- 1Laboratory for Aquaculture Biotechnology, Division of Materials Chemistry, Ruđer Bošković Institute, Zagreb, Croatia
- 2Laboratory for Chiral Technologies, Division of Organic Chemistry and Biochemistry, Ruđer Bošković Institute, Zagreb, Croatia
- 3Laboratory for Bioinformatics, Faculty of Food Technology and Biotechnology, University of Zagreb, Zagreb, Croatia
- 4Department of Chemistry and Biochemistry, Faculty of Food Technology and Biotechnology, University of Zagreb, Zagreb, Croatia
- 5The Novo Nordisk Foundation Center for Biosustainability, Technical University of Denmark, Lyngby, Denmark
- 6Kodeks Ltd., Zagreb, Croatia
Seawater is an environment in which numerous microorganisms have evolved, some with a great potential for biotechnology. In recent years, many scientists have moved away from the assumption that the origin of life was in pools of water, and instead propose that life on Earth probably originated in accumulations of warm, nutrient-rich mud. This mud, also called peloid is a rich source of organisms that, due to their adaptation to this unique environment, produce a wide variety of primary and secondary metabolites with numerous and diverse activities, including anti-cancer, anti-inflammatory, antiviral, and immunomodulatory ones. In this research, two questions were addressed using collected samples of a peloid with demonstrated healing properties. Firstly biodiversity in this ecological niche was explored in order to assess microbial communities present and secondly natural products were screened for in order to assess whether predicted activities could be linked to healing properties of the peloid. The use of peloids in medical therapy dates back to ancient times. Abiotic components such as clay and mineral water are believed to be the main contributors of the healing properties of natural peloids. The places where peloids are usually found are characteristically shallow and enclosed lagoons. The constant UV exposure and increased salt concentration classify peloid as an extreme environment. The spectrum of relief’s peloid therapy is claimed to provide ranges from purely cosmetic and skin-related to musculoskeletal and immunological problems. These claims can hardly be supported by mineral content and heat-retaining properties alone. However, organic compounds from present microorganisms as well as secondary metabolites could help explain the observed range of health benefits. The fact that the relationship between the therapeutic activity of peloids and their composition besides mineral and physicochemical properties has not been extensively studied indicates untapped biotechnological potential.
Introduction
Nin is a small town on the east coast of the Adriatic sea, founded by the Illyrian tribe Liburni in the ninth century BC. This arable land is the lowest part of the geographical region rising up to 100 m above sea level and is characterized by shallow and sandy coast, lagoons, canals, and cliffs. Until early twentieth century, a small river flowed through the Nin Bay entering the sea between Queen’s and Ždrijac beach. The river carried a mixture of organic and inorganic substances, which it had been depositing for centuries before being redirected during the Austro-Hungarian era, east of Nin. The location harboring peloid mud is positioned west of Nin. It is the largest site of medicinal mud in Croatia, approx. 120,000 m2 in size that has been used for centuries for rehabilitation and medical treatment. Ancient Romans built temples and thermal baths on this locality. Vienna Medical faculty scientists conducted the first scientific studies of this peloid during the Austro-Hungarian Monarchy, while Croatian scientists continued testing this peloid mud applicability for use in thalassotherapy in 1953. This initiated the first organized and controlled mud therapy for patients in 1965. Since 1965, over 20,000 patients have participated in mud therapy under the guidance of medical personnel, dealing with problems such as functional and rheumatic disorders, musculoskeletal system diseases, and different spine deformations as the most common ones.
The classification, composition, and usage of peloids in medicine have been subject of study for many researchers. Since the early twentieth century, there have been several changes in the peloid definition and classification. The most recent one defines peloids as matured muds or muddy dispersions composed as a complex mixture of fine granular materials of geologic and biologic origin, mixed with mineral water or seawater. Different types of organic and inorganic matter (and their mixture) are part of the peloid composition. They can occur naturally or artificially during peloid maturation thus improving the physio-chemical properties and at the same time changing the mineralogy of the solid mixture. Living microorganism, such as microalgae, cyanobacteria, bacteria and protozoa, play a distinct role in this maturation process and inorganic changes of the solid-liquid peloid mixture. Metabolic pathways of microorganisms present in the peloid depend and adapt to the inorganic and organic content of clay resulting in metabolic products that have potential to improve therapeutic usefulness of peloid (Carretero, 2020).
Sometimes, peloid material can contain hazardous material, which can be released during its application that could lead to health risks. Applied to the skin, harmful ions and other compounds can enter the bloodstream and spread through the body. Detailed research is essential to avoid potential damage and to contribute and determine the compounds related to healing properties of peloids (Cara et al., 2000; Kikouama et al., 2009). Information on the peloid microorganism community, their metabolic products, together with detailed physiochemical analyses can ultimately lead to a better understanding and help defining the potential use of peloids for therapeutic purposes and medicinal or cosmetic applications.
Pelotherapy is synonymous with the term mud therapy and includes the external use of peloid (mud) for both therapeutic and cosmetic (skincare) purposes (Veniale et al., 2004). Since the ancient times there is evidence of peloid being used for medicinal purposes. It was believed that the power of natural substances in peloids could facilitate healing of the body and despite scientific advances modern pelotherapy treatments still mostly rely on these historic practices. Mud therapies are available as medicinal and cosmetic preparations in spas, wellness centers, and for home use. The addition of hydrosols, herbs, tinctures and essential oils is believed to improve the therapeutic properties of mud. The medical condition of the patient influences peloid selection for therapy, solely on the basis of recorded historical usage. Various peloid types from all parts of the world differ in their properties due to their chemical composition and (in) organic content. Different types of clays with specific nutrients, mineral content or pH value can be mixed to address more specific conditions (Routh et al., 1996; Tateo and Summa, 2007; Gomes and de, 2018).
In general, the use of peloids is believed to promote dilution and excretion of toxic substances thus eliminating them from the body; healing different diseases like constipation, high blood pressure, water retention problems, skin diseases, arthritis, psoriasis, rheumatism, and bone or muscle traumas and ultimately resulting in a better life quality, clinical improvements and reduced need for medications (Veniale et al., 2004; Forestier et al., 2010; Espejo-Antúnez et al., 2013). The complex composition and powerful adsorptive and absorptive properties of peloids are used for a variety of cosmetics and pharmaceutical formulations. Natural peloids mature in nature for undefined periods of time, while artificial gels or pastes undergo a similar maturation process for days or even years in special tanks and under specific conditions. Both natural and artificial peloids are being applied externally for cosmetic and skin protective purposes (Halevy and Sukenik, 1998; Carretero et al., 2007; Carretero, 2020).
Materials and Methods
Determination of Physical, Chemical and Atmospheric Parameters During Peloid Sampling
Sample collection for microbiome analysis was carried out in June 2021 across the Nin peloid mud micro-location (44°14′59.2″ N, 15°10′24.5″ E). GPS location during sampling was determined using Google maps mobile phone application. Details about mud and seawater samples are shown in Supplementary Table 1. Peloid samples were collected into sterile Falcon 50 mL tubes. Seawater samples were collected in sterile Schott bottles. All samples were cooled in a styrofoam container with ice packs prior to sample storage and analysis the next day at + 4°C.
Depth of the bottom of each sampling location was measured with a simple construction ruler. pH value was determined using a handheld, portable WTW pH/Oxi 340i multiparameter meter (WTW—a Xylem Inc., brand, Weilheim in Oberbayern, Germany) equipped with Schott BlueLine 24 pH electrode (Schott, Mainz, Germany). Seawater conductivity was measured with Endress + Hauser Liquiline To GO CYM290 handheld multiparameter device equipped with Endress + Hauser Memosens CLS82D conductivity electrode (Endress + Hauser, Reinach, Switzerland). Parameters describing weather conditions such as temperature, humidity, wind, and air pressure are reported in Table 1.1

Table 1. Weather conditions during sampling of seawater and sediment on the Nin peloid mud locality (sampling performed on 30th of June, 2021).
UV index values for Nin bay area were reported as very high for entire sampling period2 and are shown in Supplementary Figure 1.
Peloid Sample Collection and Subsequent Anthropological Impact Analysis
Physico-chemical and microbiological analyses were conducted on eight water samples (S1, S2, S4, S5, S7, S8, S10, S11) and two peloid mud samples (S6, S9) in order to establish anthropological impact on the microbiological composition of Nin peloid mud and seawater, and to assess the quality of this particular mud for pelotherapy purposes. Seawater and peloid mud sample analyses were conducted by Virkom d.o.o., authorized laboratory for water and sediment quality testing (Supplementary Table 1) and sampling locations are shown in Figure 1.
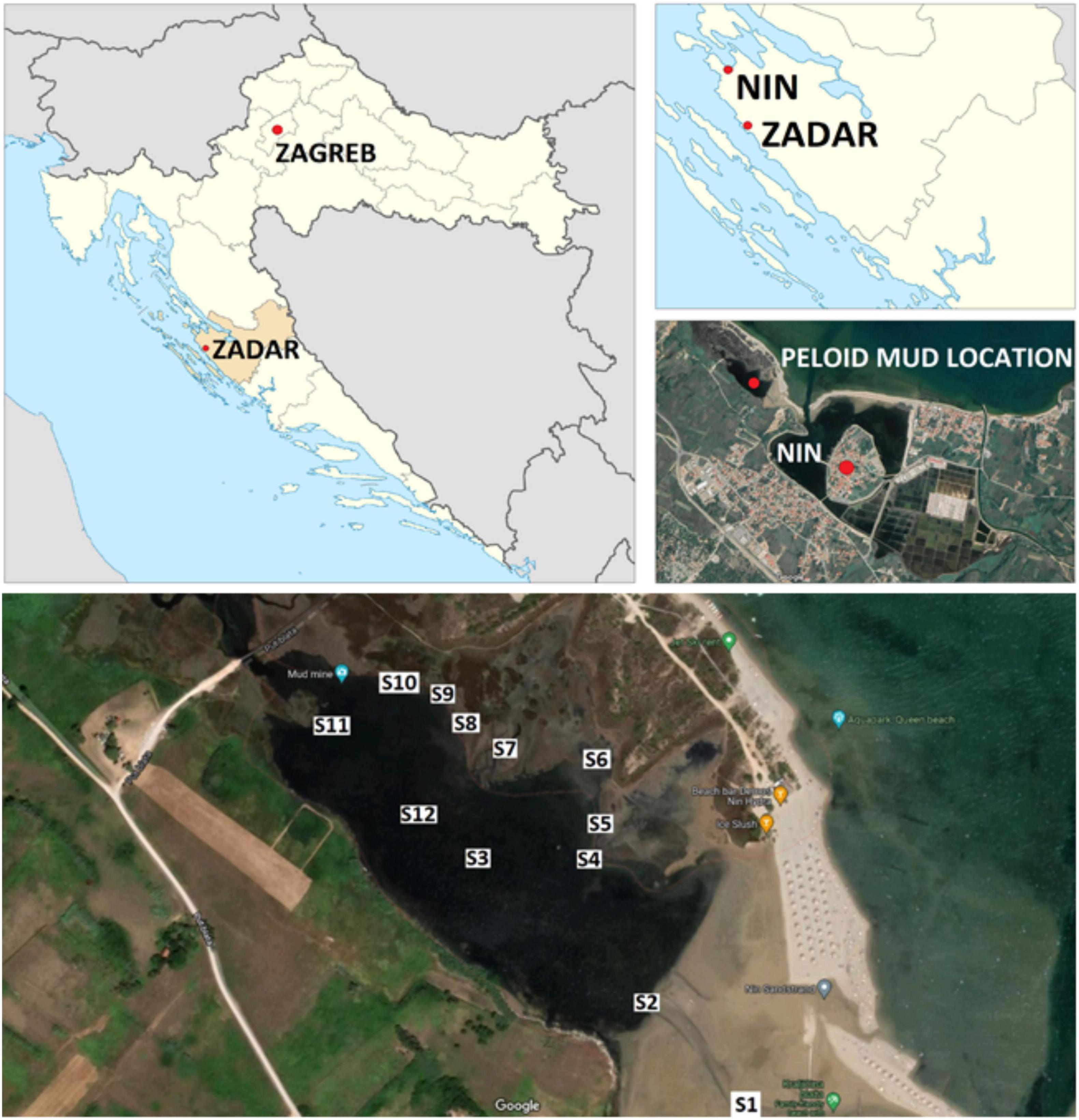
Figure 1. Sampling locations across the Nin peloid mud micro-location (44°14′59.2″ N, 15°10′24.5″ E).
Peloid Microbiome DNA Isolation, Concentration and Storage
Nine peloid mud samples (S2, S3, S4, S5, S6, S7 S8, S9, S10) were homogenized (Vortex LLG, Meckenheim, Germany) and aliquoted into 1.5 mL Eppendorf tubes using cut-tips. Seawater samples were centrifuged at + 4°C, 14,000 rpm, 15 min by using a fixed angle rotor (Eppendorf 5804R, Hamburg, Germany). Seawater centrifugation pellets were combined into composite sample (S12) in order to achieve a sufficient biomass for DNA isolation (S12). All samples were stored at –80°C (Heraeus HeraFreeze, Hanau, Germany) prior to further processing and DNA isolation.
DNA isolation was performed using two different kits: QIAmp® PowerFecal® DNA kit (Qiagen, Hilden, Germany) and DNeasy® Plant Mini kit (Qiagen, Hilden, Germany). Samples were weighed on an analytical balance (Kern ABS 120-4N, Balingen, Germany). A bead homogenizer (Tehtnica MillMix 20, Železniki, Slovenia) was used to lyse the cells, followed by DNA isolation. During the isolation procedure, manufacturer’s instructions were followed with minor modifications (in the washing step) in order to increase the DNA yield. DNA concentration was measured using a fluorometer (Quantus Fluorometer, Promega, Madison, Wisconsin, United States) and the corresponding analytical kit (QuantiFluor ONE dsDNA System Kit, Promega, Madison, Wisconsin, United States). Concentrations were expressed as ng per μL and presented in Supplementary Table 1, together with additional data on samples such as seawater content, physical parameters, location details and pH value.
Sequencing of 16S and 18S rRNA Genes and Subsequent Bioinformatics Analysis
DNA extracted using QIAmp® PowerFecal® DNA kit was used for amplicon sequencing of V3 and V4 regions of the genes coding for 16S rRNA using primer pair 341F/806R, DNA extracted using Qiagen Plant kit was used to sequence V9 region of the 18S rRNA genes using primer pair Euk1341F/EukBR. Sequencing was carried out in MRDNA laborartory (Texas, United States) using the Illumina MiSeq platform and paired-end sequencing protocol. Quantitative Insights into Microbial Ecology 2—QIIME2 (Bolyen et al., 2019) was used to perform the analysis of sequenced amplicons. Raw data, obtained from Illumina’s BaseSpace in form of demultiplexed fastq files was inspected for chimeras, denoised and inferred into amplicon sequence variants (ASVs) using DADA2 (Callahan et al., 2016). Alpha and beta diversity metrics were calculated using QIIME’s q2-diversity plugin with both datasets subsampled in order to match the sample with the lowest number of reads in the dataset. Taxonomy was assigned to ASVs using the pre-trained naive Bayes taxonomy classifier, as implemented in QIIME2. Classifier was trained on Silva database (Quast et al., 2013) release 138 with sequences clustered at 99% identity and using region matching primer pair 341F/806R for 16S data and region matching primer pair Euk1341F/EukBR for 18S data.
Sample Collection and Preparation for Bioactive Extracts for LC-Q-TOF MS2 Analysis
Peloid samples were collected on three different locations (S4, S6, S8), daytime during strongest UV radiation, and during night-time. All samples were subsequently frozen and lyophilized (main drying: 4 h, 0.1 mbar vacuum, –20°C shelf temperature; final drying: 24 h, 0.03 mbar vacuum, + 20°C shelf temperature) (Martin Christ 2–4 LSC plus, Osterode am Harz, Germany). All samples were homogenized and weighed on an analytical balance (Kern ABS 120-4N, Balingen, Germany) prior to extraction. 10 ± 0.5 g portions of lyophilized peloid mud samples (S4, S6, S8) were extracted in dichloromethane:methanol 1:1 mixture used as an extraction solvent, with the help of sonication at 35 kHz frequency for 10 min (Bandelin Sonorex, Berlin, Germany). After this extraction, samples were centrifuged at 4,000 rpm for 5 min (Tehtnica LC-320, Železniki, Slovenia) and supernatants were collected. Fresh extraction solvent was added to the peloid mud samples and the procedure was repeated twice. Collected extracts were filtered using blue band filter paper (Whatman Grade 1 filter paper, 110 mm) and Chromafil RC (45/25) syringe filters. Samples were evaporated (Büchi Labortechnik AG Rotavapor R-215, Flawil, Switzerland), resuspended with methanol to achieve a concentration of 10 mg/mL and filtered using Chromafil Xtra PTFE (20/25) syringe filters prior to LC-QTOF MS2 analysis.
LC-Q-TOF MS2 Analysis
The liquid chromatography-high-resolution mass spectrometry (LC-HRMS) was carried out using a Quadrupole Time-of-Flight (qTOF; 6546 LC/Q-TOF, Agilent, United States) equipped with an Agilent Dual Jet Stream electrospray ionization (Dual AJS ESI), coupled to an ultra-high-performance liquid chromatography instrument (UHPLC; 1290 Infinity, Agilent, United States). Extracts were separated on a ZORBAX Eclipse Plus C18 RRHD column (2.1 × 50 mm; 1.8 μm, Agilent) using gradient elution: 0–2 min 50% B, 2–12 min 50–95% B, 12–12.5 min 40% B, followed by 2 min equilibration at flow rate of 0.4 mL/min. The column was kept at 40°C and the samples at 5°C. The acquisition was performed in full scan MS mode over the m/z 100–1,700 mass range. The mass spectrometer was operated in both positive and negative ionization modes, respectively, with the following operating parameters: drying gas (N2) flow rate of 13 L/min, drying gas (N2) temperature 225°C, nebulizer 45 psig, capillary voltage 3,500 V, nozzle voltage 1,000 V, and fragmentor voltage 175 V. The Auto MS/MS mode using the acquisition rate of 2 scans/spectra and collision energy 25 V were applied to obtain the fragment ions. Accurate mass measurement was achieved by means of reference ion correction using continuous infusion of internal reference masses [M + H] + at m/z 121.0509 (purine), [M + H] + at m/z 922.0098 (HP-0921) for positive polarity and [TFA-H]—at m/z 112.9856 (TFA anion), and [M + COOH]—at m/z 966.0007 (HP-0921) for negative polarity at a flow rate 0.050 mL/min during data acquisition. MS acquisition was controlled by Agilent MassHunter Acquisition Software (V.10.1). Raw data were processed using MassHunter Qualitative Software (V.10.0.) and exported to mgf format for subsequent bioinformatics compound identification.
Results
Taxonomic Profiling of Peloid “Microbiota”
Taxonomic distribution of prokaryotic and eukaryotic communities in the peloid mud from Nin lagoon was assessed using amplicon sequencing of variable regions of 16S and 18S rRNA genes. In case of peloid mud samples, nine were collected in total, from various micro locations around the Nin lagoon (Figure 1), while the seawater samples from the lagoon entry were pooled and centrifuged in order to obtain sufficient biomass for DNA isolation. All samples were aliquoted, and DNA was extracted using two different extraction kits adapted for prokaryotes and eukaryotes, respectively. The DNA concentration of sample S4 extracts obtained by DNeasy® Plant Mini kit was deemed not sufficient and therefore this sample was omitted from 18S rRNA sequencing. In total 19 DNA extracts with sufficient amount of DNA were sequenced, 10 targeting 16S and 9 targeting 18S rRNA variable gene regions. The results of the 16S rRNA sequencing indicated that 98.56% sequences could be classified as bacteria, whereas the remaining 1.44% sequences accounted for Archaea. Rarefaction curve, which shows the number of OTUs as a function of the sequencing depth, indicates that total diversity was successfully captured, since all samples achieved saturation (data not shown). A total number of OTUs observed at the rarefied sequencing depth of 2,200 is shown in Figure 2A, indicating the most OTU rich sample was—S3 (253) and the poorest one, with lowest number of OTUs—a seawater sample S12 (85). The overall number of eukaryotic OTUs, as seen from the 18S rRNA sequencing results, is generally lower compared to prokaryote and ranges from 25 to 222 OTUs per sample. The lower species richness in case of eukaryotes is supported by Shannon diversity index (Figure 2B). A great prokaryotic diversity was also detected at the phylum level with a total of 42 observed phyla (Figure 3). Furthermore, abundance of microbial phyla was similar among seven peloid samples collected at the coastal area (S4, S5, S6, S7, S8, S9, S10). The most abundant phylum in the peloid mud found in the coastal area was Proteobacteria with the average abundance of 52.68% across seven samples. The next highly represented phyla were Bacteroidetes (16.92%) and Desulfobacteriota (10.13%) followed by Cyanobacteria (5.74%). Sample S8 showed higher abundance of the Firmicutes phylum (11.07%) in comparison to the coastal seawater samples. The peloid mud samples S2 and S3 were characterized by phyla Chloroflexi (50.29%) and Desulfobacterota (26.2%). The seawater sample (S12), exhibited lowest diversity and was dominated by Proteobacteria (71.37%) and Bacteroidetes (22.72%).
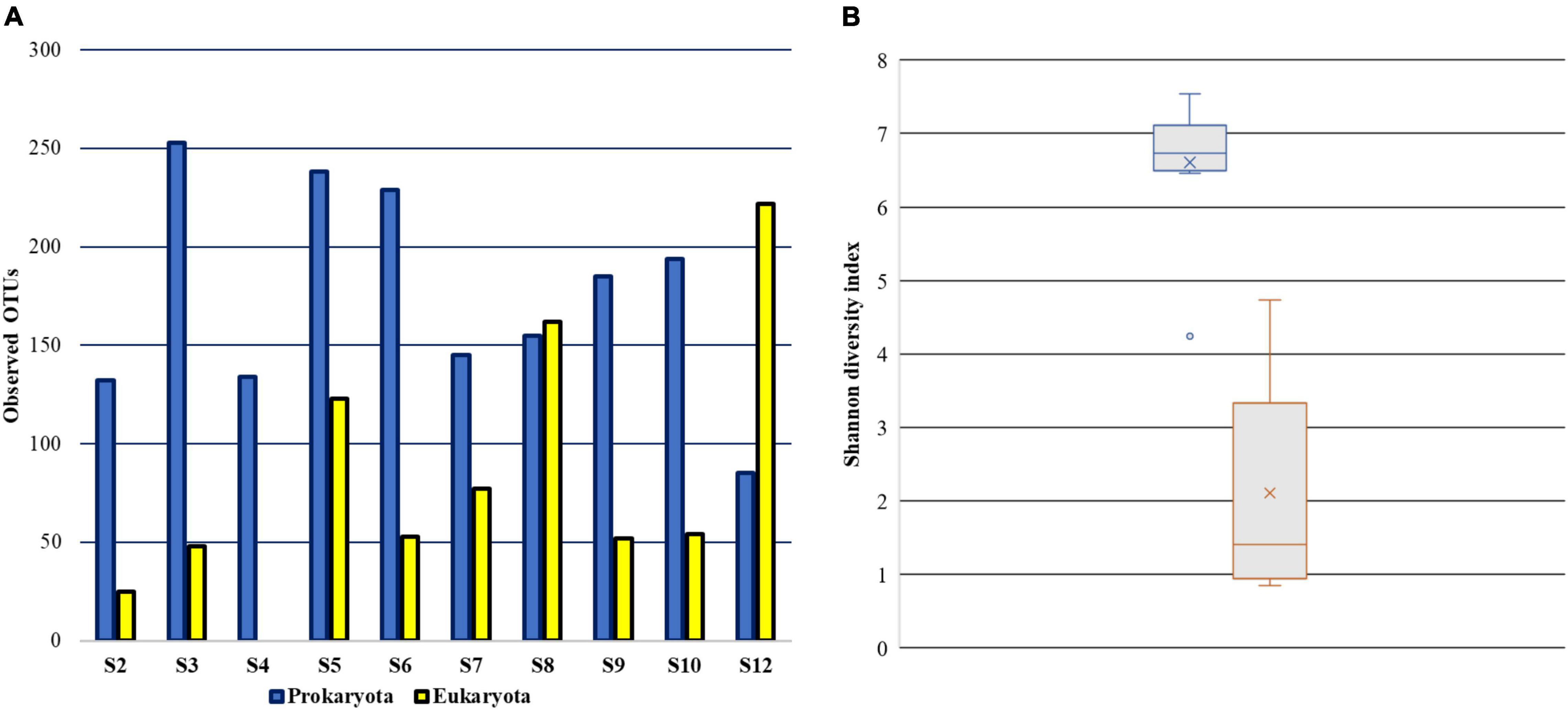
Figure 2. Alpha diversity of peloid prokaryotic and eukaryotic communities. (A) The number of observed OTUs at maximum sequencing depth (B) Shannon diversity index.
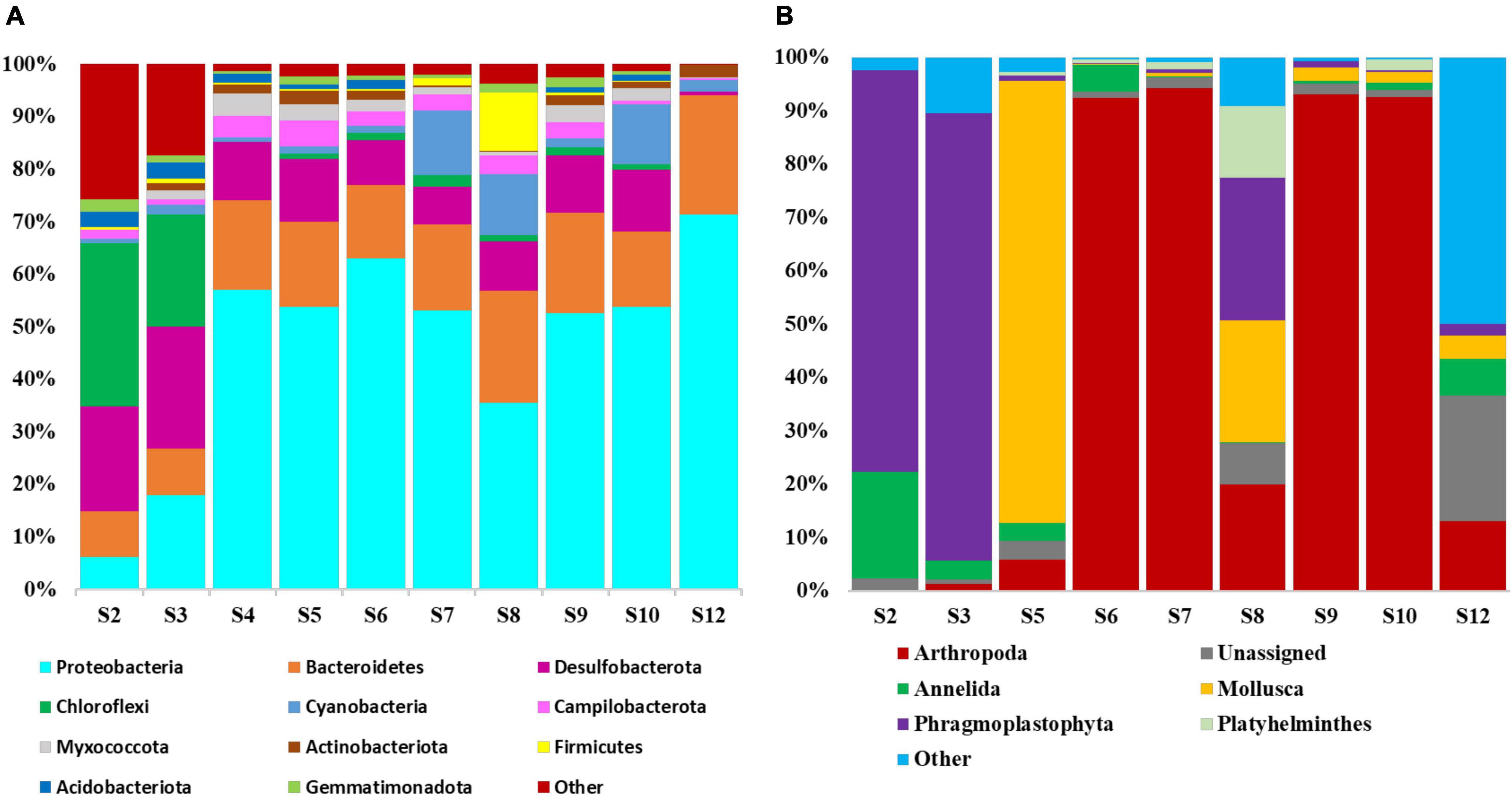
Figure 3. Relative abundance of the main (A) prokaryotic and (B) eukaryotic phyla identified in the peloid samples collected at Nin lagoon. The phyla represented by less than 1% are indicated as “other.”
Prokaryotic dataset on the class level (Figure 4) indicated that Gammaproteobacteria, Alphaproteobacteria and Bacteroidia dominated the coastal seawater peloid microbiota with an average relative abundance of 39.13, 13.55, and 16.19%, respectively. However, the most abundant classes in samples S2 and S3 were Desulfobacteria (16.10%), Anaerolineae (11.25%), Dehalococcoidia (13.81%), and Gammaproteobacteria (10.61%). In the water sample (S10), classes Alphaproteobacteria and Bacteroidia contributed to 64.55 and 22.69% of the total bacteria. Species level data revealed that in average 60.12% of the species from different genera and families have been classified as uncultured microorganisms (Supplementary Figure 2).
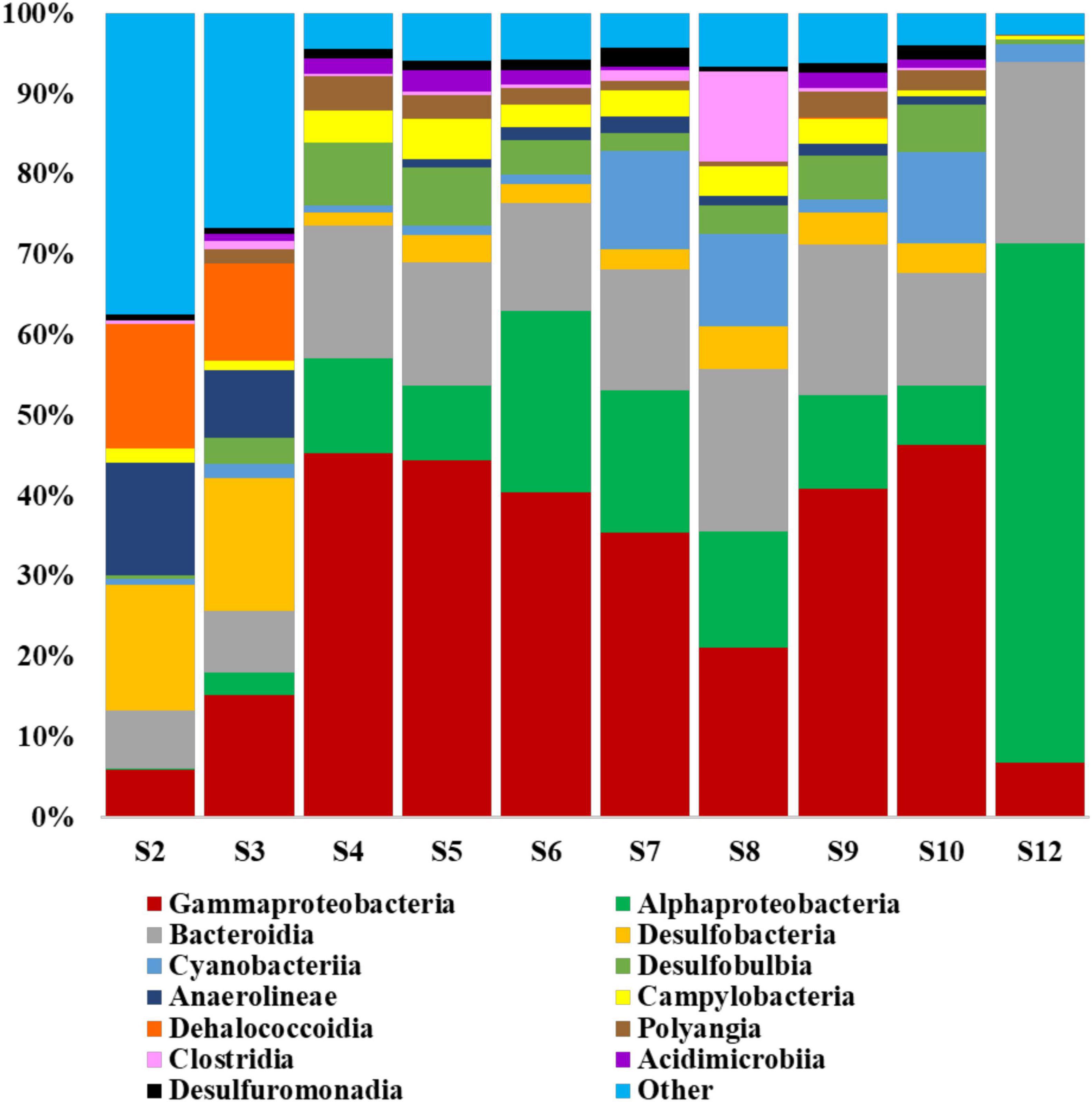
Figure 4. Relative abundance of the main prokaryotic classes as identified by 16S rRNA sequencing. The classes represented by less than 1% are indicated as “other.”
In the eukaryotic portion, based on the 18S rRNA sequencing results, the species Cythere lutea from the phylum Arthropoda was dominant in the peloid samples from coastal locations- S6, S7, S9, and S10, whereas in the sediments from S2, S3, and in the seawater sample, the species C. lutea was not detected (Figure 5). In the coastal sample S5, genus Caenogastropoda (47.39%) and species Cylichna cylindracea (34.87%), both belonging to phylum Mollusca, were most abundant. Coastal are sample S8 was characterized by genus Caenogastropoda (22.70%), species Juncus effusus (15.90%), and Limonium bicolor (10.66%). In the sediment samples S2 and S3, the species Posidonia oceanica was dominant with relative abundance of 74.3 and 83.08%, respectively. Another prominent species, found in the sediment sample S2 was Tubifex tubifex from phylum Annelida while genera Picomonas, Chrysochromulina and Copepoda prevailing in the water sample. In this latter sample, a relatively high ratio of 18S rRNA sequencing reads (23.6%) could not be assigned to any phylum from the SILVA v138 database.
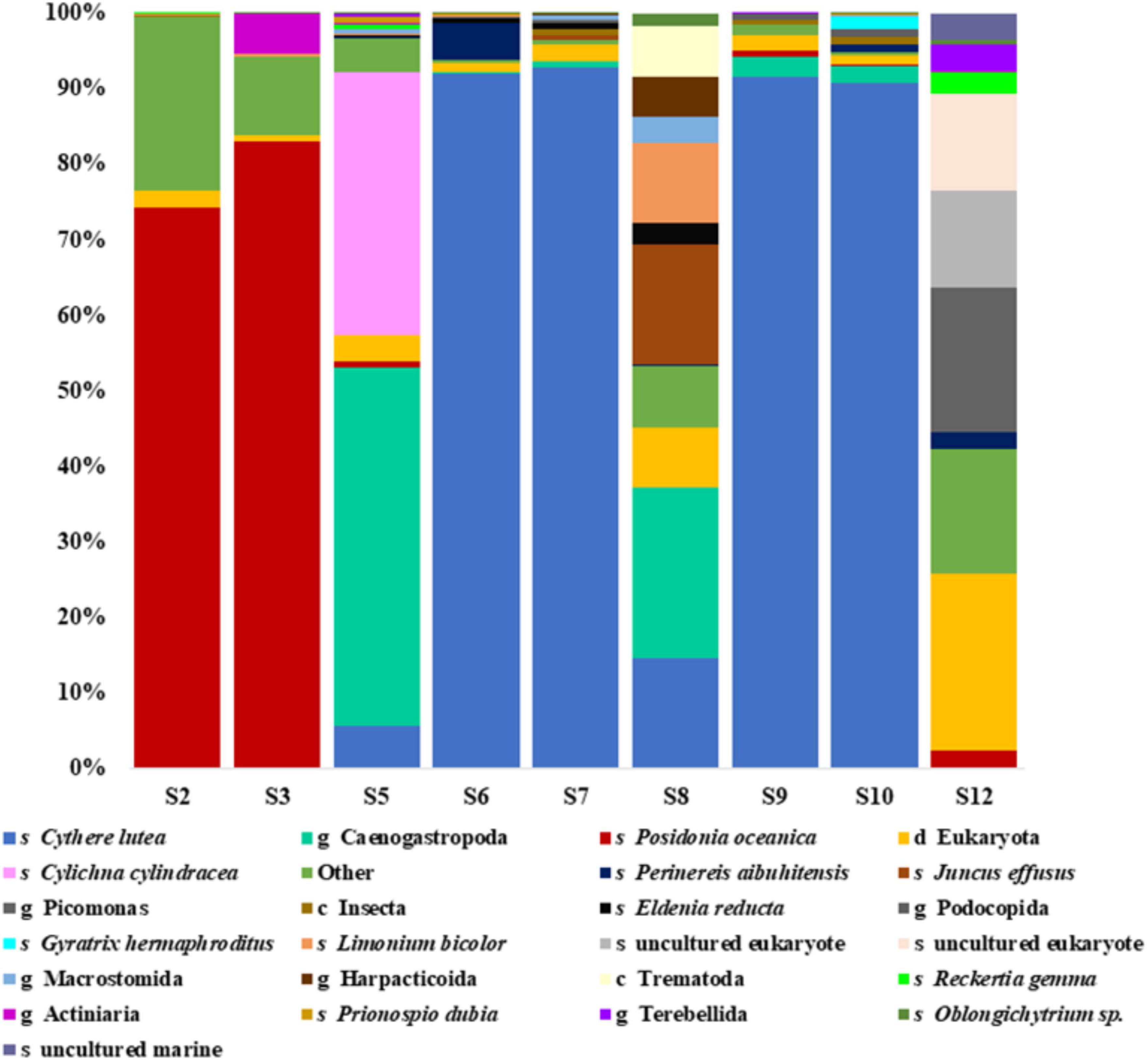
Figure 5. Relative abundances of the most prominent taxonomic ranks detected in the eukaryotic dataset as identified by 18S rRNA sequencing. The taxonomic groups represented by less than 0.1% are indicated as “other.” Letters “s, g, c, and d” indicate taxonomic ranks species, genus, class and domain, respectfully.
Peloid Natural Products Discovery Pipeline
For peloid natural product discovery we have produced 12 spectral libraries, 6 for peloid samples at nighttime and 6 for peloids sampled at daytime. Produced libraries contained a total of 14,417 individual mass spectra produced using a Quadrupole Time-of-Flight (qTOF; 6546 LC/Q-TOF, Agilent, United States) LC-HRMS. In order to identify known natural products, preferably with predetermined biological activity, which would enable us to evaluate Nin peloid mud medicinal property claims, we have used freely available COlleCtion of Open Natural prodUcTs (COCONUT).3 This is an aggregated repository of annotated and predicted natural products (NPs) collected from various open sources and providing a web interface to browse, search but also download NPs related data in order to make your own copy of a NPs repository (Sorokina et al., 2021). Downloaded version of the COCONUT repository was used to set up natural product discovery and analysis bioinformatics pipeline. Information downloaded from COCNUT was used to dereplicate spectra—a process where information on chemical compounds of known natural products is used to identify these compound in an experimental sample, based on recorded mass spectra and without having to repeat the entire isolation and structure-determination. DEREPLICATOR + algorithm was used for dereplicating mass spectra against diverse metabolites, including peptide natural products, polyketides, terpenes, benzenoids, alkaloids, flavonoids, and other classes of natural products (Mohimani et al., 2018). To be able to screen and dereplicate large numbers of recorded mass spectra, we were unable to use publicly available GNPS (Global Natural Products Social Molecular Networking) services (Wang et al., 2016)4 so therefore we have built our own dereplication server application based on the sources mentioned above. The dereplication server backend was written in Python programming language, utilizing Open Babel (O’Boyle et al., 2011; Anonymus, 2021b),5 molmass (Gohlke, 2021),6 and RDKit (Anonymus, 2021a)7 open source cheminformatics libraries. These chemical toolboxes were designed to handle numerous different formats of chemical data and, thanks to their openness, collaborative projects allowing anyone to search, convert, analyze, or store chemical data. In this case these toolboxes were used to convert 314,479 NPs present in COCONUT into molecular data and indexed libraries for DEREPLICATOR + search using mass spectra generated from peloid samples in this study. The dereplication server scheme is displayed in Figure 6.
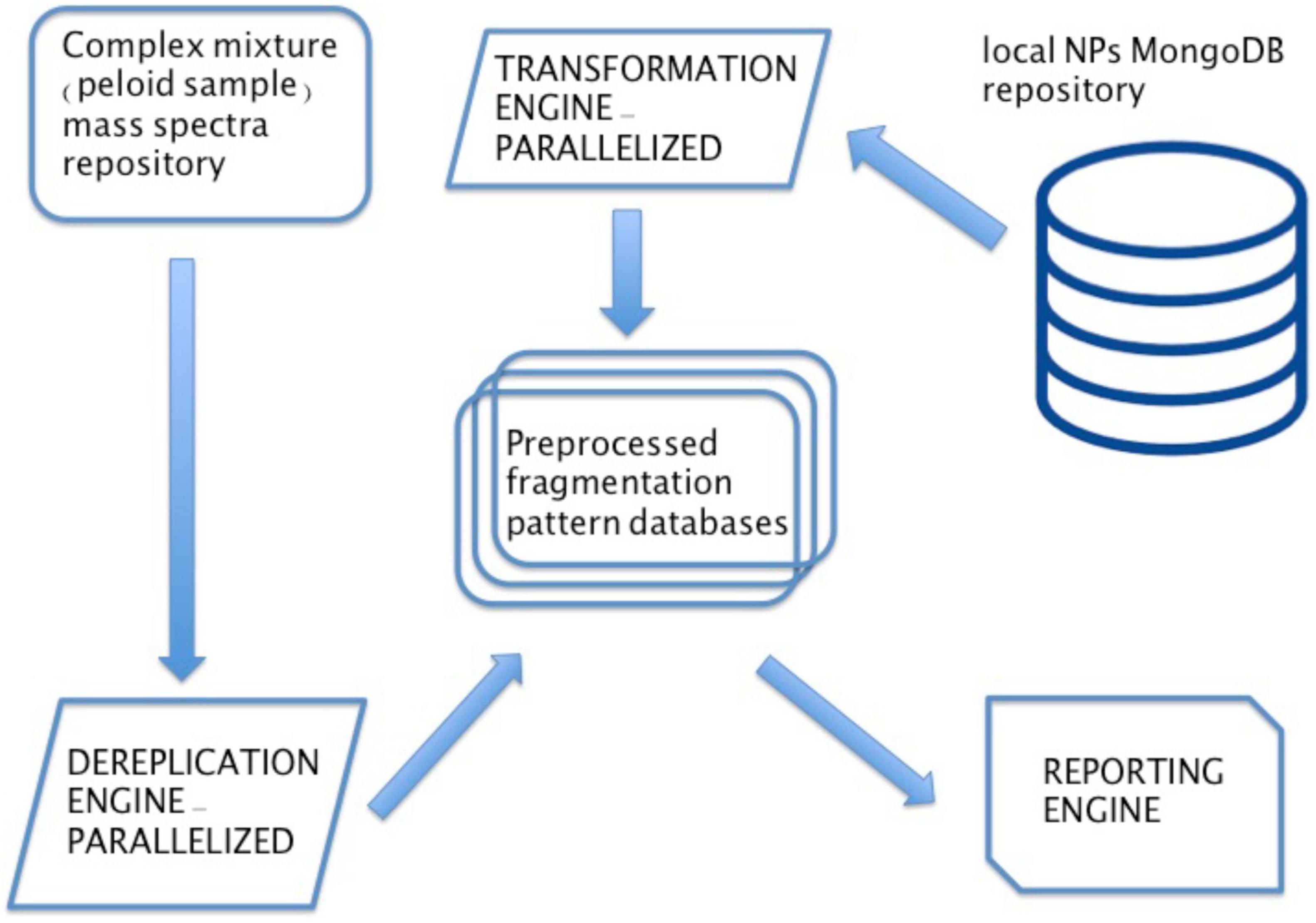
Figure 6. Dereplication server scheme. The dereplication server was set up using three distinct engines. First a transformation engine, using open source cheminformatics toolboxes to convert chemical data stored on a local instance of MongoDB NoSQL database containing entire COCONUT repository on a Ubuntu server machine. The data is transformed in a format compatible with DEREPLICATOR + algorithm version 2.5.0.-Linux, which is the core of the second component—dereplication engine. Finally a third—reporting engine traverses all of the temporary working directories and creates a user-friendly Excel table report containing all identified NPs. Custom made Python program serves as a “glue” connecting the three engines, feeding them with input/output and making both dereplication search and database fragmentation and pre-processing run in parallel based on MS1 data.
Medicinal Contribution of Microorganisms Living in an Extreme Environment
Peloid samples were used to record raw spectral data, which was processed using MassHunter Qualitative Software (V.10.0.) and exported to mgf format. Obtained sample extract chromatograms indicated abundance of compounds and little difference between UV irradiated and night-collected samples (Figure 7). Six spectral libraries were produced for daytime collected samples and six for nighttime collected samples—total of 12, which were subsequently fed into above described dereplication server pipeline for bioinformatic NPs identification. Spectral libraries were initially sorted according to MS1 parent ion masses and matched to our COCONUT derived dereplication database, tolerating product ion absolute deviation of 0.2 Da. Out of 7,208 recorded spectra within daytime collected sample libraries, 7,181 were matched to COCONUT NPs based on MS1 parent ion masses. For nighttime collected samples, 7,184 spectra were matched accordingly, out of 7,209 recorded ones. Unmatched spectra (52 in total) were all recorded in negative ion mode and correspond most likely to a single unknown compound, based on the same low-end precursor mass recorded for all 52 spectra—195.812 Da. Obtained results indicate existence of bioactive compounds within day/night samples (Supplementary Table 2) from three Nin peloid mud locations (S4, S6, S8).
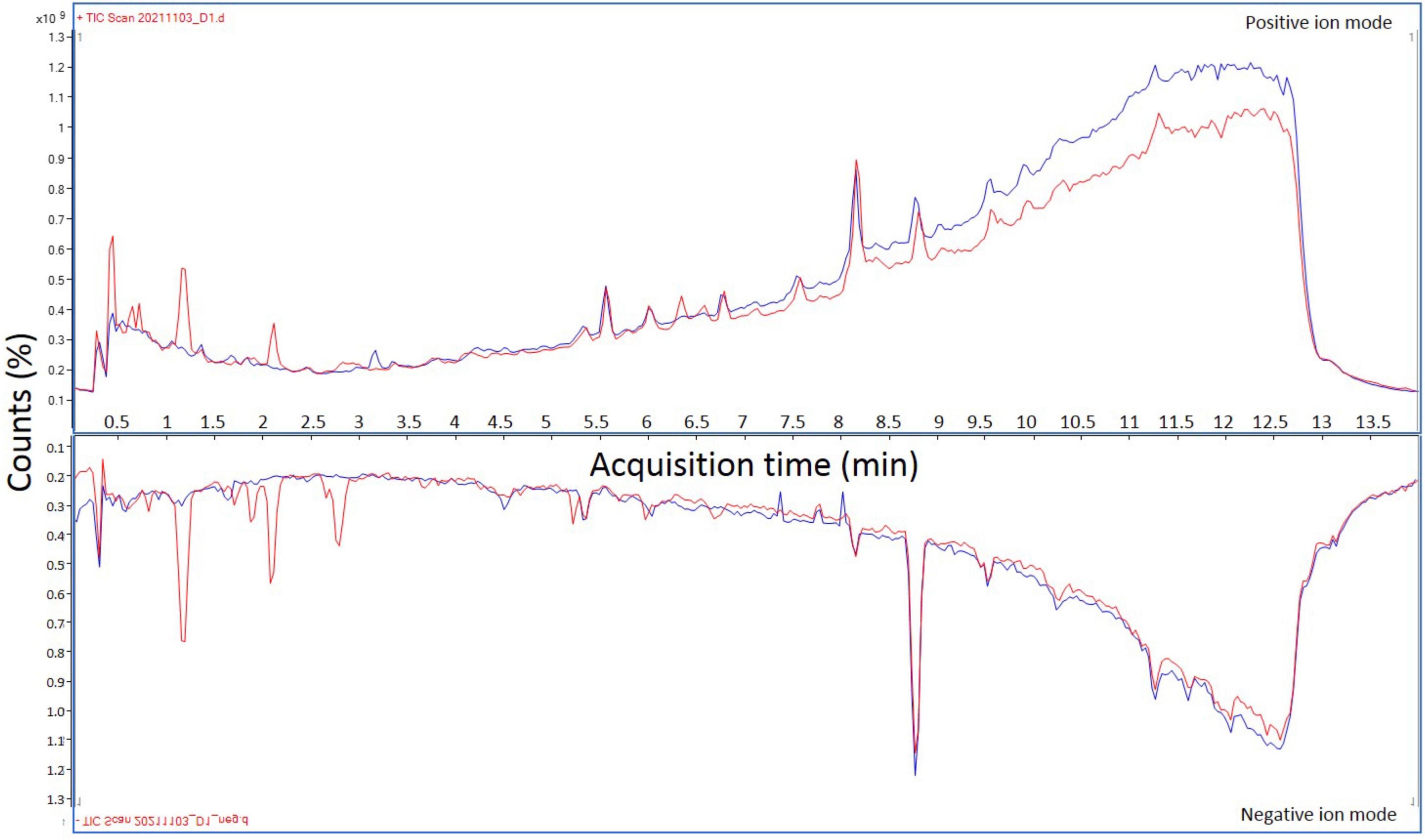
Figure 7. Overlapped representative total ion chromatograms of peloid mud extracts in both positive and negative ion modes. Total ion chromatograms corresponding to extracts derived from peloid mud collected during the strongest UV irradiation are indicated by red lines, while total ion chromatograms representing extracts derived from samples collected at night are shown in blue.
PASS (Lagunin et al., 2000) predicted bioactivities of identified NPs strongly correlate with historical notes on Nin peloid mud medicinal usage (data presented in Supplementary Table 2). From total of 24 unique NPs identified by dereplication in day and night samples, 12 of them are found in both sample types, while 6 NPs are unique for day samples with further 6 for night samples. Major predicted bioactivities indicate strong correlation with skin-related, cosmetic benefits of pelotherapy, musculoskeletal and general pain-relief alleviation related to Nin peloid contained compounds of natural origin. These predicted activities range from anti-inflammatory, antifungal, antipsoriatic, antiacne, antioxidant, antipruritic—allergic and histamine release inhibitory are the most common, to more general—anti-cancer, radioprotective, antihelmintic, antiparasitic, cholesterolemic, and antidiabetic. A lot of chemoprotectant and radioprotectant activities is most likely related to extreme conditions such as high UV irradiation during the day, combined with fluctuating hypersaline conditions resulting from water evaporation. Unfortunately, none of the dereplication-identified compounds had taxonomic information attached, so we were unable to determine their putative source. However, a significant portion of spectra (4,567 spectra from all samples—31.68% of all generated spectra) matched at least one compound in our NPs database with less than 0.002 Da difference in MS1 recorded molecular mass. These matches corresponded to 375 unique NPs for day and night samples when joined. Taxonomic distribution of organisms linked to those compounds indicate flowering plants to be the dominant source, followed by microalgae—more precisely a group of photosynthetic heterokonts (Cavalier-Smith and Chao, 2006; Riisberg et al., 2009) belonging to SAR clade that includes stramenopiles, alveolates, and Rhizaria, which are known to produce pigments with antioxidative properties (Frommolt et al., 2008). Taxonomic distribution of producer organisms was obtained using ETE3 toolkit (Huerta-Cepas et al., 2016), included in the dereplication pipeline. Although this MS1 correlation between precursor masses from peloid extracts and isotopic masses of NPs in COCONUT database is nowhere near as confirmative as dereplication it indicates an interesting find that requires further investigation. Comparison of taxonomic distribution of MS1 matches obtained in this study and taxonomic distribution of entire COCONUT NPs search space show a discrepancy when it comes to microalgae. COCONUT NPs are heavily biased in terms of taxonomy. As an example, in the current version of COCONUT Eukaryotes make up 98% of taxonomic entries with Streptophyta phylum constituting almost 96% of all Eukaryote taxonomy, followed by Bacteria with 1.5% and Archea with 0.5% of entries at highest, superkingdom level. This is expected, since flowering plants currently dominate the research of natural products with medicinal potential (Ahn, 2017; Fitzgerald et al., 2020), however, our MS1 analysis indicates somewhat different taxonomic distribution. Although all retrieved NPs were of eukaryotic source, on the level of Phylum we are seeing different picture with phylum Chlorophyta ranked as second most abundant contributor, right after Streptophyta,. This is opposed to global COCONUT NPs taxonomic distribution where Streptophyta are followed by Ascomyceta and Actinobacteria as second and third major phylum contributors of natural compounds and Chlorophyta, containing majority of known aquatic photosynthetic eukaryotic organisms, ranked as fourth. Our simple peloid MS1 correlation analysis could indicate that algae have a much greater potential when it comes to natural compounds, which is not being reflected in current NPs and genomic databases.
Discussion
Peloid is a mud, often used therapeutically, as part of “pelotherapy,” for therapeutic bathing (balneotherapy) or simply by smearing over affected body parts. It consists of humus and minerals deposited naturally by geological, biological, chemical, and physical processes. Peloid classification is not an exact science, but most sources agree that majority of peloids can be described as heterogeneous systems, with a solid phase composed from a mixture of organic and inorganic solids, suspended within a liquid phase consisting of water-solvated inorganic and organic ions or molecules (Gomes et al., 2013; Maraver et al., 2021). Medicinal use of different types of peloid in folk medicine dates to the prehistoric times. Indigenous peoples around the world still use it widely, and arguably, humans were not the ones who first discovered benefits of “mud therapy.” Different animals like elephants, pigs, rhinos, and other mammals are frequently enjoying mud baths to stay cool prevent sunburns and to remove parasites. Europe has a long tradition of using peloids for medicinal baths and wraps spanning for at least 200 years. Today medicinal usage of peloids is mostly confined to spas, which use either artificial ponds or mix clays with mineral or sea water, or to a number of natural locations such as Nin lagoon, where people apply mud freely throughout the year. Historically, peloid treatments have been used for a variety of ailments, most notably rheumatic disorders, osteoarthritis, gynecological disorders, sciatica, skin diseases, trauma, and many more.
It is difficult to disregard a long history including oral and written lore of alleged medicinal benefits of mud baths as a simple placebo, but it is also difficult to attribute all or majority of reported activities to mineral content, heat retention or “spa” relaxation effect. Peloid is a complex microbial community and presence of bacteria and other microorganisms in peloids has the potential to “boost” their therapeutic value (Pesciaroli et al., 2016). Secondary metabolites of microorganisms, such as Streptomyces species produce a plethora of compounds, such as polyketides (Caffrey, 2012), peptides (Agrawal et al., 2017), and polyketide-peptide hybrids (Du et al., 2003). Most of these compounds have been characterized with different biological activities, including antibacterial (Procópio et al., 2012; Jang et al., 2013; Sivalingam et al., 2019), antifungal (Abou-Zeid and El-Sherbini, 1974; Qi et al., 2019), anticancer (Nachtigall et al., 2011; Tan et al., 2015), and immune suppressive ones (Sehgal, 1998; Bolourian and Mojtahedi, 2018). Furthermore, microalgae (Barkia et al., 2019), diatoms (Tolomio et al., 2002), and thermophilic Cyanobacteria detected in peloids (Marcolongo et al., 2006; Kim Tiam et al., 2019; Zampieri et al., 2020) also produce compounds such as sulfoglycolipids, glycoglycerolipids known for their bioactivity.
In this study we investigated a healing mud located near the city of Nin, due to its recorded history of usage in pelotherapy. Our first goal was to evaluate the microbial community within this mud in order to assess its diversity and second to identify natural compounds exhibiting biological activity, which could be linked to alleged peloid healing properties. In the first part of the study, we had investigated peloid microbial community composition, sampled from several microlocations using amplicon sequencing of V3 and V4 regions of the gene coding for 16S rRNA for bacterial portion and V9 DNA region of the 18S rRNA gene for eukaryotic portion. Subsequent metataxonomic analysis showed, that in the case of Nin peloid, greater taxonomic diversity lies within prokaryotic community, with most abundant phylum being Proteobacteria (52.68%) followed by Bacteroidetes (16.92%), Desulfobacterota (10.13%), and Cyanobacteria (5.74%). For the eukaryotic part of the community, species Cythere lutea from the phylum Arthropoda was dominant (some samples > 90%), followed by Caenogastropoda (47.39%) and Cylichna cylindracea (34.87%), both belonging to phylum Mollusca and plant species like Juncus effusus (15.90%) and Limonium bicolor (10.66%). However, the eukaryotic composition displayed higher variance dependent on sampling micro-location. For example, in the sediment samples from the bottom of the lagoon, the algae species like Posidonia oceanica were a majority, comprising 74.3 and 83.08% of all eukaryotic amplicons. Regarding eukaryotes, it is important to note that in this study, a relatively large portion of 18S rRNA sequencing reads (23.6%) from a pooled water sample could not be assigned to any phylum from the SILVA v138 database, which is a serious limitation, potentially undermining the actual contribution of some important species such as microalgae.
In search for bioactive compounds of natural origin, DEREPLICATOR + algorithm (Mohimani et al., 2018) and COCONUT (COlleCtion of Open Natural ProdUcTs) were used. COCONUT is one of the biggest and best annotated resources for natural products (NP) available, hosting over 400,000 NPs aggregated across 52 different databases (Sorokina et al., 2021). In order to screen peloid mud for NPs, we opted for DCM-MeOH extracts of deep frozen lyophilized mud samples. These extracts were analyzed using liquid chromatography-high-resolution mass spectrometry, producing a peloid MS2 library that was dereplicated on our local server dereplication engine (Figure 6). The dereplication results indicate high portion of detected compounds to exhibit biological activities in accordance with reported historical healing properties of mud. It should be noted that we have not been able to determine the majority of compounds producing spectra in our library, which indicates latent potential in this particular healing mud. This was further confirmed by high percentage of MS1 matches to COCONUT NPs (31.68%). This MS1 analysis indicated that microalgae portion is being underrepresented in both NPs containing databases, but also in genomic databases, as suspected by our compound bioprospecting and 18S rRNA gene sequencing subsequent analysis. Using standard kits for DNA isolation and current reference databases for rRNA sequence based taxonomic analysis this study has shown that peloid mud contains great microbial biodiversity with abundance of microorganisms not represented in existing databases. Using mass spectrometry based NPs screening of deep frozen peloid mud DCM-MeOH extracts, identified compounds displayed a range of activities (Supplementary Table 3), which correlate with historical records of this particular mud healing properties.
In closing, this study provides the characterization of both prokaryotic and eukaryotic contribution to peloid. This study also reports the “biotic” part of the peloid attributed healing properties by screening for useful compounds being produced by microorganisms making up the peloid ecosystem. The fact that relatively large portion of eukaryotic amplicons could not be assigned to any phylum using current SILVA database highlights the underrepresentation of microorganism data from aquatic environments in existing databases and gives perspective for our future research. In future we would like to focus on isolation and characterization of particular bioactive compounds and cultivation of specific microorganisms responsible for their biosynthesis in order to truly tap into peloid biotechnological potential.
Data Availability Statement
The datasets presented in this study can be found in online repositories. The names of the repository/repositories and accession number(s) can be found below: http://www.ebi.ac.uk/ena/browser/view/PRJEB48902 (Amplicon sequencing data). Peloid LC-Q-TOF MS2 day/night sample analysis (12 spectral libraries in .mgf format) submitted as MassIVE dataset: MSV000088445 (ftp://MSV000088445@massive.ucsd.edu).
Author Contributions
DV and MB performed literature overview, manuscript writing, peloid sampling, physico-chemical analyses, DNA extraction, and sample preparation for downstream analyses. AS and JZ performed the study design, data analysis, drafting, revising, final approval, and handled the accountability of all aspects of the work. ES, DO, and LČ performed the QIIME analysis, assisted in sample planning and DNA isolation, and concentration. MR and RČ-R assisted in data analysis, revising, sample preparation, final approval, and were also involved in the accountability of all aspects of the work. JD and SR participated in bioinformatics analysis pipeline design and implementation and handled datasets. AH was in charge of LC-QTOF, operated the spectrometer, and optimized all relevant parameters for mass spectrometry data acquisition. All authors contributed to the article and approved the submitted version.
Funding
This study was supported by the Croatian Government and the European Union through the European Regional Development Fund: Competitiveness and Cohesion Operational Programme (KK.01.2.1.02.0015) Development of Innovative Biobased Products by in silico Assessment of Microalgae Bioactivity awarded to MR as Principal Investigator and Competitiveness and Cohesion Operational Programme (KK.01.1.1.01) The Scientific Centre of Excellence for Marine Bioprospecting–BioProCro awarded to RČ-R as Principal Investigator.
Conflict of Interest
SR was employed by the Kodeks Ltd.
The remaining authors declare that the research was conducted in the absence of any commercial or financial relationships that could be construed as a potential conflict of interest.
Publisher’s Note
All claims expressed in this article are solely those of the authors and do not necessarily represent those of their affiliated organizations, or those of the publisher, the editors and the reviewers. Any product that may be evaluated in this article, or claim that may be made by its manufacturer, is not guaranteed or endorsed by the publisher.
Supplementary Material
The Supplementary Material for this article can be found online at: https://www.frontiersin.org/articles/10.3389/fmars.2022.822139/full#supplementary-material
Footnotes
- ^ https://www.wunderground.com/weather/hr/zemunik/LDZD
- ^ https://kunden.dwd.de/uvi_de/index.jsp
- ^ https://coconut.naturalproducts.net/
- ^ https://gnps.ucsd.edu/ProteoSAFe/static/gnps-splash.jsp
- ^ http://openbabel.org/wiki/Main_Page
- ^ https://pypi.org/project/molmass/
- ^ https://www.rdkit.org/
References
Abou-Zeid, A. A., and El-Sherbini, S. H. (1974). Fermentative production of cycloheximide by Streptomyces griseus and Streptomyces noursei. J. Appl. Chem. Biotechnol. 24, 283–291. doi: 10.1002/jctb.2720240413
Agrawal, S., Acharya, D., Adholeya, A., Barrow, C. J., and Deshmukh, S. K. (2017). Nonribosomal peptides from marine microbes and their antimicrobial and anticancer potential. Front. Pharmacol. 8:828. doi: 10.3389/fphar.2017.00828
Ahn, K. (2017). The worldwide trend of using botanical drugs and strategies for developing global drugs. BMB Rep. 50, 111–116. doi: 10.5483/bmbrep.2017.50.3.221
Anonymus (2021a) RDKit: Open-Source Cheminformatics Software. Available online at: http://www.rdkit.org/ (accessed October 11, 2021). doi: 10.1353/mrw.0.0006
Anonymus (2021b). The Open Babel Package. Available online at: http://openbabel.org/ (accessed October 11, 2021).
Barkia, I., Saari, N., and Manning, S. R. (2019). Microalgae for high-value products towards human health and nutrition. Mar. Drugs 17:304. doi: 10.3390/md17050304
Bolourian, A., and Mojtahedi, Z. (2018). Immunosuppressants produced by Streptomyces: evolution, hygiene hypothesis, tumour rapalog resistance and probiotics. Environ. Microbiol. Rep. 10, 123–126. doi: 10.1111/1758-2229.12617
Bolyen, E., Rideout, J. R., Dillon, M. R., Bokulich, N. A., Abnet, C. C., Al-Ghalith, G. A., et al. (2019). Reproducible, interactive, scalable and extensible microbiome data science using QIIME 2. Nat. Biotechnol. 37, 852–857. doi: 10.1038/s41587-019-0209-9
Caffrey, P. (2012). Dissecting complex polyketide biosynthesis. Comput. Struct. Biotechnol. J. 3:e201210010. doi: 10.5936/csbj.201210010
Callahan, B. J., McMurdie, P. J., Rosen, M. J., Han, A. W., Johnson, A. J. A., and Holmes, S. P. (2016). DADA2: high-resolution sample inference from Illumina amplicon data. Nat. Methods 13, 581–583. doi: 10.1038/nmeth.3869
Cara, S., Carcangiu, G., Padalino, G., Palomba, M., and Tamanini, M. (2000). The bentonites in pelotherapy: chemical, mineralogical and technological properties of materials from Sardinia deposits (Italy). Appl. Clay Sci. 16, 117–124. doi: 10.1016/S0169-1317(99)00049-6
Carretero, M. I. (2020). Clays in pelotherapy. a review. part II: organic compounds, microbiology and medical applications. Appl. Clay Sci. 189:105531. doi: 10.1016/j.clay.2020.105531
Carretero, M. I., Pozo, M., Sánchez, C., García, F. J., Medina, J. A., and Bernabé, J. M. (2007). Comparison of saponite and montmorillonite behaviour during static and stirring maturation with seawater for pelotherapy. Appl. Clay Sci. 36, 161–173. doi: 10.1016/j.clay.2006.05.010
Cavalier-Smith, T., and Chao, E. E.-Y. (2006). Phylogeny and megasystematics of phagotrophic heterokonts (kingdom Chromista). J. Mol. Evol. 62, 388–420. doi: 10.1007/s00239-004-0353-8
Du, L., Cheng, Y. Q., Ingenhorst, G., Tang, G. L., Huang, Y., and Shen, B. (2003). Hybrid peptide-polyketide natural products: biosynthesis and prospects towards engineering novel molecules. Genet. Eng. 25, 227–267. doi: 10.1007/978-1-4615-0073-5_11
Espejo-Antúnez, L., Cardero-Durán, M. A., Garrido-Ardila, E. M., Torres-Piles, S., and Caro-Puértolas, B. (2013). Clinical effectiveness of mud pack therapy in knee osteoarthritis. Rheumatology 52, 659–668. doi: 10.1093/rheumatology/kes322
Fitzgerald, M., Heinrich, M., and Booker, A. (2020). Medicinal plant analysis: a historical and regional discussion of emergent complex techniques. Front. Pharmacol. 10:1480. doi: 10.3389/fphar.2019.01480
Forestier, R., Desfour, H., Tessier, J.-M., Françon, A., Foote, A. M., Genty, C., et al. (2010). Spa therapy in the treatment of knee osteoarthritis: a large randomised multicentre trial. Ann. Rheum. Dis. 69, 660–665. doi: 10.1136/ard.2009.113209
Frommolt, R., Werner, S., Paulsen, H., Goss, R., Wilhelm, C., Zauner, S., et al. (2008). Ancient recruitment by chromists of green algal genes encoding enzymes for carotenoid biosynthesis. Mol. Biol. Evol. 25, 2653–2667. doi: 10.1093/molbev/msn206
Gohlke, C. (2021). Molmass. Available online at: https://pypi.org/project/molmass (accessed October 11, 2021).
Gomes, C., Carretero, M. I., Pozo, M., Maraver, F., Cantista, P., Armijo, F., et al. (2013). Peloids and pelotherapy: historical evolution, classification and glossary. Appl. Clay Sci 75, 28–38. doi: 10.1016/j.clay.2013.02.008
Gomes, C., and de, S. F. (2018). Healing and edible clays: a review of basic concepts, benefits and risks. Environ. Geochem. Health 40, 1739–1765. doi: 10.1007/s10653-016-9903-4
Halevy, S., and Sukenik, S. (1998). Different modalities of spa therapy for skin diseases at the dead sea area. Arch. Dermatol. 134, 1416–1420. doi: 10.1001/archderm.134.11.1416
Huerta-Cepas, J., Serra, F., and Bork, P. (2016). ETE 3: reconstruction, analysis, and visualization of phylogenomic data. Mol. Biol. Evol. 33, 1635–1638. doi: 10.1093/molbev/msw046
Jang, K. H., Nam, S.-J., Locke, J. B., Kauffman, C. A., Beatty, D. S., Paul, L. A., et al. (2013). Anthracimycin, a potent anthrax antibiotic from a marine-derived actinomycete. Angew. Chem. Int. Ed. Engl. 52, 7822–7824. doi: 10.1002/anie.201302749
Kikouama, J. R. O., Konan, K. L., Katty, A., Bonnet, J. P., Baldé, L., and Yagoubi, N. (2009). Physicochemical characterization of edible clays and release of trace elements. Appl. Clay Sci. 43, 135–141. doi: 10.1016/j.clay.2008.07.031
Kim Tiam, S., Gugger, M., Demay, J., Le Manach, S., Duval, C., Bernard, C., et al. (2019). Insights into the diversity of secondary metabolites of Planktothrix using a biphasic approach combining global genomics and metabolomics. Toxins 11:498. doi: 10.3390/toxins11090498
Lagunin, A., Stepanchikova, A., Filimonov, D., and Poroikov, V. (2000). PASS: prediction of activity spectra for biologically active substances. Bioinformatics 16, 747–748. doi: 10.1093/bioinformatics/16.8.747
Maraver, F., Armijo, F., Fernandez-Toran, M. A., Armijo, O., Ejeda, J. M., Vazquez, I., et al. (2021). Peloids as thermotherapeutic agents. Int. J. Environ. Res. Public Health 18:1965. doi: 10.3390/ijerph18041965
Marcolongo, G., de Appolonia, F., Venzo, A., Berrie, C. P., Carofiglio, T., and Ceschi Berrini, C. (2006). Diacylglycerolipids isolated from a thermophile cyanobacterium from the Euganean hot springs. Nat. Prod. Res. 20, 766–774. doi: 10.1080/14786410500176393
Mohimani, H., Gurevich, A., Shlemov, A., Mikheenko, A., Korobeynikov, A., Cao, L., et al. (2018). Dereplication of microbial metabolites through database search of mass spectra. Nat. Commun. 9:4035. doi: 10.1038/s41467-018-06082-8
Nachtigall, J., Kulik, A., Helaly, S., Bull, A. T., Goodfellow, M., Asenjo, J. A., et al. (2011). Atacamycins A–C, 22-membered antitumor macrolactones produced by Streptomyces sp. C38. J. Antibiot. 64, 775–780. doi: 10.1038/ja.2011.96
O’Boyle, N. M., Banck, M., James, C. A., Morley, C., Vandermeersch, T., and Hutchison, G. R. (2011). Open babel: an open chemical toolbox. J. Cheminform. 3:33. doi: 10.1186/1758-2946-3-33
Pesciaroli, C., Viseras, C., Aguzzi, C., Rodelas, B., and González-López, J. (2016). Study of bacterial community structure and diversity during the maturation process of a therapeutic peloid. Appl. Clay Sci. 132, 59–67. doi: 10.1016/j.clay.2016.05.015
Procópio, R. E., Silva, I. R., Martins, M. K., Azevedo, J. L., and Araújo, J. M. (2012). Antibiotics produced by streptomyces. Braz. J. Infect. Dis. 16, 466–471. doi: 10.1016/j.bjid.2012.08.014
Qi, D., Zou, L., Zhou, D., Chen, Y., Gao, Z., Feng, R., et al. (2019). Taxonomy and broad-spectrum antifungal activity of Streptomyces sp. SCA3-4 isolated from rhizosphere soil of Opuntia stricta. Front. Microbiol. 10:1390. doi: 10.3389/fmicb.2019.01390
Quast, C., Pruesse, E., Yilmaz, P., Gerken, J., Schweer, T., Yarza, P., et al. (2013). The SILVA ribosomal RNA gene database project: improved data processing and web-based tools. Nucleic Acids Res. 41, D590–D596. doi: 10.1093/nar/gks1219
Riisberg, I., Orr, R. J. S., Kluge, R., Shalchian-Tabrizi, K., Bowers, H. A., Patil, V., et al. (2009). Seven gene phylogeny of heterokonts. Protist 160, 191–204. doi: 10.1016/j.protis.2008.11.004
Routh, H. B., Bhowmik, K. R., Parish, L. C., and Witkowski, J. A. (1996). Balneology, mineral water, and spas in historical perspective. Clin. Dermatol. 14, 551–554. doi: 10.1016/s0738-081x(96)00083-1
Sehgal, S. N. (1998). Rapamune (RAPA, rapamycin, sirolimus): mechanism of action immunosuppressive effect results from blockade of signal transduction and inhibition of cell cycle progression. Clin. Biochem. 31, 335–340. doi: 10.1016/s0009-9120(98)00045-9
Sivalingam, P., Hong, K., Pote, J., and Prabakar, K. (2019). Extreme environment streptomyces: potential sources for new antibacterial and anticancer drug leads? Int. J. Microbiol. 2019:5283948. doi: 10.1155/2019/5283948
Sorokina, M., Merseburger, P., Rajan, K., Yirik, M. A., and Steinbeck, C. (2021). COCONUT online: collection of open natural products database. J. Cheminform. 13:2. doi: 10.1186/s13321-020-00478-9
Tan, L. T.-H., Ser, H.-L., Yin, W.-F., Chan, K.-G., Lee, L.-H., and Goh, B.-H. (2015). Investigation of antioxidative and anticancer potentials of Streptomyces sp. MUM256 isolated from malaysia mangrove soil. Front. Microbiol. 6:1316. doi: 10.3389/fmicb.2015.01316
Tateo, F., and Summa, V. (2007). Element mobility in clays for healing use. Appl. Clay Sci. 36, 64–76. doi: 10.1016/j.clay.2006.05.011
Tolomio, C., Ceschi Berrini, C., de Appolonia, F., Galzigna, L., Masiero, L., Moro, I., et al. (2002). Diatoms in the thermal mud of Abano Terme, Italy (Maturation period). Algol. Stud. 105, 11–27. doi: 10.1127/algol_stud/105/2002/11
Veniale, F., Barberis, E., Carcangiu, G., Morandi, N., Setti, M., Tamanini, M., et al. (2004). Formulation of muds for pelotherapy: effects of “maturation” by different mineral waters. Appl. Clay Sci. 25, 135–148. doi: 10.1016/j.clay.2003.10.002
Wang, M., Carver, J. J., Phelan, V. V., Sanchez, L. M., Garg, N., Peng, Y., et al. (2016). Sharing and community curation of mass spectrometry data with global natural products social molecular networking. Nat. Biotechnol. 34, 828–837. doi: 10.1038/nbt.3597
Zampieri, R. M., Adessi, A., Caldara, F., Codato, A., Furlan, M., Rampazzo, C., et al. (2020). Anti-Inflammatory activity of exopolysaccharides from Phormidium sp. ets05, the most abundant cyanobacterium of the therapeutic euganean thermal muds, using the zebrafish model. Biomolecules 10:582. doi: 10.3390/biom10040582
Keywords: peloids, mass spectrometry, dereplication, health benefits, extreme environment, rRNA gene sequencing
Citation: Vadlja D, Bujak M, Čož-Rakovac R, Roje M, Čižmek L, Horvatić A, Svetličić E, Diminić J, Rakovac S, Oros D, Zucko J and Starcevic A (2022) Bioprospecting for Microorganisms in Peloids—Extreme Environment Known for Its Healing Properties. Front. Mar. Sci. 9:822139. doi: 10.3389/fmars.2022.822139
Received: 25 November 2021; Accepted: 08 February 2022;
Published: 22 March 2022.
Edited by:
Gennaro Riccio, Anton Dohrn Zoological Station, ItalyReviewed by:
Ida Orefice, Anton Dohrn Zoological Station, ItalyValeria Costantino, University of Naples Federico II, Italy
Copyright © 2022 Vadlja, Bujak, Čož-Rakovac, Roje, Čižmek, Horvatić, Svetličić, Diminić, Rakovac, Oros, Zucko and Starcevic. This is an open-access article distributed under the terms of the Creative Commons Attribution License (CC BY). The use, distribution or reproduction in other forums is permitted, provided the original author(s) and the copyright owner(s) are credited and that the original publication in this journal is cited, in accordance with accepted academic practice. No use, distribution or reproduction is permitted which does not comply with these terms.
*Correspondence: Jurica Zucko, jzucko@pbf.hr; Antonio Starcevic, astar@pbf.hr
†These authors have contributed equally to this work and share first authorship
‡These authors share last authorship