- 1Department of Invertebrate Zoology and Hydrobiology, Faculty of Biology and Environmental Protection, University of Łódź, Łódź, Poland
- 2Center for Development Cooperation in Fisheries, Institute of Marine Research, Bergen, Norway
The benthic ecology of the Gulf of Guinea is critically understudied, and knowledge about the composition and biodiversity of the tanaidacean communities remains lacking. Our analysis of tanaidacean collection from 270 quantitative samples collected using 0.1 m2 van Veen grab along the Ghanaian coast (nine transects, six stations at each transect, 25–1,000 m depth range) reveals a high species richness and very low abundance. The mean density of Tanaidacea across all the samples equals only 0.03 ± 0.55 ind./0.1 m2. A total of 87 tanaidacean species were recorded, of which only three were known for science (3.4%), emphasizing the need for intensified taxonomic effort in this region. Circa 40% of the species were singletons, 98% of the species had a total abundance lower than 10 individuals, and approximately half of the species were found only in one sample. The highest species richness was recorded in the slope (500–1,000 m), despite the elevated levels of barium and hydrocarbons at those depths. This area was also characterized by the most unique species composition. Species accumulation curves did not reach an asymptote, suggesting an undersampling of the area and a great rarity of the species. Results of the canonical correspondence analysis (CCA) and cluster analysis demonstrated a positive influence of oxygen concentration and fluorescence, particularly in the shallow shelf sites (25–50 m), which were characterized by a higher abundance of Tanaidacea. Depth zonation of tanaidacean communities with a division between shallow-water taxa (Leptocheliidae Lang, 1973 and Kalliapseudidae Lang, 1956) through families with wide bathymetric range (Pseudotanaidae Sieg, 1976, Apseudidae Leach, 1814) to the true deep-sea forms (Paranarthrurellidae Błażewicz, Jóźwiak and Frutos, 2019) is also evident. We further discuss the problems associated with multivariate analysis of highly speciose but less abundant taxa.
Introduction
The importance of the rare species in the functioning of marine benthic ecosystems and their ecological significance is the topic of renewed debate (Lyons et al., 2005; Ellingsen et al., 2007; Włodarska-Kowalczuk et al., 2012; Mouillot et al., 2013; Leroy et al., 2017; Chapman et al., 2018; Säterberg et al., 2019). The role of species with restricted ranges and/or a very small population size has often been neglected in the terrestrial and marine studies alike, while greater attention is directed to dominant species or the so-called foundation taxa (Grime, 1998; Ellison et al., 2005; Angelini et al., 2011). However, recent analysis has demonstrated that even a small size and a less abundant species may be very important for the stability of the whole ecosystem. Such species that may increase functional redundancy are an important element of trophic webs and might be crucial for ecosystem services (Ellingsen et al., 2007; Dee et al., 2019; Säterberg et al., 2019). Moreover, dominant species may only provide a short-term resistance to ecosystem changes, whereas the loss of rare species may lead to the erosion of an ecosystem functioning over longer time periods (Smith and Knapp, 2003). Leitão et al. (2016) showed that rare species disproportionately contribute to the functional structure of species assemblages. The majority of these hypotheses have as yet only been tested for specific ecosystems and for a small number of taxonomic groups. Therefore, it remains difficult to draw broader conclusions that can be applied to various terrestrial, freshwater, and marine ecosystems across spatial and temporal scales.
Studies of potentially rare and ecologically important taxa are crucial for future conservation planning and studies of ecosystem response to changes (Costello and Chaudary, 2017). Rare taxa are often difficult to study due to undersampling and the lack of taxonomic experts (Guisan et al., 2006; Jóźwiak et al., 2020). Results of the analysis on the role of rare species are often difficult to interpret and could be biased due to their extremely low abundance. Therefore, the application of modern modeling techniques and attempts to link the data on species distribution and abundance with environmental variables often does not allow for straightforward conclusions (Reiss et al., 2015).
Rare species are also considered more vulnerable to both natural and anthropogenic changes associated with climate warming, pollution events, or dynamics in natural geochemical processes (Leitão et al., 2016; Obst et al., 2018). The loss of rare species might cause a significant decline in the abundance of other trophic levels (Bracken and Low, 2012). This disparity between their potential importance and difficulties in data analysis may preclude meaningful assessments of their functional ecology.
Tanaidacean diversity is significantly understudied, with the estimated number of species an order of magnitude higher than the currently described forms (Appeltans et al., 2012). Our knowledge of this group of crustaceans is centered in taxonomy (Błażewicz-Paszkowycz et al., 2012). Few deep-sea biodiversity assessments of tanaidacean communities exist, but all demonstrate a large number of singletons and a high percentage of species new to science (Pabis et al., 2014, 2015; Błażewicz-Paszkowycz et al., 2015; Stępień et al., 2018, 2019; Błażewicz et al., 2019a; Jóźwiak et al., 2020). Even fewer studies were based on quantitative samples (Błażewicz-Paszkowycz et al., 2015; Błażewicz et al., 2019a; Jóźwiak et al., 2020), though general information like the total number of species or the total number of individuals can be also found in less targeted studies of deep-sea macrofauna (e.g., Grassle and Maciolek, 1992; Cosson et al., 1997; McCallum et al., 2015; Wilson, 2017). It is therefore possible that many rare tanaidaceans may become extinct even before they are described (Costello et al., 2013) and their functional ecology deduced.
The collective knowledge of the biology of particular tanaidacean species remains in its infancy. A majority of the species are suggested to be free-living epibenthic crustaceans feeding on detritus, though some taxa construct sediment tubes for protection (Hassack and Holdich, 1987; Błażewicz-Paszkowycz and Ligowski, 2002; Larsen, 2005). However, a variety of life strategies have been recorded within Tanaidacea. For example, representatives of Metapseudidae Lang, 1970 and Tanzanapseudidae Băcescu, 1975 inhabit coral reefs or hydroid colonies (Błażewicz-Paszkowycz et al., 2012; Jóźwiak and Błażewicz, 2021), whereas some Kalliapseudidae are associated with seagrasses (Leite, 1995). Some tanaidaceans are also trophically specialized, for example, Kalliapseudinae species are suspension-feeders, filtering food particles from the water column (Drumm and Heard, 2011), where Exspina typica is considered a parasite that digs cavities in the bodies of holothurians (Alvaro et al., 2011). Locally, tanaidacean densities as high as 53,000 ind./m2 were recorded (Dayton and Oliver, 1977), and their abundance might be a significant element of the whole community (Sokolova, 1972; McCallum et al., 2015; Golovan et al., 2019). Combined with their role as the prey of various benthic invertebrates such as polychaetes (Oliver and Slattery, 1985), decapods (Balasubramanian et al., 1979), isopods (Kneib, 1985), amphipods (Guţu and Sieg, 1999), as well as numerous species of fishes (Larsen, 2005) and even wading birds (Băcescu and Guţu, 1975), tanaidaceans may represent a group of high ecological importance.
Studies of benthic diversity of the Gulf of Guinea and the whole West African coast are still scarce and mostly focus on shallow water sites (e.g., Buchanan, 1957; Longhurst, 1958, 1959; Bassindale, 1961; Le Loeuff and Intès, 1999; Pabis et al., 2020). Recent analysis has revealed exceptionally high species richness of some benthic invertebrates, like cumaceans (Stȩpień et al., 2021) and polychaetes (Sobczyk et al., 2021). Just 10 species of Tanaidacea have previously been described in the Gulf of Guinea, whereas the number of species known from the whole west coast of Africa totals 80 (Jóźwiak et al., 2017). The Gulf of Guinea is a large marine ecosystem influenced by multiple natural factors, such as ocean currents (including Guinea Current, Benguela Current, and South Equatorial Counter Current), upwelling events, and nutrient input from land drainage. This basin is also characterized by a high diversity of habitats (Scheren et al., 2002; Ukwe et al., 2003; Ayamdoo, 2016).
The Gulf of Guinea is host to a very high diversity of benthic fauna. Yet, this ecosystem is threatened by human activities including oil excavation and the dyeing industry (Scheren et al., 2002; Ayamdoo, 2016; Sobczyk et al., 2021). In this study, we provide the first biodiversity assessment of tanaidacean communities from the West African coast based on a large set of 270 quantitative samples collected in the Gulf of Guinea. We identified the most important natural and anthropogenic factors that may shape tanaidacean communities along a depth gradient from 25 to 1,000 m along the coast of Ghana.
Materials and Methods
Study Area
The Gulf of Guinea is a large open bay on the Atlantic coast of West Africa between latitudes 58°N and 58°S and longitudes 88°W and 128°E (Ukwe et al., 2003). The main currents of this basin are the Guinea Current, by the Benguela Current, and the South Equatorial Counter Current (Schneider, 1990; Ukwe et al., 2006). The Gulf of Guinea is characterized by the occurrence of oxygen minimum zones (Levin, 2003; Levin et al., 2009) and by dynamic sedimentation phenomena associated with coastal erosion (Ukwe et al., 2003). The coastal areas of Ghana, stretched across a distance of 565 km, are located in the atypical tropical climate region (Le Loeuff and Cosel, 1998), and are characterized by high dynamics of water masses and upwellings (Djagoua et al., 2011). This part of the coastline contains no large river systems, except for the Volta River estuary, which is located in the eastern part of the coast.
Sampling
Samples were collected in October and November of 2012 in the Gulf of Guinea off Ghana from onboard the RV Dr. Fridtjof Nansen at nine transects (Figure 1 and Supplementary Appendix 1). A total of 270 samples were gathered using 0.1 m2 van Veen grab supported with the video-assisted monitoring system (VAMS) allowing for appropriate sediment penetration and avoiding any underestimations associated with sampling. For each transect, seven stations were designated from the littoral to bathyal depths (0, 25, 50, 100, 250, 500, 1,000 m). Five samples were collected at each station. The material was sieved through 0.3-mm sieves, preserved primarily in 4% formaldehyde solution, sorted, and finally transferred to 70% ethanol.
The material will be deposited at the University Museum of Bergen (Norway).
Physical and chemical properties, including temperature, conductivity, and oxygen level (Seabird 911 CTD Plus and SBE 21 Seacat thermosalinographs were used) were measured at each station. Additionally, sediment samples were collected for further laboratory analysis of sediment structure, total organic matter (TOM) content, fluorescence, level of hydrocarbons, and toxic metals. Sediment grain size was determined by mixing the sediment with water and sieving it through a 0.063-mm sieve. Larger particles were then sieved through Endecott sieves. For calculations, we used the equations of Folk and Ward (1957) and Buchanan (1984). TOM was determined as the weight loss in a 2–3 g dried sample (1,058°C for 20 h) after 2 h of combustion at 4,808°C. Petroleum hydrocarbon content was determined using a gas chromatograph with a flame ionization detector (GC/FID) according to the Intergovernmental Oceanographic Commission, Manuals and Guides No. 11, UNESCO Intergovernmental Oceanographic Commission (1982). Metals (Ba, Cd, Cr, Cu, Pb, Zn) were analyzed via inductively coupled plasma-atomic emission spectrometry (ICP-AES), whereas mercury (Hg) was determined via cold vapor atomic emission spectrometry (CVAAS) after drying, sieving, and digestion (Jarvis and Jarvis, 1992; Elezz et al., 2018).
Data Analysis
Specimens were identified to the morphospecies level (Wägele, 2005). Mean values of abundance (± 1 SD) and mean values of species richness per sample were calculated for each station (25, 50, 100, 250, 500, and 1,000 m) to investigate trends in abundance and species richness along a depth gradient of the Ghanaian shelf and slope. The Kruskal–Wallis test was used to assess statistically significant differences in tanaidacean abundance and species richness between depths. Post hoc testing was performed using Dunn’s test with the software package Statistica 13. Species Chao 1 accumulation curve averaging over 999 permutations was created using the vegan package (vegan:specaccum; Oksanen et al., 2020) in R software (R Core Team, 2019).
The number of rare species recorded in the material was assessed. Singletons were defined as species represented by only one individual in the whole material. We have also calculated the number of unique species (species found in one sample only). Additionally, we have calculated the number of species common to a given depth zone and common to different transects. In addition, bathymetric distribution of each species and family was described.
Hierarchical agglomerative clustering based on Bray–Curtis formula was used to analyze the similarity between the samples via the group average method on transformed data (presence–absence). Similarity profile routine tests (SIMPROF) with a 1% significance were also applied (clustig:simprof, clustig:simprof.plot; Clarke et al., 2008; Whitaker and Christman, 2014).
The association between tanaidacean communities and all the collected environmental factors was done in R software (R Core Team, 2019). To transform environmental data for reducing biases associated with unequal ranges of some variables (for example barium and gravel), we used Yeo-Johnson power transformation from “caret” package (caret:preProcess() function; Kuhn, 2020). Further, canonical correspondence analysis (CCA) was performed using the cca function from the vegan package (Oksanen et al., 2020) with initial 21 variables (for the full list of variables see Supplementary Appendix 2). The anova.cca function from the vegan package (Oksanen et al., 2020) was used to determine the statistically significant factors in shaping diversity on each station. By using vif function from the car package (Fox and Weisberg, 2019), we assessed the degree of multicollinearity between analyzed variables. We compared the results with Pearson correlation matrix (corrplot:corrplot; Wei and Simko, 2017; Figure 2) to exclude strongly correlated variables (r > 0.68). Variables with the highest correlation and vif value, as well as those statistically insignificant, were excluded before another ordination analysis. Four variables were included in the final version of CCA.
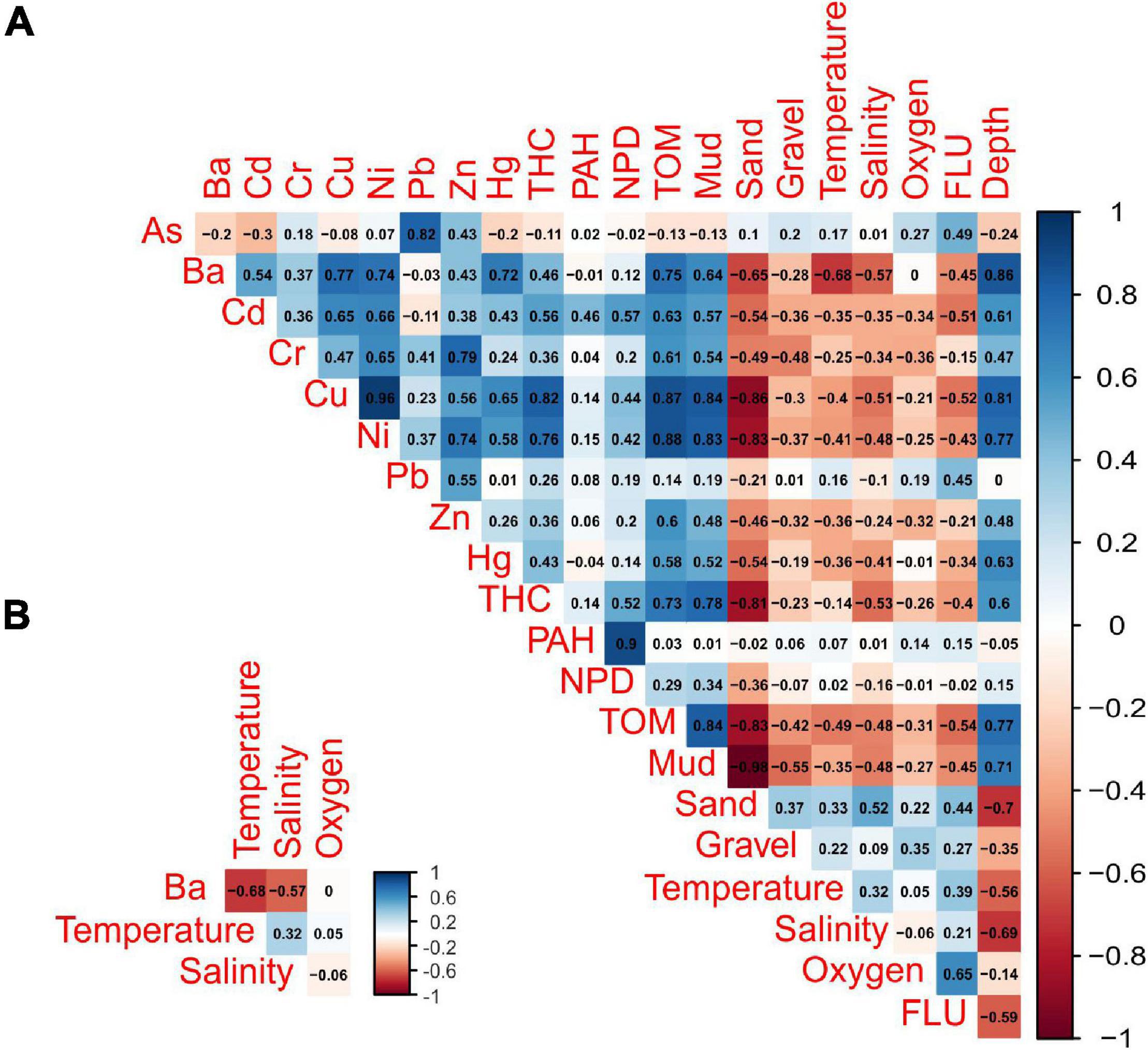
Figure 2. Pearson’s correlation matrix of environmental variables (A). Only four of the least correlated (r < 0.70) variables were used in the CCA analysis (B).
Results
Eighty-seven species (646 individuals) representing 19 families were found in the analyzed material (Table 1). Four families (Akanthophoreidae Sieg, 1986; Anarthruridae Lang, 1971; Pseudotanaidae and Typhlotanaidae Sieg, 1984) were the most speciose with 10 or more species recorded. Apseudidae (209 individuals), Leptocheliidae (95 individuals), and Parapseudidae Guţu, 1981 (90 individuals) accounted for more than 60% of tanaidacean material.
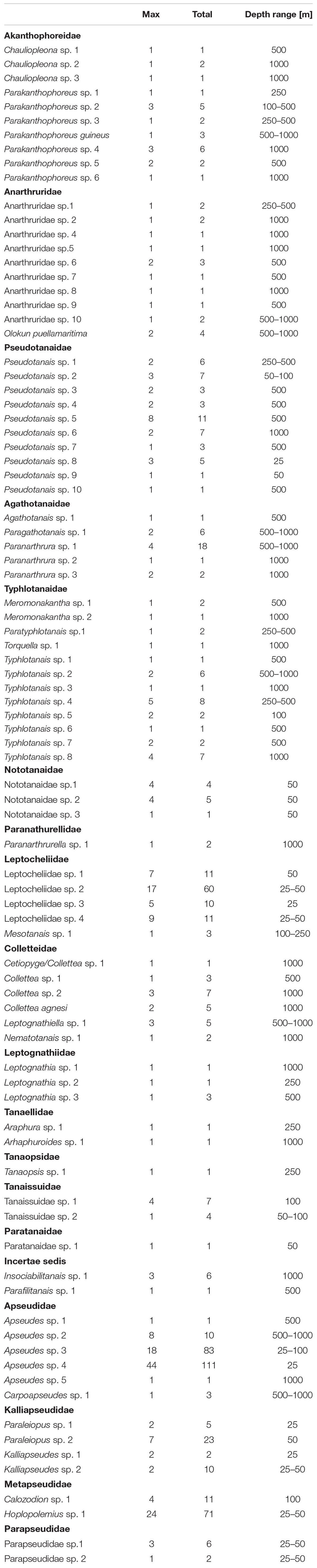
Table 1. Total abundance and maximum abundance recorded in a single sample of each species, together with depth range in the studied material.
The mean abundance of tanaidaceans from all collected samples was 0.03 ± 0.55 ind./0.1 m2. The frequency of occurrence in samples was also very low. Tanaidaceans were recorded in 113 out of 270 samples (42% of samples). Mean abundance decreased along the depth gradient up to 250 m stations (Figure 3). Then, abundance increased at 500 and 1,000 m (Figure 3), although there were no statistically significant differences between each depth zone (Kruskal–Wallis test, Dunn’s test). The material revealed large numbers of singletons and uniques, with 35 singletons (40% of all species) and 53 uniques found. Eighty-five species (98% of all species) had a total abundance lower than 10 individuals.
Mean species richness per sample (S) was greatest in the shallows (25–50 m) and on the slope (500–1,000 m). However, significant differences were only found between 50 and 250 m depth zones (Figure 4). The highest numbers of species were recorded at 500 and 1,000 m (33 and 34 species, respectively), and this area was also characterized by the highest number of species unique to those depth zones (Figure 5). Species accumulation curves did not reach the asymptotic level, which may suggest undersampling of the studied area (Figure 6). Low numbers of species were recorded despite a collection of five replicate samples at each station (Supplementary Appendix 3). The rarity and restricted distribution of taxa was also visible in the low number of species common to different depth zones (Table 2). Commonality of species between transects was also low, demonstrating a restricted spatial distribution of the majority of the species (Table 3).
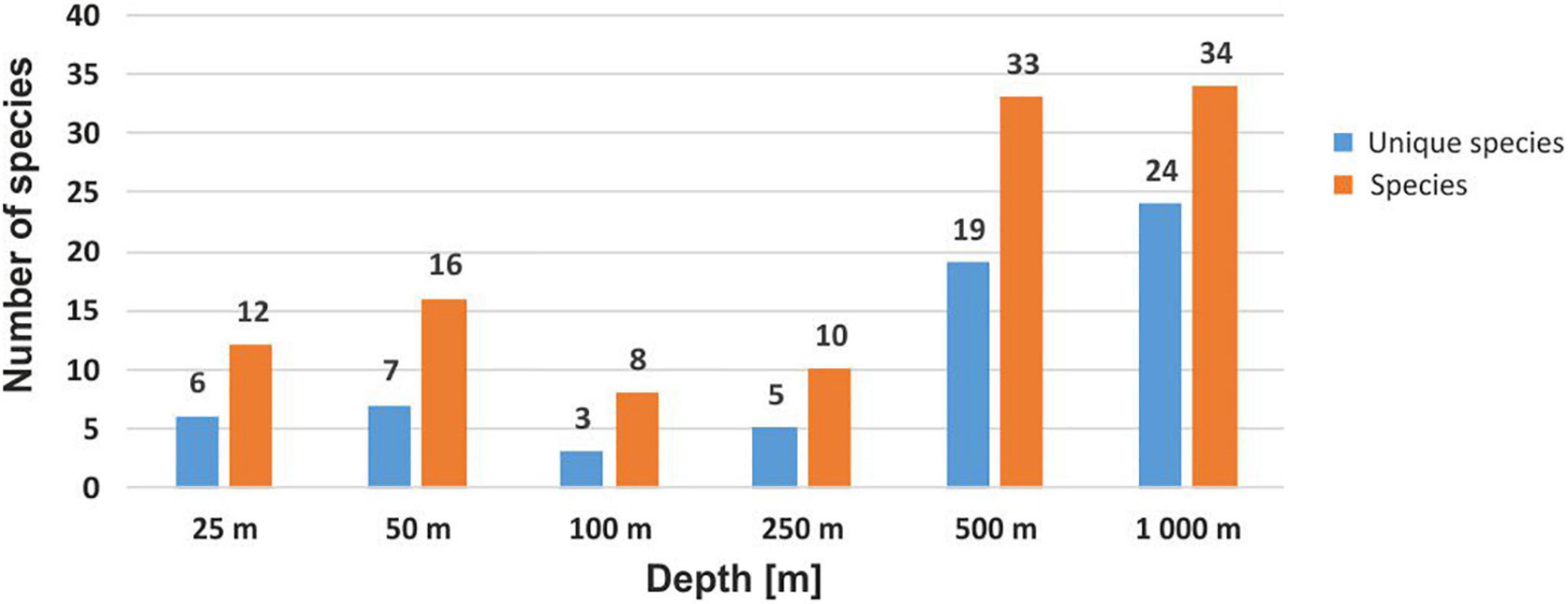
Figure 5. Total number of species recorded at each depth, together with the number of species unique to a given depth.
Only one family of tanaidaceans (Pseudotanaidae) was distributed along a whole depth gradient. We have recorded two main groups of families, one associated with shallow shelf areas (Kalliapseudidae, Parapseudidae, Metapseudidae, Leptocheliidae, Nototanaidae Sieg, 1976, and Paratanaidae Lang, 1949) and the second associated with slope depths (Agathotanaidae Lang, 1971, Colletteidae Larsen and Wilson, 2002, and Paranarthurellidae) (Table 1 and Figure 7).
Clustering analysis revealed five distinct groups supported by 1% SIMPROF (Figure 8) with a low similarity between each group (3–22%). The first group contained three stations from 250 m in which seven species were grouped within six families. This cluster was characterized by low oxygen concentration (1.4 ± 0.2). Group 2 comprised 6 stations, mostly from 500 m, and grouped 28 species within 9 families. High concentration of heavy metals such as barium (152.3 ± 115.6), chromium (60.2 ± 7.6), total hydrocarbons (13.3 ± 6.6), and organic matter (11.2 ± 3.7), as well as the domination of silty sediment were characteristic of these stations. Nine stations from the deepest sections (500–1,000 m) were grouped in cluster 3 which, in general, was characterized by a similar set of variables as cluster 2 with 31 species within 12 families. Stations from the shallowest sites were grouped as cluster 4. Twelve stations were characterized by the highest oxygen concentration (4.2 ± 0.2), temperature (24.7 ± 1.7), and the highest gravel content in the sediments (4.3 ± 6.4). Heavy metals reached the lowest concentration (e.g., barium 16.6 ± 8.3, nickel 13.3 ± 7.4), but elevated arsenic concentration (39.7 ± 55.0) at stations located at transect 4 (25 m: 106.84) and 8 (50 m: 186.78) were recorded. Despite this fact, tanaidaceans reached the highest abundance at stations located at 25 and 50 m depth. The last cluster grouped nine stations, mostly from 100 m. The group is characterized by the highest salinity (35.7 ± 0.1) and the lowest abundance of tanaidaceans.
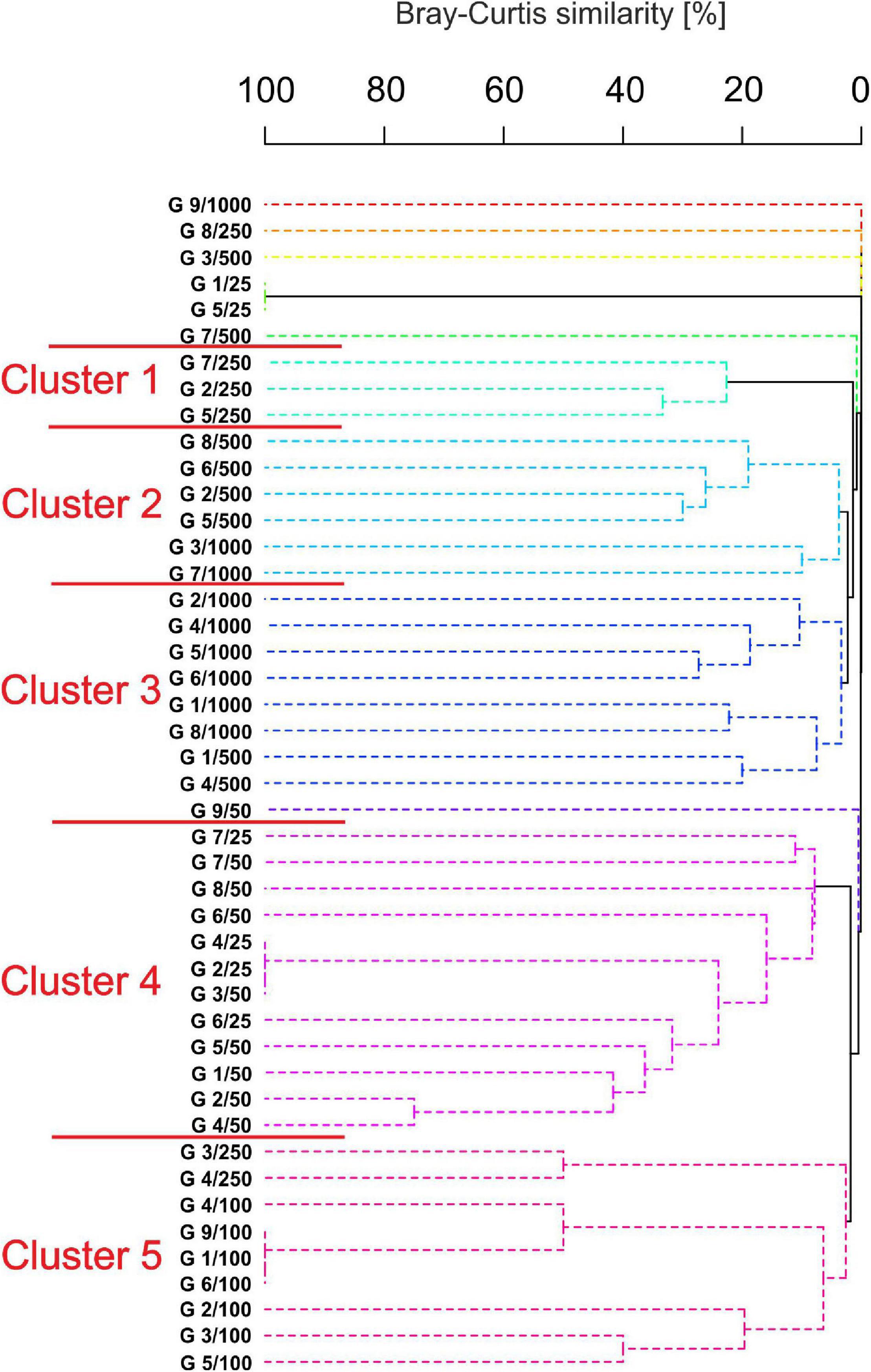
Figure 8. Dendrogram of samples for Bray Curtis similarity, square root transformed data, and group average grouping method with 1,000 iterations. (Spotted lines indicate the samples that cannot be significantly differentiated by SIMPROF).
Canonical correspondence analysis with four environmental variables: barium, oxygen concentration, salinity, and temperature revealed that species composition of tanaidaceans along the Ghanaian coast is depth-related rather than transect-specific or sediment particle size-specific (Figure 9 and Supplementary Appendix 4). All the four factors were statistically significant (Table 4). The ordination analysis clearly showed separation of each depth zone, except of 25–50 m stations which were grouped together. CCA axis 1 distinguishes the stations into two groups: the first is characterized by higher oxygen concentration at shallowest (25–50 m; mean: 4.21 ± 0.31) and the deepest stations (1,000 m; mean: 3.55 ± 0.29); and the second by a decreased oxygen level at stations at 250 m (mean: 1.53 ± 0.17) and 500 m (mean: 1.81 ± 0.30). CCA axis 2 divided stations based on a salinity, temperature, and barium gradient. Sites located at bathyal zone (500 m; mean: 87.92 ± 48.92 and 1,000 m; mean: 216.83 ± 78.56) were characterized by a higher barium concentration than those from the continental shelf (25–50 m; mean: 15.58 ± 9.44). Salinity was highest at 100 m stations and lowest at 1,000 m. Temperature decreased with depth reaching 27.49 ± 1.48 at 25 m and 14.19 ± 11.90 at 1,000 m.
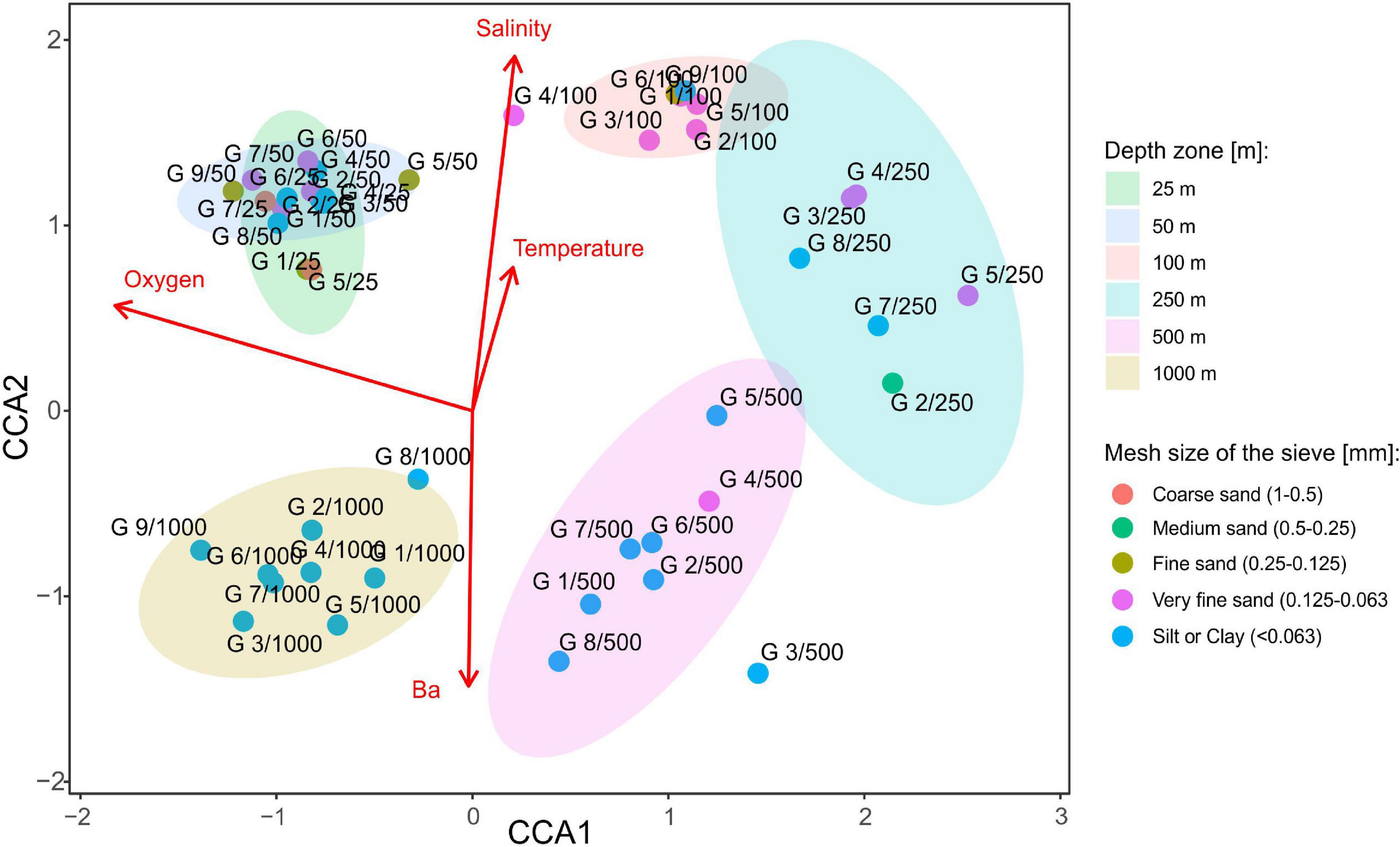
Figure 9. Canonical correspondence analysis (CCA) showing species composition differences on each sampling site with selected environmental factors as red arrows (barium, temperature, salinity, and oxygen). Sediment size is differentiated by the color of the points. In addition, to describe species composition along the depth gradient, depth zones were distinguished as ellipses with 0.95 confidence intervals. Only statistically significant variables were included into ordination analysis. See Supplementary Appendix 1 for a full list of factors.
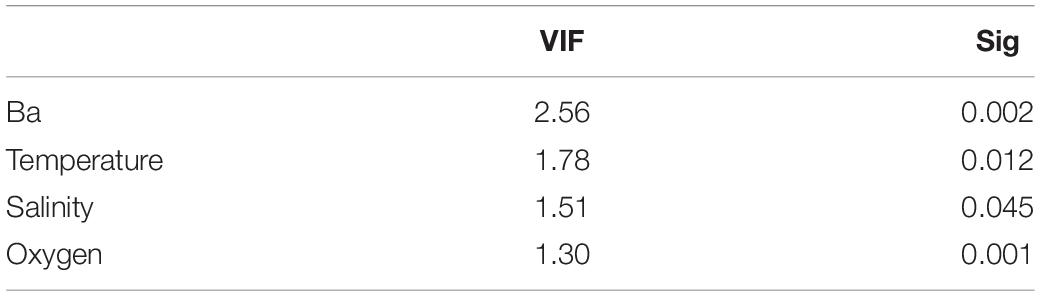
Table 4. Value of variance influence factor and statistical significance of selected variables used in final version of canonical correspondence analysis.
Discussion
Diversity Patterns Along a Depth Gradient
Our study of tanaidacean diversity in the Gulf of Guinea revealed very high species richness. With a total of 87 species of which 84 appeared to be new for science, the number of species across the whole west coast of Africa now reached totals 164 (Jóźwiak et al., 2017). Our results may be attributed to the high sampling efforts across both the continental shelf and slope depths, as each was characterized by a distinct set of species.
Despite our quantitative sampling methodology collecting the fauna at localized scales, our results are similar to the results of earlier studies based on less precise epibenthic sledge (EBS) and other trawling devices that are indiscriminately designed to collect large number of taxa from large bottom areas. Cumulatively, we have sampled 27 m2 of seabed. Comparison of this sampling area with studies based on qualitative samplers, such as the KuramBio cruise where total area sampled using EBS equaled 53,709 m2 (Jóźwiak et al., 2020) or benthic sampling in the Ross Sea, where the area of only single EBS sample varied between 247 and 1,493 m2 (Pabis et al., 2015), shows the unique character of our results. Earlier studies, based mostly on a relatively small set of several EBS samples, also demonstrated high species richness of tanaidacea, for example, Ross Sea (72 species) (Pabis et al., 2015), Amundsen Sea (37 species), Scotia Sea (51 species) (Pabis et al., 2014), and region of the Kuril-Kamchatka Trench (48 species; Błażewicz-Paszkowycz et al., 2015), at significantly lesser spatial resolutions. The scale of the sampled area is crucial for all species richness assessments because it allows for the penetration of a larger number of habitats (Błażewicz et al., 2019a). Nevertheless, a large number of species new to science and a large number of singletons were visible in all earlier tanaidacean studies (Pabis et al., 2014, 2015; Błażewicz-Paszkowycz et al., 2015; Stępień et al., 2018, 2019; Błażewicz et al., 2019a; Jóźwiak et al., 2020), although not at the scale recorded in the Gulf of Guinea.
We have recorded a striking rarity of tanaidacean species along the coast of Ghana. Despite the high sampling effort and the replicated sampling scheme, which allows for penetration of larger areas of the bottom and minimizes undersampling of species (Somerfield and Clarke, 1997; Eleftheriou and McIntyre, 2005), 40% of the species were singletons, 98% of the species had total abundance lower than 10 individuals, and approximately half of the species were unique to specific samples. Similar results were observed in deep-sea tanaidacean assemblages from other basins, for example, from the Amundsen and Scotia Seas 85 species were recorded, with about 90% species new for science, and most of the species were found only in a single sample (uniques) (Pabis et al., 2015). Similarly, studies in CCFZ area revealed 98 tanaidacean species, out of which 47 of them were singletons while 57 were uniques (Błażewicz et al., 2019a). Recorded in our studies, values of singletons and rare species are much higher than the average values recorded for various macrofaunal taxa that are most often characterized by 30–35% share of singletons (Ellingsen et al., 2007). Moreover, an earlier analysis of sampling replicability at the same station in the studies of tanaidacean fauna did not show such a strong level of rarity on a small scale (Jóźwiak et al., 2020). Some species may, however, display locally higher abundances. Such patchy distribution was recorded for Apseudes sp. 4, where 44 of the 111 individuals of this species were found in one sample (Table 1).
Very steep species accumulation curves were also present for tanaidaceans in the classic deep-sea study of Grassle and Maciolek (1992). This study was based on 233 boxcorer samples (1,500–2,500-m depth range), collected off of the North American coast. Really high abundances of tanaidaceans were rarely recorded, with the majority found in the very shallow areas, and mostly comprised a single species (Bailey-Brock, 1984; Sheridan, 1997). In the deep-sea, relatively high abundances of these crustaceans have only been recorded in some more productive sites in the northeast Atlantic (Cosson et al., 1997).
A high number of singletons is not atypical for the deep-sea, although it is often difficult to assess if the large number of singletons is due to sampling effort and methodology, or if it reflects environmentally driven patterns (Rex and Etter, 2010). The deep-sea studies generally suffer from undersampling and are subject to huge bias in biodiversity inventories, assessments of rarity, and description of distribution patterns (Jóźwiak et al., 2020; Rex and Etter, 2010). A very high number of singletons accompanied by a high share of species new to science was also recorded for other groups of peracarid crustacean, namely cumaceans and isopods (e.g., Brandt et al., 2007; Stȩpień et al., 2021). Peracarid diversity from the Southern Ocean demonstrated another interesting pattern. The majority of species were recorded in a low number of samples, but the authors suggested that they are not rare, but instead, their distribution is highly patchy (Kaiser et al., 2007). The study, however, was based on only 19 EBS samples, and this problem requires further investigation. The patterns we observed in Ghana appear different due to the large number of samples collected and high degree of replication, yet they still yielded a very high number of singletons accompanied by a large number of samples without tanaidaceans.
Unfortunately, we cannot analyze functional diversity of tanaiadaceans due to the lack of comprehensive data about their biology. It has been suggested that the majority of taxa are likely detritus feeders and that their ecological diversity seems to be low (Błażewicz-Paszkowycz et al., 2012). Conversely, a taxonomic group characterized by such exceptionally high species richness must be an important element of ecosystem functioning, suggesting a complicated set of mutual interactions between various species and their habitats (Buhl-Mortensen et al., 2010). This is poignant in a marine ecosystem like the Ghanaian coast, where even core benthic taxa like polychaetes have a relatively low abundance (Sobczyk et al., 2021). A total of 87 species of tanaiadacea is a very high number, even compared to the 251 species of polychaetes recorded in the same material (Sobczyk et al., 2021), especially given that polychaetes belong to the most dominant benthic taxa, both in terms of abundance and diversity (Rouse and Pleijel, 2001). We might speculate that even if tanaidaceans are not the most important consumers, they may comprise a major component of trophic webs as prey for predatory polychaetes, decapods, or fishes (Balasubramanian et al., 1979; Nagelkerken and van der Velde, 2004; Larsen, 2005; Jumars et al., 2015). At the same time, predatory polychaetes like Goniadidae Kinberg, 1866; Glyceridae Grube, 1850 and Polynoidae Kinberg, 1856 are among the most important groups of benthic fauna on the coast of Ghana, especially in the shallower shelf sites (Sobczyk et al., 2021) along with a high diversity of decapods (Podwysocki et al., unpublished results).
Despite their small size, tanaidaceans may be important for bioturbation of the bottom sediments, especially in the surface layers, like in the case of shallow water Apseudomorpha (Larsen, 2005), which was the dominant element of fauna in our material. Rare and small organisms are typically a minor element of the sediment reworking processes. However, in the coast of Ghana, burrowing polychaetes, the most important biotubators, were highly diverse (71 species), yet their abundance and size were low (Sobczyk et al., 2021), potentially elevating the functional importance of other taxa. The mean total abundance of macrofauna in the same set of samples was relatively low, particularly on the lower shelf and slope (Pabis et al., 2020), making tanaidaceans a proportionally important element of those communities. Polychaete functional diversity also decreased from shelf to slope (Sobczyk et al., 2021), suggesting that the role of tanaidaceans in the benthic ecosystem may increase proportionally with depth. Our results are verified by studies from the Australian continental slope, where tanaidaceans were the second most abundant group of benthic macrofauna, after polychaetes, although their abundance remained relatively low with 561 individuals collected in c. 1000.1 m2 Smith–McIntyre grab samples (McCallum et al., 2015).
Our earlier results suggested that the Gulf of Guinea may have a high ecosystem resilience due to exceptionally high diversity (Sobczyk et al., 2021; Stȩpień et al., 2021). High diversity may allow for functional redundancy within the ecosystem where multiple species play the same role (Biggs et al., 2020). This observation might be crucial in the context of strong anthropopressure recorded in the Gulf of Guinea (Scheren et al., 2002), especially with ongoing industrialization and oil excavation (Scheren et al., 2002; Ukwe et al., 2003; Ayamdoo, 2016; Sobczyk et al., 2021). Our results revealed links between tanaidacean communities and changes in environmental factors, although multivariate analysis bias on a count of low abundances cannot be discounted (Reiss et al., 2015). The similarity level recorded in the cluster analysis was very low, and results of the CCA did not demonstrate a very strong pattern. Taking into account the above mentioned problems, these results have to be treated cautiously. Therefore, the possible use of tanaidacean communities as indicators of changes in benthic ecosystem in the Gulf of Guinea is doubtful despite their importance for overall biodiversity.
Depth was an important factor in structuring tanaidacean communities along the coast of Ghana. The clear separation of the slope samples, bottom area affected by larger concentrations of barium and hydrocarbons in the sediments, was not accompanied by a decrease in abundance or species richness, unlike Cumacea and Polychaeta (Sobczyk et al., 2021; Stȩpień et al., 2021). This is somewhat surprising since barium is known for its toxic influence on benthic fauna (Olsgard and Gray, 1995; Gomez-Gesteira et al., 2003; Stark et al., 2020), However, barium and other toxic metal concentrations were similar to the background levels according to the OSPAR and KLIF (Norwegian Pollution Authority) guidelines, and the general environmental status of investigated sites was good, with only some local pollution (Iversen et al., 2011; Pabis et al., 2020; Sobczyk et al., 2021). In the case of the Ghanaian coast, toxic metals originated from oil excavation and the dyeing industry (Scheren et al., 2002; Ayamdoo, 2016; Sobczyk et al., 2021). As such, we suggest that the higher diversity recorded on the slope (Figure 3) could be explained by the fact that bathyal is a major hot spot of benthic diversity in the Worlds Ocean (Danovaro et al., 2009). This pattern is supported by earlier studies of tanaidacean communities at slope depths (Pabis et al., 2014, 2015; Błażewicz-Paszkowycz et al., 2015). This may prove important for the stability of slope ecosystems in the Gulf of Guinea, where even an elevated level of pollutants might be compensated by the presence of a large number of species. Abundant and speciose communities were also recorded at shallow shelf sites characterized by higher oxygen contents and higher fluorescence. Both factors are very important for various benthic invertebrates (Levin and Sibuet, 2012), and thus it is not surprising that they exerted a positive influence on the tanaidacean communities.
Our results demonstrate that the analysis of rare and less abundant but highly speciose taxa, such as small peracarid crustaceans, should become an important element of future studies of marine benthic ecosystems. Some studies of particular species suggest that tanaidaceans may constitute good indicators of changes in environmental conditions (Ambrosio et al., 2014). However, the analysis of their communities might prove difficult as changes in community structure are hard to detect due to a high degree of rarity and their low abundance. Therefore, studies of such small size and rare taxonomic groups might require different sampling strategies and/or the use of more specialized sampling equipment.
It is important to consider that though we sampled c. 500 km of coastline during our study, we only recorded a very low number of tanaidacean species common to different transects. Deciphering range descriptions in the case of species that likely have a small population size, patchy distribution, and low abundance is difficult. Our results strongly suggest that the majority of tanaidaceans have very restricted spatial distribution, even at a moderate scale. The restricted vertical range of many species (Table 1 and Figure 4) is not surprising and is due mostly to affinities of tanaidacean families to given depth zones that are associated with their phylogeny, for example, phylogenetically young families have colonized the deep-sea relatively recently (Błażewicz-Paszkowycz et al., 2012).
High total species richness might be also a consequence of microhabitat diversity. Previous studies demonstrate strong affinities between bottom type or complexity of habitats and composition of tanaidacean fauna (Holdich and Bird, 1985; Siciński et al., 2012). Sediment grain size was not a significant factor in the CCA analysis, although grain size is likely an important factor for small crustaceans that are associated with soft bottom ecosystems (Błażewicz-Paszkowycz et al., 2012). Significant knowledge gaps remain as surrounding links between sediment character and composition of tanaidacean fauna, whereas interpretation of our results is difficult due to the low abundances encountered.
Life history characteristics of a given species may differ strongly with environmental conditions and habitat characteristics (Rumbold et al., 2015). Ellingsen et al. (2007) postulated links between habitat diversity and degree of rarity. This may hold for the Gulf of Guinea, an area characterized by highly dynamic environmental factors and diverse habitats (Brind’Amour et al., 2009; Buhl-Mortensen et al., 2017; Pabis et al., 2020). The small size and low dispersal potential of Tanaidacea (Larsen, 2005; Błażewicz-Paszkowycz et al., 2012) strongly influence their distribution patterns. Though we do not know the habitat requirements and environmental tolerance of the particular tanaiadacean species recorded in our study, habitat-specific species are considered to be rare (Ellingsen et al., 2007). In the case of organisms with a size that often does not exceed 2 or 3 mm, the scale of the potential microhabitat diversity might be significantly reduced compared with larger taxa characterized by higher mobility and the presence of planktonic larval stages.
Families Distribution Along a Depth Gradient
Material collected along the coast of Ghana has provided a unique opportunity to analyze changes in Tanaidacea composition along a depth gradient (25–1,000 m). This is the first study of this nature since Jakiel et al. (2018) exacted tanaidacean collection from Icelandic waters, and since Pabis et al. (2015) analysed material from the Ross Sea. The former study was, however, limited only to representatives of the family Pseudotanaidae, whereas the latter analyzed samples from completely different depths (365–3,490 m). The sampling effort in both of those studies was very low. The Tanaidacea recorded in the Gulf of Guinea showed a clear transition from taxa known as typically shallow-water (Leptocheliidae and Kalliapseudidae), through families with a wide bathymetric range (Pseudotanaidae and Apseudidae) to the true deep-sea forms (Paranarthrurellidae) (Błażewicz-Paszkowycz et al., 2012).
Fourteen out of the 22 known families of suborder Tanaidomorpha were found in the Gulf of Guinea. Leptocheliidae are generally described as tanaidaceans with well-developed functional eyes and inhabiting the shelf, although the genera Bathyleptochelia and Mesotanais have been previously recorded below shelf depths (Błażewicz-Paszkowycz et al., 2012). Earlier observations fully correspond with our results: Mesotanais sp. 1 was only found at stations from 100 to 250 m depth; non-Mesotanais Leptocheliidae were collected only at 25 and 50 m. Other tanaidomorphan families found on the shelf (50 m depth) in the Gulf of Guinea include Nototanaidae and Paratanaidae, which similar to Leptocheliidae, are considered shallow-water taxa, with some deep-sea exceptions. Leptocheliidae may be a polyphyletic group, which makes comparisons of their bathymetric range with wider studies doubtful and uncertain (Błażewicz-Paszkowycz et al., 2012). In contrast, the monophyly of Tanaissuidae Bird and Larsen, 2009 is well-supported (Bird and Larsen, 2009), but the family is mostly represented by shelf taxa and some single deep-water forms, like Bathytanaissus (Bird and Holdich, 1989). In our material, this family was recorded in a depth range between 50 and 100 m.
Members of the family Tanaopsidae Błażewicz-Paszkowycz and Bamber, 2012 were recorded in the Gulf of Guinea only from one sample taken at 250 m. This family is known to have a worldwide distribution and wide bathymetric range, from intertidal to over 3,000 m, which is likely explained by its polyphyletic nature (Błażewicz-Paszkowycz and Bamber, 2012).
We also found a group of tanaidomorphan families characterized by exceptionally wide depth ranges. For example, Akanthophoreidae (100–1,000 m in Gulf of Guinea) are generally well represented in the deep-sea worldwide, but found also on the shelf (Larsen and Araújo-Silva, 2014). Anarthruridae (250–1,000 m in the Gulf of Guinea) are a well-defined, cosmopolitan family recorded primarily in the deep-sea (Larsen, 2013), albeit with some shallow water representatives (Dojiri and Sieg, 1997). In our material, this family was only present at depths below the shelf, supporting the suggested deep-water origins of this group (Błażewicz-Paszkowycz et al., 2012). Pseudotanaidae is another family with worldwide distribution and with the highest diversity recorded in the deep sea, as well as enormous depth range, from intertidal to over 7,000 m (Jakiel et al., 2018). Intriguingly, this family can be morphologically and bathymetrically divided into shallow-water forms with functional eyes and blind, deep-sea forms (Błażewicz-Paszkowycz and Bamber, 2012). Our results support those observations, as Pseudotanaidae were recorded along a full depth range (25–1,000 m), with only two species characterized by the presence of eyes found at shallowest stations, and remaining eight blind species were collected in the deep water (Table 1). Typhlotanaidae is a family of possible deep-sea origin (Błażewicz-Paszkowycz et al., 2012), that also has representatives on the shelf depths (Błażewicz-Paszkowycz, 2007; Segadilha and Lavrado, 2018). In the Gulf of Guinea typhlotanaids were primarily recorded from deeper parts of the basin (250–1,000 m), but a single species—Typhlotanais sp. 5—was found also on the shelf (100 m). Tanaellidae Larsen and Wilson, 2002 likely have a deep-sea origin, but Blażewicz-Paszkowycz and Bamber (2012) found representatives of this family in the shallow waters of Bass Strait. This family was represented exclusively by deep-sea forms in our data, namely Araphura sp. 1 recorded at 250 m and Arhaphuroides sp. 1 found at 1,000 m.
Two other families representing the suborder Tanaidomorpha (Colletteidae and Leptognathiidae Sieg, 1976) have a relatively wide depth range in the Gulf of Guinea, but representatives of both families were recorded on the slope (from 500 to 1,000 m and from 250 to 10,000 m, respectively). Those two taxa comprised very important elements of deep-sea Tanaidacea communities analyzed in earlier studies (Pabis et al., 2014, 2015; Błażewicz-Paszkowycz et al., 2015). Though wider literature indicates shallow-water records of those families, they need to be treated cautiously because both groups are clearly polyphyletic (Bird and Larsen, 2009; Błażewicz-Paszkowycz et al., 2013). The problem is even more pronounced in Leptognathiidae because the family itself, as well as the type genus Leptognathia, were treated as a repository group for decades (Bird, 2007).
The family Agathotanaidae is almost exclusively a deep-sea group, with the majority of the records from below 1,000 m depth. Only single species were found at shallower sites, e.g., Agathotanais misakiensis and A. toyoshioae from 211 to 493 and 95 m depth, respectively (Kakui and Kohtsuka, 2015). In the Gulf of Guinea, five agathotanaid species were recorded, all of which were present at the deepest stations (500 and 1,000 m). Finally, the family Paranarhrurellidae, a group that was recorded twice in our material but only at the deepest stations (1,000 m), confirms the true deep-sea origin of this family. This matches the described range of this group, starting from the bathyal and ending at hadal depths (Błażewicz et al., 2019b).
Apseudomorpha were represented in the Gulf of Guinea by only four families and most of their records are from the shelf. This observation is in accordance with the general distributional patterns of this suborder, as only one from 12 known families of Apseudomorpha (family Gigantapseudidae Kudinova-Pasternak, 1978) is considered an exclusively deep-sea taxon. The family Kalliapseudidae was found in the Gulf of Guinea at the shallowest stations (25 and 50 m), aligning our results with other studies of this group. Kalliapseudids are generally characterized as shallow marine and estuarine tanaidaceans, mainly occurring at depths higher than 200 m (Drumm and Heard, 2011). At the same depths (25–50 m), we found representatives of another typically shallow-water tropical family, the Parapseudidae Guţu, 2008. The third apseudomorphan family, the Metapseudidae, were recorded slightly deeper, from 25 to 100 m, also in accordance with previous findings (Stȩpień and Blażewicz-Paszkowycz, 2013; Jóźwiak and Błażewicz, 2021). The last family recorded on the coast of Ghana are the Apseudidae and they have a very wide and disjunct bathymetrical range. These can be differentiated into two subfamilies: firstly the Apseudinae, that group primarily shallow-water taxa and some deep-sea exceptions; and secondly, the Leviapseudinae that are composed of exclusively deep-water forms (Błażewicz-Paszkowycz et al., 2012).
Concluding Remarks
The great diversity of Tanaidacea, Cumacea (Stȩpień et al., 2021), and Polychaeta (Sobczyk et al., 2021), encountered in benthic communities to date, make the Gulf of Guinea an area of particular interest for conservation strategies in the Atlantic. The Gulf of Guinea seems to be a great natural laboratory for studies of ecosystem resilience to changes associated with pollution and climate warming. Our study establishes the region as a good model for studies of Large Marine Ecosystem response to human pressure. As a region characterized by high benthic diversity, it provides an opportunity to study links between species richness, habitat diversity, and ecosystem stability. The high number of species new to science uncovered in our materials reveal a great potential for studies related to interactions between local and regional species pools. Our results from a relatively small part of the Ghanaian coast have increased the number of Tanaidacea known from the whole west coast of Africa by over 100%, from 80 to 164 species (Jóźwiak et al., 2017), demonstrating a great need of further taxonomic studies. It emphasizes the role of taxonomic expertise as a foundation for biodiversity inventories and ecological analysis, especially in the regions that were previously neglected in studies of benthic fauna. Currently, only four species from this collection were officially described (Jóźwiak et al., 2017), but next descriptions will follow this general inventory.
Rare or narrow range species belong to the most underestimated marine invertebrates (Higgs and Attrill, 2015), and studies of their diversity should be amongst the priorities of marine science. The steep species accumulation curve encountered despite the high sampling effort further necessitates a more comprehensive evaluation of the most appropriate sampling strategies in the future monitoring of this region. To facilitate a more comprehensive taxonomic analysis, we need not only a larger number of replicated samples (Hughes et al., 2021), but also the simultaneous use of various sampling methodologies (Jóźwiak et al., 2020). The analysis of sampling efficiency and replication schemes should be prioritized in future studies to better record novel species.
Tanaidaceans belong to the most underestimated groups of benthic organisms (Appeltans et al., 2012). Despite the increased taxonomic effort in recent years (Błażewicz-Paszkowycz et al., 2012), the number of recorded new species remains much higher than the number currently described. This problem is widespread in studies of other groups of peracarid crustaceans. Kaiser et al. (2009) found that in the Amundsen Sea, 96% of collected isopods were new to science, and a large majority of those taxa are yet to be described, as in tanaidacean studies (Pabis et al., 2014, 2015; Błażewicz-Paszkowycz et al., 2015; Stępień et al., 2018, 2019; Błażewicz et al., 2019a; Jóźwiak et al., 2020). These problems must be addressed with studies of both particular taxonomic groups and particular regions of the world. Without a comprehensive taxonomic analysis, it is impossible to deduce meaningful estimates of marine benthic diversity on a global scale or to analyze the problems associated with ecosystem functioning, conservation priorities, and management strategies (Costello et al., 2012; Higgs and Attrill, 2015; Costa et al., 2020).
Data Availability Statement
The original contributions presented in the study are included in the article/Supplementary Material, further inquiries can be directed to the corresponding author/s.
Author Contributions
PJ: concept of the manuscript, taxonomic identification, statistical analysis, sampling design, and manuscript writing. KP: concept of the manuscript, statistical analysis, writing of the manuscript, sampling design, and coordination of benthic ecology in the project. RS: statistical analysis and manuscript writing. BS: sampling design, proofreading, and head of the FAO project. All authors contributed to the article and approved the submitted version.
Funding
The sampling cruise and the environmental data analysis within this program were funded by the Norwegian Agency for Development Cooperation (NORAD) (Oil for Development Program—OfD) and by the Food and Agriculture Organization of the United Nations (FAO). PJ and KP were also supported by University of Łódź.
Conflict of Interest
The authors declare that the research was conducted in the absence of any commercial or financial relationships that could be construed as a potential conflict of interest.
Publisher’s Note
All claims expressed in this article are solely those of the authors and do not necessarily represent those of their affiliated organizations, or those of the publisher, the editors and the reviewers. Any product that may be evaluated in this article, or claim that may be made by its manufacturer, is not guaranteed or endorsed by the publisher.
Acknowledgments
We would like to thank the crew of RV Fridtjof Nansen and the scientists from Ghana for their assistance in the collecting and processing of the samples. We thank to both reviewers of the manuscript, their valuable comments substantially improved the article.
Supplementary Material
The Supplementary Material for this article can be found online at: https://www.frontiersin.org/articles/10.3389/fmars.2022.779134/full#supplementary-material
References
Alvaro, M. C., Błażewicz-Paszkowycz, M., Davey, N., and Schiaparelli, S. (2011). Skin digging tanaids: the unusual parasitic behaviour of Exspina typica in Antarctic waters and worldwide deep basins. Antarct. Sci. 23, 343–348. doi: 10.1017/s0954102011000186
Ambrosio, E. S., Ferreira, A. C., and Rodrigues-Capitulo, A. (2014). The potential use of Sinelobus stanfordi (Richardson, 1901) (Crustacea, Tanaidacea) as a biological indicator of water quality in a temperate estuary of South America. Limnetica 33, 139–152.
Angelini, C., Altieri, A. H., Silliman, B. R., and Bertness, M. D. (2011). Interactions among Foundation Species and Their Consequences for Community Organization, Biodiversity, and Conservation. BioScience 61, 782–789. doi: 10.1525/bio.2011.61.10.8
Appeltans, W., Ahyong, S. T., Anderson, G., Angel, M. V., Artois, T., Bailly, N., et al. (2012). The magnitude of global marine species diversity. Cur. Biol. 22, 2189–2202. doi: 10.1016/j.cub.2012.09.036
Ayamdoo, N. A. (2016). Protecting the Gulf of Guinea in an oil boom: regulating offshore petroleum pollution in a divided world. J. World Energ. Law Bus. 619, 219–232. doi: 10.1093/jwelb/jww007
Băcescu, M., and Guţu, M. (1975). A new genus (Discapseudes n. g.) and three new species of Apseudidae (Crustacea. Tanaidacea) from the northeastern coast of South America. Zool. Meded. 49, 95–113.
Bailey-Brock, J. H. (1984). Ecology of the tube-building polychaete Diopatra leuckarti Kinberg, 1865 (Onuphidae) in Hawaii: community structure, and sediment stabilizing properties. Zool. J. Linn. Soc. 80, 191–199., doi: 10.1111/j.1096-3642.1984.tb01972.x
Balasubramanian, N. C., Lalithambika-Devi, B., and Krishnankutty, M. J. (1979). Feeding behaviour and preying efficiency of Metapenaeus dobsoni. Indian J. Mar. Sci. 8, 197–200.
Bassindale, R. (1961). On the marine fauna of Ghana. Proc. Zool. Soc. Lond. 137, 481–510. doi: 10.1111/j.1469-7998.1961.tb06086.x
Biggs, C. R., Yeager, L. A., Bolser, D. G., Bonsell, C., Dichiera, A. M., Hou, Z., et al. (2020). Does functional redundancy affect ecological stability and resilience? A review and meta-analysis. Ecosphere 11:e03184. doi: 10.1002/ecs2.3184
Bird, G. J. (2007). Families Anarthruridae Lang, 1971, Colletteidae Larsen & Wilson, 2002, and Leptognathiidae Sieg, 1976. In: Larsen, K., and M. Shimomura (eds.), Tanaidacea (Crustacea: peracarida) From Japan III. The Deep Trenches: the Kurile-Kamchatka Trench and Japan Trench. Zootaxa 1599, 61–85.
Bird, G. J., and Holdich, D. M. (1989). Tanaidacea (Crustacea) of the north-east Atlantic: the subfamily Pseudotanainae (Pseudotanaidae) and the family Nototanaidae. Zool. J. Linn. Soc. 97, 233–298.
Bird, G. J., and Larsen, K. (2009). Tanaidacean phylogeny – the second step: the basal paratanaoidean families (Crustacea: Malacostraca). Arthropod Syst. Phylogeny 67, 137–158.
Błażewicz, M., Jóźwiak, P., Jennings, R. M., Studzian, M., and Frutos, I. (2019a). Integrative systematics and ecology of a new deep-sea family of tanaidacean crustaceans. Sci. Rep. 9:18720. doi: 10.1038/s41598-019-53446-1
Błażewicz, M., Jóźwiak, P., Menot, L., and Pabis, K. (2019b). High species richness and unique composition of the tanaidacean communities associated with five areas in the Pacific polymetallic nodule fields. Prog. Oceanogr. 176:102141. doi: 10.1016/j.pocean.2019.102141
Błażewicz-Paszkowycz, M. (2007). A revision of the family Typhlotanaidae Sieg, 1984 (Crustacea: Tanaidacea) with the remarks on the Nototanaidae Sieg, 1976. Zootaxa 1598, 1–141. doi: 10.11646/zootaxa.1598.1.1
Błażewicz-Paszkowycz, M., and Ligowski, R. (2002). Diatoms as food source indicator for some Antarctic Cumacea and Tanaidacea (Crustacea). Antarct. Sci. 14, 11–15. doi: 10.1017/s0954102002000524
Błażewicz-Paszkowycz, M., Bamber, R. N., and Jóźwiak, P. (2013). Tanaidaceans (Crustacea: Peracarida) from the SoJaBio joint expedition in slope and deeper waters in the Sea of Japan. Deep Sea Res. II Top. Stud. Oceanogr. 86–87, 181–213.
Błażewicz-Paszkowycz, M., Bamber, R., and Anderson, G. (2012). Diversity of Tanaidacea (Crustacea: Peracarida) in the World’s Oceans – How Far Have We Come? PLoS One 7:e33068. doi: 10.1371/journal.pone.0033068
Błażewicz-Paszkowycz, M., Pabis, K., and Jóźwiak, P. (2015). Tanaidacean fauna of the Kuril-Kamchatka Trench and adjacent abyssal plain—abundance, diversity and rare species. Deep-Sea Res. Part II Top. Stud. Oceanogr. 86, 181–213.
Bracken, M. E., and Low, N. H. (2012). Realistic losses of rare species disproportionately impact higher trophic levels. Ecol. Lett. 15, 461–467. doi: 10.1111/j.1461-0248.2012.01758.x
Brandt, A., Brix, S., Brökeland, W., Choudhury, M., Kaiser, S., and Malyutina, M. (2007). Deep-sea isopod biodiversity, abundance, and endemism in the Atlantic sector of the Southern Ocean—Results from the ANDEEP I-III expeditions. Deep-Sea Res. Part II Top. Stud. Oceanogr. 54, 1760–1775. doi: 10.1016/j.dsr2.2007.07.015
Brind’Amour, A., Menot, L., Galeron, J., and Crassous, P. (2009). Spatial organization of a sedimentary macrobenthic community located on the West African Equatorial margin. Deep Sea Res. II Top. Stud. Oceanogr. 56, 2292–2298. doi: 10.1016/j.dsr2.2009.04.010
Buchanan, J. B. (1957). The bottom fauna communities across the continental shelf off Accra, Ghana (Gold Coast). Proc. Zool. Soc. Lond. 130, 1–56. doi: 10.1111/j.1096-3642.1958.tb00562.x
Buchanan, J. B. (1984). “Sediment analysis,” in McIntyre Methods for the Study of Marine Benthos, eds N. A. Holme and A. D. McIntyre (Oxford: Blackwell Scientific Publications), 41–65.
Buhl-Mortensen, L., Serigstad, B., Buhl-Mortensen, P., Olsen, M. N., Ostrowski, M., Błażewicz-Paszkowycz, M., et al. (2017). First observations of the structure and megafaunal community of a large Lophelia reef on the Ivorian-Ghanaian margin (the Gulf of Guinea). Deep-Sea Res. Part II Top. Stud. Oceanogr. 137, 148–156. doi: 10.1016/j.dsr2.2016.06.007
Buhl-Mortensen, L., Vanreusel, A., Gooday, A. J., Levin, L. A., Priede, I. G., Buhl-Mortensen, P., et al. (2010). Biological structures as a source of habitat heterogeneity and biodiversity on the deep ocean margins. Mar. Ecol. 31, 21–50.
Chapman, A. S. A., Tunnicliffe, V., and Bates, A. E. (2018). Both rare and common species make unique contributions to functional diversity in an ecosystem unaffected by human activities. Divers. Distrib. 24, 568–578. doi: 10.1111/ddi.12712
Clarke, K. R., Sommerfield, P. J., and Gorley, R. N. (2008). Testing of null hypotheses in explanatory community analyzes: similarity profiles and biota-environment linkage. J. Exp. Mar. Biol. Ecol. 366, 56–69. doi: 10.1016/j.jembe.2008.07.009
Cosson, N., Sibuet, M., and Galeron, J. (1997). Community structure and spatial heterogeneity of the deep-sea macrofauna at three contrasting stations in the tropical northeast Atlantic. Deep Sea Res. Part I Oceanogr. Res. Pap. 44, 247–269. doi: 10.1016/S0967-0637(96)00110-0
Costa, C., Fanelli, E., Marini, S., Danovaro, R., and Aguzzi, J. (2020). Global Deep-Sea Biodiversity Research Trends Highlighted by Science Mapping Approach. Front. Mar. Sci. 7:384. doi: 10.3389/fmars.2020.00384
Costello, M. J., and Chaudary, C. (2017). Marine biodiversity, biogeography, deep-sea gradients, and conservation. Curr. Biol. 27, 511–527. doi: 10.1016/j.cub.2017.04.060
Costello, M. J., May, R. M., and Stork, N. E. (2013). Can we name Earth’s species before they go extinct? Science 339, 413–416. doi: 10.1126/science.1230318
Costello, M. J., Wilson, S., and Houlding, B. (2012). Predicting total global species richness using rates of species description and estimates of taxonomic effort. Syst. Biol. 61:871–883. doi: 10.1093/sysbio/syr080
Danovaro, R., Canals, M., Gambi, C., Heussner, S., Lampadariou, N., and Vanreusel, A. (2009). Exploring benthic biodiversity patterns and hotspots on European margin slopes. Oceanography 22, 16–25. doi: 10.5670/oceanog.2009.02
Dayton, P. K., and Oliver, J. S. (1977). Antarctic soft bottom benthos in oligotrophic and eurythropic environments. Science 197, 55–58. doi: 10.1126/science.197.4298.55
Dee, L. E., Cowles, J., Isbell, F., Pau, S., Gaines, S. D., and Reich, P. B. (2019). When do ecosystem services depend on rare species? Trends Ecol. Evol. 34, 746–758. doi: 10.1016/j.tree.2019.03.010
Djagoua, E. V., Kassi, J. B., Mobia, B., Kouadio, J. M., Dro, C., Affian, K., et al. (2011). Ivorian and Ghanaian upwelling comparison: intensity and impact on phytoplankton biomass. Am. J. Sci. Ind. Res. 2, 740–747. doi: 10.5251/ajsir.2011.2.5.740.747
Dojiri, M., and Sieg, J. (1997). “The Tanaidacea,” in Taxonomic Atlas of the Benthic Fauna of the Santa Maria Basin and Western Santa Barbara Channel. Volume 11. The Crustacea Part 2. The Isopoda, Cumacea and Tanaidacea, eds J. A. Blake and P. H. Scott (Santa Barbara, CA: Santa Barbara Museum of Natural History), 181–278.
Drumm, D. T., and Heard, R. W. (2011). Systematic revision of the family Kalliapseudidae (Crustacea: Tanaidacea). Zootaxa 3142, 1–172. doi: 10.11646/zootaxa.3142.1.1
Eleftheriou, A., and McIntyre, A. D. (eds) (2005). Methods For The Study Of Marine Benthos, 3rd Edn. Oxford: Blackwell Science.
Elezz, A. A., Mustafa Hassan, H., Abdulla Alsaadi, H., Easa, A., Al-Meer, S., Elsaid, K., et al. (2018). Validation of total mercury in marine sediment and biological samples, using cold vapour atomic absorption spectrometry. Methods Protoc. 1:31. doi: 10.3390/mps1030031
Ellingsen, K. E., Hewitt, J. E., and Thrush, S. F. (2007). Rare species, habitat diversity and functional redundancy in marine benthos. J. Sea Res. 58, 291–301. doi: 10.1016/j.seares.2007.10.001
Ellison, A. M., Bank, M. S., Clinton, B. D., Colburn, E. A., Elliott, K., Ford, C. R., et al. (2005). Loss of foundation species: consequences for the structure and dynamics of forested ecosystems. Front. Ecol. Environ. 3, 479–486. doi: 10.2307/3868635
Folk, R. L., and Ward, W. C. (1957). Brazos River bar [Texas]; a study in the significance of grain size parameters. J. Sediment. Res. 27, 3–26. doi: 10.1306/74D70646-2B21-11D7-8648000102C1865D
Fox, J., and Weisberg, S. (2019). An {R} Companion to Applied Regression, Third edition. Thousand Oaks CA: Sage.
Golovan, O. A., Błażewicz, M., Brandt, A., Jażdżewska, A., Jóźwiak, P., Lavrenteva, A. V., et al. (2019). Diversity and distribution of peracarid crustaceans (Malacostraca) from the abyss adjacent to the Kuril-Kamchatka Trench. Mar. Biodivers. 49, 1343–1360. doi: 10.1007/s12526-018-0908-3
Gomez-Gesteira, J. L., Dauvin, J. C., and Salvande-Fraga, M. (2003). Taxonomic level for assessing oil spill effects on soft-bottom sublittoral benthic communities. Mar. Pollut. Bull. 46, 562–572. doi: 10.1016/S0025-326X(03)00034-1
Grassle, J. F., and Maciolek, N. J. (1992). Deep-sea richness, regional and local diversity estimates from quantitative bottom samples. Am. Nat. 139, 313–341. doi: 10.1086/285329
Grime, J. P. (1998). Benefits of Plant Diversity to Ecosystems: immediate, Filter and Founder Effects. J. Ecol. 86, 902–910. doi: 10.1046/j.1365-2745.1998.00306.x
Guisan, A., Broennimann, O., Engler, R., Vust, M., Yoccoz, N. G., Lehmann, A., et al. (2006). Using niche-based models to improve the sampling of rare species. Conserv. Biol. 20, 501–511. doi: 10.1111/j.1523-1739.2006.00354.x
Guţu, M., and Sieg, J. (1999). “Ordre des Tanaidaces,” in Traite de Zoologie 7(3)(A), Crustaces Pericarides, ed. J. Forest (Monaco: Mermoires de I’Institut Oceanographique), 353–389.
Hassack, E., and Holdich, D. M. (1987). The tubulicous habit amongst the Tanaidacea (Crustacea, Peracarida) with particular reference to deep-sed speckles. Zool. Sci. 16, 223–233. doi: 10.1111/j.1463-6409.1987.tb00069.x
Higgs, N. D., and Attrill, M. J. (2015). Biases in biodiversity: wide-ranging species are discovered first in the deep sea. Front. Mar. Sci. 2:61. doi: 10.3389/fmars.2015.00061
Holdich, D. M., and Bird, G. J. (1985). Tanaidacea (Crustacea) from sublittoral waters off West Scotland, including the description of two new genera. J. Nat. Hist. 20, 79–100. doi: 10.1080/00222938600770081
Hughes, A. C., Orr, M. C., Ma, K., Costello, M. J., Waller, J., Provoost, P., et al. (2021). Sampling biases shape our view of the natural world. Ecography 44, 1259–1269. doi: 10.1111/ecog.05926
Iversen, P. E., Green, A. M. V., Lind, M. J., Petersen, M. R. H. P., Bakke, T., Lichtenthaler, R., et al. (2011). Guidelines for Offshore Environmental Monitoring on the Norwegian Continental Shelf. Klif Report. Oslo: Climate and Pollution Agency.
Jakiel, A., Stȩpień, A., and Błażewicz, M. (2018). A tip of the iceberg—Pseudotanaidae (Tanaidacea) diversity in the North Atlantic. Mar. Biodivers. 48, 859–895. doi: 10.1007/s12526-018-0881-x
Jarvis, I., and Jarvis, K. E. (1992). Plasma spectrometry in the earth sciences: techniques, applications and future trends. Chem. Geol. 95, 1–33. doi: 10.1016/0009-2541(92)90041-3
Jóźwiak, P., and Błażewicz, M. (2021). Muvi schmallenbergi gen. nov., sp. nov. (Crustacea, Tanaidacea) from the southeast Australian coast, with comments on the distribution and habitat preferences of Chondropodinae. PeerJ 9:e11607. doi: 10.7717/peerj.11607
Jóźwiak, P., Janicka, M., Dębiec, P., Stępiński, A., Mielczarz, K., Serigstad, B., et al. (2017). New Tanaidacea (Crustacea: Peracarida) from the Gulf of Guinea. Mar. Biodivers. 48, 1715–1730. doi: 10.1007/s12526-017-0646-y
Jóźwiak, P., Pabis, K., Brandt, A., and Błażewicz, M. (2020). Epibenthic sled versus giant box corer – comparison of sampling gears for tanaidacean species richness assessment in the abyssal benthic ecosystem. Prog. Oceanogr. 181:102255. doi: 10.1016/j.pocean.2019.102255
Jumars, P. A., Dorgan, K. M., and Lindsay, S. M. (2015). Diet of worms emended: an update for polychaete feeding guilds. Ann. Rev. Mar. Sci. 7, 497–520. doi: 10.1146/annurev-marine-010814-020007
Kaiser, K., Barnes, D. K. A., and Brandt, A. (2007). Slope and deep-sea abundance across scales: Southern Ocean isopods show how complex the deep sea can be. Deep-Sea Res. Part II Top. Stud. Oceanogr. 54, 1776–1789. doi: 10.1016/j.dsr2.2007.07.006
Kaiser, S., Barnes, D. K., Sands, C. J., and Brandt, A. (2009). Biodiversity of an unknown Antarctic Sea: assessing isopod richness and abundance in the first benthic survey of the Amundsen continental shelf. Mar. Biodivers. 39:27. doi: 10.1007/s12526-009-0004-9
Kakui, K., and Kohtsuka, H. (2015). Two New Shallow-water Species of Agathotanais (Crustacea: Tanaidacea) from Japan. Species Divers. 20, 45–58. doi: 10.12782/sd.20.1.045
Kneib, T. (1985). Patterns in the utilisation of the intertidal salt marsh by larvae and juveniles of Fundulus heteroclits (Linneaus) and Fundulus luciae (Baird). J. Exp. Mar. Biol. Ecol. 83, 41–51. doi: 10.1016/0022-0981(84)90116-3
Larsen, K. (2013). The ANDEEP Tanaidacea (Crustacea: Peracarida) revisited II: the family Anarthruridae and family incertae sedis, with descriptions of three new species and the erection of a new genus. Zootaxa 3694, 367–380. doi: 10.11646/zootaxa.3694.4.5
Larsen, K., and Araújo-Silva, C. L. (2014). The ANDEEP Tanaidacea (Crustacea: Peracarida) revisited III: the family Akanthophoreidae. Zootaxa 3796, 237–264. doi: 10.11646/zootaxa.3796.2.2
Le Loeuff, P., and Cosel, R. (1998). Biodiversity patterns of the marine benthic fauna on the Atlantic coast of tropical Africa in relation to hydroclimatic conditions and paleogeographic events. Acta Oecol. 19, 309–321. doi: 10.1016/S1146-609X(98)80035-0
Le Loeuff, P., and Intès, A. (1999). Macrobenthic communities in the continental shelf of Côte-d’Ivoire. Seasonal and diel cycles in relation to hydroclimate. Acta Oceanol. 22, 529–550. doi: 10.1016/S0399-1784(00)87685-9
Leitão, R. P., Zuanon, J., Villéger, S., Williams, S. E., Baraloto, C., Fortunel, C., et al. (2016). Rare species contribute disproportionately to the functional structure of species assemblages. Proc. R. Soc. B 283, 494–499. doi: 10.1098/rspb.2016.0084
Leite, P. P. (1995). Seasonal and spatial distribution of Kalliapseudes schubarti Mane-Garzon (Tanaidacea, Crustacea), in the Araca region, Sao Sebastiao. Arq. Biol. Tecnol. 38, 605–618.
Leroy, B., Gallon, R., Feunteun, E., Robuchon, M., and Frédéric, Y. (2017). Cross-taxon congruence in the rarity of subtidal rocky marine assemblages: no taxonomic shortcut for conservation monitoring. Ecol. Indic. 77, 239–249. doi: 10.1016/j.ecolind.2017.02.012
Levin, L. A. (2003). Oxygen minimum zone benthos: adaptation and community response to hypoxia. Oceanogr. Mar. Biol. 41, 1–45.
Levin, L. A., and Sibuet, M. (2012). Understanding continental margin biodiversity: a new imperative. Ann. Rev. Mar. Sci. 4, 79–112. doi: 10.1146/annurev-marine-120709-142714
Levin, L., Whitcraft, C. R., Mendoza, G. F., Gonzalez, J. P., and Cowie, G. (2009). Oxygen and organic matter thresholds for benthic faunal activity on the Pakistan margin oxygen minimum zone (700—1100 m). Deep Sea Res. II Top. Stud. Oceanogr. 56, 449–471. doi: 10.1016/j.dsr2.2008.05.032
Longhurst, A. R. (1958). An Ecological Survey of the West African MarineBenthos. London: Colonial Office Fisheries Publication, 1–102.
Longhurst, A. R. (1959). Benthos densities off tropical West Africa. ICES Mar. Sci. Symp. 25, 21–28. doi: 10.1093/icesjms/25.1.21
Lyons, K. G., Brigham, C. A., Traut, B. H., and Schwartz, M. W. (2005). Rare species and ecosystem functioning. Conserv. Biol. 19, 1019–1024. doi: 10.1111/j.1523-1739.2005.00106.x
McCallum, A. W., Woolley, S., Błażewicz-Paszkowycz, M., Browne, J., Gerken, S., Kloser, R., et al. (2015). Productivity enhances benthic species richness along an oligotrophic Indian Ocean continental margin. Glob. Ecol. Biogeogr. 24, 462–471. doi: 10.1111/geb.12255
Mouillot, D., Bellwood, D. R., Baraloto, C., Chave, J., Galzin, R., Harmelin-Vivien, M., et al. (2013). Rare species support vulnerable functions in high-diversity ecosystems. PLoS Biol. 11:e1001569. doi: 10.1371/journal.pbio.1001569
Nagelkerken, I., and van der Velde, G. (2004). Relative importance of interlinked mangroves and seagrass beds as feeding habitats for juvenile reef fish on a Caribbean island. Mar. Ecol. Prog. Ser. 274, 153–159. doi: 10.3354/meps274153
Obst, M., Vicario, S., Lundin, K., Berggren, M., Karlsson, A., Haines, R., et al. (2018). Marine long-term biodiversity assessment suggests loss of rare species in the Skagerrak and Kattegat region. Mar. Biodivers. 48, 2165–2176. doi: 10.1007/s12526-017-0749-5
Oksanen, J., Blanchet, F. G., Friendly, M., Kindt, R., Legendre, P., McGlinn, D., et al. (2020). Vegan: community Ecology Package. R package version 2. 5-7. Available Online at: https://CRAN.R-project.org/package=vegan.
Oliver, J. S., and Slattery, P. N. (1985). Effects of crustacean predators on species composition and population structure of soft bodied infauna from McMurdo Sound, Antarctica. Ophelia 24, 155–175. doi: 10.1080/00785326.1985.10429725
Olsgard, F., and Gray, J. S. (1995). A comprehensive analysis of effects of offshore oil and gas exploration and production on the benthic communities of the Norwegian continental shelf. Mar. Ecol. Prog. Ser. 122, 277–306. doi: 10.3354/meps122277
Pabis, K., Błażewicz-Paszkowycz, M., Jóźwiak, P., and Barnes, D. K. A. (2014). Tanaidacea of the Amundsen and Scotia seas: unexplored diversity. Antarct. Sci. 27, 19–30. doi: 10.1017/S0954102014000303
Pabis, K., Jóźwiak, P., Lörz, A. N., Schnabel, K., and Błażewicz-Paszkowycz, M. (2015). First insights into the deep-sea tanaidacean fauna of the Ross Sea – species richness and composition across the shelf break, slope and abyss. Polar Biol. 38, 1429–1437. doi: 10.1007/s00300-015-1706-z
Pabis, K., Sobczyk, R., Siciński, J., Ensrud, T., and Serigstadt, B. (2020). Natural and anthropogenic factors influencing abundance of the benthic macrofauna along the shelf and slope of the Gulf of Guinea, a large marine ecosystem off West Africa. Oceanologia 62, 83–100. doi: 10.1016/j.oceano.2019.08.003
R Core Team (2019). R: a Language And Environment For Statistical Computing. Vienna, Austria: R Foundation for Statistical Computing.
Reiss, H., Birchenough, S., Borja, A., Buhl-Mortensen, L., Craeymeersch, J., Dannheim, J., et al. (2015). Benthos distribution modelling and its relevance for marine ecosystem management. ICES J. Mar. Sci. 72, 297–315. doi: 10.1093/icesjms/fsu107
Rex, M. A., and Etter, R. J. (2010). Deep-Sea Biodiversity; Patterns and Scale. Cambridge, MA: Harvard University Press.
Rumbold, C. E., Obenat, S. M., and Spivak, E. D. (2015). Comparison of life history traits of Tanais dulongii (Tanaidacea: Tanaididae) in natural and artificial marine environments of the south-western Atlantic. Helgol. Mar. Res. 69, 231–242. doi: 10.1007/s10152-015-0432-9
Säterberg, T., Jonsson, T., Yearsley, J., Berg, S., and Ebenman, B. (2019). A potential role for rare species in ecosystem dynamics. Sci. Rep. 9:11107. doi: 10.1038/s41598-019-47541-6
Scheren, P. A., Ibe, A. C., Janssen, F. J., and Lemmens, A. M. (2002). Environmental pollution in the Gulf of Guinea – a regional approach. Mar. Pollut. Bull. 44, 633–641. doi: 10.1016/S0025-326X(01)00305-8
Schneider, W. (1990). Field Guide to the Commercial Marine Resources of the Gulf of Guinea. Rome: Food and Agriculture Organization of the United Nations.
Segadilha, J. L., and Lavrado, H. P. (2018). Tanaidacea fauna (Peracarida, Crustacea) from the shallow sublittoral zone of Admiralty Bay, King George Island, Antarctica, with new records. Polar Biol. 41, 589–597. doi: 10.1007/s00300-017-2220-2
Sheridan, P. (1997). Benthos of adjacent mangrove, seagrass and non-vegetated habitats in Rookery Bay, Florida, USA. Estuar. Coast. Shelf Sci. 44, 455–469. doi: 10.1006/ecss.1996.0125
Siciński, J., Pabis, K., Jażdżewski, K., Konopacka, A., and Błażewicz-Paszkowycz, M. (2012). Macrozoobenthos of two Antarctic glacial coves: a comparison with non-disturbed bottom areas. Polar Biol. 35, 355–367. doi: 10.1007/s00300-011-1081-3
Smith, M. D., and Knapp, A. K. (2003). Dominant species maintain ecosystem function with non-random species loss. Ecol. Lett. 6, 509–517. doi: 10.1046/j.1461-0248.2003.00454.x
Sobczyk, R., Czortek, P., Serigstad, B., and Pabis, K. (2021). Modelling of polychaete functional diversity: a Large marine ecosystem response tomultiple natural factors and human impacts on the West African continental margin. Sci. Total Environ. 792:148075. doi: 10.1016/j.scitotenv.2021.148075
Sokolova, M. N. (1972). Trophic structure of deep-sea macrobenthos. Mar. Biol. 16, 1–12. doi: 10.1007/bf00347841
Somerfield, P. J., and Clarke, K. R. (1997). A comparison of some methods commonly used for the collection of sublittoral sediments and their associated fauna. Mar. Environ. Res. 43, 145–156.
Stark, J. S., Mohammad, M., McMinn, A., and Ingels, J. (2020). Diversity, abundance, spatial variation, and human impacts in marine meiobenthic Nematode and Copepod communities at Casey Station, East Antarctica. Front. Mar. Sci. 7:480. doi: 10.3389/fmars.2020.00480
Stȩpień, A., and Blażewicz-Paszkowycz, M. (2013). Four new species and two new genera of Metapseudidae (Crustacea: Tanaidacea: Apseudomorpha) from Australian coral reefs. Zootaxa 3717, 559–592. doi: 10.11646/zootaxa.3717.4.7
Stępień, A., Pabis, K., and Błażewicz, M. (2018). Small-scale species richness of the Great Barrier Reef tanaidaceans—results of the CReefs compared with worldwide diversity of coral reef tanaidaceans. Mar. Biodivers. 49, 1169–1185. doi: 10.1007/s12526-018-0894-5
Stępień, A., Pabis, K., and Błażewicz, M. (2019). Tanaidacean faunas of the Sea of Okhotsk and northern slope of the Kuril-Kamchatka Trench. Prog. Oceanogr. 178:102196. doi: 10.1016/j.pocean.2019.102196
Stȩpień, A., Pabis, K., Sobczyk, R., and Serigstad, B. (2021). High Species Richness and Extremely Low Abundance of Cumacean Communities Along the Shelf and Slope of the Gulf of Guinea (West Africa). Front. Mar. Sci. 8:703547. doi: 10.3389/fmars.2021.703547
Ukwe, C. N., Ibe, C. A., Alo, B. I., and Yumkella, K. K. (2003). Achieving a paradigm shift in environmental and living resources management in the Gulf of Guinea: the large marine ecosystemapproach. Mar. Pollut. Bull. 47, 219–225. doi: 10.1016/S0025-326X(02)00473-3
Ukwe, C. N., Ibe, C. A., and Sherman, K. (2006). A sixteen-country mobilization for sustainable fisheries in the Guinea Current Large Marine Ecosystem. Ocean Coast. Manag. 49, 385–412. doi: 10.1016/j.ocecoaman.2006.04.006
UNESCO Intergovernmental Oceanographic Commission (1982). The Determination of Petroleum Hydrocarbons in Sediments (Manuals and Guides No. 11, Paris). Paris: UNESCO.
Wei, T., and Simko, V. (2017). R Package “corrplot”: visualization of a Correlation Matrix (Version 0.84). Available Online at: https://guthub.com/taiyun/corrplot.
Whitaker, D., and Christman, M. (2014). Clustig: significant cluster analysis. R package version 1.1. Available Online at: https://CRAN.R-project.org/package=clustsig.
Wilson, G. D. F. (2017). Macrofauna abundance, species diversity and turnover at three sites in Clipperton-Clarion Fracture Zone. Mar. Biodivers. 47, 323–347.
Keywords: Gulf of Guinea, West Africa, continental margin, depth gradient, singletons, rare species, Tanaidacea
Citation: Jóźwiak P, Pabis K, Sobczyk R and Serigstad B (2022) A Paradise for Rare Species: Tanaidacean Fauna of the West African Continental Margin. Front. Mar. Sci. 9:779134. doi: 10.3389/fmars.2022.779134
Received: 18 September 2021; Accepted: 07 January 2022;
Published: 18 February 2022.
Edited by:
Torben Riehl, Senckenberg Museum, GermanyReviewed by:
Helena Passeri Lavrado, Federal University of Rio de Janeiro, BrazilSaskia Brix, Senckenberg Museum, Germany
Copyright © 2022 Jóźwiak, Pabis, Sobczyk and Serigstad. This is an open-access article distributed under the terms of the Creative Commons Attribution License (CC BY). The use, distribution or reproduction in other forums is permitted, provided the original author(s) and the copyright owner(s) are credited and that the original publication in this journal is cited, in accordance with accepted academic practice. No use, distribution or reproduction is permitted which does not comply with these terms.
*Correspondence: Piotr Jóźwiak, cGlvdHIuam96d2lha0BiaW9sLnVuaS5sb2R6LnBs