- 1State Key Laboratory of Tropical Oceanography, Guangdong Key Laboratory of Ocean Remote Sensing, South China Sea Institute of Oceanology, Chinese Academy of Sciences, Guangzhou, China
- 2Southern Marine Science and Engineering Guangdong Laboratory (Guangzhou), Guangzhou, China
- 3University of Chinese Academy of Sciences, Beijing, China
- 4School of Marine Science, Sun Yat-sen University, Guangzhou, China
- 5Guangdong Provincial Key Laboratory of Marine Resources and Coastal Engineering, Guangzhou, China
Plastic pollution is one of the growing environmental problems in the world currently. The situation of microplastics (MPs) in the South China Sea (SCS) is not yet fully understood. This study investigated the spatial distribution, morphological characterization, and chemical composition of MPs in surface seawater in the southwestern SCS, based on cruise data in 2018. Our analysis shows that the average abundance of surface MPs in seawater was 0.072 ± 0.053 particles/m3 and 88.4% of MPs were <2 mm. 97.3% of MPs were fibers and fragments. Polyethylene terephthalate (PET), polyvinyl chloride (PVC), and cellophane were predominant polymer components of surface MPs. Higher MPs abundance (0.083 ± 0.063 particles/m3) and bigger MPs sizes were found at surface water in Nansha Islands than in Xisha Islands (0.032 ± 0.01 particles/m3). In addition, more polymer types of MPs were found in Nansha Islands, while more MPs films were found in Xisha Islands. PET and cellophane dominated in Nansha Islands, while PVC dominated in Xisha Islands with no cellophane detected. The surface MPs were low in abundance and diverse in polymers in the southwestern SCS with apparent differences between islands and between onshore and offshore, owing to plastics wastes from vessel traffics and some inhabited islands.
Introduction
Increasing quantities of waste debris originated from marine-based sources and land-based sources due to human activities have been gathered in the worldwide marine environment. Nearly 60–80% of marine debris is plastics (UNEP, 2005; GESAMP, 2010). Due to the difficulty of degrading, the oceans finally become one of the main sinks of waste plastics debris. Increasing input of waste plastics into the oceans has posed serious threats to the marine environment (Thompson et al., 2004; Li, 2019).
Microplastics (MPs) are defined as small plastics with particle sizes < 5 mm (Thompson et al., 2004; GESAMP, 2015). This debris is regarded as a threat to marine biota (Desforges et al., 2014). It has been reported that MPs present in all the types of aquatic environments around the world (Cózar et al., 2014; Eriksen et al., 2014) such as terrestrial aquatic ecosystem (Lin et al., 2018), beaches and coastal areas (Cheung et al., 2016; Huang et al., 2021), offshore waters (Lusher et al., 2015; Reisser et al., 2015; Waller et al., 2017), sediments (Thompson et al., 2004; Qiu et al., 2015), marginal seas (Cai et al., 2018), and islands (Ding et al., 2019; Huang et al., 2019; Wang et al., 2019b). Coastal cities, ports, shipping, poorly regulated coastal landfills, and dumping sites are all the important sources of MPs worldwide (Jambeck et al., 2015; Hughes et al., 2017). Marine MPs can originate from marine-based sources or land-based sources. However, land-based sources are the primary sources of MPs (marine-based sources including fishing, aquaculture, and shipping so on; land-based sources including wastewater effluent, run-off, and rivers) (Andrady, 2011). Furthermore, MPs can easily be ingested and threatened the health of living things and the sustainable development of marine ecosystems (Vandermeersch et al., 2015; Sun et al., 2017). Being ingested, MPs can transfer toxic chemicals into organisms as a carrier, such as invertebrates, fish, birds, and mammals, which could cause adverse physical or chemical threats to the health of organisms (Teuten et al., 2007; Cole et al., 2011; Wright et al., 2013; Desforges et al., 2014; McCormick et al., 2014). Although more and more studies have been focused on the source, transport, and fate of MPs in the marine environment, the total status and mechanism are still unclear (Harris et al., 2021; Li et al., 2022).
The South China Sea (SCS) is the biggest semi-enclosed marginal seas in the west Pacific with seasonal monsoon climate. Being surrounded by several developing countries with large populations, intensive industrial, agricultural, and shipping activities have resulted in considerable anthropogenic pressure to the SCS. Most of Xisha Islands and Nansha Islands, which lie in the western and southern parts of the SCS, respectively, are original coral reefs and atolls. Due to the considerable distance from mainland China, the status of MPs pollution in Xisha Islands and Nansha Islands is still unclear (Huang et al., 2020; Tan et al., 2020). Therefore, microscopic observations and spectrum identification of MPs sampled from a cruise in the southwestern SCS were used to detect the abundance and diversity of MPs in these remote reefs and atolls and finally compare the differences of MPs pollution between Xisha Islands and Nansha Islands. The aims of this study were: (1) to examine and compare the abundances and distributions of MPs in surface water around Xisha Islands and Nansha Islands, southwestern of the SCS and (2) to discuss the potential sources of MPs in the uninhabited islands.
Materials and Methods
Fields and Sampling
The major sampling sites were around Nansha Islands and another three sampling sites were around Zhongsha Island and Xisha Islands. Among the sites in Nansha Islands, three sampling sites were around Yongshu Islands and the rest were around southern reefs (Figure 1). Twenty two surface water samples were collected using a neuston trawl with a mesh size of 167 μm and an opening diameter of 60 cm from these remote reefs and atolls between March and April 2018. The meteorological conditions were stable in the SCS during this premonsoon period (Wang et al., 2009). Details of all the sampling sites are provided in Supplementary Table 1.
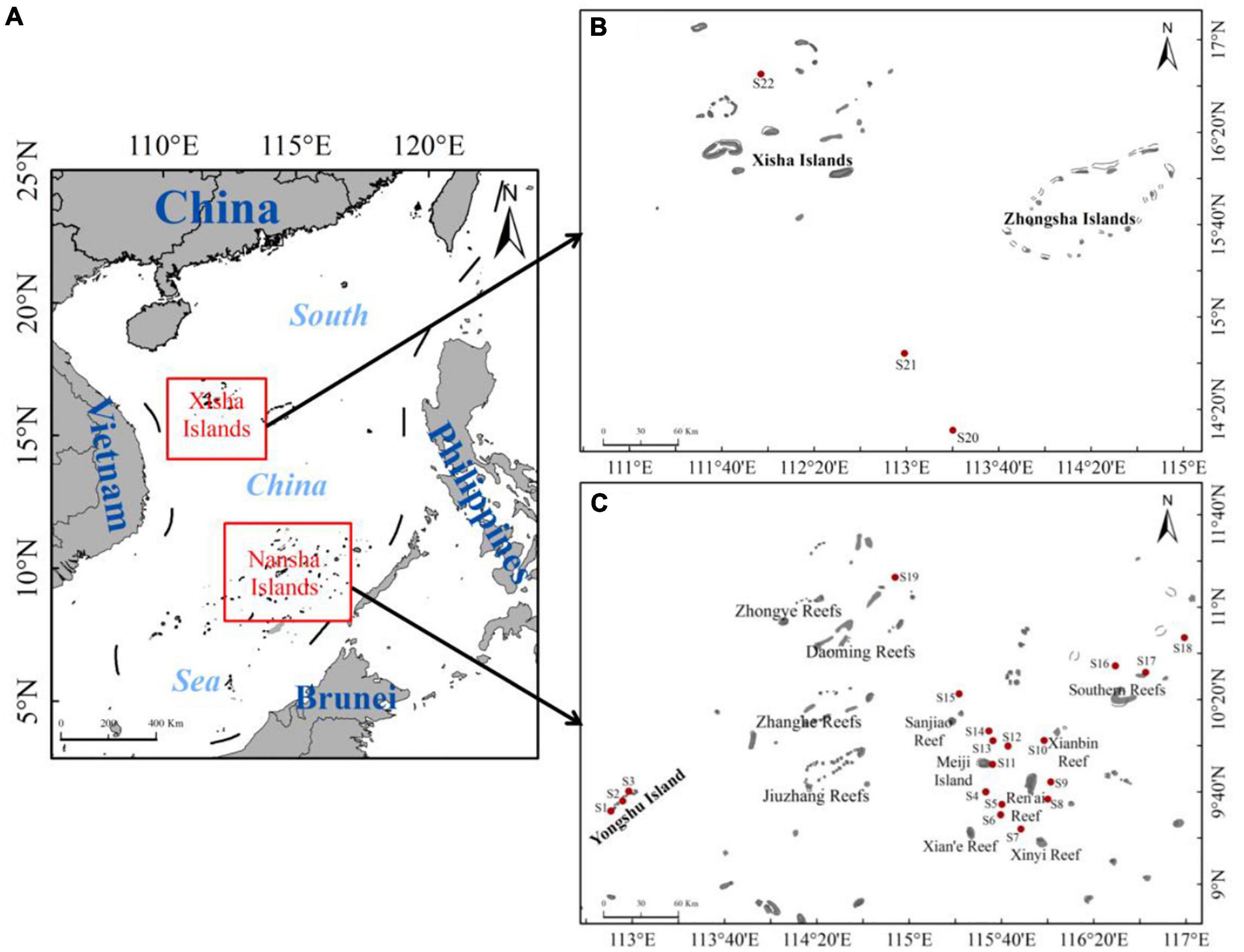
Figure 1. (A) Location of sampling sites in the South China Sea (SCS). (B) Three sampling sites near Xisha Islands. (C) Sampling sites around Nansha Islands in detail.
Extraction and Identification of Microplastics
In the laboratory, all the field samples were processed by filtration, elimination of organic matters, and refiltration (Su et al., 2016). Finally, the filter was placed into a clean petri dish and covered with aluminum foils for further observation (Lin et al., 2018). Careful visual sorting of residues is necessary to separate the plastic debris from other materials such as organic debris and other items (Hidalgo-Ruz et al., 2012). All the collected and processed filters were observed and identified carefully under a stereo light microscope (OLYMPUS SZX10, Tokyo, Japan) (Shaw and Day, 1994; Moore et al., 2002; Doyle et al., 2011). MPs images were taken with a digital camera (OLYMPUS DP80, Tokyo, Japan). The dominant color at surface of each MPs was recorded and their sizes were measured by the Image J software (1.46r, National Institutes of Health, Bethesda, Maryland, United States) (Lin et al., 2018; Tan et al., 2020). According to the external morphologies of MPs, shapes of MPs were categorized into fiber, fragment, and film. The abundance of MPs in this study was defined as the number of particles per cubic meter in surface water (particles/m3).
Every suspected MP was transferred by tweezers to the objective table and detected the composition by attenuated total reflection Fourier transformed infrared reflection (ATR-μ-FTIR, Spotlight 200i, PerkinElmer, Waltham, MA, United States). Each particle was scanned at least three times in the IR spectrum ranging from 750 to 4,000 cm–1 to obtain better spectra. The acquired spectra were compared with the standard FTIR spectrum databases using PerkinElmer Spectrum software (Waltham, Massachusetts, United States). The MPs composition was confirmed and recorded when spectral similarity was >80%.
Quality Assurance and Quality Control
According to the floatation of MPs, extensive precaution was carried out along the field sampling and sample analysis in the laboratory to restrict background pollution. The filter and trawl were rinsed with clean seawater (filtered with a 2-μm membrane filter, GF/F, Whatman) before and after each use on board. All the containers and experimental tools were rinsed three times with Milli-Q water. All the openings of the analysis devices were covered with aluminum foil to avoid cross-pollution from the air and other materials. Before use, the liquid used in the experiment was filtered through glass fiber filter (0.2 μm, GF/F, Whatman). After detecting every specimen using ATR-μ-FTIR, the objective surface was thoroughly cleaned with filtered anhydrous ethanol to avoid cross-contamination (Ding et al., 2019). A total of two blank laboratory procedures were setup, one for the laboratory filtration process (blank 1) and one for the microscopy process (blank 2). The blanks were treated with 250 ml Milli-Q water as the field samples. No MPs were found in blank 1 and only one microfiber was found in blank 2, which were subsequently identified as cotton fibers from cotton laboratory coats by μ-FTIR (Cai et al., 2018; Lin et al., 2018). In this case, our environment in the laboratory was considered clean and appropriate to carry out MPs experiments.
Statistical Analysis
All the sites plots were drawn by ArcGIS 10 software. Data analyses were performed by SPSS software version 20.0 (SPSS Incorporation, Armonk, New York, United States) and Microsoft Excel 2013. Numerical data are all presented as the mean ± SD. The differences between the abundances of MPs were performed as the one-way ANOVA (Webb et al., 2012). In all the cases, p < 0.05 was set as statistically significant.
Results
Abundance and Distribution of Microplastics
Microplastics were detected at all the sampling sites. Abundance of MPs varied widely and ranged from 0.015 to 0.239 particles/m3, with an average of 0.072 ± 0.053 particles/m3 (Figure 2A). The highest MPs abundance occurred in site S1 (0.239 particles/m3), located on the southern fringe of Yongshu Island (Figure 2C). The MPs abundances at all the three sites around Yongshu Island were much higher than the average level of all the sample sites. The MPs abundance at sampling sites S4 and S6 exceeded 0.1 particles/m3, surrounded by Ren’ai Reef, Meiji Island, and Xian’e Reef. Besides, the lowest MPs abundances were detected at S17 and S22, with 0.015 and 0.019 particles/m3, respectively. S17 is located in Southern Reefs and S22 is located in the north-central part of Xisha Islands (Figures 1A, 2B). The abundance at site S1 was almost 16 times greater than sites S17 and S22.
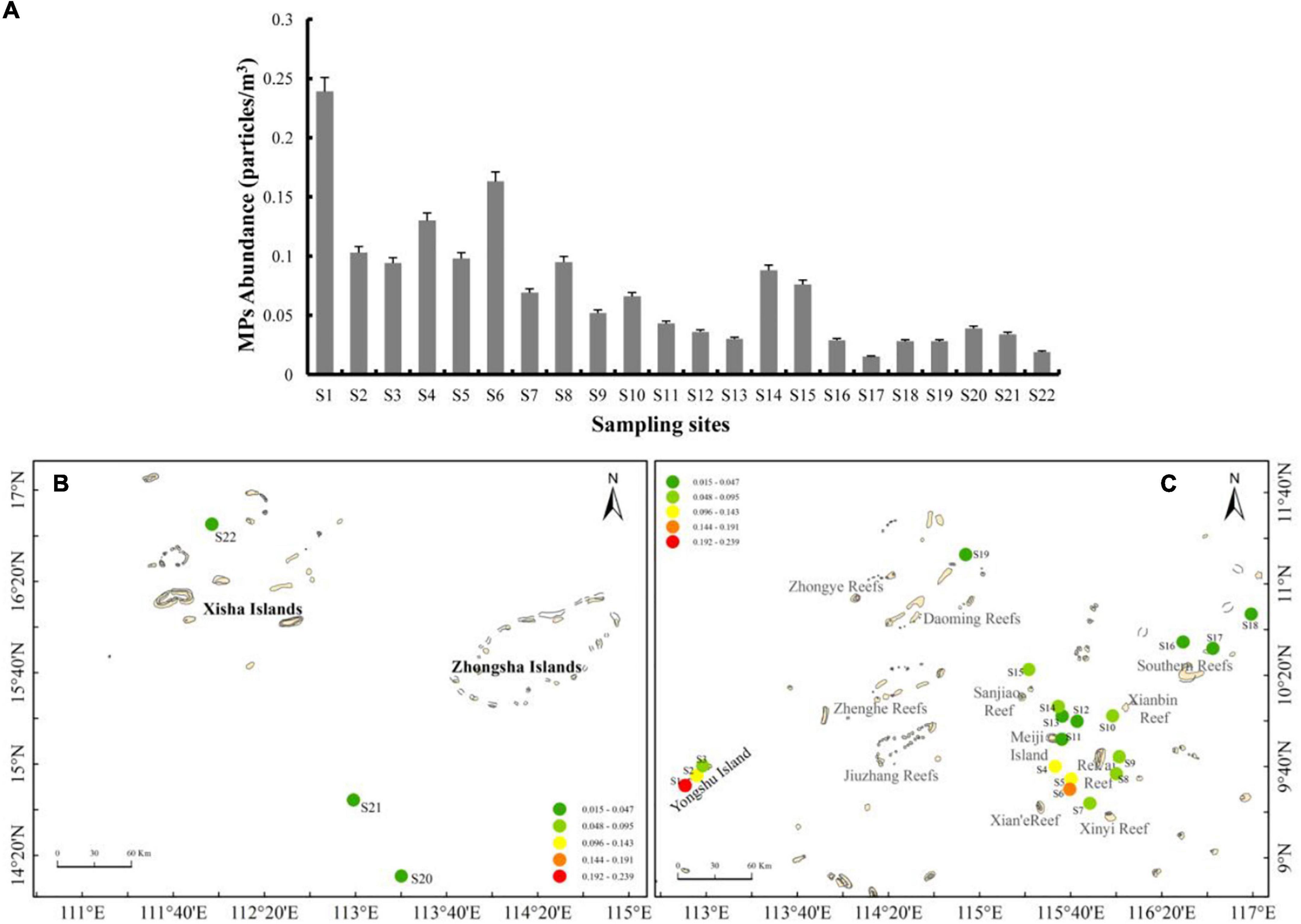
Figure 2. (A) Abundance of surface microplastics (MPs) in the sampling sites around the southwestern SCS. (B,C) Spatial distribution of MPs abundances in the surface water surrounding southwestern SCS.
There were nine sites with higher MPs abundances than the average level (S1, S2, S3, S4, S5, S6, S8, S14, and S15). All these sites are located at the east of nearest islands/reefs and all these nine sites are around Nansha Islands (Figure 2C). The MPs abundances in sites S1, S4, and S6 were 2–3 times higher than the average MPs abundances. Among these nine sites, S1, S2, and S3 are closest to Yongshu Island, where site S1 is in the south, S2 is in the east, and S3 is in the west of Yongshu Island (Figures 1C, 2C). MPs abundances of these three sites showed a decreasing trend from south to north. Sites S8 were located on the eastern waters of Meiji Island (Figure 2C). Both the Yongshu Island and Meiji Island are inhabited islands, which may account for the high MPs in these sites (Ministry of Civil Affairs of the People’s Republic of China, 2020).1
Sites S4–S15 are located around the scattered reefs in Nansha Islands such as Meiji Island and Xian’e Reef; sites S16–S18 are located in the Southern Reefs and S19 are located in the north of Daoming Reefs. The MPs abundances near these scattered reefs were higher than those near the Southern Reefs. Higher MPs abundances were mainly located in the south of reefs around Meiji Island or Ren’ai Reefs, with values larger than the average level (Figures 1C, 2C). The rest three sites, S20, S21, and S22, were all in low MPs abundances, located away from Nansha Islands and near Zhongsha Island or Xisha Islands (Figure 2B).
Surface Morphology of Microplastics: Shape, Size, and Color
In this study, three irregular shapes of MPs were observed (Figure 3), which accounted for 67.1% (fibers), 30.2% (fragments), and 2.7% (films) for all the collected MPs. The MPs fibers accounted for a much higher proportion than fragments (ANOVA, F = 8.594, p = 0.005) and films (ANOVA, F = 26.124, p < 0.001).
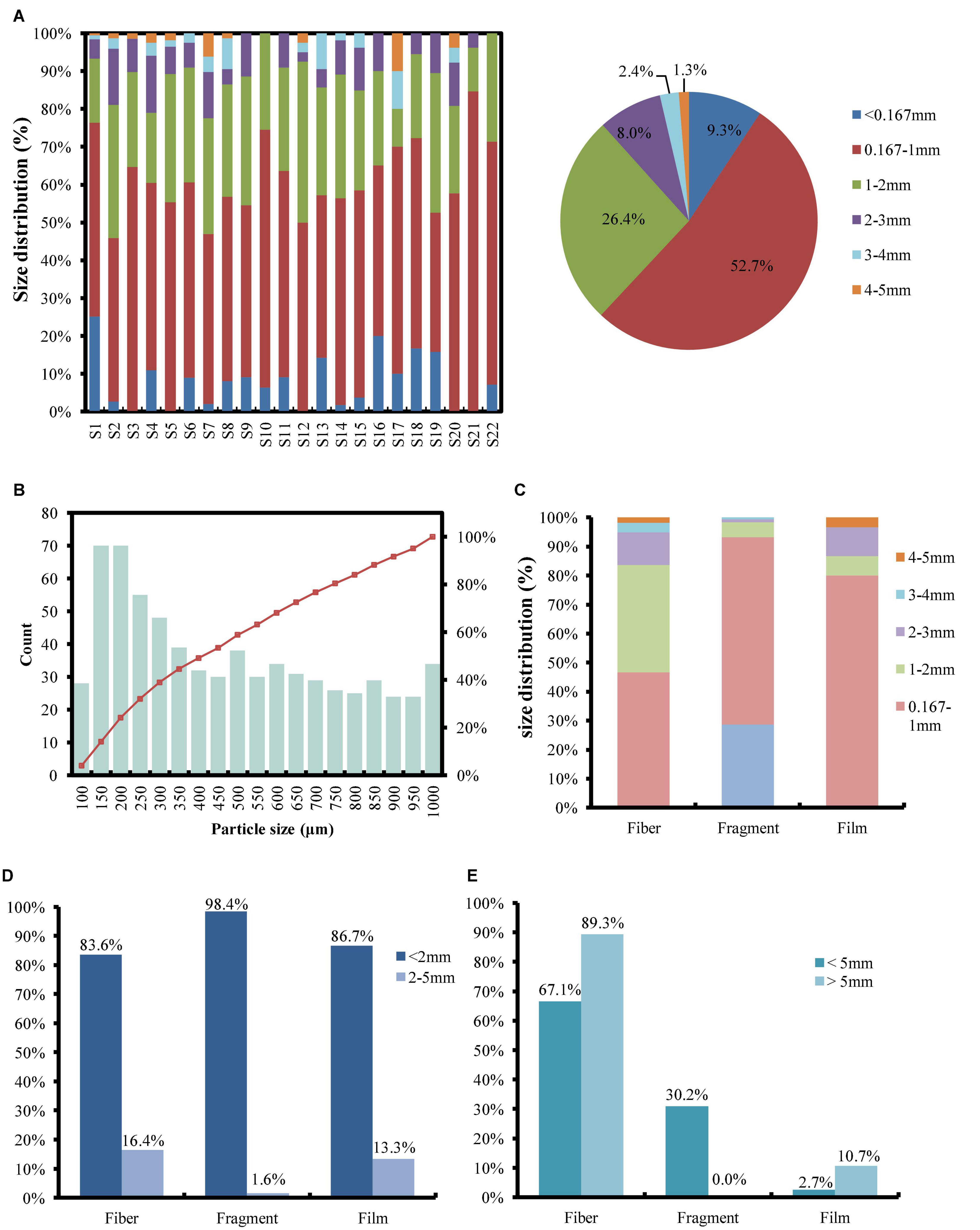
Figure 3. (A) The size distribution of surface MPs around the southwestern SCS. S20, S21, and S22 were located around Zhongsha Island and Xisha Islands. (B) Frequency distribution and cumulative curve of MPs particles <1 mm (n = 696). (C) The size distribution of different MPs shapes: fibers, fragments, and films. (D) The percentages of different shapes between MPs <2 mm and 2–5 mm. (E) The percentages of different shapes between MPs (<5 mm) and plastic debris >5 mm.
More than 80% of MPs are fibers at several sampling sites in terms of number (92% at site S2, 82.4% at site S11, 82.6% at site S13, 87.3% at site S14, 89.5% at site S19, and 86.7% at site S22) indicating that MPs fibers dominated at these sites. It should be noted that the average MPs abundances of these sites were relatively low with values < 0.1 particles/m3, except S2 and S14. MPs fragments were distributed in all the sites with percentages ranging from 6 to 50%, the highest proportion (50.2% at site S1) and the lowest proportion (6.6% at site S2) were near Yongshu Island. There were seven sites with more than 30% of MPs fragments, which were located near Yongshu Island (site S1), Ren’ai Reef and Xianbin Reef (sites S4, S6, S8, and S10), and Southern Reef (site S16 and S18). Although the fraction of films in all the three MPs shapes was only 2.7%, it distributed in ∼60% of the sampling sites. There were a few fragmentary distributions at sites S1–S9 near Yongshu Island, Meiji Island, and Ren’ai Reef, with the highest percentage at site S21 (28.6%), near Xisha Islands (Supplementary Figure 1).
The mean size of MPs particles was 991.46 ± 879.68 μm. The smallest particle size of all the MPs samples was 53.26 μm and the largest particle size of all the MPs samples was 4867.87 μm. Large plastic debris (>5 mm) was also found at more than half of the sampling sites. Their sizes ranged from 5.01 to 35.94 mm and accounted for 2.31% in the quantity of all the detected plastic debris. The MPs were categorized into six size classes based on their diameter (< 0.167, 0.167–1, 1–2, 2–3, 3–4, and 4–5 mm) (Figure 3A).
Microplastics with 0.167–1 mm accounted for the highest proportion in all the six classes (52.7%). 88.4% of the MPs were smaller than 2 mm, of which 26.4% of MPs were between 1 and 2 mm and 9.3% of MPs were < 0.167 mm (Figure 3A). The variations of MPs abundance with size < 1 mm from S1 to S22 presented a similar trend as that of total MPs abundances, with a significant positive correlation (r = 0.97, p < 0.001). Except for sites S2 and S7 with 45% of MPs < 1 mm, all the remaining sites contained 50% MPs with sizes < 1 mm. 44.5% of MPs particles with size of 0.167–1 mm were <350 μm and mainly concentrated in the range of 150–250 μm (Figure 3B). Thus, smaller-sized MPs (< 2 mm) dominated the MPs in our study area.
The population of MPs fibers with a size of 0.167–1 mm was the largest among all the MPs fibers followed by MPs fibers with the size of 1–2 mm and MPs fibers with size < 0.167 mm were low. The population of MPs fibers with size < 2 mm accounted for 83.6% of the total number of MP fibers (Figures 3C,D). ∼95% of MPs with size < 0.167 mm were MPs fragments and the diameter of MPs fragments was all < 3 mm. MPs fragments < 2 mm accounted for 98% of the total population of MPs fragments (Figure 3D). No MPs film with size < 0.167 mm was detected and 73% of MPs films were of size between 0.167 and 1 mm. Although fraction of MPs fibers in size of 4–5 mm was the lowest in the population of MPs fibers, the population of MPs fibers in size of 4–5 mm was larger than that of MPs fragments and films. In 4–5 mm, the proportion of MPs films was the highest (Figure 3C). The highest proportion of MPs particles < 2 mm was MPs fragments, followed by fibers and films (Figure 3D). Thus, size distribution of MPs fibers was the broadest and continuous, while MPs fragments and films were mainly concentrated in the size of < 2 mm. The MPs fragments were all < 5 mm compared to the other two shapes of MPs. Nearly 90% of the MPs particles > 5 mm were MPs fibers and the remaining small portion was MPs films (Figure 3E).
Several colors were observed from the surface of all the MPs samples. The dominant color of MPs is blue (45.6%), followed by black (24.2%), transparent (12.3%), red (10.1%), yellow (4.7%), green (2.9%), and white (0.2%) (Supplementary Figure 2). The blue and black MPs account for almost 70% of the observed MPs particles and the transparent and red MPs account for ∼22.4%. Transparent and yellow MPs accounted for a larger proportion of MPs > 3 mm and most of the plastic debris > 5 mm was transparent. The color distribution and proportion of MPs varied at each sampling site. Blue, black, and transparent MPs particles appeared at all the sampling sites. The proportion of white MPs particles in this study was only 0.2%, found at site S20 near Zhongsha Island and site S22 near Xisha Islands. No white MPs particle was found at all the Nansha Islands sampling sites.
The proportions of the colors in the three different shapes of MPs particles were also diverse. All the seven colors were found in MPs fibers and fragments, but black, red, and white MPs films were not detected. The prominent colors of MPs fibers were black (35%), blue (30%), and red and transparent (13%, respectively). 84.4% of MPs fragments were blue and the rest ∼15% of MPs fragments were in the other five colors. 79% of the MPs films were transparent, while green, blue, and yellow accounted for the remaining 21% (Supplementary Figure 2).
Microplastics Composition and Diversity
According to μ-FTIR results of chemical characterization identification of MPs, 26.3% of the total suspected particles were removed because the matching spectral characteristics were <80% of standard polymers. There are 27 plastic polymers detected in the rest of the samples. The components of the six conventional plastic polymers accounted for ∼75% of all the MPs pieces including polyethylene terephthalate (PET) (30.8%), polyvinyl chloride (PVC) (13.5%), cellophane (12.6%), polypropylene (PP) (8.1%), vinylidene chloride/vinyl chloride copolymer (PVDC) (Saran, 6%), and polyamide 6 (PA6) (Nylon 6, 3.4%). The other 21 polymers accounted for 25.6% of the total MPs (Figure 4A). Combining the distribution of various polymers at all the sampling sites, ∼30% of the entire PET composition were detected at site S8. In comparison, about 17% of PVC, 42% of PP, and 35% of PVDC were documented at site S6. These two sites are located near Ren’ai Reef. 22% of cellophanes were gathered at site S3 near northern Yongshu Island (Figure 4B). There is a positive correlation between MPs abundances and the diversity of polymers types (r = 0.730, p < 0.01). For example, sites with much higher MPs abundance than averaged level also contained more MPs polymer types such as S1 (0.239 particles/m3), S4 (0.13 particles/m3), and S6 (0.163 particles/m3) (Figures 4B,C). However, the MPs polymers did not present a significant spatial distribution, despite the correlation between MPs abundances and polymers types.
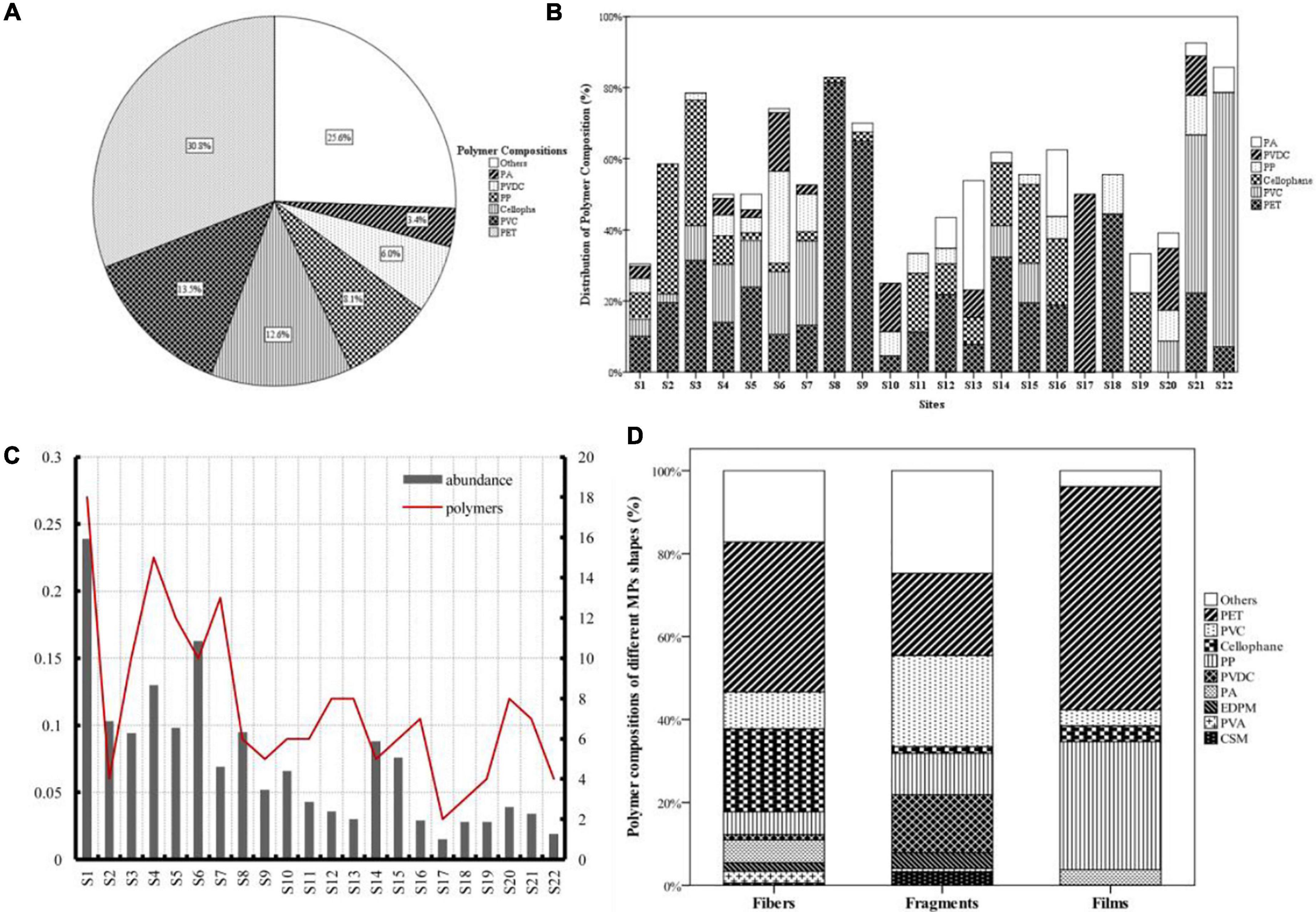
Figure 4. (A,B) The proportions of six major polymer of surface MPs around the southwestern SCS. S20, S21, and S22 were located around Zhongsha Island and Xisha Islands. (C) MPs abundances and total polymer types at all the sampling sites. (D) The proportions of different shapes of MPs polymers: fibers, fragments, and films.
Five main polymer components of the MPs fibers were PET (36.2%), cellophane (20.1%), PVC (8.9%), PP, and PA (5.5%, respectively) (Figure 4D), which are all the common plastics used in clothing fabrics, industrial fabrics, engineering, and construction. The average diameter and median diameter of MPs fibers were 1.29 and 1.065 mm, respectively, which were much bigger than that of MP fibers (<0.5 mm) discharged from wastewater treatment systems. Only 13.4% of the MPs fibers in this study were < 0.5 mm, which indicated that clothing fiber shedding was not the primary source of fibers, but fishing activities and industrial fabrics on ships along their paths were the primary sources of MPs fibers in this region.
The average diameter of MPs fragments in this study was 0.37 mm, much smaller than those of MPs fibers and films. The MPs fragments were irregular in shape and hard in texture. PVC (21.9%), PET (19.8%), PVDC (14%), and PP (9.9%) accounted for 65.6% of total MPs fragments (Figure 4D). PVC is divided into two types, rigid PVC and soft PVC. Rigid PVC is commonly used for plastic profiles, pipes, and packaging materials, while soft PVC is used for hoses, cables wires, and various molds. PET and PVDC are mostly used for disposable packaging such as beverage bottles and food packaging. The average diameter of MPs films was 1.15 mm and 74.5% of MPs films were transparent. Nearly 85% of MPs films were composed of PET (53.8%) and PP (30.8%) (Figure 4D), both of which were mainly used for electrical insulation materials and packaging. MPs fibers and fragments had a wide variety of polymer compositions. They were mostly colored, while MPs films were relatively concentrated in a smaller number of polymers and were mostly transparent.
Microplastics Pollution in Nansha Islands and Xisha Islands
The abundance of floating MPs on the sea surface of Nansha Islands (0.083 ± 0.063 particles/m3) was 2.5 times higher than that in Xisha Islands (0.032 ± 0.01 particles/m3). It was also slightly higher than the average abundance of MPs in the southwestern SCS (0.072 ± 0.053 particles/m3) in this study. MPs fibers and fragments dominated both the Xisha Islands and Nansha Islands, but percentage of MPs films in Xisha Islands (12.1%) was nearly six times higher than that in Nansha Islands (2%) (F = 46.885, p = 0.005).
A total of 88.1% of MPs were with size < 2 mm in Nansha Islands, of which 55.4% were MPs fibers, 31.1% were MPs fragments, and 1.6% were MPs films. Abundances of MPs with 2–5 mm accounted for 11.9% of total MPs, of which 11.1% were long MPs fibers. In contrast, 90.9% of MPs particles in Xisha Islands were < 2 mm with a vast majority of fibers (60.6%), while MPs fragments and MPs films together only accounted for the remaining 30.3%. MPs fibers, fragments, and films with 2–5 mm were all found in Nansha Islands, while no films larger than 2 mm were found in Xisha Islands (Table 1). In general, the diameters of MPs particles in Xisha Islands were relatively smaller, especially the MPs films which all the sizes were < 2 mm. MPs in Nansha Islands and Xisha Islands were mainly in blue, black, and transparent and the proportions of MPs in these three colors were slightly different. The blue MPs (42.7%) proportion was three times higher than that of transparent MPs (12.8%) in Nansha Islands. The ratio of MPs in these three main colors in Xisha Islands was relatively consistent and the ratio of transparent MPs in Xisha Islands (25.6%) was twice as high as that in Nansha Islands (12.8%).
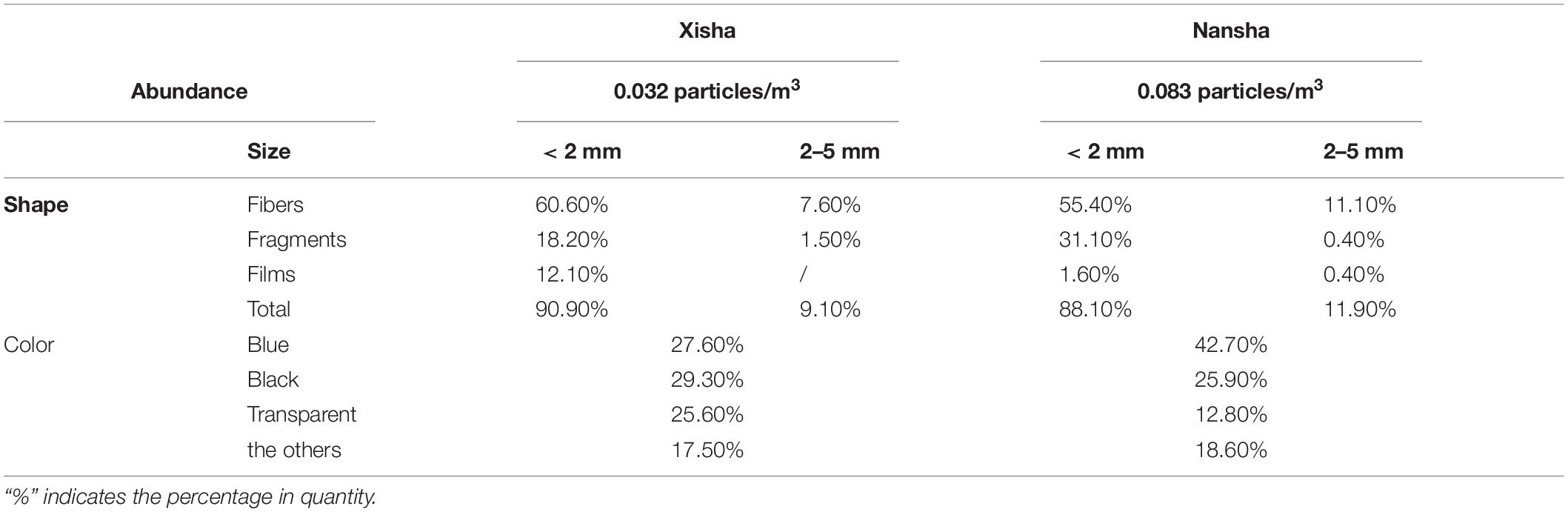
Table 1. Comparison of the abundance and external morphological characteristics of microplastics (MPs) in Xisha Islands and Nansha Islands.
The average abundances of the floating MPs of Xisha Islands and Nansha Islands were different. In addition, the MPs fibers was the dominant shape of MPs in Xisha Islands and Nansha Islands, while the proportion of MPs films was higher in Xisha Islands than that in Nansha Islands and the proportion of MPs fragments was higher in Nansha Islands than that in Xisha Islands. The MPs particles with size < 2 mm differed between Xisha Islands and Nansha Islands. There are more MPs films < 2 mm in Xisha Islands than Nansha Islands. The main components of MPs at Nansha Islands were PET (22.6%) and Cellophane (11.2%), while PVC accounted for 41.5% at Xisha Islands and no cellophane was detected.
High proportion of suspected MPs particles in Xisha Islands (85.80%) were identified as polymers, while lower in Nansha Islands (60.6%). There was dominant polymer at Xisha Islands, with PVC accounting for > 40% of the six common polymers. No dominant polymer of MPs was observed at Nansha Islands, with the total percentages of six common polymers accounted for almost 50% of total MPs (Figure 5). However, the ANOVA results showed no statistically significant difference in the polymer composition of MPs from Nansha Islands and Xisha Islands (F = 2.135, p = 0.172).
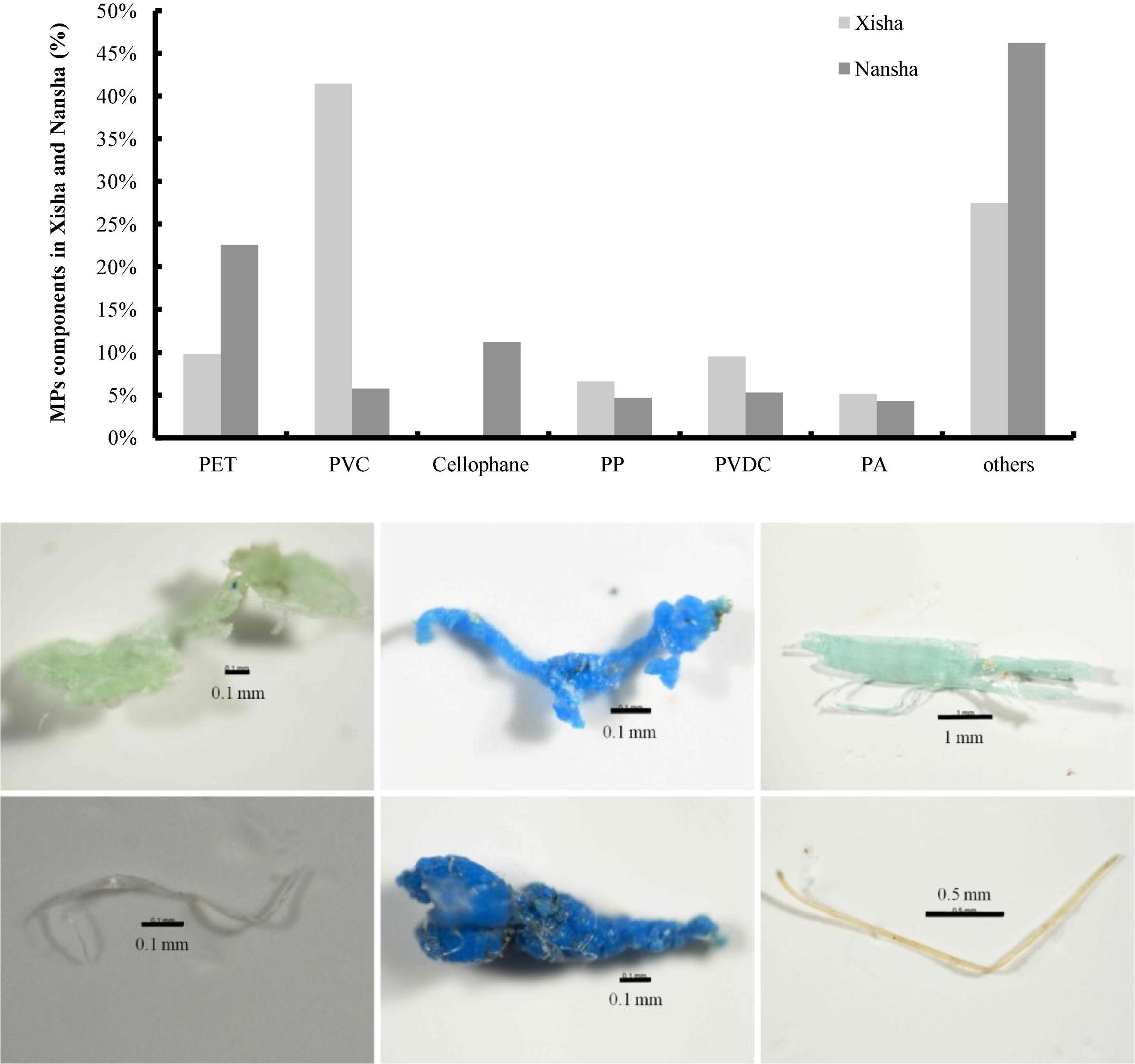
Figure 5. The proportions of six prominent MPs polymers in surface water around Xisha Islands and Nansha Islands. The figures below show each representative MPs particle of the six main polymers, the first row from left to right is polyethylene terephthalate (PET), polyvinyl chloride (PVC), and cellophane; the second row from left to right is polypropylene (PP), PVDC, and polyamide (PA). These MPs particles had a μ-FTIR spectral match of 85% or more.
Discussion
Microplastics Pollution Status in the Overall Southwestern South China Sea and Different Islands
Our results indicated varying degrees of MPs pollution in the southwestern SCS. The abundances of MPs collected by neuston trawl in this study were approximately similar to the plankton trawling sampling in previous studies (Cai et al., 2018; Wang et al., 2019b; Tan et al., 2020). The MPs abundance reported in this study was low in the global ocean (Supplementary Table 2). The MPs abundance in the southwestern SCS (0.072 ± 0.053 particles/m3) was slightly higher than the overall MPs abundance in the SCS (0.045 ± 0.093 particles/m3) (Cai et al., 2018). The average abundance of MPs in the SCS was one order of magnitude lower than that in the East China Sea and the Bohai Sea, while the East China Sea (0.167 ± 0.138 particles/m3) was about 2.3 times higher and the Bohai Sea (0.33 ± 0.34 particles/m3) was about 4.5 times higher than that in the southwestern SCS (Zhao et al., 2014; Zhang et al., 2017). Although there are lots of islands in the southwestern SCS, the input of MPs from land-based sources is limited due to sparse population, low human production, and livelihood activities (Browne et al., 2011; Cheung et al., 2016; Cai et al., 2018). Similarly, compared with the neighboring inshore waters in the western Pacific, the MPs abundance in the SCS is significantly lower than that of Korean (47 ± 192 pieces/m3) (Song et al., 2014). The current reported MPs count in Japanese inshore waters was 1.72 pieces/m3, which was calculated based on observed averaged concentration (3.7 pieces/m3) (Isobe et al., 2015). The abundance of MPs in coastal waters in the northern SCS, such as Beibu Gulf (Zhu J. et al., 2019; Huang et al., 2021), Hainan Island (Qi et al., 2020), and the Pearl River Estuary (Fu et al., 2003; Zhou et al., 2011; Lin et al., 2018), is much higher than that in our study area. These observations indicated that MPs highly pollute the densely populated offshore areas with intensive human activities. The floating MPs in seawater also show an evident influence by land-based sources. In addition, the MPs abundance in the SCS was at a low level compared to the other oceans or seas in the world such as the Mediterranean Sea (Collignon et al., 2012), Atlantic Ocean (Lusher et al., 2014; Enders et al., 2015), northern Gulf of Mexico (Di Mauro et al., 2017), and Georgia Strait, Canada (Desforges et al., 2014; Supplementary Table 2).
The initial plastic forms can determine the MPs shapes, especially when the plastics enter the oceans as wastes or litter. Aging processes such as mechanical wear and tear, photo-oxidative aging, and biological contamination then change the shape of plastic debris. Although plastics degrade as soon as they come into contact with air, sunlight, and water, it takes time due to their resistance to degrade (Andrady, 2011). After entering the marine environment, seawater properties and movements such as waves and circulation can affect the weathering and fragmentation of plastics (Ter Halle et al., 2017). The comparative analysis of MPs showed that the external morphological characteristics of MPs were significantly different between Xisha Islands and Nansha Islands. Still, the abundances and polymer compositions of MPs were not quite different. The numbers of vessel activities in inhabited islands in Xisha Islans are much higher than that in Nansha Islands, which reveal that the current land-based emissions and sea-borne vessel activities are still the primary sources of plastic polymers entering the SCS. More data are needed to further elucidate the effects of the complex circulation and seawater movements on the degradation and aging of MPs in the SCS.
Due to the tremendous spatial heterogeneity of MPs in the ocean (Suaria et al., 2020; Zambrano et al., 2020), the different numbers and distributions of sampling sites in Xisha Islands and Nansha Islands may result in differences in area-averaged MPs abundance. The one-way ANOVA showed that the difference of the average MPs abundance between Xisha Islands and Nansha Islands was within the sampling error. Indeed, adding more sampling sites near Xisha Islands will help to improve the accuracy of this conclusion.
Effect of Different Sampling and Detection Methods on the Results
Different sampling methods can lead to variations in MPs abundances such as using different sampling devices with varying sizes of mesh, using different units to represent the abundances of MPs, and also different detection guidelines that can lead to variations in MPs compositions and MPs shapes.
At present, there are two methods for collecting MPs samples in sea surface water, namely, trawl sampling and pump water sampling. The results from these two methods are different from each other. Trawl sampling was used in this study and the various mesh sizes of the trawl nets may lead to several orders of magnitude differences in the measurement results. The main sampling sites in this study were near the islands and reefs of Nansha Islands and Xisha Islands. Compared with previous studies on MPs pollution near the islands and reefs in the SCS (Supplementary Table 2), the difference in MPs abundance between trawl sampling with a diameter of mesh ranged from 160 to 333 μm was within one order of magnitude (Wang et al., 2019b; Tan et al., 2020).
In contrast, the difference in MPs abundance between pumping and trawl sampling can be as high as 5–6 orders of magnitude because the range of membrane diameters used for pumped water sampling was generally less than 50 μm (Huang et al., 2019, 2020; Nie et al., 2019; Md Amin et al., 2020). These differences indicated that most sizes of the floating MPs are smaller than 160 μm or even 100 μm. Therefore, smaller pore sizes for pumping water sampling can provide a more comprehensive picture of the abundances and contamination of floating MPs at the sea surface. This study showed that the MPs abundance near Nansha Islands (0.083 ± 0.063 particles/m3) was higher than that near Xisha Islands (0.032 ± 0.01 particles/m3) through trawl sampling. However, only the MPs abundance data from pumping water sampling (filter membrane pore size 20–45 μm) in Xisha islands can be found in previous studies. Some previous studies found that MPs abundances were higher in Nansha Islands than in Xisha Islands (Huang et al., 2019, 2020; Nie et al., 2019), but Ding et al. (2019) found that the MPs concentration in Xisha Islnads (6.3 particles/L) was higher than that in Terengganu Island (3.3 particles/L) in the southern SCS (Md Amin et al., 2020). Given high spatial and temporal heterogeneity of MPs in the environment, it requires various sample sizes and combined sampling methods to achieve statistically detectable changes in MPs abundances (Ryan et al., 2009).
In addition to the effect of different sampling methods, various detection methods will also affect MPs compositions and apparent features. For example, in some studies, the polymer identification was randomly selected from the microscopically examined suspected MPs particles (Cai et al., 2018; Lin et al., 2018; Wang et al., 2019b; Tan et al., 2020) instead of identifying all the suspected MPs, so the identification results may be biased and unable to fully reflect the real situation of the samples. In this study, all the suspected MPs were identified by μ-FTIR to avoid errors in the results caused by random sampling as much as possible. Nevertheless, there is still no adequate standard for MPs sampling and identification up to now and many efforts are needed to improve the accuracy of both the microscopic examination and μ-FTIR identification of MPs. Thus, it is necessary to develop a unified, efficient, and less error-prone standard for MPs experiments as soon as possible.
Shape recognition is another part of MPs detections. Three shapes were recognized in these MPs samples: fibers, fragments, and films. The percentages of MPs polymer were inconsistent for different shapes, with the highest percentage of 73.9% and 65.7% for MPs films and fragments, respectively. Although MPs fibers accounted for the highest total sample volume, only 38.7% of the microfibers were identified as polymers by μ-FTIR. MPs films and fragments had significantly higher polymer percentage because the surface characteristics of these two shapes of MPs were obvious and the accuracy of microscopic polymer detection was higher. It is more challenging to distinguish man-made recycled fibers, plant and animal fibers, and polymer fibers by microscope, so there is a substantial degree of uncertainty in the results of microscopic examination.
Relationship Between Each Characteristic of Microplastics and Comparisons of Their Regional Differences
In our results, the percentage of MPs fibers was the highest, the percentage of MPs fragments was the second, and the percentage of MPs films was the least. There were 88.4% of MPs with sizes smaller than 2 mm and 62% of MPs with sizes smaller than 1 mm in this study. The sharpness of the edges of plastic fragments is an indicator for the age of MPs in the sea (Shaw and Day, 1994; Hidalgo-Ruz et al., 2012; Rodríguez-Seijo and Pereira, 2016; Pan et al., 2019). In this study, there are few samples with sharp edges. MPs fibers, fragments, and films were all detected with relatively smooth edges and aged bifurcation appeared around the edge of some fibers, indicating that the aging fibers may split into much smaller microshapes.
Different proportions of MPs were in different colors and different shapes. However, the blue and black MPs were the most abundant, while 80% of MPs fragments were blue and 78.8% of MPs films were transparent. Various colors of MPs may show great similarity to the natural marine food, which may lead to ingestion by mistake by marine biota (Wright et al., 2013). Our results were inconsistent with early research (around Zhongye, Daoming, Jiuzhang, and Zhenghe Reefs) near Nansha Islands, where dominant colors were transparent and green (Tan et al., 2020). The blue and black MPs dominated our research area, which was different from the Pearl River surface water where the main colors of MPs were white and red (Lin et al., 2018). In addition, no microbeads (pellets) were observed in all the MPs samples in this study, which agreed with the results of previous studies in Pearl River (Lin et al., 2018) and in Nansha Islands (Tan et al., 2020). There were no significant spatial characteristics of different shapes of MPs. Twenty five plastic fibers and three plastic films were identified with the sizes > 5 mm (Figure 3E), which was much less than the 182 particles detected in a previous study (Tan et al., 2020).
Related findings from the other areas in the SCS showed that blue and transparent MPs were the most abundant near Zhubi Reef (Huang et al., 2019). Transparent MPs were more abundant than blue near Xisha Islands (Huang et al., 2020), while blue and white MPs were the most abundant near Nanxun Islands (Nie et al., 2019) and black MPs was the largest proportion in Haikou Bay (Qi et al., 2020). Moreover, blue MPs were the most abundant in the seabirds on Yongxing Island, followed by black MPs (Zhu C. et al., 2019). There were more transparent MPs in other areas of Nansha Islands, especially the transparent MPs films. In this study, colored MP fragments were the most abundant, followed by colored MPs fibers. The percentage of colored MPs fragments was consistent with previous studies mentioned above, except for the higher rate of transparent MPs films. Among the colored MPs, blue MPs fragments and black and red MPs fibers were the most abundant. Similar to the results of pumping water sampling (sampling mesh < 100 μm) in previous studies, this study also found a higher proportion of blue MPs particles. Results also revealed that MPs fragments had smaller particle diameters than MPs fibers and films, which may be related to long-distance transport and the greater susceptibility of hard plastics to abrasion (Tan et al., 2020).
According to Suaria et al. (2020), 92% of the floating microfibers on the sea surface were plant and animal fibers and regenerated fibers and only 8% were synthetic fibers. MPs fibers were mainly from fiber shedding during laundering of clothing, weathering, and shredding of industrial fabrics such as canvas, tarpaulins, fishing nets, and cables (Browne et al., 2011; Wang et al., 2019b). The proportion of polymers in all the three shapes of MPs particles in this study was much higher than that in global ocean surface (Suaria et al., 2020). This result may be related to the semi-enclosed characteristics of the SCS and the study of Suaria et al. (2020) is a global average abundance counting only microfibers polymers. In this study, longer MPs fibers (>2 mm) accounted for 16.4% of the total plastic fibers and shorter MPs fibers (<2 mm) accounted for the rest 83.6% of the total plastic fibers. The shorter MPs fibers may be derived from the decomposition of longer plastic fibers or atmospheric deposition because of their small size (Liu et al., 2019; Suaria et al., 2020). The large proportion of shorter MPs fibers in this study suggested that the MPs fibers in this area may have been disintegrated in the ocean or deposited from the atmosphere for a long time. The overall particles sizes of MPs fragments were small in this study, with 90% less than 1 mm in diameter and 98.4% less than 2 mm in diameter, while 86.7% of the MPs films were less than 2 mm in diameter. Irregular, hard, and millimeter-sized pieces and particles of PE and PP may originate from decomposing larger hard plastic wastes (Wang et al., 2019b).
All the common plastic polymer components were found in the sea surface floating MPs. In this study, MPs fibers and films were mostly made of PET and cellophane and MPs fragments components were the most abundant in polymers of PVC, PET, and PVDC. Compared with the results from other waters in the SCS, the primary polymer components of MPs in surface seawater were PE, urethane alkyd/Alkyd resin (UA), PET, PP, PA, and PVC (Cai et al., 2018; Wang et al., 2019b; Tan et al., 2020). The most polymer components of MPs in the sand of some island in the SCS were PP, PE, and PET (Zhang et al., 2019) and the polymers of MPs in the surface water of Xisha Islands were PET and PP (Huang et al., 2020). Compared with other seas in China, the polymers of MPs in surface water of the Bohai Sea were mainly PE, PP, PS, and PET (Zhang et al., 2017);, while as PE, PP, and cellophane in the Yellow Sea (Li and Sun, 2020) and PE, PP, and polystyrene (PS)-poly(styrene:vinyl alcohol) (VA) copolymers in the East China Sea (Liu et al., 2018). These results showed that PE and PET were the main polymer components of MPs in the surface waters of the Chinese offshore and distant seas. The common polymer components of MPs in the Pearl River were PP, PE, and PET (Lin et al., 2018); PA and cellophane (Yan et al., 2019), which was different with PVC, are the most frequently detected polymers in MPs from Changjiang Estuary (Xu et al., 2018).
Due to the still unclear spatial heterogeneity of MPs, the main polymers of MPs near the southern coast of Korea were Alkyd Paint particles (Song et al., 2014). In contrast, the main polymers of MPs in the northern Gulf of Mexico were cellophane, PE, and Alkyd resin (Di Mauro et al., 2017), which were somewhat similar to the SCS (Supplementary Table 3). These polymers can be found almost everywhere in contemporary human production and life. Cellophane is an organic cellulose-based polymer that has been used in food packaging and cigarette wrappers. It is also used as a release agent in fiberglass and rubber products and is often used as a coating in combination with synthetic polymers (Yang et al., 2015; Zhu L. et al., 2019). Synthetic plastic fibers are mainly used in garment manufacturing and production tools (fishing nets, ropes, and threads, etc.). Fibers have been documented as the leading MPs pollutants in domestic wastewater from rivers, estuaries, marine aquatic systems, and wastewater treatment plants (Wang et al., 2019b; Suaria et al., 2020). The average and median diameter of MPs fibers in this study were 1.29 and 1.065 mm, respectively, and MPs fibers discharged from wastewater treatment systems were usually < 0.5 mm (Lin et al., 2018; Wang et al., 2019a). Only 13.4% of the MPs fibers in this study were < 0.5 mm, indicating that shedding clothing fibers from wastewater was not the primary source of MPs fibers. The industrial fabrics from fishing activities and marine vessels were the primary MPs sources in the southwestern SCS. Possible sources of PA include clothing, packaging, and fishing (Yan et al., 2019). PE is widely applied in food packaging bags and agricultural film (Zhu et al., 2018). PP is generally used in fishing tools (Nie et al., 2019). In addition, the proportion of MPs fibers > 2 mm was higher in Nansha Islands than in Xisha Islands, which may imply that more fishing nets or ropes or threads aged or abandoned in Nansha Islands. These may come from intentional or unintentional actions of fishermen in this region. The difference in MPs pollution in Xisha Islands and Nansha Islands indicated the need to strengthen control of plastic waste treatment from ships and fishing activities, raise the awareness of environmental protection in people, and reduce the man-made plastic waste at sea, especially at the open sea.
Overall, this study showed a high spatial heterogeneity of MPs and there is no obvious distribution pattern in the open sea. In addition to the significant influence of MPs from land-based emissions, atmospheric deposition is also a non-negligible source for marine MPs. Indeed, more data are necessary to further understand the life cycles and detailed distributions of MPs in the SCS.
Conclusion
This study highlighted that the low MPs abundance but diverse polymer types were found in the southwestern SCS. The mixed mixture of the various MPs particles resulted from long-distance transportation. The average MPs abundance in the southwest SCS was 0.072 ± 0.053 particles/m3, more than 80% of MPs were < 2 mm, and the main polymer types were PET, PVC, and cellophane. There was spatial heterogeneity of MPs in the SCS. The MPs abundance in Nansha Islands is higher than that in Xisha Islands and the diameter of MPs in Xisha Islands is smaller than that in Nansha Islands, while the diversity of MPs polymers increased from Xisha Islands to Nansha Islands. In the southwestern SCS, MPs mainly originated from the weathering and decomposition of plastic waste from marine vessel activities and land-based sources.
In addition, the variability of external morphological characteristics of MPs in Nansha Islands and Xisha Islands may also be affected by marine environment through influencing weathering and decomposition of plastics and MPs. Different sampling and identification methods can cause significant differences in MPs abundances and characteristics. Establishing unified sampling methods and more adequate collection of in situ are crucial in eliminating artificial errors and systematic errors in future MPs studies, which will lead to a better understanding about the past and present of MPs.
Data Availability Statement
The original contributions presented in the study are included in the article/Supplementary Material, further inquiries can be directed to the corresponding authors.
Author Contributions
JY contributed to the conceptualization, methodology, investigation, writing—original draft preparation, and visualization. DT contributed to the resources, funding acquisition, project administration, conceptualization, supervision, and writing—review and editing. SW contributed to methodology, sampling, and investigation. LH contributed to the formal analysis, software, visualization, and methodology. KP contributed to the formal analysis and methodology. All authors contributed to the article and approved the submitted version of the manuscript.
Funding
This study was financially supported by grants by the Guangdong Key Special Supporting Team Project (2019BT02H594 and 2017B030301005), the National Natural Science Foundation of China (41876136), the Key Special Project of Southern Marine Science and Engineering Guangdong Laboratory (Guangzhou) (GML2019ZD0602), the Foundation of the South China Sea Institute of Ecological Environment Engineering Innovation of Chinese Academy of Sciences (ISEE2019ZR02), the Consulting Project for Academic of the Chinese Academy of Sciences: Development and Maritime Rights of Islands and Reefs in the South China Sea leading by Academician Wang Ying (2016ZWH005A-005), the National Science and Technology Fundamental Resources Investigation Program of China (2018FY100100), and The Research Fund Program of Guangdong Provincial Key Laboratory of Marine Resources and Coastal Engineering.
Conflict of Interest
The authors declare that the research was conducted in the absence of any commercial or financial relationships that could be construed as a potential conflict of interest.
Publisher’s Note
All claims expressed in this article are solely those of the authors and do not necessarily represent those of their affiliated organizations, or those of the publisher, the editors and the reviewers. Any product that may be evaluated in this article, or claim that may be made by its manufacturer, is not guaranteed or endorsed by the publisher.
Acknowledgments
We thank the Nansha Survey Cruise organized by the Academician Wang Ying, Nanjing University in March to April, 2018. We would like to thank Drs. Lang Lin, Hengxiang Li, and Prof. Xiangrong Xu of the UCAS (University of Chinese Academy of Sciences) Key Laboratory of Tropical Marine Bio-resources and Ecology, South China Sea Institute of Oceanology, Chinese Academy of Sciences, for their help and guidance during the laboratory experiments. We are thankful to Dr. Yupeng Liu of the South China Sea Institute of Oceanology, Chinese Academy of Sciences for his invaluable suggestion on writing and revising.
Supplementary Material
The Supplementary Material for this article can be found online at: https://www.frontiersin.org/articles/10.3389/fmars.2022.830318/full#supplementary-material
Footnotes
References
Andrady, A. L. (2011). Microplastics in the marine environment. Mar. Pollut. Bull. 62, 1596–1605. doi: 10.1016/j.marpolbul.2011.05.030
Browne, M. A., Crump, P., Niven, S. J., Teuten, E., Tonkin, A., Galloway, T., et al. (2011). Accumulation of microplastic on shorelines woldwide: sources and sinks. Environ. Sci. Technol. 45, 9175–9179. doi: 10.1021/es201811s
Cai, M., He, H., Liu, M., Li, S., Tang, G., Wang, W., et al. (2018). Lost but can’t be neglected: huge quantities of small microplastics hide in the South China Sea. Sci. Total Environ. 633, 1206–1216. doi: 10.1016/j.scitotenv.2018.03.197
Cheung, P. K., Cheung, L. T. O., and Fok, L. (2016). Seasonal variation in the abundance of marine plastic debris in the estuary of a subtropical macro-scale drainage basin in South China. Sci. Total Environ. 562, 658–665. doi: 10.1016/j.scitotenv.2016.04.048
Cole, M., Lindeque, P., Halsband, C., and Galloway, T. S. (2011). Microplastics as contaminants in the marine environment: a review. Mar. Pollut. Bull. 62, 2588–2597. doi: 10.1016/j.marpolbul.2011.09.025
Collignon, A., Hecq, J.-H., Glagani, F., Voison, P., Collard, F., and Goffart, A. (2012). Neustonic microplastic and zooplankton in the North Western Mediterranean Sea. Mar. Pollut. Bull. 64, 861–864. doi: 10.1016/j.marpolbul.2012.01.011
Cózar, A., Echevarria, F., Gonzalez-Gordillo, J. I., Irigoien, X., Ubeda, B., Hernandez-Leon, S., et al. (2014). Plastic debris in the open ocean. Proc. Natl. Acad. Sci. U.S.A. 111, 10239–10244. doi: 10.1073/pnas.1314705111
Desforges, J.-P. W., Galbraith, M., Dangerfield, N., and Ross, P. S. (2014). Widespread distribution of microplastics in subsurface seawater in the NE Pacific Ocean. Mar. Pollut. Bull. 79, 94–99. doi: 10.1016/j.marpolbul.2013.12.035
Di Mauro, R., Kupchik, M. J., and Benfield, M. C. (2017). Abundant plankton-sized microplastic particles in shelf waters of the northern Gulf of Mexico. Environ. Pollut. 230, 798–809. doi: 10.1016/j.envpol.2017.07.030
Ding, J., Jiang, F., Li, J., Wang, Z., Sun, C., Wang, Z., et al. (2019). Microplastics in the coral reef systems from xisha islands of south china sea. Environ. Sci. Technol. 53, 8036–8046. doi: 10.1021/acs.est.9b01452
Doyle, M. J., Watson, W., Bowlin, N. M., and Sheavly, S. B. (2011). Plastic particles in coastal pelagic ecosystems of the Northeast Pacific ocean. Mar. Environ. Res. 71, 41–52. doi: 10.1016/j.marenvres.2010.10.001
Enders, K., Lenz, R., Stedmon, C. A., and Nielsen, T. G. (2015). Abundance, size and polymer composition of marine microplastics =10μm in the Atlantic Ocean and their modelled vertical distribution. Mar. Pollut. Bull. 100, 70–81. doi: 10.1016/j.marpolbul.2015.09.027
Eriksen, M., Lebreton, L. C., Carson, H. S., Thiel, M., Moore, C. J., Borerro, J. C., et al. (2014). Plastic Pollution in the World’s Oceans: more than 5 Trillion Plastic Pieces Weighing over 250,000 Tons Afloat at Sea. PLoS One 9:e111913. doi: 10.1371/journal.pone.0111913
Fu, J., Mai, B., Sheng, G., Zhang, G., Wang, X., Peng, P., et al. (2003). Persistent organic pollutants in environment of the Pearl River Delta. Chemosphere 52, 1411–1422. doi: 10.1016/s0045-6535(03)00477-6
GESAMP (2010). Proceedings of the GESAMP International Workshop on Plastic Particles as a Vector in Transporting Persistent. Italy: FAO.
GESAMP (2015). “Sources, Fate and Effects of Microplastics in the Marine Environment: a Global Assessment. IMO/FAO/UNESCO-IOC/UNIDO/-WMO/IAEA/UN/UNEP/UNDP,” in Joint Group of Experts on the Scientific Aspects of Marine Environmental Protection Reports and Studies, ed. P. J. Kershaw (Italy: FAO).
Harris, P. T., Tamelander, J., Lyons, Y., Neo, M. L., and Maes, T. (2021). Taking a mass-balance approach to assess marine plastics in the South China Sea. Mar. Pollut. Bull. 171:112708. doi: 10.1016/j.marpolbul.2021.112708
Hidalgo-Ruz, V., Gutow, L., Thompson, R. C., and Thiel, M. (2012). Microplastics in the marine environment: a review of the methods used for identification and quantification. Environ. Sci. Technol. 46, 3060–3075. doi: 10.1021/es2031505
Huang, L., Li, Q., Xu, X., Yuan, X., Lin, L., and Li, H. (2020). Composition and distribution of microplastics in the surface seawater of Xisha Islands. Chinese. Sci. Bull. 65, 2627–2635. doi: 10.1360/tb-2020-0220
Huang, Y., Xiao, X., Effiong, K., Xu, C., Su, Z., Hu, J., et al. (2021). New Insights into the Microplastic Enrichment in the Blue Carbon Ecosystem: evidence from Seagrass Meadows and Mangrove Forests in Coastal South China Sea. Environ. Sci. Technol. 55, 4804–4812. doi: 10.1021/acs.est.0c07289
Huang, Y., Yan, M., Xu, K., Nie, H., Gong, H., and Wang, J. (2019). Distribution characteristics of microplastics in Zhubi Reef from South China Sea. Environ. Pollut. 255:113133. doi: 10.1016/j.envpol.2019.113133
Hughes, T. P., Barnes, M. L., Bellwood, D. R., Cinner, J. E., Cumming, G. S., Jackson, J. B. C., et al. (2017). Coral reefs in the Anthropocene. Nature 546, 82–90. doi: 10.1038/nature22901
Isobe, A., Uchida, K., Tokai, T., and Iwasaki, S. (2015). East Asian seas: a hot spot of pelagic microplastics. Mar. Pollut. Bull. 101, 618–623. doi: 10.1016/j.marpolbul.2015.10.042
Jambeck, J. R., Geyer, R., Wilcox, C., Siegler, T. R., Perryman, M., Andrady, A., et al. (2015). Plastic waste inputs from land into the ocean. Science 347, 768–771. doi: 10.1126/science.1260352
Li, C., Zhu, L., Wang, X., Liu, K., and Li, D. (2022). Cross-oceanic distribution and origin of microplastics in the subsurface water of the South China Sea and Eastern Indian Ocean. Sci. Total Environ. 805:150243. doi: 10.1016/j.scitotenv.2021.150243
Li, Q., and Sun, X. (2020). Progress on microplastics research in the Yellow Sea China. Anthropocene Coasts 3, 43–52. doi: 10.1139/anc-2018-0033
Li, D. (2019). Research Advance and Countermeasures on Marine Microplastic Pollution (in Chinese). Res. Environ. Sci. 32, 197–202. doi: 10.13198/j.issn.1001-6929.2018.11.25
Lin, L., Zuo, L. Z., Peng, J. P., Cai, L. Q., Fok, L., Yan, Y., et al. (2018). Occurrence and distribution of microplastics in an urban river: a case study in the Pearl River along Guangzhou City. China Sci. Total Environ. 644, 375–381. doi: 10.1016/j.scitotenv.2018.06.327
Liu, K., Wu, T., Wang, X., Song, Z., Zong, C., Wei, N., et al. (2019). Consistent Transport of Terrestrial Microplastics to the Ocean through Atmosphere. Environ. Sci. Technol. 53, 10612–10619. doi: 10.1021/acs.est.9b03427
Liu, T., Sun, X., Zhu, M., Liang, J., and Zhao, Y. (2018). Distribution and composition of microplastics in the surface water of the East China Sea (In Chinese). Oceanol. Limnol. Sin. 49, 62–69. doi: 10.11693/hyhz20170100021
Lusher, A. L., Burke, A., O’Connor, I., and Officer, R. (2014). Microplastic pollution in the Northeast Atlantic Ocean: validated and opportunistic sampling. Mar. Pollut. Bull. 88, 325–333. doi: 10.1016/j.marpolbul.2014.08.023
Lusher, A. L., Tirelli, V., O’Connor, I., and Officer, R. (2015). Microplastics in Arctic polar waters: the first reported values of particles in surface and sub-surface samples. Sci. Rep. 5:14947. doi: 10.1038/srep14947
McCormick, A., Hoellein, T. J., Mason, S. A., Schluep, J., and Kelly, J. J. (2014). Microplastic is an Abundant and Distinct Microbial Habitat in an Urban River. Environ. Sci. Technol. 48, 11863–11871. doi: 10.1021/es503610r
Md Amin, R., Shazira Sohaimi, E., Tuan Anuar, S., and Bachok, Z. (2020). Microplastic ingestion by zooplankton in Terengganu coastal waters, southern South China Sea. Mar. Pollut. Bull. 150:110616. doi: 10.1016/j.marpolbul.2019.110616
Ministry of Civil Affairs of the People’s Republic of China (2020). Announcement by the Ministry of Civil Affairs on the State Council’s approval of the establishment of a municipal district in Sansha City, Hainan Province, PRC. (In Chinese). Available online at: http://www.mca.gov.cn/article/xw/tzgg/202004/20200400026955.shtml [accessed on April 18, 2020]
Moore, C. J., Moore, S. L., Weisberg, S. B., Lattin, G. L., and Zellers, A. F. (2002). A comparison of neustonic plastic and zooplankton abundance in southern California’s coastal waters. Mar. Pollut. Bull. 44, 1035–1038. doi: 10.1016/S0025-326X(02)00150-9
Nie, H., Wang, J., Xu, K., Huang, Y., and Yan, M. (2019). Microplastic pollution in water and fish samples around Nanxun Reef in Nansha Islands. South China Sea. Sci. Total Environ. 696:134022. doi: 10.1016/j.scitotenv.2019.134022
Pan, Z., Guo, H., Chen, H., Wang, S., Sun, X., Zou, Q., et al. (2019). Microplastics in the Northwestern Pacific: abundance, distribution, and characteristics. Sci. Total Environ. 650, 1913–1922. doi: 10.1016/j.scitotenv.2018.09.244
Qi, H., Fu, D., Wang, Z., Gao, M., and Peng, L. (2020). Microplastics occurrence and spatial distribution in seawater and sediment of Haikou Bay in the northern South China Sea. Estuar. Coastal Shelf Sci. 239:106757. doi: 10.1016/j.ecss.2020.106757
Qiu, Q., Peng, J., Yu, X., Chen, F., Wang, J., and Dong, F. (2015). Occurrence of microplastics in the coastal marine environment: first observation on sediment of China. Mar. Pollut. Bull. 98, 274–280. doi: 10.1016/j.marpolbul.2015.07.028
Reisser, J., Slat, B., Noble, K., du Plessis, K., Epp, M., Proietti, M., et al. (2015). The vertical distribution of buoyant plastics at sea: an observational study in the North Atlantic Gyre. Biogeosciences 12, 1249–1256. doi: 10.5194/bg-12-1249-2015
Rodríguez-Seijo, A., and Pereira, R. (2016). “Chapter 3: morphological and physical characterization ofmicroplastics,” in Comprehensive Analytical Chemistry, Vol. 75, eds T. A. P. Rocha-Santos and A. C. Duarte (Netherlands: Elsevier), 49–65.
Ryan, P. G., Moore, C. J., van Franeker, J. A., and Moloney, C. L. (2009). Monitoring the abundance of plastic debris in the marine environment. Philos. Trans. R Soc. Lond. B Biol. Sci. 364, 1999–2012. doi: 10.1098/rstb.2008.0207
Shaw, D. G., and Day, R. H. (1994). Colour- and form-dependent loss of plastic micro-debris from the North Pacific Ocean. Mar. Pollut. Bull. 28, 39–43. doi: 10.1016/0025-326x(94)90184-8
Song, Y. K., Hong, S. H., Jang, M., Kang, J. H., Kwon, O. Y., Han, G. M., et al. (2014). Large accumulation of micro-sized synthetic polymer particles in the sea surface microlayer. Environ. Sci. Technol. 48, 9014–9021. doi: 10.1021/es501757s
Su, L., Xue, Y., Li, L., Yang, D., Kolandhasamy, P., Li, D., et al. (2016). Microplastics in Taihu Lake. China. Environ. Pollut. 216, 711–719. doi: 10.1016/j.envpol.2016.06.036
Suaria, G., Achtypi, A., Perold, V., Lee, J. R., Pierucci, A., Bornman, T. G., et al. (2020). Microfibers in oceanic surface waters: a global characterization. Sci. Adv. 6:eaay8493. doi: 10.1126/sciadv.aay8493
Sun, X., Li, Q., Zhu, M., Liang, J., Zheng, S., and Zhao, Y. (2017). Ingestion of microplastics by natural zooplankton groups in the northern South China Sea. Mar. Pollut. Bull. 115, 217–224. doi: 10.1016/j.marpolbul.2016.12.004
Tan, F., Yang, H., Xu, X., Fang, Z., Xu, H., Shi, Q., et al. (2020). Microplastic pollution around remote uninhabited coral reefs of Nansha Islands. South China Sea. Sci. Total Environ. 725:138383. doi: 10.1016/j.scitotenv.2020.138383
Ter Halle, A., Ladirat, L., Martignac, M., Mingotaud, A. F., Boyron, O., and Perez, E. (2017). To what extent are microplastics from the open ocean weathered? Environ. Pollut. 227, 167–174. doi: 10.1016/j.envpol.2017.04.051
Teuten, E. L., Rowland, S. J., Galloway, T. S., and Thompson, R. C. (2007). Potential for plastic to transport hydrohobic contaminants. Environ. Sci. Technol. 41, 7759–7764. doi: 10.1021/es071737s
Thompson, R. C., Olsen, Y., Mitchell, R. P., Davis, A., Rowland, S. J., John, A. W., et al. (2004). Lost at sea: where is all the plastic? Science 304:838. doi: 10.1126/science.1094559
Vandermeersch, G., Van Cauwenberghe, L., Janssen, C. R., Marques, A., Granby, K., Fait, G., et al. (2015). A critical view on microplastic quantification in aquatic organisms. Environ. Res. 143, 46–55. doi: 10.1016/j.envres.2015.07.016
Waller, C. L., Griffiths, H. J., Waluda, C. M., Thorpe, S. E., Loaiza, I., Moreno, B., et al. (2017). Microplastics in the Antarctic marine system: an emerging area of research. Sci. Total Environ. 598, 220–227. doi: 10.1016/j.scitotenv.2017.03.283
Wang, B., Huang, F., Wu, Z., Yang, J., Fu, X., and Kazuyoshi Kikuchi. (2009). Multi-scale climate variability of the South China Sea monsoon: a review. Dyn. Atmos. Oceans 47, 15–37. doi: 10.1016/j.dynatmoce.2008.09.004
Wang, T., Zou, X., Li, B., Yao, Y., Zang, Z., Li, Y., et al. (2019b). Preliminary study of the source apportionment and diversity of microplastics: taking floating microplastics in the South China Sea as an example. Environ. Pollut. 245, 965–974. doi: 10.1016/j.envpol.2018.10.110
Wang, T., Li, B., Zou, X., Wang, Y., Li, Y., Xu, Y., et al. (2019a). Emission of primary microplastics in mainland China: invisible but not negligible. Water Res. 162, 214–224. doi: 10.1016/j.watres.2019.06.042
Webb, H. K., Arnott, J., Crawford, R. J., and Ivanova, E. P. (2012). Plastic Degradation and Its Environmental Implications with Special Reference to Poly(ethylene terephthalate). Polymers 5, 1–18. doi: 10.3390/polym5010001
Wright, S. L., Thompson, R. C., and Galloway, T. S. (2013). The physical impacts of microplastics on marine organisms: a review. Environ. Pollut. 178, 483–492. doi: 10.1016/j.envpol.2013.02.031
Xu, P., Peng, G., Su, L., Gao, Y., Gao, L., and Li, D. (2018). Microplastic risk assessment in surface waters: A case study in the Changjiang Estuary. China, Mar. Pollut. Bull. 133, 647–654. doi: 10.1016/j.marpolbul.2018.06.020
Yan, M., Nie, H., Xu, K., He, Y., Hu, Y., Huang, Y., et al. (2019). Microplastic abundance, distribution and composition in the Pearl River along Guangzhou city and Pearl River estuary. China, Chemosphere 217, 879–886. doi: 10.1016/j.chemosphere.2018.11.093
Yang, D., Shi, H., Li, L., Li, J., Jabeen, K., and Kolandhasamy, P. (2015). Microplastic Pollution in Table Salts from China. Environ. Sci. Technol. 49, 13622–13627. doi: 10.1021/acs.est.5b03163
Zambrano, M. C., Pawlak, J. J., Daystar, J., Ankeny, M., Goller, C. C., and Venditti, R. A. (2020). Aerobic biodegradation in freshwater and marine environments of textile microfibers generated in clothes laundering: effects of cellulose and polyester-based microfibers on the microbiome. Mar. Pollut. Bull. 151:110826. doi: 10.1016/j.marpolbul.2019.110826
Zhang, L., Zhang, S., Wang, Y., Yu, K., and Li, R. (2019). The spatial distribution of microplastic in the sands of a coral reef island in the South China Sea: comparisons of the fringing reef and atoll. Sci. Total Environ. 688, 780–786. doi: 10.1016/j.scitotenv.2019.06.178
Zhang, W., Zhang, S., Wang, J., Wang, Y., Mu, J., Wang, P., et al. (2017). Microplastic pollution in the surface waters of the Bohai Sea. China. Environ. Pollut. 231, 541–548. doi: 10.1016/j.envpol.2017.08.058
Zhao, S., Zhu, L., Wang, T., and Li, D. (2014). Suspended microplastics in the surface water of the Yangtze Estuary System, China: first observations on occurrence, distribution. Mar. Pollut. Bull. 86, 562–568.
Zhou, P., Huang, C., Fang, H., Cai, W., Li, D., Li, X., et al. (2011). The abundance, composition and sources of marine debris in coastal seawaters or beaches around the northern South China Sea (China). Mar. Pollut. Bull. 62, 1998–2007. doi: 10.1016/j.marpolbul.2011.06.018
Zhu, C., Li, D., Sun, Y., Zheng, X., Peng, X., Zheng, K., et al. (2019). Plastic debris in marine birds from an island located in the South China Sea. Mar. Pollut. Bull. 149:110566. doi: 10.1016/j.marpolbul.2019.110566
Zhu, J., Zhang, Q., Li, Y., Tan, S., Kang, Z., Yu, X., et al. (2019). Microplastic pollution in the Maowei Sea, a typical mariculture bay of China. Sci. Total Environ. 658, 62–68. doi: 10.1016/j.scitotenv.2018.12.192
Zhu, L., Wang, H., Chen, B., Sun, X., Qu, K., and Xia, B. (2019). Microplastic ingestion in deep-sea fish from the South China Sea. Sci. Total Environ. 677, 493–501. doi: 10.1016/j.scitotenv.2019.04.380
Keywords: microplastics, spatial distribution, morphological characterization, chemical composition, the South China Sea
Citation: Yu J, Tang D, Wang S, He L and Pathira Arachchilage KRL (2022) Spatial Distribution and Composition of Surface Microplastics in the Southwestern South China Sea. Front. Mar. Sci. 9:830318. doi: 10.3389/fmars.2022.830318
Received: 07 December 2021; Accepted: 06 January 2022;
Published: 11 February 2022.
Edited by:
Xiaoshan Zhu, Tsinghua University, ChinaReviewed by:
Qipei Li, Hainan University, ChinaMuhammad Reza Cordova, Indonesian Institute of Sciences, Indonesia
Copyright © 2022 Yu, Tang, Wang, He and Pathira Arachchilage. This is an open-access article distributed under the terms of the Creative Commons Attribution License (CC BY). The use, distribution or reproduction in other forums is permitted, provided the original author(s) and the copyright owner(s) are credited and that the original publication in this journal is cited, in accordance with accepted academic practice. No use, distribution or reproduction is permitted which does not comply with these terms.
*Correspondence: Danling Tang, bGluZ3ppc3RkbEAxMjYuY29t; Sufen Wang, c3VmZW53YW5nMTk3NkAxMjYuY29t