- 1SARTI Research Group, Electronics Department, Universitat Politècnica de Catalunya (UPC), Vilanova i la Geltrú, Spain
- 2Department of Marine Renewable Resources, Institute of Marine Science (ICM-CSIC), Barcelona, Spain
- 3SARTI Research Group, Mathematics Department, Universitat Politècnica de Catalunya (UPC), Vilanova i la Geltrú, Spain
- 4Stazione Anton Dhorn (SZN), Naples, Italy
Behavioral rhythms are a key aspect of species fitness, since optimize ecological activities of animals in response to a constantly changing environment. Cabled observatories enable researchers to collect long-term biological and environmental data in real-time, providing relevant information on coastal fishes’ ecological niches and their temporal regulation (i.e., phenology). In this framework, the platform OBSEA (an EMSO Testing-Site in the NW coastal Mediterranean) was used to monitor the 24-h and seasonal occurrence of an ecologically iconic (i.e., top-predator) coastal fish species, the common dentex (Dentex dentex). By coupling image acquisition with oceanographic and meteorological data collection at a high-frequency (30 min), we compiled 8-years’ time-series of fish counts, showing daytime peaks by waveform analysis. Peaks of occurrence followed the photophase limits as an indication of photoperiodic regulation of behavior. At the same time, we evidenced a seasonal trend of counts variations under the form of significant major and minor increases in August and May, respectively. A progressive multiannual trend of counts increase was also evidenced in agreement with the NW Mediterranean expansion of the species. In GLM and GAM modeling, counts not only showed significant correlation with solar irradiance but also with water temperature and wind speed, providing hints on the species reaction to projected climate change scenarios. Grouping behavior was reported mostly at daytime. Results were discussed assuming a possible link between count patterns and behavioral activity, which may influence video observations at different temporal scales.
Introduction
Diel (i.e., 24-h based) and seasonal biological processes of species inhabiting temperate regions, are synchronized to changes in photoperiod length and overall levels of environmental illumination (Foster and Kreitzman, 2010; Visser et al., 2010; Helm et al., 2013; Kronfeld-Schor et al., 2013). In marine coastal fishes, the photoperiod light intensity are among the most important environmental variables controlling biological rhythms and overall phenology (Naylor, 2010). For example, environmental illumination determines the timing of activity of predators and preys, that perform their ecological tasks according to a trade-off between maximum opportunities of visual-based feeding and minimum mortality risk (Daan, 1981; Reebs, 2002; Brierley, 2014; Mittelbach et al., 2014). However, the exposure of marine costal ecosystems to solar light produces a seasonal co-variation of photoperiod length with other habitat variables that also affect biological rhythms. For example, temperature can have strong effects on fishes at day-night and seasonal scales (Reebs, 2002; López-Olmeda et al., 2006). Combined photoperiod length and temperature cycles regulate physiological processes over the day-night alternation, resulting in global growth and reproduction patterns at a seasonal level (Falcón et al., 2010; Bulla et al., 2017; Cowan et al., 2017). Nevertheless, many marine species can also follow the lunar or tidal cycle to carry out their biological processes within the lunar day of 24.8-h (Naylor, 2010). In particular, tidal rhythms in marine species were related to locomotion and reproduction (Wagner et al., 2007; Aguzzi et al., 2010).
The interaction of activity rhythms of all species within a marine community may affect the estimation of its overall biodiversity. This is particularly significant for ecologically important species, such as top-predators, that play a critical role in maintaining the structure and stability of communities and affect ecosystem functioning (Heithaus et al., 2008, 2012; Byrnes et al., 2021). Sampling should be repeated at a frequency sufficient to grasp the whole alternation between consecutive peak and through in population abundances as a product of massive rhythmic displacement (Aguzzi et al., 2015b). Moreover, that sampling has to be repeated in association with concomitant data collection to understand how photoperiod length, light intensity and other environmental variables modulate behavioral responses (Aguzzi et al., 2020d). Similar temporal effects exist on fish grouping behavior (Rodriguez-Pinto et al., 2020), whose strategy can be related to foraging, spawning and predator evasion (Ford and Swearer, 2013; Makris et al., 2019; Lear et al., 2021). Moreover, environmental modulation of grouping behavior of fish has been observed in association to photoperiod changes (Meager et al., 2012; Georgiadis et al., 2014). Changes on grouping behavior, driven by human activities such as fishing, could affect the ecosystem functioning, and have repercussions for biodiversity conservation and fisheries management strategies (Sbragaglia et al., 2021).
Data on the phenology of marine fishes, as a product of a variation in local abundances, can be studied by cabled observatories for their capability to perform high-frequency, continuous and long-lasting imaging along with a concomitant multiparametric oceanographic data acquisition (Snelgrove et al., 2014; Danovaro et al., 2017; Aguzzi et al., 2019, 2020a,2020b; Rountree et al., 2020). In particular, cabled systems have the capacity to host many environmental sensors at high resolution, collecting many habitat variables, thus giving a better instrumental field approach to fishes’ ecological niches (Hutchingson, 1957). Stand-alone or lander-based cameras are also good tools to study those aspects of species (e.g., Langlois et al., 2020; Drazen et al., 2021). But, given to energy constrains, a limited set of environmental variables is usually acquired. Each environmental variable measured by the installed sensor (e.g., essential environmental variables) can add habitat information for each imaged species (Aguzzi et al., 2020b). Time-lapse imaging studies with that technology have been efficiently used to describe diel and seasonal patterns in fish counts as a proxy for behavior rhythms, resulting in projected abundance changes at all depths of the continental margin (e.g., Juniper et al., 2013; Doya et al., 2014; Matabos et al., 2014, 2015; Milligan et al., 2020). In fact, in the marine three-dimensional scenario of the seabed and the water-column, day-night and seasonal shifts in populations bathymetric distributions, displacement ranges, and overall activity, influence the number of collectable animals into our sampling windows (e.g., Aguzzi and Company, 2010; Scapini, 2014; Chatzievangelou et al., 2021). A variation in counted animals produce changes in estimated abundances for a species in comparison to all the others (i.e., evenness; Aguzzi et al., 2015b). When rhythmic abundance changes are not carefully considered at sampling, their effect transcend to the computed biodiversity (Doya et al., 2017).
The use of cabled observatories for the monitoring of economically or ecologically important fish species is of relevance for the international conservation strategy agendas (Aguzzi et al., 2020c). Here, we used a coastal cable observatory to video-monitor the 24-h and seasonal occurrence of a top-predator, the common dentex (Dentex dentex; hereafter refers to as Dentex), at an artificial reef at high frequency over almost a decade. This species represents an iconic study case also for its value in commercial and recreational fisheries (Marengo et al., 2014; Sbragaglia et al., 2020), and a previous time-lapse study at the same artificial reef using the same cabled observatory suggested a relationship of fish presence with temperature, salinity and photoperiod length (Sbragaglia et al., 2019). Here, we moved a step forward and attempted to measure the association of count patterns over the 24-h to the photoperiod length, scaling this phenomenon over the whole seasonal cycle (i.e., photoperiodism). In doing so, we evaluated which of the measured oceanographic and meteorological variables mostly affected the reported count patterns. At the same time, we innovatively quantified the occurrence and the temporal dynamic of grouping behavior, also relating this phenomenon to the environmental variation.
Materials and Methods
The OBSEA Platform Location and Equipment
The coastal Seafloor Observatory (OBSEA1) is a cabled observatory platform located at 4 km off Vilanova i la Geltrú (Catalonia, Spain) at 20 m depth within the Colls i Miralpeix Natura 2000 area (Aguzzi et al., 2011; Del Rio et al., 2020; Figures 1A,B). The observatory is equipped with an OPT-06 Underwater IP Camera (OpticCam), which can acquire images/footages of the surrounding environment with a resolution of 640 × 480 pixels.
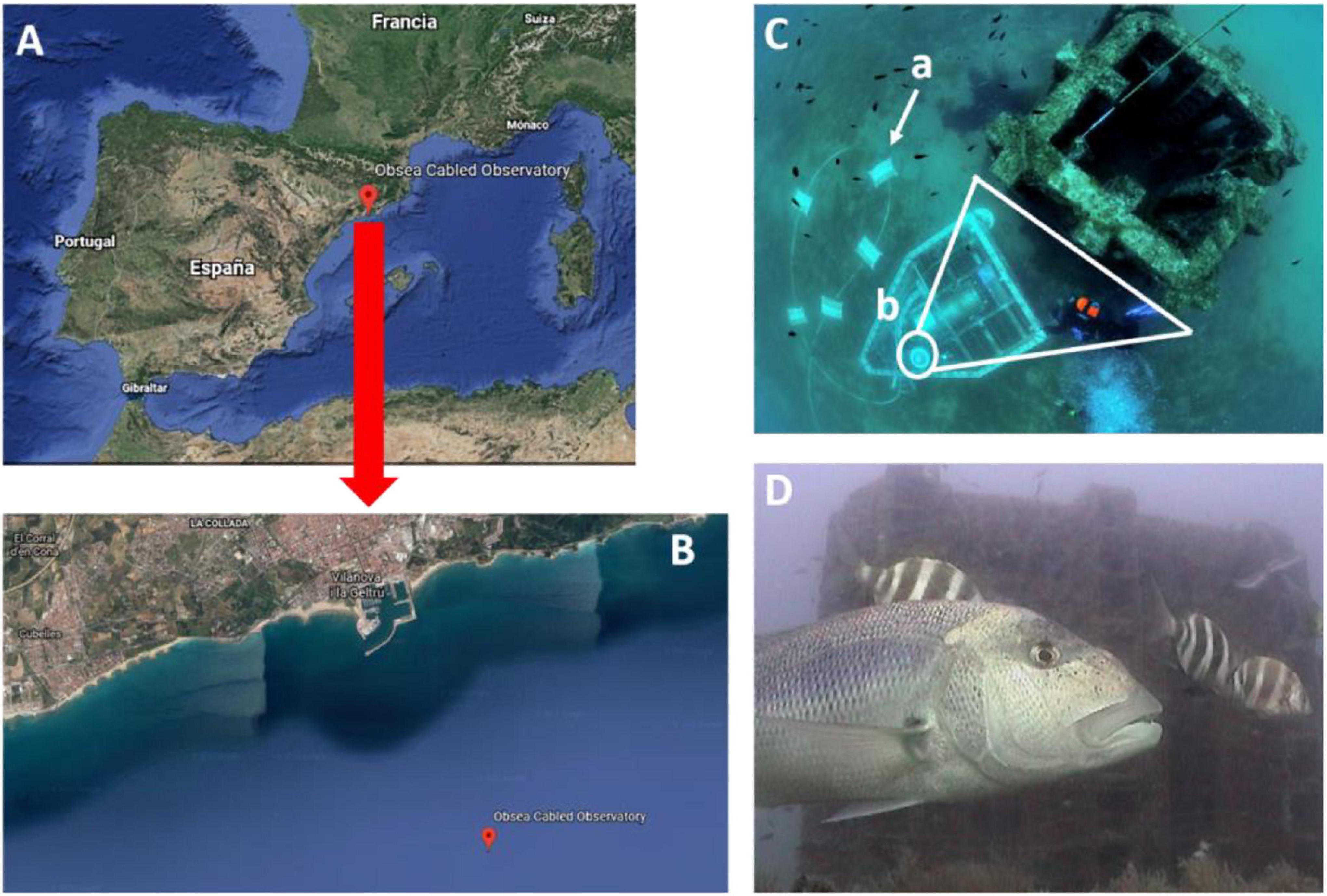
Figure 1. Location of the OBSEA video platform in the NW Mediterranean (A) with specifications for the Catalan coasts, indicating its position off the harbor of Vilanova i la Geltrú (B). The OBSEA platform is connected to shore with an Ethernet powering/data transfer cable (C.a), camera focusing on the artificial reef (C.b), where the number of individuals per photo of D. dentex can be observed and counted within a constant field of view (D).
OBSEA is also equipped with two custom developed white LEDs (2,900 lumen; color temperature of 2,700 K), located besides the camera (with an angle of 120°) at 1 m distance from each other to allow image acquisition at night (Aguzzi et al., 2011). A procedure controlling the ON-OFF status of lighting immediately before and after image acquisition, was performed because of the artificial photic footprint on species (e.g., Aguzzi et al., 2010; Matabos et al., 2011). The lights were switched ON and OFF (lasting for 3 s) by a LabView application that also controlled their white balance.
Image Acquisition, Fish Counting and Environmental Data Processing
We acquired 70,254 images with a 30 min time-lapse mode, continuously during 8 years (2012-2019), preserving the same field of view, centered on the artificial reef at 3.5 m in front of the OBSEA (see Figure 1C). Individuals of Dentex were manually counted for each image (Figure 1D) by a trained operator following procedures by Condal et al. (2012) and Aguzzi et al. (2013).
Temperature (°C), salinity (PSU), and depth (m) were measured by the CTD probe installed aside the camera (Aguzzi et al., 2011). Furthermore, we collected data of air temperature (°C), wind speed (km/h), and wind direction (deg.) from the meteorological station located on SARTI (Development Center of Remote Acquisition and Information Processing Systems) rooftop in Vilanova i la Geltrú. We also gathered sun irradiance (W/m2) and rain (mm) from the Catalan Meteorological Service station in San Pere de Ribes (6 km away from the OBSEA). Time series for all the environmental data compiled by selecting and extracting only readings contemporary to the timing of all acquired images.
We applied range filters for the fluctuation of environmental variables in order to remove out-layer data (i.e., due to instruments malfunctioning). Guillén et al. (2018) was referenced for water temperature and salinity (i.e., ranges of 11-28°C and 36.80-39.67 PSU, respectively), since authors have a 10 years’ time series of readings from a nearby station in Barcelona (Spain). For air temperature and wind speed (ranges of 3-31°C and 0-60 km/h, respectively) we used an online website2 with 30 years of hourly weather modeled data. Rain and solar irradiance were not filtered since downloaded from an Institutional and already filtered source3.
Multivariate Analysis
Prior to the multivariate analysis, we transformed the number of individuals of Dentex per photo into nominal presence/absence response variable (Zuur et al., 2007). In order to obtain an optimized model for fish presence/absence, we then executed a correlation analysis on the environmental variables, to group the highly-correlated ones, removing the lesser representative from further analyses (Zuur et al., 2007). We used a General Linear Model (GLM) and a General Additive Model (GAM) using a binomial distribution, to identify which selected environmental variables mostly affected fish presence/absence, and compared the results between those analyses. We tested both methods because we did not have a priori reason for using a particular model.
We proceeded with the same multivariate analyses to describe the grouping behavior of Dentex. In order to do so, we firstly ranked images depending on variable number of pictured individuals (i.e., starting from 1). That frequency of groups of individuals was compiled into a frequency histogram plot. Then, we transformed the number of individuals into a nominal variable for grouping or not grouping behavior (i.e., “0” when in the photo there was only one individual, and “1” there was more than one individual). Then, we added this column of values to the temporal variables (i.e., hours, months and years), to detect any temporal pattern for this social behavior and identify which environmental variables affect it. We interpreted the data based on ethological common use of the wording (as per the general definition Pitcher, 1983). Thus, we consider the occurrence of the grouping behavior as the co-presence of fishes in the same field of view of the camera.
The correlation analysis was carried out with the library “PerformanceAnalytics,” and GLM and GAM models were executed using the libraries “gdata” and “mgcv” of R software.
Time Series Analysis
In order to obtain a global overview of Dentex diel and seasonal behavioral rhythms across consecutive years, we first plotted the 8-years visual counts time series computing the means and standard errors (SE) values per each month of the time series. Temporal gaps in image acquisition were evidenced by line discontinuity. Time series analysis was performed separately for time series of fish counts and each relevant environmental variable for the presence/absence of Dentex evidenced by GLM and GAM modeling (see previous Section). All graphic outputs were again plotted in local time.
Waveform analysis was carried out to describe the diel and seasonal pattern of activity rhythm of the species. Waveforms computing was as follows: time series of visual counts were subdivided in 30 min time-series and averaged together over a standard 24-h period (i.e., 48 values per segment). A consensus averaged fluctuation over that standard 24-h period was then obtained by averaging all values of the different segments at the corresponding timings. The resulting means (± SE) were plotted to identify peaks and troughs in the waveform profile. The peaks temporal amplitude (i.e., the phase) was then computed according to the Midline Estimating Statistic of Rhythm (MESOR) method (Aguzzi et al., 2006), by re-averaging all waveform averages and the resulting value was represented as a threshold horizontal line superimposed onto the waveform plot. The Onset and Offset timings of activity (delimiting peaks intervals) were estimated by considering the first and the last waveform value above the MESOR. The peak was considered as continuous if no more than 3 values occurred below the MESOR (Aguzzi et al., 2020d). All waveform analyses were carried out using the library “ggplot2” of R software.
That waveform analysis was firstly conducted on the fish 8-years count time series and solar irradiance data, to visualize the general peaks as a proxy for the solar-driven, behaviorally induced changes in abundance as a product of behavioral activity (i.e., the photic character of the species ecological niche). Then, the same waveform analysis was repeated for each month and each season, by joining time series counts for winter (i.e., December, January, and February), spring (i.e., March, April, and May), summer (June, July, and August), and autumn (i.e., September, October, and November), to assess peaks’ timings and amplitude variations as marker of photoperiodic regulation of behavioral rhythms. Moreover, to better describe the seasonal behavior of Dentex, and its relation with the photoperiod, we plotted the mean values (± SE) and MESORs of number of counts and solar irradiance of each month of the year. Finally, the same waveform analysis was performed for those environmental variables selected by models of presence/absence data (see previous Section).
Additionally, we assessed precisely the average values of those environmental variables selected by GLM and GAM modeling for presence/absence data (see previous Section) at Dentex waveform peaks crossing MESOR (see above), in order to add information on the species multidimensional niche (sensu Pocheville, 2015). At the same time, to better describe the environmental and temporal pattern of grouping behavior, we additionally plotted conditional densities of the environmental variables selected by GLM and GAM models of grouping or not grouping behavioral data.
An integrated chart depicting the temporal relationships of waveform peaks (i.e., the phases) in fish counts and the solar irradiance was created month by month over the whole 8 years of data acquisition (Aguzzi et al., 2012, 2015a). The values of each monthly waveform were compared with the respective MESOR through an inequality function in Excel (i.e., each waveform value per 30 min automatically resulted as “major” or “minor” in relation to the MESOR). All waveforms values identified as greater than the MESOR (i.e., the peak duration) were then plotted as horizontal continuous bar per each month. That operation was repeated for solar irradiance.
Results
A total of 140257 photos should have been obtained during 8-years of monitoring (i.e., one per 30 min, from 2012 to 2019), but due to several malfunctioning problems creating gaps in the time series, we were able to analyze only 7,0254 photos (50.09% out of the total expected photos). The 95.99% of analyzed images (67438 photos) contained no Dentex (i.e., has “zero” as count value), and a few observations had high abundance (e.g., in only 5 photos there were more than 8 individuals; 0.18%).
We counted a total of 3,747 individuals of Dentex. The three months with the highest number of individuals were (Table 1): August with 649 number of individuals of Dentex (17.32%), July with 615 counts (16.41%), and finally October with 494 individuals of Dentex counted (13.18%).
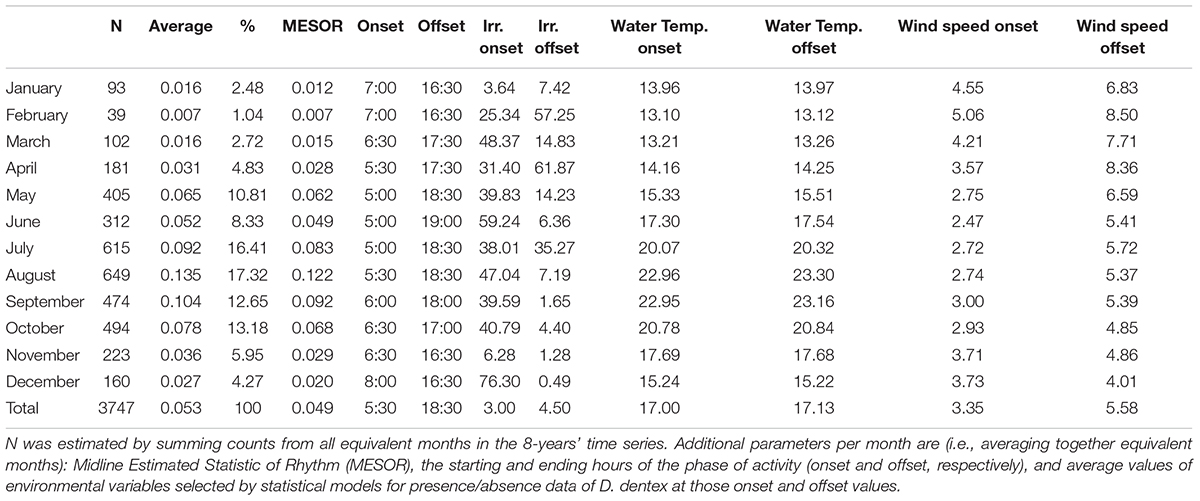
Table 1. Monthly D. dentex visual counts number (N), average and relative percentage (%) out of the total within the 2012-2019 monitoring period.
By compiling this time series into monthly estimates (± SE), we observed a consistent seasonal trend in Dentex counts (Figure 2). A major peak occurred in spring-summer and its height progressively increased over the consecutive years.
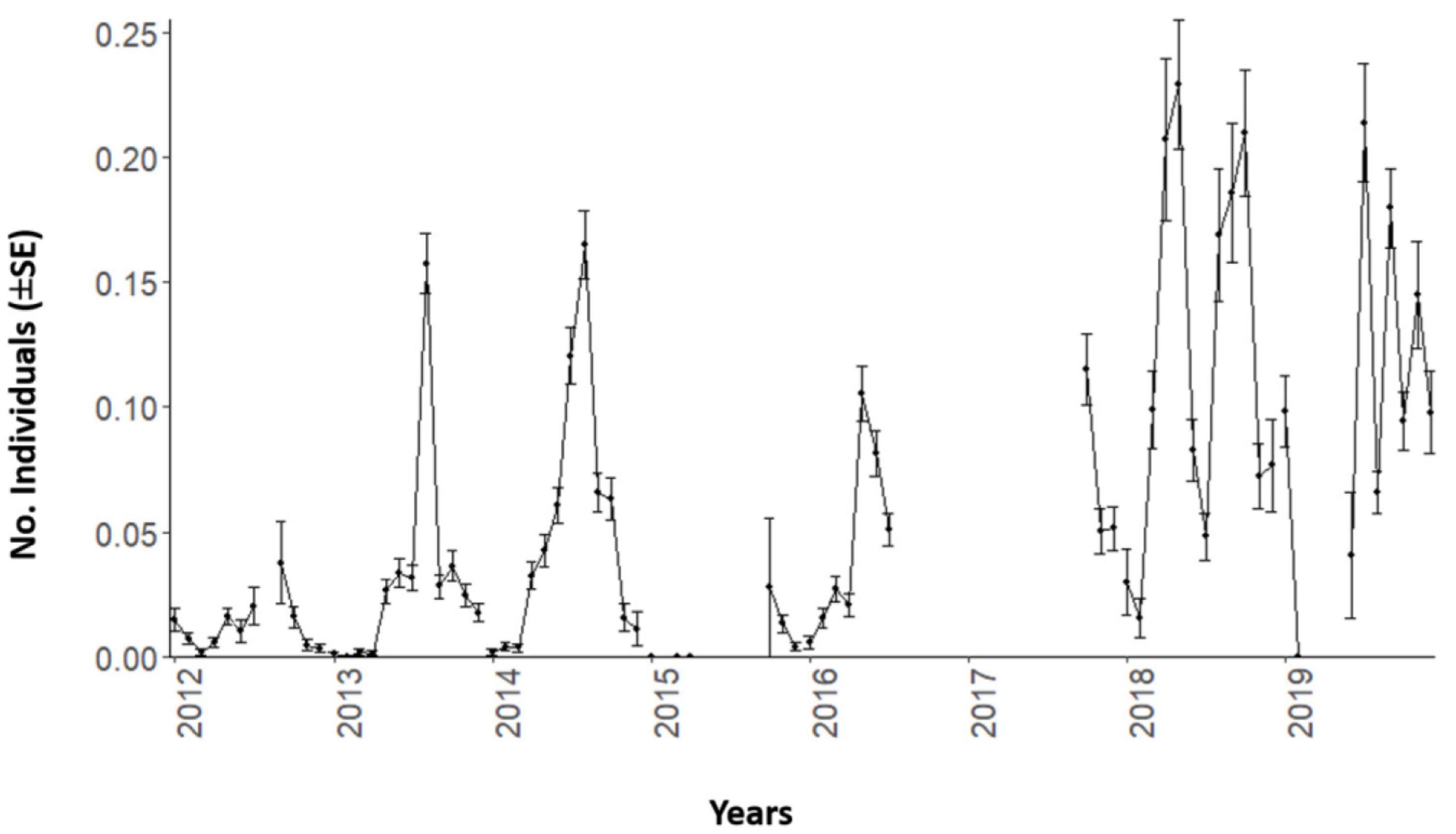
Figure 2. Mean values and standard errors (± SE) per each month of the D. dentex counts time series during 8 years (from 2012 to 2019) of monitoring at the OBSEA video platform. Temporal gaps in image acquisition are evidenced by line discontinuities.
Multivariate Statistic
From the correlation analysis among the environmental variables, we observed a significant relationship between water and air temperatures (Correlation Index = 0.69) (Figure 3). Accordingly, we removed the air temperature as explanatory variable from the further analysis. We did not eliminate water temperature because it was considered a more biologically important variable for Dentex.
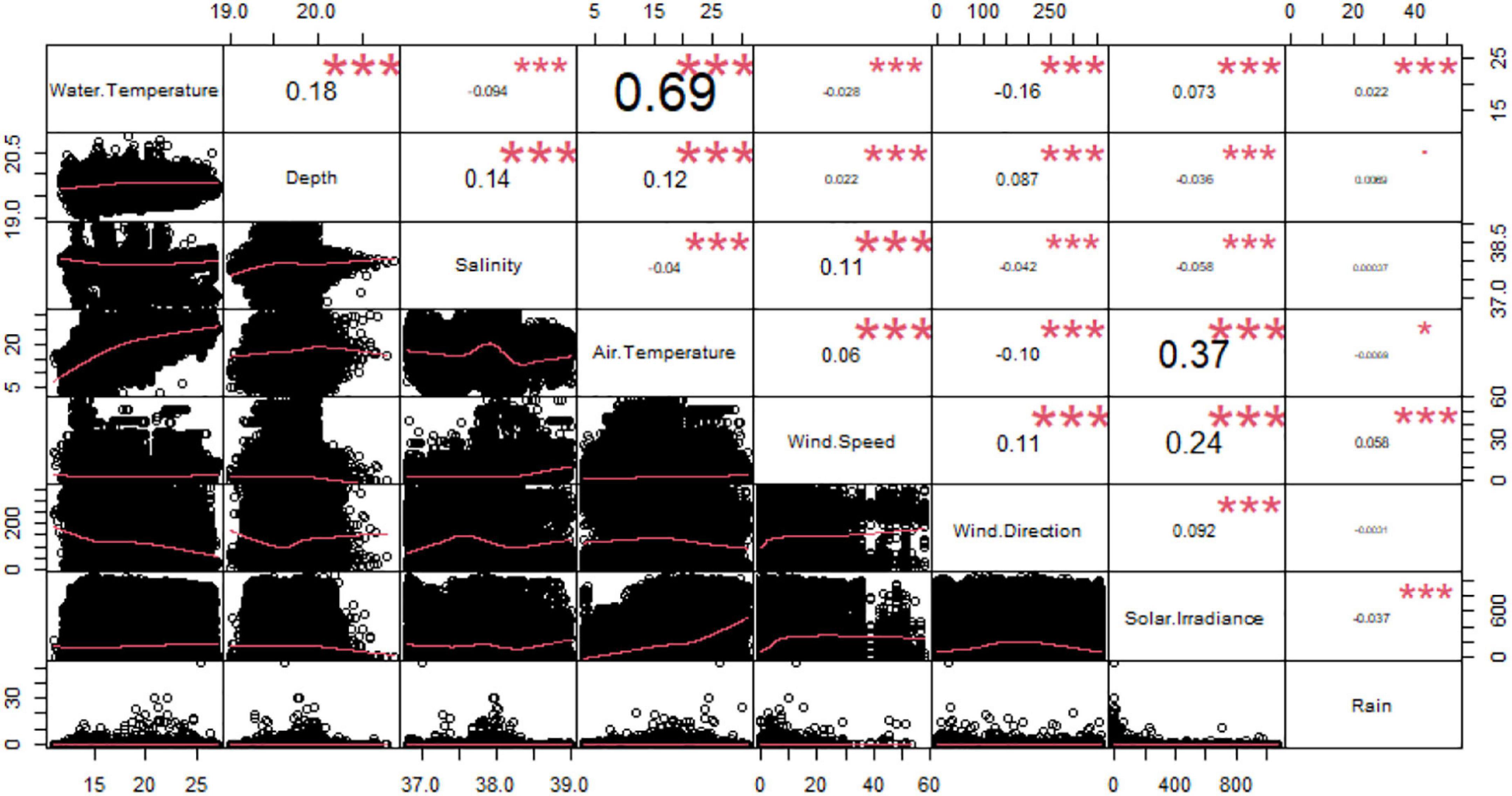
Figure 3. Correlation chart among the environmental variables. The name of each variable is shown on the diagonal. Below the diagonal the bivariate scattered plots with the fitted line in red are displayed. Above the diagonal the value of the correlation plus the significance level as stars: to p-values of 0, 0.001, 0.1, 0.05, 0.1, and 1 correspond respectively “***”, “**”, “*”, “.”, and “”.
We observed that in both GLM and GAM models on presence/absence data all the variables were significant at the 5% level, except for salinity, wind direction and rain (Supplementary Table 1). Both approaches gave a model where water temperature, wind speed and solar irradiance were selected (Table 2 and Supplementary Table 2). So, we selected these variables for the next time series analysis.
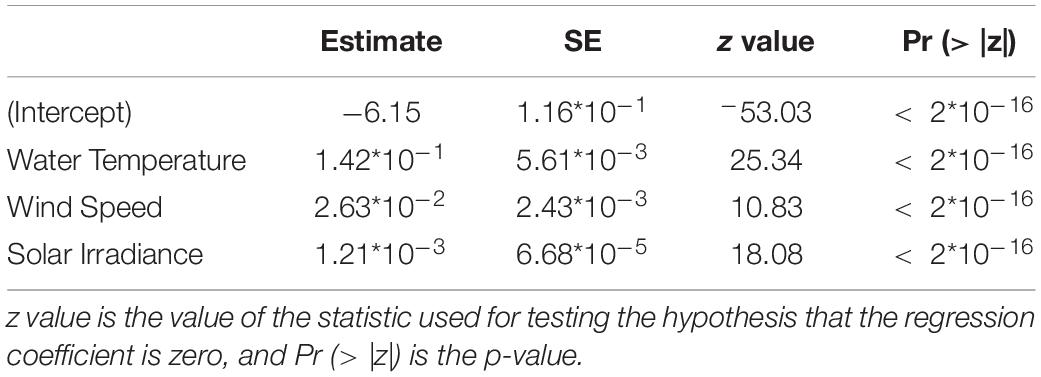
Table 2. Results from the most representative GAM modeling for the presence/absence data of D. dentex, where metrics are also indicated: SE is the Standard Error of the estimated fitted mean parameter.
To study the grouping behavior of Dentex, we computed the percentage on the total number of images where it was present (2816 photos; Figure 4). Mostly, it was observed as solitary (2231 photos; 79.23%), but more rarely it appeared in pairs or in larger groups. In particular, in 395 photos (14.03%) it occurred in pairs, in 169 photos (6%) it occurred in groups of 3-5 individuals. Finally, it was observed in groups of 6-8 or more individuals (i.e., 16 and 5 photos respectively, equal to 0.57 and 0.18%). The maximum number of individuals in a single photo has been detected during 27th July 2019 at 8:00 in a group of 11 individuals.
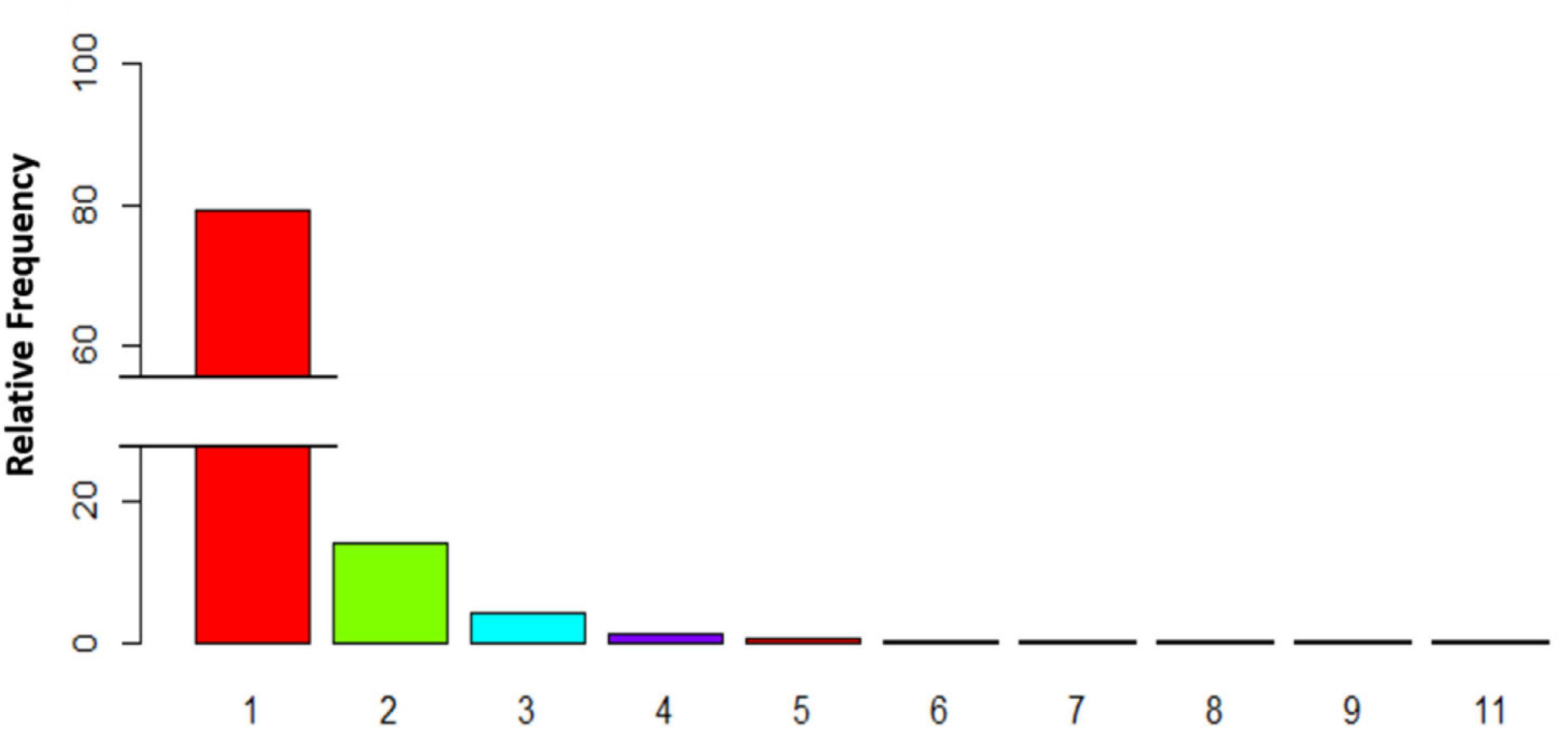
Figure 4. Histogram depicting the relative percentage of images with variable number of individuals of D. dentex, where fishes of this species were present, as a quantification of grouping behavior.
Afterward, we carried out correlation analysis between environmental and temporal variables observing that there was a significant relationship between water and air temperature (Correlation Index = 0.69) (Supplementary Figure 1). As before, we removed air temperature as explanatory variable.
Then, we performed GLM and GAM models on the grouping or not grouping behavioral data (respectively when Dentex was observed alone or in group of two or more individuals) with the selected environmental and temporal variables. In both models all the variables were significant at the 5% level, except for wind speed and direction, solar irradiance, rain and hours (Supplementary Table 3). We observed in both approaches that water temperature, solar irradiance, hours, months and years were selected as relevant variables for the grouping behavior of the Dentex (Table 3) (Supplementary Table 4). It has to be noted that solar irradiance and month were slightly less significant than the other variables regarding the p-values (respectively Pr (> | z|) = 1.27*10–02 and Pr (> | z|) = 3.82*10–03).
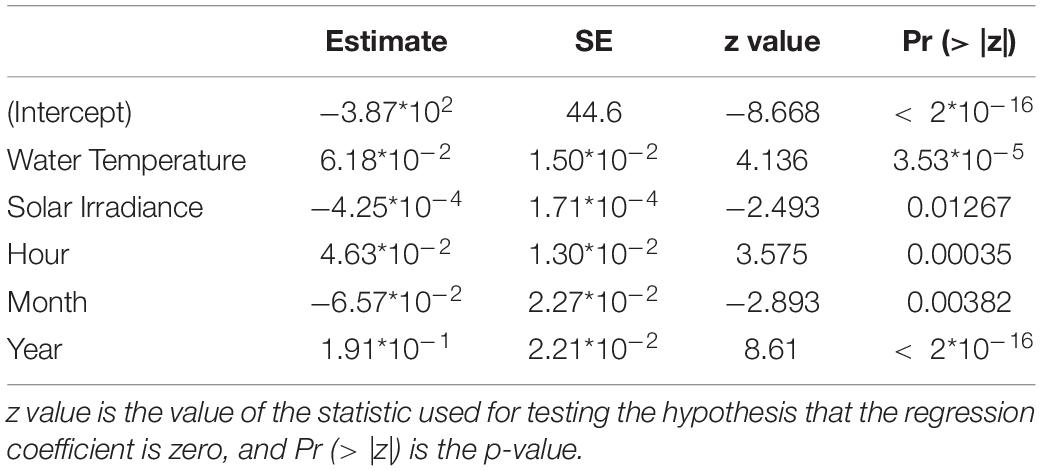
Table 3. Results from the most representative GAM modeling for the grouping or not grouping behavioral data of D. dentex, where metrics are also indicated: SE is the Standard Error of the estimated fitted mean parameter.
We decided to report only GAMs upon GLMs results for both presence/absence and grouping or not grouping behavioral data, even if the two methods obtained same outputs, because GAMs models were considered an extension of GLMs.
Diel and Seasonal Fish Count Patterns
The waveform analysis on the 8-years’ time series showed the occurrence of a solid diurnal peak, defining an increase of occurrence in the light hours (Figure 5). That waveform analysis repeated at the seasonal level (Figure 6) evidenced the photoperiodic regulation of occurrence with transient uni- and bimodality in counts peaks: crepuscular and diurnal peaks during respectively short and long photophases (i.e., autumn-winter versus spring-summer). Also peaks temporal limits are following irradiance temporal limits.
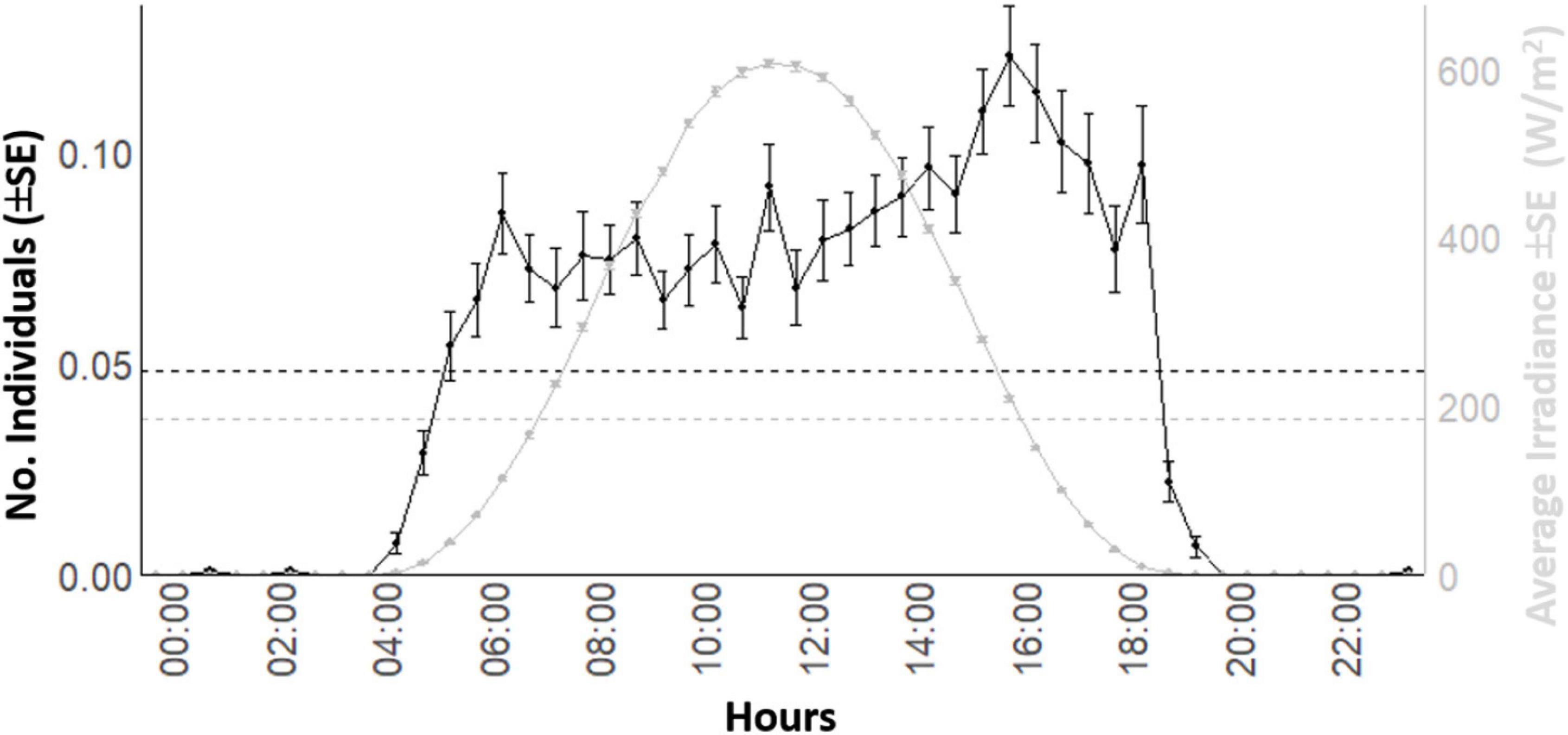
Figure 5. Global waveform analysis output plot for the D. dentex visual count and solar irradiance time series from 8 years (i.e., 2012–2019) of monitoring at the OBSEA video platform. The dashed horizontal line is the MESOR.
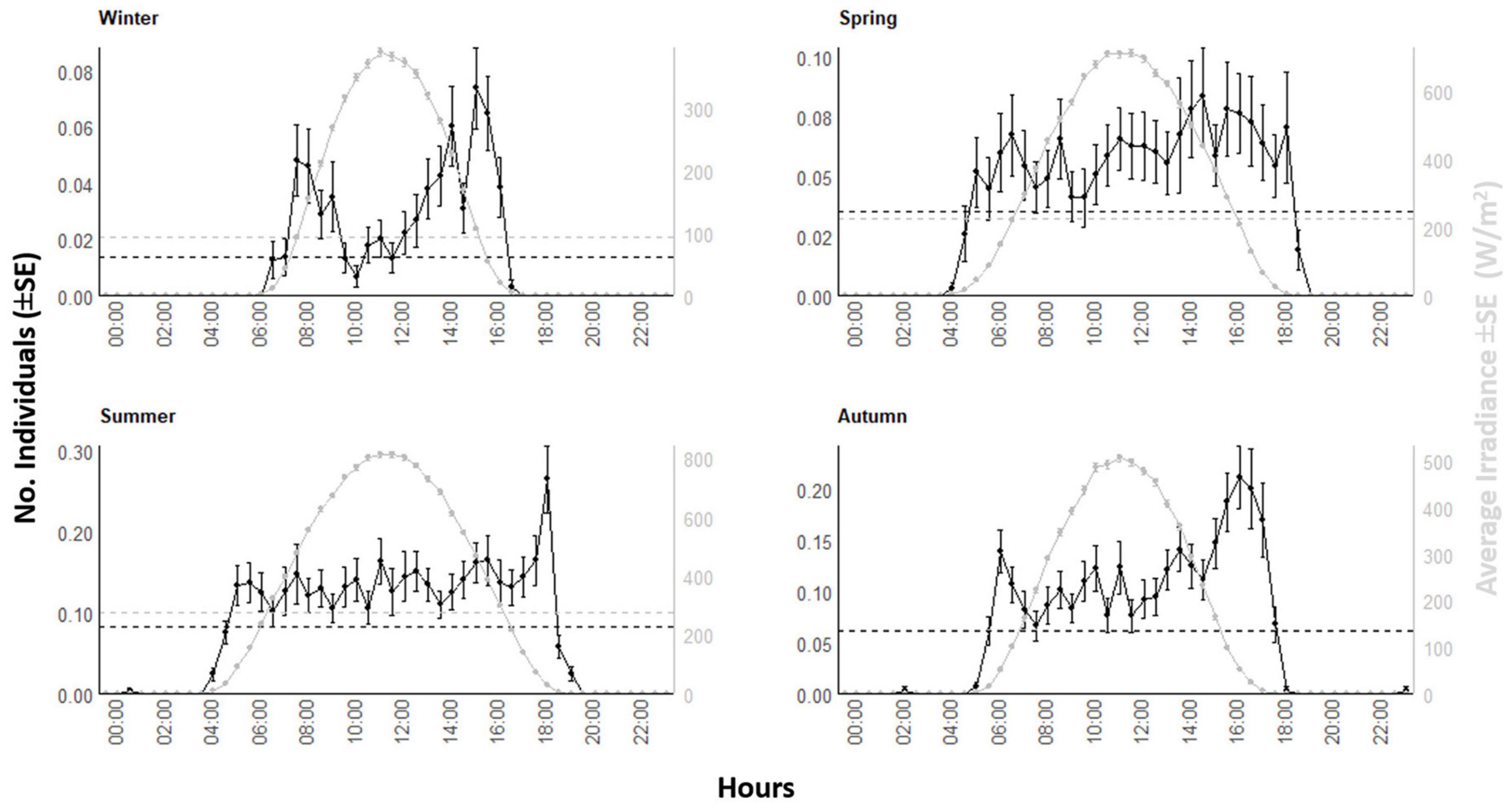
Figure 6. Waveform analysis output plots for visual counts of D. dentex and solar irradiance during different seasons (i.e., winter, spring, summer, and autumn) from 8 years (i.e., 2012–2019) of monitoring at the OBSEA video platform. The dashed horizontal line is the MESOR.
In the plotting of mean counts per month of Dentex vs. mean solar irradiance depicting the overall seasonal fluctuation trend in local abundance evidenced a general increase from winter to summer, with two peaks, a major on August and a minor on May (Figure 7). The increase of the solar irradiance follows a similar pattern but with a peak in June (Figure 7). In accordance, the waveforms MESORs values of Dentex for the different months (see Table 1) is increasing from February, when this average value is at the minimum, to August, when this average value is at the maximum (i.e., 0.007 and 0.122 individuals per photo, respectively). At the same time, the MESORs values of the solar irradiance are increasing from a minimum in December to a maximum in June (i.e., from 76.23 to 297.95 W/m2, respectively) (Table 4).
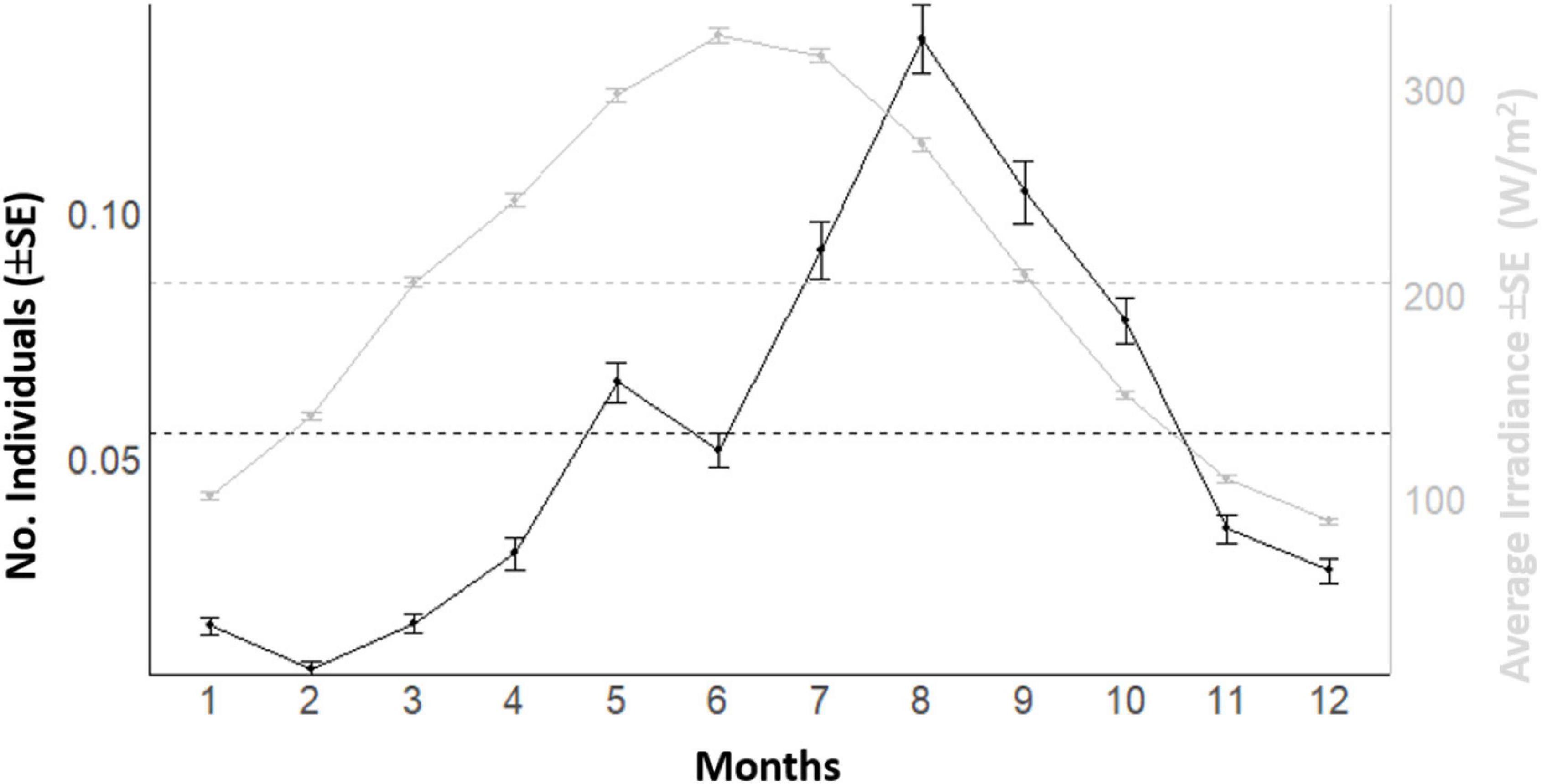
Figure 7. Plot of mean counts (± SE) per each month of the year of D. dentex visual counts and solar irradiance recorded during 8 years (i.e., 2012–2019) of observations at the OBSEA. The dashed horizontal line is the MESOR.
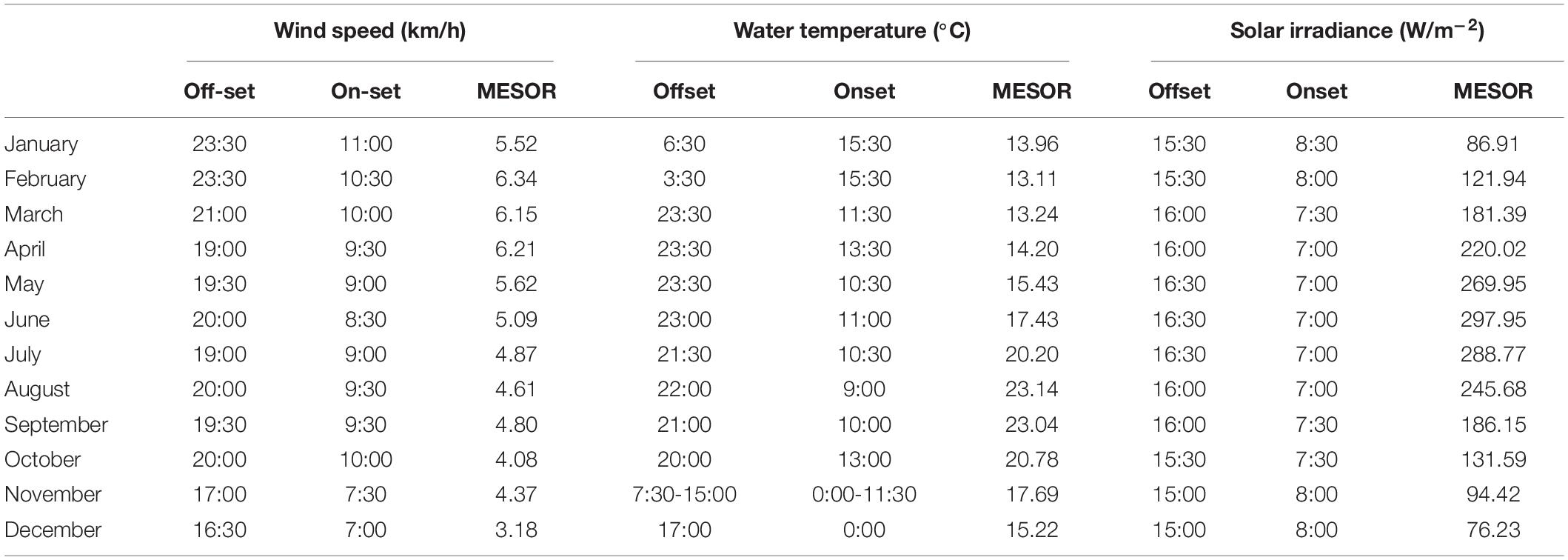
Table 4. Midline Estimated Statistic of Rhythm (MESOR), onset and offset timings (hours) per each month, within the 2012-2019 monitoring period, for the environmental variables selected by GLM and GAM modeling for presence/absence data.
In the integrated chart comparing Dentex waveforms peaks (i.e., means values higher than the MESOR as horizontal continuous band) (Figure 8 and Supplementary Figures 2, 3) we could observe counts increases form December to June, with onset and offset timings that shift form 8:00 and 16:30, to 5:00 and 19:00, respectively (see Table 1). For solar irradiance peaks amplitude also varied from December to May, with onset and offset at 8:00/15:00 and 7:00/16:30, respectively (see Table 4). The integrated chart (see Figure 8) indicated that Dentex counts followed the solar irradiance pattern, with values of onset and offset that could anticipate and are delayed to the irradiance onset of maximum 2 and 2.5-h, respectively.
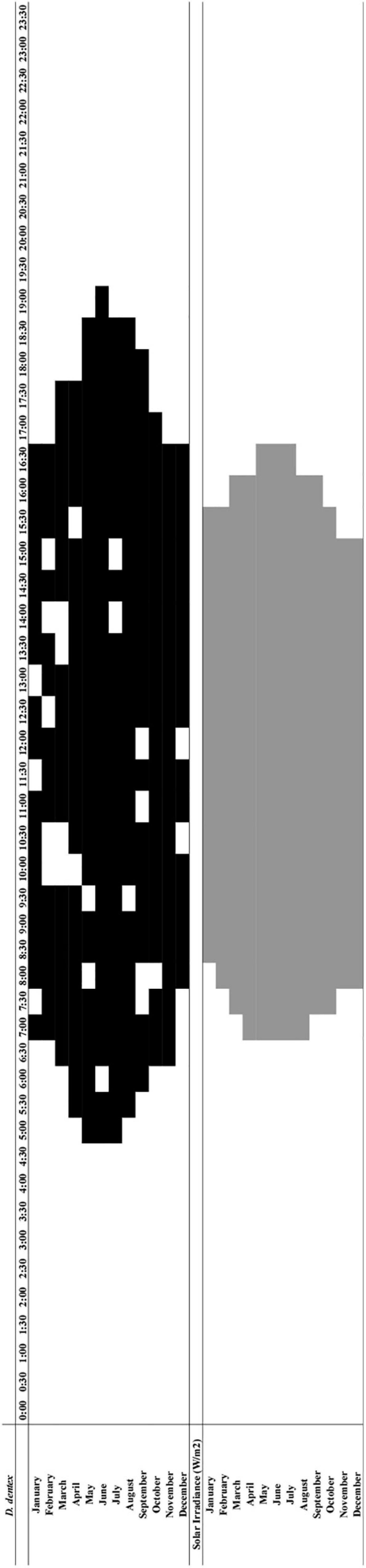
Figure 8. Integrated chart depicting the temporal relationships of D. dentex active phase between months of the year (black), and periods of the diel cycle when the solar irradiance showed significantly increased values along the different months of the year (gray).
In order to describe the photic niche of Dentex, we noted that the average values of irradiance when Dentex averaged counts start spiking (i.e., is becoming active) as the peak onset; these are between 3 and 76.3 W/m2 (see Table 1 and Supplementary Figures 2, 3). Inactivity (i.e., offset) occurs for average values of solar irradiance between 0.49 and 61.87 W/m2 (Table 1 and Supplementary Figures 2, 3).
Environmental Cycles
In Table 4, we reported MESOR values, onset and offset per each month of the year for the environmental variable previously selected by GLM and GAM models for presence/absence data (i.e., water temperature, wind speed, and solar irradiance). The temporal dynamic of those variables is described below, but not for solar irradiance that was already described (see previous Section).
The water temperature cycle (Supplementary Figures 4, 5) had a phase shift to early hours from January, with an onset and offset at 15:30 and 6:30 respectively, to December, with onset and offset at 0:00 and 17:00 respectively. Furthermore, we reported that the water temperature had a minimum and a maximum MESOR value in February and August (i.e., 13.11°C and 23.14°C, respectively). One should notice that those two months also correspond to the minimum and maximum for Dentex.
The wind speed cycle (Supplementary Figures 6, 7) followed the same pattern of solar irradiance and fish visual counts (see previous Section). Its onset anticipated its timing from January at 11:00 to June at 8:30. Whilst, the offset progressively delayed from December at 16:30 to June at 20:00. Furthermore, we noticed that wind speed has a minimum and a maximum MESOR value in December and February of 3.18 km/h and 6.34 km/h, respectively.
In order to describe the ecological niche of Dentex, we annotated the average values of the detected relevant variables from the statistical models when Dentex started and finished its active phase (i.e., onset and offset, respectively) (see Table 1 and Supplementary Figures 2–7). Indeed, when this species started to spike (i.e., is becoming active) as the peak onset, the values of water temperature and wind speed were, respectively, between 13.1-22.96°C and 2.47-5.06 km/h (see Table 1). Instead, inactivity (i.e., offset) occurs, in average values, between 13.12-23.3°C water temperature, and 4.01-8.5 km/h wind speed.
Plotting conditional densities for the most important explanatory variables of the grouping or not grouping behavioral data of Dentex (i.e., the distribution of the nominal variable for the grouping behavior of Dentex given a certain value of environmental and temporal driver) (Supplementary Figure 8), we observed that Dentex form groups during the day or at dusk and down, but not during the night. Moreover. The frequency of grouping increased along the years of observation. No particular seasonal pattern along the months of the year has been observed for grouping behavior. Furthermore, we could not obtain particular information on the relationship between grouping and the environmental variables selected by the models for this behavior.
Discussion
We described the occurrence of diel and seasonal behavioral patterns in a coastal marine top predator, Dentex, by analyzing 8-years of high-frequency and continuous time series of visual-counts plus concomitant multiparametric oceanographic and meteorological data. Firstly, we detected a relationship between fish counts and the solar irradiance as a proxy for rhythmic activity. Then, a seasonal variation in video-counts was evidenced with a major peak in August and a minor one in May, suggesting for local abundance changes, possibly linked to population dynamics (e.g., seasonal migration). Also, the species counts were significantly correlated to water temperature and wind speed. Finally, we detected the occurrence of grouping behavior correlated to solar irradiance and water temperature, suggesting an effect of the environment as a regulator of grouping behavior.
Limitations in Cabled Observatory Monitoring Strategies
Cabled observatories provide a spatially limited data acquisition (a single platform can provide a relatively narrow field of view of few m2). Another problem is that with this methodology it is not possible to separate the influence of abundance variation from activity variation, and the first one certainly affects the results of the second. Anyway, general inferences can be made on activity rhythms with spatially limited sampling windows (Hansteen et al., 1997; Refinetti et al., 2007; Bu et al., 2016; Gaudiano et al., 2021). Even trawling, which is the more spatially representative tool, is still anyway limited in comparison to the real extent of marine species distributions (Cama et al., 2011; Sonnewald and Türkay, 2012; Ünlüoğlu, 2021). Furthermore, Campos-Candela et al. (2018) recently reviewed some methods to inference abundance from visual counts with cameras stating that averaged estimates of animal density do not show any substantial improvement after an adequate sampling effort (i.e., number of cameras and deployment time).
In our monitoring, fish were observed during daytime and this could cause more observations per day in summer than in winter, being the photoperiodic difference between months the cause for an increased probability in observing fishes in a summer day rather than in a winter day. In any case, there are diurnal species that are sampled more in winter for a reason that is related to an increase in their abundance and not to the possible effect of increasing photoperiod (Condal et al., 2012; Aguzzi et al., 2015a). Here, it is difficult to methodologically distinguish this abundance/activity/photoperiod phenomenon with the present methodology.
In order to acquire more representative results on rhythmic movements and habitat use of fishes at the scale of species distribution a better spatial coverage in monitoring would be needed (Holt, 2009). Networks of cameras with synchronous image acquisition routines may be required to track the species movements across different levels of habitat heterogeneity (e.g., Doya et al., 2017; Aguzzi et al., 2020b; Rountree et al., 2020). Such a synchronous image acquisition could clarify if the peaks in video counts of Dentex in different areas are associated to a different habitat uses (e.g., preying vs. resting), and then could be used to relate this information to the activity rhythms. Inspiration on how to set the network monitoring may be drown from spatially extended surveys with camera traps, aiming at the visual census of fauna in terrestrial environments (e.g., Beaudrot et al., 2016; Norouzzadeh et al., 2018).
OBSEA data collection could be implemented with other complementary actions within the monitoring area, such as the classic visual census sampling by divers (Samoilys and Carlos, 2000; Grane-Feliu et al., 2019), and collected data could be cross-checked with information provided by telemetry. This technology allows the tracking of particular individuals over large period of times (Hussey et al., 2015; Villegas-Ríos et al., 2017; Brownscombe et al., 2020; Matley et al., 2021). Acoustic telemetry could help achieving continuous long-term tracking of single individuals to study the habitat use of fish species (Hussey et al., 2015; Dominoni et al., 2017; Lennox et al., 2017), overcoming the spatial and temporal bias of fixed-point video monitoring for a reliable evaluation of population demography and local biodiversity (Aguzzi et al., 2020a,b). It is impossible with fixed cameras imaging technologies to support for fish “site fidelity” when this area specificity is not a clear life trait of the species (e.g., territoriality, burrowing, etc.). We have no morphological tools to identify the individuals, whose position and orientation changes within the field of view. For this reason, we may need acoustic tagging coupled with imaging to enforce such a site specificity study.
Cabled observatory imaging equipment could have some monitoring footprint on coastal areas for the introduction of light at nocturnal image sampling, which can induce behavioral disturbance on the local fauna (e.g., Davies et al., 2015; Kurvers et al., 2018; Czarnecka et al., 2019; Lucena et al., 2021). Nevertheless, in our case it is unlikely that the OBSEA lightening system, active every 30 min for about 3 s, affected the reported Dentex count patterns, being the individuals of this species absent at nighttime all the yearlong (see previous Section). However, the environmental footprint of future long-term monitoring could be reduced with the use of acoustic multi-beam cameras (Aguzzi et al., 2019).
Despite the evidenced monitoring limitations, we would like to stress out that one positive aspect of cabled observatory use is the low-invasive character at data collection. For example, visual census obtained by divers implies a factor of disturbance on the organisms as human presence (Harmelin-Vivien and Francour, 1992; Januchowski-Hartley et al., 2011; Assis et al., 2013; Azzurro et al., 2013; Emslie et al., 2018; Pais and Cabral, 2018).
How to Interpret Day-Night Rhythms in Dentex Visual Counts
Counts peaks timing and amplitude followed the photophase. In our case, the interpretation of video-counts peaks in terms of increase or decrease activity should be carried out with precaution. A similar precaution is adopted when evaluating the ecological meaning of species peaks in catches or visual census; i.e., animals captures or spotting are provoked by their increased availability in the sampling area for their resting or because of their activity (Aguzzi and Bahamon, 2009; Aguzzi and Company, 2010). Notwithstanding, many species of fishes display activity rhythms (e.g., Eriksson, 1978; Muller, 1978; Helfman, 1986) that drive changes in abundance between day and night in coastal areas, as detected by different sampling systems and methodologies (e.g., Aguzzi et al., 2013; Hawley et al., 2017; Schalm et al., 2021). Diurnal, nocturnal, and crepuscular activity is often described as a product of fish behavioral response to solar irradiance variations (Helfman, 1986; Coles, 2014).
In this scenario, almost no Dentex was consistently detected at nighttime over several consecutive years. This observation suggests that video-counts peaks are a product of an increase activity at daytime. Laboratory data on fish behavior and physiology may provide a first insight on this phenomenon, assuming a link between visual counts and activity. Photoperiodic regulation of fish physiology and swimming behavior occur for the modulation that light intensity and temperature exert on the production of hormones (e.g., Pavlidis et al., 1999; Cowan et al., 2017; Sánchez-Vázquez et al., 2019). Fish melatonin measures environmental light levels and, as a result, variable rates of swimming occur (Saha et al., 2019).
A daytime activity increases for Dentex resulting in the increment of video-spotting at the OBSEA can be postulated for the following reasons. First, animals rest at nighttime within Posidonia seagrass beds (Zabala et al., 1992). Second, the species has a home range of less than 1 km2 in specific period of the year (Aspillaga et al., 2019), with the exception of moments in which a migration may follow bathymetric changes related to optimal water temperature (Aspillaga et al., 2017; see next Section). Third, Dentex is a visual predator whose prey spotting is optimized during light hours (Marengo et al., 2014).
Our data suggest an increase of activity during daytime (and consequent resting at night), which implies a visual-oriented hunting strategy as already indicated by Marengo et al. (2014). This diurnal temporal character of Dentex ecological niche (i.e., sensu Hut et al., 2012) matches the daytime video-occurrence increases of its fish preys within the Spariformes order (Morales-Nin and Moranta, 1997), that were spotted at the OBSEA (Aguzzi et al., 2013), but also observed to be present in other Mediterranean areas (D’Anna et al., 1994; Azzurro et al., 2007; Lök et al., 2008; Witkowski et al., 2016). For example, Diplodus vulgaris, Oblada melanura, and Spicara maena are preys of Dentex (Morales-Nin and Moranta, 1997) with diurnal increases in presence and activity that are sustained also at twilight conditions (Santos et al., 2002; La Mesa et al., 2013; Witkowski et al., 2016). Predators and preys seek for temporal overlapping (predators) or avoidance (preys) of their activity phases over the 24-h cycle (e.g., Kronfeld-Schor and Dayan, 2003; McCauley et al., 2012; Kerr et al., 2015; Andersen et al., 2017; Olivares et al., 2020; Priou et al., 2021).
Seasonal Fluctuation in Fish Video-Counts
Here, we reported a seasonal rhythm in visual counts of Dentex, with a significant increase in August and a second minor peak in May, as consistent across multiple years. In the past study of Sbragaglia et al. (2019) at the OBSEA, the major peak in counts of Dentex on August was detected, but not the minor one of May. This points out the strategic importance of a prolonged monitoring activity at the OBSEA. That seasonal pattern has been also detected with recreational fishing data for the Italian coasts (Sbragaglia et al., 2020).
We interpreted the first large peak of August as the product of thermocline regulation on fish behavior. Dentex shows a preference for warm suprathermoclinal waters, whose shallowest depths (i.e., between 20-30 m) are usually reached in our monitoring geographic zone (i.e., the NW Mediterranean) in July and August (Aspillaga et al., 2017). The OBSEA is placed within that depth range and this fact may explain the count increase of summer.
Another explanation could be that Dentex seasonal counts increase are synchronized upon maximum abundances of its preys (see previous Section), that augment in the OBSEA area in spring-summer; e.g., D. vulgaris from June to October, O. melanura in May and June, and S. maena from May to July (Aguzzi et al., 2015a). Seasonally synchronic abundance changes may occur between fish predators and preys (Fox and Bellwood, 2011; Bustos et al., 2015; Mishra et al., 2020; Liu et al., 2021). Possibly, the presence of artificial reef structures nearby the OBSEA attract fish preys and consequently concentrate the presence of the Dentex as well.
We observed a second, minor peak of Dentex counts in May that can be discussed in relation to the phenology of breeding. If from one side, the species migrates deeper to reproduce in areas at 40-100 m depth from March to June (Marengo et al., 2014; Grau et al., 2016), from the other we did not observe a temporally concomitant drop in counts at the OBSEA location in May (as an indication for a deeper migration of individuals in that period). Possibly, some individuals that have finished the reproduction (or with no mature gonads), return (or stay) to shallower depths for foraging. In fact, regressing ovaries in females and late developing testes of Dentex were already reported during May (Grau et al., 2016).
Species Relationship With Water Temperature and Wind Speed
The oceanographic and meteorological monitoring was dedicated to understand the species tolerance to certain ranges in the variation of selected measured habitat variables, as an indication of the effects that climate change may exert on fish’s phenology (Stevenson et al., 2015). Those ranges have a practical value for ecological monitoring, since indicate a roadmap to develop smart sampling procedures in marine species: i.e., the optimum time window when to expect a maximum presence of individuals, according to the fluctuation status of key environmental drivers (Aguzzi et al., 2020b).
Here, counts of Dentex were related to the water temperature, being the seasonal peak always reported above an averaged threshold of 13.1°C. A past study at the OBSEA with 3 years’ time-series detected the increase in number counts of Dentex above 20°C (Sbragaglia et al., 2019). This highlight the importance of pursuing the monitoring activities at the OBSEA to better characterize the environmental preference of this species.
The importance of water temperature as environmental driver has already been described in many fish species (Vinagre et al., 2016; Van Der Walt et al., 2021). Temperature deeply affects fish presence (or absence), because it influences directly species physiological performance (Cussac et al., 2009; Freitas et al., 2016; Day et al., 2018; Waldock et al., 2019). Dentex can cope with temperature range above our reported threshold, as also indicated by the current trend of geographic expansion in the North Mediterranean (Orozco et al., 2011; García-Rubies et al., 2013). We confirmed that trend by a progressive increase in counts over the years (i.e., see Figure 2), which would possibly continue in the next decade, when temperature is expected to grow in the NW Mediterranean (Bahamon et al., 2020). This indicates the value of cabled-observatory assets to disclose the occurrence of progressive trends in population shifts beyond more contingent seasonal dynamics due to the climate forcing.
We found a significant relationship between Dentex counts and wind speed. This variable affects the population distribution in some fish species (Daskalov, 2003; Teo et al., 2007; Bakun and Weeks, 2008; Selleslagh and Amara, 2008; Brander, 2010; Kuparinen et al., 2010), based on upwelling nutrient inputs (Bakun and Weeks, 2008; Bellido et al., 2008; Brander, 2010) although this phenomenon is not relevant in a shallow costal area, such as the one where the OBSEA is deployed.
The changes in wind speed and direction could also affect indirectly other environmental variables, that consequently affect the marine biota. For example, it was observed that changes in wind affected salinity in the North Sea and in the Baltic Sea (Schrum, 2001), which had negative consequences on cod recruitment in both areas (Brander, 2010). In our case, salinity was not significantly associated to counts of Dentex nor to wind. Hence, the same dynamic reported for cod recruitment in the North Sea and Baltic Sea may not be valid in our case. Notwithstanding, wind speed may resuspend and mix seabed and water column nutrients at periods of blowing, hence influencing the coastal food web with the consequent overall increase of trophism at all predator levels of the trophic food web (Bellido et al., 2008). For the overall increase in pray abundance, Dentex counts may consequently increase at moments of wind blowing.
The Grouping Behavior of the Species
We reported data on the grouping behavior of Dentex, that showed a clear 24-h modulation. Here, the formation of groups of Dentex significantly occurred more during daytime (including twilight hours) than nighttime, given the broad phase relationship between all visual counts and solar irradiance as a proxy for diurnal activity rhythms (see previous Section). Differently, no peaking was reported over different seasons. A seasonality for Dentex grouping behavior was described in rocky coastal areas for juveniles during summer (Chemmam-Abdelkader et al., 2004; Sahyoun et al., 2013). We did not observe this phenomenon, but we could not resolve if our video-monitoring were composed by individuals in this stage of development, since no tools for body sizing (e.g., lasers) were present aside the camera; however, we can assume that the majority of individuals were adults. Indeed, for adults Dentex, groups of individuals may be detected during the spawning season in spring, between 40 and 100 m depth (Marengo et al., 2014), but, given the shallower depth of OBSEA deployment, we did not observe this phenomenon (see previous Section).
The grouping behavior of Dentex was associated to solar irradiance and water temperature. Grouping has been already broadly correlated to the environmental variation in previous works for different fish species (Félix-Hackradt et al., 2010; Meager et al., 2012; Georgiadis et al., 2014; Palacios-Fuentes et al., 2020). In particular, the formation of fish groups has been related to light intensity (Meager et al., 2012; Georgiadis et al., 2014) and to water temperature (Power et al., 2000; Davoren et al., 2006; Meager et al., 2012; Palacios-Fuentes et al., 2020). Also, the weak increase of grouping behavior reported across consecutive years of observations (see Supplementary Figure 8) is likely the result of the increasing abundance of this species in the OBSEA area (see also previous Section).
Data Availability Statement
The original contributions presented in the study are included in the article/Supplementary Material, further inquiries can be directed to the corresponding author/s.
Ethics Statement
Ethical review and approval was not required for the animal study because we did not actively sampled, nor manipulated, nor killed any animals. We just performed video imaging.
Author Contributions
MF: conceptualization, formal analysis, investigation, writing-original draft, and writing-review and editing. VS: conceptualization and formal analysis. ET, JAn, IM and JP: formal analysis. JdRF: funding acquisition. MNC and DMT: data curation. JAg: conceptualization, formal analysis, and writing-review and editing. All authors gave final approval for publication.
Funding
MF was supported by an FPI pre-doctoral research fellowship (ref. PRE2018-083839). This work was developed in the framework of the Research Unit Tecnoterra (ICM-CSIC/UPC) and the following project activities: RESBIO (TEC2017-87861-R; Ministerio de Ciencia, Innovación y Universidades), JERICO-S3: (Horizon 2020; Grant Agreement no. 871153). This work used the EGI infrastructure with the dedicated support of INFN - CATANIA - STACK.
Conflict of Interest
The authors declare that the research was conducted in the absence of any commercial or financial relationships that could be construed as a potential conflict of interest.
Publisher’s Note
All claims expressed in this article are solely those of the authors and do not necessarily represent those of their affiliated organizations, or those of the publisher, the editors and the reviewers. Any product that may be evaluated in this article, or claim that may be made by its manufacturer, is not guaranteed or endorsed by the publisher.
Acknowledgments
We want to thank the members of the Development Center of Remote Acquisition and Information Processing Systems (SARTI) for the maintenance of the OBSEA seafloor platform. In particular we want to thank DMT, who is included in the RESBIO project, and MNC, Matias Carandell and Enoc Martinez, who performed the tasks for the maintenance of the OBSEA structure. We also acknowledge financial support from the Spanish Ministry of Science and Innovation (Juan de la Cierva Incorporación Research Fellowship to VS #IJC2018-035389-I), plus the funding from the Spanish government through the ‘Severo Ochoa Centre of Excellence’ accreditation (CEX20 19-000928-S).
Supplementary Material
The Supplementary Material for this article can be found online at: https://www.frontiersin.org/articles/10.3389/fmars.2022.837216/full#supplementary-material
Footnotes
References
Aguzzi, J., and Bahamon, N. (2009). Modeled day–night biases in decapod assessment by bottom trawling survey. Fish. Res. 100, 274–280. doi: 10.1016/j.fishres.2009.08.010
Aguzzi, J., Bullock, N. M., and Tosini, G. (2006). Spontaneous internal desynchronization of locomotor activity and body temperature rhythms from plasma melatonin rhythm in rats exposed to constant dim light. J. Circad. Rhyth. 4, 1–6. doi: 10.1186/1740-3391-4-6
Aguzzi, J., Chatzievangelou, D., Marini, S., Fanelli, E., Danovaro, R., Flögel, S., et al. (2019). New high-tech interactive and flexible networks for the future monitoring of deep-sea ecosystems. Environ. Sci. Technol. 53, 6616–6631. doi: 10.1021/acs.est.9b00409
Aguzzi, J., and Company, J. B. (2010). Chronobiology of deep-water decapod crustaceans on continental margins. Adv. Mar. Biol. 58, 155–225. doi: 10.1016/B978-0-12-381015-1.00003-4
Aguzzi, J., Company, J. B., Costa, C., Matabos, M., Azzurro, E., Mànuel, A., et al. (2012). Challenges to assessment of benthic populations and biodiversity as a result of rhythmic behaviour: video solutions from cabled observatories. Ocean. Mar. Biol. Ann. Rev. 50, 235–286. doi: 10.1201/b12157-6
Aguzzi, J., Sbragaglia, V., Tecchio, S., Navarro, J., and Company, J. B. (2015b). Rhythmic behavior of marine benthopelagic species and the synchronous dynamics of benthic communities. Deep Sea Res. Part I 95, 1–11. doi: 10.1016/j.dsr.2014.10.003
Aguzzi, J., Doya, C., Tecchio, S., De Leo, F. C., Azzurro, E., Costa, C., et al. (2015a). Coastal observatories for monitoring of fish behavior and their responses to environmental changes. Rev. Fish Biol. Fish. 25, 463–483. doi: 10.1007/s11160-015-9387-9
Aguzzi, J., Costa, C., Furushima, Y., Chiesa, J. J., Company, J. B., Menesatti, P., et al. (2010). Behavioral rhythms of hydrocarbon seep fauna in relation to internal tides. Mar. Ecol. Prog. Ser. 418, 47–56. doi: 10.3354/meps08835
Aguzzi, J., López-Romero, D., Marini, S., Costa, C., Berry, A., Chumbinho, R., et al. (2020d). Multiparametric monitoring of fish activity rhythms in an Atlantic coastal cabled observatory. J. Mar. Syst. 212:103424. doi: 10.1016/j.jmarsys.2020.103424
Aguzzi, J., Chatzievangelou, D., Company, J. B., Thomsen, L., Marini, S., Bonofiglio, F., et al. (2020a). The potential of video imagery from worldwide cabled observatory networks to provide information supporting fish-stock and biodiversity assessment. ICES J. Mar. Sci. 77, 2396–2410. doi: 10.1093/icesjms/fsaa169
Aguzzi, J., Chatzievangelou, D., Francescangeli, M., Marini, S., Bonofiglio, F., del Rio, J., et al. (2020b). The hierarchic treatment of marine ecological information from spatial networks of benthic platforms. Sensors 20:1751. doi: 10.3390/s20061751
Aguzzi, J., Iveša, N., Gelli, M., Costa, C., Gavrilovic, A., Cukrov, N., et al. (2020c). Ecological video monitoring of Marine Protected Areas by underwater cabled surveillance cameras. Mar. Policy 119:104052. doi: 10.1016/j.marpol.2020.104052
Aguzzi, J., Mànuel, A., Condal, F., Guillén, J., Nogueras, M., del Rio, J., et al. (2011). The new seafloor observatory (OBSEA) for remote and long-term coastal ecosystem monitoring. Sensors 11, 5850–5872. doi: 10.3390/s110605850
Aguzzi, J., Sbragaglia, V., Santamaría, G., del Río, J., Sarda, F., Nogueras, M., et al. (2013). Daily activity rhythms in temperate coastal fishes: insights from cabled observatory video monitoring. Mar. Ecol. Prog. Series 486, 223–236. doi: 10.3354/meps10399
Andersen, N. G., Lundgren, B., Neuenfeldt, S., and Beyer, J. E. (2017). Diel vertical interactions between Atlantic cod Gadus morhua and sprat Sprattus sprattus in a stratified water column. Mar. Ecol. Prog. Series 583, 195–209. doi: 10.3354/meps12319
Aspillaga, E., Bartumeus, F., Starr, R. M., López-Sanz, A., and Linares, C. (2017). Thermal stratification drives movement of a coastal apex predator. Sci. Rep. 7, 1–10. doi: 10.1038/s41598-017-00576-z
Aspillaga, E., Safi, K., Hereu, B., and Bartumeus, F. (2019). Modelling the three-dimensional space use of aquatic animals combining topography and Eulerian telemetry data. Methods Ecol. Evol. 10, 1551–1557. doi: 10.1111/2041-210X.13232
Assis, J., Claro, B., Ramos, A., Boavida, J., and Serrão, E. A. (2013). Performing fish counts with a wide-angle camera, a promising approach reducing divers’ limitations. J. Exp. Mar. Biol. Ecol. 445, 93–98. doi: 10.1016/j.jembe.2013.04.007
Azzurro, E., Aguzzi, J., Maynou, F., Chiesa, J. J., and Savini, D. (2013). Diel rhythms in shallow Mediterranean rocky-reef fishes: a chronobiological approach with the help of trained volunteers. J. Mar. Biol. Assoc. U K 93, 461–470. doi: 10.1017/S0025315412001166
Azzurro, E., Pais, A., Consoli, P., and Andaloro, F. (2007). Evaluating day–night changes in shallow Mediterranean rocky reef fish assemblages by visual census. Mar. Biol. 151, 2245–2253. doi: 10.1007/s00227-007-0661-9
Bahamon, N., Aguzzi, J., Ahumada-Sempoal, M. Á, Bernardello, R., Reuschel, C., Peters, F., et al. (2020). Stepped coastal water warming revealed by multiparametric monitoring at NW Mediterranean fixed stations. Sensors 20:2658. doi: 10.3390/s20092658
Bakun, A., and Weeks, S. J. (2008). The marine ecosystem off Peru: what are the secrets of its fishery productivity and what might its future hold? Prog. Oceanogr. 79, 290–299. doi: 10.1016/j.pocean.2008.10.027
Beaudrot, L., Ahumada, J. A., O’Brien, T., Alvarez-Loayza, P., Boekee, K., Campos-Arceiz, A., et al. (2016). Standardized assessment of biodiversity trends in tropical forest protected areas: the end is not in sight. PLoS Biol. 14:e1002357. doi: 10.1371/journal.pbio.1002357
Bellido, J. M., Brown, A. M., Valavanis, V. D., Giráldez, A., Pierce, G. J., Iglesias, M., et al. (2008). “Identifying essential fish habitat for small pelagic species in Spanish Mediterranean waters,” in Essential Fish Habitat Mapping in the Mediterranean, ed. V. D. Valavanis (Dordrecht: Springer Netherlands), 171–184. doi: 10.1007/978-1-4020-9141-4_13
Brander, K. (2010). Impacts of climate change on fisheries. J. Mar. Syst. 79, 389–402. doi: 10.1016/j.jmarsys.2008.12.015
Brierley, A. S. (2014). Diel vertical migration. Curr. Biol. 24, R1074–R1076. doi: 10.1016/j.cub.2014.08.054
Brownscombe, J. W., Griffin, L. P., Chapman, J. M., Morley, D., Acosta, A., Crossin, G. T., et al. (2020). A practical method to account for variation in detection range in acoustic telemetry arrays to accurately quantify the spatial ecology of aquatic animals. Methods Ecol. Evol. 11, 82–94. doi: 10.1111/2041-210X.13322
Bu, H., Wang, F., McShea, W. J., Lu, Z., Wang, D., and Li, S. (2016). Spatial co-occurrence and activity patterns of mesocarnivores in the temperate forests of Southwest China. PLoS One 11:e0164271. doi: 10.1371/journal.pone.0164271
Bulla, M., Oudman, T., Bijleveld, A. I., Piersma, T., and Kyriacou, C. P. (2017). Marine biorhythms: bridging chronobiology and ecology. Philosoph. R. Soc. B 372:20160253. doi: 10.1098/rstb.2016.0253
Bustos, C. A., Landaeta, M. F., Palacios-Fuentes, P., Jahnsen-Guzmán, N., and Balbontín, F. (2015). Comparing early life traits of hakes from Chilean Patagonian fjords inferred by otolith microstructure analysis. Fish. Res. 164, 35–44. doi: 10.1016/j.fishres.2014.10.016
Byrnes, E. E., Daly, R., Leos-Barajas, V., Langrock, R., and Gleiss, A. C. (2021). Evaluating the constraints governing activity patterns of a coastal marine top predator. Mar. Biol. 168, 1–15. doi: 10.1007/s00227-020-03803-w
Campos-Candela, A., Palmer, M., Balle, S., and Alós, J. (2018). A camera-based method for estimating absolute density in animals displaying home range behaviour. J. Anim. Ecol. 87, 825–837. doi: 10.1111/1365-2656.12787
Cama, A., Josa, P., Ferrer-Obiol, J., and Arcos, J. M. (2011). Mediterranean Gulls Larus melanocephalus wintering along the Mediterranean Iberian coast: numbers and activity rhythms in the species’ main winter quarters. J. Ornithol. 152, 897–907. doi: 10.1007/s10336-011-0673-6
Chatzievangelou, D., Bahamon, N., Martini, S., del Rio, Fernandez, J., Riccobene, G., et al. (2021). Integrating diel vertical migrations of bioluminescent deep scattering layers into monitoring programs. Front. Mar. Sci. 8:615. doi: 10.3389/fmars.2021.661809
Chemmam-Abdelkader, B., Kraiem, M. M., and El Abed, A. (2004). Etude de l’age et de la croissance de deux especes de dentes (Dentex dentex et de Dentex maroccanus) des cotes Tunisiennes. Bull. Inst. Natn. Scien. Tech. Mer de Salammbo 31, 43–51.
Coles, D. P. (2014). Dusk Transition in Sub-Tropical Reef Fish Communities Off of North and South Carolina. [dissertation/master’s thesis]. Ann Arbor, MI: The Graduate School of the College of Charleston.
Condal, F., Aguzzi, J., Sardà, F., Nogueras, M., Cadena, J., Costa, C., et al. (2012). Seasonal rhythm in a Mediterranean coastal fish community as monitored by a cabled observatory. Mar. Biol. 159, 2809–2817. doi: 10.1007/s00227-012-2041-3
Cowan, M., Azpeleta, C., and López-Olmeda, J. F. (2017). Rhythms in the endocrine system of fish: a review. J. Comp. Physiol. B 187, 1057–1089. doi: 10.1007/s00360-017-1094-5
Cussac, V. E., Fernández, D. A., Gómez, S. E., and López, H. L. (2009). Fishes of southern South America: a story driven by temperature. Fish Physiol. Biochem. 35, 29–42. doi: 10.1007/s10695-008-9217-2
Czarnecka, M., Kakareko, T., Jermacz, L., Pawlak, R., and Kobak, J. (2019). Combined effects of nocturnal exposure to artificial light and habitat complexity on fish foraging. Sci. Total Env. 684, 14–22. doi: 10.1016/j.scitotenv.2019.05.280
Daan, S. (1981). “Adaptive daily strategies in behavior,” in Biological rhythms, ed. J. Aschoff (Boston: Springer), 275–298. doi: 10.1007/978-1-4615-6552-9_15
D’Anna, C., Badalamenti, F., Gristina, M., and Pipitone, C. (1994). Influence of artificial reefs on coastal nekton assemblages of the Gulf of Castellammare (Northwest Sicily). Bull. Mar. Sci. 55, 418–433.
Danovaro, R., Aguzzi, J., Fanelli, E., Billet, D., Gjerde, K., Jamieson, A., et al. (2017). A new international ecosystem-based strategy for the global deep ocean. Science 355, 452–454. doi: 10.1126/science.aah7178
Daskalov, G. M. (2003). Long-term changes in fish abundance and environmental indices in the Black Sea. Mar. Ecol. Prog. Ser. 255, 259–270. doi: 10.3354/meps255259
Davies, T. W., Coleman, M., Griffith, K. M., and Jenkins, S. R. (2015). Night-time lighting alters the composition of marine epifaunal communities. Biol. Lett. 11:20150080. doi: 10.1098/rsbl.2015.0080
Davoren, G. K., Anderson, J. T., and Montevecchi, W. A. (2006). Shoal behaviour and maturity relations of spawning capelin (Mallotus villosus) off Newfoundland: demersal spawning and diel vertical movement patterns. Can. J. Fish. Aquat. Sci. 63, 268–284. doi: 10.1139/f05-204
Day, P. B., Stuart-Smith, R. D., Edgar, G. J., and Bates, A. E. (2018). Species’ thermal ranges predict changes in reef fish community structure during 8 years of extreme temperature variation. Div. Distrib. 24, 1036–1046. doi: 10.1111/ddi.12753
Del Rio, J., Nogueras, M., Toma, D. M., Martínez, E., Artero-Delgado, C., Bghiel, I., et al. (2020). Obsea: a decadal balance for a cabled observatory deployment. IEEE Access 8, 33163–33177. doi: 10.1109/ACCESS.2020.2973771
Dominoni, D. M., Åkesson, S., Klaassen, R., Spoelstra, K., and Bulla, M. (2017). Methods in field chronobiology. Philosoph. Transact. R. Soc. B 372:20160247. doi: 10.1098/rstb.2016.0247
Doya, C., Aguzzi, J., Pardo, M., Matabos, M., Company, J. B., Costa, C., et al. (2014). Diel behavioral rhythms in sablefish (Anoplopoma fimbria) and other benthic species, as recorded by the Deep-sea cabled observatories in Barkley canyon (NEPTUNE-Canada). J. Mar. Syst. 130, 69–78. doi: 10.1016/j.jmarsys.2013.04.003
Doya, C., Chatzievangelou, D., Bahamon, N., Purser, A., De Leo, F., Juniper, K., et al. (2017). Seasonal monitoring of deep-sea cold-seep benthic communities using an Internet Operated Vehicle (IOV). PLoS One 12:e0176917. doi: 10.1371/journal.pone.0176917
Drazen, J. C., Leitner, A. B., Jones, D. O., and Simon-Lledó, E. (2021). Regional variation in communities of demersal fishes and scavengers across the CCZ and Pacific Ocean. Front. Mar. Sci. 8, 1–18. doi: 10.3389/fmars.2021.630616
Emslie, M. J., Cheal, A. J., MacNeil, M. A., Miller, I. R., and Sweatman, H. P. (2018). Reef fish communities are spooked by scuba surveys and may take hours to recover. PeerJ 6:e4886. doi: 10.7717/peerj.4886
Eriksson, L. O. (1978). “Nocturnalism versus diurnalism-dualism within fish individuals,” in Rhythmic activity of fishes, ed. J. E. Thorpe (London: Academic), 69–90.
Falcón, J., Migaud, H., Munoz-Cueto, J. A., and Carrillo, M. (2010). Current knowledge on the melatonin system in teleost fish. Gen. Comp. Endocr. 165, 469–482. doi: 10.1016/j.ygcen.2009.04.026
Félix-Hackradt, F. C., Spach, H. L., Moro, P. S., Pichler, H. A., Maggi, A. S., Hostim-Silva, M., et al. (2010). Diel and tidal variation in surf zone fish assemblages of a sheltered beach in southern Brazil. Latin Am. J. Aquat. Res. 38, 447–460. doi: 10.3856/vol38-issue3-fulltext-9
Ford, J. R., and Swearer, S. E. (2013). Two’s company, three’s a crowd: Food and shelter limitation outweigh the benefits of group living in a shoaling fish. Ecology 94, 1069–1077. doi: 10.1890/12-1891.1
Foster, R. G., and Kreitzman, L. (2010). Seasons of life: the biological rhythms that enable living things to thrive and survive. New Haven, CT: Yale University Press.
Fox, R. J., and Bellwood, D. R. (2011). Unconstrained by the clock? Plasticity of diel activity rhythm in a tropical reef fish, Siganus lineatus. Funct. Ecol. 25, 1096–1105. doi: 10.1111/j.1365-2435.2011.01874.x
Freitas, C., Olsen, E. M., Knutsen, H., Albretsen, J., and Moland, E. (2016). Temperature-associated habitat selection in a cold-water marine fish. J. Anim. Ecol. 85, 628–637. doi: 10.1111/1365-2656.12458
García-Rubies, A., Hereu, B., and Zabala, M. (2013). Long-term recovery patterns and limited spillover of large predatory fish in a Mediterranean MPA. PLoS One 8:e73922. doi: 10.1371/journal.pone.0073922
Gaudiano, L., Pucciarelli, L., and Mori, E. (2021). Livestock grazing affects movements and activity pattern of Italian roe deer in Southern Italy. Eur. J. Wildlife Res. 67, 1–8. doi: 10.1007/s10344-021-01506-1
Georgiadis, M., Mavraki, N., Koutsikopoulos, C., and Tzanatos, E. (2014). Spatio-temporal dynamics and management implications of the nightly appearance of Boops boops (Acanthopterygii, Perciformes) juvenile shoals in the anthropogenically modified Mediterranean littoral zone. Hydrobiologia 734, 81–96. doi: 10.1007/s10750-014-1871-z
Grane-Feliu, X., Bennett, S., Hereu, B., Aspillaga, E., and Santana-Garcon, J. (2019). Comparison of diver operated stereo-video and visual census to assess targeted fish species in Mediterranean marine protected areas. J. Exp. Mar. Biol. Ecol. 520:151205. doi: 10.1016/j.jembe.2019.151205
Grau, A., Saborido-Rey, F., Pastor, E., Palmer, M., Massutí-Pascual, E., Quetglas, A., et al. (2016). Reproductive strategy of common dentex Dentex dentex: management implications. Mediter. Mar. Sci. 17, 552–566. doi: 10.12681/mms.1156
Guillén, J., Arin, L., Salat, J., Puig, P., Estrada, M., Palanques, A., et al. (2018). Coastal oceanographic signatures of heat waves and extreme events of dense water formation during the period 2002-2012 (Barcelona, NW Mediterranean). Sci. Mar. 82, 189–206. doi: 10.3989/scimar.04766.26A
Hansteen, T. L., Andreassen, H. P., and Ims, R. A. (1997). Effects of spatiotemporal scale on autocorrelation and home range estimators. J. Wildlife Manag. 61, 280–290. doi: 10.2307/3802583
Harmelin-Vivien, M. L., and Francour, P. (1992). Trawling or visual censuses? Methodological bias in the assessment of fish populations in seagrass beds. Mar. Ecol. 13, 41–51. doi: 10.1111/j.1439-0485.1992.tb00338.x
Hawley, K. L., Rosten, C. M., Haugen, T. O., Christensen, G., and Lucas, M. C. (2017). Freezer on, lights off! Environmental effects on activity rhythms of fish in the Arctic. Biol. Lett. 13:20170575. doi: 10.1098/rsbl.2017.0575
Heithaus, M. R., Frid, A., Wirsing, A. J., and Worm, B. (2008). Predicting ecological consequences of marine top predator declines. Trends Ecol. Evol. 23, 202–210. doi: 10.1016/j.tree.2008.01.003
Heithaus, M. R., Wirsing, A. J., and Dill, L. M. (2012). The ecological importance of intact top-predator populations: a synthesis of 15 years of research in a seagrass ecosystem. Mar. Freshw. Res. 63, 1039–1050. doi: 10.1071/MF12024
Helfman, G. S. (1986). “Fish behaviour by day, night, and twilight,” in Behaviour of Teleost Fishes, ed. T. J. Pitcher (London: Chapman and Hall), 366–387. doi: 10.1007/978-1-4684-8261-4_14
Helm, B., Ben-Shlomo, R., Sheriff, M. J., Hut, R. A., Foster, R., Barnes, B. M., et al. (2013). Annual rhythms that underlie phenology: biological time-keeping meets environmental change. Proc. R. Soc. B 280:20130016. doi: 10.1098/rspb.2013.0016
Holt, R. D. (2009). Bringing the Hutchinsonian niche into the 21st century: ecological and evolutionary perspectives. Proc. Natl. Acad. Sci. 106, 19659–19665. doi: 10.1073/pnas.0905137106
Hussey, N. E., Kessel, S. T., Aarestrup, K., Cooke, S. J., Cowley, P. D., Fisk, A. T., et al. (2015). Aquatic animal telemetry: a panoramic window into the underwater world. Science 348:6240. doi: 10.1126/science.1255642
Hut, R. A., Kronfeld-Schor, N., van der Vinne, V., and De la Iglesia, H. (2012). In search of a temporal niche: environmental factors. Prog. Brain Res. 199, 281–304. doi: 10.1016/B978-0-444-59427-3.00017-4
Hutchingson, G. E. (1957). Concluding remarks. Cold Spring Harbor Symp. 22, 415–427. doi: 10.1101/SQB.1957.022.01.039
Januchowski-Hartley, F. A., Graham, N. A., Feary, D. A., Morove, T., and Cinner, J. E. (2011). Fear of fishers: human predation explains behavioral changes in coral reef fishes. PLoS One 6:e22761. doi: 10.1371/journal.pone.0022761
Juniper, S. K., Matabos, M., Mihaly, S., Ajayamohan, R. S., Gervais, F., and Bui, A. O. (2013). A year in Barkley Canyon: a time-series observatory study of mid-slope benthos and habitat dynamics using the NEPTUNE Canada network. Deep Sea Res. Part II 92, 114–123. doi: 10.1016/j.dsr2.2013.03.038
Kerr, K. A., Cornejo, A., Guichard, F., Crespi Abril, A. C., and Collin, R. (2015). Planktonic predation risk: effects of diel state, season and prey life history stage. J. Plankt. Res. 37, 452–461. doi: 10.1093/plankt/fbv006
Kronfeld-Schor, N., Bloch, G., and Schwartz, W. J. (2013). Animal clocks: when science meets nature. Proc. R. Soc. B 280:2013135420131354. doi: 10.1098/rspb.2013.1354
Kronfeld-Schor, N., and Dayan, T. (2003). Partitioning of time as an ecological resource. Ann. Rev. Ecol. Evol. Syst. 34, 153–181. doi: 10.1146/annurev.ecolsys.34.011802.132435
Kuparinen, A., Klefoth, T., and Arlinghaus, R. (2010). Abiotic and fishing-related correlates of angling catch rates in pike (Esox lucius). Fish. Res. 105, 111–117. doi: 10.1016/j.fishres.2010.03.011
Kurvers, R. H., Drägestein, J., Hölker, F., Jechow, A., Krause, J., and Bierbach, D. (2018). Artificial light at night affects emergence from a refuge and space use in guppies. Sci. Rep. 8:14131. doi: 10.1038/s41598-018-32466-3
La Mesa, G., Consalvo, I., Annunziatellis, A., and Canese, S. (2013). Spatio-temporal movement patterns of Diplodus vulgaris (Actinopterygii, Sparidae) in a temperate marine reserve (Lampedusa, Mediterranean Sea). Hydrobiologia 720, 129–144. doi: 10.1007/s10750-013-1631-5
Langlois, T., Goetze, J., Bond, T., Monk, J., Abesamis, R. A., Asher, J., et al. (2020). A field and video annotation guide for baited remote underwater stereo-video surveys of demersal fish assemblages. Methods Ecol. Evol. 11, 1401–1409. doi: 10.1111/2041-210X.13470
Lear, K. O., Whitney, N. M., Morris, J. J., and Gleiss, A. C. (2021). Temporal niche partitioning as a novel mechanism promoting co-existence of sympatric predators in marine systems. Proc. R. Soc. B 288:20210816. doi: 10.1098/rspb.2021.0816
Lennox, R. J., Aarestrup, K., Cooke, S. J., Cowley, P. D., Deng, Z. D., Fisk, A. T., et al. (2017). Envisioning the future of aquatic animal tracking: technology, science, and application. BioScience 67, 884–896. doi: 10.1093/biosci/bix098
Liu, K., Yu, C. G., Xu, Y. J., Jiang, X. Q., Zheng, J., Yu, N. J., et al. (2021). Spatio-temporal niche of major fish species in Pishan waters off Zhejiang Province, China. J. Appl. Ecol. 32, 1069–1079. doi: 10.13287/j.1001-9332.202103.033
Lök, A., Gül, B., Ulaş, A., Düzbastılar, F. O., and Metin, C. (2008). Diel variations on the fish assemblages at artificial reefs in two different environments of the Aegean Sea (Western Coast of Turkey). Turkish J. Fish. Aquat. Sci. 8, 79–085.
López-Olmeda, J. F., Madrid, J. A., and Sánchez-Vázquez, F. J. (2006). Light and temperature cycles as zeitgebers of zebrafish (Danio rerio) circadian activity rhythms. Chronob. Internat. 23, 537–550. doi: 10.1080/07420520600651065
Lucena, M. B., Mendes, T. C., Barbosa, M. C., Cordeiro, C. A., Eggertsen, L. M., and Ferreira, C. E. (2021). Does the colors of light matter? Testing different light color in nocturnal underwater visual censuses. Mar. Env. Res. 166:105261. doi: 10.1016/j.marenvres.2021.105261
Makris, N. C., Godø, O. R., Yi, D. H., Macaulay, G. J., Jain, A. D., Cho, B., et al. (2019). Instantaneous areal population density of entire Atlantic cod and herring spawning groups and group size distribution relative to total spawning population. Fish Fish. 20, 201–213. doi: 10.1111/faf.12331
Marengo, M., Durieux, E. D., Marchand, B., and Francour, P. (2014). A review of biology, fisheries and population structure of Dentex dentex (Sparidae). Rev. Fish Biol. Fish. 24, 1065–1088. doi: 10.1007/s11160-014-9363-9
Matabos, M., Aguzzi, J., Robert, K., Costa, C., Menesatti, P., Company, J. B., et al. (2011). Multi-parametric study of behavioural modulation in demersal decapods at the VENUS cabled observatory in Saanich Inlet, British Columbia, Canada. J. Exp. Mar. Bio. Ecol. 401, 89–96. doi: 10.1016/j.jembe.2011.02.041
Matabos, M., Bui, A. O., Mihály, S., Aguzzi, J., Juniper, S. K., and Ajayamohan, R. S. (2014). High-frequency study of epibenthic megafaunal community dynamics in Barkley Canyon: a multi-disciplinary approach using the NEPTUNE Canada network. J. Mar. Syst. 130, 56–68. doi: 10.1016/j.jmarsys.2013.05.002
Matabos, M., Piechaud, N., De Montigny, F., Sarradin, P. M., and Sarrazin, J. (2015). The VENUS cabled observatory as a method to observe fish behavior and species assemblages in a hypoxic fjord, Saanich Inlet (British Columbia, Canada). Can. J. Fish. Aquat. Sci. 72, 24–36. doi: 10.1139/cjfas-2013-0611
Matley, J. K., Klinard, N. V., Martins, A. P. B., Aarestrup, K., Aspillaga, E., Cooke, S. J., et al. (2021). Global trends in aquatic animal tracking with acoustic telemetry. Trends Ecol. Evol. 2021:001. doi: 10.1016/j.tree.2021.09.001
McCauley, D. J., Hoffmann, E., Young, H. S., and Micheli, F. (2012). Night shift: expansion of temporal niche use following reductions in predator density. PLoS One 7:e38871. doi: 10.1371/journal.pone.0038871
Meager, J. J., Skjæraasen, J. E., Karlsen, O., Løkkeborg, S., Mayer, I., Michalsen, K., et al. (2012). Environmental regulation of individual depth on a cod spawning ground. Aquat. Biol. 17, 211–221. doi: 10.3354/ab00469
Milligan, R. J., Scott, E. M., Jones, D. O., Bett, B. J., Jamieson, A. J., O’brien, R., et al. (2020). Evidence for seasonal cycles in deep-sea fish abundances: a great migration in the deep SE Atlantic? J. Anim. Ecol. 89, 1593–1603. doi: 10.1111/1365-2656.13215
Mishra, P., Mohanty, A. K., Kumar Swain, R., Parganiha, A., and Pati, A. K. (2020). Circannual production rhythms of seven commercially important fishes in the Chilika lagoon. Biol. Rhyth. Res. 2020, 1–23. doi: 10.1080/09291016.2020.1750132
Mittelbach, G. G., Ballew, N. G., and Kjelvik, M. K. (2014). Fish behavioral types and their ecological consequences. Can. J. Fish. Aquat. Sci. 71, 927–944. doi: 10.1139/cjfas-2013-0558
Morales-Nin, B., and Moranta, J. (1997). Life history and fishery of the common dentex (Dentex dentex) in Mallorca (Balearic Islands, western Mediterranean). Fish. Res. 30, 67–76. doi: 10.1016/S0165-7836(96)00560-7
Muller, K. (1978). “Locomotor activity of fish and environmental oscillations,” in Rhythmic activity of fishes, ed. J. E. Thorpe (London: Academic Press), 1–19.
Norouzzadeh, M. S., Nguyen, A., Kosmala, M., Swanson, A., Palmer, M. S., Packer, C., et al. (2018). Automatically identifying, counting, and describing wild animals in camera-trap images with deep learning. Proc. Natl. Acad. Sci. 115, E5716–E5725. doi: 10.1073/pnas.1719367115
Olivares, M., Tiselius, P., Calbet, A., and Saiz, E. (2020). Non-lethal effects of the predator Meganyctiphanes norvegica and influence of seasonal photoperiod and food availability on the diel feeding behaviour of the copepod Centropages typicus. J. Plankt. Res. 42, 742–751. doi: 10.1093/plankt/fbaa051
Orozco, M. J., Lizaso, J. L. S., and Fernández, A. M. (2011). Capturas del dentón (Dentex dentex) en dos puertos del Mediterráneo Ibérico. Mediterránea 22, 212–229. doi: 10.14198/MDTRRA2011.ESP.08
Pais, M. P., and Cabral, H. N. (2018). Effect of underwater visual survey methodology on bias and precision of fish counts: a simulation approach. PeerJ 6:e5378. doi: 10.7717/peerj.5378
Palacios-Fuentes, P., Díaz-Astudillo, M., Reculé, M. A., Ojeda, F. P., and Landaeta, M. F. (2020). Presettlement schooling behaviour of a rocky fish in a shallow area. Is it related to local environmental conditions? Sci. Mar. 84, 243–252. doi: 10.3989/scimar.05043.19A
Pavlidis, M., Greenwood, L., Paalavuo, M., Mölsä, H., and Laitinen, J. T. (1999). The effect of photoperiod on diel rhythms in serum melatonin, cortisol, glucose, and electrolytes in the common dentex, Dentex dentex. Gen. Comp. Endocrinol. 113, 240–250. doi: 10.1006/gcen.1998.7190
Pitcher, T. J. (1983). Heuristic definitions of fish shoaling behaviour. Anim. Behav. 31, 611–613. doi: 10.1016/S0003-3472(83)80087-6
Pocheville, A. (2015). “The ecological niche: history and recent controversies,” in Handbook of evolutionary thinking in the sciences, eds T. Heams, P. Huneman, G. Lecointre, and M. Silberstain (Dordrecht: Springer Netherlands), 547–586. doi: 10.13140/RG.2.1.3205.8405
Power, M., Attrill, M. J., and Thomas, R. M. (2000). Temporal abundance patterns and growth of juvenile herring and sprat from the Thames estuary 1977–1992. J. Fish Biol. 56, 1408–1426. doi: 10.1111/j.1095-8649.2000.tb02153.x
Priou, P., Nikolopoulos, A., Flores, H., Gradinger, R., Kunisch, E., Katlein, C., et al. (2021). Dense mesopelagic sound scattering layer and vertical segregation of pelagic organisms at the Arctic-Atlantic gateway during the midnight sun. Prog. Oceanogr. 196:102611. doi: 10.1016/j.pocean.2021.102611
Reebs, S. G. (2002). Plasticity of diel and circadian activity rhythms in fishes. Rev. Fish Biol. Fish. 12, 349–371. doi: 10.1023/A:1025371804611
Refinetti, R., Cornélissen, G., and Halberg, F. (2007). Procedures for numerical analysis of circadian rhythms. Biol. Rhyth. Res. 38, 275–325. doi: 10.1080/09291010600903692
Rodriguez-Pinto, I. I., Rieucau, G., Handegard, N. O., and Boswell, K. M. (2020). Environmental context elicits behavioral modification of collective state in schooling fish. Animal Behaviour 165, 107–116. doi: 10.1016/j.anbehav.2020.05.002
Rountree, R. A., Aguzzi, J., Marini, S., Fanelli, E., De Leo, F. C., Del Rio, J., et al. (2020). “Towards an optimal design for ecosystem-level ocean observatories,” in Oceanography and Marine Biology, eds S. J. Hawkins, A. L. Allcock, A. E. Bates, A. J. Evans, L. B. Firth, C. D. McQuaid, et al. (Milton Park: Taylor and Francis), 79–106. doi: 10.1201/9780429351495-2
Saha, S., Singh, K. M., and Gupta, B. B. P. (2019). Melatonin synthesis and clock gene regulation in the pineal organ of teleost fish compared to mammals: similarities and differences. Gen. Comp. Endocrinol. 279, 27–34. doi: 10.1016/j.ygcen.2018.07.010
Sahyoun, R., Bussotti, S., Di Franco, A., Navone, A., Panzalis, P., and Guidetti, P. (2013). Protection effects on Mediterranean fish assemblages associated with different rocky habitats. J. Mar. Biol. Assoc. U K 93, 425–435. doi: 10.1017/S0025315412000975
Samoilys, M. A., and Carlos, G. (2000). Determining methods of underwater visual census for estimating the abundance of coral reef fishes. Env. Biol. Fish. 57, 289–304. doi: 10.1023/A:1007679109359
Sánchez-Vázquez, F. J., López-Olmeda, J. F., Vera, L. M., Migaud, H., López-Patiño, M. A., and Míguez, J. M. (2019). Environmental cycles, melatonin, and circadian control of stress response in fish. Front. Endocrinol. 10:279. doi: 10.3389/fendo.2019.00279
Santos, M. N., Monteiro, C. C., and Gaspar, M. B. (2002). Diurnal variations in the fish assemblage at an artificial reef. ICES J. Mar. Sci. 59, S32–S35. doi: 10.1006/jmsc.2001.1166
Sbragaglia, V., Correia, R. A., Coco, S., and Arlinghaus, R. (2020). Data mining on YouTube reveals fisher group-specific harvesting patterns and social engagement in recreational anglers and spearfishers. ICES J. Mar. Sci. 77, 2234–2244. doi: 10.1093/icesjms/fsz100
Sbragaglia, V., Jolles, J. W., Coll, M., and Arlinghaus, R. (2021). Fisheries-induced changes of shoaling behaviour: mechanisms and potential consequences. Trends Ecol. Evol. 10, 885–888. doi: 10.1016/j.tree.2021.06.015
Sbragaglia, V., Nuñez, J. D., Dominoni, D., Coco, S., Fanelli, E., Azzurro, E., et al. (2019). Annual rhythms of temporal niche partitioning in the Sparidae family are correlated to different environmental variables. Sci. Rep. 9, 1708. doi: 10.1038/s41598-018-37954-0
Scapini, F. (2014). Behavior of mobile macrofauna is a key factor in beach ecology as response to rapid environmental changes. Estuar. Coast. Shelf Sci. 150, 36–44. doi: 10.1016/j.ecss.2013.11.001
Schalm, G., Bruns, K., Drachenberg, N., Geyer, N., Foulkes, N. S., Bertolucci, C., et al. (2021). Finding Nemo’s clock reveals switch from nocturnal to diurnal activity. Sci. Rep. 11, 1–11. doi: 10.1038/s41598-021-86244-9
Schrum, C. (2001). Regionalization of climate change for the North Sea and Baltic Sea. Clim. Res. 18, 31–37. doi: 10.3354/cr018031
Selleslagh, J., and Amara, R. (2008). Environmental factors structuring fish composition and assemblages in a small macrotidal estuary (eastern English Channel). Est. Coast. Shelf Sci. 79, 507–517. doi: 10.1016/j.ecss.2008.05.006
Snelgrove, P. V., Thrush, S. F., Wall, D. H., and Norkko, A. (2014). Real world biodiversity–ecosystem functioning: a seafloor perspective. Trends Ecol. Evol. 29, 398–405. doi: 10.1016/j.tree.2014.05.002
Sonnewald, M., and Türkay, M. (2012). Abundance analyses of mega-epibenthic species on the Dogger Bank (North Sea): Diurnal rhythms and short-term effects caused by repeated trawling, observed at a permanent station. J. Sea Res. 73, 1–6. doi: 10.1016/j.seares.2012.05.015
Stevenson, T. J., Visser, M. E., Arnold, W., Barrett, P., Biello, S., Dawson, A., et al. (2015). Disrupted seasonal biology impacts health, food security and ecosystems. Proc. R. Soc. B 282:20151453. doi: 10.1098/rspb.2015.1453
Teo, S. L., Boustany, A. M., and Block, B. A. (2007). Oceanographic preferences of Atlantic bluefin tuna, Thunnus thynnus, on their Gulf of Mexico breeding grounds. Mar. Biol. 152, 1105–1119. doi: 10.1007/s00227-007-0758-1
Ünlüoğlu, A. (2021). Diel Variability in the Bottom-Trawl Catch Rates of Sparid Fishes in Ýzmir Bay (Central-Eastern Aegean Sea). Nat. Eng. Sci. 6, 138–154.
Van Der Walt, K. A., Porri, F., Potts, W. M., Duncan, M. I., and James, N. C. (2021). Thermal tolerance, safety margins and vulnerability of coastal species: Projected impact of climate change induced cold water variability in a temperate African region. Mar. Env. Res. 169:105346. doi: 10.1016/j.marenvres.2021.105346
Villegas-Ríos, D., Reale, D., Freitas, C., Moland, E., and Olsen, E. M. (2017). Individual level consistency and correlations of fish spatial behaviour assessed from aquatic animal telemetry. Anim. Behav. 124, 83–94. doi: 10.1016/j.anbehav.2016.12.002
Vinagre, C., Leal, I., Mendonca, V., Madeira, D., Narciso, L., Diniz, M. S., et al. (2016). Vulnerability to climate warming and acclimation capacity of tropical and temperate coastal organisms. Ecol. Indic. 62, 317–327. doi: 10.1016/j.ecolind.2015.11.010
Visser, M. E., Caro, S. P., Van Oers, K., Schaper, S. V., and Helm, B. (2010). Phenology, seasonal timing and circannual rhythms: towards a unified framework. Philosoph. Trans. R. Soc. Bo 365, 3113–3127. doi: 10.1098/rstb.2010.0111
Wagner, H. J., Kemp, K., Mattheus, U., and Priede, I. G. (2007). Rhythms at the bottom of the deep sea: cyclic current flow changes and melatonin patterns in two species of demersal fish. Deep Sea Res. Part I 54, 1944–1956. doi: 10.1016/j.dsr.2007.08.005
Waldock, C., Stuart-Smith, R. D., Edgar, G. J., Bird, T. J., and Bates, A. E. (2019). The shape of abundance distributions across temperature gradients in reef fishes. Ecol. Lett. 22, 685–696. doi: 10.1111/ele.13222
Witkowski, F., Vion, A., and Bouchoucha, M. (2016). Temporal partitioning of diurnal behavioral patterns of Coris julis and Diplodus vulgaris (Actinopterygii: Perciformes) in Mediterranean coralligenous habitats. Acta Ichthyol. Piscat. 46, 171–183. doi: 10.3750/AIP2016.46.3.02
Zabala, M., García-Rubies, A., and Corbera, J. (1992). Els peixos de les illes Medes i del litoral català: guia per observar-los al seu ambient. Badalona, ES: Escola del Mar, Centre d’Estudis Marins de Badalona.
Keywords: day-night rhythms, photoperiodism, imaging, cabled observatories, visual predator, temporal niche, habitat use, monitoring footprint
Citation: Francescangeli M, Sbragaglia V, del Rio Fernandez J, Trullols E, Antonijuan J, Massana I, Prat J, Nogueras Cervera M, Mihai Toma D and Aguzzi J (2022) Long-Term Monitoring of Diel and Seasonal Rhythm of Dentex dentex at an Artificial Reef. Front. Mar. Sci. 9:837216. doi: 10.3389/fmars.2022.837216
Received: 16 December 2021; Accepted: 16 February 2022;
Published: 18 March 2022.
Edited by:
Henry Ruhl, Central and Northern California Ocean Observing System (CeNCOOS), United StatesReviewed by:
José Lino Vieira De Oliveira Costa, University of Lisbon, PortugalPeter M. J. Herman, Delft University of Technology, Netherlands
Copyright © 2022 Francescangeli, Sbragaglia, del Rio Fernandez, Trullols, Antonijuan, Massana, Prat, Nogueras Cervera, Mihai Toma and Aguzzi. This is an open-access article distributed under the terms of the Creative Commons Attribution License (CC BY). The use, distribution or reproduction in other forums is permitted, provided the original author(s) and the copyright owner(s) are credited and that the original publication in this journal is cited, in accordance with accepted academic practice. No use, distribution or reproduction is permitted which does not comply with these terms.
*Correspondence: Marco Francescangeli, marco.francescageli@upc.edu; Jacopo Aguzzi, jaguzzi@icm.csic.es