- 1Biodiversity Research Center, Academia Sinica, Taipei, Taiwan
- 2Biodiversity Program, Taiwan International Graduate Program, Biodiversity Research Center, Academia Sinica and National Taiwan Normal University, Taipei, Taiwan
- 3Institute of Marine Environment and Resources, Vietnam Academy of Science and Technology, Haiphong, Vietnam
Examining the bacterial communities of offspring is key to understanding the establishment of coral-bacteria associations. Although high sensitivity to the environment is expected, previous studies have only examined bacterial communities of coral offspring in ex situ (laboratory) environments, not in in situ (field) environments. Here, we examined and compared the effect of ex situ and in situ environments on bacterial communities of newly released offspring (eggs and larvae) and their maternal colonies in two phylogenetically distant coral species with different reproductive modes: Dipsastraea speciosa (Scleractinia; spawner) and Heliopora coerulea (Octocorallia; brooder). Our results demonstrated that the spawning environments do not affect the bacterial composition in maternal colonies, but influence that of the offspring (eggs of D. speciosa and larvae of H. coerulea). Dominant bacterial operational taxonomic units (OTUs) varied between in situ and ex situ environments in the eggs of D. speciosa. The composition of bacterial communities among larvae of H. coerulea samples was more diverse in in situ environments than in ex situ environments. This study provides the first information on in situ bacterial communities in coral eggs and larvae and highlights their sensitivities to the local environment. Future studies must take into consideration the influence of ex situ environments on bacterial communities in coral offspring.
Introduction
Resident bacterial communities are known to profoundly influence the physiology and health of both flora and fauna (Thompson et al., 2015; Wang et al., 2017; Douglas, 2019; van Oppen and Blackall, 2019; Apprill, 2020). Likewise, in reef-building corals, recent studies have found that coral-associated bacteria play important functional roles, such as protection against pathogens and the provision of nutrients (carbon, nitrogen, and sulfur). For example, diazotrophs that inhabit within the coral tissue or skeleton (Rohwer et al., 2002; Radecker et al., 2015) provide fixed nitrogen products that are crucially limiting resources in oligotrophic coral reef waters, sustaining their high productivity (Lesser et al., 2007).
Despite the importance of coral-bacteria associations, we know very little about the mechanisms relating to the establishment. Studying microbial communities in coral offspring is key to understanding the establishment of the coral-microbe association, but nevertheless, the majority of knowledge comes from studies on coral endosymbiotic algae (Symbiodiniaceae) in coral offspring, closely related to the reproductive mode of coral host. Most brooding corals release larvae with endosymbiotic algae, whereas spawning corals release eggs without those (Bright and Bulgheresi, 2010; Hartmann et al., 2017). However, such information has not been studied well in coral-bacteria associations.
Previous studies have examined coral-associated bacteria in coral offspring (eggs and larvae) in ex situ (laboratory) environments, not in in situ (field) environments (Supplementary Table 1). This difference could lead to there being a bias of real bacterial communities in coral offspring as a few studies have demonstrated that environmental conditions are an important factor that affects the bacterial communities in coral offspring (Lema et al., 2016; Leite et al., 2017; Zhou et al., 2017; Bernasconi et al., 2019; Damjanovic et al., 2020a). In adult corals, there was a study recently published showing the difference in bacterial communities between ex situ and in situ environments (Damjanovic et al., 2020b). However, no such ex situ and in situ comparisons were studied for the bacterial communities in coral offspring, in spite of that bacteria and coral offspring are sensitive to the environment (Chan et al., 2019; Epstein et al., 2019).
Here we examined the effect of in situ and ex situ environments on bacterial communities of coral offspring and maternal corals in two coral species with different reproductive modes; Dipsastraea speciosa (Scleractinia; spawner) and Heliopora coerulea (Octocorallia; brooder). Our results indicate that bacterial communities vary between in situ and ex situ environments in coral offspring, but not in maternal corals. The results highlight the sensitivity of bacterial communities in coral offspring to the environment and bring to light a potential bias introduced by customary ex situ sampling methods that may affect studies on bacteria associated with coral offspring.
Materials and Methods
We examined bacterial communities of offspring (eggs or larvae) and maternal corals in two coral species with different reproductive modes, D. speciosa (spawner) and H. coerulea (brooder, branching morphotype; Yasuda et al., 2014). D. speciosa is hermaphroditic spawner that releases buoyant egg-sperm bundles into the water column for external fertilization during annual spawning events (Lin and Nozawa, 2017). H. coerulea is a gonochoric brooder, which releases planula larvae in early summer, that is neutral to negative buoyancy (Babcock, 1990; Harii et al., 2002). Larvae of H. coerulea are brooded for up to 2 weeks inside polyps and then moved to the surface of maternal colonies for “surface brooding” for ≈4 days before detaching for dispersal (Babcock, 1990; Harii and Kayanne, 2003). Two sampling methods were employed in this study; ex situ (laboratory) sampling and in situ (field) sampling (Table 1). Sampling was conducted at Lyudao (Green Island), Taiwan in April and May 2018 (Figure 1A).
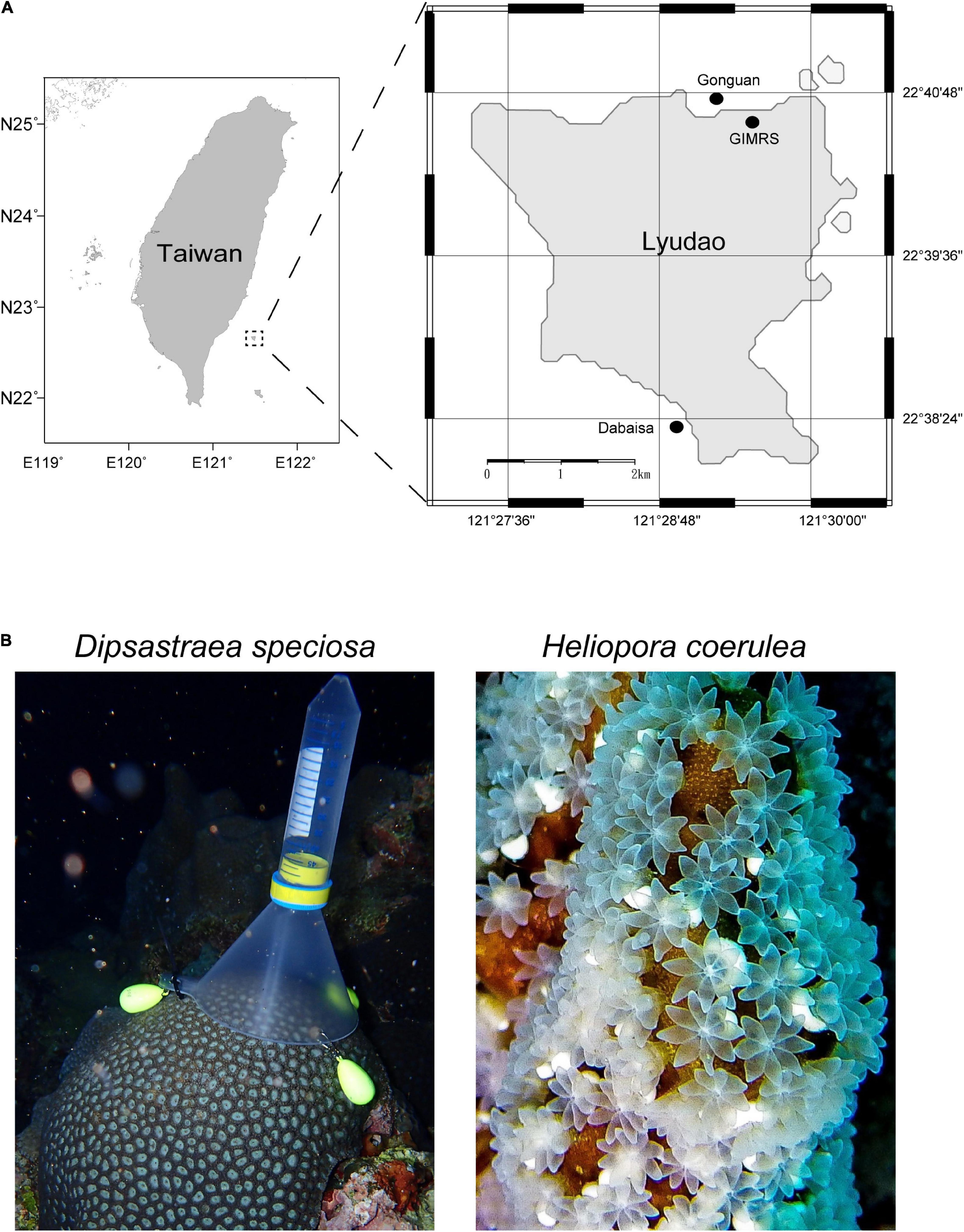
Figure 1. (A) Map of sampling sites, Gongguan and Dabaisa in Lyudao, Taiwan. GIMRS = Green Island Marine Research Station. (B) Photographs for in situ sampling for Dipsastraea speciosa (left column) and Heliopora coerulea (right column).
Ex situ Sampling
Seawater used in the experiment was collected from Gongguan and stored in a 1,500-L tank at the beginning of the experiment (April 26; 4 days before the full moon). The seawater stock tank was placed in the hallway of the Green Island Marine Research Station (GIMRS) in the shade, and seawater was aerated with air stones. Seawater from the stock tank was used for coral rearing for the ex situ sampling.
For D. speciosa, one fragment (≈10 cm in diameter) with mature pigmented eggs (violet gray) was collected from five colonies using a hammer and a chisel at 3–8 m depth in Gongguan, Lyudao on April 27 (3 days before the full moon; Figure 1A). The fragments were kept in a Gongguan harbor (≈3 m depth) for 1 day to allow for recovery and then transferred to GIMRS (Figure 1A). The five fragments were placed separately in 20-L rearing tanks with aerated filtered seawater (100 μm) in the laboratory. Seawater temperature was maintained at ≈26°C by an air-conditioner. The rearing tanks were kept under fluorescent light (6,000 lux) during daytime (6:00–18:00) and kept in darkness during nighttime (18:00–6:00) to induce spawning (Lin et al., 2021). A quarter of the seawater in the rearing tank was changed every 3 days with filtered seawater (100 μm) from the seawater stock tank.
The spawning of D. speciosa fragments was monitored daily by using a plastic cup placed upside down on the top of each fragment. When fragments spawned at ≈22:00, egg-sperm bundles were released from polyps, floated to the water surface, and gradually disintegrated into eggs and sperm. As eggs have strong buoyancy, eggs were trapped inside the reversed cup, while sperm dispersed into the water. Spawning of D. speciosa occurred on May 1 and 2 [1 and 2 days after the full moon (AFM)] and spawned eggs (≈200 eggs) were collected at 7:00 the following morning (≈9 h after spawning). Egg samples from 2 fragments were collected on the first day (sample ID: ds2l and ds4l) and others on the second day. After spawning, a small tissue sample (≈1 cm in diameter) was collected from each maternal coral (fragment).
For H. coerulea, one branch (2 cm in diameter, 5–8 cm in length) that had many brooded larvae partially emerge from polyps on the branch surface was collected from each of the five female colonies using a hammer and a chisel at 5–8 m depth at Daibaisa, Lyudao on May 1 (1 day AFM; Figure 1A). Each branch was kept in a zip-lock bag with seawater, transferred to GIMRS, and placed in a 3-L rearing tank with aerated filtered seawater (100 μm). Seawater from the 1,500-L seawater tank was used in the rearing tank, and filtered seawater was changed every 1–2 days. During the rearing period, larvae of H. coerulea gradually appeared, detached from polyps, and drifted into the water column (Harii and Kayanne, 2003). Ten to fifteen larvae from each branch that were released on May 5 (5 days AFM) were collected in the rearing tank by a dropper. After collecting larvae, a small tissue sample (≈1 cm in diameter) was collected from each branch using a nipper as a sample of the maternal colony. A 1-L seawater sample was collected from the 1,500-L seawater tank for reference of environmental microbes for the ex situ samples of both species on May 1 (1 day AFM).
In situ Sampling
The spawning timing of D. speciosa is well-documented at the study location and was expected between 21:00 and 22:00 around 5–7 days AFM from April to June (Lin and Nozawa, 2017). Colonies of D. speciosa that had matured pigmented eggs (violet gray) were selected by visually examining small fragments of colonies broken off using a hammer and a chisel at 3–7 m depth at Gongguan on May 3 (3 days AFM). A gamete trap consisting of a funnel and a 50 ml falcon tube was positioned on each of the 5 selected colonies (Figure 1B). Spawning of the colonies was monitored daily from 21:00 to 22:00 using scuba from May 3 (3 days AFM) and was observed from 21:00 to 22:00 on May 5 (5 days AFM). Egg-sperm bundles were released from colonies, floated toward the water surface, and were captured by the gamete trap (Figure 1B). Spawned gametes in the falcon tube were collected at 22:00 on the spawning night. A tissue sample was taken from the five colonies using a hammer and a chisel and kept in a zip-lock bag with seawater. A 1-L seawater sample was also collected as a sample of environmental bacteria at 3-m depth.
For H. coerulea, larvae loosely attached to the colony surface were collected in situ from five colonies at 1–3 m depth in Gongguan on May 5 (5 days AFM; Figure 1B). Larvae from each colony were collected using a dropper and kept in a 15-ml falcon tube. A tissue sample from each colony was collected using a hammer and a chisel and kept in a zip bag with seawater. One-liter seawater sample was collected as a sample of environmental bacteria at 3-m depth.
Total DNA Extraction and Amplification
All ex situ and in situ samples of gametes, larvae, and maternal coral tissue were washed twice with filtered seawater (0.22 μm), and the sperms were filtered out during washing, then coral samples were stored in 99% ethanol at −20°C at GIMRS. The seawater samples were filtered through cellulose acetate membranes with 0.2-μm pores (Aventec, Tokyo, Japan) and the membranes were stored at −20°C. All coral samples were washed with tris-EDTA (TE) buffer (10 mM Tris-HCl and 1 mM EDTA, ph 8) before DNA extraction. Egg and larval samples were frozen in liquid nitrogen and then homogenized using a sterile plastic pestle in 1.5 ml tubes. Coral tissue samples that contained skeletons were grounded using a sterile stainless mortar and pestle with liquid nitrogen. The homogenized tissues and membranes of seawater samples were suspended in TE buffer for total DNA extraction using the modified traditional cetyltrimethylammonium bromide (CTAB) method (Wilson, 2001; Hong et al., 2009).
Amplification of the 16S ribosomal RNA gene was performed by PCR using a pair of universal bacterial primers: 968F (5′-AACGCGAAGAACCTTAC-′3′) and Uni1391R (5′-ACGGGCGGTGWGTRC-3′) for the bacterial V6–V8 hypervariable regions (Nubel et al., 1996; Jorgensen et al., 2012). The PCR was performed in 50 μl reaction volumes, consisting of 2.5 U TaKaRa Ex Taq (Takara Bio, Otsu, Japan), 1X TaKaRa Ex Taq buffer, 0.2 mM deoxynucleotide triphosphate mixture (dNTP), 0.2 mM of each primer, and more than 20 ng of total DNA. The thermocycler was set to an initial step of 94°C for 3 min, 30 cycles of 94°C for 30 s, 55°C for 10 s, 72°C for 15 s, and a final extension of 72°C for 5 min. Target DNA bands (≈420 bp) were examined on 1.5% agarose gel after electrophoresis and eluted using QIAEX II Gel Extraction Kit (Qiagen, Valencia, CA, United States).
To tag each of the bacterial V6–V8 amplicon with a unique barcode sequence, each tag primer was designed with four overhanging nucleotides at 5’ ends of the common primers (Supplementary Table 2). The tagging reaction was performed with a 5-cycle PCR, each cycle was run at 94°C for 30 s, 56°C for 10 s, and 72°C for 20 s with the modified primers. End products were purified by the same gel elution method described above, and DNA concentration was determined with the Qubit dsDNA HS assay (Invitrogen, Carlsbad, CA, United States).
Sequencing and Data Processing
We pooled all V6–V8 amplicons of coral and seawater samples equally for 2 × 250 pair-end reads in Illumina NovaSeq paired-end sequencing (BIOTOOLS Co., Taipei, Taiwan). High-quality reads that were produced after raw reads were merged, sorted, and trimmed using mothur (Schloss et al., 2009) based on the following criteria: (1) reads of 350–500 bp long, (2) average quality score > 27, (3) homopolymer length < 8 bp, and (4) reads with any ambiguous base (N) removed. Then the four base tags and primer sequences were removed.
For the operational taxonomic unit (OTU) analysis, quality-filtered reads were pooled together and OTUs were assigned at 97% identity with the UPARSE pipeline (Edgar, 2013). In UPARSE, de-replication was performed and singletons were excluded (options: –derep_prefix and –minsize 2). Each OTU was classified with a bootstrap value set to 0.8 using a classifier (Wang et al., 2007) against the SILVA database (release 128) implemented in mothur. OTUs that were not assigned to a Bacteria domain or those that were assigned to Chloroplast were removed in subsequent analyses.
Data Analyses
Alpha-diversity and beta-diversity of bacterial communities were calculated after rarefying to an even 8,000 sequence depth in each sample by USEARCH (v10; options: -otutab_norm). For alpha-diversity, the Shannon index, Chao1 richness, and Species Evenness were calculated. Kruskal-Wallis tests were conducted to detect significant differences in the indexes of each coral species. Comparisons were made between the in situ and ex situ samples of eggs, larvae, and maternal colonies and between offspring (eggs or larvae) and maternal colonies within the in situ or ex situ samples. Statistical analyses were performed using R ver. 3.6.2 (R Core Team, 2019).
For beta-diversity, relative abundances of bacterial families (Supplementary Figure 1) and OTUs were calculated for individual samples (Chen et al., 2011), and then the Bray-Curtis distance was estimated between samples (Bray and Curtis, 1957). Based on the Bray-Curtis distance, the non-metric multidimensional scaling (nMDS) analysis and the agglomerative hierarchical clustering (CLUSTER) with complete-linkage were performed using the Primer 6 software (PRIMER-E, Lutton, Ivybridge, United Kingdom). nMDS plot with 95% CIs around each group was visualized with the “stat_ellipse” function in R. Significant variations between in situ and ex situ samples were examined using Analysis of Similarity (ANOSIM) in the Primer 6 software.
Data Availability Statement
The data sets generated for this study can be found in the NCBI SRA PRJNA706097.
Results
The data set of bacterial communities consisted of 2,361,933 reads from a total of 40 samples of offspring and maternal corals and three seawater samples after quality control and removal of no-Bacteria and Chloroplast OTUs. The number of reads per sample ranged between 8,277 and 179,418, with an average of 54,928 reads per sample, representing 6,601 OTUs. The data set was rarefied to the lowest number reads (8,000 reads) across samples, resulting in 5,181 OTUs for the analysis (Supplementary Figure 2 and Supplementary Table 3).
Alpha diversity of bacterial communities was compared using the Shannon, Chao1, and Evenness indices (Figure 2). There was no significant variation in alpha diversity of bacterial communities between in situ and the ex situ samples in maternal corals and offspring of each coral species (Kruskal-Wallis tests; p > 0.05). Although there was a tendency of higher alpha diversity in offspring than maternal colonies in D. speciosa and the opposite in H. coerulea, these were not statistically significant (Kruskal-Wallis tests; p > 0.05).
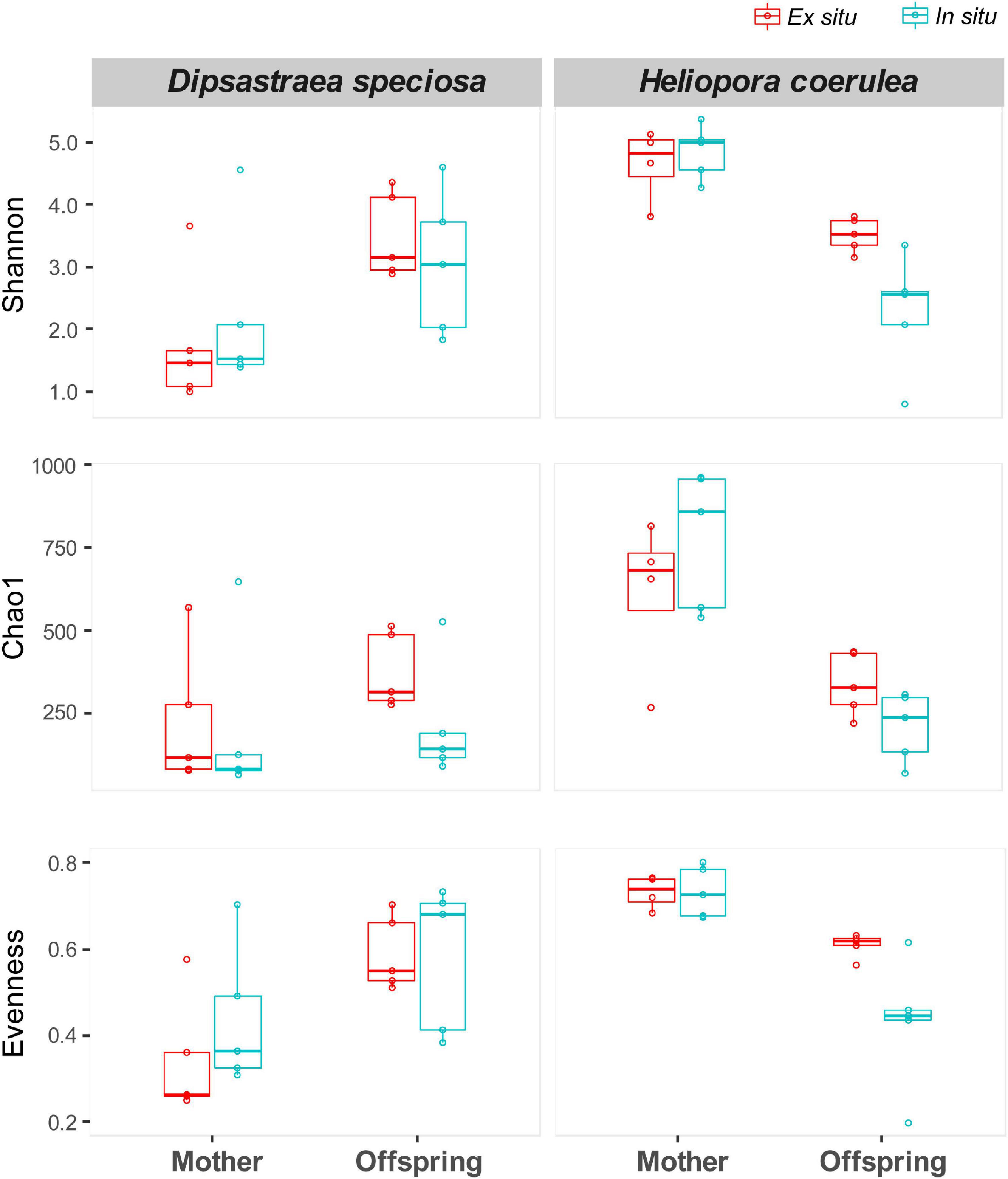
Figure 2. Alpha-diversity indices of bacterial communities (Shannon diversity, Chao 1 richness, and evenness) for the in situ (cyan) and ex situ samples (red) of maternal corals and offspring (eggs or larvae) in Dipsastraea speciosa and Heliopora coerulea. No significant variation was detected between the in situ and ex situ samples of maternal corals and offspring and between maternal corals and offspring within the in situ and ex situ samples of each species (Kruskal-Wallis test; p > 0.05).
Beta diversity of bacterial communities was grouped into three clusters; maternal corals, offspring, and seawater samples (ANOSIM, R = 0.56, p < 0.001; Figure 3A). The bacterial communities of maternal corals were clustered by species (2-way crossed ANOSIM, R = 0.97, p < 0.001), except for sample “DS4F” (Figure 3A), whereas the bacterial communities of offspring were primarily grouped by the in situ and ex situ sampling environments (2-way crossed ANOSIM, R = 0.71, p < 0.001). The nMDS analysis indicated similar patterns, with a wide distribution of in situ samples of offspring, especially for H. coerulea larvae (Figure 3B).
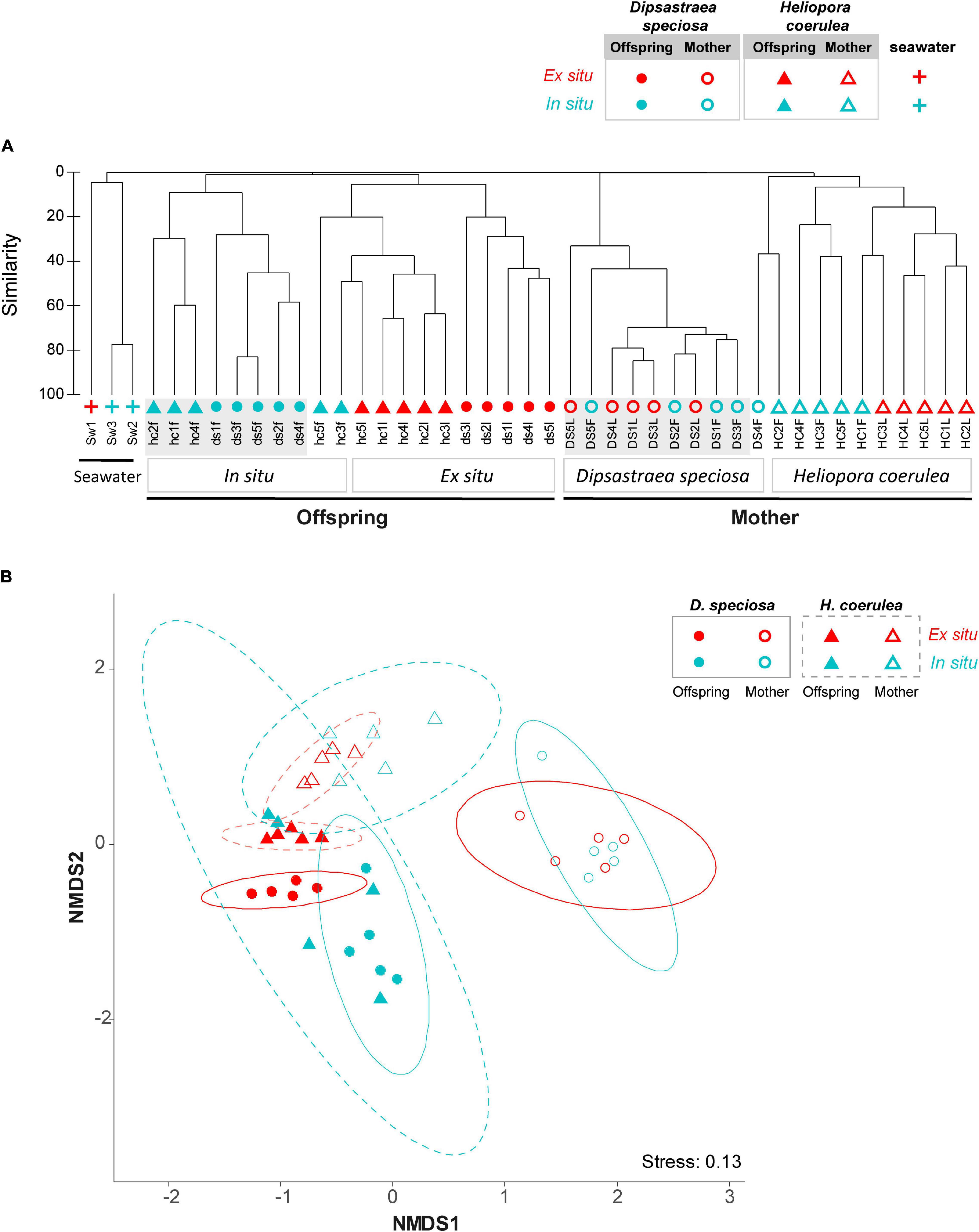
Figure 3. Bacterial community composition in (A) clustering and (B) nMDS plots. The symbols and colors denote; Dipsastraea speciosa (circles), Heliopora coerulea (triangles), and seawater (crosses); offspring (filled symbols) and maternal corals (blank symbols); in situ (cyan) and ex situ (red) environments. 95% CI ellipses are also shown in the nMDS plot for D. speciosa (solid line) and H. coerulea (dashed line). Abbreviations in sample names denote as follows; the first 2 characters represent coral species (DS for D. speciosa, HC for H. coerulea); the number (1–5) is replicate colony number; the last character is sampling method [F for the in situ (field) samples and L for the ex situ (laboratory) samples]; capital letters for maternal coral samples and small letters for offspring samples. nMDS, non-metric multidimensional scaling.
The top 30 OTUs (> 0.09% in cumulative relative abundance) of bacteria in the maternal corals and offspring were compared between the in situ and ex situ samples for each species (Figure 4 and Supplementary Table 4). D. speciosa had three OTU clusters, Groups 1–3 (Figure 4A and Supplementary Table 4A): The maternal coral samples were dominated by OTU Group 2. However, the offspring samples were dominated by OTU Group 1 for the in situ samples, and OTU Group 3 for the ex situ samples. Dominant bacterial OTUs in Group 2 were almost absent in both offspring and seawater samples, whereas some dominant OTUs in the offspring samples were detected in maternal corals.
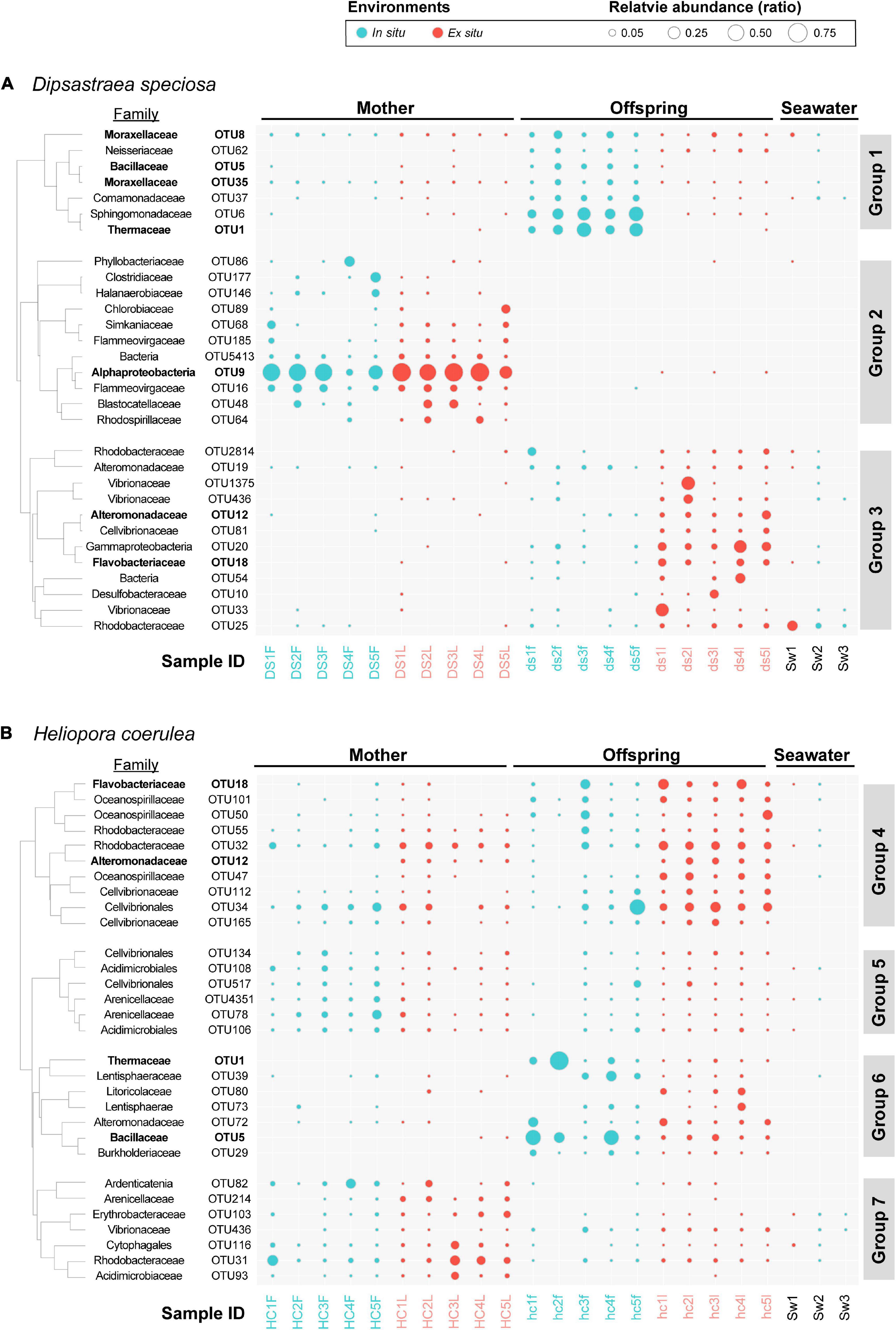
Figure 4. Bubble charts of the top 30 bacterial OTUs at the family level in (A) Dipsastraea speciosa and (B) Heliopora coerulea, with clusters based on Pearson’s correlations. Colors represent in situ (cyan) and ex situ (red) samples. Sample IDs are listed on the x-axis, and bacterial family and OTUs are shown on the y-axis. For sample IDs, refer to Figure 3 and Table 1. The size of the bubble indicates the relative abundance of OTUs in each sample. Bacterial families (OTUs) discussed in the text are highlighted in bold. OTUs, operational taxonomic units.
In H. coerulea, there were four OTU clusters, Groups 4–7 (Figure 4B and Supplementary Table 4B). The maternal corals were dominated almost evenly by OTUs in Groups 4, 5, and 7, irrespective of sampling environments. The OTUs dominated in maternal corals were also dominant in offspring, except for sample “hc2f.” The offspring samples were dominated by OTUs in all four OTU groups: OTUs in Group 6 were more abundant in in situ samples, whereas OTUs in Group 4 were more common in ex situ samples (Figure 4B). Four OTUs were detected in offspring samples of both coral species; OTU1 and OTU5 in in situ samples and OTU12 and OTU18 in ex situ samples (Figure 4).
Discussion
This study examined the effect of in situ and ex situ environments on bacterial communities of both offspring (eggs and larvae) and maternal corals in two phylogenetically distant coral species, with different reproductive modes; D. speciosa and H. coerulea. Our results demonstrate that spawning environments influence the composition of bacterial communities in offspring, but not in maternal corals. These findings are in agreement with previous studies that conclude bacterial communities in coral offspring are sensitive to local environments (Apprill et al., 2009; Zhou et al., 2017; Chan et al., 2019; Epstein et al., 2019) and highlight that sensitivity is higher than bacterial communities of their maternal colonies.
Alpha Diversity and the Winnow Process Hypothesis
While the alpha-diversity of bacterial communities was similar between the in situ and ex situ environments, they showed different trends between offspring and maternal corals in the two coral species: Alpha-diversity tended to be higher in offspring compared to maternal corals in D. speciosa, whereas this tendency was opposite in H. coerulea. The result for the spawning coral D. speciosa supports the “winnow process” hypothesis which proposes that alpha-diversity of bacterial communities is initially higher in early life stages and declines in later life (Littman et al., 2009). However, such a hypothesis could not explain the opposite trend of bacterial community for brooding coral, H. coerulea. Although Damjanovic et al. (2020a) reported higher alpha-diversity of bacterial communities in larvae than in maternal corals in brooding scleractinian coral, Pocillopora acuta, two of the five sample pairs showed similar alpha-diversity between them. Therefore, the currently limited information does not support the winnow process hypothesis for brooding corals.
Bacterial Communities in Eggs of Dipsastraea speciosa
The dominant bacterial OTUs (the top 30 OTUs) in eggs of D. speciosa were different between in situ and the ex situ environments and inconsistent with their maternal colonies. The same dominant bacterial OTUs between in situ and ex situ mother samples (Group 2 in Figure 4A) indicate that ex situ maternal colonies were not under stress, because many studies have shown that the bacterial communities in adult coral were changed under stress (Bourne et al., 2008). However, unlike maternal colonies, this stability was absent in the offspring samples, and the dominant bacterial group in the mother was not vertically transmitted to their offspring.
The dominant bacteria in in situ and ex situ offspring (Groups 1 and 3 in Figure 4A) can be detected in both maternal colonies and seawater samples, so that it is difficult to suggest that these groups were vertically transmitted from mother or horizontally transmitted from environments. Interestingly, both Group 1 and Group 3 dominant bacteria can be detected in in situ and ex situ offspring samples, but with different relative abundance.
In ex situ offspring samples, we had similar results in D. speciosa offspring with previous studies. Many of the dominant bacteria in ex situ samples (Group 3 in Figure 4A) have been previously reported from other studies which collected offspring samples under ex situ conditions; e.g., Rhodobacteraceae, Alteromonadaceae, Vibrionaceae, and Flavobacteriaceae (Apprill et al., 2009, 2012; Lema et al., 2016; Zhou et al., 2017; Bernasconi et al., 2019; Chan et al., 2019; Damjanovic et al., 2019a,2020a). Of these, Rhodobacteraceae and Alteromonadaceae have been detected in eggs of other spawning scleractinian coral species (Apprill et al., 2009; Zhou et al., 2017; Bernasconi et al., 2019; Damjanovic et al., 2019a).
In contrast, the majority of dominant bacteria detected in in situ offspring samples in the present study were newly recorded in bacterial communities in the eggs of spawning scleractinian coral species [e.g., Anoxybacillus (OTU5; family Bacillaceae), Meiothermus (OTU1; family Thermaceae), and Enhydrobacter (OTU35; family Moraxellaceae)], except Acinetobacter (OTU8; family Moraxellaceae). Acinetobacter was reported as core bacteria and suggested vertically transmitted from mother to offspring through the mucus in coral Mussismilia hispida (Leite et al., 2017). This finding indicates the bacteria group might be horizontally transmitted from the in situ environments of this study but more likely have existed already in other studies but was previously not noted due to low relative abundance in ex situ samples.
The variance in relative abundance between in situ and ex situ offspring samples may come from that the ex situ environment had smaller water volume and water exchange rate. Among ex situ offspring-dominated bacteria (Group 3), Vibrionaceae, Alteromonadaceae, and Rhodobacteraceae have been reported to be uptaken from the environment by coral recruits (Lema et al., 2016; Damjanovic et al., 2019a,b). In addition, Alteromonas (OTU19; belonged to Family Alteromonadaceae) was reported that it was released from maternal colony to seawater with offspring when spawning, and the offspring would uptake the bacteria from seawater (Ceh et al., 2013). Therefore, smaller water volume and exchange rate of the ex situ environment may increase the probability of the bacteria in seawater being uptaken by coral offspring. On the other hand, the two in situ dominated bacterial OTUs, Acinetobacter (OTU8) and Enhydrobacter (OTU35), that appeared in all the sample pairs of D. speciosa from both in situ and ex situ environments indicate that some bacteria are also vertically transmitted via mucus-covered egg-sperm bundles.
Bacterial Communities in Larvae of Heliopora coerulea
Brooding coral H. coerulea shared more common dominant bacteria between maternal colonies and their offspring samples compared to spawning coral D. speciosa (Figure 4). This may be due to the longer incubation time of brooder offspring developing to larvae inside mother than that of spawner offspring. However, in previous studies, only a small number of bacterial strains were shared between mother and their offspring in brooding scleractinian coral Pocillopora damicornis and P. acuta (Epstein et al., 2019; Damjanovic et al., 2020a). These dissimilarities are most likely caused by the super-dominant (>90% relative abundance) bacteria (taxa) in maternal colonies that were not dominant in the offspring of P. damicornis and P. acuta. H. coerulea is phylogenetically distant (Class Octocorallia) from scleractinians (Class Hexacorallia) and the phylogenetic difference might affect these results. The other possibility may come from the difference in contact time between maternal corals and offspring: Larvae of H. coerulea have a longer brooding (contact) time including the unique “surface brooding” (Babcock, 1990; Harii and Kayanne, 2003) compared to eggs of D. speciosa and larvae of P. damicornis and P. acuta (Epstein et al., 2019; Damjanovic et al., 2020a).
Although the majority of the dominant bacteria in larvae of H. coerulea was consensus with their maternal colonies and may be vertically transmitted from maternal corals (irrespective of in situ or ex situ environments), the spawning environment also affected the beta diversity of bacterial communities in the larvae. The larvae from the in situ environment had more diverse bacterial communities among samples than those from the ex situ environment (Figure 3B). The higher beta diversity of bacterial communities in the offspring from the in situ environment may indicate the prevalence of horizontal transmission of bacteria from environment to coral offspring and flexibility of bacterial communities in coral offspring in nature. Previous studies demonstrated that coral recruits under different planulation environments had different bacterial communities in four Acropora species and Pocillopora damicornis (Chan et al., 2019; Damjanovic et al., 2019b; Epstein et al., 2019). Our results further demonstrate that the spawning environment affects bacterial communities in coral offspring before the planulation stage.
Conclusion and Implication
This study provides the first report on in situ bacterial communities from coral offspring (eggs of the scleractinian coral, D. speciosa, and larvae of the octocoral, H. coerulea). We demonstrated that different environments influence bacterial communities in coral offspring, but not in maternal colonies, highlighting the sensitivity of bacterial communities to local environments for offspring compared to maternal corals. Bacterial communities between maternal colonies and offspring were more similar in H. coerulea than in D. speciosa, which suggest a higher ratio of vertically transmitted bacteria in H. coerulea, probably due to the longer contact time between offspring and maternal corals. Our findings on the sensitivity of bacterial communities in coral offspring to the local environment call attention to future studies when bacterial communities in coral offspring are sampled from ex situ environments.
Data Availability Statement
The datasets presented in this study can be found in online repositories. The names of the repository/repositories and accession number(s) can be found below: https://www.ncbi.nlm.nih.gov/, SRA PRJNA706097.
Author Contributions
YN and J-HS contributed to the study design and wrote the manuscript. C-HL, YN, C-LF, VDHD, and AJM performed the coral sampling. J-HS and C-HL performed the experiment. J-HS analyzed the data. YN and AJM contribute to the manuscript revision. All authors contributed to the article and approved the submitted version.
Funding
This work was financially supported by the internal research grant of Academia Sinica to YN.
Conflict of Interest
The authors declare that the research was conducted in the absence of any commercial or financial relationships that could be construed as a potential conflict of interest.
Publisher’s Note
All claims expressed in this article are solely those of the authors and do not necessarily represent those of their affiliated organizations, or those of the publisher, the editors and the reviewers. Any product that may be evaluated in this article, or claim that may be made by its manufacturer, is not guaranteed or endorsed by the publisher.
Acknowledgments
We would like to thank GIMRS that provided technical support in Lyudao, Taiwan. We thank J.-Y. Ding and Y.-L. Chen for their comments and suggestions.
Supplementary Material
The Supplementary Material for this article can be found online at: https://www.frontiersin.org/articles/10.3389/fmars.2022.796514/full#supplementary-material
References
Apprill, A. (2020). The role of symbioses in the adaptation and stress responses of marine organisms. Ann. Rev. Mar. Sci. 12, 291–314.
Apprill, A., Marlow, H. Q., Martindale, M. Q., and Rappe, M. S. (2009). The onset of microbial associations in the coral Pocillopora meandrina. ISME J. 3, 685–699. doi: 10.1038/ismej.2009.3
Apprill, A., Marlow, H. Q., Martindale, M. Q., and Rappe, M. S. (2012). Specificity of associations between bacteria and the coral Pocillopora meandrina during Early Development. Appl. Environ. Microbiol. 78, 7467–7475. doi: 10.1128/AEM.01232-12
Babcock, R. (1990). Reproduction and development of the blue coral Heliopora coerulea (Alcyonaria Coenothecalia). Mar. Biol. 104, 475–481.
Bernasconi, R., Stat, M., Koenders, A., Paparini, A., Bunce, M., and Huggett, M. J. (2019). Establishment of coral-bacteria symbioses reveal changes in the core bacterial community with host ontogeny. Front. Microbiol. 10:1529. doi: 10.3389/fmicb.2019.01529
Bourne, D., Iida, Y., Uthicke, S., and Smith-Keune, C. (2008). Changes in coral-associated microbial communities during a bleaching event. ISME J. 2, 350–363. doi: 10.1038/ismej.2007.112
Bray, J. R., and Curtis, J. T. (1957). An Ordination of the Upland Forest Communities of Southern Wisconsin. Ecol. Monogr. 27, 326–349.
Bright, M., and Bulgheresi, S. (2010). A complex journey: transmission of microbial symbionts. Nat. Rev. Microbiol. 8, 218–230.
Ceh, J., Kilburn, M. R., Cliff, J. B., Raina, J.-B., van Keulen, M., and Bourne, D. G. (2013). Nutrient cycling in early coral life stages: Pocillopora damicornis larvae provide their algal symbiont (symbiodinium) with nitrogen acquired from bacterial associates. Ecol. Evol. 3, 2393–2400. doi: 10.1002/ece3.642
Chan, W. Y., Peplow, L. M., Menendez, P., Hoffmann, A. A., and van Oppen, M. J. H. (2019). The roles of age, parentage and environment on bacterial and algal endosymbiont communities in Acropora corals. Mol. Ecol. 28, 3830–3843.
Chen, C. P., Tseng, C. H., Chen, C. A., and Tang, S. L. (2011). The dynamics of microbial partnerships in the coral Isopora palifera. ISME J. 5, 728–740. doi: 10.1038/ismej.2010.151
Damjanovic, K., Menéndez, P., Blackall, L. L., and van Oppen, M. J. H. (2019a). Early life stages of a common broadcast spawning coral associate with specific bacterial communities despite lack of internalized bacteria. Microb. Ecol. 79, 706–719. doi: 10.1007/s00248-019-01428-1
Damjanovic, K., van Oppen, M. J. H., Menendez, P., and Blackall, L. L. (2019b). Experimental inoculation of coral recruits with marine bacteria indicates scope for microbiome manipulation in Acropora tenuis and Platygyra daedalea. Front. Microbiol. 10:1702.
Damjanovic, K., Menéndez, P., Blackall, L. L., and van Oppen, M. J. H. (2020a). Mixed-mode bacterial transmission in the common brooding coral Pocillopora acuta. Environ. Microbiol. 22, 397–412.
Damjanovic, K., Blackall, L. L., Peplow, L. M., and van Oppen, M. J. H. (2020b). Assessment of bacterial community composition within and among Acropora loripes colonies in the wild and in capativity. Coral Reefs 39, 1245–1255. doi: 10.1007/s00338-020-01958-y
Douglas, A. E. (2019). Simple animal models for microbiome research. Nat. Rev. Microbiol. 17, 764–775. doi: 10.1038/s41579-019-0242-1
Edgar, R. C. (2013). UPARSE: Highly accurate otu sequences from microbial amplicon reads. Nat. Methods 10, 996–998. doi: 10.1038/nmeth.2604
Epstein, H. E., Torda, G., Munday, P. L., and van Oppen, M. J. H. (2019). Parental and early life stage environments drive establishment of bacterial and dinoflagellate communities in a common coral. ISME J. 13, 1635–1638. doi: 10.1038/s41396-019-0358-3
Harii, S., and Kayanne, H. (2003). Larval dispersal, recruitment, and adult distribution of the brooding stony octocoral Heliopora coerulea on Ishigaki Island, Southwest Japan. Coral Reefs 22, 188–196.
Harii, S., Kayanne, H., Takigawa, H., Hayashibara, T., and Yamamoto, M. (2002). Larval survivorship, competency periods and settlement of two brooding corals, Heliopora coerulea and Pocillopora damicornis. Mar. Biol. 141, 39–46.
Hartmann, A. C., Baird, A. H., Knowlton, N., and Huang, D. (2017). The paradox of environmental symbiont acquisition in obligate mutualisms. Curr. Biol. 27, 3711–3716e3713. doi: 10.1016/j.cub.2017.10.036
Hong, M. J., Yu, Y. T., Chen, C. A., Chiang, P. W., and Tang, S. L. (2009). Influence of species specificity and other factors on bacteria associated with the coral Stylophora pistillata in Taiwan. Appl. Environ. Microbiol. 75, 7797–7806.
Jorgensen, S. L., Hannisdal, B., Lanzen, A., Baumberger, T., Flesland, K., Fonseca, R., et al. (2012). Correlating microbial community profiles with geochemical data in highly stratified sediments from the Arctic Mid-Ocean Ridge. Proc. Natl. Acad. Sci. U. S. A. 109, E2846–E2855. doi: 10.1073/pnas.1207574109
Leite, D. C., Leao, P., Garrido, A. G., Lins, U., Santos, H. F., Pires, D. O., et al. (2017). Broadcast spawning coral Mussismilia hispida can vertically transfer its associated bacterial core. Front. Microbiol. 8:176. doi: 10.3389/fmicb.2017.00176
Lema, K. A., Clode, P. L., Kilburn, M. R., Thornton, R., Willis, B. L., and Bourne, D. G. (2016). Imaging the uptake of nitrogen-fixing bacteria into larvae of the coral Acropora millepora. ISME J. 10, 1804–1808. doi: 10.1038/ismej.2015.229
Lesser, M. P., Falcon, L. I., Rodriguez-Roman, A., Enriquez, S., Hoegh-Guldberg, O., and Iglesias-Prieto, R. (2007). Nitrogen fixation by symbiotic cyanobacteria provides a source of nitrogen for the scleractinian coral Montastraea cavernosa. Mar. Ecol. Prog. Ser. 346, 143–152.
Lin, C. H., and Nozawa, Y. (2017). Variability of spawning time (lunar day) in Acropora versus merulinid corals: a 7-yr record of in situ coral spawning in Taiwan. Coral Reefs 36, 1269–1278. doi: 10.1007/s00338-017-1622-5
Lin, C. H., Takahashi, S., Mulla, A. J., and Nozawa, Y. (2021). Moonrise timing is key for synchronized spawning in coral Dipsastraea speciosa. Proc. Natl. Acad. Sci. U. S. A. 118:34. doi: 10.1073/pnas.2101985118
Littman, R. A., Willis, B. L., and Bourne, D. G. (2009). Bacterial communities of juvenile corals infected with different Symbiodinium (dinoflagellate) clades. Mar. Ecol. Prog. Ser. 389, 45–59. doi: 10.3354/meps08180
Nubel, U., Engelen, B., Felske, A., Snaidr, J., Wieshuber, A., Amann, R. I., et al. (1996). Sequence heterogeneities of genes encoding 16S rRNAs in Paenibacillus polymyxa detected by temperature gradient gel electrophoresis. J. Bacteriol. 178, 5636–5643. doi: 10.1128/jb.178.19.5636-5643.1996
R Core Team (2019). R: A language and environment for statistical computing. Vienna: R Foundation for Statistical Computing.
Radecker, N., Pogoreutz, C., Voolstra, C. R., Wiedenmann, J., and Wild, C. (2015). Nitrogen cycling in corals: the key to understanding holobiont functioning? Trends Microbiol. 23, 490–497. doi: 10.1016/j.tim.2015.03.008
Rohwer, F., Seguritan, V., Azam, F., and Knowlton, N. (2002). Diversity and distribution of coral-associated bacteria. Mar. Ecol. Prog. Ser. 243, 1–10.
Schloss, P. D., Westcott, S. L., Ryabin, T., Hall, J. R., Hartmann, M., Hollister, E. B., et al. (2009). Introducing mothur: open-source, platform-independent, community-supported software for describing and comparing microbial communities. Appl. Environ. Microbiol. 75, 7537–7541. doi: 10.1128/AEM.01541-09
Thompson, J. R., Rivera, H. E., Closek, C. J., and Medina, M. (2015). Microbes in the coral holobiont: partners through evolution, development, and ecological interactions. Front. Cell. Infect. Microbiol. 4:176. doi: 10.3389/fcimb.2014.00176
van Oppen, M. J. H., and Blackall, L. L. (2019). Coral microbiome dynamics, functions and design in a changing world. Nat. Rev. Microbiol. 17, 557–567.
Wang, Q., Garrity, G. M., Tiedje, J. M., and Cole, J. R. (2007). Naive Bayesian classifier for rapid assignment of rRNA sequences into the new bacterial taxonomy. Appl. Environ. Microbiol. 73, 5261–5267.
Wang, Y., Kuang, Z., Yu, X., Ruhn, K. A., Kubo, M., and Hooper, L. V. (2017). The intestinal microbiota regulates body composition through NFIL3 and the circadian clock. Science 357, 912–916. doi: 10.1126/science.aan0677
Wilson, K. (2001). Preparation of genomic DNA from bacteria. Curr. Protoc. Mol. Biol. 2001, 2–4. doi: 10.1002/0471142727.mb0204s56
Yasuda, N., Taquet, C., Nagai, S., Fortes, M., Fan, T. Y., Phongsuwan, N., et al. (2014). Genetic structure and cryptic speciation in the threatened reef-building coral Heliopora coerulea along Kuroshio Current. Bull. Mar. Sci. 90, 233–255.
Keywords: bacterial communities, coral offspring, sampling method, alpha diversity, beta diversity
Citation: Shiu J-H, Lin C-H, Mulla AJ, Dang VDH, Fong C-L and Nozawa Y (2022) Bacterial Communities in Coral Offspring Vary Between in situ and ex situ Spawning Environments. Front. Mar. Sci. 9:796514. doi: 10.3389/fmars.2022.796514
Received: 17 October 2021; Accepted: 14 January 2022;
Published: 16 February 2022.
Edited by:
Nina Yasuda, University of Miyazaki, JapanReviewed by:
Chuya Shinzato, The University of Tokyo, JapanHironobu Fukami, University of Miyazaki, Japan
Cecilia Conaco, University of the Philippines Diliman, Philippines
Copyright © 2022 Shiu, Lin, Mulla, Dang, Fong and Nozawa. This is an open-access article distributed under the terms of the Creative Commons Attribution License (CC BY). The use, distribution or reproduction in other forums is permitted, provided the original author(s) and the copyright owner(s) are credited and that the original publication in this journal is cited, in accordance with accepted academic practice. No use, distribution or reproduction is permitted which does not comply with these terms.
*Correspondence: Jia-Ho Shiu, amlhc3IxOEBnbWFpbC5jb20=; Yoko Nozawa, bm96YXdheUBnYXRlLnNpbmljYS5lZHUudHc=