- 1Programa de Magister en Ciencias con Mención en Pesquerías, Departamento de Oceanografía, Universidad de Concepción, Concepción, Chile
- 2Interdisciplinary Center for Aquaculture Research (INCAR), Universidad de Concepción, Concepción, Chile
- 3Programa de Estudios Ecosistémicos del Golfo de Arauco (PREGA), Facultad de Ciencias Naturales y Oceanográficas, Universidad de Concepción, Concepción, Chile
- 4Centro de Investigación Oceanográfica COPAS Sur-Austral and COPAS COASTAL, Universidad de Concepción, Concepción, Chile
River runoff to the coastal zone is one of the most important environmental variables that influences the structure and functioning of the neritic trophic web and modulates temporal fluctuations of coastal fishery production in many ecosystems worldwide. The relationship between recruitment of anchovy (Engraulis ringens) and common sardine (Strangomera bentincki) in central-south Chile (34°–40°S) and fluctuations in river runoff was analyzed during the last two decades (1999–2018) using linear and nonlinear regression models. River runoff was also incorporated as a co-variate in Beverton-Holt (BH) stock-recruitment models. Anchovy recruitment was found to be positive and significantly associated with river runoff for all analyzed rivers both for the runoff calculated during its main reproductive period (July–October) and for each single month during this period. This detected nonlinear relationship remained significant when runoff was analyzed for the central macrozone (CMZ) and southern macrozone (SMZ). In contrast, no relationship between runoff and recruitment was detected for the common sardine in any river or macrozone (CMZ, SMZ), for each month of the main reproductive season or for the whole reproductive period (July–October). No density-dependent regulation was detected using BH stock-recruitment models for either species, with or without total freshwater input (i.e., the joint discharge of all rivers; RIVSUM index) as a covariate. Our results highlight the relevance of river runoff as a major driving factor in anchovy recruitment variability, suggesting that high (low) monthly runoff anomalies during the main reproductive period of anchovy populations markedly affect the survival of its early stages, altering recruitment success. For common sardine the runoff effect was not significant, which suggests that other oceanographic/climatic factors (wind-driven upwelling, sea surface temperature, El Niño) are more relevant in driving the variability of its recruitment than river runoff.
Introduction
Several factors are drivers of fluctuations in marine fish populations, which may be due to a combination of climate variability, population dynamics and trophic interactions with other species (Lehodey et al., 2006). Fluctuations in environmental conditions at different time and space scales affect the dynamics of fishery resources. Most fishery production worldwide occurs in coastal regions, which are largely associated with coastal upwelling, wind stress and tidal mixing, and land-based runoff including major river flows (Caddy and Bakun, 1994). River discharge appears to be one of the most important environmental variables in this regard (Drinkwater and Frank, 1994; Palomera et al., 2007; Sabates et al., 2007; Reinfelds et al., 2013); it may produce significant fluctuations in nutrient transport, primary production and fish recruitment (Quiñones and Montes, 2001; Soto-Mendoza et al., 2010; Parada et al., 2013; Bonanno et al., 2016), and consequently in the biomass of fish stocks in central-south Chile.
Variability in river runoff is associated with changes in circulation patterns and vertical stability, modifying mixing and exchange processes of nutrients in the coastal zone and accordingly modulating primary production (Anabalón et al., 2016; Gómez-Canchong et al., 2017; Jacob et al., 2018; Testa et al., 2018). Changes in freshwater flow have been linked to fishery production (e.g., Drinkwater and Frank, 1994; Quiñones and Montes, 2001; Lloret et al., 2004; Díaz-Ochoa and Quiñones, 2008; Gillson, 2011). River runoff plays a main role in physical, chemical and biological processes in coastal regions (Masotti et al., 2018; Testa et al., 2018; Saldías and Lara, 2020). Many studies report relationships between river runoff and subsequent fish landings (Quiñones and Montes, 2001; Lloret et al., 2004; Gillson et al., 2012; Meynecke et al., 2012), reproductive migrations, spawning and recruitment success (Gillson, 2011; Reinfelds et al., 2013; Walsh et al., 2013).
Small pelagic fish that inhabit coastal upwelling systems are especially sensitive to the variability of the environment during all stages of their life cycle (Cubillos et al., 2007a; Gomez et al., 2012; Checkley et al., 2017; Canales et al., 2020). Two small pelagic fish, anchovy (Engraulis ringens) and common sardine (Strangomera bentincki), are important species for both industrial and small-scale purse-seine fleets in central-south Chile (Castro and Hernández, 2000; Cubillos and Arcos, 2002; Arteaga and Cubillos, 2008). Inter-annual changes in spring upwelling intensity affect the abundance and availability of planktonic food for small pelagic fish in central-south Chile, regulating the recruitment of common sardine (Gomez et al., 2012; Parada et al., 2013). El Niño and La Niña significantly modify the neritic environment; in cold years (La Niña) there is an increase in common sardine recruitment as a result of an increase in biological production (Parada et al., 2013; Jacob et al., 2018). Variations in river discharge among years are correlated with El Niño Southern Oscillations (ENSO), since an increase in precipitation in central Chile during El Niño years leads to high river flows (Montecinos et al., 2011; Garreaud et al., 2017). Conversely, La Niña is often associated with a decrease in precipitation and low river discharge (Montecinos et al., 2011; Parada et al., 2013). During El Niño periods, the recruitment levels of common sardine decrease consistent with a decline in the availability of food for the pre-recruits and changes in the vertical structure of the oxygen concentration, generating a decrease in survival (Cubillos et al., 2001; Gomez et al., 2012).
This scenario could lead to an increase in the abundance of anchovy as a result of interaction with the less abundant cohorts of common sardine (Cubillos and Arcos, 2002; Gatica et al., 2007). However, few studies have explored the possible role of freshwater input on the dynamics of common sardine and anchovy in central-south Chile, and without conclusive results (Soto-Mendoza et al., 2010; Saavedra et al., 2014). The maximum reproductive activity of both species occurs mainly in the second semester, beginning between July and August and extending until October (Cubillos and Arcos, 2002; Cubillos et al., 2007a; Claramunt et al., 2014); this is the period when the highest river flows as well as rainfall take place in central-south Chile (Montecinos et al., 2011; Valenzuela and Garreaud, 2019).
The occurrence of large concentrations of anchovy and common sardine near the mouth of the rivers of central-south Chile was highlighted in several studies (Castro and Hernández, 2000; Cubillos et al., 2001, 2007a; Quiñones et al., 2009; Soto-Mendoza et al., 2010; Saavedra et al., 2018). Hydroacoustic assessments of both species carried out by the Chilean Institute for Fisheries Development (IFOP) also reported high acoustic densities of both resources that may be related to productivity generated by the discharge of nutrients through the rivers (Saavedra et al., 2014, 2018). Furthermore, it has been shown that changes produced by freshwater inflow into the coastal zone off central-south Chile can modify the composition of phytoplankton (Anabalón et al., 2016; Masotti et al., 2018; Testa et al., 2018), as well as primary productivity and plankton metabolism (Jacob et al., 2018), which consequently could have a cascading effect on higher trophic levels (Anabalón et al., 2016).
River runoff and precipitation in central-south Chile have a marked effect on physical, chemical and biological processes of marine ecosystems, maintaining high primary productivity rates in coastal waters (Masotti et al., 2018; Testa et al., 2018). Considering the Mega Drought (MD) that affected central-south Chile and significantly altered the spatial and temporal dynamics of runoff and precipitation levels during the 2010–2018 period (Garreaud et al., 2017, 2019; Grez et al., 2020), it is of great relevance to understand how river runoff fluctuations can influence recruitment dynamics of anchovy and common sardine, which are especially sensitive to environmental variability at all stages of their life cycle (Cubillos et al., 2007b; Yáñez et al., 2008; Gomez et al., 2012). The hypothesis of this study was formulated based on the premise that changes in freshwater input to the coastal zone at different time/spatial scales may be contributing to the triggering of marked changes in anchovy and common sardine population dynamics in central-south Chile. In consequence, we tested the hypothesis that river runoff fluctuations could affect early stages of anchovy and common sardine life cycles, especially during their main reproductive periods, resulting in significant variation in their recruitment.
Materials and Methods
Study Area
This study is focused on the coastal zone of central-south Chile (Figure 1; 33°–40°S). High abundances of anchovy and common sardine eggs have been observed in four areas within the spawning areas of this zone: (i) Punta Nugurne to Constitución (35°30′S), (ii) mouth of the Itata River to Concepción Bay (36°30′S), (iii) Arauco Gulf (37°S), and (iv) Lebú to Corral (38°20′ to 39°40′S) (Cubillos et al., 2007a; Claramunt et al., 2014; Bustos et al., 2020). The study area (SA) was separated into two macrozones: the central macrozone (CMZ) and the southern macrozone (SMZ) (Figure 1). The annual mean river discharge varied from ∼105.91 to 915.16 m3s–1 in the central macrozone, and from ∼17.42 to 522.37 m3s–1 in the southern macrozone during the study period (1999–2018; Table 1).
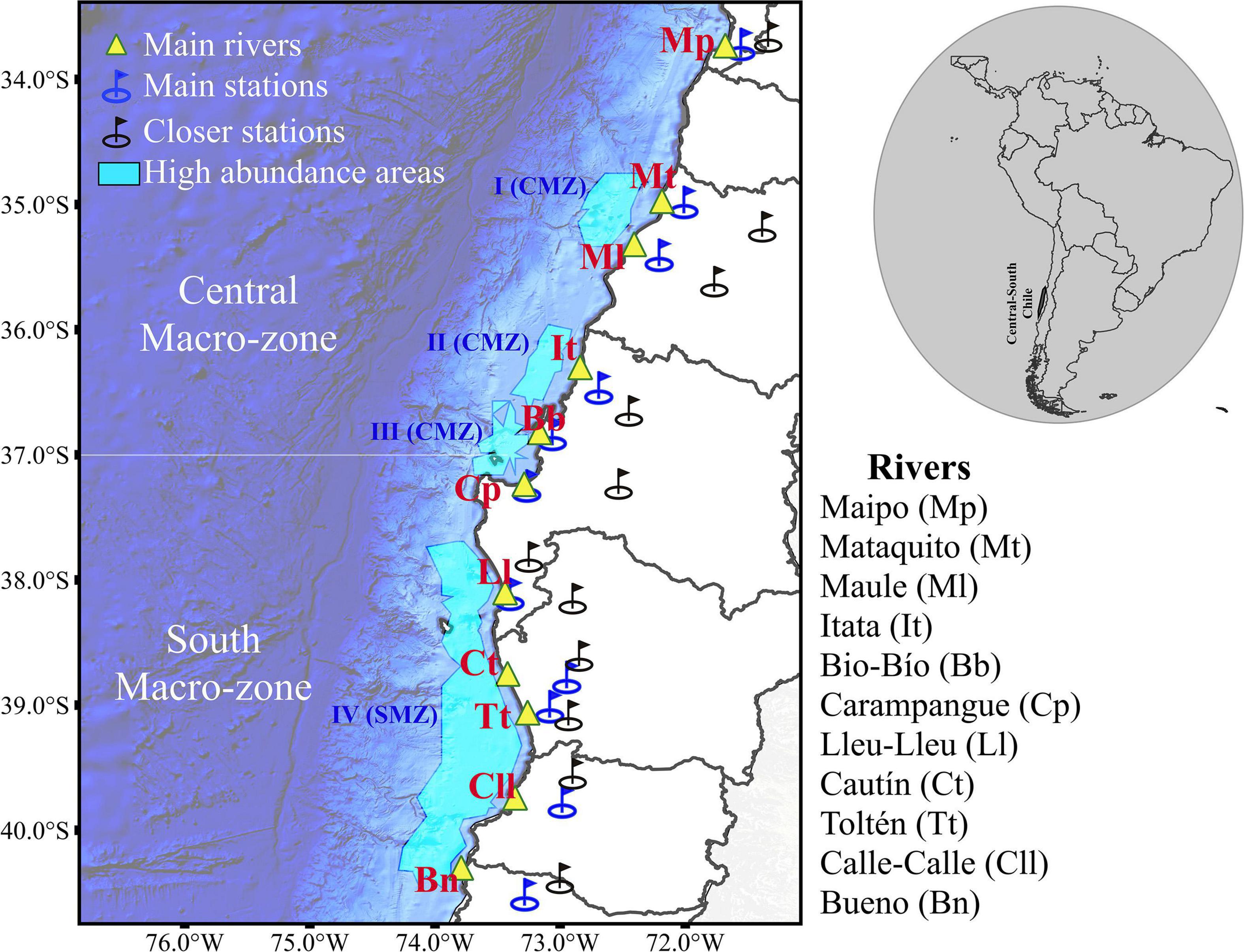
Figure 1. Map of the study area showing the geographic location of main rivers in the central and southern macrozones including river mouths (yellow triangles), selected gauging stations (blue circles) and gauging stations used to fill in missing observations (black circles). High abundance areas of anchovy and common sardine eggs are indicated with sky blue polygons from north to south as: I (Punta Nugurne to Constitución, 35°30′S), II (mouth of the Itata River to Concepcion Bay, 36°30′S), III (Arauco Gulf, 37°S), and IV (Lebú, 38°20′ to Corral, 39°40′S).
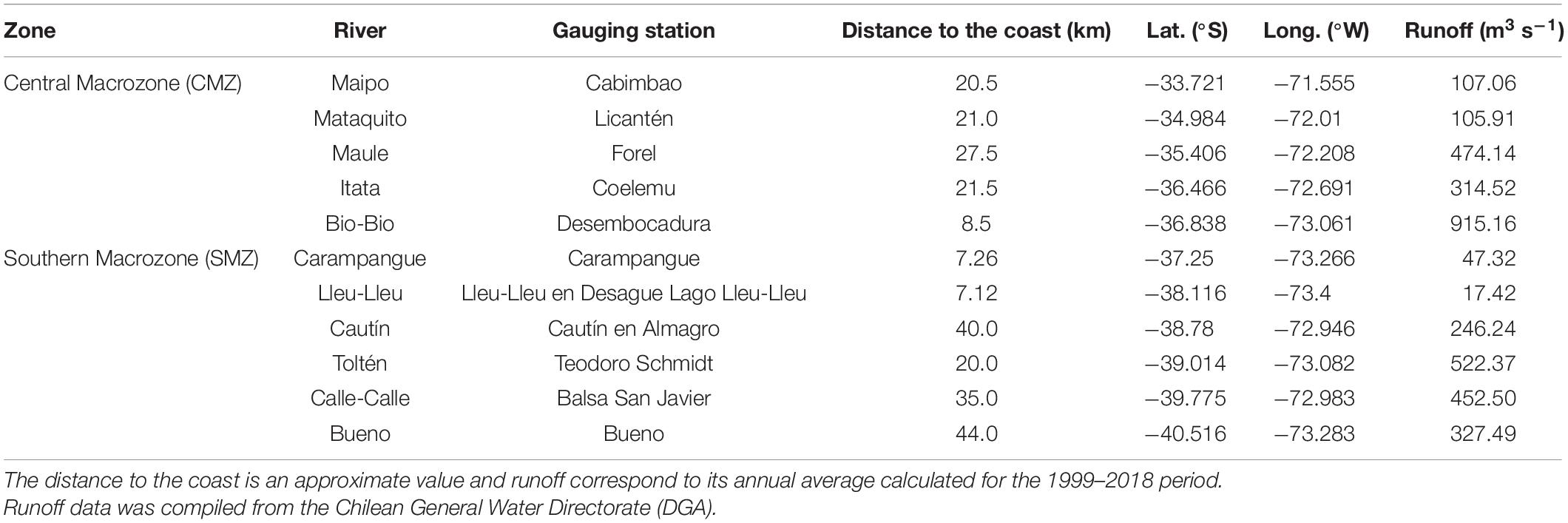
Table 1. Locations of rivers and their gauging stations for the total study area including the central and southern macrozones.
River Runoff Data
Gauging stations in the Maipo, Mataquito, Maule, Itata, Bio-Bio, Carampangue, Lleu-Lleu, Cautín, Toltén, Calle-Calle and Bueno rivers (Figure 1) located close to the coast (ca. ∼22 km on average; Table 1) were selected for further analysis. Daily runoff data from selected stations were provided by the Chilean General Water Directorate (DGA1) and the Center of Climate and Resilience Research2. Missing observations for the 1999–2018 study period (Supplementary Table 1) were filled in using linear regressions incorporating all coincident non-missing monthly data of the gauge of interest and its closest gauging station (Harvey et al., 2012; Arriagada et al., 2021; Supplementary Table 2). The consistency between the filled time series and the data obtained from the nearby gauging station was tested using double mass curve analysis (Gao et al., 2017; Pirnia et al., 2019; Supplementary Figure 1).
The seasonal dynamics of runoff of each river was determined using monthly means calculated over 20 years (1999–2018, Figure 2). Monthly means of runoff show similar behavior for all rivers, with major/minor peaks during the winter/summer seasons (Figure 2). The joint discharge of all rivers was calculated and is operationally called RIVSUM. Since analyzed rivers correspond to more than 90% of the total runoff of the study area (Alvarez-Garreton et al., 2018), RIVSUM is an indicator of total river freshwater input to the coastal zone of central-south Chile. Runoff data was analyzed monthly (i.e., period of selected months) and annually using data from each river individually (local scale) and using the RIVSUM indicator (global scale). The effect of runoff from the central macrozone (CMZ) and the southern macrozone (SMZ) on the recruitment dynamics of both species was also analyzed. Based on the life cycle of both species in central-south Chile, we selected two periods of river runoff (using RIVSUM) to determine the influence of runoff on anchovy and common sardine annual recruitment. The first period (P1) covers from July to August, which is historically considered as the main spawning period (Cubillos and Arcos, 2002); the second period (P2) extends from July to October, because marked inter-annual changes in spawning extent and intensity, showing high reproductive indicators within this second period, were detected in acoustic surveys conducted annually by the Chilean Institute for Fisheries Development (IFOP) (Supplementary Table 3).
Fishery Data
Acoustic surveys carried out by the Chilean Institute for Fisheries Development (IFOP) were conducted every summer season during the 2000–2019 period (N = 20) to estimate the biomass of recruits (in weight) and abundance (in number) of anchovy and common sardine (Supplementary Material Acoustic Surveys, Supplementary Table 3) in the central-south zone of Chile (Saavedra et al., 2014, 2018). Annual estimates of both species’ recruits and abundances were used later to determine their relationship with runoff variability. Estimates of the spawning stock biomass obtained by applying the Daily Egg Production Method (DEPM) were also available for 2002–2019 (Grendi et al., 2020). The DEPM biomass for anchovy and common sardine were used to analyze stock-recruitment relationships, incorporating river runoff variability into classical stock-recruitment (SR) Beverton-Holt (BH) models.
Effect of River Runoff on Recruitment
Regression analyses were conducted to detect the relationship (if any) between recruitment of both species and river runoff. Based on the premise that river runoff may affect early stages of fish life cycles (i.e., main spawning period), its effects would be observed later during the recruitment period. Hence regressions were always carried out lagging the recruitment time series by 1 year, because both fish species recruit to the fishery at the end of the first year of life (Arteaga and Cubillos, 2008; Claramunt et al., 2014; Saavedra et al., 2018). Recruit values for year t (Rt) were related to annual or monthly (selected months) runoff values of year t–1 (Qt–1). Bonferroni correction was used to account for multiple testing and to decrease the probability of incorrectly rejecting a null hypothesis (i.e., of making a Type I error). Adjusted p-values were calculated according to the number of hypotheses tested (Bretz et al., 2011). For example, when linear regressions between anchovy recruitment and runoff for eleven rivers were conducted (m = 11, one regression for each analyzed river), p-values were adjusted according to m.
Effect of River Runoff and Spawning Stock Biomass on Fish Recruitment
Linear and multiple linear regression analyses, with river runoff and spawning biomass as predictor variables, were conducted as exploratory analyses. Then nonlinear regression models (Seber and Wild, 2003) were used to detect any improvement in model fit over simpler linear models. An asymptotic exponential curve (Crawley, 2007), similar to the well-known Michaelis-Menten function (Ritz and Streibig, 2008) was selected in nonlinear regression models when only runoff was used to detect any effect on anchovy recruitment, because as in the Beverton-Holt fishery model, an upper limit for the dependent variable (recruitment) exists. As runoff increases, we expect a nonlinear increase in anchovy recruitment up to a maximum level, beyond which it remains constant. For common sardine, a negative exponential function (Bolker, 2008) was used because preliminary analyses revealed a slight decrease in common sardine recruitment as runoff increases. Bonferroni correction to account for multiple testing was used as applied in the previous section. Previous studies suggest that density-dependence plays a role in the regulation of the temporal dynamics of both species (Pedraza-Garcia and Cubillos, 2008; Canales et al., 2020) and for that reason, Beverton-Holt (BH) models (Beverton and Holt, 1993) were included in our analyses. BH models use a form of density-dependent compensation, which may cause the recruitment to level out (compensation) at high spawning-stock biomass. They rely on the assumption that there is competition for food and space among juveniles, and that the mortality rate of juveniles (i.e., recruitment) is thus density dependent (Hilborn and Waiters, 1992; Chen and Irvine, 2001). BH models were used to fit stock-recruitment (SR) relationships without (Eq. 1) and with (Eq. 2) river runoff as an explanatory variable, as:
where R and S correspond to recruitment (number) and spawning stock biomass (tons), respectively, a and b are the parameters of the stock-recruitment model, a being the estimated maximum number of recruits, parameter q accounts for runoff effect, and the term eε is the lognormal distribution of the errors ε. Q corresponds to river runoff (m3s–1) and is expressed in Eq. (2) as an anomaly, which is the deviation from the mean calculated for the whole time series as in Santojanni et al. (2006).
River runoff was incorporated into SR models using the P2 (July–October) period. However, analyses were also conducted that take into account monthly runoff (i.e., July, August, September, October, November, December). The months of November and December were also analyzed due the fact that it has been verified that the maximum reproductive indicators of both species can be observed until December in some years (Saavedra et al., 2018). Performance of each fitted model was estimated by model comparison using the Akaike Information Criterion (AIC) (Burnham and Anderson, 2002).
Analyses were conducted using the R statistical and programming software (R Development Core Team, 2017, version 3.5.0); the pseudo-R-squared (pR2) from non-linear models were calculated using the Nagelkerke function included in the R package “rcompanion” (Mangiafico, 2015). Stock-recruitment models without the incorporation of river runoff as a covariate were used as a comparison (null) model. Pseudo-R-squared was estimated as:
Where = log and l(0) = logL(0) correspond to the log likelihoods of fitted and null models, respectively (Nagelkerke, 1991).
Results
Effect of Single Rivers on Anchovy and Common Sardine Recruitment Using Linear and Non-linear Models
When the effect of runoff during the main reproductive period (P2) on anchovy recruitment was analyzed for each river individually using linear regression models, significant associations (p < 0.05) were detected for the Mataquito, Carampangue and Lleu-Lleu rivers (Supplementary Table 4). For common sardine, no significant associations between recruitment and runoff were found for any river (Supplementary Table 4). When this effect was analyzed for anchovy using non-linear models, parameter a was found to be significant for all rivers of the study area, and b was detected as significant for all except for the Cautín, Calle-Calle and Bueno rivers. Marked improvements over linear models were detected using pR2, which increased between 11.8 and 41.7% for the Bueno and Maule rivers, respectively (Supplementary Table 5). For common sardine, nonlinear regression models show that for all rivers parameter a was significant (p < 0.001) but b was non-significant. This means that a significant decrease in recruitment could not be detected. Improvements in model fits using pR2 were not as marked as for anchovy; their values varied between 0.002% for the Cautín River and 16% for the Itata and Carampangue rivers (Supplementary Table 6).
Effect of Total Freshwater Input (RIVSUM) on Anchovy and Common Sardine Recruitment Using Linear and Non-Linear Models
Positive/negative relationships between river runoff and recruitment of anchovy/sardine were found at different time scales when runoff was analyzed for the total study area (SA) using linear regression models. Marked and significant associations (p < 0.05) between anchovy recruitment at year t (Rt) and RIVSUMt–1 were found at monthly scales (July–August, p = 0.05, r2 = 30.4%; July–October, p < 0.05, r2 = 39.9%) (Figure 3 and Supplementary Figure 2), and also for the central macrozone (CMZ; r2 = 32.6%, p < 0.05) and southern macrozone (SMZ; r2 = 47.5%, p < 0.01). Non-significant associations were found at an annual scale (p > 0.05, r2 = 41.61%).
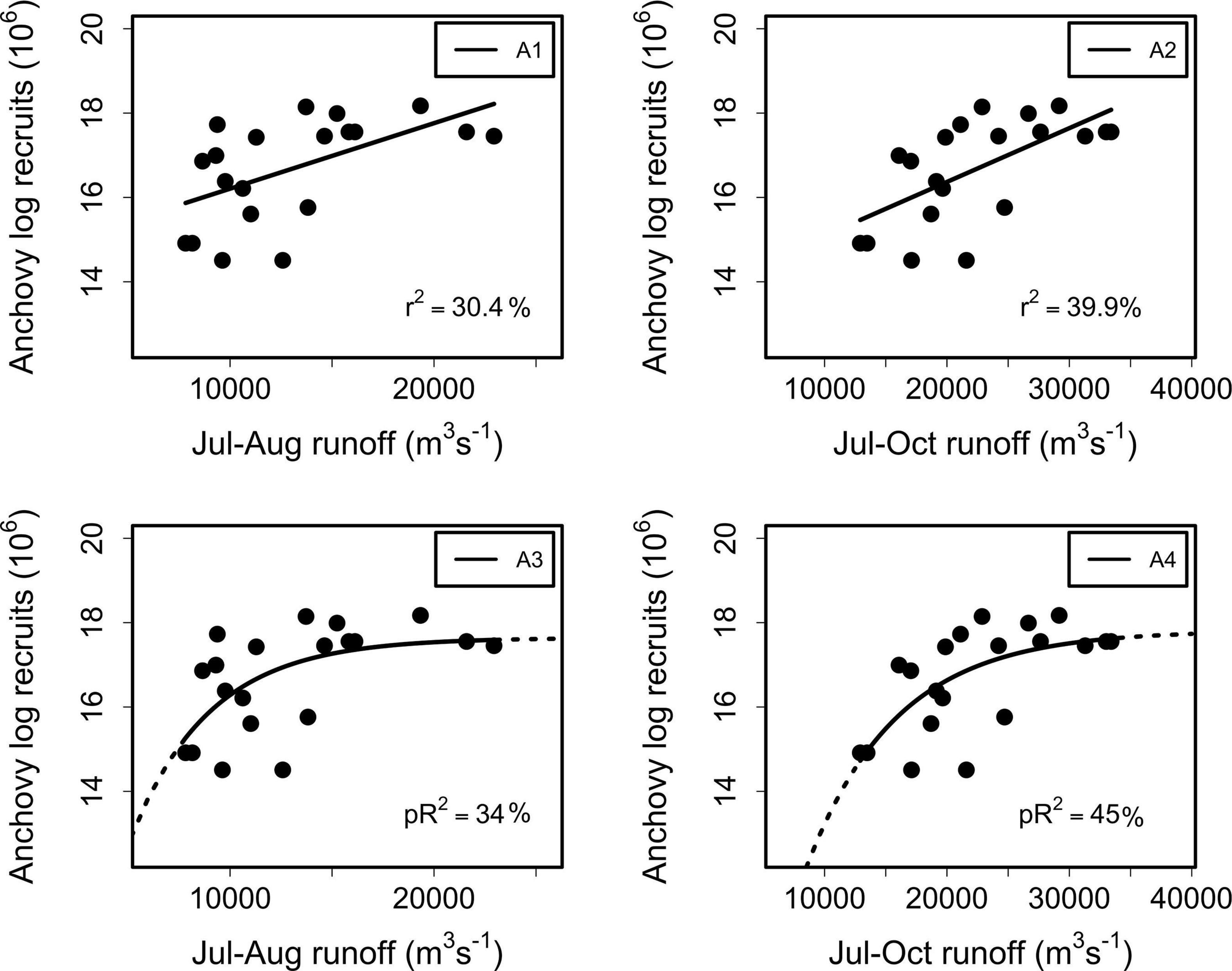
Figure 3. Linear (A1, A2) and nonlinear (A3, A4) regressions between log recruits of anchovy at year t and RIVSUMt–1 during P1 (July–August) for the total study area (left side panels) and P2 (July–October) (right side panels). For model A1 p = 0.05, and for models A2, A3 and A4 p < 0.01.
For common sardine no significant associations (p > 0.05) were found at any time or spatial scale using linear regression models (Figure 4 and Supplementary Figure 3). Further analyses using nonlinear regression models showed that anchovy recruitment was significantly associated (p < 0.01 for a, p < 0.05 for b) with RIVSUMt–1 at a monthly scale (July–December, Table 2), and during the main reproductive periods (P1, P2) for the total study area (Figure 3, Table 2, and Supplementary Figure 4).
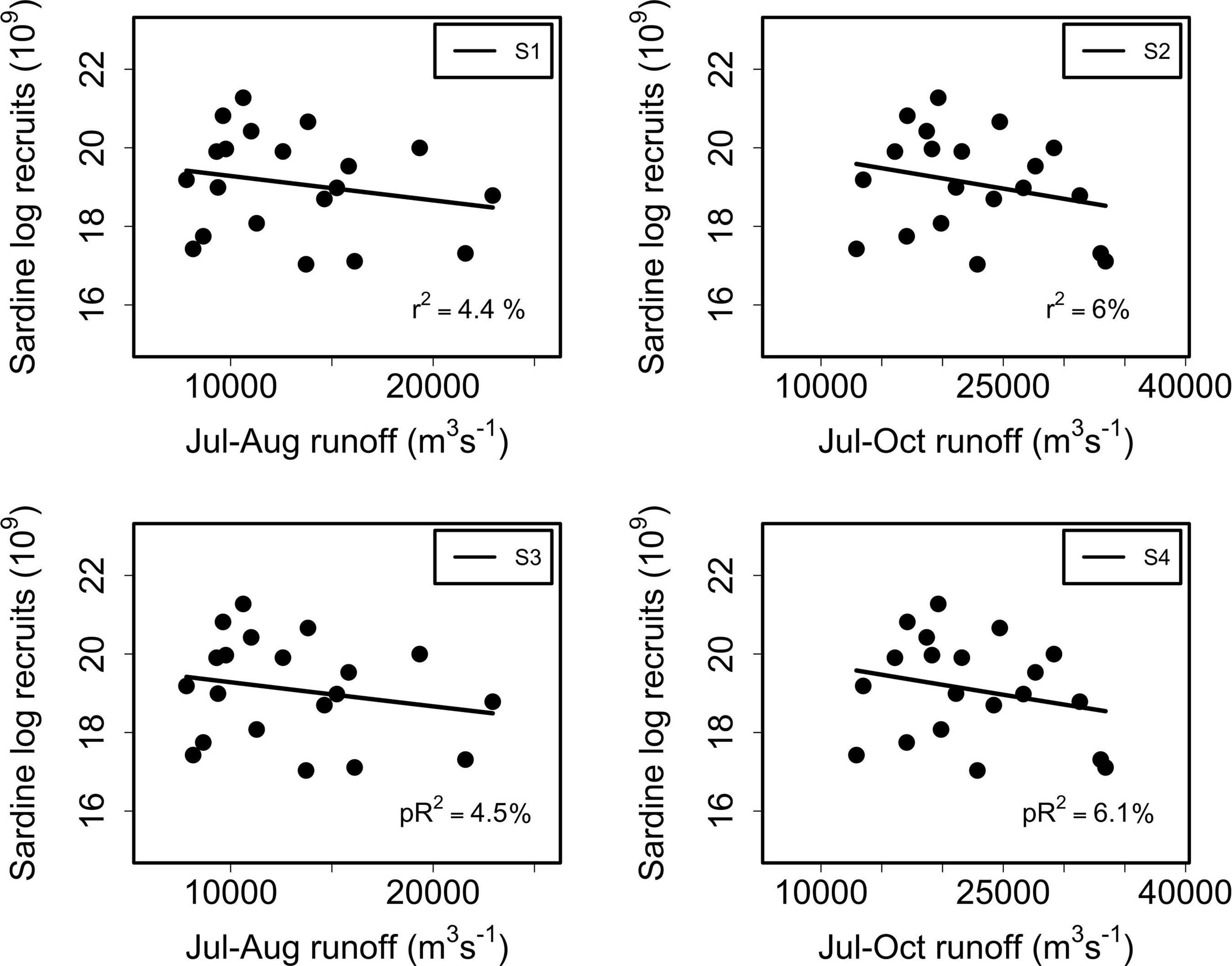
Figure 4. Linear (S1, S2) and nonlinear (S3, S4) regressions between log recruits of sardine at year t and RIVSUMt–1 during P1 (July–August) for the total study area (left side panels) and P2 (July–October) (right side panels). For all models, parameters were non-significant (p > 0.05), except for S3 and S4 for which a was significant.
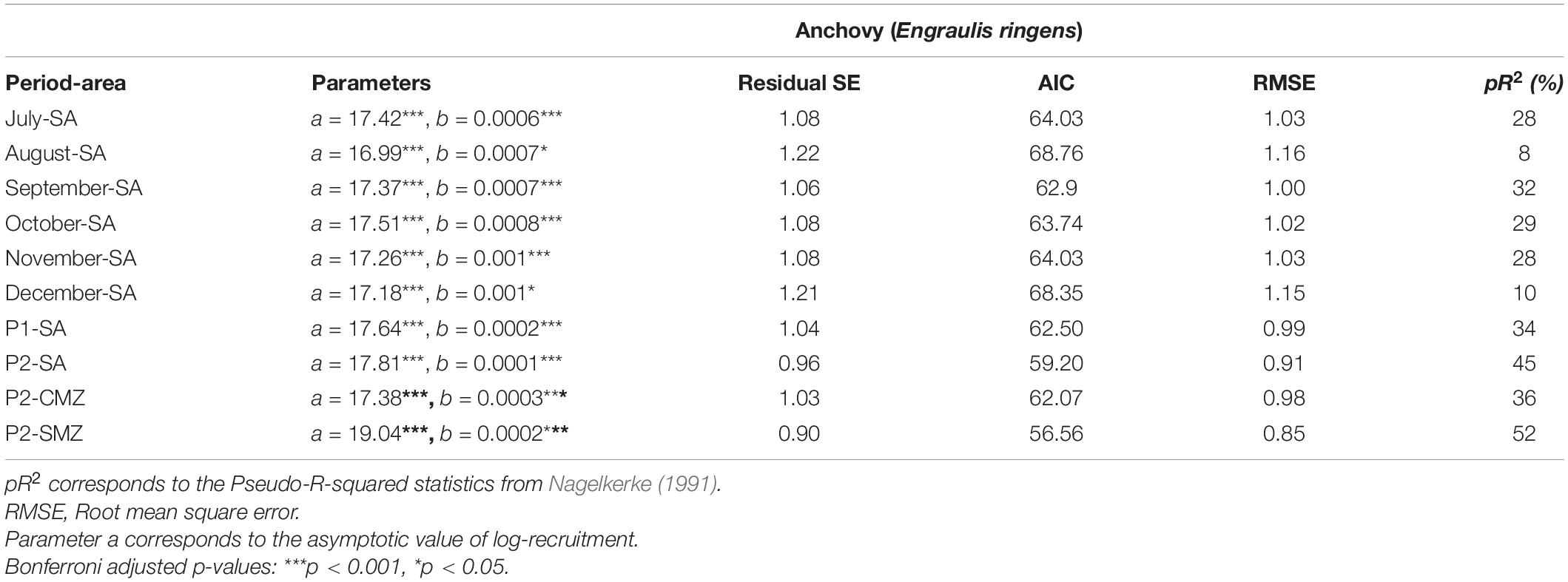
Table 2. Asymptotic exponential regression model results between anchovy recruitment and total river runoff (RIVSUMt–1) calculated for the total study area (SA), central macrozone (CMZ) and southern macrozone (SMZ) during single months or selected critical periods P1 (July–August) or P2 (July–October).
Anchovy recruitment was also significantly associated with total runoff (RIVSUMt–1) during the main reproductive period (P2) for the central (CMZ; pR2 = 36%, p < 0.001) and southern macrozones (SMZ, pR2 = 52%, p < 0.001) (Figure 5 and Table 2): a major improvement over linear regression models. Out-of-sample prediction from nonlinear models shows that as runoff increases, anchovy recruitment tends to asymptotic levels (Figures 3, 5). On the contrary, a decrease in runoff produced a marked and accelerated reduction in recruitment, especially for runoff from the central macrozone (Figure 5). This result is in agreement with those who found that environmental factors, but not density-dependence, are more relevant for the prediction of anchovy recruitment in central-south Chile (Canales et al., 2020). For common sardine, nonlinear regression parameter b was not significant (p > 0.05) for RIVSUMt–1 at a monthly and aggregated scale (P1, P2; Supplementary Figure 5) for all spatial scales (SA, CMZ, SMZ; Figure 6 and Table 3). This means that a significant decrease in common sardine recruitment cannot be detected as river runoff increases. Accordingly, small improvements in pR2 were detected (no greater than 14%) comparing nonlinear to linear regression models (Table 3). Among all nonlinear models tested for anchovy recruitment, those fitted using total runoff from the southern macrozone (SMZ) and total study area (SA) calculated during the main reproductive period (P2) show the lowest root mean square error (RMSE) (Table 2). For sardine, as expected, all nonlinear models show higher values than those obtained for anchovy (Table 3). No significant patterns are visible in autocorrelation plots of residuals from linear and nonlinear models between anchovy/sardine recruitment and RIVSUMt–1 during P1 and P2 (Supplementary Figures 6, 7), respectively. Residuals from linear and nonlinear models between anchovy/sardine recruitment and RIVSUMt–1 during P2 for the central and southern macrozones behaves as white noise. For the models applied to sardine recruitment only one coefficient at lag 7 is outside the 95% confidence intervals (Supplementary Figures 8, 9).
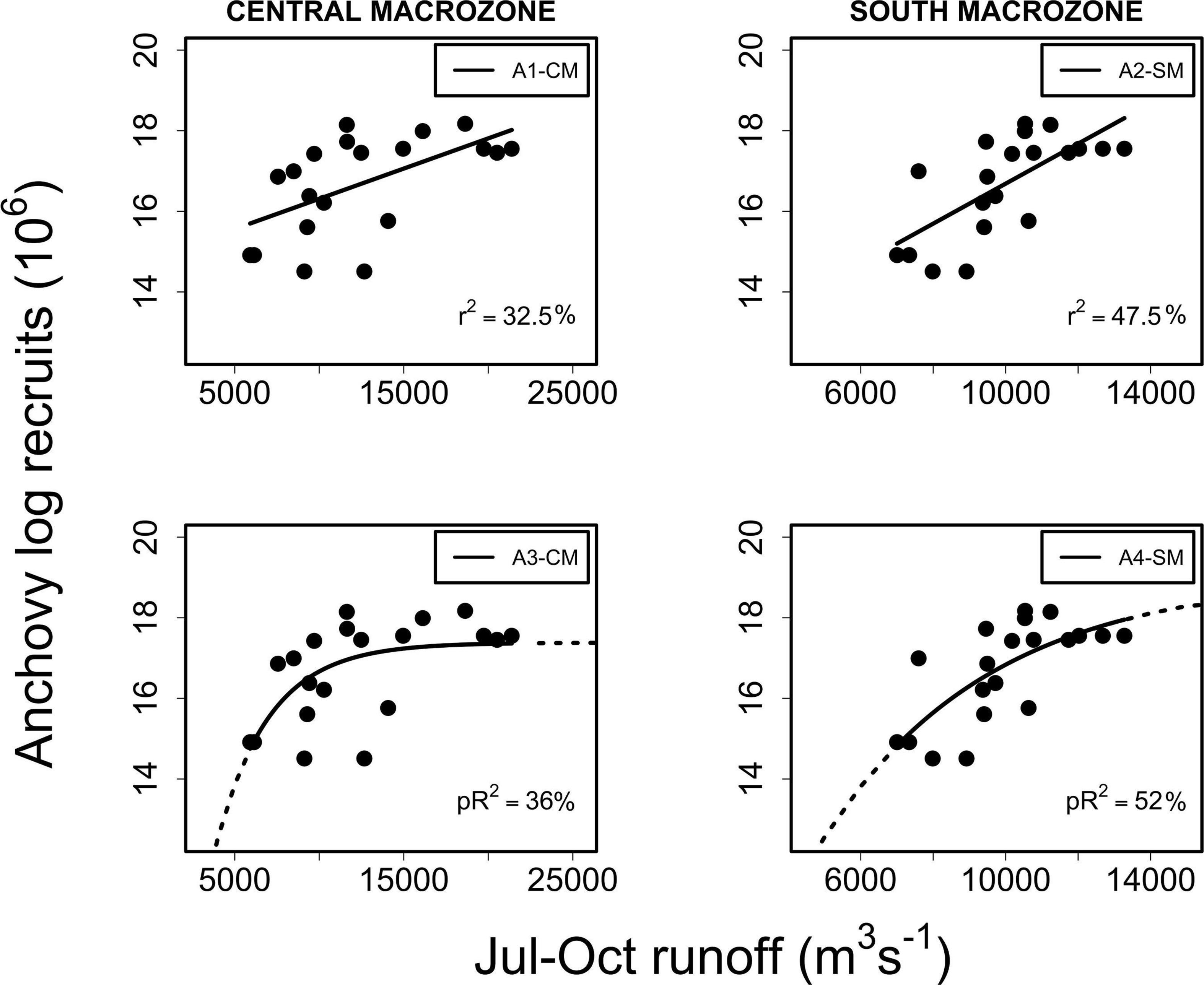
Figure 5. Linear (A1-CM, A2-SM) and nonlinear (A3-CM, A4-SM) regressions between log recruits of anchovy at year t and RIVSUMt–1 for the central macrozone (left side panels) and south macrozone (right side panels) during the main reproductive period P2 (July–October). For models A1-CM and A2-SM p < 0.01, and for models A3-CM and A4-SM p < 0.001.
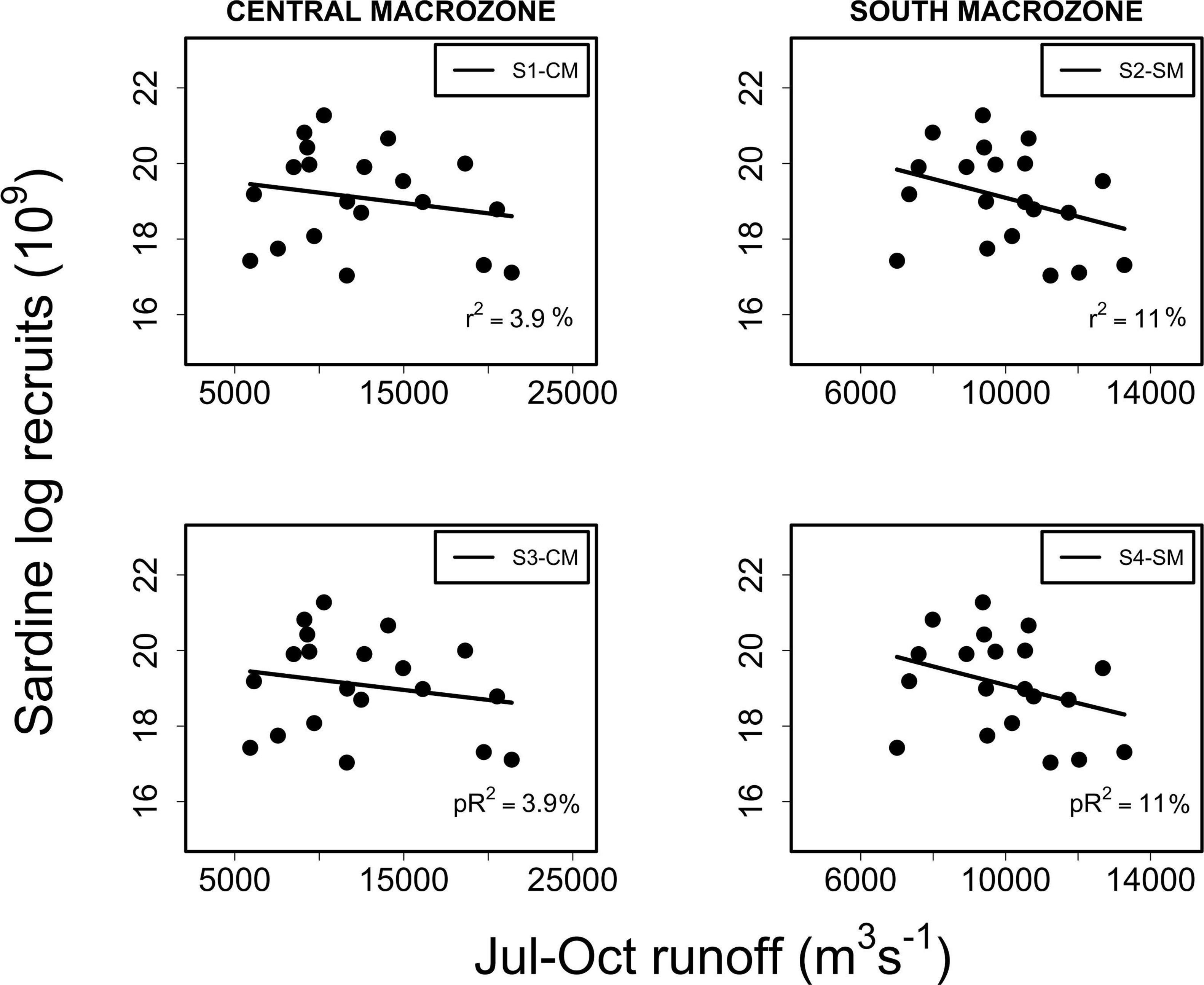
Figure 6. Linear (S1-CM, S2-SM) and nonlinear (S3-CM, S4-SM) regressions between log recruits of sardine at year t and RIVSUMt–1 for the central macrozone (left side panels) and south macrozone (right side panels) during the main reproductive period P2 (July–October). For all models p > 0.05.
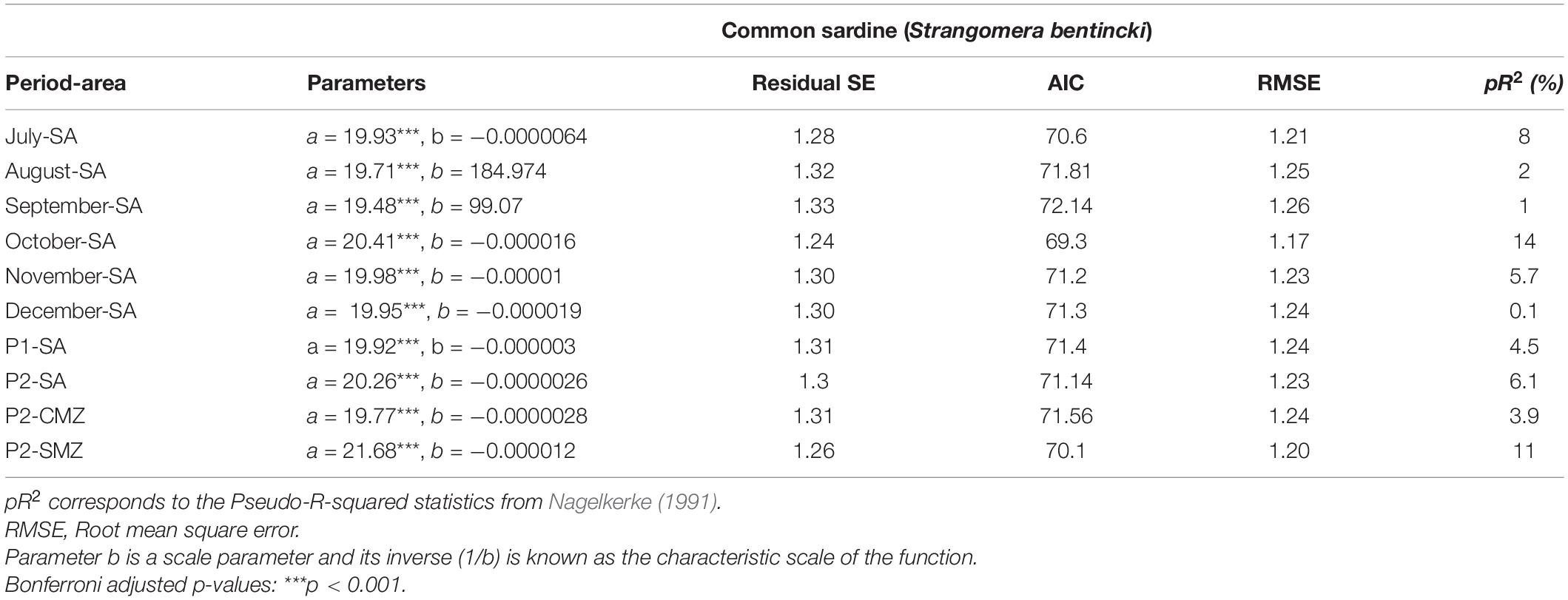
Table 3. Negative exponential regression model results between sardine recruitment and river runoff (RIVSUMt–1) calculated for the study area (SA), central macrozone (CMZ) and southern macrozone (SMZ) during single months or selected critical periods P1 (July–August) or P2 (July–October).
Linear Regression and Stock Recruitment Models With and Without Runoff as a Covariate
At a macroscale (total study area), anchovy spawning stock biomass at year t−1 (SSBt–1) was not associated (p > 0.05) with recruitment at year t (Rt) using a simple linear regression model. Spawning stock biomass was still non-significant after including it into a multiple linear regression model, and only RIVSUM at year t−1 (RIVSUMt–1) was found to be statistically significant (p < 0.05). This latter model that includes RIVSUMt–1 explains over 70% of recruitment variability (r2 = 72.41%). For common sardine, SSBt–1 and RIVSUMt–1 were not associated (p > 0.05) with recruitment (Rt) using simple and multiple linear regression models.
For anchovy and sardine, the Beverton-Holt (BH) stock-recruitment model showed non-significant (p > 0.05) parameters (a, b). Marked improvements in model fits (pR2) were detected for both species when q (associated with runoff, RIVSUMt–1) calculated during the main reproductive period P2 (July–October) was included as a covariate in the BH model; however, non-significant coefficients associated with runoff were detected (Table 4). The lowest root mean square error (RMSE) was obtained for the anchovy BH stock-recruitment model using RIVSUMt–1 as a covariate (Table 4).
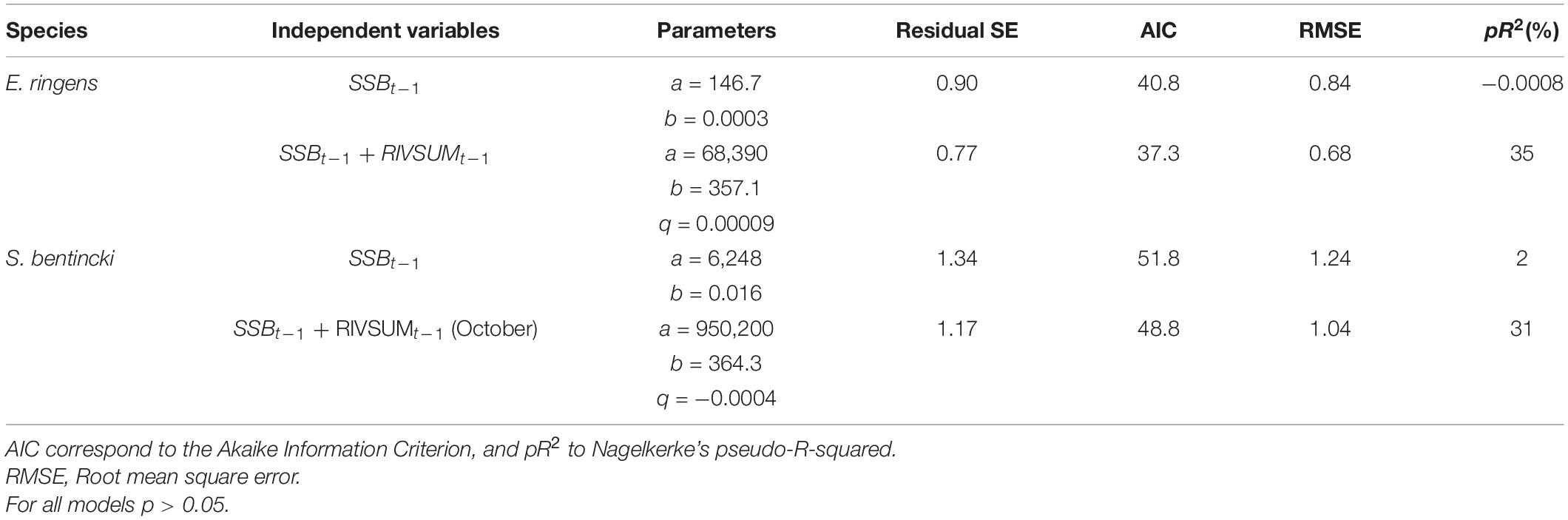
Table 4. Beverton-Holt (BH) stock-recruitment model parameters with and without runoff as a covariable for anchovy (P2, July–October runoff) and sardine (October runoff).
Observed trends in anchovy recruitment are better tracked when RIVSUMt–1 was incorporated as a covariate in the stock-recruitment (SR) model for the July–October period (P2) in comparison with fitted recruitment using the BH model (Figure 7). For common sardine, fitted recruitment using the BH model with runoff as a covariate is much closer to observed recruitment than observations fitted using only the BH model. This improvement can also be observed, for example, when RIVSUMt–1 during the month of October was included in the BH model (Figure 7). Pseudo-R2 for those models were 35% and 31% for anchovy and common sardine, respectively (Table 4). However, non-significant q associated with RIVSUMt–1 were detected at a monthly scale during the July–December period when the relationship between monthly total runoff and recruitment of both species was analyzed using the BH model with covariates (Supplementary Tables 7, 8). The incorporation of runoff in SR models led to a decrease in AIC values and consequently to an increase in model support (Table 4). These results indicate that the relationship between recruitment for anchovy and common sardine and total freshwater input is better described by a nonlinear rather than a linear model. Our results also suggest that freshwater input plays a major role for anchovy and sardine recruitment; however, BH stock recruitment models with runoff as a covariate, in the form that were parametrized in this study, are not able to detect the significant effect of runoff on recruitment.
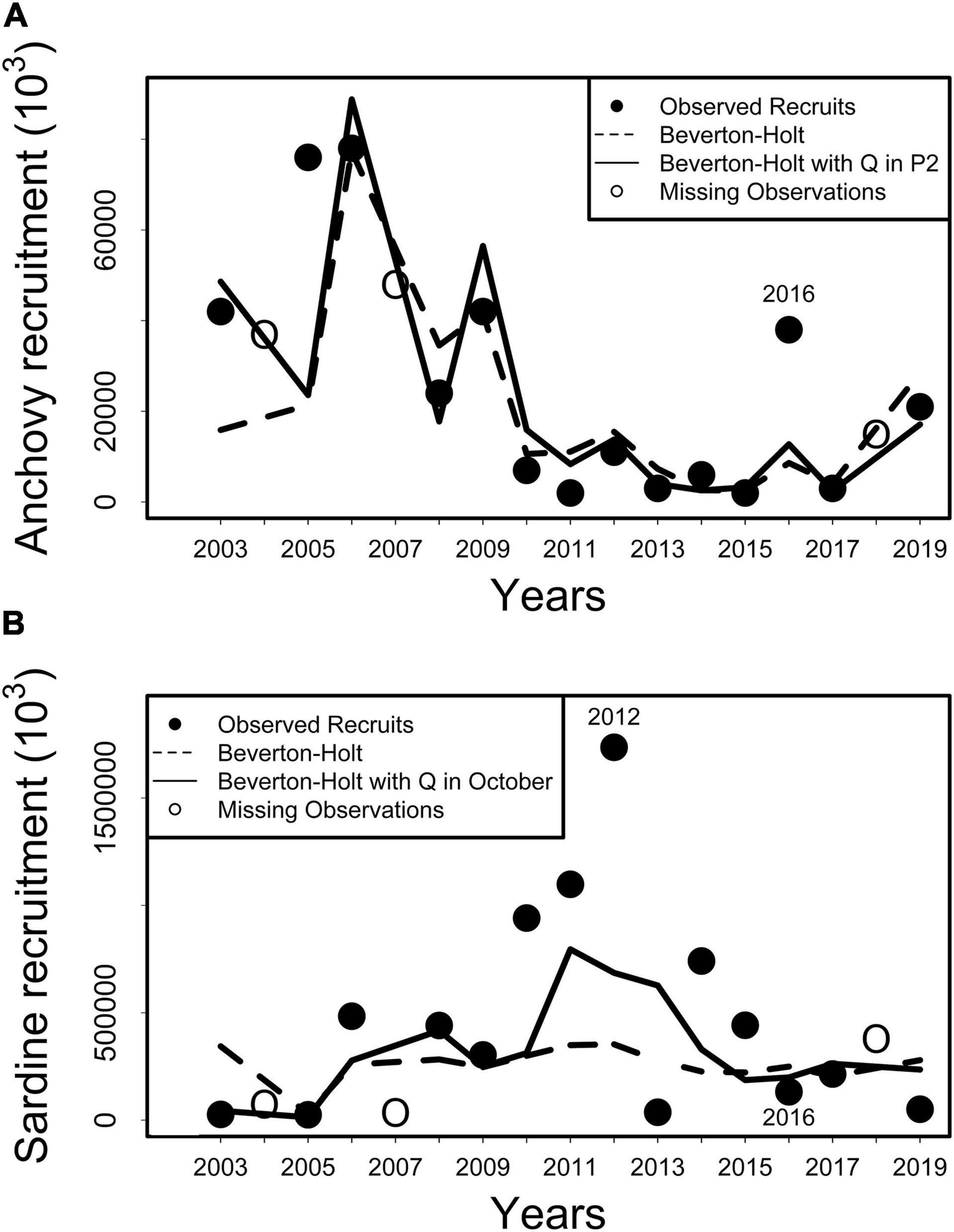
Figure 7. Observed recruits (filled black circles) and fitted observations using the Beverton-Holt stock recruitment model (dotted line) for (A) Engraulis ringens (upper panel) and (B) Strangomera bentincki (lower panel) during the 2003–2019 period. The fitted curves for stock recruitment models including lagged runoff (RIVSUMt–1) as a covariate during P2 (July–October) for anchovy and during October for sardine are indicated with a continuous line. Missing observations (empty white circles) correspond to years t for which recruitment estimates were available (2004, 2007, 2018) but spawning stock biomass (SSB) at year t–1 were not (2003, 2006, 2017). For all models Bonferroni adjusted p-values >0.05.
Discussion
Our study not only confirms but also quantifies for the first time the magnitude of the relationship between anchovy/common sardine recruitment and runoff variability in central-south Chile. Anchovy recruitment was positively affected by river runoff, whereas common sardine recruitment was not associated to freshwater input. Previous studies suggested a possible link between recruitment of both species and runoff in this area (Soto-Mendoza et al., 2010; Saavedra et al., 2014, 2018), but the effect of runoff on recruitment was not quantified. Since common sardine and anchovy are similar in size, their growth and reproduction strategies are similar; they tend to form mixed schools (Cubillos et al., 2001; Arteaga and Cubillos, 2008; Claramunt et al., 2014). Some similarities could be expected in terms of the effect of climatic or oceanographic factors on recruitment, however, our results indicate contrasting effects of freshwater input on the recruitment dynamics of these species.
Anchovy recruitment was markedly affected by runoff variability for almost all rivers of the study area, especially during its main reproductive period (July–October), while common sardine recruitment was not affected by freshwater input at this scale. The same results were obtained for both species when the effect of total freshwater input (RIVSUM) on recruitment dynamics was quantified. Anchovy recruitment was markedly affected by total freshwater input during its main reproductive period (July–August, July–October) for both macrozones (central and southern macrozones). This effect became clearer when a nonlinear asymptotic exponential curve was used to quantify the effect of runoff on recruitment (Figures 3, 5), especially for the southern macrozone (SMZ), for which a much better fit was obtained compared to a linear model (SMZ, pR2 = 52%, p < 0.001). For common sardine, no association between total freshwater input and recruitment could be detected at any spatial or temporal scale.
Effect of Individual River Runoff During Anchovy and Sardine Reproductive Periods on Recruitment
Each river makes a different contribution to recruitment variability for anchovy and common sardine. When considering runoff during the main reproductive period (P2, July–October) for the whole study area using nonlinear regression models (Supplementary Tables 5, 6), the effect was always positive for anchovy, whereas no effect was detected for common sardine.
Specifically, as in the applied nonlinear regression model approach, the asymptotic parameter a (Bolker, 2008) represents the maximum level of recruitment, and its estimate for anchovy fluctuates around 12 on a logarithmic scale; a maximum recruitment biomass close to 163,000 t could be expected when rivers of the study area reach their maximum historical runoff levels. Other factors that can influence recruitment (e.g., wind-driven upwelling) were not considered in this study, and therefore these results should be interpreted with caution, because recruitment is also affected by other multiple factors that can vary over time. Consequently, we believe that runoff can be used as a broad indicator of recruitment variability for anchovy for the whole study area, but not for common sardine.
Effect of Aggregate Runoff (RIVSUM) During Anchovy and Common Sardine Reproductive Periods on Recruitment
Our results show a marked association between anchovy recruitment and fluctuations in total river runoff (RIVSUM) during the main reproductive activity period (P2, July–October), extending also until December (Table 2). Those results support our hypothesis that river runoff is a key factor in the dynamics of anchovy recruitment, promoting optimal environmental conditions that improve development and growth of early stages of anchovy, enhancing recruitment success. A recent study found that changes in salinity have an effect on hatching and hatching enzyme expression in anchovy off central Chile, suggesting that decreases in seawater salinity (due to increased rainfall and/or river runoff) during the spawning period may increase hatching success (Castro et al., 2021). The Mega Drought (MD) that has affected central-south Chile since 2010 produced a consistent decrease in runoff (30–60% relative to historical values) and plume areas (35 to 60%) of all rivers within the central macrozone (Boisier et al., 2016; Garreaud et al., 2017; Alvarez-Garreton et al., 2018); a reduction in phytoplankton biomass was also observed in coastal areas influenced by the Maipo and Mataquito rivers (Masotti et al., 2018). Under this scenario, we hypothesize that decreased runoff values, reduced plume area and the reduction of phytoplankton biomass may have contributed to the reduction of food availability for anchovy due to a lower nutrient supply. At the same time, enhanced salinity levels (due to reduced runoff) may have contributed to reduce the hatching success of anchovy eggs.
One main mechanism that has been proposed to explain the effect of runoff on anchovy recruitment in other parts of the world is that an increase (decrease) in river runoff levels could contribute to an increase (decrease) in the spawning area and the area favorable to post-larval recruitment (Lloret et al., 2004; Santojanni et al., 2006; Martín et al., 2008). Periods of marked decrease in runoff levels could be detrimental to anchovy recruitment. Therefore, considering the current reduction of river runoff and freshwater input to the coastal zone in central-south Chile due to climate change and water use for anthropogenic activities (Garreaud et al., 2017, 2019; Grez et al., 2020), a decrease in recruitment level of anchovy is likely to occur during years of reduced runoff. Many studies have shown a relationship between freshwater input to the coastal system (through river runoff and/or precipitation) and recruitment variability of neritic fish populations (Quiñones and Montes, 2001; Lloret et al., 2004; Santojanni et al., 2006; Martín et al., 2008; Gillson, 2011; Meynecke et al., 2012). For anchovy populations, a strong correlation was detected between Ebro river runoff (Mediterranean Sea) in spring and anchovy landings 1 year later (Lloret et al., 2004), suggesting that river runoff influences spawning and the survival rate of early stages of this species. There is also evidence that an increase in runoff levels of the Po River in the Adriatic Sea (Santojanni et al., 2006) and the Rhône River in the Gulf of Lions (Catalan Coast of the northwestern Mediterranean Sea) are associated with an increase in anchovy (Engraulis encrasicolus) recruitment (Lloret et al., 2001) and landings (Martín et al., 2008), respectively.
No association was found between recruitment and river runoff during the main reproductive period for the common sardine. This does not mean that runoff plays no role in the dynamics of common sardine recruitment, but it may be more affected by other factors like sea surface temperature and density-dependent (endogenous) mechanisms (Pedraza-Garcia and Cubillos, 2008; Canales et al., 2020).
Stock Recruitment Models With and Without Runoff as a Covariate
A non-significant effect of the spawning biomass was observed on anchovy recruitment variability using a Beverton-Holt stock-recruitment (SR) model (Table 4). A positive but non-significant effect of runoff on anchovy recruitment was detected when RIVSUM for the July–October period was included as a covariate in the SR model, being the effect of SSBt–1 still non-significant (Table 4), which suggests that SSBt–1 is not a dominant factor in the regulation of anchovy recruitment. These latter results agree with the weak influence of density-dependent regulation detected for anchovy populations in central-south Chile (Pedraza-Garcia and Cubillos, 2008; Canales et al., 2020). These authors reported an explained variance of the per capita population growth rate that varied between 23% and 15%. According to Canales et al. (2020), an anchovy model with a climate effect (Southern Oscillation Index, SOI) performed better than a model using the endogenous effect alone. Considering the weak density dependence effect in anchovy populations detected in previous studies and similar results obtained in this study, we believe that river runoff plays a key role in the dynamics of anchovy recruitment variability, but we note that it is not the only factor involved in the regulation of its recruitment.
No effect of SSBt–1 on recruitment was detected for common sardine with or without RIVSUMt–1 as a covariate. It has been found that density dependence only explains 27% of common sardine biomass variability (Canales et al., 2020). Our results are generally consistent with previous studies that reported weak or absence of SR relationships in small pelagic fishes in different ecosystems as a consequence of climatic fluctuations that affect survival from hatching to recruitment, so that recruitment is largely uncoupled from spawning biomass (Szuwalski et al., 2015; Checkley et al., 2017; Hilborn et al., 2017; Canales et al., 2020). In consequence, our results support the hypothesis that fluctuations in river runoff during the main reproductive period for anchovy (P2, July–October) have an effect on the variability of its recruitment.
A fitted SR model that includes runoff as a covariate performed well in tracking anchovy recruitment variability over time except for 2016 (Figure 7). The year 2016 is the only year for the whole study period that shows an observed recruit level that is far from fitted model; in this case, observed recruits are remarkably higher than fitted recruits (Figure 7). The anchovy recruitment success for 2016 can be explained by the presence of less abundant cohorts of common sardine the same year through a biological mechanism of interaction (Cubillos and Arcos, 2002; Cubillos et al., 2006). Recruitment of common sardine appears to be negatively associated with sea surface temperature (SST) anomalies observed during its pre-recruitment period (August–December; Cubillos and Arcos, 2002) and with the SST in the El Niño 3.4 region (Gomez et al., 2012), which means that a warm (cold) habitat is unfavorable (favorable) for common sardine recruitment. A strong El Niño event that started by mid-2015 characterized by an El Niño 3.4 index that reached +2.1°C during the austral summer of 2016 (the second highest value since 1948), affected the southeast Pacific down to Patagonia (Santoso et al., 2017; León-Muñoz et al., 2018; Garreaud et al., 2019). In addition, the Southern Annular Mode (SAM) reached its highest value during the summer of 2016, producing extreme dry conditions over this area. These anomalous warm habitat conditions could have been detrimental to the survival of common sardine offspring during the pre-recruit period of 2015, as appears to have occurred during El Niño 1997–1998 (Cubillos and Arcos, 2002). Extreme positive SST anomalies could reflect changes in food availability for common sardine during its pre-recruitment period, and a small year-class of common sardine favors the offspring survival of anchovy through an opportunistic competitive advantage mechanism (Cubillos and Arcos, 2002). This scenario could explain the difference between fitted and observed values of anchovy recruitment for 2016 (Figure 7). The marked decrease in anchovy recruitment (recruitment failure) that started in 2010 and lasted for several years coincides with the beginning of the Mega Drought (MD). The MD has triggered important changes in primary productivity in the coastal zone off central-south Chile. Rivers located in the central macrozone reduce their runoff, plume areas and nutrient export by about 50% in comparison to values observed before 2010, which translates into a marked reduction in the chlorophyll pool within their plumes (Masotti et al., 2018). Drought events or reduced river runoff are commonly accompanied by changes in the abundance of neritic fisheries, which in some cases can lead to recruitment failure (e.g., Gillson et al., 2012; Costalago et al., 2018). Our results reinforce the idea that fluctuations of freshwater entry to the coastal zone due to decreased river runoff is a significant factor that affects the recruitment of anchovy.
It is important to remark that a SR model that incorporates RIVSUMt–1 performed much better than a linear multiple regression model (pR2 = 35% for anchovy), which means that a nonlinear model should be used to quantify the dynamics of anchovy recruitment, which increases and tend to an asymptote at high levels of runoff considering or not considering its spawning stock biomass.
It was found for the common sardine that positive anomalies of river runoff during October are associated with a small decrease in annual recruitment using the BH stock recruitment model. Among the mechanisms that can lead to reduced recruitment due to a marked increase in runoff in central-south Chile, Quiñones and Montes (2001) suggested that excessively high flow rates could be detrimental to the recruitment of species inhabiting estuaries (the rock cod, Eleginops maclovinus), because eggs and larvae could be transported offshore to less favorable environments. Fitted Beverton-Holt stock-recruitment models that included RIVSUMt–1 during October as a covariate performed relatively well in tracking common sardine recruitment variability at the beginning (2003–2009) and at the end (2016–2019) of the analyzed time period, but clearly runoff between 2009 and 2014 was not the main factor controlling common sardine recruitment between 2010 and 2015 (Figure 7), which can explain a p-value > 0.05 for this latter model. Abnormal large-scale climate conditions due to a combined Pacific Decadal Oscillation Index (PDO) and Regional Precipitation Index (RPI) that reached its lowest historical value since 1948 during a similar time period between 2010 and 2014 produced an extended cold and dry period that affected central-south Chile (Garreaud et al., 2019). During this period the PDO (calculated from May to September) was in a cold phase (mean PDO = −0.45), and the RPI also fluctuated around its lowest values (RPI < 0.6), which meant a rainfall deficit of 30% (Garreaud et al., 2019). This scenario, low PDO/low RPI, indicates one of the most extreme periods of the MD that manifested as an uninterrupted sequence of dry years that started in 2010, severely affecting central-south Chile (Figure 8).
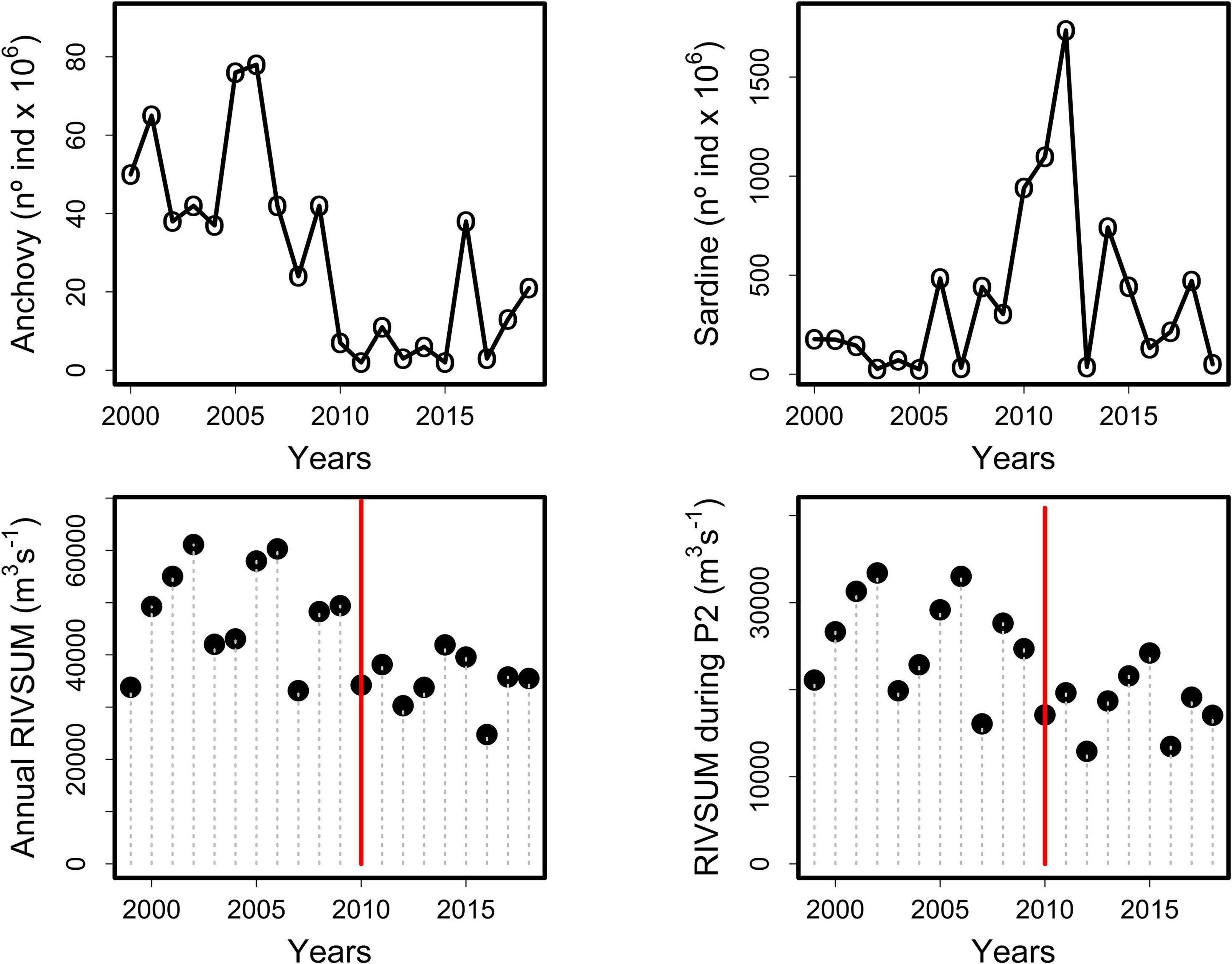
Figure 8. Annual changes in recruit abundance for anchovy (left upper plot) and common sardine (right upper plot). Total annual freshwater input (RIVSUM) for the whole study area (left bottom plot) and during the main reproductive period (P2, July to October) (right bottom plot). The red vertical bars indicate the beginning of the Mega-Drought (MD).
According to our results, the reduced runoff during this period should enhance an increase in common sardine recruitment. This tendency was generally observed in the trajectory of fitted recruitment observations using the BH model with runoff as a covariate, however, it is also clear that extremely high observed recruitment levels of common sardine (e.g., 2012) cannot be explained using only total freshwater input (Figure 7). The dynamics of common sardine may have been mostly affected by sea surface temperature (SST) associated with upwelling favorable winds during 2010–2015, and not by river runoff. There is evidence that cold (hot) habitat conditions during the pre-recruitment period are favorable (unfavorable) for common sardine growth rates (Canales et al., 2020) and recruitment (Cubillos and Arcos, 2002; Gomez et al., 2012). Under this scenario, the strong and weak La Niña of 2010 and 2011, respectively (Santoso et al., 2017) would have contributed most to the two highest common sardine recruitment observations in 2011 and 2012 (Figure 7). In contrast, the strong El Niño that started by mid-2015 (Santoso et al., 2017), and the abnormally high sea water temperatures recorded in central-south Chile during the spring of 2015 and summer of 2016 (León-Muñoz et al., 2018) would be the main cause of the low sardine recruitment observed during 2016 (Figure 7), as was reported for El Niño years of 1997 and 2002 (Gomez et al., 2012).
The MegaDrought, 25% of which has been attributed to anthropogenic climate change (Boisier et al., 2016), produced a marked reduction in rainfall/runoff levels in central-south Chile. The reduction in freshwater discharge of major rivers like the Bio-Bio and Itata rivers produced a 25–75% decrease of nitrate and phosphate export to the coastal zone–nutrients which are essential for phytoplankton growth, larval development and feeding of sardine and anchovies (Grez et al., 2020). The high rainfall variability in the study area and climate model uncertainties make it difficult to predict the response of local rainfall to anthropogenic climate forcing (Boisier et al., 2016). In this context, state-of-the-art general circulation models forced with a high emission scenario project to year 2050 a deficit of 15–20% in precipitation levels in central Chile (Collins et al., 2013). Obviously, this reduction in rainfall will reduce runoff levels accordingly, due to the strong association between annual rainfall and runoff (RIVSUM) in the study area, in which rainfall explains 84% of runoff variance. According to our results, anchovy recruitment will be reduced independently from high levels of spawning stock biomass. However, the runoff/anchovy recruitment association found in this study is only valid for the historical (1999–2018) runoff range detected for the central macrozone (∼5,900–21,380 m3/s) and southern macrozone (∼7,000–13,270 m3/s). According to out-of-sample predictions of anchovy recruitment based on runoff (Figures 3, 5), anchovy recruitment tends to decrease markedly (below these runoff levels) or to reach an asymptote (above these runoff levels), but other factors can interact with runoff or play a more relevant role than runoff in modulating recruitment outside these ranges. For sardine, spawning stock biomass and runoff levels are not relevant factors in the modulation of recruitment, and coastal chlorophyll, spring upwelling intensity and sea surface temperature appear to be determinant for the prediction of recruitment scenarios (Gomez et al., 2012).
Our results show that the magnitude of the association between anchovy recruitment and river runoff tended to be more marked for rivers whose mouths are located close to historically high egg abundance areas detected for anchovy and common sardine populations (Figure 1). This means that runoff models from rivers located at the extremes of the study area, which are the Maipo River at the northern edge of the central macrozone and the Bueno and Calle-Calle rivers at the southern edge of the southern macrozone, performed poorer (larger AIC) than models fitted to their closest rivers (smaller AIC) (Supplementary Table 5). The Maipo River mouth is located far from its closest historically high egg abundance area detected for anchovy, which extends from Punta Nugurne to Constitución (35°30′S) (Figure 1), which is probably the main cause of its reduced influence on anchovy recruitment. The Calle-Calle and Bueno river mouths are located at the southern edge of the study area, close to the end and out of the most southerly high egg abundance area that extends from Lebú to Corral (38°20′S to 39°40′S) (Figure1). Again, the distance of river mouths to high egg abundance areas detected for anchovy plays a role when trying to evaluate the magnitude of anchovy recruitment based on river runoff input to the coastal zone. There is evidence that small rivers (small runoff levels) can produce large plumes (Saldías et al., 2012, 2016), and therefore the location of their mouths in relation to high egg abundance areas could be more relevant than their runoff levels for evaluating their effect on anchovy recruitment. This could be the case for the Carampangue, Mataquito and Lleu-Lleu rivers, whose mouths are located close to high egg abundance areas of anchovy (Figure 1); their runoff levels do not exceed ca. 33% of the Itata runoff or ca. 13% of the Bio-Bio runoff (Figure 2), but their regression models performed as well or better than those applied to the Itata or Bio-Bio rivers to explain anchovy recruitment variability (Supplementary Tables 4, 5).
Early life stages of anchovy and common sardine in central-south Chile are markedly influenced by wind-driven upwelling, ENSO events, sea surface temperature and by the coastal morphology that favors the retention of their eggs and larvae (Castro and Hernández, 2000; Cubillos et al., 2001, 2006; Gomez et al., 2012). Therefore, future studies should be focused on analyzing the interaction of those factors with river runoff and also on quantifying their joint impact on anchovy recruitment variability. If the reduced runoff observed during the last decade (2010–2020) in rivers of central Chile (Alvarez-Garreton et al., 2021) persist, recruitment of anchovy populations will probably remain at low levels, stressing the health status of these populations even more.
Data Availability Statement
Publicly available datasets were analyzed in this study. This data can be found here: The fisheries data (e.g., recruitment) is provided in the Supplementary Material. The river runoff data used can be downloaded from https://snia.mop.gob.cl/BNAConsultas/reportes and from https://explorador.cr2.cl/.
Author Contributions
AZ, RQ, RM, and LC contributed to the conception and design of the study and performed the statistical analysis. AZ and RM organized the data base. AZ, RQ, and RM wrote the first draft of the manuscript. All authors contributed to manuscript revision, read, and approved the submitted version.
Funding
This research was funded by the Interdisciplinary Center for Aquaculture Research (INCAR; FONDAP-ANID Project N°15110027) and by the Programa de Estudios Ecosistémicos del Golfo de Arauco (Grant PREGA N° 4505467817; Faculty of Natural and Oceanographic Sciences of the University of Concepción) financed by Celulosa Arauco y Constitución S.A. LC was also partially funded by COPAS Sur-Austral ANID AFB170006 and COPAS COASTAL FB210021.
Conflict of Interest
The authors declare that the research was conducted in the absence of any commercial or financial relationships that could be construed as a potential conflict of interest.
Publisher’s Note
All claims expressed in this article are solely those of the authors and do not necessarily represent those of their affiliated organizations, or those of the publisher, the editors and the reviewers. Any product that may be evaluated in this article, or claim that may be made by its manufacturer, is not guaranteed or endorsed by the publisher.
Acknowledgments
The authors would like to thank two reviewers whose comments and suggestions greatly improved the manuscript.
Supplementary Material
The Supplementary Material for this article can be found online at: https://www.frontiersin.org/articles/10.3389/fmars.2022.800759/full#supplementary-material
Footnotes
References
Alvarez-Garreton, C., Boisier, J., Garreaud, R., Seibert, J., and Vis, M. (2021). Progressive water deficits during multiyear droughts in basins with long hydrological memory in Chile. Hydrol. Earth Syst. Sci. 1, 429–446. doi: 10.5194/hess-25-429-2021
Alvarez-Garreton, C., Mendoza, P. A., Pablo Boisier, J., Addor, N., Galleguillos, M., Zambrano-Bigiarini, M., et al. (2018). The CAMELS-CL dataset: catchment attributes and meteorology for large sample studies-Chile dataset. Hydrol. Earth Syst. Sci. 22, 5817–5846. doi: 10.5194/hess-22-5817-2018
Anabalón, V., Morales, C. E., González, H. E., Menschel, E., Schneider, W., Hormazabal, S., et al. (2016). Micro-phytoplankton community structure in the coastal upwelling zone off Concepción (central Chile): annual and inter-annual fluctuations in a highly dynamic environment. Prog. Oceanogr. 149, 174–188. doi: 10.1016/j.pocean.2016.10.011
Arriagada, P., Karelovic, B., and Link, O. (2021). Automatic gap-filling of daily streamflow time series in data-scarce regions using a machine learning algorithm. J. Hydrol. 598:126454. doi: 10.1016/j.jhydrol.2021.126454
Arteaga, M., and Cubillos, L. A. (2008). Análisis de los cambios de abundancia de sardina común (Strangomera bentincki): estructura año calendario versus año biológico. Lat. Am. J. Aquat. Res. 36, 137–151. doi: 10.3856/vol36-issue2-fulltext-1
Beverton, R. J. H., and Holt, S. J. (1993). On the Dynamics of Exploited Fish Populations. Dordrecht: Springer, doi: 10.1007/978-94-011-2106-4
Boisier, J. P., Rondanelli, R., Garreaud, R. D., and Muñoz, F. (2016). Anthropogenic and natural contributions to the Southeast Pacific precipitation decline and recent megadrought in central Chile. Geophys. Res. Lett. 43, 413–421. doi: 10.1002/2015GL067265
Bonanno, A., Barra, M., Basilone, G., Genovese, S., Rumolo, P., Goncharov, S., et al. (2016). Environmental processes driving anchovy and sardine distribution in a highly variable environment: the role of the coastal structure and riverine input. Fish. Oceanogr. 25, 471–490. doi: 10.1111/fog.12166
Bretz, F., Hothorn, T., and Westfall, P. (2011). Multiple Comparisons Using R. Boca Raton, FL: CRC Press.
Burnham, K. P., and Anderson, D. R. (2002). Model Selection and Multimodel Inference. A Practical Information-Theoretic Approach, 2nd Edn. Berlin: Springer.
Bustos, B., Cubillos, L. A., Claramunt, G., and Castro, L. R. (2020). Daily anchovy (Engraulis ringens) egg mortality rates in different spawning zones along the Chilean coast. Reg. Stud. Mar. Sci. 35:101200. doi: 10.1016/j.rsma.2020.101200
Caddy, J. F., and Bakun, A. (1994). A tentative classification of coastal marine ecosystems based on dominant processes of nutrient supply. Ocean Coast. Manag. 23, 201–211. doi: 10.1016/0964-5691(94)90019-1
Canales, T. M., Lima, M., Wiff, R., Contreras-Reyes, J. E., Cifuentes, U., and Montero, J. (2020). Endogenous, climate, and fishing influences on the population dynamics of small pelagic fish in the Southern Humboldt Current ecosystem. Front. Mar. Sci. 7:82. doi: 10.3389/fmars.2020.00082
Castro, L. R., and Hernández, E. (2000). Early Life Survival of the Anchoveta Engraulis ringens Off Central Chile during the 1995 and 1996 winter spawning seasons. Trans. Am. Fish. Soc. 129, 1107–1117. doi: 10.1577/1548-86592000129<1107:ELSOTA<2.0.CO;2
Castro, L. R., Morin, V., Tiznado, O., Miranda, A., Soto, S., and Gonzalez, M. (2021). Effects of salinity changes on hatching, hatching-gene expression, and hatching-enzyme expression in anchoveta Engraulis ringens eggs. Mar. Ecol. Prog. Ser. 658, 181–194. doi: 10.3354/meps.13548
Checkley, D. M., Asch, R. G., and Rykaczewski, R. R. (2017). Climate, anchovy, and sardine. Ann. Rev. Mar. Sci. 9, 469–493. doi: 10.1146/annurev-marine-122414-033819
Chen, D. G., and Irvine, J. R. (2001). A semiparametric model to examine stock–recruitment relationships incorporating environmental data. Can. J. Fish. Aquat. Sci. 58, 1178–1186. doi: 10.1139/f01-037
Claramunt, G., Cubillos, L. A., Castro, L., Hernández, C., and Arteaga, M. (2014). Variation in the spawning periods of Engraulis ringens and Strangomera bentincki off the coasts of Chile: a quantitative analysis. Fish. Res. 160, 96–102. doi: 10.1016/j.fishres.2013.09.010
Collins, M., Knutti, R., Arblaster, J., Dufresne, J.-L., Fichefet, T., Friedlingstein, P., et al. (2013). “Long-term climate change: projections, commitments and irreversibility,” in Climate Change 2013: The Physical Science Basis. Contribution of Working Group I to the Fifth Assessment Report of the Intergovernmental Panel on Climate Change, eds T. F. Stocker, D. Qin, G.-K. Plattner, M. Tignor, S. K. Allen, J. Doschung, et al. (Cambridge: Cambridge University Press), 1029–1136.
Costalago, D., Potter, P., Pattrick, P., and Strydom, N. A. (2018). Influence of environmental variables on the larval stages of anchovy, Engraulis encrasicolus, and sardine, Sardinops sagax, in Algoa Bay, South Africa. Environ. Biol. Fishes 101, 225–236. doi: 10.1007/s10641-017-0693-z
Cubillos, L. A., and Arcos, D. F. (2002). Recruitment of common sardine (Strangomera bentincki) and anchovy (Engraulis ringens) off south-central Chile in the 1990s and the impact of the 1997-1998 El Niño. Aquat. Living Resour. 15, 87–94. doi: 10.1016/S0990-7440(02)01158-0
Cubillos, L. A., Arcos, D. F., Bucarey, D. A., and Canales, M. T. (2001). Seasonal growth of small pelagic fish off Talcahuano, Chile (37°S, 73°W): a consequence of their reproductive strategy to seasonal upwelling? Aquat. Living Resour. 14, 115–124. doi: 10.1016/S0990-7440(01)01112-3
Cubillos, L. A., Castro, L., Claramunt, G., and Oyarzún, C. (2006). Evaluación del Stock Desovante de Anchoveta y Sardina Común entre la V y X Región, año 2005. Fondo de Investigación Pesquera, FIP N°2005–02, 153.
Cubillos, L. A., Ruiz, P., Claramunt, G., Gacitúa, S., Núñez, S., Castro, L. R., et al. (2007a). Spawning, daily egg production, and spawning stock biomass estimation for common sardine (Strangomera bentincki) and anchovy (Engraulis ringens) off central southern Chile in 2002. Fish. Res. 86, 228–240. doi: 10.1016/j.fishres.2007.06.007
Cubillos, L. A., Serra, R., and Fréon, P. (2007b). Synchronous pattern of fluctuation in three anchovy fisheries in the humboldt current system. Aquat. Living Resour. 20, 69–75. doi: 10.1051/alr:2007017
Díaz-Ochoa, J. A., and Quiñones, R. A. (2008). Relationship of precipitation, freshwater input, and sea level height with the abundance of the white shrimp (Litopenaeus occidentalis; Street, 1871) off Buenaventura, eastern tropical Pacific. Fish. Res. 92, 148–161. doi: 10.1016/j.fishres.2008.01.002
Drinkwater, K. F., and Frank, K. T. (1994). Effects of river regulation and diversion on marine fish and invertebrates. Aquat. Conserv. Mar. Freshw. Ecosyst. 4, 135–151. doi: 10.1002/aqc.3270040205
Gao, P., Li, P., Zhao, B., Xu, R., Zhao, G., Sun, W., et al. (2017). Use of double mass curves in hydrologic benefit evaluations. Hydrol. Process. 31, 4639–4646. doi: 10.1002/hyp.11377
Garreaud, R. D., Alvarez-Garreton, C., Barichivich, J., Pablo Boisier, J., Christie, D., Galleguillos, M., et al. (2017). The 2010-2015 megadrought in central Chile: impacts on regional hydroclimate and vegetation. Hydrol. Earth Syst. Sci. 21, 6307–6327. doi: 10.5194/hess-21-6307-2017
Garreaud, R. D., Boisier, J. P., Rondanelli, R., Montecinos, A., Sepúlveda, H. H., and Veloso-Aguila, D. (2019). The central chile mega drought (2010–2018): a climate dynamics perspective. Int. J. Climatol. 40, 421–439. doi: 10.1002/joc.6219
Gatica, C., Arteaga, M., Giacaman, J., and Ruiz, P. (2007). Tendencias en la biomasa de sardina común (Strangomera bentincki) y anchoveta (Engraulis ringens) en la zona centro-sur de Chile, entre 1991 y 2005. Investig. Mar. 35, 13–24. doi: 10.4067/s0717-71782007000100002
Gillson, J. (2011). Freshwater flow and fisheries production in estuarine and coastal systems: where a drop of rain is not lost. Rev. Fish. Sci. 19, 168–186. doi: 10.1080/10641262.2011.560690
Gillson, J., Suthers, I., and Scandol, J. (2012). Effects of flood and drought events on multi-species, multi-method estuarine and coastal fisheries in eastern Australia. Fish. Manag. Ecol 19, 54–68.
Gómez-Canchong, P. Quiñones, R. A., Neira, S., and Arancibia, H. (2017). Modelling fishery-induced impacts on the food web of the continental shelf off central-south Chile using a size-based network approach. Lat. Am. J. Aquat. Res. 45, 748–765. doi: 10.3856/vol45-issue4-fulltext-11
Gomez, F., Montecinos, A., Hormazabal, S., Cubillos, L. A., Correa-Ramirez, M., and Chavez, F. P. (2012). Impact of spring upwelling variability off southern-central Chile on common sardine (Strangomera bentincki) recruitment. Fish. Oceanogr 21, 405–414.
Grendi, C., Cubillos, L., Castro, L., Soto, S., Claramunt, G., Bonicelli, J., et al. (2020). Evaluación del Stock Desovante de Anchoveta y Sardina Común entre la Región de Valparaíso y la Región de Los Lagos, año 2019. Convenio de Desempeño 2019. Valparaíso: Instituto de Fomento Pesquero, 307.
Grez, P. W., Aguirre, C., Farías, L., Contreras-López, M., and Masotti, Í (2020). Evidence of climate-driven changes on atmospheric, hydrological, and oceanographic variables along the Chilean coastal zone. Clim. Change 163, 633–652. doi: 10.1007/s10584-020-02805-3
Harvey, C. L., Dixon, H., and Hannaford, J. (2012). An appraisal of the performance of data-infilling methods for application to daily mean river flow records in the UK. Hydrol. Res. 43, 618–636. doi: 10.2166/nh.2012.110
Hilborn, R., Amoroso, R. O., Bogazzi, E., Jensen, O. P., Parma, A. M., Szuwalski, C., et al. (2017). When does fishing forage species affect their predators? Fish. Res. 191, 211–221. doi: 10.1016/j.fishres.2017.01.008
Hilborn, R., and Waiters, C. J. (1992). “Quantitative fisheries stock assessment,” in Choice, Dynamics and Uncertainty, eds R. Hilborn and C. J. Walters (Boston, MA: Springer), 451–538.
Jacob, B. G., Tapia, F. J., Quiñones, R. A., Montes, R., Sobarzo, M., Schneider, W., et al. (2018). Major changes in diatom abundance, productivity, and net community metabolism in a windier and dryer coastal climate in the southern Humboldt Current. Prog. Oceanogr. 168, 196–209. doi: 10.1016/j.pocean.2018.10.001
Lehodey, P., Alheit, J., Barange, M., Baumgartner, T., Beaugrand, G., Drinkwater, K., et al. (2006). Climate variability, fish, and fisheries. J. Clim. 19, 5009–5030. doi: 10.1175/JCLI3898.1
León-Muñoz, J., Urbina, M. A., Garreaud, R., and Iriarte, J. L. (2018). Hydroclimatic conditions trigger record harmful algal bloom in western Patagonia (summer 2016). Sci. Rep. 8, 1–10. doi: 10.1038/s41598-018-19461-4
Lloret, J., Lleonart, J., Solé, I., and Fromentin, J. M. (2001). Fluctuations of landings and environmental conditions in the north-western Mediterranean Sea. Fish. Oceanogr 10, 33–50.
Lloret, J., Palomera, I., Salat, J., and Sole, I. (2004). Impact of freshwater input and wind on landings of anchovy (Engraulis encrasicolus) and sardine (Sardina pilchardus) in shelf waters surrounding the Ebre (Ebro) River delta (north-western Mediterranean). Fish. Oceanogr. 13, 102–110. doi: 10.1046/j.1365-2419.2003.00279x
Mangiafico, S. (2015). An R Companion for the Handbook of Biological Statistics. North Brunswick Township, NJ: Rutgers Coop. Ext.
Martín, P., Bahamon, N., Sabatés, A., Maynou, F., Sánchez, P., and Demestre, M. (2008). European anchovy (Engraulis encrasicolus) landings and environmental conditions on the Catalan Coast (NW Mediterranean) during 2000-2005. Hydrobiologia 612, 185–199. doi: 10.1007/s10750-008-9482-1
Masotti, I., Aparicio-Rizzo, P., Yevenes, M. A., Garreaud, R., Belmar, L., and Farías, L. (2018). The influence of river discharge on nutrient export and phytoplankton biomass off the Central Chile Coast (33°-37°S): Seasonal cycle and interannual variability. Front. Mar. Sci. 5:423. doi: 10.3389/fmars.2018.00423
Meynecke, J. O., Grubert, M., and Gillson, J. (2012). Giant mud crab (Scylla serrata) catches and climate drivers in Australia - A large scale comparison. Mar. Freshw. Res. 63, 84–94. doi: 10.1071/MF11149
Montecinos, A., Kurgansky, M. V., Muñoz, C., and Takahashi, K. (2011). Non-ENSO interannual rainfall variability in central Chile during austral winter. Theor. Appl. Climatol. 106, 557–568. doi: 10.1007/s00704-011-0457-1
Nagelkerke, N. J. D. (1991). A note on a general definition of the coefficient of determination. Biometrika 78, 691–692. doi: 10.1093/biomet/78.3.691
Palomera, I., Olivar, M. P., Salat, J., Sabatés, A., Colla, M., García, A., et al. (2007). Small pelagic fish in the NW Mediterranean Sea: an ecological review. Prog. Oceanogr. 74, 377–396.
Parada, C., Gatica, C., Ernst, B., Gretchina, A., Yannicelli, B., Porobic, J., et al. (2013). Variabilidad ambiental y recursos pesqueros en el Pacifico suroriental: estado de la investigacion y desafios para el manejo pesquero. Lat. Am. J. Aquat. Res. 41, 1–28. doi: 10.3856/vol41-issue1-fulltext-1
Pedraza-Garcia, M., and Cubillos, L. A. (2008). Population dynamics of two small pelagic fish in the central-south area off Chile: delayed density-dependence and biological interaction. Environ. Biol. Fish. 82, 111–122. doi: 10.1007/s10641-007-9260-3
Pirnia, A., Golshan, M., Darabi, H., Adamowski, J., and Rozbeh, S. (2019). Using the Mann–Kendall test and double mass curve method to explore stream flow changes in response to climate and human activities. J. Water Clim. Chang. 10, 725–742. doi: 10.2166/wcc.2018.162
Quiñones, R. A., Hernández, A., Carrasco, P., Araya, I., and Muñoz, H. (2009). “The fisheries of the coastal system of the Itata River Basin,” in The Hydrographic Basin of the Itata River: Scientific Contributions for its Management, eds O. Parra, J. C. Castilla, H. Romero, R. A. Quiñones, and A. Camaño (Concepción: Universidad de Concepción), 193–211.
Quiñones, R. A., and Montes, R. M. (2001). Relationship between freshwater input to the coastal zone and the historical landings of the benthic/demersal fish Eleginops maclovinus in central-south Chile. Fish. Oceanography 10, 311–328.
R Development Core Team (2017). R: A Language and Environment for Statistical Computing. Vienna: R Foundation for Statistical Computing.
Reinfelds, I. V., Walsh, C. T., Van der Meulen, D. E., Growns, I. O., and Gray, C. A. (2013). Magnitude, frequency and duration of instream flows to stimulate and facilitate catadromous fish migrations: australian bass (Macquaria novemaculeata Perciformes, Percichthyidae). River Research and Applications 527, 512–527. doi: 10.1002/rra
Ritz, C., and Streibig, J. C. (2008). Nonlinear Regression with R. Copenhagen: Springer, doi: 10.1007/978-0-387-09616-2
Saavedra, A., Catasti, V., Leiva, F., Vargas, R., Cifuentes, U., Reyes, E., et al. (2014). Evaluación Hidroacústica de los Stocks de Anchoveta y Sardina común Entre la V y X Regiones, año 2014. Informe Final FIP 2013-05. 306. Chile: Instituto de Fomento Pesquero.
Saavedra, A., Vargas, R., Catasti, V., Lang, C., Cifuentes, U., Grendi, C., et al. (2018). Evaluación Hidroacústica de los Stocks de Anchoveta y Sardina Común entre la V y X Regiones, año 2018. Informe Final.
Sabates, A., Olivar, M. P., Salat, J., Palomera, I., and Alemany, F. (2007). Physical and biological processes controlling the distribution of fish larvae in the NW Mediterranean. Prog. Oceanogr. 74, 355–376.
Saldías, G. S., and Lara, C. (2020). Satellite-derived sea surface temperature fronts in a river-influenced coastal upwelling area off central–southern Chile. Reg. Stud. Mar. Sci. 37:101322. doi: 10.1016/j.rsma.2020.101322
Saldías, G. S., Largier, J. L., Mendes, R., Pérez-Santos, I., Vargas, C. A., and Sobarzo, M. (2016). Satellite-measured interannual variability of turbid river plumes off south-central Chile: Spatial patterns and the influence of climate variability. Prog. Oceanogr. 146, 212–222. doi: 10.1016/j.pocean.2016.07.007
Saldías, G. S., Sobarzo, M., Largier, J., Moffat, C., and Letelier, R. (2012). Seasonal variability of turbid river plumes off central Chile based on high-resolution MODIS imagery. Remote Sens. Environ. 123, 220–233. doi: 10.1016/j.rse.2012.03.010
Santojanni, A., Arneri, E., Bernardini, V., Cingolani, N., Di Marco, M., and Russo, A. (2006). Effects of environmental variables on recruitment of anchovy in the Adriatic Sea. Clim. Res. 31, 181–193. doi: 10.3354/cr031181
Santoso, A., Mcphaden, M. J., and Cai, W. (2017). The defining characteristics of ENSO extremes and the strong 2015/2016 El Niño. Rev. Geophys. 55, 1079–1129. doi: 10.1002/2017RG000560
Seber, G. A. F., and Wild, C. J. (2003). Nonlinear Regression (Wiley Series in Probability and Statistics). Hoboken, NJ: Wiley.
Soto-Mendoza, S., Castro, L. R., and Llanos-Rivera, A. (2010). Variabilidad espacial y temporal de huevos y larvas de Strangomera bentincki y Engraulis ringens, asociados a la desembocadura del río Itata, Chile. Rev. Biol. Mar. Oceanogr. 45, 471–487. doi: 10.4067/S0718-19572010000300012
Szuwalski, C. S., Vert-Pre, K. A., Punt, A. E., Branch, T. A., and Hilborn, R. (2015). Examining common assumptions about recruitment: a meta-analysis of recruitment dynamics for worldwide marine fisheries. Fish Fish 16, 633–648. doi: 10.1111/faf.12083
Testa, G., Masotti, I., and Farías, L. (2018). Temporal variability in net primary production in an upwelling area off central Chile (36°S). Front. Mar. Sci. 5:179. doi: 10.3389/fmars.2018.00179
Valenzuela, R. A., and Garreaud, R. D. (2019). Extreme daily rainfall in south-central Chile and its relationship with low-level horizontal water vapor fluxes. J. Hydrometeorol. 20, 1829–1850. doi: 10.1175/jhm-d-19-0036.1
Walsh, C. T., Reinfelds, I. V., Ives, M. C., Gray, C. A., West, R. J., and Van der Meulen, D. E. (2013). Environmental influences on the spatial ecology and spawning behaviour of an estuarine-resident fish, Macquaria colonorum. Estuar. Coast. Shelf Sci. 118, 60–71. doi: 10.1016/j.ecss.2012.12.009
Yáñez, E., Hormazábal, S., Silva, C., Montecinos, A., Barbieri, M. A., Valdenegro, A., et al. (2008). Coupling between the environment and the pelagic resources exploited off northern Chile: Ecosystem indicators and a conceptual model. Lat. Am. J. Aquat. Res 36, 159–181. doi: 10.3856/vol36-issue2-fulltext-3
Keywords: anchovy, sardine, river runoff, recruitment, small pelagics, Eastern South Pacific, Chile
Citation: Zunguza A, Montes RM, Cubillos LA and Quiñones RA (2022) River Runoff as a Major Driver of Anchovy (Engraulis ringens) Recruitment but Not of Common Sardine (Strangomera bentincki) in Central-South Chile. Front. Mar. Sci. 9:800759. doi: 10.3389/fmars.2022.800759
Received: 24 October 2021; Accepted: 06 January 2022;
Published: 11 February 2022.
Edited by:
Jose Luis Iriarte, Austral University of Chile, ChileReviewed by:
Margaret Siple, National Oceanic and Atmospheric Administration (NOAA), United StatesBernardo Patti, National Research Council (CNR), Italy
Copyright © 2022 Zunguza, Montes, Cubillos and Quiñones. This is an open-access article distributed under the terms of the Creative Commons Attribution License (CC BY). The use, distribution or reproduction in other forums is permitted, provided the original author(s) and the copyright owner(s) are credited and that the original publication in this journal is cited, in accordance with accepted academic practice. No use, distribution or reproduction is permitted which does not comply with these terms.
*Correspondence: Renato A. Quiñones, cnF1aW5vbmVAdWRlYy5jbA==