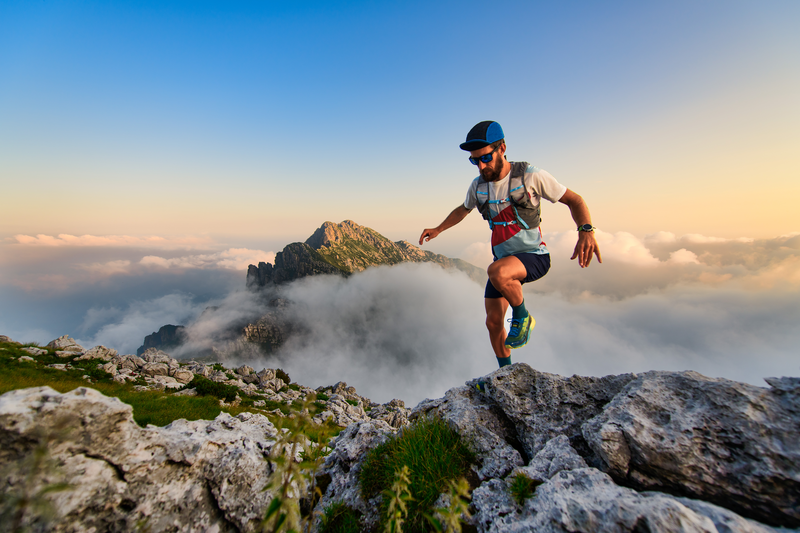
94% of researchers rate our articles as excellent or good
Learn more about the work of our research integrity team to safeguard the quality of each article we publish.
Find out more
ORIGINAL RESEARCH article
Front. Mar. Sci. , 22 February 2022
Sec. Coral Reef Research
Volume 9 - 2022 | https://doi.org/10.3389/fmars.2022.787314
Increases in sea surface temperature impact animal metabolism, which in turn could influence benthic structure and resulting algal-coral balance. We utilized a long-term coral reef dataset from the west coast of Hawai‘i Island to investigate impacts of annual positive and negative sea surface temperature anomalies (SSTA) on benthic cover [algal turf, macroalgae, crustose coralline algae (CCA), and coral], herbivore density (sea urchins, grazers, browsers, and scrapers) and the relationship between benthic cover and herbivore density. Results showed significantly lower coral cover, but higher CCA cover with positive SSTA. Additionally, the density of sea urchins, grazers and browsers increased with increasing SSTA. Warming disrupted the normal relationship between herbivores and benthic cover on reefs, particularly for grazers where higher densities were coupled with lower algal turf cover only during negative SSTA. The direction of the relationship between benthic cover and herbivore type changed with positive SSTA, where increased algal turf cover was associated with increased herbivore density. Here, herbivores are likely responding accordingly to increases in food availability due to increased metabolism under warming. Despite herbivore populations increasing in density over the past two decades, algal turf cover remains on an upward trajectory. These results indicate that warming can alter herbivore-algal dynamics, where greater herbivore densities may be required to cause a reduction in algal turf cover. Protection of herbivores in addition to reducing nutrient input onto reefs will be essential in driving a reduction in algal turf cover on Hawaiian reefs.
The worldwide loss of live coral cover and phase shifts from coral- to algal-dominated reefs has sparked various efforts to facilitate coral reef persistence and resilience (Hughes et al., 2018a; National Academies of Sciences, Engineering, and Medicine [NAS], 2019). A key indicator of coral reef health and area of research includes preventing the transition from coral to macroalgal dominance (Hughes, 1994), where overfishing of herbivorous fish and eutrophication favor algal growth (Pandolfi et al., 2005). Algae are major space competitors with corals (McCook, 2001; McManus and Polsenberg, 2004), where the control of algal cover is important in preventing suffocation of corals, allowing growth of crustose coralline algae (CCA), and providing space for juvenile corals to settle (Edmunds and Carpenter, 2001; Craggs et al., 2019). Large declines in coral cover following severe coral bleaching events provide substantial open reef substrate on dead coral colonies, which typically are rapidly colonized with early successional algal turf (Hughes et al., 2018b) and has been observed along the west coast of Hawai‘i Island (hereafter West Hawai‘i) (Kramer et al., 2016). Notably, not all algal species in Hawai‘i have a negative impact on coral, with some native species being economically valuable and culturally significant (McDermid et al., 2019).
Algal dominance is controlled through grazing by herbivorous fish and sea urchins (Carpenter and Edmunds, 2006; Johansson et al., 2010). For example, the mass mortality of the sea urchin Diadema antillarum in the 1980s helped catalyze significant increases in algal dominance in Caribbean reefs (Lessios et al., 1984; Carpenter, 1990). In general, coral reefs with lower levels of herbivorous fish show greater algal cover (Edwards et al., 2014). While many conservation efforts have focused on macroalgal control, the majority of algae on coral reefs are algal turf species (Jouffray et al., 2015; Gove et al., 2019). Algal growth is also influenced by a variety of bottom-up factors, such as light, salinity and nutrient concentration, where these impacts can sometimes outweigh those of herbivorous fish (Gilby et al., 2015).
Algal turf is often the most abundant benthic group and may be indicative of a degrading reef (Sandin et al., 2008). Close proximity of algal turf can lead to negative outcomes for coral, causing coral tissue loss (Williams et al., 2019) and algal overgrowth is a main contributor to coral mortality (Couch et al., 2014). This is particularly relevant for Hawaiian reefs, as algal turf is the dominant benthic group in over 50% of Hawaiian coral reefs (Jouffray et al., 2015). Algal turf, however, is a less clearly defined term than “macroalgae.” It encompasses a diverse algal assemblage including sparse mixed species of turf, clumps of filamentous algae, cropped, diminutive forms of various macroalgal species (less than 2 cm in height) and substrate that appears bare (Connell et al., 2014; Williams et al., 2019).
As a generalist herbivore, the collector sea urchin Tripneustes gratilla has been used as a biocontrol agent in Hawai‘i to reduce the abundance of invasive macroalgae (Neilson et al., 2018). In situ experiments with manual removal of invasive macroalgae and outplanting of T. gratilla individuals in Kāne‘ohe Bay showed increases in coral cover and CCA over the 2-year monitoring period (Neilson et al., 2018). Herbivorous fishes are also critical in sustaining coral dominance where coral reefs with greater densities of herbivorous fish, a proxy for herbivory intensity (Graham et al., 2015), often have lower levels of algal cover (Heenan and Williams, 2013). Herbivorous fishes can be classified as grazers, scrapers and browsers, which each have a different role in algal control (Green and Bellwood, 2009). Grazers feed on a combination of epilithic algal turf and sediment and are primarily represented by surgeonfishes from the genus Acanthurus in Hawai‘i (Donovan et al., 2018). Scrapers feed on epilithic algal turf and underlying substrate, removing part of the reef carbonate and contributing to bioerosion (Green and Bellwood, 2009). In Hawai‘i, scrapers primarily consist of parrotfish species from the Scarus and Chlorurus genera. Browsers mainly feed on macroalgae, removing fleshy macroalgae from the substrate (Hoey and Bellwood, 2008), mainly represented by Kyphosus (unicornfishes) and Naso (parrotfishes) genera. Herbivore management is one way to prevent algal overgrowth and boost coral resilience helping to maintain reef health (Williams et al., 2019).
Due to anthropogenic increases in CO2, the ocean is undergoing rapid changes resulting in increasing sea surface temperature among other stressors (Pörtner et al., 2019). Variations in temperature strongly impact fish biology through influencing growth, reproduction, and metabolism (Pörtner, 2002) where climate projections show species-specific increases and decreases in fish abundance (Wernberg et al., 2013, 2016; Maltby et al., 2020). In Hawai’i, increasing sea surface temperature has varying effects, where fish biomass increased for detritivores but decreased for browsers (Heenan et al., 2016). The impacts of temperature on corals are well investigated, with increased temperature being the greatest cause of bleaching and mortality (Hughes et al., 2018a). For CCA, warming generally has a positive effect on CCA cover (O’Leary and McClanahan, 2010). While warming has been shown to negatively impact macroalgal assemblages (Wernberg et al., 2013, 2016), algal turfs have been shown to increase their productivity under increased temperature (Connell and Russell, 2010; Bender et al., 2014).
Generally, a positive relationship exists between temperature and herbivorous fish grazing (Smith, 2008) because for ectotherms, their metabolic rates increase with greater temperatures resulting in greater food consumption (Brown et al., 2004). For example, a study examining grazing across a temperature gradient found that parrotfish bite rates increased with increasing temperature (Smith, 2008). If temperature influences the feeding rate of herbivores, temperature can also impact the ratio of algal consumption to algal production and therefore overall algal cover. The consequences of this herbivorous fish diet shift, plus the cumulative impacts of temperature on benthic types at the community and ecosystem level have not been explored, where warming is likely to influence variable relationships between benthic cover and herbivores.
The objectives of this study were to: (1) investigate the impacts of positive and negative sea surface temperature anomalies (SSTA) on benthic cover (algal turf, macroalgae, CCA, and coral) and herbivore density (sea urchins, grazers, browsers, and scrapers), (2) test how positive and negative SSTA affect the relationship between benthic cover and herbivore density. This allowed us to investigate how future ocean warming would impact herbivore densities in Hawai‘i, and how this might relate to top-down algal dominance, herbivore management, and future remediation efforts of degraded reefs.
The dataset utilized in this study was part of the long-term, daytime monitoring efforts of coral reefs in West Hawai‘i conducted by the Hawai‘i Division of Aquatic Resources (DAR) at 23–25 permanent sites from 1999 to 2019 spanning over 140 km of the West Hawai‘i coastline (Supplementary Figure 1). Surveys were repeated 3–6 times annually, where at each site, four replicate transects were surveyed and averaged for the final data point (individuals m–2) with annual, site-level averages used for the analysis. Each transect was 25 m long by 4 m wide, with the first two transects placed in opposing directions from a central pin, and the second two transects placed 10 m inshore and approximately parallel to the first two. Transects were located in coral reef habitats, at depths ranging from 5–15 m with a mean of 11.6 m. All species of fish and selected invertebrates were recorded along the transects.
A pair of divers each surveyed a 25 m × 2 m belt on either side of the transect line with counts combined to generate the full transect width. The divers first performed a “high” swim, swimming down the transect and recording larger mobile species, mid-water species and uncommon species. On return, the divers performed a “low” swim quantifying the remaining fishes, including cryptic species and recruits. During the “low” swim, sea urchins were also counted but only included “free” urchin species (Tripneustes gratilla, Chondrocidaris gigantea, Diadema paucispinum, Echinothrix calamaris, and Heterocentrotus mammillatus) and not rock boring species. All fishes were classified into functional groups, with herbivores further subdivided into scrapers, grazers and browsers, based on trophic classifications and diet information from Donovan et al. (2018). Only herbivorous fish species were considered in the analysis.
Co-located information on macroalgal, algal turf, hard coral, and CCA cover was available for 6 years of the DAR surveys; 2003, 2007, 2011, 2014, 2016, and 2017. Surveys were conducted following a standard operating procedure used by the Hawai‘i Department of Land and Natural Resources’ DAR (Williams et al., 2006), where photographs were used to survey coral reef benthos, taken at 1 m intervals along the length of fixed monitoring transects. DAR staff analyzed images using Coral Point Count with Extensions (Kohler and Gill, 2006) where benthic habitat cover was averaged across the four transects per site.
The United States National Oceanic and Atmosphere Administration (NOAA) Coral Reef Watch program (CRW) generates daily, satellite-derived global 5 km resolution SST dating back to 1985 (NOAA Coral Reef Watch, 2017). From the SST observations, CRW also generates sea surface temperature anomaly (SSTA) by subtracting the long-term (7 years) SST mean from the SST at that given location at that same time of year, with a positive anomaly indicating the SST is warmer than average. SSTA data were extracted for each of the survey sites from 1999 to 2019 with yearly SSTA means derived from daily SSTA measurements (Supplementary Figure 2). CRW temperature data were accessed at https://coralreefwatch.noaa.gov/product/5km/index.php#data_access.
To determine how benthic cover and herbivore densities were affected by temperature, survey data were matched with the SSTA conditions of the previous year, i.e., we expected changes in benthic cover and densities would lag behind changes in the environment. Previous research has shown that a 1-year lag in seawater temperature data (rather than current or 2-year lagged data) best explains changes in fish and benthic structures (Halford and Caley, 2009; Edmunds and Lasker, 2016; Ribeiro et al., 2018; Petraitis and Dudgeon, 2020), where 1-year lags in physical conditions are often used to assess changes in coral reef composition (Cooper et al., 2015; Donovan et al., 2021). Here we have adopted a similar approach with our analysis. If the previous yearly SSTA mean at a given survey site was positive, this was considered a “warm” year and if the SSTA was negative, this was considered a “cool” year for the analysis.
All statistical procedures were performed in R software (v 4.0.2). To assess whether benthic cover differed between positive and negative SSTA, we used bootstrapping with 10,000 iterations of site-level observations for the 6 years of benthic data to generate 95% confidence intervals (CI) for percentage benthic cover across warm and cool years. Non-overlapping 95% CIs was an indication that means were significantly different between depths (Cumming and Finch, 2005). Since herbivore counts were performed annually, to assess the impact of temperature on herbivore density, the mean annual density was compared with mean annual SSTA using Spearman’s correlations.
We used generalized additive models (GAM) to identify habitat-abundance associations and account for non-linear relationships observed between benthic cover and herbivore groups using the mgcv package in R. For these models, only fish and urchin data for which co-located benthic data were available were used (n = 148). Eight models were developed where we included five variables in each model; sea urchins, browsers, grazers, scrapers, and site. Conventional random effects can be incorporated into GAM models (Wood, 2006) where “site” was specified as a random effect in our models to account for possible spatial autocorrelation of sites and population sample bias (Zuur et al., 2009). A separate model was conducted for each benthic type – algal turf, macroalgae, CCA, and coral. To compare reefs exposed to positive and negative SSTA, we categorized all surveys into “warm” (n = 73) and “cool” (n = 75) years, performing separate analyses depending on temperature. Collinearity among variables was assessed prior to all analyses via regression analysis and using variance inflation factors (VIF) where the highest VIF for any driver was less than 2, lower than our a priori cut-off value of 3 (Zuur, 2012).
All models were performed using the following procedure. Models were fitted with a beta distribution, suitable for proportional data which cannot be modeled as binomial. Here, the percentage of benthic cover was represented as a proportion between 0 and 1. Model validation was performed by assessing the heterogeneity of the error distribution and histograms of the residuals. Given our small number of response values, we limited the number of knots to five to prevent overfitting of our models.
We used model selection and averaging procedures from the MuMIn R package (Barton, 2013) calculating Akaike’s information criterion (AIC) corrected for small sample size (AICc) (Anderson, 2008) and the AICc-based relative importance weights (w). Here, all possible models through various combinations of variables were run using the dredge procedure. P-values derived from GAM models are usually approximates and typically low, therefore, we used a weight of evidence approach to test for driver significance (Burnham and Anderson, 2002). The variable importance output gives the total weight of each driver across all possible models, that is, the summed weights across all models. As models sum to 1, values close to 1 indicate drivers that occur in large portions of the models and the higher the probability that that driver is important (Burnham and Anderson, 2002).
To display the relationships between benthic cover and each herbivore group, we used the predict procedure from the mgvc R package, to generate visualizations of smoothers of the top ranked models. The top ranked models included all models with a weight > 0.05. Model averaging generated coefficients that we then used for prediction. The predict function takes the averaged, fitted GAM model and produces predictions given model covariate values, where here the predictor variable of interest had values equally spaced between the variable’s minimum and maximum values, and all other drivers were set to their means. We used the resulting output to generate smoothers with the response of benthic cover shown against untransformed variables to ease interpretation of the effect of each herbivore group on benthic cover.
Mean coral cover was ∼37% from 2003 to 2014. In 2016, there was a large decrease where coral cover was 18.9 ± 1.4% (mean ± standard error). In 2017, coral cover dropped to a mean of 17.8 ± 1.4% (Figure 1A). Macroalgal (MA) cover was low, with values ranging from 0.2 to 1.0% of benthic cover between 2003 and 2017. Algal turf cover represented the dominant benthic class during the survey period, showing a general increase in mean cover across the permanent monitoring sites from 42.3 ± 2.3% in 2003 to 55.3 ± 2.4% in 2017. CCA cover was 8.3 ± 0.8% in 2003 and increased to 17.8 ± 2.4 in 2017 (Figure 1A).
Figure 1. Patterns of benthic cover and herbivore density over survey years. The mean density ± standard error for the 25 sites is shown for each year for benthic cover (A), sea urchins (B), scrapers and browsers (C), and grazers (D). Note, benthic analyses were only conducted for 6 years of the 20-year survey period.
From 1999 to 2019, there was a steady increase in sea urchin density across the permanent plots in West Hawai‘i, with a mean density increase of 0.15 in 1999 to 1.09 sea urchins per m2 in 2019 (Figure 1B). Scrapers showed more variable mean densities across the survey period with a mean of 0.017 ± 0.003 in 1999 and 0.024 ± 0.004 in 2019 (Figure 1C). Browsers had a mean density of 0.013 ± 0.002 in 1999 and 0.016 ± 0.002 in 2019 (Figure 1C). Grazers showed an increasing trend across the survey period with a mean density of 0.28 ± 0.02 in 1999 and 0.56 ± 0.06 in 2019 (Figure 1D).
Bootstrapped estimates of CI for benthic cover show that there was significantly higher coral cover in cool years (36%) than in warm years (24%). Comparing this result to Figure 1, the majority of this coral loss occurred between 2014 and 2016. On the other hand, there was greater CCA cover in warm years (14%) than in cool years (6%). Both algal turf and MA cover were similar between warm and cool years (Figure 2).
Figure 2. Patterns of benthic cover across warm and cold years. Mean percentage cover with 95% confidence intervals is shown for coral, turf, macroalgae (MA), and crustose coralline algae (CCA). “Warm” represents years with positive sea surface temperature anomalies where benthic data were paired with temperature data from the previous year to allow a 1-year lag for effects.
When comparing mean herbivore density and mean SSTA, there were significant, positive correlations for three of the herbivore groups: sea urchins, grazers, and browsers, where there were greater densities with increases in SSTA (Figure 3).
Figure 3. Relationships between herbivore density and sea surface temperature anomalies. Each point represents the mean density across the 25 survey sites plotted with the mean SSTA for the previous year. The Pearson correlation coefficient, r, and significance of the relationship are shown for each correlation.
When considering the relationship between algal turf cover and each herbivore group in cool years, the top models contained all factors at least once (Supplementary Table1 and Figure 4). Positive relationships were seen between algal turf cover and sea urchin and scraper densities, where algal turf cover increased with increasing herbivore density (Figure 4). A negative relationship between algal turf cover and grazer density was seen with a flatter relationship between algal turf cover and browser density. Site had the highest variable importance weight (w = 1) indicating significantly different site differences influencing the relationship between algal turf cover and herbivore group. Grazers had the second highest variable importance weight across all models (w = 0.973; Supplementary Table 1 and Figure 5) indicating that this variable was more strongly coupled to algal turf cover than other herbivore groups, which had much lower weights. Additionally, the top model containing grazers and site as the only two variables explained the greatest amount of variance (adj-R2 = 0.9).
Figure 4. The proportion of benthic cover relative to different herbivore densities across warm (red bars) and cool (blue bars) years. Relationships are separately plotted for each benthic cover type [algal turf, macroalgae, crustose coralline algae (CCA), and coral] and herbivore type (sea urchin, grazer, browser, and scraper) where survey data were matched with SSTA data from the previous year. Solid lines are smoothers of each driver, where only the drivers retained in the top models are shown, with shaded areas indicating 95% confidence bands. Proportion of benthic cover is shown on the y-axis of each plot, with the various herbivore densities on the x-axis, with increasing values toward the right edge of each plot.
Figure 5. Variable importance scores for each factor in predicting the proportion of benthic cover across warm and cool sea surface temperature anomalies. Importance scores are separated for each benthic type. The higher the number of models the factor was present in, the closer the variable importance score to 1, indicating a higher importance metric.
For MA, the top models contained all factors except site (Supplementary Table 1 and Figure 4). A negative relationship was seen between macroalgal cover and grazer density with a slightly less steep negative relationship observed between MA and sea urchin density. A slightly positive relationship of MA cover was seen with browser density until a density of ∼0.025 individuals/m2 where cover began to decrease. A flat relationship between MA cover and scraper density existed until a density of ∼0.03 individuals/m2 where cover began to increase steeply (Figure 4). Scrapers and browsers had the highest weights across all models (w = 0.990 and 0.862 respectively; Figure 5) with the top model explaining a moderate proportion of variance (adj-R2 = 0.42).
For CCA, the top models contained all factors except grazers (Supplementary Table 1 and Figure 4). Positive relationships were seen between CCA cover and both sea urchin and browser densities, with a slightly negative relationship between CCA cover and scraper density (Figure 4). Sea urchins and browsers had the highest weights across all models (w = 0.999 and 0.986 respectively; Figure 5) with the top model explaining a high amount of variance (adj-R2 = 0.65).
For coral, the top models contained all factors except browsers (Supplementary Table 1 and Figure 4). A positive relationship between coral cover and grazer density was seen with a slightly negative relationship between coral cover and scraper density. There was a steeper negative relationship between coral cover and sea urchin density (Figure 4). Similar to algal turf cover, site had the highest variable importance weight (w = 1), followed closely by sea urchins (w = 0.992) and grazers (0.938; Figure 5) with the top model explaining a very high amount of variance in coral cover (adj-R2 = 0.93).
When considering the relationship between algal turf cover and herbivore group across warm years, the top models contained all factors except site (Supplementary Table 1 and Figure 4). Positive relationships were seen between algal turf cover and sea urchin, grazer and browser densities, where algal turf cover increased with increasing herbivore density (Figure 4). A slight positive relationship was seen between algal turf cover and scrapers. Sea urchins had the highest weight across all models (w = 0.947; Figure 5) however only a low amount of variance was explained by the top model (adj-R2 = 0.23).
For MA, the top models contained all factors except site (Supplementary Table 1 and Figure 4). Positive relationships between MA and both grazer and browser densities were seen with a slightly positive relationship between MA and sea urchin density. On the other hand, a slightly negative relationship between MA and scraper density was seen (Figure 4). Browsers had the highest weight across all models (w = 0.986; Figure 5). A low amount of variance was explained by the top model (adj-R2 = 0.29).
For CCA, the top models contained all factors except site (Supplementary Table 1 and Figure 4). Positive relationships between CCA cover and both sea urchin and grazer densities were seen, with flatter relationships between CCA cover and both browser and scraper density (Figure 4). Browsers had the highest weight across all models (w = 0.999; Figure 5) where, similar to the models with algal turf and MA, only a low amount of variance was explained by the top model (adj-R2 = 0.22).
For coral, the top models contained all factors except browsers (Supplementary Table 1 and Figure 4). Negative relationships between coral cover and both sea urchin and grazer density were seen with a positive relationship between coral cover and scraper density (Figure 4). Sea urchins had the highest weight across all models (w = 1) closely followed by grazers and site (w = 0.999 for both; Figure 5) with a high amount of variance explained by the top model (adj-R2 = 0.84).
Over the past 20 years in West Hawai‘i, hard coral cover has decreased, with the steepest decline in cover after 2015. Decrease in coral cover are associated with positive SSTA, illustrating the impact of the severe marine heatwave in 2015 where nearly 50% of coral cover was lost to mortality (Kramer et al., 2016). Macroalgal cover represents a minimal benthic component of coral reefs surveyed in West Hawai‘i with no significant differences in cover between warm and cool SSTA. CCA cover has shown a consistent increase since 2011, likely driven by positive SSTA where there is significantly higher CCA cover in warm years in comparison to cool years. Positive impacts of warming on CCA cover have been noted in other studies (O’Leary and McClanahan, 2010). Algal turf cover has also increased over time, representing a mean of 55% of cover in 2017. For algal turf, there are similar amounts of cover across warm and cool years indicating that increased temperatures are not driving changes in cover, although cover is not necessarily reflective of algal productivity, which is known to increase with increased temperature (Connell and Russell, 2010; Bender et al., 2014). Algal turf cover is highly negatively correlated with coral cover (Supplementary Figure 3), where the increases in algal turf cover are likely related to the increased space available for colonization after coral death (Kramer et al., 2016), with opposite trajectories seen in algal turf cover and coral cover from 2014 onward.
For all herbivore groups, there was a higher density in 2019 in comparison to 1999, where sea urchins and grazers show the clearest upward trends over the past 20 years. For sea urchins, grazers and browsers, these increases in density are influenced by increasing temperature, with significant positive relationships between density and SSTA seen for these three groups. For scrapers, a slight positive relationship between density and SSTA was observed but this was not strong or significant. The increases in scraper density after 2015 could have been influenced by factors other than temperature. For example, a ban on the use of SCUBA gear while spearfishing in West Hawai‘i was enacted in 2013. This may have helped boost scraper densities as the scraper group is mainly composed of parrotfishes, a family often targeted by spearfishers. The increased densities of sea urchins, grazers and browsers observed with positive SSTA indicate that thermal tolerance limits have not yet been reached for these herbivores on Hawaiian reefs, although it is important to note that biomass and fish size were not assessed here. With ongoing ocean warming, it will be important to assess whether densities remain on an upward trajectory, especially if thermal tolerances are breached.
Our analyses revealed herbivore-specific relationships with benthic types, where the direction of the relationship differed depending on temperature. In cool years, algal turf cover decreased with increasing grazer density. The very high importance of grazers in the model suggests that this group of herbivores are most tightly linked to algal turf cover. These results suggest that during cool years, grazers are either inhabiting areas with lower algal turf cover, or that grazers are contributing to reductions in algal turf cover. This relationship is reversed in warm years where increases in algal turf cover become coupled with increases in grazer density, a pattern observed across all herbivore groups.
Ectotherms are physiologically plastic, likely tolerating increases in temperature through herbivory (Pörtner, 2002; Seebacher et al., 2015). Studies have shown aquatic animals increase herbivory in response to high temperatures, including zooplankton (Boersma et al., 2016), freshwater fish (Prejs, 1984), and parrotfish (Smith, 2008). Positive correlations between algal turf cover and herbivore densities have also been observed in other studies (Russ et al., 2015; Morais et al., 2020). This study shows that in warm years, herbivores are responding to spatial patterns in algal distributions where grazing surface area is a strong predictor of the abundance of herbivorous fishes (Tootell and Steele, 2016; Oakley-Cogan et al., 2020).
Similar relationships were seen with MA, where increases in MA cover were coupled with increases in grazer and browser densities in warm years. In cool years, we see a negative relationship between MA cover and grazer and browser densities, a role that has been identified across many reef systems (Williams et al., 2019), especially for browsers whose dominant food source is macroalgae (Hoey and Bellwood, 2008). With macroalgae being the dominant food source, the strong positive relationship with MA cover and highest model weight in warm years suggests that during warmer years, browsers are resource-limited and are tracking nutritional resources closely. Similar, but much weaker relationships between MA and sea urchin density were also seen. In these surveys, the dominant species of sea urchin is T. gratilla, a species which shows a greater preference for brown algae such as Turbinaria spp. (Lewis et al., 2018), which is rare on the reefs surveyed here (M. Lamson, pers. comm.). MA cover is very low in West Hawaii, which could explain the weak correlations observed here between MA and sea urchin density.
For CCA, the positive relationship between CCA cover and sea urchins across warm and cool years are steeper than any other herbivore, with sea urchins having the highest importance for explaining CCA cover. This could indicate that sea urchins are clearing some space for CCA to grow, a response also documented on other reefs (Sammarco et al., 1974; Johansson et al., 2010). Similar results in the Caribbean have been noted with CCA cover increasing with increasing grazer density due to removal of competitive fleshy algae (O’Leary and McClanahan, 2010).
For coral, we see decreases in cover with increasing sea urchin density across both warm and cold years. Whether sea urchins are causing coral cover decline or merely associating with low cover coral reefs in Hawai’i will be important to determine, especially as sea urchins show a strong upward population increase. It is important to note that of all sea urchins surveyed here, only T. gratilla shows similar abundances from day to night, while all other species show an increase in night-time surveys (Walsh et al., 2004), indicating that the reported urchin densities are likely underestimated.
On the other hand, coral cover increases with grazer density in cool years, but decreases with grazer density in warm years. A positive relationship between coral cover and scraper density emerges in warm years. Previous research has shown that highly abundant sea urchins reduce the density of coral recruits and increase the mortality of small coral fragments (Dang et al., 2020) where sea urchins prey on living coral tissue of adult colonies (Ruiz-Ramos et al., 2011). There is, however, the confounding strong correlation between algal turf and coral cover which could be influencing the negative relationships observed here, where these negative relationships could be driven by herbivores favoring nutritional resources and areas with greater algal turf rather than coral cover.
Additionally, in cool years, site variability greatly influenced algal turf cover and CCA cover, but barely in warm years, suggesting a homogenizing impact of increased temperature. For MA, site had low weight across both warm and cool models, likely due to low MA cover throughout all West Hawai’i. On the other hand, site had high weight across both warm and cool models for coral cover, suggesting important roles of local adaptation and local conditions influencing cover.
Despite the large increases in herbivore densities over the past two decades, algal turf continues to increase in cover. Herbivore abundance seems to be responding accordingly to increases in food availability where increased algal cover is driving energetic shifts towards greater herbivore populations in warm years. While algal turf cover would increase at a much greater rate without herbivores, the results suggest that current grazing levels may not be enough to cause reductions in algal turf cover, where algal production is still exceeding grazing capacity. The increased metabolism and consumption of herbivorous fish driven by warming are not outpacing algal turf productivity but is still an important mechanism contributing to control of algal turf in a warming ocean. The relationships between herbivore groups and benthic cover are conceptualized in Figure 6. A mismatch between the thermal limits of herbivorous fish and that of algal turf species could drive a greater competitive advantage for algae during warm years.
Figure 6. Changes in benthic-herbivore relationships across cool (top panel) and warm (bottom panel) years. In cool years, there is a balance between coral and algal turf cover. Increases in grazers are associated with decreased algal turf cover, while the opposite relationship is seen with sea urchins. In warm years, coral mortality opens space up for algae to colonize. Due to increases in animal metabolism and tracking of resources, there are increases in density across all herbivore groups with increases in algal turf cover. Green arrows indicate top-down control of algal turf cover or balanced relationships. Red arrows indicate insufficient top-down control of algal turf cover. Symbols courtesy of the Integration and Application Network, University of Maryland Center for Environmental Science (https://ian.umces.edu/symbols/).
Other factors are likely contributing to increases in algal turf cover, where eutrophication and sedimentation can be just as important as a reduction in herbivory in causing phase shifts (Gilby et al., 2015). Increases in nutrients and sediment loads can stimulate algal growth with bottom-up processes precipitating coral-algal phase shifts on Mesoamerican reefs despite healthy herbivore populations (Arias-Gonzalez et al., 2017). As nitrogen pollution strongly impacts Hawaiian resource fish populations, consisting of mainly herbivores (Foo et al., 2021), addressing water quality in Hawai’i should be a priority in conjunction with herbivore management. Additionally, across algal turf, MA, and CCA benthic types, the amount of variance explained by the models greatly decreased when comparing warm to cool years with a shift in the relative importance of different herbivore groups depending on temperature, indicating that during warm years, factors other than herbivory are important in driving cover. Warming reduces the predictability of relationships between benthic types and herbivore groups.
Further, there are clear differences between the impacts of herbivorous fish and sea urchins on Hawaiian coral reefs, with critical implications for herbivore management. The groups are not functionally equivalent where grazer densities are more strongly associated with decreased algal turf cover and greater sea urchin densities with increasing CCA cover. A balance of sea urchin abundance relative to herbivorous fishes will be important to ensure a positive influence of sea urchins on reef health and resilience, and prevention of high substrate erosion (McClanahan, 1995).
It is important to highlight the limitations of this study as well as critical areas of future research. Here, we generalize the impacts of warm and cool years on the benthic-herbivore relationship (Figure 6), while it is likely that both the extent of heat stress experienced and previous heatwave events would influence the trajectory of change (Maynard et al., 2008; Hughes et al., 2018b). Future studies, which monitor both benthic cover and herbivore abundance annually could utilize rates of change alongside various temperature metrics, to understand how severe heatwaves and ecological memory potentially modify the benthic-herbivore relationship.
Investigating algae-fish relationships has important consequences for local management. Our analyses show that increasing temperature disrupts the normal habitat associations of herbivore densities on coral reefs. Temperature changes should, therefore, be integrated into herbivore management strategies. The future health of coral reefs and recovery from bleaching will largely be determined by local herbivore population effectiveness in algal control (Williams et al., 2019), particularly in West Hawai‘i where algal turf already dominates reefs (Jouffray et al., 2015). Our results show that herbivore populations, despite increasing in density over the past decade, are likely impacting algal turf but not enough to cause a reduction in algal turf cover over time. Increasing temperatures may be establishing a higher threshold of herbivore densities required to cause a reduction in algal turf cover on Hawaiian reefs. In conjunction with bottom-up drivers of algal turf cover, i.e., mitigation of land-use impacts to improve water quality, algal-dominated reefs will benefit from protection measures to increase herbivore populations and to encourage the increased resilience and expansion of corals. Reducing coastal eutrophication will also be critical in mitigating future algal turf growth in a warming ocean, where herbivores are likely to be pushed outside of their optimal thermal windows.
Publicly available datasets were analyzed in this study. This data can be found here: https://www.ncei.noaa.gov/access/metadata/landing-page/bin/iso?id=gov.noaa.nodc:0164965.
Ethical review and approval was not required for the animal study because results only involved analysis of publicly available surveys of fish and coral.
SF and GA conceived the ideas for the manuscript with CT involved in the collection of the data. SF analyzed the data and led the writing of the manuscript. All authors contributed critically to drafts and the final version.
This work was supported by the Dorrance Family Foundation fund, Lenfest Ocean Program, NOAA Coral Reef Conservation Program, and the Hawaii Department of Land and Natural Resources, Division of Aquatic Resources.
The authors declare that the research was conducted in the absence of any commercial or financial relationships that could be construed as a potential conflict of interest.
All claims expressed in this article are solely those of the authors and do not necessarily represent those of their affiliated organizations, or those of the publisher, the editors and the reviewers. Any product that may be evaluated in this article, or claim that may be made by its manufacturer, is not guaranteed or endorsed by the publisher.
We thank the Hawai‘i Division of Aquatic Resources team in Kailua-Kona for collection and provision of the extensive sea urchin, fish, and benthic data. We also thank David Knapp for extracting sea surface temperature data for the analyses.
The Supplementary Material for this article can be found online at: https://www.frontiersin.org/articles/10.3389/fmars.2022.787314/full#supplementary-material
Anderson, D. R. (2008). Model Based Inference in the Life Sciences: A Primer on Evidence. New York, NY: Springer.
Arias-Gonzalez, J. E., Fung, T., Seymour, R. M., Garza-Pearez, J. R., Acosta-Gonzalez, G., Bozec, Y.-M., et al. (2017). A coral-algal phase shift in Mesoamerica not driven by changes in herbivorous fish abundance. PLoS One 12:e0174855. doi: 10.1371/journal.pone.0174855
Barton, K. (2013). MuMln: Multi-Model Inference. R Package Version 1.9.13. Available online at: https://cran.r-project.org/web/packages/MuMIn/ (accessed January, 2022).
Bender, D., Diaz-Pulido, G., and Dove, S. (2014). Warming and acidification promote cyanobacterial dominance in turf algal assemblages. Mar. Ecol. Prog. Ser. 517:271284.
Boersma, M., Mathew, K. A., Niehoff, B., School, K. L., Franco-Santos, R. M., and Meunier, C. L. (2016). Temperature driven changes in the diet preference of omnivorous copepods: no more meat when it’s hot? Ecol. Lett. 19, 45–53. doi: 10.1111/ele.12541
Brown, J. H., Gillooly, J. F., Allen, A. P., Savage, V. M., and West, G. B. (2004). Toward a metabolic theory of ecology. Ecology 85, 1771–1789.
Burnham, K. P., and Anderson, D. R. (2002). Model Selection and Multimodel Inference: A Practical Information-Theoretic Approach, 2nd Edn. New York, NY: Springer.
Carpenter, R. C., and Edmunds, P. J. (2006). Local and regional scale recovery of Diadema promotes recruitment of scleractinian corals. Ecol. Lett. 9, 271–280. doi: 10.1111/j.1461-0248.2005.00866.x
Connell, S. D., and Russell, B. D. (2010). The direct effects of increasing CO2 and temperature on non-calcifying organisms: increasing the potential for phase shifts in kelp forests. Proc. R. Soc. B. 277, 1409–1415. doi: 10.1098/rspb.2009.2069
Connell, S., Foster, M., and Airoldi, L. (2014). What are algal turfs? Towards a better description of turfs. Mar. Ecol. Prog. Ser. 495, 299–307. doi: 10.3354/meps10513
Cooper, J. K., Spencer, M., and Bruno, J. F. (2015). Stochastic dynamics of a warmer Great Barrier Reef. Ecology 96, 1802–1811. doi: 10.1890/14-0112.1
Couch, C. S., Garriques, J. D., Barnett, C., Preskitt, L., Cotton, S., Giddens, J. L., et al. (2014). Spatial and temporal patterns of coral health and disease along leeward Hawai‘i Island. Coral Reefs 33, 693–704. doi: 10.1007/s00338-014-1174-x
Craggs, J., Guest, J., Bulling, M., and Sweet, M. (2019). Ex situ co culturing of the sea urchin, Mespilia globulus and the coral Acropora millepora enhances early post-settlement survivorship. Sci. Rep. 9:129854. doi: 10.1038/s41598-019-49447-9
Cumming, G., and Finch, S. (2005). Inference by eye: confidence intervals and how to read pictures of data. Am. Psychol. 60, 170–180. doi: 10.1037/0003-066X.60.2.170
Dang, V. D. H., Fong, C.-L., Shiu, J.-H., and Nozawa, Y. (2020). Grazing effects of sea urchin Diadema savignyi on algal abundance and coral recruitment processes. Sci. Rep. 10:20346. doi: 10.1038/s41598-020-77494-0
Donovan, M. K., Burkepile, D. E., Kratochwill, C., Shlesinger, T., Sully, S., Oliver, T. A., et al. (2021). Local conditions magnify coral loss after marine heatwaves. Science 372, 977–980. doi: 10.1126/science.abd9464
Donovan, M. K., Friedlander, A. M., Lecky, J., Jouffray, J.-F., Williams, G. J., Wedding, L. M., et al. (2018). Combining fish and benthic communities into multiple regimes reveals complex reef dynamics. Sci. Rep. 8:16943. doi: 10.1038/s41598-018-35057-4
Edmunds, P. J., and Lasker, H. R. (2016). Cryptic regime shift in benthic community structure on shallow reefs in St. John, US Virgin Islands. Mar. Ecol. Prog. Ser. 559, 1–12. doi: 10.3354/meps11900
Edmunds, P., and Carpenter, R. C. (2001). Recovery of Diadema antillarum reduces macroalgal cover and increases abundance of juvenile corals on a Caribbean reef. Proc. Natl. Acad. Sci. U.S.A. 98, 5067–5071. doi: 10.1073/pnas.071524598
Edwards, C. B., Friedlander, A. M., Green, A. G., Hardt, M. J., Sala, E., Sweatman, H. P., et al. (2014). Global assessment of the status of coral reef herbivorous fishes: evidence for fishing effects. Proc. R. Soc. B 281:20131835. doi: 10.1098/rspb.2013.1835
Foo, S. A., Walsh, W. J., Lecky, J., Marcoux, S., and Asner, G. P. (2021). Impacts of pollution, fishing pressure, and reef rugosity on resource fish biomass in West Hawaii. Ecol. Appl. 31:e2213. doi: 10.1002/eap.2213
Gilby, B. L., Maxwell, P. S., Tibbetts, I. R., and Stevens, T. (2015). Bottom-up factors for algal productivity outweigh no-fishing marine protected area effects in a marginal coral reef system. Ecosystems 18, 1056–1069.
Gove, J. M., Lecky, J., Walsh, W. J., Ingram, R. J., Leong, K., Williams, I., et al. (2019). West Hawai‘i Integrated Ecosystem Assessment Ecosystem Status Report: PIFSC Special Publication, SP-19-001. Honolulu, HI: Pacific Islands Fisheries Science Center, 46. doi: 10.25923/t3cc-2361
Graham, N. A. J., Jennings, S., MacNeil, M. A., Mouillot, D., and Wilson, S. K. (2015). Predicting climate-driven regime shifts versus rebound potential in coral reefs. Nature 518, 94–97. doi: 10.1038/nature14140
Green, A. L., and Bellwood, D. R. (2009). Monitoring Functional Groups of Herbivorous Reef Fishes as Indicators of Coral Reef Resilience – A Practical Guide for Coral Reef Managers in the Asia Pacific Region. Gland: IUCN working group on Climate Change and Coral Reefs.
Halford, A. R., and Caley, M. J. (2009). Towards an understanding of resilience in isolated coral reefs. Global Change Biol. 15, 3031–3045. doi: 10.1111/j.1365-2486.2009.01972.x
Heenan, A., and Williams, I. D. (2013). Monitoring herbivorous fishes as indicators of coral reef resilience in American Samoa. PLoS One 8:e79604. doi: 10.1371/journal.pone.0079604
Heenan, A., Hoey, A. S., Williams, G. J., and Williams, I. D. (2016). Natural bounds on herbivorous coral reef fishes. Proc. R. Soc. B 283:20161716. doi: 10.1098/rspb.2016.1716
Hoey, A. S., and Bellwood, D. R. (2008). Cross-shelf variation in the role of parrotfishes on the Great Barrier Reef. Coral Reefs 27, 37–47.
Hughes, T. P. (1994). Catastrophes, phase shifts, and large-scale degradation of a Caribbean coral reef. Science 265, 1547–1551. doi: 10.1126/science.265.5178.1547
Hughes, T. P., Anderson, K. D., Connolly, S. R., Heron, S. F., Kerry, J. T., Lough, J. M., et al. (2018a). Spatial and temporal patterns of mass bleaching of corals in the anthropocene. Science 359, 80–83. doi: 10.1126/science.aan8048
Hughes, T. P., Kerry, J. T., Connolly, S. R., Baird, A. H., Eakin, M., Heron, S. F., et al. (2018b). Ecological memory modifies the cumulative impact of recurrent climate extremes. Nat. Clim. Change 9, 40–43. doi: 10.1038/s41558-018-0351-2
Johansson, C., Bellwood, D., and Depczynski, M. (2010). Sea urchins, macroalgae and coral reef decline: a functional evaluation of an intact reef system, Ningaloo, Western Australia. Mar. Ecol. Prog. Ser. 414, 65–74.
Jouffray, J.-B., Nystrom, M., Norstrom, A. V., Williams, I. D., Wedding, L. M., Kittinger, J. N., et al. (2015). Identifying multiple coral reef regimes and their drivers across the Hawai‘ian archipelago. Philos. Trans. R. Soc. Lond. B Biol. Sci. 370:20130268. doi: 10.1098/rstb.2013.0268
Kohler, K. E., and Gill, S. M. (2006). Coral Point Count with Excel extensions (CPCe): a visual basic program for the determination of coral and substrate coverage using random point count methodology. Comput. Geosci. 32, 1259–1269. doi: 10.1016/j.cageo.2005.11.009
Kramer, K. L., Cotton, S. P., Lamson, M. R., and Walsh, W. J. (2016). “Bleaching and catastrophic mortality of reef-building corals along west Hawai‘i island: findings and future directions,” in Proceedings of the 13th International Coral Reef Symposium, Honolulu, HI, 219–230.
Lessios, H. A., Robertson, D. R., and Cubit, J. D. (1984). Spread of Diadema mass mortality through the Caribbean. Science 226, 335–337. doi: 10.1126/science.226.4672.335
Lewis, L. S., Smith, J. E., and Eynaud, Y. (2018). Comparative metabolic ecology of tropical herbivorous echinoids on a coral reef. PLoS One 13:e0190470. doi: 10.1371/journal.pone.0190470
Maltby, K. M., Rutterford, L. A., Tinker, J., Genner, M. J., and Simpson, S. D. (2020). Projected impacts of warming seas on commercially fished species at a biogeographic boundary of the European continental shelf. J. Appl. Ecol. 57, 2222–2233. doi: 10.1111/1365-2664.13724
Maynard, J. A., Anthony, K. R. N., Marshall, P. A., and Masiri, I. (2008). Major bleaching events can lead to increased thermal tolerance in corals. Mar. Biol. 155, 173–182.
McClanahan, T. R. (1995). Fish predators and scavengers of the sea urchin Echinometra mathaei in Kenyan coral-reef marine parks. Env. Biol. Fish. 43, 187–193. doi: 10.1007/bf00002490
McCook, L. (2001). Competition between corals and algal turfs along a gradient of terrestrial influence in the nearshore central Great Barrier Reef. Coral Reefs 19, 419–425. doi: 10.1007/s003380000119
McDermid, K. J., Martin, K. J., and Haws, M. C. (2019). Seaweed resources of the Hawaiian Islands. Bot. Mar. 62, 443–462. doi: 10.1515/bot-2018-0091
McManus, J. W., and Polsenberg, J. F. (2004). Coral–algal phase shifts on coral reefs: Ecological and environmental aspects. Prog. Oceanogr. 60, 263–279. doi: 10.1016/j.pocean.2004.02.014
Morais, R. A., Depczynski, M., Fulton, C., Marnane, M., Narvaez, P., Huertas, V., et al. (2020). Severe coral loss shifts energetic dynamics on a coral reef. Funct. Ecol. 34, 1507–1518. doi: 10.1111/1365-2435.13568
National Academies of Sciences, Engineering, and Medicine [NAS] (2019). A Decision Framework for Interventions to Increase the Persistence and Resilience of Coral Reefs. Washington, DC: The National Academies Press.
Neilson, B., Wall, C. A., Mancini, F. T., and Gewecke, C. A. (2018). Herbivore biocontrol and manual removal successfully reduce invasive macroalgae on coral reefs. PeerJ. 6:e5332. doi: 10.7717/peerj.5332
NOAA Coral Reef Watch (2017). Updated Daily. NOAA Coral Reef Watch Version 3.0 Daily Global 5-km Satellite Virtual Station Time Series Data for Southeast Florida, March 12, 2013–March 11, 2014. College Park, MD: NOAA Coral Reef Watch.
O’Leary, J. K., and McClanahan, T. R. (2010). Trophic cascades result in large-scale coralline algae loss through differential grazer effects. Ecology 91, 3584–3597. doi: 10.1890/09-2059.1
Oakley-Cogan, A., Tebbett, S. B., and Bellwood, D. R. (2020). Habitat zonation on coral reefs: Structural complexity, nutritional resources and herbivorous fish distributions. PLoS One 15:0233498. doi: 10.1371/journal.pone.0233498
Pandolfi, J. M., Jackson, J. B., Baron, N., Bradbury, R. H., Guzman, H. M., Hughes, T. P., et al. (2005). Are U.S. coral reefs on the slippery slope to slime? Science 307, 1725–1726. doi: 10.1126/science.1104258
Petraitis, P. S., and Dudgeon, S. R. (2020). Declines over the last two decades of five intertidal invertebrate species in the western North Atlantic. Commun. Biol. 3:591. doi: 10.1038/s42003-020-01326-0
Pörtner, H. O. (2002). Climate variations and the physiological basis of temperature dependent biogeography: systemic to molecular hierarchy of thermal tolerance in animals. Comp. Biochem. Physiol. A Mol. Integr. Physiol. 132, 739–761. doi: 10.1016/s1095-6433(02)00045-4
Pörtner, H. O., Roberts, D. C., Masson-Delmotte, V., Zhai, P., Tignor, M., Poloczanska, K., et al. (2019). IPCC, 2019: IPCC Special Report on the Ocean and Cryosphere in a Changing Climate. Available online at: https://www.ipcc.ch/srocc/ (accessed September, 2021).
Prejs, A. (1984). Herbivory by temperate fresh-water fishes and its consequences. Environ. Biol. Fish. 10, 281–296. doi: 10.1007/bf00001481
Ribeiro, F. V., Sá, J. A., Fistarol, G. O., Salomon, P. S., Pereira, R. C., Souza, M. L. A. M., et al. (2018). Long-term effects of competition and environmental drivers on the growth of the endangered coral Mussismilia braziliensis (Verril, 1867). PeerJ 6:e5419. doi: 10.7717/peerj.5419
Ruiz-Ramos, D. V., Hernández-Delgado, E. A., and Schizas, N. V. (2011). Population status of the long spined urchin Diadema antillarum in Puerto Rico 20 years after a mass mortality event. Bull. Mar. Sci. 87, 113–127. doi: 10.5343/bms.2010.1038
Russ, G. R., Questel, S. L. A., Rizzari, J. R., and Alcala, A. C. (2015). The parrotfish-coral relationship: refuting the ubiquity of a prevailing paradigm. Mar. Biol. 162, 2029–2045. doi: 10.1007/s00227-015-2728-3
Sammarco, M., Lewinton, J. S., and Ogden, J. C. (1974). Grazing and control of coral reef community structured by Diadema antillarum Philippi (Echinodermata: Echinoidea): a preliminary study. J. Mar. Res. 32, 47–53.
Sandin, S. A., Smith, J. E., DeMartini, E. E., Dinsdale, E. A., Donner, S. D., Friedlander, A. M., et al. (2008). Baselines and degradation of coral reefs in the Northern Line Islands. PLoS One 3:1984. doi: 10.1371/journal.pone.0001548
Seebacher, F., White, C. R., and Franklin, C. E. (2015). Physiological plasticity increases resilience of ectothermic animals to climate change. Nat. Clim. Change 5:61. doi: 10.1038/nclimate2457
Smith, B. (2008). Temperature effects on herbivory for an Indo-Pacific parrotfish in Panamá: implications for coral–algal competition. Coral Reefs 27, 397–405. doi: 10.1007/s00338-007-0343-6
Tootell, J. S., and Steele, M. A. (2016). Distribution, behavior, and condition of herbivorous fishes on coral reefs track algal resources. Oecologia 181, 13–24. doi: 10.1007/s00442-015-3418-z
Walsh, W., Williams, I. D., Cotton, S. P., and Carman, B. (2004). Crepuscular and Night Survey Component for West Hawai‘i Aquarium Project (WHAP). NOAA Project Report. Washington, DC: NOAA, 27.
Wernberg, T., Bennett, S., Babcock, R. C., de Bettignies, T., Cure, K., Depczynski, M., et al. (2016). Climate-driven regime shift of a temperate marine ecosystem. Science 353, 169–172. doi: 10.1126/science.aad8745
Wernberg, T., Smale, D. A., Tuya, F., Thomsen, M. S., Langlois, T. J., de Bettignies, T., et al. (2013). An extreme climatic event alters marine ecosystem structure in a global biodiversity hotspot. Nat. Clim. Change 3, 78–82. doi: 10.1038/nclimate1627
Williams, I. D., Cotton, S. P., Walsh, W. J., and Stamoulis, K. A. (2006). Surveys of Benthic Reef Communities using Digital Still Photos. Division of Aquatic Resources Report. Townsville MC Qld: Australian Institute of Marine Science.
Williams, I. D., Kindinger, T. L., Couch, C. S., Walsh, W. J., Minton, D., and Oliver, T. A. (2019). Can herbivore management increase the persistence of Indo-Pacific coral reefs? Front. Mar. Sci 6:557. doi: 10.3389/fmars.2019.00557
Wood, S. N. (2006). Low rank scale invariant tensor product smooths for generalized additive mixed models. Biometrics 62, 1025–1036. doi: 10.1111/j.1541-0420.2006.00574.x
Zuur, A. F. (2012). Beginner’s Guide to Generalized Additive Models with R. Newburg: Highland Statistics Ltd.
Keywords: algal turf cover, herbivory, resilience, coral reef, macroalgae, sea surface temperature anomaly
Citation: Foo SA, Teague CH and Asner GP (2022) Warming Alters the Relationship Between Benthic Cover and Herbivores on Hawaiian Reefs. Front. Mar. Sci. 9:787314. doi: 10.3389/fmars.2022.787314
Received: 30 September 2021; Accepted: 01 February 2022;
Published: 22 February 2022.
Edited by:
Jesús Ernesto Arias González, Centro de Investigaciones y Estudios Avanzados, Instituto Politécnico Nacional de México (CINVESTAV), MexicoReviewed by:
Thibaut de Bettignies, Muséum National d’Histoire Naturelle, FranceCopyright © 2022 Foo, Teague and Asner. This is an open-access article distributed under the terms of the Creative Commons Attribution License (CC BY). The use, distribution or reproduction in other forums is permitted, provided the original author(s) and the copyright owner(s) are credited and that the original publication in this journal is cited, in accordance with accepted academic practice. No use, distribution or reproduction is permitted which does not comply with these terms.
*Correspondence: Shawna A. Foo, c2Zvb0Bhc3UuZWR1
Disclaimer: All claims expressed in this article are solely those of the authors and do not necessarily represent those of their affiliated organizations, or those of the publisher, the editors and the reviewers. Any product that may be evaluated in this article or claim that may be made by its manufacturer is not guaranteed or endorsed by the publisher.
Research integrity at Frontiers
Learn more about the work of our research integrity team to safeguard the quality of each article we publish.