- Faroe Marine Research Institute, Tórshavn, Faroe Islands
Atlantic mackerel Scomber scombrus is an important migratory fish in Faroese waters and the Northeast Atlantic at large. Similar to other fish species from marine environments, mackerel can be infected by the myxozoan parasite Kudoa thyrsites, leading to myoliquefaction that renders the fish commercially unviable. Despite the ecological and economic significance of K. thyrsites as a parasite, little is known regarding its prevalence in the Faroese mackerel fishery. Prior to analysing field samples, we examined 104 samples of Atlantic mackerel selected from a Faroese processing plant on the basis of visible soft-tissue. Using microscope smears we observed K. thyrsites in 98% of the soft-tissue mackerel specimens and a direct comparison with qPCR demonstrated strong agreement between the two techniques (Φ = 0.429, p<0.01; Fisher’s exact test). We used qPCR to analyze a total of 594 Atlantic mackerel specimens, collected from Faroese fishing grounds during 2017 and 2018. Overall prevalence was 4.1% (95% confidence interval; 2.5-5.7%) and ranged from 0-14% at different locations. Infection by K. thyrsites was documented in fish as young as 2 years (195g) and up to 14 years (615g) of age. A logistic regression model indicated the odds of infection were statistically associated with sampling location and month, but not gender, age, weight or length, although a Chi-squared test on age categories showed the oldest fish (>10 years) had highest prevalence (10.8%, CI: 4.2-17.5%). In order to investigate potential infection pathways we performed qPCR analysis on eDNA in water samples collected from the Faroese Plateau. However, we found no molecular evidence for K. thyrsites spores in Faroese plankton assemblages. Our data support the prevailing hypothesis that both the alternating invertebrate host and infection of Atlantic mackerel by K. thyrsites occurs in more southern waters.
Introduction
Fish infected with myxosporidia from the family Kudoidae (Meglitsch, 1947) show myoliquefaction, more commonly referred to as soft-tissue. The mechanism for this phenomenon has been linked to proteases, like cathepsin, which are released by the pre-sporogenic plasmodia into host muscle tissue (Henning et al., 2013). The post mortem drop in muscle pH boosts the activity of the cathepsin enzyme (Levsen, 2015) leading to further decomposition. Notably, the presence of parasite-derived proteases in fresh and frozen/thawed commercial fish species can render the filet unfit for consumption, leading to significant economic losses.
The number of documented species in the genus Kudoa has increased from 44 (Moran et al., 1999a) to close to 100 (Levsen, 2015). Myoliquefaction of numerous fish species occurs in association with the genus Kudoa across a broad range of environments. In particular, K. thyrsites exhibits a global distribution (Whipps and Kent, 2006) and has been reported in 18 different fish families, comprising 9 orders (Henning et al., 2013), it is thus of major concern for both capture fisheries and aquaculture. It has been reported in northeast Pacific Hake (Merluccius productus) (King et al., 2012), Mediterranean silver scabbardfish (Lepidopus caudatus) (Kovaleva et al., 1979; Giulietti et al., 2019) and Atlantic mackerel (Scomber scombrus) (Levsen et al., 2008). Aquaculture of Atlantic salmon (Salmo salar) in British Columbia, Canada has suffered financial losses due to K. thyrsites infection (St-Hilaire et al., 1997a, St-Hilaire et al., 1998; Moran et al., 1999b). More recently, K. islandica has been identified in spotted wolfish (Anarchicas minor), Atlantic lumpfish (Anarhichas lupus) and lumpsucker (Cyclopterus lumpus) in the North Atlantic (Kristmundsson and Freeman, 2014). Additionally, K. islandica has been found in reared lumpsucker in Norway (Alarcon et al., 2015). Currently, there are no documented cases of K. thyrsites or K. islandica in Faroese aquaculture.
Atlantic mackerel is an economically important species for the Faroe Islands, and in the Northeast Atlantic at large. Previous work has developed our understanding of mackerel in regards to feeding preferences (Langøy et al., 2012; Bachiller et al., 2016), distribution of spatial biomass (Nøttestad et al., 2016) and recent patterns of geographical expansion (Ólafsdóttir et al., 2019). Earlier work in the northern North Sea found up to 8.9% of mackerel exhibiting “soft flesh” phenomenon in association with K. thyrsites spores in muscle tissue (Levsen et al., 2008). Annual surveys of mackerel have documented prevalence of post-mortem myoliquefaction at 0.6-3.1% (n=2500) and have observed significantly lower prevalence in medium (400-600g) compared to large-sized (>600g) mackerel (Levsen, 2015, Giulietti et al. 2022).
Kudoa thyrsites is classified as a multivalvulid myxosporidia (Myxozoa) (Lom and Dyková, 2006), an obligate parasite that alternates between invertebrate and vertebrate hosts (Kent et al., 2001; Americus et al., 2020). The general biology of Myxozoa displays bi-phasic life cycles that alternate between myxospores and actinospores and is well characterized in fresh water myxozoans (Yokoyama, 2003). Actinospores are released from an invertebrate host and may attach and enter a fish host, resulting in the development of a plasmodium (Lom and Dyková, 2006; Okamura et al., 2015). The skin, fins, gills and buccal cavity have all been demonstrated as portals of entry for actinospores (triactynomyxon) of Myxobolus cerebralis (El-Matbouli et al., 1995). The release of myxospores can occur when the fish host is alive, through the egestion of phagocytosed spores as observed in common Carp (Cyprinus carpio) (Ogawa et al., 1992). In Kudoa spp., it has been suggested that post-mortem myoliquefaction may serve to facilitate myxospore release (Langdon, 1991). Most actinosporeans have been reported to infect oligochaetes (Kent et al., 2001), although polychaetes, sipunculids, bryozoans and cephalopods have been described as invertebrate hosts for myxozoans (Yokoyama, 2003). The invertebrate host for K. thyrsites infection in mackerel is unknown. Infections in aquaculture are known from exposure to infected seawater (Moran et al., 1999c; Jones et al., 2012) and PCR-based analysis of raw seawater from infection hotspots detects K. thyrsites year round (Jones et al., 2016).
In recent years, quality control procedures used at land-based fish processing plants in the Faroes have identified soft-flesh in the mackerel fishery as an economically significant problem. However, the prevalence of K. thyrsites infection in Faroese fishing grounds remains currently uncharacterised. The specific objectives of the present study were (i) compare detection of K. thyrsites with microscopic and molecular techniques as the causative agent of post-mortem myoliquefaction, (ii) determine overall prevalence and spatio-temporal variability of K. thyrsites in the commercial Faroese fishery and (iii) examine the potential occurrence of K. thyrsites in water samples in Faroese waters.
Material and Methods
Sampling of Muscle Tissue
Selected Soft-Flesh Mackerel Specimens From Production Plant
A total of 104 mackerel were obtained from a land-based commercial production plant in the Faroe Islands (Varðin Pelagic, Tvøroyri). The samples originated from the production line in August-November 2015 and were selected on the basis of those specimens that exhibited evidence of post-mortem myoliquefaction (i.e. “soft mackerel”). All samples were stored at -20°C at the Faroe Marine Research Institute until further analysis.
Mackerel Specimens From Faroese Fishery
A total of 584 mackerel were sampled from commercial trawlers and during two research trips of the Faroe Marine Research Institute during 2017 (#184) and 2018 (#400). Samples were collected from different locations from the Faroese fishery, both in Faroese and British territorial waters (Figure 1). Full details of the region and time of year samples were collected are documented in Tables 1, 2. The biological parameters sampled were pinched-tail-length (Hansen et al., 2018), ungutted weight, sex, sexual maturity and age (as inferred from annual growth increments of the otoliths). All samples were stored at -20°C prior to further analysis.
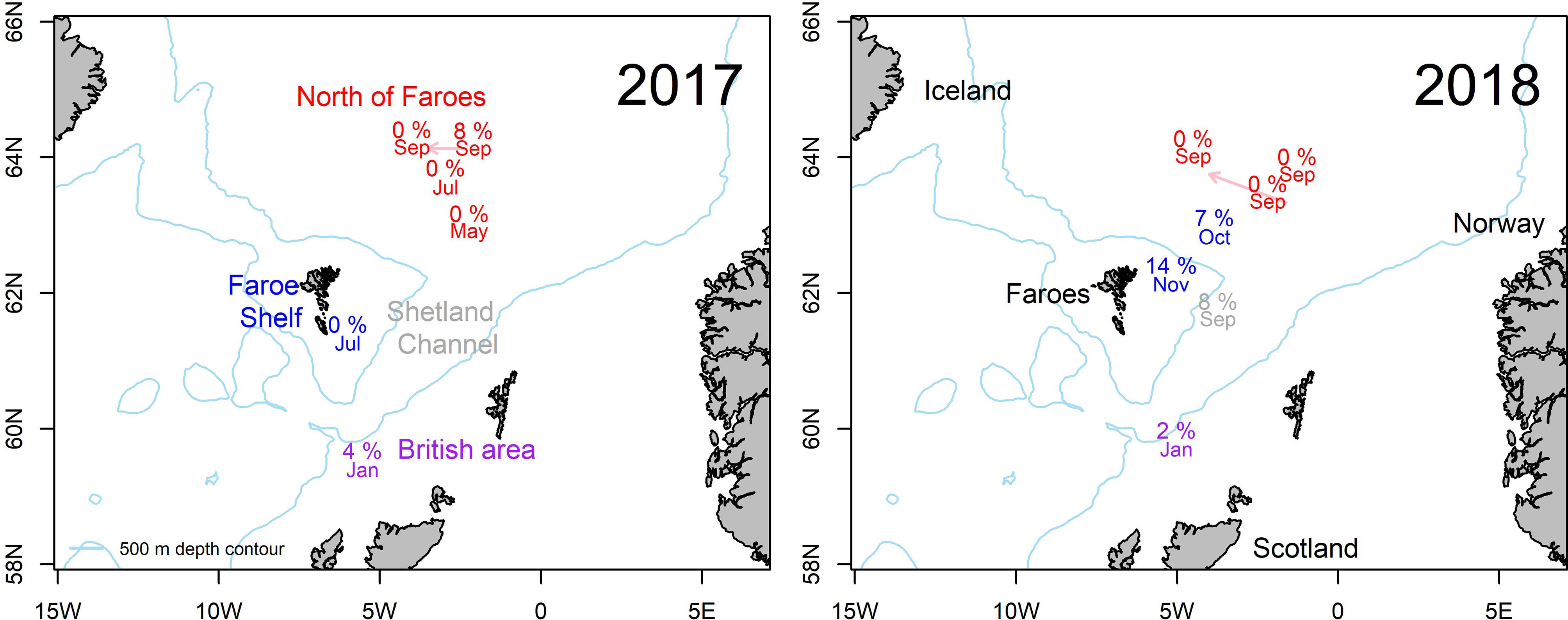
Figure 1 Map of the study area documenting sample locations. Prevalence of Kudoa thyrsites in Atlantic mackerel in 2017 (left) and 2018 (right). Month of capture and prevalence of infection is shown. Arrows indicate displacement of text label for plotting clarity. Text colours correspond to different sampling regions: North of Faroes (Red), Faroe Shelf (Blue), Shetland Channel (grey) and British Area (purple).
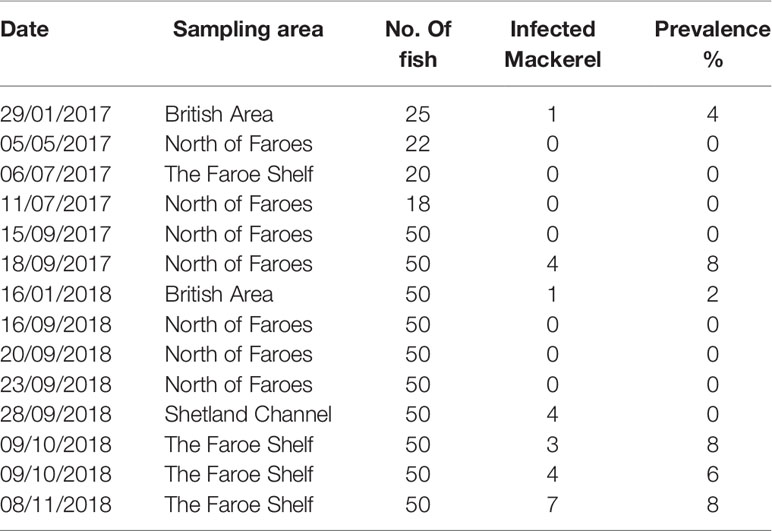
Table 1 Prevalence of Kudoa thyrsites infection in Atlantic mackerel samples collected during 2017 and 2018.
Analysis of K. thyrsites Infection
The 104 soft-flesh samples obtained from the factory processing plant were analysed using both microscope smears and qPCR detection. The 594 field samples of mackerel from the wild fishery were analysed with qPCR. Positive molecular detections were verified by microscope smears of archived muscle samples.
Microscope Smears
Specimens were defrosted at room temperature and muscle samples extracted (approx. 1.5 cm deep) from both the left and right side of the anterior dorsal fin. Muscle smears were prepared and examined by light microscopy (Optica DM-20) at 400x magnification. An approximately 1mm x 1mm piece was extracted from each muscle sample with a scalpel (chopping of muscle fibres) and forceps and used to prepare microscope wet-smears (St-Hilaire et al., 1997a; Giulietti et al., 2022). Muscle samples were subsequently stored at -20°C for molecular analysis. The left side was used for sample preparation and the right-side as a sample archive. One-hour searches were conducted on each smear for the detection of K. thyrsites spores, which were recorded photographically (Figures 2, 3). The selected smears were preserved by mounting in glycerol-gelatin (Berland, 2005). Samples were classified as infected or not-infected depending on the detection of K. thyrsites spores. K. thyrsites were clearly identified by their morphology, specifically the presence of one enlarged polar capsule (Figure 3), which are evenly sized in the recently documented K. islandica (Kristmundsson and Freeman, 2014).
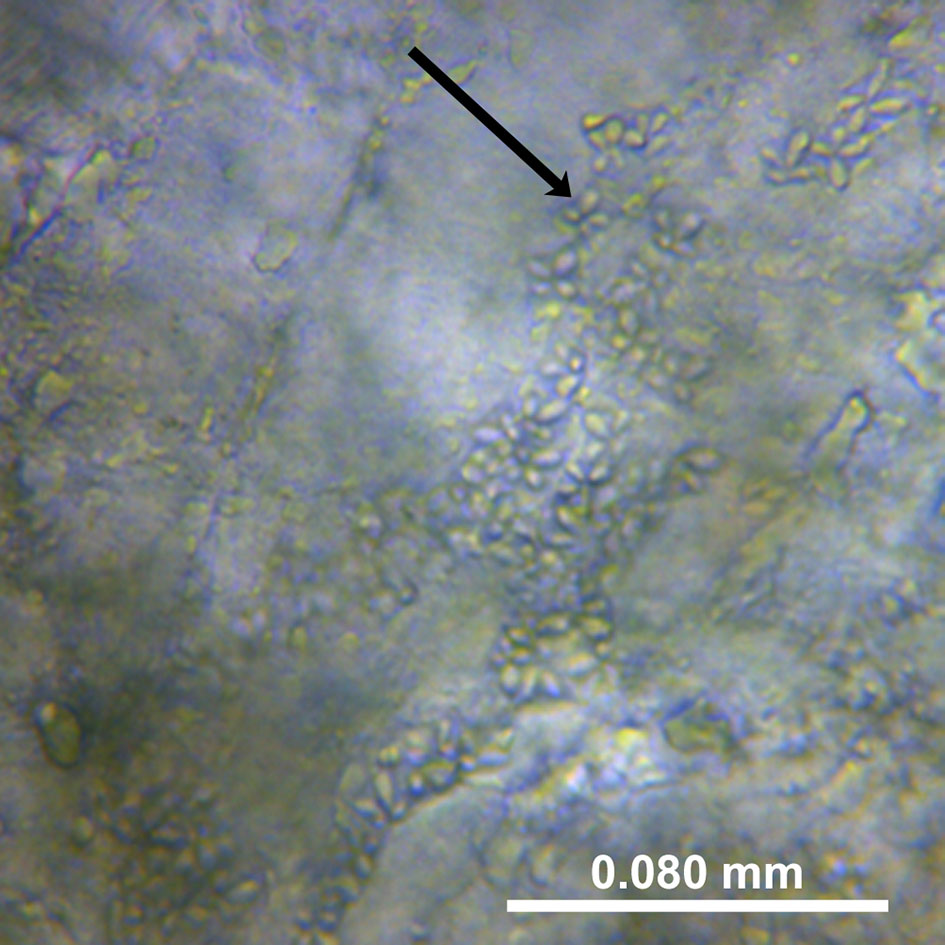
Figure 2 A photo from a wet smear of Kudoa thyrsites spores from the soft mackerels examined. The muscle is partially degraded by the cathepsin enzyme; magnification 400x.
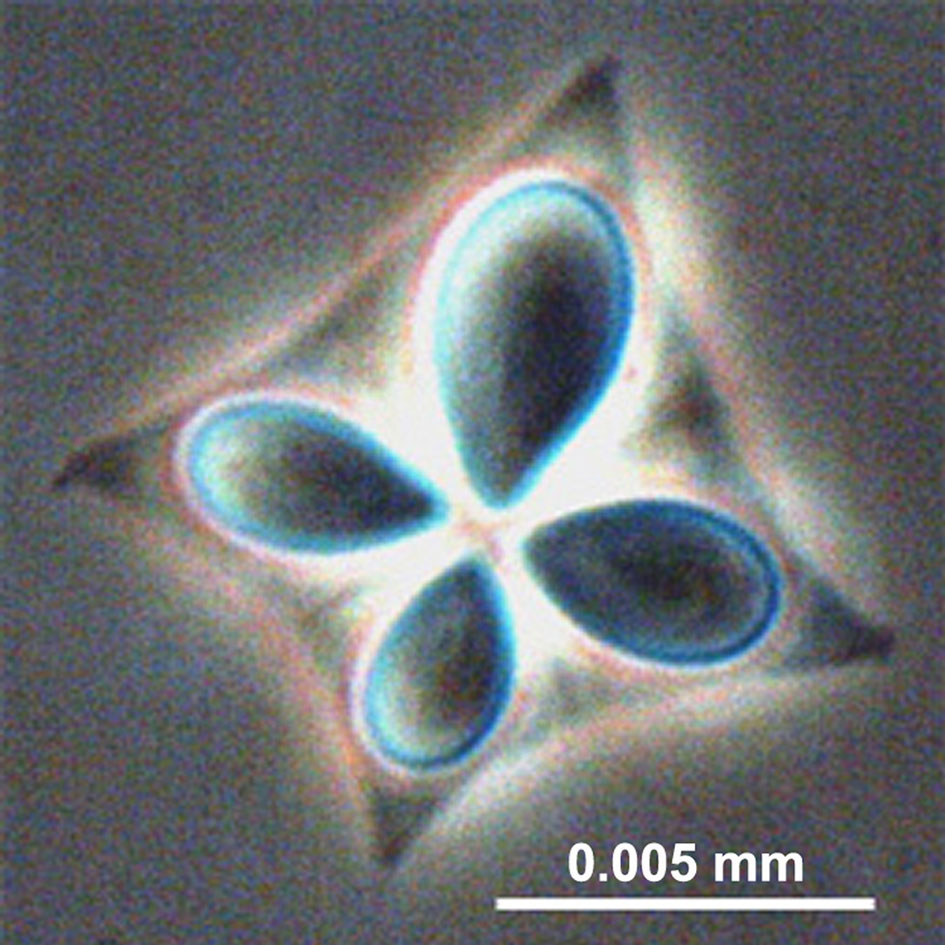
Figure 3 A single spore of Kudoa thyrsites with four nematocysts (polar capsules) clearly visible. (phase contrast. Levsen, 2015).
qPCR Detection
The three muscle tissue replicates (approx. 200 mm3) were extracted separately using a HotSHOT DNA preparation based on a previously published method (Truett et al., 2000). In brief, 50 µL of alkaline lysis buffer (25 mM NaOH, 0.2 mM disodium EDTA, pH 12) was added to a sterile 200 µL PCR tube. The muscle sub-samples were added to the tube and mashed with a sterile spatula. The lysis solution was incubated in a PCR machine preheated to 95°C for 30 min. and cooled at 4°C. Subsequently 50 µL of neutralization buffer (40 mM Tris-HCl, pH 5) was added to the tube, which was vortexed and incubated for 10-15 min. The tube was centrifuged to collect cellular debris and the supernatant removed and diluted 1:10 with nuclease-free water for subsequent PCR reactions.
Presence/absence tests were performed on the diluted lysates based on a previously published method validated for K. thyrsites (Funk et al., 2007). Each reaction well contained 4µL of QuantiTect, 0.25 µL of Kudoa Probe, 0.4 µL of Kudoa Primer- F, 0.4 µL of Kudoa Primer-R and 2.95 µL of nuclease-free water. 2 µL of DNA template was added to bring the reaction volume to 10 µL. Samples were 1:10 dilutions of the HotSHOT lysate. Kudoa Probe: VIC-TATCGCGAGAGCCGC-MGB. Kudoa F-primer: TGGCGGCCAAATCTAGGTT. Kudoa R-primer: GACCGCACACAAGAAGTTAATCC. Positive controls were included in each plate run that were obtained from a previous analysis of visibly infected tissue that yielded consistent amplification in PCR runs. The negative control replaced DNA templates with 2µL of nuclease-free water. Reactions were carried out in 96-well plates using an Applied Biosystems StepONE Plus realtime PCR platform. Samples were analysed in triplicate, corresponding to the three muscle sub-sample tissues extracted independently. The amplification profile was 50°C for 2 mins and 95°C for 15 mins then 45 cycles of 94°C for 15 s and 60°C for 1 min. The Ct value was defined as 20 times the standard deviation of the baseline fluorescent signal. Samples were classified as positive for K. thyrsites, if more than 1 of the 3 subsamples exhibited positive amplification.
Plankton Samples Collected From Faroe Plateau
Water Column Sampling
Water column samples were collected on the Faroese Shelf from January through to December (2018) with weekly to biweekly resolution (61.9703 °N. 6.8805 °W) as part of the Faroese Marine Ecosystem Observing (FAMEOS) program. Approximately 9 L of sample water were collected in acid-washed carboys following thorough rinsing (four times) with sample water. Seawater samples were collected using a pump (6m) from a land-based sampling facility connected to the fjord. The depth of the fjord is 18m and is well-mixed throughout the year due to tidal currents. A sample volume of 1.5 L replicate was collected onto 0.2 µm sterivex filters (Millipore; #SVGP01050) using a peristaltic pump and silicon tubing. Between each sampling event, the silicon tubing was stored in a solution of 10% v/v HCl. Prior to sampling, the tubing was flushed with 5 volumes of distilled water and 1 L of sample water prior to connecting sterivex tubing. Upon completion of the filtration the Sterivex filters were capped and stored at -80°C until extraction.
DNA was extracted directly from the Sterivex cartridges using a modified protocol of the Qiagen Dneasy Blood and Tissue Kit (Qiagen, #69504). Sterivex cartridges were removed from the freezer and allowed to defrost at room temperature for 20 min. A final check on residual volume was performed by expunging air through the Sterivex cartridge with a 50mL sterile syringe. The male nipple of the Sterivex cartridge was flame sealed and extraction reagents were added directly inside of the cartridge using sterile filter pipette tips. Extraction proceeded according to the manufacturer’s instructions with the following modifications. A volume of 720 μL of buffer ATL and 80 μL of proteinase K was added directly to the interior of the Sterivex cartridge. The female inlet was capped with a male luer-lock cap. The Sterivex cartridges were then placed in a rotary spinner and incubated at 56°C for 2 h. The cartridges were rotated 90° around their central axis every 30 min to ensure even coverage of the filter roll with extraction solution. The lysis solution was removed from the Sterivex cartridge using a sterile 3 mL luer-lock syringe and transferred to sterile DNase-free 2mL Eppendorf tubes. The Eppendorfs were pulse vortexed (10 s) and spun down in a mini-centrifuge. Subsequently, 600 μL of extraction solution was transferred to a clean 2 mL Eppendorf tube, followed by 600 μL of buffer AL solution. These Eppendorf tubes were pulse vortexed (10 s) to mix and centrifuged. Taking each Eppendorf in turn, 600 μL of ethanol was added followed by pulse-vortexing and centrifugation. This extraction solution was added to the Qiagen DNEasy Blood and Tissue kit spin columns in 3x ~ 600 μL aliquots. The spin column procedure followed the manufacturers instructions. DNA was eluted from the spin column in 120 μL of nuclease-free water following an incubation period of 5 min at 37°C. The flow-through from the first elution step was pipetted back onto the column for a second elution under identical conditions.
DNA concentrations in the extracts were measured with a Qubit Fluorometer and Qubit dsDNA HS assay (Q32854). Following the manufacturer’s instructions, DNA extracts were frozen at −20°C until further processing (Salter et al., 2019). Presence-absence tests for K. thyrsites were carried out according to the qPCR protocol described in section 2.2.2. Single filter replicates were analysed in triplicate on non-diluted DNA extractions. Inhibition controls were carried out on seawater samples using a commercially available assay (Techne TKIT06035). An Applied Biosystems StepOnePLUS realtime PCR platform was used for amplification. DNA extract aliquots (40 µL) were spiked with 5 µL of control template. Each PCR reaction mixture of 20 µL contained 5 µL sample (including control template), 10 µL of 2X qPCR mastermix (TKITMM01), 2 µL of control primer/probe (TaqMan hydrolysis probe) and 3 µL of nuclease-free seawater. Template and primer/probe sequences are proprietary (Techne). PCR reactions were performed under thermocycler conditions of 2 mins at 95°C and 50 cycles of 10 s at 95°C and 60 s at 60°C. Fluorogenic data was collected through the VIC channel. Inhibition was tested for in four technical PCR replicates of each sample. A comparison of Ct values between samples including internal control template and nuclease-free seawater with internal control template was used to diagnose inhibition, where a Ct shift of >3 was considered as inhibitory (Salter et al., 2019). Inhibition was not detected in any of the seawater samples.
Statistical Analysis
Mean-Square Contingency Coefficient (Φ)
Statistical agreement between the visual and molecular detection methods was determined from binomial presence-absence data and calculated from mean square contingency coefficients (e.g. Salter et al., 2019), a metric commonly referred to as Φ (Cheetham and Hazel, 1969). Visual and molecular detection rates were treated as binary variables (1 = presence and 0 = absence). The two independent detection methods were compared in a 2x2 contingency table. The phi coefficient (Φ) was calculated according to Equation 1 and can be considered as analytically equivalent to the Pearson’s product moment correlation taking values that range from -1 (negatively correlated) to 1 (positively correlated).
where a = presence-presence, b=presence-absence, c=absence-presence and d= absence-absence.
Chi-Squared and Fisher’s Exact Tests
Following the recommendation of (Rósza et al., 2000) we define prevalence as the proportion of examined individuals that tested positive for K. thyrsites. Statistical comparisons of prevalence were based on the analysis of 2x(n) contingency tables, where rows represented the binomial categories of infected or not infected and (n) the number of columns representing categorical descriptor variables (e.g. year, age class). If all cells of the contingency table had expected frequencies >5 we used a Chi-squared test, if this assumption was not met, and or the contingency table was 2x2, we used a Fisher’s exact test, significance level, α = 0.05.
Logistic Regression (Generalised Linear Model)
Statistical analyses were run in the R environment (RStudio Team, 2020) using base R and the aod package (Lesnoff and Lancelot, 2019). We used a logistic regression to model the odds of infection as a binomial outcome from a linear combination of the following predictor variables: latitude (location), time (month), age (year class), size (weight) and gender (M/F). Predictor variables were continuous, except for gender, which was included as a binary variable. Model fit was measured by examining if the model including predictor variables fits significantly better than a null model (intercept only). The test statistic compares the residual deviance of the two model versions from distributed Chi-squared values with degrees of freedom equal to the number of predictor variables in the fitted model. The statistical significance of model coefficients was examined from Chi-squared tests, significance level,ĸα = 0.05.
Prevalence Confidence Intervals
Prevalence data are presented with 95% confidence intervals, calculated according to the following Equation 2:
where p’ = x/n, x = samples positive for K. thyrsites, n = number of samples, q’ = 1-p’, α = 0.05, Zα = 1.96
Welch Two Sample t-Test
The inter-annual differences between length and age parameters of the sampled mackerel were determined using a Welch two sample t-test to account for unequal sample size/variance in 2017 and 2018.
Results
Detection of Infections in Soft-Flesh Specimens From Factory Processing
A total of 104 soft-flesh samples obtained from the factory processing plant in 2015 were examined both by microscope smears and qPCR (Table 2). The proportion of K. thyrsites spores (Figure 2) was 98% from microscope smears compared to 90% from qPCR. No samples scored positive by qPCR and negative by microscopy. In some cases it was possible to observe multiple spores in the soft-flesh mackerel specimens (Figure 3). The mean square contingency co-efficient calculated from the 2x2 contingency table was 0.43. A Fisher’s exact test also demonstrated a significant association (p<0.001) of detection outcome from the two methodological approaches.
Prevalence in Faroese Commercial Fishery
The mackerel sampled in 2017-2018 ranged from 2-14 years (Figure 4A) corresponding to a length range of 28.1-41.1 cm (Figure 4B) and weights of 190-660g (data not shown). Fish 5 years and older dominated in both 2017 (median = 6 years) and 2018 (median = 8 years). The size at age was also comparable between 2017 and 2018 (Figure 4C). Welch’s two-sample t-test showed that on average mackerel sampled in 2017 were significantly younger (p<0.001) and smaller (p<0.01) than in 2018.
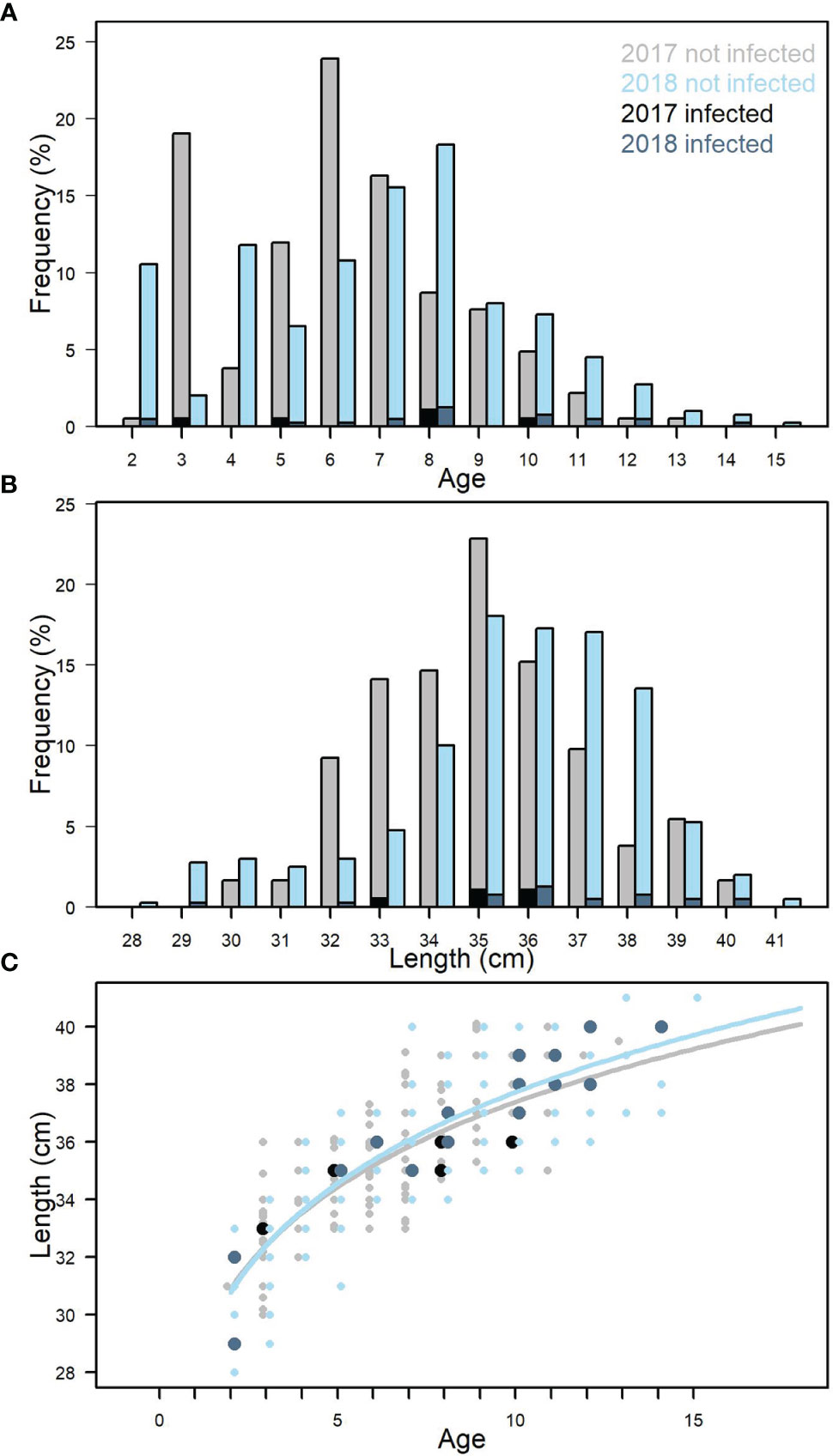
Figure 4 Age distribution for all samples from 2017 and 2018 (A). Length distribution for all samples from 2017 and 2018 (B). Length-weight relationship for all samples. 2017 and 2018 (C). Black and dark blue indicate fish infected with Kudoa thyrsites.
The overall prevalence of K. thyrsites detected by qPCR was 4.1% (95% confidence interval (CI): 2.5-5.7%). Prevalence was higher in 2018 (4.8%, CI: 2.7-6.8%) compared to 2017 (2.7%, CI: 0.4-5.1%) but the overlapping confidence intervals as well as a Chi-squared test indicated there was no significant association between sampling year and overall prevalence (p>0.05). The range in the proportion of fish infected was 0-14%, with the maximum value measured on the Faroese shelf in October 2018 (Figure 1 and Table 1). Positive PCR detection of K. thyrsites was detected in fish as young as 2 years (29.8 cm and 195g) and up to 14 years (40 cm and 595g). Two of the 24 positive PCR samples were negative for the presence of K. thyrsites spores when examined under the microscope.
A logistic regression model was used to test if the odds of prevalence could be explained by a linear combination of the following predictor variables: latitude, month, weight, age and gender. Model fit was tested by examining the residual deviance for the model including predictors with a null model (intercept only). Residual deviance was 180 on 578 degrees of freedom and null deviance was 200 on 583 degrees of freedom. The distributed Chi-squared value on 5 degrees of freedom (number of predictor variables) was 20.13, corresponding to significance level of p<0.005. The logistic regression model including predictor variables thus had a statistically significant better fit than a null model. The coefficients within the model and their statistical significance were as follows: latitude (-0.67, p<0.01), month (0.39, p<0.001), age (0.18, p>0.05), weight (-0.001, p>0.05) and gender (0.32, p>0.05). Consequently, only geographical location and month were significant predictor variables. The negative co-efficient for latitude (°N) indicates a decrease in the log odds of prevalence moving northward, whilst the positive coefficient for month indicates an increase in the log odds of prevalence later in the year.
Sampling locations were classified into different regions within the study area (see Tables 1, 2). The Faroese shelf had highest prevalence (8.2%, CI: 4.1-12.4%, n=170), followed by the British Area (2.7%, CI: 0-6.3%, n=75) and the Northern area (1.4%, CI: 1.4% 0-2.7%, n=289). A Chi-squared test of the 2x3 contingency table showed that prevalence was significantly associated with region (p<0.05). Since age was only just outside statistical significance in the logistic regression, we also examined differences categorically. The oldest fish (>10 years) had the highest prevalence (10.8%, CI: 4.2-17.5%, n= 83), followed by medium (6-9 years) fish (3.1%, CI: 1.2-5.0%, n=322) and young (2-5 years) fish (2.8%, CI: 0.4-5.2%). A Chi-squared test on the contingency table showed a significant association between age category and prevalence (p<0.01).
Seawater Analyses
Water column samples were analysed from the Faroese shelf spanning a full seasonal cycle from February-November in 2018 (Table 3). During the year, temperature ranged from 6.2-10.5°C, peaking in September. Pre-bloom chlorophyll (chl < 1 μg L-1) nitrate concentrations were 11.6 ± 0.4 μmol L-1 and drawn down to 3.6 μmol L-1 on 5th June corresponding to a first phytoplankton bloom peaking at 3.7 μg chl L-1. A second larger phytoplankton bloom (6.6 μg chl L-1) occurred on 21st August, followed by a rapid decline. DNA was successfully extracted from every sample and extract concentrations ranged from 1.2-8.4 ng μL-1 (average = 5.4 ng μL-1). All samples successfully amplified for a PCR inhibition control with Ct scores ranging from 23.16 ± 0.14 to 24.12 ± 0.17, which in all cases deviated <3 Ct from a negative control (nuclease-free water) spiked with a control template. None of the water samples were positive for K. thyrsites.
Discussion
Prevalence of K. thyrsites in Faroese Fishery
K. thyrsites was confirmed as the causative agent of myoliquefaction of Atlantic Mackerel within the Faroese wild fishery. Investigations of myoliquefaction in various fish species in Icelandic waters have identified K. islandica as the causative agent (Kristmundsson and Freeman, 2014), although it has not yet been identified in Atlantic mackerel. The morphology of K. thyrsites and K. islandica is quite distinct, where the former has one enlarged polar capsule, clearly visible in our samples (Figure 3). The vast majority of specimens obtained from a factory processing plant tested positive for K. thyrsites using both microscope smears and PCR. There was good statistical agreement between the two techniques, enabling us to apply qPCR to a large number of (>500) natural samples. It is unclear why we could not observe spores in a small fraction of the samples. There is some anecdotal evidence of spores disappearing in soft-flesh mackerel specimens (Levsen, personal communication), which could be related to spatial heterogeneity within the muscle sample itself. The focus of the present study was not to describe the intensity of infection. However, it is possible that quantifying variation of intensity across regions or age categories, along with manual muscle texture testing (Levsen et al., 2008) could explain some of the small discrepancies in detection. Future work integrating prevalence, intensity and muscle texture of K. thyrsites infected mackerel, and other Kuoda genera, is generally required (Giulietti et al. 2022).
There was spatial and temporal variability evident in the prevalence of K. thyrsites in mackerel comprising the Faroese wild fishery. Logistic regression modelling showed that both more northerly latitudes and later sampling months increased the log odds of detecting prevalence. Different regions were also statistically associated with K. thyrsites, with the shallow Faroese shelf environment displaying highest prevalence. Previous studies in the Northern North Sea have also documented temporal variability of K. thyrsites in mackerel (Levsen et al., 2008), including inter-annual differences (Levsen, 2015). Although our prevalence estimates are similar to those reported in the North Sea, we did not detect statistically significant inter-annual variability.
Separating spatial and temporal variability in migratory fish such as Atlantic mackerel remains challenging. For example in our dataset, the observed prevalence in the British Area is associated with sampling in January. However, the Faroese shelf and the area north of the Faroe islands both had sample coverage during September and October, with notably higher prevalence observed on the Faroese shelf. Specific factors that influence regional patterns of infection could be related to water mass properties characterising different oceanographic regimes. For example there are notable differences in temperature and salinity properties that comprise different water masses found to the north of the Faroe Islands, as well as there is variability in the relative composition of water masses among years (Hátún and Chafik, 2021). All of the samples collected north of Faroes are from an environment influenced by relatively cold Norwegian North Atlantic Water, while those on The Faroe Shelf are from an environment characterised by warmer and more saline waters.
Prevalence of K. thyrsites in mackerel was evident across the entire range of age classes sampled in the Faroese wild fishery. Prevalence was highest in larger and older fish (>10 years) and is consistent with studies from the North sea (Levsen, 2015). However, notably we also detected K. thyrsites in two and three year old fish, clearly demonstrating the potential for infection in younger adults. Presumably the likelihood of parasite-host encounters scale as a function of age, although migratory patterns into potentially infective areas may also contribute. Traditional stock migration patterns have shown that spawning occurs in the southern areas (close to Spain, France and Ireland) from February-July. Adult mackerel (>2-3 years) distribute to the north during the feeding migration phase in summer and can be found in an area extending from Gibraltar (36°N) up to Svalbard (78°N) to the areas east of Greenland (Jansen et al., 2016; Nøttestad et al., 2016). In late autumn the mackerel return towards the wintering areas around Shetland Isles and stay there during winter. In January the mackerel start their southward migration to the spawning grounds (Jansen et al., 2012) and we detected infection in January in the British area. Interestingly, no K. thyrsites was detected in mackerel from the Faroese Shelf late spring/early summer (May and July). The spatial and temporal patterns observed in our data appear to suggest that mackerel migrating into Faroese fishing grounds become infected in other areas. Our observations need to be interpreted cautiously within the context of the sampling regime. Further research is required to fully elucidate spatial and temporal patterns in the occurrence of infection.
Non-Detection of K. thyrsites in Seawater Samples
In the current study we did not detect the presence of K. thyrsites in Faroese shelf seawater samples. Although it is challenging to conclude absence from non-detection in PCR-assays, it seems unlikely that extraction or inhibition of seawater samples could adequately explain the ubiquitous non-detection observed in our study. In all samples tested, DNA concentrations in extracts were reasonably high and an independent extraction control confirmed there was no PCR-inhibition of seawater samples used in the present study. Furthermore, specific multiplex inhibition controls for quantitative PCR of Atlantic cod (Gadus morhua) using the same extraction method has not revealed any specific inhibition of seawater samples collected on the Faroese shelf (Salter et al., 2019). Failure of the extraction methodology to specifically extract DNA from the K. thyrsites target also seems unlikely. The Qiagen extraction kit used in this study includes a vigorous bead-beating step that has been applied previously to successfully extract K. thyrsites rDNA from seawater filters (Jones et al., 2016). The seawater samples used in this study were obtained from an archive of a coastal environmental DNA monitoring program (FAMEOS) and so spiking parallel water samples with the possible range of un-envisaged targets was not feasible. However, future studies targeting K. thyrsites in natural seawater samples may consider the addition of spores as an additional control measure.
The non-detection of K. thyrsites in annual seawater samples from the Faroese shelf support a hypothesis that Atlantic mackerel are not locally exposed to the parasite (Table 3). This is further supported by the circumstantial observation that K. thyrsites has not currently been observed in farmed Atlantic Salmon on the Faroese shelf, unlike other areas (St-Hilaire et al., 1997a; St-Hilaire et al., 1997b; St-Hilaire et al., 1997c; St-Hilaire et al., 1998; Moran et al., 1999b). The Faroese shelf is the shallowest environment in our survey area and corresponds to the highest prevalence of K. thyrsites (Table 1). Considering that practically all known life-cycles of myxosporeans involve annelid worms as an alternate host (Yokoyama, 2003), tight pelagic-benthic coupling on a tidally-mixed shelf might be considered to promote encounter rates between actinospores and migratory fish hosts. It is clear from seawater screening close to aquaculture facilities suffering from Kudoa spp. infections that myxospores can be detected in seawater samples and UV irradiation of raw seawater reduces infection (Jones et al., 2016). A seawater study of K. yasunagai in infected aquaculture areas appears to suggest some seasonality of myxospore abundances that were linked to water temperature and ecology of the alternate invertebrate host (Ishimaru et al., 2014). Our seawater survey covered the typical annual temperature range of the Faroese shelf and growth and decline of phytoplankton blooms that might influence invertebrate ecology. To the best of our knowledge our seawater survey is the first attempt to detect K. thyrsite myxospores in seawater samples in association with wild fish infections. One unexplored infection pathway is through feeding. In particular, sampling regimes that target intensive feeding in Southern spawning areas (Jansen et al., 2021) may help identify infection pathways occurring in Atlantic mackerel prior to their northward migration.
Conclusions
In the present study, we have investigated the prevalence of K. thyrsites in Atlantic mackerel in the Faroese fishery. Examination of soft-flesh (myoliquefaction) specimens obtained from a Faroese processing factory demonstrated K. thyrsites as the causative agent. We found excellent agreement between microscope smears and PCR detection of K. thyrsites, allowing us to apply more rapid molecular detection methods on a large number (>500) of mackerel specimens caught from the wild Faroese fishery. We observed infection across the entire age range (2-14 years) of mackerel and statistical analyses indicated spatial and temporal, but not inter-annual, variability in prevalence. Highest prevalence was observed on the relatively shallow, tidally mixed Faroese shelf. It was not possible to detect K. thyrsites spores in seawater samples from the Faroese shelf, despite sampling across the entire range of biogeochemical conditions occurring throughout the year. Taken together, with the absence of K. thyrsites infection in farmed salmon on the Faroese shelf, our data support the hypothesis that infection likely occurs in southern spawning areas and infected fish migrate north into the Faroese fishery. Future work investigating fish and water column samples in these southern areas could help resolve the spatial and temporal dynamics of K. thyrsites prevalence in Atlantic mackerel.
Data Availability Statement
The original contributions presented in the study are included in the article/supplementary material. Further inquiries can be directed to the corresponding author.
Ethics Statement
The samples were taken on sea onboard fishery or research vessels. The fish was cooled in RSW seawater onboard and later frozen on land. Thus all sampling occurred from already dead fish.
Author Contributions
DH and IS developed the main ideas. EH constructed the maps and histograms. IS and DH wrote the manuscript with feed-back from EH. All authors approved the submitted version.
Funding
This work was funded by Fiskivinnugransking (Fisheries Research Fund of the Faroe Islands Grant number 16/00632) and Felagið Nótaskip (Faroese Pelagic Organisation), Faroe Islands. We thank them sincerely for making this work possible. The collection of environmental DNA samples from coastal time-series was financially supported through the FAMEOS project (Granskingarráðið).
Conflict of Interest
The authors declare that the research was conducted in the absence of any commercial or financial relationships that could be construed as a potential conflict of interest.
Publisher’s Note
All claims expressed in this article are solely those of the authors and do not necessarily represent those of their affiliated organizations, or those of the publisher, the editors and the reviewers. Any product that may be evaluated in this article, or claim that may be made by its manufacturer, is not guaranteed or endorsed by the publisher.
Acknowledgments
The samples of soft mackerel came from Varðin Pelagic. Tvøroyri and the samples 2017-2018 were provided by research vessel “Magnus Heinason” and different commercial pelagic fishery vessels. Initial qPCR-analysis (the 2015 sample of soft mackerel) was done by Dr. Jonhard Eysturskarð; the PCR-analysis of the 2017-18 samples and stomach was done at Inova by Fiskaaling. Otolith work was done by the staff of Havstovan. Dr. Jan A. Jacobsen commented on the manuscript and assistant Dagunn H.J. Clementsen helped with the figures. The authors are grateful for the excellent effort of all the crew and staff involved. We acknowledge the provision of DNA archive samples from the genomic observatory FAMEOS (Faroese Marine Ecosystem Monitoring Study) (PI: Ian Salter).
References
Alarcon C. R., Lee H., Goodarzi H., Halberg N., Tavazoie S. F. (2015). N6-Methyladenosine Marks Primary microRNAs for Processing. Nature 519, 482–485. doi: 10.1038/nature14281
Americus B., Lotan T., Bartholomew J. L., Atkinson S. D. (2020). A Comparison of the Structure and Function of Nematocysts in Free-Living and Parasitic Cnidarians (Myxozoa). Int. J. Parasitol. 50, 763–769. doi: 10.1016/j.ijpara.2020.04.012
Bachiller E., Skaret G., Nøttestad L., Slotte A. (2016). Feeding Ecology of Northeast Atlantic Mackerel, Norwegian Spring-Spawning Herring and Blue Whiting in the Norwegian Sea. PloS One 11 (2), e0149238. doi: 10.1371/journal.pone.0149238
Berland B. (2005). Whole Mounts (Malaysia: Occasional Publication No. 1. Institute of Oceanography Kustem), 1–54.
Cheetham A. H., Hazel H. E. (1969). Binary (Presence–Absence) Similarity Coefficients. Paleontology. 43, 1130–1136.
El-Matbouli M., Hoffmann R. W., Mandok C. (1995). Light and Electron Microscopic Observations on the Route of Thetriactinomyxon-Sporoplasm of Myxobolus Cerebralis Fromepidermis Into Rainbow Trout Cartilage. J. Fish. Biol. 46, 919–935. doi: 10.1111/j.1095-8649.1995.tb01397.x
Funk V. A., Rapp M., Sojonky K., Jones S., Robinson J., Falkenberg and Miller K. M. (2007). Development and Validation of an RNA- and DNAbased Quantitative PCR Assay for Determination of Kudoa Thyrsites Infection Levels in Atlantic Salmon Salmo Salar. Dis. Aquat. Org. 75, 239–249. doi: 10.3354/dao075239
Giulietti L., Karlsbakk E., Cipriani P., Bao M., Storesund J. E., Marathe N. P., et al. (2022). Long-Term Investigation of the ‘Soft Flesh’ Condition in Northeast Atlantic Mackerel Induced by the Myxosporean Parasite Kudoa Thyrsites (Cnidaria, Myxozoa): Temporal Trends and New Molecular Epidemiological Observations. Fish. Res. 248, 106221. doi: 10.1016/j.fishres.2021.106221
Giulietti L., Mattiucci S., Paoletti M., Grevskott D. H., Bao M., Cipriani P., et al. (2019). Morphological and Molecular Identification of a New Kudoa Thyrsites Isolate in Mediterranean Silver Scabbardfish Lepidopus Caudatus. Dis. Aquat. Org. 132, 125–134. doi: 10.3354/dao03316
Hansen F. T., Burns F., Post S., Thygesen U. H., Jansen T. (2018). Length Measurement Methods of Atlantic Mackerel (Scomber Scombrus) and Atlantic Horse Mackerel (Trachurus Trachurus) – Current Practice, Conversion Keys and Recommendations. Fish. Res. 205, 57–64. doi: 10.1016/j.fishres.2018.04.002
Hátún H., Chafik L. (2021). And Larsen, KThe Norwegian Sea Gyre – A Regulator of Iceland-Scotland Ridge Exchanges. Front. Mar. Sci. 8, 1–15. doi: 10.3389/fmars.2021.694614
Henning S. S., Hoffmann L. C., Manley M. (2013). A Review of Kudoa-Induced Myoliquefaction of Marine Fish Species in South Africa and Other Countries. S. Afr. J. Sci. 109, 1–5. doi: 10.1590/sajs.2013/20120003
Ishimaru K., Matsuura T., Tsunemoto K., Shirakashi S. (2014). Seasonal Monitoring of Kudoa Yasunagai From Seawater and Aquaculture Water Using Quantitative PCR. Dis. Aquat. Org. 108, 45–52. doi: 10.3354/dao02702
Jansen T., Campbell A., Kelly C. J., Hátún H., Payne M. R. (2012). Migration and Fisheries of North East Atlantic Mackerel (Scomber Scombrus) in Autumn and Winter. PloS One 7, 1–9. doi: 10.1371/journal.pone.0051541
Jansen T., Post S., Kristiansen T., Oskarsson G. J., Boje J., MacKenzie B. R., et al. (2016). Ocean Warming Expands Habitat of a Rich Natural Resource and Benefits a National Economy. Ecol. Appl. 26, 2021–2032. doi: 10.1002/eap.1384
Jansen T., Slotte A., dos Santos Schmidt T. C., Sparrevohn C. R., Jacobsen J. A., Kjesbu O. S. (2021). Bioenergetics of Egg Production in Northeast Atlantic Mackerel Changes the Perception of Fecundity Type and Annual Trends in Spawning Stock Biomass. Prog. Ocean., 16, 198. doi: 10.1016/j.pocean.2021.102658
Jones S. R. M., Cho S., Nguyen J., Mahony M. (2016). Acquired Resistance to Kudoa Thyrsites in Atlantic Salmon Salmo Salar Following Recovery From a Primary Infection With the Parasite. Aquaculture 451, 457–462. doi: 10.1016/j.aquaculture.2015.10.002
Jones S. R. M., Forster I., Liao X., Ikonomou M. G. (2012). Dietary Nicarbazin Reduces Prevalence and Severity of Kudoa Thyrsites (Myxosporea: Multivalvulida) in Atlantic Salmon Salmo Salar Post-Smolts. Aquaculture 342-343, 1–6. doi: 10.1016/j.aquaculture.2012.01.033
Kent M. L., Andree K. B., Bartholomew J. L., El-Matbouli M., Desser S. S., Devlin R. H., et al. (2001). Recent Advances in Our Knowledge of the Myxozoa. J. Eukaryot. Microbiol. 48, 395–413. doi: 10.1111/j.1550-7408.2001.tb00173.x
King J. R., McFarlane G. A., Jones S. R. M., Gilmore S. R., Abbott C. L. (2012). Stock Delineation of Migratory and Resident Pacific Hake in Canadian Waters. Fish. Res. 114, 19–30. doi: 10.1016/j.fishres.2010.12.024
Kovaleva A. A., Shulman S. S., Yakovlev V. N. (1979). Myxosporidians of the Genus Kudoa (Myxosporidia. Multivalvulea) From the Atlantic Ocean Basin. Trudy. Zool. Inst. Akad. Nauk. SSSR. 78, 42–64).
Kristmundsson Á., Freeman M. A. (2014). Negative Effects of Kudoa Islandica N. Sp. (Myxosporea: Kudoidae) on Aquaculture and Wild Fisheries in Iceland. Int. J. Parasitol. 3 (2) 135–146. doi: 10.1016/j.ijppaw.2014.06.001
Langøy H., Nøttestad L., Skaret G., Broms C., Fernö A. (2012). Overlap in Distribution and Diets of Atlantic Mackerel (Scomber Scombrus), Norwegian Spring- Spawning Herring (Clupea Harengus) and Blue Whiting (Micromesistius Poutassou) in the Norwegian Sea During Late Summer. Mar. Biol. Res. 8, 442–460. doi: 10.1080/17451000.2011.642803
Langdon J. S. (1991). Myoliquefaction Post-Mortem ('Milkyflesh') Due to Kudoa Thyrsites (Gilchrist) (Myxosporea:Multivalvulida) in Mahi Mahi, Coryphaena Hippurus L. J.Fish. Dis. 14, 45–54. doi: 10.1111/j.1365-2761.1991.tb00575.x
Lesnoff M., Lancelot R. (2019) R Package Aod: Analysis of Overdispersed Data, Version 1.3.1. Available at: https://CRAN.R-project.org/package=aod.
Levsen A. (2015). A Review of Kudoa Species (Myxozoa. Multivalvulida) Affecting Seafood Quality. With Emphasis on K. Thyrsites in Atlantic Mackerel (Scomber Scombrus). J. Fish. Sci. Tech., 3–9. Nha Thrang University.
Levsen A., Jørgensen A., Mo T. A. (2008). Occurrence of Postmortem Myoliquefactive Kudoosis in Atlantic Mackerel. Scombrus Scombrus L. From the North Sea. J. Fish. Dis. 31, 601–611.
Lom J., Dyková I. (2006). Myxozoan Genera: Definition and Notes on Taxonomy, Life-Cycle Terminology and Pathogenic Species. Fol. Parasit. 53, 1–36. doi: 10.14411/fp.2006.001
Meglitsch P. A (1947). Studies on Myxosporidia from the Beaufort Region. II. Observations on Kudoa clupeidae (Hahn), gen. nov. J. Parasitol.. 33 (3), 271–277.
Moran J. W. D., Margolis L., Webster J. M., Kent M. L. (1999a). Development of Kudoa Thyrsites (Myxozoa: Myxosporidia) in Netpen-Reared Atlantic Salmon Determined by Light Microscopy and a Polymerase Chain Reaction Test. Dis. Aquat. Org. 37, 185–193. doi: 10.3354/dao037185
Moran J. W. D., Whitaker D. J., Kent M. L. (1999b). A Review of the Myxosporean Genus Kudoa Meglitsch, (1947) and its Impact on the International Aquaculture Industry and Commercial Fisheries. Aquac. 172 (1-2), 163–196. doi: 10.1016/S0044-8486(98)00437-2
Moran J. D. W., Whitaker D. J., Kent M. L. (1999c). Natural and Laboratory Transmission of the Marine Myxozoan Parasite Kudoa Thyrsites to Atlantic Salmon. J. Aquatulture Anim. Health 11, 110–115. doi: 10.1577/1548-8667(1999)011<0110:NALTOT>2.0.CO;2
Nøttestad L., Utne K. R., Óskarsson G. J., Jónsson S. T., Jacobsen J. A., Tangen Ø., et al. (2016). Quantifying Changes in Abundance. Biomass. And Spatial Distribution of Northeast Atlantic Mackerel (Scomber Scombrus) in the Nordic Seas From 2007 to 2014. ICES. J. Mar. Sci. 73 (2), 359–373. doi: 10.1093/icesjms/fsv218
Ogawa K., Delgahapitiya K. P., T. and Wakabayashi H. (1992). Histological Studies on the Host Response to Myxobolus Artus Akhmerov 1960 (Myxozoa: Myxobolidae) Infection in the Skeletal Muscle of Carp, Cyprinus Carpio L. J. Fish. Biol. 41, 363–371. doi: 10.1111/j.1095-8649.1992.tb02665.x
Okamura B., Gruhl A., Bartholomew J. L. (2015). “An Introduction to Myxozoan Evolution, Ecology and Development,” in Myxozoan Evolution, Ecology and Development, 1–20. Switzerland: Springer Link International
Ólafsdóttir A. H., Utne K. R., Jacobsen J. A., Jansen T., Óskarsson G. J., Nøttestad L., et al. (2019). Geographical Expansion of Northeast Atlantic Mackerel (Scomber Scombrus) in the Nordic Seas From 2007 to 2016 was Primarily Driven by Stock Size and Constrained by Low Temperatures. Deep-Sea. Res. Part II. 159, 152–168. doi: 10.1016/j.dsr2.2018.05.023
Rósza L., Reiczigel J., Majoros G. (2000). Quantifying Parasites in Samples of Hosts. J. Parasitol. 86, 228–232. doi: 10.1645/0022-3395(2000)086[0228:QPISOH]2.0.CO;2
Salter I., Eliasen S., Joensen M., Kristiansen R., Steingrund P., Vestergaard P. (2019). Quantitative Environmental DNA Survey Maps Regional Biomass of Atlantic Cod in Oceanic Waters. Nat. Commun. Bio. 2, 461–470. doi: 10.1038/s42003-019-0696-8
St-Hilaire S., Hill M., Kent M. L., Whitaker D. J., Ribble C. (1997b). A Comparative Study of Muscle Texture and Intensity of Kudoa Thyrsites Infection in Farm-Reared Atlantic Salmon. Salmo Salar. On the Pacific Coast of Canada. Dis. Aquat. Org. 31, 221–225. doi: 10.3354/dao031221
St-Hilaire S., Ribble C., Whitaker D. J., Kent M. L. (1997a). Evaluation of a Nondestructive Diagnostic Test for Kudoa Thyrsites in Farmed Atlantic Salmon (Salmo Salar). Aquac. 156, 139–144. doi: 10.1016/S0044-8486(97)00081-1
St-Hilaire S., Ribble C., Whitaker D. J., Kent M. L. (1997c). Prevalence of Kudoa Thyrsites Sexually Mature and Immature Pen-Reared In Atlantic Salmon (Salmo Salar) in British Columbia .Canada. Aquaculture 162, 69–77. doi: 10.1016/S0044-8486(98)00208-7
St-Hilaire S., Ribble C., Whitaker D. J., Kent M. L. (1998). Prevalence of Kudoa Thyrsites in Sexually Mature and Immature Pen-Reared Atlantic Salmon (Salmo Salar) in British Columbia, Canada. Aquaculture 162, 69–77. doi: 10.1016/S0044-8486(98)00208-7
Truett G. E., Heeger P., Mynatt R. L., Walker J. A., Warman M. L. (2000). Preparation of PCR-Quality Mouse Genomic DNA Wit Hot Sodium Hydroxide and Tris (HotSHOT). Biotechniques. 29 (1), 52–54. doi: 10.2144/00291bm09
Whipps C. M., Kent M. L. (2006). Phylogeography of the Cosmopolitan Marine Parasite Kudoa Thyrsites (Myxozoa: Myxosporea). J. Eukaryot Microbiol. 53, 364–373. doi: 10.1111/j.1550-7408.2006.00114.x
Keywords: Kudoa thyrsites, Atlantic mackerel, Faroe Islands, myoliquification, FAMEOS, Faroese Marine Ecosystem Observing Study, eDNA
Citation: Højgaard DP, í Homrum E and Salter I (2022) Prevalence of Kudoa thyrsites (Myxozoa, Multivalvulida) in Atlantic Mackerel, Scomber scombrus L., in the Vicinity of the Faroe Islands. Front. Mar. Sci. 9:818507. doi: 10.3389/fmars.2022.818507
Received: 19 November 2021; Accepted: 02 May 2022;
Published: 06 June 2022.
Edited by:
Teresa Sofia Giesta da Silva, Marine and Freshwater Research Institute, IcelandReviewed by:
Teunis Jansen, Technical University of Denmark, DenmarkSimon Jones, Department of Fisheries and Oceans, Canada
Copyright © 2022 Højgaard, í Homrum and Salter. This is an open-access article distributed under the terms of the Creative Commons Attribution License (CC BY). The use, distribution or reproduction in other forums is permitted, provided the original author(s) and the copyright owner(s) are credited and that the original publication in this journal is cited, in accordance with accepted academic practice. No use, distribution or reproduction is permitted which does not comply with these terms.
*Correspondence: Ian Salter, aWFuc0BoYXYuZm8=; Dánjal Petur Højgaard, ZGFuamFscGV0dXJoQGdtYWlsLmNvbQ==