- 1CAS Key Laboratory of Marine Ecology and Environmental Sciences, Institute of Oceanology, Chinese Academy of Sciences, Qingdao, China
- 2University of Chinese Academy of Sciences, Beijing, China
- 3Laboratory for Marine Ecology and Environmental Science, Qingdao National Laboratory for Marine Science and Technology, Qingdao, China
- 4Center for Ocean Mega-Science, Chinese Academy of Sciences, Qingdao, China
- 5Shandong Hydrobios Resources Conservation and Management Center, Yantai, China
Estuaries are nutrient-rich environments with a gradient of fresh to salt water. They support high primary productivity and an abundance of zooplankton. Estuaries are used by many fish as nursery grounds because their environmental conditions provide abundant food for larval and adult fish. Ichthyoplankton, which comprise fish eggs and larvae, are important for the recruitment of fish species. At present, there are no systematic reviews on ichthyoplankton in estuaries from a global perspective. Here, research on ichthyoplankton over the last 60 years (1951–2022) was reviewed, focusing on three aspects: evaluation of ichthyoplankton published studies, community structure, and factors affecting community structure. The results show that research on estuarine ichthyoplankton has increased, from less than 1 article per year in the 1950s to more than 30 articles per year in the 2020s. The keyword used most commonly was ‘community structure’ and ‘dynamics’ and the word used most recently was ‘climate change’. Regarding the geographical distribution of the studies carried out on estuarine ichthyoplankton, USA had the most (32.9% of all studies), followed by Australia (8.1%), South Africa (6.1%), Brazil (5.6%), Japan (5.1%), China (4.9%), Canada (4.8%), and Portugal (4.8%). Salinity and turbidity were the main factors affecting the ichthyoplankton community structure in estuaries. Climate change indirectly affected the community structure of estuarine ichthyoplankton by changing the spawning time, spawning location, and hatching time of species. The movement of spawning sites poleward and the advance of spawning time showed a consistent trend. In addition, the development of DNA barcoding techniques will be a useful supplement to traditional taxonomic methods for identifying ichthyoplankton and will provide new opportunities for the development of systematic taxonomy in this field. Our review contributes to a broader understanding of estuarine ichthyoplankton and provides theoretical support for estuarine environmental protection and the sustainable use of estuarine fishery resources.
1 Introduction
Estuaries are the most productive and nutrient-rich ecosystems on Earth. Many species complete part or all of their life history in estuaries, and they are especially important foraging and breeding habitats for many fish species (Sheaves et al., 2015; Xian et al., 2016; Lefcheck et al., 2019). However, owing to their unique geographical location, they have been subject to human development for millennia (Limburg, 1999; Lotze, 2010). At present, approximately 40% of the world’s population lives in coastal areas (Barragán and de Andrés, 2015). In some estuarine areas, the populations of more than 90% of economically important species, including fish, have been exhausted, and the overall biomass is less than 50% of the historical abundance. The main driving factors of this change are the overexploitation of resources and the loss of habitat (Lotze et al., 2006). Therefore, to improve decision-making for estuarine ecological management and sustainable use of resources, more baseline information is needed.
The ichthyoplankton stage (including fish eggs and larvae) is important for the growth and development of estuarine communities and can change quickly in response to environmental variations (Shan et al., 2004). Although this stage in the fish life history is short, it is the most vulnerable stage in the whole development cycle (Shao et al., 2001; Jiang et al., 2006a). The quantity and survival of ichthyoplankton reflects the biomass and interannual dynamics of future fish stocks (Butler et al., 2003; Song et al., 2019). It provides the basis for the recruitment of fish stocks and the sustainable use of fishery resources (Chambers and Trippel, 1997; Cao et al., 2007). Moreover, as a major predator and important consumer of planktonic (especially zooplankton) of secondary production (Monteiro et al., 2021), ichthyoplankton play an important role in the energy transfer of estuarine ecosystems (Wan and Sun, 2006). Ichthyoplankton are, thus, a key link in the aquatic food web (Wan and Jiang, 2000). Investigating and evaluating the ichthyoplankton community not only lays a foundation for understanding the status of fish stocks, determining spawning sites and spawning cycles, and clarifying fishery management, but also helps to clarify the energy flow and material circulation of estuarine ecosystems.
Owing to the limitations of previous scientific and technological methods, early research reports on estuarine ichthyoplankton were mainly descriptions of ecological habits, species composition and distribution (Jones and Menon, 1951; Chandra, 1962). After the 1970s, studies began to focus on the life history and morphological functions (Hickling, 1970), ecological habits (Blaber, 1997), species composition and distribution characteristics (Able, 1978; Melvillesmith and Baird, 1980) of estuarine ichthyoplankton. Since the 1980s, factors affecting the distribution of ichthyoplankton have been considered. For example, turbidity was found to be one of the main factors affecting their distribution (Boehlert and Morgan, 1985). Salinity was also an important environmental factor affecting the distribution of ichthyoplankton in estuaries (Locke and Courtenay, 1995; Zhang et al., 2016). In addition, the quantity and distribution of copepods, which are the main food source for ichthyoplankton, played an important role (Islam et al., 2006; Jiang et al., 2006). What’s more, the breeding migration of offshore fishes was one of the important recruitment sources for estuarine nursery areas (Schilling et al., 2021). Therefore, the distribution of ichthyoplankton in estuaries was jointly determined by a variety of environmental factors such as temperature, salinity, dissolved oxygen, food, runoff and climate (Xiao et al., 2013; Sloterdijk et al., 2017).
Human activities, such as overfishing, dam construction and environmental pollution, are also important factors affecting estuarine ichthyoplankton (Shan et al., 2004). Overfishing reduces the adult stocks, which weakens the function of estuarine nurseries (Yu et al., 2018). Dam construction changes the amount of sediment—especially fine sediment—entering the sea from the estuary, which leads to a decline in the self-purification capacity of the estuary (Yang et al., 1992). Environmental pollution, caused by the discharge of industrial wastewater and domestic sewage (Guan et al., 1992), has changed the nutritional structure of estuarine water bodies (Pombo et al., 2002), thus destroying the spawning and breeding grounds of fish (Yao, 1995). Recently, climate change has been shown to have a subtle influence on the distribution of ichthyoplankton in estuaries (Thaxton et al., 2020). For example, the increase in the sea surface temperature has led to a northward movement of spawning sites and an advance of the spawning period (Zhang Z. et al., 2020). Therefore, exploring the impact of climate change on changes in ichthyoplankton community structure in estuaries has become a new research focus.
Although ichthyoplankton play an important ecological role, identifying individual species is still difficult (Zhang et al., 2015). At present, more than 36,058 fish species have been identified as adults (Fricke et al., 2021), but only approximately 10% of these can be identified as larvae and postlarvae, while less than 10% of eggs can be identified to species (Shao et al., 2001). Owing to the lack of specific features that can be used for morphological classification, little research has been carried out on the morphological classification of ichthyoplankton (Peterson and Lietz, 2017). Generally, eggs from different species are collectively referred to as ‘fish eggs’ in the investigation and assessment of fishery resources, resulting in inaccuracies in related work such as resource assessment, population dynamics research and spawning site identification (Shao et al., 2001; Zhang et al., 2015). Understanding how to identify ichthyoplankton species efficiently and accurately is essential for fishery-related research.
Scientific and technological progress has greatly improved the accuracy of ichthyoplankton identification. For example, the development of scanning electron microscope technology allows researchers to observe the egg membrane structure of fish eggs and identify species (Gao, 2015). In recent years, DNA barcoding has become an effective method for fish identification (Hebert et al., 2003). Tautz first proposed that DNA sequences could be used for species identification or classification (Tautz et al., 2002). Subsequently, studies have found that the application of DNA barcoding can greatly improve the accuracy of species identification (Pegg et al., 2006; Valdez-Moreno et al., 2010; Ko et al., 2013; Zhou et al., 2017; Liu et al., 2021). The growth of various fish genetic sequences collected by global shared gene databases, such as DNA Data Bank of Japan, European Molecular Biology Laboratory and GenBank, has provided a substantial number of comparable adult fish homologous DNA sequences which can be used to identify ichthyoplankton, and the accuracy of DNA barcoding techniques in this field will continue to increase (Lin et al., 2018). The simplicity and efficiency of DNA barcoding is helping its growth as a new technique to study ichthyoplankton.
The aim of this review is to evaluate the status of research on ichthyoplankton in estuarine ecosystems, explore changes in the ichthyoplankton community structure and its influencing factors. The research gaps and recommendations for future research are also presented.
2 Methods
A scientometric and systematic review was carried out using the ‘Web of Science’ ‘China National Knowledge Infrastructure’, ‘PubMed’, ‘Scopus’ and ‘Engineering Village’ databases. The following terms were used to identify in the title or abstract or keywords, refine and organize existing literature: (‘ichthyoplankton’ or ‘egg’ or ‘larva’ or ‘larvae’) and (‘fish’) and (‘estuary’ or ‘estuarine’ or ‘estuaries’). The detailed search conditions used in this study and output results from each database are showed in Supplementary Materials 1 (Table S1) and Supplementary Materials 2 respectively. Papers published up to April 2022 were considered, while technical reports, academic theses, book chapters and scientific events summaries were excluded (Figure 1). A total of 2, 314 articles were retrieved from the databases. After screening and exclusion (reviews, technical reports, protocols, grey literature, did not fit the objectives, letters or short communications and duplicate articles), 836 articles were systematically analyzed according to the following parameters: 1) year of publication; 2) authors; 3) geographical location of the study (according to the location of the corresponding author); 4) subject (according to keywords); and 5) citations. The data concerning community structure (i.e., ecological categorization, species composition, temporal and spatial distribution), affecting factors (i.e., temperature, salinity, overfishing, pollution and climate changes) were also summarized.
To understand the relationship between the countries and the most influential researchers concerned with ichthyoplankton in estuarine ecology, a cluster analysis among (i) the countries and (ii) the most influential researchers in the study area were performed using the VOSviewer software (version 1.6.15; Centre for Science and Technology Studies, the Netherlands). The inclusion criteria for this analysis were that the country (occurrence) and author (citation) were recorded at least 5 time and 50 times, respectively, among the analyzed studies. The VOSviewer software generates a network whose visualization is based on nodes and connections, with the diameter of each node indicating the volume of (i) publications per country and (ii) citations of the referred author, while the distance between two nodes indicates the approximate intensity of the relationship between them (the stronger the relationship, the shorter the connection distance). Clusters are grouped by different colors (van Eck et al., 2014).
3 Evaluation of Ichthyoplankton in Estuarine Ecosystems
3.1 Historical Overview
Figure 2 represents the absolute and cumulative number of papers published until April 2022. The number of papers on estuarine ichthyoplankton increased year by year, especially after 1990s. The earliest full text in the database appeared in 1951 and it described and illustrated the larval development of the Indian shad until it became a juvenile (Jones and Menon, 1951). Figure 3 represents the clustering relationship between keywords (appearing more than 10 times). The diameter of the node indicates the volume of publications, and the connections indicate the intensity of the relationship between the countries. Colors represent the time when keywords appeared. The keyword used most was ‘community structure’ and ‘dynamics’ (the largest node diameter), and the keyword used latest was ‘climate change’ (the lightest color), mainly because the impact of climate change on estuarine ichthyoplankton is a long-term process (Zhang Z. et al., 2020). The cluster analysis with the greatest centrality among the researchers in this study area can be seen in Figure 4. The most cited authors (largest node diameter) among all the studies examined were A.K. Whitfield (n = 341; 10.94%), followed by S.J.M. Blaber (n = 162; 5.20%), K.W. Able (n = 143; 4.59%) and N.A. Strydom (n = 142; 4.56%).
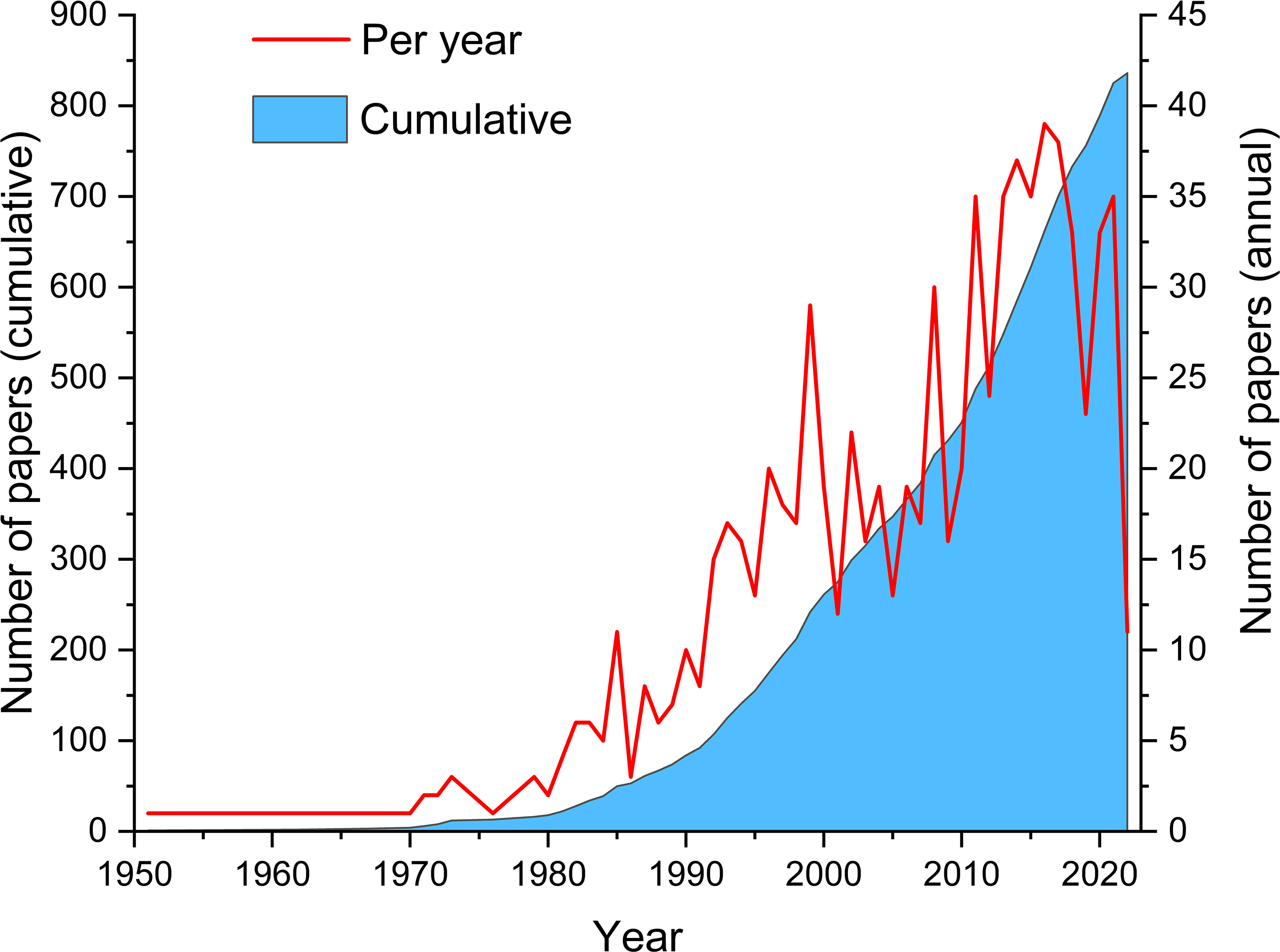
Figure 2 Number of included estuarine ichthyoplankton papers (annual and cumulative) published between 1951 and 2022.
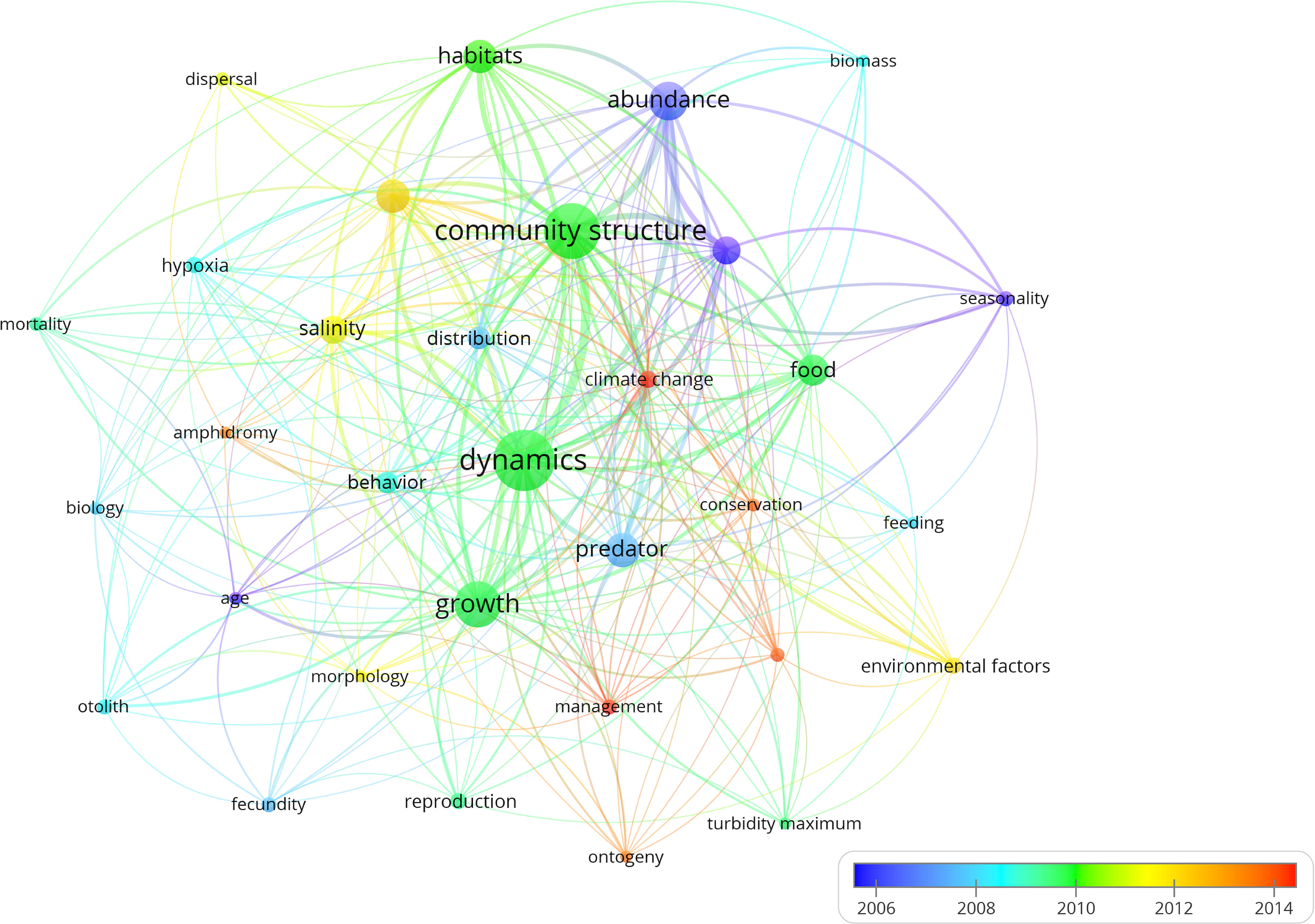
Figure 3 Cluster analysis showing the most important estuarine ichthyoplankton related keywords used in research papers a minimum of 10 times. The diameter of the node indicates the volume of occurrences and the connections indicate the intensity of the relationship between the keywords.
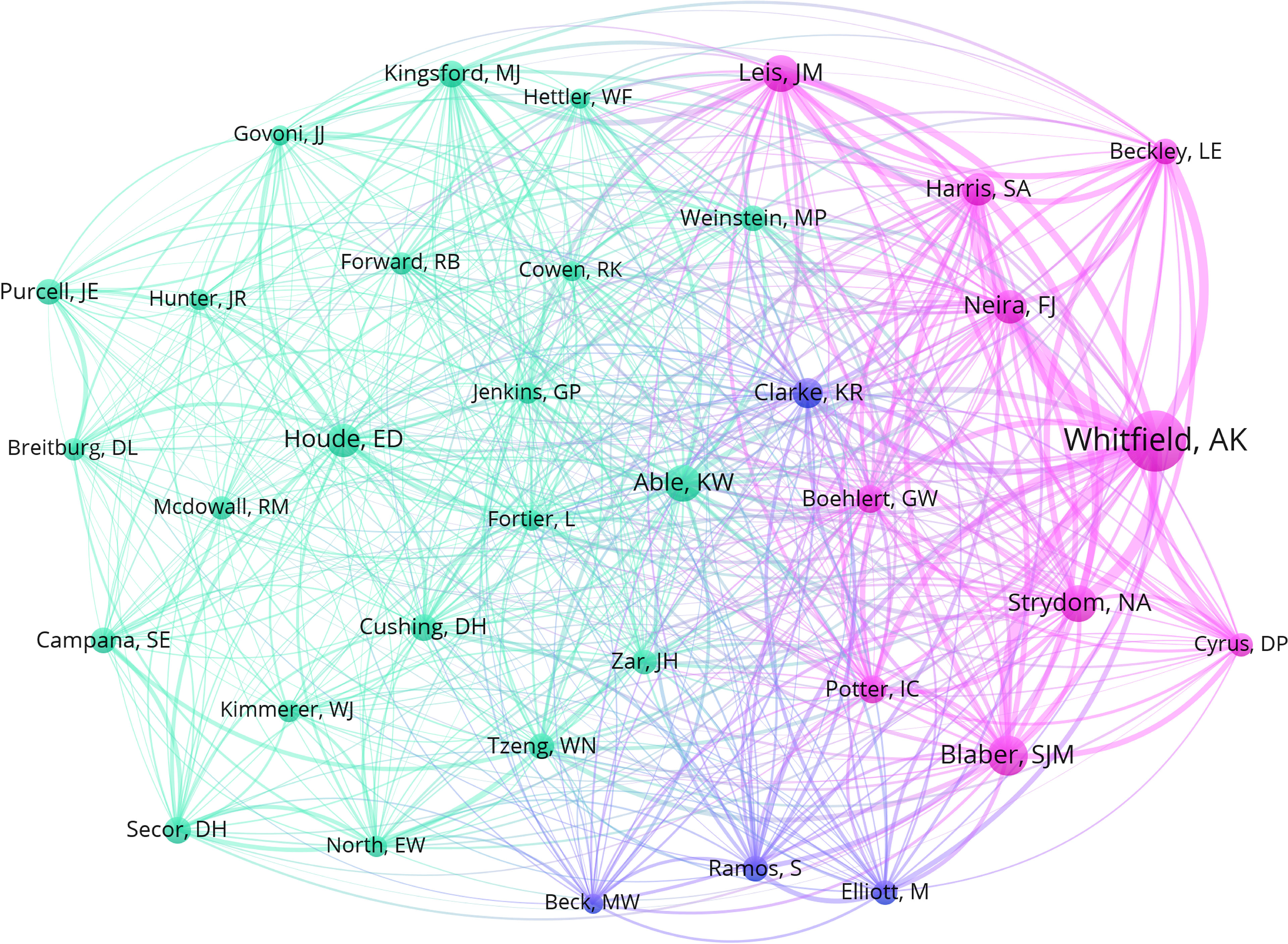
Figure 4 Cluster analysis of ichthyoplankton scientists with more than fifty citations between 1951 and 2022. The diameter of each node represents the total number of citations by each scientist and the connections indicate the intensity of collaboration between researchers.
3.2 Geographical Overview
Regarding the geographical distribution of all studies carried out on estuarine ichthyoplankton, USA stands out (32.9% of the studies), followed by Australia (8.1%), South Africa (6.1%), Brazil (5.6%), Japan (5.1%), China (4.9%), Canada (4.8%), and Portugal (4.8%) (Figure 5). Another 66 countries have articles published on the topic; however, 23 countries (34%) presented only one publication. The cluster analysis performed with these data showed a strong relationship among USA, Australia and Brazil, for which the nodes were more expansive and had stronger connectivity (Figure 6). Interestingly, among the 26 countries with more than five publications evaluating estuarine ichthyoplankton, 18 countries (69%) were classified as developed countries, suggesting a lack of investment in education, science and technology in developing countries worldwide. Figure 7 represents the research status of ichthyoplankton in estuaries around the world and systematically classifies the reports from countries with more than 30 articles (i.e., the top 10 countries with the largest number of articles). The results show that among these 10 countries, the research focus is on the impact of environmental factors on the community structure of estuarine ichthyoplankton, especially in China, France, Brazil and Portugal (accounting for more than 60% of the total number of articles published in the top 10 countries). At present, there are few studies on the impact of climate change on community structure, and the application of DNA barcoding for the identification of ichthyoplankton has not been popularized.
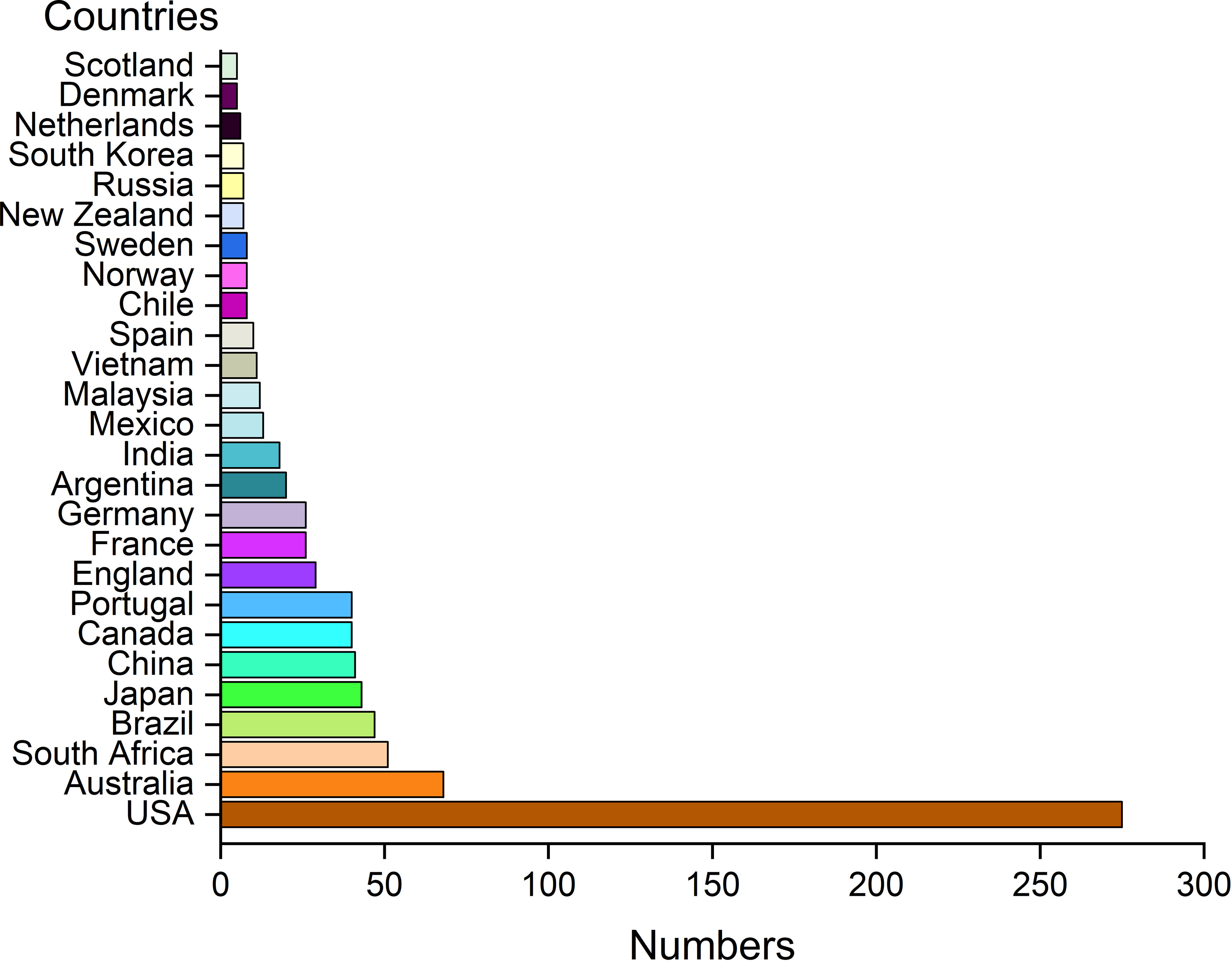
Figure 5 Number of papers per country evaluating estuarine ichthyoplankton, from 1951 to 2022, with at least five occurrences.
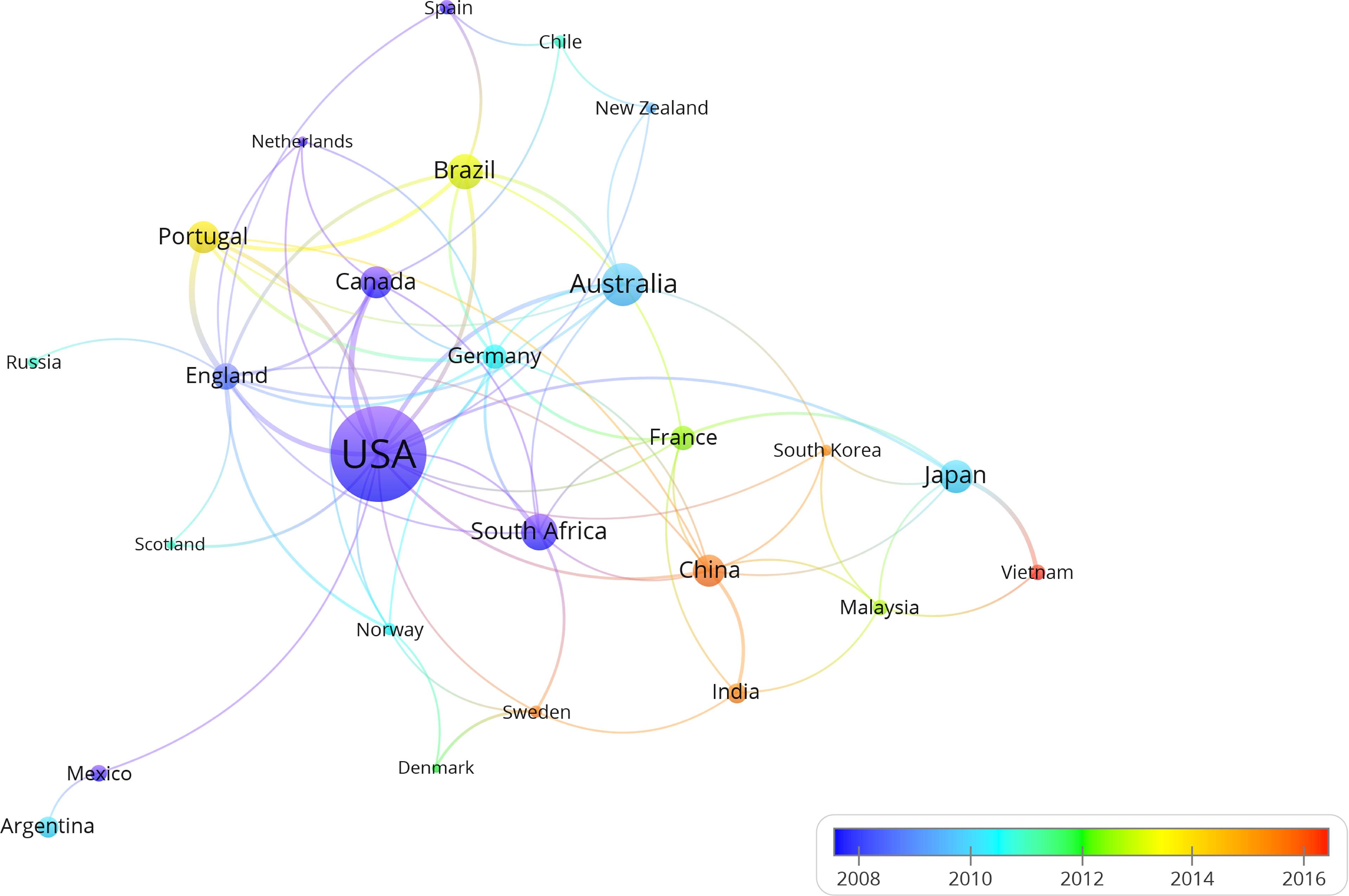
Figure 6 Cluster analysis of the countries with studies (more than 5 literatures) evaluating estuarine ichthyoplankton. The diameter of the node indicates the volume of publications and the connections indicate the intensity of the relationship between the countries.
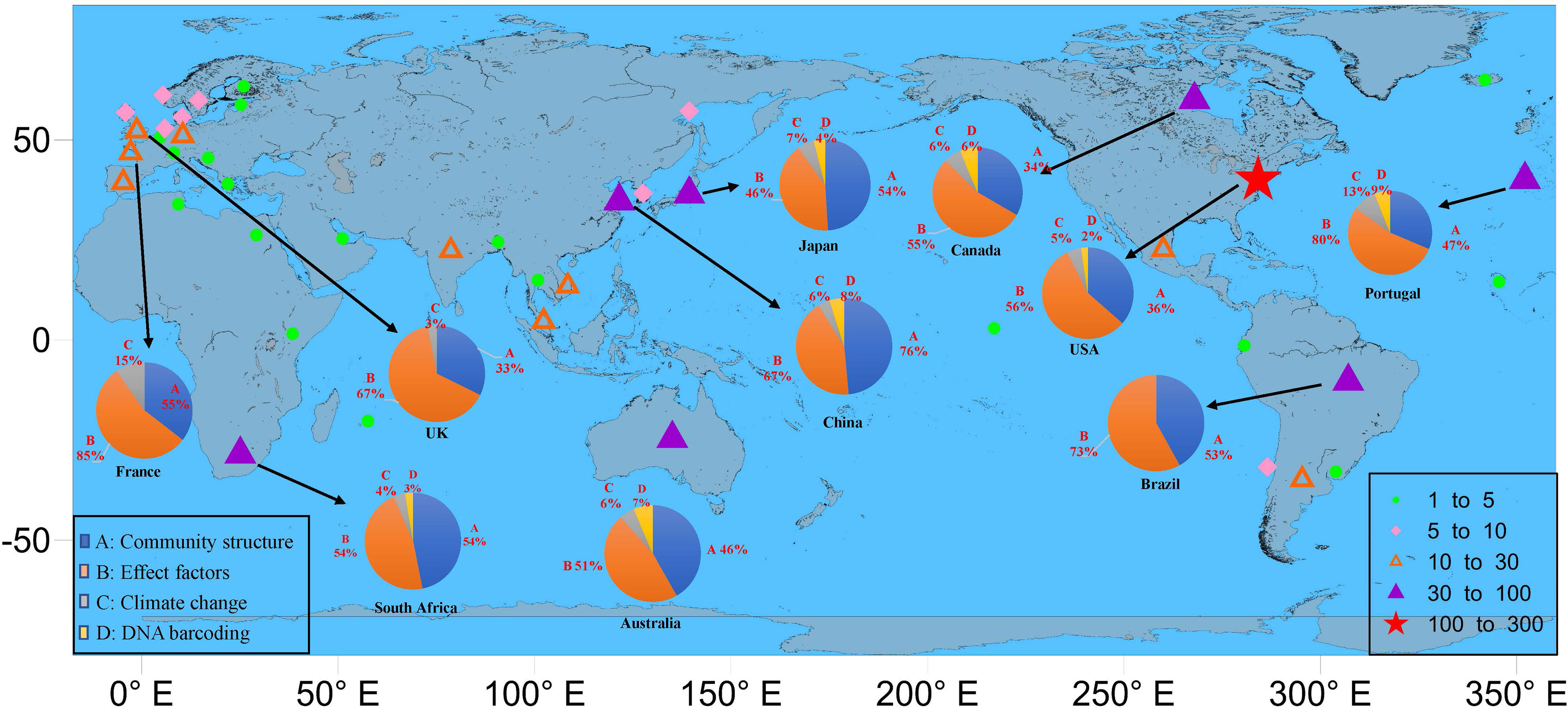
Figure 7 The research status of ichthyoplankton in estuaries around the world and systematically classifies the reports of countries with more than 30 articles (i.e. the top 10 countries with the largest number of articles).
4 Studies on the Community Structure of Estuarine Ichthyoplankton
Community structure is one of the important studies on the estuarine ichthyoplankton. In this study, the ecological categorization, species composition, temporal and spatial distribution of ichthyoplankton and the application of emerging identification methods (DNA barcoding) were classified under the broad topic of community structure. 480 of total 836 literature results were reported on community structure, including 20 on ecological categorization, 159 on species composition, 266 on temporal and spatial distribution, and 37 on application of DNA barcoding (Table 1).
4.1 The Ecological Categorization of Estuarine Ichthyoplankton
There are many biological classifications of estuarine ichthyoplankton communities in many different types of estuaries. Generally, communities can be divided into different ecological or functional groups according to different characteristics such as spawning habits, temperature and salinity tolerance, migratory habits and spatial distribution (Xian et al., 2016). For example, Yang et al. (1990) and Luo (1994) divided the ichthyoplankton in the Yangtze Estuary into four ecological categories according to the ecological habits and distribution characteristics of larvae and juvenile stages. These categories are generally recognized by other Chinese researchers (Liu et al., 2008; Zhang et al., 2015). Potter et al. (1990) and Whitfield (1989) classified ichthyoplankton into five types according to their habits and the relationship between spawning area of estuarine fish and estuaries, respectively. Islam and Tanaka (2006) classified the ichthyoplankton of the Chikugo estuary in Japan into four groups according to different types of salinity adaptation. Wang et al. (2020) classified the ichthyoplankton of Shijiu Port (China) into warm-temperature and cold-temperature species according to the parental thermophily and into demersal fish and pelagic fish according to space distribution characteristics. The first two classification methods are the most commonly used, and they both have similar classification criteria and high similarity of classification results (Table S2 in Supplementary Materials 1). However, there is no international common classification standard on the ecological categorization of estuarine ichthyoplankton. Therefore, there is a need to integrate an understanding of community structure in terms of ecological categorization to provide more comprehensive information.
4.2 Species Composition of Estuarine Ichthyoplankton
As estuaries are the sites where rivers enter the ocean and they reflect land–sea interactions (Zhuang, 2006), they are rich in nutrients and food sources, which provide suitable conditions for the breeding of fish (Huang et al., 2017). Thus, the species and number of ichthyoplankton living in an estuary are determined by the environmental characteristics and material sources (Shan et al., 2004). The specific ichthyoplankton communities formed in estuaries differ from other marine areas owing to the interaction of freshwater runoff and tidal currents (Whitfield, 1994). A common characteristic of ichthyoplankton communities in estuaries is a mix of rare and highly abundant species (Gaughan et al., 1990; Harrison and Whitfield, 1990; Pfirrmann et al., 2021). Many studies have shown that ichthyoplankton communities in warm water estuaries are dominated by Gobiidae, which live in estuaries, and Clupeidae and Engraulidae, which lay eggs seasonally in estuaries (Talbot and Able, 1984; Drake and Arias, 1991; Monteleone, 1992; Harris and Cyrus, 2000; Strydom et al., 2003; Bonecker et al., 2019; Zhang et al., 2019; Amezcua et al., 2020; Chermahini et al., 2021).
The species composition of ichthyoplankton communities varies according to the environmental conditions of each estuary (Shan et al., 2004). The Yangtze Estuary has the most comprehensively studied ichthyoplankton communities in China (Zhang et al., 2015). Since the 1980s, abundant work has been done on the community structure of ichthyoplankton in this area. By comparing the research results from the 1980s with more recent work, it is clear that the quantity of existing ichthyoplankton species has declined sharply in the Yangtze Estuary. Although Engraulidae is still the dominant species in this region, some other species, such as Sciaenidae, have begun to increase in the past decade (Table 2). These surveys show that the species composition and community structure of ichthyoplankton in the Yangtze Estuary have changed remarkably, mainly through the reduction in species numbers and the replacement of dominant species (see examples in Table 2). However, there are relatively few research reports on ichthyoplankton in other estuaries in China, except in the Jiujiang Estuary (Jiang and Chen, 1993), Taiwan (Tzeng and Wang, 1993), Laizhou Bay (Song et al., 2010), the Pearl River Estuary (Xiao et al., 2013) and the Yellow River Estuary (Bian et al., 2010; Song et al., 2019). Therefore, more attention should be paid to the study of these estuaries in the future, to understand the community structure of their ichthyoplankton.
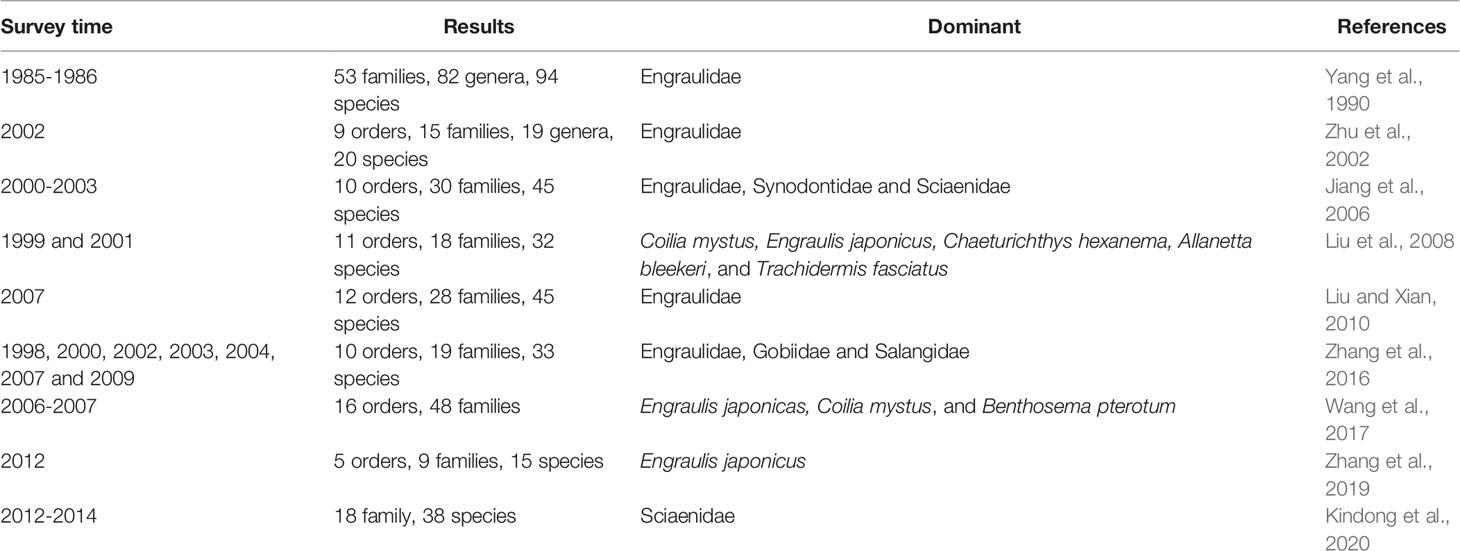
Table 2 Species composition and dominant species of ichthyoplankton in different periods in the Yangtze estuary.
The dominance of the estuarine ichthyoplankton assemblages by members of the Engraulidae, Clupeidae and Gobiidae can be attributed to these taxa spawning in estuaries and being represented mainly by preflexion and flexion larvae that are easily sampled by plankton nets. Many marine taxa that recruit into estuaries as early juveniles are seldom sampled because they tend to be able to avoid most plankton nets. So, the ichthyoplankton community structure as recorded by plankton nets is biased towards those species that are represented in estuaries by early larval stages (Table 3). Moreover, the species composition and abundance in two regions with similar environmental conditions and geographical locations is also similar (de Morais and de Morais, 1994; Korsman et al., 2017). For example, a report compared the composition of several estuarine areas in southwestern Australia and southeastern Africa, which were at similar latitudes (lower than 30°S) and flowed into the Indian Ocean, and found that Clupeidae, Mugilidae, Atherinidae and Gobiidae were important families in these areas (Potter et al., 1990). Similarly, a study comparing the composition of ichthyoplankton in seven permanently open estuaries and five temporarily open estuaries along the coastline of the Eastern Cape of South Africa found that the majority of ichthyoplankton appearing in the two types of estuaries were Clupeidae and Gobiidae (Strydom et al., 2003). New research has confirmed this conclusion (Korsman et al., 2017; Pfirrmann et al., 2021). However, other studies have reported different results. The species richness in the mangrove estuaries of the Western Indo-Pacific was higher than that of tropical Atlantic mangrove estuaries (Robertson and Alongi, 1992). The diversity of larvae in the Sine-Saloum Delta (Senegal) was lower than that of other tropical estuaries (Sloterdijk et al., 2017). This may be caused by large differences between one or more environmental conditions such as temperature, salinity or climate change. Therefore, the ichthyoplankton community structure presents particular distribution characteristics because of the differing adaptability of ichthyoplankton to diverse types of estuaries.
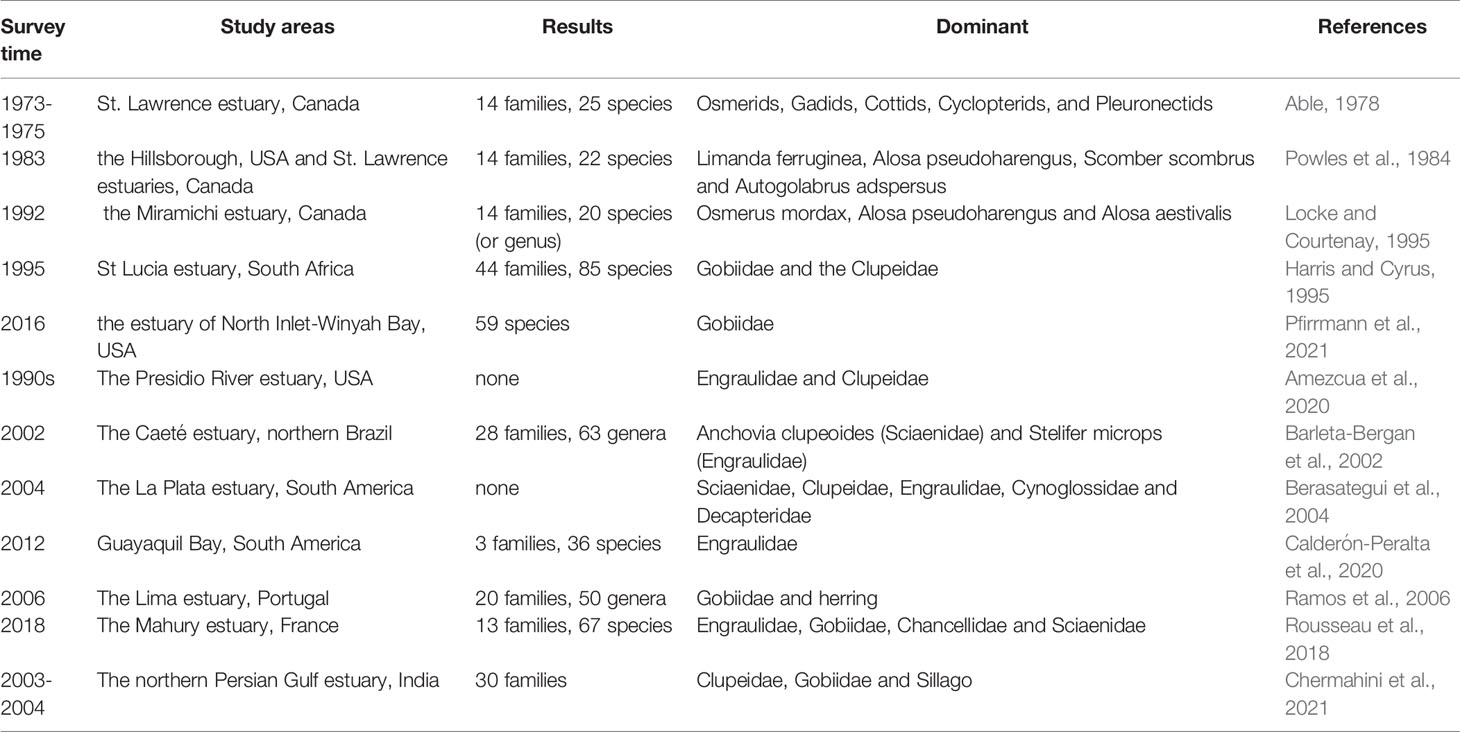
Table 3 Species composition and dominant species of ichthyoplankton in different periods and regions.
4.3 Temporal and Spatial Distribution of Ichthyoplankton in Estuaries
Estuarine areas have complex and variable physical, chemical and hydrological conditions, with environmental factors that change dramatically in time and space (Harris and Cyrus, 1995; Hettler and Hare, 1998). The estuarine ichthyoplankton communities also change in time and space in line with different fish reproductive seasons and environmental factors (Harris et al., 2001; Ren et al., 2021).
There are notable seasonal variations in the composition, abundance and spatial distribution of ichthyoplankton communities (Harris et al., 1999). Generally, the abundance of estuarine ichthyoplankton reaches its peak in spring or summer (Kindong et al., 2020; Monteiro et al., 2021), because these are the reproductive seasons for most fish (Sabates et al., 2007; Primo et al., 2012; Korsman et al., 2017). For example, in the Yangtze Estuary, fish eggs, larvae and juveniles appeared throughout the year, but mainly in spring and summer (Liu and Xian, 2010; Jiang et al., 2013). The number of fish eggs was highest in spring, while the number of larvae and juveniles was relatively small; in summer, the number of fish eggs decreased, while larvae and juveniles increased. The species composition of eggs, larvae and juveniles in different seasons was also notably different (Xian and Luo, 2015; Zhang et al., 2019). Seasonal variation and changes in the quantity of ichthyoplankton species in the Yangtze River were all restricted by changes in hydrological factors, such as runoff and interactions among the Yangtze River, the Yellow Sea cold water mass and the Taiwan warm current (Yang et al., 1990). In addition, the seasonal rhythm of variations in ichthyoplankton was also an adaptation in the distribution of dominant species in time to avoid intense competition and make full use of food resources (Shan et al., 2004). The seasonal distribution of ichthyoplankton was also closely related to environmental factors such as temperature, salinity, depth, chlorophyll a and freshwater input (Wang et al., 2017; Gao et al., 2018).
The spatial distribution of ichthyoplankton is often characterized by the heterogeneity of the horizontal distribution and vertical stratification. This is related to the ecological habits and physiological changes (such as species and development period) of larvae and juveniles, as well as to changes in the external environment (such as prey, light, runoff and tides) (Yang et al., 2006). The difference in horizontal distribution is related to variations in the species and abundance in different regions, as well as to the ecological categorization of species in different regions (Zhang et al., 2016). For example, most of the ichthyoplankton in the Yangtze Estuary in spring belong to the brackish water type, which mainly propagated in the lower Yangtze River channel and the marine area near the estuary with water temperatures of 12.0–22.0°C and salinity of 0.1–12.0 (Yang et al., 1990). In autumn, the ichthyoplankton in the Yangtze Estuary are mainly coastal species, followed by brackish water and inshore species; freshwater ichthyoplankton are the least common (Ding and Xian, 2011). In addition, the ichthyoplankton in the lower estuary mostly belong to marine spawning fish, while those in the upper estuary belong to estuarine spawning fish (Neira and Potter, 1992).
The number of ichthyoplankton species in the estuarine area decreases substantially from the downstream to the upstream. For example, it was found that the monthly average number of species in the lower reaches of the Swan Estuary in Southwestern Australia was far higher than that in the upper reaches of the estuary (Neira and Potter, 1992). However, this distribution pattern is not universal. For example, the highest abundance of ichthyoplankton was found in the upper Lima Estuary (Portugal) (Ramos et al., 2006). Similarly, in the Caeté Estuary (Brazil), the peak density of larvae and juveniles occurred in the upper estuary in the dry season, and the peak number of species occurred in the upper estuary between the dry and wet seasons (Barleta-Bergan et al., 2002). The results in the Jiulong River Estuary (China) showed that the highest number of fish eggs was in the upper estuary, and the highest number of species was in the lower estuary. The larvae and juveniles with the highest number and the greatest diversity of species were relatively concentrated in the middle of the estuary (Jiang and Chen, 1993). Therefore, the horizontal distribution pattern of ichthyoplankton in estuaries is related to both salinity and runoff (Sanvicente-Anorve et al., 2000; Costa et al., 2016).
The difference in the vertical distribution is not only related to the ecological preferences of different species but also to the water structure and fluctuation; for example, the herring family generally live in the upper and middle layer of the estuary, while Gobiidae inhabit the bottom of estuarine and coastal waters (Muhling et al., 2007). In addition to examining the vertical distribution of the ichthyoplankton community in estuaries, studies are currently focusing on individual activities such as the depth trend of some species and their diurnal periodic vertical movement. For example, the larvae of Anchoa mitchilli and Gobiosoma bosc in the Hudson estuary (America) showed periodic vertical movement behavior over the day and night (Schultz et al., 2003). The vertical migration of larvae and juveniles may be used to avoid cold and low-salinity surface water (Ramos et al., 2006). In addition, vertical migration could help the larvae and juveniles use the two-layer circulation to maintain a dynamic balance and avoid being washed out of the estuary (Weinstein et al., 1980).
4.4 Application of DNA Barcoding in Estuarine Ichthyoplankton Identification
DNA barcoding is an effective method for species identification based on molecular biology techniques. It identifies species through differences in the sequence of standardized short gene fragments (Chu et al., 2019). Identification is possible if, first, the fragment is conservative enough to be amplified in a wide range with universal primers, and second, the fragment has enough variation to distinguish the DNA sequences of different species (Hebert et al., 2003). Compared with the traditional morphological classification methods, the biggest advantage of the DNA barcoding technique is that it can accurately identify individuals at different development stages, as well as species with high morphological similarity and individuals that are difficult to distinguish owing to the lack of morphological classification data (Liu et al., 2021). The application of this technique to the identification of ichthyoplankton can solve many problems that cannot be solved by traditional morphological classification methods. For example, Bian et al. used cytochrome oxidase subunit I sequence to identify fish eggs collected from seaweed in the Yellow Sea area, and determined that they were Hyporhamphus sajori, rather than Strongylura anastomella, which has a similar egg morphology, spawning time and geographical distribution (Bian et al., 2007).
However, DNA barcoding is not applicable for the identification of all fish. Some fishes, such as Epinephelinae and Sillago, are prone to hybridization (Lin et al., 2014). Therefore, it is difficult to identify them only from the cytochrome oxidase subunit I sequence, and joint labeling with other genes is required. In addition, because the databases are incomplete, some fish records have only 16S and 12S fragments, and the cytochrome oxidase subunit I gene cannot be identified to the species level (Li et al., 2017). For some related species, DNA barcoding needs to be combined with morphology, geography and molecular genetics to identify them accurately. A combination of traditional morphological identification and DNA barcoding can improve the accuracy and efficiency of ichthyoplankton species identification (Zhang G et al., 2020; Liu et al., 2021).
In summary, the development of DNA barcoding techniques will become a powerful supplement to the traditional taxonomy of ichthyoplankton and provide new opportunities for the development of systematic taxonomy in this field. The application of DNA barcoding techniques in ichthyoplankton can address the limitations of traditional morphological classification. Because DNA barcoding is a digital format, the sample identification process can be automated and standardized, reducing the need for expert input and establishing an easy-to-use database in a short time. Both taxonomists and non-professionals will be able to find species information on ichthyoplankton rapidly through the international database and identify potential hidden species or new species. The development of DNA barcoding techniques will greatly alleviate the limitations of ichthyoplankton morphological identification and the shrinking number of traditional taxonomists.
5 Factors Influencing Ichthyoplankton Community Structure in Estuaries
The factors affecting the community structure of estuarine ichthyoplankton have been one of the most important concerns of researchers. In this study, we artificially divided the influencing factors into natural and anthropogenic factors. Natural factors include temperature, salinity, turbidity, hydrodynamic conditions, food, predators, and climate change. And the anthropogenic factors include construction of dams, overfishing and pollution. The results showed that 540 of 836 literatures were on factors affecting community structure, with temperature and salinity (310) being the most documented, followed by hydrodynamics (177) and food (128) (Table 1).
There are differing views on the reasons for changes in the ichthyoplankton community structure in estuaries. Many researchers believe that the regional change in fish communities is mainly caused by gradients of temperature, salinity and depth as well as different sediment types (Liu et al., 2008; Monteiro et al., 2021; Chermahini et al., 2021). However, other studies propose that individual behavior plays an important role in the distribution of ichthyoplankton (Costa et al., 2011; Munk et al., 2014). A few studies suggest that the spatiotemporal variation of the ichthyoplankton community is mainly related to the meteorological and seasonal characteristics of the ocean, especially in the middle latitudes (Hernández-Miranda et al., 2003; Thaxton et al., 2020). There are also reports that the horizontal distribution of larvae and juveniles in temperate coastal waters is related to the distribution of adults, as well as the location and type of spawning (floating or sinking) (Azeiteiro et al., 2006; Ribeiro et al., 2015). Other studies have found that in addition to temperature and salinity, suspended solids, suspended organic matter, chlorophyll a and runoff all affect the spatial structure of ichthyoplankton in estuaries (Faria et al., 2006; Ramos et al., 2006; Zhang et al., 2015). In addition, the annual cyclical changes of climate and hydrodynamics also have a strong impact on ichthyoplankton communities in estuaries (Sloterdijk et al., 2017; Gao et al., 2018). To review the contribution of each factor affecting the ichthyoplankton community structure in the estuary more clearly, we divided the factors into two types (natural factors and anthropogenic factors) to discussion.
5.1 Natural Factors
5.1.1 Temperature and Salinity
Temperature has a major and direct impact on fish reproduction, recruitment, physiology, growth and behavior (Sabates et al., 2006; Doring et al., 2018). These are important for the quantity and spatial distribution of ichthyoplankton (Bruno et al., 2014; Chermahini et al., 2021). Estuaries are generally shallow, and the temperature varies greatly, with both diurnal and seasonal variations. Thus, estuarine species that can adapt to a wide range of temperatures tend to be dominant (Yang et al., 2006). In temperate and subtropical estuaries, the peak of species numbers generally occurs in spring and summer (Primo et al., 2011; Wang et al., 2017). In other words, temperature affects the reproductive cycle and timing of adult fish (Holmes and Henderson, 1990; Palomera, 1992). Taking a simple example, in the Yangtze Estuary, the water temperature rose from spring (average of 19.9°C) to summer (average of 29.9°C), the food supply was plentiful, many fish spawned in the estuary, and the species and quantity of fish eggs and juveniles in the estuary increased greatly. In winter, the water temperature dropped (average of 16.6°C), the food supply was scarce, and the number of spawning fish and the species and quantity of fish eggs, larvae and juveniles was greatly reduced (Jiang and Chen, 1993). The peak abundance of larvae and juveniles usually occurred just before or after the maximum water temperature (Newton, 1996). Thus, the seasonal variation of temperature played a key role in the temporal variation of the juvenile fish community (Demirel, 2015).
Salinity is also an important environmental factor affecting estuarine organisms. For example, it affects the hatching period of fish. In some species, the hatching period is shortened with an increase in salinity, while in others it is prolonged with an increase in salinity; a few species are not affected by salinity (Wang and Zhang, 1998). Salinity not only affects the growth, development and reproduction of ichthyoplankton, but also the spatiotemporal distribution and abundance of species (Jiang et al., 2013; Lima et al., 2019). Salinity is a key abiotic factor determining the structure of the ichthyoplankton community in estuaries (Wooldridge, 1999; Campfield and Houde, 2011; Song et al., 2019). In estuarine areas, salinity is affected by runoff, wind, waves and tidal current, and has complex temporal and spatial variations. The special geographical conditions of estuaries and the inflow of potentially large amounts of fresh water determine that the salinity gradually increases with distance from the river mouth, and the different ecological categorizations and distributions of estuarine organisms often reflect the salinity gradient (Mouny and Dauvin, 2002). Generally, estuarine salinity is high in the dry season and low in the flood season. In the Caeté Estuary (Brazil), seasonal fluctuations in salinity caused changes in the number of ichthyoplankton species and community composition (Barleta-Bergan et al., 2002). Reduced salinity levels may cause a peak in the number of fish eggs and larvae, and low salinity coastal systems have a marked impact on the hatching and development of fish eggs and larvae in the Yangtze Estuary (Jiang et al., 2006). In addition, salinity can interact with organic compounds and modulate their toxicity, which is essential to ichthyoplankton growth in estuaries (Segarra et al., 2021).
5.1.2 Turbidity
Estuarine water carries substantial quantities of suspended particles, which result in high turbidity, especially when there is a large inflow of river water. Turbidity has an important impact on the temporal and spatial distribution of ichthyoplankton in estuaries (Costa et al., 2011). The most abundant species in the Large Swan Estuary (Australia) were estuarine resident larvae and juveniles because of the turbid conditions in the upper estuary (Neira and Potter, 1992). Thus, turbidity can be considered as one of the most important factors affecting the density of estuarine species (Harris and Cyrus, 1995). The maximum turbidity zone of estuaries has been widely studied because it is a universal feature of estuarine dynamics (Schubel, 1968; Jager, 1999; Islam et al., 2006). This zone also has a high proportion of food organisms and is therefore more conducive for the feeding of ichthyoplankton (Shideler and Houde, 2014). In high turbidity areas, the probability of ichthyoplankton encountering predators is also reduced, which improves the survival rate (Parrish, 1989). Many fish rely on vision to catch their prey, and the level of light in turbid water can affect the behavior and predation of larvae and juveniles (Shoji et al., 2006; Bradbury et al., 2006; Chicharo et al., 2009). Accordingly, the maximum turbidity zone of estuaries often has the highest abundance of larvae and juveniles (North and Houde, 2003). In contrast, Faria et al. found a low density and abundance of ichthyoplankton in the maximum turbidity zone of the Guadiana Estuary on the Iberian Peninsula. However, this may have been caused by: a) high turbidity hindering feeding by some species; b) large mesh sizes in the net allowing many small individuals to escape; and c) the larvae and juveniles in the maximum turbidity zone moving deeper in the water during the day when the trawl was carried out (Faria et al., 2006). The maximum turbidity zone of the estuary can change over a short time scale in some seasons owing to the injection of fresh water and the effect of wind (North and Houde, 2003); this leads to an associated change in the ichthyoplankton community.
5.1.3 Hydrodynamic Conditions
Many marine fish migrate from open ocean spawning grounds to estuarine nursery grounds in their early life cycle stage (Churchill et al., 1999; Islam et al., 2007). Because estuaries are at the confluence of rivers and the ocean, the migration of ichthyoplankton is affected by complex hydrodynamic conditions, such as river runoff, tides, water masses and ocean currents. Estuaries pose a special challenge to the migration of ichthyoplankton because the speed of river runoff is usually faster than the swimming speed of larvae and juveniles (Forward and Tankersley, 2001; Schultz et al., 2003). Freshwater injection and the tidal action of seawater were found to be the two main factors determining the physical and chemical properties of estuarine ecosystems (Yang et al., 2006). Consequently, changes in estuarine hydrodynamic conditions not only affect the migration of ichthyoplankton, but also affect their community structure and distribution.
River runoff is a key factor in adjusting the structure and function of estuarine systems (Taylor, 1982). The change in estuarine runoff can cause many effects, including floodplain development, accelerated transport of organic matter, dilution and movement of pollutants, shifts in peak salinity and the formation of density gradients, changes in the residence time of some particles and biota in the estuary (Kimmerer, 2002), changes in topography, and changes in turbidity and dissolved oxygen levels. These effects influence the abundance and distribution pattern of estuarine organisms (Drinkwater and Frank, 1994; Grange and Allanson, 1995; Chiappa-Carrara et al., 2003; Monteiro et al., 2021). The location of the spawning center in the Yangtze Estuary tends to move nearshore or offshore depending on the amount of fresh water entering the sea, salinity and transparency (Yang et al., 1990).
Runoff was the main factor affecting the abundance and distribution of ichthyoplankton in the Guadiana Estuary (Spain and Portugal) before the construction of the Alqueva Dam (Portugal) (Faria et al., 2006). Although the inflow of fresh water is basically stable year after year, it can still affect the recruitment of some species of larvae and change the composition of the ichthyoplankton community (de Morais and de Morais, 1994). The relationship between species abundance and river runoff also varies because different species have different adaptations to the flow and time of runoff (Costa et al., 2007). For example, the abundance and survival rate of several estuary-dependent species increased with the injection of fresh water (Kimmerer, 2002). Whitfield found that ichthyoplankton abundance in Eastern Cape estuaries (southern Africa) increased with increasing riverine input (Whitfield, 1994). In Japan, the runoff from Chikugo River affected the recruitment of Lateolabrax japonicus in two ways: a) recruitment was increased by increasing the temperature and accelerating the growth of larvae and juveniles; and b) high inflows of runoff reduced the possibility of migration to the estuary by dispersing larvae and juveniles in the ocean, enhanced the predation activities of other organisms by reducing turbidity, and reduced the availability of predated zooplankton (Shoji et al., 2006).
The water level and currents in estuaries fluctuate periodically owing to the influence of tides in nearby coastal waters. A substantial quantity of seawater can flow into estuarine areas driven by the wind, thus changing the original habitat conditions for estuarine organisms and affecting their community pattern (Tzeng and Wang, 1993). The resulting estuary tides are usually larger and more complex than tides in the open ocean. Under the action of tides, the salinity of estuaries increases, especially in the middle and lower reaches, and the water body is relatively stratified with a high abundance of shallow marine organisms. If the effect of flushing water is strong, the salinity of the estuary decreases, and the water is better mixed. In this case, the dominant species of the biological community are mainly estuarine species, or even freshwater species (Yang et al., 2006). According to the relationship between tidal influences and species abundance, fish could be divided into two groups: a) at low tide, they are mainly freshwater species; and b) at high tide, they are mainly marine species. In general, at high tide, more species are found in the lower reaches of the estuary because many marine species enter the estuary with the tide (‘selective tidal stream transport’), increasing the species diversity (Kingsford and Suthers, 1996; Faria et al., 2006; Primo et al., 2012).
Tides not only affect the distribution and structure of ichthyoplankton communities, but also considerably influence the migration, predation, avoidance and reproduction of larvae and juveniles (Gibson, 2003). Seasonal species can be carried from the ocean to the estuary by tidal currents, but migration to the estuary can be hindered by high-throughput runoff, especially in the surface water layer. This effect often occurs in the subtropical estuaries of South America (Garcia et al., 2003). In tidal regions, animals with weak swimming abilities may accomplish efficient directed transport by ascending in the water column during the appropriate tidal phase and holding position on the bottom during the opposite tide. The behavior of modulating horizontal transport through timed vertical migration is termed ‘selective tidal stream transport’ (Walker et al., 1978) and is used by a number of species, including ichthyoplankton, living in tidally energetic regions. The interaction between tidal currents and larval behavior (vertical migration) also plays an important role in the upwelling movement and retention of plankton in the original habitat (Chen et al., 1997). Larvae and juveniles appeared in the upper layer of a water body at high tide and in the lower layer of a water body at low tide, which indicated that the larvae and juveniles were using the tide as a retention mechanism (Islam et al., 2007). For example, in the Swartkops estuary (South Africa), early juveniles of marine species which utilize the estuary as a nursery ground, such as Rhabdosargus holubi, Liza richardsoni and Heteromycteris capensis, enter the estuary on the flood-tide and actively migrate towards the banks to prevent being swept back out to sea (Beckley, 1985). Similarly, in Swartvlei estuary (South Africa), Rhabdosargus holubi enter the estuary mainly as postlarvae, and congregate along the margins or on the bottom where water current speeds are reduced (Whitfield, 1989b).
In addition to runoff and tides, other hydrodynamic conditions also affect ichthyoplankton in estuaries. For example, the reproduction of Engraulidae is restricted by the specific geographical location of the Yangtze Estuary and the variation of water potential in various other systems, such as the Yangtze River runoff, the Kuroshio warm current and the Yellow Sea cold water mass (Wu, 1989). Upwelling can make the nearshore seawater flow toward the ocean, thus delaying the replenishment of seawater farming land to the estuary as well as reducing the species diversity in the lower estuary (Ramos et al., 2006; Valencia et al., 2019). Hydrodynamic conditions such as the Kuroshio Current and upwelling can increase food supplies for the ichthyoplankton in estuaries, thus affecting their community structure (Huang et al., 2017).
5.1.4 Food
In the early life stages after hatching, fish rely on yolk as a food resource for a very short time. After a brief period of mixed nutrition, they switch completely to external food sources. For example, the larvae of marine fish mainly feed on copepods (Dagg and Govoni, 1996), while freshwater fish mainly feed on rotifers (Wang et al., 2006). Individual fish species select different food organisms at different growth and development stages. For example, in the Yangtze Estuary (China), the larvae and juveniles of Coilia mystus feed on zooplankton such as Cladocera and Amphipoda. And then their food composition changes gradually to the eggs and juveniles of fish such as Larimichthys polyactis, Chaetorichthys stigmatias and Harpadon nehereus, shrimps, copepods and Amphipoda, and other small animals, such as squid and squilla (Wang et al., 2006). Similarly, in the Sundays Estuary (South Africa), larvae also showed changes in diet at different stages of development (Strydom et al., 2014). From the perspective of spatial distribution, larvae and juveniles prefer to live in marine areas with a stable and reliable food supply of plankton (Whitfield, 1999).
In the Mira and Guadiana Estuaries (Portugal), the spatial and diurnal distribution of larvae and juveniles was related to the vertical and horizontal migration of food organisms (Esteves et al., 2000). The species and quantity of food organisms have an important impact on the ichthyoplankton community. Larvae and juveniles were found to gather in deep areas with a high food concentration (Shideler and Houde, 2014). According to Drake et al., stepwise multiple regression analysis of the spatial and temporal distribution of Engraulis encrasicolus showed that its main food source, Mesopodopsis slabberi, could explain 45% of its change in density (Drake et al., 2007). The peak abundance of larvae and juveniles in spring and summer was related to the large-scale reproduction of zooplankton after the flood season, because a large number of zooplankton provided a good food supply for young fish larvae and juveniles (Newton, 1996). In some estuarine ecosystems, the peak abundance of larvae and juveniles coincides with the peak of phytoplankton productivity and biomass (Livingston et al., 1997; Garcia et al., 2003).
5.1.5 Predators
In estuarine and marine ecosystems, adult fish, invertebrates, mammals and some zooplankton have a strong predation effect on ichthyoplankton. The complex feeding relationship has an important impact on ichthyoplankton communities, especially abundance. In the Yangtze Estuary, the feeding relationship between planktonic copepods and fish eggs and larvae determines that changes in abundance affect the quantity of fish eggs and larvae (Jiang et al., 2006). According to Esteves et al., the abundance of larvae and juveniles in the Mira and Guadiana Estuaries (Portugal) was positively correlated with the abundance of several invertebrates that prey on fish eggs and larvae (Esteves et al., 2000). Jellyfish are important predators of larvae and juveniles, and they are also important regulators of the zooplankton community structure in several ecosystems (Pereira et al., 2014). For example, the monthly decline in ichthyoplankton community abundance in 2002 (Guadiana Estuary, Portugal) was closely related to the increase in jellyfish abundance (Faria et al., 2006).
5.2 Climate Change
Ichthyoplankton are thought to be sensitive to climate change, and a number of studies have focused on the impact of climate change on the distribution and interannual change of ichthyoplankton communities (Zhang Z. et al., 2020). Stochastic climate events (cyclones) changed the community composition of ichthyoplankton in estuaries by bringing intense rainfall, which caused the distribution and abundance of estuary resident species and marine migratory species to change substantially (Martin et al., 1992). The seasonal changes in temperature and precipitation caused by climate change were the main reasons for the temporal differences of larvae and juveniles, which strengthens the supposition that interannual climate and hydrodynamic changes have a strong impact on the composition of estuarine ichthyoplankton communities (Ramos et al., 2006).
Global warming causes changes in the temperature, salinity, dissolved oxygen and other environmental factors, resulting in changes to the temporal and spatial distribution of ichthyoplankton (Sloterdijk et al., 2017). The increase in temperature may lead to earlier fish spawning (Thaxton et al., 2020), extension of the spawning time (Primo et al., 2011; Acha et al., 2012), shortening of egg hatching time (Hassell et al., 2008), early gonadal maturation (Thaxton et al., 2020) and northward expansion of spawning areas (Auth et al., 2018; Zhang et al., 2019).
Droughts and water shortages may reduce river runoff to the extent that the salinity stratification in estuaries is reduced, resulting in a decline in fish with strong dependence on estuary conditions, such as Acanthopagrus butcheri (Williams et al., 2013). This may have a substantial impact on the development and supply of larvae and juveniles (Primo et al., 2011). Climate change also causes hypoxia and ocean acidification, which greatly reduces the survival rate of ichthyoplankton embryos (Hassell et al., 2008; Valencia et al., 2019). The impacts of climate variability on ichthyoplankton assemblages will have consequences for marine pelagic food webs and fish population biology, namely recruitment (Monteiro et al., 2021).
The El Niño–Southern Oscillation is an irregular fluctuation that can affect the entire tropical Pacific as well as the global climate. It is a manifestation of ocean–atmosphere interactions, which leads to fluctuations in the wind, rainfall, thermocline depth and circulation, and ultimately affects the biological productivity and changes the feeding and reproduction of fish, birds and mammals (Fiedler, 2002). El Niño can affect the cold water mass of the Yellow Sea and the Taiwan warm current, altering the ocean temperature, salinity and food sources, and changing the location of the central spawning grounds and hence the temporal and spatial distribution pattern of ichthyoplankton in estuaries (Shan et al., 2004). A similar conclusion was reached in a study of the Central Gulf of California (Sánchez-Velasco et al., 2004). In addition, the El Niño phenomenon can affect the rate of recruitment of larvae and juveniles to the adult fish community. In an El Niño year, the recruitment rate is higher than the average level, but in the years before and after El Niño, the recruitment rate is lower than the average level (Franco-Gordoa et al., 2004; Acha et al., 2012). Therefore, under the influence of global climate change, the community structure, distribution range and life history characteristics of ichthyoplankton will undergo major changes.
5.3 Anthropogenic Factors
5.3.1 Construction of Dams
Over the past few decades, many large-scale dam construction projects have occurred in or near rivers and estuaries. These projects play an important role in economic development and social progress, but also have a considerable impact on the ecological environment of estuaries (Burns et al., 2006). The construction of dams in the main stream of a river can change the flow pattern of the whole river, particularly the distribution of runoff and sediment downstream of the dam, which results in changes in the amount of runoff, sediment and nutrients flowing into the estuary and sea (Shan et al., 2004). This alters the hydrological dynamics and sediment deposition processes of estuaries and their adjacent coasts, thus changing the spatial pattern of ichthyoplankton in the estuary (Faria et al., 2006). For example, large-scale water conservancy projects in the main stream of the Yangtze River altered the original seasonal distribution of runoff. The flow velocity, sediment discharge, salinity distribution and bottom material conditions were all changed (Shan et al., 2004). Owing to the backflow of seawater, the salinity in the Yangtze Estuary has increased, which affects the distribution of fish species in the estuary. The distribution area of offshore species has expanded and that of estuarine fish has contracted (Xian and Luo, 2015). Owing to the increase in transparency in estuarine waters caused by the decreased inflow of sediment, areas with high phytoplankton productivity have expanded, and the position of spawning and feeding grounds of many fish has changed accordingly (Luo, 1994; Gao et al., 2018).
After the Aswan Dam was built on the Nile River in Egypt, the nutrients transported to the waters of the Nile Estuary decreased by more than 90%, resulting in the serious depletion of fishery resources in the coastal waters (Nixon, 2004). Similarly, the Alqueva Dam has affected the abundance and community structure of ichthyoplankton in the estuary by changing the river runoff (Faria et al., 2006). Thirty years after the completion of the dam in the upper Petitcodiac River in Canada, the representative anadromous species had disappeared (Aube et al., 2005).
5.3.2 Overfishing
The combination of nutrient-rich fresh and saline aquatic habitats in estuaries makes them extremely productive for fisheries; for this reason, many large fishing grounds are located near estuaries (Shan et al., 2004). In recent years, fishing intensity has increased annually owing to the modernization of fishing gear and the improvement of fishing technology, far exceeding the capacity of resource recruitment (Song et al., 2019). This has greatly impacted ichthyoplankton as the recruitment source for fisheries and has changed the community composition. Some economically valuable species have disappeared from their original habitats, fishery resources have been degraded, and many traditional fishing grounds have been become uneconomic (Hale et al., 2016). For example, in the Yangtze Estuary, overfishing of Trichiurus japonicus, Larimichthys polyactis and other important economic species has led to fish miniaturization, early maturity and a young age structure (Shan et al., 2004). The primary reason for the miniaturization of the fish community is the uncontrolled overuse of marine biological resources in coastal waters (Zhu et al., 2002).
Overfishing is the main cause of the substantial reduction of fishery resources (Song et al., 2019). Consequently, overfishing and environmental degradation lead to a loss of ecosystem resilience and integrity. Because the fishery yield is dependent on ecosystem productivity, a more unstable ecosystem leads to unpredictable changes in both quality and quantity (Ryder et al., 1981). Overfishing greatly reduces the survival rate of adult fish and affects spawning, resulting in a reduction of ichthyoplankton abundance. Therefore, under the combined effect of overfishing and environmental change, the population and community structure of ichthyoplankton in estuarine ecosystems has changed markedly (Bian et al., 2010).
5.3.3 Pollution
Human activities are highly concentrated in estuarine areas, and environmental changes have a profound impact. The development of modern industry and agriculture has led to a substantial quantity of industrial wastewater and domestic sewage being discharged into estuaries through various pathways. The discharge volume and the concentration of pollutants are increasing year by year, causing eutrophication and declining water quality of estuaries and their adjacent coastal waters.
In addition to red tides, eutrophication may also lead to oxygen deficiency or even anoxia in estuaries and nearby waters. Oxygen deficiency can destroy the biological community structure in affected waters, which changes fish food resources, including the species composition and quantity of plankton, aquatic plants, benthos and other food organisms, disrupting the food chain of fish (Liu et al., 2008). In addition, oil pollution from factories and shipping is becoming more serious. Sedimentary oil can also cause secondary pollution, leading to long-term pollution of water bodies and damaging the normal biochemical processes. This, in turn, destroys the spawning and feeding grounds of fish over a large area, damaging the self-renewal mechanism of fish resources and leading to an imbalance in fish community niches. Fish larval physiology has been changed by pollution, leading to increased tissue damage and decreased survival rates, all of which affect the structure of estuarine fishery resources (Shan et al., 2004; Bajo et al., 2020).
Microplastics (particle size < 5 mm) do great harm to juvenile fish. While ingestion of microplastics leads to intestinal obstruction of juvenile fish, which affects their feeding, microplastics also have a high capacity to absorb pollutants, which leads to the accumulation of pollutants in the body (Rodrigues et al., 2019). However, the specific impact of microplastics on the ichthyoplankton community structure of estuarine fish is unclear.
6 Conclusion
This review contributes important theoretical and practical information for estuarine environmental protection and the sustainable use of estuarine resources. We found an increase in the amount of research on estuarine ichthyoplankton over time, but it was mainly concentrated in developed countries. Salinity and turbidity are the main factors affecting changes in ichthyoplankton community structure in estuaries. Climate change indirectly affects the community structure of estuarine ichthyoplankton by altering the spawning time, spawning location and egg hatching time of adult fish. The movement of spawning sites poleward and the advance of spawning time have become a consistent trend. Although there is little research on the DNA barcoding of ichthyoplankton, evidence suggests that the wide use of DNA barcoding will provide an effective solution for the accurate identification of ichthyoplankton.
Therefore, strengthening the research on estuarine ichthyoplankton in developing countries can further deepen the understanding of estuarine ichthyoplankton ecology and help to provide references for the protection of fishery resources in these countries. The impact of climate change on ichthyoplankton in estuaries has attracted more and more attention, but its potential driving mechanism needs to be further explored. The impact of human activities (such as overfishing, environmental pollution and dam construction) on ichthyoplankton in estuaries is often ignored, and more attention should be paid to it. DNA barcoding has broad development prospects in the identification of ichthyoplankton. The identification method combining molecular and morphology may become the mainstream method in the future.
Although this study systematically reviews the research on ichthyoplankton in estuaries all over the world, there are still many limitations. On the one hand, many non-English literature sources are excluded, which leads to some deviations in the statistical results. On the other hand, this study focuses on the community structure and influencing factors of estuarine ichthyoplankton, but does not discuss its potential driving mechanism in detail. Thus, to strengthen the study of estuarine ichthyoplankton ecology, we need to fully understand the structure and function of estuarine ecosystems and the impact of human activities on estuarine resources so that we can develop protective countermeasures.
Author Contributions
HZ and YW analyzed the literatures and completed the first draft. HZ and WX provided guidance on the structure of the paper. SL provided the suggestions on this paper, and YW, CL and HZ modified the paper. All authors were involved in the revision of the original manuscript. All authors contributed to the article and approved the submitted version.
Funding
The present work was supported by National Natural Science Foundation of China (No. 41976094 and 31872568); Key Deployment Project of Center for Ocean Mega-Science, Chinese Academy of Sciences (COMS2019Q14); Youth Innovation Promotion Association CAS (No.2020211).
Conflict of Interest
The authors declare that the research was conducted in the absence of any commercial or financial relationships that could be construed as a potential conflict of interest.
The reviewer YL is currently organizing a Research Topic with the author HZ.
Publisher’s Note
All claims expressed in this article are solely those of the authors and do not necessarily represent those of their affiliated organizations, or those of the publisher, the editors and the reviewers. Any product that may be evaluated in this article, or claim that may be made by its manufacturer, is not guaranteed or endorsed by the publisher.
Acknowledgments
We thank Leonie Seabrook, PhD, from Liwen Bianji (Edanz) (www.liwenbianji.cn/), for editing the English text of a draft of this manuscript.
Supplementary Material
The Supplementary Material for this article can be found online at: https://www.frontiersin.org/articles/10.3389/fmars.2022.794433/full#supplementary-material
References
Able K. W. (1978). Ichthyoplankton of the St. Lawrence Estuary - Composition, Distribution, and Abundance. J. Fish. Res. Board Can. 35 (12), 1518–1531. doi: 10.1139/f78-241
Acha E. M., Simionato C. G., Carozza C., Mianzan H. (2012). Climate-Induced Year-Class Fluctuations of Whitemouth Croaker Micropogonias Furnieri (Pisces, Sciaenidae) in the Rio De La Plata Estuary, Argentina-Uruguay. Fish. Oceanogr. 21 (1), 58–77. doi: 10.1111/j.1365-2419.2011.00609.x
Amezcua F., Rodriguez-Preciado J. A., Calderon-Perez A., Rendon-Rodriguez S., Green L., Chazarreta C. J. (2020). Larval Fish Assemblages in Nearshore Waters of Southeast Gulf of California: Vertical and Temporal Patterns. Neotrop. Ichthyol. 18 (1), e190102. doi: 10.1590/1982-0224-2019-0102
Aube C. I., Locke A., Klassen G. J. (2005). Zooplankton Communities of a Dammed Estuary in the Bay of Fundy, Canada. Hydrobiologia 548, 127–139. doi: 10.1007/s10750-005-4730-0
Auth T. D., Daly E. A., Brodeur R. D., Fisher J. L. (2018). Phenological and Distributional Shifts in Ichthyoplankton Associated With Recent Warming in the Northeast Pacific Ocean. Glob. Change Biol. 24 (1), 259–272. doi: 10.1111/gcb.13872
Azeiteiro U. M., Bacelar-Nicolau L., Resende P., Goncalves F., Pereira M. J. (2006). Larval Fish Distribution in Shallow Coastal Waters Off North Western Iberia (NE Atlantic). Estuarine Coastal Shelf Sci. 69 (3-4), 554–566. doi: 10.1016/j.ecss.2006.05.023
Bajo B. A., Castro L. R., Costalago D. (2020). Elucidating Trophic Pathways of the Most Abundant Fish Larvae in Northern Patagonia Using δ13c and δ15n Isotopes. Mar. Ecol.: Prog. Ser. 650, 253–267. doi: 10.3354/meps13374
Barleta-Bergan A., Barletta M., Saint-Paul U. (2002). Structure and Seasonal Dynamics of Larval Fish in the Caete River Estuary in North Brazil. Estuarine Coastal Shelf Sci. 54 (2), 193–206. doi: 10.1006/ecss.2001.0842
Barragán J. M., de Andrés M. (2015). Analysis and Trends of the World’s Coastal Cities and Agglomerations. Ocean Coast. Manage. 114, 11–20. doi: 10.1016/j.ocecoaman.2015.06.004
Beckley L. E. (1985). Tidal Exchange of Ichthyoplankton in the Swartkops Estuary Mouth, South Africa. South Afr. J. Zool. 20, 15–20. doi: 10.1080/02541858.1985.11447906
Berasategui A. D., Acha E. M., Araoz N. C. F. (2004). Spatial Patterns of Ichthyoplankton Assemblages in the Rio De La Plata Estuary (Argentina-Uruguay). Estuarine Coastal Shelf Sci. 60 (4), 599–610. doi: 10.1016/j.ecss.2004.02.015
Bian X. D., Zhang X. M., Gao T. X., Wan R. J., Zhang P. D. (2010). Category Composition and Distributional Patterns of Ichthyoplankton in the Yellow River Estuary During Spring and Summer 2007. J. Fish. Sci. China 17 (4), 815–827. doi: CNKI:SUN:ZSCK.0.2010-04-024
Bian X. D., Zhang X. M., Gao T. X., Xiao Y. S., Wan R. J. (2007). Identification of Fish Eggs of Japanese Halfbeak (Hyporhamphus Sajori) Using mtDNA Sequencing. J. Ocean Univ. China 37, 111–116. doi: 10.16441/j.cnki.hdxb.2007.s1.018
Blaber S. J. M. (1997). Fish and Fisheries of Tropical Estuaries. Trans. Am. Fish. Soc. 128 (1), 183–184. doi: 10.1577/1548-8659(1999)128<0183:FAFOTE>2.0.CO;2
Boehlert G. W., Morgan J. B. (1985). Turbidity Enhances Feeding Abilities of Larval Pacific Herring, Clupea Harengus Pallasi. Hydrobiologia 123 (2), 161–170. doi: 10.1007/BF00018978
Bonecker A. C. T., de Castro M. S., Costa P. G., Bianchini A., Bonecker S. L. C. (2019). Larval Fish Assemblages of the Coastal Area Affected by the Tailings of the Collapsed Dam in Southeast Brazil. Reg. Stud. Mar. Sci. 32, 100848. doi: 10.1016/j.rsma.2019.100848
Bradbury I. R., Gardiner K., Snelgrove P. V. R., Campana S. E., Bentzen P., Guan L. (2006). Larval Transport, Vertical Distributions and Localized Recruitment in Anadromous Rainbow Smelt (Osmerus Mordax). Can. J. Fish. Aquat. Sci. 63 (12), 2822–2836. doi: 10.1139/F06-164
Bruno D. O., Delpiani S. M., Cousseau M. B., de Astarloa J. M. D., Blasina G. E., Mabragana E., et al. (2014). Ocean-Estuarine Connection for Ichthyoplankton Through the Inlet Channel of a Temperate Choked Coastal Lagoon (Argentina). Mar. Freshw. Res. 65 (12), 1116–1130. doi: 10.1071/Mf13128
Burns M. D. M., Garcia A. M., Vieira J. P., Bemvenuti M. A., Marques D., Condini V. (2006). Evidence of Habitat Fragmentation Affecting Fish Movement Between the Patos and Mirim Coastal Lagoons in Southern Brazil. Neotrop. Ichthyol. 4 (1), 69–72. doi: 10.1590/s1679-62252006000100006
Butler J. L., Jacobson L. D., Barnes J. T., Moser H. G. (2003). Biology and Population Dynamics of Cowcod (Sebastes Levis) in the Southern California Bight. Fish. Bull. 101 (2), 260–280.
Calderón-Peralta G., Ayora-Macias G., Solís-Coello P. (2020). Variación Espacio-Temporal De Larvas De Peces En El Golfo De Guayaquil, Ecuador. J. Coast. Res. 49 (1), 135–156. doi: 10.25268/bimc.invemar.2020.49.1.778
Campfield P. A., Houde E. D. (2011). Ichthyoplankton Community Structure and Comparative Trophodynamics in an Estuarine Transition Zone. Fish. Bull. 109 (1), 1–19. doi: 10.1007/s12562-010-0303-x
Cao W., Chang J., Duan Z. (2007). Fish Resources of Early Life Stages in Yangtze River (Beijing, China: Waterpower Press).
Chambers R. C., Trippel E. A. (1997). “Early Life History and Recruitment: Legacy and Challenges,” in Early Life History and Recruitment in Fish Populations (London: Chapman and Hall), 515–549. doi: 10.1007/978-94-009-1439-1_19
Chandra R. (1962). A Preliminary Account of Distribution and Abundance of Fish Larvae in Hooghly Estuary. Indian J. Fish. Sect. A. 9 (1), 48. doi: 10.1007/978-1-4020-2792-5_6
Chen Y. H., Shaw P. T., Wolcott T. G. (1997). Enhancing Estuarine Retention of Planktonic Larvae by Tidal Currents. Estuarine Coastal Shelf Sci. 45 (4), 525–533. doi: 10.1006/ecss.1996.0217
Chermahini M. A., Shabani A., Naddafi R., Ghorbani R., Rabbaniha M., Noorinejad M. (2021). Diversity, Distribution, and Abundance Patterns of Ichthyoplankton Assemblages in Some Inlets of the Northern Persian Gulf. J. Sea Res. 167, 101981. doi: 10.1016/j.seares.2020.101981
Chiappa-Carrara X., Sanvicente-Anorve L., Monreal-Gomez A., De Leon D. S. (2003). Ichthyoplankton Distribution as an Indicator of Hydrodynamic Conditions of a Lagoon System in the Mexican Caribbean. J. Plankton Res. 25 (7), 687–696. doi: 10.1093/plankt/25.7.687
Chicharo L., Faria A., Morais P., Amaral A., Mendes C., Chicharo M. A. (2009). How to Sample Larval Fish for Taxonomical and Ecophysiological Studies in Shallower Temperate Coastal Ecosystems? Cah. Biol. Mar. 50 (4), 311–318. doi: 10.1007/978-0-387-89959-6_39
Chu C., Loh K. H., Ng C. C., Ooi A. L., Konishi Y., Huang S. P., et al. (2019). Using DNA Barcodes to Aid the Identification of Larval Fishes in Tropical Estuarine Waters (Malacca Straits, Malaysia). Zool. Stud. 58, e30. doi: 10.6620/zs.2019.58-30
Churchill J. H., Forward R. B., Luettich R. A., Hench J. L., Hettler W. F., Crowder L. B., et al. (1999). Circulation and Larval Fish Transport Within a Tidally Dominated Estuary. Fish. Oceanogr. 8, 173–189. doi: 10.1046/j.1365-2419.1999.00025.x
Costa A. J. G., Costa K. G., Pereira L. C. C., Sampaio M. I., da Costa R. M. (2011). Dynamics of Hydrological Variables and the Fish Larva Community in an Amazonian Estuary of Northern Brazil. J. Coastal Res. 64, 1960–1964.
Costa M. D. P., Possingham H. P., Muelbert J. H. (2016). Incorporating Early Life Stages of Fishes Into Estuarine Spatial Conservation Planning. Aquat. Conserv. 26 (6), 1013–1030. doi: 10.1002/aqc.2584
Costa M. J., Vasconcelos R., Costa J. L., Cabral H. N. (2007). River flow Influence on the fish Community of the Tagus Estuary (Portugal). Hydrobiologia 587, 113–123. doi: 10.1007/s10750-007-0690-x
Dagg M. J., Govoni J. J. (1996). Is Ichthyoplankton Predation an Important Source of Copepod Mortality in Subtropical Coastal Waters? Mar. Freshw. Res. 47 (2), 137–144. doi: 10.1071/mf9960137
Demirel N. (2015). Ichthyoplankton Dynamics in a Highly Urbanized Estuary. Mar. Biol. Res. 11 (7), 677–688. doi: 10.1080/17451000.2015.1007873
de Morais T. A., de Morais T. L. (1994). The Abundance and Diversity of Larval and Juvenile fish in a Tropical Estuary. Estuaries 17, 216–225. doi: 10.2307/1352571
Ding Y. W., Xian W. W. (2011). Temporal and Spatial Structure of Ichthyoplankton Assemblages in the Yangtze Estuary During Autumn. J. Ocean Univ. China 41 (10), 67–74. doi: 10.16441/j.cnki.hdxb.2011.10.011
Doring J., Neumann S. I., Sloterdijk H., Ekau W. (2018). Seasonal Growth Differences of Larval Hyporhamphus Picarti (Hemiramphidae) in the Sine Saloum Estuary, Senegal. J. Appl. Ichthyol. 34 (1), 97–102. doi: 10.1111/jai.13528
Drake P., Arias A. M. (1991). Composition and Seasonal Fluctuations of the Ichthyoplankton Community in a Shallow Tidal Channel of Cadiz Bay (Sw Spain). J. Fish Biol. 39 (2), 245–263. doi: 10.1111/j.1095-8649.1991.tb04360.x
Drake P., Borlan A., Gonzalez-Ortegon E., Baldo F., Vilas C., Fernandez-Delgado C. (2007). Spatio-Temporal Distribution of Early Life Stages of the European Anchovy Engraulis Encrasicolus L. Within a European Temperate Estuary With Regulated Freshwater Inflow: Effects of Environmental Variables. J. Fish Biol. 70 (6), 1689–1709. doi: 10.1111/j.1095-8649.2007.01433.x
Drinkwater K. F., Frank K. T. (1994). Effects of River Regulation and Diversion on Marine fish and Invertebrates. Aquatic Conservation: Freshwater and Marine. Ecosystems 4, 135–151. doi: 10.1002/aqc.3270040205
Esteves E., Pina T., Chícharo M. A., Andrade J. P. (2000). The Distribution of Estuarine fish Larvae: Nutritional Condition and Co-Occurrence With Predators and Prey. Acta Oecol. 21 (3), 161–173. doi: 10.1016/S1146-609X(00)01078-X
Faria A., Morais P., Chicharo M. A. (2006). Ichthyoplankton Dynamics in the Guadiana Estuary and Adjacent Coastal Area, South-East Portugal. Estuarine Coastal Shelf Sci. 70 (1-2), 85–97. doi: 10.1016/j.ecss.2006.05.032
Fiedler P. C. (2002). Environmental Change in the Eastern Tropical Pacific Ocean: Review of ENSO and Decadal Variability. Mar. Ecol.: Prog. Ser. 244, 265–283. doi: 10.3354/meps244265
Forward R. B., Tankersley R. A. (2001). Selective Tidal–Stream Transport of Marine Animals. Oceanogr. Mar. Biol. Ann. Rev. 39, 305–353. doi: 10.1080/00049185908702346
Franco-Gordoa C., Godínez-Domíngueza E., Filonovc A. E., Tereshchenkoc I. E., Freireb J. (2004). Plankton Biomass and Larval Fish Abundance Prior to and During the El Niño Period of 1997–1998 Along the Central Pacific Coast of México. Prog. Oceanogr. 63 (3), 99–123. doi: 10.1016/j.pocean.2004.10.001
Fricke R., Eschmeyer W. N., van der R. L. (2021). “Eschmeyer's Catalog of Fishes,” in Genera, Species, References. San Francisco: California Academy of Sciences, Internet publication. Available at: http://researcharchive.calacademy.org/research/ichthyology/catalog/fishcatmain.asp.
Gao D. (2015). Applying Basic Research on the Morphological and Molecular Approaches to Identifying the Ichthyoplankton (D. Qingdao: Ocean University of China).
Gao L., Cheng F., Song Y. Q., Jiang W., Feng G. P., Luo Y. M., et al. (2018). Patterns of Larval Fish Assemblages Along the Direction of Freshwater Input Within the Southern Branch of the Yangtze Estuary, China: Implications for Conservation. J. Freshw. Ecol. 33 (1), 97–114. doi: 10.1080/02705060.2018.1426503
Garcia A. M., Vieira J. P., Winemiller K. O. (2003). Effects of 1997-1998 El Nino on the Dynamics of the Shallow-Water Fish Assemblage of the Patos Lagoon Estuary (Brazil). Estuarine Coastal Shelf Sci. 57, 489–500. doi: 10.1016/S0272-7714(02)00382-7
Gaughan D. J., Neira F. J., Beckley L. E., Potter I. C. (1990). Composition, Seasonality and Distribution of the Ichthyoplankton in the Lower Swan Estuary, South-Western Australia. Aust. J. Mar. Freshw. Res. 41 (4), 529–543. doi: 10.1071/MF9900529
Gibson R. N. (2003). Go With the flow: Tidal Migration in Marine Animals. Hydrobiologia 503, 153–161. doi: 10.1023/B:HYDR.0000008488.33614.62
Grange N., Allanson B. R. (1995). The Influence of Freshwater Inflow on the Nature, Amount and Distribution of Seston in Estuaries of the Eastern Cape, South Africa. Estuarine Coastal Shelf Sci. 40, 403–420. doi: 10.1006/ecss.1995.0028
Guan X., Du X., Lin Y., Tang R. (1992). Effects of Sewage Discharge on Fishery Water Environment in Yangtze River Estuary in Summer. Shanghai J. Water Resour. 2, 15–20. doi: CNKI:SUN:HYHJ.0.1992-04-004
Hale E. A., Park I. A., Fisher M. T., Wong R. A., Stangl M. J., Clark J. H. (2016). Abundance Estimate for and Habitat Use by Early Juvenile Atlantic Sturgeon Within the Delaware River Estuary. Trans. Am. Fish. Soc. 145 (6), 1193–1201. doi: 10.1080/00028487.2016.1214177
Harris S. A., Cyrus D. P. (1995). Occurrence of Fish Larvae in the St Lucia Estuary, Kwazulu-Natal, South Africa. S. Afr. J. Mar. Sci. 16, 333–350. doi: 10.2989/025776195784156601
Harris S. A., Cyrus D. P. (2000). Comparison of Larval Fish Assemblages in Three Large Estuarine Systems, KwaZulu-Natal, South Africa. J. Mar. Biol. 137 (3), 527–541. doi: 10.1007/s002270000356
Harris S. A., Cyrus D. P., Beckley L. E. (1999). The Larval Fish Assemblage in Nearshore Coastal Waters Off the St Lucia Estuary, South Africa. Estuarine Coastal Shelf Sci. 49 (6), 789–811. doi: 10.1006/ecss.1999.0554
Harris S. A., Cyrus D. P., Beckley L. E. (2001). Horizontal Trends in Larval Fish Diversity and Abundance Along an Ocean-Estuarine Gradient on the Northern KwaZulu-Natal Coast, South Africa. Estuarine Coastal Shelf Sci. 53 (2), 221–235. doi: 10.1006/ecss.2001.0803
Harrison T. D., Whitfield A. K. (1990). Composition, Distribution and Abundance of Ichthyoplankton in the Sundays River Estuary. S. Afr. J. Zool. 25 (3), 161–168. doi: 10.1080/02541858.1990.11448206
Hassell K. L., Coutin P. C., Nugegoda D. (2008). Hypoxia, Low Salinity and Lowered Temperature Reduce Embryo Survival and Hatch Rates in Black Bream Acanthopagrus Butcheri (Munrol 1949). J. Fish Biol. 72 (7), 1623–1636. doi: 10.1111/j.1095-8649.2008.01829.x
Hebert P. D. N., Cywinska A., Ball S., deWaard J. R. (2003). Biological Identifications Through DNA Barcodes. Philos. Trans. R. Soc. Lond. B. Biol. Sci. 270, 313–321. doi: 10.1098/rspb.2002.2218
Hernández-Miranda E., Palma A. T., Ojeda F. P. (2003). Larval fish Assemblages in Nearshore Coastal Waters Off Central Chile: Temporal and Spatial Patterns. Estuarine Coastal Shelf Sci. 56, 1075–1092. doi: 10.1016/S0272-7714(02)00308-6
Hettler W. F. Jr., Hare J. A. (1998). Abundance and Size of Larval fishes Outside the Entrance to Beaufort Inlet, North Carolina. Estuaries 21, 476–499. doi: 10.2307/1352846
Hickling C. F. (1970). A Contribution to the Natural History of the English Grey Mullets [Pisces, Mugilidae]. J. Mar. Biol. Assoc. U. K. 50 (3), 609–633. doi: 10.1017/S0025315400004914
Holmes R. H. A., Henderson P. A. (1990). High fish Recruitment in the Severn Estuary: The Effect of a Warm Year. J. Fish Biol. 36, 961–963. doi: 10.1111/j.1095-8649.1990.tb05641.x
Huang D. L., Zhang X., Jiang Z. J., Zhang J. P., Arbi I., Jiang X., et al. (2017). Seasonal Fluctuations of Ichthyoplankton Assemblage in the Northeastern South China Sea Influenced by the Kuroshio Intrusion. J. Geophys. Res. Oceans 122 (9), 7253–7266. doi: 10.1002/2017jc012906
Islam M. S., Hibino M., Tanaka M. (2006). Distribution and Diets of Larval and Juvenile fishes: Influence of Salinity Gradient and Turbidity Maximum in a Temperate Estuary in Upper Ariake Bay, Japan. Estuarine Coastal Shelf Sci. 68, 62–74. doi: 10.1016/j.ecss.2006.01.010
Islam M. S., Hibino M., Tanaka M. (2007). Tidal and Diurnal Variations in Larval fish Abundance in an Estuarine Inlet in Ariake Bay, Japan: Implication for Selective Tidal Stream Transport. Ecol. Soc Jpn. 22, 165–171. doi: 10.1007/s11284-006-0001-4
Islam M. S., Tanaka M. (2006). Spatial Variability in Nursery Functions Along a Temperate Estuarine Gradient: Role of Detrital Versus Algal Trophic Pathways. Can. J. Fish. Aquat. Sci. 63 (8), 1848–1864. doi: 10.1139/f06-086
Jager Z. (1999). Selective Tidal Stream Transport of Flounder Larvae (Platichthys Flesus L.) in the Dollard (Ems Estuary). Estuarine Coastal Shelf Sci. 49 (3), 347–362. doi: 10.1006/ecss.1999.0504
Jiang S., Chen F. (1993). Studies on Estuarine Ecology of Ichthyoplankton of Jiulong River. J. Oceanogr. Taiwan Strait 12 (4), 351–358. doi: CNKI:SUN:TWHX.0.1993-04-007
Jiang M., Shen X., Chen L. (2006). Relationship Between With Abundance Distribution of Fish Eggs, Larvae and Environmental Factors in the Changjiang Estuary and Vicinity Waters in Spring. Mar. Environ. Sci. 25 (2), 37–39. doi: 10.3969/j.issn.1007-6336.2006.02.010
Jiang M., Shen X. Q., Li L., Quan W. M. (2013). Annual Variability of Ichthyoplankton in the Yangtze Estuary of China From August 2002 to 2009. Oceanol. Hydrobiol. Stud. 42 (1), 59–69. doi: 10.2478/s13545-013-0060-4
Jiang M., Shen X. Q., Wang Y. L., Yuan Q., Chen L. F. (2006a). Species of Fish Eggs and Larvae and Distribution in Changjiang Estuary and Vicinity Waters. Acta Oceanol. Sin. 28 (2), 171–174. doi: 10.1007/978-94-017-9136-6_8
Jones S., Menon P. M. G. (1951). Observations on the Life-History of the Indian Shad, Hilsa Ilisha (Hamilton). P. Indian Natl. Sci. Ac. Sect. B. 33 (3), 101–124. doi: 10.1007/BF03049975
Kimmerer W. J. (2002). Physical, Biological, and Management Responses to Variable Freshwater Flow Into the San Francisco Estuary. Estuaries 25 (6B), 1275–1290. doi: 10.1007/BF02692224
Kindong R., Wu J., Gao C., Dai L., Tian S., Dai X., et al. (2020). Seasonal Changes in Fish Diversity, Density, Biomass, and Assemblage Alongside Environmental Variables in the Yangtze Estuary. Environ. Sci. Pollut. Res. 27 (20), 25461–25474. doi: 10.1007/s11356-020-08674-8
Kingsford M. J., Suthers I. M. (1996). The Influence of Tidal Phase on Patterns of Ichthyoplankton Abundance in the Vicinity of an Estuarine Front, Botany Bay, Australia. Estuarine Coastal Shelf Sci. 43 (1), 33–54. doi: 10.1006/ecss.1996.0056
Korsman B. M., Kimball M. E., Hernandez F. J. (2017). Spatial and Temporal Variability in Ichthyoplankton Communities Ingressing Through Two Adjacent Inlets Along the Southeastern US Atlantic Coast. Hydrobiologia 795 (1), 219–237. doi: 10.1007/s10750-017-3131-5
Ko H. L., Wang Y. T., Chiu T. S., Lee M. A., Leu M. Y., Chang K. Z., et al. (2013). Evaluating the Accuracy of Morphological Identification of Larval Fishes by Applying DNA Barcoding. PloS One 8 (1), e53451. doi: 10.1371/journal.pone.0053451
Lefcheck J. S., Hughes B. B., Johnson A. J., Pfirrmann B. W., Rasher D. B., Smyth A. R., et al. (2019). Are Coastal Habitats Important Nurseries? A Meta-Analysis. Conserv. Lett. 12 (4), e12645. doi: 10.1111/conl.12645
Lima A. R. A., Ferreira G. V. B., Barletta M. (2019). Estuarine Ecocline Function and Essential Habitats for Fish Larvae in Tropical South Western Atlantic Estuaries. Mar. Environ. Res. 151, 104786. doi: 10.1016/j.marenvres.2019.104786
Limburg K. E. (1999). Estuaries, Ecology, and Economic Decisions: An Example of Perceptual Barriers and Challenges to Understanding. Ecol. Econ. 30 (1), 185–188. doi: 10.1016/S09218009(99)00045-2
Lin S., Wang L., Zheng L., Dong Y., Liu S., Ding S. (2014). Current Status and Future Prospect of DNA Barcoding in Marine Biology. Acta Ocean. Sinica. 36 (12), 1–17. doi: 10.3969/j.issn.0253-4193.2014.12.001
Lin J., Wu H., Chen K., Du Q., Huang Z., Huang K., et al. (2018). Identification of Ichthyoplankton Using DNA Barcoding in Xiamen Bay. J. Fish. Res. 40 (5), 340–348. doi: 10.14012/j.cnki.fjsc.2018.05.002
Liu S., Xian W. (2010). Ichthyoplankton Community Structure Characteristics During Spring in Yangtze Estuary Before and After Impoundment of Three Gorges Reservoir. J. Yangtze River Sci. Res. Institute 27 (10), 82–87. doi: 10.3969/j.issn.1001-5485.2010.10.017
Liu S. D., Xian W. W., Liu D. (2008). Characteristics of Ichthyoplankton Assemblages in Yangtze Estuary and Adjacent Waters in Spring. J. Appl. Ecol. 19 (10), 2284–2292. doi: CNKI:SUN:YYSB.0.2008-10-029
Liu S., Yang Y., He Y., Ji X., Wang Y., Zhang H. (2021). Morphological Classification of Ichthyoplankton in the Changjiang River Estuary Based on DNA Barcoding. Haiyang Xuebao 43 (8), 93–104. doi: 10.12284/hyxb2021062
Livingston R. J., Niu X., Lewis F. G., Woodsum G. C. (1997). Freshwater Input to a Gulf Estuary: Long-Term Control of Trophic Organization. Ecol. Appl. 7, 277–299. doi: 10.1890/1051-0761(1997)007[0277:FITAGE]2.0.CO;2
Li Y., Zhang Y., Zhang R., Song P., Wang L., Zhang L. (2017). Identification of Several Fish Larvae Based on DNA Barcoding Int the Investigated Waters of Cangnan. J. Ocean Univ. China 36 (12), 1–17. doi: 10.16441/j.cnki.hdxb.20160343
Locke A., Courtenay S. C. (1995). Effects of Environmental-Factors on Ichthyoplankton Communities in the Miramichi Estuary, Gulf of St-Lawrence. J. Plankton Res. 17 (2), 333–349. doi: 10.1093/plankt/17.2.333
Lotze H. K. (2010). “Historical Reconstruction of Human-Induced Changes in US Estuaries,” in Oceanography and Marine Biology: An Annual Review, vol. Vol. 48 . Eds. Gibson R. N., Atkinson R. J. A., Gordon J. D. M. (Boca Raton, FL: CRC Press), 267–338.
Lotze H. K., Lenihan H. S., Bourque B. J., Bradbury R. H., Cooke R. G., Jackson J. B. C., et al. (2006). Depletion, Degradation, and Recovery Potential of Estuaries and Coastal Seas. J. Sci. 312 (5781), 1806–1809. doi: 10.1126/science.1128035
Martin T. J., Cyrus D. P., Forbes A. T. (1992). Episodic Events - the Effects of Cyclonic Flushing on the Ichthyoplankton of St-Lucia Estuary on the Southeast Coast of Africa. Neth. J. Sea Res. 30, 273–278. doi: 10.1016/0077-7579(92)90065-M
Melvillesmith R., Baird D. (1980). Abundance, Distribution and Species Composition of Fish Larvae in the Swartkops Estuary. S. Afr. J. Zool. 15 (2), 72–78. doi: 10.1080/02541858.1980.11447689
Monteiro M., Azeiteiro U. M., Martinho F., Pardal M. A., Primo A. L. (2021). Long-Term Changes of Ichthyoplankton Communities in an Iberian Estuary are Driven by Varying Hydrodynamic Conditions. J. Plankton Res. 43 (1), 33–45. doi: 10.1093/plankt/fbaa057
Monteleone D. M. (1992). Seasonality and Abundance of Ichthyoplankton in Great South Bay, New York. Estuaries 15, 230–238. doi: 10.2307/1352697
Mouny P., Dauvin J. C. (2002). Environmental Control of Mesozooplankton Community Structure in the Seine Estuary (English Channe1). Acta Oceanol. Sin. 25 (1), 13–22. doi: 10.1016/S0399-1784(01)01177-X
Muhling B. A., Beckley L. E., Olivar M. P. (2007). Ichthyoplankton Assemblage Structure in Two Meso-Scale Leeuwin Current Eddies, Eastern Indian Ocean. Deep Sea Res. Part II 54, 1113–1128. doi: 10.1016/j.dsr2.2006.05.045
Munk P., Cardinale M., Casini M., Rudolphi A. C. (2014). The Community Structure of Over-Wintering Larval and Small Juvenile Fish in a Large Estuary. Estuarine Coastal Shelf Sci. 139, 27–39. doi: 10.1016/j.ecss.2013.12.024
Neira F. J., Potter I. C. (1992). Movement of Larval Fishes Through the Entrance Channel of a Seasonally Open Estuary in Western Australia. Estuarine Coastal Shelf Sci. 35 (2), 213–224. doi: 10.1016/S0272-7714(05)80114-3
Newton G. M. (1996). Estuarine Ichthyoplankton Ecology in Relation to Hydrology and Zooplankton Dynamics in a Salt-Wedge Estuary. Mar. Freshwater Res. 47 (2), 99–111. doi: 10.1071/Mf9960099
North E. W., Houde E. D. (2003). Linking ETM Physics, Zooplankton Prey and Fish Early-Life Histories to White Perch (Morone Americana) and Striped Bass (M. Saxatilis) Recruitment Success. Mar. Ecol.: Prog. Ser. 260, 219–236. doi: 10.3354/meps260219
Palomera I. (1992). Spawning of Anchovy Engraulis Encrasicolus in the North-Western Mediterranean Relative to Hydrographic Features in the Region. Mar. Ecol.: Prog. Ser. 79, 215–223. doi: 10.3354/meps079215
Parrish J.D. (1989). Fish Communities of Interacting Shallow Water Habitats in Tropical Oceanic Regions. Mar. Ecol.: Prog. Ser. 58, 143–160.
Pegg G. G., Sinclair W. T., Briskey L., William J. A. (2006). mtDNA Barcode Identification of Fish Larvae in the Southern Great Barrier Reef, Australia. Sci. Marina 70 (S2), 7–12. doi: 10.3989/scimar.2006.70s27
Pereira R., Teodosio M. A., Garrido S. (2014). An Experimental Study of Aurelia Aurita Feeding Behaviour: Inference of the Potential Predation Impact on a Temperate Estuarine Nursery Area. Estuarine Coastal Shelf Sci. 146, 102–110. doi: 10.1016/j.ecss.2014.05.026
Peterson G. S., Lietz J. E. (2017). Identification of Ruffe Larvae (Gymnocephalus Cernua) in the St. Louis River, Lake Superior: Clarification and Guidance Regarding Morphological Descriptions. J. Great Lakes Res. 43 (1), 205–210. doi: 10.1016/j.jglr.2016.10.005
Pfirrmann B. W., Kimball M. E., Mace M. M. III, Turley B. D. (2021). Summer Ichthyoplankton Assemblage Diversity Within a Southeastern United States Estuary. Estuaries Coasts 44 (1), 253–268. doi: 10.1007/s12237-020-00777-2
Pombo L., Ellio M., Rebelo E. (2002). Changes in the Fish Faunal of the Ria De Aveiro Estuarine Lagoon (Portugal) During the Twentieth Century. J. Fish Biol. 61, 167–181. doi: 10.1111/j.1095-8649.2002.tb01769.x
Potter I. C., Beckley L. E., Whitfield A. K., Lenanton R. C. (1990). Comparisons Between the Roles Played by Estuaries in the Life Cycles of Fishes in Temperate Western Australia and Southern Africa. Environ. Biol. Fishes 28, 143–178. doi: 10.1007/BF00751033
Powles H.Auger F.Fitzgerald G. J. (1984). Nearshore Ichthyoplankton of a North Temperate Estuary. Can. J. Fish. Aquat. Sci. 41 (11), 1653–1663. doi: 10.1139/f84-204.
Primo A. L., Azeiteiro U. M., Marques S. C., Pardal M. A. (2011). Impact of Climate Variability on Ichthyoplankton Communities: An Example of a Small Temperate Estuary. Estuarine Coastal Shelf Sci. 91 (4), 484–491. doi: 10.1016/j.ecss.2010.11.009
Primo A. L., Azeiteiro U. M., Marques S. C., Re P., Pardal M. A. (2012). Seasonal, Lunar and Tidal Control of Ichthyoplankton Dynamics at the Interface Between a Temperate Estuary and Adjacent Coastal Waters (Western Portugal). Sci. Mar. 76 (2), 237–246. doi: 10.3989/scimar.03415.18A
Ramos S., Cowen R. K., Paris C., Re P., Bordalo A. A. (2006). Environmental Forcing and Larval Fish Assemblage Dynamics in the Lima River Estuary (Northwest Portugal). J. Plankton Res. 28 (3), 275–286. doi: 10.1093/plankt/fbi104
Ren P., Schmidt B. V., Fang D. A., Xu D. P. (2021). Spatial Distribution Patterns of Fish Egg and Larval Assemblages in the Lower Reach of the Yangtze River: Potential Implications for Conservation and Management. Aquat. Conserv. 31 (8), 1929–1944. doi: 10.1002/aqc.3595
Ribeiro F., Hale E., Hilton E. J., Clardy T. R., Deary A. L., Targett T. E., et al. (2015). Composition and Temporal Patterns of Larval Fish Communities in Chesapeake and Delaware Bays, USA. Mar. Ecol.: Prog. Ser. 527, 167–180. doi: 10.3354/meps11265
Robertson A. I., Alongi D. M. (1992). Tropical Mangrove Ecosystems (Washington, D.C: American Geophysical Union).
Rodrigues S. M., Almeida C. M. R., Silva D., Cunha J., Antunes C., Freitas V., et al. (2019). Microplastic Contamination in an Urban Estuary: Abundance and Distribution of Microplastics and Fish Larvae in the Douro Estuary. Sci. Total. Environ. 659, 1071–1081. doi: 10.1016/j.scitotenv.2018.12.273
Rousseau Y., Blanchard F., Gardel A. (2018). Spatiotemporal Dynamics of Larval Fish in a Tropical Estuarine Mangrove: Example of the Mahury River Estuary (French Guiana). Can. J. Fish. Aquat. Sci. 75 (2), 235–246. doi: 10.1139/cjfas-2016-0267
Ryder R. A., Kerr S. R., Taylor W. W., Larkin P. A. (1981). Community Consequences of Fish Stock Diversity. Can. J. Fish. Aquat. Sci. 38, 1856–1866. doi: 10.1139/f81-231
Sabates A., Martin P., Loret J., Raya V. (2006). Sea Warming and fish Distribution: The Case of the Small Pelagic fish, Sardinella Aurita, in the Western Mediterranean. Glob. Change Biol. 12, 2209–2219. doi: 10.1111/j.1365-2486.2006.01246.x
Sabates A., Olivar M. P., Salat J., Palomera I., Alemany F. (2007). Physical and Biological Processes Controlling the Distribution of fish Larvae in the NW Mediterranean. Prog. Oceanogr. 74, 355–376. doi: 10.1016/j.pocean.2007.04.017
Sánchez-Velasco L., Avalos-García C., Rentería-Cano M., Shirasago B. (2004). Fish Larvae Abundance and Distribution in the Central Gulf of California During Strong Environmental Changes (1997–1998 El Niño and 1998–1999 La Niña). Deep Sea Res. Part II 51 (6-9), 711–722. doi: 10.1016/j.dsr2.2004.05.021
Sanvicente-Anorve L., Flores-Coto C., Chiappa-Carrara X. (2000). Temporal and Spatial Scales of Ichthyoplankton Distribution in the Southern Gulf of Mexico. Estuarine Coastal Shelf Sci. 51 (4), 463–475. doi: 10.1006/ecss.2000.0692
Schilling H. T., Hinchliffe C., Gillson J. P., Miskiewicz A. G., Suthers I. M. (2021). Coastal Winds and Larval Fish Abundance Indicate a Recruitment Mechanism for Southeast Australian Estuarine Fisheries. Fish. Oceanogr. 31 (1), 40–55. doi: 10.1111/fog.12561
Schubel J. R. (1968). Turbidity Maximum of the Northern Chesapeake Bay. Science 161, 1013–1015. doi: 10.1126/science.161.3845.1013
Schultz E. T., Lwiza K. M. M., Fencil M. C., Martin J. M. (2003). Mechanisms Promoting Upriver Transport of Larvae of Two Fish Species in the Hudson River Estuary. Mar. Ecol.: Prog. Ser. 251, 263–277. doi: 10.3354/meps251263
Segarra A., Mauduit F., Amer N. R., Biefel F., Hladik M. L., Connon R. E., et al. (2021). Salinity Changes the Dynamics of Pyrethroid Toxicity in Terms of Behavioral Effects on Newly Hatched Delta Smelt Larvae. Toxics 9 (2), 40. doi: 10.3390/toxics9020040
Shan X. J., Xian W. W., Wu Y. F. (2004). Progress of Studies on Ichthyoplankton Ecology of Changjiang River Estuary. J. Oceanol. Limnol. 0 (4), 87–93. doi: 10.13984/j.cnki.cn37-1141.2004.04.015
Shao G., Yang R., Chen K. (2001). An Identification Guide of Marine Fish Eggs From Taiwan (M. Taibei: Academia Sinica).
Sheaves M., Baker R., Nagelkerken I., Connolly R. M. (2015). True Value of Estuarine and Coastal Nurseries for Fish: Incorporating Complexity and Dynamics. Estuaries Coasts 38 (2), 401–414. doi: 10.1007/s12237-014-9846-x
Shideler A. C., Houde E. D. (2014). Spatio-Temporal Variability in Larval-Stage Feeding and Nutritional Sources as Factors Influencing Striped Bass (Morone Saxatilis) Recruitment Success. Estuaries Coasts 37 (3), 561–575. doi: 10.1007/s12237-013-9696-y
Shoji J., Ohta T., Tanaka M. (2006). Effects of River Flow on Larval Growth and Survival of Japanese Seaperch Lateolabrax Japonicus (Pisces) in the Chikugo River Estuary, Upper Ariake Bay. J. Fish Biol. 69 (6), 1662–1674. doi: 10.1111/j.1095-8649.2006.01235.x
Sloterdijk H., Brehmer P., Sadio O., Muller H., Doring J., Ekau W. (2017). Composition and Structure of the Larval Fish Community Related to Environmental Parameters in a Tropical Estuary Impacted by Climate Change. Estuarine Coastal Shelf Sci. 197, 10–26. doi: 10.1016/j.ecss.2017.08.003
Song X. K., Liu A. Y., Yang Y. Y., Yang J. M., Ren L. H., Liu L. J., et al. (2010). Distributing of Ichthyoplankton and the Correlation With Environmental Factors in Laizhou Bay. J. Oceanol. Limnol. 41 (3), 378–385. doi: 10.11693/hyhz201003013013
Song Y. F., Zhang L. J., Luo X. X. (2019). Spatiotemporal Distribution of Fish Eggs and Larvae in the Huanghe (Yellow) River Estuary, China in 2005-2016. J. Oceanol. Limnol. 37 (5), 1625–1637. doi: 10.1007/s00343-019-8167-0
Strydom N. A., Sutherland K., Wooldridge T. H. (2014). Diet and Prey Selection in Late-Stage Larvae of Five Species of Fish in a Temperate Estuarine Nursery Area. Afr. J. Mar. Sci. 36, 85–98. doi: 10.2989/1814232X.2014.895420
Strydom N. A., Whitfield A. K., Wooldridge T. H. (2003). The Role of Estuarine Type in Characterizing Early Stage Fish Assemblages in Warm Temperate Estuaries, South Africa. Zool. Afr. 38 (1), 29–43. doi: 10.1080/15627020.2003.11657192
Talbot C. W., Able K. W. (1984). Composition and Distribution of Larval fishes in New Jersey High Marshes. Estuaries 7, 434–443. doi: 10.2307/1351624
Tautz D., Arctander P., Minelli A., Thomas R., Vogler A. (2002). DNA Points the Way Ahead in Taxonomy. Nature 418, 479. doi: 10.1038/418479a
Taylor R. H. (1982). “The St. Lucia Estuary: The Aquatic Environment: Physical and Chemical Characteristics,” in St. Lucia Research Review, Proceedings of the Natal Parks Board 1992. Ed. Taylor R. H. (South Africa: Symposium), 42–56.
Thaxton W. C., Taylor J. C., Asch R. G. (2020). Climate-Associated Trends and Variability in Ichthyoplankton Phenology From the Longest Continuous Larval Fish Time Series on the East Coast of the United States. Mar. Ecol.: Prog. Ser. 650, 269–287. doi: 10.3354/meps13404
Tzeng W. N., Wang Y. T. (1993). Hydrography and Distribution Dynamics of Larval and Juvenile Fishes in the Coastal Waters of the Tanshui River Estuary, Taiwan, With Reference to Estuarine Larval Transport. J. Mar. Biol. 116, 205–217. doi: 10.1007/BF00350010
Valdez-Moreno M., Vásquez-Yeomans L., Elías-Gutiérrez M., Natalia V., Pauly D. N. H. (2010). Using DNA Barcodes to Connect Adults and Early Life Stages of Marine Fishes From the Yucatan Peninsula, Mexico: Potential in Fisheries Management. Mar. Freshw. Res. 61 (6), 655–671. doi: 10.1071/MF09222
Valencia B., Giraldo A., Rivera-Gomez M., Izquierdo-Pena V., Cuellar-Chacon A. (2019). Effects of Seasonal Upwelling on Hydrography and Mesozooplankton Communities in a Pacific Tropical Cove Off Colombia. Rev. Biol. Trop. 67 (4), 945–962. doi: 10.15517/rbt.v67i4.35489
van Eck N. J., Waltman L., Ding Y., Wolfram D. (2014). “Visualizing Bibliometric Networks,” in Measuring Scholarly Impact: Methods and Practice. Ed. Rousseau R. (London: Springer).
Walker M. G., Jones F. R. H., Arnold G. P. (1978). Movements of Plaice (Pleuronectes-Platessa L) Tracked in Open Sea. J. Du Conseil 38 (1), 58–86. doi: 10.1093/icesjms/38.1.58
Wang J. W., Huang J., Tao H. H. (2020). Investigation on Ichthyoplankton in Offshore Area of Shijiu Port in Spring. J. Anhui Agric. Sci. 48 (21), 91–94. doi: 10.3969/j.issn.0517-6611.2020.21.024
Wang X., Ma A., Zhang X. (2006). A Review on Feeding Behavior Ecology of Early Development Stage of Marine Fish. Stud. Mar. Sin. 30 (11), 69–75. doi: 10.3969/j.issn.1000-3096.2006.11.015
Wang X. G., Sun D., Lin S. Q. (2017). The Ecological Study of Fish Larvae in the Yangtze Estuary and Adjacent Waters. J. Shanghai Fish. Univ. 26 (5), 733–742. doi: 10.12024/jsou.20170301964
Wang H. T., Zhang P. J. (1998). Effects of Environmental Conditions on Fertilized Eggs and Early Larva of Marine Fishes. Mar. Sci. 04, 50–52. doi: 10.1017/S0266078400010713
Wan R., Jiang Y. (2000). The Species and Biological Characteristics of the Eggs and Larvae of Osteichthyes in the Bohai and Yellow Sea. J. Shanghai Fish. Univ. 9 (4), 290–297. doi: 10.11964/jfc.20210512867
Wan R., Sun S. (2006). The Category Composition and Abundance of Ichthyoplankton in the Ecosystem of the Yellow Sea and the East China Sea. Curr. Zool. 52 (1), 28–44. doi: 10.3969/j.issn.1674-5507.2006.01.004
Weinstein M. P., Weiss S. L., Hodson R. G., Gerry L. R. (1980). Retention of Three Taxa of Post Larval fishes in a Intensively flushed Tidal Estuary, Cape Fear River, North Carolina. Fish. Bull. 78, 419–436.
Whitfield A. K. (1999). Ichthyofaunal Assemblages in Estuaries: A South African Case Study. Rev. Fish Biol. Fish. 9, 151–186. doi: 10.1023/A:1008994405375
Whitfield A. K. (1989). Ichthyoplankton Interchange in the Mouth Region of a Southern African Estuary. Mar. Ecol. Prog. Ser. 54, 25–33. doi: 10.3354/meps054025
Whitfield A. K. (1994). Abundance of Larval and Juvenile Marine Fishes in the Lower Reaches of 3 Southern African Estuaries With Differing Fresh-Water Inputs. Mar. Ecol.: Prog. Ser. 105 (3), 257–267. doi: 10.3354/meps105257
Williams J., Jenkins G. P., Hindell J. S., Swearer S. E. (2013). Linking Environmental Flows With the Distribution of Black Bream Acanthopagrus Butcheri Eggs, Larvae and Prey in a Drought Affected Estuary. Mar. Ecol.: Prog. Ser. 483, 273–287. doi: 10.3354/meps10280
Wooldridge T. (1999). Estuarine Zooplankton Community Structure and Dynamics. (Cambridge, U.K: Cambridge University Press).
Wu G. Z. (1989). The Ecological Characteristics of Distribution of Eggs, Larvae and Juveniles of the Engraulis Japonicus (Temminck & Schlegel) and Anchoviella Commersonii in the Changjiang River Estuary. J. Oceanol. Limnol. 3 (20), 217–229. doi: CNKI:SUN:HYFZ.0.1989-03-002
Xian W. W., Luo B. Z. (2015). Estuarine Ecology and Environment Before the Impoundment of the Three Gorges Project (Beijing: China Ocean Press).
Xian W. W., Zhang H., Liu S. D. (2016). Research Advance in Estuarine Ichthyoplankton Ecology. Stud. Mar. Sin. 00, 167–180. doi: 10.12036/hykxjk20160718001
Xiao Y. Z., Wang R., Zheng Y. J., He W. (2013). Species Composition and Abundance Distribution of Ichthyoplankton in the Pearl River Estuary. J. Trop. Oceanogr. 32 (6), 80–87. doi: 10.3969/j.issn.1009-5470.2013.06.012
Yang Y. F., Wang Q., Chen J. F., Pang S.X.J.A.E.S. (2006). Research Advance in Estuarine Zooplankton Ecology. Acta Ecol. Sin. 26 (2), 576–585. doi: 10.3321/j.issn:1000-0933.2006.02.034
Yang G., Wu J., Gao M. (1992). Effects of the Three Gorges Project on Sedimentary Structure and Geochemical Characteristics in the Yangtze Estuary. Study Marina Sin. 33, 69–108.
Yang D., Wu G., Sun J. (1990). The Investigation Ok Pelagic Eggs, Larvae and Juveniles of Fishes at the Mouth of the Changjiang River and Adjacent Areas. J. Oceanol. Limnol. 4 (21), 346–355.
Yao Y. (1995). Investigation of Petroleum Pollution in Yangtze River Estuary. J. Dalian Univ. 4 (3), 225–230. doi: CNKI:SUN:SSDB.0.1995-03-007
Yu G., Dong J., Li Y., Fu J., Li Y., Wang A. (2018). Species Composition and Protection of Fish Eggs and Larvae in the Coastal Waters in Liaodong Bay. J. Dalian Univ. 33 (3), 370–378. doi: 10.16535/j.cnki.dlhyxb.2018.03.015
Zhang Z., Mammola S., Xian W., Zhang H. (2020). Modelling the Potential Impacts of Climate Change on the Distribution of Ichthyoplankton in the Yangtze Estuary, China. Divers. Distrib. 26 (1), 126–137. doi: 10.1111/ddi.13002
Zhang H., Xian W., Liu S. (2015). Ichthyoplankton Assemblage Structure of Springs in the Yangtze Estuary Revealed by Biological and Environmental Visions. PeerJ 3, e1186. doi: 10.7717/peerj.1186
Zhang H., Xian W., Liu S. (2016). Autumn Ichthyoplankton Assemblage in the Yangtze Estuary Shaped by Environmental Factors. PeerJ 4, e1922. doi: 10.7717/peerj.1922
Zhang H., Xian W., Liu S. (2019). Seasonal Variations of the Ichthyoplankton Assemblage in the Yangtze Estuary and its Relationship With Environmental Factors. PeerJ 7, e6482. doi: 10.7717/peerj.6482
Zhang G., Zheng L., He H., Lin H., Zhan X., Li K. (2020). The Continuous Observation of Ichthyoplankton Species Composition and Diversity in Summer in Yangtze Estuary. J. Fish. Res. 42 (1), 10–21. doi: 10.14012/j.cnki.fjsc.2020.01.002
Zhou X., Guo S., Song NA, Zhang X. (2017). Identification of Cynoglossus Joyneri Eggs and Larvae by DNA Barcoding and Morphological Method. Biodiversity Sci. 25 (8), 847–855. doi: 10.17520/biods.2017094
Zhuang P. (2006). Fishes of the Yangtze Estuary. (Shanghai: Shanghai Scientific & Technical Publishers).
Keywords: estuary, environmental factors, human activities, climate change, DNA barcoding, fish eggs, community structure, larvae
Citation: Zhang H, Wang Y, Liang C, Liu S and Xian W (2022) Estuarine Ichthyoplankton Studies – A Review. Front. Mar. Sci. 9:794433. doi: 10.3389/fmars.2022.794433
Received: 13 October 2021; Accepted: 26 April 2022;
Published: 24 May 2022.
Edited by:
Çetin Keskin, Istanbul University, TurkeyReviewed by:
Alan Whitfield, South African Institute for Aquatic Biodiversity, South AfricaYuan Li, Ministry of Natural Resources, China
Jose Henrique Muelbert, Universidade Federal do Rio Grande, Brazil
Copyright © 2022 Zhang, Wang, Liang, Liu and Xian. This is an open-access article distributed under the terms of the Creative Commons Attribution License (CC BY). The use, distribution or reproduction in other forums is permitted, provided the original author(s) and the copyright owner(s) are credited and that the original publication in this journal is cited, in accordance with accepted academic practice. No use, distribution or reproduction is permitted which does not comply with these terms.
*Correspondence: Hui Zhang, emhhbmdodWlAcWRpby5hYy5jbg==; Weiwei Xian, d3d4aWFuQHFkaW8uYWMuY24=
†These authors have contributed equally to this work and share first authorship