Storm Surge Due to Climate Change in Osaka Bay, Japan. Clim. Change 169, 1–20. doi: 10.1007/s10584-021-03282-y
Holling C. S., Gunderson L. H. (2002). “Resilience and Adaptive Cycle,” in Panarchy - Understanding Transformation in Human and Natural Systems. Eds. Gunderson L. H., Holling C. S. (Washington, DC: Island Press), 25–62.
Horstman A. E. M., Wijnberg K. M., Smale A. J., Hulscher S. J. M. H., Wijnbergt K. M., Hiih H. (2009). Long-Term Coastal Management Strategies: Useful or Useless? J. Coast. Res. I, 233–237. https://www.jstor.org/stable/25737572.
Jacob C., Bernatchez P., Dupras J., Cusson M. (2021). Not Just an Engineering Problem: The Role of Knowledge and Understanding of Ecosystem Services for Adaptive Management of Coastal Erosion. Ecosyst. Serv. 51, 16. doi: 10.1016/j.ecoser.2021.101349
Jajac N., Kilič J., Rogulj K. (2019). An Integral Approach to Sustainable Decision-Making Within Maritime Spatial Planning-A DSC for the Planning of Anchorages on the Island of Šolta, Croatia. Sustain ability 11, 27. doi: 10.3390/su11010104
Jones R. N., Patwardhan A., Cohen S. J., Kumar P., Barros R., Dokken D. J., et al. (2014). ““Foundations for Decision Making,” in Climate Change 2014: Impacts, Adaptation, and Vulnerability. Part A: Global and Sectoral Aspects. Contribution of Working Group II to the Fifth Assessment Report of the Intergovernmental Panel on Climate Change, vol. 195–228. Eds. Field C. B., Barros V. R., Dokken D. J., Mach K. J., Mastrandrea M. D., Bilir T. E., et al (Cambridge, United Kingdom and New York, NY, USA: Cambridge University Press).
Koike K. (1996). The Countermeasures Against Coastal Hazards in Japan. GeoJournal 38, 301–312. doi: 10.1007/BF00204722
Koontz T. M., Gupta D., Mudliar P., Ranjan P. (2015). Adaptive Institutions in Social-Ecological Systems Governance: A Synthesis Framework. Environ. Sci. Policy 53, 139–151. doi: 10.1016/j.envsci.2015.01.003
Lima M., Coelho C., Veloso-Gomes F., Roebeling P. (2020). An Integrated Physical and Cost-Benefit Approach to Assess Groins as a Coastal Erosion Mitigation Strategy. Coast. Eng. 156, 103614. doi: 10.1016/j.coastaleng.2019.103614
Maia A., Bernardes C., Alves M. (2015). Cost-Benefit Analysis of Coastal Defenses on the Vagueira and Labrego Beaches in North West Portugal. J. Integr. Coast. Zo. Manage. 15, 81–90. doi: 10.5894/rgci521
Marie G., Bernatchez P., Fraser C., Touchette M., Papageorges S., Coulombe D., et al. (2017). L’adaptation Aux Aléas Côtiers Dans Un Contexte De Changements Climatiques: Portrait Des Besoins Exprimés Et Des Outils Proposés a L’échelle Des MRC De L’est Du Québec,“ in Chaire de Recherche en Géoscience Côtier, Laboratoire de Dynamique Et De Gestion Intégrée Des Zones Côtières (Rimouski, QC, Canada: Université du Québec à Rimouski).
Marttunen M., Lienert J., Belton V. (2017). Structuring Problems for Multi-Criteria Decision Analysis in Practice: A Literature Review of Method Combinations. Eur. J. Oper. Res. 263, 1–17. doi: 10.1016/j.ejor.2017.04.041
Maystre L. Y., Pictet J., Simos J. (1994). Méthodes Multicritères ELECTRE: Description, Conseils Pratiques Et Cas D’application a La Gestion Environnementale (PPUR presses polytechniques).
McIntosh B. S., Ascough J. C., Twery M., Chew J., Elmahdi A., Haase D., et al. (2011). Environmental Decision Support Systems (EDSS) Development - Challenges and Best Practices. Environ. Model. Software 26, 1389–1402. doi: 10.1016/j.envsoft.2011.09.009
Monterroso I., Binimelis R., Rodríguez-Labajos B. (2011). New Methods for the Analysis of Invasion Processes: Multi-Criteria Evaluation of the Invasion of Hydrilla Verticillata in Guatemala. J. Environ. Manage. 92, 494–507. doi: 10.1016/j.jenvman.2010.09.017
Morris R. L., Konlechner T. M., Ghisalberti M., Swearer S. E. (2018). From Grey to Green: Efficacy of Eco-Engineering Solutions for Nature-Based Coastal Defence. Glob. Change Biol. 24, 1827–1842. doi: 10.1111/gcb.14063
Morris R. L., Strain E. M. A., Konlechner T. M., Fest B. J., Kennedy D. M., Arndt S. K., et al. (2019). Developing a Nature-Based Coastal Defence Strategy for Australia. Aust. J. Civ. Eng. 17, 167–176. doi: 10.1080/14488353.2019.1661062
Moschella P. S., Abbiati M., Åberg P., Airoldi L., Anderson J. M., Bacchiocchi F., et al. (2005). Low-Crested Coastal Defence Structures as Artificial Habitats for Marine Life: Using Ecological Criteria in Design. Coast. Eng. 52, 1073–1087. doi: 10.1016/j.coastaleng.2005.09.014
National Research Council (2009). Informing Decisions in a Changing Climate (Washington, DC: The National Academies Press). doi: 10.17226/12626
Nicholls R. J., Cazenave A. (2010). Sea-Level Rise and Its Impact on Coastal Zones. Science (80-. ) 328, 1517–1520. doi: 10.1126/science.1185782
O’Riordan T. (2005). “Inclusive and Community Participation in the Coastal Zone: Opportunities and Dangers,” in Managing European Coasts. Eds. Vermaat J., Salomons W., Bouwer L., Turner K. (Berlin, Heidelberg: Springer Berlin Heidelberg), 173–184.
Polasky S., Carpenter S. R., Folke C., Keeler B. (2011). Decision-Making Under Great Uncertainty: Environmental Management in an Era of Global Change. Trends Ecol. Evol. 26, 398–404. doi: 10.1016/j.tree.2011.04.007
Polomé P., Marzetti S., van der Veen A. (2005). Economic and Social Demands for Coastal Protection. Coast. Eng. 52, 819–840. doi: 10.1016/j.coastaleng.2005.09.009
Ranasinghe R. (2016). Assessing Climate Change Impacts on Open Sandy Coasts: A Review. Earth-Sci.s Rev. 160, 320–332. doi: 10.1016/j.earscirev.2016.07.011
Reed M. S. (2008). Stakeholder Participation for Environmental Management: A Literature Review. Biol. Conserv. 141, 2417–2431. doi: 10.1016/j.biocon.2008.07.014
Saarikoski H., Mustajoki J., Barton D. N., Geneletti D., Langemeyer J., Gomez-Baggethun E., et al. (2016). Multi-Criteria Decision Analysis and Cost-Benefit Analysis: Comparing Alternative Frameworks for Integrated Valuation of Ecosystem Services. Ecosyst. Serv. 22, 238–249. doi: 10.1016/j.ecoser.2016.10.014
Saaty T. L. (2008). Decision Making With the Analytic Hierarchy Process. Int. J. Serv. Sci. 1, 83–98. doi: 10.1504/IJSSCI.2008.017590
Sauvé P., Bernatchez P., Glaus M. (2022). Identification of Coastal Defence Measure Best Adapted to Mitigate Hazards in Specific Coastal Systems: Development of a Dynamic Literature Meta-Analysis Methodology. J. Mar. Sci. Eng, 13. doi: 10.3390/jmse10030394
Sauvé P., Bernatchez P., Moisset S., Glaus M., Goudreault M.-O (2022). Case Studies on Coastal Defence Measures: A Meta-Analysis of Literature to Ultimately Improve Decision-Making. Manag.; submitted
Savoie-Ferron F., Goudreault M.-O., Autret R., Bandet M. (2020). “Suivi Environnemental De Travaux De Rechargement De Plage Et De La Renaturalisation D’une Plage Comme Solution D’adaptation Aux Aléas Côtiers Dans L’estuaire Et Le Golfe Du Saint-Laurent,” in Chaire de recherche en géoscience côtière, Laboratoire de dynamique et de gestion intégrée des zones côtières (Rimouski, Qc, Canada: Université du Québec à Rimouski), pp. 162. Available at: https://ldgizc.uqar.ca/Web/docs/default-source/default-document-library/savoie-ferron_etal_2020_rapport_final_recharge_corrige_cps-17-18-02.pdf?sfvrsn=60fef139_0.
Schoonees T., Gijón Mancheño A., Scheres B., Bouma T. J., Silva R., Schlurmann T., et al. (2019). Hard Structures for Coastal Protection, Towards Greener Designs. Estuaries. Coast. 42, 1709–1729. doi: 10.1007/s12237-019-00551-z
Scott J. A., Ho W., Dey P. K. (2012). A Review of Multi-Criteria Decision-Making Methods for Bioenergy Systems. Energy 42, 146–156. doi: 10.1016/j.energy.2012.03.074
Stagl S. (2006). Multicriteria Evaluation and Public Participation: The Case of UK Energy Policy. Land. Use Policy 23, 53–62. doi: 10.1016/j.landusepol.2004.08.007
Tavra M., Jajac N., Cetl V. (2017). Marine Spatial Data Infrastructure Development Framework: Croatia Case Study. ISPRS. Int. J. Geo-Info. 6, 15. doi: 10.3390/ijgi6040117
Thi Oanh P., Tamura M., Kumano N., Nguyen Q. V. (2020). Cost-Benefit Analysis of Mixing Gray and Green Infrastructures to Adapt to Sea Level Rise in the Vietnamese Mekong River Delta. Sustainability 12, 1–19. doi: 10.3390/su122410356
Trutnevyte E., Stauffacher M., Scholz R. W. (2011). Supporting Energy Initiatives in Small Communities by Linking Visions With Energy Scenarios and Multi-Criteria Assessment. Energy Policy 39, 7884–7895. doi: 10.1016/j.enpol.2011.09.038
Trutnevyte E., Stauffacher M., Scholz R. W. (2012). Linking Stakeholder Visions With Resource Allocation Scenarios and Multi-Criteria Assessment. Eur. J. Oper. Res. 219, 762–772. doi: 10.1016/j.ejor.2012.01.009
USACE. (2006). “ldquo;Planning and Design Process,” in Coastal Engineering Manual (Washington, DC, US: U.S. Army corps of Engineers), 17.
Valloni R., Ferretti O., Barsanti M., Ugolotti M. (2003). “The Morpho-Sedimentological Types of Italian Coasts and Their Susceptibility to Sea-Level Rise: A First Approximation,” in Quaternary Coastal Morphology and Sea Level Changes (Puglia, Italy: Final Conference Project IGCP 437), 5–6. Available at: http://www.igcp437finalconference.unisalento.it/AbstractBook/ValloniV_VI.pdf.
van Slobbe E., de Vriend H. J., Aarninkhof S. G. J., Lulofs K., de Vries M. B., Dircke P. (2013). Building With Nature: In Search of Resilient Storm Surge Protection Strategies. Nat. Haz. 66, 1461–1480. doi: 10.1007/s11069-013-0612-3
Vuik V., Borsje B. W., Willemsen P. W. J. M., Jonkman S. N. (2019). Salt Marshes for Flood Risk Reduction: Quantifying Long-Term Effectiveness and Life-Cycle Costs. Ocean. Coast. Manage. 171, 96–110. doi: 10.1016/j.ocecoaman.2019.01.010
Walling E., Vaneeckhaute C. (2020). Developing Successful Environmental Decision Support Systems: Challenges and Best Practices. J. Environ. Manage. 264, 16. doi: 10.1016/j.jenvman.2020.110513
Wegner G., Pascual U. (2011). Cost-Benefit Analysis in the Context of Ecosystem Services for Human Well-Being: A Multidisciplinary Critique. Glob. Environ. Change 21, 492–504. doi: 10.1016/j.gloenvcha.2010.12.008
Westmacott S. (2001). Developing Decision Support Systems for Integrated Coastal Management in the Tropics: Is the ICM Decision-Making Environment Too Complex for the Development of a Useable and Useful DSS? J. Environ. Manage. 62, 55–74. doi: 10.1006/jema.2001.0420
Wong P. P., Losada I. J., Gattuso J.-P., Hinkel J., Khattabi A., McInnes K. L., et al. (2014). “Coastal Systems and Low-Lying Areas,” in Climate Change 2014: Impacts, Adaptation, and Vulnerability. Part A: Global and Sectoral Aspects. Contribution of Working Group II to the Fifth Assessment Report of the Intergovernmental Panel on Climate Change (Cambridge, United Kingdom: Cambridge University Press), 361–409.
Wong-Parodi G., Mach K. J., Jagannathan K., Sjostrom K. D. (2020). Insights for Developing Effective Decision Support Tools for Environmental Sustainability. Curr. Opin. Environ. Sustain. 42, 52–59. doi: 10.1016/j.cosust.2020.01.005
Keywords: coastal engineering, coastal defence measure, decision-making, integrated coastal zone management (ICZM), participatory process, coastal protection, multicriteria decision analysis (MCDA), PROMETHEE
Citation: Sauvé P, Bernatchez P and Glaus M (2022) Multicriteria Decision Analysis to Assist in the Selection of Coastal Defence Measures: Involving Coastal Managers and Professionals in the Identification and Weighting of Criteria. Front. Mar. Sci. 9:845348. doi: 10.3389/fmars.2022.845348
Received: 29 December 2021; Accepted: 21 March 2022;
Published: 14 April 2022.
Edited by:
Giuseppe Barbaro, Mediterranea University of Reggio Calabria, ItalyReviewed by:
Nikša Jajac, University of Split, CroatiaJonathan Pearson, University of Warwick, United Kingdom
Copyright © 2022 Sauvé, Bernatchez and Glaus. This is an open-access article distributed under the terms of the Creative Commons Attribution License (CC BY). The use, distribution or reproduction in other forums is permitted, provided the original author(s) and the copyright owner(s) are credited and that the original publication in this journal is cited, in accordance with accepted academic practice. No use, distribution or reproduction is permitted which does not comply with these terms.
*Correspondence: Philippe Sauvé, philippe.sauve@uqar.ca