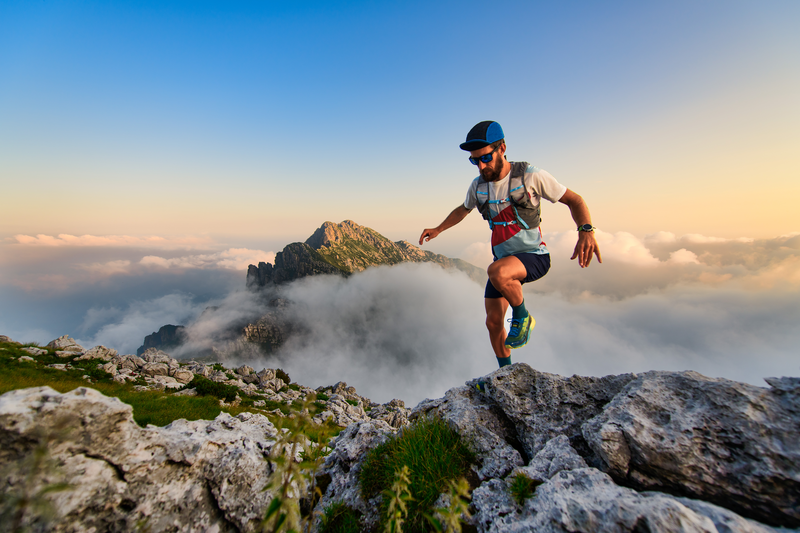
94% of researchers rate our articles as excellent or good
Learn more about the work of our research integrity team to safeguard the quality of each article we publish.
Find out more
ORIGINAL RESEARCH article
Front. Mar. Sci. , 14 February 2022
Sec. Marine Ecosystem Ecology
Volume 9 - 2022 | https://doi.org/10.3389/fmars.2022.822978
This article is part of the Research Topic Physical Drivers of Biogeographical Shifts in the Northeastern Atlantic – and Adjacent Shelves View all 19 articles
Calanus finmarchicus is a key secondary producer in the North Atlantic. Shortly prior to the spring bloom the animals ascend from diapause at depth to surface waters, where the females spawn partly, based on winter lipid reserves. C. finmarchicus eggs are an important prey of first feeding fish larvae inhabiting North Atlantic shelves during early spring and are thus essential for larval survival. Comprehensive late April surveys have been carried out on and around the Faroe shelf, which is located between the Northeast Atlantic and the Nordic Seas, for more than two decades. One aim is to investigate the critical match-mismatch between the spring bloom development, zooplankton reproduction and occurrence of first feeding fish larvae. In this study, we examine spatial and temporal changes in pre-bloom reproductive activity of C. finmarchicus on and around the shelf using a unique dataset of more than 8,000 examined females sampled during the period 1997–2020. Enhanced productivity was observed on the north-western side of the shelf, where the main flow of oceanic water to the inner permanently well mixed shelf takes place. We attribute this increased productivity to enhanced food (phytoplankton) availability in the seasonally stratified outer shelf, slightly upstream of the main egg production area. Both individual egg production rates and the fraction of spawning females declined throughout the Faroe shelf during the examined period. This decline could not be explained by the employed environmental parameters. The declining pre-bloom egg production may have consequences for first feeding fish larvae.
Calanus finmarchicus is a key secondary producer and the most studied copepod species in the North Atlantic, ranging geographically from the Gulf of Maine to the North Sea (Melle et al., 2014). Although C. finmarchicus is an oceanic copepod species, it dominates the zooplankton biomass in Faroese waters (Gaard, 1999). The species is an essential food source for a number of pelagic fish stocks, and an important prey for larvae and juveniles of benthic fish species including Faroe Plateau cod (Gadus morhua) larvae and juveniles. In particular, C. finmarchicus eggs are the dominant prey of first feeding cod larvae, which are spawned on the Faroe shelf during February-April (Gaard and Steingrund, 2001; Jacobsen et al., 2020), and they are an important prey in neighboring shelves as well (Heath and Lough, 2007). Hence, a temporal match between the abundance of larvae and C. finmarchicus eggs may be of critical importance for subsequent cod recruitment (Hjort, 1914; Cushing, 1990). One of the key life history traits for C. finmarchicus is dormancy, a strategy acquired in order to persist through seasonally adverse conditions (i.e., winter). As such, in late summer in the Northeast Atlantic pre-adult C. finmarchicus copepodites descend to deep waters within the Norwegian Sea gyre and the Atlantic subpolar gyre to overwinter in a resting state known as diapause (e.g., Heath et al., 2000).
The Faroe Islands are situated on the Iceland-Scotland ridge, which divides the mentioned gyres, and thus two major overwintering regions of C. finmarchicus (Figure 1A). Off the Faroe Shelf, the water in the upper layers (0–500 m) is dominated by relatively warm and saline Modified North Atlantic Water (MNAW) (Hansen and Østerhus, 2000), a mixture of subtropical waters and cold and low-saline, but zooplankton rich, waters from the subpolar gyre (Hátún et al., 2005, 2009). Since the MNAW-carrying currents are coming from the south-west, the temperature and salinity on the outer Faroe shelf is generally higher on the western side and lower on the eastern side. MNAW subsequently cross the Iceland-Faroe Ridge in a clock-wise path around the Faroe shelf, while they are cooled and freshened by an admixture of colder, less saline as well as zooplankton rich water from the Norwegian Sea and the East Icelandic Current (EIC) (Figure 1A; Larsen et al., 2012; Kristiansen et al., 2016). Under the MNAW layer, cold and less saline overflow water flows equator-wards from the depths of the Norwegian Sea. During winter and early spring, this overflow carries large quantities of zooplankton through the Faroe Shetland Channel and further through the Faroe Bank Channel into the North Atlantic Ocean (Heath et al., 2000). This overflow is often regarded as a main source of C. finmarchicus for the Faroe shelf (Gaard and Hansen, 2000; Jónasdóttir et al., 2008). The MNAW currents, enriched by C. finmarchicus in the north-eastern Iceland Basin, might, however, be a more direct source of this copepod for the Faroe shelf (Figure 1A). Consequently, C. finmarchicus that are advected onto the Faroe shelf may originate both from sources in the Nordic Seas (blue in Figure 1A) and sources in the Northeast Atlantic (brown in Figure 1A), where the water mass characteristics are very different. C. finmarchicus diapause duration has been shown to be significantly shorter in the Northwestern Atlantic (i.e., west of the Faroes) than in the Nordic Seas (north of the Faroes) (Melle et al., 2014).
Figure 1. Map of the study area with bottom depth contours. (A) The main warm (red arrows) and cold (blue arrows) currents influencing and carrying C. finmarchicus to the Faroe shelf (green) are displayed. C. finmarchicus originating from the Northeast Atlantic (NEA source) are colored brown, while C. finmarchicus from the Norwegian Sea (NS source) are illustrated in blue. (B) Distribution of C. finmarchicus egg production stations (blue dots) on and around the Faroe shelf 1997–2020. The green dashed line represents the approximate position of the Faroe shelf front (Larsen et al., 2009) separating the permanently mixed inner shelf (green) from the seasonally stratified outer shelf. “O” and “S” denote fixed coastal stations on the shelf. SPG, Subpolar Gyre; MNAW, Modified North Atlantic Water; EIC, East Icelandic Current; NSG, Norwegian Sea Gyre; FSC, Faroe Shetland Channel; WR, Western Region; SB, Skeivi Banki; EB, Eastern Banks.
The Faroe Shelf may be divided into exclusive domains based on oceanography (Larsen et al., 2008, 2009), and on phytoplankton (biomass and species composition) variability (Gaard, 1996; Eliasen et al., 2017). One main division is formed by the tidal front at the approximately 100–150 m bottom depth contour, which separates the permanently well mixed inner shelf from the surrounding seasonally stratified outer shelf (Figures 1B, 2A; Hansen et al., 2005; Larsen et al., 2008, 2009). The inner Faroe shelf is characterized by strong tidal currents with a clockwise residual circulation around the islands (Larsen et al., 2008). Effective winter cooling in the shallow waters and excess precipitation over land result in lower temperature and salinity during spring on the inner shelf than in the outer shelf waters (Larsen et al., 2009). This difference in hydrographic conditions between the inner and outer shelf also affects the distribution, composition and productivity of zooplankton (Gaard, 1999; Jacobsen et al., 2018). On average, the Eastern Banks (see Figure 1B) bloom first, i.e., in April and the bloom on the inner shelf typically occurs in May. In June, the outer shelf contains the highest near-surface chlorophyll concentrations. In addition to this, there is an area to the west of the shelf, the Western Region (WR) (Hátún et al., 2013; Figure 1B), where the shelf is wide, the tidal currents are weak and where early spring chlorophyll means and variances are particularly high resulting from several events of temporary stratification, before the permanent summer stratification sets in, typically in late May/early June (Eliasen et al., 2017). The main influx of oceanic and outer shelf water to the inner shelf likely takes place through the WR, via three channelized flows, whereof the influx immediately west of the Islands likely is the strongest (Hátún et al., 2013; Rasmussen et al., 2014; Figures 1B, 2A). The initiation of the spring bloom on the inner shelf usually occurs in May, but the timing as well as the magnitude is highly variable between years (Debes et al., 2008a; Eliasen et al., 2019). There is generally an inverse relationship between late winter/early spring temperature and the initiation and magnitude of the spring bloom (Hansen et al., 2005).
Figure 2. Mean (1997–2020) distribution in (A) temperature, (B) chlorophyll, (C) C. finmarchicus spawning fraction and (D) individual egg production rates (eggs female–1 day–1) in the uppermost 50 m on the Faroe Shelf in late April. The dashed curves illustrate the typical position of the tidal front between the WR and the inner shelf, and the white arrows represent the inflow region. The white full line in (C,D) shows the domain of the north-western area, averaged to produce the time series in Figure 4. Yearly distribution maps are provided in Supplementary Material.
Once in the upper layers, C. finmarchicus molt into adults and reproduction begins. Long-term monitoring of zooplankton on the Faroe shelf has shown that the abundance and biomass of C. finmarchicus fluctuates markedly between years (Gaard and Hansen, 2000; Gaard, 2003; Jacobsen et al., 2018). A part of this variation is due to changes in the copepod’s advection and productivity (Gaard, 2000), while the highly variable mid-summer abundances are likely induced by interannual variability in grazing pressure by 0-group fish (Jacobsen et al., 2019). The egg production rates (EPR) of C. finmarchicus have previously been linked to the phytoplankton spring bloom, as shown on both sides of the North Atlantic (Stenevik et al., 2007; Head et al., 2013a,b; Melle et al., 2014) as well as locally on the Faroe Shelf (Gaard, 2000; Debes et al., 2008b). Interannual variability in the timing of the spring bloom on the Faroe shelf influences reproduction, and consequently the number of generations completed (Gaard, 2000; Debes and Eliasen, 2006; Debes et al., 2008b). Local seasonal studies of C. finmarchicus EPR show that the production increases shortly after ascent in April, i.e., during the pre-bloom, then declines, before increasing again after the initiation of the spring bloom (Gaard, 2000; Debes et al., 2008b). Laboratory experiments have revealed that temperature also is a factor that may influence EPR positively, shortening the spawning intervals (Hirche et al., 1997). However, females tend to be larger in cold waters, and large females typically produce more eggs than small females (Head et al., 2013a; Melle et al., 2014). Furthermore, the initial reproduction phase, which most often occurs during the pre-bloom, is significant. Average EPR in the Northeast Atlantic during the pre-bloom correspond to approximately 10 eggs female–1 day–1 (Niehoff et al., 1999, 2011; Gislason, 2005; Stenevik et al., 2007; Jónasdóttir et al., 2008; Debes et al., 2008b; Kristiansen et al., 2021). This phase, which is likely supported by internal lipid reserves (Irigoien et al., 2008; Madsen et al., 2008), is the focus of the present paper.
There are, as previously mentioned, regional differences in the entry into and emergence from diapause (e.g., Planque et al., 1997; Kristiansen et al., 2016). How these are controlled remains largely unknown. One suggestion is that control may be via internal biological processes linked to the accumulation and utilization of lipids prior and during diapause, which are modulated by environmental conditions, e.g., temperature (Johnson et al., 2008; Head et al., 2013b; Jónasdóttir et al., 2019). Rising temperatures have caused a clear northward shift in C. finmarchicus distribution in recent decades (Reygondeau and Beaugrand, 2011). However, oceanic warming will not be uniform in the North Atlantic. Some regions will warm less than others (Drijfhout et al., 2012), while deep water warming will lag that at the surface (Li et al., 2013). In the Faroe area, observed phenology changes in C. finmarchicus around 2003–2007 resulted in earlier emergence of the individuals (Kristiansen et al., 2016; Jacobsen et al., 2018). The observed changes in this region are most likely attributed to either (1) a change in water mass distribution in the area altering the abundance and timing of the copepod and/or (2) a recent warming of the North Atlantic waters inducing earlier emergence. However, potential changes in the pre-bloom EPR have so far remained unknown.
The main objective of this paper is to explore the pre-bloom C. finmarchicus egg production on and around the Faroe shelf from 1997 to 2020, and to establish how the pre-bloom egg production responds to changes in environmental conditions. Firstly, we identify egg reproduction “hotspots” based on egg production spatial analyses. Next, we describe temporal variability in the egg production, and compare these to environmental conditions. This study provides the hitherto most comprehensive set of estimates of pre-bloom C. finmarchicus EPR in Faroese waters and perhaps all North Atlantic.
Samples for C. finmarchicus egg production measurements were collected with R/V Magnus Heinason in and around the Faroe shelf in late April during the years 1997–2020 (except for 2002 and 2010) (Table 1 and Figure 1B). Temperature and chlorophyll a (hereafter termed just chlorophyll) samples were also collected enabling examination of relationships between C. finmarchicus production and its habitat.
C. finmarchicus females, used for egg production measurements were collected with a WP-2 net with a mesh size of 200 μm and a 2 L non-filtering cod-end. The net was hauled from 50 m depth to the surface with a speed of 0.3–0.5 m s–1. Immediately after sampling, healthy females were sorted into 0.5 L plastic containers filled with 60 μm filtered seawater (one female per container) and incubated at in situ temperature (flowing ambient water) and dim light for 24 h. Each container was equipped with a “false-bottom” consisting of 300 μm mesh size net, to minimize egg cannibalism. Replicates of between 5 and 20 females were incubated at each station. Following the incubation period, the content of the chamber was filtered through a 30 μm sieve and the eggs counted under a stereomicroscope. In a subset of years, the prosome length of a portion of females was measured to the nearest 0.1 mm with an ocular micrometer (Table 1).
The CTD (Conductivity, Temperature, Depth) data used in this study is the same material as was utilized in Jacobsen et al. (2018), but updated to include 2017–2020. Temperature and fluorescence (designed to measure chlorophyll) were measured with a Seabird Electronics SBE911 plus CTD, equipped with a rosette sampler. Samples for chlorophyll measurements were taken at 5, 20, and 40 m depth at a subset of stations each year. These were analyzed spectrophotometrically according to Parsons et al. (1984). Fluorescence values from the CTD were converted to chlorophyll using linear regression analysis between fluorescence and chlorophyll from the extracted samples as described in Salter et al. (2020). No CTD data exist for 2017 and no fluorescence data exist for 2020. For each station calibrated/converted temperature and chlorophyll values were averaged over 6–50 m depth (as the uppermost 5 m are often affected by large signal-to-noise ratio), so as to be comparable to the EPR.
In addition, temperature was measured at coastal station O using Aanderaa, Sensordata followed by Starmon temperature recorders (Figure 1B). At station S samples were collected for chlorophyll measurements, which were analyzed spectrophotometrically (Parsons et al., 1984). The coastal stations are landbased monitoring sites, where temperature has been logged continuously and samples for chlorophyll have been collected weekly since the 1997 (Debes et al., 2008a; Eliasen et al., 2017). Data from stations O and S are expected to represent the entire inner shelf, since the location of the stations is where the water column is always well mixed from surface to bottom (Larsen et al., 2008). The coastal measurements are presented together with the cruise CTD data, and the coastal measurements are used when comparing environmental variables with EPR data.
EPR observations are spatio-temporally scattered. Therefore, prior to spatial analysis, stations were grouped onto a 0.125° latitude × 0.25° longitude grid. Regions with < 10 observations were then excluded before mapping temporal averages using the package “mba” in R (R Core Team, 2018). Because of the spatio-temporal scattering of observations, relationships between EPR and environmental variables were based on pre-bloom period annual means. Since the EPR data is not normally distributed, we used non-parametric tests to evaluate temporal trends and to compare means. A p-value < 0.05 was considered statistically significant.
The upper layer (6–50 m) late April average (1997–2020) temperature map in Figure 2A shows a colder inner Faroe shelf surrounded by warmer outer shelf/offshelf waters, which is caused by more effective winter cooling of the shallower water column. Intrusion of relatively warm oceanic waters onto the WR overlays the colder and denser shelf waters, and thus establishes stratification, evident as a warm tongue. The typical position of the sharp tidal front between the stratified WR and the well mixed inner shelf (evident in synoptic temperature maps, not shown) is illustrated in Figure 2A.
Chlorophyll concentrations were, on average, highest over the WR (Figure 1B) during late April, and phytoplankton from this regions appears to emanate across the tidal front and onto the inner shelf (Figure 2B). The main spring bloom initiates after April, and the chlorophyll concentrations are therefore generally low.
On average, the highest fraction of spawning females and the highest mean EPR during the pre-bloom in late April were observed in the inflow region from the WR and into the inner shelf, immediately west of the Islands (Figures 2C,D). The values are elevated immediately south of the tidal front, while the highest values are observed within the well mixed inner shelf. This stands in contrast to the lowest values in the oceanic waters over the so-called Skeivi Banki (see Figure 1B).
Out of the 8,171 females that were incubated, 5,299 laid eggs. Individual daily EPR ranged from 0 to 139 eggs female–1 day–1. 85% of the females laid less than 20 eggs, and only 0.1% or 11 females laid more than 100 eggs. Of these 11 females, the only one that was length measured was 3.1 mm, i.e., larger than average.
Upper layer (6–50 m) late April mean temperature increased steadily around the turn of the century from roughly 6.5 to 7.5°C (Figure 3A). Since 2003 the temperature has varied between approximately 7 and 8°C with no apparent trend. Variability in the average CTD temperature values sampled on the inner shelf (domain defined by the 100 m depth contour) corresponded well with measurements from coastal station O (Figure 3A).
Figure 3. (A) Temperature and (B) chlorophyll from CTD casts, averaged vertically over 6–50 m and laterally over the inner shelf (dashed black lines, domain defined by the 100 m depth contour) and from coastal stations O and S, respectively (solid black lines) in late April 1997–2020 (Jacobsen et al., 2018, updated). Vertical lines show spatial standard deviation for the CTD casts.
In most years the phytoplankton concentrations were low in late April. The variability between years was, however, pronounced and no long-term trend is discernible (Figure 3B). The start of the spring bloom (defined as the day of year when the chlorophyll concentration ≥ 1 mg m–3) had generally not occurred during the time of sampling, except in year 2000, 2008–2010, and 2018. Similar to the temperature, spatially averaged chlorophyll values sampled on the inner shelf with the CTD corresponded well with values sampled at coastal station S (Figure 3B). This means both that the station S chlorophyll data are representative for the large parts of the shelf during late April, and that bloom dynamics in the WR likely have a large impact on the observed variability.
The overall pre-bloom period mean fraction of spawning females (SF) ranged from ∼40 to 95%. The SF was highly variable between years, with peaks around the turn of the century and in 2018 (Figure 4A). An inspection of the linear regression slope between the SF and year showed a statistically significant decreasing trend on the inner shelf (rs = −0.47, p = 0.03) (Figure 4A). The decline corresponded to approximately 2% each year.
Figure 4. Mean C. finmarchicus (A) fraction of spawning females and (B) individual egg production rates (eggs female–1 day–1) on the inner Faroe shelf (solid black line, domain defined by the 100 m depth contour) and in the north-western area (dashed black line, area outlined in Figures 2C,D) in late April 1997–2020. Vertical lines show spatial standard deviation for the inner shelf.
Pre-bloom mean EPR varied from 2 to 20 eggs female–1 day–1, with peaks in 2000, 2003, 2007, and 2018 (Figure 4B). A visual examination of the mean EPR indicated higher rates during the period 1997–2007 compared with 2008–2020, which was confirmed by a Wilkoxon rank sum test (p = 0.04) (Figure 5). The mean value for the period 1997–2007 was 12.8 eggs female–1 day–1, while it was 7.1 eggs female–1 day–1 in 2008–2020. This difference in EPR was also apparent if females not producing eggs were excluded before testing (Wilkoxon rank sum test, p = 0.04).
Figure 5. Jitter and box plot of individual egg production rates (eggs female–1 day–1) on the Faroe shelf in late April during the time periods 1997–2007 and 2008–2020. The line in the middle of the boxes represents the median, and the lower and upper ends of the box are the 25 and 75% quartiles, respectively. The lower and upper whiskers are constructed according to R’s default box plot code (R Core Team, 2018). The points represent observations.
Although, EPR values in general were higher in the north-western area (domain outlined in Figures 2C,D), there was no statistical difference between pre-bloom mean values for the inner shelf and the north-western area (p > 0.1). Thus, since there is more data for the inner shelf, we use inner shelf EPR for the remainder of the paper.
The decrease in SF and EPR was apparent over most of the shelf, but particularly on the inner shelf (Figure 6).
Figure 6. Distribution of slopes of the linear regression for the change in (A) fraction of spawning females and (B) individual egg production rates (eggs female–1 day–1) as a function of year. Stars (*) indicate locations where p < 0.1.
Exploratory data analysis did not suggest that EPR was significantly positively correlated to sea temperature (at coastal station O) even when excluding years when chlorophyll concentrations exceed 1 mg m–3 (rs = 0.34, p > 0.1, Figure 7A). Nevertheless, no low values (< 5 eggs female–1 day–1) were observed at temperatures above 7°C, indicating that temperature may still have an effect.
Figure 7. Individual egg production rates (eggs females–1 day–1) on the inner shelf in late April vs. (A) temperature at station O (open circles indicate bloom years) and (B) chlorophyll at station S 1997–2020. Vertical lines indicate standard deviation.
The positive relationship between EPR and chlorophyll levels (at coastal station S) approached significance (rs = 0.39, p = 0.08, Figure 7B), and low EPR values were not observed at chlorophyll levels above 1.5 mg m–3 (i.e., in 2000 and 2018).
Pre-bloom mean female prosome length was 2.7 mm. There was a significant negative correlation between seawater temperature (6–50 m) and female size (r = −0.35, p < 0.01, Figure 8A). However, there was a significant positive correlation between size of the females and EPR (rs = 0.13, p < 0.01, Figure 8B). Since females were only length measured in a subset of study years, it could not be determined whether the mean size of the females had changed during the examined period (i.e., from 1997 to 2020).
Figure 8. (A) Mean female size (prosome length) vs. temperature (6–50 m) at a given station [PL = 3.17-0.06 (T)] and (B) individual egg production rates (eggs female–1 day–1) vs. female size [EPR = -25.61 + 12.07 (PL)].
In this article, we have investigated temporal and spatial variations in pre-bloom C. finmarchicus egg production on and around the Faroe shelf during the years 1997–2020 in relation to relevant available environmental variables. Comparisons with other field measurements indicate similar pre-bloom EPR to those reported in the literature from adjacent areas (Niehoff et al., 1999, 2011; Gislason, 2005; Stenevik et al., 2007; Jónasdóttir et al., 2008; Kristiansen et al., 2021). Enhanced SF and individual EPR were localized in the main inflow region north-west of the islands. However, both the SF and individual EPR decreased during the examined period. To our knowledge, this study is the first to report long-term changes in pre-bloom C. finmarchicus egg production in the whole North Atlantic.
The most pronounced influx of outer shelf water to the inner shelf takes place via the WR (Hátún et al., 2013; Rasmussen et al., 2014). Jacobsen et al. (2018) showed that the abundance of C. finmarchicus eggs, nauplii and copepodites in late April is highest in oceanic waters, especially over the Skeivi Banki region (Figure 1B), and much lower in the north-eastern area of the shelf. This stands in contrast to the SF and EPR discussed here, indicating that most of the overwintered C. finmarchicus that populate the shelf during the pre-bloom are presumably advected through the WR. Once on the shelf, the tidal currents distribute the individuals in an anti-cyclonic circulation pattern around the islands (Larsen et al., 2008). The relatively high abundance of phytoplankton (food) in the WR probably enhances the copepods’ reproduction leading to the relatively high EPR observed in the north-western area (Figures 2B–D). This assumption is supported by Madsen et al. (2008) who found that females residing in the south-western area of the shelf contained more food in their guts compared with their counterparts in the north-eastern area of the shelf (Madsen et al., 2008). Madsen et al. (2008) also found markedly higher wax ester content in females outside the tidal front than on the inner shelf, suggesting that females that recently have entered the inner shelf in this region, may partly fuel their egg production by their lipid store. Using numbers from Stenevik et al. (2007), the energy need of C. finmarchicus to produce an average of 10 eggs female–1 day–1 is 6.9 μg C day–1. Assuming a pre-bloom carbon to chlorophyll ratio of 49 (Meyer-Harms et al., 1999) and a C. finmarchicus filtering rate of 0.133 l day–1 (Meyer-Harms et al., 1999), this translates to a required chlorophyll concentration of 1.4 mg m–3. Late April mean chlorophyll values in the WR exceed this threshold (Figure 2B). Thus, it is feasible to assume that the increased EPR observed in the north-western area are a direct effect of increased food consumption. However, the production is clearly also partly fueled by lipid stores as the energy demand to support the observed mean EPR is in most cases higher than the concurrent chlorophyll concentrations. It is noteworthy that the productive north-western area is close to densely populated seabird cliffs [e.g., the only gannet (Morus bassanus) colony in the Faroes], and it is just upstream of the main spawning region of cod (Gaard and Steingrund, 2001; Ottosen et al., 2018).
Despite the partial spatial overlap between EPR and chlorophyll, over the temporal scale of the present study, the relationship between EPR and food availability only approached statistical significance (Figure 7B), and we found no significant temporal relationship between EPR and temperature (Figure 7A). Overall, it may be difficult to discern the effects of these factors independently in the field, where the effects of one factor may be overridden by the other. Chlorophyll concentrations on the Faroe shelf tend to decrease with increasing temperatures (Hansen et al., 2005), and this could have contributed to the apparent lack of relationship between ambient temperature and EPR and chlorophyll and EPR, respectively. Furthermore, the effect of temperature on EPR might be outweighed by the fact that female prosome length, and thus also the EPR, decrease with increasing temperature (Figure 8).
Both the SF and EPR decreased during the 24 years study period (Figures 4–6), but the decline could not be explained by the employed environmental parameters. Several potential reasons for the decline, meriting further research, are suggested below:
(i) In addition to mere food abundance, food quality (phytoplankton species composition and particle size) may affect the EPR. Diatoms, which require silicate for growth, have been shown to be positively selected by C. finmarchicus (Meyer-Harms et al., 1999). A decline in pre-bloom silicate concentrations has been observed throughout the subpolar North Atlantic during the last three decades (Hátún et al., 2017), which likely has a negative effect on diatom growth. This could have affected the pre-bloom EPR in e.g., 2008 and 2009, when chlorophyll concentrations were relatively high (>1 mg m–3), but the EPR were low (Figure 7B).
(ii) Several authors have shown a positive relationship between female size and EPR (e.g., Jónasdóttir et al., 2005; Head et al., 2013a; Melle et al., 2014). In Figure 8B we also show, that the egg production potential of C. finmarchicus on and around the Faroe shelf is influenced by the size of the female. The C. finmarchicus population on the shelf is likely a mixture of animals derived from the Iceland Basin (Hátún et al., 2016) and Norwegian Sea (Gaard and Hansen, 2000; Jónasdóttir et al., 2008; Figure 1A). Females in the Norwegian Sea are significantly larger than females from the Iceland Basin (Jónasdóttir et al., 2008; Kristiansen et al., 2021), which could lead to increased EPR by females derived from the Norwegian Sea (Kristiansen et al., 2021). Thus, the variability in EPR may reflect variability in the distribution of different C. finmarchicus populations (or water masses) surrounding the Faroe shelf.
(iii) Jacobsen et al. (2018) concluded that there had been a change in C. finmarchicus phenology in the outer Faroe shelf in 2007 resulting in earlier emergence of the population as the copeodite stage composition in late April changed from dominance of overwintered late stage (CIV-CVI) copepodites to dominance of early stage (CI-CIII) copepodites. This is the same year as the shift in EPR occurred (Figures 4B, 5). A similar change took place in 2003 north of the Faroe Islands in the south-western Norwegian Sea (Kristiansen et al., 2016). Earlier emergence would inevitably lead to an earlier peak in pre-bloom egg production. Thus, although we observe a reduction in EPR in late April, the production might only have been displaced to an earlier point in the season, i.e., a phenological shift.
(iv) Alternatively, the reduced EPR may be a direct consequence of global warming. Although, the temperature on the Faroe shelf showed no apparent temporal trend (Figure 3A), temperatures in deep waters (i.e., overwintering locations) surrounding the Faroes have increased by approximately 0.1°C (Hansen et al., 2016). Increased temperatures may lead to lower body sizes and lower internal lipid stores, affecting the EPR negatively. Unfortunately, female size was not measured on a regular basis during the present time-series study and we could not determine if there have been temporal changes in mean female length. We recommend measuring a subset of females and preserving individuals for lipid content in all future experiments.
C. finmarchicus is, as mentioned, a principal food source for several species of fish, including fish larvae that inhabit the Faroe shelf during spring and summer. In particular, studies have shown that C. finmarchicus eggs are the pre-dominant food of first feeding Faroe Plateau cod larvae during spring, i.e., in late April and early May (Gaard and Steingrund, 2001; Jacobsen et al., 2020). Thus, with regards to first feeding fish larvae spawned on the Faroe shelf in spring, a reduction or possible mismatch in prey, i.e., C. finmarchicus egg abundance and fish larval abundance could have an impact on recruitment to the cod stock. Since the Faroe shelf is situated near two major overwintering regions of C. finmarchicus—the Atlantic subpolar gyre and the Norwegian Sea gyre (Heath et al., 2000)—our findings might also be relevant for neighboring shelf ecosystems. Further studies on this important topic on the Faroe shelf should respect spatial oceanographic structures on the shelf, and emphasize on the productive inflow in the north-western area.
We here demonstrate that the Faroe shelf is not homogenous regarding pre-bloom Calanus finmarchicus egg production. On average, the highest EPR were on the north-western side of the shelf, close to the area where the phytoplankton spring bloom is initiated and to the main cod spawning grounds. This is also where the influx of oceanic waters to the inner shelf is largest. Underlying marked inter-annual variability there was a significant reduction in the fraction of spawning females and EPR on the inner shelf during the last two decades. As evident from the current study, resolving the pre-bloom C. finmarchicus egg production is not a trivial task. Neither could variability in phytoplankton biomass nor temperature fully explain the observed variability in EPR. Other factors, such as (i) declining silicate concentrations in the North Atlantic, (ii) variability in water mass distribution surrounding the Faroes, (iii) a phenology change that happened in 2007 and (iv) increased overwintering temperatures, could all have affected the EPR. Our findings might reflect conditions over large parts of the Northeastern Atlantic and the Nordic Seas.
Publicly available datasets were analyzed in this study. The CTD data used in the study can be found here: http://envofar.fo/data/index.php?dir=Hydrography%2FCRUISES&sort=N&order=A and the environmental data from station S is located at: http://envofar.fo/data/index.php?dir=Timeseries&sort=N&order=A. The original contributions presented in the study are included in the article/Supplementary Material, further inquiries can be directed to the corresponding author/s.
SJ conceived the study, did the data analyses, and led the writing process in correspondence with HH and EG. EG produced the egg production data. All authors have read, commented, and agreed to the manuscript before submission.
The authors declare that the research was conducted in the absence of any commercial or financial relationships that could be construed as a potential conflict of interest.
All claims expressed in this article are solely those of the authors and do not necessarily represent those of their affiliated organizations, or those of the publisher, the editors and the reviewers. Any product that may be evaluated in this article, or claim that may be made by its manufacturer, is not guaranteed or endorsed by the publisher.
The Supplementary Material for this article can be found online at: https://www.frontiersin.org/articles/10.3389/fmars.2022.822978/full#supplementary-material
Cushing, D. H. (1990). Plankton production and year-class strength in fish populations: an update of the Match/Mismatch hypothesis. Adv. Mar. Biol. 26, 249–293.
Debes, H. H., and Eliasen, K. (2006). Seasonal abundance, reproduction and development of four key copepod species on the Faroe shelf. Mar. Biol. Res. 2, 249–259. doi: 10.1080/17451000600798787
Debes, H. H., Gaard, E., and Hansen, B. (2008a). Primary production on the Faroe shelf: temporal variability and environmental influences. J. Mar. Syst. 74, 686–697. doi: 10.1016/j.jmarsys.2008.07.004
Debes, H. H., Eliasen, K., and Gaard, E. (2008b). Seasonal variability in copepod ingestion and egg production on the Faroe shelf. Hydrobiologia 600, 247–265. doi: 10.1007/s10750-007-9238-3
Drijfhout, S., van Oldenborgh, G. J., and Cimatoribus, A. (2012). Is a decline of AMOC causing the warming hole above the North Atlantic in observed and modeled warming patterns? J. Clim. 25, 8373–8379. doi: 10.1175/JCLI-D-12-00490.1
Eliasen, S. K., Hátún, H., Larsen, K. M. H., Hansen, B., and Rasmussen, T. A. S. (2017). Phenologically distinct phytoplankton regions on the Faroe Shelf - identified by satellite data, in-situ observations and model. J. Mar. Syst. 169, 99–110. doi: 10.1016/j.jmarsys.2017.01.015
Eliasen, S. K., Hátún, H., Larsen, K. M. H., Vang, H. B. M., and Rasmussen, T. A. S. (2019). The Faroe shelf spring bloom onset explained by a ‘Critical Volume Hypothesis.’. J. Mar. Syst. 194, 91–101. doi: 10.1016/j.jmarsys.2019.02.005
Gaard, E. (1999). The zooplankton community structure in relation to its biological and physical environment on the Faroe shelf, 1989-1997. J. Plankton Res. 21, 1133–1152. doi: 10.1093/plankt/21.6.1133
Gaard, E. (2000). Seasonal abundance and development of Calanus finmarchicus in relation to phytoplankton and hydrography on the Faroe Shelf. ICES J. Mar. Sci. 57, 1605–1611. doi: 10.1006/jmsc.2000.0963
Gaard, E. (2003). Plankton variability on the Faroe Shelf during the 1990s. ICES Mar. Sci. Symp. 219, 182–189.
Gaard, E., and Hansen, B. (2000). Variations in the advection of Calanus finmarchicus onto the Faroe Shelf. ICES J. Mar. Sci. 57, 1612–1618. doi: 10.1006/jmsc.2000.0962
Gaard, E., and Steingrund, P. (2001). Reproduction of faroe plateau cod: spawning grounds, egg advection and larval feeding. Fróðskaparrit 48, 87–103. doi: 10.1002/9781119460701.ch3
Gislason, A. (2005). Seasonal and spatial variability in egg production and biomass of Calanus finmarchicus around Iceland. Mar. Ecol. Prog. Ser. 286, 177–192. doi: 10.3354/meps286177
Hansen, B., Eliasen, S. K., Gaard, E., and Larsen, K. M. H. (2005). Climatic effects on plankton and productivity on the Faroe Shelf. ICES J. Mar. Sci. 62, 1224–1232. doi: 10.1016/j.icesjms.2005.04.014
Hansen, B., Larsen, K. M. H., Hátún, H., and Østerhus, S. (2016). A stable faroe bank channel overflow 1995-2015. Ocean Sci. 12, 1205–1220. doi: 10.5194/os-12-1205-2016
Hansen, B., and Østerhus, S. (2000). North Atlantic–Nordic Seas exchanges. Prog. Oceanogr. 45, 109–208. doi: 10.1016/S0079-6611(99)00052-X
Hátún, H., Azetsu-Scott, K., Somavilla, R., Rey, F., Johnson, C., Mathis, M., et al. (2017). The subpolar gyre regulates silicate concentrations in the North Atlantic. Sci. Rep. 7:14576. doi: 10.1038/s41598-017-14837-4
Hátún, H., Larsen, K. M. H., and Debes, H. (2013). The Western Region. Technical Report Nr.: 13- 07. Faroe Islands: Havstovan.
Hátún, H., Lohmann, K., Matei, D., Jungclaus, J. H., Pacariz, S. V., Bersch, M., et al. (2016). An inflated subpolar gyre blows life toward the northeastern Atlantic. Prog. Oceanography 147, 49–66. doi: 10.1016/j.pocean.2016.07.009
Hátún, H., Payne, M. R., Beaugrand, G., Reid, P. C., Sandø, A. B., Drange, H., et al. (2009). Large bio-geographical shifts in the north-eastern Atlantic Ocean: from the subpolar gyre, via plankton, to blue whiting and pilot whales. Prog. Oceanogr. 80, 149–162. doi: 10.1016/j.pocean.2009.03.001
Hátún, H., Sande, A. B., Drange, H., Hansen, B., and Valdimarsson, H. (2005). Ocean science: influence of the atlantic subpolar gyre on the thermohaline circulation. Science 309, 1841–1844. doi: 10.1126/science.1114777
Head, E. J. H., Harris, L. R., Ringuette, M., and Campbell, R. W. (2013a). Characteristics of egg production of the planktonic copepod, Calanus finmarchicus, in the Labrador Sea: 1997-2010. J. Plankton Res. 35, 281–298. doi: 10.1093/plankt/fbs097
Head, E. J. H., Melle, W., Pepin, P., Bagøien, E., and Broms, C. (2013b). On the ecology of Calanus finmarchicus in the Subarctic North Atlantic: a comparison of population dynamics and environmental conditions in areas of the Labrador Sea-Labrador/Newfoundland Shelf and Norwegian Sea Atlantic and Coastal Waters. Prog. Oceanogr. 114, 46–63. doi: 10.1016/j.pocean.2013.05.004
Heath, M. R., Fraser, J. G., Gislason, A., Hay, S. J., Jónasdóttir, S. H., and Richardson, K. (2000). Winter distribution of Calanus finmarchicus in the Northeast Atlantic. ICES J. Mar. Sci. 57, 1628–1635. doi: 10.1006/jmsc.2000.0978
Heath, M. R., and Lough, R. G. (2007). A synthesis of large-scale patterns in the planktonic prey of larval and juvenile cod (Gadus morhua). Fish. Oceanogr. 16, 169–185. doi: 10.1111/j.1365-2419.2006.00423.x
Hirche, H. J., Meyer, U., and Niehoff, B. (1997). Egg production of Calanus finmarchicus: effect of temperature, food and season. Mar. Biol. 127, 609–620. doi: 10.1007/s002270050051
Hjort, J. (1914). “Fluctuations in the great fisheries of northern Europe viewed in the light of biological research,” in Proceedings of the Rapports et ProcésVerbaux des Réunions du Conseil Permanent International pour l’Exploration de la Mer, Copenhagen.
Irigoien, X., Fernandes, J. A., Grosjean, P., Denis, K., Albaina, A., and Santos, M. (2008). Spring zooplankton distribution in the Bay of Biscay from 1998 to 2006 in relation with anchovy recruitment. J. Plankton Res. 31, 1–17. doi: 10.1093/plankt/fbn096
Jacobsen, S., Gaard, E., Hátún, H., Steingrund, P., Larsen, K. M. H., Reinert, J., et al. (2019). Environmentally driven ecological fluctuations on the faroe shelf revealed by fish juvenile surveys. Front. Mar. Sci. 6:559. doi: 10.3389/fmars.2019.00559
Jacobsen, S., Gaard, E., Larsen, K. M. H., Eliasen, S. K., and Hátún, H. (2018). Temporal and spatial variability of zooplankton on the Faroe shelf in spring 1997–2016. J. Mar. Syst. 177, 28–38. doi: 10.1016/j.jmarsys.2017.08.004
Jacobsen, S., Nielsen, K. K., Kristiansen, R., Grønkjær, P., Gaard, E., and Steingrund, P. (2020). Diet and prey preferences of larval and pelagic juvenile Faroe Plateau cod (Gadus morhua). Mar. Biol. 167:122. doi: 10.1007/s00227-020-03727-5
Johnson, C. L., Leising, A. W., Runge, J. A., Head, E. J. H., Pepin, P., Plourde, S., et al. (2008). Characteristics of Calanus finmarchicus dormancy patterns in the Northwest Atlantic. ICES J. Mar. Sci. 65, 339–350. doi: 10.1093/icesjms/fsm171
Jónasdóttir, S. H., Richardson, K., Heath, M. R., Ingvarsdóttir, A., and Christoffersen, A. (2008). Spring production of Calanus finmarchicus at the Iceland-Scotland Ridge. Deep Sea Res. I Oceanogr. Res. Pap. 55, 471–489. doi: 10.1016/j.dsr.2007.12.009
Jónasdóttir, S. H., Trung, N. H., Hansen, F., and Gärtner, S. (2005). Egg production and hatching success in the calanoid copepods Calanus helgolandicus and Calanus finmarchicus in the North Sea from March to September 2001. J. Plankton Res. 27, 1239–1259. doi: 10.1093/plankt/fbi091
Jónasdóttir, S. H., Wilson, R. J., Gislason, A., and Heath, M. R. (2019). Lipid content in overwintering Calanus finmarchicus across the Subpolar Eastern North Atlantic Ocean. Limnol. Oceanogr. 64, 2029–2043. doi: 10.1002/lno.11167
Kristiansen, I., Gaard, E., Hátún, H., Jónasdóttir, S. H., and Ferreira, S. A. (2016). Persistent shift of Calanus spp. in the southwestern Norwegian Sea since 2003, linked to ocean climate. ICES J. Mar. Sci. 73, 1319–1329. doi: 10.1093/icesjms/fsv222
Kristiansen, I., Jónasdóttir, S. H., Gaard, E., Eliasen, S. K., and Hátún, H. (2021). Seasonal variations in population dynamics of Calanus finmarchicus in relation to environmental conditions in the southwestern Norwegian Sea. Deep Sea Res. I Oceanogr. Res. Pap. [Epub ahead of print] doi: 10.1016/j.dsr.2021.103508
Larsen, K. M. H., Hansen, B., and Svendsen, H. (2008). Faroe shelf water. Continent. Shelf Res. 28, 1754–1768. doi: 10.1016/j.csr.2008.04.006
Larsen, K. M. H., Hansen, B., and Svendsen, H. (2009). The Faroe Shelf front: properties and exchange. J. Mar. Syst. 78, 9–17. doi: 10.1016/j.jmarsys.2009.02.003
Larsen, K. M. H., Hátún, H., Hansen, B., and Kristiansen, R. (2012). Atlantic water in the Faroe area: sources and variability. ICES J. Mar. Sci. 69, 802–808. doi: 10.1093/icesjms/fss028
Li, C., von Storch, J. S., and Marotzke, J. (2013). Deep-ocean heat uptake and equilibrium climate response. Clim. Dyn. 40, 1071–1086. doi: 10.1007/s00382-012-1350-z
Madsen, M. L., Gaard, E., and Hansen, B. W. (2008). Wax-ester mobilization by female Calanus finmarchicus (Gunnerus) during spring ascendance and advection to the Faroe Shelf. ICES J. Mar. Sci. 65, 1112–1121. doi: 10.1093/icesjms/fsn097
Melle, W., Runge, J., Head, E., Plourde, S., Castellani, C., Licandro, P., et al. (2014). The North Atlantic Ocean as habitat for Calanus finmarchicus: environmental factors and life history traits. Prog. Oceanogr. 129, 244–284. doi: 10.1016/j.pocean.2014.04.026
Meyer-Harms, B., Irigoien, X., Head, R., and Harris, R. (1999). Selective feeding on natural phytoplankton by Calanus finmarchicus before, during, and after the 1997 spring bloom in the Norwegian Sea. Limnol. Oceanogr. 44, 154–165. doi: 10.4319/lo.1999.44.1.0154
Niehoff, B., Hirche, H.-J., and Bamstedt, U. (2011). The reproduction of Calanus finmarchicus in the Norwegian Sea in spring. Sarsia 85, 15–22. doi: 10.1080/00364827.2000.10414552
Niehoff, B., Klenke, U., Hirche, H. J., Irigoien, X., Head, R., and Harris, R. (1999). A high frequency time series at Weathership M, Norwegian Sea, during the 1997 spring bloom: the reproductive biology of Calanus finmarchicus. Mar. Ecol. Prog. Ser. 176, 81–92. doi: 10.3354/meps176081
Ottosen, K. M., Steingrund, P., Magnussen, E., and Payne, M. R. (2018). Distribution and timing of spawning Faroe Plateau cod in relation to warming spring temperatures. Fish. Res. 198, 14–23.
Parsons, T. R., Maita, Y., and Lalli, C. M. (1984). A Manual of Chemical & Biological Methods for Seawater Analysis. Oxford: Pergamon Press, 115.
Planque, B., Hays, G. C., Ibanez, F., and Gamble, J. C. (1997). Large scale spatial variations in the seasonal abundance of Calanus finmarchicus. Deep Sea Res. I Oceanogr. Res. Pap. 44, 315–326. doi: 10.1016/S0967-0637(96)00100-8
R Core Team (2018). R: A Language and Environment for Statistical Computing. Vienna: R Foundation for Statistical Computing.
Rasmussen, T. A. S., Olsen, S. M., Hansen, B., Hátún, H., and Larsen, K. M. H. (2014). The Faroe shelf circulation and its potential impact on the primary production. Continent. Shelf Res. 88, 171–184. doi: 10.1016/j.csr.2014.07.014
Reygondeau, G., and Beaugrand, G. (2011). Future climate-driven shifts in distribution of Calanus finmarchicus. Glob. Change Biol. 17, 756–766. doi: 10.1111/j.1365-2486.2010.02310.x
Salter, I., Eliasen, S. K., and Jacobsen, S. (2020). Variability in the Relationship Between in Situ Fluorescence and Chlorophyll-A Concentration in Faroese Waters (2012-2019): Recommendations for Database Management. Technical Report Nr.: 20-02. Faroe Islands: Havstovan.
Keywords: Calanus finmarchicus, egg (production), temperature, chlorophyll, pre-bloom, Faroe shelf
Citation: Jacobsen S, Gaard E and Hátún H (2022) Declining Pre-bloom Calanus finmarchicus Egg Production Adjacent to Two Major Overwintering Regions in the Northeastern Atlantic. Front. Mar. Sci. 9:822978. doi: 10.3389/fmars.2022.822978
Received: 26 November 2021; Accepted: 11 January 2022;
Published: 14 February 2022.
Edited by:
Martin Edwards, Plymouth Marine Laboratory, United KingdomReviewed by:
Edward Buskey, University of Texas at Austin, United StatesCopyright © 2022 Jacobsen, Gaard and Hátún. This is an open-access article distributed under the terms of the Creative Commons Attribution License (CC BY). The use, distribution or reproduction in other forums is permitted, provided the original author(s) and the copyright owner(s) are credited and that the original publication in this journal is cited, in accordance with accepted academic practice. No use, distribution or reproduction is permitted which does not comply with these terms.
*Correspondence: Sólvá Jacobsen, c29sdmFqQGhhdi5mbw==
Disclaimer: All claims expressed in this article are solely those of the authors and do not necessarily represent those of their affiliated organizations, or those of the publisher, the editors and the reviewers. Any product that may be evaluated in this article or claim that may be made by its manufacturer is not guaranteed or endorsed by the publisher.
Research integrity at Frontiers
Learn more about the work of our research integrity team to safeguard the quality of each article we publish.