- 1Polar Science Center, Applied Physics Laboratory, University of Washington, Seattle, WA, United States
- 2Greenland Institute of Natural Resources, Nuuk, Greenland
- 3United States Geological Survey, Alaska Science Center, Anchorage, AK, United States
- 4Environment and Climate Change Canada, University of Alberta, Edmonton, AB, Canada
- 5Norwegian Polar Institute, FRAM Centre, Tromsø, Norway
- 6Natural History Museum, University of Oslo, Oslo, Norway
- 7Wildlife Research Section, Department of Environment, Government of Nunavut, Igloolik, NT, Canada
- 8Environment and Climate Change Canada, Winnipeg, MB, Canada
- 9Independent Researcher, Cooks Creek, MB, Canada
- 10All-Russian Research Institute for Environment Protection, Moscow, Russia
- 11Department of Biological Sciences, University of Alberta, Edmonton, AB, Canada
Satellite telemetry (ST) has played a critical role in the management and conservation of polar bears (Ursus maritimus) over the last 50 years. ST data provide biological information relevant to subpopulation delineation, movements, habitat use, maternal denning, health, human-bear interactions, and accurate estimates of vital rates and abundance. Given that polar bears are distributed at low densities over vast and remote habitats, much of the information provided by ST data cannot be collected by other means. Obtaining ST data for polar bears requires chemical immobilization and application of a tracking device. Although immobilization has not been found to have negative effects beyond a several-day reduction in activity, over the last few decades opposition to immobilization and deployment of satellite-linked radio collars has resulted in a lack of current ST data in many of the 19 recognized polar bear subpopulations. Here, we review the uses of ST data for polar bears and evaluate its role in addressing 21st century conservation and management challenges, which include estimation of sustainable harvest rates, understanding the impacts of climate warming, delineating critical habitat, and assessing potential anthropogenic impacts from tourism, resource development and extraction. We found that in subpopulations where ST data have been consistently collected, information was available to estimate vital rates and subpopulation density, document the effects of sea-ice loss, and inform management related to subsistence harvest and regulatory requirements. In contrast, a lack of ST data in some subpopulations resulted in increased bias and uncertainty in ecological and demographic parameters, which has a range of negative consequences. As sea-ice loss due to climate warming continues, there is a greater need to monitor polar bear distribution, habitat use, abundance, and subpopulation connectivity. We conclude that continued collection of ST data will be critically important for polar bear management and conservation in the 21st century and that the benefits of immobilizing small numbers of individual polar bears in order to deploy ST devices significantly outweigh the risks.
1 Introduction
Satellite telemetry (ST) has been widely used to determine the movements, distribution, and habitat selection of mammals, birds and fishes (Hart and Hyrenbach, 2009; Gaidet et al., 2010; Harcourt et al., 2019; Hays et al., 2019; Davidson et al., 2020; Sequeira, 2020). This technology has helped to protect populations, conserve biodiversity, ensure sustainable use, and implement ecosystem-based management by providing data on how animals use the landscape and respond to current or expected environmental changes (e.g., Block et al., 2016). ST data are particularly important for long-lived species that are mobile, difficult to observe directly, or have complex life cycles that complicate other forms of monitoring (e.g., species that feed and reproduce in different locations). In the case of the polar bear (Ursus maritimus), a marine carnivore that ranges widely across international boundaries and occurs at low densities across a vast and sparsely populated Arctic (Amstrup, 2003), ST has been a critical research tool for management, conservation, and informing international agreements on environmental protection (PBRS, 2015).
Satellite-linked radio tags (SLRTs), which transmit to polar-orbiting satellites, have been a key tool in the study of polar bears because remote habitats and harsh Arctic conditions preclude the use of other methods (e.g., very high frequency [VHF] signals) that require the transmitter and receiver to be in proximity. As early as 1965, scientists suggested that SLRTs had the potential to provide reliable location data for polar bears, regardless of season or geographic area (PBSG, 1966; Flyger and Townsend., 1968). The first successful use of ST to track polar bears was in 1977 using the NIMBUS 6 satellite system to follow three adult females in Alaska for 8-390 days (Kolz et al., 1980). In 1979, four female polar bears in Lancaster Sound, Canada (Schweinsburg and Lee, 1982) and four bears (one male and three females) in the Greenland Sea (Larsen et al., 1983) were tracked using the NIMBUS 6 system for 40-266 and 18-63 days, respectively. In these studies, the SLRTs were housed in collars that weighed approximately 5 kg and were fastened with a harness consisting of steel cables covered by plastic and rubber tubes. The cables were fastened by a magnesium bolt under the chest to allow for release when the bolt corroded.
Since those initial studies, advances in technology, attachment/release methods, and satellite systems have made SLRTs less cumbersome and improved their ability to remotely monitor polar bears, resulting in widespread and diverse use. Establishment of the Argos Data Collection and Location System in 1978 (CLS, 2016) facilitated ST as a tool for wildlife research (Fancy et al., 1988; Harris et al., 1990; Garner et al., 1994; Belikov et al., 1998; Schwartz and Arthur, 1999; Tomkiewicz et al., 2010), which for polar bears included a new ability to identify subpopulation boundaries (e.g., Bethke et al., 1996; Taylor et al., 2001; Mauritzen et al., 2002; PBSG, 2018). In the early 2000s, Global Positioning System (GPS) capability revolutionized satellite tracking by providing locations accurate to 30 m (Schwartz and Arthur, 1999; Frair et al., 2010; Tomkiewicz et al., 2010) compared to, at best, 400 m accuracy of Argos locations (Douglas et al., 2012). This allowed for fine-scale analyses of habitat use (Andersen et al., 2008). In 2011, advent of the Iridium satellite system (Iridium Satellite Communications, McLean, Virginia, USA) allowed more data to be transferred at a lower cost than the Argos system (e.g., Thomas et al., 2011; Lone et al., 2018b; Pagano et al., 2020) and enabled two-way communication with SLRTs. This allowed SLRTs to be programmed remotely (e.g., changing the duty cycle) and opened the possibility for release devices that could be triggered by the user. The addition of salt-water sensors, thermometers, accelerometers, and video cameras further extended the types of data provided by SLRTs, allowing detailed behavioral and ecological investigations (Fancy et al., 1988; Lone et al., 2018a; Pagano et al., 2018).
Most SLRTs used in polar bear research have been attached as collars, and almost exclusively to adult female bears as their neck and head morphology maximize retention (Figure 1). Subadult bears are generally not collared to avoid potential injury during growth, and adult males are not collared because their necks are larger in circumference than their heads, which causes collars to slip off. Other attachment methods including harnesses, ear-mounted transmitters (Wiig et al., 2017), glue-to-fur transmitters (Rode et al., 2014a), and subcutaneous implants (Mulcahy and Garner, 1999; Amstrup et al., 2001) have been used with limited success.
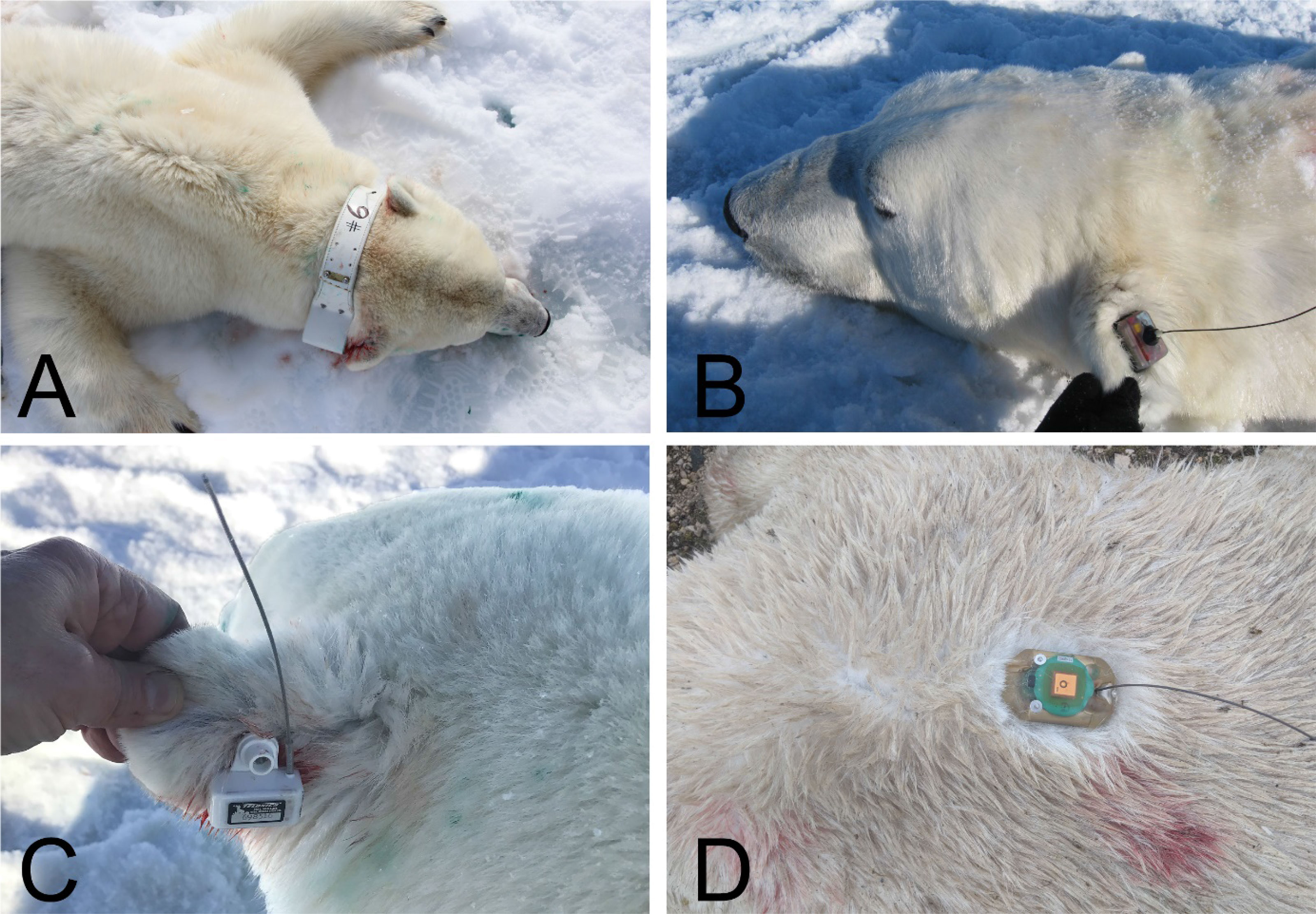
Figure 1 Different types of satellite tags (ST) on polar bears: (A): ARGOS Satellite collar (Telonics), (B): Satellite ear-attached tag (Wildlife Computers, Redmond WA), (C): Satellite ear-attached tag (Telonics), and (D): Glue-on satellite tag (Wildlife Computers, Redmond WA). Collars have been the most robust and reliable way to collect multi-year data from polar bears. Photos by Kristin Laidre (A), George Durner/USGS (C) and Karyn Rode/USFWS (B, D).
The International Association for Bear Research and Management (IBA; https://www.bearbiology.org/) and the International Union for Conservation of Nature (IUCN) Species Survival Commission (SSC) Bear Specialist Group (BSG) support science-based management and conservation of the world’s eight species of bears (IBA, 2009). These groups note that, while alternative or less-invasive methods may be useful in some circumstances, handling and instrumenting bears with SLRTs is essential to address conservation challenges of the 21st century (IBA, 2009). In recent decades, Indigenous communities in parts of the Canadian and United States Arctic have expressed concern about the application of SLRTs on polar bears. Often, opposition to chemical immobilization, handling, and collaring is based on concerns about possible negative physical and behavioral impacts to individual bears, such as collars that become too tight if the bear grows or changes body condition (ITK, 2009; Henri et al., 2010; Wong et al., 2017; AEWC, 2019), and on potential health risks to humans who consume the meat of polar bears that have been immobilized (Semple et al., 2000). In Svalbard, concerns about the appearance and possible discomfort of collars have been expressed by tourists and the general public. Despite these concerns, no negative effects have been documented in any study focused on addressing them (Messier, 2000; Semple et al., 2000; Thiemann et al., 2013; Rode et al., 2014a; see section 2.1).
For management purposes, the world’s population of an estimated ~26,000 polar bears (Regehr et al., 2016) is divided into 19 subpopulations with varying levels of immigration and emigration (PBSG, 2018). Polar bears are distributed widely across remote environments that are already rapidly changing due to sea-ice loss resulting from climate warming, the primary long-term threat to the species (Figure 2, Laidre et al., 2008; Wiig et al., 2008; Amstrup et al., 2010; Kovacs et al., 2011; Castro de la Guardia et al., 2013; Regehr et al., 2016). With an ice-free Arctic predicted to occur in the summer as early as 2034 (Peng et al., 2020), profound changes in the movements and distribution of bears are expected, with attendant challenges to their management and conservation (Atwood et al., 2015). To date, ecological and demographic changes associated with sea-ice loss have been detected for 16 of the 19 subpopulations (noting that changes for some high-latitude subpopulations have not been negative, and that some subpopulations are data deficient; PBSG, 2018). In this paper, we review available information on the effects of chemically immobilizing and attaching SLRTs to polar bears. We examine a suite of scientific areas where ST data have been critical to management and conservation, including the value of auxiliary information collected during ST deployments. We conclude by making the case that ST data from polar bears will be critical to inform management decisions in the 21st century as climate warming increasingly impacts polar bear subpopulations and as human activities in the Arctic increase. We also discuss potential ways to reduce concerns about possible effects of ST studies on polar bears.
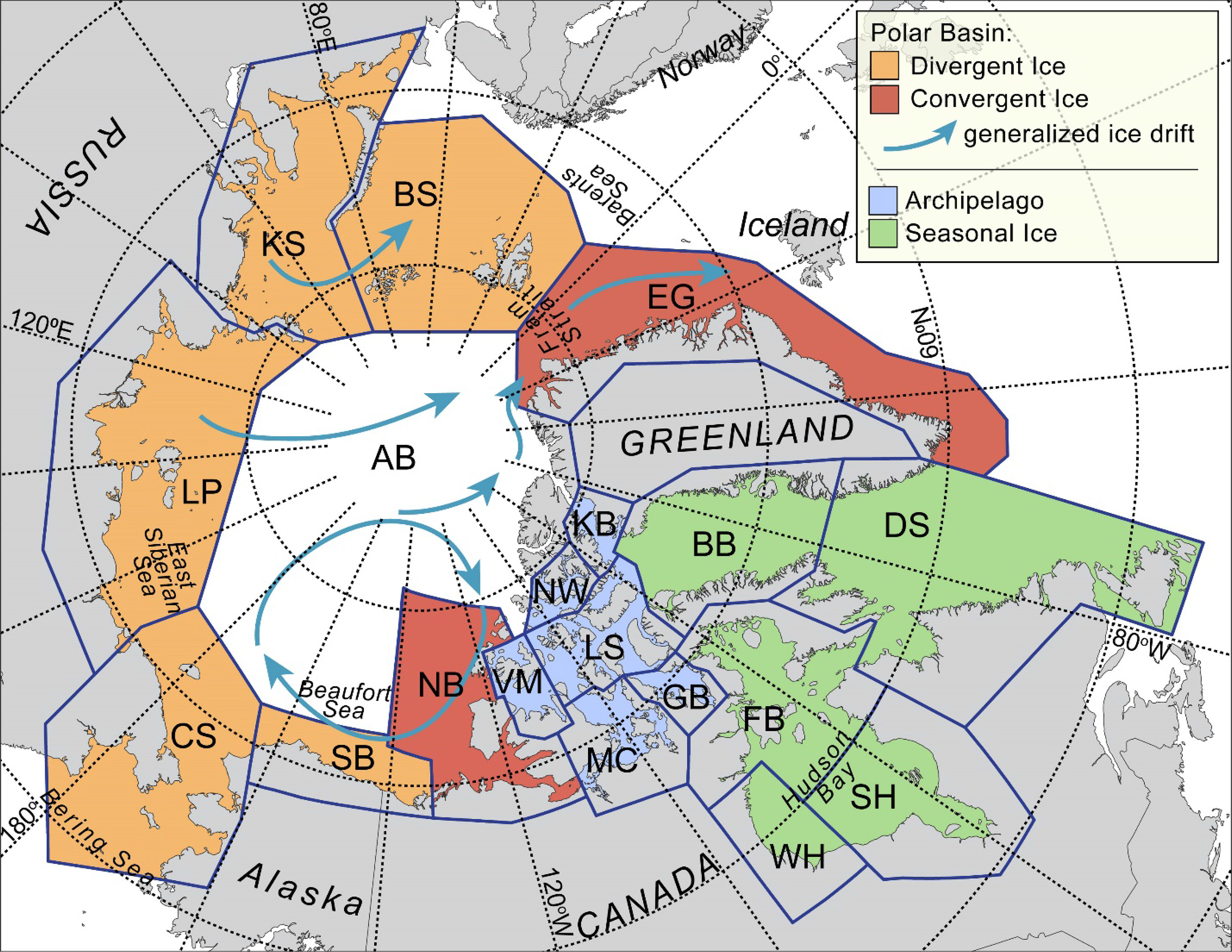
Figure 2 Polar bear ecoregions, 19 subpopulations (AB, Arctic Basin; BB, Baffin Bay; BS, Barents Sea; CS, Chukchi Sea; DS, Davis Strait; EG, East Greenland; FB, Foxe Basin; GB, Gulf of Boothia; KB, Kane Basin; KS, Kara Sea; LP, Laptev Sea; LS, Lancaster Sound; MC, M’Clintock Channel; NB, Northern Beaufort Sea; NW, Norwegian Bay; SB, Southern Beaufort Sea; SH, Southern Hudson Bay; VM, Viscount Melville Sound; WH, Western Hudson Bay), and place names mentioned in the text with arrows showing ice drift. Ecoregions as defined by Amstrup et al. (2008).
2 Background
2.1 Chemical Immobilization and SLRT Deployment
Polar bears must be chemically immobilized to deploy SLRTs (Stirling et al., 1989). Drugs are usually administered via helicopter-based darting, although in some areas bears on land are captured in culvert traps or darted from snowmobiles. Ground-darting of polar bears is relatively rare due to safety risks associated with not being able to control the animal’s movement from the time a drug is injected to the point at which the animal becomes immobilized. Bears typically remain immobilized and sedated for up to one hour, during which time researchers mark (e.g., using ear tags, tattoos, microchip) or re-identify a previously captured individual, record morphometric data, collect tissue samples, and possibly apply a SLRT.
Since 1985, the most common immobilizing drug for polar bears has been Telazol® (a 1:1 mixture of tiletamine hydrochloride and zolazepam hydrochloride; also sold as Zoletil®; Stirling et al., 1989). Telazol® has proven to be reliable with a high margin of safety (Kreeger and Arnemo, 2012). In the Southern Beaufort Sea subpopulation, the mortality rate during chemical immobilization was reduced from 1.4% to 0.1% following a switch from Sernylan to Telazol® (Thiemann et al., 2013; Rode et al., 2014a). Even prior to widespread use of Telazol®, mortality rates during capture and handling were <1% during research in Nunavut and the Northwest Territories, Canada (Messier, 2000). Notably, most deaths of anesthetized bears were due to reasons other than the choice of immobilizing agent (e.g., drowning, dart injury, or being killed by another bear while recovering from anesthesia; Messier, 2000; Rode et al., 2014a).
The persistence of Telazol® in polar bear tissues has been a concern in regions where humans consume polar bear meat. Semple et al. (2000) determined that both Telazol® and its metabolites declined to trace levels in blood serum, kidney, muscle, and adipose tissue of polar bears within 24 hours of dosing, although low levels of metabolites were detectable 11 days after capture. Ryan et al. (2009) in a study of 15 American black bears (U. americanus) detected Telazol® in muscle and kidney of one bear, and in the serum of two bears, seven days post-capture; and in the urine of one bear 14 days post-capture. Semple et al. (2000) suggested that a human consuming the meat of a previously immobilized bear would be unlikely to experience the pharmacological effects from Telazol® if it were eaten 24 hours or more post-capture. To avoid any risk of human exposure to metabolites—even though negative human health effects have not been associated with exposure—two studies have suggested avoiding consumption of harvested bear meat within 14 days post-capture (Cattet, 2003; Ryan et al., 2009). Captured polar bears are typically marked with unique numerical identifiers applied as lip tattoos and/or ear tags, and with a more visible but non-permanent mark using fur dye, which can be used to determine if and when a harvested bear was previously immobilized.
Several studies have investigated effects of capture and handling on polar bear behavior and found no long-term negative impacts. Bear movement rates and activity are generally suppressed immediately after capture. Thiemann et al. (2013) and Rode et al. (2014a) showed that polar bear activity and movement rates returned to near-normal levels within 2-3.5 days post-capture, with the majority reaching normal activity and movement within 5 days. Additionally, and importantly, direct observations of polar bears following capture as well as video-camera footage support that bears can successfully kill and eat a seal within 24 hours of capture despite reduced activity and movement (Pagano et al., 2018). Also, Stirling et al., (in press) used approximately 1,800 hours of direct visual observation of polar bears to compare the behavior of animals of the same sex- and age-classes that had, and had not, been previously immobilized. That study found that bears were more likely to sleep, and less likely to hunt, within 48 hours of capture, but no negative behavioral differences were detected beyond that period. Furthermore, Stirling et al., (in press) visually observed three adult bears that successfully killed seals within 50 hours after capture.
Other studies have investigated whether immobilization and handling have longer-term effects on polar bear body condition (i.e., fatness) and reproduction. Rode et al. (2014a) assessed 26 years of capture data in the Southern Beaufort Sea and found no directional change in body condition of 197 polar bears captured 2-10 times (1-2 captures per year). Similarly, the body condition of 309 polar bears captured twice in different years did not differ from the body condition of 734 bears at initial capture (Rode et al., 2014a). Messier (2000) also found no effect of helicopter-based capture on polar bear body condition. Litter size and mass of cubs was unaffected by single or multiple captures of the mother, and capture of the mother was not found to influence cub survival (Amstrup, 1993; Rode et al., 2014a). Further, bears that were captured as dependent young in the spring achieved greater adult body size than bears that were not captured as dependent young (Rode et al., 2014a). These results suggest that effects on activity, movement, and feeding behavior post-capture do not translate to reduced body condition or survival for most sex and age classes. One exception may be pregnant females captured just prior to denning (Lunn et al., 2004). Ramsay and Stirling (1986) and Amstrup (1993) indicated that some pregnant females captured at dens before giving birth relocated to a new den site, while Lunn et al. (2004) found that pregnant polar bears at dens seldom relocated if not disturbed by capture. Capturing females at dens is now largely avoided.
Rode et al. (2014a) also investigated the effects of collaring on polar bear activity and movements, health, and survival of dependent young. Activity and movement rates of collared bears were not different from those wearing glue-to-fur or ear-mounted transmitters, and body condition of females that had worn a collar for one year (n = 56) was similar to females that had never worn a collar (n = 238; Rode et al., 2014a) (Figure 3). Survival of cubs was similar for adult females that had, and had not, worn a collar the previous year. Currently, all handling of wildlife, including immobilization and deployment of SLRTs on polar bears, must be approved by national agencies and Institutional Animal Care and Use Committees.
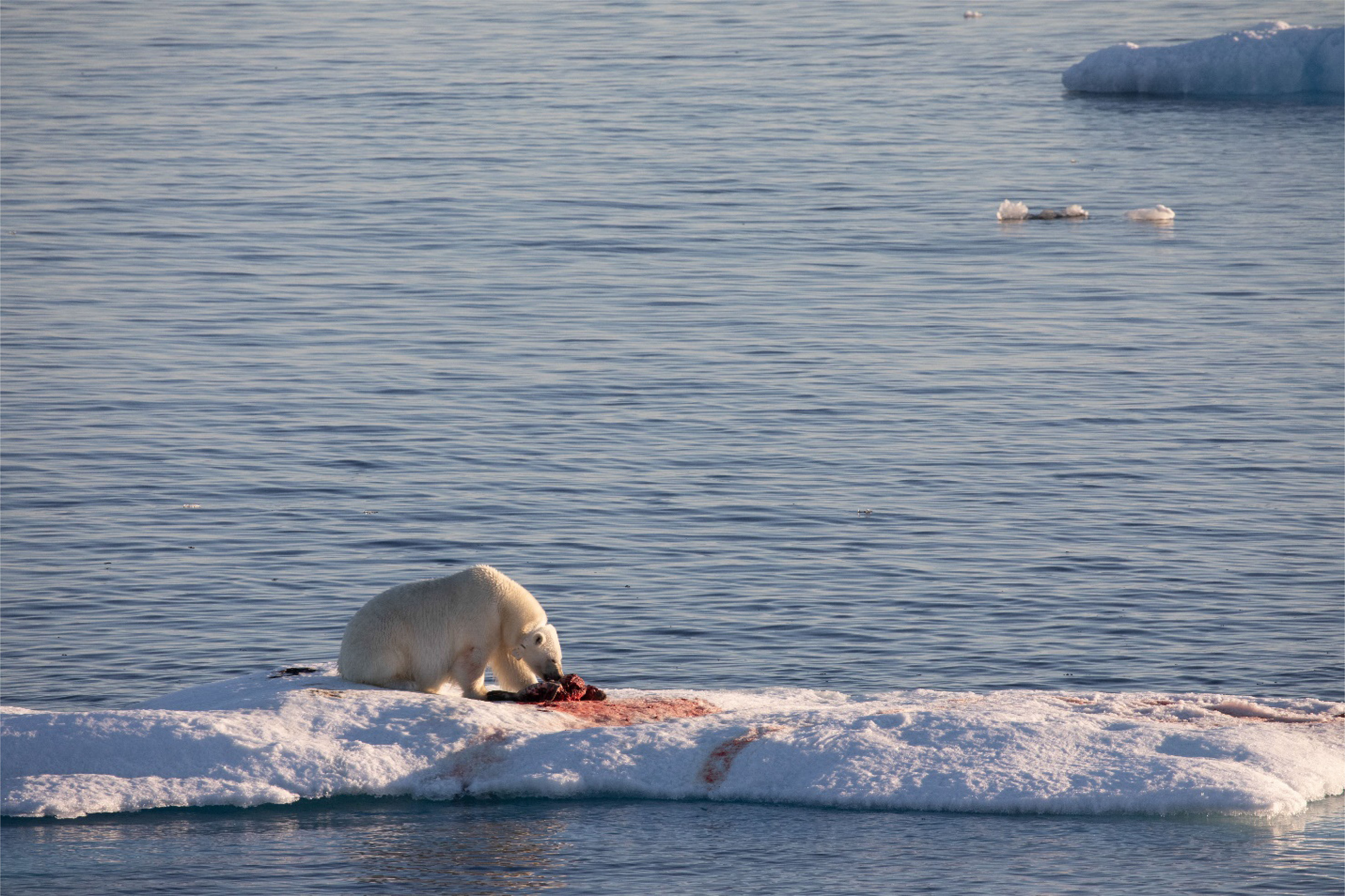
Figure 3 A satellite-collared female polar bear in East Greenland feeding on a harp seal kill in July 2019. This bear was captured, and the collar deployed two years before the photo was taken. This bear would score high on a body condition index. Several scientific studies have demonstrated that the presence of satellite telemetry devices does not impact body condition over the long term. Photo by Nick Cobbing.
2.2 SLRT Types
Various attachment types for satellite transmitters, including collars, glue-to-fur, implants, and ear tags, can be used on polar bears (Figure 1). There are trade-offs between retention, operational life, data quality, and the animal’s tolerance of the different attachment types. Collars are most frequently used because their size (currently ~1.5 kg; https://www.telonics.com/) can accommodate a battery that provides high-quality data for > 1 year (e.g., Amstrup et al., 2004). For an adult female of 200-300 kg, a collar represents 0.5-0.8% of the bear’s mass. Additionally, collars allow for the attachment of devices for monitoring behavior, such as video cameras and activity sensors with high spatial and temporal resolution (e.g., Whiteman et al., 2015; Pagano et al., 2018). Although collars are usually only deployed on adult females (Figure 4), glue-to-fur and ear-mounted transmitters can be deployed on subadults and adults of both sexes. However, low battery capacity associated with the small size of ear-mounted transmitters (≤70 g) and the propensity of bears to remove the transmitters has resulted in a mean functional life of 70 days (Wiig et al., 2017), which is too short to evaluate seasonal patterns in movements and habitat use. Additionally, Wiig et al. (2017) reported injuries to ear pinna resulting from ear-mounted transmitters being forcefully removed (e.g., during intraspecific interaction or while hunting). The use of subcutaneous SLRTs implanted in the dorsal cervical region with a percutaneous antenna was attempted on seven adult male polar bears in 1996 and 1997, with limited success (Mulcahy and Garner, 1999; Amstrup et al., 2001). There have been no attempts to use implanted transmitters on polar bears since 1997.
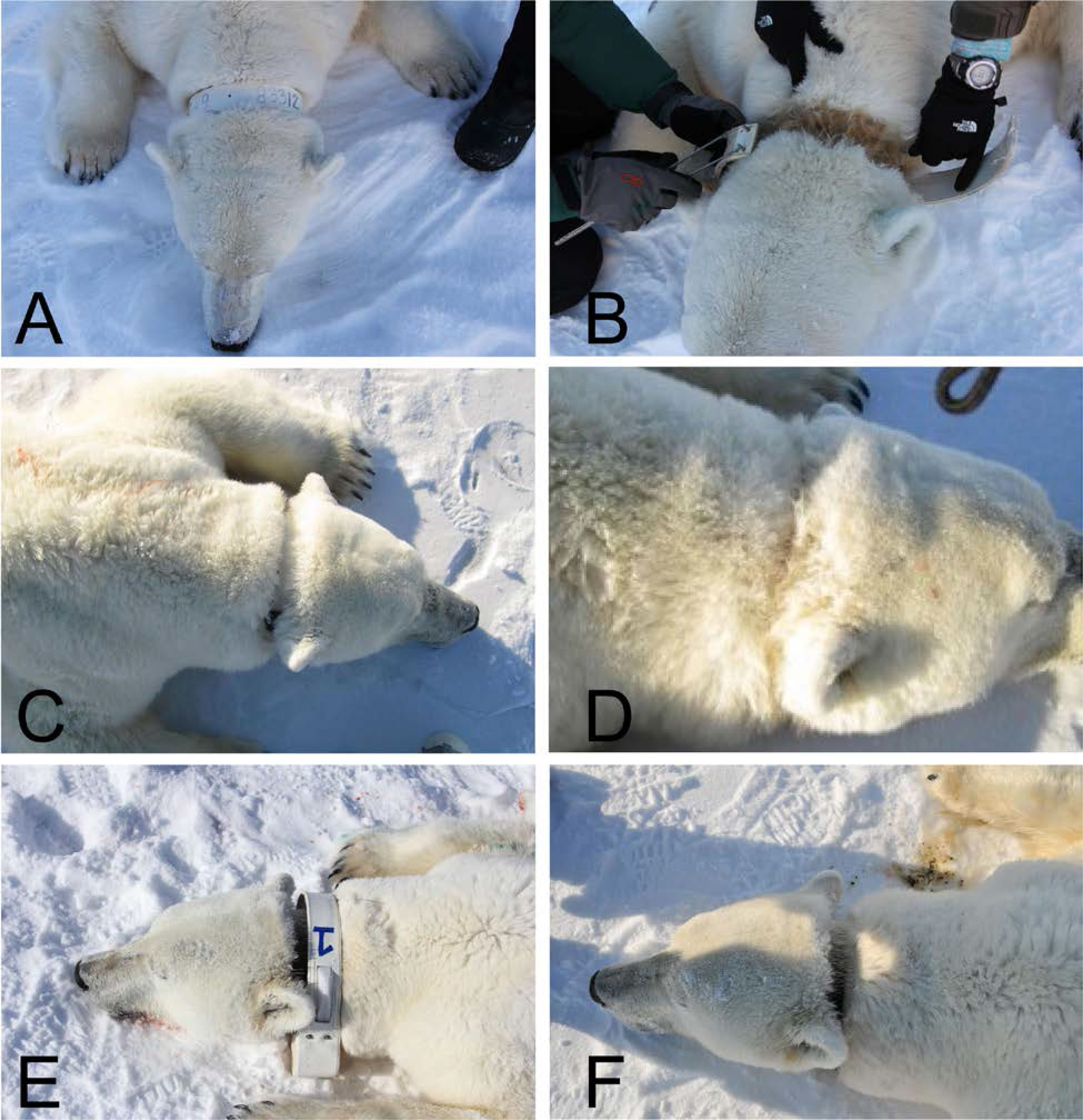
Figure 4 (A-F). Three adult female polar bears shown when satellite telemetry collar was deployed (left) and when it was removed (right). (A, B) show bear D24042 from East Greenland after one year. (C, D) show bear X35260 from Kane Basin after two years, and (E, F) show bear D7388 from Baffin Bay after four years. Satellite telemetry collars can be deployed for different durations and controlled by release mechanisms or removal upon recapture. Discoloration is matted hair under the collar.
3 A Review of the Role of ST Data in Past Polar Bear Management and Conservation
3.1 Subpopulation Delineation
Pedersen (1945) suggested that polar bears undertook long migrations around the Polar Basin, and early scientific debate focused on whether there was one or more polar bear populations across the Arctic (e.g., Lentfer, 1972; Vibe, 1976; Larsen and Stirling, 2009). Investigations using locations of captured and marked bears as well as data from VHF radio collars on adult females facilitated the delineation of multiple subpopulations resulting from seasonal fidelity to localized areas and natural obstacles to movement (e.g., land masses; Taylor and Lee, 1995). ST data have been used to quantify exchange among subpopulations (Mauritzen et al., 2001; Laidre et al., 2018a) and identify occasional long-distance movements across subpopulation boundaries of up to 5,000 km in one year (Durner and Amstrup, 1995; Bethke et al., 1996; Taylor et al., 2001; Mauritzen et al., 2002; Amstrup et al., 2004; Obbard and Middel, 2012; Scharf et al., 2019) (Figure 5). ST data also have been used to understand how bear distributions change as sea-ice loss progresses (Durner et al., 2019; Pagano et al., 2021) and ensure that management practices are applied to demographically relevant groupings of animals (e.g., Laidre et al., 2015; Lone et al., 2018a; Lone et al., 2018b). For example, Amstrup et al. (2004) used ST data to develop contours describing the probability that bears belonged to the Southern Beaufort Sea or Chukchi Sea subpopulation. Scharf et al. (2019) expanded methods for subpopulation delineation into a Bayesian framework that accounted for selection by bears of dynamic sea-ice habitats. Both ST and genetic methods (Paetkau et al., 1999; Peacock et al., 2015; Viengkone et al., 2016) have been used to understand the degree to which individual subpopulations are isolated (Bethke et al., 1996; Mauritzen et al., 2001; Taylor et al., 2001), which can affect population viability (e.g., via source-sink dynamics or small-population effects).
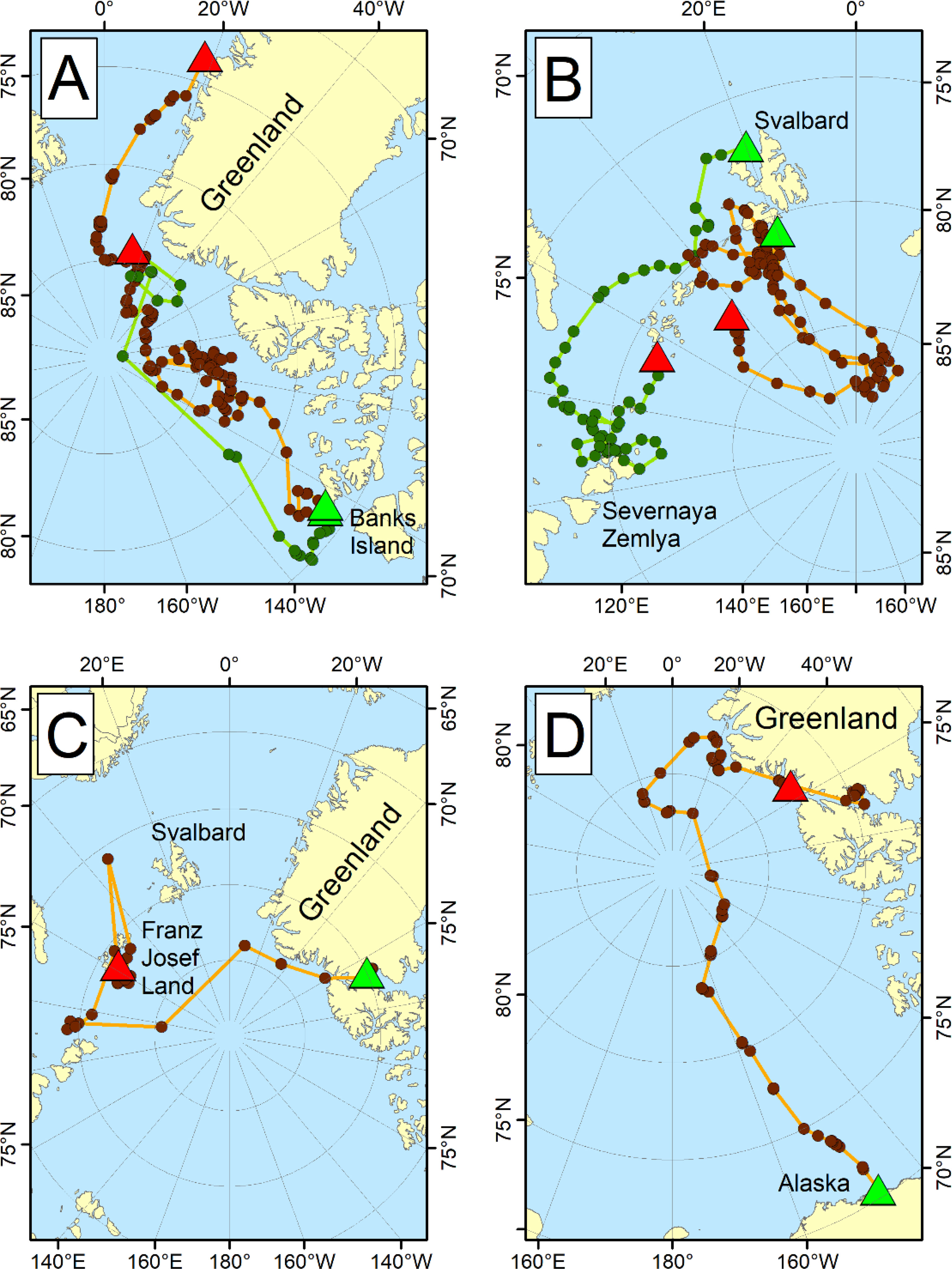
Figure 5 Long-distance movements of satellite-collared adult female polar bears across subpopulation boundaries, with green triangles showing start locations and red triangles showing end locations. Data shown for (A) two bears tagged in the Northern Beaufort Sea, one of which traveled to northern Greenland from May to October 1993 (green dots), and the other to eastern Greenland from April 1993 to February 1995 (brown dots; Lunn et al., 1995); (B) two bears tagged in the Barents Sea, one of which moved from Svalbard to Severnaya Zemlya in the Kara Sea from April to November 2013 (green dots), and another that moved from Svalbard into the Arctic Basin from April 2014 to September 2015 (brown dots; Aars, unpublished data); (C) one bear tagged in Kane Basin between April 2013 and 2015 crossed the Arctic Basin to Franz Josef Land in the Barents Sea (Laidre et al., 2020b); and (D) one bear tagged in the Southern Beaufort Sea between May 1992 and December 1993 traveled across the Arctic Basin to Greenland (Durner and Amstrup, 1995). Distances moved by polar bears in these figures are upwards of 3,500 km across the polar basin.
3.2 Abundance and Demography
Estimates of polar bear abundance are used to monitor subpopulation trend and inform management, including estimation of sustainable harvest (e.g., Lunn et al., 2016; Stapleton et al., 2016; Obbard et al., 2018; Regehr et al., 2018). Estimates of vital rates (e.g., reproduction, survival) and their relationship with environmental conditions are needed to evaluate subpopulation viability and the demographic effects of sea-ice loss (e.g., Hunter et al., 2010; Regehr et al., 2010). ST data also have been essential in many demographic analyses to clearly define demographic parameters (e.g., to distinguish between mortality and emigration) and to model nonrandom movements of bears in and out of sampling areas, which lead to substantive bias—usually negative—in parameter estimates (Regehr et al., 2009; Peñaloza et al., 2014).
For example, Regehr et al. (2018) used ST data to model the movement of bears with respect to a geographically limited sampling area in the Chukchi Sea subpopulation, leading to the first biologically plausible estimates of survival and abundance, which were subsequently used to inform harvest management (Regehr et al., 2021b). Similarly, in the Southern Beaufort Sea, ST data were used to model transitions between geographic regions (i.e., “states”) of the study area to help explain heterogeneity in recapture probabilities (Bromaghin et al., 2021). In the Barents Sea subpopulation, ST data were used to design large-scale aerial surveys (Aars et al., 2009; Aars et al., 2017) and extrapolate density estimates to areas not covered by line-transects with a ratio estimator based on the locations of collared females at the time of the survey. In Aars et al. (2017), ST data were used to estimate the number of bears that were swimming and therefore not detectable. Availability of ST data also ensured ecotype-specific movements did not result in biased abundance estimates (Aars et al., 2017).
During an assessment of the Baffin Bay and Kane Basin subpopulations, current and historical ST data showed that both subpopulations had redistributed due to sea-ice loss (Laidre et al., 2018a; Laidre et al., 2018b; Laidre et al., 2020a; Laidre et al., 2020b; Atkinson et al., 2021). Further, for Baffin Bay, ST data identified that the definition of this subpopulation had changed over time because capture-recapture studies in the 1990s did not fully sample the subpopulation’s autumn range. Without this information, comparison of abundance estimates between the 1990s and 2010s would have led to a biased assessment of subpopulation trend.
3.3 Habitat Use
ST data have been instrumental in understanding the relationships between polar bears and their habitats. Wiig (1995) noted large variation in home range size of female polar bears in the Svalbard area of the Barents Sea subpopulation, and Mauritzen et al. (2001) reported females in this subpopulation belonged to one of two ecotypes. “Coastal” bears use land in Svalbard much of the year, as well as nearby sea ice when seasonally available; whereas “pelagic” bears travel longer distances and use the marginal ice zone (MIZ) most of the year. Mauritzen et al. (2003) also found that habitat selection among Barents Sea bears depended on female reproductive status. Further, Brun et al. (2021) identified that coastal females showed high fidelity to local areas within Svalbard, with close relatives using overlapping home ranges. Similar patterns have been shown in East Greenland (Laidre et al., 2015).
Resource selection functions (RSFs, Manly et al., 2002) using ST data have identified three key physical features that define important polar bear habitat: medium to high sea-ice concentrations (Arthur et al., 1996; Ferguson et al., 2000a; Durner et al., 2004; Durner et al., 2006; Durner et al., 2009; Wilson et al., 2014; Laidre et al., 2015; McCall et al., 2016; Lone et al., 2018b), sea ice cover that exceeds 50% sea-ice concentration (Durner et al., 2004; Durner et al., 2006; Durner et al., 2009; Laidre et al., 2015; Lone et al., 2018b), and sea ice over the biologically productive continental shelves (Durner et al., 2004; Durner et al., 2006; Durner et al., 2009; Wilson et al., 2014; Lone et al., 2018b; Laidre et al., 2018b). RSFs also have identified finer-scale habitat selection based on ice type (e.g., landfast, drift ice), sea-ice stage (i.e., thickness and age; Ferguson et al., 2000a), sea-ice form (i.e., floe size; Ferguson et al., 2000a; Durner et al., 2004), chlorophyll-a concentration (Wilson et al., 2014), and proximity to glacier fronts (Freitas et al., 2012; Hamilton et al., 2017). Activity sensor data from SLRTs have been used to identify differences in activity patterns associated with habitat, such as a decline in activity when polar bears are in suboptimal habitats, likely in response to reduced prey availability (Whiteman et al., 2015; Ware et al., 2017).
For subpopulations with extensive or complete summer sea-ice melt, the distribution of ST locations relative to the timing and duration of breakup or freeze-up, average sea-ice concentration, and spatial extent of ice-free waters, have been used to infer the timing of movement of polar bears to and from land (e.g., Regehr et al., In Review; Stirling et al., 1999; Atwood et al., 2016b; McCall et al., 2016; Laidre et al., 2020a). ST data have been used to identify the environmental thresholds that trigger long-distance swimming (Pagano et al., 2012; Pilfold et al., 2017; Lone et al., 2018a), quantify the energetic costs of occupying an increasingly fractured and mobile sea-ice platform (Auger-Méthé et al., 2016; Durner et al., 2017; Lone et al., 2018a; Pagano et al., 2018; Pagano et al., 2020) (Figure 6), and compare the energetic demands on females with different habitat use strategies (Blanchet et al., 2020). For multiple subpopulations, ST data have shown an increasing frequency of short- and long-distance swims (i.e., >50 km) associated with foraging and migrating to land during the annual open-water period (Pagano et al., 2012; SWG, 2016; Pilfold et al., 2017; Lone et al., 2018a).
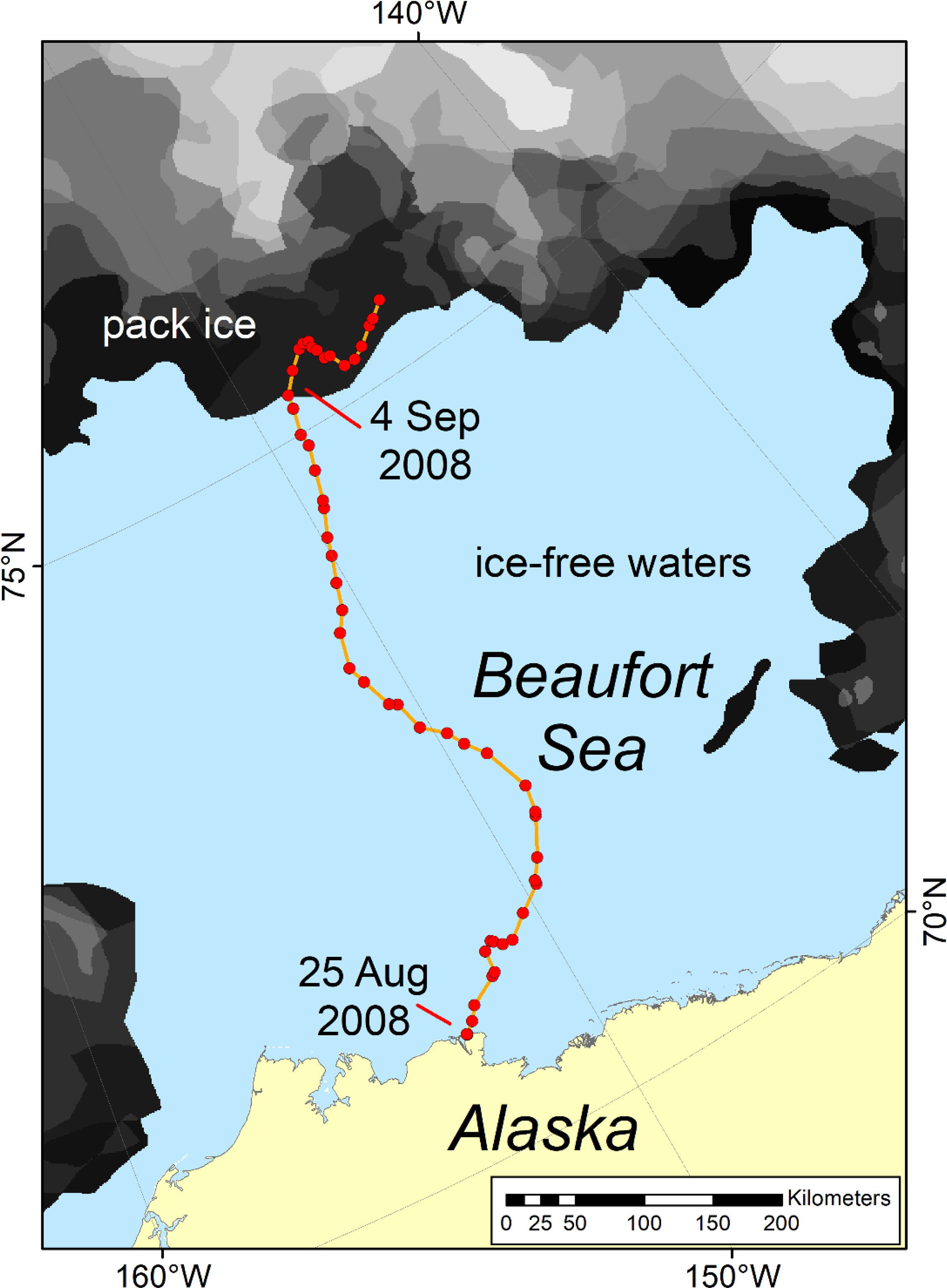
Figure 6 Track of a satellite-collared adult female polar bear that swam north from Alaska (Southern Beaufort Sea subpopulation) through ice-free waters from 25 August to 4 September 2008. For details see Durner et al. (2011).
RSFs using ST data also have been used to make inference about regions used by polar bears but not accessible to humans (e.g., Durner et al., 2009; Wilson et al., 2016; Lone et al., 2018b; Durner et al., 2019) (Figure 7), for example by extrapolating habitat-specific polar bear densities to remote areas that could not be directly sampled (Regehr et al., 2018). Also, projections of polar bear habitat to inform the decision to list polar bears as “threatened” under the U.S. Endangered Species Act relied heavily on ST data (USFWS, 2008), as did the definition of critical habitat for polar bears in the Alaskan Beaufort and Chukchi seas (Durner et al., 2009; USFWS, 2010).
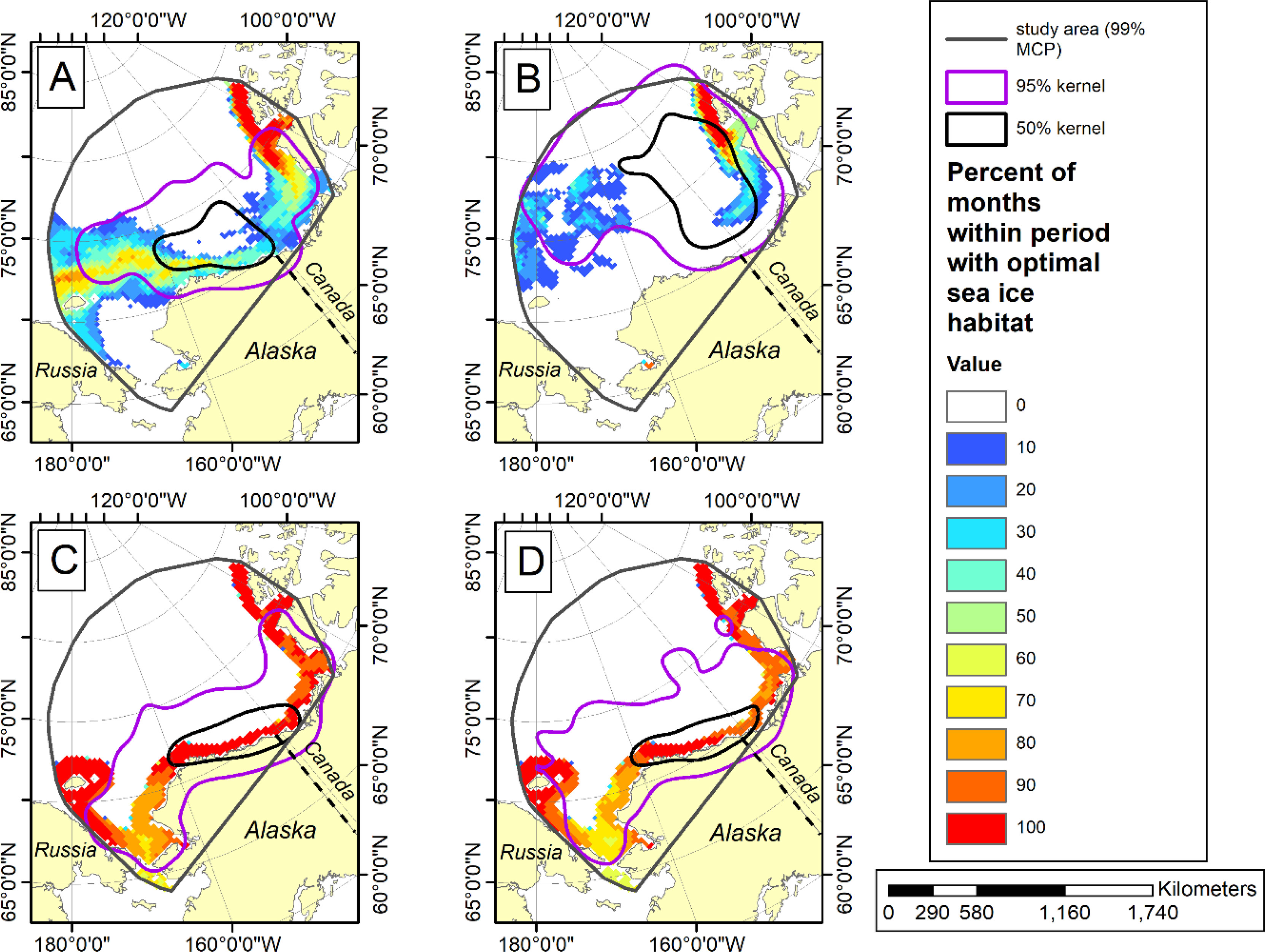
Figure 7 Optimal sea ice habitat (Durner et al., 2019) color-coded by percentage of months with optimal sea ice habitat. The 95% and 50% kernel utilization distributions (contours) show the seasonal distribution of adult female polar bears instrumented with satellite-linked collars in the Southern Beaufort Sea during minimum (summer) and maximum (winter) ice extent for two periods (1985-1995 and 2007-2016). (A) summer ice extent season for 1985-1995 and (B) for 2007-2016; (C) winter ice extent season for 1985-1995 and (D) for 2007-2016. For details see Durner et al. (2019).
3.4 Maternal Denning
General patterns in polar bear denning have been determined by combining information from scientists and Arctic Indigenous residents (e.g., Harington, 1968; Larsen, 1972; Uspenski and Kistchinski, 1972; Lentfer and Hensel, 1980; Larsen, 1985; Stishov, 1991; Stirling and Andriashek, 1992; Van de Velde et al., 2003; Derocher et al., 2011; Aars, 2013), with ST data playing a critical role in reducing bias and uncertainty around denning habitat and locations (Lunn et al., 2004; Yee et al., 2017; Escajeda et al., 2018; Durner et al., 2020). ST data have been used to identify “core” denning areas (Harington, 1968), such as Wrangel Island (Rode et al., 2018), Svalbard (Andersen et al., 2012), the Beaufort Sea (Amstrup and Gardner, 1994), the north-central region of the Canadian Arctic Archipelago (Messier et al., 1994), East Greenland (Born et al., 1997; Wiig et al., 2003; Laidre et al., 2015) and Hudson Bay (Lunn et al., 2004; Yee et al., 2017). ST data also have been combined with snow-drift models to predict optimal denning habitat (Merkel et al., 2020).
Temperature and activity sensors on ST collars (Fancy et al., 1988; Ware et al., 2015) have provided data on den entrance and emergence dates based on changes in temperature and activity (e.g., Messier et al., 1994; Wiig, 1995; Lunn et al., 2004; Fischbach et al., 2007; Andersen et al., 2012; Olson et al., 2017). This has allowed comparisons of denning phenology across the polar bear range, including identifying that polar bears in high latitudes enter dens earlier in autumn, sometimes enter temporary dens to shelter from brief periods of intense cold, and exit later in spring (Messier et al., 1994; Ferguson et al., 2000b; Escajeda et al., 2018) compared to bears at lower latitudes (Amstrup and Gardner, 1994; Lunn et al., 2004; Rode et al., 2018a). Wiig (1995) used the denning frequency of individual females in the Svalbard area over multiple years to deduce if they had reproduced successfully, which allowed estimation of reproductive rates.
ST data collected over 30 years from satellite collared bears in the Southern Beaufort Sea have revealed a shift in the distribution of maternal dens from ice to land (Fischbach et al., 2007; Olson et al., 2017) as the stability of sea ice decreased (e.g., Durner et al., 2017). Atwood et al. (2016b) examined ST data collected from 1986 to 2014 and found that the proportion of collared bears coming ashore increased from ~6% prior to 1999 to ~20% from 2000-2014 and the mean length of stay on land increased by 31 days. Further, empirical relationships between sea-ice availability and the duration and proportion of bears summering (Stirling et al., 1999; Rode et al., 2015; Atwood et al., 2016b; Cherry et al., 2016) and denning onshore (Fischbach et al., 2007; Olson et al., 2017) have been used to model the effects of changing energetics on polar bear reproduction and survival (Molnár et al., 2011; Robbins et al., 2012; Molnár et al., 2014; Molnár et al., 2020).
3.5 Nutritional Ecology and Health
Data collected when immobilizing individual polar bears to deploy SLRTs, including body measurements and samples, provide critical insights into population status and health. Studies using these data have identified mechanisms by which movements and habitat use affect body condition (Stirling et al., 1999; Laidre et al., 2020a; Laidre et al., 2020b; Blanchet et al., 2020), pathogen exposure (Atwood et al., 2017), immune function (Whiteman et al., 2019), diet (Rogers et al., 2015; Tartu et al., 2018; Boucher et al., 2019; Blévin et al., 2020) and energetics (Whiteman et al., 2015; Ware et al., 2017; Pagano et al., 2020; Blanchet et al., 2020). Further, these data have identified the mechanisms by which environmental change has affected individual health and population vital rates (e.g., Stirling et al., 1999; Rode et al., 2014b; Obbard et al., 2016; Pagano et al., 2018).
Divergent space-use strategies identified by ST, together with diet and health data obtained during captures, can be combined for understanding linkages between polar bear energetics, behavior, and pollutant and pathogen exposure (Atwood et al., 2017; Tartu et al., 2018; Blanchet et al., 2020; Blévin et al., 2020). Information on land use combined with diet and energetics studies in the Southern Beaufort Sea indicate that the majority of the subpopulation summers on the sea ice where prey access is reduced and bears are largely fasting (Whiteman et al., 2015; Atwood et al., 2016b; Ware et al., 2017) whereas a smaller subset of the population summers on shore where they benefit from access to subsistence-harvested bowhead whales (Balaena mysticetus) (Rogers et al., 2015). Identifying these divergent behaviors and their potential impacts on vital rates aids in developing and interpreting population models.
Studies have also identified regions and habitat use patterns associated with higher contaminant loads (Olsen et al., 2003; van Beest et al., 2016; Tartu et al., 2018; Blévin et al., 2020) and greater pathogen exposure (Atwood et al., 2017). For example, polychlorinated biphenyls and perfluoroalkyl substances were higher in Barents Sea polar bears that occupied larger offshore home ranges than in bears that used coastal areas in the Svalbard archipelago (Olsen et al., 2003; van Beest et al., 2016), which was further linked to variation in diet, energy needs and proximity to pollutant sources (Tartu et al., 2018; Blévin et al., 2020). In the Southern Beaufort Sea, plasma concentrations of some contaminants (i.e., chlordanes) were lower in land-based bears compared to the offshore ones (Atwood et al., 2017).
3.6 Anthropogenic Disturbance
ST data have been used in observational (Amstrup, 1993) and simulation studies (Wilson and Durner, 2020) on the response of polar bears to disturbances associated with oil and gas extraction (e.g., Amstrup et al., 2006; Wilson and Durner, 2020). In Alaska, the Arctic coastal plain and nearshore region is home to a large industrial footprint (i.e., Prudhoe Bay and Kuparuk oil fields, the National Petroleum Reserve-Alaska [NPR-A], the Beaufort Sea Oil and Gas Lease Sale Area) (Figure 8). Legislation passed in 2017 (public law no. 115-97) included a provision to open the 1002 Area of the Arctic National Wildlife Refuge (ANWR) to oil and gas exploration, which may lead to increased human activity in key denning areas of the Southern Beaufort Sea subpopulation. For several decades, records of maternal den locations in Alaska (including those from ST) have been aggregated into a database that is used by industrial operators to plan winter activities to mitigate disturbance of denned bears (Durner et al., 2020). These data also have been used to monitor the proportion of land-based dens in areas of management interest including the NPR-A, the Prudhoe Bay and Kuparuk oil fields, and in the ANWR (Atwood et al., 2020).
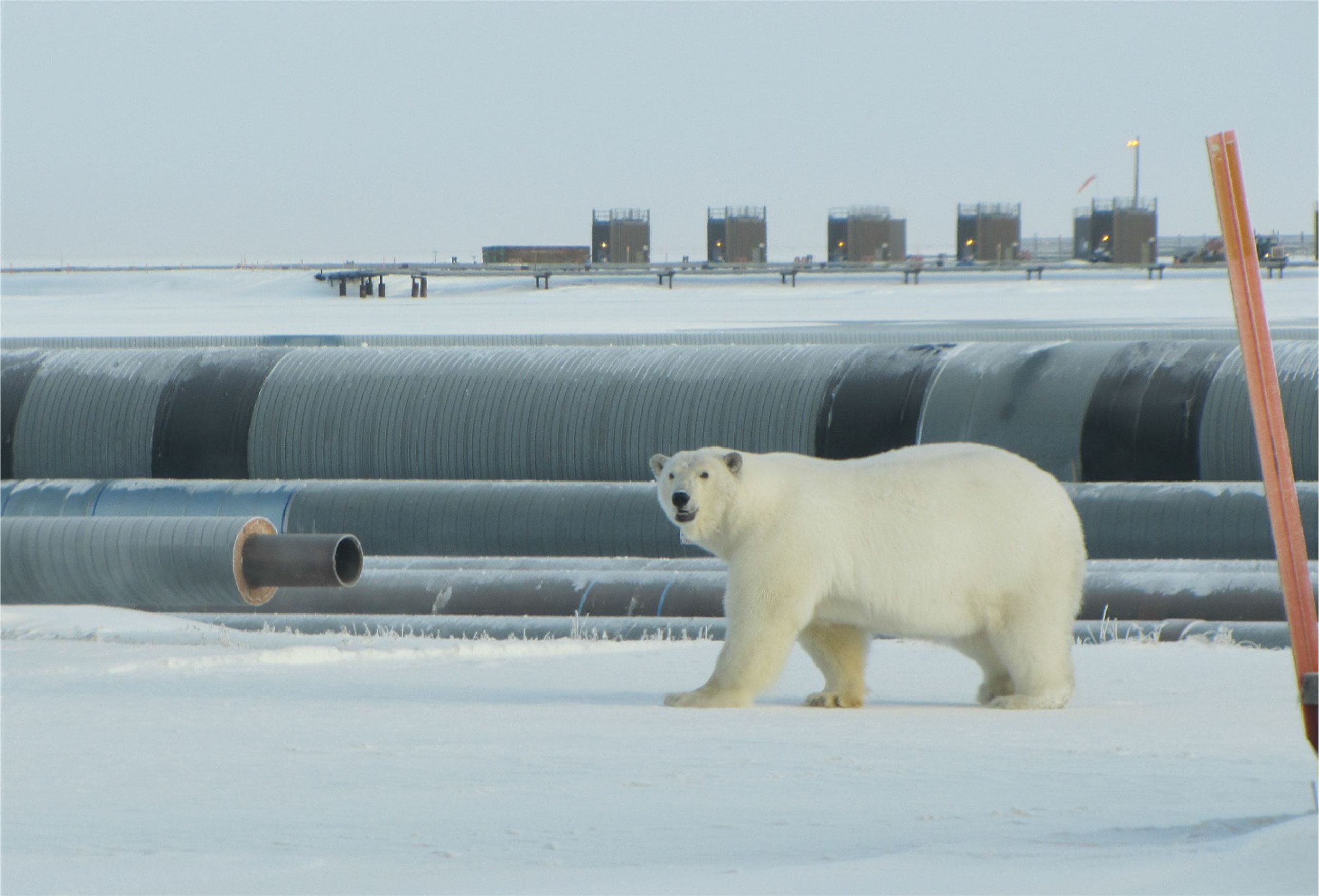
Figure 8 A polar bear walking through oilfield infrastructure near Prudhoe Bay on the Arctic coastal plain of Alaska, which is home to a large industrial footprint. Photo credit: BP Exploration (Alaska), Inc., with permission.
ST data have shown increasing overlap between human activities and polar bear maternal denning as resource exploration in the Arctic expands (Amstrup and Gardner, 1994) and more polar bears use land for summering and maternal denning (Fischbach et al., 2007; Atwood et al., 2016b). Wilson and Durner (2020) used ST data to estimate the incidental take of denning polar bears caused by winter hydrocarbon seismic surveys in ANWR, as required for compliance with the U.S. Marine Mammal Protection Act (16 U.S.C. 1361). Thirty years of ST data on den distribution, denning habitat, and den entrance and emergence dates were critical for designing seismic surveys that reduced the numbers of bears disturbed. More broadly, ST data have been used to understand and mitigate (Regehr et al., In Review; Atwood et al., 2016a) an increasing number of human-bear conflicts resulting from the loss of Arctic sea ice (Derocher et al., 2004; Schliebe et al., 2008; Towns et al., 2009; Moshøj, 2014; Wilder et al., 2017).
4 How Will Satellite Telemetrybe Crucial for Conservation in the 21st Century?
Depending on the subpopulation, scientific studies to inform polar bear management can cost upwards of 1 million US dollars for one field season (e.g., collection of 100 genetic biopsy samples from a helicopter). Conducting these studies without concurrently collecting ST data is inefficient and leaves managers without information needed to understand the ecological and demographic status of a subpopulation. The importance of ST data will grow as the Arctic changes under climate warming. Here, we discuss crucial applications of ST data in the 21st century, which include designing effective studies, estimating demographic parameters, managing harvest, designating protected areas, improving public safety, forecasting future subpopulation status, and complying with national and international protected species legislation (Figure 9).
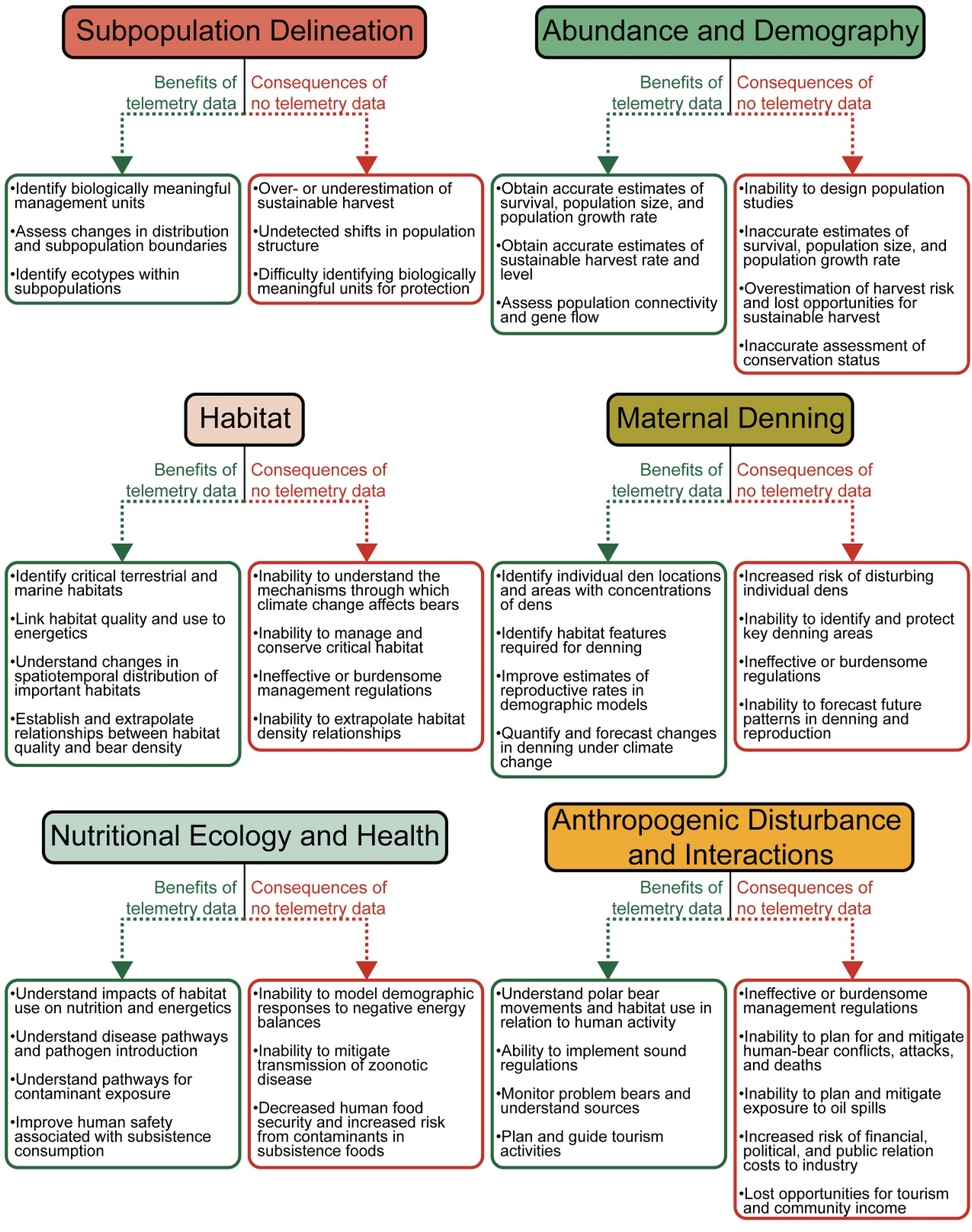
Figure 9 Overview of the uses of satellite telemetry data for conservation and management of polar bears, together with the consequences of not having satellite telemetry data.
4.1 Maximizing Data Collection Under Deteriorating Field Conditions
Climate warming—a key threat driving the need for current information on polar bears—is making scientific research more difficult. Over the last decade, the cost and risks to human safety in studying polar bears have increased. In some parts of their range, bears are less accessible during the spring and summer when research has typically occurred, due to sea ice that is too thin or unstable to work on and fly over. Seasonal windows for sampling bears on their sea-ice habitat have shrunk (Stern and Laidre, 2016) and the number of days with poor weather has increased (Hasemyer, 2021). Thus, opportunities to continue the type of long-term research programs that have been key to understanding aspects of polar bear ecology and demography to date (e.g., Bromaghin et al., 2021) are disappearing. ST will become increasingly important as future research and monitoring efforts rely on sampling smaller numbers of animals.
Additionally, in the last decade, there has been a shift away from physical-capture studies and toward less-invasive sampling methods (e.g., genetic sampling using biopsy darting, distance-sampling aerial surveys), which also facilitate the collection of fewer basic life-history data useful for demographic modeling. Thus, contemporary ST data have been, and will continue to be, critical to designing less-invasive studies as sea-ice conditions deteriorate and polar bear distribution changes.
4.2 Estimating Subpopulation Abundance When Boundaries Are Changing
The importance of ST data in demographic studies will increase as climate warming alters polar bear habitat use, distribution, and subpopulation connectivity. Due to the high mobility of polar bears and difficulty sampling remote Arctic regions, the number of bears within an area at any given time can differ from the total number of bears that use the area over multiple years (e.g., Regehr et al., 2018). Lack of ST data will likely lead to increased bias in demographic parameter estimates and increased difficulty in defining the study population and distinguishing between changes in space use and changes in the overall number of bears. Without ST data, it will not be possible to model movements in and out of the sampling area and reduce bias associated with non-random temporary emigration (Regehr et al., 2018; e.g., Figure 10). It also will not be possible to extrapolate to unsampled areas and interpret changes in estimated abundance over time (i.e., delineating true change in abundance from changes in distribution); see section 3.1.
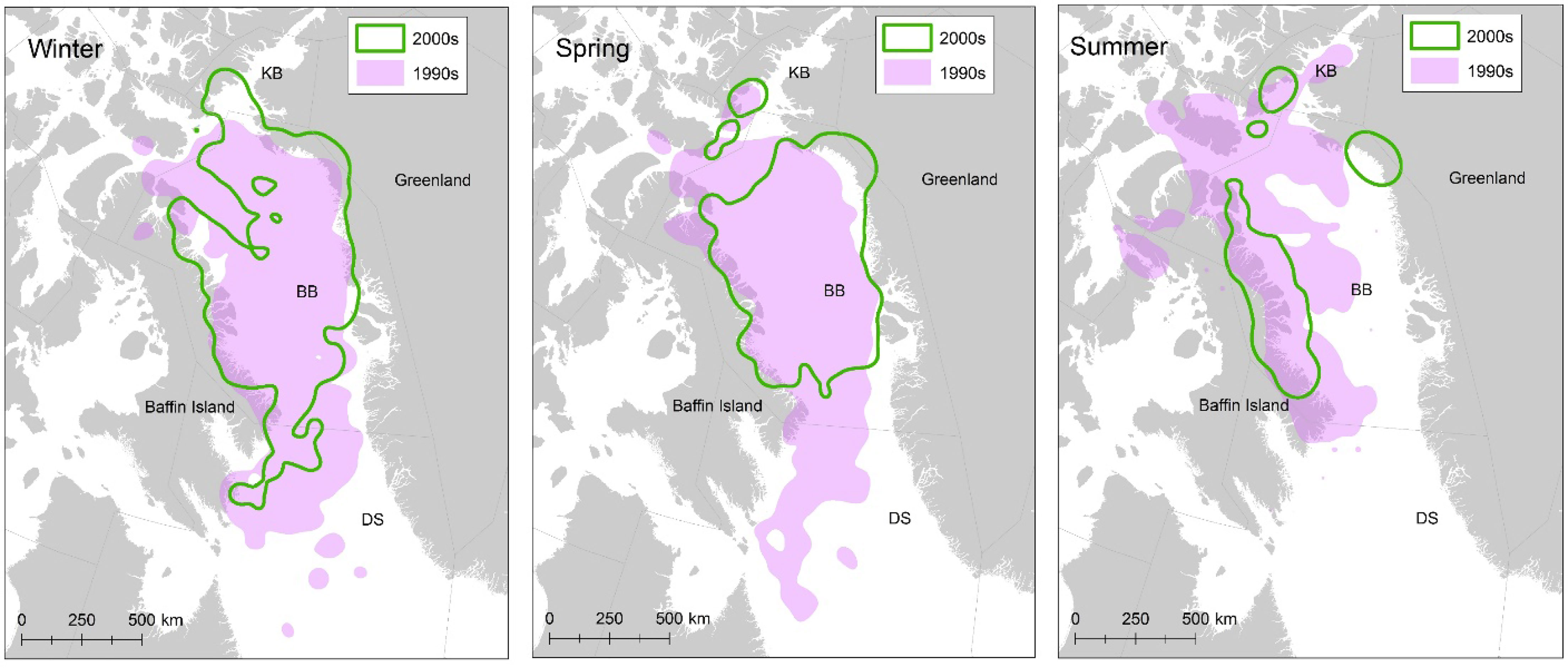
Figure 10 Satellite telemetry data allow for identifying shifts in polar bear space use over time and have proven critical to interpreting data collected during population assessments (e.g., Laidre et al., 2020a). These data from the Baffin Bay subpopulation demonstrated large shifts in seasonal space use over two decades.
Without ST data or methods to correct estimates of abundance for polar bear movements, managers can expect bears may be “double-counted” in capture-recapture studies of adjacent subpopulations that experience immigration and emigration. This can artificially inflate abundance estimates, resulting in overharvest or misapplication of policy decisions that require accurate estimates of abundance, such as negligible impact determinations under the U.S. Marine Mammal Protection Act.
4.3 Improving Harvest Management in a Warming Arctic
ST data will be integral to identifying sustainable strategies for subsistence harvest (as well as guided sport hunting, where allowed) under climate change. The biological effects of human-caused removals (i.e., the combination of subsistence harvest, defense kills, and other sources of direct human-caused mortality) on polar bear subpopulations are evaluated with demographic models that use estimates of reproduction, survival, and abundance (e.g., Taylor et al., 2008; Regehr et al., 2017). Accurate assessment of harvest effects requires the ability to identify demographically meaningful groups of bears and obtain unbiased demographic inputs which, as discussed above, often require ST data. This will become increasingly important as polar bears exhibit variable responses to climate change, which necessitates subpopulation-specific harvest assessments. For example, the previous standard application under stable environmental conditions of a 4.5% harvest rate at a 2:1 male-to-female ratio (Taylor et al., 1987; Regehr et al., 2021a; Regehr et al., 2021b) may not be supportable in the future for some subpopulations. Furthermore, modern methods to evaluate harvest use stochastic frameworks where larger uncertainty (e.g., environmental, statistical, model-based) often translates into increased risk of negative demographic effects, which can lead to lower harvest levels aimed at reducing risk. In the Southern Hudson Bay (SH) subpopulation, lack of ST data made it difficult to confirm whether declining abundance estimates, obtained from sequential aerial surveys (Obbard et al., 2015; Obbard et al., 2018), were caused by declines in polar bear numbers or changes in polar bear distribution. This uncertainty was propagated through a quantitative harvest risk assessment (Regehr et al., 2021a).
4.4 Forecasting the Future of the Species and Complying With Protected Species Legislation
ST data are needed to build RSFs (see section 3.3) that identify essential habitats and project their future availability under different greenhouse gas emission scenarios (Amstrup et al., 2010; Atwood et al., 2016a). Habitat assessments are a fundamental requirement for implementation of protected species legislation in a warming Arctic. Article II of the 1973 Agreement on the Conservation of Polar Bears requires the contracting parties to “take appropriate action to protect the ecosystems of which polar bears are a part, with special attention to habitat components…”. Furthermore, the Polar Bear Range States Circumpolar Action Plan (CAP) considered goals for defining essential habitat and multiple other agreements on polar bears (e.g., Article 2 of the U.S-Russia Polar Bear Agreement; United States T. Doc. 107-10) require the identification and conservation of key polar bear habitats. Projections of future polar bear habitats will also require ST data combined with projections of pollutant transport to and dynamics in Arctic ecosystems to predict future exposure to contaminants.
4.5 Reducing Disturbance and Human-Bear Interactions in a Warming Arctic
Sea-ice decline has led to increases in commercial shipping and made coastal and offshore industrial development more economically viable (Smith and Stephenson, 2013; Eguíluz et al., 2016). For example, the Mary River iron ore mine on Baffin Island (Nunavut, Canada) ships to worldwide markets at a rate of one freighter every 48 h from July through October and is planning for an eventual increase to 3 ships per day, during the period of sea-ice formation (Nunavut Impact Review Board, 2009; Baffinland Iron Mines Corporation, 2018). Increased shipping will lead to direct disturbances of polar bears. ST data from collared adult females will be critical to evaluate this (e.g., Born et al., 2011; Born et al., 2012).
Using ST data to establish empirical relationships between reduced sea ice and increased land use by polar bears will be important for projecting interactions with humans and developing mitigation plans. Declines in sea-ice habitat are resulting in a growing reliance on terrestrial habitat for resting during summer and fall and for maternal denning in winter. This will pose challenges to balancing industrial development and human safety with polar bear conservation (Stirling et al., 1999; Cherry et al., 2013; Rode et al., 2015; Atwood et al., 2016b). As bears spend more time on land (Regehr et al., In Review), they will become increasingly vulnerable to anthropogenic stressors, particularly those associated with industrial activities (Atwood et al., 2016a).
Several new ST applications have potential to monitor and mitigate the risk of human-polar bear conflict. For example, in Churchill, Manitoba, ear-mounted transmitters are being deployed on polar bears captured in town to determine whether those bears travel north and contribute to the increasing number of human-bear interactions in coastal communities along the Kivalliq, Nunavut coast of Hudson Bay (Government of Manitoba unpublished data). Elsewhere, real-time bear location data are being used to alert communities when bears are approaching. In Longyearbyen, Svalbard, geo-fenced ST collars have been deployed to provide alerts when collared polar bears come within a 25 km radius of the community (J. Aars, Norwegian Polar Institute, unpublished data) (Figure 11). Further, applications to deter wildlife-livestock conflict may be adapted for polar bears. For example, radio-activated guard (RAG) devices employ a scanning radio receiver to monitor the proximity of radio-collared animals to a protected area (Breck et al., 2003) and may have applications in Arctic communities and at industrial facilities. If a radio-collared animal approaches a delineated protected area, the RAG unit activates a non-lethal deterrent (e.g., strobe light, sound effects) to discourage the animal from advancing. In sum, ST represents a valuable tool for monitoring the risks that polar bears can pose to human safety and reducing conflicts through both reactive (e.g., monitoring the movement of translocated bears) and proactive (e.g., geo-fencing, RAG devices) management applications.
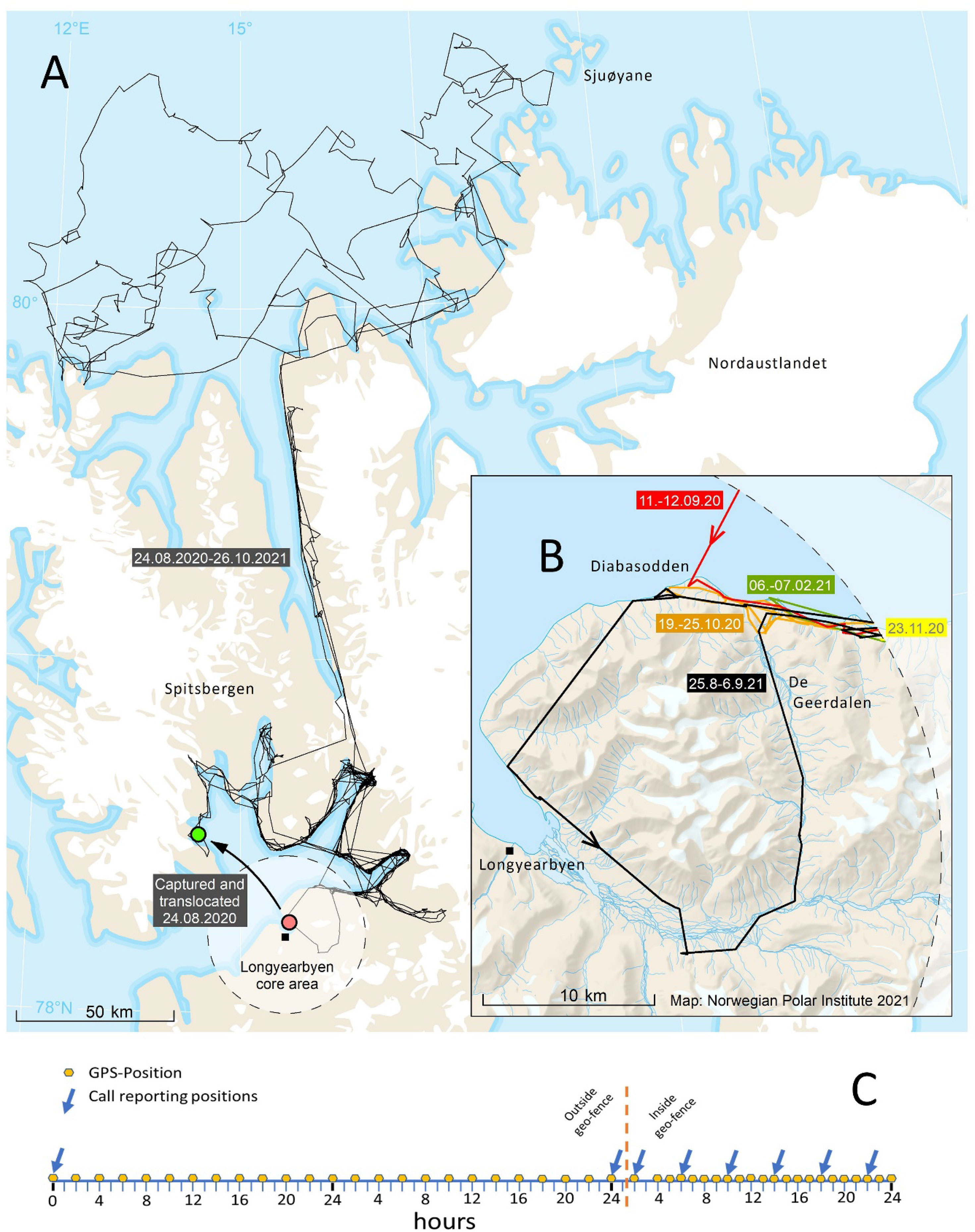
Figure 11 Movements of an adult female polar bear near Longyearbyen, the main settlement in Svalbard, in August 2021 (A, B). The Telonics iridium collar was set to collect GPS positions every other hour and report them every second day. When the collared bear moved inside a circle with radius of 25 km (‘geo-fence’) from Longyearbyen, the collar sent data more frequently (collecting a GPS location every hour and reporting every 4th hour) so the bear’s proximity to town could be monitored (C).
Additionally, in the foreseeable future, ST data are likely to be important to understanding the impacts of the fast-growing ship-based and terrestrial ecotourism industry focused on viewing polar bears in their natural habitats. Understanding movements and seasonal habitat use of bears can help guide future regulations so that ecotourism activities avoid sensitive habitats.
5 Recommendations to Facilitate Future Collection of ST Data and Reduce Concerns
In the future, managers who have access to ST data will have information that is critical to conservation of polar bears (Figure 9 and Table 1). Lack of ST data will make management decisions riskier to polar bears and to humans. Table 1 and references within illustrate the wealth of information obtained from applying SLRTs to a small number of polar bears in a subpopulation, in contrast to other monitoring methods that would require sampling a much larger number of bears. In some cases, deployment of <10 SLRTs per year, over a 3-5-year period, provided information that was necessary to design an effective study and interpret demographic results of capture-recapture studies (e.g., Baffin Bay subpopulation, Laidre et al., 2020a; Laidre et al., 2020b; Atkinson et al., 2021). In other cases, the availability of ST data was a determining factor in whether a capture-recapture study produced useful results. For example, lack of ST data for the M’Clintock Channel subpopulation led to negatively biased estimates of survival and forced analysts to estimate abundance using only two years of data in a closed-population capture-recapture model (Dyck et al., 2021). Similarly, preliminary capture-recapture analyses for the Viscount Melville subpopulation failed to produce biologically plausible results, whereas subsequent analyses that used ST data led to more accurate estimates of survival and abundance. Here we identify three methods that may facilitate collection of ST data and reduce concerns.
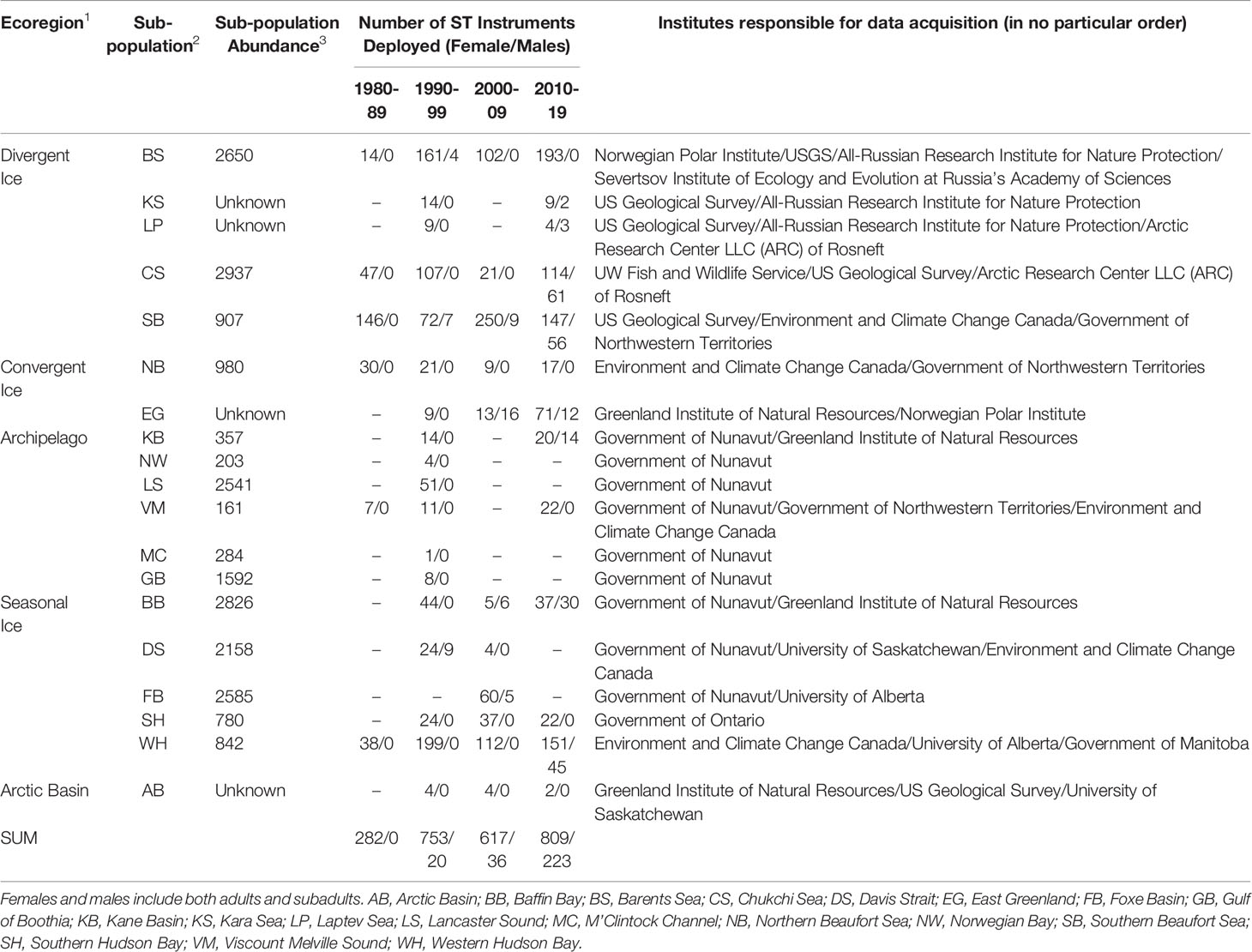
Table 1 Number of bears instrumented with satellite tags (ST) (any type) by subpopulation over the last 40 years.
5.1 Establish Common Objectives
Scientists, managers, Indigenous partners, and stakeholders should review the status of polar bear subpopulations, identify what information is most important to management and conservation, and determine appropriate methods to collect it. This requires discussing the pros and cons of all research methods and identifying the ramifications if ST data are not collected, such as increased uncertainty and bias. The discussion should extend to impacts on management decisions (e.g., regarding sustainable harvest level) and potential ramifications for resource users, regulatory entities, and others. In this manner, decisions about whether to collect ST data and the number of bears to be fitted with SLRTs can be based on a transparent assessment of the most effective research methods available to meet objectives, while recognizing approaches vary between subpopulations. We suggest that this type of front-end, collaborative discussion could lead to a better understanding of concerns around ST and help identify mutually acceptable solutions.
Open discussions should occur around the level of disturbance caused by any field study on polar bears. Even genetic capture-recapture studies, increasingly used as a less-invasive research method (e.g., Atkinson et al., 2021), involve briefly pursuing and temporarily altering the behavior of large numbers of bears from a helicopter (upwards of >1,000 bears biopsy darted in some cases, Atkinson et al., 2021). In this context, the relatively small number of bears that must be physically captured to obtain ST data (Table 1) represents a small amount of disturbance with a potentially large increase in the amount of information. In many situations, improvements to study design and parameter estimation that result from ST data mean that sample sizes of other types of data (e.g., from biopsies) can be substantively reduced while still meeting research objectives.
5.2 Use the Minimum Necessary Sample Sizes
Collar deployment should be guided by research objectives that are explicitly linked to management and conservation needs. Statistical power analyses can estimate how many representative individuals are needed to answer specific questions, while accounting for potential problems with data acquisition or tag failure. This will ensure that no more ST devices are deployed than are needed to address specific goals. One approach may be to agree upon a minimum sample size and number of sampling years needed for a robust assessment and commit to reengaging all parties prior to increasing sample sizes (e.g., Sequeira et al., 2019). Furthermore, temporal limits could be placed on the duration over which individual bears wear ST collars (e.g., Laidre et al., 2020b). A release mechanism can be programmed for collars to drop off on a specific date or bears can be recaptured and collars manually removed. These approaches require balancing multiple tradeoffs, given that sample sizes and how long individuals wear SLRTs is positively correlated with the probability that ST data will reveal individual variability in the behavior and the adaptive potential of polar bears.
5.3 Advance ST Technology
Improvements in SLRTs have the potential to alleviate concerns about effects on polar bears. Size reduction of collars, reliable release mechanisms, and tags that serve as alternatives to collars for short-term data collection, are all useful areas of development (e.g., Wiig et al., 2017). Several such efforts have occurred in recent years but continued technological advances warrant ongoing investment in methods for tracking polar bears. Although much of the current understanding of movements of polar bears comes from the deployment of ST collars on adult females, development of SLRTs that enable the tracking of adult males and juvenile bears for a year or more, would greatly improve knowledge.
Finally, we recommend continued use of field data and modern analytical methods to investigate the potential effects of chemical immobilization and collaring on polar bears. While most studies of capture effects have found no long-term impacts on fitness, the potential for such impacts should be revisited over time. Meta-analyses of data from multiple subpopulations could increase statistical power. For subpopulations with a long history of capture-recapture studies, it is possible to fit models that include “trap effects” (e.g., Regehr et al., 2007; Kéry and Schaub, 2012), which are specifically designed to identify temporary effects on reproduction, survival, or other demographic parameters following capture.
6 Conclusion
In this paper, we review how ST data have contributed to polar bear management and conservation and discuss their increasing importance to address current and future challenges. Climate warming is shifting the geographic ranges of bears, inside and outside of historical subpopulation boundaries, resulting in altered metapopulation dynamics and the appearance of bears in new areas. With an ice-free Arctic expected within decades (Wang and Overland, 2009; IPCC, 2019), it will be critical to understand how bears redistribute. Some subpopulations may become genetically or demographically isolated (Maduna et al., 2021), with ramifications for population viability (i.e., as a function of health, energetics, human-caused removals, and contaminant exposure) and sustainable use. Further, increasing development in the Arctic (e.g., new shipping routes, resource extraction) will require access to contemporary ST data to meet legal requirements for environmental assessments and monitoring. There are vast areas where few or no ST data are available from polar bears due to lack of research efforts, including most of the Russian Arctic, the Arctic Basin, and the Last Ice Area, the latter of which is predicted to be a long-term refuge for the species (Moore et al., 2019). ST data from these regions and their respective subpopulations will be critical to conservation and management of the species. In summary, ST provides critical data that allow managers to make informed management and regulatory decisions and ST will be vital to the conservation of polar bears in the 21st century.
Author Contributions
KL lead the writing with contributions from GD, NL, EVR, TA, KR, JA, HR, and ØW. All authors contributed to the article and approved the submitted version.
Funding
Authors were supported by their individual agencies. KL was supported by NASA grant 80NSSC18K1229.
Conflict of Interest
The authors declare that the research was conducted in the absence of any commercial or financial relationships that could be construed as a potential conflict of interest.
The reviewer LQ declared a past co-authorship with one of the authors [KL, EVR, and KR] to the handling Editor.
Publisher’s Note
All claims expressed in this article are solely those of the authors and do not necessarily represent those of their affiliated organizations, or those of the publisher, the editors and the reviewers. Any product that may be evaluated in this article, or claim that may be made by its manufacturer, is not guaranteed or endorsed by the publisher.
Acknowledgments
This review was a product of an international scientific research workshop (“Polar bear demography: status and future direction”, held in Lyngen, Norway, 12-14 February 2019) under the umbrella of the Polar Bear Range States Circumpolar Action Plan (CAP). Ben Cohen assisted with figures. Nick Cobbing kindly gave us permission to use his photo. Any use of trade, firm, or product names is for descriptive purposes only and does not imply endorsement by the U.S. Government. Lori Quakenbush, Anthony Pagano, and one anonymous reviewer made helpful comments.
Footnotes
- ^ As defined by Amstrup et al. (2008)
- ^ Based on deployment location
- ^ Current estimate (PBSG 2019)
References
Aars J. (2013). Variation in Detection Probability of Polar Bear Maternity Dens. Polar Biol. 36, 1089–1096. doi: 10.1007/s00300-013-1331-7
Aars J., Marques T. A., Buckland S. T., Andersen M., Belikov S., Boltunov A., et al. (2009). Estimating the Barents Sea Polar Bear Subpopulation Size. Mar. Mamm. Sci. 25, 35–52. doi: 10.1111/j.1748-7692.2008.00228.x
Aars J., Marques T. A., Lone K., Andersen M., Wiig Ø., Bardalen Fløystad I. M., et al. (2017). Polar Bear Population Structure and Trend in the Western Barents Sea. Polar Res. 36, 1374125. doi: 10.1080/17518369.2017.1374125
AEWC (2019). A Resolution Supporting Non-Invasive Research on Polar Bears (Alaska Eskimo Whaling Commission Convention Resolution). 2019-03. Available at: http://www.aewc-alaska.org/.
Amstrup S. C. (1993). Human Disturbances of Denning Polar Bears in Alaska. Arctic 46, 246–250. doi: 10.14430/arctic1349
Amstrup S. C. (2003). “Polar Bear, Ursus Maritimus”, in Wild Mammals of North America: Biology, Management, and Conservation. Eds. Feldhamer G. A., Thompson B. C., Chapman J. A. (Baltimore, MD: John Hopkins University Press), 587–610.
Amstrup S. C., DeWeaver E. T., Douglas D. C., Marcot B. G., Durner G. M., Bitz C. M., et al. (2010). Greenhouse Gas Mitigation can Reduce Sea-Ice Loss and Increase Polar Bear Persistence. Nature 468, 955–958. doi: 10.1038/nature09653
Amstrup S. C., Durner G. M., McDonald T. L., Johnson W. R. (2006). Estimating Potential Effects of Hypothetical Oil Spills on Polar Bears (Anchorage, AK: U. S. Geological Survey, Alaska Science Center). doi: 10.3133/85885
Amstrup S. C., Durner G. M., McDonald T. L., Mulcahy D. M., Garner G. W. (2001). Comparing Movement Patterns of Satellite-Tagged Male and Female Polar Bears. Can. J. Zool. 79, 2147–2158. doi: 10.1139/z01-174
Amstrup S. C., Gardner C. (1994). Polar Bear Maternity Denning in the Beaufort Sea. J. Wildl. Manage. 58, 1–10. doi: 10.2307/3809542
Amstrup S. C., Marcot B. G., Douglas D. C. (2008). “A Bayesian Network Modeling Approach to Forecasting the 21st Century Worldwide Status of Polar Bears”, in Arctic Sea Ice Decline: Observation, Projections, Mechanisms, and Implications. Eds. DeWeaver E. T., Bitz C. M., Tremblay L. B. (Washington, DC: American Geophysical Union), 213–268.
Amstrup S. C., McDonald T. L., Durner G. M. (2004). Using Satellite Radiotelemetry Data to Delineate and Manage Wildlife Populations. Wildl. Soc Bull. 32, 661–679. doi: 10.2193/0091-7648(2004)032[0661:USRDTD]2.0.CO;2
Andersen M., Derocher A. E., Wiig Ø., Aars J. (2008). Movements of Two Svalbard Polar Bears Recorded Using Geographical Positioning System Satellite Transmitters. Polar Biol. 31, 905–911. doi: 10.1007/s00300-008-0428-x
Andersen M., Derocher A. E., Wiig Ø., Aars J. (2012). Polar Bear (Ursus Maritimus) Maternity Den Distribution in Svalbard, Norway. Polar Biol. 35, 499–508. doi: 10.1007/s00300-011-1094-y
Arthur S. M., Manly B. F. J., McDonald L. L., Garner G. W. (1996). Assessing Habitat Selection When Availability Changes. Ecology 77, 215–227. doi: 10.2307/2265671
Atkinson S. N., Laidre K. L., Arnold T. W., Stapleton S., Regehr E. V., Born E. W., et al. (2021). A Novel Mark-Recapture-Recovery Survey Using Genetic Sampling for Polar Bears Ursus Maritimus in Baffin Bay. Endanger. Species Res. 46, 105–120. doi: 10.3354/esr01148
Atwood T. C., Bromaghin J. F., Patil V. P., Durner G. M., Douglas D. C., Simac K. S. (2020). Analyses on Subpopulation Abundance and Annual Number of Maternal Dens for the U.S. Fish and Wildlife Service on Polar Bears (Ursus Maritimus) in the Southern Beaufort Sea, U.S. Geological Survey Open-File Report 2020-1087. doi: 10.3133/ofr20201087
Atwood T. C., Duncan C., Patyk K. A., Nol P., Rhyan J., McCollum M., et al. (2017). Environmental and Behavioral Changes may Influence the Exposure of an Arctic Apex Predator to Pathogens and Contaminants. Sci. Rep. 7, 13193. doi: 10.1038/s41598-017-13496-9
Atwood T. C., Marcot B. G., Douglas D. C., Amstrup S. C., Rode K. D., Durner G. M., et al. (2015). Evaluating and Ranking Threats to the Long-Term Persistence of Polar Bears, U.S. Geological Survey Open-File Report 2014-1254. doi: 10.3133/ofr20141254
Atwood T. C., Marcot B. G., Douglas D. C., Amstrup S. C., Rode K. D., Durner G. M., et al. (2016a). Forecasting the Relative Influence of Environmental and Anthropogenic Stressors on Polar Bears. Ecosphere 7, e01370. doi: 10.1002/ecs2.1370
Atwood T. C., Peacock E., McKinney M. A., Lillie K., Wilson R., Douglas D. C., et al. (2016b). Rapid Environmental Change Drives Increased Land Use by an Arctic Marine Predator. PloS One 11, e0155932. doi: 10.1371/journal.pone.0155932
Auger-Méthé M., Lewis M. A., Derocher A. E. (2016). Home Ranges in Moving Habitats: Polar Bears and Sea Ice. Ecography 39, 26–35. doi: 10.1111/ecog.01260
Baffinland Iron Mines Corporation (2018). Mary River Project Phase 2 Proposal. TSD 02: Project Description (Cambridge Bay, NU). NIRB File No. 08MN053.
Belikov S. E., Garner G. W., Wiig Ø., Boltonov A. N., Gorbonov Y. A. (1998). Polar Bears of the Severnaya Zemlya Archipelago of the Russian Arctic. Ursus 10, 33–40.
Bethke R., Taylor M., Amstrup S., Messier F. (1996). Population Delineation of Polar Bears Using Satellite Collar Data. Ecol. Appl. 6, 311–317. doi: 10.2307/2269574
Blanchet M.-A., Aars J., Andersen M., Routti H. (2020). Space-Use Strategy Affects Energy Requirements in Barents Sea Polar Bears. Mar. Ecol. Prog. Ser. 639, 1–19. doi: 10.3354/meps13290
Blévin P., Aars J., Andersen M., Blanchet M.-A., Hanssen L., Herzke D., et al. (2020). Pelagic vs. Coastal – Key Drivers of Pollutant Levels in Barents Sea Polar Bears With Contrasted Space-Use Strategies. Environ. Sci. Technol. 54, 985–995. doi: 10.1021/acs.est.9b04626
Block B. A., Holbrook C. M., Simmons S. E., Holland K. N., Ault J. S., Costa D. P., et al. (2016). Toward a National Animal Telemetry Network for Aquatic Observations in the United States. Anim. Biotelemetry 4, 6. doi: 10.1186/s40317-015-0092-1
Born E. W., Laidre K. L., Dietz R., Wiig Ø. (2011). “Polar Bear Ursus Maritimus,” in Eastern Baffin Bay. A Strategic Environmental Impact Assessment of Hydrocarbon Activities. Eds. Boertmann D., Mosbech A. (Roskilde, Denmark: Scientific Report No. 9, Danish Centre for Environment and Energy), 93–101.
Born E. W., Laidre K. L., Dietz R., Wiig Ø., Andersen M. (2012). “Polar Bear Ursus Maritimus”, in The Western Greenland Sea, A Strategic Environmental Impact Assessment of Hydrocarbon Activities. Eds. Boertmann D., Mosbech A. (Roskilde, Denmark: Scientific Report No. 22, Danish Centre for Environment and Energy), 102–114.
Born E. W., Wiig Ø., Thomassen J. (1997). Seasonal and Annual Movements of Radio-Collared Polar Bears (Ursus Maritimus) in Northeast Greenland. J. Mar. Syst. 10, 67–77. doi: 10.1016/S0924-7963(96)00072-3
Boucher N. P., Derocher A. E., Richardson E. S. (2019). Space Use Patterns Affect Stable Isotopes of Polar Bears (Ursus Maritimus) in the Beaufort Sea. Polar Biol. 42, 1581–1593. doi: 10.1007/s00300-019-02546-9
Breck S. W., Williamson R., Niemeyer C., Shivik J. A. (2003). Non-Lethal Radio Activated Guard for Deterring Wolf Depredation in Idaho: Summary and Call for Research. Vertebr. Pest Conf. 20, 223–226.
Bromaghin J. F., Douglas D. C., Durner G. M., Simac K. S., Atwood T. C. (2021). Survival and Abundance of Polar Bears in Alaska’s Beaufort Sea 2001-2016. Ecol. Evol. 11, 14250–14267. doi: 10.1002/ece3.8139
Brun C., Blanchet M. A., Ims R. A., Aars J. (2021). Stability of Space Use in Svalbard Coastal Female Polar Bears: Intra-Individual Variability and Influence of Kinship. Polar Res. 40, 5355. doi: 10.33265/polar.v40.5355
Castro de la Guardia L., Derocher A. E., Myers P. G., Terwisscha van Scheltinga A. D., Lunn N. J. (2013). Future Sea Ice Conditions in Western Hudson Bay and Consequences for Polar Bears in the 21st Century. Glob. Change Biol. 19, 2675–2687. doi: 10.1111/gcb.12272
Cattet M. (2003). A CCWHC Technical Bulletin: Drug Residues in Wild Meat – Addressing a Public Health Concern. Canadian Cooperative Wildlife Health Centre (Newsletters and Publications Paper 46). Available at: https://www.ccwhc.ca/newsletters/technical_bulletin9-1.pdf.
Cherry S. G., Derocher A. E., Lunn N. J. (2016). Habitat-Mediated Timing of Migration in Polar Bears: An Individual Perspective. Ecol. Evol. 6, 5032–5042. doi: 10.1002/ece3.2233
Cherry S. G., Derocher A. E., Thiemann G. W., Lunn N. J. (2013). Migration Phenology and Seasonal Fidelity of an Arctic Marine Predator in Relation to Sea Ice Dynamics. J. Anim. Ecol. 82, 912–921. doi: 10.1111/1365-2656.12050
CLS (2016). Argos User’s Manual. Worldwide Tracking and Environmental Monitoring by Satellite (Toulousse, France: Collecte Localisation Satellites). Available at: https://www.argos-system.org/manual/index.html.
Davidson S. C., Bohrer G., Gurarie E., LaPoint S., Mahoney P. J., Boelman N. T., et al. (2020). Ecological Insights From Three Decades of Animal Movement Tracking Across a Changing Arctic. Science 370, 712–715. doi: 10.1126/science.abb7080
Derocher A. E., Andersen M., Wiig Ø., Aars J., Hansen E., Biuw M. (2011). Sea Ice and Polar Bear Den Ecology at Hopen Island, Svalbard. Mar. Ecol. Prog. Ser. 441, 273–277. doi: 10.3354/meps09406
Derocher A. E., Lunn N. J., Stirling I. (2004). Polar Bears in a Warming Climate. Int. Comp. Biol. 44, 163–176. doi: 10.1093/icb/44.2.163
Douglas D. C., Weinzierl R., Davidson S. C., Kays R., Wikelski M., Bohrer G. (2012). Moderating Argos Location Errors in Animal Tracking Data. Meth. Ecol. Evol. 3, 999–1007. doi: 10.1111/j.2041-210X.2012.00245.x
Durner G. M., Amstrup S. C. (1995). Movements of a Polar Bear From Northern Alaska to Northern Greenland. Arctic 48, 338–341. doi: 10.14430/arctic1257
Durner G. M., Amstrup S. C., Atwood T. C., Douglas D. C., Fischbach A. S., Olson J. W., et al. (2020). Catalogue of Polar Bear (Ursus Maritimus) Maternal Den Locations in the Beaufort and Chukchi Seas and Nearby Areas 1910–2018. (Reston, Virginia:U.S. Geological Survey Data Series), 1121. doi: 10.3133/ds1121
Durner G. M., Amstrup S. C., Nielson R., McDonald T. (2004). “Using Discrete Choice Modeling to Generate Resource Selection Functions for Female Polar Bears in the Beaufort Sea,” in: Ed.Huzurbazar S.. Resource Selection Methods and Applications. Proceedings of The First International Conference on Resource Selection, Laramie, Wyoming, 13–15 January 2003, 107–120. Omnipress, Madison, WI.
Durner G. M., Douglas D. C., Albeke S. E., Whiteman J. P., Amstrup S. C., Richardson E., et al. (2017). Increased Arctic Sea Ice Drift Alters Adult Female Polar Bear Movements and Energetics. Glob. Change Biol. 23, 3460–3473. doi: 10.1111/gcb.13746
Durner G. M., Douglas D. C., Atwood T. C. (2019). Are Polar Bear Habitat Resource Selection Functions Developed From 1985-1995 Data Still Useful? Ecol. Evol. 9, 8625–8638. doi: 10.1002/ece3.5401
Durner G. M., Douglas D. C., Nielson R. M., Amstrup S. C. (2006). A Model for Autumn Pelagic Distribution of Adult Female Polar Bears in the Chukchi Sea 1987-1994. Final Report to U. S. Fish and Wildlife Service (Anchorage, AK: U. S. Geological Survey, Alaska Science Center). Contract Completion Report 70181-5-N240.
Durner G. M., Douglas D. C., Nielson R. M., Amstrup S. C., McDonald T. L., Stirling I., et al. (2009). Predicting 21st-Century Polar Bear Habitat Distribution From Global Climate Models. Ecol. Monogr. 79, 25–58. doi: 10.1890/07-2089.1
Durner G. M., Whiteman J. P., Harlow H. J., Amstrup S. C., Regehr E. V., Ben-David M. (2011). Consequences of Long-Distance Swimming and Travel Over Deep-Water Pack Ice for a Female Polar Bear During a Year of Extreme Sea Ice Retreat. Polar Biol. 34, 975–984. doi: 10.1007/s00300-010-0953-2
Dyck M., Lukacs P. M., Ware J. (2021). Recovery From Reduction: The M’clintock Channel Polar Bear Subpopulation, Nunavut, Canada. Arctic 74 (4), 509–524.
Eguíluz V. M., Fernández-Gracia J., Irigoien X., Duarte C. M. (2016). A Quantitative Assessment of Arctic Shipping in 2010–2014. Sci. Rep. 6, 30682. doi: 10.1038/srep30682
Escajeda E., Laidre K. L., Born E. W., Wiig Ø., Atkinson S., Dyck M., et al. (2018). Identifying Shifts in Maternity Den Phenology and Habitat Characteristics of Polar Bears (Ursus Maritimus) in Baffin Bay and Kane Basin. Polar Biol. 41, 87–100. doi: 10.1007/s00300-017-2172-6
Fancy S. G., Pank L. F., Douglas D. C., Curby C. H., Garner G. W., Amstrup S. C., et al. (1988). Satellite Telemetry: A New Tool for Wildlife Research and Management, U. S. Fish and Wildlife Service Resource Publication No. 172.
Ferguson S. H., Taylor M. K., Messier F. (2000a). Influence of Sea Ice Dynamics on Habitat Selection by Polar Bears. Ecology 81, 761–772. doi: 10.1890/0012-9658(2000)081[0761:IOSIDO]2.0.CO;2
Ferguson S. H., Taylor M. K., Rosing-Asvid A., Born E. W., Messier F. (2000b). Relationships Between Denning of Polar Bears and Conditions of Sea Ice. J. Mammal. 81, 1118–1127. doi: 10.1644/1545-1542(2000)081<1118:RBDOPB>2.0.CO;2
Fischbach A. S., Amstrup S. C., Douglas D. C. (2007). Landward and Eastward Shift of Alaskan Polar Bear Denning Associated With Recent Sea Ice Changes. Polar Biol. 30, 1395–1405. doi: 10.1007/s00300-007-0300-4
Flyger V., Townsend M. R. (1968). The Migration of Polar Bears. Sci. Am. 218, 108–116. doi: 10.1038/scientificamerican0268-108
Frair J. L., Fieberg J., Hebblewhite M., Cagnacci F., DeCesare N. J., Pedrotti L. (2010). Resolving Issues of Imprecise and Habitat-Biased Locations in Ecological Analyses Using GPS Telemetry Data. Philos. Trans. R. Soc B 365, 2187–2200. doi: 10.1098/rstb.2010.0084
Freitas C., Kovacs K. M., Andersen M., Aars J., Sandven S., Skern-Mauritzen M., et al. (2012). Importance of Fast Ice and Glacier Fronts for Female Polar Bears and Their Cubs During Spring in Svalbard, Norway. Mar. Ecol. Prog. Ser. 447, 289–304. doi: 10.3354/meps09516
Gaidet N., Cappelle J., Takekawa J. Y., Prosser D. J., Iverson S. A., Douglas D. C., et al. (2010). Potential Spread of Highly Pathogenic Avian Influenza H5N1 by Wildfowl: Dispersal Ranges and Rates Determined From Large-Scale Satellite Telemetry. J. Appl. Ecol. 47 (5), 1147–1157. doi: 10.1111/j.1365-2664.2010.01845.x
Garner G. W., Belikov S. E., Stishov M. S., Barnes V. G., Arthur S. M. (1994). Dispersal Patterns of Polar Bears From the Denning Concentration of Wrangel Island. Int. Conf. Bear Res. Manage. 9, 401–410. doi: 10.2307/3872726
Hamilton C. D., Kovacs K. M., Ims R. A., Aars J., Lydersen C. (2017). An Arctic Predator-Prey System in Flux: Climate Change Impacts on Coastal Space Use by Polar Bears and Ringed Seals. J. Anim. Ecol. 86, 1054–1064. doi: 10.1111/1365-2656.12685
Harcourt R., Sequeira A. M. M., Zhang X., Roquet F., Komatsu K., Heupel M., et al. (2019). Animal-Borne Telemetry: An Integral Component of the Ocean Observing Toolkit. Front. Mar. Sci. 6, 326. doi: 10.3389/fmars.2019.00326
Harington C. R. (1968). Denning Habits of the Polar Bear (Ursus Maritimus Phipps) (Canadian Wildlife Service Report Series No. 5).
Harris R. B., Fancy S. G., Douglas D. C., Garner G. W., Amstrup S. C., McCabe T. R., et al. (1990). Tracking Wildlife by Satellite: Current Systems and Performance, U. S. Fish and Wildlife Service Technical Report No. 30.
Hart K. M., Hyrenbach K. D. (2009). Satellite Telemetry of Marine Megavertebrates: The Coming of Age of an Experimental Science. Endanger. Species Res. 10, 9–20. doi: 10.3354/esr00238
Hasemyer D. (2021). “Polar Bears Are Suffering From the Arctic’s Loss of Sea Ice. So Is Scientists’ Ability to Study Them,” in Climate News. Available at: https://insideclimatenews.org/news/05102021/polar-bear-science-research-arctic-sea-ice-climate-change/.
Hays G. C., Bailey H., Bograd S. J., Bowen W. D., Campagna C., Carmichael R. H., et al. (2019). Translating Marine Animal Tracking Data Into Conservation Policy and Management. Trends Ecol. Evol. 34, 459–473. doi: 10.1016/j.tree.2019.01.009
Henri D., Gilchrist H. G., Peacock E. (2010). “Understanding and Managing Wildlife in Hudson Bay Under a Changing Climate: Some Recent Contributions From Inuit and Cree Ecological Knowledge,” in A Little Less Arctic: Top Predators in the World’s Largest Northern Inland Sea. Eds. Ferguson S. H., Loseto L. L., Mallory M. L. (New York, NY: Springer), 267–289.
Hunter C. M., Caswell H., Runge M. C., Regehr E. V., Amstrup S. C., Stirling I. (2010). Climate Change Threatens Polar Bear Populations: A Stochastic Demographic Analysis. Ecology 91, 2883–2897. doi: 10.1890/09-1641.1
IBA (2009) Use of Radio-Collars in Bear Research, Management and Conservation: Joint Position Statement. Available at: https://www.bearbiology.org/wp-content/uploads/2019/05/IBA-and-BSG-position-statement-Radiocollaring-bears-06.04.2019-Final-Electronic-Version.pdf.
IPCC (2019) IPCC Special Report on the Ocean and Cryosphere in a Changing Climate”). Available at: https://www.diva-portal.org/smash/record.jsf?pid=diva2:1446300.
ITK (2009) Resolution A09-06-11: Approval of Polar Bear Research Methods. Resolution Passed on 10 June 2009 During the Annual General Meeting Held in Nain, Nunatsiavut. Available at: https://www.itk.ca/wp-content/uploads/2019/08/A09-06-11-Approval-of-Polar-Bear-Research-Methods.pdf.
Kéry M., Schaub M. (2012). Bayesian Population Analysis Using WinBUGS: A Hierarchical Perspective (San Diego, CA: Academic Press).
Kolz A. L., Lentfer J. W., Fallek H. G. (1980). “Satellite Radio Tracking of Polar Bears Instrumented in Alaska,” in A Handbook on Biotelemetry and Radio Tracking: Proceedings of an International Conference on Telemetry and Radio Tracking in Biology and Medicine, Oxford, 20-22 March 1979. Eds. Amlaner C. J. Jr., Macdonald D. W. (Oxford, UK: Pergamon Press), 743–752.
Kovacs K. M., Lydersen C., Overland J. E., Moore S. (2011). Impacts of Changing Sea-Ice Conditions on Arctic Marine Mammals. Mar. Biodivers. 41, 181–194. doi: 10.1007/s12526-010-0061-0
Kreeger T. J., Arnemo J. M. (2012). Handbook of Wildlife Chemical Immobilization, 4th ed, Published by authors.
Laidre K. L., Atkinson S., Regehr E. V., Stern H. L., Born E. W., Wiig Ø., et al. (2020a). Interrelated Ecological Impacts of Climate Change on an Apex Predator. Ecol. Appl. 30, e02071. doi: 10.1002/eap.2071
Laidre K. L., Atkinson S. N., Regehr E. V., Stern H. L., Born E. W., Wiig Ø., et al. (2020b). Transient Benefits of Climate Change for a High-Arctic Polar Bear (Ursus Maritimus) Subpopulation. Glob. Change Biol. 26, 6251–6265. doi: 10.1111/gcb.15286
Laidre K. L., Born E. W., Atkinson S. N., Wiig Ø., Anderson L. W., Lunn N. J., et al. (2018a). Range Contraction and Increasing Isolation of a Polar Bear Subpopulation in an Era of Sea Ice Loss. Ecol. Evol. 8, 2062–2075. doi: 10.1002/ece3.3809
Laidre K. L., Born E. W., Haegerty P., Wiig Ø., Stern H., Dietz R., et al. (2015). Shifts in Female Polar Bear (Ursus Maritimus) Habitat Use in East Greenland. Polar Biol. 38, 879–893. doi: 10.1007/s00300-015-1648-5
Laidre K. L., Stern H., Born E. W., Heagerty P., Atkinson S., Wiig Ø., et al. (2018b). Changes in Winter and Spring Resource Selection by Polar Bears Ursus Maritimus in Baffin Bay Over Two Decades of Sea-Ice Loss. Endanger. Species Res. 36, 1–14. doi: 10.3354/esr00886
Laidre K. L., Stirling I., Lowry L. F., Wiig Ø., Heide-Jørgensen M. P., Ferguson S. H. (2008). Quantifying the Sensitivity of Arctic Marine Mammals to Climate-Induced Habitat Change. Ecol. Appl. 18, S97–S125. doi: 10.1890/06-0546.1
Larsen T. (1972). Norwegian Polar Bear Hunt, Management and Research. Int. Conf. Bear Res. Manage. 2, 159–164. doi: 10.2307/3872579
Larsen T. (1985). Polar Bear Denning and Cub Production in Svalbard, Norway. J. Wildl. Manage. 49, 320–326. doi: 10.2307/3801524
Larsen T., Jonkel C., Vibe C. (1983). Satellite Radio-Tracking of Polar Bears Between Svalbard and Greenland. Int. Conf. Bear Res. Manage. 5, 230–237. doi: 10.2307/3872542
Larsen T. S., Stirling I. (2009). The Agreement on the Conservation of Polar Bears – Its History and Future (Norsk Polarinstitutt Rapportserie), 127.
Lentfer J. W. (1972). Polar Bear: Sea Ice Relationships. Int. Conf. Bear Res. Manage. 2, 165–171. doi: 10.2307/3872580
Lentfer J. W., Hensel R. J. (1980). Alaskan Polar Bear Denning. Int. Conf. Bear Res. Manage. 4, 101–108. doi: 10.2307/3872850
Lone K., Kovacs K. M., Lydersen C., Fedak M., Andersen M., Lovell P., et al. (2018a). Aquatic Behaviour of Polar Bears (Ursus Maritimus) in an Increasingly Ice-Free Arctic. Sci. Rep. 8, 9677. doi: 10.1038/s41598-018-27947-4
Lone K., Merkel B., Lydersen C., Kovacs K. M., Aars J. (2018b). Sea Ice Resource Selection Models for Polar Bears in the Barents Sea Subpopulation. Ecography 41, 567–578. doi: 10.1111/ecog.03020
Lunn N. J., Servanty S., Regehr E. V., Converse S. J., Richardson E., Stirling I. (2016). Demography of an Apex Predator at the Edge of Its Range – Impacts of Changing Sea Ice on Polar Bears in Hudson Bay. Ecol. Appl. 26, 1302–1320. doi: 10.1890/15-1256
Lunn N. J., Stirling I., Andriashek D. (1995). Movements and Distribution of Polar Bears in the Northeastern Beaufort Sea and Western M’Clure Strait. Final Report to the Inuvialuit Wildlife Management Advisory Committee (Edmonton, AB: Canadian Wildlife Service).
Lunn N. J., Stirling I., Andriashek D., Richardson E. (2004). Selection of Maternity Dens by Female Polar Bears in Western Hudson Bay, Canada and the Effects of Human Disturbance. Polar Biol. 27, 350–356. doi: 10.1007/s00300-004-0604-6
Maduna S. N., Aars J., Fløystad I., Klütsch C. F. C., Zeyl Fiskebeck E. M. L., Wiig Ø., et al. (2021). Sea Ice Reduction Drives Genetic Differentiation Among Barents Sea Polar Bears. Proc. R. Soc B. 288, 20211741. doi: 10.1098/rspb.2021.1741
Manly B. F. J., McDonald L. L., Thomas D. L., McDonald T. L., Erickson W. P. (2002). Resource Selection by Animals: Statistical Design and Analysis for Field Studies. 2nd edition (Dordrectht: Kluwer Academic Publishers).
Mauritzen M., Derocher A. E., Pavolova O., Wiig Ø. (2003). Female Polar Bears, Ursus Maritimus, on the Barents Sea Drift Ice: Walking the Treadmill. Anim. Behav. 66, 107–113. doi: 10.1006/anbe.2003.2171
Mauritzen M., Derocher A. E., Wiig Ø. (2001). Space-Use Strategies of Female Polar Bears in a Dynamic Sea Ice Habitat. Can. J. Zool. 79, 1704–1713. doi: 10.1139/z01-126
Mauritzen M., Derocher A. E., Wiig Ø., Belikov S. E., Boltunov A. N., Hansen E., et al. (2002). Using Satellite Telemetry to Define Spatial Population Structure in Polar Bears in the Norwegian and Western Russian Arctic. J. Appl. Ecol. 39, 79–90. doi: 10.1046/j.1365-2664.2002.00690.x
McCall A. G., Pilfold N. W., Derocher A. E., Lunn N. J. (2016). Seasonal Habitat Selection by Adult Female Polar Bears in Western Hudson Bay. Popul. Ecol. 58, 407–419. doi: 10.1007/s10144-016-0549-y
Merkel B., Aars J., Liston G. E. (2020). Modelling Polar Bear Maternity Den Habitat in East Svalbard. Polar Res. 39, 3447. doi: 10.33265/polar.v39.3447
Messier F. (2000). Effects of Capturing, Tagging, and Radio-Collaring Polar Bears for Research and Management Purposes in Nunavut and Northwest Territories (Iqaluit, NU: Government of Nunavut).
Messier F., Taylor M. K., Ramsay M. A. (1994). Denning Ecology of Polar Bears in the Canadian Arctic Archipelago. J. Mammal. 75, 420–430. doi: 10.2307/1382563
Molnár P. K., Bitz C. M., Holland M. M., Kay J. E., Penk S. R., Amstrup S. C. (2020). Fasting Season Length Sets Temporal Limits for Global Polar Bear Persistence. Nat. Clim. Change 10, 732–738. doi: 10.1038/s41558-020-0818-9
Molnár P. K., Derocher A. E., Klanjscek T., Lewis M. A. (2011). Predicting Climate Change Impacts on Polar Bear Litter Size. Nat. Commun. 2, 186. doi: 10.1038/ncomms1183
Molnár P. K., Lewis M. A., Derocher A. E. (2014). Estimating Allee Dynamics Before They can be Observed: Polar Bears as a Case Study. PloS One 9, e85410. doi: 10.1371/journal.pone.0085410
Moore G. W. K., Schweiger A., Zhang J., Steele M. (2019). Spatiotemporal Variability of Sea Ice in the Arctic’s Last Ice Area. Geophys. Res. Lett. 46 (20), 11237–11243. doi: 10.1029/2019GL083722
Moshøj C. M. (2014). On Thin Ice: Human-Polar Bear Conflicts in Ittoqqortoormiit (Copenhagen: World Wildlife Fund Verdensnaturfonden).
Mulcahy D. M., Garner G. (1999). Subcutaneous Implantation of Satellite Transmitters With Percutaneous Antennae Into Male Polar Bears (Ursus Maritimus). J. Zoo Wildl. Med. 30, 510–515.
Nunavut Impact Review Board (2009). Guidelines for the Preparation of an Environmental Impact Statement for Baffinland Iron Mines Corporation’s Mary River Project (Cambridge Bay, NU: Nunavut Impact Review Board, NIRB File No. 08MN053).
Obbard M. E., Cattet M. R. L., Howe E. J., Middel K. R., Newton E. J., Kolenosky G. B., et al. (2016). Trends in Body Condition in Polar Bears (Ursus Maritimus) From the Southern Hudson Bay Subpopulation in Relation to Changes in Sea Ice. Arctic Sci. 2, 15–32. doi: 10.1139/as-2015-0027
Obbard M. E., Middel K. R. (2012). Bounding the Southern Hudson Bay Polar Bear Subpopulation. Ursus 23, 134–144. doi: 10.2192/URSUS-D-11-00030.1
Obbard M. E., Stapleton S., Middel K. R., Thibault I., Brodeur V., Jutras C. (2015). Estimating the Abundance of the Southern Hudson Bay Polar Bear Subpopulation With Aerial Surveys. Polar Biol. 38, 1713–1725. doi: 10.1007/s00300-015-1737-5
Obbard M. E., Stapleton S., Szor G., Middel K. R., Jutras C., Dyck M. (2018). Re-Assessing Abundance of Southern Hudson Bay Polar Bears by Aerial Survey: Effects of Climate Change at the Southern Edge of the Range. Arctic Sci. 4, 634–655. doi: 10.1139/as-2018-0004
Olsen G. H., Mauritzen M., Derocher A. E., Sørmo E. G., Skaare J. U., Wiig Ø., et al. (2003). Space-Use Strategy Is an Important Determinant of PCB Concentrations in Female Polar Bears in the Barents Sea. Environ. Sci. Technol. 37, 4919–4924. doi: 10.1021/es034380a
Olson J. W., Rode K. D., Eggett D., Smith T. S., Wilson R. R., Durner G. M., et al. (2017). Collar Temperature Sensor Data Reveal Long-Term Patterns in Southern Beaufort Sea Polar Bear Den Distribution on Pack Ice and Land. Mar. Ecol. Prog. Ser. 564, 211–224. doi: 10.3354/meps12000
Paetkau D., Amstrup S. C., Born E. W., Calvert W., Derocher A. E., Garner G. W., et al. (1999). Genetic Structure of the World’s Polar Bear Populations. Mol. Ecol. 8, 1571–1584. doi: 10.1046/j.1365-294x.1999.00733.x
Pagano A. M., Atwood T. C., Durner G. M., Williams T. M. (2020). The Seasonal Energetic Landscape of an Apex Marine Carnivore, the Polar Bear. Ecology 101, e02959. doi: 10.1002/ecy.2959
Pagano A. M., Durner G. M., Amstrup S. C., Simac K. S., York G. S. (2012). Long-Distance Swimming by Polar Bears (Ursus Maritimus) of the Southern Beaufort Sea During Years of Extensive Open Water. Can. J. Zool. 90, 663–676. doi: 10.1139/z2012-033
Pagano A. M., Durner G. M., Atwood T. C., Douglas D. C. (2021). Effects of Sea Ice Decline and Summer Land Use on Polar Bear Home Range Size in the Beaufort Sea. Ecosphere 12 (10), e03768. doi: 10.1002/ecs2.3768
Pagano A. M., Durner G. M., Rode K. D., Atwood T. C., Atkinson S. N., Peacock E., et al. (2018). High-Energy, High-Fat Lifestyle Challenges and Arctic Apex Predator, the Polar Bear. Science 359, 568–572. doi: 10.1126/science.aan8677
PBSG (1966) in Proceedings of the First International Scientific Meeting on the Polar Bear at Fairbanks, Alaska, USA, 6-10 September 1965 (U. S. Department of the Interior), Resource Publication 16, The University of Alaska, International Conference Proceedings Series, No. 1.
PBSG (2018). “2016 Status Report on the World’s Polar Bear Subpopulations,” in Polar Bears: Proceedings of the Eighteenth Working Meeting of the IUCN/SSC Polar Bear Specialist Group, 7-11 June 2016, Anchorage, Alaska, Oslo, Norway. Eds. Durner G. M., Laidre K. L., York G. S. (Gland, Switzerland; Cambridge, UK: IUCN Species Survival Commission Occasional Paper No. 63), 1–32.
Peacock E., Sonsthagen S. A., Obbard M. E., Boltunov A., Regehr E. V., Ovsyanikov N., et al. (2015). Implications of the Circumpolar Genetic Structure of Polar Bears for Their Conservation in a Rapidly Warming Arctic. PloS One 10, e112021. doi: 10.1371/journal.pone.0112021
Peñaloza C. L., Kendall W. L., Langtimm C. A. (2014). Reducing Bias in Survival Under Nonrandom Temporary Emigration. Ecol. Appl. 24, 1155–1166. doi: 10.1890/13-0558.1
Peng G., Matthews J. L., Wang M., Vose R., Sun L. (2020). What do Global Climate Models Tell Us About Future Arctic Sea Ice Coverage Changes? Climate 8, 15. doi: 10.3390/cli8010015
Pilfold N. W., McCall A., Derocher A. E., Lunn N. J., Richardson E. (2017). Migratory Response of Polar Bears to Sea Ice Loss: To Swim or Not to Swim. Ecography 40, 189–199. doi: 10.1111/ecog.02109
Polar Bear Range States (PBRS) (2015) Circumpolar Action Plan: Conservation Strategy for Polar Bears. A Product of the Representatives of the Parties to the 1973 Agreement on the Conservation of Polar Bears. Available at: https://polarbearagreement.org/index.php/resources/circumpolar-action-plan/cap-2015-2025-docs/circumpolar-action-plan.
Ramsay M. A., Stirling I. (1986). Long-Term Effects of Drugging and Handling Free-Ranging Polar Bears. J. Wildl. Manage. 50, 619–626. doi: 10.2307/3800972
Regehr E. V., Ben-David M., Amstrup S. C., Durner G. M., Horne J. S. (2009). “Chapter 4. Quantifying Bias in Capture-Recapture Studies for Mobile Species: A Case Study With Polar Bears,” in Polar Bear (Ursus Maritimus) Demography in Relation to Arctic Sea Ice Decline (Laramie, WY: University of Wyoming).
Regehr E. V., Dyck M., Gilbert G., Iverson S., Lee D., Lunn N. J., et al. (2021a). Incorporating Climate Change in Harvest Risk Assessments for Polar Bears Ursus Maritimus in Southern Hudson Bay. Biol. Conserv. 258. doi: 10.1016/j.biocon.2021.109128
Regehr E. V., Hostetter N. J., Wilson R. R., Rode K. D., St. Martin M., Converse S. J. (2018). Integrated Population Modeling Provides the First Empirical Estimates of Vital Rates and Abundance for Polar Bears in the Chukchi Sea. Sci. Rep. 8, 16780. doi: 10.1038/s41598-018-34824-7
Regehr E. V., Hunter C. M., Caswell H., Amstrup S. C., Stirling I. (2010). Survival and Breeding of Polar Bears in the Southern Beaufort Sea in Relation to Sea Ice. J. Anim. Ecol. 79, 117–127. doi: 10.1111/j.1365-2656.2009.01603.x
Regehr E. V., Laidre K. L., Akçakaya H. R., Amstrup S. C., Atwood T. C., Lunn N. J., et al. (2016). Conservation Status of Polar Bears (Ursus Maritimus) in Relation to Projected Sea-Ice Declines. Biol. Lett. 12, 20160556. doi: 10.1098/rsbl.2016.0556
Regehr E. V., Laidre K. L., Atwood T., Stern H., Cohen B. (In Review). “Sea-Ice Conditions Predict Polar Bear Land Use Around Military Installations in Alaska,” in Human Wildlife Interactions. In Review.
Regehr E. V., Lunn N. J., Amstrup S. C., Stirling I. (2007). Effects of Earlier Sea Ice Breakup on Survival and Population Size of Polar Bears in Western Hudson Bay. J. Wildl. Manage. 71, 2673–2683. doi: 10.2193/2006-180
Regehr E. V., Runge M. C., Von Duyke A., Wilson R. R., Polasek L., Rode K. D., et al. (2021b). Demographic Risk Assessment for a Harvested Species Threatened by Climate Change: Polar Bears in the Chukchi Sea. Ecol. Appl., e02461. doi: 10.1002/eap.2461
Regehr E. V., Wilson R. R., Rode K. D., Runge M. C., Stern H. L. (2017). Harvesting Wildlife Affected by Climate Change: A Modelling and Management Approach for Polar Bears. J. Appl. Ecol. 54, 1534–1543. doi: 10.1111/1365-2664.12864
Robbins C. T., Lopez-Alfaro C., Rode K. D., Tøien Ø., Nelson O. L. (2012). Hibernation and Seasonal Fasting in Bears: The Energetic Costs and Consequences for Polar Bears. J. Mammal. 93, 1493–1503. doi: 10.1644/11-MAMM-A-406.1
Rode K. D., Olson J., Eggett D., Douglas D. C., Durner G. M., Atwood T. C., et al. (2018a). Den Phenology and Reproductive Success of Polar Bears in a Changing Climate. J. Mammal. 99, 16–26. doi: 10.1093/jmammal/gyx181
Rode K. D., Pagano A. M., Bromaghin J. F., Atwood T. C., Durner G. M., Simac K. S., et al. (2014a). Effects of Capturing and Collaring on Polar Bears: Findings From Long-Term Research on the Southern Beaufort Sea Population. Wildl. Res. 41, 311–322. doi: 10.1071/WR13225
Rode K. D., Regehr E. V., Douglas D. C., Durner G., Derocher A. E., Thiemann G. W., et al. (2014b). Variation in the Response of an Arctic Top Predator Experiencing Habitat Loss: Feeding and Reproductive Ecology of Two Polar Bear Populations. Glob. Change Biol. 20, 76–88. doi: 10.1111/gcb.12339
Rode K. D., Wilson R. R., Regehr E. V., St. Martin M., Douglas D. C., Olson J. (2015). Increased Land Use by Chukchi Sea Polar Bears in Relation to Changing Sea Ice Conditions. PloS One 10, e0142213. doi: 10.1371/journal.pone.0142213
Rogers M. C., Peacock E., Simac K., O’Dell M. B., Welker J. M. (2015). Diet of Female Polar Bears in the Southern Beaufort Sea of Alaska: Evidence for an Emerging Alternative Foraging Strategy in Response to Environmental Change. Polar Biol. 38, 1035–1047. doi: 10.1007/s00300-015-1665-4
Ryan C. W., Vaughan M. R., Meldrum J. B., Duncan R. B., Edwards J. W. (2009). Retention Time of Telazol in Black Bears. J. Wildl. Manage. 73, 210–213. doi: 10.2193/2008-182
Scharf H. R., Hooten M. B., Wilson R. R., Durner G. M., Atwood T. C. (2019). Accounting for Phenology in the Analysis of Animal Movement. Biometrics 75, 810–820. doi: 10.1111/biom.13052
Schliebe S., Rode K. D., Gleason J. S., Wilder J., Proffitt K., Evans T. J., et al. (2008). Effects of Sea Ice Extent and Food Availability on Spatial and Temporal Distribution of Polar Bears During the Fall Open-Water Period in the Southern Beaufort Sea. Polar Biol. 31, 999–1010. doi: 10.1007/s00300-008-0439-7
Schwartz C. C., Arthur S. M. (1999). Radio-Tracking Large Wilderness Mammals: Integration of GPS and Argos Technology. Ursus 11, 261–274.
Schweinsburg R. E., Lee L. J. (1982). Movement of Four Satellite-Monitored Polar Bears in Lancaster Sound, Northwest Territories. Arctic 35, 504–511. doi: 10.14430/arctic2357
Semple H. A., Gorecki D. K. J., Farley S. D., Ramsay M. A. (2000). Pharmacokinetics and Tissue Residues of Telazol® in Free-Ranging Polar Bears. J. Wildl. Dis. 36, 653–662. doi: 10.7589/0090-3558-36.4.653
Sequeira A. M. M. (2020). Predators on Track for Ocean Protection Around Antarctica. Nature 580, 34–35. doi: 10.1038/d41586-020-00640-1
Sequeira A. M. M., Heupel M. R., Lea M. A., Eguiluz V. M., Duarte C. M., Meekan M. G., et al. (2019). The Importance of Sample Size in Marine Megafauna Tagging Studies. Ecol. Appl. 29, e01947. doi: 10.1002/eap.1947
Smith L. C., Stephenson S. R. (2013). New Trans-Arctic Shipping Routes Navigable by Midcentury. Proc. Nat. Acad. Sci. U.S.A. 110, E1191–E1195. doi: 10.1073/pnas.1214212110
Stapleton S., Peacock E., Garshelis D. (2016). Aerial Surveys Suggest Long-Term Stability in the Seasonally Ice-Free Foxe Basin (Nunavut) Polar Bear Population. Mar. Mamm. Sci. 32, 181–201. doi: 10.1111/mms.12251
Stern H. L., Laidre K. L. (2016). Sea-Ice Indicators of Polar Bear Habitat. Cryosphere 10, 2027–2041. doi: 10.5194/tc-10-2027-2016
Stirling I., Andriashek D. (1992). Terrestrial Maternity Denning of Polar Bears in the Eastern Beaufort Sea Area. Arctic 45, 363–366. doi: 10.14430/arctic1415
Stirling I., Lunn N. J., Iacozza J. (1999). Long-Term Trends in the Population Ecology of Polar Bears in Western Hudson Bay in Relation to Climate Change. Arctic 52, 294–306. doi: 10.14430/arctic935
Stirling I., Regehr E. V., Spencer C., Burns L. E., Laidre K. L. (In Press). Comparing the Behavior of Previously Immobilized and Non-Immobilized Wild Polar Bears. Arctic 75.
Stirling I., Spencer C., Andriashek D. (1989). Immobilization of Polar Bears (Ursus Maritimus) With Telazol® in the Canadian Arctic. J. Wildl. Dis. 25, 159–168. doi: 10.7589/0090-3558-25.2.159
Stishov M. S. (1991). “Results of Aerial Counts of the Polar Bear Dens on the Arctic Coasts of the Extreme Northeast Asia,” in Polar Bears: Proceedings of the Tenth Working Meeting of the IUCN/SSC Polar Bear Specialist Group, October 25-29, Sochi, USSR. Eds. Amstrup S. C., Wiig Ø. (Gland, Switzerland; Cambridge, UK: IUCN Species Survival Commission Occasional Paper No. 7), 90–92.
SWG (2016). Re-Assessment of the Baffin Bay and Kane Basin Polar Bear Subpopulations: Final Report to the Canada-Greenland Joint Commission on Polar Bear, 31 July 2016 Nuuk, Greenland:Greenland Institute of Natural Resources.
Tartu S., Aars J., Andersen M., Polder A., Bourgeon S., Merkel B., et al. (2018). Choose Your Poison – Space-Use Strategy Influences Pollutant Exposure in Barents Sea Polar Bears. Environ. Sci. Technol. 52, 3211–3221. doi: 10.1021/acs.est.7b06137
Taylor M. K., Akeeagok S., Andriashek D., Barbour W., Born E. W., Calvert W., et al. (2001). Delineating Canadian and Greenland Polar Bear (Ursus Maritimus) Populations by Cluster Analysis of Movements. Can. J. Zool. 79, 690–709. doi: 10.1139/z01-028
Taylor M. K., DeMaster D. P., Bunnell F. L., Schweinsburg R. E. (1987). Modeling the Sustainable Harvest of Female Polar Bears. J. Wildl. Manage. 51, 811–820. doi: 10.2307/3801746
Taylor M. K., Laake J., McLoughlin P. D., Cluff H. D., Born E. W., Rosing-Asvid A., et al. (2008). Population Parameters and Harvest Risks for Polar Bears (Ursus Maritimus) of Kane Basin, Canada and Greenland. Polar Biol. 31, 491–499. doi: 10.1007/s00300-007-0375-y
Taylor M., Lee J. (1995). Distribution and Abundance of Canadian Polar Bear Populations: A Management Perspective. Arctic 48, 147–154. doi: 10.14430/arctic1236
Thiemann G. W., Derocher A. E., Cherry S. G., Lunn N. J., Peacock E., Sahanatien V. (2013). Effects of Chemical Immobilization on the Movement Rates of Free-Ranging Polar Bears. J. Mammal. 94, 386–397. doi: 10.1644/12-MAMM-A-230.1
Thomas B., Holland J. D., Minot E. O. (2011). Wildlife Tracking Technology Options and Cost Considerations. Wildl. Res. 38, 653–663. doi: 10.1071/WR10211
Tomkiewicz S. M., Fuller M. R., Kie J. G., Bates K. K. (2010). Global Positioning System and Associated Technologies in Animal Behaviour and Ecological Research. Philos. Trans. R. Soc B 365, 2163–2176. doi: 10.1098/rstb.2010.0090
Towns L., Derocher A. E., Stirling I., Lunn N. J., Hedman D. (2009). Spatial and Temporal Patterns of Problem Polar Bears in Churchill, Manitoba. Polar Biol. 32, 1529–1537. doi: 10.1007/s00300-009-0653-y
USFWS (2008). Determination of Threatened Status for the Polar Bear (Ursus Maritimus) Throughout Its Range. Final Rule. Federal Register 73 (95), 28212–28303.
USFWS (2010). Designation of Critical Habitat for the Polar Bear (Ursus Maritimus) in the United States. Final Rule. Federal Register 75 (234), 76086–76137.
Uspenski S. M., Kistchinski A. A. (1972). New Data on the Winter Ecology of the Polar Bear (Ursus Maritimus Phipps) on Wrangel Island. Int. Conf. Bear Res. Manage. 2, 181–197. doi: 10.2307/3872582
van Beest F. M., Aars J., Routti H., Lie E., Andersen M., Pavlova V., et al. (2016). Spatiotemporal Variation in Home Range Size of Female Polar Bears and Correlations With Individual Contaminant Load. Polar Biol. 39, 1479–1489. doi: 10.1007/s00300-015-1876-8
Van de Velde F., OMI, Stirling I., Richardson E. (2003). Polar Bear (Ursus Maritimus) Denning in the Area of the Simpson Peninsula, Nunavut. Arctic 56, 191–197.
Vibe C. (1976). “Preliminary Report on the First Danish Polar Bear Expedition to North East Greenland 1973,” in Polar Bears: Proceedings of the Fifth Working Meeting of the Polar Bear Specialist Group (Morges, Switzerland: IUCN Publications New Series Supplementary Paper No. 42), 74–79.
Viengkone M., Derocher A. E., Richardson E. S., Malenfant R. M., Miller J. M., Obbard M. E., et al. (2016). Assessing Polar Bear (Ursus Maritimus) Population Structure in the Hudson Bay Region Using SNPs. Ecol. Evol. 6, 8474–8484. doi: 10.1002/ece3.2563
Wang M., Overland J. E. (2009). A Sea Ice Free Summer Arctic Within 30 Years? Geophys. Res. Lett. 36, L07502. doi: 10.1029/2009GL037820
Ware J. V., Rode K. D., Bromaghin J. F., Douglas D. C., Wilson R. R., Regehr E. V., et al. (2017). Habitat Degradation Affects the Summer Activity of Polar Bears. Oecologia 184, 87–99. doi: 10.1007/s00442-017-3839-y
Ware J. V., Rode K. D., Pagano A. M., Bromaghin J., Robbins C. T., Erlenbach J., et al. (2015). Validation of Mercury Tip-Switch and Accelerometer Activity Sensors for Identifying Resting and Active Behavior in Bears. Ursus 26, 86–96. doi: 10.2192/URSUS-D-14-00031.1
Whiteman J. P., Harlow H. J., Durner G. M., Anderson-Sprecher R., Albeke S. E., Regehr E. V., et al. (2015). Summer Declines in Activity and Body Temperature Offer Polar Bears Limited Energy Savings. Science 349, 295–298. doi: 10.1126/science.aaa8623
Whiteman J. P., Harlow H. J., Durner G. M., Regehr E. V., Amstrup S. C., Ben-David M. (2019). Heightened Immune System Function in Polar Bears Using Terrestrial Habitats. Physiol. Biochem. Zool. 92, 1–11. doi: 10.1086/698996
Wiig Ø. (1995). Distribution of Polar Bears (Ursus Maritimus) in the Svalbard Area. J. Zool. Lond. 237, 515–529. doi: 10.1111/j.1469-7998.1995.tb05012.x
Wiig Ø., Aars J., Born E. W. (2008). Effects of Climate Change on Polar Bears. Sci. Prog. 91, 151–173. doi: 10.3184/003685008X324506
Wiig Ø., Born E. W., Laidre K. L., Dietz R., Jensen M. V., Durner G. M., et al. (2017). Performance and Retention of Lightweight Satellite Radio Tags Applied to the Ears of Polar Bears (Ursus Maritimus). Anim. Biotelemetry 5, 9. doi: 10.1186/s40317-017-0124-0
Wiig Ø., Born E. W., Toudal Pedersen L. (2003). Movement of Female Polar Bears (Ursus Maritimus) in the East Greenland Pack Ice. Polar Biol. 26, 509–516. doi: 10.1007/s00300-003-0513-0
Wilder J. M., Vongraven D., Atwood T., Hansen B., Jessen A., Kochnev A., et al. (2017). Polar Bear Attacks on Humans: Implications of a Changing Climate. Wildl. Soc. Bull. 41, 537–547. doi: 10.1002/wsb.783
Wilson R. R., Durner G. M. (2020). Seismic Survey Design and Effects on Maternal Polar Bear Dens. J. Wildl. Manage. 84, 201–212. doi: 10.1002/jwmg.21800
Wilson R. R., Horne J. S., Rode K. D., Regehr E. V., Durner G. M. (2014). Identifying Polar Bear Resource Selection Patterns to Inform Offshore Development in a Dynamic and Changing Arctic. Ecosphere 5, 136. doi: 10.1890/ES14-00193.1
Wilson R. R., Regehr E. V., Rode K. D., St Martin M. (2016). Invariant Polar Bear Habitat Selection During a Period of Sea Ice Loss. Proc. R. Soc B 283, 20160380. doi: 10.1098/rspb.2016.0380
Wong P. B. Y., Dyck M. G., Arviat Hunters, Trappers, Ikajutit Hunters, Trappers, Mayukalik Hunters, Trappers, Murphy R. W. (2017). Inuit Perspectives of Polar Bear Research: Lessons for Community-Based Collaborations. Polar Rec. 53, 257–270. doi: 10.1017/S0032247417000031
Keywords: climate change, conservation, movements, polar bear, radio collar, satellite, telemetry
Citation: Laidre KL, Durner GM, Lunn NJ, Regehr EV, Atwood TC, Rode KD, Aars J, Routti H, Wiig Ø, Dyck M, Richardson ES, Atkinson S, Belikov S and Stirling I (2022) The Role of Satellite Telemetry Data in 21st Century Conservation of Polar Bears (Ursus maritimus). Front. Mar. Sci. 9:816666. doi: 10.3389/fmars.2022.816666
Received: 17 November 2021; Accepted: 14 March 2022;
Published: 14 April 2022.
Edited by:
Ana M. M. Sequeira, University of Western Australia, AustraliaReviewed by:
Jan Marcin Weslawski, Institute of Oceanology (PAN), PolandLori Quakenbush, Alaska Department of Fish and Game, United States
Copyright © 2022 Laidre, Durner, Lunn, Regehr, Atwood, Rode, Aars, Routti, Wiig, Dyck, Richardson, Atkinson, Belikov and Stirling. This is an open-access article distributed under the terms of the Creative Commons Attribution License (CC BY). The use, distribution or reproduction in other forums is permitted, provided the original author(s) and the copyright owner(s) are credited and that the original publication in this journal is cited, in accordance with accepted academic practice. No use, distribution or reproduction is permitted which does not comply with these terms.
*Correspondence: Kristin L. Laidre, a2xhaWRyZUB1dy5lZHU=
†Deceased