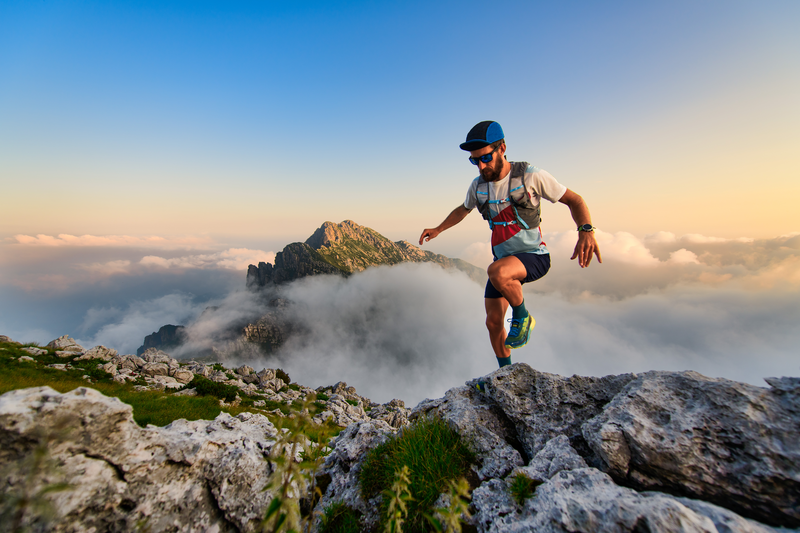
94% of researchers rate our articles as excellent or good
Learn more about the work of our research integrity team to safeguard the quality of each article we publish.
Find out more
ORIGINAL RESEARCH article
Front. Mar. Sci. , 10 March 2022
Sec. Marine Megafauna
Volume 9 - 2022 | https://doi.org/10.3389/fmars.2022.816881
This article is part of the Research Topic Tracking Marine Megafauna for Conservation and Marine Spatial Planning View all 35 articles
Understanding both the distribution and habitat use of populations through the annual cycle is vital to understanding how vulnerable species are to environmental change. However, distributions and habitat use can vary among individuals and populations, particularly in generalist species, with variation depending on external environmental factors, such as resource availability. Comprehensive information across multiple populations is important to guide spatial planning of protected areas and is increasingly available for breeding individuals, but it is still lacking for many species, particularly seabirds, during the non-breeding season, especially those with declining populations. We investigated within-species variation in migratory strategies, non-breeding habitat selection and habitat and spatial specialisation in a declining, opportunistic, generalist seabird, the European herring gull Larus argentatus, from multiple breeding colonies across northwest England and southwest Scotland using global positioning system (GPS) tracking during the non-breeding season of 2014/15. Although several individuals stayed within the area of the breeding colony, the majority of individuals migrated in a southerly direction and spent half of the annual cycle (on average 53%) away from the breeding area and kept moving through the non-breeding period. During non-breeding, herring gulls selected mainly marine intertidal, but also a range of anthropogenic terrestrial habitats. However, habitat selection differed between geographical regions, within a geographical region and among individuals. There was a generalist use of non-breeding habitats at the population level, but some habitat specialisation at the individual level that was repeatable through the non-breeding period despite individuals showing low spatial specialisation. The results highlight the importance of intertidal habitat and a mix of alternative foraging habitats in the wintering areas of herring gulls. The results also highlight that habitat selection in an opportunistic generalist can vary even between nearby regions and that appropriate conservation management plans may need to be tailored to regional differences in specific non-breeding areas.
The patterns of space use exhibited by species reflect their movement and habitat selection in response to resource availability, mediated by biotic mechanisms and intrinsic factors acting on individuals (Börger et al., 2008). This process is dynamic, as habitat selection forces and resource availability can vary through time and space (Viana et al., 2018). In temperate climes, the non-breeding period is typically characterised by reduced food productivity, leading to energetically challenging conditions, exacerbated by short days, lower temperatures, and extreme weather (Newton, 2008; Osterblom et al., 2008; Fort et al., 2009). These seasonal changes can lead to nomadic or migratory movements at the end of the breeding season away from the breeding area to avoid the seasonally deteriorating conditions. The costs of such movements in terms of time, energy, and mortality risk need to be balanced against advantages of more favourable conditions at non-breeding sites (Alerstam et al., 2003). Ultimately, the different periods of the annual cycle when individuals experience different ecological circumstances are inextricably linked, and carry-over effects between different periods can have important consequences for the individual and consequently the population (Fretwell, 1972; Marra et al., 1998, 2015). To better understand species’ and populations’ vulnerability to environmental change and anthropogenic pressures it is therefore necessary to understand habitat use of individuals during the entire annual cycle. Movements and habitat selection in the non-breeding period can be different from the breeding period as individuals are no longer constrained in space and time to a central breeding location (Phillips et al., 2009; Quillfeldt et al., 2010; Dias et al., 2011; McFarlane Tranquilla et al., 2013; Anderson et al., 2019). The rapid development of modern tracking technology (Wilson et al., 2002; Burger and Shaffer, 2008) now provides a promising approach to study individual strategies throughout the annual cycle.
Movement and habitat selection can have important conservation and spatial planning implications (Webster et al., 2002; Esler, 2014). If populations show strong migratory connectivity, that is cohesion of populations among the different phases of the annual cycle (Cohen et al., 2019), then local environmental change might only influence populations that use the affected area but not others. Within an area, reduced resource availability during the non-breeding season can increase inter- and intra-specific competition for limited resources (Schoener, 1968). For many species, intra-specific competition can be reduced through individual resource partitioning and habitat specialisation (Perry, 1996; Wikelski and Wrege, 2000; Pearson et al., 2002; Phillips et al., 2011). This will be the case for generalist species, where individuals can switch to different resources and habitats based on food availability. Although generalist species are expected to consume the most readily available resource within their foraging range (Schoener, 1971), resource selection can vary considerably at the individual level (Bolnick et al., 2003; Araújo et al., 2011). This may result in variation in space use and habitat selection between individuals of a population, leading to a population being distributed over a larger range of environments, which may buffer the population from localised environmental perturbations.
By contrast, if individuals from different breeding populations use the same non-breeding area (weak connectivity) and habitat, detrimental environmental change occurring at that location may have an effect on a wider spatial scale and can lead to population declines in all breeding populations using that location in the non-breeding period (Webster et al., 2002; Iwamura et al., 2013; Esler, 2014). To ensure suitable conservation actions, a good knowledge of the population distributions in time and space is essential. Although non-breeding distributions may be known to some extent from ring recoveries, this information can be biassed (Clark et al., 2009), and tracking studies allow seasonal movements and habitat selection to be studied in more detail. Since movement and habitat selection can vary among individuals and in space (Bonnot et al., 2015; Leclerc et al., 2016; Montgomery et al., 2018), we require multi-site studies to broaden our understanding of a species’ non-breeding distribution (Bernard et al., 2021).
Here we studied individual and spatial variation in non-breeding movements and habitat selection in a generalist species, the European herring gull Larus argentatus. In the non-breeding season herring gulls make non-extensive movements away from the breeding colony (Harris, 1964; Coulson and Butterfield, 1985; Calladine, 2002; Camphuysen et al., 2011). They are opportunistic generalists that forage year-round on a range of resources including marine (fishery discards, intertidal invertebrates such as worms, molluscs, and crustaceans) and terrestrial (natural and anthropogenic items from farmland, landfill, and built-up areas) habitats (Hunt, 1972; Götmark, 1984; Kubetzki and Garthe, 2003; Enners et al., 2018; Clewley et al., 2021). However, at the individual level herring gulls can show extensive specialism (McCleary and Sibly, 1986; Pierotti and Annett, 1991; van den Bosch et al., 2019). Herring gulls have shown dramatic population declines in Europe (e.g., Hario and Rintala, 2016; Nager and O’Hanlon, 2016), and in the United Kingdom is red-listed as a species of conservation concern (Stanbury et al., 2021). The reasons for the population declines are poorly understood (Nager and O’Hanlon, 2016). For their effective conservation management, we need more information on their habitat and resource requirements throughout the annual cycle. Using global positioning system (GPS) tracking, we recorded individual movements and habitat selection of adult herring gulls throughout the non-breeding period to investigate the distribution of multiple breeding populations and hence their migratory connectivity, and what habitats they selected in their non-breeding area. As herring gulls are opportunistic foragers exhibiting flexible foraging strategies, we expect that there is among-individual and within-season variation in space use and habitat selection. Having a better understanding of the among-population and within-species variation in movements and habitat selection and specialisation during specific periods in the annual cycle will give us important insights for spatial planning and the conservation and management of generalist species in a rapidly changing world.
Habitat selection of herring gulls during the non-breeding season was studied using data from individuals tracked from 2014 to 2015 from five colonies in two regions along the west coast of the United Kingdom: in the southwest Scotland region we tracked birds in the Southern Hebrides (Oronsay and Islay) and Firth of Clyde (Lady Isle and Pladda), and in the northwest England region from Walney (Figure 1). Between May 16 and June 6, 2014, breeding birds were captured on the nest during incubation with either a wire mesh walk-in trap (Bub, 1991) or a remote-controlled noose trap. A solar-powered GPS device (Nanofix PathTrack Ltd, Otley, United Kingdom) was attached to 50 birds across colonies, using a cross-over Teflon wing harness (see Thaxter et al., 2014). At Walney, we deployed devices with an internal antenna (combined device and harness weight: 16 g; device dimensions = 52 mm × 22 mm × 14 mm) and at all other colonies, devices with an external antenna (14 g, 41 mm × 22 mm × 14 mm). The combined device and harness weight was 1.0–1.6% of an individual’s body mass. A further 56 individuals were also caught and ringed but not tagged giving a total of 106 birds (Lady Isle: six tagged, eight controls; Pladda: six tagged, four controls; Islay: four tagged; Oronsay: 10 tagged, three controls; Walney: 24 tagged, 41 controls). All caught birds were measured [head-bill length, gonys depth, maximum wing chord (all ±1 mm), and body weight (±10 g)], marked with a metal ring and an alphanumeric colour ring enabling individual identification in the field. They were also sexed by taking a small blood sample for molecular sexing or, when blood sampling was not possible, from head plus bill length measurements (Coulson et al., 1983). For individuals where sex was determined (N = 42), the sex ratio at deployment was female-biassed (31 females and 12 males).
Figure 1. Map of the United Kingdom with the study area in the inset and the herring gull breeding colonies marked with a star. Overlaid are the 95% kernel density estimations (KDEs) of use locations during the non-breeding season (for definition see main text) for individuals from each set of colonies: Green – Southern Hebrides (Islay and Oronsay); Yellow – Firth of Clyde (Pladda and Lady Isle); and Purple – Walney, northwest England. KDEs for each region were weighted by the number of use locations included per individual.
Devices with the same harness attachment but a relatively heavier weight (maximum 2.9% of body mass) had no detectable impacts on breeding success and local return rate for up to 3 years in the closely related lesser black-backed gull Larus fuscus (Thaxter et al., 2016; Kavelaars et al., 2018). To further check whether devices and harnesses impacted the local return rates of our study birds, we monitored colour ring sightings of tagged and control birds at their breeding colonies up to 3 years after device deployment. Resighting probability did not differ between tagged and control herring gulls [Cox regression in the R-package survival (Therneau, 2020), accounting for when individuals were last seen; tagged versus control individuals: χ21 < 0.1, P = 0.825, device type with or without external antenna: χ21 = 1.8, P = 0.182, N = 106].
GPS-devices were set to record fixes throughout the day and night between March and September (the period covering the breeding season) at least every 5 min, but less frequently when the battery power was low (typical range 5–40 min). From October until February, recording intervals were increased to at least once every 30 min (Walney, Northwest England) or 20 min (all southwest Scotland colonies, where devices were also switched off between 22:00 and 04:00 to conserve battery power). On an individuals’ return to their breeding site in 2015, time-stamped GPS fixes that had been stored in the devices were downloaded remotely to a base station. Thirty-five tagged birds were re-sighted in 2015 in the colony where they were tagged the previous season and a further two tagged birds were re-sighted outside a breeding colony in a later year. Of the 35 tagged birds that were re-sighted in their colony, eight devices had failed by the time of return.
The number of daily fixes taken per individual varied through time. There were on average ca. 120 fixes per day per individual in July and August. However, due to low sunlight and short days during the winter at high latitude, which reduced solar charging of the devices, it dropped to ca. 2 fixes per day per individual in December and January. The number of fixes varied between individuals with some birds having periods of no fixes. Where periods of insufficient numbers of daily fixes in late winter coincided with the time individuals started to move back to the breeding colony, we were unable to accurately identify the end of the non-breeding period (see below). We therefore excluded data from seven returning individuals with working GPS-devices. Thus, we obtained information for the complete non-breeding period for 20 individuals with at least one GPS fix obtained on 40–100% of days (mean = 76.8%, SD = 19.4, N = 20) during the non-breeding period (see Supplementary Table 1).
The raw GPS fixes were processed to remove erroneous fixes and those during nocturnal roosting. First, erroneous locations, identified by travelling speeds exceeding 66 km h–1, the maximum reported flight speed of a herring gull (Schnell and Hellack, 1979), were removed. The accuracy of location depends on the number of satellites contacted, with GPS fixes obtained from four or more satellites having an error <20 m (G. Brodin, PathTrack pers. comm.). We therefore removed a small number of fixes obtained from fewer than four satellites. Second, as we were interested in how herring gulls associate with habitat for resource acquisition, we removed all fixes earlier than an hour after dawn and later than an hour before dusk that were likely from individuals at a nocturnal roost or commuting to or from their roost. In winter, herring gulls can leave their night-time roost during a period of 3–4 h from just before sunrise, and start returning to the roost from 3 h before sunset onward (Cooke and Ross, 1972). This left a total of 30,806 use locations.
From the processed GPS fixes we identified the start and the end of the non-breeding period from movement patterns. Movements of breeding individuals are characterised by regular daily return trips to the colony where they attend and guard territories, nests, and offspring (e.g., Bukacińska et al., 1996). Non-breeding periods can thus be defined by the end and subsequent re-initiation of regular colony attendance. We determined colony-specific polygons that defined each colony’s boundary. The last day an individual was recorded within the colony boundary before being absent from the colony for at least 10 consecutive days was classified as the start of the non-breeding period (departure date). The non-breeding period ended when the individual returned to the colony, defined as the first date that it was present within the colony boundary for at least part of each of 10 subsequent days (arrival date). The timing of breeding was similar across all colonies and therefore was not thought to affect colony arrival or departure dates among colonies.
For each GPS fix we calculated its distance from the centre of the breeding site using the distHaversine function in the R package geosphere (Hijmans, 2019a). The non-breeding day-time distributions of the tagged herring gulls were illustrated with the combined 95% (home range) kernel density estimations (KDEs) maps for all individuals from each of the three breeding locations: Southern Hebrides (Oronsay and Islay) and Firth of Clyde (Pladda and Lady Isle) in southwest Scotland, and Walney in northwest England (Figure 1). KDEs were combined for the two Southern Hebrides and two Firth of Clyde colonies due to the overlap of birds’ movements and because of the small sample sizes per colony. KDEs were calculated using a grid size of 500 m2. For each individual, the most appropriate smoothing parameter (h) was calculated using a custom function, reported for each individual in Supplementary Table 2. To avoid under- or over-smoothing, this function identified a “minimum” (or adjusted) h-reference bandwidth by searching iteratively for the smallest h over progressively smaller scales starting with the h-reference bandwidth value. The smallest h prior to the eventual break-up of the 95% KDE spatial polygons was selected to create the optimum KDE for each individual. We created combined utilization distribution (UD) kernels for each of the three locations, using the individual KDEs weighted by the number of fixes per individual. To quantify overlap between the KDEs of the three breeding locations, we calculated Bhattacharyya’s affinity index values in the R package adehabitatHR, which range from 0 (no overlap) to 1 (identical KDEs) (Bhattacharyya, 1943; Fieberg and Kochanny, 2005).
To explore daytime habitat selection in the non-breeding period, all retained GPS fixes were considered use locations, which represented places where the birds foraged or loafed, although which potentially, may have also represented some commuting flights. All use locations were compared with random points that represented the availability of different habitats in the area used by the herring gulls. To first describe the available habitat within each region at the landscape scale, which individuals could migrate to, we created a 184.73 km buffer (representing the maximum distance that tagged herring gulls moved away from their colonies, see section “Results”) around each colony. To create a similar density (2.95 available locations per 10 km2) as for the local scale (see below) we generated 31,593 random locations within the potential migration range of each colony, constant for all individuals. Herring gulls are coastal surface feeders when foraging in the marine environment, and spend most of their time at sea within 40 km of the coast (Kubetzki and Garthe, 2003; Anderson et al., 2019). Therefore, we removed available locations beyond 40 km from the coastline out to sea (13,165 available locations), giving a total of 144,800 available locations at the landscape scale.
To create available locations within the birds’ reach from use locations (local scale), we first calculated the daily distance moved by individuals during the non-breeding period as the distance between an individual’s consecutive daily centroid positions (based on all daily use locations) using the distHaversine function in the R package geosphere (Hijmans, 2019a). We then defined the 75% of the distribution of daily distances moved (7.35 km) around each use location as the area accessible to that individual on that day and generated random available locations within that range using the gBuffer function in the R package rgeos (Bivand and Rundel, 2019). To determine the optimal number of available locations required per use location, we tested the effects of different ratios of use to available, varying from 1:1 to 1:50 at intervals of 5, on the performance of the mixed effects logistic regression models (see Statistical Analysis below). For each use to available ratio we obtained the χ2-value of the habitat parameters and the model performance, measured as area under the receiver operator curve (AUC), using the R package pROC (Robin et al., 2011). The χ2- and AUC-values levelled off by the ratio of 1 use fix to 50 available locations (Supplementary Figure 2). Therefore, we used a ratio of 1:50 to be representative of habitat heterogeneity within the relatively large area an individual gull could have covered every day. At the local scale, within the accessible area of each of the 30,806 use locations, we thus generated 50 available locations.
Each use and available location was assigned to one of seven broad habitat categories (referred to as “habitat” hereafter): six known to be used by foraging herring gulls: arable, grassland, built-up, freshwater, intertidal, and marine offshore habitats (Hunt and Hunt, 1973; Götmark, 1984; Kubetzki and Garthe, 2003). All other habitats were pooled into a single “other” category that included habitats that are not typical herring gull foraging habitats (Supplementary Table 3). The habitat type at each use and available location was extracted using the raster package in R (Hijmans, 2019b) from the CORINE Land Cover 2018 classification of satellite image data (25 ha minimum mapping unit, European Environment Agency, 2019).
In order to validate that use locations did reflect habitats where herring gulls foraged, we also obtained an independent measure of habitats used for foraging by looking at the stable isotope ratios of feathers that herring gulls grow during the non-breeding period (outer primary and head feathers, Olsen and Larsson, 2004). In our study area, feather isotope ratios can discriminate between marine and terrestrial resources (O’Hanlon et al., 2017). We collected three head feathers and 10 barbs of the outermost primary (representative of the entire feather, Wiley et al., 2010) from individuals at tagging. Collected feather material was first washed in a liquid detergent (Ecover™) diluted with deionised water (approximate 1:99 dilution), then in a mixture of two parts chloroform and one part of methanol, and finally air-dried. Dried samples were homogenised and 1–2 mg of material was weighed into tin capsules, sealed and combusted to analyse for carbon, nitrogen, and sulphur isotopes by continuous-flow isotope ratio mass spectrometry [using a Elementar vario PYRO cube elemental analyser (Hanau, Germany) linked to a IsoPrime (now Elementar) VisION Mass Spectrometer (Cheadle Hulme, United Kingdom) at the National Environmental Isotope Facility in East Kilbride, United Kingdom]. The stable isotope ratios are expressed as δ13C (13C/12C), δ15N (15N/14N), and δ34S (34S/32S) in parts per thousand (‰) relative to the international references Vienna PeeDee belemnite (V-PDB) marine fossil limestone for carbon, atmospheric N2 for nitrogen, and Vienna Cañon Diablo Troilite (V-CDT) for sulphur, respectively. Measurement accuracy was ±0.1‰ for δ13C, ±0.2‰ for δ15N, and ±0.70‰ for δ34S based on the standard deviation of the repeated analyses of laboratory standards (MSAG2, methanesulfonamide and gelatine; M1, methionine and gelatin; and SAAG, sulphanilamide and gelatine) and International Standards (USGS40 Glutamic Acid and IAEA S1, S2, and S3 silver sulphides). Head and primary feather materials of each individual were analysed separately and then averaged per individual. These isotope ratios allow for a good discrimination between marine and terrestrial food sources (Hobson and Welch, 1995; Hobson et al., 2015; O’Hanlon et al., 2017). Feather stable isotopes and habitat use data were available for 10 gulls tagged in Scotland, all females. We extracted the first principal component in a principal component analysis of the three stable isotope ratios. To determine whether the proportion of use fixes in marine and intertidal habitat (from the 2014/15 non-breeding season) was related to an individual’s feather stable isotope ratio (grown during the 2013/14 non-breeding season) we performed a linear mixed-effect model, in the R package lme4 (Bates et al., 2014), with colony included as a random effect. Although the feather isotope ratio and GPS tracking data related to different, but consecutive non-breeding periods, we assumed individuals would use similar areas and habitats as herring gulls have high between-year site fidelity to non-breeding areas (Clark et al., 2016).
All statistical analyses were performed in R, Version 4.0.3 (R Development Core Team, 2020) and throughout we report means and standard deviations (SD). To test for habitat selection, we used resource selection functions (RSFs), a commonly used approach to characterise the pattern of resources that are preferentially used or avoided by animals over a period of time (Manly et al., 2002) and that enables ecological limitations and needs to be identified (Fieberg et al., 2021). The analysis considered a use/availability design where known presences (use fixes) are compared with a random sample of pseudo-absences across “available” resources (available fixes) (Boyce, 2006). Logistic regression provides a useful method to fit RSFs to quantify resource, or habitat, selection with use-available data (Johnson et al., 2006). This approach is straightforward to perform and allows the inclusion of random effects, which can help account for differences in samples sizes of use locations among individuals (Gillies et al., 2006). We estimated the relative probability of selection for different habitats using mixed effects logistic regression models for binomial data and a logit-link function (Avgar et al., 2017) in lme4 (Bates et al., 2014). Use or available was the binomial response variable (1 and 0, respectively) and habitat category was considered as the explanatory variable. Month nested within Bird ID was included as a random effect to account for the variable number of locations per bird and month. Because of the separation of the non-breeding distributions of birds from colonies in the southwest Scotland and northwest England regions (see section “Results”), we also considered region as an explanatory factor in the analyses. Of the 20 herring gulls with sufficient data, 18 were females and two were males (Supplementary Table 1). Although non-breeding movements can differ between the sexes (Catry et al., 2005), we were not able to formally compare between the sexes and pooled both sexes for the analyses. In the first instance we ran a model on all data with region (southwest Scotland and northwest England) and habitat and their interaction as fixed effects. Given the non-overlapping distribution in the non-breeding period and the difference in habitat availability between the two regions (see section “Results”), we also carried out separate RSFs for individuals from northwest England and southwest Scotland with habitat as the fixed effect. To estimate the variance explained by each model, we calculated conditional and marginal R2 values in the R package MuMIn (Barton, 2012). The marginal R2 values are associated with the fixed effects alone, while the conditional R2 values are associated with the fixed and random effects (Nakagawa and Schielzeth, 2013). Diagnostic plots were checked to ensure all model assumptions were met.
From the model outputs, we obtained relative selection strength (RSS) by exponentiating the parameter coefficients for each habitat type; the RSS value reflects how strongly each habitat type is used relative to its availability, expressed relative to a reference habitat (Avgar et al., 2017). If the confidence interval of the RSS value overlapped with 1 then there was no selection for a given habitat. An RSS value <1 indicated a habitat was used less than expected from its availability, while an RSS value >1 indicated a habitat was used more than expected from its availability. As the reference habitat category, we used the habitat “other.” The probability of use of “other” was relatively low (2.4% of use locations across both regions) which could affect the confidence interval of the RSS. However, we found the same qualitative patterns when using alternative habitats as the reference category (results not shown) and therefore we retained “other” habitat as the reference category to compare the relative extent to which the remaining six habitats were selected.
Our RSS values are averages per habitat across all individuals (Manly et al., 2002) and assume that all individuals select similarly between habitats. To explore variation in habitat selection between individuals we used an Eigenanalyses of Selection Ratios (Calenge and Dufour, 2006), carried out in the R package adehabitatHS (Calenge, 2006). Eigenanalyses maximise the differences between habitat use and availability on the first factorial axes. If all individuals select the same habitat, then all variation in selection is explained on the first factorial axis whereas if there is among-individual variation in habitat selection the explained variation is distributed across multiple axes (Calenge and Dufour, 2006). As the sum of all the proportions of habitats equals 1, the strength of habitat selection depicted in Eigenanalyses plots is scaled in comparison to all options included (Aebischer, 1993). As herring gulls in the southwest Scotland and the northwest England regions showed non-overlapping non-breeding distributions and the regions differed in habitat availability (see section “Results”), we carried out separate Eigenanalyses for the two regions.
To investigate habitat specialisation of individual gulls we calculated the proportional similarity index (PSi) per individual where a value of 0 indicates an absolute habitat specialist and 1 an absolute habitat generalist (Schoener, 1968; Bolnick et al., 2002), following the equation in Bolnick et al. (2007), using the R package RInSp (Zaccarelli et al., 2013). For each individual this was calculated for the entire non-breeding period and for each month.
Spatial specialisation in where individual gulls spent the non-breeding period can be expressed as home range area and distance moved, with spatially more specialised individuals having smaller home ranges and moving over shorter distances (van den Bosch et al., 2019). As a measure of home range area we calculated 50 and 95% minimum convex polygons (MCP) around use fixes using the mcp function in the R package adehabitatHR (Calenge, 2006) for each individual and month. MCPs were calculated only when a minimum of five individual use fixes per individual and month were available, therefore we were unable to create monthly MCPs for 5 months for three individuals (bird 12,658 in January; bird 12,696 in November; bird 12,703 in December, January, and April). We found no correlation between the number of fixes and the 50 or 95% MCP area (P > 0.45, N = 20). As a measure of distance moved, we calculated the mean distance between consecutive monthly MCP centroids, calculated using the distHaversine function in the R package geosphere (Hijmans, 2019a), for each individual. Home range area and distance moved were significantly positively correlated (50% MCP: r = 0.67, P = 0.001, N = 20; 95% MCP: r = 0.55, P = 0.01, N = 20). We therefore used the mean distance between centroids of consecutive monthly 95% MCPs as our measure of spatial specialisation. Finally, we calculated the distance between the centroid of each monthly 95% MCP for each individual and its breeding colony to explore movements during the non-breeding period.
To determine whether spatial specialisation, based on the distance between centroids of consecutive monthly 95% MCP, was related to habitat specialisation (PSi), we ran a Pearson’s correlation test. To explore the consistency of an individuals’ habitat and spatial specialisation over the non-breeding period we calculated repeatabilities of monthly PSi and distance between 95% MCP centroids of consecutive months, respectively, using the rpt.remlLMM function in the R package rptR (Schielzeth and Nakagawa, 2011).
Details on the non-breeding season of the tracked individuals by colony is summarised in Table 1. To explore differences among colonies we performed a linear model for each variable (departure date, arrival date, length of absence from colony, habitat specialisation, spatial specialisation, mean distance of monthly 95% MCP centroids to the breeding colony, and non-breeding home range size). As there was only one tracked individual from the Pladda colony we omitted this individual from these analyses (Table 1). Subsequent Tukey post-hoc pairwise comparisons were performed using the glht function in the R package multcomp (Hothorn et al., 2008) to explore which colonies differed from each other.
Table 1. Summary information on the non-breeding season of the tracked individuals by colony, including the results of linear models performed for each variable.
Tagged individual herring gulls left the breeding site over a 2.5 month period (departure dates ranged from June 30 to September 13, 2014), returned back to their breeding site between mid-January to mid-March (range from January 12 to March 19, 2015) and were absent from the breeding site on average for 193 ± 38 days (N = 20). Herring gulls from Oronsay, Southern Hebrides, departed earliest and stayed away from the colony longest, while gulls breeding at Walney, northwest England, departed latest and stayed away from the colony for the least time (Table 1 and Supplementary Table 4). For the other colonies, departure dates and length of period absent from the colony were intermediate between Oronsay and Walney (Table 1 and Supplementary Table 4). Differences in arrival date in spring were qualitatively similar among colonies but more variable between individuals (Table 1). Herring gulls moved up to 184.7 km (mean = 115.7 ± 47.3 km, range = 10.5–184.7 km, N = 20) away from their breeding site. The non-breeding KDEs of individuals tagged from the two most northerly colonies (Oronsay and Islay, Southern Hebrides) spread over a large area of the coastline of southwest Scotland and Northern Ireland and only partly overlapped with the more restricted non-breeding KDEs of Firth of Clyde (Lady Isle and Pladda) individuals (Bhattacharyya’s affinity index = 0.19). The non-breeding KDEs of individuals tagged in Walney, northwest England were further south and did not overlap with any of the individuals tagged in Scotland (Bhattacharyya’s affinity index = 0; Figure 1). Thus, the non-breeding areas of the tagged herring gulls fell into two distinct regions: one in northwest England and the other in southwest Scotland. The frequency of available habitat categories differed between the two regions (χ2-test, χ26 = 7359.5, P < 0.001) with a greater availability of intertidal and arable but lower availability of freshwater habitats in northwest England compared to southwest Scotland (Table 2).
Table 2. Composition of available habitat at the landscape scale within migration range of each herring gull colony in southwest Scotland (combined Southern Hebrides and Firth of Clyde) and in northwest England (Walney).
The most parsimonious RSF model, based on Akaike’s information criterion (AIC) values (Table 3), included the interaction between region and habitat, indicating that individuals from the two regions were selecting habitats differently. Based on the region-specific RSF models, in both northwest England and southwest Scotland, herring gulls selected (RSS > 1) intertidal, grassland, freshwater, and built-up habitats, whilst in Scotland they also selected arable habitat (Figure 2 and Supplementary Table 5). This indicates that these habitats were used more often relative to their availability compared to selection for a control group of “other” habitat. However, the rank of habitats selected by the gulls differed between the two regions. In northwest England gulls showed the strongest selection for intertidal, grassland, and freshwater habitats (Figure 2A), whilst in southwest Scotland, gulls showed the strongest selection for intertidal habitat, followed by arable and built-up habitats relative to “other” habitat (Figure 2B). Herring gulls in southwest Scotland showed a weak selection for marine habitat whilst birds in northwest England avoided (RSS < 1) this habitat. In northwest England, the gulls used arable habitat as would be expected given its availability. The proportion of each individual’s use fixes in the different habitats are given in Supplementary Table 6.
Table 3. Rank of general linear mixed effect models explaining variation in habitat use of herring gulls by habitat category (habitat) and region using Akaike’s information criterion corrected for small sample size (AICc): df is the degrees of freedom, wi is the Akaike weight, and ΔAICc is the AICc difference.
Figure 2. Resource selection strength (RSS) values with lower and upper 95% confidence intervals from the Resource Selection Function model in Supplementary Table 4 for herring gulls in (A) northwest England and (B) southwest Scotland. RSS = 1 (indicated by the dotted vertical line) indicates that use of the habitat is proportional to its availability; an RSS value with 95% confidence intervals not overlapping with the dotted vertical line show habitats that were preferred (RSS > 1) or avoided (RSS < 1) by herring gulls. The reference category was “other” habitat. Note log-scaling of the x axis.
The first principal component of the three stable isotope ratios from feathers moulted during the non-breeding season explained 76.6% of the variance and all three stable isotope ratios loaded negatively onto the first principal component (−0.52 to −0.65). The proportion of use points in marine and intertidal habitats significantly correlated with the bird’s feather stable isotope ratio (linear mixed-effect model: χ2 = 5.07, df = 1, P = 0.024, r = −0.62, N = 10, Supplementary Figure 3). This supports that the habitat use described by the tracking data indeed reflects the habitats used by gulls for consuming food (as based on the stable isotope ratios of feathers).
Habitat selection varied between individuals within regions as revealed by the Eigenanalyses of Selection Ratios that were run separately for the two regions, northwest England and southwest Scotland (Figure 3). The first factorial axis explained 76.7% of the selection ratio variance for northwest England and 49.2% of the selection ratio variance in southwest Scotland. The remaining variance on the second factorial axis thus revealed variation in habitat selection between individuals. In southwest Scotland (50.8% of the variance on the second factorial axis) some individuals showed a stronger selection for grassland and arable habitats whilst others showed a stronger selection for intertidal habitats (Figure 3B). In northwest England, the among-individual variation was less pronounced (23.3% of the variance on the second factorial axis), partly driven by one individual showing a strong selection for grassland habitat with the remaining gulls having a stronger selection for intertidal and freshwater habitats (Figure 3A).
Figure 3. Plot of Eigenanalysis of selection ratios for herring gulls using the non-breeding regions (A) northwest England and (B) southwest Scotland. The position of habitats reflects the biological significance of the first two factorial axes. The position of the arrows indicates the habitats selected by different individual gulls, with no selection in the centre of the axes. Eigenanalyses maximise the differences between habitat use and availability on the first factorial (horizontal) axes. If all individuals select the same habitat types, then all variation in selection is explained on the first factorial axis whereas the among-individual variation in habitat selection is distributed across the second factorial (vertical) axis. The first factorial axis explained 76.7% of the selection ratio variance for northwest England and 49.2% for southwest Scotland. The second factorial axis explained 23.3% of the selection ratio variance for northwest England and 50.8% for southwest Scotland.
Individual variation in habitat use was also reflected by the range of habitat specialisation shown by individuals, with PSi values ranging from 0.32 to 0.82 (median: 0.72, Supplementary Table 5). Most individuals had PSi values >0.5 and tended to be generalists in the habitats they used over the non-breeding period. Three individuals, however, had PSi <0.4 indicating some level of habitat specialisation; they included the two males and all three individuals specialised on the intertidal habitat (Supplementary Table 5). We found a low, but statistically significant repeatability in individual habitat specialisation across months (r = 0.22, 95% confidence interval = 0.05, 0.39).
Overall individuals did not migrate far from the breeding colony, with a mean distance of monthly 95% MCPs centroids from the breeding site of 57.0 km (range = 0.6–177.9 km, N = 20, Supplementary Table 1 and Supplementary Figure 4). Over the non-breeding season, the mean 95% MCP of individuals was 456 ± 400 km2. However, once in their wintering area individuals showed limited spatial specialisation (measured as the mean distance between centroids of consecutive monthly 95% MCPs) with a median value of 30.5 km. Spatial specialisation varied among individuals (range: 0.07–84.7 km) with individuals from Lady Isle, Firth of Clyde showing greater spatial specialisation than those from the other colonies (Table 1, Supplementary Table 3, and Supplementary Figure 4). This indicates that although some individuals spent the entire non-breeding season in a specific area, most individuals moved between different areas over the course of the breeding season (Supplementary Table 1). Spatial specialisation was only weakly repeatable within individuals across months (r = 0.15, 95% confidence interval = 0.00, 0.33) and was not related to habitat specialisation (r = 0.24, P = 0.31, N = 20).
Herring gulls tracked from five colonies across two geographical regions (southwest Scotland and northwest England) showed migratory connectivity at the regional level. At the local scale within southwest Scotland, however, migratory connectivity was less pronounced with overlap between the non-breeding areas of individuals from the different breeding colonies. Thus, migratory connectivity in herring gulls is scale dependent. During the non-breeding season, gulls used a range of habitats as would be expected for an opportunistic generalist. However, habitats were not used randomly based on their availability. Habitat selection differed between regions and individuals although populations from both regions strongly selected intertidal habitat suggesting this is an important habitat for wintering gulls. Individuals from the colonies considered moved around during the non-breeding season to different extents and did not show spatial specialisation. Within populations, individuals also differed in habitat selection with a moderate degree of habitat specialisation, independent of spatial specialisation. Hence, individuals sought similar resources at different locations, although given the low within-individual repeatability of habitat specialisation an individual’s resource use appeared to be flexible across time and space. Our results provide information on the distribution and habitat requirements of this generalist species during the non-breeding season and highlight considerable among- and within-population variation. The gull’s distribution over a broad range of habitats and space may help reduce competition for limited resources and buffer populations from localised anthropogenic pressures during the non-breeding season. However, our results also highlight the importance of intertidal habitats, as well as the availability of a mix of alternative habitats, for herring gulls during the non-breeding season, which should be considered in regional-specific spatial planning or conservation management for this species.
After the breeding season, the majority of herring gulls in the study area moved in a southerly direction. However, the timing of migration varied among colonies. Individuals breeding further north dispersed earlier and stayed away from the colony for longer, consistent with earlier migration at higher latitude in other migrating species (Hagan et al., 1991). We were unable to identify whether breeding attempts were successful or not for all individuals, with early departure rates likely involving failed breeders. Therefore, breeding failures may have influenced colony departure dates. Most individuals stayed away from the breeding site for roughly half of the annual cycle. Three individuals (two birds from Lady Isle, Firth of Clyde and one from Walney, northwest England) did not move further away from their breeding colony than they typically would in the breeding season (45 km, Clewley et al., 2021; O’Hanlon and Nager, unpublished data), although they stopped visiting the colony site regularly. The remaining individuals moved considerably further (up to 185 km). These migration distances were similar, or slightly shorter, to those estimated for herring gulls in the Netherlands based on ringing recoveries (Camphuysen et al., 2011), and considerably shorter than observed for American herring gulls (Larus smithsoniansus) breeding in northeastern North America (Anderson et al., 2019; Baak et al., 2021), possibly reflecting the mild oceanic climate of coastal Western Europe compared to northeastern North America.
Herring gulls breeding in northwest England and southwest Scotland spent the non-breeding period in distinct regions, suggesting migratory connectivity across geographic regions. Strong migratory connectivity across larger geographic scale has been previously found for American herring gulls (Anderson et al., 2019). However, on a finer geographical scale migratory connectivity was weaker. While the breeding home ranges of herring gulls from southwest Scotland were distinct (O’Hanlon and Nager, unpublished data) there was overlap between their non-breeding home ranges indicating weak migratory connectivity among populations at this scale. Migratory connectivity in seabirds has been shown to vary between species. For example, Merkel et al. (2021) found strong migratory connectivity in common (Uria aalge) and Brünnich’s guillemots (Uria lomvia) in the Northeast Atlantic, whereas Frederiksen et al. (2012) found weak migratory connectivity among black-legged kittiwake (Rissa tridactyla) populations wintering in the Northwest Atlantic. Here we found migratory connectivity varying within a species and depending on spatial scale, as has been shown in other species (Trierweiler et al., 2014; Phipps et al., 2019).
In general, individual herring gulls in our study area had relatively small home ranges during the non-breeding period, and which were considerably smaller than reported for American herring gulls in northeastern North America (Anderson et al., 2019), although different methods were used to calculate home ranges and therefore should be compared cautiously (Börger et al., 2008). Individuals moved relatively short distances between months once in their wintering grounds (distance moved between consecutive monthly MCPs), which varied considerably between individuals. Most individuals visited several areas with little spatial specialisation to a specific location. Movements between distinct areas within the non-breeding season has been reported for a range of North Atlantic seabirds (Amélineau et al., 2021). Herring gulls can show high between-year winter site fidelity (Ceia et al., 2014; Clark et al., 2016) and breeding birds can show high spatial site specialisation in foraging locations within years (Davis, 1975). The lack of spatial specialisation in this study could be due to fewer predictable and profitable foraging sites that are worth concentrating on during the non-breeding season, for example, fish docks (Davis, 1975). Alternatively, resources may be predictable from year-to-year, but only for short periods of time. Therefore, individuals may show short-term fidelity to multiple locations over the course of the non-breeding season. Across both regions, we observed among-colony differences in the distances moved between months with the greatest between-month movements made by individuals that bred further north in the Southern Hebrides (Oronsay and Islay). Although these individuals spent part of the non-breeding period in the same area as the Firth of Clyde (Pladda and Lady Isle) birds they moved through a wider area of the non-breeding range. This may indicate that changes in local resource availability in some areas, for example through reduced food availability or increased competition, resulted in individuals moving to new areas to find food over the course of the non-breeding season (Loretto et al., 2016). Exploring habitat specialisation alongside spatial specialisation provides complementary information on how individuals use the environment (Carneiro et al., 2017). This approach has been used to better explore spatial specialisation in other marine organisms and is relevant to species with a wide range of ecologies. Cory’s shearwaters (Calonectris borealis) (Dias et al., 2011), northern gannets (Morus bassanus) (Fifield et al., 2014), and tiger sharks (Galeocerdo cuvier) (Lea et al., 2015) have all been reported to show high spatial specialisation, or site fidelity, in winter destinations between years, although with variation between individuals. Breeding Cory’s shearwaters also show high spatial specialisation within a breeding season, possibly due to having access to sites with long-term high food availability (Navarro and González-Solís, 2009). In contrast, breeding herring gulls have been reported to show weaker spatial than habitat specialisation, but both influenced reproductive output (van den Bosch et al., 2019). The observed weak small-scale spatial specialisation within the broader wintering destination of herring gulls would be beneficial for coping with rapidly changing environments (Dias et al., 2011).
Little has previously been published on the use of habitats relative to their availability by non-breeding gulls, with fishery discards, landfill sites, and intertidal habitats all thought to be important resources for some populations during this period (Kihlman and Larsson, 1974; Monaghan, 1980; Horton et al., 1983; Hüppop and Wurm, 2000; Anderson et al., 2019). The non-breeding home range of herring gulls in our study area covered a range of different habitats and, as expected for an opportunistic forager, they used the available variety of habitats. The use of a range of habitats in non-breeding herring gulls is consistent with observations on breeding individuals, which also forage in a diversity of marine and terrestrial habitats (Harris, 1965; Hunt and Hunt, 1973; Kubetzki and Garthe, 2003; Enners et al., 2018). Individuals from many breeding colonies select intertidal habitat as their main foraging habitat (Camphuysen, 1995; Clewley et al., 2021) as did the non-breeding gulls in our study. This suggests that intertidal areas are also important foraging habitats for wintering herring gulls, at least in our study area. Foraging on intertidal resources can improve productivity of breeding herring gulls compared to other food sources, including in our study area (Pierotti and Annett, 1991; O’Hanlon et al., 2017). Intertidal prey can be richer in energy and/or nutrient content than other prey (O’Hanlon et al., 2017; but see van Donk et al., 2017), particularly in calcium content, which is important for egg formation and chick growth (Reynolds and Perrins, 2010). Similar energetic or nutritional benefits may also arise for non-breeding birds.
GPS data provided an indication of the habitats used by herring gulls during the non-breeding season. However, at the temporal resolution we obtained, the GPS data could not determine whether individuals were specifically foraging at that location and were therefore consuming resources from that habitat. Temporal resolution of data has also limited the interpretation of actual resource use of other non-breeding herring gull populations (Anderson et al., 2019). Within this study, support for the use of habitats for foraging was shown through analysis of stable isotope ratios in feathers that were grown during the non-breeding period. We found that the stable isotope ratios of feathers from a sub-sample of tagged individuals grown during the previous winter correlated with the use of marine habitat in the following winter based on GPS data. Assuming that herring gulls do have high between-year site fidelity in non-breeding areas (Ceia et al., 2014; Clark et al., 2016), and within-season consistency in habitat specialisation (our results), this indicates that the habitat use described by the tracking data did reflect the habitats used by gulls for consuming food (based on the stable isotope ratios of feathers).
At the population level, herring gulls also used grassland, arable, and built-up habitats to a greater extent than expected given their availability in the landscape. Arable and grassland habitats may be profitable to foraging gulls due to spilt grains or supplementary feeding of livestock (Drury and Smith, 1968; Mitchell et al., 2004). In the mild oceanic climate of the British Isles, soil invertebrates, in particular earthworms, are also accessible for most of the non-breeding period in these habitats (Kruuk, 1978). Earthworms can form an important part of the herring gull’s diet during the breeding season (McCleary and Sibly, 1986; Pennycott et al., 2020). Although fish and refuse are thought to be the most energy-rich and easily digestible food items for large gulls (Annett and Pierotti, 1989), preferences for arable and grassland habitat, where foraging might be more predictable and less risky (van Donk et al., 2017), might suggest that in the non-breeding period gulls adopt a more risk-averse resource utilisation strategy than when breeding.
The use of built-up areas and landfill sites by large gulls, including herring gulls, is well-documented (Kihlman and Larsson, 1974; Monaghan, 1980; Horton et al., 1983; Cook et al., 2008; Spelt et al., 2019). Although wintering herring gulls do use built-up areas, they appear to show a greater preference for other habitats and use landfill sites predominantly at high-tide when there is no access to intertidal prey (Kihlman and Larsson, 1974). The relatively frequent use of freshwater, as was also observed by Anderson et al. (2019), is likely due to gulls choosing safe areas on inland water bodies near good foraging locations to preen and roost (Clark, 2014). In addition, herring gulls are likely to require a supply of fresh water near feeding areas (Schreiber, 1967), particularly if they have been foraging in intertidal and/or offshore marine habitats. In our study area, herring gulls infrequently used offshore marine habitat within 40 km of the coast and in northwest England, in a lower proportion to its availability. This contrasts with regular observations of herring gulls offshore in the winter in this area over 20 years ago (Stone et al., 1995). This may suggest that reduced fisheries and discarding activity, at least in the Firth of Clyde (Thurstan and Roberts, 2010), may have reduced the profitability of foraging out at sea. We, however, need to acknowledge that we only observed mainly adult females and that habitat use patterns may be different for male and immature herring gulls.
Although marine intertidal habitat was the preferred habitat in both regions, the ranking of the remaining habitats differed with grassland and freshwater being the next preferred habitats in northwest England compared to arable and built-up habitat in southwest Scotland. Although the relative importance of habitats appeared to differ between the two regions, this could not be formally tested. Regional differences in what habitats were most selected were also observed in American herring gulls (Anderson et al., 2019). The differences observed in habitat selection between the regions may be due to functional responses to variation in habitat availability (Mysterud and Ims, 1998), although differences in the relative quality of habitats between regions cannot be ruled out. For instance, the availability of arable habitat was lower in southwest Scotland than northwest England. If that habitat contains important resources for herring gulls the relative selection strength might be expected to be higher where this habitat is rarer.
Within populations, individual herring gulls varied in their habitat use and their extent of habitat and spatial specialism over the non-breeding season. This pattern of intra-individual variation in habitat selection was found in two different landscapes (southwest Scotland and northwest England), although it was more pronounced in southwest Scotland. Habitat specialisation, although repeatable within individuals, was low and independent of spatial specialisation, potentially because birds moved around during the non-breeding period and flexibly used available resources at each location. These results concur with previous studies in that, although being generalists at the population level, individual gulls can be specialists (Navarro et al., 2017; van den Bosch et al., 2019). The extent of specialisation may be less pronounced in the non-breeding period than in the breeding period, potentially due to individuals no longer being constrained to a central breeding location. Non-breeding individuals may change which habitats they use if there are seasonal changes in relative resource availability in different habitats. Increased specialism can benefit individuals through increased foraging efficiency and energy intake (MacArthur and Pianka, 1958; Dukas and Kamil, 2001; van den Bosch et al., 2019), and through reducing intra-specific competition for resources (Bolnick et al., 2003; Ceia and Ramos, 2015). In this study, individuals did not specialise on one habitat over the course of the non-breeding season, except for three individuals that specialised on intertidal habitats, including the only two males in the study, but they may have reduced intra-specific competition through flexible use of a range of habitats.
It is not clear what factors influence variation in habitat selection between individual herring gulls. The characteristics of an individual may explain some of the variation in habitat selection such as age, sex, body size, or personality (e.g., Hebblewhite and Merrill, 2008; Bonnot et al., 2015; Leclerc et al., 2016; Montgomery et al., 2018). For example, smaller females and less experienced immature herring gulls might be at a competitive disadvantage compared to experienced, larger adult males, which may result in them utilising a variety of potentially less favourable habitats (Monaghan, 1980; van Donk et al., 2018). As most individuals in this study were females, the low specialisation observed may be due to these individuals being outcompeted by males, or larger wintering individuals from Scandinavia (Calladine, 2002). Understanding what factors shape variation in habitat selection can highlight a species’ potential for plastic responses to environmental change (Grist et al., 2014; Müller et al., 2014). Increased environmental heterogeneity and diversity of ecological opportunities are predicted to increase ecological segregation between conspecifics within (Robertson et al., 2015) and among populations (Rosenblatt et al., 2015). Therefore, it would be beneficial to track movements and observe behaviours of a broader section of the wintering population to improve our understanding of variation in habitat and spatial specialisation in wintering gulls.
Conditions in the non-breeding period can have an importance influence on annual survival and carry-over effects on the breeding performance of seabirds (reviewed in Strøm et al., 2021) and can be important for the regulation of seabird populations (Lack, 1966; Gaston, 2003; Sæther et al., 2016). Within this study, herring gull populations, and individuals, differed in their use of foraging habitats during the non-breeding period. Such differences can have important implications for population trends (Corbeau et al., 2021). Differences in adult survival between populations of herring gulls are hypothesised to be associated with differences in the availability of natural foraging habitats (Pons and Migot, 1995; Anderson et al., 2019). Although we do not have separate estimates of adult survival rates for northwest England and southwest Scotland, herring gull populations in the northwest England region are declining (Sellers and Shackleton, 2011) whereas populations in southwest Scotland remained relatively stable over the same period (O’Hanlon and Nager, 2018). The migratory connectivity in northwest England and southwest Scotland could be a factor in the differences in trajectories of populations at the larger regional scale. Differences in habitat availability and use during the non-breeding season may also be a factor affecting differences in population trends. Regional variation in population trends in American herring gull populations in northeastern North America had been hypothesised to be due to migratory connectivity and differences in non-breeding habitat use (Anderson et al., 2019). Despite the high degree of mixing among individuals from colonies in southwest Scotland, individuals were spread over a large area and used a variety of habitats. Consequently, localised environmental changes would be unlikely to affect all herring gull colonies in the region similarly. Indeed, although relatively stable overall, breeding populations in southwest Scotland varied in their population trajectories over a relatively small spatial scale (O’Hanlon and Nager, 2018). Part of the among-colony variation in population trajectories was linked to the availability of local foraging habitats (O’Hanlon and Nager, 2018), however carry-over effects from the non-breeding season may also have had an effect.
Herring gulls used a broad range of habitats during the non-breeding season with habitat selection differing between geographical regions and between individuals in the same region. This highlights that information on the habitat preferences of generalist species cannot be generalised, as the habitat preferences of individuals in one region may differ from those in relatively nearby regions. Although intertidal habitat was preferred, it is only available for variable periods depending on the timing of the tide and strength and direction of wind. Therefore, while this habitat provides high-quality food (Pierotti and Annett, 1991; O’Hanlon et al., 2017), alternative foraging habitats may be required. An availability of a range of habitats is thus likely to be important for herring gulls during the non-breeding season.
Identifying the intertidal areas most favourable to herring gulls and providing effective protection during the non-breeding period could benefit populations. For example, through reducing human disturbance, sustainable management of harvesting intertidal invertebrates, and managing conflict between wildlife and shellfisheries, which would likely benefit multiple species using intertidal habitats (Burton et al., 2002; Clarke et al., 2017; Clewley et al., 2021). The majority of individuals we studied tended to be habitat generalists over the non-breeding season but varied in the habitats selected indicating some level of habitat segregation, as well as spatial segregation, that might affect intra-specific competition. Availability of alternative foraging opportunities in the vicinity of favourable intertidal areas may make populations less vulnerable to temporary environmental perturbations and human pressures (Webster et al., 2002; Esler, 2014). However, individual variation in the use of habitat and space during the non-breeding season highlights that individuals within a population are not ecologically equivalent, and that intrinsic factors may drive variation in patterns of space use among individuals of a species. There is a need therefore to better understand the intrinsic drivers of habitat and spatial segregation between individuals, and identify what carry-over effects foraging on different resources during the non-breeding season may have on the following breeding season (Robb et al., 2008; Sorensen et al., 2009). A better understanding of habitat selection and its spatial variation can have important implication for implementing conservation management plans for wintering populations of generalist species of conservation concern across their annual cycle and will necessitate local or regional management plans that incorporate both marine and terrestrial environments.
The raw data supporting the conclusions of this article will be made available by the authors, without undue reservation.
The animal study was reviewed and approved by Animal Welfare and Ethical Review Body (AWERB) of the University of Glasgow. Birds were tagged under licence, with approval by the Independent Special Methods Technical Panel of the UK Ringing Scheme.
NO’H, CT, NB, and RN contributed to conception and design of the study and wrote sections of the manuscript. NO’H, CT, NC, DG, GCl, GCo, and RM collected and processed the data. NO’H performed the statistical analysis and wrote the first draft of the manuscript. All authors contributed to manuscript revision, read, and approved the submitted version.
Work in southwest Scotland was supported by the European Union’s INTERREG IVA Programme (project 2859 “IBIS”) managed by the Special EU Programmes Body and the Natural Environment Research Council and the Department for Environment, Food, and Rural Affairs (Grant Number NE/L003090/1. Marine Ecosystems Research Programme). Work in northwest England was supported by the Department of Energy and Climate Change (DECC) (now the Department for Business, Energy, and Industrial Strategy, BEIS) Offshore Energy Strategic Environmental Assessment research programme.
The authors declare that the research was conducted in the absence of any commercial or financial relationships that could be construed as a potential conflict of interest.
All claims expressed in this article are solely those of the authors and do not necessarily represent those of their affiliated organizations, or those of the publisher, the editors and the reviewers. Any product that may be evaluated in this article, or claim that may be made by its manufacturer, is not guaranteed or endorsed by the publisher.
We thank the technical support of the GPS-devices from Gary Brodin at PathTrack and Nosrat Mirzai, and Ewan Wakefield and Paul Johnston for statistical advice. We also thank John Hartley (Hartley Anderson Ltd.), Emma Cole, Mandy King, Sophie Thomas, and James Burt (DECC), and the late Mark Rehfisch (BTO) for support. Angus Lothian, Terry Southall, and the RSPB Oronsay Staff helped with fieldwork in Scotland. We also thank Cumbria Wildlife Trust and Natural England for permissions and Emily Scragg for help with fieldwork in northwest England. Thanks to the reviewers for all their constructive comments that improved the manuscript.
The Supplementary Material for this article can be found online at: https://www.frontiersin.org/articles/10.3389/fmars.2022.816881/full#supplementary-material
Aebischer, N. J. (1993). Immediate and delayed effects of a gale in late spring on the breeding of the Shag Phalacrocorax aristotelis. IBIS 135, 225–232. doi: 10.1111/j.1474-919X.1993.tb02838.x
Alerstam, T., Hedenström, A., and Åkesson, S. (2003). Long-distance migration: Evolution and determinants. Oikos 103, 247–260. doi: 10.1034/j.1600-0706.2003.12559.x
Amélineau, F., Merkel, B., Tarroux, A., Descamps, S., Anker-Nilssen, T., Bjørnstad, O., et al. (2021). Six pelagic seabird species of the North Atlantic engage in a fly-and-forage strategy during their migratory movements. Mar. Ecol. Prog. Ser. 676, 127–144. doi: 10.3354/meps13872
Anderson, C. M., Gilchrist, H. G., Ronconi, R. A., Shlepr, K. R., Clark, D. E., Weseloh, D. V. C., et al. (2019). Winter home range and habitat selection differs among breeding populations of herring gulls in eastern North America. Mov. Ecol. 7, 1–11. doi: 10.1186/s40462-019-0157-5
Annett, C. A., and Pierotti, R. (1989). Chick hatching as a trigger for dietary switching in the Western Gull. Colon. Waterbirds 12, 4–11. doi: 10.2307/1521306
Araújo, M. S., Bolnick, D. I., and Layman, C. A. (2011). The ecological causes of individual specialisation. Ecol. Lett. 14, 948–958. doi: 10.1111/j.1461-0248.2011.01662.x
Avgar, T., Lele, S. R., Keim, J. L., and Boyce, M. S. (2017). Relative Selection Strength: Quantifying effect size in habitat- and step-selection inference. Ecol. Evol. 7, 5322–5330. doi: 10.1002/ece3.3122
Baak, J. E., Mallory, M. L., Anderson, C. M., Auger-méthé, M., Ch, A., Janssen, M. H., et al. (2021). Inter-individual variation in the migratory behaviour of a generalist seabird, the herring gull (Larus smithsoniansus), from the Canadian Arctic. Anim. Migration 8, 144–155.
Bates, D., Maechler, M., Bolker, B., and Walker, S. (2014). lme4: Linear mixed-effects models using Eigen and S4. R Packag. Vers. 1, 1–6.
Bernard, A., Rodrigues, A. S. L., Cazalis, V., and Grémillet, D. (2021). Toward a global strategy for seabird tracking. Conserv. Lett. 2021, 1–15. doi: 10.1111/conl.12804
Bhattacharyya, A. (1943). On a measure of divergence between two statistical populations defined by their probability distributions. Bull. Calcutta Math. Soc. 35, 99–110.
Bivand, R., and Rundel, C. (2019). rgeos: Interface to Geometry Engine - Open Source (‘GEOS’). R package version 0.4. Vienna: R Core Team.
Bolnick, D. I., Svanbäck, R., Araújo, M. S., and Persson, L. (2007). Comparative support for the niche variation hypothesis that more generalized populations also are more heterogeneous. Proc. Natl. Acad. Sci. U S A. 104, 10075–10079. doi: 10.1073/pnas.0703743104
Bolnick, D. I., Svanbäck, R., Fordyce, J. A., Yang, L. H., Davis, J. M., Hulsey, C. D., et al. (2003). The ecology of individuals: incidence and implications of individual specialization. Am. Nat. 161, 1–28. doi: 10.1086/343878
Bolnick, D. I., Yang, L. H., Fordyce, J. A., Davis, J., and Svanback, R. (2002). Measuring individual-level resource specialization. Ecology 83, 2936–2941. doi: 10.1890/0012-9658(2002)083[2936:milrs]2.0.co;2
Bonnot, N., Verheyden, H., Blanchard, P., Cote, J., Debeffe, L., Cargnelutti, B., et al. (2015). Interindividual variability in habitat use: Evidence for a risk management syndrome in roe deer? Behav. Ecol. 26, 105–114. doi: 10.1093/beheco/aru169
Börger, L., Dalziel, B. D., and Fryxell, J. M. (2008). Are there general mechanisms of animal home range behaviour? A review and prospects for future research. Ecol. Lett. 11, 637–650. doi: 10.1111/j.1461-0248.2008.01182.x
Boyce, M. S. (2006). Scale for resource selection functions. Divers. Distrib. 12, 269–276. doi: 10.1111/j.1366-9516.2006.00243.x
Bukacińska, M., Bukaciński, D., and Spaans, A. L. (1996). Attendance and diet in relation to breeding success in Herring Gulls (Larus argentatus). Auk 113, 300–309. doi: 10.2307/4088896
Burger, A. E., and Shaffer, S. A. (2008). Application of tracking and data-logging technology in research and conservation of seabirds. Auk 125, 253–264. doi: 10.1525/auk.2008.1408
Burton, N. H. K., Armitage, M. J. S., Musgrove, A. J., and Rehfisch, M. M. (2002). Impacts of man-made landscape features on numbers of estuarine waterbirds at low tide. Environ. Manage. 30, 857–864. doi: 10.1007/s00267-002-2732-5
Calenge, C. (2006). The package “adehabitat” for the R software: A tool for the analysis of space and habitat use by animals. Ecol. Modell. 197, 516–519. doi: 10.1016/j.ecolmodel.2006.03.017
Calenge, C., and Dufour, A. B. (2006). Eigenalaysis of selection ratios from animal radio-tracking data. Ecology 87, 2349–2355. doi: 10.1890/0012-9658(2006)87[2349:eosrfa]2.0.co;2
Calladine, J. (2002). “Herring Gull Larus argentatus,” in The migration atlas: movements of the birds of Britain and Ireland, eds C. Wernham, M. Toms, J. Marchant, J. Clark, G. Siriwardena, and S. Baillie (London: T&AD Poyser), 369–372.
Camphuysen, C. J., Vercruijsse, H. J. P., and Spaans, A. L. (2011). Colony- and age-specific seasonal dispersal of Herring Gulls Larus argentatus breeding in The Netherlands. J. Ornithol. 152, 849–868. doi: 10.1007/s10336-011-0664-7
Camphuysen, K. C. J. (1995). Herring gull Larus argentatus and Lesser black-backed gull L. fuscus feeding at fishing vessels in the breeding season: competitive scavenging versus efficient flying. Ardea 83, 365–380.
Carneiro, A. P. B., Bonnet-Lebrun, A.-S., Manica, A., Staniland, I. J., and Phillips, R. A. (2017). Methods for detecting and quantifying individual specialisation in movement and foraging strategies of marine predators. Mar. Ecol. Prog. Ser. 578, 151–166. doi: 10.3354/meps12215
Catry, P., Phillips, R. A., and Croxall, J. P. (2005). Sexual segregation in birds: Patterns, processes and implications for conservation. Cambridge: Cambridge University Press, doi: 10.1017/CBO9780511525629.019
Ceia, F. R., and Ramos, J. A. (2015). Individual specialization in the foraging and feeding strategies of seabirds: a review. Mar. Biol. 162, 1923–1938. doi: 10.1007/s00227-015-2735-4
Ceia, F., Paiva, V., Fidalgo, V., Morais, L., Baeta, A., Crisóstomo, P., et al. (2014). Annual and seasonal consistency in the feeding ecology of an opportunistic species, the yellow-legged gull Larus michahellis. Mar. Ecol. Prog. Ser. 497, 273–284. doi: 10.3354/meps10586
Clark, D. E. (2014). Roosting, Site Fidelity, and Food Sources of Urban Gulls In Massachusetts: Implications For Protecting Public Water Supplies. Massachusetts, MA: University of Massachusetts.
Clark, D. E., Koenen, K. K. G., Whitney, J. J., MacKenzie, K. G., and DeStefano, S. (2016). Fidelity and persistence of Ring-Billed (Larus delawarensis) and Herring (Larus argentatus) Gulls to wintering sites. Waterbirds 39, 220–234. doi: 10.1675/063.039.sp120
Clark, J. A., Thorup, K., and Stroud, D. A. (2009). Quantifying the movement patterns of birds from ring recoveries. Ringing Migr. 24, 180–188. doi: 10.1080/03078698.2009.9674390
Clarke, L. J., Hughes, K. M., Esteves, L. S., Herbert, R. J. H., and Stillman, R. A. (2017). Intertidal invertebrate harvesting: A meta-analysis of impacts and recovery in an important waterbird prey resource. Mar. Ecol. Prog. Ser. 584, 229–244. doi: 10.3354/meps12349
Clewley, G. D., Barber, L. J., Conway, G. J., Clark, N. A., Donato, B. J., Thaxter, C. B., et al. (2021). Foraging habitat selection by breeding Herring Gulls (Larus argentatus) from a declining coastal colony in the United Kingdom. Estuar. Coast. Shelf Sci. 261:107564. doi: 10.1016/j.ecss.2021.107564
Cohen, E. B., Rushing, C. R., Moore, F. R., Hallworth, M. T., Hostetler, J. A., Ramirez, M. G., et al. (2019). The strength of migratory connectivity for birds en route to breeding through the Gulf of Mexico. Ecography 42, 658–669. doi: 10.1111/ecog.03974
Cook, A., Rushton, S., Allan, J., and Baxter, A. (2008). An evaluation of techniques to control problem bird species on landfill sites. Environ. Manage. 41, 834–843. doi: 10.1007/s00267-008-9077-7
Cooke, F., and Ross, R. K. (1972). Diurnal and seasonal activities of a post-breeding population of gulls in southeastern Ontario. Wilson Bull. 84, 164–172.
Corbeau, A., Collet, J., Pajot, A., Joo, R., Thellier, T., and Weimerskirch, H. (2021). Differences in foraging habitat result in contrasting fisheries interactions in two albatross populations. Mar. Ecol. Prog. Ser. 663, 197–208. doi: 10.3354/meps13648
Coulson, J. C., and Butterfield, J. (1985). Movements of British Herring Gulls. Bird study 32, 91–103. doi: 10.1080/00063658509476863
Coulson, J. C., Thomas, C. S., Butterfield, J. E., Duncun, N., Monaghan, P., and Shedden, C. (1983). The use of head and bill length to sex live gulls Laridae. IBIS 125, 549–557. doi: 10.1111/j.1474-919x.1983.tb03148.x
Davis, J. W. F. (1975). Specialization in feeding location by Herring Gulls. J. Anim. Ecol. 44, 795–804. doi: 10.2307/3719
Dias, M. P., Granadeiro, J. P., Phillips, R. A., Alonso, H., and Catry, P. (2011). Breaking the routine: individual Cory’s shearwaters shift winter destinations between hemispheres and across ocean basins. Proc. Biol. Sci. 278, 1786–1793. doi: 10.1098/rspb.2010.2114
Drury, W. H., and Smith, W. J. (1968). Defense of feeding areas by adult Herring Gulls and intrusion by young. Evolution 22, 193–201. doi: 10.1111/j.1558-5646.1968.tb03462.x
Dukas, R., and Kamil, A. C. (2001). Limited attention: the constraint underlying search image. Behav. Ecol. 12, 192–199. doi: 10.1093/beheco/12.2.192
Enners, L., Schwemmer, P., Corman, A. M., Voigt, C. C., and Garthe, S. (2018). Intercolony variations in movement patterns and foraging behaviors among herring gulls (Larus argentatus) breeding in the eastern Wadden Sea. Ecol. Evol. 8, 7529–7542. doi: 10.1002/ece3.4167
Esler, D. (2014). Applying metapopulation theory to conservation of migratory. Conserv. Biol. 14, 366–372. doi: 10.1046/j.1523-1739.2000.98147.x
European Environment Agency (2019). Copernicus Land Monitoring Service 2019. København: European Environment Agency.
Fieberg, J., and Kochanny, C. O. (2005). Quantifying home-range overlap: the importance of the utilization distribution. J. Wildl. Manage. 69, 1346–1359. doi: 10.2193/0022-541x(2005)69[1346:qhotio]2.0.co;2
Fieberg, J., Signer, J., Smith, B., and Avgar, T. (2021). A ‘How to’ guide for interpreting parameters in habitat-selection analyses. J. Anim. Ecol. 90, 1027–1043. doi: 10.1111/1365-2656.13441
Fifield, D. A., Montevecchi, W. A., Garthe, S., Robertson, G. J., Kubetzki, U., and Rail, J. (2014). Migratory tactics and wintering areas of Northern Gannets (Morus bassanus) breeding in North America. Ornithol. Monogr. 79, 1–63.
Fort, J., Porter, W. P., and Grémillet, D. (2009). Thermodynamic modelling predicts energetic bottleneck for seabirds wintering in the northwest Atlantic. J. Exp. Biol. 212, 2483–2490. doi: 10.1242/jeb.032300
Frederiksen, M., Moe, B., Daunt, F., Phillips, R. A., Barrett, R. T., Bogdanova, M. I., et al. (2012). Multicolony tracking reveals the winter distribution of a pelagic seabird on an ocean basin scale. Divers. Distrib. 18, 530–542. doi: 10.1111/j.1472-4642.2011.00864.x
Gaston, A. J. (2003). Synchronous fluctuations of Thick-billed Murre (Uria Lomvia) colonies in the Eastern Canadian Arctic suggest population regulation in winter. Auk 120, 362–370. doi: 10.1093/auk/120.2.362
Gillies, C. S., Hebblewhite, M., Nielsen, S. E., Krawchuk, M. A., Aldridge, C. L., Frair, J. L., et al. (2006). Application of random effects to the study of resource selection by animals. J. Anim. Ecol. 75, 887–898. doi: 10.1111/j.1365-2656.2006.01106.x
Götmark, F. (1984). Food and foraging in five European Larus gulls in the breeding season: a comparative review. Ornis Fenn. 61, 9–18.
Grist, H., Daunt, F., Wanless, S., Nelson, E. J., Harris, M. P., Newell, M., et al. (2014). Site fidelity and individual variation in winter location in partially migratory European shags. PLoS One 9:e98562. doi: 10.1371/journal.pone.0098562
Hagan, J. M., Lloyd-Evans, T. L., and Atwood, J. L. (1991). The relationship between latitude and the timing of spring migration of North American landbirds. Ornis Scand. 22, 129–136.
Hario, M., and Rintala, J. (2016). Population trends in Herring Gulls (Larus argentatus), Great Black-backed Gulls (Larus marinus) and Lesser Black-backed Gulls (Larus fuscus fuscus) in Finland. Waterbirds 39, 10–14.
Harris, M. P. (1964). Recoveries of ringed Herring Gulls. Bird Study 11, 183–191. doi: 10.1080/00063656409476068
Harris, M. P. (1965). The food of some Larus gulls. IBIS 107, 43–53. doi: 10.1111/j.1474-919X.1965.tb07280.x
Hebblewhite, M., and Merrill, E. (2008). Modelling wildlife-human relationships for social species with mixed-effects resource selection models. J. Appl. Ecol. 45, 834–844. doi: 10.1111/j.1365-2664.2008.01466.x
Hijmans, R. (2019a). geosphere: Spherical Trigonometry. R package version 1.5-10. Vienna: R Core Team.
Hobson, K. A., and Welch, H. E. (1995). Cannibalism and trophic structure in a high Arctic lake: insights from stable-isotope analysis. Can. J. Fish. Aquat. Sci. 52, 1195–1201. doi: 10.1139/f95-116
Hobson, K. A., Blight, L. K., and Arcese, P. (2015). Human-induced long-term shifts in gull diet from marine to terrestrial sources in North America’s coastal Pacific: More evidence from more isotopes (δ2H, δ34S). Environ. Sci. Technol. 49, 10834–10840. doi: 10.1021/acs.est.5b02053
Horton, N., Brough, T., and Rochard, J. B. A. (1983). The importance of refuse tips to gulls wintering in an inland area of south-east England. J. Appl. Ecol. 20, 751–765.
Hothorn, T., Bretz, F., and Westfall, P. (2008). Simultaneous inference in general parametric. Biometric J. 50, 346–363.
Hunt, G. L. (1972). Influence of food distribution and human disturbance on the reproductive success of Herring Gulls. Ecology 53, 1051–1061.
Hunt, G. L., and Hunt, M. W. (1973). Habitat partitioning by foraging gulls in Maine and Northwestern Europe. Auk 90, 827–839.
Hüppop, O., and Wurm, S. (2000). Effects of winter fishery activities on resting numbers, food and body condition of large gulls Larus argentatus and L. marinus in the south-eastern North Sea. Mar. Ecol. Prog. Ser. 194, 241–247. doi: 10.3354/meps194241
Iwamura, T., Possingham, H. P., Chadès, I., Minton, C., Murray, N. J., Rogers, D. I., et al. (2013). Migratory connectivity magnifies the consequences of habitat loss from sea-level rise for shorebird populations. Tohoku J. Exp. Med. 230:0325. doi: 10.1098/rspb.2013.0325
Johnson, C. J., Nielsen, S. E., Merrill, E. H., McDonald, T. L., and Boyce, M. S. (2006). Resource selection functions based on use–availability data: Theoretical motivation and evaluation methods. J. Wildl. Manage. 70, 347–357.
Kavelaars, M. M., Stienen, E., Matheve, H., Buijs, R. J., Lens, L., and Müller, W. (2018). GPS tracking during parental care does not affect early offspring development in lesser black-backed gulls. Mar. Biol. 165, 1–8. doi: 10.1007/s00227-018-3347-6
Kihlman, J., and Larsson, L. (1974). On the importance of refuse dumps as a food source for wintering Herring Gulls Larus argentatus. Ornis Scand. 5, 63–70.
Kruuk, H. (1978). Foraging and spatial organisation of the European Badger Meles meles. Behav. Ecol. Sociobiol. 4, 75–89.
Kubetzki, U., and Garthe, S. (2003). Distribution, diet and habitat selection by four sympatrically breeding gull species in the south-eastern North Sea. Mar. Biol. 143, 199–207. doi: 10.1007/s00227-003-1036-5
Lea, J. S. E., Wetherbee, B. M., Queiroz, N., Burnie, N., Aming, C., Sousa, L. L., et al. (2015). Repeated, long-distance migrations by a philopatric predator targeting highly contrasting ecosystems. Sci. Rep. 5:sre11202. doi: 10.1038/srep11202
Leclerc, M., Vander Wal, E., Zedrosser, A., Swenson, J. E., Kindberg, J., and Pelletier, F. (2016). Quantifying consistent individual differences in habitat selection. Oecologia 180, 697–705. doi: 10.1007/s00442-015-3500-6
Loretto, M. C., Schuster, R., and Bugnyar, T. (2016). GPS tracking of non-breeding ravens reveals the importance of anthropogenic food sources during their dispersal in the Eastern Alps. Curr. Zool. 62, 337–344. doi: 10.1093/cz/zow016
MacArthur, R. H., and Pianka, E. R. (1958). On optimal use of a patchy environment. Am. Nat. 100, 603–609.
Manly, B., McDonald, L., Thomas, D., McDonald, T., and Erickson, W. (2002). “Resource selection by animals,” in Statistical design and analysis for field studies, eds B. B. Manly, L. McDonald, and D. L. Thomas (Berlin: Springer), 1–209.
Marra, P. P., Cohen, E. B., Loss, S. R., Rutter, J. E., and Tonra, C. M. (2015). A call for full annual cycle research in animal ecology. Biol. Lett. 11:2015.0552. doi: 10.1098/rsbl.2015.0552
Marra, P., Hobson, K. A., and Holmes, R. T. (1998). Linking winter and summer events in a migratory bird by using stable-carbon isotopes. Science 282, 1884–1886. doi: 10.1126/science.282.5395.1884
McCleary, R. H., and Sibly, R. M. (1986). Feeding specialization and preference in Herring Gulls. J. Anim. Ecol. 55, 245–259.
McFarlane Tranquilla, L. A., Montevecchi, W. A., Hedd, A., Fifield, D. A., Burke, C. M., Smith, P. A., et al. (2013). Multiple-colony winter habitat use by murres Uria spp. in the Northwest Atlantic Ocean: Implications for marine risk assessment. Mar. Ecol. Prog. Ser. 472, 287–303. doi: 10.3354/meps10053
Merkel, B., Descamps, S., Yoccoz, N., Grémillet, D., Fauchald, P., Danielsen, J., et al. (2021). Strong migratory connectivity across meta-populations of sympatric North Atlantic seabirds. Mar. Ecol. Prog. Ser. 676, 173–188. doi: 10.3354/meps13580
Mitchell, P. I., Newton, S. F., Ratcliffe, N., Eds, T. E. D., Dunn, T. E., Poyser, A. D., et al. (2004). Seabird populations of Britain and Ireland: Results of the Seabird 2000 census. London: JNCC.
Monaghan, P. (1980). Dominance and dispersal between feeding sites in the Herring gull (Larus argentatus). Anim. Behav. 28, 521–527.
Montgomery, R. A., Redilla, K. M., Ortiz-Calo, W., Smith, T., Keller, B., and Millspaugh, J. J. (2018). Evaluating the individuality of animal-habitat relationships. Ecol. Evol. 2018, 10893–10901. doi: 10.1002/ece3.4554
Müller, M. S., Massa, B., Phillips, R. A., and Dell’omo, G. (2014). Individual consistency and sex differences in migration strategies of Scopoli’s shearwaters Calonectris diomedea despite year differences. Curr. Zool. 60, 631–641. doi: 10.1093/czoolo/60.5.631
Mysterud, A., and Ims, R. A. (1998). Functional responses in habitat use: availability influences relative use in trade-off. Ecology 79, 1435–1441.
Nager, R. G., and O’Hanlon, N. J. (2016). Changing numbers of three gull species in the British Isles. Waterbirds 39, 15–28.
Nakagawa, S., and Schielzeth, H. (2013). A general and simple method for obtaining R2 from generalized linear mixed-effects models. Methods Ecol. Evol. 4, 133–142. doi: 10.1111/j.2041-210x.2012.00261.x
Navarro, J., and González-Solís, J. (2009). Environmental determinants of foraging strategies in Cory’s shearwaters Calonectris diomedea. Mar. Ecol. Prog. Ser. 378, 259–267. doi: 10.3354/meps07880
Navarro, J., Grémillet, D., Ramirez, F. J., Afán, I., Bouten, W., and Forero, M. G. (2017). Shifting individual habitat specialization of a successful predator living in anthrop ogeniclandscapes. Mar. Ecol. Prog. Ser. 578, 243–251. doi: 10.3354/meps12124
O’Hanlon, N. J., and Nager, R. G. (2018). Identifying habitat-driven spatial variation in colony size of Herring Gulls Larus argentatus. Bird Study 65, 306–316. doi: 10.1080/00063657.2018.1518970
O’Hanlon, N. J., Mcgill, R. A. R., and Nager, R. G. (2017). Increased use of intertidal resources benefits breeding success in a generalist gull species. Mar. Ecol. Prog. Ser. 574, 193–210. doi: 10.3354/meps12189
Osterblom, H., Olsson, O., Blenckner, T., and Furness, R. W. (2008). Junk-food in marine ecosystems. Oikos 117, 967–977. doi: 10.1111/j.2008.0030-1299.16501.x
Pearson, D., Shine, R., and How, R. (2002). Sex-specific niche partitioning and sexual size dimorphism in Australian pythons (Morelia spilota imbricata). Biol. J. Linn. Soc. 77, 113–125. doi: 10.1046/j.1095-8312.1999.00075.x
Pennycott, T. W., Grant, D., and Nager, R. G. (2020). Earthworms in the diet of Herring Gulls Larus argentatus breeding on an off-shore island. Bird Study 67, 131–134. doi: 10.1080/00063657.2020.1743232
Perry, G. (1996). The evolution of sexual dimorphism in the lizard Anolis polylepis (Iguania): Evidence from intraspecific variation in foraging behavior and diet. Can. J. Zool. 74, 1238–1245. doi: 10.1139/z96-137
Phillips, R. A., Bearhop, S., McGill, R. A. R., and Dawson, D. A. (2009). Stable isotopes reveal individual variation in migration strategies and habitat preferences in a suite of seabirds during the nonbreeding period. Oecologia 160, 795–806. doi: 10.1007/s00442-009-1342-9
Phillips, R. A., McGill, R. A. R., Dawson, D. A., and Bearhop, S. (2011). Sexual segregation in distribution, diet and trophic level of seabirds: Insights from stable isotope analysis. Mar. Biol. 158, 2199–2208. doi: 10.1007/s00227-011-1725-4
Phipps, W. L., López-López, P., Buechley, E. R., Oppel, S., Álvarez, E., Arkumarev, V., et al. (2019). Spatial and Temporal Variability in Migration of a Soaring Raptor Across Three Continents. Front. Ecol. Evol. 7:1–14. doi: 10.3389/fevo.2019.00323
Pierotti, R., and Annett, C. A. (1991). Diet choice in the Herring Gull: constraints imposed by reproductive and ecological factors. Ecology 72, 319–328. doi: 10.2307/1938925
Pons, J.-M., and Migot, P. (1995). Life-history strategy of the herring gull: changes in survival and fecundity in a population subjected to various feeding conditions. J. Anim. Ecol. 64, 592–599. doi: 10.2307/5802
Quillfeldt, P., Voigt, C. C., and Masello, J. F. (2010). Plasticity versus repeatability in seabird migratory behaviour. Behav. Ecol. Sociobiol. 64, 1157–1164. doi: 10.1007/s00265-010-0931-2
R Development Core Team (2020). R: A Language and Environment for Statistical Computing. Vienna: R Foundation for Statistical Computing.
Reynolds, S. J., and Perrins, C. M. (2010). Dietary Calcium Availability and Reproduction in Birds. Curr. Ornithol. 2010, 31–74. doi: 10.1007/978-1-4419-6421-2
Robb, G. N., McDonald, R. A., Chamberlain, D. E., Reynolds, S. J., Harrison, T. J. E., and Bearhop, S. (2008). Winter feeding of birds increases productivity in the subsequent breeding season. Biol. Lett. 4, 220–223. doi: 10.1098/rsbl.2007.0622
Robertson, A., McDonald, R. A., Delahay, R. J., Kelly, S. D., and Bearhop, S. (2015). Resource availability affects individual niche variation and its consequences in group-living European badgers Meles meles. Oecologia 178, 31–43. doi: 10.1007/s00442-014-3202-5
Robin, X., Turck, N., Hainard, A., Tiberti, N., Lisacek, F., Sanchez, J.-C., et al. (2011). pROC: an open-source package for R and S+ to analyze and compare ROC curves. BMC Bioinformatics 12:77. doi: 10.1007/s00134-009-1641-y
Rosenblatt, A. E., Nifong, J. C., Heithaus, M. R., Mazzotti, F. J., Cherkiss, M. S., Jeffery, B. M., et al. (2015). Factors affecting individual foraging specialization and temporal diet stability across the range of a large “generalist” apex predator. Oecologia 178, 5–16. doi: 10.1007/s00442-014-3201-6
Sæther, B. E., Grøtan, V., Engen, S., Coulson, T., Grant, P. R., Visser, M. E., et al. (2016). Demographic routes to variability and regulation in bird populations. Nat. Commun. 7:ncomms12001. doi: 10.1038/ncomms12001
Schielzeth, H., and Nakagawa, S. (2011). rptR: Repeatability for Gaussian and non-Gaussian data. Vienna: R Package.
Schnell, G. D., and Hellack, J. J. (1979). Bird flight speeds in nature: Optimized or a compromise? Am. Nat. 113, 53–66. doi: 10.1086/283364
Schoener, T. W. (1968). The Anolis lizards of Bimini: Resource partitioning in a complex fauna. Ecology 49, 704–726. doi: 10.2307/1935534
Schoener, T. W. (1971). Theory of feeding strategies. Annu. Rev. Ecol. Syst. 2, 369–404. doi: 10.1146/annurev.es.02.110171.002101
Schreiber, R. W. (1967). Roosting behavior of the Herring gull in central Maine. Wilson Bull. 79, 421–431.
Sellers, R., and Shackleton, D. (2011). Numbers, distribution and population trends of large gulls breeding in Cumbria, northwest England. Seabird 24, 90–102.
Sorensen, M. C., Hipfner, J. M., Kyser, T. K., and Norris, D. R. (2009). Carry-over effects in a Pacific seabird: stable isotope evidence that pre-breeding diet quality influences reproductive success. J. Anim. Ecol. 78, 460–467. doi: 10.1111/j.1365-2656.2008.01492.x
Spelt, A., Williamson, C., Shamoun-Baranes, J., Shepard, E., Rock, P., and Windsor, S. (2019). Habitat use of urban-nesting lesser black-backed gulls during the breeding season. Sci. Rep. 9:10527. doi: 10.1038/s41598-019-46890-6
Stanbury, A., Eaton, M., Aebischer, N., Balmer, D., Brown, A., Douse, A., et al. (2021). The status of our bird populations: the fifth Birds of Conservation Concern in the United Kingdom, Channel Islands and Isle of Man and second IUCN Red List assessment of extinction risk for Great Britain. Br. Birds 114, 723–747.
Stone, C. J., Webb, A., and Tasker, M. L. (1995). The distribution of auks and Procellariiformes in north-west European waters in relation to depth of sea T. Bird Study 42, 50–56. doi: 10.1080/00063659509477148
Strøm, H., Descamps, S., Ekker, M., Fauchald, P., and Moe, B. (2021). Tracking the movements of North Atlantic seabirds: steps towards a better understanding of population dynamics and marine ecosystem conservation. Mar. Ecol. Prog. Ser. 676, 97–116. doi: 10.3354/meps13801
Thaxter, C. B., Ross-smith, V. H., Clark, J. A., Clark, N. A., Conway, G. J., Marsh, M., et al. (2014). A trial of three harness attachment methods and their suitability for long-term use on Lesser Black-backed Gulls and Great Skuas. Ringing Migr. 29, 65–76. doi: 10.1080/03078698.2014.995546
Thaxter, C. B., Ross-Smith, V. H., Clark, J. A., Clark, N. A., Conway, G. J., Masden, E. A., et al. (2016). Contrasting effects of GPS device and harness attachment on adult survival of Lesser Black-backed Gulls Larus fuscus and Great Skuas Stercorarius skua. IBIS 158, 279–290. doi: 10.1111/ibi.12340
Therneau, T. (2020). A Package for Survival Analysis in R. R package version 3.2-7. Vienna: R Core Team.
Thurstan, R. H., and Roberts, C. M. (2010). Ecological meltdown in the Firth of Clyde, Scotland: Two centuries of change in a coastal marine ecosystem. PLoS One 5:0011767. doi: 10.1371/journal.pone.0011767
Trierweiler, C., Klaassen, R. H. G., Drent, R. H., Exo, K. M., Komdeur, J., Bairlein, F., et al. (2014). Migratory connectivity and population specific migration routes in a long-distance migratory bird. Proc. R. Soc. B Biol. Sci. 281:2897. doi: 10.1098/rspb.2013.2897
van den Bosch, M., Baert, J. M., Müller, W., Lens, L., and Stienen, E. W. M. (2019). Specialization reduces foraging effort and improves breeding performance in a generalist bird. Behav. Ecol. 30, 792–800. doi: 10.1093/beheco/arz016
van Donk, S., Camphuysen, K. C. J., Shamoun-Baranes, J., Meer, J., and Van Der. (2017). The most common diet results in low reproduction in a generalist seabird. Ecol. Evol. 2017, 1–10. doi: 10.1002/ece3.3018
van Donk, S., Shamoun-Baranes, J., Bouten, W., Van Der Meer, J., and Camphuysen, K. C. J. (2018). Individual differences in foraging site fidelity are not related to time-activity budgets in Herring Gulls. IBIS 162, 429–445. doi: 10.1111/ibi.12697
Viana, D. S., Granados, J. E., Fandos, P., Pérez, J. M., Cano-Manuel, F. J., Burón, D., et al. (2018). Linking seasonal home range size with habitat selection and movement in a mountain ungulate. Mov. Ecol. 6, 1–11. doi: 10.1186/s40462-017-0119-8
Webster, M. S., Marra, P. P., Haig, S. M., Bensch, S., and Holmes, R. T. (2002). Links between worlds: unraveling migratory connectivity. Trends Ecol. Evol. 17, 76–83. doi: 10.1016/S0169-5347(01)02380-1
Wikelski, M., and Wrege, P. H. (2000). Niche expansion, body size, and survival in Galapagos marine iguanas. Oecologia 124, 107–115. doi: 10.1007/s004420050030
Wiley, A. E., Ostrom, P. H., Stricker, C. A., James, H. F., and Gandhi, H. (2010). Isotopic characterization of flight feathers in two pelagic seabirds: Sampling strategies for ecological studies. Condor 112, 337–346. doi: 10.1525/cond.2010.090186
Wilson, R. P., Grémillet, D., Syder, J., Kierspel, M. A. M., Garthe, S., Weimerskirch, H., et al. (2002). Remote-sensing systems and seabirds: Their use, abuse and potential for measuring marine environmental variables. Mar. Ecol. Prog. Ser. 228, 241–261. doi: 10.3354/meps228241
Keywords: biologging, habitat specialism, spatial specialisation, resource selection function, home range, Laridae, utilisation distribution
Citation: O’Hanlon NJ, Thaxter CB, Burton NHK, Grant D, Clark NA, Clewley GD, Conway GJ, Barber LJ, McGill RAR and Nager RG (2022) Habitat Selection and Specialisation of Herring Gulls During the Non-breeding Season. Front. Mar. Sci. 9:816881. doi: 10.3389/fmars.2022.816881
Received: 17 November 2021; Accepted: 04 February 2022;
Published: 10 March 2022.
Edited by:
Ryan Rudolf Reisinger, University of Southampton, United KingdomReviewed by:
Maelle Connan, Nelson Mandela University, South AfricaCopyright © 2022 O’Hanlon, Thaxter, Burton, Grant, Clark, Clewley, Conway, Barber, McGill and Nager. This is an open-access article distributed under the terms of the Creative Commons Attribution License (CC BY). The use, distribution or reproduction in other forums is permitted, provided the original author(s) and the copyright owner(s) are credited and that the original publication in this journal is cited, in accordance with accepted academic practice. No use, distribution or reproduction is permitted which does not comply with these terms.
*Correspondence: Nina J. O’Hanlon, bmluYS5vaGFubG9uQEJUTy5vcmc=
Disclaimer: All claims expressed in this article are solely those of the authors and do not necessarily represent those of their affiliated organizations, or those of the publisher, the editors and the reviewers. Any product that may be evaluated in this article or claim that may be made by its manufacturer is not guaranteed or endorsed by the publisher.
Research integrity at Frontiers
Learn more about the work of our research integrity team to safeguard the quality of each article we publish.