- 1College of Life Science, Huzhou University, Huzhou, China
- 2Zhejiang Provincial Key Laboratory of Aquatic Bioresource Conservation and Development Technology, Huzhou, China
- 3Nation and Local Joint Engineering Laboratory of Aquatic Animal Genetic Breeding and Nutrition, Huzhou, China
This study aimed to explore the influence of soybean meal on intestinal mucosa metabolome and signaling pathway of mirror carp (Cyprinus carpio Songpu) by integrating liquid chromatography-tandem mass spectrometry (LC-MS/MS)-based metabolomics. Fish meal (Con) was control group, soybean meal (Dou) group, AMPK activator (300 mg kg–1 Metformin, Met) and AMPK inhibitor (0.2 mg kg–1 Compound-c, c-Com) which added to soybean meal were experimental groups. The metabolome profiles of the intestinal mucosa were determined in fish fed diets Con, Dou, Met, and c-Com on 7th and 59th day. The results were shown: compared with the Con group, the weight gain rate and the specific growth rate of fish experimental groups were significantly decreased (P < 0.05), feed conversion ratio (FCR) was significantly increased (P < 0.05). Compared with the Con group, sphingosine, glycocholic acid, majorities of sugar metabolites were up-regulated, and phosphatidylcholine (PC) and lysophosphatidylcholine (LysoPC), amino acids were reduced significantly in all experiment groups (P < 0.05). Oxidized glutathione was up-regulated in Dou on 7th day, Met on 7th and 59th day (P < 0.05). ADP (adenosine diphosphate) and AMP (adenosine monophpsphate) were up-regulated in Dou, Met, c-Com on 59th day (P < 0.05). Compared with the Dou group, sphingosine was down-regulated on 7th day, up-regulated on 59th day in Met and on 7th and 59th day in c-Com (P < 0.05). Oxidized glutathione and isocitrate on 7th day, L-Valine, L-histidine, and L-isoleucine on 59th day were up-regulated in Met (P < 0.05). Nucleoside metabolites and ADP were up-regulated in c-Com on 7th day (P < 0.05). In conclusion, soybean meal influenced intestinal mucosa metabolic processes, including lipid, amino acid, sugar, apoptosis, and oxidative injury; and changed energy metabolism in intestinal mucosa, enriched in the AMPK, TOR, FoxO signaling pathway; Metformin could aggravate oxidative damage, alleciated apoptosis for the short term, and aggravate apoptosis, improve carbohydrate catabolism and amino acid anabolism for the long term; Compound-c exacerbated apoptosis. repaired oxidative damage, and enhanced nucleoside catabolism.
Introduction
Currently, the high demand and cost of fish meals entail evaluating the substitution of fish meal with soybean meal in fish diets, but the substitution of fish meal by soybean meal over a certain range could reduce the growth performance, affect the intestinal health of fish (Wang et al., 2016) and decrease the protease activities in both intestine and hepatopancreas (Lin and Luo, 2011). The replacement ratio of fish meal with soybean meal reached 20% can cause intestinal inflammation in common carp (Urn et al., 2008). Soybean meal induces Hsp70 activation by reducing intestinal p38MAPK phosphorylation of part of the intestine and elicits cell protective response (Antonopoulou et al., 2017). One of the main limiting factors in soybean meals is the presence of anti-nutritional factors that cause intestinal injury and antioxidant damage. Among them, protease inhibitors, soybean agglutinin, tannin, phytic acid, soybean antigen have a strong anti-nutritional effect. 3.5% Soya lectin (Buttle et al., 2001) significantly damaged the integrity of posterior intestinal villi in Atlantic salmon. The trypsin inhibitor (Maytorena-Verdugo et al., 2017) may have reacted with the essential group of protease, which inhibits the binding of protease with a substrate, and reduces or even prevents the activity of the protease. Tannins in the SBM diet (Becker and Makkar, 1999) can inhibit gastric enzyme activity after combination with carbohydrates. Evidence suggests that intestinal mucosal barrier dysfunction is a prerequisite for enteropathy (Turner, 2009; Bron et al., 2017). Usually, intestinal inflammation accompanies with abnormal expression of MUC-2 and tight junction proteins such as claudin-4, occluding, and ZO-1 (Hara et al., 2000; Velcich et al., 2002; Jiang et al., 2015). In addition, fish fed a high dose of soybean meal presented gross intestinal inflammation coupled with increased expression of pro-inflammatory cytokines such as IL-1β and TNF-α accompanied by the up-regulation of NF-κB (Jiang et al., 2015; Gu et al., 2016).
The metabolism and absorption of intestinal nutrients play an important role in the growth performance of fish. The integrity and proper functioning of intestinal mucosal cells are essential. Previous experiments have been confirmed, anti-nutritional factors in soybean meal lead to intestinal damage by affecting some signaling pathways. Soybean glycinin (Zhang et al., 2020) increased ROS generation related to the NOX signaling pathway in the mid and distal intestine and decreased ROS elimination capacity related to NrF2 translocation in the whole intestine of juvenile grass carp (Ctenopharyngodon idella). Glycinin (Jiang et al., 2015) and β-conglycinin (Zhang et al., 2013) of soybean can affect the gene expression of the intestinal TOR signaling pathway and thus affect protein synthesis, and reduce fish growth performance. Soybean soaking water has a function in ameliorating obesity through inhibiting lipid synthesis as well as stimulating fatty acid oxidation by elevating the levels of phosphorylation of adenosine monophosphate-activated protein kinase (AMPK) and acetyl-CoA carboxylase (ACC) in the experiment in mice (Park et al., 2015). As a cellular energy sensor, AMPK is a serine/threonine-protein kinase that affects fatty acid, cholesterol, glucose metabolism, protein synthesis, and other metabolic pathways by regulating downstream target proteins (Kahn et al., 2005). There is no direct evidence if soybean meal affects fish intestine health and energy metabolism by affecting the AMPK signaling pathway on fish. Metabolomics is the attempt to identify and quantify all endogenous small molecule metabolites in an organism or biofluid sample and is common in experimental studies of aquatic animals (Long et al., 2021; Xiang et al., 2021; Yang et al., 2021). This study is based on metabonomics to investigate the effects of soybean meal on intestinal mucosa metabolome and the influence on AMPK and other signaling pathways.
Materials and Methods
Experimental Diets, Fish Feeding, and Sampling
Metformin (97%) and compound-c [98.59%, C24H25N5O; 6-[4-(2-piperidin-1-ylethoxy) phenyl]-3-pyridine-4-ylpyrazolo [1, 5-a] pyrimidine] used in this study were purchased from Sigma-Aldrich. The ingredients and nutrient content of the experimental diets are shown in Table 1. The levels of nutrients were designed to meet the requirements of common carp (Cyprinus carpio) according to the National Research Council [NRC] (2011). Fish meal (Con) used as the dietary protein source was the control group, 40% soybean meal (Dou), 300 mg kg–1 metformin (Met) (Silva et al., 2015), and 0.2 mg kg–1 compound-c (c-Com) (Kim et al., 2011), which added to soybean meal were experimental groups. Amino acids were balanced with coated amino acids. All diets were isonitrogenous and isolipidic. All the feed ingredients were stirred evenly in the mixer. After adding lipid and water, the mixture was homogenized and extruded through a pelletizer with a 2 mm mold. The diets were air-dried at room temperature and stored at −20°C until feeding.
The juvenile mirror carps (Cyprinus carpio Songpu) used in this study were purchased from Heilongjiang River Fishery Research Institute of the Chinese Academy of Fishery Sciences. Before the trial, the fish were acclimatized to the recirculating aquaculture system for 2 weeks. Then, 348 fish with a mean initial weight of 9.51 (±0.06) g were randomly assigned to 12 aquariums (500 L water) with an initial stocking density of 29 fish per aquarium. Each feed was randomly assigned to three aquariums. In the feeding trial, each diet was fed at 5% of the bodyweight of fish twice per day for the 59 days. The water temperature and pH were 26 (±2)°C and 7.0 (±0.3), respectively, dissolved oxygen was higher than 5 mg/l. The water quality was tested using YSI Professional Plus, and one-third of water was changed a week thrice to remove solid substances and reduce ammonia concentration.
At the beginning and end of the trial, fish in each aquarium were counted and weighed. After that, 18 fish per diet were anesthetized by MS-222 (100 mg/L), the intestine was quickly removed on ice, dissected longitudinally, rinsed in cold physiological saline (0.86% NaCl solution, pH = 7.2), and the intestinal mucosa was scraped with a glass slide. The intestinal mucosas of three fishes were pooled into one sample, frozen in liquid nitrogen, and stored at −80°C. Samples were collected on the 7th (short-term) and 59th day (long-term). The following growth parameters were calculated after the feeding trial: weight gain rate (WGR), specific growth rate (SGR), feed conversion ratio (FCR), and survival rate (SR).
Non-targeted Metabolomic and Statistical Data Analysis
Metabolite extraction and liquid chromatograph-mass spectrometer analysis were performed by Shanghai Majorbio Bio-pharm Technology Co., Ltd. (Shanghai, China). Metabolite data and profilings were obtained and analyzed on the free online platform of Majorbio Cloud Platform.1 After identification and quantification, metabolites were annotated into HMDB (human metabolome database) and KEGG (Kyoto encyclopedia of genes and genomes), respectively. Differential metabolites in the mucosa of different treatments were identified using univariate analysis and multivariate statistics. Univariate analysis was performed by the methods of t-test and folded change (FC) analysis (P < 0.05; FC ≥ 1 or ≤1). Multivariate statistics were performed by Orthogonal Partial Least Squares Discriminant Analysis (OPLS-DA) with variable importance for the projection (VIP) values (≥1.0). HMDB pie chart showed the superclass of differential metabolites between groups, and KEGG was used to help in understanding the functions of metabolites. Growth performance indicators were analyzed by one-way ANOVA using SPSS IBM Statistics 25. Duncan’s analysis was used for multiple comparisons.
Results
Growth Performance
Compared with the Con group, the WGR and SGR of fish fed the soybean meal, metformin, and compound-c diets were significantly decreased (P < 0.05). FCR of fish in the experimental groups was significantly increased than those fish in the Con group (P < 0.05). There was no significantly difference in SR of fish among groups (P > 0.05, in Table 2).
Principal Component Analysis
The metabolite peaks extracted from all samples and QC samples were analyzed by principal component analysis (Figure 1). It was found that QC samples clustered together, indicating that this experiment had good repeatability, a stable system, and reliable experimental results. Con on 7th day, Dou on 7th day, and Met on 7th day were dispersed among samples, while c-Com on 7th day, Con on 59th day, Dou on 59th day, Met on 59th day, and c-Com on 59th day groups had more concentrated sample points.
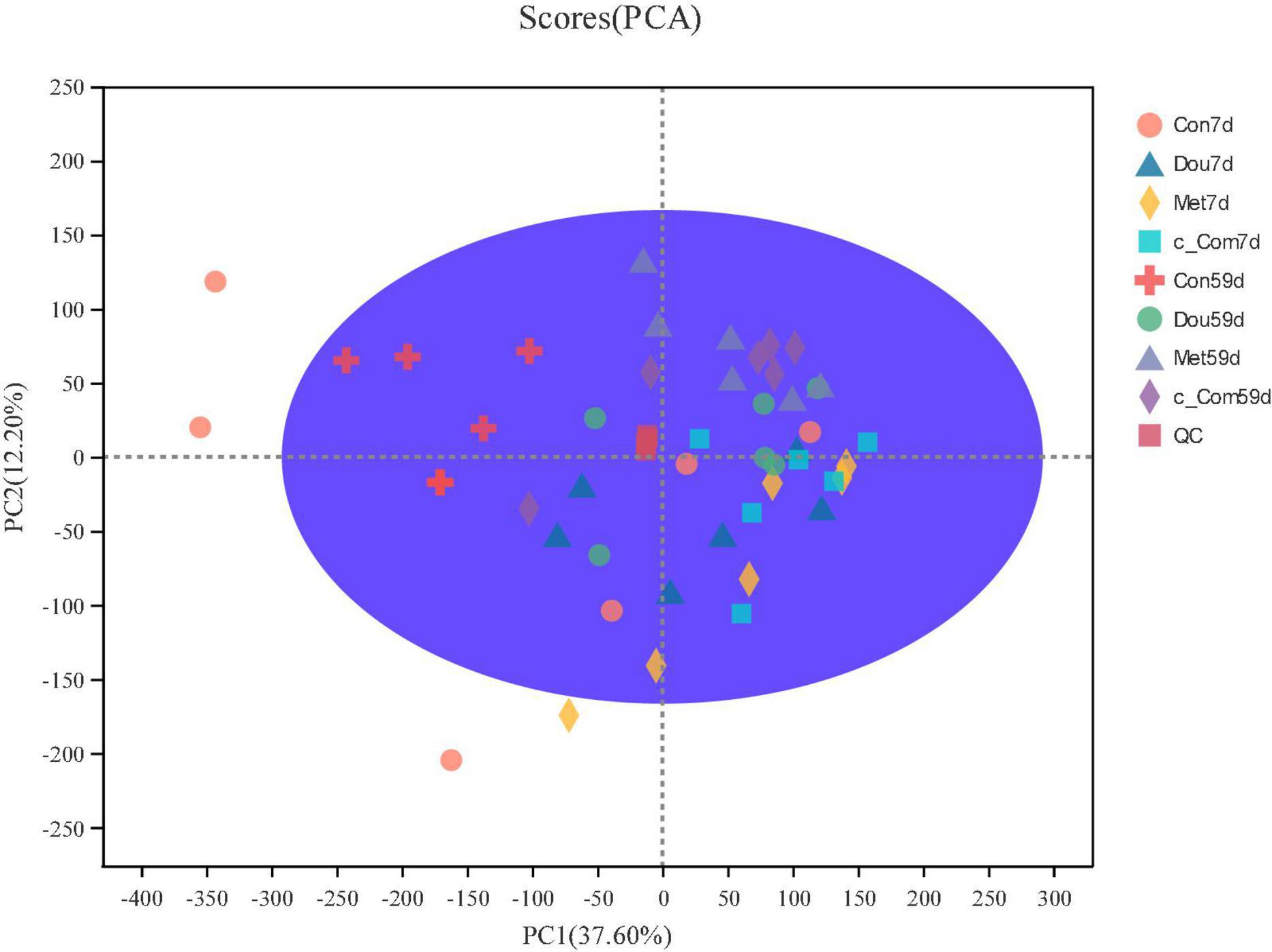
Figure 1. Primcipal component analysis score chart. After dimension reduction analysis, there are relative coordinate points on principal components P1 and P2, and the distance of each coordinate point represents the degree of aggregation and dispersion between samples. The confidence ellipse means that the “real” samples group is distributed in this region with 95% confidence.
Differential Metabolites and Differential Pathways
The identification and quantification results of metabolites showed that a total of 1,332 metabolites had been obtained, of which 1,157 and 363 were annotated into HMDB (Human Metabolome Database) and KEGG (Kyoto Encyclopedia of Genes and Genomes), respectively. Score plots of the (O) PLS-DA performed to verify the differentiated metabolites between the two groups and multivariate analysis supervision are shown in Figures 2–6. R2X (cum) and R2Y (cum) represent cumulative interpretation rates. Q2 indicates the prediction ability of the model. In all comparisons, R2Y was close to 1, indicating that the model is reliable. In Dou vs. Con, Met vs. Con, c-Com vs. Con, and c-Com on 59th day, vs. Dou on 59th day groups, Q2 > 0.5 indicated the model’s prediction ability was good. The pie chart (Figure 7) showed the HMDB superclass classification of the different metabolites between every two groups. As shown in the figure, Lipids and lipid-like molecules (purple) accounted for the largest proportion of all groups, respectively. The differential metabolites selected above were enriched into the KEGG database for analysis to obtain differential metabolic pathways. The differential metabolic pathways were sorted according to their significance (P-value) (Figure 8), and the differential metabolites involved in the differential metabolic pathways were emphatically analyzed (Supplementary Table 1).
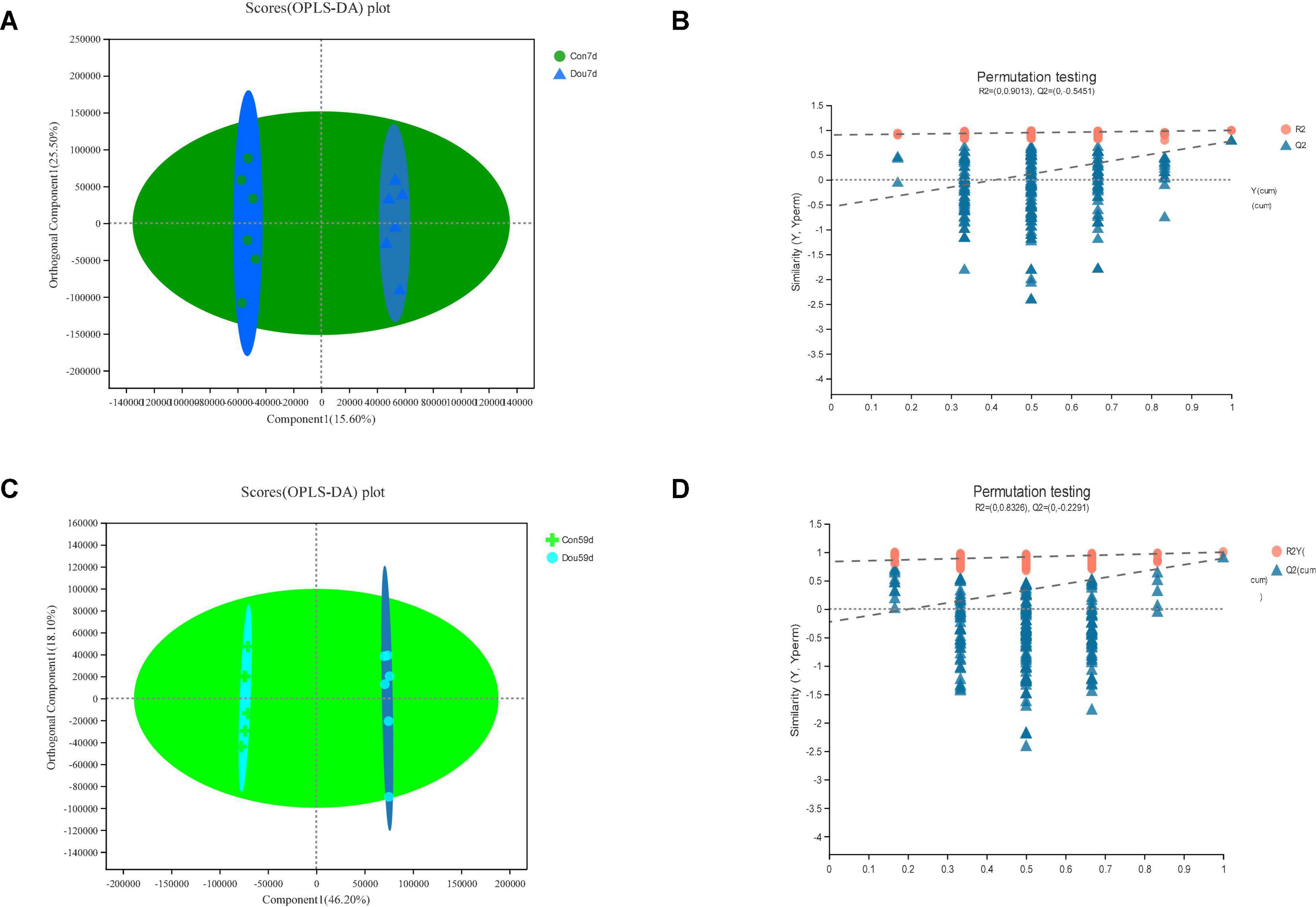
Figure 2. In Dou vs. Con, panels (A,B) for 7th day and panels (C,D) for 59th day. Panels (A,C) are OPLS-DA scores plot. Panels (B,D) are OPLA-DA permutation tests. (B) R2X (cum) = 0.571, R2Y (cum) = 0.994, Q2 = 0.777. (D) R2X (cum) = 0.705, R2Y (cum) = 0.999, Q2 = 0.89.
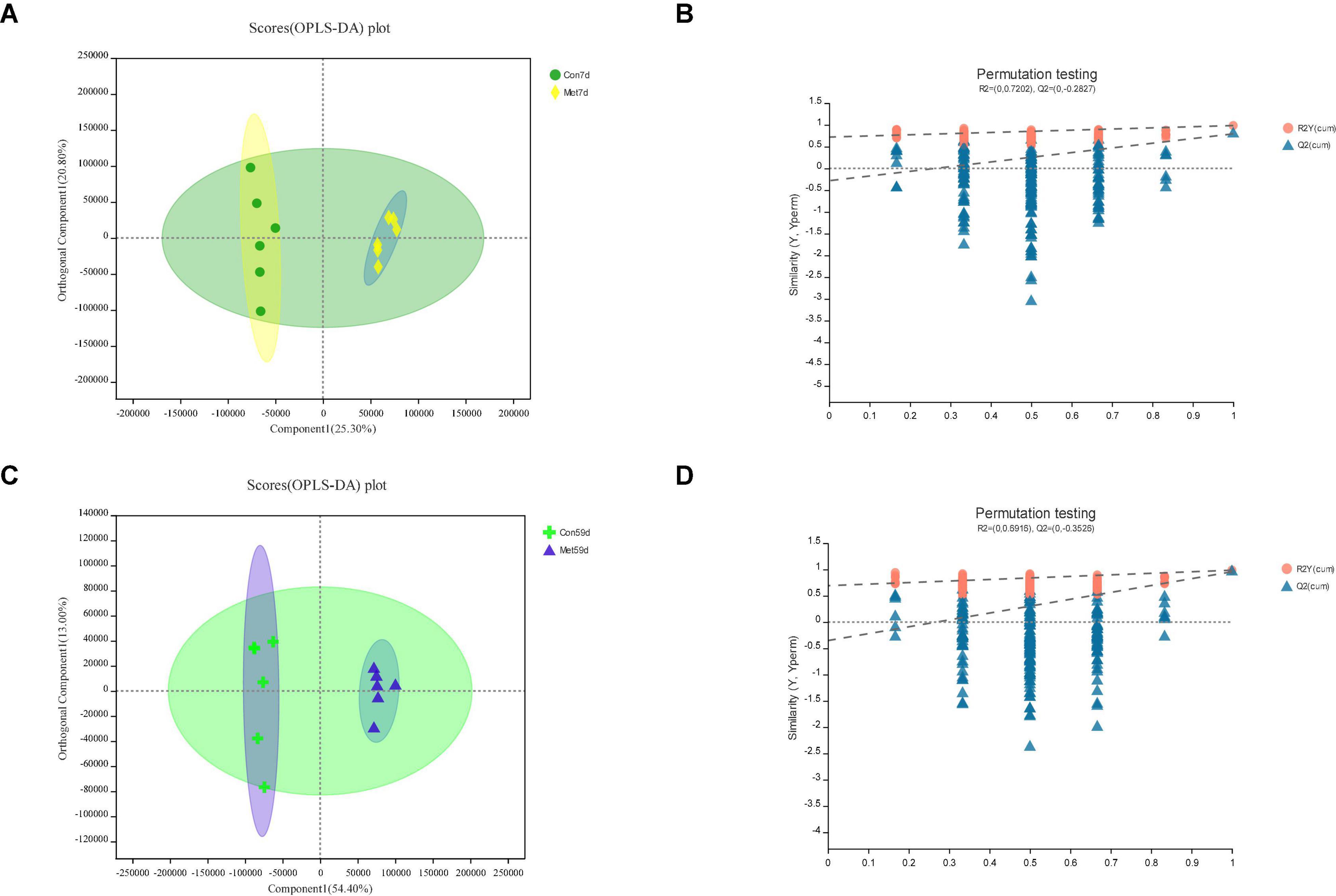
Figure 3. In Met vs. Con, panels (A,B) for 7th day and panels (C,D) for 59th day. Panels (A,C) are OPLS-DA scores plot. Panels (B,D) are OPLA-DA permutation tests. (B) R2X (cum) = 0.462, R2Y (cum) = 0.985, Q2 = 0.794. (D) R2X (cum) = 0.674, R2Y (cum) = 0.986, Q2 = 0.956.
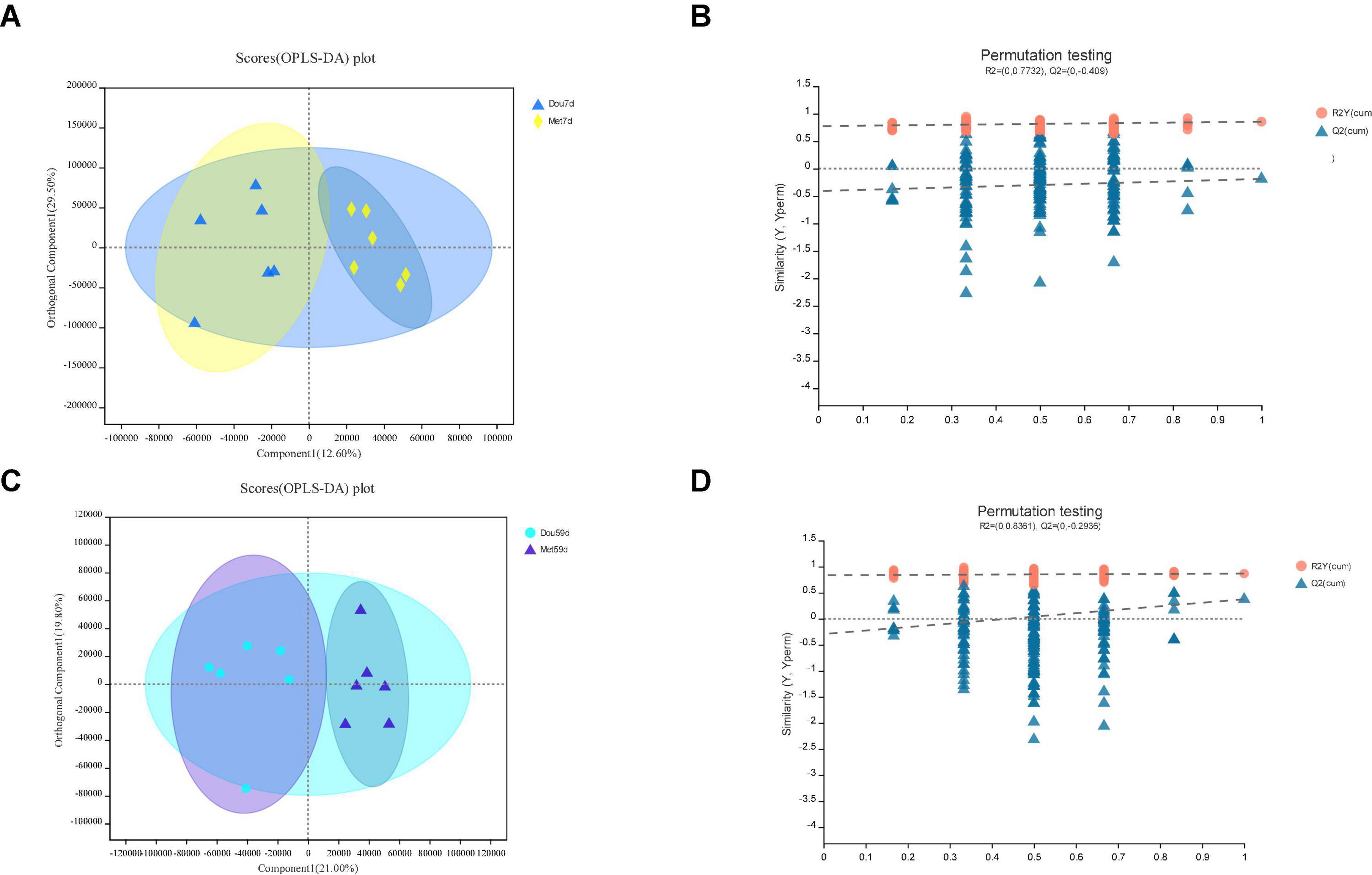
Figure 4. In Met vs. Dou, panels (A,B) for 7th day and panels (C,D) for 59th day. Panels (A,C) are OPLS-DA scores plot. Panels (B,D) are OPLA-DA permutation tests. (B) R2X (cum) = 0.421, R2Y (cum) = 0.855, Q2 = −0.188. (D) R2X (cum) = 0.408, R2Y (cum) = 0.867, Q2 = 0.374.
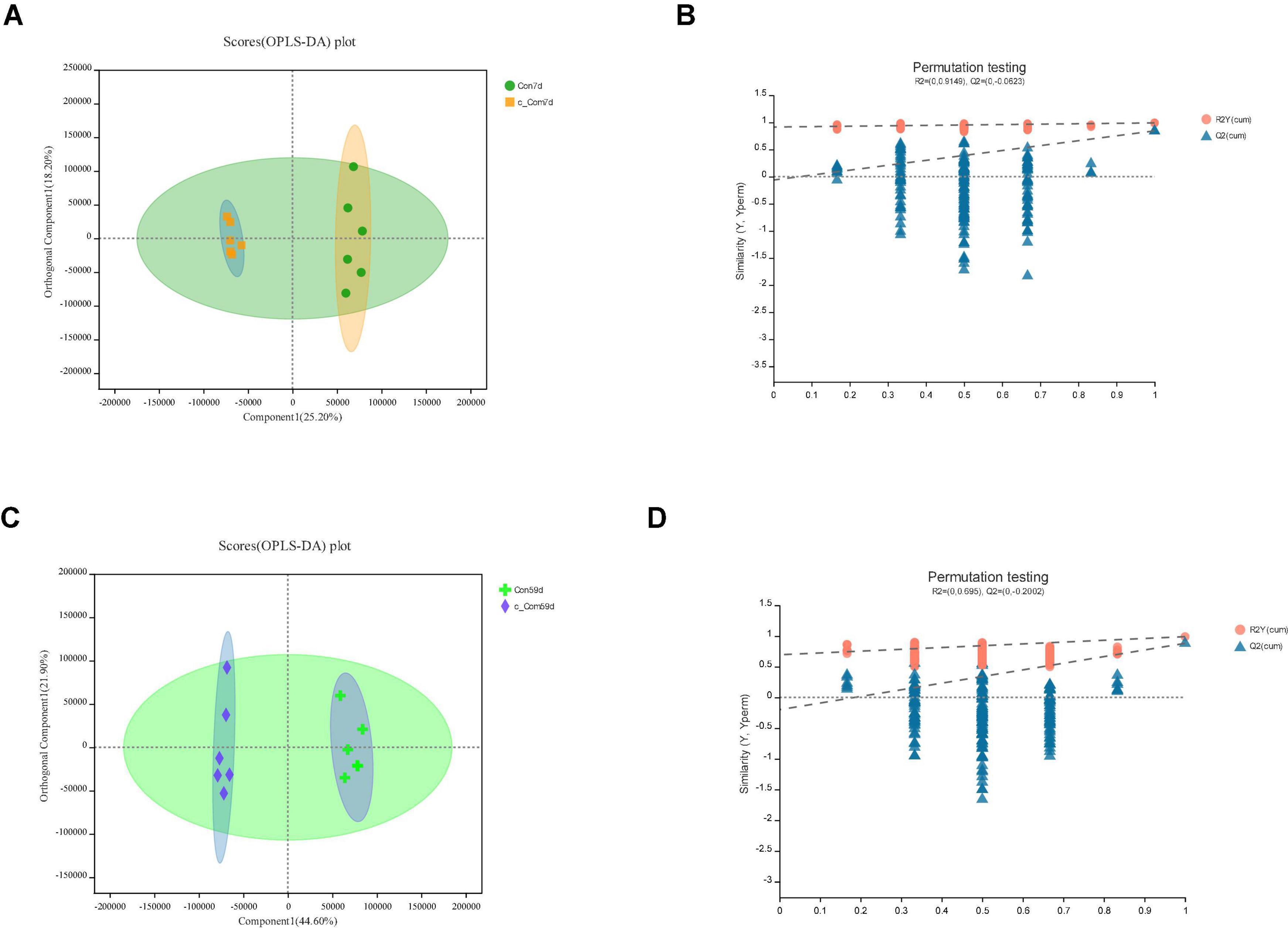
Figure 5. In c-Com vs. Con, panels (A,B) for 7th day and panels (C,D) for 59th day. Panels (A,C) are OPLS-DA scores plot. Panels (B,D) are OPLA-DA permutation tests. (B) R2X (cum) = 0.558, R2Y (cum) = 0.991, Q2 = 0.844. (D) R2X (cum) = 0.665, R2Y (cum) = 0.99, Q2 = 0.881.
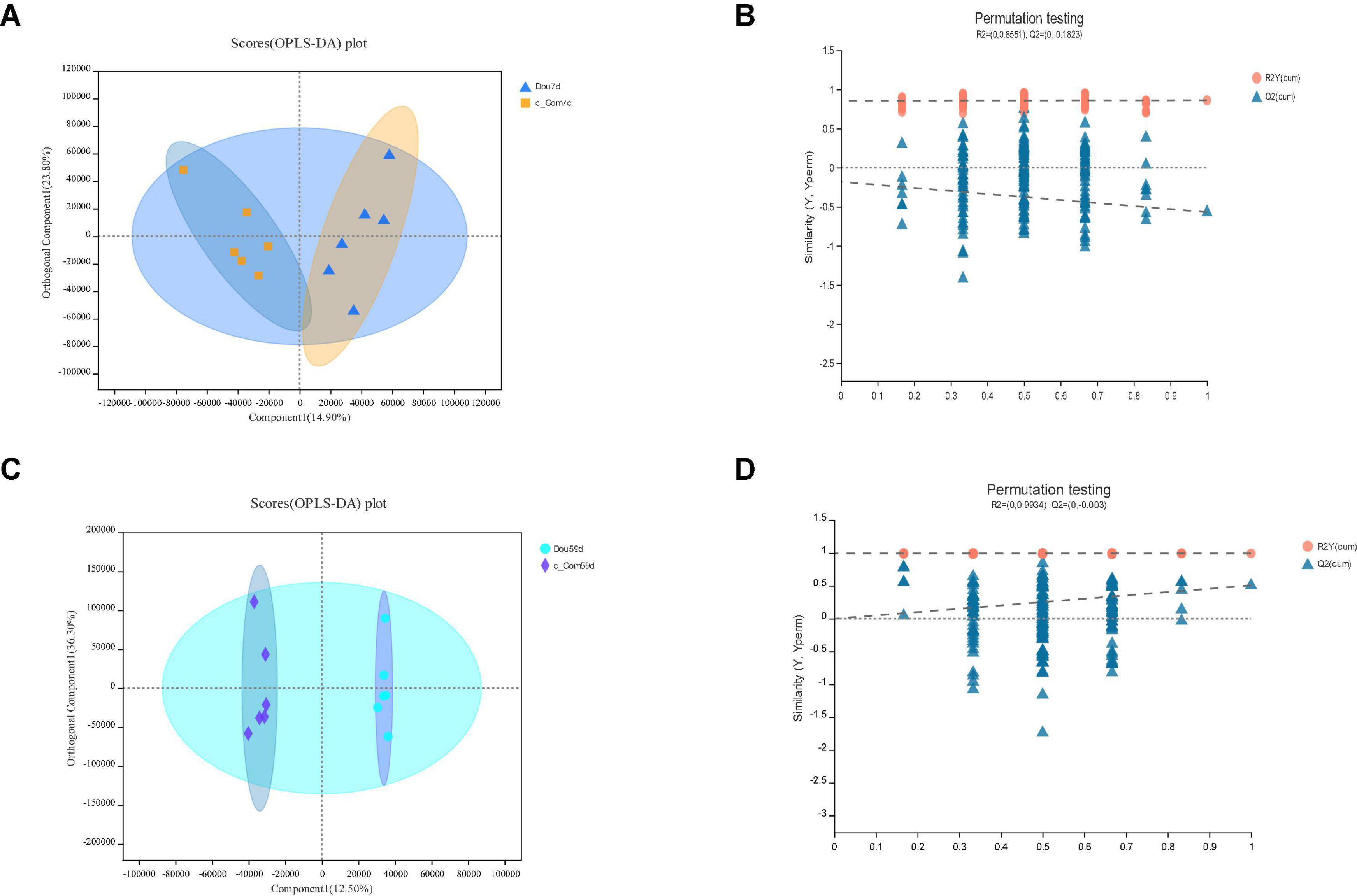
Figure 6. In c-Com vs. Dou, panels (A,B) for 7th day and panels (C,D) for 59th day. Panels (A,C) are OPLS-DA scores plot. Panels (B,D) are OPLA-DA permutation tests. (B) R2X (cum) = 0.388, R2Y (cum) = 0.86, Q2 = −0.57. D: R2X (cum) = 0.787, R2Y (cum) = 0.993, Q2 = 0.506.
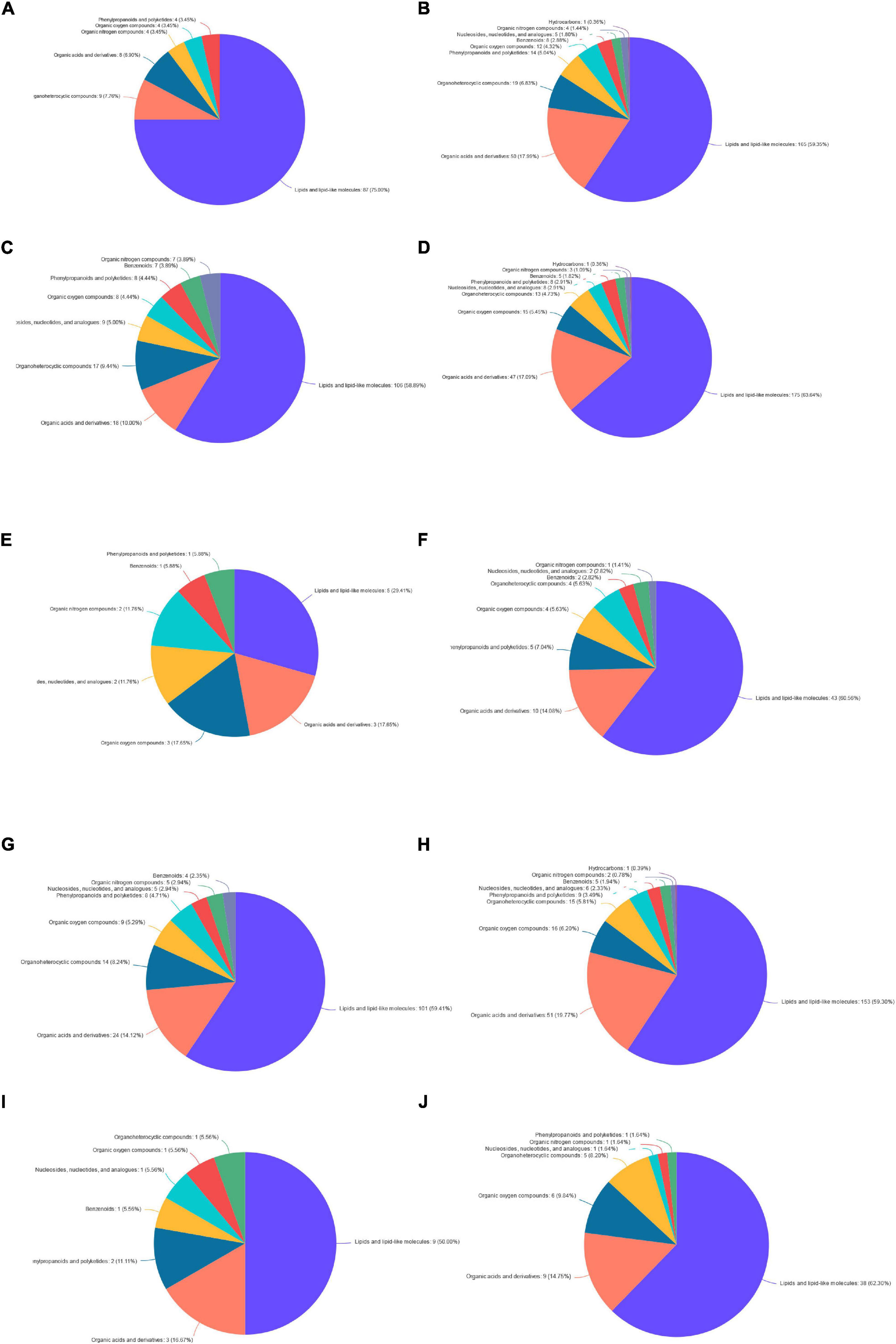
Figure 7. The pie chart. Panel (A) for Dou vs. Con on 7th day; panel (B) for Dou vs. Con on 59th day; panel (C) for Met vs. Con on 7th day; panel (D) for Met vs. Con on 59th day; panel (E) for Met vs. Dou on 7th day; panel (F) for Met vs. Dou on 59th day; panel (G) for c-Com vs. Con on 7th day; panel (H) for c-Com vs. Con on 59th day; panel (I) for c-Com vs. Dou on 7th day; panel (J) for c-Com vs. Dou on 59th day.
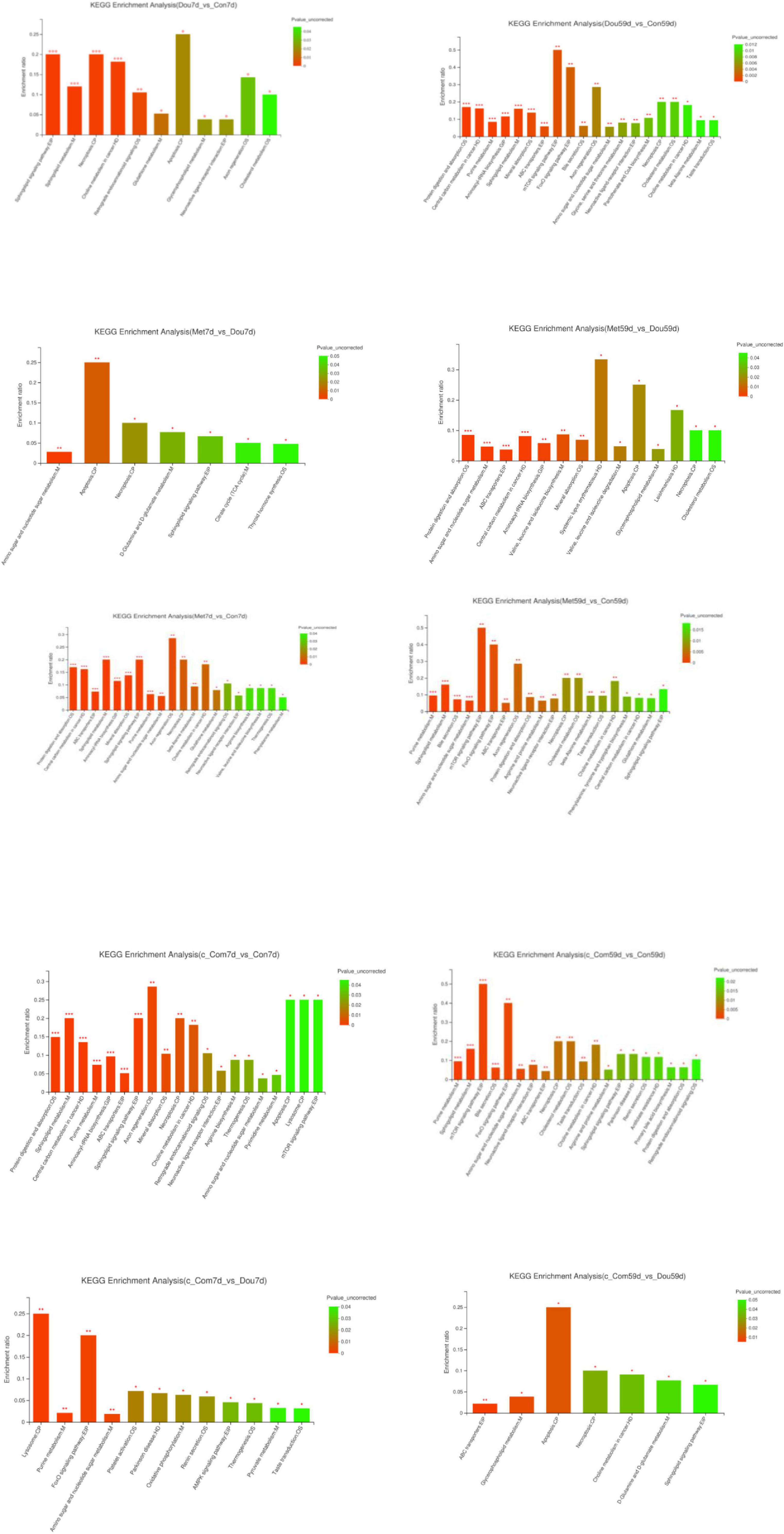
Figure 8. KEGG enrichment analysis. The abscissa represents the pathway name, and the ordinate represents enrichment rate, representing the ratio between the Metabolite number enriched in this pathway, and the Background number annotated. The higher the ratio, the greater the degree of enrichment. The color gradient of the column represents the significance of enrichment. The darker the default color is, the more significant the enrichment of the KEGG term is. In particular, P-value < 0.001 is marked as ***, P-value < 0.01 is marked as **, and P-value < 0.05 is marked as *. The following table is the same.
Dou vs. Con
On the 7th day, compared with the Con group, sphingosine was up-regulated in the Dou group (P < 0.05), which was annotated into apoptosis, sphingolipid signaling pathway, sphingolipid metabolism and necroptosis pathway and glycocholic acid in cholesterol metabolism, and most of phosphatidylcholine (PC) and lysophosphatidylcholine (LysoPC) were down-regulated (P < 0.05) in choline metabolism in cancer. On the 59th day, compared with the Con group, same with 7th day, sphingosine and glycocholic acid were up-regulated (P < 0.05), and most of PC and LysoPC were down-regulated in the Dou group (P < 0.05). Furthermore, nucleoside and sugar metabolites in purine metabolism, amino sugar, and nucleotide sugar metabolism pathways were up-regulated (P < 0.05), and amino acids enriched in central carbon metabolism in cancer, protein digestion and absorption, aminoacyl-tRNA biosynthesis, mineral absorption, ABC transporters were down-regulated in Dou group (P < 0.05). ADP and AMP enriched in the AMPK signaling pathway, mTOR signaling pathway, and FoxO signaling pathway were up-regulated (P < 0.05) in the Dou group. And taurine was up-regulated in neuroactive ligand-receptor interaction, primary bile acid biosynthesis, and taurine and hypotaurine metabolism. Taurocholic acid was down-regulated in bile secretion, cholesterol metabolism, primary bile acid biosynthesis in the Dou group on the 59th day.
Met vs. Con
On the 7th day, compared with the Con group, the addition of metformin into soybean meal up-regulated sphingosine in apoptosis, sphingolipid signaling pathway, sphingolipid metabolism, and necroptosis pathways, oxidized glutathione in glutathione metabolism, nucleoside, and sugar metabolites in purine metabolism and amino sugar and nucleotide sugar metabolism pathways (P < 0.05), down-regulated PC in choline metabolism in cancer, amino acids in ABC transporters, protein digestion and absorption, phenylalanine, tyrosine and tryptophan biosynthesis, central carbon metabolism in cancer and aminoacyl-tRNA biosynthesis and l-arginine in arginine biosynthesis and mTOR signaling pathway (P < 0.05). On the 59th day, compared with the Con group, the Met group up-regulated sphingosine, oxidized glutathione, nucleoside, and sugar metabolites (P < 0.05), and down-regulated PC and amino acids (P < 0.05), as the same with the 7th day. In addition to that, Met group up-regulated glycocholic acid (P < 0.05) and down-regulated taurocholic acid (P < 0.05) in bile secretion, cholesterol metabolism, primary bile acid biosynthesis, and up-regulated taurine (P < 0.05) in neuroactive ligand-receptor interaction, primary bile acid biosynthesis, and taurine and hypotaurine metabolism at 59th day. Furthermore, up-regulation of ADP and AMP (P < 0.05) were enriched in the AMPK signaling pathway, FoxO signaling pathway, and mTOR signaling pathway in the Met group on the 59th day.
Met vs. Dou
On the 7th day, compared with the Dou group, Met down-regulated sphingosine (P < 0.05) in apoptosis, necroptosis, and sphingolipid signaling pathway, up-regulated isocitrate (P < 0.05) in citrate cycle (TCA cycle), and up-regulated oxidized glutathione (P < 0.05) in thyroid hormone synthesis. On the 59th day, differently with 7th day, Met up-regulated sphingosine (P < 0.05) in apoptosis and necroptosis, up-regulated amino acids (P < 0.05) enriched in protein digestion and absorption, ABC transporters, central carbon metabolism in cancer, aminoacyl-tRNA biosynthesis, valine, leucine, and isoleucine biosynthesis, mineral absorption, and valine, leucine, and isoleucine degradation, increased most of the sugar metabolites (P < 0.05) in amino sugar and nucleotide sugar metabolism, and down-regulated taurocholic acid (P < 0.05) in cholesterol metabolism.
c-Com vs. Con
On both the 7th and the 59th day, compared with the Con group, the addition of compound-c into soybean meal down-regulated amino acids, l-arginine (P < 0.05), and up-regulated nucleoside and sugar metabolites (P < 0.05) in purine metabolism, amino sugar, and nucleotide sugar metabolism pathways, and up-regulated sphingosine (P < 0.05) in sphingolipid signaling pathway, sphingolipid signaling pathway, necroptosis, and apoptosis, up-regulated ADP (P < 0.05) in neuroactive ligand-receptor interaction, thermogenesis, lysosome, down-regulated most of PC and LysoPC (P < 0.05) in choline metabolism in cancer. Beyond that, long-term treatment of c-Com up-regulated ADP and AMP (P < 0.05) enriched in FoxO signaling pathway, mTOR signaling pathway, PI3K-Akt signaling pathway, and AMPK signaling pathway, and up-regulated taurine (P < 0.05) in cholesterol metabolism, primary bile acid biosynthesis, and taurine and hypotaurine metabolism, down-regulated taurocholic acid (P < 0.05) and up-regulated glycocholic acid (P < 0.05) in bile secretion, cholesterol metabolism, and primary bile acid biosynthesis.
c-Com vs. Dou
On the 7th day, compared with the Dou group, c-Com up-regulated ADP, acetyl adenylate, and UDP-D-galactose (P < 0.05), mainly enriched in the AMPK signaling pathway, purine metabolism, FoxO signaling pathway, amino sugar, nucleotide sugar metabolism, and oxidative phosphorylation. On the 59th day, c-Com upregulated sphingosine (P < 0.05) in apoptosis and necroptosis and sphingolipid signaling pathway, up-regulated LysoPC in choline metabolism in cancer (P < 0.05), increased (R)-(+)-2-Pyrrolidone-5-carboxylic acid (P < 0.05) in D-glutamine and D-glutamate metabolism, and up-regulated l-valine and xanthosine (P < 0.05) and down-regulated chitobiose and N, N’-diacetylchitobiose (P < 0.05) in ABC transporters.
Discussion
Lipid Metabolism
Sphingosine affected intestinal mucosal cells through apoptosis and necroptosis. Sphingolipids and their metabolites are important active molecules, which participate in many important signal transduction processes such as cell growth, differentiation, senescence, and programmed cell death, including many bioactive metabolites ceramide, sphinganine, sphingosine, and sphingosine-1-phosphate [SM(d18:0/14:0)]. Ceramide can be converted to sphingosine. Activated sphingosine kinase catalyzes sphingosine phosphorylation to sphingosine-1-phosphate. Both ceramide and sphingosine can act as second messengers, promoting cell death, cell senescence, and growth stagnation, while sphingosine-1-phosphate regulates signaling pathways that stimulate cell growth and inhibit programmed cell death (Petra et al., 2020). Previous studies have shown that sphingosine induces apoptosis, which depends on the activation of Caspases-3, -7, and -8 (Chang et al., 2003). Several previous studies showed that the pro-apoptotic gene caspases-3, -7, and -8 were up-regulated by high-dose soybean meal (Bakke-McKellep et al., 2007; Chen et al., 2018; Duan et al., 2019). In this study, soybean meal significantly affected the contents of sphingosine and SM(d18:0/14:0) metabolites. Adding metformin was the opposite of that of the soybean meal group, and compound-c was similar to the soybean meal group. Metformin activated AMPK through inhibiting mitochondrial complex I, which led to the inhibition of mTOR signaling, autophagy, and apoptosis activation, decreased ROS generation, DNA damage, and inflammatory response (Lv and Guo, 2020). Compound-c is the primary reagent used as an AMPK inhibitor (Dasgupta and Seibel, 2018). In previous studies, compound C induced apoptosis, but AMPK was not involved in this effect (Jin et al., 2009). Consistent with the studies mentioned above, these results indicate soybean meal-induced intestinal mucosal cell apoptosis with AMPK independence.
Phosphatidylcholine, also known as lecithin, is an important component of the cell membrane. PC and LysoPC were involved in choline metabolism in cancer in this study. This study has shown that PC and LysoPC were down-regulated by soybean meal. However, PC and LysoPC in the Met group did not change significantly compared with the Dou group. These suggested that lipid metabolism, especially PC and LysoPC metabolism in the intestinal mucosa of carp may be affected through AMPK signaling pathway.
Further more, taurine, glycocholic acid, and betaine were changed by soybean meal whether activator of inhibitor was added or not. According to Valenzuela et al. (2021), tolerance to soybean meal is associated with immune and lipid metabolism-related pathways in fish. The results of this experiment are consistent with it. Acted as an activator of AMPK, metformin administration increases the bile acid pool in the intestine, which may affect glucagon-like-peptide-1 secretion and cholesterol levels (Lv and Guo, 2020). Therefore, metformin down-regulated taurocholic acid of cholesterol metabolism with consistent function. The result indicated that soybean meal could affect lipid metabolism of intestinal mucosa of carp unrelated to AMPK signaling pathway.
Protein and Amino Acid Metabolism
Aminoacyl-tRNA synthetases (aaRS) participate in one of the most important steps of life activities–protein biosynthesis. Hence, in the intestinal mucosa, the level of amino acids enriched in the pathways can reflect the availability of amino acids. This experiment found that amino acids were down-regulated in experimental groups, enriched in protein digestion and absorption pathways, ABC transporters, central carbon metabolism in cancer, aminoacyl-tRNA biosynthesis, so soybean meal significantly reduced the amino acids content and the utilization in the carp intestines. This is the same as the results of Zhou in carps (Zhou, 2021), and the difference with the conclusion from Wei et al. (2017) that increased the utilization of amino acids in fish muscles, which the different types of fish should cause a difference. In contrast, metformin up-regulated amino acids in the result. This maybe related with that metformin activates AMPK, which leads to the inhibition of mTOR signaling, and as a result, protein synthesis is disturbed (Lv and Guo, 2020).
In addition to that, arginine plays a vital role in regulating animals’ nutritional metabolism, growth, and development (Rhoads and Wu, 2009). Arginine stimulates protein synthesis, cell proliferation, DNA synthesis, cell protection, and migration (Rhoads et al., 2004; Corl et al., 2008; Tan et al., 2010; Tan et al., 2015). Activation of the mTOR signaling pathway is an important mechanism for arginine to alleviate cell stress injury (Tan et al., 2010). Compared with Con, enriched in the mTOR signaling pathway, arginine was significantly down-regulated in experimental groups. Zhang et al. (2013) found that anti-nutritional factors in soybean decreased TOR gene expression in foregut and midgut and increased 4E-BP gene expression in the hindgut of Jian carp. This is consistent with this experiment. These suggested that soybean meal caused intestinal mucosa injury, which may be related to the down-regulation of arginine, which does not activate the mTOR signaling pathway.
Sugar Metabolism and Purine Metabolism
In addition to lipid and protein, sugar is also one of the essential energy materials in fish, which can be directly used to power the body to reduce fat and protein consumption in fish. This experiment suggested that the soybean meal group significantly raised sugar metabolites on the 59th day. This was the same as Zhou’s research in carp and the result of Wei in turbot (Scophthalmus maximus L) that glucose increased in the liver metabolites. Compared with the soybean meal group, isocitrate was significantly up-regulated and enriched in the citrate cycle in Met on 7th day, indicating regulating glucose metabolism. This result was consistent with the property that metformin can regulate the AMPK signaling pathway and further increase glucose catabolism by activating the AMPK signaling pathway (Lv and Guo, 2020). However, this phenomenon did not last for 59 days. It was speculated that soybean meal had an effect on glucose metabolism in the intestinal mucosa; as a result, there was no significant change in glucose metabolism when Met was added. Therefore, adding an activator could not enhance glucose metabolism on the 59th day. Additionally, metabolites in purine metabolic pathways were significantly increased in Dou on 59th day vs. Con on 59th day and c-Com on 7th day vs. Dou on 7th day, and not changed between Met and Dou, which indicated that both soybean meal and compound-c up-regulated the nucleotide metabolism of intestinal mucosal cells. We speculated that the mechanism of soybean meal on intestinal nucleoside metabolism might not be through the AMPK signaling pathway.
Energy Metabolism
Adenosine triphosphate box transporter (ATP-binding Cassette transporter, ABC transporter) is involved in many important physiological processes, such as nutrient intake, cellular detoxification, lipid homeostasis, signal transduction, disease virus defense, and antigen presentation (Wilkens, 2015), whose primary main function is to utilize the energy generated by ATP (adenosine triphosphate) hydrolysis to transport the substrate across the membrane against the concentration gradient (Borths et al., 2002; Locher, 2004; Kathawala et al., 2015). This experiment found down-regulated amino acids in experimental groups enriched in ABC transporters. At the same time, compared with the Con group, ADP and AMP of Dou on 59th day, Met on 7th day and 59th day and c-Com on 7th day, and 59th day were significantly increased and enriched in FoxO and AMPK signaling pathways. The content of ADP, AMP, and ATP in intestinal mucosa reflects the level of energy metabolism. Metformin acts as an AMPK activator by inhibiting mitochondrial complex I (Batandier et al., 2006; Viollet et al., 2012). Mitochondrial complex I is critical for electron transport. As a result, the production of ATP was reduced, and the intracellular concentration of ADP increased. Consequently, the cellular levels of AMP increases and ultimately activate AMPK (Viollet et al., 2012; Pryor and Cabreiro, 2015). Members of the class O of forkhead box transcription factors (FoxO) have essential roles in metabolism, cellular proliferation, stress tolerance, and probably lifespan (Weigel et al., 1989). The AKT/PKB-mediated phosphorylation of FoxO inhibits FoxO function by promoting its interaction with 14-3-3 proteins and its relocalization from the nucleus to the cytosol (Van der Horst and Burgering, 2007). Lee et al. (2017) suggested that FoxO transcription factors could feasibly act downstream of AMPK to mediate the induction of antioxidant systems in response to H2. This study suggested that soybean meal can improve ADP and AMP content then activate the AMPK and FoxO pathway.
Oxidative Damage
The soybean meal can cause intestinal oxidative damage, which has been shown in grass carp (Duan et al., 2019; Zhang et al., 2020), turbot (Chen et al., 2018), and Scophthalmus maximus L. (Gu et al., 2021). In this study, enriched in glutathione metabolism, oxidized glutathione was up-regulated in the soybean meal group on the 7th day, and the amount of oxidized glutathione in the Met group was significantly higher than that in the Dou group at 7th day. These suggested that soybean meal might cause oxidative damage to the intestinal mucosa in short-time treatment, and metformin supplementation aggravated the injury. However, in a previous study, metformin suppresses mitochondrial complex I, thereby preventing the generation of reactive oxygen species (ROS) and further decreasing DNA damage (Algire et al., 2012). The results of this experiment are inconsistent with this and require further validation.
Conclusion
In conclusion, soybean meal influenced intestinal mucosa metabolic processes, including lipid, amino acid, sugar, apoptosis, and oxidative injury; and changed energy metabolism in intestinal mucosa, enriched in the AMPK, TOR, FoxO signaling pathway; Metformin could aggravate oxidative damage, alleciated apoptosis for the short term, and aggravate apoptosis, improve carbohydrate catabolism, and amino acid anabolism for the long term; Compound-c exacerbated apoptosis. repaired oxidative damage, and enhanced nucleoside catabolism.
Data Availability Statement
The original contributions presented in the study are publicly available. This data can be found here: https://www.ebi.ac.uk/metabolights/, MTBLS4074.
Ethics Statement
The animal study was reviewed and approved by the Committee on the Ethics of Animal Experiments of Huzhou University.
Author Contributions
JZ: experimental operation, field sampling, and writing—original draft preparation. QX: conceptualization and writing—review and editing. Both authors read and approved the final manuscript.
Funding
This work was financially supported by the Natural Science Foundation of China, NSFC (31972800), and the China Agriculture Research System of MOF and MARA (CARS-45).
Conflict of Interest
The authors declare that the research was conducted in the absence of any commercial or financial relationships that could be construed as a potential conflict of interest.
Publisher’s Note
All claims expressed in this article are solely those of the authors and do not necessarily represent those of their affiliated organizations, or those of the publisher, the editors and the reviewers. Any product that may be evaluated in this article, or claim that may be made by its manufacturer, is not guaranteed or endorsed by the publisher.
Supplementary Material
The Supplementary Material for this article can be found online at: https://www.frontiersin.org/articles/10.3389/fmars.2022.844716/full#supplementary-material
Footnotes
References
Algire, C., Moiseeva, O., Deschnes-Simard, X., Amrein, L., Petruccelli, L., Birman, E., et al. (2012). Metformin reduces endogenous reactive oxygen species and associated DNA damage. Cancer Prev. Res. 5, 536–543. doi: 10.1158/1940-6207.CAPR-11-0536
Antonopoulou, E., Chouri, E., and Feidantsis, K. (2017). Effects of partial dietary supplementation of fish meal with soymeal on the stress and apoptosis response in the digestive system of common dentex (Dentex dentex). J. Biol. Res. 24, 1–10. doi: 10.1186/s40709-017-0071-1
Bakke-McKellep, A. M., Penn, M. H., Salas, P. M., Refstie, S., Sperstad, S., Landsverk, T., et al. (2007). Effects of dietary soyabean meal, inulin and oxytetracycline on intestinal microbiota and epithelial cell stress, apoptosis and proliferation in the teleost Atlantic salmon (Salmo salar L.). Br. J. Nutr. 97, 699–713. doi: 10.1017/S0007114507381397
Batandier, C., Guigas, B., Detaille, D., El-Mir, M., Fontaine, E., Rigoulet, M., et al. (2006). The ROS production induced by a reverse-electron flux at respiratory-chain complex 1 is hampered by metformin. J. Bioenerg. Biomembr. 38, 33–42. doi: 10.1007/s10863-006-9003-8
Becker, K., and Makkar, H. P. S. (1999). Effects of dietary tannic acid and quebracho tannin on growth performance and metabolic rates of common carp (Cyprinus carpio L.). Aquaculture 175, 327–335. doi: 10.1016/S0044-8486(99)00106-4
Borths, E. L., Locher, K. P., Lee, A. T., and Rees, D. C. (2002). The structure of Escherichia coli BtuF and binding to its cognate ATP binding cassette transporter. Proc. Natl. Acad. Sci. U. S. A. 99, 16642–16647. doi: 10.1073/pnas.262659699
Bron, P. A., Kleerebezem, M., Brummer, R. J., Cani, P. D., Mercenier, A., MacDonald, T. T., et al. (2017). Can probiotics modulate human disease by impacting intestinal barrier function? Br. J. Nutr. 117, 93–107. doi: 10.1017/S0007114516004037
Buttle, L. G., Burrells, A. C., and Good, J. E. (2001). The binding of soybean agglutinin (SBA) to the intestinal epithelium of Atlantic salmon, Salmo salar and Rainbow trout, Oncorhynchus mykiss, fed high levels of soybean meal. Vet. Immunol. Immunopathol. 80, 237–244. doi: 10.1016/S0165-2427(01)00269-0
Chang, H. C., Hsu, C., Hsu, H. K., and Yang, R. C. (2003). Functional role of caspases in sphingosine-induced apoptosis in human hepatoma cells. IUBMB Life 55, 403–407. doi: 10.1080/15216540310001594184
Chen, Z. C., Zhao, S. F., Liu, Y., Yang, P., Ai, Q. H., Zhang, W. B., et al. (2018). Dietary citric acid supplementation alleviates soybean meal−induced intestinal oxidative damage and micro−ecological imbalance in juvenile turbot, Scophthalmus maximus L. Aquac. Res. 49, 3804–3816. doi: 10.1111/are.13847
Corl, B. A., Odle, J., Niu, X. M., Moeser, A. J., Gatlin, L. A., Phillips, O. T., et al. (2008). Arginine activates intestinal p70S6k and protein synthesis in piglet rotavirus enteritis. J. Nutr. 138, 24–29. doi: 10.1093/jn/138.1.24
Dasgupta, B., and Seibel, W. (2018). Compound C/Dorsomorphin: its Use and Misuse as an AMPK Inhibitor. Methods Mol. Biol. 1732, 195–202. doi: 10.1007/978-1-4939-7598-3_12
Duan, X. D., Feng, L., Jiang, W. D., Wu, P., Liu, Y., Kuang, S. Y., et al. (2019). Dietary soybean β-conglycinin suppresses growth performance and inconsistently triggers apoptosis in the intestine of juvenile grass carp (Ctenopharyngodon idella) in association with ROS-mediated MAPK signalling. Aquac. Nutr. 25, 770–782. doi: 10.1111/anu.12895
Gu, M., Bai, N., Zhang, Y. Q., and Krogdahl, A. (2016). Soybean meal induces enteritis in turbot Scophthalmus maximus at high supplementation levels. Aquaculture 464, 286–295. doi: 10.1016/j.aquaculture.2016.06.035
Gu, M., Pan, S. H., Li, Q., Qi, Z. Z., Deng, W. Z., and Bai, N. (2021). Protective effects of glutamine against soy saponins-induced enteritis, tight junction disruption, oxidative damage and autophagy in the intestine of Scophthalmus maximus L. Fish Shellfish Immunol. 114, 49–57. doi: 10.1016/j.fsi.2021.04.013
Hara, A., Saegusa, M., Mitomi, H., Kurihara, M., Ishihara, K., Hotta, K., et al. (2000). Colonic mucin-carbohydrate components in colorectal tumors and their possible relationship to MUC2, p53 and DCC immunoreactivities. Pathol.-Res. Pract. 196, 159–166. doi: 10.1016/S0344-0338(00)80096-5
Jiang, W. D., Hu, K., Zhang, J. X., Liu, Y., Jiang, J., Wu, P., et al. (2015). Soyabean glycinin depresses intestinal growth and function in juvenile Jian carp (Cyprinus carpio var Jian): protective effects of glutamine. Br. J. Nutr. 114, 1569–1583. doi: 10.1017/S0007114515003219
Jin, J. F., Mullen, T. D., Hou, Q., Bielawski, J., Bielawska, A., Zhang, X. M., et al. (2009). AMPK inhibitor Compound C stimulates ceramide production and promotes Bax redistribution and apoptosis in MCF7 breast carcinoma cells. J. Lipid Res. 50, 2389–2397. doi: 10.1194/jlr.M900119-JLR200
Kahn, B. B., Alquier, T., Carling, D., and Hardie, D. G. (2005). AMP-activated protein kinase: ancient energy gauge provides clues to modern understanding of metabolism. Cell Metab. 1, 15–25. doi: 10.1016/j.cmet.2004.12.003
Kathawala, R. J., Gupta, P., Ashby, C. R., and Chen, Z. S. (2015). The modulation of ABC transporter-mediated multidrug resistance in cancer: a review of the past decade. Drug Resist. Updates 18, 1–17. doi: 10.1016/j.drup.2014.11.002
Kim, Y. M., Kim, M. Y., Kim, H. J., Roh, G. S., Ko, G. H., Seo, H. G., et al. (2011). Compound-c independent of AMPK inhibits ICAM-1 and VCAM-1 expression in inflammatory stimulants-activated endothelial cells in vitro and in vivo. Atherosclerosis 219, 57–64. doi: 10.1016/j.atherosclerosis.2011.06.043
Lee, J. A., Yang, G. B., Kim, Y. J. C., Tran, Q. H. B., Choe, W. B. D., Kang, I. B. D., et al. (2017). Hydrogen-rich medium protects mouse embryonic fibroblasts from oxidative stress by activating LKB1-AMPK-FoxO1 signal pathway. Biochem. Biophys. Res. Commun. 491, 733–739. doi: 10.1016/j.bbrc.2017.07.119
Lin, S., and Luo, L. (2011). Effects of different levels of soybean meal inclusion in replacement for fish meal on growth, digestive enzymes and transaminase activities in practical diets for juvenile tilapia, Oreochromis niloticus × O. aureus. Anim. Feed Sci. Technol. 168, 80–87. doi: 10.1016/j.anifeedsci.2011.03.012
Locher, K. P. (2004). Structure and mechanism of ABC transporters. Curr. Opin. Struct. Biol. 14, 426–431. doi: 10.1016/j.sbi.2004.06.005
Long, S. S., Dong, X. H., Tan, B. P., Zhang, S., Xie, S. W., Yang, Q. H., et al. (2021). Growth performance, antioxidant ability, biochemical index in serum, liver histology and hepatic metabolomics analysis of juvenile hybrid grouper (♀ Epinephelus fuscoguttatus × ♂ Epinephelus lanceolatus) fed with oxidized fish oil. Aquaculture 545:737261. doi: 10.1016/j.aquaculture.2021.737261
Lv, Z., and Guo, Y. (2020). Metformin and its benefits for various diseases. Front. Endocrinol. 11:191. doi: 10.3389/fendo.2020.00191
Maytorena-Verdugo, C. I., Córdova-Murueta, J. H., and García-Carreño, F. L. (2017). Peptidase compensation in the digestive system of whiteleg shrimp Penaeus vannamei against dietary Kunitz−type soybean trypsin inhibitor. Aquac. Nutr. 23, 1095–1103. doi: 10.1111/anu.12477
National Research Council [NRC] (2011). Nutrient Requirements of Fish and Shrimp. Washington, DC: The National Academies Press.
Park, Y. M., Lim, J. H., and Seo, E. W. (2015). Anti-obesity effect of by-product from soybean on mouse fed a high fat diet. Korean J. Plant Resour. 28, 168–177. doi: 10.7732/kjpr.2015.28.2.168
Petra, G., Markova, C. E. P., and Mirela, S. (2020). Targeting ceramide metabolism in hepatocellular carcinoma: new points for therapeutic intervention. Curr. Med. Chem. 27, 6611–6627. doi: 10.2174/0929867326666190911115722
Pryor, R., and Cabreiro, F. (2015). Repurposing metformin: an old drug with new tricks in its binding pockets. Biochem. J. 471, 307–322. doi: 10.1042/BJ20150497
Rhoads, J. M., Chen, W., Gookin, J., Wu, G. Y., Fu, Q., Blikslager, A. T., et al. (2004). Arginine stimulates intestinal cell migration through a focal adhesion kinase dependent mechanism. Gut 53, 514–522. doi: 10.1136/gut.2003.027540
Rhoads, J. M., and Wu, G. Y. (2009). Glutamine, arginine, and leucine signaling in the intestine. Amino Acids 37, 111–122. doi: 10.1007/s00726-008-0225-4
Silva, F. H., Alexandre, E. C., Calmasini, F. B., Calixto, M. C., and Antunes, E. (2015). Treatment with metformin improves erectile dysfunction in a murine model of obesity associated with insulin resistance. Urology 86, 423.e1–6. doi: 10.1016/j.urology.2015.04.035
Tan, B., Xiao, H., and Xiong, X. (2015). L-arginine improves DNA synthesis in LPS-challenged enterocytes. Front. Biosci. 20, 989–1003. doi: 10.2741/4352
Tan, B., Yin, Y. L., Kong, X. F., Li, P., Li, X. L., Gao, H. J., et al. (2010). L-arginine stimulates proliferation and prevents endotoxin-induced death of intestinal cells. Amino Acids 38, 1227–1235. doi: 10.1007/s00726-009-0334-8
Turner, J. R. (2009). Intestinal mucosal barrier function in health and disease. Nat. Rev. Immunol. 9, 799–809. doi: 10.1038/nri2653
Urn, P. A., Gonalves, A. A., Taverne-Thiele, J. J., Schrama, J. W., Verreth, J. A. J., and Rombout, J. H. W. M. (2008). Soybean meal induces intestinal inflammation in common carp (Cyprinus carpio L.). Fish Shellfish Immunol. 25, 751–760. doi: 10.1016/j.fsi.2008.02.013
Valenzuela, L., Pacheco, S., Rincón, G., Pavez, L., Lam, N., Hernández, A. J., et al. (2021). Intestinal transcriptome analysis reveals enrichment of genes associated with immune and lipid mechanisms, favoring soybean meal tolerance in high-growth zebrafish (danio rerio). Genes 12:700. doi: 10.3390/genes12050700
Van der Horst, A., and Burgering, B. M. T. (2007). Stressing the role of FoxO proteins in lifespan and disease. Nat. Rev. Mol. Cell Biol. 8, 440–450. doi: 10.1038/nrm2190
Velcich, A., Yang, W. C., Heyer, J., Fragale, A., Nicholas, C., Viani, S., et al. (2002). Colorectal cancer in mice genetically deficient in the mucin Muc2. Science 295, 1726–1729. doi: 10.1126/science.1069094
Viollet, B., Guigas, B., Sanz Garcia, N., Leclerc, J., Foretz, M., and Andreelli, F. (2012). Cellular and molecular mechanisms of metformin: an overview. Clin. Sci. 122, 253–270. doi: 10.1042/CS20110386
Wang, Y. Y., Yu, S. L., Wang, Y. Y., Che, J. F., Zhao, L. P., Bu, X. Y., et al. (2016). Effect of replacing fish meal with soybean meal on growth, feed utilization and nitrogen and phosphorus excretion of juvenile Pseudobagrus ussuriensis. Aquac. Res. 47, 3145–3155. doi: 10.1111/are.12765
Wei, Y., Liang, M., Mai, K., Zheng, K., and Xu, H. (2017). H-1 NMR-based metabolomics studies on the effect of size-fractionated fish protein hydrolysate, fish meal and plant protein in diet for juvenile turbot (Scophthalmus maximus L.). Aquac. Nutr. 23, 523–536. doi: 10.1111/anu.12420
Weigel, D., Jurgens, G., Kuttner, F., Seifert, E., and Jackle, H. (1989). The homeotic gene fork head encodes a nuclear protein and is expressed in the terminal regions of the Drosophila embryo. Cell 57, 645–658. doi: 10.1016/0092-8674(89)90133-5
Wilkens, S. (2015). Structure and mechanism of ABC transporters. F1000prime Rep. 7:14. doi: 10.12703/P7-14
Xiang, Q. Q., Yan, H., Luo, X. W., Kang, Y. H., Hu, J. M., and Chen, L. Q. (2021). Integration of transcriptomics and metabolomics reveals damage and recovery mechanisms of fish gills in response to nanosilver exposure. Aquat. Toxicol. 237:105895. doi: 10.1016/j.aquatox.2021.105895
Yang, B. T., Wen, B., Ji, Y., Wang, Q., Zhang, H. R., Zhang, Y., et al. (2021). Comparative metabolomics analysis of pigmentary and structural coloration in discus fish (Symphysodon haraldi). J. Proteom. 233:104085. doi: 10.1016/j.jprot.2020.104085
Zhang, J. X., Guo, L. Y., Feng, L., Jiang, W. D., Kuang, S. Y., Liu, Y., et al. (2013). Soybean β-conglycinin induces inflammation and oxidation and causes dysfunction of intestinal digestion and absorption in Fish. PLoS One 8:e58115. doi: 10.1371/journal.pone.0058115
Zhang, Y. L., Jiang, W. D., Duan, X. D., Feng, L., Wu, P., Liu, Y., et al. (2020). Soybean glycinin caused NADPH-oxidase-regulated ROS overproduction and decreased ROS elimination capacity in the mid and distal intestine of juvenile grass carp (Ctenopharyngodon idella). Aquaculture 516:734651. doi: 10.1016/j.aquaculture.2019.734651
Keywords: intestinal mucosa, metabolome, soybean meal, metformin, compound-c, carp
Citation: Zhao JY and Xu QY (2022) Influence of Soybean Meal on Intestinal Mucosa Metabolome and Effects of Adenosine Monophosphate-Activated Protein Kinase Signaling Pathway in Mirror Carp (Cyprinus carpio Songpu). Front. Mar. Sci. 9:844716. doi: 10.3389/fmars.2022.844716
Received: 28 December 2021; Accepted: 16 February 2022;
Published: 23 March 2022.
Edited by:
Kang-le Lu, Jimei University, ChinaReviewed by:
Hongyan Tian, Yancheng Institute of Technology, ChinaXiaodan Wang, East China Normal University, China
Mahmoud A. O. Dawood, Kafrelsheikh University, Egypt
Copyright © 2022 Zhao and Xu. This is an open-access article distributed under the terms of the Creative Commons Attribution License (CC BY). The use, distribution or reproduction in other forums is permitted, provided the original author(s) and the copyright owner(s) are credited and that the original publication in this journal is cited, in accordance with accepted academic practice. No use, distribution or reproduction is permitted which does not comply with these terms.
*Correspondence: Qiyou Xu, MDI2NTVAempodS5lZHUuY24=