- 1Department of Ecology, Environment and Plant Sciences, Stockholm University, Stockholm, Sweden
- 2Baltic Sea Centre, Stockholm University, Stockholm, Sweden
Eutrophication is a process that results in excessive phytoplankton blooms, which sink to the sediment and enrich the organic matter (OM). This alters the available resources to benthic organisms and may have consequences for feeding ecology and reproduction strategies of marine populations. While effects of eutrophication on biodiversity are well documented, the more subtle effects of OM on population dynamics and diet plasticity are understudied. We performed a reciprocal transfer experiment with the benthic bioindicator amphipod Monoporeia affinis from two stations in the Baltic Sea with differing sediment OM (low and high) creating four treatments (low control, low transferred, high transferred, and high control). We investigated sediment OM effects on: i) the dietary niche and organism body condition of two different life stages of M. affinis utilizing bulk stable isotopes δ13C and δ15N, and C:N ratio; and ii) M. affinis fecundity and embryo viability. There was no initial significant differences between the females from different stations in terms of δ13C, δ15N, C:N, fecundity or viable embryos. However, we found that moving females from high OM to low OM (where the low OM sediment has higher δ15N and lower δ13C) significantly depleted their 13C values, while amphipods in low OM sediment had always significantly enriched 15N regardless of female origin indicating feeding on the new sediment. Although end-of-experiment females had lower C:N than initial females, individuals in low OM sediment presented significantly higher C:N (indicating higher body condition) than those in high OM sediment. Conversely to adult amphipods, no effects of OM were seen for juveniles δ13C or δ15N, but their individual biomass was larger in high OM sediment treatments and high OM transferred to low OM sediment. Our results indicate that the low range of sediment OM tested here altered female amphipod δ13C, δ15N and C:N ratios, with those in low OM treatments having a better body condition, but those in high OM treatments had a greater reproductive success in terms of offspring biomass. Our findings suggest a tradeoff between female condition and reproduction and indicates that even relatively small levels of sediment organic enrichment will impact female condition. Our study provides valuable information useful to interpret the effects of OM on amphipod populations used as bioindicators for anthropogenic impacts.
Introduction
Anthropogenic eutrophication is an increasing worldwide problem from changed agricultural methods and food preferences, as well as combustion of fossil fuels (Rabalais et al., 2010; Malone and Newton, 2020). In marine environments, low levels of organic matter (OM) enrichment has been shown to be stimulating to biodiversity and increases biomass, especially in nutrient-limited benthic habitats (Cederwall and Elmgren, 1980; Widbom and Elmgren, 1988). However, excessive OM enrichment alters ecosystem functions through reducing biodiversity and simplifying trophic interactions (Nordström and Bonsdorff, 2017; Drylie et al., 2020; Malone and Newton, 2020). Eutrophication alters available food sources to macrofauna, by increasing phytoplankton derived OM inputs to the sediment (Griffiths et al., 2017), that in turn can also increase availability of other potential food sources for macrobenthos like nematodes and juvenile polychaetes in the meiofauna (Widbom and Elmgren, 1988; Ejdung and Elmgren, 1998; Broman et al., 2019; Ptatscheck et al., 2020). Increased resource availability can have important consequences for population growth by increasing the energy available for reproduction (van Noordwijk and de Jong, 1986; McNamara and Houston, 1996; Binzer et al., 2016), with studies showing a positive correlation between available resources and fecundity in crustaceans (Eriksson Wiklund et al., 2008; González-Ortegón et al., 2018).
One of the most eutrophic regional seas in the world is the Baltic Sea (Malone and Newton, 2020). The Baltic Sea is a well-studied eutrophic system, where nitrogen (N) and phosphorus (P) loading has increased by a factor of four and eight, respectively, since the 1950’s (Larsson et al., 1985), but inputs have been reduced and eutrophication improved in recent years (Andersen et al., 2017). To detect long-term ecological changes and assess habitat quality status of Baltic benthic ecosystems, monitoring programs regularly survey the population dynamics of organisms sensitive to stress, such as the amphipod Monoporeia affinis (Lindström). This monitoring effort includes reproductive endpoints of these amphipods as a bioindicator of exposure to contaminants and/or hypoxia (Sundelin and Eriksson, 1998; Eriksson Wiklund and Sundelin, 2001). M. affinis, one of the most abundant macrofauna in soft sediments of the northern Baltic Proper (Ankar and Elmgren, 1975; Broman et al., 2019; Raymond et al., 2021), are important bioturbators (Karlson et al., 2007) and through their role as prey for commercially important fish species represent a key link for benthic-pelagic coupling through the food web (Casini et al., 2004). M. affinis feed mostly on recently deposited OM in the spring and summer, and switch to more opportunistic deposit feeders in sediments at the other times of the year including meiofauna (Lopez and Elmgren, 1989; Viitasalo et al., 2007; Karlson et al., 2008; Hedberg et al., 2020; Ledesma et al., 2020). However, the consequences of sediment OM enrichment to M. affinis trophic ecology and dietary niche are not yet fully understood.
Ecological niche is the sum of all interactions of an organism, both biotic and abiotic (Grinnell, 1917; Elton, 1927; Vandermeer, 1972); thus, understanding an organism’s ecological niche improves our knowledge of not only its interactions with other species, but also allows for predictions on how that ecological niche will be affected by abiotic pressures, such as eutrophication. Stable isotopes are a common tool to investigate trophic (dietary) niche, as carbon (C) isotopes indicate primary production sources utilized, while N isotopes indicate relative trophic position of the organism (Peterson and Fry, 1987; Layman et al., 2007). Population trophic niche can be measured from the inter-individual variability in C and N isotopes in bivariate space (Layman et al., 2007; Jackson et al., 2011). Trophic niche is expected to expand with organic content due to the increased resource availability and diversity of the meiofauna, the prey of M. affinis, which widens the utilized C sources and can increase δ15N through addition of trophic levels (Widbom and Elmgren, 1988; Karlson et al., 2014; González-Ortegón et al., 2018; Ledesma et al., 2020). After a threshold of organic enrichment is reached, however, the M. affinis trophic niche may contract through meiofauna biodiversity loss, and thus reduction of diverse resources (González-Ortegón et al., 2018; Karlson et al., 2018). However, physiological factors can also influence the trophic niche size, through increased variability in δ13C and δ15N from starvation or metabolic alterations in different life stages (Gorokhova, 2017; Karlson et al., 2018). Additionally, the ratio of total C to N can also provide information on the physiological condition of an organism, as it is often used as a proxy for lipid content (Lehtonen, 1996; Post et al., 2007; Karlson et al., 2018), and thus through stable isotope measurements, we receive information on both resource utilization and organism condition.
Sediment OM can also have population level effects on reproductive endpoints of benthic species (Heip, 1995; Sundelin and Eriksson, 1998; Eriksson Wiklund et al., 2008; Löf et al., 2016). M. affinis have a two-year life cycle in the northern Baltic Proper, with copulation occurring in the late autumn, and offspring released the following spring (Cederwall, 1978), timed to the spring phytoplankton bloom (Sundelin, 1983; Lehtonen and Andersin, 1998; Eriksson Wiklund and Sundelin, 2001). Males die after copulation and females die within three months after the offspring release (Sundelin, 1983). Female M. affinis invest a large amount of lipids into their reproductive tissue, ensuring the survival of their offspring during the period lacking high-quality food availability, before the spring phytoplankton bloom sedimentation (Aljetlawi and Leonardsson, 2002; Aljetlawi and Leonardsson, 2003). Female M. affinis are believed to stop feeding while the eggs are developing (Lehtonen and Andersin, 1998; Eriksson Wiklund et al., 2008), which may increase both their δ13C and δ15N values (Post et al., 2007; Doi et al., 2017; Karlson et al., 2018).
Eriksson Wiklund et al. (2008) found that amphipods from the Baltic proper are likely adapted to high OM and their fecundity is reduced when transplanted to lower OM Bothnian Sea sediments. In contrast, amphipods from the Bothnian Sea benefited when exposed to higher OM, but additional abiotic differences between the Baltic proper and Bothnian Sea make teasing apart OM effects difficult. Research is largely lacking linking the more realistic effects of eutrophication (i.e. less than the10-fold increase as in Eriksson Wiklund et al., 2008) on M. affinis population dynamics, occupied dietary niche, and niche partitioning between life stages (adults vs. newly hatched juveniles).
In order to investigate the effect sediment OM content has on reproductive success, body condition and trophic niche in M. affinis recently hatched juveniles and their progenitor mothers, we designed and carried out an experiment utilizing two geographically close stations in the Baltic Sea (Baltic proper basin, in contrast to different basins in Eriksson Wiklund et al., 2008), but differing two-fold in sediment OM content. We sampled gravid females and sediment from two stations and utilized a reciprocal transplant experimental design, creating two control and two transferred treatments. We tested three hypotheses with this experiment (details in Table S1): 1. higher OM sediment content will positively affect female condition (female C:N) and nutritional status (inferred from δ13C and δ15N) due to higher resource availability, which will in turn result in higher fecundity, viable embryos (VE), juvenile biomass, and juvenile C:N; 2. higher OM sediment content will significantly: i) increase or decrease mean female and juvenile δ13C values in the direction of the sediment isotope signal, ii) increase mean female and juvenile δ15N values in the direction of the sediment isotope signal, and iii) increase female inter-individual variability in bi-variate isotope composition (measured through stable isotope range, isotope anomalies, and euclidian distance) through changes in resource availability; 3. if M. affinis do not eat while carrying eggs, we will expect a starvation effect in females visible through: i) increased δ13C and δ15N values from initial to end of experiment, and ii) lower C:N ratios in the end of the experiment compared to the initial females; and finally, 4. female and progenitor offspring δ13C and δ15N will differ significantly, with higher δ15N in females, and juvenile δ13C matching the sediment δ13C more closely than females. In all of the above hypotheses, we expect the various endpoints measured in amphipods to change more in transferred than in control treatments due to plasticity in the feeding niche. Specifically for hypothesis two, we predict that moving females from low OM sediment to high OM sediment will expand the trophic niche (larger range of δ13C and δ15N), and moving females from high OM sediment to low OM sediment will compress the trophic niche.
Materials and Methods
Sampling and Experimental Set Up
Samples were collected on 24 January 2019 outside of Askö marine station in the Baltic Sea. Benthic sled drags were taken at two stations (Low OM station 6019: latitude 58 44 32 N, longitude 17 40 58 E, depth 42 m, 1.77% C, 0.26% N; and High OM station 6022: latitude 58 44 68 N, longitude 17 48 75 E, depth 41 m, 3.7% C, 0.52% N; Figure 1). The low OM station is characterized by sandy clay sediments (i.e. larger particle sizes) and good oxygenation indicative of a transport bottom, while the high OM stations has muddy clay sediments (i.e. smaller particle sizes) and periodic hypoxia, representative of an accumulation bottom. Sediment was sieved through 1 mm to obtain individual gravid Monoporeia affinis and remove additional macrofauna, and sediment and water were saved. Materials were transported to Stockholm University, where the experimental units were set up in a 4°C constantly dark room to simulate aphotic field conditions from where the samples were collected. The sieved sediment and water were added to each individual 50 ml beaker (n = 64), with sediment around 3 cm depth (41.6 cm3 sediment volume) and a water column of around 3 cm. Four treatments were set up as follows: high control (gravid females, water, and sediment from High OM station 6022), high transferred (gravid females from Low OM station 6019 in water and sediment from High OM station 6022), low transferred (gravid females from High OM station 6022 in water and sediment from Low OM station 6019), and low control (gravid females, water, and sediment from Low OM station 6019). The experimental setup can be found in Figure 2. For comparisons between treatments, the sediment OM content is referred to as high or low, and the amphipod origin is described as control (same experimental treatment as origin) or transferred (not same experimental treatment as origin). See Figure 2 also for timeline and treatments. One gravid M. affinis was individually placed in each beaker, corresponding to 722 ind m-2, within densities previously reported for M. affinis in the area (Ankar and Elmgren, 1975; Raymond et al., 2021). Sediment was allowed to settle for 24 hours before adding oxygenation by bubbling air through a hose into the water. Water (alternating brackish and fresh) was added to each beaker when required due to evaporation, and salinity (6.9 ± 0.9) was checked to ensure no physiological stress on the amphipods.
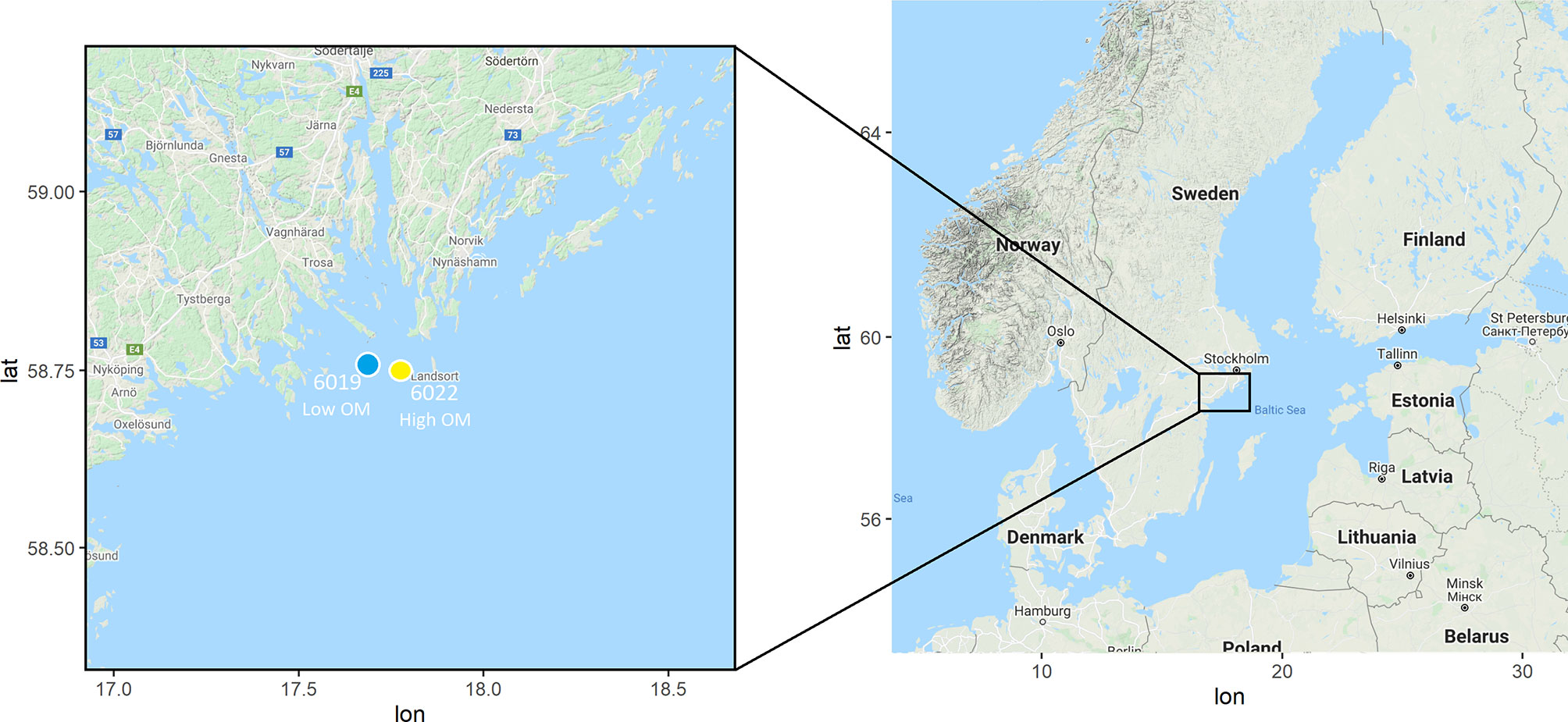
Figure 1 Map of the stations used in this experiment, located along the Swedish coast in the Baltic Proper basin of the Baltic Sea, off the research station on the island of Askö. Station 6019 represents the low organic matter (OM) station (blue), and station 6022 represents the high OM station (yellow).
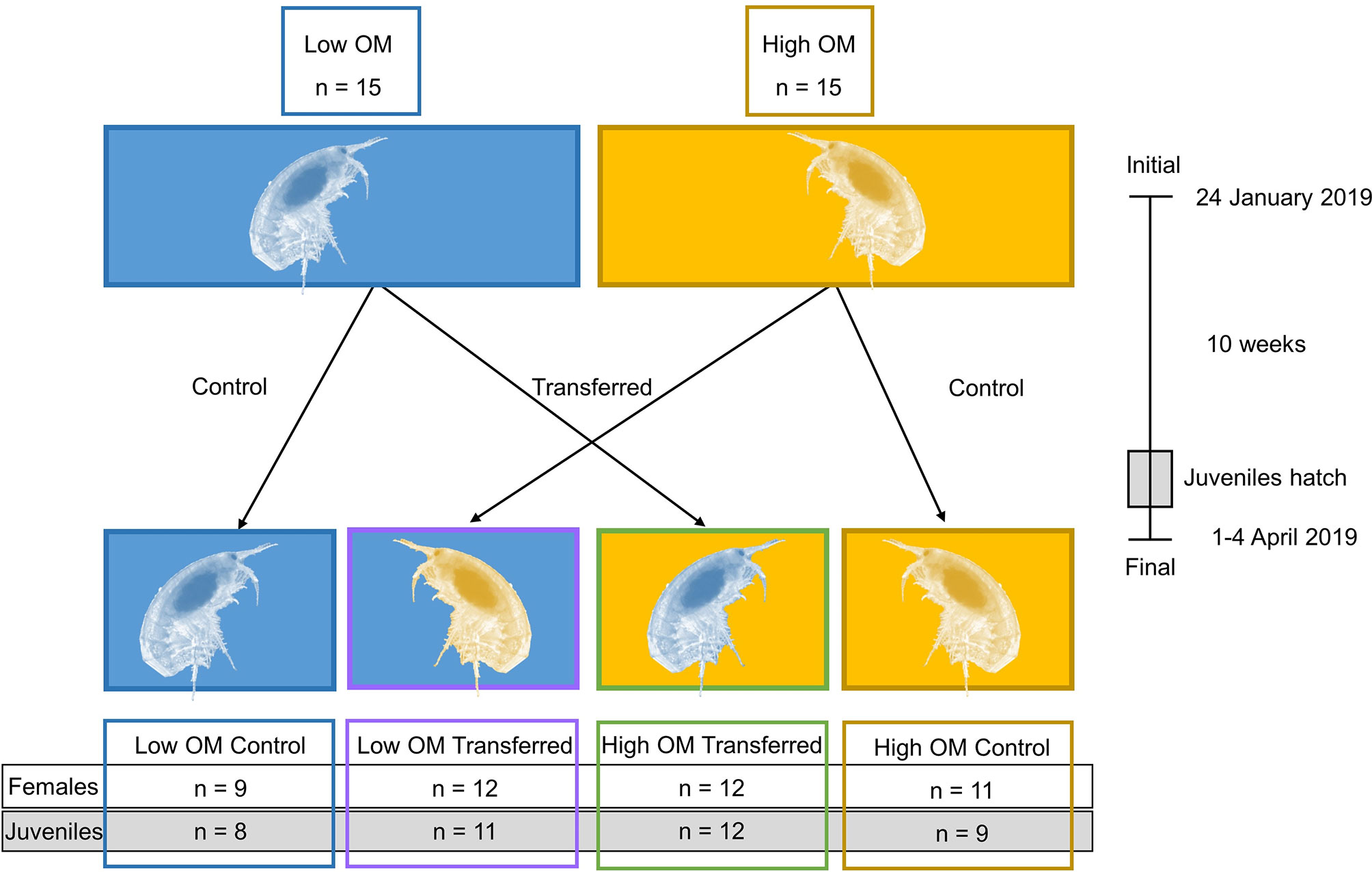
Figure 2 Experimental set-up, including number of replicates for the two time points (initial and final) and each treatment. Initial samples (n = 15 for each station) were taken from Monoporeia affinis amphipods in the low organic matter (OM) station (blue) and the high OM station (yellow). Experimental treatments were set up as follows: low OM amphipods were kept in low OM sediment (Low Control; blue); high OM amphipods were transplanted into low OM sediment (Low Transferred; purple); low OM amphipods were transplanted into high OM sediment (High Transferred; green); and high OM amphipods were kept in high OM sediment (High Control; yellow). The amphipods acclimated and eggs developed for 10 weeks, with the juveniles hatching towards the end of the experiment. Then, the experiment was ended and all amphipods were collected as final samples. The recovered number of females (n = 9-12 per treatment) and number of 13-pooled juveniles per composite sample (n = 8-12 per treatment) are indicated for each treatment along the bottom of the figure.
All initial female M. affinis samples (n = 15 from each station; initial) were collected and frozen within 24 hours of field sampling. A sediment sample was taken from each station for initial stable isotopes and preserved by freezing at -80°C until stable isotope analysis. Eggs from the initial gravid females were removed from the female and counted under a stereomicroscope (Stereo Zoom 2000, Leica) to assess individual fecundity (number of embryos per female). Embryos with aberrations were identified according to Sundelin and Eriksson (1998), and percent viable embryos (VE) were calculated as the percentage of eggs without aberrations. Number of malformed, arrested, and membrane-damaged embryos were not differentiated between. The female were frozen separately in -80°C until further processing.
The experiment ran for 10 weeks. At experiment termination (end), a subsample (1.5 ml) of sediment was taken from each microcosm and frozen at -80°C. The surviving females and hatched juveniles were collected by sieving the sediment on a sterilized 200 µm sieve with brackish water, and then washed onto a sterile petri dish with sterile MilliQ water for counting recovered juveniles under a stereomicroscope. The females and juveniles were frozen separately in -80°C until further processing (females: n = 44; juveniles: n = 40; sediment: n = 45; see Figure 2 for more details).
Stable Isotope Analysis
Animal and bulk sediment samples were analyzed for bulk C and N elemental and stable isotope analysis from the initial time point (initial; head of females and sediment) and the experiment termination (end; head of females, sediment and a subset of 13 randomly selected pooled juveniles from each replicate to reach sufficient biomass for analyses). Samples were dried in a 60°C oven overnight (or over 3 nights in the case of sediment). Each sample was weighed and packaged in a tin capsule before analyzed at the University of California-Davis Stable Isotope Facility on a PDZ Europa ANCA-GSL elemental analyzer interfaced to a PDZ Europa 20-20 isotope mass spectrometer (Sercon Ltd., Cheshire, UK). The stable isotope ratios (R) of 13C:12C and 15N:14N was calculated based on the equation:
and utilized international standards (Vienna Pee Dee Belemnite for C and atmospheric nitrogen gas for N) and are denoted by δ (‰). Standard deviation was ± 0.09 ‰ for C and ± 0.12 ‰ for N. Percent C and N were calculated, as well as C:N. Due to high lipid percentage in amphipods, δ13C values for the amphipods were corrected for high C:N ratio according to Post et al. (2007). It has been shown that percent lipid and C:N ratio correlates strongly for M. affinis (Lehtonen, 1996).
Statistics
Statistical tests were performed in R (version 3.5.0, R Core Team, 2018). When required by assumptions, data was tested for normality with a Shapiro–Wilk test, and for homogeneity of variance with a Levene’s test in the ‘car’ package. When data did not meet the assumptions of normality or homogeneity of variance, transformation was attempted, and if unsuccessful, non-parametric Kruskal-Wallis tests were performed instead of an analysis of variance (ANOVA) test. When significant ANOVA results were found, Tukey post-hoc tests were utilized to determine which variables differed significantly.
Three types of tests were here used: 1) The parametric t-tests and 2-way ANOVA to test for differences between groups and the interaction between independent variables when the data is normally distributed and the variances are homogeneous; 2) the Kruskal-Wallis tests when these assumptions are not met; 3) linear models or correlations to test the relationship between two continuous variables. A summary of the statistical tests and how they relate to the three hypotheses can be found in Table S1. Hypothesis 1 was tested with unpaired t-tests between initial stations female fecundity (number of eggs per female), viable embryos (VE), and C:N ratios. To evaluate the effect of sediment OM content on amphipod reproduction, the number of recovered juveniles (square root transformed) at the end of the experiment were tested with a 2-way ANOVA as a response variable to sediment OM content (high or low) and female origin (control or transferred) as fixed factors with interaction allowed. Still related to H1, end of experiment female C:N (proxy for female body condition), juvenile biomass per individual (as a measure female reproductive output), and juvenile C:N ratios (proxy for juvenile body condition) were each tested as dependent variables with univariate Kruskal-Wallis test with sediment OM content (high or low) and female origin (control or transferred) as explanatory variables. Juvenile biomass per individual was calculated by dividing the total dry weight by the number of pooled individuals (n=13). Finally, multiple linear regressions were used to identify significant associations between amphipod female body condition, nutritional status (inferred from isotopes values) and reproductive output when assumptions were met: i) initial female C:N & VE, ii) initial female δ15N (higher δ15N may indicate higher trophic position or deteriorated body condition) & VE, and iii) end female C:N & number of recovered juveniles per female. When assumptions of multiple linear regressions were not met, Spearman’s rank correlation was run comparing between: i) end female δ 15N & number of recovered juveniles per female, and ii) end female δ13C & number of recovered juveniles per female. Hypothesis 2 was tested by comparing initial female δ13C and δ15N (separately) with unpaired t-tests between the two stations. Continuing with H2, end of experiment female and juvenile δ13C and δ15N were tested separately as dependent variables in a 2-way ANOVA in response to sediment OM content (high or low) and female origin (control or transferred) with interaction allowed, to assess if the transfer of females to new sediment altered their isotope values. Sediment stable isotopes were also statistically tested for differences between end of experiment treatments. To evaluate inter-individual variability in bi-variate isotope composition, ranges in female δ13C and δ15N were calculated in initial stations and end of experiment treatments. Additionally, euclidian distance (ED) was calculated within each treatment for end of experiment females by the following formula.
where δ13Ca and δ15Na are δ13C and δ15N anomalies calculated by taking the difference of each individual replicate female δ13C and δ15N values from the mean δ13C and δ15N of each treatment (Karlson et al., 2018). Differences in δ13Ca between treatments at the end of experiment females were evaluated with a 2-way ANOVA in response to sediment OM content (high or low) and female origin (control or transferred) with interaction allowed. End of experiment female δ15Na and ED were evaluated separately by Kruskal-Wallis test with sediment OM content (high or low) and female origin (control or transferred) as explanatory variables. Finally, hypothesis 3 (aimed at addressing if female amphipods ate during the experiment) was tested by multiple 2-way ANOVA tests using female δ13C and δ15N as response variables to time (initial or end of experiment) and treatment (low control, low transferred, high transferred, or high control), with interaction allowed. Female C:N was tested with a Kruskal-Wallis test as a response variable to time (initial or end of experiment) to assess changes in female amphipods regarding body condition. Finally to test hypothesis 4, juvenile and end of experiment female δ13C and δ15N were tested for differences with unpaired t-tests within each treatment.
Results
We had a 97% survival of adult Monoporeia affinis during the experiment before juvenile release (i.e. two females died before releasing juveniles from eggs), and 89% of females survived after juvenile release until the experiment termination. The offspring were released from the egg sacs in mid-March to early April, with variation in time of hatching seen between individuals.
Effects of Sediment OM Content on Reproduction and C:N (Hypothesis 1)
Reproductive Endpoints
We found that initial females (i.e. females sampled from the initial stations before the experiment) at the low OM station had an average fecundity of 39 (median 40) and 91% viable embryos (VE). Females at the high OM station had an average fecundity of 43 (median 47) and 94% VE. No significant differences were detected between stations for fecundity or proportion of deformed embryos per female (Figure S1; unpaired t-test; fecundity t = 0.77, df = 28, p = 0.45; VE t = 0.81, df = 16, p = 0.43). Additionally, there was no significant differences in the homogeneity of variance between the VE of the stations (Figure S1; Levene’s test; F1,28 = 3.2, p = 0.086).
At the end of the experiment, an average of 22 juveniles (median 26) were recovered from the low control, 24 (median 22) from the high control, 30 (median 29.5) from low transferred and 32 (median 33) in high transferred. The number of juveniles recovered was not significantly different between treatments (Figure S2; 2-way ANOVA; F3,40 = 1.8, p = 0.17). There was, however, a significant difference between treatments in juvenile mean biomass (Figure 3; Kruskal-Wallis; χ2 = 8.4, df = 3, p = 0.04), with low control being significantly lower than low transferred (Independent Mann-Whitney U with Holm-corrected p-values; W = 11, p = 0.04).
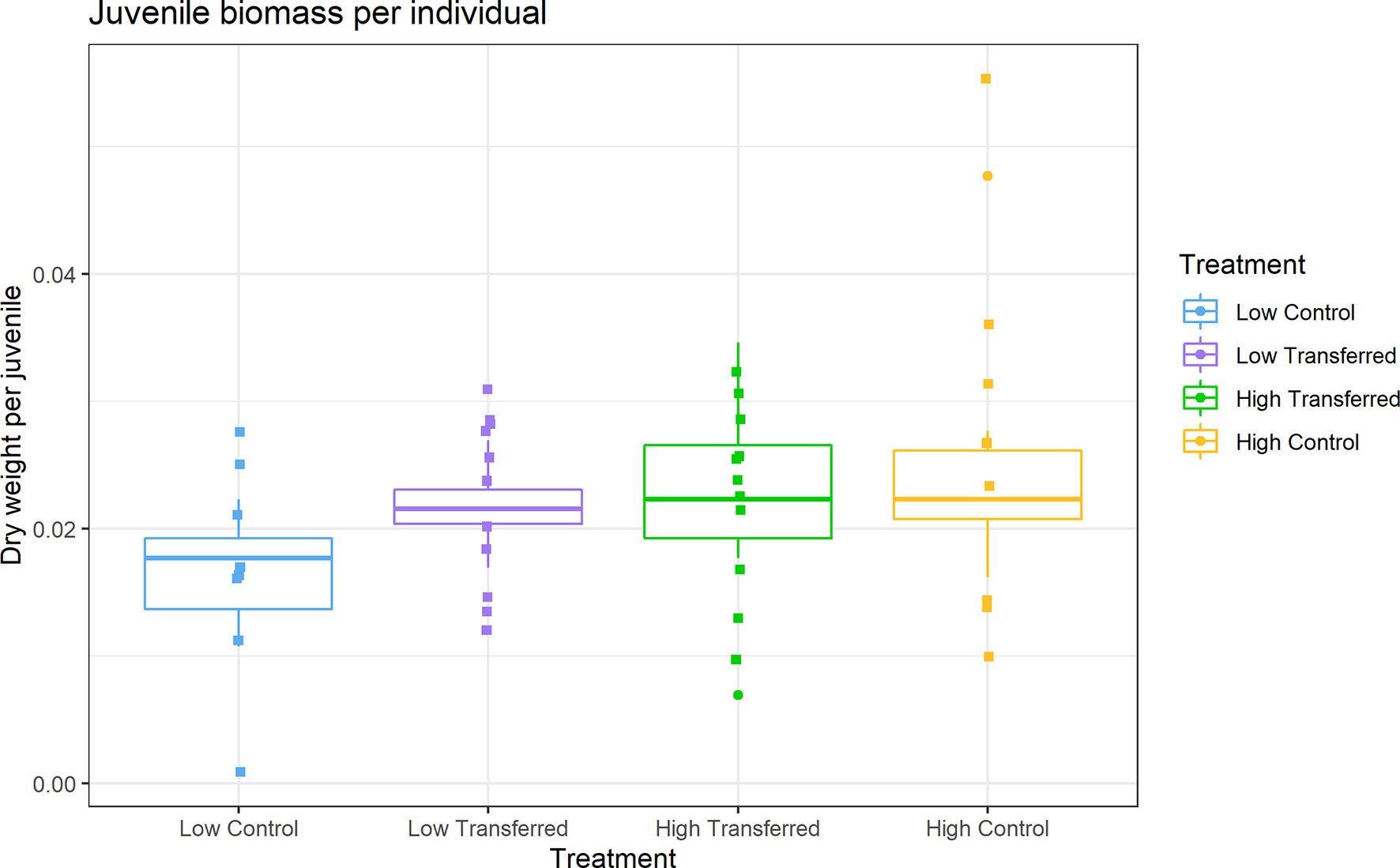
Figure 3 Juvenile biomass in dry weight (mg) per individual by treatment. See Figure 2 for experimental treatment details.
C:N Ratios
Initial female C:N content did not significantly differ between the two stations (unpaired t-test; t = -0.78, df = 27.97, p = 0.44). On the other hand, low sediment OM treatment females had a significantly higher C:N than high sediment OM females at the end of the experiment (Kruskal-Wallis; χ2 = 5.7, df = 1, p = 0.02), but female origin (control or transferred) was not significant explanatory variable for female C:N (χ2 = 0.85, df = 1, p = 0.36). Juvenile C:N was not significantly different between treatments (χ2 = 5.4, df = 3, p = 0.14).
Relationship Between Female Condition, Nutritional Status and Reproductive Endpoints
There was no significant relationship between initial female C:N and VE (Table S2; multiple linear regression; R2 = -0.054, F3,26 = 0.51, p = 0.68), or between initial female δ15N (indicative of higher trophic position or deteriorated body condition) and VE (Table S2; R2 = -0.027, F3,26 = 0.74, p = 0.54). However, multiple linear regressions between end of experiment female C:N and number of recovered juveniles per female showed a significant positive relationship (Figure 4; Table S2; R2 = 0.34, F7,36 = 4.14, p = 0.002). We found no correlation between end of experiment female δ15N and number of recovered juveniles per female (Spearman’s rank correlation; r(72) = 0.19, p = 0.22), and no correlation between end of experiment female δ13C and number of recovered juveniles per female (r(72) = -0.12, p = 0.44).
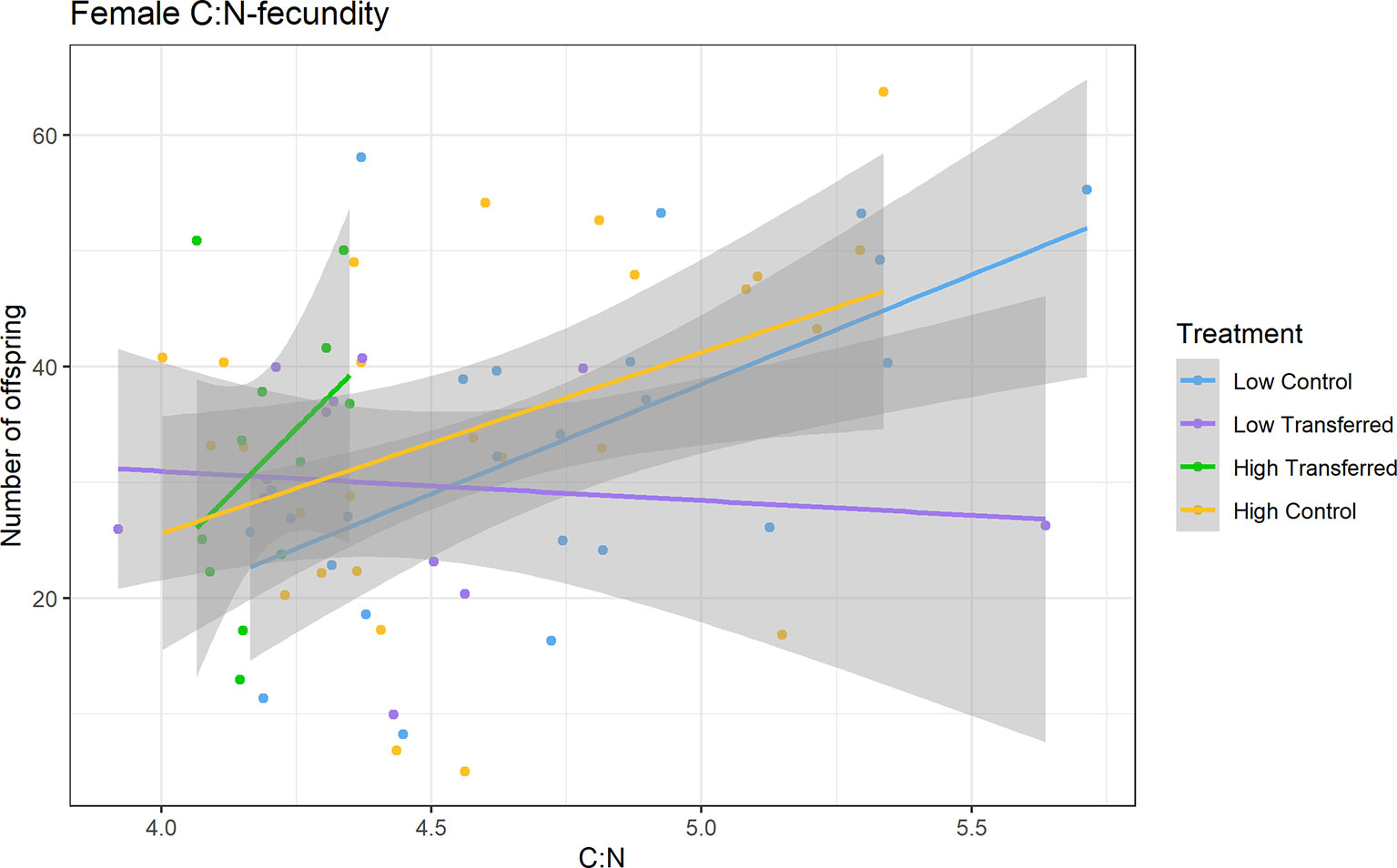
Figure 4 Linear models of female C:N ratios and fecundity (number of offspring per female) by treatment. See Figure 2 for experimental treatment details.
Effects of Sediment OM Content and Female Origin on Stable Isotope Composition of Females and Juveniles (Hypothesis 2)
Female and Juvenile Stable Isotope Composition
Initial females did not differ between stations by δ13C, but low OM females showed significantly higher δ15N than high OM females (unpaired t-test; δ13C t = -0.35, df = 26.17, p = 0.73; δ15N t = -2.51, df = 27.32, p = 0.018). Similarly, end of experiment females did not differ significantly in terms of δ13C by sediment OM content (Figure 5; high or low; 2-way ANOVA; F1,70 = 1.6, p = 0.21) or female origin (control or transferred; F1,70 = 1.6, p = 0.21). However, there was a significant interactive effect between sediment OM and female origin (F1,70 = 4.7, p = 0.03), with end of experiment females in the low transferred treatment showing significantly lower δ13C than the high control (Tukey posthoc test; p = 0.04). Sediment OM content was a significant factor (Figure 5; F1,70 = 8.9, p = 0.004) in end of experiment female δ15N, with δ15N of females in low OM treatments higher in than those in high OM treatments. There was no significant effect of female origin (F1,70 = 1.08, p = 0.3) or interaction between sediment OM content and female origin (F1,70 = 1.5, p = 0.22) on end of experiment female δ15N. Sediment isotope values differed between high OM and low OM (Figure S3; low OM sediment -24.6 δ13C, 4.08 δ15N; high OM sediment -24.07 δ13C, 4.1 δ15N). Sediment from the low OM station had significantly higher δ15N values (unpaired t-test; δ15N t = -3.55, df = 42.92, p < 0.001) and significantly lower δ13C values (Kruskal-Wallis, χ2 = 6.35, df = 1, p = 0.01) compared to sediment from the high OM station at the end of the experiment (Figure S3). Juveniles did not differ significantly in δ13C or δ15N by treatment and there was no significant interaction effects between sediment OM and female origin (Figure 5; 2-way ANOVA; δ13C F3,36 = 1.9, p = 0.17; δ15N F3,36 = 1.6, p = 0.22).
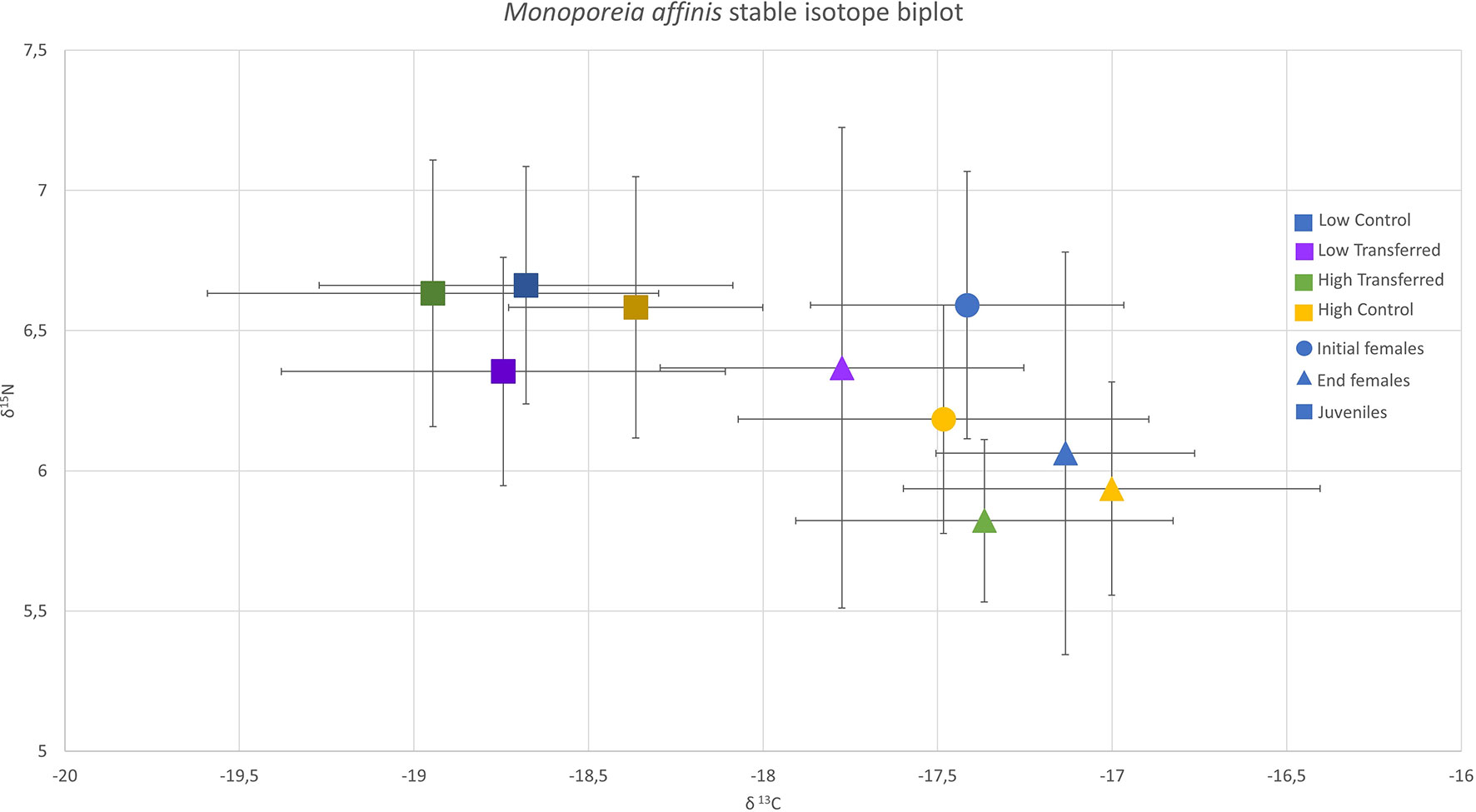
Figure 5 Stable isotope biplot (δ13C and δ15N) of Monoporeia affinis, in which the symbol represents the mean, and lines represent the standard deviation. Initial females are represented by circles, end of experiment females represented by squares, and juveniles (13-pooled individuals) are represented by triangles. See Figure 2 for experimental details.
Stable Isotope Indices
Range of female δ13C was similar between initial stations (high OM 33% larger range) and end of experiment treatments (high OM treatments 31% larger range; Table 1). Range of female δ15N was also similar between initial stations (low OM 8% larger range), but low OM sediment treatments showed 78% higher δ15N range at the end of the experiment (Table 1). Female δ13Ca did not vary significantly by sediment OM content, female origin, or interaction between the two (2-way ANOVA; sediment OM content F1,70 = 0.0, p = 1; female origin F1,70 = 0.03, p = 0.87; interaction F1,70 = 0.03, p = 0.87). Similarly, there was no significant effect of sediment OM content (Kruskal-Wallis; χ2 = 0.34, df = 1, p = 0.56) or female origin (χ2 = 0.07, df = 1, p = 0.8) on female δ15Na. Finally, euclidian distance (ED) was not significantly different between end females in the treatments by sediment OM content (Kruskal-Wallis; χ2 = 1.64, df = 1, p = 0.2) or female origin (χ2 = 2.96, df = 1, p = 0.09).

Table 1 Range in δ13C and δ15N in female Monoporeia affinis from the two initial stations before the experiment, and females after the end of the experiment in the four treatments.
Effects of Sediment OM Content and Female Origin on Female Starvation (Hypothesis 3)
Stable isotope signature of the female amphipods changed during the duration of the experiment, and this change was different among the experimental treatments. Female δ13C was not significantly different from the start of the experiment to the end (Fig 5; 2-way ANOVA; F1,68 = 0.8, p = 0.37), but was significantly different between treatments (F3,68 = 4.7, p = 0.005), with low transferred females being significantly lower compared to high control and low control. There was no significant interactive effect between treatment and time (F1,68 = 0.44, p = 0.51) on female δ13C. In addition, female δ15N was significantly higher in low OM treatments compared to high OM treatments (F3,68 = 3.4, p = 0.02), and significantly lower at the end of the experiment in comparison with the initial females (Figure 6; F1,68 = 6.9, p = 0.01), but there was no significant interactive effect between treatment and time (F1,68 = 0.8, p = 0.37). As expected, end of experiment females had significantly lower C:N ratios than initial females (χ2 = 39.52, df = 1, p < 0.001).
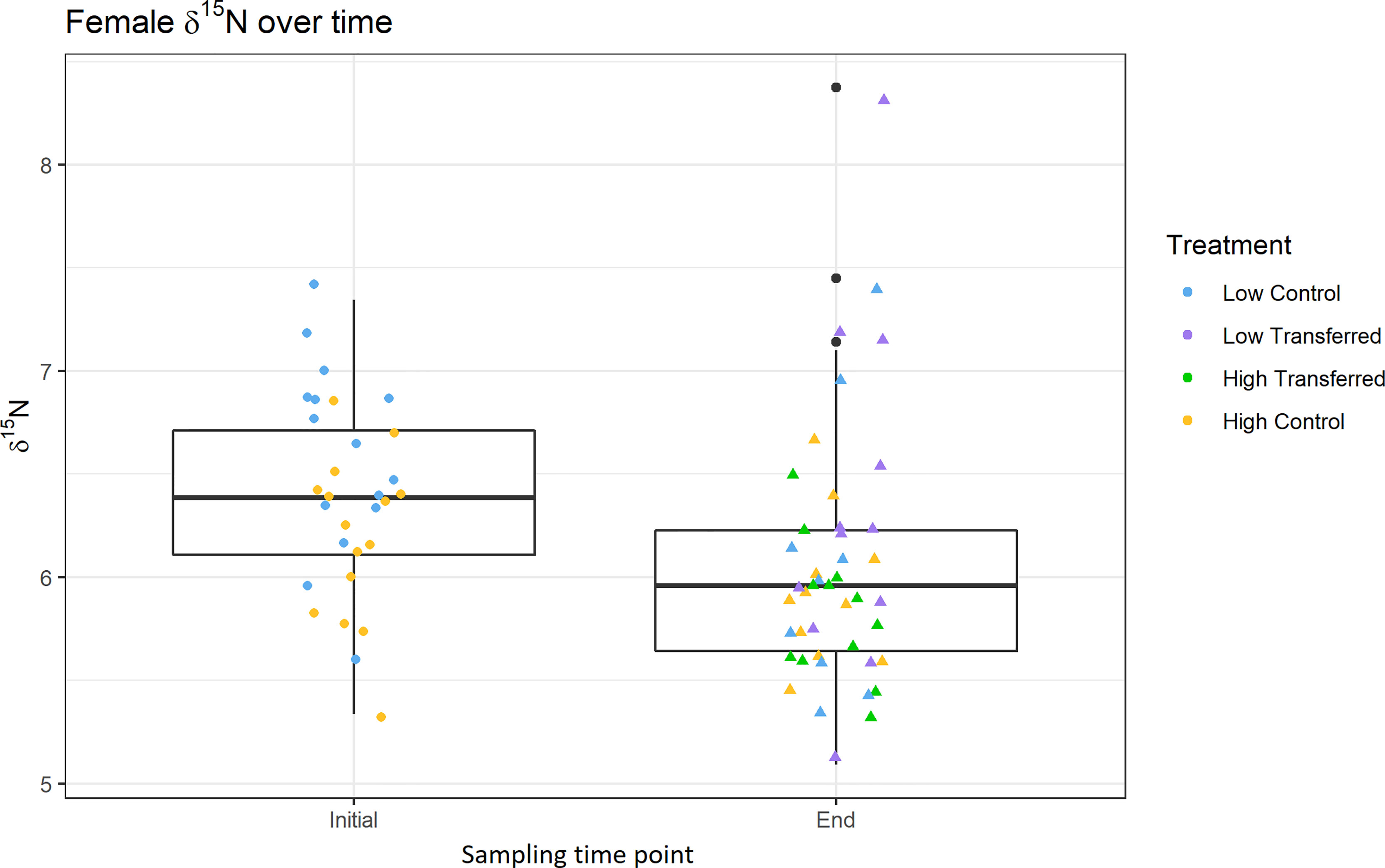
Figure 6 δ15N of females from initial samples (left) and end of experiment (right). Box plots include all treatments, points show the color of the treatment. See Figure 2 for experimental details.
Comparison Between Female and Juvenile Stable Isotopes (Hypothesis 4)
Juveniles were significantly depleted in 13C compared to end females across all treatments (Figure 5; Two-sided unpaired Student’s t-test; high control: t = 6.3, df = 16.8, p < 0.001; high transferred: t = 6.5, df = 21.3, p < 0.001; low control: t = 6.4, df = 11.5, p < 0.001; low transferred: t = 4, df = 19.4, p < 0.001). For 15N, juveniles were significantly enriched compared to end females in high OM treatments (Figure 7; high control: t = 3.4, df = 15.5, p = 0.004; high transferred: t = -5.05, df = 18.2, p < 0.001), but were not significantly different in low OM treatments (Figure 7; low control: t = 2.1, df = 13.2, p = 0.05; low transferred: t = 0.05, df = 16.02, p = 0.96).
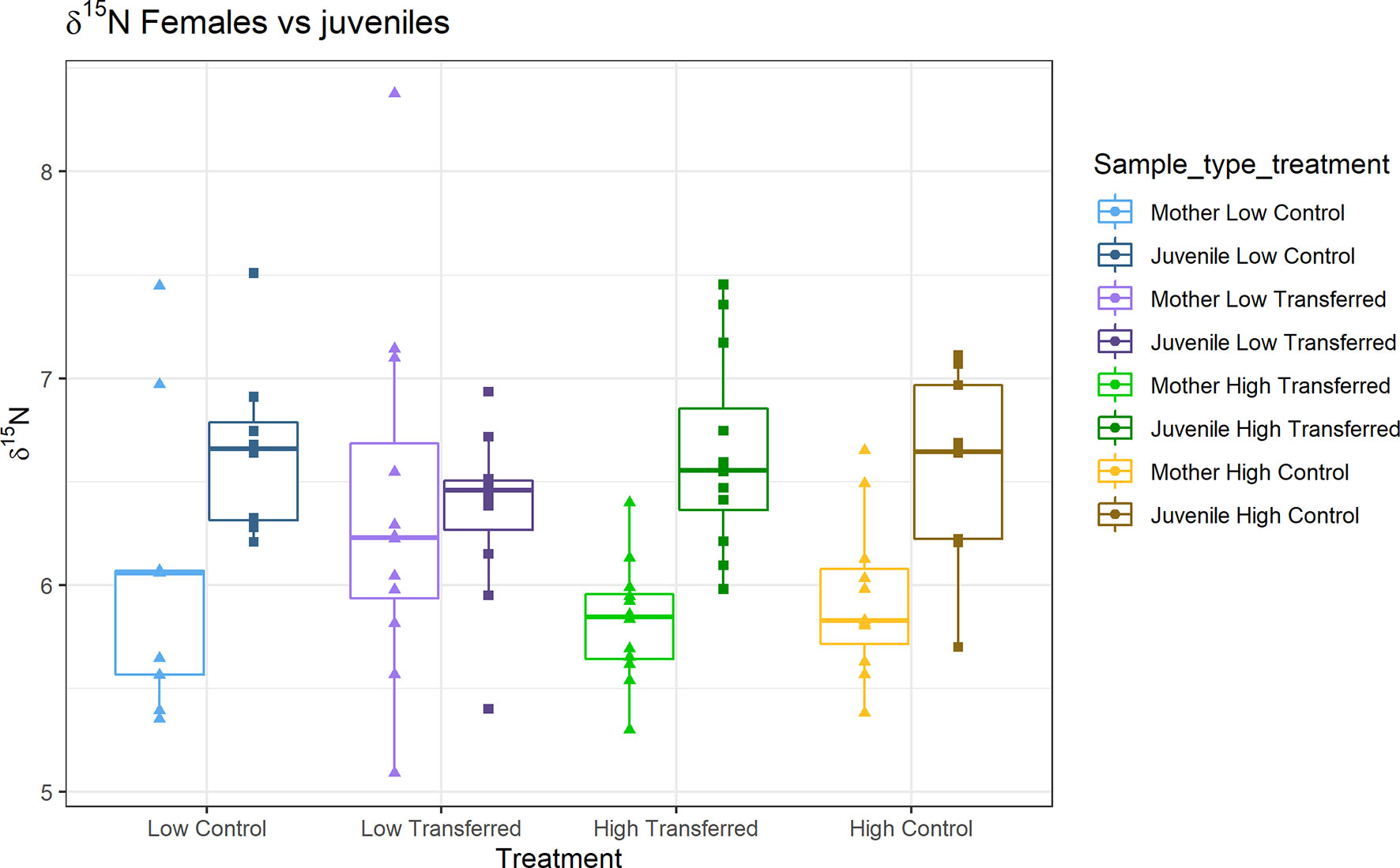
Figure 7 Female and juvenile δ15N at the end of the experiment. See Figure 2 for experimental details. The females are the lighter version of the treatment colors (left) and the corresponding juveniles are darker version of the treatment colors (right).
Discussion
This study tests how amphipods from nearby stations that differ in organic matter (OM) content respond in a reciprocal transplant experiment regarding their isotope composition and offspring survival and condition. We predicted that higher OM sediment would lead to better female body condition, which in turn would lead to more successful reproductive output (fecundity, number of juveniles, and juvenile biomass). We found mixed support for this hypothesis: number of juveniles was significantly explained by female condition in terms of C:N ratios (proxy for lipid content), supporting that better female condition is related to higher reproductive output. However, end of experiment female C:N was significantly higher in low OM sediment, refuting that female condition would be better in high OM, but offspring biomass was significantly lower in the low control treatment, supporting that high OM would be beneficial for reproductive success. Females in low OM treatments showed significantly higher δ15N compared to females in high OM treatments at the end of the experiment, indicating that they fed actively on this 15N enriched sediment, despite its low OM content. We thus reject hypothesis 3, and conclude that females do not stop feeding while carrying their offspring, as end of experiment female δ15N values do not indicate starvation, though female C:N ratios did decrease from initial to end, possibly indicating malnutrition. Juveniles show significantly lower δ13C than females in all treatments, but only juveniles in high OM treatments show significantly higher δ15N than females. We will discuss first reproductive endpoints, then dietary changes, and finally comparing the two time points and two generations of Monoporeia affinis.
Effects on Reproduction and Organism Condition (Hypothesis 1)
We found no support for a positive effect of higher sediment OM content on fecundity and viable embryos (VE) (hypothesis one), as there were no significant differences between the initial stations in terms of fecundity or VE, nor between number of hatched juveniles in the different end treatments. This could however simply be an effect of low sample size. We found similar fecundities and percent VE to previous studies (fecundity this study: 39 & 43; fecundity previous studies 29-45 in the Askö area; VE this study 91 & 94%; VE previous studies 96.4-99.7%; Elmgren et al., 1983; Sundelin, 1983; Ledesma et al., 2020). Adverse effects on fecundity at very high OM levels of more than 14% have been reported (Sundelin and Eriksson, 1998; Löf et al., 2016). However, in previous transplant experiments, Eriksson Wiklund et al. (2008) found that 10-fold higher sediment OM in the Baltic proper (5% C content compared to 0.5% in the Bothnian Sea) was not only beneficial, but critical for these amphipods to maintain fecundity. Indeed, Bothnian Sea amphipods from very low OM sediments significantly improved their fecundity when transplanted to the much higher OM Baltic proper sediment. Our results, that investigated more subtle and realistic increases in OM between two nearby stations (2-fold difference in OM and removing potential effects from differences in salinity and temperature), partly supports the positive effects of organic enrichment for offspring. Although there was no significant difference in number of offspring among our treatments, the individual mean biomass for the offspring whose mother was transplanted from low to high OM was indeed higher than in low OM. We cannot exclude that this effect is due to a somewhat earlier hatching in the high OM treatment allowing more time for feeding. Eriksson Wiklund et al. (2008) found however no effect from sediment OM on hatching time in their transplant experiment. Higher food availability in the OM treatment has therefore likely favored growth for juveniles. Another explanation could be that female amphipods can have the ability to adjust their offspring investment according to outside factors, such as food availability or exposure to contaminants (Sundelin and Eriksson, 1998; Eriksson Wiklund et al., 2008). Contaminants like polyaromatic hydrocarbons and heavy metals can accumulate and reach relatively high concentrations in some areas of the Baltic Sea Proper (Raymond et al., 2021), with reported effects on the fecundity of M. affinis (Sundelin and Eriksson, 1998; Eriksson Wiklund et al., 2008). Nevertheless, it is possible that in some cases, positive effects of higher resource availability related to moderate increases in sediment OM on fecundity may be masked by increased energy requirements for detoxification strategies (Sundelin and Eriksson, 1998; Eriksson Wiklund et al., 2008).
Organic enrichment can alter organism condition. Indeed, Eriksson Wiklund et al. (2008) found an increase in certain essential fatty acids when M. affinis were moved from low OM to high OM sediment, and a decrease in certain essential fatty acids when moved from high OM to low OM sediment. Contradicting this pattern, females in our experiment had significantly higher C:N ratios (a proxy for lipid content) in low OM sediment treatments than high OM sediment treatments, with higher C:N ratios generally indicating better body condition (Lehtonen, 1996; Drylie et al., 2020). We found that, in general, number of recovered juveniles was explained by female C:N. This agrees with theory that parents with greater resources are able to contribute more energy to reproduction (van Noordwijk and de Jong, 1986; McNamara and Houston, 1996; Marshall et al., 2010; González-Ortegón et al., 2018). Indeed, previous studies have also found positive correlations between female lipid content and number of eggs produced in amphipods (Glazier, 2000), as well as positive correlations between female shrimp trophic position and fecundity (González-Ortegón et al., 2018). The reciprocal transfer design applied here allowed a novel way to explore potential relationships between body condition and reproductive output. Females from high OM when transferred to low OM sediment showed a higher C:N ratio but lower reproductive output (Figure 2), suggesting a possible higher allocation of resources to maintain body condition in females (high C:N ratio) at the expense of lower reproductive output (biomass of juveniles). Such tradeoffs in situations of low resource availability have been found in marine crustaceans (Guisande et al., 1996) but would require further investigation for M. affinis populations.
Effects on Trophic Niche (Hypothesis 2)
Eutrophication has been shown to simplify feeding interactions (Nordström and Bonsdorff, 2017) and increase the size of the population stable isotopic niche (Wedchaparn et al., 2016). Thus, we expected an increased niche of M. affinis in sediments with higher sediment OM content. Indeed δ13C ranges were larger in females in high OM sediment treatments, with females in our low OM treatments showing a 31% lower δ13C range compared to high sediment OM females, indicating less available resources in low OM sediment, especially when females were moved from high OM sediment to low OM sediment. Accordingly, Dolédec et al. (2021) and Karlson et al. (2014) have found a positive relationship between sediment OM and niche size in freshwater benthic invertebrates and M. affinis, respectively. However, more severe levels of OM enrichment than those tested here can also lead to a temporal homogenization of prey communities, which would lead to a narrower isotopic niche of consumers (Cook et al., 2018). This was found by Karlson et al. (2018), in which M. affinis showed a narrower isotopic niche while consuming high quality diatoms as food. The ecological consequences at a population level of these changes in δ13C range are however hard to predict. Despite the larger δ13C range (indicative of greater resource diversity), females from high OM did not show higher C:N ratios, our proxy for body condition. Similarly Karlson et al. (2015), found no relationship between niche size (also measured by δ13C ranges) and C:N ratios in M. affinis, indicating that increased resources would not necessarily lead to better body condition. Additionally, the significantly more enriched 15N values of females in low OM sediment treatments than in high OM sediment treatments likely indicate a greater diversity of available resources, higher trophic position of available prey in low OM sediment, or that limited resource availability could translate into starvation effects (Gorokhova, 2017; Karlson et al., 2018). We believe either of the first two explanations are more likely, as the low OM sediment shows significantly higher δ15N than high OM sediment (Fig S3) and that females in low OM sediment had the higher C:N ratios (Figure 4).
Female and Juvenile Comparison (Hypotheses 3 and 4)
M. affinis females die soon after the offspring hatch, and it is believed that they stop eating for some time before they die (Lehtonen and Andersin, 1998; Sundelin and Eriksson, 1998), thus we predicted enriched 15N at the experiment termination. We found, however, support for females feeding, as discussed above. Female δ15N significantly decreased from initial to end of experiment samples, indicating that females were not starving, leading us to reject hypothesis 3. This feeding seems to be more visible for females from low OM sediment. While female stable isotope composition did not differ significantly between initial stations, the δ15N values from females in low OM sediment treatments were significantly enriched compared to females in high OM treatments, indicating that they actively fed on the low OM sediment which had higher δ15N values. This is further indicated by the significantly depleted 13C in low transferred females, which suggests feeding from low OM sediments with lower δ 13C values. This possible plastic response of M. affinis to sediment conditions would result in the benefit of a less severe reduction in body condition (C:N), but we observed a significantly lower C:N ratio in end females compared to initial females indicating a loss of lipids, as found previously in Lehtonen (1996). It is possible that females originally from sites with high OM require more resources to maintain their body condition, therefore increasing their feeding rate in the new sediment when transferred to low OM conditions. This could explain the more pronounced change in δ13C and δ15N seen in females in the low transfer treatment than in females in the high transfer treatment.
We found that females and their hatched juveniles differed significantly in terms of δ13C across all treatments, and in δ15N in both high OM treatments. Our findings of juveniles having enriched 15N compared to females support results by Rodil et al. (2020), who found that juveniles collected in the field showed 15N enrichment and 13C depletion from consuming reworked OM compared to adult M. affinis. However, experimental studies with radiolabeled fresh algae has shown that juveniles feed more on fresh algae than aged sediment material (Byrén et al., 2006), and other studies on crustaceans have found depleted 15N in larvae compared to adults (González-Ortegón et al., 2018), likely because quick growth and molting leads to comparatively lower fractionation (Schimmelmann, 2011; Gorokhova, 2017). Physiological effects confounding diet interpretation can therefore not be excluded; adults have higher lipid content than juveniles with will results in depleted 13C values, and food limitation can result in increased δ15N values (Doi et al., 2017; Gorokhova, 2017; Karlson et al., 2018). Furthermore, we isolated M. affinis and measured their response to sediment OM enrichment while removing the effects of interspecific competition (in the case of females) and predation, as well as preventing introduction of new food sources. It is also important to recognize the variability in isotope fractionation between life stages, nutritional status of individuals, temperature, chemical exposure, and parasites. Deciphering the mechanism behind the enriched 15N of juveniles will require more experiments. Our findings are also confounded by several other factors that can affect differences between adult and juvenile stable isotope fractionation – such as food limitation. For example, lower δ15N values of starved crustaceans can be related to re-assimilation of the nitrogen in the exoskeleton, which is isotopically light (Passano, 1960; Gorokhova, 2017), and could be an explanation for lower female δ15N. In addition, we must be cautious interpreting differences between juveniles and females in isotopic signature, as we utilized different tissues for females and juveniles. Juvenile samples were composed of 13-pooled individuals, where we utilized the entire body, while female samples consisted only of the head of a single individual. Furthermore, the difference in hatching times translates to disparities in feeding time, and potential variation for stable isotope endpoints, especially when comparing to the progenitor mothers. Hence, isotope niche comparisons cannot be reliably performed between juveniles and adults in this study.
Conclusions and Ecological Relevance
Our study provides information on the effects of OM content on amphipod diet plasticity and population dynamics, namely that M. affinis’ diet varies depending on available resources and there are potential benefits of OM enrichment for the offspring. Our results are in agreement with previous literature showing stimulation to biodiversity and increases in biomass having an effect on M. affinis at low levels of increased OM content, from 2 to 5% (Cederwall and Elmgren, 1980; Widbom and Elmgren, 1988). We found no differences in niche size that could be linked to reproductive success but our results may suggest a tradeoff between female condition and reproductive success. The results here indicate that a moderate increase of sediment OM content is potentially beneficial for reproductive success in M. affinis. Additional studies testing realistic ranges of OM enrichment and lipid analyses are required to confirm such beneficial effects, and quality of OM should also be considered in future experiments. As coastal zones face increasing OM inputs from land use changes and climate change, experiments such as this one will be important for the predictions of how populations will react, and how key species and their ecosystem functions will be subsequently impacted.
Data Availability Statement
The original contributions presented in the study are included in the article/Supplementary Material. Further inquiries can be directed to the corresponding authors.
Author Contributions
All authors contributed to conceptualization, contributed to the manuscript, and gave final approval. JAG performed the experiment, laboratory analysis, statistical analysis, and wrote the first manuscript draft. FJAN provided financial support.
Funding
Financial support was provided by the Swedish Environmental Protection Agency in collaboration with the Swedish Agency for Marine and Water Management through a Research Grant (NV-802-0151-18) to FJAN.
Conflict of Interest
The authors declare that the research was conducted in the absence of any commercial or financial relationships that could be construed as a potential conflict of interest.
Publisher’s Note
All claims expressed in this article are solely those of the authors and do not necessarily represent those of their affiliated organizations, or those of the publisher, the editors and the reviewers. Any product that may be evaluated in this article, or claim that may be made by its manufacturer, is not guaranteed or endorsed by the publisher.
Acknowledgments
We would like to acknowledge the help of Séréna Albert and the Askö team in the field, Nellie Stjärnkvist with the experiment and Johanna Hedberg with the stable isotope sample preparations. We would also like to acknowledge the International Council for the Exploration of the Seas for Early Career Scientist Support funding to JG to present this work in the 13th Baltic Sea Science Congress 2021. We are grateful to two anonymous reviewers for their comments which have greatly improved this manuscript.
Supplementary Material
The Supplementary Material for this article can be found online at: https://www.frontiersin.org/articles/10.3389/fmars.2022.789700/full#supplementary-material
References
Aljetlawi A. A., Leonardsson K. (2002). Size-Dependent Competitive Ability in a Deposit-Feeding Amphipod, Monoporeia Affinis. Oikos 97, 31–44. doi: 10.1034/j.1600-0706.2002.970103.x
Aljetlawi A. A., Leonardsson K. (2003). Survival During Adverse Seasons Reveals Size-Dependent Competitive Ability in a Deposit-Feeding Amphipod. Monoporeia affinis 7, 164–170. doi: 10.1034/j.1600-0706.2003.12183.x
Andersen J. H., Carstensen J., Conley D. J., Dromph K., Fleming-Lehtinen V., Gustafsson B. G., et al. (2017). Long-Term Temporal and Spatial Trends in Eutrophication Status of the Baltic Sea: Eutrophication in the Baltic Sea. Biol. Rev. 92, 135–149. doi: 10.1111/brv.12221
Ankar S., Elmgren R. (1975). A Survey of the Benthic Macro- and Meiofauna of the Askö - Landsort Area. Havsforskningsinst Skr. Book with ISBN 9185326054, Publisher "Contributions of the Askö Laboratory, Stockholm University" Location Trosa, Sweden 239, 257–264.
Binzer A., Guill C., Rall B. C., Brose U. (2016). Interactive Effects of Warming, Eutrophication and Size Structure: Impacts on Biodiversity and Food-Web Structure. Glob. Change Biol. 22, 220–227. doi: 10.1111/gcb.13086
Broman E., Raymond C., Sommer C., Gunnarsson J. S., Creer S., Nascimento F. J. A. (2019). Salinity Drives Meiofaunal Community Structure Dynamics Across the Baltic Ecosystem. Mol. Ecol. 28, 3813–3829. doi: 10.1111/mec.15179
Byrén L., Ejdung G., Elmgren R. (2006). Uptake of Sedimentary Organic Matter by the Deposit-Feeding Baltic Amphipods Monoporeia Affinis and Pontoporeia Femorata. Mar. Ecol. Prog. Ser. 313, 135–143. doi: 10.3354/meps313135
Casini M., Cardinale M., Arrhenius F. (2004). Feeding Preferences of Herring (Clupea Harengus) and Sprat (Sprattus Sprattus) in the Southern Baltic Sea. ICES J. Marine Sci. 61, 1267–1277. doi: 10.1016/j.icesjms.2003.12.011
Cederwall H. (1978). Long Term Fluctuations in the Macrofauna of Northern Baltic Soft Bottoms Book ISBN 9185326151, Publisher "Contributions of the Askö Laboratory, Stockholm University" Number 22 in series, Location Trosa, Sweden.
Cederwall H., Elmgren R. (1980). Biomass Increase of Benthic Macrofauna Demonstrates Eutrophication of the Baltic Sea. Ophelia Suppl. 1, 287–304.
Cook S. C., Housley L., Back J. A., King R. S. (2018). Freshwater Eutrophication Drives Sharp Reductions in Temporal Beta Diversity. Ecology 99, 47–56. doi: 10.1002/ecy.2069
Doi H., Akamatsu F., González A. L. (2017). Starvation Effects on Nitrogen and Carbon Stable Isotopes of Animals: An Insight From Meta-Analysis of Fasting Experiments. R. Soc Open Sci. 4, 170633. doi: 10.1098/rsos.170633
Dolédec S., Simon L., Blemus J., Rigal A., Robin J., Mermillod-Blondin F. (2021). Multiple Stressors Shape Invertebrate Assemblages and Reduce Their Trophic Niche: A Case Study in a Regulated Stream. Sci. Total Environ. 773, 145061. doi: 10.1016/j.scitotenv.2021.145061
Drylie T. P., Lohrer A. M., Needham H. R., Pilditch C. A. (2020). Taxonomic and Functional Response of Estuarine Benthic Communities to Experimental Organic Enrichment: Consequences for Ecosystem Function. J. Exp. Marine Biol. Ecol. 532, 151455. doi: 10.1016/j.jembe.2020.151455
Ejdung G., Elmgren R. (1998). Predation on Newly Settled Bivalves by Deposit-Feeding Amphipods: A Baltic Sea Case Study. Mar. Ecol. Prog. Ser. 168, 87–94. doi: 10.3354/meps168087
Elmgren R., Hansson S., Larsson U., Sundelin B., Boehm P. D. (1983). The “Tsesis” Oil Spill: Acute and Long-Term Impact on the Benthos. Mar. Biol. 73, 51–65. doi: 10.1007/BF00396285
Elton C. (1927) Animal Ecology (London, UK: Sidgwick and Jackson). Available at: https://press.uchicago.edu/ucp/books/book/chicago/A/bo25281897.html (Accessed December 7, 2020).
Eriksson Wiklund A., Sundelin B. (2001). Impaired Reproduction in the Amphipods Monoporeia Affinis and Pontoporeia Femorata as a Result of Moderate Hypoxia and Increased Temperature. Mar. Ecol. Prog. Ser. 222, 131–141. doi: 10.3354/meps222131
Eriksson Wiklund A.-K. E., Sundelin B., Rosa R. (2008). Population Decline of Amphipod Monoporeia Affinis in Northern Europe: Consequence of Food Shortage and Competition? J. Exp. Marine Biol. Ecol. 367, 81–90. doi: 10.1016/j.jembe.2008.08.018
Glazier D. S. (2000). Is Fatter Fitter? Body Storage and Reproduction in Ten Populations of the Freshwater Amphipod Gammarus Minus. Oecologia 122, 335–345. doi: 10.1007/s004420050039
González-Ortegón E., Vay L. L., Walton M. E. M., Giménez L. (2018). Maternal Trophic Status and Offpsring Phenotype in a Marine Invertebrate. Sci. Rep. 8, 9618. doi: 10.1038/s41598-018-27709-2
Gorokhova E. (2017). Individual Growth as a non-Dietary Determinant of the Isotopic Niche Metrics. Methods Ecol. Evol. 9, 269–277. doi: 10.1111/2041-210X.12887
Griffiths J. R., Kadin M., Nascimento F. J. A., Tamelander T., Törnroos A., Bonaglia S., et al. (2017). The Importance of Benthic-Pelagic Coupling for Marine Ecosystem Functioning in a Changing World. Glob. Change Biol. 23, 2179–2196. doi: 10.1111/gcb.13642
Grinnell J. (1917). The Niche-Relationships of the California Thrasher. Auk. 34, 427–433. doi: 10.2307/4072271
Guisande C., Sánchez J., Maneiro I., Miranda A. (1996). Trade-Off Between Offspring Number and Offspring Size in the Marine Copepod Euterpina Acutifrons at Different Food Concentrations. Mar. Ecol. Prog. Ser. 143, 37–44. doi: 10.3354/meps143037
Hedberg P., Albert S., Nascimento F. J. A., Winder M. (2020). Effects of Changing Phytoplankton Species Composition on Carbon and Nitrogen Uptake in Benthic Invertebrates. Limnol. Oceanogr. 00:lno.11617. doi: 10.1002/lno.11617
Heip C. (1995). Eutrophication and Zoobenthos Dynamics. Ophelia 41, 113–136. doi: 10.1080/00785236.1995.10422040
Jackson A. L., Inger R., Parnell A. C., Bearhop S. (2011). Comparing Isotopic Niche Widths Among and Within Communities: SIBER - Stable Isotope Bayesian Ellipses in R: Bayesian Isotopic Niche Metrics. J. Anim. Ecol. 80, 595–602. doi: 10.1111/j.1365-2656.2011.01806.x
Karlson K., Bonsdorff E., Rosenberg R. (2007). The Impact of Benthic Macrofauna for Nutrient Fluxes From Baltic Sea Sediments. AMBIO: A J. Hum. Environ. 36, 161–167. doi: 10.1579/0044-7447(2007)36[161:TIOBMF]2.0.CO;2
Karlson A. M. L., Gorokhova E., Elmgren R. (2014). Nitrogen Fixed By Cyanobacteria Is Utilized By Deposit-Feeders. PloS One 9, e104460. doi: 10.1371/journal.pone.0104460
Karlson A. M. L., Gorokhova E., Elmgren R. (2015). Do Deposit-Feeders Compete? Isotopic Niche Analysis of an Invasion in a Species-Poor System. Sci. Rep. 5, 9715. doi: 10.1038/srep09715
Karlson A. M. L., Nascimento F. J. A., Elmgren R. (2008). Incorporation and Burial of Carbon From Settling Cyanobacterial Blooms by Deposit-Feeding Macrofauna. Limnol. Oceanogr. 53, 2754–2758. doi: 10.4319/lo.2008.53.6.2754
Karlson A. M. L., Reutgard M., Garbaras A., Gorokhova E. (2018). Isotopic Niche Reflects Stress-Induced Variability in Physiological Status. R. Soc. Open Sci. 5, 171398. doi: 10.1098/rsos.171398
Larsson U., Elmgren R., Wulff F. (1985). Eutrophication and the Baltic Sea: Causes and Consequences. AMBIO 14, 9–14.
Layman C. A., Arrington D. A., Montaña C. G., Post D. M. (2007). Can Stable Isotope Ratios Provide for Community-Wide Measures of Trophic Structure? Ecology 88, 42–48. doi: 10.1890/0012-9658(2007)88[42:CSIRPF]2.0.CO;2
Ledesma M., Gorokhova E., Holmstrand H., Garbaras A., Karlson A. M. L. (2020). Nitrogen Isotope Composition of Amino Acids Reveals Trophic Partitioning in Two Sympatric Amphipods. Ecol. Evol. 00:ece3.6734. doi: 10.1002/ece3.6734
Lehtonen K. K. (1996). Ecophysiology of the Benthic Amphipod Monoporeia Affinis in an Open-Sea Area of the Northern Baltic Sea: Seasonal Variations in Body Composition, With Bioenergetic Considerations. Mar. Ecol. Prog. Ser. 143, 12. doi: 10.3354/meps143087
Lehtonen K., Andersin A. (1998). Population Dynamics, Response to Sedimentation and Role in Benthic Metabolism of the Amphipod Monoporeia Affinis in an Open-Sea Area of the Northern Baltic Sea. Mar. Ecol. Prog. Ser. 168, 71–85. doi: 10.3354/meps168071
Löf M., Sundelin B., Bandh C., Gorokhova E. (2016). Embryo Aberrations in the Amphipod Monoporeia Affinis as Indicators of Toxic Pollutants in Sediments: A Field Evaluation. Ecol. Indic. 13, 18–30, doi: 10.1016/j.ecolind.2015.05.058
Lopez G., Elmgren R. (1989). Feeding Depths and Organic Absorption for the Deposit Feeding Benthic Amphipods Pontoporera Affinis and Pontoporeia Femorata. Limnol. Oceanogr. 34, 982–991. doi: 10.4319/lo.1989.34.6.0982
Malone T. C., Newton A. (2020). The Globalization of Cultural Eutrophication in the Coastal Ocean: Causes and Consequences. Front. Mar. Sci. 7. doi: 10.3389/fmars.2020.00670
Marshall D. J., Heppell S. S., Munch S., Warner R. R. (2010). The Relationship Between Maternal Phenotype and Offspring Quality: Do Older Mothers Really Produce the Best Offspring? J. Ecol. pages 2862–2873 13. doi: 10.1890/09-0156.1
McNamara J. M., Houston A. I. (1996). State-Dependent Life Histories. Nature 380, 215–221. doi: 10.1038/380215a0
Nordström M. C., Bonsdorff E. (2017). Organic Enrichment Simplifies Marine Benthic Food Web Structure: Organic Enrichment Simplifies Food Webs. Limnol. Oceanogr. 62, 2179–2188. doi: 10.1002/lno.10558
Passano L. M. (1960). “Molting and its Control”, in The Physiology of Crustacea. Ed. Waterman T. H. (New York, NY: Academic Press), 473–536.
Peterson B. J., Fry B. (1987). Stable Isotopes in Ecosystem Studies. Annu. Rev. Ecol. Systematics 18, 293–320. doi: 10.1146/annurev.es.18.110187.001453
Post D. M., Layman C. A., Arrington D. A., Takimoto G., Quattrochi J., Montaña C. G. (2007). Getting to the Fat of the Matter: Models, Methods and Assumptions for Dealing With Lipids in Stable Isotope Analyses. Oecologia 152, 179–189. doi: 10.1007/s00442-006-0630-x
Ptatscheck C., Brüchner-Hüttemann H., Kreuzinger-Janik B., Weber S., Traunspurger W. (2020). Are Meiofauna a Standard Meal for Macroinvertebrates and Juvenile Fish? Hydrobiologia 847, 2755–2778. doi: 10.1007/s10750-020-04189-y
Rabalais N. N., Díaz R. J., Levin L. A., Turner R. E., Gilbert D., Zhang J. (2010). Dynamics and Distribution of Natural and Human-Caused Hypoxia. Biogeosciences 7, 585–619. doi: 10.5194/bg-7-585-2010
Raymond C., Gorokhova E., Karlson A. M. (2021). Polycyclic Aromatic Hydrocarbons Have Adverse Effects on Benthic Communities in the Baltic Sea: Implications for Environmental Status Assessment. Front. Environ. Sci. 9. doi: 10.3389/fenvs.2021.624658
R Core Team (2018). R: A Language and Environment for Statistical Computing (Boston, MA, USA: R Foundation for Statistical Computing). Available at: https://www.R-project.org/.
Rodil I. F., Lucena-Moya P., Tamelander T., Norkko J., Norkko A. (2020). Seasonal Variability in Benthic–Pelagic Coupling: Quantifying Organic Matter Inputs to the Seafloor and Benthic Macrofauna Using a Multi-Marker Approach. Front. Mar. Sci. 7. doi: 10.3389/fmars.2020.00404
Schimmelmann A. (2011). Carbon, Nitrogen and Oxygen Stable Isotope Ratios in Chitin. Topics Geobiol. 34, 81–103. doi: 10.1007/978-90-481-9684-5_4
Sundelin B. (1983). Effects of Cadmium on Pontoporeia Affinis (Crustacea: Amphipoda) in Laboratory Soft-Bottom Microcosms. Mar. Biol. 74, 203–212. doi: 10.1007/BF00413923
Sundelin B., Eriksson A. (1998). Malformations in Embryos of the Deposit-Feeding Amphipod Monoporeia Affinis in the Baltic Sea. Mar. Ecol. Prog. Ser. 171, 165–180. doi: 10.3354/meps171165
Vandermeer J. H. (1972). “Niche Theory,” in Annual Review of Ecology and Systematics. (CA, USA: Annual Reviews), vol. 3.
van Noordwijk A. J., de Jong G. (1986). Acquisition and Allocation of Resources: Their Influence on Variation in Life History Tactics. Am. Nat. 128, 137–142. doi: 10.1086/284547
Viitasalo S., Katajisto T., Viitasalo M. (2007). Bioturbation Changes the Patterns of Benthic Emergence in Zooplankton. Limnol. Oceanogr. 52, 2325–2339. doi: 10.4319/lo.2007.52.6.2325
Wedchaparn O., Zhao L., Fan Y., He D., Zhang H., Ivan L. N., et al. (2016). Comparison of the Trophic Niches Between Two Planktivorous Fishes in Two Large Lakes Using Stable Isotope Analysis. Biochem. Systematics Ecol. 68, 148–155. doi: 10.1016/j.bse.2016.07.007
Keywords: stable isotopes, organic matter content, Monoporeia affinis, isotopic niche, Baltic Sea
Citation: Garrison JA, Karlson AML and Nascimento FJA (2022) Amphipod Isotope Composition, Condition and Reproduction in Contrasting Sediments: A Reciprocal Transfer Experiment. Front. Mar. Sci. 9:789700. doi: 10.3389/fmars.2022.789700
Received: 05 October 2021; Accepted: 02 May 2022;
Published: 30 May 2022.
Edited by:
Nadezhda Berezina, Zoological Institute (RAS), RussiaReviewed by:
Kyung-Hoon Shin, Hanyang University, South KoreaNataliia Kalinkina, Institute of Water Problems of the North Karelian Research Centre (RAS), Russia
Copyright © 2022 Garrison, Karlson and Nascimento. This is an open-access article distributed under the terms of the Creative Commons Attribution License (CC BY). The use, distribution or reproduction in other forums is permitted, provided the original author(s) and the copyright owner(s) are credited and that the original publication in this journal is cited, in accordance with accepted academic practice. No use, distribution or reproduction is permitted which does not comply with these terms.
*Correspondence: Julie A. Garrison, julie.garrison@su.se; Agnes M. L. Karlson, agnes.karlsson@su.se
†These authors have contributed equally to this work and share last authorship