- 1College of Marine Sciences, Shanghai Ocean University, Shanghai, China
- 2School of Marine Sciences, University of Maine, Orono, ME, United States
- 3Pacific Islands Fisheries Science Center, National Oceanic and Atmospheric Administration, Honolulu, HI, United States
- 4MARBEC, IRD, CNRS, Université de Montpellier, Sète, France
- 5College of Fisheries, Ocean University of China, Qingdao, China
Hatchery programs are commonly used to enhance fishery stocks, while the efforts to minimize potential negative ecological impacts have grown in recent years. In China, Fenneropenaeus chinensis is a fast-growing, short-lived shrimp species with a high commercial value. F. chinensis fishery is heavily dependent on the hatchery program. We evaluated the trade-off between economic profits and ecological impacts of F. chinensis hatchery program in the Jiaozhou Bay of China. The total length of released individuals was 1.4 cm. The results showed that artificially released F. chinensis individuals experienced high predation pressure during the first 2 weeks. The economic profit peaked when 198 million individuals were released. The modeled hatchery program yielded a lower proportion of individuals with the increasing amount of F. chinensis release. The temporally uniform hatchery release was more efficient than other hatchery release scenarios (e.g., increasing the released amount year by year) in a long-term hatchery program. F. chinensis had the negative impacts on two large predatory fishes. Large fishes recovered at a slower rate than small fishes after the F. chinensis release stopped. Reducing fishing pressure could offset negative impacts of F. chinensis release on large fishes. The study indicates that the effectiveness of F. chinensis release cannot be enhanced by simply increasing the released amount. A long-term F. chinensis hatchery program with a large released amount may present additional challenges for managing natural resources in an ecosystem context.
Introduction
The production of global capture fisheries has remained stable over the last 10 years (FAO, 2020). The depletion of fish stocks has been increasingly reported in many marine ecosystems (Link and Watson, 2019). In addition to controlling catch and fishing effort, hatchery programs have been widely applied to fisheries aiming to improve the health of threatened or endangered natural resources (Taylor et al., 2017; Lorenzen et al., 2021). Globally, hatchery programs, such as pink salmon in Prince William Sound and European eel in River Ceira, have been practiced for several decades (Amoroso et al., 2017; Félix et al., 2020). The hatchery programs’ socio-economic benefits have been perceived such as seafood revenue and harvest opportunities for fishermen (Kitada, 2018). Many hatchery species, such as Pacific salmon, are recognized as keystone species in local ecosystems (Tatara and Berejikian, 2012). Hatchery-released individuals can mitigate the fishing pressure to the wild populations and help maintain the stability of local food webs (Anderson et al., 2020).
There are several risks associated with hatchery programs in a marine ecosystem. A large number of released hatchery individuals not only reduce the genetic diversity (Bell et al., 2005; Hagen et al., 2020), but also result in the competition of food and habitats with wild populations (Kitada, 2020), leading to calling for reducing the negative ecological impacts of hatchery programs (Osborne et al., 2020). A large number of released fish can affect the local trophic interactions. The combined hatchery and wild populations may temporarily exceed the local carrying capacity. This can deteriorate the status of the targeted stocks (Tatara and Berejikian, 2012). The cost and benefit of hatchery releases play an important role in the decision-making process. An increasing number of studies are now focusing on balancing economic profits and ecosystem impacts (Camp et al., 2017).
Many stocks of commercial importance are declining along the Chinese coast due to prolonged high fishing pressure (Liang and Pauly, 2020). Fenneropenaeus chinensis is a short-lived, fast-growing shrimp with a high commercial value in Chinese waters. Most of Fenneropenaeus chinensis individuals will die after spawning, and the span life is less than 2 years (Wang et al., 2006). High fishing pressure threatens the sustainable exploitation of F. chinensis stock. The hatchery program, which releases hatchery F. chinensis individuals into the ecosystem, has been developed and implemented over the past decades to rebuild the stock and maintain fishery productivity (Su et al., 2020). Hatchery programs cover the water areas from the East China Sea to the Bohai Sea. F. chinensis releases have been found to increase incomes and create employment opportunities for local residents. However, hatchery programs seemed to fail to prevent the decline of the wild population.
Hatchery release strategies need to consider release size, release habitat, release season, density-dependent effects, and predation processes (Bell et al., 2005). Single-species approaches have been developed to evaluate the profit benefits and risks (Lorenzen, 2005; Ye et al., 2005). Taylor and Suthers (2008) used the Predatory Impact Model to evaluate the optional release strategies associated with prey-predator relationships. In China, F. chinensis hatchery programs are now more likely to enhance the fishery production rather than to rebuild the wild population (Wang et al., 2006). F. chinensis fishery has become increasingly dependent on hatchery releases. For this reason, the number of released F. chinensis has increased every year. But the recapture rates are lower than 10% in many places (Wang et al., 2014). In addition to economic profits, there is a need to identify whether the released F. chinensis individuals influence the stability of local food webs. Studies applied the static food web model Ecopath to estimate the maximum ecological carrying capacity of F. chinensis releases (Lin et al., 2013, 2018; Wang et al., 2016). These studies are inadequate for advising to implement F. chinensis releases in a dynamic perspective. Moreover, the changes of trophic interactions caused by fishing may complicate the management of F. chinensis releases.
Responsible approach to marine stock enhancement requires a set of principles to ensure success and avoid mistakes (Blankenship and Leber, 1995; Lorenzen et al., 2010). Management strategy evaluation (MSE) can facilitate fisheries management achieving by assessing candidate strategies (Punt et al., 2016). Ecosystem models are recognized as a necessary complement to support managing fisheries at an ecosystem level (Hilborn, 2011). In this study, we use a published ecosystem model OSMOSE-JZB (Object-oriented Simulator of Marine ecOSystEms) to evaluate the impacts of F. chinensis release in Jiaozhou Bay, China in a dynamic perspective. OSMOSE-JZB can simulate the seasonal variations in Jiaozhou Bay ecosystem (Xing et al., 2017) and inform fisheries management using simulation (Xing et al., 2020a,2021a,2021b). The objectives of this study are to (1) analyze the economic profit with increasing F. chinensis releases; (2) investigate ecological impacts of F. chinensis release; and (3) evaluate fishing impacts on the F. chinensis release and ecosystem. The study can help us better understand economic profits and ecological impacts of hatchery programs.
Materials and Methods
Study Area
Jiaozhou Bay is a semi-closed bay located in the southeast of Shandong peninsula, China (Figure 1). The study area ranges from 35.95°N to 36.30°N in latitude and from 120.04°E to 120.45°E in longitude. Historically, Jiaozhou Bay supported highly productive fisheries. The local food web has become more vulnerable due to long-term overfishing (Ma et al., 2018). A 3-month fishing closure has been implemented for several decades. It seems not to prevent the stock decline. Jiaozhou Bay is an important nursing ground for F. chinensis. F. chinensis hatchery program launched by Chinese government chooses the Jiaozhou Bay as a release site.
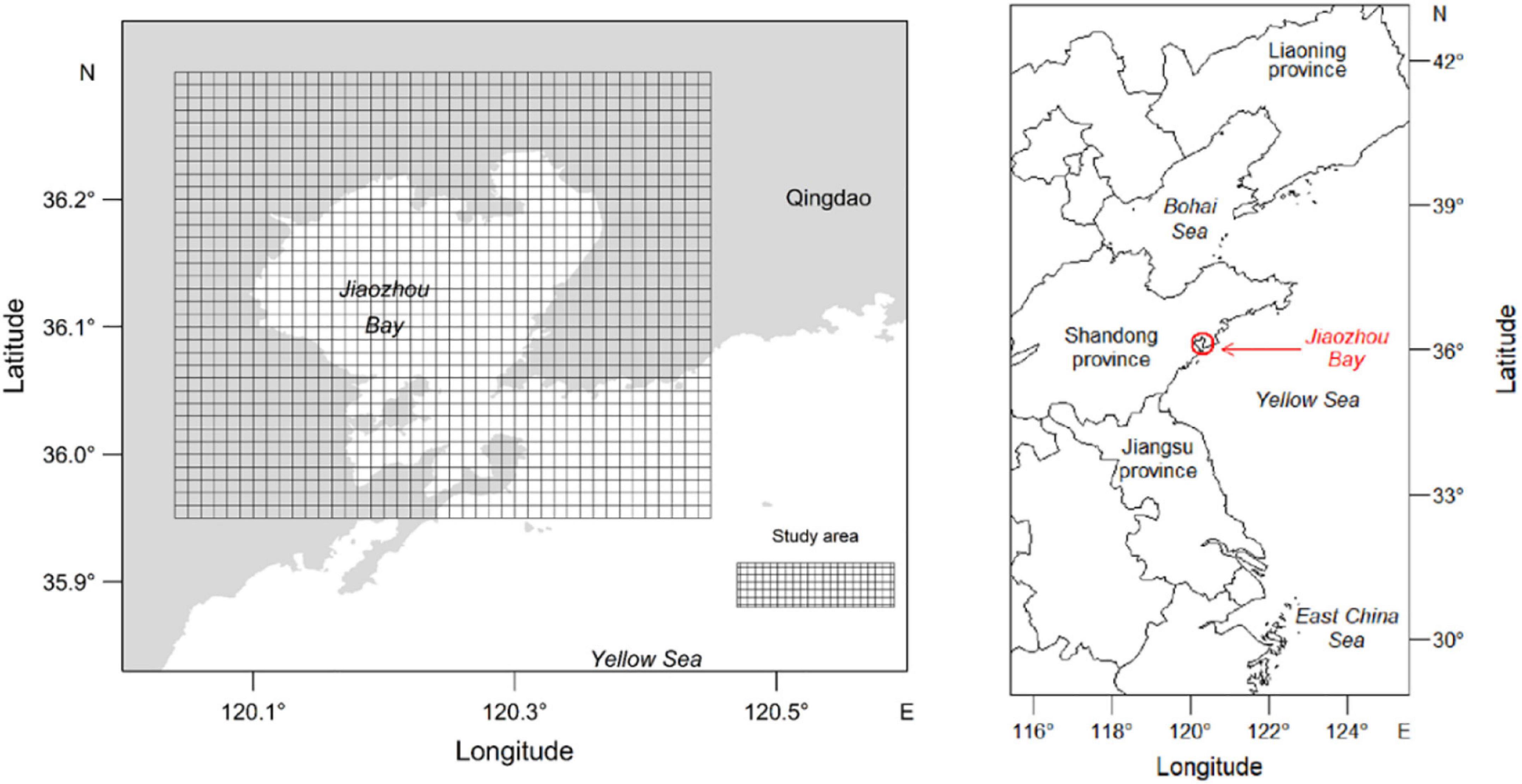
Figure 1. Fenneropenaeus chinensis hatchery program implements in the Jiaozhou Bay, China. Study area is shown on the left. Chinese water area ranging from East China Sea to Bohai Sea is shown on the right.
Operating Model
The ecosystem model OSMOSE-JZB is developed to simulate the food web in the Jiaozhou Bay during 2011 (Xing et al., 2017). The fishes and invertebrate species simulated by the model account for over 83% of the total community biomass from the survey data. The model has been calibrated by an evolutionary algorithm (EA). The modelled food web did not include F. chinensis as the biomass (<1 ton) was much less than other species. In this study, we used the published OSMOSE-JZB model to investigate the commercial profits and ecosystem impacts of releasing F. chinensis into the Jiaozhou Bay. The descriptions of model structure and introducing F. chinensis into the OSMOSE-JZB model are shown as below.
Object-Oriented Simulator of Marine ecOSystEms-JZB Model
Object-oriented simulator of marine ecOSystEms-JZB model consists of a biogeochemistry model FVCOM-NEMURO (Finite Volume Coastal Ocean Model-North Pacific Ecosystem Model Used for Regional Oceanography) and a multispecies individual-based model OSMOSE (Xing et al., 2017). The modelled food web contains low trophic level (LTL) groups and high trophic level (HTL) groups (Table 1). The data were collected from the bottom trawl conducted in February, May, August, and November of 2011. There are 24 times steps (numbered as 0 to 23) in a simulated year. There are 5 LTL groups (i.e., Plk0 to Plk4) which are small phytoplankton (PS), large phytoplankton (PL), small zooplankton (ZS), large zooplankton (ZL), and predatory zooplankton (ZP) that fed on other plankton. Spatio-temporal distributions of LTL groups are simulated by FVCOM-NEMURO model and used as prey fields for high trophic level groups. In other words, LTL groups cannot fed on HTL groups. The FVCOM-NEMURO model forced the OSMOSE model via the predation processes. The parameter “Plank.access” (i.e., the proportion of LTL biomass available to HTL groups) is used to determine the proportion of total LTL biomass available to HTL groups. The description of FVCOM-NEMURO model is presented in Xing et al. (2017).
Object-oriented simulator of marine ecOSystEms model simulates the whole life cycle of HTL groups from egg to adult stage (Shin and Cury, 2001). There are 14 HTL groups (i.e., SP0 to SP13) including 7 fishes, 2 crabs, 5 shrimp species, and 2 cephalopods. Fish schools (i.e., “super-individual”) are the basic modelled unit of carrying out ecological processes. One HTL group consists of fish schools characterized by size, weight, age, and geographical location (Shin and Cury, 2001). Major ecological processes carried out at a time step include spatial movement, predation process, growth, reproduction, various mortality sources, and migration:
Spatial Movement
At the beginning of a time step, the diffusions of fish schools are initiated by “random walk” processes. Fish schools can distribute over the cells within a two-dimensional gridded map of the study area, which consists of 1,435 square grids (0.01° × 0.01°). The parameter “Random walk range” controls the range of moving for fish schools and is set based on the swimming ability of HTL groups.
Predation Process
The model assumes the predation processes are opportunistic and size-based (Shin and Cury, 2004). The predators can only obtain the prey species which are within the suitable size range and stay in the same cell. A matrix is used to define the probability of encounter for each species in the water column. The prey biomass available to the predators are calculated as Eqs. S1, S2 (Supplementary Table 6).
Growth
The growth depends on the amount of foods obtained from predation processes. Individuals will starve or stop growing as a result of lack of food. The somatic growth follows the von Bertalanffy model. The individual weight at a given size is calculated by the weight-length relationship. The maximum rate of ingestion r defines the maximum intake food for a fish school. It is set to 3.5 g⋅g–1⋅year–1 for each HTL group (Grüss et al., 2015). The predation efficiency ξ judges whether a fish school obtains enough food. The critical predation efficiency ξcrit for each HTL group is set to 0.57 (Shin and Cury, 2004). The starvation mortality is calculated as Eq. S3 (Supplementary Table 6).
Reproduction
The reproduction processes are related to spawning stock biomass, relative fecundity, and the percentage of eggs produced at each time step during spawning season. The amount of spawned eggs is calculated as Eq. S7 in Supplementary Table 6.
Various Mortality Sources
There are five mortality sources: predation mortality (Mpredation), starvation mortality (Mstarvation), fishing mortality (Mfishing), larval mortality (Mlarval), and additional natural mortality (Mnatural). Mlarval represents high mortality for the stage from eggs to first feeding larvae. Some species do not spawn in the simulated area. Thus, their Mlarval values were set to Null. Mnatural represents the mortality sources which are not explicitly represented in the model such as disease. The model calculates mortality rates using a stochastic mortality algorithm that makes all mortality unbiased. Mpredation and Mstarvation are calculated by the model in predation processes. In contrast to Mpredation and Mstarvation, the other three mortality types (Mfishing, Mlarval, and Mnatural) are set to constant values. There is a seasonal closure implemented from June 1st to September 1st. Mfishing of each HTL group is uniform during the fishing season. The minimum catch size was set to 5 cm, meaning only individuals larger than 5cm can be harvested.
Migration
High trophic level groups are further categorized into resident species and migratory species (Table 1). The model allows migratory species to migrate into/out of the simulated area at a given time step.
The OSMOSE-JZB model parameters are categorized into two types: (1) “known parameter” which can be obtained directly from survey data and relevant studies and (2) “unknown parameter” whose value needs to estimated by the model calibration. There are five “unknown parameters” that are the proportion of LTL biomass available to HTL groups (Plank.access), flux biomass of migratory species (Bioflux), larval mortality (Mlarval), relative fecundity (RF), and fishing mortality (Mfishing). The evolutionary algorithm (EA) developed by Oliveros-Ramos et al. (2017) estimated the values of “unknown parameters” sequentially along multiple calibration phases. A likelihood-based objective function measured the model fitness by comparing the predicted biomass with observed biomass. The OSMOSE-JZB model performance has been validated and evaluated by Xing et al. (2017). Supplementary Material summarized the information of main parameters for species diffusions (Supplementary Table 1), predation processes (Supplementary Table 2), growth (Supplementary Table 4), reproduction (Supplementary Table 4), various mortality sources (Supplementary Table 3), and migration (Supplementary Table 1). The information of LTL groups, such as size range and trophic level, are shown in Supplementary Table 5. The detailed descriptions of OSMOSE-JZB model structure, parameterization, and model calibration can be found in Xing et al. (2017).
Introducing Fenneropenaeus chinensis Into the Object-Oriented Simulator of Marine ecOSystEms-JZB Model
Fenneropenaeus chinensis is a short-lived, fast-growing shrimp and does not spend the full life cycle in the Jiaozhou Bay. They come into the Jiaozhou Bay during spring and migrate out after August (Yang et al., 2020). Wang et al. (2014) conducted the survey of the F. chinensis hatchery release implemented in 2012. There were 90 million F. chinensis individuals at the size of 1.4 cm released into Jiaozhou Bay on May 22, 2012. The total amount of F. chinensis metapopulation [a combination of hatchery individuals (2.427 million) and wild individuals (0.108 million)] was 2.535 million at the beginning of August, while wild individuals made up less than 4.3% (Wang et al., 2014). The F. chinensis wild population was not included in our model as its population was very small. The released amount is lower than those in 1980s [1985 (120 million), 1986 (200 million), 1988 (180 million), and 1989 (98 million)] (Liu et al., 1993).
In the study, we attempted to introduce the hatchery-released F. chinensis into the OSMOSE-JZB model. The configurations of wild HTL groups (i.e., SP0 to SP13) were kept the same to that set in our previous study (Xing et al., 2017). Similarly, the parameters of F. chinensis (SP14) were categorized into (1) “known parameters” which were set based on survey data and relevant studies and (2) “unknown parameters” which were estimated by the model calibration. The model released 90 million F. chinensis individuals at the size of 1.4 cm into the Jiaozhou Bay at the end of May (time step 9). Then, F. chinensis would stay in the simulated area until the end of August (time step 15). The artificially released F. chinensis was not in the simulated area during other time periods (time steps 0∼8 and 16∼23). Jiaozhou Bay is much smaller than other ecosystems that its water area is less than 500 km2. F. chinensis could distribute over the simulated area. The F. chinensis diffusions were initiated by “random walk” processes. The “Random walk range” of F. chinensis was set the same to other shrimp species (SP1 and SP12) modelled in the OSMOSE-JZB (Supplementary Table 1).
Fenneropenaeus chinensis predation processes were opportunistic and size-based. The maximum rate of ingestion r and critical predation efficiency ξcrit were set to 3.5 g⋅g–1⋅year–1 and 0.57, respectively (Xing et al., 2017). The minimum and maximum prey-predator size ratios were set based on the relevant studies and shown in Supplementary Table 2. The F. chinensis growth was dependent on foods obtained in predation processes. The calculation of available prey biomass for F. chinensis was the same to other HTL groups. The newly released F. chinensis individuals were younger than the age at sexual maturity (13 to 14 months). The wild individuals was not included in the model as the small population size. Therefore, our model did not consider the reproduction of F. chinensis.
Mpredation and Mstarvation of F. chinensis were calculated by the model in predation processes rather than be set to constant values. Mlarval of F. chinensis was set to null as the model did not simulate the life stage before the first feeding larvae. 3-month seasonal fishing closure (June 1st to August 31st, time steps 10∼15) can protect F. chinensis from fishing. The size of F. chinensis at the time step 9 (the end of May) was smaller than the minimum catch size (5 cm). F. chinensis did not stay in the simulated area since the time step 16 (beginning of September). Thus, Mfishing of F. chinensis was set to zero.
Only the Mnatural was the “unknown parameters” of F. chinensis which was estimated by the model calibration. In contrast to other HTL groups (i.e., SP0 to SP13), Mnatural of F. chinensis was divided into two parts: Mnatural–P1 and Mnatural–P2 described high mortality in the first half month of releasing (time step 9) and low mortality during the following 3 months (time steps 10∼15), respectively. Mnatural–P2 was uniform over the time steps 10∼15. Only F. chinensis Mnatural values (Mnatural–P1 and Mnatural–P2) were estimated from the model calibration by fitting the predicted biomass and abundance to the survey data reported by Wang et al. (2014). The parameters of movement, predation, growth, and migration for F. chinensis were set based on the relevant studies (see Supplementary Tables 1-4).
We developed an evolutionary algorithm (EA) to find the optimal combination of Mnatural–P1 and Mnatural–P2 generation by generation (Supplementary Figure 1). The sum of relative estimation error for biomass and abundance (i.e., sumREE) was used to measure the model fitness. The sumREE was calculated as Eq. S8 (Supplementary Table 6). A lower value of sumREE indicated that the predictions fitted the survey data better. The predictions were F. chinensis biomass and abundance at the time step 14 (first half of August). The survey data were F. chinensis biomass and abundance collected on August 11 of 2012 which were 47.194 t and 2.427 million individuals, respectively (Wang et al., 2014). The key steps of model calibration include: (1) selecting candidate Mnatural–P1 and Mnatural–P2 (Selection); (2) creating the parameter combinations (Crossover); (3) running the model with different parameter combinations (Offspring); (4) finding the optimal combination of Mnatural–P1 and Mnatural–P2 with the lowest sumREE (Evaluation); and (5) executing the next generation (Stopping criterion). Additionally, we made the sensitivity analysis on Mnatural–P1 and Mnatural–P2 to further solidify our study. The descriptions of model calibration and sensitivity analysis can be found in the Supplementary Materials.
Simulation Design
We designed four scenarios (i.e., S0 to S3) in the study:
(1) Scenario S0 simulated releasing 90 million F. chinensis individuals at the size of 1.4 cm (total length) into Jiaozhou Bay. The hatchery program was implemented for 1 year (Figure 2A);
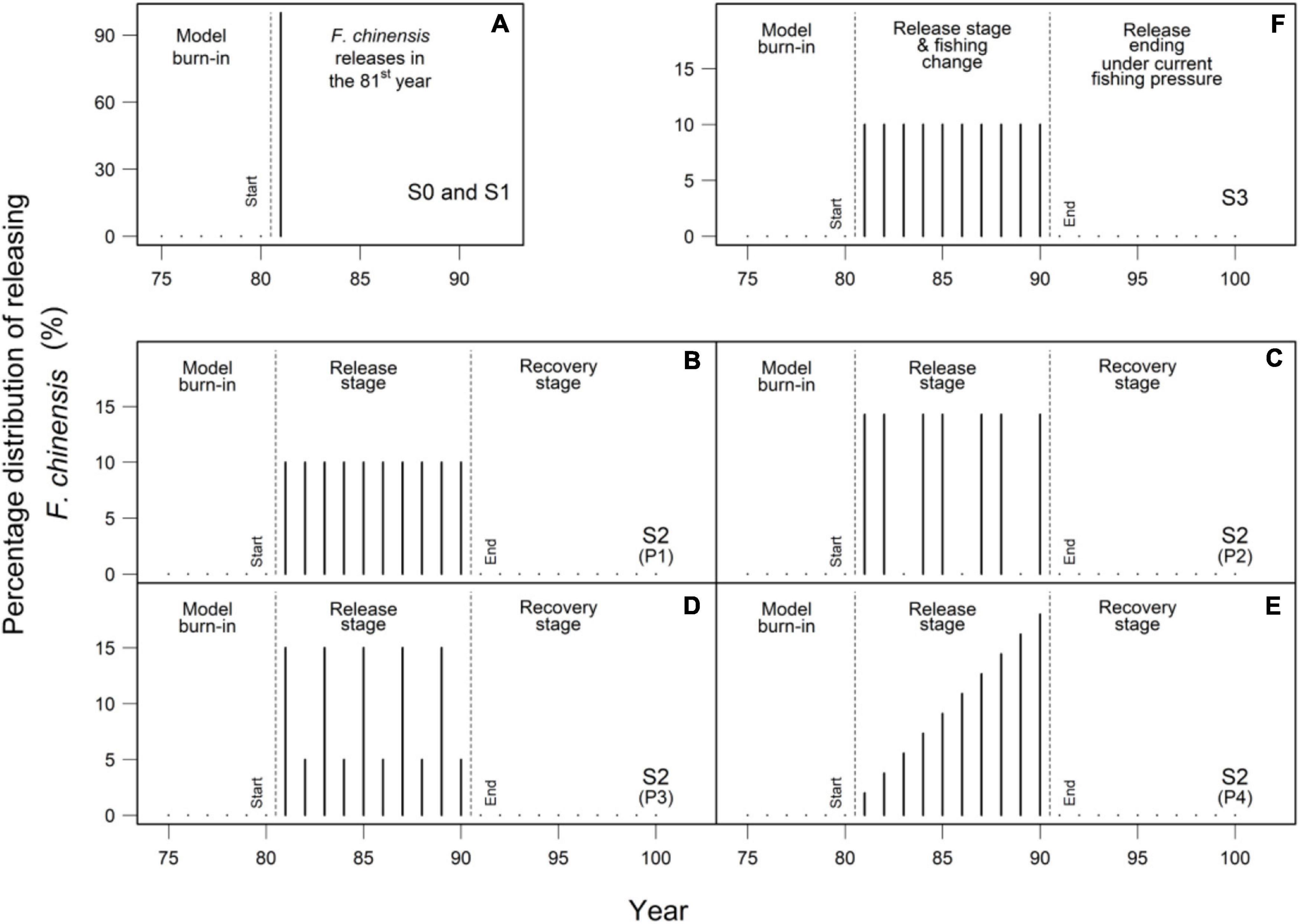
Figure 2. Percentage distribution of releasing F. chinensis in the scenarios S0 and S1 (A), S2 (B–E), and S3 (F). The sum of percentages in the release stage was equal to 100%.
(2) Scenario S1 focused on the economic benefits and ecological impacts in the 1-year F. chinensis release (Figure 2A). The amount of released F. chinensis individuals varied at 15 levels that were set from 0.2 to 3.0 times of the current level (the annual released amount was 90 million) with a step of 0.2;
(3) Scenario S2 evaluated the economic benefits and ecological impacts of a long-term releasing program in a dynamic perspective. The 12 F. chinensis release strategies were implemented in the first 10 years (release stage), and then stopped for the next 10 years (recovery stage). The total amount of released F. chinensis individuals were set at 180 million (Rx0.2, 10 × 0.2 × 90 × 106), 900 million (Rx1, 10 × 1 × 90 × 106), and 1980 million (Rx2.2, 10 × 2.2 × 90 × 106). There were four different F. chinensis releasing patterns (P1 to P4). The pattern P1 released F. chinensis following a uniform distribution (Figure 2B). The pattern P2 released F. chinensis for 2 years and then stopped for 1 year to provide a temporal buffer for the non-released species (Figure 2C). The cycle was executed in the first 10 years. The pattern P3 released F. chinensis at a high level in odd years and at a low level in 7 years (Figure 2D). The pattern P4 released F. chinensis in an incremental manner (Figure 2E);
(4) Scenario S3 investigated the responses of F. chinensis and community of wild species to the changes in fishing pressure. There were two stages (20 years) simulated in the S3. In the first stage (first 10 years), the three F. chinensis release strategies under three fishing scenarios were implemented for 10 years. The total amount of released F. chinensis was set at 180 million (Rx0.2, 10 × 0.2 × 90 × 106), 900 million (Rx1, 10 × 1 × 90 × 106), and 1980 million (Rx2.2, 10 × 2.2 × 90 × 106). F. chinensis releases followed a uniform distribution over 10 years (Figure 2F). Three levels of fishing pressure were considered: 0.8 (Fx0.8, low level), 1 (Fx1, current level), and 1.2 (Fx1.2, high level) times of current fishing mortality rates, which were identical to the setting used in the initial OSMOSE-JZB model (Xing et al., 2017). In the second stage (next 10 years), the F. chinensis release stopped and fishing mortality was set the same to the initial OSMOSE-JZB model (i.e., the current fishing pressure).
All simulations experienced 80 years of burn-in period that ran with the same configuration of the initial OSMOSE-JZB model built by Xing et al. (2017). The F. chinensis release scenarios started since the 81st year in each scenario. For the scenario S0 and S1, the model ran another 1 year (81st year) after the 80 years burn-in period. For the scenarios S2 and S3, the model ran 20 years (from 81st to 100th year) after the burn-in period. The initial OSMOSE-JZB model ran for 100 years to compare with the scenarios S2 and S3 as the reference state that the F. chinensis release did not occur. Every simulation was repeated 100 times due to the model stochasticity. The model outputs were the average of the 100 simulation runs.
Impact Evaluation
In the scenario S0, we described seasonal variations of F. chinensis population in terms of biomass, abundance, mean trophic level, predation mortality, and size structure. In the scenario S1, the modeled data at the end of August (time step 15) were used to quantify the hatchery program’s economic benefits. The indicators were the estimated biomass, abundance, commercial value (ECV), economic profit (EP, the difference between ECV and charge of releasing F. chinensis (Cost)), and the ratio of ECV and Cost (REC). In the scenario S2, the economic profit measured the economic benefit from F. chinensis releases. In the scenario S3, the total EP (i.e., the sum of EP in the release stage) indicated the effectiveness of F. chinensis releases. The relative biomass (Bio81/Bio80, Bio90/Bio80, and Bio100/Bio80) evaluated the responses of wild species (SP0 to SP13) to long-term F. chinensis releases in the scenarios S2 and S3. Bio80 was the simulated wild species biomass in the 80th year that F. chinensis did not release. Bio81 was the simulated wild species biomass in the 81st year that was the 1st year of F. chinensis release. Bio90 was the simulated wild species biomass in the 90th year that was the last year of F. chinensis release. Bio100 was the simulated wild species biomass in the 100th year that was the last year of recovery stage. The indicators used in the study were summarized in Table 2.
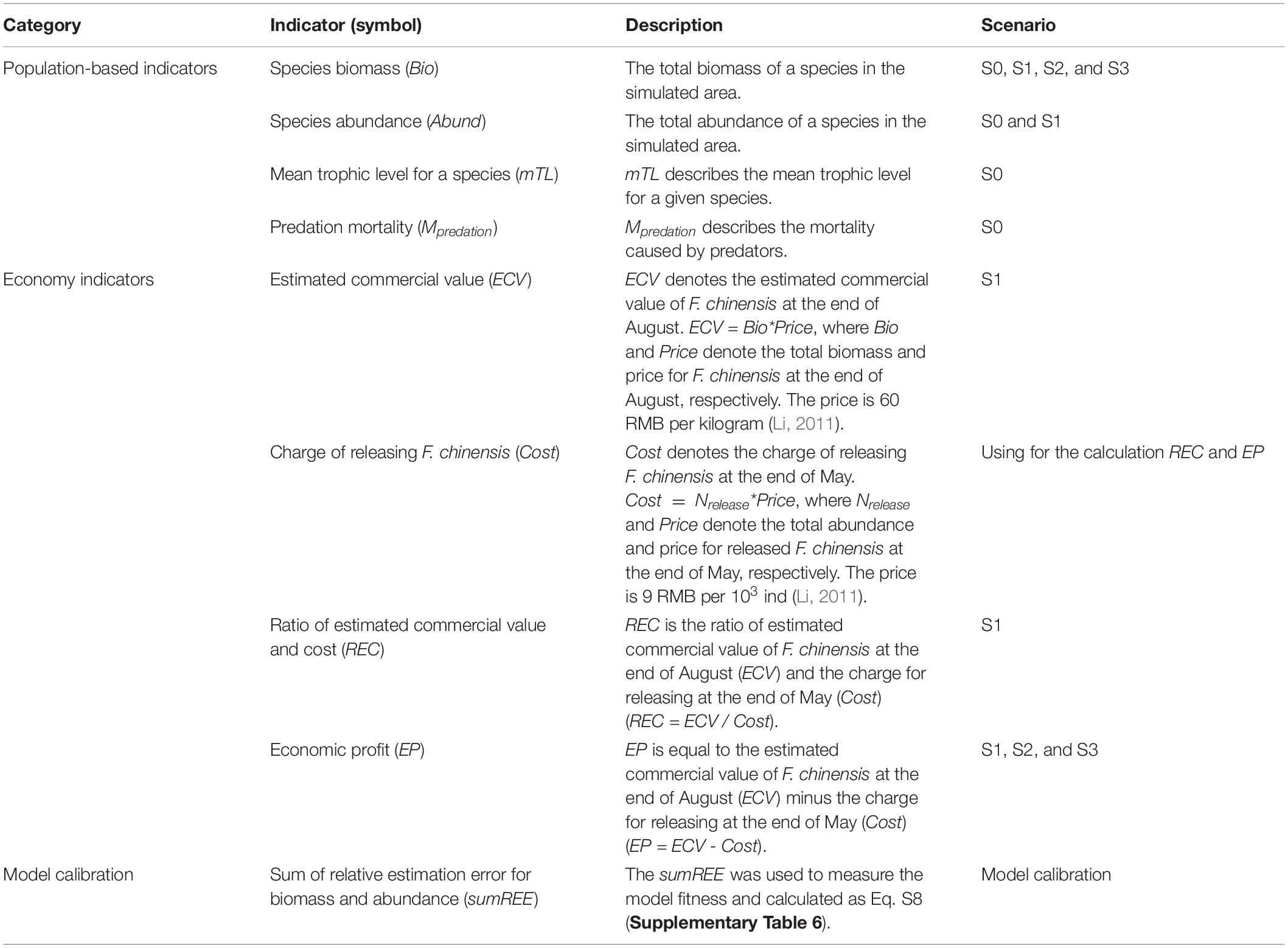
Table 2. Definitions of indicators in the scenarios S0 to S3, model performance evaluation, and model calibration.
Results
Seasonal variations appeared in biomass, abundance, mean trophic level, predation mortality, and size structure for F. chinensis (Figure 3). F. chinensis biomass increased slowly after the second half of July and peaked in the second half of August (Figure 3A). F. chinensis abundance showed the largest decline during the 1st month after the release (Figure 3B). The trophic level of F. chinensis increased rapidly before the first half of July and then remained stable (Figure 3C). F. chinensis experienced high predation mortality (higher than 0.4) during the first half month of release (Figure 3D). In July and August, the dominant size group of F. chinensis was 10–16 cm, which accounted for over 60% of the total biomass (Figure 3E).
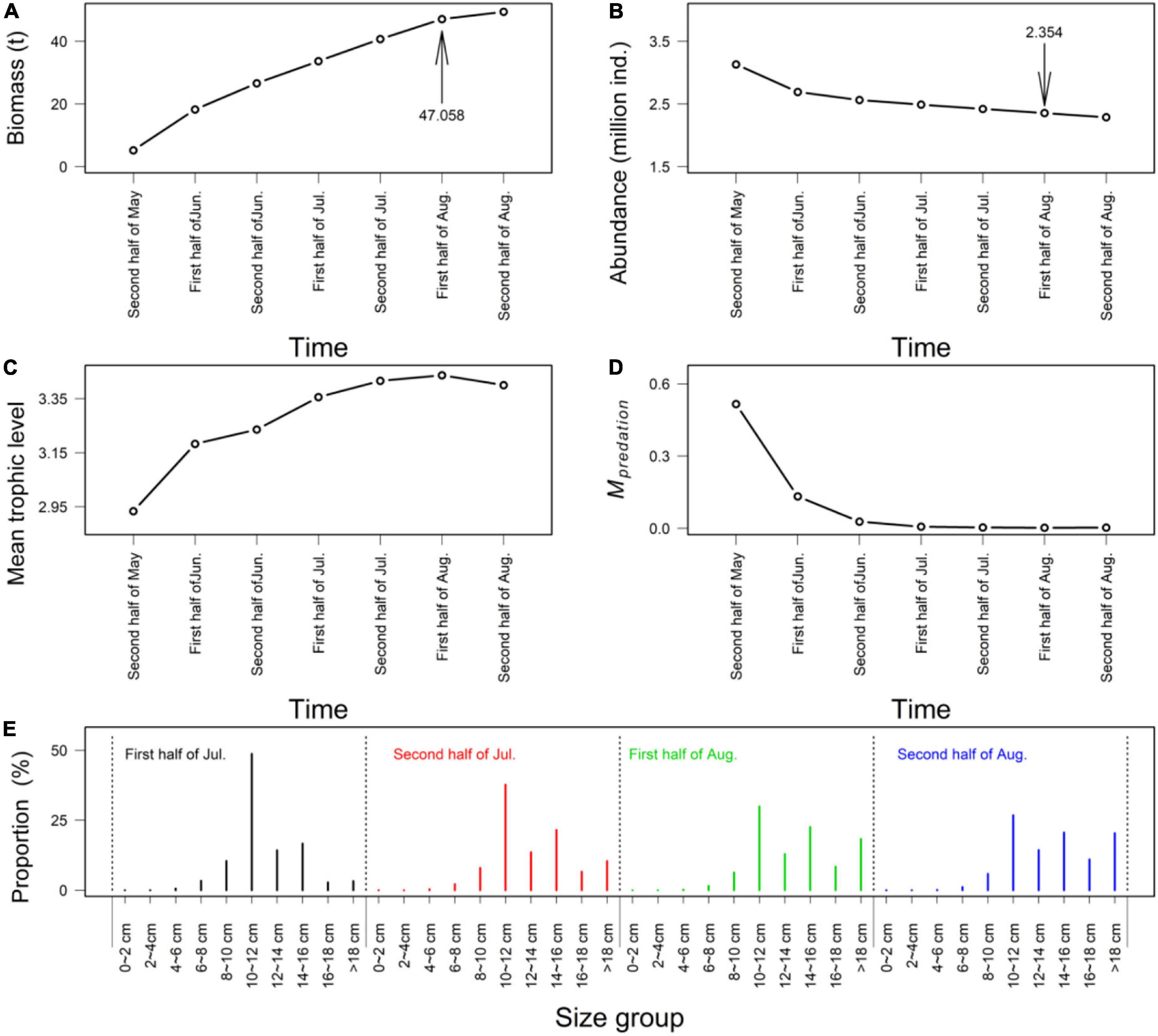
Figure 3. The outputs of biomass (A), abundance (B), mean trophic level (C), predation mortality (D), and mean size of population (E) for F. chinensis in 2012. (A–D) showed the variations of biomass, abundance, metatrophic level, and predation mortality from the end of May (time step 9) and the end of August (time step 15). (E) showed the variations of size structure in population within July and August (time steps 12∼15).
The scenario S1 simulated 1-year F. chinensis hatchery program under different released amounts. In this scenario, the estimated biomass and estimated commercial value (ECV) of F. chinensis was highest when 234 million F. chinensis individuals (Multiplier = 2.6) were released (Figures 4A,C). The abundance increased with the increase of released amount (Figure 4B). The economic profit (EP) increased rapidly when the annual released amount increased from 18 million (Multiplier = 0.2) to 90 million (Multiplier = 1.0). The EP increased slightly when the released amount was between 90 million and 144 million, and then declined when the released amount was higher than 198 million (Multiplier = 2.2) (Figure 4D). The ratio of ECV and Cost (REC) was highest with the annual released amount of 18 million (Multiplier = 0.2), and then decreased rapidly along with increasing released amount (Figure 4E). The proportion of individuals larger than 10 cm exceeded 85% when the released amount was between 18 million to 108 million (Multiplier = 1.2). There was a downward trend for the proportion of individuals larger than 10 cm with increasing released F. chinensis individuals (Figure 4F).
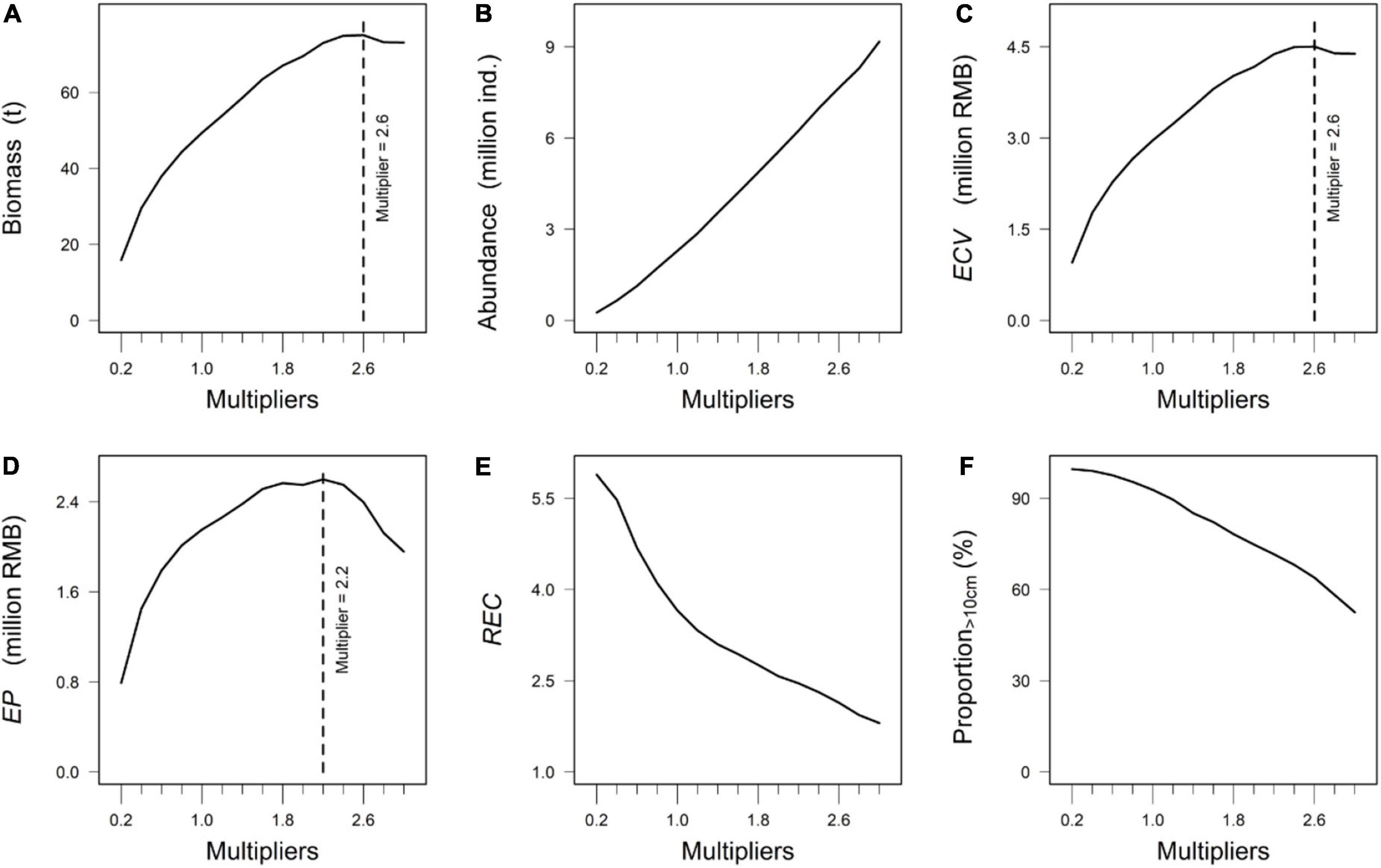
Figure 4. The outputs of estimated biomass, abundance, and commercial value (ECV) in S1 were shown on the top (A–C). The outputs of economic profit (EP), ratio of estimated commercial value (ECV) and charge of releasing F. chinensis (Cost) (REC), and proportion of individuals larger than 10 cm at the end of August in S1 were shown on the bottom (D–F). The calculation of indicators were shown in Table 2.
The scenario S2 simulated a long-term hatchery program that F. chinensis releases implemented for 10 years and then stopped during following 10 years. In the scenario S2, the sum of economic profit in the release stage (i.e., total EP) was highest under P1 and lowest under P2 especially when the 10-year released amount was more than 900 million (Rx1). The sum of economic profit under P2 to P4 declined when the 10-year released amount increased from 900 million to 1980 million (Rx2.2). The annual economic profit (i.e., EP) did not exceed 2.8 million RMB despite increasing the released F. chinensis amount (Figure 5A). The relative changes of two large fish groups (SP6 and SP11) biomass were bigger than other wild species. The biomass of two large fishes at the end of recovery stage (Bio100) were lower than those before F. chinensis released (Bio80). It suggested that they did not recover to the previous levels. The large amount of F. chinensis (Rx2.2) had negative impacts on small shrimps (SP1). In contrast to the two large fishes, the biomass of small shrimps could rose back to the status before releasing. It suggested large fishes recovered slowly compared to small shrimps. Additionally, F. chinensis releases seemed not to influence other wild species (Figure 5B).
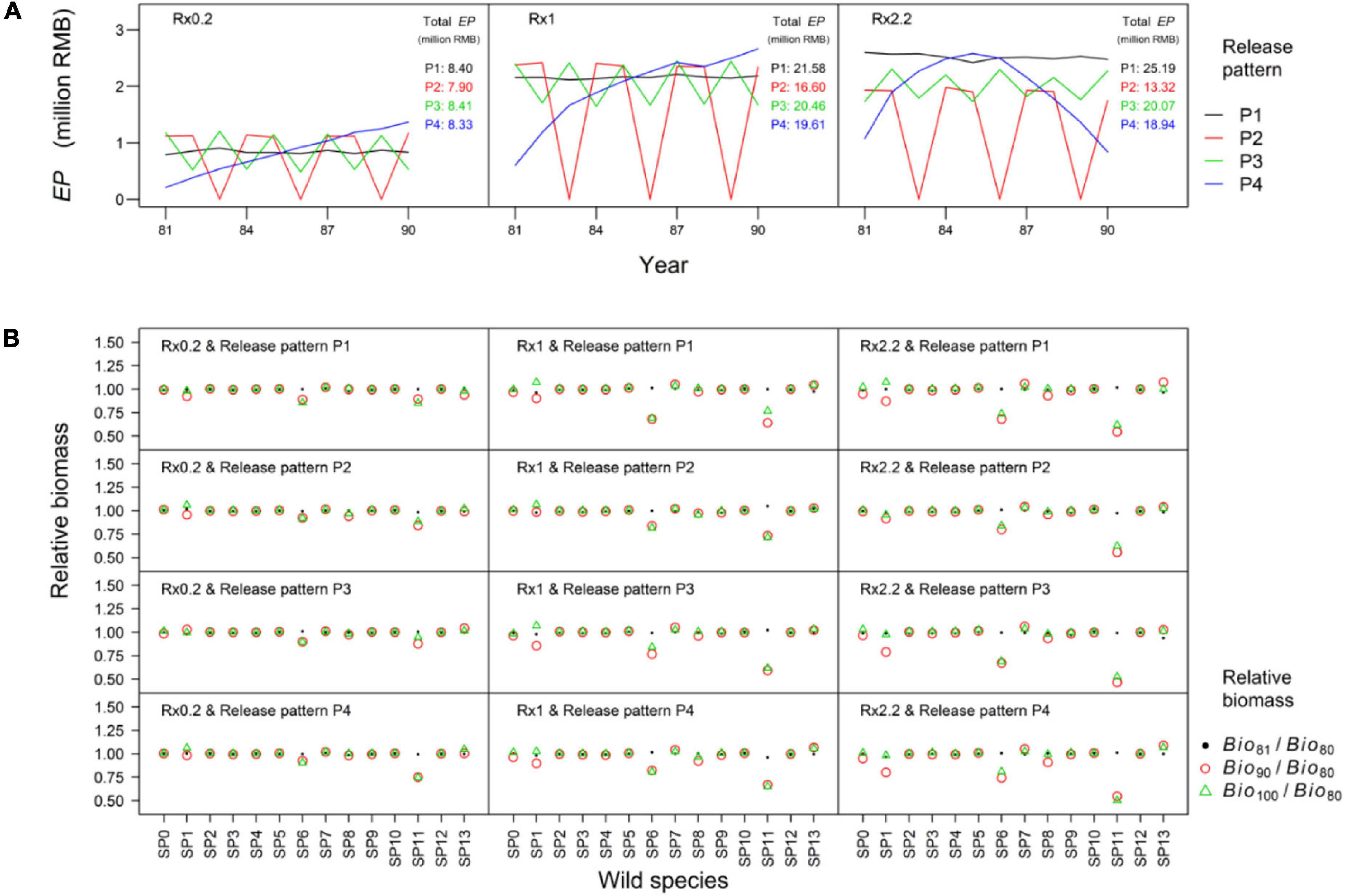
Figure 5. The economic profit (EP) of F. chinensis release (A) and variations of wild species biomass (B) in S2. The F. chinensis release implemented from 81st year to 90th year. The total EP was the sum of EP (economic profit) in the release stage and presented in the figures. The calculation of indicators were shown in Table 2. The Bio80, Bio81, Bio90, and Bio100 were wild species biomass (i.e., SP0 to SP13) in the 80th, 81st, 90th, and 100th year, respectively.
The scenario S3 simulated a long-term hatchery program under different fishing pressure. For this scenario, the sum of economic profit had small changes after fishing changed. The sum of economic profit increased when released amount increased (Figure 6A). Fishing had more negative impacts on the two large fishes (SP6 and SP11) than other spices. The Bio90/Bio80 values for Sebastes schlegelii (SP6) were more than 1.2. It suggested that reducing fishing pressure could offset the negative impact of F. chinensis release. The Bio90/Bio80 of Johnius belangerii (SP11) under the medium (Rx1) and high (Rx2.2) levels of F. chinensis releases were less than 1. It suggested the impacts of F. chinensis release were bigger than fishing when the released amount was large (Figure 6B).
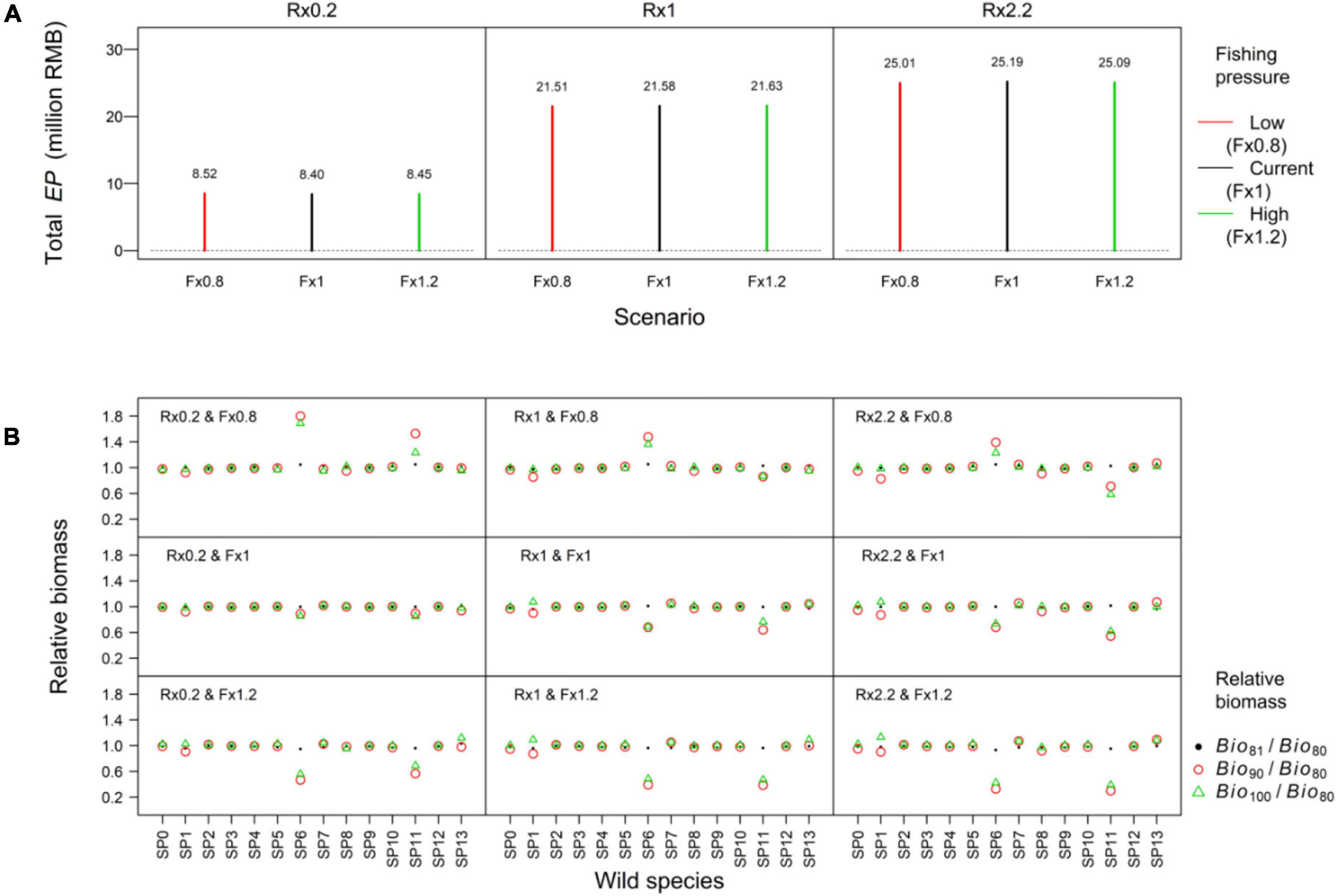
Figure 6. The sum of economic profit in the release stage (Total EP) (A) and variations of wild species biomass (B) in S3. The F. chinensis release implemented and fishing changed since 81st year. Since 91st year, the F. chinensis release stopped and fishing mortality rates of HTL groups were set the same to that in Xing et al. (2017) (current fishing pressure level). The Bio80, Bio81, Bio90, and Bio100 were wild species biomass in the 80th, 81st, 90th, and 100th year, respectively. The calculation of indicators were shown in Table 2.
Discussion
The study provided the insights of F. chinensis population dynamics. Mortality of fish populations is strongly density-dependent in the early stages of the life cycle (Lorenzen and Camp, 2019). High Mnatural–P1 value suggested most of the released F. chinensis juveniles died within the first 2 weeks. One reason is that high density has the negative impacts on the postrelease survival (Schloesser et al., 2021). Another possible reason is that some individuals died during the transportation process. Changes in environmental factors influenced F. chinensis especially for the species’ locomotive ability (Li et al., 2019). The mean trophic level of F. chinensis was lower than 2.95 at their initial stage of inhabiting the Jiaozhou Bay. They could not feed on large prey as they were too small (Figure 3C). The mean trophic level (TL) was 3.35 after June similar to the results of stable carbon and nitrogen isotopes (TL = 3.27) in the Laizhou Bay, which is located in the north of the Shandong Peninsula (Li et al., 2021). Similar to Jiaozhou Bay, Laoshan Bay is a small bay located in the south of the Shandong Peninsula. Yuan et al. (2015) analyzed the survey data of F. chinensis release in the Laoshan bay during 2012. They reported that the size range of F. chinensis was 10.3∼12.7 cm on July 13, 11.4∼15.7 cm on August 1, and 13.1∼17.2 cm on August 17. Our predicted F. chinensis size was similar to Yuan et al. (2015) in July but smaller in August (Figure 3E). Intense shellfish culture reduced the ecosystem productivity in Jiaozhou Bay (Han et al., 2017). F. chinensis individuals needed more food to support the growth in August compared to July. Therefore, F. chinensis growth was slower in August (Figure 3E). The F. chinensis predation mortality was higher within the 1st month in Jiaozhou Bay than in other periods (Figure 3D) due to small F. chinensis individuals were more susceptible to predation.
Releasing hatchery individuals has become the preferred approach to enhance fishery productivity. High population density may limit individual growth as a result of competition of food and habitat (Kitada, 2018). The economic profit (i.e., EP) peaked when the annual released F. chinensis were 198 million (Figure 4B). However, the proportion of individuals which were larger than 10 cm (approximately 72%) at the end of August was much lower than scenarios whose annual released amount was below 90 million (Figure 4D). This may reduce the prospective benefit of F. chinensis release to the fishery production. The results highlighted the population density should consider in a long-term F. chinensis hatchery program. For example, the sum of economic profit in the release stage was maximized under the uniform release (P1), especially when the total released amount was large (Figure 5A). We found annual economic profit could not exceed 2.8 million RMB regardless of the increasing released amount of F. chinensis (Figure 5A). Therefore, we recommend that the annual released amount should be lower than 90 million individuals per year since the economic profit is high and individual size is large. The pattern P1 seemed to be a preferable release strategy that can avoid excessive release in a long-term hatchery program compared to other release patterns.
Historically, the F. chinensis metapopulation of wild individuals and artificially released individuals was up to 242 t in August (Wu et al., 1997). It exceed over four times of our optimal F. chinensis biomass. Intensive shellfish aquaculture especially for Ruditapes philippinarum culture is an apparent reason for Jiaozhou Bay failing to provide abundant food for F. chinensis growth as before. The shrimp biomass decreased from 4.70 t/km2 in 1982 to 0.38 t/km2 in 2015, while cultured R. philippinarum biomass increased from 25 t/km2 to 100 t/km2 (Ma et al., 2018). R. philippinarum culture had marked negative impacts on shrimp as they consumed a large amount of phytoplankton (Han et al., 2018). Shellfish aquaculture is important for local residents that creates billions of income about 230 million dollars per year (Han et al., 2017). It is difficult to remove shellfish aquaculture from the Jiaozhou Bay. Future F. chinensis release strategy should consider the impact of shellfish aquaculture such as food competition.
A responsible approach to stock enhancement requires to assess and manage ecological impacts (Lorenzen et al., 2010). 1-year F. chinensis release had small impacts on wild species (Figures 5, 6). The impact on other species is mainly attributed to (1) F. chinensis is a low-trophic level shrimp feeding on small size prey and influenced wild species mainly via food competition; (2) F. chinensis stayed in the simulated area less than 4 months; and (3) Ecopath model studies evaluated the ecological carrying capacity of F. chinensis biomass were 0.85 t/km2 in Haizhou Bay (Wang et al., 2016), 2.95 t/km2 in Laizhou Bay (Lin et al., 2013), and 0.70 t/km2 in Bohai Sea (Lin et al., 2018). The F. chinensis density in our study was much lower than those in the Ecopath model studies. The simulations highlighted that the negative impacts of a long-term F. chinensis release on two large fishes (S. schlegelii and J. belangerii) should be considered (Figure 5B). The prey biomass to S. schlegelii and J. belangerii were abundant in the Jiaozhou Bay (Xing et al., 2017). The increase of F. chinensis biomass made a very small contribution to the growth of S. schlegelii and J. belangerii. S. schlegelii and J. belangerii spawned in the late spring and summer. F. chinensis biomass reached the peck in August (Figure 3). Large biomass of F. chinensis resulted in a strong food competition with S. schlegelii and J. belangerii juveniles (Xing et al., 2021a). Ecological processes can influence stock enhancements and fisheries (Johnston et al., 2018). The effectiveness of F. chinensis release seemed not to change when fishing pressure increased or declined by 20% (Figure 6A). Predator pressure to F. chinensis was low since June due to their large body size (Figures 3D,E). We found that managing fishing effort could offset the negative impacts of F. chinensis release (Figure 6B). It was noteworthy that F. chinensis resulted in a bigger decline of large fishes when fishing pressure was high.
Additional aspects should be considered using our approach to advise management. The release size can influence the effectiveness of stock enhancement and wild populations (Garlock et al., 2019). The sensitivity analysis highlighted the accuracy of estimation for short-term post-release mortality (Mnatural–P1, the mortality of F. chinensis at the initial stage of release). Moreover, reducing Mnatural–P1 could increase F. chinensis biomass (Supplementary Figure 3). Studies have demonstrated large release size can improve the effectiveness of stock enhancement (Hamasaki and Kitada, 2006; Ye et al., 2005). The cost of hatchery program will increase as the individuals at a large size have a higher price (Li, 2011). Future study will consider releasing the F. chinensis individuals at other body sizes. In addition to the release size, short-term post-release mortality is dependent on the season and release method (Leber et al., 1997; Hervas et al., 2010). Given the food web of Jiaozhou Bay has seasonal variations, the timing of F. chinensis release should be considered in the management. The economy indicators need to further improve in co-operating into fluctuations in market price and transport charge. Climate change can influence the population processes such as growth and reproduction (Szuwalski and Hollowed, 2016; Martino et al., 2019). Understanding the impact of environmental changes and shellfish culture is important for the decision-making process. Ecosystem models are usually “data-hungry,” and imprecise parameters will bias model outputs (Xing et al., 2020b). Although we compared our results to other studies, inadequate data certainly increase model uncertainty. It is difficult to involve all species in the modelled food web. This is a potential source of bias to our model predictions.
Conclusion
We evaluated the F. chinensis release in terms of economic profits and ecological impacts. Our findings highlight that the effectiveness of F. chinensis release cannot be improved by simply increasing the released amount. F. chinensis release gradually influences non-released species instead of instantaneously resulting in a big change of fish community. The potential negative impact of a long-term F. chinensis release on the ecosystem should be considered in the decision-making process. We encourage implementing the hatchery program in a dynamic perspective and ecosystem consideration instead of simply emphasizing socio-economic benefits. Our modeling approach can be applied to other ecosystems and promote implementing hatchery programs in an ecosystem context.
Data Availability Statement
The raw data supporting the conclusions of this article will be made available by the authors, without undue reservation.
Author Contributions
LX contributed to the conceptualization, carried out the data curation, formal analysis, and software, performed the methodology, and wrote the original draft. YC contribute to the conceptualization, performed the methodology, supervised the data, and wrote, reviewed, and edited the manuscript. KT performed the methodology and wrote, reviewed, and edited the manuscript. NB performed the methodology and carried out the software. YR carried out the data curation and funding acquisition and supervised the data. All authors contributed to the article and approved the submitted version.
Funding
This work was funded by the National Key R&D Program of China (2018YFD0900904 and 2018YFD0900906) and the Impact and Response of Antarctic Seas to Climate Change (IRASCC2020–2022).
Conflict of Interest
The authors declare that the research was conducted in the absence of any commercial or financial relationships that could be construed as a potential conflict of interest.
Publisher’s Note
All claims expressed in this article are solely those of the authors and do not necessarily represent those of their affiliated organizations, or those of the publisher, the editors and the reviewers. Any product that may be evaluated in this article, or claim that may be made by its manufacturer, is not guaranteed or endorsed by the publisher.
Supplementary Material
The Supplementary Material for this article can be found online at: https://www.frontiersin.org/articles/10.3389/fmars.2022.789805/full#supplementary-material
References
Amoroso, R. O., Tillotson, D. M., and Hilborn, R. (2017). Measuring the net biological impact of fisheries enhancement: pink salmon hatcheries can increase yield, but with apparent costs to wild populations. Can. J. Fish. Aquat. Sci. 74, 1233–1242. doi: 10.1139/cjfas-2016-0334
Anderson, J. H., Warheit, K. I., Craig, B. E., Seamons, T. R., and Haukenes, A. H. (2020). A Review of Hatchery Reform Science in Washington State. Washington, DC: Washington Department of Fish and Wildlife.
Bell, J. D., Rothlisberg, P. C., Munro, J. L., Loneragan, N. R., Nash, W. J., Ward, R. D., et al. (2005). Restocking and stock enhancement of marine invertebrate fisheries. Adv. Mar. Biol. 49, 1–353. doi: 10.1016/S0065-2881(05)49010-0
Blankenship, H. L., and Leber, K. M. (1995). A responsible approach to marine stock enhancement. Am. Fish. Soc. Symp. 15, 167–175. doi: 10.1016/j.marenvres.2020.104957
Camp, E. V., Larkin, S. L., Ahrens, R. N. M., and Lorenzen, K. (2017). Trade-offs between socio-economic and conservation management objectives in stock enhancement of marine recreational fisheries. Fish. Res. 186, 446–459. doi: 10.1016/j.fishres.2016.05.031
FAO (2020). The state of world fisheries and aquaculture 2020: Sustainability in action. Rome: Food and Agriculture Organization of the United Nations.
Félix, P. M., Costa, J. L., Monteiro, R., Castro, N., Quintella, B. R., Almeida, P. R., et al. (2020). Can a restocking event with European (glass) eels cause early changes in local biological communities and its ecological status? Glob. Ecol. Conserv. 21:e00884. doi: 10.1016/j.gecco.2019.e00884
Garlock, T. M., Camp, E. V., and Lorenzen, K. (2019). Efficacy of largemouth bass stock enhancement in achieving fishery management objectives in Florida. Fish. Res. 213, 180–189. doi: 10.1016/j.fishres.2019.01.010
Grüss, A., Schirripa, M. J., Chagaris, D., Drexler, M., Simons, J., Verley, P., et al. (2015). Evaluation of the trophic structure of the West Florida Shelf in the 2000 using the ecosystem model OSMOSE. J. Mar. Syst. 144, 30–47. doi: 10.1016/j.jmarsys.2014.11.004
Hagen, I. J., Ugedal, O., Jensen, A. J., Lo, H., Holthe, E., Bjøru, B., et al. (2020). Evaluation of genetic effects on wild salmon populations from stock enhancement. ICES J. Mar. Sci. 2020:fsaa235. doi: 10.1093/icesjms/fsaa235
Hamasaki, K., and Kitada, S. (2006). A review of kuruma prawn Penaeus japonicus stock enhancement in Japan. Fish. Res. 80, 80–90. doi: 10.1016/j.fishres.2006.03.018
Han, D., Chen, Y., Zhang, C., Ren, Y., Xu, B., and Xue, Y. (2018). Evaluation of effects of shellfish aquaculture and capture fishery on a semi-closed bay ecosystem. Estuar. Coast. Shelf S. 207, 175–182. doi: 10.1016/j.ecss.2018.04.005
Han, D., Chen, Y., Zhang, C., Ren, Y., Xue, Y., and Wang, R. (2017). Evaluating impacts of intensive shellfish aquaculture on a semi-closed marine ecosystem. Ecol. Modell. 359, 193–200. doi: 10.1016/j.ecolmodel.2017.05.024
Hervas, S., Lorenzen, K., Shane, M. A., and Drawvridge, M. A. (2010). Quantitative assessment of a white seabass (Atractoscion nobilis) stock enhancement program in California: Post-release dispersal, growth and survival. Fish. Res. 105, 237–243. doi: 10.1016/j.fishres.2010.06.001
Hilborn, R. (2011). Future directions in ecosystem based fisheries management?: A personal perspective. Fish. Res. 108, 235–239. doi: 10.1016/j.fishres.2010.12.030
Johnston, F. D., Allen, M. S., Beardmore, B., Riepe, C., Pagel, T., Hühn, D., et al. (2018). How ecological processes shape the outcomes of stock enhancement and harvest regulations in recreational fisheries. Ecol. Appl. 28, 2033–2054. doi: 10.1002/eap.1793
Kitada, S. (2018). Economic, ecological and genetic impacts of marine stock enhancement and sea ranching: A systematic review. Fish Fish. 19, 511–532. doi: 10.1111/faf.12271
Kitada, S. (2020). Lessons from Japan marine stock enhancement and sea ranching programmes over 100 years. Rev. Aquacult. 12, 1944–1969. doi: 10.1111/raq.12418
Leber, K. M., Blankenship, H. L., Arce, S. M., and Brennan, N. P. (1997). Influence of release season on size-dependent survival of cultured striped mullet, Mugil cephalus, in a Hawaiian estuary. Fish. Bull. 95, 267–279.
Li, F., Cong, X., and Zhang, X. (2021). Niches of four large crustacean species in Laizhou Bay. J. Fish. China 45, 1384–1394. doi: 10.11964/jfc.20200812378
Li, J., Li, W., and Zhang, X. (2019). Effects of dissolved oxygen, starvation, temperature, and salinity on the locomotive ability of juvenile Chinese shrimp Fenneropenaeus chinensis. Ethol. Ecol. Evol. 31, 155–172. doi: 10.1080/03949370.2018.1526215
Li, X. (2011). Releasing proliferation impact assessment of Chinese shrimp in the southern Shandong Peninsula (Master Thesis). China: Chinese Academy of Agricultural Sciences.
Liang, C., and Pauly, D. (2020). Masking and unmasking fishing down effects: The Bohai Sea (China) as a case study. Ocean. Coast. Manag. 184:105033. doi: 10.1016/j.ocecoaman.2019.105033
Lin, Q., Li, X., Li, Z., and Jin, X. (2013). Ecological carrying capacity of Chinese shrimp stock enhancement in Laizhou Bay of East China based on Ecopath model. Chin. J. Appl. Ecol. 24, 1131–1140. doi: 10.13287/j.1001-9332.2013.0269
Lin, Q., Shan, X., Wang, J., and Li, Z. (2018). Changes in Chinese shrimp (Fenneropenaeus chinensis) carrying capacity of the Bohai Sea. Prog. Fish. Sci. 39, 19–29. doi: 10.19663/j.issn2095-9869.20170908001
Link, J. S., and Watson, R. A. (2019). Global ecosystem overfishing: clear delineation within real limits to production. Sci. Adv. 5:eaav0474. doi: 10.1126/sciadv.aav0474
Liu, R., Cui, Y., and Xu, F. (1993). Recapture rate of released shrimp Penaeus chinensis in resource enhancement experiment in Jiaozhou Bay, Yellow Sea. Oceanologia et limnologia sinica 24, 137–142.
Lorenzen, K. (2005). Population dynamics and potential of fisheries stock enhancement: practical theory for assessment and policy analysis. Philos. Trans. R. Soc. Lond. B Biol. Sci. 360, 171–189. doi: 10.1098/rstb.2004.1570
Lorenzen, K., and Camp, E. V. (2019). Density-dependence in the life history of fishes: when is a fish recruited? Fish. Res. 217, 5–10. doi: 10.1016/j.fishres.2018.09.024
Lorenzen, K., Lebber, K. M., Loneragan, N. R., Schloesser, R. W., and Taylor, M. D. (2021). Developing and integrating enhancement strategies to improve and restore fisheries. Bull Mar. Sci. 97, 475–487. doi: 10.5343/bms.2021.0036
Lorenzen, K., Leber, K. M., and Blankenship, H. L. (2010). Responsible approach to marine stock enhancement: an update. Rev. Fish. Sci. 18, 189–210. doi: 10.1080/10641262.2010.491564
Ma, M., Xu, S., Xu, Y., Zhang, K., Yuan, W., and Chen, Z. (2018). Comparative study of Jiaozhou Bay ecosystem based on an Ecopath model. J. Fish. Sci. China 25, 413–422. doi: 10.3724/SP.J.1118.2018.17335
Martino, J. C., Fowler, A. J., Doubleday, Z. A., Grammer, G. L., and Gillanders, B. M. (2019). Using otolith chronologies to understand long-term trends and extrinsic drivers of growth in fisheries. Ecosphere 10:e02553. doi: 10.1002/ecs2.2553
Oliveros-Ramos, R., Verley, P., Echevin, V., and Shin, Y. J. (2017). A sequential approach to calibrate ecosystem models with multiple time series data. Prog. Oceanogr. 151, 227–244. doi: 10.1016/j.pocean.2017.01.002
Osborne, M. J., Dowling, T. E., Scribner, K. T., and Turner, T. F. (2020). Wild at heart: Programs to diminish negative ecological and evolutionary effects of conservation hatcheries. Biol. Conserv. 251:108768. doi: 10.1016/j.biocon.2020.108768
Punt, A. E., Butterworth, D. S., de Moor, C. L., De Oliveira, J. A. A., and Haddon, M. (2016). Management strategy evaluation: best practices. Fish Fish. 17, 303–334. doi: 10.1111/faf.12104
Schloesser, R. W., Leber, K. M., Brennan, N. P., and Caldentey, P. (2021). A novel approach to estimate postrelease survival for estuarine fishes. Bull Mar. Sci. 97, 575–598. doi: 10.5343/bms.2020.0040
Shin, Y. J., and Cury, P. (2001). Exploring fish community dynamics through size-dependent trophic interactions using a spatialized individual-based model. Aquat. Living Resour. 14, 65–80. doi: 10.1016/S0990-7440(01)01106-8
Shin, Y. J., and Cury, P. (2004). Using an individual-based model of fish assemblages to study the response of size spectra to changes in fishing. Can. J. Fish. Aquat. Sci. 61, 414–431. doi: 10.1139/f03-154
Su, S., Tang, Y., Chang, B., Zhu, W., and Chen, Y. (2020). Evolution of marine fisheries management in China from 1949 to 2019: How did China get here and where does China go next? Fish Fish. 21, 435–452. doi: 10.1111/faf.12439
Szuwalski, C. S., and Hollowed, A. B. (2016). Climate change and non-stationary population processes in fisheries management. ICES J. Mar. Sci. 73, 1297–1305. doi: 10.1093/icesjms/fsv229
Tatara, C. P., and Berejikian, B. A. (2012). Mechanisms influencing competition between hatchery and wild juvenile anadromous Pacific salmonids in fresh water and their relative competitive abilities. Environ. Biol. Fish. 94, 7–19. doi: 10.1007/s10641-011-9906-z
Taylor, M. D., Chick, R. C., Lorenzen, K., Agnalt, A., Leber, K. M., Blankenship, H. L., et al. (2017). Fisheries enhancement and restoration in a changing world. Fish. Res. 186, 407–412. doi: 10.1016/j.fishres.2016.10.004
Taylor, M. D., and Suthers, I. M. (2008). A predatory impact model and targeted stock enhancement approach for optimal release of mulloway (Argyrosomos japonicus). Rev. Fish. Sci. 16, 125–134. doi: 10.1080/10641260701727293
Wang, Q., Zhuang, Z., Deng, J., and Ye, Y. (2006). Status of enhancement, restocking and translocation operations for the shrimp Penaeus chinensis in China after 20 years of releases. Fish. Res. 80, 67–79. doi: 10.1016/j.fishres.2006.03.015
Wang, T., Zhang, H., Zhang, H., and Zhang, S. (2016). Ecological carrying capacity of Chinese shrimp stock enhancement in Haizhou Bay of east China based on Ecopath model. J. Fish. Sci. China 23, 965–975. doi: 10.3724/SP.J.1118.2016.15348
Wang, W., Zhang, K., Luo, K., Xiao, G., Song, W., Kong, J., et al. (2014). Assessment of recapture rates after hatchery release of Chinese shrimp Fenneropenaeus chinensis in Jiaozhou Bay and Bohai Bay in 2012 using pedigree tracing based on SSR markers. Fish. Sci. 80, 749–755. doi: 10.1007/s12562-014-0743-9
Wu, Y., Zhang, B., Sun, D., and Huang, B. (1997). Assessment on invertebrate abundance in May and August in the Jiaozhou Bay. Shand. Fish. 4, 8–11. doi: 10.1016/j.scitotenv.2021.145936
Xing, L., Zhang, C., Chen, Y., Shin, Y. J., Verley, P., Yu, H., et al. (2017). An individualbased model for simulating the ecosystem dynamics of Jiaozhou Bay, China. Ecol. Modell. 360, 120–131. doi: 10.1016/j.ecolmodel.2017.06.010
Xing, L., Chen, Y., Zhang, C., Li, B., Shin, Y. J., and Ren, Y. (2020a). Evaluating impacts of pulse fishing on the effectiveness of seasonal closure. Acta. Oceanol. Sin. 39, 89–99. doi: 10.1007/s13131-020-1536-x
Xing, L., Chen, Y., Zhang, C., Li, B., Tanaka, K. R., Boenish, R., et al. (2020b). Evaluating impacts of imprecise parameters on the performance of an ecosystem model OSMOSE-JZB. Ecol. Modell. 419:108923. doi: 10.1016/j.ecolmodel.2019.108923
Xing, L., Chen, Y., Boenish, R., Tanaka, K. R., Barrier, N., and Ren, Y. (2021a). Evaluating the impacts of fishing and migratory species in a temperate bay of China using the ecosystem model OSMOSE-JZB. Fish. Res. 243:106051. doi: 10.1016/j.fishres.2021.106051
Xing, L., Chen, Y., Li, B., Tanaka, K. R., Boenish, R., Ren, Y., et al. (2021b). Evaluating impacts of trophic interactions on the effectiveness of single-species fisheries management. Front. Mar. Sci. 8:698991. doi: 10.3389/fmars.2021.698991
Yang, T., Ren, J. S., Kooijman, S. A. L. M., Shan, X., and Gorfine, H. (2020). A dynamic energy budget model of Fenneropenaeus chinensis with applications for aquaculture and stock enhancement. Ecol. Modell. 431:109186. doi: 10.1016/j.ecolmodel.2020.109186
Ye, Y., Loneragan, N., Die, D., Watson, R., and Harch, B. (2005). Bioeconomic modelling and risk assessment of tiger prawn (Penaeus esculentus) stock enhancement in Exmouth Gulf, Australia. Fish. Res. 73, 231–249. doi: 10.1016/j.fishres.2004.12.004
Keywords: OSMOSE-JZB model, Fenneropenaeus chinensis release, economic profits, ecological impacts, Jiaozhou Bay
Citation: Xing L, Chen Y, Tanaka KR, Barrier N and Ren Y (2022) Evaluating the Hatchery Program of a Highly Exploited Shrimp Stock (Fenneropenaeus chinensis) in a Temperate Marine Ecosystem. Front. Mar. Sci. 9:789805. doi: 10.3389/fmars.2022.789805
Received: 05 October 2021; Accepted: 07 February 2022;
Published: 03 March 2022.
Edited by:
Huang Wei, Second Institute of Oceanography, Ministry of Natural Resources, ChinaReviewed by:
Andrew M. Scheld, Virginia Institute of Marine Science, College of William & Mary, United StatesNeil R. Loneragan, Murdoch University, Australia
Copyright © 2022 Xing, Chen, Tanaka, Barrier and Ren. This is an open-access article distributed under the terms of the Creative Commons Attribution License (CC BY). The use, distribution or reproduction in other forums is permitted, provided the original author(s) and the copyright owner(s) are credited and that the original publication in this journal is cited, in accordance with accepted academic practice. No use, distribution or reproduction is permitted which does not comply with these terms.
*Correspondence: Yiping Ren, cmVueWlwQG91Yy5lZHUuY24=