- 1Key Laboratory of Aquatic Product Processing, Ministry of Agriculture and Rural Affairs, South China Sea Fisheries Research Institute, Chinese Academy of Fishery Sciences, Guangzhou, China
- 2Tropical Fisheries Research and Development Center, South China Sea Fisheries Research Institute, Chinese Academy of Fishery Sciences/Sanya Tropical Fisheries Research Institute, Sanya, China
- 3Guangdong Provincial Key Laboratory of Improved Variety Reproduction in Aquatic Economic Animals, Institute of Aquatic Economic Animals, School of Life Sciences, Sun Yat-Sen University, Guangzhou, China
- 4Southern Marine Science and Engineering Guangdong Laboratory, South China Sea Fisheries Research Institute, Zhanjiang, China
A 6-week feeding trial was carried out to investigate whether dietary L-carnitine supplementation could alleviate the adverse effects of high-fat diet on the growth, antioxidation and lipid metabolism of Trachinotus ovatus. Four isonitrogenous experimental diets were formulated supplementing with or without L-carnitine (low fat diet (D1): 13% lipid; high fat diet (D2): 20% lipid; D3: 20% lipid with 0.02% L-carnitine; D4: 20% lipid with 0.06% L-carnitine). The final body weight, weight gain rate, specific growth ratio and feed intake in fish fed with D2 diet were significantly lower than that of fish fed with D1, D3 and D4 diets (P < 0.05). The weight gain rate and specific growth ratio of D2 diet were as low as 167.21% and 2.41% initial body weight/d, respectively. However, experimental diets were unable to change the feed conversion ratio and survival rate of fish (P > 0.05). The lowest value of crude protein and the highest value of crude lipid of whole-body were found in fish fed with D2 diet (P < 0.05), which were 31.35% and 17.41% wet weight respectively. Meantime, the level of crude lipid of whole-body in fish fed with D3 and D4 diets was significantly higher than that of fish fed with D2 diet (P < 0.05). Besides, there were significantly higher levels of triglyceride and total cholesterol in the D2 diet when compared to the other diets (P < 0.05). Additionally, the superoxide dismutase activity, malondialdehyde content and the mRNA levels of manganese superoxide dismutase and glutathione peroxidase of the liver in fish fed with D2 diet were significantly higher than those fed with D1, D3 and D4 diets (P < 0.05). The mRNA levels of carnitine palmitoyl transferase 1 and peroxisome proliferator-activated receptors-alpha of the liver in fish fed with D2 diet were significantly higher than those fed with D1, D3 and D4 diets (P < 0.05). In conclusion, L-carnitine supplementation in high-fat diet improved the growth performance and health of T. ovatus by promoting lipid hydrolysis, improving cholesterol transport and antioxidant capacity. Therefore, we recommended the 0.02% addition level of L-carnitine for T. ovatus diet.
Introduction
In animals, L-carnitine is synthesized from essential amino acids lysine and methionine supported by vitamin C and other secondary chemical compounds produced in the body (Sabzi et al., 2017). Its main function is as a cofactor to transport long-chain unsaturated fatty acids to mitochondria matrix for β-oxidation to generate energy (Chen et al., 2020). Thus, L-carnitine plays an important regulatory role in energy metabolism and lipid metabolism (Selcuk et al., 2010; Chen et al., 2020). Although organisms can synthesize L-carnitine, dietary supplementation is usually required when the lipid concentration in the body exceeds the synthetic ability of organisms (Li et al., 2019). Besides, the L-carnitine synthesis capacity of juvenile fish is limited due to the mechanism of L-carnitine synthesis is under developed (Li et al., 2019). Therefore, L-carnitine is considered to be an essential nutrient for animals under specific conditions.
In view of the key regulatory role of L-carnitine in metabolism, the addition of L-carnitine to fish feed has drawn extensive attention to its potential to improve growth, feed efficiency, and reduce body-fat deposition. L-carnitine supplementation has been proved to increase the yield of cultured fish, which promoted weight gain and feed efficiency and decreased lipid deposition (Desai et al., 2010; Haji-abadi et al., 2010; Ozorio et al., 2010 Zheng et al., 2014). However, some studies showed contradictory results. Dietary supplementation with L-carnitine has no effect on growth performance and feed efficiency of several species of cultured fish, but altered the lipid metabolism in liver (Gaylord and Gatlin, 2000; Yang et al., 2009; Selcuk et al., 2010; Ozorio et al., 2012). The above results indicated that the effects of dietary L-carnitine supplementation on fish are species-specific. In addition, the contradictory results may also be different from the growth stages, feed composition (lipid, protein, lysine and L-carnitine content) and environmental conditions (water quality and water temperature) (Ozorio et al., 2012; Li et al., 2019).
The protein content of aquatic feed is the most important factor affecting fish growth and feed costs. Dietary lipid is the second important nutrient after protein for aquatic animals and play a key regulatory role in maintaining growth and normal physiological function of fish. It is well known that marine carnivorous fish preferentially use protein as energy source, and lipids are the main source of dietary energy and essential fatty acids (López et al., 2009). Therefore, partial replacement of protein with lipid is one of the main strategies to reduce feed costs. Within a certain range, increasing the dietary lipid level can not only improve feed efficiency, but also spare protein (Schuchardt et al., 2008; López et al., 2009). However, excessive lipid levels in the diet might cause adverse effects on fish, such as poor growth performance, abnormal lipid metabolism, oxidative stress and immunosuppression (Guo et al., 2019; Xie et al., 2020; Xu et al., 2021).
Trachinotus ovatus is widely distributed in southern China, Southeast Asia, Japan and Australia (Zhao et al., 2021). This species is popular because of its high nutritional value, rapid growth and delicious meat. Due to the increasing market demand, T. ovatus has become a vital economically marine fish widely cultured in the southern coast of China. However, in order to reduce the culturing cost, high-fat diet has been widely used to feed T. ovatus in practical aquaculture conditions, which causes high mortality and economic losses. Therefore, in this study, L-carnitine was supplemented in the high-fat diet to study its effect on growth, antioxidant capacity, body composition and lipid metabolism of T. ovatus. The results obtained in this study can provide reference for the research and development of high-fat diet formulas for T. ovatus.
Materials and Methods
Experimental Diets
The formulation, proximate composition and nutrient levels of experimental diets were shown in Table 1. Four isonitrogenous experimental diets were performed as following: low fat diet (D1) contained 13% lipid level; high fat diet (D2) contained 20% lipid level; D3 diet contained 20% lipid with 200 mg/kg L-carnitine; D4 diet contained 20% lipid with 600 mg/kg L-carnitine. The experimental diets were prepared and manufactured according to the procedure described by Zhao et al. (2020). Briefly, all dry ingredients were finely ground and thoroughly mixed. Then soybean lecithin and fish oil were gradually added and mixed constantly. Subsequently, 50 mL of water for each 100 g of the mixture was slowly blended. The 2.5-mm-diameter puffed pellets were produced by using a puffing apparatus (Institute of Chemical Engineering, South China University of Technology, Guangdong, China). The puffed pellets were oven-dried at 60°C to approximately 10% moisture and then stored at -20°C until feeding.
Experimental Fish and Feeding Management
Juvenile T. ovatus were obtained from a commercial farm (Lingshui, Hainan, China). The feeding experiment was performed in the Bay (Lingshui, Hainan, China) using sixteen seawater cages (1.0 m × 1.0 m × 1.5 m). Prior to the feeding experiment, fish were fed the D1 group diet for 2 weeks to acclimate to the experimental conditions and facilities. At the beginning of the feeding experiment, a total of 320 healthy fish (average body weight 7.60 ± 0.06 g) were distributed randomly into sixteen seawater cages at 20 fish per cage. The fish were slowly hand-fed to apparent satiation two times daily at 08:00 and 16:00, and the feeding experiment lasted for six-weeks. Feed consumption, fish death amounts and weight were recorded for each sea cage every day. After six-weeks feeding experiment, all survival fish were starved for 24 h, and then weighed in batches after anesthesia (MS-222, Sigma, St Louis, MO, USA).
Sample Collection
After six-weeks feeding experiment, all survived fish were starved for 24 h and anesthetized (MS-222, Sigma, St Louis, MO, USA). Then, four fish from each cage were randomly collected and frozen in liquid nitrogen, and then stored at - 80 °C for whole body composition analysis. Four individuals from each cage were randomly collected for obtaining the blood sample. Serum was obtained based on procedures of Li et al. (2014). Briefly, the blood was collected from the tail sinus with 1 ml sterile syringes and then centrifuged (5000 rpm, 10 min) at 4°C. Then, the serum was separated and stored in the liquid nitrogen for analysis of the haematological biochemical index. The livers were removed from six fish per cage, and immediately frozen in liquid nitrogen and stored at - 80 °C for enzyme activity and RNA expression analysis.
Chemical Analysis of the Experimental Diets and Whole body
The crude lipid, crude protein and moisture of experimental diets and whole body were determined and analyzed according to the standard procedures of AOAC (1995). Briefly, crude protein content was detected using the Kjeldahl method (1030-Auto-analyzer, Tecator), crude lipid was determined by the Soxhlet extractor method (Soxtec System HT6, Tecator), moisture was analysed by drying in an oven at 105°C to acquire dry weight and ash was examined after combustion in a muffle furnace at 550°C for 24 h.
Survival Rate and Growth Performance
During the feeding experiment, the number of dead fish were recorded to calculate the survival rate. The weight gain rate (WGR), feed conversion ratio (FCR), specific growth ratio (SGR) and feed intake (FI) were calculated as follows: WGR (%) = 100 × (final body weight - initial body weight)/initial body weight; SGR (% initial body weight/day) = 100 × (Ln final mean weight - Ln initial mean weight)/number of days; FCR = dry feed intake/(final body weight - initial body weight); FI (g/fish) = dry feed intake/number of fish in each cage].
Serum Parameters Assays
Serum levels of triglyceride (TG) and total cholesterol (T-CHO) were determined on Chemray 240 automatic chemistry analyser (Rayto Life Science Co., Ltd.) with the commercial kits (Huili Biotech Co., Ltd.).
Antioxidant Enzyme Activity Analysis
Liver samples were homogenized in ice-cold phosphate buffer (pH 6.4, 1:10 dilution). Afterward, the above homogenate was centrifuged (10 min, 4°C, 1200 g) to collect supernatant for antioxidant enzyme activity analysis. Superoxide dismutase (SOD)(A001-1) activities and malondialdehyde (MDA) (A003-1) contents in liver were determined following the instructions of the reagent kits (Nanjing Jiancheng Bioengineering Institute, Nanjing, China). SOD was determined by the xanthine oxidase method. One unit of enzyme activity was defined as the amount of enzyme exhibiting 50% inhibition of the SOD per milligram of protein in l mL solution. MDA was determined using the thiobarbituric acid method.
Total RNA Extraction and Gene Expression Analysis
Liver RNA extraction and real-time quantitative PCR analysis were performed according to our previously published methods (Zhao et al., 2020). Briefly, the livers from each cage were pooled for the isolation of total RNA using Trizol® reagent (Invitrogen, USA). 1% agarose gel electrophoresis and spectrophotometer (NanoDrop 2000, Thermo Fisher, United States) were used to ascertain the quality and quantity of RNA. Then, cDNA was synthesized using PrimeScript™ RT reagent Kit with gDNA Eraser (Takara, Dalian, China) following the manufacturer’s instructions. Primers for real-time quantitative PCR were shown in Table 2. Real-time quantitative PCR assays were quantified on the Light Cycler 480 (Roche Applied Science, Basel Switzerland) using an SYBR® Premix Ex TaqTM II (Takara, Dalian, China). The real-time PCR conditions were as follows: 95°C for 1 min, followed by 40 amplification cycles at 95°C for 5 s, 60°C for 15 s and 72°C for 20s. The relative expression levels of target genes were quantified using 2-ΔΔCt method.
Statistical Analysis
All data in this study were shown as means ± standard error (SE). All data were analyzed in SPSS 22.0 (SPSS, Chicago, IL, USA) and followed by one-way analysis of variance (ANOVA) as well as Duncan’s multiple range test. A P-value < 0.05 was deemed to be statistically significant.
Results
Growth Performance and Feed Utilization
The growth performance (WGR, SGR), SR and FCR of T. ovatus were shown in Table 3. The final body weight (FBW), WGR and SGR of fish fed with D2 diet were significantly lower than that of other diet groups (P < 0.05). Dietary L-carnitine supplementation was unable to change the FCR of fish (P > 0.05), however, FI in fish fed with D2 diet was significantly lower than those fed with D1, D3 and D4 diets (P < 0.05). The SR was 90% approximately, and there was no significant difference among all diet treatments (P > 0.05).
Whole-Body Composition
As showed in Table 4, experimental diets significantly altered the whole-body composition of T. ovatus. The lowest value of crude protein was found in fish fed with D2 diet, and significantly lower than those fed the D1, D3 and D4 diets (P < 0.05). Meantime, fish fed with D2 diet showed higher crude lipid and significantly higher than those fed with D1, D3 and D4 diets (P < 0.05). However, there was no significant difference in the moisture among all diet treatments (P > 0.05).
Antioxidant Capacity
Antioxidant enzyme activities and expression levels of antioxidant related-genes were presented in Figures 1, 2, respectively.
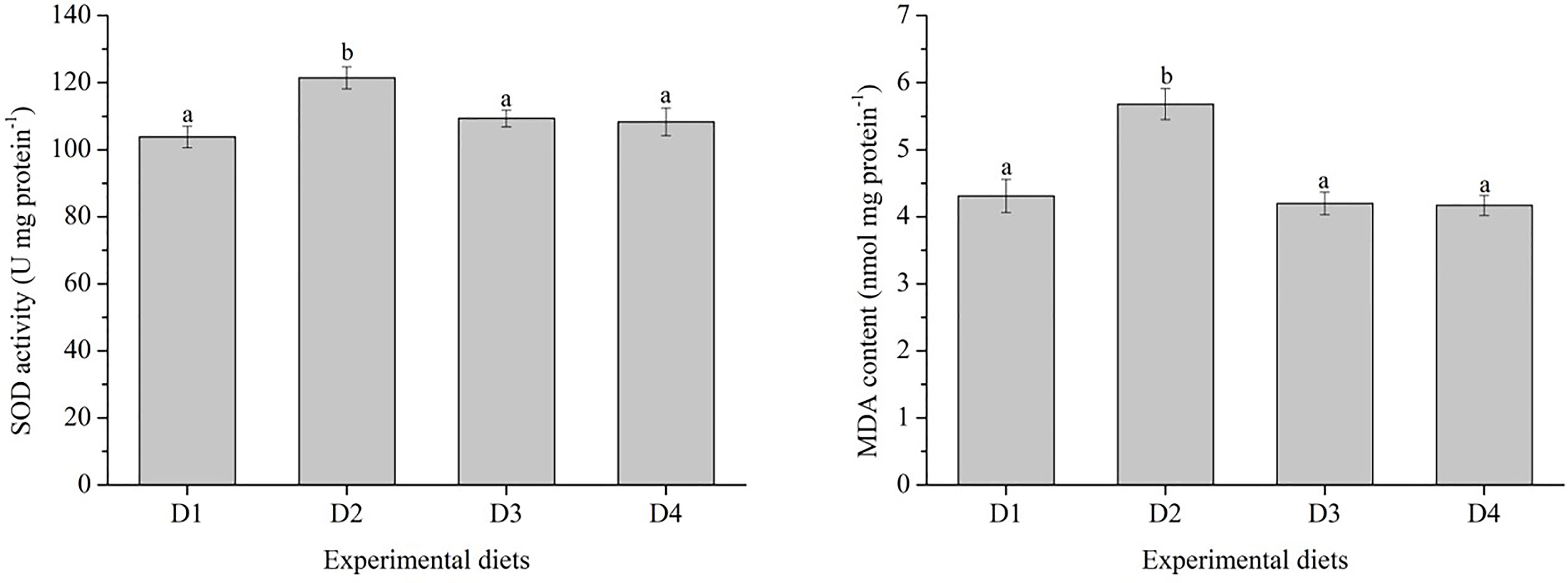
Figure 1 Specific activities of liver antioxidant enzymes of Trachinotus ovatus fed experimental diets for 56 days. Different superscript letters in the same row means the significant difference at P < 0.05. SOD, superoxide dismutase; MDA, malondialdehyde.
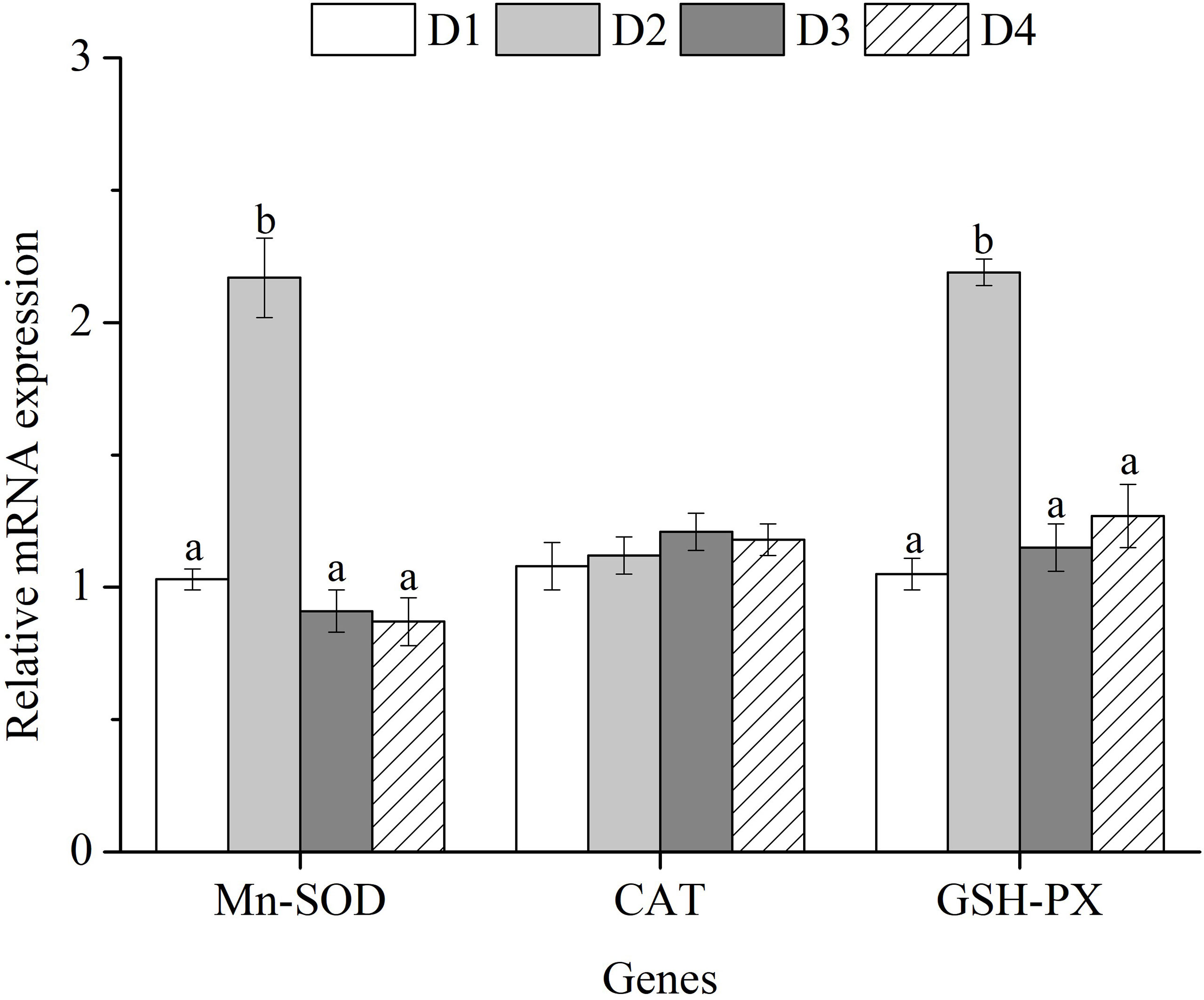
Figure 2 The expression profiles of antioxidant-related genes in the liver of Trachinotus ovatus fed experimental diets for 56 days. Different superscript letters in the same row means the significant difference at P < 0.05. Mn-SOD, manganese superoxide dismutase; CAT, catalase; GSH-PX, glutathione peroxidase.
The SOD activity and MDA content in fish fed with D2 diet were significantly higher than those fed with D1, D3 and D4 diets (P < 0.05) (Figure 1).
The mRNA levels of manganese superoxide dismutase (Mn-SOD) and glutathione peroxidase (GSH-PX) in fish fed with D2 diets were significantly higher than those fed with D1, D3 and D4 diets (P < 0.05). However, there was no significant difference in the mRNA level of catalase (CAT) among all diet treatments (P > 0.05) (Figure 2).
Serum Parameters
Higher levels of TG and T-CHO were found in D2 diet feeding fish than that of the other group (P < 0.05) (Figure 3).
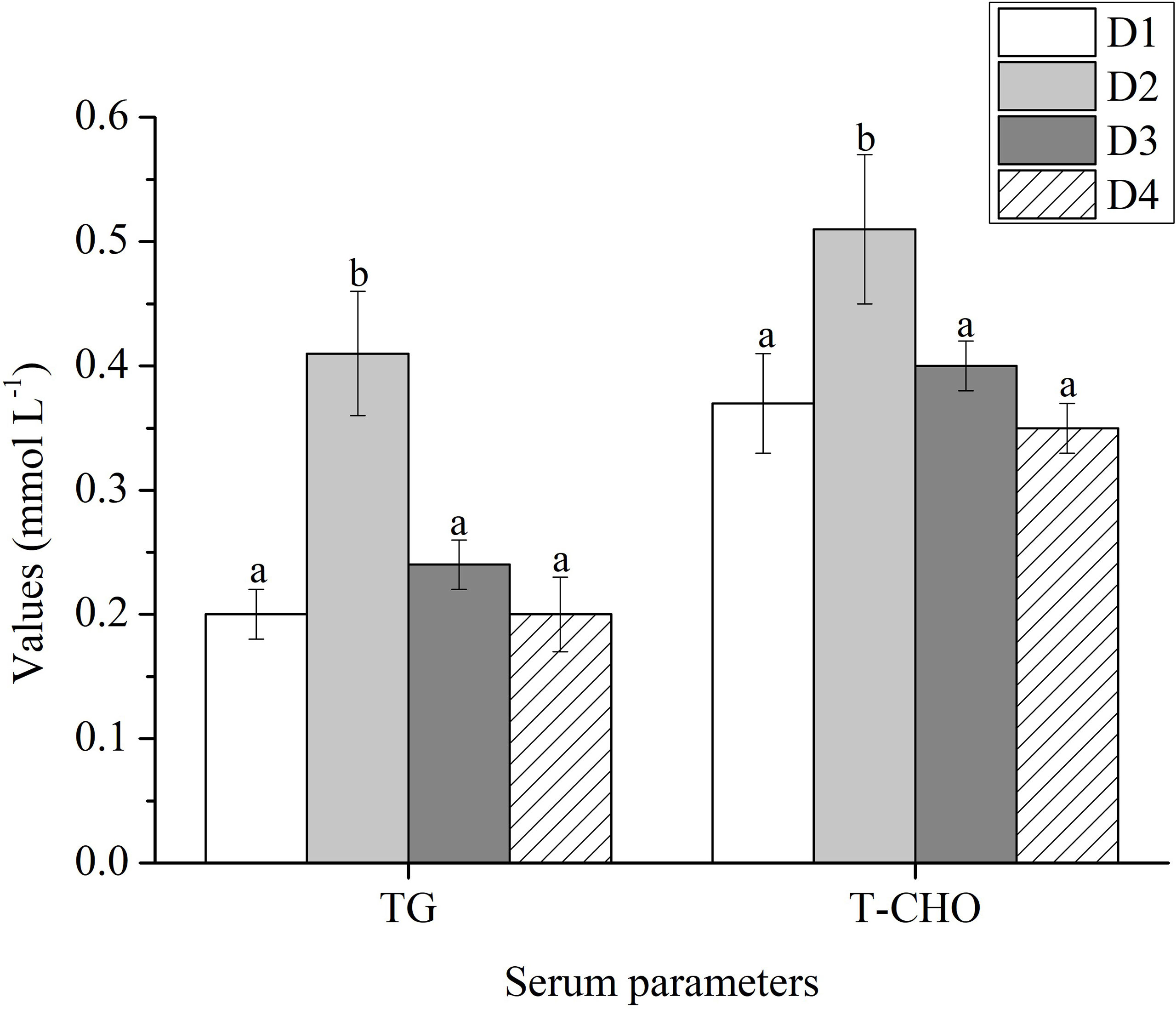
Figure 3 Serum parameters of Trachinotus ovatus fed experimental diets for 56 days. Different superscript letters in the same row means the significant difference at P < 0.05. TG, triglyceride (mmol L-1); T-CHO, total cholesterol (mmol L-1).
Expression Levels of Genes Related to Lipid Metabolism
The mRNA levels of carnitine palmitoyl transferase 1 (CPT1) and peroxisome proliferator-activated receptors-alpha (PPARα) in fish fed with D2 diet were significantly higher than those fed with D1, D3 and D4 diets (P < 0.05) (Figure 4).
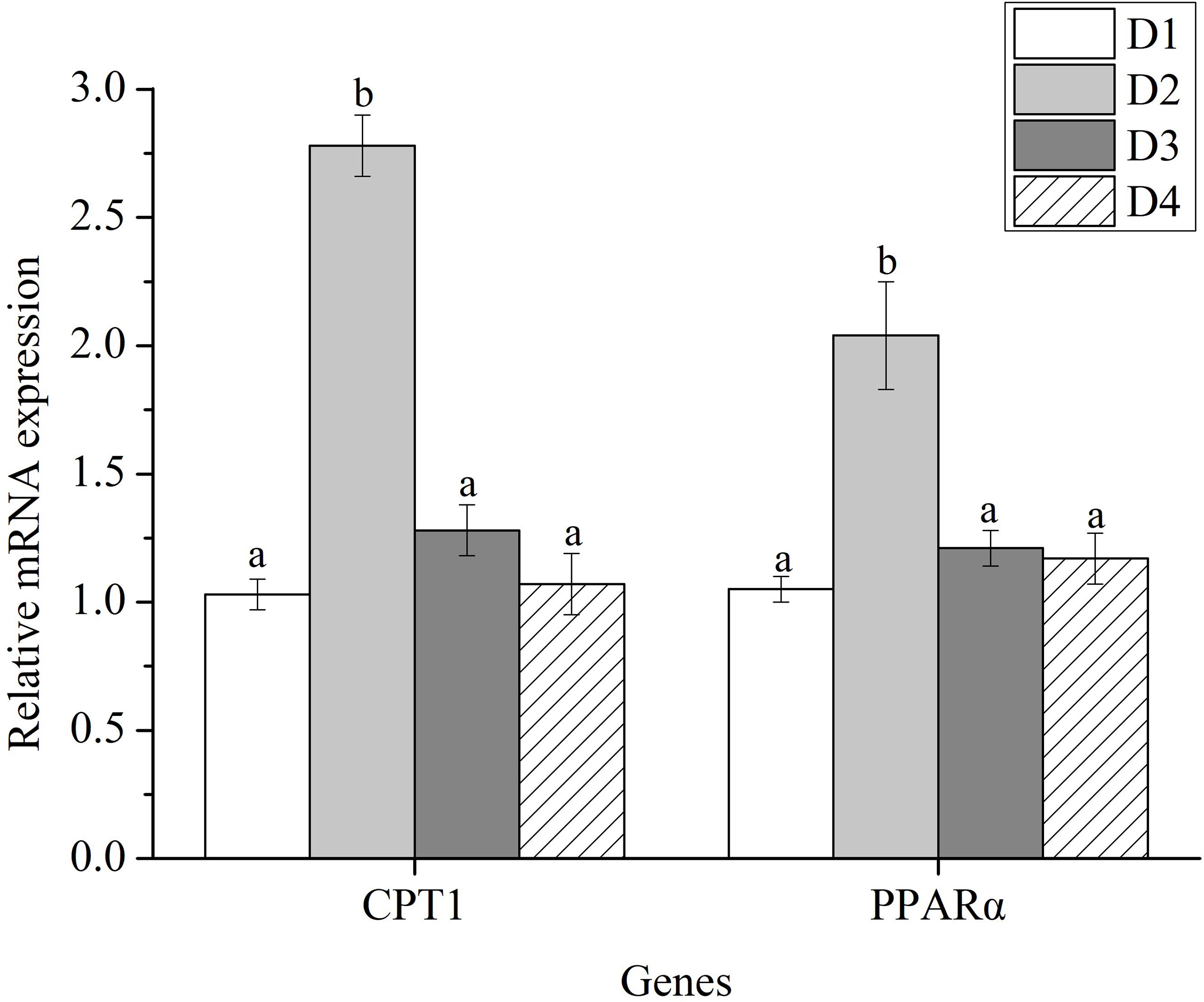
Figure 4 The expression profiles of lipolysis-related genes in the liver of Trachinotus ovatus fed experimental diets for 56 days. Different superscript letters in the same row means the significant difference at P < 0.05. CPT1, carnitine palmitoyl transferase 1; PPARα, peroxisome proliferator-activated receptors-alpha.
Discussion
In this study, the results showed that the growth performance (WGR and SGR) of T. ovatus exposed to a high-fat diet without L-carnitine (D2 diet) was significantly lower than that of the low-lipid diet (D1 diet), indicating that excessive lipid intake reduced the growth performance of T. ovatus. Similarly, the poor growth performance caused by high-fat diet has been reported in studies on Atractoscion nobilis (López et al., 2006), Ctenopharyngodon idellus (Li et al., 2016), Micropterus salmoides (Zhou et al., 2020) and T. ovatus (Fang et al., 2021). The poor growth of fish caused by high-fat diet is mainly attributed to lower feed intake and metabolic disorders caused by lipid deposition in the liver (Wang et al., 2005; Song et al., 2009; Ding et al., 2020). Similar results were obtained in this study. T. ovatus fed the high-fat diet without L-carnitine (D2 diet) showed lower FI, which can be used to explain that the high-fat diet reduced the growth of T. ovatus. Meantime, the present results showed that the growth performance (WGR and SGR) and FI of T. ovatus in the low-lipid group (D1) was similar to that in the high-lipid supplemented with L-carnitine groups (D3 and D4), which demonstrated that dietary L-carnitine supplementation was helpful to alleviate the adverse effects caused by high-fat diet on the growth of T. ovatus. Normal physiological functions are essential for the growth of fish, and L-carnitine can alleviate the adverse effects of high-fat diet on the growth of fish may be attributed to its ability to promote lipid hydrolysis, improve lipid utilization and depress lipid peroxidation (Dikel et al., 2010; Sabzi et al., 2017; Li et al., 2019).
Regarding whole-body composition, the present results indicated that T. ovatus fed high-fat diet (D2) obtained higher lipid and lower protein concentration in whole-body than T. ovatus fed low-lipid diet (D1). Similar results have been reported in studies on T. ovatus (Fang et al., 2021), Scortum barcoo (Song et al., 2009) and Salmo salar (Bjerkeng et al., 1997). Excessive lipid intake by fish resulted in excessive fat deposition in visceral cavity and tissues, which not only has an adversely impact on the growth and metabolism function of fish, but also reduce the commercial value of the fish products (Bjerkeng et al., 1997). In the present study, it was found that the whole-body protein was significantly increased and the whole-body lipid was significantly reduced in the high-fat diet supplemented with L-carnitine groups (D3 and D4) when compared to the high-lipid group (D2). The present results indicated that the addition of L-carnitine in high-fat diet was helpful to reduce whole-body lipid concentration and promote protein deposition. Dietary L-carnitine supplementation can promote the oxidative metabolism of lipids, thereby reducing the accumulation of lipids in the body (Yang et al., 2012). Similarly, dietary supplementation with L-carnitine can promote protein deposition and decrease whole-body lipid concentration, which has been reported in studies on C. carpio (Sabzi et al., 2017), Bidyanus bidyanus (Yang et al., 2012), Oncorhynchus mykiss (Haji-abadi et al., 2010) and hybrid tilapia (Yang et al., 2009). However, some studies conversely found that dietary L-carnitine supplementation did not alter the lipid content of fish, such as Dicentrarchus labrax (Dias et al., 2001) and Morone saxatilis male × M. chrysops female (Gaylord and Gatlin, 2000). Species differences may be an important reason for inconsistent results. In addition, different experimental results may also be related to fish growth stage, feed formula and environmental factors. At present, it is not easy to explain the causal mechanisms of the effects of dietary L-carnitine supplementation on body composition of fish.
TG and T-CHO concentrations in the serum of T. ovatus were significantly higher in the high-fat diet group (D2) than in the low-lipid diet group (D1). These results were in accordance with data in C. carpio (Sabzi et al., 2017), B. bidyanus (Yang et al., 2012) and Ctenopharyngodon Idella (Du et al., 2006). The results showed that high-fat diet could promote the deposition of TG and T-CHO in blood. However, this study results found that L-carnitine supplementation in high-fat diet (D3 and D4) significantly reduced the contents of TG and T-CHO in serum compared to the high-fat diet without L-carnitine supplementation (D2). Dietary L-carnitine supplementation decreased the concentrations of TG and T-CHO in plasma have also been found in D. labrax (Santulli et al., 1988), hybrid tilapia (Yang et al., 2009) and C. carpio (Sabzi et al., 2017). The results indicated that dietary L-carnitine supplementation could reduce intravascular cholesterol and triglyceride deposition and improve fish health by increasing the transport capacity of cholesterol.
Additionally, Gou et al. (2016) reported that the addition of L-carnitine in the diet promoted the lipid hydrolysis capacity of the liver by increasing the activity of lipoprotein lipase and lipase. Similar results were obtained in this study, the present results found that the mRNA levels of CPT1 and PPARα in fish fed with high-fat diet (D2) were significantly higher than those fed with low-lipid (D1) and high-lipid with L-carnitine supplementation (D3 and D4) diets. The main function of CPT1 is to transport long-chain fatty acids to the mitochondrial region for β-oxidation (Chen et al., 2020). PPARα can catalyse the hydrolysis of lipoproteins and participate in lipolysis (Pawlak et al., 2015). The results indicated that T. ovatus responded to the adverse effects of high-fat diet by increasing the expression of lipid hydrolysis related genes (CPT1 and PPARα), which was helpful to promote lipid hydrolysis and reduce lipid deposition in the liver. However, the addition of L-carnitine in the high-fat diet decreased the expression levels of CPT1 and PPARα compared to the high-fat diet group. The results demonstrated that L-carnitine promoted liver lipolysis and improved liver health, so it did not induce the expression of CPT1 and PPARα to deal with the adverse effects of liver lipid deposition. Similar conclusions have also been reported by Chen et al. (2020), their study found that dietary L-carnitine supplementation could enhance lipid metabolism and decrease lipid deposition in the whole-body of fish.
For fish, high-fat diet intake is easy to cause lipid accumulation and peroxidation in liver (Zhou et al., 2020; Fang et al., 2021). In response to lipid deposition and peroxidation, cells can produce reactive oxygen species (ROS) to reduce oxidative stress and damage, but excessive production of ROS will destroy the integrity of organelles and cause damage to cells and tissues (Zhao et al., 2020; Zhou et al., 2020). SOD is an important enzymatic antioxidant and GSH-PX is a non-enzymatic antioxidant in cellular antioxidant system. SOD and GSH-PX can alleviate cells from oxidative stress by scavenging ROS (Fang et al., 2021). MDA is the final product of lipid peroxidation, and its accumulation may cause cell toxicity and accelerate cell and tissue damage (Liu et al., 2021). The present results found that T. ovatus fed with high-fat diet (D2) showed higher SOD activity, MDA content and mRNA levels of Mn-SOD and GSH-PX, and significantly higher than those fed with low-lipid (D1) and high-lipid with L-carnitine supplementation (D3 and D4) diets. The results indicated that high-fat diet without L-carnitine supplementation led to lipid deposition and peroxidation, which activated the antioxidant system of T. ovatus to alleviate the oxidative pressure. However, the increase of SOD activity and mRNA levels of Mn-SOD and GSH-PX did not eliminate excessive ROS, thus maintaining the MDA content at a high level in the high-lipid group. Previous studies have reported that dietary L-carnitine supplementation inhibited lipid peroxidation and improved fish resistance to oxidative stress by increasing the activities of antioxidant enzymes (Mohseni and Ozorio, 2014; Sabzi et al., 2017; Zhou et al., 2020). Similarly, the present results found that T. ovatus fed with high-fat diet with L-carnitine supplementation showed lower SOD activity, MDA content and mRNA levels of Mn-SOD and GSH-PX when compared to the high-fat diet group. Above results demonstrated that T. ovatus were not exposed to oxidative stress caused by high-fat diet, which may be attributed to L-carnitine supplementation promoting lipid hydrolysis, improving cholesterol transport and scavenging excessive ROS. Therefore, T. ovatus did not need to produce SOD and induce the expression of Mn-SOD and GSH-Px to deal with the oxidative stress caused by high-fat diet intake.
Conclusion
In conclusion, L-carnitine supplementation in high-fat diet can alleviate the adverse effects of high-fat diet on the growth performance of T. ovatus. Besides, dietary L-carnitine supplementation can also improve the health of T. ovatus by promoting lipid hydrolysis, improving cholesterol transport and antioxidant capacity.
Data Availability Statement
The original contributions presented in the study are included in the article/supplementary material. Further inquiries can be directed to the corresponding author.
Ethics Statement
The animal study was reviewed and approved by the care and use of laboratory animals in Sun Yat-sen University and the National Institutes of Health Guide for Care and Use of Laboratory Animals. Written informed consent was obtained from the owners for the participation of their animals in this study.
Author Contributions
The authors thank the participants who gave their time to the trial. WZ, JW and JNdesigned the study. XC carried out the rearing work and measured experimental parameters. WZ and XC analyzed the results and wrote the paper with contributions from the other authors. All authors contributed to the article and approved the submitted version.
Funding
The study was supported by the National Natural Science Foundation of China (32172984); the Southern Marine Science and Engineering Guangdong Laboratory funds (ZJW-2019-06); China-asean Maritime Cooperation Fund Project “China-Asean Modern Marine Fisheries Technology R&D and Industrialization Demonstration”; Central Public-interest Scientific Institution Basal Research Fund, CAFS (2020TD55); Central Public-interest Scientific Institution Basal Research Fund, South China Sea Fisheries Research Institute, CAFS (2021XK02, 2021SD09).
Conflict of Interest
The authors declare that the research was conducted in the absence of any commercial or financial relationships that could be construed as a potential conflict of interest.
Publisher’s Note
All claims expressed in this article are solely those of the authors and do not necessarily represent those of their affiliated organizations, or those of the publisher, the editors and the reviewers. Any product that may be evaluated in this article, or claim that may be made by its manufacturer, is not guaranteed or endorsed by the publisher.
References
AOAC. (1995). Official Methods of Analysis of AOAC, International (Washington: Association of Official Analytical Chemist International).
Bjerkeng B., Refstie S., Fjalestad K. T., Storebakken T., Rdbotten M., Roem A. J. (1997). Quality Parameters of the Flesh of Atlantic Salmon (Salmo Salar) as Affected by Dietary Fat Content and Full-Fat Soybean Meal as a Partial Substitute for Fish Meal in the Diet. Aquaculture 157, 297–309. doi: 10.1016/S0044-8486(97)00162-2
Chen Y., Sun Z., Liang Z., Xie Y., Luo Q., Zhu J., et al. (2020). Effects of Dietary Fish Oil Replacement by Soybean Oil and L-Carnitine Supplementation on Growth Performance, Fatty Acid Composition, Lipid Metabolism and Liver Health of Juvenile Largemouth Bass, Micropterus Salmoides. Aquaculture 516, 734596. doi: 10.1016/j.aquaculture.2019.734596
Desai A. S., Singh R. K., Sapkale P. H., Patil S. D. (2010). Effects of Feed Supplementation With L-Carnitine on Growth and Body Composition of Asian Catfsh, Clarias Batrachus Fry. J. Appl. Anim. Res. 38, 153–157. doi: 10.1080/09712119.2010.10539502
Dias J., Arzel J., Corraze G., Kaushik J. (2001). Effects of Dietary L-Carnitine Supplementation on Growth and Lipid Metabolism in European Seabass (Dicentrarchus Labrax). Aquac. Res. 32, 206–215. doi: 10.1046/j.1355-557x.2001.00016.x
Dikel S., Ünalan B., Eroldoğan O. T., Hunt A.Ö. (2010). Effects of Dietary L-Carnitine Supplementation on Growth, Muscle Fatty Acid Composition and Economic Profit of Rainbow Trout (Oncorhynchus Mykiss). Turk. J. Fish. Aquat. Sci. 10, 173–180. doi: 10.4194/trjfas.2010.0203
Ding T., Xu N., Liu Y., Du J., Xiang X., Xu D., et al. (2020). Effect of Dietary Bile Acid (BA) on the Growth Performance, Body Composition, Antioxidant Responses and Expression of Lipid Metabolism-Related Genes of Juvenile Large Yellow Croaker (Larimichthys Crocea) Fed High-Fat Diets. Aquaculture 518, 734768. doi: 10.1016/j.aquaculture.2019.734768
Du Z. U., Clouet P., Zheng W. H., Degrace P., Tian L. X., Liu Y. J. (2006). Biochemical Hepatic Alterations and Body Lipid Composition in the Herbivorous Grass Carp (Ctenopharyngodon Idella) Fed High-Fat Diets. Br. J. Nutr. 95, 905–915. doi: 10.1079/bjn20061733
Fang H. H., Xie J. J., Zhao W., Liu Z. L., Liu Y. J., Tian L. X., et al. (2021). Study Supplementation of Astaxanthin in High-Fat Diet on Growth Performance, Antioxidant Ability, Anti-Inflammation, Non-Specific Immunity and Intestinal Structure of Juvenile Trachinotus Ovatus. Aquac. Nutr. 27, 2575–2586. doi: 10.1111/anu.13386
Gaylord T. G., Gatlin D. M. (2000). Effects of Dietary Carnitine and Lipid on Growth and Body Composition of Hybrid Striped Bass (Morone Chrysops ♀× MSaxatilis ♂)Fish Physiol. Biochem 22, 297–302. doi: 10.1023/A:1007843223107
Gou G., Jiang M., Wen H., Wu F., Liu W., Tian J. (2016). Effects of Dietary L-Carnitine Supplementation on Growth, Hepatic Lipid Metabolism and Antioxidant Ability in GIFT, Oreochromis Niloticus. Fresh. Fish. 46, 81–88. doi: 10.13721/j.cnki.dsyy.20160505.013
Guo J., Zhou Y., Zhao H., Chen W., Chen Y., Lin S. (2019). Effect of Dietary Lipid Level on Growth, Lipid Metabolism and Oxidative Status of Largemouth Bass, Micropterus Salmoides. Aquaculture 506, 394–400. doi: 10.1016/j.aquaculture.2019.04.007
Haji-abadi S. M. A. J., Soofiani N. M., Sadeghi A. A., Chamani M., Riazi G. H. (2010). Effects of Supplemental Dietary L-Carnitine and Ractopamine on the Performance of Juvenile Rainbow Trout, Oncorhynchus Mykiss. Aquac. Res. 41, 1582–1591. doi: 10.1111/j.1365-2109.2009.02462.x
Li L., Limbu S. M., Ma Q., Chen L., Zhang M., Du Z. (2019). The Metabolic Regulation of Dietary L-Carnitine in Aquaculture Nutrition: Present Status and Future Research Strategies. Rev. Aquac. 11, 1228–1257. doi: 10.1111/raq.12289
Liu Z., Zhao W., Hu W., Zhu B., Xie J., Liu Y., et al. (2021). Lipid Metabolism, Growth Performance, Antioxidant Ability and Intestinal Morphology of Rainbow Trout (Oncorhynchus Mykiss) Under Cage Culture With Flowing Water Were Affected by Dietary Lipid Levels. Aquac. Rep. 19, 100593. doi: 10.1016/j.aqrep.2021.100593
Li M., Wu W., Zhou P., Xie F., Zhou Q., Mai K. (2014). Comparison Effect of Dietary Astaxanthin and Haematococcus Pluvialis on Growth Performance, Antioxidant Status and Immune Response of Large Yellow Croaker Pseudosciaena Crocea. Aquaculture 434, 227–232. doi: 10.1016/j.aquaculture.2014.08.022
Li A., Yuan X., Liang X. F., Liu L., Li J., Li B., et al. (2016). Adaptations of Lipid Metabolism and Food Intake in Response to Low and High Fat Diets in Juvenile Grass Carp (Ctenopharyngodon Idellus). Aquaculture 457, 43–49. doi: 10.1016/j.aquaculture.2016.01.014
López L. M., Durazo E., Viana M. T., Drawbridge M., Bureau D. P. (2009). Effect of Dietary Lipid Levels on Performance, Body Composition and Fatty Acid Profile of Juvenile White Seabass, Atractoscion Nobilis. Aquaculture 289, 101–105. doi: 10.1016/j.aquaculture.2009.01.003
López L. M., Torres A. L., Durazo E., Drawbridge M., Bureau D. P. (2006). Effects of Lipid on Growth and Feed Utilization of White Seabass (Atractoscion Nobilis) Fingerlings. Aquaculture 253, 557–563. doi: 10.1016/j.aquaculture.2005.08.007
Mohseni M., Ozorio R. O. S. (2014). Effects of Dietary L-Carnitine Level on Growth Performance, Body Composition and Antioxidant Status Beluga (Huso Huso L. 1758). Aquac. Nutr. 20, 477–485. doi: 10.1111/anu.12100
Ozorio R. O. A., Escorcio C., Bessa R. J. B., Ramos B., Goncalves J. F. M. (2012). Comparative Effects of Dietary L-Carnitine Supplementation on Diploid and Triploid Rainbow Trout (Oncorhynchus Mykiss). Aquac. Nutr. 18, 189–201. doi: 10.1111/j.1365-2095.2011.00888.x
Ozorio R. O. A., Van Ginneken V. J. T., Bessa R. J. B., Verstegen M. W. A., Verreth J. A. J., Huisman B. A. (2010). Effects of Exercise on L-Carnitine and Lipid Metabolism in African Catfsh (Clarias Gariepinus) Fed Different Dietary L-Carnitine and Lipid Levels. Br. J. Nutr. 103, 1139–1150. doi: 10.1017/S0007114509993035
Pawlak M., Lefebvre P., Staels B. (2015). Molecular Mechanism of Pparα Action and Its Impact on Lipid Metabolism, Inflammation and Fibrosis in Non-Alcoholic Fatty Liver Disease. J. Hepatol. 62, 720–733. doi: 10.1016/j.jhep.2014.10.039
Sabzi E., Mohammadiazarm H., Salati A. P. (2017). Effect of Dietary L-Carnitine and Lipid Levels on Growth Performance, Blood Biochemical Parameters and Antioxidant Status in Juvenile Common Carp (Cyprinus Carpio). Aquaculture 480, 89–93. doi: 10.1016/j.aquaculture.2017.08.013
Santulli A., Modica A., Curatolo A., D'Amelio V. (1988). Carnitine Administration to Sea Bass (Dicentrarchus Labrax L.) During Feeding on a Fat Diet: Modification of Plasma Lipid Levels and Lipoprotein Pattern. Aquaculture 68, 345–351. doi: 10.1016/0044-8486(88)90248-7
Schuchardt D., Vergara J. M., Fernández-Palacios H., Kalinowski C. T., Hernández-Cruz C. M., Izquierdo M. S., et al. (2008). Effects of Different Dietary Protein and Lipid Levels on Growth, Feed Utilization and Body Composition of Red Porgy (Pagrus Pagrus) Fingerlings. Aquac. Nutr. 14, 1–9. doi: 10.1111/j.1365-2095.2007.00484.x
Selcuk Z., Tiril S. U., Alagil F., Belen V., Salman M., Cenesiz S., et al. (2010). Effects of Dietary L-Carnitine and Chromium Picolinate Supplementations on Performance and Some Serum Parameters in Rainbow Trout (Oncorhynchus Mykiss). Aquacult. Int. 18, 213–221. doi: 10.1007/s10499-008-9237-z
Song L. P., An L., Zhu Y. A., Li X., Wang A. Y. (2009). Effects of Dietary Lipids on Growth and Feed Utilization of Jade Perch, Scortum Barcoo. J. World Aquac. Soc 40, 266–273. doi: 10.1111/j.1749-7345.2009.00249.x
Tan X., Sun Z., Huang Z., Zhou C., Lin H., Tan L., et al. (2017). Effects of Dietary Hawthorn Extract on Growth Performance, Immune Responses, Growth- and Immune-Related Genes Expression of Juvenile Golden Pompano (Trachinotus Ovatus) and Its Susceptibility to Vibrio Harveyi Infection. Fish Shellfish Immunol. 70, 656–664. doi: 10.1016/j.fsi.2017.09.041
Wang J. T., Liu Y. J., Tian L. X., Mai K. S., Du Z. Y., Wang Y., et al. (2005). Effect of Dietary Lipid Level on Growth Performance, Lipid Deposition, Hepatic Lipogenesis in Juvenile Cobia (Rachycentron Canadum). Aquaculture 249, 439–447. doi: 10.1016/j.aquaculture.2005.04.038
Xie J., Liao S., Wang R., He X., Fang H., Zhuang Z., et al. (2020). Molecular Cloning, Functional Characterization and Expression Analysis of P65 Subunit of Golden Pompano (Trachinotus Ovatus) and Response to High Fat Diet and LPS Administration. Aquaculture 514, 734508. doi: 10.1016/j.aquaculture.2019.734508
Xu P., Lin H., Wang R., Yu W., Zhou C., Tan X., et al. (2021). Effects of Dietary Lipid Levels on Growth Performance, Plasma Biochemistry, Lipid Metabolism and Intestinal Microbiota of Juvenile Golden Pompano (Trachinotus Ovatus). Aquac. Nutri. 27, 1683–1698. doi: 10.1111/anu.13307
Yang S. D., Liu F. G., Liou C. H. (2012). Effects of Dietary L-Carnitine, Plant Proteins and Lipid Levels on Growth Performance, Body Composition, Blood Traits and Muscular Carnitine Status in Juvenile Silver Perch (Bidyanus Bidyanus). Aquaculture 342-343, 48–55. doi: 10.1016/j.aquaculture.2012.02.002
Yang S. D., Wen Y. C., Liuo C. H., Liu F. G. (2009). Influence of Dietary L-Carnitine on Growth, Biological Traits and Meat Quality in Tilapia. Aquac. Res. 40, 1374–1382. doi: 10.1111/j.1365-2109.2009.02234.x
Zhao W., Fang H. H., Gao B. Y., Dai C. M., Liu Z. Z., Zhang C. W., et al. (2020). Dietary Tribonema Sp. Supplementation Increased Growth Performance, Antioxidant Capacity, Immunity and Improved Hepatic Health in Golden Pompano (Trachinotus Ovatus). Aquaculture 529, 735667. doi: 10.1016/j.aquaculture.2020.735667
Zhao W., Fang H., Liu Z., Chen J., Zhang C., Gao B., et al. (2021). Responses in Growth Performance, Enzymatic Activity, Immune Function and Liver Health After Dietary Supplementation ofPorphyridium Sp. In Juvenile Golden Pompano (Trachinotus Ovatus). Aquac. Nutr. 27, 679–690. doi: 10.1111/anu.13214
Zheng J., Luo Z., Zhuo M., Pan Y., Song Y., Hu W., et al. (2014). Dietary L-Carnitine Supplementation Increases Lipid Deposition in the Liver and Muscle of Yellow Catfsh (Pelteobagrus Fulvidraco) Through Changes in Lipid Metabolism. Br. J. Nutr. 112, 698–708. doi: 10.1017/S0007114514001378
Keywords: L-Carnitine, growth performance, antioxidation, lipid metabolism, Trachinotus ovatus
Citation: Chen X, Niu J, Wang J and Zhao W (2022) Effects of L-carnitine Supplementation in High-Fat Diet on Growth, Antioxidant Capacity and Lipid Metabolism of Golden Pompano (Trachinotus ovatus). Front. Mar. Sci. 9:831153. doi: 10.3389/fmars.2022.831153
Received: 08 December 2021; Accepted: 23 March 2022;
Published: 13 April 2022.
Edited by:
Hüseyin Sevgili, Hüseyin Sevgili, TurkeyReviewed by:
Mansour Torfi Mozanzadeh, South Iran Aquaculture Research Center, IranOğuz Taşbozan, Cukurova University, Turkey
Copyright © 2022 Chen, Niu, Wang and Zhao. This is an open-access article distributed under the terms of the Creative Commons Attribution License (CC BY). The use, distribution or reproduction in other forums is permitted, provided the original author(s) and the copyright owner(s) are credited and that the original publication in this journal is cited, in accordance with accepted academic practice. No use, distribution or reproduction is permitted which does not comply with these terms.
*Correspondence: Jun Wang, anVubnl3YW5nQDE2My5jb20=; Wei Zhao, end0am54eXNjQDE2My5jb20=