- 1Key Laboratory of Mariculture, Ministry of Education, Ocean University of China, Qingdao, China
- 2Function Laboratory for Marine Fisheries Science and Food Production Processes, Qingdao National Laboratory for Marine Science and Technology, Qingdao, China
- 3Research Office, Homey Group Co., Ltd., Rongcheng, China
A two-stage diet-switch experiment was conducted to examine the hypothesis that the changes in digestive enzyme activities of sea cucumber (Apostichopus japonicus) induced by historic diets might persist in the regenerated intestines. In stage I, A. japonicus were treated with two different diets for 56 days, including diet A with 11% crude protein, 1% crude lipid, and 40% carbohydrate, and diet B with 18% crude protein, 2% crude lipid, and 35% carbohydrate. In stage II, each treatment was subjected to evisceration with 0.35M KCl or not (eviscerated and non-eviscerated groups), half of which were then switched to different diets from diet A to B or vice versa for 112 days. The persistence of digestive enzyme activities was evaluated by measuring the changes in digestive enzyme activities before and after evisceration. In stage I, diets B and A increased trypsin and amylase activities, respectively. In stage II, the higher trypsin activities were observed in eviscerated and non-eviscerated A. japonicus that had consumed diet B in stage I. The higher amylase activities were observed only in eviscerated A. japonicus that had consumed diet A in stage I. It indicated that the historic diets showed long-term effects on the digestion of A. japonicus, which led to the persistence of changes in both trypsin and amylase activities in intestines, especially in the regenerated intestines. In addition, the specific growth rates (SGRs) and metabolic rates (MRs) of A. japonicus were affected by the long-term effects of historic diets. Meanwhile, the relationships between enzymic activities, SGRs, and MRs were observed in A. japonicus, indicating that the historic diets could produce long-term effects on the growth and metabolism of A. japonicus through their long-term effects of historic diets on digestive enzyme activities. In conclusion, the present study showed that the changes in digestive enzyme activities induced by different diets in stage I could persist in the intestines and regenerated intestines, leading to long-term effects of historic diets on the growth and metabolism of A. japonicus.
Introduction
The stimulus of historic nutrition can induce temporary or long-term changes in digestive ability and subsequent growth potential and metabolic status in later life of organisms (Patel and Srinivasan, 2002; Vera et al., 2017). This phenomenon originating from mammals is termed nutritional programming (Symonds et al., 2009). In recent decades, nutritional programming has been widely studied and reported in numerous aquaculture species (Fang et al., 2014; Gong et al., 2015; Moghadam et al., 2015; Balasubramanian et al., 2016). For instance, early feeding diets enriched in plant-based lipids negatively influenced the growth and survival of gilthead seabream (Sparus aurata) in later life (Turkmen et al., 2015). An acute glucose stimulus during the first feeding period induced the long-term repression of weight gains and disturbed the gluconeogenesis regulation of Siberian sturgeon (Acipenser baerii) (Gong et al., 2015). Fang et al. (2014) reported that the activities of amylase were significantly enhanced in adult zebrafish (Danio rerio) fed a high-carbohydrate diet than those fed a low-carbohydrate diet in the yolk-sac larval stage, indicating the long-term effects of early high-carbohydrate diets on carbohydrate digestion of zebrafish. Similar observations were also detected in Atlantic salmon (Salmo salar) (Vera et al., 2017) and rainbow trout (Oncorhynchus mykiss) (Balasubramanian et al., 2016).
So far, studies about the long-term effects induced by historic diets remain limited in aquatic invertebrates, especially for those with the regeneration ability. Sea cucumber (Apostichopus japonicus) is considered an economically important species (Yang et al., 2015). It has been widely cultured along the coastal regions of several Asian countries for centuries, including China, Japan, Korea, and Russia, because of its high nutritional and medicinal value (Xia et al., 2015a). In 2011, the annual production of A. japonicus in the world was more than 138,000 tons, more than 90% of which originated from aquaculture in China. Until 2021, A. japonicas in China achieved nearly 200,000 tons of annual production (Ministry of Agriculture and Rural Affairs of People’s Republic of China, 2011, 2021). With the expansion of A. japonicus cultivation, more artificial diets were applied in commercial aquaculture to improve their production (Ying et al., 2009; Liao et al., 2015b). Because the detritus of macroalgae and sea mud in the sediment are the main food sources for A. japonicus, sea mud and powdered macroalgae are usually used as the main component of artificial diets (Ying et al., 2010; Sudong et al., 2012). Besides, Xia et al. (2015b) found that A. japonicus fed a diet with fish meal showed the best growth performance, indicating the important effect of animal-source protein on A. japonicus. The optimum protein level of diet fed to A. japonicus was 18–24%; hence, fish meal is used as the dietary component to improve the protein level of artificial diets (Zhu et al., 2005; Liao et al., 2015a). Numerous studies reported that the digestive physiology of A. japonicus would be affected by different diets, suggesting the flexibility of digestive physiology (Liao et al., 2015b; Wen et al., 2016b). In addition, other fascinating biological behaviors were observed in A. japonicus, such as autolysis, aestivation, evisceration, and regeneration (Wang et al., 2015; Ru et al., 2019). Evisceration involves various complex physiological processes and results in the expulsion of the digestive tracts and other viscera (García-Arrarás et al., 1998; Ding et al., 2019). Following evisceration, the new intestine is regenerated from the residual coelomic epithelial cells through the processes of dedifferentiation, migration, redifferentiation, and division (Leibson, 1992; García-Arrarás and Greenberg, 2001; Zang et al., 2012). The persistence of the digestive physiology for the regenerated intestines of A. japonicus after evisceration, that is, whether the digestive ability of the regenerated intestine is affected by the historic digestive status before evisceration, remains unclear.
Digestion is one of the crucial metabolic processes in animals because it determines the availability of nutrient requirements (Gisbert et al., 2009). The whole digestive process primarily relies on the types and activities of digestive enzymes (Sveinsdóttir et al., 2006; Nazemroaya et al., 2015). Trypsin is an important serine alkaline protease responsible for protein digestion (Mir et al., 2018). Previous studies examined the trypsin activities to assess the digestion ability of aquatic organisms under different nutritional conditions, such as European sea bass (Dicentrarchus labrax) (Parma et al., 2019), olive flounder (Paralichthys olivaceus) (Bae et al., 2020), rainbow trout (Kasiga et al., 2020), and sea cucumber (Bai et al., 2015; Wen et al., 2016b). In addition, amylase and lipase are crucial digestive enzymes that can reveal the absorptive capability for dietary carbohydrates and lipids, respectively (Hidalgo et al., 1999; Liao et al., 2015b; Xia et al., 2015a). Previous studies demonstrated that the digestive enzymes, including trypsin, amylase, and lipase, could respond to different diets, which is termed as enzymatic plasticity (Sabat et al., 1999; Liu and Wang, 2007). For example, Bolasina et al. (2006) reported that the changes in diets led to subsequent variations in the trypsin and lipase activities during the ontogenetic development of Japanese flounder (P. olivaceus). European sea bass consuming a microparticulate diet showed higher trypsin and amylase activities compared with those consuming natural prey (Infante Zambonino and Cahu, 1994). The adjustment of enzymatic plasticity is crucial for organisms to maintain an appropriate level of digestive ability in response to different environmental conditions and physiological statuses (Fu et al., 2006; Garland, 2011; Kasiga et al., 2020).
Carbon stable isotope analysis is routinely employed to quantify food assimilation, providing details of time-integrated food (Kürten et al., 2013; Vander Zanden et al., 2015; Wen et al., 2016a). The turnover of stable isotope in organisms is generally driven by both growth and metabolism (Macavoy et al., 2006; Tarboush et al., 2006). With known growth performance in terms of specific growth rate (SGR), the metabolic rate (MR) can be estimated based on the simulation of isotopic turnover curves, and the metabolic status of the organism is thus evaluated with stable isotope analysis (Buchheister and Latour, 2010; Antonio and Richoux, 2016). The objectives of the present study were to investigate the long-term effects of historic diets on the digestive enzyme activities of regenerated intestines of A. japonicus after evisceration and assess the persistence of digestive physiology and subsequent effects on the growth and metabolism of A. japonicus by means of diet switch and stable isotope analysis.
Materials and Methods
Diet Preparation
Numerous studies reported that diets consisting of sea mud, fish meal, and macroalgae, including S. thunbergii, Ulva lactuca, and Gracilaria lemaneiformis, could meet the nutritional requirements and lead to the rapid growth of A. japonicus (Ying et al., 2009; Sun et al., 2012; Wen et al., 2016a). Meanwhile, two different types of diets were formulated based on previous studies for the following feed experiment, to avoid the effects of dietary additives on the digestive enzyme activities (Shi et al., 2015; Wen et al., 2016a), and identified as diet A and diet B. Diet A comprised sea mud and S. thunbergii. Sea mud was collected from the intertidal zone (Qingdao, Shandong province, China), and S. thunbergii was obtained from Xiaoheishan Island (Yantai, Shandong province, China). Besides these contents, commercial fish meal (Qingdao Seven Good Biological Technology Co., Ltd.) was added to diets denoted as diet B. The ingredients of these diets were ground, sieved through a 0.25-mm mesh, mixed adequately, slightly watered, stirred, and extruded into pellets (diameter = 1.8 mm) using a feed processing machine (Tairun Equipment, Jining, China). The pelleted diets were dried at 60°C for 48 h and stored at –20°C for the feeding experiment. The concentrations of nitrogen and carbon elements in diets were measured using an elemental analyzer (Vario EL III; Elementar Co., Germany), and the content of crude protein was estimated as nitrogen concentration multiplied by 6.25. The crude lipid content was analyzed using the Soxhlet extraction method with petroleum ether (Luque De Castro and Priego-Capote, 2010). For ash content measurement, dried diets were combusted at 500°C for 6 h in a muffle furnace (Nabertherm, Germany). Carbohydrate = 100 - crude protein - crude lipid – ash (Seo et al., 2011; Xia et al., 2015a; Hassaan et al., 2018). The crude protein, lipid, and carbohydrate contents of diet A versus diet B were 10.88 vs. 17.67%, 1.07 vs. 1.65%, and 40.41 vs. 34.55%, respectively. The detailed ingredients and nutritional composition of these diets are shown in Table 1.
Diet-Switch Experiment
A total of 300 A. japonicus juveniles were obtained from Tianheng aquaculture company (Qingdao, Shandong province, China) with an average body weight of 8.80 ± 0.21 g. These individuals were acclimated to the experimental conditions for 2 weeks. Then, the feeding experiment was conducted for two different stages (stage I and stage II). In stage I, all A. japonicus were transferred to 2 tanks [2 m × 1.5 m × 0.8 m (L × W × H)], resulting in 150 individuals in each tank. They were classified into two groups, which were fed diet A (group A) and diet B (group B) for 56 days, respectively. Three individuals in each group were randomly sampled and quickly dissected for intestines and body walls after freeze anesthesia at six time points, including days 0, 7, 14, 28, 42, and 56, during the period of the feeding experiment in stage I. The intestines and body walls were immediately stored at −80°C until assayed for digestive enzyme activities and the carbon stable isotope. All A. japonicus were starved for 24 h prior to sampling.
Further, 120 individuals in each tank were transferred to 12 glass aquariums [70 cm × 40 cm × 40 cm (L × W × H)] for a further 112-day feeding experiment (stage II). These aquariums from each tank were classified into two groups (six aquariums in each group), which were then subjected to evisceration by the intra-coelomic injection of 0.35M KCl (eviscerated group) or not (non-eviscerated group). The eviscerated A. japonicus from groups A and B were named as groups AE and BE while the non-eviscerated individuals from groups A and B were named as groups AN and BN, respectively. Each non-eviscerated and eviscerated group was further divided into two groups (three aquariums in each group), of which one was fed the same diets as in stage I and the other was treated with diets switching from diet A to B or vice versa. Group AN, BN, AE, and BE treated with diet switch or not were renamed as ANA, ANB, BNA, BNB, AEA, AEB, BEA, and BEB, respectively (Figure 1).
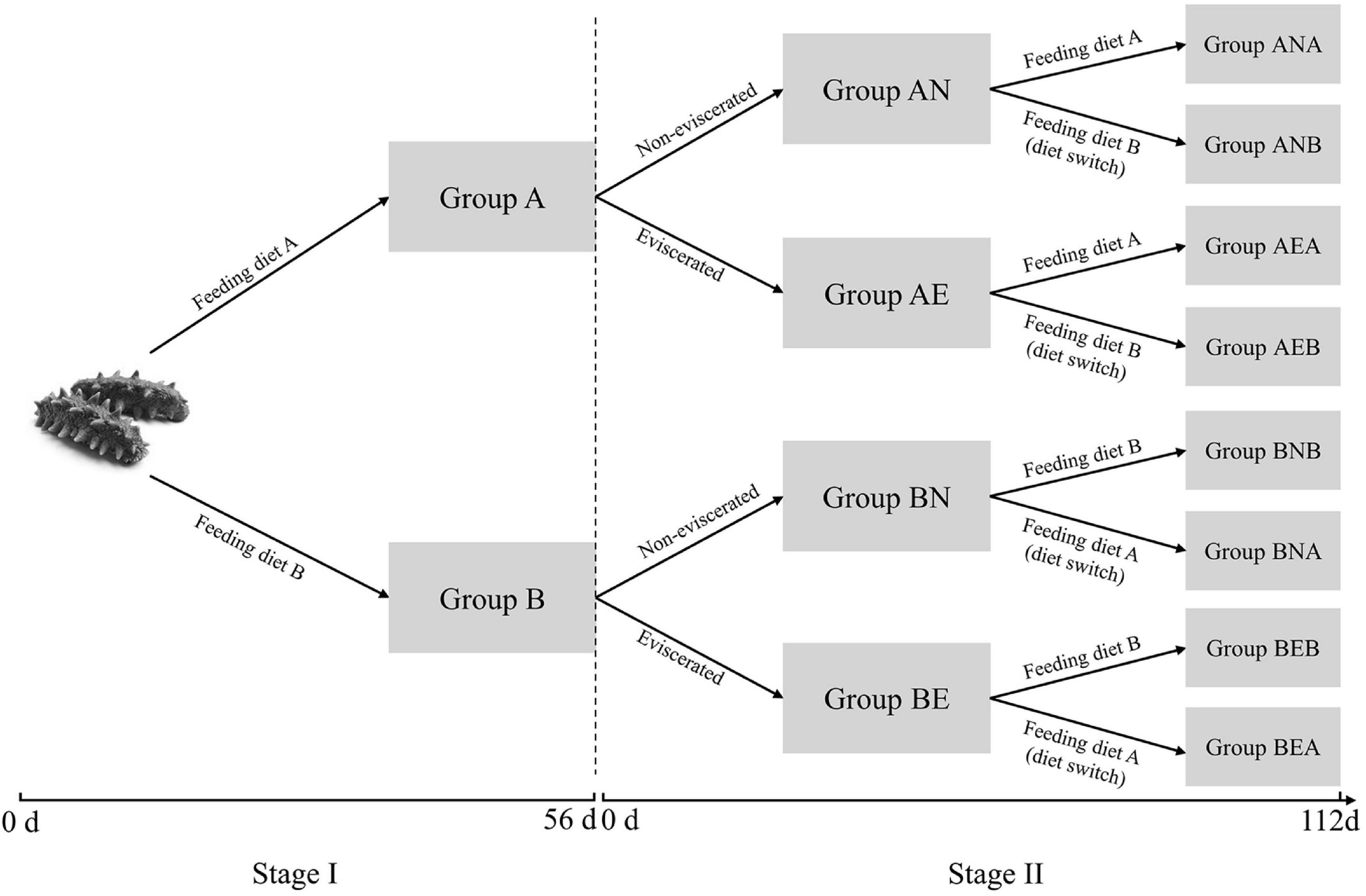
Figure 1. Schematic presentation of diet-switch experiment in A. japonicus. The whole experiment was carried out for 168 days and classified into two stages. In stage I, A. japonicus were randomly assigned to group A and group B, which were fed diet A and diet B for 56 days, respectively. In stage II, each group was subjected to evisceration or not (eviscerated and non-eviscerated), and switched to different diets from diet A to B or vice versa for 112 days.
Finally, three individuals in each non-eviscerated group, including groups ANA, ANB, BNA, and BNB, were sampled on days 7, 14, 28, 49, 70, 91, and 112 in stage II. Because of 21-day intestine regeneration, the sample collections in eviscerated groups (AEA, AEB, BEA, and BEB) were conducted only on days 21, 28, 35, 49, 70, 91, and 112. The sampling tissues and method in stage II were the same as those in stage I. In addition, the initial and final weights of A. japonicus were measured in both stages I and II for the following calculation of the body weight gain (BWG), SGR, and growth rate constant (k).
During the whole experiment, A. japonicus were fed once a day at 15:00 with a daily ration of 5% wet body weight, based on which the individuals were under the apparent satiation. The residual feed and feces were cleaned by siphoning every day. The temperature was constantly kept at 16.5 ± 0.5°C, the salinity varied between 29 and 31 ppt, the levels of dissolved oxygen were greater than 6.0 mg/L, and a normal photoperiod 14:10 h (L/D) was applied. The water environmental conditions were kept relatively constant for each aquarium during the experiment.
Determination of Enzymatic Activity
The intestinal tissues were homogenized for 2 min in a pre-cooled homogenization buffer using a tissue grinder (JXFSTPRP-CL-24; ShangHaiJingXin Co., China). The supernatants were obtained after centrifugation at 20,000g for 25 min at 4°C, pipetted into clean centrifuge tubes, and immediately stored at 4°C until analysis (less than 12 h).
The protein concentration, trypsin activities, amylase activities, and lipase activities in the intestines of A. japonicus were determined using the standard kits (A080-2, C016, A045-4, and A054-2-1) from the Nanjing Jiancheng Bioengineering Institute (Nanjing, China) (Xionggao et al., 2014; Liao et al., 2015b; Ye et al., 2019). The experimental procedures were conducted following the manufacturer’s protocols. Both the trypsin and amylase activities were tested using a spectrophotometer (UV5100, METASH, Shanghai, China). The protein concentrations and lipase activities were determined using a microplate reader (Synergy Mx, Bio Tek, VT, United States). The specific trypsin and amylase activities were expressed as trypsin and amylase unit per mg protein, respectively. The specific lipase activity was expressed as lipase unit per g protein.
Growth Performance
The BWG (%) of A. japonicus was calculated as (Liao et al., 2015b):
where IBW and FBW are the initial body weight and final body weight, respectively.
The SGR of A. japonicus was calculated as (Giri et al., 2013):
where Wt and W0 are the final and initial weights of individuals, respectively, and t is the duration of the experiment.
Carbon Stable Isotope Analysis
The diets and body walls of A. japonicus were freeze-dried for 48 h to constant weight and homogenized. A stable isotope mass spectrometer (EA-IRMS, Thermo Finnigan MAT Delta-plus) was used to determine the ratio of carbon isotope (δ13C) with the accuracy of ± 0.1‰.
The ratio of carbon stable isotope is generally defined as follows (Peterson and Fry, 1987):
where R is the ratio of 13C and 12C.
The stable isotope turnover model is defined as follows (Hesslein et al., 1993):
where Ct represents the carbon stable isotope ratio of biological tissue on day t. Cf represents the ratio of stable isotope when diets and tissue turnover reach a balance after dietary transformation. C0 represents the carbon stable isotope ratio of the initial biological tissue. k is the growth rate constant, and m is the metabolism rate constant.k+ m is the isotopic turnover rate.
The growth rate constant (k) was calculated as follows (Buchheister and Latour, 2010):
where Wf and Wi represent the final and initial weights of A. japonicus, respectively, and t is the duration of the experiment in days.
The isotopic turnover rate (k+ m) was obtained by fitting the exponential model to match the observed isotopic data, and the metabolism rate constant (m) was thus obtained by means of k+ m minus k (Winter et al., 2019).
Statistical Analysis
Significant differences in nutritional composition between diet A and diet B were determined using the Student’s t- test. For stage I, the differences in the digestive enzyme activities between groups fed different diet types and between sampling times were compared with a two-way analysis of variance (ANOVA) and the differences in the FW, BWG, SGRs, and MRs at the end of stage I between different diet types were compared with the Student’s t- test. For stage II, a four-way ANOVA was applied to explore the effects of diet in stage I, diet in stage II, evisceration, and sampling time on the digestive enzyme activities, while a three-way ANOVA was used to compare the differences in FW, BWG, SGRs, and MRs at the end of stage II between diet in stage I, diet in stage II and evisceration. For ANOVA, if the interaction of main factors was detected, a one-way ANOVA or the Student’s t- test was conducted to examine the effects of one main factor at the specific level of the other main factor(s). The factors detected to be significant by ANOVAs were further analyzed using a Bonferroni multiple comparison procedure, which adjusted the observed significance level by multiplying it by the number of comparisons being made (Zar, 1999; Norusis, 2008). Pearson correlation analysis was used to examine the relationships of SGRs and MRs to the digestive enzyme activities. A P value of 0.05 was used as the significance level. Before statistical analyses, raw data were diagnosed for the normality of distribution and homogeneity of variance with the Kolmogorov–Smirnov test and Levene’s test, respectively. The statistical analysis was conducted using IBM SPSS Statistics (Version 25) and Origin (Version 9.1) software.
Results
Enzymatic Activities
The digestive enzyme activities of A. japonicus fed different diets in stage I are presented in Figure 2. Similar trends of trypsin activities of A. japonicus were observed in groups A and B (Figure 2A). Specifically, the trypsin activities of A. japonicus in groups A and B first increased, reaching the peak on day 42 and then decreased on day 56. A. japonicus fed diet B showed significantly higher trypsin activities than those fed diet A in stage I (P < 0.05). The amylase activities of A. japonicus in group A showed an abrupt increase in the first 7 days and then gradually decreased until day 28, followed by an increasing trend to the end of stage I (Figure 2B). The amylase activities of A. japonicus in group B decreased first and then showed a slight fluctuation until the end of stage I. Meanwhile, the amylase activities of A. japonicus in group A were higher than those of individuals in group B (P < 0.05). As shown in Figure 2C, the lipase activities of A. japonicus in group A quite fluctuated throughout stage I, with the highest value on day 28 and the lowest on day 42. For A. japonicus in group B, an increase in the lipase activity was observed before 7 days. After gradually decreasing between day 7 and day 42, a further increase in lipase activities was observed until the end of stage I. A. japonicus fed diet A showed higher lipase activities than those fed diet B from day 14 to the end of stage I.
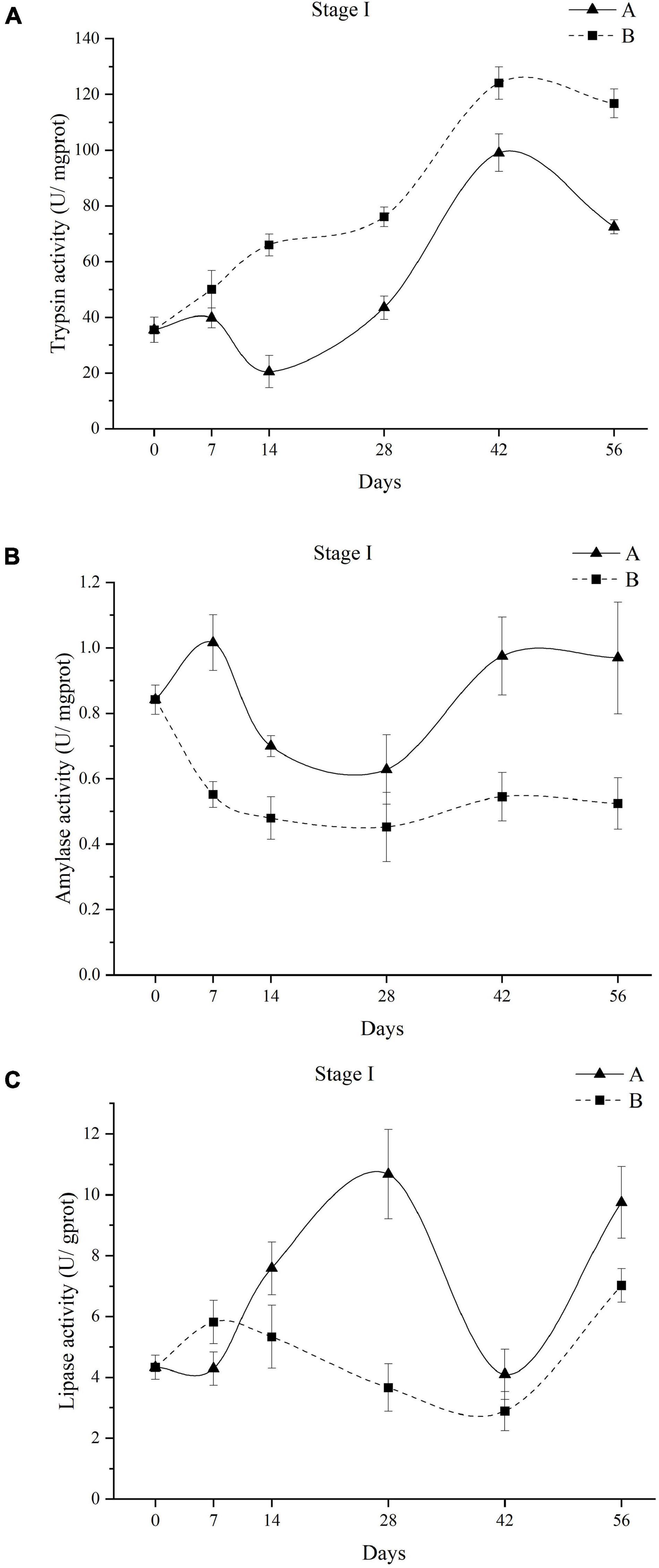
Figure 2. Changes in trypsin activities of A. japonicus feeding different diets in stage I (A); Changes in amylase activities of A. japonicus feeding different diets in stage I (B). Changes in lipase activities of A. japonicus feeding different diets in stage I (C). Data are presented as mean ± SD (n = 3).
As shown in Figure 3A, the individuals in the non-eviscerated and eviscerated groups presented similar trends. A. japonicus in group BNA showed significantly higher trypsin activities than those in group ANA, except on days 28, 49, and 91 (P < 0.05, Figure 3A). The trypsin activities of A. japonicus in group BEA were significantly higher than those of individuals in group AEA (P < 0.05, Figure 3A), although this difference was non-significant on day 70. The trypsin activities of A. japonicus in group BEA increased rapidly and returned to non-eviscerated levels in less time than the activities of those in group AEA. As shown in Figure 3B, little changes were found in trypsin activities of A. japonicus in groups ANB and BNB. In contrast, the trypsin activities in eviscerated groups, particularly group BEB, greatly changed. A. japonicus in groups BNB and BEB exhibited significantly higher trypsin activities than those in groups ANB and AEB (P < 0.05). For eviscerated A. japonicus, the trypsin activities in group BEB increased rapidly and took 28 days to reach non-eviscerated levels, while the trypsin activities in group AEB returned to non-eviscerated levels after 35 days.
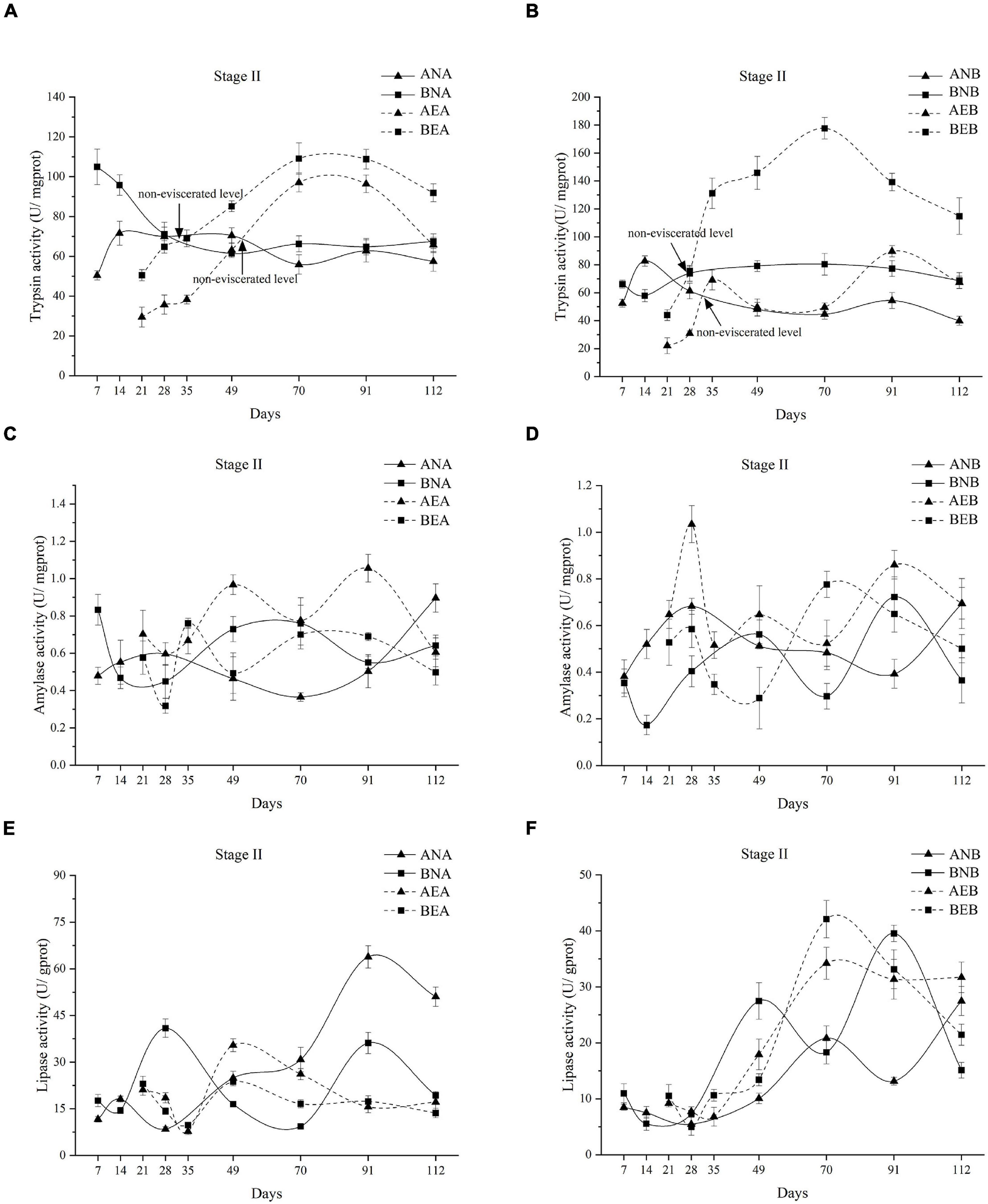
Figure 3. Changes in trypsin activities of A. japonicus feeding different diets in stage II (A,B). Non-eviscerated levels of trypsin activities indicate the intersection of the eviscerated group and its corresponding non-eviscerated group. Changes in amylase activities of A. japonicus feeding different diets in stage II (C,D). Changes in lipase activities of A. japonicus feeding different diets in stage II (E,F). Data are presented as mean ± SD (n = 3).
Dramatic fluctuations were observed in the amylase activities of A. japonicus in stage II (Figures 3C,D). Significant differences were found in amylase activities between A. japonicus in group ANA and BNA on days 7, 49, 70, and 112, but the amylase activities of A. japonicus in group ANA were higher than those of individuals in group BNA only on day 112 (P < 0.05, Figure 3C). For eviscerated A. japonicus, significantly higher amylase activities were observed in group AEA than in group BEA on days 28, 49, and 91 (P < 0.05, Figure 3C). As shown in Figure 3D, the amylase activities of A. japonicus in group ANB were higher than those of individuals in group BNB on days 14, 28, 70, and 112 (P < 0.05). A. japonicus in group AEB exhibited higher amylase activities than those in group BEB except on days 21 and 70 (P < 0.05).
The lipase activities of A. japonicus fed diet A in stage II fluctuated; only the lipase activities of A. japonicus in group ANA gradually increased during stage II (Figure 3E). A. japonicus in group ANA showed significantly higher lipase activities than those in group BNA from day 49 to the end of stage II (P < 0.05, Figure 3E). For eviscerated A. japonicus, similar values of lipase activities were observed in groups AEA and BEA during stage II except for days 28, 49, and 70, with higher lipase activities in group AEA than in group BEA (P < 0.05, Figure 3E). As shown in Figure 3F, the lipase activities of all A. japonicus fed diet B in stage II increased during the whole stage II. Notably, the lipase activities of A. japonicus in group ANB were significantly higher than those of individuals in group BNB only on day 112 (P < 0.05). The higher lipase activities were found in A. japonicus in group AEB than those in group BEB only on days 28 and 112 (P < 0.05).
Growth Performance
The FBW and BWG of A. japonicus in stage I are shown in Table 2. A. japonicus in group B showed significantly higher FBW and BWG than those in group A (P < 0.05). As shown in Table 3, diet in stage I, diet in stage II, and evisceration showed an influence on the FBW and BWG of A. japonicus, but their interaction did not affect the FBW and BWG. A. japonicus fed diet B, regardless of the stage, exhibited significantly higher FBW and BWG compared with those fed diet A in stage I or II (P < 0.05). The FBW of non-eviscerated A. japonicus was significantly higher rather than that of eviscerated A. japonicus, while the BWG of non-eviscerated A. japonicus was significantly lower than that of eviscerated A. japonicus (P < 0.05). The growth rates of A. japonicus in stage I are presented in Table 4. A. japonicus in group B showed a significantly higher growth rate than those in group A (P < 0.05). The growth performance of A. japonicus in stage II is shown in Table 4. The growth rates of A. japonicus in groups BNA, BNB, BEA, and BEB were higher than those of individuals in groups ANA, ANB, AEA, and AEA, respectively, despite non-significant differences in A. japonicus fed the same diet in stage II (P > 0.05).
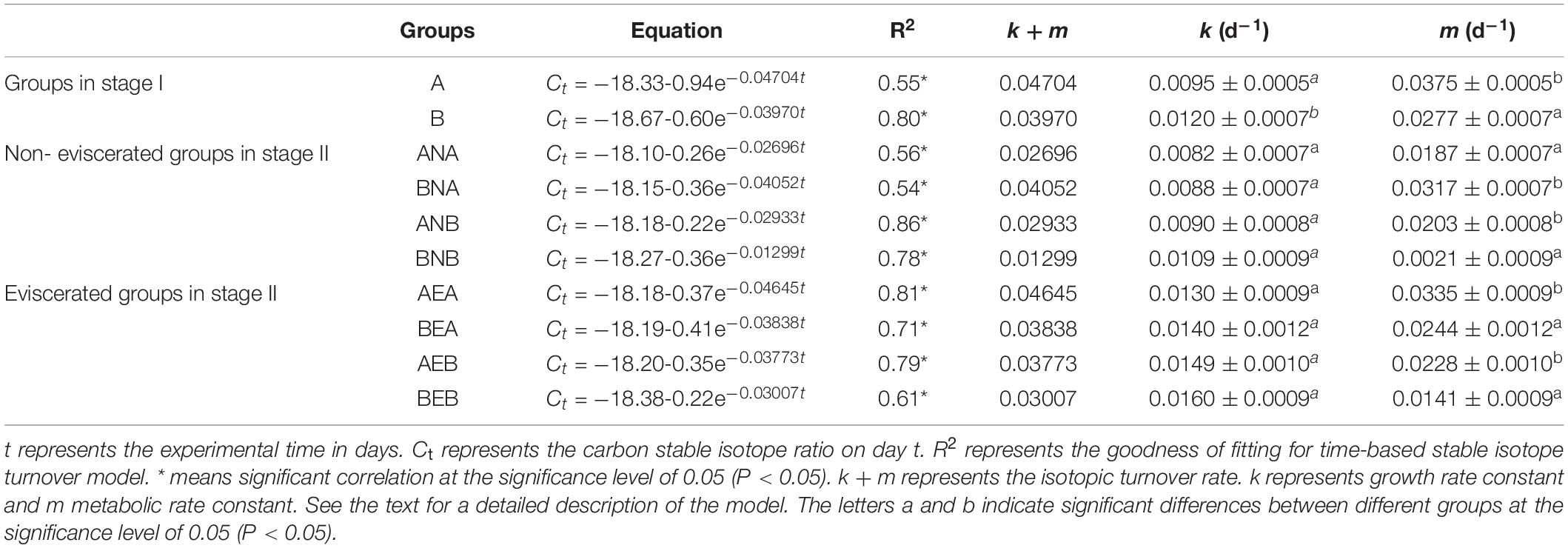
Table 4. Summary of time-based stable isotope turnover models and related growth and metabolism parameters for body walls of A. japonicus.
Time-Dependent Isotopic Turnover and Metabolism
The carbon isotope ratios of diet A and B were measured as –17.97 ± 0.06 and –18.09 ± 0.05, respectively. The trends of carbon isotope ratios in the body walls of A. japonicus were determined and are visualized in Figure 4. In stage I, group A not only exhibited higher carbon isotope ratios but also responded more quickly than group B (Figure 4A). In stage II, different trends of carbon isotope ratios were observed in different groups, which were caused by the historic and new diets (Figures 4B–E). However, the carbon isotope ratios of A. japonicus fed diet A in stage I were always higher than those of individuals fed diet B in stage I. The changes in 13C were modeled as functions of time (time models). The related parameters for different groups are presented in Table 4. In stage I, the higher MR was observed in A. japonicus in group A than in group B (P < 0.05). In stage II, A. japonicus in groups ANB, AEA, and AEB exhibited significantly higher MR than in groups BNB, BEA, and BEB, respectively (P < 0.05).
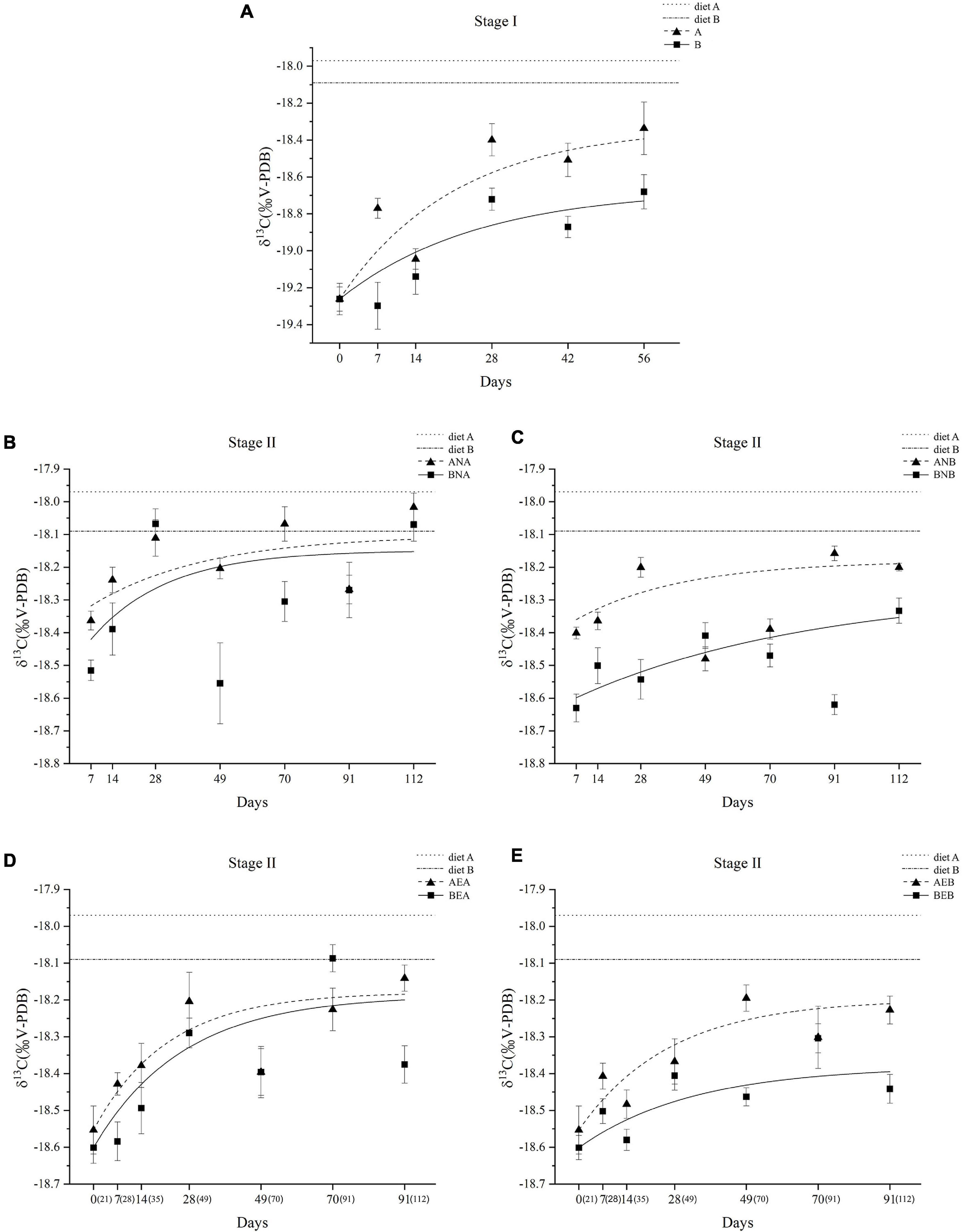
Figure 4. Changes in observed δ13C values of body walls of A. japonicus in stage I (A) Changes in observed δ13C values of body walls of A. japonicus in stage II (B-E). Data are presented as mean ± SD (n = 3).
Relationships Between Enzymic Activities, SGRs, and MRs
Linear regression was applied to examine the relationship between enzymic activities, SGRs, and MRs (Figure 5). A positive relationship was identified between the trypsin activities and SGRs (P < 0.05), but a non-significant relationship was found between trypsin activities and MRs (Figures 5A,B). The amylase activities of A. japonicus negatively correlated with the SGRs but positively correlated with the MRs of A. japonicus (P < 0.05, Figures 5C,D). No significant relationships between lipase activities, SGRs, and MRs were observed in A. japonicus (Figures 5E,F).
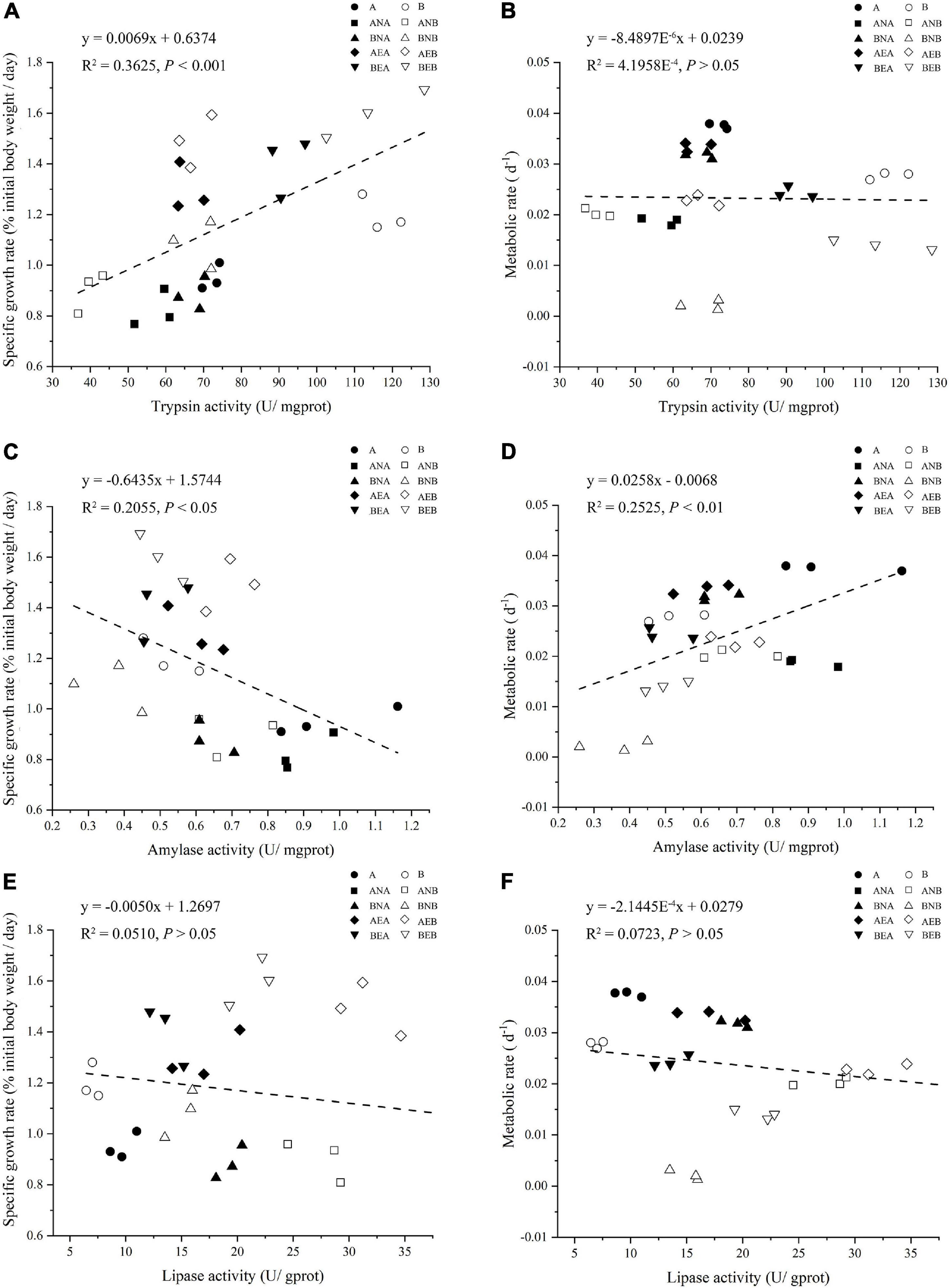
Figure 5. Relationships between trypsin activities, specific growth rates, and metabolic rates of A. japonicus (A,B); Relationships between amylase activities, specific growth rates, and metabolic rates of A. japonicus (C,D); Relationships between lipase activities, specific growth rates, and metabolic rates of A. japonicus (E,F).
Discussion
The present study explored the changes in the digestive enzyme activities, SGRs, and MRs of A. japonicus resulting from diet switch before and after evisceration. It revealed that the short-term plasticity of trypsin and amylase in response to historic diets would persist in non-regenerated and regenerated intestines, suggesting the long-term effect of historic diets on the digestion of A. japonicus. The correlation between the digestive enzyme activities, SGRs, and MRs suggested the effects of the changes in enzymatic activities induced by different diets on the SGR and MR of A. japonicus. This study provided a comprehensive view of the persistence of the plasticity of digestive enzymes in regenerated intestines and revealed the long-term effects of historic diets on digestion, growth, and metabolism of A. japonicus.
In stage I, enzymatic activities induced by distinct diets were different. High trypsin and amylase activities were observed in A. japonicus fed diet B and A. japonicus fed diet A, respectively. It was concluded that the activities of trypsin and amylase were positively affected by the dietary protein and carbohydrate contents, respectively, which were largely consistent with previous studies about white shrimp (Litopenaeus vannamei) (Muhlia-Almazan et al., 2003), sea bass (Peres et al., 1996), and large yellow croaker (Pseudosciaena Crocea) (Yu et al., 2012). And Xia et al. (2015a) also reported that the amylase activity of A. japonicus increased with the elevating dietary carbohydrate levels from 25.61 to 45.31%. However, the lipase activities of A. japonicus in group B fed a lipid-rich diet (diet B) were lower than those of group A fed a low-lipid diet (diet A), which was contrary to the previous study that the increasing lipase activities of A. japonicus were accompanied by the enhanced dietary lipid levels (Liao et al., 2015a). The positive relationship between lipase activities and dietary lipid content in that study was determined based on the changes in lipase activities of A. japonicus fed the isonitrogenous diet. Thus, in the present study, the changes in lipase activities of A. japonicus fed different diets might be not only affected by dietary lipids but also other dietary nutrients, which was furtherly supported by previous findings that the dietary proteins, carbohydrates, and lipids had interactive effects on the lipase activities (Xia et al., 2015a; Huang et al., 2019). According to the changes in digestive enzyme activities induced by different diets, great plasticity of the digestive enzymes under exposure to different diets was found in A. japonicus in stage I.
In stage II, although A. japonicus in groups BNA and ANA, groups BNB and ANB, groups BEA and AEA, as well as groups BEB and AEB were fed the same diet, higher trypsin activities were observed in groups BNA, BNB, BEA, and BEB than in groups ANA, ANB, AEA, and AEB, respectively. It suggested that the plasticity of trypsin induced by diet in stage I could persist in the non-regenerated and regenerated intestines. The persistence of plasticity was also detected in the amylase activities of eviscerated A. japonicus. In detail, A. japonicus in group AEA showed higher amylase activities than those in group BEA despite all individuals fed diet A in stage II. Also, A. japonicus in group AEB showed higher amylase activities than those in group BEB despite individuals fed diet B in stage II. The persistence of digestion plasticity has already been reported at molecular levels by Geurden et al. (2007), who found that the early hyperglucidic diet could rapidly increase the expression of α-amylase of larvae rainbow trout, and for juveniles subjected to the challenge test with a 25% dextrin diet, the higher expression of α-amylase was observed in rainbow trout that experienced the hyperglucidic stimulus compared with those fed a hypoglucidic diet at first feeding, suggesting that short-term digestive plasticity of fish resulting from dietary carbohydrates in the early feeding stage would persist in the later life. Therefore, in the present study, it was hypothesized that the genes related to trypsin and amylase activities could be affected by diet in stage I and subsequently regulated the trypsin activities and amylase activities in stage II. However, further studies need to be conducted to prove this hypothesis. Additionally, the lipase activities of A. japonicus exhibited no persistence of plasticity, which might be explained by the poor demand of dietary lipids and low lipase activities of A. japonicus (Liao et al., 2015a; Ye et al., 2019).
Changes in the digestive enzyme activities of A. japonicus before and after evisceration indicated the long-term effects of historic diets on digestion; the long-term effects of diets have been reported by numerous studies. For example, rainbow trout fry fed a plant-based diet for a short time enhanced the acceptance and use of a plant-based diet during their later life (Geurden et al., 2013; Balasubramanian et al., 2016). Besides, the early hyperglucidic diet stimuli showed long-term effects on carbohydrate digestion of rainbow trout in later life, which resulted in the persistence of amylase plasticity of the organism (Geurden et al., 2007). Similarly, in the present study, the long-term effects of diet in stage I on trypsin and amylase activities led to the persistence of these two enzymatic activities of A. japonicus. In addition, the trypsin activities of eviscerated A. japonicus fed diet B in stage I returned to the non-eviscerated levels in less time than those fed diet A in stage I, regardless of which diet was fed to A. japonicus in stage II. This finding suggested that the high-protein diet (diet B) in stage I could accelerate the recovery of trypsin activities of eviscerated A. japonicus, which further confirmed the positive long-term effects of historic diets on the digestion of A. japonicus. Moreover, the trypsin activities of non-eviscerated A. japonicus in stage II were affected by the historic diets in stage I, while for eviscerated A. japonicus, both trypsin and amylase activities were affected by diet in stage I, suggesting that the digestion of regenerated intestines was more susceptible to the long-term effect of historic diets compared with that of non-regenerated intestines.
For the growth performance of A. japonicus in stage I, A. japonicus in group B possessed higher FBW, BWG, and SGR than those in group A. Previous studies documented that adequate dietary protein contributed to the growth of A. japonicus (Seo et al., 2011; Li et al., 2021). For MR in stage I, A. japonicus fed diet B exhibited lower MR than those fed diet A, which might be related to the low carbohydrate content of diet B. Increased MR in response to high-carbohydrate diets was observed in the present study, which was consistent with that in Southern catfish (Silurus meridionalis) and black carp (Mylopharyngdon piceus Richardson) (ShiJian and XieXiao, 2007; Cai et al., 2010). Moreover, the negative relationship between MR and SGR of A. japonicus was reported by Xia et al. (2015a), in which A. japonicus fed a diet consisting of 75% S. thunbergii and 25% soybean meal showed higher MR but lower SGR than those of A. japonicus fed a diet consisting of 100% S. thunbergii. It suggested that the growth rate of A. japonicus would be restricted when more energy was provided to meet metabolic requirements.
In stage II, eviscerated A. japonicus exhibited higher BWG but lower FBW compared with the non-eviscerated A. japonicus. It was consistent with the previous finding that the growth performance would be rapidly improved after the intestinal regeneration of A. japonicus, although the body weights of eviscerated A. japonicus could not exceed that of non-eviscerated individuals due to huge loss weights caused by evisceration (Zang et al., 2012). The growth performance of A. japonicus in stage II was affected by not only evisceration but also the diet types in different stages. Diet B as diet in stages I or II would enhance FBW, BWG, and SGRs of all A. japonicus in stage II. Especially for A. japonicus fed diet B in stage II, it was more beneficial for their subsequent growth performance if diet B was included in stage I. However, for non-eviscerated or eviscerated groups, the differences in SGRs of A. japonicus fed the same diet in stage II were non-significant. It suggested that A. japonicus showed similar SGRs when they consumed the same diet, and the SGRs of A. japonicus were slightly affected by the long-term effects of the historic diets.
The changes in MRs of A. japonicus fed the same diet in stage II indicated that the MRs of A. japonicus seemed to be affected by diet in stage I, which was in agreement with previous findings that the nutritional history had major impacts on metabolic processes to improve fish performance (Vera et al., 2017). Besides, Lage et al. (2020) also demonstrated that the early dietary restriction resulted in a long-term modification of the metabolism of white shrimp by regulating the mRNA levels. In addition, the enzymatic activities are important for digesting and assimilating nutrients and are closely associated with growth performance and metabolic status (Sunde et al., 2001; Rungruangsak-Torrissen et al., 2006). In the present study, the SGRs and MRs of A. japonicus were positively affected by trypsin and amylase activities, respectively, which were consistent with the positive relationship between trypsin activities and body weight observed in Atlantic salmon (Rungruangsak-Torrissen et al., 2006). Besides, Rungruangsak-Torrissen et al. (2006) and Murashita et al. (2013) reported that the changes in digestive enzyme activities could be applicable as the indicators for growth and metabolic studies of fish to reflect the growth and metabolism during the development of organisms. Therefore, in the present study, based on the relationship between digestive enzyme activities, growth, and metabolism, the historic diets could produce long-term effects on the growth and metabolism of A. japonicus by affecting the enzyme activities in stage II.
In summary, the persistence of digestive enzyme activities induced by historic diets was found in non-eviscerated and eviscerated A. japonicus, indicating the long-term effects of historic diets on the digestion of A. japonicus. The digestion of regenerated intestines was more susceptible to the long-term effects of historic diets than those of non-regenerated intestines. The changes in SGRs and MRs, as well as their relationships with digestive enzyme activities, revealed that the historic diets showed long-term effects on the growth and metabolism of A. japonicus through long-term effects of historic diets on digestive enzyme activities. Further studies are required to investigate the molecular mechanism of the persistence of digestive enzyme activities and the long-term effects of historic diets on A. japonicus.
Data Availability Statement
The raw data supporting the conclusions of this article will be made available by the authors, without undue reservation.
Author Contributions
XL was in charge of feeding experiments, data analysis, and manuscript writing. QG and YT were in charge of experimental guidance and manuscript revision. YM, YX, and YC were in charge of feeding experiments. All authors designated in this manuscript actively participated in this research.
Funding
This study presented in the manuscript was funded by the National Natural Science Foundation of China (Grant No. 31672657) and Taishan Industrial Leading Talent Program (Project No. LJNY201710).
Conflict of Interest
YS and WJ were employed by Homey Group Co., Ltd.
The remaining authors declare that the research was conducted in the absence of any commercial or financial relationships that could be construed as a potential conflict of interest.
Publisher’s Note
All claims expressed in this article are solely those of the authors and do not necessarily represent those of their affiliated organizations, or those of the publisher, the editors and the reviewers. Any product that may be evaluated in this article, or claim that may be made by its manufacturer, is not guaranteed or endorsed by the publisher.
Acknowledgments
Thanks to Tianheng aquaculture company for the supply of sea cucumber. Meanwhile, thanks to the postgraduates of the Function Laboratory for Marine Fisheries Science and Food Production Processes for their assistance in sample collection.
References
Antonio, E. S., and Richoux, N. B. (2016). Influence of diet on the metabolic turnover of stable isotope ratios and fatty acids in the omnivorous shrimp Palaemon peringueyi. Mar. Biol. 163, 1–12. doi: 10.1007/s00227-016-2930-y
Bae, J., Hamidoghli, A., Won, S., Choi, W., Lim, S., Kim, K., et al. (2020). Evaluation of seven different functional feed additives in a low fish meal diet for olive flounder, Paralichthys olivaceus. Aquaculture 525:e735333. doi: 10.1016/j.aquaculture.2020.735333
Bai, Y., Zhang, L., Liu, S., Ru, X., Xing, L., Cao, X., et al. (2015). The effect of salinity on the growth, energy budget and physiological performance of green, white and purple color morphs of sea cucumber, Apostichopus japonicus. Aquaculture 437, 297–303. doi: 10.1016/j.aquaculture.2014.12.020
Balasubramanian, M. N., Panserat, S., Dupont-Nivet, M., Quillet, E., Montfort, J., Le Cam, A., et al. (2016). Molecular pathways associated with the nutritional programming of plant-based diet acceptance in rainbow trout following an early feeding exposure. BMC Genomics 17:449. doi: 10.1186/s12864-016-2804-1
Bolasina, S., Pérez, A., and Yamashita, Y. (2006). Digestive enzymes activity during ontogenetic development and effect of starvation in Japanese flounder, Paralichthys olivaceus. Aquaculture 252, 503–515. doi: 10.1016/j.aquaculture.2005.07.015
Buchheister, A., and Latour, R. J. (2010). Turnover and fractionation of carbon and nitrogen stable isotopes in tissues of a migratory coastal predator, summer flounder (Paralichthys dentatus). Can. J. Fish. Aquat. Sci. 67, 445–461. doi: 10.1139/F09-196
Cai, C. F., Ye, Y. T., Chen, L. Q., Qin, J. G., and Wang, Y. L. (2010). Oxygen consumption and ammonia excretion of black carp (Mylopharyngdon piceus Richardson) and allogynogenetic crucian carp (Carassius auratus gibelio female x Cyprinus carpio male) fed different carbohydrate diets. Fish Physiol. Biochem. 36, 1191–1198. doi: 10.1007/s10695-010-9398-3
Ding, K., Zhang, L., Sun, L., Lin, C., Feng, Q., Zhang, S., et al. (2019). Transcriptome analysis provides insights into the molecular mechanisms responsible for evisceration behavior in the sea cucumber Apostichopus japonicus. Comp. Biochem. Physiol. D-Genomics Proteomics 30, 143–157. doi: 10.1016/j.cbd.2019.02.008
Fang, L., Liang, X., Zhou, Y., Guo, X., He, Y., Yi, T., et al. (2014). Programming effects of high-carbohydrate feeding of larvae on adult glucose metabolism in zebrafish, Danio rerio. Br. J. Nutr. 111, 808–818. doi: 10.1017/S0007114513003243
Fu, X., Xue, C., Miao, B., Li, Z., and Gao, X. (2006). Distribution and seasonal activity variation of proteases in digestive tract of sea cucumber Stichopus japonicus. Fish. Sci. 72, 1130–1132. doi: 10.1111/j.1444-2906.2006.01268.x
García-Arrarás, J. E., Estrada-Rodgers, L., Santiago, R., Torres, I. I., Díaz-Miranda, L., and Torres-Avillán, I. (1998). Cellular mechanisms of intestine regeneration in the sea cucumber, Holothuria glaberrlma Salenka (Holothuroidea: Echinodermata). J. Exp. Zool. 281, 288–304. doi: 10.1002/(SICI)1097-010X(19980701)281:43.0.CO;2-K
García-Arrarás, J. E., and Greenberg, M. J. (2001). Visceral regeneration in holothurians. Microsc. Res. Tech. 55, 438–451. doi: 10.1002/jemt.1189
Garland, T. (2011). The flexible phenotype: A Body-Centred integration of ecology, physiology, and behaviour. Anim. Behav. 82, 609–610. doi: 10.1016/j.anbehav.2011.06.012
Geurden, I., Aramendi, M., Zambonino-Infante, J., and Panserat, S. (2007). Early feeding of carnivorous rainbow trout (Oncorhynchus mykiss) with a hyperglucidic diet during a short period: Effect on dietary glucose utilization in juveniles. Am. J. Physiol. Regul. Integr. Comp. Physiol. 292, R2275–R2283. doi: 10.1152/ajpregu.00444.2006
Geurden, I., Borchert, P., Balasubramanian, M. N., Schrama, J. W., Dupont-Nivet, M., Quillet, E., et al. (2013). The positive impact of the Early-Feeding of a Plant-Based diet on its future acceptance and utilisation in rainbow trout. PLoS One 8:e83162. doi: 10.1371/journal.pone.0083162
Giri, S. S., Sukumaran, V., and Oviya, M. (2013). Potential probiotic Lactobacillus plantarum VSG3 improves the growth, immunity, and disease resistance of tropical freshwater fish, Labeo rohita. Fish Shellfish Immunol. 34, 660–666. doi: 10.1016/j.fsi.2012.12.008
Gisbert, E., Gimenez, G., Fernandez, I., Kotzamanis, Y., and Estevez, A. (2009). Development of digestive enzymes in common dentex Dentex dentex during early ontogeny. Aquaculture 287, 381–387. doi: 10.1016/j.aquaculture.2008.10.039
Gong, G., Xue, M., Wang, J., Wu, X., Zheng, Y., Han, F., et al. (2015). The regulation of gluconeogenesis in the Siberian sturgeon (Acipenser during)affected later in life by a short-term high-glucose programming during early life. Aquaculture 436, 127–136. doi: 10.1016/j.aquaculture.2014.10.044
Hassaan, M. S., Mahmoud, S. A., Jarmolowicz, S., El-Haroun, E. R., Mohammady, E. Y., and Davies, S. J. (2018). Effects of dietary baker’s yeast extract on the growth, blood indices and histology of Nile tilapia (Oreochromis niloticus L.) fingerlings. Aquac. Nutr. 24, 1709–1717. doi: 10.1111/anu.12805
Hesslein, R. H., Hallard, K. A., and Ramlal, P. (1993). Replacement of Sulfur, Carbon, and Nitrogen in Tissue of Growing Broad Whitefish (Coregonus nasus) in Response to a Change in Diet Traced by \u03b4 34 S, \u03b4 13 C, and \u03b4 15 N. Can. J. Fish. Aquat. Sci. 50, 2071–2076. doi: 10.1139/f93-230
Hidalgo, M. C., Urea, E., and Sanz, A. (1999). Comparative study of digestive enzymes in fish with different nutritional habits. Proteolytic and amylase activities. Aquaculture 170, 267–283. doi: 10.1016/S0044-8486(98)00413-X
Huang, Q., Qin, D., Tan, B., Du, T., Yang, Y., Yang, Q., et al. (2019). The optimal dietary protein level of juvenile silver sillago Sillago sihama at three dietary lipid levels. Aquac. Res. 51, 816–827. doi: 10.1111/are.14431
Infante Zambonino, J. L., and Cahu, C. (1994). Development and response to a diet change of some digestive enzymes in sea bass (Dicentrarchus labrax) larvae. Fish Physiol. Biochem. 12, 399–408. doi: 10.1007/BF00004304
Kasiga, T., White, B. M., Bruce, T. J., and Brown, M. L. (2020). Effect of fish meal replacement with Carinata Brassica carinata in low animal protein diets of rainbow trout Oncorhynchus mykiss (Walbaum) on trypsin activity, protein and amino acid digestibility and bioavailability. Aquac. Res. 51, 2134–2149. doi: 10.1111/are.14564
Kürten, B., Painting, S. J., Struck, U., Polunin, N. V. C., and Middelburg, J. J. (2013). Tracking seasonal changes in North Sea zooplankton trophic dynamics using stable isotopes. Biogeochemistry 113, 167–187. doi: 10.1007/s10533-011-9630-y
Lage, L. P. A., Weissman, D., Serusier, M., Putrino, S. M., Baron, F., Guyonvarch, M., et al. (2020). Long-term impact of a 4-day feed restriction at the protozoea stage on metabolic gene expressions of whiteleg shrimp (Litopenaeus vannamei). PeerJ 8:e8715. doi: 10.7717/peerj.8715
Leibson, N. L. (1992). Regeneration of digestive tube in holothurians Stichopus japonicus and Eupentacta fraudatrix. Monogr. Dev. Biol. 23, 51–61.
Li, B. S., Han, X. J., Wang, J. Y., Song, Z. D., Sun, Y. Z., Wang, S. X., et al. (2021). Optimal dietary methionine requirement for juvenile sea cucumber Apostichopus japonicus selenka. Aquac. Res. 52, 1348–1358. doi: 10.1111/are.14989
Liao, M., Ren, T., He, L., Han, Y., and Jiang, Z. (2015b). Optimum dietary proportion of soybean meal with fish meal, and its effects on growth, digestibility, and digestive enzyme activity of juvenile sea cucumber Apostichopus japonicus. Fish. Sci. 81, 915–922. doi: 10.1007/s12562-015-0916-1
Liao, M., Ren, T., Chen, W., Han, Y., Liu, C., Jiang, Z., et al. (2015a). Effects of dietary lipid level on growth performance, body composition and digestive enzymes activity of juvenile sea cucumber, Apostichopus japonicus. Aquac. Res. 48, 92–101. doi: 10.1111/are.12864
Liu, Q. S., and Wang, D. H. (2007). Effects of diet quality on phenotypic flexibility of organ size and digestive function in Mongolian gerbils (Meriones unguiculatus). J. Comp. Physiol. B 177, 509–518. doi: 10.1007/s00360-007-0149-4
Luque De Castro, M. D., and Priego-Capote, F. (2010). Soxhlet extraction: Past and present panacea. J. Chromatogr. A 1217, 2383–2389. doi: 10.1016/j.chroma.2009.11.027
Macavoy, S. E., Arneson, L. S., and Bassett, E. (2006). Correlation of metabolism with tissue carbon and nitrogen turnover rate in small mammals. Oecologia 150, 190–201. doi: 10.1007/s00442-006-0522-0
Ministry of Agriculture and Rural Affairs of People’s Republic of China (2011). China Fisheries statistical Yearbook. Beijing, China: China Agriculture Press.
Ministry of Agriculture and Rural Affairs of People’s Republic of China (2021). China Fisheries statistical Yearbook. Beijing, China: China Agriculture Press.
Mir, I. N., Srivastava, P. P., Bhat, I. A., Muralidhar, A. P., Varghese, T., Gireesh-Babu, P., et al. (2018). Expression and activity of trypsin and pepsin during larval development of Indian walking catfish (Clarias magur). Aquaculture 491, 266–272. doi: 10.1016/j.aquaculture.2018.03.049
Moghadam, H., Mørkøre, T., and Robinson, N. (2015). Epigenetics—potential for programming fish for aquaculture? J. Mar. Sci. Eng. 3, 175–192. doi: 10.3390/jmse3020175
Muhlia-Almazan, A., Garcia-Carreno, F. L., Sanchez-Paz, J. A., Yepiz-Plascencia, G., and Peregrino-Uriarte, A. B. (2003). Effects of dietary protein on the activity and mRNA level of trypsin in the midgut gland of the white shrimp Penaeus vannamei. Comp. Biochem. Physiol. B 135, 373–383. doi: 10.1016/S1096-4959(03)00091-5
Murashita, K., Furuita, H., Matsunari, H., Yamamoto, T., Awaji, M., Nomura, K., et al. (2013). Partial characterization and ontogenetic development of pancreatic digestive enzymes in Japanese eel Anguilla japonica larvae. Fish Physiol. Biochem. 39, 895–905. doi: 10.1007/s10695-012-9749-3
Nazemroaya, S., Yazdanparast, R., Nematollahi, M. A., Farahmand, H., and Mirzadeh, Q. (2015). Ontogenetic development of digestive enzymes in Sobaity sea bream Sparidentex hasta larvae under culture condition. Aquaculture 448, 545–551. doi: 10.1016/j.aquaculture.2015.06.038
Norusis, M. (2008). SPSS statistics 17.0: Guide to data analysis. Upper Saddle River: Prentice-Hall.
Parma, L., Yufera, M., Navarro-Guillen, C., Moyano, F. J., Soverini, M., D’Amico, F., et al. (2019). Effects of calcium carbonate inclusion in low fishmeal diets on growth, gastrointestinal pH, digestive enzyme activity and gut bacterial community of European sea bass (Dicentrarchus labrax L.) juveniles. Aquaculture 510, 283–292. doi: 10.1016/j.aquaculture.2019.05.064
Patel, M. S., and Srinivasan, M. (2002). Metabolic programming: Causes and consequences. J. Biol. Chem. 277, 1629–1632. doi: 10.1074/jbc.R100017200
Peres, A., Cahu, C. L., Infante, J., LeGall, M. M., and Quazuguel, P. (1996). Amylase and trypsin responses to intake of dietary carbohydrate and protein depend on the developmental stage in sea bass (Dicentrarchus labrax) larvae. Fish Physiol. Biochem. 15, 237–242. doi: 10.1007/BF01875574
Peterson, B., and Fry, B. (1987). Stable isotopes in ecosystem studies. Annu. Rev. Ecol. Syst. 18, 293–320. doi: 10.1146/annurev.es.18.110187.001453
Ru, X., Zhang, L., Li, X., Liu, S., and Yang, H. (2019). Development strategies for the sea cucumber industry in China. J. Oceanol. Limnol. 37, 300–312. doi: 10.1007/s00343-019-7344-5
Rungruangsak-Torrissen, K., Moss, R., Andresen, L. H., Berg, A., and Waagbo, R. (2006). Different expressions of trypsin and chymotrypsin in relation to growth in Atlantic salmon (Salmo salar L). Fish Physiol. Biochem. 32, 7–23. doi: 10.1007/s10695-005-0630-5
Sabat, P., Lagos, J. A., and Bozinovic, F. (1999). Test of the adaptive modulation hypothesis in rodents: Dietary flexibility and enzyme plasticity. Comp. Biochem. Physiol. A Mol. Integr. Physiol. 123, 83–87. doi: 10.1016/S1095-6433(99)00042-2
Seo, J. Y., Shin, I. S., and Lee, S. M. (2011). Effect of dietary inclusion of various plant ingredients as an alternative for Sargassum thunbergii on growth and body composition of juvenile sea cucumber Apostichopus japonicus. Aquac. Nutr. 17, 549–556. doi: 10.1111/j.1365-2095.2010.00849.x
Shi, C., Dong, S., Pei, S., Wang, F., Tian, X., and Gao, Q. (2015). Effects of diatom concentration in prepared feeds on growth and energy budget of the sea cucumber Apostichopus japonicus (Selenka). Aquac. Res. 46, 609–617. doi: 10.1111/are.12206
ShiJian, F., and XieXiao, J. (2007). Effect of Dietary Carbohydrate Levels on Routine Metabolic Rate in Silurus meridionalis Chen. J. Anhui Agric. Sci. 35, 7857–7859. doi: 10.13989/j.cnki.0517-6611.2007.25.094
Sudong, X., Hongsheng, Y., Yong, L., Shilin, L., Yi, Z., and Lili, Z. (2012). Effects of different seaweed diets on growth, digestibility, and ammonia-nitrogen production of the sea cucumber Apostichopus japonicus (Selenka). Aquaculture 338, 304–308. doi: 10.1016/j.aquaculture.2012.01.010
Sun, Z. L., Gao, Q. F., Dong, S. L., Shin, P., and Wang, F. (2012). Estimates of carbon turnover rates in the sea cucumber Apostichopus japonicus (Selenka) using stable isotope analysis: The role of metabolism and growth. Mar. Ecol. Prog. 457, 101–112. doi: 10.3354/meps09760
Sunde, J., Taranger, G. L., and Rungruangsak-Torrissen, K. (2001). Digestive protease activities and free amino acids in white muscle as indicators for feed conversion efficiency and growth rate in Atlantic salmon (Salmo salar L). Fish Physiol. Biochem. 25, 335–345. doi: 10.1023/A:1023233024001
Sveinsdóttir, H., Thorarensen, H., and Gudmundsdóttir, Á (2006). Involvement of trypsin and chymotrypsin activities in Atlantic cod (Gadus morhua) embryogenesis. Aquaculture 260, 307–314. doi: 10.1016/j.aquaculture.2006.06.009
Symonds, M. E., Sebert, S. P., Hyatt, M. A., and Budge, H. (2009). Nutritional programming of the metabolic syndrome. Nat. Rev. Endocrinol. 5, 604–610. doi: 10.1038/nrendo.2009.195
Tarboush, R. A., MacAvoy, S. E., Macko, S. A., and Connaughton, V. (2006). Contribution of catabolic tissue replacement to the turnover of stable isotopes in Danio rerio. Can. J. Zool. 84, 1453–1460. doi: 10.1139/z06-136
Turkmen, S., Luis Castro, P., Jose Caballero, M., Maria Hernandez-Cruz, C., Saleh, R., Jesus Zamorano, M., et al. (2015). Nutritional stimuli of gilthead seabream (Sparus aurata) larvae by dietary fatty acids: Effects on larval performance, gene expression and neurogenesis. Aquac. Res. 48, 202–213. doi: 10.1111/are.12874
Vander Zanden, M. J., Clayton, M. K., Moody, E. K., Solomon, C. T., and Weidel, B. C. (2015). Stable isotope turnover and Half-Life in animal tissues: A literature synthesis. PLoS One 10:e0116182. doi: 10.1371/journal.pone.0116182
Vera, L. M., Metochis, C., Taylor, J. F., Clarkson, M., Skjaerven, K. H., Migaud, H., et al. (2017). Early nutritional programming affects liver transcriptome in diploid and triploid Atlantic salmon, Salmo salar. BMC Genomics 18:886. doi: 10.1186/s12864-017-4264-7
Wang, T., Sun, L., and Chen, M. (2015). Aestivation and regeneration. Dev. Aquac. Fish. Sci. 39, 177–209. doi: 10.1016/B978-0-12-799953-1.00011-8
Wen, B., Gao, Q., Dong, S., Hou, Y., Yu, H., and Li, W. (2016b). Effects of dietary inclusion of benthic matter on feed utilization, digestive and immune enzyme activities of sea cucumber Apostichopus japonicus (Selenka). Aquaculture 458, 1–7. doi: 10.1016/j.aquaculture.2016.01.028
Wen, B., Gao, Q., Dong, S., Hou, Y., and Yu, H. (2016a). Uptake of benthic matter by sea cucumber Apostichopus japonicus (Selenka): Insights from carbon stable isotopes and fatty acid profiles. J. Exp. Mar. Biol. Ecol. 474, 46–53. doi: 10.1016/j.jembe.2015.10.003
Winter, E. R., Nolan, E. T., Busst, G. M. A., and Britton, J. R. (2019). Estimating stable isotope turnover rates of epidermal mucus and dorsal muscle for an omnivorous fish using a diet-switch experiment. Hydrobiologia 828, 245–258. doi: 10.1007/s10750-018-3816-4
Xia, B., Gao, Q., Wang, J., Li, P., Zhang, L., and Zhang, Z. (2015a). Effects of dietary carbohydrate level on growth, biochemical composition and glucose metabolism of juvenile sea cucumber Apostichopus japonicus (Selenka). Aquaculture 448, 63–70. doi: 10.1016/j.aquaculture.2015.05.038
Xia, B., Wang, J., Gao, Q., Sun, Y., Zhang, L., Ma, J., et al. (2015b). The nutritional contributions of dietary protein sources to tissue growth and metabolism of sea cucumber Apostichopus japonicus (Selenka): Evidence from nitrogen stable isotope analysis. Aquaculture 435, 237–244. doi: 10.1016/j.aquaculture.2014.09.042
Xionggao, S., Xiaoqin, L., Xiangjun, L., Chonggui, T., Bo, L., Xianqi, C., et al. (2014). The improvement of growth, digestive enzyme activity and disease resistance of white shrimp by the dietary citric acid. Aquac. Int. 22, 1823–1835. doi: 10.1007/s10499-014-9785-3
Yang, H., Yuan, X., Zhou, Y., Mao, Y., Zhang, T., and Liu, Y. (2015). Effects of body size and water temperature on food consumption and growth in the sea cucumber Apostichopus japonicus (Selenka) with special reference to aestivation. Aquac. Res. 36, 1085–1092. doi: 10.1111/j.1365-2109.2005.01325.x
Ye, Z., Hui, L., Qing, W., and Bingjun, L. (2019). The influence of three antibiotics on the growth, intestinal enzyme activities, and immune response of the juvenile sea cucumber Apostichopus japonicus selenka. Fish Shellfish Immunol. 84, 434–440. doi: 10.1016/j.fsi.2018.10.022
Ying, L., Shuanglin, D., Xiangli, T., Fang, W., and Qinfeng, G. (2009). Effects of dietary sea mud and yellow soil on growth and energy budget of the sea cucumber Apostichopus japonicus (Selenka). Aquaculture 286, 266–270. doi: 10.1016/j.aquaculture.2008.09.029
Ying, L., Shuanglin, D., Xiangli, T., Fang, W., and Qinfeng, G. (2010). The effect of different macroalgae on the growth of sea cucumbers (Apostichopus japonicus Selenka). Aquac. Res. 41, e881–e885. doi: 10.1111/j.1365-2109.2010.02582.x
Yu, H., Ai, Q., Mai, K., Ma, H., Cahu, C. L., and Infante, J. L. Z. (2012). Effects of dietary protein levels on the growth, survival, amylase and trypsin activities in large yellow croaker, Pseudosciaena Crocea R., Larvae. Aquac. Res. 43, 178–186. doi: 10.1111/j.1365-2109.2011.02814.x
Zang, Y., Tian, X., Dong, S., and Dong, Y. (2012). Growth, metabolism and immune responses to evisceration and the regeneration of viscera in sea cucumber, Apostichopus japonicus. Aquaculture 358, 50–60. doi: 10.1016/j.aquaculture.2012.06.007
Zar, J. H. (1999). Biostatistical Analysis (Fourth Edition). Fundamentals of air pollution. Englewood Cliffs: Prentice Hall international.
Keywords: trypsin activity, amylase activity, specific growth rate, metabolic rate, carbon stable isotope
Citation: Li X, Gao Q, Tian Y, Mei Y, Xu Y, Cao Y, Dong S, Zhou Y, Sun Y and Ju W (2022) Persistence of Digestive Enzyme Activities of Sea Cucumber (Apostichopus japonicus): Evidence From Diet Switch After Evisceration. Front. Mar. Sci. 9:843612. doi: 10.3389/fmars.2022.843612
Received: 26 December 2021; Accepted: 07 February 2022;
Published: 24 February 2022.
Edited by:
Sílvia Lourenço, Escola de Turismo e Tecnologia Marítima, Instituto Politécnico de Leiria, PortugalReviewed by:
Bin Xia, Qingdao Agricultural University, ChinaMansour Torfi Mozanzadeh, South Iran Aquaculture Research Center, Iran
Ana Pombo, Polytechnic Institute of Leiria, Portugal
Copyright © 2022 Li, Gao, Tian, Mei, Xu, Cao, Dong, Zhou, Sun and Ju. This is an open-access article distributed under the terms of the Creative Commons Attribution License (CC BY). The use, distribution or reproduction in other forums is permitted, provided the original author(s) and the copyright owner(s) are credited and that the original publication in this journal is cited, in accordance with accepted academic practice. No use, distribution or reproduction is permitted which does not comply with these terms.
*Correspondence: Qinfeng Gao, cWZnYW9Ab3VjLmVkdS5jbg==