- BioCost Research Group, Facultad de Ciencias and Centro de Investigaciones Científicas Avanzadas, Universidad de A Coruña, A Coruña, Spain
Kelps dominate rocky shores in temperate latitudes, acting as important foundation species. Recently, kelp forests have received growing attention for their decline around the world. Climate change is one of the main drivers of their deforestation, both through abiotic stress and/or distortion of biotic interactions. In NW Spain, golden kelp (Laminaria ochroleuca) forests have recently declined in some areas due to excessive grazing. To further investigate the mechanisms behind this decline, a thorough 1-year seasonal demographic study was carried out on eight golden kelp reefs: four healthy and four degraded by herbivory. Kelps covered the full range of size classes throughout the year on healthy reefs. By contrast, only small, newly recruited kelps were found on degraded reefs in spring-summer, because excessive herbivory in autumn-winter caused a total collapse of kelp populations on a reef-scale (i.e., all kelps reduced to inviable stubs with no blade and no growth meristem). Bite marks in kelps covering all size classes showed that fishes, instead of invertebrates, were the culprits of the excessive herbivory. Recent studies, mostly conducted in the Indo-Pacific, also found that fish herbivory, alone or in combination with abiotic stressors, can maintain a canopy-free state in kelp reefs. To the best of our knowledge, this is the first time that fish is also shown to cause the collapse of entire kelp forests on a reef scale in the temperate Atlantic. The implications of this finding for the management and potential recovery of degraded reefs are discussed.
Introduction
Climate change is behind the redistribution of many species on a global scale, and consequently shifts community structure and ecosystem functioning (Pecl et al., 2017; Gilson et al., 2021). In marine environments, poleward range shifts have been documented for several species in response to rising seawater temperatures (Lima et al., 2007; Sorte et al., 2010; Poloczanska et al., 2013). These range shifts are especially noticeable in temperate-subtropical transition zones where species of both climatic regimes co-exist (Horta e Costa et al., 2014; Troast et al., 2020; Smith et al., 2021). On a community level, the ecological impact of these shifts may be more widespread when they involve foundation or habitat-forming species (Zarnetske et al., 2012).
Kelps (brown seaweeds of the order Laminariales) are foundation species that dominate large portions of the subtidal of temperate and subpolar rocky shores worldwide, being substratum for several sessile animals and algae, and habitat for mobile organisms (Mann, 1982; Steneck et al., 2002). Kelp forest distribution, structure and productivity are influenced by abiotic (e.g., temperature, light, substrata, sedimentation, nutrients, water motion, and salinity) and biotic (e.g., grazing, competition, and disease) factors (Dayton, 1985; Bekkby et al., 2014; Smale, 2020). Although kelp forests dominate cold-water coastal zones, they have also been found at low latitudes thanks to cool ocean currents toward the equator or upwelling, but here their resilience is lower because of physiological adjustment to higher temperatures (Wernberg et al., 2010). Thus, the low-latitude limits of kelp ranges may be more susceptible to shifts in their distribution and abundance due to climate change, through gradual warming and/or extreme heatwave events, combined with other physiological stressors (e.g., low nutrient availability) (Burdett et al., 2019). In mid-latitudes away from the range limits, kelps may be more susceptible to biotic pressures such as herbivory (Steneck et al., 2002).
Range shifts as a consequence of water warming have been documented for kelps worldwide, while some cold-temperate kelps have contracted their area of distribution, others with warm-temperate affinities have expanded it poleward (Smale et al., 2020). On the one hand, range contractions have been predicted and/or observed for several kelps in both hemispheres, e.g., Laminaria hyperborea (Gunnerus) Foslie and Saccharina latissima (Linnaeus) C.E. Lane, C. Mayes, Druehl & G.W. Sounders in the Atlantic (Araújo et al., 2016; Casado-Amezúa et al., 2019), Macrocystis pyrifera (Linnaeus) C.Agardh and Ecklonia spp. in the Pacific (Tanaka et al., 2012; Kumagai et al., 2018; Butler et al., 2020), and Ecklonia radiata (C. Agardh) J. Agardh in the Indian Ocean (Wernberg et al., 2016). On the other hand, the warm-temperate Laminaria ochroleuca Bachelot Pylaie has both increased in abundance at its northern range limit and expanded its range poleward (Smale et al., 2014; Schoenrock et al., 2019).
The Atlantic coast of Europe is a hotspot of warming, particularly the southernmost Iberian Peninsula (Lima and Wethey, 2012). However, water warming is not uniform along the coasts of the peninsula, being greater toward the eastern Cantabrian Sea (southern part of the Bay of Biscay) than in the western Atlantic Iberia, despite their close latitudes (Gómez-Gesteira et al., 2008). This difference is due to the periodic upwelling events that cool the waters off the western Atlantic Iberia coast in spring-summer while increasing nutrient availability (Fraga, 1981). Upwelling events are less common on the western Cantabrian Sea (Llope et al., 2006; Alvarez et al., 2011), possibly explaining why water warming appears to have driven the decline of some seaweeds on the Cantabrian coast, while its impact is not so clear on the west. For example, the golden kelp Laminaria ochroleuca is a perennial seaweed that ranges from Morocco to Great Britain, being the northwest coast of the Iberian Peninsula its range-center (Birkett et al., 1998). Occasional small deep waters populations have also been found in the southwest Mediterranean (Algeria, Sea of Alboran), of the Straits of Messina and Azores (Drew, 1974; Tittley and Neto, 2000; Flores-Moya, 2012; Tempera et al., 2021). Like other Laminariales, its life cycle alternates between a haploid microscopic stage (gametophyte), and a diploid macroscopic one (sporophyte). This kelp reaches maximum biomass values in summer, and the fertile tissue develops in the distal part of the sporophyte blade. At maturity, the latter disintegrates releasing the haploid zoospores. Fertile golden kelp plants are found year round, but sorus production peaks in spring (March) and, mostly, summer (July) (Izquierdo, 1998). The decline of this kelp has been documented at its low latitude range limits, retracting northwards along Portugal (Tuya et al., 2012) and westward on the north of the Iberian Peninsula (Fernández, 2011, 2016; Díez et al., 2012; Voerman et al., 2013). Storm damage aside (Pereira et al., 2017), rising water temperatures are considered the main culprit for the decline of L. ochroleuca in these areas (Pereira et al., 2011; Biskup et al., 2014). Accordingly, the rocky shore of the northwest Iberian Peninsula appears as a potential refuge for L. ochroleuca against warming, since seawater temperature in the region has remained within its optimal range (<20°C) (tom Dieck, 1992; Izquierdo, 1998; Franco et al., 2016). However, a decrease in the abundance of some golden kelp populations has recently been documented in this region, both in the intertidal (Piñeiro-Corbeira et al., 2016; Barrientos et al., 2020) and in the subtidal (Piñeiro-Corbeira et al., in press). In particular, L. ochroleuca used to form stable canopy forests within the limits of a Marine Protected Area (MPA, Islas Atlánticas de Galicia National Park) (Seoane-Camba and Campo Sancho, 1968; Varela, 2000; Vilas Paz et al., 2014; Piñeiro-Corbeira et al., in press), but recent studies show that these canopy forests have disappeared due to excessive grazing (Barrientos and Piñeiro-Corbeira, under review).
The importance of consumer control by macro and mesograzers over primary producers such as seaweeds and seagrasses has been extensively tested in the world’s oceans (Poore et al., 2012). Although some studies have argued that no latitudinal gradient seems to be present in the effect of herbivory on primary producers (Poore et al., 2012), others indicate that consumer pressure is higher in the tropics (Longo et al., 2018). In particular, herbivorous fish are recognized as important components of tropical assemblages, as they keep coral reefs free of canopy algae by reducing their competition (Bellwood et al., 2004; Steneck et al., 2017). In comparison, algae-consuming fishes have a limited presence in temperate latitudes (Meekan and Choat, 1997; Floeter et al., 2005), and invertebrate mesograzers in general, and sea urchins in particular, have often been considered one of the main causes of occasional kelp eradication in these regions (Estes and Palmisano, 1974; Dayton, 1975; Buschmann et al., 2004; Krumhansl and Scheibling, 2011; Filbee-Dexter and Scheibling, 2014; Ling et al., 2015; O’Brien and Scheibling, 2016; Gutow et al., 2020). However, both past (Choat, 1982; Andrew and Jones, 1990; Jones and Andrew, 1990) and more recent studies (Vergés et al., 2009; Taylor and Schiel, 2010; Basford et al., 2015; Steneck et al., 2017) show that herbivorous fish may also be important components on temperate reefs despite having less abundance and biomass than in low-latitudes (Holland et al., 2020). A higher fish herbivory pressure may be an indirect consequence of climate change in temperate regions. On the one hand, water warming has increased the feeding rate on kelp reefs and forest of other brown seaweeds by some herbivorous fish native to temperate zones (Gianni et al., 2017). On the other hand, warm-water fishes have been driven by currents and overwinter into cold-water ecosystems in recent times, and their occurrence is predicted to increase with ocean warming and marine heatwaves, adding new herbivory pressure (Vergés et al., 2014a; Basford et al., 2015; Smith et al., 2016, 2021; Beck et al., 2017). One of the best studied examples of warm-water fish range expansion into temperate regions (tropicalization) linked to the disappearance of kelp forests occurred in Western Australia (Wernberg et al., 2012). A marine heatwave induced a phase shift from an E. radiata canopy forest to a low-biomass algal turf along several kilometers of coastline, and has been maintained ever since by a combination of native and tropical fish herbivory (Bennett et al., 2015; Zarco-Perello et al., 2017; Zarco-Perello et al., 2021). Similarly, but not requiring a marine heatwave, the decline of E. radiata forest in eastern Australia has been attributed to enhanced fish herbivory as an indirect consequence of water warming (Vergés et al., 2016). In temperate western Japan, herbivorous fish, together with other biotic and abiotic factors, have also been considered as one of the causes for the decline of kelp beds (e.g., Saccharina sp., Ecklonia sp., Eisenia sp.) or for the maintenance of poorly vegetated areas known as “isoyake” (Fujita, 2010; Yamaguchi, 2010). Experimental studies in the Mediterranean Sea also concluded that fish herbivory by range-shifting and native species can turn canopy forest (i.e., Cystoseira sp.) into barrens (Vergés et al., 2014b).
Here, the agents and demographic impact of the excessive herbivory detected in golden kelp reefs of Islas Atlánticas de Galicia National Park are investigated. A thorough 1-year seasonal study was carried out to compare the demography of golden kelp reefs inside (degraded by excessive herbivory) and outside (healthy canopy forests) the MPA. Emphasis was also placed on collecting morphological evidence to elucidate the consumers behind the decimation of golden kelp forests. Accordingly, grazing marks on blades of a large number of L. ochroleuca plants covering the entire individual size range found at each reef were quantified and categorized. Initially, sea urchins seemed an unlikely candidate to explain the excessive grazing given that, as many other invertebrates of commercial interest (Piñeiro-Corbeira et al., 2018; Barrientos et al., 2019), they are heavily exploited in northwest Spain and, astonishingly, their exploitation includes the MPA and neighboring areas (Catoira Gómez, 2009; Fernández-Boán et al., 2012). To our knowledge, no previous study has provided such a detailed individual-based assessment of demographic damage caused by herbivores on wild kelp populations.
Materials and Methods
Sampling Site
The study was carried out at eight subtidal Laminaria ochroleuca rocky reefs within a large ria (Ría de Vigo), in the northwest of the Iberian Peninsula. Four sites were degraded reefs located within the borders of the Islas Atlánticas National Park (heredafter referred to as degraded reefs), spread along the landward side of the Cíes archipelago. The other four were nearby (<10 km) healthy reefs outside the park limits and located on the both northern and southern shores of the ria (Figure 1). These sites were selected based on previous knowledge of the study area, and had similar conditions in terms of depth (8 m on average, according to the tide), wave exposure (semi-sheltered), substrate (rocky or rocky with sand), temperature (between 13 and 17°C, according to the season), salinity (29–36 S/m), and nitrates and phosphates concentrations (18.39–0.03 μmol⋅l–1 and 0.05–0.86 μmol⋅l–1, respectively) (Supplementary Figure 1).
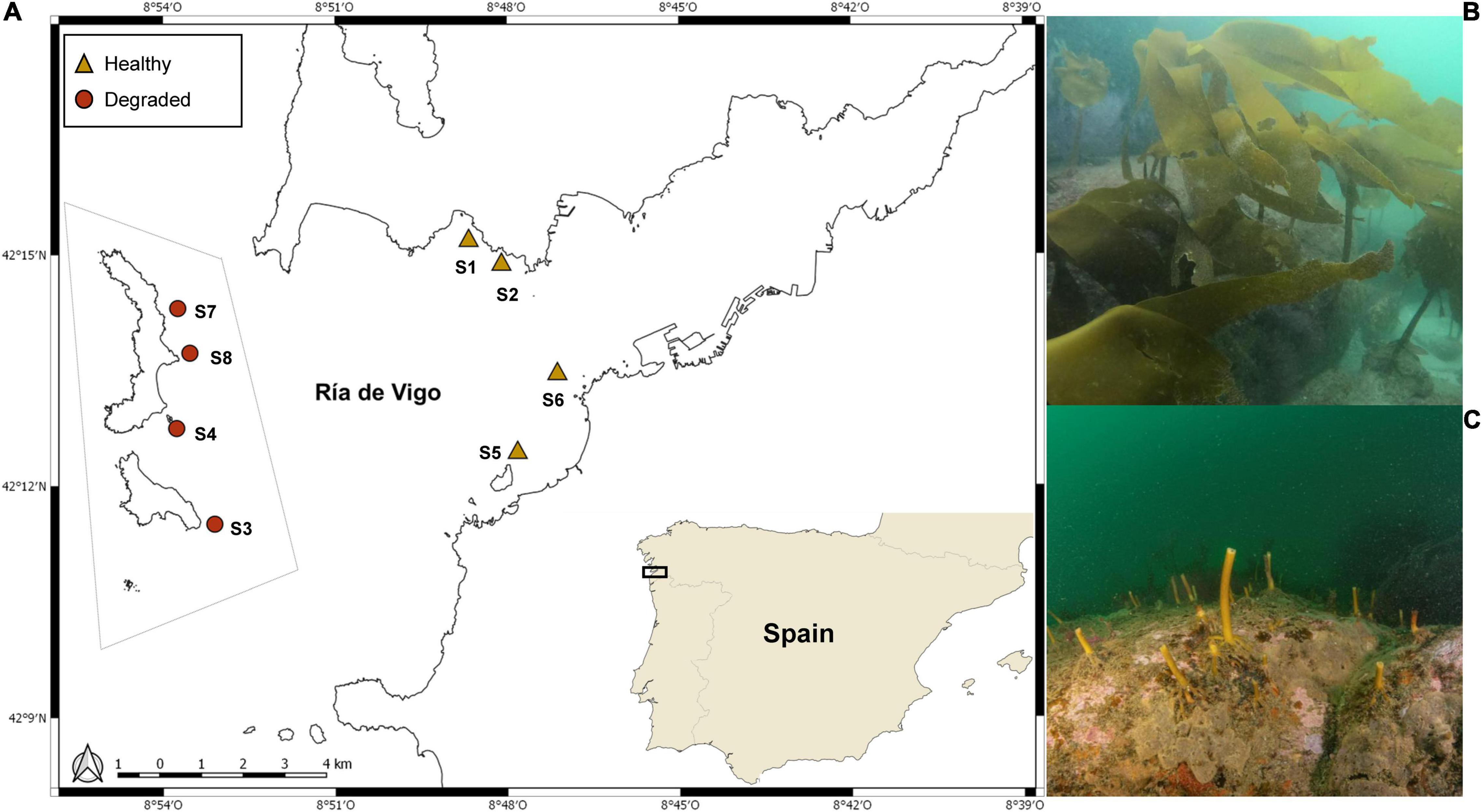
Figure 1. Laminaria ochroleuca. (A) Map showing the location of the eight kelp reefs selected in the study. Symbols indicate healthy (triangles) and degraded (circles) golden kelp reefs in Ría de Vigo, NW Spain. The dashed gray line shows the limits of Islas Atlánticas National Park (MPA). Underwater photograph from kelp reefs in winter showing: (B) well-developed kelp reef and (C) overgrazed kelp reef. Photograph credits: Manuel E. García.
Kelp Population Structure
From summer 2019 to spring 2020, seasonal sampling surveys were conducted in which every L. ochroleuca sporophyte (from recruits to adults) found on five haphazardly placed 0.25 m2 quadrats on each reef (n = 1,973 for the entire study) was collected. Once in the laboratory, three morphological traits were immediately recorded for each plant: wet weight, blade area, and diameter at the basis of the stipe. Stipe diameter was measured with a caliper and served as a proxy for size class because (a) it increases as the plant grows, (b) it does not undergo seasonal fluctuations, and (c) it is readily recorded on most of the plants collected provided they were not reduced to just a holdfast by herbivory. The one-side blade area was measured on size-calibrated digital photographs of each L. ochroleuca blade with the help of the image analysis software ImageJ (National Institutes of Health, United States). Individual counts, wet weights and blade areas were used to obtain population estimates of abundance (number of individuals per m2), biomass (kg WW per m2), and Lamina area index (LAI). Additionally, individuals were sorted into 1-mm stipe diameter size-classes to estimate abundance, biomass and LAI for each class in order to provide a detailed demographic description of the population structure (Lüning, 1969). Abundance and biomass estimates discriminated between viable plants and “stubs” (i.e., ecologically dead plants that had lost their growth meristem due to herbivory, Andrew and Jones, 1990).
Herbivory Intensity on Kelp
To assess seasonal changes in the intensity of herbivory pressure on kelp reefs, an Herbivory Pressure Index (HPI) was designed in which each kelp was assigned to one of the following seven categories: HPI-0 (no bite marks), HPI-1 (<25% of the blade edge with bite marks), HPI-2 (25–50% of blade edge with bite marks), HPI-3 (50–75% of blade edge with bite marks), HPI-4 (75–100% of blade edge with bite marks), HPI-5 (stipe and holdfast only, no growth meristem left, bite marks on stipe apex), and HPI-6 (holdfast only with bite marks on apex). Additionally, kelps in HPI categories 1–4 were further examined to discriminate between bite marks distinctively caused by fish (clear crescent and/or circular shaped marks) and those caused by invertebrates (irregularly serrated marks) (Figure 2). The relative abundance of both types of marks along the blade edge was used to assign each kelp to one of the following seven categories of an Invertebrate/Vertebrate Ratio (IVR): IVR-1 (0:100 ratio), IVR-2 (5:95), IVR-3 (25:75), IVR-4 (50:50), IVR-5 (75:25), IVR-6 (95:5), and IVR-7 (100:0) (Supplementary Figure 2). All assignments to both HPI and IVR categories were done by a single researcher to avoid observer biases. Before any assignment, the accuracy of the procedure was assessed by a blind self-calibration exercise in which HPI and IVR categories were assigned to a set of plants whose bite marks had been measured with the help of ImageJ.
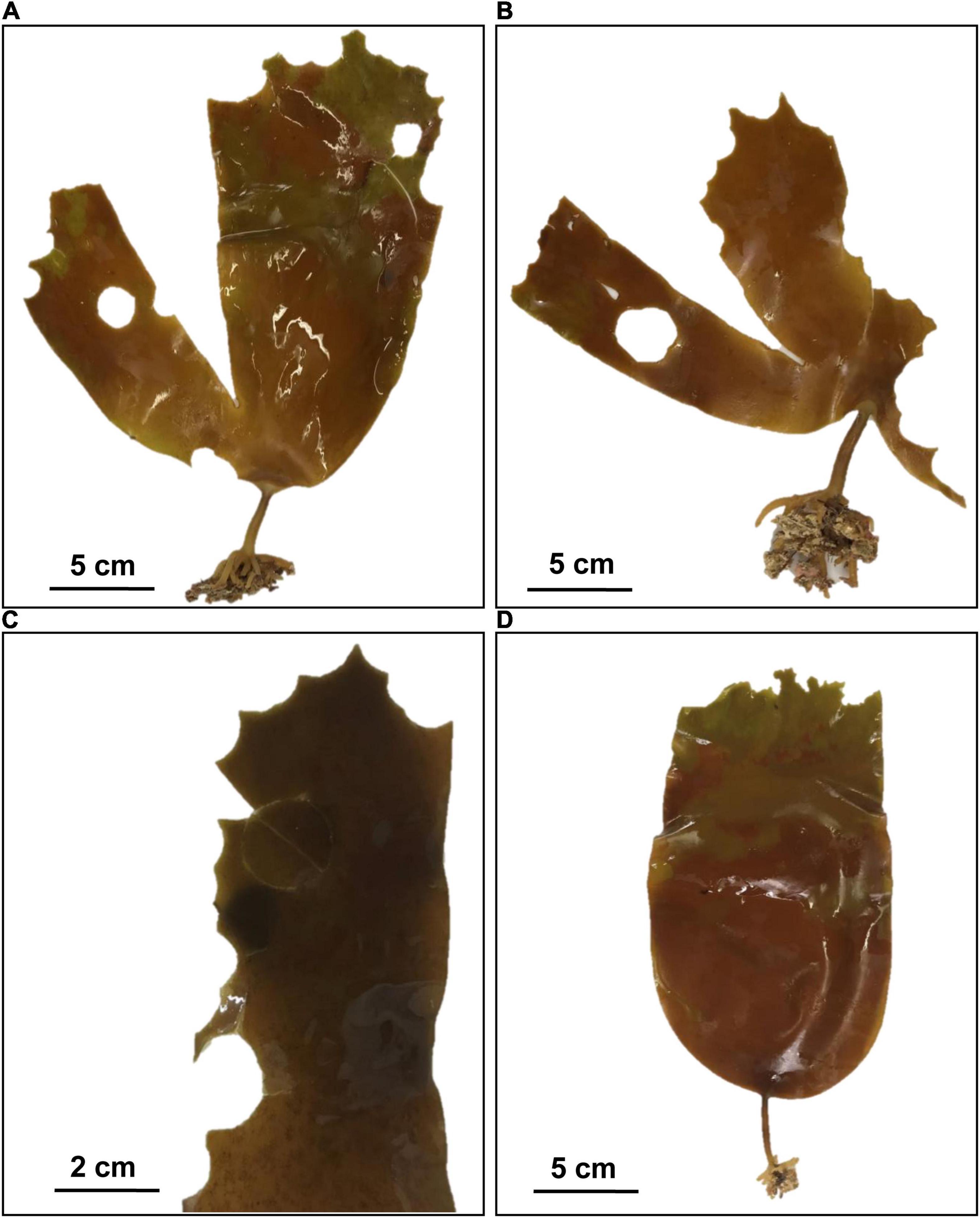
Figure 2. Laminaria ochroleuca. Examples of (A–C) clear crescent and circular shaped fish bite marks and (D) irregular serrated sea urchin bite marks on golden kelps.
Macro-Herbivore Abundance
Macro-herbivore abundance was visually assessed seasonally between autumn 2019 and spring 2020 at the eight reefs. On each reef, all fish were counted along seven randomly oriented 25 × 4 m belt transects with the help of video recordings (Franco et al., 2017). Fishes were categorized according to trophic affinity (Rodríguez Solórzano et al., 1983; Miller and Loates, 1997; Muus et al., 1998; Froese and Pauly, 2019), and only seaweed-eating fish, both omnivorous and herbivorous, were considered for this study. On the other hand, three sea urchin species which were found living in the area (Paracentrotus lividus, Sphaerechinus granularis and Echinus esculentus) were counted along seven 25 × 2 m transects.
Statistical Analysis
To model the effect of the conservation Status of the reef (fixed categorical covariate with two levels: degraded and healthy) on individual (size as stipe diameter, wet weight, and blade area) and population (density, biomass, and LAI) attributes of kelp, generalized linear mixed-effects models (GLMM) were used which included Site (individual and population attributes) and sampling Quadrat (individual attributes only) as random effects. Due to the lack of any viable kelps in degraded reefs in winter, separate models were fitted to each season rather than a combined analysis of the whole data set. Depending on the nature of the data (counts, continuous variables), several error terms and link functions were tested with and without the dispersion parameter modeled as a function of Status. The best-fit model for each variable and season was determined by chi-square tests of Akaike Information Criterion (AIC). Model fitting was conducted with package “glmmTMB” (Bolker, 2021) of R 4.1.1 software with RStudio interface (R Core Team, 2021), while model assumptions were verified by plotting residuals vs. fitted values, vs. each covariate in the model, and with simulation-based approaches that create readily interpretable scales (quantile) residuals (Dunn and Smyth, 1996; Gelman and Hill, 2006) available in the “DHARMa” package (Hartig, 2021). Wald χ2 statistics, least-squared means, and pairwise tests were computed with the help of packages “car,” “effects,” “emmeans” (Fox and Weisberg, 2019; Lenth, 2021). GLMMs were also used to model the abundance of both seaweed-eating fish and sea urchins, this time with Conservation Status (categorical with two levels: degraded and healthy) and Season (categorical with three levels: autumn, winter, and spring) as fixed covariates, and Site as a random effect. Again, several error terms appropriate for count data (Poisson, Quasi–Poisson, negative binomial) were tested with a log link. Model selection, validation and inference followed the same procedures and used the same R-packages described for kelp data.
The influence of conservation Status (fixed factor with two levels: healthy vs. degraded) and Season (fixed factor with four levels: summer, autumn, winter, and spring) on the frequency of kelps assigned to each category of HPI (fixed factor with seven levels, one per HPI category) was tested with a 3-way general loglinear model. Loglinear models are typically used for analyzing contingency tables, and treat cell frequencies as counts distributed as a Poisson random variable modeled with a log-link. In our case, maximum likelihood estimation of model parameters was done with the Newton-Raphson method, and a significant 3-way interaction was interpreted as evidence that the frequency of the various categories of HPI differed between healthy and degraded reefs and that their differences changed with Season. To test whether there was a significant 3-way interaction, both a full model with the three factors and all their interactions, and a reduced model derived from it after removing the higher order 3-way interaction were fitted. These two models were compared (Chi-squared test) to assess whether omitting the 3-way interaction significantly worsened the fit. If so, separate loglinear models were fitted to the frequencies recorded on each season (i.e., 2-way contingency tables with Status and HPI as fixed factors) to test for the influence of Status on the frequency of the various categories of HPI with a Chi-squared test. When significant, Chi-squared tests were followed by odds ratio tests for each HPI category to interpret the lack of independence in the corresponding contingency table. Loglinear models (3-way and 2-way) and odds ratio tests were likewise used to test for the independence between the effects of conservation Status and Season on Invertebrate/Vertebrate Ratio (IVR) frequencies (fixed factor with seven levels, one per IVR category) following the same procedure described above for HPI. Loglinear model fitting and testing was performed with the help of IBM SPSS Stastistics v27 package while odds ratio tests were computed following Agresti (1996) as described by Quinn and Keough (2002).
Results
Kelp Population Structure
Survey data showed markedly contrasting seasonal demographic patterns between Laminaria ochroleuca populations on healthy and degraded reefs. Both mean individual size (as stipe diameter) and density differed between healthy and degraded reefs, particularly in summer and winter (Supplementary Table 1). Plants covered a much wider range of individual sizes in healthy reefs (Figure 3), with larger plants reaching stipe diameters close to 30 mm all year-round. Additionally, both mean individual size and density changed little over the year on healthy reefs (least-squared mean values between 20 and 40 plants/m2), except for a mild drop in mean size spring when a large number of recently recruited plants (stipe diameter ≤ 1 mm) were added to the populations and the mean density increased up to 60.5 ± 16.30 plants/m2 (Figures 4A,B). By contrast, the largest individuals found on degraded reefs barely reached 10 mm, and this only occurred in autumn. These populations were exclusively composed of plants recruited in the year, and mean individual size increased, while density decreased, as they grew from summer to autumn. By winter, all the populations in degraded reefs had collapsed and no viable individual was found at any of the sampled sites, being the degraded reefs reoccupied at very high densities (42.0 ± 17.90 plants/m2) by very small (0.65 ± 0.119 mm stipe diameter) newly recruited plants by the following spring.
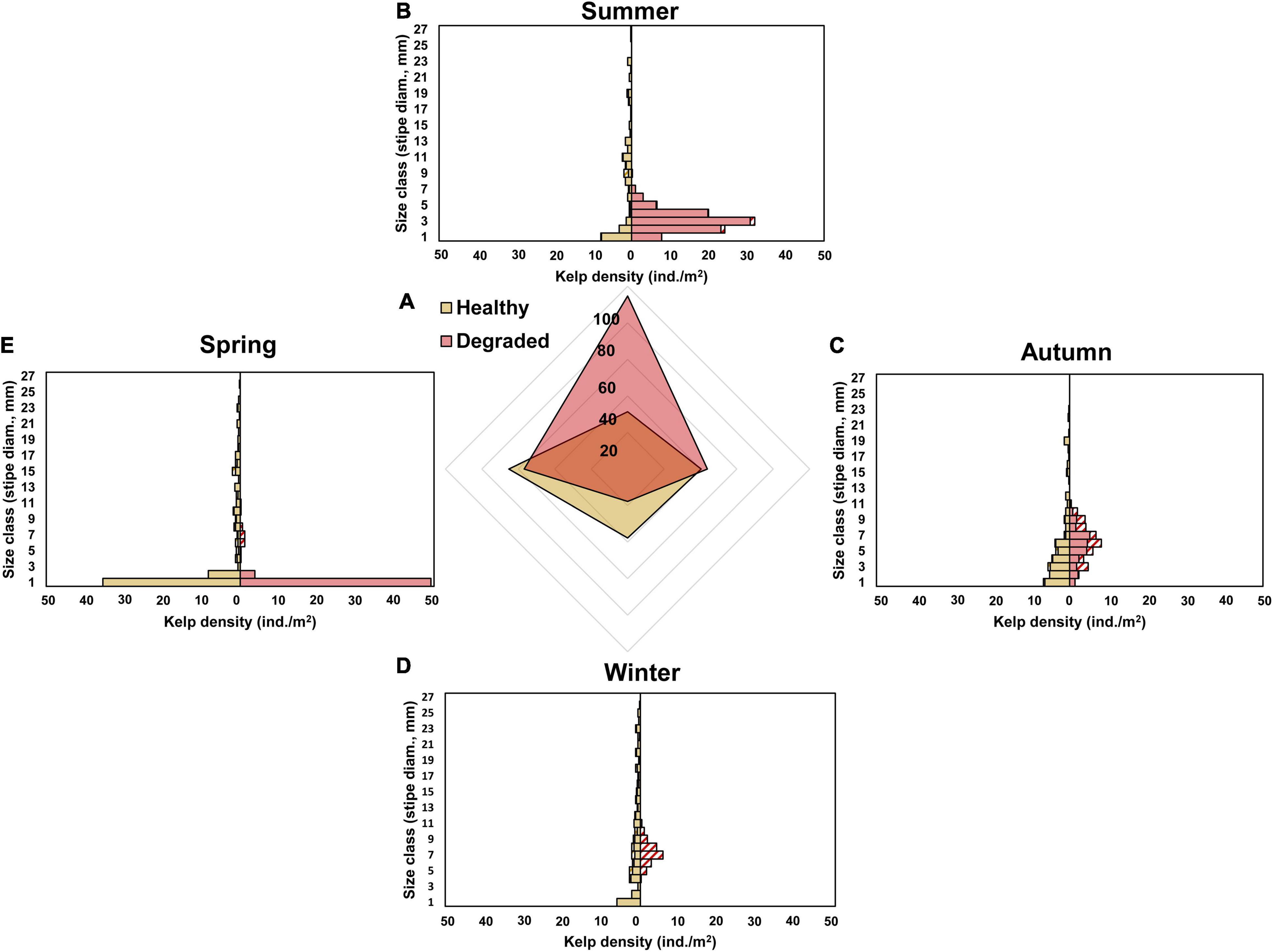
Figure 3. Laminaria ochroleuca. Seasonal changes in the density (as number of individuals per m2) recorded on healthy and degraded golden kelp reefs. Abundance estimates shown for (A) the whole population and (B–E) for kelps separated into size classes according to stipe diameter (stipe diameter recorded just above the holdfast). The radar plot (A) shows seasonal averages for healthy (light shading) and degraded (dark shading) reefs (four sampling sites for each reef type, five sampling quadrats per site); plot axes point to the corresponding season of the year. Striped bars in panels (B–E) are ecologically dead plants that lost their growth meristem due to herbivorism.
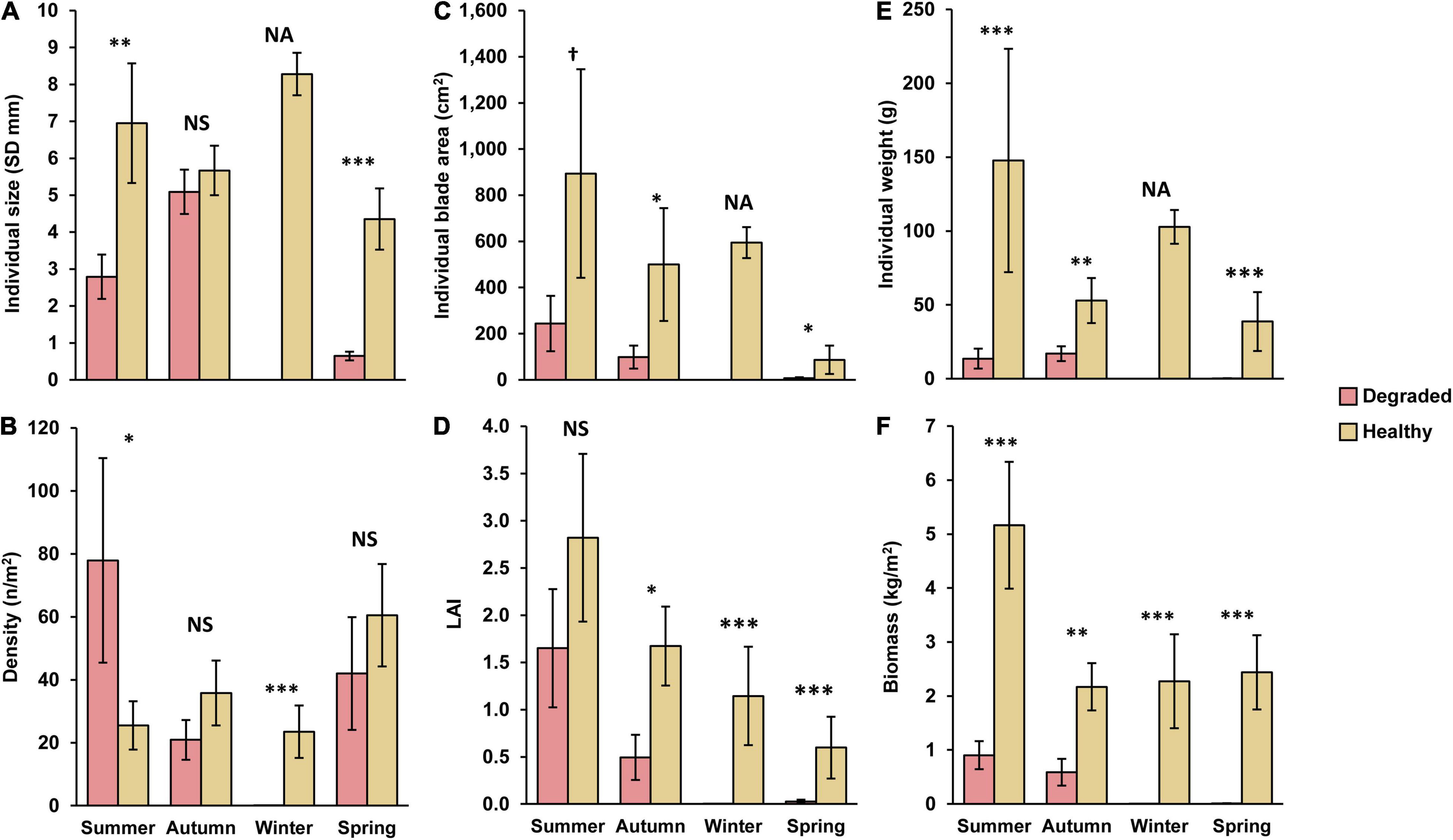
Figure 4. Laminaria ochroleuca. Influence of conservation status (healthy vs. degraded golden kelp reefs) on (A,C,E) individual (size as stipe diameter SD, blade area, weight) and (B,D,F) population (density, Leaf Area Index, biomass) attributes. Values are least-squares means (and SE) from a generalized linear mixed model GLMM. df for individual-based means are 597 (summer), 313 (autumn), 154 (winter), and 562 (spring). df for population-based means are 35 (summer), 36 (autumn), 19 (winter), and 35 (spring). Significant differences between healthy and degraded kelp reefs are shown for each season based on Wald χ2 tests of conservation Status as fixed term fitted in a GLMM with Site (all variables) and sampling Quadrat (individual-based variables only) as random effects. †P < 0.1; *P < 0.5; **P < 0.01; ***P < 0.001. NS = non-significant. NA = GLMM not applicable to winter populations because no viable plant was found on degraded reefs. A detailed summary of each GLMM can be found in the Supplementary Material.
The differences between healthy and degraded reefs were even more pronounced when populations and individuals were compared in terms of biomass and of photosynthetic surface area. The mean individual blade surface was consistently and significantly higher on healthy than on degraded reefs throughout the year (Figure 4C), and followed the seasonal fluctuations typical of a perennial plant whose blade plays a reproductive role, meaning that its sizes decreases drastically each year after spore release. In our case, blade development peaked in summer (mean individual blade area = 894 ± 452.0 cm2), decreasing afterward in autumn and winter as individuals matured and their blade degraded (500 ± 244.4 and 594 ± 67.0 cm2, respectively; Figure 4C). In spring, mean blade area in healthy reefs reached a low (26 ± 7.7 cm2) due as populations combined adult plants at different stages of blade re-growth with a large number of recently recruited plants with tiny blades (Supplementary Figure 3). The seasonal changes in individual blade size had a distinct effect on the capacity of kelp populations for light harvesting as LAI followed a neat gradual decreasing trend from a summer peak (2.82 ± 0.889) to a spring low (0.60 ± 0.328; Figure 4D). In comparison, the photosynthetic surface area in degraded reefs attained some development only in summer and autumn, but its values were just 20–27% (mean individual blade area) or 30–60% (LAI) of those recorded on healthy reefs. The rest of the year, the photosynthetic surface was minimal (spring) or zero (winter) either because the populations contained only new recruits (spring) or because there were no viable individuals at all (winter).
Like the photosynthetic surface area, kelp biomass was significantly higher in healthy than in degraded reefs throughout the year. The changes in mean individual size and blade development described above meant that mean plant weight in healthy reefs ranged from 147.8 ± 75.65 g WW in summer to 38.7 ± 19.93 g WW in spring (Figure 4E), with the largest plants reaching weights ranging from 1.27 kg in summer to 0.62 kg in autumn. Meanwhile, plant weight was, on average, from 67.8% (autumn) to 99.4% (spring) smaller in degraded than in healthy reefs, and the largest plants never exceeded 122 g WW. Unsurprisingly, the population biomass followed the same pattern, being largely stable in healthy reefs for most of the year (autumn-spring range for mean biomass: 2.17–2.44 kg/m2, Figure 4F and Supplementary Figure 4), and only showing a marked increase in summer when mean biomass per square meter doubled (5.16 ± 1.174 kg/m2) due to the complete development of blades in most plants. By contrast, the mean biomass on degraded reefs barely reached 1 kg per square meter (0.90 ± 0.247 kg/m2) in summer, lowering to 0.009 ± 0.0046 kg/m2 in spring and to zero in winter.
Herbivory Intensity on Kelp
The frequency of the various categories of the herbivory pressure index (HPI) differed between healthy and degraded reefs, and the 3-way general loglinear model showed that their differences varied with season (χ2 = 274.31, p-value < 0.001 for the HPI × Status × Season interaction) (Figure 5 and Table 1A). On healthy reefs, the partition of the kelps among the various categories of HPI remained remarkably stable throughout the year, with most kelps assigned to HPI-1 (<25% of the blade edge with herbivory marks): from 59.26% in winter to 72.82% in autumn. The second most frequent category after HPI-1, but at some distance, was HPI-0 (no bite markings), which accounted for 12% of the plants from summer to winter, and up to 23.3% in spring. On the other hand, the categories with the plants most severely damaged by herbivores (HPI-5 and 6) where the loss of blade and growth meristem implies that these plants are ecologically dead, never exceeded 13.8% (winter).
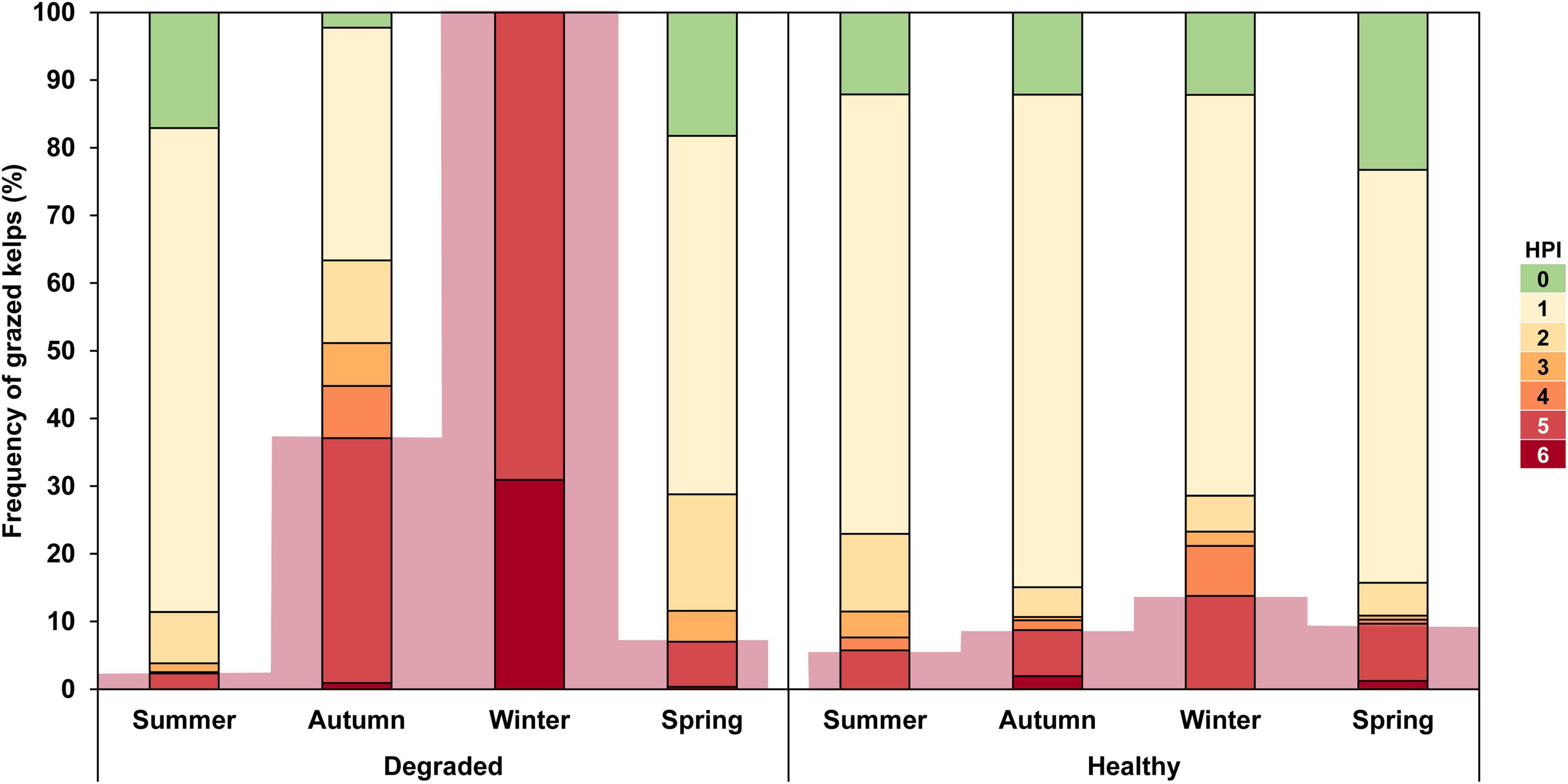
Figure 5. Laminaria ochroleuca. Seasonal changes in the frequency of Herbivory Pressure Index (HPI) categories in kelps sampled on degraded (n = 1,090) and healthy (n = 883) golden kelp reefs. HPI-0 = no bite marks, HPI-1 ≤ 25% of the blade edge with bite marks, HPI-2 = 25–50% of the blade edge with bite marks, HPI-3 = 50–75% of the blade edge with bite marks, HPI-4 = 75–100% of blade edge with bite marks, HPI-5 = stipe and holdfast only, HPI-6 = holdfast only. Light-red background shading highlights changes in the frequency of ecologically dead kelps (i.e., downgraded to a meristem-less stage by herbivory).
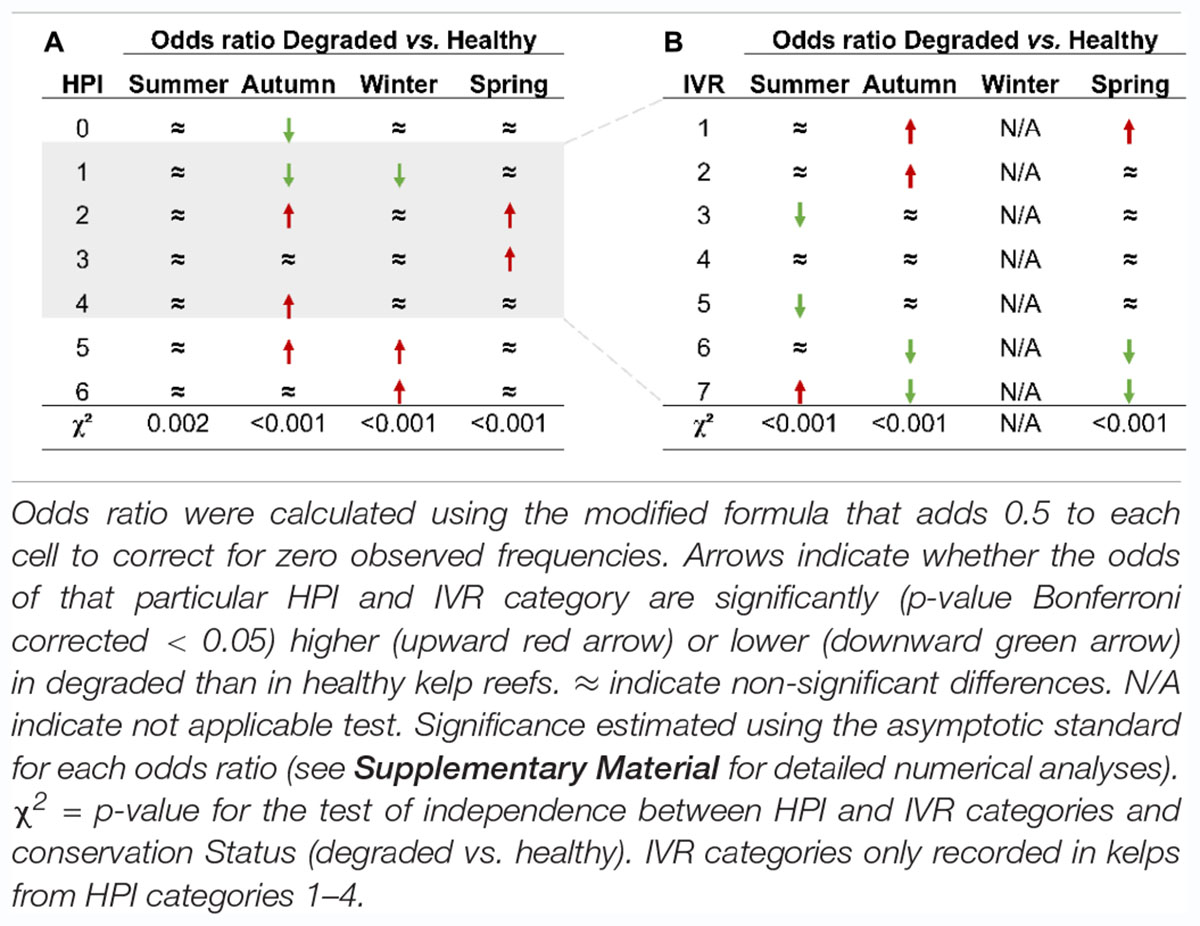
Table 1. Ratio test for the odds of each (A) herbivory pressure index (HPI) and (B) invertebrate/vertebrate ratio (IVR) category on degraded vs. healthy golden kelp reefs.
Unlike the stability of healthy reefs, the frequency of the various categories of HPI varied greatly throughout the year on degraded reefs. Furthermore, odds ratio tests showed significant differences between healthy and degraded reefs for many categories of HPI in all seasons, although the differences between healthy and degraded reefs were distinctly more pronounced in winter (χ2 = 215.69, p-value < 0.001, Supplementary Table 2) and autumn (χ2 = 114.25, p-value < 0.001) than in spring (χ2 = 39.34, p-value < 0.001) or summer (χ2 = 18.40, p-value = 0.002). In fact, the pattern of damage found on degraded reefs in summer and spring shared many similarities with the pattern observed on healthy reefs: a predominance of HPI-1 and a very low frequency of extremely damaged plants (HPI-5 and 6) (Figure 5 and Table 1A). This pattern changed considerably in autumn and winter, when few (36.65% in autumn) or none (winter) of the kelps showed little damage by herbivores. Indeed, the odds of encountering a kelp with little (HPI-1) or no damage (HPI-0) on degraded reefs was often significantly lower than on healthy reefs (Supplementary Table 2). In parallel with the decline of damage-free kelps, the relative abundance of ecologically dead kelps increased sharply in degraded reefs in autumn, when 36.2% belonged to HPI-5 and 0.9% to HPI-6, and especially in winter when all the plants belonged to either HPI-5 (69.1%) and HPI-6 (30.9%). As a result, the odds of finding an HPI-5 kelp on degraded reefs increased significantly in autumn (7.52 times greater than in healthy reefs, p-value < 0.0001) and in winter (12.95 times greater, p-value < 0.0001) while the chances of finding an HPI-6 kelp in winter was 167.70 times higher in degraded than in healthy reefs (p-value < 0.0001).
As for the agents of herbivory damage, the invertebrate/vertebrate ratio (IVR) index was applied to the 1,383 partially damaged plants with clear herbivory marks on their blade (i.e., HPI categories 1–4). Again, the frequency of the different IVR categories varied significantly with the conservation status and with season (χ2 = 98.211, p-value < 0.001 for the IVR x conservation Status x Season interaction) (Figure 6). On healthy reefs, the allocation of kelps to IVR categories was uniform throughout the year with over 60% of plants in IVR categories 6 and 7, i.e., plants where all or nearly all (>95%) the damage was identified as being caused by invertebrates. This pattern was likewise applicable to degraded reefs, but only in summer when an overwhelming majority of plants with clear herbivory marks on their blade were allocated to IVR-6 and 7 (86.1%). In fact, the odds of finding a kelp in the IVR-7 category were significantly higher (3.80 times greater, p-value < 0.0001) on degraded than on healthy reefs (Table 1B and Supplementary Table 2). Nonetheless, since most of the partially damaged summer plants belonged to HPI-1 (i.e., <25% blade edge damaged), the net damaged caused by the invertebrates on degraded kelp populations could be regarded as moderate. Summer aside, most of the damaged caused by herbivores on degraded reefs was readily identified as the distinctive fish bite marks. Thus, IVR categories 1 and 2 became predominant in autumn (25.4% and 38.8%, respectively) and the odds of a kelp being assigned to them on degraded reefs were 3.80 (IVR-1, p-value < 0.001) and 5.26 (IVR-2, p-value < 0.0001) times greater than on healthy reefs. Autumn gave way to a winter in which all plants were reduced to non-viable stumps by herbivores, and where the lack of blade did not allow an assignment to any IVR category. In spring, with the recruitment of new plants on degraded reefs, fish damage was again predominant, with 61.5% of plants assigned to IVR categories 1 and 2. This was very different from the situation recorded in healthy reefs at the same season and made the odds of belonging to IVR-1 5.25 times higher on degraded than on healthy reefs.
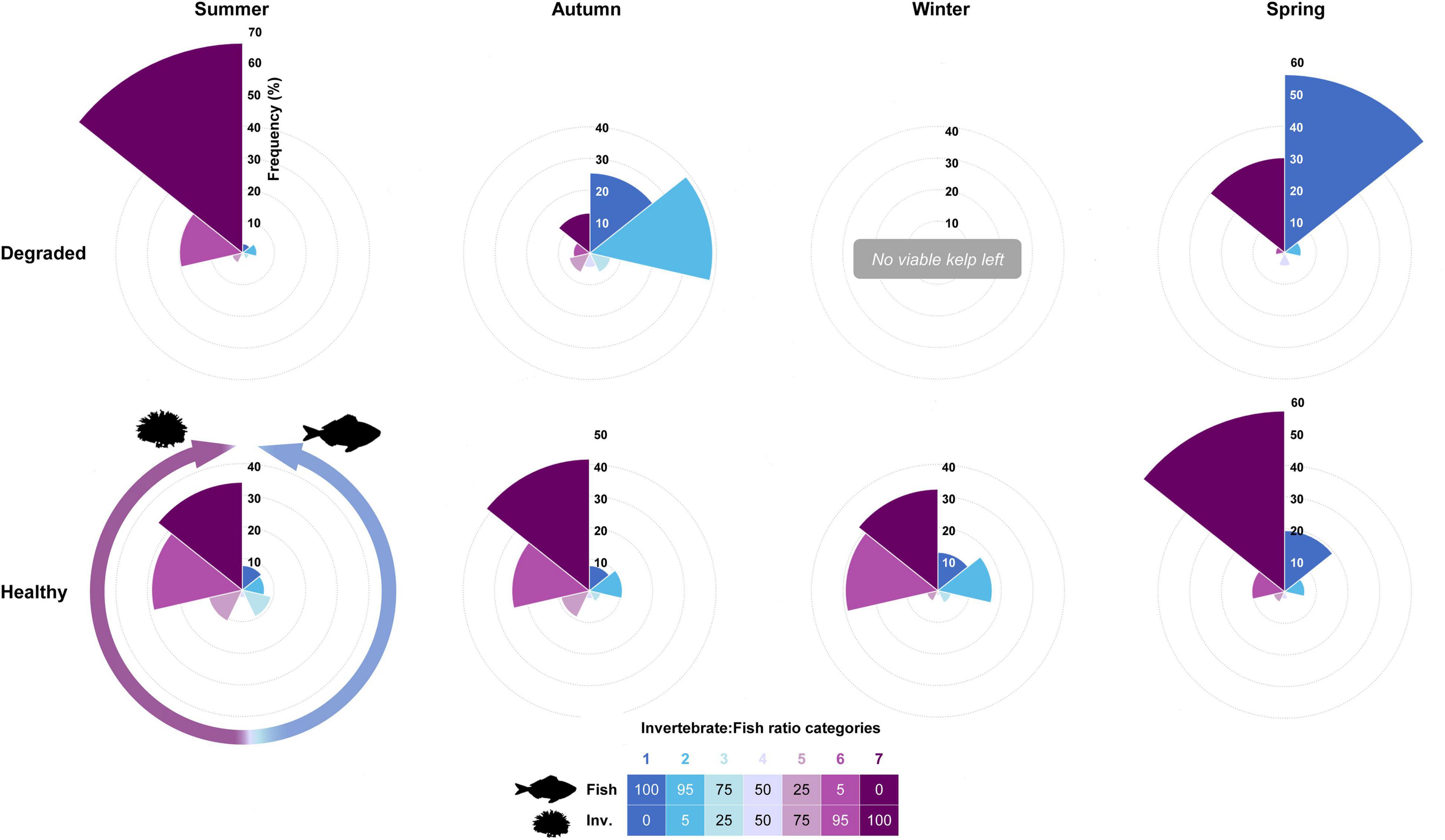
Figure 6. Laminaria ochroleuca. Seasonal changes in the relative herbivory pressure by fishes and invertebrates. Frequency of Invertebrate/Vertebrate Ratio (IVR) categories recorded in kelps from HPI categories 1–4 sampled on degraded (n = 729) and healthy (n = 654) reefs (see text and Figure 5 for a detailed explanation of HPI categories). IVR is based on the relative length of herbivory marks by fishes and invertebrates found along the blade edge, assigning each kelp to one out of seven categories of Invertebrate: Fish ratios: IVR-1 (0:100 ratio, dark blue), IVR-2 (5:95), IVR-3 (25:75), IVR-4 (50:50), IVR-5 (75:25), IVR-6 (95:5), and IVR-7 (100:0, dark purple).
Abundance of Macro-Grazers
Between autumn 2019 and spring 2020, we conducted a total of 168 quantitative fish and sea urchin transects at the four degraded (n = 84) and the four healthy reefs (n = 84), with an average depth of 8.1 ± 1.52 m (mean ± SD). From the 20 species of fish recorded (Supplementary Table 3), only 5 included seaweeds in their diet and were selected for further analyses: four omnivorous (Boops boops, Diplodus vulgaris, Diplodus sargus, and Spondyliosoma cantharus), and one herbivorous (Sarpa salpa) (Figure 7A and Supplementary Table 3). A GLMM analysis of the combined abundances of the five fish revealed no interaction between reef Status and Season. Consequently, a reduced model with no interaction was fitted to the abundance data using Site (reef) as a random covariate, a Quasi–Poisson error distribution, and a log link (Table 2). The GLMM indicated that the conservation status had a significant effect on the abundance of seaweed-eating fish (p-value = 0.0305), and model inferences show that, on average, they were 3.58 times more abundant on degraded than on healthy reefs (model-estimated means ± SE: 6.4 ± 2.63 ind./100 m2 and 1.8 ± 0.90 ind./100 m2 for healthy and degraded reefs, respectively). Interestingly, the only herbivorous fish recorded in our study (S. salpa) was detected exclusively on degraded reefs (Figure 7A) and only in the cooler seasons (autumn, winter). Like B. boops, S. salpa typically occur in very large schools.
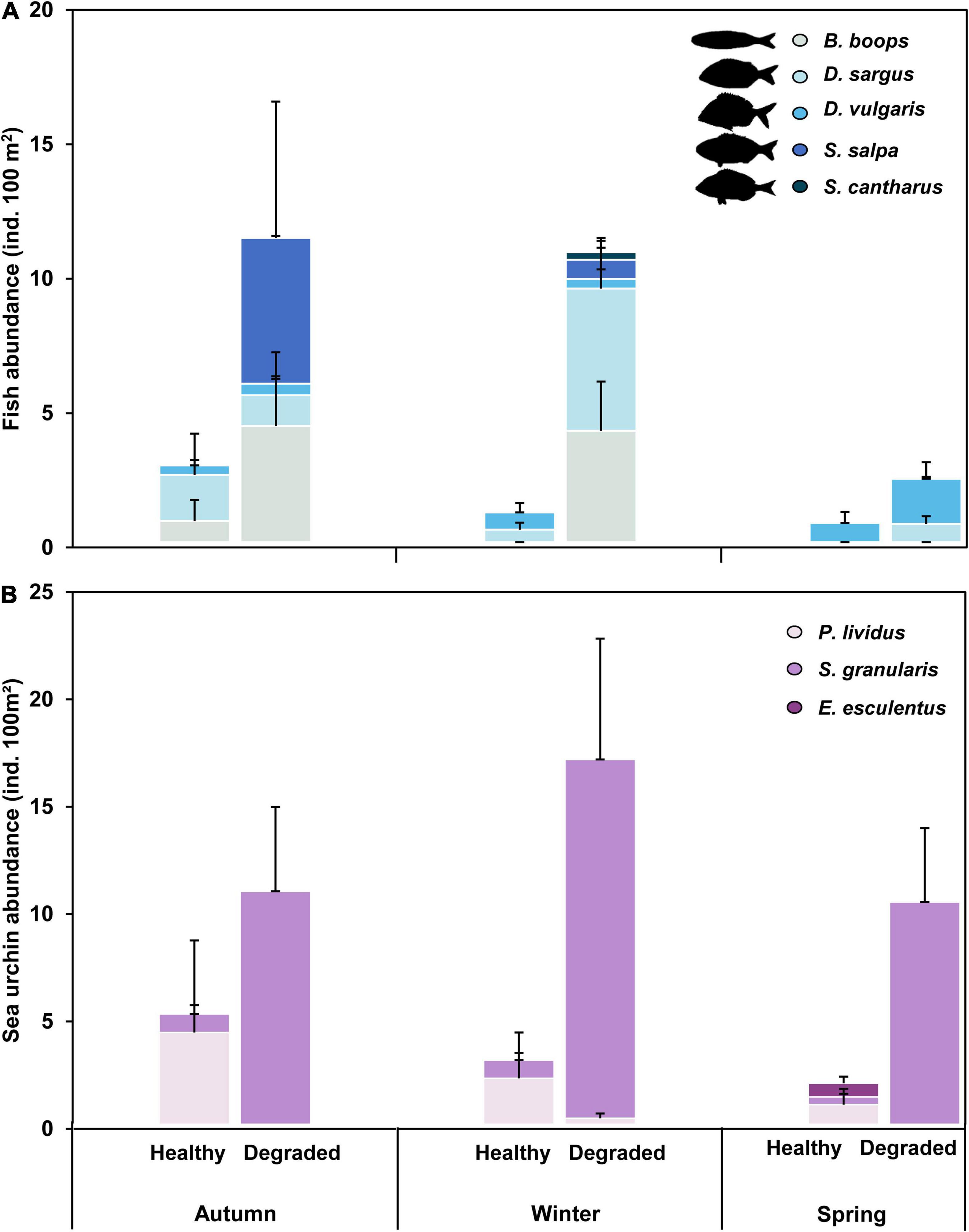
Figure 7. Abundance (mean + SE) of (A) fish and (B) sea urchins on healthy and degraded golden kelp reefs in autumn, winter and spring. Only fish that included seaweeds in their diet shown.
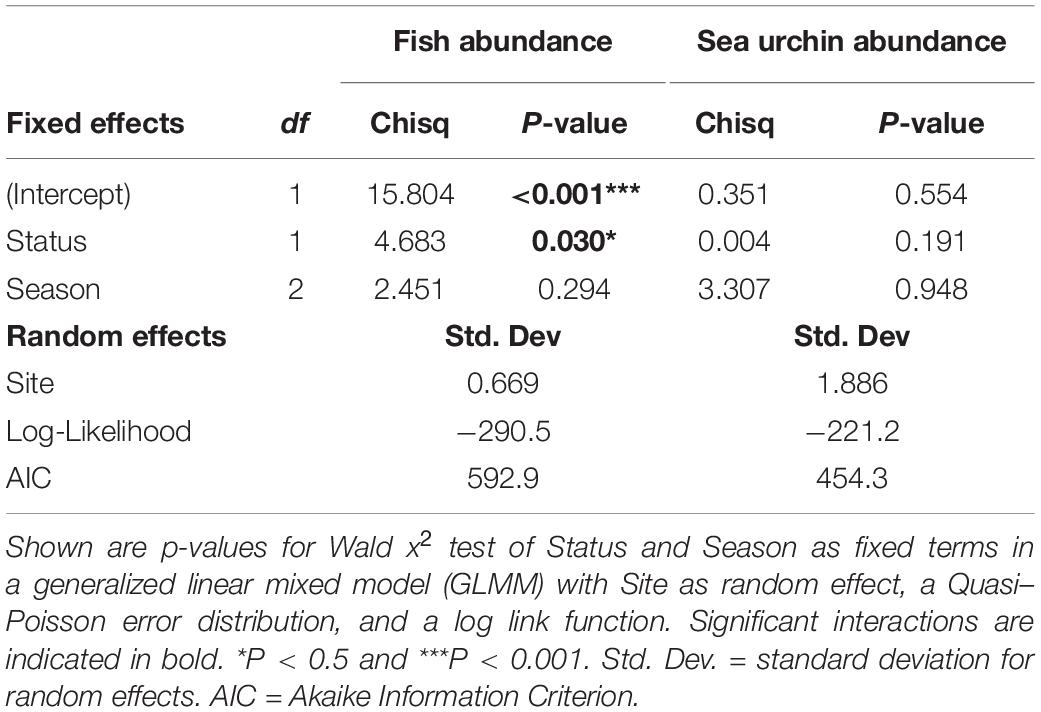
Table 2. Influence of conservation Status (fixed covariate with two levels: healthy vs. degraded) and Season (fixed covariate with three levels: autumn, winter, and spring) on the abundance of seaweed-eating fish and sea urchins.
We found three species of sea urchin at the sampling reefs: Paracentrotus lividus, Echinus esculentus and Sphaerechinus granularis (Figure 7B). As found for fish abundances, no significant interaction between Status and Season was detected by a preliminary GLMM analysis; consequently, a simpler reduced model with no interaction was fitted to sea urchin data. GLMM results for the reduced model revealed that, although mean sea urchins abundance was higher on degraded reefs, the difference was non-significant (Table 2). This was likely linked to the strong spatial variability among reefs found in the sea urchin data (χ2 likelihood ratio test for random factor Site = 120.01, p-value < 0.0001), which makes the mean values for each Status somewhat misleading. Thus, the mean abundances estimated for each conservation Status depended mostly on only two sites (one degraded, S8, and one healthy, S2) where sea urchins were particularly abundant, while these invertebrates were notably scarce or even totally absent at the remaining six sites (Supplementary Table 4).
Discussion
Until now, the northwest Spain could be thought of as a likely refuge for golden kelp forest against warming due to the cooling influence of the upwelling events off its coasts, especially compared to the warmer surrounding shorelines (Southern Portugal, Cantabrian Sea) (Fernández, 2011; Tuya et al., 2012; Voerman et al., 2013). However, our study shows that meeting the thermal requirements of kelp is not a guarantee of survival, as biotic interactions may also be as decisive as abiotic environmental conditions. Water warming cannot be the immediate cause of the decline of the kelp populations investigated in Ría de Vigo, as reefs located a few km away under similar abiotic conditions (temperature, salinity, nutrients, light, and wave exposure) followed contrasting demographic trajectories. Instead, it is overgrazing that is preventing some kelp beds from regaining the healthy forests with large adult kelp that used to occupy those reefs some years ago. This is clearly evidenced by our Herbivory Pressure Index, which shows how the herbivory damage gradually increased in autumn and, especially in winter, on degraded reefs relative to healthy reefs. Thus, the frequency of still viable but severely damaged kelp (i.e., HPI categories 2–4) increased on degraded reefs relative to healthy reefs in autumn, leading to a population collapse in winter when all kelps on degraded reefs had been reduced by herbivores to meristem-less stubs, thereby forcing a recolonization of those reefs by new recruits the following spring. A pattern that, although our data only covers one seasonal cycle, has been recurrent in the area in recent years (C. Piñeiro-Corbeira personal observation).
Herbivory pressure leading to kelp forest collapse is not unusual in temperate latitudes. However, it has been mainly attributed to sea urchins. Indeed, the involvement of sea urchins in kelp collapse has been thoroughly studied in temperate areas in the past (Estes and Palmisano, 1974; Dayton, 1975), but also in the present linked to climate change (Carnell and Keough, 2019; Christie et al., 2019). Depending on the environment (e.g., food availability, predator abundance, and diseases), sea urchins may vary their densities or even their behavior with consequences for kelps and other canopy-forming seaweeds (Mann, 2000). Thus, predator release (e.g., from finfish, crabs, lobsters, and mammals) has allowed sea urchin populations to proliferate, resulting in the collapse of kelp forest (Steneck and Johnson, 2014). In other cases, some sea urchins shift their behavior from a cryptic life in crevices sheltered from predators, when drift kelps and detritus are available, to emerge in dense aggregations that directly consume large macroalgae and new recruits when drift is scarce (Mann, 2000). While the first behavior is compatible with seaweed canopies, the second can lead to a shift to an alternate stable-state known as “sea urchin barrens,” with consequences for ecosystem function (Mann, 2000; Filbee-Dexter and Scheibling, 2014; Edwards et al., 2020). In some cases, the shift to a barren state occurred after another event of reduced kelp abundance, as was observed with Strongylocentrotus franciscanus and Strongylocentrotus purpuratus in Macrocystis pyrifera forest in California (Foster and Schiel, 2010), or with Heliocidaris erythrogramma in Ecklonia radiata forest in Australia (Kriegisch et al., 2019). In our study, signs of sea urchin browsing on kelps (irregular serrated bite marks) were evident on the blade edge of some recruit and adult kelps on both degraded and healthy reefs year round. However, IVR estimates show that sea urchin bite marks prevailed over fish bite marks only in reefs and/or at times when most kelps were little or no damaged by herbivores (i.e., HPI 0-1), i.e., healthy reefs year round and degraded reefs only in summer. This means that sea urchins had a low net population impact on kelp reefs, and rules out sea urchins as a potential agent of the kelp collapse recorded in degraded reefs. A low impact of sea urchin browsing on kelp was also suggested for the nearby coast of Portugal (Franco et al., 2015), and seems likewise consistent with the overall low abundance of sea urchins detected in our study. In this regard, Galicia (NW Spain) is a major sea urchin-producer in Spain and, although regulated, commercial exploitation has shown signs of over-exploitation, especially at local level (Fernández Pulpeiro et al., 2007; Casal et al., 2020).
Our results support that fish herbivory, rather than sea urchins, is the main cause of the inability of L. ochroleuca to recover the canopy state on degraded reefs in Ría de Vigo. Crescent shaped bite marks, unmistakably caused by fish, were found in kelp reefs year-round regardless of their conservation status. In most occasions (i.e., healthy reefs year-round, degraded reefs in summer) fish bite marks were considerably less frequent than sea urchin bites. However, fish bite marks became predominant on degraded reefs in autumn (IVR categories 1–2), coinciding with a massive bite damage on those reefs (i.e., 50% of kelp in HPI categories ≥ 3) and just before the winter collapse. Moreover, the meristem-less stubs found on degraded reefs in winter showed bite marks that matched the clean cuts made by fish, reinforcing the notion that it was the fish that consumed the kelp. While herbivorous fish are known to play a major role in controlling algal growth in tropical regions (Bellwood et al., 2004; Steneck et al., 2017), fish in temperate regions are more often linked to regulating the abundance of lower herbivores, sea urchins included. Thus, when temperate fish have been shown to play a role in the shift between kelp and barren states, they have historically been associated with the maintenance of a canopy state rather than its collapse (Steneck and Johnson, 2014). More recently, however, it has been shown that herbivorous fish can also have a negative impact on temperate canopies. Studies conducted in the Indo-Pacific (Western and Eastern Australia, New Zealand, and Japan) found that fish herbivory acted in combination with abiotic stressors (e.g., warming, waves) to maintain a canopy-free state (Smale and Wernberg, 2013; Bennett et al., 2015; Vergés et al., 2016; Zarco-Perello et al., 2017). In other studies, again mostly conducted in the Indo-Pacific (Jones and Andrew, 1990; Taylor and Schiel, 2010; but see Vergés et al., 2014b for a study in the Mediterranean), fish herbivores alone caused the decline of kelp and/or functionally similar canopy seaweeds (e.g., fucoids), even in the absence of external perturbations. In the Atlantic, although former exclusion experiments showed that herbivores (fish included) can drive kelp recruits into hiding in crevices under intense herbivory (Franco et al., 2015), this is the first time that fish is shown to cause the collapse of entire kelp forests on a reef scale, leaving no surviving kelp at all. Unlike some of studies conducted in the Indo-Pacific, there is no reason to suppose that fish in NW Spain is maintaining a deforested state caused by an external agent. A general warming trend as observed in many other parts of the world is discernible in NW Spain but decadal time series of relevant environmental factors such as water and air temperature, marine heat waves, wave intensity and nutrient concentrations show no evidence of pulse perturbations (Piñeiro-Corbeira et al., 2016; Barrientos et al., 2020). Alternatively, fish herbivory alone may be the cause of the current canopy-free state found in the degraded reefs. Although the degraded reefs in our study lacked large kelps, data from healthy reefs show that fish are capable of completely consuming the blade and growth meristem of these large individuals (striped bars for healthy reefs in Supplementary Figure 4), turning them into non-viable stumps that eventually die and fall off. Therefore, it does seem feasible that fish alone could collapse an entire kelp forest, juveniles and adults included. In this regard, monitoring surveys of the healthy reefs are still in progress to see if, in the near future, they will be degraded by herbivory alone. Should this be the case, it may have implications for the predictions about the future distribution of kelps in a short-medium term climate change scenario. It has been predicted that kelps are likely to disappear from the Lusitanian region due to water warming (Brodie et al., 2014). However, we are already observing the collapse of kelp forests due to herbivory, even in the absence of direct physiological stress caused by temperature.
Previous studies on temperate reefs considered the impact of both native temperate fish (Andrew and Jones, 1990; Vergés et al., 2009; Gianni et al., 2017) and non-native tropical herbivorous fish that recently expanded their range and/or abundance into temperate latitudes due to global warming (Basford et al., 2015; Bennett et al., 2015; Zarco-Perello et al., 2017; Agostini et al., 2021). In our case, it seems highly unlikely that the collapse of kelp forests in recent years can be attributed to the arrival of non-native fish, as all fish species recorded in the study area were historically present in the region. This included the salema, Sarpa salpa, the only obligate herbivorous fish in the region, and the most likely candidate for causing the collapse of the kelp forests in our study. On the one hand, its bite corresponds to the size and shape of the distinctive markings found on our kelp (perfectly circular holes, clean crescent-shaped cuts) (Boudouresque and Meinesz, 1982; Francesch, 2008; García, 2008; Franco et al., 2015). On the other hand, its abundance, as well as that of other omnivorous fishes, was higher on degraded reefs than on healthy ones, especially in autumn and winter when a peak in fish grazing pressure was detected in our study. Salema is an abundant and important warm-temperate macrograzer in the Mediterranean Sea, and its distribution range also includes the Western Indian (from Mozambique to Cape of Good Hope) and the Eastern Atlantic (from Bay of Biscay to Cape of Good Hope, including Azores, Canary Islands, and Cape Verde Islands) Oceans (Froese and Pauly, 2019). Its distribution, biology, and feeding habits (particularly over seagrasses) have been widely studied in the Mediterranean (Jadot et al., 2006; Buñuel et al., 2020a,b). By comparison, its Atlantic populations have received less attention, possibly because of the limited economic value of this fish (but see Méndez Villamil et al., 2002; and Paiva et al., 2018) and although the native range of salema extends northwards to the warm-climate Bay of Biscay, the specific environmental conditions of the eastern coasts of NW Spain do not seem to have been particularly suitable for this species (Kesner-Reyes et al., 2020). Nonetheless, reports by local fishermen suggest that this fish may have become more abundant, and its individuals larger, in and around the Ria de Vigo in recent years (Piñeiro-Corbeira et al., in press), seemingly coinciding with the recent collapse of kelp forests in this area. Moreover, landings data show that this fish was rarely caught in the area before 2004, while from 2006 onward 10--35 tonnes are usually caught each year.1 The potential drivers of this alleged increase remain unassessed. Nonetheless, since salema has a clear affinity for warm-temperate conditions, it cannot be ruled out that it may have been favored by the moderate water warming recorded in this area in recent decades (especially in autumn) (Piñeiro-Corbeira et al., 2016; Barrientos et al., 2020). If proven, we could be facing another example of biotic multipliers of the effects of climate change (sensu Zarnetske et al., 2012), as found in other cases where global warming favored an increase in herbivory pressure on kelp forests, either by sea urchins or by fish (Johnson et al., 2011; Wernberg et al., 2012).
The gregarious behavior of S. salpa, sometimes forming sizeable schools (Verlaque, 1990), could also explain the temporal pattern of damage seen in our study on degraded reefs where the collapse of kelp forests occurs rapidly in the transition from autumn to winter. Intense reef-wide damage in such a short time requires an herbivore capable of increasing its consuming pressure on kelp considerably in a likewise short time, something that seems easier to achieve for a gregarious and highly mobile species such as salema. Moreover, landings data show that, although salema is occasionally captured in Ria de Vigo throughout the year, the largest catches typically occur between July and November, thereby coinciding with the time frame when kelp damage is greatest. Studies in the Mediterranean show that schools are highly length-assorted, and that body length is positively correlated with school size (Buñuel et al., 2020a). Furthermore, salema in the Mediterranean shifts its feeding strategy as it grows, with larger schools made of larger individuals showing a potentially cooperative strategy. As a result, schools made of large individuals as those typically fished in NW Spain (pers. obs.), can very quickly cause intense damage on the vegetation. Further studies would be required to assess whether a similar shift in feeding behavior with body size is likewise applicable to the case of Atlantic populations of salema. Meanwhile, high mobility and large schools might also explain the spatial pattern of damage seen in NW Spain, where deforested reefs are located within a few kilometers of healthy canopy kelp forests, if the chances that a reef might be deforested depended on the movement patterns of salema’s schools. In this regard, the degraded reefs detected so far were all located on the islands just off the entrance of the ria, while healthy reefs were found somewhat further inland, what might be indicative of a preference by salema for the outer reaches of the ria. Nevertheless, given that there is, on average, six times less kelp biomass on degraded than on healthy reefs at the time of the summer peak, it seems plausible that salema may spread its impact to new healthy reefs in the coming years, should the biomass now found in degraded reefs be insufficient to sustain its populations. In this regard, monitoring of healthy reefs in future years could detect whether such spatial spread of damage is occurring.
Conclusion
This study reveals, for the first time, that herbivorous fish are able to lead to an annual collapse of kelp forests in the temperate Atlantic. That the degradation of Atlantic kelp forests on NW Spain is driven by fishes, rather than by sea urchins as typical seen in many other temperate latitudes, is a challenge from a management perspective. Attempts have been made to reduce the impact of herbivorous fish through exclusion devices with some success in short-term experiments, but their long-term implementation and scaling up to management-relevant sizes remains unresolved (Bennett et al., 2017). Alternatively, transplanting adult kelps or seeding very young plants have been explored as restoration tools to combat kelp forest decline with mixed results (Fredriksen et al., 2020). However, these bottom-up approaches seem appropriate for degraded reefs where propagule supply is an issue, while there is evidence that they are inappropriate when herbivory is strong (Carney et al., 2005; Vergés et al., 2020). Therefore, perhaps the only alternative to recover kelp canopy forests in NW Spain may be the removal of the herbivore that is preventing this recovery. In the case of deforestations caused by sea urchins, there is evidence that a massive removal of sea urchins can revert sea urchin barrens to a canopy kelp forest (Leinaas and Christie, 1996; Steneck et al., 2013), although the trajectory toward reversion may also depend on the characteristics of the community that has replaced the kelp forest (e.g., a crustose coralline assemblage or a fleshy turf) (Filbee-Dexter and Wernberg, 2018). However, sea urchins are more easily susceptible to removal from deforested areas than fish because they are slow and sedentary animals, which makes it easier to capture them and to delimit the population to be targeted. In the case of fish, on the other hand, it will be necessary to determine the actual home-range of schools involved in the deforestation, in order to assess the feasibility of their removal.
Data Availability Statement
The raw data supporting the conclusions of this article will be made available by the authors, without undue reservation.
Author Contributions
SB: conceptualization, methodology, field sampling, formal analysis, visualization, and writing-original draft preparation. CP-C: conceptualization, methodology, field sampling, formal analysis, visualization, project administration, supervision, and assistance in manuscript writing. RB: funding acquisition, project administration, conceptualization, methodology, formal analysis, visualization, assistance in manuscript writing, and supervision. All authors revised and approved the manuscript.
Funding
This research was funded by the “Fundación Biodiversidad y el Ministerio de Transición Ecológica y el Reto Demográfico.” BioCost Research Group acknowledges funding from Xunta de Galicia (grants ED431D 2017/2020 and ED431B 2018/49 of “Axudas para a consolidación e estructuración de unidades de investigación competitivas do SUG” program). SB received financial support from the Xunta de Galicia and the European Union (European Social Found – ESF) (grant ED481A-2018/101). CP-C received financial support from Xunta de Galicia “Axudas de apoio á etapa de formación posdoutoral “(grant ED481B-2021/095)”.
Conflict of Interest
The authors declare that the research was conducted in the absence of any commercial or financial relationships that could be construed as a potential conflict of interest.
Publisher’s Note
All claims expressed in this article are solely those of the authors and do not necessarily represent those of their affiliated organizations, or those of the publisher, the editors and the reviewers. Any product that may be evaluated in this article, or claim that may be made by its manufacturer, is not guaranteed or endorsed by the publisher.
Supplementary Material
The Supplementary Material for this article can be found online at: https://www.frontiersin.org/articles/10.3389/fmars.2022.817021/full#supplementary-material
Footnotes
References
Agostini, S., Harvey, B. P., Milazzo, M., Wada, S., Kon, K., Floc’h, N., et al. (2021). Simplification, not “tropicalization”, of temperate marine ecosystems under ocean warming and acidification. Glob. Chang. Biol. 27, 4771–4784. doi: 10.1111/gcb.15749
Agresti, A. (1996). An Introduction to Categorical Data Analysis. Hoboken, NJ: John Wiley & Sons, Inc.
Alvarez, I., Gomez-Gesteira, M., deCastro, M., Lorenzo, M. N., Crespo, A. J. C., and Dias, J. M. (2011). Comparative analysis of upwelling influence between the western and northern coast of the Iberian Peninsula. Cont. Shelf Res. 31, 388–399. doi: 10.1016/j.csr.2010.07.009
Andrew, N., and Jones, G. (1990). Patch formation by herbivorous fish in a temperate Australian kelp forest. Oecologia 85, 57–68. doi: 10.1007/BF00317343
Araújo, R. M., Assis, J., Aguillar, R., Airoldi, L., Bárbara, I., Bartsch, I., et al. (2016). Status, trends and drivers of kelp forests in Europe: an expert assessment. Biodivers. Conserv. 25, 1319–1348. doi: 10.1007/s10531-016-1141-7
Barrientos, S., Barreiro, R., Cremades, J., and Pineiro-Corbeira, C. (2020). Setting the basis for a long-term monitoring network of intertidal seaweed assemblages in northwest Spain. Mar. Environ. Res. 160:105039. doi: 10.1016/j.marenvres.2020.105039
Barrientos, S., Barreiro, R., Olmedo, M., and Piñeiro-Corbeira, C. (2019). Can patch size and patch distance improve the recolonization of mussel-seed beds exploited for aquaculture? Aquat. Conserv. Mar. Freshw. Ecosyst. 29, 1897–1908. doi: 10.1002/aqc.3191
Basford, A. J., Feary, D. A., Truong, G., Steinberg, P. D., Marzinelli, E. M., and Vergés, A. (2015). Feeding habits of range-shifting herbivores: tropical surgeonfishes in a temperate environment. Mar. Freshw. Res. 67, 75–83. doi: 10.1071/MF14208
Beck, H. J., Feary, D. A., Nakamura, Y., and Booth, D. J. (2017). Temperate macroalgae impacts tropical fish recruitment at forefronts of range expansion. Coral Reefs 36, 639–651. doi: 10.1007/s00338-017-1553-1
Bekkby, T., Rinde, E., Gundersen, H., Norderhaug, K. M., Gitmark, J. K., and Christie, H. (2014). Length, strength and water flow: relative importance of wave and current exposure on morphology in kelp Laminaria hyperborea. Mar. Ecol. Prog. Ser. 506, 61–70. doi: 10.3354/meps10778
Bellwood, D. R., Hughes, T. P., Folke, C., and Nyström, M. (2004). Confronting the coral reef crisis. Nature 429, 827–833. doi: 10.1038/nature02691
Bennett, S., Wernberg, T., and de Bettignies, T. (2017). Bubble curtains: herbivore exclusion devices for ecology and restoration of marine ecosystems? Front. Mar. Sci. 4:302. doi: 10.3389/fmars.2017.00302
Bennett, S., Wernberg, T., Harvey, E. S., Santana-Garcon, J., and Saunders, B. J. (2015). Tropical herbivores provide resilience to a climate-mediated phase shift on temperate reefs. Ecol. Lett. 18, 714–723. doi: 10.1111/ele.12450
Birkett, D. A., Maggs, C. A., Dring, M. J., and Boaden, P. J. S. (1998). Infralittoral Reef Biotipes with Kelp Species. An Overview of Dynamic and Sensivity Characteristics for Conservation Management of Marine SAC’s. Scottish Association of Marine Science: UK Marine SACs Project, Vol. VII., Oban: The Scottish Association for Marine Science.
Biskup, S., Bertocci, I., Arenas, F., and Tuya, F. (2014). Functional responses of juvenile kelps, Laminaria ochroleuca and Saccorhiza polyschides, to increasing temperatures. Aquat. Bot. 113, 117–122. doi: 10.1016/j.aquabot.2013.10.003
Bolker, B. (2021). Getting Started with the glmmTMB package. Software. Vienna: R Foundation for Statistical Computing.
Boudouresque, C.-F., and Meinesz, A. (1982). Découverte de l’herbier de Posidonie. Cahiers du Parc National de. Cah. Parc Nation Port-Cros, Fr. 4, 1–79.
Brodie, J., Williamson, C. J., Smale, D. A., Kamenos, N. A., Mieszkowska, N., Santos, R., et al. (2014). The future of the northeast Atlantic benthic flora in a high CO2 world. Ecol. Evol. 4, 2787–2789. doi: 10.1002/ece3.1105
Buñuel, X., Alcoverro, T., Pagès, J. F., Romero, J., Ruiz, J. M., and Arthur, R. (2020a). The dominant seagrass herbivore Sarpa salpa shifts its shoaling and feeding strategies as they grow. Sci. Rep. 10:10622. doi: 10.1038/s41598-020-67498-1
Buñuel, X., Alcoverro, T., Romero, J., Arthur, R., Ruiz, J. M., Pérez, M., et al. (2020b). Warming intensifies the interaction between the temperate seagrass Posidonia oceanica and its dominant fish herbivore Sarpa salpa. Mar. Environ. Res. 165:105237. doi: 10.1016/j.marenvres.2020.105237
Burdett, H. L., Wright, H., and Smale, D. A. (2019). Photophysiological responses of canopy-forming kelp species to short-term acute warming. Front. Mar. Sci. 6:516. doi: 10.3389/fmars.2019.00516
Buschmann, A. H., García, C., Espinoza, R., Filún, L., and Vásquez, J. A. (2004). “Sea urchin (Loxechinus albus) and kelp (Macrocystis pyrifera) in protected areas,” in Sea urchins Fisheries and Ecology, eds J. Lawrence and O. Guzmán (Lancaster, PA: DEStech Publications), 120–130.
Butler, C. L., Lucieer, V. L., Wotherspoon, S. J., and Johnson, C. R. (2020). Multi-decadal decline in cover of giant kelp Macrocystis pyrifera at the southern limit of its Australian range. Mar. Ecol. Prog. Ser. 653, 1–18. doi: 10.3354/meps13510
Carnell, P. E., and Keough, M. J. (2019). Reconstructing historical marine populations reveals major decline of a kelp forest ecosystem in Australia. Estuar. Coast. 42, 765–778. doi: 10.1007/s12237-019-00525-1
Carney, L. T., Waaland, J. R., Klinger, T., and Ewing, K. (2005). Restoration of the bull kelp Nereocystis luetkeana in nearshore rocky habitats. Mar. Ecol. Prog. Ser. 302, 49–61. doi: 10.3354/meps302049
Casado-Amezúa, P., Araújo, R., Bárbara, I., Bermejo, R., Borja, Á, Díez, I., et al. (2019). Distributional shifts of canopy-forming seaweeds from the Atlantic coast of Southern Europe. Biodivers. Conserv. 28, 1151–1172. doi: 10.1007/s10531-019-01716-9
Casal, G., Fernández-Boán, M., Fernández, N., Freire, J., and Fernández, L. (2020). Spatial structure and abundance of the sea urchin Paracentrotus lividus in subtidal fishing grounds of the Galician coast (NW- Spain). Estuar. Coast. Shelf Sci. 239:106753. doi: 10.1016/j.ecss.2020.106753
Catoira Gómez, J. L. (2009). Cultivo y Gestión del Erizo de Mar (Paracentrotus lividus, Lamarck, 1816): Informe Final. Madrid: Ministerio de Medio Ambiente y Medio Rural y Marino.
Choat, J. H. (1982). Fish feeding and the structure of benthic communities in temperate waters. Annu. Rev. Ecol. Evol. Syst. 13, 423–449. doi: 10.1146/annurev.es.13.110182.002231
Christie, H., Gundersen, H., Rinde, E., Filbee-Dexter, K., Norderhaug, K. M., Pedersen, T., et al. (2019). Can multitrophic interactions and ocean warming influence large-scale kelp recovery? Ecol. Evol. 9, 2847–2862. doi: 10.1002/ece3.4963
Dayton, P. K. (1975). Experimental studies of algal canopy interactions in a sea otter-dominated kelp community at Amchitka Island, Alaska. Fish. Bull. 73, 230–237.
Dayton, P. K. (1985). Ecology of kelp communities. Annu. Rev. Ecol. Evol. Syst. 16, 215–245. doi: 10.1146/annurev.es.16.110185.001243
Díez, I., Muguerza, N., Santolaria, A., Ganzedo, U., and Gorostiaga, J. M. (2012). Seaweed assemblage changes in the eastern Cantabrian Sea and their potential relationship to climate change. Estuar. Coast. Shelf Sci. 99, 108–120. doi: 10.1016/j.ecss.2011.12.027
Drew, E. A. (1974). An ecological study of Laminaria ochroleuca Pyl. growing below 50 metres in the Straits of Messina. J. Exp. Mar. Biol. Ecol. 15, 11–24. doi: 10.1016/0022-0981(74)90059-8
Dunn, P. K., and Smyth, G. K. (1996). Randomized quantile residuals. J. Comput. Graph. Stat. 5, 236–244. doi: 10.2307/1390802
Edwards, M., Konar, B., Ju-Hyoung, K., Gabara, S., Genoa, S., McHugh, T., et al. (2020). Marine deforestation leads to widespread loss of ecosystem function. PLoS One 15:e0226173. doi: 10.1371/journal.pone.0226173
Estes, J. A., and Palmisano, J. F. (1974). Sea otters: their role in structuring nearshore communities. Science 185, 1058–1060. doi: 10.1126/science.185.4156.1058
Fernández Pulpeiro, E., Lustres, V., Brea, E., Sestelo, M., Souto, J., and Pazos, M. (2007). Recartografado e Dinámica de Poboacións de Especies de Invertebrados Mariños Asociadas a Substratos Rochosos da Costa da Morte. Santiago de Compostela: Universidad de Santiago de Compostela.
Fernández, C. (2011). The retreat of large brown seaweeds on the north coast of Spain: the case of Saccorhiza polyschides. Eur. J. Phycol. 46, 352–360. doi: 10.1080/09670262.2011.617840
Fernández, C. (2016). Current status and multidecadal biogeographical changes in rocky intertidal algal assemblages: the northern Spanish coast. Estuar. Coast. Shelf Sci. 171, 35–40. doi: 10.1016/j.ecss.2016.01.026
Fernández-Boán, M., Fernández, L., and Freire, J. (2012). History and management strategies of the sea urchin Paracentrotus lividus fishery in Galicia (NW Spain). Ocean Coast. Manag. 69, 265–272. doi: 10.1016/j.ocecoaman.2012.07.032
Filbee-Dexter, K., and Scheibling, R. E. (2014). Sea urchin barrens as alternative stable states of collapsed kelp ecosystems. Mar. Ecol. Prog. Ser. 495, 1–25. doi: 10.3354/meps10573
Filbee-Dexter, K., and Wernberg, T. (2018). Rise of turfs: a new battlefront for globally declining kelp forests. BioScience 68, 64–76. doi: 10.1093/biosci/bix147
Floeter, S. R., Behrens, M. D., Ferreira, C. E. L., Paddack, M. J., and Horn, M. H. (2005). Geographical gradients of marine herbivorous fishes: patterns and processes. Mar. Biol. 147, 1435–1447. doi: 10.1007/s00227-005-0027-0
Flores-Moya, A. (2012). “Warm temperate seaweed communities: a case study of deep water kelp forests from the Alboran Sea (SW Mediterranean Sea) and the Strait of Gibraltar,” in Seaweed biology, eds C. Wiencke and K. Bischof (Cham: Springer), 315–327. doi: 10.1007/978-3-642-28451-9_15
Foster, M. S., and Schiel, D. R. (2010). Loss of predators and the collapse of southern California kelp forests (?): alternatives, explanations and generalizations. J. Exp. Mar. Biol. Ecol. 393, 59–70. doi: 10.1016/j.jembe.2010.07.002
Fraga, F. (1981). “Upwelling off the Galician coast, northwest Spain,” in Coastal and Estuarine Sciences 1, ed. F. A. Richards (Washington, DC: American Geophysical Union), 176–182. doi: 10.1016/j.marpolbul.2006.03.011
Francesch, O. (2008). Laminarias y Sabogas. Wordpress [Online]. Available online at: https://oscar2007.wordpress.com/ (accessed October 27, 2020).
Franco, J. N., Tuya, F., Bertocci, I., Martínez, B., and Arenas, F. (2016). Eco-physiological response of the kelp Laminaria ochroleuca to varying temperature and nutrients: implications under climate change. Front. Mar. Sci. 3:106. doi: 10.3389/conf.FMARS.2016.04.00106
Franco, J. N., Wernberg, T., Bertocci, I., Duarte, P., Jacinto, D., Vasco-Rodrigues, N., et al. (2015). Herbivory drives kelp recruits into ‘hiding’ in a warm ocean climate. Mar. Ecol. Prog. Ser. 536, 1–9. doi: 10.3354/meps11445
Franco, J. N., Wernberg, T., Bertocci, I., Jacinto, D., Maranhão, P., Pereira, T., et al. (2017). Modulation of different kelp life stages by herbivory: compensatory growth versus population decimation. Mar. Biol. 164, 164. doi: 10.1007/s00227-017-3196-8
Fredriksen, S., Filbee-Dexter, K., Norderhaug, K. M., Steen, H., Bodvin, T., Coleman, M. A., et al. (2020). Green gravel: a novel restoration tool to combat kelp forest decline. Sci. Rep. 10:3983. doi: 10.1038/s41598-020-60553-x
Froese, R., and Pauly, D. (2019). FishBase. World Wide Web electronic Publication [Online]. Available online at: www.fishbase.org (accessed October 27, 2020).
Fujita, D. (2010). Current status and problems of isoyake in Japan. Bull. Fish. Res. Agen 32, 33–42.
García, R. (2008). La laminaria, un alga básica para el ecosistema, desaparece en Galicia. La Voz Galicia Nov. 6, 2008.
Gelman, A., and Hill, J. (2006). Data analysis using Regression and Multilevel/Hierarchical Models. New York, NY: Cambridge university press.
Gianni, F., Bartolini, F., Pey, A., Laurent, M., Martins, G. M., Airoldi, L., et al. (2017). Threats to large brown algal forests in temperate seas: the overlooked role of native herbivorous fish. Sci. Rep. 7:6012. doi: 10.1038/s41598-017-06394-7
Gilson, A. R., Smale, D. A., and O’Connor, N. (2021). Ocean warming and species range shifts affect rates of ecosystem functioning by altering consumer–resource interactions. Ecology 102:e03341. doi: 10.1002/ecy.3341
Gómez-Gesteira, M., deCastro, M., Alvarez, I., and Gómez-Gesteira, J. L. (2008). Coastal sea surface temperature warming trend along the continental part of the Atlantic Arc (1985–2005). J. Geophys. Res. 113:C04010. doi: 10.1029/2007JC004315
Gutow, L., Poore, A. G. B., Díaz Poblete, M. A., Villalobos, V., and Thiel, M. (2020). Small burrowing amphipods cause major damage in a large kelp. Proc. R. Soc. Lond. B Biol. Sci. 287:20200330. doi: 10.1098/rspb.2020.0330
Hartig, F. (2021). DHARMa: Residual Diagnostics for Hierarchical (Multi-Level/Mixed) Regression Models. R Package Version 0.4.4.
Holland, M. M., Smith, J. A., Everett, J. D., Vergés, A., and Suthers, I. M. (2020). Latitudinal patterns in trophic structure of temperate reef-associated fishes and predicted consequences of climate change. Fish Fish. 21, 1092–1108. doi: 10.1111/faf.12488
Horta e Costa, B., Assis, J., Franco, G., Erzini, K., Henriques, M., Gonçalves, E. J., et al. (2014). Tropicalization of fish assemblages in temperate biogeographic transition zones. Mar. Ecol. Prog. Ser. 504, 241–252. doi: 10.3354/meps10749
Izquierdo, J. L. (1998). Estudio Biosistemático del Género Laminaria SECT. Digitatae (Laminariales, Phaeophyceae) en las Costas Atlánticas de la Península Ibérica. Doctoral thesis. Madrid: Universidad Complutense de Madrid.
Jadot, C., Donnay, A., Acolas, M. L., Cornet, Y., and Bégout Anras, M. L. (2006). Activity patterns, home-range size, and habitat utilization of Sarpa salpa (Teleostei: Sparidae) in the Mediterranean Sea. ICES J. Mar. Sci. 63, 128–139. doi: 10.1016/j.icesjms.2005.06.010
Johnson, C. R., Banks, S. C., Barrett, N. S., Cazassus, F., Dunstan, P. K., Edgar, G. J., et al. (2011). Climate change cascades: shifts in oceanography, species’ ranges and subtidal marine community dynamics in eastern Tasmania. J. Exp. Mar. Biol. Ecol. 400, 17–32. doi: 10.1016/j.jembe.2011.02.032
Jones, G. P., and Andrew, N. L. (1990). Herbivory and patch dynamics on rocky reefs in temperate Australasia: the roles of fish and sea urchins. Austral. Ecol. 15, 505–520. doi: 10.1111/j.1442-9993.1990.tb01474.x
Kesner-Reyes, K., Garilao, C., Kaschner, K., Barile, J., and Froese, R. (2020). “AquaMaps: algorithm and data sources for marine organisms,” in FishBase, World Wide Web electronic Publication [online], eds R. Froese and D. Paul Available online at: www.fishbase.org (accessed June 1, 2021).
Kriegisch, N., Reeves, S. E., Flukes, E. B., Johnson, C. R., and Ling, S. D. (2019). Drift-kelp suppresses foraging movement of overgrazing sea urchins. Oecologia 190, 665–677. doi: 10.1007/s00442-019-04445-6
Krumhansl, K. A., and Scheibling, R. E. (2011). Spatial and temporal variation in grazing damage by the gastropod Lacuna vincta in Nova scotian kelp beds. Aquat. Biol. 13, 163–173. doi: 10.3354/ab00366
Kumagai, N. H., García Molinos, J., Yamano, H., Takao, S., Fujii, M., and Yamanaka, Y. (2018). Ocean currents and herbivory drive macroalgae-to-coral community shift under climate warming. Proc. Natl. Acad. Sci. U.S.A. 115, 8990–8995. doi: 10.1073/pnas.1716826115
Leinaas, H. P., and Christie, H. (1996). Effects of removing sea urchins (Strongylocentrotus droebachiensis): stability of the barren state and succession of kelp forest recovery in the east Atlantic. Oecologia 105, 524–536. doi: 10.1007/BF00330016
Lenth, R. V. (2021). Emmeans: Estimated Marginal Means, Aka Least-Squares Means. R Package Version 1.7.0.
Lima, F. P., and Wethey, D. S. (2012). Three decades of high-resolution coastal sea surface temperatures reveal more than warming. Nat. Commun. 3:704. doi: 10.1038/ncomms1713
Lima, F. P., Ribeiro, P., Queiroz, N., Hawkins, S. J., and Santos, A. M. (2007). Do distributional shifts of northern and southern species of algae match the warming pattern? Glob. Change Biol. 13, 2592–2604. doi: 10.1111/j.1365-2486.2007.01451.x
Ling, S. D., Scheibling, R. E., Rassweiler, A., Johnson, C. R., Shears, N., Connell, S. D., et al. (2015). Global regime shift dynamics of catastrophic sea urchin overgrazing. Philos. Trans. R. Soc. Lond. B Biol. Sci. 370, 20130269. doi: 10.1098/rstb.2013.0269
Llope, M., Anadón, R., Viesca, L., Quevedo, M., González-Quirós, R., and Stenseth, N. C. (2006). Hydrography of the southern Bay of Biscay shelf-break region: integrating the multiscale physical variability over the period 1993–2003. J. Geophys. Res. Oceans 111:C09021. doi: 10.1029/2005JC002963
Longo, G. O., Hay, M. E., Ferreira, C. E. L., and Floeter, S. R. (2018). Trophic interactions across 61 degrees of latitude in the Western Atlantic. Glob. Ecol. Biogeogr. 28, 107–117. doi: 10.1111/geb.12806
Lüning, K. (1969). Standing crop and leaf area index of the sublittoral Laminaria species near Helgoland. Mar. Biol. 3, 282–286. doi: 10.1007/BF00360961
Mann, K. H. (1982). Ecology of Coastal Waters: A Systems Approach. Great Britain: University of California Press.
Mann, K. H. (2000). Ecology of Coastal Waters: With Implications for Management. USA. Oxford: Blackwell Science, Inc.
Meekan, M. G., and Choat, J. H. (1997). Latitudinal variation in abundance of herbivorous fishes: a comparison of temperate and tropical reefs. Mar. Biol. 128, 373–383. doi: 10.1007/s002270050103
Méndez Villamil, M., Lorenzo, J. M., Pajuelo, J. G., Ramos, A., and Coca, J. (2002). Aspects of the life history of the salema, Sarpa salpa (Pisces, Sparidae), off the Canarian Archipelago (central-east Atlantic). Environ. Biol. Fishes 63, 183–192. doi: 10.1023/A:1014216000459
Miller, P. J., and Loates, M. J. (1997). Peces de España y de Europa. Barcelona: Ediciones Omega, S.A.
Muus, B., Nielsen, J., Dahlstrom, P., and Nyström, B. (1998). Peces de mar del Atlántico y del Mediterráneo. Guía de Identificación. Barcelona: Ediciones Omega, S.A.
O’Brien, J. M., and Scheibling, R. E. (2016). Nipped in the bud: mesograzer feeding preference contributes to kelp decline. Ecology 97, 1873–1886. doi: 10.1890/15-1728.1
Paiva, R. B., Neves, A., Sequeira, V., Vieira, A. R., Costa, M. J., and Gordo, L. S. (2018). Age, growth and reproduction of the protandrous hermaphrodite fish, Sarpa salpa, from the Portuguese continental coast. J. Mar. Biolog. Assoc. U. K. 98, 269–281. doi: 10.1017/S0025315416001405
Pecl, G. T., Araújo, M. B., Bell, J. D., Blanchard, J., Bonebrake, T. C., Chen, I.-C., et al. (2017). Biodiversity redistribution under climate change: impacts on ecosystems and human well-being. Science 355:eaai9214. doi: 10.1126/science.aai9214
Pereira, T. R., Engelen, A. H., Pearson, G. A., Serrão, E. A., Destombe, C., and Valero, M. (2011). Temperature effects on the microscopic haploid stage development of Laminaria ochroleuca and Sacchoriza polyschides, kelps with contrasting life histories. Cah. Biol. Mar. 52, 395–403.
Pereira, T. R., Engelen, A. H., Pearson, G. A., Valero, M., and Serrão, E. A. (2017). Population dynamics of temperate kelp forests near their low-latitude limit. Aquat. Bot. 139, 8–18. doi: 10.1016/j.aquabot.2017.02.006
Piñeiro-Corbeira, C., Barreiro, R., and Cremades, J. (2016). Decadal changes in the distribution of common intertidal seaweeds in Galicia (NW Iberia). Mar. Environ. Res. 113, 106–115. doi: 10.1016/j.marenvres.2015.11.012
Piñeiro-Corbeira, C., Barrientos, S., Barreiro, R., Aswani, S., Pascual-Fernández, J., and De la Cruz-Modino, R. (in press). “Can local knowledge of Small-scale fishers be used to monitor and assess changes in marine ecosystems in a European context?,” in Exploring the Multiple Values of Nature: Connecting Ecosystems and People across Landscapes, eds I. Misiune, D. Depellegrin, and L. Egarter Vigl (Basingstoke: Springer Nature).
Piñeiro-Corbeira, C., Barrientos, S., Olmedo, M., Cremades, J., and Barreiro, R. (2018). By-catch in no-fed aquaculture: exploiting mussel seed persistently and extensively disturbs the accompanying assemblage. ICES J. Mar. Sci. 75, 2213–2223. doi: 10.1093/icesjms/fsy107
Poloczanska, E. S., Brown, C. J., Sydeman, W. J., Kiessling, W., Schoeman, D. S., Moore, P. J., et al. (2013). Global imprint of climate change on marine life. Nat. Clim. Chang. 3, 919–925. doi: 10.1038/nclimate1958
Poore, A. G. B., Campbell, A. H., Coleman, R. A., Edgar, G. J., Jormalainen, V., Reynolds, P. L., et al. (2012). Global patterns in the impact of marine herbivores on benthic primary producers. Ecol. Lett. 15, 912–922. doi: 10.1111/j.1461-0248.2012.01804.x
Quinn, G. P., and Keough, M. J. (2002). Experimental Design and Data Analysis for Biologists. Cambridge: Cambridge University Press.
R Core Team (2021). R: A Lenguaje and Environment for Statistical Computing. Vienna: R Foundation for statisical computing.
Rodríguez Solórzano, M., Devesa Regueiro, S., and Soutullo Garrido, L. (1983). Guía dos Peixes de Galicia. Vigo: Editorial Galaxia.
Schoenrock, K. M., O’Callaghan, T., O’Callaghan, R., and Krueger-Hadfield, S. A. (2019). First record of Laminaria ochroleuca Bachelot de la Pylaie in Ireland in Béal an Mhuirthead, county Mayo. Mar. Biodivers. Rec. 12:9. doi: 10.1186/s41200-019-0168-3
Seoane-Camba, J., and Campo Sancho, J. (1968). Resultados de una primera exploración algológica con escafandra autónoma en la Ría de Vigo. Public. Técnicas Junta Estud. Pesca 7, 333–344.
Smale, D. A. (2020). Impacts of ocean warming on kelp forest ecosystems. New Phytol. 225, 1447–1454. doi: 10.1111/nph.16107
Smale, D. A., and Wernberg, T. (2013). Extreme climatic event drives range contraction of a habitat-forming species. Proc. R. Soc. Lond. B Biol. Sci. 280:20122829. doi: 10.1098/rspb.2012.2829
Smale, D. A., Epstein, G., Hughes, E., Mogg, A. O. M., and Moore, P. J. (2020). Patterns and drivers of understory macroalgal assemblage structure within subtidal kelp forests. Biodivers. Conserv. 29, 4173–4192. doi: 10.1007/s10531-020-02070-x
Smale, D. A., Wernberg, T., Yunnie, A. L. E., and Vance, T. (2014). The rise of Laminaria ochroleuca in the Western English Channel (UK) and comparisons with its competitor and assemblage dominant Laminaria hyperborea. Mar. Ecol. 36, 1033–1044. doi: 10.1111/maec.12199
Smith, S. M., Fox, R. J., Donelson, J. M., Head, M. L., and Booth, D. J. (2016). Predicting range-shift success potential for tropical marine fishes using external morphology. Biol. Lett. 12:20160505. doi: 10.1098/rsbl.2016.0505
Smith, S. M., Malcolm, H. A., Marzinelli, E. M., Schultz, A. L., Steinberg, P. D., and Vergés, A. (2021). Tropicalisation and kelp loss shift trophic composition and lead to more winners than losers in fish communities. Glob. Change Biol. 27, 2537–2548. doi: 10.1111/gcb.15592
Sorte, C. J. B., Williams, S. L., and Carlton, J. T. (2010). Marine range shifts and species introductions: comparative spread rates and community impacts. Glob. Ecol. Biogeogr. 19, 303–316. doi: 10.1111/j.1466-8238.2009.00519.x
Steneck, R. S., and Johnson, C. (2014). “Kelp forests: Dynamic patterns, processes, and feedbacks,” in Marine Community Ecology and Conservation, eds M. D. Bertness, J. F. Bruno, B. R. Silliman, and J. J. Stachowicz (Sunderland, MA: Sinauer), 315–336. doi: 10.1111/ele.13828
Steneck, R. S., Bellwood, D. R., and Hay, M. E. (2017). Herbivory in the marine realm. Curr. Biol. 27, R484–R489. doi: 10.1016/j.cub.2017.04.021
Steneck, R. S., Graham, M. H., Bourque, B. J., Corbett, D., Erlandson, J. M., Estes, J. A., et al. (2002). Kelp forest ecosystems: biodiversity, stability, resilience and future. Environ. Conserv. 29, 436–459. doi: 10.1017/S0376892902000322
Steneck, R. S., Leland, A., McNaught, D. C., and Vavrinec, J. (2013). Ecosystem flips, locks, and feedbacks: the lasting effects of fisheries on Maine’s kelp forest ecosystem. Bull. Mar. Sci. 89, 31–55. doi: 10.5343/bms.2011.1148
Tanaka, K., Taino, S., Haraguchi, H., Prendergast, G., and Hiraoka, M. (2012). Warming off southwestern Japan linked to distributional shifts of subtidal canopy-forming seaweeds. Ecol. Evol. 2, 2854–2865. doi: 10.1002/ece3.391
Taylor, D. I., and Schiel, D. R. (2010). Algal populations controlled by fish herbivory across a wave exposure gradient on southern temperate shores. Ecology 91, 201–211. doi: 10.1890/08-1512.1
Tempera, F., Milla-Figueras, D., Sinde-Mano, A. L., Atchoi, E., and Afonso, P. (2021). Range extension of mesophotic kelps (Ochrophyta: Laminariales and Tilopteridales) in the central north Atlantic: opportunities for marine forests research and conservation. J. Phycol. 57, 1140–1150. doi: 10.1111/jpy.13162
Tittley, I., and Neto, A. I. (2000). A provisional classification of algal-characterised rocky shore biotopes in the Azores. Hydrobiologia 440, 19–25. doi: 10.1023/A:1004172321900
tom Dieck, I. (1992). North Pacific and North Atlantic digitate Laminaria species (Phaeophyta): hybridization experiments and temperature responses. Phycologia 31, 147–163. doi: 10.2216/i0031-8884-31-2-147.1
Troast, B., Paperno, R., and Cook, G. S. (2020). Multidecadal shifts in fish community diversity across a dynamic biogeographic transition zone. Divers. Distrib. 26, 93–107. doi: 10.1111/ddi.13000
Tuya, F., Cacabelos, E., Duarte, P., Jacinto, D., Castro, J. J., Silva, T., et al. (2012). Patterns of landscape and assemblage structure along a latitudinal gradient in ocean climate. Mar. Ecol. Prog. Ser. 466, 9–19. doi: 10.3354/meps09941
Varela, C. L. (2000). Estudio de las Poblaciones Infralitorales de Laminaria ochroleuca y L. hyperborea (Laminariales. Phaeophyta). Master thesis. A Coruña: Universidade da Coruña.
Vergés, A., Alcoverro, T., and Ballesteros, E. (2009). Role of fish herbivory in structuring the vertical distribution of canopy algae Cystoseira spp. in the Mediterranean Sea. Mar. Ecol. Prog. Ser. 375, 1–11. doi: 10.3354/meps07778
Vergés, A., Campbell, A. H., Wood, G., Kajlich, L., Eger, A. M., Cruz, D., et al. (2020). Operation Crayweed: ecological and sociocultural aspects of restoring Sydney’s underwater forests. Ecol. Manag. Restor. 21, 74–85. doi: 10.1111/emr.12413
Vergés, A., Doropoulos, C., Malcolm, H., Skye, M., Garcia-Pizá, M., Marzinelli, E., et al. (2016). Long-term empirical evidence of ocean warming leading to tropicalization of fish communities, increased herbivory, and loss of kelp. Proc. Natl. Acad. Sci. U.S.A. 113, 13791–13796.
Vergés, A., Steinberg, P. D., Hay, M. E., Poore, A. G., Campbell, A. H., Ballesteros, E., et al. (2014a). The tropicalization of temperate marine ecosystems: climate-mediated changes in herbivory and community phase shifts. Proc. Biol. Sci. 281:20140846. doi: 10.1098/rspb.2014.0846
Vergés, A., Tomas, F., Cebrian, E., Ballesteros, E., Kizilkaya, Z., Dendrinos, P., et al. (2014b). Tropical rabbitfish and the deforestation of a warming temperate sea. J. Ecol. 102, 1518–1527. doi: 10.1111/1365-2745.12324
Verlaque, M. (1990). Relations entre Sarpa salpa (Linnaeus, 1758) (Téléostéen, Sparidae), les autres poissons brouteurs et le phytobenthos algal méditérranéen. Oceanol. Acta 13, 373–388.
Vilas Paz, A., Gamallo Liste, B., Framil Barreiro, J., Fernández Bouzas, J. A., Sanz Ochoa, K., Lois Silva, M., et al. (2014). Guía de Visita-Parque Nacional Marítimo-Terrestre de las Islas Atlánticas de Galicia. Madrid: Organismo Autónomo Parques Nacionales.
Voerman, S. E., Llera, E., and Rico, J. M. (2013). Climate driven changes in subtidal kelp forest communities in NW Spain. Mar. Environ. Res. 90, 119–127. doi: 10.1016/j.marenvres.2013.06.006
Wernberg, T., Bennett, S., Babcock, R. C., de Bettignies, T., Cure, K., Depczynski, M., et al. (2016). Climate-driven regime shift of a temperate marine ecosystem. Science 353, 169–172. doi: 10.1126/science.aad8745
Wernberg, T., Smale, D. A., Tuya, F., Thomsen, M. S., Langlois, T. J., de Bettignies, T., et al. (2012). An extreme climatic event alters marine ecosystem structure in a global biodiversity hotspot. Nat. Clim. Change 3:78. doi: 10.1038/nclimate1627
Wernberg, T., Thomsen, M. S., Tuya, F., Kendrick, G. A., Staehr, P. A., and Toohey, B. D. (2010). Decreasing resilience of kelp beds along a latitudinal temperature gradient: potential implications for a warmer future. Ecol. Lett. 13, 685–694. doi: 10.1111/j.1461-0248.2010.01466.x
Yamaguchi, A. (2010). Biological aspects of herbivorous fishes in the coastal areas of western Japan. Bull. Fish. Res. Agen. 32, 89–94.
Zarco-Perello, S., Bosch, N. E., Bennett, S., Vanderklift, M. A., and Wernberg, T. (2021). Persistence of tropical herbivores in temperate reefs constrains kelp resilience to cryptic habitats. J. Ecol. 109, 2081–2094. doi: 10.1111/1365-2745.13621
Zarco-Perello, S., Wernberg, T., Langlois, T. J., and Vanderklift, M. A. (2017). Tropicalization strengthens consumer pressure on habitat-forming seaweeds. Sci. Rep. 7:820. doi: 10.1038/s41598-017-00991-2
Keywords: fish herbivory, kelp decline, Laminaria ochroleuca, rocky shores, temperate reef
Citation: Barrientos S, Piñeiro-Corbeira C and Barreiro R (2022) Temperate Kelp Forest Collapse by Fish Herbivory: A Detailed Demographic Study. Front. Mar. Sci. 9:817021. doi: 10.3389/fmars.2022.817021
Received: 17 November 2021; Accepted: 03 January 2022;
Published: 31 January 2022.
Edited by:
Stelios Katsanevakis, University of the Aegean, GreeceReviewed by:
Stein Fredriksen, University of Oslo, NorwayJason Michael Hall-Spencer, University of Plymouth, United Kingdom
Copyright © 2022 Barrientos, Piñeiro-Corbeira and Barreiro. This is an open-access article distributed under the terms of the Creative Commons Attribution License (CC BY). The use, distribution or reproduction in other forums is permitted, provided the original author(s) and the copyright owner(s) are credited and that the original publication in this journal is cited, in accordance with accepted academic practice. No use, distribution or reproduction is permitted which does not comply with these terms.
*Correspondence: Sara Barrientos, sara.barrientos@udc.es