- 1Department of Biosciences, Faculty of Science and Engineering, Swansea University, Swansea, United Kingdom
- 2Cultech Ltd., Port Talbot, United Kingdom
- 3AB Agri Ltd., Peterborough, United Kingdom
Probiotic use in aquaculture settings can be an approach for disease control and dietary supplementation. We assessed the antagonistic effect of culture supernatants of lactic acid bacteria on the growth of known shrimp pathogens, Vibrio (Listonella) anguillarum, Vibrio alginolyticus, and V. harveyi, using a quantitative microplate bioassay. Supernatants from Lactobacillus curvatus subsp. curvatus, L. plantarum, and Pediococcus acidolactici significantly inhibited the growth of these vibrios. The active component(s) were heat stable (> 100°C) and resistant to freeze-thawing. Most of this inhibitory activity was brought about by the production of an acid pH; however, there was evidence for other factors playing a role. In the search for novel probiotic bacteria, an organism was isolated from the gastrointestinal tract of healthy whiteleg shrimp (Penaeus vannamei)—identified tentatively as Carnobacterium maltaromaticum. This isolate, however, had less potent vibriocidal activity than the lactic acid bacteria and reduced shrimp survival at a dose of 1 × 107 bacteria/shrimp. During a 28-day feeding trial, juvenile P. vannamei fed with L. plantarum supplemented diets showed no gross changes in growth parameters compared with the control. We suggest that lactic acid bacteria could be incorporated into biofloc formulations to purge the growth of pathogenic vibrios in pond settings, rather than being fed directly to shrimp.
Introduction
Shellfish production is of increasing importance globally to provide a food source for human populations. Shrimp are the main cultured crustaceans with whiteleg (Pacific white) shrimp Penaeus (Litopenaeus) vannamei constituting the largest—4.97 million metric tons yielded in 2018 (FAO, 2020). Shrimp aquaculture has been plagued by disease and epizootics caused by bacterial (e.g., vibrio) and viral (e.g., white spot syndrome virus) pathogens over the last few decades (Naylor et al., 2021; Dhar et al., 2022; Rowley, 2022). To counter these, improvements in shrimp health have been pursued including development of antimicrobials but with their adverse consequences for the development of antibiotic resistance (Chi et al., 2017; Thornber et al., 2020; Sharma et al., 2021), vaccines (Rowley and Pope, 2012; Amatul-Samahah et al., 2020), biofloc technology (Avnimelech, 2015), and pre/pro-biotics (see Ringø et al., 2020; Butt et al., 2021; Knipe et al., 2021 for recent reviews). In terms of probiotics, research has focused on either seeking beneficial bacteria found naturally in the gastrointestinal (GI) tract of shrimp or employing existing probiotics used in humans including lactic acid-producing bacteria (Kesarcodi-Watson et al., 2008; Ringø et al., 2020).
Lactic acid bacteria are a heterogeneous group of Gram-positive, rod-shaped, non-spore-forming, bacteria that ferment carbon sources to produce organic acids including lactic acid. This group includes a wide range of genera including Lactobacillus, Lactococcus, Leuconostoc, Oenococcus, Pediococcus, Streptococcus, and Weissella (Cohen et al., 2008). These are found in a wide range of environments and in association with both animals and plants. In humans and other terrestrial mammals, they are found on the skin, and in the oral cavity, the gastrointestinal (GI) tract, and the vagina (Barbés, 2008). Lactic acid bacteria are important probiotics in the manufacture of fermented foods. In terms of their potential as probiotics, key species include Lactobacillus plantarum, L. casei, L. acidophilus, L. brevis, L. rhamnosus, and Pediococcus acidilactici (e.g., Gareau et al., 2010; Holzapfel and Wood, 2014; Deng et al., 2022). Members of the genus Lactobacillus have been extensively studied with respect to their potential as probiotics to maintain both human (e.g., Gareau et al., 2010; Blum et al., 2022) and animal health (e.g., Ringø et al., 2020; Deng et al., 2022). They produce a wide range of metabolites including bacteriocins (e.g., plantaricin), bacteriocin-like factors, lactic acid and hydrogen peroxide that kill or inhibit the growth of bacteria including those that may be potentially pathogenic to the host (Corr et al., 2007). Furthermore, in some animals, lactobacilli readily adhere to the epithelial cells that line the GI tract, which facilitates their colonization (Altermann et al., 2005).
An increasing number of studies are investigating the potential of lactic acid bacteria as probiotics for shellfish including shrimp (see recent reviews by Ringø et al., 2020; Knipe et al., 2021; Naiel et al., 2021). For example, Castex et al. (2008) explored the commercial application of P. acidilactici (Bactocell®) on the GI tract microbiota of Penaeus stylirostris and found that the hepatosomatic index was higher in the probiotic group compared to controls, coupled with significant reductions in both total bacterial and vibrio counts after probiotic administration. Similarly, summer syndrome caused by Vibrio nigripulchritudo was significantly reduced for shrimp in the probiotic group, which demonstrates the efficacy of probiotics in reducing pathogenic bacteria in culture settings. In other studies, short term administration of L. plantarum or L. acidophilus in combination with Saccharomyces cerevisiae in the diet of P. vannamei also act as general immune stimulants that lead to resistance to bacterial infection (e.g., Chiu et al., 2007; Pooljun et al., 2020). Synbiotic preparations of pre- and pro-biotics based on L. plantarum have shown promising potential as feed supplements to improve the growth/of P. vannamei (Kuo et al., 2021; Prabawati et al., 2022).
Herein, we set out to examine the potential of lactic acid bacteria as probiotics for shrimp with reference to their antagonistic behavior toward several Vibrio spp. considered to be pathogens of these animals. In response to evidence that probiotic administration results in significant improvement on growth and feed conversion (e.g., Balcázar et al., 2007), we also investigated whether delivery of a commercial strain of L. plantarum used in probiotic preparations for humans in feed has any effect on the growth potential of P. vannamei. Finally, we also sought lactic acid bacteria found naturally in the GI tract of juvenile shrimp as putative probiotics for later studies.
Materials and Methods
Source of Potential Probiotics for Penaeus vannamei
Commercially Available Lactic Acid Bacteria
Three species of lactic acid bacteria commonly used as probiotics in animal studies were selected for screening for anti-Vibrio activity, namely, Lactobacillus plantarum (NCIMB 30280), Pediococcus acidilactici (NCIMB 8018), and Lactobacillus curvatus subsp. curvatus (NCIMB 9716). L. plantarum (from Cultech Ltd., Baglan, United Kingdom), P. acidolactici, and L. curvatus were stored on slopes of de Man, Rogosa, and Sharpe (MRS) agar (Difco, Becton Dickinson & Co., Oxford, United Kingdom) and grown aerobically on MRS medium at 30°C when required.
Natural Microbiota of Healthy Shrimp
The microbiota of healthy post-larvae and gastrointestinal tract microflora of juvenile P. vannamei were screened for potential novel Lactobacillus-like probiotic bacterial strains. Six post-larval and juvenile shrimp of sizes 0.5 ± 0.1 and 8 ± 0.5 g, respectively, were sampled. Animals were obtained from the Centre for Sustainable Aquatic Research facility at Swansea University. Whole post-larval shrimp homogenate was prepared (after washing with sterile 3% NaCl [w/v] solution) as their size made removing intact hind gut and hepatopancreas challenging. Juvenile shrimp were dissected aseptically with samples of whole mid-hind gut, including feces, and hepatopancreas taken. Biofilm swabs from the surfaces of shrimp rearing tanks were also tested. Samples were placed in tubes containing 500 μl of sterile 3% NaCl solution before homogenization. Dilutions of the homogenates were performed followed by spread plating on MRS agar (plus 2% NaCl). Plates were incubated under aerobic and anaerobic conditions at 37°C for 72 h. All visually distinct colonies were streaked onto fresh plates. Gram staining and gross colony morphology of isolates were recorded. Initial identification of those Gram-positive isolates obtained from MRS agar plates displaying anti-Vibrio activity was made using the API 50 CHL sugar fermentation test (BioMérieux United Kingdom Ltd., Basingstoke, United Kingdom) as per the manufacturer’s instructions.
Screening Panel of Putative Bacterial Pathogens of Shrimp
Vibrio spp. were selected based on prior evidence of pathogenicity against crustaceans, particularly shrimp: Vibrio harveyi (NCIMB 1280), V. alginolyticus (NCIMB 1339), and V. anguillarum (NCIMB 829). All cultures were sourced from NCIMB Ltd. (Aberdeen, United Kingdom) and maintained on tryptic soy agar (TSA) plus 2% NaCl slopes.
Cell-Free Culture Supernatant Antagonism Assay
This qualitative technique determined whether the cell-free culture supernatant of potential probiotics exhibited any antagonistic activity toward Vibrio spp. Tryptic soy agar plates (+ 2% NaCl) and tryptic soy broth (TSB, + 2% NaCl) were used to culture the vibrios. Cell-free culture supernatant obtained from incubations of the potential probiotic isolates was tested for antagonistic activity against these bacteria. The strains of lactic acid bacteria were cultured in MRS broth at 30°C for 24 h, and subsequently, they were centrifuged (6,000 × g, 10 min at 25°C) and the supernatant was filter sterilized (0.22 μm). Supernatants were stored at 4°C for no more 24 h prior to assay commencement. V. harveyi and V. anguillarum were grown in TSB (plus 2% NaCl) at 25°C for 18 h and adjusted to ca. 2 × 109 total bacteria.ml–1. Culture (100 μl) was then evenly spread on each plate and 12 equidistant, 4 mm diameter wells were punched into the agar. Forty microliters of the potential probiotic cell-free culture supernatant was added to each well in triplicate (technical replicates). A negative control of the appropriate uninoculated broth was added to the remaining three wells. Each plate was run in duplicate and incubated at 25°C for 24 and 48 h.
The effects of varying the incubation period were assessed by using cell-free supernatant produced from culture samples extracted daily over 7 days. To assess the heat stability of putative anti-Vibrio factors, the activity of cell-free culture supernatant (24 h culture) was determined after exposure to temperatures of 65 and 100°C. In addition, to check their stability, the supernatants were also subjected to several cycles of freeze-thawing at –80°C.
Quantification of Microbial Growth Inhibition by Cell-Free Culture Supernatants
The ability of lactic acid bacteria culture supernatants to inhibit the bacterial growth of pathogenic vibrios was quantified using a microplate-based assay. Crustacean pathogens V. harveyi and V. alginolyticus were cultured in TSB (plus 2% NaCl) at 25°C for 12 h, and 10 ml aliquots were centrifuged (1,000 × g for 5 min at 25°C), washed twice in 3% NaCl solution, and adjusted to ca. 1 × 109 cells ml–1.
Cell-free culture supernatants (pH 4.0) of both the commercially available lactobacilli and shrimp isolates were prepared as stated above. Aliquots of these supernatants were also adjusted to pH 6.2 (that of uninoculated MRS broth) and were tested alongside the original supernatants (pH 4.0). Fifty microliters of V. harveyi or V. alginolyticus suspension were incubated with 100 μl of cell-free culture supernatant at 25°C with shaking for 30 min, in flat-bottomed, 96 well plates. All combinations of cell-free culture supernatant and Vibrio were included and run in triplicate on each plate, alongside controls [cell-free culture supernatants and pathogen suspensions were replaced by sterile 3% NaCl solution (w/v)]. A second 96-well plate was prepared with 200 μl of sterile TSB (+ 2% NaCl) per well. Post incubation, 50 μl from each well of the first plate was transferred aseptically to the corresponding wells of the second plate and the OD550 was recorded at 60 min intervals over a 24 h period (at 25°C).
Assessment of Pathogenicity of the Carnobacterium maltaromaticum Isolate Toward Penaeus vannamei
To ascertain if C. maltaromaticum was pathogenic to shrimp (and hence its suitability as a probiotic), post larval shrimp were challenged in vivo (see section “Results”). Shrimp weighing 1.0 ± 0.2 g (mean ± S.D., two groups each of 10 animals) were housed in two, 30 l tanks within a closed re-circulating system. Animals of the first group were administered an intramuscular injection of 100 μl sterile 3% NaCl solution, between the second and third pleomeres, and the remaining group was injected with 100 μl C. maltaromaticum suspension (∼1 × 107 viable bacteria per shrimp). No feed was administered during this trial, and the animals were checked at 12 h intervals for mortality changes. At 24, 72, and 96 h post-injection, two shrimp from each group were sacrificed for bacteriology. The small size of the animals made obtaining hemolymph via needle impractical and so the euthanized animals were bisected transversely at the join between the cephalothorax and first abdominal segment (pleomere). The cut surface of the abdominal section was touched onto two MRS agar (plus 2% NaCl) plates, the ca. 50 μl of hemolymph deposited was spread aseptically, and the plates were incubated at 25°C (72 h) before being checked for growth. Twenty-four hours after the end of the trial, samples of the tank’s surface biofilm were taken and plated (MRS agar plus 2% NaCl) to ascertain whether suspected C. maltaromaticum had become a component of the system microbiota.
Lactobacillus plantarum Feed Trial in Penaeus vannamei
Two groups each containing 54 post-larval P. vannamei (0.5 ± 0.05 g) were used to assess the short term (28 day) effects of the oral administration of L. plantarum on growth, feed conversion ratio (FCR) and shrimp survival. Each diet group was housed over three randomly assigned, 30 l tanks (18 animals per tank). One group was fed the control diet and the second group received the same diet top-coated with L. plantarum culture. A commercial shrimp maturation diet (Dragon Feeds; 42% protein, 10% lipid, 1 mm pellets) was used as the basis for both treatments. In the case of the L. plantarum supplemented diet, a powdered mix of lyophilized L. plantarum culture (Cultech Ltd., Baglan, United Kingdom) at a concentration of ca. 1.6 × 1011 CFU g–1 in skimmed milk powder was top coated onto a commercial diet such that it contained a mass of the probiotic mixture equivalent to 1% (w/w) of the total (final) mass of feed. The viability of L. plantarum in the final feed was estimated by spread plating techniques as ca. 2 × 108 CFU g–1. The control feed was coated by the same method and contained the equivalent amount of skimmed milk powder. All feed was stored in sealed containers at 4°C until required. Fresh feed was produced halfway through the trial.
Animals were fed twice daily with the feed amount equivalent to ca. 7% of tank biomass per day. Prior to feeding, fecal matter and uneaten feed were removed from the tanks, the unconsumed feed was collected separately from the feces, dried at RT and weighed. Five randomly selected animals from each tank were weighed weekly and survival was monitored daily. These values were used to estimate the biomass of each tank and thus the amount of feed required for the subsequent 7 days. Accurate tank biomass determinations were made, via a total count and batch weighing of the animals, at the start of the trial, at the midpoint, and at the end of the trial (28 days). From these data, average values for each tank for individual animal weight and growth rates were determined. Furthermore, to estimate FCR the amount of feed consumed was calculated by subtracting the amount of un-consumed feed from the amount administered.
Statistical Analyses
Analysis of variance (ANOVA) together with a Bonferroni multiple comparisons post hoc test was used to determine potential differences between bacterial growth curves. This followed the determination of normal distribution of the data via the application of a Kolmogorov–Smirnov test. Survival data were assessed using a log-rank (Mantel-Cox) curve comparison test. All values are shown as arithmetic means ± 1 standard error of the mean (S.E.M). The reader should refer to figure and table descriptors for respective sample (n) sizes.
Results
Antagonistic Behavior of Cell-Free Supernatants of Lactobacillus plantarum, Pediococcus acidolactici, and Lactobacillus curvatus subsp. curvatus
The cell-free culture supernatants of L. plantarum and P. acidilactici inhibited the growth of V. harveyi and V. anguillarum producing 1.5–3 mm diameter clearance zones around wells (Figure 1A). The supernatant of L. curvatus subsp. curvatus, however, produced only weak, intermittent interference of V. anguillarum growth. Consequently, it was decided not to undertake further testing of L. curvatus subsp. curvatus. The ability of L. plantarum and P. acidilactici cell-free culture supernatants to inhibit the growth of V. harveyi and V. anguillarum was undiminished by heating or freeze-thawing (Figure 1B). Interestingly, cell-free culture supernatants of L. plantarum or P. acidilactici collected over the 7 day growth period produced zones of pathogen inhibition comparable with the 24 h samples.
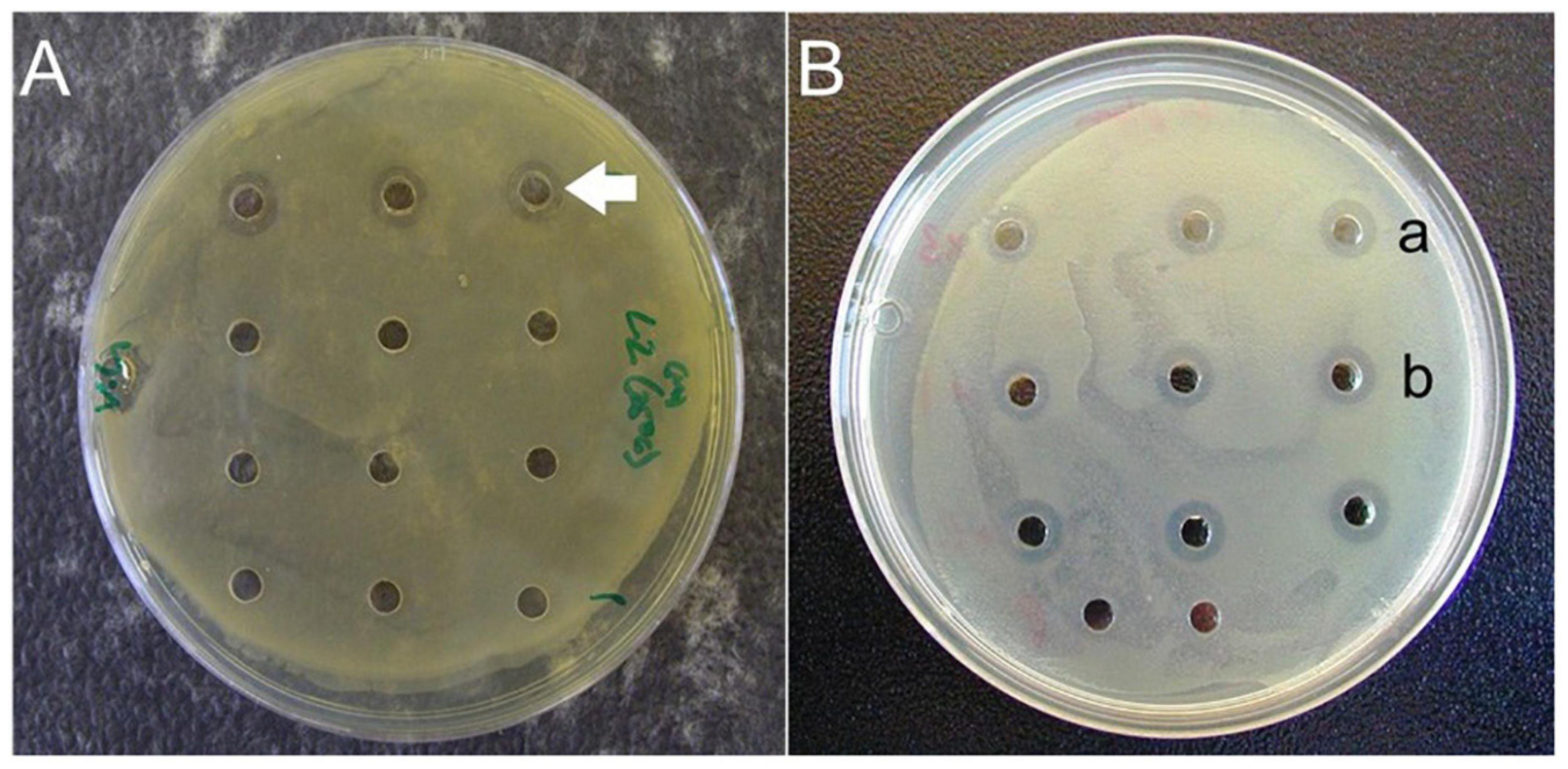
Figure 1. (A) Cell-free culture supernatant antagonistic activity assay against Vibrio harveyi. Positive results observed using cell-free supernatant from Lactobacillus plantarum (unlabeled arrow). Supernatant in the remaining wells (from other isolates from shrimp GI tracts) displayed no antagonistic activity. (B). Cell-free culture supernatant antagonistic activity assay against V. alginolyticus. Positive results observed using cell-free supernatant from L. plantarum subjected to freezing/thawing (row a) and heating (row b).
A microplate reader-based assay was used to fully quantify the interaction between culture supernatants of L. plantarum and P. acidolactici with vibrios. Cell-free culture supernatant obtained from L. plantarum and P. acidilactici inhibited the growth of both V. harveyi and V. alginolyticus over a 24 h period (Figures 2A–D). No detectable bacterial growth was observed in any of the wells containing unaltered cell-free culture supernatant at pH 4. Growth did, however, occur in wells when the pH of the cell-free supernatant was adjusted to 6.2 for both lactic acid bacteria. This growth was, however, less than that observed in the appropriate positive controls (Vibrio plus MRS broth; pH 6.2). In the case of pH adjusted L. plantarum culture supernatant, V. harveyi displayed a slight, but not statistically significant decline in growth/cell number after 15 h, when compared with the positive (bacteria-only) control. The effects were more pronounced for V. alginolyticus, where growth was significantly lower over the incubation period in wells containing the pH adjusted cell-free supernatant (pH 6.2) in comparison with that in the bacteria-only control (Figure 2B). The pH adjusted cell-free culture supernatants of P. acidilactici and L. plantarum appeared equally ineffective in inhibiting the growth of V. harveyi (Figures 2A,C). However, both were more effective in their inhibition of V. alginolyticus producing statistically significant growth inhibition (Figures 2B,D).
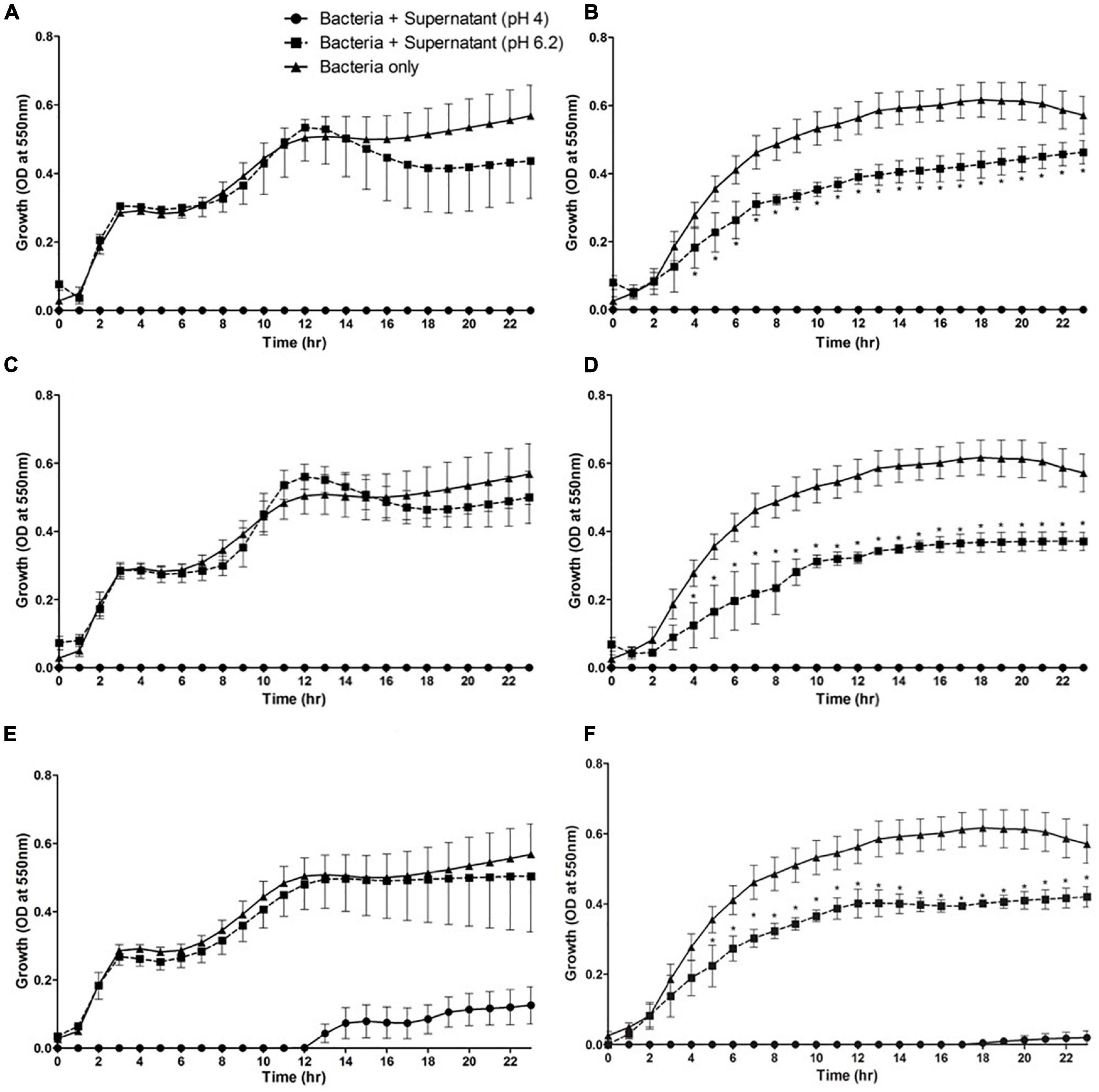
Figure 2. Growth profiles of pathogenic Vibrio species in the presence of culture supernatants from lactic acid bacteria in vitro. Vibrio harveyi (A,C,E) and V. alginolyticus (B,D,F) were incubated in the presence of culture supernatant from Lactobacillus plantarum (A,B), Pediococcus acidilactici (C,D), and Carnobacterium maltaromaticum (E,F). Mean values ± S.E.M, n = 5 (per bacterium, 15 in total), *P < 0.05 compared to bacteria alone control and cell-free supernatant of the respective lactic acid bacterium at pH 6.2.
Screening of the Microbiota of Penaeus vannamei for Potential Novel Lactobacilli-Like Probiotic Bacteria
The isolates from P. vannamei (Table 1) represent all the colonies observed growing on MRS plates. As the gross morphology of these colonies was highly uniform, all were screened for vibriocidal activity. This screening yielded only two isolates that exhibited potential antagonistic activity toward the selected target Vibrio spp. (V. harveyi and V. alginolyticus) but both had no apparent activity against V. anguillarum. The isolates were tentatively identified using the API 50 CHL (V5.1) sugar fermentation test as the lactic acid bacterium, Carnobacterium maltaromaticum (97.7% match). The bacteria isolated on MRS media under anaerobic conditions (see Table 1) failed to grow after exposure to aerobic conditions and therefore were not tested for anti-Vibrio antagonistic behavior.
As was the case with L. plantarum and P. acidilactici, the cell-free culture supernatants of C. maltaromaticum isolates were found to be acidic (ca. pH 4) and initially inhibited the growth of both V. harveyi and V. alginolyticus in the microplate assay (Figures 2E,F). This inhibition was not total, however, with vibrio growth occurring after 12 h in the case of V. harveyi and 18 h in the case of V. alginolyticus. Adjusting the cell-free culture supernatant pH to 6.2 for C. maltaromaticum did not interfere with the growth of V. harveyi but reduced the growth rate of V. alginolyticus in relation to the bacteria only control (Figure 2F). Cell-free supernatants of the isolates were found to have no demonstrable inhibitory activity against V. anguillarum when using zone of inhibition assays (not shown).
Carnobacterium maltaromaticum Challenge of Shrimp
Exposure of shrimp to 1 × 107 CFUs of C. maltaromaticum significantly (P = 0.0039) decreased survival (60%) over 96 h (Figure 3). Tissue from C. maltaromaticum-inoculated shrimp on MRS agar produced large numbers of colonies similar in shape and morphology to the inoculum, implying this bacterium was not effectively cleared by the host’s immune defenses. Biofilm samples collected from the tanks containing the C. maltaromaticum administered animals were positive for the presence of lactic acid bacteria 24 h after the end of the trial but no further identification of these was carried out and so their identity could be unrelated to the challenge bacteria.
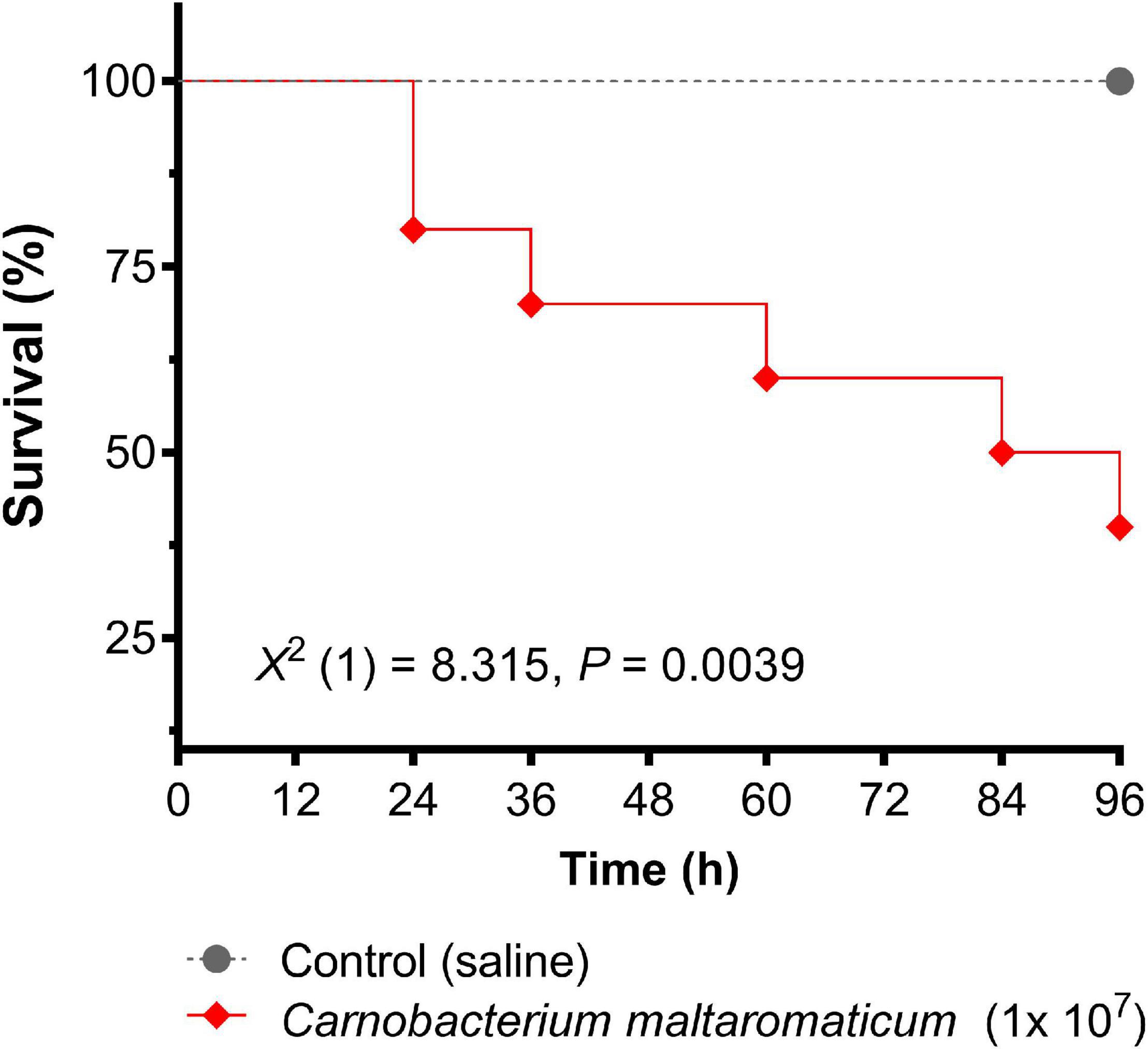
Figure 3. Survival of whiteleg shrimp, Penaeus vannamei, over 96 h post inoculation with Carnobacterium maltaromaticum. Each shrimp was administered 1 × 107 bacteria via intramuscular injection (n = 10 per group, 20 in total). Data were analyzed using the log-rank (Mantel–Cox) curve comparison test.
Diet Trials Using Lactobacillus plantarum
No measurable beneficial effect was observed in shrimp fed with L. plantarum supplemented diets compared with those fed control diets without probiotic. No statistically significant differences were observed between the control and L. plantarum supplemented diet groups for any of the performance parameters assessed (P > 0.05; Table 2).

Table 2. Performance data obtained for post-larval Penaeus vannamei fed a commercial shrimp maturation diet and the equivalent diet supplemented with Lactobacillus plantarum, for 28 days.
Discussion
In the last twenty years an increasing number of studies show improvements in growth, digestive enzymes and survival after bacterial challenge, changes in the gut microbiome, and enhanced immune capacity of P. vannamei following feeding with lactic acid bacteria alone or in combination with prebiotics, other probiotics, or immune stimulants (Chiu et al., 2007, 2021; Vieira et al., 2008; Kongnum and Hongpattarakere, 2012; Nguyen et al., 2018; Zheng et al., 2018, 2020; Du et al., 2020; Pooljun et al., 2020; Kuo et al., 2021; Prabawati et al., 2022). Most studies report enhanced growth in the presence of probiotics (e.g., Chiu et al., 2021; Kuo et al., 2021), others (Bernal et al., 2017; Nguyen et al., 2018) including our current study, did not find evidence of such improvements. We observed no changes in the recorded parameters (e.g., growth rate, FCR; Table 2) between the control and L. plantarum diet groups over the 28 day feeding period. It could be argued that the benefits of such probiotic feed may be cumulative and gradual, and therefore, would only become apparent over longer term administration, however, other workers using the same species of shrimp and a variety of probiotic bacteria (Bacillus subtilis, Pseudomonas aestumarina, Roseobacter gallaeciensis, and V. alginolyticus) have reported clear benefits in growth parameters over a similar short period (e.g., Balcázar et al., 2007). It could also be argued that the optimal environmental conditions in our recirculating aquaculture system (e.g., temperature, oxygenation, and waste product removal) and formulated shrimp diets produced the ideal growth rates that could not be further improved because of probiotic incorporation. Hence, it would be useful to repeat these trials under field conditions in commercial production sites where our environmental conditions may not be readily maintained.
Our study has shown that all three species of lactic acid bacteria tested (L. plantarum, P. acidolactici, and L. curvatus) exhibit antagonistic activity to V. alginolyticus and V. harveyi in the form of inhibitory compounds released into the culture media. The main active component in the inhibitory activity displayed by the lactic acid bacteria was probably the acidic pH. In addition to this, however, there appeared to be secondary component(s) involved in this inhibition. For instance, the pH adjusted (pH 6.2) cell-free culture supernatant of all three strains still significantly inhibited the growth of V. alginolyticus compared to the bacteria-only controls. Although the inhibition exhibited was considerably reduced compared to that of the original acidic supernatants, the presence of a secondary inhibitory component separate to organic acids was suggested. Indeed, strains of all three species of the lactic acid bacteria employed in the current study have been found to produce antimicrobial peptides or bacteriocins in addition to lactic acid (Suma et al., 1998; Blom et al., 2001; Jamuna and Jeevaratnam, 2004; Martin-Visscher et al., 2008; Funck et al., 2020). Furthermore, Corr et al. (2007) highlighted the importance of bacteriocin production as an explanation for the antagonistic behavior of lactic acid bacteria in the murine GI tract. In this model, these authors showed that strains of Lactobacillus salivarius that can produce bacteriocins were able to protect mice against Listeria monocytogenes while strains unable to produce these factors had no such ability.
The cell-free culture supernatant of the putative C. maltaromaticum isolate displayed less inhibitory ability toward potential pathogens than that of L. plantarum or P. acidilactici. Despite this, the fact that it is isolated from the host species, and therefore potentially a constituent of the normal GI tract microbiota, made it worthy of further evaluation. A fundamental question when selecting a potential probiotic is whether it is of benefit to the host organism (Fuller, 1992). Consequently, any candidate micro-organism displaying significant pathogenicity toward the host could not reasonably be forwarded for further study as a probiotic (Decamp and Moriarty, 2006; Kesarcodi-Watson et al., 2008). Our initial susceptibility studies showed that injection of the C. maltaromaticum-like isolate from shrimp resulted in a cumulative mortality of 64% after a high challenge dose of ca. 1 × 107 viable bacteria shrimp–1. For that reason, together with its limited anti-vibrio activity, we decided not to pursue it further in additional feeding trials.
Whether C. maltaromaticum, L. plantarum and P. acidilactici could maintain their inhibitory potential in vivo within the shrimp’s GI tract and benefit the host is an important question. Unless they multiply and colonize the gut, then there is arguably little chance that the anti-vibrio effect seen in the in vitro assays would be replicated in vivo without constant feeding. Many of the feeding trials of fish and shellfish with lactic acid bacteria suggest that these bacteria do not readily colonize the GI tract of such animals. For instance, Castex et al. (2008) were unable to find viable P. acidilactici in the shrimp GI tract within 24 h post-feeding. In another experiment where the authors took hourly samples post-feeding, they found a rapid reduction in P. acidilactici numbers. Similarly, Kesselring et al. (2019) fed shrimp the commercial probiotic mix, AquaStar® (a mixture of P. acidilactici, L. reuteri, Enterococcus faecium, and Bacillus subtilis) but the beneficial effects of this required continuous or pulse-feeding. Overall, however, despite the apparent lack of long-term colonization by some lactic acid bacteria of the GI tract of shellfish, such as shrimp, there are data implying that such bacteria do act to alter the microbiome (Zheng et al., 2020; Chiu et al., 2021). For example, Zheng et al. (2020) found that diets supplemented with culture supernatants without viable L. plantarum were able to modulate the gastrointestinal bacterial microbiome toward the presence of beneficial genera potentially improving the digestive activity and health of such animals. Other approaches of adding probiotics to the rearing water holding shrimp may provide a practical way around the problem of the need for constant feeding with probiotic-containing diets. One such approach could involve the incorporation of probiotic bacteria into the uni- and multi-cellular organisms in biofloc technology that has recently been studied with other bacterial probiotics (Panigrahi et al., 2020).
Conclusion
Lactic acid bacteria tested herein produced inhibitory factors that interfered with the growth of several pathogenic vibrios in vitro. A short-term trial feeding L. plantarum to juvenile shrimp was without significant effects on the growth and survival of such animals. Such results highlight that bacteria screened as potential probiotics using in vitro assays do not always demonstrate growth-promoting capacities in vivo. Other mechanisms, such as the inclusion of probiotic bacteria in shrimp rearing ponds as part of biofloc technology—recently studied by Panigrahi et al. (2020) and Flores-Valenzuela et al. (2021)—may be an avenue worth pursuing.
Data Availability Statement
The raw data supporting the conclusions of this article will be made available by the authors, without undue reservation.
Author Contributions
JT, IL, and AR designed the study with the assistance of all other authors. JT, IL, CC, and AR analyzed the data. AR, CC, and SP acquired the funding. All authors either drafted or edited the manuscript.
Funding
JT was supported by the European Social Fund doctoral training grant. AR and CC were supported by the BBSRC/NERC ARCH-UK network grant (BB/P017215/1).
Conflict of Interest
MW and SP were employed by the company Cultech Ltd. IL is employed by the company AB Agri Ltd., but not at the time the study was conducted.
The remaining authors declare that the research was conducted in the absence of any commercial or financial relationships that could be construed as a potential conflict of interest.
Publisher’s Note
All claims expressed in this article are solely those of the authors and do not necessarily represent those of their affiliated organizations, or those of the publisher, the editors and the reviewers. Any product that may be evaluated in this article, or claim that may be made by its manufacturer, is not guaranteed or endorsed by the publisher.
Acknowledgments
The technical assistance of Alex Keay and Craig Pooley is gratefully acknowledged.
References
Altermann, E., Russell, W. M., Azacarate-Peril, M. A., Barrangou, R., Buck, B. L., McAuliffe, O., et al. (2005). Complete genome sequence of the probiotic lactic acid bacterium Lactobacillus acidophilus NCFM. Proc. Natl. Acad. Sci. U.S.A. 102, 3906–3912. doi: 10.1073/pnas.0409188102
Amatul-Samahah, M. A., Omar, W. H. H. W., Ikhsan, N. F. M., Azmai, M. N. A., and Ina-Salwany, M. Y. (2020). Vaccination trials against vibriosis in shrimp: A review. Aquaculture Rep. 18:100471. doi: 10.1016/j.aqrep.2020.100471
Avnimelech, Y. (2015). Biofloc Technology - a Practical Guide Book, 3rd. Edn. Baton Rouge: The World Aquaculture Society.
Balcázar, J. L., Rojas-Luna, T., and Cunningham, D. P. (2007). Effect of the addition of four potential probiotic strains on the survival of Pacific white shrimp (Litopenaeus vannamei) following immersion challenge with Vibrio parahaemolyticus. J. Invertebr. Pathol. 96, 147–150. doi: 10.1016/J.JIP.2007.04.008
Barbés, C. (2008). “Lactobacilli,” in Therapeutic Microbiology: Probiotics and Related Strategies, eds J. Versalovic and M. Wilson (Washington: American Society of Microbiology Press), 19–33.
Bernal, M. G., Marrero, R. M., Campa-Córdova, A. I., and Mazón-Suástegui, J. M. (2017). Probiotic effect of Streptomyces strains alone or in combination with Bacillus and Lactobacillus in juveniles of the white shrimp Litopenaeus vannamei. Aquacult. Int. 25, 927–939. doi: 10.1007/s10499-016-0085-y
Blom, H., Katla, T., Nissen, H., and Helge, H. (2001). Characterization, production and purification of Carnocin H, a bacteriocin produced by Carnobacterium 377. Curr. Microbiol. 43, 227–231. doi: 10.1007/s002840010292
Blum, J., Silva, M., Byrne, S. J., Butler, C. A., Adams, G. G., Reynolds, E. C., et al. (2022). Temporal development of infant oral microbiome. Crit. Rev. Microbiol 11, 1–13. doi: 10.1080/1040841X.2021.2025042
Butt, U. D., Lin, N., Akter, N., Siddiqui, T., Li, S., and Wu, B. (2021). Overview of latest developments in the role of probiotics, prebiotics and synbiotics in shrimp aquaculture. Fish Shellfish Immunol. 114, 263–281. doi: 10.1016/j.fsi.2021.05.003
Castex, M., Chim, L., Pham, D., Lemaire, P., Wabete, N., Nicolas, J.-L., et al. (2008). Probiotic P. acidilactici application in shrimp Litopenaeus stylirostris culture subject to vibriosis in New Caledonia. Aquaculture 275, 182–193. doi: 10.1016/j.aquaculture.2008.01.011
Chi, T. T. K., Clausen, J. H., Van, P. T., Tersbøl, B., and Dalsgaard, A. (2017). Use practices of antimicrobials and other compounds by shrimp and fish farmers in Northern Vietnam. Aquaculture Rep. 7, 40–47. doi: 10.1016/j.aqrep.2017.05.003
Chiu, C.-H., Guu, Y.-K., Liu, C.-H., Pan, T.-M., and Cheng, W. (2007). Immune responses and gene expression in white shrimp Litopenaeus vannamei, induced by Lactobacillus plantarum. Fish Shellfish Immunol. 23, 364–377. doi: 10.1016/j.fsi.2008.12.003
Chiu, S. T., Chu, T.-W., Simangunsong, T., Ballantyne, R., Chiu, C.-S., and Liu, C.-H. (2021). Probiotic. Lactobacillus pentosus BD6 boost the growth and health status of white shrimp, Litopenaeus vannamei via oral administration. Fish Shellfish Immunol. 117, 124–135. doi: 10.1016/j.fsi.2021.07.024
Cohen, D. P. A., Vaughan, E. E., de Vos, W. M., and Zoetendal, E. G. (2008). “Proteomic approaches to study lactic acid bacteria,” in Therapeutic Microbiology: Probiotics and Related Strategies, eds J. Versalovic and M. Wilson (Washington, USA: American Society of Microbiology Press), 205–221. doi: 10.1128/9781555815462.ch16
Corr, S. C., Riedel, C. U., O’Toole, P. W., and Gahan, C. G. M. (2007). Bacteriocin production as a mechanism for the antiinfective activity of Lactobacillus salivarius UCC118. Proc. Natl. Acad. Sci. U.S.A. 104, 7617–7621. doi: 10.1073/pnas.0700440104
Decamp, O., and Moriarty, D. J. W. (2006). Probiotics as alternative to antimicrobials: Limitations and potential. J. World Aquaculture Soc. 37, 60–62.
Deng, Z. X., Hou, K. W., Zhao, J. C., and Wang, H. F. (2022). The probiotic properties of lactic acid bacteria and their applications in animal husbandry. Curr. Microbiol. 79:22. doi: 10.1007/s00284-021-02722-3
Dhar, A. K., Cruz-Flores, R., and Bateman, K. S. (2022). “Viral diseases of crustaceans,” in Invertebrate Pathology, eds A. F. Rowley, C. J. Coates, and M. M. A. Whitten (Oxford: Oxford University Press), 368–399.
Du, Y., Wang, B., Jiang, K., Wang, M., Zhou, S., Liu, M., et al. (2020). Exploring the influence of the surface proteins on probiotic effects performed by Lactobacillus pentosus HC-2 using transcriptome analysis in Litopenaeus vannamei midgut. Fish Shellfish Immunol. 87, 853–870. doi: 10.1016/j.fsi.2019.02.027
Flores-Valenzuela, E., Miranda-Baeza, A., Rivas-Vega, M. E., Miranda-Arizmendi, V., Beltrán-Ramírez, O., and Emerenciano, M. G. C. (2021). Water quality and productive response of Litopenaeus vannamei reared in biofloc with addition of commercial strains of nitrifying bacteria and Lactobacillus rhamnosus. Aquaculture 542:736869. doi: 10.1016/j.aquaculture.2021.736869
Fuller, R. (1992). “History and development of probiotics,” in Probiotics the Scientific Basis, ed. R. Fuller (London: Chapman & Hall), 1–8. doi: 10.1007/978-94-011-2364-8_1
Funck, G. D., Marques, J., de, L., Dannenberg, G., da, S., Cruxen, C. E., et al. (2020). Characterization, toxicity and optimization for the growth and production of bacteriocin-like substances by Lactobacillus curvatus. Probiotics Antimicr. Prot. 12, 91–101. doi: 10.1007/s12602-019-09531-y
Gareau, M. G., Sherman, P. M., and Walker, W. A. (2010). Probiotics and the gut microbiota in intestinal health and disease. Nat. Rev. Gastroenterol. Hepatol. 7, 503–514. doi: 10.1038/nrgastro.2010.117
Holzapfel, W. H., and Wood, B. J. B. (2014). Lactic Acid Bacteria; Biodiversity and Taxonomy. (New York: John Wiley & Sons) doi: 10.1002/9781118655252
Jamuna, M., and Jeevaratnam, K. (2004). Isolation and partial characterization of bacteriocins from Pediococcus species. Appl. Microbiol. Biotechnol. 65, 433–439. doi: 10.1007/s00253-004-1576-8
Kesarcodi-Watson, A., Kaspar, H., Lategan, M. J., and Gibson, L. (2008). Probiotics in aquaculture: The need, principles and mechanisms of action and screening processes. Aquaculture 274, 1–14. doi: 10.1016/j.aquaculture.2007.11.019
Kesselring, J. C., Gruber, C., Standen, B., and Wein, S. (2019). Continuous and pulse-feeding application of multispecies probiotic bacteria in whiteleg shrimp. Litopenaeus vannamei. J. World Aquacult. Soc. 50, 1123–1132. doi: 10.1111/jwas.12640
Knipe, H., Temperton, B., Lange, A., Bass, D., and Tyler, C. R. (2021). Probiotics and competitive exclusion of pathogens in shrimp. Rev. Aquacult. 13, 324–352. doi: 10.1111/raq.12477
Kongnum, K., and Hongpattarakere, T. (2012). Effect of Lactobacillus plantarum isolated from digestive tract of wild shrimp on growth and survival of white shrimp (Litopenaeus vannamei) challenged with Vibrio harveyi. Fish Shellfish Immunol. 32, 170–177. doi: 10.1016/j.fsi.2011.11.008
Kuo, H.-W., Chang, C.-C., and Cheng, W. (2021). Synbiotic combination of prebiotic, cacao pod husk pectin and probiotic, Lactobacillus plantarum, improve the immunocompetence and growth of Litopenaeus vannamei. Fish Shellfish Immunol. 118, 333–342. doi: 10.1016/j.fsi.2021.09.023
Martin-Visscher, L. A., van Belkum, M. J., Garneau-Tsodikova, S., Whittal, R. M., Zheng, J., McMullen, L. M., et al. (2008). Isolation and characterization of carnocyclin A, a novel circular bacteriocin produced by Carnobacterium maltaromaticum UAL307. Appl. Environ. Microbiol. 74, 4756–4763. doi: 10.1128/AEM.00817-08
Naiel, M. A. E., Farag, M. R., Gewida, A. G. A., et al. (2021). Using lactic acid bacteria as an immunostimulant in cultured shrimp with special reference to Lactobacillus spp. Aquacult. Int. 29, 219–231. doi: 10.1007/s10499-020-00620-2
Naylor, R. L., Hardy, R. W., Buschmann, A. H., Bush, S. R., Cao, L., Künger, D. H., et al. (2021). A 20-year retrospective review of global aquaculture. Nature 591, 551–563. doi: 10.1038/s41586-021-03308-6
Nguyen, T. T. G., Nguyen, T. C., Leekriangsak, M., Pham, T. T., Pham, Q. H., Lueangthuwapranit, C., et al. (2018). Promotion of Lactobacillus plantarum on growth and resistance against hepatopancreatic necrosis disease pathogens in white-leg shrimp (Litopenaeus vannamei). Thai J. Vet. Med. 48, 19–28.
Panigrahi, A., Das, R. R., Sivalumar, M. R., Saravanan, A., Sudheer, N. S., et al. (2020). Bio-augmentation of heterotrophic bacteria in biofloc system improves growth, survival, and immunity of Indian white shrimp Penaeus indicus. Fish Shellfish Immunol. 98, 477–487. doi: 10.1016/j.fsi.2020.01.021
Pooljun, C., Daorueang, S., Weerachatyanukul, W., Direkbusarakom, S., and Jariyapong, P. (2020). Enhancement of shrimp health and immunity with diets supplemented with combined probiotics: application to Vibrio parahaemolyticus infections. Dis. Aquat. Org. 140, 37–46. doi: 10.3354/dao03491
Prabawati, E., Hu, S.-Y., Chiu, S.-T., Balantyre, R., Risjani, Y., and Liu, C.-H. (2022). A synbiotic containing prebiotic prepared from a by-product of king oyster mushroom, Pleurotus eryngii and probiotic, Lactobacillus plantarum incorporated in diet to improve the growth performance and health status of shrimp, Litopenaeus vannamei. Fish Shellfish Immunol. 120, 155–165. doi: 10.1016/f.fsi.2021.11.031
Ringø, E., Van Doan, H., Lee, S., and Kyu Song, S. (2020). Lactic acid bacteria in shellfish: Possibilities and challenges. Rev. Fish. Sci. Aquaculture 28, 139–169. doi: 10.1080/23308249.2019.1683151
Rowley, A. F. (2022). “Bacterial diseases of crustaceans,” in Invertebrate Pathology, eds A. F. Rowley, C. J. Coates, and M. M. A. Whitten (Oxford: Oxford University Press), 400–435. doi: 10.1093/oso/9780198853756.003.0015
Rowley, A. F., and Pope, E. C. (2012). Vaccines and crustacean aquaculture - A mechanistic exploration. Aquaculture 334, 1–11. doi: 10.1016/j.aquaculture.2011.12.011
Sharma, L., Nagpal, R., Jackson, C. R., Patel, D., and Singh, P. (2021). Antibiotic-resistant bacteria and gut microbiome communities associated with wild-caught shrimp from the United States versus imported farm-raised retail shrimp. Sci. Rep. 11:3356. doi: 10.1038/s41598-021-82823-y
Suma, K., Misra, M. C., and Varadaraj, M. C. (1998). Plantaricin LP84, a broad spectrum heat-stable bacteriocin of Lactobacillus plantarum NCIM 2084 produced in a simple glucose broth medium. Int. J. Food Microbiol. 40, 17–25. doi: 10.1016/s0168-1605(98)00010-5
Thornber, K., Verner-Jeffreys, D., Hinchliffe, S., Rahman, M. M., Bass, D., and Tyler, C. R. (2020). Evaluating antimicrobial resistance in the global shrimp industry. Rev. Aquacult. 12, 966–986. doi: 10.1111/raq.12367
Vieira, F. D. N., Neto, C. C. B., Mouriño, J. L. P., Jatobá, A., Ramirez, C., Martins, M. L., et al. (2008). Time-related action of Lactobacillus plantarum in the bacterial microbiota of shrimp digestive tract and its action as immunostimulant. Pesquisa Agropecuária Brasileira 43, 763–769.
Zheng, X., Duan, Y., Dong, H., and Zhang, J. (2020). The effect of Lactobacillus plantarum administration on the intestinal microbiota of white leg shrimp Penaeus vannamei. Aquaculture 526:735331. doi: 10.1016/j.aquaculture.2020.735331
Keywords: aquaculture, shellfish health, disease, biofloc, competitive exclusion, Carnobacterium maltaromaticum, Vibrio harveyi, Vibrio alginolyticus
Citation: Thompson J, Weaver MA, Lupatsch I, Shields RJ, Plummer S, Coates CJ and Rowley AF (2022) Antagonistic Activity of Lactic Acid Bacteria Against Pathogenic Vibrios and Their Potential Use as Probiotics in Shrimp (Penaeus vannamei) Culture. Front. Mar. Sci. 9:807989. doi: 10.3389/fmars.2022.807989
Received: 02 November 2021; Accepted: 07 February 2022;
Published: 28 February 2022.
Edited by:
Ying Liu, Dalian Ocean University, ChinaReviewed by:
Vikash Kumar, Central Inland Fisheries Research Institute (ICAR), IndiaJude Juventus Aweya, Shantou University, China
Copyright © 2022 Thompson, Weaver, Lupatsch, Shields, Plummer, Coates and Rowley. This is an open-access article distributed under the terms of the Creative Commons Attribution License (CC BY). The use, distribution or reproduction in other forums is permitted, provided the original author(s) and the copyright owner(s) are credited and that the original publication in this journal is cited, in accordance with accepted academic practice. No use, distribution or reproduction is permitted which does not comply with these terms.
*Correspondence: Christopher J. Coates, Yy5qLmNvYXRlc0Bzd2Fuc2VhLmFjLnVr; Andrew F. Rowley, YS5mLnJvd2xleUBzd2Fuc2VhLmFjLnVr