- 1Department of Geology, Faculty of Natural Sciences and Engineering, University of Ljubljana, Ljubljana, Slovenia
- 2Joint Research Centre, European Commission, Brussels, Belgium
- 3Department of Geology, Faculty of Science, University of Zagreb, Zagreb, Croatia
The Bay of Koper is influenced by agricultural, urban, and port activities, therefore pollution from trace metals is a concern. A total of 20 sediment samples obtained from four 10-cm sediment cores were analyzed. Element concentration in the sediment of the bay was determined spatially and temporally from the recent surface to depth. The results were correlated with the composition and diversity of the benthic foraminiferal assemblages. Major element concentrations indicate natural lithogenic origin (which is also confirmed by mineralogical features). The benthic foraminiferal assemblages in sediment samples, although mainly composed of representatives of the Rotaliida, show moderate to high species diversity and are dominated by the pollution tolerant species Ammonia pakinsoniana, Haynesina sp., Valvulineria bradyana and the non-keel Elphidium sp. and subordinated by Ammonia tepida and Haynesina depressula. Canonical correspondence analysis (CCA) on foraminiferal species and trace element concentrations shows a possible control of some potential toxic elements (i.e., Cu, Ni, Pb, Zr, Cr, As) on the diversity and taxonomic composition of foraminiferal assemblages. Nevertheless, foraminiferal diversity and dominance in the bay are related to sediment characteristics such as sediment grain size, and the amount of terrigenous inflow rather than to the element concentrations of sediments. This study evaluated ecological conditions by using the Foram-AMBi and EcoQS indices. The values of the Foram-AMBI index reflect the good to moderate quality of ecological conditions, whereas high to poor ecological statuses were interpreted by calculating EcoQS.
Introduction
The Adriatic Sea is one of the largest recent epicontinental seas in the world and a young marine ecosystem that was subject to various natural and anthropogenic processes during the Holocene: marine transgression (Trincardi et al., 1994; Correggiari et al., 1996), regional climate fluctuations (Giani et al., 2012; Appiotti et al., 2014), and urbanization and pollution (Lotze et al., 2006; Cozzi and Giani, 2011). The Bay of Koper in southern part of the Gulf of Trieste (north-eastern Adriatic Sea), represents an area of particular interest, where the most recent anthropogenic pressure was generated by industrial and domestic activities along the coast. The Bay of Koper is characterized by the Port of Koper, one of the most important ports in the northern Adriatic. Through its activities, the port covers a wide range of freight transport (Cepak and Marzi, 2009) and in recent years also international passenger transport, organized in 12 dedicated terminals along 2 piers. The port’s annual cargo throughput is growing since the 90s of the 20th century and has already counts some 20 million tonnes per year in 2015. With its activities, the port represents a potential source of pollution for different environmental matrices: air, water, sediments, and soil. Therefore, several studies have been carried out on this issue (Žitnik et al., 2005; David et al., 2007; Cepak and Marzi, 2009; Zuin et al., 2009; Mladenović et al., 2013; Zupančič and Skobe, 2014; Rogan Šmuc et al., 2018). The results of above mentioned studies show that the ecological quality status of the seafloor sediments and water is better than in other ports of the Eastern Adriatic, that the accumulation of pollutants in the sediments is less significant due to the sedimentological characteristics (i.e., mineralogy, grain size) and the advective transport of particles.
In addition, industrial and municipal wastewater damage the coastal area (the amount of wastewater rises considerably during the summer months). Rivers (especially those along the southern Slovenian coast) also contribute by delivering sediment loads from their catchments (Cozzi et al., 2020). Due to generally superficial clockwise (Malačič and Petelin, 2001) sea currents, the bay may also be threatened by pollutants from the northern parts of the Gulf of Trieste, which is also highly industrialized and urbanized (Adami et al., 1996; Barbieri et al., 1999; Cibic et al., 2008; Cepak and Marzi, 2009; Aquavita et al., 2010). All of these anthropogenic pressures may lead to the accumulation of pollutants in the coastal environment and affect the natural conditions of the area (Dassenakis et al., 2003).
Recent decades have seen increased concern over the management and protection of these nearshore marine areas, and considerable efforts have been made to develop tools to assess their environmental and ecological status (Borja et al., 2008; Birk et al., 2012). The main objective of these efforts is to use the pollutants, physico-chemical parameters, and biological elements of the ecosystem to assess the status in an integrated manner (Borja et al., 2008). Among the biological indicators, benthic species are preferred due to their main characteristics: reduced motility, high diversity, and a life span that allows for the monitoring of the short, medium or long-term effects of any discharged substances (Solis-Weiss et al., 2007 and references therein). The resulting species composition, replacements, eliminations, diversity, or changes in abundance may indicate the recent history of events affecting the area (Solis-Weiss et al., 2001).
Benthic foraminifera – protozoans that live as epiphytic, epifaunal, or infauna on soft sediments from transitional and coastal environments to the deep sea – are useful ecological indicators of depositional dynamics owing to their abundance and adaptations to different environmental conditions (i.e., Kaminski et al., 2002; Oldfield et al., 2003; Morigi et al., 2005; Bouchet et al., 2007; Gooday et al., 2021; Romano et al., 2021). The usefulness of foraminifera for such studies stems from their short life cycles, high biodiversity, and the ecological preferences of known species (Murray, 2006). Because benthic foraminifera are small and abundant compared to other hard-shelled taxa, they are easy to collect, and small sample sizes can provide a reliable database for statistical analysis (Frontalini and Coccioni, 2008). They are among the best tools to study human-induced marine degradation over long periods of time using sediment cores (Yasuhara et al., 2012). Several ecological studies on the effects of element content (trace metals) on modifications and changes in foraminifera (Jorissen, 1987; Alve, 1991, 1995; Yanko et al., 1998; Angel et al., 2000; Coccioni, 2000; Debenay et al., 2001; Armynot du Châtelet et al., 2004; Bergin et al., 2006; Burone et al., 2006; Ferraro et al., 2006; Frontalini and Coccioni, 2008; Frontalini et al., 2009; Rumolo et al., 2009; Vidović et al., 2009, 2014, 2016; Caruso et al., 2011; Yasuhara et al., 2012; Popadić et al., 2013; Romano et al., 2016) have been made, many of them in gulfs with port activities. Studies in the Gulf of Trieste show a minor increase in foraminiferal diversity in areas with intensive agricultural and maricultural activities (Vidović et al., 2016). Higher concentrations of trace elements due to industrial activities in the Gulf of Rijeka resulted in the dominance of stress-tolerant epifaunal foraminiferal species (Popadić et al., 2013).
Studies of benthic foraminifera in the Adriatic Sea have shown that organic matter is an important controlling factor for assemblage abundance and diversification (Jorissen, 1987, 1988; Coccioni et al., 2009; Melis et al., 2019). Thus, the foraminiferal distribution pattern follows the granulometric characteristics of the seafloor sediments (Jorissen, 1987, 1988).
In last decades, the sediment in the Gulf of Trieste has been intensively studied in numerous environmental studies focused on elemental (heavy metal) distribution in relation to anthropogenic impacts (Donazzolo et al., 1981; Brambati and Catani, 1988; Hohenegger et al., 1988, 1993; Faganeli et al., 1991; Ogorelec et al., 1991; Adami et al., 1996; Colizza et al., 1996; Covelli and Fontolan, 1997; Barbieri et al., 1999; Horvat et al., 1999; Covelli et al., 2006, 2012; Cibic et al., 2008; Aquavita et al., 2010; Vidović et al., 2016; Melis et al., 2019). Some studies showed positive correlations between abundances of tolerant taxa (especially Ammonia spp., Bolivina spp. and Bulimina spp.) and increased concentrations of Ni, Zn, and As (Frontalini and Coccioni, 2008; Melis et al., 2019). Sediment from Bay of Koper were classified as silty clay, rich in foraminiferal and molluscan fragments (Ogorelec et al., 1987, 1991; Rogan Šmuc et al., 2018). The grain-size of the sediment increases toward the central part of the bay (Ogorelec et al., 1987). Most of trace elements (such us Cr, Cu, Zn, Cd, Ni) are following the organic matter content in sediment which was shown with the same distribution pattern of these two parameters (Ogorelec et al., 1987; Faganeli et al., 1991). The contents of trace elements in the surface sediments of the Bay of Koper are comparable to the geochemical background of this area (Ogorelec et al., 1987). To date, there is no study in the Bay of Koper that evaluates the impact of the anthropogenic influence on benthic foraminifera and geochemical proxies.
The integrative approach we propose to assess the potential anthropogenic impacts (port activities, industry, municipal activities, tourism) on the Bay of Koper is based on the benthic foraminifera assemblages. The study is focused on the spatio-temporal comparison of mineralogical and geochemical properties (element concentrations) of sediments and benthic foraminifera from four sites in the bay with different degrees of anthropogenic load. This work contributes to detect anthropogenic stress against a background of natural conditions in the southern part of the Gulf of Trieste. For ecological condition evaluation, Foram-AMBi and EcoQS indices were calculated.
Study Area
The Bay of Koper on the Northern Adriatic Sea (Figure 1A) constitutes the enclosed south-eastern part of the Gulf of Trieste. The bay covers approximately 35 km2 and stretches from Izola in the south to the cliffs of Debeli rtič in the north. Two rivers, the Rižana and the Badaševica, flow into the bay.
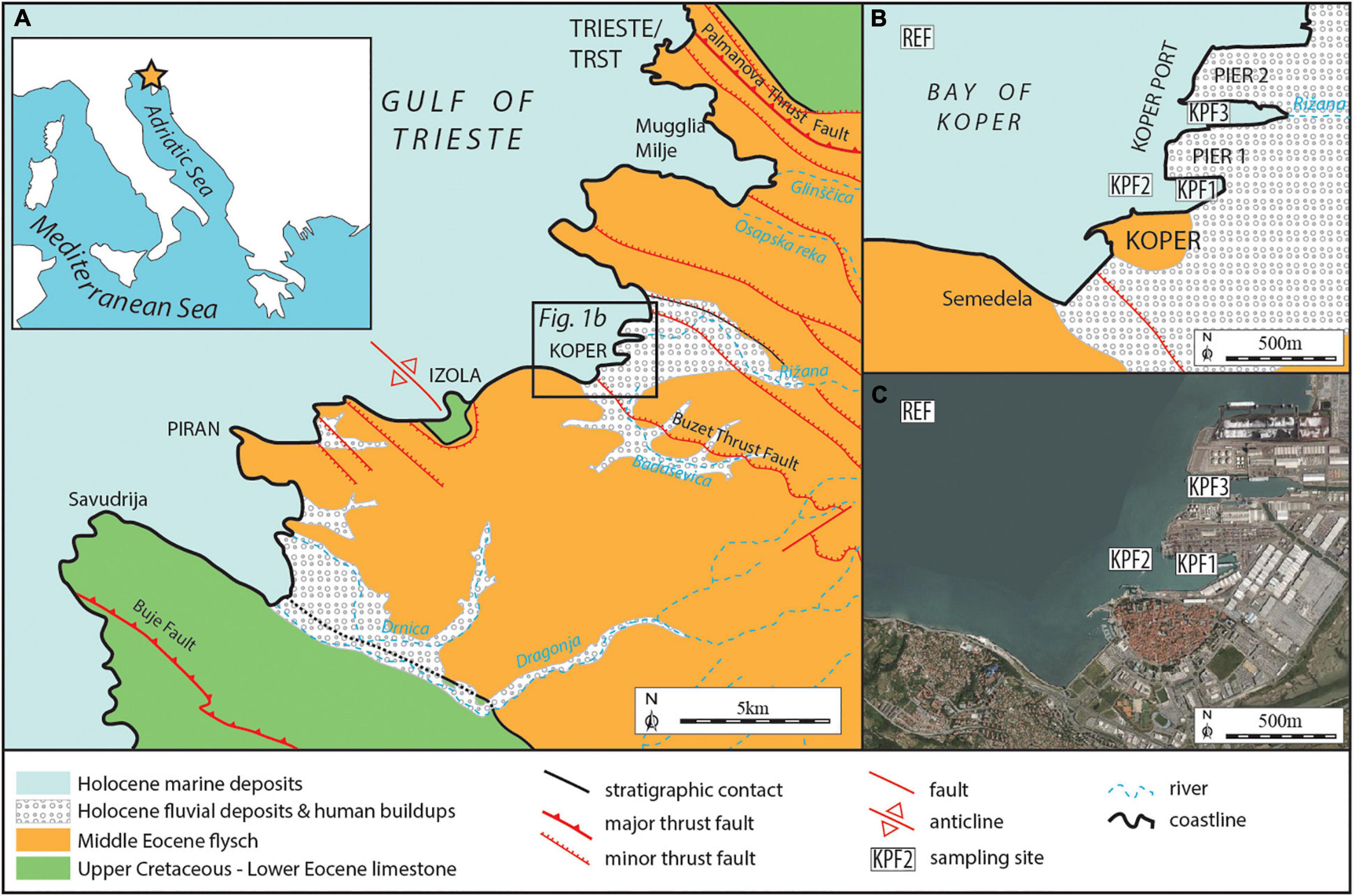
Figure 1. (A) Geographical and geological map of investigated area. (B) Enlarged boxed area from Figure 1A with sampling locations. (C) Bay of Koper with sampling locations (http://gis.arso.gov.si/atlasokolja/).
The Gulf of Trieste/Trst coast geologically consists mainly of Middle Eocene Flysch, whereas Cretaceous – Early Eocene limestone outcrops in its southern part along the Istra Peninsula, in the core of the Izola Anticline and north of the city of Trieste/Trst (Figures 1A,B; Pleničar et al., 1973; Pavšič and Peckmann, 1996; Placer et al., 2004, 2010; Vrabec and Rožič, 2014).
The base rock below Gulf of Trieste/Trst, and in areas north of the gulf, is covered by Pliocene-Pleistocene fluvial deposits (mainly sand, gravel, and silty clays) occasionally interrupted by marine and brackish sediments. These do not outcrop but are covered by two types of Holocene deposits. The first are fluvial/deltaic deposits found on the mainland: coarse-grained, carbonate-siliciclastic Soča/Isonzo River sediments characterize the northern rim of the gulf, whereas to the south the Rižana and Dragonja rivers contribute fine siliciclastic material with brackish environments at river mouths (Pleničar et al., 1973). The second are marine deposits found on the sea bottom in the gulf. These are fine-grained clastic sediments (sandy silt, clayish silt, silt and silty sands) with common foraminifera, bivalve and gastropod shells (Ogorelec et al., 1987, 1991, 1997). The Holocene transgression is dated with the radiocarbon method at 8.270 ± 50 yr BP - 9.160 ± 120 yr BP (Ogorelec et al., 1981; Covelli et al., 2006). The thickness of the marine sedimentary cover varies from 0 meters in coastal areas to several tens of meters in the central part of the gulf (Romeo, 2009; Slavec, 2012; Vrabec et al., 2014; Trobec, 2015; Trobec et al., 2018). Specific sub-recent sedimentary environments are represented by the Sečovlje and Strunjan Salinas (Ogorelec et al., 1981).
Materials and Methods
Sampling
Sediment samples were collected at four sites in Koper Bay during the summer of 2014 (Figures 1B,C). Three sampling sites were located in the Port of Koper, KPF1 (13°44021′E, 45°33,108′N, water depth 13.6 m) and KPF2 (13°43′51.10″E, 45°33′5.67″N, water depth 13 m) in the part of the port with the highest activity, and KPF3 (13°44,318′E, 45°33,489′N, water depth 13.2 m) near the outflow of Rižana River. The reference sampling sites REF (13°42,321′E, 45°34,320′N, water depth 18.5 m) were positioned outside the port near the anchorage in the fairway to the port and have been considered as reference sampling sites by the National Institute of Biology for decades.
The sediment samples were taken with a gravity sampler using plastic cores (10 cm diameter) to avoid metal contaminations. Two cores were collected at each location, one core was dedicated to meiofaunal analysis and other was used for geochemical and mineralogical studies. The upper 10 cm of the sediment was immediately sliced into 2-cm thick depth intervals and marked with numbers/1 = 0–2cm,/2 = 2–4cm,/3 = 4–6cm,/4 = 6–8cm,/5 = 8–10cm. The sediment samples were stored in clean plastic bags and immediately frozen. The sediment samples derived from the cores dedicated for the geochemical and mineralogical analysis were freeze-dried (lyophilized) until a constant weight was achieved. The samples were further homogenized and crushed to a fine powder using an agate mortar.
Mineralogical Analyses
The general mineral composition of the sediment samples was measured by X-ray powder diffraction using a Philips PW3710 X-ray diffractometer with CuKα1 radiation and a secondary graphite monochromator. The data was collected at 40 kV with a current of 30 mA at a speed of 3.4° 2θ per minute in a range from 2 to 70° (2θ). Oriented clay mineral aggregate was also performed using a household kitchen blender, an ultrasonic bath, ultracentrifugation, and the common glass slide method. In order to show the extent of expansion and/or concentration in the d-spacing that would indicate certain clay minerals, the oriented samples were air dried, glycolated with ethylene glycol, and heated to 550°C for 2 h. Diffraction patterns were identified using X’Perth Highscore Plus 4.6 diffraction software using the PAN-ICSD database and the full pattern fit method (Rietveld) for the quantitative mineral phase analysis.
Geochemical Analyses
Multi-elemental analyses of total elemental concentrations were performed using a portable handheld ThermoFisher Niton XL3t-GOLDD 900S-He X-ray fluorescence (XRF) analyzer. Approximately 3 g of powdered sediment samples were pressed into pellets using stainless steel capsules, a hammer and a pellet press tool. Two factory setting modes were used during the measurements: “Mining” mode for major elements and “Soil” mode for trace elements. When measuring with using “Mining” mode, helium (He) gas was purged in the analyzer, allowing better detection of light elements (Mg, Si, Al, S, and P). The measurement time on each sample was 210 s in “Mining” mode and 180 s in “Soil” mode. The accuracy and precision of the sediment analyses were evaluated using the pre-calibrated 24 reference standards (NIST, USGS) and the NIST-1d (limestone) and NIST-88b (dolomitic limestone) standards at the beginning and end of the measurement. According to the 2 replicate measurements of sediment samples and measured references analytical quality was satisfactory for almost all elements.
Foraminiferal Analyses
The sediment samples from the cores dedicated for foraminiferal analyses were stained in a 70% (ethanol/2g L– 1) Rose Bengal mixture (Walton, 1952). To obtain a good staining, the samples were treated with Rose Bengal for at least two weeks (Schönfeld et al., 2012). Specimens were considered “alive” when all chambers expect the last one or two were well stained (Caulle et al., 2015). In the laboratory, the samples were wet sieved, repeatedly rinsed with fresh water, through a 63 μm mesh sieve, and dried at room temperature. From each sample, using the Reich microsplitter, at least living + dead 200 - 300 foraminiferal tests (Murray, 2006; Martínez-Colón et al., 2018) were picked. The study of foraminiferal assemblages was carried out on the total assemblage instead of on the living assemblage. The total population is a more reliable indicator of assemblages because all of the seasonal variations are integrated into it, and no seasonal variation of living species will be overemphasized (Scott and Medioli, 1980). Considering the sedimentation rate of about 0.25 cm/year for the area in the immediate vicinity of the sampling area (Ogorelec et al., 1997), the collected cores probably provide information for the last 4-5 decades.
The specimens were examined under a stereomicroscope and identified according to the generic taxonomy Loeblich and Tappan (1987) and to species level when possible (due to size < 63 μm), following studies on Mediterranean and Adriatic foraminifera (Cimerman and Langer, 1991; Sgarrella and Moncharmont Zei, 1993; Ćosović et al., 2011; Hayward et al., 2021) and the species names follow the World Modern Foraminiferal Database (World Register of Marine Species, 2021).
Elpidium specimens that lack elements to be classified at the species level, were divided into two groups based on different life-strategies (have a keel or not, being infaunal and epifaunal, after Murray, 2006; Vidović et al., 2009, 2016).
Species with relative abundances greater than 10% are considered abundant, those between 5 and 10% are considered common, and those below 5% are considered rare (Lo Giudice Cappelli and Austin, 2019). For all studied assemblages, species diversity, expressed as Species richness (S), the Fisher α-index, the Shannon H-index (which considers both the abundance and evenness of species), and dominance (Simpson and Berger-Parker indices) were calculated. Species diversity is affected by salinity (Murray, 1991), values of Fisher α-index > 5 indicate normal marine salinity, while hyposaline and hypersaline conditions are characterized by low species diversity (index values < 5). These indices were computed for all assemblages using free statistical software PAST (PALentological Statistic; version 2.14; (Hammer et al., 2001).
The Foram-AMBI index was calculated (Foram-AMBI = [(0 *%GRI) + (1.5 *%GRII) + (3 *%GRIII) + (4.5 + %GRIV) + (6 *%GRV)]/100) according to Borja et al. (2000). Foraminiferal species are grouped in five ecological groups according to their sensitivity to organic matter enrichment (from the most sensitive GRI to the 1st order opportunists GRV, Table 1). For the calculation only species assigned to the ecological groups (Alve et al., 2016; Jorissen et al., 2018; Dubois et al., 2021; Parent et al., 2021) were used. The unassigned species are excluded from the data set before the proportion is calculated (Borja et al., 2000, 2008). The proportion of the five groups sums up to 1. The index ranges from 0 (100% of species GRI) to 6 (100% of species GRV) reflecting the degree of pollution and considers an individual species sensitivity to environmental stressor.
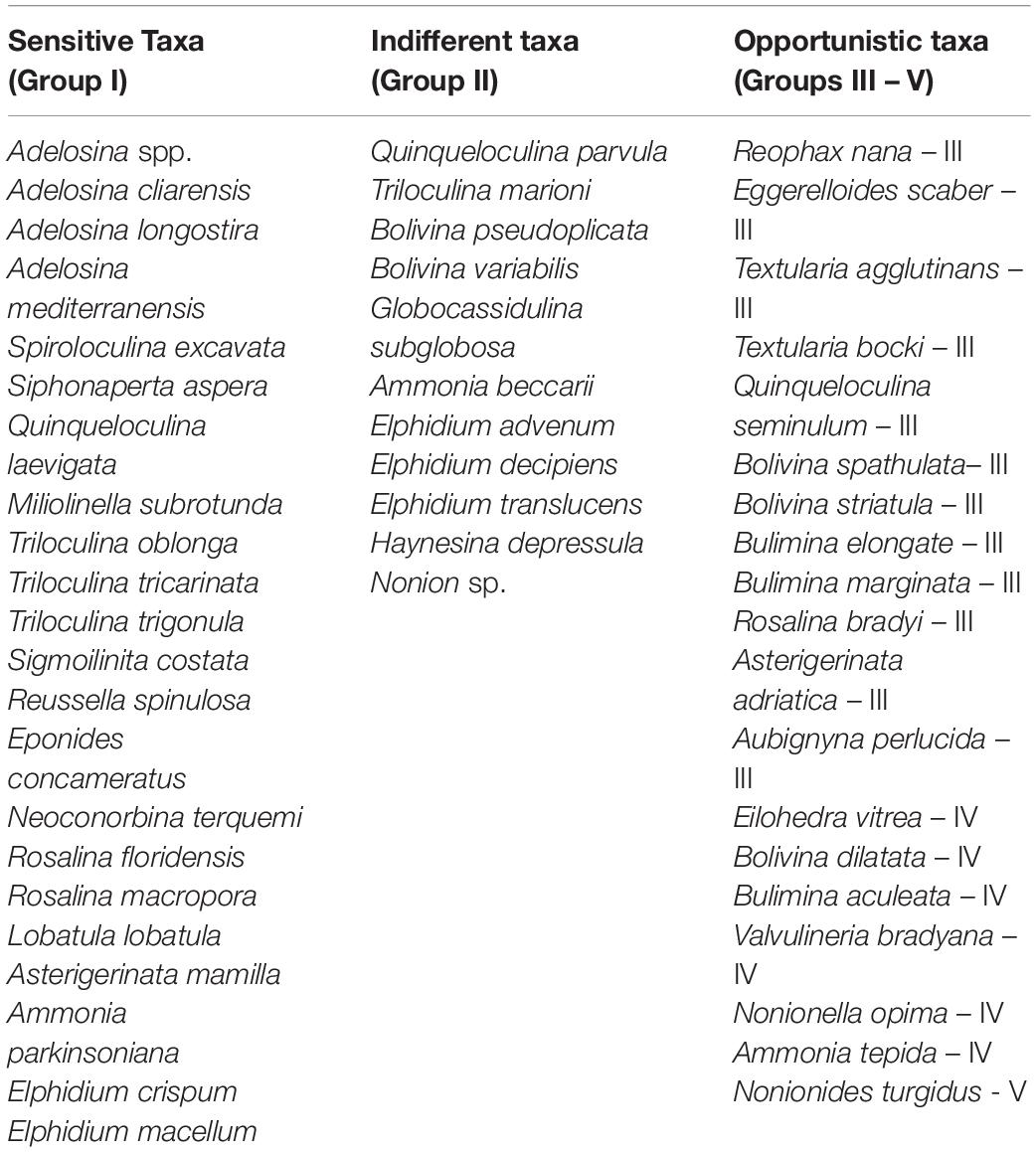
Table 1. The list of 51 identified taxa that have been assigned to ecological groups from the most sensitive to organic enrichment (Group 1) to the 1st order opportunists (Group V) for the Foram-AMBI calculation.
Five EcoQS categories (high, good, moderate, poor or bad) classify coastal waterbodies according to ecological quality statuses. The EcoQS was reconstructed (Bouchet et al., 2012) by applying diversity index exp (H’bc). Species diversity index H’ was calculated using the SpadeR program (version 2016, Chao and Shen, 2003; Chao et al., 2016; El Kateb et al., 2020). The classes boundaries are from Bouchet et al. (2012) and Hess et al. (2020). Sample KPF3/3 which contained < 100 foraminiferal tests were excluded from calculation).
Statistical Analyses
Statistica 13.3 software was used for basic statistical analyses (median, min., max.) for both mineralogical and geochemical data (Supplementary Tables 1–3). Canonical correspondence analysis (CCA) was applied to construct site/species matrix for 31 environmental variables (10 from X-ray diffraction analyses and 21 from the X-ray fluorescence analyses) and to identify correlations within and between the data sets. The free statistical software PAST package (Moore and Reynolds, 1997) was used for these analyses.
The strength of the correlation between the variables was measured using the correlation coefficient “r”, also known as Pearson’s r or the product-moment correlation coefficient. The values of the correlation coefficient r range from zero, indicating no correlation between variables, to + 1, indicating full positive or −1, indicating full negative correlations (Hammer et al., 2001). The Spearman Rank Order correlation coefficient was measured between the multivariate components (CCA) with the highest percentage of variance (axes 1 and 2) and the mineralogical and geochemical data.
To better define the relationships between the variability of foraminiferal species and environmental conditions, statistical analyses (canonical correspondence analysis, CCA) of the total foraminiferal assemblages were carried out using the PAST program package (Hammer et al., 2001). Prior to the analyses an additive logarithmic transformation log (x + 1) was performed on standardized data (relative abundances of foraminiferal species) in order to reduce the significance of extreme values, reduce the contributions of common species, increase the contributions of rare species and normalize the data (Hammer and Harper, 2006).
Results
Mineralogy of Sediment
Mineralogical analysis of the sediments of the Bay of Koper revealed the following mineral composition (Figure 2 and Supplementary Table 1): quartz (median 39.9%), calcite (median 29.4%), illite/muscovite (median 25.1%), Na-Ca-plagioclase (median 3.9%), dolomite (median 1.1%), chlorite group (median 0.6%), pyrite (median 0.4%) and kaolinite (median 0.1%). Certain differences in mineral composition between the sampling sites were found. Higher concentrations of minerals characteristic of siliciclastic rocks (quartz, plagioclase, muscovite) were encountered at coastal sites (KPF1, KPF2, KPF3). The offshore reference site (REF) was characterized by elevated concentrations of carbonate minerals (calcite, dolomite) were measured.
Geochemistry of Sediment
The results for major, minor and trace elements in sediment samples are presented in Supplementary Tables 2, 3. The highest concentrations of major elements in sediment (Figure 3) show Si with higher concentrations in samples near the coast (KPF1, KPF2 and KPF3; 21.3-22.1%) and lower concentrations at offshore reference sites (REF; 19.7%). A reverse trend was observed for Ca (7.6-8.3% near the coast, 9.4% offshore) and Mg (1.5-2.0% near the coast, 2.11% offshore). Other major and minor elements (Si, Al, Fe, K, Ti, Mn, Cr) show a similar composition at all sampling sites with rather minimal differences (differences < 1%) at site KPF3 at the Rižana River inflow. Concentrations of elements over the entire depth in the sediment are practically constant (differences < 0.5%).
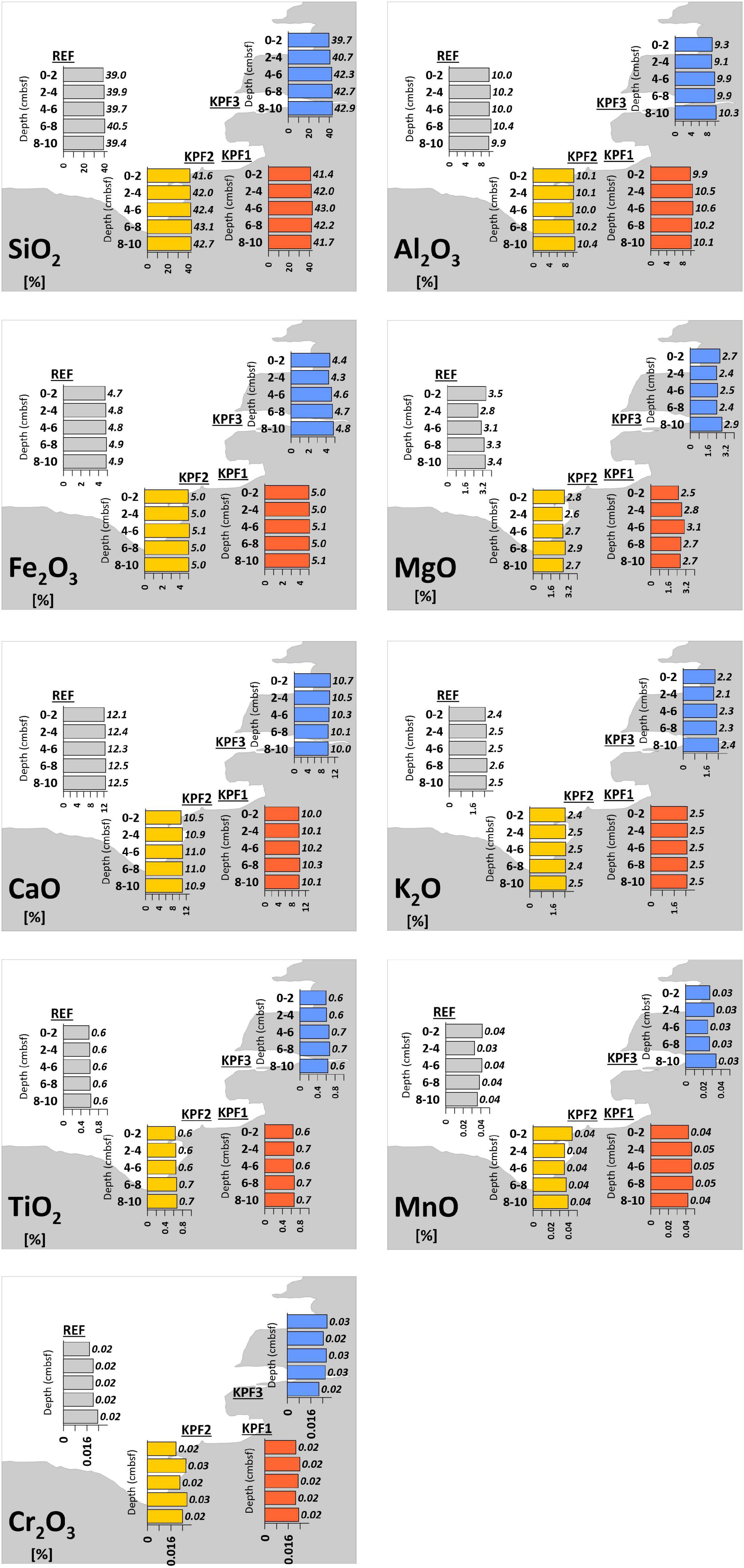
Figure 3. Major oxides concentrations (%) of sediments of Bay of Koper (cmbsf = cm below sediment floor).
In general, concentrations of trace elements (As, Cr, Cu, Nb, Ni, Th, Zn, and Zr) were higher at coastal sampling sites (KPF1 and KPF2 in basin I, KPF3 in basin II) and lower at the reference site in the bay (REF) (Figure 4). For As, Ni, Nb and Th, the higher concentrations were observed at sites KPF1 or KPF2 in basin I and for Cr, Cu, Zr and Zn at site KPF3, where the Rižana River flows into the sea. Concentrations of other trace elements (Pb, Rb, Sr and V) were higher at the reference location (REF) and lower at coastal sites. The trace elements do not show any significant changes and trends at sediment depth (Figure 4).
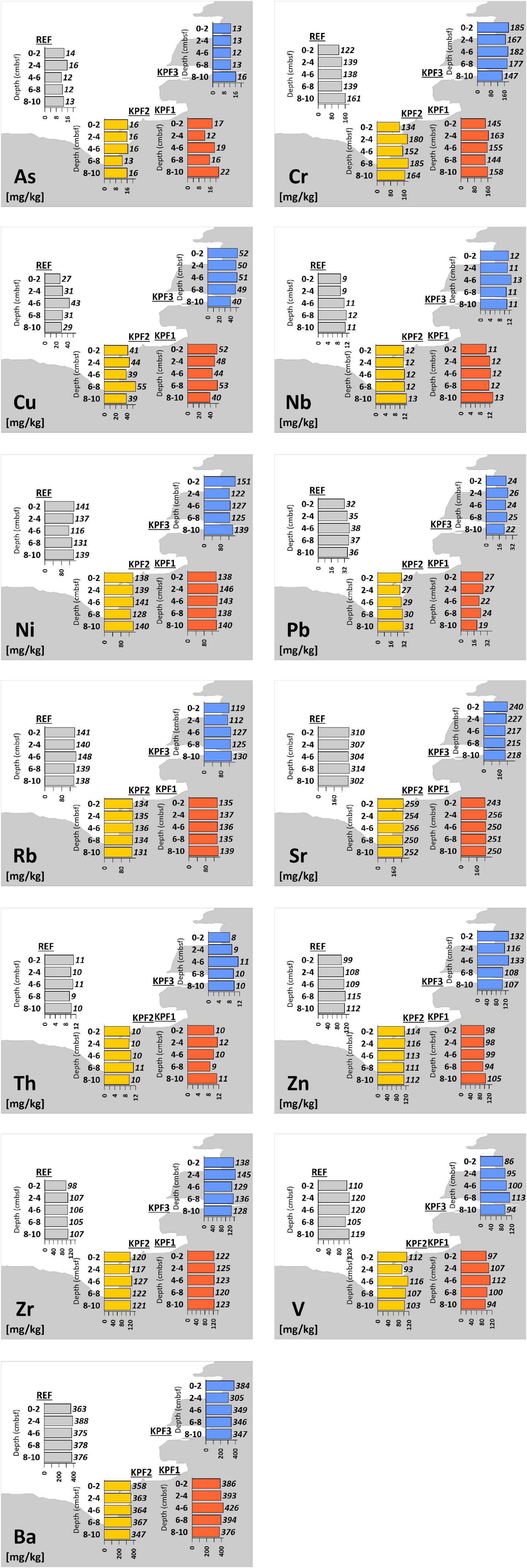
Figure 4. Concentrations of trace elements (mg/kg) of sediments of Bay of Koper (cmbsf = cm below sediment floor).
Foraminifera
Diversity and Dominance
A total of 83 species belonging to 57 genera were identified (Supplementary Table 4), of which 18 taxa were agglutinated, 69 perforate-hyaline and 31 porcelaneous. Most foraminifera were rare and contributed less than 5% (Table 2) to the assemblages or were found in only a few samples (Supplementary Table 4). The low number of foraminiferal tests (N = 12) in the depth interval of 6–8 cm KPF3/3 (Table 3) excludes this interval from statistical interpretation. Unidentifiable miliolid fragments and reworked Eocene planktonic foraminifera were also listed (Supplementary Table 4) but are not included in any analysis.
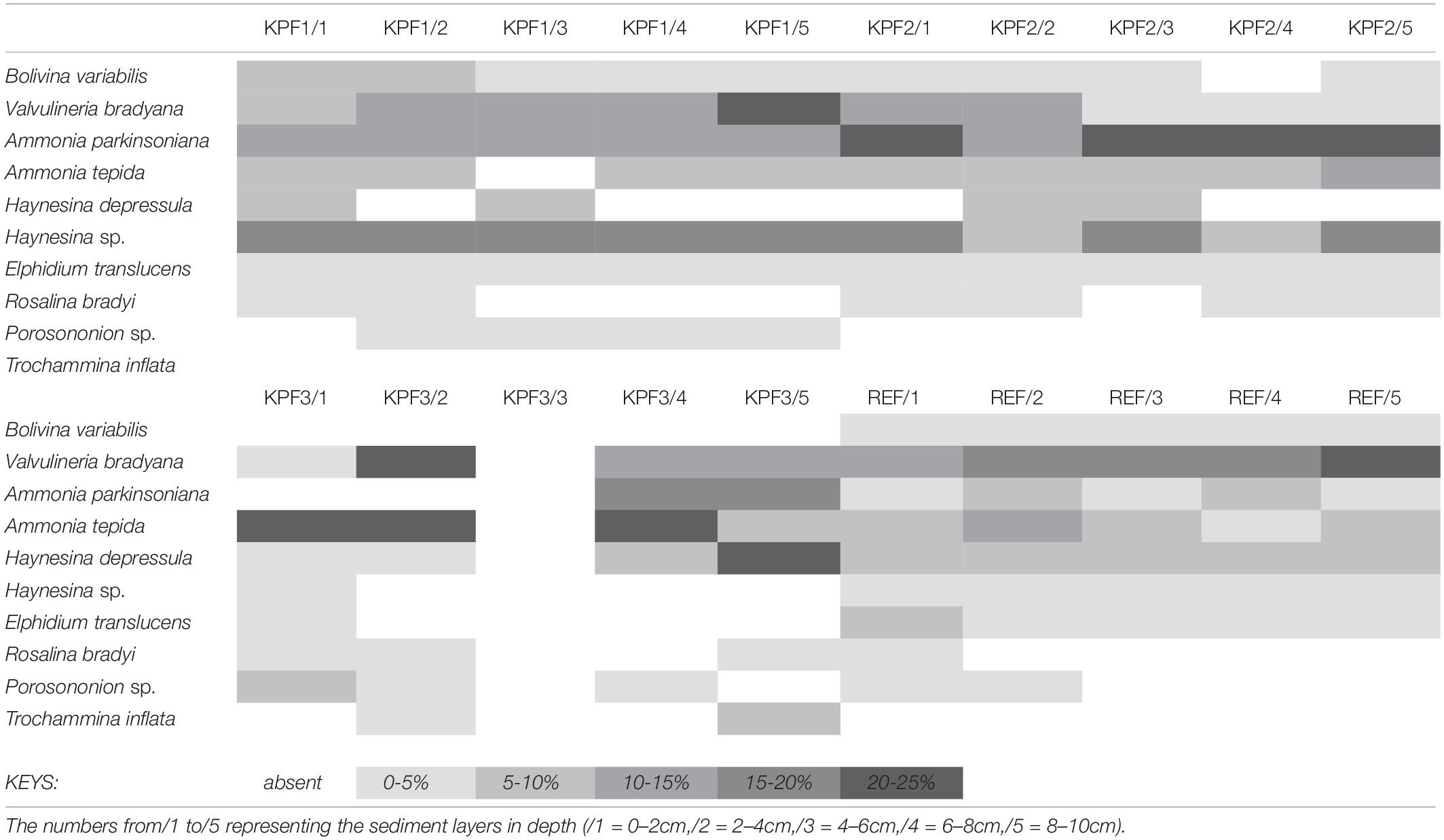
Table 2. Comparative status of the abundant and common species (> 5%) of total assemblages in at least one of studied interval and sample.
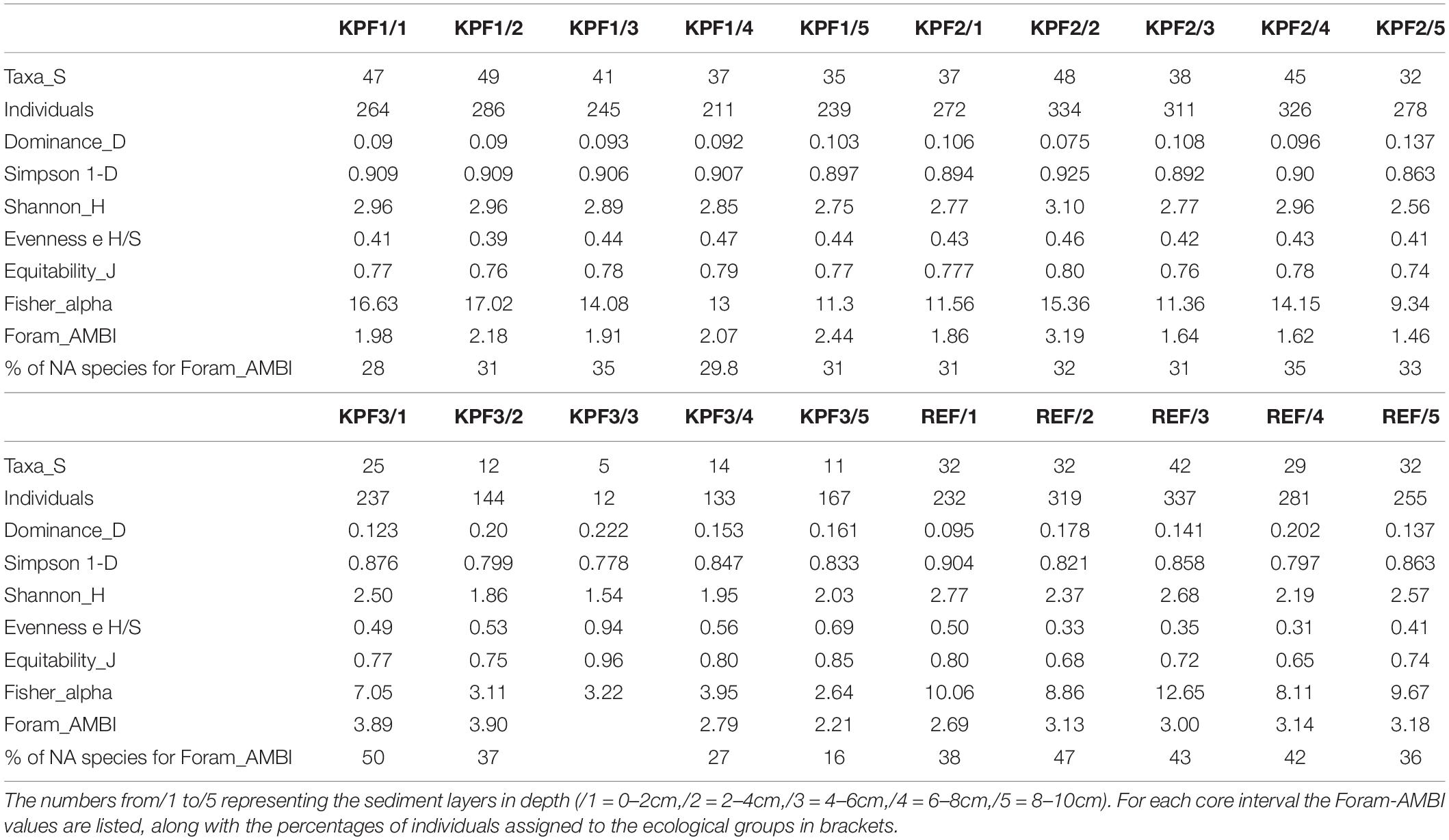
Table 3. Number of taxa (Species richness), number of individuals, diversity indices from standardized aliquots for total assemblages from four locations (KPF1, KPF2, KPF3 and REF) from the Bay of Koper.
The species richness (S) of the total assemblages (Table 3 and Supplementary Table 4) ranges from 5 (KPF3/3) to 49 (KPF1/2). The number of species decreased from the top to the bottom of each studied core. The Shannon H-index (Table 3) ranges from 1.5 to 3.1, with the highest values indicated in the central parts of the cores. The Simpson 1-D values (Table 3) remained constant throughout each core. The values of Fisher’s alpha index (Table 3) varied between stations (from 2.6 at station KPF3 to 17.0 at station KPF1) and along each core (exhibiting a down-core decreasing trend).
The percentage of tolerant species (Foram-AMBI assigned species; Table 3) varies among stations and also along individual core. The highest percentage of tolerant species is at REF (from 32.7% REF/1 to 47.4% at REF/5), and the lowest at KPF2 (from 21.2% to 27.6% at KPF2/2). At stations KPF1 and KPF2, the proportion of tolerant species within the total foraminiferal assemblages decreases downcore. The lowest values of the Foram-AMBI index are at KPF 2 (ranging from 1.46 to 3.19, Tables 3, 4), followed by stations KPF1 (1.92-2.44) and REF with values between 2.6 and 3.2, while station KPF3 has the highest value (up to 3.9). The values of EcoQS indicate poor status in the REF1-REF3 samples and KPF2/1 and KPF2/5 and bad status for KPF1station, older part of the REF station and middle part of the KPF2 (Table 4).
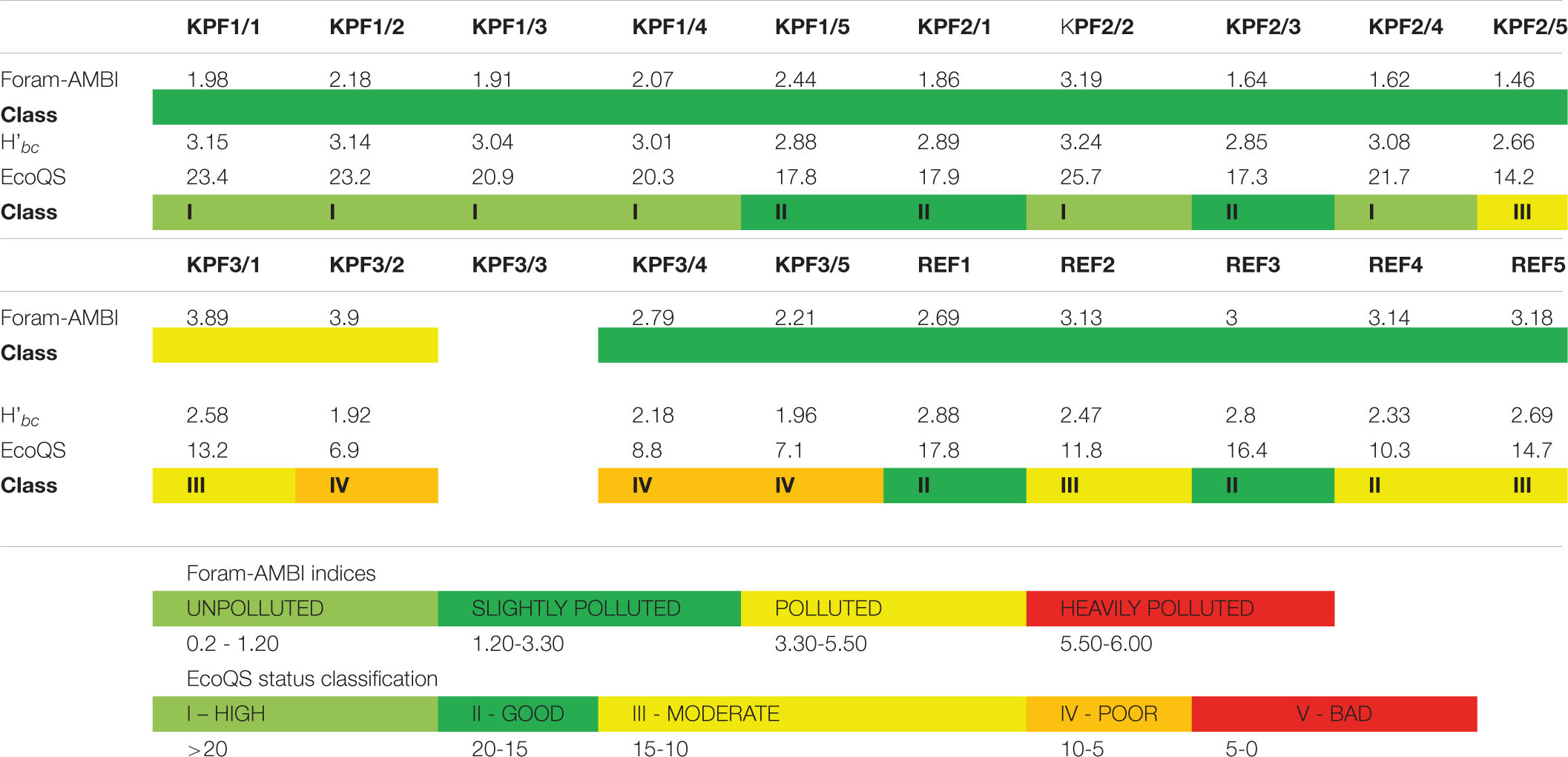
Table 4. Foram-AMBI indices and ecological quality statuses EcoQS at each studied interval obtained from the study of total assemblages.
Foraminiferal Distribution
The distribution of foraminiferal species in total assemblages showed variations between stations, while changes along the core at each station are less obvious (Figure 5 and Supplementary Table 4).
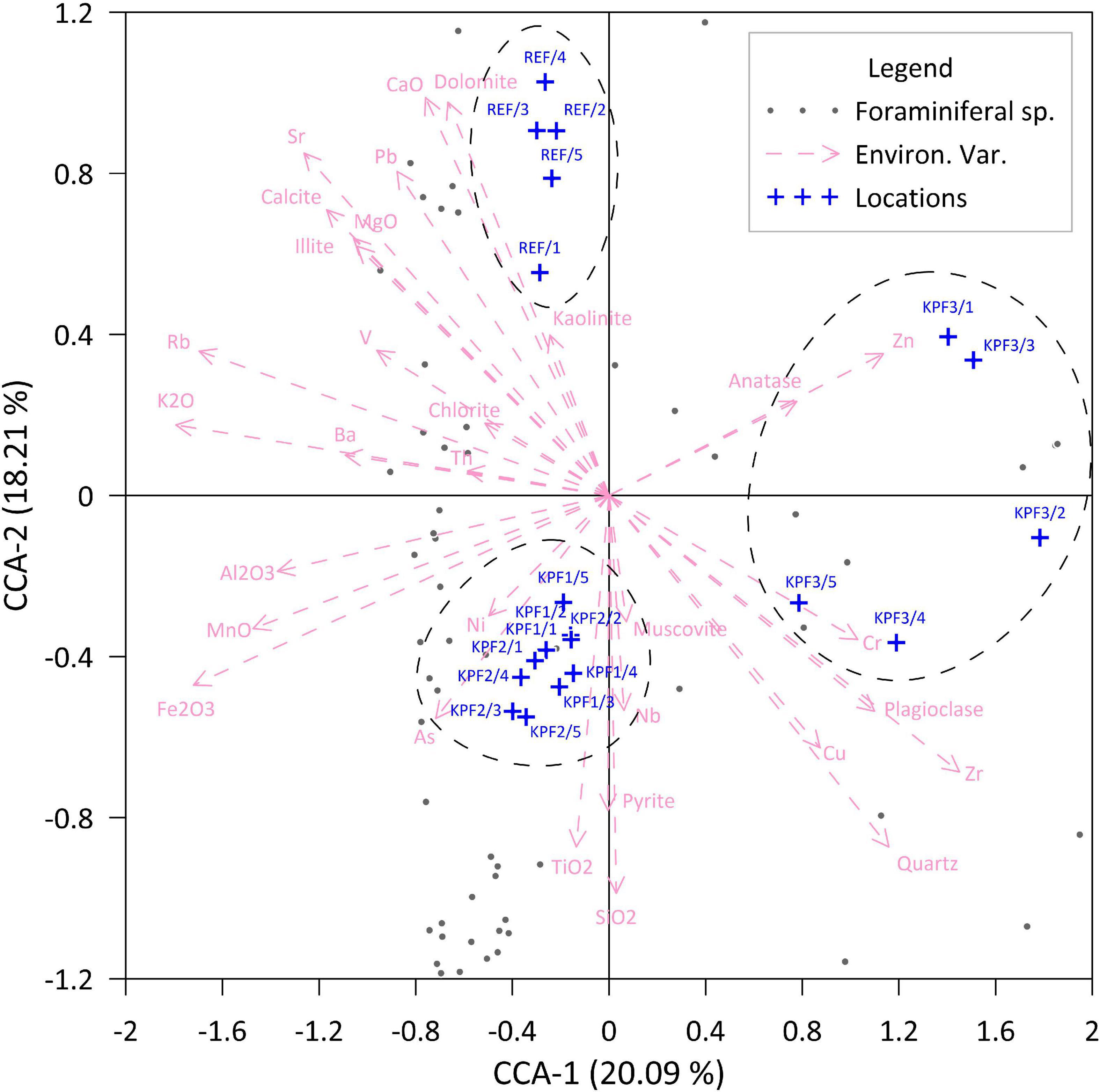
Figure 5. Canonical correspondence analyses (CCA) of total foraminiferal assemblages, and mineralogical and geochemical data in sediment samples from four locations (KPF1, KPF2, KPF3, and REF) from Bay of Koper.
At station KPF1, infaunal, planispiral Haynesina sp. (Figures 6A,B) and trochospiral A. parkinsoniana (Figure 6F) dominated with relative frequencies of 15.5–16.7% and 11.5–18.9%, while the other species contributing > 10% were V. bradyana (9.0-18.4%), H. depressula (2.9–8.7%), A. tepida (Figures 6D,E,G; 1.6–9.6%), B. variabilis (1.4–5.3%) and Elphidium translucens (2.8–4.0%). Miliolids constituted between 6.2 and 9.5% and agglutinated foraminifera between 1.2 and 3.4% to total assemblage.
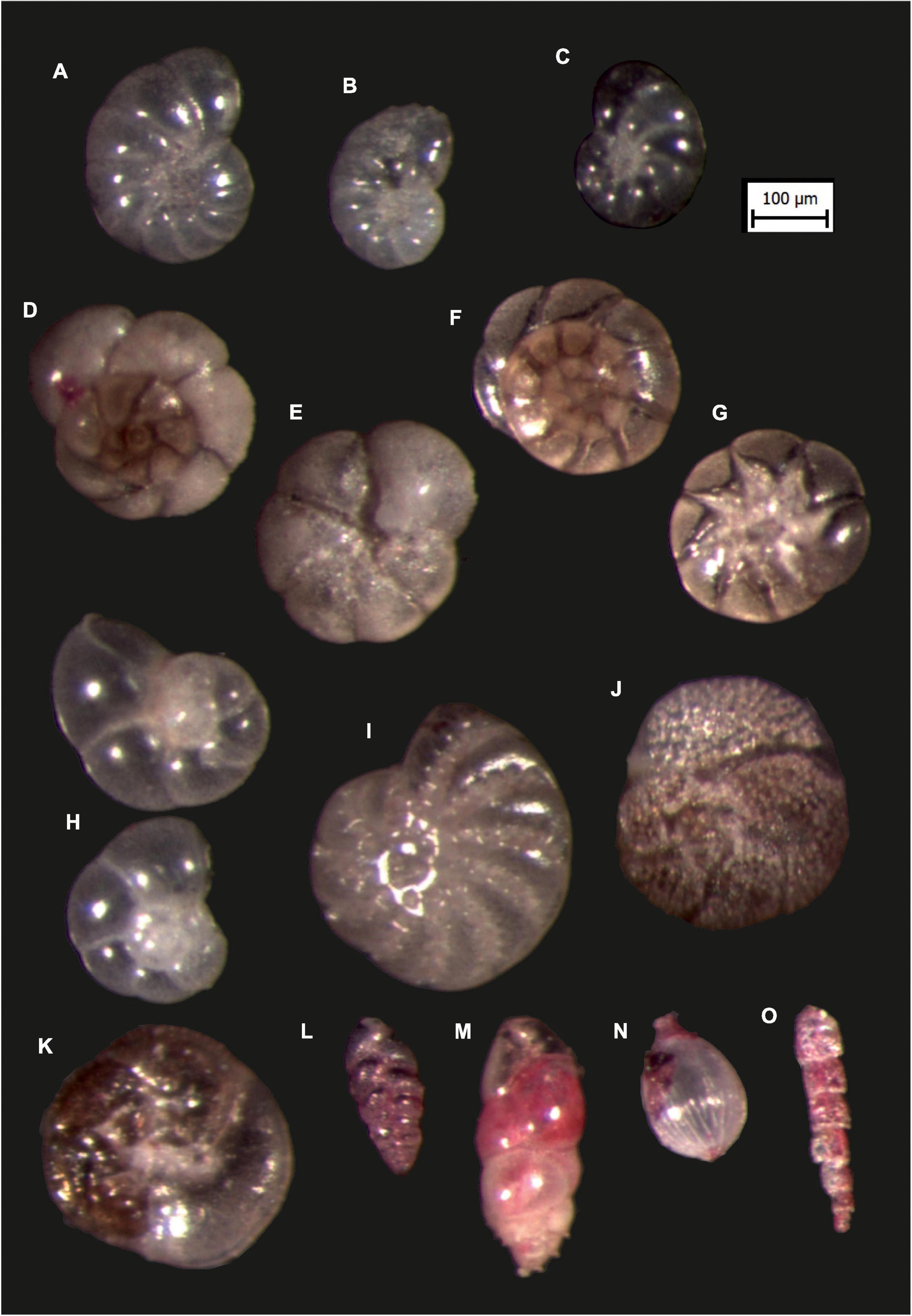
Figure 6. Microphoto images (using Olympus U-TV1XC camera) of the selected foraminiferal species: (A) Haynesina depressula (Walker and Jacob, 1798), KPF1/5. (B) Haynesina depressula (Walker and Jacob, 1798), KPF1/5. (C) Haynesina depressula (Walker and Jacob, 1798), KPF2/5. (D) Ammonia tepida (Cushman, 1926): spiral side, KPF1/2, (E) Ammonia tepida (Cushman, 1926): umbilical side, KPF1/2. (F) Ammonia parkinsoniana (d’ Orbigny, 1839): spiral side, KPF1/2. (G) Ammonia parkinsoniana (Cushman, 1926): umbilical side, KPF1/2. (H) Aubignyna perlucida (Heron-Allen and Earland, 1913): KPF2/2. (I) Elphidium sp., non-keeled, KPF1/4. (J) Rosalina bradyi (Cushman, 1926): spiral side, KPF1/1. (K) Rosalina bradyi (Cushman, 1926): umbilical side, KPF1/1. (L) Bolivina variablis (Williamson, 1858), KPF1/5. (M) Bulimina elongata, d’ Orbigny, 1826, KPF1/3. (N) Lagena striata (d’ Orbigny, 1839), KPF1/3. (O) Reophax sp., KPF1/1.
The three co-dominant species of the foraminiferal assemblages from station KPF2 were A. parkinsoniana (18.15–28.77%), Haynesina sp. (Figure 6C; 8.63–17.63%) and V. bradyana (5.04–12.13%). Individuals of A. tepida and H. depressula were subordinate, but still contributed significantly to the assemblages (5.83–11.15% and 2.45–6.25%, respectively). Agglutinated and porcelaneous foraminifera were rare throughout the core, with the only representatives of M. subrotunda (1.44–2.21%), Sigmolilinita sp. (1.23–3.86%) and P. lecalvezae showing slightly greater abundances.
Significant decrease in the diversity of foraminiferal taxa were found in the sediments collected at station KPF3. The dominant species were A. tepida (5.99–24.7%) and V. bradyana (7.06–21.43%), while the subordinate species was H. depressula (4.7–28.14%). H. depressula was rare in the upper part (< 5%) and, then became more frequent until it reached the highest frequency at the bottom of the core. Non-keeled Elphidium sp. and Porosononion sp. occurred in significant amounts in the first two centimeters of the core, accounting for 10.59% and 9.02% of the assemblage, respectively. Large amounts of miliolid fragments and significant occurrences of reworked Eocene planktonic foraminifera (including pollen grains) characterized the station, especially the 4–6 cm depth interval (KPF3/3).
In the core of the REF station, tests of non-keeled Elphidium sp. predominated (20.69% in REF/1 and 38.79% in REF/4). Moreover, individuals of V. bradyana (Figure 6H) were subordinate with constant abundance throughout the core (14.66% in the depth interval of 0–2 cm to 20.39% in the depth interval of 8–10 cm). H. depressula (5.64–9.05%) and A. tepida (4.63–10.34%) were regularly present throughout the core, except for A. tepida, which was more abundant in the depth interval of 2–4 cm. The agglutinated foraminifera Textularia bocki contributed significantly to the assemblage (4.98% in REF/4).
Results of Statistical Analyses
Canonical correspondence analysis (CCA) of total species abundances (response) with the gradient of environmental variables (mineralogy and geochemistry) from four stations grouped the samples into three main groups: the first included the samples from stations KPF1, KPF2, the second included the samples from the REF site, while the third included only the samples from station KPF3 (Figure 5).
In addition, the CCA analyses show that stations KPF1 and KPF2 correlate with pyrite, As, Ni, Ti and Si, the REF station correlates with higher carbonates contents (calcite, dolomite), illite, kaolinite, Sr and Pb, and station KPF3 correlates with plagioclase, muscovite, quartz, Cu, Cr and Zn.
The measure of similarity accounted for the eigenvectors CCA-1 and CCA-2 is 20.09 and 18.21% respectively.
Correlation analysis of multivariate foraminiferal data (CCA axis 1 and 2) of the samples from all 4 stations with geochemical data did not show a strong or significant relationship between the community and the environmental parameters observed. However, due to the strong influence of station KPF3 on the plotting of the samples in multivariate space, a further correlation with geochemical results was carried out, now only on the CCA foraminiferal data of the other three stations. This analysis produced the following results: significant positive correlation of the CCA-1 axis with S and Zr, a strong positive correlation with Cu and Cr, as well as a significant negative correlation of the same axis with Fe, Mg, Ca, Rb, Sr, Pb and V. A significant positive correlation of the CCA-2 axis appears with Ca, Rb and Sr, and a strong positive correlation with Mg, Pb and Zn, while a significant negative correlation with Si, Fe and Ti is indicated (Table 5).

Table 5. Spearman Rank Order correlations analysis between total foraminiferal assemblages and geochemical data from three (KPF1, KPF2 and REF) sampling stations (marked correlations are significant at p < 0.05).
Discussion
Based on study of four sediment cores we investigated the impact of increasing shipping and human-induced activities (i.e., industry, tourism) in the Bay of Koper on the geochemical element composition of sediments and benthic foraminiferal assemblages over the past 40 - 50 years. The study of the composition and diversity of foraminiferal assemblages were used to describe the ecological conditions by using Foram-AMBI and EcoQS indices.
Environmental Characterization Based on Mineralogical and Geochemical Data
The mineralogical composition and the content of major elements (Figure 3) reflect the geological characteristics of the wider area of the Gulf of Trieste/Trst coast. Higher concentrations of minerals characteristic of siliciclastic rocks (quartz, plagioclase, muscovite; Figure 2 and Supplementary Table 1) can be explained by the geological siliciclastic properties of the hinterland of the bay, which is more influenced along the coast (KPF1-KPF3). The significant trend of increasing sediment carbonate content toward the central part of the bay can be observed, which is attributed to the considerably higher proportion of carbonate skeletons of various organisms in this part of the bay (REF). The same trend was already described by Ogorelec et al. (1987). Similar pattern was also indicated by the content level of major elements, with higher Si and Al levels along the coast, and higher Ca and Mg levels in the inner part of the bay, which can be also explained by the geogenic origin of the hinterland. The authigenic mineral pyrite indicates the reduction conditions in the sediment. Ogorelec et al. (1987) describe the reduction conditions already a few centimeters below the surface, which is favorable for pyrite formation. Slightly lower levels of Fe at the Rižana outflow (KPF3) can be explained by the stronger inflow of oxygenated river water into the bay and the oxidizing environment of the sedimentation (Ogorelec et al., 1987).
The concentrations of trace elements (As, Cr, Cu, Nb, Ni, Pb, Rb, Sr, Th, Zn, V, Ba) show spatial differences between the sampling sites, indicating a non-point source of potential pollutants into the environment. In general, As, Ni, Nb, and Th are highest at KPF1, Cr, Cu, Zn, and Zr at KPF3 and Pb, Rb, Sr, and V at the REF site. In addition, the Spearman correlation analyses confirms strong or moderately positive correlations between trace elements at the respective sampling site. The statistical grouping of the sites (Figure 5) can be explained by the geogenic origin, the different basic mineralogical characteristics of the sediment (carbonates at REF and siliciclastic at KPF1-KPF3) and the higher inflow of river water into the bay (between KPF3 and other sites). Although the minor positive signal of trace element concentrations might be recognized in the inner part of the port of Koper (KPF1 and KPF2), the values are generally within the background geological levels, which is in accordance with analyses previously published on the Bay of Koper (Ogorelec et al., 1987, 1991; Rogan Šmuc et al., 2018). Contrary some trace elements (i.e., As, Cd, Cr, Cu, Ni, Zn) show higher concentrations compared to other locations in the Adriatic Sea (Adami et al., 1996; Aquavita et al., 2010). Concentrations of elements over the entire depth in the sediment are practically constant which is certainly also affected by bioturbation.
While the element concentration in the sediment were described to be controlled by the natural conditions (mineralogical, grain size, organic matter) these should be considered in background evaluation as the basis for the assessment of anthropogenic pollution (Coccioni et al., 2009).
The Response of Foraminiferal Assemblages
The foraminiferal species from studied samples (one replica, Table 2), including the most common (A. tepida, A. parkinsoniana, V. bradyana, Haynesina sp., H. depressula, B. variabilis and Elphidium spp. (keeled and no-keeled) are characteristic of infra-circalittoral of Mediterranean and northern Adriatic (Jorissen, 1987; Hohenegger et al., 1988, 1993; Cimerman and Langer, 1991; Sgarrella and Moncharmont Zei, 1993; Coccioni et al., 2009; Vidović et al., 2009, 2014; Ćosović et al., 2011; Popadić et al., 2013; Jorissen et al., 2018; Melis et al., 2019).
Foraminiferal diversity parameters show clustering of stations, KPF1, KPF2 and REF with higher values and KPF3 has lower values. This trend is clearly visible in the multivariate biplots (Figure 5). Knowing that low diversity is related to highly variable conditions and/or stressful environmental conditions, we assume that the position of station KPF3 off the coast of the Rižana River caused a decrease in diversity (Table 3). The freshwater influence is confirmed by: (i) the significant content of reworked Eocene planktonic foraminifera (Supplementary Table 4, reaching frequency of 82% of total skeletal grains in sample KPF 3/3) transported as sediment load from the Eocene Flysch in the hinterland (Pleničar et al., 1973); (ii) the abundance of species A. tepida and H. depressula (including Haynesina sp.), known for their tolerance to salinity fluctuations, and their ability to survive in nutrient-rich conditions off river mounts (Murray, 2006; Barbieri et al., 2019). In coastal environments, parameters such as active bioturbation, current and wave regimes, and biotic interactions may be important to the distribution of benthic foraminifera (Murray, 2006 and references therein). Although almost all identified benthic species are considered opportunistic taxa, we hypothesize that river flow and sediment surge have caused certain physical disturbances in benthic microhabitats that some species cannot tolerate (elphidiids and infaunal representatives).
Several studies on the influence of trace metal concentrations on the distribution of foraminifera typically indicate a negative correlation between trace metal concentrations and foraminiferal diversity and abundance (Alve, 1991; Sharifi et al., 1991; Yanko et al., 1999; Coccioni, 2000; Carnahan et al., 2008; Frontalini and Coccioni, 2008; Coccioni et al., 2009; Frontalini et al., 2009; Li et al., 2013; Martins et al., 2013). Our results show that the highest values of species diversity were found in sediments with the highest concentration of Fe and Mn (KPF1 station). Also, no negative correlation was found between foraminiferal diversity and concentrations of Ni and Pb. It must be emphasized that some discrepancies were observed in the correlation of Fe concentrations with species diversity at certain depth-interval levels at each station. This may be due to sediment mixing at depth due to bioturbation and sediment mixing in Bay of Koper caused by waves induced by strong winds (Leder et al., 1998). Carnahan et al. (2008) showed that abundance of Ammonia decreases with increasing Pb concentrations, and Elphidium specimens are more numerous when the environment is enriched with Fe. Our data shows no correlation between the abundance of representatives of genera Ammonia and Elphidium (non-keeled in particular, sensu Murray, 2006; Vidović et al., 2009, 2014) and the concentration of Fe and Pb at each station (all depth intervals). The distribution of trace metals showed that As and Rb have similar distributions along the core, while concentrations of Pb, Cu, and Cr increase slightly downwards from the core, with these conditions associated with reduced biodiversity. Nevertheless, we can conclude that no significant changes occurred in the overall composition of the assemblages. The representatives of genus Ammonia are tolerant to trace metal pollution (Armynot du Châtelet et al., 2004; Ferraro et al., 2006; Frontalini and Coccioni, 2008). In particular, A. tepida is tolerant of elevated trace metal pollution (Armynot du Châtelet et al., 2004; Barbieri et al., 2019) and the species abundance increases with increases in Ni, Cr, Cd, As, and Hg concentrations (Frontalini and Coccioni, 2008; Melis et al., 2019). As an opportunistic feeder A. tepida showed a strong response to phytodetrital input (Wukovits et al., 2018), and recorded phytoalgal bloom in the Northern Adriatic could cause the species abundance (Cabrini et al., 2012; Cozzi et al., 2020). The positive response of representatives of the genus Ammonia at KPF 3 and KPF 2 to slightly elevated concentrations of trace elements (but within normal levels) is consistent with the previous study in Gulf of Trieste (Melis et al., 2019). In general, a comparison of the distribution of the most abundant species indicates a moderate supply of organic matter at all sites because H. depressula in a case of increased organic supplies (Jorissen et al., 2018) would disappear. The positive correlation between abundance of H. depressula and Zn concentration (Melis et al., 2019) was recorded at KPF2.
The Foram-AMBI index reflects changes in organic matter concentrations (Jorissen et al., 2018; Parent et al., 2021). The presence and abundance of organic matter are related to mud content in sediments, and consequently affect the concentration of oxygen and pollutants (El Kateb et al., 2020). The calculated Foram-AMBI index values (Table 4) imply from good and slightly polluted (KPF1 and KPF2 assemblages) to moderate and polluted (KPF3 and REF assemblages) conditions. Our results are consistent with previous studies (Coccioni, 2000; Frontalini and Coccioni, 2008; Coccioni et al., 2009; Frontalini et al., 2009) suggesting that the decrease in diversity of foraminiferal assemblages correlates with the increase in abundance of tolerant species. The percentage of unassigned species ranged from 29 – 36% in KPF1, 30-34% in KPF2 and 38-43% in REF, which is well above the quality assurance threshold of 20% unassigned (Alve et al., 2016). Because of the high number of unassigned species, these indices are used to get trends in changes in the ecological status of stations only. For the REF station, the moderate to good EcoQS ecological status (Table 4) comes from the position of the station with ship traffic, where ballast water is openly discharged into the environment. Our study questioned the status of the REF site as the referral for further investigations of benthic habitats. The diversified assemblages of opportunistic detritivores (Supplementary Table 4) in the bay showed high (KPF1) and good to high (KPF2) ecological quality statuses (Table 4). The unstable condition at the KPF3 station due to vicinity of river input, resulted with moderate to poor ecological status. At all studies cores the better ecological quality for benthic foraminifera were observed in younger intervals, because of proliferation of opportunistic foraminifera.
Investigating anthropogenic impacts on foraminiferal abundances and taxonomic composition of assemblages in different enclosed shallow water settings (lagoons) in the Adriatic, especially in the Northern Adriatic (Donnici and Serandrei Barbero, 2002; Frontalini and Coccioni, 2008; Bergamin et al., 2009; Coccioni et al., 2009; Frontalini et al., 2009; Frontalini and Coccioni, 2011; Vidović et al., 2016; Bouchet et al., 2018; Barbieri et al., 2019; Melis et al., 2019), and in the Mediterranean Sea (Türkmen et al., 2011; El-Gamal et al., 2012; Dimiza et al., 2016; Jorissen et al., 2018) have recently intensified. Our results fit the distribution of benthic foraminifera recorded in these studies. Representatives of the stress-tolerant genus Ammonia dominate the sediments of most lagoons. In the studied samples the values of the Foram-AMBI indices, although considered with caution, indicate a good to moderate ecological conditions, which does not suggest the highest organic concentrations. It seems that for the dominance of A. tepida in the assemblages, together with Haynesina sp. and H. depressula the following factors are important: river inflow, eutrophication of the area (Giani et al., 2012), anthropogenic pressure and shallow-water and enclosed morphology of the area. Epiphytic forms which were common until the 1990s (Murray, 2006), are less common due to the disappearance of vegetation cover (Li et al., 2013).
Considering the sedimentary rates in the area (Ogorelec et al., 1997), it seems that more or less similar geochemical conditions prevailed in the last decades, despite the increased anthropogenic pressure from the activities in the Port of Koper, which favored the development of opportunistic benthic foraminifera. Normal marine conditions continued to prevail, reflected in moderate diversity along the shallow sedimentary cores. According to our interpretation, the environmental characteristics that contribute most to the distribution of foraminifera in Bay of Koper are shallow-water, semi-enclosed area with freshwater inflow, limited enrichment with trace elements despite anthropogenic pressure and low to moderate concentration of organic matter.
Conclusion
Coastal environments are often interesting for environmental studies in the light of various anthropogenic pressures. In Gulf of Trieste, and consequently in Bay of Koper, numerous investigations were done also due to specific natural characteristic of this area. Besides the intensive urbanization, tourism, and agriculture of the wider area, the port activity in the Bay of Koper represents an important factor in the study of marine environmental pollution. The main aim of this paper is to highlight, by means of statistical analysis, the correlation between the foraminiferal parameters (diversity, relative abundance and absolute abundance) and the possible natural and anthropogenic stressors due to intensify anthropogenic pressure in last three decades in the bay.
A total of 20 sediment samples (one replica) were analyzed at four sampling sites with a varying degree of anthropogenic input. The mineralogical characteristics and major element concentrations indicate the characteristics of a natural lithogenic origin, siliciclastic rocks on the coastline, and more carbonate components in the inner part of the bay. The trace metals, whose concentrations are higher at the location inside the port and at the inflow of the Rižana River (values of Fisher index, As, Cr, Cu, Nb, Ni, Zn, Zr, Th), can be of anthropogenic input. However, no significantly increased concentrations of elements were observed, which means that the pollution of trace metal is still negligible.
The total benthic foraminiferal assemblages of moderate diversity are composed of opportunistic species. Ammonia parkinsoniana, Haynesina sp., Valvulineria bradyana and the non-keel Elphidium sp. dominate, while A. tepida and Haynesina depressula are subordinate. A. tepida proved to be the most tolerant taxa, whereas H. depressula, could be considered a less tolerant species, as it benefitted from the less stressful conditions documented at the KPF1 and KPF2 sites. Infaunal life strategists fit well with fluctuations in salinity caused by river supply (KPF3) and the possible higher amount of organic matter. The general geochemical preferences of dominant species are consistent with those from other studies.
The values of the Foram-AMBI index imply good to moderate ecological quality of the studied area in the Bay of Koper, with the most polluted area near to river mouth (KPF 3). The application of the index has to be treated with caution in order to assess the ecological conditions, as more than 20% of the species present are not assigned to any ecological group. The calculated ecological quality status (EcoQS) classified KPF 1 and KPF2 stations as of high and good conditions, REF as moderate to good, and KPF3 as of moderate to bad ecological conditions for benthic foraminifera.
Data Availability Statement
The datasets presented in this study can be found in online repositories. The names of the repository/repositories and accession number(s) can be found in the article/Supplementary Material.
Author Contributions
PŽR and JV: conceptualization. PŽR, JV, VĆ, and MD: methodology. JV and MD: software. PŽR, JV, VĆ, AH, and MD: formal analysis. PŽR, JV, and VĆ: investigation. PŽR and JV: writing—original draft preparation. PŽR, VĆ, MD, and BR: writing—review and editing. MD and BR: visualization. All authors have read and agreed to the published version of the manuscript.
Funding
This research was financially supported by the Ministry of Higher Education, Science, and Technology of the Republic of Slovenia (Slovenian Research Agency); Program Group Geoenvironment and geomaterials (P1-0195) and the project record of environmental change and human impact in Holocene sediments, Gulf of Trieste (J1-1712). Sediment sampling was performed by the technical support of the National Institute of Biology, Marine Biology Station Piran. The last part of the work was executed in the frame of the Croatian Science Foundation project IP–2019–04-5775 BREEMECO. For grammar corrections we would like to thank Jeff Bickert. Many thanks also to the reviewers who make the manuscript significantly upgraded.
Conflict of Interest
The authors declare that the research was conducted in the absence of any commercial or financial relationships that could be construed as a potential conflict of interest.
Publisher’s Note
All claims expressed in this article are solely those of the authors and do not necessarily represent those of their affiliated organizations, or those of the publisher, the editors and the reviewers. Any product that may be evaluated in this article, or claim that may be made by its manufacturer, is not guaranteed or endorsed by the publisher.
Supplementary Material
The Supplementary Material for this article can be found online at: https://www.frontiersin.org/articles/10.3389/fmars.2022.812622/full#supplementary-material
References
Adami, G., Barbieri, P., Campisi, B., Predonzani, S., and Reisenhofer, E. (1996). Anthropogenic heavy metal distribution in sediments from an area exposed to industrial pollution (Harbour of Trieste, Northern Adriatic Sea). Boll. Della Soc. Adriat. Sci. 77, 5–18.
Alve, E. (1991). Benthic foraminifera in sediment cores reflecting heavy metal pollution in Sorfjord, Western Norway. J. Foraminifer. Res. 21, 1–19. doi: 10.2113/gsjfr.21.1.1
Alve, E. (1995). Benthic foraminiferal responses to estuarine pollution: a review. J. Foraminifer. Res. 25, 190–203. doi: 10.2113/gsjfr.25.3.190
Alve, E., Korsun, S., Schönfeld, J., Dijkstra, N., Golikova, E., Hess, S., et al. (2016). Foram-AMBI: a sensitivity index based on benthic foraminiferal faunas from North-East Atlantic and Arctic fjords, continental shelves and slopes. Mar. Micropaleontol. 122, 1–12. doi: 10.1016/j.marmicro.2015.11.001
Angel, D. L., Verghese, S., Lee, J. J., Saleh, A. M., Zuber, D., Lindell, D., et al. (2000). Impact of a net cage fish farm on the distribution of benthic foraminifera in the northern Gulf of Eilat (Aqaba, Red Sea). J. Foraminifer. Res. 30, 54–65. doi: 10.2113/0300054
Appiotti, F., Krželj, M., Russo, A., Feretti, M., Bastianini, M., and Marincioni, F. (2014). A multidisciplinary study on the effects of climate change in the northern Adriatic Sea and the Marche region. Reg. Environ. Change 14, 2007–2024. doi: 10.1007/s10113-013-0451-5
Aquavita, A., Predonzani, S., Mattassini, G., Rossin, P., Tamberlich, F., Falomo, J., et al. (2010). Heavy metals contents and distribution in coastal sediments of the gulf of Trieste (Northern Adriatic Sea, Italy). Water Air Soil Pollut. 211, 95–111. doi: 10.1016/j.jes.2017.12.009
Armynot du Châtelet, E., Debenay, J. P., and Soulard, R. (2004). Foraminiferal Proxies from pollution monitoring in moderately polluted harbours. Environ. Pollut. 127, 27–40. doi: 10.1016/s0269-7491(03)00256-2
Barbieri, G., Rossi, V., Vaiani, S. C., and Horton, B. P. (2019). Benthic ostracoda and foraminifera from the North Adriatic Sea (Italy, Mediterranean Sea): a proxy for the epositional characterization of river-influenced shelves. Mar. Micropaleontol. 153:101772. doi: 10.1016/j.marmicro.2019.101772
Barbieri, P., Adami, G., Predonzani, S., and Edoardo, R. (1999). Heavy metals in surface sediments near urban and industrial sewage discharges in the Gulf of Trieste. Toxicol. Environ. Chem. 71, 105–114. doi: 10.1080/02772249909358785
Bergamin, L., Romano, E., Finois, M. G., Venti, F., Bianchi, J., Colasanti, A., et al. (2009). Benthic foraminifera from the coastal zone of Baia (Naples, Italy): assemblage distribution and modification as tools from environmental characterisation. Mar. Pollut. Bull. 59, 234–244. doi: 10.1016/j.marpolbul.2009.09.015
Bergin, F., Kucuksezgin, F., Uluturham, E., Barut, I. F., Meric, E., Avsar, N., et al. (2006). The response of benthic foraminifera and ostracoda to heavy metal pollution in Gulf of Izmir (Eastern Aegean Sea). Estuar. Coast. Shelf Sci. 66, 368–386. doi: 10.1016/j.ecss.2005.09.013
Birk, S., Bonne, W., Borja, A., Brucet, S., Courrat, A., Poikane, S., et al. (2012). Three hundred ways to assess Europe’s surface water: an almost complete overview of biological methods to implement the Water Framework Directive. Ecol. Indic. 18, 31–41. doi: 10.1016/j.ecolind.2011.10.009
Borja, A., Bricker, S. B., Dauer, D. M., Demetriades, N. T., Ferreira, J. G., Forbes, A. T., et al. (2008). Overview of integrative tools and methods in assessing ecological integrity in estuarine and coastal systems worldwide. Mar. Pollut. Bull. 56, 1519–1537. doi: 10.1016/j.marpolbul.2008.07.005
Borja, A., Franco, J., and Pérez, V. (2000). A marine biotic index to establish the ecological quality of soft-bottom benthos within European estuarine and coastal environments. Mar. Pollut. Bull. 40, 1100–1114. doi: 10.1016/S0025-326X(03)00090-0
Bouchet, V. M. P., Alve, E., Rygg, B., and Telford, R. J. (2012). Benthic foraminifera provide a promising tool for ecological quality assessment of marine waters. Ecol. Indic. 23, 66–75. doi: 10.1016/j.ecolind.2012.03.011
Bouchet, V. M. P., Debenay, J.-P., Sauriau, P.-G., Radford-Knoery, J., and Soletchnik, P. (2007). Effects of short-term environmental disturbances on living benthic foraminifera during the Pacific oyster summer mortality in the Marennes-Oléron Bay (France). Mar. Environ. Res. 64, 358–383. doi: 10.1016/j.marenvres.2007.02.007
Bouchet, W. M. P., Goberville, E., and Frontalini, F. (2018). Benthic foraminifera to assess ecological quality statuses in Italian transitional waters. Ecol. Indic. 84, 130–139. doi: 10.1016/j.ecolind.2017.07.055
Brambati, A., and Catani, G. (1988). Le coste e I fondali del Golfo di Trieste dall’Isonzo a Punta Sottile: aspetti geologici, geomorfologici, sedimentologici e geotecnici. Hydrores Trieste 6, 13–28.
Burone, L., Venturini, N., Sprechmann, P., Valente, P., and Muniz, P. (2006). Foraminiferal responses to polluted sediments in the Montevideo coastal zone, Uruguay. Mar. Pollut. Bull. 52, 61–73. doi: 10.1016/j.marpolbul.2005.08.007
Cabrini, M., Fornasaro, D., Cossarini, G., Lipizer, M., and Virgilio, D. (2012). Phytoplankton temporal changes in a coastal northern Adriatic site during the last 25 years. Estuar. Coast. Shelf Sci. 115, 113–124. doi: 10.1016/j.ecss.2012.07.007
Carnahan, E. A., Hoare, A. M., Hallock, P., Lidz, B. H., and Reich, C. D. (2008). Distribution of heavy metals and foraminiferal assemblages in sediments of Biscayne Bay, Florida, USA. J. Coast. Res. 24, 159–169. doi: 10.2112/06-0666.1
Caruso, A., Cosentino, C., Tranchina, L., and Brai, M. (2011). Response of benthic foraminifera to heavy metal contamination in marine sediments (Sicilian coasts, Mediterranean Sea). Chem. Ecol. 27, 9–30. doi: 10.1080/02757540.2010.529076
Caulle, C., Mojtahid, M., Gooday, A. J., Jorissen, F. J., and Kitazato, H. (2015). Living (Rose – Bengal – stained) foraminiferal fauna along a strong bottom-water oxygen gradient on the Indian margin (Arabian Sea). Biogeosciences 12, 5005–5012. doi: 10.5194/bg-12-5005-2015
Cepak, F., and Marzi, B. (2009). Environmental impacts of the Port of Koper. Varst. Narave 22, 97–116.
Chao, A., Ma, K. H., Hsieh, T. C., and Chun-Hou, C. (2016). Introduction to Online Program Spader (Species-Richness Prediction and Diversity Estimation in R). Available Online at: https://chao.shinyapps.io/SpadeR/, version [accessed September 2016].
Chao, A., and Shen, T. J. (2003). Nonparametric estimation of Shannon’s index of diversity when there are unseen species in sample. Environ. Ecol. Stat. 10, 429–443.
Cibic, T., Acquavita, A., Aleffi, F., Bettoso, N., Blasutto, O., De Vittor, C., et al. (2008). Integrated approach to sediment pollution: a case study in the Gulf of Trieste. Mar. Pollut. Bull. 56, 1650–1667. doi: 10.1016/j.marpolbul.2008.05.009
Cimerman, F., and Langer, M. R. (1991). Mediterranean Foraminifera. Razred za Naravoslovne Vede, Classis IV: Historia Naturalis, Dela-Opera 30. Ljubljana: Slovenian academy of sciences and arts, 119.
Coccioni, R. (2000). “Benthic foraminifera as bioindicators of heavy metal pollution: a case study from the Goro Lagoon (Italy),” in Environmental Micropaleontology, ed. R. Martin (New York, NY: Kluwer Academic), 71–103. doi: 10.1007/978-1-4615-4167-7_4
Coccioni, R., Frontalini, F., Marsili, A., and Mana, D. (2009). Benthic foraminifera and trace element distribution: a case-study from the heavily polluted lagoon of Venice (Italy). Mar. Pollut. Bull. 59, 257–267. doi: 10.1016/j.marpolbul.2009.08.009
Colizza, E., Fontolan, G., and Brambati, A. (1996). Impact of a coastal disposal site for inert wastes on the physical marine environment, Barcola-Bovedo, Trieste, Italy. Environ. Geol. 27, 270–285. doi: 10.1007/bf00766697
Correggiari, A., Field, M., and Trincardi, F. (1996). “Late quaternary large dunes on the sediment-starved Adriatic shelf,” in Geology of Siliciclastic Shelf Seas, Vol. 117, eds M. De Batist and P. Jacob (London: Geological Society), 155–169. doi: 10.1144/gsl.sp.1996.117.01.09
Ćosović, V., Zavodnik, D., Borčić, A., Vidović, J., Deak, S., and Moro, A. (2011). A checklist of foraminifera of the eastern shelf of the Adriatic Sea. Zootaxa 3035, 1–56. doi: 10.11646/zootaxa.3035.1.1
Covelli, S., and Fontolan, G. (1997). Application of a normalization procedure in determining regional geochemical baselines: Gulf of Trieste, Italy. Environ. Geol. 30, 34–45. doi: 10.1007/s002540050130
Covelli, S., Fontolan, G., Faganeli, J., and Ogrinc, N. (2006). Anthropogenic markers in the Holocene stratigraphic sequence of the Gulf of Trieste (northern Adriatic Sea). Mar. Geol. 230, 23–51.
Covelli, S., Langone, L., Aquavita, A., Piani, R., and Emili, A. (2012). Historical flux of mercury associated with mining and industrial sources in the Murano and Grado Lagoon (northern Adriatic Sea). Estuar. Coast. Shelf Sci. 113, 7–19. doi: 10.1016/j.ecss.2011.12.038
Cozzi, S., Cabrini, M., Kralj, M., De Vittor, C., Celio, M., and Giani, M. (2020). Climatic and anthropogenic impacts on environmental conditions and phytoplankton community in gulf of Trieste (Northern Adriatic Sea). Water 12:2652. doi: 10.3390/w12092652
Cozzi, S., and Giani, M. (2011). River water and nutrient discharges in the northern Adriatic Sea: current importance and long-term changes. Cont. Shelf Res. 31, 1881–1893. doi: 10.1016/j.csr.2011.08.010
Cushman, J. A. (1926). The foraminifera of the Velasco shale of the Tampico Embayment. Bull. Am. Assoc. Pet. Geol. 10, 581–612. doi: 10.1306/3D932728-16B1-11D7-8645000102C1865D
Dassenakis, M., Adrianos, H., Depiazi, G., Konstantas, A., Karabela, M., Sakellari, A., et al. (2003). The use of various methods for the study of metal pollution in marine sediments, the case of Euvoikos Gulf, Greece. Appl. Geochem. 18, 781–794. doi: 10.1016/s0883-2927(02)00186-5
David, M., Gollasch, S., Cabrini, M., Perkoviè, M., Bošnjak, D., and Virgilio, D. (2007). Results from the first ballast water sampling study in the Mediterranean Sea – the Port of Koper study. Mar. Pollut. Bull. 54, 53–65. doi: 10.1016/j.marpolbul.2006.08.041
Debenay, J. P., Tsakiridis, E., Soulard, R., and Grossel, H. (2001). Factors determining the distribution of foraminiferal assemblages in Port Joinville Harbor (Ile d’Yeu, France): the influence of pollution. Mar. Micropaleontol. 43, 75–118. doi: 10.1016/s0377-8398(01)00023-8
Dimiza, M. D., Triantaphyllou, M. V., Koukousioura, O., Hallock, P., Simboura, N., Karageorgis, A. P., et al. (2016). The foram stress index: a new tool for environmental assessment of soft-bottom environments using benthic foraminifera. A case study from the Saronikos Gulf, Greece, Eastern Mediterranean. Ecol. Indic. 60, 611–621. doi: 10.1016/j.ecolind.2015.07.030
Donazzolo, R., Hieke Merlin, O., MenegazzoVitturi, I., Orio, A. A., Pavoni, B., Perin, G., et al. (1981). Heavy metal contamination in surface sediments from the Gulf of Venice, Italy. Mar. Pollut. Bull. 12, 417–425. doi: 10.1016/0025-326x(81)90160-0
Donnici, S., and Serandrei Barbero, R. (2002). The benthic foraminiferal communities of the northern Adriatic continental shelf. Mar. Micropalaeontol. 44, 93–123. doi: 10.1016/s0377-8398(01)00043-3
d’ Orbigny, A. D. (1826). Tableau méthodique de la classe des céphalopodes. Ann. Sci. Nat. 7, 245–314.
Dubois, A., Barras, C., Pavard, J.-C., Donnay, A., Béatrix, M., and Bouchet, V. M. P. (2021). Distribution patterns of benthic foraminifera in fish farming areas (Corsica, France): implications for the Implementation of benthic Indices in Biomonitoring Studies. Water 13:2821. doi: 10.3390/w13202821
El Kateb, A., Stalder, C., Martinez-Colon, M., Mateu-Vinces, G., Francescangeli, F., Coletti, G., et al. (2020). Foraminiferal-based biotic indices to assess the ecological quality status of the Gulf of Gabes (Tunisia): present limitation and future perspective. Ecol. Indic. 111:105962. doi: 10.1016/j.ecolind.2019.105962
El-Gamal, A. A., Peterson, R. N., and Burnett, W. C. (2012). Detecting freshwater inputs via groundwater discharge to Marina Lagoon, Mediterranean Coast, Egypt. Estuar. Coast. 35, 1486–1499. doi: 10.1007/s12237-012-9539-2
Faganeli, J., Planinc, R., Pezdic, J., Smodis, B., Stegnar, P., and Ogorelec, B. (1991). Marine geology of the gulf of Trieste (North Adriatic): geochemical aspects. Mar. Geol. 99, 93–108. doi: 10.1016/0025-3227(91)90085-i
Ferraro, L., Sprovieri, M., Alberico, I., Lirer, F., Prevedello, L., and Marsella, E. (2006). Benthic foraminifera and heavy metals distribution: a case study from the Naples Harbour (Tyrrhenian Sea, Southern Italy). Environ. Pollut. 142, 274–284. doi: 10.1016/j.envpol.2005.10.026
Frontalini, F., Buosi, C., Da Pelo, S., Coccioni, R., Cherchi, A., and Bucci, C. (2009). Benthic foraminifera as bio-indicators of trace element pollution in the heavily contaminated Santa Gilla Lagoon (Cagliari, Italy). Mar. Pollut. Bull. 58, 858–877. doi: 10.1016/j.marpolbul.2009.01.015
Frontalini, F., and Coccioni, R. (2008). Benthic foraminifera for heavy metal pollution monitoring: a case study from the central Adriatic Sea coast of Italy. Estuar. Coast. Shelf Sci. 76, 404–417. doi: 10.1016/j.ecss.2007.07.024
Frontalini, F., and Coccioni, R. (2011). Benthic foraminifera as bioindicators of pollution: a review of Italian research over the last three decades. Rev. Micropaléontol. 54, 115–127. doi: 10.1016/j.revmic.2011.03.001
Giani, M., Djakovac, T., Degobbis, D., Cozzi, D., Solidoro, C., and Fonda-Umani, S. (2012). Recent changes in the marine ecosystems of the northern Adriatic Sea. Estuar. Coast. Shelf Sci. 115, 1–13. doi: 10.1016/j.ecss.2012.08.023
Gooday, A. J., Lejzerowicz, F., Goineau, A., Holzmann, M., Kamenskaya, O., Kitizato, H., et al. (2021). The biodiversity and distribution of abyssal benthic foraminifera and their possible ecological roles: a synthesis across the clarion-clipperton zone. Front. Mar. Sci. 8:634726. doi: 10.3389/fmars.2021.634726
Hammer, Ø., and Harper, D. A. T. (2006). Paleontological Data Analysis. Hoboken, NJ: Wiley Online Library.
Hammer, Ø., Harper, D. A. T., and Ryan, P. D. (2001). PAST: paleontological statistics software package for education and data analysis. Palaeontol. Electronica 4, 1–9.
Hayward, B. C., Holzmann, M., Pawlowski, J., Parker, H. J., Kaushik, T., Toyofuku, M. S., et al. (2021). Molecular and morphological taxonomy of living Ammonia and related taxa (Foraminifera) and their biogeography. Micropaleontology 67, 109–313.
Heron-Allen, E., and Earland, A. (1913). Clare island survey: part 64. Foraminifera. Proc. R. Ir. Acad. 31, 1–188.
Hess, S., Alve, E., Andersen, T. J., and Joranger, T. (2020). Defining ecological reference conditions in naturally stressed environments – how difficult is it? Mar. Environ. Res. 156:104885. doi: 10.1016/j.marenvres.2020.104885
Hohenegger, J., Piller, W. E., and Baal, C. (1988). Reason for spatial micro distribution of foraminifers in an intertidal pool (northern Adriatic Sea). Mar. Ecol. 10, 43–78. doi: 10.1111/j.1439-0485.1989.tb00065.x
Hohenegger, J., Piller, W. E., and Baal, C. (1993). Horizontal and vertical spatial micro distribution of foraminifers in the shallow subtidal Gulf of Trieste, northern Adriatic Sea. J. Foraminifer. Res. 23, 79–101. doi: 10.2113/gsjfr.23.2.79
Horvat, M., Covelli, S., Faganeli, J., Logar, M., Mandic, V., and Rajar, R. (1999). Mercury in contaminated coastal environments; a case study: the Gulf of Trieste. Sci. Total Environ. 237, 43–56. doi: 10.1016/s0048-9697(99)00123-0
Jorissen, F., Nardelli, M. P., Almodgi-Labin, A., Barras, C., Bergamin, L., Bicchi, E., et al. (2018). Developing foram-AMBI for biomonitoring in the Mediterranean: species assignments to ecological categories. Mar. Micropaleontol. 140, 33–45. doi: 10.1016/j.marmicro.2017.12.006
Jorissen, F. J. (1987). The distribution of benthic foraminifera in the Adriatic Sea. Mar. Micropaleontol. 12, 21–48. doi: 10.1016/0377-8398(87)90012-0
Jorissen, F. J. (1988). Benthic foraminifera from the Adriatic Sea: principles of phenotypic variation. Utrecht Micropaleontol. Bull. 37, 1–174.
Kaminski, M. A., Aksu, A. E., Box, M., Hiscott, R. N., Filipescu, S., and Al-Salameen, M. (2002). Late glacial to holocene benthic foraminifera in the Marmara Sea: implications for Black Sea Mediterranean Sea connections following the last deglaciation. Mar. Geol. 190, 165–202. doi: 10.1016/s0025-3227(02)00347-x
Leder, N., Smirčić, A., and Vilibić, I. (1998). Extreme values of surface wave heights in the Northern Adriatic. Geofizika 15, 1–13.
Li, T., Xiang, R., and Li, T. (2013). Benthic foraminiferal assemblages and trace metals reveal the environment outside the Pearl River Estuary. Mar. Pollut. Bull. 75, 114–125. doi: 10.1016/j.marpolbul.2013.07.055
Lo Giudice Cappelli, E., and Austin, W. E. N. (2019). Size matters: analyses of benthic foraminiferal assemblages across differing size fraction. Front. Mar. Sci. 6:752. doi: 10.3389/fmars.2019.00752
Loeblich, A. R., and Tappan, H. (1987). Foraminiferal Genera and their Classification, Vol. 2. New York, NY: Van Nostrand Reinhold, 970.
Lotze, H. K., Lenihan, H. S., Bourque, B. J., Bradbury, R. H., Cooke, R. G., Kay, M. C., et al. (2006). Depletion, degradation, and recovery potential of estuaries and coastal seas. Science 312, 1806–1809. doi: 10.1126/science.1128035
Malačič, V., and Petelin, B. (2001). “Chapter 6, regional studies,” in Physical Oceanography of the Adriatic Sea, Past, Present, Future, eds B. Cushman-Roisin, M. Gačić, P. M. Poulain, and A. Artegiani (Amsterdam: Kluwer Academic), 167–181.
Martínez-Colón, M., Hallock, P., Green-Ruíz, C. R., and Smoak, J. M. (2018). Benthic foraminifera as bioindicators of potentially toxic element (PTE) pollution: torrecillas lagoon (San Juan Bay Estuary), Puerto Rico. Ecol. Indic. 89, 516–527. doi: 10.1016/j.ecolind.2017.10.045
Martins, V. A., Frontalini, F., Tramonte, K. M., Figueira, R. C., Miranda, P., Sequeira, C., et al. (2013). Assessment of the health quality of Ria de Aveiro (Portugal): heavy metals and benthic foraminifera. Mar. Pollut. Bull. 70, 18–33. doi: 10.1016/j.marpolbul.2013.02.003
Melis, R., Celio, M., Bouchet, V., Varagona, G., Bazzaro, M., Crosera, M., et al. (2019). Seasonal response of benthic foraminifera to anthropogenic pressure in two stations of the Gulf of Trieste (northern Adriatic Sea, Italy): the marine protected area of Miramare versus the Servola water sewage outfall. Mediterr. Mar. Sci. 20, 120–141.
Mladenović, A., Pogačnik, Ž, Milačič, R., Petkovšek, A., and Cepak, F. (2013). Dredger mud from the Port of Koper – civil engineering applications. Mater. Technol. 47, 353–356.
Moore, D. M., and Reynolds, R. C. Jr. (1997). X-Ray Diffraction and the Identification and Analysis of Clay Minerals, 2nd Edn. Oxford: Oxford University Press, 332–378.
Morigi, C., Jorissen, F. J., Fraticelli, S., Horton, B. P., Principi, M., Sabbatini, A., et al. (2005). Benthic foraminiferal evidence for the formation of the Holocene mud-belt and bathymetrical evolution in the central Adriatic Sea. Mar. Micropaleontol. 57, 25–49. doi: 10.1016/j.marmicro.2005.06.001
Murray, J. W. (1991). Ecology and Paleoecology of Benthic Foraminifera. London: Longman Harlow, 397.
Murray, J. W. (2006). Ecology and Applications of Benthic Foraminifera. Cambridge: Cambridge University Press, 426.
Ogorelec, B., Faganeli, J., Mušiè, M., and Èermelj, G. (1997). Reconstruction of paleoenvironment in the Bay of Koper: (Gulf of Trieste, northern Adriatic). Ann. Ser. Hist. Nat. 7, 187–200.
Ogorelec, B., Mišiè, M., and Faganeli, J. (1991). Marine geology of the Gulf of Trieste (northern Adriatic): sedimentological aspects. Mar. Geol. 99, 79–92. doi: 10.1016/0025-3227(91)90084-h
Ogorelec, B., Mišiè, M., Faganeli, J., Stegnar, P., Vrišer, B., and Vukoviè, A. (1987). Recentni sediment Koprskega zaliva. Geologija 30, 87–121.
Ogorelec, B., Mišiè, M., Šercelj, A., Cimerman, F., Faganeli, J., and Stegnar, P. (1981). Sediment seèoveljske soline (Sediment of the salt marsh of Sečovlje). Geologija 24, 179–216.
Oldfield, F., Asioli, A., Accorsi, C. A., Mercuri, A. M., Juggins, S., Langone, L., et al. (2003). A high resolution late Holocene palaeo environmental record from the central Adriatic Sea. Quat. Sci. Rev. 22, 319–342. doi: 10.1016/s0277-3791(02)00088-4
Parent, B., Hyams-Kaphzan, O., Barras, C., Lubinevsky, H., and Jorissen, F. (2021). Testing foraminiferal environmental quality indices along a well-defined organic matter gradient in the Eastern Mediterranean. Ecol. Indic. 125:107498. doi: 10.1016/j.ecolind.2021.107498
Pavšič, J., and Peckmann, J. (1996). Stratigraphy and sedimentology of the Piran flysch area (Slovenia). Annales 9, 123–138.
Placer, L., Košir, A., Popit, T., Šmuc, A., and Juvan, G. (2004). The buzet thrust fault in istria and overturned carbonate megabeds in the Eocene flysch in the Dragonja Valley (Slovenia). Geologija 47, 193–198. doi: 10.5474/geologija.2004.015
Placer, L., Vrabec, M., and Celarc, B. (2010). Osnove razumevanja tektonske zgradbe NW Dinaridov in polotoka Istre. Geologija 53, 55–86. doi: 10.5474/geologija.2010.005
Pleničar, M., Polšak, A., and Šikić, D. (1973). Osnovna Geološka karta SFRJ. L 33-88, Trst [Kartografsko gradivo]. 1:100.000. Beograd: Zvezni Geološki Zavod.
Popadić, A., Vidović, J., Ćosović, V., Medaković, D., Dolenec, M., and Felja, I. (2013). Impact evaluation of the industrial activities in the Bay of Bakar (Adriatic Sea, Croatia): recent benthic foraminifera and heavy metals. Mar. Pollut. Bull. 76, 333–348. doi: 10.1016/j.marpolbul.2013.09.039
Rogan Šmuc, N., Dolenec, M., Kramar, S., and Mladenović, A. (2018). Heavy metal signature and environmental assessment of nearshore sediments: port of Koper (Northern Adriatic Sea). Geoscience 8:398. doi: 10.3390/geosciences8110398
Romano, E., Bergamin, L., Ausili, A., Magni, M. C., and Gabellini, M. (2016). Evolution of the anthropogenic impact in the Augusta Harbor (Eastern Sicily, Italy) in the last decades: benthic foraminifera as indicator of the environmental status. Environ. Sci. Pollut. Res. 23, 10514–10528. doi: 10.1007/s11356-015-5783-x
Romano, E., Bergamin, L., Di Bella, L., Frezza, V., Pierfranceschi, G., Marassich, A., et al. (2021). Benthic foraminifera as environmental indicators in extreme environments: the marine cave of Bue Marino (Sardinia, Italy). Ecol. Indic. 120:106977. doi: 10.1016/j.ecolind.2020.106977
Romeo, R. (2009). Studio Geofisico Integrato ad Alta Risoluzione dei Depositi Marini e Della Struttura del Substrato Della Riviera di Miramare (Golfo di Trieste). Ph.D. thesis. Trieste: University of Trieste.
Rumolo, P., Salvagio Manta, D., Sprovieri, M., Coccioni, R., Ferraro, L., and Marsella, E. (2009). Heavy metals in benthic foraminifera from the highly polluted sediments of the Naples harbor (Southern Tyrrhenian Sea, Italy). Sci. Total Environ. 407, 5795–5820. doi: 10.1016/j.scitotenv.2009.06.050
Schönfeld, J., Alve, E., Geslin, E., Jorissen, F., Korsun, S., and Spezzaferri, S. (2012). The FOBIMO (foraminiferal bio-monitoring) initiative — towards a standardised protocol for soft-bottom benthic foraminiferal monitoring studies. Mar. Micropaleontol. 94, 1–13. doi: 10.1016/j.marmicro.2012.06.001
Scott, D. B., and Medioli, F. (1980). Quantitative studies of marsh foraminiferal distributions in Nova Scotia and comparison with those in other parts of the world: implications for sea-level studies. Spec. Publ. Cushman Found. Foraminifer. Res. 17, 1–58.
Sgarrella, F., and Moncharmont Zei, M. (1993). Benthic foraminifera of the Gulf of Naples (Italy): systematics and autoecology. Boll. Della Soc. Paleontol. Ital. 32, 145–264.
Sharifi, A. R., Crouda, I. W., and Austin, R. L. (1991). Benthic foraminifera as pollution indicators in Southampton Water, Southern England, UK. J. Micropaleontol. 10, 109–113. doi: 10.1144/jm.10.1.109
Slavec, P. (2012). Analiza Morfologije Morskega dna Slovenskega Morja = Slovenian Seafloor Morphology analysis. Master thesis. Ljubljana: University of Ljubljana, 58.
Solis-Weiss, V., Aleffi, F., Bettoso, N., Rossin, P., and Orel, G. (2007). The benthic macrofauna at the outfalls of the underwater sewage discharges in the Gulf of Trieste (northern Adriatic Sea). Ann. Ser. Hist. Nat. 17, 1–16.
Solis-Weiss, V., Rossin, P., Aleffi, F., Bettoso, N., Orel, G., and Vriser, B. (2001). “Gulf of Trieste: sensitivity areas using benthos and GIS techniques,” in Proceedings of the MEDCOAST 01, Hammamet.
Trincardi, F., Correggiari, A., and Roveri, M. (1994). Late-quaternary transgressive erosion and deposition in a modern continental shelf: the Adriatic semi enclosed basin. Geomar. Lett. 14, 41–51. doi: 10.1007/bf01204470
Trobec, A. (2015). Raziskave Zgradbe Sedimentnega Morskega dna v Strunjanskem Zalivu s Podpovršinskim Sonarjem = Investigation of sea Bottom Sediment Structure in the Strunjan Bay with Sub-Bottom Sonar Profiler. Master thesis. Ljubljana: University of Ljubljana, 63.
Trobec, A., Busetti, M., Zgur, F., Baradello, L., Babich, A., Cova, A., et al. (2018). Thickness of marine Holocene sediment in the Gulf of Trieste (northern Adriatic Sea). Earth Syst. Sci. Data 10, 1077–1092. doi: 10.5194/essd-10-1077-2018
Türkmen, M., Türkmen, A., and Tepe, Y. (2011). Comparison of metals in tissues of fish from Paradeniz Lagoon in the coastal area of Northern East Mediterranean. Bull. Environ. Contam. Toxicol. 87, 381–385. doi: 10.1007/s00128-011-0381-1
Vidović, J., Ćosović, V., JuraČić, M., and Petricioli, D. (2009). Impact of fish farming on foraminiferal community, Drvenik Veliki Island, Adriatic Sea, Croatia. Mar. Pollut. Bull. 58, 1297–1309. doi: 10.1016/j.marpolbul.2009.04.031
Vidović, J., Dolenec, M., Dolenec, T., Karamarko, V., and Žvab Rožič, P. (2014). Benthic foraminifera assemblages as elemental pollution bioindicator in marine sediments around fish farm (Vrgada Island, Central Adriatic, Croatia). Mar. Pollut. Bull. 83, 198–213. doi: 10.1016/j.marpolbul.2014.03.051
Vidović, J., Nawrot, R., Gallmetzer, I., Haselmair, A., Tomašových, A., Stachowitch, M., et al. (2016). Anthropogenically inducted environmental changes in the Northeastern Adriatic Sea in the last 400 years (Panzano Bay, Gulf of Trieste). Biogeosciences 13, 5965–5981. doi: 10.5194/bg-13-5965-2016
Vrabec, M., Busetti, M., Zgur, F., Facchin, L., Pelos, C., Romeo, R., et al. (2014). “Refleksijske seizmiène raziskave v slovenskem morju SLOMARTEC 2013,” in Raziskave s Podroèja Geodezije in Geofizike 2013: Zbornik del, ed. M. Kuhar (Ljubljana: Fakulteta za Gradbeništvo in Geodezijo), 97–101.
Vrabec, M., and Rožič, B. (2014). “Strukturne in sedimentološke posebnosti obalnih klifov. In: 4. slovenski geološki kongres, Ankaran, 8.-10. Oktober 2014,” in Povzetki in Ekskurzije = Abstracts and Field Trips, eds B. Rožiè, T. Verbovšek, and M. Vrabec (Ljubljana: Naravoslovnotehniška Fakulteta), 84–91.
Walton, W. R. (1952). Techniques for recognition of living foraminifera, contribution Cushman foundation. J. Foraminifer. Res. 3, 56–60.
Walker, G., and Jacob, E. (1798). “An arrangement and description of minute and rare shells,” in Essays on the Microscope. The Second Edition, with Considerable Additions and Improvements, eds G. Adams and F. Kanmacher (London: Dillon & Keating), 629–645.
Williamson, W. C. (1858). On the Recent Foraminifera of Great Britain. London: The Ray Society, 1–107.
World Register of Marine Species (2021). World Register of Marine Species. Available Online at: http://www.marinespecies.org [accessed November 8, 2021].
Wukovits, J., Oberrauch, M., Enge, A. J., and Heinz, P. (2018). The distinct roles of two intertidal foraminiferal species in phytodetrital carbon and nitrogen fluxes – results from laboratory feeding experiments. Biogeosciences 15, 6185–6198. doi: 10.5194/bg-15-6185-2018
Yanko, V., Ahmad, M., and Kaminski, M. (1998). Morphological deformities of benthic foraminiferal tests in response to pollution by heavy metals: implications for pollution monitoring. J. Foraminifer. Res. 28, 177–200.
Yanko, V., Arnold, A. J., and Parker, W. C. (1999). “Effects of marine pollution on benthic foraminifera,” in Modern Foraminifera, ed. B. K. Sen Gupta (Dordrecht: Kluwer Academic Publishers), 217–235. doi: 10.1007/0-306-48104-9_13
Yasuhara, M., Hunt, G., Breitburg, D., Tsujimoto, A., and Katsuki, K. (2012). Human-induced marine ecological degradation: micropaleontological perspectives. Ecol. Evol. 2, 3242–3268. doi: 10.1002/ece3.425
Žitnik, M., Jakomin, M., Pelicon, P., Rupnik, Z., Simèiè, J., Burdar, M., et al. (2005). Port of Koper – elemental concentration in aerosols by PIXE. X-Ray Spectrom. 34, 330–334. doi: 10.1002/xrs.828
Zuin, S., Belac, E., and Marzi, B. (2009). Life cycle assessment of ship-generated waste management of Luka Koper. Waste Manag. 29, 3036–3046. doi: 10.1016/j.wasman.2009.06.025
Keywords: marine sediments, major, minor and trace elements, benthic foraminifera, geoenvironmental assessment, Bay of Koper, Northern Adriatic
Citation: Žvab Rožič P, Vidović J, Ćosović V, Hlebec A, Rožič B and Dolenec M (2022) A Multiparametric Approach to Unravelling the Geoenvironmental Conditions in Sediments of Bay of Koper (NE Adriatic Sea): Indicators of Benthic Foraminifera and Geochemistry. Front. Mar. Sci. 9:812622. doi: 10.3389/fmars.2022.812622
Received: 10 November 2021; Accepted: 28 February 2022;
Published: 04 April 2022.
Edited by:
Martina Orlando-Bonaca, Marine Biology Station Piran, National Institute of Biology, SloveniaReviewed by:
Luciana Ferraro, Institute of Marine Science (CNR), ItalyAlessandra Asioli, Institute of Marine Science (CNR), Italy
Copyright © 2022 Žvab Rožič, Vidović, Ćosović, Hlebec, Rožič and Dolenec. This is an open-access article distributed under the terms of the Creative Commons Attribution License (CC BY). The use, distribution or reproduction in other forums is permitted, provided the original author(s) and the copyright owner(s) are credited and that the original publication in this journal is cited, in accordance with accepted academic practice. No use, distribution or reproduction is permitted which does not comply with these terms.
*Correspondence: Petra Žvab Rožič, petra.zvab@ntf.uni-lj.si