- 1Department of Marine Biotechnology, Stazione Zoologica Anton Dohrn, Naples, Italy
- 2Department of Marine Animal Conservation and Public Engagement, Stazione Zoologica Anton Dohrn, Calabria Marine Centre, Amendolara, Italy
- 3Department of Integrated Marine Ecology, Stazione Zoologica Anton Dohrn, Naples, Italy
- 4Aquatic Chemical Ecology, Biocenter, Institute of Zoology, University of Cologne, Köln, Germany
- 5Animal Ecology I, University of Bayreuth, Bayreuth, Germany
- 6Department Aquatic Ecosystem Analysis and Management, Helmholtz Centre for Environmental Research—UFZ, Magdeburg, Germany
- 7Department River Ecology, Helmholtz Centre for Environmental Research—UFZ, Magdeburg, Germany
- 8Department of Marine Biotechnology, Ischia Marine Centre, Stazione Zoologica Anton Dohrn, Punta San Pietro, Naples, Italy
- 9Department of Integrated Marine Ecology, Ischia Marine Centre, Stazione Zoologica Anton Dohrn, Punta San Pietro, Naples, Italy
- 10Ecosustainable Marine Biotechnology Department, Shimoda Marine Research Center, University of Tsukuba, Shizuoka, Japan
Communication among marine organisms are generally based on production, transmission, and interpretation of chemical cues. Volatile organic compounds (VOCs) can act as infochemicals, and ocean acidification can alter their production in the source organisms as well as the interpretation of the information they drive to target organisms. Two diatoms (Cocconeis scutellum var. parva and Diploneis sp.) and a macroalga (Ulva prolifera), all common epiphytes of Posidonia oceanica leaves, were isolated and cultured at two pH conditions (8.2 and 7.7). Their biomass was collected, and the VOCs produced upon wounding were extracted and analyzed using gas chromatography. Chemotactic reactions of invertebrates triggered by VOCs were tested using a static choice experimental arena and a flow-through flume system. Odor choice experiments were performed on several invertebrates associated with P. oceanica meadows to investigate the modification of behavioral responses due to the growth of algae in acidified environments. Complex patterns of behavioral responses were recorded after exposure to algal VOCs. This study demonstrated that a) ocean acidification alters the bouquet of VOCs released by diatoms and macroalgae and b) these compounds act as infochemicals and trigger peculiar behavioral responses in benthic invertebrates. In addition, behavioral responses are species-specific, dose-dependent, and are modified by environmental constraints. In fact, the static diffusion in choice arenas produced different responses as compared to flow-through flume systems. In conclusion, we demonstrate that in future marine environments higher CO2 concentrations (leading to a pH 7.7 by the end of this century) will modify the production of VOCs by micro- and macroalgae as well as the recognition of these infochemicals by marine invertebrates.
Introduction
Marine organisms use chemical cues to interact and communicate within environments suffused with information produced by prey, predators, competitors, and conspecifics (Brönmark and Hansson, 2000; Pohnert et al., 2007; Zupo and Maibam, 2011; Mutalipassi et al., 2019, 2021; Zupo et al., 2019). This communication has evolved from bacteria to plants and animal to be considered a biological machineries able to sense and process key information (Vos et al., 2006). The term “infochemical” refers to compounds that bring an information possibly received and interpreted by various species living in the closer environment (Vet and Dicke, 1992; Zupo et al., 2019).
Such chemical cues may play a key role in the ecology of organisms, both vertebrates, and invertebrates, associated with aquatic environments (Derby and Sorensen, 2008). Various factors influence the production of infochemicals. Local ecological conditions and their temporal variations can affect the concentration and the composition of infochemicals produced by micro- and macro-algae, and the same factors can change the response of target organisms due to alteration of the perceptive abilities of individual invertebrate species (Wyatt et al., 2014). Marine invertebrates rely upon chemical cues to communicate and find food sources, and these chemical stimuli produce complex behavioral responses of invertebrates, due to individual variability and stochastic factors that can influence the organism’s chemotaxis (Fink, 2007; Zupo et al., 2016). In addition, “odor recognition” has not evolved for feeding purposes only (Jones and Flynn, 2005) but it is adopted by various species to interact with the surrounding environment. For example it is used to detect the presence of possible predators, to avoid hazards (Maibam et al., 2014), or to identify suitable habitats (Watson and Ridal, 2004; Fink, 2007). Various infochemicals can trigger different species-specific reactions according to the receivers’ roles in the food webs (Zupo et al., 2016). Among infochemicals, volatile organic compounds (VOCs) are low molecular weight compounds with a high structural diversity in aquatic ecosystems, quickly transferred among organisms, acting as kairomones (interspecific communication), pheromones (intraspecific communication), or as activated defenses (Fink, 2007; Mutalipassi et al., 2021). VOCs derived from the wounding of diatoms are important infochemicals, especially for the vagile fauna associated with Posidonia oceanica meadows (Jüttner et al., 2010). Volatile infochemicals can be interpreted by several species as a warning signal, indicating the presence of a predator or a toxic compound, triggering an avoidance or escape reaction, or they can be interpreted as an attractive signal, indicating a food source, the presence of possible mates, or a host location (Jüttner et al., 2010; Zupo et al., 2016). The information is carried according to the characteristics and the concentration of the informational molecules and by the concentration of infochemical diffused in the water; this means that various concentrations of the same VOC may trigger contrasting reactions in target organisms (Maibam et al., 2015; Zupo et al., 2015). In addition, co-evolutionary constraints cause, as consequence, that invertebrates associated with seagrass meadow should be a) able to identify native “odors,” originally present in their specific habitat in comparison to a bouquet of various VOCs, and b) unable to identify and interpret “odors” typically produced by organisms living in different habitats (Jüttner et al., 2010).
A change in secondary metabolites produced by algae upon wounding may play a key role in shaping food webs and affecting the small-scale structure of the temporal and spatial distribution of invertebrates (Vos et al., 2006). Several organisms evolved the ability to recognize VOCs generated during the wounding (Fink, 2007), but the perception of these infochemicals could be altered in an acidified environment (Hall-Spencer et al., 2008; Munday et al., 2009, 2010; Zupo et al., 2015). The rise in atmospheric carbon dioxide, forecasted to result in a future decrease of 0.43 points of pH in the next century (Caldeira and Wickett, 2003, 2005; Gattuso and Hansson, 2011; Pachauri et al., 2014), is causing changes in the chemistry of oceans. Still, it may affect physiological processes and metabolism, influencing the production of secondary metabolites, VOCs, and other infochemicals (Poore et al., 2013). Similarly, global changes may interfere with the functionality of the chemical receptors of invertebrates (Maibam et al., 2015) and the chemotactic reactions of invertebrates, with important consequences on plant-animal interactions. These relationships may have dramatic effects on the structure of animal communities associated with key aquatic environments (Knutzen, 1981).
Metabolic changes due to high concentrations of CO2 influence plant-animal communications and may cause a misunderstanding in the interpretation of the information, as it was previously demonstrated both in benthic (Zupo et al., 2015, 2016; Mutalipassi et al., 2019) and in planktonic organisms (Havas and Rosseland, 1995; Maibam et al., 2015). In parallel, algae living in an acidified environment may modify the patterns of production of secondary metabolites (eventually playing the role of infochemicals), and this additional factor should be taken into consideration to understand “wrong” responses of target organisms (Poore et al., 2013; Jin et al., 2020). To understand these fundamental aspects within the complex ecology of the seagrass P. oceanica (L.) Delile, 1813 and the relationship among VOCs, vagile epifauna, and seawater acidification, we investigated the behavioral responses of invertebrates associated with P. oceanica meadows, exposed at current (8.2) and acidified (7.7) pH to volatile infochemicals produced by three sympatric algae, Cocconeis scutellum var. parva (Grunow) Cleve 1895, Diploneis sp. (C. G. Ehrenberg) P.T. Cleve 1894 and Ulva prolifera (O. F. Müller) J. Agardh 1883, cultured at normal (8.2) and acidified pH (7.7). The two diatoms are among the most abundant micro- epiphytes recorded on the P. oceanica leaves (Ruocco et al., 2019; Mutalipassi et al., 2020) while Ulva prolifera is a ubiquitous macroalga and it is present also as an epiphyte of P. oceanica leaves (Zupo, 2000; Piazzi et al., 2016; Zupo et al., 2016). This macroalga has been selected also because it represents an interesting model in ocean acidification studies, due to its physiological responses in higher CO2 environments (Liu et al., 2012; Xu and Gao, 2012; Zhang et al., 2012; Zupo et al., 2016) and because climate change has increased the frequency of massive proliferation of this genus in other areas of the globe, known as “green tide” (Smetacek and Zingone, 2013; Gao et al., 2016). In addition, all the chosen algae may be present in the diet of the target invertebrates selected for this experimental research (Naylor, 1955a,b; Zupo et al., 2007; Leidenberger et al., 2012).
In particular, we selected the crustacean decapod Hippolyte inermis Leach, 1816 (both adults and juveniles), the crustacean isopod Idotea balthica (Pallas, 1772; both adults and juveniles), and two mollusc species belonging to the family Rissoidae, namely Alvania lineata Risso, 1826 and Rissoa italiensis Verduin, 1985.
Rissoidae are an important taxon within the class Gastropoda, with 28 genera and 318 species. They are mainly marine and represent an important component of aquatic food webs, being nourishment for other benthic invertebrates, birds, and demersal fish (Warén, 1996). They are considered herbivore-deposit feeders, with R. italiensis typical of shallow beds at the depths of 1–3 m (Gambi et al., 1992) and A. lineata distributed at intermediate depths from 3 to 10 m (Milazzo et al., 2000) living close to the basal part of P. oceanica leaves (Russo et al., 1984).
H. inermis, a decapod crustacean living in P. oceanica, is a model organism to investigate plant-animal interactions, apoptosis, and chemical ecology issues (Zupo, 1994; Zupo et al., 2016; Mutalipassi et al., 2018). Natural populations of this shrimp undergo a process of sex reversal triggered by an apoptogenic compound produced by diatoms of the genus Cocconeis, and this mechanism has a stabilizing effect on the shrimp’s natural populations (Zupo, 2000; Zupo et al., 2014). The apoptosis of the androgenic gland induces the destruction of their testis and triggers the production of early females, increasing the reproductive fitness of the species (Zupo and Messina, 2007). It has been demonstrated that H. inermis can detect and recognize volatile compounds produced by epiphytes living in their habitat, probably due to co-evolutionary processes (Fink, 2007; Maibam et al., 2014; Zupo et al., 2016).
The euryhaline crustacean isopod I. balthica is reported from a large geographical area ranging from the Northern Atlantic and the Baltic Sea to the Mediterranean and Black Sea (Salemaa, 1985), although the specific status of the Mediterranean populations is under evaluation (Natal, 2015), and generally occurs in shallow vegetated habitats. It features an opportunistic feeding behavior, which may undergo ontogenetic, seasonal, sex-related, and geographical variations (Merilaita and Jormalainen, 2000; Böstrom and Mattila, 2005; Bell and Sotka, 2012; Leidenberger et al., 2012; Leidenberger, 2013). In the Mediterranean Sea, this species is often associated with P. oceanica litter (Dimech et al., 2006; Sturaro et al., 2010) where it plays a role in the degradation processes (Wittmann et al., 1981). However, I. balthica is able to consume live leaves, although it prefers algal material, as Ulvaceae (Lorenti and Fresi, 1982), seemingly due to lower abundance of deterrent compounds and to a higher tissue toughness compared with seagrass leaves. In idoteids, the grazing activity is facilitated by the presence of large molar processes in each mandible and of heavily chitinized structures for crushing, biting, and scraping (Naylor, 1955b). Behavioral tests were performed by comparing the reactions shown in two different choice tests, i.e., in static chambers (Zupo et al., 2016; Mutalipassi et al., 2020) and straight flow-through flume systems (Voigt and Atema, 1996; Atema et al., 2002), in order to detect various types of reactions of invertebrates both in flume conditions and in static conditions. The research aimed at checking: 1) if micro and macroalgae may change their production of VOCs in an acidified world and 2) if ocean acidification may modify the communication within species associated with the same ecosystem.
Materials and Methods
Collection, Treatment, and Rearing of Algae and Invertebrates
Seagrass leaves were collected in a meadow off Lacco Ameno d’Ischia (Gulf of Naples, Italy). Epiphytes were isolated under a stereomicroscope and thalli of U. prolifera were detached using forceps. Macroalgal thalli were transferred to sterilized Petri dishes and cultured in Guillard’s f/2 medium without silica (Sigma-Aldrich, Milan, Italy). Axenic conditions of algal cultures were weekly checked under light microscopy by examining the cultures and removing other epiphytes.
Two benthic diatoms, namely C. scutellum var. parva and Diploneis sp. were obtained from the diatom collection at Stazione Zoologica and clones were cultured in axenic cultures in multi-wells filled with Guillard’s f/2 medium with silica (Sigma-Aldrich, Milan, Italy). Micro- and macro-algae principal cultures were kept under controlled conditions (18°C, 12/12h photoperiod) in a thermostatic chamber at an average irradiance of 100 μmol photons m–2 s–1 (Raniello et al., 2007).
Ad hoc designed photo-bioreactors were used to culture C. scutellum var. parva, Diploneis sp., and U. prolifera at pH 7.7 and 8.2, in three replicates for each species and each pH, as described by Mutalipassi et al. (2019). About 35,000 cells. mm–2 of C. scutellum var. parva, Diploneis sp., and 5 grams (FW) of U. prolifera were inoculated in each photo-bioreactor according to the experimental plan. The pH of the medium remained constant during the experiments, due to an automatized system that allows small additions of CO2, immediately dissolved by the activity of a pump. The presence of a pump, as well as the high sensitivity of the used instruments, avoided water stratification and excessive additions of carbon dioxide.
Invertebrates were collected in fall. Vagile invertebrates were collected in a seagrass meadow off Lacco Ameno d’Ischia (Gulf of Naples, Italy) by horizontally towing a plankton trawl over the P. oceanica canopy. Samples were immediately sorted on boat to separate the main taxa, discarding superfluous organisms. Target species were identified in the laboratory and sorted according to the size. Organisms were reared in 2-L aerated vessels containing 1.5 L of filtered seawater and fed on epiphytised leaves of P. oceanica. Prior to starting the experiment, animals were starved 24 h to increase their food choice motivation (Moelzner and Fink, 2014), and individuals tested in acidified conditions were gradually adapted to the pH regime before to starting choice experiments (Zupo et al., 2016). Organisms used for choice tests were released after each experiment.
Water Chemistry
Water samples were collected from each photo-bioreactor every 3 days to analyze seawater carbonates (Supplementary Table 1). Salinity was measured using an electronic probe for saltwater (HI-96822, Hanna Instruments, Woonsocket, Rhode Island, United States); pHNBS and total alkalinity were measured using a mini-titrator water analyzer (HI-84531-02, Hanna Instruments). Pump and pH probe calibrations were performed using a pump calibration standard (HI-84531-55) and three calibration solutions (pH 4.01, 7.01, and 8.30). Both the salinity probe and the mini-titrator are provided with a temperature compensating feature. Water samples were analyzed within 1 h after the sampling. The partial pressure of carbon dioxide was elaborated using the CO2Sys EXCEL Macro (Pierrot et al., 2006).
Analysis of Volatile Organic Compounds
On the 16th day of culture, diatoms were collected by scraping the internal surface of photo-bioreactors using a steel blade. The material collected in each photo-bioreactor was washed out using 100 ml of filtered seawater and transferred into a clean Erlenmeyer flask. The biomass collected from each photo-bioreactor was then centrifuged (10 min at 1,500 g at 4°C), and the supernatant was discharged. The vessels containing the diatom biomass were weighed and immediately frozen (–20°C) up to their transfer for VOCs extraction and chromatographic analyses.
Macroalgae, as well, at the end of the grow-out period (16 days) were collected by clean forceps, mopped on paper and their fresh weight was recorded prior to be transferred into 50 ml tubes to be frozen (–20°C) until their use for VOCs extraction and chromatographic analyses as described by Moelzner and Fink (2014). To simulate wounding and production of odors, 80, 70, and 200 mg (dry mass) of Diploneis sp., C. scutellum var. parva, and U. prolifera, respectively, for each pH condition, were transferred into 100 ml flasks and activated by thawing them in 25% NaCl (Fink et al., 2006a). The cell crushing led to the activation of a lipoxygenase cascade and the production of VOCs (Jüttner, 2005). Closed-loop stripping (45 min at 22°C) was used to concentrate VOCs and samples were then absorbed on a cartridge filled with 150 mg of Tenax TA VOC adsorbent (Supelco, Bellefonte, PA, United States) as described by Jüttner (1988). For later use in the behavioral assays, the Tenax cartridges were eluted with 6 mL of diethyl-ether as described by Fink et al. (2006b); the eluates were collected in glass tubes, and the ether was gently evaporated in a stream of nitrogen gas (N2, grade 5.0) and the residue was re-dissolved in 100 microliters of pure ethanol. Control samples were obtained using a similar procedure applied to filtered seawater (Moelzner and Fink, 2014). Sodium chloride and all solvents were of analytical grade, and they were obtained from VWR, Darmstadt, Germany. The produced VOC solutions were stored at –80°C. For the VOC analyses, separate VOC extractions were thermally desorbed from Tenax TA at 240°C with helium as carrier gas (15 ml/min) and transferred in split injection mode onto a capillary column (DB 1301, 30 m length, 0.25 mm i.d., 0.25 μm film thickness; J&W Scientific, Folsom, CA, United States) of an Agilent 7890A gas chromatograph combined with an Agilent 5975C single-quadrupole mass spectrometer (Agilent, Waldbronn, Germany). Helium was used as transfer and carrier gas. Volatile Organic algal compounds were separated and analyzed with 3-hexanone as an internal standard as described by Moelzner and Fink (2014). All substances that appeared in each run of one algal treatment and did not appear in the analytical blank measurements were listed if mass-spectra and retention times were consistent (Supplementary Tables 4–6). The peak areas of the internal standard do not appear in the table of detected compounds. Peak areas of each substance were normalized to the internal standard to correct for variances in trapping material and desorption. Unfortunately to technical problems with the thermo-desorption unit, one sample each of U. prolifera and of C. scutellum var. parva was lost. Differences in volatile composition were plotted in a non-metric multidimensional scaling (NMDS) based on Bray-Curtis dissimilarities (Minchin, 1987) utilizing the R, version 4.0.5 (R Core Team, 2019) package vegan version 2.5.7 (Oksanen et al., 2015).
Preparation of Agarose Gels
Agarose gels for the static chamber experiments (Table 1) were prepared by dissolving 1.2 g of agarose (Sigma A-9045) in 200 mL of filtered and sterilized seawater to obtain a 0.06% agarose gel. The agarose solution was heated (80°C), mixed until transparent, and small quantities of 0.1 M NaOH and 0.1 M of HCl were added to adjust the pH to a value of 8.2 and 7.7, respectively, according to the treatment.
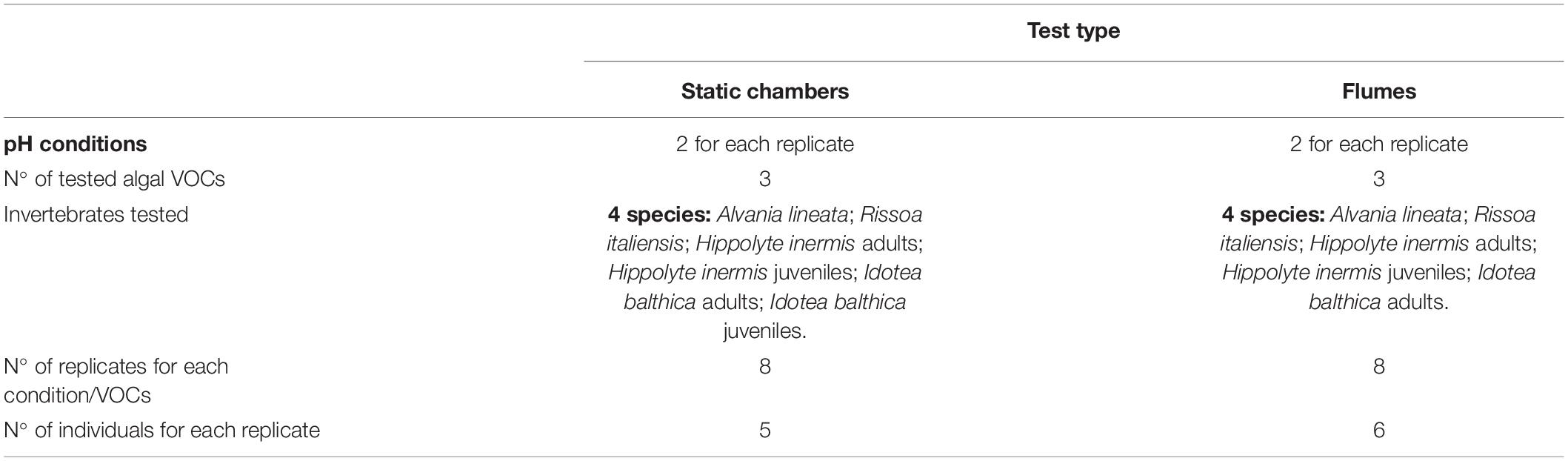
Table 1. Experimental plan of behavioral tests for the three algae and four species considered. The number of replicates, treatments, and pH levels are shown in the table.
Control and VOC gels were prepared by including 250, 25, and 2.5 μL of, respectively, control VOCs and algal VOCs ethanol solution. Algal VOCs and control VOCs were poured into the agar solutions at room temperature, just before gelling (Zupo et al., 2016; Mutalipassi et al., 2020), and allowed to gel in a refrigerator at 5°C for 2 h prior to be used for the bioassays. Gels were cut into blocks of 0.5 cm3 using clean glass coverslips, before presenting them in the static choice experimental arenas (Jüttner et al., 2010). Thus, volumes of 1, 0.1, and 0.01 μL of VOCs-solution were transferred via an agar block into a 200 mL medium (Zupo et al., 2015).
For the flume experiments (Table 1), three solutions were prepared for each micro- and macro-alga and each pH condition of cultures. We used, for each species and condition, three doses of VOCs to obtain a comparable volume/volume ratio, in the testing media, as compared to the ones adopted in static chamber experiments, considering that laminar flows are at least unperturbed in the testing area (Voigt and Atema, 1996; Mueller et al., 2014; Jutfelt et al., 2017).
These three concentrations, named “low,” “medium” and “high,” respectively, were chosen to simulate the VOCs released by diatoms or macroalgae growing on 5, 50, and 500 mm2 of Posidonia leaf, respectively (Maibam et al., 2014; Mutalipassi et al., 2020). The three surfaces taken into consideration correspond to the surface that a small grazer, a large grazer, and a herbivorous fish might wound, respectively (Buia et al., 1992, 2000; Maibam et al., 2014; Mutalipassi et al., 2020).
Tests on Invertebrates
Odor choice experiments were performed to test the effect of plant bouquets of odors on the crustaceans H. inermis (adult and juvenile individuals), I. balthica (adults and juveniles), and on adults of the mollusks A. lineata and R. italiensis. As aforementioned, two different tests were performed (Table 1) in a static choice experimental arena (Jüttner et al., 2010) and a dynamic flow-through flume system (Atema et al., 2002). In both test systems, the behavioral responses of invertebrates were assayed at ambient (pH 8.2) and acidified (pH 7.7) seawater pH conditions.
Static Choice Experimental Arena
Experimental arenas were set into Petri dishes (14 cm diameter) each containing 200 mL of filtered seawater (approx. 1 cm height of water in each Petri dish) to reduce the chance of introducing extraneous odors. Eight replicates for each treatment were performed in a room at 18°C with a shaded light source, to minimize phototactic reactions. The surface of Petri dishes was divided into five sectors (Figure 1A) corresponding to a VOC gradient as previously described (Jüttner et al., 2010; Zupo et al., 2016; Mutalipassi et al., 2020). At the start of an experimental trial, five individuals were positioned at the center of each arena. The chemotactic reactions of tested individuals were recorded four times, every 5 min (Jüttner et al., 2010), by visually checking and recording the number of individuals present in each sector. Eight independent replicate assays were conducted for each combination of algal species (3), algal culture pH condition (2), invertebrate (6), and invertebrate test pH (2).
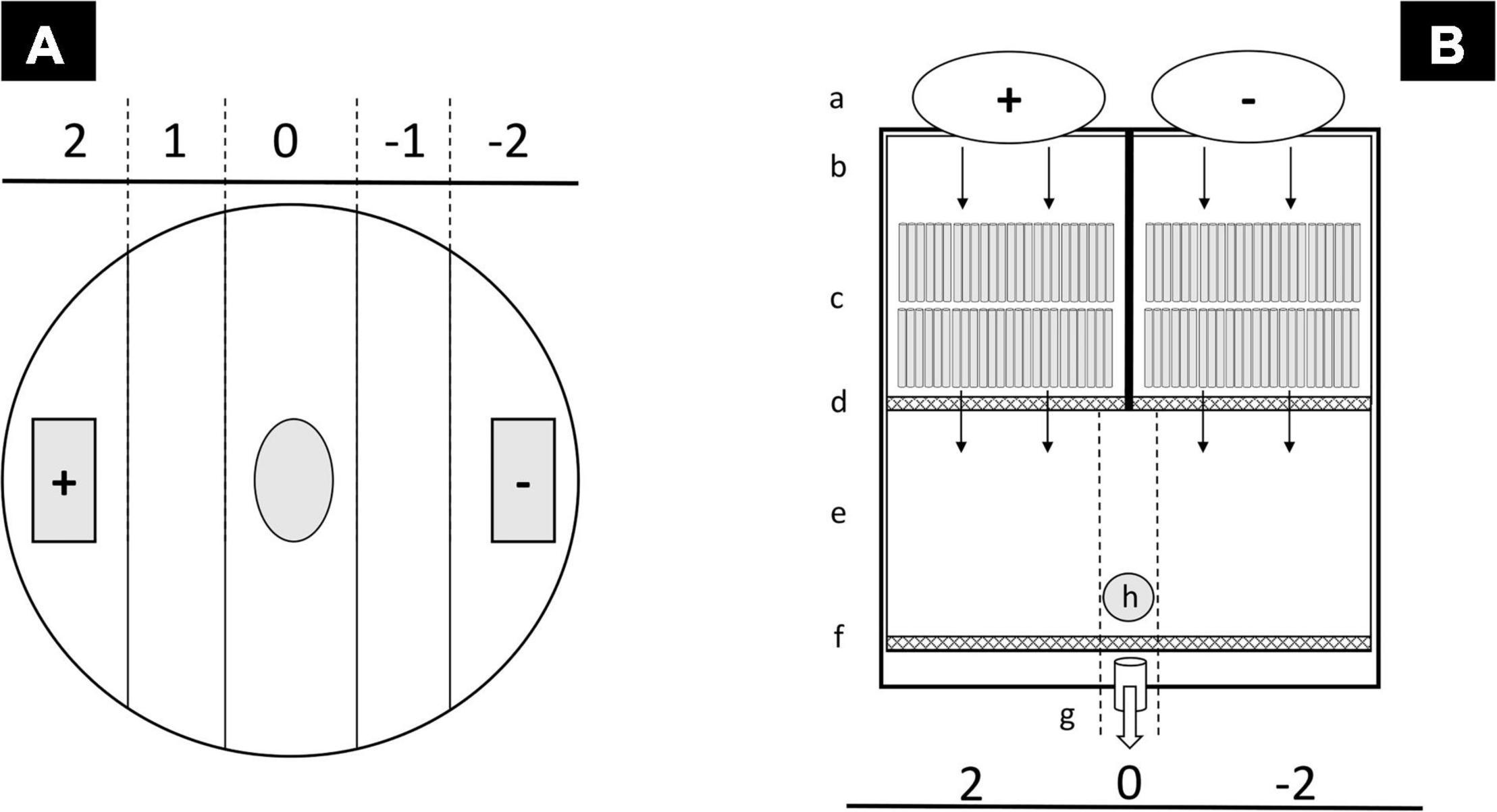
Figure 1. Schematic representation of static choice experimental arena (A) and flow-through flume system (B). In static choice experimental arena (A), volatile organic compound (VOC)-supplemented agarose was positioned in the (+) square, while the control agarose was positioned in the (–) square. Number of individuals in each sector was recorded at each timepoint. Individuals were positioned in the center of the “0” area. In flow-through flume system (B), (VOC)-supplemented solution (a) was dropped in water inflow compartments (b); fixed straws reduce the turbulence of the flux (c); two fine mesh (0.5 mm) nets (d and f) contain animals in test arena (e). A pipe allows the flux of water to be drained (g). Individuals were positioned in the center of the test area (h).
Flow-Through Flume System
Linear flow-through flumes (Voigt and Atema, 1996; Atema et al., 2002; Jutfelt et al., 2017) were used to test the effect of VOCs dissolved into a low-speed water flow. Flume systems were built using gray glass to avoid the influences of light on behavioral choices (Figure 1B). They were divided by two grids (0.1 mesh) in a straws area, an experimental arena, and an overflow area (Figure 1B). Experimental arenas had a length of 8 by 14 cm (width). The water level was 3 cm, and the total volume was 900 mL. The result was a non-mixing flow of water. It contained on a side the algal VOCs and on the opposite side the control VOCs (Kroon, 2011). Two storage tanks were filled with 200 mL of the above-described VOCs and the control solutions respectively. They were positioned on the two opposite corners of the straw areas, and water streams were kept divided and parallel by using plastic straws fixed over the bottom. The liquid was discharged by an overflow placed at the other side of the flumes. The velocity of the liquid flow was 5 cm min–1; at this speed, the containers were emptied within 5 min after the start of each test. Invertebrates were free to move and choose one of the two sides of the container (VOCs or control). Six individuals, for each species, age, and for each treatment were tested in each of eight replicates (Table 1). Just before the start of each test, the VOCs containers were filled up with either the appropriate VOCs (+) or the control (–) stock solutions. At the start of experiments, test animals were placed in a central rectangular starting zone. The number of individuals present on each side of the experimental arena was recorded at 2, 3, 4, and 5 min. As above, we conducted eight independently replicated assays for each combination of algal species (3), algal culture pH condition (2), invertebrate (6) and invertebrate test pH (2).
Statistical Analyses of Behavioral Assays
The significance of differences in the distribution of individuals between the positive (VOC areas) and negative (control areas) in 8 trials for each assay (according to species and pH regimes) was tested by means of repeated-measures ANOVA using Prism 7 (GraphPad software; summary of results available in Supplementary Table 2). In each sector of the arenas, the mean number of individuals was calculated, for each assay, at each of four experimental times. An attraction-repulsion (A/R) index was calculated for each test according to the following equations (Jüttner et al., 2010):
Equation (1) was adapted for flow-through flume system:
Where N2, N–2, N1, N–1 are the number of individuals in sector +2, –2, +1, –1, respectively (the latter two only in static arenas).
The attraction-repulsion indices obtained for each time point (i.e., after 0, 5, 10, 15, and 20 min) were used to calculate a linear regression coefficient for each test; the slope of each equation indicates the tendency of a given species to move toward positive, negative, or neutral areas. The slopes of linear regressions indicate the tendency to move toward a target, not the time spent by individuals in each sector. In addition, to determine sector preferences during the experimental time, we computed a second index, called “integral index” (INT), adopting the following equation (Jüttner et al., 2010):
where are the attraction-repulsion index for each time-lapse. A positive integral index indicates a longer permanence in the positive sectors, a negative value a higher permanence in negative sectors, values close to 0 indicate that individuals were not moving, or they showed random movements in the two directions.
The scores obtained for the attraction-repulsion indices and for the integral index, for each test, were organized in an orthogonal coordinate system to summarize the results. Species with positive integral index and negative slope (first sector) show a preference for the volatile organic compound but a tendency to move toward the control; species in the second sector, with both positive values, were attracted by the odor and were moving toward the positive zones. Species in the third sector (positive slope, negative INT) were moving toward the VOCs source, but remained most of the time in the negative sectors; finally, clusters in the fourth sector indicate a high repulsive tendency (negative slope, negative INT). The scores obtained for the attraction-repulsion indices, for each time and target organism, has been analyzed using TWO ways ANOVA with Tukey’s multiple comparisons test to identify significance of differences among behavioral responses to algae odor bouquets at each concentration and cultured condition (resume available in Supplementary Table 3).
Results
Impact of Seawater pH on Algal Volatile Organic Compounds Bouquets
The NMDS ordination (based on Bray-Curtis dissimilarities of normalized peak areas) gave a clear indication of consistent alterations in the algal VOC bouquets with seawater pH levels during growth (Figures 2–4). We were able to separately quantify 28 distinct VOC peaks for U. prolifera (Figure 2), 36 for Diploneis sp. (Figure 3), and 31 for C. scutellum var. parva (Figure 4). The loss of one replicate analysis of both U. prolifera and C. scutellum var. parva (each at pH 8.2) prevented a quantitative statistical analysis of the VOC bouquets. Due to a lack of authentic reference compounds, no unequivocal identification of those algal VOCs was possible beyond a preliminary assignment (Supplementary Tables 4–6) that requires confirmation in further studies. Nevertheless, our analyses give clear indications for altered VOC bouquets within the same algal isolates due to simulated seawater acidification (Figures 2–4).
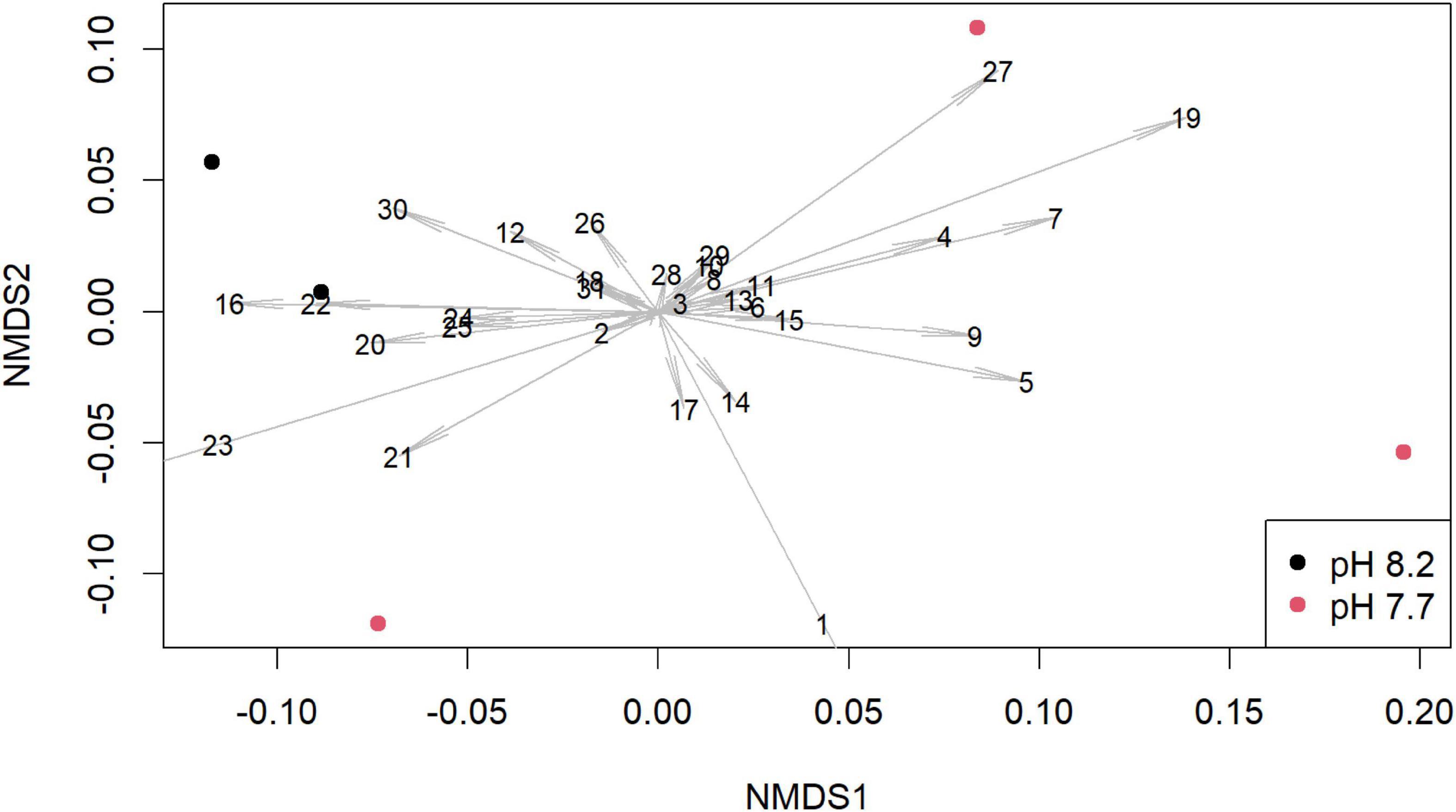
Figure 2. Non-metric multidimensional scaling (NMDS) plot of the abundance of volatile organic compounds (VOCs) normalized to internal standard from Cocconeis scuteullum var. parva depending on culture pH level. Dots indicate independently replicate and separately analyzed cultures of the same algal isolate, distances between dots indicate Bray-Curtis dissimilarities, arrow lengths indicate the contribution of the respective peak to the dissimilarity, numbers indicate the peak-identities (see Supplementary Table 4).
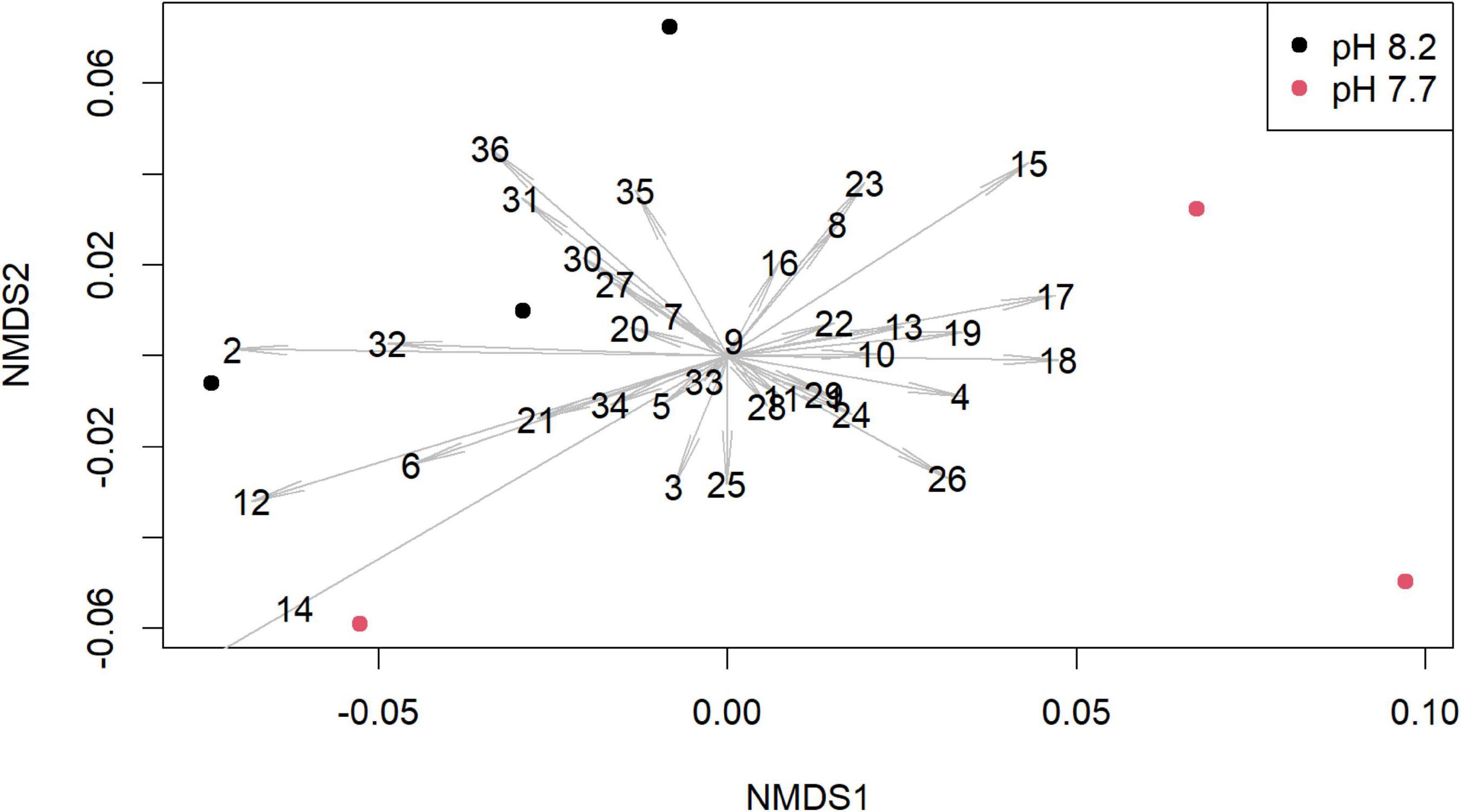
Figure 3. Non-metric multidimensional scaling (NMDS) plot of the abundance of volatile organic compounds (VOCs) normalized to internal standard from Diploneis sp. depending on culture pH level. Dots indicate independently replicate and separately analyzed cultures of the same algal isolate, distances between dots indicate Bray-Curtis dissimilarities, arrow lengths indicate the contribution of the respective peak to the dissimilarity, numbers indicate the peak-identities (see Supplementary Table 5).
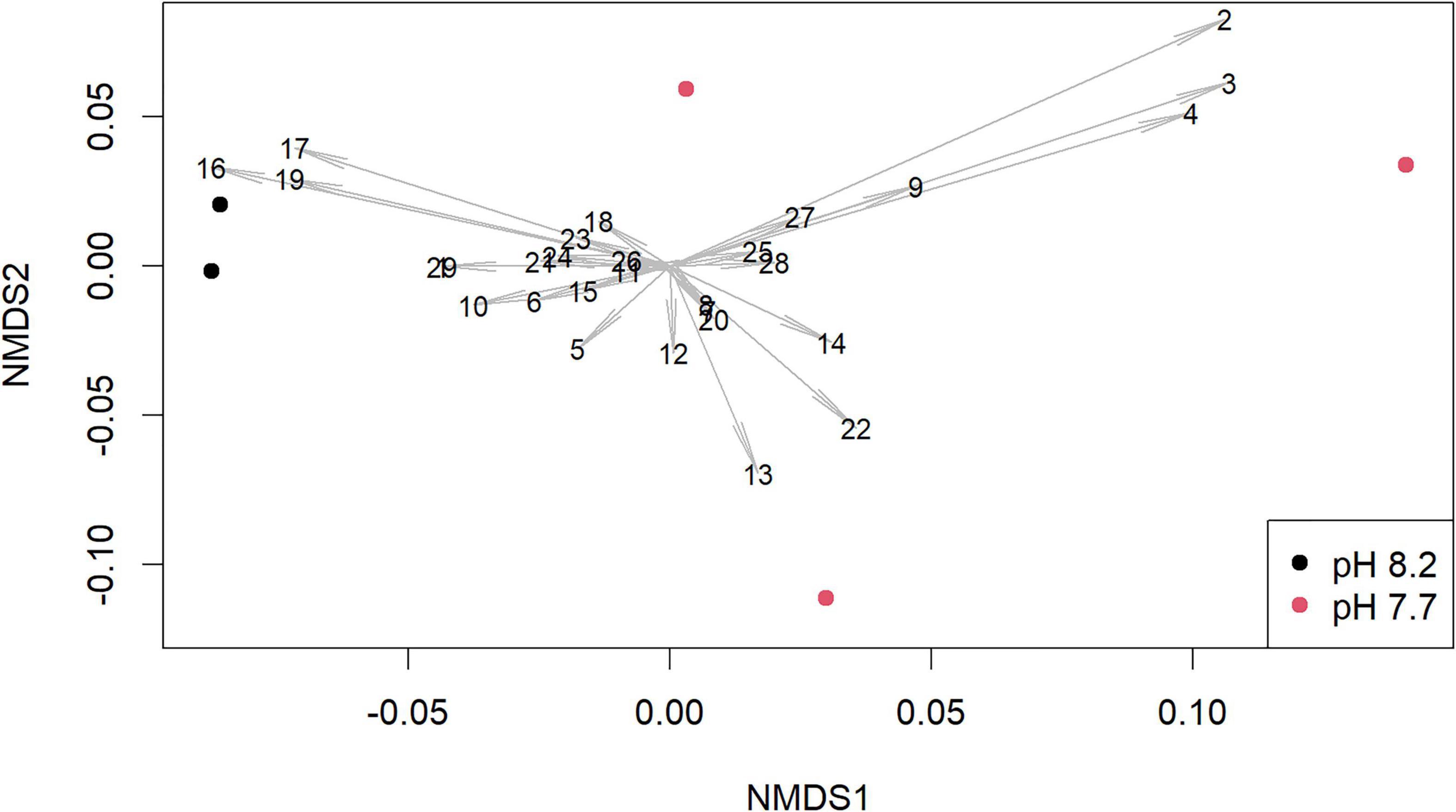
Figure 4. Non-metric multidimensional scaling (NMDS) plot of the abundance of volatile organic compounds (VOCs) normalized to internal standard from Ulva prolifera depending on culture pH level. Dots indicate independently replicate and separately analyzed cultures of the same algal isolate, distances between dots indicate Bray-Curtis dissimilarities, arrow lengths indicate the contribution of the respective peak to the dissimilarity, numbers indicate the peak-identities (see Supplementary Table 6).
Odor Choice Experiments
A complex pattern of chemotactic reactions was shown by the considered invertebrates according to algal species and pH conditions. In addition, in some cases, the behavioral reactions to VOCs differed in static arenas and flow-through flumes.
A. lineata was able to identify various odor bouquets produced by tested algae at different concentration and culture methods. In static chambers, they identified the infochemicals produced by C. scutellum var. parva and U. prolifera cultured at normal pH and administered at low, medium and high concentrations (One-way ANOVA; Supplementary Table 2). In the same conditions, they were able to identify VOCs produced by C. scutellum and U. prolifera at medium concentration and acidified culture condition, and by Diploneis only at medium concentration and normal pH condition. In flumes, Alvania was able to identify VOCs produced by Ulva, Cocconeis and Diploneis cultured at various conditions (Figure 5; One-way ANOVA; Supplementary Table 2). Alvania individuals were attracted by C. scutellum var. parva VOCs (cultured at normal pH) and administered at low and medium concentrations, (CLN, CMN; Figure 5). Contrasting responses to high concentrations of C. scutellum var. parva VOCs (normal conditions, CHN) were observed, with an attraction in static chambers and a repulsion in flumes. Medium and high concentrations of U. prolifera VOCs produced a repulsion in both test chambers at pH 8.2 (UMN, UHN) while the same species cultured and administered at pH 7.7, triggered attraction at medium concentration of VOCs (UMA) in static chamber and, in flow-through flumes, attraction at low concentration (ULA) and repulsion at high concentration (UHA; Figure 5). In addition, differences in static chambers and flumes were observed in the reactions to VOCs produced at normal and acidified conditions by C. scutellum var. parva and U. prolifera. In the static chambers (Figure 5A), A. lineata showed a tendency to move through low concentrations of VOCs produced by Diploneis sp., cultured at pH 8.2 (DLN), and C. scutellum var. parva (CLN), in both testing conditions. Differences in the position of individuals in response to the combination of various odor bouquets have been demonstrated (Tukey’s post hoc; Supplementary Table 3). In particular, reactions to medium concentration of VOCs of C. scutellum at pH 7.7 (CMA) were statistically different from reactions triggered by VOCs of C. scutellum cultured at pH 8.2 (CLN, CMN, CHN), in static chamber. On the contrary, in flumes, these differences were confirmed only in the combination of C. scutellum at medium concentration, at acidified pH (CMA) vs. C. scutellum at low concentration at normal pH (CLN). Diploneis sp. in flow-through testing chamber triggers no differences in the position of individuals except for the combination between medium concentration at normal pH (DMN) vs. high concentration at acidified pH (DHA). The repulsive behavior of Alvania lineata individuals vs. VOCs of U. prolifera especially when cultured at normal pH, were confirmed by Tukey’s post hoc (Supplementary Table 3).
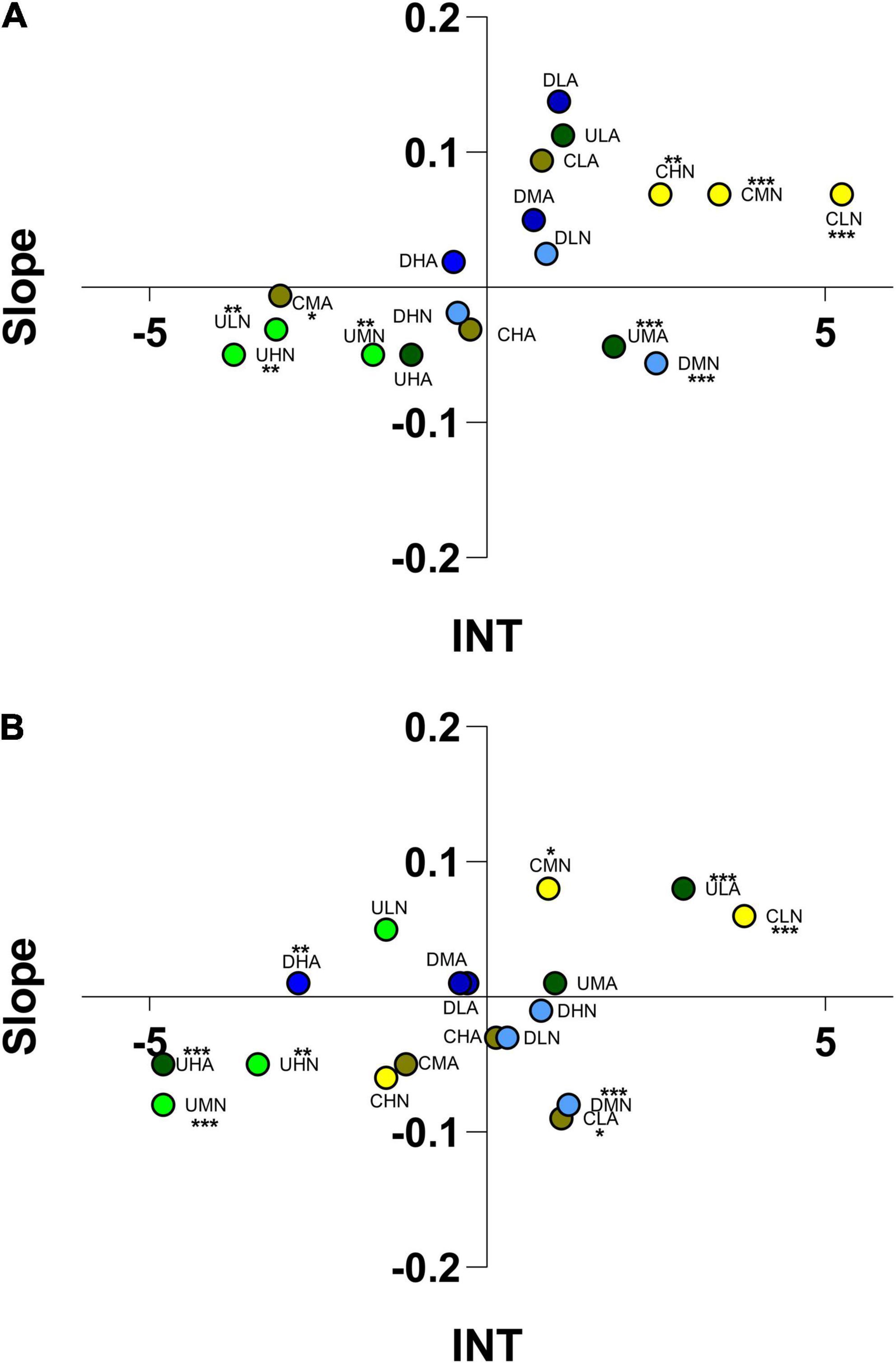
Figure 5. Ordination into 1 of 4 sectors of Alvania lineata according to the scores reached for “Slope” and “INT” (integral time) in static arenas (A) and flow-through flumes (B). The letters indicate the algae considered (C, Cocconeis scutellum var. parva; D, Diploneis sp.; U, Ulva prolifera), the concentration of VOCs (L, low; M, medium; H, high), and the culture and test conditions (N, normal conditions; A, acidified conditions). Asterisks indicate the significance of individuals’ position in the space.
In R. italiensis, an attractive behavior toward acidified C. scutellum var. parva and Diploneis sp. VOCs was observed in both static chamber and flume. The position of Rissoa italiensis individuals triggered by VOCs of C. scutellum cultured at pH 7.7 (CLA, CMA, CHA) is statistically different by the behaviors triggered by VOCs of the same algae cultured at normal pH (Tukey’s post hoc; Supplementary Table 3), that was ignored in static chambers (One-way ANOVA: Supplementary Table 2) and triggered repulsion at high and medium concentration (CMN, CHN) in flow-through flumes (Figure 6; Tukey’s post hoc; Supplementary Table 3). Rissoa italiensis exhibited significant reactions to the VOCs of C. scutellum (One-way ANOVA; Supplementary Table 2) as well as to the VOCs produced by Diploneis sp. at ambient pH, that triggered repulsion, both in static chambers and flumes. In addition, acidified Diploneis sp. VOCs triggered attraction in static chambers and, at medium concentration, in flow-through flumes (Figure 6). Differences between position of individuals triggered by Diploneis sp. cultured at normal pH and acidified pH were statistically significant, and these differences are more evident in static chamber, where tested individuals were able to identify (One-way ANOVA; Supplementary Table 2) but also to react specifically to VOCs administered at all concentrations and pH (Tukey’s post hoc; Supplementary Table 3). Rissoa italiensis was repelled by U. prolifera VOCs in normal pH conditions at high concentration (UHN), in both odor choice tests, and was slightly attracted by high concentrations of VOCs of U. prolifera cultured in acidified medium (UHA), in flumes. The responses to VOCs of U. prolifera cultured in normal pH conditions increased linearly in flumes: at low concentration of VOCs (ULN), R. italiensis spent most of the experimental time in positive sectors, at medium concentration (UMN), the source of the odor was about to be ignored, and at high concentrations (UHN) a repulsion behavior was observed. Statistics confirmed that U. prolifera cultured at normal pH and administered at high concentration (UHN) produced a pattern of responses that is statistically different from the responses produced by organisms to the other odor bouquets produced by the same macroalga (Tukey’s post hoc; Supplementary Table 3).
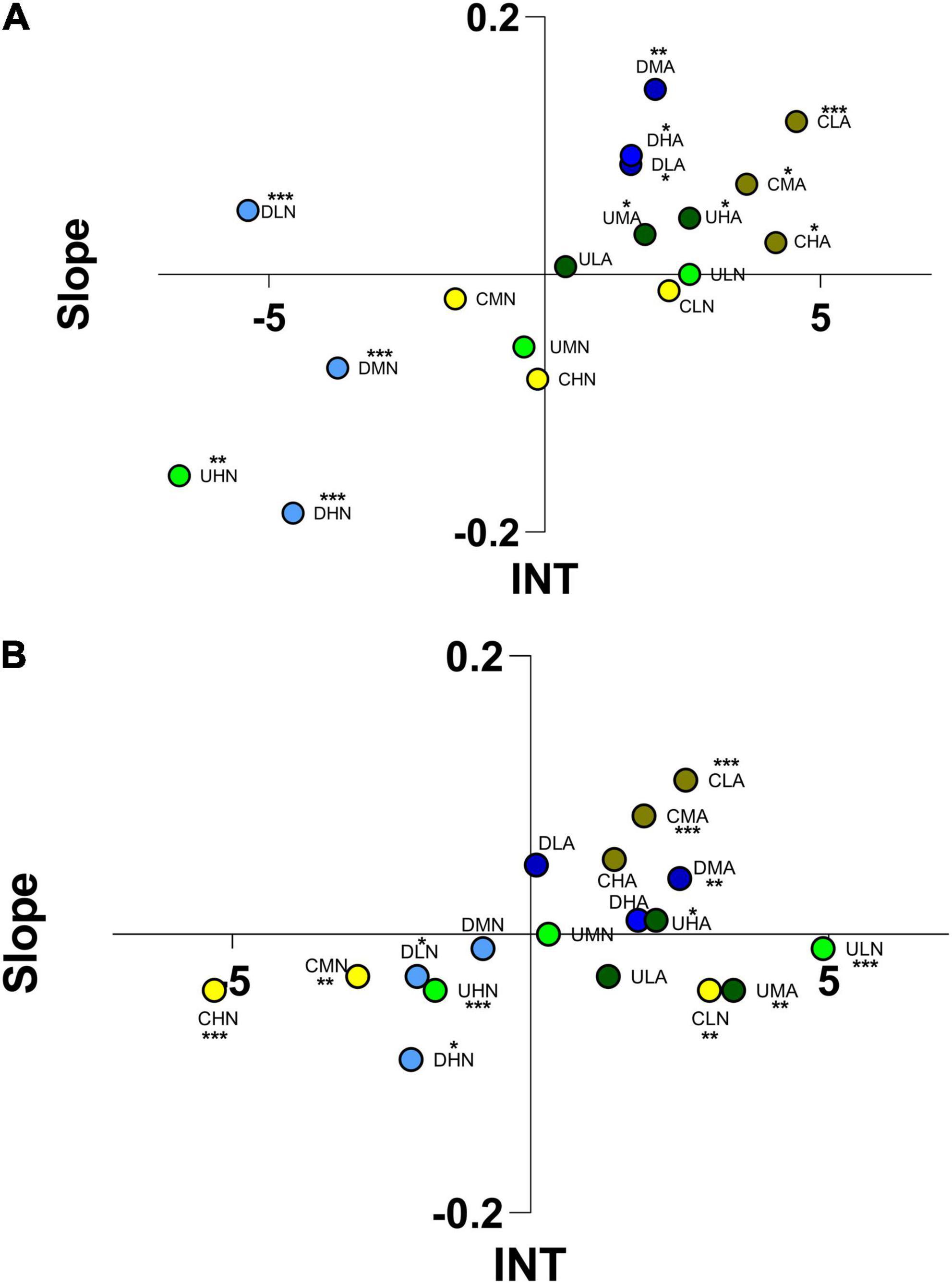
Figure 6. Ordination into 1 of 4 sectors of Rissoa italiensis according to the scores reached for “Slope” and “INT” (integral time) in static arenas (A) and flow-through flumes (B). The letters indicate the algae considered (C, Cocconeis scutellum var. parva; D, Diploneis sp.; U, Ulva prolifera), the concentration of VOCs (L, low; M, medium; H, high), and the culture and test conditions (N, normal conditions; A, acidified conditions). Asterisks indicate the significance of individuals’ position in the space.
Adult individuals of H. inermis were attracted, with differences between the two odor choice methods, by low concentrations of VOCs produced by Diploneis sp. (acidified conditions; DLA) and U. prolifera (both pH and test conditions; ULN, ULA) and repelled by higher concentrations of VOCs produced by the same algae (DHN, DHA, UHN, UHA, UMA) as well as by VOCs produced by C. scutellum var. parva (CHN, CMA, CHA in static chambers; CLN, CMN, CHN, CLA, CMA, CHA in flumes; Figure 7). Adults of H. inermis were able to identify VOCs produced by Cocconeis species at different concentrations, especially in flumes (One-way ANOVA; Supplementary Table 2), and different patterns of repulsive reactions were exhibited in response to VOCs produced in acidified environments vs. ambient pH ones (Tukey’s post hoc; Supplementary Table 3). Low concentration of VOCs of U. prolifera (ULN, ULA) produced a statistically significant pattern of reactions if compared to high concentration of VOCs produced at normal and acidified pH and to medium concentration VOCs produced at pH 7.7 by the same macroalga (Tukey’s post hoc; Supplementary Table 3).
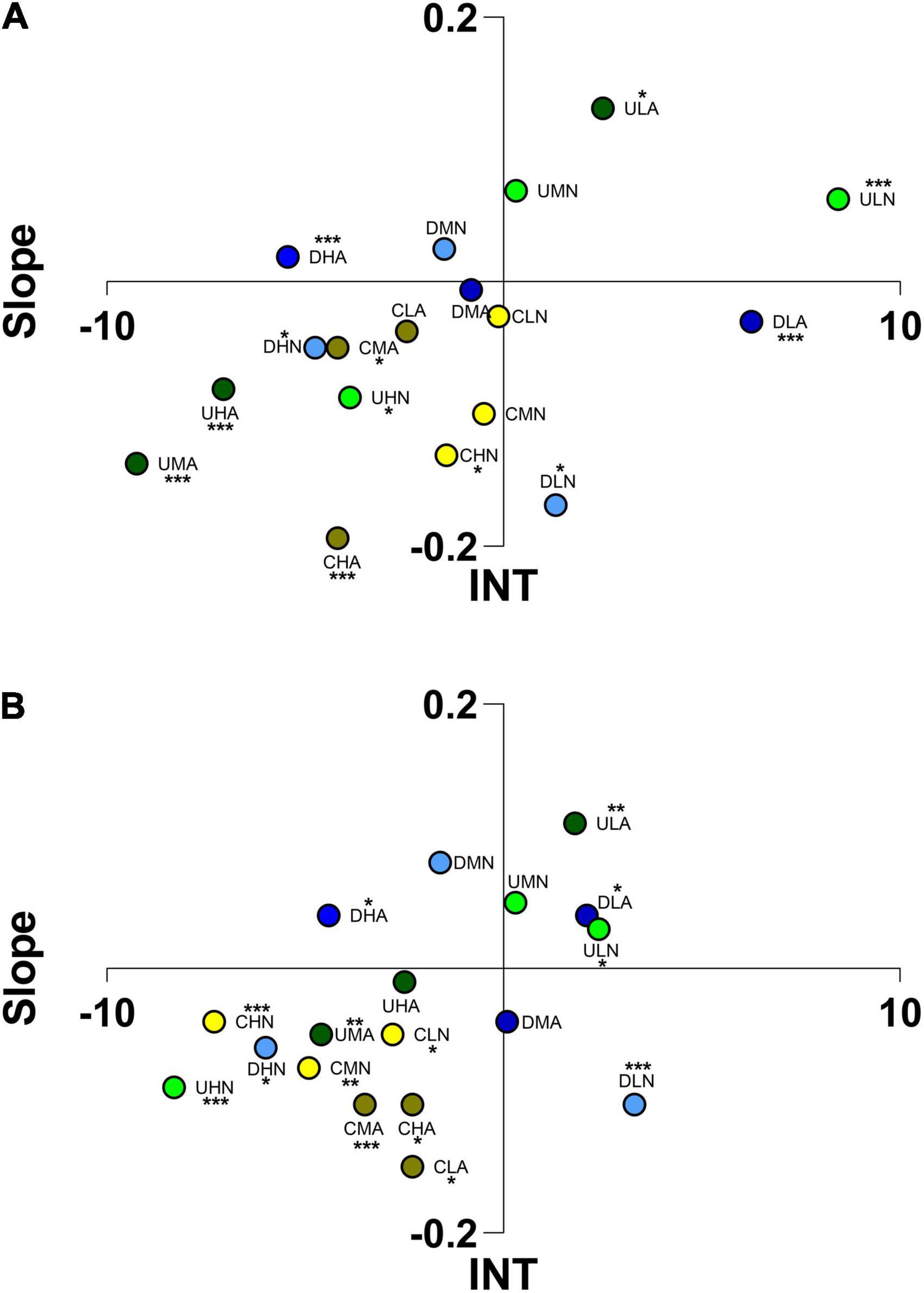
Figure 7. Ordination into 1 of 4 sectors of Hippolyte inermis adults according to the scores reached for “Slope” and “INT” (integral time) in static arenas (A) and flow-through flumes (B). The letters indicate the algae considered (C, Cocconeis scutellum var. parva; D, Diploneis sp.; U, Ulva prolifera), the concentration of VOCs (L, low; M, medium; H, high), and the culture and test conditions (N, normal conditions; A, acidified conditions). Asterisks indicate the significance of individuals’ position in the space.
In contrast, behavioral reactions shown by juveniles of H. inermis were different: juveniles were attracted by C. scutellum var. parva at low and medium concentrations (CLN, CMN, CLA) and by Diploneis sp. (DLN; Figure 8) both in static chambers and flumes, with significant differences in the distribution of individuals compared with responses triggered by odor bouquets administered at different concentration or culture conditions (Tukey’s post hoc; Supplementary Table 3). Individuals spent most of the time in positive sectors of the choice arenas at low concentrations of U. prolifera VOCs (ULN) that was statistically different compared with the responses triggered by other U. prolifera odor bouquets (Tukey’s post hoc; Supplementary Table 3). These odor bouquets, at the administered concentrations, were identified by juveniles of this target species (One-way ANOVA, S2). High concentrations of VOCs of the three algae, at both culture conditions, as well as Diploneis sp. and U. prolifera at the medium concentration (ambient and acidified pH) produced a repulsive response in both odor choice chambers.
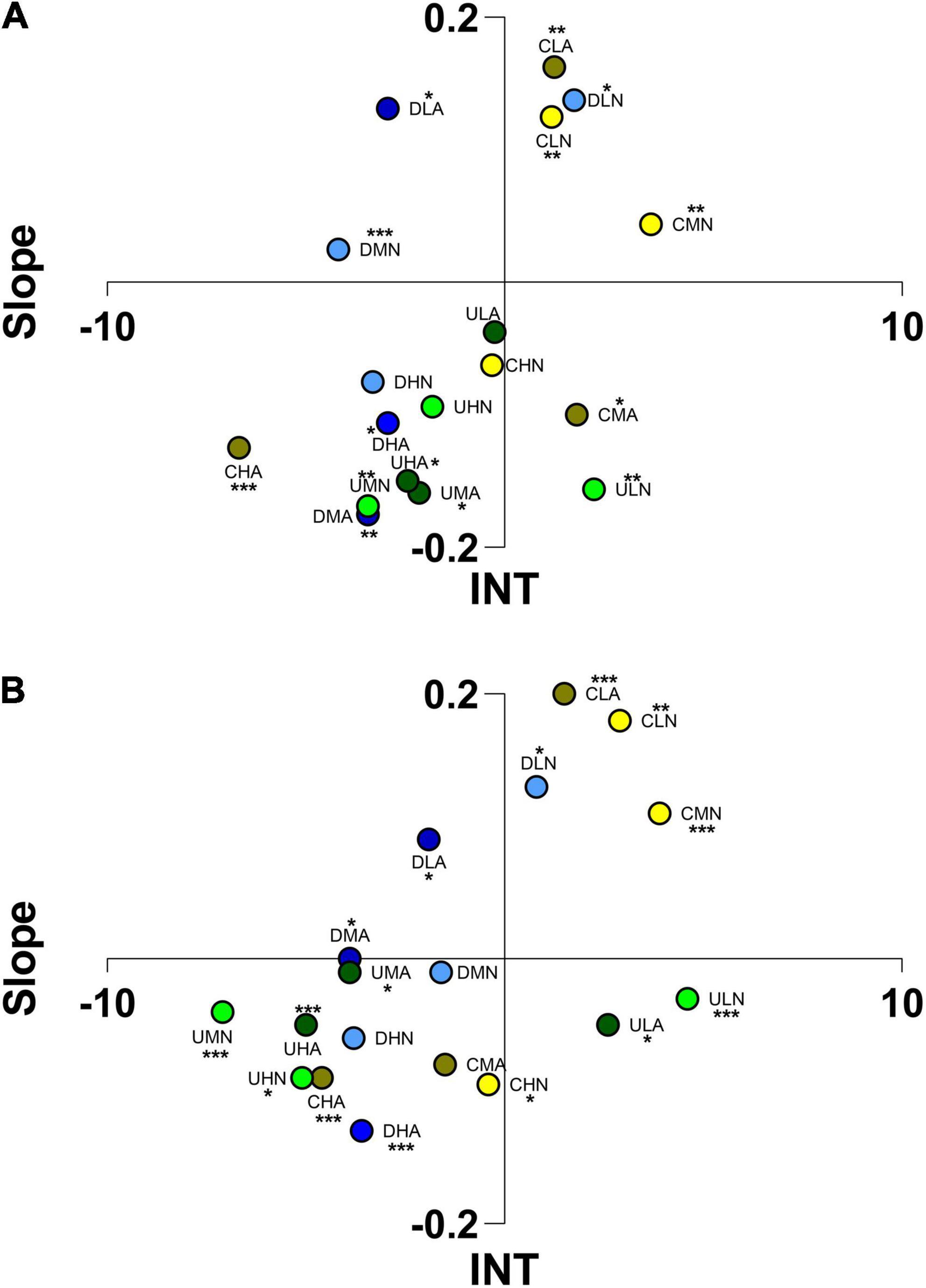
Figure 8. Ordination into 1 of 4 sectors of Hippolyte inermis juveniles according to the scores reached for “Slope” and “INT” (integral time) in static arenas (A) and flow-through flumes (B). The letters indicate the algae considered (C, Cocconeis scutellum var. parva; D, Diploneis sp.; U, Ulva prolifera), the concentration of VOCs (L, low; M, medium; H, high), and the culture and test conditions (N, normal conditions; A, acidified conditions). Asterisks indicate the significance of individuals’ position in the space.
In static chambers, adults of I. balthica were attracted at medium and low concentration of VOCs produced by C. scutellum var. parva cultured at acidified conditions (CLA, CMA), with statistical differences vs. medium and high concentrations of VOCs of Cocconeis cultured at ambient pH (CMN, CHN; Figure 9A; Tukey’s post hoc; Supplementary Table 3). In flow-through flumes, adults of I. balthica spent most of the experimental time in negative sectors when exposed to Cocconeis VOCs, and differences among VOCs concentrations and culture conditions were not significant (Figure 9B; Tukey’s post hoc; Supplementary Table 3). High concentrations of VOCs of Diploneis sp. (DHN, DHA) produced repulsion both in static chambers and flumes, with behaviors triggered by the same VOCs administered at low and medium concentrations significantly different (DLN, DMN, DLA, DMA; Tukey’s post hoc; Supplementary Table 3).
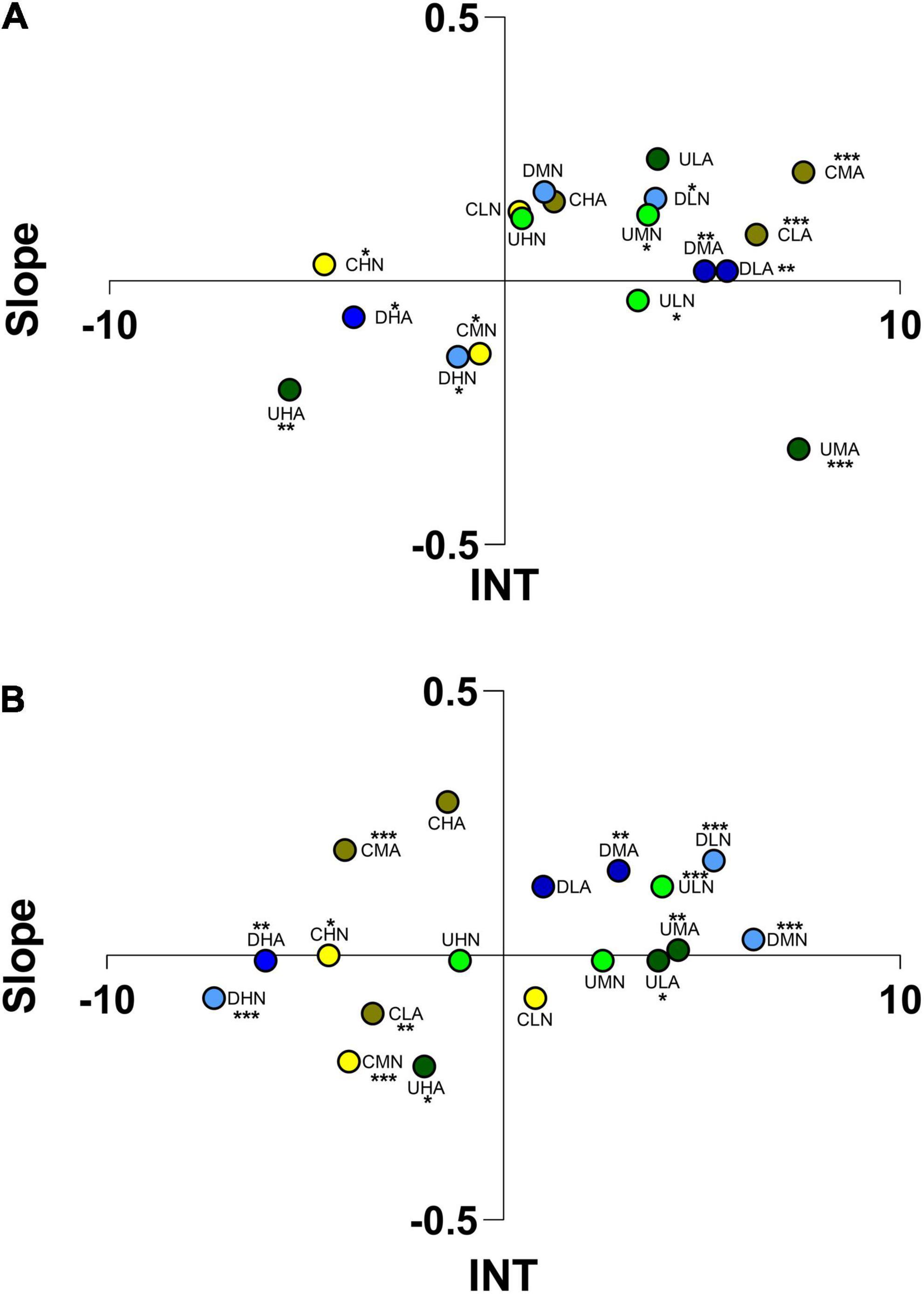
Figure 9. Ordination into 1 of 4 sectors of Idotea balthica adults according to the scores reached for “Slope” and “INT” (integral time) in static arenas (A) and flow-through flumes (B). The letters indicate the algae considered (C, Cocconeis scutellum var. parva; D, Diploneis sp.; U, Ulva prolifera), the concentration of VOCs (L, low; M, medium; H, high), and the culture and test conditions (N, normal conditions; A, acidified conditions). Asterisks indicate the significance of individuals’ position in the space.
Ulva VOCs produced an opposite behavioral reaction: at high concentrations, I. balthica were not able to identify VOCs produced at ambient pH (UHN) but were repelled by VOCs produced in acidified conditions (UHA) while lower concentrations of U. prolifera VOCs primed an attractive reaction (ULN, ULA The reactions to VOCs at high concentration produced by U. prolifera cultured at acidified conditions (UHA) and to the VOCs of the same algae administered at lower concentration, were significantly different in both pH conditions (UMN, ULN, ULA, UMA; Tukey’s post hoc; Supplementary Table 3).
Due to the unique behavior of I. balthica juveniles, it was impossible to perform odor choice experiments in flow-through flumes. Juveniles of this isopod exhibited ability to climb glass walls, plastic nets and to pass below the plastic frames used to divide the different areas of the flume. In static arenas (Figure 10), juveniles of I. balthica were attracted by C. scutellum var. parva cultured at normal pH (CLN, CMN) and they exhibitd scarce tendency to identify the VOCs produced by the same alga cultured and tested in acidified conditions. In addition, they were able to identify Diploneis VOCs administered at normal and low concentration (DLN, DLA, DMN), although no significant differences were found among different treatments (Tukey’s post hoc; Supplementary Table 3).
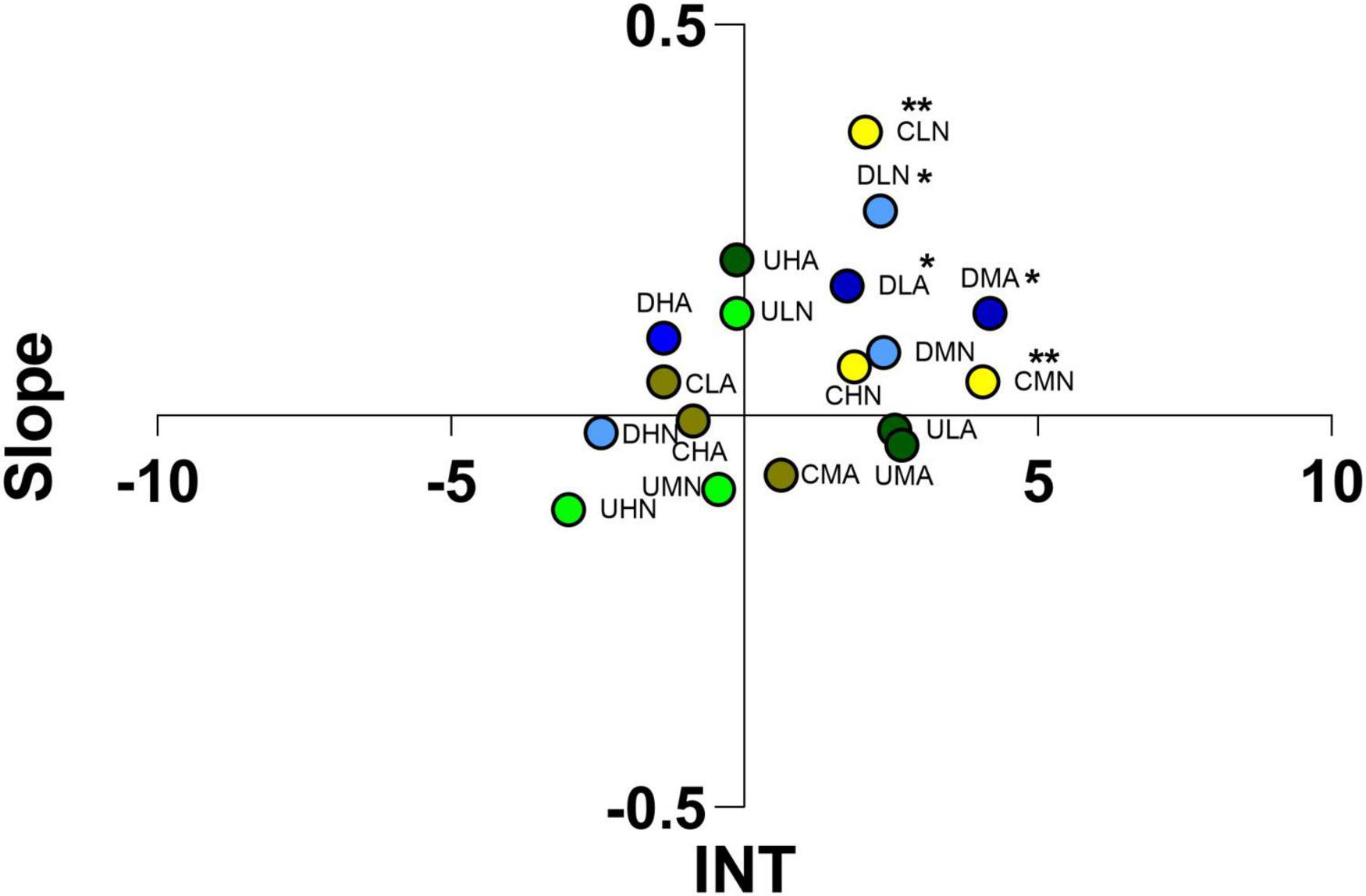
Figure 10. Ordination into 1 of 4 sectors of Idotea balthica juveniles according to the scores reached for “Slope” and “INT” (integral time) in static arenas. The letters indicate the algae considered (C, Cocconeis scutellum var. parva; D, Diploneis sp.; U, Ulva prolifera), the concentration of VOCs (L, low; M, medium; H, high), and the culture and test conditions (N, normal conditions, A, acidified conditions). Asterisks indicate the significance of individuals’ position in the space.
Discussion
Algae produce a wide spectrum of VOCs including terpenoids, furans, sulfur compounds, alkanes, alcohols, aldehydes, ketones, and esters (Walsh et al., 1998; Zuo et al., 2012b). Even closely related algae produce rather different and often species-specific VOC patterns (Fink et al., 2006a). In this study, we investigated for the first time how ocean acidification can influence the production of VOCs by various algae and how the recognition of infochemicals by associated invertebrates may vary consequently. Not surprisingly, we found differences in the VOC bouquets between the macroalga U. prolifera and the two benthic diatoms, also when comparing the two diatoms themselves (Diploneis sp. and C. scutellum var. parva). Previous studies focused on the changes in the VOCs production of macro- and microalgae in response to environmental factors, such as temperature, light, nutrition condition, water flow, and other abiotic factors (Bonsang et al., 2010; Zuo et al., 2012a; Xu et al., 2017; Ye et al., 2018). Altered behavioral responses under simulated ocean acidification had previously been investigated in assays at different pH levels for the invertebrates, but with algae grown under standard (ambient) pH conditions only, thus ignoring the potential of algal culture pH to alter the VOC composition (Maibam et al., 2015; Zupo et al., 2015; Mutalipassi et al., 2020). Differentially expressed compounds impact the bouquet composition and they are, in some cases, recognized as infochemicals by invertebrates (Moelzner and Fink, 2014). While the majority of studies on VOCs as attractants and repellents are related to the need to fight agricultural crop pests (Sarles et al., 2015; Himanen et al., 2017), a few studies have investigated the behavioral responses of invertebrates to algal VOCs in marine environments (Maibam et al., 2015; Zupo et al., 2015; Mutalipassi et al., 2020).
Our analyses of the VOC bouquets of the macroalga U. prolifera and the two benthic diatoms Diploneis sp. and C. scutellum var. parva grown under ambient (8.2) or acidified (7.7) pH conditions via GC-MS confirmed that shifts in culture pH by half a pH unit do indeed cause marked alterations in the algal VOC bouquets. It is thus not surprising that our subsequent behavioral assays indicated that invertebrate responses depend on the VOCs quantities offered, that different organisms react differently to algal VOCs, and that ocean acidification may change the relationships between algae and associated invertebrates in the P. oceanica communities. Ocean acidification, in fact, should be considered as a key factor to the ecology of plant-animal interactions that affect both emitters and receivers in the infochemical communication in aquatic environments. The VOC perception by receivers could be altered by ocean acidification due to modification in receptors’ conformation as demonstrated in rockfishes (Hamilton et al., 2014). In contrast, emitters undergo metabolic modifications in high CO2 conditions, leading to different VOC bouquets. In this study, indeed, we have shown that the pattern of VOCs production can be altered by the pH of the algal growth medium.
As regards the observed differences in the behavioral responses to various concentrations of infochemicals, they are quite common in this kind of study. A bouquet of volatile compounds may trigger contrasting and sometimes opposite responses, according to doses (Mutalipassi et al., 2019, 2020).
We performed each test in a static choice chamber (Zupo et al., 2015) and in flumes (Voigt and Atema, 1996; Atema et al., 2002) to investigate the relation between flume speed and behavioral reactions, to check whether flume speed is an important factor and whether it can interfere with experimental conclusions. Contrasting responses, eventually produced by the two experimental systems, may be explained by a diverse interpretation of the same bouquet in two conditions. It has been demonstrated that the spatial and temporal distribution of infochemicals in marine environments is shaped primarily by specific environmental conditions, such as hydrodynamics (Kozlowski et al., 2001). In static chambers, tested organisms may perceive the gradients of odor concentration as a linear function that spreads homogeneously from the source until saturation of the experimental arena (Voigt and Atema, 1996; Maibam et al., 2015). Previous studies demonstrated, using Kriging spatial interpolators, that odors released in the static chamber can be described by isolines through linear gradients from the VOCs releasing point to the negative control area (Maibam et al., 2015). For these reasons, static testing chamber could be more suitable for mimicking environments characterized by low hydrodynamic forces, where the interspace between the source of chemical cues and the receiver tends to become saturated quickly. This is the case of infochemical communications in small dimensional scale interactions, low hydrodynamic habitats, or confined environments. Differently, in the flumes, individuals perceive odors as pulse over time mimicking highly turbulent environments (Voigt and Atema, 1996). In these environments, characterized by high hydrodynamic forces, odor dispersal causes intermittency in the chemical signal even when the source emits continuously. Therefore, animals that use chemical cues to localize odor sources must overcome signal intermittency. Static and flume testing chambers are designed to test, respectively: (a) chemotaxis without the interference of turbulence, in which behavioral reaction are triggered only by chemical cues, and (b) eddy-chemotaxis, in which organisms track odors and turbulence simultaneously, using internal chemical and hydrodynamic fine structure of odor plume to localize the VOCs source (Kozlowski et al., 2001; Gardiner and Atema, 2007). In our investigation, the INT-Slope values obtained from the different combinations of “source organisms/VOCs concentration/pH value/target species” are kept in the same sector both in static chambers and flumes for all the three tested concentrations. In these cases, the results provided by the two testing methods are comparable, and this could indicate that no influences are evidenced in the identification and interpretation of chemical cues. In several cases, distributed along the different target-organisms, odors that trigger behavioral reactions in one testing chamber (as confirmed by statistical analysis) lose the carried information when administered using the other testing chamber. In most cases, these lacks in infochemical-triggered behavioral reactions have been observed especially in flumes, as in the case of several VOCs administered to A. lineata (CHN, CMA, UMA, ULN; Figure 5). In contrast, investigations on adult individuals of I. balthica evidenced different behavioral reactions considering the same combinations in the two testing chambers (Figure 9). In fact, turbulence deeply affected the perception or the interpretation of C. scutellum VOCs in adult isopods. However, this was observed only in an acidified environment, in the case of low and medium concentration VOCs (CLA, CMA) that are kept in completely different sectors with both methods. No differences were observed in behavioral reactions triggered by VOCs produced and administered at ambient pH (CMN, CHN). The differences in the behavioral reactions using the two testing chambers belong to a still unexplored field and could open new research topics leading to better understanding of VOC perception in marine environments under a range of hydrodynamic patterns. Thus, the reactions recorded by invertebrates in this study should be carefully interpreted due to the difficulties to relate the tested concentrations of VOCs with natural phenomena.
The results from our investigations suggest that the highest doses/concentrations of VOCs, as compared to medium and low concentrations of each odor bouquets, produce a more repulsive reaction in marine invertebrates, regardless of the life stage (i.e., larval vs. adult). This can probably be explained by a dose-dependent interpretation of the message transmitted by VOCs. As mentioned above, VOCs may bring a clear “danger” message in those cases, signaling the presence of a predator or a potentially dangerous larger grazer (Mutalipassi et al., 2020). The identification of a high concentration of VOCs is, indeed, compatible only with the presence of large-sized organisms, such as one or more fishes, that are wounding epiphytic algae over the canopy of P. oceanica (Zupo et al., 2015). For these reasons, infochemicals that at low concentration may indicate the presence of a food source, might at higher concentrations be interpreted as a “run-away” message (Jüttner et al., 2010).
These different behaviors should be linked to the peculiar physiology and life strategy of each tested organism, with respect to the differences in the attractiveness/repulsion for different invertebrate species. Hence, the same bouquet of odor may bring contrasting information to different organisms according to their ecology and trophic role (Sbarbati and Osculati, 2006). For example, a high concentration of U. prolifera VOCs (normal conditions) produced a repulsion in adults of H. inermis and a strong attraction in adults of I. balthica, while the VOCs produced by C. scutellum var. parva induced an attraction in A. lineata and repulsion in R. italiensis. The different localization of the two gastropods along the leaves of P. oceanica may explain the opposing reactions observed. Rissoa italianensis lives on leaves in shallow beds (1–3 m) which are heavily epiphytized by filamentous and encrusting macrophytes, while A. lineata lives at intermediate depths (3–10 m) on leaves that are generally covered by diatoms (Gambi et al., 1992; Mazzella et al., 1992). Consequently, the higher concentration of VOCs may be interpreted as a different signal representing an attractant for invertebrates that need to maximize the search for food, such as I. balthica (an invertebrate characterized by high motile activity), and a repellent for other invertebrates (Fink, 2007), for which mimicry and defense represent the most important behavioral constraints (Lamberti et al., 1995), such as H. inermis. Indeed, since Hippolytidae are exposed to high predation pressure, their behavior is deeply influenced by the need to avoid predation (Zupo, 1994; Bedini et al., 1997; Zupo and Nelson, 1997) and consequently, the diffusion of a large quantity of wound-activated VOCs may indicate the presence of predators chewing parts of P. oceanica and its epiphytes. Idotea balthica, living in low-energy P. oceanica detritus characterized by a high amount of poorly digestible structural carbohydrates (Sturaro et al., 2010), evolved a different interpretation of the odor, giving priority to the exploration of the area for a possible food source over the need to escape from possible predators. This species is probably especially accustomed to the odor of chewed algae and, as demonstrated for other species (Fink et al., 2006a), it is probably attracted by other animals grazing in the same area following an odor patch.
The reactions of invertebrates observed during the experiment are not only species-specific to the VOCs produced by different algae but, in addition, that behavioral response can change according to the life stages of the organism considered. Indeed, differences in the attractive responses were observed between adults and juveniles both in H. inermis and I. balthica. Hippolyte inermis adults were attracted by U. prolifera at low (ambient and acidified pH) and medium concentration (ambient pH) and by Diploneis sp. (acidified pH). A reverse pattern of responses was observed in juveniles of the same species that showed an attraction for VOCs produced by C. scutellum var. parva at low concentration and medium concentration (ambient pH) as well as by Diploneis sp. at low concentration (ambient pH). Contrasting responses were observed in adults and juveniles of I. balthica too: adults were repelled by C. scutellum var. parva and attracted by U. prolifera, while the juveniles were attracted by VOCs from the diatom and repelled by those from the macroalga.
Larvae and juveniles of invertebrates and vertebrates use chemical cues to detect and reach the most favorable microhabitat (Herrnkind and Butler, 1986; Wethey, 1986; Pawlik, 1992; Ben-Tzvi et al., 2008, 2010), and the selection of a protected and preferred microhabitat is a key process to ensure successful recruitment. Our results indicate contrasting behavioral responses between juveniles and adults. In fact, while juveniles of both crustacean species were attracted by diatom bouquets, especially at low concentrations, in adults belonging to the same species repulsive responses have been observed, especially related to C. scutellum var. parva VOCs. Previous investigations demonstrated that adult H. inermis is strongly repelled by VOCs produced by Cocconeis (Jüttner et al., 2010). In addition, VOCs produced by this diatom attracted juveniles of H. inermis. We can thus hypothesize that these VOCs act as infochemicals and are an indicator of the “preferred” habitat, in this case, the epiphytised P. oceanica leaves (Zupo et al., 2019). The same behavioral response, observed in juveniles of I. balthica, may be explained with the search for food sources, but not with the search for the correct habitat, because I. balthica hatch as fully functional juveniles, lacking a free-swimming larval phase (Strong, 1978; Strong and Daborn, 1979; Tuomi et al., 1988; Fava et al., 1992). For this reason, freshly hatched juveniles are born in the mother’s environment, such as in leaf debris, rich in shelters, where the species’ peculiar mimicry is fully functional and efficient (Toonen et al., 2007; Camur-Elipek, 2009).
The behavioral responses to VOCs produced at ambient and acidified pH lead to contrasting responses within the same species. In fact, A. lineata and R. italiensis showed opposite pattern of responses when exposed to VOCs produced at different pH. In H. inermis and I. balthica adults, ocean acidification had a relatively lower impact on odor recognition, because only a few behavioral differences were observed. Differences were observed in the response to VOCs from C. scutellum var. parva produced and tested at acidified and ambient pH: in every tested organism, except for H. inermis adults, ocean acidification produced an alteration of behavioral responses. In both crustacean species, juveniles responded being attracted to VOCs produced and tested at ambient pH and repelled by C. scutellum var. parva VOCs produced and tested at acidified conditions. Cocconeis scutellum var. parva is a benthic diatom that does not have antimitotic compounds, and it is not toxic for benthic invertebrates (Zupo, 2000; Zupo and Messina, 2007). Nevertheless, it triggers specific physiological reactions and induces the sex reversal in H. inermis (Zupo et al., 2007). The relationship between Cocconeis diatoms and H. inermis suggested to be part of a peculiar co-evolutive process through competitive trophic interaction that allows the shrimp to stabilize natural populations (Zupo, 2020). In fact, it has been demonstrated that, in addition to co-evolutionary relationships in the frame of mutual cooperation between species, adaptations may lead to different degrees of advantages for two co-evolving organisms. In the case of Hippolyte-Cocconeis, shrimp populations are stabilized due to the effect of a very specific apoptogenic compound produced by the diatom (Zupo, 2020). The opposite reactions of H. inermis, exhibited in response to VOCs of C. scutellum var. parva cultured at acidified pH may produce a deep alteration of the coevolution of shrimp and diatom in P. oceanica meadows.
It is important to investigate seawater pH-dependent physiological changes in all components of the marine food webs, rather than just in animals (Maibam et al., 2015; Zupo et al., 2015; Mutalipassi et al., 2020) to understand potential consequences of global changes in general and ocean acidification in particular. Here we demonstrated that seawater acidification alters the bouquet of volatile infochemicals liberated by various benthic algae. These changes in algal VOC bouquets could subsequently be linked to alterations in the foraging behavior of invertebrates that use those algae as both habitat and food resources. This highlights that holistic approaches and experiments addressing ocean acidification effects across multiple trophic levels are required to understand the consequences and impacts of ocean acidification. This investigation is the first addressing these issues across multiple trophic levels with organisms from a shared habitat, and it provides a crucial advancement for the mechanistic understanding of the consequences of ocean acidification. Even though this will require further studies, it is a major step forward in this research area and encourages to apply such multi-trophic perspectives when investigating ocean acidification or other anthropogenic stressors on ecological interactions.
Conclusion
In conclusion, we confirmed that diatoms and macroalgae both produce VOCs acting as infochemicals for the invertebrates associated with the leaf stratum of P. oceanica. The conveyed information is dose-dependent, producing variable responses according to VOC concentrations. In addition, the responses are species-specific, and strictly related to the ecological strategies of each species. Finally, our data indicate that the increasing levels of CO2 forecasted for the next decades will modify the production and the recognition of infochemicals by animal communities associated to P. oceanica meadows. The field of VOCs’ chemical ecology is still poorly explored and exhibits unsolved questions (Fink, 2007). These results add knowledge to a relatively undiscovered topic, evidencing an intricate web of reactions that appears to be strongly influenced and altered by ocean acidification. For these reasons, a research effort is needed to disentangle chemical signals behind plant-animal interactions, to understand how chemical communications may occur in the marine environment and how they are modified by chemical environments.
Data Availability Statement
The original contributions presented in the study are included in the article/Supplementary Material, further inquiries can be directed to the corresponding author/s.
Ethics Statement
All animal experiments were carried in accordance with the EU Directive 2010/63/EU and The Code of Ethics of the World Medical Association (Declaration of Helsinki) for animal experiments.
Author Contributions
MM, VM, VZ, EE, and PF conceived and designed this study. MM, VM, and FG collected and sorted the animals for the study. MM and VM isolated and cultured micro and macroalgae used in this manuscript, with the taxonomical identification made by LP, and collected biometric data and managed experimental animals in the facility. MM, VM, FG, LP, ML, and MB performed the behavioral experiments. MM, VM, EE, PF, MS, and VZ reviewed and analyzed the data. MS, PF, and EE performed biochemical analysis and statistics on biochemical data. The manuscript was written by MM and VM, with the contribution of MS for the biochemical sections and under the supervision of PF, EE, and VZ. All authors analyzed interpreted the data, reviewed, and approved the manuscript.
Funding
This research was partially sustained by an Open University Ph.D. project funded by Stazione Zoologica Anton Dohrn. VM was funded by the Project Acid.it “Science for mitigation and adaptation policies to ecological and socio-economic impacts of acidification on Italian seas”, supervised by VZ and performed in cooperation with OGS Institute (Italy), and by the German Research Foundation (DFG), projects EL179/13-1 and FI 1548/9-1.
Conflict of Interest
The authors declare that the research was conducted in the absence of any commercial or financial relationships that could be construed as a potential conflict of interest.
Publisher’s Note
All claims expressed in this article are solely those of the authors and do not necessarily represent those of their affiliated organizations, or those of the publisher, the editors and the reviewers. Any product that may be evaluated in this article, or claim that may be made by its manufacturer, is not guaranteed or endorsed by the publisher.
Acknowledgments
MM performed research in the frame of an Open University Ph.D. Programme funded by the SZN, under the supervision of VZ Sampling operations were conducted on board the R/V Phoenicia property of Stazione Zoologica Anton Dohrn, operated by Cpt. Vincenzo Rando. Two reviewers helped to improve the presentation of the manuscript.
Supplementary Material
The Supplementary Material for this article can be found online at: https://www.frontiersin.org/articles/10.3389/fmars.2022.809702/full#supplementary-material
Supplementary Table 1 | Seawater carbonate chemistry variables (mean values ± standard deviation) of Cocconeis scutellum var. parva, Diploneis sp., and Ulva prolifera cultures at the two pH conditions (pH 7.7 and 8.2). pHNBS, measured pH (NBS scale); TA, total alkalinity; pCO2 = CO2 partial pressure.
Supplementary Table 2 | Resume of the significance of differences in the distribution of individuals between the positive (VOC) and negative (Control) areas according to species and pH regimes. Analysis performed using ANOVA repeated-measures.
Supplementary Table 3 | Resume of the results of TWO ways ANOVA analysis with Tukey’s multiple comparisons test to identify significance of differences among behavioral responses to algae odor bouquets at each concentration and cultured condition.
Supplementary Table 4 | Putative substances identified by GC-MS in extracts of Cocconeis scutellum var. parva kept at two different ambient pH conditions. Retention times and peak areas obtained in two or three replicate analyses are given. Analysis and identification were carried out as described by Moelzner and Fink (2014).
Supplementary Table 5 | Putative substances identified by GC-MS in extracts of Diploneis sp. kept at two different ambient pH conditions. Retention times and peak areas obtained in two or three replicate analyses are given. Analysis and identification were carried out as described by Moelzner and Fink (2014).
Supplementary Table 6 | Putative substances identified by GC-MS in extracts of Ulva prolifera kept at two different ambient pH conditions. Retention times and peak areas obtained in two or three replicate analyses are given. Analysis and identification were carried out as described by Moelzner and Fink (2014).
References
Atema, J., Kingsford, M. J., and Gerlach, G. (2002). Larval reef fish could use odour for detection, retention and orientation to reefs. Mar. Ecol. Prog. Ser. 241, 151–160. doi: 10.3354/meps241151
Bedini, R., Canali, M. G., and Baldi, C. (1997). Mimetismo criptico nei crostacei della prateria a Posidonia oceanica (L.) Delile. Biol. Mar. Mediterr. 4, 353–355.
Bell, T. M., and Sotka, E. E. (2012). Local adaptation in adult feeding preference and juvenile performance in the generalist herbivore Idotea balthica. Oecologia 170, 383–393. doi: 10.1007/s00442-012-2302-3
Ben-Tzvi, O., Abelson, A., Polak, O., and Kiflawi, M. (2008). Habitat selection and the colonization of new territories by Chromis viridis. J. Fish Biol. 73, 1005–1018. doi: 10.1111/j.1095-8649.2008.02003.x
Ben-Tzvi, O., Tchernov, D., and Kiflawi, M. (2010). Role of coral-derived chemical cues in microhabitat selection by settling Chromis viridis. Mar. Ecol. Prog. Ser. 409, 181–187.
Bonsang, B., Gros, V., Peeken, I., Yassaa, N., Bluhm, K., Zoellner, E., et al. (2010). Isoprene emission from phytoplankton monocultures: the relationship with chlorophyll-a, cell volume and carbon content. Environ. Chem. 7, 554–563. doi: 10.1071/EN09156
Böstrom, C., and Mattila, J. (2005). Effects of isopod grazing: an experimental comparison in temperate (Idotea balthica, Baltic Sea, Finland) and subtropical (Erichsonella attenuata). Crustaceana 78, 185–200. doi: 10.1163/1568540054020541
Brönmark, C., and Hansson, L. A. (2000). Chemical communication in aquatic systems: an introduction. Oikos 88, 103–109.
Buia, M. C., Gambi, M. C., and Zupo, V. (2000). Structure and functioning of Mediterranean seagrass ecosystems: an overview. Biol. Mar. Mediterr. 7, 167–190.
Buia, M. C., Zupo, V., and Mazzella, L. (1992). Primary production and growth dynamics in Posidonia oceanica. Mar. Ecol. 13, 2–16. doi: 10.1111/j.1439-0485.1992.tb00336.x
Caldeira, K., and Wickett, M. (2005). Ocean model predictions of chemistry changes from carbon dioxide emissions to the atmosphere and ocean. J. Geophys. Res. C Oceans 110, 1–12. doi: 10.1029/2004JC002671
Caldeira, K., and Wickett, M. E. (2003). Anthropogenic carbon and ocean pH. Nature 425:365. doi: 10.1038/425365a
Camur-Elipek, B. (2009). Observations with a different approach to morphological colour variation in an isopod population (Crustacea: Isopoda). Biologia 64, 124–129. doi: 10.2478/s11756-009-0023-9
Derby, C. D. C. D., and Sorensen, P. W. P. W. (2008). Neural processing, perception, and behavioral responses to natural chemical stimuli by fish and crustaceans. J. Chem. Ecol. 37, 898–914.
Dimech, M., Borg, J. A., and Schembri, P. J. (2006). Motile macroinvertebrate assemblages associated with submerged Posidonia oceanica litter accumulations. Biol. Mar. Mediterr. 13, 130–133.
Fava, G., Zangaglia, A., and Cervelli, M. (1992). Ecology of Idotea baltica (pallas) populations in the lagoon of venice. Oceanol. Acta 15, 651–660.
Fink, P. (2007). Ecological functions of volatile organic compounds in aquatic systems. Mar. Freshw. Behav. Physiol. 40, 155–168. doi: 10.1080/10236240701602218
Fink, P., Von Elert, E., and Jüttner, F. (2006a). Oxylipins from freshwater diatoms act as attractants for a benthic herbivore. Arch. Hydrobiol. 167, 561–574. doi: 10.1127/0003-9136/2006/0167-0561
Fink, P., Von Elert, E., and Jüttner, F. (2006b). Volatile foraging kairomones in the littoral zone: attraction of an herbivorous freshwater gastropod to algal odors. J. Chem. Ecol. 32, 1867–1881.
Gambi, M. C., Lorenti, M., Russo, G. F., Scipione, M. B., and Zupo, V. (1992). Depth and seasonal distribution of some groups of the vagile fauna of the Posidonia oceanica leaf stratum: structural and trophic analyses. Mar. Ecol. 13, 17–39. doi: 10.1111/j.1439-0485.1992.tb00337.x
Gao, G., Liu, Y., Li, X., Feng, Z., and Xu, J. (2016). An ocean acidification acclimatised green tide alga is robust to changes of seawater carbon chemistry but vulnerable to light stress. PLoS One 11:e0169040. doi: 10.1371/journal.pone.0169040
Gardiner, J. M., and Atema, J. (2007). Sharks need the lateral line to locate odor sources: rheotaxis and eddy chemotaxis. J. Exp. Biol. 210, 1925–1934.
Gattuso, J.-P., and Hansson, L. (2011). “Ocean acidification: background and history,” in Ocean Acidification, eds J.-P. Gattuso and L. Hansson (Oxford: Oxford University Press), 1–20.
Hall-Spencer, J. M., Rodolfo-Metalpa, R., Martin, S., Ransome, E., Fine, M., Turner, S. M., et al. (2008). Volcanic carbon dioxide vents show ecosystem effects of ocean acidification. Nature 454, 96–99. doi: 10.1038/nature07051
Hamilton, T. J., Holcombe, A., and Tresguerres, M. (2014). CO2-induced ocean acidification increases anxiety in rockfish via alteration of GABA receptor functioning. Proc. Biol. Sci. 281:20132509. doi: 10.1098/rspb.2013.2509
Havas, M., and Rosseland, B. O. (1995). Response of zooplankton, benthos, and fish to acidification: an overview. Water Air Soil Pollut. Focus 85, 51–62. doi: 10.1007/BF00483688
Herrnkind, W. F., and Butler, M. J. IV (1986). Factors regulating postlarval settlement and juvenile microhabitat use by spiny lobsters Panulirus argus. Mar. Ecol. Prog. Ser. 34, 23–30.
Himanen, S. J., Li, T., Blande, J. D., and Holopainen, J. K. (2017). “Volatile organic compounds in integrated pest management of Brassica oilseed crops,” in Integrated management of insect pests on canola and other Brassica oilseed crops, ed. G. V. P. Reddy (Wallingford: CABI Publishing), 281–294. doi: 10.1079/9781780648200.0281
Jin, P., Hutchins, D. A., and Gao, K. (2020). The impacts of ocean acidification on marine food quality and its potential food chain consequences. Front. Mar. Sci. 7:543979. doi: 10.3389/fmars.2020.543979
Jones, R. H., and Flynn, K. J. (2005). Nutritional status and diet composition affect the value of diatoms as copepod prey. Science 307, 1457–1459. doi: 10.1126/science.1107767
Jutfelt, F., Sundin, J., Raby, G. D., Krång, A.-S., and Clark, T. D. (2017). Two-current choice flumes for testing avoidance and preference in aquatic animals. Methods Ecol. Evol. 8, 379–390. doi: 10.1111/2041-210X.12668
Jüttner, F. (1988). Quantitative analysis of volatile organic-compounds. Methods Enzymol. 167, 609–616.
Jüttner, F. (2005). Evidence that polyunsaturated aldehydes of diatoms are repellents for pelagic crustacean grazers. Aquat. Ecol. 39, 271–282. doi: 10.1007/s10452-005-3419-9
Jüttner, F., Messina, P., Patalano, C., and Zupo, V. (2010). Odour compounds of the diatom Cocconeis scutellum: effects on benthic herbivores living on Posidonia oceanica. Mar. Ecol. Prog. Ser. 400, 63–73. doi: 10.3354/meps08381
Knutzen, J. (1981). Effects of decreased pH on marine organisms. Mar. Pollut. Bull. 12, 25–29. doi: 10.1016/0025-326X(81)90136-3
Kozlowski, C., Yopak, K., Voigt, R., and Atema, J. (2001). An initial study on the effects of signal intermittency on the odor plume tracking behavior of the American lobster, Homarus americanus. Biol. Bull. 201, 274–276.
Kroon, F. J. (2011). A fluviarium with controlled water velocity and quality for preference - avoidance experiments with fish and invertebrates. Limnol. Oceanogr. Methods 1, 39–44. doi: 10.4319/lom.2011.1.39
Lamberti, G. A., Gregory, S. V., Ashkenas, L. R., Li, J. L., Steinman, A. D., and McIntire, C. D. (1995). Influence of grazer type and abundance on plant-herbivore interactions in streams. Hydrobiologia 306, 179–188.
Leidenberger, S. (2013). Adaptation to the Baltic Sea - the Case of Isopod Genus Idotea. Ph.D Thesis. Göteborg: University of Gothenburg.
Leidenberger, S., Harding, K., and Jonsson, P. R. (2012). Ecology and distribution of the isopod genus Idotea in the baltic sea: key species in a changing environment. J. Crustacean Biol. 32, 359–381. doi: 10.1163/193724012X626485
Liu, H. P., Chen, R. Y., Zhang, Q. X., Wang, Q. Y., Li, C. R., Peng, H., et al. (2012). Characterization of two isoforms of antiliopolysacchride factors (Sp-ALFs) from the mud crab Scylla paramamosain. Fish. Shellfish Immunol 33, 1–10. doi: 10.1016/j.fsi.2012.03.014
Lorenti, M., and Fresi, E. (1982). Grazing of Idotea baltica Basteri on Posidonia oceanica: preliminary observations. CIESM 28e Congr. Ass. Cannes Comit. Benthos 28, 147–148.
Maibam, C., Fink, P., Romano, G., Buia, M. C., Butera, E., and Zupo, V. (2015). Centropages typicus (Crustacea, Copepoda) reacts to volatile compounds produced by planktonic algae. Mar. Ecol. 36, 819–834. doi: 10.1111/maec.12254
Maibam, C., Fink, P., Romano, G., Buia, M. C., Gambi, M. C., Scipione, M. B., et al. (2014). Relevance of wound-activated compounds produced by diatoms as toxins and infochemicals for benthic invertebrates. Mar. Biol. 161, 1639–1652. doi: 10.1007/s00227-014-2448-0
Mazzella, L., Buia, M. C., Gambi, M. C., Lorenti, M., Russo, G., Scipione, M. B., et al. (1992). “Primary production of Posidonia oceanica and the vagile fauna of leaf stratum: a spatio temporal analysis in a meadow off the island of Ischia (Gulf of Naples, Italy),” in Space and Time Series Data Analysis in Coastal Benthic Ecology, ed. B. F. Keegan (Brussels: Commission of the European Communities), 519–539.
Merilaita, S., and Jormalainen, V. (2000). Different roles of feeding and protection in diel microhabitat choice of sexes in Idotea baltica. Oecologia 122, 445–451. doi: 10.1007/s004420050965
Milazzo, M., Chemello, R., Badalamenti, F., and Riggio, S. (2000). Molluscan assemblages associated with photophilic algae in the marine reserve of Ustica Island (Lower Tyrrhenian Sea, Italy). Ital. J. Zool. 67, 287–295. doi: 10.1080/11250000009356326
Minchin, P. R. (1987). An evaluation of the relative robustness of techniques for ecological ordination. Vegetatio 69, 89–107. doi: 10.1007/bf00038690
Moelzner, J., and Fink, P. (2014). The smell of good food: volatile infochemicals as resource quality indicators. J. Anim. Ecol. 83, 1007–1014. doi: 10.1111/1365-2656.12220
Mueller, C. E., Larsson, A. I., Veuger, B., Middelburg, J. J., and Van Oevelen, D. (2014). Opportunistic feeding on various organic food sources by the cold-water coral Lophelia pertusa. Biogeosciences 11, 123–133. doi: 10.5194/bg-11-123-2014
Munday, P. L., Dixson, D. L., Donelson, J. M., Jones, G. P., Pratchett, M. S., Devitsina, G. V., et al. (2009). Ocean acidification impairs olfactory discrimination and homing ability of a marine fish. Proc. Natl. Acad. Sci. U.S.A. 106, 1848–1852. doi: 10.1073/pnas.0809996106
Munday, P. L., Dixson, D. L., Mccormick, M. I., Meekan, M., Ferrari, M. C. O., and Chivers, D. P. (2010). Replenishment of fish populations is threatened by ocean acidification. Proc. Natl. Acad. Sci. U.S.A. 107, 12930–12934.
Mutalipassi, M., Fink, P., Maibam, C., Porzio, L., Buia, M. C., Gambi, M. C., et al. (2020). Ocean acidification alters the responses of invertebrates to wound-activated infochemicals produced by epiphytes of the seagrass Posidonia oceanica. J. Exp. Mar. Bio. Ecol. 530–531:151435. doi: 10.1016/j.jembe.2020.151435
Mutalipassi, M., Maibam, C., and Zupo, V. (2018). The sex change of the caridean shrimp Hippolyte inermis Leach: temporal development of the gonopore morphology. Zoomorphology 137, 377–388. doi: 10.1007/s00435-018-0405-z
Mutalipassi, M., Mazzella, V., and Zupo, V. (2019). Ocean acidification influences plant-animal interactions: the effect of Cocconeis scutellum parva on the sex reversal of Hippolyte inermis. PLoS One 14:e0218238. doi: 10.1371/journal.pone.0218238
Mutalipassi, M., Riccio, G., Mazzella, V., Galasso, C., Somma, E., Chiarore, A., et al. (2021). Symbioses of cyanobacteria in marine environments : ecological insights and biotechnological perspectives. Mar. Drugs 19:227. doi: 10.3390/md19040227
Natal, A. I. L. (2015). Phylogeny of the Marine Isopod Genus Idotea in the Northeast Atlantic Ocean and Mediterranean Sea. Ph.D Thesis. Porto: Porto University.
Naylor, E. (1955a). The comparative external morphology and revised taxonomy of the British species of Idotea. J. Mar. Biol. Assoc. U. K. 34, 467–493.
Naylor, E. (1955b). The diet and feeding mechanism of Idotea. J. Mar. Biol. Assoc. U. K. 34, 347–355. doi: 10.1017/S0025315400008766
Oksanen, J., Blanchet, F. G., Kindt, R., Legendre, P., Minchin, P. R., O’Hara, R. B., et al. (2015). Vegan: community ecology package. R package vegan, vers. 2.2-1. Worl. Agro. Cent 3, 7–81.
Pachauri, R. K., Allen, M. R., Barros, V. R., Broome, J., Cramer, W., Christ, R., et al. (2014). “Climate change 2014: synthesis report,” in Contribution of Working Groups I, II and III to the Fifth Assessment Report of the Intergovernmental Panel on Climate Change, eds R. Pachauri and L. Meyer (Geneva: IPCC), doi: 10.1046/j.1365-2559.2002.1340a.x
Pawlik, J. R. (1992). “Chemical ecology of the settlement of benthic marine-invertebrates,” in Oceanography and Marine Biology: An Annual Review ed. UCL Press (London: Aberdeen University Press), 273–335.
Piazzi, L., Balata, D., and Ceccherelli, G. (2016). Epiphyte assemblages of the Mediterranean seagrass Posidonia oceanica: an overview. Mar. Ecol. 37, 3–41. doi: 10.1111/maec.12331
Pierrot, D., Lewis, E., and Wallace, D. W. R. (2006). CO2SYS DOS Program Developed for CO2 System Calculations. ORNL/CDIAC-105. Oak Ridge, TN: Oak Ridge National Laboratory.
Pohnert, G., Steinke, M., and Tollrian, R. (2007). Chemical cues, defence metabolites and the shaping of pelagic interspecific interactions. Trends Ecol. Evol. 22, 198–204. doi: 10.1016/j.tree.2007.01.005
Poore, A. G. B. B., Graba-Landry, A., Favret, M., Sheppard Brennand, H., Byrne, M., Dworjanyn, S. A., et al. (2013). Direct and indirect effects of ocean acidification and warming on a marine plant-herbivore interaction. Oecologia 173, 1113–1124. doi: 10.1007/s00442-013-2683-y
R Core Team (2019). R: A Language and Environment for Statistical Computing. Vienna: R Foundation for Statistical Computing.
Raniello, R., Iannicelli, M. M., Nappo, M., Avila, C., and Zupo, V. (2007). Production of Cocconeis neothumensis (Bacillariophyceae) biomass in batch cultures and bioreactors for biotechnological applications: light and nutrient requirements. J. Appl. Phycol. 19, 383–391. doi: 10.1007/s10811-006-9145-4
Ruocco, N., Cavaccini, V., Caramiello, D., Ianora, A., Fontana, A., Zupo, V., et al. (2019). Noxious effects of the benthic diatoms Cocconeis scutellum and Diploneis sp. on sea urchin development: morphological and de novo transcriptomic analysis. Harmful Algae 86, 64–73. doi: 10.1016/j.hal.2019.05.009
Russo, G. F., Fresi, E., Vinci, D., and Chessa, L. A. (1984). “Mollusk syntaxon of foliar stratum along a depth gradient in a Posidonia oceanica (L.) Delile meadow: diel variability,” in International Workshop on Posidonia oceanica Beds, eds C. F. Boudouresque, A. Jeudy de Grissac, and J. Olivier (Marseille: GIS Posidonie), 303–310.
Salemaa, H. (1985). Karyological studies in Idotea spp (Isopoda, Valvifera). Crustaceana 48, 74–87. doi: 10.1163/156854085X00738
Sarles, L., Verhaeghe, A., Francis, F., and Verheggen, F. J. (2015). Semiochemicals of Rhagoletis fruit flies: potential for integrated pest management. Crop Protect. 78, 114–118. doi: 10.1016/j.cropro.2015.09.001
Sbarbati, A., and Osculati, F. (2006). Allelochemical communication in vertebrates: Kairomones, Allomones and Synomones. Cells Tissues Organs 183, 206–219.
Smetacek, V., and Zingone, A. (2013). Green and golden seaweed tides on the rise. Nature 504, 84–88.
Strong, K. W. (1978). Breeding and bionomics of Idotea baltica (Pallas)(Crustacea: Isopoda). Proc. Natl. Acad. Sci. U.S.A. 28, 217–230.
Strong, K. W., and Daborn, G. R. (1979). Growth and energy utilisation of the intertidal isopod Idotea baltica (Pallas) (Crustacea: Isopoda). J. Exp. Mar. Biol. Ecol. 41, 101–123. doi: 10.1016/0022-0981(79)90046-7
Sturaro, N., Caut, S., Gobert, S., Bouquegneau, J. M., and Lepoint, G. (2010). Trophic diversity of idoteids (Crustacea, Isopoda) inhabiting the Posidonia oceanica litter. Mar. Biol. 157, 237–247. doi: 10.1007/s00227-009-1311-1
Toonen, R. J., Wetzer, R., Daley, S., and Wares, J. P. (2007). An evaluation of cryptic lineages of Idotea balthica (Isopoda: Idoteidae): morphology and microsatellites. J. Crustacean Biol. 27, 643–648. doi: 10.1651/S-2825R.1
Tuomi, J., Jormalainen, V., and Ilvessalo, H. (1988). Growth, food consumption and reproductive tactics of the aquatic isopod Idotea baltica. Ann. Zool. Fennici 25, 145–151.
Vet, L. E. M., and Dicke, M. (1992). Ecology of infochemical use by natural enemies in a tritrophic context. Annu. Rev. Entomol. 37, 141–172. doi: 10.1146/annurev.en.37.010192.001041
Voigt, R., and Atema, J. (1996). Chapter 12 orientation of marine invertebrates to odor sources. Tested Stud. Lab. Teach. 18, 213–230.
Vos, M., Vet, L. E. M., Wäckers, F. L., Middelburg, J. J., Van Der Putten, W. H., Mooij, W. M., et al. (2006). Infochemicals structure marine, terrestrial and freshwater food webs: implications for ecological informatics. Ecol. Inform. 1, 23–32. doi: 10.1016/j.ecoinf.2005.06.001
Walsh, K., Jones, G. J., and Dunstan, R. H. (1998). Effect of high irradiance and iron on volatile odour compounds in the cyanobacterium Microcystis aeruginosa. Phytochemistry 49, 1227–1239. doi: 10.1016/S0031-9422(97)00943-6
Warén, A. (1996). Ecology and systematics of the North European species of Rissoa and Pusillina (Prosobranchia: Rissoidae). J. Mar. Biol. Assoc. U. K. 76, 1013–1059. doi: 10.1017/S0025315400040947
Watson, S. B., and Ridal, J. (2004). Periphyton: a primary source of widespread and severe taste and odour. Water Sci. Technol. 49, 33–39. doi: 10.2166/wst.2004.0527
Wethey, D. S. (1986). Ranking of settlement cues by barnacle larvae: influence of surface contour. Bull. Mar. Sci. 39, 393–400.
Wittmann, K., Mazzella, L., Fresi, E., and Napoli, S. Z. (1981). Age specific patterns of leaf growth: their determination and importance for epiphytic colonization in Posidonia oceanica (L.) Delile. Rapp. Commission Internat. Mer Mediterr, 2, 189–192.
Wyatt, T. D., Hardege, J. D., and Terschak, J. (2014). Ocean acidification foils chemical signals. Science 346:176. doi: 10.1126/science.346.6206.176-a
Xu, J., and Gao, K. (2012). Future CO2-induced ocean acidification mediates the physiological performance of a green tide alga. Plant Physiol. 160, 1762–1769. doi: 10.1104/pp.112.206961
Xu, Q., Yang, L., Yang, W., Bai, Y., Hou, P., Zhao, J., et al. (2017). Volatile organic compounds released from Microcystis flosaquae under nitrogen sources and their toxic effects on Chlorella vulgaris. Ecotoxicol. Environ. Saf. 135, 191–200. doi: 10.1016/j.ecoenv.2016.09.027
Ye, C., Yang, Y., Xu, Q., Ying, B., Zhang, M., Gao, B., et al. (2018). Volatile organic compound emissions from Microcystis aeruginosa under different phosphorus sources and concentrations. Phycological Res. 66, 15–22. doi: 10.1111/pre.12201
Zhang, D., Yao, Z., Lai, Q., and Lin, J. (2012). Effects of sex change on number of olfactory chemoreceptors in Lysmata shrimp. J. Shellfish Res. 31, 861–865. doi: 10.2983/035.031.0334
Zuo, Z., Zhu, Y. R., Bai, Y. L., and Wang, Y. (2012b). Volatile communication between Chlamydomonas reinhardtii cells under salt stress. Biochem. Syst. Ecol. 40, 19–24. doi: 10.1016/j.bse.2011.09.007
Zuo, Z., Zhu, Y., Bai, Y., and Wang, Y. (2012a). Acetic acid-induced programmed cell death and release of volatile organic compounds in Chlamydomonas reinhardtii. Plant Physiol. Biochem. 51, 175–184. doi: 10.1016/j.plaphy.2011.11.003
Zupo, V. (1994). Strategies of sexual inversion in Hippolyte inermis Leach (Crustacea, Decapoda) from a Mediterranean seagrass meadow. J. Exp. Mar. Biol. Ecol. 178, 131–145. doi: 10.1016/0022-0981(94)90229-1
Zupo, V. (2000). Effect of microalgal food on the sex reversal of Hippolyte inermis (Crustacea: Decapoda). Mar. Ecol. Prog. Ser. 201, 251–259. doi: 10.3354/meps201251
Zupo, V. (2020). “Co-evolution of the shrimp Hippolyte inermis and the Diatoms Cocconeis spp. in Posidonia oceanica: sexual adaptations explained by ecological fitting,” in Co-Evolution of Secondary Metabolites, eds J. M. Mérillon and K. Ramawat (Cham: Springer), 135–147. doi: 10.1007/978-3-319-96397-6_27
Zupo, V., and Maibam, C. (2011). “Ecological role of benthic diatoms as regulators of invertebrate physiology and behaviour,” in Diatoms: Classification, Ecology and Life Cycle, ed. J. C. Compton (New York, NY: Nova Publisher), 149–168.
Zupo, V., and Messina, P. (2007). How do dietary diatoms cause the sex reversal of the shrimp Hippolyte inermis Leach (Crustacea, Decapoda). Mar. Biol. 151, 907–917. doi: 10.1007/s00227-006-0524-9
Zupo, V., and Nelson, W. G. (1997). Influence of light on the spatial distribution and the ecology of Hippolyte zostericola (Smith)(Decapoda: Natantia) in the Indian River Lagoon (Florida, USA). Biol. Mar. Mediterr. 4, 156–166.
Zupo, V., Juttner, F., Maibam, C., Butera, E., Blom, J. F., Juttner, F., et al. (2014). Apoptogenic metabolites in fractions of the benthic diatom Cocconeis scutellum parva. Mar. Drugs 12, 547–567. doi: 10.3390/md12010547
Zupo, V., Maibam, C., Buia, M. C., Gambi, M. C., Patti, F. P., Scipione, M. B., et al. (2015). Chemoreception of the seagrass Posidonia oceanica by benthic invertebrates is altered by seawater acidification. J. Chem. Ecol. 41, 766–779. doi: 10.1007/s10886-015-0610-x
Zupo, V., Messina, P., Buttino, I., Sagi, A., Avila, C., Nappo, M., et al. (2007). Do benthic and planktonic diatoms produce equivalent effects in crustaceans? Mar. Freshw. Behav. Physiol. 40, 169–181. doi: 10.1080/10236240701592930
Zupo, V., Mutalipassi, M., Fink, P., and Di Natale, M. (2016). Effect of ocean acidification on the communications among invertebrates mediated by plant-produced volatile organic compounds. Glob. Ecol. Biogeogr. 1, 012–018. doi: 10.17352/gje.000002
Keywords: VOCs, chemotaxis, Cocconeis scutellum, Diploneis, Ulva prolifera, behavioral responses, volatile organic compound, Posidonia oceanica
Citation: Mutalipassi M, Mazzella V, Schott M, Fink P, Glaviano F, Porzio L, Lorenti M, Buia MC, von Elert E and Zupo V (2022) Ocean Acidification Affects Volatile Infochemicals Production and Perception in Fauna and Flora Associated With Posidonia oceanica (L.) Delile. Front. Mar. Sci. 9:809702. doi: 10.3389/fmars.2022.809702
Received: 05 November 2021; Accepted: 20 January 2022;
Published: 01 March 2022.
Edited by:
Bin Wu, Zhejiang University, ChinaReviewed by:
Jiajun Wu, City University of Hong Kong, Hong Kong SAR, ChinaUlrich Hoeger, Johannes Gutenberg University Mainz, Germany
Copyright © 2022 Mutalipassi, Mazzella, Schott, Fink, Glaviano, Porzio, Lorenti, Buia, von Elert and Zupo. This is an open-access article distributed under the terms of the Creative Commons Attribution License (CC BY). The use, distribution or reproduction in other forums is permitted, provided the original author(s) and the copyright owner(s) are credited and that the original publication in this journal is cited, in accordance with accepted academic practice. No use, distribution or reproduction is permitted which does not comply with these terms.
*Correspondence: Mirko Mutalipassi, bWlya28ubXV0YWxpcGFzc2lAc3puLml0; Eric von Elert, ZXZlbGVydEB1bmkta29lbG4uZGU=; orcid.org/0000-0001-7758-716X; Valerio Zupo, dmFsZXJpby56dXBvQHN6bi5pdA==; dnp1cG9Ac3puLml0; orcid.org/0000-0001-9766-8784
†These authors have contributed equally to this work and share first authorship
‡These authors have contributed equally to this work and share the last authorship