- 1Institute of Marine Research, Bergen, Norway
- 2Norwegian Institute for Nature Research (NINA), Trondheim, Norway
During the last decades, many wild Atlantic salmon populations have declined dramatically. One hypothesis for an observed reduction in salmon marine growth and survival is reduced abundance of prey. However, the effect of spatial and temporal variation in marine prey abundance on post-smolt feeding conditions is poorly understood. Here we use stomach content data from 2572 salmon post-smolts sampled during 25 years in the Northeast Atlantic Ocean to examine spatial and temporal changes in diet and stomach fullness. Sandeel larvae west of Scotland and Ireland and in the northern North Sea, herring larvae in the eastern part of the Norwegian Sea, and amphipods in the western part of the Norwegian Sea were particularly important prey species. There was a reduction of fish larvae in the post-smolt stomachs over a large geographic area when comparing the period 1995–2004 to 2008–2019. This may be result of a bottom-up driven process, as increasing extent of Arctic Water masses and zooplankton abundance were positively correlated with post-smolt stomach fullness in the Norwegian Sea. Furthermore, the interspecific competition for fish larvae between post-smolts and mackerel may have increased with a larger mackerel stock expanding the feeding migrations northwards since 2007.
Introduction
Since the 1960s, many wild Atlantic salmon populations have declined dramatically, both because of human impacts in rivers and coastal areas, and ecosystem effects in the ocean causing increased mortality in the marine phase (Parrish et al., 1998; Otero et al., 2011; Forseth et al., 2017; ICES, 2021a). Individual growth during the marine phase has been reduced in many regions, concurring with lower marine survival (Friedland et al., 2000; Jonsson and Jonsson, 2004; Todd et al., 2008; Jonsson et al., 2016). Several causes for the reduction in growth and survival have been suggested, spanning from increased numbers and spatial shifts of predators, or reduced prey availability due to interspecific competition, to anthropogenic activity such as fish farming (e.g., Parrish et al., 1998; Jonsson et al., 2016; Vollset et al., 2016; Thorstad et al., 2021). The vast ocean areas used by Atlantic salmon as marine feeding grounds, and the lack of annual ocean surveys targeting Atlantic salmon, have limited the possibilities of detailed studies addressing the different hypotheses on why marine growth and survival have declined. There is large regional and temporal variation in marine growth and survival of Atlantic salmon (ICES, 2021a; Vollset et al., 2022), further complicating such studies.
Despite being one of the world’s most studied fish species, detailed knowledge of the ocean distribution of Atlantic salmon has been limited. Recent studies based on advances in genetic and electronic tagging methods have shown how Atlantic salmon post-smolts (the life stage when they have entered the marine environment for the first time and until the end of the first winter in the sea) and adults use large areas of the North Atlantic Ocean, and how salmon from different regions vary in their migration patterns and distribution (Strøm et al., 2017; Bradbury et al., 2021; Gilbey et al., 2021; Rikardsen et al., 2021). The increased knowledge on the oceanic distribution of Atlantic salmon has provided new opportunities to analyze and understand the causality underlying the variation in marine growth and survival. The distribution of post-smolts from different European regions in the northeast-Atlantic Ocean was recently mapped (Gilbey et al., 2021). The area west of Ireland and Scotland and further north into the Norwegian Sea is an important migratory pathway for post-smolts in the Northeast Atlantic. These results form an important basis for studies aiming at addressing how regional mechanisms affect post-smolt growth and survival in the Northeast Atlantic.
The first months at sea are thought to be a critical period for the overall marine growth, survival and time spent at sea of Atlantic salmon (McCarthy et al., 2008; Trehin et al., 2021). For post-smolts sampled in the Norwegian Sea, there was a drop in condition factor in the period 2004–2012 and the condition factor remained low thereafter. The drop in condition factor was associated with reduced stomach fullness, indicating poorer prey availability and feeding opportunities during this period (Utne et al., 2021a). A reduced growth of Atlantic salmon from several populations based on scale analyses has also been shown during this period (Jensen et al., 2012; Trehin et al., 2021; Vollset et al., 2022). The observed reduction in stomach fullness, condition factor and individual growth may explain the reduced marine survival after 2004. The marine survival has in general decreased since the 1960s (Parrish et al., 1998) but information about post-smolt size and feeding conditions in the Northeast-Atlantic prior to 1995 is limited. The range of prey species consumed by post-smolts during their first summer in the ocean, and which prey species were the most important food items, have been identified (e.g., Rikardsen et al., 2004; Haugland et al., 2006; Utne et al., 2021a). However, these data have not been used to address how the post-smolt diet may have changed in time and space, and potentially contributed to the reduced condition and growth of Atlantic salmon at sea. Combining knowledge of migratory pathways and distribution of post-smolts with data on the abiotic and biotic conditions along the migration route, make it possible to study how prey availability and other factors affect post-smolts within this geographic region. We hypothesize that the marine feeding conditions for post-smolt in parts of the Northeast Atlantic became poorer in the period 2004–2012, and that this was linked to large-scale ecosystem changes, since there was a synchronous reduction in condition factor, stomach fullness and growth in Atlantic salmon from many European populations.
The main objective of the present study was to investigate if there has been a reduction of post-smolt prey availability within geographic regions along the migratory routes in the Northeast-Atlantic, and if so, to identify the mechanisms causing this reduction. The approach is to estimate (1) geographic and temporal variation in consumption of important prey species and prey groups, (2) the geographic variation in post-smolt stomach fullness in the period 2008–2019 compared to the period 1995–2004, 3) how changes in the marine environment have affected post-smolt feeding conditions. We link data on post-smolt diet in the Northeast Atlantic during 1995–2019 with data on hydrographic conditions, prey abundance and potential competitors.
Materials and Methods
Biological Sampling
Atlantic salmon post-smolts were caught in trawl surveys conducted west of Ireland and Scotland, in the northern North Sea, and in the Norwegian Sea in May-August during 1995–2019 (Figure 1A). The total sample consisted of 2,572 post-smolts caught during a total of 40 surveys (Supplementary Table 1). These include dedicated salmon surveys targeting areas where salmon were known to migrate, surveys mapping the geographic distribution of salmon, and ecosystem surveys targeting other pelagic species but catching salmon post-smolts as bycatch. In all surveys, a pelagic trawl towed at the surface at 3–5 knots (5.6–9.3 km/h) with additional floats was used. Two large floats attached on each side and a float in the center kept the trawl at the surface during towing. More information about surveys objectives, trawling and biological sampling is given by Utne et al. (2021a). Salmon larger than 35 cm before August and larger than 40 cm in August (Gilbey et al., 2021) were excluded from further analyses to ensure that the dataset only included post-smolts.
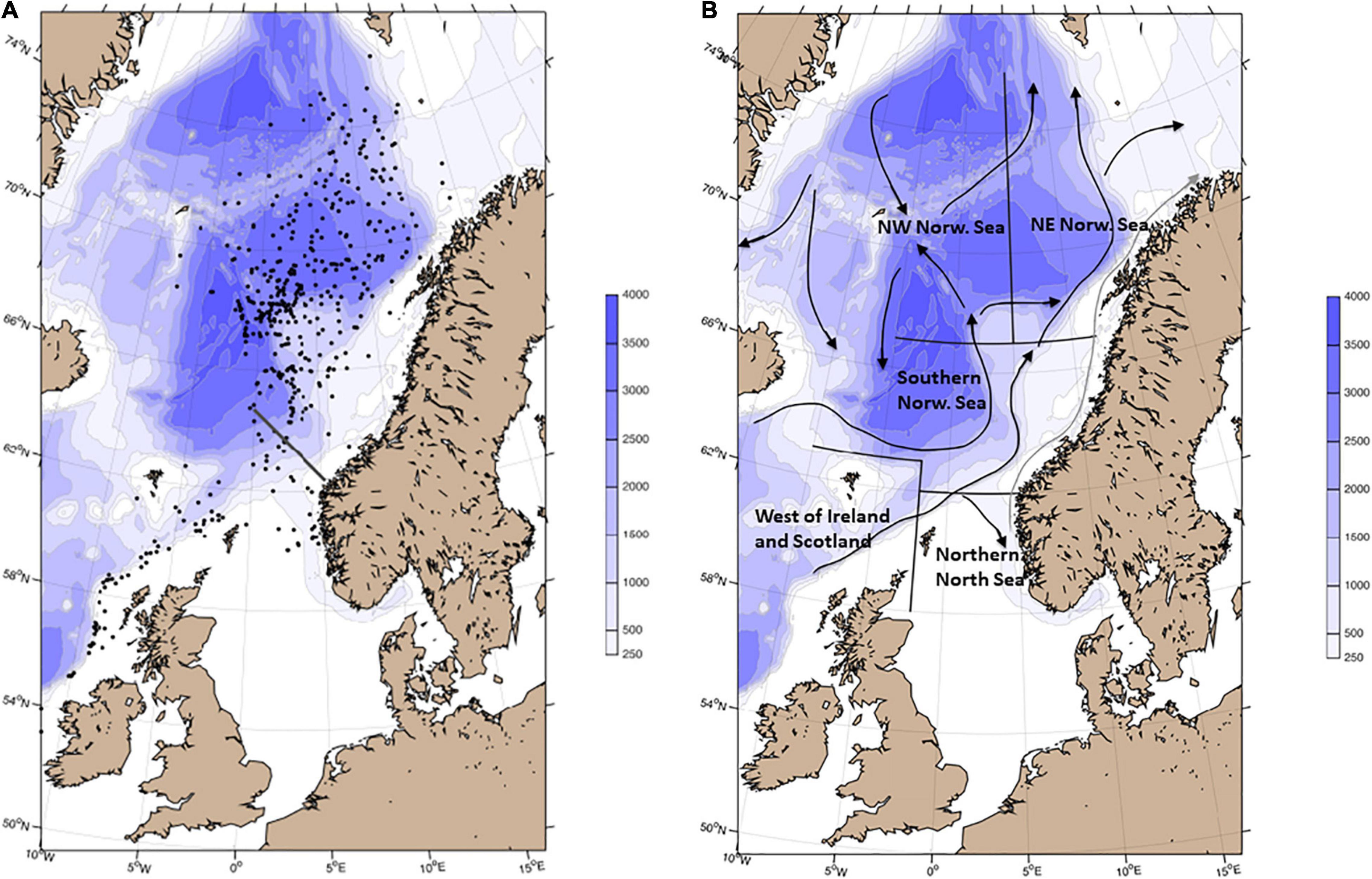
Figure 1. (A) The geographic distribution of sampled post-smolts. Each circle represents a location where post-smolts were sampled, but the number of individuals within each location vary from one to tens of post-smolts. Black solid line off the Norwegian coast around 63°N represents the survey transect measuring hydrography and sampling zooplankton (Svinøy section). (B) Map of the study area with the different sub-regions addressed in the analyses, and arrows representing the general currents in the Northeast Atlantic.
Fork length to nearest half centimeter and weight to nearest gram were recorded for all sampled post-smolts. Stomachs were removed from the fish on deck and frozen for later analyses of diet. The stomach content was identified to species level when possible, or to closest family if too digested for species identification. Prey identification was based on visual identification of body morphology and species-specific characteristics such as spines, number of legs, pigmentation, etc. The weight of all unique prey groups in each stomach was recorded for all samples. Prey species and groups from each stomach were dried separately at 70°C for more than 24 h, and dry weight was recorded. The feeding ratio (FR) is an estimate of the dry weight of the stomach content relative to the fish dry weight. It is a snapshot of the stomach content at the time of sampling. The feeding ratio was calculated following Eq. 1:
where mf is the mass (g) of the post-smolt and ms is the mass (g) of the stomach content. FRfish and FRzoo are equivalent to FR where ms is the mass of fish or zooplankton in the stomach, respectively.
Regional and Temporal Variation in Diet
To understand spatial and temporal variation in the post-smolt diet, the data sampled along the post-smolt migration routes in the northeast Atlantic region, as identified by Gilbey et al. (2021), were divided into five regions. The diet of post-smolts sampled in each region is presented on an annual basis. The rationale for the separation of subregions was differences in depth (shelf, slope, or deep basin areas), ocean current systems, and dominating water masses. The subregions are: (1) West of Ireland and Scotland (west of 2°W and south of 63°N), where post-smolts migrate along the narrow shelf area dominated by warm Atlantic water flowing northwards. (2) Northern North Sea, which is a shallow shelf area (east of 2°W d south of 62°N). This is the migration pathway for salmon originating from rivers with outlets into the North Sea. Fish from Ireland, western parts of Scotland and countries further south can also migrate through the northwestern part of this area. (3) Southern Norwegian Sea (between 62 and 67°N). The migratory pathway for post-smolts in this region is along the slope on the eastern side of the Norwegian Sea in the warm Atlantic water flowing northwards. (4) Northwestern Norwegian Sea (north of 67°N and west of 5°E). A general pattern is that post-smolts when reaching the Vøring platau at 67°N either migrate northwestward or follow the slope further northeastward, although the migration pattern seems to vary greatly in this region. The northwestward migration starts in a region of the western branch of the Atlantic water, the Norwegian Atlantic Front Current (NwAFC), and continue toward Jan Mayen (Figure 1B). (5) Northeastern Norwegian Sea (north of 67°N and east of 5°E). Individuals following the eastern branch of the Atlantic water, the Norwegian Atlantic Slope Current (NwASC), will mainly be distributed in the northeastern Norwegian Sea.
Geographic and Temporal Variation in Stomach Fullness and Consumption of Important Prey
One objective was to identify geographic variation in stomach fullness, here expressed as FRzoo and FRfish, and if these indices changed with time. Secondly, we wanted to identify geographic variation in the probability to consume important prey species. The following prey species/groups were included in the analyses, as they have been identified as important prey groups in earlier studies (Haugland et al., 2006; Utne et al., 2021a): Ammodytes spp. larvae (hereafter referred to as sandeel), Clupea harengus larvae (hereafter referred to as herring larvae), Sebastidae and Myctophidae larvae (hereafter referred to as mesopelagic fish larvae), amphipods, euphausiids, and copepods. The number of samples from each year varied, and some years with few or no samples limited the possibility to perform a temporal analysis of geographic differences in feeding ratio or the probability to consume important prey. The data set was therefore aggregated into two time periods for geographical analyses of stomach fullness: 1995–2004 (high condition factor period) and 2008–2019 (low condition factor period) (Utne et al., 2021a). There were no data available for the years 2005–2007. A division into finer time periods was initially tested but rejected due to limited sample size and poor model fit to data for some of the periods.
Geographic interpolated maps of FRzoo, FRfish, and six prey species/groups were produced with generalized additive modeling (GAM) (Hastie and Tibshirani, 1990). This modeling approach interpolates with a smoothing function. Smoothing regression techniques are data-driven and do not force the shape of the response to any parametric form. FRfish and FRzoo had skewed distributions with a high proportion of zero values, and a two-component hurdle model was therefore applied for these response variables. The hurdle model combined two models: (1) A binomial (empty/not empty stomachs) model, and (2) a positive biomass model for the stomach content, for which the data was modeled with a gamma distribution. A Tweedie distribution with log-link was tested for these response variables, but the model fits were not found satisfactory.
For estimating the probability of consuming different prey items, a binomial model was applied, where the response variable was presence/absence of respective prey in the stomachs. From 2012 and onward, all fish larvae were identified to teleosti and not to species or family level. Only years with fish larvae identified to species or family level were included in the models for prey presence. In the applied models,
r is the response for fish i, s1 is a two-dimension smoothing term used for latitude and longitude, year is included as a random effect variable, and ε is a random normal distributed error term with variance according to the distribution of the response variable. For presence/absence of prey, a logit-link was applied, while a log-link was used for FRfish and FRzoo. The parsimony principle was used to select the model with the lowest Akaike Information Criterion (AIC) (Burnham and Anderson, 2002). GAMs were fitted using the R statistical programming v.4.1.0 environment with package mgcv v. 1.8-35 (Wood, 2011). All model assumptions were visually assessed using Q–Q plots and residual variation vs. fitted values and leverage.
A reduced feeding ratio for post-smolts sampled in the Norwegian Sea in the period 2003–2012 have previously been presented (Utne et al., 2021a) based on the same dataset applied in the analyses presented here. Here we use the data in new analyses to understand the geographic variation in feeding ratio within the Northeast Atlantic, and if this has changed over time. The dataset is also, for the first time, used to explore where the different prey groups are commonly found in post-smolt stomachs.
Linking Post-smolt Feeding to Data on Environmental Conditions and Abundance of Prey and Potential Competitors
The final objective of this study was to understand if changes in post-smolt stomach fullness could be explained by changes in environmental conditions, prey abundance, or interspecific competition. For the Norwegian Sea, there are sufficient data from post-smolt stomach samples, prey abundance and environmental data to test this statistically. For the region west of Ireland and Scotland and the northern North Sea, the data are not sufficient to support statistical analyses, but relevant data are presented and evaluated qualitatively.
For the Norwegian Sea ecosystem, historic recruitment of Norwegian-Spring-Spawning Herring (NSSH) for the years 1995–2018 as estimated by stock assessment (ICES, 2020a) was used as a proxy for potential availability of fish larvae available as prey for post-smolts in each year. Standing biomass of zooplankton in May was included as an index of interannual variability in zooplankton abundance. This zooplankton index was calculated from plankton net (WP2) hauls from 200 m depth to the surface at stations with fixed spacing covering the majority of the Norwegian Sea. More information about the zooplankton index is given by ICES (2021b). Mackerel can be a potential competitor for post-smolt prey (Utne et al., 2021b). The spawning stock biomass for Northeast Atlantic mackerel estimated from the analytic assessment (ICES, 2021c) was therefore included in the analyses. Two indices of water circulation for the Norwegian Sea were retrieved from ICES (2021b), which were maximum salinity of the Atlantic Water masses (ATLsmax) and proportion of arctic water masses ARCprop. Both indices were measured at a hydrographic transect in the southern Norwegian Sea in May (Svinøy section, Figure 1A). The central part of this transect covers the core of the warm and saline Atlantic Water flowing northward in the Norwegian Sea. ARCprop represents the westernmost part of the transect, which is influenced by Arctic Water from the Iceland and Greenland seas (Figure 1B). ATLsmax is the maximum salinity measured along the transect, while ARCprop is the proportion of the transect with salinity < 34.9‰ and water temperature < 4°C.
For the region west of Scotland and Ireland, historic recruitment of blue whiting for the years (1995–2018) as estimated by stock assessment (ICES, 2020a) was included in the analyses. Total annual commercial landings were used as a proxy of sandeel abundance, because historic estimates of recruitment or stock size were not available for the regions west Scotland (ICES sandeel region 6a), around Shetland (ICES sandeel region 7), and northern North Sea (ICES sandeel region 5). Total annual commercial landings in these regions give a qualitative representation of sandeel abundance in the years 1995–2018 (ICES, 2020b), with the assumption that the landings reflect stock abundance.
The indices are presented with loess smoothing overlaying the annual point values to show the general trend in temporal development. Loess smoothing is a non-parametric technique that fits a smooth curve through a scatter plot by using local weighted regression, which was done with the R-package “stats” (R Core Team, 2020) using span equal to 0.75 and 2nd degree polynomials.
To test if post-smolt feeding the Norwegian Sea was affected by changes in the environmental variables, a two-step analysis was conducted. In the first part of the analyses, GAMs were applied to estimate annual indices of post-smolt feeding ratio of fish and zooplankton (FRfish and FRzoo). This was necessary to standardize the response variable and eliminate bias from uneven sampling effort. Initial data exploration revealed that the feeding ratios were zero-inflated with a few high values, and a two-step hurdle model was therefore applied. Presence/absence of the response variable was modeled with a binomial distribution with a logit-link. The positive FR observations were modeled with a gamma distribution with a log-link function. The full models prior to model selection takes the form
where FR is either FRfish and FRzoo, αis the model intercept, s1 is a two-dimension smoothing term used for latitude and longitude, s2 and s3 are one-dimensional smoothing terms where the number of splines are restricted to 5, doy is the day number of the year, fr is FRfish when FRzoo is the response variable and FRzoo when FRfish is the response variable, year is modeled with a fixed effect, i represents fish number and ε is a normal distributed random error term. Model fitting was done in R (R Core Team, 2020) using the mgcv package (Wood, 2011). Model evaluation followed the general description as described for the geographic interpolation.
In the second part of the analyses, Spearman rank tests were used to calculate the correlation between annual indices of post-smolt feeding and environmental variables.
Results
Regional and Temporal Variation in Post-smolt Diet
A regional presentation of post-smolt diet and stomach fullness (Figure 2) shows that sandeel was the most important prey item around the British Isles. In the Norwegian Sea, fish larvae dominated the diet, especially in the southern and eastern region. The proportion of amphipods in the post-smolt diet increased further north and west, and this prey was also important for post-smolts in the Norwegian Sea. Detailed patterns for each region are described in the following.
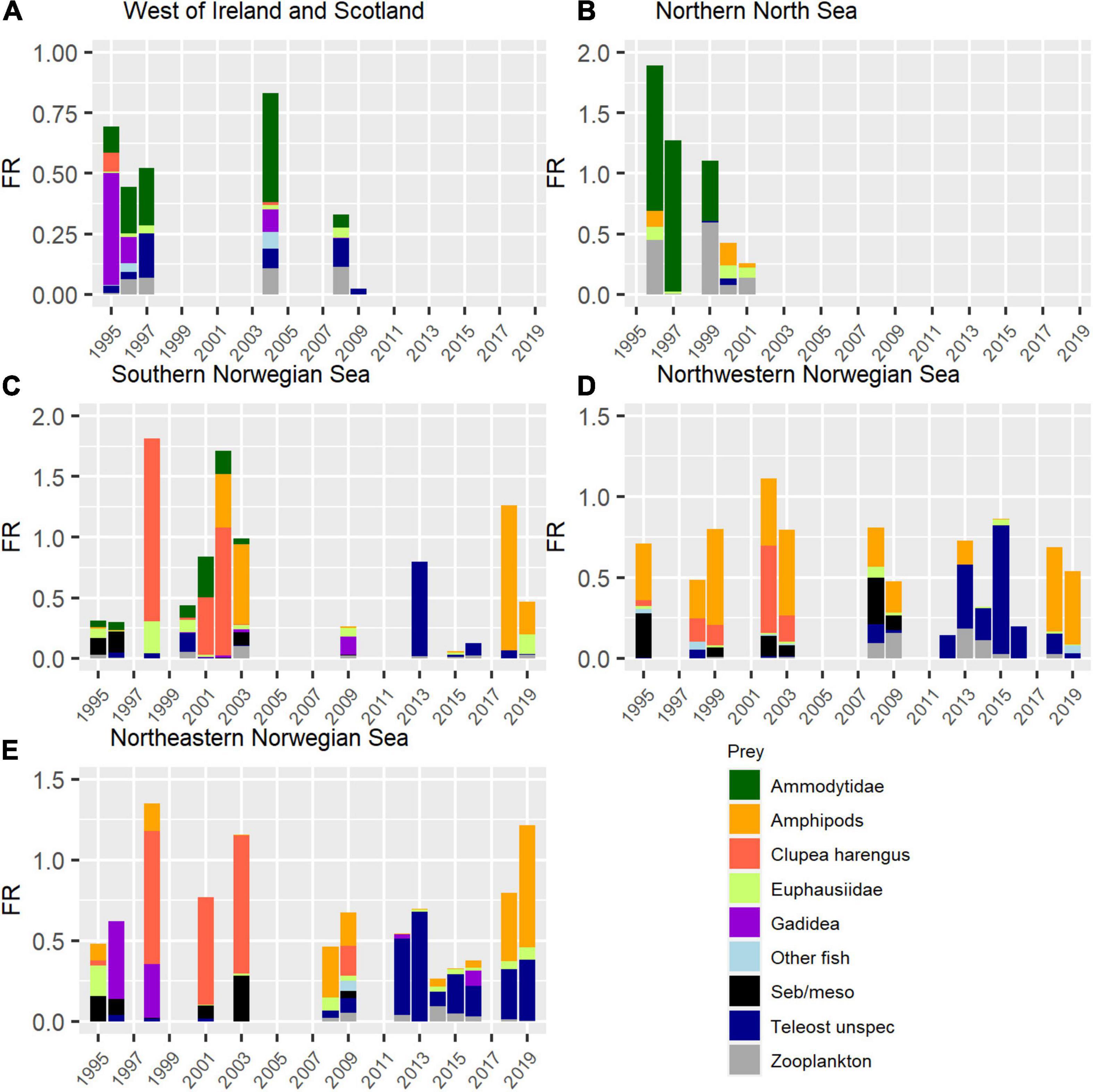
Figure 2. Histograms of annual average FR values for different regions, where each annual value split into prey groups (by weight). The regions are (A) West of Ireland and Scotland, (B) Northern North Sea, (C) Southern Norwegian Sea, (D) Northwestern Norwegian Sea, and (E) Northeastern Norwegian Sea. The figure was produced with the use of the following R-packages: ggplot2 (Wickham, 2016) and gridExtra (Auguié, 2017).
West of Ireland and Scotland
The dominating prey were sandeel and fish from the cod family (Gadidae) (Figure 2A). The years with the lowest FR values were 2008 and 2009, which were the last 2 years with data sampling in the region. In contrast to previous years, gadoids and sandeel were in general absent from the diet these 2 years, even though the small proportion of unidentified fish larvae could belong to these groups.
Northern North Sea
The dominating prey were sandeel larvae and various zooplankton species (Figure 2B). Both euphausiids and amphipods were present but did not dominate the diet. The years with the lowest FR values were 2000 and 2001, which were the two last years with data sampling in the region. In contrast to previous years, sandeel or any other fish larvae were not present in the salmon stomachs these 2 years.
Southern Norwegian Sea
The most common prey varied between years, but herring larvae and amphipods dominated the diet in years with high FR in this region (Figure 2C). Both these prey species have large interannual variation, varying from close to absent to being the most common prey in the region. Amphipods reappeared in the diet in 2018 and 2019 after being absent during 2008–2016. Larvae of sandeel, mesopelagic fish and fish from the cod family (Gadidae), as well as euphausiids and other zooplankton were also consumed by post-smolts in the southern Norwegian Sea, although the importance of each of these prey groups was relatively small.
Northwestern Norwegian Sea
Amphipods were, as in the southern Norwegian Sea, an important part of post-smolt diet in the Northwestern Norwegian Sea (Figure 2D). The exception was the period 2012–2016, when amphipods were absent or a very limited part of the diet in this region. The fish larvae consumed were herring or mesopelagic fish larvae.
Northeastern Norwegian Sea: The diet was dominated by fish larvae in this region (Figure 2E). Herring larvae dominated the diet in years with the highest post-smolt feeding ratios (1998, 2001, and 2003). Zooplankton did not dominate the diet in this region, but the strong appearance of amphipods seen further south and west in 2018 and 2019 was also apparent here. Larvae of gadoids or mesopelagic fish were also an important part of the diet.
Geographic Distribution of Prey in Post-smolt Stomachs
Sandeel was part of the diet of post-smolts west of Ireland, around Shetland and in the northern North Sea (Figure 3A). Sandeel larvae were also found in stomachs at the boundary between the North Sea and the southern Norwegian Sea, but not close to the Norwegian coast or further north in the Norwegian Sea.
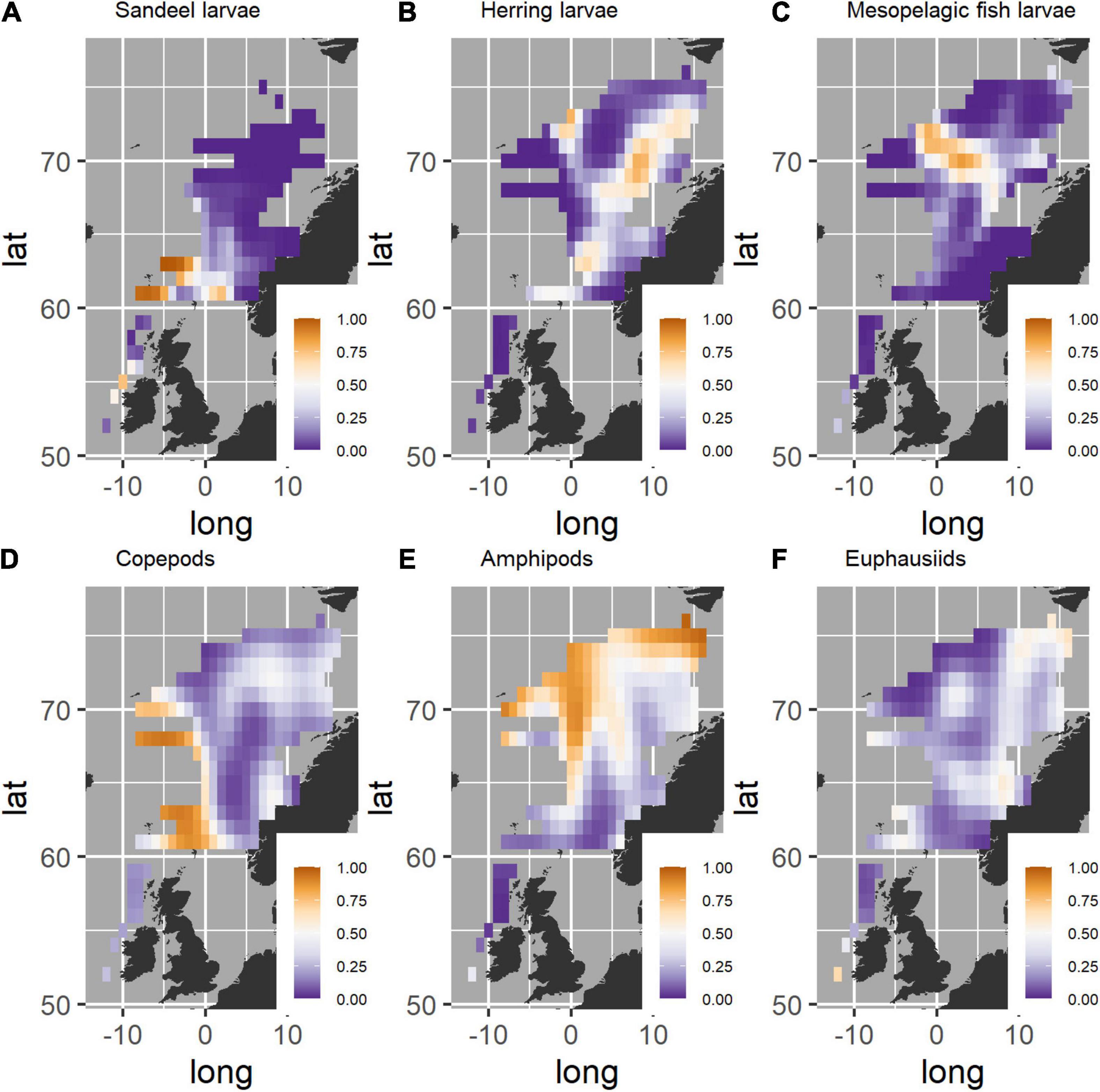
Figure 3. Geographic variation in probability of finding different prey in post-smolt stomachs in areas of the Northeast Atlantic Ocean. A value of 1 indicates that the prey was found in all post-smolt stomachs in the region, while 0 indicates that the prey was not found in any post-smolt stomachs. (A) sandeel larvae, (B) herring larvae, (C) mesopelagic fish larvae, (D) copepods, (E) amphipods, (F) euphausiids. The figure was produced with the use of the following R-packages: ggplot2 (Wickham, 2016) and gridExtra (Auguié, 2017).
Herring larvae (Figure 3B) were consumed over a large area from west and north of Shetland to the eastern part of the Norwegian Sea off the Norwegian coast from around 62 to 75 °N. The highest probability of finding herring larvae in post-smolt stomachs was in the northeastern part of the Norwegian Sea outside the Norwegian continental and the Barents Sea shelf.
Larvae of mesopelagic fish (Figure 3C) were consumed throughout the Norwegian Sea. The probability of finding larvae of mesopelagic fish in post-smolt stomachs was higher in central areas of the Norwegian Sea in the region from the Vøring platau and toward Jan Mayen than in the remaining Norwegian Sea. Larvae of mesopelagic fish were not found in post-smolt stomachs in the North Sea and only to a limited extent west of Ireland and around Scotland.
Copepods were consumed over the entire study area (Figure 3D). The region with the highest probability of finding copepods in post-smolts stomachs was in the western part of the Norwegian Sea, where almost all salmon post-smolts had consumed copepods.
Amphipods were consumed over large parts of the Norwegian Sea, but was most commonly found in stomachs sampled in the central, northern and western parts (Figure 3E). Amphipods were not consumed west of Ireland and Scotland, and only to a limited extent in the northern North Sea and in the eastern Norwegian Sea.
Euphausiids were consumed over large parts of the sampled region (Figure 3F). This includes west of Ireland, north of Scotland and the eastern part of the Norwegian Sea from around 60 °N and up to Svalbard at 76 °N. Euphausiids were also consumed in central parts of the Norwegian Sea. Euphausiids were not found in the stomachs in the northwestern area along the Polar Front between the Lofoten Basin and the Greenland Basin.
Geographic Variation in Stomach Fullness in 1995–2004 Compared to 2008–2019
Fish larvae were consumed by post-smolts over most of the sampled area west of Ireland, north of Scotland, the northern North Sea and throughout the Norwegian Sea during the first time period (1995–2004). The areas with the highest FRfish, with average values well exceeding 1.0, were found west of Ireland, in the northern North Sea, in the southern Norwegian Sea, and in the northeastern part of the Norwegian Sea (Figure 4A). In the second period (2008–2019), the FRfish was lower than in the first period in all regions, with the largest decrease west of Ireland and in the northeastern Norwegian Sea (Figures 4B,C).
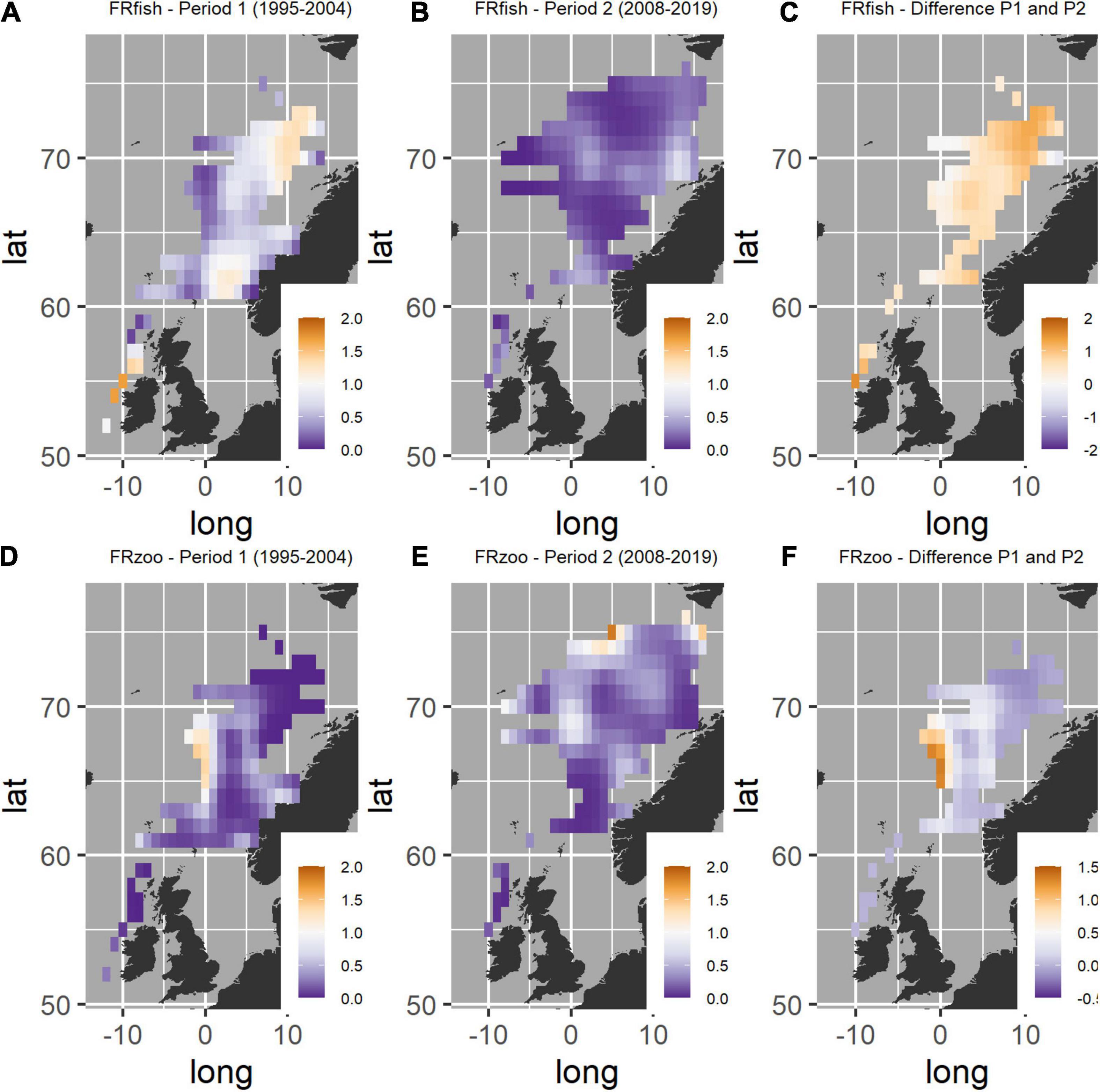
Figure 4. Geographic variation in feeding ratio (FR) estimated by using a hurdle model in areas of the Northeast Atlantic Ocean. (A) FRfish in period 1 (1995–2004), (B) FRfish in period 2 (2008–2019), (C) difference in FRfish between period 1 and 2 where positive values indicate that the FRfish was highest in period 1, (D) FRzoo in period 1, (E) FRzoo in period 2, and (F) difference in FRzoo between period 1 and 2 where positive values indicate higher values in period 1. The figure was produced with the use of the following R-packages: ggplot2 (Wickham, 2016) and gridExtra (Auguié, 2017).
Zooplankton were consumed over large parts of the Norwegian Sea. The highest FRzoo values were in the western part of the Norwegian Sea in the first period (1995–2004) (Figure 4D), and in the northern Norwegian Sea in the second period (2008–2019) (Figure 4E). Comparisons of FRzoo between the two periods were restricted to areas that were sampled in both periods, thereby excluding the northernmost Norwegian Sea in the comparison. There was a pattern with lower values in the second than the first period in the western region of the Norwegian Sea around 64–71°N (Figure 4F). For the other regions, the area west of Ireland and the central and eastern Norwegian Sea, there were only minor differences between the two periods, and the difference varied between positive and negative values.
Linking Post-smolt Feeding to Data on Environmental Conditions and Abundance of Prey and Potential Competitors
Post-smolt FRfish in the Norwegian Sea was positively correlated with the extent of Arctic Water (ARCprop), and negatively correlated with the spawning stock biomass of mackerel (Table 1). FRfish was not correlated with properties of the Atlantic Water nor to the zooplankton abundance index for the Norwegian Sea. There was a positive correlation between FRfish and herring recruitment strength, although not statistically significant with the selected threshold of 0.05 for the p-values (p = 0.064, Table 1).
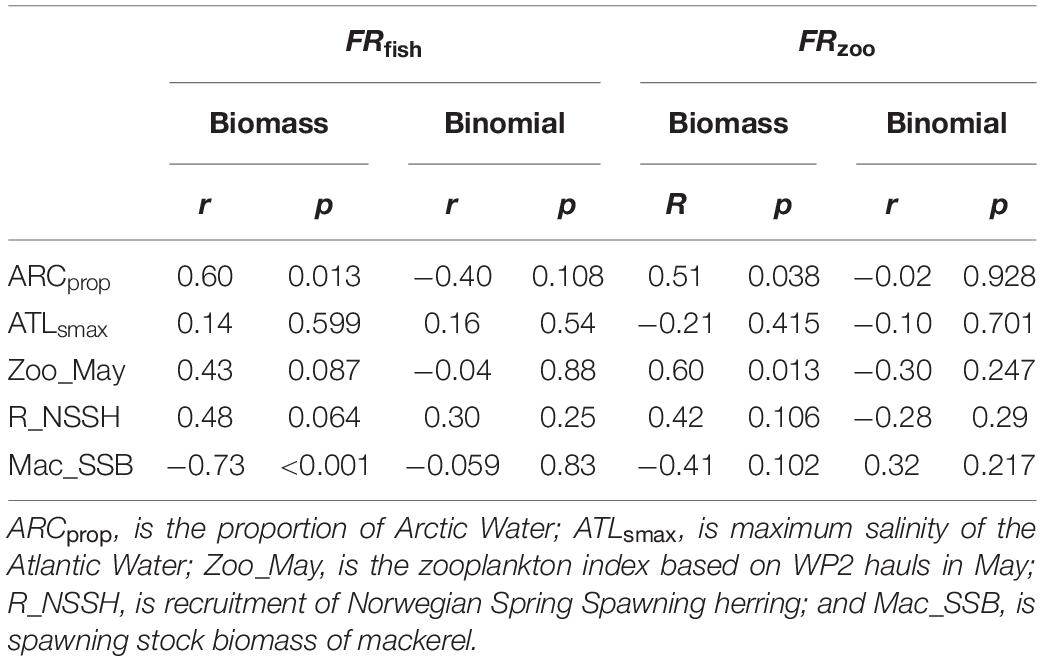
Table 1. Metrics of Spearman’s correlations (r-rank correlation coefficient and p-value) between post-smolt feeding ratios and environmental variables for the Norwegian Sea.
Post-smolt FRzoo in the Norwegian Sea was positively correlated with the zooplankton abundance index for the Norwegian Sea (Table 1). There was also a significant correlation between FRzoo and the inflow of arctic water masses, although this correlation coefficient was lower than for the zooplankton index. There was no significant correlation between FRzoo and properties of the Atlantic water masses nor to the spawning stock biomass of mackerel.
The probability of finding zooplankton or fish larvae in the post-smolt stomachs, as estimated by using the binomial model, was not correlated to the indices for water masses ARCprop or ATLsmax, the plankton index, spawning stock biomass of mackerel or fish larvae indices.
Discussion
Post-smolt diet and stomach fullness in the Northeast Atlantic changed considerably over the 25-year study period. There was a reduction of fish larvae in the post-smolt stomachs over a large geographic area when comparing the periods 1995–2004 and 2008–2019. We identified the geographic distribution of prey organisms dominating in the post-smolt diet. Sandeel west of Scotland and Ireland and in the northern North Sea, herring in the eastern part of the Norwegian Sea and amphipods in the western part of the Norwegian Sea were particularly important prey species. Reduced proportion of Arctic Water and reduced zooplankton abundance were the major changes affecting post-smolt feeding conditions in the Norwegian Sea. Furthermore, the results indicate that the collapse of several sandeel populations in the Northeast-Atlantic and poor recruitment of NSS-herring since year 2004 have reduced prey availability for post-smolts. The analyses presented here show how these changes have affected salmon feeding conditions by reducing the availability of important prey for salmon, and is an important step further to understand the mechanisms behind reduced marine growth and survival of Atlantic salmon.
Reduced feeding of post-smolts during 2008–2019, as indicated by a reduction of prey in post-smolt stomachs in the Norwegian Sea, was positively correlated with a reduction of Arctic Water in the Norwegian Sea. The large-scale changes to water circulation affected the plankton production and species composition in the Norwegian Sea, and thereby changed the productivity of the ecosystem (Skagseth et al., this issue). These changes in the ecosystem most likely affected the abundance of prey important for salmon through bottom-up processes, as shown in the present study. The findings are furthermore supported by a drop in marine growth for Norwegian salmon occurring simultaneously as the Norwegian Sea ecosystem changed (Vollset et al., 2022). Analogous ecosystem changes have been shown to impact feeding conditions in the Northwest Atlantic Ocean, where capelin is an important prey species for Atlantic salmon. Mills et al. (2013) showed how changing hydrographic conditions in the northwest Atlantic ecosystem in the 1990s propagated to higher trophic levels by affecting species composition and abundance of plankton, as well as the planktivorous fish capelin. A reduction in capelin size and energy density due to the changes in the ecosystem reduced the quality of capelin as prey for salmon (Renkawitz et al., 2015). Previous studies have demonstrated a correlation between reduced return rates of salmon in the northeast Atlantic to large scale oceanographic changes such as the AMO index or the subpolar gyre, or to water temperature or plankton production south of the Norwegian Sea (e.g., Beaugrand and Reid, 2003, 2012; Friedland et al., 2009; Almodovar et al., 2019). The changes to the plankton community seen in the North Sea and west of Ireland and Scotland (Beaugrand and Reid, 2003, 2012), with a reduction of total copepods and euphausiids, may have continued into the southern Norwegian Sea with the retraction of the inflow of arctic water from the west. There are also studies that show how salmon prey have declined in abundance correlated to changes in water circulation and increased water temperatures. One example is the correlation between primary production, fish recruitment and warmer water in the North Sea in the years 1988–2013 (Capuzzo et al., 2018). Along the Norwegian coast, changes in the water temperature have been linked to lower zooplankton abundance and reduced recruitment of NSSH (Toresen et al., 2019).
Most likely, both global and regional changes in ocean circulation systems and environmental conditions affect salmon feeding conditions, and global and regional changes are often partly correlated. The inflow of Arctic Water into the Norwegian Sea with the East Icelandic Current partly follows the same decadal trend as the subpolar Gyre, which regulates the amount of Arctic Water being transported northwards west of the British Iles (ICES, 2021b). The positive effect of a strong subpolar gyre, which increase the flow of arctic water into the Faroe Iceland ridge, impacts the calanoid copepods in this region (Hatun et al., 2009). Hence, the inflow of Arctic Water into the Norwegian Sea is not only regulated by the East Iceland Current, but also by large scale mixing of Sub-Tropical and Sup-Polar water masses in the Atlantic Ocean. The observed changes in prey availability presented here spans over the period 1995–2019, and no comparable information is available for the years prior to 1995. It is therefore unknown whether the apparent good feeding conditions for post-smolts in the period 1995–2004 are representative for previous decades which had higher return rates of salmon than recent years. However, the East Icelandic Current transporting Arctic Water into the Norwegian sea was unusually strong and cold in the 1960s (Scarnecchia, 1984). Even though the intensity of this current became gradually reduced, the Norwegian Sea was greatly influenced by Arctic Water during the 1970s and 1980s (Dickson and Østerhus, 2007). This indicates that prey availability for post-smolt in the Norwegian Sea could have been high in the decades prior to 1995, even though for instance herring larvae were absent in the period 1960–1982 due to the collapse of NSS-herring stock (ICES, 2020a).
This study showed that reduced abundance of important prey targeted by Atlantic salmon post-smolts has had a negative impact on the feeding conditions for post-smolts in the northeast Atlantic, irrespective of the underlying mechanisms. Sandeel dominated the diet of post-smolts in the late 1990s in several regions, including the area west of Scotland and in the northern North Sea. Sampling west of Scotland in the 1980s also confirmed the importance of sandeel as prey in this region (Morgan et al., 1986). Sandeel larvae became absent in the post-smolt diet in several regions after 2004, which seemed to be linked to a general reduction of the stomach fullness of salmon post-smolts. The decline in sandeel abundance is reflected in a reduction in the sandeel fisheries. The sandeel fishery around Shetland decreased gradually in the early 2000s and came to a full stop in 2003 (Figures 5A–C). The sandeel fishery in the eastern part northern North Sea and in the area west of Scotland decreased in the late 1990s, until a stop in 2004. Except for some minor catches in 2007, there have not been catches of sandeel from these regions after 2003 and 2004. There is no indication of increased abundance of sandeel at the traditional important sandeel banks in the Northern North Sea, according to annual surveys (ICES, 2020b).
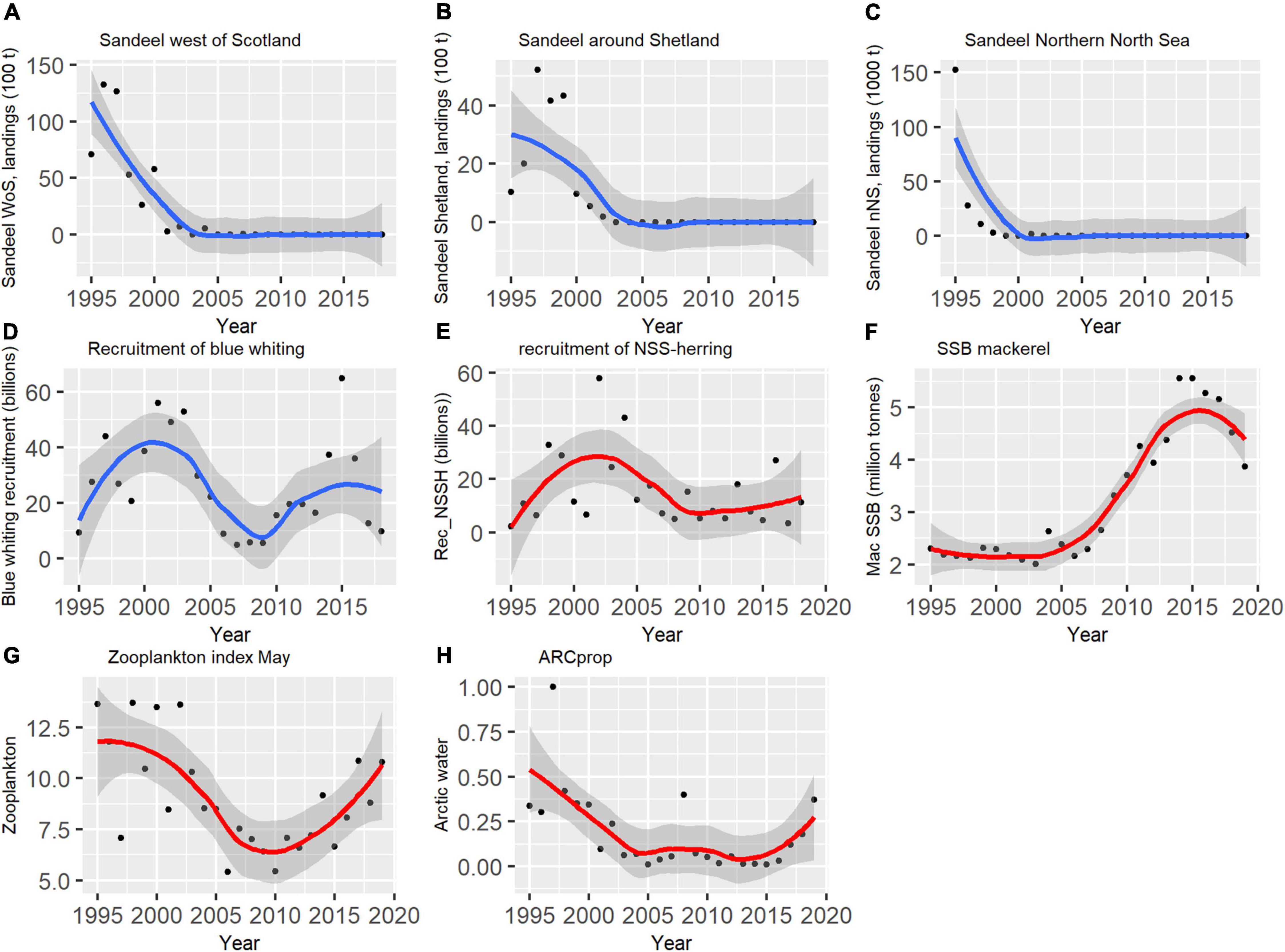
Figure 5. Temporal development of prey abundance and environmental indices potentially important for post-smolts in the Northeast-Atlantic. Indices represented with a blue line is south of the Norwegian Sea, while red lines represent indices for the Norwegian Sea. (A) Sandeel landings west of Scotland (woS), (B) sandeel landings around Shetland, (C) sandeel landings in the northern North Sea, (D) recruitment of blue whiting, (E) recruitment of Norwegian spring-spawning herring (NSSH), (F) spawning stock biomass mackerel (G) zooplankton index May, (H) index for the proportion of arctic water (ARCprop), and (I) salinity of Atlantic water (ATLsmax). The figure was produced with the use of the following R-packages: ggplot2 (Wickham, 2016) and cowplot (Wilke, 2020).
The disappearance of sandeel larvae available for post-smolts west of Scotland can potentially be compensated by abundant larvae of gadoids in this area in years when gadoid larvae are abundant (Figure 5D). The gadoids identified in post-smolts stomachs in this region were most likely blue whiting (Micromesistius poutassou), since this species has previously been abundant in post-smolt stomachs (Holst et al., 1996), and because of the geographic overlap between blue whiting larvae (Bailey, 1982) and salmon post-smolts in the region. Recruitment of blue whiting was strong in the years 1995–2004. This was followed by a period of poor recruitment until 2010 and onward, when recruitment again increased (ICES, 2020a). Hence, blue whiting may be important for post-smolt feeding west of Ireland and Scotland in years with strong recruitment, but there is a lack of post-smolt data from this region during the recent years.
The results in this study show that herring larvae were an important part of the post-smolt diet in large parts of the Norwegian Sea, and especially in the northeastern region. This result supports the findings by Haugland et al. (2006), who reported that post-smolt stomach fullness increase in years with strong recruitment of herring. Norwegian spring-spawning herring (NSSH) has a recruitment pattern with occasional very strong year-classes (Figure 5E), but in most years the larvae survival is low (Skagseth et al., 2015). This means that the abundance of herring larvae available for post-smolts, and other predators in the ecosystem feeding on herring larvae (Skjoldal et al., 2004), is highly variable between years. There has not been any strong year-classes of herring since 2004 (ICES, 2020a). Poor survival in the first larvae stage, increased predation on the larvae along the Norwegian coast or in the Norwegian Sea, or geographic shifts in spawning locations may have affected the abundance of herring larvae available for post-smolts. There was a clear reduction in zooplankton biomass along the drifts route for NSSH larvae after 2004 (Toresen et al., 2019). This indicates that the poor recruitment is mainly regulated by prey abundance and a potential mismatch between herring larvae and their prey (Toresen et al., 2019). After 2007, the abundance of mackerel has increased in the Norwegian Sea (Figure 5F; Nøttestad et al., 2016). Mackerel feed on herring larvae when they have the opportunity (Skaret et al., 2015). The increased abundance of mackerel may also have contributed to reduced survival of herring larvae (Toresen et al., 2019) and therefore contributed to a lower abundance of larvae available for post-smolts.
Competition with mackerel cannot explain the reduction in Atlantic salmon survival during last decades (Utne et al., 2021b), but the negative correlation between mackerel spawning stock biomass and biomass of fish larvae in salmon post-smolt stomachs in the present study suggests that competition with mackerel for fish larvae may have a negative effect on post-smolt feeding conditions. However, in the Norwegian Sea there was no indication of a competition between mackerel and Atlantic salmon post-smolts for zooplankton, as there was no correlation between mackerel biomass and the biomass of zooplankton in post-smolt stomachs. This is not surprising, considering the two species mainly target different zooplankton species (Utne et al., 2021b). As an alternative explanation to competition between mackerel and Atlantic salmon post-smolts for fish larvae, the negative correlation between mackerel biomass and biomass of fish larvae in post-smolt stomachs may not be due to competition, but caused by changes in water circulation and environmental conditions affecting both species simultaneously, as the mechanisms affecting mackerel recruitment are not properly understood.
The positive correlation between the Norwegian Sea zooplankton abundance index (Figure 5G) and post-smolt stomach fullness of zooplankton shows a direct link between the abundance of zooplankton and post-smolt feeding. Although fish larvae are the preferred prey for post-smolts, a large proportion of the diet is zooplankton (Rikardsen et al., 2004; Utne et al., 2021a). Especially amphipods are an important part of the diet (Utne et al., 2021a), although the results presented here show a large spatial and temporal variation in biomass of amphipods in the diet. The northwestern Norwegian Sea toward the Polar Front is a region where amphipods dominated in post-smolts stomachs, but amphipods were an important part of the diet over a wider geographic area in some years. The variation in biomass of amphipods in post-smolt stomachs probably reflects temporal and spatial variation in amphipod abundance, but the dynamics of amphipods in the Norwegian Sea is not known. The sudden increase of amphipods in the post-smolt diet throughout the Norwegian Sea in 2018 and 2019 coincides with the increased proportion of Arctic Water in the Norwegian Sea (Figure 5H). Zooplankton abundance in the sea is normally bottom-up driven, but with potential top-down regulation by pelagic fish (Huse et al., 2012). Water transport, vertical wind mixing, water temperature and other hydrographic conditions impact zooplankton abundance (Skjoldal et al., 2004; Assmus et al., 2009), often in ways that are not fully understood. In addition, the East Icelandic Current transports copepods into the southwestern Norwegian Sea. Since 2004 there was a sudden drop in the strength of this current, which reduced the transport of copepods into the Norwegian Sea (Kristiansen et al., 2019). This reduced advection of Arctic copepod into the Norwegian Sea may have affected the smolt feeding negatively. This is further supported by the fact that post-smolt in the Southwestern Norwegian Sea had the largest drop in stomach fullness of zooplankton from the years 1995–2004 to 2008–2019.
There has been a period from the early 2000s with less available marine prey for Atlantic salmon post-smolts in a large geographic area in the Northeast Atlantic Ocean. Changes in hydrography and zooplankton abundance in the Norwegian Sea, and recruitment of important fish stocks in the Northeast Atlantic have contributed to the decline in available prey for Atlantic salmon. The effects of this reduction in prey abundance may also have been amplified by increased interspecific competition for fish larvae with mackerel in the 2008–2019 period. The long-term trend in available prey for post-smolts in periods before 1995 is unknown, since we lack data from earlier periods. There may be some recent signals of improved feeding conditions in some regions of the Northeast Atlantic Ocean. A high proportion of Arctic Water into the Norwegian Sea is associated with good post-smolt feeding conditions, and the increased proportion of Arctic Water in 2018 and 2019 compared to the period 2005–2016 may continue due to the temporal autocorrelation in proportions of water masses in the Norwegian Sea. The stop in the sandeel fishery in the early 2000’s both in the North Sea and west of Ireland and Scotland also sets the ground for a recovery of sandeel in this region if the environmental conditions turn favorable. Identifying important feeding areas for salmon and making the management decisions necessary to improve and maintain healthy and diverse ecosystems in these areas are important steps toward maintaining Atlantic salmon population. A recovery of reduced Atlantic salmon populations will require healthy and productive natal river systems, and reducing major threats from human activities in coastal areas. However, for this long-distant migrating species, also the marine feeding habitat is of major importance. Knowledge of, and willingness to preserve, marine ecosystems is necessary to ensure healthy Atlantic salmon populations across Europe.
Data Availability Statement
Publicly available datasets were analyzed in this study. This data can be found here: http://metadata.nmdc.no/metadata-api/landingpage/5cc7cbf7d8f56eebaacb5bc8b44dda4d.
Author Contributions
KU, VW, and ØS delineated the study. WM and CB sampled parts of the data. VW, ET, WM, and KU retrieved funding for the study. KU analyzed the data. KU, ET, VW, ØS, WM, and CB wrote the manuscript. All authors contributed to the article and approved the submitted version.
Funding
This study was funded by the Research Council of Norway as part of project 280308 SeaSalar, and the European Commission under the 7th Framework Program, Grant Agreement no. 212529 (Salsea-Merge).
Conflict of Interest
The authors declare that the research was conducted in the absence of any commercial or financial relationships that could be construed as a potential conflict of interest.
Publisher’s Note
All claims expressed in this article are solely those of the authors and do not necessarily represent those of their affiliated organizations, or those of the publisher, the editors and the reviewers. Any product that may be evaluated in this article, or claim that may be made by its manufacturer, is not guaranteed or endorsed by the publisher.
Acknowledgments
We thank all people who assisted gathering the data presented, including the skippers and crew onboard the vessels collecting samples.
Supplementary Material
The Supplementary Material for this article can be found online at: https://www.frontiersin.org/articles/10.3389/fmars.2022.824614/full#supplementary-material
References
Almodovar, A., Ayllon, D., Nicola, G. G., Jonsson, B., and Elvira, B. (2019). Climate-driven biophysical changes in feeding and breeding environments explain the decline of southernmost European Atlantic salmon populations. Can. J. Fish. Aquat. Sci. 76, 1581–1595. doi: 10.1139/cjfas-2018-0297
Assmus, J., Melle, W., Tjostheim, D., and Edwards, M. (2009). Seasonal cycles and long-term trends of plankton in shelf and oceanic habitats of the Norwegian Sea in relation to environmental variables. Deep Sea Res. II Top. Stud. Oceanogr. 56, 1895–1909. doi: 10.1016/j.dsr2.2008.11.004
Bailey, R. S. (1982). The population biology of blue whiting in the North-Atlantic. Adv. Mar. Biol. 19, 257–355. doi: 10.1016/B978-0-12-381015-1.00002-2
Beaugrand, G., and Reid, P. C. (2003). Long-term changes in phytoplankton, zooplankton and salmon related to climate. Glob. Change Biol. 9, 801–817. doi: 10.1046/j.1365-2486.2003.00632.x
Beaugrand, G., and Reid, P. C. (2012). Relationships between North Atlantic salmon, plankton, and hydroclimatic change in the Northeast Atlantic. ICES J. Mar. Sci. 69, 1549–1562. doi: 10.1093/icesjms/fss153
Bradbury, I. R., Lehnert, S. J., Messmer, A., Duffy, S. J., Verspoor, E., Kess, T., et al. (2021). Range-wide genetic assignment confirms long-distance oceanic migration in Atlantic salmon over half a century. ICES J. Mar. Sci. 78, 1434–1443. doi: 10.1093/icesjms/fsaa152
Burnham, K. P., and Anderson, D. R. (2002). Model Selection and Multimodel Inference: A Practical Information-Theoretic Approach. New York, NY: Springer-Verlag, 488.
Capuzzo, E., Lynam, C. P., Barry, J., Stephens, D., Forster, R. M., Greenwood, N., et al. (2018). A decline in primary production in the North Sea over 25 years, associated with reductions in zooplankton abundance and fish stock recruitment. Glob. Change Biol. 24, 352–364. doi: 10.1111/gcb.13916
Dickson, B., and Østerhus, S. (2007). One hundred years in the Norwegian Sea. Nor. Geogr. Tidsskr. 61, 56–75. doi: 10.1080/00291950701409256
Forseth, T., Barlaup, B. T., Finstad, B., Fiske, P., Gjoaester, H., Falkegard, M., et al. (2017). The major threats to Atlantic salmon in Norway. ICES J. Mar. Sci. 74, 1496–1513. doi: 10.1093/icesjms/fsx020
Friedland, K. D., Hansen, L. P., Dunkley, D. A., and MacLean, J. C. (2000). Linkage between ocean climate, post-smolt growth, and survival of Atlantic salmon (Salmo salar L.) in the North Sea area. ICES J. Mar. Sci. 57, 419–429. doi: 10.1006/jmsc.1999.0639
Friedland, K. D., MacLean, J. C., Hansen, L. P., Peyronnet, A. J., Karlsson, L., Reddin, D. G., et al. (2009). The recruitment of Atlantic salmon in Europe. ICES J. Mar. Sci. 66, 289–304. doi: 10.1093/icesjms/fsn210
Gilbey, J., Utne, K. R., Wennevik, V., Beck, A. C., Kausrud, K., Hindar, K., et al. (2021). The early marine post-smolt distribution of Atlantic salmon in the NE Atlantic: a genetically informed stock-specific synthesis. Fish Fish. 22, 1274–1306. doi: 10.1111/faf.12587
Hastie, T. J., and Tibshirani, R. J. (1990). Generalized Additive Models. London: Chapman & Hall/CRC, 335.
Hatun, H., Payne, M. R., Beaugrand, G., Reid, P. C., Sando, A. B., Drange, H., et al. (2009). Large bio-geographical shifts in the north-eastern Atlantic Ocean: from the subpolar gyre, via plankton, to blue whiting and pilot whales. Prog. Oceanogr. 80, 149–162. doi: 10.1016/j.pocean.2009.03.001
Haugland, M., Holst, J. C., Holm, M., and Hansen, L. P. (2006). Feeding of Atlantic salmon (Salmo salar L.) post-smolts in the Northeast Atlantic. ICES J. Mar. Sci. 63, 1488–1500. doi: 10.1016/j.icesjms.2006.06.004
Holst, J. C., Hansen, L. P., and Holm, M. (1996). Observations of Abundance, Stock Composition, Body Size and Food of Postsmolts of Atlantic Salmon in the NE Atlantic During Summer: ICES CM Documents;1996/M:4. Burnaby, BC: ICES, 16.
Huse, G., Holst, J. C., Utne, K., Nøttestad, L., Melle, W., Slotte, A., et al. (2012). Effects of interactions between fish populations on ecosystem dynamics in the Norwegian Sea – results of the INFERNO project Preface. Mar. Biol. Res. 8, 415–419. doi: 10.1080/17451000.2011.653372
ICES (2020a). Working Group on Widely Distributed Stocks (WGWIDE). ICES Document, Vol. 82. Burnaby, BC: ICES, 1019.
ICES (2020b). Herring Assessment Working Group for the Area South of 62° N (HAWG), Vol. 2. Burnaby, BC: ICES, 1054.
ICES (2021a). Working group on North Atlantic Salmon (WGNAS). ICES Sci. Rep. 3:407. doi: 10.17895/ices.pub.7923
ICES (2021b). Working group on the integrated assessments of the Norwegian Sea (WGINOR; outputs from 2020 meeting). ICES Sci. Rep. 3:114.
Jensen, A. J., Maoileidigh, N. O., Thomas, K., Einarsson, S. M., Haugland, M., Erkinaro, J., et al. (2012). Age and fine-scale marine growth of Atlantic salmon post-smolts in the Northeast Atlantic. ICES J. Mar. Sci. 69, 1668–1677. doi: 10.1093/icesjms/fss086
Jonsson, B., and Jonsson, N. (2004). Factors affecting marine production of Atlantic salmon (Salmo salar). Can. J. Fish. Aquat. Sci. 61, 2369–2383. doi: 10.1139/f04-215
Jonsson, B., Jonsson, N., and Albretsen, J. (2016). Environmental change influences the life history of salmon Salmo salar in the North Atlantic Ocean. J. Fish Biol. 88, 618–637. doi: 10.1111/jfb.12854
Kristiansen, I., Hatun, H., Petursdottir, H., Gislason, A., Broms, C., Melle, W., et al. (2019). Decreased influx of Calanus spp. into the south-westem Norwegian Sea since 2003. Deep Sea Res. I Oceanogr. Res. Pap. 149:103048. doi: 10.1016/j.dsr.2019.05.008
McCarthy, J. L., Friedland, K. D., and Hansen, L. P. (2008). Monthly indices of the post-smolt growth of Atlantic salmon from the Drammen River, Norway. J. Fish Biol. 72, 1572–1588. doi: 10.1111/j.1095-8649.2008.01820.x
Mills, K. E., Pershing, A. J., Sheehan, T. F., and Mountain, D. (2013). Climate and ecosystem linkages explain widespread declines in North American Atlantic salmon populations. Glob. Change Biol. 19, 3046–3061. doi: 10.1111/gcb.12298
Morgan, R. I. G., Greenstreet, S. P. R., and Thorpe, J. E. (1986). First Observations on Distribution, Food and Fish Predators of Postsmolt Atlantic Salmon, Salmo salar, in the Outer Firth of Clyde. ICES C.M., 1986/M: 8. Burnaby, BC: ICES.
Nøttestad, L., Utne, K. R., Oskarsson, G. J., Jonsson, S. T., Jacobsen, J. A., Tangen, O., et al. (2016). Quantifying changes in abundance, biomass, and spatial distribution of Northeast Atlantic mackerel (Scomber scombrus) in the Nordic seas from 2007 to 2014. ICES J. Mar. Sci. 73, 359–373. doi: 10.1093/icesjms/fsv218
Otero, J., Jensen, A. J., L’Abee-Lund, J. H., Stenseth, N. C., Storvik, G. O., and Vollestad, L. A. (2011). Quantifying the ocean, freshwater and human effects on year-to-year variability of one-sea-winter Atlantic Salmon Angled in multiple Norwegian Rivers. PLoS One 6:e24005. doi: 10.1371/journal.pone.0024005
Parrish, D. L., Behnke, R. J., Gephard, S. R., McCormick, S. D., and Reeves, G. H. (1998). Why aren’t there more Atlantic salmon (Salmo salar)? Can. J. Fish. Aquat. Sci. 55, 281–287. doi: 10.1139/d98-012
R Core Team (2020). R: A Language and Environment for Statistical Computing. Vienna: R Foundation for Statistical Computing.
Renkawitz, M. D., Sheehan, T. F., Dixon, H. J., and Nygaard, R. (2015). Changing trophic structure and energy dynamics in the Northwest Atlantic: implications for Atlantic salmon feeding at West Greenland. Mar. Ecol. Prog. Ser. 538, 197–211. doi: 10.3354/meps11470
Rikardsen, A. H., Haugland, M., Bjorn, P. A., Finstad, B., Knudsen, R., Dempson, J. B., et al. (2004). Geographical differences in marine feeding of Atlantic salmon post-smolts in Norwegian fjords. J. Fish Biol. 64, 1655–1679. doi: 10.1111/j.0022-1112.2004.00425.x
Rikardsen, A. H., Righton, D., Strom, J. F., Thorstad, E. B., Gargan, P., Sheehan, T., et al. (2021). Redefining the oceanic distribution of Atlantic salmon. Sci. Rep. 11:12266. doi: 10.1038/s41598-021-91137-y
Scarnecchia, D. L. (1984). Climatic and oceanic variations affecting yield of Icelandic stocks of Atlantic salmon (Salmo-Salar). Can. J. Fish. Aquat. Sci. 41, 917–935. doi: 10.1139/f84-107
Skagseth, Ø., Broms, C., Gundersen, K., Hátún, H., Kristiansen, I., and Larsen, K. M. H. (this issue). Arctic and Atlantic waters in the Norwegian Basin, Between Year Variability and Potential Ecosystem Implication.
Skagseth, O., Slotte, A., Stenevik, E. K., and Nash, R. D. M. (2015). Characteristics of the Norwegian coastal current during years with high recruitment of Norwegian Spring Spawning Herring (Clupea harengus L.). PLoS One 10:e0144117. doi: 10.1371/journal.pone.0144117
Skaret, G., Bachiller, E., Langoy, H., and Stenevik, E. K. (2015). Mackerel predation on herring larvae during summer feeding in the Norwegian Sea. ICES J. Mar. Sci. 72, 2313–2321. doi: 10.1093/icesjms/fsv087
Skjoldal, H. R., Sætre, R., Fernø, A., Misund, O. A., and Røttingen, I. (2004). The Norwegian Sea Ecosystem. Trondheim: Tapir Academic Press, 559.
Strøm, J. F., Thorstad, E. B., Chafe, G., Sorbye, S. H., Righton, D., Rikardsen, A. H., et al. (2017). Ocean migration of pop-up satellite archival tagged Atlantic salmon from the Miramichi River in Canada. ICES J. Mar. Sci. 74, 1356–1370. doi: 10.1093/icesjms/fsw220
Thorstad, E. B., Bliss, D., Breau, C., Damon-Randall, K., Sundt-Hansen, L. E., Hatfield, E., et al. (2021). Atlantic salmon in a rapidly changing environment-Facing the challenges of reduced marine survival and climate change. Aquat. Conserv. Mar. Freshw. Ecosyst. 31, 2654–2665. doi: 10.1002/aqc.3624
Todd, C. D., Hughes, S. L., Marshall, C. T., Maclean, J. C., Lonergan, M. E., and Biuw, E. M. (2008). Detrimental effects of recent ocean surface warming on growth condition of Atlantic salmon. Global Change Biol. 14, 958–970. doi: 10.1111/j.1365-2486.2007.01522.x
Toresen, R., Skjoldal, H. R., Vikelao, F., and Martinussen, M. B. (2019). Sudden change in long-term ocean climate fluctuations corresponds with ecosystem alterations and reduced recruitment in Norwegian spring-spawning herring (Clupea harengus, Clupeidae). Fish Fish. 20, 686–696. doi: 10.1111/faf.12369
Trehin, C., Rivot, E., Lamireau, L., Meslier, L., Besnard, A. L., Gregory, S. D., et al. (2021). Growth during the first summer at sea modulates sex-specific maturation schedule in Atlantic salmon. Can. J. Fish. Aquat. Sci. 78, 659–669. doi: 10.1139/cjfas-2020-0236
Utne, K. R., Pauli, B. D., Haugland, M., Jacobsen, J. A., Maoileidigh, N. O., Melle, W., et al. (2021a). Poor feeding opportunities and reduced condition factor for salmon post-smolts in the Northeast Atlantic Ocean. ICES J. Mar. Sci. 78, 2844–2857. doi: 10.1093/icesjms/fsab163
Utne, K. R., Thomas, K., Jacobsen, J. A., Fall, J., Maoileidigh, N. O., Broms, C. T., et al. (2021b). Feeding interactions between Atlantic salmon (Salmo salar) postsmolts and other planktivorous fish in the Northeast Atlantic. Can. J. Fish. Aquat. Sci. 78, 255–268. doi: 10.1139/cjfas-2020-0037
Vollset, K. W., Barlaup, B. T., Mahlum, S., Bjorn, P. A., and Skilbrei, O. T. (2016). Estimating the temporal overlap between post-smolt migration of Atlantic salmon and salmon lice infestation pressure from fish farms. Aquac. Environ. Interact. 8, 511–525. doi: 10.3354/aei00195
Vollset, K. W., Urdal, K., Utne, K. R., Thorstad, E. B., Sægrov, H., Raunsgard, A., et al. (2022). Ecological regime shift in the Northeast Atlantic Ocean revealed from the unprecedented reduction in marine growth of Atlantic salmon. Sci. Adv. 8, doi: 10.1126/sciadv.abk2542
Wickham, H. (2016). ggplot2: Elegant Graphics for Data Analysis. New York, NY: Springer-Verlag. doi: 10.1007/978-3-319-24277-4
Keywords: Norwegian Sea, fish larvae, zooplankton, mackerel, oceanography, diet
Citation: Utne KR, Skagseth Ø, Wennevik V, Broms CT, Melle W and Thorstad EB (2022) Impacts of a Changing Ecosystem on the Feeding and Feeding Conditions for Atlantic Salmon During the First Months at Sea. Front. Mar. Sci. 9:824614. doi: 10.3389/fmars.2022.824614
Received: 29 November 2021; Accepted: 22 February 2022;
Published: 15 March 2022.
Edited by:
Alberto Basset, University of Salento, ItalyReviewed by:
Daniel James Madigan, University of Windsor, CanadaJohn Kocik, Northeast Fisheries Science Center, United States
Copyright © 2022 Utne, Skagseth, Wennevik, Broms, Melle and Thorstad. This is an open-access article distributed under the terms of the Creative Commons Attribution License (CC BY). The use, distribution or reproduction in other forums is permitted, provided the original author(s) and the copyright owner(s) are credited and that the original publication in this journal is cited, in accordance with accepted academic practice. No use, distribution or reproduction is permitted which does not comply with these terms.
*Correspondence: Kjell Rong Utne, a2plbGwucm9uZy51dG5lQGhpLm5v