- 1South China Sea Institute of Planning and Environmental Research, State Oceanic Administration, Guangzhou, China
- 2Key Laboratory of Marine Environmental Survey Technology and Application, Ministry of Natural Resources of the People’s Republic of China (MNR), Guangzhou, China
- 3State Key Laboratory of Tropical Oceanography, South China Sea Institute of Oceanology, Chinese Academy of Sciences, Guangzhou, China
The classifications and associated characteristics of marine heatwaves (MHWs) in the northern South China Sea (SCS) have been investigated. Based on the ratio of the area with MHWs to the total area of the northern SCS, the MHWs are divided into three categories, i.e., narrow coverage, moderate coverage, and wide coverage. Different kinds of MHWs (i.e., narrow coverage, moderate coverage, and wide coverage) contribute around 64, 28, and 8% to the total days with MHWs, respectively. Narrow coverage MHW scatters over the northern SCS continental shelf, and moderate (wide) coverage MHWs are concentrated east of Hainan Island (mid of northern SCS continental shelf). Wide coverage MHWs contribute more than 50% to the total MHW cumulative sea surface temperature (SST) anomalies. The cumulative days and temperature anomalies of all kinds of MHWs exhibit significant long-term trends and decadal variability.
Introduction
Marine heatwave (MHW) is defined as an anomalously warm, discrete, and prolonged event, which has drawn great attention due to its extraordinary influences on ecosystem structure and functioning (Wernberg et al., 2013; Hobday et al., 2016; Gao et al., 2020). Many MHW events have been recorded, and they mainly occur off the coast (Garrabou et al., 2009; Feng et al., 2013; Mills et al., 2013; Bond et al., 2015; Oliver et al., 2017; Schlegel et al., 2017; Benthuysen et al., 2018; Tan and Cai, 2018; Gao et al., 2020). As a consequence of climate change, the frequency and intensity of MHWs in the world oceans are increasing (Hobday et al., 2018). Both the sea surface heat flux and the oceanic process can contribute to the generation and evolution of MHWs, and their contributions vary in different oceans (Chen et al., 2014; Schlegel et al., 2017). Like the northeast Pacific MHWs, the importance of the contribution of sea surface heat flux and vertical entrainment varies at different developmental stages (Chen et al., 2021a,b).
The South China Sea (SCS) is the largest semi-enclosed marginal sea, which connects the North Pacific and the East Indian Ocean. Due to the East Asian monsoon, SCS upper layer circulation exhibits distinct seasonal shifts (Qu et al., 2000; Gan et al., 2006; Wang et al., 2020a,b, c). Driven by the northeasterly winter (southwest summer) monsoon, a mature basin-scale cyclonic (anticyclonic) circulation is formed. Its sea surface temperature (SST) also presents a significant seasonal cycle, and air-sea heat flux plays a leading role while ocean circulation advection plays a secondary role (Qu, 2001). The SCS temperature interannual variability and long-term change have been widely studied, and the circulation advection effect is one of the major contributions (Wang, 2002; Liu et al., 2004, 2014; Xiao et al., 2018, 2020; Wang et al., 2021). Additionally, the surface heat fluxes are also key factors modulating the SST anomalies in the SCS (Wu et al., 2014; Wang and Wu, 2021). However, the short-term extreme event-based research still lacks, and the short-term extreme events actually exert a more important influence on ecosystems due to substantially higher temperatures than normal temperature (Smith, 2011; Li et al., 2019), such as harmful algae blooms, which are closely related to extreme warm events or MHWs (Cai et al., 2016, 2017). Under global warming, there is a long-term increase of MHWs in marginal seas in China (Yao et al., 2020).
Recently, summer MHWs in the SCS have been reported (Yao and Wang, 2021). Under the influence of intensification of the western North Pacific subtropical high, upwelling in the mid-western SCS weakens and then causes basin-wide severe summer MHWs. Due to the MHWs in the SCS, the symbiotic relationship between corals and algae has been disrupted (Hughes et al., 2017; Eakin et al., 2019). Then, the coral reefs in the SCS sharply declined (Zhang et al., 2017). Extreme warming of SCS can also modulate the Southern China winter rainfall (Wang et al., 2018).
Marine heatwaves in the SCS have not been widely studied earlier. Therefore, a detailed examination of the MHWs in the northern SCS is presented in this study, which will add to our knowledge of extreme events in the SCS and carry implications for ecological studies in the SCS.
Data and Methods
Data
The SST data are obtained from the United States National Oceanic and Atmospheric Administration (NOAA) Optimum Interpolation SST (OISST) version 2 (Reynolds et al., 2007), which is a daily and 0.25 × 0.25° gridded product from Advanced Very High-Resolution Radiometer (AVHRR) satellite data, with bias correction using ship and buoy data. The period 1982–2019 is used in this study. Since the SST anomaly basically obeys the Gaussian distribution, the raw SST data are utilized.
Definition of Indices
Following the study by Hobday et al. (2016), an MHW event is defined as warming exceeding the threshold lasting at least for 5 persistent days. The threshold is defined as the 90th percentile of the samples within an 11−day window centered on a specific day in all years. To measure the influence period of an MHW, a metric of duration was utilized. The duration is defined as the days between the start and end dates, and the intensity is the average of daily intensity anomalies relative to the climatology.
The northern SCS continental shelf is a whole area of shelf, geographically. However, MHW events do not fully occupy the entire continental at the same time. To evaluate its influence, its space occupancy and associated characteristics need to be known. The probability of space occupancy (PSO) is defined as the percentage of MHW area in the total area in the northern SCS continental shelf every day (bounded by the 500 m-isobath, 110°E, and 24°N). The probability of occurrence with different PSOs is defined as the ratio of days within a range of PSO (5% is utilized in this article) to the total days with MHWs. Narrow coverage MHW day is defined as the PSO smaller than 20%, and moderate coverage MHW day is between 20 and 50%, and wide coverage MHW day is larger than 50%.
Characteristics of Marine Heatwaves in the Northern South China Sea
Marine heatwave occurs most frequently to the east of Hainan Island with 2.2–2.4 events per year, and secondarily along the coast of Guangdong and west of Luzon Strait with 1.9–2.2 events per year (Figure 1A). On the western margin of SCS, the duration of MHW is the longest, ranging from 12 to 14 days (Figure 1B). On the northern SCS continental shelf, the duration of MHW is between 10 and 11 days. Although there was a spatial difference in the MHW frequency and duration distribution, the differences were not significant. The mean intensity of MHW exhibits a belt distribution parallel to the coast of Guangdong, declining outward sea (Figure 1E). On the northern SCS continental shelf, the mean intensity of MHW is higher than 1.5°C. In the open sea, the MHW is mainly below 1.5°C, except for a 1.6°C area west of the Luzon Strait.
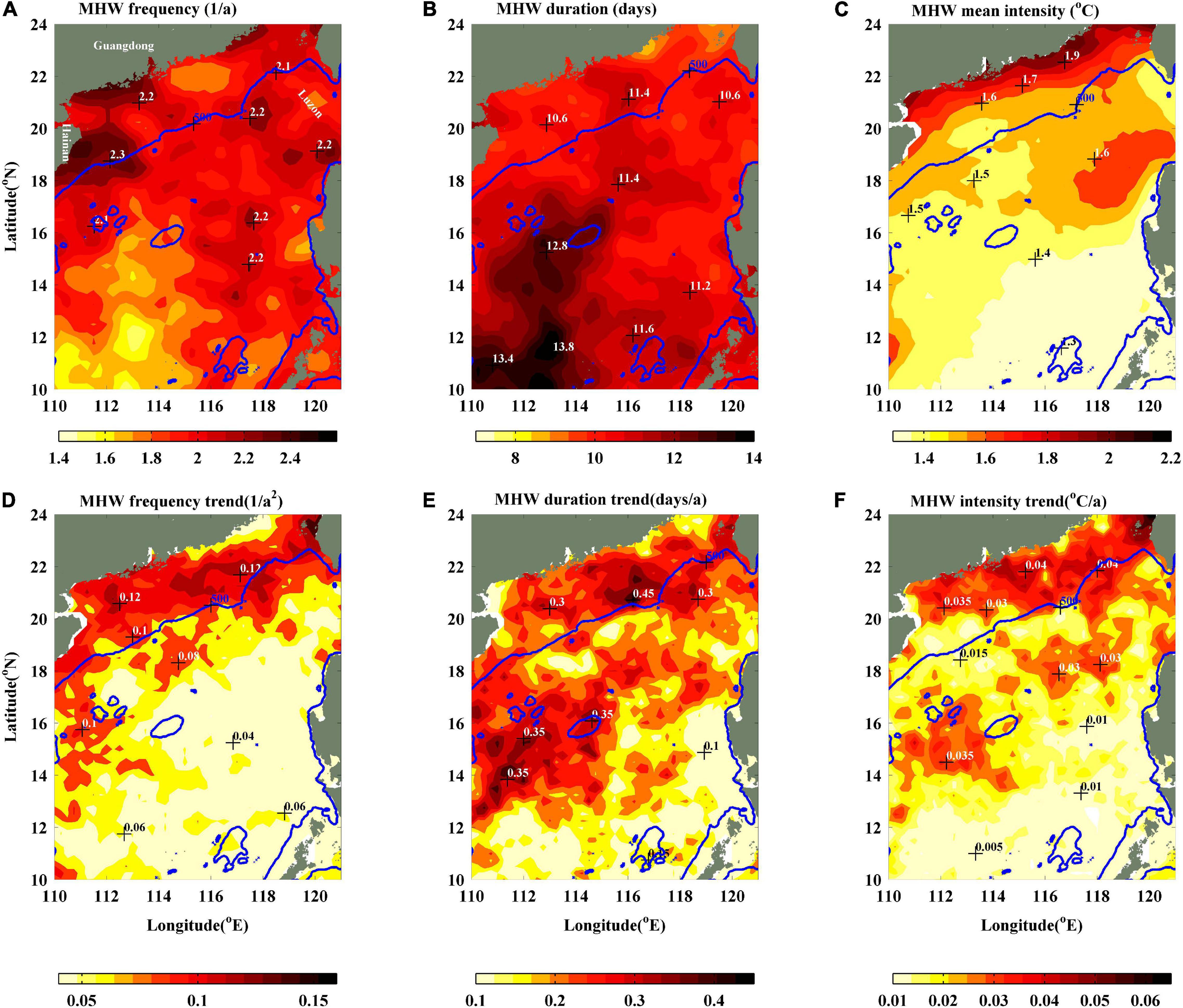
Figure 1. Spatial distributions of (A) the number of marine heatwaves (MHWs) in each year, (B) average MHW duration, and (C) average MHW intensity in the South China Sea during the period of 1982–2018. (D–F) Are the same as (A–C), but for their trends.
The significant long-term trend of MHW occurrence and intensity mainly centers in the northern SCS continental shelf and the western margin (Figures 1D–F). On the northern SCS continental shelf, the number of MHW occurrences increases by 0.1–0.14 per year. In the open sea, the long-term trend is mainly less than 0.06 per year. On the northern SCS continental shelf and the western margin, the long-term duration trend is 0.3–0.4 d/a. The enhancement of MHW intensity is also mainly in the northern SCS continental shelf with an amplitude of around 0.04°C/a.
Narrow coverage MHWs dominate the northern SCS continental shelf, contributing around 64% to the total MHW days (Figure 2A). Moderate coverage MHWs occupy around 28%, and wide coverage is only around 8%. These results mean that the MHWs are mainly scattered in the northern SCS continental shelf. The average intensities of narrow- and moderate-coverage MHWs are equivalent and are both significantly lower than those of wide coverage MHWs (Figure 2B). The distribution of different MHW SDs is similar to that of average intensity (Figure 2C).
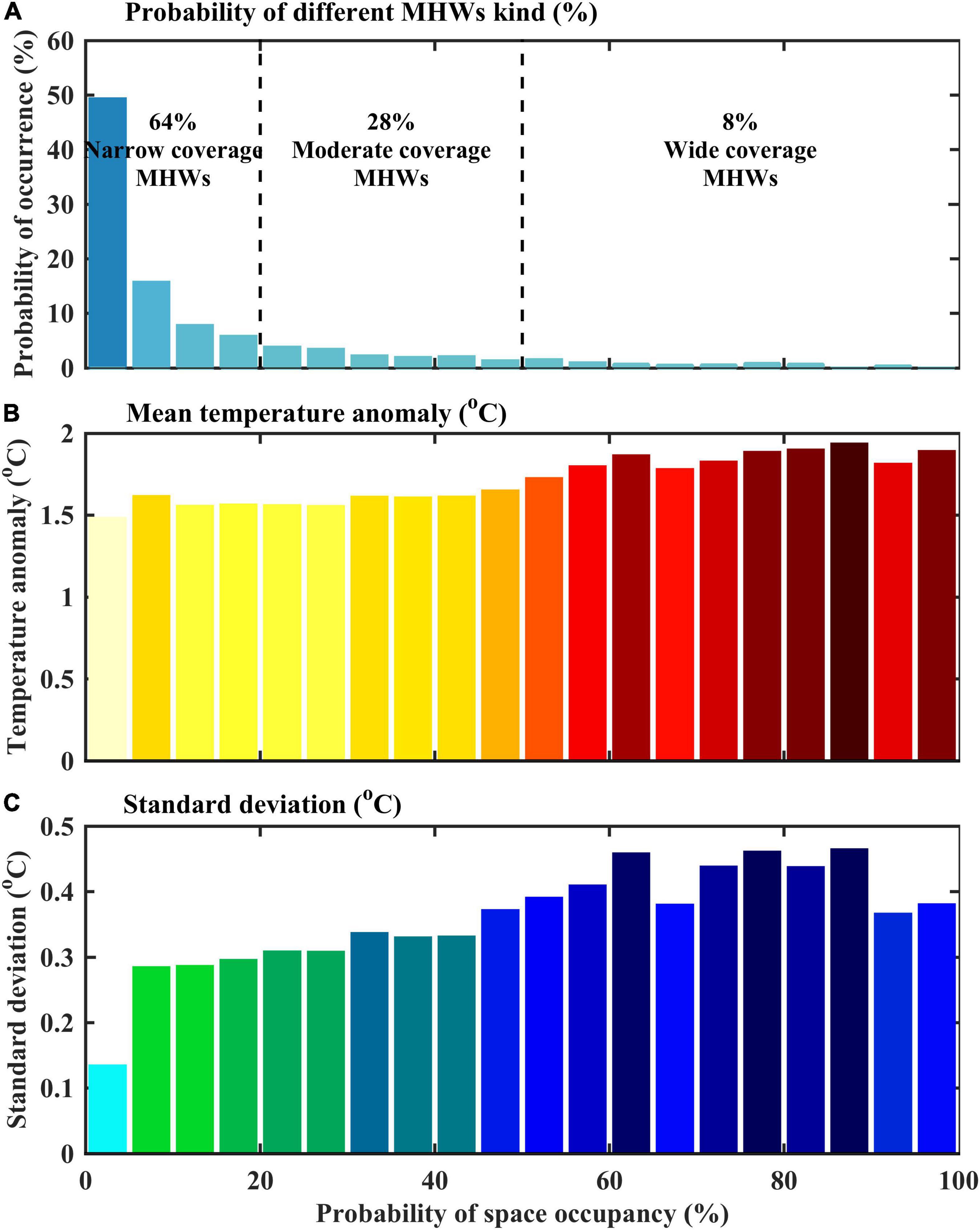
Figure 2. (A) Occurrence probability of different MHWs, which is classified by the ratio of the area with MHWs to the total area of the northern South China Sea continental shelf bounded by the 500 m isobaths (see the text for the details of the definition). (B) Average sea surface temperature (SST) anomalies of different MHWs. (C) Average spatial SD of different MHWs.
The probability of MHW occurrence is utilized to analyze the distribution of different kinds of MHWs (Figure 3). The wide coverage MHWs mainly occupy the mid-northern SCS continental shelf (Figure 3A). The moderate coverage MHWs are mainly concentrated at the east of Hainan Island (Figure 3B). The distribution of narrow coverage MHWs is very scattered, with a relatively large value region in the eastern and western parts (Figure 3C). Although the wide coverage MHW percentages are very low (Figure 2A), the contribution of this kind of MHWs to the cumulative SST anomalies is the largest with approximately 50% at the mid-northern SCS continental shelf (Figure 3D). The contribution of moderate coverage MHWs ranges from ∼30 to 40% (Figure 3E). The contribution of narrow coverage MHWs is also concentrated at the eastern and western northern SCS continental shelf with about 40% (Figure 3F).
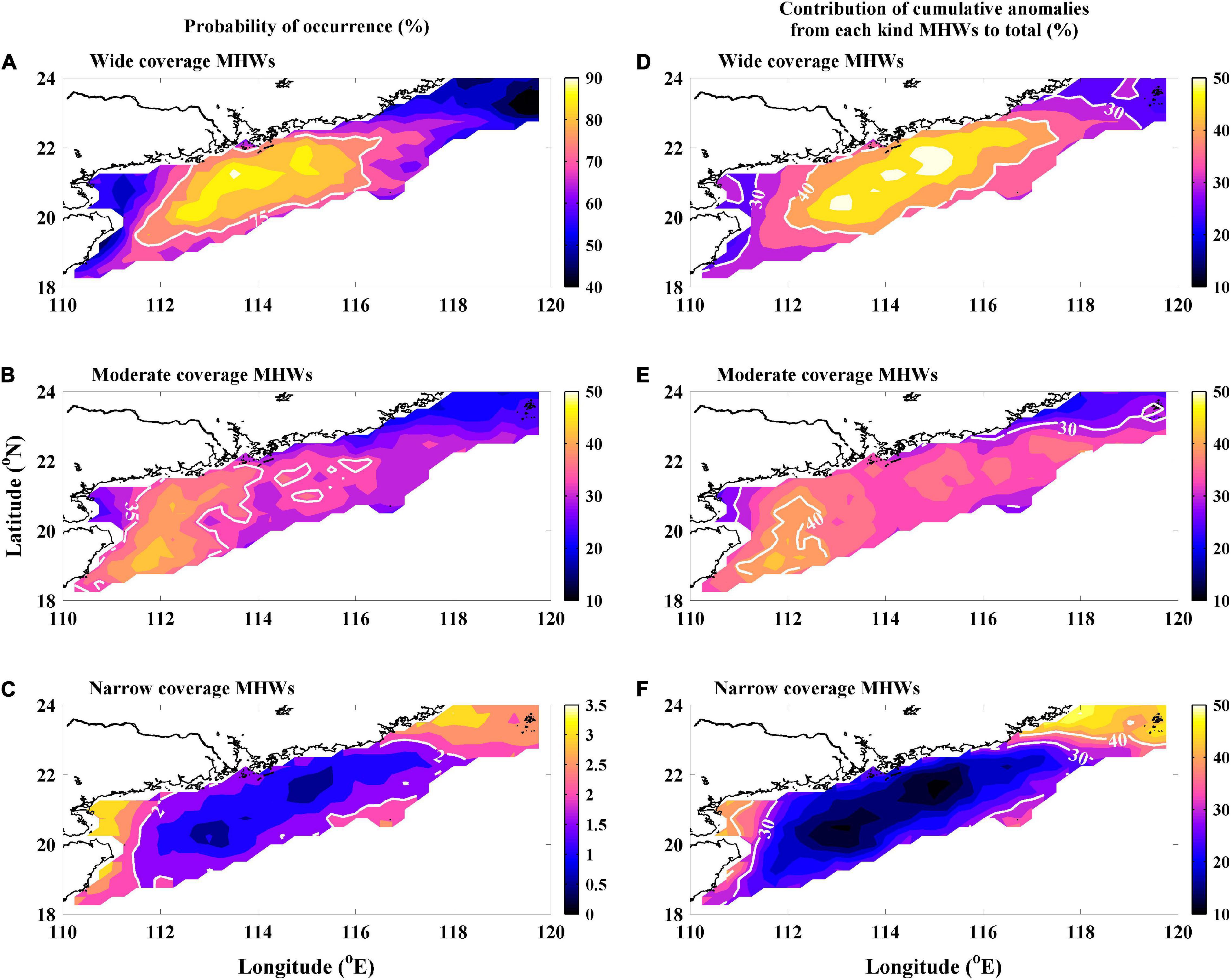
Figure 3. (A) Distribution of the ratio of the days with warming at each point to the total days for wide coverage MHWs (the warming area is larger than 50% in the northern South China Sea continental shelf). (B,C) Are the same as (A), but for moderate coverage MHWs (the ratio of warming area is between 20 and 50%) and narrow coverage MHWs (the ratio of warming area is less than 20%). (D–F) Are the same as (A–C), but for the cumulative SST anomalies.
The spatial distributions of the average intensity of all kinds of MHWs are similar to the total intensity (Figures 1C, 4A–C). The band-like average intensity decreases from the coast to the open sea. The average intensity of wide coverage MHWs is the largest, followed by moderate- and narrow-coverage MHWs. The SD of all kinds of MHWs is large at the eastern northern SCS continental shelf and small at the western SCS (Figures 4D–F).
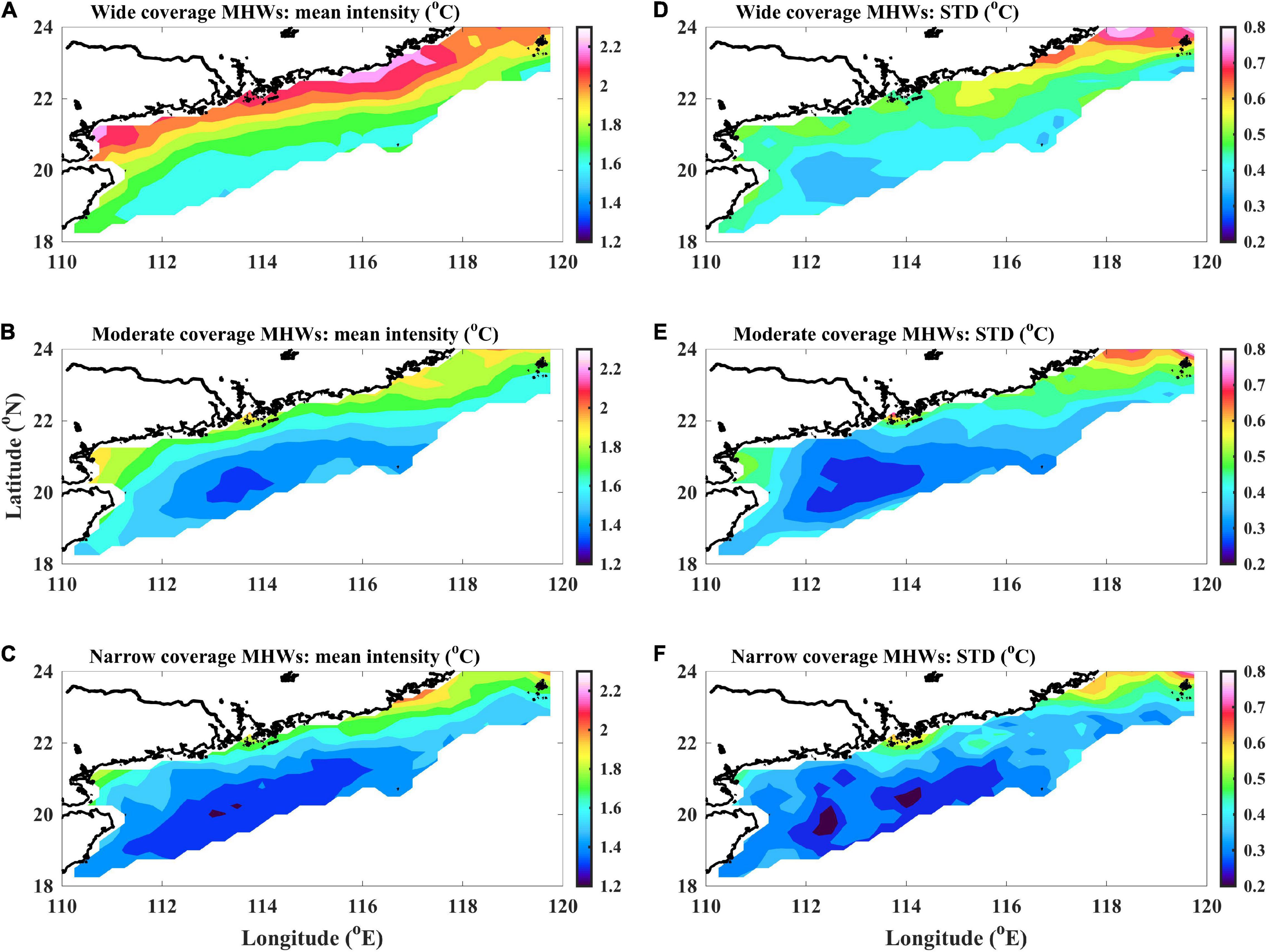
Figure 4. (A) Distribution of the average intensity of wide coverage MHWs. (B,C) Are the same as (A), but for moderate coverage MHWs and narrow coverage MHWs. (D–F) Are the same as (A–C), but for the SD of SST anomalies.
Variability of Different Kinds of Marine Heatwaves in the Northern South China Sea
There was an increasing long-term trend of cumulative days for all kinds of MHWs (Figure 5). In the 1980s, no wide coverage MHWs and rare moderate coverage MHWs occurred (Figures 5A,B). After the 1980s, the cumulative days of these two kinds of MHWs both increased rapidly and presented significant decadal variability. For the narrow coverage MHWs, decadal variance is also present (Figure 5C).
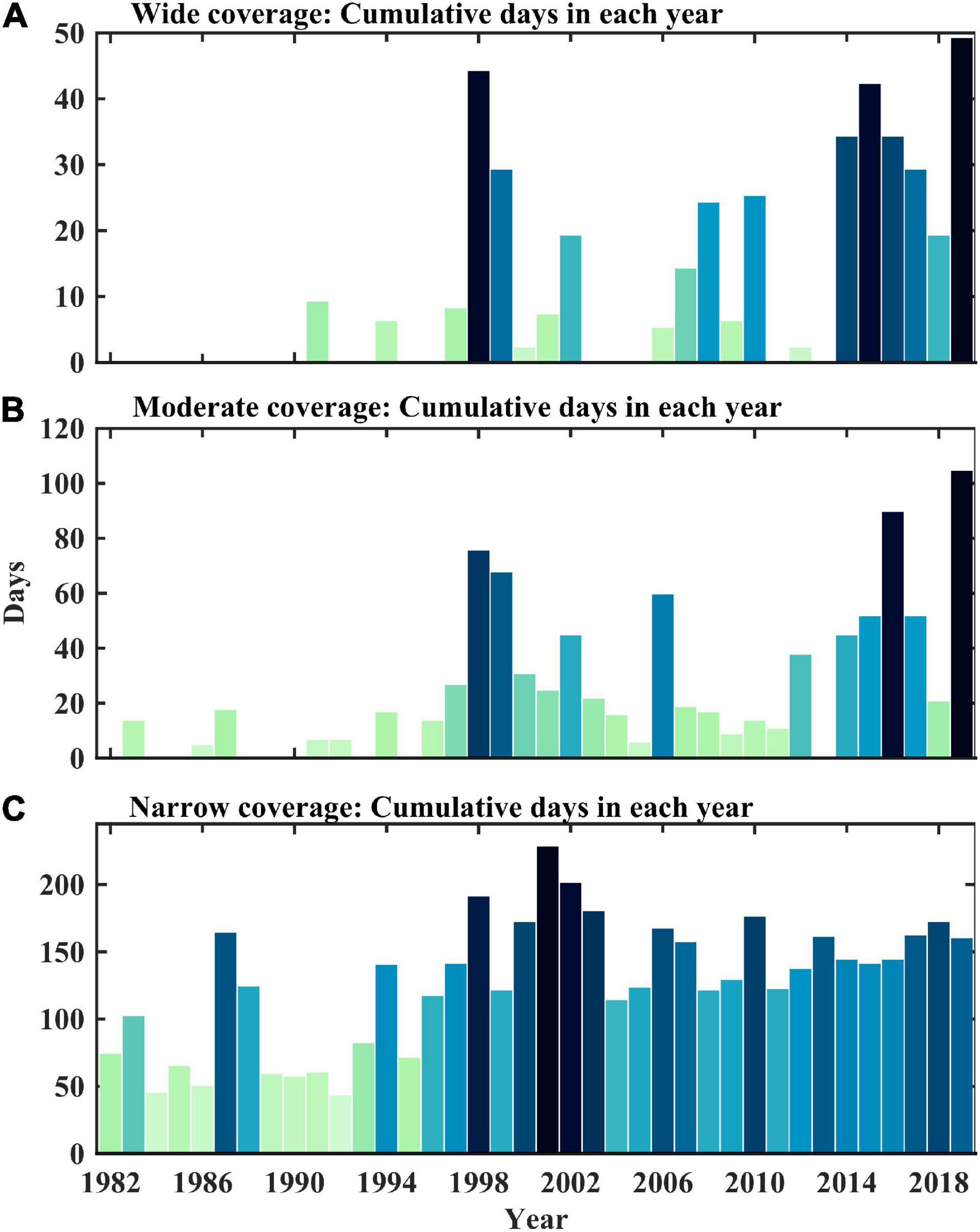
Figure 5. (A) Cumulative days for the wide coverage MHWs in the northern South China Sea continental shelf each year. (B,C) Are the same as (A), but for moderate coverage MHWs and narrow coverage MHWs.
The average intensity of all kinds of MHWs does not present a significant long-term trend or decadal variability (Figures 6A–C). However, the yearly cumulative SST anomalies still present a significant long-term trend and decadal variability (Figures 6D–F) induced by the variability in yearly cumulative days (Figure 5).
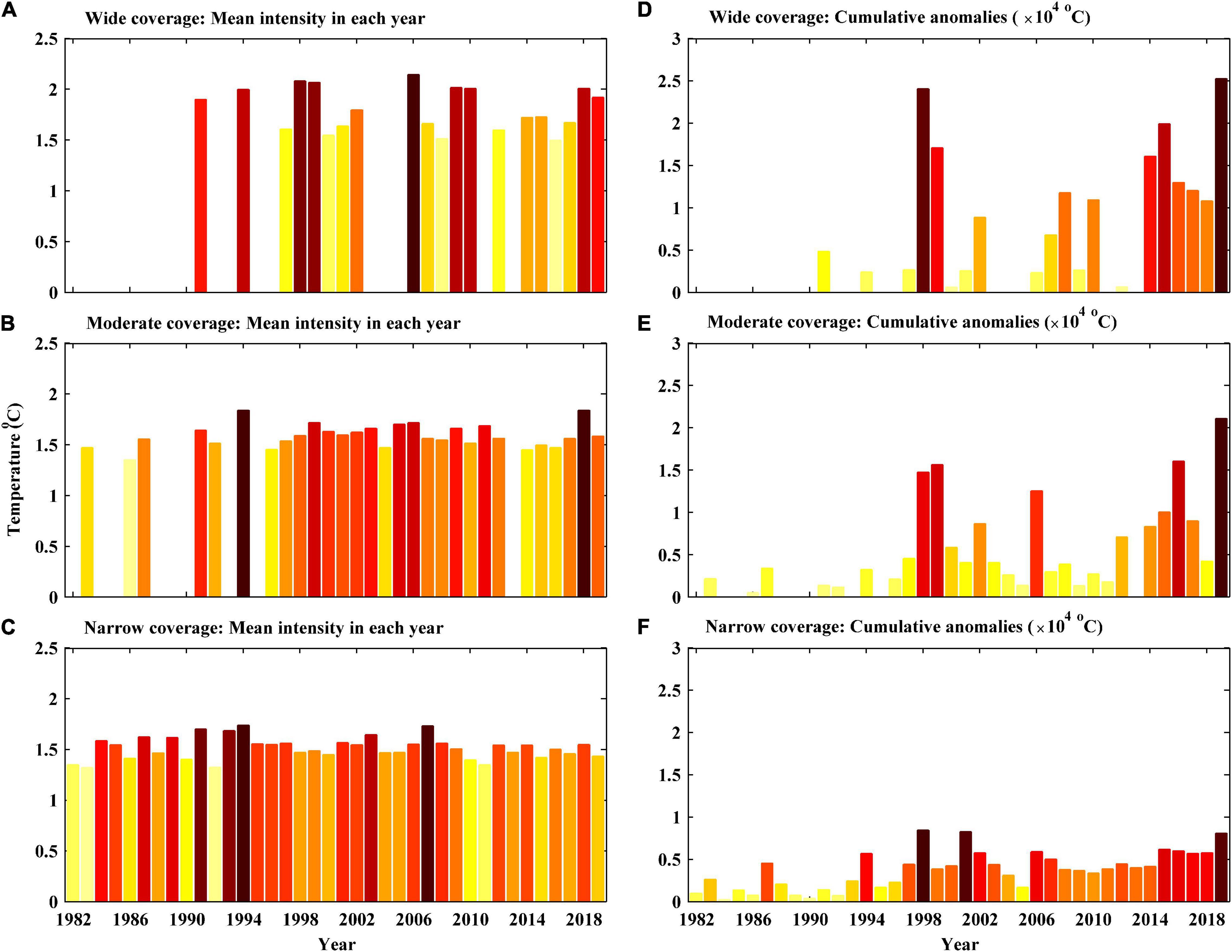
Figure 6. (A) Average intensity for the wide coverage MHWs in the northern South China Sea continental shelf each year. (B,C) Are the same as (A), but for moderate coverage MHWs and narrow coverage MHWs. (D–F) Are the same as (A–C), but for the cumulative SST anomalies in each year.
Conclusion
Based on the long time series of SST, the features of MHWs in the northern SCS continental shelf are studied. Using the daily ratio of the area with MHWs to the total area of the northern SCS continental shelf, the MHWs are divided into three categories, i.e., narrow coverage, moderate coverage, and wide coverage.
The occurrence days of narrow coverage MHWs contribute around 64% to the total days with MHWs. This kind of MHW is scattered over the northern SCS continental shelf, with a lower temperature anomaly and SD. Moderate coverage MHWs occupy around 28%, with a slightly higher mean temperature anomaly than narrow coverage MHWs. This kind of MHW is mainly concentrated at the east of Hainan Island. The wide coverage MHWs contribute around 8%, mainly occupying the mid of northern SCS continental shelf. However, the contribution of this category of MHW to the cumulative SST anomalies is largest with around 50% at the mid of the northern SCS continental shelf.
The cumulative days and temperature of all kinds of MHWs exhibit significant long-term trends and decadal variability. However, their mean intensities in each year do not present such significant variability.
Many factors, such as the air-sea heat flux, ocean currents, and large-scale air-sea interaction, could modulate the generation and evolution of MHWs. Their variability and spatial distribution potentially contribute to the spatial characteristics of MHWs. Therefore, the classifications and characteristics of MHWs may be potentially affected by these factors. The details of the relationship between them remain to be revealed in the future.
Data Availability Statement
The original contributions presented in the study are included in the article/supplementary material, further inquiries can be directed to the corresponding author/s.
Author Contributions
YW analyzed and wrote the manuscript. JZ and ZW collected the data and analyzed the variability. SL, ST, FY, QC, and YH participated in the discussion and analysis. All authors contributed to the article and approved the submitted version.
Funding
This study was supported by the State Key Laboratory of Tropical Oceanography, South China Sea Institute of Oceanology, Chinese Academy of Sciences (LTO2113), the Marine Economic Development Project of Guangdong Province (GDNRC [2020]050), the Open Foundation of Key Laboratory of Technology for Safeguarding of Maritime Rights and Interests and Application, SOA (SCS1501), and the Natural Science Foundation of Guangdong Province (2021A1515012538).
Conflict of Interest
The authors declare that the research was conducted in the absence of any commercial or financial relationships that could be construed as a potential conflict of interest.
Publisher’s Note
All claims expressed in this article are solely those of the authors and do not necessarily represent those of their affiliated organizations, or those of the publisher, the editors and the reviewers. Any product that may be evaluated in this article, or claim that may be made by its manufacturer, is not guaranteed or endorsed by the publisher.
Acknowledgments
The OISST data for this article are available at http://apdrc.soest.hawaii.edu/data/data.php.
References
Benthuysen, J. A., Oliver, E. C. J., Feng, M., and Marshall, A. G. (2018). Extreme marine warming across tropical Australia during austral summer 2015-16. J. Geophys. Res.: Oceans 123, 1301–1326. doi: 10.1002/2017JC013326
Bond, N. A., Cronin, M. F., Freeland, H., and Mantua, N. (2015). Causes and impacts of the 2014 warm anomaly in the NE Pacific. Geophys. Res. Lett. 42, 3414–3420. doi: 10.1002/2015GL063306
Cai, R., Tan, H., and Kontoyiannis, H. (2017). Robust surface warming in offshore China seas and its relationship to the east Asian monsoon wind field and ocean forcing on interdecadal time scales. J. Climate 30, 8987–9005. doi: 10.1175/JCLI-D-16-0016.1
Cai, R., Tan, H., and Qi, Q. (2016). Impacts of and adaptation to inter-decadal marine climate change in coastal China seas. Int. J. Climatol. 36, 3770–3780. doi: 10.1002/joc.4591
Chen, K., Gawarkiewicz, G. G., Lentz, S. J., and Bane, J. M. (2014). Diagnosing the warming of the Northeastern US Coastal Ocean in 2012: a linkage between the atmospheric jet stream variability and ocean response. J. Geophys. Res.: Oceans 119, 218–227. doi: 10.1002/2013JC009393
Chen, Z., Shi, J., and Li, C. (2021a). Two types of warm blobs in the Northeast Pacific and their potential effect on the El Niño. Int. J. Climatol. 41, 2810–2827. doi: 10.1002/joc.6991
Chen, Z., Shi, J., Liu, Q., Chen, H., and Li, C. (2021b). A persistent and intense marine heatwave in the Northeast Pacific during 2019–2020. Geophys. Res. Lett. 48:e2021GL093239. doi: 10.1029/2021GL093239
Eakin, C. M., Sweatman, H. P., and Brainard, R. E. (2019). The 2014–2017 global-scale coral bleaching event: insights and impacts. Coral Reefs 38, 539–545. doi: 10.1007/s00338-019-01844-2
Feng, M., McPhaden, M. J., Xie, S.-P., and Hafner, J. (2013). La Niña forces unprecedented Leeuwin current warming in 2011. Sci. Rep. 3:1277. doi: 10.1038/srep01277
Gan, J., Li, H., Curchitser, E., and Haidvogel, D. (2006). Modeling South China Sea circulation: response to seasonal forcing regimes. J. Geophys. Res. 111:C06034. doi: 10.1029/2005JC003298
Gao, G., Marin, M., Feng, M., Yin, B., Yang, D., Feng, X., et al. (2020). Drivers of marine heatwaves in the East China Sea and the South Yellow Sea in three consecutive summers during 2016–2018. J. Geophys. Res.:Oceans 125:e2020JC016518. doi: 10.1029/2020JC016518
Garrabou, J., Coma, R., Bensoussan, N., Bally, M., Chevaldonne, P., Cigliano, M., et al. (2009). Mass mortality in Northwestern Mediterranean rocky benthic communities: effects of the 2003 heat wave. Global Change Biol. 15, 1090–1103. doi: 10.1111/j.1365-2486.2008.01823.x
Hobday, A. J., Alexander, L. V., Perkins, S. E., Smale, D. A., Straub, S. C., Oliver, E. C., et al. (2016). A hierarchical approach to defining marine heatwaves. Prog. Oceanography 238:227. doi: 10.1016/j.pocean.2015.12.014
Hobday, A. J., Oliver, E. C. J., Sen Gupta, A., Benthuysen, J. A., Burrows, M. T., Donat, M. G., et al. (2018). Categorizing and naming marine heatwaves. Oceanography 31, 162–173. doi: 10.5670/oceanog.2018.205
Hughes, T. P., Kerry, J. T., Álvarez-Noriega, M., Álvarez-Romero, J. G., Anderson, K. D., Baird, A. H., et al. (2017). Global warming and recurrent mass bleaching of corals. Nature 543, 373–377. doi: 10.1038/nature21707
Li, Y., Ren, G., Wang, Q., and You, Q. (2019). More extreme marine heatwaves in the China Seas during the global warming hiatus. Environ. Res. Lett. 14:104010. doi: 10.1088/1748-9326/ab28bc
Liu, Q., Jiang, X., Xie, S. P., and Liu, W. T. (2004). A gap in the Indo–Pacific warm pool over the South China Sea in boreal winter. J. Geophys. Res. 109:C07012. doi: 10.1029/2003JC002179
Liu, Q., Wang, D., Wang, X., Shu, Y., Xie, Q., and Chen, J. (2014). Thermal variations in the South China Sea associated with eastern and central Pacific El Niño and their mechanisms. J. Geophys. Res. 119, 8955–8972. doi: 10.1002/2014JC010429
Mills, K. E., Pershing, A. J., Brown, C. J., Yong, C., Fu-Sung, C., and Holland, D. S. (2013). Fisheries management in a changing climate lessons from the 2012 ocean heat wave in the Northwest Atlantic. Oceanography 26, 191–195. doi: 10.5670/oceanog.2013.27
Oliver, E. C. J., Benthuysen, J. A., Bindoff, N. L., Hobday, A. J., Holbrook, N. J., Munday, C. N., et al. (2017). The unprecedented 2015/16 Tasman Sea marine heatwave. Nat. Commun. 8:16101. doi: 10.1038/ncomms16101
Qu, T. (2001). Role of ocean dynamics in determining the mean seasonal cycle of the South ChinaSea surface temperature. J. Geophys. Res.106–C 4, 6943–6955. doi: 10.1029/2000JC000479
Qu, T., Mitsudera, H., and Yamagata, T. (2000). Intrusion of the North Pacific waters into the South China Sea. J. Geophys. Res. 105, 6415–6424. doi: 10.1029/1999JC900323
Reynolds, R. W., Smith, T. M., Liu, C., Chelton, D. B., Casey, K. S., and Schlax, M. G. (2007). Daily high-resolution-blended analyses for sea surface temperature. J. Clim. 20, 5473–5496. doi: 10.1175/2007JCLI1824.1
Schlegel, R. W., Oliver, E. C. J., Perkins-Kirkpatrick, S., Kruger, A., and Smit, A. J. (2017). Predominant atmospheric and oceanic patterns during coastal marine heatwaves. Front. Mar. Sci. 4:323. doi: 10.3389/fmars.2017.00323
Smith, M. D. (2011). The ecological role of climate extremes: current understanding and future prospects. J. Ecol. 99, 651–655. doi: 10.1111/j.1365-2745.2011.01833.x
Tan, H., and Cai, R. (2018). What caused the record-breaking warming in East China seas during August 2016? Atmospheric Sci. Lett. 19:e853. doi: 10.1002/asl.853
Wang, C. (2002). Atmospheric circulation cells associated with theEl Niño–Southern Oscillation. J. Clim. 15, 399–419. doi: 10.1175/1520-0442(2002)015<0399:ACCAWT>2.0.CO;2
Wang, Q., Cai, W., Zeng, L., and Wang, D. (2018). Nonlinear meridional moisture advection and the ENSO-southern China rainfall teleconnection. Geophys. Res. Lett. 45, 4353–4360. doi: 10.1029/2018GL077446
Wang, Q., Wang, Y. X., Sui, J. P., Zhou, W. D., and Li, D. N. (2021). Effects of weak and strong winter currents on the thermal state of the South China Sea. J. Climate 34, 313–325. doi: 10.1175/JCLI-D-19-0790.1
Wang, Q., Zeng, L., Shu, Y., Liu, Q., Zu, T., Li, J., et al. (2020a). Interannual variability of South China Sea winter circulation: response to Luzon Strait transport and El Niño wind. Climate Dynamics 54, 1145–1159. doi: 10.1007/s00382-019-05050-2
Wang, Q., Zeng, L., Chen, J., He, Y., Zhou, W., and Wang, D. (2020b). The linkage of Kuroshio intrusion and mesoscale eddy variability in the northern South China Sea: subsurface speed maximum. Geophys. Res. Lett. 47:e2020GL087034. doi: 10.1029/2020GL087034
Wang, Q., Zhou, W. D., Zeng, L., Chen, J., He, Y. K., and Wang, D. X. (2020c). Intraseasonal variability of cross-slope flow in the northern South China Sea. J. Phys. Oceanogr. 50, 2071–2084. doi: 10.1175/JPO-D-19-0293.1
Wang, Z.-Z., and Wu, R. (2021). Individual and combined impacts of ENSO and East Asian winter monsoon on the South China Sea cold tongue intensity. Climate Dynamics 56, 3995–4012. doi: 10.1007/s00382-021-05682-3
Wernberg, T., Smale, D. A., Tuya, F., Thomsen, M. S., Langlois, T. J., deBettignies, T., et al. (2013). An extreme climatic event alters marine ecosystem structure in a global biodiversity hotspot. Nat. Climate Change 3, 78–82. doi: 10.1038/nclimate1627
Wu, R., Chen, W., Wang, G., and Hu, K.-M. (2014). Relative contribution of ENSO and East Asian winter monsoon to the South China Sea SST anomalies during ENSO decaying years. J. Geophys. Res. 119, 5046–5064. doi: 10.1002/2013JD021095
Xiao, F., Wang, D., and Yang, L. (2020). Can tropical Pacific winds enhance the footprint of the Interdecadal Pacific Oscillation on the upper-ocean heat content in the South China Sea? J. Clim. 33, 4419–4437. doi: 10.1175/JCLI-D-19-0679.1
Xiao, F., Zeng, L., Liu, Q., Zhou, W., and Wang, D. (2018). Extreme subsurface warm events in the South China Sea during 1998/99 and 2006/07: Observations and mechanisms. Clim. Dyn. 50, 115–128. doi: 10.1007/s00382-017-3588-y
Yao, Y., and Wang, C. (2021). Variations in summer marine heatwaves in the South China Sea. J. Geophys. Res.: Oceans 126: e2021JC017792. doi: 10.1029/2021JC017792
Yao, Y., Wang, J., Yin, J., and Zou, X. (2020). Marine heatwaves in China’s marginal seas and adjacent offshore waters: Past, Present, and Future. J. Geophys. Res.: Oceans 125:e2019JC015801. doi: 10.1029/2019JC015801
Keywords: northern South China Sea, marine heatwaves, classification of MHWs, long-term trends and decadal variability, probability of space occupancy
Citation: Wang Y, Zeng J, Wei Z, Li S, Tian S, Yang F, Chen Q and He Y (2022) Classifications and Characteristics of Marine Heatwaves in the Northern South China Sea. Front. Mar. Sci. 9:826810. doi: 10.3389/fmars.2022.826810
Received: 02 December 2021; Accepted: 04 January 2022;
Published: 03 February 2022.
Edited by:
Wei-Bo Chen, National Science and Technology Center for Disaster Reduction (NCDR), TaiwanCopyright © 2022 Wang, Zeng, Wei, Li, Tian, Yang, Chen and He. This is an open-access article distributed under the terms of the Creative Commons Attribution License (CC BY). The use, distribution or reproduction in other forums is permitted, provided the original author(s) and the copyright owner(s) are credited and that the original publication in this journal is cited, in accordance with accepted academic practice. No use, distribution or reproduction is permitted which does not comply with these terms.
*Correspondence: Jisheng Zeng, 969735@qq.com; Zheng Wei, 155050074@qq.com