- 1State Key Laboratory of Water Environment Simulation and School of Environment, Beijing Normal University, Beijing, China
- 2Yellow River Estuary Wetland Ecosystem Observation and Research Station, Ministry of Education, Dongying, China
- 3Tang Scholar, Beijing Normal University, Beijing, China
While nutrient enrichment and herbivory have been well recognized as the main driving factors of seagrass meadow fragmentation and degradation, there is limited understanding of how their relative importance shifts across large spatial scales where environmental factors such as turbidity can vary. In this study, a field control experiment was conducted in two Zostera japonica meadows distributed on the two banks of the Yellow River Estuary with different turbidity, to investigate the combined effects of nutrient enrichment and herbivory on seagrass and macroalgae. Our results showed that turbidity had the mediating force of shifting the relative importance of nutrient enrichment and herbivory to seagrass and macroalgae. While herbivory played a vital role in maintaining the balance between the two primary producers in a turbid environment, nutrient enrichment tended to offset herbivory-induced biomass loss by promoting seagrass growth in a less turbid system. Additionally, two potential mechanisms that might regulate the responses of seagrasses and macroalgae to nutrient enrichment and herbivory under different turbidity are proposed. On the one hand, turbidity might mediate the feeding preference of herbivores. On the other hand, nutrient enrichment favors the growth of opportunistic macroalgae over seagrass in turbid systems. Our study emphasizes the mediating force of turbidity on seagrass ecosystems, and provides references for the protection and restoration of seagrass meadows under multiple environmental stressors, and prompts further studies on the feedback between sediment dynamics and seagrass meadows in the context of ecogeomorphology.
Introduction
Seagrass meadows are among the most widely distributed coastal ecosystems in the world, and play a crucial role in the transitional zone between land and sea (Du et al., 2020; Orth et al., 2020). Seagrass ecosystems are recognized as the most productive ecosystems with an annual carbon sequestration potential as high as 43.9 ± 12.1 MtC yr–1 (Bertram et al., 2021), and provide US$100 million per year to the world’s blue economy (Stockbridge et al., 2020). However, in recent years, the structure and function of seagrass ecosystems have been threatened by multiple global stressors caused by human activities and climate change, resulting in pervasive and continuous degradation of seagrass meadows across the world (Waycott et al., 2009). Environmental changes such as warming (Repolho et al., 2017), eutrophication (Ontoria et al., 2019), sea-level rise (Albert et al., 2017) pose critical threats to the species composition, distribution, and productivity of seagrass ecosystems. Subject to the short or long-term effects of multiple environmental stressors, the response patterns of seagrass ecosystems need further studies.
Nutrient enrichment and herbivory have been recognized as the main global stressors that cause fragmentation and degradation of seagrass meadows (Leoni et al., 2008). It was found that eutrophication overrides warming as a dominant stressor for temperate seagrass meadows (Mvungi and Pillay, 2019). Many studies have explored the negative effects of nutrient enrichment on the structure and function of the seagrass ecosystem (Schmidt et al., 2012; Wang et al., 2020). For example, nutrient enrichment could cause epiphytic algae or macroalgae bloom (Leston et al., 2008; Archer et al., 2018), which leads to nutrient competition, algal shading, or direct toxicity of sulfide accumulation on seagrass (Darnell and Dunton, 2017; Viana et al., 2020). In parallel, it is also established that herbivory acts as a natural disturbance with a relatively high impact on seagrass. To some extent, herbivory significantly decreases seagrass biomass and structural complexity of canopy (Moran and Bjorndal, 2005; Fourqurean et al., 2010; Scott et al., 2021), and has long-term impacts on nitrogen and organic matter reserves of tissues (Moran and Bjorndal, 2007). Meanwhile, the selective grazing behavior of herbivores regulates the competition between seagrass and algae, and further affects the dynamics of the food web (Ebrahim et al., 2014; Fourqurean et al., 2019).
In addition to their separate effects, nutrient enrichment and herbivory interact in a variety of ways in seagrass communities (York et al., 2012; Whalen et al., 2013; Carr and Boyer, 2014). Numerous manipulative experiments have explored the combined effects of nutrient enrichment and herbivory as bottom-up and top-down controls on seagrass ecosystem. However, these experiments were mostly conducted in distinct natural habitats with wide-ranging varieties in latitude, trophic level, etc., occasionally leading to contrasting findings (Hughes et al., 2004; Duffy et al., 2015). For example, some studies showed that nutrient enrichment overrides top-down control on the shift of the dominant species from seagrass to algae (Hughes et al., 2004; Östman et al., 2016), while other studies revealed insignificant nutrient effects but strong top-down control on seagrass growth (Heck et al., 2000; Campbell et al., 2018). It is increasingly recognized that the separate or combined effects of top-down and bottom-up controls are context-dependent and vary across environmental gradients, thus emphasizing the mediating forces of other environmental factors of the habitat (Burkepile and Hay, 2006; Baden et al., 2010; Listiawati and Kurihara, 2021).
Regarding the environmental mediation of top-down and bottom-up controls on seagrass ecosystems, previous studies have revealed the importance of temperature as a mediating factor. For instance, Duffy et al. (2015) reported that similar to species richness, the effect of grazers controlling algae increased with temperature. Temperature-induced changes in the herbivore functional composition were further found to alter the relative importance of nutrients (Campbell et al., 2018). In addition to temperature, turbidity has been found to be one of the key factors driving the changes in the spatiotemporal distribution of seagrass (Garrido et al., 2013; Yamamoto et al., 2019). A short-term increase in turbidity would lead to long-term effects on the survival of seagrass (Li et al., 2021). Elevated turbidity is associated with a lower nutrient removal capacity of seagrass by reducing the intensity of light and photosynthesis (Bulmer et al., 2018). High turbidity is also related to high suspended sediment concentration which may carry extra nutrients available for algae growth in source-limited systems, and potentially enhance interspecific competition between primary producers (Schallenberg and Burns, 2004; Vieira et al., 2020). Furthermore, herbivory was found to be increased in turbid waters due to the reduced efficiency of predators (Savic et al., 2018). However, whether turbidity could play explicit mediating roles on bottom-up and top-down controls such as nutrient enrichment and herbivory needs to be examined.
Yellow River is one of the sandiest rivers in the world, with an annual sediment load of approximately 1.6 billion tons, creating a highly turbid Yellow River Estuary (YRE) (Wang et al., 2015; Yu et al., 2017). In this study, a field control experiment was conducted at the north and south banks of the YRE to investigate the combined effects of nutrient enrichment and herbivory on seagrass ecosystems under different turbidity. We hypothesized that turbidity had the mediating effect on shifting the relative importance of nutrient enrichment and herbivory on seagrass. In testing the hypothesis we aim to explore: (1) the effects of nutrient enrichment, herbivory and their interactions on the growth of two primary producers, i.e., seagrass and macroalgae; (2) the relative importance of nutrient enrichment and herbivory to the growth of seagrass under different turbidity; (3) potential mechanisms of turbidity that might regulate the responses of seagrasses and macroalgae to nutrient enrichment and herbivory. Our study emphasizes the mediating force of turbidity on seagrass ecosystems, and provides references for the protection and restoration of seagrass meadows under multiple environmental stressors, and prompts further studies on the feedback between sediment dynamics and seagrass meadows in the context of ecogeomorphology.
Materials and Methods
Study Sites
The Yellow River Estuary is located on the southern bank of the Bohai Sea and the western part of the Laizhou Bay in Dongying City, Shandong Province, China (Figure 1). The tide is irregular semidiurnal, with spring and neap tidal ranges of 1.06–1.78 m and 0.46–0.78 m, respectively. Seagrass meadows in the YRE are dominated by the temperate seagrass Zostera japonica, which are distributed along the intertidal zones on the north and south banks of the estuary, and adjacent to the saltmarsh habitat of Spartina alterniflora. Zostera japonica and green macroalgae Ulva intestinalis (hereafter referred to as “seagrass” and “macroalgae,” respectively) are the dominant primary producers of the seagrass meadows, which provide habitat for herbivores and predators. The dominant herbivores in the seagrass meadows are mainly intermediate consumers such as fishes and macrobenthic invertebrate, including small-sized herbivores such as small shrimps, Mollusca and Polychaetes (1∼3 cm in size), median-sized herbivores such as herbivorous crabs (3∼10 cm in size) and large-sized herbivores such as herbivorous fishes (> 10 cm).
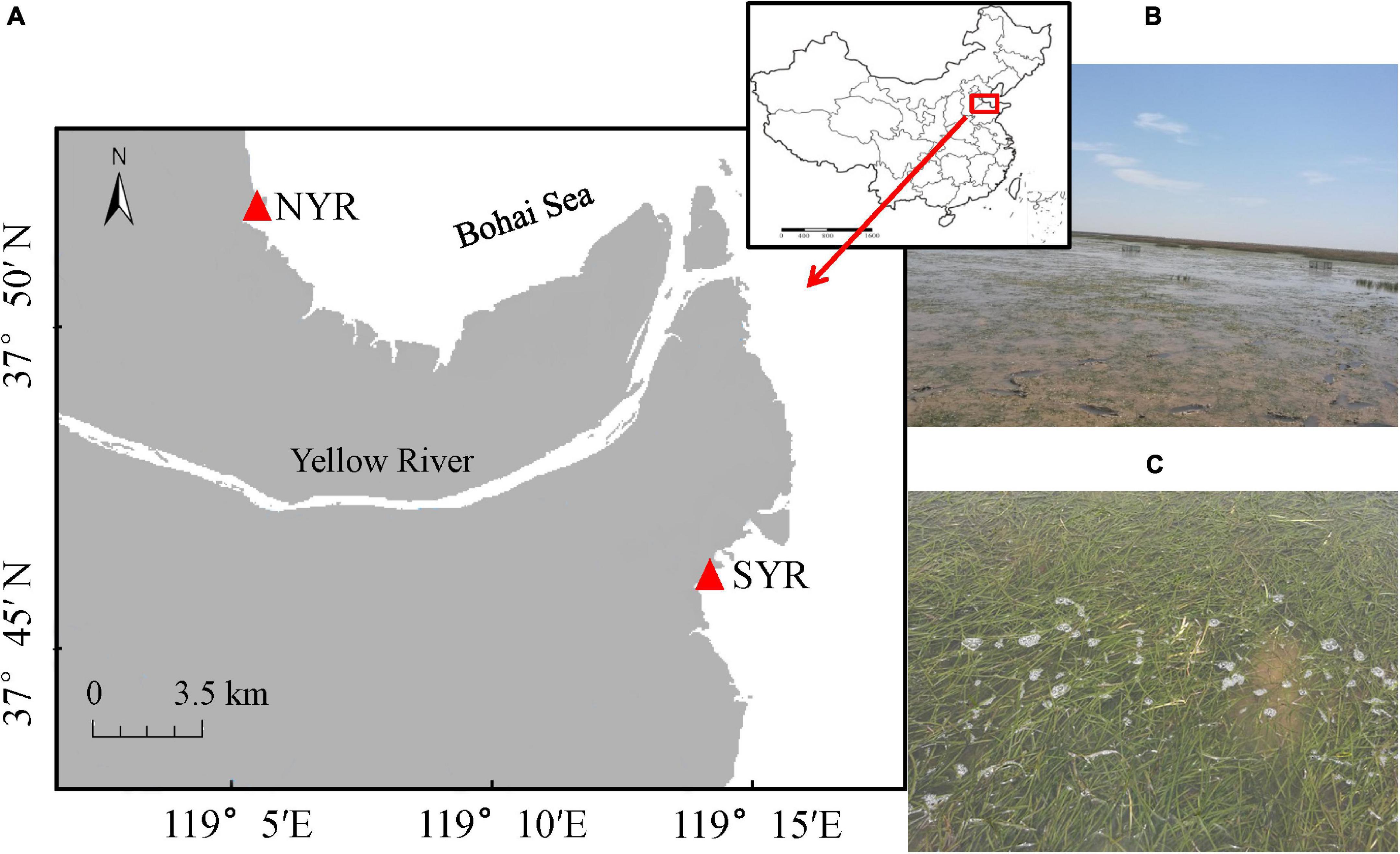
Figure 1. Schematic diagram of the Zostera japonica meadows in the Yellow River Estuary: (A) location of the two study sites; (B) a illustrating photograph of the seagrass meadow in the north bank of the Yellow River Estuary (NYR); (C) a illustrating photograph of the seagrass meadow in the south bank of the Yellow River Estuary (SYR).
Two study sites were selected on the north and south banks (NYR, SYR) of the river mouth which were both nutrient-limited systems with different water turbidity. Due to the prevailing estuarine circulation, a large amount of sediment is transported to the south bank of the estuary, especially during the Water-Sediment Regulation Scheme (WSRS) period, i.e., a 1-month period during the flood season every summer during which artificial flood pulses are created through dam regulation to mitigate the siltation both in the Xiaolangdi Reservoir and the lower reaches, and restore ecohydrological conditions downstream (Wang et al., 2017). As a result, significant differences in water turbidity are created between the two banks. Therefore, the seagrass meadows in the south bank are exposed to more turbidity than those in the north bank. Meanwhile, all meadows in the YRE are distributed in nutrient-limited areas with low nitrogen and phosphorus content in the water column, rendering them ideal for testing the interactive effects of nutrient enrichment and herbivory under different turbidity. NYR was located at 37°50′56.4″ N, 119°05′49.20″ E, where the water turbidity ranged from 11.7 to 25.4 NTU; SYR was located at 37°44′20.40″ N, 119°15′10.79″ E, where the water turbidity ranged from 50.80 to 90.36 NTU. The experiment was run for 120 days, from May to September in 2018, during which the salinity of the seawater ranged from 25.8 to 26.6 PPT, the temperature of the seawater ranged from 14.6 to 32.9°C, and the pH ranged from 8.04 to 8.25. Salinity was measured by a portable salinity meter (AZ8371, Shanghai Shuangxun Electronic, China), and temperature, pH and turbidity were measured by a multiparameter water quality probe (YSI Pro1020, RAE Systems, United States). No significant differences were found between the two sites during the experimental period except for the turbidity.
Experimental Design
Experiments of the same design were replicated in the two sites. We conducted a fully crossed 2 × 4 factorial design with two treatments of nutrients (nutrient enrichment or control) and four treatments of herbivory (seagrass only, crab inclusion, small and median-sized herbivores inclusion or all herbivores inclusion) to obtain different levels of herbivory intensity. The 8 treatments (n = 4 for each treatment) were randomly distributed across 32 plots at each site, totaling 64 experimental plots for the two sites. Experiment plots (1 m × 1 m × 1 m) were set up in areas without crab burrowing holes to eliminate the interference of crab, and were separated by a minimum distance of 10 m to prevent any nutrient transfer between each other (Campbell et al., 2018; Yan et al., 2020). Each plot was made of four metal reinforcing rods to fix the position.
Seagrass only plots (S) were set up by enclosing plots in cube-shaped cages made of plastic extruded nets (1 cm mesh size) to prevent almost all the herbivory effects, and the nets were inserted 10 cm deep into the bottom sediment to prevent the entry of burrowing crabs. Crab inclusion plots (S + CR) were of similar construction to S plots, but the nets were parallel with the bottom sediment surface to allow the entry of burrowing crabs. Small and median-sized herbivore inclusion plots (S + MH) were enclosed in plastic extruded nets (3 cm mesh size), and the nets were raised 2 cm above the bottom surface to allow the entry of all the dominant small and median-sized herbivores, but prevent large herbivores. All herbivore inclusion plots (S + LH) were of similar construction, but had the two side panels parallel to the primary flow direction removed to allow the entry of all herbivores.
Nutrient enrichment plots received 500 g of slow-release Osmocote fertilizer (N:P = 14:14) enclosed in eight nylon mesh bags. Four of the bags were buried 5 cm under the four corners of the plot, and the other four bags were tethered in the middle of the metal rod of the plot to simulate changing nutrient enrichment under tidal fluctuations. Each bag was replaced every 4 weeks to ensure consistent nutrient delivery (Mutchler and Hoffman, 2017; Archer et al., 2018).
Plot Sampling
After 120 days, all the macroalgae and seagrasses of each plot were collected, washed on the spot, and brought back to the laboratory. We selected five seagrass shoots of each plot to assess seagrass morphological characteristics such as leaf length, leaf width, rhizome length, and number of leaves. Each seagrass shoot was subsequently divided into aboveground (leaf) and belowground (rhizome and root) samples. The seagrass and macroalgae samples were dried with an oven (60°C, 48 h), and weighed for dry mass per m2. The total biomass of seagrass was the sum of the above- and below-ground biomass.
Herbivory Intensity
Seagrass only plots (S) were considered to have no herbivory, and the herbivory intensities of other treatments were calculated per plot by subtracting the seagrass biomass of each treatment (S + CR, S + MH, S + LH) from the biomass of seagrass only (S) treatment, all for nutrient control treatment (natural conditions). Herbivory intensities were divided into aboveground, belowground, and total herbivory intensities as the biomass.
Statistical Analyses
A Principal Component Analysis (PCA) was performed for each site to obtain a multi-metric index (MI) for seagrass growth among treatments (Garcia-Marin et al., 2013; Bourque and Fourqurean, 2014). The PCA was used to reduce data complexity and reveal variables that explained maximum variability in the seagrass growth characteristics. Six variables were included in the PCA analysis, i.e., aboveground biomass, belowground biomass, leaf length, leaf width, rhizome length and number of leaves.
Data were subject to normality (Shapiro-Wilk test) and homogeneity of variance checks (Levene’s test) before analyses. Firstly, three-way ANOVA was used to examine the interactive effect of nutrient enrichment, herbivory and site (turbidity) on the biomass of macroalgae, above/belowground biomass and morphological characteristics of seagrass. Secondly, a two-way Analysis of Variance (ANOVA) followed by Tukey HSD post-hoc test was used to assess differences in the biomass of macroalgae, above/belowground biomass, morphological characteristics and multi-metric index of seagrass among nutrient enrichment and herbivory treatments in the two sites. One-way ANOVA was also used to compare the difference of herbivory intensity between the various herbivory treatments, in the above-, belowground and total parts, respectively. Data went through logarithm, square root or sine/cosine transformations when necessary to meet homogeneity of variance. The significance level was P < 0.05 (all variables were expressed as mean ± standard error). All statistical analyses were conducted using SPSS 20.0.
Results
Herbivory Intensity
Herbivory intensity is defined as the seagrass biomass difference between the various herbivory treatments and the seagrass only treatment without nutrient enrichment. Our results showed that the belowground herbivory intensity was consistently higher than aboveground throughout all herbivory treatments in the NYR, whereas the opposite was found in the SYR, and the total herbivory intensity was consistently higher throughout all herbivory treatments in the NYR than SYR (Figure 2). Herbivory intensity of S + CR treatment was significantly lower in aboveground, belowground and total biomass than other treatments in the NYR, while S + MH (small- and median-sized herbivore inclusion) and S + LH treatments (additional large-sized herbivore) showed no significant differences (P < 0.05), which reflected the dominance of small- and median-sized herbivores. There was no significant difference in the herbivory intensity among the various herbivory treatments in the SYR, except for the total biomass of the S + CR treatment.
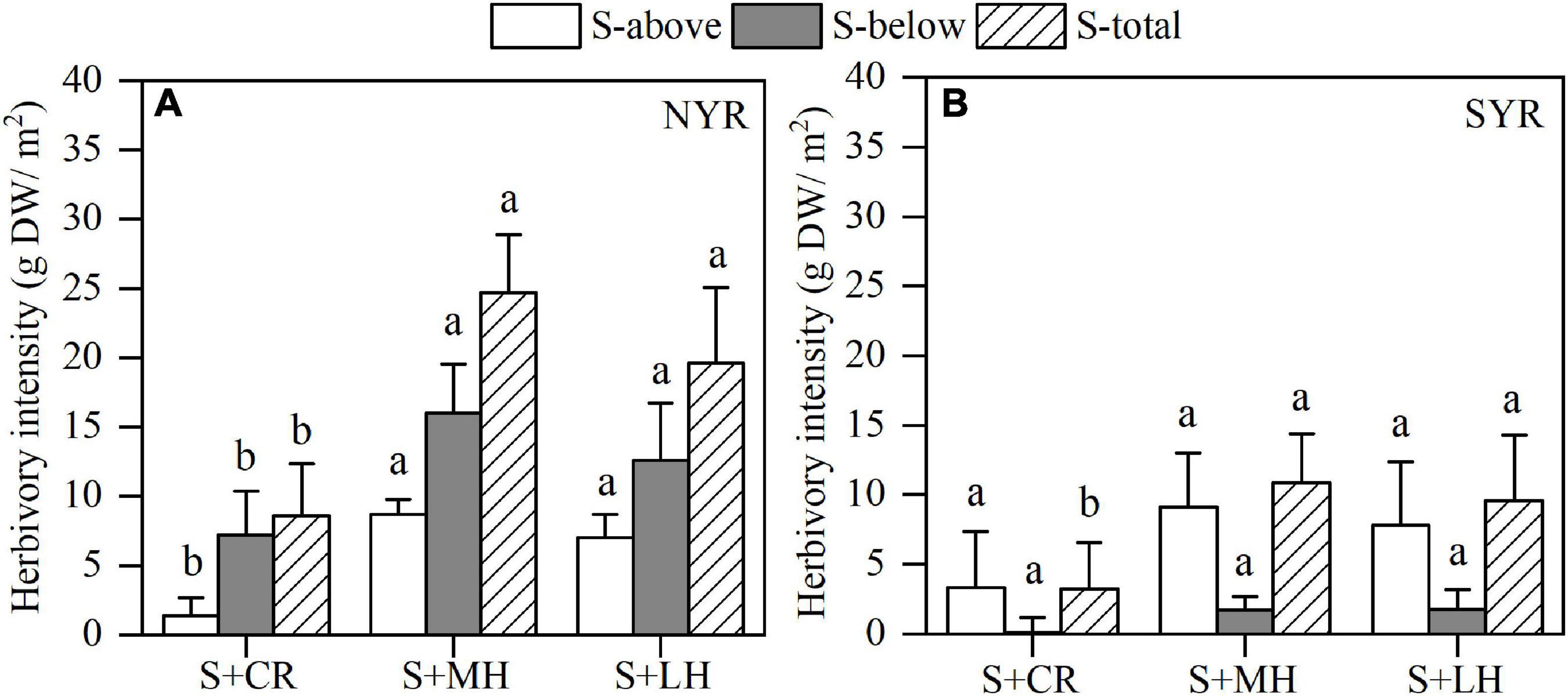
Figure 2. Herbivory intensity of aboveground (S-above), belowground (S-below), and total parts (S-total) of seagrass among different herbivory treatments in the NYR (A) and SYR (B) (mean ± SE, n = 4). Within each parts of seagrass, bars sharing a letter are not significantly different from one another (p > 0.05).
Seagrass Growth Index
In the NYR, PCA extracted two principal components that together described 60.24% of the variation in the original data, and PC1 explained 36.64% of the variation in the data set (Supplementary Table 1). In the SYR, two principal components together described 62.81% of the variation in the original data, and PC1 explained 36.81% of the variation in the dataset (Supplementary Table 2). MI of each plot was calculated by the equations shown in Table 1. Nutrient enrichment significantly affected MI (P < 0.01) in the NYR, while herbivory (P < 0.05) and nutrient enrichment × herbivory interactions (P < 0.01) had a significant effect on MI in the SYR (Supplementary Tables 4, 5).

Table 1. Equations for the multi-metric index (MI) values of each site obtained from the eigenvectors of Supplementary Tables 1, 2.
Seagrass Response to Nutrient Enrichment and Herbivory
Nutrient enrichment × Site (Turbidity) interactions were detectable on seagrass biomass (above- and belowground) and morphological characteristics (leaf width and rhizome length), while Herbivory × Site (Turbidity) interactions were only observed on number of leaves (P < 0.05; Supplementary Table 3), which suggested a rather strong interplay between turbidity and nutrient enrichment. Nutrient enrichment without herbivory decreased seagrass above- and belowground biomass in both sites (S plots; Figures 3A,C). When nutrient enrichment acted alongside herbivory treatment, however, the response of seagrass contrasted in the two sites. In the less turbid NYR, nutrient enrichment significantly increased seagrass belowground biomass (P < 0.01; Figure 3C and Supplementary Table 4). On the contrary, in the more turbid SYR, the above- and belowground biomass of seagrass did not show a significant response to nutrient enrichment (P > 0.05; Figures 3B,D and Supplementary Table 5), and the herbivory effect tended to dominate (P < 0.01; Supplementary Table 5). Positive nutrient enrichment effect on seagrass biomass manifested in the increase of rhizome length and number of leaves in the NYR (P < 0.05; Figures 4B,C and Supplementary Table 4), albeit a decline in leaf length (P < 0.05; Figure 4A and Supplementary Table 4). Similar to biomass, morphological characteristics in the SYR did not show a significant response to nutrient enrichment (P > 0.05; Supplementary Table 5), except for the reduction of rhizome length (P < 0.05; Figure 4D and Supplementary Table 5).
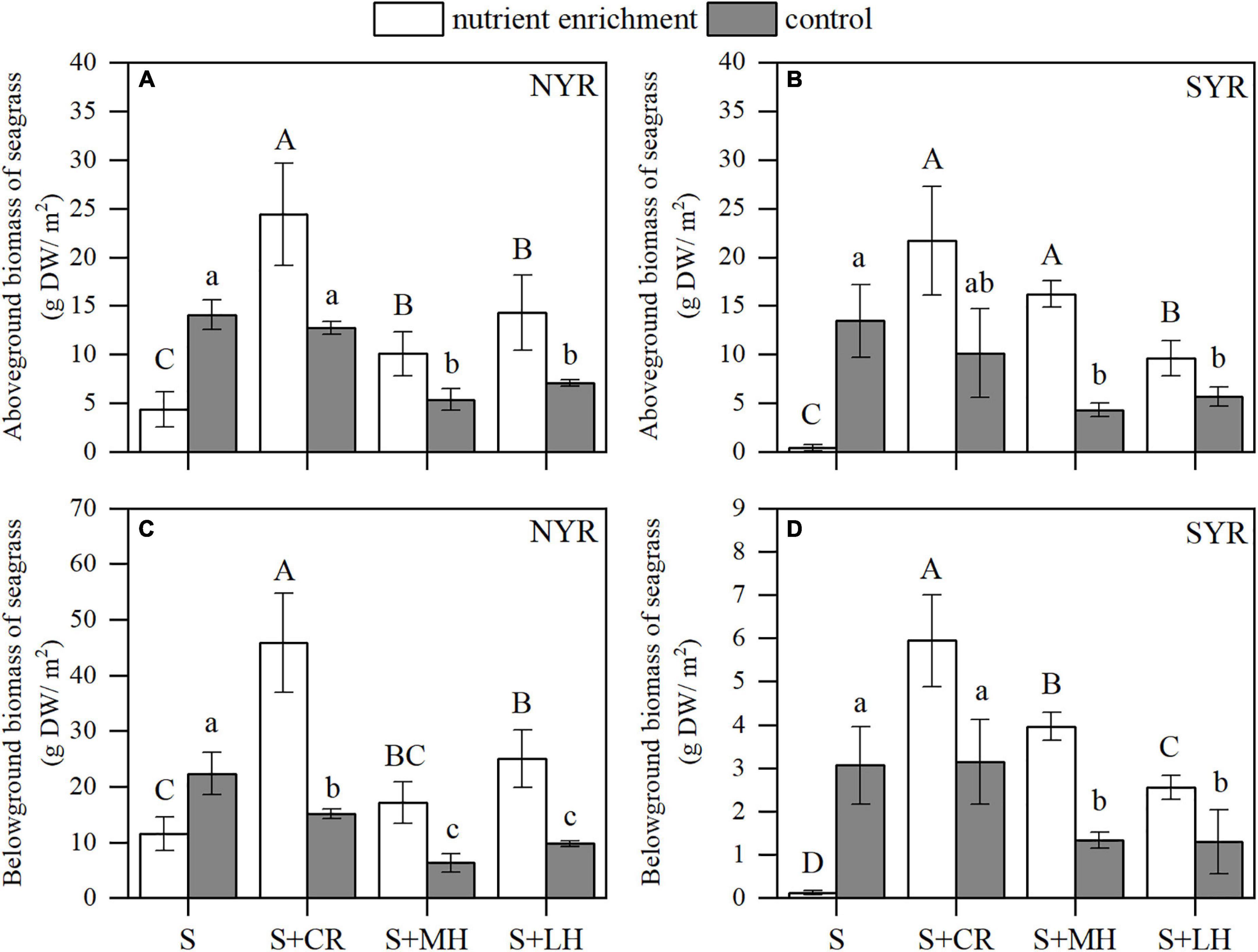
Figure 3. Effects of nutrient enrichment and herbivory on seagrass biomass in the NYR and SYR (mean ± SE, n = 4). (A) Aboveground biomass of seagrass in the NYR; (B) aboveground biomass of seagrass in the SYR; (C) belowground biomass of seagrass in the NYR; (D) belowground biomass of seagrass in the SYR. Within nutrient treatments (enrichment or control), bars sharing a letter are not significantly different from one another (p > 0.05).
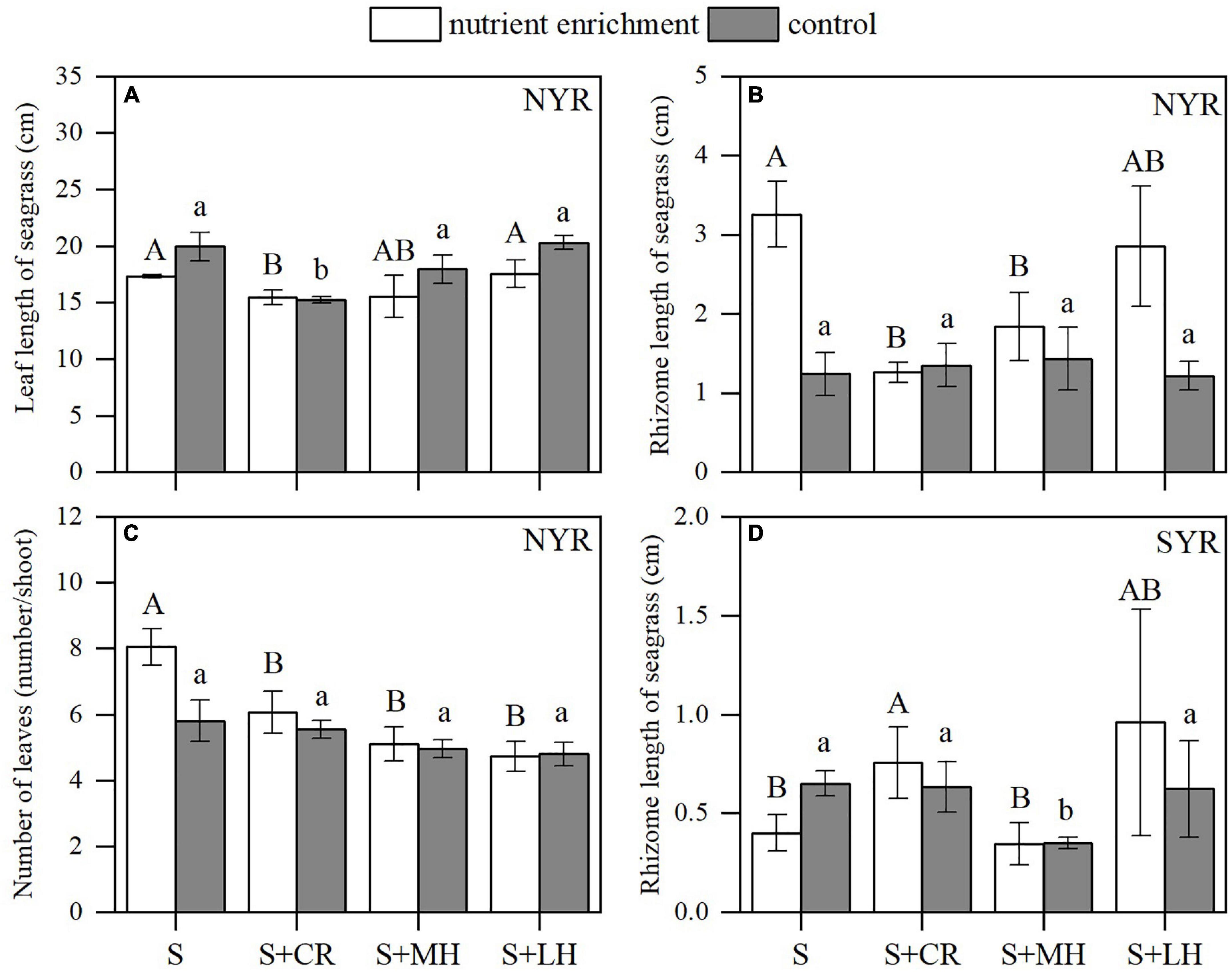
Figure 4. Effects of nutrient enrichment and herbivory on the morphological characteristics of seagrass (mean ± SE, n = 4). (A) Leaf length in the NYR; (B) rhizome length in the NYR; (C) number of leaves in the NYR; (D) rhizome length in the SYR. Within nutrient treatments (enrichment or control), bars sharing a letter are not significantly different from one another (p > 0.05).
Generally, herbivory without nutrient enrichment decreased the above- and belowground biomass of seagrass with the maximum biomass attained in the seagrass only plots (S) in both sites (Figure 3). As herbivory intensity increased, seagrass biomass declined further. Herbivory combined with nutrient enrichment resulted in the maximum biomass attained in the plots with crab inclusion (S + CR), and the minimum in the seagrass only plots (S) where the competitor macroalgae appeared to be dominant as a result of the nutrient enrichment (Figure 3). This is especially prominent in the more turbid SYR site where the seagrass almost died off under nutrient enrichment without herbivory (Figures 3B,D). The negative effect of herbivory was detectable on the leaf length (P < 0.05) and number of leaves (P < 0.01) of seagrass in the NYR (Figure 4). Herbivorous crabs had a significant contribution to the decline of leaf length and leaf numbers as shown in the minimum leaf length and leaf numbers for the crab inclusion (S + CR) plots.
Macroalgae Response to Nutrient Enrichment and Herbivory
Nutrient enrichment without herbivory increased 161.42 and 207.83% of the macroalgae biomass in the NYR and SYR, respectively (S plots; Figure 5). Herbivory × Site (Turbidity) and Nutrient enrichment × Herbivory × Site (Turbidity) interactions were detectable on macroalgae biomass (P < 0.001; Supplementary Table 3), which indicated the regulation of turbidity on herbivory effect as well as Nutrient enrichment × Herbivory interaction. Herbivory had a significant negative effect on the biomass of macroalgae in both sites regardless of nutrient treatments (P < 0.001; Supplementary Tables 4, 5). However, the effect of inclusion of different body-sized herbivores was only detectable under nutrient enrichment in more turbid SYR, which was evidence for the mediating effect of turbidity on herbivory combined with nutrient enrichment.
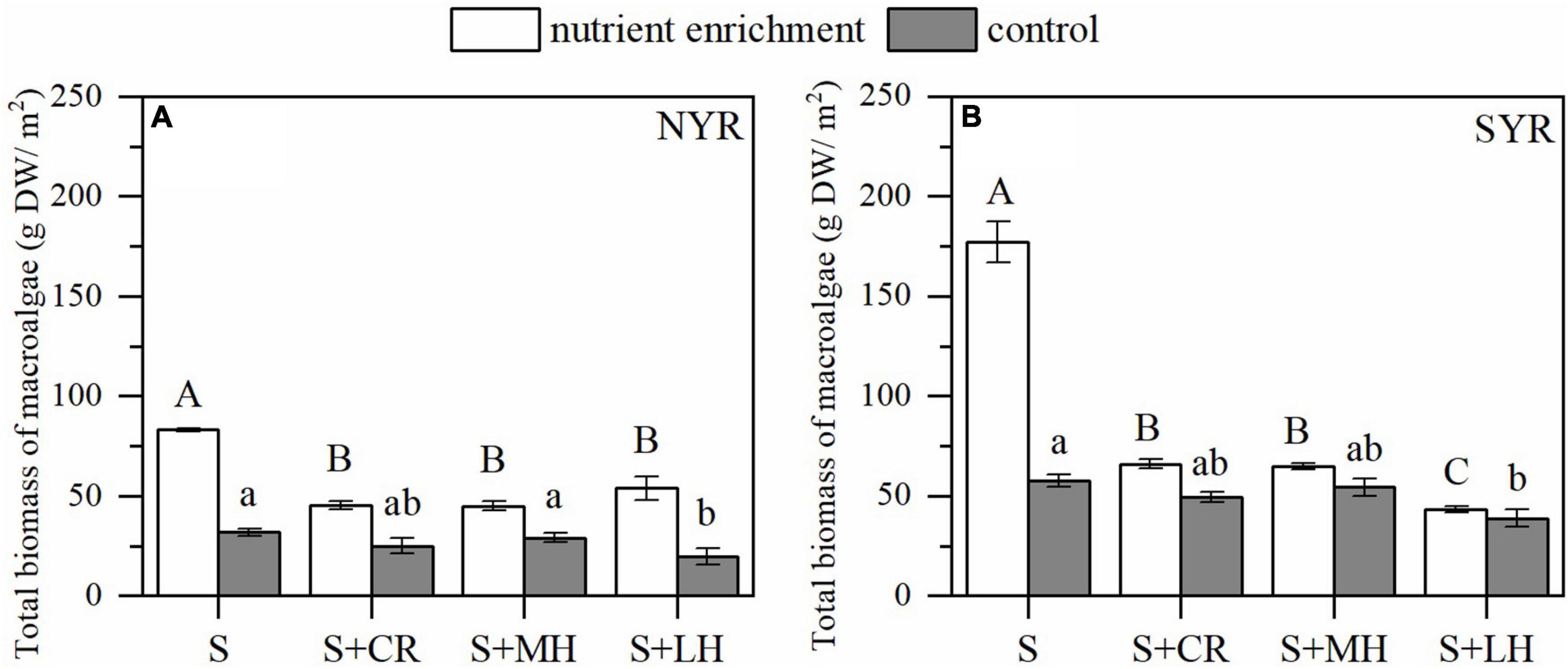
Figure 5. Effects of nutrient enrichment and herbivory on the total biomass of macroalgae in the NYR (A) and SYR (B) (mean ± SE, n = 4). Within nutrient treatments (enrichment or control), bars sharing a letter are not significantly different from one another (p > 0.05).
Discussion
In coastal ecosystems, seagrass meadows are threatened by multiple short or long-term environmental stressors. While numerous studies have explored the effects of nutrient enrichment and herbivory on seagrass ecosystem structure and functioning, there is limited understanding of how their relative importance shifts across large spatial scales where environmental factors such as turbidity can vary significantly. Our study showed that turbidity mediated the nutrient enrichment effect on seagrass biomass and morphological characteristics, as well as the herbivory effect on macroalgae as the main competitive primary producer. In the less turbid site, nutrient enrichment alone suppressed seagrass biomass but promoted macroalgae growth. At the same time, herbivory without nutrient enrichment had a significant negative effect on the biomass of seagrass and macroalgae. When nutrient enrichment acted alongside herbivory, it significantly promoted seagrass above- and belowground biomass and resulted in the increase of rhizome length and number of leaves. Yet, the effect of inclusion of different body-sized herbivores on seagrass and macroalgae was not obvious. In the more turbid site, nutrient enrichment or herbivory alone induced similar effects as in the less turbid site. Notably, under nutrient enrichment alone, excessive macroalgae significantly suppressed seagrass growth and even led to seagrass mortality. When nutrient enrichment acted alongside herbivory, seagrass biomass as well as morphological characteristics (except for rhizome length) did not exhibit significant change. The herbivory effect tended to dominate where low intensity herbivory could suppress macroalgae proliferation due to the increased herbivory pressure and selective-grazing on macroalgae. And the effect of inclusion of different body-sized herbivores on seagrass and macroalgae was detectable.
Nutrient enrichment could facilitate the growth of seagrass as reported in many previous studies in the resource-limited system (Heck et al., 2000; Yan et al., 2020). In the less turbid NYR, nutrient enrichment increased rhizome length and thus significantly increased belowground biomass, while aboveground biomass did not increase as much as the belowground parts, e.g., leaf width showed no response and leaf length even decreased. This may be attributed to the fact that seagrass preferred to absorb nitrogen and phosphorus by belowground parts from sediment pore water (Archer et al., 2018; Ruocco et al., 2018). Another factor was the higher macroalgae cover on the aboveground parts which limited nutrient and other resource availability to seagrass, thereby reducing the seagrass aboveground biomass (Han and Liu, 2014; Han et al., 2016). The similar effect of macroalgae was also observed on seagrass biomass in the SYR where both above- and belowground parts did not exhibit significant increase under nutrient enrichment. In the SYR, rhizome length also declined under nutrient enrichment, again presumably due to the nutrient-enhanced macroalgae competition (Wang et al., 2021). Previous studies have reported the fast-growing capacity of opportunistic macroalgae under nutrient enrichment (Glasby, 2012; Schmidt et al., 2012). Our study suggested nutrient enrichment promoted the proliferation of macroalgae to possess a competitive advantage against seagrass in a more turbid system. Previous studies also highlighted the negative effect of turbidity on seagrass nutrient uptake due to its high sensitivity to light reduction (Bulmer et al., 2018).
Herbivory plays a vital role in maintaining the balance between the two primary producers, especially in high turbidity systems. Nutrient enrichment without herbivory led to a dramatic decline or even death of seagrass, especially in the SYR with high turbidity where macroalgae exhibited fast-growing capacity. The presence of herbivores could counteract the negative effect of nutrient enrichment on the competition between seagrass and macroalgae. The herbivory effect on macroalgae between crab inclusion treatment and other treatments (additional small and median-sized herbivores or large-sized herbivores inclusion) did not differ significantly in the NYR, which showed that herbivorous crabs tended to be the dominant grazer of macroalgae (Figure 5). While in the SYR, other herbivores such as small-sized shrimps, Mollusca and Polychaetas as well as large herbivorous fishes were also found to preferentially feed on macroalgae under nutrient enrichment. It might be due to the long-term training for herbivores in high turbidity environments to form selective-grazing on nutrient-enriched food, which has also been recognized in other seagrass systems (Klumpp et al., 1993; Hays, 2005). Nutrient-induced herbivory of macroalgae was also evidenced by the overall reduced herbivory intensity on seagrass in the SYR than NYR (Figure 1).
Overall, the relative importance of nutrient enrichment and herbivory was shifted under the mediating effects of turbidity. Herbivory played a vital role in maintaining the balance between the two primary producers in high turbidity systems. While in a less turbid system, nutrient enrichment tended to offset the herbivory-induced biomass loss by promoting seagrass growth. The combined effect of nutrient enrichment and herbivory on seagrass and macroalgae in high and low turbidity systems are summarized in the schematic diagram shown in Figure 6. The responses of seagrasses and macroalgae to nutrient enrichment and herbivory varying under different turbidity suggested two potential mechanisms. Firstly, turbidity could mediate the grazing pressure and preference of herbivores. In a high turbidity environment, the majority of the different body-sized herbivores preferred to utilize the fast-growing and nutrient-enriched plant such as macroalgae, whereas herbivores tended to feed on seagrass in less turbid environments. Our results showed that herbivory had a significant effect on the multi-metric index of seagrass (Supplementary Table 5). And the significant effects of herbivory-site (turbidity) and herbivory-nutrient enrichment-site (turbidity) interactions on macroalgae biomass could also be evidence for this potential mechanism (Supplementary Table 3). The positive effect of turbidity to promote selective-grazing on macroalgae has been reported in previous studies conducted in seagrass meadow or other aquatic systems such as rivers (Ruiz and Romero, 2003; Savic et al., 2018). The positive effect of turbidity on herbivory could be attributed to the cascading effect of predators on herbivore, i.e., the reduced efficiency of predators caused by suspended sediment in turbid rivers (Savic et al., 2018). The selective-grazing of herbivores on algae in turbid waters could arise from the fast-growing ability as well as the distribution of algae covering the aboveground parts of seagrass (Ruiz and Romero, 2003; Savic et al., 2018). Therefore, the presence of herbivores is essential for the maintenance of ecosystem health in high turbidity sites.
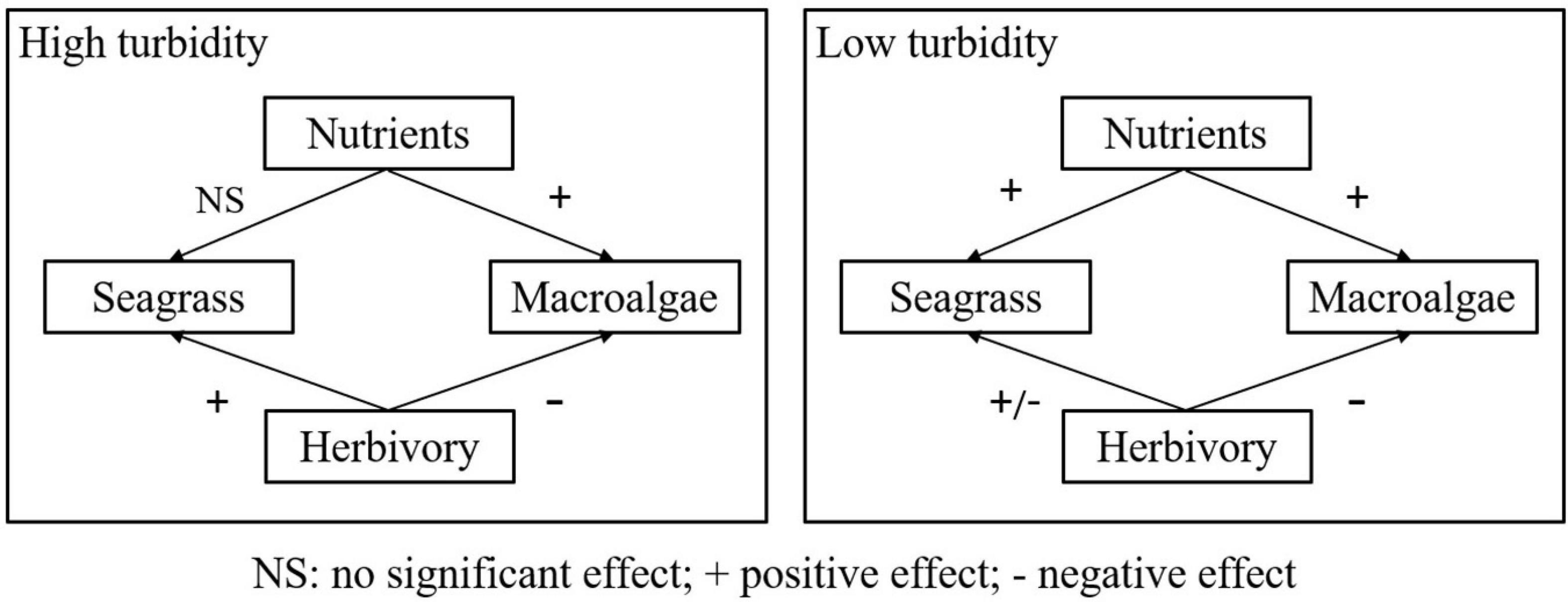
Figure 6. A schematic diagram summarizing the combined effect of nutrient enrichment and herbivory on seagrass and macroalgae in high and low turbidity systems.
The other potential mechanism was that nutrient enrichment favored the growth of opportunistic macroalgae over seagrass in a high turbidity system. Under nutrient enrichment alone, macroalgae suppressed seagrass and caused die off in the more turbid SYR, and yet the biomass of seagrass did not show a significant response to nutrient enrichment when combined with herbivory. On the contrary, in the less turbidity NYR, nutrients showed a significant effect on the biomass as well as the multi-metric index of seagrass (Supplementary Table 4). This potential mechanism was also evident in the effects of nutrient enrichment-site (turbidity) interactions on seagrass above- and belowground biomass (Supplementary Table 3). Previous studies found that turbidity had significant negative effect on seagrass nutrient uptake due to its high sensitivity to light reduction (Bulmer et al., 2018). Meanwhile, the contribution of algae loads to light reduction on seagrass was also found to be critical in turbid waters (Ow et al., 2020). Thus, macroalgae has the superiority in competing for nutrients against seagrass in turbid system. It suggested that to some extent, nutrient enrichment had a positive effect on seagrass meadows under natural herbivory conditions in a less turbid environment, while in the more turbid system, it mainly caused negative effect and needs to be closely monitored.
This article provides field evidence for the mediating forces of turbidity on the combined effect of nutrient enrichment and herbivory. Herbivory played a vital role in maintaining the balance between seagrass and macroalgae in high turbidity systems. However, the importance of herbivores may have been underestimated in the context of coastal eutrophication management, especially in high turbidity systems where small increases in nutrients could have tremendous effects on the growth of seagrass. In a less turbid system, nutrient enrichment had a positive effect on seagrass meadows under natural herbivory conditions. Our study emphasizes the mediating force of turbidity on seagrass ecosystems, and provides references for the regulation of nutrient supply and herbivore exclusion in systems with varying turbidity. Many estuarine seagrass ecosystems face increasing hydrological alterations caused by human activities and climate change, which further alters sediment dynamics including sedimentation, resuspension, etc., and induces water turbidity and bed level changes (Palinkas and Koch, 2012; João et al., 2013). Feedback between seagrass meadows and sediment dynamics has been recognized as being vital to seagrass restoration (Suykerbuyk et al., 2016; Zhu et al., 2021), further studies are thus needed to investigate the influence of sediment dynamics on the distribution pattern and ecogeomorphological processes of seagrass meadows.
Data Availability Statement
The raw data supporting the conclusions of this article will be made available by the authors, without undue reservation.
Author Contributions
JB, JY, and XW: conceptualization. XW: data analysis and writing—original draft preparation. DS and BC: writing—review and editing. DS: supervision. All authors contributed to the article and approved the submitted version.
Funding
This research was funded by the National Key R&D Program of China (2019YFE0121500), the Key Project of National Natural Science Foundation of China (51639001 and U1901212), the Joint Funds of the National Natural Science Foundation of China (U2006215), and the National Natural Science Foundation of China (51909005).
Conflict of Interest
The authors declare that the research was conducted in the absence of any commercial or financial relationships that could be construed as a potential conflict of interest.
Publisher’s Note
All claims expressed in this article are solely those of the authors and do not necessarily represent those of their affiliated organizations, or those of the publisher, the editors and the reviewers. Any product that may be evaluated in this article, or claim that may be made by its manufacturer, is not guaranteed or endorsed by the publisher.
Acknowledgments
The staffs of the Yellow River Estuary Management Station, Yellow River Delta National Nature Reserve Administration Bureau, are also gratefully acknowledged for their assistance in this study.
Supplementary Material
The Supplementary Material for this article can be found online at: https://www.frontiersin.org/articles/10.3389/fmars.2022.787041/full#supplementary-material
References
Albert, S., Saunders, M. I., Roelfsema, C. M., Leon, J. X., Johnstone, E., Mackenzie, J. R., et al. (2017). Winners and losers as mangrove, coral and seagrass ecosystems respond to sea-level rise in Solomon Islands. Environ. Res. Lett. 12:094009.
Archer, S. K., Hensel, E., and Layman, C. A. (2018). Ambient nutrient availability drives the outcome of an interaction between a sponge (Halichondria melanadocia) and seagrass (Thalassia testudinum). J. Exp. Mar. Biol. Ecol. 503, 86–91. doi: 10.1016/j.jembe.2018.02.005
Baden, S., Boström, C., Tobiasson, S., Arponen, H., and Moksnes, P.-O. (2010). Relative importance of trophic interactions and nutrient enrichment in seagrass ecosystems: a broad-scale field experiment in the Baltic-Skagerrak area. Limnol. Oceanogr. 55, 1435–1448. doi: 10.4319/lo.2010.55.3.1435
Bertram, C., Quaas, M., Reusch, T. B. H., Vafeidis, A. T., Wolff, C., and Rickels, W. (2021). The blue carbon wealth of nations. Nat. Clim. Change 11:886. doi: 10.1038/s41558-021-01089-4
Bourque, A. S., and Fourqurean, J. W. (2014). Effects of common seagrass restoration methods on ecosystem structure in subtropical seagrass meadows. Mar. Environ. Res. 97, 67–78. doi: 10.1016/j.marenvres.2014.03.001
Bulmer, R. H., Townsend, M., Drylie, T., and Lohrer, A. M. (2018). Elevated Turbidity and the Nutrient Removal Capacity of Seagrass. Front. Mar. Sci. 5:462. doi: 10.3389/fmars.2018.00462
Burkepile, D. E., and Hay, M. E. (2006). Herbivore VS Nutrient Control of Marine Primary Producers: context-Dependent Effects. Ecology 87, 3128–3139. doi: 10.1890/0012-9658(2006)87[3128:hvncom]2.0.co;2
Campbell, J. E., Altieri, A. H., Johnston, L. N., Kuempel, C. D., Paperno, R., Paul, V. J., et al. (2018). Herbivore community determines the magnitude and mechanism of nutrient effects on subtropical and tropical seagrasses. J. Ecol. 106, 401–412. doi: 10.1111/1365-2745.12862
Carr, L. A., and Boyer, K. E. (2014). Variation at multiple trophic levels mediates a novel seagrass-grazer interaction. Mar. Ecol. Prog. Ser. 508, 117–128. doi: 10.3354/meps10855
Darnell, K. M., and Dunton, K. H. (2017). Plasticity in turtle grass (Thalassia testudinum) flower production as a response to porewater nitrogen availability. Aquat. Bot. 138, 100–106. doi: 10.1016/j.aquabot.2017.01.007
Du, J., Hu, W., Nagelkerken, I., Sangsawang, L., Loh, K. H., Ooi, J. L.-S., et al. (2020). Seagrass meadows provide multiple benefits to adjacent coral reefs through various microhabitat functions. Ecosyst. Health. Sustainabilit. 6:1812433. doi: 10.1080/20964129.2020.1812433
Duffy, J. E., Reynolds, P. L., Boström, C., Coyer, J. A., Cusson, M., Donadi, S., et al. (2015). Biodiversity mediates top–down control in eelgrass ecosystems: a global comparative-experimental approach. Ecol. Lett. 18, 696–705. doi: 10.1111/ele.12448
Ebrahim, A., Olds, A. D., Maxwell, P. S., Pitt, K. A., Burfeind, D. D., and Connolly, R. M. (2014). Herbivory in a subtropical seagrass ecosystem: separating the functional role of different grazers. Mar. Ecol. Prog. Ser. 511, 83–91. doi: 10.3354/meps10901
Fourqurean, J. W., Manuel, S., Coates, K. A., Kenworthy, W. J., and Smith, S. R. (2010). Effects of excluding sea turtle herbivores from a seagrass bed: overgrazing may have led to loss of seagrass meadows in Bermuda. Mar. Ecol. Prog. Ser. 419, 223–232. doi: 10.3354/meps08853
Fourqurean, J. W., Manuel, S. A., Coates, K. A., Massey, S. C., and Kenworthy, W. J. (2019). Decadal Monitoring in Bermuda Shows a Widespread Loss of Seagrasses Attributable to Overgrazing by the Green Sea Turtle Chelonia mydas. Estuar. Coast. 42, 1524–1540. doi: 10.1007/s12237-019-00587-1
Garcia-Marin, P., Cabaco, S., Hernandez, I., Vergara, J. J., Silva, J., and Santos, R. (2013). Multi-metric index based on the seagrass Zostera noltii (ZoNI) for ecological quality assessment of coastal and estuarine systems in SW Iberian Peninsula. Mar. Pollut. Bull. 68, 46–54. doi: 10.1016/j.marpolbul.2012.12.025
Garrido, M., Lafabrie, C., Torre, F., Fernandez, C., and Pasqualini, V. (2013). Resilience and stability of Cymodocea nodosa seagrass meadows over the last four decades in a Mediterranean lagoon. Estuar. Coast. Shelf Sci. 130, 89–98. doi: 10.1016/j.ecss.2013.05.035
Glasby, T. M. (2012). Caulerpa taxifolia in seagrass meadows: killer or opportunistic weed? Biol. Invasions. 15, 1017–1035. doi: 10.1007/s10530-012-0347-1
Han, Q., and Liu, D. (2014). Macroalgae blooms and their effects on seagrass ecosystems. J. Ocean. U. China. 13, 791–798. doi: 10.1007/s11802-014-2471-2
Han, Q., Soissons, L. M., Bouma, T. J., van Katwijk, M. M., and Liu, D. (2016). Combined nutrient and macroalgae loads lead to response in seagrass indicator properties. Mar. Pollut. Bull. 106, 174–182. doi: 10.1016/j.marpolbul.2016.03.004
Hays, C. G. (2005). Effect of nutrient availability, grazer assemblage and seagrass source population on the interaction between Thalassia testudinum (turtle grass) and its algal epiphytes. J. Exp. Mar. Biol. Ecol. 314, 53–68. doi: 10.1016/j.jembe.2004.08.017
Heck, K. L. Jr., Pennock, J. R., Valentine, J. F., Coen, L. D., and Sklenar, S. A. (2000). Effects of nutrient enrichment and small predator density on seagrass ecosystems: an experimental assessment. Limnol. Oceanogr. 45, 1041–1057.
Hughes, A. R., Bando, K. J., Laura, F. R., and Susan, L. W. (2004). Relative effects of grazers and nutrients on seagrasses: a meta-analysis approach. Mar. Ecol. Prog. Ser. 282, 87–99.
João, D. L. E. S., Ana, A., Ana Isabel, L., and João Miguel, D. (2013). Turbidity under changing physical forcing over two contrasting locations of seagrass meadows. J. Coastal Res. 65, 2023–2028. doi: 10.2112/SI65-342.1
Klumpp, D. W., Salita-Espinosa, J. T., and Fortes, M. D. (1993). Feeding ecology and trophic role of sea urchins in a tropical seagrass community. Aquat. Bot. 45, 205–229. doi: 10.1016/0304-3770(93)90022-O
Leoni, V., Vela, A., Pasqualini, V., Pergent-Martini, C., and Pergent, G. (2008). Effects of experimental reduction of light and nutrient enrichments (N and P) on seagrasses: a review. Aquat. Conserv. Mar. Freshwat. Ecosyst. 18, 202–220. doi: 10.1002/aqc.842
Leston, S., Lillebø, A., and Pardal, M. (2008). The response of primary producer assemblages to mitigation measures to reduce eutrophication in a temperate estuary. Estuar. Coast. Shelf. S. 77, 688–696. doi: 10.1016/j.ecss.2007.11.002
Li, C., Zhang, Y.-H., Wu, X.-X., Jiang, Y.-S., Li, W.-T., and Zhang, P.-D. (2021). Changes in survival and growth in response to different combinations of turbidity and duration in eelgrass Zostera marina plants. Estuar. Coast. Shelf. S. 249, 107108. doi: 10.1016/j.ecss.2020.107108
Listiawati, V., and Kurihara, H. (2021). Ocean warming and acidification modify top-down and bottom-up control in a tropical seagrass ecosystem. Sci. Rep. 11:13605. doi: 10.1038/s41598-021-92989-0
Moran, K. L., and Bjorndal, K. A. (2005). Simulated green turtle grazing affects structure and productivity of seagrass pastures. Mar. Ecol. Prog. Ser. 305, 235–247. doi: 10.3354/meps305235
Moran, K. L., and Bjorndal, K. A. (2007). Simulated green turtle grazing affects nutrient composition of the seagrass Thalassia testudinum. Mar. Biol. 150, 1083–1092. doi: 10.1007/s00227-006-0427-9
Mutchler, T., and Hoffman, D. K. (2017). Response of seagrass (Thalassia testudinum) metrics to short-term nutrient enrichment and grazing manipulations. J. Exp. Mar. Biol. Ecol. 486, 105–113. doi: 10.1016/j.jembe.2016.09.015
Mvungi, E. F., and Pillay, D. (2019). Eutrophication overrides warming as a stressor for a temperate African seagrass (Zostera capensis). PLoS One 14:e0215129. doi: 10.1371/journal.pone.0215129
Ontoria, Y., Gonzalez-Guedes, E., Sanmartí, N., Bernardeau-Esteller, J., Ruiz, J. M., Romero, J., et al. (2019). Interactive effects of global warming and eutrophication on a fast-growing Mediterranean seagrass. Mar. Environ. Res. 145, 27–38. doi: 10.1016/j.marenvres.2019.02.002
Orth, R. J., Lefcheck, J. S., McGlathery, K. S., Aoki, L., Luckenbach, M. W., Moore, K. A., et al. (2020). Restoration of seagrass habitat leads to rapid recovery of coastal ecosystem services. Sci. Adv. 6:eabc6434. doi: 10.1126/sciadv.abc6434
Östman, Ö, Eklöf, J., Eriksson, B. K., Olsson, J., Moksnes, P. O., Bergström, U., et al. (2016). Top-down control as important as nutrient enrichment for eutrophication effects in North Atlantic coastal ecosystems. J. Appl. Ecol. 53, 1138–1147. doi: 10.1111/1365-2664.12654
Ow, Y. X., Ng, K. J., Lai, S., Yaakub, S. M., and Todd, P. (2020). Contribution of epiphyte load to light attenuation on seagrass leaves is small but critical in turbid waters. Mar Freshwater Res. 71, 929–934. doi: 10.1071/MF19178
Palinkas, C. M., and Koch, E. W. (2012). Sediment accumulation rates and submersed aquatic vegetation (SAV) distributions in the mesohaline Chesapeake Bay, USA. Estuar Coast. 35, 1416–1431. doi: 10.1007/s12237-012-9542-7
Repolho, T., Duarte, B., Dionísio, G., Paula, J. R., Lopes, A. R., Rosa, I. C., et al. (2017). Seagrass ecophysiological performance under ocean warming and acidification. Sci. Rep. 7:41443. doi: 10.1038/srep41443
Ruiz, J. M., and Romero, J. (2003). Effects of disturbances caused by coastal constructions on spatial structure, growth dynamics and photosynthesis of the seagrass Posidonia oceanica. Mar. Pollut. Bull. 46, 1523–1533. doi: 10.1016/j.marpolbul.2003.08.021
Ruocco, M., Marin-Guirao, L., Ravaglioli, C., Bulleri, F., and Procaccini, G. (2018). Molecular level responses to chronic versus pulse nutrient loading in the seagrass Posidonia oceanica undergoing herbivore pressure. Oecologia 188, 23–39. doi: 10.1007/s00442-018-4172-9
Savic, A., Pesic, V., Dordevic, M., Randelovic, V., Juskovic, M., and Andrzej, G. (2018). Effects of nutrients and turbidity on grazer-periphyton interactions: a case study from the Nisava River, Balkan Peninsula. North-West. J. Zool. 14, 226–231.
Schallenberg, M., and Burns, C. W. (2004). Effects of sediment resuspension on phytoplankton production: teasing apart the influences of light, nutrients and algal entrainment. Freshw. Biol. 49, 143–159. doi: 10.1046/j.1365-2426.2003.01172.x
Schmidt, A. L., Wysmyk, J. K., Craig, S. E., and Lotze, H. K. (2012). Regional-scale effects of eutrophication on ecosystem structure and services of seagrass beds. Limnol. Oceanogr. 57, 1389–1402. doi: 10.4319/lo.2012.57.5.1389
Scott, A. L., York, P. H., and Rasheed, M. A. (2021). Herbivory Has a Major Influence on Structure and Condition of a Great Barrier Reef Subtropical Seagrass Meadow. Estuar. Coast. 44, 506–521. doi: 10.1007/s12237-020-00868-0
Stockbridge, J., Jones, A. R., and Gillanders, B. M. (2020). A meta-analysis of multiple stressors on seagrasses in the context of marine spatial cumulative impacts assessment. Sci. Rep. 10, 1–11. doi: 10.1038/s41598-020-68801-w
Suykerbuyk, W., Bouma, T. J., Govers, L. L., Giesen, K., de Jong, D. J., Herman, P., et al. (2016). Surviving in changing seascapes: sediment dynamics as bottleneck for long-term seagrass presence. Ecosystems 19, 296–310.
Viana, I. G., Moreira-Saporiti, A., and Teichberg, M. (2020). Species-Specific Trait Responses of Three Tropical Seagrasses to Multiple Stressors: the Case of Increasing Temperature and Nutrient Enrichment. Front. Plant Sci. 11:571363. doi: 10.3389/fpls.2020.571363
Vieira, R., Martin, A., Engelen, A. H., Thomsen, M. S., and Arenas, F. (2020). Interactive effects of co-occurring anthropogenic stressors on the seagrass, Zostera noltei. Ecol. Indic. 109:105780. doi: 10.1016/j.ecolind.2019.105780
Wang, H., Wu, X., Bi, N., Li, S., Yuan, P., Wang, A., et al. (2017). Impacts of the dam-orientated water-sediment regulation scheme on the lower reaches and delta of the Yellow River (Huanghe): a review. Global. Planet. Change 157, 93–113. doi: 10.1016/j.gloplacha.2017.08.005
Wang, L., Tomas, F., and Mueller, R. S. (2020). Nutrient enrichment increases size of Zostera marina shoots and enriches for sulfur and nitrogen cycling bacteria in root-associated microbiomes. FEMS. Microbiol. Ecol. 96:fiaa129. doi: 10.1093/femsec/fiaa129
Wang, S., Fu, B., Piao, S., Lü, Y., Ciais, P., Feng, X., et al. (2015). Reduced sediment transport in the Yellow River due to anthropogenic changes. Nat. Geosci. 9, 38–41. doi: 10.1038/ngeo2602
Wang, X., Yan, J., Bai, J., Shao, D., and Cui, B. (2021). Effects of interactions between macroalgae and seagrass on the distribution of macrobenthic invertebrate communities at the Yellow River Estuary, China. Mar. Pollut. Bull. 164:112057. doi: 10.1016/j.marpolbul.2021.112057
Waycott, M., Duarte, C. M., Carruthers, T. J., Orth, R. J., Dennison, W. C., Olyarnik, S., et al. (2009). Accelerating loss of seagrasses across the globe threatens coastal ecosystems. Proc. Natl. Acad. Sci. 106, 12377–12381. doi: 10.1073/pnas.0905620106
Whalen, M. A., Duffy, J. E., and Grace, J. B. (2013). Temporal shifts in top-down vs. bottom-up control of epiphytic algae in a seagrass ecosystem. Ecology 94, 510–520. doi: 10.1890/12-0156.1
Yamamoto, T., Malingin, M. A. C. L., Marivic Pepino, M., Yoshikai, M., Campos, W., Miyajima, T., et al. (2019). Assessment of coastal turbidity improvement potential by terrigenous sediment load reduction and its implications on seagrass inhabitable area in Banate Bay, central Philippines. Sci. Total. Environ. 656, 1386–1400. doi: 10.1016/j.scitotenv.2018.11.243
Yan, J., Heide, T., Cui, B., Bai, J., Ysebaert, T., and Koppel, J. (2020). A healthy trophic structure underlies the resistance of pristine seagrass beds to nutrient enrichment. Limnol. Oceanogr. 65, 2748–2756. doi: 10.1002/lno.11545
York, P. H., Kelaher, B. P., Booth, D. J., and Bishop, M. J. (2012). Trophic responses to nutrient enrichment in a temperate seagrass food chain. Mar. Ecol. Prog. Ser. 449, 291–296. doi: 10.3354/meps09541
Yu, X., Xie, X., and Meng, S. (2017). Modeling the Responses of Water and Sediment Discharge to Climate Change in the Upper Yellow River Basin, China. J. Hydrol. Eng. 22:05017026. doi: 10.1061/(ASCE)HE.1943-5584.0001590
Keywords: seagrass meadow, turbidity, the Yellow River Estuary, nutrient enrichment, herbivory
Citation: Wang X, Bai J, Yan J, Cui B and Shao D (2022) How Turbidity Mediates the Combined Effects of Nutrient Enrichment and Herbivory on Seagrass Ecosystems. Front. Mar. Sci. 9:787041. doi: 10.3389/fmars.2022.787041
Received: 30 September 2021; Accepted: 24 January 2022;
Published: 10 February 2022.
Edited by:
Zeng Zhou, Hohai University, ChinaReviewed by:
Quan-Xing Liu, East China Normal University, ChinaWenWen Liu, Xiamen University, China
Copyright © 2022 Wang, Bai, Yan, Cui and Shao. This is an open-access article distributed under the terms of the Creative Commons Attribution License (CC BY). The use, distribution or reproduction in other forums is permitted, provided the original author(s) and the copyright owner(s) are credited and that the original publication in this journal is cited, in accordance with accepted academic practice. No use, distribution or reproduction is permitted which does not comply with these terms.
*Correspondence: Dongdong Shao, ZGRzaGFvQGJudS5lZHUuY24=