- 1Department of Biosciences, Ecology and Evolutionary Biology, Rice University, Houston, TX, United States
- 2Department of Biological Sciences, Clemson University, Clemson, SC, United States
Marine diseases have caused large scale decreases in coral cover across the Caribbean and are unfortunately projected to increase as sea surface temperatures rise. Identifying the main drivers of disease transmission is essential for our understanding and response to diseases in the future. This study investigates the effects of direct-contact, waterborne, Symbiodiniaceae composition, and butterflyfish foraging on the transmission rates of stony coral tissue loss disease (SCTLD) across Montastraea cavernosa (MCAV) coral fragments. M. cavernosa fragments were placed in direct contact with diseased Orbicella and Montastraea colonies with the purpose of creating disease MCAV fragments for experimental trials with butterflyfish. Three treatments were used to investigate waterborne transmission (control), mechanically injury to a healthy coral (limited), and direct contact feeding across a diseased and healthy coral (unlimited). After the experimental trials, the composition of Symbiodiniaceae was analyzed for every MCAV fragment. Direct contact transmission took on average 3.9 days in 2019 and 11.9 days in 2020 with significantly quicker rates of transmission between donor diseased Orbicella and Montastraea than Montastraea to Montastraea. The composition of Symbiodiniaceae differed significantly between the fragments used in 2019 (dominated by Durusdinium) than in 2020 (dominated by Cladocopium spp.). The limited treatment had the quickest time to transmission compared to the unlimited and control treatments. Symbiodiniaceae differences between 2019 and 2020 might explain differences in transmission rates and overall susceptibility between the years. The species of Symbiodiniaceae may play a role in the susceptibility of corals to the transmission of SCTLD. Additionally, we also have some suggestive evidence that butterflyfish do not directly increase infection rates, but instead might be increasing infection recovery.
Introduction
Coral reefs in the Caribbean are experiencing numerous anthropogenic disturbances including warming sea surface temperature, ocean acidification, and nutrient pollution that are threatening the ecosystems as well as the organisms that inhabit it (Gardner et al., 2005; Miller et al., 2009; Mumby and Steneck, 2011). As a result of these stressors, there has been a dramatic increase in the diversity, frequency, and virulence of hard coral pathogens that target Scleractinian hard corals, the foundation species of coral reefs (Harvell et al., 2002; Altizer et al., 2013; Burge and Hershberger, 2020). The first hard coral disease was recorded in the 1970s and since then, over 70% of all disease reports are from the Caribbean with over 15 types of diseases impacting 118 hard and 22 soft corals (Green and Bruckner, 2000); dubbing the Caribbean as a “disease hot spot” (Hayes and Goreau, 1998; Weil et al., 2006; Harvell et al., 2007). Coral diseases have had significant effects on hard coral with particular diseases decreasing live coral cover by over 60%, with some specific species of coral experiencing losses near 90% (Miller et al., 2009).
Marine diseases are naturally occurring in the environment, but with ongoing and forecasted climate-driven changes, disease outbreaks are expected to become more frequent (Harvell et al., 2002; Altizer et al., 2013; Burge and Hershberger, 2020) and may follow other disturbance events, such as bleaching (Weil et al., 2006; Brandt and McManus, 2009; Miller et al., 2009). Several studies have focused on the effects of individual and combined perturbations on hard corals and discovered that corals exposed had significantly higher disease prevalence than hard corals that remained unexposed to stressors (Vega Thurber et al., 2014; Maher et al., 2019). Additionally, there is an increasing diversity of pathogens, taking numerous forms (viruses, bacteria, parasites, dinoflagellate, among others), that can target a wide range of hosts making intervention methods difficult (Walton et al., 2018; Bateman et al., 2020). With coral being vital to coral reef ecosystems, there have been tremendous efforts to understand the mechanisms behind disease virulence.
The most recent disease to affect the Caribbean is stony coral tissue loss disease (SCTLD) which was first detected near Virginia Key, FL in 2014 and has since spread to other parts of the Caribbean (Precht et al., 2016; Alvarez-Filip et al., 2019; Weil et al., 2019). SCTLD has affected more than 20 different hard coral species and, in some circumstances, can cause a coral colony to have 100% mortality in a matter of months (Lunz et al., 2017; Florida Keys National Marine Sanctuary, 2018). Studies suggest that this disease is waterborne and may be moving between reefs via ocean currents and direct-contact transmission accounting for the rapid spread of SCTLD (Bruckner et al., 1997; Walton et al., 2018; Aeby et al., 2019; Muller and van Woesik, 2020; Rosales et al., 2020; Sharp et al., 2020). Although SCTLD can be completely detrimental to live coral cover, there has been some variation in overall virality of SCTLD with some coral colonies experiencing effects ranging from 100% mortality to complete recovery from SCTLD in the field (Landsberg et al., 2018; Aeby et al., 2019; Florida Keys National Marine Sanctuary, 2018; Muller and van Woesik, 2020; Noonan and Childress, 2020; Ushijima et al., 2020). Additionally, there are results that suggest that corals can experience a change in resistance or recovery to SCTLD (Paul et al., 2019; Meiling et al., 2020). This variation in virulence has led researchers to look at external drivers that may be influencing SCTLD spread and recovery.
The presence and foraging habits of corallivores, like butterflyfish, has been previously observed to facilitate disease spread (Aeby and Santavy, 2006; Pratchett et al., 2006; Cole et al., 2009; Raymundo et al., 2009; Bruckner and Bruckner, 2016; Nicolet et al., 2018a,b). By inflicting mechanical injury on coral polyps, corallivores provide a mechanism for infection (Aeby and Santavy, 2006; Bruckner and Bruckner, 2016). There is also evidence that disease could spread through corallivore feces (Pratchett et al., 2013; Grupstra et al., 2020). Alternatively, some studies have suggested that corallivores are actually beneficial to hard corals and can ameliorate disease by the removal of diseased tissue (Cole et al., 2009; Raymundo et al., 2009; Noonan and Childress, 2020).
Other laboratory studies have found no evidence of corallivores contributing to disease transmission (Nicolet et al., 2018a,b) and recommend that alternative processes like the composition of Symbiodiniaceae, the symbiotic dinoflagellates within coral tissue, control the susceptibility of coral to disease (Baker et al., 2004; Iglesias-Prieto et al., 2004; Berkelmans and van Oppen, 2006; Jones et al., 2008; Correa and Baker, 2009). And this relationship has been observed with numerous diseases and pathogenic strains like Vibrio spp. (Correa and Baker, 2009; Rouzé et al., 2016). However, the group of Symbiodiniaceae that is most beneficial for a coral’s holobiont has been variable across studies. Clearly additional research is needed to understand the potential role that corallivores and Symbiodiniaceae may have on disease outbreaks (Aronson and Precht, 2001; Bruckner and Bruckner, 2016).
This study sought to investigate the influence of Symbiodiniaceae and the foureye butterflyfish, Chaetodon capistratus, foraging on the rate of SCTLD transmission and recovery in a laboratory experiment. We predicted that the species of Symbiodiniaceae plays a strong role in direct contact transmission and that the foureye butterflyfish plays a strong role in the transmission of disease. We also predicted that hard corals in treatments with foureye butterflyfish would have slower rates of SCTLD transmission compared to corals that were in the treatment with no foureye butterflyfish. This is based on observations made in the field where the recovery of hard corals to SCTLD in the middle Florida Keys that were observed to be predated on by foureye butterflyfish (Noonan and Childress, 2020). However, we did predict that SCTLD transmission would be quickest in our direct contact transmission trials. Through this experiment, we hope to be able to determine which factors have the most influence on SCTLD transmission in a laboratory setting. This study will provide insight into whether butterflyfish or Symbiodiniaceae played a larger role in the rapid spread of SCTLD across the Caribbean.
Materials and Methods
Coral Collection and Disease Transmission
To study the transmission of SCLTD, healthy fragments of Montastraea cavernosa (fMCAV) (n = 41) were donated by Florida Fish and Wildlife Conservation Commission’s coral nursery near Marathon, FL in June 2019 and again in June 2020. It is unknown whether the corals fragments collected in 2020 were from the same colony than fragments collected in 2019. Each fMCAV was approximately 3–4 cm in diameter containing 6–12 polyps. These fragments were photographed, measured, tagged, and housed in a single 135-gal tank that had continuously running seawater at the Keys Marine Laboratory (KML) in Layton, FL. Active SCTLD infected corals of three species, Montastraea cavernosa (ddMCAV) (n = 6), Orbicella faveolata (ddOFAV) (n = 8), and Orbicella annularis (ddOANN) (n = 3), were identified as actively infected by SCTLD and collected by collaborators on actively infected reefs off of Looe Key in the Florida Keys in 2019 and on actively infected reefs offshore of Hollywood, FL in 2020. The diseased colonies were cut from naturally occurring live corals from Broward county, FL. For the purposes of this study, only diseased colonies showing the characteristics of SCTLD were collected.
Diseased colonies were transported to KML where they were photographed, measured, tagged, and housed in two 40-gal tanks. Every coral (fMCAV, ddMCAV, ddOFAV, ddOANN) was allowed an acclimation period of at least 72 h before the contact-based transmission study or the experimental trials began. All tanks used in this experiment were a part of a well seawater system that treats and releases well water into a thermally and pH controlled flowthrough system. All experiments were conducted under normal photoperiod with temperatures ranging from 23.68 to 32.81 degrees Celsius with an average temperature of 26.61 degrees Celsius.
Once acclimated, about half of the fMCAV (n = 22) were randomly selected and transferred into the 40-gal flowthrough tanks with the diseased coral colonies. They were randomly placed in direct contact with the active disease margin on one of the ddMCAV, ddOFAV, or ddOANN colonies (Figure 1). All fMCAV and diseased colonies were photographed and monitored for disease presence daily for the duration of the experimental trials. A coral would be considered infected or diseased when there were signs of subacute to acute tissue loss with distinct paling of tissue. When a fMCAV was showing signs of SCTLD it was randomly paired with a healthy fMCAV and allocated to 1 of 3 experimental treatments described below.
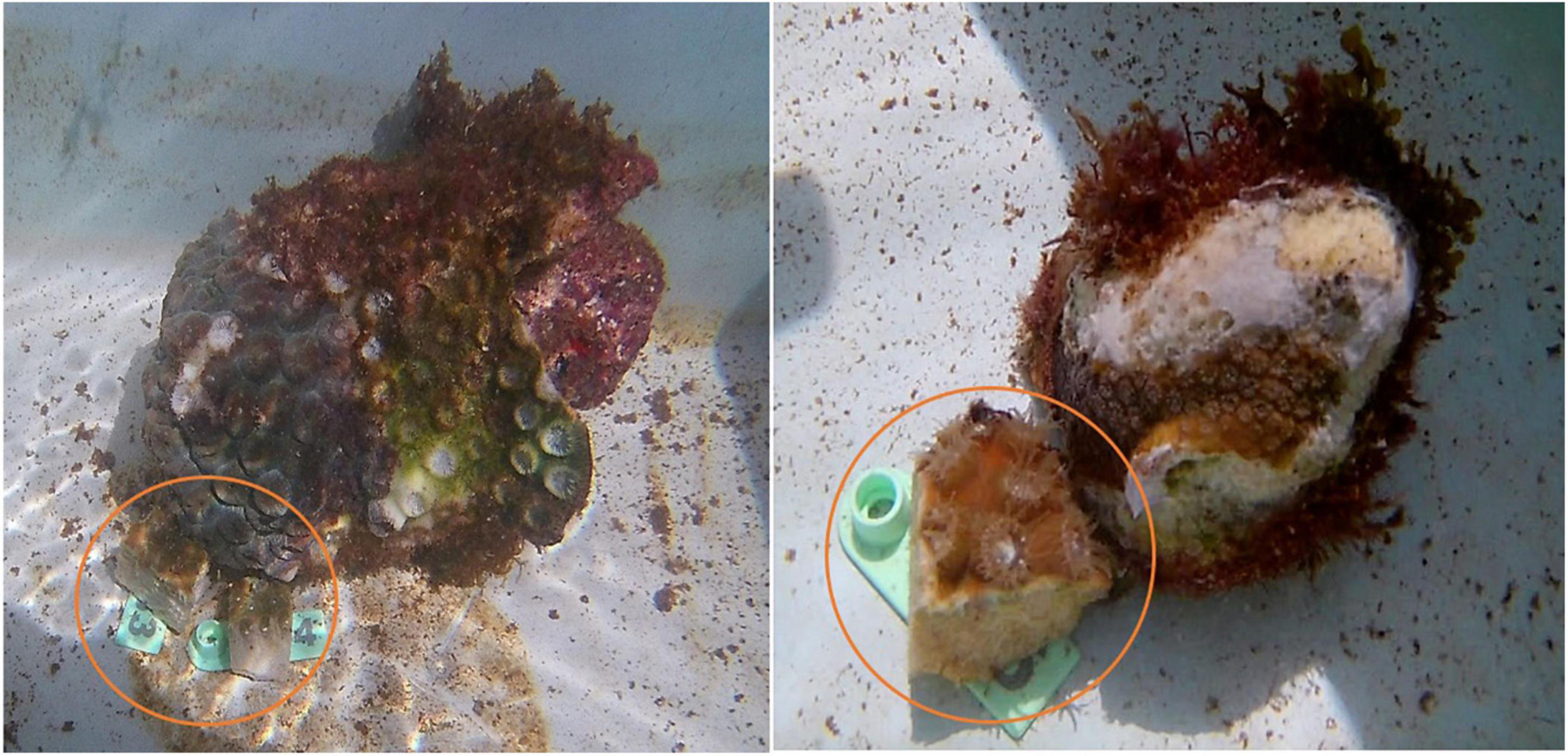
Figure 1. The coral fragments circled in orange are Montastraea cavernosa fragments (fMCAV) that were placed in direct contact with a donor diseased corals to induce infection and study direct contact transmission rates.
Transmission Experiment With Chaetodon capistratus
To investigate the role foureye butterflyfish may be having on SCTLD transmission, a laboratory experiment was conducted at the Keys Marine Lab in Layton, FL using Chaetodon capistratus (n = 13). The foureye butterflyfish was used for this study because they can be found in high abundances around active SCTLD corals and were observed to feed on diseased tissue in the field (Noonan and Childress, 2020). Adult individuals of C. capistratus (total length 7.6–10.3 cm) were collected using hand nets by Dynasty Marine Associates near Marathon, FL and transported in a live well to KML. Individuals were placed into their own 40-gal tank where they acclimated to laboratory conditions for 48 h prior to the transmission experiment. C. capistratus were fed brine shrimp and coral fragments daily for the extent of the experimental trials and were held in a running seawater system with the same environmental parameters described above.
Three treatments (control, limited fish access, unlimited fish access) were used to investigate the transmission rates of SCTLD across fMCAV (Figure 2A). In the control treatment there was a diseased and healthy fMCAV with no foureye butterflyfish. This treatment tested disease transfer through the process of waterborne transmission alone. In the limited fish access treatment, the newly diseased fMCAV was covered with a Vexar cage therefore limiting the introduced foureye butterflyfish to feed only on the healthy coral fragment. This treatment allowed us to examine the role mechanical injury from active feeding, in addition to waterborne transmission, had on disease transmission. In the unlimited fish access treatment, the foureye butterflyfish had complete access to both the healthy and diseased fMCAV; this allowed us to test whether back and forth contact, on top of mechanical injury and waterborne transmission, increased transmission rates.
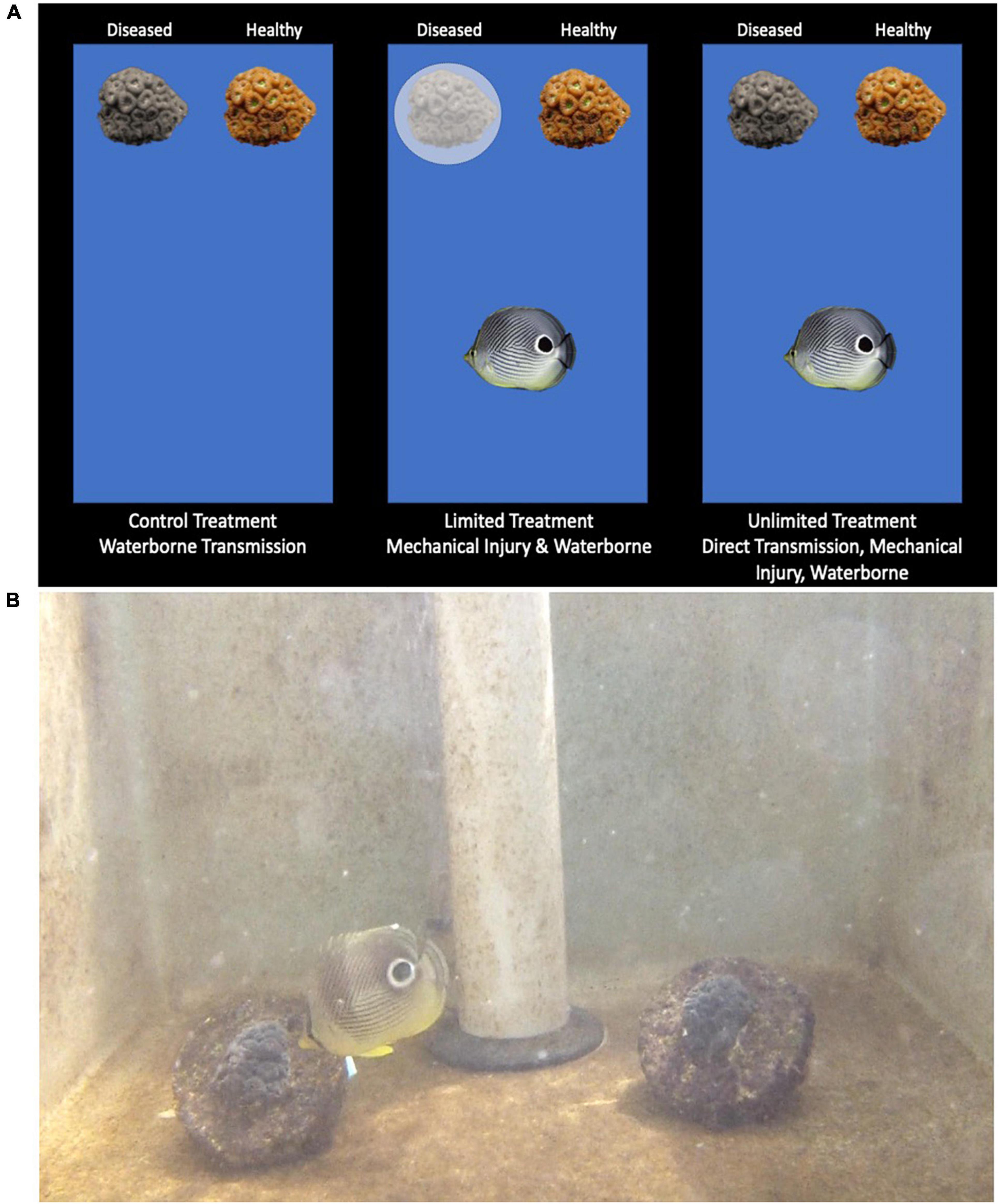
Figure 2. Three treatments were used for the transmission experiment: control (waterborne), limited (mechanical injury and waterborne), and unlimited (direct feeding transfer, mechanical injury, waterborne) (A). Foureye butterflyfish feeding on the diseased coral fragment in the unlimited treatment (B).
In the limited and unlimited treatment, the diseased and healthy fMCAV were placed behind a barrier in a 40-gal tank with a foureye butterflyfish. The barrier was lifted and the foureye was allowed to forage on the experimental fragment(s) for a total of an hour a day (Figure 2B). Note: There was one foureye butterflyfish in the limited fish access treatment that found a way to move the cage over the diseased coral for 3 out of the 5 days adding diseased bites to the analysis for the limited treatment trials. The foraging behaviors of the butterflyfish during that 1 h period were recorded using an in situ GoPro camera and video recordings were analyzed using Behavioral Observation Research Interactive Software (BORIS). Bite location (healthy coral, diseased coral, elsewhere in tank), bite rate (bites/hour), and overall activity pattern (time spent swimming, resting, out of view) were estimated for each 1-h video. The observer only counted bites when the mouth of the foureye butterflyfish and the substrate were clearly visible, underestimating the actual bite rates. After the foraging hour, the barrier separating the fish from the coral fragments was reinstalled to ensure no further foraging on the corals would occur during the time the camera was turned off. Each coral fragment from the control, limited, and unlimited treatments were photographed and monitored to track the presence or absence of SCTLD. After 5 days of daily exposure to butterflyfish foraging, the healthy and diseased fMCAVs were placed back into a single 40-gal holding tank and monitored for an additional 7 days to assess disease recovery.
After the coral disease transmission study, the foureye butterflyfish were placed with a single donor diseased coral (ddMCAV, ddOFAV, or ddOANN) daily for 5 days to observe individual activity patterns and feeding preferences to assess variation due to butterflyfish personality differences. A donor diseased coral was placed into a 40-gal tank with one of the foureye butterflyfish where the butterflyfish was filmed foraging for 1 h with an in situ GoPro camera to later be analyzed using BORIS software. We measured bites rates, activity patterns, and preferences for particular coral tissue types (healthy, diseased, dead skeleton, dead overgrown skeleton). Each diseased coral colony was photographed and analyzed using ImageJ software to calculate the percentage of healthy, diseased, and coral skeleton each day.
Symbiodiniaceae Collection and ITS2 Library Preparation for Amplicon Sequencing
One polyp was biopsied from each fMCAV and each donor diseased coral colony using a 60 ml syringe (with a blunt-tipped needle). A total of 43 fMCAV, 3 ddMCAV, 4 ddOFAV, and 2 ddOANN were sampled. 1 ddOFAV and 3 ddMCAV tissue samples were not collected because the colony had experienced 100% mortality by the end of the experiment. The needle was inserted into a single corallite and the plunger was pulled out, sucking the polyp tissue into the syringe. The syringe tip was then placed into a test tube and the tissue was expelled by depressing the syringe plunger. The test tube was then filled with ethanol and stored in a –20 degree Celsius freezer until it was transported back to Clemson University where it was then stored in a –80 degree Celsius freezer.
Sample DNA was extracted using the Qiagen DNeasy PowerBiofilm Kit (Qiagen, United States) as directed by the manufacturer. The Symbiodiniaceae ITS-2 region was amplified from each sample using the primer pair SYM_VAR_5.8S2 and SYM_VAR_REV. These primers and PCR conditions are described previously in Hume et al. (2018). Custom barcoded primers were designed with the ITS2 primers using the protocol from Kozich et al. (2013). After PCR amplification the samples were run on a 1.5% agarose gel to confirm the amplicon size. The concentration of Symbiodiniaceae DNA after PCR amplification was determined using Qubit 3.0® (Thermo Fisher Scientific, United States). All libraries were diluted to 5 ng/μL prior to pooling. After pooling samples were denatured and diluted according to the Illumina denature and dilute libraries guide.1
Symbiodiniaceae Illumina Sequencing, Sequence Analysis, and Taxonomic Classification
Symbiodiniaceae ITS2 amplicons were sequenced using a V2 500-cycle kit (250-basepair paired-end reads) on a MiSeq (Illumina, San Diego, CA). Demultiplexed paired-end reads were imported into QIIME 2 for sequence quality control and analysis (Bolyen et al., 2018). Removal of low-quality sequences, chimeric sequences, and clustering into amplicon sequence variants (ASVs) was completed using the DADA2 program in QIIME2 (Callahan et al., 2016). Taxonomic assignment of the Symbiodiniaceae spp. was completed using the RefSeq file from the SymPortal database developed by Hume et al. (2019). The RefSeq file was converted into a QIIME2 artifact and a custom QIIME2 database was made. The database was trained using the naive-Bayes machine-classifier in QIIME2 prior to taxonomic classification of reads.
Statistical Analyses
Bite rates were calculated as the number of bites taken on the different substrates divided by the length of the video recording. Bite rates were estimated for each individual video recording with the donor diseased corals (n = 56) and for the limited fish access (n = 6) and unlimited fish access (n = 7) treatments. Activity patterns were estimated by dividing the time spent swimming or resting by the total length of the video recording that the butterflyfish was present (in view of the camera). To estimate the foraging preferences of the butterflyfish, the Strauss’ linear resource selection index (L) was used. This index allowed us to assess an individual’s selection for diseased tissue in their proportion of total bites and whether they significantly preferred or avoided diseased tissue based on the frequency of bites relative to the available surface area of diseased tissue, healthy tissue, dead skeleton, dead overgrown skeleton, or another location in the tank. This index is calculated using the formula:
where i is the focal substrate type (diseased tissue), ri is the proportion of bites taken on diseased tissue, and pi is the proportion of diseased tissue available on the diseased coral colony (Strauss, 1979). This index varies between –1 and 1 with a strong preference for diseased tissue being 1 and a strong preference for healthy tissue being –1. To calculate and compare the bite rates among treatments, t-tests and a single-factor ANOVA was used.
The QIIME2 pipeline (version 2021.4) was used to calculate alpha and beta diversity (Bolyen et al., 2018). The ITS2 reads were rarefied to an even sampling depth of 58,059 reads per sample. Alpha diversity was calculated in QIIME2 using the Shannon Index (Shannon, 1948). A nonparametric Kruskal-Wallis test was used to determine statistical significance. P-values were adjusted using Benjamini and Hochberg FDR correction (Benjamini and Hochberg, 1995). The Bray Curtis Index was used to calculate beta diversity using QIIME2 (Bray and Curtis, 1957). A PERMANOVA was performed to determine statistical differences in beta diversity. P-values were adjusted using Benjamini and Hochberg FDR correction. The Phyloseq package (version 1.26.1) was used to generate alpha diversity, beta diversity, and relative abundance plots (McMurdie and Holmes, 2013).
T-tests were used to assess differences in time-to-infection within fMCAV with the donor diseased corals in the contact-based experiments. A Mantel-Cox Proportion Hazards test was used to track disease transmission in the three treatments (control—no fish, limited fish access, unlimited fish assess). A Mantel-Cox test is a non-parametric test that compares the rate of transmission between treatments, the outcome of the treatments (no transmission or transmission to the “healthy” fMCAV), and the time to the outcome (Aeby et al., 2019). The butterflyfish impact on disease transmission data was analyzed using a logistic regression analysis, where the state of the fMCAV after 5 days of exposure (binomial: diseased or healthy) was the response variable, and the factors in the model were treatment (limited fish access vs. unlimited fish access) and an individual fish’s behavioral attributes (bite rate, Strauss’ index, stationary behavior). Infection status of each coral fragment (infected, uninfected) and end of experiment status of each coral fragment (diseased, healed, resistant) were compared by experimental treatment (control, limited, unlimited) for each Symbiodiniaceae genotype (Breviolum, Cladocopium spp., Durusdinium) using a log-linear (three-way) contingency table analysis. These analyses were completed using JMP software.
Results
Disease Transmission by Direct Contact
Montastraea cavernosa fragments (fMCAV) were highly susceptible to contact-based transmission from the donor diseased Orbicella (ddOANN and ddOFAV) colonies with 3 of the fMCAV having disease lesions within the first 24 h in the summer of 2019. For the rest of the fMCAV in contact with ddOANN or ddOFAV, transmission took an average of 2.5 days. Donor diseased Montastraea cavernosa (ddMCAV) also transmitted disease to fMCAV but at a significantly slower rate than ddOANN or ddOFAV (t = 2.36, df = 1.7, p = 0.007) with an average rate to infection at 5.3 days. The overall rate of transmission in 2020 was 5.75 days slower than transmission in 2019, however, there were 3 fMCAV in 2020 that were showing signs of infection within the first 24 h. Similar to 2019, Orbicella in 2020 transferred disease significantly faster than ddMCAV (t = 2.26, df = 1.9, p = 0.022), with an average rate of infection 7.4 days sooner than ddMCAV. Additionally, there were 2 fMCAVs in contact with ddMCAV that never contracted the disease. For both years, there was a significant effect of donor diseased coral species on transmission rates with ddOANN and ddOFAV transferring disease 6.8 days faster than ddMCAV (t = 2.12, df = 1.16, p = 0.002). There was also a significant effect of year on the rate of transmission for the contact-based transmissions between our donor diseased colonies and fMCAV with quicker rates of transmission occurring in 2019 (t = 2.13, df = 1.15, p = 0.007).
Individual Variation in Butterflyfish Foraging Behavior
Observations of foureye butterflyfish feeding on donor diseased corals revealed consistent individual differences in their activity patterns, bite rates, and preferences for different coral tissue types. There was a significant effect of coral species on the number of bites taken by individuals (F = 9.11, df = 1.2, p < 0.001), with more bites occurring on ddOFAV than ddOANN or ddMCAV. Fish paired with ddOANN spent significantly more time stationary than when paired with ddOFAV or ddMCAV (F = 5.73, df = 1.2, p = 0.006). There was a significant difference between the number of bites taken on the different substrate and tissue types (F = 11.45, df = 4.28, p < 0.001), with more bites being taken off the tank than any coral tissue types (Supplementary Table 1). When evaluating bites on coral tissue types, there were significantly more bites being taken from healthy coral tissue than the other tissue types (F = 11.06, df = 3.22, p < 0.001). Bite rates from personality assessments ranged from 0.11 to 6.93 with an average rate of 3.85 bites/min. Strauss index ranged from –0.0025 to 0.4233 with an average index of 0.1426 showing no strong preference for diseased over healthy coral. The size of individual foureye butterflyfish (Chaetodontidae) ranged from 7.6 to 10.3 cm with a mean length of 9.22 cm. Size was not correlated with either bite rate or activity-stationary behavior but was positively correlated with the Strauss index (F = 12.45, df = 58, P = 0.0008).
Disease Transmission by Butterflyfish
During the butterflyfish disease transmission experiment, butterflyfish swam on average 60.34% of the total time, rested against the tank for 7.73% of the time, and were out of view for 31.92% of the time. The percent of time swimming ranged from 0.0 to 100%, time spent resting ranged from 0.0 to 87.0%, and out of view from 0.0 to 100%. For the limited treatment, there was an average of 46.57 bites on the healthy coral, 8.7 bites on the disease coral, and 145.13 bites elsewhere in the tank (other) (Figure 3). Bites on the healthy coral ranged from 0 to 266, bites on the diseased coral ranged from 0 to 90, and bites on other ranged from 0 to 436. In the limited treatment, 23.2% of all bites were taken on the healthy coral and 72.4% on other. For the unlimited treatment, there was an average of 17.23 bites on the healthy coral, 11.67 bites on the disease coral, and 67.83 bites on other. Bites on the healthy coral ranged from 0 to 114, bites on the diseased coral ranged from 0 to 85, and bites on other ranged from 0 to 332. In the unlimited treatment 17.8% of all bites were taken on the healthy coral, 12.1% on the diseased coral, and 70.1% on other.
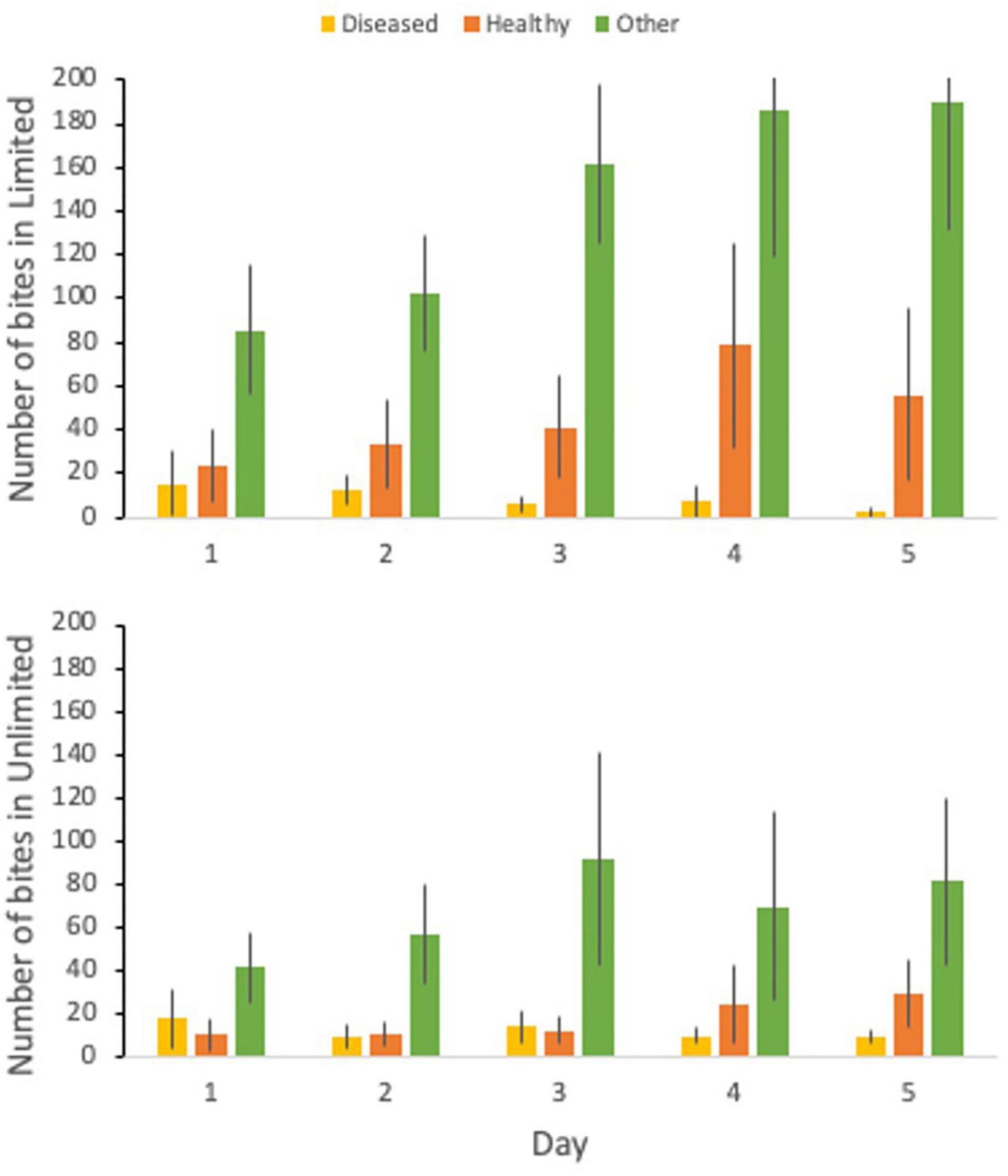
Figure 3. The number of bites taken by C. capistratus on the diseased coral (yellow), healthy coral (orange), and elsewhere in the tank (green) for the 5-day trial for the limited (top) and unlimited (bottom) treatments.
There was a significant difference between the total number of bites (t = 2.00, df = 57, p < 0.001) between the two treatments with the limited treatment having double the amount of bites than the unlimited treatment. There was also a significant difference between the bite rate (t = 2.00, df = 56, p < 0.001) between the limited and unlimited treatment. The number of bites on healthy (t = 2.03, df = 37, p = 0.052) or diseased (t = 2.00, df = 58, p = 0.54) coral between the two treatments was not significantly different. However, there was a significant difference between the number of bites taken elsewhere in the tank (t = 2.01, df = 54, p = 0.004) with the limited treatment having over two times the number of bites than the unlimited treatment.
Disease transmission increased over the 5 day transmission experiment and the rate of transmission was faster in the limited fish access treatments than in the control and unlimited fish access treatment (χ2 = 5.85, p = 0.054) (Figure 4). The control and unlimited treatments had similar rates of infection: the control treatment had 0% of the corals infected on day 3 but by day 5 50% of the corals were infected; the unlimited treatment had 14% of the corals infected by day 3 but held steady until day 5 where 43% of fragments became infected. Contrarily, by day 3 of the limited treatments, 50% of our healthy corals were infected and by day 5 84% of the coral fragments were diseased. Corals in the limited treatment were two and a half times more likely to pick up disease than in the control with a risk ratio of 0.4 (95% confidence interval, 0.16–1.03) or the unlimited treatment with a risk ratio of 2.5 (95% confidence interval, 1.02–6.13). There was an effect of year on the days to transmission (χ2 = 7.22, p = 0.007) but did not change the overall effect of treatment (χ2 = 5.34, p = 0.069) (Figure 4).
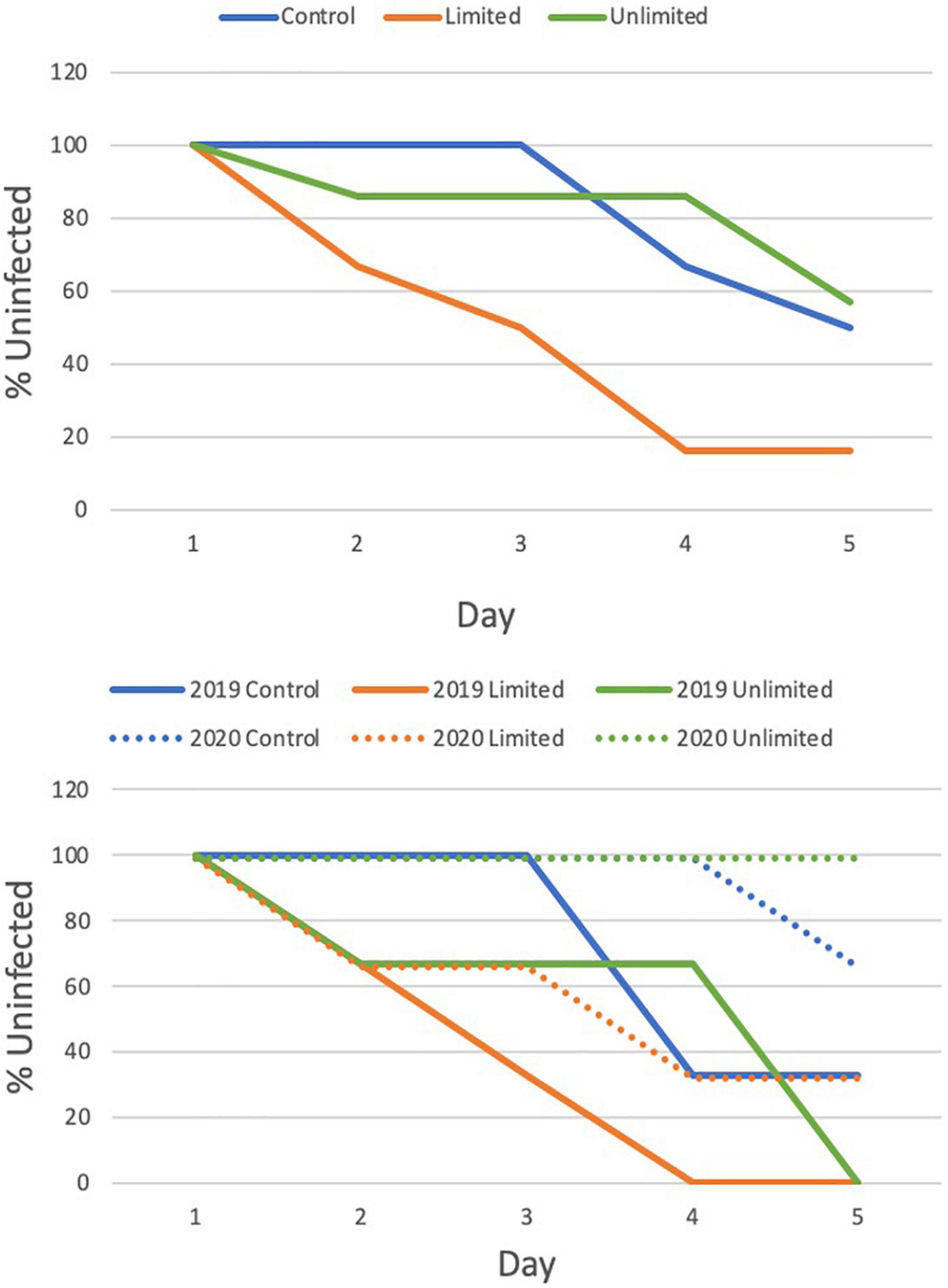
Figure 4. Cox Proportional Hazards test to evaluate the effect of treatment (top) and by year (bottom) on the transmission of Stony coral tissue loss disease to healthy M. cavernosa fragments.
A week after the transmission trials, there was 0% mortality for the fMCAV in 2019 and 2020, however, we did see significant variation in the amount of fMCAV that remained diseased between the 2 years (t = 2.07, df = 1.23, p < 0.001) (Supplementary Table 2). In 2019, 66.7% remained diseased after a week while 33.3% showed no signs of active disease and were considered healed (Supplementary Table 3). In 2020, only 4.0% remained diseased a week after the trial and 56.0% were healed. The other 40.0% never showed signs of disease throughout the trials or for the week after and were classified as resistant.
Symbiodiniaceae ITS2 Analysis
A total of 14,313,403 reads were generated after Illumina sequencing. The minimum number of reads per sample was 125,500 and the maximum number of reads per samples was 512,974. The median number of reads per sample was 265,344. After sequence quality control, paired-end read joining, and chimera removal a total of 10,112,769 high-quality sequences remained. In 2019, healthy Montastraea cavernosa fragments (fMCAV) were composed almost entirely of Durusdinium with only two fragments (2019-MCAV-82 and 2019-MCAV-85) having small concentrations of Cladocopium spp. (Figure 5A). In 2020, fMCAV were composed entirely of Cladocopium spp. Significant differences in Shannon diversity were observed in the samples from different years (H = 22.25; p < 0.001) (Figure 5B). There were also significant differences in beta diversity of Symbiodiniaceae spp. collected from coral fragments in years 2019 and 2020 (p = 0.001) (Figure 5C). Donor diseased corals had variable compositions of Symbiodiniaceae ranging from fully Cladocopium spp. or Breviolum to a combination of Breviolum, Cladocopium spp., and Durusdinium (Figure 5A).
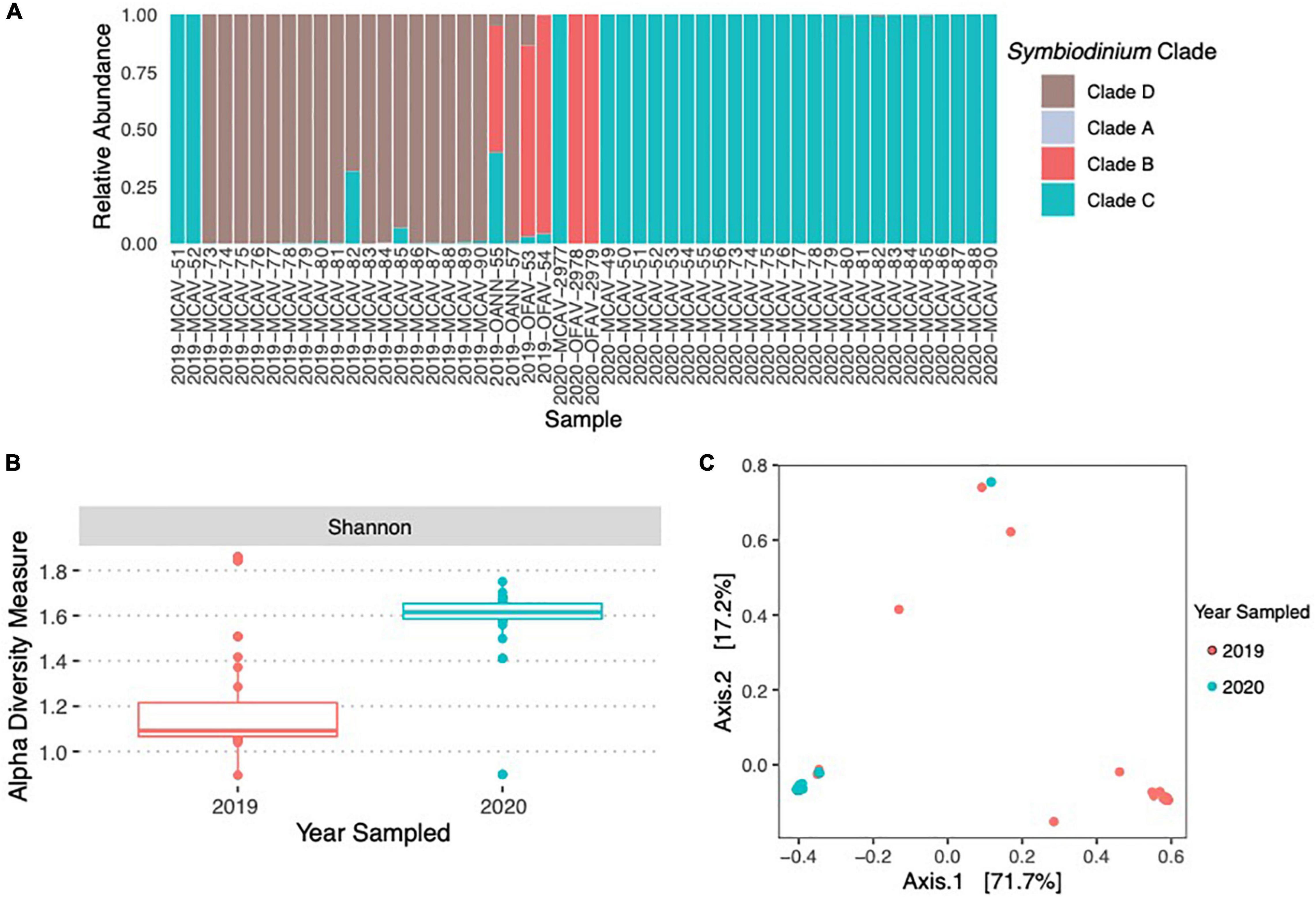
Figure 5. Relative abundance of Symbiodiniaceae species across the donor diseased corals and the coral fragments used in the experimental trials in 2019 and 2020 (A). There were significant differences in the alpha diversity of Symbiodiniaceae using Shannon Diversity Index between the 2 years of the experimental trials (B). There were also significant differences between the community compositions of Symbiodiniaceae between the samples used in 2019 and 2020 (C).
Since the coral fragments used in 2019 and those used in 2020 differed in the species of Symbiodiniaceae present, we also conducted a log-linear (three-way) contingency table analysis to see if species of Symbiodiniaceae (or year) interacted with experimental treatment in either the initial (infected or uninfected) or end status of infection (diseased, healed, or resistant). Species was far more important in infection status and end status than experimental treatment (Figure 6 and Table 1). However, there was a significant interaction between Symbiodiniaceae species and experimental treatment. Those corals in treatments where butterflyfish were allowed to feed directly on the disease corals (unlimited) tended to show increased frequency of healing over those fragments in either control conditions (no butterflyfish) or limited conditions (butterflyfish present but unable to feed directly on diseased corals) (Figure 6 and Table 1).
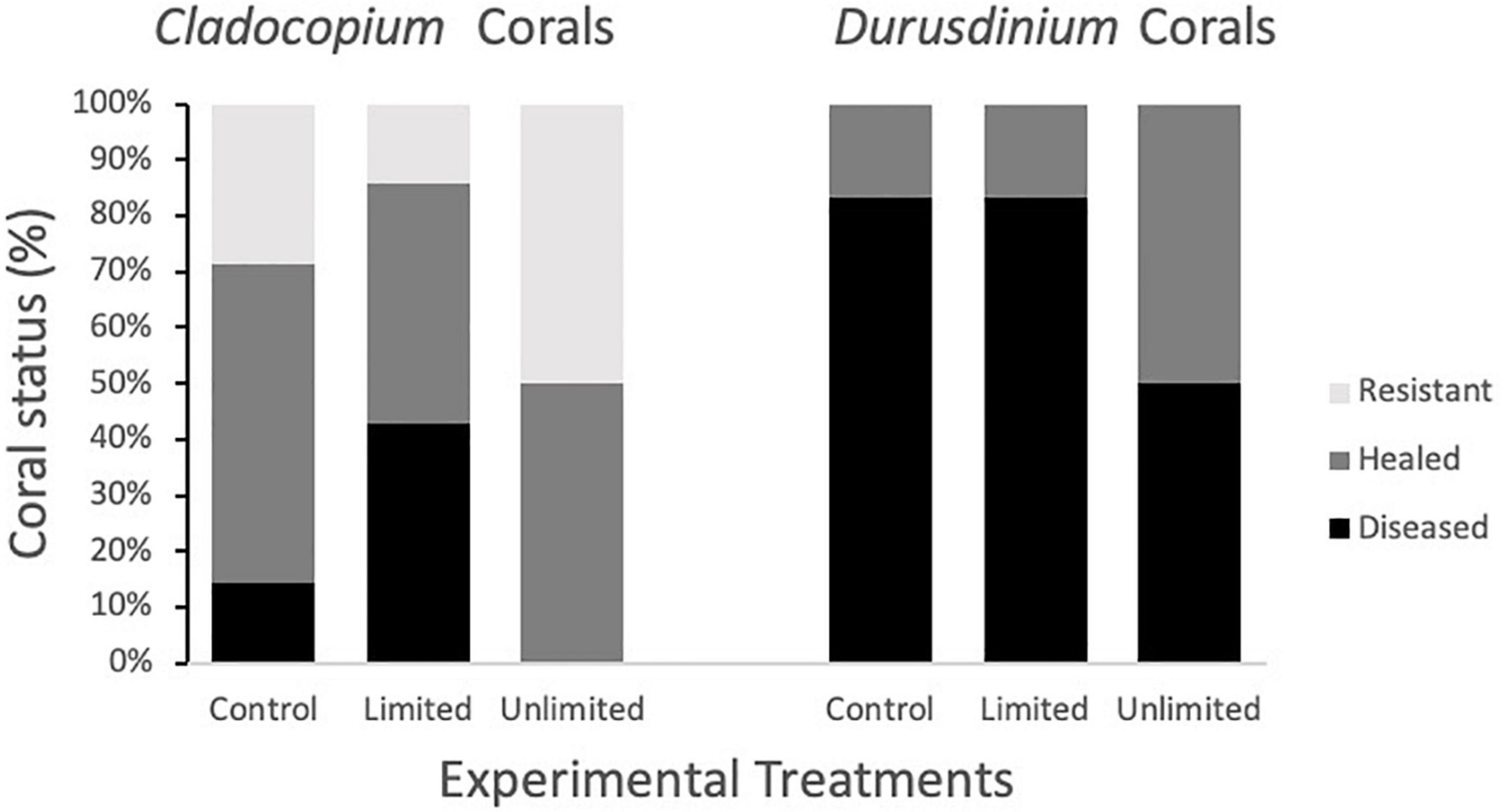
Figure 6. Coral fragment status at the end of the study as a function of experimental treatment (control, limited, unlimited) and presence of Cladocopium spp. vs. Durusdinium.
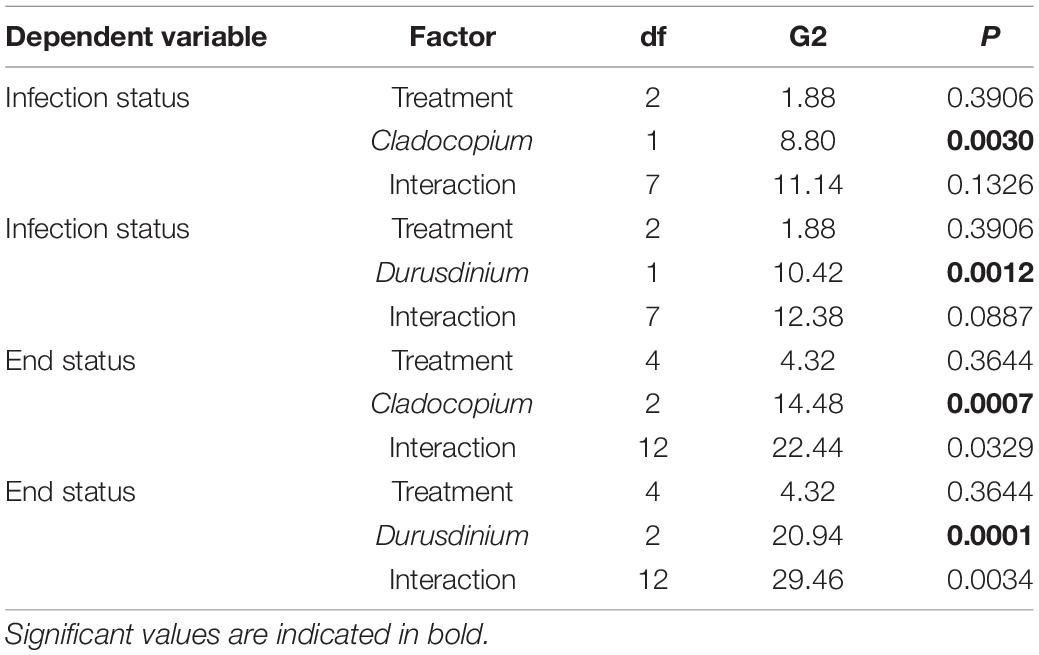
Table 1. Three way log-linear contingency table analysis of treatment (control, limited, unlimited) and species of Symbiodiniaceae (Cladocopium or Durusdinium present) on the infection status (infected, uninfected) or end of experiment status (diseased, healed, resistant).
Discussion
Our rate of contact-based transmission was quicker than has been observed previously (Aeby et al., 2019; Dobbelaere et al., 2020) with 27% of the M. cavernosa fragments (fMCAV) having disease lesions within the first 24 h. The remaining fMCAVs had variable rates to infection. M. cavernosa is known to be susceptible to SCLTD, but transmission rates have been widely variable (Aeby et al., 2019; Meiling et al., 2020). Previous transmission studies have found that donor diseased corals with acute lesions were more successful at transferring disease than corals with subacute lesions (Aeby et al., 2019). In our study, the M. cavernosa donor diseased corals (ddMCAV) had acute lesions and, therefore, were expected to transfer disease the quickest but the Orbicella donors with subacute lesions transferred disease 6.8 days faster. Although the lesion stage (subacute vs. acute) did not have the expected effect, previous studies have also found that interspecific transmission (MCAV to OFAV) is often quicker than intraspecific transmission (MCAV to MCAV) (Aeby et al., 2019) and offers support for our findings that Orbicella donor diseased corals transmitted the disease quickest to fMCAV than the ddMCAV to fMCAV. These results also build on the previous “diagnosis” that SCTLD is a generalist pathogen and can transfer between species easily (Walton et al., 2018; Muller and van Woesik, 2020).
Based on previous observations of butterflyfish foraging on diseased corals in the field (Aeby, 2002; Chong-Seng et al., 2011; Nicolet et al., 2018a; Noonan and Childress, 2020), we expected foureye butterflyfish to preferentially feed on the disease margins on the donor diseased corals and on the diseased corals used in the unlimited treatments. When observed with the donor diseased corals, there was a significant difference in the number of bites taken on the different tissue types, but most bites were taken from the healthy coral tissue instead of the diseased or coral skeleton. There could be more nutritional advantages to feeding on healthy coral tissue over other tissue types, but an analysis of nutritional content of the microbial community needs to be investigated further (Chong-Seng et al., 2011). There is also the possibility that the “healthy” tissues might have had disease present but were not showing visible lesions altering butterflyfishes selection of one coral tissue over another.
In the transmission experiment, butterflyfish showed no preference for either the healthy or diseased coral, and actually preferred to feed on the tank more than the coral. Foureye butterflyfish are facultative corallivores (Birkeland and Neudecker, 1981; Gore, 1984; Lasker, 1985; Neudecker, 1985; Pitts, 1991; Bellwood et al., 2010), however, we found it surprising how often the butterflyfish were feeding around the tank instead of on the corals themselves. We suspect that disease tissues sloughed off the corals, were no longer connected to the stinging defenses of the coral itself, making it the easiest food resource available. With this logic though, the sloughing diseased tissue that was barely attached to the coral should have been the next preferred food item, however, that was not what we observed. There is the potential that the bites around the tank could have been focused on other food types like algae or polychaetes but needs further investigation. Although the specific content around the tank has yet to be analyzed, this could be an important finding since there has been recent evidence that the bacteria, Rhodobacterales and Rhizobiales, present in SCTLD may be transmitting through the sediment (Rosales et al., 2020). If butterflyfish are selectively feeding on sloughed tissue that has settled on the substrate, they may be decreasing the number of free bacteria or pathogens available for the future infection of adjacent healthy hosts.
Time to infection was slower in the treatment trials than the contact-based transmission trials which follows previous observations that direct contact between corals is the quickest way for disease to be transmitted (Aeby et al., 2019; Muller and van Woesik, 2020). In general, there was no significant effect of treatment on the transmission of disease to the healthy fragments after 5 days, although we found that the limited treatment had the quickest rate of transmission compared to the unlimited or control treatments. These results suggest that the foureye butterflyfish is not serving as a primary vector for disease transmission, similar to the foureye butterflyfishes role with black band disease outbreaks (Aeby and Santavy, 2006).
Algal symbionts, like Symbiodiniaceae, have also been identified to be important in the functional responses of coral to environmental stressors (Baker et al., 2004; Iglesias-Prieto et al., 2004; Berkelmans and van Oppen, 2006; Jones et al., 2008), like coral disease (Correa and Baker, 2009; Rouzé et al., 2016). In our study, fMCAVs collected in 2019 were composed of mostly Durusdinium Symbiodiniaceae with only 2 fragments having a small composition of Cladocopium spp. as well. fMCAVs from 2020 were composed entirely of Cladocopium spp. These results may suggest that fragments in 2020 were fragmented from a different coral colony than fragments collected in 2019. There was a significant effect of year on the rate of transmission in both the contact-based transmission study and the butterflyfish treatments with disease transmitting significantly quicker in 2019 than in 2020. This may suggest that corals harboring Durusdinium may be more susceptible to disease than coral composed of Cladocopium spp., however, this study did not directly address this hypothesis. Although many studies support that the species of Symbiodiniaceae can have an effect on a coral’s fitness, there is variation in which species of Symbiodiniaceae is most beneficial to a coral based on the disease present (Correa and Baker, 2009; Rouzé et al., 2016). Our study suggests that corals that are harboring Durusdinium may be more susceptible to infection by SCTLD. Alternatively, the difference among years could be a result of inherent genetic resistance to SCTLD within the populations collected in 2020 that have been exposed to SCTLD in the field since 2014.
In addition to the differences in infection rates between the years, we also observed differences in recovery rates with corals from 2020 having quicker rates of recovery after initial SCTLD infection. We also observed an effect of treatment on the recovery rates of corals. For both Cladocopium spp. and Durusdinium, but especially for Durusdinium harboring corals, unlimited treatments showed more healing than for the other two treatments. Fifty percent of corals were healed in the unlimited treatment where 0% of the corals from the control treatment and 16% of corals from the limited treatment healed. This suggests that butterflyfish may be helping Durusdinium harboring corals to recover faster even though they are more susceptible to disease initially. This effect is not as significant for Cladocopium spp. corals because they are resistant to infection even without the presence of butterflyfish. Previous laboratory transmission studies have also found that many corals were able to ameliorate the disease in 2020 (Meiling et al., 2020) which was not consistently seen in studies conducted prior to 2020 (Aeby et al., 2019). Alternatively, it is possible that the pathogen infecting the corals collected in 2020 was less virulent than that experienced by the corals in 2019.
We believe there may be some underlying factors that could have contributed to the transmission of SCLTD that were outside of the parameters collected in this study. We do not know the genotypic history of our coral fragments that could have compromised our hosts (Lafferty and Holt, 2003; Lesser et al., 2007; Muller et al., 2008; Muller and van Woesik, 2012; Brandt et al., 2013) or increased resistance (Mullen et al., 2004; Gochfeld and Aeby, 2008; Shore-Maggio et al., 2015). The fecal excretion of corallivores has also been suggested to play a role in disease transmission (Ezzat et al., 2020; Grupstra et al., 2020). Sediment samples were collected in 2019 and will be used to estimate the composition of diseased or symbiotic materials that may be in foureye feces or free living in other biotic matter. In addition to the tests conducted, we wanted to run statistics to analyze whether butterflyfish or species of Symbiodiniaceae had a larger influence on the transmission of SCTLD in our laboratory experiment. Unfortunately, the lack of variation of Symbiodiniaceae species within a year and the small sample size limited our ability to run analyses that could determine rankings of importance/effect.
Symbiodiniaceae spp. may play a role in the susceptibility of corals to the transmission of SCTLD. However, while our sample sizes are small, we do also have some suggestive evidence that butterflyfish may not directly increase infection rates, but instead might be important in the process of disease recovery. This would be consistent with our original supposition that butterflyfish may be a net positive benefit to corals infected with SCTLD (Noonan and Childress, 2020). This study also identifies that behavioral differences among individuals plays an important role in foraging behavior and explains the range of results from butterflyfish increasing disease spread to ameliorating it (Aeby and Santavy, 2006; Cole et al., 2008; Chong-Seng et al., 2011; Raj et al., 2016; Nicolet et al., 2018a,b). We believe that butterflyfish may have had an influence on the recovery of some coral colonies from SCTLD across the middle Florida Keys and further studies involving coral diseases should consider corallivores as a significant contributor to coral recovery.
Data Availability Statement
The datasets presented in this study can be found in online repositories. The names of the repository/repositories and accession number(s) can be found below: NCBI (accession: PRJNA778185).
Ethics Statement
The animal study was reviewed and approved by the Clemson Universities Institutional Animal Care and Use Committee.
Author Contributions
KT and MC: conceptualization. KT and LO’C: methodology, investigation, resources, and writing—original draft preparation. KT, LO’C, and MC: formal analysis and supervision. KT, LO’C, and KM: data curation. KT, KM, LO’C, and MC: writing—review and editing. KT: funding acquisition. All authors have read and agreed to the published version of the manuscript.
Funding
This research was funded by the American Museum of Natural History Lerner-Gray Fund for Marine Research, the American Society of Ichthyologists and Herpetologists Raney Award, Clemson BioSci Grants in Aid of Research, the South Carolina Space Grant Consortium Kathryn D. Sullivan Earth and Marine Science Fellowship (#2014105), Sigma Xi Grants-in-Aid of Research, and Clemson University.
Conflict of Interest
The authors declare that the research was conducted in the absence of any commercial or financial relationships that could be construed as a potential conflict of interest.
Publisher’s Note
All claims expressed in this article are solely those of the authors and do not necessarily represent those of their affiliated organizations, or those of the publisher, the editors and the reviewers. Any product that may be evaluated in this article, or claim that may be made by its manufacturer, is not guaranteed or endorsed by the publisher.
Acknowledgments
This research was made possible by Florida Fish and Wildlife Conservation Commission (SAL-19-2168-SRP) and Clemson Universities Institutional Animal Care and Use Committee (AUP 2019-045). We thank to Kylie Smith and Kerry Maxwell from FWC and Karen Neely, Brian Walker, and Hunter Noren from Nova Southeastern for donating the coral for our transmission experiment; Cindy Lewis and Josh Farmer from the Keys Marine Laboratory in Layton, FL for their never-ending wisdom; and Emma Crowfoot for assisting with data processing and analysis.
Supplementary Material
The Supplementary Material for this article can be found online at: https://www.frontiersin.org/articles/10.3389/fmars.2022.800423/full#supplementary-material
Footnotes
- ^ https://support.illumina.com/content/dam/illumina-support/documents/documentation/system_documentation/miseq/miseq-denature-dilute-librariesguide-15039740-10.pdf
References
Aeby, G. (2002). Trade-offs for the butterflyfish, Chaetodon multicinctus, when feeding on coral prey infected with trematode metacercariae. Behav. Ecol. Sociobiol. 52, 158–165. doi: 10.1007/s00265-002-0490-2
Aeby, G. S., and Santavy, D. L. (2006). Factors affecting susceptibility of the coral Montastraea faveolata to black-band disease. Mar. Ecol. Prog. Ser. 318, 103–110. doi: 10.3354/meps318103
Aeby, G. S., Ushijima, B., Campbell, J. E., Jones, S., Williams, G. J., Meyer, J. L., et al. (2019). Pathogenesis of a tissue loss disease affecting multiple species of corals along the Florida Reef Tract. Front. Mar. Sci. 6:678. doi: 10.3389/fmars.2019.00678
Altizer, S., Ostfeld, R. S., Johnson, P. T., Kutz, S., and Harvell, C. D. (2013). Climate change and infectious diseases: from evidence to a predictive framework. Science 341, 514–519. doi: 10.1126/science.1239401
Alvarez-Filip, L., Estrada-Saldívar, N., Pérez-Cervantes, E., Molina-Hernández, A., and González-Barrios, F. J. (2019). A rapid spread of the stony coral tissue loss disease outbreak in the Mexican Caribbean. PeerJ 7:e8069. doi: 10.7717/peerj.8069
Aronson, R. B., and Precht, W. F. (2001). “White-band disease and the changing face of Caribbean coral reefs,” in The Ecology and Etiology of Newly Emerging Marine Diseases, ed. J. W. Porter (Dordrecht: Springer), 25–38. doi: 10.1007/978-94-017-3284-0-2
Baker, A. C., Starger, C. J., McClanahan, T. R., and Glynn, P. W. (2004). Corals’ adaptive response to climate change. Nature 430:741. doi: 10.1038/430741a
Bateman, K. S., Feist, S. W., Bignell, J. P., Bass, D., and Stentiford, G. D. (2020). “Marine pathogen diversity and disease outcomes,” in Marine Disease Ecology, eds D. C. Behringer, K. D. Lafferty, and B. R. Silliman (Oxford: Oxford University Press), 3–44.
Bellwood, D. R., Klanten, S., Cowman, P. F., Pratchett, M. S., Konow, N., and Van Herwerden, L. (2010). Evolutionary history of the butterflyfishes (f: Chaetodontidae) and the rise of coral feeding fishes. J. Evol. Biol. 23, 335–349. doi: 10.1111/j.1420-9101.2009.01904.x
Benjamini, Y., and Hochberg, Y. (1995). Controlling the false discovery rate: a practical and powerful approach to multiple testing. J. R. Stat. Soc. Ser. B 57, 289–300. doi: 10.1111/j.2517-6161.1995.tb02031.x
Berkelmans, R., and van Oppen, M. J. H. (2006). The role of zooxanthellae in the thermal tolerance of corals: a ‘nugget of hope’ for coral reefs in an era of climate change. Proc. R. Soc. Lond. B Biol. Sci. 273, 2305–2312. doi: 10.1098/rspb.2006.3567
Birkeland, C., and Neudecker, S. (1981). Foraging behavior of two Caribbean chaetodontids: Chaetodon capistratus and C. aculeatus. Copeia 198, 169–178.
Bolyen, E., Rideout, J. R., Dillon, M. R., Bokulich, N. A., Abnet, C., Al-Ghalith, G. A., et al. (2018). QIIME 2: reproducible, interactive, scalable, and extensible microbiome data science. PeerJ Prepr. 6:e27295v2. doi: 10.7287/peerj.preprints.27295v2
Brandt, M. E., and McManus, J. W. (2009). Disease incidence is related to bleaching extent in reef-building corals. Ecology 90, 2859–2867. doi: 10.1890/08-0445.1
Brandt, M. E., Smith, T. B., Correa, A. M. S., and Vega-Thurber, R. (2013). Disturbance driven colony fragmentation as a driver of a coral disease outbreak. PLoS One 8:e57164. doi: 10.1371/journal.pone.0057164
Bray, J. R., and Curtis, J. T. (1957). An ordination of the upland forest communities of southern Wisconsin. Ecol. Monogr. 27, 326–349. doi: 10.2307/1942268
Bruckner, A. W., and Bruckner, R. J. (2016). “Mechanical lesions and corallivory,” in Diseases of Coral, eds C. M. Woodley, C. A. Downs, A. W. Bruckner, J. W. Porter, and S. B. Galloway (Hoboken, NJ: John Wiley & Sons), 242–265. doi: 10.1002/9781118828502.ch17
Bruckner, A. W., Bruckner, R. J., and Williams, E. H. (1997). Spread of a black band disease epizootic through the coral reef system in St. Ann’s Bay, Jamaica. Bull. Mar. Sci. 61, 919–928.
Burge, C. A., and Hershberger, P. K. (2020). “Climate change can drive marine disease,” in Marine Disease Ecology, eds D. C. Behringer, B. R. Silliman, and K. D. Lafferty (Oxford: Oxford University Press), 83–94. doi: 10.1093/oso/9780198821632.003.0005
Callahan, B. J., McMurdie, P. J., Rosen, M. J., Han, A. W., Johnson, A. J. A., and Holmes, S. P. (2016). DADA2: high-resolution sample inference from Illumina amplicon data. Nat. Methods 13, 581–583. doi: 10.1038/nmeth.3869
Chong-Seng, K. M., Cole, A. J., Pratchett, M. S., and Willis, B. L. (2011). Selective feeding by coral reef fishes on coral lesions associated with brown band and black band disease. Coral Reefs 30, 473–481. doi: 10.1007/s00338-010-0707-1
Cole, A. J., Pratchett, M. S., and Jones, G. P. (2008). Diversity and functional importance of coral-feeding fishes on tropical coral reefs. Fish Fish. 9, 286–307. doi: 10.1098/rspb.2017.0906
Cole, A. J., Seng, K. M. C., Pratchett, M. S., and Jones, G. P. (2009). Coral-feeding fishes slow progression of black-band disease. Coral Reefs 28:965. doi: 10.1007/s00338-009-0519-3
Correa, A. M. S., and Baker, A. C. (2009). Understanding diversity in coral-algal symbiosis: a cluster-based approach to interpreting fine-scale genetic variation in the genus Symbiodiniaceae. Coral Reefs 28, 81–93. doi: 10.1007/s00338-008-0456-6
Dobbelaere, T., Muller, E. M., Gramer, L. J., Holstein, D. M., and Hanert, E. (2020). Coupled epidemio-hydrodynamic modeling to understand the spread of a deadly coral disease in Florida. Front. Mar. Sci. 7:1016. doi: 10.3389/fmars.2020.591881
Ezzat, L., Lamy, T., Maher, R. L., Munsterman, K. S., Landfield, K. M., Schmeltzer, E. R., et al. (2020). Parrotfish predation drives distinct microbial communities in reef-building corals. Anim. Microb. 2:5. doi: 10.1186/s42523-020-0024-0
Florida Keys National Marine Sanctuary (2018). Florida Reef Tract Corals Disease Outbreak: Disease. Available online at: https://floridakeys.noaa.gov/coraldisease/disease.html (accessed March 8, 2019).
Gardner, T. A. I, Cote, M., Gill, J. A., Grant, A., and Watkinson, A. R. (2005). Hurricanes and caribbean coral reefs: impacts, recovery patterns, and role in long-term decline. Ecology 86, 174–184. doi: 10.1890/04-0141
Gochfeld, D., and Aeby, G. (2008). Antibacterial chemical defenses in Hawaiian corals provide possible protection from disease. Mar. Ecol. Prog. Ser. 362, 119–128. doi: 10.3354/meps07418
Gore, M. A. (1984). Factors affecting the feeding behavior of a coral reef fish, Chaetodon capistratus. Bull. Mar. Sci. 35, 211–220.
Green, E. P., and Bruckner, A. W. (2000). The significance of coral disease epizootiology for coral reef conservation. Biol. Conserv. 96, 347–361. doi: 10.1016/s0006-3207(00)00073-2
Grupstra, C., Rabbitt, K. M., Howe-Kerr, L. I., and Correa, A. (2020). Fish predation on corals promotes the dispersal of coral symbionts. Anim Microbiome 3:25. doi: 10.1186/s42523-021-00086-4
Harvell, C. D., Jordan-Dahlgren, E., Merkel, S., and Rosenberg, E. (2007). Coral disease, environmental drivers, and the balance between coral and microbial associates. Oceanography 20, 172–195. doi: 10.5670/oceanog.2007.91
Harvell, C. D., Mitchell, C. E., Ward, J. R., Altizer, S., Dobson, A. P., Ostfeld, R. S., et al. (2002). Climate warming and disease risks for terrestrial and marine biota. Science 296, 2158–2162. doi: 10.1126/science.1063699
Hayes, R. L., and Goreau, N. I. (1998). The significance of emerging diseases in the tropical coral reef ecosystem. Rev. Biol. Trop. 46(Suppl. 5), 173–185.
Hume, B. C. C., Smith, E. G., Ziegler, M., Warrington, H. J. M., Burt, J. A., LaJeunesse, T. C., et al. (2019). SymPortal: a novel analytical framework and platform for coral algal symbiont next-generation sequencing ITS2 profiling. Mol. Ecol. Resour. 19, 1063–1080. doi: 10.1111/1755-0998.13004
Hume, B. C. C., Ziegler, M., Poulain, J., Pochon, X., Romac, S., Boissin, E., et al. (2018). An improved primer set and amplification protocol with increased specificity and sensitivity targeting the Symbiodiniaceae ITS2 region. PeerJ 6:e4816. doi: 10.7717/peerj.4816
Iglesias-Prieto, R., Beltran, V. H., LaJeunesse, T. C., Reyes-Bonilla, H., and Thome, P. E. (2004). Different algal symbionts explain the vertical distribution of dominant reef corals in the eastern Pacific. Proc. R. Soc. Lond. B Biol. Sci. 271, 1757–1763. doi: 10.1098/rspb.2004.2757
Jones, A. M., Berkelmans, R., van Oppen, M. J. H., Mieog, J. C., and Sinclair, W. (2008). A community change in the algal endosymbionts of a scleractinian coral following a natural bleaching event: field evidence of acclimatization. Proc. R. Soc. Lond. B Biol. Sci. 275, 1359–1365. doi: 10.1098/rspb.2008.0069
Kozich, J. J., Westcott, S. L., Baxter, N. T., Highlander, S. K., and Schloss, P. D. (2013). Development of a dual-index sequencing strategy and curation pipeline for analyzing amplicon sequence data on the miseq illumina sequencing platform. Appl. Environ. Microbiol. 79, 5112–5120. doi: 10.1128/AEM.01043-13
Lafferty, K. D., and Holt, R. D. (2003). How should environmental stress affect the population dynamics of disease? Ecol. Lett. 6, 654–664. doi: 10.1046/j.1461-0248.2003.00480.x
Landsberg, J. H., Kiryu, Y., Wilson, P., Perry, N., Waters, Y., and Huebner, L. (2018). “Disease lab analysis updates,” in Powerpoint Presentation Presented to the Florida Coral Disease Advisory Counsil in December 2018.
Lasker, H. R. (1985). Prey preferences and browsing pressure of the butterflyfish Chaetodon capistratus on Caribbean gorgonians. Mar. Ecol. Progr. Ser. Oldendorf 21, 213–220. doi: 10.3354/meps021213
Lesser, M. P., Bythell, J. C., Gates, R. D., Johnstone, R. W., and Hoegh-Guldberg, O. (2007). Are infectious diseases really killing corals? Alternative interpretations of the experimental and ecological data. J. Exp. Mar. Bio. Ecol. 346, 36–44. doi: 10.1016/j.jembe.2007.02.015
Lunz, K., Landsberg, J., Kiryu, Y., and Brinkhuis, V. (2017). Investigation of the Coral Disease Outbreak Affecting Scleractinian Corals of the Florida Reef Tract. Miami, FL: Florida DEP, 19.
Maher, R. L., Rice, M. M., McMinds, R., Burkepile, D. E., and Thurber, R. V. (2019). Multiple stressors interact primarily through antagonism to drive changes in the coral microbiome. Sci. Rep. 9:6834. doi: 10.1038/s41598-019-43274-8
McMurdie, P. J., and Holmes, S. (2013). Phyloseq: an r package for reproducible interactive analysis and graphics of microbiome census data. PLoS One 8:e61217. doi: 10.1371/journal.pone.0061217
Meiling, S., Smith, T. B., Muller, E., and Brandt, M. E. (2020). 3D photogrammetry reveals dynamics of stony coral tissue loss disease (SCTLD) lesion progression across a thermal stress event. Front. Mar. Sci. 7:1128. doi: 10.3389/fmars.2020.597643
Miller, J., Muller, E., Rogers, C., Waara, R., Atkinson, A., Whelan, K. R. T., et al. (2009). Coral disease following massive bleaching in 2005 causes 60% decline in coral cover on reefs in the US Virgin Islands. Coral Reefs 28:925. doi: 10.1007/s00338-009-0531-7
Mullen, K. M., Peters, E. C., and Harvell, C. D. (2004). “Coral resistance to disease,” in Coral Health and Disease, eds E. Rosenberg and Y. Loya (Berlin: Springer).
Muller, E. M., and van Woesik, R. (2012). Caribbean coral diseases: primary transmission or secondary infection? Glob. Chang. Biol. 18, 3529–3535. doi: 10.1111/gcb.12019
Muller, E. M., Rogers, C. S., Spitzack, A. S., and Van Woesik, R. (2008). Bleaching increases likelihood of disease on Acropora palmata (Lamarck) in Hawksnest Bay, St John, US Virgin Islands. Coral Reefs 27, 191–195. doi: 10.1007/s00338-007-0310-2
Muller, E., and van Woesik, R. (2020). Spatial epidemiology of the stony-coral-tissueloss- disease in Florida. Front. Mar. Sci. 7:163. doi: 10.3389/fmars.2020.00163
Mumby, P. J., and Steneck, R. S. (2011). “The resilience of coral reefs and its implications for reef management,” in Coral Reefs: An Ecosystem in Transition, eds Z. Dubinsky and N. Stambler (New York, NY: Springer Science+Business Media).
Neudecker, S. (1985). “Foraging patterns of chaetodontid and pomacanthid fishes at St. Croix (US Virgin Islands),” in Proceedings of the 5th Int. Coral Reef Congr, Vol. 5, Tahiti, PA, 415–420.
Nicolet, K. J., Chong-Seng, K. M., Pratchett, M. S., Willis, B. L., and Hoogenboom, M. O. (2018a). Predation scars may influence host susceptibility to pathogens: evaluating the role of corallivores as vectors of coral disease. Sci. Rep. 8:5258. doi: 10.1038/s41598-018-23361-y
Nicolet, K. J., Hoogenboom, M. O., Pratchett, M. S., and Willis, B. L. (2018b). Selective feeding by corallivorous fishes neither promotes nor reduces progression rates of black band disease. Mar. Ecol. Progr. Ser. 594, 95–106. doi: 10.3354/meps12525
Noonan, K. R., and Childress, M. J. (2020). Association of butterflyfishes and stony coral tissue loss disease in the Florida Keys. Coral Reefs 39, 1581–1590. doi: 10.1007/s00338-020-01986-8
Paul, V. J., Ushijima, B., and Aeby, G. (2019). Studies of the Ecology and Microbiology of Florida’s Coral Tissue Loss Diseases. Miami, FL: Florida DEP.
Pitts, P. A. (1991). Comparative use of food and space by three Bahamian butterflyfishes. Bull. Mar. Sci. 48, 749–756.
Pratchett, M. S., Berumen, M. L., and Kapoor, B. G. (Eds.) (2013). Biology of Butterflyfishes. Boca Raton, FL: CRC Press.
Pratchett, M. S., Wilson, S. K., and Baird, A. H. (2006). Declines in the abundance of Chaetodon butterflyfishes following extensive coral depletion. J. Fish Biol. 69, 1269–1280. doi: 10.1111/j.1095-8649.2006.01161.x
Precht, W. F., Gintert, B. E., Robbart, M. L., Fura, R., and van Woesik, R. (2016). Unprecedented disease-related coral mortality in southeastern Florida. Sci. Rep. 6:31374. doi: 10.1038/srep31374
Raj, K. D., Aeby, G. S., Mathews, G., Bharath, M. S., Rajesh, S., Laju, R. L., et al. (2016). “Patterns in the abundance of fish and snail corallivores associated with an outbreak of acute tissue loss disease on the reefs of Vaan Island in the Gulf of Mannar, India,” in Proceedings of the 13th international coral reef symposium at Honolulu, Hawaii, from 19th–24th June, Honolulu, HI.
Raymundo, L. J., Halford, A. R., Maypa, A. P., and Kerr, A. M. (2009). Functionally diverse reef-fish communities ameliorate coral disease. Proc. Natl. Acad. Sci. U.S.A. 106, 17067–17070. doi: 10.1073/pnas.0900365106
Rosales, S. M., Clark, A. S., Huebner, L. K., Ruzicka, R. R., and Muller, E. M. (2020). Rhodobacterales and Rhizobiales are associated with stony coral tissue loss disease and its suspected sources of transmission. Front. Microbiol. 11:681. doi: 10.3389/fmicb.2020.00681
Rouzé, H., Lecellier, G., Saulnier, D., and Berteaux-Lecellier, V. (2016). Symbiodiniaceae clades A and D differentially predispose Acropora cytherea to disease and Vibrio spp. colonization. Ecol. Evol. 6, 560–572. doi: 10.1002/ece3.1895
Shannon, C. E. (1948). A mathematical theory of communication. Bell Sys. Tech. J. 27, 379–423. doi: 10.1002/j.1538-7305.1948.tb01338.x
Sharp, W. C., Shea, C. P., Maxwell, K. E., Muller, E. M., and Hunt, J. H. (2020). Evaluating the small-scale epidemiology of the stony-coral-tissue-loss-disease in the middle Florida Keys. PLos One 15:e0241871. doi: 10.1371/journal.pone.0241871
Shore-Maggio, A., Runyon, C. M., Ushijima, B., Aeby, G. S., and Callahan, S. M. (2015). Differences in bacterial community structure in two color morphs of the Hawaiian reef coral Montipora capitata. Appl. Environ. Microbiol. 81, 7312–7318. doi: 10.1128/AEM.01935-15
Strauss, R. E. (1979). Reliability estimates for Ivlev’s electivity index, the forage ratio, and a proposed linear index of food selection. Trans. Am. Fish. Soc. 108, 344–352.
Ushijima, B., Meyer, J. L., Thompson, S., Pitts, K., Marusich, M. F., Tittl, J., et al. (2020). Disease diagnostics and potential coinfections by Vibrio coralliilyticus during an ongoing coral disease outbreak in Florida. Front. Microbiol. 11:569354. doi: 10.3389/fmicb.2020.569354
Vega Thurber, R. L., Burkepile, D. E., Fuchs, C., Shantz, A. A., McMinds, R., and Zaneveld, J. R. (2014). Chronic nutrient enrichment increases prevalence and severity of coral disease and bleaching. Glob. Change Biol. 20, 544–554. doi: 10.1111/gcb.12450
Walton, C. J., Hayes, N. K., and Gilliam, D. S. (2018). Impacts of a regional, multi-year, multi-species coral disease outbreak in Southeast Florida. Front. Mar. Sci. 5:323. doi: 10.3389/fmars.2018.00323
Weil, E., Hernández-Delgado, E., Gonzalez, M., Williams, S., Suleimán-Ramos, S., Figuerola, M., et al. (2019). Spread of the new coral disease “SCTLD” into the Caribbean: implications for Puerto Rico. Reef Encount. 34, 38–43.
Keywords: coral disease, SCTLD, transmission, butterflyfish, Symbiodiniaceae, coral recovery
Citation: Titus K, O’Connell L, Matthee K and Childress M (2022) The Influence of Foureye Butterflyfish (Chaetodon capistratus) and Symbiodiniaceae on the Transmission of Stony Coral Tissue Loss Disease. Front. Mar. Sci. 9:800423. doi: 10.3389/fmars.2022.800423
Received: 23 October 2021; Accepted: 09 February 2022;
Published: 21 March 2022.
Edited by:
William F. Precht, Dial Cordy and Associates, Inc., United StatesReviewed by:
Adán Guillermo Jordán-Garza, Universidad Veracruzana, MexicoGretchen Goodbody-Gringley, Central Caribbean Marine Institute, Cayman Islands
Copyright © 2022 Titus, O’Connell, Matthee and Childress. This is an open-access article distributed under the terms of the Creative Commons Attribution License (CC BY). The use, distribution or reproduction in other forums is permitted, provided the original author(s) and the copyright owner(s) are credited and that the original publication in this journal is cited, in accordance with accepted academic practice. No use, distribution or reproduction is permitted which does not comply with these terms.
*Correspondence: Kara Titus, a3JuNEByaWNlLmVkdQ==